- 1Centre for Sustainable Aquatic Ecosystems, Harry Butler Institute, Murdoch University, Perth, WA, Australia
- 2Environmental and Conservation Sciences, College of Science, Health, Engineering and Education, Murdoch University, Perth, WA, Australia
- 3Department of Primary Industries and Regional Development, Western Australian Fisheries and Marine Research Laboratories, Perth, WA, Australia
Portunid crabs contribute to significant commercial and recreational fisheries globally and are commonly fished in estuaries and/or marine embayments, which are amongst the most degraded of all aquatic ecosystems. Portunus armatus were collected seasonally between April and February from five locations across three systems in temperate south-western Australia. The dietary composition of crabs was quantified and compared between two estuaries (Peel-Harvey and Swan-Canning) and a sheltered marine embayment (Cockburn Sound) containing three distinct habitats: shallow seagrass, shallow sand and deep sand. Overall, crabs ingested large volumes of bivalves (both live organisms and dead shell), polychaetes, crustaceans (e.g., amphipods, small decapods), and smaller volumes of teleosts, echinoderms and plant material (seagrass, algae). Analysis of Similarities showed that dietary composition varied significantly among the five locations (two estuaries and three habitats within Cockburn Sound) and seasons, with greater location than seasonal differences in the two estuaries. Diets were most distinct in the Cockburn Sound seagrass due to greater volumes of decapods and teleosts and smaller volumes of bivalve shell consumed in this habitat. Crabs from both estuaries consumed greater quantities of bivalves than those from Cockburn Sound. Seasonal differences in both estuaries were greatest between summer and winter, with a more diverse range of prey and large quantities of bivalves ingested in summer, whereas small bivalves and bivalve shell in the Peel-Harvey and polychaetes and other crustaceans in the Swan-Canning, were consumed in greater quantities in winter. The summer diet in the Peel-Harvey Estuary in the current study was compared to that 20 years previous and with documented change in the benthic macroinvertebrate fauna. Currently, crabs consume smaller volumes of high-calorie prey, i.e., polychaetes, small bivalves and teleosts, and instead ingest greater proportions of calcareous material than previously. This marked shift in dietary composition parallels changes in benthic macroinvertebrates in the Peel-Harvey Estuary. Overall, prey availability appears to be the major factor influencing the spatial and temporal differences in P. armatus diets in these three coastal systems.
Introduction
Portunid crabs contribute to significant commercial and recreational fisheries globally and are commonly fished in estuaries and/or marine embayments, which are amongst the most anthropogenically degraded of all aquatic ecosystems (Tweedley et al., 2016a). Blue swimmer crabs (BSC) of the Portunus pelagicus species complex (sensu Lai et al., 2010) are distributed and caught commercially and recreationally throughout Australia and south-east Asia to the Persian Gulf in Oman (Lai et al., 2010). They are of high economic and social value to local communities, with the majority of catch taken from protected coastal embayments (<20 m depth) and estuaries that are inherently subject to environmental change and highly variable catch rates (Johnston et al., 2020a,b). Fisheries for BSC have shown declines in a number of regions including Indonesia (Prince et al., 2020) and Australia (Johnston et al., 2011a,b; Harris et al., 2012; Chandrapavan et al., 2019). These declines have been linked to fishing pressure and environmental perturbations, such as extreme heat events and flooding in Australia. Within Australia, the total commercial catch of Portunus armatus (formerly Portunus pelagicus) was ∼2,000 t in 2017, ∼0.5% of the global BSC catch (FAO, 2020; Johnston et al., 2020a). Portunus armatus is also an iconic recreational species and, in Western Australia, is the most captured species by boat-based recreational fishers (Ryan et al., 2019).
In south-western Australia, P. armatus supports important recreational and commercial fisheries in the Peel-Harvey Estuary (PHE), Swan Canning Estuary (SCE) and prior to its closure in 2014, Cockburn Sound (CS) (Johnston et al., 2014, 2020b; Obregon et al., 2020). The commercial catch of P. armatus in the West Coast Bioregion of Western Australia, now comprises of catches mainly from the PHE and SCE was 75.2 t in 2017, but historically, has been as high as 360 t when catches in CS peaked in the late 1990s (Johnston et al., 2020b, 2021). The total boat-based recreational catch in the West Coast Bioregion for 2017/18 was estimated to be 54 t, ∼42% of the total crab catch in this bioregion (Ryan et al., 2019). Recent reductions in total catch of crabs in this bioregion are attributed to the decline and closure of the CS fishery initially in 2006 (Johnston et al., 2011a,b). This fishery reopened in 2009, but stocks declined again, and the fishery has remained closed since 2014 and exhibits little sign of recovery (Johnston et al., 2020b). The demise of the CS fishery demonstrates the need to better understand how variable environmental conditions influence P. armatus population dynamics and the ecosystem that supports the population, particularly prey abundance.
Although species in the genus Portunus are found in estuaries and coastal marine environments, they do not complete the whole of their life-cycle within estuaries (Potter et al., 2015a,b). Typically, they enter estuaries as juveniles, remaining there for extended periods before migrating into marine environments to release their eggs (Potter et al., 1983; Metcalf et al., 1995; Potter and de Lestang, 2000; de Lestang et al., 2003). In the PHE, P. armatus is found in greatest densities in salinities above 30 and leaves the estuary during winter months when salinities are lower (following rainfall and river flow) to release their eggs in the adjacent marine embayment (Potter et al., 1983; de Lestang et al., 2003). The productivity of the estuarine environment clearly influences the growth and reproduction of P. armatus populations in south-western Australia. The Peel-Harvey and Swan-Canning estuaries have experienced increased urbanization, eutrophication, marinisation and more frequent hypoxic events over the last 40 years (Hallett et al., 2018; Valesini et al., 2019; Rose et al., 2020), leading to marked changes in the composition of the benthic macroinvertebrate and fish fauna in these estuaries (Tweedley et al., 2014; Potter et al., 2016; Valesini et al., 2017). The nearby sheltered coastal marine embayment of CS has also been altered by anthropogenic change, initially through industrial development and nutrient discharge which led to an ∼75% loss of seagrass from the late 1960s to early 1980s (Cambridge and McComb, 1984; Cambridge et al., 1986; Kendrick et al., 2002).
Portunid crabs are opportunistic predators and feed on a wide range of benthic macroinvertebrates, e.g., bivalves, gastropods, polychaetes, and small crustaceans, as well as teleosts and small volumes of algae and seagrass (Laughlin, 1982; Williams, 1982; Edgar, 1990; Stoner and Buchanan, 1990; Hsueh et al., 1992; de Lestang et al., 2000). Variations in dietary composition of portunids depend largely on prey availability (Williams, 1982), which can vary spatially between marine and estuarine ecosystems and habitats (Bloomfield and Gillanders, 2005; Tweedley et al., 2015) and temporally (Kalejta and Hockey, 1991). Ontogeny and molt stage also influence the diet of portunid crabs both directly, through prey selection (Stoner and Buchanan, 1990; Hsueh et al., 1992; de Lestang et al., 2000; Josileen, 2011), and indirectly, by influencing crab behavior and habitat usage and therefore prey availability (Hines et al., 1987; Shirley et al., 1990; Wolcott and Hines, 1990). As crabs grow, they predate on larger, more mobile prey, while the consumption of calcareous material is greater prior to and after molting compared to a more diverse diet during the inter-molt phase (de Lestang et al., 2000). Third party certification by organizations such as the Marine Stewardship Council (MSC) provides independent verification of the sustainability of fisheries resources. These assessments examine stock dynamics of the target species and the effects of fishing on the ecosystem and are underpinned by important biological data on growth, mortality, reproductive output and ecosystem food webs. The latter highlights the importance of dietary studies for providing information on food webs and identifying potential changes associated with either fishing or environmental change (Maschette et al., 1990).
The overall aim of this study was to determine if the diet of P. armatus differed between five locations: two estuaries (PHE, SCE) and the three habitats present within a sheltered marine embayment (CS—shallow seagrass, shallow sand and deep sand). Seasonal differences in P. armatus diet were also investigated in the two estuaries, where greater numbers of samples were taken throughout the year than in CS. Finally, decadal changes in diet of crabs in the PHE were explored by comparing historical data from 20 years ago (November to April of 1995/96 and 1996/97 in de Lestang et al., 2000), to current data (January 2017). Any dietary shifts were related to changes in the benthic community documented by Wildsmith et al. (2009) over these two decades.
Materials and Methods
Site Description
The SCE and PHE and the sheltered marine embayment of CS are located within 70 km of each other along the lower west coast of Western Australia (Figure 1). This region experiences a Mediterranean climate, comprising cool wet winters and hot dry summers with 60–80% of rainfall occurring between May and September (Crisp et al., 2018; Hallett et al., 2018). The region is microtidal, with a spring tidal range of only ∼0.6–0.8 m (Valesini et al., 2014). The SCE, bordered by the city of Perth (population of ∼2 million), is 50 km long with a surface area of ∼55 km2 and remains permanently open to the Indian Ocean. The estuary comprises a narrow entrance channel that opens into two basins and the tidal portions of the Swan and Canning rivers. Although more than half of the estuary is shallow, i.e., <2 m in depth, it reaches a maximum depth of ∼20 m in the entrance channel. The seagrass Zostera muelleri is present in the entrance channel and Halophila ovalis forms extensive beds in shallow parts of the basins (Hogan-West et al., 2019).
