- 1Mississippi Laboratories, Southeast Fisheries Science Center, National Marine Fisheries Service, National Oceanic and Atmospheric Administration, Pascagoula, MS, United States
- 2Louisiana Department of Wildlife and Fisheries, New Orleans, LA, United States
- 3Center for Fisheries Research and Development, Gulf Coast Research Laboratory, The University of Southern Mississippi, Ocean Springs, MS, United States
- 4Greater Atlantic Regional Fishery Office, National Marine Fisheries Service, National Oceanic and Atmospheric Administration, Gloucester, MA, United States
- 5School of Natural Resources, University of Missouri, Columbia, MO, United States
In the northern Gulf of Mexico (GOM), whale sharks (Rhincodon typus) form large aggregations at continental shelf-edge banks during summer; however, knowledge of movements once they leave aggregation sites is limited. Here we report on the seasonal occurrence of whale sharks in the northern GOM based on over 800 whale shark sightings from 1989 to 2016, as well as the movements of 42 whale sharks tagged with satellite-linked and popup satellite archival transmitting tags from 2008 to 2015. Sightings data were most numerous during summer and fall often with aggregations of individuals reported along the continental shelf break. Most sharks (66%) were tagged during this time at Ewing Bank, a known aggregation site off the coast of Louisiana. Whale shark track duration ranged from three to 366 days and all tagged individuals, which ranged from 4.5 to 12.0 m in total length, remained within the GOM. Sightings data revealed that whale sharks occurred primarily in continental shelf and shelf-edge waters (81%) whereas tag data revealed the sharks primarily inhabited continental slope and open ocean waters (91%) of the GOM. Much of their time spent in open ocean waters was associated with the edge of the Loop Current and associated mesoscale eddies. During cooler months, there was a net movement southward, corresponding with the time of reduced sighting reports. Several sharks migrated to the southwest GOM during fall and winter, suggesting this region could be important overwintering habitat and possibly represents another seasonal aggregation site. The three long-term tracked whale sharks exhibited interannual site fidelity, returning one year later to the vicinity where they were originally tagged. The increased habitat use of north central GOM waters by whale sharks as summer foraging grounds and potential interannual site fidelity to Ewing Bank demonstrate the importance of this region for this species.
Introduction
Our understanding of the ecology of large marine vertebrates has increased significantly in recent years due to advances in bio-logging technology to study their movements, behavior, physiology and habitat preferences (Hart and Hyrenbach, 2009; Jaine et al., 2014; Chmura et al., 2018; Whitford and Klimley, 2019). Highly migratory fishes, such as pelagic sharks, have been particularly difficult to study due to the complexity of their ecology and the concealing nature and remoteness of their environment. Satellite transmitters have successfully been used to track movements of pelagic sharks across ocean basins and has become a common tool for researchers (Hammerschlag et al., 2011; Hussey et al., 2015). Moreover, this technology has been widely used to monitor habitat use patterns (Hussey et al., 2015), as well as address inter-disciplinary ecological and resource management questions involving foraging ecology (Papastamatiou et al., 2010; Hammerschlag et al., 2011; Jaine et al., 2014), migratory behavior (Lea et al., 2015; Doherty et al., 2017a; Skomal et al., 2017) and overlap in species distribution and fishery exploitation areas (Graham et al., 2016; Queiroz et al., 2016; Calich et al., 2018).
The whale shark, Rhincodon typus (Smith, 1828), is a large-bodied, filter feeding species with a circumglobal distribution in warm temperate and tropical marine waters (Compagno, 2001; Jaffa and Taher, 2007). Although whale sharks are known to use the entire water column, from the surface down to over 2000 m (Tyminski et al., 2015), they are thought to spend most of their time in the top 100 m (Wilson et al., 2006; Rowat and Gore, 2007; Tyminski et al., 2015). This primarily epipelagic behavior has allowed for observations by commercial mariners, offshore industry employees, and recreational boaters providing much of the occurrence and distribution data for whale sharks worldwide (Gudger, 1934; Silas, 1986; Taylor, 1996; Colman, 1997; Stevens, 2007). Whale shark occurrence has been correlated with high abundance of prey in coastal areas (Heyman et al., 2001; Rohner et al., 2015, 2018) and productive frontal zones and large-scale spawning events in offshore waters (Hoffmayer et al., 2005; de la Parra Venegas et al., 2011; Ryan et al., 2017).
The majority of whale shark sightings are of solitary individuals (Colman, 1997; Hoffmayer et al., 2005; Rowat and Brooks, 2012); however, whale sharks can also be found in aggregations in certain regions (e.g., Rowat and Brooks, 2012). By studying whale shark occurrence reports, scientists have been able to identify relatively predictable spatiotemporal aggregation patterns at 12 locations, worldwide (Graham and Roberts, 2007; Rowat and Brooks, 2012; Sequeira et al., 2013; Berumen et al., 2014). The purpose of these aggregations is not completely understood, but they typically occur in areas of high localized productivity (Stevens, 2007; Sequeira et al., 2013) and are generally dominated by juvenile males (Rowat et al., 2009; Rowat and Brooks, 2012; Norman et al., 2017a). This high predictability of occurrences has led to commercial whale shark ecotourism in several of these locations, including waters off Australia, the Philippines, Mozambique, Seychelles, Djibouti, Belize, Gulf of California, Holbox, Bay Islands of Honduras, the Red Sea, and the Arabian Gulf (Rowat and Brooks, 2012; Fox et al., 2013; Robinson et al., 2013; Berumen et al., 2014; Rohner et al., 2015), and has allowed access for researchers to study this enigmatic species.
Knowledge on movement and habitat use patterns for whale sharks remains fragmented despite the growing number of tagging studies in recent years. Only a limited number of tagging studies have been performed in regions outside known aggregation sites (Rowat and Brooks, 2012; Sequeira et al., 2013), typically due to the prohibitive costs of vessel operations and spotter planes, which are critical to locating these animals due to sporadic sightings and their epipelagic nature (Colman, 1997). Most studies to date described short-term movements with mean track durations of ∼ 90 days (e.g., Sequeira et al., 2013), and only a few recent studies investigated seasonal changes in habitat use (Berumen et al., 2014; Robinson et al., 2017; Diamant et al., 2018; Cochran et al., 2019). Due to their migratory nature and longevity, there remains a need for long-term studies documenting connectivity into other areas (Sequeira et al., 2013; Andrzejaczek et al., 2016; McKinney et al., 2017; Norman et al., 2017a; Araujo et al., 2019) in order to facilitate international management on this species.
Several molecular studies have revealed that whale sharks form a single global population, however, there is evidence suggesting the Atlantic Ocean population is genetically different from the Indo-Pacific Ocean populations (Castro et al., 2007; Schmidt et al., 2009; Vignaud et al., 2014). Early whale shark reports from the western Atlantic Ocean primarily focused on their occurrence (Gudger, 1918, 1934; Bigelow and Schroeder, 1948; Baughman and Springer, 1950; Breuer, 1954); however, more recent studies have documented aggregations (Graham and Roberts, 2007; de la Parra Venegas et al., 2011; Fox et al., 2013; Cárdenas-Palomo et al., 2014) and connectivity between the Gulf of Mexico (GOM) and Caribbean Sea (Hueter et al., 2013; McKinney et al., 2017; Norman et al., 2017a; Rooker et al., 2019). McKinney et al. (2017) utilizing photo-identification to document whale shark connectivity in the western central Atlantic Ocean, and reported movements between Honduras, Belize, and Mexico with limited connectivity between these regions and the northern GOM. In the largest whale shark satellite tagging study to date in the Atlantic Ocean, Hueter et al. (2013) deployed 35 satellite tags on sharks off the Yucatan Peninsula and documented movement into the northern GOM, the Caribbean Sea, and one shark moving into the South Atlantic Ocean. Additional tagging studies in this region should help to further define whale shark movement patterns and connectivity to other regions.
Unlike other locations throughout the world, the northern GOM is one of the few areas where whale sharks rarely occur in coastal waters, but rather form aggregations along the continental shelf edge (Hoffmayer et al., 2005, 2007; Burks et al., 2006; McKinney et al., 2012, 2017). The nearly exclusive pelagic nature of whale sharks in this region has made it difficult to gather information on their occurrence, and much of the existing information was collected opportunistically (Springer, 1957; Hoffman et al., 1981; Hoffmayer et al., 2005). Beginning in 2003, researchers at The University of Southern Mississippi developed the Northern Gulf of Mexico Whale Shark Sightings Survey (NGWSSS) to gain a better understanding of whale shark occurrence and distribution patterns in the northern GOM (Hoffmayer et al., 2005). This effort has resulted in the long-term monitoring of a predictable, seasonal whale shark aggregation site in the GOM, which facilitated the deployment of satellite tags to track their movements. This study combines multiple tag technologies along with sightings data to further elucidate whale shark movements and habitat use patterns in the GOM.
Materials and Methods
Whale Shark Sightings Data
Details of whale shark sightings reported (2003–2016) to the NGWSSS were obtained from research trips, aerial surveys, recreational and commercial fishers, recreational divers, and various sources within the oil and gas industry (e.g., platform personnel, helicopter pilots) (Hoffmayer et al., 2005)1. Information requested from individuals who encountered whale sharks included date, location, direction and distance from a coastal landmark, or identifier number of specific offshore petroleum platforms, number of individuals, estimated total length (TL) of observed sharks, and observed behavior (e.g., swimming, feeding). Follow-up emails and phone calls were conducted to determine the validity of the reports and to request video and photos. Additional sightings data from National Marine Fisheries Service (NMFS) aerial surveys (1989–1998; n = 81 sightings) and the Wildbook for Whale Shark Photo-Identification Database2 from the northern GOM (area 2e; 1999–2016; n = 104) were included. Since the majority of the sightings had no associated effort data, we utilized these data as presence only.
All sightings data were combined and plotted in Quantum GIS 2.18 (QGIS Developmental Team 2016) using the global WGS 1984 PDC Mercator projection to investigate seasonal patterns of occurrence and distribution. Seasons were defined using the meteorological definition as spring (March–May), summer (June–August), fall (September–November), and winter (December–February). Seasonal utilization distributions (UDs) were calculated using a fixed kernel density estimator, which was weighted based on the number of sharks reported at each encounter, and analyses were conducted using the KS package (Duong, 2007) in R statistical software (R Core Team, 2016). Total usage space (95%), representing the entire activity space (Burt, 1943), and core usage (50%), representing concentrated use (Worton, 1989), were calculated from the UDs. Any duplication of a single sighting event (i.e., more than one report of the same shark at the same place and time) was removed from the dataset so that the weighing reflected the number of individuals during a sighting event and was not impacted by the number of people observing the sharks.
