- 1George S. Wise Faculty of Life Sciences, School of Zoology, Tel Aviv University, Tel Aviv, Israel
- 2The Steinhardt Museum of Natural History, Israel National Center for Biodiversity Studies, Tel Aviv University, Tel Aviv, Israel
Determining demosponge reproductive strategies is essential to understanding their ecology and life history, as well as for the management of benthic marine environments. This is especially important in mesophotic ecosystems, which have been suggested to serve as a refuge for shallow-water populations and for which knowledge is lacking. Here we compared the reproductive strategies of two common Mediterranean demosponges species: Chondrosia reniformis and Axinella polypoides, which can be found in both shallow and mesophotic habitats along the Israeli coast. Samples were collected over 2 years, via SCUBA diving from the shallow coast (2–32 m) and Remotely Operated Vehicle from the mesophotic sponge grounds (95–120 m). A. polypoides and C. renifornis differed in oocyte morphology and development, but both demonstrated reproductive plasticity with regard to temperature and depth: temperature appears to regulate their reproduction in the shallow water, as both species exhibited seasonality; whereas, in the mesophotic zone, where the temperature amplitude is much smaller, seasonality was not observed for either species. Furthermore, in the mesophotic zone, C. reniformis exhibited low fecundity and probably invests more in asexual reproduction by budding. Mesophotic A. polypoides, in contrast, exhibited enhanced and continuous asynchronous sexual reproduction year-round. Our findings suggest that reproduction plasticity may be a general rule for sponge species that inhabit different habitats; such plasticity could allow sponges to thrive in different environmental conditions. These findings further emphasize the importance of protecting the mesophotic sponge grounds, which can serve as a refuge in the face of the adverse impact of anthropogenic disturbances and rising seawater temperatures.
Introduction
Demosponges comprise the most diverse class in the phylum Porifera (Hooper and Van Soest, 2002; Morrow and Cárdenas, 2015). They play an important role as environmental engineers (Kenchington et al., 2013; Gerovasileiou et al., 2016), especially in temperate and cold seas (Bo et al., 2012; Beazley et al., 2013). This holds particularly true for the Mediterranean Sea, where demosponges often dominate the benthic fauna (Voultsiadou, 2009; Coll et al., 2010). In recent years mesophotic sponge grounds have been discovered along the Israeli coast of the Mediterranean Sea. In these habitats, sponges grow in high density, accounting for ∼80% of the total live coverage (Idan et al., 2018). They thereby increase structural complexity and provide a settlement substrate for benthic invertebrates, as well as a refuge from predators, in addition to serving as spawning and nursery grounds (Bo et al., 2012; Gerovasileiou et al., 2016).
Due to their distance from land, it was suggested that mesophotic ecosystems are not influenced by anthropogenic activities or natural disturbances (Hinderstein et al., 2010; Locker et al., 2010). Thus, the mesophotic ecosystems have been considered to constitute a refuge for many shallow-water species, as spawning grounds and a source of larvae contributing to species resilience (Hinderstein et al., 2010; Kahng et al., 2010; Smith et al., 2010). Despite the lack of knowledge regarding fundamental aspects of sponge biology and ecology in the mesophotic ecosystems (Maldonado et al., 2017; Cerrano et al., 2019), it has been suggested that mesophotic communities to a great extent represent an extension of the shallow-water ones (Lesser et al., 2009). Such a connection is probably directly dependent on the degree of larval dispersal and gene flow between the two zones, and the reproductive strategies of the relevant sponges (Lesser et al., 2009; Kahng et al., 2010).
Determining the reproductive strategies of sponges is essential to understanding their ecology, life history, and populations and community dynamics, as well as for the management of benthic marine environments. Sexual reproduction in demosponges is diverse, as different species can be either gonochoric, sequential, or simultaneous hermaphrodites, and can also be either brooders (viviparity) or broadcasters (oviparity) (Maldonado, 2006). The majority of the oviparous sponges are gonochoristic (Sará et al., 1992; Ereskovsky, 2010). The onset of gametogenesis, as well as spawning, can be triggered by various stimuli, such as water temperature, lunar phase, photoperiod, and nutrient flux (Elvin, 1976; Simpson, 1984; Witte, 1996; Usher et al., 2004; Riesgo et al., 2007; Riesgo and Maldonado, 2008). Reproduction within a limited time-frame also leads to synchronization in gametogenesis (Usher et al., 2004; Maldonado and Riesgo, 2009b). In Demosponges, male gametes usually derive from choanocytes, whereas the origin of oocytes is more complicated, as in some cases, they can originate from amoebocytes, and in others from choanocytes (Sará et al., 1992; Ereskovsky, 2010). While oogenesis takes several months, studying spermatogenesis is more difficult as it is a quick process that usually occurs within less than a month (Riesgo, 2007; Ereskovsky, 2010).
In the mesophotic zone, many of the above-mentioned stimuli are stable and constant (Kress et al., 2014). Accordingly, we might expect the reproductive patterns of species that inhabit a wide depth range to display a change with depth, as has been demonstrated for some species (Witte, 1996). Other species may follow their regular seasonal cycles, as the shallow-water populations do. Such cycles have been attributed to seasonal changes in food source availability; more precisely—POC flux (Witte, 1996). To the best of our knowledge, only one study to date has compared the reproduction of sponges from different depths. Although that study was limited to the shallow zone (0–30 m), differences in fecundity were found (Meroz-Fine et al., 2005).
Along the Israeli coast, 12 sponge species can be found in both shallow and mesophotic habitats (Idan et al., 2018). This provided us with the opportunity to study and compare the reproduction strategies of two such species: Chondrosia reniformis and Axinella polypoides (Idan et al., 2018). These species were chosen as they are the only ones that are abundant in both habitats.
C. reniformis is a massive lobate, smooth sponge, common in the Mediterranean and Eastern Atlantic Ocean, found in shallow and mesophotic habitats (Figures 1A,B). It is known from shallow habitats in the Mediterranean as a gonochoric broadcaster (Riesgo and Maldonado, 2008; Di Camillo et al., 2012). Its reproductive cycle seems to vary among areas, from seasonal to continuous, with a peak during summer (Riesgo and Maldonado, 2008; Di Camillo et al., 2012; Yaniv, 2013). A. polypoides, or the Common Antlers Sponge, is an erect, branching species. It is also a species common throughout the Mediterranean and in the eastern Atlantic Ocean, but in spite of its abundance, its reproductive cycle has not yet been described (Figures 1C,D).
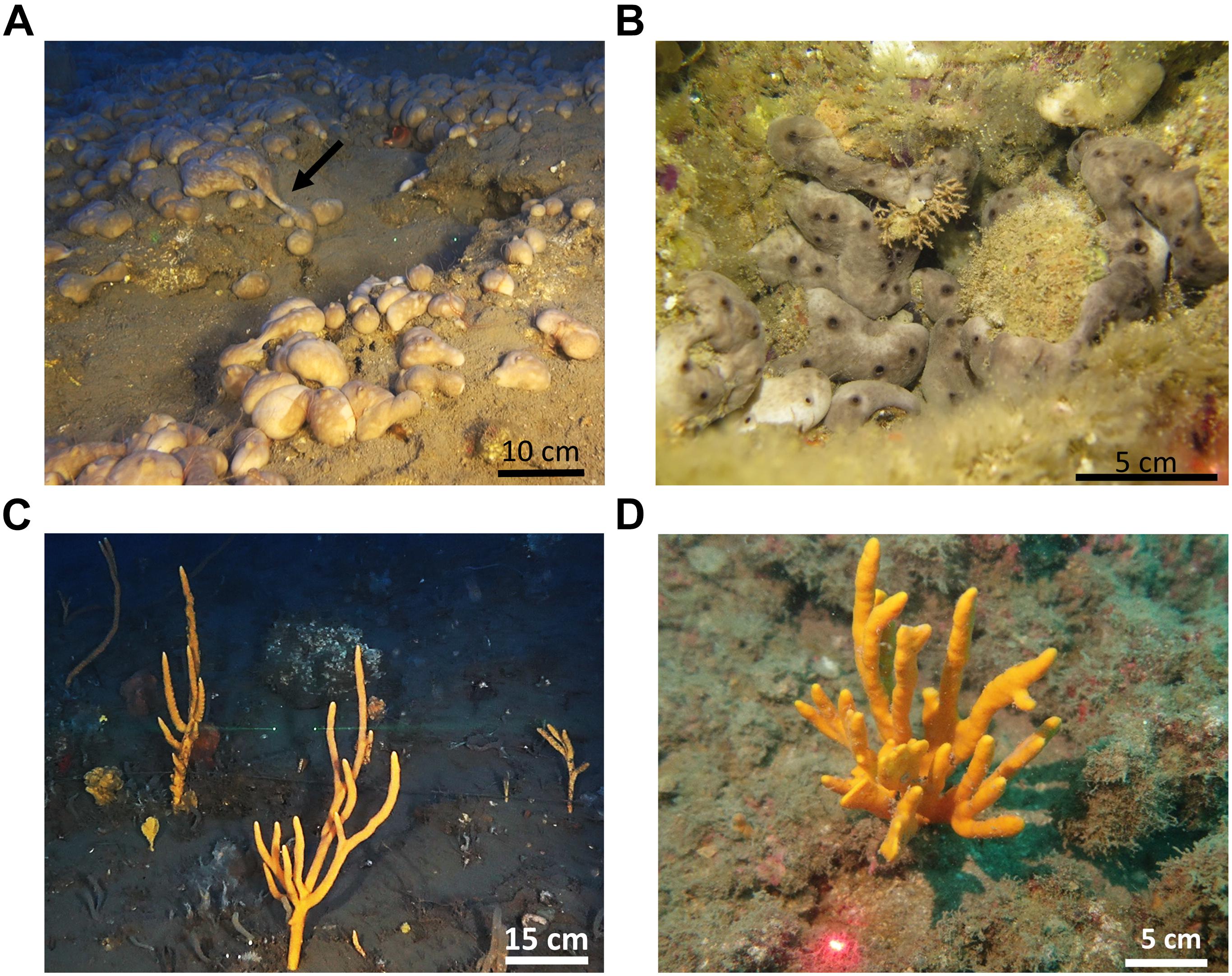
Figure 1. Growth forms of Chondrosia reniformis and Axinella polypoides in the study sites. (A) Extensive open area in the mesophotic habitat (arrow marks a creeping event); (B) A small crevice in the shallow water (3 m) inhabited by many specimens. (C) Average size specimens in the mesophotic habitat; (D). The largest specimen found in the shallow water (30 m).
