- 1Institute for Marine and Antarctic Studies, University of Tasmania, Hobart, TAS, Australia
- 2Australian Antarctic Division, Department of Agriculture, Water and the Environment, Kingston, TAS, Australia
- 3British Antarctic Survey, Natural Environment Research Council, Cambridge, United Kingdom
- 4CSIRO Oceans and Atmosphere, Hobart, TAS, Australia
- 5Sydney Institute of Marine Science, Mosman, NSW, Australia
- 6Department of Primary Industries, Parks, Water and Environment, Hobart, TAS, Australia
Climate change, fisheries and invasive species represent three pervasive threats to seabirds, globally. Understanding the relative influence and compounding nature of marine and terrestrial threats on the demography of seabird communities is vital for evidence-based conservation. Using 20 years of capture-mark-recapture data from four sympatric species of albatross (black-browed Thalassarche melanophris, gray-headed T. chrysostoma, light-mantled Phoebetria palpebrata and wandering Diomedea exulans) at subantarctic Macquarie Island, we quantified the temporal variability in survival, breeding probability and success. In three species (excluding the wandering albatross because of their small population), we also assessed the influence of fisheries, oceanographic and terrestrial change on these rates. The Southern Annular Mode (SAM) explained 20.87–29.38% of the temporal variability in survival in all three species and 22.72–28.60% in breeding success for black-browed and gray-headed albatross, with positive SAM events related to higher success. The El Niño Southern Oscillation (ENSO) Index explained 21.14–44.04% of the variability in survival, with higher survival rates following La Niña events. For black-browed albatrosses, effort in south-west Atlantic longline fisheries had a negative relationship with survival and explained 22.75–32.21% of the variability. Whereas increased effort in New Zealand trawl fisheries were related to increases in survival, explaining 21.26–28.29 % of variability. The inclusion of terrestrial covariates, reflecting extreme rainfall events and rabbit-driven habitat degradation, explained greater variability in trends breeding probability than oceanographic or fisheries covariates for all three species. These results indicate managing drivers of demographic trends that are most easily controlled, such as fisheries and habitat degradation, will be a viable option for some species (e.g., black-browed albatross) but less effective for others (e.g., light-mantled albatross). Our results illustrate the need to integrate fisheries, oceanographic and terrestrial processes when assessing demographic variability and formulating the appropriate management response.
Introduction
Climate-driven changes in the marine ecosystem affect animal populations in complex ways (Constable et al., 2014), yet understanding how populations respond to environmental change is vital for predicting their viability (Vargas et al., 2007). In the Southern Ocean, climate change is driving regional trends in sea ice extent and seasonality (Stammerjohn et al., 2012), causing major oceanic fronts to move southwards (Sokolov and Rintoul, 2009), increasing wind intensity (Turner et al., 2009) and enhancing eddy activity (Meredith and Hogg, 2006). Furthermore, large-scale climate cycles such as the Southern Annular Mode (SAM) and the El Niño Southern Oscillation (SOI) are also influenced by climate change, with demographic consequences predicted for multiple species (Forcada and Trathan, 2009).
For marine predators, changes in physical oceanographic and atmospheric conditions may influence individual foraging efficiency by affecting the accessibility and predictability of prey (Lea et al., 2006), or the energetic cost of reaching foraging areas (Weimerskirch et al., 2012). However, these changes are occurring simultaneously with fisheries that overlap with the foraging habitat of numerous seabird species (Tuck et al., 2003). Through incidental mortality (bycatch) (Anderson et al., 2011), discarding of non-target catch and offal (Bartumeus et al., 2010) and increased competition for resources (Cury et al., 2011), fisheries can influence survival or induce changes in foraging behavior that affects breeding frequency or success. For small populations, simultaneous negative effects on both survival and reproductive output can drive rapid declines and lead to extirpation (Weller et al., 2014).
Information about the processes that influence demographic variability is fundamental to evidence-based conservation. However, for species such as albatross that are highly philopatric, long-ranging, pelagic foragers, it can be difficult to quantify these processes. Albatrosses have a delayed onset of maturity (typically > 10 years), after which breeding occurs annually or biennially (Jouventin and Dobson, 2002). Adult survival is high, with individuals of some species living up to 50 years (Weimerskirch et al., 1987). This means any increase in mortality or decrease in reproductive output has considerable consequences for population viability, even if these take some time to become visible in the population (Phillips et al., 2016).
Decreases in adult survival due to fisheries bycatch are the greatest contributor to the rapid, widespread declines of several albatross populations (Tuck et al., 2001; Phillips et al., 2016). Implementation of technological solutions that mitigate bycatch, such as the use of exclusion devices (Maree et al., 2014), integrated-weight lines (Robertson et al., 2010) or underwater line-setters (Gilman et al., 2003) have slowed or stopped declines, with minor increases at some colonies (Inchausti and Weimerskirch, 2001; Robertson et al., 2014). However, as their distributions often span multiple fisheries management boundaries, there is wide variation in the implementation of mitigation measures (Phillips et al., 2016).
Albatrosses also face terrestrial pressures including habitat degradation and effects of invasive species. Introduced mammals are particularly problematic for seabirds through predation (Cuthbert and Hilton, 2004), competition for habitat (Brothers and Bone, 2008) or habitat degradation (Cleeland et al., 2020).
With different life-history traits, each albatross species within a community will respond differently to environmental variation, be it marine or terrestrial. Integrated studies addressing the effects of both at-sea (e.g., oceanographic and fisheries) and terrestrial factors (e.g., habitat quality, weather and invasive species) on the demographic rates of multiple species allow rates to be compared and contrasted, providing a comprehensive understanding of albatross demography. Quantification of marine and terrestrial influences provides the evidence base to support conservation action by reducing uncertainty. Specifically, it allows the identification of drivers that are more easily managed (e.g., invasive species, fisheries), and which buffer against those that are not (e.g., climate change).
Four species of Southern Ocean albatross breed at Macquarie Island; black-browed Thalassarche melanophris, gray-headed Thalassarche chrysostoma, light-mantled Phoebetria palpebrata, and wandering albatrosses Diomedea exulans. With the exception of light-mantled albatrosses, these populations are small (< 80 breeding pairs), and therefore at risk of local extinction. At Macquarie Island, declines in adult survival of wandering albatrosses have been linked to bycatch in tuna longline fisheries (de la Mare and Kerry, 1994; Terauds et al., 2006), although no such links have been established for black-browed or gray-headed albatrosses (Terauds et al., 2005). More recently, declines in the breeding probability of black-browed, gray-headed and light-mantled albatrosses have been attributed to wide-scale habitat degradation caused by invasive rabbits Oryctolagus cuniculus; for black-browed albatrosses, the combination of heavy rainfall events and rabbit density also caused substantial reductions in breeding success (Cleeland et al., 2020).
To understand the influence of at-sea and terrestrial factors on demography of the Macquarie Island albatross community, we aimed to: (1) determine annual trends in survival, breeding probability and breeding success of each species; (2) compare and contrast demographic variability across species, and; (3) assess the relative influence of various environmental processes on demography.
Materials and Methods
Field Methods
We analyzed 20 years (1995–2014) of capture-mark-recapture data from four albatross species breeding at subantarctic Macquarie Island (54.6° S, 158.9° E). There are approximately 40, 80 and five breeding pairs of black-browed, gray-headed and wandering albatrosses, respectively, at Macquarie Island (DPIPWE, 2014). In contrast, there are approximately 2150 breeding pairs of light-mantled albatross (DPIPWE, 2014). All accessible albatross chicks of each species were banded with stainless steel leg bands between 1995 and 2014 (seasons 1994/95 and 2013/14), except for light-mantled albatross, where this applied only to chicks within seven study areas (∼10% of total island breeding population). From 1994 to 2003, any unbanded adults that could be captured safely were also banded. Checks in early incubation allowed breeding adults to be identified, and at the end of chick-rearing to band chicks and quantify fledging rates. Any banded nonbreeding adults were also recorded. All field procedures were approved by the Tasmanian Government Department of Primary Industries, Parks, Water and Environment’s Animal Ethics Committee and were carried out in accordance with the approved guidelines (permit number TFA9195).
Demographic Analysis
Demographic parameters – survival (Φ), return (r), breeding probability (β), breeding success (γ), and detection probability (p) – were estimated using a hidden Markov model framework, in which it was assumed individuals transition between different states (e.g., breeder, nonbreeder, etc.; see Supplementary Figure 1a) over time, with transitions between states described by a Markov chain. The state of an individual was assumed to be observed indirectly by means of an event (hereafter referred to as an encounter). Encounters were assumed to be dependent on the true, but unobserved, state of the individual. Encounter histories for each individual were used to fit a population-level model, with model parameters related to environmental parameters as described below. Models were fitted in the E-Surge 1.9.0 software (Choquet et al., 2009b) using maximum likelihood estimation.
Individual encounter histories (total birds: black-browed, n = 225; gray-headed, n = 513; light-mantled, n = 1215; and wandering albatross, n = 78) consisted of four observable adult states (successful breeder, failed breeder, nonbreeder and breeder with uncertain breeding outcome) and three unobservable states (post-successful breeder, post-failed breeder, post non-breeder) (Supplementary Figure 1a). The unobservable post-breeding states were included to account for differences in year-to-year transitional breeding probability (ψ) generated by biennial breeding behavior (Barbraud and Weimerskirch, 2012). A small proportion of breeding outcomes were unknown, as the nest was not revisited late in the breeding season (1.5%, 1.1%, 1.3%, and 2.3% of observations for black-browed, gray-headed, light-mantled and wandering albatrosses). State uncertainty was incorporated in an event matrix, allowing the likelihood of being a successful breeder to be assessed (Gimenez et al., 2012).
To remain tractable but functional due to the small population sizes, the analysis was restricted to adult demographic parameters by suppressing all chick, juvenile and pre-breeding states before the first breeding attempt. It was also assumed demographic parameters were not affected by age, experience, sex or quality. Further, we expected similar survival, regardless of state. For gray-headed, light-mantled and wandering albatrosses (biennial breeders) the probabilities of return and breeding for successful breeders were estimated separately from those of failed and nonbreeders for the time-dependent models (Supplementary Table 1). In contrast, estimates for black-browed albatrosses (annual breeders) were derived using a time-dependent (t) model for all demographic parameters. For all species, time-dependent parameters were retained when modeling the demographic parameter of interest.
Nest accessibility for gray-headed and light-mantled albatrosses was affected by severe habitat degradation due to heavy rabbit grazing during the 2000s, influencing detection probability (p), and so rabbit density was included as a covariate, accordingly (Supplementary Table 1). Detection probabilities were also estimated separately for breeders and nonbreeders because bands are easier to read on the former (Supplementary Figure 2). Complete model input notation, probability estimation and transition matrix structures are provided in Supplementary Figure 1, Supplementary Table 1, and Supplementary Appendix 1.
