- 1Department of Biology, Norwegian University of Science and Technology, Trondheim, Norway
- 2National Institute of Aquatic Resources, Technical University of Denmark, Lyngby, Denmark
Several aspects of the biology of European eel (Anguilla anguilla) larvae are still unknown; particularly, information about their functional development and feeding is sparse. In the present study, we histologically characterize the digestive system of wild caught specimens of European eel leptocephalus larvae. The aim was to provide more understanding about how food may be ingested and mechanically processed in the leptocephalus larvae, and to discuss this in the context of its hypothesized feeding strategy. Larvae were caught in the Sargasso Sea during the “Danish Eel Expedition 2014” with the Danish research vessel Dana. The larval sizes ranged from 7.0 to 23.3 mm standard length (SL) at catch. We found that the mouth/pharynx, especially the anterior esophagus, was surrounded by a multi-layered striated muscle tissue and that the epithelium in the mouth/pharynx had a rough filamentous surface, followed by epithelial columnar cells with multiple cilia in the anterior esophagus. This suggests an expandable pharynx/esophagus, well-suited for the transportation of ingested food and likely with a food-crushing or grinding function. The digestive tract of the larvae consisted of a straight esophagus and intestine ventrally aligned within the larval body, and its length was linearly correlated to the larval length (SL). The length of the intestinal part constituted up to 63% of the total length of the digestive tract. The intestinal epithelium had a typical absorptive epithelium structure, with a brush border and a well-developed villi structure. Some cilia were observed in the intestine, but any surrounding muscularis was not observed. The liver was observed along the posterior part of the esophagus, and pancreatic tissue was located anterior to the intestine. Our findings support the hypothesis that the eel leptocephalus may ingest easily digestible gelatinous plankton and/or marine snow aggregates. The muscular esophagus and the ciliated epithelium appear sufficient to ensure nutrient transport and absorption of the ingested food through the digestive tract.
Introduction
The European eel (Anguilla anguilla) is a catadromous fish whose lifecycle has fascinated scientists since Aristotle (384–322 BC; Cresswell, 1883). After an extensive migration across the Atlantic Ocean, the European eel spawns in the Sargasso Sea (Schmidt, 1923; Munk et al., 2010; Righton et al., 2016; Miller et al., 2019). From here, their leptocephalus larvae drift back to the coasts of Europe and North Africa, where they metamorphose into juvenile glass eels and enter coastal or freshwater habitats. The larval stage is assumed to last for about 1–2 years (van Ginneken and Maes, 2005; Bonhommeauz et al., 2009; Munk et al., 2010). However, many aspects of their life cycle and the biology of the early life stages are still scarcely described from nature (Righton et al., 2016; Miller et al., 2020).
The evolutionary adaption of a leptocephalus larval stage is unique to the fishes of the superorder Elopomorpha, which consists of 25 families of fish and more than 1000 species found all over the world (McCleave and Miller, 1994; Greenwood et al., 1996; Chen et al., 2014). Despite their widespread distribution and occasionally large abundances, little is known about the characteristics and adaptations of the leptocephalus larvae to especially their foraging habits (Miller, 2009; Miller et al., 2018). Leptocephalus larvae differ from other pelagic marine fish larvae in several aspects. They are generally of quite large size with a relatively small and thin head (lepto = thin, cephalus = head), and their bodies are transparent and laterally compressed into their characteristic leaf-like appearance (Miller, 2009). Their bodies encompass a gelatinous matrix, mainly consisting of glycosaminoglycans (GAG), commonly referred to as the GAG-layer (Pfeiler, 1991). The outer body possesses numerous chloride cells, which accommodate osmoregulation within the GAG tissue (Lee et al., 2013) and the GAG layer further provides buoyancy and structural support for the leptocephalus larvae (Smith, 2005; Tsukamoto et al., 2009). The GAG-layer makes up most of the larval body, and the external layer of the larvae is only a few cell layers thick (Miller, 2009). The GAG not only provides structural support, but it also functions as an energy storage, which is used during the transformation into the juvenile glass eel (Kawakami et al., 2009; Tsukamoto et al., 2009). The GAG matrix gives the larva a unique ability to achieve a larger body size without the energetic expense of increased number of cells and organ tissues (Bishop and Torres, 1999). The metabolism of leptocephalus larvae has indeed been found to be relatively low (Pfeiler and Govoni, 1993; Bishop and Torres, 1999).
The natural diet and feeding habits of leptocephalus larvae are frequently addressed, and two hypotheses concerning the primary food source are currently put forward. One hypothesis suggests gelatinous plankton as an important part of the diet. This is based on recent studies using rRNA sequencing and DNA-sequence analyses of gut content of European eel larvae which showed a dominance of Hydrozoa taxa (phylum Cnidaria), suggesting that gelatinous plankton is an important part of the diet for these leptocephalus larvae (Riemann et al., 2010; Ayala et al., 2018; Miller et al., 2020). Further, studies on vertical distribution in the area of European eel spawning (Castonguay and McCleave, 1986) showed correspondence between vertical patterns in distribution of the leptocephalus larvae and of small hydrozoans, supporting this proposed predator-prey linkage (Munk et al., 2018). Another hypothesis proposes that marine snow particles, i.e., flocculated dead organic matter could be major components of the leptocephalus diet, (Miller et al., 2011, 2018, 2020). This is supported by isotope studies indicating low trophic position of gut contents (Miller et al., 2012) and by visual observations of the contents showing discarded larvacean houses, as well as plankton fecal pellets which could be part of the marine snow (Mochioka and Iwamizu, 1996; Riemann et al., 2010). No live specimens of eel larvae have been observed or uncovered from the Sargasso Sea, but from laboratory culture experiments it has been shown how young eel larvae utilize a feeding posture into a tense sigmoid body curve from where it can launch toward food particles (Butts et al., 2016). European eel larvae initiating exogenous feeding in culture have been described (Sørensen et al., 2016), but only one successful study of larval early feed uptake is known (Butts et al., 2016). The Japanese eel can, however, be reared on diets based on shark egg-yolk and krill hydrolysates to adult sizes (Tanaka et al., 2003; Okamura et al., 2013).
Most marine pelagic fish larvae have a simple intestinal digestive system at the start of exogenous feeding, with a short esophagus and gut (Kjørsvik et al., 2004; Zambonino Infante et al., 2008). During larval growth and development, the gut becomes further elongated and strengthened, the surface absorption increases, and uptake of nutrients becomes more efficient. The liver, gallbladder, and pancreas are functional when the larvae begin exogenous feeding (Hoehne-Reitan and Kjørsvik, 2004). The stomach is usually not fully functional before the juvenile stage (Lazo et al., 2011).
The structure of the digestive tract has been described for the leptocephalus (Otake, 1996) and the pre-leptocephalus stages of A. japonica (Ozaki et al., 2006), as well as for yolk-sac larvae of the European eel (Mazurais et al., 2013). Three-dimensional reconstruction of European eel larvae based on sectioned first feeding larvae furthermore demonstrated that biting force and particle clearing matched only soft smaller food items (Bouilliart et al., 2015). With such specific exceptions however, European eel larvae seems to develop in line with the general ontogeny of marine fish. In the present study, we outline the basic morphological characteristics of the digestive system of European eel leptocephalus larvae based on histological examination of wild caught specimens from the Sargasso Sea. Our specific aims were to establish the background for evaluating how food may be ingested and mechanically processed in eel leptocephalus larvae, and to avail insight into possible feeding patterns and the functional characteristics of their digestive system.
Materials and Methods
Collection and Preparation of Eel Larvae
European eel larvae (N = 21 larvae) were collected at four different locations (Figure 1) in the Sargasso Sea in March-April 2014 during the research cruise Danish Eel Expedition 2014: Sargasso Eel (see Ayala and Munk, 2018). All sampled anguillid leptocephalus larvae were photographed on a stage micrometer glass slide (Nikon Nikon, Tokyo, Japan) using a DSLR camera (Nikon D70 Nikon D70; Nikon, Tokyo, Japan) attached via C-mount to stereo dissection microscope (Olympus SZ40 Olympus Optical, Tokyo, Japan). The leptocephali were identified to species Anguilla anguilla or A. rostrata by counting the myomeres following the guidelines of Smith (1979). An overlap in number of myomeres, i.e., ∼111 occurs between the two species, which is why only larvae exceeding this quantity of myomeres where used in this study. Images of each larva were used to measure standard length (SL), calibrating and measuring individual images using Fiji software package. Standard length (SL) was measured from the upper jaw (excluding protruding teeth) following the notochord to its posterior tip (Sørensen et al., 2016). Sampled larvae were euthanized in an overdose of tricaine methanesulfonate (MS222, Finquel, Argent Chemical Laboratories Inc., Redmond, United States), fixed in 2.5% formaldehyde and 2.5% glutaraldehyde in 0.11 M hepes buffer (0.5% sucrose, pH 7.4), and stored cold (4°C) until further processing immediately after shipment to the Norwegian University of Science and Technology (NTNU) in Trondheim. Selected A. anguilla larvae were processed and analyzed at the histological laboratory at the Centre of Fisheries and Aquaculture (NTNU Sealab). The fixative was chosen as it preserves tissue suitable for electron microscopy studies.
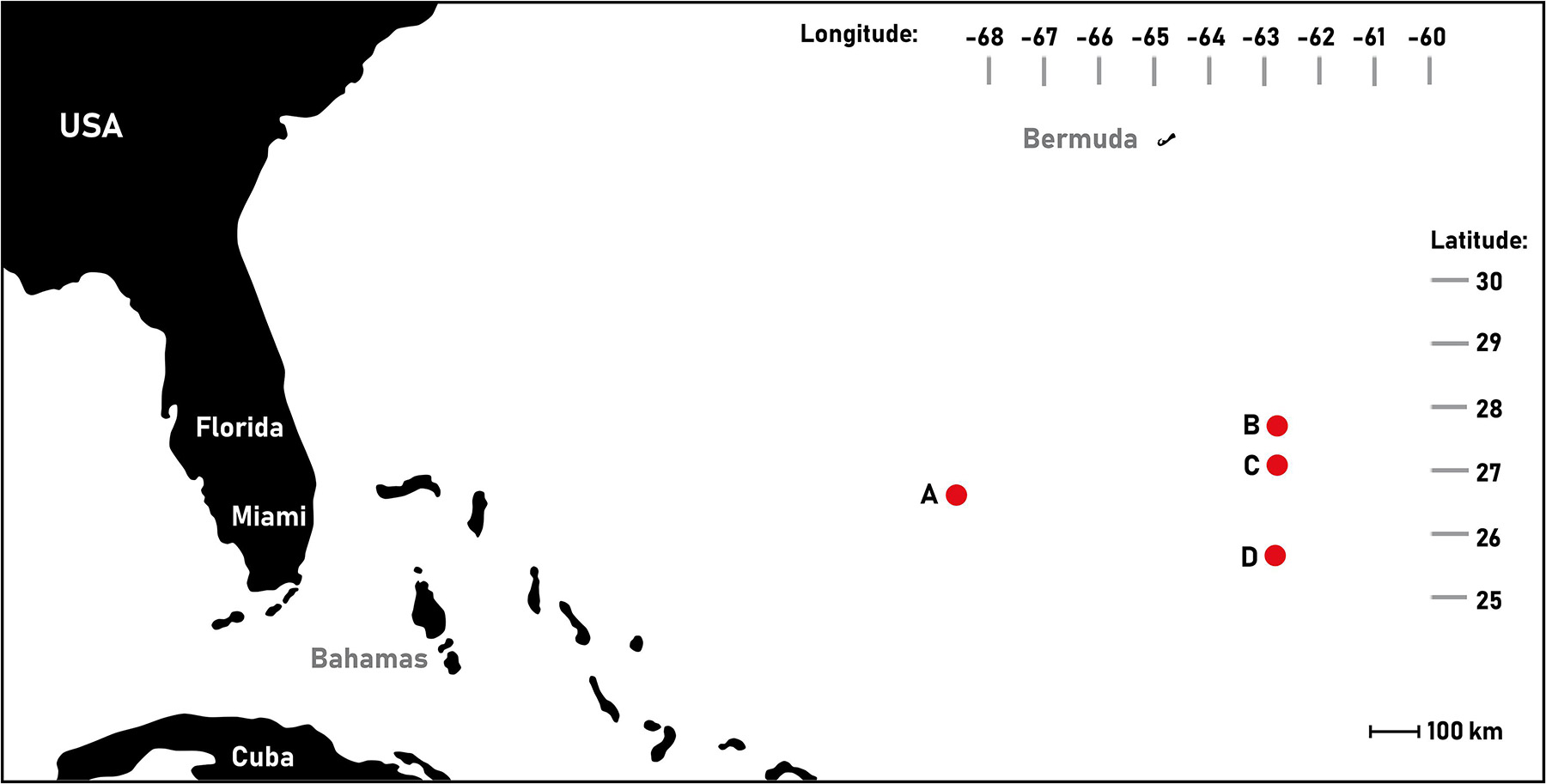
Figure 1. Sampling locations of European eel (Anguilla anguilla) leptocephalus larvae in the Sargasso Sea. This map was created using BatchGeo (07.04.2015, https://batchgeo.com/). (A) West, (B) North east, (C) Mid-east, and (D) Southeast.
