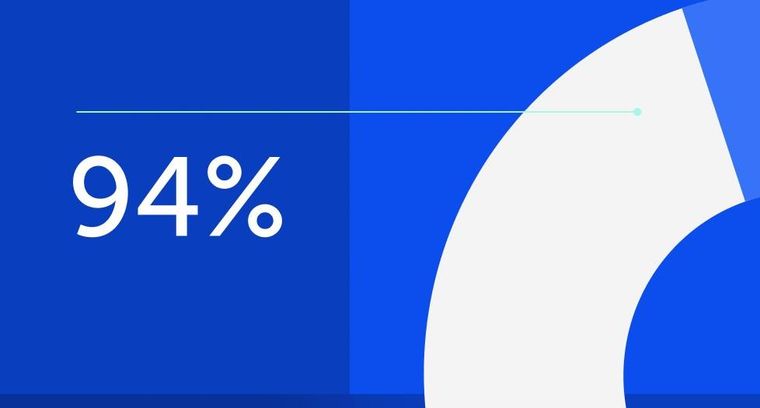
94% of researchers rate our articles as excellent or good
Learn more about the work of our research integrity team to safeguard the quality of each article we publish.
Find out more
ORIGINAL RESEARCH article
Front. Mar. Sci., 08 December 2020
Sec. Deep-Sea Environments and Ecology
Volume 7 - 2020 | https://doi.org/10.3389/fmars.2020.603593
This article is part of the Research TopicDeep-sea Sponge Ecosystems: Knowledge-based Approach Towards Sustainable Management and ConservationView all 29 articles
Sponges hold a key role in benthic environments, and specifically in the Mediterranean Sea. Past events of mass mortality in sponge communities have been linked to extended periods of high-temperature anomalies, yet it is unknown how a gradual change, such as the constant rise in global seawater temperatures, will affect biodiversity. Here we present a case study of Agelas oroides, a common massive sponge in the Mediterranean Sea, found at a wide depth range of 1–150 m. Last documented in the 1970s, A. oroides was considered lost from the Israeli coastal fauna. However, its recent rediscovery in mesophotic depths, where environmental conditions are stable, provided an opportunity to examine whether it can survive the present conditions in the shallow Israeli coast – where temperatures increased by 3°C during the past 60 years, while the nutrients concentration decreased following the damming of the Nile River. To test this hypothesis, A. oroides individuals were collected during winter from mesophotic sponge grounds (100–120 m) and transplanted to a shallow rocky habitat (10 m). Control individuals were transplanted back to the mesophotic habitat. Sponge survival, temperature, and nutrient concentrations were measured in both habitats. The shallow-transplanted sponges’ survival decreased only when the ambient temperature exceeded 28°C. In contrast, the control group at the mesophotic depth, where the temperature never rose above 20°C, survived the duration of the experiment. Our findings suggest that a prolonged period of high temperatures may constitute a major factor in A. oroides survival and disappearance from the Israeli shallow habitats.
Porifera (sponges) form the oldest metazoan phylum and have demonstrated resilience and survival under various environmental conditions over different geological eras (Feuda et al., 2017). Nonetheless, environmental conditions are considered a key factor in determining the dynamics of sponge spatial distribution, with temperature playing a central role (Garrabou et al., 2009, 2019; Di Camillo et al., 2013). For example, Mediterranean sponge diversity has been shown to follow an NNW-SSE gradient that correlates with an increase in water temperature and salinity and a decrease in nutrient concentration (Voultsiadou, 2009). In addition, several studies demonstrated that extended periods of high-temperature anomalies during the summer resulted in mass mortality in sponge communities (Garrabou et al., 2009, 2019; Di Camillo et al., 2013; Ereskovsky et al., 2019). Furthermore, recent studies found that elevated temperatures led to significant physiological responses in several sponge species, such as a decrease in buoyant weight, prevalence of diseases, and an increase in mortality (Webster, 2007; Massaro et al., 2012; Bennett et al., 2017; Laffy et al., 2019; Beepat et al., 2020).
The present case-study species, Agelas oroides (Schmidt, 1864), is a sponge common to the Mediterranean Sea, at both shallow (<40 m) and mesophotic (40–150 m) depths (Voultsiadou, 2009; Topaloglu and Evcen, 2014; Costa et al., 2018; Grenier et al., 2018). It inhabits sheltered habitats with low light intensity (i.e., entrances to caves, crevices, or mesophotic habitats) (Tsurnamal, 1968; Gerovasileiou and Voultsiadou, 2012; Gerovasileiou et al., 2017; Grenier et al., 2018). It has an important ecological role, increasing the structural complexity of its surroundings and consequently providing a habitat for the invertebrates and fish that dwell in and on it (Bo et al., 2012; Beazley et al., 2013; Kenchington et al., 2013; Gerovasileiou et al., 2016). A. oroides has also proven to be an important source of many new natural products, some with demonstrated bioactivity, e.g., oroidin (Sauleau et al., 2017; Kovalerchik et al., 2020). A. oroides is thus of considerable interest in the ecological and biotechnological context and a meaningful study object.
It is therefore of concern that the distribution range of A. oroides in the Mediterranean appears to have decreased over time. Historically, A. oroides was considered abundant in the shallow water of the Israeli Mediterranean coast. Although few specimens were collected from mesophotic depths in the 1970s, A. oroides was last reported from the shallow Israeli coast in the 1960s (Tsurnamal, 1968). Since then, it has been regarded as lost from the Israeli sponge fauna. The reason for its regional disappearance is unclear, but over the past 60 years, seawater temperature along the Israeli Mediterranean coast has risen by over 3°C during the summer, presently surpassing temperatures of 30°C (Lipkin and Safriel, 1971; Shaltout and Omstedt, 2014; Ozer et al., 2017). An alternative explanation may relate to the construction of the Aswan High Dam in 1965 that limited the discharge of the Nile River to the overflow of the dam only (Oren, 1969). This limitation dramatically reduced the primary source of nutrient flow into the south Eastern Mediterranean (Oren, 1969; Azov, 1991), further lowering nutrients concentration and resulting in ultra-oligotrophic conditions in the Levant Basin (Krom et al., 2010; Kress et al., 2014; Ozer et al., 2017). Therefore, these two factors together (temperature and nutrient concentration) are the most likely causes for the disappearance of local A. oroides populations. As seawater temperature continues to rise and the ecology of the oceans is changing, it is essential to understand the interaction with other environmental factors and potential effects on biodiversity.