To test for goodness-of-fit, encounter histories were transformed to a single-state and assessed using U-Care 2.3.2 software, including tests for transience (Choquet et al., 2009a). Tests for trap-dependence (Test 2: 2.CT + 2.Cl) were excluded since the model structure accounted for differences in breeding probability based on previous breeding states; characteristic of species that defer breeding in some years (Barbraud and Weimerskirch, 2012). Correction for lack of fit within the populations was applied using an over-dispersion factor (ĉ; Lebreton et al., 1992).
A sequence of models was fitted for each species to disentangle the various influences on demography. First, models were fit using each predictor variable in turn. Significant covariates were then grouped into models that included fisheries, oceanographic or terrestrial covariates, to provide an integrated overview of their effects on demographic rates. Finally, all significant covariates were integrated into a global model to determine the total demographic variability that could be explained by the covariates. Prior to their inclusion in the models environmental covariates were tested for collinearity using a Pearson’s correlation matrix in R (R Core Team, 2016). Variables with a correlation coefficient of > 0.8 were excluded from the demographic models.
The significance of environmental covariates for each demographic parameter was evaluated using analysis of deviance tests (ANODEV), and the strength of the effect ascertained by considering the total variation explained by the covariates (Grosbois et al., 2008). This analysis quantifies how much of the annual variation in a demographic parameter can be explained by annual environmental covariates and therefore does not express a direct link between environmental drivers and population level outcomes.
Wandering albatrosses were excluded from the environmental analysis due to their small population size. Base models described the total variation explained by the covariates (%R2). In addition, because long-term temporal trends in the covariate and demographic rate can obscure their relationship, detrended models that provide a measure of short-term variation were implemented, which described the total variation explained by the covariates when temporal trends were removed (detrended %R2). The base and detrended models are regarded as explaining long-term and short-term variability, respectively. Model selection was based on Akaike’s information criterion (AIC), whereby the model with the lowest AIC (where ΔQAIC > 2) was preferred (Burnham and Anderson, 2002).
Covariates
Fisheries and oceanographic covariates were taken from the foraging distribution of each species determined by previous tracking studies (Figure 1; Cleeland et al., 2019). Fisheries covariates represent monthly totals, whereas each oceanographic covariate consisted of a time series of values averaged from daily and monthly data to give an annual mean. The temporal range of each environmental covariate was aligned to the appropriate stage of the breeding cycle for each species (Supplementary Table 2).
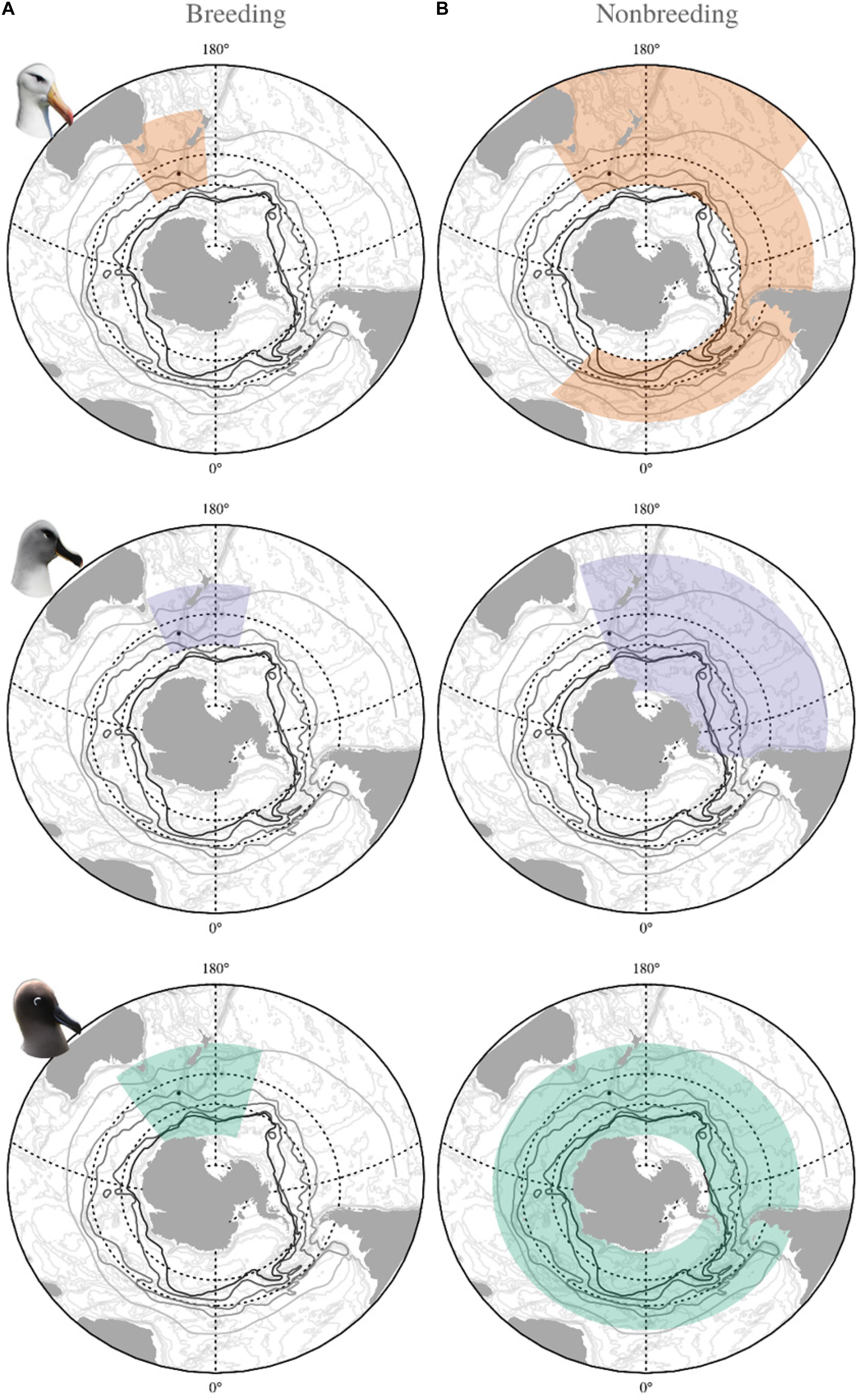
Figure 1. Spatial extent of oceanographic covariates incorporated in the demographic modeling of black-browed (orange), gray-headed (purple) and light-mantled (green) albatross from Macquarie Island during (A) breeding, and (B) nonbreeding periods.
Oceanographic covariates were obtained as monthly sea ice extent (ice), sea surface temperature (sst), sea surface height anomaly (ssha), wind speed (wind), meridional wind (wind_v) and zonal wind (wind_u). For albatrosses, these oceanographic parameters may directly affect the energetic costs of flight to foraging grounds, or indirectly by influencing prey availability. Sea ice conditions around Antarctica are tightly coupled with primary productivity and the abundance of salps and krill (Loeb et al., 1997). Furthermore, the influence of sea surface temperature on foraging conditions is known demographic parameters and population growth rate in albatrosses (Jenouvrier et al., 2018). Similarly, sea surface height anomalies provide a measure of eddy activity, particularly at frontal regions between cold and warm eddies, and are known to aggregate prey and influence foraging conditions for seabirds (Bost et al., 2009).
Large-scale climate indices – the Southern Annular Mode (SAM) and Southern Oscillation Index (SOI) – were also included in the models. Both the SAM and SOI influence seabird demography by modifying ocean circulation patterns, which in turn influences primary production (Trathan et al., 2007). Furthermore, changes in the Southern Ocean wind regime, associated with the SAM, can have a direct effect on the energetic cost of flight and therefore foraging (Weimerskirch et al., 2012). As temporal lags are likely to exist between changes in SAM and SOI and the responses of prey populations and the vital rates of seabirds, biannual lags from 2 to 3 years were tested for SAM (Barbraud and Weimerskirch, 2003) and half to one and a half years for SOI (Lovenduski and Gruber, 2005).
Fisheries effort within the breeding and nonbreeding distributions was included in models of black-browed and gray-headed albatross survival. These species commonly associate with vessels and are killed incidentally by fishing gear (Phillips et al., 2016). Fisheries effort was a proxy for elevated mortality risk, although discards provide a food resource, from which the benefit may outweigh the negative effects of bycatch (Granadeiro et al., 2014). Fisheries covariates were excluded from models for light-mantled albatrosses because they are not regularly caught as bycatch and are thought to be competitively excluded from attending fisheries vessels (Phillips et al., 2005). Fisheries covariates comprised monthly trawl and longline effort at 5 × 5 degree resolution obtained from regional fisheries management organizations in the form of the number of hooks set, or of hours trawled. Effort data included in the modeling was limited to national and international fisheries with reported evidence of albatross attendance at or interactions with vessels within each fleet.
Terrestrial covariates from Cleeland et al. (2020) were included in the global breeding probability and breeding success models to evaluate the relative importance of conditions at the breeding colony. These comprised rabbit density (a proxy for nesting habitat degradation), and extreme rainfall events during early chick-rearing. The latter represents the number of days in January of rainfall in the 80th percentile of the long-term average and is tested on breeding success to capture the potential these events have on reducing survival of young chicks at the nest.
Results
Comparative Demographic Rates
The Macquarie Island albatross species have differing long-term population trends: black-browed and light-mantled albatrosses are increasing, wandering albatrosses are decreasing, and gray-headed albatrosses are stable (Figure 2). Of these, black-browed albatrosses had the lowest adult survival (Φ = 0.914 ± 0.007) and breeding success (γ= 0.516 ± 0.015) (Table 1), and wandering albatrosses had the lowest breeding probability (β = 0.738 ± 0.039). A negative trend in adult survival, seen in the slope of the linear model, was only evident for wandering albatrosses (Φ = −0.56 ± 0.29) (Supplementary Table 3). Black-browed, gray-headed and wandering albatross breeding probability decreased during the study period (β = −0.89 ± 0.15, β = −1.17 ± 0.18 and β = −0.62 ± 0.22). Modeled breeding success of the four species showed no trend between 1995 and 2014.
