- 1Centro Oceanográfico de Málaga, Instituto Español de Oceanografía, Málaga, Spain
- 2Unidad Mérida, Centro de Investigación y de Estudios Avanzados del IPN, Cordemex, Mexico
- 3Marine Laboratory Aberdeen, Marine Scotland Science, Aberdeen, United Kingdom
The influence of hydrochemistry and trophic conditions on the coastal zooplankton community’s biomass and metabolic activities was investigated along the Spanish Mediterranean coastal waters, from Algeciras Bay to Barcelona, from autumn 2011 to autumn 2012. Two hydrographic regions were differentiated: NW Alboran (ALB) and W Mediterranean (MED). Zooplankton metabolism was assessed from measurements of the electron transport system (ETS) and aminoacyl-tRNA synthetases (AARS) activities, as proxies for potential respiration and somatic growth, respectively. Zooplankton showed three to fivefold higher biomass in ALB than in MED during autumn 2011 and spring 2012. However, in autumn 2012, a drastic decrease in biomass standing stock was observed in ALB, with no significant differences between the two regions. This biomass depletion event was not associated with environmental variables, food availability or zooplankton metabolic rates, but coincided with a twofold peak of Sardina pilchardus landings in ALB. A reduced standing stock coupled with high zooplankton growth rates suggests mortality by predation as the main cause for the low zooplankton biomass typically observed in MED, and in ALB during autumn 2012.
Introduction
The Mediterranean Sea is characterized by a high biodiversity compared to its size (Bianchi and Morri, 2000), and is mainly considered an oligotrophic basin (Siokou-Frangou et al., 2010). Some of the most productive areas of the Mediterranean Sea are located in its westernmost basin, where the Atlantic water inflow through the Strait of Gibraltar fuels high variability in both hydrological and physico-chemical conditions (Mercado et al., 2007; Mazzocchi et al., 2014; Yebra et al., 2017b; Gómez-Jakobsen et al., 2019). Mesoscale dynamics in the Alboran Sea, originated by this Atlantic jet, largely conditions the temporal and spatial distribution patterns and metabolism of the planktonic communities (Lafuente et al., 1998; Yebra et al., 2018), as it affects the distribution of temperature, salinity, oxygen concentration and underwater luminic field (Parrilla et al., 1994; Hirst and Lucas, 1998; Hernández-León et al., 2010; Teuber et al., 2013). Accordingly, several studies have revealed a strong coupling between the composition and metabolism of phytoplankton and zooplankton and the presence of upwelling, eddies, and fronts in the Spanish Mediterranean waters (Gaudy and Youssara, 2003; Alcaraz et al., 2007; Mercado et al., 2014; Yebra et al., 2018). Biotic factors such as prey availability and abundance of predators also determine zooplankton population dynamics, modulating their growth and mortality (Lin et al., 2013; Maar et al., 2014). Moreover, changes in hydrology modifying the mesoscale dynamics in the western Mediterranean waters would affect the distribution and abundance of the zooplankton communities via direct effects on their metabolism and/or by affecting different trophic levels of the food web. Such variations may be responsible for the increased frequency of jellyfish blooms (Bernard et al., 2011; Brotz and Pauly, 2012; Falkenhaug, 2014) or the decrease in the small pelagic fish (SPF) stocks observed in the Alboran and NW Mediterranean (MED) basins (Van Beveren, 2015; Brosset et al., 2016, 2017; Albo-Puigserver et al., 2020), with notable socioeconomic impacts on tourism and fisheries. Despite the suspected importance of zooplankton in the Spanish Mediterranean (Mercado et al., 2007; Saraux et al., 2019; Yebra et al., 2019), studies focused on determining which specific factors drive the variability patterns of zooplankton biomass and metabolic rates in these coastal waters are scarce (Herrera et al., 2014; Yebra et al., 2017b, 2018). Mercado et al. (2007) found that inter-annual changes in zooplankton abundance were correlated to dinoflagellates abundance in the northwestern Alboran Sea coast. In the same region, Yebra et al. (2017b) observed that phytoplankton abundance was the main driver of zooplankton metabolism changes. However, mechanisms regulating zooplankton communities across the Mediterranean coast may vary since other factors such as the abundance of potential predators (e.g., SPFs) may also widely differ (FAO, 2016). To our knowledge, no previous surveys analyze the coastal zooplankton communities across multiple basins of the Mediterranean Sea at once.
Field assessments of zooplankton metabolism are also scarce probably due to drawbacks associated with classical methodologies. For instance, the artificial cohort (Kimmerer and McKinnon, 1987) or the egg production rate methods (Berggreen et al., 1988) require the collection and incubation of living organisms during oceanographic cruises, which can be logistically challenging. On-board incubation experiments are usually conducted on a single species (hence not covering the whole community) and are quite time-consuming (taking from hours to weeks) (Kobari et al., 2019). These limitations hamper the acquisition of field metabolic rates at the same time and spatial scales as other variables such as zooplankton biomass or taxonomic composition, restricting the extrapolation of results to natural conditions at sea. To overcome these issues, several indirect methods have been developed based on biochemical indices to assess the metabolic rates of zooplankton communities. These methods have the advantage that they do not require incubation of living organisms, as they can be snap-frozen on board research vessels and stored for later laboratory analyses (Yebra et al., 2017a). This facilitates the acquisition of such data at a spatiotemporal resolution comparable to environmental variables. In this work, we estimated zooplankton potential respiration as electron transport system (ETS) activity (Packard et al., 1971) and somatic growth as aminoacyl-tRNA synthetases (AARS) activity (Yebra and Hernández-León, 2004). We present the first comprehensive study of the factors driving the spatiotemporal variability of the Spanish Mediterranean coastal zooplankton communities, from Algeciras Bay to Barcelona. This pioneer study combines the assessment of zooplankton biomass and metabolic rates together with their potential prey and predators’ abundance in the field, improving our knowledge on the potential mechanisms driving the decline of small pelagic fisheries in the MED Sea.
Materials and Methods
Sampling
Three oceanographic cruises were carried out along the Spanish Mediterranean coast (Figure 1), in autumn 2011 (21st November to 14th December), spring 2012 (6–20th March), and autumn 2012 (30th November to 15th December), on board R/V Odón de Buen (autumn 2011) and R/V Emma Bardán (spring and autumn 2012). During the cruises, 10 transects, consisting of four stations each located at 0.5, 1, 3, and 10 km distance from the coastline, were sampled perpendicular to the coast (Figure 1). Stations depth ranged from 6 to 372 m (Supplementary Table S1). Samplings were carried out during morning hours to minimize the possible differences among stations due to the diel variability. A Seabird25 CTD was used to obtain vertical profiles of temperature and salinity along the water column. Due to a breakdown and change of R/V some very shallow stations could not be sampled during the spring 2012 cruise.
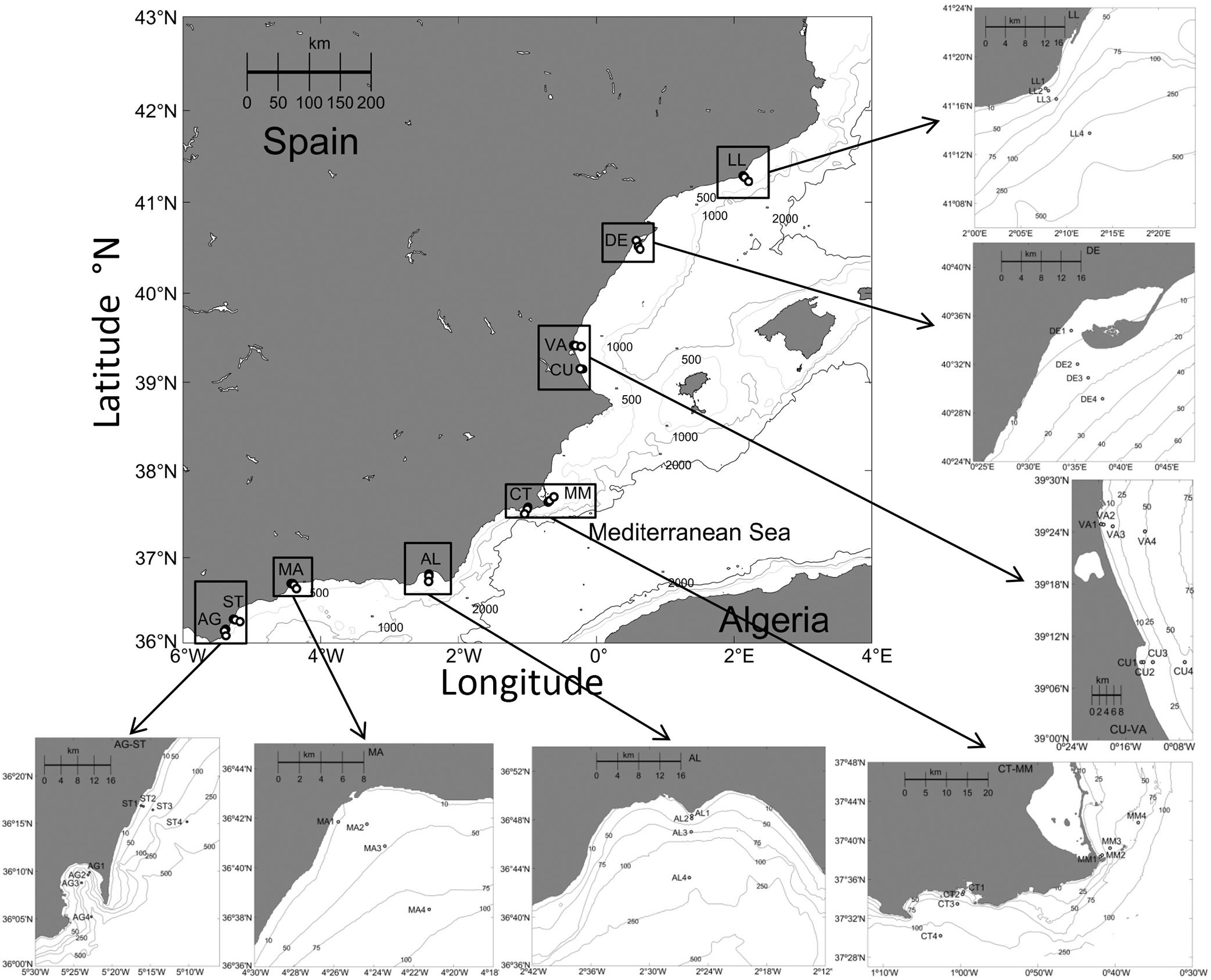
Figure 1. Transects location map in the W Mediterranean Sea (AG: Algeciras, AL: Almería, CT: Cartagena, CU: Cullera, DE: Delta del Ebro, LL: Llobregat, MA: Málaga, MM: Mar Menor, ST: Sotogrande, VA: Valencia).
Chlorophyll a
Niskin bottles (10 L) were used to collect water samples from surface to 40 m depth at 10 m intervals and an additional bottle was deployed near the bottom at each station. When bottom depth was less than 10 m, surface and near-bottom bottles were deployed. For the analysis of chlorophyll a (Chl a), 1 L of seawater was filtered through a GF/F Whatman filter (pore size 0.7 μm). The filters were immediately frozen at −20°C until their transfer to the laboratory. The concentration of Chl a (μg⋅L–1) was determined by spectrophotometry after extracting the pigments in 90% acetone overnight at 4°C (SCOR-UNESCO, 1966). Chl a data are presented as water column average from surface to 40 m depth or to near the bottom when stations were shallower than 40 m. Additionally, satellite Chl a concentrations (30 days moving mean) in the NW Alboran (ALB) Sea from January 2011 to December 2012 was calculated from daily images of MODIS-Aqua. The latter were processed as described in Gómez-Jakobsen et al. (2018), including the use of the SMED algorithm specifically developed for the study area.
