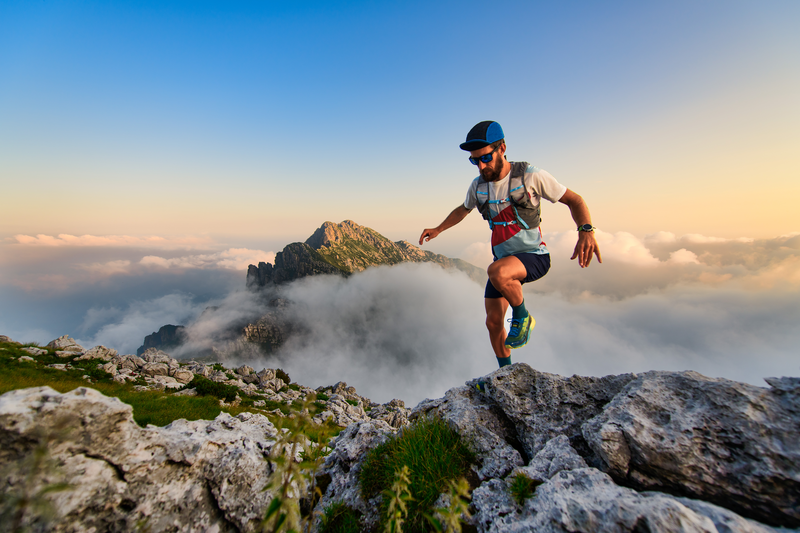
94% of researchers rate our articles as excellent or good
Learn more about the work of our research integrity team to safeguard the quality of each article we publish.
Find out more
ORIGINAL RESEARCH article
Front. Mar. Sci. , 12 March 2021
Sec. Marine Fisheries, Aquaculture and Living Resources
Volume 8 - 2021 | https://doi.org/10.3389/fmars.2021.618910
Environmental conditions can strongly influence the growth performance of pearl oysters and affect pearl farm production schedules. Growth and condition index (CI) of two age cohorts of Pteria penguin were measured for 13 months to investigate differences in growth performance between four culture sites within the northern (Vava’u) and southern (Tongatapu) island groups of the Kingdom of Tonga. Environmental conditions were also measured at culture sites and used to explore potential effects on oyster growth and condition. Between island groups, growth performance of P. penguin was superior at northern sites and was most strongly related to higher water temperatures at these sites. Within the southern island group, growth performance varied significantly between sites and may be driven by differences in wave energy. Monthly growth rates (GM) of P. penguin also showed significant temporal variation related to age and environmental conditions. This study demonstrated significant variation in the growth performance of P. penguin at latitudinal and local scales and suggests that in oligotrophic marine environments with minimal terrestrial inputs, such as Tonga, water temperature and wave exposure may be the primary environmental conditions influencing the growth performance of P. penguin. This study therefore recommends that optimal culture sites for P. penguin in Tonga are characterized primarily by warmer water temperatures (25–30°C) and low wave exposure (<15 joules m2 day–1). Culture of P. penguin at sites with more suitable environmental conditions enables pearl production to begin up to 34.2 % (6.5 months) earlier than at less-suitable sites and this may greatly influence mabé pearl farm profitability and feasibility.
The winged pearl oyster, Pteria penguin (Röding 1798), occurs in a diverse range of marine environments, from the east coast of Africa, throughout Asia and northern Australia, and the western Pacific (Wada and Tëmkin, 2008). Pteria penguin is widely cultured for the production of high-quality mabé pearls (half-pearls) at both commercial and subsistence scales, supporting a range of pearl-based livelihoods (Southgate et al., 2008, 2019; Johnston et al., 2019). The Kingdom of Tonga is the leading producer of mabé pearls in the south Pacific (Johnston et al., 2019) and has experienced rapid industry development sustained by recently improved hatchery, husbandry, and pearl-culture methods (Wassnig and Southgate, 2012; Southgate et al., 2016; Gordon et al., 2018, 2019, 2020). Subsistence-level mabé pearl farming has considerable potential for livelihood support (Yamamoto and Tanaka, 1997; Anon, 2018; Johnston et al., 2019), with small-scale farms capable of generating annual profits of more than twice the average income in Tonga (Johnston et al., 2020). Currently, mabé pearl farms are distributed among the three main island groups of Tonga (Johnston et al., 2019) which span three degrees of latitude (∼300 km) and vary in environmental conditions (Smallhorn-West et al., 2020). To date, no studies have investigated large-scale spatial variation in growth performance of P. penguin, yet such information is vital to inform pearl farm site selection to maximize pearl farm productivity, profitability, and associated livelihood benefits (Pouvreau and Prasil, 2001; Gaertner-Mazouni and Le Moullac, 2016; Gueguen et al., 2016).
Mabé pearl culture, like round pearl culture, utilizes oysters of a specific minimum size for pearl production (Gervis and Sims, 1992; Taylor and Strack, 2008). The initial phase of mabé pearl production therefore involves a non-productive culture period of around 1–3 years where wild-collected, or hatchery-produced, P. penguin are cultured to pearl-production size (100–140 mm dorso-ventral height, DVH) (Kripa et al., 2008; Milione and Southgate, 2012; Gordon et al., 2019, 2020). Appropriately sized oysters are then implanted for pearl production by attaching hemispherical nuclei to the inner nacreous surfaces of pearl oyster shells (Haws et al., 2006; Kishore et al., 2015). Successive layers of nacre (mother of pearl) are subsequently deposited over the nuclei (Taylor and Strack, 2008) to produce mabé pearls with commercial nacre thickness within 9–12 months (Kishore et al., 2015; Gordon et al., 2018, 2019). Maximizing the growth performance of P. penguin up to pearl-production size will therefore reduce the non-productive culture period and overall production time, thereby increasing pearl farm profitability and feasibility (Johnston and Hine, 2015; Johnston et al., 2020).
