- 1Biodiversity and Reef Conservation Laboratory, Unidad Académica de Sistemas Arrecifales, Instituto de Ciencias del Mar y Limnología, Universidad Nacional Autónoma de México, Puerto Morelos, Mexico
- 2Parque Nacional Arrecifes de Cozumel, Comisión Nacional de Áreas Naturales Protegidas, Cozumel, Mexico
In the Caribbean, disease outbreaks have emerged as significant drivers of coral mortality. Stony Coral Tissue Loss Disease (SCTLD) is a novel white plague-type disease that was first reported off the Florida coast in 2014. This disease affects >20 coral species and is spreading rapidly throughout the Caribbean. In December 2018, SCTLD reached southwestern (SW) Cozumel, one of the healthiest reef systems in the Caribbean. In this study, we integrate data from multiple survey protocols conducted between July 2018 and April 2020 to track the progression of the outbreak in SW Cozumel and to quantify the impacts of SCTLD on coral communities and the benthic composition of reefs. Given that the SCTLD outbreak coincided with a period of prolonged thermal stress that concluded in widespread coral bleaching in autumn 2019, we also investigated whether this event further exacerbated coral mortality. Our findings show that SCTLD spread throughout SW Cozumel in only 2 months and reached a peak after only 5 months. By the summer of 2019, most of the afflicted corals were already dead. Species of the families Meandrinidae, Faviinae, and Montastraeidae showed 33–95% mortality. The widespread coral die-off caused an overall loss of 46% in coral cover followed by a rapid increase of algae cover across all surveyed reefs that persisted until at least April 2020. In November 2019, more than 15% of surveyed coral colonies were bleached. However, we did not find that bleaching further increased coral mortality at either the colony or the community level, which suggests that the coral communities were able to recover from this event despite still being affected by the disease. In conclusion, SCTLD is radically changing the ecology of coral reefs by decimating the populations of several key reef-builders and reconfiguring the benthic assemblages. The actions needed to restore coral populations have to be accompanied by stringent controls related to the effects of climate change, coastal development, and wastewater treatment to improve coral conditions and ecosystem resilience.
Introduction
Coral reefs have shifted away from coral dominance over the past few decades, which has compromised ecosystem stability and reduced their capacity to support important ecosystem services and biodiversity (Hughes et al., 2017; Perry and Alvarez-Filip, 2019). Climate-driven thermal stress has become a major force reshaping coral reef communities, threatening the persistence of coral-dominated reefs across the tropics (Hoegh-Guldberg, 1999; Hughes et al., 2018; Oliver et al., 2018). However, coral loss may also occur in response to other stressors or to multiple stressors that act simultaneously, and at times synergistically, to increase coral mortality or limit coral growth and reproduction (Darling et al., 2010; Ban et al., 2014; Elías-Ilosvay et al., 2020).
In particular, disease outbreaks have emerged as significant causes of coral die-off in the Caribbean region, with devastating effects on key reef-building species (Gladfelter, 1982; Weil, 2004; Harvell et al., 2007). Recent studies have shown that the increases in both the frequency and intensity of coral disease outbreaks are often related to multiple pressures, such as rising sea surface temperatures and decreased seawater quality (Randall and Van Woesik, 2017). For example, numerous studies have documented that coral disease outbreaks, especially those related to white plague disease, occur either simultaneously or following thermally induced coral bleaching events (Cróquer and Weil, 2009; Rogers et al., 2009; Precht et al., 2016; Gintert et al., 2019). This is because abnormally warm temperatures may increase the abundance and virulence of pathogens and/or immunocompromised coral hosts (Sokolow, 2009; Reed et al., 2010; Maynard et al., 2015; Pinzón et al., 2015). In consequence, increasing thermal stress has facilitated the occurrence of outbreaks, particularly in areas in which corals are abundant (Bruno et al., 2007). Both diseases and bleaching events are thus key drivers for triggering mass coral mortality in the Caribbean (Cróquer and Weil, 2009; Eakin et al., 2010), which has contributed to important shifts in assemblage composition and to long-term phase-shifts in some coral reefs.
Stony coral tissue loss disease (SCTLD) is an unprecedented white plague-type disease first observed off the Florida coast in 2014 (Precht et al., 2016). Since then, outbreaks of the disease have been confirmed in the Western Caribbean, the Greater Antilles, the Bahamian Archipelago, and the Eastern Caribbean (Alvarez-Filip et al., 2019; Weil et al., 2019; Kramer et al., 2020). This disease affects at least 20 species of scleractinian corals, including major reef-building species and species listed as endangered on the Red List of the International Union for Conservation of Nature (FDEP, 2018; Alvarez-Filip et al., 2019). When corals become infected, multifocal acute lesions can arise that quickly progress throughout the colony, resulting in total mortality in most cases. The exact putative agent is still unknown, although the characterizations of the microbial communities of the lesions and the responses to antibiotic treatments suggest that SCTLD is associated with bacteria of the Flavobacteriales, Rhodobacterales, and Rhizobiales orders (Aeby et al., 2019; Meyer et al., 2019). Moreover, the disease is highly contagious, and at least in Florida, SCTLD has followed a linear spread pattern, moving slightly faster to the north than to the south. This progression may possibly have been due to the influence of currents (Muller et al., 2020) and sediments, which have been identified as possible sources of transmission (Aeby et al., 2019; Muller et al., 2020; Rosales et al., 2020).
In a decadal study in Florida, thermal-stress events were found to be responsible for coral bleaching and associated coral diseases (van Woesik and McCaffrey, 2017). In that region, a warm winter and summer appeared at the onset of widespread coral bleaching in 2014, which likely enhanced the severity of SCTLD (Manzello, 2015; Precht et al., 2016; Walton et al., 2018; Gintert et al., 2019). However, although the onset of SCTLD may have co-occurred with high water temperatures, which may have in turn helped the emergence of SCTLD, these temperatures did not necessarily facilitate its spread (Muller et al., 2020). Furthermore, in the Mexican Caribbean, it has been observed that a decrease in water temperatures during winter did not slow the progression of SCTLD (Alvarez-Filip et al., 2019), which was further supported by findings from the Florida Reef Tract that suggested that water temperature did not affect either disease prevalence or virulence (Aeby et al., 2019). Moreover, a trade-off between disease and bleaching susceptibility in coral species has been observed (Shore-Maggio et al., 2018), suggesting that the impacts from bleaching events and diseases are not necessarily synergistic.
In addition to thermal stress, other environmental stressors may have influenced the spread of SCTLD, as the prevalence and progression of the disease have been found to vary between sites, suggesting a potential role of local-scale habitat variation in environmental conditions, such as nutrient concentrations, turbidity, and temperature variability (Rippe et al., 2019). Recent studies have found that the presence of certain bacteria associated with soil, human, and animal gut consortia may be a contributing factor to SCTLD, implicating surface runoff and/or fecal contamination in the spread of the disease (Iwanowicz et al., 2020). Further, the linear spread of SCTLD along the Florida Reef Tract (Muller et al., 2020) indicates that water currents may play an important role in disease transmission and geographic spread, as the causative agent of SCTLD might have been transported to the first-infected colonies within sediments resuspended by dredging operations (Dobbelaere et al., 2020).
A rigorous evaluation throughout the Mexican Caribbean determined that the disease afflicted all mainland reefs surveyed along the shore within a few months, as the onset of SCTLD in this region occurred in summer 2018; however, due to the high variability in disease prevalence among these sites, a geographical gradient was not evident (Alvarez-Filip et al., 2019). So far, SCTLD may have considerably altered the structure of the coral communities and decreased their physical functionality in the region (Alvarez-Filip et al., 2019; Estrada-Saldívar et al., 2020). In contrast to what was observed in the mainland reefs, SCTLD was first observed in December 2018 on the western coast of Cozumel Island. This allowed for the implementation of preventative measures by the managers of the Arrecifes de Cozumel National Park and allies in the tourism sector to monitor the disease. These reefs constitute some of the most popular diving sites for tourists in the Caribbean as well as being important sites for Caribbean cruises, and thus Cozumel receives thousands of tourists each year that generate an annual estimated income of US$ 304 million dollars (CONANP-GIZ, 2017).
The reefs in southwestern (SW) Cozumel are among the most complex and best-conserved reef systems in the Caribbean, as they present high coral cover and high diversity when compared to those of other reefs in the region (Bozec et al., 2015; Martínez-Rendis et al., 2020; McField et al., 2020). Although the coral reefs in Cozumel have exhibited increases in macroalgae cover, they have simultaneously shown increases in coral cover over the last decade, suggesting that they are highly resilient despite growing anthropogenic threats (Contreras-Silva et al., 2020). Although the SW Cozumel reefs were among the last to be affected by the SCTLD outbreak, as the first report of the disease was in Paraiso reef (located near the northwestern boundary of the Arrecifes de Cozumel marine protected area, MPA; Figure 1) on December 5, 2018, SCTLD spread very rapidly across the 20 km of SW Cozumel during the winter. The disease outbreak remained throughout 2019, affecting coral communities and coinciding with a severe thermal stress event that peaked in autumn 2019. In this study, we first set out to describe the timing of the spread of SCTLD and its impacts on the coral communities within the reefs of the Cozumel MPA. Second, we assessed the consequences of coral die-off on the composition of the benthic community by quantifying changes in the cover of corals and algae. Lastly, we evaluated whether coral mortality was further exacerbated by thermal stress.
