- 1Department of Aquatic Resources Management, Faculty of Fisheries and Marine Sciences, IPB University, Bogor, Indonesia
- 2Environmental Research Center, IPB University, Bogor, Indonesia
- 3Center for Coastal and Marine Resources Studies, IPB University, Bogor, Indonesia
- 4Department of Aquatic Product Technology, Faculty of Fisheries and Marine Sciences, IPB University, Bogor, Indonesia
- 5Faculty of Fisheries and Marine Sciences, IPB University, Bogor, Indonesia
- 6Aquaculture and Sea-Ranching, Faculty of Agriculture and Environmental Sciences, University of Rostock, Rostock, Germany
- 7Department of Biology – Genetics, Ecology and Evolution, Aarhus University, Aarhus, Denmark
The paper aims to elucidate the physico-chemical characteristics of the shell of mangrove horseshoe crabs (Carcinoscorpius rotundicauda) and determine the compilation matrix for the first time. The shell composition matrix of C. rotundicauda has never been studied in detail before, especially the shape of the foam, the chemical composition, the functional groups and the mechanical-physical and thermal properties of the shell. Based on this study, the shell structure of the mangrove horseshoe crab has the potential to be used as the base structure for developing bio-foam insulator material in the future. Therefore, the shell of mangrove horseshoe crabs has a unique natural structure in the form of foam. Its robust and elastic structure has the potential for further development for new marine biomaterials. The formation and composition of horseshoe crab shells foam are also believed to be multifunctional in mobility, used for defense mechanisms and thermal stability. The horseshoe crab samples were collected from Pacitan coastal waters, East Java, Indonesia. The research was conducted using physico-chemical and mechanical-physical analysis. The scanning electron microscopy was used in order to clarify the physico-chemical characteristics. The measurements of the mechanical-physical characteristics included density, unit cell size, and water absorption. The tensile strength and compressive strength were analyzed based on the American Society for Testing Material. Thermal resistance was measured by thermal gravimetric analysis. The results showed that the horseshoe crab shells have a unique structure, where chitin, protein and some minerals are the main chemical elements. The combination and major constituents of the horseshoe crab shell material provide strong and plastic mechanical properties with a maximum tensile strength of 60.46 kPa and maximum compressive strength of 110.55 kPa, water absorption of 0.01195 ± 0.001% and a density value of 0.1545 ± 0.011 g/cm3 as well as the capability to withstand thermal loads with peak decomposition values of 267.4–823.2°C and thermal stability of 60.59%. Using natural marine biomaterials in the future will be beneficial because it leaves no harmful residues and therefore has environmental advantages and at the same time, it is also more cost-effective.
Introduction
In the book “Biological Materials of Marine Origin: Vertebrates” Ehrlich (2010) states that the sea is a material source with great potential for humans in future life. A wide variety and uniqueness characterize marine materials due to marine life diversity; they are conveyed through composition, structure and chemical elements. The engineering of materials originating from the universe evolution for millions of years makes a variety of materials extremely complicated and unique (Jabbari et al., 2014). Additionally, Wegst and Ashby (2004) and Meyers et al. (2008) showed that biological materials are multifunctional because they combine biology, mechanics, and several other functions. Biological materials can even be categorized as polymers, inorganic materials, concentrates, adhesives, and composites (Meyers et al., 2008). The development of marine biological materials is rapidly growing due to the application potential in various life and medical sciences.
Horseshoe crabs are an ancient marine species with attractive functional shapes and behavior. They have descended from Trilobites (Subphylum Trilobitomorpha), which have lived for 445 million years (Chen et al., 2010). Genetically, this species is quite close to Pycnogonida (sea spiders) and Arachnida (spiders, scorpions, harvestmen, mites, etc.) (Edgecombe and Legg, 2014; Giribet, 2018). Kassim et al. (2018) reported that horseshoe crabs (Carcinoscorpius rotundicauda) have a body-forming and protective shell with a strong elastic structure. It is formed by the evolutionary process of the hardened material (Joffe et al., 1975).
Indonesia is known to harbor three species of horseshoe crabs, i.e., Tachypleus gigas (Müller, 1785), Tachypleus tridentatus (Leach, 1819) and C. rotundicauda (Latreille, 1802) (Mashar et al., 2017; John et al., 2018a, b). Usually, C. rotundicauda, known as the mangrove horseshoe crab, lives in muddy mangrove ecosystems and warm-soft sandy estuaries. In Indonesia, the mangrove horseshoe crabs can be easily found along North Java coastal waters, such as in Indramayu, Subang, Semarang, Demak, Rembang, Sumenep, Bangkalan, Probolinggo, Gresik, and Lamongan. However, the horseshoe crab is experiencing pressure and conservation problems, so it needs research as a baseline for future management (Wang et al., 2020). Current studies on horseshoe crabs in Indonesia cover their biological aspects, such as taxonomy and morphology (Meilana et al., 2016; Sumarmin et al., 2017; Erwyansyah et al., 2018; Aini et al., 2020), reproductive biology (Eidman et al., 1997), feeding habits (Nuraisah et al., 2020), and habitat distribution (Mashar et al., 2017; Erwyansyah et al., 2018; John et al., 2018a, b) solely.
Inspired by nature (bio-inspiration), further materials from horseshoe crabs can be developed in the future, such as different biomaterials like, marine medicines, antioxidants, antifouling and antibacterial materials, etc. (Ismail et al., 2011; Pati et al., 2020). But for this further use, the physico-chemical characteristic informations of the horseshoe crab shells becomes important in the creation of environmentally friendly advanced materials. Mechanical studies on the hard shell (solid cuticle) characteristics of arthropods have been of engineers and biologist interest for five decades (Jensen and Weis-Fogh, 1962; Hepburn and Ball, 1973; Hepburn and Joffe, 1974a, b; Joffe and Hepburn, 1974; Gadgey and Bahekar, 2017). Nevertheless, the compilation matrix of the shell of horseshoe crabs has never been studied before. Additionally, studies are developing regarding horseshoe crab blood benefits and use as a modern pharmaceutical ingredient (Krisfalusi-Gannon et al., 2018).
Chen et al. (2008a) described that a shell of horseshoe crabs consists of 50–60 nm chitin fibers arranged in parallel and layers (nanofibers of chitosan layers). Characteristics of functional groups in the shells of horseshoe crabs show that the shells consist of hydroxyl groups, secondary amides, asymmetric C–H, C–H alkanes, C=O secondary amides and C-aliphatic ether (Kassim et al., 2018). Briefly, the arthropods exoskeleton consists mainly of chitin and is multifunctional, such as supporting the body, resisting mechanical loads, protecting harsh environmental conditions and resistance to desiccation (Neville, 1975; Vincent, 1991, 2002; Vincent and Wegst, 2004; Sanchez et al., 2005; Meyers et al., 2008). Therefore, this study aimed to elucidate the physico-chemical characteristics of the shell of mangrove horseshoe crabs (C. rotundicauda) for the first time. The new information on the shells composition matrix of C. rotundicauda has never been studied in detail before, especially the shape of the foam, the chemical composition, the functional groups and the mechanical-physical and thermal properties of these shells are a novelty in this baseline study. Based on this study, the shell structure of the mangrove horseshoe crab has the potential to be used as the base structure for developing bio-foam insulator material in the future, such as insulation materials in buildings, vehicle interiors and as blocking materials to block the flow of heat energy.
Materials and Methods
Research Location and Examined Crabs
Horseshoe crab samples were taken from local fishermen at coastal waters of Pacitan, north part of East Java – Indonesia, in April and June 2018. Laboratory work was carried out in January–July 2019 in three laboratories, namely Laboratory of Preservation and Diversification of Aquatic Products, Department of Aquatic Products Technology – Faculty of Fisheries and Marine Sciences, IPB University, Center for Research and Development of Postharvest Agriculture in Cimanggu in Bogor, and Physics Research Center, Indonesian Institute of Sciences in Serpong, Indonesia.
The morphometric measurement of 20 examined crabs C. rotundicauda consisted of a length profile of the main body parts, namely prosoma = 65 ± 0.41 mm, ophistoma = 51.15 ± 1.06 mm, and telson = 135 ± 4.32 mm. The frontal margins of the C. rotundicauda have a rounded shape with a flat front view and a length of 113.80 ± 1.06 mm. The pedipalps show an identical shape to the pedipalp size = 5.36 ± 0.21 mm and 5.37 ± 0.21 mm. The telson (cross-section) was of triangular shape with obtuse angles, a width of 4.57 ± 0.38 mm and a height of 5.45 ± 0.19 mm. The genital operculum had a round shape with a V pattern and at the posterior end were marginal spines have a uniform shape between the left and right side and size that is marginal right spines have a length of 7.37 ± 0.18 mm to 11.33 ± 0.19 mm and left 7.38 ± 0.20 mm to 11.32 ± 0.18 mm. The total length of C. rotundicauda was 259 ± 4.12 mm. The specimens used were males (10) and females (10). The morphometric data can be seen on Table 1.
