- 1Department of Anatomy and Embryology, Faculty of Veterinary Medicine, Kafrelsheikh University, Kafr El Sheikh, Egypt
- 2Department of Physiology, Faculty of Veterinary Medicine, Kafrelsheikh University, Kafr El Sheikh, Egypt
- 3Department of Fish Diseases and Management, Sakha Aquaculture Research Unit, Central Laboratory for Aquaculture Research, A.R.C., Giza, Egypt
- 4Department of Biotechnology, College of Science, Taif University, Taif, Saudi Arabia
- 5Department of Fish Diseases and Management, Faculty of Veterinary Medicine, Kafrelsheikh University, Kafr El Sheikh, Egypt
- 6Department of Animal and Aquatic Sciences, Faculty of Agriculture, Chiang Mai University, Chiang Mai, Thailand
- 7Innoviative Agriculture Research Center, Faculty of Agriculture, Chiang Mai University, Chiang Mai, Thailand
- 8Department of Animal Production, Faculty of Agriculture in Cairo, Al-Azhar University, Cairo, Egypt
- 9Department of Husbandry and Animal Wealth Development, Faculty of Veterinary Medicine, Mansoura University, Mansoura, Egypt
- 10Department of Animal Production, Faculty of Agriculture, Kafrelsheikh University, Kafr El Sheikh, Egypt
Aeromonas hydrophila infection is one of the major diseases that cause inflammation and immunodepression in aquatic animals. Likewise, waterborne toxins are known for their negative impact on the immunity and antioxidant responses of aquatic organisms. In this study, Lactobacillus plantarum (LP20) was incorporated in Nile tilapia, Oreochromis niloticus, diets (50 mg/kg) to investigate its role in the antioxidant capacity, immunity, and anti-inflammation induced by deltamethrin (DLM) toxicity (96-h LC50 was 14.9 μg/L) and A. hydrophila (LD50, 2 × 108 CFU/ml). One hundred and twenty healthy fish with an initial weight of 28.21 ± 1.34 g were allotted in 12 glass aquaria (60 L) and divided randomly into four groups (triplicates, 10 fish per aquarium). The control fish neither received LP20 nor were exposed to DLM. The second group of fish was fed the control diet and subjected to DLM (DLM group). The third group of fish was provided with LP20 without DLM toxicity (LP20 group), and the fourth group of fish was fed LP20 and subjected to DLM (DLM + LP20 group). After 30 days, fish were intraperitoneally injected with A. hydrophila. Ten days post infection, the survival rate was lower in fish exposed to DLM than those exposed to LP20. Further, fish fed on LP20 had elevated serum total protein (sTP), albumin (ALB), globulin (GLB), phagocytic index (PI), phagocytic (PA), and lysozyme activities (LZM), but they had lower urea, uric acid bilirubin, creatinine, glucose, aspartate aminotransferase (AST), alkaline phosphatase (ALP), and alanine aminotransferase (ALT) activities after 24 h, 48 h, and 1 week post A. hydrophila challenge. However, fish exposed to DLM had lower sTP, ALB, GLB and higher urea, uric acid, bilirubin, creatinine, glucose, ALT, AST, and ALP after 24 h, 48 h, and 1 week post A. hydrophila challenge. Markedly, after the A. hydrophila challenge, fish exposed to DLM + LP20 displayed upregulated levels of superoxide dismutase (SOD), catalase (CAT), and glutathione peroxidase (GPx) genes. The transcription of interferon gamma (IFN-γ), interleukin 12 (IL-12), and tumor necrosis factor alpha (TNF-α) was upregulated in DLM and DLM + LP20 groups. Fish exposed to DLM downregulated interleukin 8 (IL-8) gene expression after A. hydrophila challenge. Further, fish displayed upregulation of heat shock protein 70 (HSP70) gene expression after DLM toxicity. Besides, fish exposed to DLM toxicity and A. hydrophila infection had severe inflammatory features in the liver, spleen, gills, and intestine, while dietary LP20 relieved the inflammatory features. In summary, dietary LP20 relieves Nile tilapia from oxidative stress, immunosuppression, and inflammation induced by DLM and A. hydrophila infection.
Introduction
Aquaculture production has expanded recently as a primary source of animal protein with feasible cost compared to the other animal protein sources (Dawood, 2021). This activity requires many factors to ensure its success, including the availability of seeds, technical skills, optimal feed requirements, and reasonable environmental conditions (Wu et al., 2019). In closed and indoor aquaculture systems, all factors can be controlled to avoid stressful conditions that could impair aquatic organisms’ performance; however, in outdoor systems, the farming conditions cannot be fully controlled (Zaki et al., 2020). Stressful environmental conditions (e.g., high or low water temperature, high stocking rate, malnutrition, and infectious diseases) weaken the organism’s immune system, increase attack by infectious invaders on the body, and induce severe mortality (Shourbela et al., 2021). Pesticide and insecticide derivatives resulting from the agriculture sector pollute the drainage water likely to be used for the rearing of aquatic animals (El Megid et al., 2020; Abdo et al., 2021). The remaining derivatives generally accumulate in the fish body and impair growth performances and health condition. Deltamethrin (DLM) is one of the common pyrethroid insecticides regularly used in agriculture-related activities (Bradbury and Coats, 1989). It weakens the immune system of fish and makes them vulnerable to bacterial pathogens (Eder et al., 2007). Aeromonas hydrophila is a bacterial pathogen that widely attacks aquatic organisms, especially when they suffer from stressful conditions, including water pollution (Fernandes et al., 2019). A. hydrophila infection is increasing in cultured organisms because of the rising natural resistance to pathogens due to the excessive use of antibiotics, resulting in severe fish farming hazards (Ahmadifar et al., 2019). A. hydrophila can survive in fresh or brackish water under aerobic and anaerobic environments, which threatens the aquaculture industry (Chen et al., 2018). A. hydrophila induces hemorrhagic disease, ulcerative syndrome, and motile Aeromonas septicemia in Nile tilapia (Oreochromis niloticus), usually resulting in high mortalities (Nicholson et al., 2020). Although antibiotics are suggested to enhance the resistance of aquatic organisms against infectious diseases, they have other side effects (e.g., weakening the natural immunity, reducing the resistance to pathogenic bacteria, and indirectly impacting the human body) (Dawood et al., 2021; Limbu et al., 2021). Thus, several countries have banned chemotherapies and encourage farmers to use alternative friendly substances (Chen et al., 2020).
The concept of using functional additives is practiced for its positive impact on aquatic animal efficiency (Ringo et al., 2018; Dawood et al., 2020a). Probiotics can enhance the variety of intestinal microbiota, compete for harmful microorganisms, facilitate absorption of nutrients through the intestinal villi, and activate the local intestinal immunity, increasing innate immunity (Dawood et al., 2018; Ringø et al., 2020). Generally, bacteria, including Lactobacillus plantarum, Lactococcus lactis, Enterococcus faecium, Bacillus subtilis, and Saccharomyces cerevisiae, have been utilized as probiotics for aquatic animals (Newaj-Fyzul et al., 2014). Among them, L. plantarum (LP20) recently has attracted extensive attention as a probiotic in feed industries due to its non-toxic, significant stress resistance (low pH and bile salt resistance) (Ismaeil et al., 2020), beneficial effect on the hosts (Van Nguyen et al., 2019), and stability under processing conditions (Dawood et al., 2016). Interestingly, LP20 has been known to serve as a protector against DLM toxicity. For instance, LP20 inclusion in the Nile tilapia diet improved immune and antioxidant defenses to ambient DLM toxicity via anti-inflammatory impact in the gills, liver, spleen, and intestinal tissues (Dawood et al., 2020b). Further, dietary LP20 protected Nile tilapia from ammonium chloride toxicity and Streptococcus agalactiae infection (Van Nguyen et al., 2019).
Nile tilapia is a freshwater species and widely cultured in several countries due to its fast growth rate, high market need, and reasonable prices (FAO, 2018). However, in some countries (e.g., Egypt), the effluent water from the agriculture sector is usually used in Nile tilapia farming ponds. The drainage water is rich in pesticides and insecticides that negatively affect fish performance. Concurrently, the current study investigated the impact of LP20 in the alleviation of oxidative injury, immunosuppression, and inflammation generated by DLM and A. hydrophila in Nile tilapia.
Materials and Methods
Experimental Design
Fish were obtained from Tahoun farm located in the Kafr El Sheikh governorate and transferred to the Sakha Aquaculture Research Unit, Kafr El Sheikh, Egypt. After accommodation for 7 days (with basal diet), 120 healthy fish with an initial weight of 28.21 ± 1.34 g were allocated into 12 glass aquaria (60 L) and divided into four groups: hereafter, control, DLM, LP20, and DLM + LP20 in triplicates (10 fish per aquarium) where all aquaria were allocated randomly without locating aquaria from the same group beside each other.
Two sets of diets were created by supplementing the basal diet with 0 or 50 mg LP20/kg (L. plantarum, 2 × 1011 CFU/g, House Wellness Foods Corp., Itami, Japan) (Dawood et al., 2020d); then 30–40% water was added. The LP20 was added to the fish oil and well mixed and then added to the remaining ingredients and mixed again. A meat mincer subsequently pelleted the dough through a die of 1 to 2 mm. The pellets were air-dried at room temperature for 24 h and finally kept at 4°C for the trial. The prepared diet composition was checked by using the standard method (AOAC, 2007). The formulation and chemical composition of the basal diet are provided as a Table 1. The prepared diets were fed to Nile tilapia for 30 days. Fish were fed the test diets at 3% of the total biomass twice (at 08:00 and 16:00) daily. The LP20 dose was chosen based on the report done by Dawood et al. (2020d).
The DLM was obtained from the Kafr El-Zayat Company for Chemicals and Pesticides, Egypt, with 98.5% purity. Then, DLM was dissolved in ethanol to have a concentration of 14.5 μg/L. The 96-h LC50 was 14.5 μg/L, and the lower and upper values were 14.1 and 14.9 μg/L, respectively, within the 95% confidence limits as previously reported by Cengiz et al. (2017) and Golow and Godzi (1994). A stock solution was prepared by diluting DLM with acetone to give a dosing concentration of 14.9 μg/L. One third of the water in each aquarium was changed daily and replaced with the prepared DLM solution to keep the DLM level constant during the trial (14.9 μg/L). The trial was conducted under a natural light-and-dark cycle (12 h:12 h). The water temperature was 21 ± 2°C, while pH was 7.1 ± 0.8, dissolved oxygen was 6.5 ± 0.5 mg/L, and total ammonia was 0.23 ± 0.03 mg/L. After 30 days, all fish were euthanized by tricaine methanesulfonate (100 mg/L), and then the liver, gills, intestine, and spleen tissues were taken from three fish per aquarium for histopathological analysis (using 40% ethyl alcohol).
Aeromonas hydrophila Infection and Sampling
The A. hydrophila inoculum was generously supplied by the Department of Fish Diseases and Management, Faculty of Veterinary Medicine, Kafrelsheikh University, Egypt. The A. hydrophila inoculum was made by culturing the organism using tryptic soy agar (TSA) at 37°C for 24 h with continuous shaking (250 rpm). The LD50 (lethal dose, the dose which kills 50% of the injected fish) was assessed before the final challenge up to 10 days by following Reed and Muench (1938). The bacterial fluid was diluted in phosphate-buffered saline (PBS, pH 7.2) and adjusted to a concentration of 2 × 108 CFU/ml.
After the DLM exposure trial (30 days), the remaining fish in each aquarium (seven fish per aquarium, triplicates per group) were intraperitoneally injected with 0.2 ml/fish of (2.8 × 106 CFU) A. hydrophila, according to Li et al. (2011) and Ran et al. (2018). Fish were offered feed at 2% of the bodyweight daily for 10 days. The challenged fish were observed daily, and all mortalities were recorded when fish showed no movement for 1 h (Lu et al., 2019). To confirm that the death was caused by A. hydrophila, the clinical–pathological features were observed, including swimming abnormalities, pale gills, bloat, skin ulcerations, skin ulcers, septicemia, and hemorrhagic features. The daily relative percentage of survival was calculated (Saputra et al., 2016). After A. hydrophila challenge, all fish were anesthetized using 150 mg/L of MS222 (Argent Laboratories, Redmond, Washington). Blood samples were taken (1 ml from three fish per aquarium at each sampling point) from the caudal vein using disposable 3-cc syringes and 21-gauge needles (AMECO, Cairo, Egypt). The blood was collected after 24 h, 48 h, and 1 week using EDTA-coated vials, while non-coated vials were utilized for serum collection. Blood samples were kept for 2 h and then centrifuged at 3,500 × g for 15 min at 4°C to obtain the serum. The serum was maintained at −20°C for further evaluation. The challenge with A. hydrophila lasted until the 10th day, and then the fish (six fish per group) were dissected to collect the liver, gills, intestine, and spleen tissues for gene expression (kept at −80°C) and histopathological analysis (using 40% ethyl alcohol).
