- Alaska Fisheries Science Center, National Marine Fisheries Service, Juneau, AK, United States
The evolution of fisheries science and management toward an ecosystem perspective necessitates the meaningful incorporation of human dimensions. Whereas great strides have been made over the last several decades at moving toward ecosystem based fisheries management (EBFM), largely through the development of integrated ecosystem assessments (IEAs), the inclusion of human dimensions into these efforts has often been fragmentary and, in juxtaposition to the biophysical dynamics, sometimes even seemingly superficial. This presents a great challenge to the accuracy and applicability of these results, as the lack of appropriate incorporation of humans can be problematic in terms of both social and biophysical consequences. This study systematically documents current social science understanding of the multiple human dimensions that should be incorporated within ecosystem assessments and the overall approach to each of these within IEAs and other EBFM efforts. These dimensions include the multi-faceted nature of human well-being, heterogeneity in human well-being derived from fisheries, adaptive behaviors, and cumulative effects. The systematic inclusion of these dimensions into IEAs is then laid out in a conceptual framework that details how a perturbation reverberates through a fisheries system and the iterative approach that should be undertaken to understand its impacts on human dimensions. This framework is supplemented with a data collection scheme that is intended to facilitate operationalization. The detailed examination of incorporating human dimensions within IEAs presented in this study should further resonate with other ecosystem assessment efforts, providing not just ample evidence of the need for moving beyond simplistic assumptions of human homogeneity but a means of systematically integrating a more realistic and representative perspective.
Introduction
The movement toward ecosystem based fisheries management (EBFM) is guided by a growing awareness that a holistic perspective, inclusive of various biophysical components as well as complex human dimensions, can improve the efficacy of policy making by understanding the tradeoffs between management objectives and integrating cumulative impacts on key components of marine systems (Link and Browman, 2014). The transition toward EBFM represents a positive step toward integrating multiple ecosystem components and scientific disciplines. However, the complexity of how humans interact with, derive value from, and respond to changes in their marine ecosystems are still largely diluted in these efforts that disproportionately focus on biophysical complexity.
Perhaps the most prevalent mechanism toward guiding the development of EBFM [and ecosystem based management (EBM) more broadly] is the formulation of integrated ecosystem assessments (IEAs), which are an approach to synthesizing and organizing existing scientific knowledge to evaluate ecosystem status with respect to multiple objectives (Levin et al., 2009; International Council for the Exploration of the Sea [ICES], 2017). The IEA process has been conceptualized as a five-step, feedback loop that includes scoping, indicator selection, risk analysis, management strategy evaluation, and continued monitoring (Levin et al., 2009). The inaugural, scoping step of IEAs often includes the development of conceptual models, which represent qualitative, directional linkages amongst biophysical and human components in an ecosystem and are intended to integrate different forms of scientific and stakeholder knowledge (Ogden et al., 2005; Levin et al., 2016). Qualitative network models (QNMs) are mathematical representations used to operationalize conceptual models, which examine the qualitative impacts of a perturbation on an ecosystem through dynamic simulations (Dambacher et al., 2009).
While great progress has been made toward EBFM across the world, the inclusion of humans within IEAs has been fragmentary and, in juxtaposition to the biophysical dynamics that are examined, sometimes seemingly superficial and often antagonistic in terms of the relationships identified between humans and nature (Kittinger et al., 2012; Levin et al., 2016). This may be due to issues with model stability when QNMs are operationalized, and the inherent focus on biophysical dynamics as perturbations in these models, which drive the inclusion of complex ecological linkages that leave limited room for human dimensions (Melbourne-Thomas et al., 2012; Wildermuth et al., 2017). Furthermore, much of what facilitates the utilization of QNMs to model complex ecosystems – variable reduction (eliminating variables that do not meet some significance criteria or qualitatively aggregating them), as well as linearity and equilibrium assumptions – also understandably hinders their capacity to reflect the real world (Dambacher et al., 2009). This may be a greater impediment to integrating humans into these models than biophysical dynamics alone due to the inherent heterogeneity of human populations, the complexity of how they interact with fisheries, and their capacity to adapt over a much shorter timeframe than is true for many components of natural systems. However, a poor understanding of social systems can lead to management choices that increase social conflict, diminish adaptive capacity, undermine trust between managers and stakeholders, and decrease resilience while limiting the accuracy of predictions about human responses (Hall-Arber et al., 2009; Lord et al., 2009). Although it should be noted that there are multiple mechanisms for integrating human dimensions into fisheries management beyond quantitative ecosystem assessments, including indicators (Szymkowiak and Kasperski, 2020), social impact assessments (Hall-Arber et al., 2009), and analyses of management outcomes (Szymkowiak and Kasperski, 2020), this study focuses on ecosystem assessments specifically due to their increasing utilization as part of the shift toward EBFM.
This study systematically documents social science understanding of various human dimensions that should be incorporated within ecosystem assessment frameworks, the current overall approach to each of these within IEAs and other EBFM efforts, as well as examples from the literature of how the incorporation of these elements could be enhanced (see section “Human Dimensions for Inclusion in Ecosystem Assessments”). Ecosystem assessment frameworks, hereinafter ecosystem assessments, include a range of tools intended to holistically examine ecosystems, from IEAs to mechanistic or other models. The Section “Bridging the Human Dimensions Gap in Fisheries Ecosystem Assessments” presents a conceptual framework for integrating human dimensions into ecosystem assessments. The framework is supplemented with a data collection scheme that is intended to facilitate operationalization (see section “Data Collection Framework”), which has been a missing component of many established frameworks, representing a key limitation to meaningfully incorporating humans into ecosystem assessments. The Section “Integrating Human Dimensions Data Into Ecosystem Assessments” discusses how those data components may be integrated into ecosystem assessments given the scope of current modeling frameworks in this area.
Human Dimensions for Inclusion in Ecosystem Assessments
Human interactions with, and benefits from, fisheries participation are rich and diverse, highly responsive to changes in the fisheries system, and affected by a multitude of co-occurring, iterative, or otherwise asynchronous factors. The multifaceted nature of human dimensions needs to be incorporated within ecosystem assessments to reflect these realities and improve predictions. The following sections explore these dimensions, including the varied components of well-being that individuals derive from fisheries (see section “Multifaceted Nature of Well-Being”), the heterogeneity of how that well-being is distributed across populations (see section “Heterogeneity in Well-Being Derived From Fisheries”), adaptive responses to degradation in well-being (see section “Adaptive Behavior”), and the cumulative effects of iterative adaptations or perturbations (see section “Cumulative Effects”). These are summarized in Table 1 along with the current social science knowledge and prevalent ways in which these dynamics are being addressed within ecosystem assessments.
Multifaceted Nature of Well-Being
Well-being is conceptualized as a state when basic needs are met and humans and communities can pursue their goals and enjoy a satisfactory quality of life (Breslow et al., 2016). The concept encompasses everything from material needs and physical health to social relations, culture and aesthetics, mental health, and connection to the environment. Among social scientists the conceptualization of well-being is increasingly guiding the development of frameworks intended to holistically examine the effects of natural resource management policies on human communities (Breslow et al., 2016; Leong et al., 2019).
Within fisheries there is a vibrant body of literature documenting the multifaceted dimensions of well-being that people derive from fisheries, which are summarized in Table 2. Researchers have shown that fishing is associated with, amongst others, a connection to the water, family heritage, a sense of community, and identity (Hall-Arber et al., 2009; Holland et al., 2019; Szymkowiak, 2020a). Indeed fishing is often described as a way of life rather than an occupation by both fishers and their families, embedded with a sense of pride associated with their resilience (Britton, 2012; Szymkowiak, 2020a). Despite evidence of the diversity of well-being that people derive from fisheries, ecosystem assessments have largely focused on economic benefits, or at most a highly condensed subset of well-being components including equity measured with respect to gear conflicts, livelihood measured with respect to employment and revenues, family connection and physical safety measured with respect to time at sea (Fulton et al., 2014, 2018; DePiper et al., 2017; Zador et al., 2017; Gaichas et al., 2018; Okamoto et al., 2020).
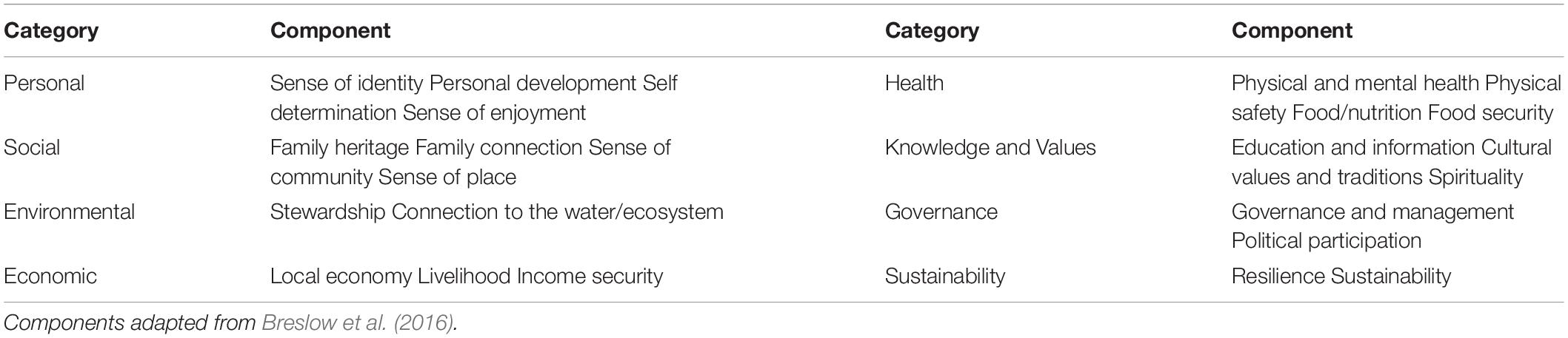
Table 2. Well-being categories and components associated with fisheries participation from the literature.
