- 1Departamento de Ecología y Territorio, Facultad de Estudios Ambientales y Rurales, Pontificia Universidad Javeriana, Bogotá, Colombia
- 2Applied Ocean Sciences, Fairfax Station, VA, United States
- 3Fundación Macuáticos Colombia, Medellín, Colombia
- 4Neural and Cognitive Plasticity Lab, Department of Psychology, State University of New York at Buffalo, Buffalo, NY, United States
- 5R&E Ocean Community Conservation Foundation, Oakville, ON, Canada
Soundscapes with minimal anthropogenic noise sources are key for the survival and effective communication of marine mammals. The Gulf of Tribugá is part of the breeding ground for humpback whale Stock G. Currently, no large-scale infrastructure exists on the Gulf's coastline, making it an area with high biodiversity and little anthropogenic noise. Whale-watching is one of the few human activities that contributes to the soundscape. By Morro Mico, on the southern limit of the Utría Natural National Park, an Ecological Acoustic Recorder (EAR, Oceanwide Science Institute) was deployed in the Gulf to record samples of acoustic activity from October to November 2018. It recorded for 10-min intervals with 20-min lapses for a duty cycle of 33.3%. One of the common peak frequencies of humpback whale song units from these recordings was used as input to an acoustic propagation model using the parabolic equation to simulate the communication space of a humpback whale when zero, one, and two boats are present. GPS positions of theodolite data from various whale watching scenarios in the Gulf were used to inform the models. Model results indicate that humpback whale song communication space could be reduced by as much as 63% in the presence of even one whale-watching boat. The boats traveling through the Gulf are the same as those used in whale-watching, and their engine noise while passing Morro Mico coincided with song structural and temporal changes observed in the acoustic data. Combining in situ data with acoustic models can advance the understanding of the spatio-temporal acoustic reactions of whales when their vocalizations are masked by boat noise. This project serves as an approximation of how humpback whale Stock G may respond to whale-watching vessel noise in the Gulf of Tribugá.
1. Introduction
Anthropogenic noise has increased in recent years as a result of human population growth, transportation network expansion, urbanization, and resource extraction (Shannon et al., 2016). In the ocean, anthropogenic noise has also increased as shipping lanes have expanded (McDonald et al., 2006; Hildebrand, 2009). Non-shipping human-generated noise sources such as seismic surveys, pile driving for off-shore construction, and military activities also contribute to underwater noise (Tougaard et al., 2009; Wright, 2014). As a result, human-generated noise often overlaps (masks) biological sounds in time and frequency, which could mean a loss of communication space for marine fauna (Cholewiak et al., 2018). For the purposes of this paper, masking is defined as a situation when noise interferes with an animal's ability to detect, interpret, and/or discriminate a sound (Fletcher and Munson, 1937). Because of masking release strategies (Erbe et al., 2016), this is not always <0 dB signal-to-noise ratio (SNR) over the same frequencies at the same time. However, we use “masked” as one of the SNR range labels in the model output and this will be discussed more thoroughly in section 3.2.
Acoustic signals are important for marine animals because they facilitate biological and ecological processes such as navigation, communication, and habitat selection (Haver et al., 2019). Marine mammals rely on the ability to detect meaningful signals from conspecifics, echoes from prey, or natural sounds that facilitate navigation, socializing, and foraging (McDonald et al., 2008). Since acoustic signals are the primary modality used by these animals, increasing noise pollution could affect their behavior, potentially reducing their access to important habitats, interfering with finding mates, reducing protective contact calling with offspring, or detecting prey. Furthermore, sustained acoustic pollution has been shown to increase stress hormone levels, causing harm by compromising the immune system and affecting an organism's health and reproductive success in the long term (Fair and Becker, 2000; McDonald et al., 2008; Rolland et al., 2012).
Humpback whales (Megaptera novaeangliae) are found in all major oceans and migrate long distances, depending on highly productive feeding grounds in high-latitude areas during the summer months for sustenance, and spending the winter months in low-latitude breeding grounds giving birth to their young and mating (Clapham, 1996, 2000). They are known for their evolving vocal display called “song,” which is one of the most complex acoustic displays in the animal kingdom (Payne and McVay, 1971; Stimpert et al., 2012). Song is performed by males, predominantly on the breeding grounds (Stimpert et al., 2012), but also heard along migration routes and on the feeding grounds (Mattila et al., 1987; McSweeney et al., 1989; Clapham and Mattila, 1990; Clapham and Mead, 1999; Norris et al., 1999; Herman, 2017). Although the function of song is still a source of debate among experts, one possibility states that it could attract females to singers in a lekking arena or mediate interactions between males, making it particularly important for breeding functions (Herman, 2017).
Global humpback whale breeding areas have been impacted by noise pollution from human activities (Au and Green, 2000) like whale-watching tourism (Parsons, 2012) and shipping (Hildebrand, 2009; Tsujii et al., 2018). Boat noise has been found to reduce communication space, leading to chronic effects on populations (Putland et al., 2018). Marine shipping, particularly large ocean container ships, hydrocarbon transport, and cruise ships, is a recognized and persistent anthropogenic source of low-frequency ocean noise, contributing to the masking of essential sounds produced and heard by marine mammals and fish (Weilgart, 2007; Hatch et al., 2008; Hildebrand, 2009; Erbe, 2012; McKenna et al., 2012; Merchant et al., 2014; Williams et al., 2015). In this study's field site only small vessels that are commonly used in transport, artisanal fishing, and whale-watching are present (Rueda, 1997), so this manuscript will focus on masking effects from such vessels.
When vessel traffic noise and humpback whale song are both primary contributors to the soundscape, like in the Colombian Pacific during breeding season, overlap in time and frequency exists between the two sound sources (Heenehan et al., 2019). Humpback whales have been documented to decrease the number of bottom-feeding events per dive and reduce feeding dive descent rate as the intensity of ship noise increases, indicating that ship noise can impact foraging rates and efficiency (Blair et al., 2016). Furthermore, boat direction, speed, and passing frequency are correlated with changes in humpback whale behaviors like respiration rate, diving, swimming speed, communication, and social interaction (Bauer and Herman, 1986; Au and Green, 2000; Scheidat et al., 2004; Sousa-Lima and Clark, 2008; Stamation et al., 2010). Some animals respond to band-limited noise by changing the frequencies of their vocalizations to shift away from the potential effects of masking (Tyack, 2008; Kaplan and Mooney, 2015). In addition to correlating with detrimental behavioral changes and masking song, fishing and tourism-based whale-watching vessels have also been shown to mask the social sounds used by humpback whale competitive groups and mother and calf pairs (Cholewiak et al., 2018).
Along the coast of the Eastern Tropical Pacific Ocean, whale watching activities occur during the humpback whale breeding season because their exciting surface behaviors, like breaching, are easily visible for tourists to view (Darling and Berube, 2001). The effects of noise from whale-watching vessels have been researched in several areas of the world (Parsons, 2012). In South America, studies have found a variety of responses of humpback whales to whale-watching activity, but most are limited to visual observations of surface behaviors (Scheidat et al., 2004; Sousa-Lima and Clark, 2009; Avila et al., 2015). Other studies investigated the whales' interactions with noise generated by humans (Sousa-Lima and Clark, 2008). There is currently a lack of studies on acoustic reactions of humpback whales to whale-watching vessels on the South American Pacific Coast. More specifically, no acoustic studies of the effects of whale-watching on humpback whales have been conducted on the Pacific Coast of Colombia where the breeding ground is surrounded by a coastline not heavily populated by human infrastructure.
The region of Chocó, Colombia, is sparsely populated with small artisanal fishing villages and few roads. The only way to arrive to this Northwestern Colombian coastal area is by small aircraft over an Andean Corridor from Medellín or Bogotá or by overnight boat from Buenaventura. This creates an ecotourism haven. The region is also void of shipping lanes. The Gulf of Tribugá, located in the northern Colombian Pacific, is part of the breeding grounds for humpback whales belonging to the breeding Stock G between the months of May and December (Avila et al., 2020) (Figure 1). It is an opportunistic location to study the effects of whale-watching boats since, compared to other coastal areas, it contains a small variety and a low density of motorized vessels. Families use either dug out canoes or small fiberglass boats with outboard motors to travel between villages, fish, and provide SCUBA and/or whale-watching tours. Once a week a fuel boat (about 40 m in length) transits the Gulf on Thursdays, and for a few months in the spring and summer a couple of shrimping boats sit off-shore harvesting. Aside from these few larger vessels, nearly all motorized vessel noise in the Gulf of Tribugá is from the same boats being used in whale-watching activities. Whale-watching is one of the primary ecotourism activities in Chocó, providing a large portion of available jobs to the surrounding communities (Hoyt and Iñíguez, 2008). Furthermore, the whale-watching industry in this area is offered by the community (Velandia and Díaz, 2016), and it is still in its infancy compared to many other areas where humpback whales breed. Despite the relatively small operational capacity, it is possible that noise from these whale-watching vessels still cause any of the aforementioned adverse effects on nearby humpback whales and negatively affect their singing behavior (Sousa-Lima and Clark, 2008).
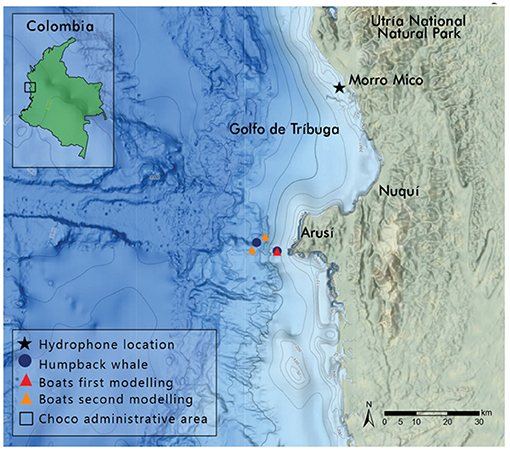
Figure 1. Map of recording site: Morro Mico, Chocó, Colombia. Triangles mark the locations of boats in both models; circles mark the locations of humpback whales in both models. Boats and whales were the source locations in the models. A star marks the deployment location of the EAR (color online).