Satellite Tagging
Satellite tags were opportunistically deployed on whale sharks from 2008 to 2014 in the northern GOM from the DeSoto Canyon to the Flower Gardens Banks National Marine Sanctuary. The primary site for tag deployment was Ewing Bank, a topographic feature ∼130 km south of Cocodrie, Louisiana (Figure 1). A spotter plane provided aerial support for the at-sea research team by covering a 52 km2 radius around the targeted search area. Divers and vessel crew estimated the total length (TL) of the individual whale sharks to the nearest 0.5 m and sex was determined visually by the presence or absence of claspers. Satellite tags tethered to a titanium anchor dart (64 mm long × 16 mm wide × 1 mm thick) were implanted at the base of the dorsal fin using a 2-m pole spear (Ray Odor, Lutz, FL, United States) with a modified tagging tip. A rubber stopper was used on the tagging tip to ensure the tag anchor would not penetrate the skin deeper than 10 cm. The GPS location and time of tag deployment were recorded.
Two different satellite tag types were deployed in this study: satellite-linked towable Smart Position and Temperature Transmitting (SPOT; model SPOT-253, Wildlife Computers, Inc.) and popup satellite archival transmitting (PSAT; model X-Tag, Microwave Telemetry, Inc.; model MK10-PAT, Wildlife Computers, Inc.) tags. A Wildlife Computers, Inc. titanium anchor dart was used with each tag type, however, the tether setup was different for each tag type. We used a 1.5 m of 1.9 mm braided Honeywell Spectra fiber line tied to the tag and the titanium dart using a Palomar knot that was sealed with Krazy Glue instant adhesive (High Point, NC, United States). For the PAT tags, a 15 cm segment of heat shrink-wrapped (3.2 mm Ancor Marine grade heat shrink tubing, Marinco, Menomonee Falls, WI, United States) 1.8 mm monofilament line (136 kg test extra hard Hi-Catch Momoi Fishing Net, Mfg. Co., Ltd., Ako City, Hyogo prefecture, Japan) was attached to the tag and the anchor using stainless steel sleeves (168-2-VB4, Nicopress Products, Cleveland, OH, United States). In addition, the Wildlife Computers, Inc. RD1800 release device was attached to the MK10-PAT tags to sever the monofilament if the whale shark swam below 1800 m depth to prevent the tag from being crushed at extreme depth. The PSATs were attached with a 15 cm length of 2.0 mm 7 × 7 stainless steel cable/1.8 mm monofilament line connected with a stainless steel sleeve and coated in 3.2 mm heat shrink tubing. The monofilament end of the tag and the stainless steel cable was affixed to the titanium anchor using Nicopress stainless steel sleeves. On the PSAT tags, a constant pressure release mechanism was enabled to trigger tag detachment from sharks if depth variations <2 m were maintained for a period of 96 h. All tags were painted with antifouling paint; the X-Tags were treated by the manufacturer, while the MK10-PAT and SPOT tags were painted with gray transducer paint (Pettit Marine Paint, Kop-Coat Marine Group, Rockaway, NJ, United States) to deter growth of epibionts and to minimize attempted predation on the tag (Robinson et al., 2017). For analysis, sharks were separated by sex and categorized immature (<8.0 m TL) or mature (>8.0 m TL) following Norman and Stevens (2007).
Geolocation and Behavior Estimation
PSAT Tags
We used a hidden Markov model (HMM), to generate daily location and behavioral state estimates from PSAT data using the R package HMMoce3, following methods by Braun et al. (2018a; 2018b). The HMM uses a two-step Bayesian state-space approach to estimate the joint probability distribution of location and behavior at each daily point. First, the model estimates daily observation-based location likelihoods generated by matching of in situ light-based longitude, sea surface temperature (SST), depth-temperature profiles, and ocean heat content (OHC) data collected from the tags to available time-synoptic oceanographic data. Daily location likelihood surfaces are generated across standard depth levels associated with each oceanographic product, and an overall likelihood is generated by combining the individual profile likelihoods for each depth level. Light-based longitude likelihoods were derived using longitude estimates provided from manufacturer-specific post-processing software (e.g., GPE2 software, Wildlife Computers, Inc.). Daily in situ SST estimates were compared to remotely sensed SST from daily, optimally−interpolated SST fields (OI−SST, 0.25° resolution; Reynolds et al., 2007; Banzon et al., 2016) to generate SST likelihoods. Modeled depth-temperature products from the HYbrid Coordinate Ocean Model (HYCOM, 0.08° resolution; Chassignet et al., 2007) at standard depth levels were compared to daily depth-temperature bins from tag data to estimate depth-temperature likelihoods. While Wildlife Computers tags provided binned temperature and depth data, which could be used in the HMMoce Package directly, temperature and depth time series data provided by Microwave Telemetry tags were first converted to depth-temperature bins using the R package RchivalTag (Bauer et al., 2015). Ocean heat content (OHC) was calculated by integrating the heat content of the water column above the minimum daily temperature recorded by the tag (Luo et al., 2015), and HYCOM fields, and these were used to generate an OHC likelihood. Start and end locations were considered known in all cases and model runs.
Next, the model infers the probability density of daily locations and behaviors by fitting an underlying Brownian movement model to the daily likelihood surfaces. The model allows for switching between two behavioral states, nominally migratory and resident, which are characterized by high and low rates of diffusion, respectively (Braun et al., 2018a). Daily model outputs included a daily location estimate, hereafter referred to as most probable track (MPT) locations, and the probability of a whale shark being in the resident behavioral state. Behavioral state probabilities range from 0 to 1, and we considered a whale shark resident when resident behavior probability was ≥0.7, and migratory when resident probability was ≤0.3. Behavioral state was defined as unknown if resident probabilities were between 0.3 and 0.7. Once MPTs were generated, cumulative track distances (km) were calculated using great circle distance (km) between MPT daily locations using the Fields package (Nychka et al., 2015) in R. Daily rates of movement were calculated for each track day by calculating the great circle distance between locations.
Towed SPOT Tags
The towed SPOT tags used standard Doppler-based geolocation to track the position of tagged whale sharks. For a detailed description of location estimate accuracy, see Hearn et al. (2013). Position estimates were filtered using a Douglas Filter applied in Movebank4. This tool is based on a maximum redundant distance (MRD) filter and removes unrealistic locations. The MRD radius was set to 200 km. Although SPOT tags can provide multiple locations per day, a single location was selected for each track day for these tag types to be consistent with data derived from other tag types used in the study. If multiple locations were available in a given track day, the location with the best location class was selected. If more than one location of a similar class was present within a day, the initial location for that class was selected. We attempted to apply a hierarchical behavioral state switching state-space model based on the first-difference correlated random walk model of Jonsen et al. (2005) and Jonsen (2016) to SPOT tag data to reconstruct tracks and identify behavioral states using the bsam package (Jonsen et al., 2020) in R. However, due to low sample size (n < 200 daily locations), short track durations and large temporal gaps between locations, we were not able to get models to converge or to return reasonable model outputs.
Seasonal Distribution and Habitat Use
To make inferences on seasonal distribution of whale sharks in the GOM, the HMM model output was used to generate a seasonal residency distribution (RD) for each PSAT-tagged shark. A RD is conceptually similar to a UD, and represents the expected residence time of an animal within each grid cell over a given time interval based on the posterior distribution of location estimates within that period (Pedersen et al., 2011). Because the models for each animal were fit over the same spatial grid, we integrated RDs for all sharks to develop population-level RDs. The advantage of calculating RDs based on modeled location estimates was that, unlike kernel UD estimates of space use based on MPT locations alone, it implicitly accounts for location uncertainty and correlation to adjacent time periods (Braun et al., 2018b). To illustrate whale shark use of continental shelf (0–200 m), slope (201–2500 m), and open ocean (>2500 m) regions (Wicksten and Packard, 2005) throughout the annual cycle, we extracted the depth from the General Bathymetric Chart of the Oceans (GEBCO) gridded bathymetry data (Gebco Compilation Group, 2020) at each estimated MPT location and at each daily SPOT tag location. We then created monthly kernel density plots of whale shark depth distributions.
Behavioral Analysis
We used results of the HMM to assess how whale shark movement behavior changed seasonally and as a function of water depth. For these analyses we considered days in which the HMM was able to confidently assign a movement state as either resident or migratory. To model seasonal changes in behavior we used logistic regression to model the probability of resident behavior as a function of two harmonic variables that allowed behavior to change in a non-linear fashion;
where m = month (1–12; Byrne et al., 2019). To account for individual variation, we included shark ID as a random effect. To evaluate support for seasonal variation we calculated Akaike’s Information Criterion (AIC) for this model and compared it to AIC of an intercept-only model, which would represent constant behavior. We considered a seasonal change in behavior to be supported if the AIC of the seasonal model was >2 AIC units less than the intercept-only model (Burnham and Anderson, 2002).
We predicted that resident behavior was more likely when whale sharks were in shallow waters associated with the continental shelf and shelf-edge. We assessed the effect of depth on resident behavior using mixed-effects logistic regression, with water depth included as a fixed effect and whale shark ID as a random effect to account for individual variation. We used a multiple imputation approach to account for location uncertainty in whale shark daily locations. To accomplish this, we sampled 50 locations (with replacement) from the daily RD of each shark, and extracted the depth at each sampled location. This provided us with 50 data sets of whale shark depth and behavior, where each data set represented a different realization of whale shark daily locations pulled from the probability distribution of a shark’s daily location as determined by the HMM. We applied the mixed-effects logistic regression model to each of the 50 imputed data sets and pooled results across models using functions in the mice package (van Buuren and Groothuis-Oudshoorn, 2011) in R. We examined the pooled fixed-effects parameter estimates and considered depth to have a significant effect on behavior if the 95% confidence interval for the estimated effect of depth did not cross 0. Because of the large depth range used by whale sharks, we rescaled depth by dividing all values by 100 to assure model convergence.
