- 1U.S. Geological Survey, Leetown Science Center, Kearneysville, WV, United States
- 2U.S. Fish and Wildlife Service, Maryland Fish and Wildlife Conservation Office, Annapolis, MD, United States
- 3U.S. Geological Survey, Alabama Cooperative Fish and Wildlife Research Unit, Auburn University, Auburn, AL, United States
- 4Alabama Cooperative Fish and Wildlife Research Unit, School of Forestry and Wildlife Sciences, Auburn University, Auburn, AL, United States
In the U.S., 525,000 horseshoe crabs (Limulus polyphemus) per year have been captured during 2013–2017, brought to biomedical facilities, and bled to produce Limulus amebocyte lysate (LAL), then mostly released to the area of capture. The Atlantic States Marine Fisheries Commission estimates short-term bleeding-induced mortality to be 15% (4% to 30%), resulting in mortality of approximately 78,750 horseshoe crabs annually in recent years comprising a minor portion (<13%) of the up to one million annual coastwide landings dominated by harvest for bait. However, the long-term effect of bleeding for LAL on annual survival and spawning behavior is unknown; thus, results from short-term studies alone might underestimate bleeding effects at the population level. To address this knowledge gap, we analyzed data from the U.S. Fish and Wildlife horseshoe crab tagging database to estimate the differences in survival and recapture rates of bled and not bled horseshoe crabs tagged in the same years and geographic area. Contrary to expectation, survival was not lower for bled crabs compared to unbled crabs. Differences varied, but survival estimates tended to be higher for bled crabs than for unbled crabs. However, biomedical culling and selection for younger or healthier animals could have resulted in biomedically tagged individuals representing a healthier subset of the overall population with subsequent higher survival. Furthermore, the tagging analysis revealed a post-bleeding reduction in capture probability, which could indicate decreased spawning activity, evident in males more than females. Continued tagging of bled and unbled crabs in the same geographic area while recording age class and sex will contribute to the further resolution of LAL production’s effect on horseshoe crab populations.
Introduction
Horseshoe crabs are the sole remaining representatives of the order of Xiphosura, with the four extant species of horseshoe crabs closely resembling their fossilized relatives from hundreds of million years ago, indicative of extraordinary evolutionary success (Rudkin and Young, 2009; BłaŻejowski, 2015). However, modern stressors associated with expanding harvest and habitat loss threaten horseshoe crabs’ current status (Berkson and Shuster, 1999; Anderson and Shuster, 2003; Smith et al., 2016; John et al., 2018). Commercial harvest of horseshoe crabs is for bait in the U.S., human consumption in Asia, and biomedical use in the U.S. and Asia (Atlantic States Marine Fisheries Commission [ASMFC], 2019; Laurie et al., 2019). The International Union for Conservation of Nature (IUCN) Red List ranks are Vulnerable for the American horseshoe crab (Limulus polyphemus), Endangered for the Asian species Tachypleus tridentatus, and Data Deficient for the two remaining Asian species (T. gigas and Carcinoscorpius rotundicauda) (Smith et al., 2016; Laurie et al., 2019).
Biomedical companies produce an assay from the hemolymph (blood) of adult L. polyphemus in the U.S. and T. tridentatus in Asia (Levin et al., 2003). The generic source of the hemolymph names the assay – LAL for Limulus amebocyte lysate and TAL for Tachypleus amebocyte lysate (Krisfalusi-Gannon et al., 2018). The lysate test detects minute quantities of bacterial endotoxin in injectable medicine or implantable devices (Levin et al., 2003). In the U.S., over 525,000 horseshoe crabs per year on average between 2013–2017 have been captured and brought to biomedical facilities and bled to produce LAL (Schmidtke et al., 2018). Although most bled horseshoe crabs are released, mortality and potential behavioral effects from hemolymph harvest are sources of uncertainty in stock assessments (Atlantic States Marine Fisheries Commission [ASMFC], 2019). The importance of LAL to human health and the need to understand LAL-harvest effects on horseshoe crabs are heightened by the potential demand to test coronavirus vaccines for bacterial contamination (Smithsonian Magazine, 8 June 2020, https://www.smithsonianmag.com/smart-news/race-coronavirus-vaccine-runs-horseshoe-crab-blood-180975048/; National Geographic, 2 July 2020, https://www.nationalgeographic.com/animals/2020/07/covid-vaccine-needs-horseshoe-crab-blood/#close).
To minimize bleeding-induced mortality due to hemolymph harvest, the Atlantic States Marine Fisheries Commission (ASMFC), which governs interstate fisheries along the Atlantic coast of the U.S., recommended best management practices (BMPs) for the biomedical bleeding process to mitigate for post-bleeding effects (Atlantic States Marine Fisheries Commission [ASMFC], 2011). The BMPs considered all steps of the process, including capturing and collecting, transport, holding, bleeding, and returning to the sea. The 41 recommended practices comprise trawl-tow times during capture, environmental conditions during transport and holding, bleeding procedures, return to the sea, monitoring, and reporting. The practices are not mandated by ASMFC, but states where the LAL process occurs can stipulate that BMPs are conditions for collection permit issuance. For example, in Maryland a chain of custody is required from harvest until release with records for number of animals, mortalities, and temperatures during transport and holding; animals must be returned to sea within 48 h (Steve Doctor, Maryland Department of Natural Resources).
Multiple studies have assessed the short-term effects of biomedical bleeding (cf Rudloe, 1983; Hurton and Berkson, 2006; Leschen and Correia, 2010; John et al., 2011; Owings et al., 2019), but long-term effects remain understudied. The short-term studies, which examine effects over weeks or months, found that post-bleeding mortality depended on the percent volume of hemolymph removed and animal handling before, during, and after the bleeding procedure. A meta-analysis of 47 bleeding-mortality studies estimated an acute post-bleeding mortality rate of 15% with a 95% confidence interval of 4% to 30% (Atlantic States Marine Fisheries Commission [ASMFC], 2019). The objective of most such studies focused on survival, but some observed post-bleeding effects on behavior as well (Kurz and James-Pirri, 2002; Anderson et al., 2013; Owings et al., 2019). Anderson et al. (2013) found that bleeding caused reduced activity and physiological changes potentially altering immune function. Owings et al. (2019) tracked 28 telemetry-tagged horseshoe crabs, 14 unbled (control), and 14 bled, and found that bled animals had less frequent spawning bouts than control animals and remained in deeper waters than control animals, but significant impacts lasted less than two weeks post bleeding.
In contrast to the numerous studies of short-term bleeding effects, studies of bleeding effects on long-term survival are lacking. A paucity of data for tracking post-bleeding fate beyond weeks or months has been an overriding reason for the lack of attention to long-term survival effects. The inability to maintain adult horseshoe crabs in captivity for long periods contributes to the absence of long-term studies of post-bleeding survival (Carmichael and Brush, 2012). The exception was a tagging data analysis conducted by Butler (2012), who found evidence of a positive effect of biomedical bleeding on annual survival, but the tag releases were not geographically constrained to control for regional differences in survival.
To address this fundamental knowledge gap and expand on the work of Butler (2012), we used capture-recapture models to analyze data from the U.S. Fish and Wildlife (USFWS) horseshoe crab tagging database to compare annual survival rates of adult horseshoe crabs based on bleeding status within the same geographic area and years. Since 1999, the USFWS has established standardized tagging protocols, facilitated tag reporting, and managed a coastwide tagging database. We focused on analyzing the USFWS database on the Delaware Bay population within the estuary and adjacent coastal waters, where there has been consistent tagging of crabs that had been bled or not bled. Our working hypothesis was that – all else equal – bled horseshoe crabs would have lower annual survival and spawning rates than not-bled (unbled) crabs due to the stress of capture and bleeding. Because most tag recaptures occur during spawning when the animal is accessible to observation, we hypothesized that lower spawning rates of bled crabs would appear as lower tag-recapture rates or capture probability estimates.
Materials and Methods
The USFWS provides tags to qualified tagging program coordinators and manages a centralized tagging database of tag releases and recaptures. The tagging program must demonstrate consistent tagging and recapture effort over multiple years, adhere to a standardized protocol, and promptly submit tagging records. Two biomedical companies, Lonza Walkersville, Inc., (hereafter Lonaz) and Wako Chemical (hereafter Wako), have tagged crabs after bleeding them for LAL; this represents the only consistent tagging effort of bled crabs in the USFWS database. Both companies captured crabs in coastal Delaware to Virginia, which extends from the mouth of Delaware Bay south to Virginia Beach (Figure 1).