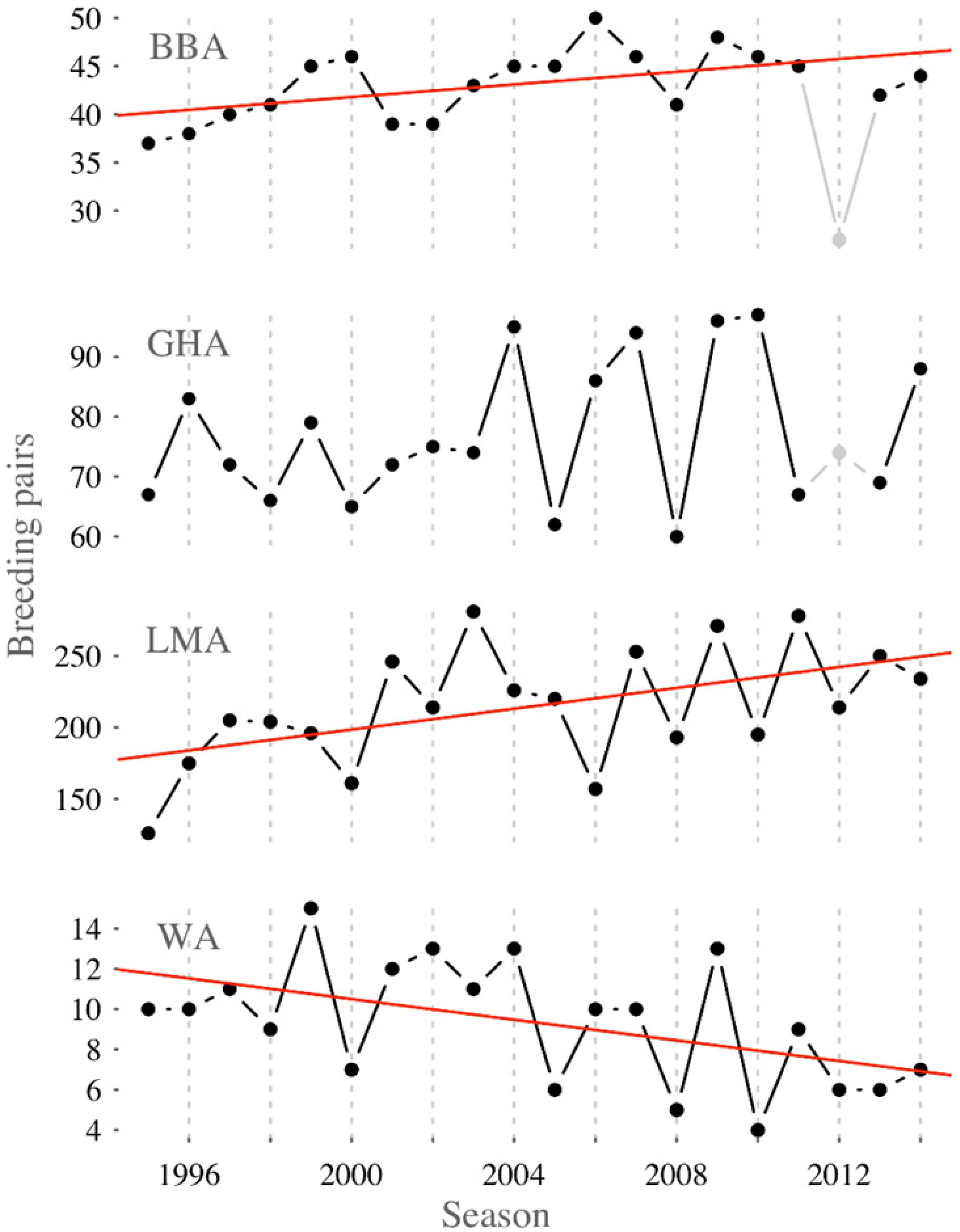
Figure 2. Trends in breeding pairs of black-browed (BBA, F1,17 = 6.88, p = 0.018, R2 = 0.25), gray-headed (GHA, F1,17 = 1.55, p = 0.229, R2 = 0.03), light-mantled (LMA, F1,18 = 6.62, p = 0.019, R2 = 0.23) and wandering albatross (WA, F1,18 = 5.84, p = 0.027, R2 = 0.20) at Macquarie Island in 1995 to 2014. Note that 1) detection probability of black-browed and gray-headed albatross was considerably lower in 2012 than other years and so this point was not included in the linear fit, and 2) the number of light-mantled albatross breeding pairs, represent those within seven study areas and approximately ∼10% of the total population.
Influence of Fisheries on Survival
Longline fishing effort in the south-west and south Atlantic Ocean explained some of the variability in declines in adult survival of black-browed albatrosses. Specifically, higher effort in Chilean (1995–2009), Falklands and ICCAT longline fisheries were associated with a decrease in survival, explaining 22.75–32.21% of the short- and long-term variability (Figure 3). Whereas trawl fishing effort in New Zealand waters was linked to higher survival of black-browed and gray-headed albatrosses and explained 21.26–28.29% of variability. For black-browed albatrosses, combined fisheries covariates explained 19.66% of short-term, and 45.34% of the long-term variability in survival (Table 2).
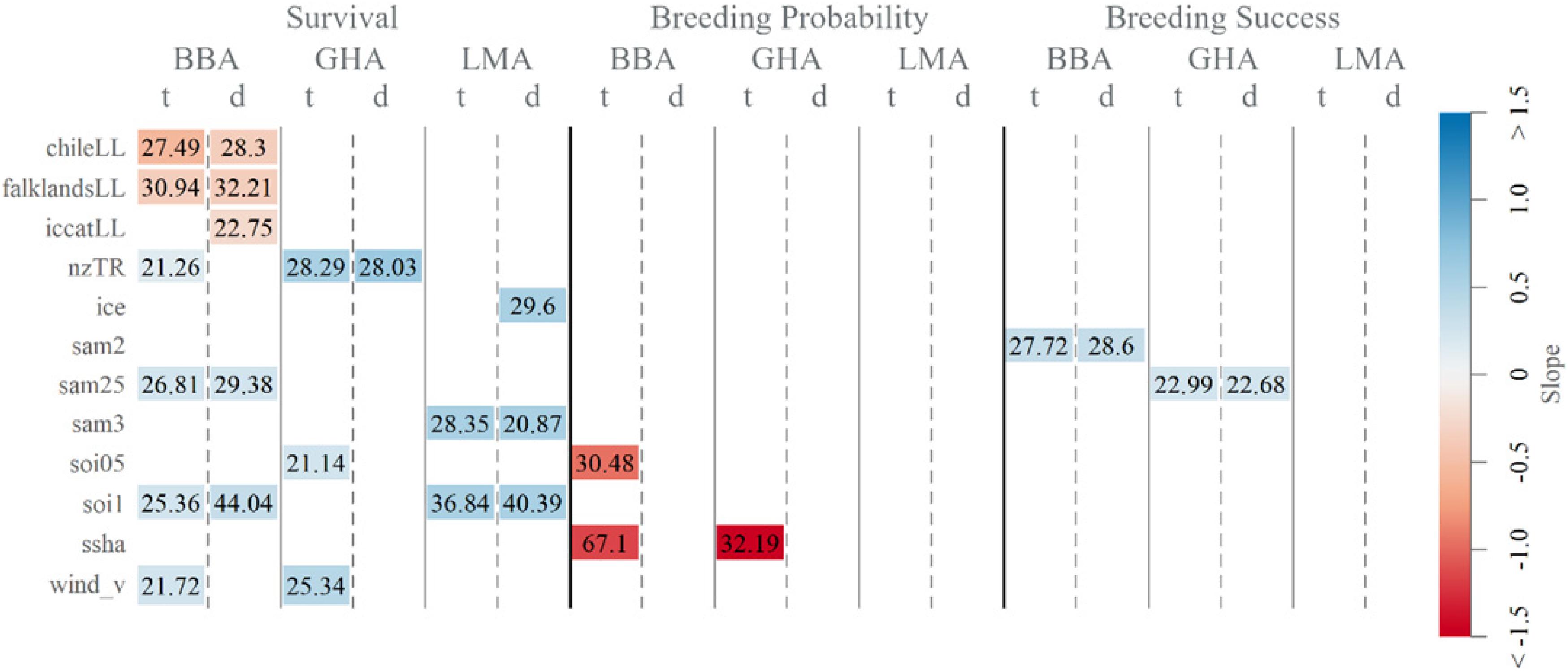
Figure 3. Deviance explained (%R2) and direction of slope of individual significant fisheries and oceanographic covariates (from Supplementary Table 2) included in survival, breeding probability and breeding success time-dependent base (t) and linear detrended (d) models of black-browed (BBA), gray-headed (GHA) and light-mantled (LMA) albatross at Macquarie Island.
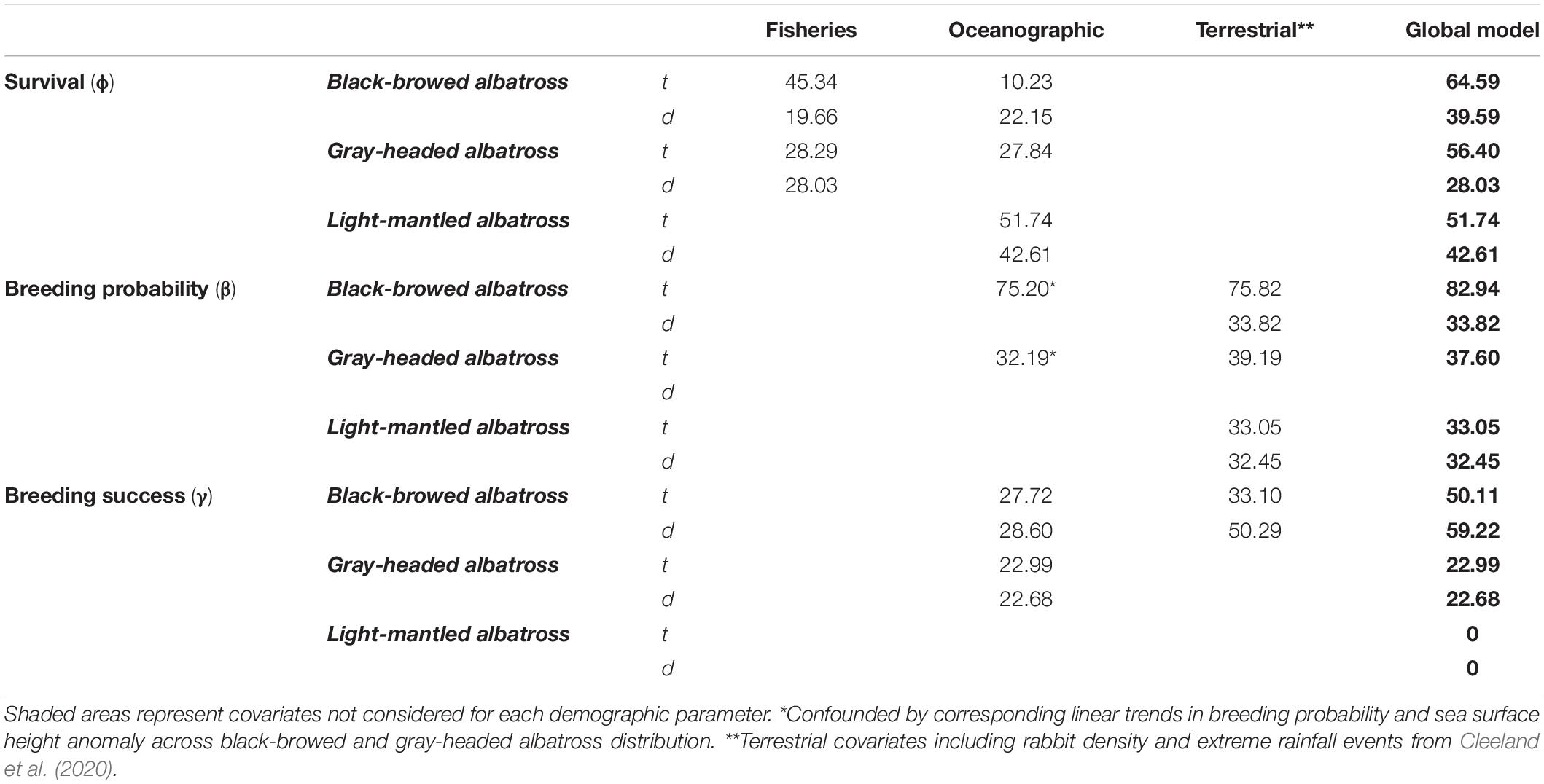
Table 2. Time-dependent (t) base and detrended (d) models with combined significant oceanographic, fisheries and terrestrial covariates explaining (%R2) Macquarie Island albatross demographic rates.