The recent rediscovery of dense populations of A. oroides in Israel’s mesophotic sponge grounds with environmental conditions markedly different from those along the shallow coast (Idan et al., 2018) has provided the opportunity to test the hypothesis that shallow-water temperature and nutrient levels have adverse effects on A. oroides and could limit its distribution along the Israeli coast. To assess the effect of habitat-related environmental factors on the growth and survival of A. oroides along the Israeli coast, an experiment was conducted in which sponges from the mesophotic sponge ground were transplanted to a shallow rocky habitat, and others returned to mesophotic depths as controls.
Mesophotic sponges were collected at a depth of 100–120 m from a site that is part of a submerged sandstone ridge off the coast of Herzliya along the Israeli Mediterranean coast (32.17710°N, 034.63306°E, WGS-84). Sponges were transplanted from this mesophotic site to a rocky, shallow habitat in 10 m depth off the coast of Mikhmoret (32.42854°N, 034.87313°E, WGS-84), where A. oroides used to grow in the 1960s both under overhangs and on exposed rocks (Tsurnamal, 1968).
Sponges were collected during two expeditions onboard the R/V Mediterranean Explorer (using a Remotely Operated Vehicle (ROV; ECA-Robotics H800). This ROV is equipped with a five-function-manipulator, a full high-definition camera, and two parallel laser beams for scale. The sampled sponges were brought on board in a collection basket and immediately placed in a seawater flow tank, in which they were individually numbered and attached to settlement tiles (environmental cement® 20 cm × 20 cm × 5 cm) using coated electric wire. Twenty A. oroides individuals were collected from the mesophotic site between January and March 2018, of which fourteen were transplanted to 10 m depth at Mikhmoret, while six sponges served as a control group and were transplanted back to the mesophotic site.
On the day of collection, the specimens were transferred to running seawater tanks at the Ruppin Academic Center’s research facility in Mikhmoret. The following morning the sponges, still maintained unexposed to air, were transferred by SCUBA diving to the transplantation site. Most of the sponge specimens (n = 11) were placed (attached to tiles) in plastic crates (55 cm × 15 cm × 29 cm) that had been bolted to the rocky substrate prior to the translocation (Figure 1A). The crates were selected to allow low light and moderate water flow conditions through a mesh-like structure at the top and large holes on the sides. These conditions are naturally favored by A. oroides (Tsurnamal, 1968; Gerovasileiou and Voultsiadou, 2012; Gerovasileiou et al., 2017). The crates were cleaned of fouling organisms and algae weekly to ensure the holes stayed open, enabling water flow, and that other filter-feeders are not growing in the crates. Three specimens were attached to the crates’ exterior (Supplementary Table 1) in order to control for possible effects of the specimens being inside the plastic crates. As a control for handling and translocating the sponges, six individuals were placed with their tiles on two metal trays onboard the R/V and then positioned by ROV back on their original rocky substrate at the mesophotic site (Figure 1B), where A. oroides grows abundantly (Idan et al., 2018). The control sponges were not placed inside of crates so that in situ visual inspections of them would be possible without bringing them on board and because there was no need to cover them from direct light in the mesophotic zone. The metal trays were chosen in order to provide a sonar signature for easier location in the following visits, and since the sponges were attached to tiles, there was no physical contact between them and the metal. The location of the trays was marked on a high-resolution bathymetric map using the HYPACK® navigational system, which allowed us to return to the trays and monitor the control sponges. The choice of an open tray was made so that we can monitor the appearance of control sponges. All sponges were left to acclimate until April 1st, 2018.
Figure 1. Transplanted A. oroides. (A) specimens transplanted to the shallow site placed both inside and outside the plastic crates; (B) specimens transplanted to the deep site, placed on a metal tray.
Throughout the experiment (April to August 2018), the transplanted sponges were monitored twice a month for viability and growth. The examined parameters were: ectosome integrity, state of oscula (open or closed); water pumping.
Pumping, which indicates that the sponge is active, was visualized by releasing fluorescein-dyed seawater (fluorescein, 100 mg/L) next to the sponge and documenting if it flowed out of the oscula (Yahel et al., 2005). All parameters were measured at the transplantation site (underwater) with minimal handling of the sponges.
In order to estimate individual growth, sponge volume was assessed using 3D photogrammetry. Six randomly chosen individuals were monitored throughout the experiment. They were photographed from multiple angles twice a month for 3D model reconstruction, beginning on April 12th (Lavy et al., 2015; Olinger et al., 2019). The 3D models were reconstructed using Agisoft PhotoScan v1.4 (Agisoft, 2017) and Metashape v1.5 (Agisoft, 2019) professional edition softwares. Sponges were transferred with their tiles, without direct handling of the sponge and placed on a “Lazy Susan” turntable marked with scale bars and place-markers (Figure 2A). The cameras (Canon G16 or G12) were positioned ∼20 cm from the turntable, and the turntables were rotated to capture all possible perspectives of the specimens, rulers, and markers. For each model, 75–160 images were imported to the software and then automatically aligned (high accuracy, key point limit 100,000, tie point limit 20,000). The next step was generating a dense point cloud (high quality, depth filtering mild). Upon completion, a mesh (surface type arbitrary, face count high, calculated vortex colors enabled) was created through interpolation of the points in the dense cloud, and finally, the texture was added (mapping mode generic, blending mode average). Manual proofing was performed for each step to ensure maximal accuracy. To scale the model, markers were placed on at least two scale bars (Figure 2B). This stage was performed manually for each image, after which the base and turntable were erased, the remaining gaps were closed, and the volume was assessed.
Figure 2. Creating a 3D model of the transplanted A. oroides: (A) specimen #18 placed on the turntable. Markers and scale bars in the image were used for the alignment stage and later enabled volume assessment. (B) 3D model of specimen #18; the blue flags are the manually placed markers.
The growth rate (%) of each individual was calculated using the following formula:
Where Vt is the volume of the sponge measured at time t, Vt–1 is the volume of the sponge measured in the previous time point, and n is the number of days passed between t and t–1.
