- 1Taskforce Salmon Lice, Department of Biology, Norwegian University of Science and Technology, Trondheim, Norway
- 2Cell Molecular Biology and Genomics Group, Department of Biology, Norwegian University of Science and Technology, Trondheim, Norway
- 3Cellular and Molecular Imaging Core Facility, Department of Clinical and Molecular Medicine, Norwegian Institute of Science and Technology, Trondheim, Norway
Phenylalanine hydroxylase (PAH) is a crucial enzyme involved in tyrosine biosynthesis, having roles in neurological and physiological processes. The purpose of PAH has received little attention in crustaceans despite extensive investigations in other arthropods. Here, we characterize the PAH gene for the first time in the parasite Lepeophtheirus salmonis, a copepod that is responsible for huge economic losses in salmonid fish farming. Phylogenetic and sequence analyses confirmed that LsPAH is closely related to the metazoan PAH with conserved ACT regulatory and catalytic domains. Temporal expression patterns revealed that LsPAH is expressed throughout all developmental stages peaking during the copepodite stages, suggesting an essential role in developmental physiology. We used RNAi to knockdown LsPAH expression in the nauplius I stage to study developmental function during the larval stages. PAH knockdown impaired larval development, molting and swimming ability with severe morphological defects. This study provides insight into the role of PAH in copepods and demonstrates the importance of this metabolic gene in salmon louse growth and development.
Introduction
The marine ectoparasite of Atlantic salmon (Salmo salar), the salmon louse (Lepeophtheirus salmonis), causes substantial economic loss in salmon aquaculture and represents a significant threat to wild fish populations in both the North Pacific and North Atlantic (Pike, 1989; Torrissen et al., 2013). The feeding behavior of these parasites and aggregation of the lice on fish causes lesions, anemia, osmoregulatory imbalance, secondary infections and can lead to mortality (Pike and Wadsworth, 1999; Wagner et al., 2008). The adult female lice produce egg strings that hatch to larvae (Johnson and Albright, 1991) that are planktonic and spread by water mixing and currents and can infest fish both in farms and wild salmonids (Kristoffersen et al., 2014, 2018). There are multiple chemical treatments for the louse control in aquaculture, but the majority of these methods are losing their effect due to resistant lice (Aaen et al., 2015). Several different mechanical treatment methods have also been developed, which pose different welfare challenges for the fish (Overton et al., 2019). A better understanding of the biology of the louse is crucial for the development of new treatment methods. The physiology of lice comprises several important biological systems that are governed by various genes and proteins. Various treatment methods target these genes and proteins necessary in different biological processes such as neuromodulation, body formation, molting, embryonic development, and more. Finding ways to inhibit development and molting could help decrease the lice infestation in farmed fish. Identifying key genes involved in the growth, development, and molting of lice could be the additional step to aid the ongoing research to overcome this problem.
Phenylalanine hydroxylase (PAH) enzyme belongs to the aromatic amino acid hydroxylase family and catalyzes the conversion of phenylalanine to tyrosine in a tetrahydrobiopterin (BH4)-dependent reaction (Erlandsen et al., 2002). The PAH gene, also known as Henna in fruit flies, has been studied in mammals since late 1950s (Kaufman, 1993). PAH is a rate-limiting enzyme in the catabolism of phenylalanine and its failure leads to the deficiency of catecholamine neurotransmitter derivatives of tyrosine (Landvogt et al., 2008; Sawin et al., 2014). PAH is present in liver for removal of excess L-Phenylalanine to prevent the neurotoxic effect of hyperphenylalaninemia, however, also maintaining the level of this essential proteinogenic amino acid by preventing full catabolism (Flydal and Martinez, 2013). The studies in Drosophila, silkworm, pea aphids, and mosquitoes have revealed the association of the PAH in cuticular coloration and sclerotization that is vital in the reconstruction and remodeling of the internal structures that occurs during metamorphosis (Infanger et al., 2004; Chen et al., 2013; Fuchs et al., 2014; Simonet et al., 2016). The tanning of egg chorion and melanotic encapsulation of parasitic organisms are other general PAH related functions (Mito et al., 2010). The role of PAH in Acyrthosiphon pisum (pea aphid), Caenorhabditis elegans (roundworm), and Drosophila melanogaster (fruit fly) has been elucidated, where this gene plays a crucial role in melanization, immune stimulation and embryonic development (Morales et al., 1990; Calvo et al., 2008; Simonet et al., 2016). Moreover, functional studies of PAH in insects have provided an extensive amount of data on post-embryonic development and adult physiological processes. PAH inactivation in pea aphids has shown reduced fecundity and adult lifespan (Simonet et al., 2016). Similarly, PAH knockdown in Rhodnius prolixus produced interference with embryonic development and egg hatching, demonstrating an essential role in insect development (Sterkel and Oliveira, 2017). The role of PAH in the copepod salmon louse has not been investigated yet. However, various genes and pathways associated with the growth and development of salmon lice have been studied. RNAi induced myosupressin deficiency results in molting defects (Komisarczuk et al., 2019), ecdysone receptor, and retinoid X receptor knockdown have shown inhibition of molting from nauplii II to copepodite stage (Sandlund et al., 2016). The knockdown of glutamine frutcose-6-phosphate aminotransferase and chitin synthase 1 and 2 showed irregularities in copepodite shape and swimming defects (Braden et al., 2020).
Understanding and unraveling biochemical pathways essential for the growth and development of salmon lice is a key step in fight against the parasite. Here we focus on the molting and development of the salmon louse in planktonic stages. The molting and growth of salmon louse have been well studied, but the molecular mechanisms and critical genes involved in this process are poorly understood. In this study, we aimed to downregulate the LsPAH gene activity using RNAi, and show how this affects molting, development and swimming performance of the salmon louse.
Materials and Methods
Gene Identification and Characterization
The full-length PAH transcript sequence was assembled from RNA-seq data available in the NCBI Sequence Read Archive database (SRR6832868) and a partial sequence available at Licebase1. A full-length PAH cDNA sequence is also available at Ensembl Metazoa (EMLSAT00000009260). The PAH sequence was validated by PCR performed on cDNA derived from L. salmonis.
Phylogenetic analysis was performed using Mega7 software (Kumar et al., 2016). A Maximum Likelihood method (ML) based on the Le_Gascuel_2008 model (Le and Gascuel, 2008) was used to infer the phylogenetic relationships. Initial tree(s) for the heuristic search were obtained automatically by applying Neighbor-Join and BioNJ algorithms to a matrix of pairwise distances estimated using a JTT model, and then selecting the topology with superior log likelihood value. A discrete Gamma distribution was used to model evolutionary rate differences among sites [4 categories (+G, parameter = 1.1380)]. The rate variation model allowed for some sites to be evolutionarily invariable [(+I), 16.2857% sites]. The tree is drawn to scale, with branch lengths measured in the number of substitutions per site. The analysis involved 26 amino acid sequences. All positions with less than 80% site coverage were eliminated. There were a total of 425 positions in the final dataset.
Life Stage Expression
For stage specific expression levels of the gene of interest, salmon lice of all eight life stages were collected. The egg strings were hatched in the flow-through incubator and planktonic stages of salmon lice were collected. For parasitic stages, eight Atlantic salmon (approximately 200 g) were transferred into a 400L tank and allowed to acclimate for 3 days. The fish were infected with copepodites (Hamre et al., 2009) and the parasitic stages of samples were collected at time points, according to growth based on temperature at 8°C as previously described (Hamre et al., 2019). The following life stages and number of animals were collected for 4 biological replicates, flash frozen in liquid nitrogen and stored at −80°C until further analysis. Each biological replicate contains nauplius I (n = 50), nauplius II (n = 50), planktonic copepodite (n = 50), chalimus I (n = 5), chalimus II (n = 5), preadult I male (n = 1) and female (n = 1), preadult II male (n = 1) and female (n = 1), adult male (n = 1) and adult female (n = 1). RNA was isolated using Qiagen RNeasy Plus Mini kit (Qiagen, Hilden, Germany) and cDNA was synthesized using the QuantiTect Reverse Transcription Kit (Qiagen, Hilden, Germany) following manufacturer’s instruction. Gene expression was determined using qPCR.