Association With Oceanographic Features
Since many whale sharks used open ocean waters of the GOM, we used plots of remotely sensed sea surface height (SSH) to potentially identify localized productivity features such as currents, fronts, and eddies. Whale shark track locations were overlaid onto maps of SSH during July, September, and October 2014, and February 2015 to gain a better understanding of why they were utilizing these areas. These months and years were chosen to due to the high number of track locations during this time and variability in the position of the Loop Current and associated mesoscale features. To visualize SSH, we used gridded daily absolute dynamic topography (ADT) data from June 2009 to August 2015 provided by the Copernicus Marine and Environment Monitoring Service (CMEMS)5 and were used to form daily SSH maps for the GOM. Anti-cyclonic eddies (ACE, warm core) and cyclonic eddies (CE, cold core) were identified based on SSH; ACE were defined by having higher SSH than surrounding waters, whereas CEs were defined by having lower SSH values than the surrounding water (Faghmous et al., 2012). Since we were dealing with daily SSH maps, we selected the mid-point of the month (e.g., 15th) to be the best presentation for that month. This allowed us to investigate whether open ocean whale shark movements were associated with the Loop Current and associated mesoscale features.
Results
Sightings Data
The combination of the NMFS (n = 81), Wildbook for Whale Shark (n = 104), and NGWSSS (n = 637) datasets resulted in 822 whale shark sightings entries from 1989 to 2016, with the vast majority of the sightings (82%) occurring from 2007 to 2016. As various sources contributed to the database (e.g., aerial surveys, recreational and commercial fishers, recreational divers, and petroleum industry personnel), no effort data were recorded with the sightings and no density or abundance estimates could be generated. Overall, whale sharks were reported from continental shelf and slope waters throughout the northern GOM with most reported observations occurring from the DeSoto Canyon to waters off Corpus Christi, TX, with some of the highest concentrations occurring in areas in the north central (NC) GOM, in waters surrounding Ewing Bank, and offshore of Tampa Bay. Eighty-one percent of the sightings locations occurred in continental shelf and shelf edge waters with only 19% of the sightings occurring over continental slope and open ocean waters.
Most whale shark sightings (94%) occurred from May to November, with peak sightings in August (Figure 2). During spring, the largest core use area occurred in continental slope waters of the NC GOM, with smaller core use areas off the central Florida coast and shelf edge waters off central Texas (Figure 3A). During summer there was a relatively tight core use area found along the continental shelf edge near Ewing Bank, with smaller concentrations occurring off northwest Florida (Figure 3B). During fall, the majority of the core use areas occurred in continental shelf edge waters extending from the Mississippi River Delta eastward to the DeSoto Canyon (Figure 3C). Only 18 (2.2%) whale shark sightings were reported during winter with almost the entire core use areas during this season in continental edge/slope waters of the NC GOM (Figure 3D).
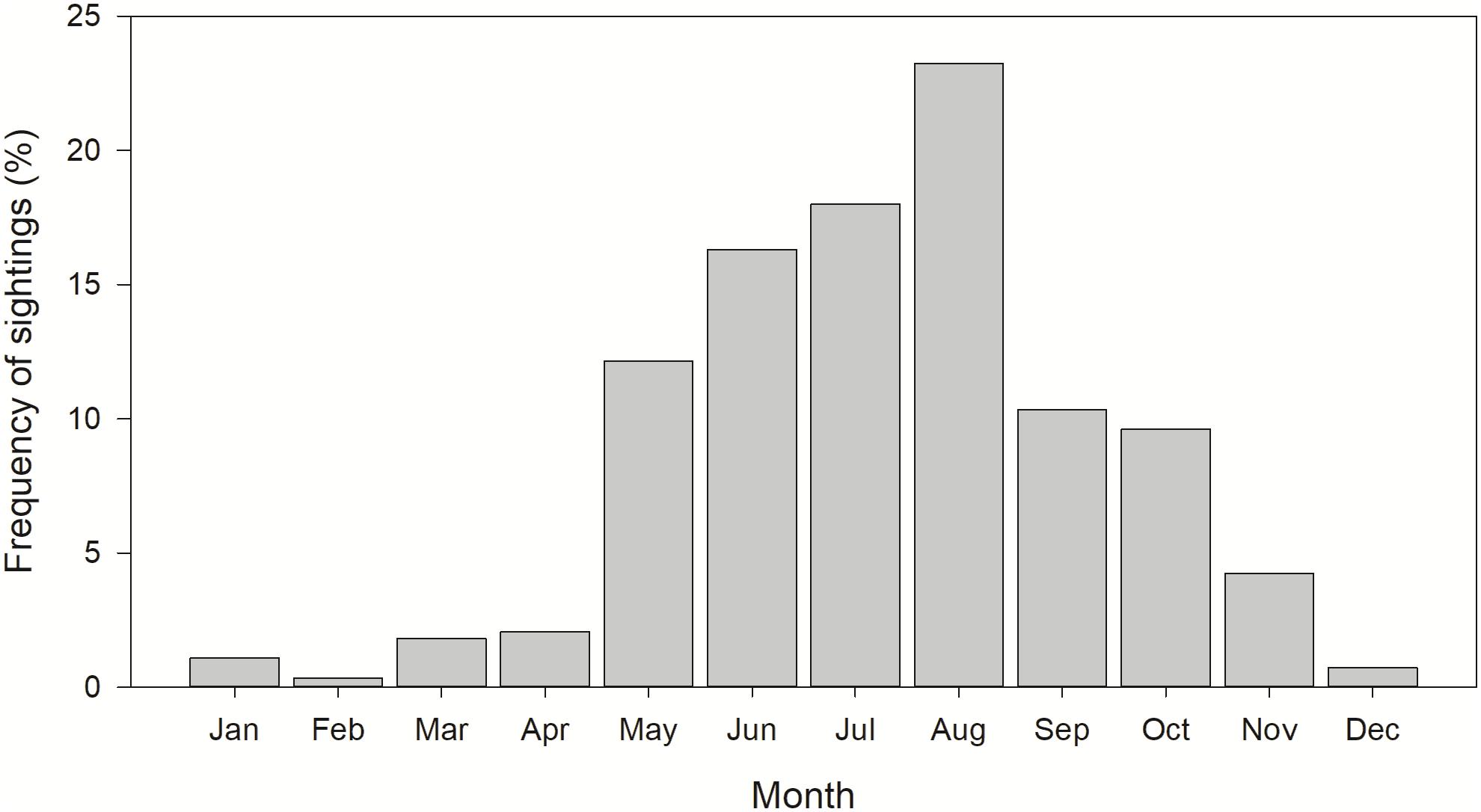
Figure 2. Monthly distribution of whale shark, Rhincodon typus, sightings reported in the northern Gulf of Mexico from 1989 to 2016. n = 822.
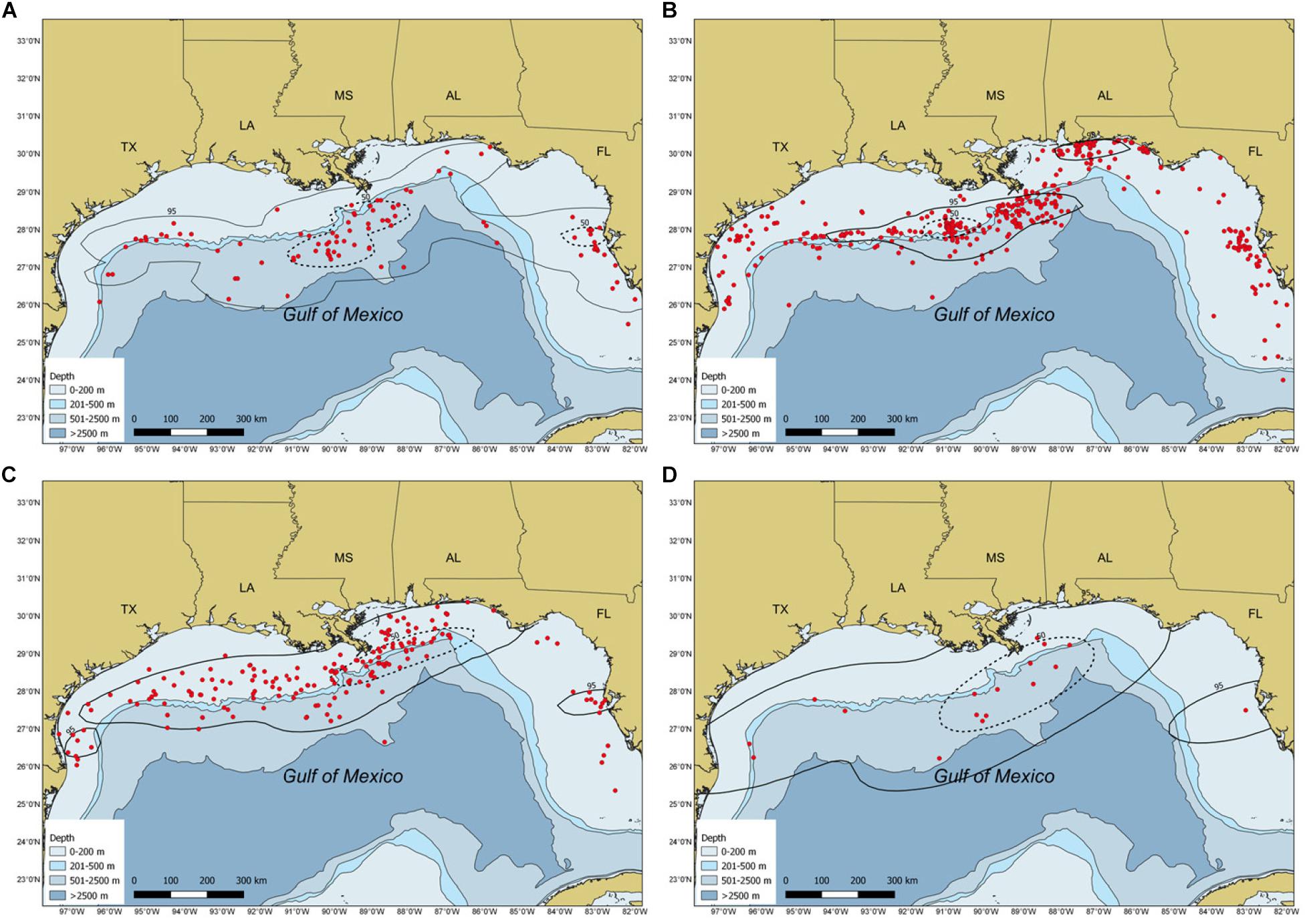
Figure 3. Maps depicting sighting locations (red circles) and weighed kernel utilization distributions of whale sharks, Rhincodon typus, in the Gulf of Mexico by season: (A) spring, (B) summer, (C) fall, and (D) winter from 1989 to 2016. The 95% (solid black line) and 50% (dashed black line) contours are presented. Circles represent both individual and aggregation sightings.
