Commentary: Combining Ecosystem and Single-Species Modeling to Provide Ecosystem-Based Fisheries Management Advice Within Current Management Systems
- 1Institute of Marine Research, Bergen, Norway
- 2National Marine Fisheries Service, Southeast Fisheries Science Center, National Oceanic and Atmospheric Administration (NOAA) Beaufort Laboratory, Beaufort, NC, United States
- 3Scottish Association for Marine Science, Scottish Marine Institute, Oban, United Kingdom
- 4Marine Institute, Oranmore, Ireland
- 5Department of Fisheries Biology, Humboldt State University, Arcata, CA, United States
- 6IFAS Nature Coast Biological Station, University of Florida, Cedar Key, FL, United States
- 7Maine Department of Marine Resources, State of Maine, West Boothbay Harbor, ME, United States
- 8Atlantic States Marine Fisheries Commission, Arlington, VA, United States
- 9Fisheries and Aquatic Ecosystems Branch, Agri-Food and Biosciences Institute, Belfast, United Kingdom
- 10National Marine Fisheries Service, Office of Science and Technology, Marine Ecosystems Division, Silver Spring, MD, United States
Although many countries have formally committed to Ecosystem-Based Fisheries Management (EBFM), actual progress toward these goals has been slow. This paper presents two independent case studies that have combined strategic advice from ecosystem modeling with the tactical advice of single-species assessment models to provide practical ecosystem-based management advice. With this approach, stock status, reference points, and initial target F are computed from a single-species model, then an ecosystem model rescales the target F according to ecosystem indicators without crossing pre-calculated single-species precautionary limits. Finally, the single-species model computes the quota advice from the rescaled target F, termed here Feco. Such a methodology incorporates both the detailed population reconstructions of the single-species model and the broader ecosystem perspective from ecosystem-based modeling, and fits into existing management schemes. The advocated method has arisen from independent work on EBFM in two international fisheries management systems: (1) Atlantic menhaden in the United States and (2) the multi species fisheries of the Irish Sea, in the Celtic Seas ecoregion. In the Atlantic menhaden example, the objective was to develop ecological reference points (ERPs) that account for the effect of menhaden harvest on predator populations and the tradeoffs associated with forage fish management. In the Irish Sea, the objective was to account for ecosystem variability when setting quotas for the individual target species. These two exercises were aimed at different management needs, but both arrived at a process of adjusting the target F used within the current single-species management. Although the approach has limitations, it represents a practical step toward EBFM, which can be adapted to a range of ecosystem objectives and applied within current management systems.
Introduction
Incorporating ecosystem information into fisheries management [i.e., Ecosystem Approach to Fisheries Management (EAFM) and Ecosystem-Based Fisheries Management (EBFM)] is an acknowledged goal of many nations based upon an agreed code of conduct for responsible fisheries management (FAO, 1995, FAO, 2008, NOAA, 2016). For simplicity, we will use the term EBFM throughout as it captures EAFM as well. EBFM has been identified as an approach to meet multiple fisheries and living resources legislative mandates (EU, 2008, EU, 2013; NOAA, 2016). Actual progress toward implementing EBFM is just beginning to be evident (e.g., Townsend et al., 2019), with some examples including limited ecosystem interactions (Marshall et al., 2019). However, current fisheries management is still largely based around single species stock assessments (Skern-Mauritzen et al., 2016). This limited progress can partly be attributed to the limitations of the modeling tools available to support management (Plagányi, 2007; Townsend et al., 2008) and also the structural inertia in the management systems themselves (Christie, 2005; Marshak et al., 2017) including a requirement to fit into existing regulatory frameworks.
Science has made progress in assessing and modeling ecosystems to inform fisheries management. Integrated Ecosystem Assessments (IEAs) are an approach to ecosystem-based management that aim to integrate components of an ecosystem, including humans, into the decision-making process so that managers can balance trade-offs and determine what management decisions are more likely to achieve objectives (Levin et al., 2009; Harvey et al., 2017). While IEAs are intended to form part of the advice process (ICES, 2019b), they have often tended to exist as standalone overviews, rather than being fully integrated into the advice-giving process (Cormier et al., 2017). Where they are integrated into the advice, they are typically used to provide context for the single species advice, rather than directly impacting tactical management. In the US, the National Oceanic and Atmospheric Administration (NOAA) Fisheries IEA program has been used to develop Ecosystem Status Reports that are frequently presented to Regional Fisheries Management Councils to provide an ecosystem context for management decision making (Slater et al., 2017). In Europe, the International Council for the Exploration of the Seas (ICES) produces Ecosystem Overviews (EOs), which are advice products with the same aim of providing ecosystem information to public authorities with competence for marine management, including the European Commission.
The use of ecosystem models, rather than an ecosystem status assessment, has the potential to provide more appropriate and quantitative inputs to the single species stock assessment models currently used to support advice. Full ecosystem models are now available for a number of ecoregions, for example, using both the Atlantis (Fulton et al., 2011) and Ecopath with Ecosim (EwE; Christensen and Walters, 2004) ecosystem modeling tools, as well as customized model sets developed for a specific region (e.g., Alaska Climate Integrated Modeling—ACLIM project; Hollowed et al., 2020). These models track the flow of energy through an entire ecosystem and allow the modeler to choose where to aggregate and where to have detail on a single species. Thus, they allow focus on species of interest while incorporating the entire ecosystem. However, while these models are used to give strategic information to managers about overall and long-term trends, they have not been previously considered suitable for tactical management use because of their inherent complexity and structural uncertainty (Collie et al., 2016). In this context, “tactical advice” comprises evaluation of stock status and setting of Total Allowable Catches (TACs), and other management measures such as spatio-temporal closures or gear regulation, while strategic management covers overall management objectives, socio-economic considerations, and selection of Harvest Control Rules.
While most single species assessments do not include any ecosystem considerations, beyond allowing for annually varying parameters such as weight-at-age or mortality, progress has been made in incorporating environmental fluctuations (Marshall et al., 2019). These include salmon forecasts (Satterthwaite et al., 2020), red tide (Sagarese et al., 2015) and variable predation, e.g., Atlantic mackerel Scomber scombrus (Moustahfid et al., 2009); NEA cod Gadus morhua, and capelin Mallotus villosus (Skern-Mauritzen et al., 2016), North Sea (ICES, 2020a), and Baltic (ICES, 2020b) assessments. The inclusion of environmental factors and predation allows for the formal inclusion of a limited range of ecological considerations, which can drive natural mortality and impact estimated productivity via recruitment or growth. Even when single species assessments include ecological considerations in some form, the main purpose of single species stock assessments is providing biological reference points, stock status determination, and forecasts of quotas or TAC. The advice from stock assessments are intended to be precautionary in the sense of attempting to avoid overfishing and hence stock collapse; meaning that the management based on the forecast TAC should limit the risk of population collapse and recruitment overfishing to within predefined limits. Bridging the gap between single species assessments and including broad ecosystem information into quota setting management has, thus far, proven elusive (Townsend et al., 2019).