External Measurements and Morphology
Fixed larvae were transferred to buffer before being digitally photographed using a stereo microscope (Leica MZ75, Leica Microsystems, Germany) and camera (Carl Zeiss Microscopy Axiocam ERc5s. Zeiss Inc., Germany). Measurements were performed on imaged larvae using the software ImageJ (NIH, United States). Standard length (SL) was measured as described above; the esophagus length (EL) was measured from the beginning of the esophagus (approximately behind the eye) until the transition zone (see Figure 2). Intestinal length (IL) was measured from the transition zone to the anus. Total digestive tract length was measured from the beginning of the esophagus to the anus. Measured areas were defined after investigating the morphological structure of the digestive tract from histological sections. The external morphology of the larvae was also described, focusing on the appearance of the digestive system and potential presence of food particles in the digestive tract. The individual larval SL from the live and fixed larvae is listed in Table 1. The average larval shrinkage for all fixed larvae was 6.6%, with a mean shrinkage of 2.4% for the larvae processed further for histology (Table 1). All descriptions of SL throughout this study are from fixed larvae unless otherwise stated.
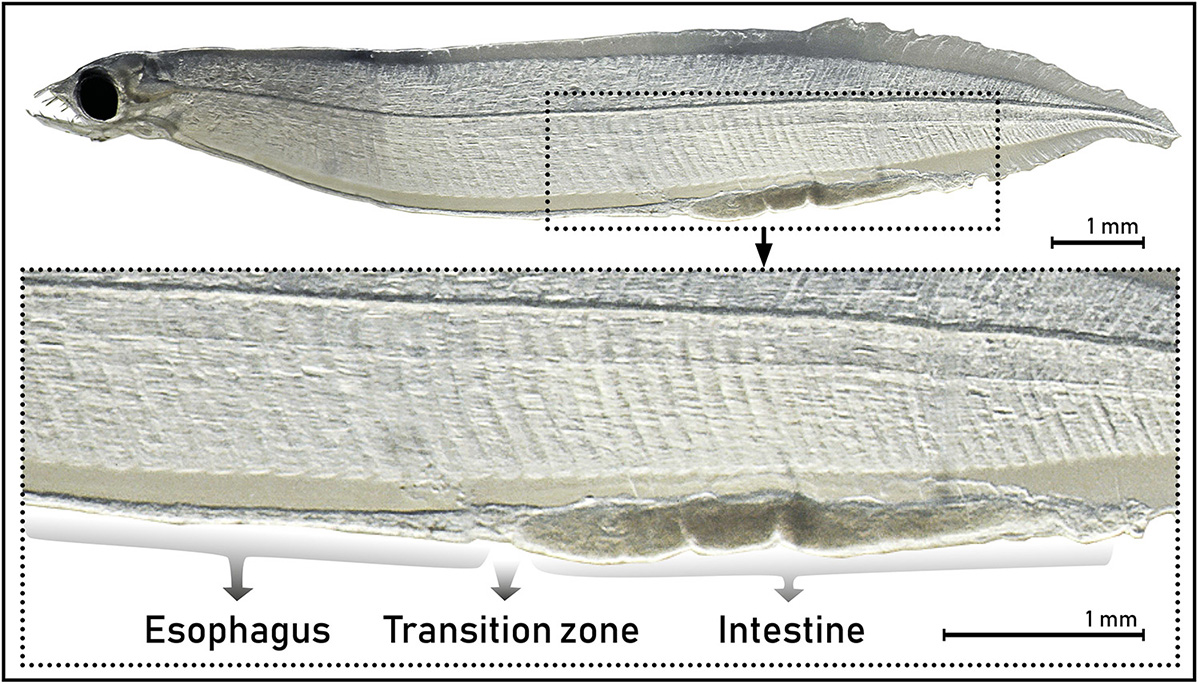
Figure 2. External appearance of the digestive tract of leptocephalus larvae of the European eel (Anguilla anguilla) (L10, SL 11.17) showing the difference between the two areas of the digestive tract, the esophagus and the intestine, and the transition zone between these.
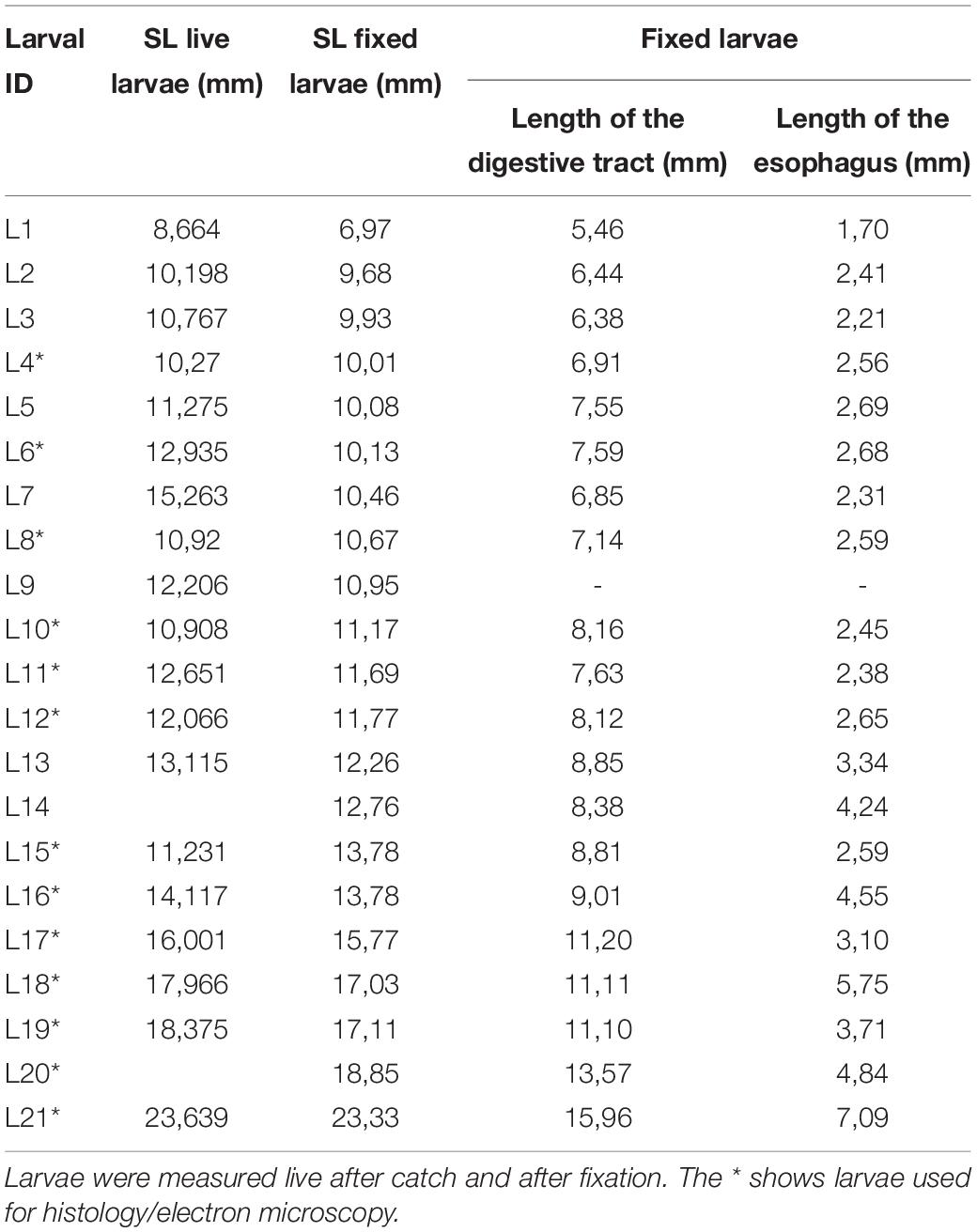
Table 1. External measurements (standard length, SL) of sampled leptocephalus larvae of the European eel (Anguilla anguilla) in the Sargasso Sea.
Morphology and Histology of the Digestive System
Of the 21 larval specimens selected, the 10 best-preserved larvae were used for histological analysis, selecting a broad range of sizes (8.6–23.7 mm SL fresh caught). The largest (and best preserved) larva was also used for transmission electron microscopy.
Histology
Before embedding in epoxy resin (EPON, Sigma-Aldrich, United States), the leptocephalus larvae were cut into three evenly sized parts, which were post-fixed in a mixture consisting of 3% potassium ferrocyanide (KFC, Sigma-Aldrich, United States) and 4% osmium (Carl Roth, Germany) for 1 h, and then bulk-stained for 1.5 h with 1.5% uranyl acetate (VWR Chemicals, United States) in distilled water. The larvae were dehydrated in ethanol and propylene oxide (Acros Organics, Belgium), before embedding in EPON.
Transverse sections (1μm) were produced on a Leica Reichert Ultracut microtome (Leica Microsystems, Germany). Sections were produced of the mouth/pharynx and of the esophagus, in the transition area between esophagus and intestine, in the intestine and at the anus (see Figure 2). One larva was also sectioned longitudinally. The sections were stained in Toluidine blue (Sigma-Aldrich, United States), mounted on glass slides with Neomount (VWR Chemicals, United States) and a coverslip, and photographed using a Zeiss Axioskop 2 plus microscope (Zeiss Inc., Germany), equipped with a Nikon DS-5 M camera (Nikon Corp., Japan). Photographs of the sections were processed with the open software ImageJ (Wayne Rasband, NIH, Bethesda, MD, United States), using a Wacom Cintiq 24HD drawing board (Wacom Technology Corporation, United States) and ImageJ (NIH, United States). For higher quality images, sections were scanned with a NanoZoomer (Model SQ C13140-21, Hamamatsu Photonics, Japan).
Transmission Electron Microscopy (TEM)
Sections for TEM were contrasted with 2% lead citrate and observed in a JEOL 1200EX electron microscope.