Blood Examination
Serum total protein (sTP) were assessed using a biuret test by following Doumas et al. (1981), and albumins were determined by following Dumas and Biggs (1972), while globulin concentration was assessed mathematically. Activities of aspartate aminotransferase (AST), alkaline phosphatase (ALP), and alanine aminotransferase (ALT) were evaluated calorimetrically at a wavelength of 540 nm (Reitman and Frankel, 1957). Serum creatinine, uric acid, urea, and bilirubin were calorimetrically assessed, following Heinegård and Tiderström (1973) and Coulombe and Favreau (1963). Blood cortisol levels (MG/100 ml) were ascertained using enzymatic colorimetric analysis with glucose oxidase and 4-aminoantipyrine (GOD/PAP) kits bought from BioMérieux (France) (Trinder, 1969). Leukocyte phagocytic index (PI) and phagocytic activity (PA) were examined following the method of Cai et al. (2004). The lysozyme activity (LZM) of sera was assayed according to the method described by Demers and Bayne (1997).
Gene Transcription
Reverse-transcription polymerase chain reaction (RT-PCR) analysis of mRNA expression for different genes and β-actin (as an internal reference for normalization of gene expression data) was performed using the primers shown in Table 2 (Ming et al., 2013; Pang et al., 2013; Chen et al., 2016; Xie et al., 2016; Ting et al., 2018). The total RNA was extracted from the liver samples utilizing TRIzol reagents (iNtRON Biotechnology) following the manufacturer’s protocol. The quality of the extracted RNA was confirmed with 2% agarose electrophoresis. The quality and quantity of the extracted RNA were determined by NanoDrop (Quawell, United States). Samples with 1.8 or more A260/A280 RNA were used. Two micrograms of total RNA was reverse-transcribed using a cDNA synthesis kit (Bioline, United Kingdom) directed by the manufacturer. Real-time PCR amplifications were performed using a SensiFAST SYBR Lo-ROX kit (Bioline) in 20 μl reaction mixtures containing 2 μl of cDNA, the gene-specific primers (0.5 μM each), and SYBR (10 μl). The thermal cycling conditions were initial denaturation at 95°C for 10 min, followed by 40 cycles at 95°C for 15 s, annealing temperature as presented in Table 2, and 60°C for 1 min. All genes were tested in triplicates, and the fold change method was used to calculate (2–ΔΔ CT) by following Livak and Schmittgen (2001).
Histopathology
The histopathological examination for liver, gills, intestine, and spleen tissues was adopted, according to Abumandour and Gewaily (2016). The sections were taken with a Leica rotatory microtome (RM 20352035; Leica Microsystems, Wetzlar, Germany) and stained with hematoxylin and eosin. Afterward, the tissue sections were examined using a BX50/BXFLA microscope (Olympus, Tokyo, Japan).
Statistical Analysis
The normality and homogeneity distribution of all the collected data (biochemical, immunological, relative gene expression, and survival rate) were verified by Shapiro–Wilk and Levene tests, respectively. Results are presented as means with the standard error of means, and they were assessed by one-way analysis of variance (ANOVA) using SPSS 22.0 (SPSS version 22, SPSS Inc., IL, United States). Duncan’s multiple-range test was used to ascertain differences among treatments with significance set at P < 0.05.
Results
Survival Rate Post Aeromonas hydrophila Challenge
The mortalities were delayed to the second-day post-challenge in all groups except for the LP20 treatment, which showed the beginning of mortality on the fifth day. After 10 days, the survival rate was lower in Nile tilapia exposed to DLM toxicity compared to those subjected to LP20 treatment (Figure 1). Fish in control, LP20, and LP20 + DLM treatments had higher survival rates than fish in the DLM treatment (P < 0.05).
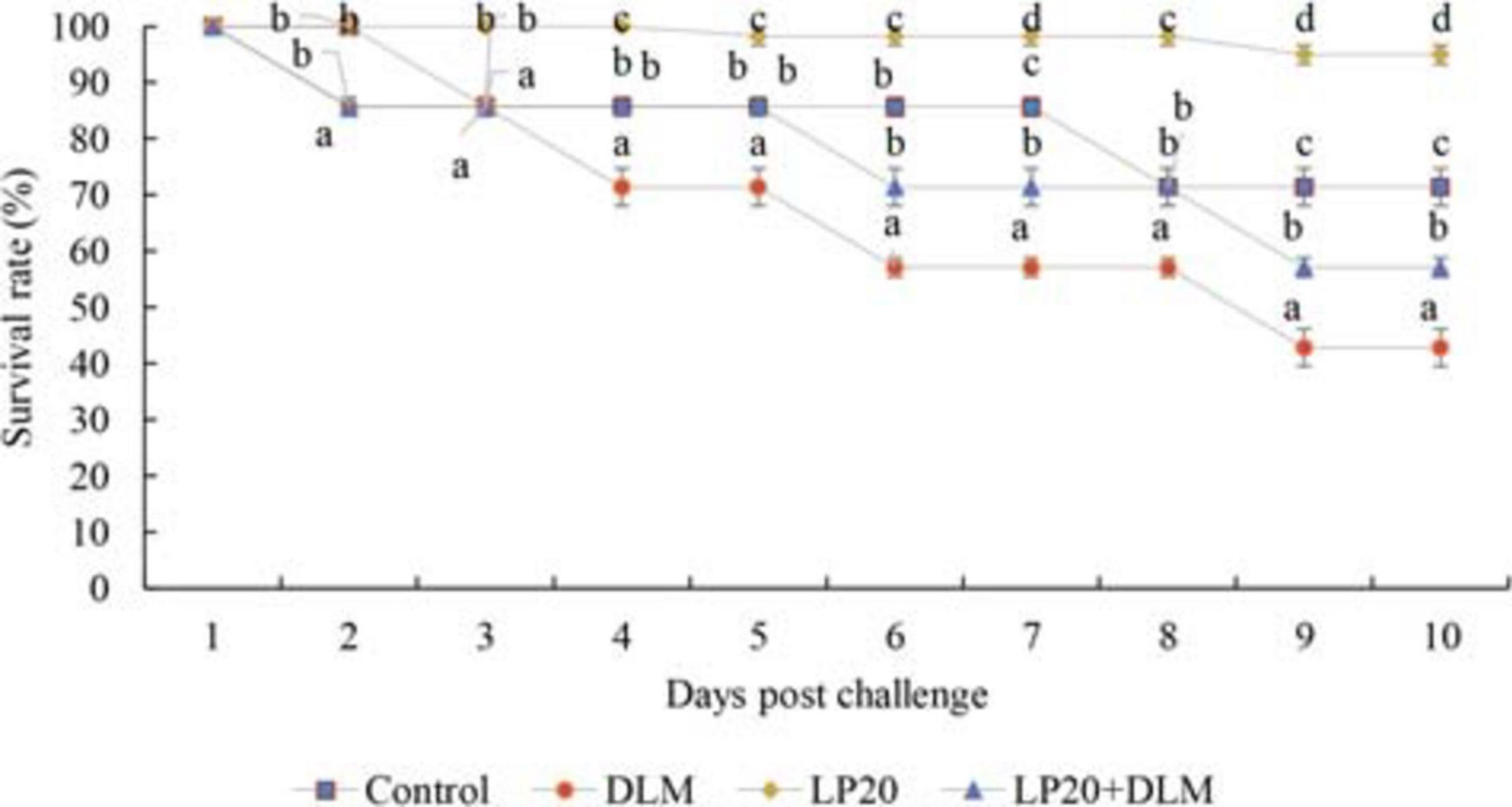
Figure 1. The survival rate of Nile tilapia exposed to DLM and challenged with Aeromonas hydrophila and fed diets with or without LP20 for 30 days. The survival rate was calculated according to the Kaplan–Meier method on a daily basis until the 10th day post challenge and expressed as mean ± SE from triplicate groups. Different letters on each period indicate significant difference P < 0.05.
Blood Measurements
Nile tilapia fed LP20 had higher total protein, albumin, and globulin but lower urea, uric acid, bilirubin, creatinine, glucose, ALT, AST, and ALP compared to the others at 24 h, 48 h, and 1 week post A. hydrophila infection (Table 3). Conversely, fish exposed to DLM had lower total protein, albumin, and globulin but higher urea, uric acid, bilirubin, creatinine, glucose, ALT, AST, and ALP than fish fed dietary LP20 (P < 0.05). Interestingly, LP20 decreased the uric acid, urea, creatinine, cortisol, ALP, ALT, and AST but increased total protein, albumin, and globulin in Nile tilapia fed LP20 and exposed to DLM (DLM + LP20) compared to fish subjected to DLM especially at 48 h and 1 week post A. hydrophila challenge (Table 3). Fish in the control and DLM treatments had increased uric acid, creatinine, bilirubin, ALT, and AST levels at 48 h and 1 week post A. hydrophila challenge. However, fish fed LP20 had reduced uric acid, creatinine, bilirubin, ALT, and AST levels.
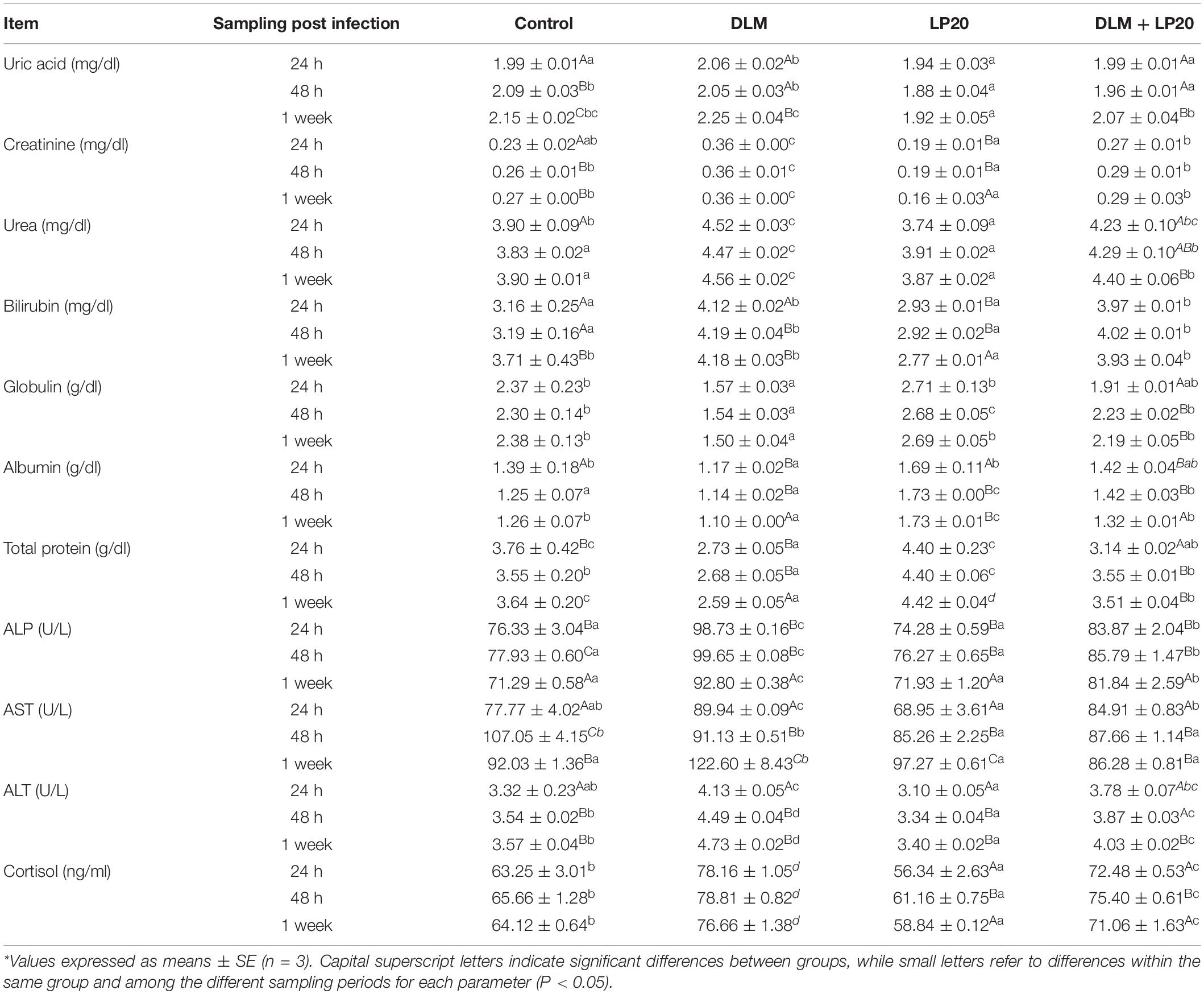
Table 3. Blood biochemical parameters of Nile tilapia exposed to deltamethrin (DLM) and challenged with Aeromonas hydrophila and fed diets with or without LP20 for 30 days.