Characterization of the Shell
The research was conducted with physico-chemical and mechanical-physical analysis. The preparation of 20 horseshoe crabs consisted of two main activities; separating the shell/exoskeleton part from the non-shell part and cleaning the shell part from dirt. Cleaning the shell was done using distilled water followed by soaking it into acetone (ratio 1:2) for 24 h to remove impurities. The characterization of 20 horseshoe crab shells began with visualization of the shell and the microstructure analysis of the shell cross-section, referring to the identification technique of Chen et al. (2008a) using scanning electron microscopy (SEM).
The composition of the constituents was identified based on the chemical composition, included analysis of Energy Dispersive X-ray (EDX; Tanasi et al., 2017), water content (AOAC, 2005; item 934.01), protein content (AOAC, 2005; item 955.04), fat content (AOAC, 2005; item 972.28), ash content (AOAC, 2005; item 938.08) and functional groups using FT-IR (Fourier Transform-Infrared) spectrophotometer (ASTM, 2013).
The number of replications for SEM and FT-IR was two for each method used. SEM analysis observed a visual appearance of a cross-sectional incision of a horseshoe crab shell opisthosoma with a structure forming a foam unit cell size of ±70 μm consist of an intermediate layer and an interior core. FT-IR analysis was conducted to determine the sample’s chemical structure and the possibilities of interaction between components. Sample preparation by method KBr pellets was a mixture of solid samples with KBr powder (5–10% of the sample KBr powder). The homogeneous mixture was formed by KBr pellets (pills KBr) with the Mini Hand Press. Once formed, the Pill was ready for analysis. The sample was 2.5 × 2.5 cm2 mounted on the IR card. Spectrum infrared waves were fired through the sample placed in between the spectrophotometer electrode and passed to the computer. Data obtained as a percentage of the absorbance value, with the spectrum’s measurement at wave number range 4000–400 cm–1. The results obtained were in the form of a spectrum that appears on the computer.
Scanning electron microscopy- energy dispersive X-ray analysis of horseshoe crab shell was cut crosswise and then was put in microscope slides. The shell structure was then observed using a light polarizing microscope with a magnification of 40 × 10 and photographed using dino lite and saved in JPEG format. XRD analysis, the type of phase and the crystallinity of the petrol shell were analyzed using X-Ray Diffraction (XRD) (PANalytical). Operational conditions equipment namely using a Cu-Kα radiation beam with an angle of 2θ, 20° to 60° and 0.1° resolution, which was operated with an X-ray diffractometer according to ASTM (2008). The sample was prepared and as much as 2 mg were placed in a holder measuring 2 × 2 cm2 on the diffractometer. The voltages are used at 40 kV and the generator current was 30 mA. The starting corner was taken at 5° and the final angle at 80° with a 4° per minute reading speed. The result was a graph of the identified phases based on the intensity and angle of two thetas formed. Determination of the phase refers to the Joint Committee on Powder Diffraction Standard (JCPDS) and calculates the crystallinity degree.
The measurements of the mechanical-physical characteristics included density, unit cell size, and water absorption. It was done following the protocol according to Schmidt and Laurindo (2009), while the tensile strength and compressive strength were analyzed based on ASTM (1991). Thermal resistance was measured by thermal gravimetric analysis (TGA; Klamczynski et al., 2007).
The number of replicates used for physico-chemical analyses was two replicates per method. The testing machine clamped tensile strength, the two ends of the sample. The “start” button was turned on, and the tool pulled the sample to break and note the tensile strength (F) and length after breaking up. After the next sheet was tested, the material’s maximum stress could withstand up to its breaking point. Compressive strength, extrudates 2 cm long, was placed on a flat disk. Then, the extrudate was pressed using another dish at a rate of 1 cm per minute. The compression value divided the applied force (kN) value distributed by the surface area sample tested. A high value indicates that the sample was relatively hard, whereas a low value indicates that the sample was soft and easy to compress. As much as 1 g of starch was added to 10 g of distilled water and then stirred for 30 s. Water absorption analysis, the mixture was allowed to stand for 30 min and then centrifuged at a speed of 4,000 rpm for 30 min. Density analysis, the sample was cut into 2 × 2 cm sizes. The mass was known by weighting the pieces with analytical balances. Calculating volume was done by multiplying the length, width and thickness of the piece.
Results
Visual Appearance and Structure of the Horseshoe Crab Shell
The horseshoe crab shell cross-section shows that the cavity structure with strut forming foam created the inside of the horseshoe crab shell, and the strut seems to provide even redistribution of pressure to all parts of the shell. Struts are hollow walls that form open foam cells in the horseshoe crab shell. Struts are described as steel poles that support a bridge but have a stochastic or irregular shape. Cavity cell unit sizes range between ±70 μm (Figure 1). Struts on the inside of the shell form irregular angles and has the function as a reinforcement system for the horseshoe crab shell.
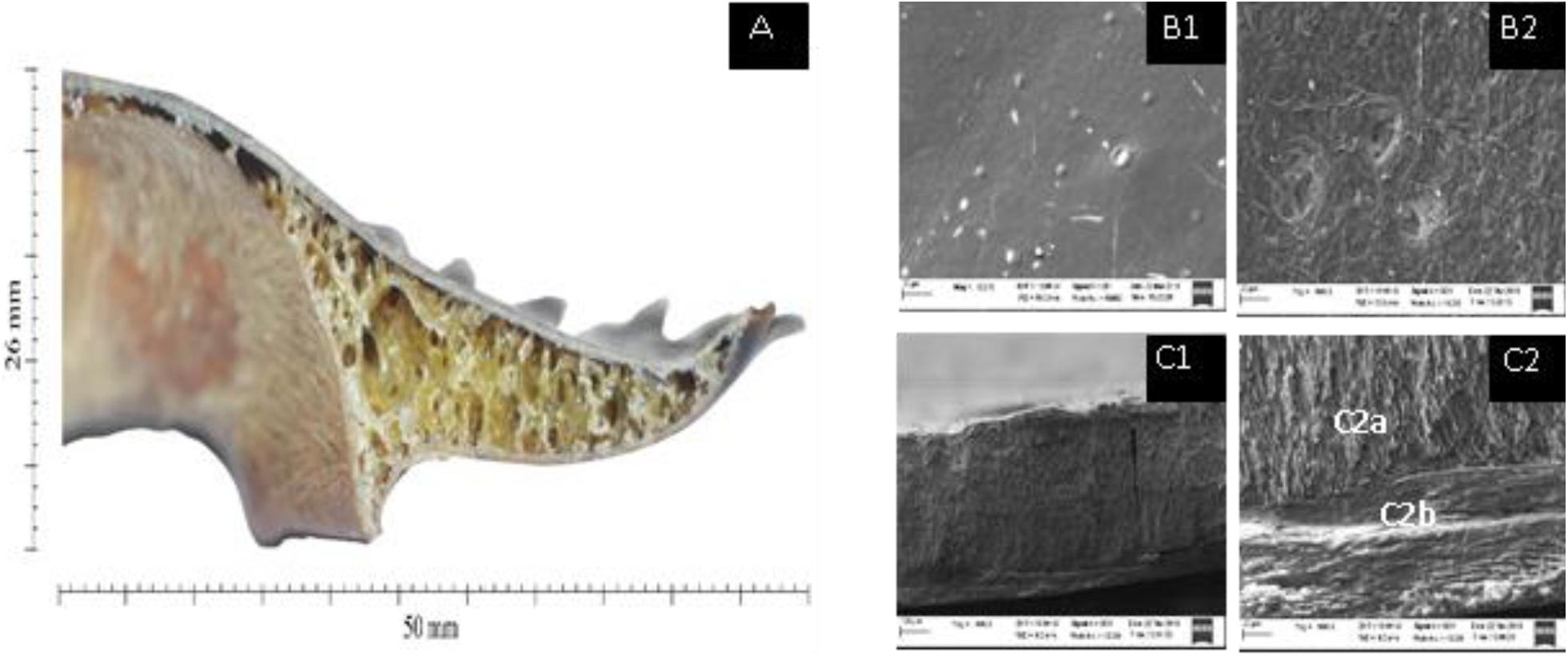
Figure 1. Visual appearance of the incision through the opisthosoma of the shell of mangrove horseshoe crabs (Carcinoscorpius rotundicauda) with a foam-shaped structure of ±70 μm (A). The microstructure of the horseshoe crab shell using SEM 500× magnification at the top (B1) and bottom (B2). 100× magnification of transverse incision (C1). 500× magnification of transverse incision (C2) showing intermediate layer (C2a) and interior core (C2b).