Effect of Oceanographic Factors
Effects of oceanographic covariates and large-scale climate indices on albatross demographic rates differed among the species. Sea ice extent explained 29.60% of the short -term variability in light-mantled albatross survival, with greater sea ice extent corresponding to increased survival (Figure 3). Increased meridional (southerly) winds were associated with higher survival of black-browed and gray-headed albatrosses. Sea surface height anomaly explained 67.10% and 32.19% of the long-term variability in black-browed and gray-headed albatross breeding probability. This correlation is not particularly informative and appears to be a result of strong linear trends in both breeding probability and sea surface height anomaly.
The SOI was positively associated with survival for all three species, whereas SAM was positively associated with survival of black-browed and light-mantled albatrosses. With a two-and-a-half-year lag, the SAM explained 29.38 and 26.81% of the short-term and long-term in black-browed albatross survival. With a longer lag time of 3 years, the SAM explained 28.35 and 20.87% of the short and long-term variability, respectively, in light-mantled albatross survival. Breeding success of black-browed and gray-headed albatrosses showed a positive association with SAM with two and two-and-a-half year lags.
A positive association between SOI and adult survival was detected for all species. For black-browed and light-mantled albatrosses, higher survival correlated with higher SOI values with a 1-year lag and explained 44.04 and 40.39% of the short-term variability and 25.36 and 36.84% of the long-term variability, respectively. Survival in gray-headed albatrosses responded more quickly, with a 6-month lag explaining 21.14% of long-term variability. Black-browed albatross breeding probability was negatively related to SOI with a 6-month lag, describing 30.48% of variability.
The most influential oceanographic covariates were combined in models for each species and described 42.61 and 51.74% of the short and long-term variation in light-mantled albatross survival (Table 2). Oceanographic factors were not strongly correlated with survival in black-browed albatrosses (short-term variability: R2 = 22.15%, long-term variability: R2 = 10.23%) or gray-headed albatrosses (long-term variability: R2 = 27.84%). Combined sea surface height anomaly and SOI (6-month lag) explained 75.20% of the long-term variability in breeding probability of black-browed albatrosses.
Global Models and Terrestrial Effects
Global models integrated all influential fisheries, oceanographic and terrestrial covariates (Figure 4). As shown by Cleeland et al. (2020), habitat degradation caused by heavy rabbit grazing is strongly correlated with the breeding probability of all three species. In this study, we see terrestrial covariates from Cleeland et al. (2020) best explain variability in breeding probability over time when compared to fisheries and oceanographic covariates (Table 2). For black-browed albatrosses, fisheries variables best explained the variability in survival (short-term variability: R2 = 19.66%, long-term variability: R2 = 45.34%), whereas terrestrial variables best explained breeding success (short-term variability: R2 = 50.29%, long-term variability: R2 = 33.10%). The remaining global models included oceanographic and terrestrial factors as correlates of breeding success. All model parameters are described in Appendix S2.
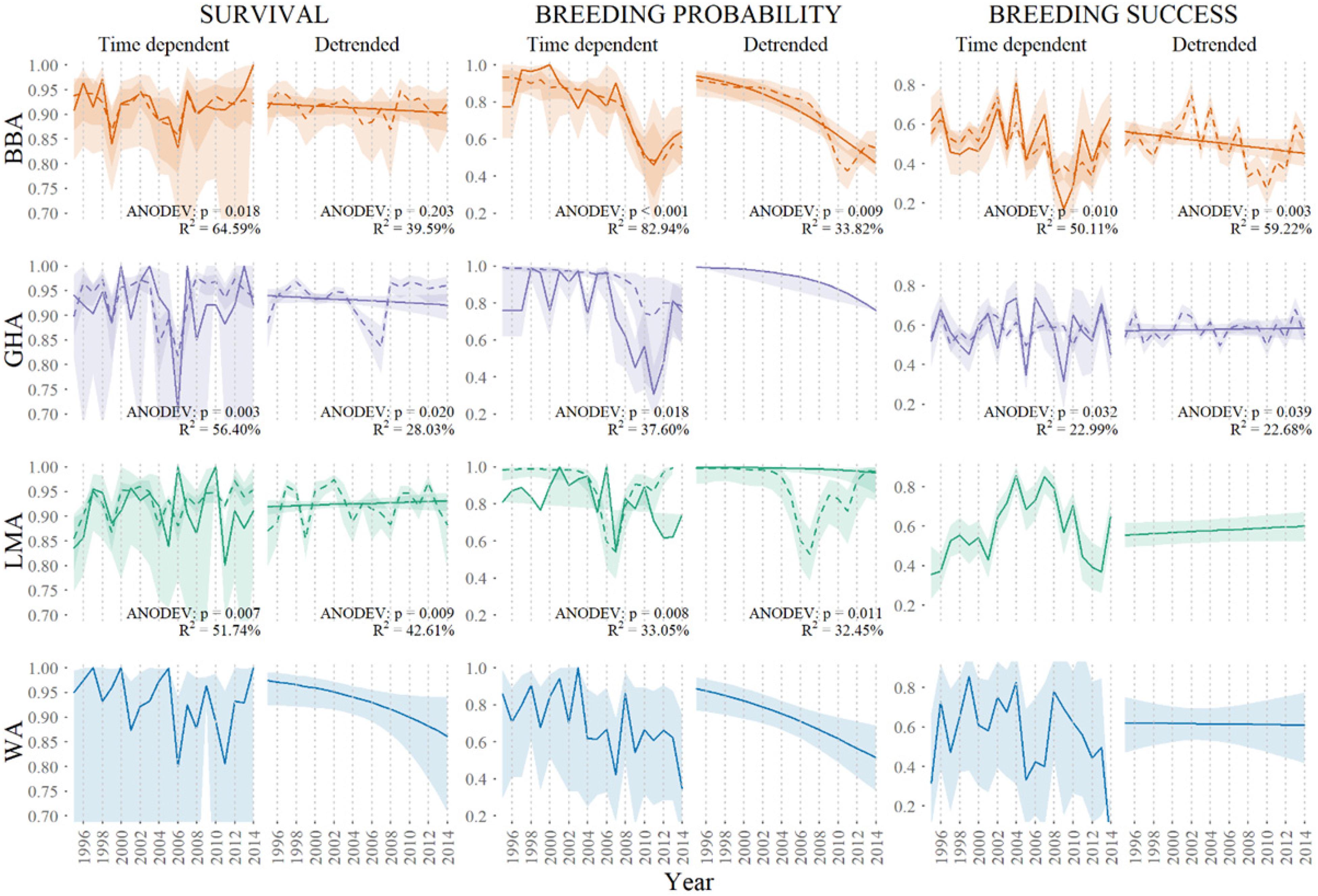
Figure 4. Global integrated models showing annual variation in demographic rates of adult black-browed (BBA, orange), gray-headed (GHA, purple) and light-mantled (LMA, green) albatross at Macquarie Island, modeled as time-dependent and linear detrended (solid colored lines) and as a function of combined significant covariates (broken colored lines) including 0.95 confidence intervals.
Discussion
Understanding the extrinsic drivers of demographic variability is critical to assess population viability, particularly in small populations where small decreases in vital rates can have a considerable impact on the population. However, the natural demographic stochasticity of small populations makes it challenging to identify the key factors contributing to demographic variability.
Species-Specific Demographic Estimates
Notwithstanding the overall similarity in survival patterns, there were important species-specific differences in survival patterns. Adult survival of black-browed albatrosses was constant throughout the study period, continuing the pattern reported between 1975 and 2000 (Terauds et al., 2005). Furthermore, the mean was within the range of values for this species at Îles Kerguelen (90.6 ± 0.5% to 94.3 ± 1.4%; Weimerskirch and Jouventin, 1998; Pardo et al., 2013), higher than survival at South Georgia during a similar period (89.3 ± 1.2% and 90.9%; Arnold et al., 2006; Pardo et al., 2017), but lower than that at Falklands (94.2%; Catry et al., 2011). Similarly, breeding success was higher than at South Georgia (Croxall et al., 1998) and lower than at the Falkland Islands (Catry et al., 2011).
Survival in gray-headed albatrosses at Macquarie Island declined by 3.4% from the first assessment between 1975 and 2000 (96.7 ± 1.1%; Terauds et al., 2005), and was lower than the long-term average at South Georgia of 95.2 ± 2.9% (Pardo et al., 2017), where the population is in rapid decline (Poncet et al., 2017), and at Campbell Island (95.3 ± 0.9%; Waugh et al., 1999). In contrast, breeding success for the Macquarie Island population (57.7 ± 1.3%) was considerably higher than Campbell Island (39.7%; Waugh et al., 1999), and that at South Georgia 30.0 ± 17.4%; Pardo et al., 2017). Estimates of gray-headed albatross survival and breeding success are highly variable in the Southern Ocean, suggesting factors that influence survival may originate from local or regional sources such as density-dependent processes and individual fisheries.