Gene Knockdown
The RNAi technique was used to knockdown the PAH gene in salmon lice following the protocol as previously described (Eichner et al., 2014). A 547 bp region of salmon lice PAH gene was amplified using the following primers (LsTyrFW1 and LsTyrRw1, Supplementary Table 1) and cloned into pCRTM4-TOPO® TA vector (Thermo Fisher Scientific) for the addition of T7 promoter. For control, a gene encoding leucine-rich repeat protein kinase in Arabidopsis (LRK) without any sequence similarity to the salmon lice genome was used. In vitro double stranded RNA (dsRNA) was synthesized using the T7 RiboMAXTM Express RNAi System (Omega Bio-Tek, United States), following the manufacturer’s instruction. The dsRNA products were purified using the RNeasy Plus Mini kit (QIAGEN, Hilden, Germany) assessed by Nanodrop spectrophotometer and quality controlled using an Agilent Bioanalyzer (Agilent Technologies, Santa Clara, CA, United States). Four groups of 30 Nauplius I were exposed to 2–4 μg of PAH dsRNA (dsPAH: 2 μg and dsPAH 2X: 4 μg) and 2 μg of non-related LRK dsRNA in 100 μl of sea water, respectively. Nauplii were exposed to dsRNA for 16 h. Two control groups were used: negative control (without any dsRNA) and larvae exposed to a non-related LRK dsRNA. The whole experiment was performed at 9°C. The gene knockdown was assessed by phenotypic changes and quantitative PCR for all treatment groups. The samples were flash frozen in liquid nitrogen and stored at −80°C for RNA extraction and gene expression analysis. The whole experiment was repeated twice with the same outcome.
Quantitative RT-PCR (qPCR)
Transcription levels were monitored by qPCR using salmon louse elongation factor 1α (ELf1α) (Frost and Nilsen, 2003) and ADP, ATP carrier protein 3 (ADT3) as reference genes. The qPCR was performed using SYBR green assays and melting curve analyses were carried out to check for primer-dimers or non-specific amplifications. Thermal cycling was performed on Roche Lightcycler® 96 system in 20 μl reactions under standard conditions. Relative expression of the target gene was quantified with the 2–ΔΔCT method (Schmittgen and Livak, 2008) using the geometric average of expression levels of ELf1α and ADT3 genes for normalization. T-tests were performed on qBase + software, version 3.2 (Biogazelle, Zwijnaarde, Belgium)2 to determine if controls and RNAi groups were differentially expressed with p-value of 0.05 as a threshold.
Cuticle Morphology and Staining
The specimens were imaged using Zeiss Axio Zoom.V16 (Carl Zeiss GmbH, Jena, Germany) and the length was measured from head to end of abdomen using ImageJ (Schneider et al., 2012). The length was analyzed by one-way ANOVA followed by post hoc multiple comparison test conducted in R (R Core Team, 2019) and figures were produced using the package ggplot2 (Wickham, 2016). For cuticle staining and imaging, the samples were fixed and stored in 10% (w/v) formaldehyde in Phosphate buffered saline prior to analysis. The specimens were thoroughly washed in distilled water and then Congo red (Sigma Aldrich, Steinheim, Germany) solution (1.5 mg/ml H2O) was added and the specimens were stained at room temperature for 24 h, transferred to distilled water and left for 5 min. The specimens were thoroughly washed several times with distilled water and then mounted in ibiTreat microwells on a thin layer of agarose for imaging. The specimens were imaged using a Zeiss LSM 880 Airyscan (Carl Zeiss GmbH, Jena, Germany) equipped with a Plan-Apochromat 10x/0.45 and a Plan-Apochromat 20x/0.8 objective. Congo red stain was excited by the 561 nm laser line and emission was detected in the range 587–731 nm.
Results
Sequence Analysis
The salmon louse PAH cDNA sequence was verified by amplification of cDNA using specific primers. We confirmed the cDNA and exon-intron structure by aligning the transcript sequence with L. salmonis genome sequence EMLSAG00000009260 (LsalAtl2s)3. This showed that the gene is composed of 7 exons with a non-coding first exon. The mapping of RNAseq reads from the Sequence Read Archive (SRA) at NCBI to the genome sequence indicated that the splice acceptor in intron 1 is located 42 nucleotides downstream of what is predicted in the EMBL gene model (EMLSAT00000009260) (Supplementary Figure 1). This transcript was found at a very low frequency in adult stages and indicates a rare spliced variant. The ORF is translated into a 451 amino acid long polypeptide with a predicted molecular mass of 51.8 kDa and an isoelectric point of 5.6. The structure of the protein was predicted using the Phyre2 server. Similar to other characterized PAH proteins, LsPAH contains a putative amino acid binding site in the conserved ACT regulatory domain (Figure 1, yellow), a conserved tetrahydrobiopterin (BH4) cofactor binding site (Figure 1, cyan), a metal-binding site for iron atoms (Figure 1, green) and a substrate-binding pocket (Figure 1, pink). The L. salmonis full-length protein BLAST search indicated 88% identity with PAH in Caligus rogercresseyi, 61% identity with Henna in Acanthaster planci and 63% identity in Drosophila melanogaster. Phylogenetic analysis revealed that LsPAH belongs to the arthropod PAH family. The outcome was a particular clustering of LsPAH with crustaceans and more specifically with copepods (Figure 2).
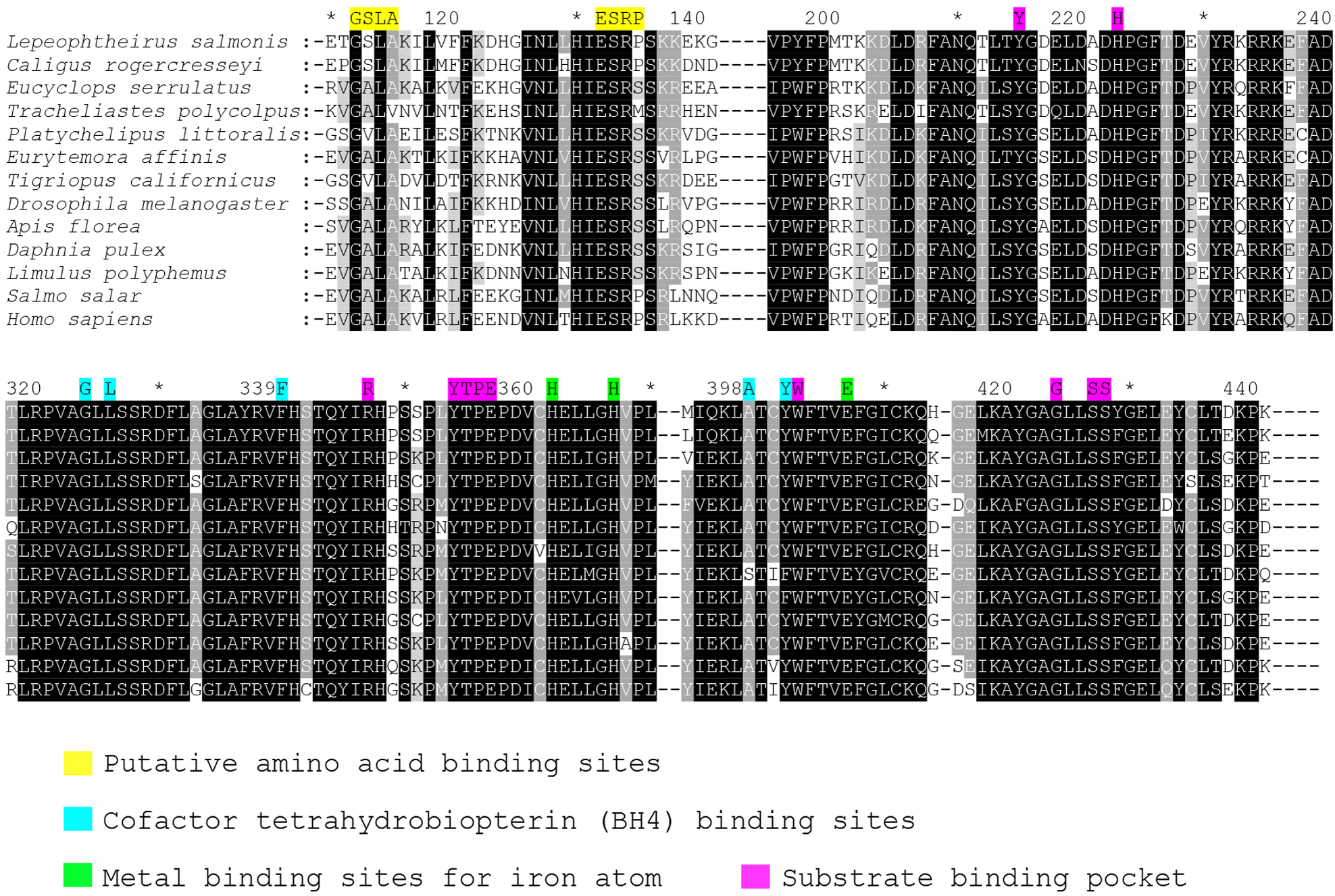
Figure 1. Sequence alignment of PAH proteins. A partial sequence alignment of PAH protein from various organisms. The conserved binding domains are indicated by different colors.