Several large-scale efforts over the last several years have been undertaken by social scientists to systematically document the linkages between human well-being and its interconnectedness with marine ecosystem use, which should serve as foundational tools for understanding the multifaceted nature of human well-being (Breslow et al., 2016; Díaz et al., 2018; Allison et al., 2020). The framework developed by Breslow et al. (2016) decomposes human well-being into four major constituents – conditions, connections, capabilities, and cross-cutting domains – that capture human needs being met, social connections, the capacity to pursue goals, and a collective satisfactory quality of life. This framework essentially groups the well-being components documented by the other aforementioned efforts (Díaz et al., 2018; Allison et al., 2020) into four constituents which may facilitate their incorporation into efforts, especially wherein social science expertise is limited regarding the complexity of these relationships. The incorporation of this framework by EBFM practitioners can readily advance the inclusion of a comprehensive understanding of human well-being, by spanning the full scope of well-being components as well as their connections.
Heterogeneity in Well-Being Derived From Fisheries
Across human populations, there are substantial differences in access to resources due to the intersectionality of social and political variables such as race, gender, and class, the combination of which can magnify and create unique forms of discrimination (Crenshaw, 1989). Within fisheries this is manifest in distributional differences in who derives, what types of, and how much well-being from fisheries (Coulthard, 2012; Allison et al., 2020). For example, those with lower socioeconomic status may have fewer options for diversifying incomes; thus the economic welfare they derive from fisheries may have greater value to them (Cinner et al., 2009).
In addition to differentiated access, demographics have been shown to shape values and well-being priorities. These differences are in turn reflected in heterogeneity in how well-being is derived from fisheries across populations. For example, researchers have demonstrated gender differences in well-being priorities, risk thresholds and occupational identities, which affect how individual participants and families respond to fishery changes (Coulthard and Britton, 2015; Kawarazuka et al., 2017). Others have documented the importance of cultural keystone species that are particularly salient in terms of shaping cultural identity and spirituality for some ethnic groups (Field, 2008; Thornton and Kitka, 2015).
In cases where ecosystem assessments seek to incorporate well-being components beyond just economic benefits, the efforts often fall short in terms of integrating the heterogeneity in how those components are derived and prioritized across populations. Even when the authors acknowledge the complexity and heterogeneity of well-being components across populations, they are forced to represent them as monolithic nodes, due to a variety of issues including a lack of data at appropriate spatial and temporal scales and presumably in part due to the focus of the models on biophysical dynamics (Harvey et al., 2016). Others model diverse well-being components but strictly on singular groups of direct commercial fisheries participants, omitting other user groups (Fulton et al., 2014, 2018).
However, some work has been done to try to integrate well-being heterogeneity into ecosystem assessments, which can inform how such efforts can be extended into the future. In the payments for ecosystem services literature, researchers have disaggregated groups of individuals for the purposes of identifying potential winners and losers in examining trade-offs in ecosystem services (Daw et al., 2011). Within the fisheries literature, researchers have incorporated heterogeneity in terms of well-being tradeoffs across user groups by focusing on communities of practice, defined as a collective group of fishers using the same gear type to target the same species (Zador et al., 2017). Others in fisheries have incorporated multiple user groups and diverse well-being components within risk assessments, relying on established indicators of social vulnerability, fishing dependence, and gentrification pressure at the ecosystem level in addition to metrics of commercial revenue, revenue diversity, shoreside support, and recreational angler days (Gaichas et al., 2018). Still others have explored fisheries benefits across diverse user groups using metrics of access and social relationships (Okamoto et al., 2020).
Adaptive Behavior
Fishermen employ diverse adaptation strategies over varying time frames in response to fisheries perturbations (Cinti et al., 2010; Abbott and Haynie, 2012; Szymkowiak, 2020a). For example, short-term adaptations to changes in stock composition may include behavioral changes on the water like targeting different areas, which evolve into cooperative arrangements between fishermen targeted at reducing fishing costs and ultimately into developing niche markets or custom processing arrangements intended to increase the value generated from each fish (Szymkowiak, 2020a; Szymkowiak and Rhodes-Reese, 2020). Incorporating these responses into ecosystem assessments is critical for accurate predictions, with examples in the literature of how misunderstood or unacknowledged fisher responses to changing conditions have led to unintended, negative consequences like increased bycatch due to the displacement of fishing effort following area closures or resource overuse associated with license permitting systems (Cinti et al., 2010; Abbott and Haynie, 2012).
Adaptive strategies can also have varied implications for overall well-being due to inter- and intra-personal tradeoffs (Coulthard, 2012; Szymkowiak, 2020a). Whereas adaptations are often employed to buffer against income losses, the chosen strategy may imply, for example, greater time away from family or increasing shoreside employment, which alter other well-being components (Coulthard, 2012; Coulthard and Britton, 2015; Szymkowiak, 2020a). Therefore, adaptive behavior mediates not just human interactions with and impacts on fisheries systems but has profound implications for how these choices affect overall human well-being, which need to be incorporated into ecosystem assessments (Kittinger et al., 2012).
The incorporation of fisher adaptive behavior into ecosystem assessments has largely relied on rational choice theory, which in its basic form assumes perfect rationality, homogeneity and single-minded utility maximization (Becker, 1976). In essence the modeler assumes individuals rank their options based on the expected mean value of the outcome and the anticipated variability in it (van Putten et al., 2013). Researchers employ a number of proxies for economic returns and income variability to incorporate this strategy into models, as documented by van Putten et al. (2013).
Yet empirical evidence has demonstrated the heterogeneity of human decision-making in general, which includes suboptimal choices and deviance from decision-making pathways and diverse well-being priorities (Beratan, 2007; Ariely, 2009). Ecosystem assessments should therefore also account for response diversity within human populations, as fishers adapt to constraints in fisheries systems with strategies that reflect their individualized economic, cultural, and social realities (Salas and Gaertner, 2004; van Putten et al., 2013). Indeed there are numerous factors underlying the diversity of fisher responses to changes that have been documented, including identity, knowledge, beliefs, perceptions of risk, family connections, and sense of community (Salas and Gaertner, 2004; Holland et al., 2019). These factors have implications for not just how fishers will respond to changes within the fisheries system but also, for example, how they may shift into other sectors and how that in turn may affect their overall well-being (Pollnac and Poggie, 2008).
One of the most common ways for fishermen to adapt to fishing variability is by diversifying their fishing portfolio (Holland and Kasperski, 2016; Cline et al., 2017). Increasing research on fisheries connectivity – the networks of alternative fisheries in which groups of fishermen are participating – points to the need to understand these connections in order to improve ecosystem assessments and predict fishers’ adaptive responses (Addicott et al., 2018; Yletyinen et al., 2018; Kroetz et al., 2019). This includes the potential for policy or ecosystem changes to result in spillover effects into other fisheries, which while potentially mitigating economic losses can also increase fishing pressure on other fisheries (Addicott et al., 2018; Kroetz et al., 2019). Furthermore, changing behaviors in response to ecological or management shifts can increase bycatch levels of non-target species that also have ecosystem implications (Szymkowiak and Rhodes-Reese, 2020).
A number of mechanisms have been developed in the literature that could serve to facilitate the incorporation of human adaptive behavior into ecosystem assessments. Adaptations are integrated as responses to impacts within the Driver-Pressure-State-Impact-Response (DPSIR) model that incorporates humans as pressures on ecosystems and wherein the responses are integrated as feedbacks on those pressures (Bowen and Riley, 2003; Levin et al., 2008). In the modified EBM-DPSER model, ecosystem services replace impacts to depict how ecosystem functions produce various services from which humans derive well-being (Kelble et al., 2013). However, the application of this framework has thus far not included heterogeneous adaptations among individual actors, which may be out of the scope of ecosystem assessments intended to capture numerous ecosystem services, but could be more readily applied in contexts wherein only a single service, such as fisheries, is being examined.
Another tool, agent-based modeling (ABM), is increasingly applied in the land management and environmental science literature for coupled socio-ecologic systems to incorporate heterogeneity in individual decision making through both cognitive strategies (learning) and preferences (Filatova et al., 2013). In this approach, researchers employ a diverse suite of tools to derive both learning typologies and preferences, including literature reviews, surveys, and expert opinion (Hunt et al., 2007: Murray-Rust et al., 2011). The potential application of ABM in fisheries is now receiving greater attention with respect to understanding interactions and feedbacks, incorporating cognitive and behavioral sciences, and projecting the human dimensions impacts of management (Burgess et al., 2018).
Another way of potentially including adaptive behavior into ecosystem assessments is to conceptualize decision-making with respect to household utility or welfare (Milner-Gulland, 2011). The household unit is often organized around non-economic concerns and family interest, distinguishing it from a business or firm structured to maximize profits (Durrenberger, 1994; Marks, 2012). Participation in fisheries has been shown to affect the character of families, necessitating adaptability and fluidity that is translated into a family value system, which contributes to personal and family identities (Britton, 2012; Marks, 2012; Szymkowiak, 2020a). In turn, this can drive how the family unit adapts to fishery changes, e.g., shifting labor, expenditures, and investments, which can reverberate throughout fishery systems in ways that defy expectations based on individual-level predictions (Marks, 2012; Szymkowiak, 2020a).