The Gulf of Tribugá is one of the most biodiverse areas in the world and has complex climatic, geological, and biological history. The acoustic environment is relatively less intense than three other marine mammal sanctuary locations along the Pacific Coast: south of Cabo Pulmo, México (Seger, 2016), in Laguna San Ignacio, México (Seger et al., 2015), and in Glacier Bay, Alaska (Seger et al., 2012). Furthermore, artisanal fishing and whale-watching efforts are regulated and no shipping lanes are nearby. Acoustic data from these locations and the Gulf of Tribugá were processed in previous work using Ulysses software (written by Drs. Aaron Thode and Jit Sarkar) by calculating the hourly-averaged 1st percentile of sound pressure levels (SPLs) (dB re 1 μPa2/Hz) in the 0–6250 Hz bandwidth. Comparing the 1st percentile minima, the Gulf of Tribugá was 26 dB re 1 μPa2/Hz lower than south of Cabo Pulmo, 37 dB re 1 μPa2/Hz lower than in Laguna San Ignacio, and 30 dB re 1 μPa2/Hz lower than in Glacier Bay National Park (Seger, 2020; Seger et al., 2020). Sediment type, recording depth, and sound speed profiles are different among these locations, but boat activity and vessel sizes are similar in all of them. Whether a result of boat activity or propagation environments, the Gulf of Tribugá has less intense 1st percentile SPL minima than the other locations. The primary acoustic components in the Gulf of Tribugá's noise budget are snapping sounds from shrimp, seasonal humpback whale song, and noise produced by small boats (Rey et al., 2019). Therefore, Tribugá is an area where the primary source of acoustic disturbance is the noise from small boats that are used in whale-watching, artisanal fishing, and/or transportation for local families (Velandia and Díaz, 2016).
This could soon change because a mega marine port construction project has been proposed. It was denied permits once on Sept 29, 2020, but reapplication may occur. As a result, there is no set start date of construction. If constructed, the port would severely impact the local soundscape, raising underwater noise from relatively low levels to higher levels more like those around other large ports. The construction process and the resulting greater abundance of large vessels would increase noise disturbance quickly and permanently alter the acoustic environment. Since it is possible that humpback whales in the Gulf of Tribugá are accustomed to lower background noise and wider communication space than even the other sanctuary areas mentioned above, new transient vessel sounds from a shipping lane could have a larger impact on them in terms of increased SPLs than in other locations.
This project on the potential acoustic effects of whale-watching on humpback whale song is part of a larger effort (The PHySIColombia Project) to document the baseline soundscape in a place with relatively low levels of anthropogenic noise as a Before-After Control-Impact (BACI) study. Results from this project are representative of the effects whale-watching boats may have had on humpback whales in an earlier era when large vessel traffic was less expansive than it is in today's global shipping economy. Using acoustic propagation models to understand the effects of noise on marine mammals is not new, but is usually applied in the Northern Hemisphere for shipping lanes and pile driving (Chen et al., 2017; Heaney et al., 2020).
The specific goals for this paper were to (a) model and map a representative noise field from whale watching boats in the Gulf of Tribugá, (b) quantify communication space reduction of a typical humpback whale song caused by whale-watching boats and (c) analyze changes in acoustic features of humpback whale song in the presence of boat noise. It is intended for these results to serve two purposes. First, the mapped models of acoustic propagation will introduce the parabolic equation as a tool for visually understanding the sound levels that humpback whales receive from different configurations of whale watching boats in very specific acoustic environments in South America. Second, any effects on the singing behavior of humpback whales, or lack thereof, while in the presence of whale-watching boats can serve as a “baseline reference” for other studies where whale-watching activities occur more often, in higher densities, and/or in the presence of larger vessel activities.
2. Materials and Methods
Methods included two components. The first was a preliminary acoustic field modeling component using source positions taken from theodolite data collected near Arusí (Figure 1) to fulfill goals (a) and (b). The second was a data-analysis component using acoustic data collected at Morro Mico to fulfill goal (c). The modeling component quantified how much the Stock G humpback whale's song could be experiencing acoustic masking from whale-watching boats and its methods will be discussed first. Validating the amount of area over which whales in Stock G would have to adapt to this whale-watching boat noise, the data-analysis component evaluated one structural and two temporal song changes when boat engines were present and will be discussed second. For this paper, “structural changes” are defined as large scale changes visible when viewing several minutes of a spectrogram, like unit changes or starting and stopping singing. “Temporal changes” are defined as changes in the length or spacing of units, and only units were considered in this analysis.
2.1. Theodolite Data Collection
A common whale-watching destination within the Gulf of Tribugá is off Arusí, toward Cabo Corrientes. Ecohotel Punta Brava is located on a cliff-side where R&E Ocean Community Conservation used a land-based theodolite station (5°29'1”N, 77°32'14”W). Theodolite observations were performed throughout the month of September 2019. Whale-watching activities were recorded for up to 10 h each day, weather permitting.
The theodolite station was positioned 23.5 m above sea level (a.s.l.), which allowed for long-range observations and monitoring of several whale groups simultaneously. The focal plane of the digital theodolite was measured as 25 m a.s.l. (including the height of the theodolite and tripod). Once a whale or group of whales entered the study area, they were tracked using the methods described in Würsig et al. (1991). The vertical and horizontal positions of whales and whale-watching boats were systematized using the open source theodolite tracking software Pythagoras (Gailey, 2002). The program converted the vertical and horizontal positions to GPS coordinates. Once the data were recorded, they were exported to an excel sheet.
2.2. Acoustic Propagation Modeling
Three general steps were used to model potential masking conditions of boat noise over humpback whale song. First, a model of the sound pressure level field of whale song was calculated from transmission loss (TL) fields computed with the acoustic source at the whale's location (from the theodolite data) and spreading over part of the Gulf of Tribugá. This and all following acoustic TL models were calculated for 350 Hz, which is a common peak frequency used in Stock G humpback whale song (Perazio and Mercado, 2018) and is also a peak frequency in whale watching boat noise. (These sound pressure level fields are most often called received levels (RL) in sonar literature.)
Second, noise fields were modeled for (1) a typical ambient noise level, and (2) a typical whale-watching boat traveling at a slow speed (Erbe, 2002). For the boat RL field, TL was computed over the same area of the Gulf with the source located at the boat(s) GPS location(s) in the theodolite data. This RL field for the boat was combined with the typical background noise level to complete a model of noise in the area. Third, the signal-to-noise ratio (SNR) between these whale communication and noise models were calculated for two scenarios: when one and two whale-watching boats were near a whale in the theodolite dataset. Planview (bird's-eye view) maps of these TL and SNR models illustrate the spatial variations of song and boat noise and depict how audible song could be throughout much of the Gulf of Tribugá when competing with noise from boat engines. The SNR planview maps for each scenario were brought into Esri's ArcGIS Pro for further analysis.
The acoustic propagation model used to compute TL given bathymetry, sound speed profile, sediment type, and source and receiver depths and positions was the parabolic equation (PE) formulation. The PE model solves the wave equation using a paraxial approximation and is well-suited for range-dependent environments at frequencies up to about 1 kHz (Heaney et al., 2020). A more detailed explanation of these models can be found in Heaney et al. (2020). The specific model implementation in use is Seahawk, a C language port of RAM (Collins, 1995) developed by Richard Campbell (RAM stands for “Range-dependent Acoustic Model”). It is based on the latest techniques in PE modeling such as the split step Padé solution which allows for large range steps and high angle acoustic propagation support.
Environmental inputs to the acoustic model were representative of the two scenarios being modeled for the Gulf of Tribugá. A common peak frequency of humpback whale song in the area (Perazio and Mercado, 2018) was used in the model: 350 Hz. This is also a common frequency in boat engine noise so masking in this frequency is highly likely. Whale song and boat noise were both assumed to be omnidirectional. Even though some research suggests that whale songs may have some level of directionality (Pack et al., 2003), a tested model to represent this is not yet available for input into the PE model. The source depth for modeled whales was 5 m and the source depth for modeled outboard motors of the boats was 2 m. The receiver depth for both the whale and boat models was 5 m (as if another whale near the surface was receiving song, or the whale being watched was receiving boat noise, respectively). The key differences between the whale and the boat models were their source positions in the Gulf of Tribugá and their source depths.
In the acoustic model, the ocean bottom was modeled as a sediment with mean grain size of 2ϕ (a coarse sandy sediment) on the Wentworth chart scale because the local SCUBA company said that the area is mostly sandy in their experience. By default, Seahawk uses a two layer model of the environment, consisting of separate water and sediment profiles defined independently. The sediment is treated as an acoustically thick halfspace, implemented as 20 wavelengths at the given frequency, containing an exponential absorptive sponge along the bottom of the sediment layer. The absorptive sponge layer ensures that sound will not reflect off the edge of the computational domain. The thickness of the water column is determined by the bathymetry and (optionally) sea surface height. The sediment was characterized by a single parameter, the log mean grain size, which is used along with the local sound speed at the water-sediment interface to determine the depth-dependent sound speed, density, and attenuation within the sediment layer (Hamilton, 1980). This single-parameter bottom characterization is useful because the log grain size parameter maps reasonably well (Wentworth, 1922) to the bottom types indicated on nautical charts. This model is an approximation of real-world conditions as the ocean bottom often contains multiple layers of sediments and is also inhomogeneous. However, if sediment data became available, the sediment model could be improved with more spatial dependence, both across the model domain and in depth.
TL fields for all the whale and boat positions were calculated over the same 150 km by 150 km area centered on the whales' GPS location from Sept 5, 2019, with computed 2,280 radials (azimuths) interpolated onto a output grid of 1,024 pixels in width. This area covers the north to south extent of the Gulf of Tribugá. TL is the pressure level field of an acoustic source with unit amplitude. The models of the whales' and boats' acoustic amplitudes will be explained in more detail during discussion of the SONAR equation.