Zooplankton
Zooplankton sampling was carried out by vertical hauls with a WP2-double net of 200 μm mesh size. Samples were fractionated on deck with sieves to obtain size classes of 200–500, 500–1000, and >1000 μm. Each fraction was quickly rinsed with filtered seawater on the corresponding mesh (to remove phytoplankton and smaller than desired zooplankton) and frozen in liquid nitrogen for later measurement of ETS activity, AARS activity, and protein content.
In the laboratory, frozen zooplankton samples were homogenized before the assays with Tris-HCl buffer (20 mM, pH 7.8) in an ice bath. Aliquots of each zooplankton sample homogenate were taken to determine ETS and AARS activities, and protein content. Homogenates were centrifuged (10 min, 8000 r/min, 0°C) and the AARS activity, as a proxy for somatic growth, was assessed on 250 μL of supernatant following Yebra and Hernández-León (2004) modified by Yebra et al. (2011). Enzyme activity was monitored for 10 min at 25°C. AARS activities were calculated as in Herrera et al. (2017) and corrected for the in situ field temperature by applying an activation energy of 8.57 kcal⋅mol–1 (Yebra et al., 2005) to the Arrhenius equation. Then, ETS activity, as proxy for potential respiration, was assayed on 100 μL of sample supernatant following Owens and King (1975). INT [2-(p-iodophenyl)-3-(p-nitrophenyl)-5-phenyl tetrazolium chloride] formation was read continuously for 8 min at 20°C as in Packard and Christensen (2004). ETS activity was calculated as in Maldonado et al. (2012) and corrected for the in situ field temperature using the Arrhenius equation with an activation energy of 15 kcal⋅mol–1 (Packard et al., 1975). Finally, zooplankton biomass was assessed as protein concentration (mg protein⋅m–3) following the bicinchoninic acid (BCA) assay (Smith et al., 1985), using a Pierce BCA protein assay kit and bovine serum albumin (BSA) as standard. In order to compare metabolic rates among stations and cruises, ETS and AARS activities were biomass standardized by dividing each sample enzyme activity by its protein content; in Section “Results,” these variables are always expressed in protein-specific units (hereafter spETS and spAARS).
Small Pelagic Fishes
We gathered published and unpublished landings and landings per unit effort (LPUE) data of the commercially exploited SPF species Sardina pilchardus (Torres et al., 2019a, b) and Engraulis encrasicolus (Giráldez et al., 2019b, c) caught by the Spanish purse seine fisheries in ALB and MED from 2004 to 2018. Other SPF species as Sardinella aurita, Trachurus mediterraneaus, Trachurus trachurus, Trachurus picturatus, Scomber scombrus, and Scomber colias are also captured by these purse seiners (SIRENO database, Spanish Institute of Oceanography, IEO). However, these species are not the primary objective of the fishery and are sometimes captured on demand, either as bait or food for larger fishes. Data on each SPF species distribution and maximum contribution to the fisheries catches were obtained from November to December cruises within the ECOMED pelagic acoustic surveys, from 2004 to 2009, covering our study area depth range from 30 to 200 m (PELMED project, IEO).
Statistical Analyses
Pearson correlations were performed to assess the relationships between the variables analyzed. Student’s tests were performed to determine differences (p < 0.05) between hydrographical zones and years for each variable. Pearson correlations were carried out to explore the relationship between SPF LPUE and zooplankton mean biomass per transect. These analyses were performed with the software package Statistica 7 (Statsoft, Inc., 1984–2005). In order to assess the factors controlling the distribution of zooplankton during each cruise, principal component analyses (PCAs) were performed. The PCA was conducted on the environmental variables and biomass of the different fractions of zooplankton by using the R-packages FactoMineR and factoextra (Lê et al., 2008). The representation quality of the variables on the two first principal components was assessed from cos2. The most significant associations among variables within the two first principal components were determined by analyzing their contributions and the statistical significance of the correlations.
Results
Hydrography
During autumn 2011, averaged sea surface temperature (SST, 0–5 m) varied between 15.6 (DE) and 18.9°C (AL) (Figure 2A). Averaged surface salinity (SSS, 0–5 m) ranged from 36.4 (ST) to 38.0 (LL) (Figure 2B). In spring 2012, surface temperature ranged from 13.1 (LL) to 15.6°C (DE) and salinity varied from 36.8 (MA) to 38.3 (LL). Temperature and salinity correlated positively in autumn 2011 (r = 0.47, p = 0.002, Supplementary Table S2) but negatively in spring 2012 (r = −0.55, p = 0.000), while no relationship was obtained in autumn 2012. Both variables were significantly different in the westernmost stations (AG to MA) compared to the easternmost stations (AL to LL) during both autumn samplings. In spring, salinity was also significantly different between ALB and MED but temperature differences were not significant (Table 1). Based on these results, we defined two zones within our study area: ALB coast (AG to MA transects, hereafter ALB) and MED coast (AL to LL transects, hereafter MED). The hydrographic zone ALB coincides with the western part of the FAO CGPM Geographical Sub-Area GSA01 (Northern Alboran Sea) whereas the hydrographic zone MED includes the FAO GSA06 (Northern Spain) and the eastern part of GSA01 (AL-CT). SST during autumn 2012 was significantly lower than in 2011, either in ALB (15.95 ± 0.34 vs. 16.56 ± 0.26°C) and in MED (15.30 ± 1.08 vs. 17.37 ± 1.04°C, Table 1).
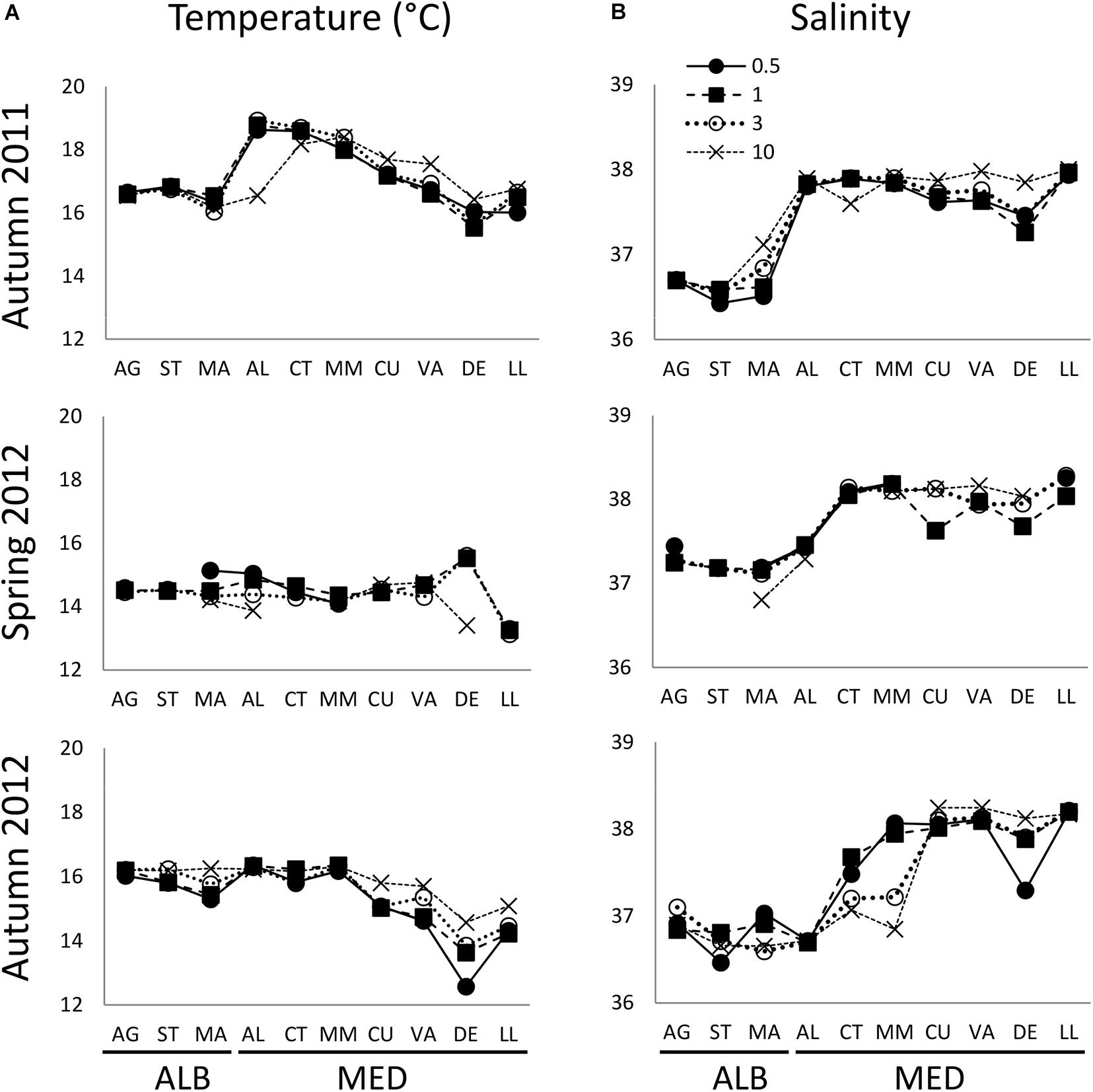
Figure 2. Average sea surface (0–5 m) (A) temperature (°C) and (B) salinity distribution from AG to LL at 0.5, 1, 3, and 10 km distance from the coast in autumn 2011, spring 2012, and autumn 2012. Transect codes as in Figure 1.
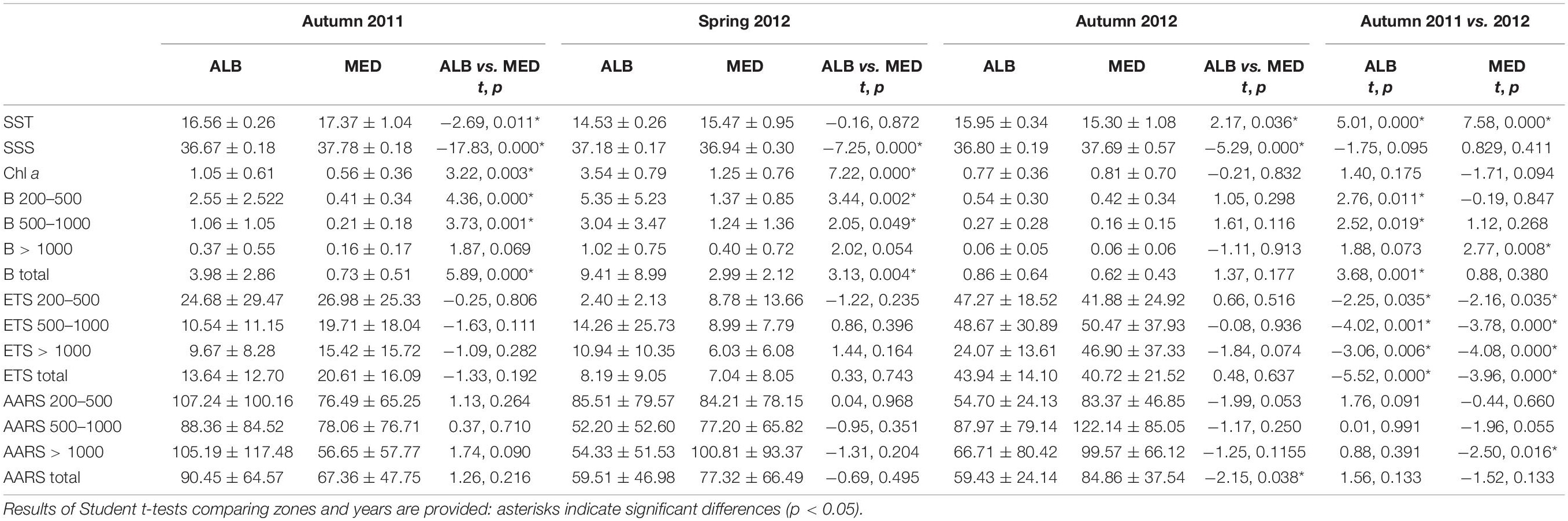
Table 1. Mean values (±SD) of sea surface temperature (SST, °C), salinity (SSS), total chlorophyll a (Chl a, μg⋅L–1), size-fractionated (200–500, 500–1000, >1000 μm), and total (>200 μm) zooplankton biomass (B, mg protein⋅m–3), spETSsitu (μL O2⋅mg prot–1⋅h–1), and spAARSsitu (nmol PPi⋅mg prot–1⋅h–1) activities at each hydrographic zone during autumn 2011, spring 2012, and autumn 2012.