Single species models have some key advantages over ecosystem models in estimating stock status and providing management advice, while ecosystem models are complementary in a management setting by quantifying broader ecological processes and uncertainties. Single species models focus on a smaller set of processes—population dynamics and harvest dynamics, and data-collection programs have largely been structured to address those interactions. This narrower focus makes them easier to create, update, and review. Single-species models are typically designed to track the details of fluctuations in a stock over time, while ecosystem models have often only been designed to represent overall trends. Single species assessments are designed to produce quantified estimates of the probability of stock collapse under different fishing pressures, while ecosystem models were not designed with this intention in mind. This last point is critical because many management systems legally require that fishing be “precautionary”—i.e., to keep the risk of stock collapse below a predefined level [The UN Fish Stocks Agreement UN FSA, 1995; Council Regulation 2019/124 (EU, 2019); Magnuson Stevens Fishery Conservation and Management Act (MSA), 2014]. However, while such single-species models can incorporate limited ecosystem or multispecies information, they cannot incorporate full ecosystem dynamics. Ecosystem models can explain some of the uncertainty that cannot be explained within single-species models, through attribution to ecological influences (trophic dynamics and/or environmental variability). Therein lies their value, because variability that can be explained and potentially managed can result in beneficial modifications to precautionary measures. However, given the complexity of ecosystem models and the single species governance structure of most fisheries management systems, it is difficult to see how ecosystem models could be used alone to duplicate the operational strengths of single species models in the foreseeable future. In theory, the two approaches are entirely complimentary, with single species models making implicit assumptions about how vital rates change (or remain constant) over time and ecological models being explicit about the influences of the surrounding environment (e.g., habitat, water quality, predator-prey abundances) on stock dynamics. A key challenge to moving EBFM forward is therefore to find ways of incorporating more ecosystem realism into the advice-giving process by combining the different skills and strengths of the two modeling approaches. Bridging the gap between single species assessments to include broader ecosystem information into actual management advice has, thus far, not been widely adopted (Townsend et al., 2019).
In this paper, we present two independent case studies that provided tactical management advice through the combined use of EwE models and single species assessments in two different settings: (1) Atlantic menhaden on the East Coast of the United States (Anstead et al., 2020; Drew et al., 2020; Chagaris et al., this volume) and (2) commercial fish stocks in the Irish Sea (Bentley et al., this volume). In both cases, an approach was developed which allowed ecosystem modeling results to be incorporated into the existing management structure, while retaining the single species assessment models used to support existing management. In both cases multiple different models (including ecosystem and multispecies models) were considered, and the EwE considered most appropriate given the management aims and state of model developments. Based on the success of these two case studies, we propose using ecosystem or multispecies models to adjust single species reference points to account for ecosystem understanding when providing management advice. We term the revised target fishing mortality Feco to highlight the ecosystem component of the advice. The approach is well suited to account for ecosystem considerations within existing single species management frameworks.
Case Study: Atlantic Menhaden
Atlantic menhaden Brevoortia tyrannus are distributed broadly along the U.S. Atlantic coast and are an important forage fish for a suite of predators that support valuable recreational fisheries. Atlantic menhaden are also harvested commercially using purse seines, processed into animal feed and vitamin supplements, and used for bait. Menhaden landings peaked during the 1950s at around 600,000 mt/yr and currently average about 180,000 mt/yr at a value of 39.4 million $USD, making it the largest fishery by tonnage on the U.S. East Coast. For nearly 20 years, the Atlantic States Marine Fisheries Commission (1981), the interstate fisheries management organization responsible for regulating the menhaden fishery, has pursued ecosystem approaches to managing this fishery. Since the first Atlantic States Marine Fisheries Commission (1981), managers have acknowledged the role of menhaden as forage for several recreationally important species (striped bass Morone saxatilis, bluefish Pomatomus saltatrix, and weakfish Cynoscion regalis) as well as marine mammals and birds. In the 2000s, several vocal environmental and sport-fishing groups were advocating for strict limits on the menhaden fishery, while the fishing industry pushed back. During this time, ASMFC engaged in ecosystem and multispecies modeling efforts to try to understand the potential impact of the menhaden fishery on its predators. However, as management objectives were unclear, modeling efforts were not well focused.
In 2010, ASFMC determined that ecological reference points (ERPs) that account for the dietary needs of menhaden’s predators were needed. Managers and stakeholders were concerned that recent declines in several predator stocks also managed by the ASMFC were linked to insufficient prey and wanted quantitative reference points that accounted for menhaden’s role as a forage fish to use for determining stock status and setting quotas. In 2012, ASMFC adopted single species reference points that provided more protection for spawning stock biomass than the previous target and threshold and established the first coastwide TAC for Atlantic menhaden to bring F below the new threshold. ASMFC also established the Ecological Reference Points Work Group (ERP WG), a technical workgroup comprised of scientists from state, federal, and academic agencies focused on developing ERPs for use in management. The ERP WG expanded on a previous set of ecosystem/multispecies models, but progress was hindered because management objectives had not been made explicit in an ecosystem context. To address this issue, ASMFC convened a workshop in 2015 to identify fundamental ecosystem management objectives for menhaden. Participants of the workshop included ASMFC managers, scientists, and stakeholders representing the menhaden fisheries, predator recreational fisheries, and environmental advocacy groups. The identified ecosystem objectives included: sustaining menhaden to provide for fisheries, sustaining menhaden to provide for predators, and providing stability for all types of fisheries. In addition to the stakeholder-oriented objective workshop, all ERP WG meetings were open to the public with regular opportunities for stakeholders to comment and ask questions.
With clear objectives, the ERP WG was able to more thoroughly evaluate models that could address the ecosystem objectives and develop approaches for establishing ERPs. The WG explored a suite of models ranging from simple to complex, including two extended surplus production models, a multi-species statistical catch-at-age model, and two EwE models of differing taxonomic and trophic complexity. These models were developed, evaluated, and vetted for management through a review process simultaneous with the menhaden single species stock assessment. Ultimately, an EwE model referred to as the Northwest Atlantic Continental Shelf-Model of Intermediate Complexity for Ecosystem Assessment (NWACS-MICE) was selected as the recommended tool to develop ERPs, based on its performance and its ability to inform the ecosystem management objectives identified by the ASMFC (Chagaris et al., this volume). The NWACS-MICE model focused on menhaden and four of its key predators that support commercial and recreational fisheries and are also managed by ASMFC—striped bass, bluefish, weakfish, and spiny dogfish Squalus acanthias. Each of these key species, except for spiny dogfish, were modeled with at least two age classes (juveniles and adults) to capture ontogenetic changes in trophic interactions. The model also included alternative forage fish, Atlantic herring Clupea harengus and anchovies Anchoa spp., and aggregated biomass pools for benthic invertebrates, zooplankton, phytoplankton, and detritus, for a total of 15 different species/functional groups and age classes in the model. The NWACS-MICE Ecosim model was calibrated to time series of relative abundance and catch from 1985 to 2017.