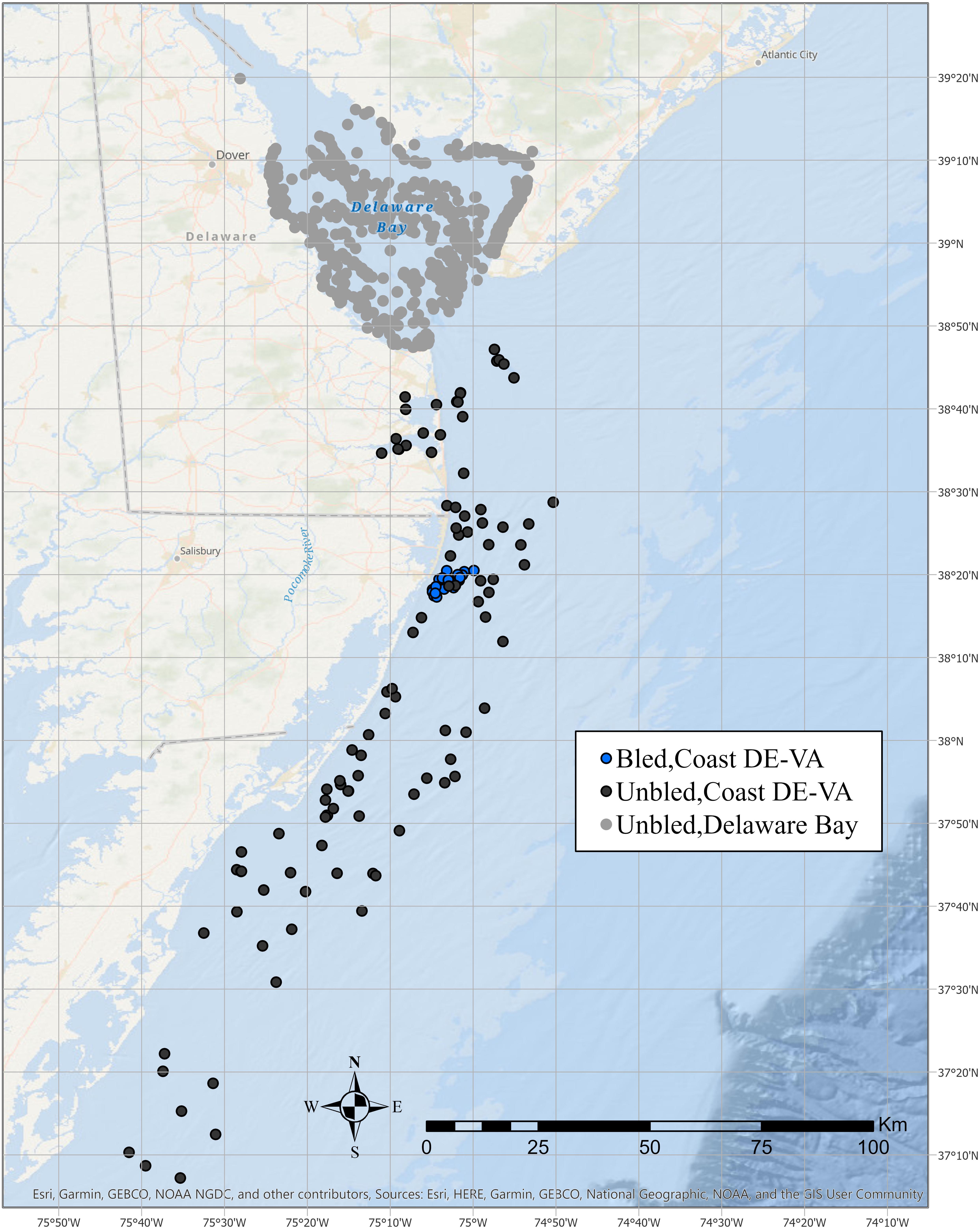
Figure 1. Tag release locations in coastal Delaware to Virginia and Delaware Bay for adult horseshoe crabs that were bled for LAL or not bled.
The two biomedical companies have followed standard protocol but tagged at different levels of effort (personal communication: Sasha Charleron, Salisbury Site Manager, Lonza Walkersville, Inc., and Christina Lecker, Plant Manager, Wako Chemical). The majority (96%) of tagged and bled crabs came from Lonza Walkersville, Inc., (Walkersville, MD, United States) with the remaining fraction tagged by Wako Chemicals. Lonza tagged approximately 3,000 per year starting in 1999 and tagged every day that bleeding operation occurs from June to November. Wako started tagging in 2008, tagged approximately 75 per year before 2012, and have tagged approximately 300 per year since 2012. No intentional sorting of animals occurred before selection for tagging, although only mature animals, as evidenced by amplexus scars in females or claspers in males, were tagged. Tag placement followed the USFWS tagging protocol. Tagged animals were treated the same as other bled animals during holding and transport.
We could not determine with certainty the bleeding status of crabs tagged by an entity other than a biomedical company. However, evidence suggests that a tagged crab is unlikely to have been bled unless it was tagged by a biomedical company. The number of crabs bled coastwide in Massachusetts, the Delaware Bay states, and South Carolina is approximately 2% of the adult population abundance only in the Delaware Bay area (0.5 million divided by 30 million in 2017; Atlantic States Marine Fisheries Commission [ASMFC], 2019), suggesting that the crabs not tagged by a biomedical company, by chance alone, were not bled. The more relevant number of crabs bled within the Delaware Bay region is much lower than the coastwide number but is considered confidential business information and not publicly available (Schmidtke et al., 2018).
A consistent minimum tagging effort was an important consideration in the selection of data for the analyses. Although >1,000 bled horseshoe crabs have been tagged in most years since 1999 within coastal Delaware to Virginia, tagging of unbled crabs within the coastal area had remained below 1,000 until 2008 (Table 1). In contrast, the number tagged within the Delaware Bay exceeded 5,000 several years before 2008 (Figure 1, Table 1). ASMFC defines the Delaware Bay population to include adults that spawn at least once in the Delaware Bay estuary (Atlantic States Marine Fisheries Commission [ASMFC], 2019). Because there is considerable movement between the Atlantic Ocean off the mouth of Delaware Bay, the coastal embayments in Delaware, Maryland, and Virginia, and Delaware Bay estuary (Swan, 2005; King et al., 2015; McGowan, 2018), these areas are considered to be part of the Delaware Bay population (Atlantic States Marine Fisheries Commission [ASMFC], 2019).
We proceeded with two analyses – one geographically expansive, which included tags released in Delaware Bay or coastal Delaware to Virginia from 1999 to 2017, and one geographically restrictive, which included tags released only in coastal Delaware to Virginia from 2008 to 2017. The geographically extensive analysis allowed for the most years in the comparison. The geographically restrictive analysis, which includes the regional coastline except for Delaware Bay proper, had the tightest focus on the area where animals were harvested for LAL production. Expanding the analysis to include Delaware Bay and coastal Delaware to Virginia areas from 1999 to 2017 provided a sample of 174,936 animals tagged with sex and bleeding status: 33,883 unbled females, 32,310 bled females, 72,216 unbled males, 36,098 bled males, and 429 with unknown sex. The disposition of recaptures included alive (75%), dead (16%), and unknown (9%) status. Among the bled animals, 1,283 (4%) females and 2,217 (6%) males were recaptured at least once; among the unbled animals, 2,525 (7%) females and 7,217 (10%) males were recaptured at least once. Restricting the analysis to coastal Delaware to Virginia from 2008 to 2017 provided a sample of 63,903 animals tagged with sex and bleeding status: 7,194 unbled females, 18,454, bled females, 17,311 unbled males, 20,846 bled males, and 98 with unknown sex. Among the bled animals, 752 (4%) females and 1,186 (6%) males were recaptured at least once; among the unbled animals, 287 (4%) females and 1,144 (7%) males were recaptured at least once. We used both mark-recapture modeling and relative risk estimation to infer bleeding effects on survival and recapture.
A description of the sampling and modeling process for capture-recapture studies can help illustrate how the parameters, i.e., probabilities of survival and capture, are related to the observed tag releases and recaptures. Sampling begins when an animal is caught, tagged, released, and available for recapture in future encounters. The tagged animal confronts alternative fates (Figure 2, Table 2). The animal could survive to the next year, die, or emigrate permanently from the study area. If the animal survives, then it could be captured or not captured. Survival is the biological parameter of interest. Capture probability is a function of sampling design and effort, catchability of the species, and environmental conditions at the time of sampling. For example, most horseshoe crab tags are recaptured on the spawning beaches; thus, decreased spawning activity reduces capture probability. To estimate survival, the probability of capture must also be estimated because otherwise these parameters are confounded. That is why raw frequencies of recapture cannot be used to infer survival. Despite accounting for capture probability (p), there is an additional complication; mortality remains confounded with permanent emigration from the study area. Thus, we qualify the parameter for survival by referring to it as ‘apparent survival’ (φ). Because permanent emigration from the Delaware Bay population is infrequent (Swan, 2005), apparent survival closely approximates actual survival for the geographically expansive analysis.
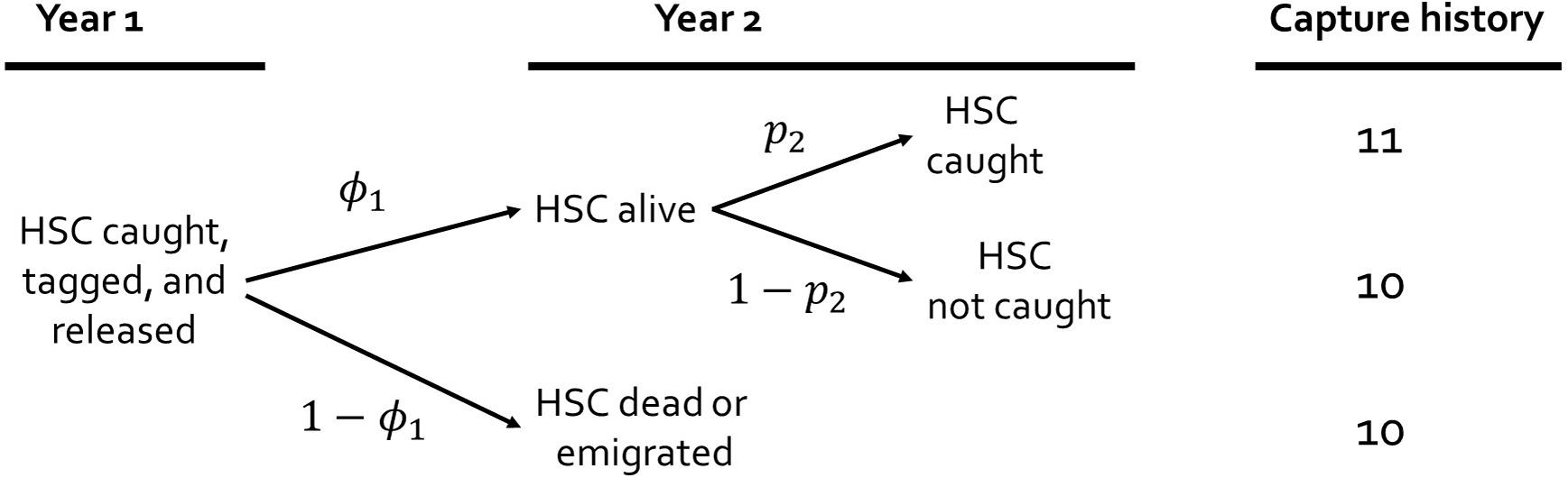
Figure 2. Diagram illustrating the capture-recapture sampling process over two years. The parameters represent the apparent survival (φ) and capture probabilities (p). The capture histories represent capture by ‘1’ and not capture by ‘0.’