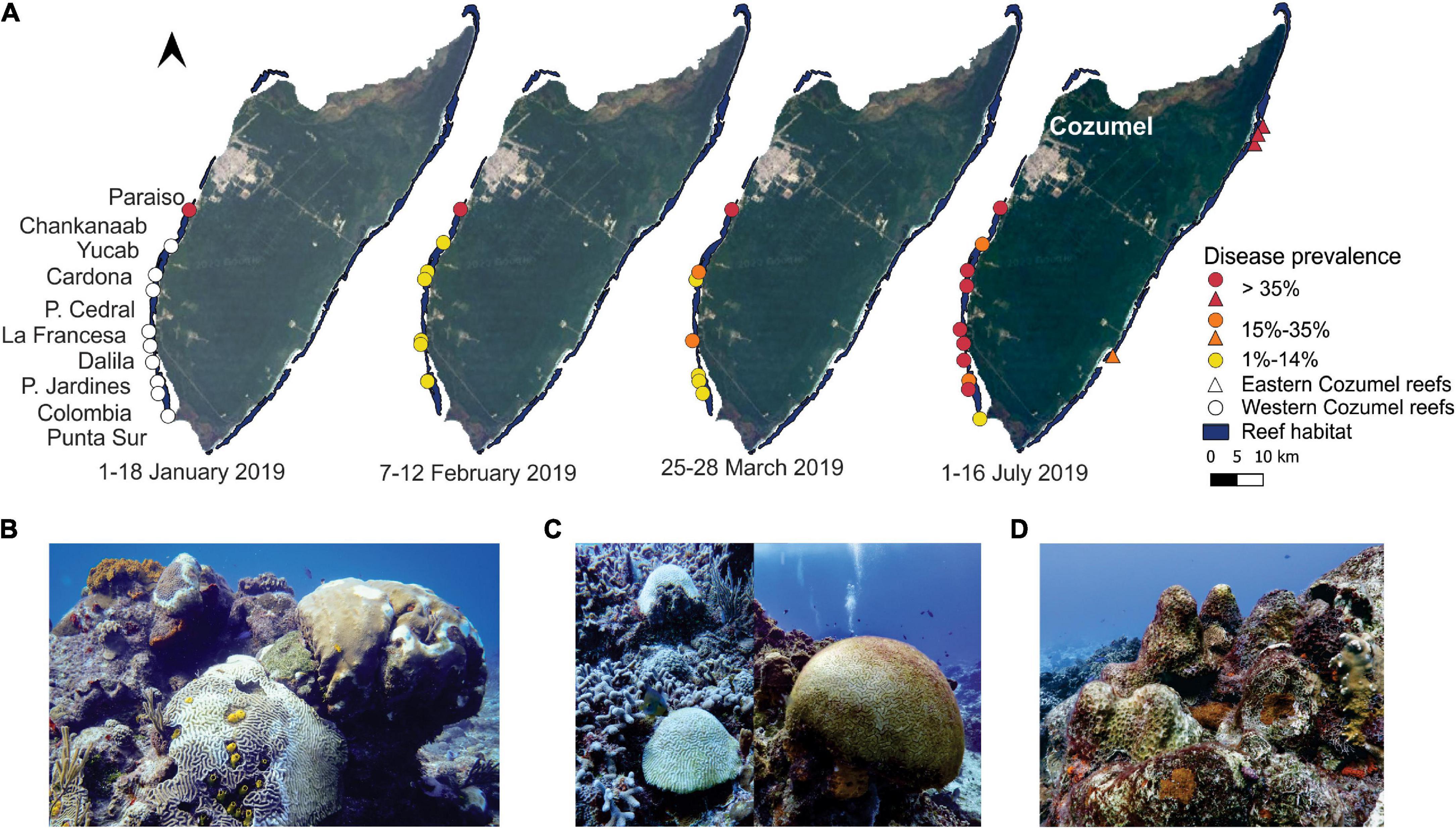
Figure 1. Progression of the Stony Coral Tissue Loss Disease (SCTLD) in southwestern (SW) Cozumel from January to March 2019. (A) Cozumel maps with the locations of the various surveyed reefs. The colors represent SCTLD prevalence for highly susceptible species. The triangles represent the reefs found in eastern Cozumel, which presented SCTLD as of September 2018 (Alvarez-Filip et al., 2019). The white circles represent the reefs studied from July 2018 to April 2020, which did not present SCTLD in January. (B) Different coral colonies of highly susceptible species with SCTLD found in Paraiso in January 2019. (C) Colonies with recent mortality: two bare (white coral skeleton) of Pseudodiploria strigosa (left), and one colony of Diploria labyrinthiformis covered by a thin layer of filamentous algae (right). All colonies were found in Paso del Cedral reef in April 2019. (D) Standing dead coral (old mortality) colonies of Montastraea cavernosa with overgrowth of cyanobacterial and filamentous algal mats found in Colombia reef in November 2019. Photo credits: Lorenzo Alvarez-Filip.
Materials and Methods
Study Area
The shelf of SW Cozumel (leeward portion) is structured into three terraces, which are often covered by sediment, bordered by distinct steps on which reef formations are found (Muckelbauer, 1990). On the edge of the terraces, at approximately 5, 10, and 20 m below sea level, a series of discontinuous reef formations that follow the contour of the edge are present (Jordán-Dahlgren, 1988; Muckelbauer, 1990). These border reefs follow a marked north-south gradient of increasing structural complexity and habitat diversity (Muckelbauer, 1990). The area is influenced by the Yucatán Current, which travels from south to north, and by the presence of temporal and tide-induced coastal counter currents (Chávez et al., 2003). The leeward coast is protected from high wave action, except in autumn when strong northern and north-western winds blow (Muckelbauer, 1990). These reefs have been protected since 1980 when the western coast of Cozumel was declared a refuge zone for the Protection of Marine Flora and Fauna. In 1996, this system was upgraded to a national park (total area of11,987.87 ha expanding to the southeast, south, and southwest of the island) under the administration of the National Commission of Natural Protected Areas (CONANP).
Field Surveys and Analyses
In this study, we used three complementary approximations to assess the spread and impacts of the SCTLD outbreak. First, to track the spread of the disease across the MPA, we conducted prospective surveys during February and March 2019 in collaboration with Park Authorities and local dive shops. Second, to assess the impacts of SCTLD and bleaching, from July 2018 to April 2020, we surveyed the coral communities of six reef sites during seven different periods in addition to four reef sites over a single period. Lastly, to estimate changes in the cover of corals, algae, and other benthic components, we conducted four benthic surveys between July 2018 and April 2020.
Prospective Surveys
From February 7, 2019, to March 27, 2019, one diver trained in coral disease identification (i.e., O.O. R-G) joined commercial diving trips (that consisted of drift dives) to survey 10 sites that dive shops use for recreational activities. During each trip, two sites were surveyed. At each site, the diver swam in a straight line at an average depth of 18 m during the first dive and 10 m during the second dive. All coral colonies that were found within 4 m across the field of vision of the diver were registered, and the following data were recorded: species identity, the presence of SCTLD, and the percentage of recent mortality for each diseased colony. The diver estimated the percentage of recent mortality, considering the entire flat surface. To confirm the sampling points, geographical coordinates were captured at the entry and exit of each dive.
Coral Community Surveys
To evaluate the coral communities, we used both bar drop and belt transect methods (Lang et al., 2012; Searle et al., 2014). The bar drop method was employed in January 2019, April 2019, and April 2020 (Paraiso, Paso del Cedral, and Colombia sites). The belt transect method was used in July 2018 (Paraiso, Chankanaab, Yucab, Paso del Cedral, Dalila, and Colombia), July 2019 (Paraiso, Chankanaab, Yucab, Paso del Cedral, Dalila, Colombia, La Francesa, and Palancar Jardines), August 2019 (Cardona and Punta Sur sites), and October and November 2019 (Paraiso, Paso del Cedral, and Colombia). Surveys were conducted between 4 and 14 m depth (Supplementary Table S1). Different methodologies were utilized because of logistic and time restrictions; however, the information is largely comparable as both methods provide detailed colony data. The main difference between these two methods is that the bar drop method allows for large areas to be surveyed, although it is not possible to estimate the total surveyed area.
For the bar drop method, 2–5 surveyors used a 1-m PVC tube that was marked every 25 cm. Each surveyor swam in a straight line holding the bar perpendicular to the swimming transect. Every three kick-cycles (i.e., a full downward and upward push for each leg), the bar was placed straight down onto the substrate. Coral colonies ≥4 cm under the mark were recorded, and if a mark did not fall directly on top of a coral, the condition of the nearest coral colony (within a 12.5-cm diameter) was then recorded (Searle et al., 2014). For the belt transect method, we followed the methodology of the Atlantic and Gulf Rapid Reef Assessment (AGRRA V5.5; Lang et al., 2012). Belt transects were haphazardly placed within the reef structure, and all corals (≥4 cm) found within the 1-m band were registered and measured (fragments were registered but not measured). In July 2018 (prior to SCTLD), an average of 5.1 (4–6) belt transects (10 × 1 m) were surveyed in each reef, whereas in the 2019 surveys, the effort increased considerably with an average of 9 (4–13) belt transects. Given the low number of transects conducted in July 2018, it is probable that some uncommon or rare species were underrepresented, although this would only make our findings more conservative for those species.
For both methods, the following data were recorded for each coral colony within the transect: species identity, colony size (maximum diameter, diameter perpendicular to the maximum diameter, and height), percentage of bleaching, percentage of mortality (recent, transient, and old), and the presence of SCTLD or other diseases (Lang et al., 2012). For this study, we also recorded colonies with 100% mortality for which mortality could be attributed to SCTLD (i.e., recent mortality was still evident). Mortality was deemed to be recent when the superficial structure of the colonies was bare (white coral skeleton) or covered by a thin layer of sediment or filamentous algae, indicating that the soft tissue had died within a time frame of hours to weeks (e.g., Figure 1C). Only 68 (out of 10,687) colonies were recorded to have been affected by other diseases, none with evidence of rapid disease progression or severe coral mortality. Therefore, we assumed that coral mortality was produced by SCTLD because this disease has a high rate of lethality and velocity of dispersion and progression (Precht et al., 2016; Muller et al., 2020). Given that bleaching was also observed during some surveys, we also identified four possible causes associated with recent mortality: bleaching (the colony only presented signs of bleaching), bleaching and SCTLD (the colony presented both signs of SCTLD and bleaching), SCTLD (the colony had only signs of SCTLD), and unconfirmed mortality, which was used for completely dead colonies (i.e., no visible signs of bleaching or SCTLD). During the surveys, to differentiate SCTLD from bleaching, we carefully observed if the colony presented live tissue. SCTLD kills the living tissue of the colony, and as it progresses, leaves a band of exposed skeleton (recent mortality). When a colony presented signs of bleaching, the remaining tissue had a pale or transparent color that was still visible.