Sponges from the control group were monitored every 3 months due to the high cost and technical difficulty of the expeditions. Volume and pumping were not measured for the control group due to the technical incompatibility of the ROV for such tasks.
In all of the specimens, part of the sponge was damaged by the ROV manipulator at the time of collection, and shortly after transplantation, the damaged part became necrotic; sponge #19 is shown as an example (Figure 3). In both shallow and mesophotic habitats, all specimens regenerated by creating a new body wall underneath the necrotic area and eventually detaching the necrotic part (Figure 3, 14/06/18). The part that was detached was measured and subtracted from the volume estimation, before its detachment, in order to compare the results accurately.
Figure 3. Sponge #19 throughout the experiment: dates and seawater temperatures are noted on each image. The part of the sponge damaged by the ROV during collection became necrotic (circled) on 13/03 – 29/05. Later (14/06), the necrotic part detached, and a new ectosome is visible. On 09/07, the sponge stopped pumping seawater. By 27/07, the sponge was dead, and on the 07/08, only the skeleton remained.
All environmental parameters in both habitats were measured in morning times (7:30–9:00 AM) unless mentioned otherwise.
Water samples were collected from both the mesophotic and shallow habitats in order to compare concentrations of particulate organic carbon (POC), total organic carbon (TOC), and total nitrogen (TN). In the mesophotic habitat, water samples were collected every 3 months using 12 L Niskin bottles placed on a typical rosette sampler (Baetge et al., 2020). The bottles were lowered while open and then closed at approximately one meter above the bottom. For each parameter, at each date, duplicate samples were taken from two separate Niskin bottles. At the shallow site, water was collected during SCUBA dives every 2–4 weeks using a single Niskin bottle, which was closed approximately one meter above the bottom.
Collection and processing followed a previously established protocol (Morganti et al., 2016; Baetge et al., 2020). Samples were collected directly from the Niskin bottles into pre-combusted 40 mL glass EPA vials with PTFE lined septa. Each vial was washed twice in sample water, then 20 ml of sample water were collected per vial, and 80 μl of HCL 32% were added. These samples were frozen onboard the R/V in dry ice and stored at −80°C (Morganti et al., 2016). Samples were analyzed at the Carlson Microbial Oceanography Lab at the Marine Science Institute, UC, Santa Barbara.
GF/F filters were pre-combusted at 400°C for 4 h and kept in a dry and clean environment prior to the expedition. Water samples were pre-filtered through 80 μm plankton mesh to avoid large particles that could block the filter and which are not utilized by sponges. Duplicates of ∼4 L from the two Niskin bottles were filtered on each mesophotic expedition, at three consecutive days for each expedition. In the shallow water, duplicates of ∼3 L were filtered at least once a month. As GF/F filters can become blocked with particles, the exact amount of water filtered was documented to obtain an accurate estimation of the POC concentration (Morganti et al., 2016). Filters were frozen immediately after filtration, and at least 24 h before analysis, the filters were dried in an oven (60°C). Samples were then analyzed using a TOC analyzer (Shimadzu Instruments), where the Carbon is oxygenized at high temperatures (800–900°C) to create CO2. The CO2 creates a signal which is then detected by Infra-Red sensors. The surface of that signal is proportional to the amount of Carbon.
Water temperature at the mesophotic sponge ground was measured every three months from January until November 2018, using a CTD mounted on the rosette sampler, creating a temperature profile of the water column. In the shallow habitat, the water temperature was monitored continuously from April to August 2018, using two HOBO Pendant® data loggers (Onset Computer Corporation, Bourne, MA, United States). In order to monitor the temperature and light inside and outside the crates, one of the loggers was placed on the inside of a crate and the other on its exterior.
The experiment lasted four months (April–August 2018) and ended when the last of the specimens transplanted to the shallow site had died. All sponges from the mesophotic control group survived the entire duration of the experiment and beyond.
Water temperature in the shallow habitat increased gradually throughout the experiment (April-August 2018) from 20 to 30.5°C (Figure 4A). No difference in temperature was measured between the loggers placed inside the crates and those placed on the crats’ exterior (Supplementary Figure 1). In contrast, over the same period, the temperature remained relatively stable in the mesophotic habitat below the seasonal thermocline (Figure 4B), decreasing by 2.4°C, from 19.5 to 17.1°C (Figure 4A).
Figure 4. Temperature regime measured from January to November 2018. (A) The temperature at the mesophotic sponge ground (100 m depth; black triangles) and at the shallow transplantation site (10 m depth; gray circles). The temperature was measured with CTD in both habitats. In addition, from April to November, the temperature in the shallow water was measured four times a day with a HOBO logger. (B) Temperature profile at the mesophotic sponge ground showing seasonal thermocline (measured with CTD).
The range of nutrient concentrations (POC, TOC, and TN) in the shallow habitat was 4.3–17.8, 92.0–159.3, and 5.0–8.0 μM, respectively (Figure 5). These concentrations were higher and showed greater variability than those in the mesophotic habitat (3.1–7.7, 68.3–101.8, and 3.7–5.8 μM, respectively; Figure 5). Differences in TOC and TN concentrations between the mesophotic and shallow water were small, with higher values in the shallow water. POC concentration at the shallow site had a greater variation between sampling dates.
Figure 5. Concentrations of organic carbon and total nitrogen at the mesophotic and transplantation sites over time (01–10/2018). At 10 m, the first three dates have only one sample for TN and TOC, and the same for the first two dates for POC. (A) Total nitrogen (TN; μM), (B) Total organic carbon (TOC; μM), and (C) Particulate organic carbon (POC; μM).
At the end of June, 3.5 months since the sponges’ transplantation, 86% of the sponges at the shallow depth were still alive (i.e., their ectosome was intact and their oscula were open), and 64–86% of them were observed to be pumping at each visit (Figure 6). After the temperature at the shallow site had risen to over 28°C in July, the sponges were still alive, but all pumping had ceased (Figure 6). Two weeks later (July 27th), only one specimen was still alive while the rest were visibly dead (i.e., their ectosome was completely fragmented). By August, all the sponges at the shallow site had died, and the experiment ended (Figure 6). The survival patterns of the sponges that had been mounted inside and outside the crates were similar (Supplementary Table 1). In contrast, all the sponges in the mesophotic control group survived until the end of the experiment and were observed alive more than 5 months later (Figure 7, Supplementary Figure 2).
