- 1Department of Medicine, Duke University Medical Center, Durham, NC, United States
- 2Division of Marine Conservation, Nicholas School of the Environment, Duke University, Durham, NC, United States
- 3Duke Cancer Institute, Duke University Medical Center, Durham, NC, United States
- 4Department of Orthopaedics, Duke University Medical Center, Durham, NC, United States
- 5Department of Chemistry, Duke University, Durham, NC, United States
Marine ecosystems contain over 80% of the world’s biodiversity, and many of these organisms have evolved unique adaptations enabling survival in diverse and challenging environments. The biodiversity within the world’s oceans is a virtually untapped resource for the isolation and development of novel compounds, treatments, and solutions to combat human disease. In particular, while over half of our anti-cancer drugs are derived from natural sources, almost all of these are from terrestrial ecosystems. Yet, even from the limited analyses to date, a number of marine-derived anti-cancer compounds have been approved for clinical use, and several others are currently in clinical trials. Here, we review the current suite of marine-derived anti-cancer drugs, with a focus on how these compounds act upon the hallmarks of cancer. We highlight potential marine environments and species that could yield compounds with unique mechanisms. Continued exploration of marine environments, along with the characterization and screening of their inhabitants for unique bioactive chemicals, could prove fruitful in the hunt for novel anti-cancer therapies.
Introduction
Cancer is a disease found in nearly all multicellular organisms (Aktipis et al., 2015). For most organisms, cancer is strongly associated with aging (Aunan et al., 2017). As individuals age, mutations accumulate within somatic cells of the body, giving rise to dysfunctional cells that forego their roles as members of a multicellular organism and activate functions, such as unregulated growth and invasion, which work against the health of the organism. In addition to uncontrolled growth, cancer displays several known “hallmarks” – phenotypic characteristics that are nearly universal across cancers. These hallmarks, described in two landmark papers by Hanahan and Weinberg, are genomic instability and mutation, sustained proliferative signaling, evading growth suppressors, enabling replicative immortality, resisting cell death, inducing angiogenesis, reprogramming of energy metabolism, tumor-promoting inflammation, avoiding immune destruction, and activating invasion and metastasis (Hanahan and Weinberg, 2000, 2011).
Decades-long research efforts into the fundamentals of cancer and its hallmarks have led to substantial improvements in clinical outcomes for patients with several cancer types through the development of new diagnostics and therapeutics. However, many of these new technologies also come at a tremendous cost. For example, in the U.S., the national expenditure on cancer totaled more than $147.3 billion in 2017, with some patients spending as much as $10,000 a month on their cancer treatments. Moreover, for many patients, even the best and most costly therapies extend survival by only a few months. Continued efforts are urgently needed to identify promising new therapies to treat cancer.
Cancer therapies can be broadly segregated into two general classes: (1) naturally derived compounds and (2) synthetic compounds. Within each of these classes are two subcategories: small molecules and biologics. Small molecules are low molecular weight compounds that can readily enter cells to elicit a biological effect. Biologics, on the other hand, are larger biomolecules, such as monoclonal antibodies or small RNAs, which often cannot penetrate cells and/or require a delivery system to enter the cell membrane and exert their effects (Mocsai et al., 2014). Over three-fourths of all anti-cancer compounds are derived from natural sources (Newman and Cragg, 2020). For example, paclitaxel and docetaxel, two of the primary chemotherapy agents used to treat breast, prostate, and other cancers, were discovered and purified from naturally occurring plant taxanes (Verweij et al., 1994). Further chemical diversification of these natural plant taxanes has led to potent derivatives with unique properties, such as cabazitaxel.
Interestingly, while the majority of approved therapeutics are derived from natural sources, almost all of these are from terrestrial ecosystems. Hundreds of thousands of natural compounds have been discovered for a variety of uses, with over 100,000 from plants alone. In contrast, in the past three decades, over 2,000 marine-derived compounds have shown a wide range of application (Hu et al., 2015).
Despite the lower number of compounds identified from the marine environment, the enormous volume of the ocean comprises 99% of the total living space on earth and is home to 80% of the world’s species (Malve, 2016). In addition to its species richness, the ocean is also comprised of extraordinary environmental diversity, with temperatures that span from −2°C to over 400°C (Mullineaux et al., 2018), pressures from 1 to over 1000 atm (Trenberth et al., 1987), salinities from 0 to over 36 ppt (Srokosz and Banks, 2019), from anoxic to normoxic, bright light to no light, and chemicals including H2S, CH4 (Lyman and Fleming, 1940), heavy metals, allelopathic defenses, and anthropogenic pollutants. Based on this environmental diversity, the earth’s marine-dwelling inhabitants contain a potential treasure trove of anti-cancer therapeutics.
Although extraordinary potential still lies unexplored within the oceans, many marine-derived compounds that have been discovered have been discovered possess extremely potent anti-cancer properties (Montaser and Luesch, 2011). These compounds are derived from diverse organisms, act through multiple molecular mechanisms, and collectively target numerous cancer hallmarks. In this review, we discuss natural products or compounds directly derived from those natural products from marine macroorganisms with known activity against the hallmarks of cancer. While a number of reviews have exhaustively cataloged the suite of marine natural products with anti-cancer activity, we focus here on the cancer hallmarks as a framework to understand the spectrum of anti-cancer activity from marine-derived sources. We highlight key features of these potential therapies and their sources, and pinpoint strategies to maximize future efforts to identify novel compounds from the marine environment.
Marine-Derived Cancer Therapies Show Activity Spanning the Hallmarks of Cancer
Over 40 years of active research in marine natural products has identified a host of bioactive compounds. We focused on literature that makes explicit reference to one or more of the cancer hallmarks. Analysis of these marine-derived anti-cancer compounds across the cancer hallmarks reveals that most marine-derived therapies act on the specific hallmark of resisting cell death. Out of the 42 compounds reviewed here, including those that act against more than one hallmark, 31 act on the resisting cell death hallmark (Figure 1A). The majority of drugs we categorized under this hallmark affect microtubule dynamics and induce apoptosis through cell cycle arrest. The next largest category of 11 compounds represents drugs that inhibit angiogenesis (Figure 1A). Most of these drugs act by suppressing vascular endothelial growth factor (VEGF) signaling. Interestingly, nine compounds target multiple hallmarks (Figures 1B,C). All other hallmarks are targeted by at least one marine-derived compound, with the exception of the genomic instability and mutation hallmark.
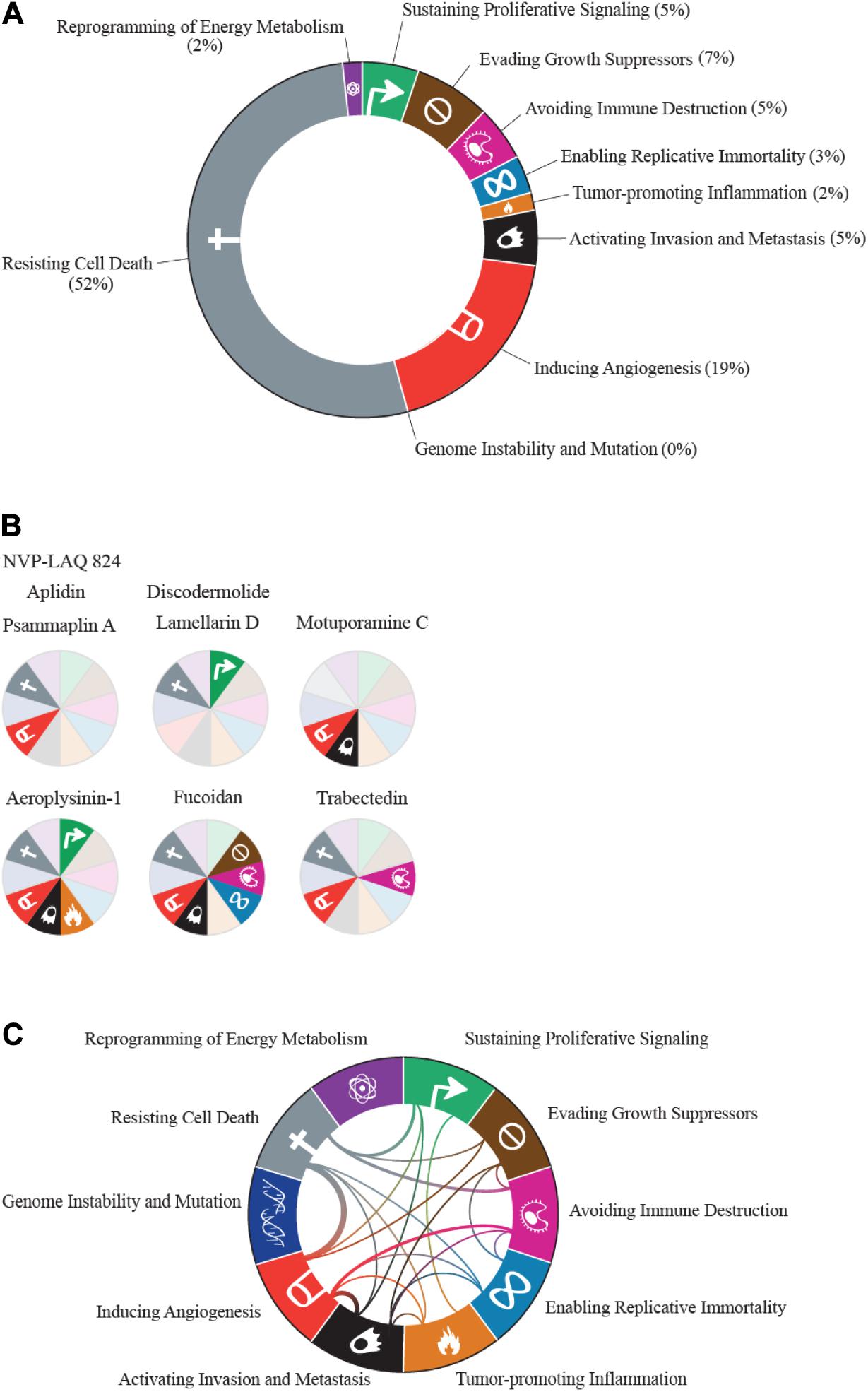
Figure 1. The hallmarks of cancer, adapted from Hanahan and Weinberg (2011), visualized according to marine drug representation. (A) All proportions were calculated based on the number of drugs classified under each hallmark. Drugs that were categorized under more than one hallmark were counted multiple times. (B) Representation of compounds that are classified under multiple hallmarks. Hallmarks that the compound acted on are represented in brighter colors with hallmark symbols. Compounds that have the same classifications are represented with the same wheel. (C) Connections between hallmarks that share at least one compound. Thicker lines represent more compounds shared between the hallmarks.
This variation in coverage across the cancer hallmarks is likely due to several factors. For one, bias in the methods used to evaluate anti-cancer properties is very likely, with the vast majority of research directed toward rapid assays for biological activity, such as proliferation, apoptosis, and cytotoxicity. Studies designed to interrogate hallmarks related to immune function, metabolism, and invasion/metastasis often require more complex, costly, and time-consuming experiments. Integrating existing statistical frameworks to predict mechanism of action (Huang et al., 2006) with a panel of simple assays to test newly isolated compounds for activity across the spectrum of cancer hallmarks would aid in identifying compounds with unique activities. In addition to the potential bias in assays used, it is also likely that many marine-derived compounds evolved to be toxins (Haefner, 2003), which may explain the increased representation of agents that target cell death pathways.
Among the compounds reviewed in this paper (see Figure 2), 19 have been tested in clinical trials, with six United States Food and Drug Administration (US FDA) approvals (eribulin, trabectedin, brentuximab vedotin, polatuzumab vedotin, cytarabine, and aplidin) and one approval in Australia (aplidin). All of these tested compounds target the resisting cell death cancer hallmark. Below we summarize specific marine-derived compounds and their known mechanisms of action across the cancer hallmarks.
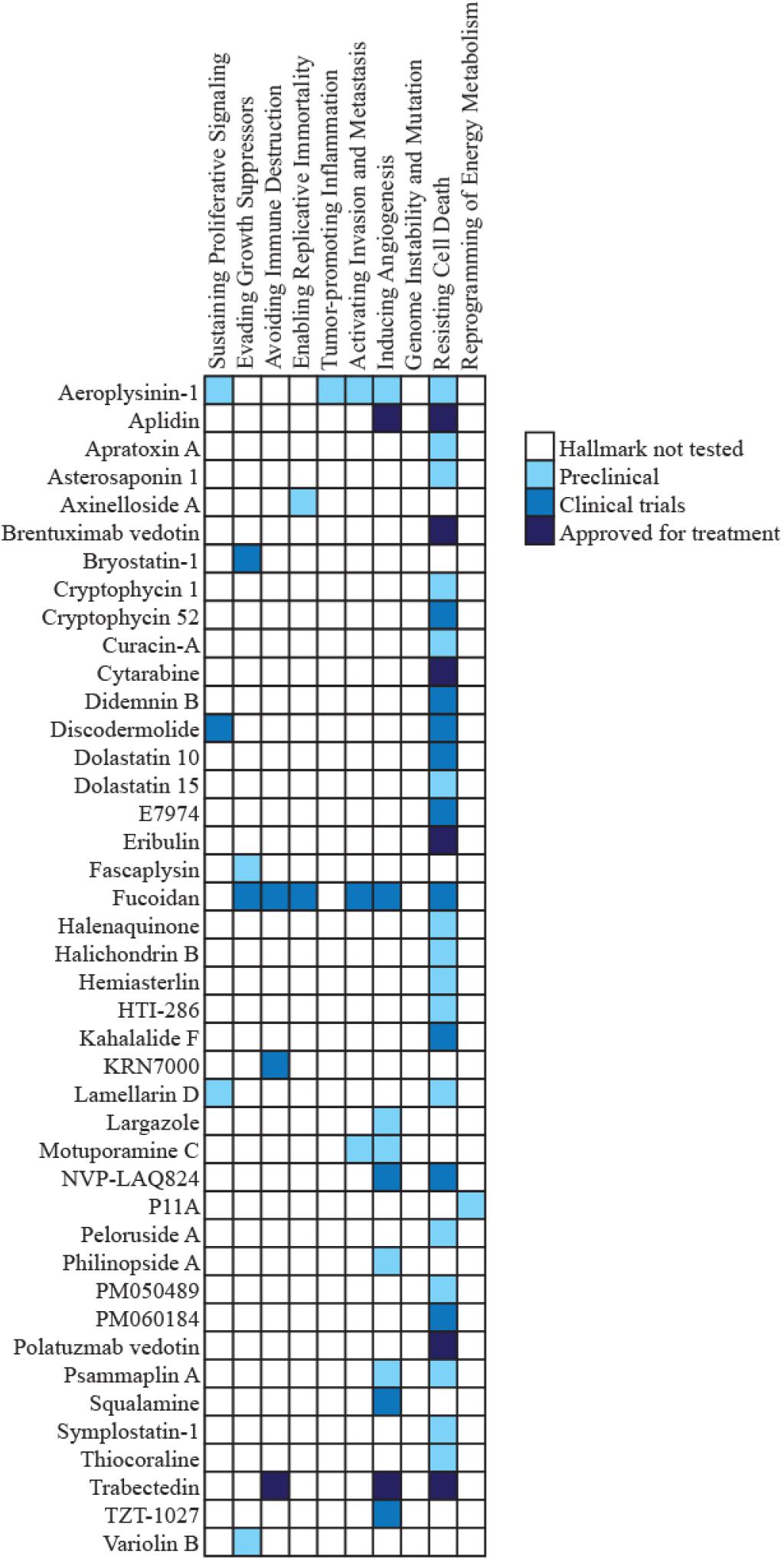
Figure 2. Clinical trial status of marine-derived compounds. The heat map depicts the clinical trial status of each of the compounds discussed in this review, categorized by hallmark. Compounds that were categorized under more than one hallmark have the same color for clinical trial status across those hallmarks. Hallmarks that were not tested for the compound are colored white.