Using the data obtained from the coral surveys, we then calculated SCTLD prevalence for each site, time period, and coral species. To evaluate disease prevalence, we took into account diseased and recently deceased colonies to prevent underestimating the effects of the disease, as has been done in other similar studies (Miller et al., 2016; Neely, 2018; Alvarez-Filip et al., 2019; Gintert et al., 2019; Estrada-Saldívar et al., 2020). Siderastrea siderea showed different signs of infection (termed white-blotch syndrome in some studies; Gintert et al., 2019; Weil et al., 2019); however, we considered that this species was affected by SCTLD, as both the timing of when signs appeared and the disease progression were similar to what we observed for other species in Cozumel and elsewhere in the Mexican Caribbean (Alvarez-Filip et al., 2019). To provide a clearer picture of the magnitude of the outbreak in Cozumel, we focused on exploring geographical and temporal trends for the most highly susceptible species, which were considered to be those with more than 10% prevalence (considering diseased and dead colonies). The highly susceptible species were identified using data obtained in July and August of 2019, as this was when the disease was most evident across all reefs sites and more data was available (10 reef sites, 86 transects). Although Orbicella franksi did not meet this criterion, it was considered to be a highly susceptible species because it is a species that has been highly affected in the rest of the Mexican Caribbean due to SCTLD. We also calculated the percentage of bleached colonies for each period and the percentage of colonies with recent mortality, considering all coral species.
Disease Progression
We used data obtained from the prospective surveys and the coral community surveys to create a rough timeline of the spatial progression of SCTLD across the Cozumel MPA. For this, we used four periods that corresponded to the first observation of the outbreak to the time that it was present across the entire study area: (1) January 1–18, (2) February 7–12, (3) March 25–28, (4) and July 1–16. As described above, we calculated SCTLD prevalence for each site and period using only highly susceptible species. For this, we also considered diseased and recently deceased colonies, in which mortality was attributable to SCTLD. Due to logistic limitations, not all sites were surveyed during each period (Supplementary Table S1).
Benthic Surveys
Benthic cover surveys were performed according to the Mesoamerican Barrier Reef System protocol (Almada-Villela et al., 2003) in July 2018 and July 2019 (Paraiso, Chankanaab, Yucab, Paso del Cedral, Dalila, and Colombia; Figure 1), November 2019 (Paraiso, Yucab, Paso del Cedral, Dalila, and Colombia), and April 2020 (Paraiso, Paso del Cedral, and Colombia). This method has been used to monitor coral reefs in Cozumel over the last two decades. At each site, six 30-m long transects were haphazardly located parallel to the coast. Each transect was surveyed using the point intercept method (every 25 cm) to determine the benthic component underneath the transect. For this study, we estimated the percentage cover of hard corals and algae by using the proportion of points under the line represented by each category. The latter category included fleshy macroalgae, calcareous macroalgae, cyanobacterial mats, and turf or filamentous algae, as all these groups can rapidly colonize the free space left by recently deceased coral tissue. Although point intercept transects are not a direct measure of the spatial coverage of benthic components, this approximation has proven useful to represent reef condition and to track temporal changes; it is also widely used in research and monitoring protocols (Hill and Wilkinson, 2004; Magdaong et al., 2014; Williams et al., 2017). In addition, the point intercept method has been shown to provide very consistent data when comparing among methods that use a two-dimensional approach (e.g., photoquadrants or videotransects; Nadon and Stirling, 2006; Jokiel et al., 2015).
We compared the percentage cover of corals and algae between July 2018 (before the outbreak) and July 2019 for each reef and for Cozumel as a whole by pooling the data from the six reefs. For this, a univariate comparison of coral and algae cover for all the periods and sites was performed using an analysis of variance (ANOVA), t-tests, or non-parametric equivalents (Mann-Whitney U test) based on an assessment of normality and homogeneity of variance using the Shapiro-Wilk test. In addition to testing for significant differences between these two periods, we calculated the percentage of change. We also tested for possible changes in dominance between coral and macroalgae cover between July 2018 and April 2020. For this, we estimated the ratio between the percentage cover of coral and algae, always using the lowest value of these two coverages as the denominator and the highest value as the numerator. In this way, we could always yield the proportion in relation to the dominant group.
Seawater Temperature
The potential effect of thermal stress was assessed from June 2018 to May 2020 with two different sources of information. First, the temperature at 8 m depth of the Cardona reef (20.41195 N, -87.02052 W) was measured with a Hobo UA-001-08 (Onset, Bourne, United States). The temperature was recorded every 30 min with a resolution of 0.10°C and an accuracy of ±0.53°C. Second, we obtained night sea surface temperature (NSST), Weekly HotSpots (WHS), and degree heating weeks (DHW) data for Cozumel from the SIMAR database (Sistema de Información y Análisis Marino Costero; Cerdeira-Estrada et al., 2018) using data from the Cozumel virtual buoy (20.575163 N, -86.840117 W), which obtains information from the OSTIA (Operational Sea Surface Temperature and Sea Ice Analysis) and GHRSST-MUR (Group for High Resolution Sea Surface Temperature-Multi-Scale Ultra-High-Resolution) products. The Ocean Forecasting Research and Development group, as part of the European Union Global Monitoring for Environment and Security (GMES) MyOcean project, produced the OSTIA data. The GHRSST-MUR data was produced by the GHRSST, Jet Propulsion Laboratory (JPL), the Physical Oceanography Distributed Active Archive Center (PO.DAAC), and the National Aeronautics and Space Administration (NASA; Cerdeira-Estrada et al., 2018). DHW is calculated by summing WHS of 1°C or greater from the preceding 12-week period and is expressed as °C-weeks (Cerdeira-Estrada et al., 2018). The WHS are positive only-anomalies derived by subtracting the mean W-NSST from a specific week minus the maximum of the adjusted monthly climatology of the night sea surface temperature (Cerdeira-Estrada et al., 2018). The WHS measures the magnitude of the thermal, whereas DHW provides a measure of accumulated thermal stress experienced by corals and is a better predictor of bleaching (Liu et al., 2014; Cerdeira-Estrada et al., 2018).
Results
The SCTLD outbreak spread rapidly throughout the reefs along the southwestern coast of Cozumel. The disease was first observed in the Paraiso reef in late December 2018 (B.Q.G, pers. Comm.; Figure 1A). During our survey on January 18, 2019, Paraiso was found to be the only site with clear evidence of SCTLD, and disease prevalence was already high with 59.6% of the colonies of highly susceptible species already presenting either partial or full recent mortality. This shows that it only took 2 or 3 weeks from when the disease was first observed (mid-late December 2018) until it was reported in the rest of the reef sites. Given the quickness of the spread, we were not able to identify a clear geographical dispersion pattern (e.g., north to south); however, by the first weeks of February, the disease was already present in all reefs in the southwestern portion of the Cozumel MPA. Nonetheless, with the exception of Paraiso, SCTLD prevalence was relatively low (5%). The species that showed early signs of the disease in the newly afflicted reefs were Eusmilia fastigiata, Dichocoenia stokesii, Colpophyllia natans, Pseudodiploria strigosa, and Meandrina spp. As the weeks passed, disease prevalence in highly susceptible species increased gradually across all reef sites, until July 2019, when all the reefs had a large number of either dead colonies or colonies with SCTLD (Figure 1A). The common scenario of the Cozumel reefs from January to June 2019 was the presence of a high abundance of diseased colonies (Figure 1B). After which, the number of recently dead colonies increased until the end of the year (Figure 1C). Furthermore, we observed many dead colonies that had growing macroalgae, cyanobacteria, and/or filamentous algal mats (Figure 1D).
The host range for SCTLD was very high in Cozumel. We identified 15 highly susceptible species for which at least 10% of the colonies that were observed in July and August 2019 were either infected or already dead (Figure 2A). However, the greatest susceptibility was observed with the species in the Meandrinidae family (70% disease prevalence), followed by those of the Faviinae subfamily (61% disease prevalence), and the Montastraeidae family (50% disease prevalence; Figure 2A). In July 2018, before the SCTLD outbreak, 98% of the coral colonies of highly susceptible species were healthy, whereas only 2% were afflicted by diseases other than white plague-type diseases (i.e., black band, dark spot, and Caribbean yellow band diseases). However, from January 2019 and throughout the rest of the year, disease prevalence and mortality considerably increased (Figure 2B). Although we did not register Dendrogyra cylindrus colonies in our transects, we observed that colonies of this species were severely affected by the disease (e.g., Figure 2C).