Scenarios #1 and #2 from the theodolite data were on September 5, 2019 (10:20 a.m.), and September 20, 2019 (10:10 a.m.), respectively. On September 5th, a single whale was being watched by one boat; on September 20th, a single whale was being watched by two boats. Additionally, the Sept 5th sighting occurred closer to shore (by 10.5 km) over a water column depth of 139 m and the September 20th scenario was over a water column depth of 933 m.
The SONAR equation used to model these two scenarios was the passive version:
where SNR is signal to noise ratio, SL is source level, TL is transmission loss, and NL is noise level. The threshold of SNR = 0 was selected to indicate a whale's song being audible over ambient sound levels (+SNR) or not (–SNR). Source levels chosen for the models were based on literature. Au et al. (2006) provided a range of SLs for each unit of humpback whale song in Hawaii. Their unit labeled E2 was noted as the most common unit in the songs in the Gulf of Tribugá data analyzed for this study, so the same SLrms of 153 dB 1 μPa @ 1 m was used to represent Stock G song in our modeling. Erbe (2002) estimated the SLs of several types of whale-watching boats in Australia during whale-watching activities for orcas, and extrapolated their 10 knot and 20 knot SL measurements to other speeds. To keep our models conservative, the slowest speed in Erbe (2002) from a similar style vessel to those used in the Gulf was chosen for our model input: 145 dB re 1 μPa @ 1 m. All inputs to the PE model and planview map grids are summarized in Table 1.
NL was included in the models for both scenarios when boat(s) were present and absent. The NL for boats being present was the incoherent sum of the RL for the boat or boats and a background ambient noise level of 48 dB re 1 μPa (Huertas et al., 2019; Rey et al., 2019), taken from measurements with conditions which best matched conditions during the study period. In the case of boats being absent, the NL was only the background ambient noise level.
The RL grids were imported into Esri's ArcGIS Pro and transformed to an area preserving the projected coordinate system to minimize bias. For each of the two scenarios, the areas covered under three SNR intervals were computed: <0 dB (masked), 0–10 dB (unreliable audibility), and >10 dB (audible). The area (km2) that these SNR intervals occupied in the models was calculated for both scenarios in the presence and absence of boats and compared.
Some assumptions needed to be made that these SNR planview maps of whale song, given surrounding boat noise from whale-watching vessels, provided evidence that a whale's communication space could have been reduced from engine noise throughout the Gulf of Tribugá. The following assumptions were made to validate the comparisons of modeling results in the area near Arusí with the data collection area near Morro Mico:
1. The acoustic propagation environment in both Arusí and Morro Mico are similar because they are in the same small Gulf, have similar bottom sediments, similar depths, similar bathymetries, and the boats and whales occupy similar distances to the coast. (Additional models from other projects have produced similar TL maps along the Gulf's coastline.)
2. The most common kind of boat used throughout the Gulf for whale-watching, transportation, and artisanal fishing are fiberglass hulls with outboard motors. Other types of vessels are relatively rare. Therefore, the boats passing by and milling around the recorder, if not engaged in whale-watching, would likely still be the same kind of boats used in whale-watching activities.
3. When boats engage in whale-watching activities, they have to approach the whales and usually do so at a typical travel speed. Sound from the approach will reach the whale and have masking effects before the boat reaches its watching point and slows down or stops.
4. Individual whales are able to, and do, traverse the distance between Morro Mi7o and Arusí in under a day's time. The whales whose songs have been analyzed from Morro Mico are capable of traveling to Arusí, so the whales used to generate our maps are from the same population (Stock G) as those recorded.
5. Acoustic data from 2018 would adequately represent boat noise in 2019. Boat traffic in the Gulf has no documented annual cycle and the artisanal fishing lifestyle (the main boat traffic contributor) has been consistent for decades.
2.3. Acoustic Data Collection
Acoustic data were collected at Morro Mico (Figure 1), from October to November 2018 (5°52.101'N, 77°19.007'W) with an Ecological Acoustic Recorder (EAR) from Oceanwide Science Institute. Morro Mico is an island in the region of Chocó that lies along the southern border of Utría National Natural Park. The EAR located there was programmed to record for 10 min at 30-min intervals for a duty cycle of 33.3%. The sampling rate was 15.625 kHz.
2.4. Acoustic Data Analysis
Acoustic data from the EAR at Morro Mico were downloaded and converted to .wav files using custom Matlab code (L. Munger). The hydrophones on the EARs had a sensitivity of –193.5 dB re 1 V/Pa with a 47.5 dB preamp. No additional gains were used. A total of 1681 10-min samples were obtained over 37 days. Data were manually analyzed every 3 h in Raven Pro 1.5 software, identifying times when acoustic sources fell into two groups: (1) small boat engine activity with whale song and (2) only humpback whale singing. A total of 67 files from the dataset were used: 33 of them contained humpback whale song and no boat activity and 34 contained both boat and song activity.
In only the 34-file set, humpback whale song structure before and after each full boat pass in a file was compared. Whether or not a qualitative difference in the song structure was observed between these two times was recorded “yes” or “no” to create a binary response variable. To consider something a qualitative change in song structure the criteria included: a different unit, a difference in bandwidth if the unit or phrase was the same, singing activity stopping, or singing activity starting. Unclassifiable changes occurred when boat noise was too loud to allow for unit identification, a boat pass continued beyond the end of the recording, or the sound of breathing whales replaced song.
For both sets of files, two quantitative measures were taken from the only or most apparent song in the file. In the set of files with boats, as many units as possible were measured even during the loudest boat activity to prevent large gaps accidentally being measured where units could be fully masked. The inability to separate boat noise frequency characteristics from song units they were superimposed on is the primary reason that only temporal features were measured and compared. These temporal measures were unit duration (time from the start to the end of a unit, in seconds) and inter-unit interval (IUI, time between consecutive song units, in seconds) (Mercado et al., 2005). All units found in each of the files were selected, for a total of 3,027 units from songs in files with boats present and 5,023 units from files without boats. Distributions of both temporal variables were then compared between when boats were and were not present to determinate changes on a larger time scale, as opposed to structural changes in which immediate pattern changes were observed.
2.5. Statistical Analysis
The null hypothesis that was tested for song structure stated that a whale would not change the structure of its song any more often than random (50% of the time) during a boat pass. The alternative hypothesis was that a whale would change the structure of its song more often than random (>50% of the time) during a boat pass.
The null hypothesis that was tested for temporal song features stated that there would be no significant difference in the length of song units or IUIs when boats were present vs. when boats were not present. The first alternative hypothesis was that average song unit length from files with boats present would be different (longer or shorter) than average song unit length from files without boats present. The second alternative hypothesis was that the average IUI length from files with boats present would be different (longer or shorter) than the average IUI length from files without boats present.
Normality distributions of song unit length and IUI length boat/non-boat pairs were examined with the Shapiro-Wilk test and homogeneity of variances were examined with the F-test. They all exhibited non-normality even after data transformation. Therefore, the non-parametric Wilcoxon rank sum test was chosen to determine if there was a significant difference between song unit lengths and IUI lengths in the presence or absence of boats (Stewart-Oaten, 1995; Marques De Sá, 2007). The level of statistical significance was set as less than 5% (p < 0.05). All statistical analyses were conducted in R version 3.5.2.
3. Results
3.1. Acoustic Propagation Modeling - SNR Planview Maps
RL (Received Level) and SNR (signal-to-noise ratio) planview maps of a hypothetically singing humpback whale from scenario #1 (1 whale, 1 boat) and scenario #2 (1 whale, 2 boats) illustrate key features about the acoustic environment of a humpback whale under undisturbed and whale-watching conditions. First, the propagation of sound emitted by any source, boat or whale, is shaped by different water depths and distances to shore (Figures 2, 4 top subplots). These observations about depth and coastal proximity match two of the three key parameters in environmental sensitivity analyses from pile driving propagation modeling along the east coast of the United States (Heaney et al., 2020), and temperature (the third parameter) in the Gulf of Tribugá would change less than at higher latitudes.
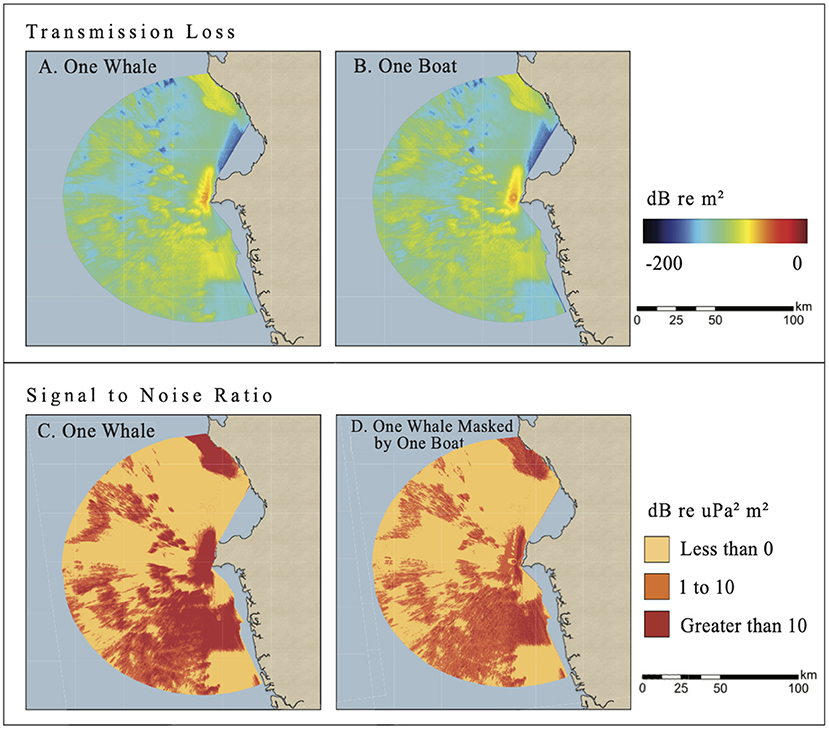
Figure 2. Planview maps for the Sept 5, 2019, scenario #1 of continuous TL values of whale song (top left) and boat noise (top right) illustrate sound transmission loss near Arusí. Blue is less audible while red is more audible. Planview maps as binned SNR values better visualize the area of relatively higher SNR communication space when only ambient noise (bottom left) vs. one boat (bottom right) is present. Red indicates very audible areas of the whale song, orange indicates unreliably audible areas, and yellow indicates masked areas. Source level of the whale's hypothetical song was 153 dB re 1 μPa @ 1 m, which would be dark red in the top left map (color online).