Fish fed dietary LP20 had higher LZM (Figure 2A), PA activities (Figure 2B), and PI (Figure 2C) than fish exposed to DLM at 24 h, 48 h, and 1 week post A. hydrophila challenge (P < 0.05). Fish fed LP20 and exposed to DLM (DLM + LP20) had similar LZM with the control at 24 and 48 h post A. hydrophila challenge, whereas after 1 week of A. hydrophila challenge, the LZM was reduced (Figure 2A). PA and PI displayed similar results at 24 h post challenge. However, at 48 h and 1 week post A. hydrophila challenge, Nile tilapia fed LP20 and exposed to DLM (DLM + LP20) had higher PA (Figure 2B) and PI (Figure 2C) than those under DLM toxicity. Fish fed the control diet had reduced LZM at 48 h and 1 week post A. hydrophila challenge compared to fish fed LP20. Also, PA was substantially increased in fish fed LP20 diet at 48 h and 1 week post A. hydrophila challenge.
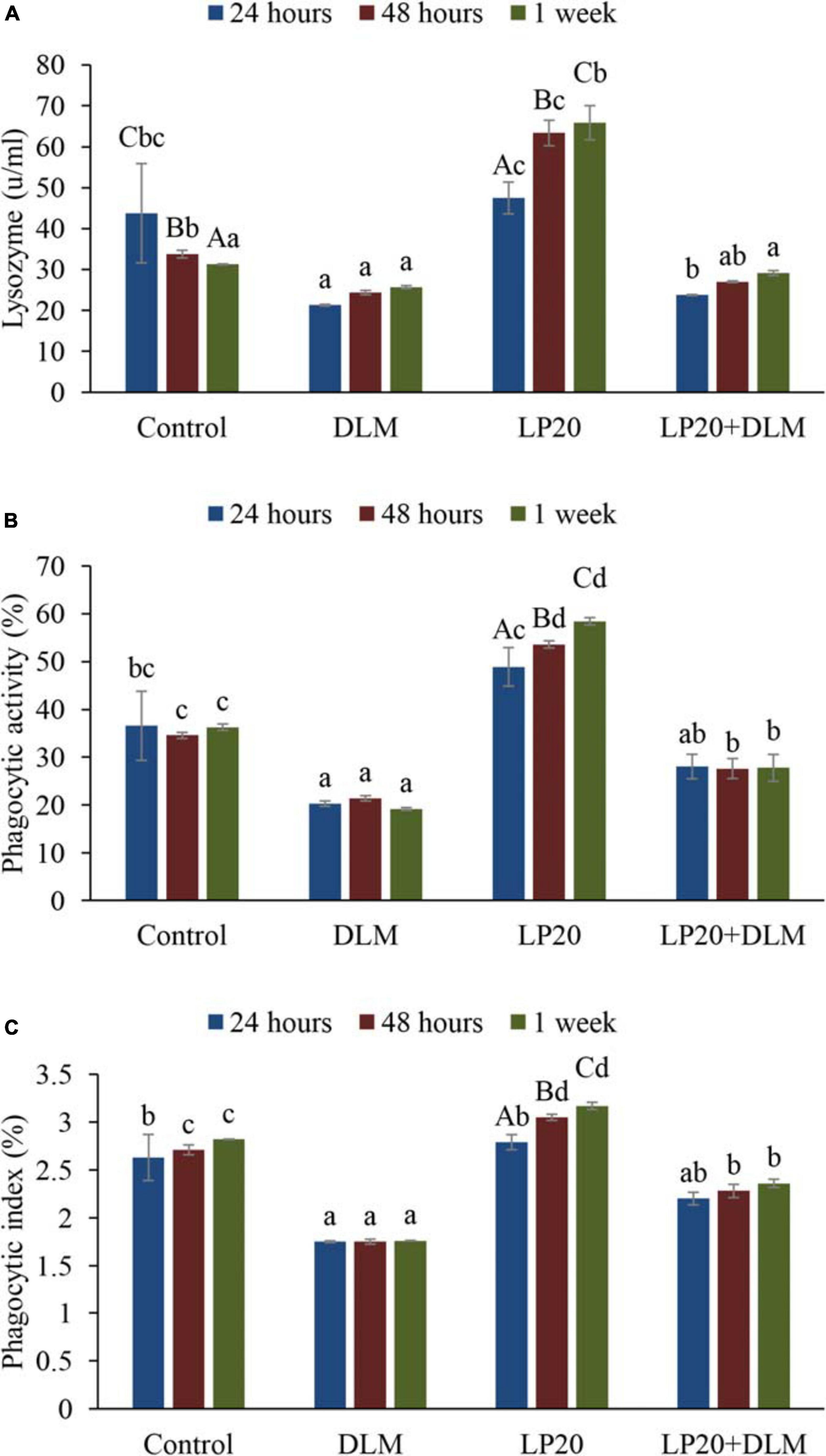
Figure 2. Blood lysozyme activity (A), phagocytic activity (B), and phagocytic index (C) of Nile tilapia exposed to DLM and challenged with Aeromonas hydrophila and fed diets with or without LP20 for 30 days. Values are expressed as mean ± SE from triplicate groups (nine fish per group). Bars with capital superscript letters indicate significant differences between groups, while those with small letters refer to differences within the same group and among the different sampling periods for each parameter (P < 0.05).
Gene Transcription Profile
After the A. hydrophila challenge, fish exposed to DLM + LP20 displayed upregulated quantities of superoxide dismutase (SOD), catalase (CAT), and glutathione peroxidase (GPx) genes (Figure 3). Besides, fish exposed to DLM and DLM + LP20 showed downregulated interferon gamma (IFN-γ), interleukin 12 (IL-12), and tumor necrosis factor alpha (TNF-α) gene expressions (Figures 4A,C,D). However, IL-8 was downregulated by DLM after the A. hydrophila challenge (Figure 4B). The transcription of HSP70 displayed increased expression under DLM toxicity (Figure 5).
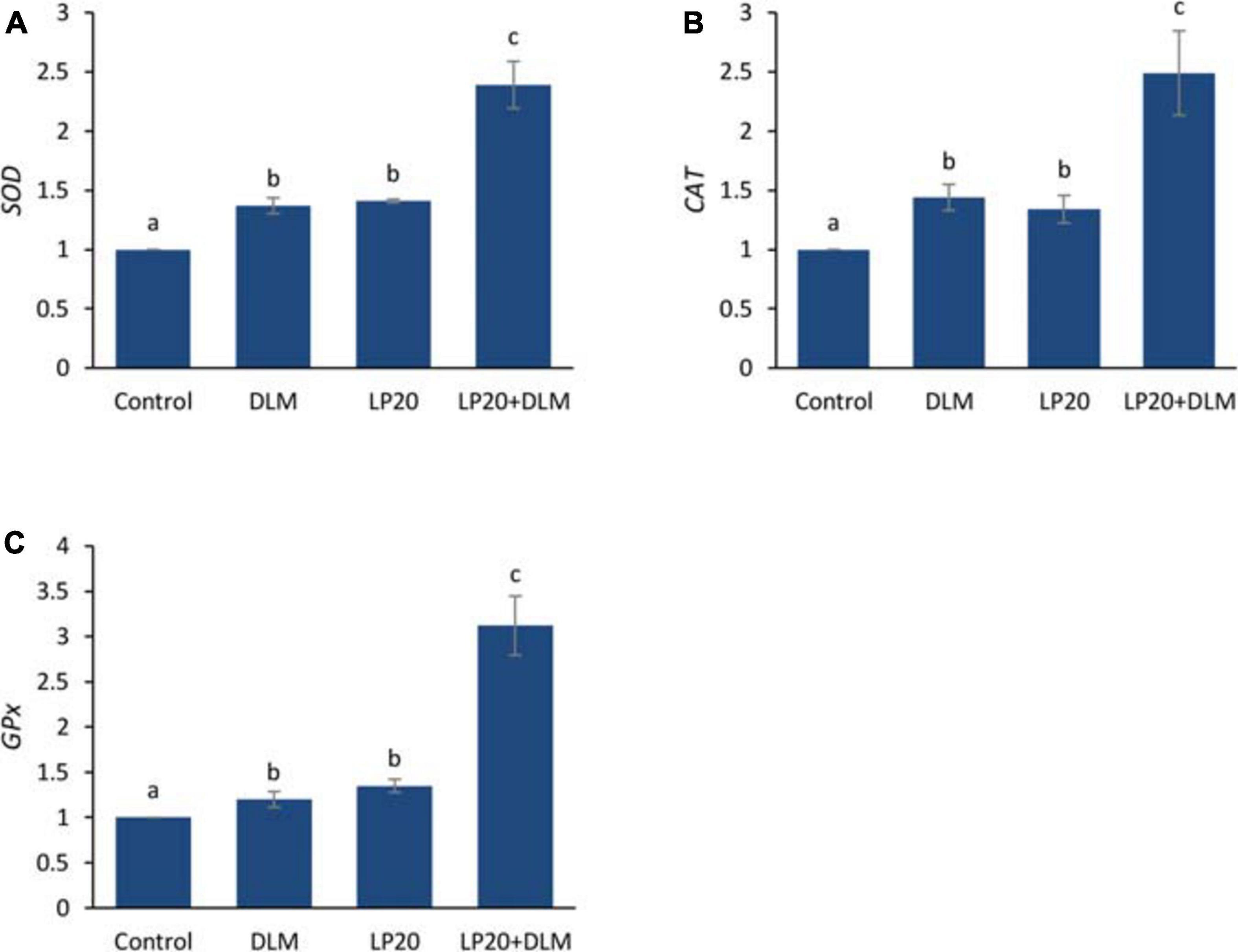
Figure 3. Relative expression of antioxidative genes (SOD, CAT, and GPx) of Nile tilapia exposed to DLM and challenged with Aeromonas hydrophila and fed diets with or without LP20 for 30 days. Values are expressed as mean ± SE from triplicate groups (six fish per group). Bars with different letters indicate significant difference from the control group (P < 0.05).
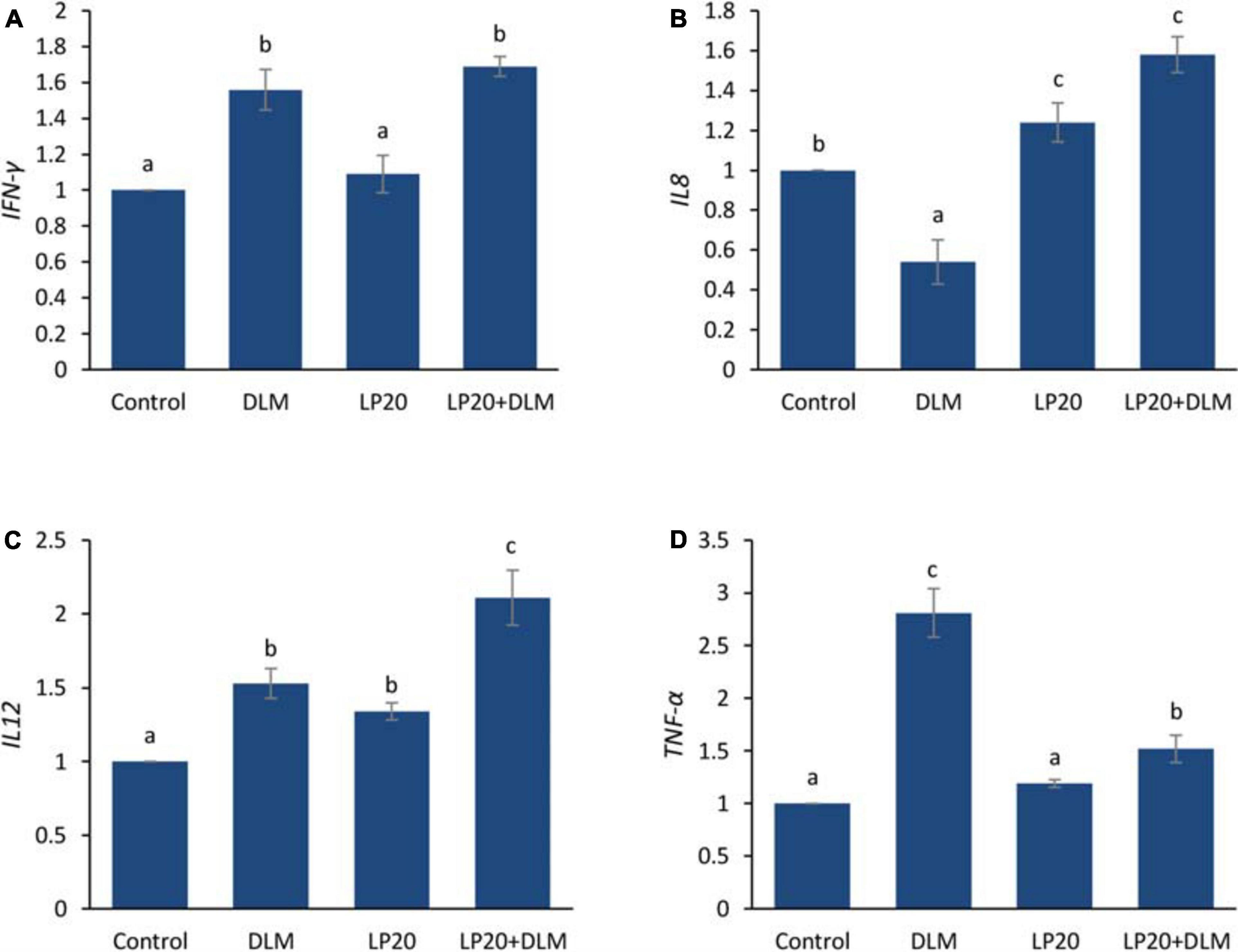
Figure 4. Relative expression of immune-related genes (IFN-γ, IL-8, IL-12, and TNF-α) of Nile tilapia exposed to DLM and challenged with A. hydrophila and fed diets with or without LP20 for 30 days. Values are expressed as mean ± SE from triplicate groups (six fish per group). Bars with different letters indicate significant difference from the control group (P < 0.05).