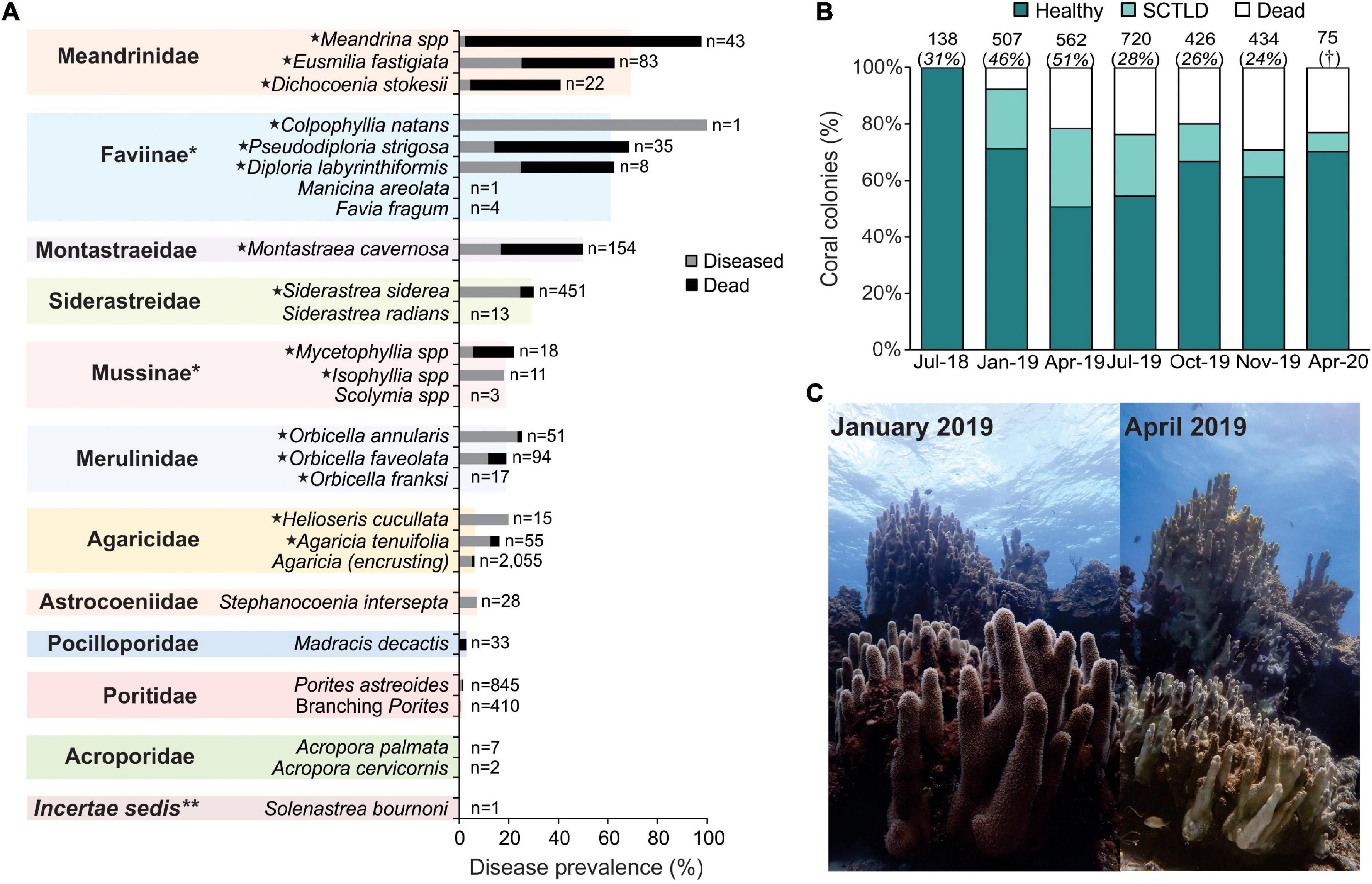
Figure 2. Prevalence of the Stony Coral Tissue Loss Disease (SCTLD) for reefs in southwestern (SW) Cozumel. (A) Prevalence of disease for all coral species and coral families across the reefs surveyed between July and August 2019 in SW Cozumel. The total number of colonies (n) is shown at the end of each bar. For this figure, we included coral colonies with total mortality but for which death could be attributable to SCTLD. The highly susceptible species are marked with a star. *Subfamilies of the Faviidae family; **Solenastrea bournoni does not have a family classification according to Budd et al. (2012). Branching Porites includes P. porites, P. furcata, and P. divaricata. (B) Proportion of healthy, afflicted, and dead colonies of highly susceptible species from July 2018 to April 2020. The total number of colonies, including all species, is shown above each bar, and the percentage that represents the colonies of highly susceptible species is shown in brackets. †For April 2020, the survey was only focused on highly susceptible species. (C) Colony of Dendrogyra cylindrus affected by SCTLD at Colombia reef at 7 m depth. In January 2019, before the outbreak reached the site, the colony was completely alive, but by April 2019, most of the tissue of the colony was lost. This colony measured 6 m in diameter (longest axis) and nearly 4 m in height. Photo credits: Lorenzo Alvarez-Filip.
The overall coral cover of the six reefs surveyed to explore changes in the benthic composition decreased significantly from 26.35 ± 2.21 (mean ± SE) in July 2018 to 14.71 ± 1.49 in July 2019 (Mann-Whitney, U = 273, Z = 3.98, P = 6.6717E-05), whereas algae cover increased significantly from 40.92 ± 2.74 to 50.64 ± 2.23 (Mann-Whitney, U = 411, Z = 2.51, P = 0.01). For each reef site, the same pattern was observed for both coral and algae cover (Figures 3A,B). Coral cover presented an overall loss of 46%, with the highest losses recorded in Paraiso (80%; Figure 3C). Likewise, a notable increase in overall algae cover of up to 34% was observed. Chankanaab was the only reef site in which algae cover did not increase, which was likely because the reef already had very high algae cover prior to the SCTLD outbreak (60%; Figure 3B). In July 2018, a clear dominance of algae over coral cover was not observed, with transects in some reefs (e.g., Colombia and Paso del Cedral) having between 2- to 10-fold more coral cover than that of algae (Figure 3D). However, in July and November 2019, a great majority of transects had a higher proportion of algae than corals, with some transects in the Paraiso reef reaching 30-fold more algae cover than that of corals (Figure 3B). By April 2020, no transects had a higher proportion of corals compared to that of algae (Figure 3D).
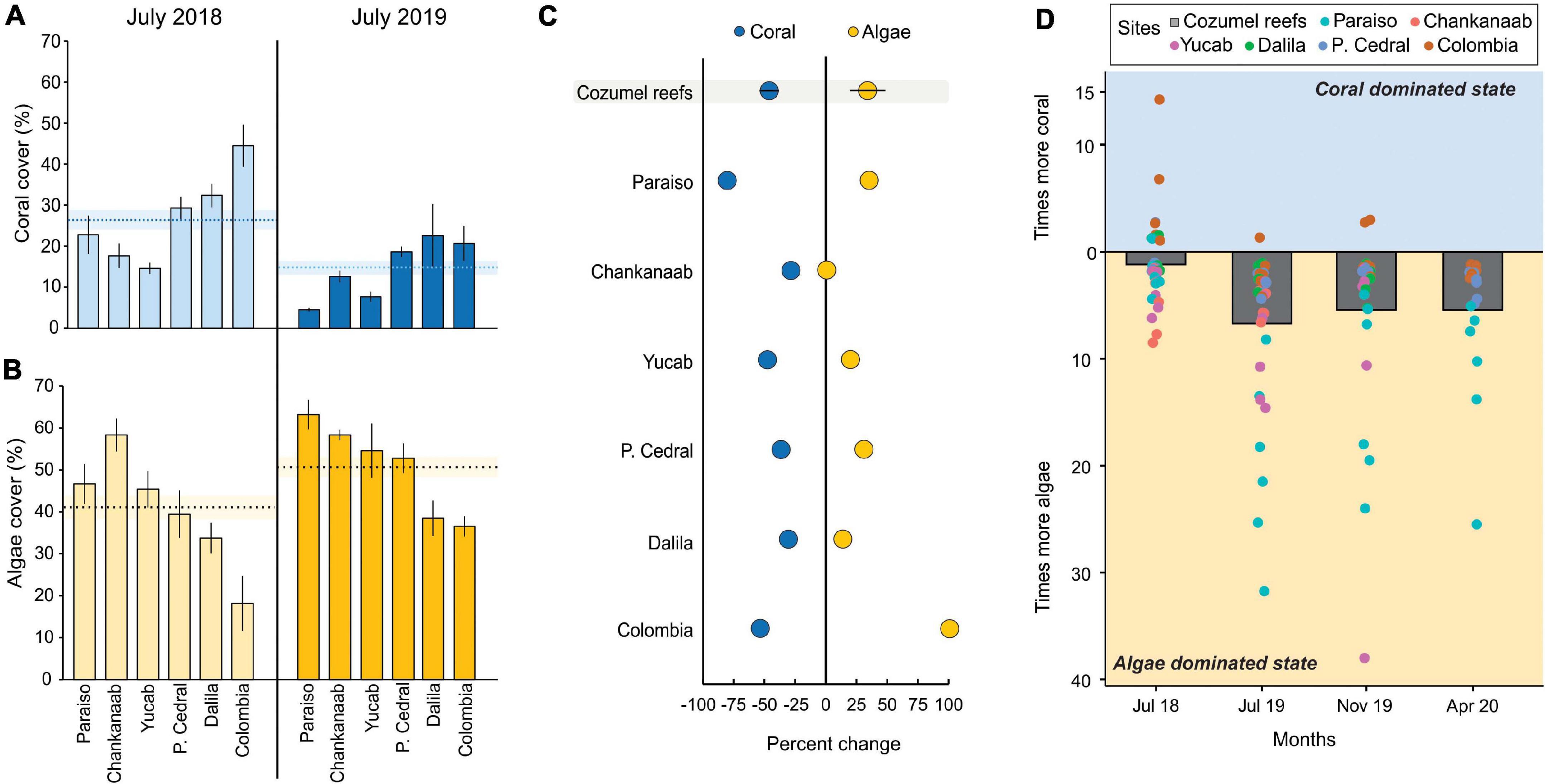
Figure 3. Benthic cover changes for six reefs in southwestern (SW) Cozumel. (A) Coral cover and (B) algae cover in July 2018 and 2019. The dotted lines represent the mean cover for all reefs, and shaded lines are the standard error. (C) The rate of change of coral and macroalgae cover between July 2018 and 2019 for each site and the mean, including all Cozumel reefs (n = 6). (D) Dominant states of algae and corals for July 2018, July 2019, November 2019, and April 2020. Each dot represents a transect for each site, and the bars are the pooled data for Cozumel. For (A–C), the error bands correspond to the standard error of the mean.
Bleaching began to be observed on the southwestern reefs of Cozumel in July 2019 (Figure 4A); however, the effects of sustained thermal stress became evident in October and November 2019 when a rapid increase in the percentage of bleached colonies was observed for all species (Figure 4B), which coincided with the period in which the accumulation of thermal stress exceeded 4 DHW (°C-weeks; Figure 4A). Furthermore, it is likely that the thermal stress was even higher than what was derived from SST given that between August and October 2019, the underwater temperature was considerably higher (+0.5°C) than the satellite derived SST (Figure 4A). An analysis of variance yielded significant differences in coral cover among surveyed periods [F(3, 102) = 8.83, p < 0.01], but the post-hoc Tukey test showed that coral cover was only significantly different between July 2018 and the rest of the surveyed periods (Figure 4B). This suggests that the increase of bleached colonies observed in October and November 2019 did not further decline coral cover.