In scenario #1, the whale's communication space model without boat noise spread along-shore (solid orange-yellow oval, Figure 2 top left) and spread more effectively to the south and west than to the north. The SNR model shows that in about half of the area, the song's sound energy would be masked (<0 dB, yellow, Figure 2 bottom left) without competing boat noise but from natural transmission loss and ambient noise. Once noise from a single boat was included in the model (Figure 2 top right), the whale's communication space was reduced further (Figure 2 bottom right), particularly in the areas that were audible with just the presence of ambient noise (SNR >10 dB) (red, Figure 2, bottom subplots).
The change in area covered by these three SNR threshold ranges in the Gulf was calculated and compared between a hypothetical whale singing in ambient noise conditions to a hypothetical whale song competing with noise from a single boat (Table 2 and Figure 3). Without boat noise the hypothetical whale song would have been very audible (SNR >10 dB) in 2,306 km2 of the total 10,255 km2 of the Gulf where sound energy propagated. By comparison, noise from one boat reduced the very audible area (>10 dB SNR) to 859 km2, or a 63% reduction. With boat noise, the area of the Gulf where the whale's song was unreliably audible increased 35%, and 588 more square km were masked (an 11% increase). The presence of one boat did not mask much more communication space for the humpback whale song than just ambient noise, but it did decrease the very audible area by more than half.
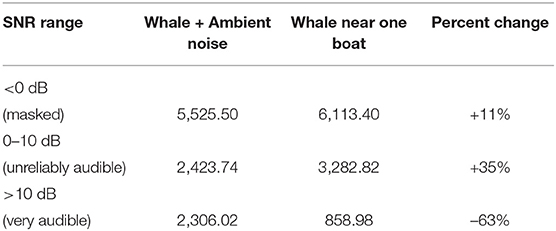
Table 2. Area (km2) that three SNR-binned audibility ranges occupy within the simulation for the September 5, 2019, scenario #1 (single whale alone with just ambient noise vs. single whale in close proximity to a single boat plus ambient noise).
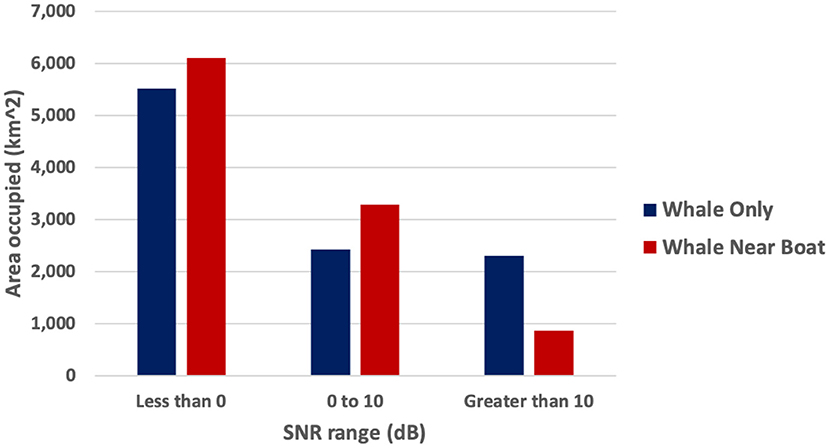
Figure 3. Sept 5, 2019, scenario #1. Comparison bar plots of the area occupied by the three SNR bins of the hypothetical whale song when only ambient noise (blue/left bars) and one boat (red/right bars) was present (color online).
In scenario #2, the whale's and boats' acoustic fields spread more evenly in all directions than in scenario #1 (Figure 4 top subplots). The SNR-binned subplots for scenario #2 (Figure 4 bottom subplots) showed that the regions where the whale song with only ambient noise was modeled as very audible (4,901 km2) shrunk to 1,375 km2 (a 72% decrease) in the presence of the two boats. The masked regions increased 79% in the presence of the two boats. Noise from two boats almost doubled the masked area as compared to just ambient noise, and only about one quarter of the very audible area remained. Percent changes in very audible, unreliably audible, and masked areas are presented for scenario #2 in Table 3 and Figure 5).
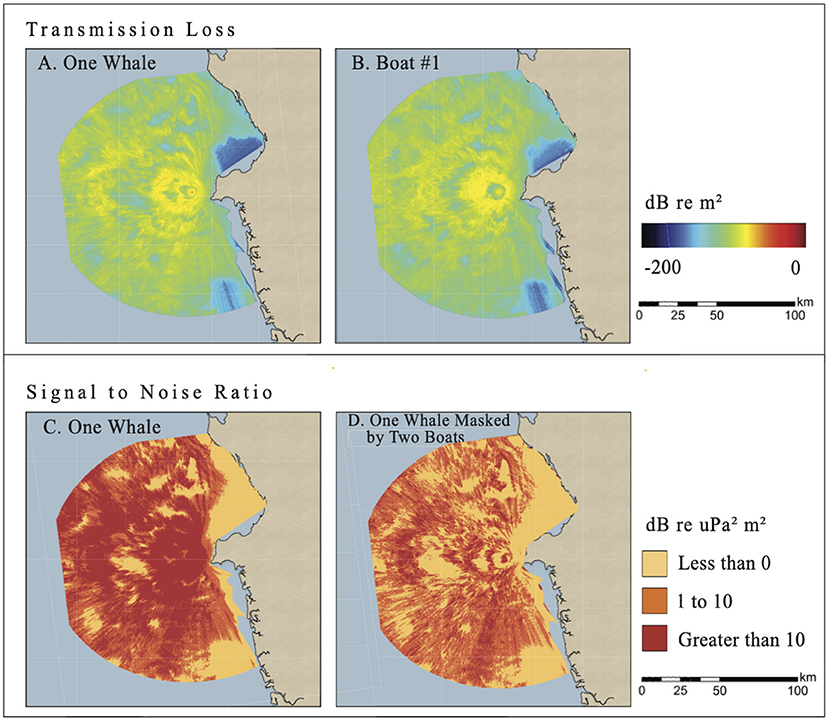
Figure 4. Planview maps for the Sept 20, 2019, scenario #2 of continuous TL values of whale song (top left) and noise from one of the two boats (top right) near Arusá. Blue is less audible while red is more audible. In the bottom panels, planview maps of binned SNR values better visualize the area of relatively higher SNR communication space when only ambient noise (bottom left) vs. two boats (bottom right) are present. Red indicates very audible areas of the whale song, orange indicates unreliably audible areas, and yellow indicates masked areas(color online).
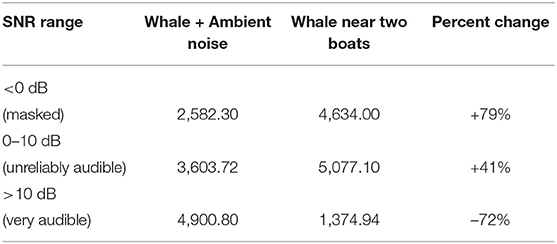
Table 3. Area (km2) that three SNR-binned audibility ranges occupy within the simulation for the September 20, 2019, scenario #2 (single whale alone with ambient noise vs. single whale in close proximity to two boats plus ambient noise).
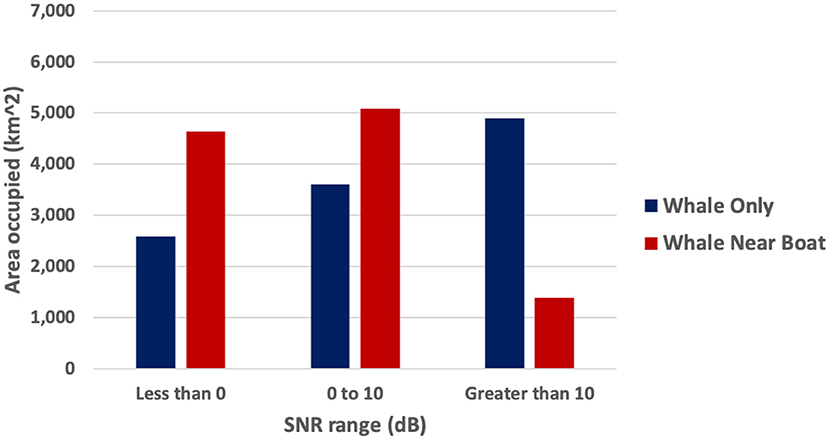
Figure 5. Sept 20, 2019, scenario. #2, side-by-side bar plots of the area covered by each SNR bin of the hypothetical whale song when only ambient noise (blue/left bars) and two boats (red/right bars) were present (color online).
A few comparisons to scenario #1 illustrate the effect of source positions and the extra boat. The source level position of the whale in scenario #1 was more affected by transmission loss and ambient noise, having more than twice the naturally masked area without any effect of boat noise than scenario #2 (5,526 km2 compared to 2,582 km2). Scenario #2's model results had 1.5 times more area of the Gulf where SNR is unreliably audible than in scenario #1 (5,077 km2 compared to 3,282 km2). Noise from two boats decreased the very audible area (>10 dB SNR) 9% more than the noise from just one boat. Finally, the scenario #2 model indicates that it would take more than two boats to mask (make SNR <0 dB) the entire communication space of a humpback whale song in the Gulf.