The cryptic colouration of light-mantled albatrosses, dispersed distribution of nests, and difficulty in accessing sites on cliffs and steep slopes result in few capture-mark-recapture studies of this species (Schoombie et al., 2016). Survival of light-mantled albatrosses at Macquarie Island (92.4%, 1995–2014) was considerably lower than at the Îles Crozet (97.3%, 1967–1995), whereas breeding success was higher at Macquarie (57.1% in 1995–2014 vs 40.9% in 1980–2005 at Îles Crozet; Delord et al., 2008).
The wandering albatross population at Macquarie Island has declined to an average of only five breeding pairs each year (DPIPWE, 2014). Its long-term persistence seems unlikely given the negative trend in adult survival and breeding probability, and the lack of immigrants from elsewhere (Terauds et al., 2006). The decline in breeding probability may be amplified by reduced survival, as it will be more difficult for widowed birds to find a new mate, particularly given the strong male-biased sex ratio (Supplementary Figure 3, Mills and Ryan, 2005). Due to the small population size we could not test for relationships between vital rates and fisheries effort or oceanographic change, but the decline of wandering albatrosses at Macquarie Island has previously been linked to tuna longline fisheries (de la Mare and Kerry, 1994). Nonetheless, this population has recovered from critically low numbers in the past. Harvesting by humans in the 18th century reduced the population to one or two breeding pairs in 1911 (Cumpston, 1968). Subsequently the number of breeding pairs fluctuated from 28 in 1968 to two in 1985, before reaching 15 in 1999 (Terauds et al., 2006). Similarly, the Amsterdam albatross Diomedea amsterdamensis increased from five to 31 breeding pairs between 1983 and 2001 (Rivalan et al., 2010). Thus, recovery capacity may not be constrained by genetic bottlenecks, as albatrosses in general show surprisingly low genetic variation (Milot et al., 2007).
Influence of Fisheries on Survival
Bycatch in longline and trawl fisheries is the greatest contributor to declines in albatross populations in the Southern Ocean (Phillips et al., 2016). Our study found a relationship between fishing effort and survival in black-browed albatrosses, with higher mortality rates associated with greater longline effort in the south-west Atlantic, a known wintering region for this population (Cleeland et al., 2019). Bycatch rates in longline fisheries that operate without mitigation measures in the southwest Atlantic ranged from as high as 0.276 black-browed albatrosses/1000 hooks in the Uruguayan tuna fishery (2004–2007) to 0.03 to 0.05 birds/1000 hooks in the Chilean artisanal hake Merluccius australis and Patagonian toothfish Dissostichus eleginoides fisheries between 1998 and 2002 (Moreno et al., 2006; ICCAT, 2010; Jiménez et al., 2010). From 2006, Chilean industrial longliners converted fishing methods to eliminate seabird bycatch in the Patagonian toothfish fishery (Robertson et al., 2014). Effort data in some fisheries, including those within the Argentinean EEZ, were not publicly available and so could not be included in our models. However, high levels of black-browed albatross bycatch occur in Argentinean longline fisheries, with estimates of 0.010 ± 0.001 birds/1000 hooks caught in 2001–2010 (Favero et al., 2013). Similarly, high mortality of black-browed albatross (0.237 birds/haul, 2008–2010) is recorded in the Argentinean demersal trawl hake M. hubbsi fishery (Tamini et al., 2015). The negative relationship between black-browed albatross survival and southwest Atlantic fisheries indicates a direct link, with high bycatch rates of black-browed albatrosses reported there previously (Favero et al., 2013; Yeh et al., 2013).
Survival of black-browed and gray-headed albatrosses at Macquarie Island was related to effort in New Zealand trawl fisheries, but with higher effort associated with increased survival. Attendance of both species at vessels is common within these fisheries, particularly over the Campbell Plateau (Richard et al., 2011), a known foraging area for these populations (Cleeland et al., 2019). Furthermore, they are known scavengers of discards from squid trawlers in this region, with black-browed albatrosses attending in high numbers during the pre-laying and early incubation periods (Petyt, 1995). High attendance, bycatch in New Zealand trawl fisheries is low (0.05 captures per 100 tows per year) (Abraham and Thompson, 2015). Previous findings from Îles Kerguelen have shown discarding practices can influence demographic rates with higher breeding success of black-browed albatrosses attributed to the availability of discards during periods of high fishing effort (Rolland et al., 2008). Furthermore, breeding success is higher at colonies where fisheries discards are detected in the diet of black-browed albatrosses (McInnes et al., 2017). For black-browed albatrosses breeding at Macquarie Island, fish species found in their diet (including Coryphaenoides spp., Cubiceps caeruleus, Halargyreus johnsonii, Lampris immaculatus, Microstoma spp., Muraenolepididae and Paranotothenia magellanica) are bycatch species in New Zealand deep-water trawl fisheries and are subject to discarding (Anderson, 2017; McInnes et al., 2017). The results from this study represent the first indication that discarding practices may directly influence albatross survival by providing an abundant and predictable supplement to natural food resources.
Effect of Oceanographic Factors
The demographic rates of albatrosses at Macquarie Island were correlated with both the SAM and SOI. The SAM has complex and lagged effects on physical oceanography and biological productivity (Marshall, 2003; Lefebvre and Goosse, 2005). Higher values for the SAM index are associated with fluctuations in penguin populations in the east Antarctic (Ainley et al., 2005), reduced krill densities at South Georgia (Fielding et al., 2014) and improved maternal condition of southern elephant seals Mirounga leonina at Macquarie Island (McMahon et al., 2017). However, the underlying mechanisms and ecological consequences are not clear, especially for wide-ranging species such as albatrosses.
High SAM indices correspond to stronger winds closer to the pole and were associated with increased survival of black-browed and light-mantled albatrosses and increased breeding success of black-browed and gray-headed albatrosses at Macquarie Island following a lag of 2 to 3 years. During positive phases of the SAM, surface waters within the Antarctic Zone are colder, have increased upwelling, iron transport, and surface chlorophyll. In contrast, in the Subantarctic Zone, a deeper mixed layer results in reduced surface productivity (Lovenduski and Gruber, 2005). Surface productivity in the Antarctic Zone is transported to lower latitudes through Ekman processes (Lovenduski and Gruber, 2005) and may provide better foraging for albatrosses breeding at Macquarie Island after a 2 to 3 year lag. Positive SAM indices also lead to increased eddy kinetic energy following a 2 to 3 year lag, due to the time taken for stronger wind forcing to influence the Antarctic Circumpolar Current (Meredith and Hogg, 2006). Nonbreeding black-browed and gray-headed and breeding light-mantled albatrosses utilize regions of higher eddy kinetic energy (Cleeland et al., 2019), which tend to aggregate prey (Bost et al., 2009; Scales et al., 2014). Nutrient retention and enrichment of surface waters through upwelling and mixing at mesoscale cyclonic eddy features support higher phytoplankton and prey concentrations relative to surrounding waters (Sokolov and Rintoul, 2007).
A positive relationship between SOI and survival was detected in all three species tested. Positive SOI (La Niña) periods are related to higher productivity in the eastern Pacific boundary currents (Barber and Chavez, 1983; Behrenfeld et al., 2001) and improved hatching success of Indian yellow-nosed albatrosses Thalassarche carteri at Amsterdam Island, and breeding success of black-browed albatrosses at Îles Kerguelen (Rolland et al., 2008, 2009). A positive SOI may enhance biological productivity and increase recruitment of mid-trophic prey species (Waluda et al., 1999). El Niño conditions in the Pacific sector covaried positively with a negative SAM during our study period, making it difficult to disentangle the influence of these two large-scale climate cycles (Stammerjohn et al., 2008).
Breeding light-mantled albatrosses forage along the Subantarctic Front near Macquarie Island, and to the south in Antarctic waters (∼65°S) at the southern boundary of the Antarctic Circumpolar Current (Cleeland et al., 2019). They have the most southerly foraging distribution of all Macquarie Island species, which may increase their responsiveness to variability in sea ice concentration and primary productivity on shorter timescales, although the proximal mechanisms are unclear. Survival of this species was positively related to sea ice extent, which may represent improved foraging conditions in more southerly waters (Loeb et al., 1997). In their known foraging habitat off east Antarctica, the abundance of Antarctic krill Euphasia superba is found in greater densities in areas of greatest annual sea-ice cover (Nicol et al., 2000). Furthermore, snow petrels Pagodroma nivea have also been found to show improved demographic responses to more extensive sea cover, including increased breeding success and fledgling body condition (Barbraud and Weimerskirch, 2001). For light-mantled albatross, an ice independent species, the relationship between sea ice conditions and survival is interesting given the potential for population level consequences due to recent and rapid sea ice loss around Antarctica (Parkinson, 2019).
The positive response of black-browed and gray-headed albatross survival to stronger southerly winds, may be due to the influence of wind on the energetics of flight. The relatively high wing loading of gray-headed albatrosses means stronger winds improve flight efficiency by increasing glide ratios and reducing the cost of reaching foraging areas (Pennycuick, 1982). For black-browed albatrosses, more southerly winds would act similarly, supporting efficient northward flight to foraging areas. In the Indian Ocean, body condition and breeding success of wandering albatrosses improved with increasing wind speed, related to the SAM (Weimerskirch et al., 2012). Furthermore, Pardo et al. (2017) showed a strong positive effect of wind patterns on adult and juvenile survival of wandering albatrosses at South Georgia. Predictions of increasingly positive SAM and subsequent strengthening of circumpolar winds may be beneficial for most albatross species at Macquarie Island, except for the light-mantled albatross.
Global Models and Terrestrial Effects
In this study we found the threat of fisheries to survival was generally more influential than changes in oceanographic factors for black-browed and gray-headed albatrosses. This is supported by the historical evidence of fisheries-related incidental mortality and new evidence of dietary linkages with discarding practices for these species (Tuck et al., 2003; McInnes et al., 2017). It is expected that albatrosses, as long-lived, wide-ranging pelagic foragers, can buffer against oceanographic variability that may influence the accessibility and predictability of prey resources. Therefore, it is noteworthy we detected significant relationships between survival and oceanographic change, suggesting its influence on individual fitness has population level consequences. This is particularly the case for black-browed albatross, where short-term variability in survival is slightly better described by oceanographic variability. Oceanographic factors explained greater variability in survival for light-mantled albatrosses than any other species. The foraging behavior of light-mantled albatrosses shows some degree of plasticity, with individuals showing ‘flexible faithfulness’ with year-to-year differences in foraging sites, but consistent use of oceanographic features including anticyclonic eddies and frontal regions (Carpenter-Kling et al., 2020). This suggests the magnitude and predictability of dynamic oceanographic features is likely have a pronounced influence on foraging outcomes, and thus has repercussions on the survival of light-mantled albatrosses.