Sustaining Proliferative Signaling
Healthy tissues carefully regulate cell division by controlling the release of growth-promoting signals that help determine precise timings in cycles of cell division (Jones and Kazlauskas, 2001). Cell cycle regulators only allow cell growth and division when all cellular requirements are met (Schafer, 1998). Unlike healthy cells, cancer cells co-opt growth signals to generate autocrine signals to divide or have dysfunctional receptors that are hypersensitive to growth signals (Hanahan and Weinberg, 2011). Imbued with these dysfunctional growth signaling properties, the cancer cells ultimately grow and divide more frequently than their neighboring normal cells, overtaking the space and resources available.
Inhibiting Epidermal Growth Factor Receptor (EGFR)
Some marine-derived drugs combat unchecked proliferation by preventing cell division through targeting of receptor-mediated growth signaling. For example, aeroplysinin-1 is a brominated tyrosine metabolite first isolated from the marine sponge Verongia aerophoba originally collected from Capo Caccia (Fattorusso et al., 1972; Ciminiello et al., 1997). Aeroplysinin-1 is a cytostatic agent, which inhibits the growth of cells without killing them (Kreuter et al., 1989, 1990). Aeroplysinin-1 leads to growth arrest by inhibiting epidermal growth factor receptor (EGFR) phosphorylation and preventing downstream EGFR-mediated signaling (Kreuter et al., 1990).
Inducing Senescence
A group of growth-arresting marine compounds is the lamellarin family of alkaloids first isolated from the mollusk Lamellaria sp. found in Koror, Palau (Andersen et al., 1985). Lamellarins induce cancer cell growth arrest and subsequent cell death. The most notable of the lamellarins is lamellarin D, which has antiproliferative activity in cancer cells through a variety of mechanisms, including induction of senescence (Bailly, 2015). Ballot et al. (2014) reported that lamellarin D induces senescence in P388 mouse leukemia and HBL cutaneous melanoma cells by arresting them in the G2 phase of the cell cycle. Like lamellarin D, another compound that induces cell cycle arrest in G2 is discodermolide. Discodermolide is a polyketide first isolated from the sponge Discodermia dissoluta found at Lucaya, Grand Bahama Island (Gunasekera et al., 1990). Discodermolide stabilizes microtubules, which leads to cell cycle arrest and the induction of senescence (ter Haar et al., 1996; Klein et al., 2005).
Evading Growth Suppressors
In addition to growth promoting signals, normal cells use cell cycle checkpoints to limit cell division. One class of cell cycle checkpoint molecules is the cyclin-dependent kinases (CDKs). CDKs act as gatekeepers at key points in the cell cycle. In healthy cells, CDKs regulate whether a cell can progress to the next stage based on environmental and intracellular conditions (Canavese et al., 2012). A number of CDK inhibitors are currently approved for treatment of breast cancer (Schettini et al., 2018), and clinical trials of CDK inhibitors are completed or ongoing for liposarcoma (Dickson et al., 2013, 2016), non-small cell lung cancer (Gopalan et al., 2014), gastric and esophageal cancer (Karasic et al., 2020), ovarian cancer (Konecny et al., 2016), and prostate cancer1 (NCT02905318).
Inhibiting Cyclins and Cyclin-Dependent Kinases
One marine-derived compound with known anti-CDK activity is fucoidan. Fucoidan is a sulfated polysaccharide that is a cell wall constituent first isolated in many types of brown seaweed, including Laminaria spp. (Bird and Haas, 1931). Fucoidan causes G1 arrest in 5637 and T-24 human bladder cancer cells as well as MCF-7 human breast cancer cells, which is mediated by downregulation of CDK2 and CDK4 (Banafa et al., 2013; Cho et al., 2014). Like fucoidan, fascaplysin also directly affects CDKs. It is a bis-indole alkaloid first isolated from the sponge Fascaplysinopsis Bergquist sp. in Ndravuni, Fiji (Roll et al., 1988; Hamilton, 2014). Fascaplysin inhibits CDK4 and induces G1 arrest. Fascaplysin also induces the build-up of reactive oxygen species and induces apoptosis (Soni et al., 2000; Hamilton, 2014). Another marine-derived CDK inhibitor, variolin B, is a pyridopyrrolopyrimidine alkaloid first extracted from the sponge Kirkpatrickia varialosa found in Cape Armitage in McMurdo Sound, Antarctica (Perry et al., 1994). Variolin B inhibits the activity of CDK1-cyclin B, CDK2-cyclin A, and CDK2/cyclin E complexes, causing HCT-116 human colon adenocarcinoma and A2780 human ovarian carcinoma cells to arrest in G2 and undergo apoptosis (Simone et al., 2005). One compound that inhibits cyclins is bryostatin-1, a PKC agonist. It is a macrocyclic lactone first isolated from Bugula neritina in the Gulf of Mexico (Pettit et al., 1982). Bryostatin-1 activates GSK3β, which induces the degradation of cyclin D1. This results in the induction of cell cycle arrest in the G2 phase (Wang et al., 2019). Bryostatin-1 has been tested in phase II clinical trials, but did not progress due to minimal activity (Raghuvanshi and Bharate, 2020). However, it is an effective anti-cancer agent when administered in combination with other drugs (Raghuvanshi and Bharate, 2020), such as dolastatin 10 or paclitaxel (Kortmansky and Schwartz, 2003; Kollar et al., 2014).
Resisting Cell Death
Apoptosis is a type of programmed cell death that is tightly regulated by multiple signaling cascades. The process can be initiated by either extrinsic or intrinsic pathways. In the extrinsic pathway, an extracellular ligand activates a death receptor that induces a signaling cascade to trigger cell death (Galluzzi et al., 2018). In the intrinsic pathway, mitochondrial instability induces mitochondrial outer membrane permeabilization through the B-cell lymphoma 2 (BCL2) apoptosis regulatory protein family (Galluzzi et al., 2018). Apoptosis occurs naturally within populations of normal cells to regulate cell number and control tissue and organ patterning, such as during embryonic development (Elmore, 2007). Tumor cells evade apoptosis in multiple ways, including loss of function in tumor suppressor genes and increased expression of anti-apoptotic regulators (Fernald and Kurokawa, 2013). By preventing apoptosis, cancer cells are able to survive for longer periods of time, which could lead to the acquisition of additional genetic and epigenetic lesions that contribute to the development of therapy resistance and metastasis.
Inducing Apoptosis Through Cell Cycle Arrest
Apoptosis is intimately linked to cell cycle regulation. Many genes involved in cell cycle progression are also involved in initiating apoptosis, and disrupting the cell cycle can trigger apoptosis (Evan et al., 1995). Drugs developed from marine sources combat the anti-apoptotic effects of cancer cells by inducing cell death in a variety of ways. Several of these compounds act by targeting cell cycle arrest, which subsequently induces apoptosis. One example of a class of compounds that inhibits cell cycle function is dolastatins. Dolastatins were first extracted from the sea hare, Dolabella auricularia, collected from the eastern coast of Mauritius (Pettit et al., 1993). Fifteen structurally unique dolastatins were identified (Pettit et al., 1989, 1993), of which the most active were dolastatins 10 and 15. Although dolastatin 10 is a pentapeptide and dolastatin 15 is a depsipeptide, both are thought to bind to the vinca domain of tubulin and interfere with tubulin polymerization, disrupting mitotic cell division (Bai et al., 1990, 1992). Interfering with the formation and function of microtubules triggers the intrinsic mitochondrial apoptosis pathway (Bai et al., 1992; Bhalla, 2003). Like dolastatin 10, a variety of other marine-derived compounds exhibit similar ability to induce apoptosis (Table 1). Dolastatin 10 reached Phase II clinical trials in multiple cancers (Vaishampayan et al., 2000; Margolin et al., 2001; Saad et al., 2002; Hoffman et al., 2003; Kindler et al., 2005; Perez et al., 2005), but despite its favorable toxicity profile, it failed to show efficacy in any of these solid tumors.
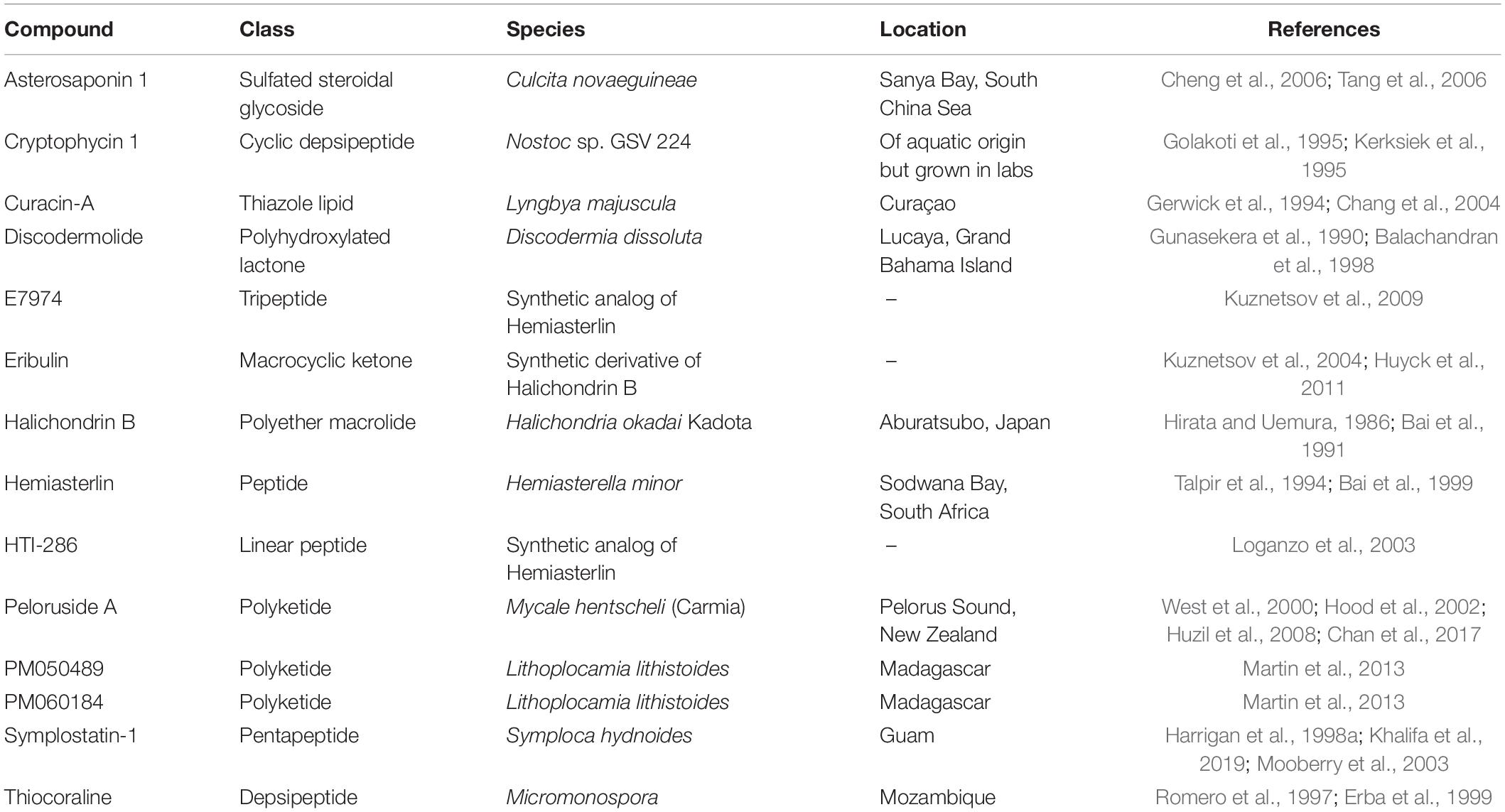
Table 1. Compounds classified under the resisting cell death hallmark that have a mechanism involving microtubules and cell cycle arrest to induce apoptosis.
Subsequent studies have developed antibody-drug conjugates using dolastatin 10 derivatives. In brentuximab vedotin, the dolastatin 10 analog monomethyl auristatin E (MMAE) is covalently bound to an antibody against CD30, an activator of B and T cells (Muta and Podack, 2013), to make an antibody-drug conjugate (Younes et al., 2010). While antibodies against CD30 were not significantly effective against lymphomas by themselves, the addition of MMAE greatly increased its efficacy (Younes et al., 2010). In March 2018, brentuximab vedotin was approved by the FDA for the treatment of Hodgkin’s lymphoma2. Another similar antibody-drug conjugate is polatuzumab vedotin. This conjugate is comprised of MMAE covalently bound to an antibody against CD79b, a component of B cell receptors (Deeks, 2019). The antibody helps guide the drug to its target cells, where the drug is internalized and induces apoptosis (Deeks, 2019). This form of targeting and drug delivery greatly reduces the toxicity to normal cells, so long as the antibody target is highly specific to a target on tumor cells. Polatuzumab vedotin was approved for treatment of relapsed/refractory diffuse large B-cell lymphoma in June 2019 (Deeks, 2019).
Other marine-derived compounds induce cell-cycle arrest and apoptosis through mechanisms that are independent of the disruption of microtubule dynamics. For example, the disulfide bromotyrosine derivative, psammaplin A, first isolated from the sponge Psammaplin aplysilla in the Kingdom of Tonga (Quinoa and Crews, 1987), is a histone deacetylase inhibitor. In Ishikawa endometrial cancer cells, psammaplin A induces cell cycle arrest and apoptosis through the downregulation of cyclins and CDKs in a p53-independent manner (Ahn et al., 2008). The cinnamic hydroxamic acid NVP-LAQ824 is a synthetic derivative of psammaplin A and is also a histone deacetylase inhibitor (Atadja et al., 2004). It activates the p21 promoter, selectively causes cell cycle arrest at the G2/M phase, and induces apoptosis in H1299, HCT116, A549, DU145, PC3, and MDA435 cancer cells (Atadja et al., 2004). NVP-LAQ824 successfully passed Phase I clinical trials for advanced solid tumors (de Bono et al., 2008). Aplidin, which was first isolated from ascidian Aplidium albicans in the Mediterranean Sea (Erba et al., 2002), is a cyclic depsipeptide that causes cell cycle arrest in G1/G2 through an unknown mechanism (Erba et al., 2002). In addition, the cyclodepsipeptide apratoxin A first extracted from the cyanobacterium Lyngbya majuscula found in Finger’s Reef, Guam induces apoptosis through cell cycle arrest (Luesch et al., 2001, 2006). Paatero et al. (2016) showed that apratoxin A specifically prevents protein translocation by inhibiting Sec61α, and thus blocks biogenesis.
Among these compounds, discodermolide (Mita et al., 2004) and E7974 (Rocha-Lima et al., 2012) reached Phase I clinical trials. PM060184 is in Phase II (see footnote 1, NCT03427268). Eribulin is FDA-approved for the treatment of metastatic breast cancer and liposarcoma, and aplidin is FDA-approved to treat multiple myeloma.
Inducing Apoptosis Through Replication, Translation, or Signaling Inhibition
While some marine-derived compounds act by inducing apoptosis through cell cycle arrest, other compounds induce apoptosis by targeting molecules that either activate apoptosis directly or are essential for cell survival. For example, didemnins are a class of depsipeptides that were first extracted from the Caribbean tunicate, Trididemnum solidum (Rinehart et al., 1981a). Among these peptides, didemnin B was the most potent (Rinehart et al., 1981a). Didemnin B inhibits protein synthesis by preventing eukaryotic elongation factor 2 (EEF2)-dependent translation elongation (Li et al., 1984; SirDeshpande and Toogood, 1995). Didemnin B reached Phase II clinical trials for the treatment of small cell lung cancer, but failed to show efficacy (Shin et al., 1994).