3.2. Effect of Noise From Boats on Structural Aspects of Humpback Whale Song
A total of 126 boat passes occurred within the set of 34 files with whale and boat activity. Qualitative analysis showed that song structure changed during a boat pass 58 times. Some of the song structure changes observed were: different unit used after a boat passed (often a different unit with a higher bandwidth than before), vocal activity stopped (Figure 6), and vocal activity started. However, song structure did not change 55 times during a boat pass and 13 times the change could not be classified (n/a) as pertaining to song structure. Therefore, song structural changes did not occur significantly more often than random (50% of the time) when a boat passed.
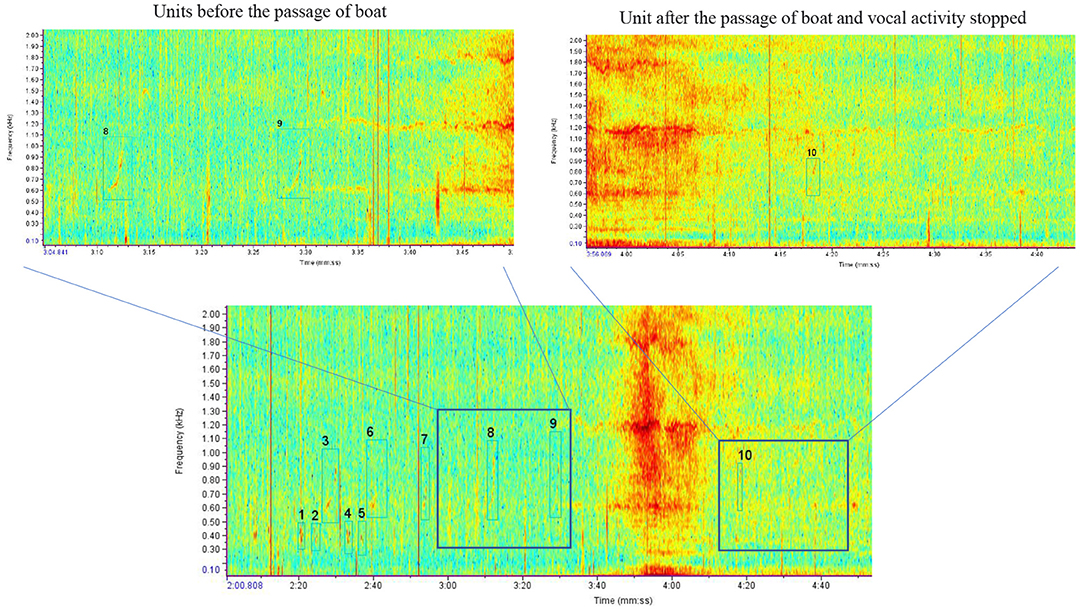
Figure 6. Example of structural change in a song: stopped singing. At the beginning of the spectrogram, a phrase change happened to the song denoted by the 1, 2, and 3 (AAB) boxes (blue) until 3:40 when the boat pass began. During boat noise, units were difficult to identify. At 4:20 when the boat pass ended, the final unit able to be found (box 10) matched unit B of the phrase from before (boxes 3, 6, 7, 8, and 9). No more singing was heard/seen for the remainder of the 10-min recording (color online).
3.3. Effect of Noise From Boats on Temporal Aspects of Humpback Whale Song
When distributions of song unit length in the presence and absence of boats (Figures 7A,B) were compared with a Wilcoxon rank sum test, there was a statistically significant difference between the ranks of their medians (p = 6.627e-06). The average song unit length and standard deviation was 1.1 ± 1.3 s with a median of 0.8 s when boats were present vs. 1.2 ± 1.1 s with a median of 0.9 s when they were absent. The presence of boat noise coincided with shorter and more variable song unit lengths in humpback whale songs in the Gulf of Tribugá.
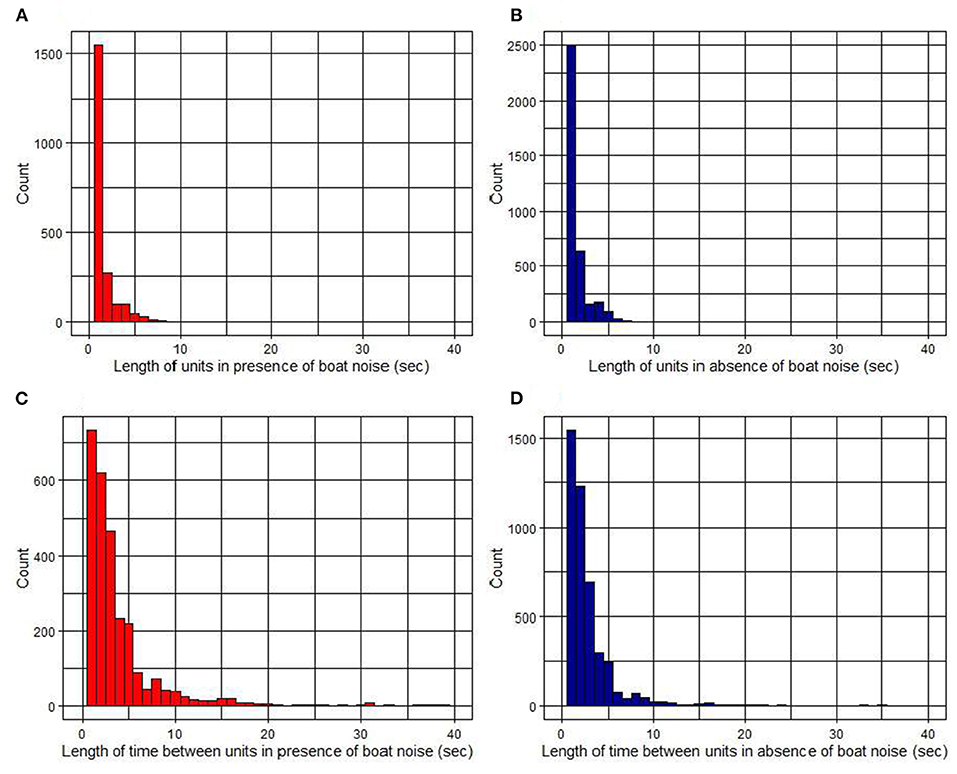
Figure 7. Temporal difference in units and unit spacing in the presence and absence of boat noise: (A) length of song units in presence of boat noise and (B) absence of boat noise (C); length of IUIs in the presence of boat noise and (D) absence of boat noise.
When the distributions of the IUI length in the presence and absence of boats (Figures 7C,D) were compared with a Wilcoxon rank sum test the ranks of their medians were also significantly different (p = 2.2e-16). The average IUI length was 4.3 ± 9.1 s with a median of 2.4 s when boats were present vs. 2.6 ± 4.5 s with a median of 1.8 s when they were absent. The presence of boat noise coincided with longer and more variable IUIs in humpback whale songs in the Gulf of Tribugá.
The rate of the singing under boat presence and absence conditions was also calculated. There were 3,026 units sung in the 340 min of files with boats and 5,023 units sung in the 330 min of files without boats present. Dividing the total number of units by the total time yielded rates of 8.9 units/minute sung when boat noise was present compared to 15.2 units/minute sung when boat noise was absent. The apparent slower singing rate in the presence of boats is likely partially due to units being masked by boats, so fewer were able to be detected during manual analysis to be included in a rate calculation. This bias in this song rate metric will be discussed later.
4. Discussion
Boat-based whale-watching is one of the activities with the highest tourism demand in the Colombian Pacific. It has become very economically important in the last decade, especially in places like the Gulf of Tribugá where it is a stable seasonal job for local fishermen (Velandia and Díaz, 2016). It is reasonable to assume that boats within whale-watching distance of a humpback whale along the coast of the Gulf of Tribugá could mask the acoustic field of a whale if it is trying to sing or produce social calls. It is likely that masking of the song by the boat noise occurs not only when the whale-watching vessel is close, but also when it is farther away, like during an approach. Adaptive strategies to reduce the effects of this masking could be pursued by the whale, but at present there is an incomplete understanding of what these strategies are and how they are used.
The propagation modeling SNR planview maps, bar plots, and tables illustrate how far a hypothetical whale song could transmit through its habitat with only ambient noise as compared to in the presence of noise from one or two boats used in whale-watching activities. The audibility regions in the models were not meant to exactly represent song detectability, but rather serve as a visualization tool to capture two uncertainties. First, fine details of the environment such as the bottom sediment properties and the complete ocean temperature and salinity across the study area are not well known and lack in situ measurements. But if these details were known, the audibility results could shift. Second, these models do not include noise contributions due to wind and waves. If wind picks up or a storm passes, ambient noise levels would rise from more crashing waves and rain drops. A change in sea state from 1 to 5 can lead to an increase in background noise levels of 10 dB re 1μPa or more. It is possible that the unreliably audible areas would become masked under poor weather conditions.
While negative SNR represents masked space in our models, marine mammals do exhibit strategies to reduce the effects of this masking (Erbe et al., 2016), thus a 0 dB SNR threshold indicating masking is debatable. While we assume here that SNR values above 10 dB are loud enough for a signal to be heard over ambient noise under the typical variations in environmental and propagation conditions, a model validation study with higher resolution environmental inputs and a Monte Carlo estimate of SNR variability would be better for establishing such thresholds. Such an estimate would be based on the uncertainties in the model input parameters such as diurnal and seasonal variations in the sound speed profile, fluctuating ambient noise levels, a variety of sediment types across the ocean floor, and/or frequency dependence of the humpback whale song and boat engine noise overlap.
Such sophisticated models, despite being in development for future work, were beyond the purpose of this project. The purpose of these models was to validate that (1) sound from boats and whales both travel many kilometers in the Gulf of Tribugá and (2) one or two whale-watching boats could reduce the communication space of a singing humpback whale. With this validation, it could be assumed that if the EAR deployed by Morro Mico recorded both whale song and boat noise, then the whale could likely detect the boat and the whale's communication space was likely reduced by the boat. Therefore, the whales that were recorded at Morro Mico likely experienced environmental pressure to change their acoustic behavior if deemed adaptive.