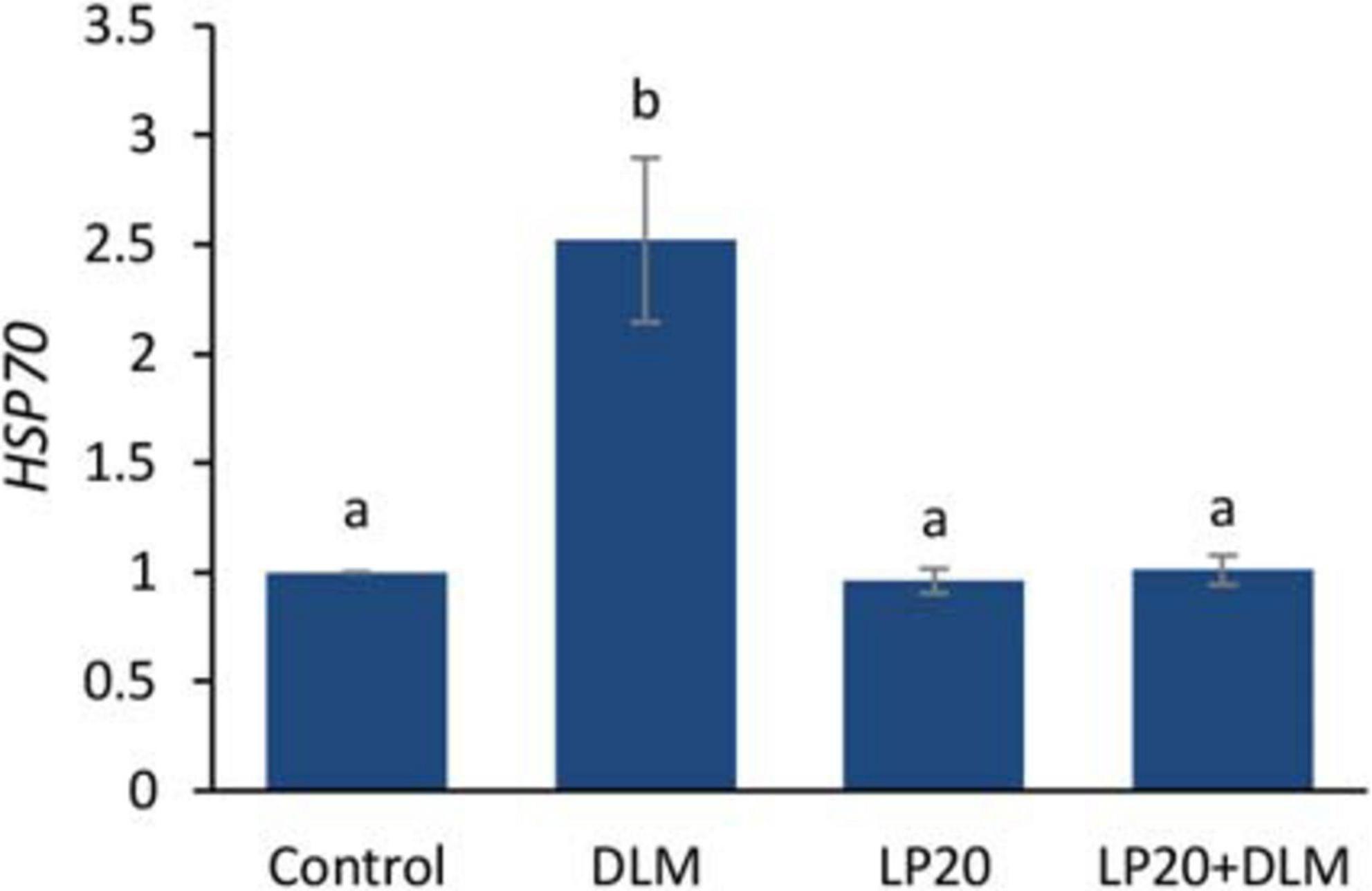
Figure 5. Relative expression of heat shock protein 70 (HSP70) gene of Nile tilapia exposed to DLM and challenged with Aeromonas hydrophila and fed diets with or without LP20 for 30 days. Values are expressed as mean ± SE from triplicate groups (nine fish per group). Bars with different letters indicate significant difference from the control group (P < 0.05).
Histopathology
The gills of fish fed with the control diet exhibited integral epithelium with copious mucous cells (Figure 6A). After A. hydrophila infection (Figure 6B), the blood vessels were slightly congested, and the apical ends of the secondary filaments were slightly dilated. The DLM toxicity produced telangiectasis, engorged blood supply, and inflammatory cell infiltration (Figure 6C). After infection (Figure 6D), the secondary epithelium presented deterioration, inflammatory edema, and increased invasion of leukocytes. In the LP20 treatment (Figure 6E), the gills were similar to the control treatment in addition to abundant immune cells. After infection (Figure 6F), the epithelium remained intact; however, the immune cells decreased in number. In the co-treated fish (DLM + LP20) (Figure 6G), the epithelium looked intact with mononuclear cell permeation. After A. hydrophila infection (Figure 6H), there was a slight telangiectasis with a little infiltration of immune cells.
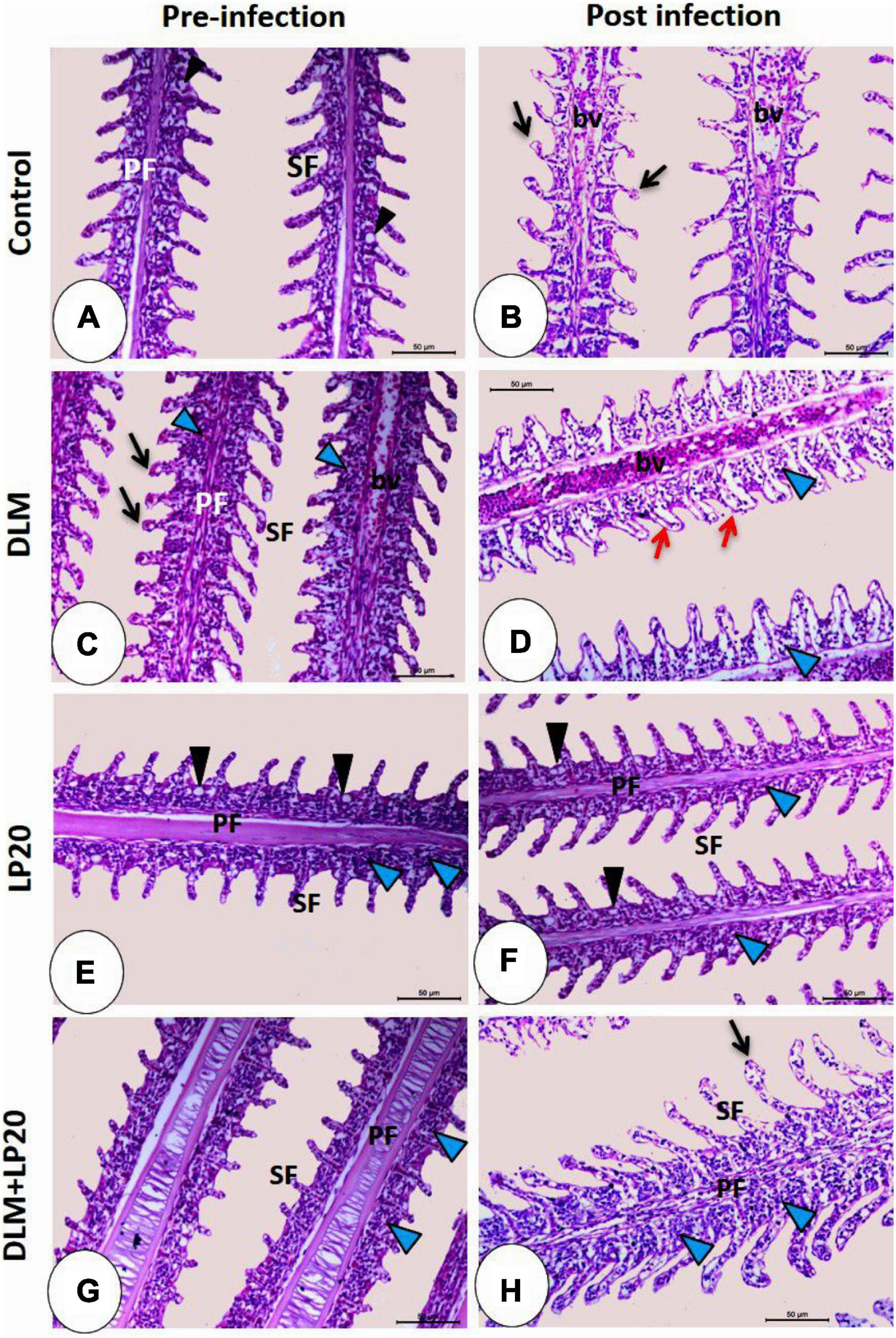
Figure 6. Histomicrograph of gills of Nile tilapia subjected to four treatments (control, DLM, LP20, and DLM + LP20) before (A,C,E,G) and after (B,D,F,H) infection. In panels (A,E,G), the gills show normal histological structures including primary filaments (PF), secondary filaments (SF), and mucous cells (black arrowhead) between the secondary filaments. The toxic effect of DLM (C) causes telangiectasia (black arrow) of the secondary filaments, congestion of blood vessels (bv) of primary filaments, and leukocytic cell infiltration (blue arrowhead). In the post-infected groups, the histopathological effect became more severe in the DLM group [the red arrow in panel (D) refers to degeneration and separation of the secondary epithelium] followed by the control group, while panels (F,H) showed normal histological structures due to the effect of LP20. Stain H&E. Bar = 100 μm.
The intestinal villi of the control fish exhibited normal structure and were formed of integral absorptive cells with goblet cells in between (Figure 7A). Post A. hydrophila infection, the intestine displayed stunted villi with degenerated and desquamated enterocytes (Figure 7B). DLM caused congestion of the apical part of the villi with augmented goblet cells (Figure 7C). Post A. hydrophila infection, there were stunted villi with severe edema, degeneration, and desquamation of enterocytes in addition to lymphoepithelium in the intact region of the villi (Figure 7D). In the LP20 fish (Figure 7E), the intestinal structure was similar to the control fish with increased length of intestinal villi and lymphoepithelium. After infection, the intestinal mucosa remained intact with some vacuolation and degeneration (Figure 7F). In the fish co-treated with DLM + LP20, the villi exposed some degeneration with desquamation in the apical region (Figure 7G). After infection, the apical part of dwarfed villi was congested while the connective tissue cores of villi were invaded with lymphocytes that migrated in the epithelium, making the lymphoepithelium (Figure 7H).