Pearl oyster growth rates (GM) are influenced by environmental factors including water temperature, food availability, turbidity, salinity, pH, current speed, and wave energy (Gervis and Sims, 1992; Lucas, 2008; Adzigbli et al., 2019). Of these, the variables considered to have the greatest influence on growth performance are water temperature (del Rio-Portilla et al., 1992; Mills, 2000; Yamamoto, 2000; Le Moullac et al., 2016) and food availability (Yukihira et al., 1998, 1999, 2006) because of their acute effects on metabolic rate and scope for growth (Numaguchi, 1994; Yukihira et al., 2000). Variations in water temperature or food availability have been related to growth performance of Pinctada margaritifera (Linnaeus 1758) (Pouvreau et al., 2000; Pouvreau and Prasil, 2001), Pinctada maxima (Jameson 1901) (Lee et al., 2008; Kvingedal et al., 2010), Pinctada fucata (Gould 1850) (Tomaru et al., 2002), and P. penguin (Milione and Southgate, 2011, 2012). Current speed and wave energy also strongly influence pearl oyster growth via their effects on food renewal, waste removal, and physical stability of oysters (Lucas, 2008; Kishore et al., 2014). Extensive study of P. margaritifera in French Polynesia showed that growth performance of this species was highest at culture sites with water temperatures between 21 and 30°C, high rates of food renewal, and high levels of particulate organic matter (Pouvreau et al., 2000; Pouvreau and Prasil, 2001). Similar national comparisons do not exist for P. penguin, although Milione et al., 2011; Milione and Southgate, 2012 indicated that the survival and GM of P. penguin was higher at turbid inshore sites than at offshore reef sites. Recruitment patterns of P. penguin also suggest that this species has a higher tolerance to turbidity and that it differs from P. margaritifera and P. maxima in its response to environmental factors (Yukihira et al., 2006; Kishore et al., 2018).
This study examined spatio-temporal variation in the growth performance and condition of two age cohorts of P. penguin between distant sites in the northern and southern island groups of the Kingdom of Tonga. We also describe variation in marine environmental variables between sites and examine their relationship with growth performance and condition of P. penguin. Our ultimate aim was to identify ranges of key environmental variables where oyster growth performance was optimized so that we could assess the implications for mabé pearl farm site selection and production schedules.
This study was conducted from September 2018 to November 2019 at two sites in the northern island group (Vava’u, 18°39’ S, 174°00’ W) and southern island group (Tongatapu, 21°07’ S, 175°11’ W) of the Kingdom of Tonga, separated by just under three degrees of latitude (∼250 km) (Figure 1A). All sites had a depth of 10–20 m but were characterized by differing environmental conditions, exposure to terrestrial inputs and wave energy (Smallhorn-West et al., 2020). Within Vava’u, the Vaipua site was located closer to terrestrial inputs from the Taoa estuary, while the Utulei site was located within Neiafu harbor. In Tongatapu, the Sopu site was exposed to higher wave energy than the Pangaimotu site, and was located further from inputs from Fanga’uta lagoon (Kaly et al., 2000; Smallhorn-West et al., 2020).
Figure 1. (A) Location of Pteria penguin culture sites in the Kingdom of Tonga: (1) Vaipua, (2) Utulei, in Vava’u, (3) Sopu, and (4) Pangaimotu in Tongatapu. Gray and green regions represent land and reefs, respectively. (B) “Young” Pteria penguin oysters housed in culture cylinders beside a multi-parameter sonde suspended from a pearl longline in Tonga [adapted from Gordon et al. (2020)].
Pteria penguin used in this study were hatchery-cultured at the Ministry of Fisheries (MoF) Aquaculture Center in Sopu using standard MoF hatchery, grow-out, and stock-maintenance procedures (Southgate et al., 2016; Gordon et al., 2020). Two age cohorts of oysters were selected: (1) “young” oysters, 0.7 years old with a mean (±SE) DVH and wet mass (WM) of 34.2 ± 0.3 mm and 5.7 ± 0.2 g, respectively; and, (2) “old” oysters, 2.7 years old with a mean DVH and WM of 89.9 ± 0.5 mm and 70.8 ± 1.6 g, respectively, at the start of the study. “Young” oysters represented the standard size of oysters received by pearl farmers from the MoF nursery and “old” oysters represented those around 12 months immature of pearl-production size (Gordon et al., 2017, 2020).
Oysters were cleaned, measured and individually numbered, before being attached to ropes with fishing line to form “chaplets” (Figure 1B; Southgate, 2008; Gordon et al., 2020). “Young” oysters were distributed between 24 chaplets, each comprising 12 pairs of oysters (n = 576), and “old” oysters between 36 chaplets of seven pairs of oysters (n = 504). Pairs of “young” and “old” oysters were spaced on chaplets at a distance of 150 and 250 mm, respectively (Gordon et al., 2020). Resulting chaplets were held for 1 month within trays suspended at a depth of 5 m from a submerged longline at Sopu, to allow recovery from drilling (September-October 2018; Gordon et al., 2020). After 1 month, chaplets were removed from the trays and oysters were measured. Six chaplets of “young” oysters and nine chaplets of “old” oysters were then transported to each culture site (site n = 270). Oyster chaplets were secured inside 2-m-long culture cylinders constructed of 40 mm pore-size galvanized wire mesh (Gordon et al., 2020; Figure 1B) and deployed to pearl longlines. Culture cylinders were suspended at a depth of 5 m and spaced at a distance of 500 mm on pearl longlines at Vava’u and Tongatapu sites (Figure 1).
Culture cylinders were cleaned and oyster survival and shell dimensions measured every three to 5 weeks for 13 months, with the exception of April to May 2019 (specifically, days 215–265) when weather conditions prevented data collection once at Vava’u sites and twice at Tongatapu sites. At each site, 20 pairs of “young” and 20 pairs of “old” oysters (site n = 80, total n = 320) were randomly selected and were repeatedly measured for DVH, shell thickness (ST) and WM, and photographed following Gordon et al. (2017, 2020). Shell dimensions were measured to ±0.1 mm using Vernier calipers, and WM was determined to ±0.1 g using an electronic balance. At each sampling event, three additional pairs of “young” and “old” oysters were haphazardly selected and harvested for assessment of condition index (CI) (site n = 12, total n = 624) using the “dry tissue mass : dry shell mass” ratio method described by Walne and Mann (1975) and Lucas and Beninger (1985). Oysters sampled for CI were dissected and tissues dried at 60°C to a constant mass in a drying oven (Freites et al., 2017).