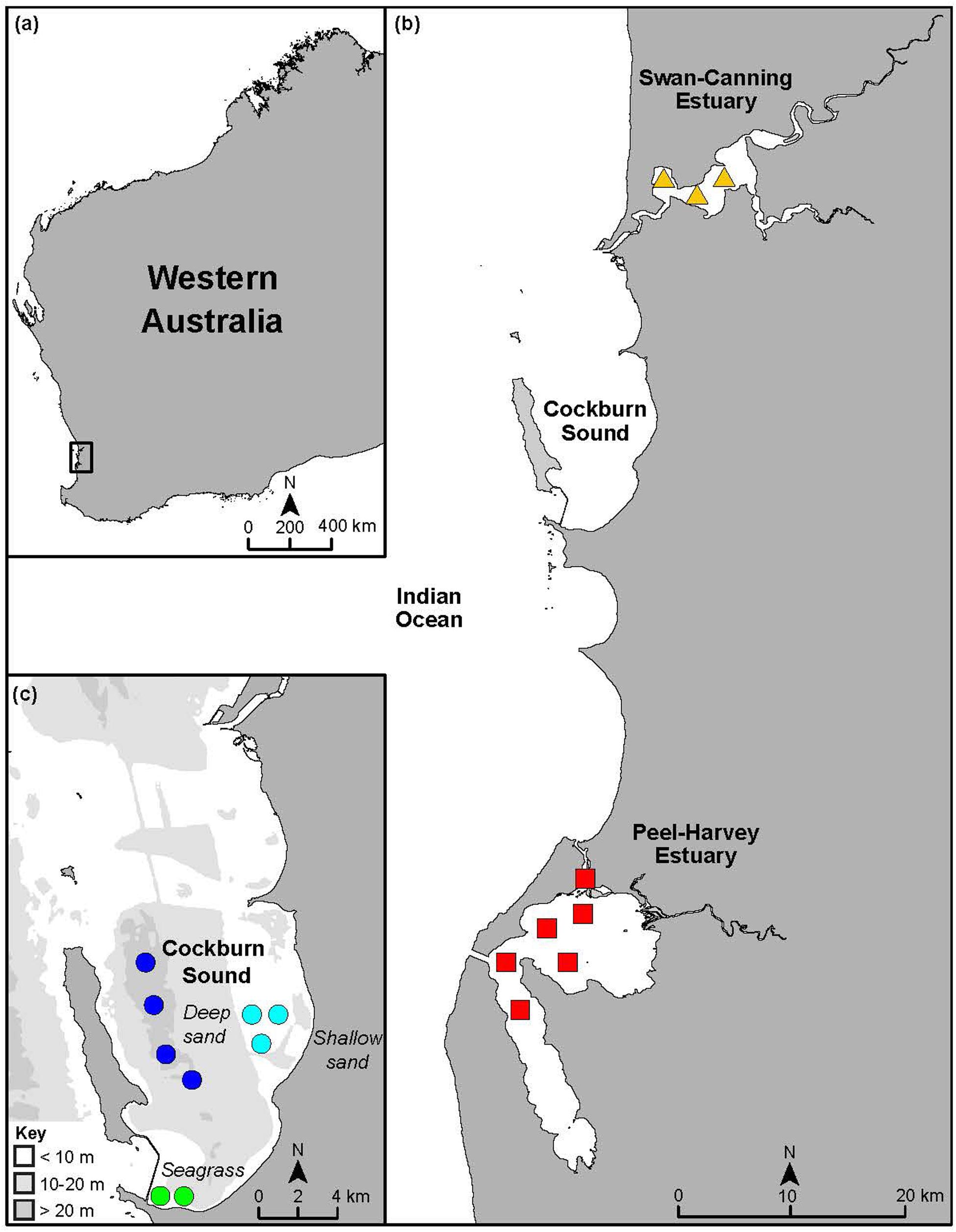
Figure 1. Maps showing (a) the location of the study area in Western Australia, (b) sampling areas in the Peel-Harvey and Swan-Canning estuaries and the marine embayment of Cockburn Sound and (c) a bathymetric map showing sampling areas in Cockburn Sound and the three habitats it contains. Symbols denote the spatial areas from which samples of Portunus armatus were collected in each system and are not reflective of replicate sites.
The PHE is the largest estuary in the region, covering an area of ∼133 km2. It consists of two large, shallow (mainly < 2 m deep) basins, which are linked to the ocean by a natural and an artificial entrance channel, and tidal portions of three rivers. Seagrasses, such as Ruppia megacarpa and H. ovalis, are present in each basin (Valesini et al., 2019). Cockburn Sound is a sheltered marine embayment, ∼100 km2 in area, located ∼25 km south of the SCE (Wakefield et al., 2013). It has a sandy, deep central basin (∼20 m deep) that is surrounded by shallow flats. Seagrasses (mainly Posidonia sinuosa and Posidonia australis) once covered most of these flats but are now restricted to small areas in the southern end of the embayment, with the remainder now bare sand (Kendrick et al., 2002).
Water temperature in the deeper waters of the two estuaries reaches a summer maxima of ∼25°C and a minima of ∼16 (SCE) and ∼14 (PHE) °C in winter (Potter et al., 2016; Poh et al., 2019). Summer temperatures in CS, are slightly cooler than in the two estuaries ranging from 22.7 to 23.9°C (Marks et al., 2020). Salinities in the downstream regions of the SCE and PHE, where crabs were sampled (Figure 1), are relatively stable compared to regions further upstream, rarely declining to < 30, but can become slightly hypersaline in summer (37 in the SCE, 40 in PHE) (Potter et al., 2016; Poh et al., 2019).
Sample Collection, Processing, and Data Treatment
A wide size range of inter-molt and pre-molt P. armatus were collected from multiple sites within the SCE (∼5–15 m deep) and PHE (∼2–3 m deep) and several sites within each of the three habitats in CS, i.e., shallow seagrass (CSSg ∼2–5 m deep), shallow sand (CSS ∼6–12 m deep) and deep sand (CSD ∼18–23 m deep; Figure 1). The CSSg habitat is located in the southern end of CS, about 8 km from the CSS habitat and 6 km from the CSD habitat.
Crabs were sampled seasonally between April 2016 and February 2017 using otter trawls in CS, SCE and PHE (two trawl nets: 5 m head rope, 51 mm wing mesh and 25 mm cod-end mesh vs. 4.5 m head rope, 25 mm wing mesh and 9 mm cod-end mesh), both towed from a ∼7 m long research vessel), gill nets in PHE (8 × 20 m panels of varying mesh size, ranging from 35 to 127 mm) and seine nets in CSSg (21.5 m net with two 10 m wing panels of 9 mm mesh and 1.5 m bunt of 3 mm mesh with a vertical drop of 1.5 m). All sampling took place after nightfall, except otter trawls in the PHE, which were deployed during the day. Crabs were euthanised in an ice slurry after capture and frozen as soon as possible to stop digestion.
In the laboratory, each crab was measured to the nearest 1 mm carapace width (CW), had its sex and molt stage recorded and its proventriculus, containing the cardiac and pyloric stomachs, removed and preserved in 70% ethanol. Crabs were classified as either small (<60 mm CW) or large (≥60 mm CW), due to large changes in the diet of P. armatus above and below this size reported from previous studies (e.g., Edgar, 1990; de Lestang et al., 2000). The proventriculus of each individual was cut open and the fullness of the foregut scored on a scale of 0 (empty) to 10 (fully distended). The items from each cardiac stomach were examined using a dissecting microscope with reflected light and identified to the lowest taxon possible. Each item was then allocated to a broader taxonomic classification (dietary category, see categories in Table 1)—small bivalves were those with a shell length of < 5 mm (mainly Arthritica semen), large bivalves were ≥ 5 mm (mainly Spisula trigonella); other crustaceans included caprellid, corophiid, and gammarid amphipods, other crabs and crustacean exoskeleton material. The frequency of occurrence (%F) was recorded and the percentage volumetric contribution to the total volume of the stomach contents (%V) determined visually for each prey item and prey category. Mollusc shell fragments were examined in detail for attached soft tissues and weathering of the edges (smooth or sharp etc.) to determine if they had been consumed as live individuals or as dead shell material. The %V, %F and stomach fullness data from all crabs with non-empty stomachs were averaged across crabs in each system (PHE, SCE, and CS) and compiled in a dietary table. This summarizes the dietary data at low taxonomic resolution and also broader dietary categories that are used in subsequent analyses, based on those used by de Lestang et al. (2003) in their study of diets of P. armatus in the PHE and the Leschenault Estuary, 80 km further south.
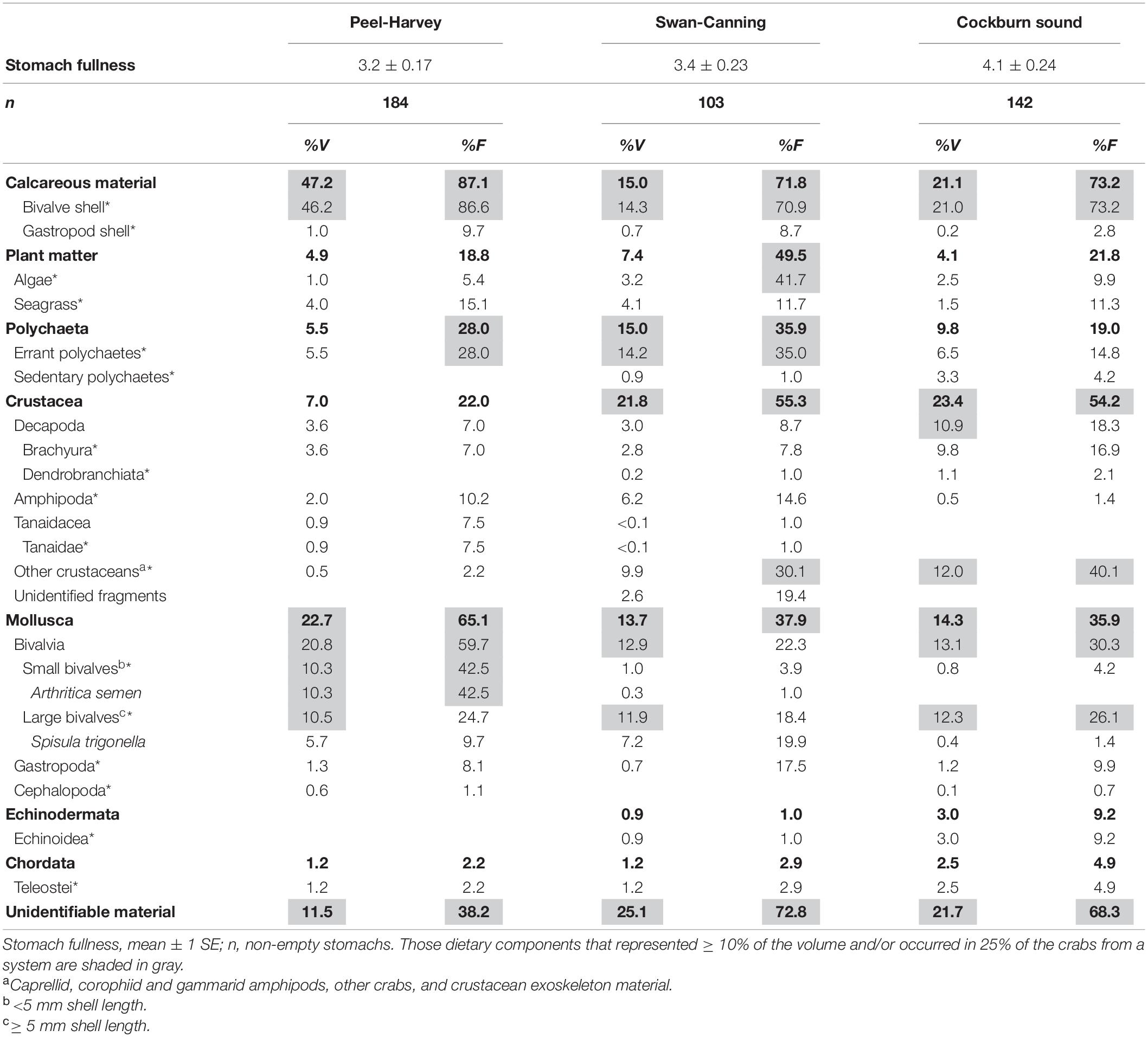
Table 1. Volumetric contribution (%V) and frequency of occurrence (%F) of major taxa (boldface), dietary categories (*) and dietary items to the dietary composition of all inter-molt and pre-molt Portunus armatus of all sizes in the three systems: Peel-Harvey and Swan-Canning estuaries and Cockburn Sound (three locations combined).
Dietary data for P. armatus collected from the PHE in the current study were compared with those collected 20 years earlier in 1995–97 by de Lestang et al. (2000). Those latter data were extracted from Figure 1 of de Lestang et al. (2000) for inter-molt crabs ≥ 60 mm CW, caught between November and April. For comparisons between current and historical data, only data from inter-molt crabs collected in January 2017 (summer) were used and the prey categories used in the current study were modified to match those of the earlier study.