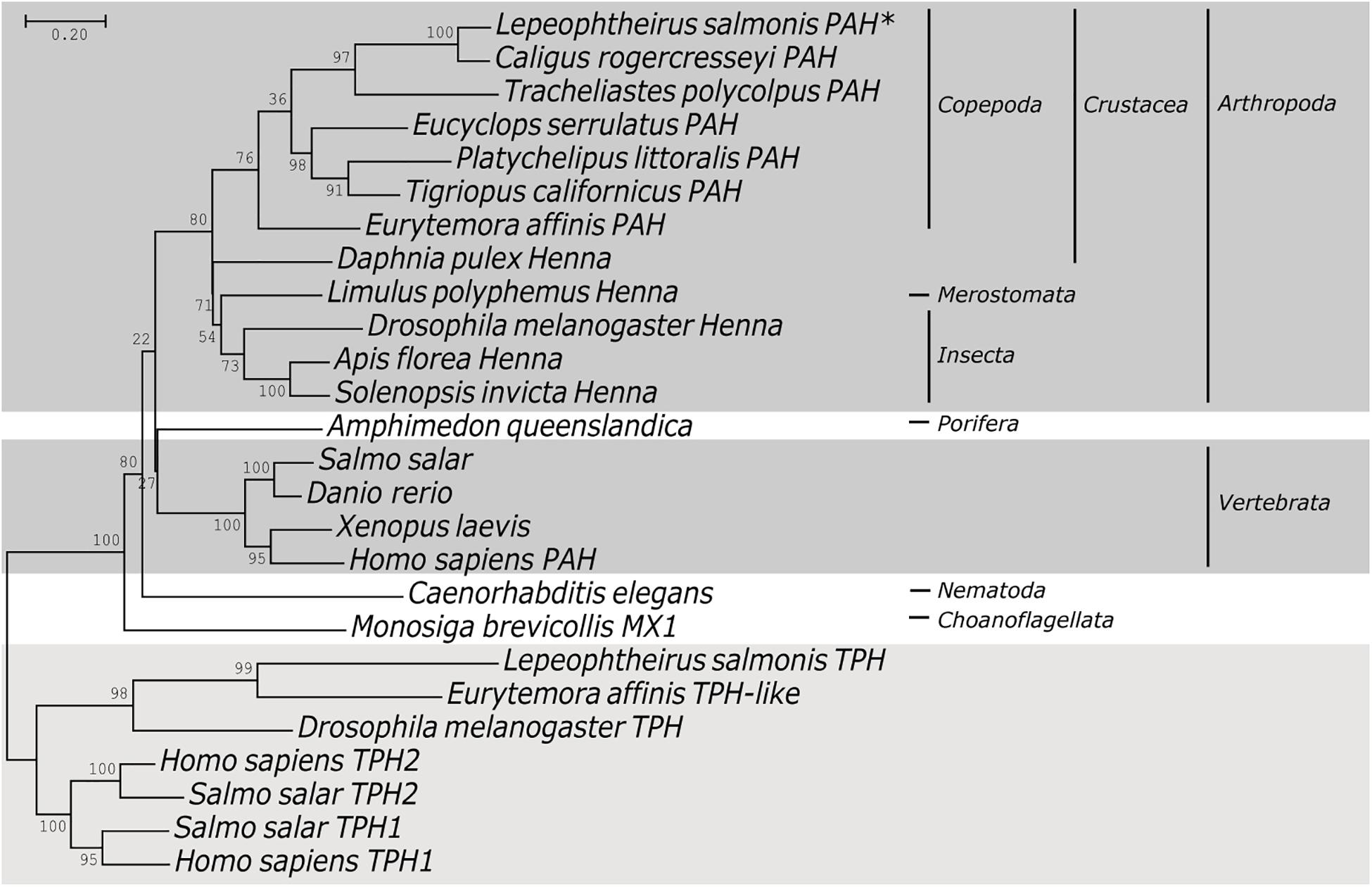
Figure 2. Phylogenetic tree of PAH proteins. Phylogenetic analysis of phenylalanine hydroxylase (PAH) among different organisms. A Maximum Likelihood tree was generated using MEGA7, with bootstrap values from 1000 replications. The sequences used for the construction of phylogenetic trees are listed in Supplementary Table 2.
Developmental Stage Gene Expression
Analysis of transcript levels using qPCR indicated that LsPAH was expressed at all developmental stages of salmon lice (Figure 3). Expression levels of LsPAH was highest in copepodites followed by adult males and adult females. The expression levels of LsPAH was relatively invariant in all other stages, with the lowest expression levels found in nauplius I.