Chlorophyll a
Chl a presented higher and more variable values in spring than in both autumns (Figure 3). Chl a concentrations were significantly higher in ALB than in MED both in autumn 2011 and spring 2012 (Table 1). In autumn 2012, mean Chl a concentration in ALB was lower than the previous year, but not significantly, and no differences in Chl a were found between the two hydrological zones (Table 1). In autumn 2012, we also observed relatively high Chl a values (2–3 μg L–1) in the most coastal station of DE and along the entire AL transect. In all cruises, Chl a concentration was negatively correlated with salinity (r = −0.47 to −0.59, p < 0.002). Chl a was significantly correlated with temperature only during autumn 2011 (r = −0.46, p = 0.003, Supplementary Table S2). Satellite Chl a concentration in ALB during the month prior the samplings was higher in autumn 2012 (ca. 0.5 mg⋅m–3) than in autumn 2011 (ca. 0.4 mg⋅m–3) when the pigment concentration did not depart significantly from the value obtained the month prior to the spring 2012 sampling (Supplementary Figure S1).
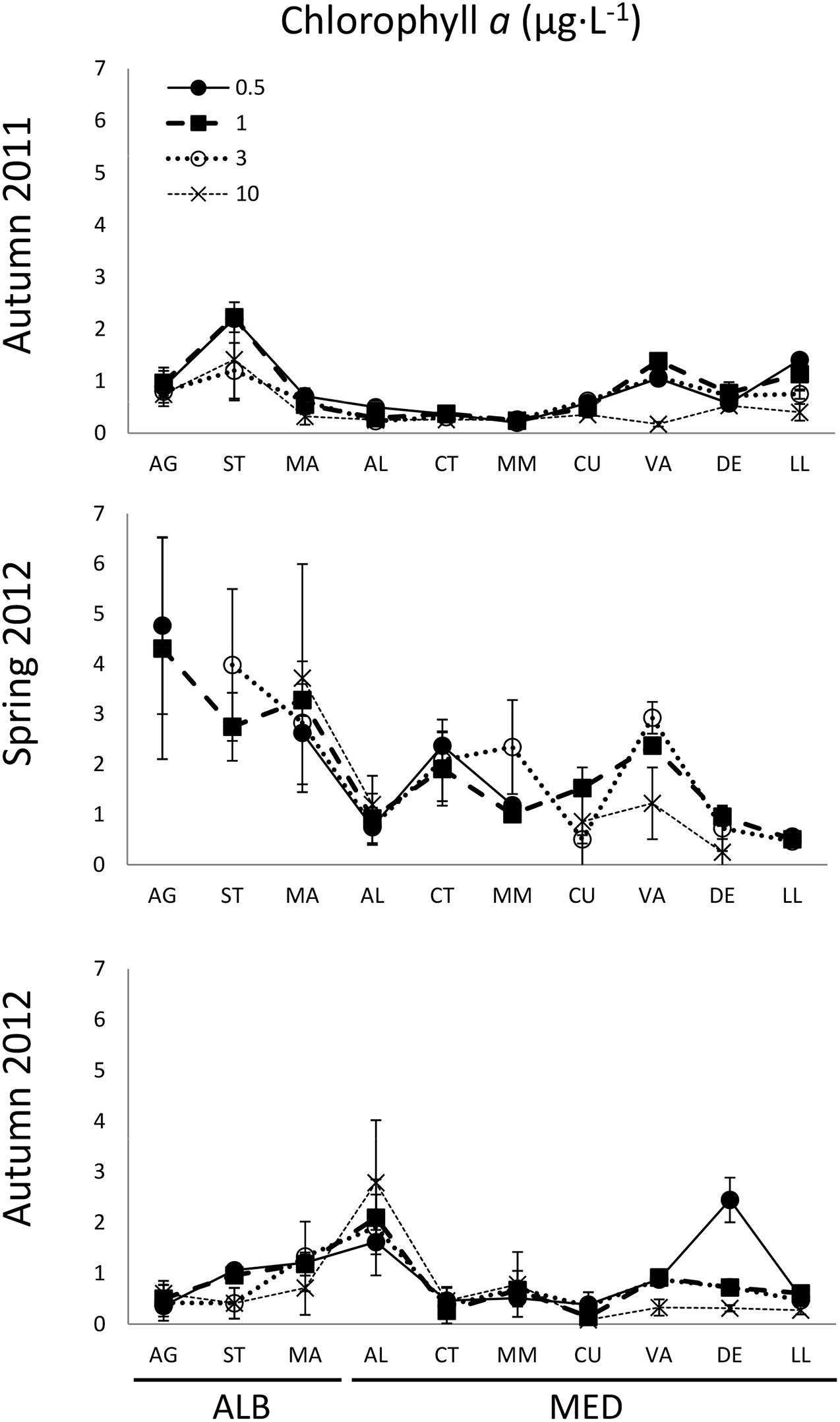
Figure 3. Mean (±SD) chlorophyll a (μg⋅L−1) distribution from AG to LL at 0.5, 1, 3, and 10 km distance from the coast in autumn 2011, spring 2012, and autumn 2012. Transect codes as in Figure 1.
Zooplankton Biomass
In autumn 2011, total zooplankton biomass was significantly higher in ALB than in MED (Table 1), showing peaks at the more coastal stations of ST and MA and decreasing values toward MED (Figure 4). Averaged total biomass recorded in ALB was fivefold higher (3.98 ± 2.86 mg prot⋅m–3) than in MED (0.73 ± 0.51 mg prot⋅m–3). This difference was driven by the 200–1000 μm fraction (Table 1). In 2012, the total zooplankton biomass mean was higher in spring than in autumn (Table 1), both in ALB (9.41 ± 8.99 mg prot⋅m–3) and MED (2.99 ± 2.12 mg prot⋅m–3), with a maximum value in ST (23.71 mg prot⋅m–3, Figure 4). As in autumn 2011, significant differences between ALB and MED were observed in the <1000 μm zooplankton biomass fractions and in the total biomass (Table 1). Opposite to this pattern, in autumn 2012 no significant differences in zooplankton biomass were found between the two zones (Table 1). Total zooplankton biomass mean in ALB (0.86 ± 0.64 mg prot⋅m–3) was significantly lower than in autumn 2011, but in MED there were no differences between autumns (0.62 ± 0.43 mg prot⋅m–3, Table 1). Coast to offshore decreasing gradients in total biomass were observed in all sampling areas during autumn 2011 and spring 2012, in contrast with autumn 2012, when biomass minima were obtained in shallow stations of ALB (Figure 4). Total zooplankton biomass did not correlate with its community spETS rates in any cruise (p > 0.05, Supplementary Table S2). Zooplankton biomass and spAARS activities were correlated only in spring 2012 (r = −0.60, p = 0.000).
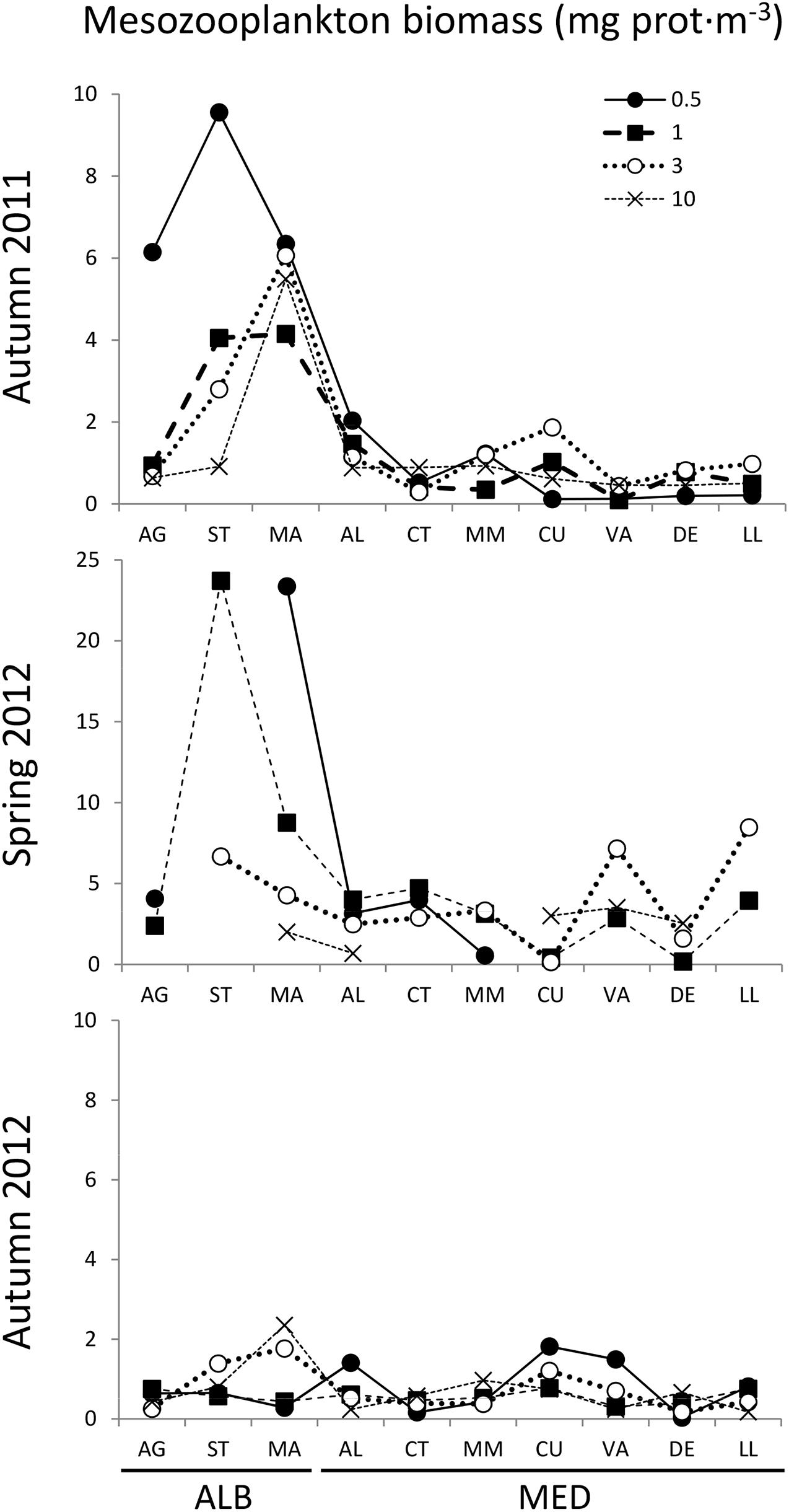
Figure 4. Total mesozooplankton biomass (>200 μm, mg prot⋅m−3) distribution from AG to LL at 0.5, 1, 3, and 10 km distance from the coast in autumn 2011, spring 2012, and autumn 2012. Transect codes as in Figure 1. Note differences in the y-axis scale among plots.
Zooplankton Metabolism
Specific ETS and AARS activities presented no significant differences between ALB and MED regions during the study period (Table 1). In autumn 2011, we observed peaks of spETS activity in both regions, with minimum values in MA and AL (Figure 5A). In contrast, in autumn 2012, spETS distribution was highly variable, with mean values significantly higher than in 2011 for all the size fractions (Table 1). The lowest zooplankton specific ETS rates were found in spring (Table 1). Opposite to spETS, specific AARS activity was higher in MA and AL during autumn 2011 and spring 2012, but not in autumn 2012 (Figure 5B). However, there were no differences in spAARS between autumn 2011 and 2012, except for the >1000 μm fraction in MED (Table 1). Enzyme activities did not present a clear coastal-offshore gradient as occurred with biomass, although rates were higher in the coastal stations (0.5 and 1 km) than in the offshore ones (10 km distance to the shore, Figure 5). There were no significant correlations between spETS or spAARS and environmental variables in any of the three cruises (p > 0.05, Supplementary Table S2).