Another mechanism of inducing apoptosis is by disrupting pro-survival signaling cascades. The pentacyclic polyketide halenaquinone was first extracted from the sponge Xestospongia exigua found in Palau (Roll et al., 1983). Halenaquinone inhibits phosphoinositide 3-kinase (PI3K) and induces apoptosis in PC12 adrenal phaeochromocytoma cells (Fujiwara et al., 2001). Aeroplysinin-1, in addition to its effects on multiple hallmarks (Figure 1B), induces cytotoxic effects by inhibiting β-catenin signaling (Park et al., 2016).
Other compounds induce apoptosis by interacting with DNA in unique ways, such as trabectedin and cytarabine. Trabectedin is a tetrahydroisoquinoline alkaloid first isolated from the Caribbean tunicate Ecteinascidia turbinata (Rinehart et al., 1981b, 1991; D’Incalci and Galmarini, 2010). By binding to the minor groove of DNA, it causes DNA to bend at the major groove. The binding of trabectedin interferes with other factors that interact with DNA, such as transcription factors and DNA repair machinery, and ultimately results in induction of apoptosis (D’Incalci and Galmarini, 2010). Moreover, trabectedin can cause DNA strands to break by interacting with transcription-coupled nucleotide excision repair machinery (Takebayashi et al., 2001). Cytarabine, a synthetic analog of naturally occurring spongothymidine, also interferes with DNA replication (Bergmann and Feeney, 1951; Jimenez et al., 2018). This compound inserts into newly replicating DNA in place of nucleotides, which prevents elongation of the new daughter strand, and induces apoptosis (Iacobini et al., 2001; Jimenez et al., 2018). Cytarabine is FDA-approved for the treatment of leukemia and lymphoma.
Inducing Apoptosis Through Mitochondrial Interactions
In healthy cells, apoptosis can be initiated through mitochondrial instability. Outer mitochondrial membrane permeabilization releases cytochrome c into the cytoplasm where it activates apoptosis-inducing caspases (Lopez and Tait, 2015). Lamellarin D induces mitochondrial permeability by opening pores in the inner mitochondrial membrane, which leads to caspase activation and subsequent apoptosis (Kluza et al., 2006). Aplidin also disturbs mitochondrial permeability in addition to its ability to induce cell cycle arrest. It activates the Fas/CD95 pathway, which leads to mitochondria-mediated apoptosis in AML HL-60, T-lymphoid Jurkat, and HEL cells (Gajate et al., 2003).
Cancer cells overcome apoptotic signaling by upregulating anti-apoptotic proteins, such as BCL-2, to inhibit mitochondrial permeabilization (Lopez and Tait, 2015), or by inhibiting caspase-mediated downstream targets (Lopez and Tait, 2015). Compounds directed toward overcoming these resistance mechanisms by targeting multiple pathways or apoptosis mechanisms could represent promising candidates to treat apoptosis-resistant cancers. For example, some cryptophycins, first extracted from cyanobacteria Nostoc sp. GSV 224 (Golakoti et al., 1995), have anti-cancer properties. Treatment of human H460 non-small cell lung carcinoma cells with the depsipeptide cryptophycin 52 induces phosphorylation of BCL-2 and causes apoptosis in lung carcinoma cells (Lu et al., 2001). Cryptophycin 52 reached Phase II clinical trials. Similarly, fucoidan, in addition to targeting other hallmarks (Figure 1B), induces apoptosis by targeting parallel apoptotic-inducing pathways, including release of cytochrome c from mitochondria (Xue et al., 2012), and activation of caspases 3, 7, 8, and 9 (Kim et al., 2010; Xue et al., 2017).
Inducing Cell Death by Necrosis
While apoptosis is a well-studied mechanism of cell death, numerous mechanisms and pathways of cell death exist (Galluzzi et al., 2018). One of these mechanisms, necrosis, involves vacuolization and autolysis of cells in response to extreme stress. Necrosis can occur either as a result of ATP depletion or through specific signaling pathways (Edinger and Thompson, 2004). One drug that can induce cancer cell necrosis is kahalalide F, a peptide first isolated from the sea slug, Elysia rufescens, in Black Point, O’ahu, Hawaii (Hamann et al., 1996; Becerro et al., 2001). Experiments with HeLa cells suggested that kahalalide F targets lysosomes, which results in extreme vacuolization and swelling (Garcia-Rocha et al., 1996). Moreover, PC3, DU1445, LNCaP, SKBR-3, BT474, MCF7, MDA-MB-231, and LoVo cells treated with kahalalide F die from a combination of swelling, karyolysis, and necrosis (Suarez et al., 2003; Molinski et al., 2009). Kahalalide Freached Phase II clinical trials for melanoma, but showed no clinical benefit (Martin-Algarra et al., 2009).
Enabling Replicative Immortality
Normal cells have a limited number of cell divisions before they either die or enter senescence (Hayflick, 1992). This limit is driven by telomere shortening at each cell division (Allsopp et al., 1995), ultimately leading to crisis and death (Muraki et al., 2012). Cancer cells, however, enable replicative immortality, often through alterations in telomere maintenance, such as re-activation of telomerase or the alternative lengthening of telomeres pathway (Muraki et al., 2012).
Drugs That Inhibit Telomerase
Axinelloside A, a sulfated lipopolysaccharide first isolated from the sponge Axinella infundibula near Shikine-jima Island (Warabi et al., 2005), inhibits telomerase activity with an IC50 of 400 nM (Warabi et al., 2005). Given the potency of axinelloside A and its mechanism of telomerase inhibition, subsequent efforts have established a scalable system to develop compounds similar to the structure of axinelloside A (Guang et al., 2017). Like axinelloside A, fucoidan, in addition to its other mechanisms of cancer cell inhibition (Figure 1B), also inhibits telomerase activity by reducing human telomerase reverse transcriptase (hTERT) expression (Han et al., 2017).
Inducing Angiogenesis
Tissues require sufficient blood supply to transport oxygen, nutrients, and waste products (Potente et al., 2011). Under normal circumstances, the growth of new blood vessels, known as angiogenesis, only occurs in certain conditions, such as wound healing, menstruation, and embryonic development (Tonini et al., 2003). Cancer cells induce angiogenesis, which provides nutrient supplies to sustain tumor growth. One of the main activators of angiogenesis is VEGF (Carmeliet, 2005; Goel and Mercurio, 2013). VEGF signals to its receptor, VEGFR, which induces signaling and gene expression pathways related to cell survival, actin reorganization, cytoskeletal rearrangement, migration, and vascular cell permeability.
Inhibiting Vascular Endothelial Growth Factor (VEGF)
A number of compounds affect tumor vasculature through VEGF inhibition. The cyclodepsipeptide TZT-1027, a synthetic derivative of dolastatin 10, causes necrosis by damaging tumor vasculature (Otani et al., 2000). TZT-1027 enhances vascular permeability, resulting in the accumulation of red blood cells and damage to tumor vasculature (Watanabe et al., 2007). Damage to the vasculature causes the depletion of oxygen and nutrients to the tumor, resulting in necrosis-mediated cell death (Natsume et al., 2003). However, a Phase II clinical trial of TZT-1027 in non-small cell lung cancer was inconclusive (Riely et al., 2007). Similarly, largazole, first extracted from cyanobacteria of Symploca sp. in Key Largo, Florida Keys (Taori et al., 2008), is a cyclodepsipeptide that inhibits VEGF signaling. Largazole rapidly metabolizes into a thiol in the cell and inhibits histone deacetylases (Ying et al., 2008). It also inhibits VEGF and its receptor, thus inhibiting angiogenesis and inducing apoptosis (Liu et al., 2013). Like largazole, Motuparamine C also affects VEGF signaling (Roskelley et al., 2001). It is an alkaloid first extracted from the sponge Xestospongia exigua in Motupore Island, Papua New Guinea (Williams et al., 1998).
Interestingly, several compounds that affect VEGF signaling also affect the hallmark of resisting cell death, including aplidin, trabectedin, NVP-LAQ824, and fucoidan. A schematic representation of all drugs that inhibit multiple hallmarks is provided in Figure 1B. Aplidin prevents angiogenesis by inhibiting VEGF signaling. It also blocks VEGF secretion and inhibits the formation of capillary-like structures (Broggini et al., 2003; Taraboletti et al., 2004). Trabectedin inhibits VEGF mRNA expression, inhibits the activity of cytokines, and induces the activity of anti-angiogenic cytokines, such as tissue inhibitor metalloproteinase 1 (TIMP-1) and Serpin E1 (Atmaca and Uzunoglu, 2014). NVP-LAQ824 downregulates the expression of VEGF in vivo in mouse models (Qian et al., 2004). In a similar manner, philinopside A affects VEGF signaling through the VEGF receptor. Philinopside A is a sulfated triterpene glycoside first extracted from the sea cucumber, Pentacta quadrangulari, found in the South China Sea (Tong et al., 2005; Aminin et al., 2015). Fucoidan, which acts across multiple hallmarks (Figure 1B), including resisting cell death, evading growth suppressors, and enabling replicative immortality, also prevents angiogenesis by inhibiting VEGF and suppressing neovascularization (Xue et al., 2012; Senthilkumar et al., 2013).
Inhibiting Angiogenesis Through Other Mechanisms
In addition to direct inhibition or downregulation of VEGF signaling/expression, a number of compounds suppress angiogenesis through alternative mechanisms. For example, squalamine is an aminosterol first isolated in a dogfish shark, Squalus acanthias, from off the New England Coast (Moore et al., 1993). Squalamine was originally identified as an antibiotic and fungicide, but was later found to inhibit neovascularization by suppressing mitogen-induced proliferation of endothelial cells that form blood vessels (Sills et al., 1998). It was successful in a Phase I/II trial for non-small cell lung cancer (Herbst et al., 2003). By contrast, psammaplin A, in addition to its role in suppressing cell death resistance, also inhibits aminopeptidase N, a galectin 3 binding partner that induces endothelial cell migration (Yang et al., 2007).
While squalamine and psammaplin A have defined mechanisms of action, other compounds, such as aeroplysinin-1, have unknown mechanisms of anti-angiogenic activity. Aeroplysinin-1 caused severe disorganization in existing blood vessels and inhibition of new blood vessel growth in the areas where the drug was applied (Rodriguez-Nieto et al., 2002). Moreover, blood vessels outside the area of application grew around the site (Rodriguez-Nieto et al., 2002). Aeroplysinin-1 also affects the sustaining proliferative signaling and resisting cell death hallmarks (Figure 1B).
Activating Invasion and Metastasis
The cause of death for nearly all patients with solid tumors is by metastatic spread of their cancer to a new site in the body. Metastasis is often a multi-step process involving detachment from neighboring tumor cells, invasion, intravasation, dissemination, extravasation, seeding, and colonization. Drugs that target one or more steps in this process have potential to prolong the lives of patients with advanced, metastatic disease.
One marine-derived compound with potential anti-metastatic capability is motuparamine C. Motuparamine C inhibits the migratory ability of cancer cells (Roskelley et al., 2001) by inducing actin filament disassembly, ultimately resulting in a decrease in cancer cell invasion (Roskelley et al., 2001). Aeroplysinin-1 downregulates integrin β1, preventing cell migration and adhesion (Bechmann et al., 2018). Another potential anti-metastatic agent is fucoidan, which targets multiple cancer hallmarks (Figure 1B). Fucoidan inhibits cancer cell invasion and metastasis through numerous mechanisms, including preventing interactions between tumor cells and fibronectin within the extracellular matrix, interfering with tumor cell-platelet interactions, and inhibiting invasion through suppression of matrix metallopeptidase 2 (MMP-2) (Liu et al., 2005; Cumashi et al., 2007; Lee et al., 2012; Atashrazm et al., 2015). Fucoidan also prevents epithelial-mesenchymal transition (Senthilkumar et al., 2013), a key process in metastasis of many solid tumors in which epithelial-like cancer cells undergo a reversible phenotypic transition to a more invasive, mesenchymal-like state (Jolly et al., 2017, 2019). Fucoidan is currently in Phase II clinical trials for metastatic colorectal cancer (see footnote 1, NCT04066660).
Genome Instability and Mutation
For normal cells to become cancerous, they must acquire mutations that enable the traits of the cancer hallmarks. These new mutations can lead to tumor formation if they favor long-term proliferation and allow cells to dominate their environment (Hanahan and Weinberg, 2011). Mutations in DNA repair machinery or telomeres are particularly harmful to healthy cells and can increase the rate of mutations, and thus the likelihood of developing cancer (Kinzler and Vogelstein, 1997). Unfortunately, no marine-derived compounds have been identified to date that can target cells with dysfunctional DNA repair machinery.
Reprogramming of Energy Metabolism
Metabolic reprogramming by cancer cells has been recognized as a key hallmark for almost a century, with landmark discoveries by Otto Warburg in the 1920s showing that cancer cells can utilize aerobic glycolysis to produce ATP even in oxygen-rich conditions (Warburg et al., 1927; Liberti and Locasale, 2016). Renewed focus on this cancer hallmark has also revealed numerous other metabolic alterations and has pinpointed how these alterations affect both gene expression and interactions between cancer cells and the tumor microenvironment (Pavlova and Thompson, 2016).
The compound P11A is a streptodepsipeptide that was first isolated from the actinobacterium, Streptomyces S11-23B in the East China Sea (Ye et al., 2017). It counteracts alterations in metabolism by downregulating genes involved in glycolysis and glutaminolysis in glioma cells, including HK2, PFKFB3, PKM2, GLS, and FASN (Ye et al., 2017).
Tumor-Promoting Inflammation
The relationship between inflammation and cancer is complex. The inflammatory response can be either a cancer-protective or cancer-enabling feature. For example, inflammation at the sites of solid tumors has been shown to promote tumorigenesis and aid cancer cells in the process of gaining the characteristics of other hallmarks (Qian and Pollard, 2010). Moreover, factors secreted by immune cells can increase mutation rates in cancer cells, thus enabling cancer cells to take on characteristics of other hallmarks (Grivennikov et al., 2010). Aeroplysinin-1 has the potential to inhibit inflammation by decreasing the levels of total reactive oxygen species (Garcia-Vilas et al., 2018), which may make this compound a promising agent to reduce the tumor-promoting effects of inflammation.
Evading Immune Destruction
The immune system is critically important for surveilling and eliminating precancerous and cancerous cells. Cancer cells evade immune surveillance by (1) escaping immune recognition and (2) inducing immune suppression through the secretion of immunosuppressive ligands (Gonzalez et al., 2018). The burgeoning area of immuno-oncology seeks to activate a patient’s immune system by targeting tumor-immune interactions to eradicate cancer cells. Interestingly, several marine-derived compounds act on the immune system to inhibit cancer, making these compounds promising agents for use as single agents or in combination with existing immunotherapies.
For example, KRN7000 is a synthetic analog of agelasphin-9b, which was extracted from the sponge Agelas mauritianus (Haefner, 2003). KRN7000 is a potent immunostimulant that induces lymphocyte proliferation (Morita et al., 1995), enhances invariant natural killer cell activity (Tashiro, 2012), and inhibits both tumor growth (Morita et al., 1995) and metastasis in mouse models (Yamaguchi et al., 1996). Natural killer cell activity is stimulated by interaction of KRN7000 with the CD1d receptor (Nicol et al., 2000). In a Phase I-II study of KRN7000-pulsed peripheral blood mononuclear cells in non-small cell lung cancer, KRN7000 stimulation was associated with increased production of interferon gamma and prolonged median survival times in a subset of patients (Motohashi et al., 2009). Since the discovery of KRN7000, a number of analogs and modifications have been reported that have increased immunostimulatory effects (Shiozaki et al., 2013; Tashiro et al., 2013).