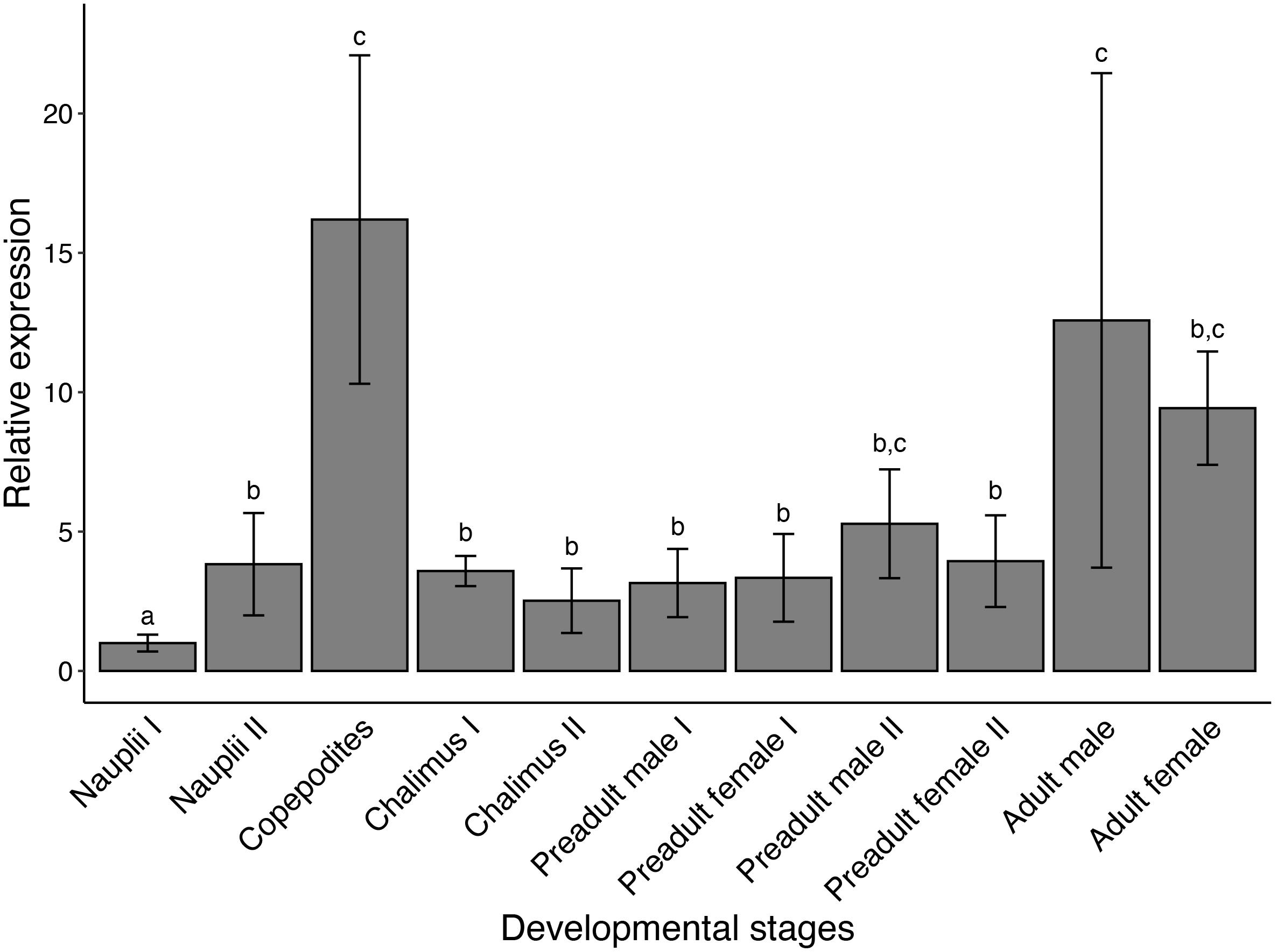
Figure 3. Developmental expression profile of LsPAH. Expression of the transcript levels of LsPAH in salmon louse developmental stages relative to transcript level in the nauplius I. Error bars represent the SE (n = 4 independent biological replicates). Each biological replicate contained 50 individuals for planktonic life-stages (nauplii I, nauplii II, and copepodites), five individuals for chalimus I and chalimus II and one individual each for pre-adult and adult life-stages. Data were analyzed using qBaseplus+ and one-way ANOVA was performed. Life stages labeled with different letters are significantly different from each other (p < 0.001).
RNAi Mediated Knockdown of LsPAH
Validation of the knockdown of LsPAH by RNAi was done using qPCR. Knockdown was assessed in copepodites 181 h post-hatching at 9°C. A significant reduction in the amount of transcripts was measured after the exposure to LsPAH dsRNA compared to the control groups (Figure 4A). The expression of genes downstream in the pathway leading to the production of the catecholamines was also assessed by qPCR (Figure 4B). There were no significant changes between the control and treatment groups in these downstream genes. There was no significant regulation in Pale, DOPA decarboxylase, Phenylethanolamine N-methyltransferase, Dopamine hydroxylase, and NBAD hydrolase in all treatment groups.
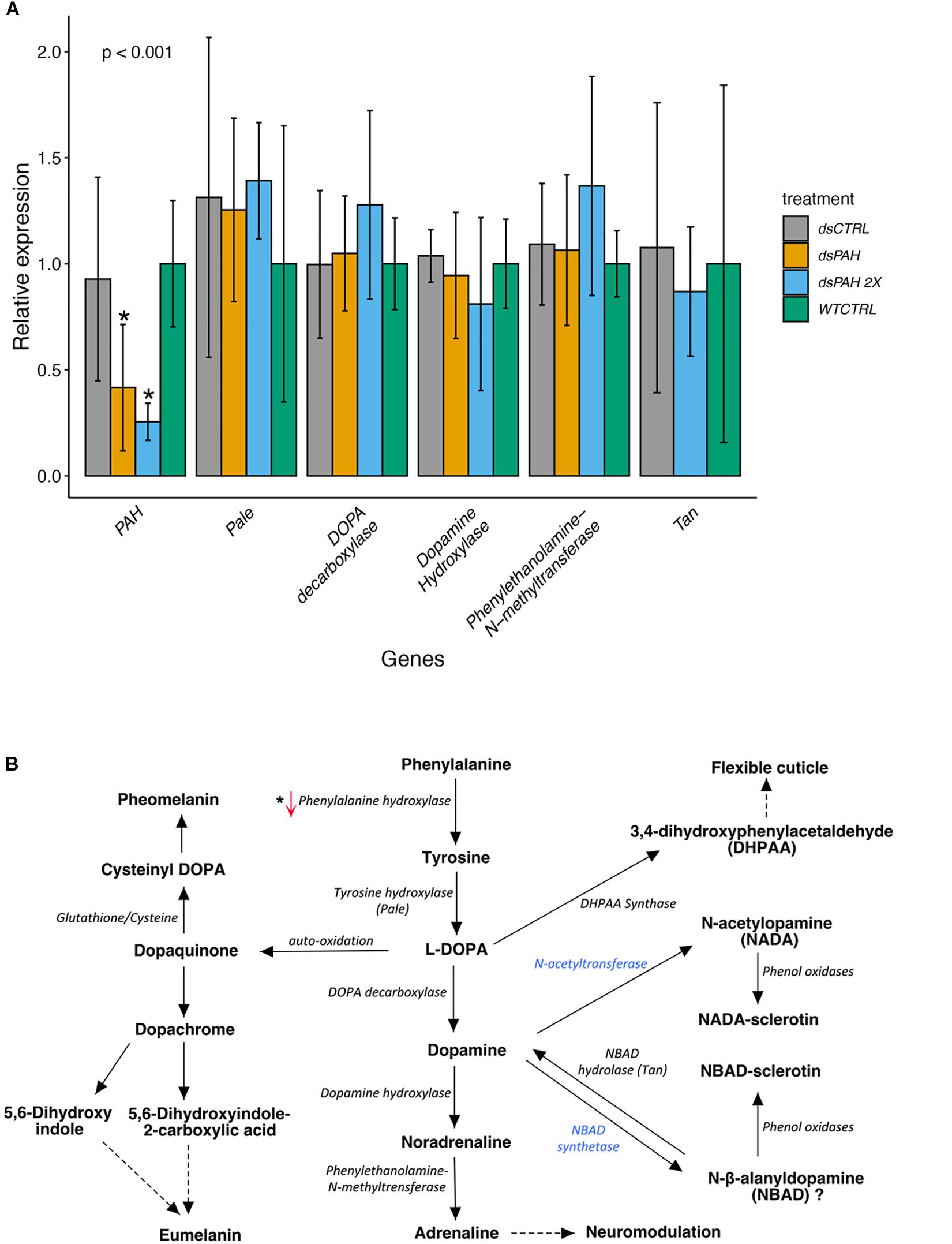
Figure 4. Gene expression of various genes following dsRNA treatment. (A) RT-qPCR determination of the various genes in Phenylalanine metabolism pathway. The expression level of LsPAH, LsPale and other genes involved in catecholamine synthesis pathway of dsRNA treated larvae, relative to expression level of the dsRNA controls. The Elf1α and ADT3 genes were used for data normalization. The data were analyzed with one-way ANOVA for the downregulation and statistically significant results are marked with asterisk (p < 0.001). dsPAH, dsRNA Phenylalanine hydroxylase; dsCTRL, non-specific dsRNA and WTCTRL, Wildtype on seawater only. Each treatment contained 5 to 8 biological replicates. (B) The metabolic pathway of phenylalanine metabolism. The gene changes in transcriptional levels are marked by upward and downward arrows for upregulation and downregulation, respectively. Blue color indicates the absence of the gene in L. salmonis.