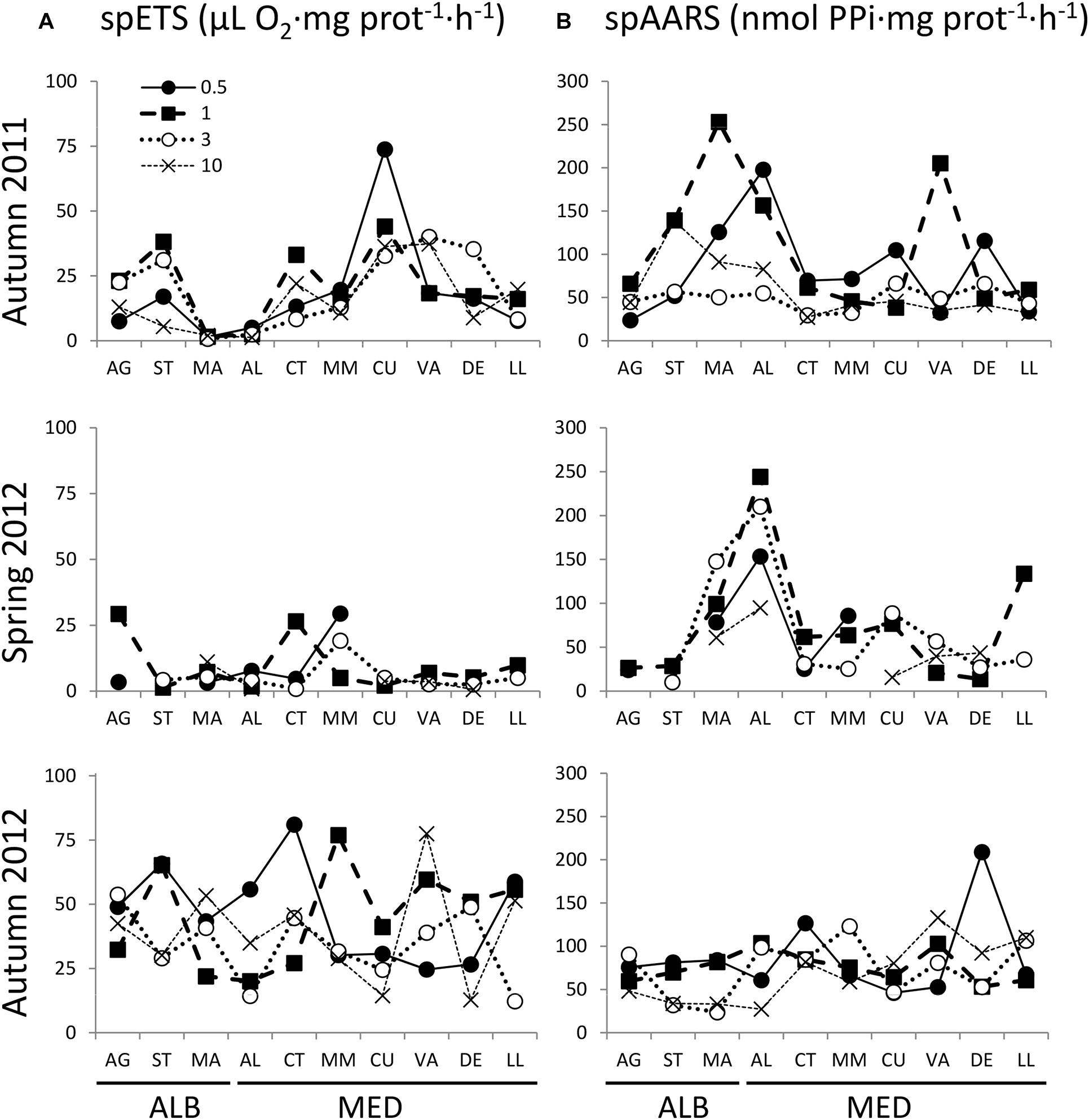
Figure 5. Mesozooplankton community (A) spETSsitu (μL O2⋅mg prot−1⋅h−1) and (B) spAARSsitu (nmol PPi⋅mg prot−1⋅h−1), from AG to LL at 0.5, 1, 3, and 10 km distance from the coast in autumn 2011, spring 2012, and autumn 2012. Transect codes as in Figure 1.
SPF Biomass
Autumn captures of anchovy in the N Alboran Sea (GSA01) were at its lowest in 2012, whereas sardine landings were significantly higher in 2012 with respect to the previous year (Figure 6). Combining Spanish purse seine landings of sardine and anchovy from 2004 to 2018, we observed that total annual landings in GSA06 were between 2.3 and 6.1 folds higher than in GSA01, being threefold higher in 2011–2012 (Figure 7A). Also, LPUE were 1.4 to 3.0 folds lower in GSA01 than in GSA06 from 2008 to 2018, with a mean ratio of 1.8 during our study (Figure 7B). Spatial distribution of sardine and anchovy LPUE along the Spanish coast during our study (November 2011 and 2012, and March 2012) presented maximum values within ALB (ST and MA), followed by AL and LL in MED (Figure 8B). Unfortunately, for several locations within MED (CU, VA, DE), there were no purse seine fisheries coinciding with our samplings. The lack of purse seiners in these locations indicates areas of very low abundance of SPF, with the exception of MA, where a voluntary closed season of the fishery in March 2012 led to the data gap in spring 2012.
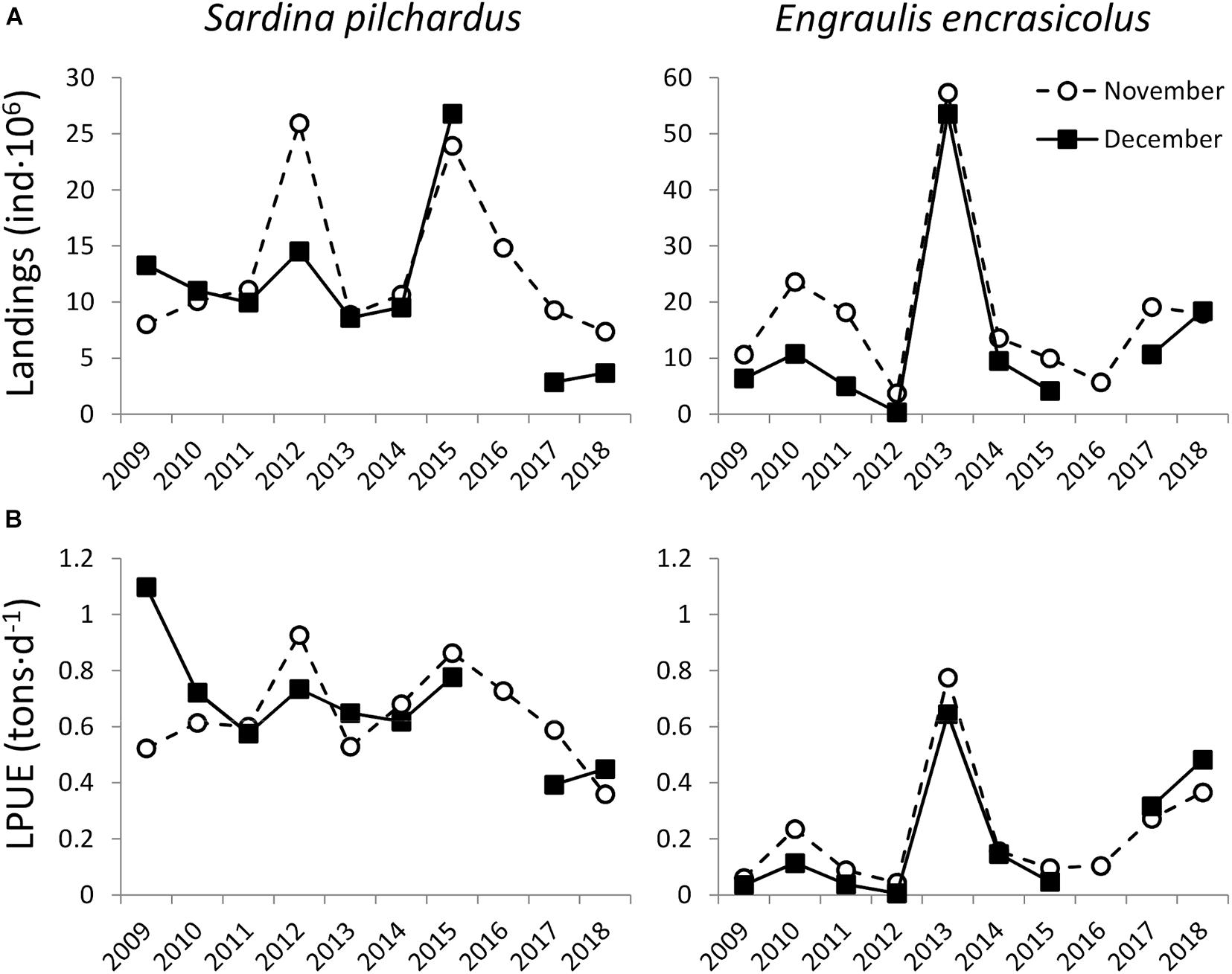
Figure 6. Interannual variability (2009–2018) in European sardine (Sardina pilchardus) and European anchovy (Engraulis encrasicolus) (A) landings (ind⋅106) and (B) LPUE (tons⋅d−1) in GSA01 during November (circles) and December (squares).
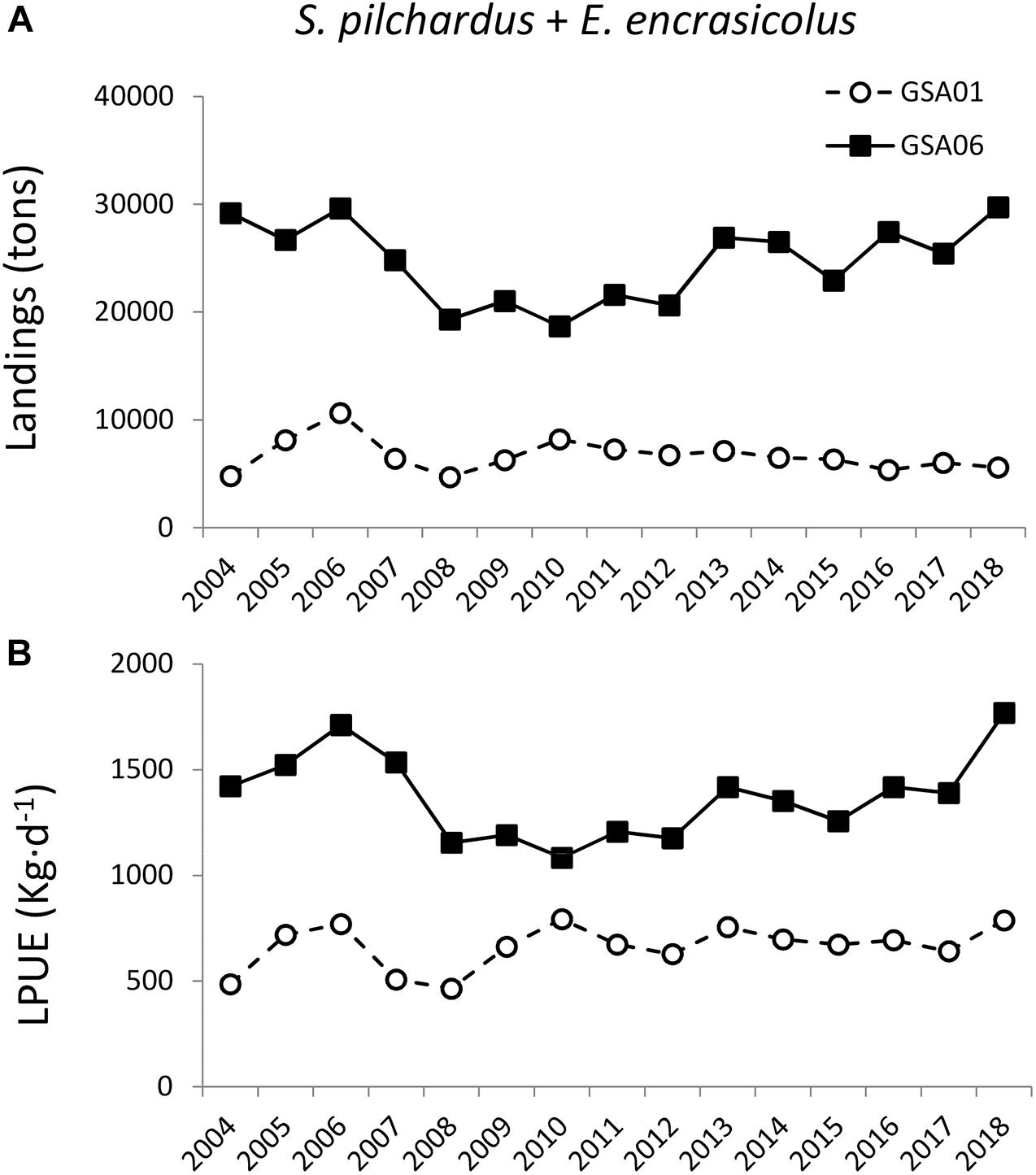
Figure 7. Interannual variability (2004–2018) of combined European sardine (Sardina pilchardus) and European anchovy (Engraulis encrasicolus) (A) landings (tons) and (B) LPUE (kg⋅d−1) in GSA01 and GSA06.