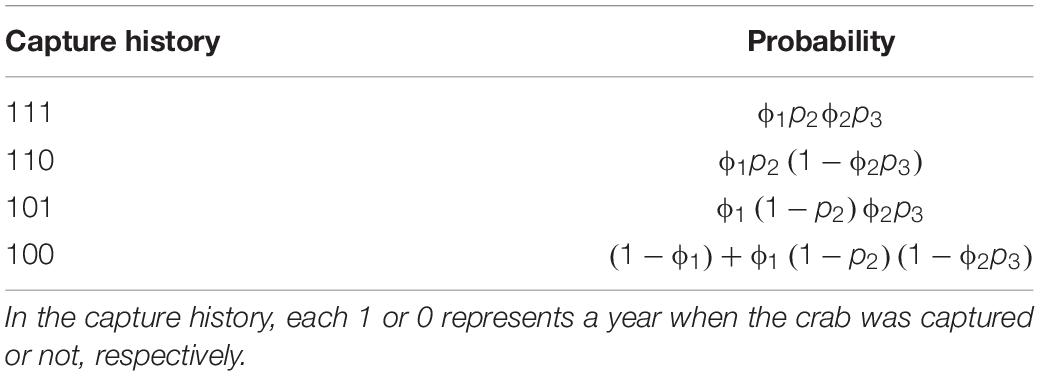
Table 2. Example capture histories and their expected probabilities as a function of apparent survival (ϕi) and capture probability (pi) for a 3-year tagging study.
The statistical theory and methods underlying inference from capture-recapture studies are well-developed (Pollock et al., 1990; Nichols, 1992; White and Burnham, 1999). The capture-recapture data form a capture history, where ‘1’ indicates the animal was captured and ‘0’ indicates the animal was not caught. Expectations for the possible capture histories are functions of the underlying probabilities for apparent survival and capture probabilities (Table 2). Capture probability and survival are not mechanistically correlated. Thus, low capture probability can be coincident with high survival or high capture probability with low survival because different processes determine capture as opposed to survival. The statistical approach to estimation is analogous to fitting a regression model by comparing model expectations to observed data to solve for the model that best fits the data. In the capture-recapture case, the observed frequencies of the capture histories are compared to the expected capture probabilities (Table 2), and the model fitting optimization estimates survival and capture using maximum likelihood methods (Pollock et al., 1990).
We fit Cormack-Jolly-Seber (CJS) models using program MARK (Pollock et al., 1990; White and Burnham, 1999) to capture histories from horseshoe crabs tagged and released in the Delaware Bay population. Models were fit using Jeff Laake’s RMark v2.2.6 (R version 3.4.0, R Core Team, 2017) to manage input for and output from program MARK (White and Burnham, 1999). The CJS models estimated apparent survival and capture probability, including sex, bleeding status, and time as possible covariates using a logit link (Pollock, 2002). Different relationships between the covariates and the parameters (survival and capture probabilities) defined alternative models. For example, in one model, bleeding status affected survival, and in an alternative model, survival was unaffected by bleeding status. The covariates’ significance was inferred by ranking the candidate models based on the Akaike Information Criteria (AIC) and using the minimum AIC to identify the best model (Burnham and Anderson, 1998). The AIC implemented in program MARK, denoted by AICc, is adjusted for small samples.
The models allowed for year-specific survival and capture probabilities. We found that year-specific survival was not estimable for many years based on zero or uninformative standard errors. Thus, we binned the years into periods defined by 2, 3, or 4 consecutive years and added those models to the candidate set, thereby estimating average annual survival within the binned periods. We used model selection (AICc) to determine which binning period best fit the data. The inference from all models relate to annual survival.
While the focus was on annual survival, we were also interested in capture probability because of the potential for an indirect impact on spawning behavior. Either beachcombers or spawning surveyors reported most (69%) recaptures in the USFWS database. But only spawning adults are found on the beach, and a decline in spawning activity would reduce capture probability. Mature males can migrate to the beach exhibiting spawning behavior as individuals or in amplexus with a mature female (Brockmann et al., 2015). Thus, a change in capture probability is a reasonable proxy for a change in spawning behavior. The capture probability for unbled crabs can be considered the baseline and the ratio of capture probabilities for unbled to bled crabs can be used to indicate a potential change in spawning activity. We calculated relative risk to infer differences in capture probability between bled and unbled individuals (Agresti, 1990; Van Sickle et al., 2006). Relative risk (RR) was calculated as RR = pu/pb, where capture probability for unbled crabs (pu) was divided by capture probability for bled crabs (pb) controlling for same year and sex. The variance of relative risk was var(RR) = var(pu) + var(pb)−2var(pu)var(pb)corr(pu,pb), and approximate 95% confidence interval (CI) was A CI for RR entirely above 1 implies capture probability was significantly lower for bled than unbled; a CI entirely below 1 implies capture probability was significantly higher for bled than unbled; and a CI that includes 1 implies capture probability was not different for bled and unbled animals.
Our analytical approach using tag and relative risk analyses controlled for sex, time, and geographic area while comparing survival and capture probability between bled and unbled crabs. Age would have been a logical covariate because adult survival declines with age; for example, the probability of stranding increases by age class (Smith et al., 2010), and stranding is the primary source of natural mortality among adults (Botton and Loveland, 1989). However, information on age was not available consistently enough to be included as a covariate. Age cannot be determined directly for horseshoe crabs, but age can be classified based on shell condition (Shuster, 2009). Thus, we compared age-class distribution across bleeding status for the subset of years when tagging programs recorded age class.
Results
For the geographic-expansive analysis covering the Delaware Bay population from 1999 to 2017, the best models included covariate effects on survival due to sex, bleeding, and time (Table 3). The top model with the most empirical support (i.e., with the minimum AICc) included interactive effects on apparent survival from sex, bleeding status, and time binned into 3-year periods. The ΔAICc for the 2nd best model was 40.20, indicating the top model fits the best among the models considered.
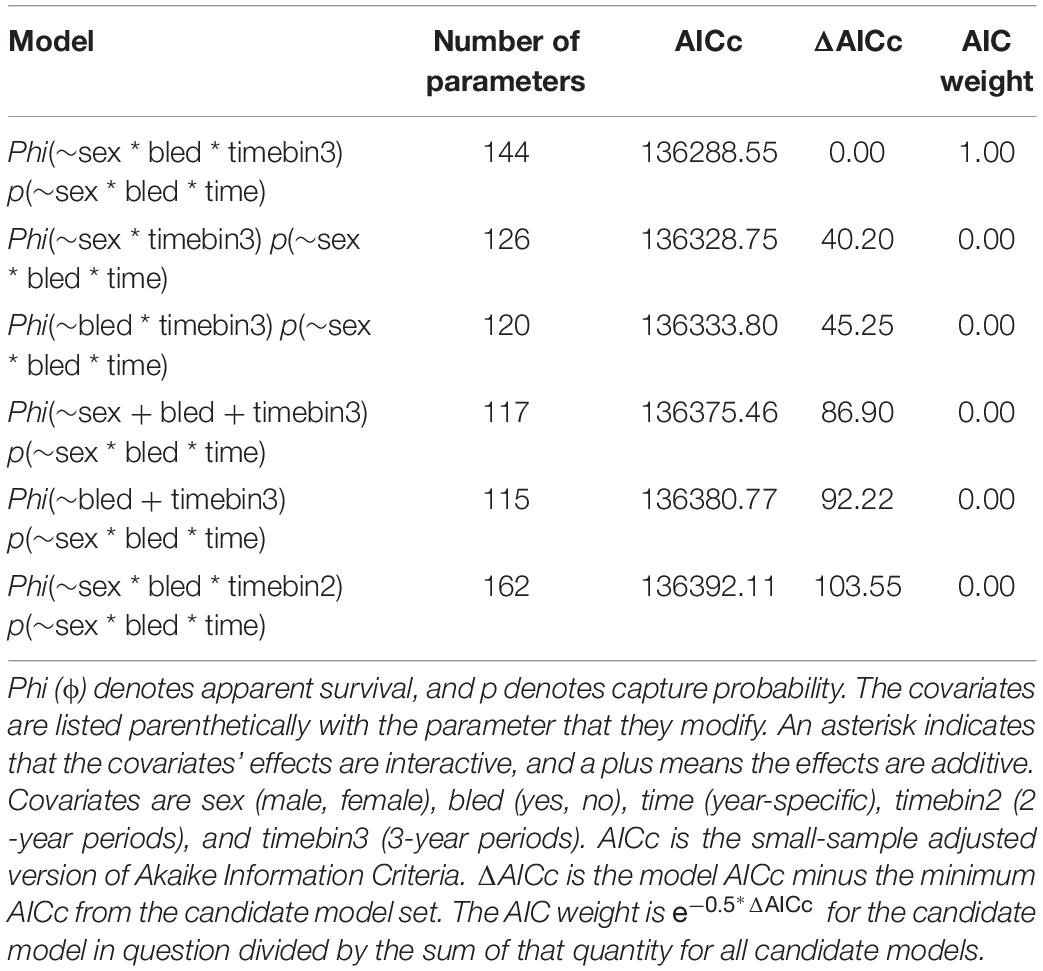
Table 3. Statistics for the top 6 candidate models fit to the capture histories for horseshoe crabs tagged in the Delaware Bay population (Delaware Bay and coastal Delaware to Virginia, cf Figure 1) from 1999 to 2017.
Survival differed by bleeding status (bled vs. unbled) in some years, but not consistently (Table 4, Figure 3). The point estimates for survival among males tended to be higher for bled crabs than for unbled crabs, but the pattern was mixed among females. The average survival was 0.69 and 0.71 for unbled males and females, respectively, and 0.72 and 0.68 for bled males and females.