Striped bass are arguably the most important nearshore gamefish on the U.S. East Coast and a major menhaden predator. The NWACS-MICE and the full-complexity NWACS EwE models both found striped bass to be the predator most sensitive to menhaden harvest, such that measures to ensure healthy striped bass populations would extend to other predators in the system. Striped bass were also found to be overfished and experiencing overfishing in their most recent single species stock assessment, and efforts are underway to reduce F and allow the stock to rebuild. Therefore, the ERPs were established based on the equilibrium tradeoff relationship between menhaden F and striped bass biomass, when striped bass are fished at their target F. Projection scenarios were run for 40 years over a range of fishing mortality rates (F) on menhaden and striped bass. Surface plots were generated from the terminal year equilibrium biomass to reveal the tradeoff between harvesting menhaden and enhancing predator populations. The ERP Ftarget and Fthreshold were defined as the Atlantic menhaden F that sustained striped bass at their biomass target or threshold, respectively, over the long term. The ERP Ftarget was 40% lower than the single species Ftarget and the ERP Fthreshold was 30% lower than its single species counterpart.
Atlantic menhaden are managed with a coastwide TAC, but the NWACS-MICE Ecosim model does not capture the short term variability exhibited by menhaden, especially with regards to recruitment, and it is not well suited to provide advice on the TAC (Chagaris et al. this volume, Drew et al. this volume). In contrast, the single species stock assessment model includes variability in recruitment during the projection period, but does not provide long-term strategic advice on ecosystem impacts. Therefore, the WG recommended a combination of the NWACS-MICE EwE model and the single species stock assessment model to set the TAC under the new ERPs. Menhaden yield streams for 2021–2022 were estimated through projections from the single species model as the annual yield with a 50% probability of exceeding the ERP Ftarget.
Case Study: Irish Sea
The fisheries of the Irish Sea have shown dramatic changes over the last 50 years. At the start of this period, the fishery was finfish dominated, consisting predominantly of cod, whiting Merlangius merlangus, and Atlantic herring. Since 1970, landings have declined by 97% for cod, by 88% for whiting, and by 81% for Atlantic herring. Over the same period, invertebrate landings increased, mainly composed of the Norway lobster Nephrops norvegicus, (+ 56%), crabs (+ 78%), and scallops (+ 34%). Landings of Nephrops remained relatively stable from the 1980s to the mid-2000s, with some declines evident since then (ICES, 2019a). From the early 2000s, management measures were implemented, mainly for the recovery of cod stocks. These included closed seasons, closed areas, and gear restrictions for 1999–2016, and multi-annual selection of TACs, restriction of effort, technical measures (more selective gears), control and enforcement, as well as structural and market measures from 2017 to the present. Combined, this resulted in declines in fishing effort by around 90% since 2003 for both the whitefish otter trawlers and the beam trawlers. Effort by the trawlers targeting Nephrops declined by around 30%. The main purpose of this management approach was to improve the cod stock, but it was not successful; there has been no recovery to the reference maximum sustainable yield (MSY) biomass level. Although there is some evidence of improvement in biomass in recent years, it appears to have declined again since 2015. Additionally, whiting has failed to recover to previous stock levels. For other stocks, namely plaice, haddock, and Atlantic herring, there has been stock recovery since the early 2000s, likely helped by the substantial effort reductions.
In 2014, the North Western Waters Advisory Council (NWWAC; an EU mandated fisheries stakeholder forum for both industry and environmental groups) asked ICES to investigate why the substantial effort reductions had not helped with recovery of cod, whiting, and sole, and if the lack of recovery could be linked to environmental factors. Based on this request, ICES set up a benchmark workshop series (WKIrish: ICES, 2016b) to examine the single species stock assessments and the possibility of ecosystem drivers having a role in the changes. The first part of the work involved a wide-ranging scoping workshop involving scientists and fishery stakeholders, this was followed by an assessment benchmark process developing and improving the single species stock assessment data and methodology, as many of the assessments were unreliable (ICES, 2017). Later, and where this paper is focused, the workshops focused on developing a suite of ecosystem models to explore the system as a whole. The aim was to develop the models in explicit collaboration with the NWWAC stakeholders for the Irish Sea, and involve a range of possible modeling approaches. These included a “Length-based Multispecies analysis by numerical simulation”—LeMans, multispecies mixed fisheries model (Thorpe and De Oliveira, 2019), MoSES (a Model for the Simulation of Ecological Systems) developed for the Irish Sea (ICES, 2020c), and an EwE model (Bentley et al., 2020). The LeMans and MoSES models were not fully operational in time for the final workshop, but the EwE model was available for operational advice, and thus, the EwE modeling approach is described in this paper.
The EwE model included 41 functional groups, including the commercial species as adults and juveniles, as well as other groups ranging from detritus, discards, and primary producers to mammals and seabirds (Bentley et al., 2020). The different commercial fleets were included with their effort, as well as temperature, top-down (e.g., predation) and bottom-up (e.g., primary production) interactions, and the North Atlantic Oscillation (NAO) anomaly, all of which were identified as significant drivers of historic biomass and catch trends. A key element of the work was the continuous involvement of the stakeholders (both industry and environmental bodies) along with stock assessment scientists responsible for the key commercial stocks. The stakeholders were able to provide pivotal information for the diets of many key species in the model, particularly for 1973, the start year for the model, identifying 80 links of which 30 were previously unknown to the scientists from stomach content records (Bentley et al., 2019a). They also provided critical information on effort trends by gear, starting well before formal records that begin in 2003 (Bentley et al., 2019b).
The key end result was that significant ecosystem drivers for stock production were identified for four species (ICES, 2020c). Significant ecosystem drivers were identified through a hypothesis testing process that evaluated goodness of fit with and without ecosystem drivers included. Both cod and whiting were strongly influenced by sea surface temperature with a 3 year lag, thus linking to recruitment. Atlantic herring had a strong link to large zooplankton abundance. Nephrops were linked to the abundance of predators at trophic level 4 and above. For sole, plaice, and haddock, no convincing ecological indicators (i.e., possessing both strong correlation and mechanism of effect) were identified.
The stock assessment scientists brought the key understanding of the assessment process, and, critically, because they understood the existing management system they brought information on how the ecosystem knowledge could be formulated to make it accessible to the process of providing policy advice, particularly in relation to MSY fishing mortality that determines TACs and quotas. The interdisciplinary approach combined the expertise of three types of experts: ecological modelers, stock assessors, and stakeholders to determine which results had the potential to become operational advice with relevance at the science-policy interface.