At each site, water temperature (°C), salinity (ppt), pH, turbidity (nephelometric turbidity units, NTU), and chlorophyll content (μg L–1) were measured using submerged multi-parameter sondes (YSI 6920-2, Xylem, Australia) and current speed (ms–1) was measured using drag-tilt current meters (Marotte HS, Marine Geophysics Lab, Australia) deployed between culture cylinders (Figure 1B). Sondes and current meters were downloaded, cleaned, and checked for functioning at each sampling event and recalibrated as required. Daily rainfall data (mm) were obtained from the Tonga Meteorological Service for Tongatapu and Vava’u. Mean wave energy (joules m2 day–1) was calculated for each site from spatial layers provided by Smallhorn-West et al. (2020).
To compare the shell dimensions of P. penguin between culture sites and ages over time, generalized additive models (GAMs) were fit to mean DVH, ST, and WM values. Total growth (GT) of P. penguin over the 13-month culture period was also calculated for DVH, ST, and WM as: GT = Gn−G1, where Gn = shell dimensions at final sampling, and G1 = shell dimensions at deployment to culture sites. Effects of site and oyster age cohort on GT of DVH, ST, and WM were examined using generalized linear models (GLM) based on a Gaussian distribution. To compare site production schedules, the age of “young” P. penguin at pearl-production sizes of 100 mm DVH (T100) (Kripa et al., 2008; Milione and Southgate, 2012) and 140 mm DVH (T140) (Gordon et al., 2019) were predicted from GAMs fit to mean DVH.
Spatio-temporal variation in environmental variables was examined using GAMs fit to mean values for each sampling period. Site-related environmental variation was also examined using principal component analysis (PCA) on summarized and scaled environmental data. Environmental data recorded 6 h post-deployment and 3 h pre-retrieval of sondes and current meters were discarded to minimize error. Outliers caused by probe malfunctioning or interference by fouling were removed or transformed in accordance with recommendations by sonde manufacturers and technicians (Xylem, Australia).
Spatio-temporal variation in GM of DVH, WM, ST, and CI between cohorts was examined using GAMs fit to raw observations. Relationships between environmental variables and GM of DVH, WM, ST, and CI were also described using GAMs using separate splines for sites and age cohorts. Environmental variables that did not contribute substantially (by AIC) to model fit were removed from GAMs and were not described in partial plots. GM of P. penguin shell dimensions for each sampling period were calculated as: GM = [(GT−GT–1) ÷ D] × 30, where GT = shell measurement of the current sampling; GT–1 = shell measurement of the previous sampling, and D = number of days between sampling events, for DVH, ST, and WM. Condition index was calculated as per Walne and Mann (1975):
The relative quality of all models was assessed using Akaike information criterion values corrected for small sample-sizes (AICc). Visual assessments of diagnostic plots were used to validate models and data transformed and/or outliers removed as required to improve diagnostics and improve compliance with model assumptions. All analyses were completed in R (R Core Team, 2019) using base R “lme4” (Bates et al., 2015), “mgcv” (Wood, 2017), and “MUMIn” (Barton, 2019) packages.
In general, mean shell dimensions of P. penguin showed minimal variation between sites until after approximately day 212 (April 2019), when values tended to trend significantly higher at northern sites (Vaipua and Utulei) than at southern sites Pangaimotu, then Sopu (Figure 2). GT in WM of both age cohorts was significantly higher at northern sites than southern sites, while GT in DVH was also higher at northern sites for “young” oysters (Table 1). All GT models confirmed an interaction between site and age cohort, with GT tending to be highest at Utulei for “young” oysters but highest at Vaipua for “old” oysters (Tables 1, 2). After 13-months culture at experimental sites, “young” oysters at Utulei had mean shell dimensions larger than, or comparable to, “old” oysters cultured at Sopu and had a mean DVH 27.2 % larger than “young” oysters at Sopu (Figure 2 and Table 1). “Young” oysters at Utulei reached minimum pearl-production size (T100 and T140) faster than at all other sites and were projected to reach T140 up to 34.2 % (6.5 months) earlier than oysters cultured at Sopu, the poorest culture site (Table 3). Oyster survival in the month following chaplet construction was 92.4 and 97.4 % for “young” and “old” oysters, respectively, while survival at all sites for the following 13-month culture period was >97.0 % for both age cohorts.
Figure 2. Spatio-temporal variation in Pteria penguin shell dimensions over time: (A) dorso-ventral height (DVH); (B) shell thickness (ST): and, (C) wet mass (WM). Splines represent GAM model predictions ±95% confidence intervals. Where one spline falls within the error envelope of another, there is no significant difference in mean measurements between those splines for that time period.
Table 1. Final shell measurements and total growth (GT) of Pteria penguin over 13-months culture at experimental sites.
Table 2. Outputs of generalized linear models of total growth (GT) in dorso-ventral height (DVH), shell thickness (ST), and wet mass (WM) of Pteria penguin between sites and age cohorts.
Table 3. Age of “young” Pteria penguin at a minimum pearl-production size of 100 and 140 mm dorso-ventral height (T100 and T140, respectively) at experimental sites.
Culture sites showed strong inter-island variation in mean water temperature, rainfall and salinity but minimal intra-island variation in environmental variables (Figures 3A–G). Principal component analysis indicated clear separation of samples from northern (Vava’u) and southern (Tongatapu) island groups along PC1 (accounting for 34.3 % of variation), but not within islands (Figure 3H). Northern sites were characterized by higher water temperature (25–30°C), chlorophyll content (0.5–2.5 μg L–1), rainfall (5–30 mm day–1) and current (0.25–0.75 ms–1), and lower salinity (33–35 ppt), turbidity (0–2 NTU), and pH (8.0–8.3) compared to southern sites (Figure 3H). Mean water temperature was significantly higher (by around 2°C) at northern sites (25–30°C) than at southern sites (23–28°C), but did not differ within islands (Figure 3A). Water temperature also showed strong seasonal trends, with maximum and minimum water temperatures occurring in February to March (days 138–197), and August to October (days 317–391), respectively (Figure 3A). Mean daily rainfall in northern sites was significantly higher than in southern sites from November to February (days 46–138) but did not differ significantly for the remainder of the year (Figure 3B). Salinity was significantly lower at northern sites than southern sites for the majority of the study period and was lower at Utulei than Vaipua from January to April (days 107–212) (Figure 3C).
Figure 3. Temporal variation in environmental conditions between culture sites (mean ± SE): (A) temperature; (B) daily rainfall; (C) salinity; (D) pH; (E) turbidity; (F) chlorophyll; (G) current speed; and (H) principle component analysis (PCA) biplot of environmental data showing vector loadings. Splines represent GAM model predictions ± 95% confidence intervals. Where one spline falls within the error envelope of another, there is no significant difference in mean measurements between those splines for that time period. Missing data was due to instrument malfunction.