Statistical Analyses
All analyses were conducted using routines in the PRIMER v7 (Clarke and Gorley, 2015) and the PERMANOVA+ add-on (Anderson et al., 2008). The spatial variable used for all testing was Location, which had five levels, i.e., the PHE, the SCE (estuaries) and the three habitats within the marine embayment CS, namely, deep sand (CSD), shallow sand (CSS), and shallow seagrass (CSSg). Initially, exploratory analyses were carried out to investigate the influence of sampling method i.e., day-time trawling and night-time gill netting, on the composition of the stomach contents of crabs ≥ 60 mm CW in the PHE. One-way Analysis of Similarities (ANOSIM) (Clarke and Green, 1988) found no significant difference (R = 0.036, P = 13.1%) in dietary composition (square root transformed %V and Bray-Curtis resemblance matrix) and the dietary data were therefore pooled across methods. The significance of sex and molt stage were also examined in a four-way PERMANOVA of the %V data by Location, Season, Molt Stage and Sex. Neither Molt Stage (P = 47.1%), Sex (P = 45.7%) nor any of their interactions significantly affected dietary composition (P = 26.9–92.6%). Thus, Molt Stage and Sex were removed from all subsequent analyses.
Individual crabs whose stomachs contained contents were sorted into random groups of two to five crabs within each Location × Season combination depending on the number of crabs within the particular combination of factors (de Lestang et al., 2000; Greenwell et al., 2019). The %V data were averaged across these groups because of the potentially low number of different taxa in each stomach. All multivariate analyses were run on the 17 broader dietary categories identified with a ∗ in Table 1. The prey category “unidentified organic material” was removed and the data re-standardized. These %V data were then averaged across crabs within each random group and square root transformed.
Spatial Differences in Diet
The pre-treated data collected from each of the five locations (PHE, SCE, CSSg, CSSS, CSSD) in summer, when ≥ 17 crabs had prey items in their stomachs at each location (Supplementary Table 1), were used to construct a Bray-Curtis resemblance matrix and subjected to one-way ANOSIM to determine whether dietary composition differed significantly among locations (P < 5%). This matrix was then subjected to the bootstrap averages routine (Clarke and Gorley, 2015) to bootstrap those samples in metric multidimensional space. A total of 120 averages of repeated bootstrap samples (bootstrapped averages) for each location were used to construct a mMDS ordination plot. A point representing the group average, and the shaded 95% bootstrap region, were superimposed on each plot.
When a significant difference among the dietary compositions of locations was detected, Similarity Percentages (SIMPER; Clarke and Gorley, 2015) was used to identify those categories that typified the diet of crabs in a location and those that were responsible for distinguishing between each pair of groups. A shade plot, derived from the square-root transformed data averaged across replicates within each Location, was constructed and used to visualize trends. This plot is a simple visualization of the frequency matrix, where a white space for a dietary category demonstrates that particular prey was not collected, while the depth of shading from gray to black is linearly proportional to the contribution of the dietary category to %V (Clarke et al., 2014). Dietary categories (y-axis) and locations (x-axis) are arranged in an optimal order, based on seriation.
Seasonal Differences in Diet
The extent of any seasonal changes in dietary composition was then determined for data from the PHE and SCE as ≥ 17 crabs had prey items in their stomachs in each season. Although data were obtained for each season in the PHE, data from autumn were excluded from the initial PERMANOVA test below as no data were available for autumn in the SCE. Pre-treated dietary data from the PHE and SCE in winter, spring and summer were used to construct a Bray-Curtis resemblance matrix and subjected to two-way PERMANOVA to determine if there was a significant Location × Season interaction. As a significant interaction was detected (P = 0.1%), separate one-way ANOSIM tests were run for each estuary to determine if dietary composition differed significantly among seasons – including all four seasons for the PHE. Note that both PERMANOVA and ANOSIM tests have been applied; PERMANOVA to determine the significance of main effects and their interactions and ANOSIM to determine both the magnitude (R statistic value which ranges from ∼0 to 1) and significance value (P as a %) of significant terms identified in the PERMANOVA (following Lek et al., 2011). Bootstrapped mMDS ordination plots were constructed for each estuary using the methodology explained above, as was a shade plot, based on data averaged across each Location × Season combination. When significant differences were found, the SIMPER routine was run to determine which dietary categories typified and distinguished different groups.
Results
A total of 630 intermolt and premolt crabs were collected and their cardiac stomachs examined, of which 429 contained food (Supplementary Table 1) and 367 of these crabs (85.5%) were ≥ 60 mm CW. The mean CW (±1 SE) of crabs ≥ 60 mm ranged from 90.5 ± 3.16 mm in the SCE (n = 80) to 104.6 ± 2.83 mm in CSS (n = 106). The percentage of crabs in inter-molt ranged from 51 to 67% in the Peel-Harvey Estuary (PHE) and Swan-Canning Estuary (SCE) but was 90% in CSS.
Overall Dietary Composition
The mean stomach fullness of all crabs (range from 0 to 10) was greatest in CS (4.06 ± 0.24 and lowest in the PHE (3.20 ± 0.17; Table 1). Mollusc shell was by far the most commonly observed dietary category occurring in > 70% of crabs from each system and contributing 47, 21, and 15% of the total volume in the PHE, CS and SCE, respectively (Table 1). Bivalves, especially small bivalves (e.g., Arthritica semen, <5 mm shell length), were also consumed more frequently and in greater volumes in the PHE (%F = 60%, %V = 21%) than in the SCE or CS (%F≈25%, %V≈13% in both). Conversely, the volumetric consumptions of other crustaceans (1.4%) and unidentifiable organic material (10.0%) were lower in the PHE than in the SCE (%V = 12.5, %F = 24.9%) and CS (%V = 12.0, %F = 20.0%; Table 1). Polychaetes and plant material were also frequently consumed by crabs in all three systems and with amphipods, were consumed in the greatest volumes by crabs in the SCE, where their respective volumes were 15.0, 7.5, and 6.4%. Crabs in CS consumed greater volumes of decapods (10.9%), specifically brachyurans (9.8%), and, to a lesser extent, teleosts (2.5%) than crabs in either estuary (Table 1).
In summer, the diet of crabs in all five locations i.e., PHE, SCE, CSSg, CSS, CSD, was dominated by mollusc shell (12–42%), with bivalves making a greater contribution in the two estuaries (>30%) than in all locations within the marine embayment of CS (<19%), where decapods and teleosts made greater contributions (Figure 2A). Decapods contributed relatively large volumes to the diets in all CS locations, especially CSSg (44%), and the PHE (>6%), but little was consumed in the SCE (1%). Other crustaceans were consumed in greater volumes in all CS locations and SCE (>7%) than in PHE (1%; Figure 2A). The total number of prey items recorded in summer was greater in the PHE (22) and SCE (18) than in each of the three CS habitats (=16 across all three habitats in CS).
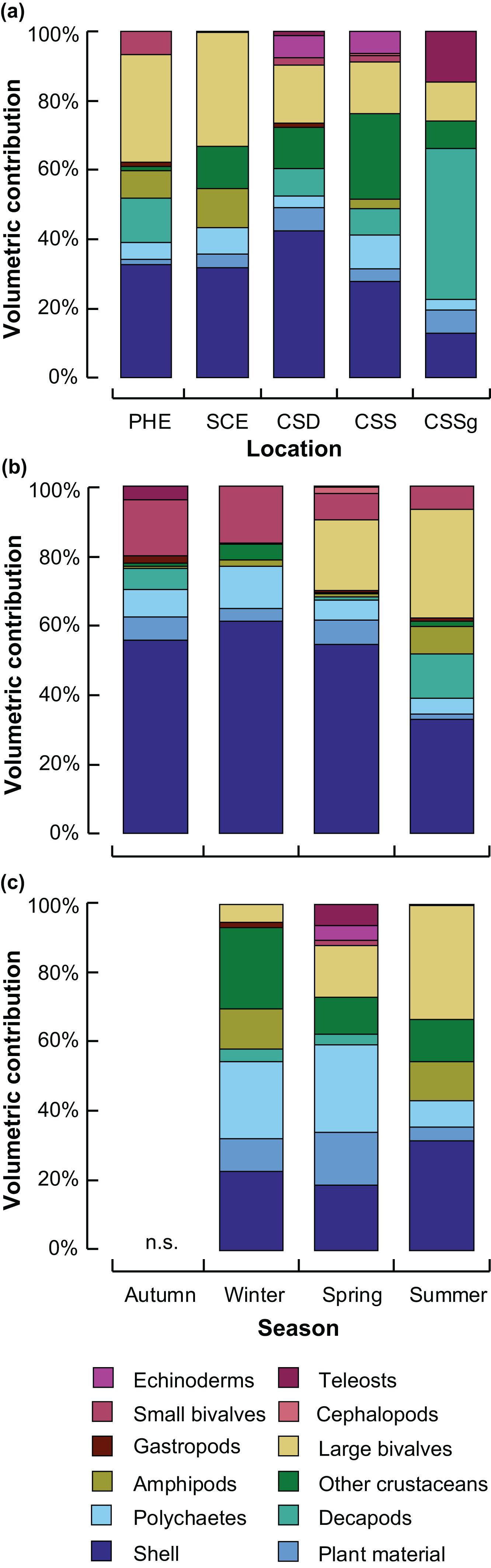
Figure 2. Stacked bar graphs showing the mean percentage volumetric contribution of the predominant prey categories to the dietary composition of Portunus armatus in (a) each location in the Austral summer and each season within the (b) Peel-Harvey (PHE) and (c) Swan-Canning (SCE) estuaries. CSD = Cockburn Sound Deep sand; CSS = Cockburn Sound Shallow sand; CSSg = Cockburn Sound Seagrass. n.s. = not sampled.
Mollusc shell was by far the dominant prey item in all seasons in the PHE (∼55%), except summer (32%), when large bivalves were consumed in similar volumes (31%) and decapods (12%) and amphipods (8%) in greater volumes than all other seasons (Figure 2B). Large bivalves (e.g., Spisula trigonella and unidentified bivalves ≥ 5 mm shell length, Table 1) were also consumed in considerable volumes in spring (20%). Although small bivalves and polychaetes were consumed in all seasons in the PHE, they made a greater contribution in autumn and winter than in summer and spring. The number of prey items recorded in summer (22), spring and autumn (both 21) were more than double that of winter (10).
Crabs in the SCE also consumed a considerable volume of mollusc shell in all seasons (19–32%), but to a lesser extent than in the PHE, and consumption was greatest in summer (Figure 2C). The ingestion of large bivalves in the SCE, like the PHE, was also greatest in summer (33%), while that of polychaetes and plant material was smaller in summer than winter or spring. In the SCE, crabs consumed other crustaceans in greater volumes in winter than spring or summer and the volumetric contribution of amphipods in spring was negligible compared to winter and summer (Figure 2C). A greater number of prey items was recorded in the SCE in summer (18) and spring (22) than in winter (13).
Spatial Differences in Summer Diet Among Locations
One-way ANOSIM demonstrated that the dietary composition of large crabs collected in summer varied significantly among the five locations (Global R = 0.347, P = 0.1%). All pairwise combinations of locations differed significantly, except CSD vs. CSS (R = −0.029, P = 55.6%) and CSD vs. SCE (R = 0.148, P = 11.2%; Supplementary Table 2). This is shown on the mMDS plot where the 95% bootstrapped regions of all locations are spatially separated, except those for CSD and CSS, which overlap substantially (Figure 3). The greatest separation was between the CSSg on the right hand side of the plot and all other locations and pairwise R values for comparisons involving this location were greater (all R > 0.500, P < 0.8%) than for all other comparisons (all R < 0.431; Supplementary Table 2). This is due to the significantly lower contribution of bivalve shell to the diet in CSSg than all other locations, where SIMPER analysis found it was a typifying dietary item (Figure 4). Conversely crabs in CSSg consumed greater amounts of brachyurans and teleosts than crabs at all other locations. The next most distinct diets were in the PHE and SCE where crabs consumed greater volumes of large bivalves and bivalve shell than at the three habitats in CS. Significant differences in dietary composition between these two estuaries (R = 0.221, P = 3.1%) were due to the greater volumes of small bivalves and brachyurans in the PHE than in the SCE (Figure 4).
