- 1Ocean and Ecosystem Sciences Division, Fisheries and Oceans Canada, Dartmouth, NS, Canada
- 2Department of Biology, Dalhousie University, Halifax, NS, Canada
The United Nations Convention on Biological Diversity was established in 1993. Canada is a signatory nation that has adopted, and exceeded, the UN Aichi biodiversity target to protect 10% of coastal and marine areas through marine protected areas or “other effective area-based conservation measures” (OECMs) by 2020. However, the science of OECMs as contributors to biodiversity conservation is relatively young and their definition and efficacy testing continue to evolve. Here, we examine whether areas closed to fishing on the Scotian Shelf in Atlantic Canada, where the groundfish community had collapsed in the early 1990s, have the potential to serve as OECMs for groundfish recovery. Using long-term research survey data, we show that three long-term area-based fishing fleet closures did not enhance per capita population growth rates of the majority of 24 common groundfish species. At a regional scale, 10 out of 24 species are currently at less than 50% of their pre-collapse (1979–1992) biomass, reflecting a sustained diminished productivity, even though fishing mortality has been drastically reduced through a moratorium in 1993. Additional measures are needed to protect severely depleted groundfish, especially when the causes of continued diminished productivity are still largely unresolved. The importance of OECMs as a risk-averse approach toward sustainability is globally accepted and they can be considered a tool toward the overarching UN Sustainable Development Goals (SDG-14). Our study provides further impetus toward articulating the criteria of OECMs and improving their design, monitoring, and testing, while placing OECMs within the broader context of sustainable ecosystem-based management.
Introduction
The United Nations (UN) Convention on Biological Diversity (CBD) was established in 1993 to promote the conservation of biodiversity, sustainable use of resources and equitable access to those resources1. The UN declared 2011–2020 the decade of Biodiversity prompting the 196 signatory parties toward specific targets by the end of the decade. Canada is a signatory nation, and committed to the UN Aichi Biodiversity Target 11 of the Strategic Plan for Biodiversity, 2011–2020 to protect 10% of coastal and marine areas through marine protected areas (MPAs) or ‘‘other effective area-based conservation measures’’ (OECMs) by 20202. To date, Canada has protected 13.81% of its national waters and plans to protect 30% by 2030 through the establishment of MPAs and OECMs3. OECMs have the potential to contribute toward the larger UN 2030 Agenda that has set 17 Sustainable Development Goals (SDGs)4. Specifically, OECMs can help to operationalize the ocean-related UN SDG-14 (Diz et al., 2018). However, the science of OECMs as contributors to biodiversity conservation is relatively young; their definition and effectiveness in terms of reaching conservation benefits has rarely been tested.
In a fisheries context, area-based closures can restrict fishing activities through limiting certain gear, seasons, or target species and have been routinely used as part of fisheries management. Thus, these areas may be considered as OECMs and count toward Aichi targets. Management objectives can differ among different OECMs, but are generally designed to protect a certain life stage, species, or population. As we end the UN decade of Biodiversity, and move toward the UN 2030 Agenda, it is timely to consider how to ensure these area-based fisheries closures are useful to the broader goals of conservation of biodiversity and sustainable use of resources.
Closed areas exist within a dynamic environment, including ecological, environmental, and fishery regulation changes (Kerr et al., 2019). It is challenging to quantify their efficacy (Halpern et al., 2004; Ahmadia et al., 2015) especially when a directed monitoring strategy is not implemented (Halpern, 2003). The closure design process should clearly identify the goals, monitoring strategies, and the indicators required to ensure the goals are achieved, but these are often excluded from the process (Pomeroy et al., 2005; Gill et al., 2017). When appropriate monitoring is in place, closed areas can be successfully designed, and modified over time, to meet their objectives (e.g., Lipcius et al., 2003). In addition to regular monitoring, targeted studies can be used to test efficacy (e.g., Lambert et al., 2006). If the proper design planning is not performed there are a host of potential issues that could lead to an ineffective closure or a closure that may actually cause more harm than having done nothing; these issues are often compounded by a lack of resources (Agardy et al., 2011; Gill et al., 2017). It follows that similar challenges face the testing of potential OECMs, which may be especially difficult in regions that have experienced a collapse of the groundfish community (Petrie et al., 2009).
In Atlantic Canada, both foreign and domestic fleets had access to valuable groundfish species such as Atlantic cod (Gadus morhua) and haddock (Melanogrammus aeglefinus) until 1977 (Frank et al., 2000). With the implementation of Canada’s 200-mile Exclusive Economic Zone (EEZ) in 1977, foreign fleets were excluded from major fishing grounds. From 1978–1985, a period of recovery was noted for many species yet domestic fleets ramped up fishing effort from 1986–1993 (Rose, 2007). By 1992–1993, cod was over-fished throughout the Atlantic basin resulting in widespread cod fishing moratoria in the early 1990’s throughout most of the region5. Overfishing effects extended beyond cod. Most of the groundfish community on the eastern Scotian Shelf had collapsed by the early 1990s (Bundy, 2005; Frank et al., 2005). In the post-collapse era, from 1993 to the early 2000s, changes in the community structure became evident across the Scotian Shelf (Bundy, 2005; Shackell and Frank, 2007; Shackell et al., 2012b) including a transition to invertebrate-dominated fisheries (Frank et al., 2005; Anderson et al., 2008), a widespread decline in body size of groundfish (Fisher et al., 2010; Shackelll et al., 2010; Shackell et al., 2012a, b; Charbonneau et al., 2019), an increase in diversity of small-bodied fish in the absence of large predators (Ellingsen et al., 2015; Stortini et al., 2018), and an increase in beta diversity across the landscape (Shackell et al., 2012b; Ellingsen et al., 2015). These ecosystem changes have persisted (Bundy et al., 2019).
In this context, the objective of our study was to examine whether three relatively large long-term fleet closed areas across the Scotian Shelf have enabled some recovery and influenced population growth rates of 24 common groundfish species. These fleet closures have been in place for over 30 years with specific objectives related to the protection of groundfish stocks but without a dedicated monitoring strategy to assess their efficacy. As a result, they are not routinely tested for meeting their original objectives. As an example, one of the closures studied herein had an objective of reducing juvenile haddock mortality, but the area was closed only after an intense period of fishing exploitation and was not fully effective in meeting its objective (Frank et al., 2000). It is currently considered an OECM and may have had unintended benefits for other species beyond the original objectives (Fisher and Frank, 2002). For closures such as these, both positive and negative unintended consequences are commonplace (Murawski et al., 2000; Agardy et al., 2011; O’Keefe et al., 2014; Dureuil et al., 2018).
First, we provide a regional overview of species status on the Scotian Shelf scale. We then divide the shelf into three sub-regions corresponding to the locations of the three long-term closed areas, and compare per capita population growth rates within open and closed areas and over time. Our results show that these three area closures did not enhance population growth rates of the majority of 24 common groundfish species, most likely because of their sustained, largely unresolved, diminished productivity since the widespread community collapse in the early 1990s. The importance of OECMs as a risk-averse approach toward sustainability is widely accepted and our study provides further impetus toward articulating the criteria of OECMs and improving their design, monitoring, and testing (Fox et al., 2012), while placing OECMs within the broader context of sustainable ecosystem-based management. Without evaluation, it is difficult to determine whether/or how area-based closures could be improved as OECMs, and it is especially difficult if other ecosystem-based measures are not fully applied across the region.
Materials and Methods
Data
We used data collected from 1979 to 2017 by Fisheries and Oceans Canada (DFO) as part of the annual Summer Research Vessel (RV) trawl survey. The RV survey did not cover the entire Scotian Shelf in 2018, so the 2018/19 data were excluded from this study. The RV survey takes place across the Scotian Shelf region during two 2-week trips between July and August (Ricard and Shackell, 2013). The survey uses a Western IIA bottom trawl and stratified sampling. Strata are identified as areas of similar depth across the Scotian Shelf. The number of sets sampled is proportional to the area of the stratum (Shackell and Frank, 2007). Measurements of biomass/abundance for each species were standardized in the RV dataset to account for differences in tow duration and speed to be representative of 1.75 nautical miles of tow distance. Biomass/abundance data were corrected for length differences in catchability (“q”-corrected) (Dempsey et al., 2019).