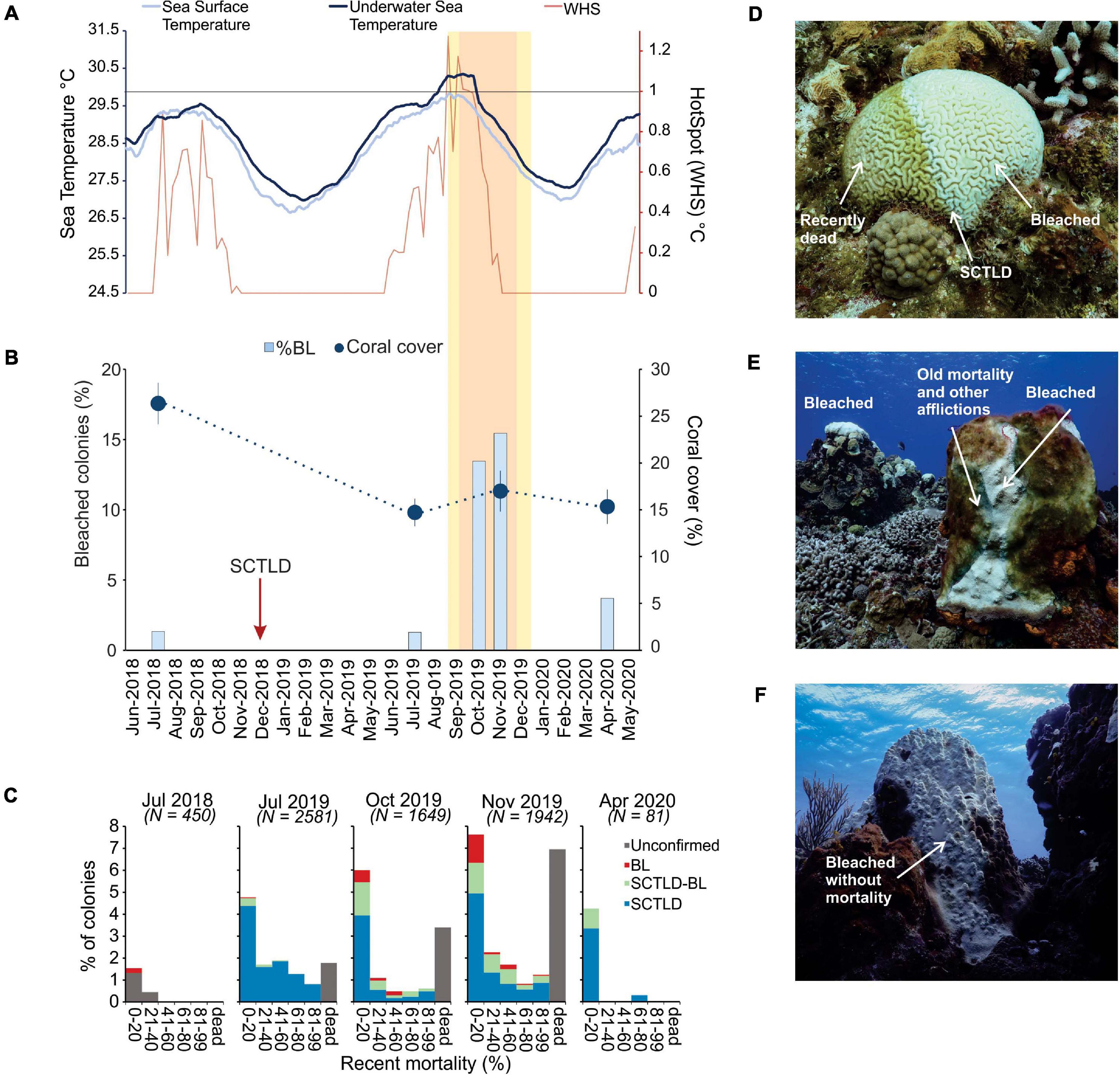
Figure 4. Bleaching and temperature trends for the reefs in southwestern (SW) Cozumel. (A) Thirty-day average of underwater sea temperature in dark blue. Thirty-day average of the daily night-time sea surface temperature in light blue. Weekly HotSpots (WHS) in red. Yellow (1–4°C-weeks) and orange (4–8°C-weeks) boxes indicate periods of prolonged accumulated thermal stress represented by the degree heating weeks. (B) Percentage of bleached colonies for the months of July 2018, July 2019, October 2019, November 2019, and April 2020 and the overall average (±SE) coral cover for all species. In October 2019, only the coral community surveys were conducted (see details in section “Materials and Methods”). (C) Percentage of colonies for each recent mortality category. (D) Diploria labyrinthiformis with lesions due to SCTLD (center of the colony), recent mortality covered by thin layer of filamentous algae, and bleached living coral tissue. (E) Colony of Orbicella faveolata with lesions of SCTLD and 100% bleaching (BL). (F) Colony of O. faveolata that presented 90% bleaching but not mortality or signs of the disease. In (C) BL = new mortality only associated with bleaching, SCTLD-BL = mortality associated with BL and SCTLD [depicted in (D) and (F)], SCTLD = mortality only associated with SCTLD, and unconfirmed indicates that it was not possible to associate mortality to either BL or SCTLD (e.g., colony completely dead). Photo credits: Lorenzo Alvarez-Filip.
Despite widespread coral bleaching, coral mortality resulting from bleaching was low (Figure 4C). Throughout the study period, the largest proportions of colonies with recent mortality were those with clear signs of being afflicted by SCTLD. A relatively small proportion of colonies (usually with a low percentage of tissue mortality) were afflicted by either bleaching and SCTLD or by only bleaching (Figures 4C–F). Overall, in October and November, the mortality associated with bleaching was found in 1–2% of the coral colonies, while the vast majority of bleaching was found near the lesions left by SCTLD (where other stressors, such as cyanobacteria, were also present; Figures 4D,E). Even in colonies that were not visually afflicted by SCTLD and that presented 100% bleaching, no evidence of tissue mortality was observed (Figure 4F). By April 2020, coral mortality (including total colony mortality) decreased significantly, and only a small percentage of colonies showed signs of SCTLD or recent mortality.
Discussion
In 2019, SCTLD disrupted coral reefs located along the leeward coast of Cozumel. The afflicted colonies observed during the surveys presented similar signs to those of colonies that had been previously reported in 2014 in Florida and to those of colonies that were later found elsewhere in the Mexican Caribbean (Precht et al., 2016; Alvarez-Filip et al., 2019). The disease was first observed in the northernmost site (i.e., Paraiso reef), but then it rapidly spread throughout SW Cozumel, causing significant coral mortality and a loss of coral cover. This rapid spread in Cozumel corresponds to what has been observed for the rest of the Mexican Caribbean, in which SCTLD was found to spread along ∼ 400 km (linear) of coastline in less than a year (Alvarez-Filip et al., 2019). The increase in coral mortality left available substrate for other organisms to colonize and grow, and in this study, a significant increase in algae cover was found after the outbreak. However, although coral diseases have been widely related to thermal stress events (Rogers et al., 2009; Randall and Van Woesik, 2017), our study indicates that the severe thermal stress of autumn 2019 did not enhance coral mortality nor did the number of diseased colonies increase.
The means by which SCTLD arrived in SW Cozumel remains uncertain, particularly because Cozumel is separated from the mainland by a deep-water channel in which the strong northward Yucatan Current passes, acting as a barrier to biological connections. However, we can speculate on three possible causes of the outbreak that are not mutually exclusive. First, given that SCTLD was present on the eastern coast of Cozumel since at least September 2018 (Alvarez-Filip et al., 2019; Figure 1), it is possible that the outbreak circled the island, aided by the predominant currents that run from south to north (Alcérreca-Huerta et al., 2019). Another potential source for the outbreak may be that recreational divers or divemasters brought the disease to SW Cozumel from the mainland. Recreational diving is a common activity across the Mexican Caribbean and it is common for divers to travel among multiple locations within a single trip. As such, divers could have brought the disease with them via their diving gear (e.g., NOAA, 2019). Lastly, it is feasible that the high influx of ships arriving to Cozumel served as a vector for the disease. Cozumel has three piers that receive more than one thousand cruise ships a year, and one of the most important cruise ship piers is located near Paraiso reef (where the disease was first reported; Figure 1A). The other two piers are located to the north within a few km of Paraiso reef. In addition, Cozumel has two piers (also 3–5 km to the north of Paraiso) that serve to connect the mainland with the island. Ferries depart from and arrive to the island in a semi-continuous manner. Ballast water from commercial ships has been linked to the transmission of SCTLD across the Caribbean region (Baigent, Unpublished), and other coral epizootics have also been heavily associated with shipping vectors, predominantly through ballast water discharges of commercial sea traffic (Aguirre-Macedo et al., 2008; Khandeparker and Anil, 2017).
Once the SCTLD outbreak reached the reefs in SW Cozumel, it spread very rapidly. Assuming that subsequent infections arose from Paraiso reef, our observations suggest that it only took ∼ 25 days for the disease to spread across the 20-km reef system of SW Cozumel (January 18, 2019, to February 12, 2019; Figure 1). This is a rate of progression of nearly 1 km d–1, which is considerably faster than what has been reported in Florida (∼ 0.1 km d–1; Muller et al., 2020). As previously mentioned, SW Cozumel hosts an important influx of divers that usually visit at least two reefs per day over multiple days. This results in hundreds of divers moving between reefs across the 20-km length of the MPA each day. Given that dive gear can harbor and proliferate pathogenic bacteria, which may survive for extended periods and can be transferred among reefs (NOAA, 2019), by remaining damp and trapping water, it is plausible that if equipment was not rigorously disinfected between dives, divers may have helped to spread the disease throughout the MPA. In addition, strong coastal currents and eddies that are formed when oceanic currents approach the coast (Alcérreca-Huerta et al., 2019) can also aid in the rapid waterborne transmission of SCTLD across and between reef sites. Regardless of whether the disease was rapidly transported by divers and/or currents, once SCTLD arrived to the reefs, it is possible that the abundant and diverse coral reef communities that were present in most sites also aided in rapid within-site transmission.
Nearly a third of all Caribbean coral species are known to be affected by SCTLD. In addition to all species that are well-known to be afflicted by this disease (FDEP, 2018; Alvarez-Filip et al., 2019), we also found species that have not yet been reported as being highly susceptible to SCTLD, such as Helioseris cucullata, Isophyllia rigida, and Agaricia tenuifolia (Figure 2A), probably because these are common species in the area. Yet, the overall prevalence and mortality observed for highly susceptible species in SW Cozumel is similar to what has been reported elsewhere in Mexico, in Florida, and in other regions of the Caribbean (Precht et al., 2016; Alvarez-Filip et al., 2019; Gintert et al., 2019; Roth et al., 2020). Similar to what was observed in Florida, we also observed that some of the most susceptible species disappeared from long-term monitoring sites (e.g., Meandrina spp.).