Results
External Measurements and Morphology
The larval standard length (SL) ranged from 6.97 to 23.33 mm (Table 1). The digestive tract appeared as a long, straight tube with no folding, with two morphologically distinct areas (Figure 2). Anteriorly, the digestive tract was thin and with an even width. After a transition zone (Figure 2), there was a sharp increase in the width of the digestive tract, and here the gall bladder was visible in some larvae. Based on the histological sections, the anterior area to this zone was identified as the esophagus and the posterior area as the intestine. The length of the digestive tract was on average 69 ± 0.9% (mean ± SE) of the SL of the leptocephalus larva, and of this 37 ± 1.6% (mean ± SE) was the esophagus with the remaining 63% being intestine. The total length of the digestive tract and the total length of the esophagus were positively linearly correlated to the larval standard length (Figure 3). The digestive tract of most larvae was opaque. A few individuals (L1 SL 6.97 mm, L10 SL 11.17 mm, and L9 SL 10.95 mm) had transparent digestive tracts, with well-defined particles visible in the intestinal lumen (Figure 4).
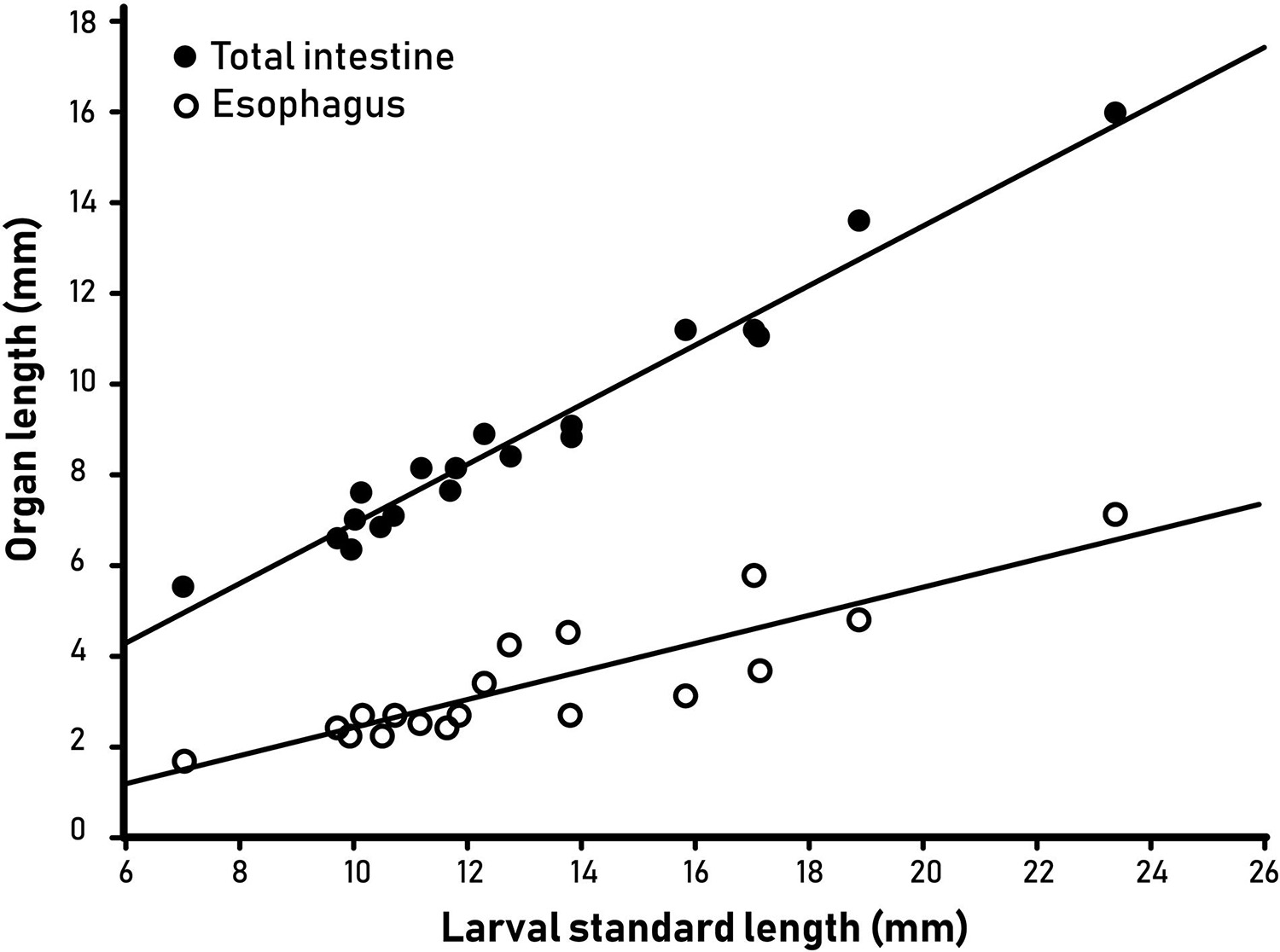
Figure 3. Length of the total intestine and esophagus of leptocephalus larvae of the European eel (Anguilla anguilla) plotted versus larval standard length.
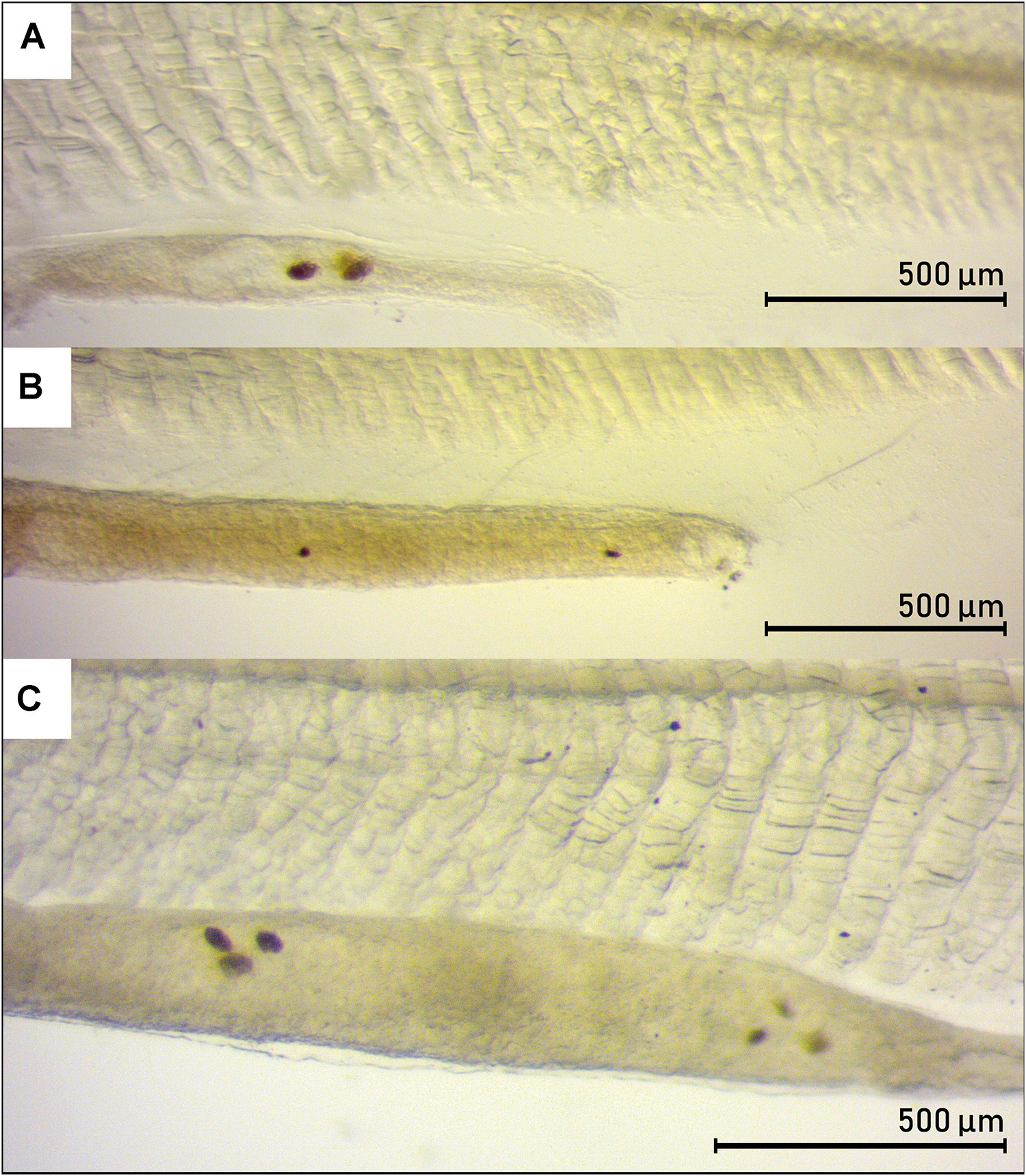
Figure 4. Presence of food-like particles in the intestine of three leptocephalus larvae of the European eel (Anguilla anguilla). (A) L9 (SL 10.95 mm), 4.0x. (B) L10 (SL 11.17 mm), 4.0x. (C) L1 (SL 6.97 mm), 5.0x.
Histology of the Digestive Tract
The internal morphology of the digestive tract of the leptocephalus larvae is described below in the anatomical order: mouth, esophagus, transition zone, intestine, and anus. Morphological/histological descriptions are mainly based on sections observed from the largest larva (L21, SL = 23.33), as this larva was especially well preserved.
Mouth and Pharynx
The “mouth/pharynx” epithelium was visible from just behind the eyes (Figure 5A, longitudinal section) and backwards (Figure 5C, transverse section). The stratified, squamous epithelium had small filaments projecting from the surface, and it was surrounded by a one-cell layer of striated skeletal muscle (Figures 5B,D). This squamous epithelium was found for all size groups, and in all areas of the mouth/pharynx, and it was most prominent in the area above the heart and close to the opercular cavity.