All data processing and analyses were completed using R (R Core Team, 2016). We selected the most common (24) groundfish species (Table 1). Of these, redfish (Sebastes spp.) were not consistently identified to species level and therefore the group consists of the two species Sebastes fasciatus (Acadian redfish) and S. mentella (Beaked redfish). Twelve of these species have been assessed for their risk of extinction by the Committee on the Status of Wildlife in Canada (COSEWIC), an independent advisory body that provides advice for listing decisions under the Canadian Species at Risk Act SARA. Two of those were deemed not at risk. Only one species has been listed under SARA (Table 1).
Long-Term Area-Based Fleet Closures
Three long-term area-based fleet closures, and corresponding “open” areas, on the western, central and eastern Scotian shelf were selected for analysis (Figure 1 and Supplementary Table 1). These fleet closures are directed toward exclusion of the groundfish fleet and are open to other fishing fleets, such as pelagic longline and scallop dredging. The timing and duration of seasonal closures may have varied a little but generally, on the western shelf, Brown’s Bank has been seasonally closed from February 1st to June 15th to groundfish fishing since 1970/72 in order to protect haddock spawning aggregations (Halliday, 1988). On the central shelf, the “Haddock Box” has been closed to mobile bottom fishing gears since 1987 and to all groundfish fishing (including fixed gear) in 1993 in order to protect the banks as a spawning and nursery area for haddock. All other forms of fishing are allowed in the Haddock Box (Frank et al., 2000). On the eastern shelf, there is a seasonal closure from November 1st to April 30th for fixed gears, and January 1st to April 30th for mobile bottom gear. This closure has been in place since 1993 to reduce the exploitation of southern Gulf cod during migrations (Chouinard, 2000).
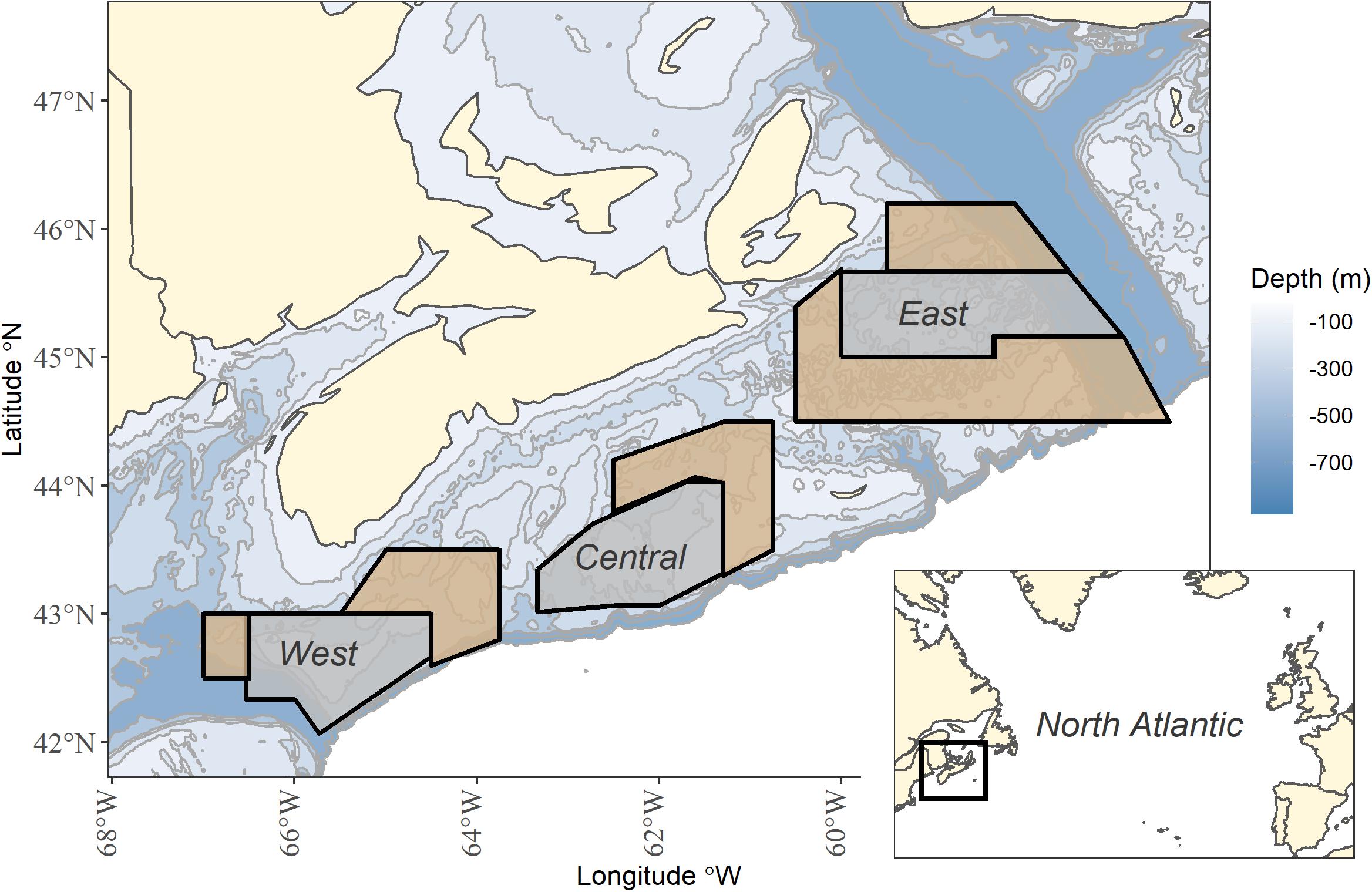
Figure 1. Long-term fleet closures (gray) and adjacent open areas (tan) in the west, central and east on the Scotian Shelf, Canada. Map inset shows study location within the North Atlantic.
Approach to Comparing Closed and Open Areas
Given the ecological changes due to the collapse of the groundfish community, we divided the time series into three periods: pre-collapse period (1979–1992), post-collapse (1993–2005) and recent (2006–2017). First, we provide a regional overview of species status during post-collapse and recent periods, relative to the pre-collapse period. Then we sub-divide the shelf into sub-regions, west, central and east, each of which contains a fleet area closure. These sub-regions generally correspond to oceanographic thermal zones, ranging from colder in the east to warmer in the west. Within each sub-region, we designated areas adjacent to each closed area as “open.” Differences in habitat can result in differences in communities rendering it difficult to compare fleet-closed areas because the open areas are not strict controls. Therefore, we experimented with the selection of open areas by searching for comparable community dominance structures, comparable levels of species biomass and similar depths between fleet-closed and open areas in the pre-collapse period (1979–1992). Species rankings were comparable but not identical between fleet-closed and open areas. Average biomass for each species in the pre-collapse period was comparable between fleet-closed and open areas for the majority of species in all sub-regions; however, the confidence intervals of average biomass did not overlap for various dominant species (Supplementary Figure 1). The exercise of choosing “open” areas reinforced that it is always difficult to define an “open” area as a control treatment (Kerr et al., 2019) but open areas are included in this analysis for the sake of design. We also acknowledge that groundfish are mobile. The RV survey occurs during the summer and while summer distribution patterns are evident (e.g., Horsman and Shackell, 2009; Ricard and Shackell, 2013). there have been no formal analyses to gauge the range of seasonal movements within and among the majority of species (Smith et al., 2015). We interpret the results acknowledging groundfish mobility, and discuss the consequences for closed area design. All analyses accounted for the stratification sampling design and differences in total area.
Analytical Approach
The overall goal was to determine whether three long-term area-based closures across the Scotian Shelf in Atlantic Canada enhanced per capita population growth rates of 24 common groundfish species. The first step was to estimate the per capita rate of change of all species in two management types (open and fleet-closed), in three sub-regions (west, central, and east) and in three periods [pre-collapse (1979–1992), post-collapse (1993–2005), recent (2006–2017)]. We focused on biomass (B) as it is a key indicator of ecosystem structure and function (Bundy et al., 2019). We used an exponential growth model to estimate per capita rate of change (Keith et al., 2015). We fit a hierarchical model where the rate of change in the response variable (BM: natural logged biomass) was calculated for each species in each unique combination of period (P), management type (M), and sub-region (S) as described above. The Year coefficient (i.e., the slope) represents the per capita growth rate for each species for each combination of PMS, which was treated as a random effect.