Nesting habitat quality (indicated by rabbit density) was the most influential determinant of the breeding probability of all three species tested, due to overgrazing and widescale slope erosion. With terrestrial factors accounting for most of the variability in breeding probability other environmental drivers are potentially masked from detection. However, this result indicates that habitat restoration at the colony is likely to lead to positive demographic and population-level responses.
The global models show terrestrial covariates are the most influential for breeding success of black-browed albatrosses, whereas for gray-headed albatrosses only oceanographic factors are identified as important. Where over 50% of demographic variability in breeding success could be described for black-browed albatrosses, no factors were detected for light-mantled albatrosses. Despite accounting for biennial breeding in the models, it is possible that this strategy influences our ability to detect significant relationships as the factors that influence breeding success may be more complex and operate over larger spatial and temporal scales.
Our study quantifies the links (e.g., demographic models) between ongoing environmental drivers and adult survival and reproductive success thereby providing information central to developing evidence-based conservation strategies and management plans. Of the three species examined in this study, the gray-headed albatross is the most threatened making it a high priority for management action. Furthermore, our results identify species for which there is no single dominant environmental driver and where multiple, targeted conservation efforts may be required to achieve long-term improvements in demographic outcomes. For black-browed albatrosses on Macquarie Island, changes in fisheries and breeding habitat quality had the greatest influence on survival, breeding probability and breeding success; factors for which mitigation strategies already exist (Croxall et al., 2012; Phillips et al., 2016). The removal of rabbits from Macquarie Island (Springer, 2016) and current bycatch mitigation in the south-west Atlantic (Otley et al., 2007; Moreno et al., 2008) may account for the increasing population of black-browed albatrosses.
By identifying common demographic drivers amongst species this study supports the development of management actions that potentially benefit all three species. Specifically, for all three species, processes on land affected breeding probability, and consequently the recent removal of rabbits from Macquarie Island is likely to ameliorate their negative effects on vegetation stability and improve reproductive output.
Understanding the demographic drivers of wild populations is difficult due to the multitude of interacting factors that can affect survival and reproductive performance. However, a full and complete understanding is not necessarily a prerequisite for management action. In some cases, a particular management intervention might buffer the influence of one driver on the population by addressing the impact of another (Pascoe et al., 2011). This is helpful when management solutions that address a single threat are more logistically practical, economically feasible or effective over more appropriate time and spatial scales than for other threats. Furthermore, intermediary management action may provide reprieve for vulnerable populations until advances in conservation technology or policy can be made. Such options are becoming exceedingly important in the face of rapid and pervasive impacts of climate change. For gray-headed and light-mantled albatrosses, there are currently few options to directly mitigate the effects of climate on their survival and breeding success. The buffer due to the eradication is therefore likely to provide important benefits, at least in the short term. For globally threatened species such as albatross that cross multiple international boundaries and are widely distributed throughout the high seas, such action may be vital.
Data Availability Statement
The datasets presented in this study can be found in online repositories. The names of the repository/repositories and accession number(s) can be found below: The long-term albatross mark-recapture dataset used in this study is housed by the Marine Conservation Branch within the Department of Primary Industries, Parks Water and Environment, Tasmania, and by the Australian Antarctic Data Centre under the Australian Antarctic program data policy (https://data.aad.gov.au/aadc/about/data_policy.cfm).
Ethics Statement
The animal study was reviewed and approved by the Tasmanian Government Department of Primary Industries, Parks, Water and Environment’s Animal Ethics Committee (permit number TFA9195).
Author Contributions
JC, DP, and MH conceived and designed the study. CM and M-AL developed research concepts. RA, GT, and BR provided access to the data. JC and BR performed data management and data quality assurance tasks. JC conducted the statistical analysis and modeling work with support from DP and RP. JC took the lead in writing the manuscript. All authors performed ecological interpretation of results and contributed substantially to manuscript revisions.
Funding
The Australian Antarctic Division through the Australian Antarctic Science Program (Project numbers: 751, 2569, 4112) has supported this research at Macquarie Island. JC was supported by a Scientific Committee for Antarctic Research Fellowship and an Antarctic Science Bursary.
Conflict of Interest
The authors declare that the research was conducted in the absence of any commercial or financial relationships that could be construed as a potential conflict of interest.
Acknowledgments
We would like to thank Rosemary Gales for her commitment to albatross monitoring at Macquarie Island over the last two decades and Aleks Terauds for valuable guidance. We also thank Sally McCarthy and John Garvey (AFMA); Marco Favero and Wieslawa Misiak (ACAP); Barbara Wienecke, Jonathan Barrington and Juan Carlos Quiroz Espinosa and Dirk Welsford (AAD); Joost Pompert (FIFD); David Ramm (CCAMLR); Nick Vogel (IATTC); Carlos Palma (ICCAT); Christopher Dick (NZ-MPI); and Craig Loveridge (SPRFMO) for contribution of fisheries data. The content of this manuscript has been published in part as part of the thesis of Cleeland (2017).
Supplementary Material
The Supplementary Material for this article can be found online at: https://www.frontiersin.org/articles/10.3389/fmars.2021.578144/full#supplementary-material
References
Abraham, E. R., and Thompson, F. N. (2015). Captures of All Birds in Trawl Fisheries, in the New Zealand Exclusive Economic Zone, from 2002–03 to 2013–14 [Online]. Available online at: https://data.dragonfly.co.nz/psc/v20150002/birds/trawl/all-vessels/eez/all/ (accessed April 31, 2017).
Ainley, D. G., Clarke, E. D., Arrigo, K., Fraser, W. R., Kato, A., Barton, K. J., et al. (2005). Decadal-scale changes in the climate and biota of the Pacific sector of the Southern Ocean, 1950s to the 1990s. Antarct. Sci. 17, 171–182. doi: 10.1017/s0954102005002567
Anderson, O. F. (2017). Fish and Invertebrate Bycatch in New Zealand Deepwater Fisheries from 1990–91 until 2013–14. New Zealand Aquatic Environment and Biodiversity Report No. 181. Wellington, NZ: Ministry for Primary Industries New Zealand Government.
Anderson, O. R. J., Small, C. J., Croxall, J. P., Dunn, E. K., Sullivan, B. J., Yates, O., et al. (2011). Global seabird bycatch in longline fisheries. Endanger. Species Res. 14, 91–106. doi: 10.3354/esr00347
Arnold, J. M., Brault, S., and Croxall, J. P. (2006). Albatross populations in peril: a population trajectory for Black-browed Albatrosses at South Georgia. Ecol. Appl. 16, 419–432. doi: 10.1890/03-5340
Barber, R. T., and Chavez, F. P. (1983). Biological consequences of El Niño. Science 222, 1203–1210.
Barbraud, C., and Weimerskirch, H. (2001). Contrasting effects of the extent of sea-ice on the breeding performance of an antarctic top predator, the snow petrel pagodroma nivea. J. Avian Biol. 32, 297–302. doi: 10.1111/j.0908-8857.2001.320402.x
Barbraud, C., and Weimerskirch, H. (2003). Climate and density shape population dynamics of a marine top predator. Proc. R. Soc. B Biol. Sci. 270, 2111–2116. doi: 10.1098/rspb.2003.2488
Barbraud, C., and Weimerskirch, H. (2012). Estimating survival and reproduction in a quasi-biennially breeding seabird with uncertain and unobservable states. J. Ornithol. 152, 605–615. doi: 10.1007/s10336-011-0686-1
Bartumeus, F., Giuggioli, L., Louzao, M., Bretagnolle, V., Oro, D., and Levin, S. A. (2010). Fishery discards impact on seabird movement patterns at regional scales. Curr. Biol. 20, 215–222. doi: 10.1016/j.cub.2009.11.073
Behrenfeld, M. J., Randerson, J. T., McClain, C. R., Feldman, G. C., Los, S. O., Tucker, C. J., et al. (2001). Biospheric primary production during an ENSO transition. Science 291, 2594–2597. doi: 10.1126/science.1055071
Bost, C. A., Cotte, C., Bailleul, F., Cherel, Y., Charrassin, J. B., Guinet, C., et al. (2009). The importance of oceanographic fronts to marine birds and mammals of the southern oceans. J. Mar. Syst. 78, 363–376. doi: 10.1016/j.jmarsys.2008.11.022
Brothers, N., and Bone, C. (2008). The response of burrow-nesting petrels and other vulnerable bird species to vertebrate pest management and climate change on sub-Antarctic Macquarie Island. Pap. Proc. R. Soc. Tasman. 142, 123–148. doi: 10.26749/rstpp.142.1.123
Burnham, K. P., and Anderson, D. R. (2002). Model Selection and Multimodel Inference: A Practical Information-Theoretic Approach. New York, NY: Springer-Verlag.
Carpenter-Kling, T., Reisinger, R. R., Orgeret, F., Connan, M., Stevens, K. L., Ryan, P. G., et al. (2020). Foraging in a dynamic environment: response of four sympatric sub-Antarctic albatross species to interannual environmental variability. Ecol. Evol. 10, 11277–11295. doi: 10.1002/ece3.6766
Catry, P., Forcada, J., and Almeida, A. (2011). Demographic parameters of black-browed albatrosses Thalassarche melanophris from the Falkland Islands. Polar Biol. 34, 1221–1229. doi: 10.1007/s00300-011-0984-3
Choquet, R., Lebreton, J. D., Gimenez, O., Reboulet, A. M., and Pradel, R. (2009a). U-CARE: utilities for performing goodness of fit tests and manipulating CApture-REcapture data. Ecography 32, 1071–1074. doi: 10.1111/j.1600-0587.2009.05968.x
Choquet, R., Rouan, L., and Pradel, R. (2009b). “Program E-SURGE: a software application for fitting multievent models,” in Modeling Demographic Processes in Marked Populations, eds D. L. Thomson, E. G. Cooch, and M. J. Conroy (Boston, MA: Springer US), 845–865. doi: 10.1007/978-0-387-78151-8_39
Cleeland, J. B. (2017). Factors that Drive Demographic Change in a Community of Albatrosses. Ph.D. Thesis, University of Tasmania, Hobart, TAS.
Cleeland, J. B., Alderman, R., Bindoff, A., Lea, M. A., McMahon, C. R., Phillips, R. A., et al. (2019). Factors influencing the habitat use of sympatric albatrosses from Macquarie Island, Australia. Mar. Ecol. Prog. Ser. 609, 221–237. doi: 10.3354/meps12811
Cleeland, J. B., Pardo, D., Raymond, B., Terauds, A., Alderman, R., McMahon, C. R., et al. (2020). Introduced species and extreme weather as key drivers of reproductive output in three sympatric albatrosses. Sci. Rep. 10:8199.