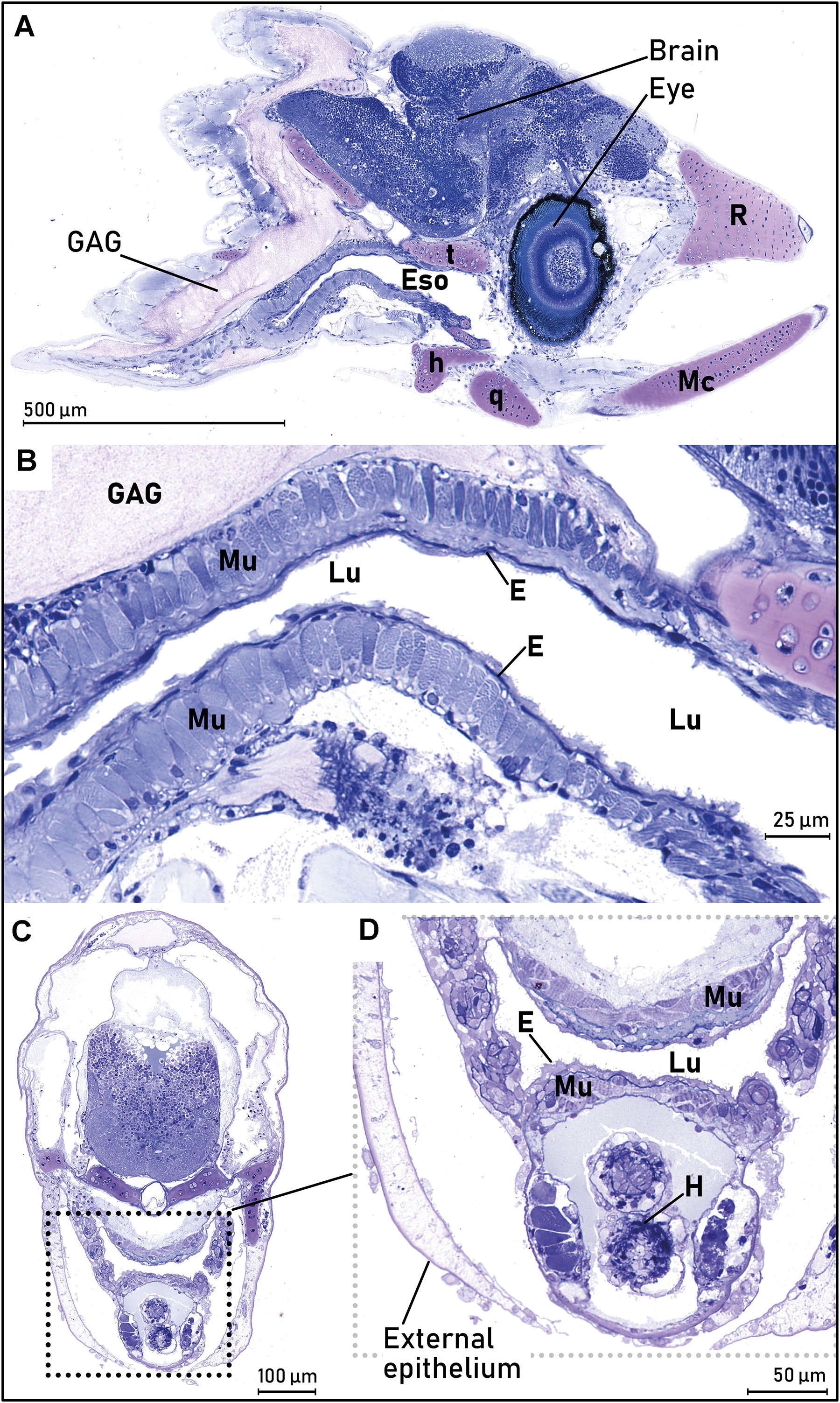
Figure 5. The mouth/pharynx of the European eel (Anguilla anguilla) leptocephalus larvae. (A) Longitudinal section of the larval head, illustrating the mouth/pharynx and esophagus (Eso) where the stratified squamous epithelium (E) covered a layer of striated muscle (Mu), visible from behind the eyes. The cartilage structure of the larval jaw bones (Mc, q, r, t) and the anterior part of the glycosaminoglycan (GAG) layer is also visible. (B) Higher magnification of same larval section as in (A), with more details of the epithelium structure. The striated squamous epithelium (E) covered a layer of striated muscle (Mu), visible from behind the eyes. The cartilage structure of the larval jaw bones (Me, q, r, t) and the anterior part of the glycosaminoglycan (GAG) layer is also visible. (C) Overview of sectional area of pharynx. Cross section of eel larval head, through the heart (behind the eyes). The squared dotted lines illustrate the esophagus shown in (D). (D) Close up of esophagus/pharynx, showing the rough, squamous epithelium (E) and the striated muscle (Mu). Abbreviations: E, epithelium; Eso, esophagus; GAG, glycosaminoglycan; H, heart; h, hyomandibulare; Lu, lumen; Mc, Meckel’s cartilage; Mu, striated muscle; q, quadratum; r, rostrum; t, trabeculum cranii.
Esophagus
In all larvae, a thick muscularis surrounding the epithelium of the anterior esophagus was observed. A thin mucosa and the same rough-textured epithelium as observed in the mouth could be seen. The stratified, squamous epithelium in the anterior esophagus of the largest larva (L21) initially appeared as a folded, expandable tissue (Figure 6A), and it was surrounded by a lamina propria, multiple layers of striated muscle, and an outer serosa (Figure 6A). The stratified squamous epithelium that was observed in the mouth was still present, and the head kidney with glomerulus, Bowman’s space and nephric tubules were observed (Figure 6B). For the smallest larvae it was difficult to determine the presence of serosa in the anterior esophagus, but it was observed in the two largest larvae. Compared to the smaller larvae, the two largest larvae had a more organized and diverse morphology of the tissue in the anterior esophagus, the epithelium appeared more organized, and mucus cells were observed in the epithelium.
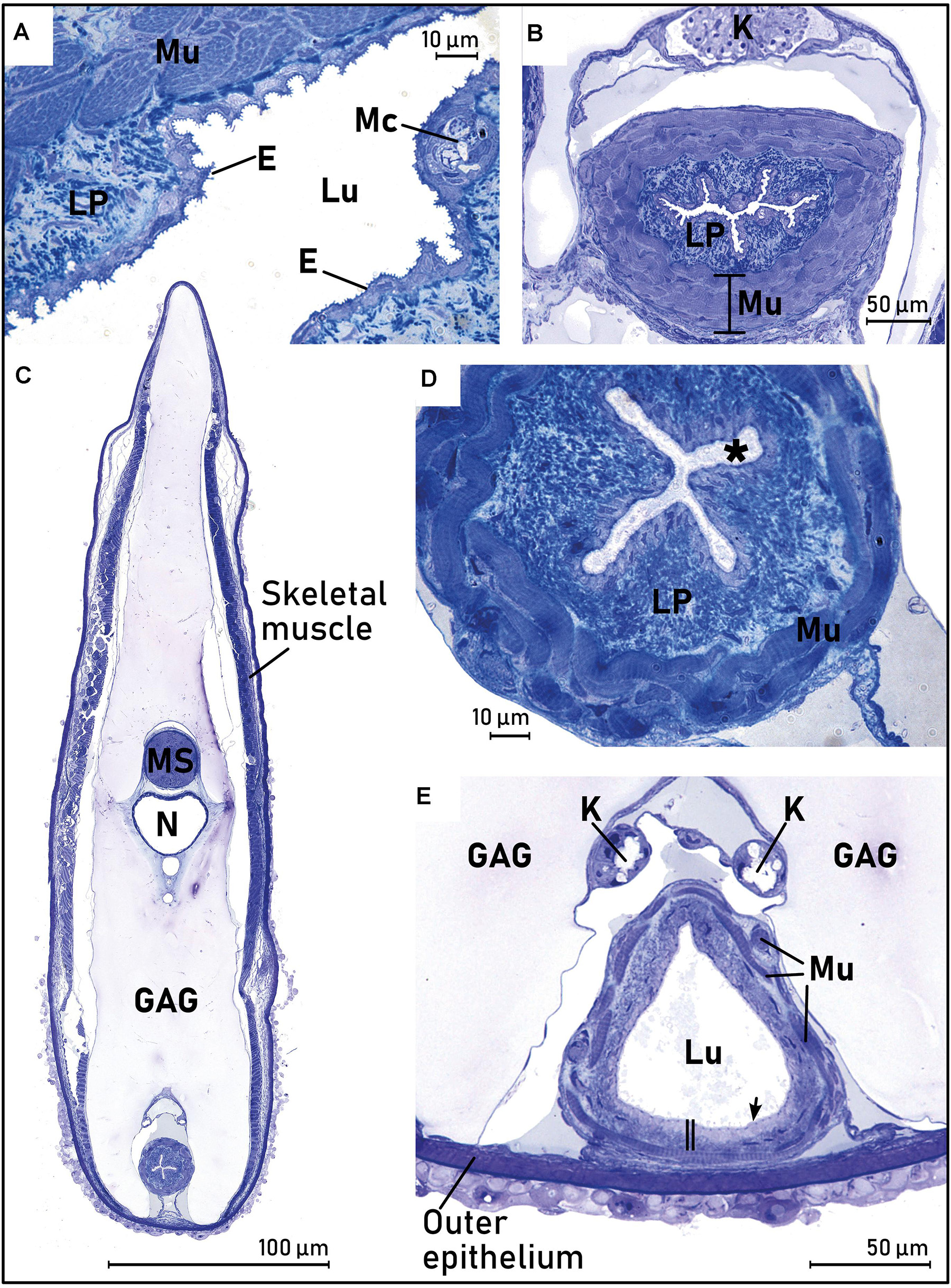
Figure 6. Cross sections of the anterior esophagus (Eso) of the largest sampled leptocephalus larvae of the European eel (Anguilla anguilla) (L21, SL = 23.33), demonstrating the rapid changes in morphology that occurs along the anterior esophagus tissue. (A) Close up of the beginning of pharynx/esophagus, with the circular and longitudinal striated skeletal muscle layers (Mu) surrounding the rough, squamous surface epithelium (E), mucus cells (MC), and the beginning of a lamina propria (LP). (B) Section through the “head kidney” with glomerulus, Bowman’s space and nephric tubules (K), 200 μm further behind (A), with the multilayered striated muscle (Mu) and a rough surface epithelium. (C) Overview 600 μm behind (A). (D) Close up of the larva in (C), where the surface epithelium is now ciliated (*), the fibroelastic lamina propria (LP) is well developed, and the multilayered striated muscle (Mu) is organized into circular and longitudinal muscle bundles. (E) Overview 800 μm behind (A), with a larger lumen (Lu), ciliated surface epithelium (arrow), the lamina propria appeared thinner (vertical double line bar), and the striated muscle bundles are thinner and more fragmented. Abbreviations: *, ciliated surface epithelium; E, Epithelium; Eso, esophagus; GAG, glycosaminoglycan; K, head kidney (6B) or kidney/nephric ducts (6E); LP, lamina propria; Lu, lumen; Mu, striated muscle.
A section 600 μm further into the esophagus from that illustrated in Figures 6A,B, demonstrated that the stratified, squamous epithelium was replaced by a pseudostratified, ciliated columnar epithelium, the folding of the esophageal epithelium increased, the lamina propria was more developed, and the surrounding multilayered striated muscle was more “multidirectional” (Figures 6C,D). The mucosa had similar thickness on the ventral and dorsal side. Nephric (kidney) ducts with a distinct ciliated lumen could be seen dorsally to the esophagus (Figure 6C). About 200 μm further into the digestive tract, the esophagus lumen opened to a wider and more triangular lumen with a ciliated epithelium (Figure 6E), the lamina propria was thinner, and the striated muscle layer was now a single non-continuous band of muscle bundles, compared to the more anterior esophagus. In this area, no folding of the epithelium was observed. Goblet cells containing mucus with a granular appearance were present, especially on the ventral side of the esophagus. The epithelium was heavily ciliated (Figure 6E) in all larvae investigated. Renal arteries were observed, stretching from the nephric ducts and up to the blood vessels at the notochord.
In all larvae, the liver appeared ventrally to the esophagus, approximately from the middle of the esophagus length (Figure 7A). Mid-esophagus was oval in shape, with taller epithelial cells that were seemingly ciliated, and with much thinner lamina propria and striated muscle-layer compared to the anterior esophagus. This morphology was observed for the remaining part of the esophagus and into the transition area. A clearly distinct submucosa was not observed in any parts of the esophagus, and the serosa was thin across the whole esophageal area.