Like KRN7000, fucoidan also improves the activity of the immune system against cancer cells. As mentioned above, fucoidan has demonstrated activity across multiple cancer hallmarks (Figure 1B). In addition to its roles across these hallmarks, fucoidan also works broadly as an immune stimulant across multiple immune subsets, including decreasing neutrophil apoptosis, activation of splenic dendritic and T-cells (Zhang et al., 2015), and activation of natural killer cells (Ale et al., 2011).
Trabectedin, which is approved for the treatment of soft tissue sarcoma, has been shown to have anti-tumor efficacy by inducing cell death of monocytes. The selective induction of apoptosis in monocytes is due to the differential expression of signaling tumor necrosis factor-related apoptosis-inducing ligand (TRAIL) receptors on monocytes and decoy receptor expression in other immune subsets, such as neutrophils and lymphocytes (Germano et al., 2013). Trabectedin increased the number of infiltrating T-lymphocytes in a spontaneous model of osteosarcoma metastasis, many of which expressed programmed cell death-1 (PD-1) (Ratti et al., 2017). Combination with an anti-PD-1 therapeutic antibody led to significantly increased control of osteosarcoma growth (Ratti et al., 2017), highlighting the importance of testing biologically informed immunotherapy combinations with these marine-derived agents.
Compound Classification by Common Mechanisms
The compounds discussed in this review were broadly classified by mechanism of action in Figure 3. Of these, the largest group of compounds with a common mechanism inhibits the activity of microtubules (Figure 3A). These compounds predominantly belong to the peptide and polyketide classes. There were a similar number of compounds that inhibit the growth of blood vessels and interfere with the cell cycle (Figures 3B,C). Compounds that inhibit new blood vessel growth are more diverse in their structures (Figure 3B) while those that interfere with the cell cycle are mostly alkaloids and peptides (Figure 3C).
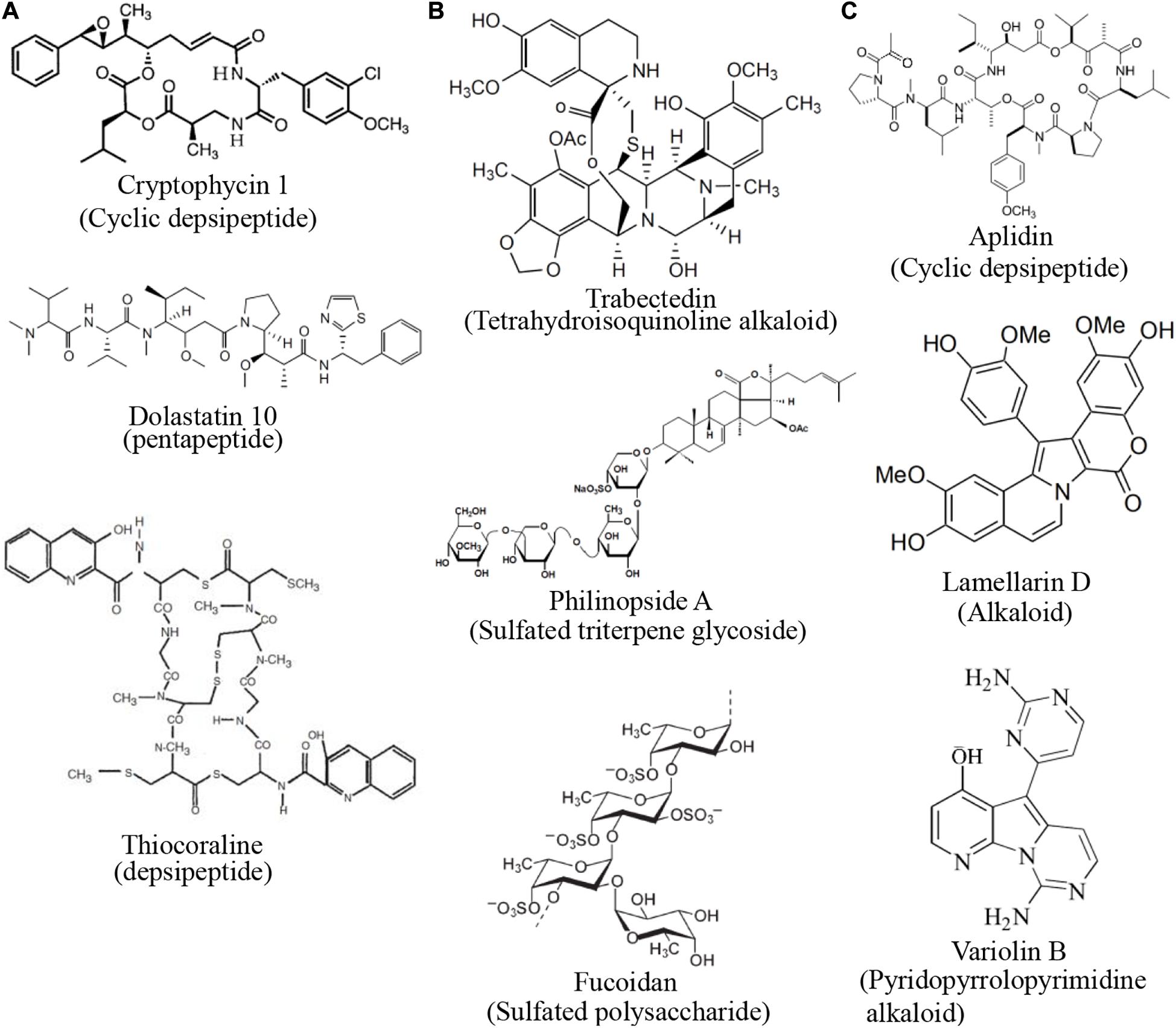
Figure 3. Structures of compounds with common mechanisms. (A) Some compounds that inhibit the activity of microtubules (B) Some compounds that inhibit blood vessel growth (C) Some compounds that interfere with the cell cycle. Structure images were taken from cited journals.
Biogeography of Marine Species That Are Sources of Anti-Cancer Compounds
The marine organisms that harbor anti-cancer chemicals span many phyla, but share some phenotypic characteristics. Many of these organisms lack significant structural defenses and have evolved the ability to create or harness secondary metabolites to reduce predation by being unpalatable or toxic. Many are benthic and either sessile, like sponges (phylum Porifera), bryozoans (phylum Bryozoa), and tunicates (phylum Chordata) or slow moving, like sea hares and sea slugs (phylum Mollusca) and sea cucumbers (phylum Echinodermata) (see Table 2 for examples). Sponges and cnidarians have long been used as sources of marine chemicals and are the two most common marine macrofaunal taxa from which bioactive compounds have been isolated (Mehbub et al., 2014). Indeed, soft, sessile, and slow marine species of any taxa are good candidates for the presence of novel metabolites to test, since they often lack other means of avoiding predation or foraging. The annual reviews of these compounds by Carroll et al. (2019, 2020) provide a comprehensive overview of compounds, their origins, and biological activities.
Not all novel compounds are necessarily created by the macrofauna from which they were isolated. Many come from symbiotic bacteria, like Candidatus Endobugula sertula, the symbiont in Bugula neritina that produces bryostatin-1 (Davidson et al., 2001). Others come from the microorganisms an animal consumes. For example, Luesch et al. (2001) proposed that dolastatin 10 may not be produced by the sea hare Dolabella auricularia, but rather by the cyanobacteria that it consumes. Likewise, the sea slug Elysia rufescens accumulates high concentrations of the anti-cancer compound Kahalalide F by consuming algae and their associated bacteria (Zan et al., 2019).
However, the interaction between the sea hare and the cyanobacterium is important, since the sea hare is responsible for accumulating the compound at higher concentrations in its tissues, facilitating identification and isolation. Thus, marine bacteria, fungi, algae, and the species that host them or eat them represent worthwhile candidates for further investigation.
Like the diversity of organisms that contain anti-cancer compounds, the habitats from which marine anti-cancer chemicals have been isolated are also diverse. These habitats span the globe, with the majority located near coastal areas or small islands in the tropical Pacific Ocean (Figure 4A). This, like most historical marine research, is likely due to ease of access to species at those locations. Estuarine and coastal environments are often home to vibrant communities of the taxa outlined above. Tropical coral reefs and shallow fouling communities are amongst the most studied and can have high abundance, biodiversity, and species richness with plentiful predators. These biological factors likely contribute to evolution of defensive chemicals in soft bodied organisms (Bakus, 1981).
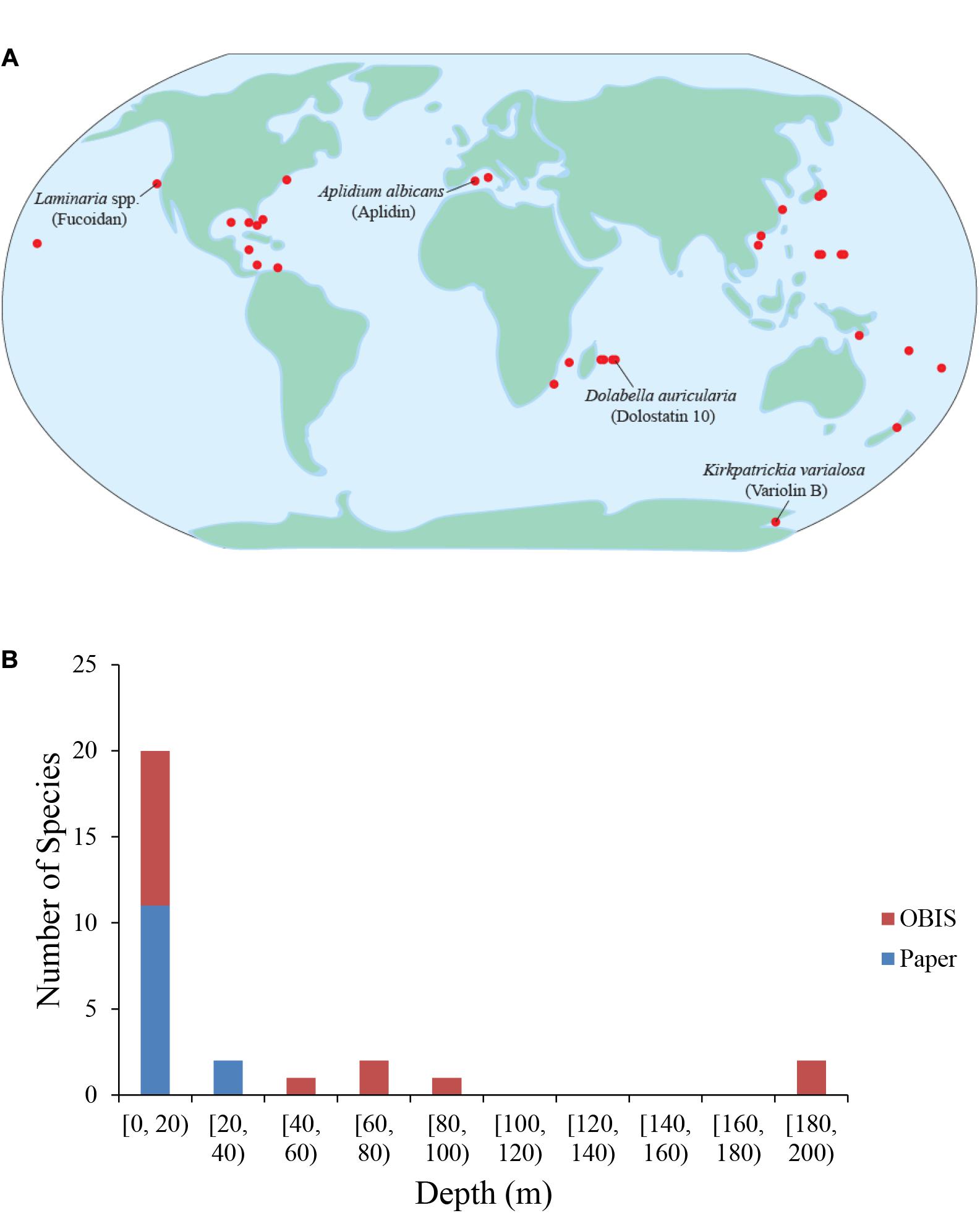
Figure 4. Geographic and depth distribution of known marine drug sources. (A) Each dot represents one marine source for one drug. No synthetic compounds were included. Some species and their compounds are labeled. (B) Depths of the marine drug source species at times of collection. The red color depicts species whose depth was determined by data from the Ocean Biogeographic Information System (OBIS), while the blue color depicts species whose depth was reported in the original papers describing the characterization/extraction/etc. of the compound (indicated as “Paper”).
Very few chemical isolations have been reported from the open ocean or in extreme environments, which is likely the result of substantial barriers associated with exploring these environments. While the barriers to exploration are often substantial, extreme environments can provide the biological, chemical, and/or physical challenges that might lead to the evolution of compounds with anti-cancer properties, and may be prime locations in which to search. One such extreme environment is Antarctica with extremes in day length and ocean temperatures normally below 0°C and a variety of known physiological adaptations. In addition, Antarctica is also home to abundant benthic communities composed of soft-bodied species likely because of a lack of large crustacean predators (Aronson and Blake, 2001). Interesting examples of compounds isolated from the cold waters of Antarctica include Variolin B, which was isolated from the sponge Kirkpatrickia variolosa, and displays pro-apoptotic activity and inhibits CDK activity (Simone et al., 2005), and Palmerlolide A and other macrolides isolated from the tunicate Synoicum adareanum, with cytotoxicity against melanoma cells by inhibiting vacuolar ATPase (Diyabalanage et al., 2006; Noguez et al., 2011).
Another extreme environment that is a promising location in which to search for anti-cancer compounds is the deep sea. The deep sea is the largest environment on Earth. With its decreasing sunlight and available organic carbon and increasing hydrostatic pressure with depth, the deep sea pelagic and benthic realms are full of novel biological adaptations. An analysis of the sampling depths across identified species and compounds reviewed herein reveals that nearly all reported isolations thus far have taken place between the surface and approximately 200 m (Figure 4B). The lack of isolations at depths deeper than 200 m is likely due to the difficulties associated with sampling at greater depths and/or the inability to recollect promising organisms. The deep-sea floor is commonly home to sea cucumbers, and rocky outcrops are often the sites of deep-sea coral reefs, many of them made of soft corals. In the deep-sea, other even more extreme environments exist that add toxic chemicals to the physical challenges. Deep-sea hydrothermal vents and cold seeps host abundant endemic communities dependent on organic carbon from hydrogen sulfide- or methane-based chemosynthesis (Van Dover, 2000). These environments are so toxic that often only endemic species can survive. Often, these species either utilize the abundant sulfide available or employ detoxification strategies to remove it from their systems (Zierenberg et al., 2000). Some species from these deep-sea environments that are particularly promising with respect to anti-cancer compounds are those that produce chemical deterrents to render them unpalatable as food (Kicklighter et al., 2004). Interestingly, all the species in the study that employed chemical defenses contained chemoautotrophic bacteria, which emphasizes the importance of symbiotic relationships with bacteria and the role their hosts play in accumulating these chemicals.
The sea also contains many hypoxic areas that support organisms with adaptations to low-oxygen conditions, which may promote the adaptation of compounds with unique properties. Hypoxic zones are home to microbes, invertebrates, and fish with adaptations for survival under chronic hypoxic stress (Childress and Seibel, 1998). Adaptations to these oxygen-poor zones include smaller and thinner bodies, more efficient respiratory surface area, and symbiosis with sulfide-oxidizing organisms (Levin, 2003). Deep bodies of water, like the Black Sea and many high-latitude fjords, with limited deep circulation often become hypoxic or anoxic at depth. The hypoxic marine ecosystems that are easiest to access are muddy, coastal benthic areas. The interstitial water within the sediments is decreasingly oxygenated with depth, yet can be full of organisms across the spectrum of taxa. Bivalved molluscs (e.g., clams and mussels) often close their valves for hours at a time and transition to anaerobic metabolism for the duration (McMahon, 1988). The adaptations observed throughout marine hypoxic environments are often unique to these locations, which increases the probability that species living in such environments utilize unique compounds.