Materials and Methods
Study Site and Sampling
For a comparison of the reproduction patterns between the mesophotic and shallow populations, samples of Axinella polypoides and Chondrosia reniformis were collected from both habitats over the course of 2 years (January 2017–January 2019; Figure 2). The mesophotic site is located approximately 16 km off the Israeli coast of Herzliya (32.17710°N, 034.63306°E WGS-84) at a depth of 95–120 m; and the shallow-water site is located off the coast of Sedot Yam (32.529633°N, 034.858553°E WGS-84). The distance between the shallow and mesophotic sites is ∼40 km (Figure 2). In the shallow-water, the sponges were collected monthly by SCUBA diving, C. reniformis at a depth of 2–4 m, and A. polypoides at a depth of 32–28 m (n = 8–11, per species per month). C. reniformis was quite rare in the 28–32 m deep habitat, while A. polypoides is rarely found in depths shallower than 27 m in the Israeli coast. Sponge sampling at the mesophotic site was conducted approximately every 3 months (n = 1–6, per species per sampling date), using a remotely operated vehicle (ROV; ECA-Robotics H800 ROV) onboard the R/V Mediterranean Explorer. At the mesophotic site, the temperature was measured using a CTD, and at the shallow site using diving computers. Vouchers of the collected material were deposited at the Steinhardt Museum of Natural History at Tel Aviv University.
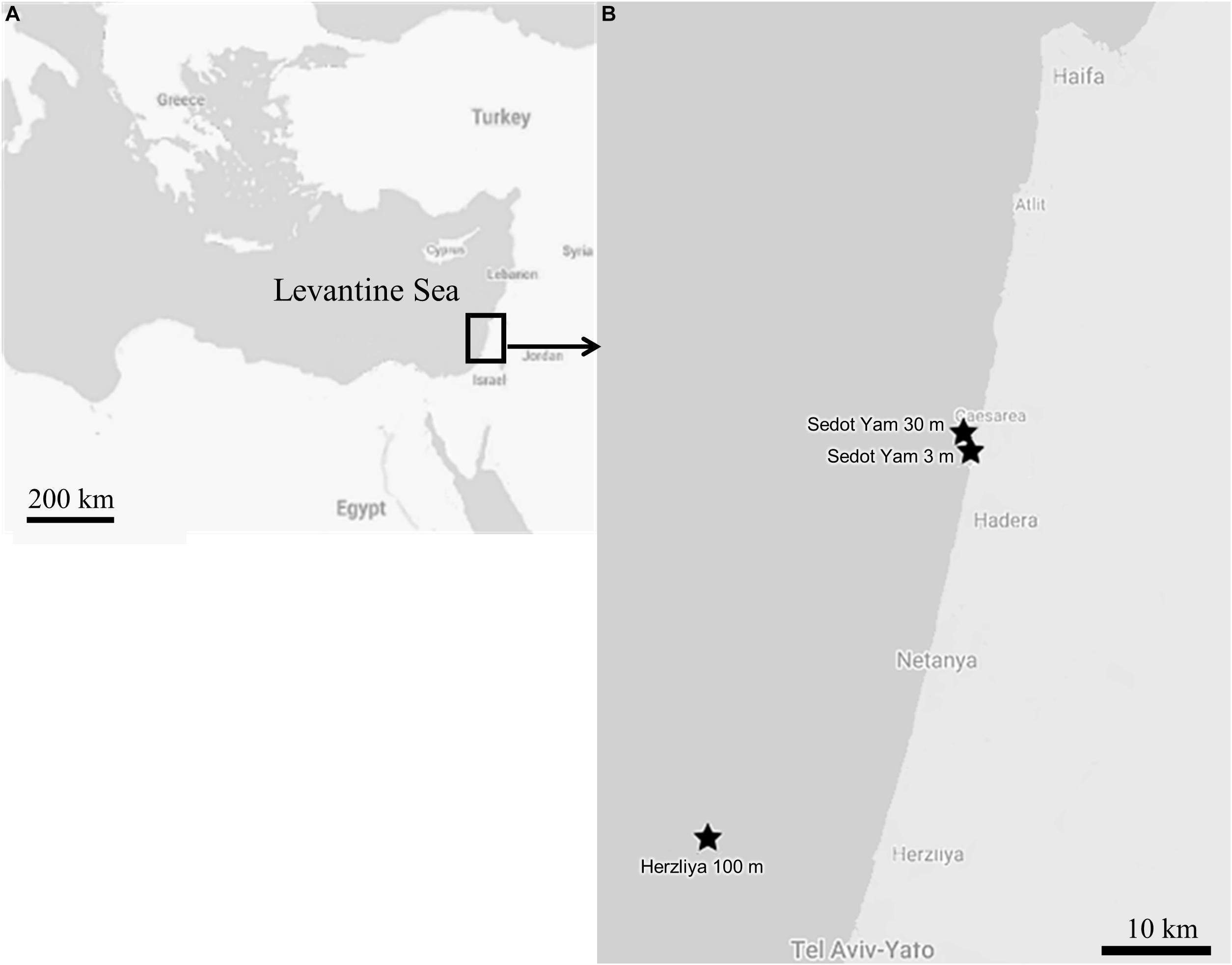
Figure 2. The study area: (A) Map of the Levantine Sea. (B) Map of the sampling sites along the Israeli coast.
Histologic Preparations
The collected specimens were photographed in situ (underwater) and in the lab following collection. A 2 cm3 piece of sponge was cut from the interior part of each sponge. Areas of the collagenous mesohyl were avoided as it had been previously shown that no reproductive elements are present in these sections (Riesgo and Maldonado, 2008). The tissue samples were then fixed in 2.5% glutaraldehyde (in filtered seawater) for at least 24 h, rinsed three times in artificial seawater, followed by dehydration (35, 50, and 70% ethanol), and preserved in 70% ethanol. The rest of the sponge was preserved in absolute ethanol to enable molecular analysis if needed. In A. polypoides, skeletal spicules were dissolved by desilicification with 4.8% hydrofluoric acid for 24 h and then rinsed three times in 70% ethanol. The C. reniformis samples were not treated with acid because they lack spicules.
The samples were cast in paraffin blocks and cut into 7 μm thick sections using a Shandon Lipshaw M1R microtome. The sections were placed on charged microscope slides, dried, and stained with Hematoxylin and 1% Eosin. The tissue sections were observed (3–5 sections per specimen) with a Zeiss Axioskop microscope equipped with a ZEISS Axiocam 105 color camera (Carl Zeiss, Oberkochen, Germany). Oocyte size was measured and recorded using ZEN lite software to determine gametogenesis (gamete growth) and timing of gamete release.
Data Analysis
The average abundance of oocytes (values ± SD), and their maximal diameter, were calculated monthly for both species for specimens from the shallow site, and approximately every 3 months for those from the mesophotic site. The abundance of oocytes per mm2 was obtained by counting the number of oocytes in 30 consecutive frames (with no overlap) and dividing this number by the size of a single frame (in mm2). For maximal diameter, 30 oocytes were measured per sponge. If fewer than 30 oocytes were found, the maximal number found was recorded. Statistical analysis was performed using R (R Core Team, 2020) and RStudio (R Studio Team, 2020) package stats (R Core Team et al., 2018).
All data were found to be normally distributed using the Shapiro-Wilk’s test. Linear regression models were used to test the relationship between temperature and oocyte diameter (C. reniformis), and the relationship between temperature and oocyte abundance and the proportion of reproducing individuals (A. polypoides). The analyzed data included all months in which the sponges reproduced.
A two-tailed Student’s t-test was performed to compare mean oocyte abundance per specimen and oocyte size at the same developmental stage in C. reniformis. In A. polypoides, the standard deviations of oocyte diameter per specimen were compared between shallow and mesophotic, for samples collected at the same sampling dates, using a two-tailed Student’s t-test.
Results
Chondrosia Reniformis
A total of 246 individuals were collected from the mesophotic and shallow habitats. The individuals ranged in length from 2 to 12 cm. No male reproductive elements were recorded from the mesophotic zone during this study; while in the shallow zone, only three specimens containing spermatocytes were found in June 2017.
During the reproduction period in the shallow zone, 32% of the individuals (17/53) contained oocytes, and in June 2017 (peak reproduction period), 70% of the individuals contained reproductive elements (three individuals contained spermatocytes and four contained oocytes; Figure 3A). In the mesophotic zone, in contrast, over the research period 14% of the individuals (5/36) contained oocytes, with a maximum of 40% of individuals containing oocytes in October 2017 (n = 5, Figure 3B). Oocytes were observed throughout the mesohyl, with the exception of the ectosome and areas with a high concentration of collagen. Oocyte shapes ranged from round to oval. Around fully developed oocytes, nurse cells and cytoplasmic bridges (Figures 4A–C) were apparent (Figures 4B,C). In sponges from the shallow zone, oocyte development seemed to roughly follow the increase in water temperature (Figure 3A), while in sponges from the mesophotic zone, only mid-developed oocytes were observed (Figures 4D, 3B). When spermatocytes were observed, they were seen occupying a substantial part of the choanosome, and only a few choanocyte chambers could be observed in these specimens (Figure 4E).