Constable, A. J., Melbourne-Thomas, J., Corney, S. P., Arrigo, K. R., Barbraud, C., Barnes, D. K. A., et al. (2014). Climate change and Southern Ocean ecosystems I: how changes in physical habitats directly affect marine biota. Glob. Chang. Biol. 20, 3004–3025.
Croxall, J. P., Butchart, S. H. M., Lascelles, B., Stattersfield, A. J., Sullivan, B., Symes, A., et al. (2012). Seabird conservation status, threats and priority actions: a global assessment. Bird Conserv. Int. 22, 1–34. doi: 10.1017/s0959270912000020
Croxall, J., Prince, P., Rothery, P., and Wood, A. (1998). “Population changes in albatrosses at South Georgia,” in Albatross Biology and Conservation, eds G. Robertson and R. Gales (Chipping Norton, NSW: Surrey Beattie), 69–83.
Cumpston, J. S. (1968). “Macquarie Island,” in Australian National Antarctic Research Expeditions Reports, ed. M. R. O Millett (Melbourne, VIC: Australian Antarctic Division, Department of External Affairs).
Cury, P. M., Boyd, I. L., Bonhommeau, S., Anker-Nilssen, T., Crawford, R. J. M., Furness, R. W., et al. (2011). Global seabird response to forage fish depletion – one-third for the birds. Science 334, 1703–1706. doi: 10.1126/science.1212928
Cuthbert, R., and Hilton, G. (2004). Introduced house mice Mus musculus: a significant predator of threatened and endemic birds on Gough Island, South Atlantic Ocean? Biol. Conserv. 117, 483–489. doi: 10.1016/j.biocon.2003.08.007
de la Mare, W. K., and Kerry, K. R. (1994). Population dynamics of the wandering albatross (Diomedea exulans) on Macquarie Island and the effects of mortality from longline fishing. Polar Biol. 14, 231–242. doi: 10.1007/bf00239171
Delord, K., Besson, D., Barbraud, C., and Weimerskirch, H. (2008). Population trends in a community of large Procellariiforms of Indian Ocean: potential effects of environment and fisheries interactions. Biol. Conserv. 141, 1840–1856. doi: 10.1016/j.biocon.2008.05.001
DPIPWE (2014). The Conservation and Status of Albatrosses and Giant Petrels on Macquarie Island: Report on the 2013/14 Field Season. Hobart, TAS: Australian Government, Department of Primary Industries Parks Water and Environment.
Favero, M., Blanco, G., Copello, S., Pon, J. P. S., Patterlini, C., Mariano-Jelicich, R., et al. (2013). Seabird bycatch in the Argentinean demersal longline fishery, 2001-2010. Endanger. Species Res. 19, 187–199. doi: 10.3354/esr00478
Fielding, S., Watkins, J. L., Trathan, P. N., Enderlein, P., Waluda, C. M., Stowasser, G., et al. (2014). Interannual variability in Antarctic krill (Euphausia superba) density at South Georgia, Southern Ocean: 1997-2013. ICES J. Mar. Sci. 71, 2578–2588. doi: 10.1093/icesjms/fsu104
Forcada, J., and Trathan, P. N. (2009). Penguin responses to climate change in the Southern Ocean. Glob. Chang. Biology 15, 1618–1630. doi: 10.1111/j.1365-2486.2009.01909.x
Gilman, E., Boggs, C., and Brothers, N. (2003). Performance assessment of an underwater setting chute to mitigate seabird bycatch in the Hawaii pelagic longline tuna fishery. Ocean Coast. Manag. 46, 985–1010. doi: 10.1016/j.ocecoaman.2003.12.001
Gimenez, O., Lebreton, J. D., Gaillard, J. M., Choquet, R., and Pradel, R. (2012). Estimating demographic parameters using hidden process dynamic models. Theor. Popul. Biol. 82, 307–316. doi: 10.1016/j.tpb.2012.02.001
Granadeiro, J. P., Brickle, P., and Catry, P. (2014). Do individual seabirds specialize in fisheries’ waste? The case of black-browed albatrosses foraging over the Patagonian Shelf. Anim. Conserv. 17, 19–26. doi: 10.1111/acv.12050
Grosbois, V., Gimenez, O., Gaillard, J. M., Pradel, R., Barbraud, C., Clobert, J., et al. (2008). Assessing the impact of climate variation on survival in vertebrate populations. Biol. Rev. 83, 357–399. doi: 10.1111/j.1469-185x.2008.00047.x
ICCAT (2010). ICCAT By-Catch Database [Online]. Available online at: https://www.iccat.int/en/bycatch.html (accessed November 07, 2016)
Inchausti, P., and Weimerskirch, H. (2001). Risks of decline and extinction of the endangered Amsterdam albatross and the projected impact of long-line fisheries. Biol. Conserv. 100, 377–386. doi: 10.1016/s0006-3207(01)00044-1
Jenouvrier, S., Desprez, M., Fay, R., Barbraud, C., Weimerskirch, H., Delord, K., et al. (2018). Climate change and functional traits affect population dynamics of a long-lived seabird. J. Anim. Ecol. 87, 906–920. doi: 10.1111/1365-2656.12827
Jiménez, S., Abreu, M., Pons, M., Ortiz, M., and Domingo, A. (2010). Assessing the impact of the pelagic longline fishery on albatrosses and petrels in the southwest Atlantic. Aquat. Living Resour. 23, 49–64. doi: 10.1051/alr/2010002
Jouventin, P., and Dobson, F. S. (2002). Why breed every other year? The case of albatrosses. Proc. R. Soc. B Biol. Sci. 269, 1955–1961. doi: 10.1098/rspb.2002.2080
Lea, M. A., Guinet, C., Cherel, Y., Duhamel, G., Dubroca, L., Pruvost, P., et al. (2006). Impacts of climatic anomalies on provisioning strategies of a Southern Ocean predator. Mar. Ecol. Prog. Ser. 310, 77–94. doi: 10.3354/meps310077
Lebreton, J. D., Burnham, K. P., Clobert, J., and Anderson, D. R. (1992). Modeling survival and testing biological hypotheses using marked animals: a unified approach with case studies. Ecol. Monogr. 62, 67–118. doi: 10.2307/2937171
Lefebvre, W., and Goosse, H. (2005). Influence of the Southern annular mode on the sea ice-ocean system: the role of the thermal and mechanical forcing. Ocean Sci. 1, 145–157. doi: 10.5194/os-1-145-2005
Loeb, V., Siegel, V., Holm-Hansen, O., Hewitt, R., Fraser, W., Trivelpiece, W., et al. (1997). Effects of sea-ice extent and krill or salp dominance on the Antarctic food web. Nature 387, 897–900. doi: 10.1038/43174
Lovenduski, N. S., and Gruber, N. (2005). Impact of the Southern annular mode on Southern Ocean circulation and biology. Geophys. Res. Lett. 32:L11603.
Maree, B. A., Wanless, R. M., Fairweather, T. P., Sullivan, B. J., and Yates, O. (2014). Significant reductions in mortality of threatened seabirds in a South African trawl fishery. Anim. Conserv. 17, 520–529. doi: 10.1111/acv.12126
Marshall, G. J. (2003). Trends in the Southern annular mode from observations and reanalyses. J. Clim. 16, 4134–4143. doi: 10.1175/1520-0442(2003)016<4134:titsam>2.0.co;2
McInnes, J. C., Jarman, S. N., Lea, M. A., Raymond, B., Deagle, B. E., Phillips, R. A., et al. (2017). DNA metabarcoding as a marine conservation and management tool: a circumpolar examination of fishery discards in the diet of threatened albatrosses. Front. Mar. Sci. 4:277. doi: 10.3389/fmars.2017.00277
McMahon, C. R., Harcourt, R. G., Burton, H. R., Daniel, O., and Hindell, M. A. (2017). Seal mothers expend more on offspring under favourable conditions and less when resources are limited. J. Anim. Ecol. 86, 359–370. doi: 10.1111/1365-2656.12611
Meredith, M. P., and Hogg, A. M. (2006). Circumpolar response of Southern Ocean eddy activity to a change in the Southern annular mode. Geophys. Res. Lett. 33:L16608.
Mills, M. S. L., and Ryan, P. G. (2005). Modelling impacts of long-line fishing: what are the effects of pair-bond disruption and sex-biased mortality on albatross fecundity? Anim. Conserv. 8, 359–367. doi: 10.1017/s1367943005002386
Milot, E., Weimerskirch, H., Duchesne, P., and Bernatchez, L. (2007). Surviving with low genetic diversity: the case of albatrosses. Proc. R. Soc. B Biol. Sci. 274, 779–787. doi: 10.1098/rspb.2006.0221
Moreno, C. A., Arata, J. A., Rubilar, P., Hucke-Gaete, R., and Robertson, G. (2006). Artisanal longline fisheries in Southern Chile: lessons to be learned to avoid incidental seabird mortality. Biol. Conserv. 127, 27–36. doi: 10.1016/j.biocon.2005.07.011
Moreno, C. A., Castro, R., Mújica, L. J., and Reyes, P. (2008). Significant conservation benefits obtained from the use of a new fishing gear in the Chilean Patagonian toothfish fishery. CCAMLR Sci. 15, 79–91.
Nicol, S., Pauly, T., Bindoff, N. L., Wright, S., Thiele, D., Hosle, G. W., et al. (2000). Ocean circulation off east Antarctica affects ecosystem structure and sea-ice extent. Nature 406, 504–507. doi: 10.1038/35020053
Otley, H. M., Reid, T. A., and Pompert, J. (2007). Trends in seabird and Patagonian toothfish Dissostichus eleginoides longliner interactions in Falkland Island waters, 2002/03 and 2003/04. Mar. Ornithol. 35, 47–55.
Pardo, D., Barbraud, C., Authier, M., and Weimerskirch, H. (2013). Evidence for an age-dependent influence of environmental variations on a long-lived seabird’s life-history traits. Ecology 94, 208–220. doi: 10.1890/12-0215.1
Pardo, D., Forcada, J., Wood, A. G., Tuck, G. N., Ireland, L., Pradel, R., et al. (2017). Additive effects of climate and fisheries drive catastrophic declines in an albatross community. Proc. Natl. Acad. Sci. U.S.A. 144, 10829–10837.