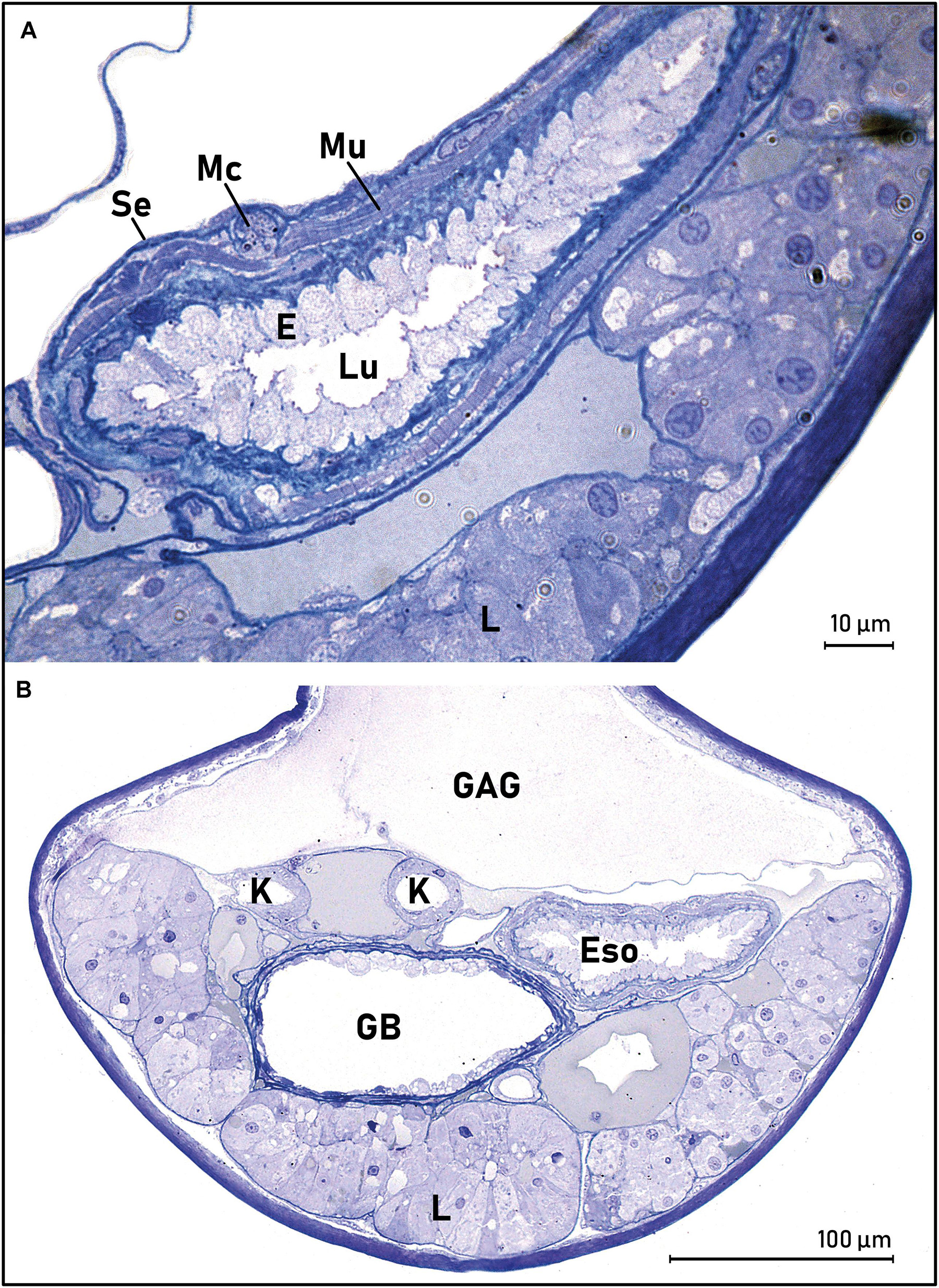
Figure 7. Middle part of esophagus (A) and transition area between the esophagus and intestine of leptocephalus larvae of the European eel (B) (Anguilla anguilla) (L21, SL = 23.33). (A) The esophagus with a thick surface epithelium (E), striated muscularis externa (Mu), and serosa (Se). The liver (L) is also present. (B) The liver is present, esophagus (Eso), kidneys (K), and the gall bladder (GB). The glycosaminoglycan layer (GAG) is visible above the digestive tract. Abbreviations: E, epithelium; Eso, esophagus; GAG, glycosaminoglycan; GB, gall bladder; K, kidney/nephric ducts; L, liver; LP, lamina propria; Lu, lumen; Mu, striated muscle; Se, serosa.
Transition Between Esophagus and Intestine
The transition area between the esophagus and intestine could be identified both by the characteristic external morphology (Figure 2), and by the appearance of the liver and gall bladder in the histological sections (Figures 7A,B). The esophageal morphology was the same as the morphology more anteriorly: tall epithelial cells with some mucus cells containing granular mucus, a thin lamina propria, a thin, more continuous muscle layer, and a thin serosa.
The liver was located on the ventral side of the digestive tract. The structure of the liver consisted of basic lobules of hepatocytes, with some extra-cellular vacuoles or deposits in between the lobules (Figure 7B). Transmission electron microscopy showed that the hepatocytes were characterized by a nucleus, dense structures of smooth and rough endoplasmic reticulum, the so-called space of Disse (perisinusoidal space between the hepatocyte and a sinusoid), and some smaller vacuoles were observed in the hepatic cells (Figure 8). A few structures resembling granules of glycogen could also be seen, but clear glycogen deposits were not observed in the hepatocytes. The gall bladder was located dorsally to the liver (Figure 7B). Three basic histological layers of the gall bladder were observed: an innermost epithelium, a layer of smooth muscle and a thin serous membrane. The gall bladder had a thin wall and large lumen. The nephric (kidney) ducts and a blood vessel, probably the hepatic vein, were observed dorsally to the gall bladder Figure 7B). Pancreatic tissue was observed laterally to the liver, identified by the location of nuclei, the rosette-like organization that is characteristic for pancreas, and with zymogen granulae. The pancreatic tissue appeared very small compared to the liver size and was easiest observed in longitudinal sections (not shown in the figures).
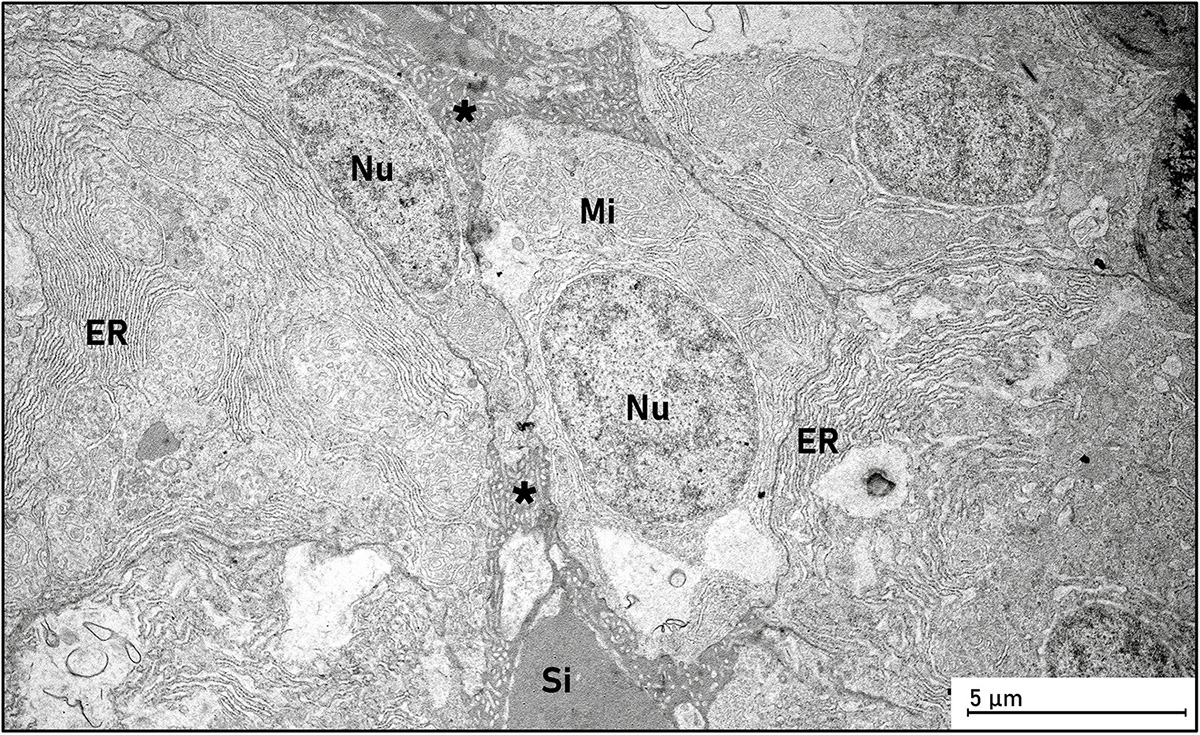
Figure 8. Transmission electron micrograph of the liver in a European eel leptocephalus larva (Anguilla anguilla). The hepatocytes were characterized by a nucleus (Nu), numerous mitochondria (Mi), dense structures of smooth and rough endoplasmic reticulum (ER), and the perisinusoidal space (space of Disse; *) was observed between the hepatocytes and a sinusoid (Si). Abbreviations: *, space of Disse; ER, endoplasmic reticulum; Mi, mitochondria; Si, sinusoid.
Intestine
The characteristics of the intestine are mainly based on sections from the largest larva (L21), due to poorly preserved tissue morphology in the smaller larvae. The intestine had a uniform morphology throughout its whole length, apart from at the hindgut/anus area. The nephric ducts were located dorsally to the intestine; from the mid-intestine and backwards only one duct was visible (Figure 9A, the urinary tract), leading to the urinary bladder located dorsally from the hindgut part (Figure 9B). The intestine had numerous villi (Figure 9A), and consisted of tall, absorptive, columnar epithelial cells, a non-conspicuous lamina propria, and an outer serosa. The columnar enterocytes in the epithelium had large nuclei with regular alignment in the basal part of the cells, and microvilli covered the luminal side of the epithelial cells (Figures 9A, 10A). The enterocytes were characterized by oblong mitochondria and much endoplasmic reticulum (ER), and some absorptive vacuoles were observed in the cytoplasm (Figures 9A, 10A). The dorsal part of the epithelium was less folded, and some cells were ciliated in this area (Figures 9A, 10B). Nutrient-like transport could be seen in between the intestinal epithelial cells (Figure 10A). Some lighter cells in the epithelium resembled goblet cells (not shown). No distinct muscularis externa was observed in any part of the intestine.