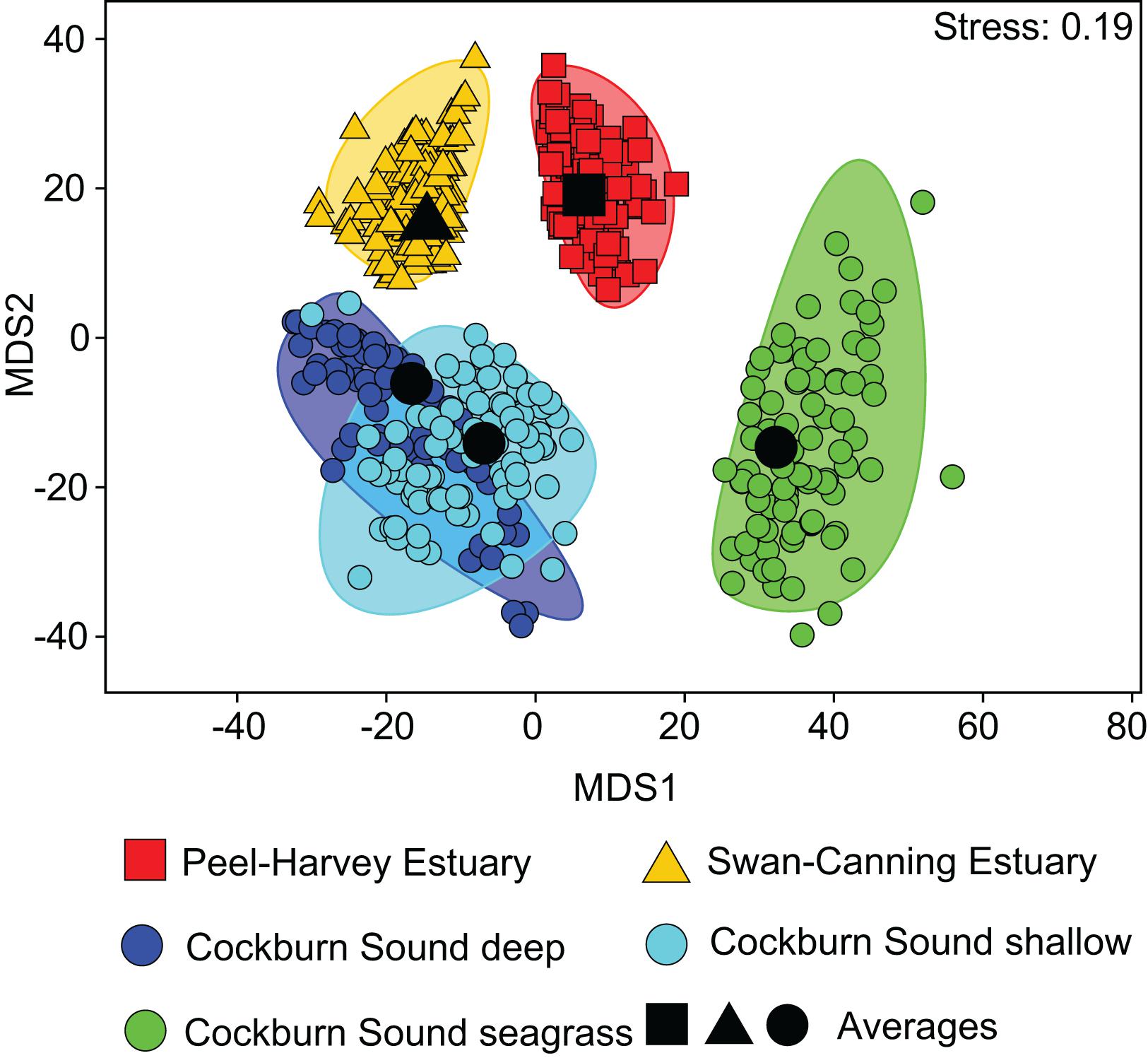
Figure 3. Two-dimensional metric Multidimensional Scaling (mMDS) ordination plot constructed from bootstrap averages of the dietary composition of Portunus armatus in each location during the Austral summer. Group averages (larger black symbols) and approximate 95% region estimates fitted to the bootstrap averages (shaded areas) are shown.
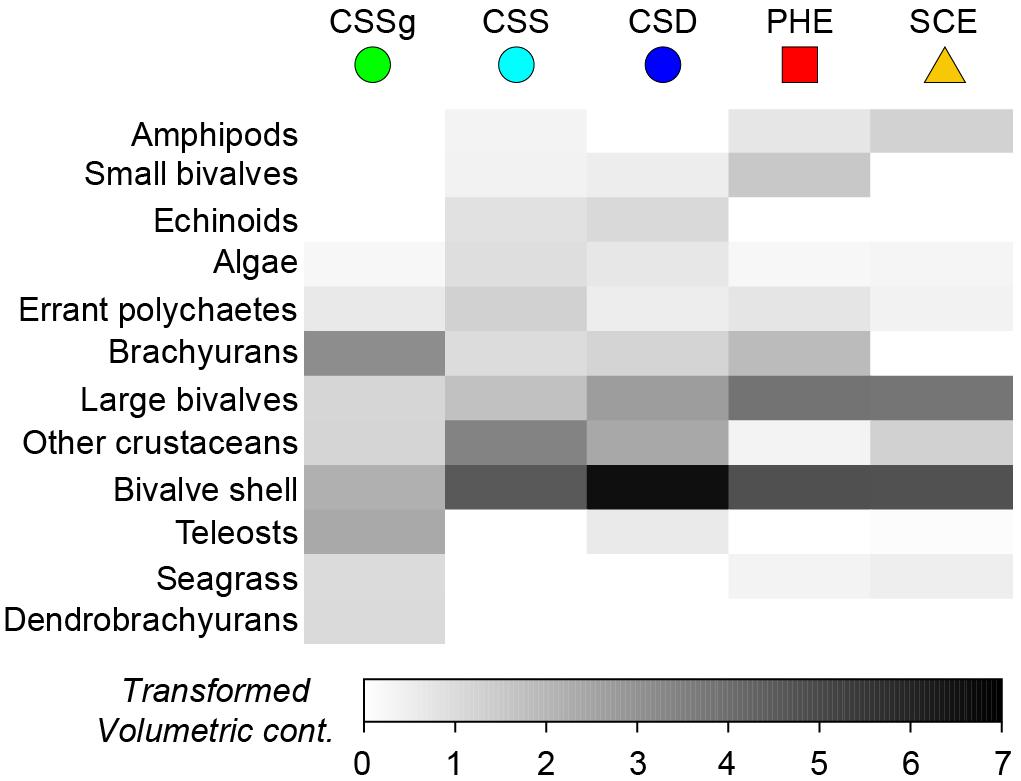
Figure 4. Shade plot showing the square-root transformed volumetric contribution of the 12 most important dietary categories to the dietary composition of Portunus armatus each location in the Austral summer. PHE = Peel-Harvey Estuary, SCE = Swan-Canning Estuary, CSD = Cockburn Sound Deep sand, CSS = Cockburn Sound Shallow sand and CSSg = Cockburn Sound Seagrass.
Seasonal Differences in Diet in the Peel-Harvey and Swan-Canning Estuaries
One-way ANOSIMs showed that dietary composition differed significantly among seasons in each estuary, but was of greater magnitude in the PHE (Global R = 0.495, P = 0.1%) than the SCE (Global R = 0.281, P = 0.1%; Figure 5). Within the PHE, the greatest differences were between summer and winter (R = 0.933, P = 0.2%) and summer and autumn (R = 0.795, P = 0.1%). This is shown on the seasonal mMDS by the large separation between the bootstrap regions for summer and both autumn and winter (Figure 5A). SIMPER identified greater consumption of large bivalves in summer and of bivalve shell and small bivalves in winter and autumn as distinguishing the diets in these seasons (Figure 6). Less pronounced differences occurred between spring and summer (R = 0.634, P = 0.2%), spring and winter (R = 0.611, P = 0.1%) and spring and autumn (R = 0.473, P = 0.1%). In spring, the consumption of large bivalves, bivalve shell and small bivalves was intermediate between those of summer and autumn or winter (Figure 6). Diets did not differ significantly between autumn and winter (R = −0.033, P = 55.5%).
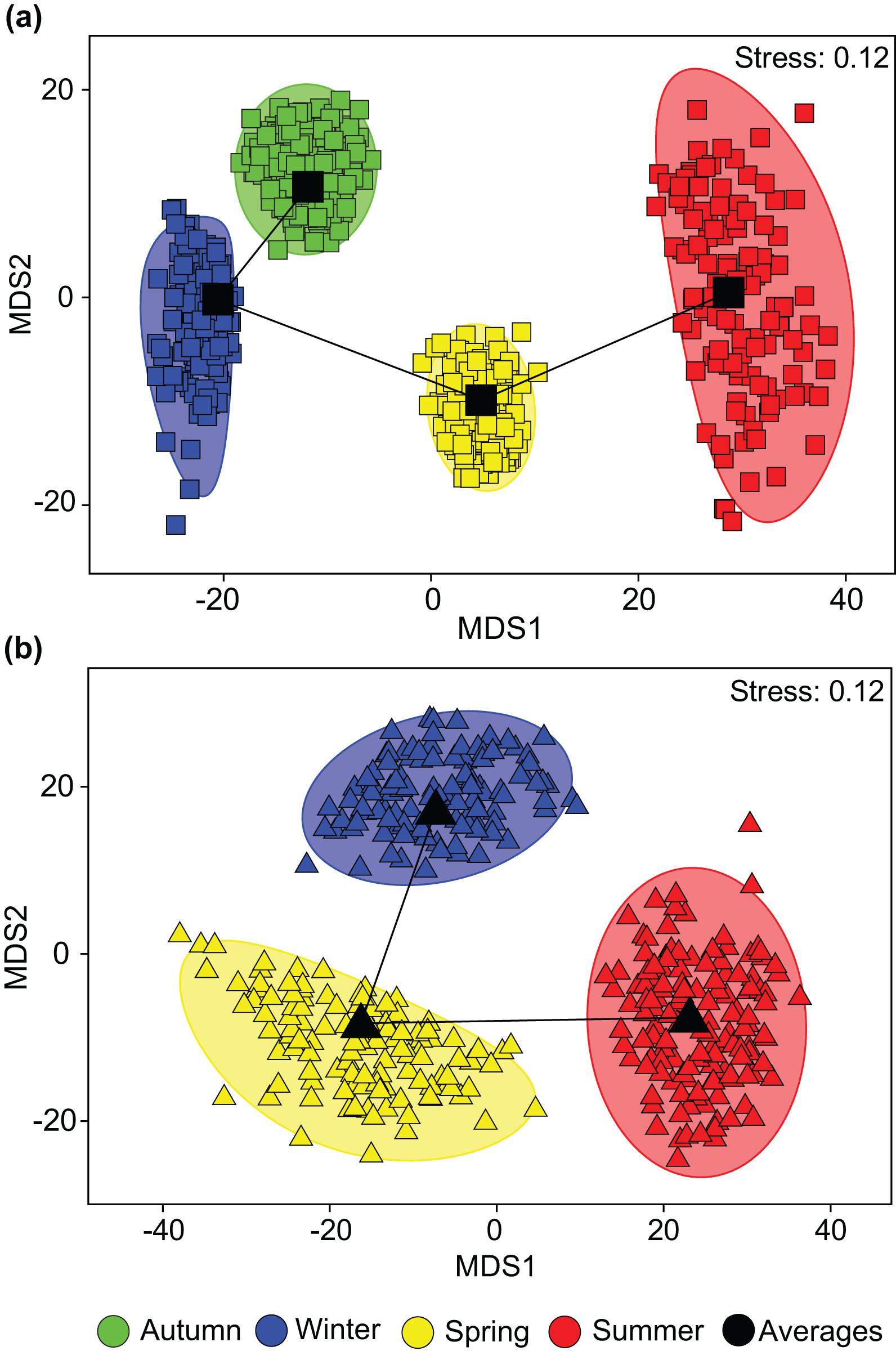
Figure 5. Two-dimensional metric Multidimensional Scaling (mMDS) ordination plot constructed from bootstrap averages of the dietary composition of Portunus armatus in the (a) Peel-Harvey and (b) Swan-Canning estuaries during each season. Group averages (larger black symbols) and approximate 95% region estimates fitted to the bootstrap averages (shaded areas) are provided.