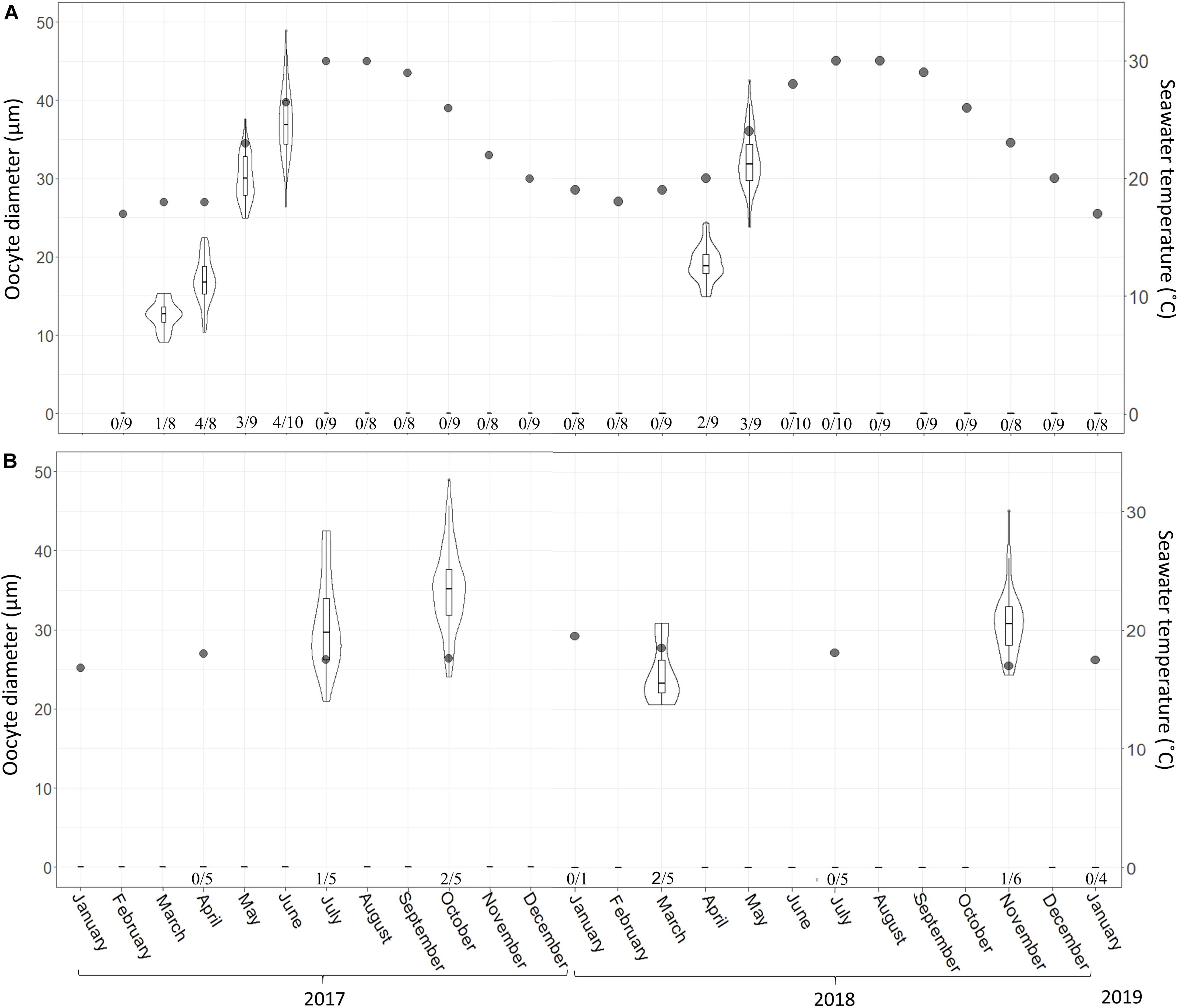
Figure 3. C. reniformis oocyte diameter and seawater temperature at 3 m (A) and 100 m (B) depth between February 2017–January 2019 and January 2017–January 2019, respectively. Shallow-water samples were collected monthly; mesophotic samples were collected seasonally. An arrow marks the month when spermatocytes were found. Number below bars is the number of individuals containing oocytes out of total number of individuals sampled. The violin plot shows size distribution, and the box plot within the violin shows quartiles and median.
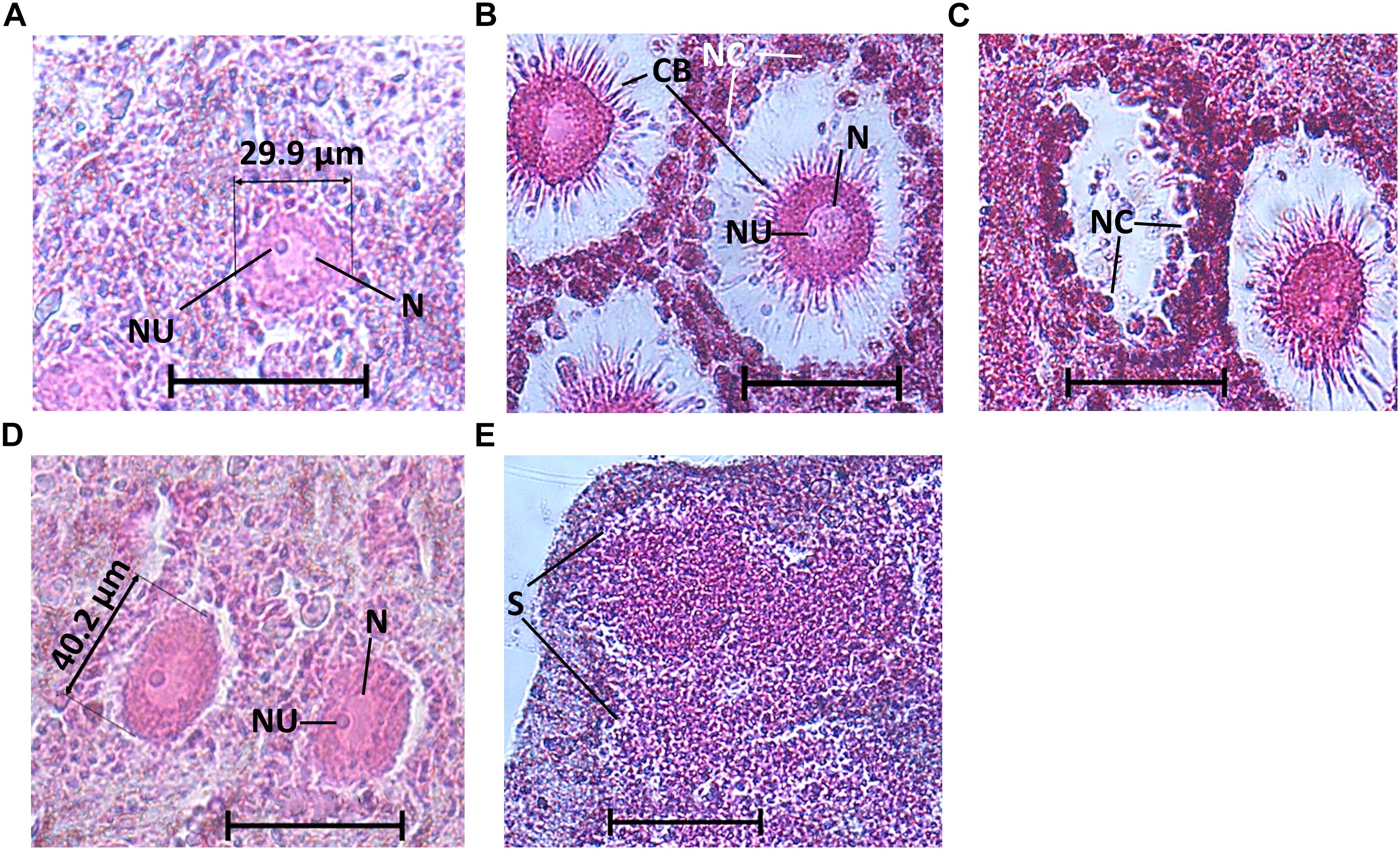
Figure 4. C. reniformis oogenesis and spermatogenesis. (A) Mid-developed oocytes of a shallow-water C. reniformis, (B) fully developed oocytes of a shallow-water C. reniformis with nurse cells, nucleus, nucleolus, and cytoplasmic bridges, (C) a hollow space, from where an oocyte was presumably released, while nurse cells are still visible. (D) Mid-developed oocytes of a mesophotic C. reniformis with nucleus and nucleolus. (E) Spermatocytes. Scale bar is 50 μm. N, nucleus; NU, nucleolus; CB, cytoplasmic bridges; NC, nurse cells; S, spermatocytes.
In the shallow habitat, the gametogenesis of C. reniformis extended from March to June in 2017 and from April to May in 2018. With the increase in temperature, we observed an increase in both oocyte diameter (from a mean ± SD of 12.5 ± 1.8 μm, n = 30 oocytes, to 35.6 ± 4.4 μm, n = 90 oocytes, in 2017 and 19.01 ± 2.17 μm, n = 53 oocytes, to 32.4 ± 3.6 μm, n = 73 oocytes, in 2018; Figure 3A and Supplementary Table 1), and abundance (from 0.88 ± 0.09 oocytes/mm2 to 25.45 ± 1.71 in 2017 and from 5.8 ± 0.51 oocytes/mm2 to 13.07 ± 3.52 in 2018; Figure 5A and Supplementary Table 2). With the exception of a decrease in abundance in June 2017, when hollow spaces, presumably reflecting where oocytes had been released, appeared in the histological preparations (Figure 4C). In contrast, in the mesophotic zone, where the temperature remained stable (16.8–19.5°C, Supplementary Table 3), a low abundance of oocytes was observed for all sampling dates (0.11 ± 0.04–6.97 ± 1.1 oocytes/mm2, similar to the lower values of the shallow zone, Supplementary Table 4), and oocyte diameter displayed less variability of size (21–49 μm, Supplementary Table 5).