Although we did not register colonies of D. cylindrus, one of the species that is most affected by SCTLD (Alvarez-Filip et al., 2019; Neely and Lewis, 2020), an extensive effort (led by the Arrecifes de Cozumel National Park and local brigades) identified eight colonies of D. cylindrus within the boundaries of the MPA (one shown in Figure 2C). Four of these colonies received treatment with antibiotics with the intention of halting disease progression (sensu Neely et al., 2020). By summer 2020, of the eight initially observed colonies, four were dead (two of which received treatment), two colonies were kept alive with continuous antibiotic treatment, and only two remained alive with no visible signs of infection without receiving any treatment (B.Q.G, pers. comm.). These last two colonies were located very close to the rocky shore in shallow water (1–2 m) and far from diving activities and thus were probably not influenced by the main currents and eddies that connect the main reef system of Cozumel (Alcérreca-Huerta et al., 2019).
The biological consequences of the severe mortality of some coral species, such as Meandrina spp. and D. cylindrus, are yet to be fully understood, but it is likely that this outbreak has resulted in the isolation of the remaining colonies, which will reduce or halt the capacity for sexual reproduction in these species. The persistence of these reproduction dynamics may lead to an Allee effect, a positive relationship between the per capita population growth rate and density, which is hypothesized to increase extinction rates when population size or density falls below a certain threshold (Knowlton, 1992). While treatment with antibiotics (or probiotics) seems to have aided some colonies, both in Cozumel and elsewhere (Neely et al., 2020; Paul et al., 2020), a priority for future research must be to assess the plausibility of conducting these kinds of interventions over periods of months to years as they are labor intensive and expensive. In particular, it is necessary to evaluate whether treated corals are able to recover and/or sustain basic biological and physiological processes, such as growth and sexual reproduction. Moreover, it is necessary to evaluate the consequences of widespread application of antibiotics in coral reef systems.
Severe ecological changes in the benthic composition of Cozumel reefs were caused by the extensive tissue mortality produced by SCTLD, reducing competition for substrate, and opening substrate for colonization of other reef benthic organisms. Before the outbreak, the mean coral cover in SW Cozumel reached 26%, although some reefs located further to the south (e.g., Colombia) had mean coral covers >45% (Figure 3) that resembled the historical baselines for Cozumel and the Caribbean region (Fenner, 1988; Jackson et al., 2014; Contreras-Silva et al., 2020). After the outbreak, live coral cover decreased by half, and filamentous algae rapidly colonized the newly available substrate left by dead coral tissue (Figure 1D and Figure 3). Coral mortality resulting from SCTLD is clearly consistent with the side-in model of reef degradation that invokes perturbations causing coral mortality as the primary factor favoring the rapid colonization of available space by non-accretional organisms (Precht et al., 2020). Furthermore, it may also prevent coral recruitment and thus the recovery of populations that are highly affected by SCTLD (e.g., Birrell et al., 2005; Arnold et al., 2010; Brandt et al., 2019; Tebbett and Bellwood, 2019); a scenario that may worsen if stressors become more frequent and/or severe. In addition, the loss of highly susceptible coral species may also trigger an abrupt decrease in the accretion (growth) of the reef framework (Estrada-Saldívar et al., 2020), as most of the highly susceptible species to SCTLD are also important reef builders. A shift in the composition of reef-building corals and reef structure may not only compromise ecosystem resilience through a reduction in functional traits (González-Barrios et al., 2020; Molina-Hernández et al., 2020) but may also affect many other reef-associated species (Idjadi and Edmunds, 2006; Rogers et al., 2014). Given the short-temporal scale of our study, we cannot conclude that the drastic changes observed in the ecological communities will lock Cozumel reefs into low-carbonate production and high algae dominated states. This is because reef dynamics are driven by a complex network of processes and interactions, such as coral recovery or competition for space among other benthic organisms with rapid rates of colonization, such as incrusting sponges or tunicates, which needs to be investigated further. Therefore, a management and research priority for the near future is to continue conducting surveys in these reefs and in many others afflicted by SCTLD in the Caribbean to explore the long-term ecological implications of coral mortality resulting from SCTLD.
Our findings show that neither disease prevalence nor the rate of tissue loss in infected colonies increased as water temperatures warmed. This coincides with what was found in Florida, where disease onset co-occurred with high water temperatures that may have aided the emergence of SCTLD (Precht et al., 2016; van Woesik and McCaffrey, 2017; Walton et al., 2018), but thermal stress may not have necessarily prolonged its spread (Muller et al., 2020). This suggests that SCTLD is not directly influenced by thermal stress and that other factors, such as currents, water quality, or sedimentation may also enhance the severity of the disease (Dobbelaere et al., 2020; Muller et al., 2020; Iwanowicz et al., 2020). However, it is important to highlight that the pathogens associated with SCTLD may be opportunistic, infecting corals that are already vulnerable and stressed by temperature, sedimentation, or water pollution (Meyer et al., 2019). In addition, thermal stress influences key physiological functions in corals, such as reproduction, growth, and calcification, all of which are essential for corals to survive and recover (Baird and Marshall, 2002; Manzello, 2010). Thus, although there is no clear evidence of a relationship between thermal stress and SCTLD, it is likely that the predicted increase in thermal stress in Caribbean reefs may reduce the capacity of populations of highly susceptible species to recover from SCTLD and may even further exacerbate coral mortality. Our findings suggest that in addition to thermal stress, it is necessary to analyze the role of other chronic sources of stress (e.g., decreasing seawater quality) to better understand the response of scleractinian corals to increasingly variable environmental conditions.
Although SCTLD had severe negative consequences on coral communities of Cozumel reefs, it also triggered a coordinated and dedicated collaborative effort between academics, MPA authorities, and members of the tourism sector to track the disease and raise awareness among the Cozumel community. Of particular importance for this study was the support of the local dive shops and divemasters that facilitated the initial surveys that were conducted to track the progression of SCTLD (January-March 2019). It is well-known that involving local communities in monitoring efforts builds public support for management initiatives (Conrad and Hilchey, 2011). In our experience, this collaboration resulted in the generation of human resources in the form of dive masters and local divers, who have continued to report to the MPA authorities while participating in citizen science surveys and educating tourists about coral disease and the importance of conserving coral reefs. Public awareness of the catastrophic coral mortality event rose to such an extend within the MPA advisory board that in the summer of 2019, a temporary closure was implemented in which tourist and recreational activities in some of the southern reefs of the MPA were restricted. These actions were intended to give the reefs an opportunity to rest and to recover from SCTLD (CONANP, 2019). These actions later became a program that stipulates rotating temporal closures across all dive sites in the MPA.
In Cozumel, as well as in many other areas afflicted by SCTLD, a growing interest in engaging in proactive activities, such as treating colonies with antibiotics, rescuing colonies of susceptible species, and collecting and conserving genetic material, has emerged (Neely et al., 2020). We believe a robust scientific framework should support all of these activities; however, it is also fundamental that a better understanding is cultivated concerning the putative infectious agents and environmental conditions that favored the rapid appearance and dispersion of SCTLD. In addition, it is important to be aware that the palliative measures used to ameliorate the damage caused by SCTLD will only succeed if the chronic threats to reefs, such as poor water quality, pollution, and the effects of climate change, are controlled. Joint efforts to develop better management actions may help to improve the environmental conditions for coral regrowth while enhancing the recovery and resilience of these ecosystems.
Data Availability Statement
All datasets generated for this study are included in the article/Supplementary Material, further inquiries can be directed to the corresponding author/s.
Author Contributions
NE-S and LA-F conceived and wrote the initial draft. NE-S, LA-F, EP-C, OOR-G, and BAQ-G collected the data. NE-S and EP-C conducted the data analysis. All authors read and approved the content of the submitted version.
Funding
This study was supported by the Comisión Nacional de Áreas Naturales Protegidas of Mexico (PROREST/CER/56/2019) and the Mexican Council of Science and Technology (CONACyT, PDC-247104).
Conflict of Interest
The authors declare that the research was conducted in the absence of any commercial or financial relationships that could be construed as a potential conflict of interest.
Acknowledgments
We want to thank the different tourism service providers, namely Careyitos, Cozumel SCUBA planet, and Dive with Martin, for their support during the field trips of February and March 2019. We also thank A. Molina-Hernández, I. Cruz-Ortega, E. Hevia del Puerto, I. Moreno-Rodríguez, and N. Hernández-Palacios for greatly assisting with data collection and logistics.
Supplementary Material
The Supplementary Material for this article can be found online at: https://www.frontiersin.org/articles/10.3389/fmars.2021.632777/full#supplementary-material
References
Aeby, G. S., Ushijima, B., Campbell, J. E., Jones, S., Williams, G. J., Meyer, J. L., et al. (2019). Pathogenesis of a tissue loss disease affecting multiple species of corals along the Florida Reef tract. Front. Mar. Sci. 6:678. doi: 10.3389/fmars.2019.00678
Aguirre-Macedo, M. L., Vidal-Martinez, V. M., Herrera-Silveira, A. J., Valdés-Lozano, D. S., Herrera-Rodríguez, M., and Olvera-Novoa, M. A. (2008). Ballast water as a vector of coral pathogens in the Gulf of Mexico: the case of the cayo arcas coral reef. Mar. Pollut. Bull. 56, 1570–1577. doi: 10.1016/j.marpolbul.2008.05.022
Alcérreca-Huerta, J. C., Encarnacion, J. I., Ordoñez-Sánchez, S., Callejas-Jiménez, M., Barroso, G. G. D., Allmark, M., et al. (2019). Energy yield assessment from ocean currents in the insular shelf of Cozumel Island. J. Mar. Sci. Eng. 7:147. doi: 10.3390/jmse7050147
Almada-Villela, P. C., Sale, P. F., Gold-Bouchot, G., and Kjerfve, B. (2003). Manual de Métodos Para el Programa de Monitoreo Sinóptico del SAM. Belice: Coastal Resources Multi-complex Building.