Based on the various microstructure views (shooting angle) of the shells, see Figures 1A–C, it can be seen that the horseshoe crab shell has a smooth appearance at the top and a rough texture as a link to the foam buffer at the bottom. The transverse incision of the horseshoe crabs shell shows two firm lines showing that the shell is composed of three main layers, which are arranged parallel to each shell, covering the exterior shell, intermediate layer and interior core.
Chemical Composition of the Horseshoe Crab Shell
The chemical composition shows that the shells’ main components are protein and crude fiber, i.e., 68.02 and 24.17%, respectively (Table 2). This component may be formed during the ecdysis or molting process. The composition of the shell matrix through observations with EDX (Figure 2 and Table 3) shows that the horseshoe crab shell is dominated by carbon (53.54%), oxygen (31.13%), and nitrogen (14.49%). Other constituent elements are chlorine (0.5%), sulfur (0.29%), phosphorus (0.09%), calcium (0.06%), potassium (0.05%), and silicon (0.03%).
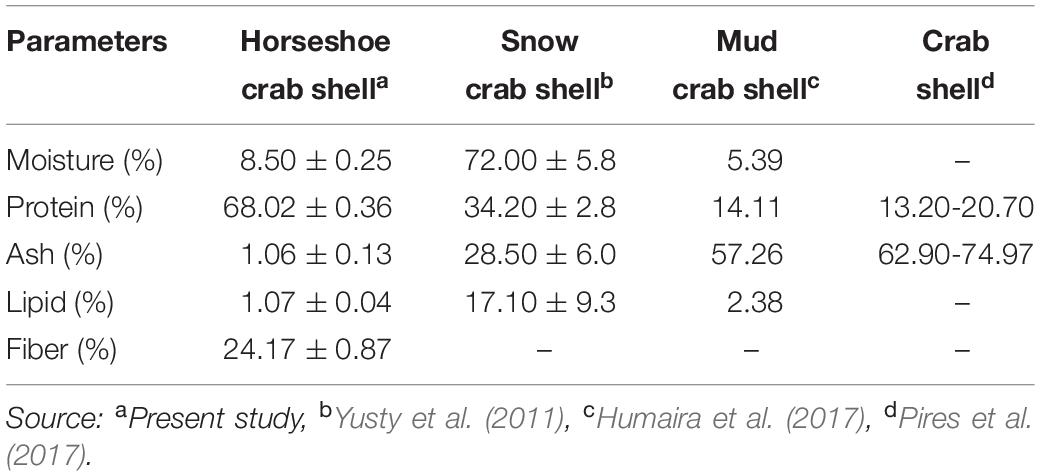
Table 2. Chemical composition of mangrove horseshoe crab (Carcinoscorpius rotundicauda) and some crustacean shells.
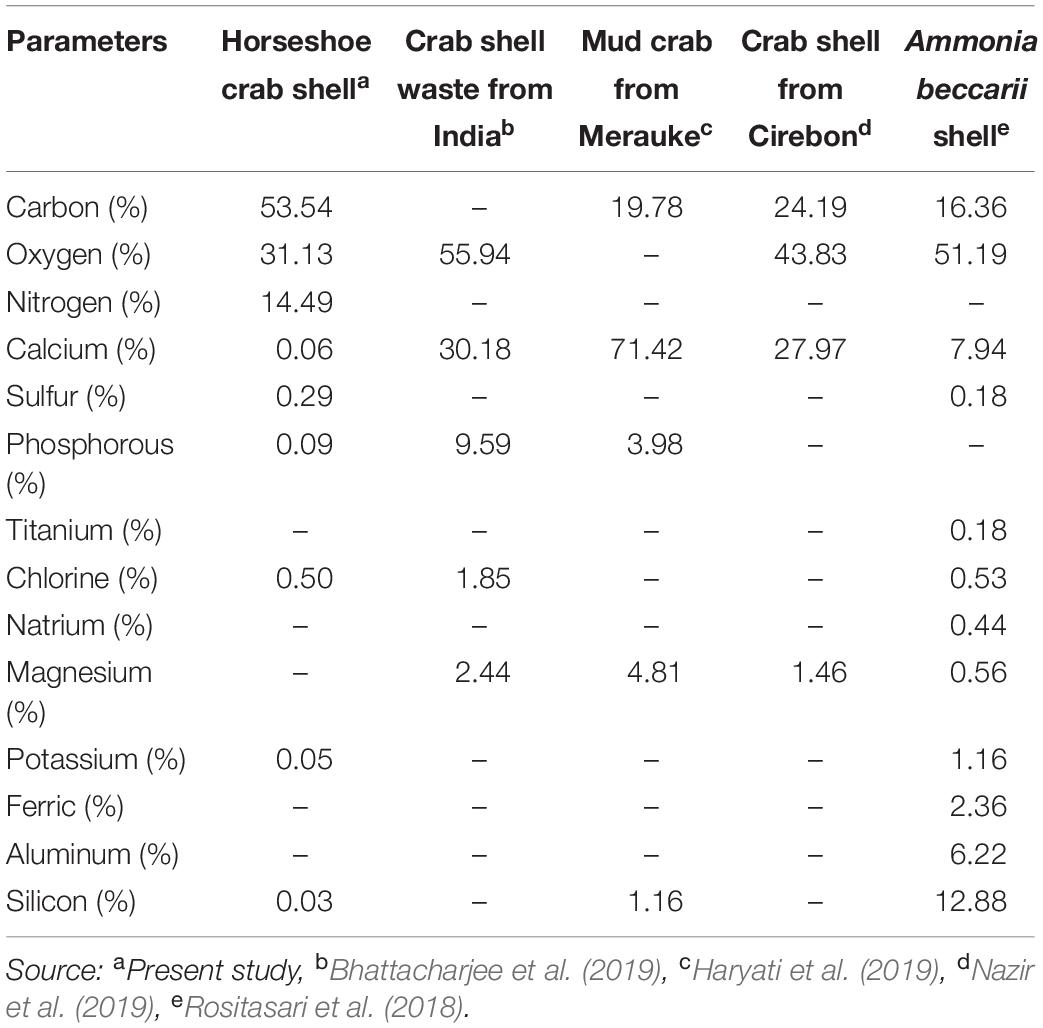
Table 3. Element levels of the shells in mangrove horseshoe crabs (Carcinoscorpius rotundicauda) and some other crustaceans.
The Horseshoe Crab Functional Shell Groups
The absorbent spectrum of horseshoe crabs shell shows the absorption of O–H stretching groups at 3475 cm–1, N–H stretching groups at 3249–3236 cm–1, C–H groups at 2,908–2,866 cm–1, amide II groups at 1564–1488 cm–1, groups of amide III at 1328–1274 cm–1and C–O groups at 1081–1029 cm–1 (Table 4). A comparison of different absorbent spectra from studies on the shells performed on different organisms showed insignificant values and still in the same structure and functional groups (Table 4).
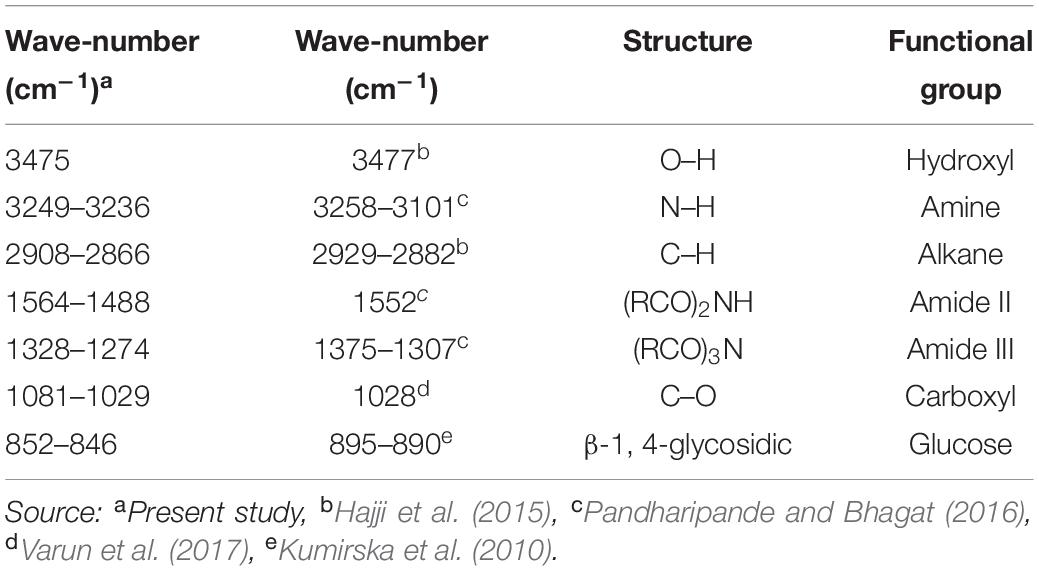
Table 4. Identification of functional groups and bonds based on the peak FTIR on horseshoe crab shell and other crab shells.