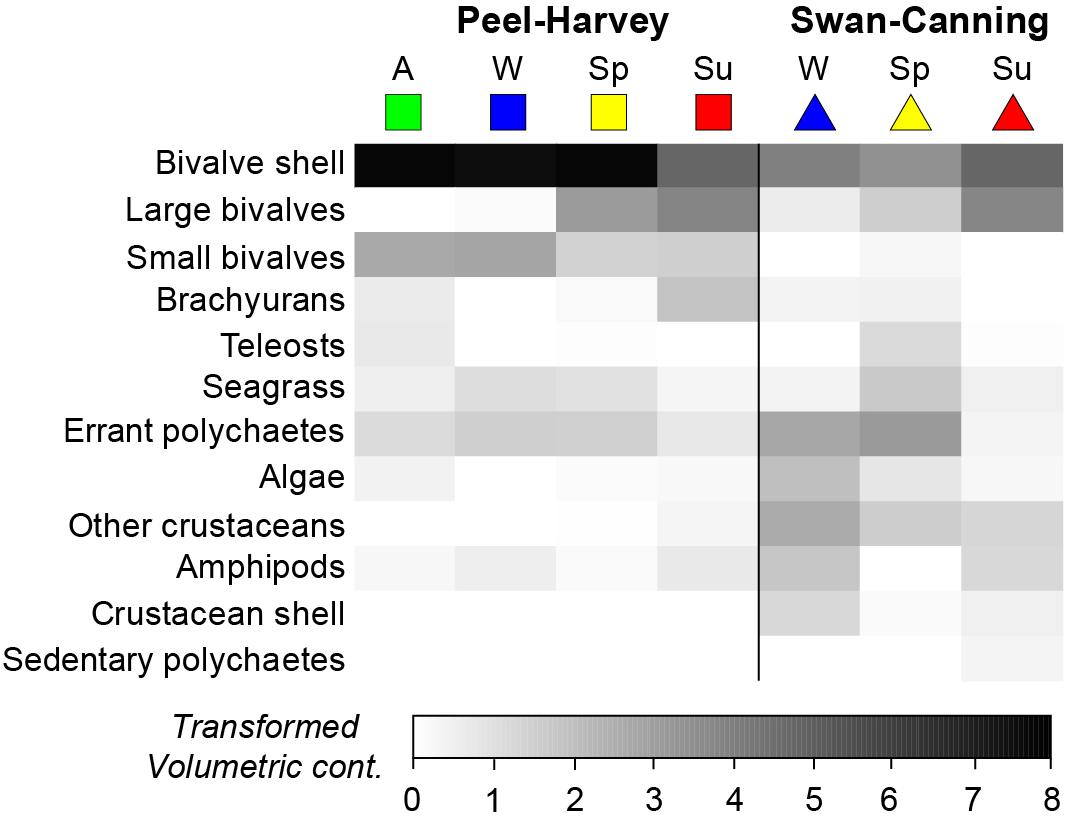
Figure 6. Shade plot showing the square-root transformed volumetric contribution of the 12 most important dietary categories to the dietary composition of Portunus armatus in each Austral season in the Peel-Harvey and Swan-Canning estuaries. A = Autumn, W = Winter, Sp = Spring, Su = Summer.
In the SCE, the greatest pairwise difference in diet was also between summer and winter (R = 0.376, P = 0.5%), as shown on the mMDS plot by the slightly greater separation of these two seasons (Figure 5B). The diet in summer also differed from that in spring (R = 0.272, P = 0.6%) and SIMPER identified the greater relative consumption of large bivalves and bivalve shell in summer (vs. spring only) and polychaetes in winter and spring (Figure 6). The spring vs. winter comparison also showed significant differences (R = 0.257, P = 2.6%), with SIMPER identifying that polychaetes and seagrass were consumed in greater volumes in spring and other crustaceans in winter. Overall, amphipods were also consumed in greater volumes in winter than spring, but as the contribution varied among crabs in winter, this dietary category was not selected by SIMPER as distinguishing the diet between seasons.
Decadal Changes in Dietary Composition in the Peel-Harvey Estuary
The mean stomach fullness of inter-molt crabs in January 2017 was 4.2 ± 0.4, similar to that of individuals collected between November and April of 1995/96 and 1996/97 (4.5 ± 0.1). Dietary composition in 2017 comprised mainly large bivalves, calcareous material and decapods, each > 15% (Figure 7). In contrast, the historic contribution of each of these items did not exceed 7%, and polychaetes and small bivalves contributed the greatest volumes to the historical diets (∼30 and 20%, respectively, Figure 7). The volumes of teleosts, tanaids, amphipods and plant material were also lower in 2017 than 20 years earlier, particularly for teleosts and tanaids that were not recorded in the 2017 diets. It should be noted that the number of stomachs examined in the summer of 2017 (27) was fewer than the past study (251). However, prey accumulation curves for the current study showed the number of prey items was reaching an asymptote (data not shown).
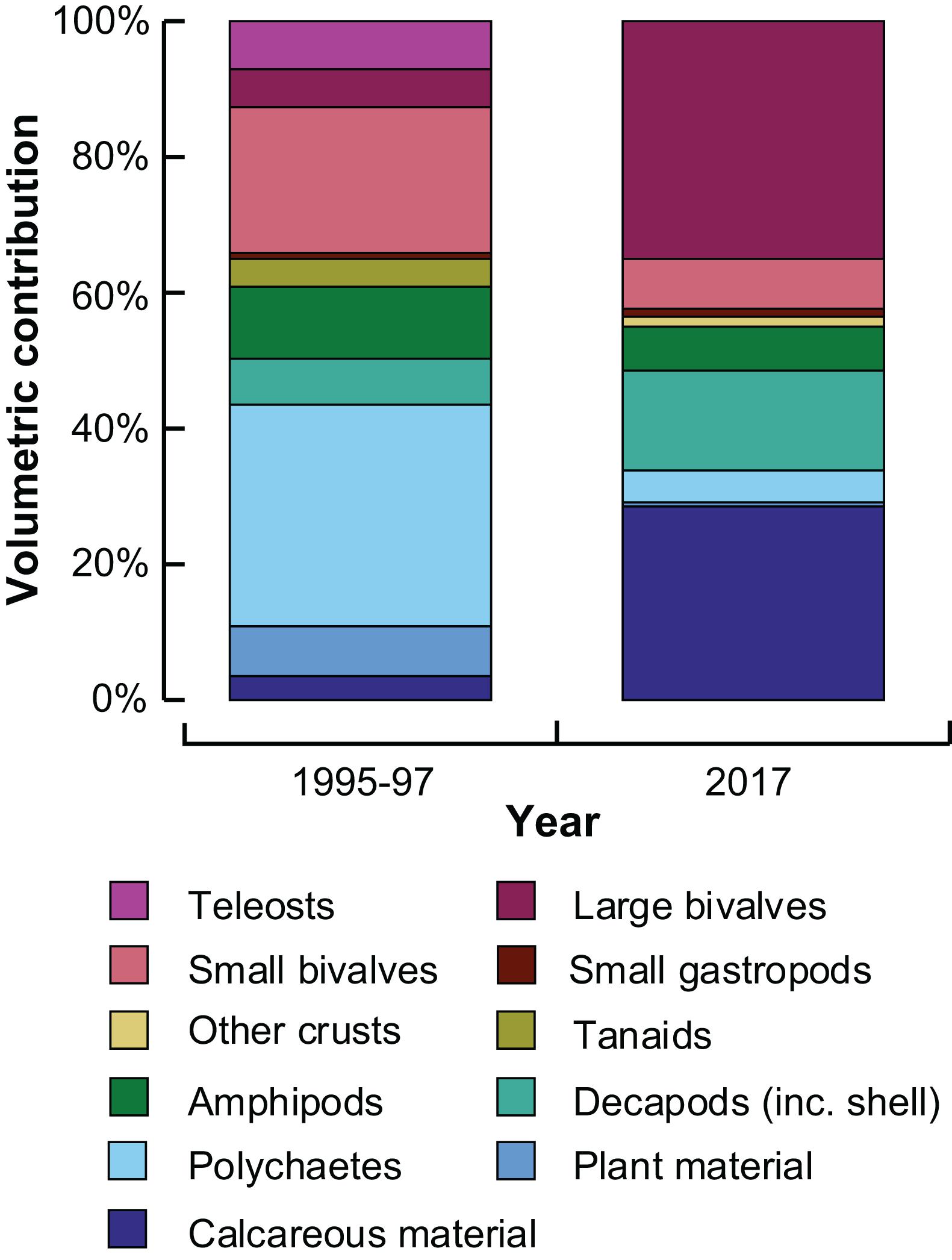
Figure 7. Stacked bar graphs showing the mean percentage volumetric contribution of prey categories to the dietary composition of large (≥60 mm carapace width) inter-moult Portunus armatus in the Peel-Harvey Estuary collected between November and April of 1995/96 and 1996/97 (de Lestang et al., 2000) and in January 2017.
Discussion
The current study determined the dietary composition of the blue swimmer crab Portunus armatus in five locations, i.e., two estuaries (Peel-Harvey and Swan-Canning) and three habitats in the sheltered marine embayment of Cockburn Sound (seagrass, shallow sand and deep sand). The dominant prey items were shelled molluscs, polychaetes and small crustaceans, which is consistent with previous studies of P. armatus in south-western Australia (de Lestang et al., 2000), the central west coast of Western Australia (Edgar, 1990) and Moreton Bay, Queensland (Williams, 1982). The extensive sampling of the three systems in different seasons enabled the diets to be compared statistically among the estuarine and marine locations and between seasons within the two estuaries. Such a comparison has not been attempted previously for this species (Williams, 1982; Edgar, 1990; de Lestang et al., 2000) or, to our knowledge, for other portunid species, such as the Blue Crab Callinectes sapidus. Our results show that in summer, the dietary composition differed markedly among the marine habitats of CS and estuarine waters of the PHE and SCE. Bivalve consumption was greater in estuaries whereas decapod and teleost consumption was greater in marine waters. Within CS, the seagrass location (CSSg) had the most distinct diet due to the lower volumes of mollusc shell and greater volumes of brachyurans and teleosts consumed. Dietary composition varied seasonally within the PHE and SCE with major differences between summer and winter in the PHE and summer and winter/spring in the SCE.