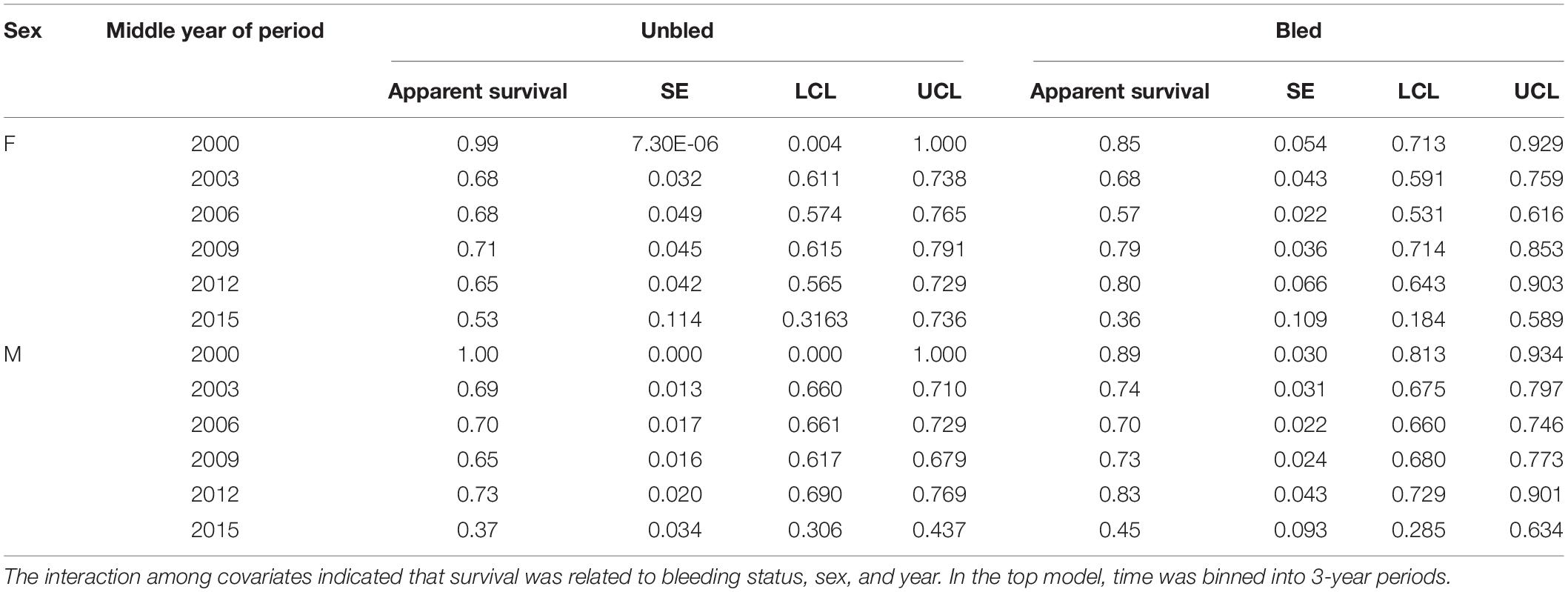
Table 4. Estimates of apparent survival, standard error (SE), and 95% confidence limits (LCL, UCL) for the top model for tagged horseshoe crabs released in Delaware Bay population (Delaware Bay and coastal Delaware to Virginia regions, cf Table 3 and Figure 1) from 1999 to 2017.
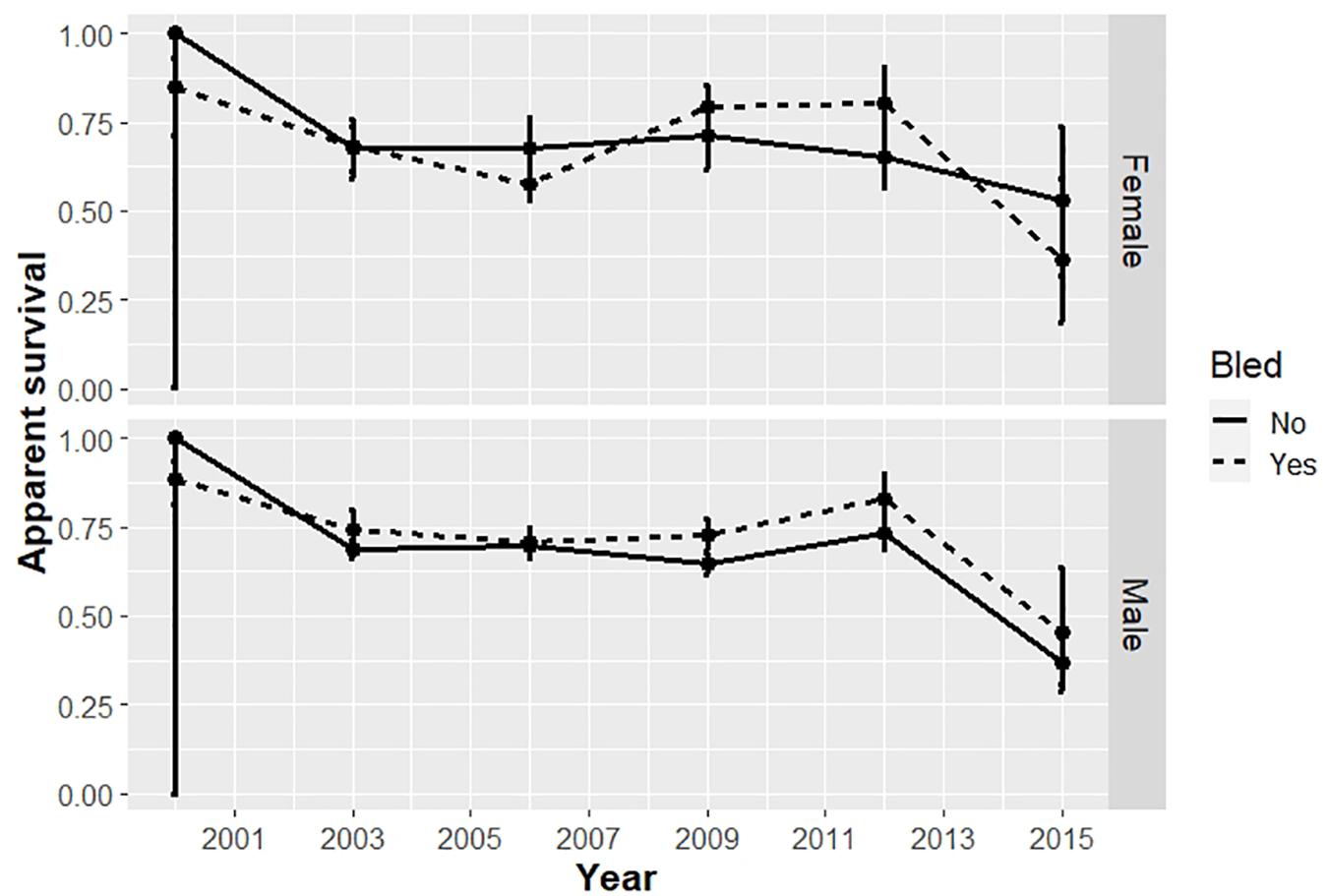
Figure 3. Apparent survival of horseshoe crabs tagged and released in the Delaware Bay population, including Delaware Bay and coastal Delaware to Virginia areas (cf Figure 1) from 1999 to 2017. Estimates with 95% confidence intervals from the candidate model with minimum AICc, which binned time into 3-year periods, are plotted for adult males and females.
For the analysis restricted to the coastal area from 2008 to 2017, the best models included covariates effects on survival due to bleeding and time, but not sex (Table 5). The top model with the most empirical support (i.e., with the minimum AICc) included bleeding status and time binned into 2-year periods. The ΔAICc for the 2nd best model was 6.86 indicating the top model fit best of the models considered. Estimates of annual survival were higher for bled than unbled crabs, although confidence intervals overlapped in most years (Table 6; Figure 4). Survival declined steeply in the last period (2014 to 2016) for both bled and unbled crabs (Figure 4). The average survival was 0.63 and 0.75 for unbled and bled animals excluding the last period, which could be an anomaly, and 0.51 and 0.68 for unbled and bled animals including the last period.
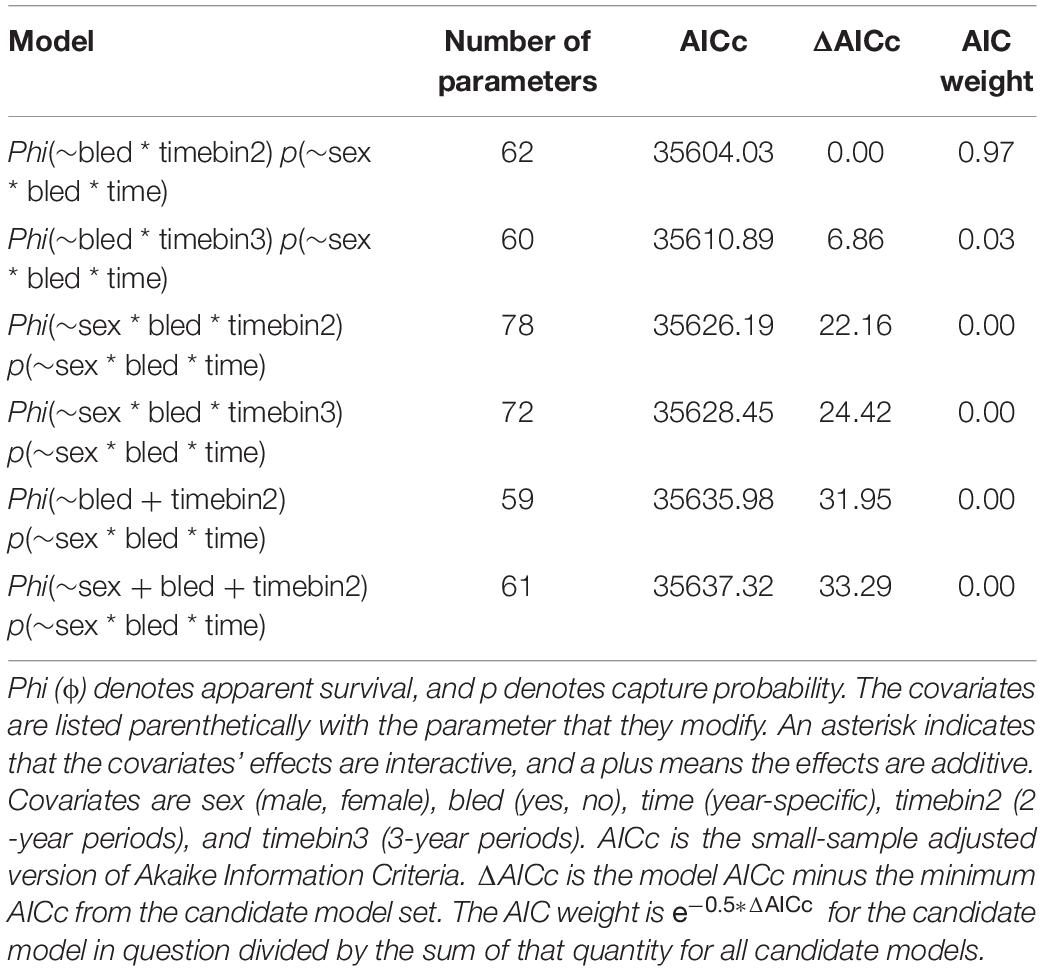
Table 5. Statistics for the top 6 candidate models fit to the capture histories for horseshoe crabs tagged in coastal Delaware to Virginia (cf Figure 1) from 2008 to 2017.