Cumulative Effects
Fishers are often responding to perturbations that are occurring synchronously or in sequence that compounds or otherwise relates their effects. These interactions can take many different forms and have been differentially characterized as synergistic or antagonistic, multiplicative, additive, mitigative, or dominant (Crain et al., 2008; Halpern et al., 2008). Yet these types of cumulative effects on human dimensions are generally not considered in ecosystem assessments and have only minimally been considered with respect to the effects on biophysical components of ecosystems (Hobday et al., 2011; Kelble et al., 2013). Given that the cumulative effects of perturbations could imply that a chosen management alternative or new stressor is much more detrimental than if considered in isolation, the omission of these types of effects from ecosystem assessments can impede the utility of their results.
The coupled effects of various management regimes within the same fisheries system have been documented with respect to adverse cumulative social impacts. While fisheries diversification is historically a common strategy employed by fishermen to mitigate revenue losses from any one fishery, the increasing institution of limited entry and catch share programs throughout fisheries systems has restricted the capacity of fishermen to readily switch target fisheries. Murray et al. (2010) and Stoll et al. (2016) document the cumulative effects of lost diversification opportunities through piecemeal fisheries regulations, which have increased uncertainty and undermined the adaptive capacity of fishermen due to the coupling of institutional constraints and investments of fishers in these institutions. Both studies also relay the potential negative implications on the ecological dimensions of the system from this degraded flexibility due to increased non-compliance and continued prosecution of the target resource even in the face of declining abundance. By effectively creating winners and losers in fishing communities, some management regimes may also undermine interpersonal relationships, shared value systems, trust, and cooperation within communities (McCay, 2004; Ringer et al., 2018). In turn, this may further decrease adaptive capacity by undermining social capital and social memory, leaving the system more vulnerable to future threats (Tuler et al., 2008; Murray et al., 2010; Ringer et al., 2018).
Whereas cumulative effects of fisheries perturbations on human populations have not been integrated into ecosystem assessments, there have been a number of social science studies examining such impacts (Tuler et al., 2008; Murray et al., 2010; Ringer et al., 2018). Due in part to the difficulty of parsing out impacts from any given perturbation, these studies have employed ethnographic research techniques. Ethnographic tools allow researchers to describe the experience of cumulative impacts in a way that reflects the voices of the stakeholders and allows the researchers to capture impacts that may have occurred at different temporal and spatial scales. However, ethnographic studies are highly resource intensive with results that may not apply across multiple contexts, limiting the scope of how they can be integrated into ecosystem assessments. This presents an inherent tradeoff, given the rich contextual information that is often developed with ethnographic studies, which researchers need to consider in light of the spatial scale that they are seeking to examine.
Another potential means of conceptualizing cumulative effects is through utilizing fisheries connectivity networks to understand compounding impacts within fishing portfolios (Addicott et al., 2018; Yletyinen et al., 2018; Kroetz et al., 2019). Connectivity networks apply the principles of social network analysis to examine fishing strategies (groups of permits or species that are similarly targeted by fisheries participants) in order to examine, for example, potential spillover effects from management changes into other fisheries, how a perturbation may reverberate through the system, and tradeoffs between localized and system-wide risks. Similarly, connectivity networks could be employed to understand whether individuals or communities are experiencing multiple stressors across the fisheries in which they are participating and thus simulate cumulative effects.
Bridging the Human Dimensions Gap in Fisheries Ecosystem Assessments
The multiple facets and dynamics of human well-being, adaptations, and interactions within fisheries systems outlined in Section “Human Dimensions for Inclusion in Ecosystem Assessments” can be integrated into ecosystem assessments through a conceptual framework that is elucidated below. This framework builds on previous efforts of scientists to inform an understanding of the human dimensions of ecological and management changes, including that of Ostrom (2009) and McGinnis and Ostrom (2014) on coupling socio-ecologic systems (SES); Pollnac et al. (2006) on a social impact assessment model; causal models used to diagram stressors, response processes, and well-being impacts (Tuler et al., 2008; Lord, 2011); and a conceptual model of the California Current (Levin et al., 2016). These previous efforts are coupled with the human well-being framework developed by Breslow et al. (2016) to incorporate a comprehensive understanding of how humans derive well-being from fisheries.
The framework, presented in Figure 1 and described below, details an iterative approach to integrating the multifaceted human dimensions discussed throughout Section “Human Dimensions for Inclusion in Ecosystem Assessments” into ecosystem assessments. Although the presentation below focuses on evaluating perturbation impacts, the same approach can be utilized to examine differential effects of management options, as in the context of management strategy evaluation. Beginning with the development of an integrated baseline model (element 1) that accounts for the intersection of demographics, well-being priorities, and mixed fishing portfolios, the framework guides how multifaceted and heterogeneous well-being should be addressed in ecosystem assessments to meaningfully incorporate the complexity of human dimensions. The framework then informs how to examine the impacts of perturbations on human dimensions, which alters how individuals participate in fisheries and the well-being they derive from them based on their demographics, well-being priorities, and fishing portfolios (element 2). The deterioration of well-being triggers adaptive responses (element 3), which are informed by, again, the intersection of demographics, fishing portfolios, and well-being. The adaptations themselves are associated with tradeoffs in inter- and intra-personal well-being, which in turn trigger further adaptive responses, represented by the two-sided arrow between adaptive behavior and cumulative well-being effects. The totality of altered well-being due to the perturbation and the adaptations that are undertaken alter baseline conditions (element 4), affecting who participates in which fisheries, how, and the well-being that they derive from that participation. Each of these elements (1–4) is associated with a set of distinct data components that are examined in detail in Section “Data Collection Framework.”
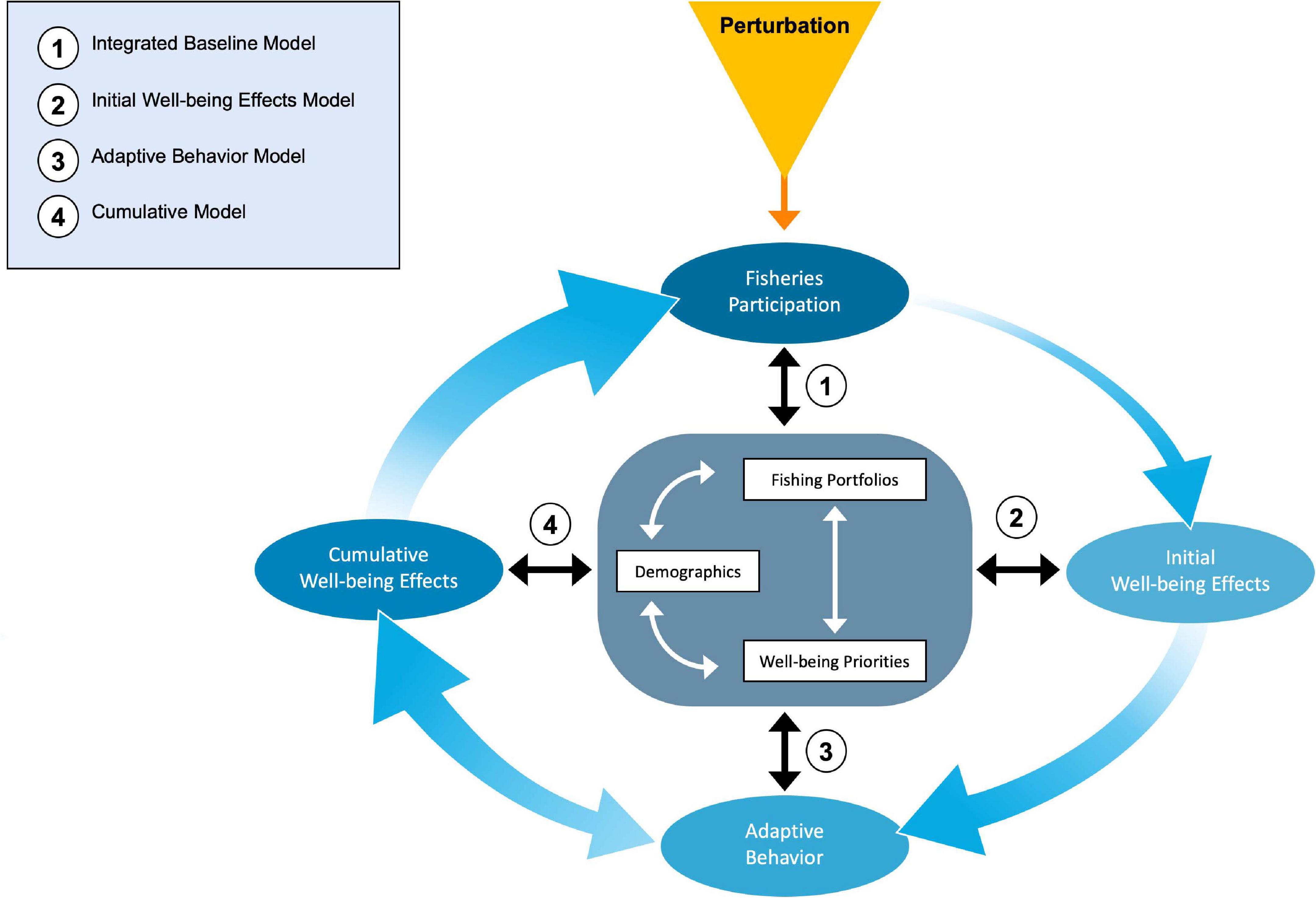
Figure 1. Conceptual framework for integrating human dimensions into ecosystem assessments, including integrated baseline, initial well-being effects, adaptive behaviors, and cumulative impacts that need to be considered in response to a perturbation.