The end products were recommendations for target Fs within the pretty-good-yield ranges that have been adopted for many stocks in the EU. ICES provides precautionary FMSY ranges (FMSY upper and FMSY lower) that are derived to deliver no more than a 5% reduction in long-term yield compared with MSY for selected stocks (Hilborn, 2010; ICES, 2016a, 2019b; Rindorf et al., 2017). Using the identified indicator for each stock, the Ftarget value was scaled linearly within the range (FMSY lower, FMSY upper) according to the current value of the indicator within the historical range during the model tuning period. For example, if the indicator identified for a particular stock was 80% of the way to the most favorable value observed over the model period, then Ftarget was adjusted to 80% of the way to the higher FMSY upper value. This linear scaling was chosen for simplicity given that this is a new approach, but in principle more complex relationships would be possible, and likely appropriate. Single species FMSY and associated quotas were adjusted for cod and whiting based on sea surface temperature, herring F, and quotas were adjusted according to empirical estimates of zooplankton abundance, and Nephrops F and quota was adjusted based on combined biomass of predators. Full details are in Bentley et al. (2020). This allows the ecosystem understanding to be incorporated within the existing single stock management framework, and critically, within the FMSY ranges that have already been identified as being precautionary. On this basis, ecosystem information can be used to set F within those ranges, and within the management advice paradigm.
Synthesis
Although the groups that completed the two case studies described above worked independently and with different management aims, the result was a common modeling approach for addressing EBFM issues. In the Irish Sea, the focus was on identifying ecological drivers acting on the stocks, whereas in the US, the focus was on the menhaden stock as a driver in the ecosystem through trophic interactions. In both situations, a mechanism of adjusting the Ftarget to produce a revised Feco was identified as an efficient method for incorporating ecological information into the stock assessment process.
The Models
Both case studies used a multi-model approach to evaluate the objectives as specified by managers and stakeholders, but ultimately settled on a final ecosystem model developed using EwE. The multi-model approach allowed for comparisons across model types, model assumptions, and for the evaluation of the models to address management needs. For the menhaden case study, the simplified EwE model was chosen as it could be used to address the management objectives while limiting overall complexity. That is, the EwE models captured the effects of top-down predation on menhaden and bottom-up effects of menhaden biomass on the growth and mortality of its predators. For the Irish Sea, the EwE model identified environmental drivers of key species to determine likely causes for failed recovery.
Both EwE models were developed following best practices (e.g., Link, 2010; Heymans et al., 2016), which included (1) tailoring the models to the questions they were intended to answer, (2) testing the uncertainty in input data, (3) making ecologically justified choices during model construction, and (4) seeking quality assurance through external review. Such rigorous approaches improve model credibility within the research community and with advisory bodies and external reviewers who are familiar with the methodology, leading to positive steps toward the application of EBFM (Townsend et al., 2019). Conceptually, the analysis could be repeated using any ecosystem modeling platform, provided that the model represents the key ecological processes with sufficient detail to address the management question.
The Method
The workflow involved in this method is summarized in Figure 1. Note that all of the steps involving the single species model are those conducted in the current management systems (e.g., ICES, 2019b), only the involvement of the ecosystem model to adjust Ftarget is novel. In both cases, the ecosystem models were initiated in response to some management question that was driven by stakeholder input. For Atlantic menhaden, the primary issue driving model development pertained to concerns raised by stakeholders over many years about the impact that menhaden harvest has on their predators. In the Irish Sea, the issue at hand centered on environmental explanations for poor recovery of several managed fish stocks following concerted effort reductions.
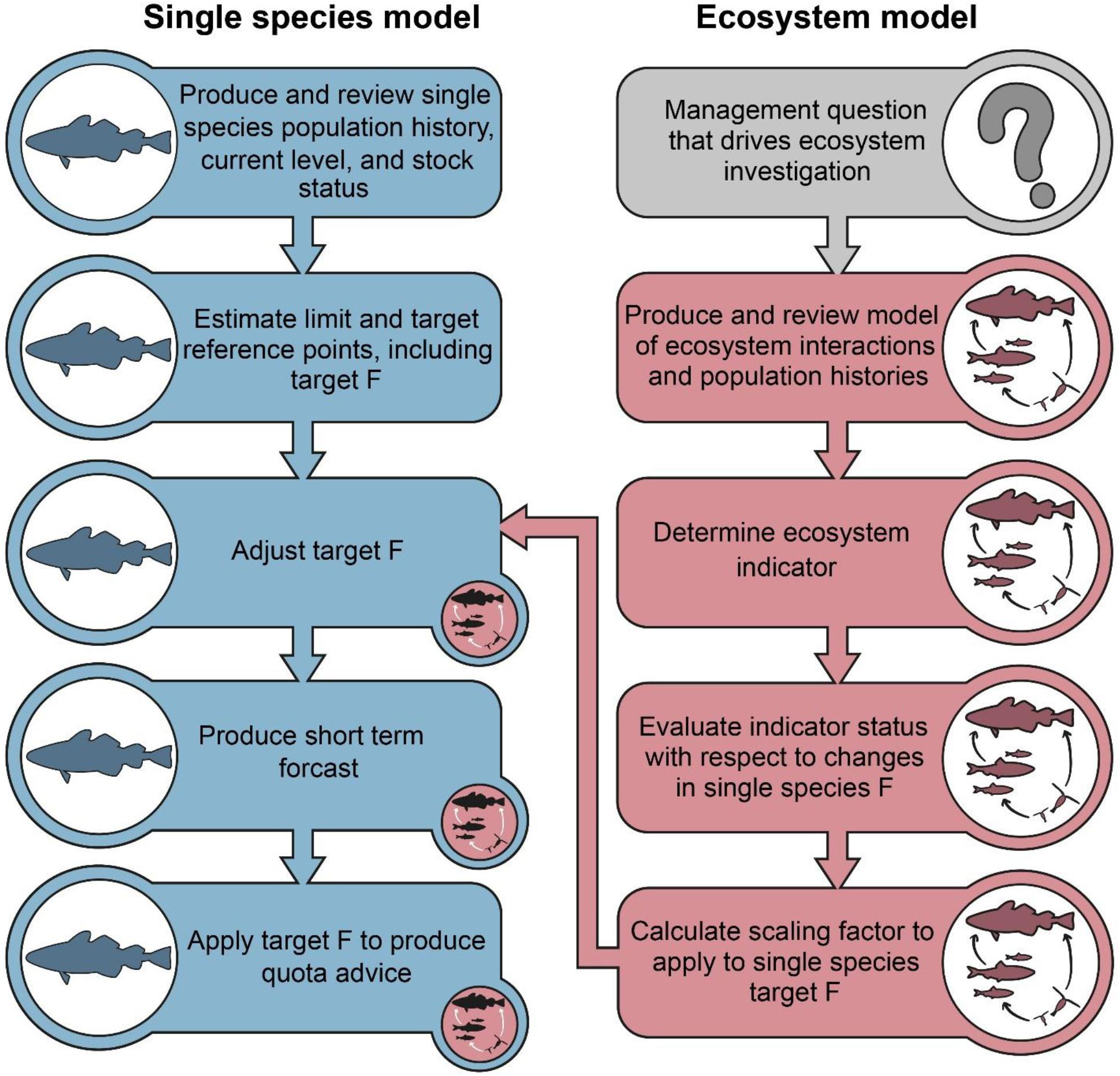
Figure 1. Flow chart outlining the steps in advice giving involved in the proposed method, with the input of the ecosystem modeling to the single species advice highlighted.