Turbidity, chlorophyll, pH, and current speed showed substantial temporal variation over the study period but little systematic variation between sites (Figure 3). Sopu experienced a peak in turbidity, chlorophyll, pH, and current speed during January to February, also coinciding with the occurrence of three severe tropical depressions (Figures 3D–G; Tonga Meteorological Service, 2019). Turbidity, pH, and current speed also generally showed lower temporal variation at northern sites than at southern sites (Figures 3D,E,G). Turbidity, chlorophyll, and current speed were generally low at all sites, with mean values below 2.0 NTU, 3.0 μg L–1 and 0.1 ms–1, respectively, over the study period (Figures 3E–G). Mean wave energy (±SE) was 17–90 times higher at Sopu than all other culture sites (181.0 ± 1.3, 10.7 ± 0.7, 6.3 ± 0.5, and 2.0 ± 0.0 joules m2 day–1, at Sopu, Pangaimotu, Utulei, and Vaipua, respectively; Smallhorn-West et al., 2020).
Monthly growth rate (GM) and CI of P. penguin varied significantly by culture site, age cohort and over time (Figure 4). GM of DVH and ST tended to decrease with time and were significantly higher at northern than southern sites for “young” oysters, and lower at Sopu than all other sites for “old” oysters, for most months (Figures 4A–D). GM of WM increased with time to a peak around July to October (days 278–381) before decreasing toward November (day 412 onward), and was significantly higher at northern than southern sites from April (day 212) onward (Figures 4E,F). Condition index generally increased with time but with minimal variation between sites and ages, with the exception of higher CI of young oysters cultured at Utulei (Figures 4G,H). Acute declines were observed in CI and GM of DVH and ST, in February (days 138–166) and June-July (days 258–288) and in CI and GM of WM in October to November 2019 (days 381–417) (Figure 4). In general, GM of shell dimensions and CI had positive relationships with water temperature, rainfall and chlorophyll content (Figure 5). GM of DVH was highest at an intermediate turbidity while GM of WM had a positive relationship with turbidity. GM of shell dimensions and CI did not show clear directional relationships with salinity, pH or current speed (Figure 5).
Figure 4. Spatio-temporal trends in mean (±SE) monthly growth rate (GM) and condition index (CI) of Pteria penguin: (A,B) dorso-ventral height (DVH); (C,D) shell thickness (ST); (E,F) wet mass (WM); and (G,H) CI. Splines represent GAM model predictions ±95% confidence intervals. Where one spline falls within the error envelope of another, there is no significant difference in measurements between those splines for that time period.
Figure 5. Partial plots from GAMs showing relationships between environmental variables and monthly growth rate (GM) in dorsoventral height (DVH), shell thickness (ST), wet mass (WM) and condition index (CI) of Pteria penguin. GAMs included variables culture site, age cohort and time and were used to adjust general relationships shown by partial plots. Splines represent GAM model predictions ±95% confidence intervals.
This study demonstrated significant variation in the growth performance of P. penguin at latitudinal and local scales. Between island groups in Tonga, growth performance of P. penguin was highest at northern sites (Vava’u) and was most strongly related to higher water temperatures at these sites. Within the southern island group, growth performance was significantly higher at the Pangaimotu site than at Sopu but was not clearly related to differences in water quality measured in this study and may be driven by differences in wave energy. Monthly growth rate of P. penguin shell dimensions also showed significant temporal variation in the form of general age-related trends interspersed by acute declines. In the sections that follow we discuss the implications of these results for mabé pearl farm site selection and production schedules.
Variation in P. penguin growth between island groups was most strongly related to water temperature, with both being significantly higher at northern sites. This positive relationship is symptomatic of the profound effect water temperature has on metabolic rate and related physiological processes of pearl oysters (Yukihira et al., 2000; Lucas, 2008) and is in keeping with results of previous aquarium and field-based studies (del Rio-Portilla et al., 1992; Mills, 2000; Yamamoto, 2000). Aquarium-based studies by Li et al. (2009, 2011) indicated that P. penguin has a relatively high tolerance to high water temperatures, experiences peak absorption efficiency and clearance and filtration rates at 28–29°C, and shows only slight declines in these metrics at 32°C. Elevated water temperatures at northern culture sites in Tonga (∼25–30°C) may therefore enable P. penguin to live at close to peak metabolic rate for the majority of the year, without exceeding the species’ upper thermal limits. Inversely, cooler temperatures in the southern island group (23–28°C) likely result in suppressed metabolic rates for the majority of the year and account for relatively poor growth performance of P. penguin at southern sites.
Northern sites were also characterized by lower salinity, turbidity, and pH, and higher chlorophyll content, rainfall, and current than at southern sites; however, these variables were likely to be only weak drivers of P. penguin growth. While all of these variables have been shown to influence the growth performance of pearl oysters (Gervis and Sims, 1992; Lucas, 2008; Adzigbli et al., 2019), at low levels and/or in systems with low variability, their effects may be substantially weaker. For example, while a positive relationship between turbidity and growth performance of P. penguin has been reported in northern Australia (Milione and Southgate, 2011, 2012), this trend was not detected in the present study. These findings are likely due to the low mean turbidity at all sites in the present study, which were comparable to offshore sites of the Great Barrier Reef (0.3–1.0 NTU) and were substantially lower than at near-shore sites (regularly > 100 NTU) shown to yield higher growth performance by P. penguin (Orpin et al., 2004; Milione and Southgate, 2012). Similarly, although salinity showed substantial inter-island variation in this study, the salinity range recorded (32–35 ppt) was very close to the species’ optimal range (Li et al., 2011) and was therefore unlikely to account for the observed growth trends.
Taken together, these results therefore indicate that in oligotrophic marine environments with minimal terrestrial inputs, such as in Tonga, water temperature may be the primary water quality factor influencing growth performance of P. penguin. Large-scale geographic trends in water temperature may therefore be a useful predictor of potential suitability and productivity of P. penguin culture sites in other island groups of Tonga.