Where the response variable is normally distributed around the mean μ and variance σ2, the expected mean is μ and variance σ2, and the random effect PMS is normally distributed around a mean of 0 and variance of δ2. We used a Bayesian framework package rstanarm in R that enables posterior distribution estimates through the use of simulations, using Markov chain Monte Carlo (MCMC) and the No-U Turn Sampling (NUTS) algorithm (Goodrich et al., 2018). We used four initialized Markov chains with 2,000 iterations (warmup period = 1,000). As diagnostics, we visually inspected posterior distributions and judged whether all chains had converged by using the diagnostics (potential scale reduction factor), which is a ratio indexing the between/within chain variance of model parameters and is ideally < 1.01 (Goodrich et al., 2018). We also monitored estimates of the effective sample size Neff, the effective number of simulation draws, which is rarely equal to, but should approach, the number of iterations. We then derived median and credible intervals of per capita rate of change from the Bayesian posterior distributions for each species, management type and period combination. The second step was to formally compare recent median per capita rates of change for each species between area management type. For each species, the median estimate of the per capita rate of change estimated in the first analysis were used as a response variable to examine the influence of Period (P), management type (M), and Sub-region (S).
We used a weighted regression where the weights were the inverse of the squared standard error from the per capita rates of change estimated in the first analysis; this accounts for the estimated error for the response variable. This use of weights is comparable to using a Bayesian approach with a non-informative prior. The full model included the 3-way interaction between Period, Management, and Sub-region, AIC model selection indicated that the preferred model was a simple intercept only model (i.e., none of the covariates in the model had a significant influence on the per-capita rates of change).
Results
Regional Species Status
Compared to the pre-collapse biomass, the recent biomass status of 7 (29%) out of 24 common groundfish species across the region is more than 1.25, indicating these species biomasses have increased by more than 25% since the pre-collapse period (Figure 2). In contrast, the recent biomass ratio of 11 (46%) species is less than 0.75 and for 9 of those species, this represents a further decline from the post-collapse period (Figure 2). Of the 12 species that had a biomass ratio less than 0.75 in the post-collapse period, 10 have shown no evidence for recovery in the recent era [halibut (Hippoglossus hippoglossus) and pollock (Pollachius virens) being the exceptions]. The majority of species that had a biomass ratio of > 0.75 in the post-collapse period also had a biomass ratio > 0.75 in the recent period (11/12 species, monkfish is the exception); species that were increasing or not declining significantly in the post-collapse period continued to do well in the recent period. Of the 10 active COSEWIC-assessed species, the current status of two species, spiny dogfish and redfish, are at or above one of their pre-collapse biomass, smooth skate (Raja senta) is currently 0.75, while four species, American plaice (Hippoglossoides platessoides), white hake (Urophycis tenuis), winter skate (Raja ocellata), Atlantic cod (Gadus morhua) are less than 0.5, and three species, thorny skate (Raja radiata, Atlantic wolfish (Anarhichas lupus) and cusk (Brosme brosme) are less than 0.25 of the pre-collapse biomass. In most cases, the current status represented a further decline from the post-collapse period. The post-collapse and recent status of abundance differs from that of biomass, where the abundance of several species is higher in the recent period. The contrast in biomass and abundance patterns reflects the decline in average body size (Supplementary Figure 1).
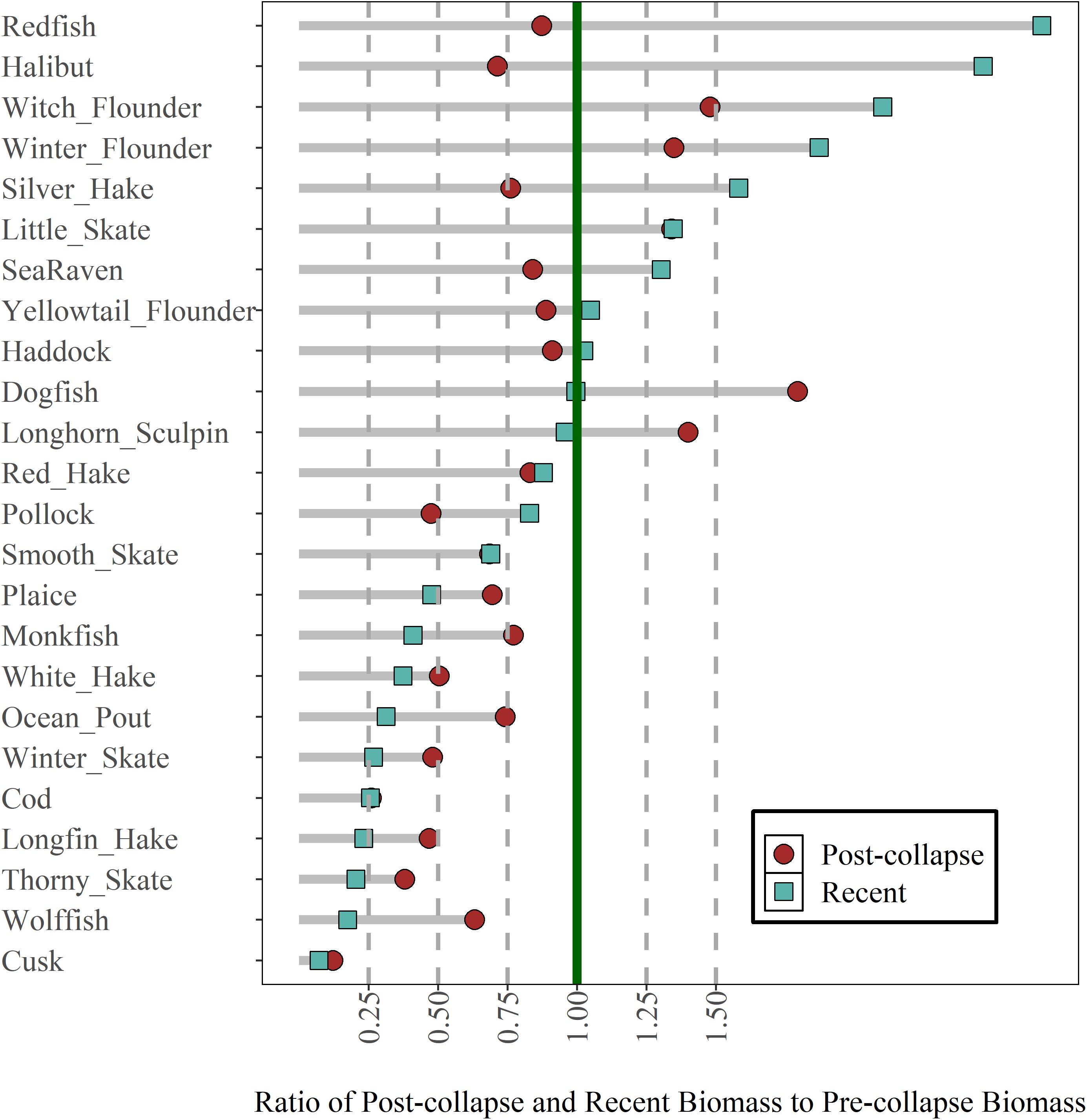
Figure 2. Regional post-collapse and recent biomass status of 24 groundfish species scaled relative to the pre-collapse period. Species are ordered based on recent status (squares). See Table 1 for species full names.
Over the three periods, 9–11 species were dominant and accounted for 95% of the total regional biomass (Figure 3). There have been regional-scale changes in the community composition over time but most of the species that were dominant in the pre-collapse period, have remained dominant, including cod and American plaice (Hippoglossoides platessoides) (Supplementary Table 1) both of which had continued to decline from the post-collapse to the most recent period. Two species, white hake (Urophycis tenuis) and thorny skate (Raja radiata), that were in the dominant species group during the first two periods are no longer in the most recent period, accounting for smaller proportions of the regional biomass. Three of the seven species that have a recent biomass status more than 1.25 (Figure 2) were not dominant in the pre-collapse period, and continue to account for a relatively low proportion of the total regional biomass [halibut, sea raven (Hemitripterus americanus) and little skate (Raja erinacea)].