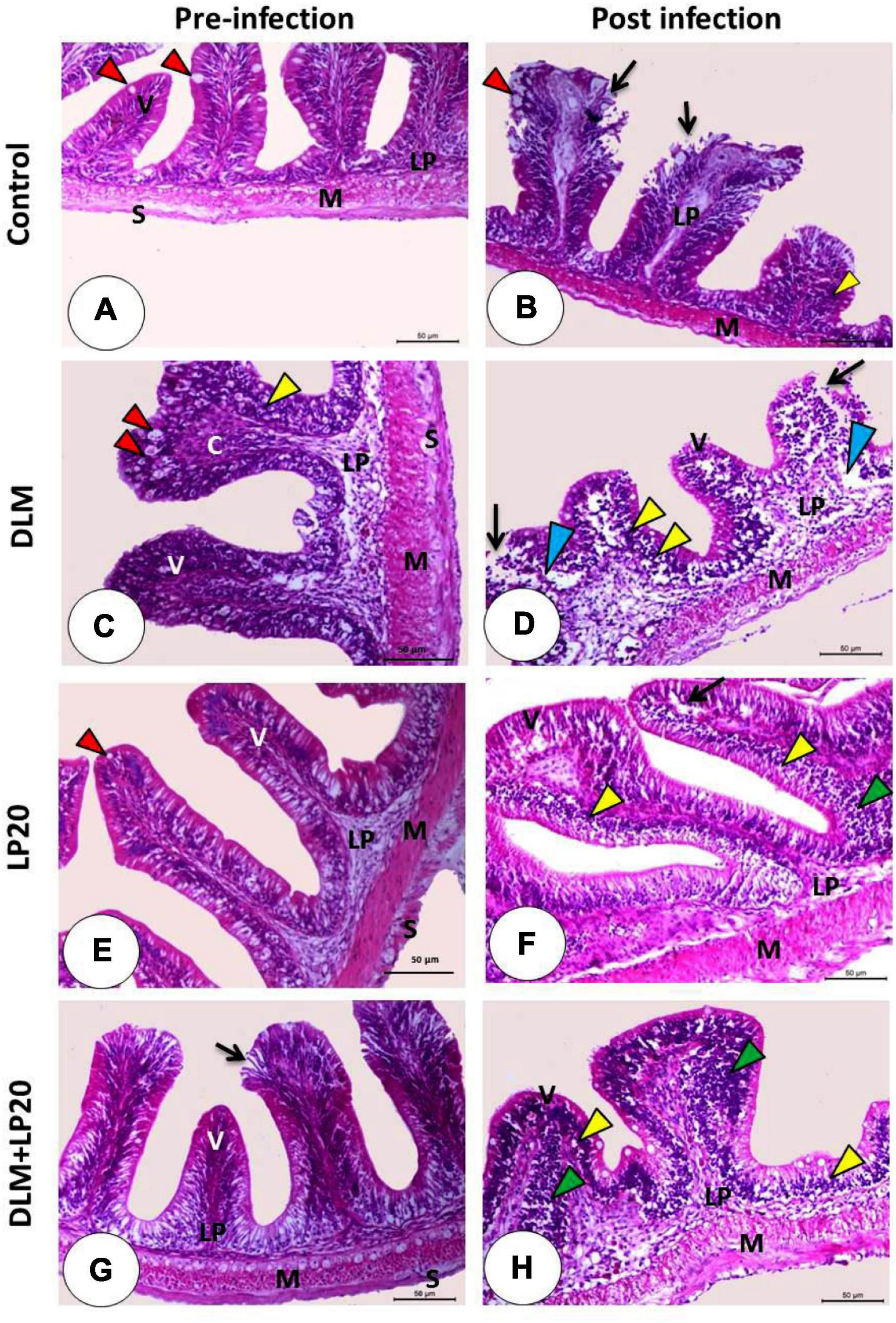
Figure 7. Histomicrograph of the intestine of Nile tilapia subjected to four treatments (control, DLM, LP20, and DLM + LP20) before infection (A,C,E,G) and after (B,D,F,H) infection. Panel (A) shows normal histological structures including the intestinal villi (V) lined by enterocytes with goblet cells (red arrowhead), lamina propria submucosa (LP), tunica muscularis (M), and tunica serosa (S), while the post-infected group (B) shows degeneration and necrosis of the apical half of the villi with desquamated enterocytes (black arrow) with lymphoepithelium (yellow arrowhead). The toxic effect of DLM (C) causes congestion of the apical part of the villi with increased goblet cells (red arrowhead) and lymphoepithelium (yellow arrowhead), while the post-infected group (D) shows severe edema (blue arrowhead), degeneration and desquamation of enterocytes (black arrow), and lymphoepithelium (yellow arrowhead). The LP20 group shows increased length of the villi, while the post-infected group (F) shows intact villi with pyknotic nuclei of the apical enterocyte (black arrow) with lymphoepithelium (yellow arrowhead) and immune cell infiltration (green arrowhead). In panel (G), the intestinal villi are intact and appeared normal except some degeneration and desquamation in the apical region of the villi (black arrow), while the post-infected group (H) shows stunted growth with lymphoepithelium (yellow arrowhead) and mononuclear cells (green arrowhead). Stain H&E. Bar = 100 μm.
The hepatopancreas in the control and LP20-fed fish (Figures 8A,E) revealed intact hepatocytes that organized in cords separated by blood sinusoids and typical pancreatic acini. The infection triggered fatty degeneration of hepatocytes, congestion of blood sinusoids, and slight degeneration of pancreatic cells (Figure 8B). The post-infected LP20-fed fish presented minor congestion of blood sinusoids (Figure 8F). The DLM-exposed fish revealed deterioration of hepatic and pancreatic cells and the appearance of melanomacrophages (Figure 8C). After infection, the liver was similar to that of fish exposed to DLM in addition to dilated blood sinusoids, lymphocytic permeation, and increased melanomacrophages (Figure 8D). The hepatopancreas in DLM + LP20 fish (Figure 8G) revealed protuberant lymphocyte infiltration. After infection, a slight fatty degeneration of the hepatic cells appeared with slightly congested blood sinusoids (Figure 8H).
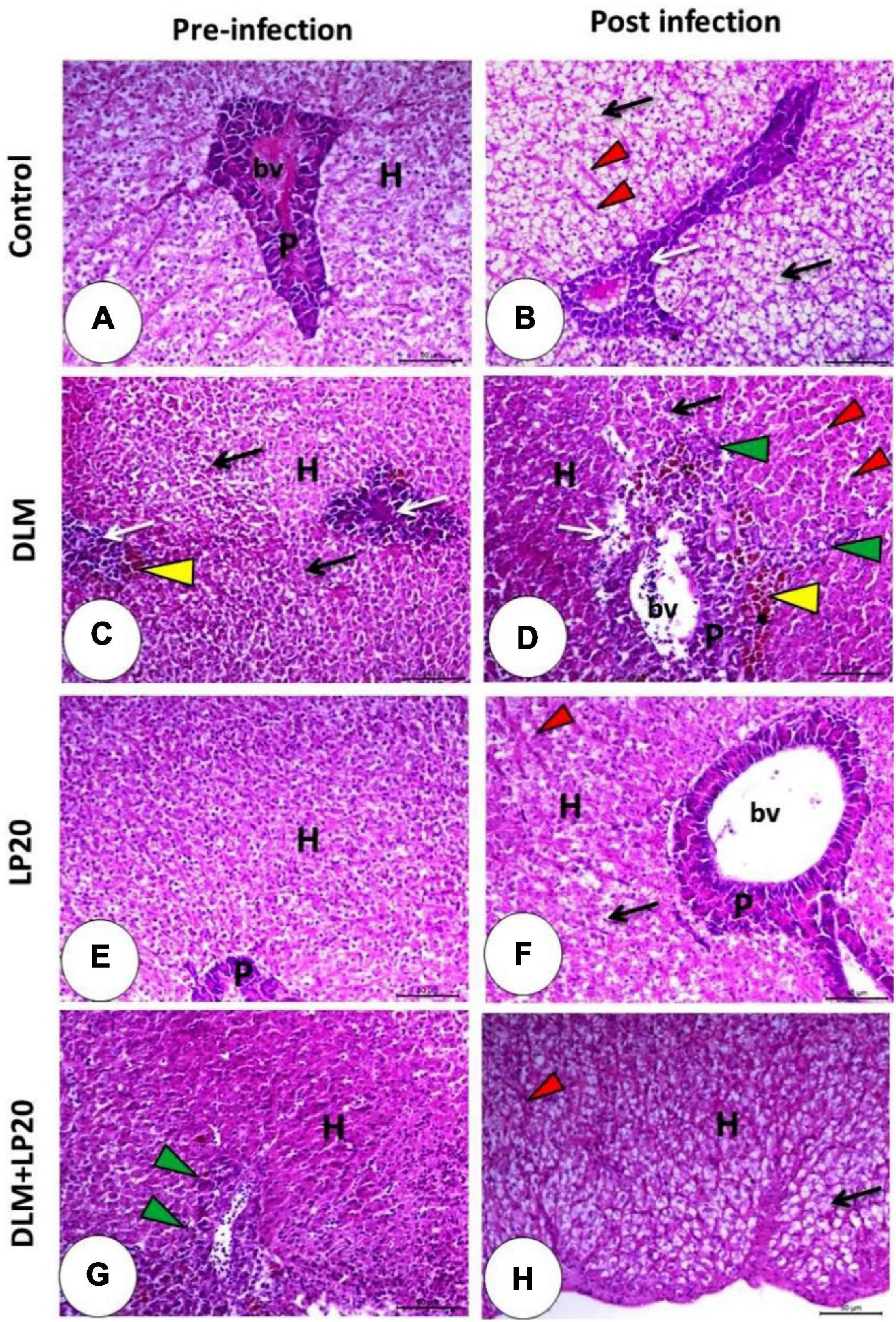
Figure 8. Histomicrograph of the liver of Nile tilapia subjected to four treatments (control, DLM, LP20, and DLM + LP20) before (A,C,E,G) and after (B,D,F,H) infection. Panel (A) shows intact hepatocytes that arranged in cords separated by blood sinusoids (H), and the pancreatic acini appeared normal with a basophilic basal part and eosinophilic apical part (P). Panel (B) shows fatty degeneration of hepatocytes, congested blood sinusoids (red arrowhead), and degenerated pancreatic cells (white arrow). The toxic effect of DLM (C) causes degeneration of hepatocytes with pyknotic nuclei (black arrow), degenerated pancreatic cells (white arrow), and melanomacrophages (yellow arrowhead). The DLM post-infected group shows degeneration of hepatic (black arrow) and pancreatic (white arrow) cells, dilated blood sinusoids (red arrowhead), melanomacrophage center (yellow arrowhead), and leukocytic infiltration (green arrowhead). LP20 improves the normal appearance of the hepatopancreas (E), while post-infection decreases histopathological deviation except slight degeneration of hepatocytes (black arrow). In panel (G,H), the hepatopancreas retains its normal structure in either the hepatic (H) or pancreatic part (P) with immune cell infiltration (green arrowhead) before infection and slight fatty degeneration (black arrow) post infection. Stain H&E. Bar = 100 μm.
The histological image of the spleen in both control and LP20-fed fish (Figures 9A,E) displayed regular red and white pulps and normal blood sinusoids. The melanomacrophage center was conspicuous in the LP20-fed fish (Figure 9E). After infection occurred in both treatments (Figures 9B,F), the melanomacrophages were increased, but the degenerated cells were obvious only in the post-infected control fish (Figure 9B). The DLM toxicity triggered a severe lymphocytic infiltration with dispersed melanomacrophages (Figure 9C). The infection of the DLM-exposed fish instigated severe deterioration of splenic cells and edema. The melanomacrophage centers were also present (Figure 9D). The DLM + LP20 treatment (Figure 9G) revealed a little disintegration with apparent melanomacrophage centers, but after infection, there were minor vascular congestion, edema, and lymphocytic infiltration (Figure 9H).