RNAi Mediated Knockdown of LsPAH Leads to Developmental Defects, Molting Arrest, and Decreased Swimming Performance
All treatment with dsPAH produced larvae with a distinct phenotype differing from the control groups (Figure 5A). The dsPAH treated nauplii were not able to completely molt to copepodite, and their heads were enlarged (when observed at the same time in development). The average length of the larvae measured after 181 h showed a significantly decreased body length of treatment groups compared to control groups (Figure 5B) and molting flaws (Figure 5C).
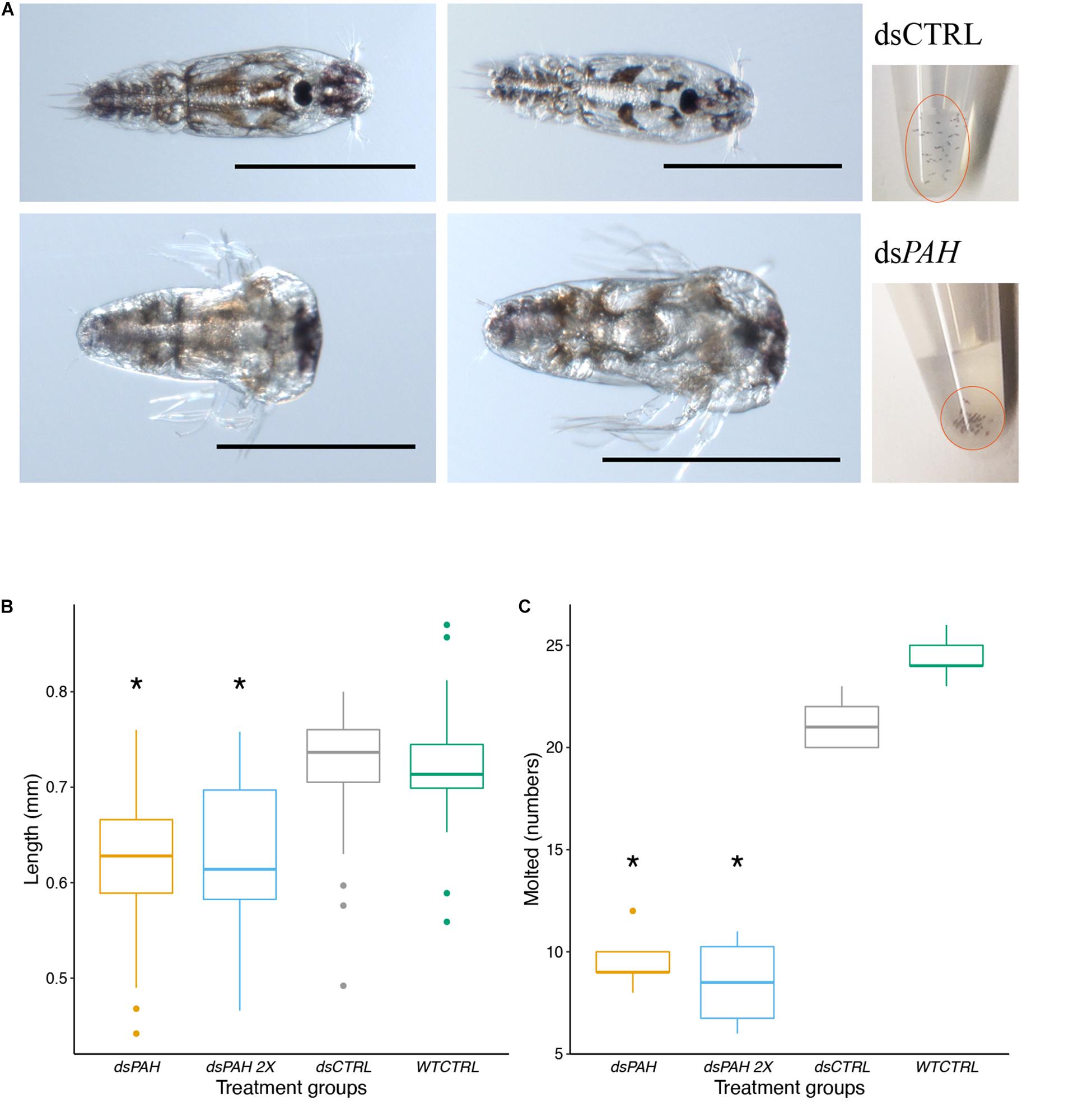
Figure 5. Larvae phenotypes induced by LsPAH RNAi. (A) The dsPAH treated larvae (lower panel) were not able to molt to copepodite or molted to a defective copepodite with an enlarged head. After 16 h treatment, dsPAH treated nauplii were lethargic and clustered in the bottom of the tube whereas mock dsCTRL (upper panel) were swimming freely and dispersed to the top level of water column. Scale bars = 500 μm. (B) The variation in larval length in relation to phenotype. The results are displayed as boxplot, n ≥ 34 individuals per treatment. Data were analyzed by one-way ANOVA followed by a post hoc multiple comparison test (Tukey’s HSD test; p < 0.001). (C) Comparison of the nauplii that molted completely to copepodite in various RNAi treatments. The results are reported as boxplot, n ≥ 5 for each group containing 30 individuals. Data were analyzed by one-way ANOVA followed by a post hoc multiple comparison test (Tukey’s HSD test; p < 0.001). The significant differences compared to controls are denoted with an asterisk.
dsPAH knockdown produced larvae with developmental defects. Knockdown larvae had asymmetric body formation during the developmental stages compared to control treatments (Figure 6). The nauplii are equipped with three exterior appendages anteriorly on both sides, and these appendages were bent and deformed in the dsPAH treated nauplii when compared to control lice. In underdeveloped copepodites, the loss of symmetry was still present, and the segmentation of cephalothorax was irregular (Figure 6D). Additionally, for the dsPAH treatment phenotype, none of the external appendages from the anterior segment could be extracted from the shed exuvia during ecdysis.