Alvarez-Filip, L., Estrada-Saldívar, N., Pérez-Cervantes, E., Molina-Hernández, A., and González-Barrios, F. J. (2019). A rapid spread of the stony coral tissue loss disease outbreak in the mexican caribbean a rapid spread of the stony coral tissue loss disease outbreak in the Mexican Caribbean. PeerJ 7:e8069. doi: 10.7717/peerj.8069
Arnold, S. N., Steneck, R. S., and Mumby, P. J. (2010). Running the gauntlet: inhibitory effects of algal turfs on the processes of coral recruitment. Mar. Ecol. Prog. Ser. 414, 91–105. doi: 10.3354/meps08724
Baird, A. H., and Marshall, P. A. (2002). Mortality, growth and reproduction in scleractinian corals following bleaching on the Great Barrier Reef. Mar. Ecol. Prog. Ser. 237, 133–141. doi: 10.3354/meps237133
Ban, S. S., Graham, N. A. J., and Connolly, S. R. (2014). Evidence for multiple stressor interactions and effects on coral reefs. Glob. Chang. Biol. 20, 681–697. doi: 10.1111/gcb.12453
Birrell, C. L., McCook, L. J., and Willis, B. L. (2005). Effects of algal turfs and sediment on coral settlement. Mar. Poll. Bull. 51, 408–414. doi: 10.1016/j.marpolbul.2004.10.022
Bozec, Y.-M., Alvarez-Filip, L., and Mumby, P. J. (2015). The dynamics of architectural complexity on coral reefs under climate change. Glob. Chang. Biol. 21, 223–235. doi: 10.1111/gcb.12698
Brandt, M. E., Olinger, L. K., Chaves-Fonnegra, A., Olson, J. B., and Gochfeld, D. J. (2019). Coral recruitment is impacted by the presence of a sponge community. Mar. Biol. 166:49. doi: 10.1007/s00227-019-3493-3495
Bruno, J. F., Selig, E. R., Casey, K. S., Page, C. A., Willis, B. L., Harvell, C. D., et al. (2007). Thermal stress and coral cover as drivers of coral disease outbreaks. PLoS Biol. 5:e124. doi: 10.1371/journal.pbio.0050124
Budd, A. F., Fukami, H., Smith, N. D., and Knowlton, N. (2012). Taxonomic classification of the reef coral family Mussidae (Cnidaria: Anthozoa: Scleractinia). Zool. J. Linn. Soc. 166, 465–529. doi: 10.1111/j.1096-3642.2012.00855.x
Cerdeira-Estrada, S., Martell-Dubois, R., Valdéz-Chavarin, J., Rosique-de la Cruz, L., Perera-Valderrama, S., López-Perea, J., et al. (2018). Sistema de Información y Análisis Marino-Costero (SIMAR). México: CONABIO.
Chávez, G., Candela, J., and Ochoa, J. (2003). Subinertial flows and transports in Cozumel Channel. J. Geophys. Res. Ocean 108, 1–11. doi: 10.1029/2002jc001456
CONANP (2019). Cozumel Protege y Conserva sus Arrecifes de Coral Para el Futuro. Available online at: https://www.gob.mx/conanp/prensa/cozumel-protege-y-conserva-sus-arrecifes-de-coral-para-el-futuro-222281
CONANP-GIZ (2017). Valoración de los Servicios Ecosistémicos del Parque Nacional Arrecifes de Cozumel y Área de Protección de Flora y Fauna Isla Cozumel. Ciudad de México: CONANP-GIZ.
Conrad, C. C., and Hilchey, K. G. (2011). A review of citizen science and community-based environmental monitoring: issues and opportunities. Environ. Monit. Assess. 176, 273–291. doi: 10.1007/s10661-010-1582-1585
Contreras-Silva, A., Tilstra, A., Migani, V., Thiel, A., Pérez-Cervantes, E., Estrada-Saldívar, N., et al. (2020). A meta-analysis to assess long-term spatiotemporal changes of benthic coral and macroalgae cover in the Mexican caribbean. Sci. Rep. 10, 1–12. doi: 10.1038/s41598-020-65801-65808
Cróquer, A., and Weil, E. (2009). Changes in Caribbean coral disease prevalence after the 2005 bleaching event. Dis. Aquatic Organ. 87, 33–43. doi: 10.3354/dao02164
Darling, E. S., Mcclanahan, T. R., and Côté, I. M. (2010). Combined effects of two stressors on Kenyan coral reefs are additive or antagonistic, not synergistic. Conserv. Lett. 3, 122–130. doi: 10.1111/j.1755-263X.2009.00089.x
Dobbelaere, T., Muller, E. M., Gramer, L., and Holstein, D. (2020). Report on the potential origin of the SCTLD in the Florida Reef Tract. Tallahassee, FL: Florida Department of Environmental Protection.
Eakin, C. M., Morgan, J. A., Heron, S. F., Smith, T. B., Liu, G., Alvarez-Filip, L., et al. (2010). Caribbean corals in crisis: record thermal stress, bleaching, and mortality in 2005. PLoS One 5:e13969. doi: 10.1371/journal.pone.0013969
Elías-Ilosvay, X. E., Contreras-Silva, A. I., Alvarez-Filip, L., and Wild, C. (2020). Coral reef recovery in the Mexican Caribbean after 2005 mass coral mortality—potential drivers. Diversity 12:338. doi: 10.3390/d12090338
Estrada-Saldívar, N., Molina-Hernández, A., Pérez-Cervantes, E., Medellín-Maldonado, F., González-Barrios, F. J., and Alvarez-Filip, L. (2020). Reef-scale impacts of the stony coral tissue loss disease outbreak. Coral Reefs 39, 861–866. doi: 10.1007/s00338-020-01949-z
Fenner, D. P. (1988). Some leeward reefs and corals of Cozumel. Mexico. Bull. Mar. Sci. 42, 133–144.
Gintert, B. E., Precht, W. F., Fura, R., Rogers, K., Rice, M., Precht, L. L., et al. (2019). Regional coral disease outbreak overwhelms impacts from local dredge project. Environ. Monit. Assess. 191, 1–39. doi: 10.1007/s10661-019-7767-7767
Gladfelter, W. B. (1982). White-band disease in Acropora palmata: implications for the structure and growth of shallow reefs. Bull. Mar. Sci. 32, 639–643.
González-Barrios, F. J., Cabral-Tena, R., and Alvarez-Filip, L. (2020). Recovery disparity between coral cover and the physical functionality of reefs with impaired coral assemblage. Glob. Chang. Biol. 27, 640–651. doi: 10.1111/gcb.15431
Harvell, C. D., Jordán-Dahlgren, E., Merkel, S., Rosenberg, E., Raymundo, L., Smith, G., et al. (2007). Coral diseases, environmental drivers and the balance between corals and microbial associates. Oceanography 20, 172–195. doi: 10.5670/oceanog.2007.91
Hill, J., and Wilkinson, C. (2004). Methods for Ecological Monitoring Of Coral Reefs. Townsville, TSV: Australian Institute of Marine Science. doi: 10.1017/CBO9781107415324.004
Hoegh-Guldberg, O. (1999). Climate change, coral bleaching and the future of the world’s coral reefs. Mar. Freshw. Res. 50, 839–866. doi: 10.1071/MF99078
Hughes, T. P., Barnes, M. L., Bellwood, D. R., Cinner, J. E., Cumming, G. S., Jackson, J. B. C., et al. (2017). Coral reefs in the Anthropocene. Nature 546, 82–90. doi: 10.1038/nature22901
Hughes, T. P., Kerry, J. T., Baird, A. H., Connolly, S. R., Dietzel, A., Eakin, C. M., et al. (2018). Global warming transforms coral reef assemblages. Nature 556, 492–496. doi: 10.1038/s41586-018-0041-42
Idjadi, J. A., and Edmunds, P. J. (2006). Scleractinian corals as facilitators for other invertebrates on a Caribbean reef. Mar. Ecol. Prog. Ser. 319, 117–127. doi: 10.3354/meps319117
Iwanowicz, D. D., Schill, W. B., Woodley, C. M., Bruckner, A. W., Neely, K., and Briggs, K. M. (2020). Exploring the stony coral tissue loss disease bacterial pathobiome. bioRxiv [preprint].
Jackson, J. B. C., Donovan, M. K., Cramer, K. L., Lam, V., and Lam, W. (2014). “Status and trends of caribbean coral reefs: 1970–2012,” in Glob. Coral Reef Monit. Network (Switz., Gland: IUCN), 306.
Jokiel, P. L., Rodgers, K. S., Brown, E. K., Kenyon, J. C., Aeby, G., Smith, W. R., et al. (2015). Comparison of methods used to estimate coral cover in the Hawaiian Islands. PeerJ 3:e954. doi: 10.7717/peerj.954
Jordán-Dahlgren, E. (1988). Arrecifes profundos de la Isla de Cozumel, Mexico. An. del Inst. Mexico: Ciencias del Mar y Limnol. - UNAM.
Khandeparker, L., and Anil, A. C. (2017). “Global concerns of ship’s ballast water mediated translocation of bacteria,” in Marine Pollution and Microbial Remediation, eds M. M. Naik and S. K. Dubey (Singapore: Springer), 255–262. doi: 10.1007/978-981-10-1044-6_16
Knowlton, N. (1992). Thresholds and multiple stable states in coral reef community dynamics. Integr. Comp. Biol. doi: 10.1093/icb/32.6.674
Kramer, P. R., Roth, L., and Lang, J. (2020). Map of Coral Cover of Susceptible Coral Species to SCTLD. Redlands, CA: ArcGIS
Lang, J. C., Marks, K. W., Kramer, P. R., and Kramer, P. A. (2012). Protocolos AGRRA 5.5. 1–44. Available online at: http://www.healthyreefs.org/cms/wpcontent/uploads/2013/07/Protoc.-AGRRA-V5.5-FINAL-Espanol.pdf (accessed September 10, 2020).