In a few areas of the models were the hypothetical whale songs masked by the noise from one or two whale-watching type boats, but more boat activity could create more expansive areas of masking. If the planned mega maritime port is built in the Gulf of Tribugá, boat noise will increase from more and larger vessels. It will be important to predict how much masking the noise from shipping lanes could have on humpback whale song. This project was the first step in understanding how one or two small vessels can reduce humpback whale communication space so the models can be further developed to predict the effects from more and larger vessels in the Gulf of Tribugá.
Since the recorded ambient sound levels here (Huertas et al., 2019; Rey et al., 2019), even with boats, are relatively lower than other humpback whale and gray whale breeding and feeding grounds in the Pacific Ocean (Seger, 2020), the Gulf of Tribugá is representative of a low disturbance “baseline” region. More heavily trafficked whale-watching areas in the world could mask whale song more and correlate with more extreme acoustic behavioral changes.
Despite this relatively low anthropogenic disturbance, two temporal aspects of singing activity were significantly different when boat noise was present compared to when it was absent. The fewer number of longer units sung in the presence of boats at longer IUIs could be a strategy to escape the masking. The longer IUIs during boat passes could also be a result of some units being completely masked in the spectrograms and therefore missed during analysis. We believe this happened rarely, but could have affected the results nonetheless.
The mean of the IUIs in the presence of boat noise was 4.3 s, but was 2.6 s (about half as long) in the absence of boat noise. Another study found average IUIs in humpback whale song ranging from 0.51 to 2.37 s (Handel et al., 2009). The IUIs in the songs recorded from the Gulf of Tribugá were longer than this range regardless of whether boat noise was present or absent. These results extend this previously published range of IUIs, but whether due to natural population differences or interruptions from boat noise is unclear.
The results also showed that (a) IUI length was more variable (larger standard deviation) than unit length regardless of whether boats were present or not and (b) the variability (standard deviation) in IUI length was larger in the presence of boat noise than in its absence. Singing tempo in the Gulf of Tribugá, therefore, was more governed by IUI length and was faster when boat noise was absent. This is contrary to previous work suggesting that humpback whales use unit length instead of IUI length to adjust song tempo (Schneider and Mercado, 2018) and that they use highly consistent unit and IUI durations for specific unit types in large-scale time (Mercado et al., 2005; Au et al., 2006). These more variable IUI and unit length results are plausible considering that songs have a naturally high level of intra-individual variability (Schneider and Mercado, 2018) are there were likely many individual whales' songs captured in our data. Or, comparing these results to previous work from other geographical regions simply documents natural differences across populations.
No common “boat-noise adapted” phrase was anecdotally noted during analyses, but doing a correlation test of which units and phrases were used during boat noise presence vs. absence would be an important next step. Not all song units are the same length. It is possible that the Stock G humpback whales are not lengthening units when boat noise is present, but rather are switching to a phrase which has naturally longer units in it. The whales could also be constantly morphing unit patterns in predictable ways (Mercado and Perazio, 2021) and these adjustments may happen more quickly in the presence of boat noise. The IUI and unit lengths in our results indicate that singing whales waited longer to sing each unit, and when they did sing, the unit was more likely to be longer. Certain phrases might be easier to use in this way than in others. This slowing down of song to possibly sing at more strategic intervals is limited, however. As the IUIs approach many seconds, and possibly minutes, long the song structure would break down. At some threshold this “slower tempo” strategy will no longer create a song, but rather a set of random calls without a phrasing or thematic structure, and a different strategy would need to be implemented. It is unclear whether the information contained in a quicker or slower song is as efficient or as comprehensible to the receiving individual as a song at “normal” speed.
Humpback whale song length as a whole is a summary of complex behavior that likely provides a relatively easy measure of response to potential disturbance (Fristrup et al., 2003). According to Fristrup et al. (2003) the increased duration of the songs might also be related to the density of the local whale population. If the local population and social activities increase, the duration of the song (and thus the duration of phrases and IUIs within that song) could also increase. This project only analyzed the clearest song in each file and did not analyze any background songs. Therefore it is possible that the longer IUIs observed during boat noise were actually a coincidental response to more nearby conspecifics.
Longer IUIs have one additional advantage in noisy conditions. They may cause the entire song to last long enough to still be present after a boat has passed. The whales could be conserving energy by singing less often while boat noise is present, under the assumption that eventually the boat will pass and then the song can return to a normal pace using a normal amount of metabolic energy. One study supports this interpretation. Miller et al. (2000) found that humpback whales sang longer songs during low frequency active sonar transmission to compensate for the acoustic interference (Miller et al., 2000).
Responding to boat noise is not the only thing that may alter humpback whale song, and some of the variability might be natural. Humpback whales tend to stop singing when they join another whale that is not singing (Tyack, 1981). Also, the location of the individual (either in deep or shallow waters) or the presence of predators could change the intensity of the vocalization, the frequencies, or the length of songs (Au et al., 2006; Guazzo et al., 2020). In these contexts, the consistency of performance may in turn be an indicator of fitness (Thompson, 1983) where it would be strategic to show high redundancy in call behavior under typical conditions as a strategy to increase detectability by congeners (Erbe et al., 2016). Furthermore, it has been found that humpback whales are capable of increasing the source levels of their song units (Guazzo et al., 2020). But even with several singers, the combined effort may not compensate for or supersede the same noise level as that in a very disturbed environment, which still results in a suboptimal communication space (Guazzo et al., 2020).
For future research, the examination of other variables is suggested, such as environmental or biological cycles, different types of noise sources like rain, wind, fish choruses, or natural physical drivers of ambient sound levels (Wenz, 1962; Clark et al., 2009). Natural or biological sounds could also create masking effects similar to that of boat noise. Furthermore, the analysis of the shipping lane masking models, currently ongoing as part of an undergraduate thesis project, and other effects such as stress levels (Rolland et al., 2012) of humpback whales that are exposed to boat noise would be informative. Since noise is a potential stressor, adapting one's song may not reduce or compensate for stress, but could exacerbate it, and prolonged stress could cause serious health problems (Erbe, 2012).
Another suggestion is to carry out a much more exhaustive estimate of the spatial overlap of boats and whales in the Gulf of Tribugá. Because the whale-watching type boats do not transmit AIS signals, there are no ship track data like those for larger ships to use as acoustic source locations in propagation models. But it is possible to place more hydrophones in several places around the Gulf of Tribugá to determine roughly where and how many boats are producing noise at several locations at any one time. Studies of temporal overlap with boat noise and whale song are also important. In this project, IUI and unit length metrics were more robust than singing rate in measuring temporal changes in humpback whale song since boat masking likely excluded units that the analysts could not see through boat noise. Therefore, future analysis should include denoising the data first if metrics like singing rate are included. For the time being, in the Gulf of Tribugá, whale watching activities occur between 8 a.m. and 5 p.m. and humpback whale singing peaks outside of these hours (Rey et al., 2019). Therefore boat noise overlaps less with song during peak singing activity and the humpback whales in Stock G still have relatively quieter night times during which to sing. If the port is built in Tribugá, though, this refuge time would no longer exist since merchant ships transit into and out of ports even in the night.
5. Conclusions
This project focused on the possible impacts that whale watching may have in one place with relatively low levels of anthropogenic noise (Gulf of Tribugá) in the world. For this, propagation models depicted and quantified the areas of the Gulf of Tribugá over which whale-watching boat noise could affect the song of humpback whales from Stock G. The statistical analyses of actual behavioral quantification in the absence and presence of noise from whale-watching type boats indicated that two of the three null hypotheses were rejected: song unit and IUI lengths were not the same when boat noise was and was not present. Rather, the presence of boat noise was coincident with longer and more variable song unit and IUI lengths in humpback whale songs.
This project did not attempt to control for all of the discussed potential behavioral and environmental variables that could have influenced song unit and IUI length instead of boat noise. This was partially because one hydrophone prevents much of the analysis, like source level determination, that would require an array of hydrophones. Mostly, however, this paper's goals were to (1) predict the masking potential of whale-watching boats in a relatively undisturbed breeding ground, (2) quantify the likely area of masking from whale-watching boat noise in the Gulf of Tribugá specifically, and (3) explore some fundamental observations about singing behavior changes in the presence of whale-watching boat noise that could be easily reproduced by students in remote field sites with access to only one hydrophone. The fact that singing behavior changed significantly over two temporal variables in the presence of boat noise in a place where anthropogenic noise is low means that even the places that may be assumed as good representations of baseline environments are not immune to the effects of disturbance. As the scientific community attempts to compare the effects of pollution on wildlife, seemingly pristine environments usually serve as controls. These results show that such control environments are not pure controls.
Data Availability Statement
The raw data supporting the conclusions of this article will be made available by the authors, without undue reservation.
Ethics Statement
Ethical review and approval was not required for the animal study because no testing was done on humpback whales, nor were boats by the researchers used to approach the whales to sample acoustic and observational data.
Author Contributions
KS, CP, and NB-A contributed to conception and design of the study and performed the fieldwork to collect the acoustic data. AV collected and processed the theodolite data. JB and KS performed the propagation modeling. SR analyzed and mapped the propagation modeling results. MR-B and LH-A analyzed the acoustic data, performed the statistical analyses, and maintained the shared database of results. MR-B and LH-A also wrote the first draft of the manuscript. KS, JB, SR, and AV wrote sections of the manuscript. AL-A served as main advisor to the two student authors and KS served as their topic advisor. All authors contributed to manuscript revision, read, and approved the submitted version.
Funding
This work was supported by Fulbright Colombia, Rufford Small Grants for Nature Conservancy, and IDEA WILD.
Conflict of Interest
KS was employed by Applied Ocean Sciences.
The remaining authors declare that the research was conducted in the absence of any commercial or financial relationships that could be construed as a potential conflict of interest.