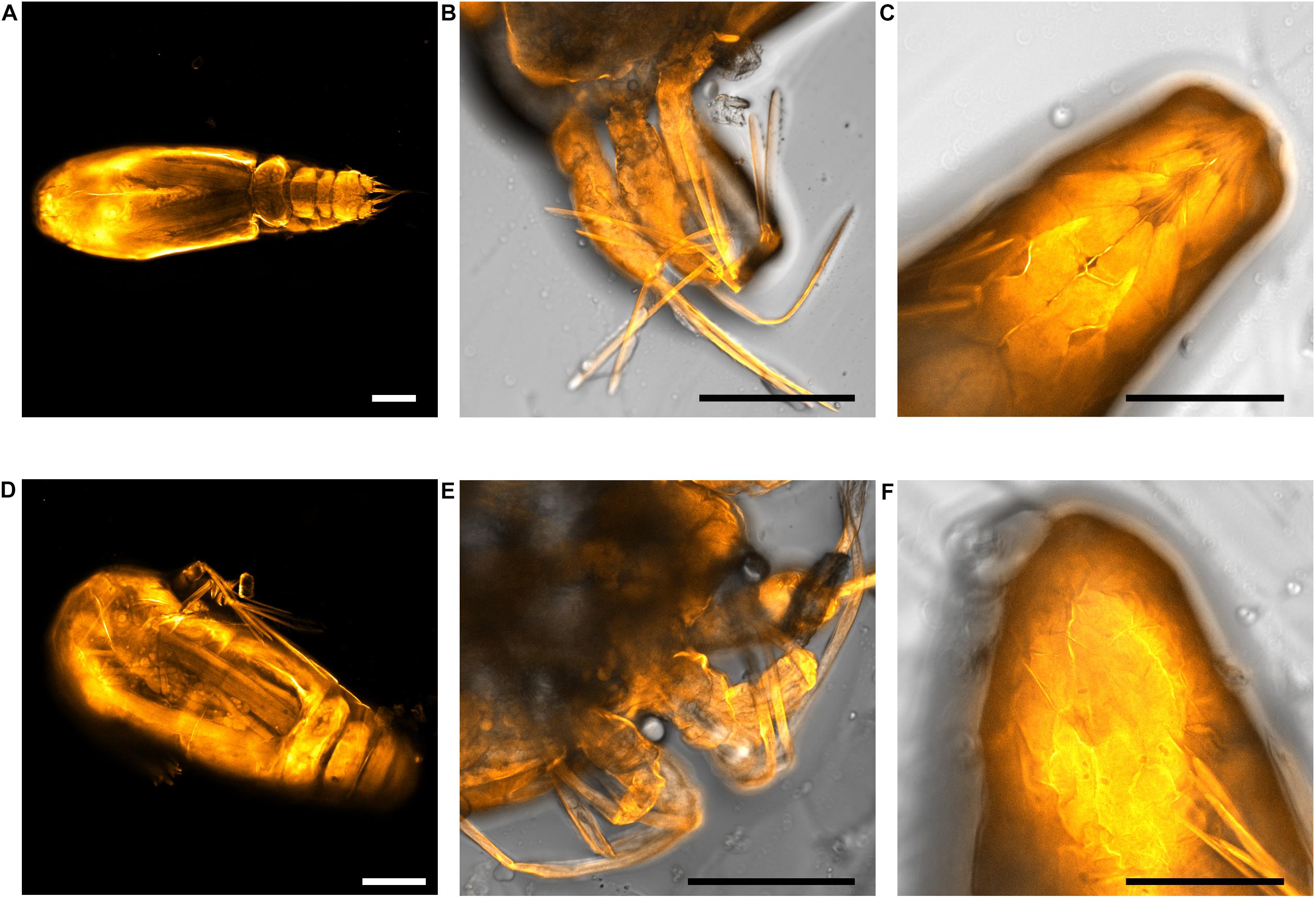
Figure 6. Cuticle staining of lice larvae. Upper panel: dsCTRL control, and lower panel: dsPAH treatment. (A,D) Copepodites treated with dsPAH revealed the unorganized internal structure and developmental defects compared to control. The cephalothorax is not uniformly segmented and is poorly formed in dsPAH treated copepodites. (B,E) The external appendages in nauplius II were distorted in dsPAH treatment compared to the control. (C,F) The posterior segment of the nauplius II just after molting was symmetrically arranged, with a centrally positioned hindgut, but this symmetry was absent in treated individuals. Scale bars = 100 μm.
The loss of function phenotype related to swimming behavior was observed at the end of the treatment period. The dsPAH treatment group had reduced mobility and sunk to the bottom of the eppendorf tube in contrast to the mock control and wild type nauplii when observed at the end of the 16 h dsRNA soaking period (Figure 5A). The control groups showed normal movements and swimming freely. In contrast, the treatment groups were lethargic and clustered at the bottom of the flow-through wells on each daily observation after the soaking period.
Discussion
In this study, we report the effect of downregulating the single copy gene LsPAH that is responsible for phenylalanine hydroxylase activity in salmon lice. Lower LsPAH activity is expected to result in reduced metabolism of the amino acid phenylalanine and affect tyrosine production. Downregulation of the LsPAH activity therefore provides an opportunity for functional analysis of disrupted phenylalanine catabolism (Simonet et al., 2016). We demonstrate a direct effect of LsPAH knockdown on the molting and development of the salmon lice.
LsPAH is expressed throughout the salmon louse lifecycle, reaching the highest expression during the copepodite stage followed by the adult stage. In Drosophila, the expression of PAH was reported to increase throughout embryonic and larval development stages with peak level occurring at pupation (Morales et al., 1990; Bel et al., 1992). The developmental expression pattern of the LsPAH in salmon lice is indicative of a vital role during the larval stage and molting. Using a RNAi based approach focusing on the nauplius stages, we demonstrated an essential role of LsPAH in molting and swimming behavior. Further examination of the larvae demonstrated that the LsPAH knockdown interferes with the ability to move, and the abnormal enlargement of the head impairs molting into the copepodite from nauplius II.
Previous studies have shown that the PAH inactivation disrupted melanin associated processes in egg, larval and adult stages and embryonic development. For example, in a holometabolous insect, Acyrthosiphon pisum, the PAH knockdown resulted in reduced adult lifespan and fecundity and impaired embryonic development in the offspring (Simonet et al., 2016). We observed similar developmental defects in salmon louse. In Bombyx mori, the PAH knockdown resulted in the failed coloration of the neonatal larvae (Chen et al., 2013). Similarly, the oviposition rate, chorion maturity, and egg hatchability were reduced in mosquitoes and silkworm following the PAH inactivation (Chen et al., 2013; Fuchs et al., 2014). In C. elegans, blister 3 (bli-3) mutants that lack dual oxidase catalyzing the tyrosine cross-linking in stabilization of cuticle, the double mutant bli-3 and pah-1 had severe phenotype changes with variable growth problems, cuticle abnormalities, growth arrest and incomplete molting with a dumpy body shape (Calvo et al., 2008). The currently known sclerotization model in arthropods is a complex process, which includes the hydroxylation of tyrosine to 3,4-dihydroxyphenylalanine (DOPA), which by decarboxylation is changed to dopamine, a compound of central significance to both sclerotization and melanin formation (Andersen, 2012). In Drosophila, the derivatives of dopamine are essential in the hardening of the cuticle. Insects produce from eumelanin, pheomelanin and dopamine derivatives like N-acetyldopamine (NADA) and N-β-alanyldopamine (NBAD), precursors for the protein crosslinking and hardening of cuticle (Sugumaran, 1998, 2009; Wittkopp and Beldade, 2009). In addition, the oxidized NADA and NBAD quinones, go through isomerization to quinone methides and crosslinking reactions with cuticle proteins side chains (most likely histidyl residues) for cuticle sclerotization (Kramer et al., 2001). Interestingly, from extensive analysis, we found that the proteins vital in the synthesis of NADA and NBAD, N-acetyltransferase and NBAD synthetase, respectively, were missing in salmon lice. However, NBAD hydrolase that hydrolyzes NBAD back to dopamine was present but no significant regulation was observed. A protein (DHPAA synthase) in Aedes aegypti, similar to DOPA decarboxylase, catalyzes the L-Dopa to 3,4-dihydroxyphenylacetaldehyde (DHPAA). It was suggested that DHPAA synthase has a role in the formation of flexible cuticle through the reactive DHPAA-mediated protein crosslinking reactions (Vavricka et al., 2011). So far, there have been no published studies of the PAH functions in copepods.
The reduced swimming ability and developmental defects could be related to reduced levels of tyrosine. Tyrosine deficiency leads to changes in metabolic derivatives like catecholamines and melanin, which are important in the formation of exoskeleton, eye and body pigmentation and neurotransmission (Wright, 1987; True, 2003; Christensen et al., 2005; Vavricka et al., 2014). Since the arthropod exoskeleton is a prerequisite for locomotor apparatus formation and other body segments, this may explain the observed molting difficulties during formation of the new exoskeleton. In nematodes, it was indicated that the PAH might have a role in supplying L-Tyr for forming L-Tyr cross links between cuticle proteins for mechanical stability (Loer et al., 1999; Calvo et al., 2008). We propose that the reduced swimming activity, molting defects and developmental deformation observed in salmon louse larvae are directly related to the exoskeleton deformation caused by the lack of tyrosine, and/or its metabolic derivatives. The external appendages were distorted in the LsPAH downregulated nauplii. Similar phenotypes were observed in double knockdown of ecdysone receptor and retinoid X receptor with developmental defects in salmon louse (Sandlund et al., 2016). The defects in locomotor apparatus due to exoskeleton deformation could explain the decreased swimming capacity. Furthermore, the increase in phenylalanine level in the larvae, that could be toxic for their development, as shown in phenylketonuria (PKU) in mammalian models (Williams et al., 2008). PAH is a rate-limiting enzyme in the catabolism of phenylalanine and its failure results in a subsequent deficiency of catecholamine neurotransmitter derivatives of tyrosine (i.e., dopamine, noradrenaline and adrenaline) leading to neuromodulation complications (Landvogt et al., 2008; Sawin et al., 2014) that could explain the reduction in movements and swimming performance.