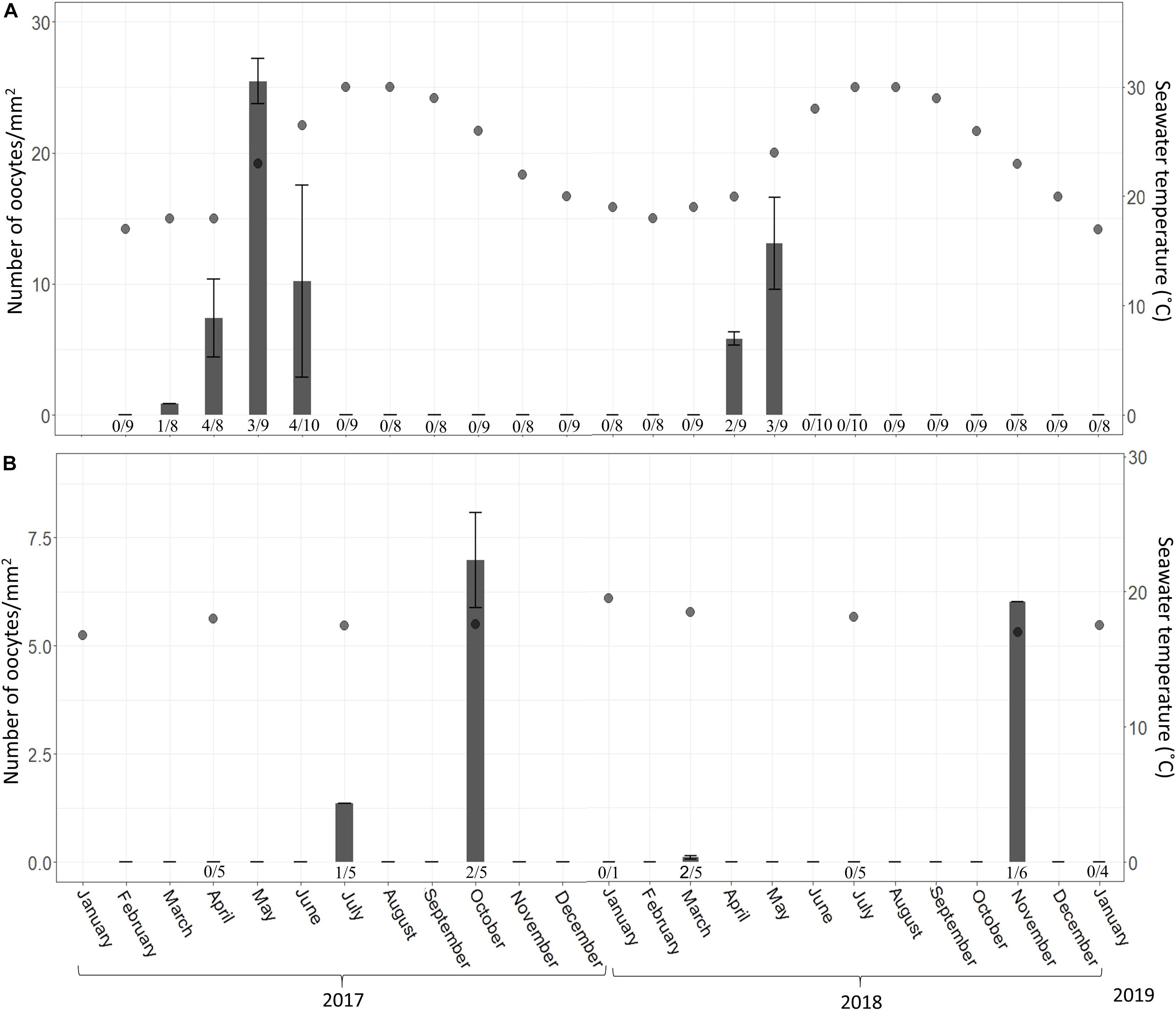
Figure 5. C. reniformis oocyte abundance (# oocytes per mm2 ± SD) at 3 m (A) and 100 m (B) between February 2017–January 2019 and January 2017–January 2019, respectively. Shallow-water samples were collected monthly; mesophotic samples were collected seasonally. An arrow marks the month when spermatocytes were found. Number below bars is the number of individuals containing oocytes out of total number of individuals collected.
In the shallow zone, a significant relationship was found between oocyte development and temperature. This relationship appears to explain 78% of the variance in oocyte diameter (Figure 2; Linear Regression, R2 = 0.78, F = 62.87, df = 16, p < 0.001) and about a quarter of the variance in oocyte abundance (number of oocytes/mm2; Figure 5; Linear regression, R2 = 0.23, F = 5.47, df = 14, p = 0.03). In the mesophotic zone, while a significant relationship between oocyte diameter and temperature was found, it explained merely 2% of the variance (Figure 3, Linear regression, R2 = 0.02, F = 4.02, df = 155, p = 0.046). No particular trend was found in regard to the relationship between oocyte abundance (number of oocytes/mm2) and temperature (Figure 5; Linear regression, R2 = 0.4, F = 4.34, df = 4, p = 0.1). The overall abundance of oocytes was significantly lower than that in the shallow zone (Figure 5; Student’s t-test: t = 2.813, df = 18.715, p = 0.01122).
Axinella Polypoides
A total of 265 individuals were collected from the shallow and mesophotic populations. The individuals collected from the shallow zone ranged in height from 5 to 15 cm, and in the mesophotic zone, they ranged from 30 to 60 cm in height. No male reproductive elements were recorded during this study from either habitat. In the shallow zone, 21% of the individuals (52\222) contained oocytes, and in the mesophotic zone, ca. 60% (26\43) contained oocytes. Oocytes were observed throughout the mesohyl, with the exception of the sponge axis, which is comprised mainly of dense spongin fibers. Oocyte shape was roughly oval with well-defined borders, but occasionally appeared amorphous, possibly due to physical constraints by other structures in the sponge (i.e., choanocyte chambers, spongin fibers, and canals; Figure 6). Oocyte diameter in the shallow-zone sponges ranged from 24 to 112 μm (Figure 7A and Supplementary Table 6); and in the mesophotic zone from 26 to 120 μm, throughout the study period (Figure 7B and Supplementary Table 7). Oocyte size variation in each month was high, but in the mesophotic zone the variation was generally significantly higher than in the shallow zone (mean standard deviation 16.9 ± 2 and 12.3 ± 3.8, respectively, Student’s t-test, t = 2.55, df = 7.55, p = 0.035). This variation is mostly attributed to within-specimen differences. Apart from the differences in size, no other morphological differences could be seen between oocytes from different individuals or dates (Figure 6).
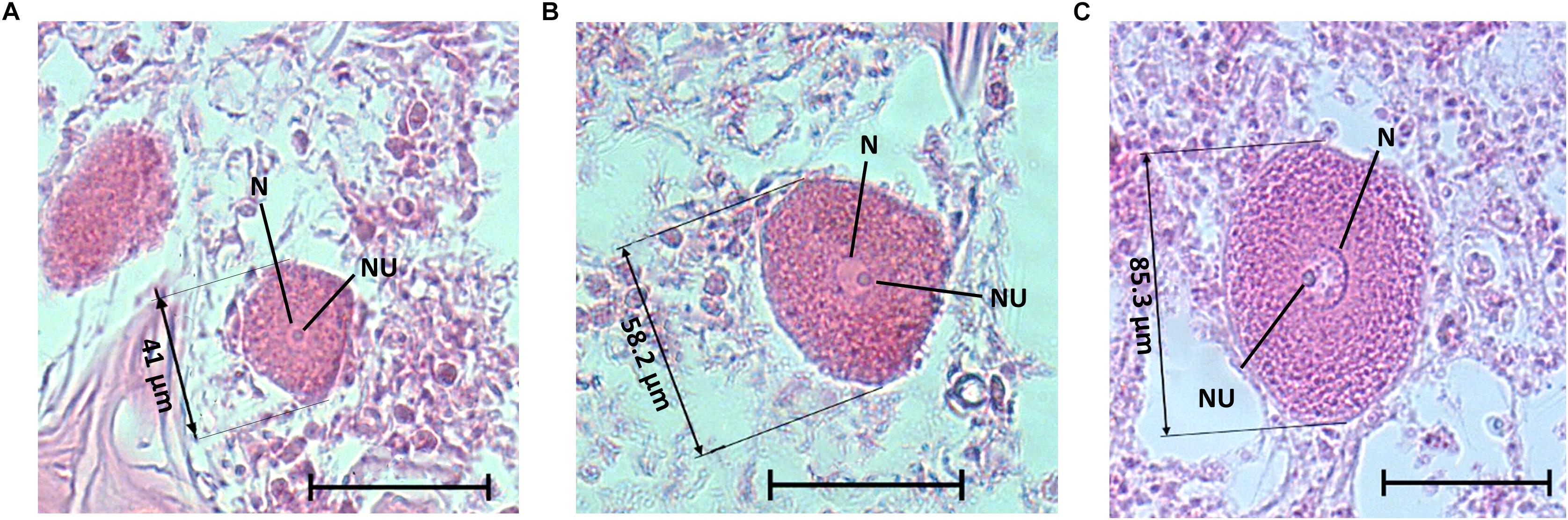
Figure 6. A. polypoides oocytes of different sizes from the shallow water, with nucleus, nucleolus, and size of the oocyte. (A) Oocyte from A. polypoides sampled in June 2017, (B) Oocyte from A. polypoides sampled in August 2017, (C) Oocyte from A. polypoides sampled in September 2017. Scale bar is 50 μm. N, nucleus; NU, nucleolus.