Next, both case studies produced and reviewed a suite of modeling approaches designed around, and in response to, the management questions. The approaches taken by ASMFC and ICES to complete this step were similar in several ways. Both case studies assembled technical workgroups that worked closely with stock assessment modelers and both models were developed through a series of workshops and meetings that were open and transparent to the public. This process is described in further detail below. An ensemble approach was initially taken that ultimately led to the selection of a single model for management use. For Atlantic menhaden, the final model was selected by the workgroup and then put forward for review by a panel of independent experts, that were also reviewing the menhaden stock assessment. In the Irish Sea, the EwE model, which had also been reviewed by the workgroup and a panel of independent experts, was selected as fitting to the observational data, and being the most operationally ready model from the ensemble being developed.
An important step in the process is the determination of the ecosystem indicator to be used to adjust F. Two different approaches were taken in these case studies. For the Irish Sea, the goal was to determine potential environmental drivers of managed fish stocks so that harvest rates could be adjusted accordingly. The ecosystem indicators identified for commercial stocks in the Irish Sea included sea surface temperature, zooplankton abundance, and predator abundance. For Atlantic menhaden, the ecosystem indicator was quite different, being a property of one of the stocks rather than an environmental variable. Here, menhaden were treated as a driver on predator populations that could be manipulated by managers and the ecosystem indicator was the biomass of their most sensitive predator, striped bass. The goal in this case was to ensure sufficient food availability to allow the projected biomass of striped bass to recover under scenarios of reduced fishing pressure. These two examples serve to highlight the flexibility of this approach—either environmental or inter-species interactions can be accommodated by adjusting target F in the way described.
The method of adjusting the Ftarget is not specified in Figure 1, because as the examples above demonstrate the adjustment will depend on the needs of the particular case. However, this method does not involve directly transferring a value of Ftarget from the ecosystem model to the single species model, which should minimize errors arising from different estimates of biomass levels in the different models. Rather, it applies a scaling factor to the Ftarget from the single-species model, thus ensuring that the resulting Ftarget is compatible with the single-species F reference points from the assessment. In the Irish Sea, Feco was specific for each species and based on a linear scalar to the environmental driver, constrained within defined targets and limits. In the menhaden example, Feco was established based on the response of a single predator. Note also that because this link simply involves rescaling the single species Ftarget value, a full coupling (two-way interaction) between the single species and ecosystem model is not required.
Finally, the assessment model must then run a short-term forecast (typically 1–3 years) with the given Ftarget to produce quota advice for the coming year or years. The proposed method adjusts the Ftarget based on ecosystem information as can be seen in the examples described above. The method does not otherwise alter the existing harvest control rule methodology, and the revised Ftarget is constrained to not exceed the existing Flim or breach precautionary guidelines.
The strength of this approach is that the assessment and management of fish stocks remains with the single species assessment models and within the current management structure as much as possible. The stock history, status, reference point calculation, initial estimate of target F, and the translation of the final target into quota advice all remain within the realm of the single-species assessment model. As a result, the advice system is familiar to managers and stakeholders, so these first steps toward EBFM are less onerous and daunting than what managers, stakeholders, and scientists might have expected. Only the adjustment of the target F is influenced by the ecosystem modeling. This also implies that no change is required to the existing assessment model. This draws on the strengths of existing single species stock assessments, while broadening the management approach to include ecosystem considerations. Essentially, the traditional single stock assessment recognizes that stock status can change in response to fishing mortality, and intrinsic population dynamics. The ecosystem model recognizes that stock status can change in response to extrinsic ecosystem factors. The proposed approach then modulates the target F from the stock assessment model with the status of the indicator(s) identified by the ecosystem model. In addition, the management framework currently in place can remain and the method simply fits within the existing structures. The management regime itself can, of course, continue to evolve and can change with time as the needs arise, but no fundamental change is required before implementing this method. The proposed method allows the key driver(s) for a particular stock to be considered without requiring that all possible drivers be included. Finally, because the method adjusts Ftarget to produce an Feco, with the constraint that Feco remains at or below existing Fthreshold levels, the risk of stock collapse is no higher, and potentially lower, than under current single species assessments and management.
Apart from the step involving adjusting the Ftarget, this workflow is exactly as is currently done for single species management advice; and thus, offers an easy and straight-forward transition to implementing EBFM.
The Process
Often, the way science is conducted is equally as important as its context when it comes to operationalizing advice (Österblom et al., 2020). To this end, the similarities shared by the United States Menhaden and Irish Sea case studies do not just lie in the nature of their approach, but also in the way the approaches were developed. Both case studies were developed at the science-policy interface where they addressed specific questions linked to impending decisions, which managers and stakeholders were already invested in. This streamlined the integration of ecosystem information into their respective advice structures by allowing ecosystem researchers to become familiar with the advice framework and management needs. Equally, the scientists linked to the advisory process (the stock assessors) were able to become accustomed to the ecosystem research, and importantly, for limitations to be made explicit and expectations to be managed throughout. Furthermore, the work was truly transdisciplinary in nature, where scientists that do not normally work together (stock assessors and ecological modelers) joined with stakeholders, advisors, and managers to co-produce a conceptual, theoretical, and methodological innovation that integrates knowledge between disciplines, to produce a practical and applicable solution. The integration of the stakeholders into this process, in both cases, was particularly important, and it is rare to see them actively engaging with a complex ecosystem model such as EwE. It is anticipated that the development of modeling and management will be an iterative process, with further refinements in the future.
In both case studies, there was strong and motivated stakeholder involvement. Critically, that involvement led to co-designed elements, e.g., question formulation, food web, and effort trajectory construction in WKIrish and specifying objectives of interest in the menhaden case study, as well as interrogation of the model results with the scientists. Equally, both case studies included those involved advising on the management of the stocks. For WKIrish, the team included those involved in the stock assessment and advice process, up to, but not directly including the managers themselves. In the EU, the next step in further developing the process would be to include policy-makers, at the national and EU ministerial level; however, that presents a substantial challenge. For the menhaden case study, the team included scientists that considered public comment and input during the model development process and met regularly with managers to report on progress and receive input. In the US case, an immediate next step would be a risk-based decision-making process to set a TAC for 2021–2022 based on the accepted ERPs. In the US case, a broader next step would be to start integrating policy decisions across management bodies for menhaden and its key predators instead of this approach being contained solely within the menhaden management arena.