Satellite Tags
From 2008 to 2014, 50 satellite tags were deployed on 48 individual whale sharks, including two individuals that were double tagged with SPOT and PSAT tags (Supplementary Tables 1, 2). The majority of the tags (33 of 50) were deployed on individuals near Ewing Bank (Figure 1), while another 15 individuals were tagged in waters south of the Mississippi Canyon, and two individuals were tagged in waters surrounding the DeSoto Canyon (Figure 1). Thirty-five tags were deployed during summer (70%) and 15 tags were deployed during fall (30%). Estimated sizes of tagged sharks ranged from 4.5 to 12.0 m TL (mean 7.8 ± 0.3 m). Seven females (range 6.0 to 11.0 m TL, mean 8.8 ± 0.6 m TL) and 35 males (range 4.5 to 12.0 m TL, mean 7.7 ± 0.3 m TL) were tagged, while sex was unable to be confidently assigned for eight sharks (range 6.0 to 9.0 m TL, mean 7.0 ± 0.6 m TL).
Thirty-two PSAT tags were deployed: 14 MK10-PAT (range 9 to 150 days, mean: 83.2 ± 15.0 days, 5 failures) and 18 X-Tag (range 6 to 366 days, mean: 144.6 ± 31.4 days, 1 failure, Supplementary Table 1). Typically, light-based longitude and HYCOM likelihoods were used in HMM geolocation models, however, if the modeled track possessed large gaps then SST or OHC likelihoods were used in combination with light-based longitude to produce the MPT (Supplementary Table 1). Behavioral states were assigned for 3,124 whale shark MPT locations from 23 sharks using the HMM, with resident, migratory, and unknown behavior assigned for 15.3, 12.4, and 72.4% of the locations, respectively. We suspect that difficulties in confidently assigning behavioral states as resident or migratory were a result of large location uncertainty relative to whale shark movement capacity. In addition to the PSAT tags, 18 SPOT tags (range 3 to 250 days, mean: 58.8 ± 15.6 days 0 failures, Supplementary Table 2) were deployed on whale sharks. Overall, location data were received from 44 of the 50 tags with a mean tag duration of 97.0 ± 15.0 days (Supplementary Tables 1, 2). The 44 tags were deployed for a total of 4,266 days and reported 3,334 days (78.2%) of geolocation data.
Seasonal Residency Distribution
All sharks remained in the GOM with the majority of the locations (∼91%) occurring over continental slope and open ocean waters (Figure 4). Mean daily rates of individual whale shark movements ranged from 3.8 to 52.3 km/day, with an overall mean rate of 20.5 ± 1.6 km/d (Supplementary Tables 1, 2). Seasonal RDs indicate a summer core use area along the continental shelf edge, slope, and open ocean waters from the DeSoto Canyon region west to Ewing Bank, with 88.1% of the locations occurring in the northern GOM (Figure 5A). During fall, the 23 tagged sharks exhibited no clear pattern in habitat use areas with most sharks widely distributed throughout the GOM (Figure 5B). There was a general southern shift in whale shark distribution in late fall and winter (November–January), corresponding to the greatest observations of tagged whale sharks in the southern GOM (Figure 5E). In fact, about 29% of the MPT locations occurred in the southern GOM, with ∼90% occurring during late fall. During winter, the largest proportion of MPT locations occurred in the southern GOM, and less than five locations occurred in continental shelf waters of the northern GOM (Figure 5C). The 14 tagged sharks during winter exhibited core use areas in the central and SW GOM over continental slope and open ocean waters (Figure 5C). During spring, MPT locations were only provided from seven tagged sharks and over 94% of the locations occurred over slope and open ocean waters of the GOM, with the core use area in the central GOM (Figure 5D). Roughly 20% of the MPT locations occurred in the southern GOM with most of those locations (∼60%) occurring in early spring. In addition to the seasonal residency distribution, the latitudinal density plot of all shark locations revealed a similar movement pattern into the southern GOM from September to March, with an uncharacteristic peak in locations at 23°N in April. However, it should be noted that a single shark heavily influenced this peak (Figure 5E).
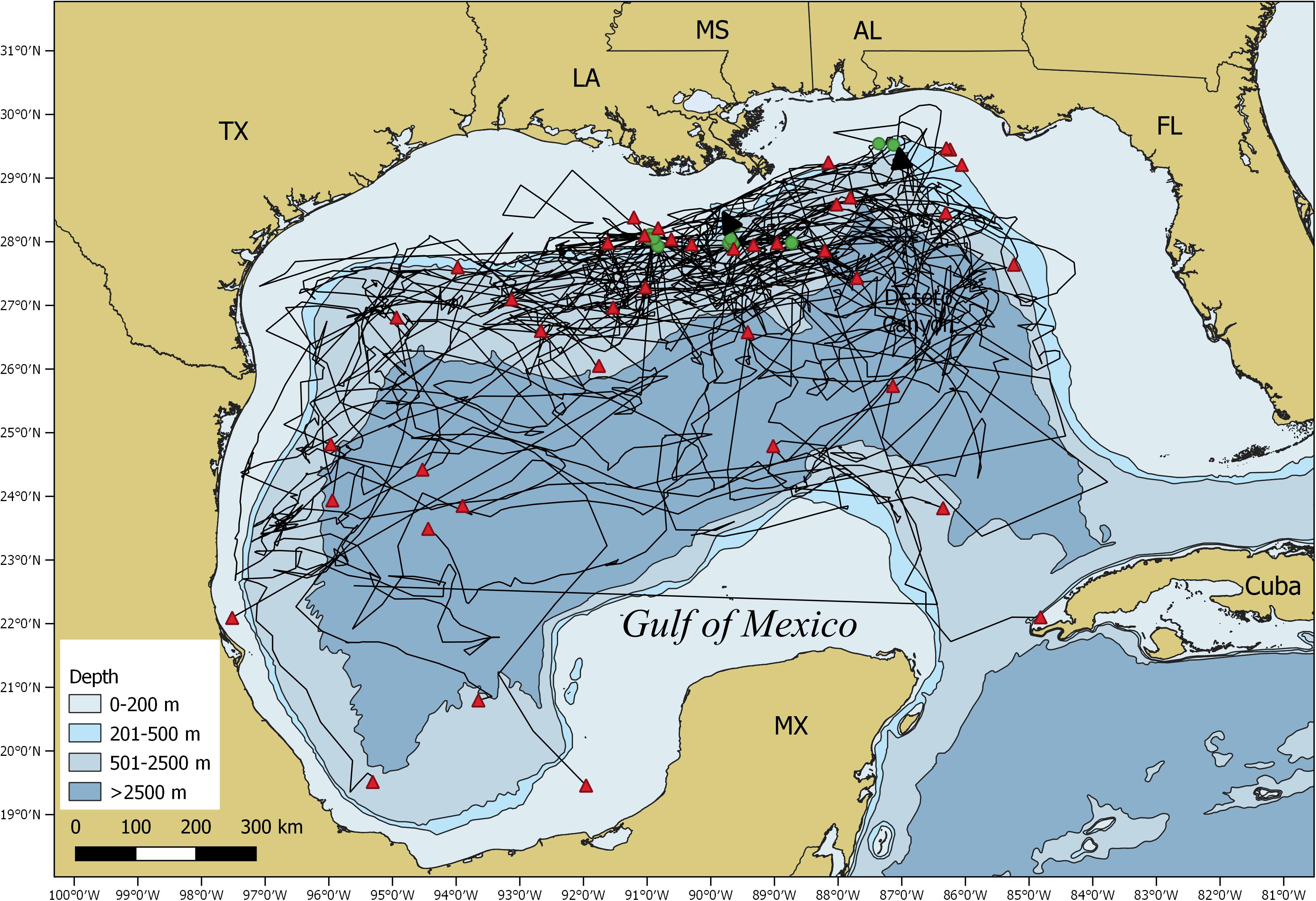
Figure 4. Map of the Gulf of Mexico showing satellite tag based movement trajectories (black lines) of all individual tagged whale sharks, Rhincodon typus, along with the tagging (green circles) and popup (red triangles) locations.
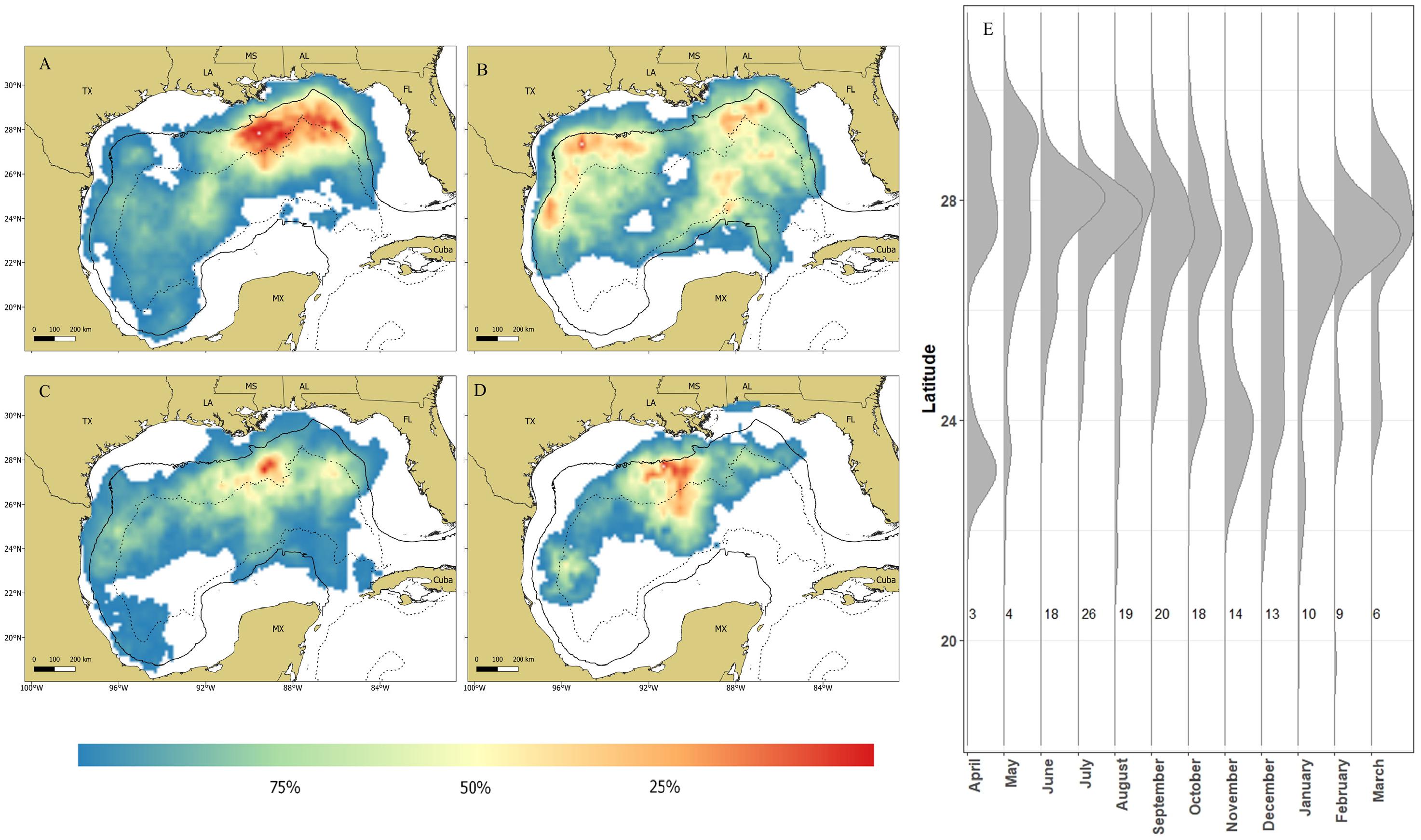
Figure 5. Maps depicting residency distributions of whale sharks, Rhincodon typus, based on most probable track locations in the Gulf of Mexico by season; (A) summer, (B) fall, (C) winter, and (D) spring. The black solid line represents the 200m isobaths and the black dashed line represents the 2,500 isobath, separating the continental shelf, slope, and open ocean water of the Gulf of Mexico. The latitudinal density plot (E) shows the latitudinal distribution of PSAT tagged whale sharks in the northern Gulf of Mexico from 2008–2014. Each vertical line represents a month of the year and the numbers to the right of each line indicate the number of individuals tracked during that month.