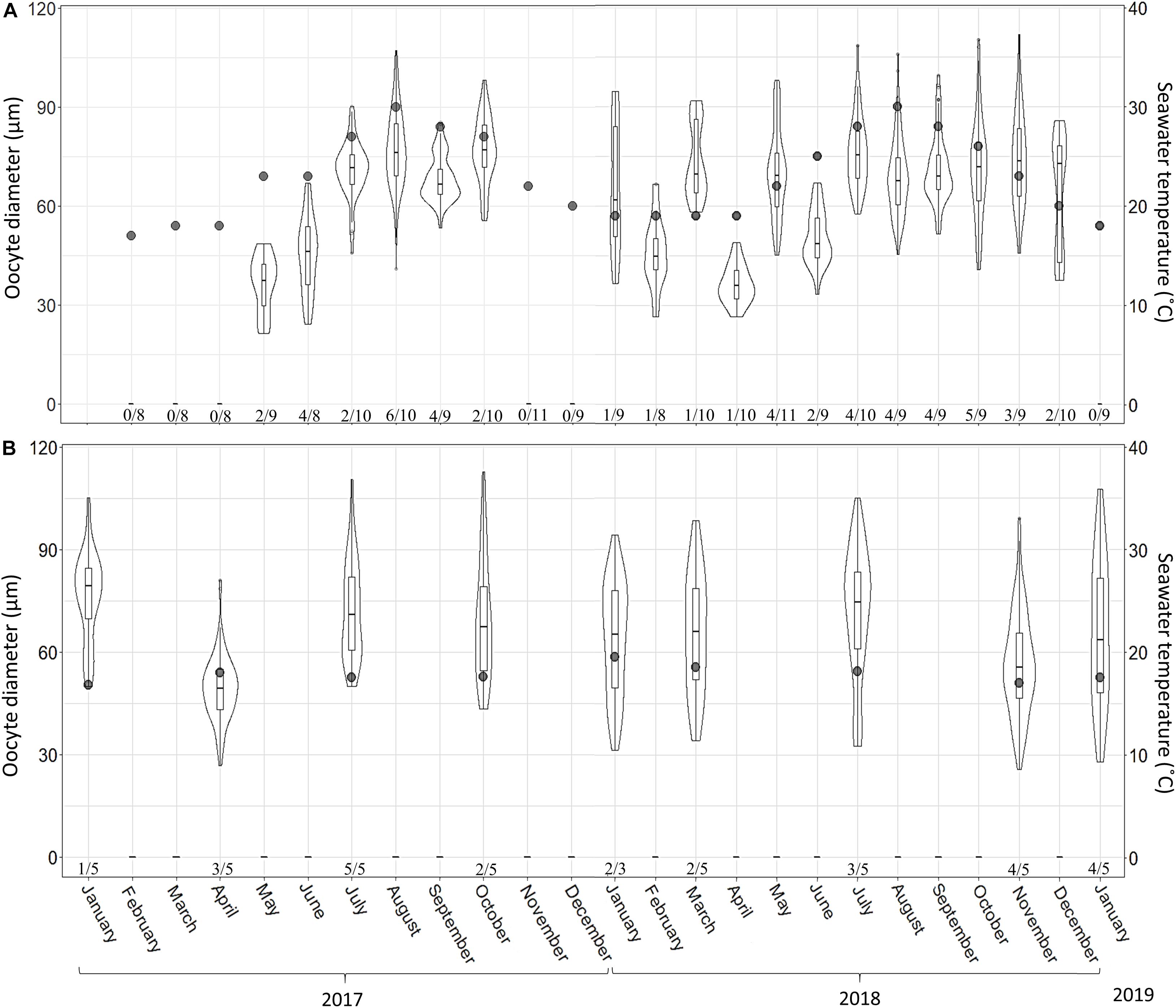
Figure 7. A. polypoides oocyte diameter at 30 m (A) and 100 m (B) depth between February 2017–January 2019 and January 2017–January 2019, respectively. Shallow-water samples were collected monthly; mesophotic samples were collected seasonally. Maximal oocyte diameter was measured (30 oocytes were measured per sponge; if 30 oocytes were not found, the maximal number found was noted). Number below bars is the number of individuals containing oocytes out of total number of individuals collected. Violin plot shows size distribution, and the box plot within the violin shows quartiles and median.
In the shallow zone, oocyte abundance followed the temperature pattern: increasing with temperature rise and decreasing when the temperature dropped. For example, the mean number of oocyte per mm2 increased from 0.28 in May 2017 to 7.04 in July 2017 and then decreased to 0.25 in February 2018 (Figure 8A and Supplementary Table 8; Linear regression: R2 = 0.47, F = 19.5, p < 0.001). The abundance of oocytes in the mesophotic zone appeared to be rather constant (0.5–2.5 oocyte per mm2) along with the constant temperature (Figure 8B and Supplementary Table 9), with the exception of July 2017, during which a higher abundance was observed. The proportion of reproducing individuals in the shallow zone also varied in accordance with temperature fluctuations (Figures 7, 8). The strong positive relationship explains approximately 70% of this variance (Linear regression: R2 = 0.69, F = 49.7, p < 0.0001).
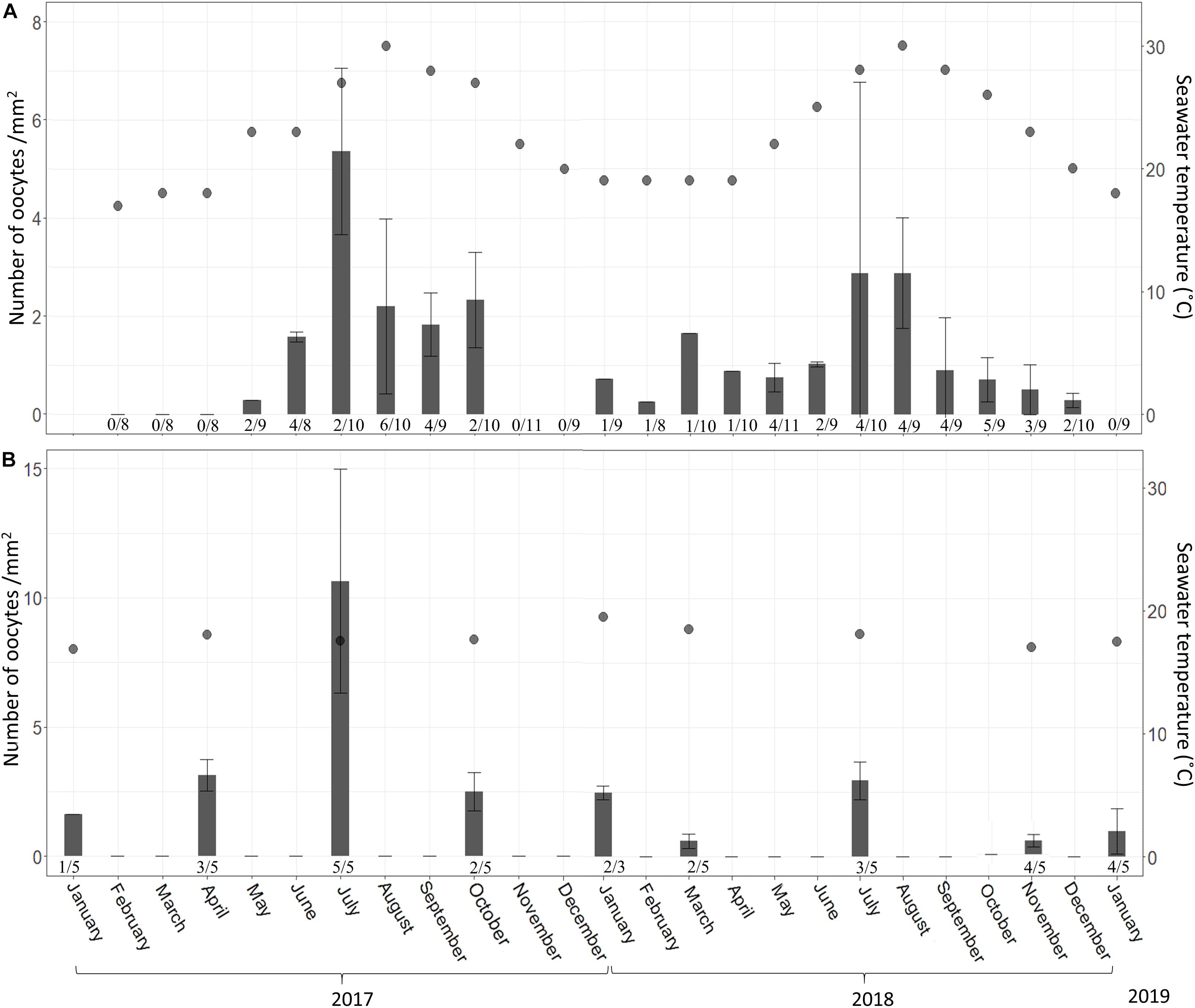
Figure 8. A. polypoides oocyte abundance (# oocytes per mm2 ± SD) at 30 m (A) and 100 m (B) depth between February 2017–January 2019 and January 2017–January 2019, respectively. Shallow-water samples were collected monthly; mesophotic samples were collected seasonally. Number below bars is the number of individuals containing oocytes out of total number of individuals collected.
Discussion
A. polypoides and C. reniformis are two of the few species that span the entire depth range between 15–130 and 0.5–130 m depth, respectively, along the Israeli coast of the Mediterranean. Here we examined the differences in the reproductive cycles of these two common species, between the mesophotic and the shallow habitats, which have markedly different abiotic conditions (i.e., constant vs. dynamic temperature and light regimes, respectively). Both genera are known to be oviparous (Alvarez and Hooper, 2002; Maldonado and Riesgo, 2009b; Di Camillo et al., 2012), but knowledge regarding the two species’ reproductive cycles has been published only for C. reniformis in the shallow water (Riesgo and Maldonado, 2008; Di Camillo et al., 2012). This study is the first to report the reproduction patterns of mesophotic populations and compare them with those of shallow-water populations.
Chondrosia Reniformis
The pattern of oocyte development and abundance observed in C. reniformis in the shallow habitat revealed seasonality. Oocyte abundance increased with rising seawater temperature, with a peak in May (when the temperature reached 23°C). The decrease in oocyte abundance in June may be related to the release of fully developed oocytes into the environment, in agreement with the pattern reported for this species in other studies in the Mediterranean (Riesgo and Maldonado, 2008; Yaniv, 2013). Spermatogenesis in C. reniformis appears to be rapid and has been documented to last less than a month, and it usually coincides with the peak of oogenesis around July and August (Riesgo and Maldonado, 2008; Di Camillo et al., 2012; Yaniv, 2013). In the present study, spermatocytes were observed only in June 2017, probably synchronized with the final developmental stage of the oocytes (Figure 3A).