Developing an Integrated Baseline Model
The foundational component of the framework is an integrated baseline model that captures how individuals relate to their fisheries at equilibrium. The conceptual framework captures these relationships through the convergence of demographics, fishing portfolios, and well-being priorities that drives meaning for fisheries participants and how they derive value from this participation, captured in element 1 in Figure 1. The convergence represented in the central rectangle in Figure 1 combines the SES framework of Ostrom (2009) and McGinnis and Ostrom (2014) and the well-being framework of Breslow et al. (2016) to demonstrate how social demographics, knowledge systems, norms, and shared histories can interplay to inform fishing portfolios and the well-being that is derived from fisheries participation – forming the integrated baseline model, element 1.
The heterogeneity in well-being that individuals derive from fisheries described in Section “Heterogeneity in Well-Being Derived From Fisheries” is in part determined by demographics and in turn shapes fisheries participation. For example, women are universally largely represented in nearshore fisheries that allow them to navigate the often dueling roles of fisheries participation and childcare (Kleiber et al., 2015; Szymkowiak, 2020b). These responsibilities drive the composition of their fishing portfolios; in turn, the local availability of nearshore fisheries may affect the presence of fishing women and other demographics in communities, much like local fisheries drive fleet composition (Salmi and Sonck-Rautio, 2018; Szymkowiak, 2020b). Similarly because demographics can shape well-being priorities (e.g., occupational identities and cultural values and traditions), those differentiated priorities can affect the demographic composition of communities and their fishing portfolios.
Although there is overlap between the types of values that individuals derive from subsistence, commercial, and recreational fishing (Biedenweg et al., 2014; Biedenweg, 2017), these practices are generally differentially motivated by well-being components such as food provisioning, sharing, livelihood, and outdoor enjoyment (Schumann and Macinko, 2007; Poe et al., 2014). The derivation and prioritization of well-being components can also be specific to target species, in turn shaping fishing portfolios and participant demographics. For example, one species may have greater economic value while another allows families to fish together because it is prosecuted under conditions wherein children can be onboard; these dynamics will affect who participates in these fisheries and how (Szymkowiak, 2020b). There are also inherent spatial and temporal dimensions to fisheries participation, which affect how well-being is derived (e.g., increased safety risk with offshore, winter fisheries in some regions) (Szymkowiak, 2020b). The pursuit of cultural keystone species for some groups can shape diverse fishing portfolios even within the same geographic community (Field, 2008; Thornton and Kitka, 2015). Thus, how specific well-being components are derived from fisheries and in turn prioritized are particular to the fishing portfolio, in terms of both the fishery type (commercial, subsistence, or recreational), the gear type, and the species. The response of individuals to perturbation is then driven by how the intersection of demographics, fishing portfolios, and well-being priorities informs the well-being that is derived from fisheries.
Social systems, institutions, and governance have important implications for determining who participates in fisheries and how, and the mechanisms that they have available to respond to change (Pollnac et al., 2006; Ostrom, 2009; McGinnis and Ostrom, 2014). The effects of these authorities and networks are captured implicitly in each of the elements – integrated baseline conditions, initial well-being effects, adaptive behaviors, and cumulative effects – in terms of how they manifest themselves. Therefore, the framework also does not devise a means of gathering data specific to this dimension, but rather acknowledges that these broader social drivers will have effects that are captured in the other data elements identified.
Incorporating Perturbations and Initial Well-Being Outcomes
When a perturbation occurs in a marine ecosystem it can have a diversity of implications on a fisheries system. For example, the marine heatwave that occurred in the Northeast Pacific from 2013 to 2015 has been implicated in severe downturns in some species, like Pacific cod, while also contributing to unprecedented recruitment in sablefish (Barbeaux et al., 2019; Hanselman et al., 2019). For the directed commercial sablefish fishery in Alaska the influx of sablefish bodes well for harvests in future years, but in the meantime the large numbers of small fish are causing average dockside prices to plummet (NPFMC, 2019). Concurrently, the trawl fleet which targets a mix of groundfish species in Alaska is witnessing record high incidental catch levels of sablefish, which could ultimately affect their capacity to harvest their directed groundfish allocations if the sablefish harvests become too high. Thus the same ecological perturbation has a mixture of impacts in this case depending on target species and fleet, including altering fish availability, stock distributions and compositions.
The ways in which perturbations manifest themselves in ecological changes then drive impacts on fisheries participation, which have well-being implications that are mediated by again the convergence of demographics, fishing portfolios and well-being priorities – represented by element 2 in Figure 1, initial well-being model. For example, in the case of small sablefish abundance noted above, both the directed sablefish fleet and the trawl fleet that catch sablefish incidentally are expending increasing effort on avoiding small sablefish. This has resulted in more time on the water for the fleets, which leads to rising fishing costs and implies reduced earnings, which may be buffered by diverse fishing portfolios (Szymkowiak and Rhodes-Reese, 2020). More days at sea can also have a myriad of other well-being implications associated with, for example, fatigue and safety on the water, missed family events and adverse impacts on social connections, but the severity of those effects are also going to depend on the person’s demographics and well-being priorities (Szymkowiak, 2020a).
Developing an Adaptive Behavior Model
Ultimately changes to well-being associated with altered fisheries participation will drive adaptive behaviors as actors try to mitigate adverse effects. In the next step of the conceptual framework (element 3 in Figure 1, adaptive behavior model), the convergence of demographics, fisheries portfolios, and well-being priorities informs the distribution of adaptive behavior. For instance, younger individuals often have greater occupational mobility and are therefore more likely to shift their labor into other industries in response to fisheries declines, while older individuals may have greater access to capital and be able to diversify their fisheries portfolios (Tuler et al., 2008; Szymkowiak, 2020a). Fishing participants who are married or have a life partner may have a financial buffer due to pluriactivity, or income generated from multiple sources, that can facilitate continued fisheries participation even in the face of revenue declines (Marks, 2012; Szymkowiak, 2020a). Demographics may also have implications for how well-being priorities affect the choice of adaptive strategy. For instance, women have been shown to value income security, safety, and time with family more than a fishing identity, which can imply that in the face of fishery downturns women may differentially opt for exiting or pluriactivity rather than prevailing in the fishery (Coulthard and Britton, 2015; Kawarazuka et al., 2017).
The adaptive responses of individuals can also have implications for fishing portfolios, well-being priorities, and some demographic characteristics – a feedback that is represented in the framework. Some of the manifestations of adaptive choices will be evident in fishing portfolios and fishery behaviors including entry/exit and diversification patterns, altered spatial distributions, days-at-sea, permit stacking, and leasing (Lord, 2011; Szymkowiak, 2020a). Some of these responses are in turn also associated with feedback to the biophysical components of ecosystem assessments due to, for example, changes in target species, spatial and temporal distribution of fishing effort, gear types and bycatch. Other adaptive choices may be evidenced in shifting personal demographics, such as geographic relocation/residency, changes in employment status, or socioeconomic status due to declining fishing revenues (Murray et al., 2010; Lord, 2011).
Modeling Cumulative Effects
The ultimate step in the conceptual framework is to identify the totality of the effects of the dynamics assessed in each iteration of the process on the well-being of the individuals under consideration. This necessitates evaluating well-being outcomes as the cumulative impacts of adaptive responses and their effects on demographics, fishing portfolios, and well-being priorities, which is captured in element 4 in Figure 1, cumulative model.
Tradeoffs in well-being components associated with adaptive choices imply a reduction in some components in order to maintain others. For example, researchers have documented the potential strain on individuals from some adaptive behaviors intended to maintain fishing revenues, including extended time out at sea, pluriactivity, fishing further or in unknown fishing grounds (Coulthard and Britton, 2015; Szymkowiak, 2020a). These behaviors can alter various well-being components by, for instance, increasing risky behavior, undermining personal or family identities, and straining family relationships to the point of dissolution (ibid.). In other cases, a prioritization of family time and risk avoidance can result in an individual shifting some of their labor into onshore employment rather than increasing fishing effort that in turn may imply a tradeoff in other uses of their time and a renegotiation of their occupational identity (Marks, 2012; Coulthard and Britton, 2015).
As individuals adapt and experience the well-being effects of their strategies they will respond with further adaptations, which is represented with a two-sided arrow between adaptations and cumulative impacts. In essence adaptation is an iterative and responsive process that will integrate learning, as well as well-being effects and prioritization. By capturing the iterative adaptation process and feedback with well-being effects, the framework inherently encapsulates cumulative well-being effects. Given the often sequential nature of perturbations that fishing participants are responding to, cumulative impacts can also be conceptualized as changes to baseline conditions inclusive of changes in the availability of adaptive strategies – represented in Figure 1 with an arrow from cumulative effects back to fisheries participation. For example, decreasing opportunities for fisheries diversification associated with the institution of limited entry and catch share programs have shifted adaptive responses in some fisheries toward pluriactivity, altered succession, and increasing value strategies like direct marketing and value added products (Murray et al., 2010; Stoll et al., 2016; Szymkowiak, 2020a). In the conceptual framework such systemic impacts of previous perturbations (in this case, management changes) would imply a new equilibrium state upon which the effects of the next perturbation would be modeled.
Data Collection Framework
The intent of developing this conceptual framework is also to identify the types of data that are needed to systematically inform an understanding of how a perturbation will reverberate throughout a fisheries system. These effects are mapped both in terms of multifaceted impacts on human dimensions as well as on biophysical components, including through changes in fishing behavior that can affect target species, area, and bycatch. The data collection framework necessitates gathering information relevant to each of the elements of the conceptual framework at the individual level to capture the heterogeneity of human well-being derived from fisheries, of adaptations, and of well-being effects of adaptive behaviors. Table 3 details data components and example elements relevant to each of the steps of the conceptual framework in Figure 1.