Summary
As fisheries management agencies evolve toward EBFM, the modeling approaches used to inform decision-making must also evolve. Previously, single-species model systems had co-evolved with single-species management and data collection systems so that they were well adapted to address the specific management issues and concerns for a given stock. Ecosystem models generally account for a broader set of ecological interactions influencing fish stocks but usually have less resolution on specific population dynamics, thus they are well-suited to complement single-species models. Combined single-species with ecosystem or multispecies modeling tools can address the standard fisheries management objectives as well as a broader suite of ecosystem-oriented objectives (such as trade-offs between fisheries for different stocks or responding to changing environmental conditions) that arise with increased EBFM implementation. The method of adjusting the target F to produce a Feco is sufficiently flexible to be used by many ecoregions; to address multiple different purposes, goals, or objectives; and fits within existing management schemes. By only adjusting the existing Ftarget, this method avoids directly transferring values between different models, and by remaining within existing limit reference points the method imposes no additional risk of stock collapse. The two examples presented here represent different goals using the same process. By fitting to ranges of Ftarget which come close to delivering MSY (as in the Irish Sea case), this approach maintained existing good yield criteria as was desired while also incorporating ecosystem information. Alternatively, as shown in the Atlantic menhaden case, the method allowed for changes in Ftarget on forage fish to support predators in a manner that draws upon best available scientific information and incorporates knowledge of the changing ecosystem. In both cases the adjustment was made without increasing the risk of stock collapse beyond that already included in the existing management system. Clearly, there will be different requirements when implementing EBFM in different fisheries and management contexts, and meeting the needs of managers and stakeholders in a given situation is likely to be critical to adoption in management (Townsend et al., 2019). Management Strategy Evaluations (MSEs) give one potential framework to explore alternative applications of this approach.
The proposed method for computing an Feco does have some limitations in that it may not necessarily include the full suite of pertinent predator and prey interactions or environmental factors, and it does not require that multi-species trajectories be linked to the single species projections when producing quota advice at the end of the process. It could be argued that the proposed approach is still quite limited in the value it gains from the ecosystem model. The ecosystem model itself is not used directly. It is used to suggest which ecosystem indicators are critical (e.g., water temperature in the WKIrish case, or predator stock biomass in the menhaden case) or to identify the predator-prey tradeoff relationship. These are empirical values that can be determined in any given year without running the ecosystem model, or in the case of menhaden, are long term equilibrium reference points. This could be considered as taking a lot of effort for a very constrained outcome. However, this approach provides a quantitative, mechanistic link between ecosystem indicators and appropriate harvest levels, instead of the ad hoc buffer approach of solely relying on indicators. The models could be used much more extensively, e.g., linking in elements of spatial distribution, growth, predation, food web effects etc. or by optimizing fishing mortality rates to achieve some broader management objective. More importantly, they have the potential to integrate numbers of pressures and drivers to indicate the likely short-term future of the stock. The approach of extending the single species assessment in this manner relies on there being sufficient data and process understanding to construct a meaningful ecosystem model.
Implementing “full” EBFM would require considering all major species and other ecosystem components interacting together and would allow for trade-offs and interactions between different fisheries and management strategies. For example, it may well be sustainable to fish a species above its long-term single species reference level (Fthreshold) in a situation where predator biomass is low or where environmental conditions are favorable. In principle, management objectives could allow for a Ftarget level being adjusted not just above the single species Ftarget, but also above the single species limit reference points, but one question that would arise is how to identify a precautionary target fishing level. The inability to identify the precautionary limit reference point leads back to the difficulties of using ecosystem models to directly inform tactical fisheries management advice. Therefore, exceeding the single species precautionary limits would require a separate analysis that this did not involve an excessive risk of inducing stock collapse. For true EBFM, one would also ideally want to allow for variations of other fish stocks and environmental conditions to have an impact on the single species stock assessment model and short-term forecast for each stock. Such a “fully coupled” system would allow for a more detailed multispecies analysis and support trade-offs between a wider range of stocks. How to achieve this is currently an active field of research (e.g., Gaichas et al., 2017; Holsman et al., 2020). Within the framework proposed here, such a system could be constructed through a tighter coupling of one or more single-species models and the ecosystem model. However, the method presented here represents a clear step forward in integrating ecosystem information into the advice-giving process, allowing ecosystem information to directly affect tactical quota-setting advice. The existence of two very different examples shows that it is a flexible and powerful approach, which can efficiently and effectively address a number of current needs in fisheries advice.
In conclusion, the very simplicity of the Feco method presented here makes it viable for immediate implementation. We do not claim that this is the only solution to implementing EBFM, nor that it can address every possible issue. Nevertheless, the method here represents a valuable and flexible step forward in practical, operational EBFM, which can be directly implemented within existing management frameworks.
Data Availability Statement
The original contributions presented in the study are included in the article/supplementary material, further inquiries can be directed to the corresponding author/s.
Author Contributions
DH led and coordinated the writing of the manuscript, with assistance from AS. All authors were involved in the underlying research and writing the manuscript.
Conflict of Interest
The authors declare that the research was conducted in the absence of any commercial or financial relationships that could be construed as a potential conflict of interest.
Funding
The Atlantic menhaden work was supported by National Oceanic and Atmospheric Administration Award No. NA15NMF4740069 and Lenfest Ocean Program grants nos. 00025536 and 00032187, and thanks all of the members of the ASMFC Menhaden Technical Committee and the ERP WG for their critical contributions to model development and helpful discussions. We acknowledge the members of the ICES Benchmark Workshop WKIrish for their participation and collaboration, and the NWWAC and BIM for facilitating the meetings. The EwE modeling work was carried out with the support of the Marine Institute and funded under the Marine Research Sub-programme by the Irish Government (Grant-Aid Agreement No. CF/16/08). DP was supported by the Science Foundation Ireland (www.sfi.ie) Investigator Programme (grant no. 14/IA/2549), and DR by Project FishKOSM funded by the Department of Agriculture, Food and the Marine’s Competitive Research Funding programmes. DH acknowledges support from the Institute of Marine Research strategic project Reduced Uncertainty in Stock Assessment (REDUS). Open access funding was provided by the Institute of Marine Research, Norway.
References
Anstead, K., Drew, K., Chagaris, D., Cieri, M., Schueller, A., McNamee, J., et al. (2020). The Past, Present, and Future of Forage Fish Management: a Case Study of Atlantic Menhaden. Front. Mar. Sci. Vol..