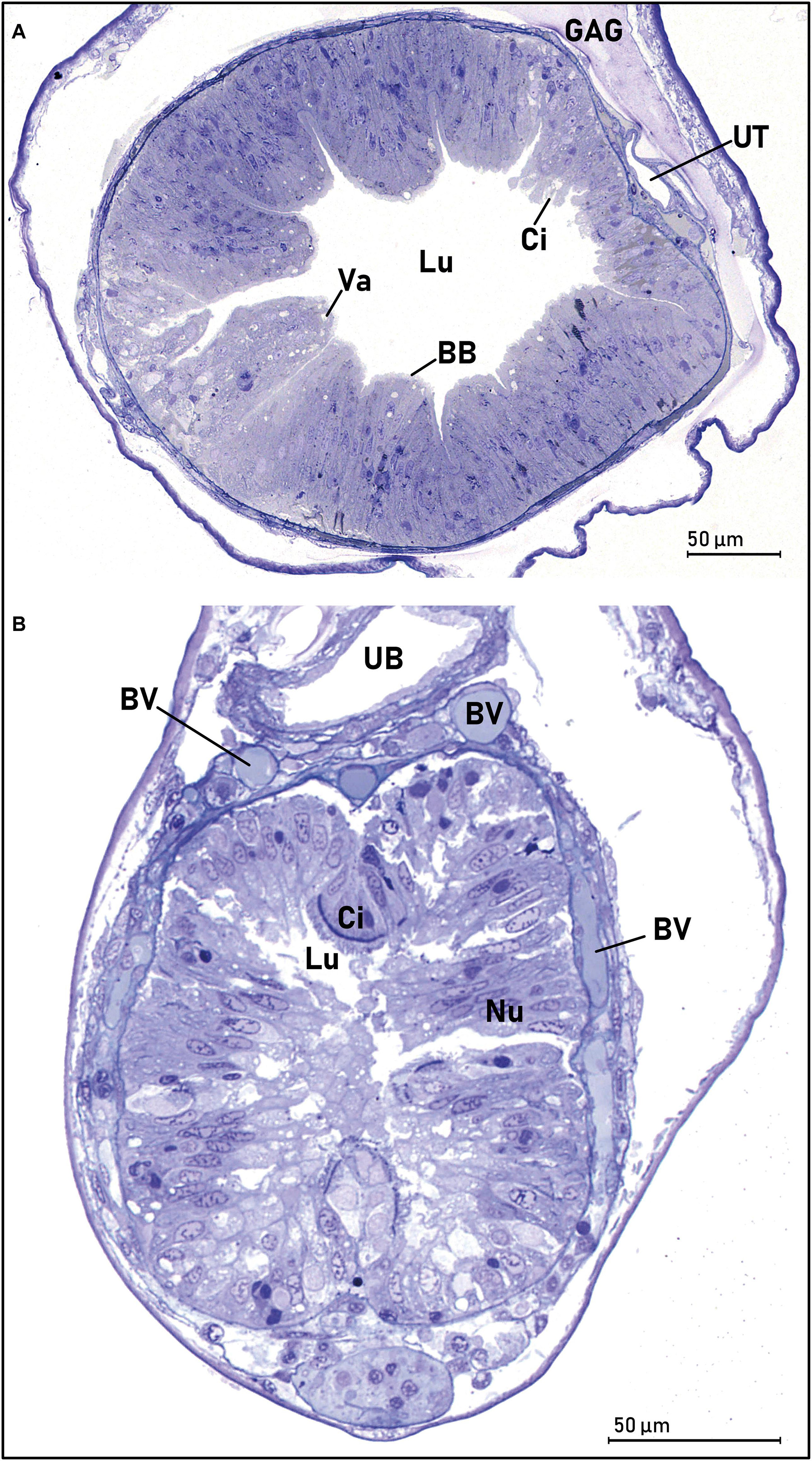
Figure 9. Transverse sections of the middle intestine (A) and the hindgut/anus (B) of a European eel larva (Anguilla anguilla) (L21, SL = 23.33). (A) The lumen (Lu) is wide, and the mucosa is folded in several villi, with an absorptive epithelium of oblong columnar cells with a microvilli brush border (BB). The dorsal part had a thinner intestinal wall, where some scattered cilia were observed. Some absorptive vacuoles were observed especially in the apical part of the enterocytes (Va). The kidney/nephric tubules were united to form a urinary tract (UT). (B) The thick intestinal wall of the hindgut/anus, consisting of columnar epithelium and the microvilli brush border. There was a very distinct area of ciliated (Ci) cells in the dorsal part of the gut epithelium. The urine bladder (UB) is visible dorsally to the intestine. The “submucosal” part of the hindgut was very vascular, and several blood vessels (BV) were visible. Abbreviations: BB, microvilli brush border; BV, blood vessels; Ci, ciliated cells; Lu, lumen; Nu, nucleus; UB, urine bladder; UT, urinary tract; Va, vacuoles.
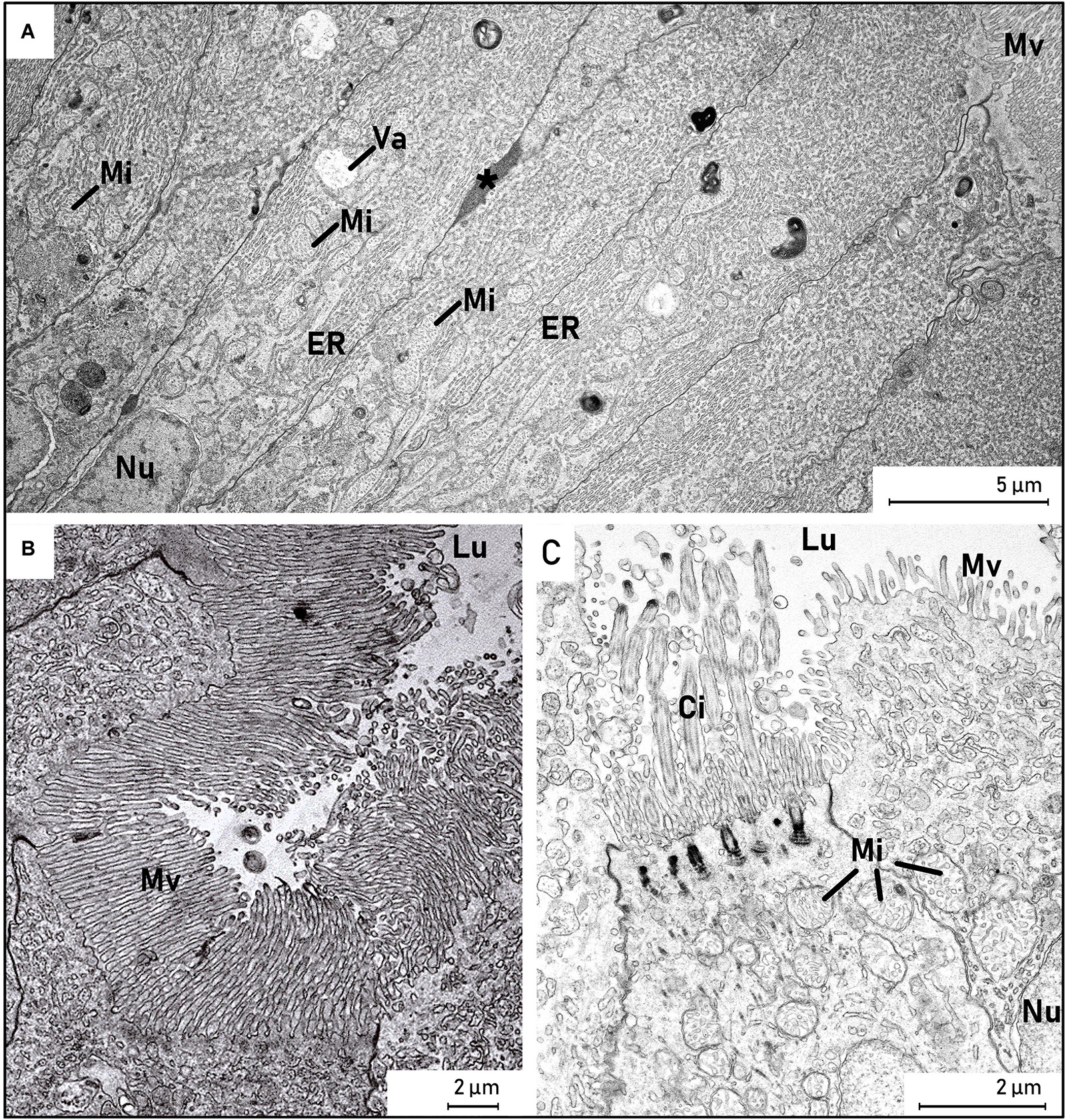
Figure 10. Transmission electron micrograph of enterocytes from a European eel larva (Anguilla anguilla) (L21, SL = 23.33). (A,B) The apical part of the columnar enterocytes in the epithelium had large nuclei (Nu) with regular alignment in the basal part of the cells, and microvilli (MV) covered the luminal side of the epithelial cells. The enterocytes were characterized by oblong mitochondria (Mi) and much endoplasmic reticulum (ER), and some absorptive vacuoles (Va) were observed in the cytoplasm. Nutrient-like transport could be seen in between the intestinal epithelial cells (*). (C) The dorsal part of the intestinal epithelium, where some cells were ciliated (Ci). Abbreviations: (*), lipid-like transport; Ci, cilia; ER, endoplasmic reticulum; Lu, lumen; Mi, mitochondria; Mv, microvilli; Nu, nucleus; Va, vacuole.
Hindgut/Anus
The most distinct morphological features of the hindgut/anus were the narrowing of the lumen, a more even thickness of the mucosa and an appearance of goblet cells. Cilia were observed in the dorsal part of the epithelium, and the urinary bladder was visible dorsally to the intestine (Figure 9B). The tissue surrounding the anus was heavily vascularized and several blood vessels were observed. No distinct pinocytotic activity was observed, but the hindgut tissue was poorly preserved in all larvae.
Discussion
Our new findings about the functional muscular morphology of the pharynx/esophagus in early leptocephalus larvae seem important for understanding how these larvae ingest their feed. The filamentous squamous epithelium combined with the strong multiple layers of striated muscle that we observed in the anterior esophagus of European eel larvae, has not previously been described in Japanese eel or in other fish larvae. However, we have also recently observed a similar muscular part of the esophagus in samples from young Anguilla japonica leptocephalus (Kjørsvik and Bardal, unpublished). In larger A. japonica leptocephalus (44.0 mm TL), a circular smooth muscle layer under the epithelium in the foregut epithelial cells was observed by Otake (1996), and this was not found in either the mid or hindgut of these larvae. The muscular pharynx/esophagus should make these small larvae able to ingest directly the calycophoran siphonophores that are the most abundant gelatinous zooplankton in the southern Sargasso Sea (Ayala et al., 2018; Lüskow et al., 2019), and which are the main type of siphonophore sequences found in both the leptocephalus gut contents and in marine snow particles (Ayala et al., 2018; Lundgreen et al., 2019).
The digestive tract of the European eel leptocephalus larva is a long straight tube, with no coiling, which concur with observations from other species of leptocephalus larvae (Miller, 2009). The leptocephalus larvae used in the present study were in relatively early stages, but also for larger/older leptocephalus larvae the gut is described as relatively straight (for Arisoma balearum, Hulet, 1978). When Anguilla anguilla larvae are metamorphosing into glass-eel, the digestive tract becomes more complex, but is basically still a straight tube (Ciccotti et al., 1993), and not until later stages, a distinct stomach and more curved intestine is seen (Kužir et al., 2012). The division into esophagus, intestine and anus described here, corresponds to findings for the Japanese eel larvae (A. japonica); but for this species it was referred to as foregut, midgut and hindgut, respectively (Otake, 1996). As we observed for the European eel larvae, goblet cells were similarly found in highest numbers in the esophagus for Japanese eel; lower numbers were found in the intestine (Otake, 1996). Further, the absence of an intestinal muscularis mucosa and muscularis externa in the European eel larvae is consistent with observations of Japanese eel larvae (Otake, 1996).
The well-developed muscularis externa and lamina propria-tissues observed in the anterior esophagus in the European eel larvae suggests that larvae have a very flexible and expandable esophagus, and they should be able to perform powerful muscle contractions. The ciliated epithelium would furthermore aid the transport of material along the digestive tract, lubricated by the mucus cells. The esophagus was found to be remarkably long, covering about 35% of the length of the digestive tract. In non-leptocephalus pelagic marine fish larvae that are preying upon zooplankton, e.g., cod larvae, the esophagus is very short compared to the main intestinal part of the digestive tract (e.g., Kjørsvik et al., 1991). The growth of the intestine and the epithelium surface is also very fast, as described in cod (Wold et al., 2008).