Figure 6. Survival of Agelas oroides specimens (%) and water temperature (°C) at the shallow transplantation site. The percentage of survival was calculated as the number of live sponges (not necessarily pumping water) out of the total number of sponges; The percentage of pumping specimens was calculated separately.
Figure 7. Agelas oroides mesophotic control group survival (% specimens) and seawater temperature (°C) at the Herzliya mesophotic sponge ground.
The growth pattern of the sponges appeared to follow an optimum curve (Figure 8). Within 1.5 months, i.e., from 12/04 – 29/05/2018, the shallow site sponges grew on average 0.19% a day, increasing the volume of the sponges by an average of 9.2% ± 2.5 when temperatures reached 24°C. As the temperature continued to rise, we observed a decline in sponges’ volume until the end of the experiment, reaching a minimum of −1.7% ± 2.6 of the original volume. The change in sponge volume was not constant: initially, the growth rate was 0.11% a day, and it increased as the temperature rose. Between 15/05 and 29/05, the growth rate reached an average of 0.43% a day. Then the volume decreased at a rather constant rate (0.23–0.26% a day) between 29/05 and 26/06.
Figure 8. Percentage change in sponge volume throughout the experiment for A. oroides specimens transplanted to a shallow site n = 6.
Agelas oroides is a common sponge in shallow waters throughout the Mediterranean (Carteron, 2002; Voultsiadou, 2005, 2009; Lejeusne et al., 2010; Topaloglu and Evcen, 2014; Costa et al., 2018). In the past, it was recorded as a common species in Israel’s coastal water (Tsurnamal, 1968). However, our findings suggest that the current distribution of A. oroides might be limited by the increasing water temperatures along the shallow Israeli coast. During the experiment, all A. oroides individuals transplanted from mesophotic to shallow water died within 4 months. In contrast, the specimens that were transplanted back to the mesophotic sponge ground survived well past the end of the experiment. The shallow habitat differed from the mesophotic habitat in many aspects that could affect the survival of the transplanted individuals: Differences in concentrations of TOC, TN, and POC were small but higher at the shallow site compared to the mesophotic sponge grounds. These findings are consistent with the results of other studies showing that nutrient levels at the Mediterranean mesophotic depths are usually lower than in the shallow water near the shore because of the run-off of freshwater from land and re-suspension of sediments due to wave action (Santinelli, 2015). While A. oroides cannot survive in the shallow local site, it thrives at other shallow locations such as Western Mediterranean, with similar DOC and POC concentrations (Morganti et al., 2017, 2019). Therefore, excess of nutrients, or nutrients limitation, were ruled out as a limiting factor. By placing the sponges inside large crates, we were able to control for other environmental parameters, such as light and water flow regime. Thus, the crates provided the sponges in shallow water conditions closer to their natural habitat, i.e., lower water motion and light intensities (Tsurnamal, 1968; Gerovasileiou et al., 2017). In the current study, the majority of sponges were vital (i.e., ectosome intact, open oscula, and pumping water) throughout the first 2.5 months of the experiment.
Moreover, the sponges seemed to have recovered from the initial damage caused by the ROV collection, rebuilding their body wall and detaching the necrotic parts – indicating that the conditions at the time of the experiment onset were suitable for their survival. In addition, all sponges increased in size during the first part of the experiment. Only when water temperature reached 28°C did the sponges begin to show signs of stress, observed by a decrease in body size and cessation of pumping. Although variations in sponge pumping are well documented as a normal phenomenon (Hoffmann et al., 2008; Lavy et al., 2016; Kumala et al., 2017), the prolonged arrest of pumping is a clear sign of sponge stress, eventually leading to its mortality (Massaro et al., 2012). Many marine organisms, including sponges, are known to be adversely affected by rising temperatures (Webster, 2007; Webster et al., 2008a; Coma et al., 2009; Garrabou et al., 2009, 2019; Cebrian et al., 2011; Pairaud et al., 2014; Laffy et al., 2019), and past events of sponge mass-mortality in the Western Mediterranean have been linked to events of unusually high temperatures (Garrabou et al., 2009, 2019; Lejeusne et al., 2010; Cebrian et al., 2011; Di Camillo et al., 2013; Ledda et al., 2014; Ereskovsky et al., 2019). It has been suggested that sponges in coral reefs are more resilient than corals to the rise in seawater temperatures, and some argue that they may outcompete corals in such cases (Bell et al., 2013, 2018). Others, however, claim that synergistic effects with additional factors (e.g., ocean acidification, reduction in nutrients, and pollution) will adversely affect their populations (Bennett et al., 2017; Lesser and Slattery, 2020). The effect of rising temperatures may be direct, by causing stress to the sponges themselves – evident by the expression of heat-shock protein (Itskovich et al., 2018), or indirect, by affecting the composition of the microbiota inhabiting the sponges (Webster et al., 2008a,b), in some cases creating favorable conditions for opportunistic or pathogenic microorganisms (Webster et al., 2008a; Laffy et al., 2019). And indeed, with the increase in seawater temperatures worldwide, there were many reports of an increase in sponge diseases and a decline in their populations (Webster et al., 2008a).
Analysis of changes in the hard-bottom community over a period of 25 years at the Ligurian Sea showed that sponges maintained their overall coverage, although this region suffered from severe urbanization of the coastline, pollution, fishing impact, and was exposed to global warming (Bertolino et al., 2016). Specifically, A. oroides showed some coverage increase (though the trend was not significant), which might suggest that this species could be resilient to temperature elevation. However, in spite of the reported seawater warming, the temperatures in the Ligurian Sea remained lower than those of the Levantine Sea and probably did not reach the threshold temperature for A. oroides survivorship.