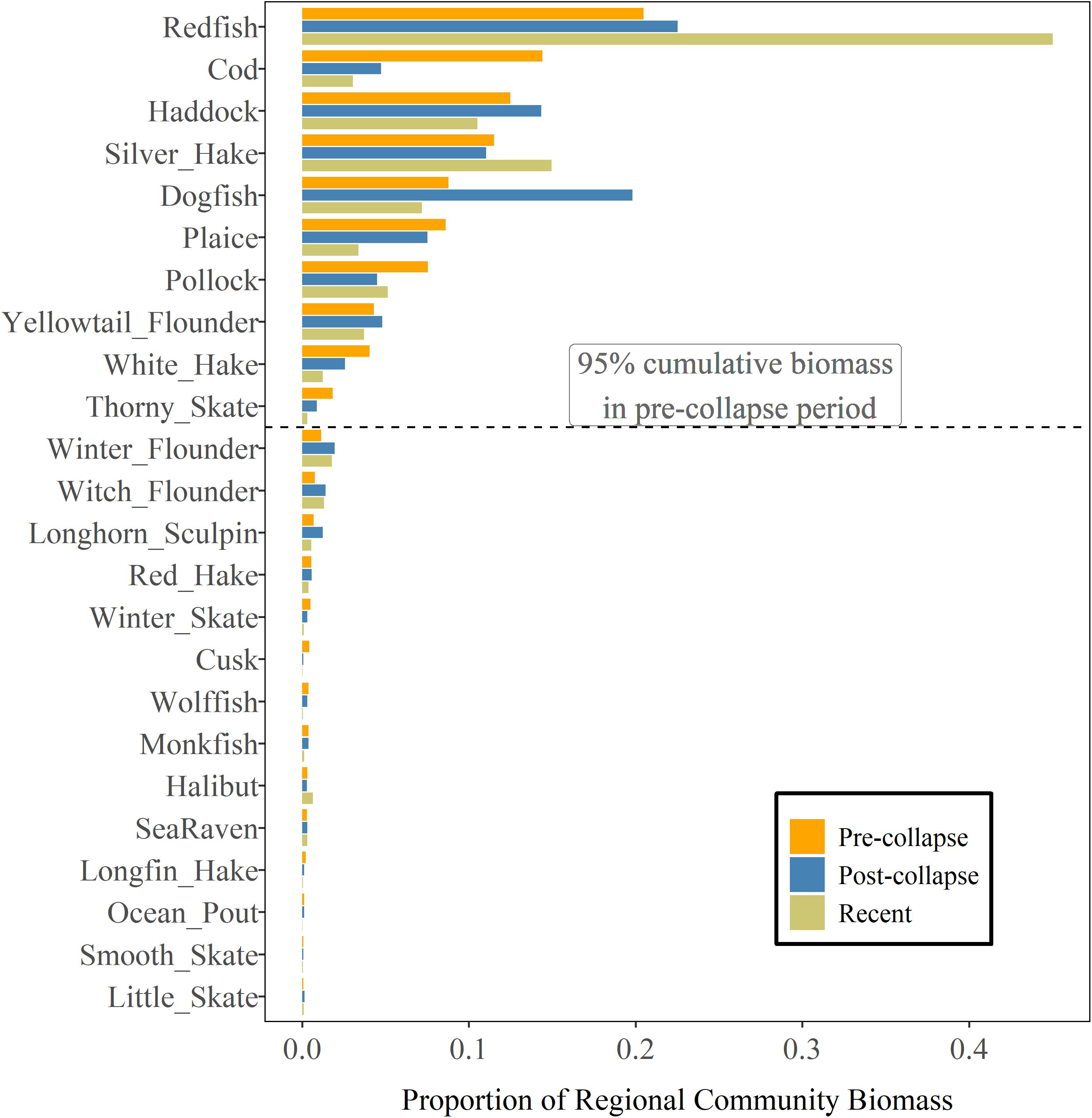
Figure 3. Regional groundfish community structure as indexed by the proportion of regional community biomass on the Scotian Shelf during pre-collapse (1979–1992), post-collapse (1993–2005) and most recent periods (2006–2017). Species are ordered by their proportion of total biomass during the pre-collapse period.
Sub-Regional Rates of Change in Closed and Open Areas
In a stable community, various species increase while others decrease and average biomass remains constant over time; it follows that individual species rates of change would be distributed around an average of zero (Shackell and Frank, 2007; Pedersen et al., 2020). In this study, the expectation is that per capita rates of change would be higher in closed areas if closed areas conferred an advantage, especially for depleted species. Specifically, there should be an increase in per capita growth rate upon closure. This is not applicable in the western sub-region, which was closed in 1972 whereas areas in the central and east were closed in 1993. For all areas, we expect higher rates in fleet-closed areas than in open areas upon closure.
At a community level, per capita rates of change did not differ significantly between fleet closed and open areas, and further, all the community level per capita rates of change were estimated to be negative in the central and eastern sub-regions (Figure 4). Note that the 95% CI’s generally cross 0 indicating that a number of species growth rates are positive despite the general decline at a community level. In the western sub-region, the per capita rates of change inside the fleet closed area in the post-collapse and recent periods are the only positive mean estimates observed, again the 95% confidence intervals do cross 0 indicating that a significant number of species are not experiencing growth in the western closure (Figure 4). Overall, positive growth rates did not translate into a significant effect of management type, period, or sub-region (Adj. R2 0.0023, F-statistic: 1.508 on 2 and 393 DF, p-value: 0.2226; Figure 4).
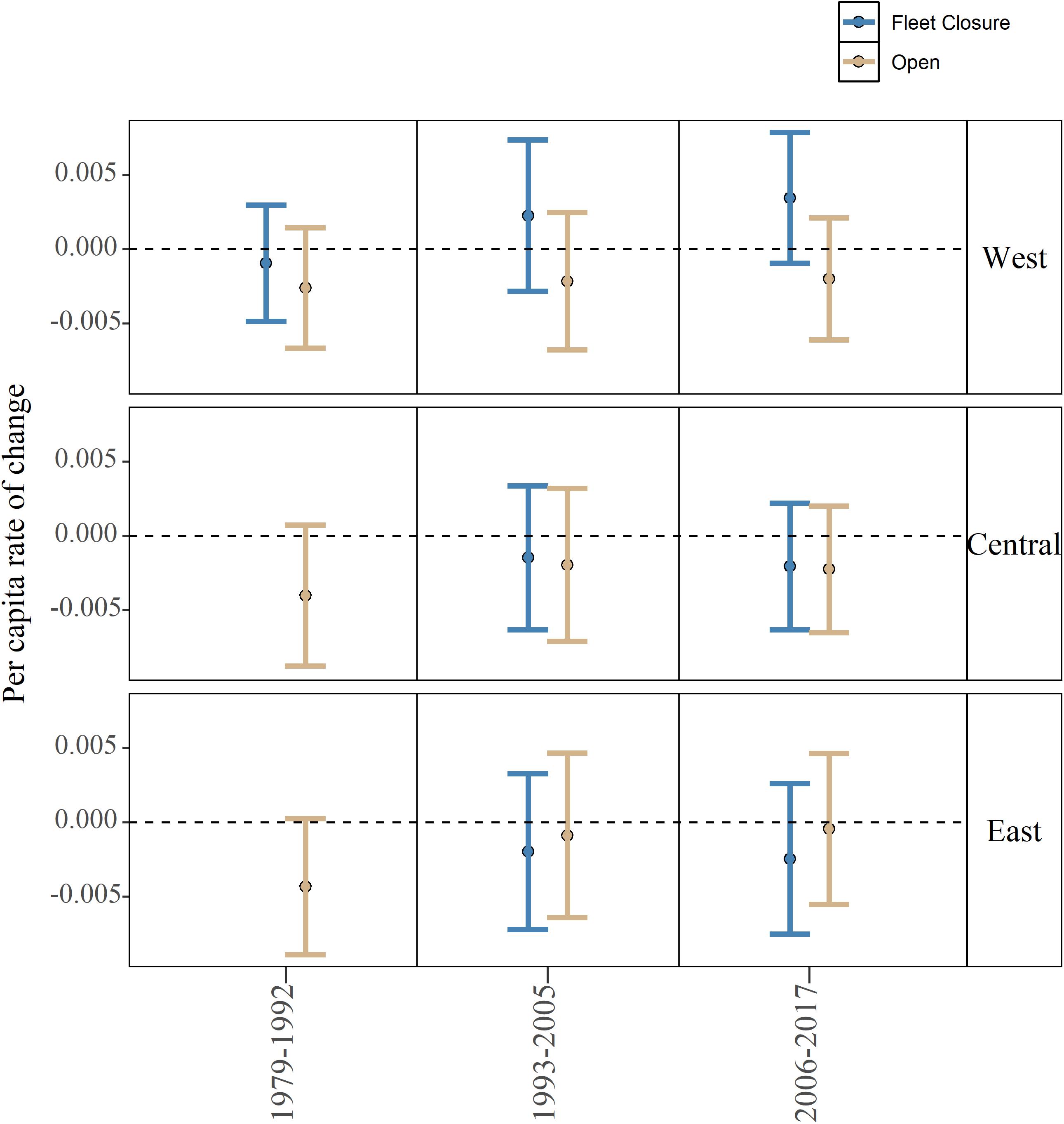
Figure 4. Model estimated community level per capita rate of change for each period (1979–1992, 1993–2005, and 2006–2017), management type (closed and open), and sub-region (west, central, and east). Error bars represent 95% confidence intervals. Note that in 1979–1992, closures for the east and central sub-regions were not yet in place.