The function of the characteristic esophagus epithelium could be to aid crushing or grinding of ingested food particles. The teeth of the European eel larvae are very long but fragile and protrude from the mouth, and they seem to only have a grasping function, as biting and chewing force is limited based on 3D reconstruction of jaw bone, ligament and muscle structure (Appelbaum and Riehl, 1993; Bouilliart et al., 2013, 2015). In the latter studies the larvae were found to possess a very low theoretical bite force of 65μN and limited ability to move the jaws, which has led to the assumption that the larvae feed on small and soft food particles (Bouilliart et al., 2013, 2015). Such weak biting force together with our findings, suggests a clear grinding or crushing function in the anterior esophagus, and may explain how ingested food is prepared for assimilation, and why gut content in wild caught leptocephalus larvae is quite homogeneous with only very few recognizable particles (Miller et al., 2019). Such mass of unidentifiable gut contents was also observed in the larvae from the present study. In fish larvae, ingested food particles are transported into the intestine (stomach is not yet developed) and exposed to digestive pancreatic enzymes in the intestinal lumen and at the intestinal brush border, resulting in a further degraded mixture and absorption by the intestinal epithelial cells (Tanaka, 1969; Lazo et al., 2011). Proteins are incompletely digested in the lumen, are absorbed by the enterocytes in the hindgut by pinocytosis and further digested by the intracellular lysosomal enzymes (Govoni et al., 1986). Recent studies on laboratory hatched European eel larvae defined the developmental time to the first feeding stage to around 10-12 days post hatch, depending on the temperature (Politis et al., 2017). The activation of genes for the mechanisms regulating feeding, such as appetite stimulators and inhibitors (orexigenic and anorexigenic factors), was then upregulated and revealed the transition to exogenous feeding (Politis et al., 2018). The development of the digestive function in the eel larvae was demonstrated through expression of genes encoding the main digestive enzymes, where transcript levels of protein digestive enzymes were higher than those of carbohydrate and lipid digesting enzymes, indicating a nutritional predisposition for proteins during this life stage (Politis et al., 2018). Here, carbohydrate hydrolysis within the first-feeding window might reflect a primary mode of digestion, while the importance of lipid hydrolysis is reflected by the high capacity of eel larvae to assimilate and metabolize dietary phospholipids (Lund et al., 2021). In our study, we observed nutrient absorptive processes in the middle intestine, although the hindgut was too poorly preserved to observe any possible pinocytotic activity.
We only observed zymogen granules in a relatively small pancreatic tissue, thus there was no clear evidence for high synthesis of pancreatic digestive enzymes as basis for digestion of prey items. This indirectly supports the proposals that a large part of the larval diet is covered by easily digested food items such as gelatinous plankton (Ayala et al., 2018) and/or marine snow characterized by partly hydrolyzed or microbial degraded organic matter (Miller et al., 2012, 2018). In a recent review on leptocephali feeding based on field and laboratory studies, Tsukamoto and Miller (2021) suggested that leptocephali have a feeding strategy based on consuming particles that can be categorized as marine snow, and that small leptocephali are also capable of consuming soft-bodied organisms if they have the opportunity. Such feeding preferences may also explain why contents in the gut of leptocephalus larvae are generally found as an unidentifiable mass, often flowing out of the gut, where only few particles dispersed are occasionally observed (Mochioka and Iwamizu, 1996; Miller, 2009; Miller et al., 2018).
The intestinal epithelium had a typical structure of absorptive digestive cells. As for Japanese eel (Otake, 1996), we did not observe submucosa and muscularis externa in the intestine and anus, only in the esophagus. However, fish larvae usually have a well-developed muscularis externa in the intestine and anus, in order to churn and mix the food particles and to aid absorption, thus pushing the food through the tube (Lazo et al., 2011; Govoni et al., 1986). Muscularis externa is also present in the adult eel intestine (Clarke and Witcomb, 1980). The late development of a muscularis externa in the European eel larvae might be due to lower enzymatic digestive processes in the intestine than in other pelagic fish larvae, and thus a churning of prey would be less important in the intestinal part of the digestive tract. We also found ciliated cells throughout the length of the digestive tract, which also are observed in other marine pelagic yolk-sac larvae such as cod (Morrison, 1993) and halibut (Kjørsvik and Reiersen, 1992). Thus, the muscular esophagus, the ciliated epithelium, and the absorptive enterocytes, could ensure the necessary nutrient transport and absorption of the ingested food through the digestive tract.
Conclusion
The digestive system of the European eel leptocephalus larva appears to be similar to that of the Japanese eel, and both species apparently diverge significantly in their general development of the digestive tract, compared to non-leptocephalus pelagic marine fish larvae. An important characteristic of the European eel larvae was the muscular pharynx/esophagus, possessing an initial rough stratified squamous surface epithelium suggested here to exert a food-grinding or crushing function. This unique frontal part is followed by a heavily ciliated epithelial surface in most of the esophagus. This potential crushing function of the esophagus should be seen in the context of the long and protruding teeth of the leptocephalus larvae, which likely are used for grasping food items, rather than biting or chewing. Thus, it might be necessary for other parts of the digestive tract to contribute to mechanical degradation and digestion of ingested particles. The intestinal epithelium had a typical absorptive structure, with some ciliated cells present throughout the length of the intestine. No muscularis externa was observed in the intestine. We also found some evidence for a rather low digestive enzyme synthesis in the exocrine pancreas. Hence our findings support the hypothesis that the eel leptocephalus ingests easily digestible items such as gelatinous plankton (Ayala et al., 2018) or marine snow partly degraded by microbial processes (Miller et al., 2012). The muscular esophagus and the ciliated epithelium appear sufficient to ensure nutrient transport and absorption of the ingested food through the digestive tract.
Data Availability Statement
The raw data supporting the conclusions of this article will be made available by the authors, without undue reservation.
Ethics Statement
Ethical review and approval was not required for this animal study in accordance with the local legislation and institutional requirements.
Author Contributions
PM and SS obtained funding for the cruise participation and conducted the sampling. HK, TB, and EK conducted the histological analysis. All authors wrote and revised the manuscript.
Funding
The Danish Eel Expedition 2014 was supported by the Carlsberg Foundation, Denmark (Grant number: 2012_01_0272) and the Danish Centre for Marine Research (Grant number: 2013_02). Larval preservation and identification was funded by the Innovation Fund Denmark, via EEL-HATCH – Eel Hatchery Technology for a Sustainable Aquaculture (Grant reference no. 11-2013-3). The histological study was funded by the Norwegian University of Science and Technology.
Conflict of Interest
HK did the histological study as her MSc-project at NTNU, and she is now employed at BioMar AS.
The remaining authors declare that the research was conducted in the absence of any commercial or financial relationships that could be construed as a potential conflict of interest.
The reviewer RH declared a past co-authorship with several of the authors, SS and PM, to the handling editor.
Acknowledgments
We are especially grateful to the crew at the Danish research vessel Dana for help and support throughout the Sargasso Sea cruise “The Danish Eel Expedition 2014” and, in particular, the team sorting all ichthyoplankton catches, identifying candidate Anguilla sp. for further analysis, including the work presented herein.
References
Appelbaum, S., and Riehl, R. (1993). Scanning electron microscopic observations on the head morphology of seven different leptocephali belonging to six eel families (Anguilliformes). Helgol. Meeresunters 47, 113–124. doi: 10.1007/bf02366187
Ayala, D. J., Munk, P., Lundgreen, R. B. C., Traving, S. J., Jaspers, C., Jørgensen, T. S., et al. (2018). Gelatinous plankton is important in the diet of European eel (Anguilla anguilla) larvae in the Sargasso Sea. Sci. Rep. 8:6156.
Ayala, J. D., and Munk, P. (2018). Growth rate variability of larval European eels (Anguilla anguilla) across the extensive eel spawning area in the southern Sargasso Sea. Fish. Oceanogr. 27, 525–535. doi: 10.1111/fog.12273
Bishop, R. E., and Torres, J. J. (1999). Leptocephalus energetics: metabolism and excretion. J. Exp. Biol. 202, 2485–2493.
Bonhommeauz, S., Blanke, B., Treguier, A. M., Grima, N., Rivot, E., Vermard, Y., et al. (2009). How fast can the European eel (Anguilla anguilla) larvae cross the Atlantic ocean? Fish. Oceanogr. 18, 371–385. doi: 10.1111/j.1365-2419.2009.00517.x
Bouilliart, M., Tomkiewicz, J., Lauesen, P., and Adriaens, D. (2013). Exploring the feeding mystery of leptocephalus larvae: a mouth full of teeth. Commun. Agric. Biol. Sci. 78, 52–53.
Bouilliart, M., Tomkiewicz, J., Lauesen, P., De Kegel, B., and Adriaens, D. (2015). Musculoskeletal anatomy and feeding performance of pre-feeding engyodontic larvae of the European eel (Anguilla anguilla). J. Anat. 227, 325–340. doi: 10.1111/joa.12335
Butts, I. A. E., Sørensen, S. R., Politis, S. N., and Tomkiewicz, J. (2016). First-feeding by European eel larvae: a step towards closing the life cycle in captivity. Aquaculture 464, 451–458. doi: 10.1016/j.aquaculture.2016.07.028
Castonguay, M., and McCleave, J. D. (1986). Vertical distributions, diel and ontogenetic vertical migrations and net avoidance of leptocephali of Anguilla and other common species in the Sargasso Sea. J. Plankton Res. 9, 195–214.
Chen, J. N., López, J. A., Lavoué, S., Miya, M., and Chen, W. J. (2014). Phylogeny of the Elopomorpha (Teleostei): evidence from six nuclear and mitochondrial markers. Mol. Phylogenet. Evol. 70, 152–161. doi: 10.1016/j.ympev.2013.09.002
Ciccotti, B. E., Macchi, E., Rossi, A., Cataldi, E., and Cataudella, S. (1993). Glass eel (Anguilla anguilla) acclimation to freshwater and seawater: morphological changes of the digestive tract. J. Appl. Ichthyol. 9, 74–81. doi: 10.1111/j.1439-0426.1993.tb00528.x
Clarke, A. J., and Witcomb, D. M. (1980). A study of the histology and morphology of the digestive tract of the common eel (Anguilla anguilla). J. Fish Biol. 16, 159–170. doi: 10.1111/j.1095-8649.1980.tb03695.x
Govoni, J. J., Boehlert, G. W., and Watanabe, Y. (1986). The physiology of digestion in larvae. Environ. Biol. Fishes 16, 59–77.
Greenwood, P. H., Rosen, D. E., Weitzman, S. H., and Myers, G. S. (1996). Phyletic studies of teleostean fishes, with a provisional classification of living forms. Bull. Am. Museum Natural History 131, 339–456.
Hoehne-Reitan, K., and Kjørsvik, E. (2004). “Functional development of the exocrine pancreas and liver in teleost fish,” in The Development of Form and Function in Fishes, and the Question of Larval Adaptation, ed. J. J. Govoni (Bethesda, MD: American Fisheries Society).
Hulet, W. H. (1978). Structure and functional development of the eel leptocephalus Ariosoma balearicum (De La Roche, 1809). Philosophical Trans. R. Soc. London. B Biol. Sci. 282, 107–138. doi: 10.1098/rstb.1978.0010
Kawakami, Y., Oku, H., Nomura, K., Gorie, S., and Ohta, H. (2009). Metabolism of glycosaminoglycan during metamorphosis in the Japanese Conger eel, Conger myriaster. Res. Lett. Biochem. 2009:251731.
Kjørsvik, E., and Reiersen, A. L. (1992). Histomorphology of the early yolk-sac larvae of the Atlantic halibut (Hippoglossus hippoglossus L.) - an indication of the timing of functionality. J. Fish Biol. 41, 1–19. doi: 10.1111/j.1095-8649.1992.tb03166.x
Kjørsvik, E., Pittman, K., and Pavlov, D. (2004). “From fertilization to the end of metamorphosis – functional development,” in Culture of Cold-Water Marine Fish, eds E. Moksness, E. Kjørsvik, and Y. Olsen (Oxford: Blackwell Publishing). doi: 10.1201/9781003064565-1
Kjørsvik, E., van der Meeren, T., Kryvi, H., Arnfinnson, J., and Kvenseth, P. G. (1991). Early development of the digestive tract of cod larvae, Gadus morhua L., during start-feeding and starvation. J. Fish Biol. 38, 1–15. doi: 10.1111/j.1095-8649.1991.tb03086.x
Kužir, S., Gjurčević, E., Nejedli, S., Baždarić, B., and Kozarić, Z. (2012). Morphological and histochemical study of intestine in wild and reared European eel (Anguilla anguilla L.). Fish Physiol. Biochem. 38, 625–633. doi: 10.1007/s10695-011-9543-7
Lazo, J. P., Darias, M. J., and Gisbert, E. (2011). “Ontogeny of the digestive tract,” in Larval Fish Nutrition, ed. G. J. Holt (Oxford: Blackwell Publishing), 5–46.