A. oroides was last observed at the shallow Israeli coast in 1967 (Tsurnamal, 1968). Since then, the temperature in the shallow waters of the Eastern Mediterranean has risen during the summer by over three degrees, from a maximum of 28.4°C in the 1960s to 31.5°C in present times (Lipkin and Safriel, 1971; Shaltout and Omstedt, 2014; Ozer et al., 2017). In this study, the daily temperature amplitude was around 28°C for 3 weeks (Figures 4A, 6), in which time most of the sponges have stopped pumping. About a week later, the temperature has surpassed 29°C, and no pumping activity was detected. The sponges, however, still appeared alive (by their ectosomes’ integrity). The ability of sponges to withstand short-term (several days) thermal stress was also demonstrated for the Mediterranean sponge Spongia officinalis (Koutsouveli et al., 2020). That work described the mechanism of genetic expression involved in heat-tolerance and adaptation of S. officinalis.
Although we have no specific information about heat-tolerance or intolerance of A. oroides, it was shown that a heat-dependent prevalence of disease in different sponges was positively correlated with the duration for which they were exposed to extreme temperature (Erwin et al., 2012; Laffy et al., 2019). Similarly, the heatwave in the year 2003, in which Mediterranean A. oroides populations suffered from mass mortality, lasted ∼100 days (Garrabou et al., 2009; Marbà et al., 2015). In 1999, on the other hand, a high-temperature anomaly of 27°C that lasted ∼30 days was reported to cause a decline of some sponge populations in the Mediterranean. A. oroides did not appear to be affected (Cerrano et al., 2000; Marbà et al., 2015; Bertolino et al., 2016).
These observations imply that not only high temperature but its duration may affect sponges’ survival. At the present study site, seawater temperature in the summer of 2018 remained above 28°C for almost 3 months and reached a maximum of 31.4°C at 10 m depth (Figures 4, 5). This suggests that a prolonged period of time during which the temperature surpasses 28°C may constitute a threshold too high for A. oroides survival and that it constitutes one of the main factors affecting the disappearance of A. oroides from the Israeli shallow waters. Another support for this hypothesis is the fact that throughout the experiment, the temperature at the mesophotic control site never surpassed 19.5°C, and the control sponges remained alive (Supplementary Figure 2). In the past, A. oroides did face temperatures surpassing this threshold along the shallow Israeli coast (28.4°C). However, the sponges were only exposed to these conditions for a short time. While sponges may have the capacity to acclimate to such conditions Guzman and Conaco (2016) and Koutsouveli et al. (2020), in long-term exposure, the damage caused by the thermal stress may accumulate and lead to the sponge death.
While other abiotic conditions that were not within the scope of this study (such as the presence of anthropogenic pollutants) may contribute to shaping sponge communities, our findings are in congruence with the notion that low sponge richness in the shallow water of this easternmost part of the Mediterranean Sea, could be attributed to temperature (Voultsiadou, 2005, 2009; Bianchi, 2007). Finally, while sea temperature continues to rise, it is expected that benthic communities in shallow, coastal waters, will likely change and result in a disappearance of temperature-sensitive species (Webster et al., 2013). A loss of habitat-engineering sponge species may have a profound impact on the ecosystem and other organisms that depend on these sponges [e.g., (Herrnkind et al., 1997; Peterson et al., 2006)]. Our present findings suggest that the mesophotic sponge grounds, in which environmental conditions are much more stable, could provide a refuge for some species like A. oroides that may gradually be displaced from shallow water in a warming climate (Webster et al., 2013). Moreover, because the abiotic conditions in the Levantine Sea are changing faster, and the temperature is rising (Marbà et al., 2015), the status of sponge communities there may provide us with a possible prediction for other areas of the Mediterranean Sea as water temperatures continue to increase.
The original contributions presented in the study are included in the article/Supplementary Material, further inquiries can be directed to the corresponding author/s.
TI conceived of the study, designed the study, carried out the field work, carried out the data and statistical analyses and drafted the manuscript. LG participated in collection of field data and data analysis and helped draft the manuscript. SS participated in the design of the study, participated in field work, and critically revised the manuscript. MI helped design the study, coordinated the study, and critically revised the manuscript. All authors gave final approval for publication and agreed to be held accountable for the work performed therein.
The study was supported by the following institutions and grants: The Israeli Ministry of Science, Technology, and Space (The Program for the Development of Scientific and Technological Infrastructure, grant 3-10343 to MI); The Israeli Ministry of National Infrastructure, Energy and Water (grant 217-17-020 to MI); and partially supported by the ISF-NSFC joint research program (grant no. 2577/18 to MI). TI was supported by the Shulamit Aloni Fellowship from the Israeli Ministry of Science, Technology, and Space.
The authors declare that the research was conducted in the absence of any commercial or financial relationships that could be construed as a potential conflict of interest.
The NGO EcoOcean for providing the facility for marine research onboard the R/V Mediterranean Explorer and for the operation of the ROV. Oded Ezra and Itai Katzman, who piloted the ROV. The students of Micha Ilan’s group who volunteered for sponge collections during the many dives and cruises onboard the research vessel. Prof. Gitai Yahel helped to plan the experiment, and the students and faculty of the Ruppin Academic Centre who assisted with dives and workspace, and lastly, Reuben Rosenblatt, the diving officer, for his support. Naomi Paz, who edited the manuscript. ECOncrete® for the generous donation of the environmental cement tiles. Collection permit was given by the Israel Nature and Parks Authority.
The Supplementary Material for this article can be found online at: https://www.frontiersin.org/articles/10.3389/fmars.2020.603593/full#supplementary-material
Supplementary Figure 1 | Temperature measured every 6 h by HOBO loggers placed inside and outside the crates at the translocation site off the coast of Mikhmoret at a depth of 10 m.
Supplementary Figure 2 | Control specimens of A. oroides in October 2018, sponges seem alive.
Supplementary Table 1 | Sponges in the transplantation experiment. Treatment 2 indicates whether the sponge was attached inside or outside a box. Initially seven sponges were attached outside the crates; two died shortly after transplantation and one was lost in a storm.
Agisoft, L. (2019). Agisoft metashape user manual, Professional edition, Version 1.5, Agisoft LLC. St. Petersburg, Russia: Agisoft.