The reproductive cycle in C. reniformis is believed to be governed by temperature. Oogenesis starts soon after the water temperature begins to rise, and the oocytes are released before the temperature peak (Riesgo and Maldonado, 2008; Yaniv, 2013). In another study, oocytes were found year-round, with maximum abundance in August, when the temperature was highest (Di Camillo et al., 2012). Thus, in the present study we expected the individuals from the mesophotic habitat, where the temperature is relatively constant and much below summer temperature in the shallow water (16.5–20°C), to exhibit a different pattern of the reproductive cycle. Indeed, the pattern observed in the mesophotic habitat markedly differed from the shallow one. The mean oocyte diameter in the mesophotic zone was larger (32.6 ± 5.8, range 20–49 μm) than in the shallow water (27.1 ± 9.3, range 9–49 μm; current study; Yaniv, 2013), and closer to what is known from the Adriatic Sea (32.6 ± 8.6 μm, range 20–75 μm; Di Camillo et al., 2012) and the Western Mediterranean (average not specified, range 35–65 μm; Riesgo and Maldonado, 2008; Di Camillo et al., 2012). Moreover, maximal oocyte diameter in the mesophotic specimens were similar to those of the fully developed oocytes from the shallow water (49 μm). Still, their morphology differed, indicating that the former were not yet mature, as nurse cells and cytoplasmic bridges were not evident in the mesophotic oocytes (Riesgo and Maldonado, 2008). It stands to reason that a higher sampling effort (i.e., monthly) would have also yielded mature oocytes.
The abundance of mesophotic oocytes was lower than in the shallow habitat’s specimens, and similar to what is known from the above-noted studies from the Adriatic and the Western Mediterranean Sea (Riesgo and Maldonado, 2008; Di Camillo et al., 2012).
The intriguing phenomenon of low male ratio (and no males in A. polypoides) was documented in many sponge studies (Ayling, 1980; Corriero et al., 1998; Mercurio et al., 2007; Leong and Pawlik, 2011; Ereskovsky et al., 2017); the absence of males might be due to a very short period of spermatogenesis, as was previously shown for several sponge species (Ayling, 1980; Fromont and Bergquist, 1994; Riesgo and Maldonado, 2008; Maldonado and Riesgo, 2009a; Ereskovsky, 2010).
While in some species asexual reproduction, usually by forming of gemmules, is associated with stressful environmental conditions (Fell, 1992; Ereskovsky, 2010), fragmentation and budding represents an important reproductive strategy for many species (Wulff, 1991; Corriero et al., 1996; Tsurumi and Reiswig, 1997; Maldonado and Uriz, 1999). including C. reniformis (Riesgo and Maldonado, 2008).
In C. reniformis, asexual reproduction is achieved by creeping and budding (Di Camillo et al., 2012; Fassini et al., 2012). Asexual reproduction may explain how, this is the most abundant sponge species in the mesophotic zone, densely covering large areas, thriving on every surface and even growing over other sponges, despite the lower fecundity (Idan et al., 2018; Figure 1A). This may indicate that C. reniformis prefers asexual over sexual reproduction in the colder and more stable conditions of the mesophotic zone. The phenomenon of high rates of asexual reproduction in stable environmental conditions was also shown for Chondrilla spp. (Zilberberg et al., 2006).
In the shallow habitat, where less favorable conditions exist and C. reniformis distribution is limited to semi-dark and patchy areas, such as crevices and overhangs (Wilkinson and Vacelet, 1979; Figure 1B), sexual reproduction may be more beneficial as the sponge larva are mobile which allow them to find suitable patches to settle (Leong and Pawlik, 2010). Additionally, the genetic diversity produced by sexual reproduction may elevate its fitness allowing C. reniformis in the shallow habitat to cope with the harsher and more variable conditions there (Hoekstra, 2005; Leong and Pawlik, 2010).
Axinella Polypoides
This is the first detailed description of a true Axinellid’s (Gazave et al., 2010) reproduction cycle.
A. polypoides demonstrated a seasonal pattern of reproduction in the shallow habitat. Both the proportion of reproducing individuals and oocyte abundance increased from early winter to mid-summer, and thereafter decreased, probably due to release of the oocytes. The above-mentioned pattern has been observed in most of the studied sponges in the Mediterranean Sea (Elvin, 1976; Fell, 1976; Meroz-Fine et al., 2005; Riesgo and Maldonado, 2008; Maldonado and Riesgo, 2009a; Di Camillo et al., 2012), with temperature thought to be one of the main factors regulating sponge reproduction (Elvin, 1976; Fell, 1976; Simpson, 1984; Maldonado and Riesgo, 2009a). In contrast, A. polypoides from the mesophotic habitat appeared to be reproducing year-round: on every sampling date oocytes were found in at least some of the specimens, including in January and April 2017 and January 2019 when the adjacent shallow-water individuals were not found to be reproducing. Only a few sponge species in the Mediterranean have been reported to reproduce year-round (Riesgo et al., 2007; Maldonado and Riesgo, 2008; Riesgo and Maldonado, 2008; Di Camillo et al., 2012). However, this phenomenon was described only in one locality: the northern Adriatic Sea, whereas in other parts of the Mediterranean, the reproduction of the same species was described as seasonal (Riesgo and Maldonado, 2008; Di Camillo et al., 2012). Our present results indicate that, compared to shallow-water populations, mesophotic A. polypoides sponges invest more in sexual reproduction, as reflected in the higher proportion of reproducing individuals (20–100%) and a more substantial variation in oocyte diameter. Our findings demonstrate that the variance in size of the oocytes in A. polypoides is an indicator of their developmental stage, suggesting a greater asynchronization of reproduction both within the population and among individuals in the mesophotic habitat. It has been postulated that the continuous oogenesis found in the northern Adriatic could be due to the high availability of food, which enables sponges to invest their energy surplus in oocyte production (Di Camillo et al., 2012). While the differences in nutrient availability between the Israeli mesophotic and shallow habitats are negligible (Idan, 2020), we suggest that the stability of other environmental conditions in the mesophotic zone could allow A. polypoides to invest its energy surplus in reproduction, thus, explaining the higher reproduction effort there. Moreover, it appears that the Israeli shallow habitat represents the edge of distribution of A. polypoides, as it is scarcer and individuals are much smaller there than in the mesophotic habitat (Figures 1C,D) and other parts of the Mediterranean.
In summary, while the reproduction of C. reniformis and A. polypoides differed in many aspects, such as oocyte abundance and morphology, both species appear to possess a degree of plasticity in their reproduction strategies with regard to temperature and depth. Temperature would seem to regulate the reproduction of both species in the shallow water, whereas, in the mesophotic zone, the temperature amplitude is much smaller and thus, other factors may be at play. Although both the studied species are oviparous and gonochoristic, they employ different reproductive strategies in the different habitats. C. reniformis exhibited low fecundity in the mesophotic zone, and probably invested more in asexual reproduction by budding and fragmentation. Asexual reproduction in C. reniformis is considered highly effective, since fragmented individuals quickly regenerate their body wall and attach themselves to the substrate (Parma et al., 2007; Di Camillo et al., 2012; Fassini et al., 2012). While reproduction by means of fragmentations is known to be the primary mode of recruitment in some rope-like and branching sponges (Wulff, 1991, 1995; Tsurumi and Reiswig, 1997), in A. polypoides, in contrast, fragments do not seem to re-attach to the substrate, even though they maintain vital signs (i.e., open oscula, and preserved ectosome integrity; Idan unpubl.). Consequently, even though stable conditions favor asexual reproduction by fragmentation, this strategy is not well suited for A. polypoides. Instead, it exhibits a shift from seasonal reproduction toward continuous sexual reproduction.
The results of this study, as well as other studies comparing sponge reproduction in different habitats (e.g., Meroz-Fine et al., 2005; Whalan et al., 2007; Maria et al., 2013; Zarrouk et al., 2013), suggest that reproduction plasticity may be a general rule for sponge species that grow in diverse habitats, allowing sponges to thrive along a wide range of environmental conditions.
Globally, shallow reefs (including sponges), are facing the deleterious effects of rising seawater temperatures (Garrabou et al., 2009, 2019; Cerrano et al., 2019; Ereskovsky et al., 2019). The deep reef refuge hypothesis suggests that the upper mesophotic reefs (30–60 m) may serve as a larval source for the adversely impacted shallow reefs (Lesser et al., 2009; Semmler et al., 2017). However, this hypothesis has been rejected for some coral species, as recent studies have demonstrated a reduced fecundity in mesophotic corals (Feldman et al., 2018; Shlesinger et al., 2018; Shlesinger and Loya, 2019) as well as low larval connectivity (Bongaerts et al., 2010, 2017). Although our current knowledge of the reproductive strategies and connectivity of mesophotic sponges, and especially of lower mesophotic communities, is very limited (Maldonado et al., 2017; Cerrano et al., 2019; Pomponi et al., 2019), our findings, along with those of other recent studies, suggest that mesophotic sponge grounds may act as a deep refuge for some sponge species (Meroz-Fine et al., 2005; Idan et al., 2018; Cerrano et al., 2019; Pomponi et al., 2019; Idan, 2020). As different species may exhibit diverse adaptations to environmental conditions that can affect their larval behavior and ability to settle in shallower habitats, the deep refuge hypothesis should be considered with respect to mesophotic sponge communities. Understanding the role of mesophotic sponge grounds as potential source of larvae is especially important, in face of the anthropogenic disturbances and rise in seawater temperatures, that are already affecting sponges in the shallow Israeli coast (Idan et al., 2018; Idan, 2020).
Data Availability Statement
The original contributions presented in the study are included in the article/Supplementary Material, further inquiries can be directed to the corresponding author/s.
Author Contributions
TI conceived of the study, designed the study, carried out the fieldwork, carried out the data and statistical analyses and drafted the manuscript. LG participated in collection of field data and data analysis and helped draft the manuscript. SS participated in the design of the study, participated in fieldwork, and critically revised the manuscript. IB prepared most of the histological sections and helped with data analysis. MI helped design the study, coordinated the study, and critically revised the manuscript. All authors gave final approval for publication and agreed to be held accountable for the work performed therein.