Conclusion
With their diverse and highly complex habitats and lifestyles, marine species represent a vast, largely unexplored source of potential anti-cancer agents. Despite the current successes and enormous potential of exploring the marine chemical landscape, several barriers must be overcome before additional, substantial progress can be made. First, improvements in synthesis, isolation chemistry, and production of synthetic derivatives are needed to address the high complexity of many of these compounds, which often have multiple chiral centers. Indeed, with thousands of these bioactive compounds having been isolated and tested over the years, the few compounds that have entered clinical trials were among the rare minority that were abundant enough or simple enough to isolate for testing. Second, there is an urgent need to conserve and preserve marine natural habitats, both for its inherent and anthropocentric value: Innovations in synthesis and isolation must be combined with sustainable efforts to increase sampling across more diverse and extreme marine environments. Third, the development of a platform to analyze newly isolated compounds for activity across the hallmarks of cancer would provide a robust and rapid system to identify new anti-cancer therapies with enhanced specificity to target multiple hallmarks and hallmarks of advanced disease, such as metastasis and immune evasion. Encouragingly, among the compounds already discovered, they collectively target nearly all of the hallmarks of cancer, mirroring the substantial diversity inherent within the marine ecosystem. With many marine species yet to be discovered, countless novel compounds may exist with the potential to enhance and complement the existing arsenal of anti-cancer therapeutics.
Author Contributions
DD, JS, SK, ES, and CR conceived and designed the study. DD, BT, SK, and ES performed the literature review. DD, BT, JO, SK, ES, MD-D, TS, DR, WE, CR, and JS wrote the manuscript. All the authors contributed to the article and approved the submitted version.
Funding
The authors acknowledge support from the Nicholas Institute and the Duke University Nicholas School of the Environment.
Conflict of Interest
The authors declare that the research was conducted in the absence of any commercial or financial relationships that could be construed as a potential conflict of interest.
Supplementary Material
The Supplementary Material for this article can be found online at: https://www.frontiersin.org/articles/10.3389/fmars.2020.614766/full#supplementary-material
Footnotes
References
Ahn, M. Y., Jung, J. H., Na, Y. J., and Kim, H. S. (2008). A natural histone deacetylase inhibitor, Psammaplin A, induces cell cycle arrest and apoptosis in human endometrial cancer cells. Gynecol. Oncol. 108, 27–33. doi: 10.1016/j.ygyno.2007.08.098
Aktipis, C. A., Boddy, A. M., Jansen, G., Hibner, U., Hochberg, M. E., Maley, C. C., et al. (2015). Cancer across the tree of life: cooperation and cheating in multicellularity. Philos. Trans. R. Soc. Lond. B Biol. Sci. 370, 20140219. doi: 10.1098/rstb.2014.0219
Ale, M. T., Maruyama, H., Tamauchi, H., Mikkelsen, J. D., and Meyer, A. S. (2011). Fucoidan from Sargassum sp. and Fucus vesiculosus reduces cell viability of lung carcinoma and melanoma cells in vitro and activates natural killer cells in mice in vivo. Int. J. Biol. Macromol. 49, 331–336. doi: 10.1016/j.ijbiomac.2011.05.009
Allsopp, R. C., Chang, E., Kashefi-Aazam, M., Rogaev, E. I., Piatyszek, M. A., Shay, J. W., et al. (1995). Telomere shortening is associated with cell division in vitro and in vivo. Exp. Cell Res. 220, 194–200. doi: 10.1006/excr.1995.1306
Aminin, D. L., Menchinskaya, E. S., Pisliagin, E. A., Silchenko, A. S., Avilov, S. A., and Kalinin, V. I. (2015). Anticancer activity of sea cucumber triterpene glycosides. Mar. Drugs 13, 1202–1223. doi: 10.3390/md13031202
Andersen, R. J., Faulkner, D. J., Cun-heng, H., Van Duyne, G. D., and Clardy, J. (1985). Metabolites of the marine prosobranch mollusc Lamellaria sp. J. Am. Chem. Soc. 107, 5492–5495. doi: 10.1021/ja00305a027
Aronson, R. B., and Blake, D. B. (2001). Global climate change and the origin of modern benthic communities in Antarctica. Am. Zool. 41, 27–39. doi: 10.1093/icb/41.1.27
Atadja, P., Gao, L., Kwon, P., Trogani, N., Walker, H., Hsu, M., et al. (2004). Selective growth inhibition of tumor cells by a novel histone deacetylase inhibitor, NVP-LAQ824. Cancer Res 64, 689–695. doi: 10.1158/0008-5472.can-03-2043
Atashrazm, F., Lowenthal, R. M., Woods, G. M., Holloway, A. F., and Dickinson, J. L. (2015). Fucoidan and cancer: a multifunctional molecule with anti-tumor potential. Mar. Drugs 13, 2327–2346. doi: 10.3390/md13042327
Atmaca, H., and Uzunoglu, S. (2014). Anti-angiogenic effects of trabectedin (Yondelis; ET-743) on human breast cancer cells. Eur. Cytokine Netw. 25, 1–7. doi: 10.1684/ecn.2014.0347
Aunan, J. R., Cho, W. C., and Soreide, K. (2017). The biology of aging and cancer: a brief overview of shared and divergent molecular hallmarks. Aging Dis. 8, 628–642. doi: 10.14336/ad.2017.0103
Bai, R., Durso, N. A., Sackett, D. L., and Hamel, E. (1999). Interactions of the sponge-derived antimitotic tripeptide hemiasterlin with tubulin: comparison with dolastatin 10 and cryptophycin 1. Biochemistry 38, 14302–14310. doi: 10.1021/bi991323e
Bai, R., Friedman, S. J., Pettit, G. R., and Hamel, E. (1992). Dolastatin 15, a potent antimitotic depsipeptide derived from Dolabella auricularia. Interaction with tubulin and effects of cellular microtubules. Biochem. Pharmacol. 43, 2637–2645. doi: 10.1016/0006-2952(92)90153-a
Bai, R., Pettit, G. R., and Hamel, E. (1990). Dolastatin 10, a powerful cytostatic peptide derived from a marine animal. Inhibition of tubulin polymerization mediated through the vinca alkaloid binding domain. Biochem. Pharmacol. 39, 1941–1949. doi: 10.1016/0006-2952(90)90613-p
Bai, R. L., Paull, K. D., Herald, C. L., Malspeis, L., Pettit, G. R., and Hamel, E. (1991). Halichondrin B and homohalichondrin B, marine natural products binding in the vinca domain of tubulin. Discovery of tubulin-based mechanism of action by analysis of differential cytotoxicity data. J. Biol. Chem. 266, 15882–15889.
Bailly, C. (2015). Anticancer properties of Lamellarins. Mar. Drugs 13, 1105–1123. doi: 10.3390/md13031105
Bakus, G. J. (1981). Chemical defense mechanisms on the Great Barrier Reef, Australia. Science 211, 497–499. doi: 10.1126/science.7455691
Balachandran, R., ter Haar, E., Welsh, M. J., Grant, S. G., and Day, B. W. (1998). The potent microtubule-stabilizing agent (+)-discodermolide induces apoptosis in human breast carcinoma cells–preliminary comparisons to paclitaxel. Anticancer Drugs 9, 67–76. doi: 10.1097/00001813-199801000-00008
Ballot, C., Martoriati, A., Jendoubi, M., Buche, S., Formstecher, P., Mortier, L., et al. (2014). Another facet to the anticancer response to lamellarin D: induction of cellular senescence through inhibition of topoisomerase I and intracellular Ros production. Mar. Drugs 12, 779–798. doi: 10.3390/md12020779
Banafa, A. M., Roshan, S., Liu, Y. Y., Chen, H. J., Chen, M. J., Yang, G. X., et al. (2013). Fucoidan induces G1 phase arrest and apoptosis through caspases-dependent pathway and ROS induction in human breast cancer MCF-7 cells. J. Huazhong Univ. Sci. Technol. Med. Sci. 33, 717–724. doi: 10.1007/s11596-013-1186-8
Becerro, M. A., Goetz, G., Paul, V. J., and Scheuer, P. J. (2001). Chemical defenses of the sacoglossan mollusk Elysia rufescens and its host Alga bryopsis sp. J. Chem. Ecol. 27, 2287–2299.
Bechmann, N., Ehrlich, H., Eisenhofer, G., Ehrlich, A., Meschke, S., Ziegler, C. G., et al. (2018). Anti-tumorigenic and anti-metastatic activity of the sponge-derived marine drugs Aeroplysinin-1 and Isofistularin-3 against Pheochromocytoma In Vitro. Mar. Drugs 16, 172. doi: 10.3390/md16050172
Bergmann, W., and Feeney, R. J. (1951). Contributions to the Study of Marine Products. XXXII. The Nucleosides of Sponges. I. J. Org. Chem. 16, 981–987. doi: 10.1021/jo01146a023
Bhalla, K. N. (2003). Microtubule-targeted anticancer agents and apoptosis. Oncogene 22, 9075–9086. doi: 10.1038/sj.onc.1207233
Bird, G. M., and Haas, P. (1931). On the nature of the cell wall constituents of Laminaria spp. Mannuronic acid. Biochem. J. 25, 403–411. doi: 10.1042/bj0250403
Broggini, M., Marchini, S. V., Galliera, E., Borsotti, P., Taraboletti, G., Erba, E., et al. (2003). Aplidine, a new anticancer agent of marine origin, inhibits vascular endothelial growth factor (VEGF) secretion and blocks VEGF-VEGFR-1 (flt-1) autocrine loop in human leukemia cells MOLT-4. Leukemia 17, 52–59. doi: 10.1038/sj.leu.2402788
Canavese, M., Santo, L., and Raje, N. (2012). Cyclin dependent kinases in cancer: potential for therapeutic intervention. Cancer Biol. Ther. 13, 451–457. doi: 10.4161/cbt.19589
Carmeliet, P. (2005). VEGF as a key mediator of angiogenesis in cancer. Oncology 69(Suppl. 3), 4–10. doi: 10.1159/000088478
Carroll, A. R., Copp, B. R., Davis, R. A., Keyzers, R. A., and Prinsep, M. R. (2019). Marine natural products. Nat. Prod. Rep. 36, 122–173.
Carroll, A. R., Copp, B. R., Davis, R. A., Keyzers, R. A., and Prinsep, M. R. (2020). Marine natural products. Nat. Prod. Rep. 37, 175–223.
Chan, A., Gilfillan, C., Templeton, N., Paterson, I., Northcote, P. T., and Miller, J. H. (2017). Induction of accelerated senescence by the microtubule-stabilizing agent peloruside A. Invest. New Drugs 35, 706–717. doi: 10.1007/s10637-017-0493-5
Chang, Z., Sitachitta, N., Rossi, J. V., Roberts, M. A., Flatt, P. M., Jia, J., et al. (2004). Biosynthetic pathway and gene cluster analysis of curacin A, an antitubulin natural product from the tropical marine cyanobacterium Lyngbya majuscula. J. Nat. Prod. 67, 1356–1367. doi: 10.1021/np0499261
Cheng, G., Zhang, X., Tang, H. F., Zhang, Y., Zhang, X. H., Cao, W. D., et al. (2006). Asterosaponin 1, a cytostatic compound from the starfish Culcita novaeguineae, functions by inducing apoptosis in human glioblastoma U87MG cells. J. Neurooncol. 79, 235–241. doi: 10.1007/s11060-006-9136-y
Childress, J. J., and Seibel, B. A. (1998). Life at stable low oxygen levels: adaptations of animals to oceanic oxygen minimum layers. J. Exp. Biol. 201, 1223–1232.
Cho, T. M., Kim, W. J., and Moon, S. K. (2014). AKT signaling is involved in fucoidan-induced inhibition of growth and migration of human bladder cancer cells. Food Chem. Toxicol. 64, 344–352. doi: 10.1016/j.fct.2013.12.009
Ciminiello, P., Fattorusso, E., Forino, M., Magno, S., and Pansini, M. (1997). Chemistry of verongida sponges VIII-bromocompounds from the mediterranean sponges Aplysina aerophoba and Aplysina cavernicola. Tetrahedron 53, 6565–6572. doi: 10.1016/s0040-4020(97)00311-6
Cumashi, A., Ushakova, N. A., Preobrazhenskaya, M. E., D’Incecco, A., Piccoli, A., Totani, L., et al. (2007). A comparative study of the anti-inflammatory, anticoagulant, antiangiogenic, and antiadhesive activities of nine different fucoidans from brown seaweeds. Glycobiology 17, 541–552.
Davidson, S. K., Allen, S. W., Lim, G. E., Anderson, C. M., and Haygood, M. G. (2001). Evidence for the biosynthesis of bryostatins by the bacterial symbiont “Candidatus Endobugula sertula” of the bryozoan Bugula neritina. Appl. Environ. Microbiol. 67, 4531–4537.
Davis, J., Fricke, W. F., Hamann, M. T., Esquenazi, E., Dorrestein, P. C., and Hill, R. T. (2013). Characterization of the bacterial community of the chemically defended Hawaiian sacoglossan Elysia rufescens. Appl. Environ. Microbiol. 79, 7073–7081.
de Bono, J. S., Kristeleit, R., Tolcher, A., Fong, P., Pacey, S., Karavasilis, V., et al. (2008). Phase I pharmacokinetic and pharmacodynamic study of LAQ824, a hydroxamate histone deacetylase inhibitor with a heat shock protein-90 inhibitory profile, in patients with advanced solid tumors. Clin. Cancer Res. 14, 6663–6673.
Deeks, E. D. (2019). Polatuzumab vedotin: first global approval. Drugs 79, 1467–1475. doi: 10.1007/s40265-019-01175-0
Dickson, M. A., Schwartz, G. K., Keohan, M. L., D’Angelo, S. P., Gounder, M. M., Chi, P., et al. (2016). Progression-free survival among patients with well-differentiated or dedifferentiated liposarcoma treated with CDK4 inhibitor palbociclib: a phase 2 clinical trial. JAMA Oncol. 2, 937–940. doi: 10.1001/jamaoncol.2016.0264
Dickson, M. A., Tap, W. D., Keohan, M. L., D’Angelo, S. P., Gounder, M. M., Antonescu, C. R., et al. (2013). Phase II trial of the CDK4 inhibitor PD0332991 in patients with advanced CDK4-amplified well-differentiated or dedifferentiated liposarcoma. J. Clin. Oncol. 31, 2024–2028. doi: 10.1200/JCO.2012.46.5476
D’Incalci, M., and Galmarini, C. M. (2010). A review of trabectedin (ET-743): a unique mechanism of action. Mol. Cancer Ther. 9, 2157–2163. doi: 10.1158/1535-7163.MCT-10-0263
Diyabalanage, T., Amsler, C. D., McClintock, J. B., and Baker, B. J. (2006). Palmerolide A, a cytotoxic macrolide from the antarctic tunicate Synoicum adareanum. J. Am. Chem. Soc. 128, 5630–5631. doi: 10.1021/ja0588508
Edinger, A. L., and Thompson, C. B. (2004). Death by design: apoptosis, necrosis and autophagy. Curr. Opin. Cell Biol. 16, 663–669. doi: 10.1016/j.ceb.2004.09.011
Elmore, S. (2007). Apoptosis: a review of programmed cell death. Toxicol. Pathol. 35, 495–516. doi: 10.1080/01926230701320337
Erba, E., Bassano, L., Di Liberti, G., Muradore, I., Chiorino, G., Ubezio, P., et al. (2002). Cell cycle phase perturbations and apoptosis in tumour cells induced by aplidine. Br. J. Cancer 86, 1510–1517. doi: 10.1038/sj.bjc.6600265
Erba, E., Bergamaschi, D., Ronzoni, S., Faretta, M., Taverna, S., Bonfanti, M., et al. (1999). Mode of action of thiocoraline, a natural marine compound with anti-tumour activity. Br. J. Cancer 80, 971–980. doi: 10.1038/sj.bjc.6690451
Evan, G. I., Brown, L., Whyte, M., and Harrington, E. (1995). Apoptosis and the cell cycle. Curr. Opin. Cell Biol. 7, 825–834. doi: 10.1016/0955-0674(95)80066-2
Fattorusso, E., Minale, L., and Sodano, G. (1972). Aeroplysinin-1, an antibacterial bromo-compound from the sponge Verongia aerophoba. J. Chem. Soc. Perkin 1, 16–18. doi: 10.1039/p19720000016
Fernald, K., and Kurokawa, M. (2013). Evading apoptosis in cancer. Trends Cell Biol. 23, 620–633. doi: 10.1016/j.tcb.2013.07.006
Fujiwara, H., Matsunaga, K., Saito, M., Hagiya, S., Furukawa, K., Nakamura, H., et al. (2001). Halenaquinone, a novel phosphatidylinositol 3-kinase inhibitor from a marine sponge, induces apoptosis in PC12 cells. Eur. J. Pharmacol. 413, 37–45. doi: 10.1016/S0014-2999(00)00944-4
Gajate, C., An, F., and Mollinedo, F. (2003). Rapid and selective apoptosis in human leukemic cells induced by Aplidine through a Fas/CD95- and mitochondrial-mediated mechanism. Clin. Cancer Res. 9, 1535–1545.