In summary, downregulation of the salmon lice PAH indicates an important role in formation of the exoskeleton of the naupliar stages. Morphological changes of the body parts of the lice nauplii results in reduced swimming capacity and a termination of development from nauplii II to copepodite.
Data Availability Statement
The datasets presented in this study can be found in online repositories. The names of the repository/repositories and accession number(s) can be found in the article/Supplementary Material.
Ethics Statement
The experiments were conducted according to Norwegian regulations on animal research. A laboratory strain of L. salmonis (LsGulen) was raised on Atlantic salmon in NTNU SeaLab in tanks with seawater as previously described (Hamre et al., 2009).
Author Contributions
PG and PW conceived and designed the study, and analyzed the data. PG performed the experiments and wrote the manuscript, with input from AMB, ASB, PW, and YO. BS and AS performed imaging. ASB participated in the design and coordination of the project. AMB and YO provided access to crucial research components. All authors have reviewed and approved the manuscript.
Conflict of Interest
The authors declare that the research was conducted in the absence of any commercial or financial relationships that could be construed as a potential conflict of interest.
Funding
This study was part of the project “Taskforce Salmon Lice” at NTNU funded by the salmon industry in Mid-Norway, the Norwegian Seafood Research Fund (project number 901241), and NTNU (https://www.ntnu.edu/oceans/taskforce).
Acknowledgments
The authors would like to thank Torfinn Sparstad for the technical help in FUGE lab, and Reidun Vadla and Stine Østerhus for the help in lice lab.
Supplementary Material
The Supplementary Material for this article can be found online at: https://www.frontiersin.org/articles/10.3389/fmars.2020.608463/full#supplementary-material
Footnotes
References
Aaen, S. M., Helgesen, K. O., Bakke, M. J., Kaur, K., and Horsberg, T. E. (2015). Drug resistance in sea lice: a threat to salmonid aquaculture. Trends Parasitol. 31, 72–81. doi: 10.1016/j.pt.2014.12.006
Andersen, S. O. (2012). “6 - Cuticular sclerotization and tanning,” in Insect Molecular Biology and Biochemistry, ed. L. I. Gilbert (San Diego: Academic Press), 167–192. doi: 10.1016/B978-0-12-384747-8.10006-6
Bel, Y., Bruce Jacobson, K., Silva, F. J., and Ferré, J. (1992). Developmental and biochemical studies on the phenylalanine hydroxylation system in Drosophila melanogaster. Insect Biochem. Mol. Biol. 22, 633–638. doi: 10.1016/0965-1748(92)90041-C
Braden, L., Michaud, D., Igboeli, O. O., Dondrup, M., Hamre, L., Dalvin, S., et al. (2020). Identification of critical enzymes in the salmon louse chitin synthesis pathway as revealed by RNA interference-mediated abrogation of infectivity. Int. J. Parasitol. 50, 873–889. doi: 10.1016/j.ijpara.2020.06.007
Calvo, A. C., Pey, A. L., Ying, M., Loer, C. M., and Martinez, A. (2008). Anabolic function of phenylalanine hydroxylase in Caenorhabditis elegans. FASEB J. 22, 3046–3058. doi: 10.1096/fj.08-108522
Chen, P., Li, L., Wang, J., Li, H., Li, Y., Lv, Y., et al. (2013). BmPAH catalyzes the initial melanin biosynthetic step in Bombyx mori. PLoS One 8:e71984. doi: 10.1371/journal.pone.0071984
Christensen, B. M., Li, J., Chen, C. C., and Nappi, A. J. (2005). Melanization immune responses in mosquito vectors. Trends Parasitol. 21, 192–199. doi: 10.1016/j.pt.2005.02.007
Eichner, C., Nilsen, F., Grotmol, S., and Dalvin, S. (2014). A method for stable gene knock-down by RNA interference in larvae of the salmon louse (Lepeophtheirus salmonis). Exp. Parasitol. 140, 44–51. doi: 10.1016/j.exppara.2014.03.014
Erlandsen, H., Kim, J. Y., Patch, M. G., Han, A., Volner, A., Abu-Omar, M. M., et al. (2002). Structural comparison of bacterial and human iron-dependent phenylalanine hydroxylases: similar fold, different stability and reaction rates. J. Mol. Biol. 320, 645–661. doi: 10.1016/S0022-2836(02)00496-5
Flydal, M. I., and Martinez, A. (2013). Phenylalanine hydroxylase: function, structure, and regulation. IUBMB Life 65, 341–349. doi: 10.1002/iub.1150
Frost, P., and Nilsen, F. (2003). Validation of reference genes for transcription profiling in the salmon louse, Lepeophtheirus salmonis, by quantitative real-time PCR. Vet. Parasitol. 118, 169–174. doi: 10.1016/j.vetpar.2003.09.020
Fuchs, S., Behrends, V., Bundy, J. G., Crisanti, A., and Nolan, T. (2014). Phenylalanine metabolism regulates reproduction and parasite melanization in the malaria mosquito. PLoS One 9:e84865. doi: 10.1371/journal.pone.0084865
Hamre, L., Bui, S., Oppedal, F., Skern-Mauritzen, R., and Dalvin, S. (2019). Development of the salmon louse Lepeophtheirus salmonis parasitic stages in temperatures ranging from 3 to 24°C. Aquac. Environ. Interact. 11, 429–443. doi: 10.3354/aei00320
Hamre, L. A., Glover, K. A., and Nilsen, F. (2009). Establishment and characterisation of salmon louse (Lepeophtheirus salmonis (Krøyer 1837)) laboratory strains. Parasitol. Int. 58, 451–460. doi: 10.1016/j.parint.2009.08.009
Infanger, L. C., Rocheleau, T. A., Bartholomay, L. C., Johnson, J. K., Fuchs, J., Higgs, S., et al. (2004). The role of phenylalanine hydroxylase in melanotic encapsulation of filarial worms in two species of mosquitoes. Insect Biochem. Mol. Biol. 34, 1329–1338. doi: 10.1016/j.ibmb.2004.09.004
Johnson, S. C., and Albright, L. J. (1991). Development, growth, and survival of Lepeophtheirus Salmonis (Copepoda: Caligidae) under laboratory conditions. J. Mar. Biol. Assoc. U. K. 71, 425–436. doi: 10.1017/S0025315400051687
Kaufman, S. (1993). The phenylalanine hydroxylating system. Adv. Enzymol. Relat. Areas Mol. Biol. 67, 77–264. doi: 10.1002/9780470123133.ch2
Komisarczuk, A. Z., Kongshaug, H., Li, M., and Nilsen, F. (2019). RNAi mediated myosuppressin deficiency affects muscle development and survival in the salmon louse (Lepeophtheirus salmonis). Sci. Rep. 9, 1–18. doi: 10.1038/s41598-019-43515-w
Kramer, K. J., Kanost, M. R., Hopkins, T. L., Jiang, H., Zhu, Y. C., Xu, R., et al. (2001). Oxidative conjugation of catechols with proteins in insect skeletal systems. Tetrahedron 57, 385–392. doi: 10.1016/S0040-4020(00)00949-2
Kristoffersen, A. B., Jimenez, D., Viljugrein, H., Grøntvedt, R., Stien, A., and Jansen, P. A. (2014). Large scale modelling of salmon lice (Lepeophtheirus salmonis) infection pressure based on lice monitoring data from Norwegian salmonid farms. Epidemics 9, 31–39. doi: 10.1016/j.epidem.2014.09.007