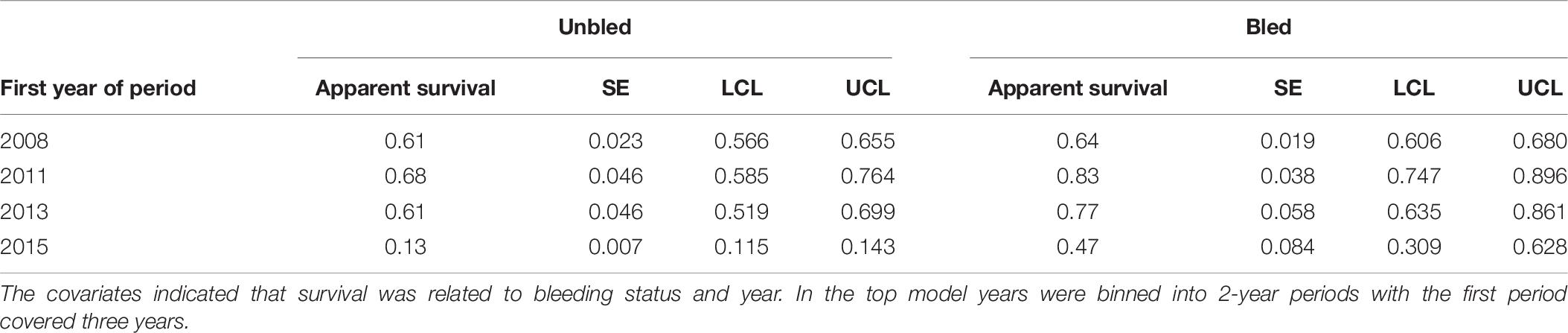
Table 6. Estimates of apparent survival, standard error (SE), and 95% confidence limits (LCL, UCL) for the top model for tagged horseshoe crabs released in coastal Delaware to Virginia from 2008 to 2017.
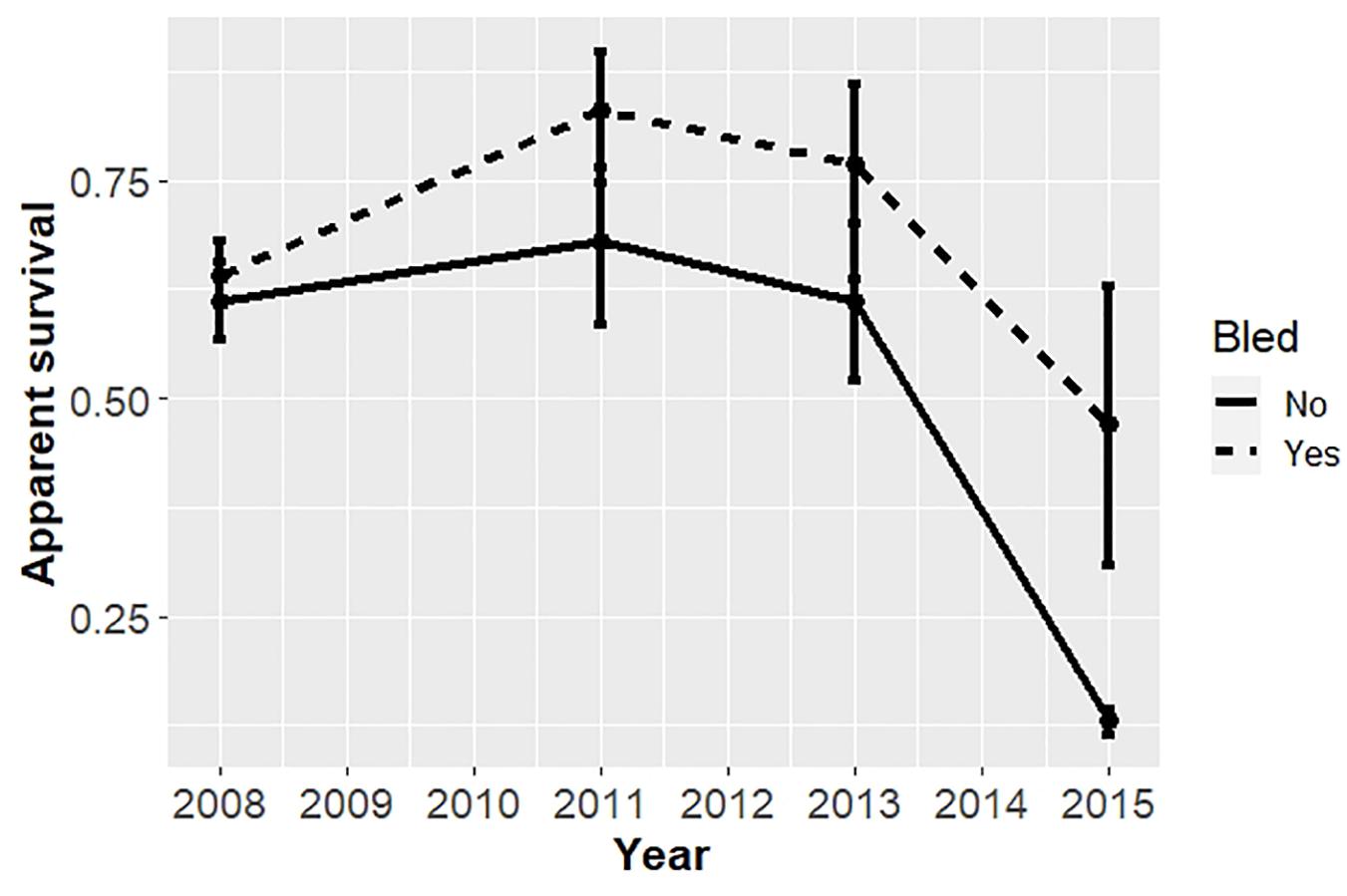
Figure 4. Apparent survival of horseshoe crabs tagged and released in the coastal Delaware to Virginia areas (cf Figure 1) from 2008 to 2017. Estimates with 95% confidence intervals from the candidate model with minimum AICc, which binned time into 2-year periods, are plotted.
Although age is a potentially significant covariate of survival, the tagging database did not consistently include age class. Lonza recorded age class for 12,496 bled crabs released from 1999 to 2002 and in 2004. Age class was recorded sporadically for 41,562 unbled crabs: 36,138 released in Delaware Bay between 2003 and 2005, 4,811 released in coastal Delaware to Virginia between 2013 and 2017, and 613 released over years and areas. Nevertheless, a composite view of age distributions of tagged crabs indicates selection for young adults among bled crabs (Figure 5).
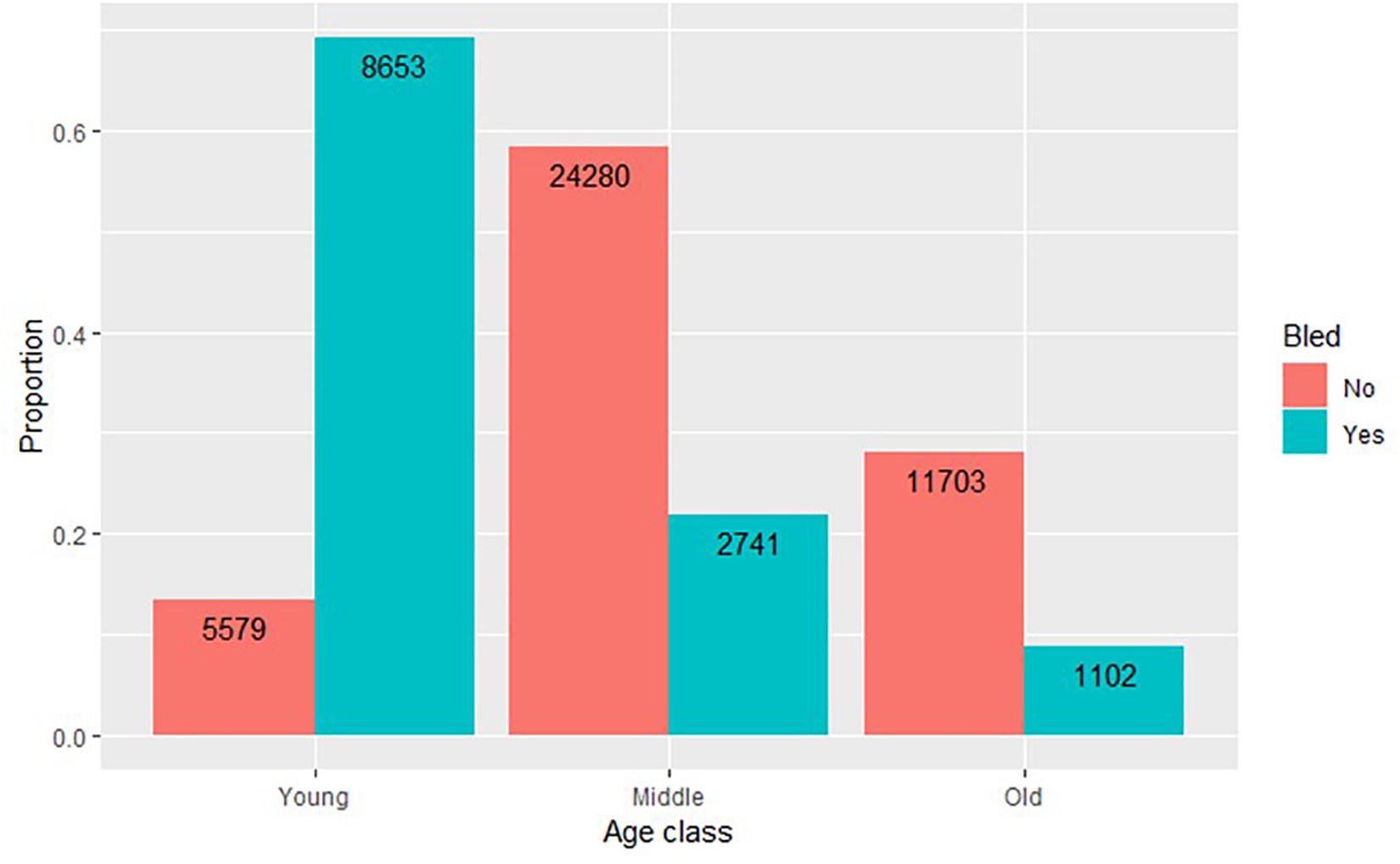
Figure 5. Age distribution for tagged horseshoe crabs. Age was recorded sporadically. Bled crabs were aged only from 1999 to 2002 and in 2004. Most unbled crabs were aged in Delaware Bay from 2003 to 2005 and in coastal areas from 2013 to 2017.