When investigating regional use of the GOM (e.g., shelf, slope, and open ocean waters) by sex and maturity state by month, females used continental slope and open ocean waters almost exclusively, whereas some mature males occurred over continental shelf and slope waters during summer and fall, while other mature males remained in slope and open ocean waters throughout the year (Figure 6). Conversely, immature males spent the majority of their time in continental shelf and slope waters, only occurring in a few locations over open ocean waters (Figure 6).
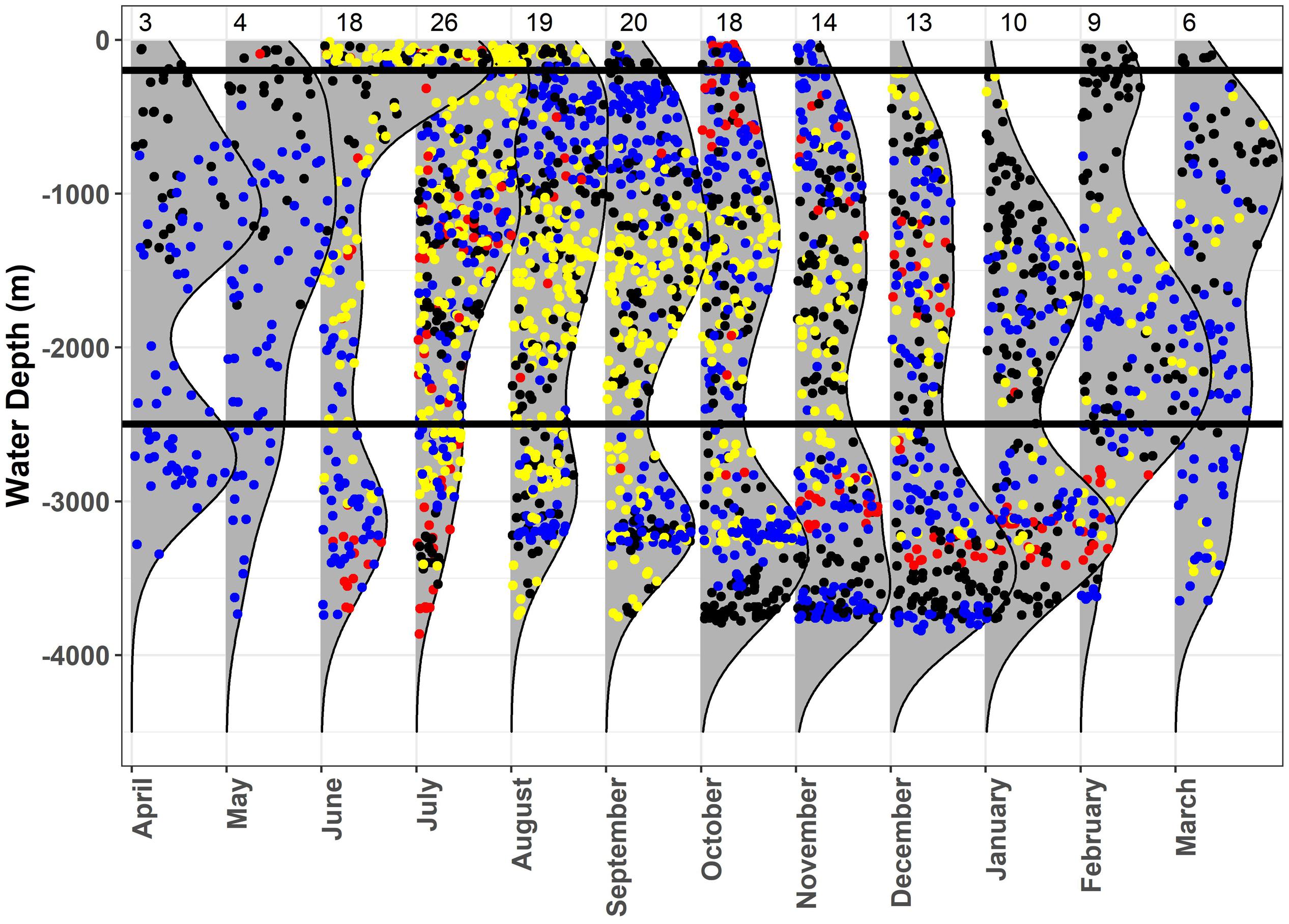
Figure 6. Monthly kernel density plots of water depth at the most probable location estimates for whale sharks, Rhincodon typus, in the Gulf of Mexico. Immature males (yellow), adult males (blue), and females (red) are represented. The black horizontal lines designate the separation between the three regions: shelf (0–200 m), slope (200–2500 m), and open ocean (>2500 m). Sample size of tagged sharks is shown at the top of the plot for each month.
Short-Term Deployments (<30 Days)
Short-term tracking data ranging from three to 29 days (mean = 15.2 ± 2.2 days) were reported from 14 of the 42-tagged sharks (33.3%), with nine of the 14 tags (64.3%) being towed SPOT tags (Supplementary Tables 1, 2). The corresponding distance traveled over this time ranged from 33 to 836 km (mean = 225 ± 63.3 km). All but two of the 14 sharks were tagged at Ewing Bank (85.7%) during summer, and the majority of the movements were within 140 km of the tagging locations (Supplementary Figure 1A).
Long-Term Deployments (<30 Days)
The duration of the 28 remaining satellite tagged sharks ranged from 30 to 366 days and their movements occurred throughout the GOM (Supplementary Figures 1–3). As such, we grouped their subsequent movements into two broad categories: short (remaining in northern GOM) and long (leaving northern GOM) distance movements. The short distance movements describe the individuals that exhibited residency to the northern GOM; whereas the long distance movements were further subdivided into movements to the southeast (SE) and southwest (SW) GOM.
Residency to the Northern GOM
Seven of the 42-tagged sharks (16.7%) remained in the northern GOM throughout their tracks, which ranged in duration from 41 to 81 days (mean 61.3 ± 5.6 days; Supplementary Tables 1, 2). All of these sharks were tagged at Ewing Bank during summer, and all ventured less than 600 km from their tagging location (Supplementary Figure 1B). Six sharks exhibited a net eastward movement with tracks terminating from 162 to 558 km east of Ewing Bank (Supplementary Figure 1B).
Movements to the Southeastern GOM
Six of the 42-tagged sharks (14.3%) moved into the SW GOM but never left the GOM during the duration of their tracks (Supplementary Figure 2), which ranged from 52 to 253 days (mean 130.7 ± 29.3 days; Supplementary Tables 1, 2) and covered distances that ranged from 637 to 6,029 km (mean 2,466.2 ± 857.5 km; Supplementary Tables 1, 2). The majority of the tags (66.7%) popped up over continental slope waters of the GOM, however, one track ended over continental shelf waters of the northeast GOM and one track terminated over coastal waters of western Cuba in the Golfo de Guanahacabibes, (Supplementary Figure 2). Several sharks used the shelf edge/slope waters of the eastern GOM, in the DeSoto Canyon region, before moving south (Supplementary Figure 2).
Movements to the Southwestern GOM
Fifteen of the 42 tagged sharks (35.7%) moved into the SW GOM, but never left the GOM during the duration of their tracks (Supplementary Figure 3), which ranged from 38 to 367 days (mean 176.8 ± 30.1 days; Supplementary Tables 1, 2), and covered from 1,192 to 11,057 km (mean 4,502.1 ± 888.1 km; Supplementary Tables 1, 2). Similar to the sharks that moved to the SE GOM, most of the tracks (80.0%) terminated over continental slope and open ocean waters of the GOM (Supplementary Figure 3). Seven sharks spent the majority of their time in northern GOM waters but did spend some time in the northern portion of the SW GOM (Supplementary Figure 3A). The eight other tagged sharks spent limited time in the northern GOM and made directed movements into the SW GOM (Supplementary Figure 3B). Two different movement patterns were observed with four sharks moving east initially in late summer/early fall before moving to the SW GOM, whereas the other four sharks began their southwest movement from the tagging sites in late summer/early fall and followed a path along the continental shelf edge/slope into the SW GOM (Supplementary Figure 3B).