Azov, Y. (1991). Eastern mediterranean—a marine desert? Mar. Poll. Bull. 23, 225–232. doi: 10.1016/0025-326x(91)90679-m
Baetge, N., Graff, J. R., Behrenfeld, M. J., and Carlson, C. A. (2020). Net community production, dissolved organic carbon accumulation, and vertical export in the western north atlantic. Front.Mar. Sci. 7:227. doi: 10.3389/fmars.2020.00227
Beazley, L. I., Kenchington, E. L., Murillo, F. J., and Sacau, M. D. (2013). Deep-sea sponge grounds enhance diversity and abundance of epibenthic megafauna in the northwest atlantic. Ices J. Mar. Sci. 70, 1471–1490. doi: 10.1093/icesjms/fst124
Beepat, S. S., Davy, S. K., Woods, L., and Bell, J. J. (2020). Short-term responses of tropical lagoon sponges to elevated temperature and nitrate. Mar. Environ. Res. 157:104922. doi: 10.1016/j.marenvres.2020.104922
Bell, J. J., Bennett, H. M., Rovellini, A., and Webster, N. S. (2018). Sponges to be winners under near-future climate scenarios. Bioscience 68, 955–968. doi: 10.1093/biosci/biy142
Bell, J. J., Davy, S. K., Jones, T., Taylor, M. W., and Webster, N. S. (2013). Could some coral reefs become sponge reefs as our climate changes? Global Change Biol. 19, 2613–2624. doi: 10.1111/gcb.12212
Bennett, H. M., Altenrath, C., Woods, L., Davy, S. K., Webster, N. S., and Bell, J. J. (2017). Interactive effects of temperature and pCO 2 on sponges: from the cradle to the grave. Global Change Biol. 23, 2031–2046. doi: 10.1111/gcb.13474
Bertolino, M., Betti, F., Bo, M., Cattaneo-Vietti, R., Pansini, M., Romero, J., et al. (2016). Changes and stability of a Mediterranean hard bottom benthic community over 25 years. J. Mar. Biol. Ass. U K. 96:341. doi: 10.1017/s0025315415001186
Bianchi, C. N. (2007). Biodiversity issues for the forthcoming tropical mediterranean Sea. Hydrobiologia 580:7. doi: 10.1007/978-1-4020-6156-1_1
Bo, M., Bertolino, M., Bavestrello, G., Canese, S., Giusti, M., Angiolillo, M., et al. (2012). Role of deep sponge grounds in the mediterranean sea: a case study in southern Italy. Hydrobiologia 687, 163–177. doi: 10.1007/978-94-007-4688-6_14
Carteron, S. (2002). Etude taxonomique des spongiaires du Liban. Stage de Maîtrise. Marseille, France: Centre d’Océanologie de Marseille.
Cebrian, E., Uriz, M. J., Garrabou, J., and Ballesteros, E. (2011). Sponge mass mortalities in a warming mediterranean sea: are cyanobacteria-harboring species worse off? PloS One 6:e20211. doi: 10.1371/journal.pone.0020211
Cerrano, C., Bavestrello, G., Bianchi, C. N., Cattaneo-Vietti, R., Bava, S., Morganti, C., et al. (2000). A catastrophic mass-mortality episode of gorgonians and other organisms in the ligurian sea (north-western mediterranean), summer 1999. Ecol. Lett. 3, 284–293. doi: 10.1046/j.1461-0248.2000.00152.x
Coma, R., Ribes, M., Serrano, E., Jiménez, E., Salat, J., and Pascual, J. (2009). Global warming-enhanced stratification and mass mortality events in the mediterranean. Proc. Nat. Acad. Sci. 106, 6176–6181. doi: 10.1073/pnas.0805801106
Costa, G., Giussani, V., Kletou, D., Kleitou, P., Pansini, M., Setti, A., et al. (2018). A first preliminary study of the shallow water sponge fauna from cyprus island (eastern mediterranean). Zootaxa 4450, 594–596. doi: 10.11646/zootaxa.4450.5.7
Di Camillo, C. G., Bartolucci, I., Cerrano, C., and Bavestrello, G. (2013). Sponge disease in the adriatic sea. Mar. Ecol. 34, 62–71. doi: 10.1111/j.1439-0485.2012.00525.x
Ereskovsky, A., Ozerov, D. A., Pantyulin, A. N., and Tzetlin, A. B. (2019). Mass mortality event of white sea sponges as the result of high temperature in summer 2018. Polar Biol. 42, 2313–2318. doi: 10.1007/s00300-019-02606-0
Erwin, P. M., Pita, L., López-Legentil, S., and Turon, X. (2012). Stability of sponge-associated bacteria over large seasonal shifts in temperature and irradiance. Appl. Environ. Microbiol. 78, 7358–7368. doi: 10.1128/aem.02035-12
Feuda, R., Dohrmann, M., Pett, W., Philippe, H., Rota-Stabelli, O., Lartillot, N., et al. (2017). Improved modeling of compositional heterogeneity supports sponges as sister to all other animals. Curr. Biol. 27, 3864–3870. doi: 10.1016/j.cub.2017.11.008
Garrabou, J., Coma, R., Bensoussan, N., Bally, M., Chevaldonné, P., Cigliano, M., et al. (2009). Mass mortality in northwestern mediterranean rocky benthic communities: effects of the 2003 heat wave. Global Change Biol. 15, 1090–1103. doi: 10.1111/j.1365-2486.2008.01823.x
Garrabou, J., Gómez-Gras, D., Ledoux, J.-B., Linares, C., Bensoussan, N., López-Sendino, P., et al. (2019). Collaborative database to track mass mortality events in the mediterranean sea. Front. Mar. Sci. 6:707. doi: 10.3389/fmars.2019.00707
Gerovasileiou, V., Chintiroglou, C. C., Konstantinou, D., and Voultsiadou, E. (2016). Sponges as “living hotels” in mediterranean marine caves. Sci. Marina 80, 279–289. doi: 10.3989/scimar.04403.14b
Gerovasileiou, V., Dimitriadis, C., Arvanitidis, C., and Voultsiadou, E. (2017). Taxonomic and functional surrogates of sessile benthic diversity in mediterranean marine caves. PloS One 12:e0183707. doi: 10.1371/journal.pone.0183707
Gerovasileiou, V., and Voultsiadou, E. (2012). Marine caves of the mediterranean Sea: a sponge biodiversity reservoir within a biodiversity hotspot. PloS One 7:e39873. doi: 10.1371/journal.pone.0039873
Grenier, M., Ruiz, C., Fourt, M., Santonja, M., Dubois, M., Klautau, M., et al. (2018). Sponge inventory of the french mediterranean waters, with an emphasis on cave-dwelling species. Zootaxa 4466, 205–228. doi: 10.11646/zootaxa.4466.1.16