Mechanical-Physical and Thermal Characteristics of Shells
The mechanical-physical characteristics of the shell of mangrove horseshoe crabs (C. rotundicauda) have a density of 0.1545 ± 0.011 g/cm3, a water absorption of 0.01195 ± 0.001%, the tensile strength of 0.06 mPa and a compressive strength of 0.11 mPa (Table 5). As shown in Table 5, the value of the mechanical-physical characteristics of the mangrove horseshoe crab (C. rotundicauda) is smaller than in some other crustaceans shells. Mechanical-physical has a correlation characteristic that tends to be positive toward the elongation value (Figures 3A,B). In contrast to the thermal characteristics, the mass negatively correlates with temperature (Figure 3C).
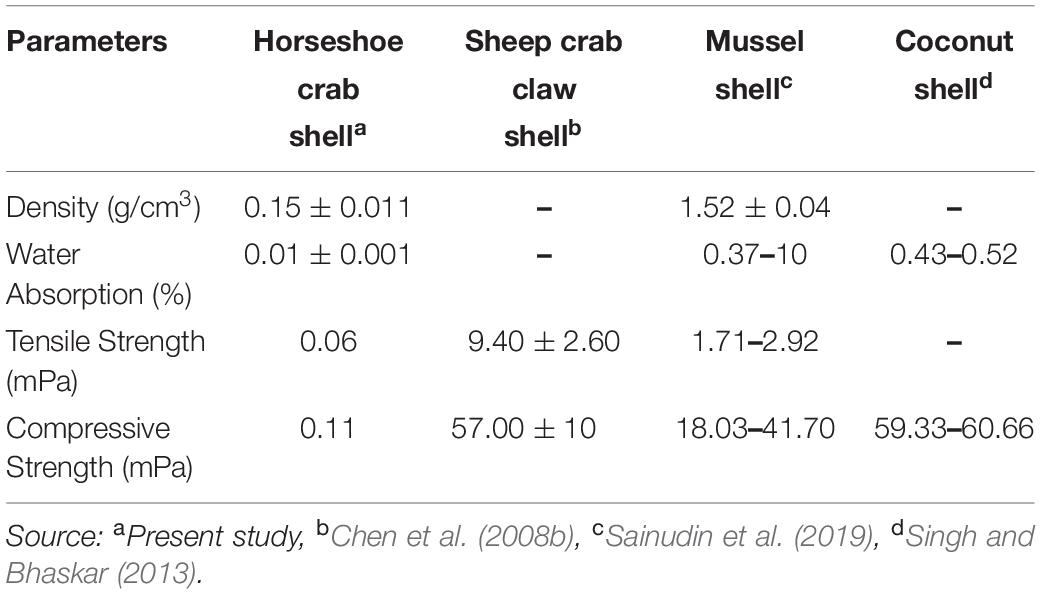
Table 5. Mechanical-physical characteristics of the mangrove horseshoe crab (Carcinoscorpius rotundicauda) and some other crustaceans shells.
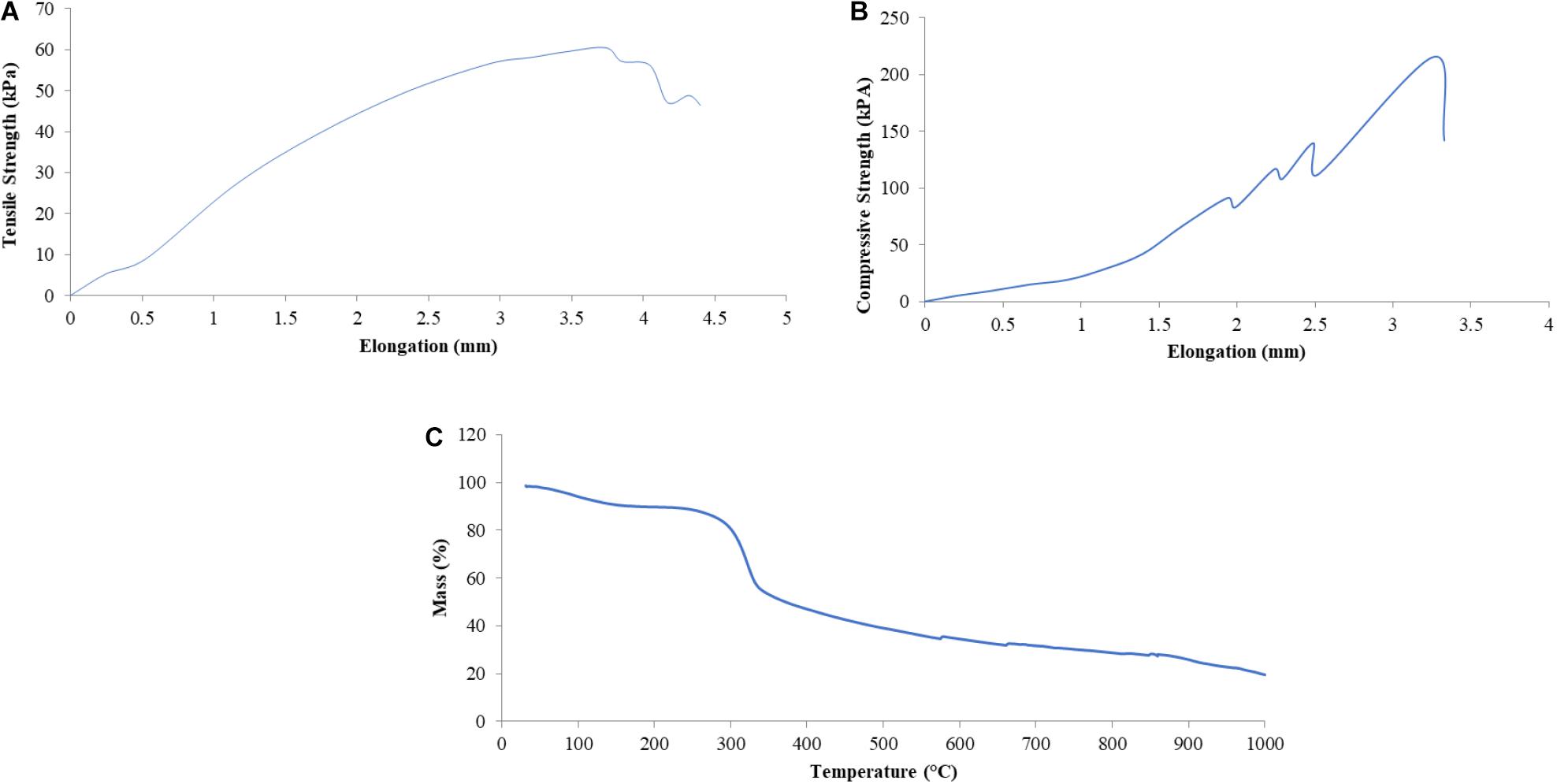
Figure 3. Some mechanical-physical characteristics [Tensile Strength (A), Compressive Strength (B)] and Thermal Resistance (C) of the shell of mangrove horseshoe crabs (Carcinoscorpius rotundicauda).
Discussion
The shell composition matrix of C. rotundicauda has never been studied in detail before, especially the shape of the foam, the chemical composition, the functional groups and the mechanical-physical and thermal properties of the shells give a unique insight which can be used in future. Each species in nature appears in various forms; this form is usually influenced by its unique surrounding environment and evolutionary processes (Jabbari et al., 2014). The shell of mangrove horseshoe crabs has a natural structure in arranging of a very strong foam, especially the β-1, 4-glycosidic (chitin) structure, which forms foam and provides resistance to structural damage and thermal degradation is of high value for future natural materials. Due to this very strong and plastic structure, it has the potential to be further developed for new marine biomaterials. The combination of constituent elements, such as polysaccharides and minerals in the shell of C. rotundicauda needs to be studied through a biomimetic approach as a reference for creating new materials with the same properties from synthetic materials that do not explore excessively against one particular species. The formation and composition of the natural material from the horseshoe crab shell foam are believed to be multifunctional in mobility, used for defense mechanisms and thermal stability. In the future, materials that are strong and able to withstand heat loads can be used as insulation materials in buildings to play a role in reducing thermal loads significantly and effectively to the energy needs of buildings or vehicle interiors and even spacecrafts. Thermal insulation as a building installation element can block the flow of heat energy through energy-saving mechanisms by reducing heat loss and recovery, controlling surface temperature, preventing steam flow, preventing water condensation on cold surfaces and increasing the efficiency of heating-cooling operations. To use natural marine biomaterials will be beneficial because it leaves no harmful residues and therefore has environmental advantages (Silva et al., 2012) and is also more cost-effective (Pati et al., 2020).