Kristoffersen, A. B., Qviller, L., Helgesen, K. O., Vollset, K. W., Viljugrein, H., and Jansen, P. A. (2018). Quantitative risk assessment of salmon louse-induced mortality of seaward-migrating post-smolt Atlantic salmon. Epidemics 23, 19–33. doi: 10.1016/j.epidem.2017.11.001
Kumar, S., Stecher, G., and Tamura, K. (2016). MEGA7: molecular evolutionary genetics analysis version 7.0 for bigger datasets. Mol. Biol. Evol. 33, 1870–1874. doi: 10.1093/molbev/msw054
Landvogt, C., Mengel, E., Bartenstein, P., Buchholz, H. G., Schreckenberger, M., Siessmeier, T., et al. (2008). Reduced cerebral fluoro-L-dopamine uptake in adult patients suffering from phenylketonuria. J. Cereb. Blood Flow Metab. 28, 824–831. doi: 10.1038/sj.jcbfm.9600571
Le, S. Q., and Gascuel, O. (2008). An improved general amino acid replacement matrix. Mol. Biol. Evol. 25, 1307–1320. doi: 10.1093/molbev/msn067
Loer, C. M., Davidson, B., and McKerrow, J. (1999). A phenylalanine hydroxylase gene from the nematode C. elegans is expressed in the hypodermis. J. Neurogenet. 13, 157–180. doi: 10.3109/01677069909083472
Mito, T., Nakamura, T., and Noji, S. (2010). Evolution of insect development: to the hemimetabolous paradigm. Curr. Opin. Genet. Dev. 20, 355–361. doi: 10.1016/j.gde.2010.04.005
Morales, G., Requena, J., Jimenez-Ruiz, A., Lopez, M. C., Ugarte, M., and Alonso, C. (1990). Sequence and expression of the Drosophila phenylalanine hydroxylase mRNA. Gene 93, 213–219. doi: 10.1016/0378-1119(90)90227-I
Overton, K., Dempster, T., Oppedal, F., Kristiansen, T. S., Gismervik, K., and Stien, L. H. (2019). Salmon lice treatments and salmon mortality in Norwegian aquaculture: a review. Rev. Aquac. 11, 1398–1417. doi: 10.1111/raq.12299
Pike, A. W. (1989). Sea lice - Major pathogens of farmed atlantic salmon. Parasitol. Today 5, 291–297. doi: 10.1016/0169-4758(89)90020-3
Pike, A. W., and Wadsworth, S. L. (1999). Sealice on salmonids: their biology and control. Adv. Parasitol. 44, 233–337. doi: 10.1016/s0065-308x(08)60233-x
Sandlund, L., Nilsen, F., Male, R., and Dalvin, S. (2016). The ecdysone receptor (EcR) is a major regulator of tissue development and growth in the marine salmonid ectoparasite, Lepeophtheirus salmonis (Copepoda, Caligidae). Mol. Biochem. Parasitol. 208, 65–73. doi: 10.1016/j.molbiopara.2016.06.007
Sawin, E. A., Murali, S. G., and Ney, D. M. (2014). Differential effects of low-phenylalanine protein sources on brain neurotransmitters and behavior in C57Bl/6-Pahenu2 mice. Mol. Genet. Metab. 111, 452–461. doi: 10.1016/j.ymgme.2014.01.015
Schmittgen, T. D., and Livak, K. J. (2008). Analyzing real-time PCR data by the comparative C(T) method. Nat. Protoc. 3, 1101–1108. doi: 10.1038/nprot.2008.73
Schneider, C. A., Rasband, W. S., and Eliceiri, K. W. (2012). NIH Image to ImageJ: 25 years of image analysis. Nat. Methods 9, 671–675. doi: 10.1038/nmeth.2089
Simonet, P., Gaget, K., Parisot, N., Duport, G., Rey, M., Febvay, G., et al. (2016). Disruption of phenylalanine hydroxylase reduces adult lifespan and fecundity, and impairs embryonic development in parthenogenetic pea aphids. Sci. Rep. 6, 1–12. doi: 10.1038/srep34321
Sterkel, M., and Oliveira, P. L. (2017). Developmental roles of tyrosine metabolism enzymes in the blood-sucking insect Rhodnius prolixus. Proc. R. Soc. B Biol. Sci. 284:2607. doi: 10.1098/rspb.2016.2607
Sugumaran, M. (1998). Unified mechanism for sclerotization of insect cuticle. Adv. Insect Physiol. 27, 229–334. doi: 10.1016/s0065-2806(08)60014-4
Sugumaran, M. (2009). Complexities of cuticular pigmentation in insects. Pigment Cell Melanoma Res. 22, 523–525. doi: 10.1111/j.1755-148X.2009.00608.x
Torrissen, O., Jones, S., Asche, F., Guttormsen, A., Skilbrei, O. T., Nilsen, F., et al. (2013). Salmon lice - impact on wild salmonids and salmon aquaculture. J. Fish Dis. 36, 171–194. doi: 10.1111/jfd.12061
True, J. R. (2003). Insect melanism: the molecules matter. Trends Ecol. Evol. 18, 640–647. doi: 10.1016/j.tree.2003.09.006
Vavricka, C., Han, Q., Huang, Y., Erickson, S. M., Harich, K., Christensen, B. M., et al. (2011). From L-dopa to dihydroxyphenylacetaldehyde: a toxic biochemical pathway plays a vital physiological function in insects. PLoS One 6:e016124. doi: 10.1371/journal.pone.0016124
Vavricka, C. J., Han, Q., Mehere, P., Ding, H., Christensen, B. M., and Li, J. (2014). Tyrosine metabolic enzymes from insects and mammals: a comparative perspective. Insect Sci. 21, 13–19. doi: 10.1111/1744-7917.12038
Wagner, G. N., Fast, M. D., and Johnson, S. C. (2008). Physiology and immunology of Lepeophtheirus salmonis infections of salmonids. Trends Parasitol. 24, 176–183. doi: 10.1016/j.pt.2007.12.010
Williams, R. A., Mamotte, C. D. S., and Burnett, J. R. (2008). Phenylketonuria: an inborn error of phenylalanine metabolism. Clin. Biochem. Rev. 29, 31–41.
Wittkopp, P. J., and Beldade, P. (2009). Development and evolution of insect pigmentation: genetic mechanisms and the potential consequences of pleiotropy. Semin. Cell Dev. Biol. 20, 65–71. doi: 10.1016/j.semcdb.2008.10.002
Keywords: RNA interference, phenylalanine hydroxylase, salmon lice, molting, larval development
Citation: Guragain P, Sporsheim B, Skjesol A, Båtnes AS, Olsen Y, Bones AM and Winge P (2020) Phenylalanine Hydroxylase RNAi Knockdown Negatively Affects Larval Development, Molting and Swimming Performance of Salmon Lice. Front. Mar. Sci. 7:608463. doi: 10.3389/fmars.2020.608463
Received: 22 September 2020; Accepted: 09 November 2020;
Published: 03 December 2020.
Edited by:
Enric Gisbert, Institute of Agrifood Research and Technology (IRTA), SpainReviewed by:
Manuel Manchado, Andalusian Institute for Research and Training in Agriculture, Fisheries, Food and Ecological Production (IFAPA), SpainSimon G. Webster, Bangor University, United Kingdom
Copyright © 2020 Guragain, Sporsheim, Skjesol, Båtnes, Olsen, Bones and Winge. This is an open-access article distributed under the terms of the Creative Commons Attribution License (CC BY). The use, distribution or reproduction in other forums is permitted, provided the original author(s) and the copyright owner(s) are credited and that the original publication in this journal is cited, in accordance with accepted academic practice. No use, distribution or reproduction is permitted which does not comply with these terms.
*Correspondence: Per Winge, cGVyLndpbmdlQG50bnUubm8=