Spatial Variation in Diet
The diet of crabs in summer differed significantly among the five locations (two estuaries and three habitats within CS), with the difference in diet between marine and estuarine waters attributed to a greater consumption of amphipods and small and large bivalves in the PHE and SCE and of decapods, teleosts and echinoderms in the sandy habitats of CS (CSD and CSS). These differences likely reflect differences in prey availability as amphipods (Corophium minor and Grandidierella propodentata) and bivalves (small—Arthritica semen and large—Sanguinolaria biradiata) that are relatively abundant in the PHE, SCE and other estuaries of south-western Australia (Wildsmith et al., 2009, 2011; Tweedley et al., 2012). In contrast, the stenohaline echinoderms are only found in the downstream areas of estuaries, where salinities are essentially marine throughout the year (Tweedley et al., 2015). The benthic macroinvertebrate assemblages within CS have not been widely studied, so comparisons of prey availability cannot be made among systems. However, echinoderms were one of the most commonly caught bycatch taxa in trawl net sampling in CSD and CSS, but less so in the seagrass habitat (CSSg, Sampey and Fromont, 2011), where they were not consumed. Teleosts were common at all locations.
The diets of crabs sampled in summer from the seagrass meadows of CSSg were unique and differed from crabs in all other locations because of a greater consumption of decapods (including smaller P. armatus) and teleosts, and a lesser consumption of molluscs. This presumably reflects a greater abundance of these prey types within the seagrass meadows of CS than the nearby sand habitats of the shallow and deeper waters. Seagrasses are known nursery areas for juvenile fish and invertebrates, including portunid crabs (Perkins-Visser et al., 1996; Hovel and Lipcius, 2001; Heck et al., 2003). In CS, Vanderklift and Jacoby (2003) found that the abundance and biomass of fishes was greater in areas with seagrass detritus. Furthermore, Edgar (1990) found that crab diets differed between those collected in vegetated and unvegetated marine habitats in marine waters off Cliff Head, Western Australia, ∼320 km north of CS. He concluded that prey availability and ontogeny were significant contributing factors to dietary differences, as different sized crabs inhabited vegetated and unvegetated habitats. The size distributions of crabs were similar in the three habitats of CS which means that the major dietary differences found in our study are probably due to a unique assemblage of prey in CSSg. Knowledge of prey availability in CS may provide an insight into why P. armatus stocks have not recovered despite the closure of the fishery (Johnston et al., 2020a). Furthermore, the disparity in diets between seagrass and sand habitats in CS highlights the importance of conserving and expanding the remaining seagrass meadows to promote a greater prey abundance for consumer species such as P. armatus.
Differences in diet between the PHE and SCE, contrast with the findings of de Lestang et al. (2000), who found no difference in diet between crabs in the PHE and the nearby Leschenault Estuary. However, the Peel-Harvey and Leschenault estuaries are more similar in geomorphology and salinity regimes than the PHE and SCE, with the former systems having extensive, large shallow basins with short, narrow entrance channels (e.g., Loneragan et al., 1986; Veale et al., 2014; Potter et al., 2016). In contrast, the SCE, has a deeper basin and a wider, deeper entrance channel (Brearley, 2005). The area where most crabs were caught in the SCE is much deeper (>10 m) than the shallow waters (<2.5 m) in the PHE and bottom salinities rarely fall below 30 in these waters (Crisp et al., 2018). Dietary differences between the PHE and SCE were smaller in summer than the other seasons, when salinities in both estuaries are closest to those in marine waters. The greater divergence of crab diets between these estuaries in the other seasons is likely related to the contrasting trends in mollusc shell consumption in each estuary, i.e., comparable in summer, but far greater in the PHE in winter and spring.
Seasonal Variation in Diet and Prey Availability
Marked seasonal variations in the diet of P. armatus were noted within the PHE and SCE. The seasonal variation was greater in the PHE than SCE, probably related to the much larger seasonal change in bottom salinities in the PHE, and the resultant greater corresponding change in the benthic macrofauna, than in the deeper more stenohaline waters of the SCE. Seasonal variation in crab diet has not been previously examined in Western Australia, but a study by Williams (1982) on the east coast of Australia found no significant seasonal changes in the diet of juvenile P. armatus in marine waters and concluded that diet is largely dependent on the availability of prey. Seasonal changes in diet have been noted in Callinectes spp. (e.g., Paul, 1981; Laughlin, 1982; Rosas et al., 1994) and are generally attributed to changes in prey availability, rather than changes in feeding behavior.
Seasonal changes in benthic faunal assemblages of potential P. armatus prey items have been documented in the PHE (Valesini et al., 2009; Wildsmith et al., 2009; Rose et al., 2019) and SCE (Valesini et al., 2009; Wildsmith et al., 2011, 2017). Seasonal differences in diet in the current study were greatest between spring/summer vs. autumn/winter in both estuaries, which is consistent with changes in the benthic macroinvertebrates of the lower reaches of both estuaries (Valesini et al., 2009). Furthermore, the abundance of small bivalves, namely Arthitica semen, and large bivalves, such as Sanguinolaria biradiata, were greatest in autumn/winter and spring, respectively (Wildsmith et al., 2017), and reflects the volumetric consumption of these taxa.
Changes to the benthic environment, whether natural (e.g., seasonal fluctuations in salinity) or anthropogenic (e.g., eutrophication and shoreline modification), influence the composition of the benthic macroinvertebrate assemblages in a system (Tweedley et al., 2014) and therefore the diet of P. armatus. The opening of the Dawesville Channel in the PHE in 1994 increased salinity through greater marine flushing and tidal exchange (Valesini et al., 2019) resulting in changes in benthic macroinvertebrate composition (Wildsmith et al., 2009) and likely the diet of P. armatus in the estuary. Changes to the estuaries and embayments in which P. armatus is commercially or recreationally fished must be considered when assessing and managing stocks, because of their possible effects on prey availability and therefore diet and the subsequent potential impacts on growth and natural mortality. Altered growth rates and natural mortality will influence commercial and recreational catches and catch rates and flow through to changes in productivity of the fisheries P. armatus supports. These factors need to be considered when managing stocks as changes may not be related to fishing pressure, rather to environmentally driven changes in the system. Teasing these effects apart is crucial for MSC certification of fisheries stocks and the renewal of certification for the P. armatus fisheries in the PHE.
Decadal Changes in Diet and Prey Availability
The dietary composition of inter-molt P. armatus in the PHE in the summer of 2017 differed greatly from the summer ∼20 years previously (de Lestang et al., 2000). In 2017, the diet was dominated by large bivalves, calcareous material and decapods and decapod shell, which were far less abundant in the historical diet. Furthermore, numerous items consumed in abundance in the 1990s were found in much smaller volumes in 2017, including small bivalves (predominantly A. semen), polychaetes, amphipods and tanaids. These changes between decades largely reflect those recorded in the benthic macroinvertebrate faunas between 1986/87 and 2003/04, with significant decreases in the abundance of A. semen, the polychaete Capitella spp. and the tanaid Tanais dulongii (Wildsmith et al., 2009). The abundance of the polychaete Simplisetia aequisetis and the amphipods Corophium minor and G. propodentata also declined significantly over the same period (Wildsmith et al., 2009). The decreased abundance of these taxa may be caused by the increased frequency of hypoxic events in the PHE (Valesini et al., 2019), following which crustaceans and large polychaetes, such as C. aequisetis, disappear (Tweedley et al., 2016b).
Despite decreases in abundance of benthic macroinvertebrates in the PHE (Wildsmith et al., 2009), the mean stomach fullness of inter-molt P. armatus in 2017 was similar to that recorded in 1995–97 (de Lestang et al., 2000). However, the much greater volumetric contribution of calcareous material (mainly mollusc shell) to the diet of crabs in 2017, is consistent with observed declines in the overall abundance of benthic macroinvertebrates between 1986/87 and 2003/04 (Wildsmith et al., 2009). Calcareous material is important for replenishing calcium carbonate in the exoskeleton of decapods after molting, but the increase in consumption seen in the current study likely constitutes a reduction in the calorific value of the diet of inter-molt crabs (de Lestang et al., 2000). If the current diet has a lower calorific value than the historical diet, it is likely to adversely effect growth and egg production and may also lead to reduced carrying capacity of the estuary.
The consumption of mollusc shell in the current study, particularly in the PHE, is greater than that recorded previously for P. armatus in the PHE and other marine systems of Western Australia (Edgar, 1990; de Lestang et al., 2000) and the east coast of Australia (Williams, 1982). Three possible reasons for this are: (1) molluscs are now lower in abundance than in the 1990s so crabs compensate by consuming more shell fragments; (2) recently molted crabs ingest large volumes of calcareous material, e.g., mollusc shell (de Lestang et al., 2000), to facilitate the recalcification of their exoskeleton, and (3) a proportion of the mollusc shell may have been ingested as whole animals rather than shell. However, the digestion of soft tissues was reduced in our study by freezing crabs immediately after capture and shell fragments were examined for attached soft tissues and edge weathering to determine whether shells had been ingested alive. The lesser volumes of mollusc shell in CS and the SCE suggest that the high volumes of mollusc shell consumed in the PHE are due, to the first two reasons above i.e., change in prey abundance and retention of shell during early inter-molt.
Other Factors Influencing Diet
The diet of P. armatus did not differ between the sexes, a finding consistent with other studies of P. armatus (Williams, 1982; de Lestang et al., 2000), C. sapidus and Callinectes similis (Hsueh et al., 1992). Nor did diet differ between crabs in inter-molt and pre-molt. This contrasts with de Lestang et al. (2000), who found that pre-molt P. armatus had lower stomach fullness than the intermolt crabs, possibly due to the cessation of feeding through pre-molt, and that pre-molt and recently molted crabs consumed greater volumes of calcareous material than inter-molt crabs. Williams (1982) also found that recently molted P. armatus consumed greater volumes of calcareous material than inter-molt crabs.
This study was unable to test for ontogenetic shifts in the dietary composition of P. armatus because of the low number of crabs < 60 mm CW (62 crabs). Ontogenetic changes in diet have been recorded in crabs from the PHE (de Lestang et al., 2000) and Cliff Head (Edgar, 1990), with larger crabs (≥ 60 mm CW) consuming larger and more mobile prey, such as polychaetes, decapods and teleosts than smaller crabs. In the PHE, the shift in diet was attributed to ontogenetic changes in prey selection because a wide size range of crabs is found in the shallow waters of this estuary (Potter et al., 1983; de Lestang et al., 2000). At Cliff Head, the dietary shift between small and larger crabs was likely to also be influenced by differences in the habitats occupied by small and large individuals, with small crabs found in shallow waters and larger ones in deeper waters (Edgar, 1990). Although dietary changes have been documented between size categories of larger crabs (i.e., between 60 and 159 mm CW), they were far smaller than those between crabs < and ≥ 60 mm CW (de Lestang et al., 2000).