While there is no significant effect of fleet closure at the community level, there may be single species that have benefited, for example, winter flounder (Pseudopleuronectes americanus), little skate (Raja erinacea) and longhorn sculpin (Myoxocephalus octodecemspinosus) in the west (Supplementary Figure 4). However, there is no systematic pattern across species within sub-regions. For species in a depleted state, we assume there is no density dependent response that may confound a response, yet still, there is no difference between management types (Figure 5). Overall, there is little evidence that closed areas have enhanced groundfish recovery.
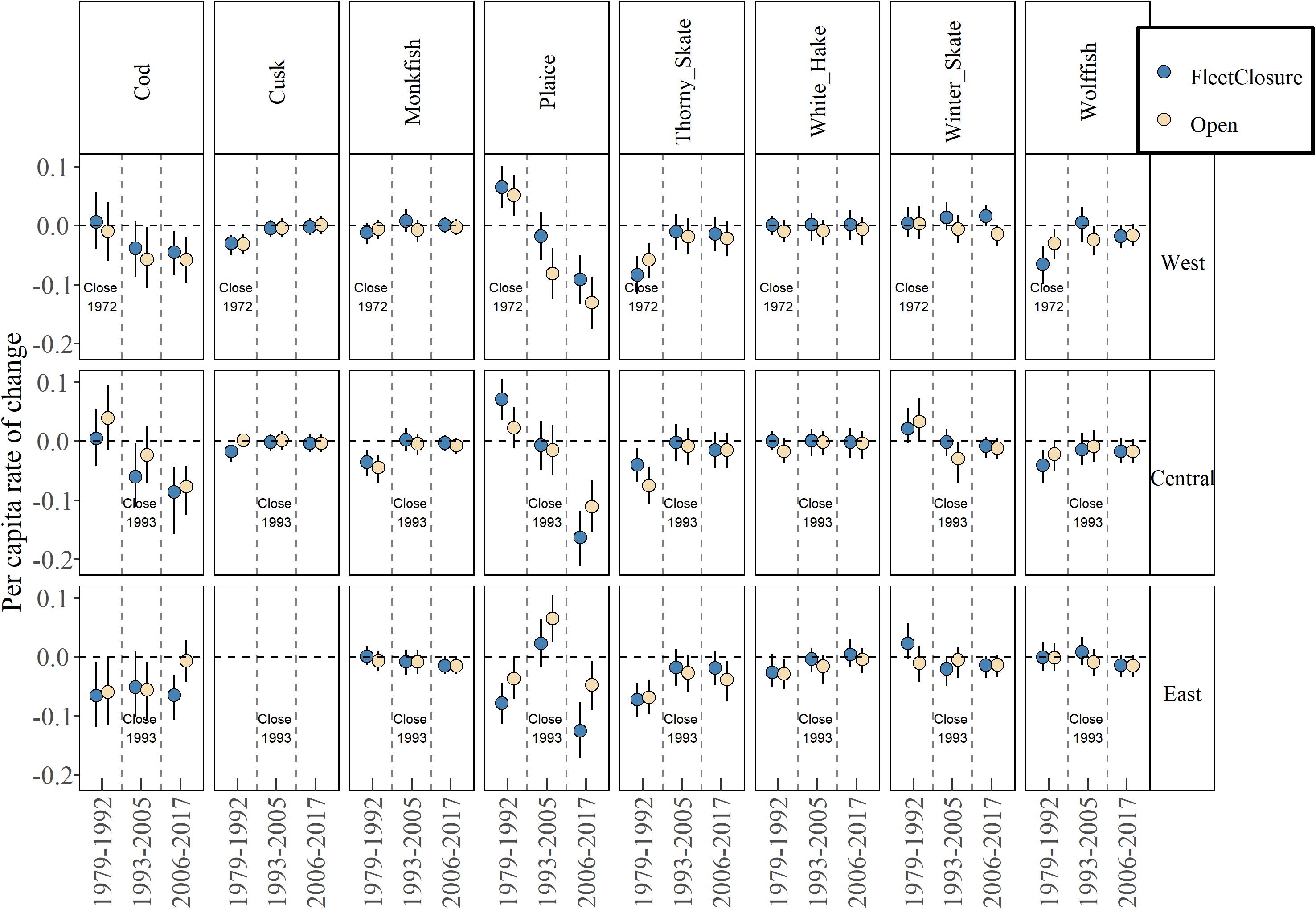
Figure 5. Median estimate and 80% credible intervals of per capita rate of change for eight severely depleted species in fleet-closed and open areas in three periods. See Supplementary Figure 4 for all species and Table 1 for species full names.
Discussion
Our analysis indicates that at the community level, including severely depleted species, there is no evidence that fleet closed areas on the Scotian Shelf have had any influence on per capita rate of changes after the collapse of the groundfish community in the early 1990s. The majority of 24 common groundfish species had similar population rates of change within and outside fleet-closed areas. At the broader regional scale, the ratio of recent to pre-collapse biomass of 46% of the species examined herein is less than 0.75 and for nine of those species, this represents a further decline from the post-collapse period. In addition, 70% of active COSEWIC-assessed species are currently less than half of their pre-collapse biomass and in most cases, have further declined from the post-collapse period. Overall, our analysis suggests that the three fleet closed areas we investigated on the Scotian Shelf had minimal conservation benefit for the depleted groundfish community, and emphasizes the need for additional measures to protect them, especially when the causes of continued diminished productivity are still largely unresolved.
There are several factors that provide context for our results, principally, historical depletion, density-dependent dispersal, groundfish mobility and/or the level of historical depletion both during and prior to the establishment of the fleet closures. From 1974–1992, the annual proportion of cod biomass removed by the fishery was 0.51 on the Scotian Shelf (Petrie et al., 2009). This exploitation rate has been argued to represent a comparable removal rate for other dominant targeted groundfish that spatially overlap with cod because the predominant fishing gear is non-selective (Shackell et al., 2005; Petrie et al., 2009). The overall effect was a collapse of many large-bodied, long lived species of the groundfish community by the early 1990s (Bundy, 2005; Frank et al., 2007). There have also been broad-scale declines in body size on the Scotian Shelf (e.g., Shackelll et al., 2010; Shackell et al., 2012b; Bundy et al., 2019), reflective of global trends where the average weight of 75% of stocks (n = 55) is currently below average (Charbonneau et al., 2019). Recovery time is related to the magnitude of initial population decline as well as the species’ body size and age at maturity (Hutchings and Baum, 2005); severely depleted formerly large-bodied species can take up to 30 years to recover (Kuparinen et al., 2014) or longer if there are Allee effects (Keith et al., 2015). In this context, it is reasonable that fleet-closed areas would not enhance community-level per capita rates of change given the severity of historical declines and how slow recovery can be for these species in the presence of an Allee effect (Kuparinen et al., 2014).