Seasonal Movements
During this study, we were able to tag 11 whale sharks on a single day, 10 July 2014 (Figure 7), where six tags remained deployed longer than 7 months, and three that were retained for the full one-year duration (Supplementary Table 1). This situation provided a unique subset of tagging data to examine individual variation in seasonal movements. During summer, nine sharks remained in the northern GOM, with seven of the sharks using similar areas throughout this time period (Figure 7A). During this time, two sharks moved southwest to continental slope waters near the Mexico-Texas border and continental slope/open ocean waters of the SW GOM, respectively (Figure 7A). Three tags popped off during summer. During fall, all eight sharks remained initially in the eastern GOM, and three sharks moved to the west, following the shelf edge and over open ocean waters (Figure 7B). The remaining sharks moved south with one shark moving the farthest south before the track terminated near the western tip of Cuba (Figure 7B). During winter, five of the six-tagged sharks associated with continental slope and open ocean waters of the NC GOM (Figures 7C,D). One shark remained exclusively in the southern GOM during this time. During spring, there was a high degree of overlap among sharks in the NC GOM (Figure 7E). One shark moved from the southern GOM toward the NC GOM to an area utilized by three other sharks (Figure 7E). One shark moved southeast toward northwestern Cuba, where the track terminated (Figure 7E). All three tagged sharks that were tracked into the following summer spent time near Ewing Bank (Figure 7F), where they had been initially tagged one year earlier.
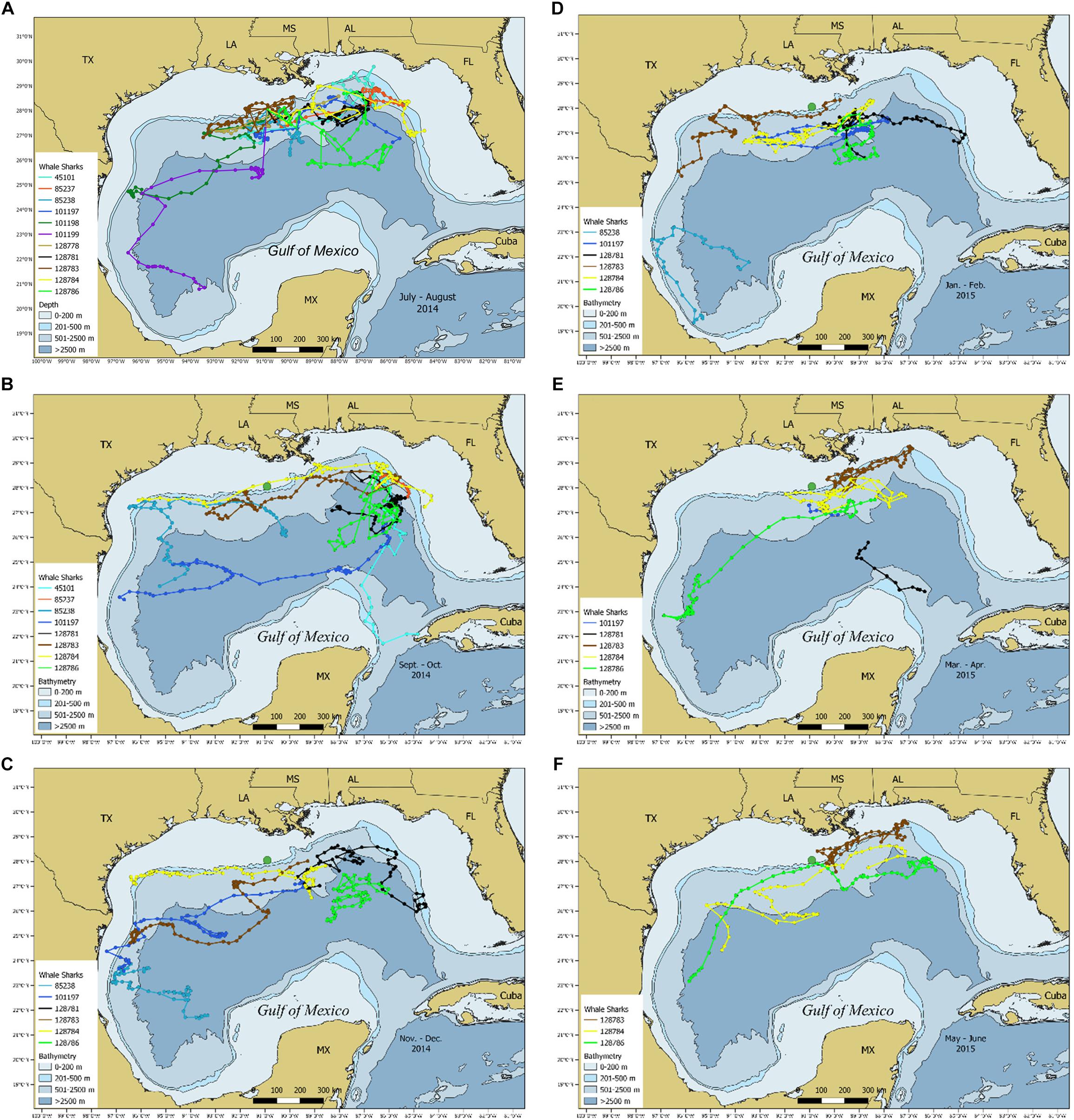
Figure 7. Maps depicting temporal changes in the most probable tracks for whale sharks, Rhincodon typus, tagged on 10 July 2014. Tracks range from (A) July–August 2014, (B) September–October 2014, (C) November–December 2015, (D) January–February 2015, (E) March–April 2015, and (F) May–June 2015. Large green circle indicates tagging location of Ewing Bank.
Behavioral Analyses
A total of 477 and 387 daily locations confidently classified as resident or migratory behavior, respectively, were used to model whale shark behavior. The model that allowed behavior to change seasonally was well supported relative to the model of constant behavior (ΔAIC = 7.86). Model results suggest the probability of resident behavior peaked during late spring and early summer and that whale sharks were less likely to engage in resident behavior during late fall and early winter (Figure 8). There was a negative effect of water depth on probability of resident behavior (β = −0.04, 95% CI: −0.06 – −0.03, Table 1). Probability of resident behavior was greatest in continental shelf and shelf-edge waters and decreased as depth increased with the lowest probabilities occurring in the open ocean waters of the GOM (Figure 8).
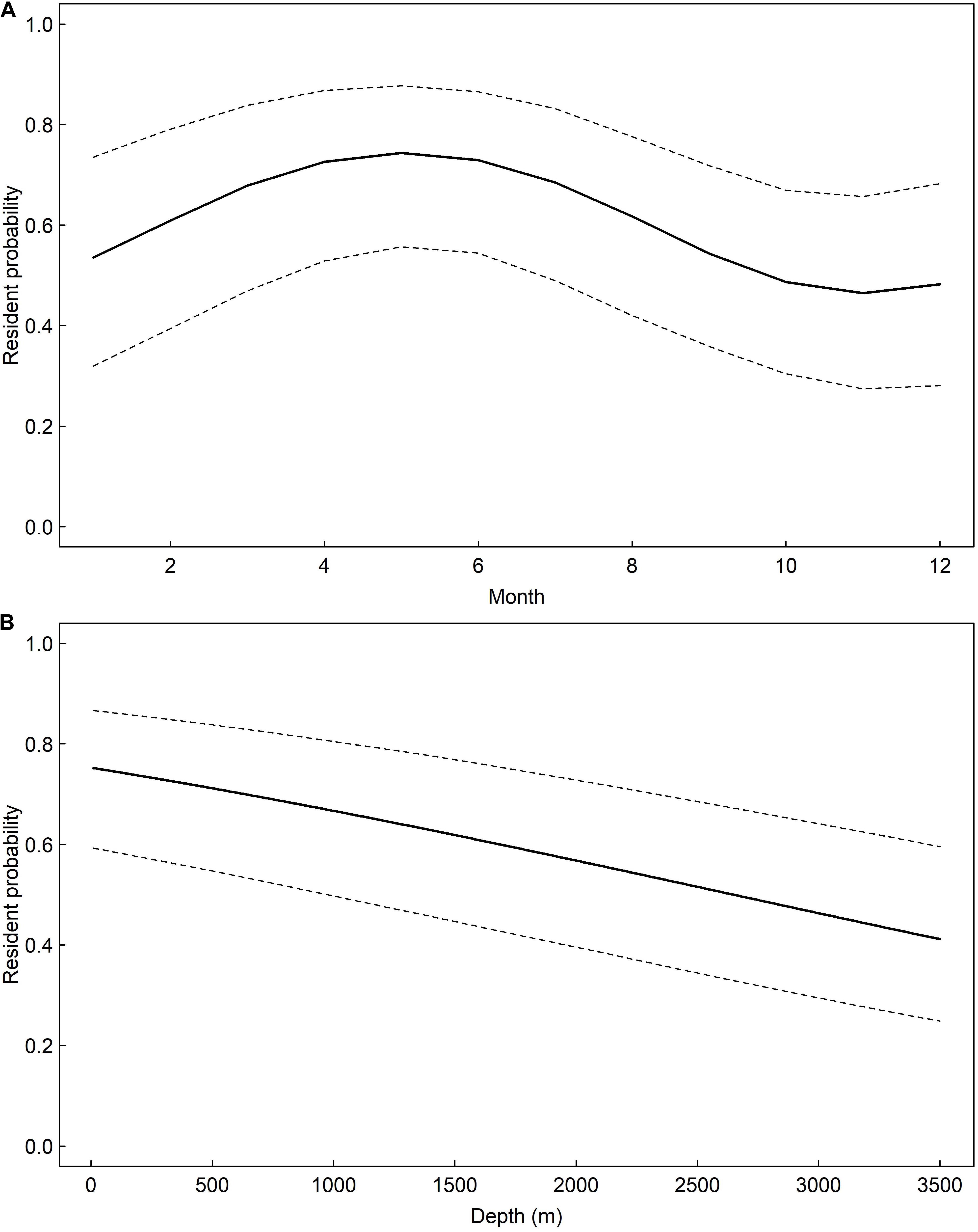
Figure 8. Predicted probability, and associated 95% confidence intervals, of whale shark, Rhincodon typus, resident state probability in the Gulf of Mexico seasonally (A) and as a function of water depth (B) based on mixed-effects logistic regression models.