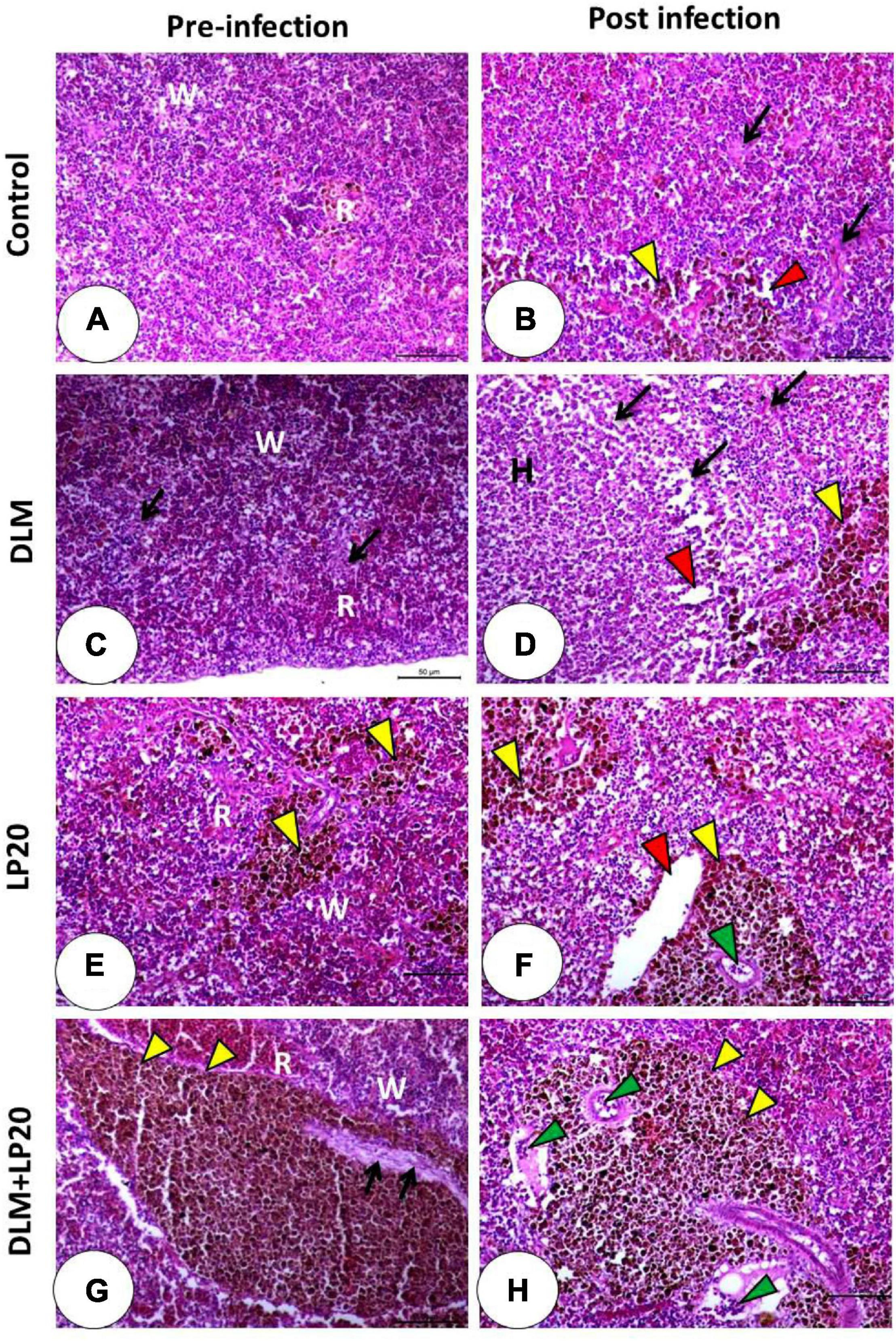
Figure 9. Histomicrograph of the spleen of Nile tilapia subjected to four treatments (control, DLM, LP20, and DLM + LP20) before (A,C,E,G) and after (B,D,F,H) infection. The control group (A) shows normal architecture of red (R) and white (W) bulbs and normal blood sinusoids, while the post-infected group (B) shows degenerated cells (black arrow), dilated blood sinusoids (red arrowhead), and melanomacrophages (yellow arrowhead). DLM (C) shows distributed degeneration (black arrow) in both white and red bulbs, while the post-infected DLM group (D) shows degeneration (black arrow), dilated blood sinusoids (red arrowhead), and melanomacrophage center. LP20 (E) shows normal architecture with distributed melanomacrophage centers (yellow arrowhead), while the post-infected group (F) shows normal red and white bulbs with dilated blood vessels (red arrowhead) and leukocytic infiltration (green arrowhead). LP20 added with DLM (G) shows increased melanomacrophage centers (yellow arrowhead) and slight degeneration (black arrow), while the post-infected group shows leukocytic infiltration (green arrowhead) besides the increased melanomacrophage centers (yellow arrowhead). Stain H&E. Bar = 100 μm.
Discussion
The obtained results showed that dietary L. plantarum (LP20) allowed Nile tilapia to overcome the negative impacts induced by DLM toxicity and A. hydrophila infection. On the 10th day post A. hydrophila challenge, fish fed with control, LP20, and DLM + LP20 displayed higher survival rates than fish exposed to DLM. The study indicates that LP20 is a crucial factor for protecting Nile tilapia from A. hydrophila infection in the presence of DLM toxicity. No earlier reports examined the role of LP20 against A. hydrophila in fish; however, Van Nguyen et al. (2019) stated that Nile tilapia fed LP20 exhibited lower mortality when infected with S. agalactiae. Probiotics in their heat-killed form enhances the intestinal immunity through the adhesion in the innate defense system and increase in the colonization of beneficial bacteria in the gastrointestinal tract (GIT) (Dawood et al., 2020c), which compete with the pathogenic bacteria and in turn increase the resistance against pathogens (Liu et al., 2016; Van Nguyen et al., 2019).
During exposure to toxins, the kidney loses its function by lowering glomerular filtration and catabolic protein rates followed by unregulated secretion of urea, uric acid, and creatinine (Jyothi and Narayan, 1999; Akturk et al., 2006). In this study, DLM toxicity and A. hydrophila infection caused the high secretion of urea, uric acid, creatinine, albumin, ALT, AST, and ALP. Nevertheless, Nile tilapia fed LP20 showed regulated levels of these values, ensuring the healthy status of the renal and hepatic tissues in fish fed LP20. Similarly, Nile tilapia exposed to DLM showed high activities of ALT, AST, and ALP (Dawood et al., 2020d). Concurrently, blood total protein and globulin were reduced by DLM and A. hydrophila but improved by LP20. The obtained results illustrated the importance of using LP20 to enhance the immune capacity of Nile tilapia under toxicity conditions (Ellis et al., 1981).
Exposure of fish to DLM also increased the level of cortisol, while feeding with LP20 presented reduced levels. The cortisol hormone can alleviate the stress effect through the secretion of glucose as a supplier of energy to manage the side effects of stress. Therefore, the increased cortisol level indicates stress induced by DLM toxicity in Nile tilapia, while LP20 lowered the stressful effect. Similarly, fish fed LP20 displayed increased resistance against stress via modulating the secretion of the cortisol hormone (Van Nguyen et al., 2019; Dawood et al., 2020d).
Serum PI, PA, and LZM were enhanced by LP20, while DLM decreased the activities at 24 h, 48 h, and 1 week post A. hydrophila challenge. Similarly, probiotics increased significantly the LZM of serum in fish (Chen et al., 2015). Furthermore, Van Nguyen et al. (2019) and Dawood et al. (2020d) observed that PA and LZM levels were elevated in LP20-fed fish. Concurrent with these results, fish delivered LP20 had upregulated IL-8 and IL-12 gene expressions, but fish exposed to DLM displayed downregulated IL-8 and IL-12 gene expressions. The improved PA and LZM levels and IL-8 and IL-12 gene expressions probably occurred because of the role of LP20 on macrophages through continuous feeding, which led to the accumulation of nutrients in the fish and, in turn, to the activation of the macrophages to produce lysozyme and interleukins (Xu et al., 2016). Besides, fish fed LP20 and exposed to DLM and A. hydrophila infection had enhanced IFN-γ gene expression that can be considered a pro-inflammatory cytokine regulated by an IL-12 precursor (Striz et al., 2014). Concurrently, Dawood et al. (2020d) reported upregulated IFN-γ gene expression in fish fed LP20 and exposed to DLM, referring to a pro-inflammatory potential of LP20 to alleviate the impairment induced by DLM and A. hydrophila. The functionality of LP20 as an immunostimulant agent is probably attributed to its high content of peptidoglycan and lipopolysaccharides, which can promote T lymphocytes (Hasan et al., 2019). Therefore, the reduced mortality rates of fish exposed to DLM and infected with A. hydrophila is associated with the enhanced immunity and pro-inflammatory cytokines by dietary LP20.
The overexpression of HSP70 is a stress response (Jun et al., 2015). The existing study demonstrated that the HSP70 transcription was increased in Nile tilapia subjected to DLM. At the same time, LP20 resulted in low expression of HSP70, which verifies that LP20 acts efficiently in balancing HSP70 in Nile tilapia under DLM toxicity and A. hydrophila infection. The toxicity with DLM and infection with A. hydrophila induced severe inflammatory characteristics in the intestine, liver, gills, and spleen of Nile tilapia, as shown in the histopathological study. This inflammation is also confirmed through the upregulation of the inflammatory genes (TNF-α and IFN-γ) and stress-involved gene (HSP70). Xenobiotics and toxins are primarily absorbed by the gills, and the skin of the fish body then can reach the remaining tissues (Ahmadifar et al., 2020). Once the intestine is attacked by the toxicity of such toxins (e.g., DLM), it will lose its function in absorbing nutrients, and the local immunity will be suppressed (Kim and Ho, 2010; Gipson, 2016). The liver detoxifying the toxins from the body of fish and the overdose of toxicity induce impaired liver function and inflammation (Groschwitz and Hogan, 2009; Ma et al., 2015). The spleen also showed inflammatory features caused by DLM and A. hydrophila infection, which can be attributed to the suppressed immunity in the spleen. Attractively, the incorporation of LP20 in Nile tilapia diets resulted in alleviating the inflammation in the gills, intestine, liver, and spleen. These results soundly supported the potential influence of LP20 to enhance the immunity and anti-inflammation caused by DLM and A. hydrophila infection in Nile tilapia. LP20 has abundant peptidoglycans that induce an immunostimulant role in the intestinal barriers (Dawood et al., 2016). Consequently, damage of the intestinal mucosal barrier owing to DLM toxicity and A. hydrophila infection may be one cause of the tissue lesions detected in the liver, spleen, gills, and intestine tissues of the Nile tilapia. Overproduction of reactive oxygen species (ROS) is also expected to impair the tissues of Nile tilapia body, which has been confirmed by the histopathological study in the current study (Rjeibi et al., 2016). However, LP20 feeding helped in protecting fish gills, intestine, spleen, and liver tissues, maybe by alleviating the role of ROS via enhanced antioxidative and proinflammatory responses. The SOD, CAT, and GPx genes are related to the capacity of fish to scavenge the excessive ROS, which generates during stress, toxicity, and outbreaks (Aliko et al., 2018; Dawood et al., 2020e). The toxicity with DLM decreased SOD, CAT, and GPx gene transcription, while LP20 feeding increased SOD, CAT, and GPx gene expressions under A. hydrophila infection. The results can explain the improved antioxidant status in the liver of DLM-stressed Nile tilapia treated with LP20 in this experiment. The cross talk between the antioxidant status and immunity is the main reason to increase fish resistance against DLM toxicity and A. hydrophila infection. In this sense, the inclusion of LP20 boosted the resistance of Nile tilapia against DLM toxicity by increasing the transcription of immune-related genes (Petit and Wiegertjes, 2016; Dawood et al., 2019; El-Murr et al., 2019). In this regard, Nile tilapia fed LP20 showed enhanced immunity and antioxidant capacity to cope with DLM toxicity (Dawood et al., 2020d). However, the present study investigated the role of LP20 in fish under DLM toxicity and A. hydrophila infection.
The obtained results reflected the harmful impact of DLM on blood health and inflammatory features, which can be attributed to the effects of DLM in breaking down the ability of blood cells to carry oxygen to the whole-body tissues. Correspondingly, fish are suffering from anemia, hemolysis, oxidative stress, and lipid peroxidation. More specifically, dietary LP20 supported Nile tilapia during DLM toxicity by reducing the oxidative stress and inflammation and increasing its ability to carry oxygen to reduce hemolysis. Concurrent with the present study, Dawood et al. (2020d) stated that LP20 mitigated the impacts of DLM on Nile tilapia via improving the immune and anti-inflammatory responses. Based on that, fish displayed improved resistance against A. hydrophila infection.
The nervous system (brain) is the target/prime organ affected by DLM toxicity (Saoudi et al., 2017). Although DLM pesticide is known for the induction of several neurotoxic effects by inhibiting acetylcholinesterase activity or incrementing acetylcholine in the brain of several fish species (Ajima et al., 2017; Singh et al., 2018), in the present study, the toxic impacts of DLM were tested in the gills, intestine, liver, and spleen tissues due to the challenge with A. hydrophila, which reduces the targeted immunity tissues. The combined stressors of DLM toxicity and A. hydrophila challenge suggested that other tissues rather than the brain can be impaired, which was very clear in the present study.