The growth performance of P. penguin also varied significantly between sites within island groups, but was not clearly related to environmental variation measured in this study. In the southern island group, growth performance of P. penguin was significantly lower at Sopu than at the Pangaimotu site, while in the northern island group site-related variation in growth was weaker and showed an interaction with age cohort. Although intra-island trends in P. penguin growth were not clearly related to water quality parameters measured in this study, they may be driven by site wave energy. The site supporting the worst oyster performance, Sopu, experiences 17–90 times higher wave energy than the Vaipua, Utulei or Pangaimotu sites (Smallhorn-West et al., 2020) and showed moderate movement of oysters in cylinders during periods of strong wave energy. Increased physical agitation of pearl oysters can increase byssal secretion (Taylor et al., 1997; Kishore et al., 2014) and reduce pearl quality (Kishore and Southgate, 2016) and may account for poorer growth performance of P. penguin cultured at Sopu than at Pangaimotu. Sopu was also the only site to record acute peaks in pH, turbidity, chlorophyll and current speed coinciding with a series of severe tropical depressions that tracked through Tonga in February 2019 (Tonga Meteorological Service, 2019). This suggests that oysters cultured at the Sopu site may also be more exposed to the effects of seasonal disturbance events than oysters at the other culture sites used in this study.
Results of this study suggest that while water temperature is the most important large-scale consideration for P. penguin culture-site selection in Tonga, wave exposure and vulnerability to disturbance events could be important local considerations. In addition to its’ effects on growth performance, sites with high wave exposure may also experience faster deterioration of infrastructure and equipment, and a lower ease of operation (Southgate, 2008). These factors can increase operating risks and maintenance and labor costs and thereby reduce mabé pearl farm profitability and feasibility (Johnston and Hine, 2015; Johnston et al., 2020).
Temporal trends in growth rate and CI of P. penguin in this study were strongly related to time and environmental variation. Monthly growth rate of DVH and ST of P. penguin tended to decrease with time, while CI and GM of WM tended to increase with time. These trends are typical of age-related changes in growth over the lifespan of pearl oysters, which are characterized by initial exponential growth, followed by a shallower increase to near maximum size (Gervis and Sims, 1992; Southgate and Lucas, 2003). Monthly growth trends also reflected a tendency for P. penguin to shift from a low WM:DVH ratio (<1:1) below around 90 mm DVH to a high WM:DVH ratio (>1:1) above this size (Milione et al., 2011; Gordon et al., 2017). Increases in GM and CI of P. penguin also mirrored increases in water temperature and rainfall from October to January (days 15–107), but declined sharply in February (days 138–166) following a series of severe tropical depressions (Tonga Meteorological Service, 2019). This acute decline in GM and CI of P. penguin was likely related to stress and a probable spawning event prior to, or triggered by, the disturbance (Southgate, 2008; Milione and Southgate, 2012). Acute declines in GM and CI of P. penguin in June-July (days 258–288) and October to November (days 381–417) were not associated with disturbances but may indicate the occurrence of additional spawning events. It is also notable that GM of P. penguin was similar at all sites until the February disturbance (days 147–151), after which oysters at northern sites showed better recovery of GM than oysters at southern sites, with this difference persisting for the remainder of the study. Site-related variation in recovery of P. penguin was also reported by Milione and Southgate (2012) and suggests environmental conditions may also determine whether disturbance events have additional chronic impacts on P. penguin growth.
All culture sites in this study yielded >97 % survival of P. penguin and produced GM higher than, or comparable to, those reported by previous studies at similar latitudes in north Queensland and China (Beer, 1999; Fu et al., 2001, 2007; Liang et al., 2001; Gu et al., 2009, 2013; Milione and Southgate, 2011, 2012; summarized in Gordon et al., 2020). These results indicate that the ranges of all environmental variables examined in the study are suitable for the culture of P. penguin. While all sites were suitable for P. penguin culture, higher water temperatures and lower wave energy in the northern island group (Vava’u) resulted in the best growth performance at these sites and are therefore recommended as the preferred location for culture of P. penguin in Tonga. This study therefore recommends that optimal culture sites for P. penguin in Tonga are primarily characterized by warm water temperatures (25–30°C) and low wave exposure (<15 joules m2 day–1).
Pteria penguin cultured at sites with more suitable environmental conditions reached pearl production size up to 34.2 % (6.5 months) earlier than oysters cultured at less suitable sites. This difference in GM would enable mabé pearl production to begin substantially sooner at more suitable culture sites and could have profound effects on production schedules, farm profitability and feasibility (Saidi et al., 2017; Johnston et al., 2020). Results of this study therefore highlight the impact of both large-scale and local environmental conditions on mabé pearl farm productivity and feasibility. Future research should now assess the effects of environmental conditions not only on growth performance, but also on mabé pearl production and quality in Tonga to enable full systematic industry recommendations to be made. This study provides vital information to inform future mabé pearl farm site selection, marine spatial planning and economic analyses to ensure continued sustainable expansion of the mabé pearl sector in Tonga and culture of P. penguin in the Pacific.
The raw data supporting the conclusions of this article will be made available by the authors, without undue reservation.
SG: conceptualization, methodology, formal analysis, investigation, writing–original draft, visualization, and project administration. MW: methodology, resources, and project administration. PS-W: methodology and writing–review and editing. SM and TH: resources. DS: methodology, formal analysis, supervision, and writing–review and editing. PS: conceptualization, methodology, funding acquisition, supervision, and writing–review and editing. All authors contributed to the article and approved the submitted version.
This study was jointly funded by the University of the Sunshine Coast Graduate Research School (grant number: 1.035.06782) and the Australian Centre for International Agricultural Research (ACIAR) and was conducted as part of ACIAR Project FIS/2016/126 “Half pearl industry development in Tonga and Vietnam” led by PCS at the University of the Sunshine Coast.
The authors declare that the research was conducted in the absence of any commercial or financial relationships that could be construed as a potential conflict of interest.
The authors would like to thank staff of the Aquaculture Division of the Ministry of Fisheries Tonga, Eileeni Fonua, Tonga Tuiano, Siaosi Vi, Siaosi Fonua, Tevita Havea, Setaleki Mafi, Meletoli Fa’anunu, Angelica Mafileo, Manasea Fosita, Viliami Ofa, Ioane Finau, Vetekina Pau’u, and Australian Volunteers for International Development (AVI) Emma Krusic, Phillip Brady, Aiden Mellor, and Jason Sheehan as well as researcher Liliani Hughes for technical assistance.