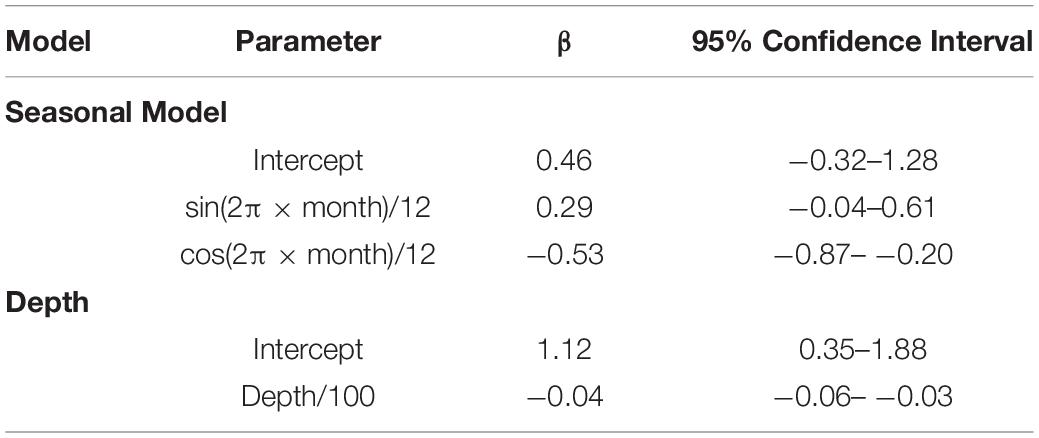
Table 1. Fixed-effects parameter estimates (β) and 95% confidence intervals from mixed-effects logistic regression models of the effects of seasonality and water depth on the probability of PSAT tagged whale sharks, Rhincodon typus (n = 22), exhibiting resident behavior in the Gulf of Mexico.
Association With Oceanographic Features
During July 2014, data from 10 PSAT tags provided 220 MPT locations (Figure 9A), with most of these locations appearing to be associated with a recently formed Loop Current eddy and two smaller anti-cyclonic, warm core eddies to the east and west (Figure 9A). PSAT data from eight whale sharks tracked during September 2014 resulted in 227 MPT locations. The majority of these locations occurred on the eastern edge of the Loop Current and an anti-cyclonic, warm core eddy just off the continental shelf edge in the western GOM (Figure 9B). Similar to September 2014, many of the 203 MPT locations available for October 2014 were on the periphery of the Loop Current and anti-cyclonic warm core eddies in the western GOM (Figure 9C). Only five whale sharks provided 143 MPT locations during February 2015, however, most of those locations were in proximity to the northern and western edge of the Loop Current (Figure 9D). In total, many of the MPT locations in these four examples were associated with the Loop Current and associated anti-cyclonic, warm core eddies (Figure 9).
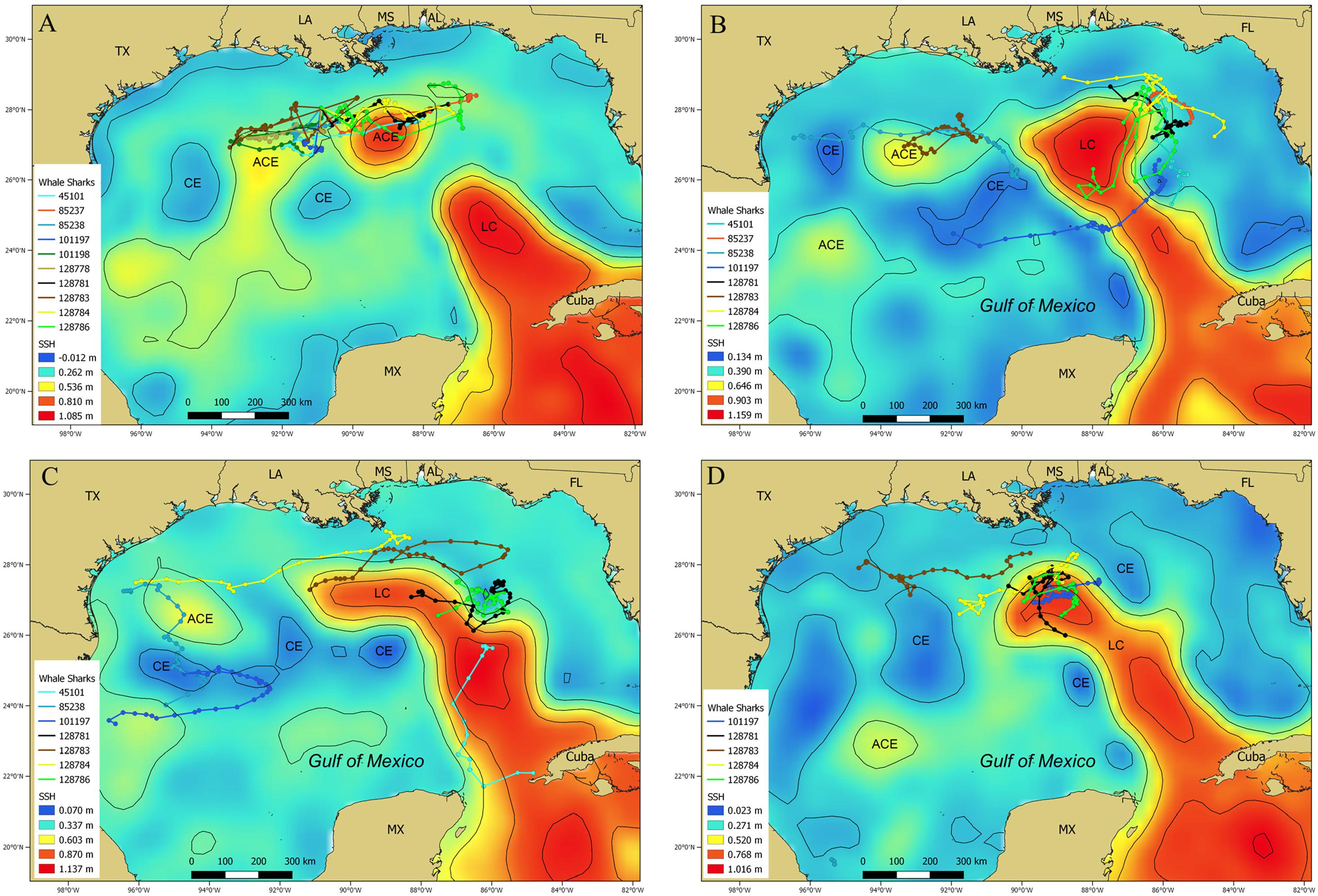
Figure 9. Maps depicting sea surface height (m) showing the Loop Current and warm core and cold core eddies in the Gulf of Mexico with whale shark, Rhincodon typus, most probable track locations overlaid during (A) July 2014, (B) September 2014, (C) October 2014, and (D) February 2015. Most of the locations from multiple individuals are associated with the Loop Current or other warm core eddies. Contour lines (black lines) are depicted at 0.2 m height increments. The Loop Current (LC), anti-cyclonic eddies (ACE), and cyclonic eddies (CE) are indicated on the map.
Discussion
Seasonal Occurrence, Distribution and Movements
This study represents the largest whale shark movement study to date in the Atlantic Ocean, with data collected from 42-tagged sharks and two decades of sightings reports. It was apparent from the temporal periodicity of sightings and MPT locations that continental shelf edge/slope and open ocean waters in the northern GOM were important habitats for whale sharks year-round. However, the highest use of northern GOM waters coincided with the warmest time of year in this region (i.e., summer and early fall). This increased habitat use of northern GOM water during summer was supported by the behavioral state models as well, which revealed that resident/foraging behavior was more likely occurring during summer over shallower continental shelf waters (Figure 8). The increased probability of foraging behavior in continental shelf waters of the NC GOM during summer was most likely related to the increased localized productivity during this time. Certain features, such as the Mississippi River Plume, upwelling along continental shelf edges, and convergence zones, occur in this region during summer and are conducive environments for plankton, a main prey source for whale sharks (Le Fevre, 1986; Richards et al., 1993; Ryan et al., 1999; Wilson et al., 2001; Hoffmayer et al., 2005; McKinney et al., 2012). These findings are consistent with other studies that have documented whale sharks association with areas of high productivity and abundant prey (Colman, 1997; Brunnschweiler et al., 2009; de la Parra Venegas et al., 2011; Berumen et al., 2014; Robinson et al., 2017). The environmental and biological conditions in the NC GOM during summer appear to allow for the occurrence of a large number of whale sharks, including the presence of a seasonal whale shark large aggregation site at Ewing Bank. Outside of this time of increased habitat use of the NC GOM, whale sharks tended to redistribute themselves throughout the GOM. The overall trend in habitat use was characterized by southerly movements into offshore waters of the central and southern GOM during late fall and early winter, and northerly movements back to the northern GOM during late winter and spring (Figure 5). These offshore movements likely explain the reduced number of sightings data during the winter and spring months (∼10% of sightings occur during this time). In addition, the behavioral state models revealed a higher probability of migratory behavior during fall, winter and early spring while individuals primarily inhabited deeper continental slope and open ocean waters of the GOM (Figure 8). These seasonal offshore movements away from known foraging areas is supported by the other whale shark tagging study in the region by Hueter et al. (2013), who reported whale shark movements from the southern GOM, with similar timings of movements away from the summer aggregation site during fall. Further supporting this is that as prey abundance at localized seasonal aggregation sites wanes, whale sharks leave these areas to undergo broad scale movements in search of other foraging opportunities (de la Parra Venegas et al., 2011; Hueter et al., 2013; Araujo et al., 2018). The ultimate purpose of the movements away from the northern GOM during fall and winter is unknown, however, as observed in other regions these movements could represent searching for additional foraging opportunities and possibly movements to more productive overwintering grounds in the GOM.
Overwintering Habitat
Interestingly, 11 of the 20 (55%) sharks tagged during fall and 11 of the 14 (79%) tagged sharks during winter spent time in the southern GOM in the current study (Supplementary Figures 2, 3), with 12 of the 17 (71%) sharks spending time in the SW GOM. This corresponded to a lack of sightings in the northern GOM during those seasons. Similarly, several tagged whale sharks from the Hueter et al. (2013) study moved from the Yucatan Peninsula into the SW GOM during fall and winter. Taken together, these data suggest the southern GOM, and more specifically SW GOM, may be suitable overwintering habitat and possibly indicate an unknown seasonal aggregation site during this time. Other large pelagic fishes, such as blue marlin, Makaira nigricans, Atlantic Bluefin tuna, Thunnus thynnus, and dusky sharks, Carcharhinus obscurus, have been shown to use the SW GOM as overwintering grounds (Kraus et al., 2011; Hoffmayer et al., 2014; Rooker et al., 2019) in the same vicinity where some whale sharks tagged in this study overwintered. Since little effort has been invested in studying whale sharks in the southern GOM, outside the Yucatan Peninsula aggregation site, future work should focus on identifying other areas in the region, such as the SW GOM, as potentially important seasonal habitat for the GOM population.