Liu, G., Heron, S. F., Mark Eakin, C., Muller-Karger, F. E., Vega-Rodriguez, M., Guild, L. S., et al. (2014). Reefscale thermal stress monitoring of coral ecosystems: new 5-km global products from NOAA coral reef watch. Remote Sens. 6, 11579–11606. doi: 10.3390/rs61111579
Magdaong, E. T., Fujii, M., and Yamano, H. (2014). Long-term change in coral cover and the effectiveness of marine protected areas in the Philippines?: a meta-analysis. Hydrobiologia 733, 5–17. doi: 10.1007/s10750-013-1720-1725
Manzello, D. P. (2010). Coral growth with thermal stress and ocean acidification: lessons from the eastern tropical Pacific. Coral Reefs 29, 749–758. doi: 10.1007/s00338-010-0623-624
Manzello, D. P. (2015). Rapid recent warming of coral reefs in the Florida keys. Sci. Rep. 5, 1–10. doi: 10.1038/srep16762
Martínez-Rendis, A., Acosta-González, G., and Arias-González, J. E. (2020). A spatio-temporal long-term assessment on the ecological response of reef communities in a Caribbean marine protected area. Aquat. Conserv. Mar. Freshw. Ecosyst. 30, 273–289. doi: 10.1002/aqc.3263
Maynard, J. A., Van Hooidonk, R., Eakin, C. M., Puotinen, M., Garren, M., Williams, G., et al. (2015). Projections of climate conditions that increase coral disease susceptibility and pathogen abundance and virulence. Nat. Clim. Chang 5, 688–694. doi: 10.1038/nclimate2625
McField, M., Kramer, P., Giró-Petersen, A., Soto, M., Drysdale, I., Craig, N., et al. (2020). Available online at: https://www.agrra.org/wpcontent/uploads/2020/04/2020_Report_Card_MAR-HRI.pdf Mesoamerican Reef Report Card (accessed September 10, 2020).
Meyer, J. L., Castellanos-Gell, J., Aeby, G. S., Häse, C., Ushijima, B., and Paul, V. J. (2019). Microbial community shifts associated with the ongoing stony coral tissue loss disease outbreak on the Florida Reef Tract. Front. Microbiol. 10:2244. doi: 10.3389/fmicb.2019.02244
Miller, M. W., Karazsia, J., Groves, C. E., Griffin, S., Moore, T., Wilber, P., et al. (2016). Detecting sedimentation impacts to coral reefs resulting from dredging the Port of Miami, Florida USA. PeerJ 4:e2711. doi: 10.7717/peerj.2711
Molina-Hernández, A., González-Barrios, F. J., Perry, C. T., and Álvarez-Filip, L. (2020). Two decades of carbonate budget change on shifted coral reef assemblages: are these reefs being locked into low net budget states?: Caribbean reefs carbonate budget trends. Proc. R. Soc. B Biol. Sci. 287, 1–9. doi: 10.1098/rspb.2020.2305rspb20202305
Muckelbauer, G. (1990). The shelf of Cozumel, Mexico: topography and organisms. Facies 23, 201–239. doi: 10.1007/BF02536714
Muller, E. M., Sartor, C., Alcaraz, N. I., and van Woesik, R. (2020). Spatial epidemiology of the stony-coral-tissue-loss disease in Florida. Front. Mar. Sci. 7:163. doi: 10.3389/fmars.2020.00163
Nadon, M. O., and Stirling, G. (2006). Field and simulation analyses of visual methods for sampling coral cover. Coral Reefs 25, 177–185. doi: 10.1007/s00338-005-0074-5
Neely, K. (2018). Surveying the Florida Keys Southern Coral Disease Boundary. Tallahassee Fl: Florida DEP.
Neely, K. L., Macaulay, K. A., Hower, E. K., and Dobler, M. A. (2020). Effectiveness of topical antibiotics in treating corals affected by stony coral tissue loss disease. PeerJ 8:e9289. doi: 10.1101/870402
Neely, K. L., and Lewis, C. L. (2020). Rapid population decline of the pillar coral dendrogyra cylindrus along the florida reef tract. bioRxiv [preprint]. doi: 10.1101/2020.05.09.085886
Oliver, E. C. J., Donat, M. G., Burrows, M. T., Moore, P. J., Smale, D. A., Alexander, L. V., et al. (2018). Longer and more frequent marine heatwaves over the past century. Nat. Commun. 9:1324. doi: 10.1038/s41467-018-03732-3739
Paul, V. J., Ushijima, B., Pitts, K., and Garg, N. (2020). Development of Probiotics and Alternative Treatments for Stony Coral Tissue Loss Disease. Tallahassee, FL: Florida Department of Environmental Protection.
Perry, C. T., and Alvarez-Filip, L. (2019). Changing geo-ecological functions of coral reefs in the Anthropocene. Funct. Ecol. 33, 976–988. doi: 10.1111/1365-2435.13247
Pinzón, J. H., Kamel, B., Burge, C. A., Harvell, C. D., Medina, M., Weil, E., et al. (2015). Whole transcriptome analysis reveals changes in expression of immune-related genes during and after bleaching in a reef-building coral. R. Soc. Open Sci. 2:140214. doi: 10.1098/rsos.140214
Precht, W. F., Aronson, R. B., Gardner, T. A., Gill, J. A., Hawkins, J. P., Hernández-Delgado, E. A., et al. (2020). The timing and causality of ecological shifts on Caribbean reefs. Adv. Mar. Biol. 87, 331–360. doi: 10.1016/bs.amb.2020.08.008
Precht, W. F., Gintert, B. E., Robbart, M. L., Fura, R., and van Woesik, R. (2016). Unprecedented disease-related coral mortality in Southeastern Florida. Sci. Rep. 6:31374. doi: 10.1038/srep31374
Randall, C. J., and Van Woesik, R. (2017). Some coral diseases track climate oscillations in the Caribbean. Sci. Rep. 7:5719. doi: 10.1038/s41598-017-05763-5766
Reed, K. C., Muller, E. M., and Van Woesik, R. (2010). Coral immunology and resistance to disease. Dis. Aquat. Organ. 90, 85–92. doi: 10.3354/dao02213
Rippe, J. P., Kriefall, N. G., Davies, S. W., and Castillo, K. D. (2019). Differential disease incidence and mortality of inner and outer reef corals of the upper Florida Keys in association with a white syndrome outbreak. Bull. Mar. Sci. 95, 305–316. doi: 10.5343/bms.2018.0034
Rogers, A., Blanchard, J. L., and Mumby, P. J. (2014). Vulnerability of coral reef fisheries to a loss of structural complexity. Curr. Biol. 24, 1000–1005. doi: 10.1016/j.cub.2014.03.026
Rogers, C. S., Muller, E. M., Spitzack, T., and Miller, J. (2009). Extensive coral mortality in the US Virgin Islands in 2005/2006: a review of the evidence for synergy among thermal stress, coral bleaching and disease. Caribb. J. Sci. 45, 204–214. doi: 10.18475/cjos.v45i2.a8
Rosales, S. M., Clark, A. S., Huebner, L. K., Ruzicka, R. R., and Muller, E. M. (2020). Rhodobacterales and Rhizobiales are associated with stony coral tissue loss disease and its suspected sources of transmission. Front. Microbiol. 11:681. doi: 10.3389/fmicb.2020.00681
Roth, L., Kramer, P. R., Doyle, E., and O’Sullivan, C. (2020). Caribbean SCTLD Dashboard. Redlands, CA: ArcGIS
Searle, L., Chapman, J., Carne, L., and Arnold, S. (2014). Belize Coral Bleaching Response and Management Plan 2008-2013: An Overview of the Response, Management Activities and Reccomendations. Sakamkam: ECOMAR.
Shore-Maggio, A., Callahan, S. M., and Aeby, G. S. (2018). Trade-offs in disease and bleaching susceptibility among two color morphs of the Hawaiian reef coral. Montipora capitata. Coral Reefs 37, 507–517. doi: 10.1007/s00338-018-1675-1670
Sokolow, S. H. (2009). Effects of a changing climate on the dynamics of coral infectious disease: a review of the evidence. Dis. Aquat. Organ. 87, 5–18. doi: 10.3354/dao02099
Tebbett, S. B., and Bellwood, D. R. (2019). Algal turf sediments on coral reefs: what’s known and what’s next. Mar. Pollut. Bull. 149:110542. doi: 10.1016/j.marpolbul.2019.110542
van Woesik, R., and McCaffrey, K. R. (2017). Repeated thermal stress, shading, and directional selection in the Florida reef tract. Front. Mar. Sci. 4:182. doi: 10.3389/fmars.2017.00182
Walton, C. J., Hayes, N. K., and Gilliam, D. S. (2018). Impacts of a regional, multi-year, multi-species coral disease outbreak in Southeast Florida. Front. Mar. Sci. 5:323. doi: 10.3389/fmars.2018.00323
Weil, E. (2004). “Coral reef diseases in the wider Caribbean,” in Coral Health and Disease, eds E. Rosenberg and Y. Loya (Berlin: Springer), doi: 10.1007/978-3-662-06414-6_2
Weil, E., Hernández-delgado, E. A., Gonzalez, M., Williams, S., Suleimán-Ramos, S., Figuerola, M., et al. (2019). Spread of the new coral disease “SCTLD” into the Caribbean?: implications for Puerto Rico. Reef Encount. 34, 38–43.
Keywords: SCTLD, disease outbreak, phase-shift, bleaching, coral mortality, white plague, thermal stress
Citation: Estrada-Saldívar N, Quiroga-García BA, Pérez-Cervantes E, Rivera-Garibay OO and Alvarez-Filip L (2021) Effects of the Stony Coral Tissue Loss Disease Outbreak on Coral Communities and the Benthic Composition of Cozumel Reefs. Front. Mar. Sci. 8:632777. doi: 10.3389/fmars.2021.632777
Received: 24 November 2020; Accepted: 09 March 2021;
Published: 30 March 2021.
Edited by:
Jesús Ernesto Arias González, Instituto Politécnico Nacional de México (CINVESTAV), MexicoReviewed by:
Ernesto Weil, University of Puerto Rico at Mayagüez, Puerto RicoCraig W. Osenberg, University of Georgia, United States
Copyright © 2021 Estrada-Saldívar, Quiroga-García, Pérez-Cervantes, Rivera-Garibay and Alvarez-Filip. This is an open-access article distributed under the terms of the Creative Commons Attribution License (CC BY). The use, distribution or reproduction in other forums is permitted, provided the original author(s) and the copyright owner(s) are credited and that the original publication in this journal is cited, in accordance with accepted academic practice. No use, distribution or reproduction is permitted which does not comply with these terms.
*Correspondence: Lorenzo Alvarez-Filip, lorenzo@cmarl.unam.mx