Extensive information about this species is published on population structure and morphometry (Koichi Sekiguchi and Shuster, 2009), genetic diversity (Meilana et al., 2016), reproductive system (Brockmann and Smith, 2009), medical applications (Krisfalusi-Gannon et al., 2018), and distribution (Mashar et al., 2017). Horseshoe crabs have a body like a coconut shell, brownish, long spines on the back and hard carapace texture. The shells cover and protect the internal organs of the body (Pratiwi, 1993). The shell hardness seems to be due to naturally occurring constituent elements formed several times during molting (Cousteau, 1975) or through sclerotization. According to Sugumaran (2010), sclerotization is a hardening process of a new cuticle exoskeleton when molting. It involves reactive catecholamine derivatives, which are produced endogenously with structural proteins and chitin fibers. Sclerotization is also known to stabilize the cuticle matrix protein to make it stiffer, harder, more insoluble and more resistant to degradation (Klowden, 2008).
Foam on the horseshoe crabs shell is only found in certain parts, namely the posterior corner of the operculum and all opisthosoma parts. It is concentrated in the marginal spines growth area. The shell structure of horseshoe crabs, which is strengthened by the strut to distribute the pressure evenly to the whole carapace, is believed to have a function as a component for mobility and defense of the horseshoe crab.
The exterior shell forms a Bouligand structure that provides rigidity to the outer side of the shell. The structure is commonly found in arthropod shells (Gadgey and Bahekar, 2017). The structure was proved to repeat, forming the exocuticle and endocuticle (Bouligand, 1972; Giraud-Guille, 1984, 1990; Roer and Dillaman, 1984; Weiner and Addadi, 1997). The intermediate layer consists of a vertical laminate with a thickness of 2–3 μm, which produces elastic characteristics and connects the exterior shell to the interior core. The interior core has a supporting foam structure and a porous shape with a stochastic-patterned strut. Forming elements and the foam structure shape are thought to function to provide mechanical strength and thermal stability to the shell. Theoretically, porous structures with strut nuclei are at least seven times stronger than similar open-cell porous; the width of the cavity in the struts further reduces the weight of the shell (Sullivan et al., 2017). In addition to the layer structure of the horseshoe crabs shell, the shell’s elasticity and thermal stability are believed to be due to the constituent material presence, i.e., chitin or minerals. Horseshoe crab shells (C. rotundicauda) in dry powder have high protein levels, while crude fiber, water, ash and fat are deficient. Generally, the percentage of protein on horseshoe crab shells is 1.99-5.15 times higher than in snow crab shells (34.20%), mud crab shells (14.11%), and crab shells (13.20%) (Table 2). This component affects the element composition of carbon in the shell. The carbon content of C. rotundicauda is also 2.21–3.27 times higher than in some other crustaceans, such as crab shell waste from India (0%) (Bhattacharjee et al., 2019), mud crab from Merauke (19.78%) (Haryati et al., 2019), crab shell from Cirebon (24.19%) (Nazir et al., 2019), and Ammonia beccarii shell (16.36%) (Rositasari et al., 2018; Table 3). According to Cardenas et al. (2003), carbon, oxygen and nitrogen atoms in arthropod shells are generally formed from organic chitin compounds and proteins or inorganic salts such as CaCO3. Existing phosphorus is in the form of phosphate ions (PO43–), associated with Ca2+ ions to form calcium phosphate, which functions to control the physico-chemical properties of the shell (Bentov et al., 2016).
Sclerotization studies have shown that the marine arthropod shell mineral elements act as nuclei to produce cross-bonds between chitin and protein forming composites. A study conducted by Andersen (2010) has shown that sclerotization produces diverse characteristics with varying thickness, stiffness, elasticity and color specifications. The sclerotization results in the shell are used as protection against environmental changes, like pressure and temperature variations (Cardenas et al., 2003).
Uptake at the wave number 3475 cm–1 indicated O–H functional groups. The hydroxy group (O–H) indicates the presence of hydrogen bonds in the sample. Hydrogen bonds produce bands with significant widening. The hydroxy group is one of the most dominant and essential groups for the actual characterization of compounds. The hydroxy group, which is at wave-number 3,400–3,200 cm–1, is a normal polymeric O–H group in the form of stretching (Coates, 2006). The absorption band at the wave-number 3141–3080 cm–1 indicates the N–H stretching functional group.
Furthermore, Coates (2006) added that the N–H group indicated amine components in the sample. The absorption band at wave-numbers of 2,908–2,866 cm–1 indicated the existence of C–H functional groups. Coates (2006) explained that the C–H group located in the absorption band 2,900–2,880 cm–1 belonged to the methylated saturated aliphatic compound. This compound was derived from methane compounds. Also, this compound has four single bonds and one of them is a hydrogen compound. Absorption at wave-numbers 1,564–1,488 cm–1 and 1,328–1,274 cm–1 indicated the presence of amide II and amide III functional groups. Prabu and Natarajan (2012) explained that the amide group’s presence was related to protein compounds in the sample. Absorption of amide groups II and III is ascribed to the N-acetyl or N-methyl groups. Carbohydrates are thought to be the main constituent of the shell acetyl polymer group. The absorption band at wave-number 1,081–1,029 cm–1 indicates the presence of C–O functional groups. Coates (2006) explains that the C–O group indicates a primary alcohol component in the shell.
The shell of horseshoe crabs has its main constituent in chitin polysaccharides with DD% 5.83. Natural chitin is formed mainly as α-chitin and β-chitin (Brunner et al., 2009). The chitin infrared spectrum displays a series of narrow absorption bands, typical of polysaccharide crystal samples. Aung et al. (2018) found a functional group in the FT-IR analysis of crab shells in the form of amide, carbonyl and hydroxyl groups. Pandharipande and Bhagat (2016) stated that the peak of chitin in crab shells from the Nagpur fish market, India was at 3,431–3,430 cm–1 (O–H), 3,258–3,101 cm–1 (N–H stretching), 1,552 cm–1 (amide II), and 1,375–1,307 cm–1 (amide III). While the peak of chitin on crab shells in the Sfax market, Tunisia was shown at 3447 cm–1 (O–H), 3,267–3,104 cm–1 (N–H stretching) and 2,929–2,882 cm–1 (asymmetrical and symmetrical C–H) (Hajji et al., 2015). The peak of chitin in crab shell samples from the Russel fish market, India was at 3,443 cm–1 (O–H stretching), 3,107 cm–1 (N–H stretching), 1,558 cm–1 (amide II), 1,314 cm–1 (amide III), 1,028 cm–1 (C–O stretching) (Varun et al., 2017). Another sign that the compound contains chitin are bending vibrations in the absorption of wave number 852–846 cm–1, which indicates the presence of β-14-glycosidic bonds. Kumirska et al. (2010) explained that the C-H deformation of the β-1.4-glycosidic bond causes a shift in the β-chitin band. Demir et al. (2016) also explained that the wavelength value of 894.91 cm–1 represented the stretching of the bonding ring for the β-1.4-glycosidic bond on chitin of the shell of blue crabs (Callinectes sapidus).
Referring to the previous visual appearance, the unit size of the shell cells was around 70 μm. Low densities can be caused by the proportion of empty spaces (Yunita and Mahyudin, 2017). Hussein et al. (2011) stated that low-density values were seen from the porous shape of the shell of horseshoe crabs support, which is not uniform due to the agglomeration of the constituent material.
Space on the composite allows water flow to enter capillarization, which results in high water absorption strength (Yunita and Mahyudin, 2017). However, the constituent material in chitin polysaccharides influenced the low water absorption capacity of horseshoe crab shells. Kumar et al. (2016) explained that the main components of the shells are chitin. Chitin chains between one and another are bound by a powerful hydrogen bond between the N-H group of one chain and the C=O group of another adjacent chain. This hydrogen bonding causes chitin to not dissolve in water and to form fibers (fibrils).
The horseshoe crab shell tensile strength produces a robust and plastic pattern with a maximum stress of 60.46 kPa, indicated by the tensile stress and long elongation (Figure 3A). Hepburn et al. (1975) elucidated that shell tensile strength is influenced by the polymer of its constituent elements, namely chitin. Chitin is able to form the horseshoe crab shell layer’s entire structure, namely Bouligand, laminate and support system (strut), which provides tensile and compressive strength. As the horseshoe crab shells are composed primarily of chitin and also protein, so chitin affords a tensile strength that is useful in curvature. In contrast, proteins may exist as reinforcement for the inorganic phase and might give rise to prestressed material types (Gadgey and Bahekar, 2017). Prestressed material is concrete that is given internal compressive stress such that it can eliminate the tensile stress caused by external loads to a certain extent. The shell water content also influences tensile strength; dry shells show a lower value of flexibility, strength and toughness than the wet shells (Gadgey and Bahekar, 2017).