Capture probability tended to be higher for unbled than bled crabs, but the difference depended on sex (Tables 7, 8). For females in the Delaware Bay population, the relative risk was significantly above 1 in about half (56%) of the years (Table 7). For males in the Delaware Bay population, relative risk exceeded 1 in all years. For females in coastal Delaware to Virginia, relative risk trended from being below 1 in 2009–2011 and not different from 1 in 2012 to remaining above 1 in 2013–2016 (Table 8). For males in coastal Delaware to Virginia, the relative risk was significantly >1 except for one year (2010) early in the time series. Capture probability was high for unbled crabs in 2016 and 2017, particularly in coastal Delaware to Virginia (Table 8), corresponding to the years with low survival (Figure 4).
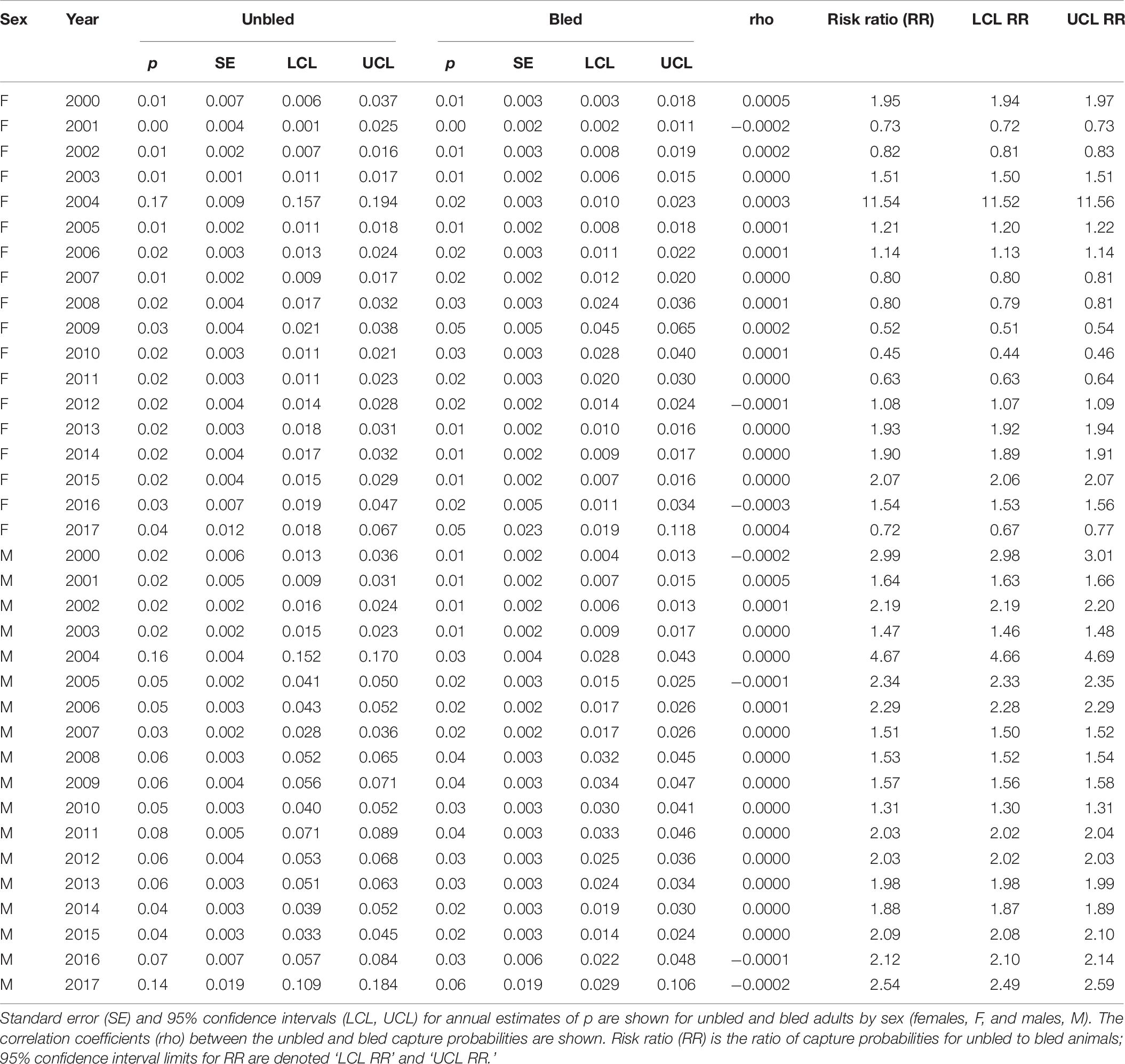
Table 7. Capture probability (p) for the top model for tagged horseshoe crabs released in the Delaware Bay population (Delaware Bay and coastal Delaware to Virginia regions) from 1999 to 2017.
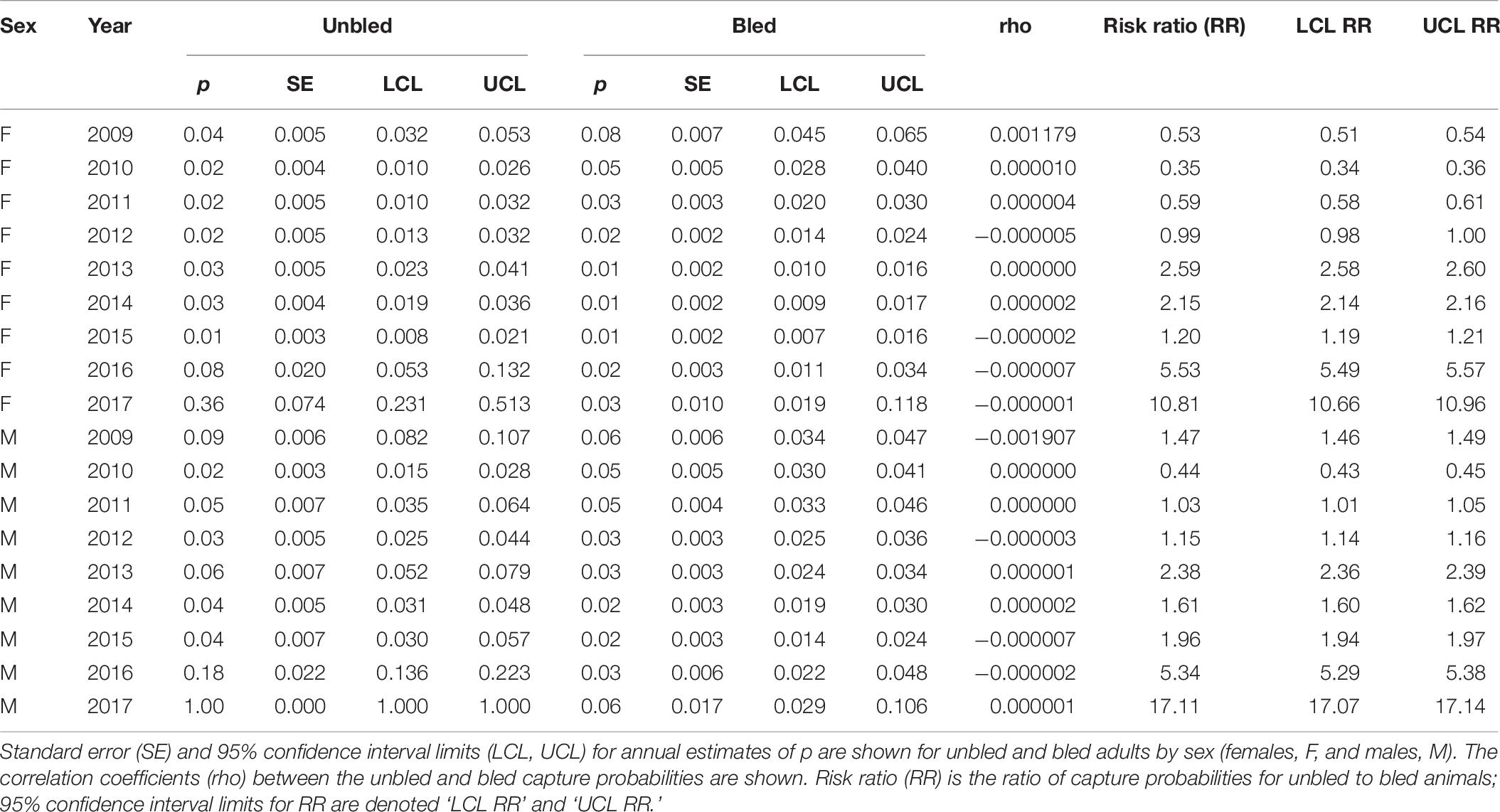
Table 8. Capture probability (p) for the top model for tagged horseshoe crabs released in coastal Delaware to Virginia from 2008 to 2017.