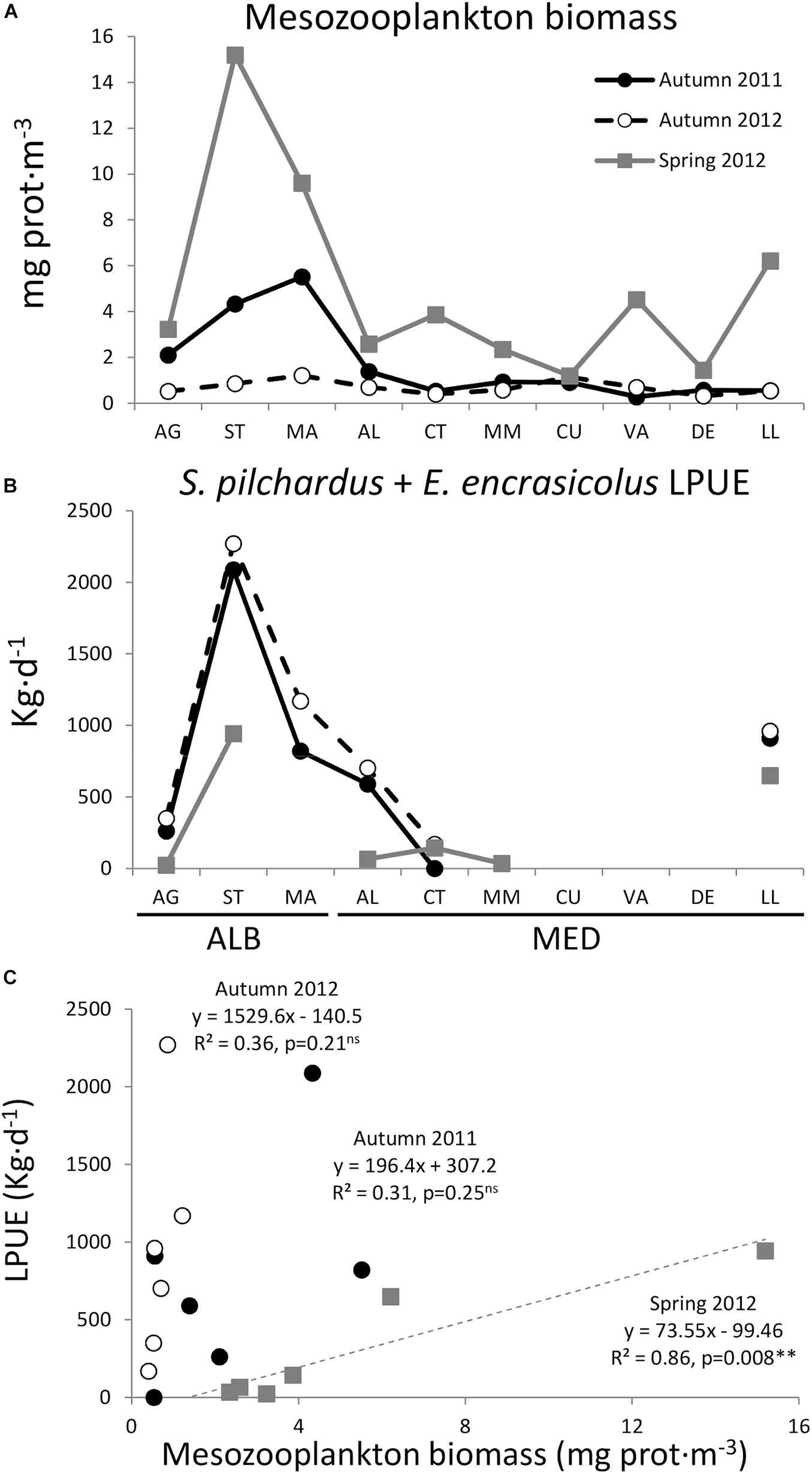
Figure 8. Distribution from AG to LL of (A) mean mesozooplankton biomass (>200 μm, mg prot⋅m−3), (B) combined European sardine (Sardina pilchardus) and European anchovy (Engraulis encrasicolus) LPUE (kg⋅d−1), and (C) Pearson correlations between zooplankton biomass and SPF LPUE in autumn 2011, spring 2012, and autumn 2012. ns: p > 0.05, ∗∗p ≤ 0.01.
Zooplankton Driving Factors
The relationships between environmental variables and zooplankton biomasses were assessed by means of PCAs conducted either on the whole pooled dataset and for each cruise separately. All variables contributed significantly to the first principal component (PC1) extracted when all cruises were analyzed together (Figure 9A), although the biological variables were the main contributors. Therefore, this component grouped the samples mainly depending on the zooplankton and phytoplankton biomass (Chl a). The second PC was mainly contributed by temperature and salinity, indicating that, overall, these hydrological variables had a less important role in determining the distribution of plankton biomass. This overall pattern was slightly modified when the data from each survey were analyzed separately. Biomass of the 200 μm zooplankton fraction, salinity, and Chl a were the main variables contributing to the first component extracted from the PCA conducted with the autumn 2011 data (Figure 9B). Accordingly, salinity correlated negatively with total zooplankton biomass in autumn 2011 (r = −0.66, p = 0.000). This PC1 discriminated the samples obtained in ALB from those collected in MED, suggesting that the correlation between biomass distribution and SSS is due to geographical distribution patterns rather than to a direct effect of the environmental variables on zooplankton growth. The zooplankton fraction larger than 1000 μm was the most important variable contributing to PC2, which discriminated samples mainly according to their latitudinal position; SST and SSS did not contributed significantly to this PC. In autumn 2012, the main variables contributing to PC1 (which also discriminated the samples from ALB and MED) were the three zooplankton size fractions and SSS (Figure 9D). SST and Chl a were the only variables that significantly contributed to PC2, indicating that these variables had a less important role in determining the distribution of zooplankton biomass. This lack of correlation between SST and zooplankton biomass distribution is also clearly shown in the biplot of the PCA performed with the spring 2012 samples (Figure 9C). This PCA also shows that Chl a and zooplankton biomass were weakly correlated. As in autumn 2011, spring PCA discriminated some samples collected at ALB featuring high biomass of zooplankton.
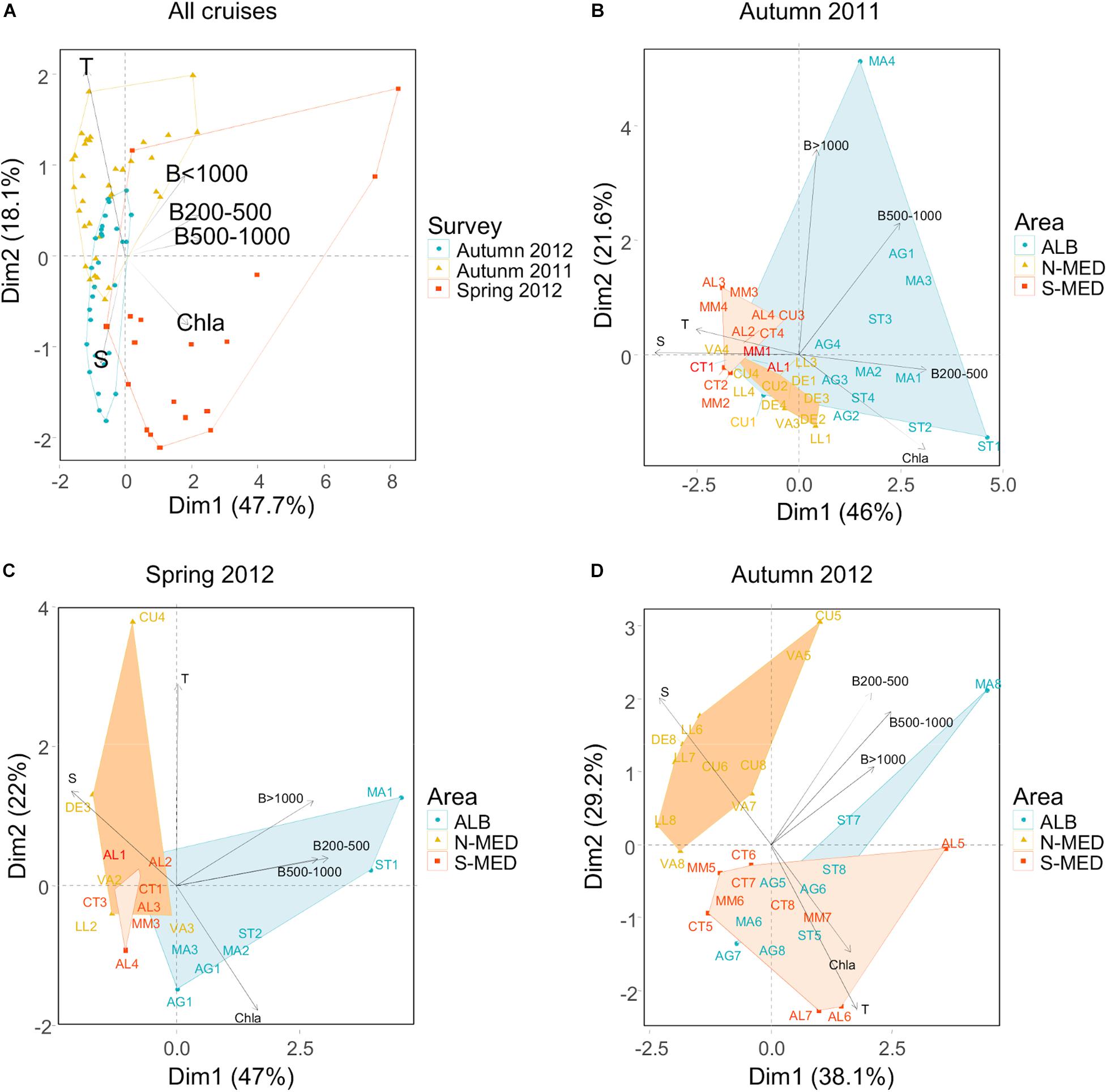
Figure 9. Scores biplot for the environmental variables (temperature, salinity, chlorophyll a, and zooplankton biomass) and samples of the two first principal components (PC1 and PC2) obtained with the PCAs performed for (A) the overall pool of samples (all cruises), (B) autumn 2011, (C) spring 2012, and (D) autumn 2012. The total variance explained for each PC is shown as well as the clusters of samples obtained in ALB and the northern (N-MED) and southern parts (S-MED) of MED.