Conclusion
Overall, prey availability appears to have had the major influence on spatial and temporal differences in the diet of P. armatus in south-western Australia. Changes to the benthic environment, whether natural or anthropogenic, influence the composition of the benthic macroinvertebrate assemblage of a system and therefore the prey availability and diet of P. armatus. When assessing and managing important fishery stocks such as P. armatus in the PHE, SCE and CS, dietary changes must be considered due to their potential subsequent impacts on growth, natural mortality and overall fishery productivity. Fishery managers need to consider that variability in fishery production may not be related to fishing pressure, and that other factors, such as changes in food sources may be having a significant influence on production. Information on prey abundance and feeding is also an important component of ecosystem-based fisheries management and gaining third party accreditation (e.g., Marine Stewardship Council) certification for sustainable fisheries. Consideration of these external factors on target species is becoming increasingly important for scientists and managers to understand the mechanisms affecting the sustainability of fisheries resources, particularly in dynamic estuarine and nearshore environments.
Data Availability Statement
The raw data supporting the conclusions of this article will be made available by the authors, without undue reservation, to any qualified researcher.
Author Contributions
TC designed the study, collected the data, processed and analyzed the data, and wrote the first draft of the manuscript and revised subsequent versions. JT designed the study, analyzed the multivariate statistics, discussed findings, and wrote sections of the manuscript and edited it. DJ designed the study, provided logistical support, discussed findings, and edited the manuscript. NL designed the study, discussed findings, and revised the manuscript. All authors gave final approval of the version to be published and agree to be accountable for all aspects of the work.
Funding
This research was funded by Department of Primary Industries and Regional Development, Western Australia, and Murdoch University.
Conflict of Interest
The authors declare that the research was conducted in the absence of any commercial or financial relationships that could be construed as a potential conflict of interest.
Acknowledgments
This project was originally undertaken as an Honours thesis at Murdoch University, Western Australia, in collaboration with the Department of Primary Industries and Regional Development (DPIRD) (Campbell, 2017). We thank many people for their assistance with sample collection, including Brian Poh, Kyle Hodson, Dr. Chris Hallett, Dr. Peter Coulson, and Dr. Alan Cottingham from Murdoch University and their funders (ARC Project LP150100451), and Josh Baker, Rachel Marks, David Harris and Chris Marsh from (DPIRD). David Harris also kindly assisted with the preparation of Figure 1. We also thank the examiners of the original thesis, Drs. Simon de Lestang and Peter Coulson, for their constructive comments.
Supplementary Material
The Supplementary Material for this article can be found online at: https://www.frontiersin.org/articles/10.3389/fmars.2021.564695/full#supplementary-material
References
Anderson, M. J., Gorley, R. N., and Clarke, K. R. (2008). PERMANOVA+ for PRIMER: Guide to Software and Statistical Methods. Plymouth: PRIMER-E.
Bloomfield, A. L., and Gillanders, B. M. (2005). Fish and invertebrate assemblages in seagrass, mangrove, saltmarsh, and nonvegetated habitats. Estuaries 28, 63–77. doi: 10.1007/bf02732754
Brearley, A. (2005). Swanland: The Estuaries and Coastal Lagoons of South-Western Australia. Perth, WA: UWA Publishing.
Cambridge, M. L., Chiffings, A. W., Brittan, C., Moore, L., and McComb, A. J. (1986). The loss of seagrass in Cockburn Sound, Western Australia. II. Possible causes of seagrass decline. Aquat. Bot. 24, 269–285. doi: 10.1016/0304-3770(86)90062-8
Cambridge, M. L., and McComb, A. J. (1984). The loss of seagrasses in Cockburn Sound, Western Australia. I. The time course and magnitude of seagrass decline in relation to industrial development. Aquat. Bot. 20, 229–247. doi: 10.1016/0304-3770(84)90089-5
Campbell, T. I. (2017). Dietary Composition of the Blue Swimmer Crab, Portunus armatus, and Life History Characteristics of Related Species. Honours thesis. Murdoch WA: Murdoch University.
Chandrapavan, A., Caputi, N., and Kangas, M. I. (2019). The decline and recovery of a crab population from an extreme marine heatwave and a changing climate. Front. Mar. Sci. 6:510. doi: 10.3389/fmars.2019.00510
Clarke, K. R., and Green, R. H. (1988). Statistical design and analyses for a ‘biological effects’ study. Mar. Ecol. Prog. Ser. 46, 213–226. doi: 10.3354/meps046213
Clarke, K. R., Tweedley, J. R., and Valesini, F. J. (2014). Simple shade plots aid better long-term choices of data pre-treatment in multivariate assemblage studies. J. Mar. Biol. Assoc. U. K. 94, 1–16. doi: 10.1017/s0025315413001227
Crisp, J. A., Loneragan, N. R., Tweedley, J. R., D’Souza, F. M., and Poh, B. (2018). Environmental factors influencing the reproduction of an estuarine penaeid population and implications for management. Fish. Manag. Ecol. 25, 203–219. doi: 10.1111/fme.12278
de Lestang, S., Hall, N. G., and Potter, I. C. (2003). Reproductive biology of the blue swimmer crab (Portunus pelagicus, Decapoda: Portunidae) in five bodies of water on the west coast of Australia. Fish. Bull. 101, 745–757.
de Lestang, S., Platell, M. E., and Potter, I. C. (2000). Dietary composition of the blue swimmer crab Portunus pelagicus L. Does it vary with body size and shell state and between estuaries? J. Exp. Mar. Biol. Ecol. 246, 241–257. doi: 10.1016/s0022-0981(99)00181-1
Edgar, G. J. (1990). Perdator-prey interactions in seagrass beds. II. Distribution and diet of the blue manna crab Portunus pelagicus Linaeus at Cliff Head, Western Australia. J. Exp. Mar. Biol. Ecol. 139, 23–32. doi: 10.1016/0022-0981(90)90035-b
Greenwell, C. N., Loneragan, N. R., Admiraal, R., Tweedley, J. R., and Wall, M. (2019). Octopus as predators of abalone on a sea ranch. Fish. Manag. Ecol. 26, 108–118. doi: 10.1111/fme.12328
Hallett, C. S., Hobday, A. J., Tweedley, J. R., Thompson, P. A., McMahon, K., and Valesini, F. J. (2018). Observed and predicted impacts of climate change on the estuaries of south-western Australia, a Mediterranean climate region. Reg. Environ. Change 18, 1357–1373. doi: 10.1007/s10113-017-1264-8
Harris, D., Johnston, D., Sporer, E., Kangas, M., Felipe, N., and Caputi, N. (2012). Biology and management of a multi-sector blue swimmer crab fishery in a subtropical embayment - Shark Bay, Western Australia. Mar. Freshw. Res. 63, 1165–1179. doi: 10.1071/mf12038
Heck, K. L., Hays, G., and Orth, R. J. (2003). Critical evaluation of the nursery role hypothesis for seagrass meadows. Mar. Ecol. Prog. Ser. 253, 123–136. doi: 10.3354/meps253123
Hines, A. H., Lipcius, R. N., and Haddon, A. M. (1987). Population dynamics and habitat partitioning by size, sex, and molt stage of blue crabs Callinectes sapidus in a sub-estuary of central Chesapeake Bay. Mar. Ecol. Prog. Ser. 36, 55–64. doi: 10.3354/meps036055
Hogan-West, K., Tweedley, J. R., Coulson, P. G., Poh, B., and Loneragan, N. R. (2019). Abundance and distribution of the non-indigenous Acentrogobius pflaumii and native gobiids in a temperate Australian estuary. Estuar. Coasts 42, 1612–1631. doi: 10.1007/s12237-019-00571-9
Hovel, K. A., and Lipcius, R. N. (2001). Habitat fragmentation in a seagrass landscape: patch size and complexity control blue crab survival. Ecology 82, 1814–1829. doi: 10.1890/0012-9658(2001)082[1814:hfiasl]2.0.co;2
Hsueh, P. W., McClintock, J. B., and Hopkins, T. S. (1992). Comparative-study of the diets of the blue crabs Callinectes-similis and C-sapidus from a mud-bottom habitat in Mobile-Bay. Alabama. J. Crust. Biol. 12, 615–619. doi: 10.1163/193724092x00094
Johnston, D., Chandrapavan, A., Wise, B., and Caputi, N. (2014). Assessment of Blue Swimmer Crab Recruitment and Breeding Stock Levels in the Peel-Harvey Estuary and Status of the Mandurah to Bunbury Developing Crab Fishery. Fisheries Research Report No. 258. Perth WA: Department of Fisheries.
Johnston, D., Harris, D., Caputi, N., de Lestang, S., and Thomson, A. (2011a). Status of the Cockburn Sound Crab Fishery. Fisheries Research Report No. 219. Perth WA: Department of Fisheries.
Johnston, D., Harris, D., Caputi, N., and Thomson, A. (2011b). Decline of a blue swimmer crab (Portunus pelagicus) fishery in Western Australia-History, contributing factors and future management strategy. Fish. Res. 109, 119–130. doi: 10.1016/j.fishres.2011.01.027
Johnston, D., Marks, R., and Grounds, G. (2020a). “West coast blue swimmer crab resource status report 2019,” in Status Reports of the Fisheries and Aquatic Resources of Western Australia 2018/19: The State of the Fisheries, eds D. J. Gaughan and K. Santoro (South Perth WA: Department of Primary Industries and regional Development), 42–48.
Johnston, D., Yeoh, D., Harris, D., and Fisher, E. (2020b). Blue Swimmer Crab (Portunus armatus) Resource in the West Coast Bioregion, Western Australia. Part 1: Peel-Harvey Estuary, Cockburn Sound and Swan-Canning Estuary. Fisheries Research Report No. 307. South Perth WA: Department of Primary Industries and Regional Development.
Johnston, D., Yeoh, D., and Harris, D. (2021). Environmental drivers of commercial blue swimmer crab (Portunus armatus) catch rates in Western Australian fisheries. Fish. Res. 235:105827. doi: 10.1016/j.fishres.2020.105827
Josileen, J. (2011). Food and feeding of the blue swimmer crab, Portunus pelagicus (Linnaeus, 1758) (Decapods, Brahcyura) along the coast of Mandapam, Tamil Nadu, India. Crustaceana 84, 1169–1180. doi: 10.1163/001121611x590111
Kalejta, B., and Hockey, P. A. (1991). Distribution, abundance and productivity of benthic invertebrates at the Berg River estuary, South Africa. Estuar. Coast. Shelf Sci. 33, 175–191. doi: 10.1016/0272-7714(91)90005-v
Kendrick, G. A., Aylward, M. J., Hegge, B. J., Cambridge, M. L., Hillman, K., Wyllie, A., et al. (2002). Changes in seagrass coverage in Cockburn Sound, Western Australia between 1967 and 1999. Aquat. Bot. 73, 75–87. doi: 10.1016/s0304-3770(02)00005-0
Lai, J. C., Ng, P. K., and Davie, P. J. (2010). A revision of the Portunus pelagicus (Linnaeus, 1758) species complex (Crustacea: Brachyura: Portunidae), with the recognition of four species. Raffles Bull. Zool. 58, 199–237.
Laughlin, R. A. (1982). Feeding habits of the blue crab, Callinectes sapidus Rathbun, in the Apalachicola Estuary, Florida. Bull. Mar. Sci. 32, 807–822.