Galluzzi, L., Vitale, I., Aaronson, S. A., Abrams, J. M., Adam, D., Agostinis, P., et al. (2018). Molecular mechanisms of cell death: recommendations of the Nomenclature Committee on Cell Death 2018. Cell Death Differ. 25, 486–541. doi: 10.1038/s41418-017-0012-4
Garcia-Rocha, M., Bonay, P., and Avila, J. (1996). The antitumoral compound Kahalalide F acts on cell lysosomes. Cancer Lett. 99, 43–50. doi: 10.1016/0304-3835(95)04036-6
Garcia-Vilas, J. A., Martinez-Poveda, B., Quesada, A. R., and Medina, M. A. (2015). Aeroplysinin-1, a sponge-derived multi-targeted bioactive marine drug. Mar. Drugs 14, 1. doi: 10.3390/md14010001
Garcia-Vilas, J. A., Martinez-Poveda, B., Quesada, A. R., and Medina, M. A. (2018). (+)-Aeroplysinin-1 modulates the redox balance of endothelial cells. Mar. Drugs 16, 316. doi: 10.3390/md16090316
Germano, G., Frapolli, R., Belgiovine, C., Anselmo, A., Pesce, S., Liguori, M., et al. (2013). Role of macrophage targeting in the antitumor activity of trabectedin. Cancer Cell 23, 249–262. doi: 10.1016/j.ccr.2013.01.008
Gerwick, W. H., Proteau, P. J., Nagle, D. G., Hamel, E., Blokhin, A., and Slate, D. L. (1994). Structure of Curacin A, a novel antimitotic, antiproliferative, and brine shrimp toxic natural product from the marine cyanobacterium Lyngbya majuscula. J. Org. Chem. 59, 1243–1245. doi: 10.1021/jo00085a006
Goel, H. L., and Mercurio, A. M. (2013). VEGF targets the tumour cell. Nat. Rev. Cancer 13, 871–882. doi: 10.1038/nrc3627
Golakoti, T. O., Heltzel, C. E., Husebo, T. L., Jensen, C. M., Larsen, L. K., Patterson, G. M. L., et al. (1995). Structure determination, conformational analysis, chemical stability studies, and antitumor evaluation of the Cryptophycins. Isolation of 18 new analogs from Nostoc sp. Strain GSV 224. J. Am. Chem. Soc. 117, 12030–12049. doi: 10.1021/ja00154a002
Gonzalez, H., Hagerling, C., and Werb, Z. (2018). Roles of the immune system in cancer: from tumor initiation to metastatic progression. Genes Dev. 32, 1267–1284. doi: 10.1101/gad.314617.118
Gopalan, P. K., Pinder, M. C., Chiappori, A., Ivey, A. M., Villegas, A. G., and Kaye, F. J. (2014). A phase II clinical trial of the CDK 4/6 inhibitor palbociclib (PD 0332991) in previously treated, advanced non-small cell lung cancer (NSCLC) patients with inactivated CDKN2A. J. Clin. Oncol. 32, 8077–8077. doi: 10.1200/jco.2014.32.15_suppl.8077
Grivennikov, S. I., Greten, F. R., and Karin, M. (2010). Immunity, inflammation, and cancer. Cell 140, 883–899. doi: 10.1016/j.cell.2010.01.025
Guang, J., Rumlow, Z. A., Wiles, L. M., O’Neill, S., and Walczak, M. A. (2017). Sulfated liposaccharides inspired by telomerase inhibitor axinelloside A. Tetrahedron Lett. 58, 4867–4871. doi: 10.1016/j.tetlet.2017.11.038
Gunasekera, S. P., Gunasekera, M., Longley, R. E., and Schulte, G. K. (1990). Discodermolide: a new bioactive polyhydroxylated lactone from the marine sponge Discodermia dissoluta. J. Org. Chem. 55, 4912–4915. doi: 10.1021/jo00303a029
Haefner, B. (2003). Drugs from the deep: marine natural products as drug candidates. Drug Discov. Today 8, 536–544. doi: 10.1016/S1359-6446(03)02713-2
Hamann, M. T., Otto, C. S., Scheuer, P. J., and Dunbar, D. C. (1996). Kahalalides: bioactive peptides from a marine mollusk elysia rufescens and its algal diet Bryopsis sp.(1). J. Org. Chem. 61, 6594–6600. doi: 10.1021/jo960877+
Hamilton, G. (2014). Cytotoxic effects of fascaplysin against small cell lung cancer cell lines. Mar. Drugs 12, 1377–1389. doi: 10.3390/md12031377
Han, M. H., Lee, D. S., Jeong, J. W., Hong, S. H., Choi, I. W., Cha, H. J., et al. (2017). Fucoidan Induces ROS-dependent apoptosis in 5637 human bladder cancer cells by downregulating telomerase activity via inactivation of the PI3K/Akt signaling pathway. Drug Dev. Res. 78, 37–48. doi: 10.1002/ddr.21367
Hanahan, D., and Weinberg, R. A. (2000). The hallmarks of cancer. Cell 100, 57–70. doi: 10.1016/S0092-8674(00)81683-9
Hanahan, D., and Weinberg, R. A. (2011). Hallmarks of cancer: the next generation. Cell 144, 646–674. doi: 10.1016/j.cell.2011.02.013
Harrigan, G. G., Luesch, H., Yoshida, W. Y., Moore, R. E., Nagle, D. G., Paul, V. J., et al. (1998a). Symplostatin 1: a dolastatin 10 analogue from the marine cyanobacterium Symploca hydnoides. J. Nat. Prod. 61, 1075–1077. doi: 10.1021/np980321c
Harrigan, G. G., Yoshida, W. Y., Moore, R. E., Nagle, D. G., Park, P. U., Biggs, J., et al. (1998b). Isolation, structure determination, and biological activity of dolastatin 12 and lyngbyastatin 1 from Lyngbya majuscula/Schizothrix calcicola cyanobacterial assemblages. J. Nat. Prod. 61, 1221–1225. doi: 10.1021/np9801211
Hayflick, L. (1992). Aging, longevity, and immortality in vitro. Exp. Gerontol. 27, 363–368. doi: 10.1016/0531-5565(92)90066-9
Herbst, R. S., Hammond, L. A., Carbone, D. P., Tran, H. T., Holroyd, K. J., Desai, A., et al. (2003). A phase I/IIA trial of continuous five-day infusion of squalamine lactate (MSI-1256F) plus carboplatin and paclitaxel in patients with advanced non-small cell lung cancer. Clin. Cancer Res. 9, 4108–4115.
Hirata, Y., and Uemura, D. (1986). Halichondrins—antitumor polyether macrolides from a marine sponge. Pure Appl. Chem. 58, 701–710. doi: 10.1351/pac198658050701
Hoffman, M. A., Blessing, J. A., Lentz, S. S., and Gynecologic Oncology Group, S. (2003). A phase II trial of dolastatin-10 in recurrent platinum-sensitive ovarian carcinoma: a Gynecologic Oncology Group study. Gynecol. Oncol. 89, 95–98. doi: 10.1016/S0090-8258(03)00007-6
Hood, K. A., West, L. M., Rouwe, B., Northcote, P. T., Berridge, M. V., Wakefield, S. J., et al. (2002). Peloruside A, a novel antimitotic agent with paclitaxel-like microtubule- stabilizing activity. Cancer Res. 62, 3356–3360.
Hu, Y., Chen, J., Hu, G., Yu, J., Zhu, X., Lin, Y., et al. (2015). Statistical research on the bioactivity of new marine natural products discovered during the 28 years from 1985 to 2012. Mar. Drugs 13, 202–221. doi: 10.3390/md13010202
Huang, R., Wallqvist, A., and Covell, D. G. (2006). Assessment of in vitro and in vivo activities in the National Cancer Institute’s anticancer screen with respect to chemical structure, target specificity, and mechanism of action. J. Med. Chem. 49, 1964–1979. doi: 10.1021/jm051029m
Huyck, T. K., Gradishar, W., Manuguid, F., and Kirkpatrick, P. (2011). Eribulin mesylate. Nat. Rev. Drug Discov. 10, 173–174. doi: 10.1038/nrd3389
Huzil, J. T., Chik, J. K., Slysz, G. W., Freedman, H., Tuszynski, J., Taylor, R. E., et al. (2008). A unique mode of microtubule stabilization induced by peloruside A. J. Mol. Biol. 378, 1016–1030. doi: 10.1016/j.jmb.2008.03.026
Iacobini, M., Menichelli, A., Palumbo, G., Multari, G., Werner, B., and Del Principe, D. (2001). Involvement of oxygen radicals in cytarabine-induced apoptosis in human polymorphonuclear cells. Biochem. Pharmacol. 61, 1033–1040. doi: 10.1016/S0006-2952(01)00548-2
Jimenez, P. C., Wilke, D. V., and Costa-Lotufo, L. V. (2018). Marine drugs for cancer: surfacing biotechnological innovations from the oceans. Clinics 73, e482s. doi: 10.6061/clinics/2018/e482s
Jolly, M. K., Somarelli, J. A., Sheth, M., Biddle, A., Tripathi, S. C., Armstrong, A. J., et al. (2019). Hybrid epithelial/mesenchymal phenotypes promote metastasis and therapy resistance across carcinomas. Pharmacol. Ther. 194, 161–184. doi: 10.1016/j.pharmthera.2018.09.007
Jolly, M. K., Ware, K. E., Gilja, S., Somarelli, J. A., and Levine, H. (2017). EMT and MET: necessary or permissive for metastasis? Mol. Oncol. 11, 755–769. doi: 10.1002/1878-0261.12083
Jones, S. M., and Kazlauskas, A. (2001). Growth factor-dependent signaling and cell cycle progression. Chem. Rev. 101, 2413–2423. doi: 10.1021/cr000101f
Karasic, T. B., O’Hara, M. H., Teitelbaum, U. R., Damjanov, N., Giantonio, B. J., d’Entremont, T. S., et al. (2020). Phase II trial of Palbociclib in patients with advanced esophageal or gastric cancer. Oncologist doi: 10.1634/theoncologist.2020-0681 [Epub ahead of print].
Kerksiek, K., Mejillano, M. R., Schwartz, R. E., Georg, G. I., and Himes, R. H. (1995). Interaction of cryptophycin 1 with tubulin and microtubules. FEBS Lett. 377, 59–61. doi: 10.1016/0014-5793(95)01271-0
Khalifa, S. A. M., Elias, N., Farag, M. A., Chen, L., Saeed, A., Hegazy, M. F., et al. (2019). Marine natural products: a source of novel anticancer drugs. Mar. Drugs 17, 491. doi: 10.3390/md17090491
Kicklighter, C. E., Fisher, C. R., and Hay, M. E. (2004). Chemical defense of hydrothermal vent and hydrocarbon seep organisms: a preliminary assessment using shallow-water consumers. Mar. Ecol. Prog. Ser. 275, 11–19. doi: 10.3354/meps275011
Kim, E. J., Park, S. Y., Lee, J. Y., and Park, J. H. (2010). Fucoidan present in brown algae induces apoptosis of human colon cancer cells. BMC Gastroenterol. 10:96. doi: 10.1186/1471-230X-10-96
Kindler, H. L., Tothy, P. K., Wolff, R., McCormack, R. A., Abbruzzese, J. L., Mani, S., et al. (2005). Phase II trials of dolastatin-10 in advanced pancreaticobiliary cancers. Invest. New Drugs 23, 489–493. doi: 10.1007/s10637-005-2909-x
Kinzler, K. W., and Vogelstein, B. (1997). Cancer-susceptibility genes. Gatekeepers and caretakers. Nature 386, 761–763. doi: 10.1038/386761a0
Klein, L. E., Freeze, B. S., Smith, A. B. III, and Horwitz, S. B. (2005). The microtubule stabilizing agent discodermolide is a potent inducer of accelerated cell senescence. Cell Cycle 4, 501–507. doi: 10.4161/cc.4.3.1550
Kluza, J., Gallego, M. A., Loyens, A., Beauvillain, J. C., Sousa-Faro, J. M., Cuevas, C., et al. (2006). Cancer cell mitochondria are direct proapoptotic targets for the marine antitumor drug lamellarin D. Cancer Res. 66, 3177–3187. doi: 10.1158/0008-5472.CAN-05-1929
Kollar, P., Rajchard, J., Balounova, Z., and Pazourek, J. (2014). Marine natural products: bryostatins in preclinical and clinical studies. Pharm. Biol. 52, 237–242. doi: 10.3109/13880209.2013.804100
Konecny, G. E., Hendrickson, A. E. W., Jatoi, A., Burton, J. K., Paroly, J., Glaspy, J. A., et al. (2016). A multicenter open-label phase II study of the efficacy and safety of palbociclib a cyclin-dependent kinases 4 and 6 inhibitor in patients with recurrent ovarian cancer. J. Clin. Oncol. 34, 5557–5557. doi: 10.1200/JCO.2016.34.15_suppl.5557
Kortmansky, J., and Schwartz, G. K. (2003). Bryostatin-1: a novel PKC inhibitor in clinical development. Cancer Invest. 21, 924–936. doi: 10.1081/CNV-120025095
Kreuter, M. H., Bernd, A., Holzmann, H., Muller-Klieser, W., Maidhof, A., Weissmann, N., et al. (1989). Cytostatic activity of aeroplysinin-1 against lymphoma and epithelioma cells. Z. Naturforsch. C. J. Biosci. 44, 680–688. doi: 10.1515/znc-1989-7-822
Kreuter, M. H., Leake, R. E., Rinaldi, F., Muller-Klieser, W., Maidhof, A., Muller, W. E., et al. (1990). Inhibition of intrinsic protein tyrosine kinase activity of EGF-receptor kinase complex from human breast cancer cells by the marine sponge metabolite (+)-aeroplysinin-1. Comp. Biochem. Physiol. B 97, 151–158. doi: 10.1016/0305-0491(90)90194-X
Kuznetsov, G., TenDyke, K., Towle, M. J., Cheng, H., Liu, J., Marsh, J. P., et al. (2009). Tubulin-based antimitotic mechanism of E7974, a novel analogue of the marine sponge natural product hemiasterlin. Mol. Cancer Ther. 8, 2852–2860. doi: 10.1158/1535-7163.MCT-09-0301
Kuznetsov, G., Towle, M. J., Cheng, H., Kawamura, T., TenDyke, K., Liu, D., et al. (2004). Induction of morphological and biochemical apoptosis following prolonged mitotic blockage by halichondrin B macrocyclic ketone analog E7389. Cancer Res. 64, 5760–5766. doi: 10.1158/0008-5472.CAN-04-1169
Le, V. H., Inai, M., Williams, R. M., and Kan, T. (2015). Ecteinascidins. A review of the chemistry, biology and clinical utility of potent tetrahydroisoquinoline antitumor antibiotics. Nat. Prod. Rep. 32, 328–347. doi: 10.1039/C4NP00051J
Lee, H., Kim, J. S., and Kim, E. (2012). Fucoidan from seaweed Fucus vesiculosus inhibits migration and invasion of human lung cancer cell via PI3K-Akt-mTOR pathways. PLoS One 7:e50624. doi: 10.1371/journal.pone.0050624
Levin, L. A. (2003). Oxygen minimum zone benthos: adaptation and community response to hypoxia. Oceanogr. Mar. Biol. 41, 1–45.