Temperature did not correlate with zooplankton metabolic rates in any of the cruises (p > 0.05, Supplementary Table S2). In autumn 2011, Chl a was positively correlated with total zooplankton biomass (r = 0.37, p = 0.018). In contrast, Chl a did not correlate with zooplankton spETS or spAARS rates (p > 0.05, Supplementary Table S2). Note that there were significant correlations between metabolic rates and the scores of the samples for PC1 or PC2 obtained from the PCAs described above.
Zooplankton mean biomass per transect (Figure 8A) showed a positive significant correlation with combined sardine and anchovy LPUE (Figure 8B), as proxy of SPF biomass, in spring 2012. However, the correlations were weak and non-significant in autumn 2011 and 2012, and the relationships were different for each cruise (Figure 8C).
Discussion
Hydrographic Zonation
Based on salinity and temperature, we defined two zones within our study area: ALB and MED. This coastal zonation is consistent with previous studies showing significant differences in plankton productivity between NW and NE Alboran coastal waters (Mercado et al., 2016; Yebra et al., 2017b; Quintanilla et al., 2020). Differences between zones are driven by the lower temperature and salinity of the surface ALB Sea waters, compared to the rest of the MED Sea. This spatial pattern is due to the inflow of Atlantic surface waters through the Strait of Gibraltar, which are colder and less saline than the Mediterranean waters. The Alboran Sea is a transition zone between the Atlantic Ocean and the Mediterranean Sea (Heburn and La Violette, 1990; Viúdez et al., 1998; Vargas-Yañez et al., 2002). The influx of Atlantic surface waters and outflow of deep Mediterranean waters through the Strait of Gibraltar promotes intense heterogeneous gradients. In the eastern limit of the Alboran basin (Almeria transect, AL), we observed a steep difference in temperature and salinity coinciding with the probable location of the Almeria-Oran front (Viúdez et al., 1998). This front is originated by the subduction of the Mediterranean waters from the East (denser) under the Atlantic origin surface waters from the West (Parrilla and Kinder, 1987; Echevarría et al., 2002; Mercado et al., 2005).
During autumn, temperature and salinity differences between the ALB basin and the MED Sea were marked, as the largest inflow of Atlantic water occurs during this seasonal period. However, in spring, salinity in Alboran increased with respect to autumn, due to vertical mixing and upwelling of Mediterranean water (Lafuente et al., 1998). Within the MED, northern waters presented lower autumn temperatures than the southern masses, as a result of the increasing latitude. It is worth noting that Delta del Ebro (DE) stations showed particularities, due to the influence of the Ebro river discharge in the coastal area. The riverine discharge modified the coastal hydrography, with low salinity throughout the year, and promoted increased coastal Chl concentration in autumn 2012. During that cruise, relatively high Chl levels were also recorded in Almeria Bay, coupled with lower than expected surface salinities from Almeria to Mar Menor offshore waters. However, these differences were not reflected in the zooplankton community biomass as indicated by the PCAs.
Zooplankton in the MED Coast
The Mediterranean Sea is characterized for being an oligotrophic basin except for some areas including the Spanish NW Mediterranean that is relatively productive (Estrada and Margalef, 1988; Siokou-Frangou et al., 2010). We observed lower chlorophyll concentrations in MED waters than in ALB throughout the year (except in autumn 2012), with maxima in spring due to the breaking of the thermocline and subsequent vertical mixing of deep waters, which load the surface layer with nutrients (Estrada, 1996). The coastal current running NE–SW (Salat, 1996) presents a permanent slope density front at its outer margin (Castellón et al., 1990) in which offshore edge the generally cyclonic circulation promotes upwelling of high salinity waters. Also, the presence of submarine canyons modifies the front, advecting nutrient-enriched deep waters to the coast (Alvarez et al., 1996). In autumn 2011 and spring 2012, we found lower zooplankton biomass in MED than in ALB, possibly due to the typically more oligotrophic conditions. The limitation of phytoplankton growth by nutrients would in turn affect the zooplankton growth and therefore its biomass standing stock is expected to be low. The zooplankton biomass recorded in our study agrees with the range of values previously observed in these waters (Champalbert, 1996; Calbet et al., 2001; Alcaraz et al., 2007), showing the expected seasonal variability with high biomass following the spring phytoplankton bloom. However, the differences in biomass between MED and ALB were not reflected in the metabolism of the zooplankton community, as their biomass-specific ETS and AARS activities were similar in both regions. Also, in Almeria (AL) spring growth rates were higher than expected by looking at the Chl a concentrations. This might be due to the positive effect of turbulence originated by the Almeria-Oran front (Gaudy and Youssara, 2003). The significantly lower biomass and Chl a found in MED compared to ALB during autumn 2011 and spring 2012 support the assumption that low zooplankton biomass in MED is due to food limitation. Moreover, in autumn 2012, when Chl a was similar in ALB and MED, the zooplankton biomass was also low in both areas. In this sense, it is worth noting that mesozooplankton prey mostly on microzooplankton, rather than on phytoplankton, especially in oligotrophic environments where heterotrophic protists dominate (Calbet, 2008). Nevertheless, a delayed effect of Chl a on zooplankton would be expected. However, zooplankton respiration and growth rates were as high in MED as in the more eutrophic waters of ALB, indicating that zooplankton metabolism was not limited in MED. Our results are in agreement with previous studies showing high field zooplankton respiration rates in the region (Alcaraz et al., 2007; Herrera et al., 2014). Similarly, Minutoli and Guglielmo (2009) found increasing ETS activities from West (ALB) to East (MED), suggesting that temperature differences between regions played an important role in shaping metabolic rates. During our study, averaged surface temperatures were higher in MED than in ALB, except during autumn 2012 when they were lower. Nevertheless, respiration rates were similar between regions through the study, and were higher in autumn 2012 than in 2011 despite that temperature decreased in 2012 (Sánchez et al., 2017). The PCA further supported the absence of relationships between environmental variables and zooplankton biomass. These results suggest that other factors not considered so far must play an important role precluding the development of larger zooplankton stocks in the NW Mediterranean coastal waters.
Zooplankton in the ALB Coast
Opposite to MED, plankton productivities in ALB are known to be among the highest of the Mediterranean Sea (Mercado et al., 2007; Yebra et al., 2017b) favored by the wind-driven coastal upwelling and the front system in the north of the Western Anticyclonic Gyre (WAG, Sarhan et al., 2000). During our study, Chl a values were high and the spring bloom was prominent. Zooplankton biomass in autumn 2011 and spring 2012 was within the range of expected values for the region (Sampaio de Souza et al., 2005; Quintanilla et al., 2015; Yebra et al., 2017b), and higher than in other areas of the Spanish Mediterranean (Camiñas, 1983; García and Camiñas, 1985; Champalbert, 1996). However, in autumn 2012, zooplankton biomass was significantly lower than expected and comparable to MED typical values.
Several factors may explain the low zooplankton biomass observed in autumn 2012 in ALB. A significant decrease in sea surface temperature was recorded that year (Sánchez et al., 2017). In autumn 2012, mean surface temperature in ALB was 0.6°C lower than in 2011, and much lower than typical values for that season (18.2°C; Sampaio de Souza et al., 2005). However, zooplankton respiration rates were higher in 2012 than in 2011 and their growth rates did not present significant differences between years. Therefore, the decrease in temperature would not explain a reduced biomass development of the zooplankton community during autumn 2012. In addition, as observed in the MED, there were no differences in Chl a concentrations between the two autumns. Besides, satellite Chl a in ALB showed higher concentrations in autumn 2012 than in 2011, prior and during the cruises, indicating that the low zooplankton biomass in ALB during 2012 was not due to food limitation. Finally, the possibility of a top-down control of the zooplankton biomass by predators was considered. The bay of Malaga, where the decrease in zooplankton biomass was more intense, is an important fish spawning area, with high larval and juvenile concentration of SPF such as the European sardine (S. pilchardus) and European anchovy (E. encrasicolus). Unfortunately, there are no continued complete records of field SPF abundances in the region covering our study period (Giráldez et al., 2019b; Torres et al., 2019a). The SPF monitoring surveys sample transects from 30–200 m depth. However, acoustic data show that most of SPF schools are concentrated in the shallow waters of the narrow continental shelf (0–50 m), thus field SPF populations are not entirely accounted for Giráldez et al. (2019a). Alternatively, the adult landings of both species were used as an indicator of potential concentration of zooplankton predators in the area. European sardine begins to reproduce in autumn (Abad and Giráldez, 1993). The peak of sardine captures in the weeks prior to the autumn 2012 cruise (Figure 6) was mainly composed of individuals younger than one year (class year 0) but that were already starting to reproduce (SIRENO database, IEO). Sardines predominantly reproduce in autumn and are known to prey preferentially on zooplankton (mainly copepods, Morote et al., 2010; Yebra et al., 2019). Thus, we suggest that the four to fivefold lower mesozooplankton biomass observed in autumn 2012 may be consequence of a high mortality by predation of SPFs, namely, European sardine, on small crustaceans (mostly within the 200–1000 μm size fraction). Although other SPF species may also prey on zooplankton during autumn, S. pilchardus was the most abundant in that period. Our results showed that the low zooplankton production observed in ALB in autumn 2012 was not caused by a growth limitation driven by environmental factors. Instead, we propose that most of the zooplankton production would have been consumed by upper trophic levels (i.e., top-down control), resulting in unexpected low biomass in the ALB coastal waters.
Zooplankton Standing Stock Control in the Western Mediterranean Coastal Waters
Assuming that a twofold increase in abundance of a single predator species caused the significant decrease in the coastal zooplankton standing stock during autumn 2012 in ALB, could the recurrently low zooplankton biomass observed in MED be also driven by SPF top-down control? To address this question, the landings of potential predators in both areas were analyzed. Landings of S. pilchardus and E. encrasicolus account from 40–53 up to 75–90% of the total SPF landings, in GSA01 and GSA06, respectively (Giráldez et al., 2019a). Furthermore, species captured with purse seine in the Mediterranean Spanish waters are known to be preferentially zooplanktivorous during their entire life cycle (Supplementary Table S3), and their distribution (i.e., bottom depth and distance to the coast) matches that of our plankton samplings (Supplementary Table S3). Based on this information, we used combined European sardine and European anchovy landings and LPUE as indicator of these species abundance in our study area. Given that in either regions studied the fishing gear is common and that there are no fishing quotas in the Mediterranean Sea, we assumed that LPUE data from both GSAs are comparable. Thus, we observed that the field SPF abundance in GSA06 has been consistently double than in GSA01 for the past decade (Figure 7). This higher predation pressure in GSA06 would explain the recurrently low zooplankton biomass and abundance recorded in the NW waters compared to the SW basin (Siokou-Frangou et al., 2010 and the references therein).
This study assessed zooplankton metabolic rates rather than biomass stock and/or abundance alone. This approach has allowed, for the first time in the NW Mediterranean, to discern the influence of environmental factors on the two components of zooplankton production separately (namely, biomass and growth rate). Previous studies have shown a good correlation between Chl a and mesozooplankton biomass in the Alboran Sea (Yebra et al., 2017b). Both our environmental and metabolic results indicate that zooplankton growth and production rates were not limited in the Spanish Mediterranean coastal waters. Instead, we hypothesize that mortality by predation of SPF zooplanktivorous species may be the main factor controlling zooplankton biomass accumulation. This is in agreement with previous works in the NW Mediterranean showing that the seasonal phytoplankton bloom had little effect on zooplankton abundance (Calbet et al., 2001; Fernández de Puelles et al., 2007). Also, the latter authors proposed the high concentration of sardine larvae in winter as one of the main causes explaining the low zooplankton numbers found in the bay of Blanes.