Parkinson, C. L. (2019). A 40-y record reveals gradual Antarctic sea ice increases followed by decreases at rates far exceeding the rates seen in the Arctic. Proc. Natl. Acad. Sci. U.S.A. 116, 14414–14423. doi: 10.1073/pnas.1906556116
Pascoe, S., Wilcox, C., and Donlan, C. J. (2011). Biodiversity offsets: a cost-effective interim solution to seabird bycatch in fisheries? PLoS One 6:e25762. doi: 10.1371/journal.pone.0025762
Pennycuick, C. J. (1982). The flight of petrels and albatrosses (Procellariiformes), observed in South Georgia and its vicinity. Philos. Trans. R. Soc. B Biol. Sci. 300, 75–106. doi: 10.1098/rstb.1982.0158
Petyt, C. (1995). Behaviour of seabirds around fishing trawlers in New Zealand subantarctic waters. Notornis 42, 99–115.
Phillips, R. A., Gales, R., Baker, G. B., Double, M. C., Favero, M., Quintana, F., et al. (2016). The conservation status and priorities for albatrosses and large petrels. Biol. Conserv. 201, 169–183. doi: 10.1016/j.biocon.2016.06.017
Phillips, R. A., Silk, J. R. D., and Croxall, J. P. (2005). Foraging and provisioning strategies of the light-mantled sooty albatross at South Georgia: competition and co-existence with sympatric pelagic predators. Mar. Ecol. Prog. Ser. 285, 259–270. doi: 10.3354/meps285259
Poncet, S., Wolfaardt, A. C., Black, A., Browning, S., Lawton, K., Lee, J., et al. (2017). Recent trends in numbers of wandering (Diomedea exulans), black-browed (Thalassarche melanophris) and grey-headed (T. chrysostoma) albatrosses breeding at South Georgia. Polar Biol. 40, 1347–1358. doi: 10.1007/s00300-016-2057-0
R Core Team (2016). R: A Language and Environment for Statistical Computing. Vienna: R Foundation for Statistical Computing.
Richard, Y., Abraham, E. R., and Berkenbusch, K. (2011). Counts of Seabirds Around Commercial Fishing Vessels within New Zealand Waters. Wellington: Unpublished report held by the Department of Conservation.
Rivalan, P., Barbraud, C., Inchausti, P., and Weimerskirch, H. (2010). Combined impacts of longline fisheries and climate on the persistence of the Amsterdam Albatross Diomedia amsterdamensis. Ibis 152, 6–18. doi: 10.1111/j.1474-919x.2009.00977.x
Robertson, G., Candy, S. G., Wienecke, B., and Lawton, K. (2010). Experimental determinations of factors affecting the sink rates of baited hooks to minimize seabird mortality in pelagic longline fisheries. Aquat. Conserv. 20, 632–643. doi: 10.1002/aqc.1140
Robertson, G., Moreno, C., Arata, J. A., Candy, S. G., Lawton, K., Valencia, J., et al. (2014). Black-browed albatross numbers in Chile increase in response to reduced mortality in fisheries. Biol. Conserv. 169, 319–333. doi: 10.1016/j.biocon.2013.12.002
Rolland, V., Barbraud, C., and Weimerskirch, H. (2008). Combined effects of fisheries and climate on a migratory long-lived marine predator. J. Appl. Ecol. 45, 4–13. doi: 10.1111/j.1365-2664.2007.01360.x
Rolland, V., Barbraud, C., and Weimerskirch, H. (2009). Assessing the impact of fisheries, climate and disease on the dynamics of the Indian yellow-nosed Albatross. Biol. Conserv. 142, 1084–1095. doi: 10.1016/j.biocon.2008.12.030
Scales, K. L., Miller, P. I., Embling, C. B., Ingram, S. N., Pirotta, E., and Votier, S. C. (2014). Mesoscale fronts as foraging habitats: composite front mapping reveals oceanographic drivers of habitat use for a pelagic seabird. J. R. Soc. Interface 11, 1–9.
Schoombie, S., Crawford, R. J. M., Makhado, A. B., Dyer, B. M., and Ryan, P. G. (2016). Recent population trends of sooty and light-mantled albatrosses breeding on Marion Island. Afr. J. Mar. Sci. 38, 119–127. doi: 10.2989/1814232x.2016.1162750
Sokolov, S., and Rintoul, S. R. (2007). On the relationship between fronts of the Antarctic circumpolar current and surface chlorophyll concentrations in the Southern Ocean. J. Geophys. Res. 112, 1–17. doi: 10.1007/s13131-012-0248-2
Sokolov, S., and Rintoul, S. R. (2009). Circumpolar structure and distribution of the antarctic circumpolar current fronts: 2. Variability and relationship to sea surface height. J. Geophys. Res. 114, 1–15. doi: 10.1007/s13131-012-0248-2
Springer, K. (2016). Methodology and challenges of a complex multi-species eradication in the sub- Antarctic and immediate effects of invasive species removal. N. Z. J. Ecol. 40, 273–278. doi: 10.20417/nzjecol.40.30
Stammerjohn, S. E., Martinson, D. G., Smith, R. C., Yuan, X., and Rind, D. (2008). Trends in Antarctic annual sea ice retreat and advance and their relation to El Niño-Southern Oscillation and Southern annular mode variability. J. Geophys. Res. 113, 1–20.
Stammerjohn, S., Massom, R., Rind, D., and Martinson, D. (2012). Regions of rapid sea ice change: an inter-hemispheric seasonal comparison. Geophys. Res. Lett. 39:L06501.
Tamini, L. L., Chavez, L. N., Góngora, M. E., Yates, O., Rabuffetti, F. L., and Sullivan, B. (2015). Estimating mortality of black-browed albatross (Thalassarche melanophris, Temminck, 1828) and other seabirds in the Argentinean factory trawl fleet and the use of bird-scaring lines as a mitigation measure. Polar Biol. 38, 1867–1879. doi: 10.1007/s00300-015-1747-3
Terauds, A., Gales, R., and Alderman, R. (2005). Trends in numbers and survival of Black-browed (Thalassarche melanophrys) and Grey-headed (T. chrysostoma) Albatrosses breeding on Macquarie Island. Emu 105, 159–167. doi: 10.1071/mu05026
Terauds, A., Gales, R., Baker, G. B., and Alderman, R. (2006). Population and survival trends of Wandering Albatrosses (Diomedea exulans) breeding on Macquarie Island. Emu 106, 211–218. doi: 10.1071/mu06007
Trathan, P. N., Forcada, J., and Murphy, E. J. (2007). Environmental forcing and Southern Ocean marine predator populations: effects of climate change and variability. Philos. Trans. R. Soc. B Biol. Sci. 362, 2351–2365. doi: 10.1098/rstb.2006.1953
Tuck, G. N., Polacheck, T., and Bulman, C. M. (2003). Spatio-temporal trends of longline fishing effort in the Southern Ocean and implications for seabird bycatch. Biol. Conserv. 114, 1–27. doi: 10.1016/s0006-3207(02)00378-6
Tuck, G. N., Polacheck, T., Croxall, J. P., and Weimerskirch, H. (2001). Modelling the impact of fishery by-catches on albatross populations. J. Appl. Ecol. 38, 1182–1196. doi: 10.1046/j.0021-8901.2001.00661.x
Turner, J., Chenoli, S. N., Abu Samah, A., Marshall, G., Phillips, T., and Orr, A. (2009). Strong wind events in the Antarctic. J. Geophys. Res. Atmos. 114, 1–25. doi: 10.11646/zootaxa.3625.1.1
Vargas, F. H., Lacy, R. C., Johnson, P. J., Steinfurth, A., Crawford, R. J. M., Dee Boersma, P., et al. (2007). Modelling the effect of El Niño on the persistence of small populations: the Galápagos penguin as a case study. Biol. Conserv. 137, 138–148.
Waluda, C. M., Trathan, P. N., and Rodhouse, P. G. (1999). Influence of oceanographic variability on recruitment in the Illex argentinus (Cephalopoda: Ommastrephidae) fishery in the South Atlantic. Mar. Ecol. Prog. Ser. 183, 159–167. doi: 10.3354/meps183159
Waugh, S. M., Weimerskirch, H., Moore, P. J., and Sagar, P. M. (1999). Population dynamics of Black-browed and Grey-headed Albatrosses Diomedea melanophrys and D. chrysostoma at Campbell Island, New Zealand, 1942-96. Ibis 141, 216–225. doi: 10.1111/j.1474-919x.1999.tb07544.x
Weimerskirch, H., and Jouventin, P. (1998). “Changes in population sizes and demographic parameters of six albatross species breeding on the French sub-Antarctic islands,” in Albatross Biology and Conservation, eds G. Robertson and R. Gales (Chipping Norton: Surrey Beatty & Sons), 84–91.
Weimerskirch, H., Clobert, J., and Jouventin, P. (1987). Survival in five southern albatrosses and its relationship with their life history. J. Anim. Ecol. 56, 1043–1055. doi: 10.2307/4965
Weimerskirch, H., Louzao, M., De Grissac, S., and Delord, K. (2012). Changes in wind pattern alter albatross distribution and life-history traits. Science 335, 211–214. doi: 10.1126/science.1210270
Weller, F., Cecchini, L. A., Shannon, L., Sherley, R. B., Crawford, R. J. M., Altwegg, R., et al. (2014). A system dynamics approach to modelling multiple drivers of the African penguin population on Robben Island, South Africa. Ecol. Modell. 277, 38–56. doi: 10.1016/j.ecolmodel.2014.01.013
Keywords: climate change, fisheries, invasive species, multi-event models, reproductive success, seabirds, Southern Ocean, survival
Citation: Cleeland JB, Pardo D, Raymond B, Tuck GN, McMahon CR, Phillips RA, Alderman R, Lea M-A and Hindell MA (2021) Disentangling the Influence of Three Major Threats on the Demography of an Albatross Community. Front. Mar. Sci. 8:578144. doi: 10.3389/fmars.2021.578144
Received: 30 June 2020; Accepted: 03 February 2021;
Published: 23 February 2021.
Edited by:
Alida Bundy, Bedford Institute of Oceanography (BIO), CanadaReviewed by:
Catherine Sarah Longo, Marine Stewardship Council (MSC), United KingdomStephani Zador, Alaska Fisheries Science Center (NOAA), United States
Copyright © 2021 Cleeland, Pardo, Raymond, Tuck, McMahon, Phillips, Alderman, Lea and Hindell. This is an open-access article distributed under the terms of the Creative Commons Attribution License (CC BY). The use, distribution or reproduction in other forums is permitted, provided the original author(s) and the copyright owner(s) are credited and that the original publication in this journal is cited, in accordance with accepted academic practice. No use, distribution or reproduction is permitted which does not comply with these terms.
*Correspondence: Jaimie B. Cleeland, amFpbWllLmNsZWVsYW5kQGdtYWlsLmNvbQ==