Adzigbli, L., Wang, Z., Zhao, Z., Yang, C., Li, J., and Deng, Y. (2019). Growth in pearl oysters: a review of genetic and environmental influences. Aquac. Res. 51, 18–28. doi: 10.1111/are.14365
Anon (2018). Kingdom of Tonga National Aquaculture Management and Development Plan 2018-2022. Nuku’alofa: Tonga Ministry of Fisheries, Pacific Community.
Barton, K. (2019). MuMIn: Multi-Model Inference. R package Version 1.43.6. Available online at: https://CRAN.R-project.org/package=MuMIn (accessed June 1, 2019).
Bates, D., Maechler, M., Bolker, B., and Walker, S. (2015). Fitting linear mixed-effects models using lme4. J. Stat. Soft. 67, 1–48. doi: 10.18637/jss.v067.i01
Beer, A. C. (1999). Larval culture, spat collection and juvenile growth of the winged pearl oyster Pteria penguin. Pearl Oyster Inf. Bull. 13, 34–35.
del Rio-Portilla, M. A., Re-Araujo, A. D., and Voltolina, D. (1992). Growth of the pearl oyster Pteria sterna under different thermic and feeding conditions. Mar. Ecol. Prog. Ser. 89, 221–227. doi: 10.3354/meps089221
Freites, L., Rojas, M., Dale, A. W., Márquez, A., Revilla, J., Troccoli, L., et al. (2017). Growth and survival of the winged oyster Pteria colymbus in suspended culture: influence of environmental factors associated to upwelling periods. Aquac. Int. 25, 1653–1666. doi: 10.1007/s10499-017-0141-2
Fu, S., Deng, C., Liang, F., Huang, H., and Xie, R. (2007). Study on techniques of round-pearl and artificial cultivation in Pteria penguin (roding). J. Guangdong Ocean Univ. 27, 34–37.
Fu, S., Deng, C., and Xie, R. (2001). Experiment on the grow out of pearl oyster Pteria penguin with columnar cages. T. Ocean Limn. 4, 20–22.
Gaertner-Mazouni, N., and Le Moullac, G. (2016). Toward sustainable pearl-oyster culture, in a changing environment: recent findings and issues from the POLYPERL project (2012-2015). Estuar. Coast. Shelf. Sci. 182, 227–228. doi: 10.1016/j.ecss.2016.11.001
Gervis, M. H., and Sims, N. A. (1992). The Biology and Culture of Pearl Oysters (Bivalvia: Pteriidae). London: ICLARM.
Gordon, S. E., Malimali, S., Wingfield, M., Kurtböke, D. I., and Southgate, P. C. (2019). Effects of nucleus position, profile and arrangement on the quality of mabé pearls produced by the winged pearl oyster Pteria penguin. Aquaculture. 498, 109–115. doi: 10.1016/j.aquaculture.2018.08.055
Gordon, S. E., Malimali, S., ‘Akau’ola, A., Wingfield, M., Kishore, P., and Southgate, P. C. (2018). Using microradiography to assess nacre thickness of mabé pearls: technique suitability and insights. Aquaculture 492, 195–200. doi: 10.1016/j.aquaculture.2018.04.016
Gordon, S. E., Ngaluafe, P., Wingfield, M., and Southgate, P. C. (2017). Morphometric relationships and shellform of cultured winged pearl oysters (Pteria penguin) in Tonga. J. Shellfish. Res. 36, 677–682. doi: 10.2983/035.036.0317
Gordon, S. E., Wingfield, M., Malimali, S., Halafihi, T., and Southgate, P. C. (2020). Assessing protective cylinders for chaplet-based culture of the winged pearl oyster Pteria penguin. J. Shellfish Res. 39, 59–66. doi: 10.2983/035.039.0106
Gu, Z., Jun, W., Xin, Z., Yaohua, S., and Wang, A. (2009). Growth of cultured wing pearl oyster (Pteria penguin) in Li’an Lagoon. Hainan. Oceanol. Limnol. Sin. 40, 423–429.
Gu, Z., Jun, W., Xin, Z., Yaohua, S., and Wang, A. (2013). Growth of winged pearl oyster (Pteria Penguin) juveniles: a comparison of wire collector culture and circle net culture. J. Shellfish Res. 32, 671–674. doi: 10.2983/035.032.0307
Gueguen, Y., Gaertner-Mazouni, N., Lo, C., and Le Moullac, G. (2016). Integrated management of pearl culture in French Polynesia in the context of global change: synopsis of existing results. Estuar. Coast. Shelf. Sci. 182, 229–234. doi: 10.1016/j.ecss.2016.07.010
Haws, M., Ellis, S., and Ellis, E. P. (2006). Producing Half-Pearls (Mabé). Zanzibar: Western Indian Ocean Marine Science Association.
Johnston, W., Gordon, S. E., Wingfield, M., Hine, D., and Southgate, P. C. (2020). Economic feasibility of small-scale mabé pearl production in Tonga using the winged pearl oyster Pteria penguin. Aquac. Rep. 17:100347. doi: 10.1016/j.aqrep.2020.100347
Johnston, W., and Hine, D. (2015). Building Business Skills and Community Based Industry Development in the Pacific. Tongan Workshops 2014/15 (Vava’u and Nuku’alofa). Canberra, ACT: Australian Centre for International Agricultural Research.
Johnston, W., Hine, D., and Southgate, P. C. (2019). Overview of the development and modern landscape of marine pearl culture in the South Pacific. J. Shellfish Res. 38, 499–518. doi: 10.2983/035.038.0301
Kaly, U., Fakatava, T., Lepa, S. T., Matoto, L., Ngaluafe, P. F., Palaki, A., et al. (2000). Status of Fanga’uta Lagoon, Tonga: Monitoring of Water Quality and Seagrass Communities 1998-2000. Scientific Monitoring Report #1. Nuku‘alofa: Tonga National Monitoring Team.