If the MED region ecosystem is at its maximum carrying capacity, and the plankton production is rapidly consumed, any variation in the planktonic community may trigger changes in the entire food web. Brosset et al. (2016, 2017) and Saraux et al. (2019) proposed that the recent decrease in condition and size of anchovy and sardine in the NW Mediterranean would be driven by changes in the zooplankton communities (bottom-up control). Our work, proposing a top-down control hypothesis, adds further evidence of the strong link between coastal zooplankton and SPF species. Therefore, small changes in the Mediterranean zooplankton communities may have a significant impact on the success of commercially important SPF species and vice versa (Mercado et al., 2007; Quintanilla et al., 2015, 2020; Basilone et al., 2020). Observations indicate that the zooplankton biomass remains higher in ALB than in MED (Yebra, unpubl. data), and that SPF stocks decline started later in ALB than in MED (Brosset et al., 2017). These facts would suggest that the intense hydrodynamics in the Alboran Sea may allow a higher carrying capacity of this basin and/or a higher plasticity and resilience of the pelagic populations of both plankton and SPF than in the NW Mediterranean. Concomitant monitoring of the planktonic communities and their predators is required to elucidate further the relationship between coastal zooplankton variability and SPF success in these waters. This approach would provide essential information to be incorporated into management models of small pelagic fisheries in the Western Mediterranean Sea.
Data Availability Statement
The original datasets presented in this article are available by request to the corresponding author. The SPF dataset is available by request to the Spanish Institute of Oceanography. Requests to access the datasets should be directed to LY.
Author Contributions
LY and JM designed the study. LY, SP, PL, SS, and FG-J performed the sampling and sample analyses. LY, EE, and JM performed the data analyses. AG and PT performed SPF data collection and analyses. LY and EE wrote the first version of the manuscript. All authors contributed significantly to revisions and improvements of the final manuscript.
Funding
The research cruises and samples analyses were supported by the Spanish Ministry of Agriculture, Food and Environment (2–3 ESMAREU), the Ministry of Economy and Competitivity (TROFOALBORAN project, CTM2009-07776/MAR) and the Spanish Institute of Oceanography (IEO). ECOMED surveys were co-funded by the European Union through the European Maritime and Fisheries Fund (EMFF) within the IEO National Program of collection, management, and use of data in the fisheries sector and support for scientific advice regarding the Common Fisheries Policy.
Conflict of Interest
The authors declare that the research was conducted in the absence of any commercial or financial relationships that could be construed as a potential conflict of interest.
Acknowledgments
We thank the crew and colleagues working on board RV Odón de Buen and RV Emma Bardán during the cruises. A. Benedicto, A. Pisarek, and A. Molenda contributed to zooplankton biochemical assays during their UCA and ERASMUS+ traineeships. We also thank the editor and two referees for their comments on the manuscript.
Supplementary Material
The Supplementary Material for this article can be found online at: https://www.frontiersin.org/articles/10.3389/fmars.2020.608690/full#supplementary-material
References
Abad, R., and Giráldez, A. (1993). Reproducción, factor de condición y talla de primera madurez de la sardina, Sardina pilchardus (walb.), del litoral de málaga, mar de alborán (1989 a 1992). Bol. Inst. Esp. Oceanogr. 8, 145–155.
Albo-Puigserver, M., Sánchez, S., Coll, M., Bernal, M., Sáez-Liante, R., Navarro, J., et al. (2020). Year-round energy dynamics of sardine and anchovy in the north-western mediterranean sea. Mar. Environ. Res. 159:105021. doi: 10.1016/j.marenvres.2020.105021
Alcaraz, M., Calbet, A., Estrada, M., Marrasé, C., Saiz, E., and Trepat, I. (2007). Physical control of zooplankton communities in the catalan sea. Progr. Oceanog. 74, 294–312. doi: 10.1016/j.pocean.2007.04.003
Alvarez, A., Tintoré, J., and Sabatés, A. (1996). Flow modification and shelf-slope exchange induced by a submarine canyon off the northeast Spanish coast. J. Geophys. Res. 101, 12043–12055. doi: 10.1029/95JC03554
Basilone, G., Ferreri, R., Barra, M., Bonanno, A., Pulizzi, M., Gargano, A., et al. (2020). Spawning ecology of the European anchovy (Engraulis encrasicolus) in the strait of sicily: linking variations of zooplankton prey, fish density, growth, and reproduction in an upwelling system. Progr. Oceanog. 184:102330. doi: 10.1016/j.pocean.2020.102330
Berggreen, U., Hansen, B., and Kiørboe, T. (1988). Food size spectra, ingestion and growth of the copepod during development: implications for determination of copepod production Acartia tonsa. Mar. Biol. 99, 341–352. doi: 10.1007/bf02112126
Bernard, P., Berline, L., and Gorsky, G. (2011). Long term (1981-2008) monitoring of the jellyfish Pelagia noctiluca (Cnidaria, Scyphozoa) on mediterranean coasts (principality of monaco and french riviera). J. Oceanog. Res. Data 4, 1–10.
Bianchi, C. N., and Morri, C. (2000). Marine biodiversity of the mediterranean sea: situation, problems and prospects for future research. Mar. Pollut. Bull. 40, 367–376. doi: 10.1016/S0025-326X(00)00027-8
Brosset, P., Fromentin, J.-M., Van Beveren, E., Lloret, J., Marques, V., Basilone, G., et al. (2017). Spatio-temporal patterns and environmental controls of small pelagic fish body condition from contrasted mediterranean areas. Progr. Oceanogr. 151, 149–162. doi: 10.1016/j.pocean.2016.12.002
Brosset, P., Le Bourg, B., Costalago, D., Bănaru, D., Van Beveren, E., Bourdeix, J.-H., et al. (2016). Linking small pelagic dietary shifts with ecosystem changes in the gulf of lions. Mar. Ecol. Prog. Ser. 554, 157–171. doi: 10.3354/meps11796
Brotz, L., and Pauly, D. (2012). Jellyfish populations in the mediterranean sea. Acta Adriat. 53, 211–230.
Calbet, A. (2008). The trophic roles of microzooplankton in marine systems. ICES J. Mar. Sci. 65, 325–331. doi: 10.1093/icesjms/fsn013
Calbet, A., Garrido, S., Saiz, E., and Alcaraz, M. (2001). Annual zooplankton succession incoastal NW Mediterranean waters: the importance of the smaller size fractions. J. Plankton Res. 23, 319–331. doi: 10.1093/plankt/23.3.319
Camiñas, J. A. (1983). Datos preliminares sobre la biomasa zooplanctónica en el sector noroccidental del mar de Alboran. Bol. Inst. Esp. Oceanogr. 1, 1–18.
Castellón, A., Font, J., and García, E. (1990). The liguro-provençal-catalan current (NW mediterranean) observed by doppler profiling in the Balearic Sea. Sci. Mar. 54, 269–276.
Champalbert, G. (1996). Characteristics of zooplankton standing stock and communities in the Western Mediterranean sea: relations to hydrology. Sci. Mar. 60, 97–113.
Echevarría, F., Lafuente, J. G., Bruno, M., Gorsky, G., Goutx, M., González, N., et al. (2002). Physical-biological coupling in the Strait of Gibraltar. Deep Sea Res. II 49, 4115–4130. doi: 10.1016/S0967-0645(02)00145-5
Estrada, M., and Margalef, R. (1988). Supply of nutrients to the Mediterranean photic zone along a persistent front. Oceanol. Acta 9, 133–142.
Falkenhaug, T. (2014). Review of jellyfish blooms in the Mediterranean and black sea. Mar. Biol. Res. 10, 1038–1039. doi: 10.1080/17451000.2014.880790
Fernández de Puelles, M. L., Alemany, F., and Jansá, J. (2007). Zooplankton time-series in the Balearic sea (Western Mediterranean): variability during the decade 1994–2003. Prog. Oceanog. 74, 329–354. doi: 10.1016/j.pocean.2007.04.009
García, A., and Camiñas, J. A. (1985). Coastal variability of zooplankton biomass in the northwestern sector of the Alboran Sea. Rapp. Comm. Int. Mer Médit. 29, 309–310.
Gaudy, R., and Youssara, F. (2003). Variations of zooplankton metabolism and feeding in the frontal area of the Alboran Sea (Western Mediterranean) in winter. Oceanol. Acta 26, 179–189. doi: 10.1016/S0399-1784(02)00005-1
Giráldez, A., Torres, P., Ramírez, J. G., Iglesias, M., González, M., and Ventero, A. (2019b). “Anchovy (Engraulis encrasicolus) stock assessment in the GFCM GSA01, Northern Alboran sea,” in Paper Presented at the GFCM. Working Group on Stock Assessment of Small Pelagic Species, Roma.
Giráldez, A., Torres, P., Ramírez, J. G., Iglesias, M., González, M., and Ventero, A. (2019c). “Anchovy (Engraulis encrasicolus) stock assessment in the GFCM GSA06, Northern Spain,” in Paper Presented at the GFCM. Working Group on Stock Assessment of Small Pelagic Species, Roma.
Giráldez, A., Torres, P., and Iglesias, M. (2019a). “In depth analysis of issues with acoustic surveys in GSA01. benchmark sardine in the Alboran sea,” in Paper Presented at the GFCM. Working Group on Stock Assessment of Small Pelagic Species, Roma.