Acknowledgments
We would like to thank the people of Chocó, Colombia, for opening their homes to our research efforts. To Checa and Cruz, thank you for being our fearless captain and keeping us housed and fed. Thank you to Marc Lammers and the Oceanwide Science Institute for loaning us the EARs. To Lili (Kákiri Estacinó de Buceo) and Emiro, thank you for the SCUBA adventures and rescuing our gear when the weather did not cooperate. We would not have been able to finish all the fieldwork without the boat assistants Meyo, Cotito, and Elian. The fieldwork would not have been possible without the approval of local councils Consejo Comunitario Los Riscales and Consejo Comunitario Coquí. We are grateful for the endless hours we suspect the reviewers spent in improving this paper. Especially to Juana, your memory will resonate in our hearts for years to come. You truly were the mastermind for the first year's success and we will never forget your contributions to our work and to our lives. This manuscript is dedicated to you.
References
Au, W., and Green, M. (2000). Acoustic interaction of humpback whales and whale-watching boats. Mar. Environ. Res. 49, 469–481. doi: 10.1016/S0141-1136(99)00086-0
Au, W. W., Pack, A. A., Lammers, M. O., Herman, L. M., Deakos, M. H., and Andrews, K. (2006). Acoustic properties of humpback whale songs. J. Acoust. Soc. Am. 120, 1103–1110. doi: 10.1121/1.2211547
Avila, I. C., Correa, L. M., and Parsons, E. (2015). Whale-watching activity in bahía málaga, on the pacific coast of colombia, and its effect on humpback whale (megaptera novaeangliae) behavior. Tour. Mar. Environ. 11, 19–32. doi: 10.3727/154427315X14398263718394
Avila, I. C., Dormann, C. F., García, C., Payán, L. F., and Zorrilla, M. X. (2020). Humpback whales extend their stay in a breeding ground in the tropical eastern pacific. ICES J. Mar. Sci. 77, 109–118. doi: 10.1093/icesjms/fsz251
Bauer, G., and Herman, L. (1986). Effects of Vessel Traffic on the Behaviour of Humpback Whales in Hawaii. rep. from kewalo basin mar. mamm. lab., univ. hawaii, honolulu, for us natl. Honolulu, HI: Mar. Fish. Serv.
Blair, H. B., Merchant, N. D., Friedlaender, A. S., Wiley, D. N., and Parks, S. E. (2016). Evidence for ship noise impacts on humpback whale foraging behaviour. Biol. Lett. 12:20160005. doi: 10.1098/rsbl.2016.0005
Chen, F., Shapiro, G. I., Bennett, K. A., Ingram, S. N., Thompson, D., Vincent, C., et al. (2017). Shipping noise in a dynamic sea: a case study of grey seals in the celtic sea. Mar. Pollut. Bull. 114, 372–383. doi: 10.1016/j.marpolbul.2016.09.054
Cholewiak, D., Clark, C. W., Ponirakis, D., Frankel, A., Hatch, L. T., Risch, D., et al. (2018). Communicating amidst the noise: modeling the aggregate influence of ambient and vessel noise on baleen whale communication space in a national marine sanctuary. Endanger.Species Res. 36, 59–75. doi: 10.3354/esr00875
Clapham, P. (2000). The Humpback Whale: Seasonal Feeding and Breeding in a Baleen Whale. Cetacean Societies: Field Studies of Dolphins and Whales, eds J. Mann, R. C. Connor, P. L. Tyack, and H. Whitehead, (Chicago; London: The University of Chicago Press), 173–196.
Clapham, P. J. (1996). The social and reproductive biology of humpback whales: an ecological perspective. Mammal Rev. 26, 27–49.
Clapham, P. J., and Mattila, D. K. (1990). Humpback whale songs as indicators of migration routes. Mar. Mammal Sci. 6, 155–160.
Clark, C. W., Ellison, W. T., Southall, B. L., Hatch, L., Van Parijs, S. M., Frankel, A., et al. (2009). Acoustic masking in marine ecosystems: intuitions, analysis, and implication. Mar. Ecol. Prog. Ser. 395, 201–222. doi: 10.3354/meps08402
Collins, M. D. (1995). User's Guide for Ram Versions 1.0 and 1.0 p. Washington, DC: Naval Research Lab.
Darling, J. D., and Berube, M. (2001). Interactions of singing humpback whales with other males. Mar. Mammal Sci. 17, 570–584. doi: 10.1111/j.1748-7692.2001.tb01005.x
Erbe, C. (2002). Underwater noise of whale-watching boats and its effects on killer whales orcinus orca), based on an acoustic impact model. Mar. Mammal Sci. 18, 394–418. doi: 10.1111/j.1748-7692.2002.tb01045.x
Erbe, C. (2012). “Effects of underwater noise on marine mammals,” in The Effects of Noise on Aquatic Life. (New York, NY: Springer), 17–22.
Erbe, C., Reichmuth, C., Cunningham, K., Lucke, K., and Dooling, R. (2016). Communication masking in marine mammals: a review and research strategy. Mar. Pollut. Bull. 103, 15–38. doi: 10.1016/j.marpolbul.2015.12.007
Fair, P. A., and Becker, P. R. (2000). Review of stress in marine mammals. J. Aquat. Ecosyst. Stress Recov. 7, 335–354. doi: 10.1023/A:1009968113079
Fletcher, H., and Munson, W. A. (1937). Relation between loudness and masking. J. Acoust. Soc. Am. 9, 1–10.
Fristrup, K. M., Hatch, L. T., and Clark, C. W. (2003). Variation in humpback whale (megaptera novaeangliae) song length in relation to low-frequency sound broadcasts. J. Acoust. Soc. Am. 113, 3411–3424. doi: 10.1121/1.1573637
Gailey, G. (2002). A note on a computer-based system for theodolite tracking of cetaceans. J. Cetacean Res. Manage. 4, 213–218.
Guazzo, R. A., Helble, T. A., Alongi, G. C., Durbach, I. N., Martin, C. R., Martin, S. W., and Henderson, E. E. (2020). The lombard effect in singing humpback whales: source levels increase as ambient ocean noise levels increase. J. Acoust. Soc. Am. 148, 542–555. doi: 10.1121/10.0001669
Hamilton, E. L. (1980). Geoacoustic modeling of the sea floor. J. Acoust. Soc. Am. 68, 1313–1340. doi: 10.1121/1.385100
Handel, S., Todd, S. K., and Zoidis, A. M. (2009). Rhythmic structure in humpback whale (megaptera novaeangliae) songs: preliminary implications for song production and perception. J. Acoust. Soc. Am. 125, EL225–EL230. doi: 10.1121/1.3124712
Hatch, L., Clark, C., Merrick, R., Van Parijs, S., Ponirakis, D., Schwehr, K., et al. (2008). Characterizing the relative contributions of large vessels to total ocean noise fields: a case study using the gerry e. studds stellwagen bank national marine sanctuary. Environ. Manage. 42, 735–752. doi: 10.1007/s00267-008-9169-4
Haver, S. M., Fournet, M. E., Dziak, R. P., Gabriele, C., Gedamke, J., Hatch, L. T., et al. (2019). Comparing the underwater soundscapes of four us national parks and marine sanctuaries. Front. Mar. Sci. 6:500. doi: 10.3389/fmars.2019.00500
Heaney, K. D., Ainslie, M. A., Halvorsen, M. B., Seger, K. D., Müller, R. A. J., Nijhof, M. J. J., et al. (2020). A Parametric Analysis and Sensitivity Study of the Acoustic Propagation for Renewable Energy Sources. Report by CSA Ocean Sciences Inc. for US Department of the Interior, Bureau of Ocean Energy Management, Office of Renewable Energy Programs. OCS Study BOEM 11, 165.
Heenehan, H., Stanistreet, J. E., Corkeron, P. J., Bouveret, L., Chalifour, J., Davis, G. E., et al. (2019). Caribbean sea soundscapes: monitoring humpback whales, biological sounds, geological events, and anthropogenic impacts of vessel noise. Front. Mar. Sci. 6:347. doi: 10.3389/fmars.2019.00347
Herman, L. M. (2017). The multiple functions of male song within the humpback whale (megaptera novaeangliae) mating system: review, evaluation, and synthesis. Biol. Rev. 92, 1795–1818. doi: 10.1111/brv.12309
Hildebrand, J. A. (2009). Anthropogenic and natural sources of ambient noise in the ocean. Mar. Ecol. Prog. Ser. 395, 5–20. doi: 10.3354/meps08353
Hoyt, E., and Iñíguez, M. (2008). Estado del avistamiento de cetáceos en américa latina. Chippenham: WDCS, 60.
Huertas, L. V., Rey, M. P., Seger, K. D., Perazio, C. E., Botero, N., Gonzalez, V., and Luna-Acosta, A. (2019). A comparison of humpback whale singing and ambient noise cycles in the gulf of tribugá, chocó, Colombia. J. Acoust. Soc. Am. 146, 2896–2896. doi: 10.1121/1.5137048
Kaplan, M. B., and Mooney, T. A. (2015). Ambient noise and temporal patterns of boat activity in the US Virgin Islands National Park. Mar. Pollut. Bull. 98, 221–228. doi: 10.1016/j.marpolbul.2015.06.047
Marques De Sá, J. P. (2007). Applied Statistics Using SPSS Statistica, Matlab and R. Berlin; Heidelberg; New York, NY: Springer Publishing Company, Incorporated.
Mattila, D. K., Guinee, L. N., and Mayo, C. A. (1987). Humpback whale songs on a North Atlantic feeding ground. J. Mammal. 68, 880–883.