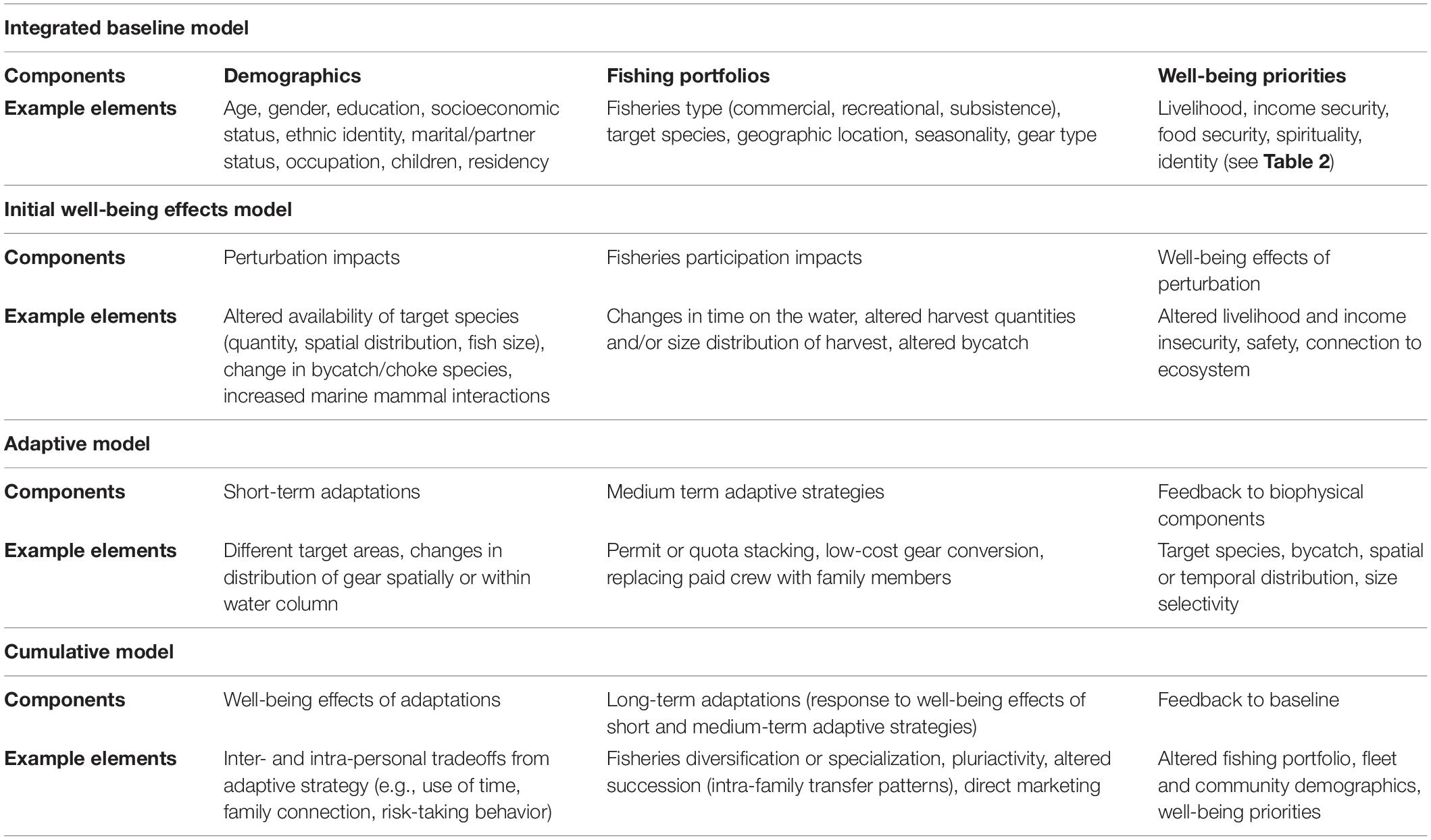
Table 3. Components and example elements of data needed for the human dimensions conceptual framework.
The integrated baseline model components include data about individual demographics, fishing portfolios, and well-being priorities, as they affect baseline fisheries participation levels and dynamics. Researchers should collect information relative to how individual demographics like age and gender affect the type of fisheries people participate in (e.g., commercial, recreational, or subsistence) and their target species, and in turn the types of well-being that they prioritize and associate with their fisheries (e.g., livelihood or food security). These elements together inform how fisheries participation manifests itself across fisheries, fleets, and fishing communities.
For the initial well-being effects model, data is gathered about the perturbation impacts, which are mapped to fisheries participation impacts and the resultant initial effects on well-being. For example, changes to the size composition of the sablefish stock in Alaska have led to increasing time on the water for the directed fleet to harvest its quotas, decreasing livelihood benefits from this fishery. While these impacts are generally conceptualized at an aggregated fleet-wide level, for the purposes of this conceptual framework, researchers should gather information on individualized well-being impacts from the perturbation relative to demographics, fisheries portfolios and well-being impacts. For example, fishermen’s age could affect how decreasing dockside prices associated with small fish ultimately impact livelihood, because in this fishery there are intergenerational differences in terms of whether fishermen own their quotas outright or are paying off loans for them (Ringer et al., 2018). Whereas lower dockside prices would imply decreased earnings for both, the implications for ultimate livelihood would presumably be more adverse for those who have to use some of their revenue to make loan payments.
For the adaptive model, data components include short and medium-term adaptations and their linkages to biophysical model components. Continuing with the Alaska sablefish example, the preponderance of small fish has caused the directed commercial fleet to harvest in deeper waters to target larger fish, increasing their rockfish bycatch; however, because this tactic has not fully resolved harvests of small fish individuals are also employing cost sharing strategies like aggregating quota from multiple shareholders on vessels (Szymkowiak and Rhodes-Reese, 2020). As with the previous elements, the adaptive model similarly requires data gathering to link individual demographics, fishing portfolios, and well-being priorities to adaptive behaviors. For example, married fishermen or those with life partners may be able to engage their partner to substitute a paid crewmember to reduce fishing costs and that choice would be informed by not just their desire to minimize adverse livelihood impacts but may also reflect other well-being priorities like family connection.
The choice of adaptations manifest in well-being impacts that are captured in the cumulative model. The cumulative model includes data components specific to the well-being effects of adaptations and how those effects than guide further, longer-term adaptive behaviors that ultimately compound well-being impacts and result in a changed baseline model. For example, the adaptation of using a family member in lieu of a paid crewmember in response to rising fishing costs implies tradeoffs in other uses of that family member’s time (e.g., paid shoreside work, leisure, child care), which may not be suitable in the long-run. Thus fishermen will employ further adaptive behaviors to mitigate such tradeoffs, for example investing in the capacity to direct market their fish to receive a higher price or diversifying their fishing portfolios. Information needs to be gathered about both these initial adaptive well-being effects and longer-term adaptive behaviors in order to understand how they may compound well-being effects and alter baseline fishing participation. Furthermore, similar to the data collection for the other elements, information for the components of cumulative impacts (well-being effects of adaptations, long-term adaptations, and feedback to baseline needs) needs to be collected relative to demographics, fishing portfolios, and well-being priorities. Direct marketing is a strategy that may more readily be applied by young people who have social media savvy while older individuals diversify fishing portfolios (Szymkowiak, 2020a), differences that may ultimately manifest themselves in intergenerational differences in fisheries participation (Ringer et al., 2018).
Integrating Human Dimensions Data Into Ecosystem Assessments
The data components detailed in Section “Data Collection Framework” are generally not available for fisheries systems across the world, with highly limited information even on the baseline components such as the demographics and fishing portfolios of individuals participating in fisheries (Kleiber et al., 2015; Szymkowiak, 2020b). Therefore, a comprehensive operationalization of the conceptual framework outlined in Figure 1 and a thorough and holistic understanding of how a perturbation courses through a fisheries system necessitates the data components described above. The level of detail provided by this data collection scheme affords modelers the latitude to incorporate human dimensions information with precision, accuracy, and spatial variability similar to what is available for some biophysical marine data. In the absence of a comprehensive data collection, existent information sources could be used to provide some information relative to the data components outlined in the conceptual framework. For example, meta-analysis of the literature about human responses to fisheries perturbations could provide a range of potential adaptations and the uncertainties around them, as could examination of historical harvest and permit data, although the latter would be limited to fisheries-specific adaptations. However, only the comprehensive data collection outlined above would provide the individual-level information required to couple with the biophysical dynamics in ecosystem assessments and develop robust analyses of perturbation impacts or management options.
Researchers will need to explore the most effective mechanisms for coupling the human dimensions presented in this paper and ecological models given their research questions; two such approaches are explored herein. Current end-to-end marine fisheries ecosystem models link human dimensions to ecological models through the use of dynamic fishing fleet sub-models that are driven largely by fish prices and catches (Haynie and Pfeiffer, 2013; Audzijonyte et al., 2019; Holsman et al., 2019). Fleets may be determined on the basis of fishing portfolios or catch composition, often in terms of some threshold for the majority of their landings or clustering algorithms (Yletyinen et al., 2018; Kroetz et al., 2019). The integration of the individual-level data generated from the conceptual framework described in Figure 1 to these dynamic fleet models can be thought of as developing probabilistic relationships of adaptive behavior based on the demographics of individual participants within those fleets. The most highly probable adaptive strategies based on choice models of individual behavior could then be included as scenarios in these end-to-end models (Holsman et al., 2020). Cumulative impacts could then be captured through iterative adaptation processes that reflect the probability of adaptive choices based on fleet demographics. What is lost in this process is a nuanced understanding of how well-being priorities and impacts are driving those choices, which are captured only implicitly by observing their probabilities. Furthermore, because these dynamic fleet models largely focus on economic outcomes, other cumulative well-being impacts would also not be readily captured except in instances wherein other well-being components could be linked to fishing behavior that is captured in these models [e.g., examining safety outcomes associated with spatial fleet distribution or other fishing effort metrics like days-at-sea (Fulton et al., 2018)].