Guzman, C., and Conaco, C. (2016). Gene expression dynamics accompanying the sponge thermal stress response. PloS One 11:e0165368. doi: 10.1371/journal.pone.0165368
Herrnkind, W. F., Butler, Iv, M. J., Hunt, J. H., and Childress, M. (1997). Role of physical refugia: implications from a mass sponge die-off in a lobster nursery in florida. Mar. Freshwater Res. 48, 759–770. doi: 10.1071/mf97193
Hoffmann, F., Røy, H., Bayer, K., Hentschel, U., Pfannkuchen, M., Brümmer, F., et al. (2008). Oxygen dynamics and transport in the mediterranean sponge aplysina aerophoba. Mar. Biol. 153, 1257–1264. doi: 10.1007/s00227-008-0905-3
Idan, T., Shefer, S., Feldstein, T., Yahel, R., Huchon, D., and Ilan, M. (2018). Shedding light on an east-mediterranean mesophotic sponge ground community and the regional sponge fauna. Mediterr. Mar. Sci. 19, 84–106. doi: 10.12681/mms.13853
Itskovich, V., Shigarova, A., Glyzina, O., Kaluzhnaya, O., and Borovskii, G. (2018). Heat shock protein 70 (Hsp70) response to elevated temperatures in the endemic baikal sponge lubomirskia baicalensis. Ecol. Indi. 88, 1–7. doi: 10.1016/j.ecolind.2017.12.055
Kenchington, E., Power, D., and Koen-Alonso, M. (2013). Associations of demersal fish with sponge grounds on the continental slopes of the northwest atlantic. Mar. Ecol. Prog. Series 477, 217–230. doi: 10.3354/meps10127
Koutsouveli, V., Manousaki, T., Riesgo, A., Lagnel, J., Kollias, S., Tsigenopoulos, C. S., et al. (2020). Gearing up for warmer times: transcriptomic response of spongia officinalis to elevated temperatures reveals recruited mechanisms and potential for resilience. Front. Mar. Sci. 6:786. doi: 10.3389/fmars.2019.00786
Kovalerchik, D., Singh, R. P., Schlesinger, P., Mahajni, A., Shefer, S., Fridman, M., et al. (2020). Bromopyrrole alkaloids of the sponge agelas oroides collected near the israeli mediterranean coastline. J. Nat. Prod. 83, 374–384. doi: 10.1021/acs.jnatprod.9b00863
Kress, N., Gertman, I., and Herut, B. (2014). Temporal evolution of physical and chemical characteristics of the water column in the easternmost levantine basin (eastern mediterranean sea) from 2002 to 2010. J. Mar. Sys. 135, 6–13. doi: 10.1016/j.jmarsys.2013.11.016
Krom, M., Emeis, K., and Van Cappellen, P. (2010). Why is the eastern mediterranean phosphorus limited? Prog. Oceano. 85, 236–244. doi: 10.1016/j.pocean.2010.03.003
Kumala, L., Riisgård, H. U., and Canfield, D. E. (2017). Osculum dynamics and filtration activity in small single-osculum explants of the demosponge halichondria panicea. Mar. Ecol. Prog. Series 572, 117–128. doi: 10.3354/meps12155
Laffy, P. W., Botté, E. S., Wood-Charlson, E. M., Weynberg, K. D., Rattei, T., and Webster, N. S. (2019). Thermal stress modifies the marine sponge virome. Environ. Microbiol. Rep. 11, 690–698. doi: 10.1111/1758-2229.12782
Lavy, A., Eyal, G., Neal, B., Keren, R., Loya, Y., and Ilan, M. (2015). A quick, easy and non-intrusive method for underwater volume and surface area evaluation of benthic organisms by 3D computer modelling. Methods Ecol. Evol. 6, 521–531. doi: 10.1111/2041-210x.12331
Lavy, A., Keren, R., Yahel, G., and Ilan, M. (2016). Intermittent hypoxia and prolonged suboxia measured in situ in a marine sponge. Front. Mar. Sci. 3:263. doi: 10.3389/fmars.2016.00263
Ledda, F. D., Pronzato, R., and Manconi, R. (2014). Mariculture for bacterial and organic waste removal: a field study of sponge filtering activity in experimental farming. Aquacul. Res. 45, 1389–1401. doi: 10.1111/are.12084
Lejeusne, C., Chevaldonné, P., Pergent-Martini, C., Boudouresque, C. F., and Perez, T. (2010). Climate change effects on a miniature ocean: the highly diverse, highly impacted mediterranean sea. Trends Ecol. Evol. 25, 250–260. doi: 10.1016/j.tree.2009.10.009
Lesser, M. P., and Slattery, M. (2020). Will coral reef sponges be winners in the Anthropocene? Global Change Biol. 26, 3202–3211. doi: 10.1111/gcb.15039
Lipkin, Y., and Safriel, U. (1971). Intertidal zonation on rocky shores at mikhmoret (mediterranean, israel). J. Ecol. 59, 1–30. doi: 10.2307/2258448
Marbà, N., Jordà, G., Agustí, S., Girard, C., and Duarte, C. M. (2015). Footprints of climate change on mediterranean sea biota. Front. Marine Sci. 2:56. doi: 10.3389/fmars.2015.00056
Massaro, A. J., Weisz, J. B., Hill, M. S., and Webster, N. S. (2012). Behavioral and morphological changes caused by thermal stress in the Great Barrier Reef sponge Rhopaloeides odorabile. J. Exp. Mar. Biol. Ecol. 416, 55–60. doi: 10.1016/j.jembe.2012.02.008
Morganti, T., Coma, R., Yahel, G., and Ribes, M. (2017). Trophic niche separation that facilitates co-existence of high and low microbial abundance sponges is revealed by in situ study of carbon and nitrogen fluxes. Limnol. Oceano. 62, 1963–1983. doi: 10.1002/lno.10546
Morganti, T., Yahel, G., Ribes, M., and Coma, R. V. (2016). VacuSIP, an improved inex method for in situ measurement of particulate and dissolved compounds processed by active suspension feeders. J. Visual. Exp.: JoVE 114, 1–14. doi: 10.1007/978-3-319-17001-5_18-1
Morganti, T. M., Ribes, M., Yahel, G., and Coma, R. (2019). Size is the major determinant of pumping rates in marine sponges. Front. Physiol. 10:1474. doi: 10.3389/fphys.2019.01474
Olinger, L. K., Scott, A. R., Mcmurray, S. E., and Pawlik, J. R. (2019). Growth estimates of caribbean reef sponges on a shipwreck using 3D photogrammetry. Sci. Rep. 9, 1–12.