Our approach explicitly compared per capita rates of change in fleet-closed and open areas assuming no density dependent response. We assumed that rates would be higher in closed areas if they conferred an advantage. However, the metric of rate of change would be affected if a species disperses in response to increased density inside a closed area. If a given species dispersed outside the closed area in response to increasing biomass, we would see no change within the closed area (e.g., Kerr et al., 2019). For species increasing in biomass across the region there might be a lagged response between the closed and open areas. However, there is likely much less of a density dependent response for species in a depleted state yet still, there was no difference between management types for depleted species.
If fishing mortality is high outside closed areas, closures will have limited effect if animals migrate beyond the closures borders (Kenchington, 2017), either through shelf-scale migrations or smaller scale seasonal migrations. Regarding shelf-scale migrations, marine species with wide geographic ranges and high dispersal rates are assumed to represent a panmictic population, and thus assumed to migrate long distances. Major oceanographic features and processes could predispose the possibility of local adaptation; banks have gyres that serve to retain larvae, and basins serve as barriers. The physiography of the Scotian Shelf is consistent with genetic differences in cod found at the bank scale, where sample sites included two of the three closed areas studied herein, which is consistent with natal fidelity (Ruzzante et al., 1998), and supported by tagging analyses (Shackell et al., 1997). The study led Ruzzante et al. (1998) to propose that oceanographic structure could inform expectations of possible sub-regional spatial structure in other species on the Scotian Shelf, regardless of level of gene flow. If we accept that there is sub-regional spatial structure on the Scotian Shelf, we still lack a good understanding of the extent and distance of seasonal migrations within sub-regions. To date, we recognize there can be seasonal movement or migration outside of the closed area, and that it may vary among species. In a situation in which species of conservation concern migrate outside the OECM and into a region of high mortality, the ability of an OECM to protect these species is questionable (Kenchington, 2017). In this case the OECM either would need to be increased in size, or the OECM should focus on sessile benthic communities and benthic habitat. Our results also further reinforce that OECM cannot be effective alone, and what happens outside OECMs has to be sustainable.
Globally, there remains the need to improve the scientific evidence to establish, monitor and quantify the conservation benefits of new OECMs, as well as to increase the effectiveness of existing OECMs (Laffoley et al., 2017; De Santo, 2018). Otherwise, counting ineffective OECMs toward Aichi Target 11 will interfere with the global intent and goal of conserving biodiversity (MacKinnon et al., 2015). Regarding criteria, the UN CBD member parties adopted voluntary guidance in the identification of OECMs in 2018. In the Canadian process, ecologically-based criteria are used to identify OECMs (Kenchington et al., 2016) and seasonal closures were not considered to be categorized as OECMs from the outset. The central fleet-closed area is currently identified as an OECM that has a primary conservation objective to ‘‘support productivity objectives for groundfish species’’6. It is also an area of high groundfish larval diversity (Shackell and Frank, 2000), containing patches of structurally complex benthic habitats (Rincon and Kenchington, 2016) as well as a core nursery area for juvenile halibut (Boudreau et al., 2017). The central fleet-closed area has the potential to act as an OECM and efforts are underway to set up a monitoring framework (Martin King, DFO, Dartmouth, NS, Canada, pers. comm.). Our analysis provides some important context to that discussion.
Given an area fits OECM ecological criteria, it remains difficult to test the efficacy of closed areas in a dynamic environment (Halpern et al., 2004; Ahmadia et al., 2015; Kerr et al., 2019) although novel approaches have recently been developed (Keith et al., 2020). Here we focused on the groundfish community using an examination of per capita growth rates as an “appropriate and meaningful indicator” (sensu Ahmadia et al., 2015) for a formerly over-fished system. For other objectives, other indicators may be more appropriate including biodiversity, age structure, body size or integrity of benthic habitat. Once OECMs are selected, the objectives of OECMs will prescribe the monitoring approach and indicator selection, and the task of doing so is getting easier as the field of protected area impact evaluation continues to grow (Ferraro and Pressey, 2015; Fraschetti et al., 2018; Donald et al., 2019).
Currently, the fleet closures studied herein cannot be considered OECMs for the groundfish community, either as primary or secondary biodiversity objectives, because the effects of historical over-fishing and diminished productivity cannot be counteracted by closed areas. Area-based fishery protection cannot enhance groundfish recovery in isolation of a broader conservation approach especially if the redistribution of fishing effort caused by area closures results in higher bycatch amounts in the larger ecosystem (e.g., Babcock et al., 2005; Hoos et al., 2019). Globally, area-based biodiversity conservation efforts can tend to drift away from the larger ecological goal of sustainability (Halpern et al., 2010). There have been repeated calls to re-integrate area-based biodiversity conservation and protection into fisheries management, so that area-based protection is considered a cornerstone of a sustainable ecosystem-based management approach and marine spatial planning (Foley et al., 2010; Halpern et al., 2010; Rees et al., 2020). The link between conservation and sustainability was recently promoted on a global scale by Diz et al. (2018) who argued that OECMs can help to operationalize the UN Sustainable Development Goal (SDG-14) which aims to “conserve and sustainably use the oceans, seas and marine resources for sustainable development.” This underscores the global need to better understand and improve the possible contribution of OECMs toward ocean sustainability. Our study provides further impetus toward articulating the criteria of OECMs, improving their design, monitoring and testing and importantly, placing OECMs within the context of sustainable ecosystem-based management.
Data Availability Statement
The raw data supporting the conclusions of this article will be made available by the authors, without undue reservation.
Ethics Statement
The animal study was reviewed and approved by Fisheries and Oceans, Canada.
Author Contributions
NS, DK, and HL contributed to the development of the concept and writing of this manuscript. NS led and DK contributed to the data analysis. All authors contributed to the article and approved the submitted version.
Funding
This research was funded by Fisheries and Oceans Canada.
Conflict of Interest
The authors declare that the research was conducted in the absence of any commercial or financial relationships that could be construed as a potential conflict of interest.
Acknowledgments
We would like to thank former Dalhousie University student Elizabeth Nagel for her initial interest and exploratory work on this topic. We are also grateful to the reviewers for their critique and insight which greatly improved the manuscript.
Supplementary Material
The Supplementary Material for this article can be found online at: https://www.frontiersin.org/articles/10.3389/fmars.2021.612859/full#supplementary-material
Footnotes
- ^ https://www.cbd.int/convention/articles/default.shtml?a=cbd-01
- ^ https://www.cbd.int/sp/targets/
- ^ https://www.dfo-mpo.gc.ca/oceans/conservation/plan/index-eng.html
- ^ https://www.un.org/sustainabledevelopment/
- ^ http://www.dfo-mpo.gc.ca/decisions/fm-2017-gp/atl-18-eng.htm
- ^ https://www.dfo-mpo.gc.ca/oceans/oeabcm-amcepz/refuges/index-eng.html
References
Agardy, T., di Sciara, G. N., and Christie, P. (2011). Mind the gap: addressing the shortcomings of marine protected areas through large scale marine spatial planning. Mar. Policy 35, 226–232. doi: 10.1016/j.marpol.2010.10.006
Ahmadia, G. N., Glew, L., Provost, M., Gill, D., Hidayat, N. I., Mangubhai, S., et al. (2015). Integrating impact evaluation in the design and implementation of monitoring marine protected areas. Philos. Trans. R. Soc. B Biol. Sci. 370:20140275. doi: 10.1098/rstb.2014.0275
Anderson, S. C., Lotze, H. K., and Shackell, N. L. (2008). Evaluating the knowledge base for expanding low-trophic-level fisheries in Atlantic Canada. Can. J. Fish. Aquat. Sci. 65, 2553–2571. doi: 10.1139/f08-156
Babcock, E. A., Pikitch, E. K., Mcallister, K., Apostolaki, P., and Santora, C. (2005). A perspective on the use of spatialized indicators for ecosystem-based fishery management through spatial zoning. ICES J. Mar. Sci. 62, 469–476. doi: 10.1016/j.icesjms.2005.01.010
Boudreau, S. A., Shackell, N. L., Carson, S., and den Heyer, C. E. (2017). Connectivity, persistence, and loss of high abundance areas of a recovering marine fish population in the Northwest Atlantic Ocean. Ecol. Evol. 7,, 9739–9749. doi: 10.1002/ece3.3495
Bundy, A. (2005). Structure and functioning of the eastern Scotian Shelf ecosystem before and after the collapse of groundfish stocks in the early 1990s. Can. J. Fish. Aquat. Sci. 62, 1453–1473. doi: 10.1139/f05-085
Bundy, A., Gomez, C., and Cook, A. M. (2019). Scrupulous proxies: defining and applying a rigorous framework for the selection and evaluation of a suite of ecological indicators. Ecol. Indic. 104, 737–754. doi: 10.1016/j.ecolind.2019.01.031
Charbonneau, J. A., Keith, D. M., and Hutchings, J. A. (2019). Trends in the size and age structure of marine fishes. ICES J. Mar. Sci. 76, 938–945. doi: 10.1093/icesjms/fsy180
Chouinard, G. A. (2000). Report of the Cod Mixing Workshop; 16-17 October 2000. Available online at: https://waves-vagues.dfo-mpo.gc.ca/Library/252370.pdf (accessed August 28, 2020).