Horseshoe crab shells produce a rigid pattern with a maximum compressive strength of 110.55 kPa, indicated by gentle compressive stress with short elongation (Figure 3B). This value is lower than the compressive strength of sheep or spider crab shells. According to Lombardi et al. (2013), the compressive strength value is influenced by the shell density and thickness and the method used. The density and compressive strength of the shell is directly proportional. The shape of the shell also affects the compressive strength value. The sample curvature can distribute the load throughout the structure resulting in a hard and rigid structure. The compressive strength is also influenced by water content and material density. Materials with high water content will reduce the fragile effect (Gadgey and Bahekar, 2017). However, high density can increase strength due to material density (Lombardi et al., 2013).
Thermal stability is produced by horseshoe crab shells of 60.59% in this study. Their decomposition forms a multi-stage decomposition curve, which means it has undergone three decomposition processes. The first decomposition occurred at a temperature range of 254.7–275.6°C with a peak of decomposition at 267.4°C, the second decomposition occurred at a temperature range of 428.6–448.1°C with a peak of decomposition at 435.9°C and the third decomposition occurred at 811.4–835.8°C with a peak of decomposition at 823.2°C. The first and second decomposition peaks (Figure 3C) indicated the degradation of organic compounds in the horseshoe crab shells, such as chitin and protein. Stawski et al. (2008) explained that the temperature of 300–460°C is the temperature range of the occurrence of thermal degradation of chitin polysaccharides from shrimp, crab, krill and squid. The mass loss at temperatures between 200 and 550°C is the temperature range for the degradation of organic and amine groups (Loganathan et al., 2017). Though, the third peak was due to the mineral content in the shell. He et al. (2014) stated the thermal decomposition of calcium carbonate occurs at temperatures around 700°C, with a very large mass reduction approaching 44%, which corresponded to the theoretical decomposition of calcium carbonate. From a thermal point of view, Gbenebor et al. (2016) experimented on the decomposition of crab and shrimp shells according to temperature, and they found the decomposition occurred in three stages. The first decrease occurred at a temperature of 60–110°C due to eliminating water with other volatile components. The second decrease occurred at 316–397°C due to chitin compounds in crab shells and at 321–397°C in shrimp shells. The third reduction of 30.93% in crab shells at 682–729°C and 18.85% in shrimp shells at 665–713°C was caused by the decomposition of CaCO3 to CaO and CO2. The thermal stability of chitin from crabs was 244% and from shrimp 112.5%. The percentage of CaCO3 elimination can increase thermal stability. These results show that the higher CaCO3 content in the crustacean exoskeleton will reduce the thermal stability of chitin. A shell with a lower CaCO3 content will produce more stable chitin. Acetyl (CH3CO) in the acetamide group (CH3CONH2) of the chitin structure provides resistance to structural damage and thermal degradation.
Conclusion
We herewith determined for the first time the compilation matrix and further physico-chemical characteristics of the shell of mangrove horseshoe crabs (C. rotundicauda). The formation and composition of the natural material from the horseshoe crab shell foam are believed to be multifunctional in mobility, used for natural defense mechanisms and thermal stability. The shell of mangrove horseshoe crabs has a hollow structure (foam) with the main chemical elements of chitin, thus providing mechanical-physical properties tensile and compressive strength of the ramps and long elongation. By combining with water absorption strength, density and thermal resistance, the shell structure of the mangrove horseshoe crab has the potential to be used as the base structure to develop bio-foam insulator material (i.e., as thermal insulators), especially the β-1, 4-glycosidic (chitin) structure which forms foam and provides resistance to structural damage and thermal degradation. This is of high value for future materials, for example, as a strong and heat resistant bio-foam insulation material, such as insulation materials in buildings, vehicle interiors, and as blocking material, to block the flow of heat energy against heat loss. Furthermore, biomaterials have a smaller environmental impact than synthetic materials, and here, we expect an improved cost-efficiency. Also, the presented study results can be used as basic information for bio-foam insulator material development, as suggested in the future.
Data Availability Statement
The original contributions presented in the study are included in the article/supplementary material, further inquiries can be directed to the corresponding author/s.
Author Contributions
YW, BR, and NI: conceptualization, methodology, investigation, analysis, resources, data curation visualization and writing – original draft. SK, PF, and FK: supervision and writing – review and editing. All authors contributed to the article and approved the submitted version.
Funding
This research was partially funded by a grant from the Demand-Driven Research Fund (DDRF) No. B-5063/IPK.2/KS.02/III/2019 for YW.
Conflict of Interest
The authors declare that the research was conducted in the absence of any commercial or financial relationships that could be construed as a potential conflict of interest.
Acknowledgments
We want to thank Miss Rani Nuraisah and Naila K. Aini for sample collection.
References
Aini, N. K., Mashar, A., Maduppa, H. H., and Wardiatno, Y. (2020). Genetic diversity of horseshoe crabs (Carcinoscorpius rotundicauda and Tachypleus gigas) in demak, madura and balikpapan waters based on random amplified polymorphic DNA marker. J. Nat. Resour. Environ. Manag. 10, 124–137. doi: 10.29244/jpsl.10.1.124-137
Andersen, S. O. (2010). Insect cuticular sclerotization: a review. Insect Biochem. Mol. Biol. 40, 166–178. doi: 10.1016/j.ibmb.2009.10.007
AOAC (2005). Official Methods of Analysis of the Association of Official Analytical Chemist, 18th Edn. Virginia: The Association of Official Analytical of Chemist (AOAC), inc.
ASTM (1991). ASTM D790M: Standard Test Methods for Flexural Properties of Unreinforced and Reinforced Plastics and Electrical Insulating Material. Philadelphia: American Society for Testing Material (ASTM).
ASTM (2008). ASTM D934: Standard Practices for Identification of Crystalline Compounds in Water Formed Deposits by X-Ray Diffraction. Pennsylvania: American Society for Testing Material (ASTM).
ASTM (2013). ASTM E1252: Standard Practice for General Techniques for Obtaining Infrared Spectra for Qualitative Analysis. Pennsylvania: American Society for Testing Material (ASTM).
Aung, K. P., Win, S. Z., and Thu, S. L. (2018). Study on chitin extraction from crab shells waste. Int. J. Sci. Eng. Appl. 7, 437–441. doi: 10.7753/ijsea0711.1007
Bentov, S., Aflalo, E. D., Tynyakov, J., Glazer, L., and Sagi, A. (2016). Calcium phosphate mineralization is widely applied in crustacean mandibles. Sci. Rep. 6:22118.
Bhattacharjee, B. N., Mishra, V. K., Rai, S. B., Parkash, O., and Kumar, D. (2019). Structure of apatite nanoparticles derived from marine animal (crab) shells: an environment-friendly and cost-effective novel approach to recycle seafood waste. ACS Omega 4, 12753–12758. doi: 10.1021/acsomega.9b00134
Bouligand, Y. (1972). Twisted fibrous arrangements in biological materials and cholesteric meso phases. Tissue Cell 4, 189–217. doi: 10.1016/s0040-8166(72)80042-9
Brockmann, H. J., and Smith, M. D. (2009). “Reproductive competition and sexual selection in horseshoe crabs,” in Biology and Conservation of Horseshoe Crabs, eds J. T. Tanacredi, M. L. Botton, and D. Smith (Boston, MA: Springer).
Brunner, E., Ehrlich, H., Schupp, P., Hedrich, R., Hunoldt, S., Kammer, M., et al. (2009). Chitin based scaffolds are an integral part of the skeleton of the marine demosponge Ianthella basta. J. Struc. Biol. 168, 539–547. doi: 10.1016/j.jsb.2009.06.018
Cardenas, G., Cabrera, G., Taboada, E., and Miranda, S. P. (2003). Chitin characterization by SEM, FTIR, XRD, and 13C cross polarization/mass angle spinning NMR. J. Appl. Polym. Sci. 94, 1877–1885.
Chen, P. Y., Lin, A. Y. M., Lin, Y. S., Seki, Y., Stokes, A. G., Peyras, J., et al. (2008a). Structure and mechanical properties of selected biological materials. J. Mech. Behav. Biomed. Mater. 1, 208–226. doi: 10.1016/j.jmbbm.2008.02.003
Chen, P. Y., Lin, A. Y. M., McKittrick, J., and Meyers, M. A. (2008b). Structure and mechanical properties of crab exoskeletons. Acta Biomaterialia 4, 587–596. doi: 10.1016/j.actbio.2007.12.010
Chen, Y., Lau, C. W., Cheung, S. G., Ke, C. H., and Shin, P. K. S. (2010). Enhanced growth of juvenile Tachypleus tridentatus (Chelicerata: Xiphosura) in the laboratory: a step towards population restocking for conservation of the species. Aquat. Biol. 11, 37–46. doi: 10.3354/ab00289
Coates, J. (2006). “Interpretation of infrared spectra, a practical approach,” in Encyclopedia of Analytical Chemistry: Applications, Theory and Instrumentation, ed. R. A. Meyers (Hoboken, NJ: John Wiley & Sons, Ltd).