Oren, O. (1969). Oceanographic and biological influence of the suez canal, the nile and the aswan dam on the levant basin. Prog. Oceano. 5, 161–167. doi: 10.1016/0079-6611(69)90038-x
Ozer, T., Gertman, I., Kress, N., Silverman, J., and Herut, B. (2017). Interannual thermohaline (1979–2014) and nutrient (2002–2014) dynamics in the levantine surface and intermediate water masses. se mediterranean sea. Global Planetary Change 151, 60–67. doi: 10.1016/j.gloplacha.2016.04.001
Pairaud, I. L., Bensoussan, N., Garreau, P., Faure, V., and Garrabou, J. (2014). Impacts of climate change on coastal benthic ecosystems: assessing the current risk of mortality outbreaks associated with thermal stress in NW mediterranean coastal areas. Ocean Dyn. 64, 103–115. doi: 10.1007/s10236-013-0661-x
Peterson, B. J., Chester, C. M., Jochem, F. J., and Fourqurean, J. W. (2006). Potential role of sponge communities in controlling phytoplankton blooms in florida bay. Mar. Ecol. Prog. Series 328, 93–103. doi: 10.3354/meps328093
Santinelli, C. (2015). “DOC in the Mediterranean Sea,” in Biogeochemistry of marine dissolved organic matter (second edition), eds D. A. Hansell and C. A. Carlson, (Pisa: Elsevier), 579–608. doi: 10.1016/b978-0-12-405940-5.00013-3
Sauleau, P., Moriou, C., and Al Mourabit, A. (2017). Metabolomics approach to chemical diversity of the mediterranean marine sponge agelas oroides. Nat. Prod. Res. 31, 1625–1632. doi: 10.1080/14786419.2017.1285298
Schmidt, O. (1864). Supplement der Spongien des Adriatischen Meeres: enthaltend die Histologie und systematische Ergänzungen. Leipzig: Engelmann.
Shaltout, M., and Omstedt, A. (2014). Recent sea surface temperature trends and future scenarios for the mediterranean sea. Oceanologia 56, 411–443. doi: 10.5697/oc.56-3.411
Topaloglu, B., and Evcen, A. (2014). Updated checklist of sponges (porifera) along the coasts of Turkey. Turkish J. Zool. 38, 665-676.
Tsurnamal, M. (1968). Studies on the porifera of the mediterranean Littoral of Israel. Ph.D, Thesis. Jerusalem: The Hebrew University.
Voultsiadou, E. (2005). Demosponge distribution in the eastern mediterranean: a NW-SE gradient. Helgoland Mar. Res. 59, 237–251. doi: 10.1007/s10152-005-0224-8
Voultsiadou, E. (2009). Reevaluating sponge diversity and distribution in the mediterranean sea. Hydrobiologia 628, 1–12. doi: 10.1007/s10750-009-9725-9
Webster, N., Pantile, R., Botte, E., Abdo, D., Andreakis, N., and Whalan, S. (2013). A complex life cycle in a warming planet: gene expression in thermally stressed sponges. Mol. Ecol. 22, 1854–1868. doi: 10.1111/mec.12213
Webster, N. S. (2007). Sponge disease: a global threat? Environ. Microbiol. 9, 1363–1375. doi: 10.1111/j.1462-2920.2007.01303.x
Webster, N. S., Cobb, R. E., and Negri, A. P. (2008a). Temperature thresholds for bacterial symbiosis with a sponge. ISME J. 2, 830–842. doi: 10.1038/ismej.2008.42
Webster, N. S., Xavier, J. R., Freckelton, M., Motti, C. A., and Cobb, R. (2008b). Shifts in microbial and chemical patterns within the marine sponge aplysina aerophoba during a disease outbreak. Environ. Microbiol. 10, 3366–3376. doi: 10.1111/j.1462-2920.2008.01734.x
Keywords: transplantation, mesophotic, sponge ground, 3D modeling, nutrients, marine ecology, temperature
Citation: Idan T, Goren L, Shefer S and Ilan M (2020) Sponges in a Changing Climate: Survival of Agelas oroides in a Warming Mediterranean Sea. Front. Mar. Sci. 7:603593. doi: 10.3389/fmars.2020.603593
Received: 07 September 2020; Accepted: 13 November 2020;
Published: 08 December 2020.
Edited by:
Shirley A. Pomponi, Florida Atlantic University, United StatesReviewed by:
Giorgio Bavestrello, University of Genoa, ItalyCopyright © 2020 Idan, Goren, Shefer and Ilan. This is an open-access article distributed under the terms of the Creative Commons Attribution License (CC BY). The use, distribution or reproduction in other forums is permitted, provided the original author(s) and the copyright owner(s) are credited and that the original publication in this journal is cited, in accordance with accepted academic practice. No use, distribution or reproduction is permitted which does not comply with these terms.
*Correspondence: Tal Idan, dGFsaWRhbkBtYWlsLnRhdS5hYy5pbA==; dGFsdHVsLmlkYW5AZ21haWwuY29t
Disclaimer: All claims expressed in this article are solely those of the authors and do not necessarily represent those of their affiliated organizations, or those of the publisher, the editors and the reviewers. Any product that may be evaluated in this article or claim that may be made by its manufacturer is not guaranteed or endorsed by the publisher.
Research integrity at Frontiers
Learn more about the work of our research integrity team to safeguard the quality of each article we publish.