Kishore, P., Hunter, J., Zeng, C., and Southgate, P. C. (2014). The effects of different culture apparatuses and current velocities on byssus production by the black-lip pearl oyster, Pinctada margaritifera. Aquaculture 434, 74–77. doi: 10.1016/j.aquaculture.2014.07.027
Kishore, P., and Southgate, P. C. (2016). The effect of different culture methods on the quality of round pearls produced by the black-lip pearl oyster Pinctada margaritifera (Linnaeus, 1758). Aquaculture 451, 65–71. doi: 10.1016/j.aquaculture.2015.08.031
Kishore, P., Southgate, P. C., Seeto, P., and Hunter, J. (2015). Factors influencing the quality of half- pearls (mabé) produced by the winged pearl oyster, Pteria penguin (Röding, 1758). Aquac. Res. 46, 769–776. doi: 10.1111/are.12228
Kishore, P., Vuibeqa, G. B., and Southgate, P. C. (2018). Developing a national spat collection program for pearl oysters in the Fiji Islands supporting pearl industry development and livelihoods. Aquac. Rep. 9, 46–52. doi: 10.1016/j.aqrep.2017.12.004
Kripa, V., Abraham, K. J., Libini, C. L., Velayudhan, T. S., Radnhakrishnan, P., Mohamed, K. S., et al. (2008). Production of design mabé pearls in the black-lipped pearl oyster, Pinctada margaritifera, and the winged pearl oyster, Pteria penguin, from Andaman and Nicobar Islands, India. J. World Aquac. Soc. 39, 131–137. doi: 10.1111/j.1749-7345.2007.00143.x
Kvingedal, R., Evans, B. S., Lind, C. E., Taylor, J. J., Dupont-Nivet, M., and Jerry, D. R. (2010). Population and family growth response to different rearing location, heritability estimates and genotype environment interaction in the silver-lip pearl oyster (Pinctada maxima). Aquaculture. 304, 1–6. doi: 10.1016/j.aquaculture.2010.02.035
Le Moullac, G., Soyez, C., Latchere, O., Vidal-Dupiol, J., Fremery, J., Saulnier, D., et al. (2016). Pinctada margaritifera responses to temperature and pH: acclimation capabilities and physiological limits. Estuar. Coast. Shelf Sci. 182, 261–269. doi: 10.1016/j.ecss.2016.04.011
Lee, A. M., Williams, A. J., and Southgate, P. C. (2008). Modelling and comparison of growth of the silver-lip pearl oyster Pinctada maxima (Jameson)(Mollusca: Pteriidae) cultured in West Papua. Indonesia. Mar. Fresh. Res. 59, 22–31. doi: 10.1071/MF07112
Li, Z., Liu, Z., and Deng, H. (2011). Effects of temperature and salinity on clearance rate, filtration rate and absorption efficiency of Pteria penguin. J. Fish. China (Shuichan Xuebao). 35, 96–103.
Li, Z., Liu, Z., Xie, L., and Liang, J. (2009). Oxygen consumption and ammonia-N excretion rates of Pteria penguin. Acta Oceanol. Sin. 31, 129–135.
Liang, F., Mao, Y., and Yu, X. (2001). A preliminary observation on the growth of the artificial spats of Pteria penguin. J. Zhanjiang Ocean Univ. 21, 6–9.
Lucas, A., and Beninger, P. G. (1985). The use of physiological condition indices in marine bivalve aquaculture. Aquaculture 44, 187–200. doi: 10.1016/0044-8486(85)90243-1
Lucas, J. S. (2008). “Environmental influences,” in The Pearl Oyster, eds P. C. Southgate and J. S. Lucas (Oxford: Elsevie), 187–230. doi: 10.1016/B978-0-444-52976-3.00006-1
Milione, M., Saucedo, P., and Southgate, P. C. (2011). Sexual development, sex ratio and morphometrics of Pteria penguin (Bivalvia: Pteriidae), in north-eastern Australia. Moll. Res. 31, 30–36.
Milione, M., and Southgate, P. C. (2011). Environmental conditions and culture method effects on growth and survival of juvenile winged pearl oyster Pteria Penguin. J. Shellfish Res. 30, 223–229. doi: 10.2983/035.030.0205
Milione, M., and Southgate, P. C. (2012). Growth of the winged pearl oyster, Pteria penguin, at dissimilar sites in northeastern Australia. J. Shellfish Res. 31, 13–20. doi: 10.2983/035.031.0102
Mills, D. (2000). Combined effects of temperature and algal concentration on survival, growth and feeding physiology of Pinctada maxima (Jameson) spat. J. Shellfish Res. 19, 159–166.
Numaguchi, K. (1994). Effects of water temperature on the filtration rate of the Japanese pearl oyster Pinctada fucata martensii. J. Jap. Aquac. Soc. (Suisanzoshoku). 42, 1–6.
Orpin, A. R., Ridd, P. V., Thomas, S., Anthony, K. R., Marshall, P., and Oliver, J. (2004). Natural turbidity variability and weather forecasts in risk management of anthropogenic sediment discharge near sensitive environments. Mar. Poll. Bull. 49, 602–612. doi: 10.1016/j.marpolbul.2004.03.020
Pouvreau, S., and Prasil, V. (2001). Growth of the black-lip pearl oyster, Pinctada margaritifera, at nine culture sites of French Polynesia: synthesis of several sampling designs conducted between 1994 and 1999. Aquat. Living Resour. 14, 155–163. doi: 10.1016/S0990-7440(01)01120-2
Pouvreau, S., Tiapari, J., Gangnery, A., Lagarde, F., Garnier, M., Teissier, H., et al. (2000). Growth of the black-lip pearl oyster, Pinctada margaritifera, in suspended culture under hydrobiological conditions of Takapoto lagoon (French Polynesia). Aquaculture 184, 133–154. doi: 10.1016/S0044-8486(99)00319-1
R Core Team (2019). R: A language and Environment for Statistical Computing. Vienna: R Foundation for Statistical Computing.
Saidi, I., Johnston, W., and Southgate, P. C. (2017). Potential profitability of pearl culture in coastal communities in Tanzania. Aquac. Rep. 5, 10–17. doi: 10.1016/j.aqrep.2016.11.003
Smallhorn-West, P. F., Gordon, S. E., Dempsey, A. C., Purkis, S., Malimali, S., Halafihi, T., et al. (2020). Tongan socio-environmental spatial layers for marine ecosystem management. Pac. Conserv. Biol. 27, 86–92. doi: 10.1071/PC19032
Southgate, P. C. (2008). “Pearl oyster culture,” in The Pearl Oyster, eds P. C. Southgate and J. S. Lucas (Oxford: Elsevier), 231–272. doi: 10.1016/B978-0-444-52976-3.00007-3
Southgate, P. C., Beer, A. C., and Ngaluafe, P. (2016). Hatchery culture of the winged pearl oyster, Pteria penguin, without living micro-algae. Aquaculture 451, 121–124. doi: 10.1016/j.aquaculture.2015.09.007
Southgate, P. C., Kishore, P., Sharma, S., and Simos, T. (2019). Empowering women through pearl industry-based livelihoods in Fiji. SPC Women Fish. Info. Bull. 29, 24–29.