Lek, E., Fairclough, D. V., Platell, M. E., Clarke, K. R., Tweedley, J. R., and Potter, I. C. (2011). To what extent are the dietary compositions of three abundant, co-occurring labrid species different and related to latitude, habitat, body size and season? J. Fish Biol. 78, 1913–1943. doi: 10.1111/j.1095-8649.2011.02961.x
Loneragan, N. R., Potter, I. C., Lenanton, R. C. J., and Caputi, N. (1986). Spatial and seasonal differences in the fish fauna of the shallows in a large Australian estuary. Mar. Biol. 92, 575–586. doi: 10.1007/bf00392517
Marks, R., Hesp, S. A., Johnston, D., Denham, A., and Loneragan, N. R. (2020). Temporal changes in the growth of a crustacean species, Portunus armatus, in a temperate marine embayment: evidence of density dependence. ICES J. Mar. Sci. 77, 773–790. doi: 10.1093/icesjms/fsz229
Maschette, D., Fromont, J., Platell, M. E., Coulson, P. G., Tweedley, J. R., Potter, I. C., et al. (2020). Characteristics and implications of spongivory in the Knifejaw Oplegnathus woodwardi (Waite) in temperate mesophotic waters. J. Sea Res. 157:101847. doi: 10.1016/j.seares.2020.101847
Metcalf, K. S., Van Montfrans, J., Lipcius, R. N., and Orth, R. J. (1995). Settlement indices for blue crab megalopae in the York River, Virginia: temporal relationships and statistical efficiency. Bull. Mar. Sci. 57, 781–792.
Obregon, C., Tweedley, J. R., Loneragan, N. R., and Hughes, M. (2020). Different but not opposed: perceptions between fishing sectors on the status and management of a crab fishery. ICES J. Mar. Sci. 77, 2354–2368. doi: 10.1093/icesjms/fsz225
Paul, R. K. (1981). Natural diet, feeding and predatory activity of the crabs Callinectes arcuatus and Callinectes toxotes (Decapoda, Brachyura, Portunidae). Mar. Ecol. Prog. Ser. 6, 91–99. doi: 10.3354/meps006091
Perkins-Visser, E., Wolcott, T. G., and Wolcott, D. L. (1996). Nursery role of seagrass beds: enhanced growth of juvenile blue crabs (Callinected sapidus Rathbun). J. Exp. Mar. Biol. Ecol. 198, 155–173. doi: 10.1016/0022-0981(96)00014-7
Poh, B., Tweedley, J. R., Chaplin, J. A., Trayler, K. M., Crisp, J. A., and Loneragan, N. R. (2019). Influence of physio-chemical and biotic factors on the distribution of a penaeid in a temperate estuary. Estuar. Coast. Shelf Sci. 218, 70–85. doi: 10.1016/j.ecss.2018.12.003
Potter, I. C., Chrystal, P. J., and Loneragan, N. R. (1983). The biology of the blue manna crab Portunus pelagicus in an Australian estuary. Mar. Biol. 78, 75–85. doi: 10.1007/bf00392974
Potter, I. C., and de Lestang, S. (2000). Biology of the blue swimmer crab Portunus pelagicus in Leschnault Estuary and Koombana Bay, south-western Australia. J. R. Soc. W A. 83, 443–458.
Potter, I. C., Tweedley, J. R., Elliot, M., and Whitfield, A. K. (2015a). The ways in which fish use estuaries: a refinement and expansion of the guild approach. Fish Fish. 16, 230–239. doi: 10.1111/faf.12050
Potter, I. C., Warwick, R. M., Hall, N. G., and Tweedley, J. R. (2015b). “The physio-chemical characteristics, biota and fisheries of estuaries,” in Freshwater Fisheries Ecology, ed. J. F. Craig (West Sussex: John Wiley & Sons, Ltd), 48–79. doi: 10.1002/9781118394380.ch5
Potter, I. C., Veale, L. J., Tweedley, J. R., and Clarke, K. R. (2016). Decadal changes in the ichthyofauna of a eutrophic estuary following a remedial engineering modification and subsequent environmental shifts. Estuar. Coast. Shelf Sci. 181, 345–363. doi: 10.1016/j.ecss.2016.08.023
Prince, J., Creech, S., Madduppa, H., and Hordyk, A. (2020). Length based assessment of spawning potential ratio in data-poor fisheries for blue swimming crab (Portunus spp.) in Sri Lanka and Indonesia: implication for sustainable management. Reg. Stud. Mar. Sci. 36:101309. doi: 10.1016/j.rsma.2020.101309
Rosas, C., Lazaro-Chavez, E., and Buckle-Ramirez, F. (1994). Feeding habits and food niche segregation of Callinectes sapidus, C. rathbunae and C. similis in a subtropical coastal lagoon of the Gulf of Mexico. J. Crust. Biol. 14, 371–382. doi: 10.1163/193724094x00344
Rose, T. H., Tweedley, J. R., Warwick, R. M., and Potter, I. C. (2019). Zooplankton dynamics in a highly eutrophic microtidal estuary. Mar. Pollut. Bull. 142, 433–451. doi: 10.1016/j.marpolbul.2019.03.047
Rose, T. H., Tweedley, J. R., Warwick, R. M., and Potter, I. C. (2020). Influences of microtidal regime and eutrophication on estuarine zooplankton. Estuar. Coast. Shelf Sci. 238:106689.
Ryan, K. L., Hall, N. G., Lai, E. K., Smallwood, C. B., Tate, A., Taylor, S. M., et al. (2019). Statewide Survey of Boat Based Recreational Fishing in Western Australia 2017/18. Fisheries Research Report No. 297. South Perth, WA: Department of Primary Industries and Regional Development.
Sampey, A., and Fromont, J. (2011). Demersal and epibenthic fauna in a temperate marine embayment, Cockburn sound, Western Australia: determination of key indicator species. J. R. Soc. W. A. 94, 1–18.
Shirley, M. A., Hines, A. H., and Wolcott, T. G. (1990). Adaptive significance of habitat selection by molting adult blue crabs Callinectes sapidus (Rathbun) within a subestuary of central Chesapeake Bay. J. Exp. Mar. Biol. Ecol. 140, 107–119.
Stoner, A. W., and Buchanan, B. A. (1990). Ontogeny and overlap in the diets of four tropical Callinectes species. Bull. Mar. Sci. 46, 3–12.
Tweedley, J. R., Warwick, R. M., Clarke, K. R., and Potter, I. C. (2014). Family-level AMBI is valid for use in north-eastern Atlantic but not for assessing the health of microtidal Australian estuaries. Estuar. Coast. Shelf Sci. 141, 85–96.
Tweedley, J. R., Warwick, R. M., and Potter, I. C. (2015). Can biotic indicators distinguish between natural and anthropogenic environmental stress in estuaries? J. Sea Res. 102, 10–21.
Tweedley, J. R., Warwick, R. M., and Potter, I. C. (2016a). “The contrasting ecology of temperate macrotidal and microtidal estuaries,” in Oceanography and Marine Biology: An Annual Review, eds R. N. Hughes, D. J. Hughes, I. P. Smith, and A. C. Dale (Boca Raton, FL: CRC Press), 73–171.
Tweedley, J. R., Hallett, C. S., Warwick, R. M., Clarke, K. R., and Potter, I. C. (2016b). The hypoxia that developed in a microtidal estuary following an extreme storm produced dramatic changes in the benthos. Mar. Freshw. Res. 67, 327–341.
Tweedley, J. R., Warwick, R. M., Valesini, F. J., Platell, M. E., and Potter, I. C. (2012). The use of benthic macroinvertebrates to establish a benchmark for evaluating the environmental quality of microtidal, temperate southern hemisphere estuaries. Mar. Pollut. Bull. 64, 1210–1221.
Valesini, F. J., Coen, N. J., Wildsmith, M. D., Hourston, M., Tweedley, J. R., Hallett, C. S., et al. (2009). Relationships between fish faunas and habitat type in south-western Australian estuaries. Project 2004/045. Draft Final Report for Fisheries Research and Development Corporation. Perth: Murdoch University.
Valesini, F. J., Cottingham, A., Hallett, C. S., and Clarke, K. R. (2017). Interdecadal changes in the community, population and individual levels of the fish fauna of an extensively modified estuary. J. Fish Biol. 90, 1734–1767.
Valesini, F. J., Hallett, C. S., Hipsey, M. R., Kilminster, K. L., Huang, P., and Hennig, K. (2019). “Peel-harvey estuary, Western Australia,” in Coasts and Estuaries: The Future, eds E. Wolanski, J. W. Day, M. Elliot, and R. Ramachandran (Amsterdam: Elsevier), 103–120.
Valesini, F. J., Tweedley, J. R., Clarke, K. R., and Potter, I. C. (2014). The importance of regional, system-wide and local spatial scales in structuring temperate estuarine fish communities. Estuar. Coasts 37, 525–547.
Vanderklift, M. A., and Jacoby, C. A. (2003). Pattern in fish assemblages 25 years after major seagrass loss. Mar. Ecol. Prog. Ser. 247, 225–235.
Veale, L. J., Tweedley, J. R., Clarke, K. R., Hallett, C. S., and Potter, I. C. (2014). Characteristics of the ichthyofauna of a temperate microtidal estuary with a reverse salinity gradient, including inter-decadal comparisons. J. Fish Biol. 85, 1320–1354.
Wakefield, C. B., Lewis, P. D., Coutts, T. B., Fairclough, D. V., and Langlois, T. J. (2013). Fish assemblages associated with natural and anthropogenically-modified habitats in a marine embayment: comparison of baited videos and opera-house traps. PLoS One 8:e59959. doi: 10.1371/journal.pone.0059959
Wildsmith, M. D., Rose, T. H., Potter, I. C., Warwick, R. M., and Clarke, K. R. (2011). Benthic macroinvertebrates as indicators of environmental deterioration in a large microtidal estuary. Mar. Pollut. Bull. 62, 525–538.
Wildsmith, M. D., Rose, T. H., Potter, I. C., Warwick, R. M., Clarke, K. R., and Valesini, F. J. (2009). Changes in the benthic macroinvertebrate fauna of a large microtidal estuary following extreme modifications aimed and reducing eutrophication. Mar. Pollut. Bull. 58, 1250–1262.
Wildsmith, M. D., Valesini, F. J., and Robinson, S. F. (2017). The value of enduring environmental surrogates as predictors of estuarine benthic macroinvertebrate assemblages. Estuar. Coast. Shelf Sci. 197, 159–172.
Williams, M. J. (1982). Natural food and feeding in the commercial sand crab Portunus pelagicus Linnaeus, 1766 (Crustacea: Decapoda: Portunidae) in Moreton Bay, Queensland. J. Exp. Mar. Biol. Ecol. 59, 165–176.
Keywords: dietary composition, Portunidae, Portunus armatus, Portunus pelagicus, seagrass, seasonal, inter-decadal, Western Australia
Citation: Campbell TI, Tweedley JR, Johnston DJ and Loneragan NR (2021) Crab Diets Differ Between Adjacent Estuaries and Habitats Within a Sheltered Marine Embayment. Front. Mar. Sci. 8:564695. doi: 10.3389/fmars.2021.564695
Received: 22 May 2020; Accepted: 19 January 2021;
Published: 18 February 2021.
Edited by:
Maria Lourdes D. Palomares, University of British Columbia, CanadaReviewed by:
Claudio Vasapollo, Independent Researcher, Ancona, ItalyM. Cristina Mangano, University of Naples Federico II, Italy
Copyright © 2021 Campbell, Tweedley, Johnston and Loneragan. This is an open-access article distributed under the terms of the Creative Commons Attribution License (CC BY). The use, distribution or reproduction in other forums is permitted, provided the original author(s) and the copyright owner(s) are credited and that the original publication in this journal is cited, in accordance with accepted academic practice. No use, distribution or reproduction is permitted which does not comply with these terms.
*Correspondence: Neil R. Loneragan, N.Loneragan@murdoch.edu.au