Cousteau, J. (1975). The Ocean World of Jacques Cousteau: Attack and Defense. Berlin: The Danbury Press.
Demir, D., Öfkeli, F., Ceylan, S., and Karagülle, N. B. (2016). Extraction and characterization of chitin and chitosan from blue crab and synthesis of chitosan cryogel scaffolds. J. Turk. Chem. Soc. Sect. A Chem. 3, 131–144.
Edgecombe, G. D., and Legg, D. A. (2014). Origins and early evolution of Arthropods. Palaeontology 57, 457–468. doi: 10.1111/pala.12105
Ehrlich, H. (2010). Biological Materials of Marine Origin: Vertebrates. New York, NY: Springer Publishing Company.
Eidman, M., Mayunar, and Redjeki, S. (1997). Pematangan gonad mimi ranti, Carcinoscorpius rotundicauda (Latreille) dan mimi bulan Tachypleus gigas (Müller) dengan berbagai jenis pakan. J. Ilmu Ilmu Perairan Dan Perikanan Indonesia 5, 1–6.
Erwyansyah, Wardiatno, Y., Kurnia, R., and Butet, N. A. (2018). Kepastian taksonomi dan sebaran belangkas Tachypleus tridentatus Leach 1819 di perairan balikpapan timur. J. Ilmu Teknologi Kelautan Tropis 10, 547–559. doi: 10.29244/jitkt.v10i3.21917
Gadgey, K. K., and Bahekar, D. A. (2017). Investigation of mechanical properties of crab shell: a review. Int. J. Latest Trends Eng. Technol. 8, 268–281.
Gbenebor, O. P., Adeosun, S. O., Lawal, G. I., and Jun, S. (2016). Role of CaCO3 in the physicochemical properties of crustacean-sourced structural polysaccharides. Mater. Chem. Phys. 184, 203–209. doi: 10.1016/j.matchemphys.2016.09.043
Giraud-Guille, M. M. (1984). Fine structure of the chitin–protein system in the crab cuticle. Tissue Cell 16, 75–92. doi: 10.1016/0040-8166(84)90020-x
Giraud-Guille, M. M. (1990). Chitin crystals in arthropod cuticles revealed by diffraction contrast transmission electron microscopy. J. Struct. Biol. 103, 232–240. doi: 10.1016/1047-8477(90)90041-a
Giribet, G. (2018). Current views on chelicerate phylogeny—a tribute to peter weygoldt. Zool. Anzeiger 273, 7–13. doi: 10.1016/j.jcz.2018.01.004
Hajji, S., Bellaaj, O. G., Younes, I., Jellouli, K., and Nasri, M. (2015). Chitin extraction from crab shells by Bacillus bacteria. Biological activities of fermented crab supernatants. Int. J. Biol. Macromol. 79, 167–173. doi: 10.1016/j.ijbiomac.2015.04.027
Haryati, E., Dahlan, K., Togibasa, O., and Dahlan, K. (2019). Protein and minerals analyses of mangrove crab shells (Scylla serrata) from Merauke as a foundation on bio-ceramic components. J. Phys.: Conf. Ser. 1204:012031. doi: 10.1088/1742-6596/1204/1/012031
He, X. Y., Jun, W., Ramdani, N., and Liu, W. (2014). Effect of crab shell particles on the thermomechanical and thermal properties of polybenzoxazine matrix. Mater. Design 61, 1–7. doi: 10.1016/j.matdes.2014.04.058
Hepburn, H. R., and Ball, A. (1973). On the structure and mechanical properties of beetle shells. J. Mat. Sci. 8, 618–623. doi: 10.1007/bf00561216
Hepburn, H. R., and Joffe, I. (1974a). Hardening of locust sclerites. J. Insect Physiol. 20, 631–635. doi: 10.1016/0022-1910(74)90183-8
Hepburn, H. R., and Joffe, I. (1974b). Locust solid cuticle-a time sequence of mechanical properties. J. Insect Physiol. 20, 497–506. doi: 10.1016/0022-1910(74)90158-9
Hepburn, H. R., Joffe, I., Green, N., and Nelson, K. J. (1975). Mechanical properties of a crab shell. Comp. Biochem. Physiol. A 50, 551–554. doi: 10.1016/0300-9629(75)90313-8
Humaira, S., Karnila, R., and Loekman, S. (2017). Komposisi kimia tepung cangkang kepiting bakau (Scylla serrata). J. Online Mahasiswa Bidang Perikanan dan Ilmu Kelautan 5, 1–8.
Hussein, A. A., Salim, R. D., and Sultan, A. A. (2011). Water absorption and mechanical properties of high-density polyethylene/egg shell composite. J. Basrah Res. 37, 36–42.
Ismail, N., Nadzari, F., Huda, I., Nazeerah, A. G., Taib, M. A. R., Chatterji, A., et al. (2011). “Potential of antibacterial and antifouling from horseshoe crabs, Tachypleus gigas and Carcinoscorpius rotundicauda against gram-positive and negative bacteria,” in Proceedings of the Universiti Malaysia Terengganu 10th International Annual Symposium (UMTAS 2011), (Terengganu: Kuala Terengganu).
Jabbari, E., Kim, D. H., Lee, L. P., Ghaemmaghami, A., and Khademnhosseini, A. (2014). Handbook of Biomimetics and Bioinspiration Biologically-Driven Engineering of Materials, Processes, Devices and Systems. Singapore: World Scientific.
Jensen, M., and Weis-Fogh, T. (1962). Biology and physics of locust flight. V. Strength and elasticity of locust cuticle. ıPhilos. Trans. R. Soc. B 245, 137–169. doi: 10.1098/rstb.1962.0008
Joffe, I., and Hepburn, H. R. (1974). A simple low-cost tensometer for bio-materials testing. Experientia 30, 113–114. doi: 10.1007/bf01921633
Joffe, I., Hepburn, H. R., and Anderson, S. O. (1975). On the mechanical properties of Limulus solid cuticle. J. Comp. Physiol. 101, 147–160. doi: 10.1007/bf00694155
John, B. A., Nelson, B. R., Sheikh, H. I., Cheung, S. G., Wardiatno, Y., Dash, B. P., et al. (2018a). A review on fisheries and conservation status of Asian horseshoe crabs. Biodivers. Conserv. 27, 3573–3598. doi: 10.1007/s10531-018-1633-8
John, B. A., Nelson, B., Sheikh, H., Cheung, S., Wardiatno, Y., Dash, B. P., et al. (2018b). Correction to: a review on fisheries and conservation status of Asian horseshoe crabs. Biodivers. Conserv. 27:3845. doi: 10.1007/s10531-018-1650-7
Kassim, Z., Wan Murni, W. N. K., Razak, M. R. M., Wan Omar, W. S., and Adam, S. B. (2018). Chitosan isolated from horseshoe crab Tachypleus gigas from the Malay Peninsula. Orient. J. Chem. 34, 928–933. doi: 10.13005/ojc/340242
Klamczynski, A., Wood, D., and Holtman, K. (2007). Cellulose fiber reinforced starch-based foam composites. J. Biobased Mater. Biol. 1, 360–366. doi: 10.1166/jbmb.2007.010
Koichi Sekiguchi, and Shuster, C. N. (2009). “Limits on the global distribution of horseshoe crabs (Limulacea): lessons learned from two lifetimes of observations: asia and America,” in Biology and Conservation of Horseshoe Crabs, eds J. T. Tanacredi, M. L. Botton, and D. Smith (Boston, MA: Springer).
Krisfalusi-Gannon, J., Ali, W., Dellinger, K., Robertson, L., Brady, T. E., Goddard, M. K. M., et al. (2018). The role of horseshoe crabs in the biomedical industry and recent trends impacting species sustainability. Front. Mar. Sci. 5:185. doi: 10.3389/fmars.2018.00185
Kumar, V., Roy, S., Sahoo, A. K., and Kumar, V. (2016). Horseshoe crabs: biomedical importance and its potential use in developing health-care products. Indian J. Geo Mar. Sci. 45, 1234–1244.
Kumirska, J., Czerwicka, M., Kaczyński, Z., Bychowska, A., Brzozowski, K., Thöming, J., et al. (2010). Application of spectroscopic methods for structural analysis of chitin and chitosan. Mar. Drugs 8, 1567–1636. doi: 10.3390/md8051567
Loganathan, S., Babu, V. R., Mishra, R. K., and Pugazhenthi, G. (2017). Thermal and Rheological Measurement Techniques for Nanomaterials Characterization, Edition: 1. Amsterdam: Elsevier.