Funding
The study was supported by the Israeli Ministry of Science, Technology, and Space (The Program for the Development of Scientific and Technological Infrastructure, grant no. 3-10343 to MI); the Israeli Ministry of National Infrastructure, Energy and Water (grant no. 217-17-020 to MI); and partially supported by the ISF-NSFC joint research program (grant no. 2577/18 to MI). TI was supported by the Shulamit Aloni fellowship from the Israeli Ministry of Science, Technology, and Space.
Conflict of Interest
The authors declare that the research was conducted in the absence of any commercial or financial relationships that could be construed as a potential conflict of interest.
Acknowledgments
The NGO EcoOcean for providing the facility for marine research onboard the R/V Mediterranean Explorer and for the operation of the ROV. Oded Ezra and Itai Katzman who piloted the ROV. The students of MI’s group who volunteered for sponge collections during the many dives and cruises on-board the research vessel. Rahaf NGO that provided the boat for the shallow water dives. The undergraduate students, Daniel Raizman, Gal Kaplan, and Erica Manor, for their help preparing the samples. Naomi Paz who edited the manuscript. Collection permit was given by the Israel Nature and Parks Authority.
Supplementary Material
The Supplementary Material for this article can be found online at: https://www.frontiersin.org/articles/10.3389/fmars.2020.610565/full#supplementary-material
References
Alvarez, B., and Hooper, J. N. (2002). “Family axinellidae carter, 1875,” in Systema Porifera, eds R. W. M. van Soest and J. Hooper (Berlin: Springer), 724–747. doi: 10.1007/978-1-4615-0747-5_80
Ayling, A. L. (1980). Patterns of sexuality, asexual reproduction and recruitment in some subtidal marine Demospongiae. Biol. Bull. 158, 271–282. doi: 10.2307/1540854
Beazley, L. I., Kenchington, E. L., Murillo, F. J., and Sacau, M. D. (2013). Deep-sea sponge grounds enhance diversity and abundance of epibenthic megafauna in the Northwest Atlantic. ICES J. Mar. Sci. 70, 1471–1490. doi: 10.1093/icesjms/fst124
Bo, M., Bertolino, M., Bavestrello, G., Canese, S., Giusti, M., Angiolillo, M., et al. (2012). Role of deep sponge grounds in the Mediterranean Sea: a case study in southern Italy. Hydrobiologia 687, 163–177. doi: 10.1007/978-94-007-4688-6_14
Bongaerts, P., Ridgway, T., Sampayo, E., and Hoegh-Guldberg, O. (2010). Assessing the ‘deep reef refugia’ hypothesis: focus on Caribbean reefs. Coral Reefs 29, 309–327. doi: 10.1007/s00338-009-0581-x
Bongaerts, P., Riginos, C., Brunner, R., Englebert, N., Smith, S. R., and Hoegh-Guldberg, O. (2017). Deep reefs are not universal refuges: reseeding potential varies among coral species. Sci. Adv. 3:e1602373. doi: 10.1126/sciadv.1602373
Cerrano, C., Bastari, A., Calcinai, B., Di Camillo, C., Pica, D., Puce, S., et al. (2019). Temperate mesophotic ecosystems: gaps and perspectives of an emerging conservation challenge for the Mediterranean Sea. Eur. Zool. J. 86, 370–388. doi: 10.1080/24750263.2019.1677790
Coll, M., Piroddi, C., Steenbeek, J., Kaschner, K., Lasram, F. B., Aguzzi, J., et al. (2010). The biodiversity of the mediterranean sea: estimates, patterns, and threats. PLoS One 5:e11842. doi: 10.1371/journal.pone.0011842
Corriero, G., Liaci, L. S., Marzano, C. N., and Gaino, E. (1998). Reproductive strategies of Mycalecontarenii (Porifera: Demospongiae). Mar. Biol. 131, 319–327. doi: 10.1007/s002270050325
Corriero, G., Sarà, M., and Vaccaro, P. (1996). Sexual and asexual reproduction in two species of Tethya (Porifera: Demospongiae) from a Mediterranean coastal lagoon. Mar. Biol. 126, 175–181. doi: 10.1007/bf00347442
Di Camillo, C., Coppari, M., Bartolucci, I., Bo, M., Betti, F., Bertolino, M., et al. (2012). Temporal variations in growth and reproduction of Tedania anhelans and Chondrosia reniformis in the North Adriatic Sea. Hydrobiologia 687, 299–313. doi: 10.1007/978-94-007-4688-6_25
Elvin, D. W. (1976). Seasonal growth and reproduction of an intertidal sponge, Haliclona permollis (Bowerbank). Biol. Bull. 151, 108–125. doi: 10.2307/1540709
Ereskovsky, A., Ozerov, D. A., Pantyulin, A. N., and Tzetlin, A. B. (2019). Mass mortality event of White Sea sponges as the result of high temperature in summer 2018. Polar Biol. 42, 2313–2318. doi: 10.1007/s00300-019-02606-0
Ereskovsky, A. V. (2010). The Comparative Embryology of Sponges. Cham: Springer Science & Business Media.
Ereskovsky, A. V., Geronimo, A., and Pérez, T. (2017). Asexual and puzzling sexual reproduction of the Mediterranean sponge Haliclona fulva (Demospongiae): life cycle and cytological structures. Invertebr. Biol. 136, 403–421. doi: 10.1111/ivb.12195
Fassini, D., Parma, L., Wilkie, I. C., Bavestrello, G., Bonasoro, F., and Carnevali, M. D. C. (2012). Ecophysiology of mesohyl creep in the demosponge Chondrosia reniformis (Porifera: Chondrosida). J. Exp. Mar. Biol. Ecol. 428, 24–31. doi: 10.1016/j.jembe.2012.05.025
Feldman, B., Shlesinger, T., and Loya, Y. (2018). Mesophotic coral-reef environments depress the reproduction of the coral Paramontastraea peresi in the Red Sea. Coral Reefs 37, 201–214. doi: 10.1007/s00338-017-1648-8
Fell, P. E. (1976). The reproduction of Haliclona loosanoffi and its apparent relationship to water temperature. Biol. Bull. 150, 200–210. doi: 10.2307/1540468
Fell, P. E. (1992). “Porifera,” in Reproductive Biology of Invertebrates, eds K. G. Adiyodi and R. G. Adiyodi (Oxford: Oxford & IBH publishing co. pvt. ltd), 1–44.
Fromont, J., and Bergquist, P. (1994). Reproductive biology of three sponge species of the genus Xestospongia (Porifera: Demospongiae: Petrosida) from the Great Barrier Reef. Coral Reefs 13, 119–126. doi: 10.1007/bf00300772
Garrabou, J., Coma, R., Bensoussan, N., Bally, M., Chevaldonné, P., Cigliano, M., et al. (2009). Mass mortality in Northwestern Mediterranean rocky benthic communities: effects of the 2003 heat wave. Glob. Change Biol. 15, 1090–1103. doi: 10.1111/j.1365-2486.2008.01823.x
Garrabou, J., Gómez-Gras, D., Ledoux, J.-B., Linares, C., Bensoussan, N., López-Sendino, P., et al. (2019). Collaborative database to track mass mortality events in the Mediterranean Sea. Front. Mar. Sci. 6:707. doi: 10.3389/fmars.2019.00707
Gazave, E., Carteron, S., Chenuil, A., Richelle-Maurer, E., Boury-Esnault, N., and Borchiellini, C. (2010). Polyphyly of the genus Axinella and of the family Axinellidae (Porifera: Demospongiaep). Mol. Phylogenet. Evol. 57, 35–47. doi: 10.1016/j.ympev.2010.05.028
Gerovasileiou, V., Chintiroglou, C. C., Konstantinou, D., and Voultsiadou, E. (2016). Sponges as “living hotels” in Mediterranean marine caves. Sci. Mar. 80, 279–289. doi: 10.3989/scimar.04403.14b
Hinderstein, L., Marr, J., Martinez, F., Dowgiallo, M., Puglise, K., Pyle, R., et al. (2010). Theme section on “Mesophotic coral ecosystems: characterization, ecology, and management”. Coral Reefs 29, 247–251. doi: 10.1007/s00338-010-0614-5
Hooper, J. N., and Van Soest, R. W. (2002). “Class demospongiae sollas, 1885,” in Systema Porifera, eds R. W. M. van Soest and J. Hooper (Springer), 15–51. doi: 10.1007/978-1-4615-0747-5_3
Idan, T. (2020). Mesophotic sponge grounds in the Levant basin: Community composition and dynamics. Ph.D. thesis, Tel Aviv University, Tel Aviv-Yafo.
Idan, T., Shefer, S., Feldstein, T., Yahel, R., Huchon, D., and Ilan, M. (2018). Shedding light on an East-Mediterranean mesophotic sponge ground community and the regional sponge fauna. Mediterranean Mar. Sci. 19, 84–106. doi: 10.12681/mms.13853
Kahng, S., Garcia-Sais, J., Spalding, H., Brokovich, E., Wagner, D., Weil, E., et al. (2010). Community ecology of mesophotic coral reef ecosystems. Coral Reefs 29, 255–275. doi: 10.1007/s00338-010-0593-6
Kenchington, E., Power, D., and Koen-Alonso, M. (2013). Associations of demersal fish with sponge grounds on the continental slopes of the northwest Atlantic. Mar. Ecol. Prog. Ser. 477, 217–230. doi: 10.3354/meps10127
Kress, N., Gertman, I., and Herut, B. (2014). Temporal evolution of physical and chemical characteristics of the water column in the Easternmost Levantine basin (Eastern Mediterranean Sea) from 2002 to 2010. J. Mar. Syst. 135, 6–13. doi: 10.1016/j.jmarsys.2013.11.016
Leong, W., and Pawlik, J. R. (2010). Fragments or propagules? Reproductive tradeoffs among Callyspongia spp. from Florida coral reefs. Oikos 119, 1417–1422. doi: 10.1111/j.1600-0706.2010.18092.x
Leong, W., and Pawlik, J. R. (2011). Comparison of reproductive patterns among 7 Caribbean sponge species does not reveal a resource trade-off with chemical defenses. J. Exp. Mar. Biol. Ecol. 401, 80–84. doi: 10.1016/j.jembe.2011.02.043
Lesser, M. P., Slattery, M., and Leichter, J. J. (2009). Ecology of mesophotic coral reefs. J. Exp. Mar. Biol. Ecol. 375, 1–8.