Conclusion
Nile tilapia exposed to DLM toxicity and A. hydrophila infection had suppressed immunity and antioxidant capacity. Moreover, the histopathological study revealed that DLM toxicity and A. hydrophila infection induced inflammatory alterations in the gills, intestine, spleen, and liver tissues. Dietary LP20 alleviated the inflammatory features caused by DLM toxicity and A. hydrophila by regulating immune-related genes.
Data Availability Statement
The raw data supporting the conclusions of this article will be made available by the authors, without undue reservation.
Ethics Statement
The study was ethically approved by the Committee of Faculty of Agriculture, Kafrelsheikh University, Egypt.
Author Contributions
MG, MD, MA-K, MS, FF, MA, and EM: conceptualization. MG, MD, MA-K, MS, and FF: formal analysis. MD, MA-E, AA, and EM: funding acquisition. MG, MD, MA-K, MS, FF, HD, and EM: investigation. MD, MA-K, and AE: methodology. MD and EM: project administration and supervision. MG, MD, and AE: writing—original draft.
Funding
This work was supported by Taif University Researchers Supporting Project number (TURSP-2020/57), Taif University, P.O. Box 11099, Taif 21944, Saudi Arabia. This research work was partially supported by Chiang Mai University.
Conflict of Interest
The authors declare that the research was conducted in the absence of any commercial or financial relationships that could be construed as a potential conflict of interest.
References
Abdo, S. E., Gewaily, M. S., Abo-Al-Ela, H. G., Almeer, R., Soliman, A. A., Elkomy, A. H., et al. (2021). Vitamin C rescues inflammation, immunosuppression, and histopathological alterations induced by chlorpyrifos in Nile tilapia. Environ. Sci. Pollut. Res. doi: 10.1007/s11356-021-12711-5
Abumandour, M., and Gewaily, M. S. (2016). Morphological studies on the gills of Puffer Fish (Lagocephalus sceleratus, Gmelin, 1789). Intern. J. Morphol. 34, 817–829. doi: 10.4067/s0717-95022016000300001
Ahmadifar, E., Kalhor, N., Dawood, M. A., Ahmadifar, M., Moghadam, M. S., Abarghouei, S., et al. (2020). Effects of polystyrene microparticles on inflammation, antioxidant enzyme activities, and related gene expression in Nile tilapia (Oreochromis niloticus). Environ. Sci. Pollut. Res. doi: 10.1007/s11356-020-11731-x
Ahmadifar, E., Moghadam, M. S., Dawood, M. A. O., and Hoseinifar, S. H. (2019). Lactobacillus fermentum and/or ferulic acid improved the immune responses, antioxidative defence and resistance against Aeromonas hydrophila in common carp (Cyprinus carpio) fingerlings. Fish Shellf. Immunol. 94, 916–923. doi: 10.1016/j.fsi.2019.10.019
Ajima, M. N. O., Pandey, P. K., Kumar, K., and Poojary, N. (2017). Neurotoxic effects, molecular responses and oxidative stress biomarkers in Nile tilapia, Oreochromis niloticus (Linnaeus, 1758) exposed to verapamil. Compar. Biochem. Physiol. Part C Toxicol. Pharmacol. 196, 44–52. doi: 10.1016/j.cbpc.2017.03.009
Akturk, O., Demirin, H., Sutcu, R., Yilmaz, N., Koylu, H., and Altuntas, I. (2006). The effects of diazinon on lipid peroxidation and antioxidant enzymes in rat heart and ameliorating role of vitamin E and vitamin C. Cell Biol. Toxicol. 22, 455–461. doi: 10.1007/s10565-006-0138-5
Aliko, V., Qirjo, M., Sula, E., Morina, V., and Faggio, C. (2018). Antioxidant defense system, immune response and erythron profile modulation in gold fish, Carassius auratus, after acute manganese treatment. Fish Shellf. Immunol. 76, 101–109. doi: 10.1016/j.fsi.2018.02.042
Bradbury, S. P., and Coats, J. R. (1989). Comparative toxicology of the pyrethroid insecticides. Rev. Environ. Contaminat. Toxicol. 108, 133–177. doi: 10.1007/978-1-4613-8850-0_4
Cai, W.-Q., Li, S.-F., and Ma, J.-Y. (2004). Diseases resistance of Nile tilapia (Oreochromis niloticus), blue tilapia (Oreochromis aureus) and their hybrid (female Nile tilapia×male blue tilapia) to Aeromonas sobria. Aquaculture 229, 79–87. doi: 10.1016/s0044-8486(03)00357-0
Cengiz, E. I., Bayar, A. S., Kızmaz, V., Başhan, M., and Satar, A. (2017). Acute toxicity of deltamethrin on the fatty acid composition of phospholipid classes in liver and gill tissues of Nile tilapia. Intern. J. Environ. Res. 11, 377–385. doi: 10.1007/s41742-017-0034-2
Chen, J., Sun, R., Pan, C., Sun, Y., Mai, B., and Li, Q. X. (2020). Antibiotics and food safety in aquaculture. J. Agric. Food Chem. 68, 11908–11919. doi: 10.1021/acs.jafc.0c03996
Chen, N., Jiang, J., Gao, X., Li, X., Zhang, Y., Liu, X., et al. (2018). Histopathological analysis and the immune related gene expression profiles of mandarin fish (Siniperca chuatsi) infected with Aeromonas hydrophila. Fish Shellf. Immunol. 83, 410–415. doi: 10.1016/j.fsi.2018.09.023
Chen, X.-M., Lu, H.-M., Niu, X.-T., Wang, G.-Q., and Zhang, D.-M. J. F. (2015). Enhancement of secondary metabolites from Bacillus Licheniformis XY-52 on immune response and expression of some immune-related genes in common carp, Cyprinus carpio. Fish Shellf. Immunol. 45, 124–131. doi: 10.1016/j.fsi.2015.02.019
Chen, Y.-B., Hu, J., Lyu, Q.-J., Liu, L.-J., Wen, L.-F., Yang, X.-K., et al. (2016). The effects of Natucin C-Natucin P mixture on blood biochemical parameters, antioxidant activity and non-specific immune responses in tilapia (Oreochromis niloticus). Fish Shellf. Immunol. 55, 367–373. doi: 10.1016/j.fsi.2016.06.016
Coulombe, J. J., and Favreau, L. (1963). A new simple semimicro method for colorimetric determination of urea. Clin. Chem. 9, 102–108. doi: 10.1093/clinchem/9.1.102
Dawood, M. A. O, Abdel-Razik, N. I., Gewaily, M. S., Sewilam, H., Paray, B. A., Soliman, A. A., et al. (2020a). β-Glucan improved the immunity, hepato-renal, and histopathology disorders induced by chlorpyrifos in Nile tilapia. Aquac. Rep. 18:100549. doi: 10.1016/j.aqrep.2020.100549
Dawood, M. A. O., AbdEl-kader, M. F., Moustafa, E. M., Gewaily, M. S., and Abdo, S. E. (2020b). Growth performance and hemato-immunological responses of Nile tilapia (Oreochromis niloticus) exposed to deltamethrin and fed immunobiotics. Environ. Sci. Pollut. Res. 27, 11608–11617. doi: 10.1007/s11356-020-07775-8
Dawood, M. A. O., Abo-Al-Ela, H. G., and Hasan, M. T. (2020c). Modulation of transcriptomic profile in aquatic animals: probiotics, prebiotics and synbiotics scenarios. Fish Shellf. Immunol. 97, 268–282. doi: 10.1016/j.fsi.2019.12.054
Dawood, M. A. O., Moustafa, E. M., Gewaily, M. S., Abdo, S. E., AbdEl-kader, M. F., SaadAllah, M. S., et al. (2020d). Ameliorative effects of Lactobacillus plantarum L-137 on Nile tilapia (Oreochromis niloticus) exposed to deltamethrin toxicity in rearing water. Aquat. Toxicol. 219:734571.
Dawood, M. A. O., Zommara, M., Eweedah, N. M., Helal, A. I., and Aboel-Darag, M. A. (2020e). The potential role of nano-selenium and vitamin C on the performances of Nile tilapia (Oreochromis niloticus). Environ. Sci. Pollut. Res. 27, 9843–9852. doi: 10.1007/s11356-020-07651-5
Dawood, M. A., Koshio, S., and Esteban, M. Á (2018). Beneficial roles of feed additives as immunostimulants in aquaculture: a review. Rev. Aquac. 10, 950–974. doi: 10.1111/raq.12209
Dawood, M. A. O., Koshio, S., Ishikawa, M., and Yokoyama, S. (2016). Immune responses and stress resistance in red sea bream, Pagrus major, after oral administration of heat-killed Lactobacillus plantarum and vitamin C. Fish Shellf. Immunol. 54, 266–275.
Dawood, M. A. O. (2021). Nutritional immunity of fish intestines: important insights for sustainable aquaculture. Rev. Aquac. 13, 642–663. doi: 10.1111/raq.12492
Dawood, M. A. O., El Basuini, M. F., Zaineldin, A. I., Yilmaz, S., Hasan, M. T., Ahmadifar, E., et al. (2021). Antiparasitic and antibacterial functionality of essential oils: an alternative approach for sustainable aquaculture. Pathogens 10:185. doi: 10.3390/pathogens10020185
Dawood, M. A. O., Magouz, F. I., Salem, M. F. I., and Abdel-Daim, H. A. (2019). Modulation of digestive enzyme activity, blood health, oxidative responses and growth-related gene expression in GIFT by heat-killed Lactobacillus plantarum (L-137). Aquaculture 505, 127–136. doi: 10.1016/j.aquaculture.2019.02.053
Demers, N. E., and Bayne, C. J. (1997). The immediate effects of stress on hormones and plasma lysozyme in rainbow trout. Dev. Compar. Immunol. 21, 363–373. doi: 10.1016/s0145-305x(97)00009-8
Doumas, B. T., Bayse, D. D., Carter, R. J., Peters, T., and Schaffer, R. (1981). A candidate reference method for determination of total protein in serum. I. Development and validation. Clin. Chem. 27, 1642–1650. doi: 10.1093/clinchem/27.10.1642
Dumas, B. T., and Biggs, H. G. (1972). Standard Methods of Clinical Chemistry. New York, NY: Academic Press.
Eder, K. J., Köhler, H.-R., and Werner, I. (2007). Pesticide and pathogen: heat shock protein expression and acetylcholinesterase inhibition in juvenile Chinook salmon in response to multiple stressors. Environ. Toxicol. Chem. 26, 1233–1242. doi: 10.1897/05-462r2.1
El Megid, A. A., Abd Al Fatah, M. E., El Asely, A., El Senosi, Y., Moustafa, M. M. A., and Dawood, M. A. O. (2020). Impact of pyrethroids and organochlorine pesticides residue on IGF-1 and CYP1A genes expression and muscle protein patterns of cultured Mugil capito. Ecotoxicol. Environ. Saf. 188:109876. doi: 10.1016/j.ecoenv.2019.109876
Ellis, A. E., Hastings, T. S., and Munro, A. L. S. (1981). The role of Aeromonas salmonicida extracellular products in the pathology of furunculosis. J. Fish Dis. 4, 41–51. doi: 10.1111/j.1365-2761.1981.tb01108.x
El-Murr, A. E. I., El Hakim, Y. A., Neamat-Allah, A. N. F., Baeshen, M., and Ali, H. A. (2019). Immune-protective, antioxidant and relative gene expression impacts of β-glucan against fipronil toxicity in Nile tilapia, Oreochromis niloticus. Fish Shellf. Immunol. 94, 427–433. doi: 10.1016/j.fsi.2019.09.033
FAO (2018). Food and Agriculture Organization of the United Nations. Aquaculture Department, The State of World Fisheries and Aquaculture. Rome: FAO.
Fernandes, D. C., Eto, S. F., Funnicelli, M. I. G., Fernandes, C. C., Charlie-Silva, I., Belo, M. A. A., et al. (2019). Immunoglobulin Y in the diagnosis of Aeromonas hydrophila infection in Nile tilapia (Oreochromis niloticus). Aquaculture 500, 576–585.
Gipson, I. K. (2016). Goblet cells of the conjunctiva: a review of recent findings. Prog. Retin. Eye Res. 54, 49–63. doi: 10.1016/j.preteyeres.2016.04.005
Golow, A. A., and Godzi, T. A. (1994). Acute toxicity of deltamethrin and dieldrin to Oreochromis niloticus (LIN). Bull. Environ. Contamin. Toxicol. 52, 351–354.