Discussion
Short-term mortality and behavioral effects due to bleeding for LAL production have been well-established (Rudloe, 1983; Kurz and James-Pirri, 2002; Hurton and Berkson, 2006; Leschen and Correia, 2010; Anderson et al., 2013; Owings et al., 2019). With limited exception (Butler, 2012), long-term bleeding effects have not been studied. Until recently, tagging programs lacked enough recaptures of bled and unbled animals required to overcome the low and variable recapture rates (<10%) for tagged horseshoe crabs. The USFWS horseshoe crab tagging database, started in 1999, provides an opportunity to examine bleeding effects over years rather than weeks or months.
In our analysis of the USFWS tagging data, the working hypotheses were that – all else equal – bled crabs survive at a lower annual rate and exhibit lower capture probabilities than unbled crabs. Our results showed mixed support for these hypotheses. There was no evidence that bleeding reduced survival based on multiple years of tagging contrary to our expectations. The geographically expansive analysis revealed a mixed pattern for female survival and a slightly higher survival for bled male crabs (Figure 3). The analysis restricted to coastal areas revealed consistently higher survival for bled than unbled crabs (Figure 4). However, capture probability was lower for bled crabs especially for males based on a higher relative risk of capture for unbled crabs.
Our comparison of survival rates between unbled and bled crabs assumed that all other relevant factors were equal. We accounted for geography at the regional scale and controlled for time and sex. However, differences in geography at a subregional scale and demographic characteristics of bled versus unbled crabs could have confounded differences in survival. Releases for bled crabs were concentrated around Ocean City, Maryland, near the LAL bleeding facilities, while unbled crabs came from the broad region encompassing the Delaware Bay population (Figure 1). Horseshoe crabs were collected for LAL production from multiple locations mostly by trawl and released near the facility after bleeding. But tagged animals were given a year to mix with the population before recaptures were noted. Horseshoe crabs in the Delaware Bay population are mobile and highly migratory (Swan, 2005: McGowan, 2018), and behavioral effects due to bleeding occur over weeks (Owings et al., 2019), so we expect that the bled crabs mixed readily with the general population during the first year at large after release.
Demographic differences in age distribution could confound survival comparisons between bled and unbled crabs. Biomedical companies cull injured crabs and crabs with a well-worn carapace and release them without bleeding or tagging, causing the demographics of bled crabs to differ from unbled (Atlantic States Marine Fisheries Commission [ASMFC], 2011). Available data revealed that biomedical companies select for younger animals for bleeding (Figure 5). Using carapace wear indicative of age class (Shuster, 2009), Brockmann et al. (2015) reviewed the evidence that young males have been found to be more vigorous than older males as shown by higher activity, quicker amplexus with mature females, longer duration of amplexus, and higher sperm concentration. Stranding mortality during spawning, which is a primary source of natural mortality (Botton and Loveland, 1989), increases with age (Penn and Brockmann, 1995; Smith et al., 2010). Overall, we expect young animals to survive at a higher rate than older animals. Continued tagging of bled and unbled in the same geographic area with consistent recording of age class and sex will help further resolve how age interacts with post-bleeding mortality. Shuster (2009) outlined a protocol for determining age class, which could be used for standardized monitoring.
The numerous tag recaptures on beaches supports a change in capture probability being indicative of a change in spawning activity, and our tagging analysis affirmed a post-bleeding effect on capture probability and thus potentially on spawning activity. The effect was more apparent in males than in females. Capture probability for unbled male crabs was roughly twice that for bled male crabs (median relative risk was 2.03 and 1.60 for Delaware Bay and coastal Delaware to Virginia, respectively). The difference in capture probability between unbled and bled female crabs was smaller compared to males (median relative risk was 1.11 and 1.20 for Delaware Bay and coastal Delaware to Virginia, respectively). It was important to control for sex in the comparison between bled and unbled because males spend more time on spawning beaches, and thus have higher capture probability, than females (Smith et al., 2010). Also note that although bled crabs are released from trawls while unbled crabs are released from both trawls and beaches, the first recorded recapture is the year after release, which allows time for the tagged animals to mix and distribute with the population. Future studies may consider incorporating a temporal component to this assessment under the hypothesis that the effects of bleeding on spawning behavior may dissipate over time.
Although our research focused on the contrast between bled and unbled crabs, the analysis revealed a reduction in survival during recent years, which has potential management implications. The drop in survival appears for both bled and unbled crabs so the exploration of causes is outside the scope of this paper. However, potential causes include temporal changes in mortality or emigration. Alternatively, the drop could be an artifact of estimates for the last years of the study being based on less data. In part to resolve the nature and source of the apparent drop in survival in recent years, we are conducting an analysis using multistate models (Bopp et al., 2019) using data up to 2019 in relation to harvest regulations.
The recent ASMFC stock assessment concluded that the short-term bleeding effect resulting in mortality of approximately 78,750 annually comprising a minor portion (<13%) of the up to one million annual coastwide landings dominated by harvest for bait and <1% of adult abundance in Delaware Bay (Atlantic States Marine Fisheries Commission [ASMFC], 2019). The male to female operational sex ratio (i.e., the ratio on the spawning beach) in 2017 was 5.2 in the Delaware Bay population and has increased in recent years (Atlantic States Marine Fisheries Commission [ASMFC], 2019) despite a male dominated bait harvest that greatly exceeds biomedical harvest. The ASMFC concluded that biomedical harvest has not significantly affected the Delaware Bay population’s fishing mortality or dynamics.
Two effects of bleeding have management implications: (1) the effects on individual behavior and mortality and (2) the effect on population status. Effects on individual behavior and mortality are well-established but mitigated through best practices, to some extent. However, results from the tagging-data analysis and the recent stock assessment do not indicate an effect of LAL production on the Delaware Bay population’s status. A population impact would require a significant effect on enough individuals relative to the population abundance. The ASMFC stock assessment found that Limulus’s biomedical harvest had not limited population growth or abundance in the Delaware Bay (Atlantic States Marine Fisheries Commission [ASMFC], 2019). Adult horseshoe crab abundance in the Delaware Bay area in 2017 was 30,852,000 comprising 8,665,000 females and 22,187,000 males (Hata and Hallerman, 2018). By comparison, bait landings from the Delaware Bay population were 339,976 (10,136 females and 329,840 males), which is only 1.1% (0.1% females and 1.5% males) of population abundance (Atlantic States Marine Fisheries Commission [ASMFC], 2019). The coastwide mortality from biomedical harvest from Massachusetts, the mid-Atlantic states, and South Carolina in 2017 was 78,750 (<13% of coastwide bait landings), and biomedical mortality from Delaware Bay would be fewer (Atlantic States Marine Fisheries Commission [ASMFC], 2019). Thus, ASMFC’s findings are not surprising given that the total harvest was less than 1.4% of abundance and biomedical mortality is a minor fraction of total harvest.
Our analysis detected a reduced relative risk for bled crabs to be recaptured, which could indicate reduced spawning activity and, in turn, reduced population productivity. An effect at the population level would require sufficient numbers relative to abundance to reduce their fecundity or spawning activity. Changes in population productivity for horseshoe crabs in the Delaware Bay area have not been detected but productivity is very difficult to measure in this species (Atlantic States Marine Fisheries Commission [ASMFC], 2019). Furthermore, current evidence reveals that the effects at the individual level are short-lived (Owings et al., 2019) or, in the long term, modest (based on this tagging analysis), and the number affected is small relative to the population size (Atlantic States Marine Fisheries Commission [ASMFC], 2019). Thus far, the evidence does not support that LAL-production has impacted the Delaware Bay population status. However, continued monitoring is warranted as the demand for LAL might increase.
Data Availability Statement
The original contributions presented in the study are included in the article/Supplementary Material, further inquiries can be directed to the corresponding author/s.
Author Contributions
All co-authors approved the publication and contributed to the concept and design of the analysis and the writing. DS and JN conducted the analyses. DS was the lead writer. All authors contributed to the article and approved the submitted version.
Funding
Funding for this study was provided by the U.S. Fish and Wildlife Service, Atlantic States Marine Fisheries Commission and the U.S. Geological Survey.
Conflict of Interest
The authors declare that the research was conducted in the absence of any commercial or financial relationships that could be construed as a potential conflict of interest.
Acknowledgments
We thank the many organizations and volunteers who contribute to the U.S. Fish and Wildlife Service horseshoe crab tagging database each year. Funding for this study was provided by the U.S. Fish and Wildlife Service, Atlantic States Marine Fisheries Commission and the U.S. Geological Survey. John Sweka and Jim Lyons provided helpful comments on earlier drafts. Any use of trade, product, website, or firm names in this publication is for descriptive purposes only and does not imply endorsement by the U.S. Government.
Supplementary Material
The Supplementary Material for this article can be found online at: https://www.frontiersin.org/articles/10.3389/fmars.2020.607668/full#supplementary-material
References
Anderson, L. I., and Shuster, C. N. Jr. (2003). “Throughout geologic time: where have they lived?,” in The American Horseshoe Crab, eds C. N. Shuster, Jr. R. B. Barlow, and H. J. Brockmann (Cambridge, MA: Harvard University Press), 189–223.
Anderson, R. L., Watson, W. H.III., and Chabot, C. C. (2013). Sublethal behavioral and physiological effects of the biomedical bleeding process on the American horseshoe crab, Limulus polyphemus. Biol. Bull. 225, 137–151. doi: 10.1086/bblv225n3p137
Atlantic States Marine Fisheries Commission [ASMFC] (2011). Horseshoe Crab Biomedical Ad-Hoc Working Group Report. Arlington, VA: Atlantic States Marine Fisheries Commission.
Atlantic States Marine Fisheries Commission [ASMFC] (2019). Horseshoe Crab Stock Assessment Report. Arlington, VA: Atlantic States Marine Fisheries Commission, 316.