McDonald, M. A., Hildebrand, J. A., and Wiggins, S. M. (2006). Increases in deep ocean ambient noise in the Northeast Pacific West of San Nicolas Island, California. J. Acoust. Soc. Am. 120, 711–718. doi: 10.1121/1.2216565
McDonald, M. A., Hildebrand, J. A., Wiggins, S. M., and Ross, D. (2008). A 50 year comparison of ambient ocean noise near san clemente island: A bathymetrically complex coastal region off Southern California. J. Acoust. Soc. Am. 124, 1985–1992. doi: 10.1121/1.2967889
McKenna, M. F., Ross, D., Wiggins, S. M., and Hildebrand, J. A. (2012). Underwater radiated noise from modern commercial ships. J. Acoust. Soc. Am. 131, 92–103. doi: 10.1121/1.3664100
McSweeney, D. J., Chu, K. C., Dolphin, W. F., and Guinee, L. N. (1989). North pacific humpback whale songs: a comparison of southeast alaskan feeding ground songs and hawaiian wintering ground songs. Mar. Mammal Sci. 5, 139–148. doi: 10.1111/j.1748-7692.1989.tb00328.x
Mercado, E. III., Herman, L. M., and Pack, A. A. (2005). Song copying by humpback whales: themes and variations. Anim. Cogn. 8, 93–102. doi: 10.1007/s10071-004-0238-7
Mercado, E. III., and Perazio, C. E. (2021). Similarities in composition and transformations of songs by humpback whales (megaptera novaeangliae) over time and space. J. Comp. Psychol. 135, 28–50. doi: 10.1037/com0000268
Merchant, N. D., Pirotta, E., Barton, T. R., and Thompson, P. M. (2014). Monitoring ship noise to assess the impact of coastal developments on marine mammals. Mar. Pollut. Bull. 78, 85–95. doi: 10.1016/j.marpolbul.2013.10.058
Miller, P. J., Biassoni, N., Samuels, A., and Tyack, P. L. (2000). Whale songs lengthen in response to sonar. Nature 405, 903. doi: 10.1038/35016148
Norris, T. F., Mc Donald, M., and Barlow, J. (1999). Acoustic detections of singing humpback whales (megaptera novaeangliae) in the eastern north pacific during their northbound migration. J. Acoust. Soc. Am. 106, 506–514. doi: 10.1121/1.427071
Pack, A. A., Potter, J., Herman, L., Hoffmann-Kuhnt, M., and Deakos, M. (2003). Determining Source Levels Sound Fields and Body Sizes of Singing Humpback Whales (megaptera novaeangliae) in the Hawaiian Winter Ground. Technical report, Dolphin Inst, Honolulu HI.
Parsons, E. (2012). The negative impacts of whale-watching. J. Mar. Biol. 2012:807294. doi: 10.1155/2012/807294
Perazio, C. E., and Mercado, E. III. (2018). Singing humpback whales megaptera novaeangliae favor specific frequency bands. Proc. Meet. Acoust. 35:010004. doi: 10.1121/2.0001029
Putland, R. L., Merchant, N. D., Farcas, A., and Radford, C. A. (2018). Vessel noise cuts down communication space for vocalizing fish and marine mammals. Glob. Change Biol. 24, 1708–1721. doi: 10.1111/gcb.13996
Rey, M. P., Huertas, L. V., Seger, K. D., Botero-Acosta, N., and Luna-Acosta, A. (2019). Diagnóstico del ciclo de canto de las ballenas jorobadas (Megaptera novaeangliae) en el golfo de tribugó, pacífico norte colombiano. SENALMAR. (Barranquilla).
Rolland, R. M., Parks, S. E., Hunt, K. E., Castellote, M., Corkeron, P. J., Nowacek, D. P., et al. (2012). Evidence that ship noise increases stress in right whales. Proc. R. Soc. B Biol. Sci. 279, 2363–2368. doi: 10.1098/rspb.2011.2429
Rueda, X. (1997). The impacts of tourism on local communities and the environment in Colombia's Pacific coast (Ph.D. thesis), Cambridge, MA: Massachusetts Institute of Technology.
Scheidat, M., Castro, C., Gonzalez, J., and Williams, R. (2004). Behavioural responses of humpback whales (megaptera novaeangliae) to whalewatching boats near isla de la plata, Machalilla National Park, Ecuador. J. Cetacean Res. Manage. 6, 63–68.
Schneider, J. N., and Mercado, E. III. (2018). Characterizing the rhythm and tempo of sound production by singing whales. Bioacoustics 28, 239–256. doi: 10.1080/09524622.2018.1428827
Seger, K. D. (2016). Ambient acoustic environments and cetacean signals: baseline studies from humpback whale and gray whale breeding grounds (Ph.D. thesis). UC San Diego, San Diego, CA, United States.
Seger, K. D. (2020). Recording a Pre-disturbed Ecosystem: the State of the Gulf of Tribugó, Colombia, Before Port Construction. UNH CARE Seminar Series.
Seger, K. D., Botero-Acosta, N., Perazio, C. E., Palanca, M., Huertas, L. V., Rey, M. P., et al. (2020). Executive Summary of the Acoustic Environment in the Gulf of Tribuga. Seattle, WA: PHySIColombia.
Seger, K. D., Thode, A., and Gabriele, C. (2012). “Two acoustic environments in glacier bay National Park,” Poster session presented at American Cetacean Society Conference (San Diego, CA).
Seger, K. D., Thode, A. M., Swartz, S. L., and Urbán R, J. (2015). The ambient acoustic environment in laguna san ignacio, baja California sur, Mexico. J. Acoust. Soc. Am. 138, 3397–3410. doi: 10.1121/1.4935397
Shannon, G., McKenna, M. F., Angeloni, L. M., Crooks, K. R., Fristrup, K. M., Brown, E., et al. (2016). A synthesis of two decades of research documenting the effects of noise on wildlife. Biol. Rev. 91, 982–1005. doi: 10.1111/brv.12207
Sousa-Lima, R. S., and Clark, C. W. (2008). Modeling the effect of boat traffic on the fluctuation of humpback whale singing activity in the abrolhos National Marine Park, Brazil. Can. Acoust. 36, 174–181.
Sousa-Lima, R. S., and Clark, C. W. (2009). Whale sound recording technology as a tool for assessing the effects of boat noise in a Brazilian marine park. Park Sci. 26, 59–63.
Stamation, K. A., Croft, D. B., Shaughnessy, P. D., Waples, K. A., and Briggs, S. V. (2010). Behavioral responses of humpback whales (megaptera novaeangliae) to whale-watching vessels on the southeastern coast of Australia. Mar. Mammal Sci. 26, 98–122. doi: 10.1111/j.1748-7692.2009.00320.x
Stimpert, A. K., Peavey, L. E., Friedlaender, A. S., and Nowacek, D. P. (2012). Humpback whale song and foraging behavior on an antarctic feeding ground. PLoS ONE 7:e51214. doi: 10.1371/journal.pone.0051214
Thompson, T. J. (1983). Temporal characteristics of humpback whale (Megaptera novaeangliae) songs (Ph.D. thesis), University of Rhode Island, Kingston, RI.
Tougaard, J., Carstensen, J., Teilmann, J., Skov, H., and Rasmussen, P. (2009). Pile driving zone of responsiveness extends beyond 20 km for harbor porpoises (phocoena phocoena (l.)). J. Acoust. Soc. Am. 126, 11–14. doi: 10.1121/1.3132523
Tsujii, K., Akamatsu, T., Okamoto, R., Mori, K., Mitani, Y., and Umeda, N. (2018). Change in singing behavior of humpback whales caused by shipping noise. PLoS ONE 13:e0204112. doi: 10.1371/journal.pone.0204112
Tyack, P. (1981). Interactions between singing hawaiian humpback whales and conspecifics nearby. Behav. Ecol. Sociobiol. 8, 105–116.
Tyack, P. L. (2008). Implications for marine mammals of large-scale changes in the marine acoustic environment. J. Mammal. 89, 549–558. doi: 10.1644/07-MAMM-S-307R.1
Velandia, M., and Díaz, J. (2016). Atlas marino-costero del pacífico norte colombiano. Bogotá: Fundación MarViva.
Weilgart, L. S. (2007). The impacts of anthropogenic ocean noise on cetaceans and implications for management. Can. J. Zool. 85, 1091–1116. doi: 10.1139/Z07-101
Wentworth, C. K. (1922). A scale of grade and class terms for clastic sediments. J. Geol. 30, 377–392.
Wenz, G. M. (1962). Acoustic ambient noise in the ocean: spectra and sources. J. Acoust. Soc. Am. 34, 1936–1956.
Williams, R., Wright, A. J., Ashe, E., Blight, L., Bruintjes, R., Canessa, R., et al. (2015). Impacts of anthropogenic noise on marine life: publication patterns, new discoveries, and future directions in research and management. Ocean Coast. Manage. 115, 17–24. doi: 10.1016/j.ocecoaman.2015.05.021
Keywords: Gulf of Tribugá, Colombia, inter-unit interval, whale-watching, humpback whale song, masking, propagation modeling
Citation: Rey-Baquero MP, Huertas-Amaya LV, Seger KD, Botero-Acosta N, Luna-Acosta A, Perazio CE, Boyle JK, Rosenthal S and Vallejo AC (2021) Understanding Effects of Whale-Watching Vessel Noise on Humpback Whale Song in the North Pacific Coast of Colombia With Propagation Models of Masking and Acoustic Data Observations. Front. Mar. Sci. 8:623724. doi: 10.3389/fmars.2021.623724
Received: 30 October 2020; Accepted: 12 February 2021;
Published: 25 March 2021.
Edited by:
Peter Corkeron, New England Aquarium, United StatesReviewed by:
Timothy J. Rowell, National Oceanic and Atmospheric Administration (NOAA), United StatesGail Schofield, Queen Mary University of London, United Kingdom
Copyright © 2021 Rey-Baquero, Huertas-Amaya, Seger, Botero-Acosta, Luna-Acosta, Perazio, Boyle, Rosenthal and Vallejo. This is an open-access article distributed under the terms of the Creative Commons Attribution License (CC BY). The use, distribution or reproduction in other forums is permitted, provided the original author(s) and the copyright owner(s) are credited and that the original publication in this journal is cited, in accordance with accepted academic practice. No use, distribution or reproduction is permitted which does not comply with these terms.
*Correspondence: Kerri D. Seger, a2Vycmkuc2VnZXJAYXBwbGllZG9jZWFuc2NpZW5jZXMuY29t