An alternative to attempting to integrate complex human dimensions within end-to-end models may be to develop conceptual models of fully integrated systems that use the relationships established between variables in Figure 1 to build fuzzy cognitive maps (FCMs). FCMs are conceptual models where the linkages between components have strengths, in addition to directionality and sign (positive or negative). The strengths of the relationships are most often the degree to which the two concepts are related although it can also convey the certainty of the relationship (Carley and Palmquist, 1992; Özesmi and Özesmi, 2004). FCMs are most often developed in a participatory context wherein stakeholders can establish relationships between variables and provide weights for them that are then aggregated into social cognitive maps in a number of possible ways including adding, or using the medians or means of strengths (Özesmi and Özesmi, 2004). Similarly to QNMs, FCMs can be perturbed with an increase in any given component(s), simulating a shock to the system (Özesmi and Özesmi, 2004). Such a perturbation would imply well-being outcomes and ensuing adaptive behavior, translating elements 2 and 3 in Figure 1 into components and linkages within a FCM, which has been explored in other contexts examining disaster impacts on socioecological systems (Furman et al., 2021). Because FCMs do not readily capture temporal dynamics, multiple models exploring iterative adaptation processes and well-being outcomes would likely be necessary to examine cumulative impacts. The nature of how FCMs are developed with stakeholder engagement provides numerous benefits in terms of buy-in, comprehensibility, and capacity to capture diverse forms of knowledge and linkages, with increasing research exploring methods for testing, calibrating, and validating FCMs (Jetter and Kok, 2014; Alibage et al., 2012).
The key linkages between the ecosystem and human dimensions components of ecosystem assessments are how perturbations reverberate through the system to ecological components that humans interact with or rely upon, such as target commercial, recreational, or subsistence fish species. The nexus in end-to-end models is then how harvests change in response to variables like abundance, spatial distribution, or distribution within the water column (Haynie and Pfeiffer, 2013). In FCMs these same variables may be conceptualized as affecting other well-being components such as increasing gear conflicts between different fleets and safety implications. The issue with including the varied human dimensions within both of these modeling frameworks is in maintaining model stability or convergence with the addition of numerous parameters. To the extent that ecological and human dimensions can be decoupled in socioecological system models to examine human responses and well-being impacts doing so could provide for the inclusion of more human dimensions variables. Such a separation could be possible if, for instance, probabilistic relationships can be developed between a set of perturbations that have similar ecological impacts and their human dimensions outcomes. However, it should be noted that ethnographic studies often inherently include all of the human dimensions described in this study, providing rich contextual information describing human connections to their ecosystem, how people make choices about responding to system perturbations, and how those choices in turn affect their well-being. The necessity of the data collection framework described herein rests on the assumption that there are often multiple constraints to conducting such studies across various spatial scales. Furthermore, the length of time needed to conduct ethnographic studies may not be well matched to ecosystem assessments which increasingly focus on understanding probabilistic human responses to quickly evolving ecological changes in order to facilitate adaptive management.
Ecosystem assessments have largely relied on homogeneous assumptions about the benefits that people derive from fisheries and their adaptations to fisheries perturbations. Such assumptions belie the differentiated values that individuals associate with fisheries and the ways in which they will therefore respond to disturbances. In turn, the outputs of these assessments may misrepresent the value systems of groups of individuals, subjugating them to those of the broader population. This is coupled with the systematic ignorance of cumulative effects within ecosystem assessments, which mostly examine impacts occurring within a vacuum of a singular perturbation. Yet fisheries participants are often responding to multiple stimuli that may be occurring simultaneously or asynchronously with effects that take on a number of different relationships and which may, in turn, limit their adaptive choices and capacities. Although such decreased system resilience may be inferred from the ways in which individuals choose to adapt, there is an implicit danger in assuming this reduced capacity is exogenous rather than an outcome of repeated perturbations.
The conceptual framework developed in this study serves to systematically address these human dimensions gaps in ecosystem assessments and frame a more comprehensive understanding of not just the effects of perturbations on people but on the biophysical components of ecosystems as well. The associated data collection scheme details a mechanism for operationalization, linking individual characteristics to behavioral choices and well-being outcomes. While ecosystem modelers struggle to balance capturing system complexity with model stability and interpretability, the simplification of these frameworks should not be at the cost of meaningfully integrating human dimensions. The alternative is potentially developing assessments that misrepresent human interactions with ecosystems and thus undermine their own utility in informing management.
Data Availability Statement
The original contributions presented in the study are included in the article/supplementary material, further inquiries can be directed to the corresponding author.
Author Contributions
MS devised the project and its main conceptual ideas, conducted the literature review, developed the conceptual framework and data collection scheme, developed the manuscript, revised the manuscript, and read and approved the submitted version.
Conflict of Interest
The author declares that the research was conducted in the absence of any commercial or financial relationships that could be construed as a potential conflict of interest.
Acknowledgments
The author would like to thank Sarah Gaichas, Stephen Kasperski, and the reviewers of this journal for their thoughtful comments on this work. The views and opinions expressed in this manuscript are the authors’ own, and do not necessarily reflect those of the National Oceanic and Atmospheric Administration or the U.S. Department of Commerce.
References
Abbott, J. K., and Haynie, A. C. (2012). What are we protecting? fisher behavior and the unintended consequences of spatial closures as a fishery management tool. Ecol. Appl. 22, 762–777. doi: 10.1890/11-1319.1
Addicott, E. T., Kroetz, K., Reimer, M. N., Sanchirico, J. N., Lew, D. K., and Hutteman, J. (2018). Identifying the potential for cross-fishery spillovers: a network analysis of Alaskan permitting patterns. Can. J. Fish. Aquatic Sci. 76, 56–68. doi: 10.1139/cjfas-2017-0550
Alibage, A., Jetter, A. J., Aminpour, P., Gray, S. A., ScAbbott, J. K., and Haynie, A. C. (2012). What are we protecting? fisher behavior and the unintended consequences of spatial closures as a fishery management tool. Ecol. Appl. 22, 762–777. doi: 10.1890/11-1319.1
Allison, E. H., Kurien, J., Ota, Y., Adhuri, D. S., Bavinck, J. B., Cisneros-Montemayor, A. M., et al. (2020). The Human Relationship with Our Ocean Planet. Washington, DC: World Resources Institute.
Ariely, D. (2009). Predictably Irrational, Revised and Expanded Edition: the Hidden Forces That Shape Our Decisions. New York, NY: HarperCollins Publishers.
Audzijonyte, A., Pethybridge, H., Porobic, J., Gorton, R., Kaplan, I., and Fulton, E. A. (2019). AtlAntis: a spatially explicit end-to-end marine ecosystem model with dynamically integrated physics, ecology and socio-economic modules. Methods Ecol. Evol. 10, 1814–1819. doi: 10.1111/2041-210x.13272
Barbeaux, S., Aydin, K., Fissel, B., Holsman, K. K., Laurel, B., Palsson, W., et al. (2019). Assessment of the Pacific cod stock in the Gulf of Alaska. Anchorage, AK: North Pacific Fishery Management Council.
Becker, G. (1976). The Economic Approach to Human Behavior. Chicago, IL: The University of Chicago Press.
Beratan, K. (2007). A cognition-based view of decision processes in complex social–ecological systems. Ecol. Soc. 12:27.
Biedenweg, K. (2017). A comparative study of human well-being indicators across three puget sound regions. Reg. Soc. Nat. Resources 30, 362–376. doi: 10.1080/08941920.2016.1209606
Biedenweg, K., Hanein, A., Nelson, K., Stiles, K., Wellman, K., Horowitz, J., et al. (2014). Developing human wellbeing indicators in the Puget Sound: focusing on the watershed scale. Coastal. Manag. 42, 374–390. doi: 10.1080/08920753.2014.923136
Bowen, R. E., and Riley, C. (2003). Socio-economic indicators and integrated coastal management. Ocean Coastal Manag. 46, 299–312. doi: 10.1016/s0964-5691(03)00008-5
Breslow, S. J., Sojka, B., Barnea, R., Basurto, X., Carothers, C., Charnley, S., et al. (2016). Conceptualizing and operationalizing human wellbeing for ecosystem assessment and management. Environ. Sci. Pol. 66, 250–259. doi: 10.1016/j.envsci.2016.06.023
Britton, E. (2012). Women as agents of wellbeing in Northern Ireland’s fishing households. Maritime Stud. 11:16.