Atlantic States Marine Fisheries Commission. (1981). Fishery Management Plan for Atlantic Menhaden. Fisheries Report No. 2. Arlington, VA: Atlantic States Marine Fisheries Commission.
Bentley, J. W., Hines, D. E., Borrett, S. R., Serpetti, N., Hernandez-Milian, G., Fox, C., et al. (2019a). Combining scientific and fishers’ knowledge to co-create indicators of food web structure and function. ICES J. Mar. Sci. 76, 2218–2234. doi: 10.1093/icesjms/fsz121
Bentley, J. W., Serpetti, N., Fox, C. J., Heymans, J. J., and Reid, D. G. (2020). Retrospective analysis of the influence of environmental drivers on commercial stocks and fishing opportunities in the Irish Sea. Fisheries Oceanogr. 29, 415–435. doi: 10.1111/fog.12486
Bentley, J. W., Serpetti, N., Fox, C., Heymans, J. J., and Reid, D. G. (2019b). Fishers’ knowledge improves the accuracy of food web model predictions. ICES J. Mar. Sci. 76, 897–912. doi: 10.1093/icesjms/fsz003
Christensen, V., and Walters, C. J. (2004). Ecopath with Ecosim: methods, capabilities and limitations. Ecol. Model. 172, 109–139. doi: 10.1016/jecolmodel.2003.09.003
Christie, D. R. (2005). Implementing an Ecosystem Approach to Ocean Management: An Assessment of Current Regional Governance Models Ocean Ecosystem Management: Challenges and Opportunities for Regional Ocean Governance. Duke Environ. Law Policy Forum 16, 117–142. doi: 10.1163/9789004389984_005
Collie, J. S., Botsford, L. W., Hastings, A., Kaplan, I. C., Largier, J. L., Livingston, P. A., et al. (2016). Ecosystem models for fisheries management: finding the sweet spot. Fish Fisheries 17, 101–125. doi: 10.1111/faf.12093
Cormier, R., Kelble, C., Anderson, M., Allen, J., Grehan, A., and Gregersen, Ó (2017). Moving from ecosystem-based policy objectives to operational implementation of ecosystem-based management measures. ICES J. Ma. Sci. 74, 406–413. doi: 10.1093/icesjms/fsw181
Drew, K., Cieri, M., Schueller, A., Buchheister, A., Chagaris, D., Nesslage, G., et al. (2020). Balancing Model Complexity, Data Requirements, and Management Objectives in Developing Ecological Reference Points for Atlantic Menhaden. Front. Mar. Sci. Vol.
EU (2008). Directive 2008/56/EC of the European Parliament and of the Council of 17 June 2008 establishing a framework community action in the field of marine environmental policy (Marine Strategy Framework Directive). Belgium: European Union. 19–40.
EU (2013). Regulation (EU) No 1380/2013 of the European Parliament and of the Council of 11 December 2013 on the Common Fisheries Policy, amending Council Regulations (EC) No 1954/2003 and (EC) No 1224/2009 and repealing Council Regulations (EC) No 2371/2002 and (EC). Belgium: European Union. 354, 22–61.
EU (2019). Council Regulation (EU) 2019/124 of 30 January 2019 fixing for 2019 the fishing opportunities for certain fish stocks and groups of fish stocks, applicable in Union waters and, for Union fishing vessels, in certain non-Union waters.ST/15733/2018/INIT OJ L 29, 31.1.2019. Belgium: European Union. 2019, 1–166.
FAO (2008). Fisheries management. 2. The ecosystem approach to fisheries. 2.1 Best practices in ecosystem modelling for informing an ecosystem approach to fisheries. Rome: FAO, 78.
Fulton, E. A., Link, J. S., Kaplan, I. C., Savina-Rolland, M., Johnson, P., Ainsworth, C., et al. (2011). Lessons in modelling and management of marine ecosystems: the Atlantis experience. Fish Fisheries 12, 171–188. doi: 10.1111/j.1467-2979.2011.00412.x
Gaichas, S. K., Fogarty, M., Fay, G., Gamble, R., Lucey, S., and Smith, L. (2017). Combining stock, multispecies, and ecosystem level fishery objectives within an operational management procedure: simulations to start the conversation. ICES J. Mar. Sci. 74, 552–565. doi: 10.1093/icesjms/fsw119
Harvey, C. J., Kelble, C. R., and Schwing, F. B. (2017). Implementing “the IEA”: using integrated ecosystem assessment frameworks, programs, and applications in support of operationalizing ecosystem-based management. ICES J. Mar. Sci. 74, 398–405. doi: 10.1093/icesjms/fsw201
Heymans, J. J., Coll, M., Link, J. S., Mackinson, S., Steenbeek, J., Walters, C., et al. (2016). Best practice in Ecopath with Ecosim food-web models for ecosystem-based management. Ecol. Model. 331, 173–184. doi: 10.1016/j.ecolmodel.2015.12.007
Hilborn, R. (2010). Pretty good yield and exploited fishes. Mar. Policy 34, 193–196. doi: 10.1016/j.marpol.2009.04.013
Hollowed, A. B., Holsman, K. K., Haynie, A. C., Hermann, A. J., Punt, A. E., Aydin, K., et al. (2020). Integrated Modeling to Evaluate Climate Change Impacts on Coupled Social-Ecological Systems in Alaska. Front. Mar. Sci. 6:775. doi: 10.3389/fmars.2019.00775
Holsman, K. K., Haynie, A. C., and Hollowed, A. B. (2020). Ecosystem-based fisheries management forestalls climate-driven collapse. Nat. Commun. 11:4579. doi: 10.1038/s41467-020-18300-3
ICES (2016a). EU request to ICES to provide FMSY ranges for selected stocks in ICES subareas 5 to 10. Available online at https://www.ices.dk/sites/pub/Publication%20Reports/Advice/2016/Special_Requests/EU_FMSY_ranges_for_selected_Western_Waters_Stocks.pdf (accessed date 5, February 2016).
ICES (2016b). Report of the Benchmark Workshop on Sharing Information on the Irish Sea Ecosystem, Stock Assessments and Fisheries Issues, and Scoping Needs for Assessment and Management Advice (WKIrish1). Dublin, Ireland. ICES CM 2015/BSG:01, 37.
ICES (2017). Report of the Benchmark Workshop on the Irish Sea Ecosystem (WKIrish3), Galway, Ireland. ICES CM 2017/BSG:01, 220.
ICES (2019a). Working Group for the Celtic Seas Ecoregion (WGCSE). ICES Sci. Rep. 1:1604. doi: 10.17895/ices.pub.4982
ICES (2019b). ICES advice basis – including precautionary approach and reasoning. Available online at https://www.ices.dk/sites/pub/Publication%20Reports/Advice/2019/2019/Introduction_to_advice_2019.pdf (accessed date 20, December 2019).