Groschwitz, K. R., and Hogan, S. P. (2009). Intestinal barrier function: molecular regulation and disease pathogenesis. J. Allergy Clin. Immunol. 124, 3–22. doi: 10.1016/j.jaci.2009.05.038
Hasan, M. T., Jang, W. J., Lee, B.-J., Kim, K. W., Hur, S. W., Lim, S. G., et al. (2019). Heat-killed Bacillus sp. SJ-10 probiotic acts as a growth and humoral innate immunity response enhancer in olive flounder (Paralichthys olivaceus). Fish Shellf. Immunol. 88, 424–431. doi: 10.1016/j.fsi.2019.03.018
Heinegård, D., and Tiderström, G. (1973). Determination of serum creatinine by a direct colorimetric method. Clin. Chim. Acta 43, 305–310. doi: 10.1016/0009-8981(73)90466-x
Ismaeil, H., Abdo, W., Amer, S., Tahoun, A., Massoud, D., Zanaty, E., et al. (2020). Ameliorative effect of heat-killed Lactobacillus plantarum L.137 and/or Aloe vera against colitis in mice. Processes 8:225. doi: 10.3390/pr8020225
Jun, Q., Hong, Y., Hui, W., Didlyn, K. M., Jie, H., and Pao, X. (2015). Physiological responses and HSP70 mRNA expression in GIFT tilapia juveniles, Oreochromis niloticus under short-term crowding. Aquac. Res. 46, 335–345. doi: 10.1111/are.12189
Jyothi, B., and Narayan, G. (1999). Certain pesticide-induced carbohydrate metabolic disorders in the serum of freshwater fish Clarias batrachus (Linn.). Food Chem. Toxicol. 37, 417–421. doi: 10.1016/s0278-6915(99)00020-4
Kim, Y. S., and Ho, S. B. (2010). Intestinal goblet cells and mucins in health and disease: recent insights and progress. Curr. Gastroenterol. Rep. 12, 319–330. doi: 10.1007/s11894-010-0131-2
Li, J., Ni, X. D., Liu, Y. J., and Lu, C. P. (2011). Detection of three virulence genes alt, ahp and aerA in Aeromonas hydrophila and their relationship with actual virulence to zebrafish. J. Appl. Microbiol. 110, 823–830. doi: 10.1111/j.1365-2672.2011.04944.x
Limbu, S. M., Chen, L.-Q., Zhang, M.-L., and Du, Z.-Y. (2021). A global analysis on the systemic effects of antibiotics in cultured fish and their potential human health risk: a review. Rev. Aquac. 13, 1015–1059.
Liu, W., Ran, C., Liu, Z., Gao, Q., Xu, S., Ringø, E., et al. (2016). Effects of dietary Lactobacillus plantarum and AHL lactonase on the control of Aeromonas hydrophila infection in tilapia. Microbiologyopen 5, 687–699. doi: 10.1002/mbo3.362
Livak, K. J., and Schmittgen, T. D. (2001). Analysis of relative gene expression data using real-time quantitative PCR and the 2– ΔΔCT method. Methods 25, 402–408. doi: 10.1006/meth.2001.1262
Lu, D.-L., Ma, Q., Wang, J., Li, L.-Y., Han, S.-L., Limbu, S. M., et al. (2019). Fasting enhances cold resistance in fish through stimulating lipid catabolism and autophagy. J. Physiol. 597, 1585–1603. doi: 10.1113/jp277091
Ma, H.-D., Wang, Y.-H., Chang, C., Gershwin, M. E., and Lian, Z.-X. (2015). The intestinal microbiota and microenvironment in liver. Autoimmun. Rev. 14, 183–191. doi: 10.1016/j.autrev.2014.10.013
Ming, C., Rui, W., Liping, L., Huang, T., Weiyi, H., Jian, L., et al. (2013). Sequence and evolution differences of Oreochromis niloticus CXC contribute to the diversification of cellular immune responses in tilapias with treatment of Streptococcus iniae. J. Anim. Vet. Adv. 12, 303–311.
Newaj-Fyzul, A., Al-Harbi, A., and Austin, B. (2014). Developments in the use of probiotics for disease control in aquaculture. Aquaculture 431, 1–11. doi: 10.1016/j.aquaculture.2013.08.026
Nicholson, P., Mon-on, N., Jaemwimol, P., Tattiyapong, P., and Surachetpong, W. (2020). Coinfection of tilapia lake virus and Aeromonas hydrophila synergistically increased mortality and worsened the disease severity in tilapia (Oreochromis spp.). Aquaculture 520:734746. doi: 10.1016/j.aquaculture.2019.734746
Pang, J.-C., Gao, F.-Y., Lu, M.-X., Ye, X., Zhu, H.-P., and Ke, X.-L. (2013). Major histocompatibility complex class IIA and IIB genes of Nile tilapia Oreochromis niloticus: genomic structure, molecular polymorphism and expression patterns. Fish Shellf. Immunol. 34, 486–496. doi: 10.1016/j.fsi.2012.11.048
Petit, J., and Wiegertjes, G. F. (2016). Long-lived effects of administering β-glucans: Indications for trained immunity in fish. Dev. Compar. Immunol. 64, 93–102. doi: 10.1016/j.dci.2016.03.003
Ran, C., Qin, C., Xie, M., Zhang, J., Li, J., Xie, Y., et al. (2018). Aeromonas veronii and aerolysin are important for the pathogenesis of motile aeromonad septicemia in cyprinid fish. Environ. Microbiol. 20, 3442–3456. doi: 10.1111/1462-2920.14390
Reed, L. J., and Muench, H. (1938). A simple method of estimating fifty percent end points. Am. J. Epidemiol. 27, 493–497. doi: 10.1093/oxfordjournals.aje.a118408
Reitman, S., and Frankel, S. (1957). A colorimetric method for the determination of serum glutamic oxalacetic and glutamic pyruvic transaminases. Am. J. Clin. Pathol. 28, 56–63. doi: 10.1093/ajcp/28.1.56
Ringo, E., Hossein, S., Ghosh, K., Doan, H. V., Beck, B. R., and Song, S. (2018). Lactic acid bacteria in finfish–an update. Front. Microbiol. 9:1818. doi: 10.3389/fmicb.2018.01818
Ringø, E., Van Doan, H., Lee, S. H., Soltani, M., Hoseinifar, S. H., Harikrishnan, R., et al. (2020). Probiotics, lactic acid bacteria and bacilli: interesting supplementation for aquaculture. J. Appl. Microbiol. 129, 116–136. doi: 10.1111/jam.14628
Rjeibi, I., Ben Saad, A., and Hfaiedh, N. (2016). Oxidative damage and hepatotoxicity associated with deltamethrin in rats: the protective effects of Amaranthus spinosus seed extract. Biomed. Pharmacother. 84, 853–860. doi: 10.1016/j.biopha.2016.10.010
Saoudi, M., Badraoui, R., Bouhajja, H., Ncir, M., Rahmouni, F., Grati, M., et al. (2017). Deltamethrin induced oxidative stress in kidney and brain of rats: protective effect of Artemisia campestris essential oil. Biomed. Pharmacother. 94, 955–963. doi: 10.1016/j.biopha.2017.08.030
Saputra, F., Shiu, Y.-L., Chen, Y.-C., Puspitasari, A. W., Danata, R. H., Liu, C.-H., et al. (2016). Dietary supplementation with xylanase-expressing B. amyloliquefaciens R8 improves growth performance and enhances immunity against Aeromonas hydrophila in Nile tilapia (Oreochromis niloticus). Fish Shellf. Immunol. 58, 397–405. doi: 10.1016/j.fsi.2016.09.046
Shourbela, R. M., Khatab, S. A., Hassan, M. M., Van Doan, H., and Dawood, M. A. O. (2021). The effect of stocking density and carbon sources on the oxidative status, and nonspecific immunity of Nile tilapia (Oreochromis niloticus) reared under biofloc conditions. Animals 11:184. doi: 10.3390/ani11010184
Singh, S., Tiwari, R. K., and Pandey, R. S. (2018). Evaluation of acute toxicity of triazophos and deltamethrin and their inhibitory effect on AChE activity in Channa punctatus. Toxicol. Rep. 5, 85–89. doi: 10.1016/j.toxrep.2017.12.006
Striz, I., Brabcova, E., Kolesar, L., and Sekerkova, A. (2014). Cytokine networking of innate immunity cells: a potential target of therapy. Clin. Sci. 126, 593–612. doi: 10.1042/cs20130497
Ting, C.-H., Chen, Y.-C., and Chen, J.-Y. (2018). Nile tilapia fry fed on antimicrobial peptide Epinecidin-1-expressing Artemia cyst exhibit enhanced immunity against acute bacterial infection. Fish Shellf. Immunol. 81, 37–48. doi: 10.1016/j.fsi.2018.07.008
Trinder, P. (1969). Determination of glucose in blood using glucose oxidase with an alternative oxygen acceptor. Ann. Clin. Biochem. 6, 24–27. doi: 10.1177/000456326900600108
Van Nguyen, N., Onoda, S., Van Khanh, T., Hai, P. D., Trung, N. T., Hoang, L., et al. (2019). Evaluation of dietary Heat-killed Lactobacillus plantarum strain L-137 supplementation on growth performance, immunity and stress resistance of Nile tilapia (Oreochromis niloticus). Aquaculture 498, 371–379. doi: 10.1016/j.aquaculture.2018.08.081
Wu, F., Yang, C., Wen, H., Zhang, C., Jiang, M., Liu, W., et al. (2019). Improving low-temperature stress tolerance of tilapia, Oreochromis niloticus: a functional analysis of Astragalus membranaceus. J. World Aquac. Soc. 50, 749–762. doi: 10.1111/jwas.12586
Xie, S., Zhou, W., Tian, L., Niu, J., and Liu, Y. (2016). Effect of N-acetyl cysteine and glycine supplementation on growth performance, glutathione synthesis, anti-oxidative and immune ability of Nile tilapia, Oreochromis niloticus. Fish Shellf. Immunol. 55, 233–241. doi: 10.1016/j.fsi.2016.05.033
Xu, H.-J., Jiang, W.-D., Feng, L., Liu, Y., Wu, P., Jiang, J., et al. (2016). Dietary vitamin C deficiency depresses the growth, head kidney and spleen immunity and structural integrity by regulating NF-κB, TOR, Nrf2, apoptosis and MLCK signaling in young grass carp (Ctenopharyngodon idella). Fish Shellf. Immunol. 52, 111–138. doi: 10.1016/j.fsi.2016.02.033
Zaki, M. A. A., Alabssawy, A. N., Nour, A. E.-A. M., El Basuini, M. F., Dawood, M. A. O., Alkahtani, S., et al. (2020). The impact of stocking density and dietary carbon sources on the growth, oxidative status and stress markers of Nile tilapia (Oreochromis niloticus) reared under biofloc conditions. Aquac. Rep. 16:100282. doi: 10.1016/j.aqrep.2020.100282
Keywords: multi stressors, Lactobacillus plantarum, Nile tilapia, anti-inflammatory, histopathology
Citation: Gewaily MS, Shukry M, Abdel-Kader MF, Alkafafy M, Farrag FA, Moustafa EM, Doan HV, Abd-Elghany MF, Abdelhamid AF, Eltanahy A and Dawood MAO (2021) Dietary Lactobacillus plantarum Relieves Nile Tilapia (Oreochromis niloticus) Juvenile From Oxidative Stress, Immunosuppression, and Inflammation Induced by Deltamethrin and Aeromonas hydrophila. Front. Mar. Sci. 8:621558. doi: 10.3389/fmars.2021.621558
Received: 26 October 2020; Accepted: 16 February 2021;
Published: 22 March 2021.
Edited by:
Lingling Wang, Dalian Ocean University, ChinaReviewed by:
Amit Kumar Sinha, University of Arkansas at Pine Bluff, United StatesSamwel Mchele Limbu, University of Dar es Salaam, Tanzania
Copyright © 2021 Gewaily, Shukry, Abdel-kader, Alkafafy, Farrag, Moustafa, Doan, Abd-Elghany, Abdelhamid, Eltanahy and Dawood. This is an open-access article distributed under the terms of the Creative Commons Attribution License (CC BY). The use, distribution or reproduction in other forums is permitted, provided the original author(s) and the copyright owner(s) are credited and that the original publication in this journal is cited, in accordance with accepted academic practice. No use, distribution or reproduction is permitted which does not comply with these terms.
*Correspondence: Mahmoud A. O. Dawood, mahmouddawood55@gmail.com; Hien Van Doan, hien.d@cmu.ac.th