Burgess, M. G., Carrella, E., Drexler, M., Axtell, R. L., Bailey, R. M., Watson, J. R., et al. (2018). Opportunities for agent-based modeling in fisheries social science. SocArXiv [preprint]. doi: 10.31235/osf.io/gzhm5
Carley, K., and Palmquist, M. (1992). Extracting, representing, and analyzing mental models. Soc. Forces 70, 601–636. doi: 10.1093/sf/70.3.601
Cinner, J. E., Daw, T., and McClanahan, T. R. (2009). Socioeconomic factors that affect artisanal fishers’ readiness to exit a declining fishery. Conserv. Biol. 23, 124–130. doi: 10.1111/j.1523-1739.2008.01041.x
Cinti, A., Shaw, W., Cudney-Bueno, R., and Rojo, M. (2010). The unintended consequences of formal fisheries policies: social disparities and resource overuse in a major fishing community in the Gulf of California. Mexico. Mar. Pol. 34, 328–339. doi: 10.1016/j.marpol.2009.08.002
Cline, T. J., Schindler, D. E., and Hilborn, R. (2017). Fisheries portfolio diversification and turnover buffer Alaskan fishing communities from abrupt resource and market changes. Nat. Commun. 8:14042. doi: 10.1038/ncomms14042
Coulthard, S. (2012). Can we be both resilient and well, and what choices do people have? Incorporating agency into the resilience debate from a fisheries perspective. Ecol. Soc. 17:4.
Coulthard, S., and Britton, E. (2015). Waving or drowning: an exploration of adaptive strategies amongst fishing households and implications for wellbeing outcomes. Sociol. Ruralis 55, 275–290. doi: 10.1111/soru.12093
Crain, C. M., Kroeker, K., and Halpern, B. S. (2008). Interactive and cumulative effects of multiple human stressors in marine systems. Ecol. Lett. 11, 1304–1315. doi: 10.1111/j.1461-0248.2008.01253
Crenshaw, K. (1989). Demarginalizing the Intersection of Race and Sex: A Black Feminist Critique of Antidiscrimination Doctrine, Feminist Theory and Antiracist Politics. Chicago, IL: University of Chicago Legal Forum, 139–168.
Dambacher, J. M., Gaughan, D. J., Rochet, M.-J., Rossignol, P. A., and Trenkel, V. M. (2009). Qualitative modelling and indicators of exploited ecosystems. Fish Fish. 10, 305–322. doi: 10.1111/j.1467-2979.2008.00323.x
Daw, T. I. M., Brown, K., Rosendo, S., and Pomeroy, R. (2011). Applying the ecosystem services concept to poverty alleviation: the need to disaggregate human well-being. Environ. Conserv. 38, 370–379. doi: 10.1017/s0376892911000506
DePiper, G. S., Gaichas, S. K., Lucey, S. M., Pinto, da Silva, P., Anderson, M. R., et al. (2017). Operationalizing integrated ecosystem assessments within a multidisciplinary team: lessons learned from a worked example. ICES J. Mar. Sci. 74, 2076–2086. doi: 10.1093/icesjms/fsx038
Díaz, S., Pascual, U., Stenseke, M., Martín-López, B., Watson, R. T., Molnár, Z., et al. (2018). Assessing nature’s contributions to people. Science 359, 270–272.
Durrenberger, E. P. (1994). Shrimpers, processors, and common property in Mississippi. Hum. Organ. 53:74. doi: 10.17730/humo.53.1.2736220061197n06
Field, L. (2008). Abalone Tales: Collaborative Explorations of Sovereignty and Identity in Native California. Durham, NC: Duke University Press Books.
Filatova, T., Verburg, P. H., Parker, D. C., and Stannard, C. A. (2013). Spatial agent-based models for socio-ecological systems: challenges and prospects. Environ. Modell. Software 45, 1–7. doi: 10.1016/j.envsoft.2013.03.017
Fulton, E. A., Punt, A. E., Dichmont, C. M., Harvey, C. J., and Gorton, R. (2018). Ecosystems say good management pays off. Fish Fish. 20, 66–96. doi: 10.1111/faf.12324
Fulton, E. A., Smith, A. D., Smith, D. C., and Johnson, P. (2014). An integrated approach is needed for ecosystem based fisheries management: insights from ecosystem-level management strategy evaluation. PLoS One 9:e84242. doi: 10.1371/journal.pone.0084242
Furman, K. L., Payam, A., Gray, S. A., and Scyphers, S. B. (2021). Mental models for assessing coastal social-ecological systems following disasters. Mar. Pol. 125:104334. doi: 10.1016/j.marpol.2020.104334
Gaichas, S. K., DePiper, G. S., Seagraves, R. J., Muffley, B. W., Sabo, M., Colburn, L. L., et al. (2018). Implementing ecosystem approaches to fishery management: risk assessment in the US Mid-Atlantic. Front. Mar. Sci. 5:442. doi: 10.3389/fmars.2018.00442
Hall-Arber, M., Pomeroy, C., and Conway, F. (2009). Figuring out the human dimensions of fisheries: illuminating models. Mar. Coastal Fish. Dynam. Manag. Ecosystem Sci. 1, 300–314. doi: 10.1577/C09-006.1
Halpern, B. S., Walbridge, S., Selkoe, K. A., Kappel, C. V., Micheli, F., D’Agrosa, C., et al. (2008). A global map of human impact on marine ecosystems. Science 319, 948–952.
Hanselman, D., Rodgveller, C., Fenske, K. H., Shotwell, K., Echave, K. B., Malecha, P. W., et al. (2019). “Assessment of the sablefish stock of Alaska,” in Stock Assessment and Fishery Evaluation Report for the Groundfish Fisheries of the Gulf of Alaska and Bering Sea/Aleutian Islands Area, eds B. E. Fissel, M. Dalton, and R. G. Felthoven (Anchorage, AK: North Pacific Fishery Management Council).
Harvey, C. J., Reum, J. C. P., Poe, M. R., Williams, G. D., and Kim, S. J. (2016). Using conceptual models and qualitative network models to advance integrative assessments of marine ecosystems. Coastal Manag. 44, 486–503. doi: 10.1080/08920753.2016.1208881
Haynie, A. C., and Pfeiffer, L. (2013). Climatic and economic drivers of the Bering Sea walleye pollock (Theragra chalcogramma) fishery: implications for the future. Can. J. Fish. Aquatic Sci. 70. 6, 841–853. doi: 10.1139/cjfas-2012-0265
Hobday, A. J., Smith, A. D. M., Stobutzki, I. C., Bulman, C., Daley, R., Dambacher, J. M., et al. (2011). Ecological risk assessment for the effects of fishing. Fish. Res. 108, 372–384.
Holland, D. S., Abbott, J. K., and Norman, K. E. (2019). Fishing to live or living to fish: job satisfaction and identity of west coast fishermen. Ambio 49, 628–639. doi: 10.1007/s13280-019-01206-w
Holland, D. S., and Kasperski, S. (2016). The impact of access restrictions on fishery income diversification of us west coast fishermen. Coastal Manag. 44, 452–463. doi: 10.1080/08920753.2016.1208883
Holsman, K. K., Elliott, L. H., Alan, H., Sophie, G., Anne, H., Bograd, S. J., et al. (2019). Towards climate resiliency in fisheries management. ICES J. Mar. Sci. 76, 1368–1378.
Holsman, K. K., Haynie, A. C., Hollowed, A. B., Reum, J. C. P., Aydin, K., Hermann, A. J., et al. (2020). Ecosystem-based fisheries management forestalls climate-driven collapse. Nat. Commun. 11:4579. doi: 10.1038/s41467-020-18300-3
Hunt, L., Kushneriuk, R., and Lester, N. (2007). Linking agent-based and choice models to study outdoor recreation behaviours: a case of the landscape fisheries model in Northern Ontario. Can. For. Snow Landsc. Res. 81, 163–174.
International Council for the Exploration of the Sea [ICES] (2017). Interim Report of the ICES/PICES/PAME Working Group on Integrated Ecosystem Assessment (IEA) for the Central Arctic Ocean. Seattle, DC: Steering group on Integrated Ecosystem Assessments ICESCM.
Jetter, A. J., and Kok, K. (2014). Fuzzy cognitive maps for futures studies—a methodological assessment of concepts and methods. Futures 61, 45–57. doi: 10.1016/j.futures.2014.05.002
Kawarazuka, N., Locke, C., McDougall, C., Kantor, P., and Morgan, M. (2017). Bringing analysis of gender and social–ecological resilience together in small-scale fisheries research: challenges and opportunities. Ambio 46, 201–213. doi: 10.1007/s13280-016-0814-5
Kelble, C. R., Loomis, D. K., Lovelace, S., Nuttle, W. K., Ortner, P. B., Fletcher, P., et al. (2013). The EBM-DPSER conceptual model: integrating ecosystem services into the DPSIR framework. PLoS One 8:e70766. doi: 10.1371/journal.pone.0070766
Kittinger, J., Finkbeiner, E., Glazier, E., and Crowder, L. (2012). Human dimensions of coral reef social-ecological systems. Ecol. Soc. 17:17.