ICES (2020a). Baltic Fisheries Assessment Working Group (WGBFAS). ICES Sci. Rep. 2:643. doi: 10.17895/ices.pub.6024
ICES (2020b). Working Group on the Assessment of Demersal Stocks in the North Sea and Skagerrak (WGNSSK). ICES Sci. Rep. 2:1140. doi: 10.17895/ices.pub.6092
ICES (2020c). Workshop on an Ecosystem Based Approach to Fishery Management for the Irish Sea (WKIrish6; outputs from 2019 meeting). ICES Sci. Rep. 2:32. doi: 10.17895/ices.pub.5551
Levin, P. S., Fogarty, M. J., Murawski, S. A., and Fluharty, D. (2009). Integrated Ecosystem Assessments: Developing the Scientific Basis for Ecosystem-Based Management of the Ocean. PLoS Biol. 7:e1000014. doi: 10.1371/journal.pbio.1000014
Link, J. S. (2010). Adding rigor to ecological network models by evaluating a set of pre-balance diagnostics: a plea for PREBAL. Ecol. Model. 221, 1580–1591. doi: 10.1016/j.ecolmodel.2010.03.012
Marshak, A. R., Link, J. S., Shuford, R., Monaco, M. E., Johannesen, E., Bianchi, G., et al. (2017). International perceptions of an integrated, multi-sectoral, ecosystem approach to management. ICES J. Mar. Sci. 74, 414–420. doi: 10.1093/icesjms/fsw214
Marshall, K. N., Koehn, L. E., Levin, P. S., Essington, T. E., and Jensen, O. P. (2019). Inclusion of ecosystem information in US fish stock assessments suggests progress toward ecosystem-based fisheries management. ICES J. Mar. Sci. 76, 1–9. doi: 10.1093/icesjms/fsy152
Moustahfid, H., Link, J. S., Overholtz, W. J., and Tyrrell, M. C. (2009). The advantage of explicitly incorporating predation mortality into age-structured stock assessment models: an application for Atlantic mackerel. ICES J. Mar. Sci. 66, 445–454. doi: 10.1093/icesjms/fsn217
Magnuson Stevens Fishery Conservation and Management Act (MSA) (2014). The Magnuson-Stevens Fishery Conservation and Management Act (16 U.S.C. 1801 - 1891(d)).
NOAA (2016). Ecosystem Based Fisheries Management Policy of the National Marine Fisheries Service, Policy. Silver Spring, MD: NOAA, 01–120.
Österblom, H., Cvitanovic, C., van Putten, I., Addison, P., Blasiak, R., Jouffray, J. B., et al. (2020). Science-Industry Collaboration: Sideways or Highways to Ocean Sustainability? One Earth 3, 79–88. doi: 10.1016/j.oneear.2020.06.011
Plagányi, ÉE. (2007). Models for an Ecosystem Approach to Fisheries. FAO Fisheries Technical Paper. No. 477. Rome: FAO.
Rindorf, A., Dichmont, C. M., Levin, P. S., Mace, P., Pascoe, S., Prellezo, R., et al. (2017). Food for thought: pretty good multispecies yield. ICES J. Mar. Sci. 74, 475–486. doi: 10.1093/icesjms/fsw071
Sagarese, S. R., Bryan, M. D., Walter, J. F., Schirripa, M., Grüss, A., and Karnauskas, M. (2015). Incorporating ecosystem considerations within the Stock Synthesis integrated assessment model for Gulf of Mexico Red Grouper (Epinephelus morio). SEDAR42-RW-01. North Charleston, SC: SEDAR, 27.
Satterthwaite, W. H., Andrews, K. S., Burke, B. J., Gosselin, J. L., Greene, C. M., Harvey, C. J., et al. (2020). Ecological thresholds in forecast performance for key United States West Coast Chinook salmon stocks. ICES J. Mar. Sci. 77, 1503–1515. doi: 10.1093/icesjms/fsz189
Skern-Mauritzen, M., Ottersen, G., Handegard, N., Huse, G., Dingsør, G., Stenseth, N. C., et al. (2016). Ecosystem processes are rarely included in tactical fisheries management. Fish Fisheries 17, 165–175. doi: 10.1111/faf.12111
Slater, W. L., DePiper, G., Gove, J. M., Harvey, C. J., Hazen, E. L., Lucey, S. M., et al. (2017). Challenges, Opportunities and Future Directions to Advance NOAA Fisheries Ecosystem Status Reports (ESRs): Report of the National ESR Workshop NOAA Technical Memorandum NMFS-F/SPO-174, 74. Washington, DC:NOAA.
Thorpe, R. B., and De Oliveira, J. A. A. (2019). Comparing conceptual frameworks for a fish community MSY (FCMSY) using management strategy evaluation — an example from the North Sea. ICES J. Mar. Sci. 76, 813–823. doi: 10.1093/icesjms/fsz015
Townsend, H. M., Link, J. S., Osgood, K. E., Gedamke, T., Watters, G. M., Polovina, J. J., et al. (2008). Report of the National Ecosystem Modeling Workshop (NEMoW). Silver Spring, MD: NOAA.
Townsend, H., Harvey, C. J., de Reynier, Y., Davis, D., Zador, S. G., Gaichas, S., et al. (2019). Progress on Implementing Ecosystem-Based Fisheries Management in the United States Through the Use of Ecosystem Models and Analysis. Front. Mar. Sci. 6:641. doi: 10.3389/fmars.2019.00641
Keywords: fisheries advice, ecosystem modeling, precautionary advice, EBFM, EAFM, MSY
Citation: Howell D, Schueller AM, Bentley JW, Buchheister A, Chagaris D, Cieri M, Drew K, Lundy MG, Pedreschi D, Reid DG and Townsend H (2021) Combining Ecosystem and Single-Species Modeling to Provide Ecosystem-Based Fisheries Management Advice Within Current Management Systems. Front. Mar. Sci. 7:607831. doi: 10.3389/fmars.2020.607831
Received: 18 September 2020; Accepted: 07 December 2020;
Published: 08 January 2021.
Edited by:
Cosimo Solidoro, National Institute of Oceanography and Experimental Geophysics (OGS), ItalyReviewed by:
Andre Eric Punt, University of Washington, United StatesElizabeth A. Fulton, Commonwealth Scientific and Industrial Research Organization (CSIRO), Australia
Copyright © 2021 Howell, Schueller, Bentley, Buchheister, Chagaris, Cieri, Drew, Lundy, Pedreschi, Reid and Townsend. This is an open-access article distributed under the terms of the Creative Commons Attribution License (CC BY). The use, distribution or reproduction in other forums is permitted, provided the original author(s) and the copyright owner(s) are credited and that the original publication in this journal is cited, in accordance with accepted academic practice. No use, distribution or reproduction is permitted which does not comply with these terms.
*Correspondence: Daniel Howell, ZGFuaWVsLmhvd2VsbEBoaS5ubw==