Lombardi, S. A., Chon, G. D., Lee, J. J. W., Lane, H. A., and Paynter, K. T. (2013). Shell hardness and compressive strength of the eastern oyster, Crassostrea virginica, and the Asian oyster, Crassostrea ariakensis. Biol. Bull. 225, 175–183. doi: 10.1086/bblv225n3p175
Mashar, A., Butet, N. A., Juliandi, B., Qonita, Y., Hakim, A. A., and Wardiatno, Y. (2017). Biodiversity and distribution of horseshoe crabs in northern coast of Java and southern coast of Madura. IOP Conf. Ser. Earth Environ. Sci. 54:012076. doi: 10.1088/1755-1315/54/1/012076
Meilana, L., Wardiatno, Y., Butet, N. A., and Krisanti, M. (2016). Karakter morfologi dan identifikasi molekuler dengan marka gen COI pada mimi (Tachypleus gigas) di perairan Utara Pulau Jawa. J. Ilmu dan Teknol. Kelautan Tropis 8, 145–158.
Meyers, M. A., Chen, P. Y., Lin, A. Y. M., and Seki, Y. (2008). Biological materials: structure and mechanical properties. Prog. Mater. Sci. 53, 1–206.
Nazir, M., Rahmawati, T., and Dara, F. (2019). Synthesis and characterization of biochar from crab shell by pyrolysis. IOP Conf. Ser.: Mater. Sci. Eng. 553:012031. doi: 10.1088/1757-899x/553/1/012031
Nuraisah, R., Aini, N. K., Mashar, A., Zairion, Z., Hastuti, Y. P., Funch, P., et al. (2020). Food habit of horseshoe crabs, Tachypleus gigas (Müller, 1785) and Carcinoscorpius rotundicauda (Latreille, 1802) in Balikpapan coastal waters, East kalimantan. J. Nat. Resour. Environ. Manag. 10, 153–162. doi: 10.29244/jpsl.10.2.153-162
Pandharipande, S. L., and Bhagat, P. H. (2016). Synthesis of chitin from crab shells and its utilization in preparation of nanostructured film. Int. J. Sci. Eng. Technol. Res. 5, 1378–1383.
Pati, S., Chatterji, A., Dash, B. P., Raveen Nelson, B., Sarkar, T., Shahimi, S., et al. (2020). Structural characterization and antioxidant potential of chitosan by gamma-irradiation from the carapace of horseshoe crab. Polymers 12:2361. doi: 10.3390/polym12102361
Pires, C., Marques, A., Carvalho, M. L., and Batista, I. (2017). Chemical characterization of Cancer pagurus, Maja squinado, Necora puber and Carcinus maenas shells. Poult. Fish. Wildl. Sci. 5:181.
Prabu, K., and Natarajan, E. (2012). Isolation and FTIR spectroscopy characterization of chitin from local sources. Adv. Appl. Sci. Res. 3, 1870–1875.
Roer, R., and Dillaman, R. (1984). The structure and calcification of the crustacean cuticle. Am. Zool. 24, 893–909. doi: 10.1093/icb/24.4.893
Rositasari, R., Suratno, and Yogaswara, D. (2018). Determination on the chemical composition of Ammonia beccarii shell using SEM and EDX: Preliminary study of benthic foraminifera capacity in response to anthropogenic metal contamination in coastal areas. IOP Conf. Ser.: Earth Environ. Sci. 118:012056. doi: 10.1088/1755-1315/118/1/012056
Sainudin, M. S., Othman, N. H., and Shahidan, S. (2019). Performance of concrete containing mussel shell (Pernaviridis) ash under effect of sodium chloride curing. IOP Conf. Ser.: Mater. Sci. Eng. 601:012033. doi: 10.1088/1757-899x/601/1/012033
Sanchez, C., Arribart, H., and Giraud-Guille, M. M. (2005). Biomimetism and bioinspiration as tools for the design of innovative materials and systems. Nat. Mat. 4, 277–288. doi: 10.1038/nmat1339
Schmidt, V. C. R., and Laurindo, J. (2009). Water absorption and mechanical properties of starch foam trays impregnated with starch acetate. Braz. J. Food Technol. 12, 34–42. doi: 10.4260/bjft2009260700005
Silva, T. H., Alves, A., Ferreira, B. M., Oliveira, J. M., Reys, L. L., Ferreira, R. J. F., et al. (2012). Materials of marine origin: a review on polymers and ceramics of biomedical interest. Int. Mater. Rev. 57, 276–306. doi: 10.1179/1743280412y.0000000002
Singh, V. K., and Bhaskar, J. (2013). Water absorption and compressive properties of coconut shell particle reinforced-epoxy composite. J. Mater. Environ. Sci. 4, 113–118.
Stawski, D., Rabief, S., Herczyska, L., and Draczyski, Z. (2008). Thermogravimetric analysis of chitins of different origin. J. Therm. Anal. Colorim. 93, 489–494. doi: 10.1007/s10973-007-8691-6
Sugumaran, M. (2010). Chemistry of cuticular sclerotization. Adv. In Insect Phys. 39, 151–209. doi: 10.1016/b978-0-12-381387-9.00005-1
Sullivan, T. N., Wang, B., Espinosa, E. D., and Meyers, M. A. (2017). Extreme lightweight structures: avian feathers and bones. Mater. Today 20, 377–391. doi: 10.1016/j.mattod.2017.02.004
Sumarmin, R., Razak, A., and Fajri, M. I. (2017). Morfometri kepiting tapal kuda dari daerah sungai nipah dan air bangis sumatera barat. J. Biosains 1, 24–32.
Tanasi, D., Greco, E., Di Tullio, V., Capitani, D., Gullì, D., and Ciliberto, E. (2017). 1H-1H NMR 2D-TOCSY, ATR FT-IR and SEM-EDX for the identification of organic residues on Sicilian prehistoric pottery. Microchem. J. 135, 140–147. doi: 10.1016/j.microc.2017.08.010
Varun, K., Senani, S., Kumar, N., Gautam, M., Gupta, R., and Gupta, M. (2017). Extraction and characterization of chitin, chitosan and chitooligosaccharides from crab shell waste. Indian J. Anim. Res. 51, 1066–1072.
Vincent, J. F. V. (2002). Arthropod cuticle: a natural composite shell system. Composites Part A Appl. Sci. Manuf. 33, 1311–1315. doi: 10.1016/s1359-835x(02)00167-7
Vincent, J. F. V., and Wegst, U. G. K. (2004). Design and mechanical properties of insect cuticle. Arthropod. Struct. Dev. 33, 187–199. doi: 10.1016/j.asd.2004.05.006
Wang, C.-C., Kwan, K. Y., Shin, P. K. S., Cheung, S. G., Itaya, S., Iwasaki, Y., et al. (2020). Future of Asian horseshoe crab conservation under explicit baseline gaps: A global perspective. Glob. Ecol. Conserv. 24:e01373. doi: 10.1016/j.gecco.2020.e01373
Wegst, U. G. K., and Ashby, M. F. (2004). The mechanical efficiency of natural materials. Philos. Mag. 84, 2167–2186. doi: 10.1080/14786430410001680935
Weiner, S., and Addadi, L. (1997). Design strategies in mineralized biological materials. J. Mater. Chem. 7, 689–702. doi: 10.1039/a604512j
Yunita, D., and Mahyudin, A. (2017). Pengaruh persentase serat bambu terhadap sifat fisik dan mekanik papan beton ringan. J. Fisika Unand 6, 348–354. doi: 10.25077/jfu.6.4.348-354.2017
Keywords: chitin, mechanical properties, shell structure, thermal stability, Chelicerata
Citation: Wardiatno Y, Riyanto B, Iskandar NA, Kleinertz S, Funch P and Kurniawan F (2021) A New Marine Biomaterial: The Shell of Mangrove Horseshoe Crabs, Carcinoscorpius rotundicauda (Latreille, 1802) Emphasizing Its Physico-Chemical Characteristics. Front. Mar. Sci. 8:612016. doi: 10.3389/fmars.2021.612016
Received: 05 October 2020; Accepted: 13 January 2021;
Published: 05 February 2021.
Edited by:
Siu Gin Cheung, City University of Hong Kong, Hong KongReviewed by:
Angela Cuttitta, National Research Council (CNR), ItalyAdrianna Ianora, University of Naples Federico II, Italy
Copyright © 2021 Wardiatno, Riyanto, Iskandar, Kleinertz, Funch and Kurniawan. This is an open-access article distributed under the terms of the Creative Commons Attribution License (CC BY). The use, distribution or reproduction in other forums is permitted, provided the original author(s) and the copyright owner(s) are credited and that the original publication in this journal is cited, in accordance with accepted academic practice. No use, distribution or reproduction is permitted which does not comply with these terms.
*Correspondence: Yusli Wardiatno, eXVzbGlAYXBwcy5pcGIuYWMuaWQ=