De Santo, E. M. (2018). Implementation challenges of area-based management tools (ABMTs) for biodiversity beyond national jurisdiction (BBNJ). Mar. Policy 97, 34–43. doi: 10.1016/j.marpol.2018.08.034
Dempsey, D., Cook, A., Gomez, C., and Bundy, A. (2019). Marindicators: Marine Ecosystem and Fishing Pressure Indicators. R Packag. version 1.0.0.
Diz, D., Johnson, D., Riddell, M., Rees, S., Battle, J., Gjerde, K., et al. (2018). Mainstreaming marine biodiversity into the SDGs : the role of other effective area-based conservation measures (SDG 14. 5). Mar. Policy 93, 251–261. doi: 10.1016/j.marpol.2017.08.019
Donald, P. F., Couturier, A. R., Jathar, G., Buchanan, G. M., Kingston, N., Balmford, A., et al. (2019). The prevalence, characteristics and effectiveness of aichi target 11’s “other effective area-based conservation measures” (OECMs) in key biodiversity areas. Convers. Lett. 12:e12659.
Dureuil, M., Boerder, K., Burnett, K. A., Froese, R., and Worm, B. (2018). Elevated trawling inside protected areas undermines conservation outcomes in a global fishing hot spot. Science 362, 1403–1407. doi: 10.1126/science.aau0561
Ellingsen, K. E., Anderson, M. J., Shackell, N. L., Tveraa, T., Yoccoz, N. G., and Frank, K. T. (2015). The role of a dominant predator in shaping biodiversity over space and time in a marine ecosystem. J. Anim. Ecol. 84, 1242–1252. doi: 10.1111/1365-2656.12396
Ferraro, P. J., and Pressey, R. L. (2015). Measuring the difference made by conservation initiatives: protected areas and their environmental and social impacts. Philos. Trans. R. Soc. B Biol. Sci. 370:20140270. doi: 10.1098/rstb.2014.0270
Fisher, J. A. D., and Frank, K. T. (2002). Changes in finfish community structure associated with an offshore fishery closed area on the Scotian Shelf. Mar. Ecol. Prog. Ser. 240, 249–265. doi: 10.3354/meps240249
Fisher, J. A. D., Frank, K. T., and Leggett, W. C. (2010). Breaking bergmann’s rule: truncation of northwest atlantic marine fish body sizes. Ecology 91, 2499–2505. doi: 10.1890/09-1914.1
Foley, M. M., Halpern, B. S., Micheli, F., Armsby, M. H., Caldwell, M. R., Crain, C. M., et al. (2010). Guiding ecological principles for marine spatial planning. Mar. Policy 34, 955–966. doi: 10.1016/j.marpol.2010.02.001
Fox, H. E., Mascia, M. B., Basurto, X., Costa, A., Glew, L., Heinemann, D., et al. (2012). Reexamining the science of marine protected areas: linking knowledge to action. Conserv. Lett. 5, 1–10. doi: 10.1111/j.1755-263x.2011.00207.x
Frank, K. T., Petrie, B., and Shackell, N. L. (2007). The ups and downs of trophic control in continental shelf ecosystems. Trends Ecol. Evol. 22, 236–242. doi: 10.1016/j.tree.2007.03.002
Frank, K. T., Petrie, B., Choi, J. S., and Leggett, W. C. (2005). Trophic cascades in a formerly cod-dominated ecosystem. Science 308, 1621–1623. doi: 10.1126/science.1113075
Frank, K. T., Shackell, N. L., and Simon, J. E. (2000). An evaluation of the Emerald/Western Bank juvenile haddock closed area. ICES J. Mar. Sci. 57, 1023–1034. doi: 10.1006/jmsc.2000.0587
Fraschetti, S., Pipitone, C., Mazaris, A. D., Rilov, G., Badalamenti, F., Bevilacqua, S., et al. (2018). Light and shade in marine conservation across European and contiguous seas. Front. Mar. Sci. 5:420.
Gill, D. A., Mascia, M. B., Ahmadia, G. N., Glew, L., Lester, S. E., Barnes, M., et al. (2017). Capacity shortfalls hinder the performance of marine protected areas globally. Nature 543, 665–669. doi: 10.1038/nature21708
Goodrich, B., Gabry, J., and Ali, I. (2018). Rstanarm: Bayesian Applied Regression Modeling Via Stan. R Package Version 2.17.4.
Halliday, R. G. (1988). Use of seasonal spawning area closures in the management of haddock fisheries in the Northwest Atlantic. NAFO Sci. Counc. Stud. 12, 27–36.
Halpern, B. S. (2003). The impact of marine reserves: do reserves work and does reserve size matter? Ecol. Appl. 13, 117–137. doi: 10.1890/1051-0761(2003)013[0117:tiomrd]2.0.co;2
Halpern, B. S., Gaines, S. D., and Warner, R. R. (2004). Confounding effects of the export of production and the displacement of fishing effort from marine reserves. Ecol. Appl. 14, 1248–1256. doi: 10.1890/03-5136
Halpern, B. S., Lester, S. E., and Mcleod, K. L. (2010). Placing marine protected areas onto the ecosystem- based management seascape. Proc. Natl. Acad. Sci. U. S. A. 107, 18312–18317. doi: 10.1073/pnas.0908503107
Hoos, L. A., Buckel, J. A., Boyd, J. B., Loeffler, M. S., and Lee, L. M. (2019). Fisheries management in the face of uncertainty : designing time-area closures that are effective under multiple spatial patterns of fishing effort displacement in an estuarine gill net fishery. PLoS One 14:e0211103. doi: 10.1371/journal.pone.0211103
Horsman, T. L., and Shackell, N. L. (2009). Atlas of important habitat for key fish species of the Scotian Shelf, Canada. Can. Tech. Rep. Fish. Aquat. Sci. 2835:viii+82.
Hutchings, J. A., and Baum, J. K. (2005). Measuring marine fish biodiversity: temporal changes in abundance, life history and demography. Philos. Trans. R. Soc. B Biol. Sci. 360, 315–338. doi: 10.1098/rstb.2004.1586
Keith, D. M., Sameoto, J. A., Keyser, F. M., and Ward-paige, C. A. (2020). Evaluating socio-economic and conservation impacts of management : a case study of time- area closures on Georges Bank. PLoS One 15:e0240322. doi: 10.1371/journal.pone.0240322
Keith, D., Akçakaya, H. R., Butchart, S. H. M., Collen, B., Dulvy, N. K., Holmes, E. E., et al. (2015). Temporal correlations in population trends : conservation implications from time-series analysis of diverse animal taxa. Biol. Conserv. 192, 247–257. doi: 10.1016/j.biocon.2015.09.021
Kenchington, E., McLean, S., and Rice, J. C. (2016). Considerations for identification of effective area-based conservation measures. DFO Can. Sci. Advis. Sec. Res. Doc. 2016/020. v + 53 p.