Locker, S., Armstrong, R., Battista, T., Rooney, J., Sherman, C., and Zawada, D. (2010). Geomorphology of mesophotic coral ecosystems: current perspectives on morphology, distribution, and mapping strategies. Coral Reefs 29, 329–345. doi: 10.1007/s00338-010-0613-6
Maldonado, M. (2006). The ecology of the sponge larva. Can. J. Zool. 84, 175–194. doi: 10.1139/z05-177
Maldonado, M., Aguilar, R., Bannister, R. J., Bell, J. J., Conway, K. W., Dayton, P. K., et al. (2017). “Sponge grounds as key marine habitats: a synthetic review of types, structure, functional roles, and conservation concerns,” in Marine Animal Forests: The Ecology of Benthic Biodiversity Hotspots, eds S. Rossi, L. Bramanti, A. Gori, and C. O. Saco del Valle (Cham: Springer International Publishing), 145–183. doi: 10.1007/978-3-319-21012-4_24
Maldonado, M., and Riesgo, A. (2008). Reproductive output in a Mediterranean population of the homosclerophorid Corticium candelabrum (Porifera, Demospongiae), with notes on the ultrastructure and behavior of the larva. Mar. Ecol. 29, 298–316. doi: 10.1111/j.1439-0485.2008.00244.x
Maldonado, M., and Riesgo, A. (2009a). Gametogenesis, embryogenesis, and larval features of the oviparous sponge Petrosia ficiformis (Haplosclerida, Demospongiae). Mar. Biol. 156, 2181–2197. doi: 10.1007/s00227-009-1248-4
Maldonado, M., and Riesgo, A. (2009b). Reproduction in the phylum Porifera: a synoptic overview. Treballs de la Societat Catalana de Biologia 59, 29–49.
Maldonado, M., and Uriz, M. J. (1999). Sexual propagation by sponge fragments. Nature 398, 476–476. doi: 10.1038/19007
Maria, M., Giuseppe, C., Miriam, G., Rossella, B., and Gaino, E. (2013). Sexual reproduction in Sarcotragus spinosulus from two different shallow environments. Mar. Ecol. Evol. Perspect. 34, 394–408. doi: 10.1111/maec.12039
Mercurio, M., Corriero, G., and Gaino, E. (2007). A 3-year investigation of sexual reproduction in Geodia cydonium (Jameson 1811)(Porifera, Demospongiae) from a semi-enclosed Mediterranean bay. Mar. Biol. 151, 1491–1500. doi: 10.1007/s00227-006-0584-x
Meroz-Fine, E., Shefer, S., and Ilan, M. (2005). Changes in morphology and physiology of an East Mediterranean sponge in different habitats. Mar. Biol. 147, 243–250. doi: 10.1007/s00227-004-1532-2
Morrow, C., and Cárdenas, P. (2015). Proposal for a revised classification of the Demospongiae (Porifera). Front. Zool. 12:7. doi: 10.1186/s12983-015-0099-8
Parma, L., Fassini, D., Bavestrello, G., Wilkie, I. C., Bonasoro, F., and Carnevali, M. C. (2007). “Ecology and physiology of mesohyl creep in Chondrosia reniformis,” in Porifera Research: Biodiversity, Innovation, Sustainability. Proc. 7th International Sponge Symposium, (Rio de Janeiro: Museu Nacional), 503–508.
Pomponi, S. A., Diaz, M. C., Van Soest, R. W., Bell, L. J., Busutil, L., Gochfeld, D. J., et al. (2019). “Sponges,” in Mesophotic Coral Ecosystems, eds K. A. Puglise, Y. Loya, and T. C.L. Bridge (Cham: Springer), 563–588.
R Core Team (2020). R: A Language and Environment for Statistical Computing (Version 4.02)[Software package]. Vienna: R Foundation for Statistical Computing.
R Core Team, Maintainer, Suggests, M., and Matrix, S. (2018). Package stats. The R Stats Package. Vienna: R Foundation for Statistical Computing.
Riesgo, A., and Maldonado, M. (2008). Differences in reproductive timing among sponges sharing habitat and thermal regime. Invertebr. Biol. 127, 357–367. doi: 10.1111/j.1744-7410.2008.00128.x
Riesgo, A., Maldonado, M., and Durfort, M. (2007). Dynamics of gametogenesis, embryogenesis, and larval release in a Mediterranean homosclerophorid demosponge. Mar. Freshw. Res. 58, 398–417. doi: 10.1071/mf06052
Riesgo, A. G. (2007). Sexual Reproduction in Demosponges: Ecological and Evolutive Implications/Reproducción sexual en Demosponjas: Implicaciones ecológicas y evolutivas. Ph.D. thesis, Universidad de Barcelona, Barcelona.
R Studio Team (2020). R Studio: Integrated Development for R (version 1.1. 463)[Computer Software]. Boston: RStudio, Inc.
Semmler, R. F., Hoot, W. C., and Reaka, M. L. (2017). Are mesophotic coral ecosystems distinct communities and can they serve as refugia for shallow reefs? Coral Reefs 36, 433–444. doi: 10.1007/s00338-016-1530-0
Shlesinger, T., Grinblat, M., Rapuano, H., Amit, T., and Loya, Y. (2018). Can mesophotic reefs replenish shallow reefs? Reduced coral reproductive performance casts a doubt. Ecology 99, 421–437. doi: 10.1002/ecy.2098
Shlesinger, T., and Loya, Y. (2019). “Sexual reproduction of scleractinian corals in mesophotic coral ecosystems vs. shallow reefs,” in Mesophotic Coral Ecosystems, eds K. A. Puglise, Y. Loya, and T. C.L. Bridge (Cham: Springer), 653–666. doi: 10.1007/978-3-319-92735-0_35
Simpson, T. L. (ed.) (1984). “Gamete, embryo, larval development,” in The Cell Biology of Sponges, (Cham: Springer), 341–413. doi: 10.1007/978-1-4612-5214-6_7
Smith, T., Blondeau, J., Nemeth, R., Pittman, S., Calnan, J., Kadison, E., et al. (2010). Benthic structure and cryptic mortality in a Caribbean mesophotic coral reef bank system, the Hind Bank Marine Conservation District, US Virgin Islands. Coral Reefs 29, 289–308. doi: 10.1007/s00338-009-0575-8
Tsurumi, M., and Reiswig, H. M. (1997). Sexual versus asexual reproduction in an oviparous rope-form sponge, Aplysina cauliformis (Porifera; Verongida). Invertebr. Reproduct. Dev. 32, 1–9. doi: 10.1080/07924259.1997.9672598
Usher, K. M., Sutton, D. C., Toze, S., Kuo, J., and Fromont, J. (2004). Sexual reproduction in Chondrilla australiensis (Porifera: demospongiae). Mar. Freshw. Res. 55, 123–134. doi: 10.1071/mf03058
Voultsiadou, E. (2009). Reevaluating sponge diversity and distribution in the Mediterranean Sea. Hydrobiologia 628, 1–12. doi: 10.1007/s10750-009-9725-9
Whalan, S., Battershill, C., and De Nys, R. (2007). Variability in reproductive output across a water quality gradient for a tropical marine sponge. Mar. Biol. 153, 163–169. doi: 10.1007/s00227-007-0792-z
Wilkinson, C. R., and Vacelet, J. (1979). Transplantation of marine sponges to different conditions of light and current. J. Exp. Mar. Biol. Ecol. 37, 91–104. doi: 10.1016/0022-0981(79)90028-5
Witte, U. (1996). Seasonal reproduction in deep-sea sponges-triggered by vertical particle flux? Mar. Biol. 124, 571–581. doi: 10.1007/bf00351038
Wulff, J. (1995). Effects of a hurricane on survival and orientation of large erect coral reef sponges. Coral Reefs 14, 55–61. doi: 10.1007/bf00304073
Wulff, J. L. (1991). Asexual fragmentation, genotype success, and population dynamics of erect branching sponges. J. Exp. Mar. Biol. Ecol. 149, 227–247. doi: 10.1016/0022-0981(91)90047-z
Yaniv, M.-T. (2013). Life Cycle, Abundance and Distribution of the Mediterranean sponge Chondrosia reniformis. M.Sc. thesis, Tel Aviv University, Tel Aviv-Yafo.
Zarrouk, S., Ereskovsky, A. V., Mustapha, K. B., Abed, A. E., and Pérez, T. (2013). Sexual reproduction of Hippospongia communis (Lamarck, 1814)(Dictyoceratida, Demospongiae): comparison of two populations living under contrasting environmental conditions. Mar. Ecol. 34, 432–442. doi: 10.1111/maec.12043
Keywords: Chondrosia reniformis, Axinella polypoides, oogenesis, temperature, sexual reproduction, asexual reproduction (budding)
Citation: Idan T, Goren L, Shefer S, Brickner I and Ilan M (2020) Does Depth Matter? Reproduction Pattern Plasticity in Two Common Sponge Species Found in Both Mesophotic and Shallow Waters. Front. Mar. Sci. 7:610565. doi: 10.3389/fmars.2020.610565
Received: 26 September 2020; Accepted: 16 November 2020;
Published: 08 December 2020.
Edited by:
Ellen Kenchington, Bedford Institute of Oceanography (BIO), CanadaReviewed by:
Cecilia Conaco, University of the Philippines Diliman, PhilippinesThierry Perez, UMR 7263 Institut Méditerranéen de Biodiversité et d’Écologie Marine et Continentale (IMBE), France
Copyright © 2020 Idan, Goren, Shefer, Brickner and Ilan. This is an open-access article distributed under the terms of the Creative Commons Attribution License (CC BY). The use, distribution or reproduction in other forums is permitted, provided the original author(s) and the copyright owner(s) are credited and that the original publication in this journal is cited, in accordance with accepted academic practice. No use, distribution or reproduction is permitted which does not comply with these terms.
*Correspondence: Tal Idan, talidan@mail.tau.ac.il; taltul.idan@gmail.com