Gómez-Jakobsen, F. J., Mercado, J. M., Tovar-Salvador, M. L., Cortés, D., Yebra, L., Salles, S., et al. (2018). New algorithms for estimating chlorophyll-a in the Spanish waters of the Western Mediterranean sea from multiplatform imagery. Int. J. Remote Sens. 39, 8837–8858. doi: 10.1080/01431161.2018.1500048
Gómez-Jakobsen, F. J., Mercado, J. M., Cortés, D., Yebra, L., and Salles, S. (2019). A first description of the summer upwelling off the Bay of Algeciras and its role in the northwestern Alboran sea. Estuar. Coast. Shelf Sci. 225:106230. doi: 10.1016/j.ecss.2019.05.012
Heburn, G. W., and La Violette, P. E. (1990). Variations in the structure of the anticyclonic gyres found in the Alboran Sea. J. Geophys. Res. 95, 1599–1613. doi: 10.1029/jc095ic02p01599
Hernández-León, S., Franchy, G., Moyano, M., Menéndez, I., Schmoker, C., and Putzeys, S. (2010). Carbon sequestration and zooplankton lunar cycles: could we be missing a major component of the biological pump? Limnol. Oceanogr. 55, 2503–2512. doi: 10.4319/lo.2010.55.6.2503
Herrera, A., Gómez, M., Packard, T. T., and Fernández, and de Puelles, M. L. (2014). Zooplankton biomass and electron transport system activity around the Balearic Islands (Western Mediterranean). J. Mar. Syst. 138, 95–103. doi: 10.1016/j.jmarsys.2014.06.006
Herrera, I., López-Cancio, J., Yebra, L., and Hernández-León, S. (2017). The effect of a strong warm winter on subtropical zooplankton biomass and metabolism. J. Mar. Res. 75, 557–577. doi: 10.1357/002224017822109523
Hirst, A. G., and Lucas, C. H. (1998). Salinity influences body weight quantification in the scyphomedusa Aurelia aurita: important implications for body weight determination in gelatinous zooplankton. Mar. Ecol. Prog. Ser. 165, 259–269. doi: 10.3354/meps165259
Kimmerer, W. J., and McKinnon, A. D. (1987). Growth, mortality, and secondary production of the copepod Acartia tranteri in Westernport bay, Australia. Limnol. Oceanogr. 32, 14–28. doi: 10.4319/lo.1987.32.1.0001
Kobari, T., Sastri, A. R., Yebra, L., Liu, H., and Hopcroft, R. R. (2019). Evaluation of trade-offs in traditional methodologies for measuring metazooplankton growth rates: assumptions, advantages and disadvantages for field applications. Progr. Oceanogr. 178:102137. doi: 10.1016/j.pocean.2019.102137
Lafuente, J. G., Cano, N., Vargas, M., Rubín, J. P., and Hernández-Guerra, A. (1998). Evolution of the Alboran Sea hydrographic structures during July 1993. Deep Sea Res. I 45, 39–65. doi: 10.1016/S0967-0637(97)00216-1
Lê, S., Josse, J., and Husson, F. (2008). FactoMineR: an r package for multivariate analysis. J. Stat. Softw. 25, 1–18. doi: 10.18637/jss.v025.i01
Lin, K. Y., Sastri, A. R., Gong, G. C., and Hsieh, C. H. (2013). Copepod community growth rates in relation to body size, temperature, and food availability in the East China sea: a test of metabolic theory of ecology. Biogeosciences 10, 1877–1892. doi: 10.5194/bg-10-1877-2013
Maar, M., Rindorf, A., Friis Møller, E., Christensen, A., Madsen, K. S., and van Deurs, M. (2014). Zooplankton mortality in 3D ecosystem modelling considering variable spatial-temporal fish consumptions in the North sea. Prog. Oceanogr. 124, 78–91. doi: 10.1016/j.pocean.2014.03.002
Maldonado, F., Packard, T. T., and Gómez, M. (2012). Understanding tetrazolium reduction and the importance of substrates in measuring respiratory electron transport activity. J. Exp. Mar. Biol. Ecol. 43, 110–118. doi: 10.1016/j.jembe.2012.08.010
Mazzocchi, M. G., Siokou, I., Tirelli, V., Bandelj, V., Fernandez, de Puelles, M. L., et al. (2014). Regional and seasonal characteristics of epipelagic mesozooplankton in the Mediterranean sea based on an artificial neural network analysis. J. Mar. Syst. 135, 64–80. doi: 10.1016/j.jmarsys.2013.04.009
Mercado, J. M., Cortés, D., García, A., and Ramírez, T. (2007). Seasonal and inter-annual changes in the planktonic communities of the northwest Alboran Sea (Mediterranean sea). Prog. Oceanogr. 74, 273–293. doi: 10.1016/j.pocean.2007.04.013
Mercado, J. M., Ramírez, T., Cortés, D., Sebastián, M., and Vargas, M. (2005). Temporal changes of the phytoplankton communities in an upwelling area of the Alborán sea. Sci. Mar. 69, 451–465. doi: 10.3989/scimar.2005.69n4451
Mercado, J. M., Sala, I., Salles, S., Cortés, D., Ramírez, T., Liger, E., et al. (2014). Effects of community composition and size structure on light absorption and nutrient uptake of phytoplankton in contrasting areas of the Alboran sea. Mar. Ecol. Prog. Ser. 499, 47–64. doi: 10.3354/meps10630
Mercado, J. M., Gómez-Jakobsen, F., Cortés, D., Yebra, L., Salles, S., León, P., et al. (2016). A method based on satellite imagery to identify spatial units of eutrophication management. Remote Sens. Environ. 186, 123–134. doi: 10.1016/j.rse.2016.08.011
Minutoli, R., and Guglielmo, L. (2009). Zooplanktonrespiratory electron transport system (ETS) activity in the Mediterranean sea: spatial and dielvariability. Mar. Ecol. Prog. Ser. 381, 199–211. doi: 10.3354/meps07862
Morote, E., Olivar, M. P., Villate, F., and Uriarte, I. (2010). A comparison of anchovy (Engraulis encrasicolus) and sardine (Sardina pilchardus) larvae feeding in the Northwest Mediterranean: influence of prey availability and ontogeny. ICES J. Mar. Sci. 67, 897–908. doi: 10.1093/icesjms/fsp302
Owens, T. G., and King, F. D. (1975). The measurement of respiratory electron-transport-system activity in marine zooplankton. Mar. Biol. 30, 27–36. doi: 10.1007/BF00393750
Packard, T. T., Healy, M. L., and Richards, F. A. (1971). Vertical distribution of the activity of therespiratory electron transport system in marine plankton. Limnol. Oceanogr. 16, 60–70. doi: 10.4319/lo.1971.16.1.0060
Packard, T. T., and Christensen, J. P. (2004). Respiration and vertical carbon flux in the gulf of maine water column. J. Mar. Res. 62, 93–115. doi: 10.1357/00222400460744636
Packard, T. T., Devol, A., and King, F. (1975). The effect of temperature on the respiratory electron transport system in marine plankton. Deep Sea Res. 22, 237–249. doi: 10.1016/0011-7471(75)90029-7
Parrilla, G., and Kinder, T. H. (1987). Oceanografía física del mar de Alborán. Boletín del Inst. Esp. Oceanogr. 4, 133–166.
Parrilla, G., Lavín, A., Bryden, H., García, M., and Millard, R. (1994). Rising temperatures in the subtropical North Atlantic ocean over the past 35 years. Nature 369, 48–51. doi: 10.1038/369048a0
Quintanilla, J. M., Laiz-Carrión, R., Uriarte, A., and García, A. (2015). Influence of trophic pathways on daily growth patterns of western Mediterranean anchovy Engraulis encrasicolus larvae. Mar. Ecol. Prog. Ser. 531, 263–275.
Quintanilla, J. M., Laiz-Carrión, R., García, A., Quintanilla, L. F., Cortés, D., Gómez-Jakobsen, F., et al. (2020). Early life trophodynamic influence on daily growth patterns of the Alboran sea sardine (Sardina pilchardus) from two distinct nursery habitats (bays of málaga and almería) in the western Mediterranean Sea. Mar. Environ. Res. 162:105195. doi: 10.1016/j.marenvres.2020.105195
Salat, J. (1996). Review of hydrographic environmental factors that may influence anchovy habitats in Northwestern Mediterranean. Sci. Mar. 60(Suppl. 2), 21–32.
Sampaio de Souza, C., Mafalda, P., Salles, S., Ramírez, T., Cortés, D., et al. (2005). Tendencias estacionales y espaciales en la comunidad mesozooplanctónica en una serie temporal plurianual en el noroeste del Mar de Alborán. España. Rev. Biol. Mar. Oceanogr. 40, 45–54. doi: 10.4067/S0718-19572005000100005
Sánchez, E., Rodríguez-Fonseca, B., Bladé, I., Brunet, M., Aznar, R., Cacho, I., et al. (2017). Progress in Detection and Projection of Climate Change in Spain Since the 2010 CLIVAR-Spain Regional Climate Change Assessment Report. Qingdao: CLIVAR Exchanges
Saraux, C., Van Beveren, E., Brosset, P., Queiros, Q., Bourdeix, J.-H., Dutto, G., et al. (2019). Small pelagic fish dynamics: a review of mechanisms in the gulf of lions. Deep Sea Res. Part II Top. Stud. Oceanogr. 159, 52–61. doi: 10.1016/J.DSR2.2018.02.010
Sarhan, T., García Lafuente, J., Vargas, M., Vargas, J. M., and Plaza, P. (2000). Upwelling mechanisms in the northwestern Alborán Sea. J. Mar. Syst. 23, 317–331. doi: 10.1016/S0924-7963(99)00068-8
Smith, P. K., Krohn, R. I., Hermanson, G. T., Mallia, A. K., Gartner, F. H., Provenzano, M. D., et al. (1985). Measurement of protein using bicinchoninic acid. Anal. Biochem. 150, 76–85. doi: 10.1016/0003-2697(85)90442-7
Siokou-Frangou, I., Christaki, U., Mazzocchi, M. G., Montresor, M., Ribera, D., Alcala, M., et al. (2010). Plankton in the open mediterranean sea: a review. Biogeosciences 7, 1543–1586. doi: 10.5194/bg-7-1543-2010
Teuber, L., Schukat, A., Hagen, W., and Auel, H. (2013). Distribution and ecophysiology of calanoid copepods in relation to the oxygen minimum zone in the Eastern Tropical Atlantic. PLoS One 8:e77590. doi: 10.1371/journal.pone.0077590
Torres, P., Giráldez, A., Ramírez, J. G., Iglesias, M., González, M., and Ventero, A. (2019a). “Sardine (Sardina pilchardus) stock assessment in the GFCM GSA01, Northern Alborán Sea,” in Paper Presented at GFCM. Working Group on Stock Assessment of Small Pelagic Species, Roma.
Torres, P., Giráldez, A., Ramírez, J. G., Iglesias, M., González, M., and Ventero, A. (2019b). “Sardine (Sardina pilchardus) stock assessment in the GFCM GSA06, Northern Spain,” in Paper Presented at GFCM. Working Group on Stock Assessment of Small Pelagic Species, Roma.
Van Beveren, E. (2015). Population Changes in Small Pelagic Fish of the Gulf of Lions: a Bottom-up Control? Ph.D thesis, University of Montpellier, France.
Vargas-Yañez, M., Plaza, F., Garcia-Lafuente, J., Sarhan, T., Vargas, J. M., and Vélez-Belchi, P. (2002). About the seasonal variability of the Alboran Sea circulation. J. Mar. Syst. 35, 229–248. doi: 10.1016/s0924-7963(02)00128-8
Viúdez, A., Pinot, J. M., and Haney, R. L. (1998). On the upper layer circulation in the Alboran sea. J. Geophys. Res. 103, 21653–21666. doi: 10.1029/98JC01082
Yebra, L., Berdalet, E., Almeda, R., Pérez, V., Calbet, A., and Saiz, E. (2011). Protein and nucleic acid metabolism as proxies for growth and fitness of Oithona davisae (Copepoda, Cyclopoida) early developmental stages. J. Exp. Mar. Bio. Ecol. 406, 87–94. doi: 10.1016/j.jembe.2011.06.019
Yebra, L., Harris, R. P., and Smith, T. (2005). Comparison of five methods for estimating growth of Calanus helgolandicus later developmental stages (CV-CVI). Mar. Biol. 147, 1367–1375. doi: 10.1007/s00227-005-0039-39
Yebra, L., and Hernández-León, S. (2004). Aminoacyl-tRNA synthetases activity as a growth index in zooplankton. J. Plankton Res. 26, 351–356. doi: 10.1093/plankt/fbh028
Yebra, L., Putzeys, S., Cortés, D., Mercado, J. M., Gómez-Jakobsen, F., León, P., et al. (2017b). Trophic conditions govern summer zooplankton production variability along the SE Spanish coast (SW Mediterranean). Estuar. Coast. Shelf Sci. 187, 134–145. doi: 10.1016/j.ecss.2016.12.024
Yebra, L., Kobari, T., Sastri, A. R., Gusmão, F., and Hernández-León, S. (2017a). Advances in biochemical indices of zooplankton production. Adv. Mar. Biol. 76, 157–240. doi: 10.1016/bs.amb.2016.09.001
Yebra, L., Herrera, I., Mercado, J. M., Cortés, D., Gómez-Jakobsen, F., Alonso, A., et al. (2018). Zooplankton production and carbon export flux in the western Alboran sea gyre (SW Mediterranean). Prog. Oceanog. 167, 64–77. doi: 10.1016/j.pocean.2018.07.009
Keywords: AARS activity, ETS activity, western Mediterranean Sea, zooplankton production, small pelagic fish landing data, zooplankton metabolism, zooplankton biomass, Alboran Sea
Citation: Yebra L, Espejo E, Putzeys S, Giráldez A, Gómez-Jakobsen F, León P, Salles S, Torres P and Mercado JM (2020) Zooplankton Biomass Depletion Event Reveals the Importance of Small Pelagic Fish Top-Down Control in the Western Mediterranean Coastal Waters. Front. Mar. Sci. 7:608690. doi: 10.3389/fmars.2020.608690
Received: 21 September 2020; Accepted: 04 December 2020;
Published: 23 December 2020.
Edited by:
Chiara Piroddi, Joint Research Centre, ItalyReviewed by:
Marta Albo-Puigserver, Consejo Superior de Investigaciones Científicas (CSIC), SpainGualtiero Basilone, Istituto per l’Ambiente Marino Costiero (IAMC), Italy
Copyright © 2020 Yebra, Espejo, Putzeys, Giráldez, Gómez-Jakobsen, León, Salles, Torres and Mercado. This is an open-access article distributed under the terms of the Creative Commons Attribution License (CC BY). The use, distribution or reproduction in other forums is permitted, provided the original author(s) and the copyright owner(s) are credited and that the original publication in this journal is cited, in accordance with accepted academic practice. No use, distribution or reproduction is permitted which does not comply with these terms.
*Correspondence: Lidia Yebra, bGlkaWEueWVicmFAaWVvLmVz