Lee, K. M., Yamada, Y., Okamura, A., Tsukamoto, K., and Kaneko, T. (2013). Hyposmoregulatory ability and ion-and water-regulatory mechanisms during the leptocephalus stages of Japanese eel Anguilla japonica. Fish. Sci. 79, 77–86. doi: 10.1007/s12562-012-0576-3
Lund, I., Reis, D. B., Tomkiewicz, J., Benini, E., Pérez, J. A., Kottmann, J. S., et al. (2021). Assessment of lipid uptake and fatty acid metabolism of European eel larvae (Anguilla anguilla) determined by 14C in vivo incubation. Aquaculture 531:735858. doi: 10.1016/j.aquaculture.2020.735858
Lundgreen, R. B. C., Jaspers, C., Traving, S., Ayala, D. J., Lombard, F., Grossart, H. S., et al. (2019). Eukaryotic and cyanobacterial communities associated with marine snow particles in the oligotrophic Sargasso Sea. Sci. Rep. 8:8891.
Lüskow, F., Neitzel, P., Miller, M. J., Jaspers, C., Marohn, L., Wysujack, K., et al. (2019). Distribution and abundance of net-captured calycophoran siphonophores and other gelatinous zooplankton in the Sargasso Sea European eel spawning area. Mar. Biodivers. 49, 2333–2349. doi: 10.1007/s12526-019-00971-x
Mazurais, D., Kjørsvik, E., World, P. A., Politis, S. N., Cahu, C., Tomkiewicz, J., et al. (2013). Biochemical, histological and molecular study of digestive tract development in European eel larvae (Anguilla anguilla) prior to exogenous feeding. Best Poster Award, Aquaculture Europe 2013, Trondheim, Norway, 10.08-12.08. Available online at: https://www.aquaeas.eu/uncategorised/422-ae2013-poster-awards (accessed March 5, 2021).
McCleave, J. D., and Miller, M. J. (1994). Spawning of Conger oceanicus and Conger triporiceps (Congridae) in the Sargasso Sea and subsequent distribution of leptocephali. Environ. Biol. Fishes 39, 339–355. doi: 10.1007/bf00004803
Miller, M. J. (2009). Ecology of anguilliform leptocephali: remarkable transparent fish larvae of the ocean surface layer. Aqua Bio Sci. Monogr. 2, 1–94.
Miller, M. J., Chikarashi, Y., Ogawa, N. O., Yamada, Y., Tsukamoto, K., and Ohkouchi, N. (2012). A low trophic position of Japanese eel larvae indicates feeding on marine snow. Biol. Lett. 9, 1–5. doi: 10.2331/suisan.51.1
Miller, M. J., Hanel, R., Feunteun, E., and Tsukamoto, K. (2020). The food source of Sargasso Sea leptocephali. Mar. Biol. 167:57.
Miller, M. J., Marohn, L., Wysujack, K., Freese, M., Pohlmann, J., Westerberg, H., et al. (2018). Morphology and gut contents of anguillid and marine eel larvae in the Sargasso Sea. Zoologischer Anzeiger 279, 138–151. doi: 10.1016/j.jcz.2019.01.008
Miller, M. J., Otake, T., Aoyama, J., Wouthuyzen, S., Suharti, S., Sugeha, H. Y., et al. (2011). Observations of gut contents of leptocephali in the North Equatorial Current and Tomini Bay, Indonesia. Coast. Mar. Sci. 35, 277–288.
Miller, M. J., Westerberg, H., Sparholt, H., Wysujack, K., Sørensen, S. R., Marohn, L., et al. (2019). Spawning by the European eel across 2000 km of the Sargasso Sea. Biol. Lett. 15:20180835. doi: 10.1098/rsbl.2018.0835
Mochioka, N., and Iwamizu, M. (1996). Diet of anguilloid larvae: leptocephali feed selectively on larvacean houses and fecal pellets. Mar. Biol. 125, 447–452.
Morrison, C. M. (1993). Histology of the Atlantic cod, Gadus morhua: an Atlas. part four: eleutheroembryo and larva. Can. Special Publication Fish. Aquat. Sci. 119:496.
Munk, P., Hansen, M. M., Maes, G. E., Nielsen, T. G., Castonguay, M., Riemann, L., et al. (2010). Oceanic fronts in the Sargasso Sea control the early life and drift of Atlantic eels. Proc. R. Soc. London Biol. Sci. 277, 3593–3599. doi: 10.1098/rspb.2010.0900
Munk, P., Nielsen, T. G., Jaspers, C., Ayala, D. J., Tang, K. W., Lombard, F., et al. (2018). Vertical structure of plankton communities in areas of European eel larvae distribution in the Sargasso Sea. J. Plankton Res. 40, 362–375. doi: 10.1093/plankt/fby025
Okamura, A., Yamada, Y., Horie, N., Mikawa, N., Tanaka, S., Kobayashi, H., et al. (2013). Hen egg yolk and skinned krill as possible foods for rearing leptocephalus larvae of Anguilla japonica. Aquac. Res. 44, 1531–1538. doi: 10.1111/j.1365-2109.2012.03160.x
Otake, T. (1996). Fine structure and function of the alimentary canal in Leptocephali of the Japanese Eel Anguilla japonica. Fish. Sci. 62, 28–34. doi: 10.2331/fishsci.62.28
Ozaki, Y., Tanaka, H., Kagawa, H., Ohta, H., Adachi, S., and Yamauchi, K. (2006). Fine structure and differentiation of the alimentary canal in captive-bred Japanese eel Anguilla japonica preleptocephali. Fish. Sci. 72, 13–19. doi: 10.1111/j.1444-2906.2006.01110.x
Pfeiler, E. (1991). Glycosaminoglycan composition of anguilliform and elopiform leptocephali. J. Fish Biol. 38, 533–540. doi: 10.1111/j.1095-8649.1991.tb03140.x
Pfeiler, E., and Govoni, J. J. (1993). Metabolic rates in early life history stages of Elopomorph fishes. Biol. Bull. 185, 277–283. doi: 10.2307/1542007
Politis, S. N., Mazurais, D., Servili, A., Zambonino-Infante, J. L., Miest, J. J., Sørensen, S. R., et al. (2017). Temperature effects on gene expression and morphological development of European eel, Anguilla anguilla larvae. PLoS One 12:e0182726. doi: 10.1371/journal.pone.0182726
Politis, S. N., Sørensen, S. R., Mazurais, D., Servili, A., Zambonino-Infante, J. L., Miest, J. J., et al. (2018). Molecular ontogeny of first-feeding European eel larvae. Front. Physiol. 9:1477.
Riemann, L., Alfredsson, H., Hansen, M. M., Als, T. D., Nielsen, T. G., Munk, P., et al. (2010). Qualitative assessment of the diet of European eel larvae in the Sargasso Sea resolved by DNA barcoding. Biol. Lett. 6, 819–822. doi: 10.1098/rsbl.2010.0411
Righton, D., Westerberg, H., Feunteun, E., Økland, F., Gargan, P., Amilhat, E., et al. (2016). Empirical observations of the spawning migration of European eels: the long and dangerous road to the Sargasso Sea. Sci. Adv. 2:e1501694. doi: 10.1126/sciadv.1501694
Schmidt, J. (1923). The breeding places of the eel. Philos. Trans. R. Soc. B 211, 179–208. doi: 10.1098/rstb.1923.0004
Smith, D. G. (1979). Guide to the Leptocephali (Elopiformes, Anguilliformes, and Notacanthiformes), Vol. 55. Washington, DC: National Oceanic and Atmospheric Administration.
Smith, D. G. (2005). Learning from leptocephali. Environ. Biol. Fishes 74, 397–400. doi: 10.1007/s10641-005-2921-1
Sørensen, S. R., Tomkiewicz, J., Munk, P., Butts, I. A., Nielsen, A., Lauesen, P., et al. (2016). Ontogeny and growth of early life stages of captive-bred European eel. Aquaculture 456, 50–61. doi: 10.1016/j.aquaculture.2016.01.015
Tanaka, H., Kagawa, H., Ohta, H., Unuma, T., and Nomura, K. (2003). The first production of glass eel in captivity: fish reproductive physiology facilitates great progress in aquaculture. Fish Physiol. Biochem. 28, 493–497. doi: 10.1023/b:fish.0000030638.56031.ed
Tanaka, M. (1969). Studies on the structure and function of the digestive system in teleost larvae. II. characteristics of the digestive system in larvae at the stage of first feeding (in Japanese, with English abstract). Jpn. J. Ichthyol. 16, 41–49.
Tsukamoto, K., and Miller, M. J. (2021). The mysterious feeding ecology of leptocephali: a unique strategy of consuming marine snow materials. Fish. Sci. 87, 11–29. doi: 10.1007/s12562-020-01477-3
Tsukamoto, K., Yamada, Y., Okamura, A., Kaneko, T., Tanaka, H., Miller, M. J., et al. (2009). Positive buoyancy in eel leptocephali: an adaptation for life in the ocean surface layer. Mar. Biol. 156, 835–846. doi: 10.1007/s00227-008-1123-8
van Ginneken, V. J. T., and Maes, G. E. (2005). The European eel (Anguilla anguilla, Linnaeus), its lifecycle, evolution and reproduction: a literature review. Rev. Fish Biol. Fish. 15, 367–398. doi: 10.1007/s11160-006-0005-8
Wold, P. A., Hoehne-Reitan, K., Rainuzzo, J., and Kjørsvik, E. (2008). Allometric growth and functional development of the gut in developing cod (Gadus morhua L.) larvae. J. Fish Biol. 72, 1637–1658. doi: 10.1111/j.1095-8649.2008.01831.x
Zambonino Infante, J. L., Gisbert, E., Sarasquete, C., Navarro, I., Gutiérres, J., and Cahu, C. L. (2008). “Ontogeny and physiology of the digestive system of marine fish larvae,” in Feeding and Digestive Functions of Fishes, eds J. E. P. Cyrino, J. P. Bureau, and B. G. Kapoor (Boca Raton: Taylor and Francis Company), 281–348. doi: 10.1201/b10749-8
Keywords: European eel, histology, morphology, Sargasso Sea, digestive system, food ingestion, muscular esophagus
Citation: Knutsen HR, Sørensen SR, Munk P, Bardal T and Kjørsvik E (2021) Digestive Tract and the Muscular Pharynx/Esophagus in Wild Leptocephalus Larvae of European Eel (Anguilla anguilla). Front. Mar. Sci. 8:545217. doi: 10.3389/fmars.2021.545217
Received: 27 March 2020; Accepted: 09 March 2021;
Published: 08 April 2021.
Edited by:
Carlos Alfonso Alvarez-González, Universidad Juárez Autónoma de Tabasco, MexicoReviewed by:
Enric Gisbert, Institute of Agrifood Research and Technology (IRTA), SpainReinhold Hanel, Thünen Institute of Fisheries Ecology, Germany
Copyright © 2021 Knutsen, Sørensen, Munk, Bardal and Kjørsvik. This is an open-access article distributed under the terms of the Creative Commons Attribution License (CC BY). The use, distribution or reproduction in other forums is permitted, provided the original author(s) and the copyright owner(s) are credited and that the original publication in this journal is cited, in accordance with accepted academic practice. No use, distribution or reproduction is permitted which does not comply with these terms.
*Correspondence: Elin Kjørsvik, elin.kjorsvik@ntnu.no
†Present address: Helene Rønquist Knutsen, BioMar AS, Trondheim, Norway