Berkson, J., and Shuster, C. N. Jr. (1999). The horseshoe crab: the battle for a true multiple-use resource. Fisheries 24, 6–10. doi: 10.1577/1548-8446(1999)024<0006:thctbf>2.0.co;2
BłaŻejowski, B. (2015). “The oldest species of the genus,” in Changing Global Perspectives on Horseshoe Crab Biology, eds R. H. Carmichael, M. L. Botton, P. K. S. Shin, and S. G. Cheung (New York, NY: Springer), 3–14. doi: 10.1007/978-3-319-19542-1_1
Bopp, J. J., Sclafani, M., Smith, D. R., McKown, K., Sysak, R., and Cerrato, R. M. (2019). Geographic-specific capture-recapture models reveal contrasting migration and survival rates of adult horseshoe crabs (Limulus polyphemus). Estuar. Coasts 42, 1570–1585. doi: 10.1007/s12237-019-00595-1
Botton, M. L., and Loveland, R. E. (1989). Reproductive risk: high mortality associated with spawning by horseshoe crabs (Limulus polyphemus) in Delaware Bay, USA. Mar. Biol. 101, 143–151. doi: 10.1007/bf00391453
Brockmann, H. J., Johnson, S. L., Smith, M. D., and Sasson, D. A. (2015). “Mating tactics of the American horseshoe crab,” in Changing Global Perspectives on Biology, Conservation and Management of Horseshoe Crabs, eds R. H. Carmichael, M. L. Botton, P. K. S. Shin, and S. G. Cheung (New York, NY: Springer), 321–351. doi: 10.1007/978-3-319-19542-1_19
Burnham, K. P., and Anderson, D. R. (1998). Model Selection and Multimodel Inference: A Practical Information-Theoretic Approach, 2nd Edn, New York, NY: Springer.
Butler, C. A. (2012). Estimation of Survival of the Atlantic Horseshoe Crab, Limulus polyphemus, by Analysis of Mark-Recapture Data with Tag Loss. Doctoral thesis, Auburn University, Auburn, AL.
Carmichael, R. H., and Brush, E. (2012). Three decades of horseshoe crab rearing: a review of conditions for captive growth and survival. Rev. Aquacult. 4, 32–43. doi: 10.1111/j.1753-5131.2012.01059.x
Hata, D., and Hallerman, E. (2018). Results of the 2019 Horseshoe Crab Trawl Survey: Report to the Atlantic States Marine Fisheries Commission Horseshoe Crab and Delaware Bay Ecology Technical Committees. Arlington, VA: ASMFC.
Hurton, L., and Berkson, J. (2006). Potential causes of mortality for horseshoe crabs (Limulus polyphemus) during the biomedical bleeding process. Fish. Bull. 104, 293–298.
John, B. A., Jalal, K. C. A., Zaleha, K., Armstrong, P., and Kmaruzzaman, B. Y. (2011). Effects of blood extraction on the mortality of Malaysian horseshoe crabs (Tachypleus gigas). Mar. Freshw. Behav. Physiol. 44, 321–327. doi: 10.1080/10236244.2011.642505
John, B. A., Nelson, B. R., Sheikh, H. I., Cheung, S. G., Wardiatno, Y., Dash, B. P., et al. (2018). A review on fisheries and conservation status of Asian horseshoe crabs. Biodivers. Conserv. 27, 3573–3598. doi: 10.1007/s10531-018-1633-8
King, T. L., Eackles, M. S., Aunins, A. W., Brockmann, H. J., Hallerman, E., and Beaver, B. L. (2015). “Conservation genetics of the American horseshoe crab (Limulus polyphemus): allelic diversity, zones of genetic discontinuity, and regional differentiation,” in Changing Global Perspectives on Horseshoe Crab Biology, Conservation And Management, eds R. H. Carmichael, M. L. Botton, P. K. S. Shin, and S. G. Cheung (New York, NY: Springer), 65–96. doi: 10.1007/978-3-319-19542-1_4
Krisfalusi-Gannon, J., Ali, W., Dellinger, K., Robertson, L., Brady, T. E., Goddard, M. K., et al. (2018). The role of horseshoe crabs in the biomedical industry and recent trends impacting species sustainability. Front. Mar. Sci. 5:185. doi: 10.3389/fmars.2018.00185
Kurz, W., and James-Pirri, M. J. (2002). The impact of biomedical bleeding on horseshoe crab, Limulus Polyphemus, movement patterns on Cape Cod, Massachusetts. Mar. Freshw. Behav. Physiol. 35, 261–268. doi: 10.1080/1023624021000019315
Laurie, K., Chen, C. P., Cheung, S. G., Do, V., Hsieh, H., John, A., et al. (2019). Tachypleus tridentatus. IUCN Red List Threat. Species 2019:e.T21309A149768986.
Leschen, A. S., and Correia, S. J. (2010). Mortality in female horseshoe crabs (Limulus polyphemus) from biomedical bleeding and handling: implications for fisheries management. Mar. Freshw. Behav. Physiol. 43, 135–147. doi: 10.1080/10236241003786873
Levin, J., Hochstein, H. D., and Novitsky, T. J. (2003). “Clotting cells and Limulus amebocyte lysate: an amazing analytical tool,” in The American Horseshoe Crab, eds C. N. Shuster, Jr. R. B. Barlow, and H. J. Brockmann (Cambridge, MA: Harvard University Press), 310–340.
McGowan, A. (2018). Horseshoe crab (Limulus polyphemus) movements following tagging in the Delaware Inland Bays, USA. Estuar. Coasts 41, 2120–2127. doi: 10.1007/s12237-018-0406-7
Owings, M., Chabot, C., and Watson, W. I. I. I. (2019). Effects of the biomedical bleeding process on the behavior of the American horseshoe crab, Limulus polyphemus, in its natural habitat. Biol. Bull. 236, 207–223. doi: 10.1086/702917
Penn, D., and Brockmann, H. J. (1995). Age-biased stranding and righting in horseshoe crabs, Limulus polyphemus. Anim. Behav. 49, 1531–1539. doi: 10.1016/0003-3472(95)90074-8
Pollock, K. H. (2002). The use of auxiliary variables in capture-recapture modelling: an overview. J. Appl. Statist. 29, 85–102. doi: 10.1080/02664760120108430
Pollock, K. H., Nichols, J. D., Brownie, C., and Hines, J. E. (1990). Statistical inference for capture-recapture experiments. Wildlife Monogr. 107, 3–97.
R Core Team (2017). R: A Language and Environment for Statistical Computing. Vienna, Austria: R Foundation for Statistical Computing. Available online at: https://www.R-project.org/
Rudkin, D. M., and Young, G. M. (2009). “Horseshoe crabs – an ancient ancestry revealed,” in Biology and Conservation of Horseshoe Crabs, eds J. T. Tanacredi, M. L. Botton, and D. R. Smith (New York, NY: Springer), 25–44.
Rudloe, A. (1983). The effect of heavy bleeding on mortality of the horseshoe crab, Limulus polyphemus, in the natural environment. J. Invertebr. Pathol. 42, 167–176. doi: 10.1016/0022-2011(83)90059-9
Schmidtke, M., Eyler, S., Michels, S., Wright, C., and Ebbin, S. (2018). Review of the Atlantic States Marine Fisheries Commission fishery Management Plan for Horseshoe Crab (Limulus polyphemus): 2017 Fishing Year. Arlington, VA: Atlantic States Marine Fisheries Commission.
Shuster, C. N. Jr. (2009). “Public participation in studies in horseshoe crab populations,” in Biology and Conservation of Horseshoe Crabs, eds J. T. Tanacredi, M. L. Botton, and D. R. Smith (New York, NY: Springer), 585–594. doi: 10.1007/978-0-387-89959-6_38
Smith, D. R., Brockmann, H. J., Beekey, M., King, T. L., Millard, M. J., and Zaldívar Rae, J. (2016). Conservation status of the American horseshoe crab (Limulus polyphemus): a regional assessment. Rev. Fish Biol. Fish. 27, 135–175. doi: 10.1007/s11160-016-9461-y
Smith, D. R., Brousseau, L. J., Mandt, M. T., and Millard, M. J. (2010). Age and sex specific migration timing and frequency of horseshoe crab spawning in Delaware Bay: insights from a large-scale radio telemetry array. Curr. Zool. 56, 563–574. doi: 10.1093/czoolo/56.5.563
Swan, B. L. (2005). Migrations of adult horseshoe crabs, Limulus polyphemus, in the Middle Atlantic Bight: a 17-year tagging study. Estuaries 20, 28–40. doi: 10.1007/bf02732751
Van Sickle, J., Stoddard, J. L., Paulsen, S. G., and Olsen, A. R. (2006). Using relative risk to compare the effects of aquatic stressors at a regional scale. Environ. Manag. 38, 1020–1030. doi: 10.1007/s00267-005-0240-0
Keywords: Limulus polyphemus, Limulus amebocyte lysate, LAL, Delaware Bay, tagging analysis, survival, spawning activity, horseshoe crab
Citation: Smith DR, Newhard JJ, McGowan CP and Butler CA (2020) The Long-Term Effect of Bleeding for Limulus Amebocyte Lysate on Annual Survival and Recapture of Tagged Horseshoe Crabs. Front. Mar. Sci. 7:607668. doi: 10.3389/fmars.2020.607668
Received: 17 September 2020; Accepted: 01 December 2020;
Published: 21 December 2020.
Edited by:
Christopher C. Chabot, Plymouth State University, United StatesReviewed by:
Winsor Hays Watson, University of New Hampshire, United StatesDaniel Sasson, South Carolina Department of Natural Resources, United States
Copyright © 2020 Smith, Newhard, McGowan and Butler. This is an open-access article distributed under the terms of the Creative Commons Attribution License (CC BY). The use, distribution or reproduction in other forums is permitted, provided the original author(s) and the copyright owner(s) are credited and that the original publication in this journal is cited, in accordance with accepted academic practice. No use, distribution or reproduction is permitted which does not comply with these terms.
*Correspondence: David R. Smith, ZHJzbWl0aEB1c2dzLmdvdg==