Southgate, P. C., and Lucas, J. S. (2003). “Reproduction, life cycles and growth,” in Aquaculture: Farming Aquatic Animals and Plants, eds J. S. Lucas and P. C. Southgate (Ofxord: Blackwell Science), 111–122.
Southgate, P. C., Strack, E., Hart, A., Wada, K. T., Monteforte, M., Cariño, M., et al. (2008). “Exploitation and culture of major commercial species,” in The Pearl Oyster, eds P. C. Southgate and J. S. Lucas (Oxford: Elsevier), 303–355. doi: 10.1016/B978-0-444-52976-3.00009-7
Taylor, J., and Strack, E. (2008). “Pearl production,” in The Pearl Oyster, eds P. C. Southgate and J. S. Lucas (Oxford: Elsevier), 273–302. doi: 10.1016/B978-0-444-52976-3.00008-5
Taylor, J. J., Rose, R. A., and Southgate, P. C. (1997). Byssus production in six age classes of the silver-lip Pearl oyster, Pinctada maxima (Jameson). Oceanograph. Lit. Rev. 12:1540.
Tomaru, Y., Kumatabara, Y., Kawabata, Z., and Nakano, S. (2002). Effect of water temperature and chlorophyll abundance on shell growth of the Japanese pearl oyster, Pinctada fucata martensii, in suspended culture at different depths and sites. Aquac. Res. 33, 109–116. doi: 10.1046/j.1365-2109.2002.00653.x
Tonga Meteorological Service (2019). Tropical Depression Forecast Track Map, Tropical Depression TD06F, TD08F, TD10F. Tropical Cyclone Warming Centre fua’amotu. Ministry of Meterology, Energy, Information, Disaster Management, Environment, Communications and Climate Change (MEIDECC). Available online at: http://www.met.gov.to/ (accessed February 20, 2019).
Wada, T. K., and Tëmkin, I. (2008). “Taxonomy and phylogeny,” in The Pearl Oyster, eds P. C. Southgate and J. S. Lucas (Oxford: Elsevier), 37–75. doi: 10.1016/B978-0-444-52976-3.00002-4
Walne, P. R., and Mann, R. (1975). “Growth and biochemical composition in Ostrea edulis and Crassostrea gigas,” in Proceeding of the 9th European Marine Biology Symposium, ed. H. Barnes (Aberdeen: Aberdeen University Press), 587–607.
Wassnig, M., and Southgate, P. C. (2012). Effects of settlement cues on behaviour and substrate attachment of hatchery reared winged pearl oyster (Pteria penguin) larvae. Aquaculture 344, 216–222. doi: 10.1016/j.aquaculture.2012.03.020
Wood, S. N. (2017). Generalized Additive Models: An Introduction with R, 2nd Edn. london: Chapman and Hall. doi: 10.1201/9781315370279
Yamamoto, K. (2000). Effects of water temperature on respiration in the pearl oyster Pinctada fucata martnsii. J. Jap. Aquac. Soc. (Suisanzoshoku) 48, 47–52.
Yamamoto, T., and Tanaka, H. (1997). Potential of Commercial Development of Mabé Pearl farming in Vava’u islands, Kingdom of Tonga (field document no. 5) (South Pacific Aquaculture Development Project Phase II) (GCP/RAS/116/JPN). Suva: Food and Agriculture Organization of the United Nations.
Yukihira, H., Klumpp, D. W., and Lucas, J. S. (1998). Comparative effects of microalgal species and food concentration on suspension feeding and energy budgets of the pearl oysters Pinctada margaritifera and P. maxima (Bivalvia: Pteriidae). Mar. Ecol. Prog. Ser. 171, 71–84. doi: 10.3354/meps171071
Yukihira, H., Klumpp, D. W., and Lucas, J. S. (1999). Feeding adaptations of the pearl oyster Pinctada margaritifera and P. maxima to variations in natural particulates. Mar. Ecol. Prog. Ser. 182, 161–173. doi: 10.3354/meps182161
Yukihira, H., Lucas, J. S., and Klumpp, D. W. (2000). Comparative effects of temperature on suspension feeding and energy budgets of the pearl oysters Pinctada margaritifera and P. maxima. Mar. Ecol. Prog. Ser. 195, 179–188. doi: 10.3354/meps195179
Keywords: Pteria penguin, pearl oyster, growth, productivity, environmental variables
Citation: Gordon SE, Wingfield M, Smallhorn-West PF, Malimali S, Halafihi T, Schoeman DS and Southgate PC (2021) Spatio-Temporal Variation in Growth Performance and Condition of the Winged Pearl Oyster Pteria penguin. Front. Mar. Sci. 8:618910. doi: 10.3389/fmars.2021.618910
Received: 19 October 2020; Accepted: 15 February 2021;
Published: 12 March 2021.
Edited by:
Morten Omholt Alver, Norwegian University of Science and Technology, NorwayReviewed by:
Luis Freites, instituto Oceanográfico de Venezuela, VenezuelaCopyright © 2021 Gordon, Wingfield, Smallhorn-West, Malimali, Halafihi, Schoeman and Southgate. This is an open-access article distributed under the terms of the Creative Commons Attribution License (CC BY). The use, distribution or reproduction in other forums is permitted, provided the original author(s) and the copyright owner(s) are credited and that the original publication in this journal is cited, in accordance with accepted academic practice. No use, distribution or reproduction is permitted which does not comply with these terms.
*Correspondence: Sophie E. Gordon, c29waGllLmdvcmRvbkByZXNlYXJjaC51c2MuZWR1LmF1
Disclaimer: All claims expressed in this article are solely those of the authors and do not necessarily represent those of their affiliated organizations, or those of the publisher, the editors and the reviewers. Any product that may be evaluated in this article or claim that may be made by its manufacturer is not guaranteed or endorsed by the publisher.
Research integrity at Frontiers
Learn more about the work of our research integrity team to safeguard the quality of each article we publish.