Li, L. H., Timmins, L. G., Wallace, T. L., Krueger, W. C., Prairie, M. D., and Im, W. B. (1984). Mechanism of action of didemnin B, a depsipeptide from the sea. Cancer Lett. 23, 279–288. doi: 10.1016/0304-3835(84)90095-8
Liberti, M. V., and Locasale, J. W. (2016). The warburg effect: how does it benefit cancer cells? Trends Biochem. Sci. 41, 211–218. doi: 10.1016/j.tibs.2015.12.001
Lichota, A., and Gwozdzinski, K. (2018). Anticancer activity of natural compounds from plant and marine environment. Int. J. Mol. Sci. 19, 3533. doi: 10.3390/ijms19113533
Liu, J. M., Bignon, J., Haroun-Bouhedja, F., Bittoun, P., Vassy, J., Fermandjian, S., et al. (2005). Inhibitory effect of fucoidan on the adhesion of adenocarcinoma cells to fibronectin. Anticancer Res. 25, 2129–2133.
Liu, Y., Wang, Z., Wang, J., Lam, W., Kwong, S., Li, F., et al. (2013). A histone deacetylase inhibitor, largazole, decreases liver fibrosis and angiogenesis by inhibiting transforming growth factor-beta and vascular endothelial growth factor signalling. Liver Int. 33, 504–515. doi: 10.1111/liv.12034
Loganzo, F., Discafani, C. M., Annable, T., Beyer, C., Musto, S., Hari, M., et al. (2003). HTI-286, a synthetic analogue of the tripeptide hemiasterlin, is a potent antimicrotubule agent that circumvents P-glycoprotein-mediated resistance in vitro and in vivo. Cancer Res. 63, 1838–1845.
Lopez, J., and Tait, S. W. (2015). Mitochondrial apoptosis: killing cancer using the enemy within. Br. J. Cancer 112, 957–962. doi: 10.1038/bjc.2015.85
Lu, K., Dempsey, J., Schultz, R. M., Shih, C., and Teicher, B. A. (2001). Cryptophycin-induced hyperphosphorylation of Bcl-2, cell cycle arrest and growth inhibition in human H460 NSCLC cells. Cancer Chemother. Pharmacol. 47, 170–178. doi: 10.1007/s002800000210
Luesch, H., Chanda, S. K., Raya, R. M., DeJesus, P. D., Orth, A. P., Walker, J. R., et al. (2006). A functional genomics approach to the mode of action of apratoxin A. Nat. Chem. Biol. 2, 158–167. doi: 10.1038/nchembio769
Luesch, H., Moore, R. E., Paul, V. J., Mooberry, S. L., and Corbett, T. H. (2001). Isolation of dolastatin 10 from the marine cyanobacterium Symploca species VP642 and total stereochemistry and biological evaluation of its analogue symplostatin 1. J. Nat. Prod. 64, 907–910. doi: 10.1021/np010049y
Malve, H. (2016). Exploring the ocean for new drug developments: marine pharmacology. J. Pharm. Bioallied. Sci. 8, 83–91. doi: 10.4103/0975-7406.171700
Margolin, K., Longmate, J., Synold, T. W., Gandara, D. R., Weber, J., Gonzalez, R., et al. (2001). Dolastatin-10 in metastatic melanoma: a phase II and pharmokinetic trial of the California Cancer Consortium. Invest. New Drugs 19, 335–340. doi: 10.1023/A:1010626230081
Martin, M. J., Coello, L., Fernandez, R., Reyes, F., Rodriguez, A., Murcia, C., et al. (2013). Isolation and first total synthesis of PM050489 and PM060184, two new marine anticancer compounds. J. Am. Chem. Soc. 135, 10164–10171. doi: 10.1021/ja404578u
Martin-Algarra, S., Espinosa, E., Rubio, J., Lopez Lopez, J. J., Manzano, J. L., Carrion, L. A., et al. (2009). Phase II study of weekly Kahalalide F in patients with advanced malignant melanoma. Eur. J. Cancer 45, 732–735. doi: 10.1016/j.ejca.2008.12.005
McMahon, R. F. (1988). Respiratory response to periodic emergence in intertidal molluscs. Am. Zool. 28, 97–114. doi: 10.1093/icb/28.1.97
Mehbub, M. F., Lei, J., Franco, C., and Zhang, W. (2014). Marine sponge derived natural products between 2001 and 2010: trends and opportunities for discovery of bioactives. Mar. Drugs 12, 4539–4577. doi: 10.3390/md12084539
Mita, A., Lockhart, A. C., Chen, T. L., Bochinski, K., Curtright, J., Cooper, W., et al. (2004). A phase I pharmacokinetic (PK) trial of XAA296A (Discodermolide) administered every 3 wks to adult patients with advanced solid malignancies. J. Clin. Oncol. 22, 2025. doi: 10.1200/jco.2004.22.90140.2025
Mocsai, A., Kovacs, L., and Gergely, P. (2014). What is the future of targeted therapy in rheumatology: biologics or small molecules? BMC Med. 12:43. doi: 10.1186/1741-7015-12-43
Molinski, T. F., Dalisay, D. S., Lievens, S. L., and Saludes, J. P. (2009). Drug development from marine natural products. Nat. Rev. Drug Discov. 8, 69–85. doi: 10.1038/nrd2487
Montaser, R., and Luesch, H. (2011). Marine natural products: a new wave of drugs? Future Med. Chem. 3, 1475–1489. doi: 10.4155/fmc.11.118
Mooberry, S. L., Leal, R. M., Tinley, T. L., Luesch, H., Moore, R. E., and Corbett, T. H. (2003). The molecular pharmacology of symplostatin 1: a new antimitotic dolastatin 10 analog. Int. J. Cancer 104, 512–521. doi: 10.1002/ijc.10982
Moore, K. S., Wehrli, S., Roder, H., Rogers, M., Forrest, J. N. Jr., McCrimmon, D., et al. (1993). Squalamine: an aminosterol antibiotic from the shark. Proc. Natl. Acad. Sci. U.S.A. 90, 1354–1358. doi: 10.1073/pnas.90.4.1354
Morita, M., Motoki, K., Akimoto, K., Natori, T., Sakai, T., Sawa, E., et al. (1995). Structure-activity relationship of alpha-galactosylceramides against B16-bearing mice. J. Med. Chem. 38, 2176–2187. doi: 10.1021/jm00012a018
Motohashi, S., Nagato, K., Kunii, N., Yamamoto, H., Yamasaki, K., Okita, K., et al. (2009). A phase I-II study of alpha-galactosylceramide-pulsed IL-2/GM-CSF-cultured peripheral blood mononuclear cells in patients with advanced and recurrent non-small cell lung cancer. J. Immunol. 182, 2492–2501. doi: 10.4049/jimmunol.0800126
Mullineaux, L. S., Metaxas, A., Beaulieu, S. E., Bright, M., Gollner, S., Grupe, B. M., et al. (2018). Exploring the ecology of deep-sea hydrothermal vents in a metacommunity framework. Front. Mar. Sci. 5:49. doi: 10.3389/fmars.2018.00049
Muraki, K., Nyhan, K., Han, L., and Murnane, J. P. (2012). Mechanisms of telomere loss and their consequences for chromosome instability. Front. Oncol. 2:135. doi: 10.3389/fonc.2012.00135
Muta, H., and Podack, E. R. (2013). CD30: from basic research to cancer therapy. Immunol. Res. 57, 151–158. doi: 10.1007/s12026-013-8464-1
Natsume, T., Watanabe, J., Koh, Y., Fujio, N., Ohe, Y., Horiuchi, T., et al. (2003). Antitumor activity of TZT-1027 (Soblidotin) against vascular endothelial growth factor-secreting human lung cancer in vivo. Cancer Sci. 94, 826–833. doi: 10.1111/j.1349-7006.2003.tb01526.x
Newman, D. J., and Cragg, G. M. (2020). Natural products as sources of new drugs over the nearly four decades from 01/1981 to 09/2019. J. Nat. Prod. 83, 770–803. doi: 10.1021/acs.jnatprod.9b01285
Nicol, A., Nieda, M., Koezuka, Y., Porcelli, S., Suzuki, K., Tadokoro, K., et al. (2000). Human invariant valpha24+ natural killer T cells activated by alpha-galactosylceramide (KRN7000) have cytotoxic anti-tumour activity through mechanisms distinct from T cells and natural killer cells. Immunology 99, 229–234. doi: 10.1046/j.1365-2567.2000.00952.x
Noguez, J. H., Diyabalanage, T. K., Miyata, Y., Xie, X. S., Valeriote, F. A., Amsler, C. D., et al. (2011). Palmerolide macrolides from the Antarctic tunicate Synoicum adareanum. Bioorg. Med. Chem. 19, 6608–6614. doi: 10.1016/j.bmc.2011.06.004
Otani, M., Natsume, T., Watanabe, J. I., Kobayashi, M., Murakoshi, M., Mikami, T., et al. (2000). TZT-1027, an antimicrotubule agent, attacks tumor vasculature and induces tumor cell death. Jpn. J. Cancer Res. 91, 837–844. doi: 10.1111/j.1349-7006.2000.tb01022.x
Paatero, A. O., Kellosalo, J., Dunyak, B. M., Almaliti, J., Gestwicki, J. E., Gerwick, W. H., et al. (2016). Apratoxin kills cells by direct blockade of the Sec61 protein translocation channel. Cell Chem. Biol. 23, 561–566. doi: 10.1016/j.chembiol.2016.04.008
Paerl, H. W., Otten, T. G., and Joyner, A. R. (2016). Moving towards adaptive management of cyanotoxin-impaired water bodies. Microb. Biotechnol. 9, 641–651. doi: 10.1111/1751-7915.12383
Page, M., West, L., Northcote, P., Battershill, C., and Kelly, M. (2005). Spatial and temporal variability of cytotoxic metabolites in populations of the New Zealand sponge Mycale hentscheli. J. Chem. Ecol. 31, 1161–1174. doi: 10.1007/s10886-005-4254-0
Park, S., Kim, J. H., Kim, J. E., Song, G. Y., Zhou, W., Goh, S. H., et al. (2016). Cytotoxic activity of aeroplysinin-1 against colon cancer cells by promoting beta-catenin degradation. Food Chem. Toxicol. 93, 66–72. doi: 10.1016/j.fct.2016.04.019
Pavlova, N. N., and Thompson, C. B. (2016). The emerging hallmarks of cancer metabolism. Cell Metab. 23, 27–47. doi: 10.1016/j.cmet.2015.12.006
Perez, E. A., Hillman, D. W., Fishkin, P. A., Krook, J. E., Tan, W. W., Kuriakose, P. A., et al. (2005). Phase II trial of dolastatin-10 in patients with advanced breast cancer. Invest. New Drugs 23, 257–261. doi: 10.1007/s10637-005-6735-y
Perry, N. B., Ettouati, L., Litaudon, M., Blunt, J. W., and Munro, M. H. G. (1994). Alkaloids from the Antarctic Sponge Kirkpatrickia varialosa. Part 1: variolin B, A new antitumour and antiviral compound. Tetrahedron 50, 3987–3992. doi: 10.1016/S0040-4020(01)89673-3
Pettit, G. R., Herold, C. L., Doubek, D. L., and Herald, D. L. (1982). Isolation and structure of bryostatin l. J. Am. Chem. Soc. 104, 6846–6848. doi: 10.1021/ja00388a092
Pettit, G. R., Kamano, Y., Dufresne, C., Cerny, R. L., Herald, C. L., and Schmidt, J. M. (1989). Isolation and structure of the cytostatic linear depsipeptide dolastatin 15. J. Org. Chem. 54, 6005–6006. doi: 10.1021/jo00287a003
Pettit, G. R., Kamano, Y., Herald, C. L., Fujii, Y., Kizu, H., Boyd, M. R., et al. (1993). Isolation of Dolastatins 10-15 from the marine mollusk Dolabella auricularia. Tetrahedron 49, 9151–9170. doi: 10.1016/0040-4020(93)80003-C
Potente, M., Gerhardt, H., and Carmeliet, P. (2011). Basic and therapeutic aspects of angiogenesis. Cell 146, 873–887. doi: 10.1016/j.cell.2011.08.039
Qian, B. Z., and Pollard, J. W. (2010). Macrophage diversity enhances tumor progression and metastasis. Cell 141, 39–51. doi: 10.1016/j.cell.2010.03.014
Qian, D. Z., Wang, X., Kachhap, S. K., Kato, Y., Wei, Y., Zhang, L., et al. (2004). The histone deacetylase inhibitor NVP-LAQ824 inhibits angiogenesis and has a greater antitumor effect in combination with the vascular endothelial growth factor receptor tyrosine kinase inhibitor PTK787/ZK222584. Cancer Res. 64, 6626–6634. doi: 10.1158/0008-5472.CAN-04-0540
Quinoa, E., and Crews, P. (1987). Phenolic constituents of Psammaplysilla. Tetrahedron Lett. 28, 3229–3232. doi: 10.1016/S0040-4039(00)95478-9
Raghuvanshi, R., and Bharate, S. B. (2020). Preclinical and clinical studies on bryostatins, a class of marine-derived protein Kinase C Modulators: a mini-review. Curr. Top. Med. Chem. 20, 1124–1135. doi: 10.2174/1568026620666200325110444
Ratti, C., Botti, L., Cancila, V., Galvan, S., Torselli, I., Garofalo, C., et al. (2017). Trabectedin overrides osteosarcoma differentiative block and reprograms the tumor immune environment enabling effective combination with immune checkpoint inhibitors. Clin. Cancer Res. 23, 5149–5161. doi: 10.1158/1078-0432.CCR-16-3186
Riely, G. J., Gadgeel, S., Rothman, I., Saidman, B., Sabbath, K., Feit, K., et al. (2007). A phase 2 study of TZT-1027, administered weekly to patients with advanced non-small cell lung cancer following treatment with platinum-based chemotherapy. Lung Cancer 55, 181–185. doi: 10.1016/j.lungcan.2006.10.002
Rinehart, K. L. Jr., Gloer, J. B., Hughes, R. G. Jr., Renis, H. E., McGovren, J. P., Swynenberg, E. B., et al. (1981a). Didemnins: antiviral and antitumor depsipeptides from a caribbean tunicate. Science 212, 933–935. doi: 10.1126/science.7233187
Rinehart, K. L. Jr., Holt, T. G., Fregeau, N. L., Stroh, J. G., Keifer, P. A., Sun, F., et al. (1991). Ecteinascadins 729, 743, 745, 759A, 759B, and 770: potent antitumor agents from the caribbean tunicate Ecteinascidia turbinata. J. Org. Chem. 55, 4512–4515. doi: 10.1021/jo00302a007
Rinehart, K. L., Shaw, P. D., Shield, L. S., Gloer, J. B., Harbour, G. C., Koker, M. E. S., et al. (1981b). Marine natural products as sources of antiviral, antimicrobial, and antineoplastic agents. Pure Appl. Chem. 53, 795–817. doi: 10.1351/pac198153040795
Rocha-Lima, C. M., Bayraktar, S., Macintyre, J., Raez, L., Flores, A. M., Ferrell, A., et al. (2012). A phase 1 trial of E7974 administered on day 1 of a 21-day cycle in patients with advanced solid tumors. Cancer 118, 4262–4270. doi: 10.1002/cncr.27428
Rodriguez-Nieto, S., Gonzalez-Iriarte, M., Carmona, R., Munoz-Chapuli, R., Medina, M. A., and Quesada, A. R. (2002). Antiangiogenic activity of aeroplysinin-1, a brominated compound isolated from a marine sponge. FASEB J. 16, 261–263. doi: 10.1096/fj.01-0427fje
Roll, D. M., Ireland, C. M., Lu, H. S. M., and Clardy, J. (1988). Fascaplysin, an unusual antimicrobial pigment from the marine sponge Fascaplysinopsis sp. J. Org. Chem. 53, 3276–3278. doi: 10.1021/jo00249a025
Roll, D. M., Scheuer, P. J., Matsumoto, G. K., and Clardy, J. (1983). Halenaquinone, a pentacyclic polyketide from a marine sponge. J. Am. Chem. Soc. 105, 6177–6178. doi: 10.1021/ja00357a049
Romero, F., Espliego, F., Perez Baz, J., Garcia de Quesada, T., Gravalos, D., de la Calle, F., et al. (1997). Thiocoraline, a new depsipeptide with antitumor activity produced by a marine Micromonospora. I. Taxonomy, fermentation, isolation, and biological activities. J. Antibiot. (Tokyo) 50, 734–737. doi: 10.7164/antibiotics.50.734
Roskelley, C. D., Williams, D. E., McHardy, L. M., Leong, K. G., Troussard, A., Karsan, A., et al. (2001). Inhibition of tumor cell invasion and angiogenesis by motuporamines. Cancer Res. 61, 6788–6794.