Kleiber, D., Harris, L. M., and Vincent, A. C. J. (2015). Gender and small-scale fisheries: a case for counting women and beyond. Fish Fish. 16, 547–562. doi: 10.1111/faf.12075
Kroetz, K., Reimer, M. N., Sanchirico, J. N., Lew, D. K., and Huetteman, J. (2019). Defining the economic scope for ecosystem-based fishery management. Proc. Natl. Acad. Sci. U S A. 116, 4188–4193. doi: 10.1073/pnas.1816545116
Leong, K. M., Wongbusarakum, S., Ingram, R. J., Mawyer, A., and Poe, M. (2019). Improving representation of human well-being and cultural importance in conceptualizing the West Hawai’i ecosystem. Front. Mar. Sci. 6:231. doi: 10.3389/fmars.2019.00231
Levin, P. S., Breslow, S. J., Harvey, C. J., Norman, K. C., Poe, M. R., Williams, G. D., et al. (2016). Conceptualization of social-ecological systems of the California current: an examination of interdisciplinary science supporting ecosystem-based management. Coastal Manag. 44, 397–408. doi: 10.1080/08920753.2016.1208036
Levin, P. S., Fogarty, M. J., Matlock, G. C., and Ernst, M. (2008). Integrated ecosystem assessments. developing the scientific basis for ecosystem-based management of the Ocean. PLoS Biol. 7:e1000014. doi: 10.1371/journal.pbio.1000014
Levin, P. S., Fogarty, M. J., Murawski, S. A., and Fluharty, D. (2009). Integrated ecosystem assessments: developing the scientific basis for ecosystem-based management of the ocean. PLoS Biol. 7:e14. doi: 10.1371/journal.pbio.1000014
Link, J. S., and Browman, H. I. (2014). Integrating what? Levels of marine ecosystem-based assessment and management. ICES J. Mar. Sci. 71, 1170–1173. doi: 10.1093/icesjms/fsu026
Lord, F. (2011). Understanding social impacts by using new variables and a causal model diagram in New England fisheries. Impact Assess. Project Appraisal 29, 59–68. doi: 10.3152/146155111X12913679730476
Lord, F., Tuler, S., Webler, T., and Dow, K. (2009). A Case Study Into the Human Dimensions of the Bouchard-120 Oil Spill in Buzzards Bay. Massachusetts, MA: Greenfield.
Marks, B. (2012). The political economy of household commodity production in the Louisiana shrimp fishery. J. Agrarian Change 12, 227–251. doi: 10.1111/j.1471-0366.2011.00353.x
McCay, B. (2004). ITQs and community: an essay on environmental governance. Rev. Agricul. Resource Econ. 33, 162–170. doi: 10.1017/s1068280500005748
McGinnis, M. D., and Ostrom, E. (2014). Social-ecological system framework: initial changes and continuing challenges. Ecol. Soc. 19:30. doi: 10.5751/ES-06387-190230
Melbourne-Thomas, J., Wotherspoon, S., Raymond, B., and Constable, A. (2012). Comprehensive evaluation of model uncertainty in qualitative network analyses. Ecol. Monographs 82, 505–519. doi: 10.1890/12-0207.1
Milner-Gulland, E. J. (2011). Integrating fisheries approaches and household utility models for improved resource management. Proc. Natl. Acad. Sci. U S A. 108, 1741–1746. doi: 10.1073/pnas.1010533108
Murray, G., Johnson, T., McCay, B. J., Danko, M., St. Martin, K., and Takahashi, S. (2010). Creeping enclosure, cumulative effects and the marine commons of New Jersey. Int. J. Commons 4, 367–389. doi: 10.18352/ijc.148
Murray-Rust, D., Dendoncker, N., Dawson, T., Acosta, L., Karali, E., Guillem, E., et al. (2011). Conceptualising the analysis of socio-ecological systems through ecosystem services and agent-based modeling. J. Land Use Sci. 6, 83–99. doi: 10.1080/1747423X.2011.558600
NPFMC (2019). Discussion Paper: Sablefish Discard Allowance. D2 Small Sablefish Discarding. Available online at: https://meetings.npfmc.org/CommentReview/DownloadFile?p=af8355e5-8e81-4165-b20e-2ce11cade94d.pdf&fileName=D2%20Small%20Sablefish%20Discarding%20Discussion%20Paper.pdf (accessed September 2020).
Ogden, J. C., Davis, S. M., Jacobs, K. J., Barnes, T., and Fling, H. E. (2005). The use of conceptual ecological models to guide ecosystem restoration in South Florida. Wetlands 25, 795–809. doi: 10.1672/0277-5212(2005)025[0795:tuocem]2.0.co;2
Okamoto, D. K., Poe, M. R., Francis, T. B., Punt, A. E., Levin, P. S., Shelton, A. O., et al. (2020). Attending to spatial social–ecological sensitivities to improve trade-off analysis in natural resource management. Fish Fish. 21, 1–12. doi: 10.1111/faf.12409
Ostrom, E. (2009). A general framework for analyzing sustainability of social-ecological systems. Science 325, 419–422. doi: 10.1126/science.1172133
Özesmi, U., and Özesmi, S. L. (2004). Ecological models based on people’s knowledge: a multi-step fuzzy cognitive mapping approach. Ecol. Modell. 176, 43–64. doi: 10.1016/j.ecolmodel.2003.10.027
Poe, M., Norman, K. C., and Levin, P. S. (2014). Cultural dimensions of socioecological systems: key connections and guiding principles for conservation in coastal environments. Conserv. Lett. 7, 166–175. doi: 10.1111/conl.12068
Pollnac, R. B., Abbott-Jamieson, S., Smith, S., Miller, M. L., Clay, P. M., and Oles, B. (2006). Toward a model for fisheries social impact assessment. Mar. Fish. Rev. 68, 1–18.
Pollnac, R. B., and Poggie, J. J. (2008). Happiness well-being and psycho-cultural adaptation to the stresses associated with marine fishing. Hum. Ecol. Rev. 15, 194–200.
Ringer, D., Carothers, C., Donkersloot, R., Coleman, J., and Cullenberg, P. (2018). For generations to come? the privatization paradigm and shifting social baselines in kodiak, Alaska’s commercial fisheries. Mar. Pol. 98, 97–103. doi: 10.1016/j.marpol.2018.09.009
Salas, S., and Gaertner, D. (2004). The behavioural dynamics of fishers: management implications. Fish Fish. 5, 153–167. doi: 10.1111/j.1467-2979.2004.00146.x
Salmi, P., and Sonck-Rautio, K. (2018). Invisible work, ignored knowledge? changing gender roles, division of labor, and household strategies in Finnish small-scale fisheries. Mar. Stud. 17, 213–221. doi: 10.1007/s40152-018-0104-x
Schumann, S., and Macinko, S. (2007). Subsistence in coastal fisheries policy: what’s in a word? Mar. Pol. 31, 706–718. doi: 10.1016/j.marpol.2006.12.010
Stoll, J. S., Beitl, C. M., and Wilson, J. A. (2016). How access to Maine’s fisheries has changed over a quarter century: the cumulative effects of licensing on resilience. Global Environ. Change 37, 79–91. doi: 10.1016/j.gloenvcha.2016.01.005
Szymkowiak, M. (2020a). Adaptations and well-being: Gulf of Alaska fishing families in a changing landscape. Ocean Coastal Manag. 197:105321. doi: 10.1016/j.ocecoaman.2020.105321
Szymkowiak, M. (2020b). Genderizing fisheries: assessing over thirty years of women’s participation in Alaska fisheries. Mar. Pol. 15:103846. doi: 10.1016/j.marpol.2020.103846
Szymkowiak, M., and Kasperski, S. (2020). Sustaining an Alaska coastal community: integrating place based well-being indicators and fisheries participation. Coastal Manag. 49, 107–131. doi: 10.1080/08920753.2021.1846165
Szymkowiak, M., and Rhodes-Reese, M. (2020). Adaptive behaviors to marine ecosystem shifts: examining fishermen’s strategies in response to abundant juvenile sablefish in Alaska. Front. Mar. Sci. 7:602281. doi: 10.3389/fmars.2020.602281
Thornton, T. F., and Kitka, H. (2015). An indigenous model of a contested pacific herring fishery in Sitka. Alaska. Int. J. Appl. Geospatial Res. 6, 94–117. doi: 10.4018/ijagr.2015010106
Tuler, S., Agyeman, J., Pinto, da Silva, P., LoRusso, K. R., and Kay, R. (2008). Assessing vulnerabilities: integrating information about driving forces that affect risks and resilience in fisheries communities. Hum. Ecol. Rev. 15, 171–184. doi: 10.1002/9780470995693.ch10
van Putten, I. E., Gorton, R. J., Fulton, E. A., and Thebaud, O. (2013). The role of behavioural flexibility in a whole of ecosystem model. ICES J. Mar. Sci. 70, 150–163. doi: 10.1093/icesjms/fss175
Wildermuth, R. P., Fay, G., and Gaichas, S. (2017). Structural uncertainty in qualitative models for ecosystem-based management of Georges Bank. Can. J. Fish. Aquatic Sci. 75, 1635–1643. doi: 10.1139/cjfas-2017-0149
Yletyinen, J., Hentati-Sundberg, J., Blenckner, T., and Bodin, Ö (2018). Fishing strategy diversification and fishers’ ecological dependency. Ecol. Soc. 23:28.
Keywords: socio-ecologic systems, integrated ecosystem assessments, ecosystem based fisheries management (EBFM), human dimensions, well-being, adaptation, cumulative impact
Citation: Szymkowiak M (2021) A Conceptual Framework for Incorporating Human Dimensions Into Integrated Ecosystem Assessments. Front. Mar. Sci. 8:617054. doi: 10.3389/fmars.2021.617054
Received: 13 October 2020; Accepted: 10 March 2021;
Published: 31 March 2021.
Edited by:
M. Veronica Morales-Zarate, Centro de Investigación Biológica del Noroeste (CIBNOR), MexicoReviewed by:
Andrés M. Cisneros-Montemayor, University of British Columbia, CanadaOscar Sosa-Nishizaki, Center for Scientific Research and Higher Education in Ensenada (CICESE), Mexico
Copyright © 2021 Szymkowiak. This is an open-access article distributed under the terms of the Creative Commons Attribution License (CC BY). The use, distribution or reproduction in other forums is permitted, provided the original author(s) and the copyright owner(s) are credited and that the original publication in this journal is cited, in accordance with accepted academic practice. No use, distribution or reproduction is permitted which does not comply with these terms.
*Correspondence: Marysia Szymkowiak, bWFyeXNpYS5zenlta293aWFrQG5vYWEuZ292