Kenchington, T. J. (2017). Implications of fish migration and fishing mortality for marine protected area design. Fish Fish. 18, 160–170. doi: 10.1111/faf.12144
Kerr, L. A., Kritzer, J. P., and Cadrin, S. X. (2019). Strengths and limitations of before – after – control – impact analysis for testing the effects of marine protected areas on managed populations. ICES J. Mar. Sci. 76, 1039–1051. doi: 10.1093/icesjms/fsz014
Kuparinen, A., Keith, D. M., and Hutchings, J. A. (2014). Allee effect and the uncertainty of population recovery. Conserv. Biol. 28, 790–798. doi: 10.1111/cobi.12216
Laffoley, D., Dudley, N., Jonas, H., MacKinnon, D., MacKinnon, K., Hockings, M., et al. (2017). An introduction to ‘other effective area - based conservation measures’ under Aichi Target 11 of the Convention on Biological Diversity : origin, interpretation and emerging ocean issues. Aquat. Conserv. Mar. Freshw. Ecosyst. 27, 130–137. doi: 10.1002/aqc.2783
Lambert, D. M., Lipcius, R. N., and Hoenig, J. M. (2006). Assessing effectiveness of the blue crab spawning stock sanctuary in Chesapeake Bay using tag-return methodology. Mar. Ecol. Prog. Ser. 321, 215–225. doi: 10.3354/meps321215
Lipcius, R. N., Stockhausen, W. T., Seitz, R. D., and Geer, P. J. (2003). Spatial dynamics and value of a marine protected area and corridor for the blue crab spawning stock in Chesapeake Bay. Bull. Mar. Sci. 72, 453–469.
MacKinnon, D., Lemieux, C. J., Beazley, K., Woodley, S., Helie, R., Perron, J., et al. (2015). Canada and aichi biodiversity target 11: understanding ‘other effective area-based conservation measures’ in the context of the broader target. Biodivers. Conserv. 24, 3559–3581. doi: 10.1007/s10531-015-1018-1
Murawski, S. A., Brown, R., Lai, H. L., Rago, P. J., and Hendrickson, L. (2000). Large-scale closed areas as a fishery-management tool in temperate marine systems: the Georges Bank experience. Bull. Mar. Sci. 66, 775–798.
O’Keefe, C., Cadrin, S., and Stokesbury, K. (2014). Evaluating effectiveness of time/area closures, quotas/caps, and fleet communications to reduce fisheries bycatch. ICES J. Mar. Sci. 71, 1286–1297. doi: 10.1093/icesjms/fst063
Pedersen, E. J., Koen-Alonso, M., and Tunney, T. D. (2020). Detecting regime shifts in communities using estimated rates of change. ICES J. Mar. Sci. 77, 1546–1555. doi: 10.1093/icesjms/fsaa056
Petrie, B., Frank, K. T., Shackell, N. L., and Leggett, W. C. (2009). Structure and stability in exploited marine fish communities: quantifying critical transitions. Fish. Oceanogr. 18, 83–101. doi: 10.1111/j.1365-2419.2009.00500.x
Pomeroy, R. S., Watson, L. M., Parks, J. E., and Cid, G. A. (2005). How is your MPA doing? a methodology for evaluating the management effectiveness of marine protected areas. Ocean Coast. Manag. 48, 485–502. doi: 10.1016/j.ocecoaman.2005.05.004
R Core Team (2016). R: a Language and Environment for Statistical Computing. Vienna: R Foundation for Statistical Computing.
Rees, S. E., Sheehan, E. V., Stewart, B. D., Clark, R., Appleby, T., Attrill, M. J., et al. (2020). Emerging themes to support ambitious UK marine biodiversity conservation. Mar. Policy 117:103864. doi: 10.1016/j.marpol.2020.103864
Ricard, D., and Shackell, N. L. (2013). Population status (abundance / biomass, geographic extent, body size and condition), important habitat, depth, temperature and salinity preferences of marine fish and invertebrates on the Scotian Shelf and Bay of Fundy. Can. Tech. Rep. Fish. Aquat. Sci. 3012:viii+180.
Rincon, B., and Kenchington, E. L. (2016). Influence of benthic macrofauna as a spatial structuring agent for juvenile haddock (Melanogrammus aeglefinus) on the Eastern Scotian Shelf, Atlantic Canada. PLoS One 11:e0163374. doi: 10.1371/journal.pone.0163374
Rose, G. A. (2007). Cod- the Ecological History of the North Atlantic Fisheries. Canada: Breakwater books.
Ruzzante, D. E., Taggart, C. T., and Cook, D. (1998). A nuclear DNA basis for shelf- and bank-scale population structure in northwest atlantic cod (Gadus morhua): labrador to Georges Bank. Mol. Ecol. 7, 1663–1680. doi: 10.1046/j.1365-294x.1998.00497.x
Shackell, N. L., Bundy, A., Nye, J. A., and Link, J. S. (2012a). Common large-scale responses to climate and fishing across Northwest Atlantic ecosystems. ICES J. Mar. Sci. 69, 151–162. doi: 10.1093/icesjms/fsr195
Shackell, N. L., Fisher, J. A. D., Frank, K. T., and Lawton, P. (2012b). Spatial scale of similarity as an indicator of metacommunity stability in exploited marine systems. Ecol. Appl. 22, 336–348. doi: 10.1890/10-2093.1
Shackell, N. L., and Frank, K. T. (2000). Larval fish diversity on the Scotian Shelf. Canadian Journal of Fisheries and Aquatic Sciences, 57, 1747–1760. doi: 10.1139/f00-111
Shackell, N. L., Frank, K. T., and Brickman, D. W. (2005). Range Contraction may not always predict core areas: an example from marine fish. Ecol. Appl. 15, 1440–1449. doi: 10.1890/04-0642
Shackell, N. L., Stobo, W. T., Frank, K. T., and Brickman, D. (1997). Growth of cod (Gadhus morhua) estimated from mark-recapture programs on the Scotian Shelf and adjacent areas. ICES J. Mar. Sci. 54, 383–398. doi: 10.1006/jmsc.1996.0173
Shackell, N., and Frank, K. (2007). Compensation in exploited marine fish communities on the Scotian Shelf, Canada. Mar. Ecol. Prog. Ser. 336, 235–247. doi: 10.3354/meps336235
Shackelll, N. L., Frank, K. T., Fisher, J. A. D., Petrie, B., and Leggett, W. C. (2010). Decline in top predator body size and changing climate alter trophic structure in an oceanic ecosystem. Proc. R. Soc. B 277, 1353–1360. doi: 10.1098/rspb.2009.1020
Smith, C. D., Serdynska, A. R., King, M. C., and Shackell, N. L. (2015). Spring, summer and fall distribution of common demersal fishes on the Scotian Shelf between 1978 and 1985. Can. Manuscr. Rep. Fish. Aquat. Sci. 3068, 4–38.
Keywords: marine protected areas, marine spatial planning, groundfish recovery, groundfish collapse, UN SDG 14
Citation: Shackell NL, Keith DM and Lotze HK (2021) Challenges of Gauging the Impact of Area-Based Fishery Closures and OECMs: A Case Study Using Long-Standing Canadian Groundfish Closures. Front. Mar. Sci. 8:612859. doi: 10.3389/fmars.2021.612859
Received: 30 September 2020; Accepted: 15 March 2021;
Published: 06 April 2021.
Edited by:
Romuald Lipcius, College of William & Mary, United StatesReviewed by:
Marco Andrello, Istituto per lo studio degli impatti Antropici e Sostenibilità in ambiente marino (CNR), ItalyLaura Airoldi, University of Padova Chioggia Hydrobiological Station, Italy
Copyright © 2021 Shackell, Keith and Lotze. This is an open-access article distributed under the terms of the Creative Commons Attribution License (CC BY). The use, distribution or reproduction in other forums is permitted, provided the original author(s) and the copyright owner(s) are credited and that the original publication in this journal is cited, in accordance with accepted academic practice. No use, distribution or reproduction is permitted which does not comply with these terms.
*Correspondence: Nancy L. Shackell, bmFuY3kuc2hhY2tlbGxAZGZvLW1wby5nYy5jYQ==