Rust, M., Helfrich, E. J. N., Freeman, M. F., Nanudorn, P., Field, C. M., Ruckert, C., et al. (2020). A multiproducer microbiome generates chemical diversity in the marine sponge Mycale hentscheli. Proc. Natl. Acad. Sci. U.S.A. 117, 9508–9518. doi: 10.1073/pnas.1919245117
Saad, E. D., Kraut, E. H., Hoff, P. M., Moore, D. F. Jr., Jones, D., Pazdur, R., et al. (2002). Phase II study of dolastatin-10 as first-line treatment for advanced colorectal cancer. Am. J. Clin. Oncol. 25, 451–453. doi: 10.1097/00000421-200210000-00005
Schafer, K. A. (1998). The cell cycle: a review. Vet. Pathol. 35, 461–478. doi: 10.1177/030098589803500601
Schettini, F., De Santo, I., Rea, C. G., De Placido, P., Formisano, L., Giuliano, M., et al. (2018). CDK 4/6 inhibitors as single agent in advanced solid tumors. Front. Oncol. 8:608. doi: 10.3389/fonc.2018.00608
Senthilkumar, K., Manivasagan, P., Venkatesan, J., and Kim, S. K. (2013). Brown seaweed fucoidan: biological activity and apoptosis, growth signaling mechanism in cancer. Int. J. Biol. Macromol. 60, 366–374. doi: 10.1016/j.ijbiomac.2013.06.030
Shin, D. M., Holoye, P. Y., Forman, A., Winn, R., Perez-Soler, R., Dakhil, S., et al. (1994). Phase II clinical trial of didemnin B in previously treated small cell lung cancer. Invest. New Drugs 12, 243–249. doi: 10.1007/BF00873966
Shiozaki, M., Tashiro, T., Koshino, H., Shigeura, T., Watarai, H., Taniguchi, M., et al. (2013). Synthesis and biological activity of hydroxylated analogues of KRN7000 (alpha-galactosylceramide). Carbohydr. Res. 370, 46–66. doi: 10.1016/j.carres.2013.01.010
Sills, A. K. Jr., Williams, J. I., Tyler, B. M., Epstein, D. S., Sipos, E. P., Davis, J. D., et al. (1998). Squalamine inhibits angiogenesis and solid tumor growth in vivo and perturbs embryonic vasculature. Cancer Res. 58, 2784–2792.
Simone, M., Erba, E., Damia, G., Vikhanskaya, F., Di Francesco, A. M., Riccardi, R., et al. (2005). Variolin B and its derivate deoxy-variolin B: new marine natural compounds with cyclin-dependent kinase inhibitor activity. Eur. J. Cancer 41, 2366–2377. doi: 10.1016/j.ejca.2005.05.015
SirDeshpande, B. V., and Toogood, P. L. (1995). Mechanism of protein synthesis inhibition by didemnin B in vitro. Biochemistry 34, 9177–9184. doi: 10.1021/bi00028a030
Soni, R., Muller, L., Furet, P., Schoepfer, J., Stephan, C., Zumstein-Mecker, S., et al. (2000). Inhibition of cyclin-dependent kinase 4 (Cdk4) by fascaplysin, a marine natural product. Biochem. Biophys. Res. Commun. 275, 877–884. doi: 10.1006/bbrc.2000.3349
Storey, M. A., Andreassend, S. K., Bracegirdle, J., Brown, A., Keyzers, R. A., Ackerley, D. F., et al. (2020). Metagenomic exploration of the marine sponge mycale hentscheli uncovers multiple polyketide-producing bacterial symbionts. mBio 11, e02997-19. doi: 10.1128/mBio.02997-19
Suarez, Y., Gonzalez, L., Cuadrado, A., Berciano, M., Lafarga, M., and Munoz, A. (2003). Kahalalide F, a new marine-derived compound, induces oncosis in human prostate and breast cancer cells. Mol. Cancer Ther. 2, 863–872.
Takebayashi, Y., Pourquier, P., Zimonjic, D. B., Nakayama, K., Emmert, S., Ueda, T., et al. (2001). Antiproliferative activity of ecteinascidin 743 is dependent upon transcription-coupled nucleotide-excision repair. Nat. Med. 7, 961–966. doi: 10.1038/91008
Talpir, R., Benayahu, Y., Kashman, Y., Pannell, L., and Schleyer, M. (1994). Hemiasterlin and geodiamolide TA; two new cytotoxic peptides from the marine sponge Hemiasterella minor (Kirkpatrick). Tetrahedron Lett. 35, 4453–4456. doi: 10.1016/S0040-4039(00)73382-X
Tang, H. F., Yi, Y. H., Li, L., Sun, P., Zhang, S. Q., and Zhao, Y. P. (2006). Asterosaponins from the starfish Culcita novaeguineae and their bioactivities. Fitoterapia 77, 28–34. doi: 10.1016/j.fitote.2005.07.009
Taori, K., Paul, V. J., and Luesch, H. (2008). Structure and activity of largazole, a potent antiproliferative agent from the Floridian marine cyanobacterium Symploca sp. J. Am. Chem. Soc. 130, 1806–1807. doi: 10.1021/ja7110064
Taraboletti, G., Poli, M., Dossi, R., Manenti, L., Borsotti, P., Faircloth, G. T., et al. (2004). Antiangiogenic activity of aplidine, a new agent of marine origin. Br. J. Cancer 90, 2418–2424. doi: 10.1038/sj.bjc.6601864
Tashiro, T. (2012). Structure-activity relationship studies of novel glycosphingolipids that stimulate natural killer T-cells. Biosci. Biotechnol. Biochem. 76, 1055–1067. doi: 10.1271/bbb.120072
Tashiro, T., Nakagawa, R., Shigeura, T., Watarai, H., Taniguchi, M., and Mori, K. (2013). RCAI-61 and related 6’-modified analogs of KRN7000: their synthesis and bioactivity for mouse lymphocytes to produce interferon-gamma in vivo. Bioorg. Med. Chem. 21, 3066–3079. doi: 10.1016/j.bmc.2013.03.028
ter Haar, E., Kowalski, R. J., Hamel, E., Lin, C. M., Longley, R. E., Gunasekera, S. P., et al. (1996). Discodermolide, a cytotoxic marine agent that stabilizes microtubules more potently than taxol. Biochemistry 35, 243–250. doi: 10.1021/bi9515127
Tong, Y., Zhang, X., Tian, F., Yi, Y., Xu, Q., Li, L., et al. (2005). Philinopside A, a novel marine-derived compound possessing dual anti-angiogenic and anti-tumor effects. Int. J. Cancer 114, 843–853. doi: 10.1002/ijc.20804
Tonini, T., Rossi, F., and Claudio, P. P. (2003). Molecular basis of angiogenesis and cancer. Oncogene 22, 6549–6556. doi: 10.1038/sj.onc.1206816
Trenberth, K. E., Christy, J. R., and Olson, J. G. (1987). Global atmospheric mass, surface pressure, and water vapor variations. J. Geophys. Res. 92, 14815–14826. doi: 10.1029/JD092iD12p14815
Vaishampayan, U., Glode, M., Du, W., Kraft, A., Hudes, G., Wright, J., et al. (2000). Phase II study of dolastatin-10 in patients with hormone-refractory metastatic prostate adenocarcinoma. Clin. Cancer Res. 6, 4205–4208.
Van Dover, C. L. (2000). The Ecology of Deep-Sea Hydrothermal Vents. Princeton, NJ: Princeton University Press.
Verweij, J., Clavel, M., and Chevalier, B. (1994). Paclitaxel (Taxol) and docetaxel (Taxotere): not simply two of a kind. Ann. Oncol. 5, 495–505. doi: 10.1093/oxfordjournals.annonc.a058903
Wang, J., Wang, Z., Sun, Y., and Liu, D. (2019). Bryostatin-1 inhibits cell proliferation of hepatocarcinoma and induces cell cycle arrest by activation of GSK3beta. Biochem. Biophys. Res. Commun. 512, 473–478. doi: 10.1016/j.bbrc.2019.03.014
Warabi, K., Hamada, T., Nakao, Y., Matsunaga, S., Hirota, H., van Soest, R. W., et al. (2005). Axinelloside A, an unprecedented highly sulfated lipopolysaccharide inhibiting telomerase, from the marine sponge, Axinella infundibula. J. Am. Chem. Soc. 127, 13262–13270. doi: 10.1021/ja052688r
Warburg, O., Wind, F., and Negelein, E. (1927). The metabolism of tumors in the body. J. Gen. Physiol. 8, 519–530. doi: 10.1085/jgp.8.6.519
Watanabe, J., Endo, Y., Shimada, N., Natsume, T., Sasaki, T., and Kobayashi, M. (2007). Antiangiogenic activity of TZT-1027 (soblidotin) on chick chorioallantoic membrane and human umbilical vein endothelial cells. In Vivo 21, 297–304.
West, L. M., Northcote, P. T., and Battershill, C. N. (2000). Peloruside A: a potent cytotoxic macrolide isolated from the new zealand marine sponge Mycale sp. J. Org. Chem. 65, 445–449. doi: 10.1021/jo991296y
Williams, D. E., Lassota, P., and Andersen, R. J. (1998). Motuporamines A–C, cytotoxic alkaloids isolated from the marine sponge Xestospongia exigua (Kirkpatrick). J. Org. Chem. 63, 4838–4841. doi: 10.1021/jo980355p
Xue, M., Ge, Y., Zhang, J., Wang, Q., Hou, L., Liu, Y., et al. (2012). Anticancer properties and mechanisms of fucoidan on mouse breast cancer in vitro and in vivo. PLoS One 7:e43483. doi: 10.1371/journal.pone.0043483
Xue, M., Ji, X., Xue, C., Liang, H., Ge, Y., He, X., et al. (2017). Caspase-dependent and caspase-independent induction of apoptosis in breast cancer by fucoidan via the PI3K/AKT/GSK3beta pathway in vivo and in vitro. Biomed. Pharmacother. 94, 898–908. doi: 10.1016/j.biopha.2017.08.013
Yamaguchi, Y., Motoki, K., Ueno, H., Maeda, K., Kobayashi, E., Inoue, H., et al. (1996). Enhancing effects of (2S,3S,4R)-1-O-(alpha-D-galactopyranosyl)-2-(N-hexacosanoylamino) -1,3,4-octadecanetriol (KRN7000) on antigen-presenting function of antigen-presenting cells and antimetastatic activity of KRN7000-pretreated antigen-presenting cells. Oncol. Res. 8, 399–407.
Yang, E., Shim, J. S., Woo, H. J., Kim, K. W., and Kwon, H. J. (2007). Aminopeptidase N/CD13 induces angiogenesis through interaction with a pro-angiogenic protein, galectin-3. Biochem. Biophys. Res. Commun. 363, 336–341. doi: 10.1016/j.bbrc.2007.08.179
Ye, X., Anjum, K., Song, T., Wang, W., Liang, Y., Chen, M., et al. (2017). Antiproliferative cyclodepsipeptides from the marine actinomycete Streptomyces sp. P11-23B downregulating the tumor metabolic enzymes of glycolysis, glutaminolysis, and lipogenesis. Phytochemistry 135, 151–159. doi: 10.1016/j.phytochem.2016.12.010
Ying, Y., Taori, K., Kim, H., Hong, J., and Luesch, H. (2008). Total synthesis and molecular target of largazole, a histone deacetylase inhibitor. J. Am. Chem. Soc. 130, 8455–8459. doi: 10.1021/ja8013727
Younes, A., Bartlett, N. L., Leonard, J. P., Kennedy, D. A., Lynch, C. M., Sievers, E. L., et al. (2010). Brentuximab vedotin (SGN-35) for relapsed CD30-positive lymphomas. N. Engl. J. Med. 363, 1812–1821. doi: 10.1056/NEJMoa1002965
Zan, J., Li, Z., Tianero, M. D., Davis, J., Hill, R. T., and Donia, M. S. (2019). A microbial factory for defensive kahalalides in a tripartite marine symbiosis. Science 364, eaaw6732. doi: 10.1126/science.aaw6732
Zhang, W., Oda, T., Yu, Q., and Jin, J. O. (2015). Fucoidan from Macrocystis pyrifera has powerful immune-modulatory effects compared to three other fucoidans. Mar. Drugs 13, 1084–1104. doi: 10.3390/md13031084
Keywords: cancer hallmarks, natural products, marine biodiversity, cancer therapy, marine medicine
Citation: Dayanidhi DL, Thomas BC, Osterberg JS, Vuong M, Vargas G, Kwartler SK, Schmaltz E, Dunphy-Daly MM, Schultz TF, Rittschof D, Eward WC, Roy C and Somarelli JA (2021) Exploring the Diversity of the Marine Environment for New Anti-cancer Compounds. Front. Mar. Sci. 7:614766. doi: 10.3389/fmars.2020.614766
Received: 07 October 2020; Accepted: 10 December 2020;
Published: 21 January 2021.
Edited by:
Anthony Lee Dellinger, Kepley BioSystems Inc., United StatesReviewed by:
Deborah Mosca, Independent Consultant, Wilmington, NC, United StatesGiovanna Romano, Anton Dohrn Zoological Station, Italy
Rob Keyzers, Victoria University of Wellington, New Zealand
Copyright © 2021 Dayanidhi, Thomas, Osterberg, Vuong, Vargas, Kwartler, Schmaltz, Dunphy-Daly, Schultz, Rittschof, Eward, Roy and Somarelli. This is an open-access article distributed under the terms of the Creative Commons Attribution License (CC BY). The use, distribution or reproduction in other forums is permitted, provided the original author(s) and the copyright owner(s) are credited and that the original publication in this journal is cited, in accordance with accepted academic practice. No use, distribution or reproduction is permitted which does not comply with these terms.
*Correspondence: Jason A. Somarelli, amFzb24uc29tYXJlbGxpQGR1a2UuZWR1