The seasonal decline in sightings during winter and early spring could have also been related to changes in whale shark behavior, with the sharks moving to deeper waters farther offshore, but not necessarily leaving the northern GOM. Cagua et al. (2015) and Norman et al. (2017b) both used acoustic telemetry to monitor fine-scale habitat use patterns and revealed whale sharks were year-round residents off Mafia Island, Tanzania and Ningaloo Reef, Australia, yet there were no sightings outside the peak tourist season at both locations. Those authors determined sharks were using deeper, offshore waters during the time of no sightings, most likely in response to changes in prey distributions. Another possible explanation for the limited number of sightings reported during winter and spring could be the result of seasonally adverse weather conditions in the GOM, which typically result in a reduced number of boaters on the water during this time. However, we relied heavily on the offshore oil and gas industry for sightings throughout the year whose presence is not affected by weather conditions during this time. Over the course of this study, the oil and gas industry provided 127 whale shark sightings, with the lowest number of sightings occurring during winter (n = 4, 3%) and spring (n = 25, 20%), despite no reduction in the number of personnel on the water (Hoffmayer, unpub. data). Not all whale sharks overwintered in the southern GOM as three of the 14 tagged sharks moved to offshore waters but never left the northern GOM, suggesting that a portion of the population overwinters in the northern GOM. Since long-term tag retention can be challenging (i.e., minimal long-term tag retention), future work combining the use of acoustic and fin-mounted satellite-linked tags deployed on whale sharks during late fall in the northern GOM would provide additional insight into overwintering habitat for this species.
GOM Residency
The long-term tracked whale sharks exhibited interannual residency, returning to the vicinity of the Ewing Bank aggregation site one year later after demonstrating a total track displacement distance of 9,305–11,057 km. In addition to the satellite tracking data, photo identification data has revealed five individuals that have shown interannual residency to Ewing Bank, ranging anywhere between one and 4 years later (Hoffmayer, unpublished data). This movement back to the summer aggregation site demonstrates a high level of site fidelity to Ewing Bank and the importance of this area to the biology and ecology of this species in the GOM. Reynolds et al. (2017) studying the movements of whale sharks off Ningaloo Reef, Australia were the first to document homing movements with some sharks moving long distances away from Ningaloo Reef, then returning interannually. Cochran et al. (2019) also reported homing migratory movement of whale sharks to and from known aggregation sites in the Red Sea, using a variety of methods, including visual surveys, passive acoustics and satellite telemetry. Additionally, McKinney et al. (2017), using photo-ID reported 90% of the re-sighted whale sharks were individuals observed at least one year later at the same aggregation site in the western Central Atlantic Ocean where they were originally identified. Thus, it has been documented that although whale sharks may move away from seasonal aggregation sites, they have a high affinity to return to those areas.
Even though several of the tagged sharks were tracked longer than 6 months, there was no evidence of departure from the GOM; however, multiple seasonal cross-basin movements were observed. Considering the large number of tagged individuals and the fact that some whale sharks have been shown to make large-scale seasonal movements (Hueter et al., 2013; Hearn et al., 2016; Araujo et al., 2018; Diamant et al., 2018), it was surprising that no individuals made movements outside the GOM. One possible explanation for this residency to the GOM could be related to tagging location. Coastal pelagic and highly migratory fishes tagged near the Yucatan Channel or Straits of Florida (the two immigration/emigration points for the GOM) show highly variable movements, whereas species tagged in the NC and NW GOM appear to have movements restricted to the GOM. For example, Luo et al. (2020) studying movements of tarpon, Megalops atlanticus, in the GOM, reported that individuals tagged in the SE GOM near the Straits of Florida showed variable movements between the GOM and United States east coast; whereas other individuals tagged in the western GOM primarily remained in the GOM. Similarly, Hueter et al. (2013) tagged 35 whale sharks in waters surrounding the Yucatan Peninsula and reported a variety of movements into the GOM, Caribbean Sea, Straits of Florida, and South Atlantic Ocean. Tagging studies of other large pelagic fishes including blue marlin, and dusky, bull, Carcharhinus leucas, scalloped hammerhead, Sphyrna lewini, and tiger, Galeocerdo cuvier, sharks exhibited similar patterns of residency in the GOM (Carlson et al., 2010; Kraus et al., 2011; Hoffmayer et al., 2014; Wells et al., 2018; Ajemian et al., 2020). Therefore, the tagging location may influence whether an individual whale shark migrates out of the GOM and into other adjacent regions.
The high level of residency in the GOM observed in this study corresponded to the lack of connectivity observed between the Ewing Bank and the Yucatan Peninsula aggregation sites. Evidence of whale shark population connectivity between the known large aggregation sites (e.g., northern GOM and Honduras, Seychelles and Tanzania, etc.) is limited. Regional comparisons of spot patterns on whale sharks within the western Indian Ocean indicated no movements among Seychelles, Djibouti, Mozambique and Tanzania, suggesting that major “known” aggregation sites in the region do not have individuals transiting among them (Brooks et al., 2010). Based on over 16 years of whale shark photo identification data, McKinney et al. (2017) revealed connectivity among individuals from aggregations in Honduras, Belize, and Mexico, however, this was likely the direct result of the close proximity of the aggregation locations. Connectivity between the United States and other regions had a lag time between sightings of greater than one year. The lack of connectivity in the present study could be because whale shark migratory patterns occur over a multi-year scale. Sequeira et al. (2013) reviewed available whale shark movement studies and presented a conceptual movement model suggesting a possible 2 to 4 year migration cycle. Wilson et al. (2006) also suggested that whale shark movements were most likely multi-year as data were not consistent with the hypothesis of sharks returning to Ningaloo Reef after a single year. The current maximum duration of one year for PSAT tags used in the current study may not be adequate to record certain aspects of whale shark migratory behavior.
Size Distribution
In this study, both juvenile and adult whale sharks were found to use open ocean habitat, however, adult males and females used deeper, offshore waters compared to the immature males. Whale sharks have been reported to show an ontogenetic shift in habitat use, with juveniles primarily utilizing nearshore, shallow, continental shelf waters, while adults tend to use deeper, offshore, open ocean waters (Rowat and Brooks, 2012; Sequeira et al., 2013; Ramírez-Macías et al., 2017). In a tagging study conducted in the southern GOM, Hueter et al. (2013) reported similar habitat use patterns with juvenile sharks using shallower waters than larger females that utilized more offshore waters. Ketchum et al. (2013) and Ramírez-Macías et al. (2017) reported whale shark size segregation in the Gulf of California, where smaller juveniles tended to aggregate in coastal waters and adults, occurred almost exclusively in oceanic waters and suggested that juveniles were foraging on abundant prey resource found in coastal waters. There are several possible explanations for ontogenetic shifts in habitat use, including thermoregulatory behavior, reproductive requirements in females, and changes in diet (Hueter et al., 2013). Hearn et al. (2016) observed a similar pattern in habitat use and suggested an ontogenetic dietary shift in adult whale sharks results in a closer association with offshore frontal zones. Since most whale shark aggregations occur in coastal waters (Rowat and Brooks, 2012) this use of more offshore waters by adults would explain why immature sharks dominate the aggregations. This use of shallower continental shelf habitat for juvenile whale sharks is not exclusive as several studies, including the current study, have reported juveniles to use offshore waters as well (Hsu et al., 2007; Araujo et al., 2018; Diamant et al., 2018). Additional tracking and dietary studies on juvenile and adult whale sharks will be instrumental in better describing stage-specific movement patterns.
Association With Oceanographic Features
Using sightings reports, satellite telemetry and spatial analyses, our study revealed that whale sharks not only occupy continental shelf and slope waters, but also used open ocean waters of the GOM. In fact, the majority (∼90%) of the MPT locations occurred over continental slope and open ocean waters. This increased use of open ocean waters was presumably related to broad scale movements to other regions of the GOM and exploitation of ephemeral hotspots of productivity, such as convergence zones, the edge of the Loop Current, and associated ACE, for foraging opportunities. During 2014–2015, an average of 80% of the MPT locations in open ocean GOM waters occurred in proximity to these features (Figure 9). Although the location of the Loop Current can vary on short time scales (e.g., days to weeks), it represents a relatively consistent area of productivity in the GOM, whereas mesoscale ACEs typically split from the Loop Current at irregular intervals and slowly move toward the western GOM (Chen et al., 2015; Dufois et al., 2016).
Even though ACEs are often associated with low productivity (i.e., low chlorophyll-a concentrations), studies have shown the eddies can have large concentrations of diatoms and other phytoplankton, resulting in enhanced epipelagic productivity as well as increased mesopelagic community biomass (McGillicuddy et al., 2007; Gaube et al., 2014; Fennell and Rose, 2015; Pascual et al., 2015). While our understanding of the influence of ACEs on whale sharks is limited, these features appear to provide enhanced foraging opportunities in the otherwise oligotrophic open ocean environment for at least two species: the blue shark, Prionace glauca, and the white shark, Carcharodon carcharias (Gaube et al., 2018; Braun et al., 2019). Additionally, reef manta rays, Manta alfredi, have been shown to exploit mesoscale eddies in offshore waters of the Great Barrier Reef for foraging purposes and further corroborate the importance of these spatially explicit features to highly migratory species (Jaine et al., 2014). Another large filter feeding elasmobranch, the basking shark, Cetorhinus maximus, is known to forage along thermal fronts and actively select the most profitable plankton patches (Sims and Quayle, 1998) and make large horizontal movements to discrete productivity hotspots along shelf edge habitats (Sims et al., 2003; Doherty et al., 2017a, b; Braun et al., 2018b). There are also several examples of whale sharks using mesoscale features for foraging while in the offshore environment, including boundary currents in the northern Pacific Ocean (Hsu et al., 2007), frontal zones in the Gulf of California (Ramírez-Macías et al., 2017), and continental shelf edge upwellings in the northern GOM (McKinney et al., 2012; Hueter et al., 2013). These mesoscale features, like convergence zones and ACEs, could prove to be critical habitat for whale sharks in offshore waters of the GOM. Further study of this association with open ocean mesoscale features is warranted, specifically using habitat modeling to explore the relationship between environmental variables and more accurate tracking locations using satellite-linked tags (i.e., fin-mounted SPOT tags).
Conclusion and Recommendations
Prior to this study, whale sharks were known to form large aggregations at continental shelf edge banks during summer in the northern GOM (Hoffmayer et al., 2005, 2007; McKinney et al., 2012), however, knowledge of their movements and habitat use patterns outside this region was limited. The sightings, satellite tracking and behavioral state model data presented in the current study further supports the fact that the continental shelf/slope waters of the NC GOM are an important foraging habitat for whale sharks during summer. During other seasons, the overall trend in habitat use was characterized by southerly movements into offshore waters of the central and southern GOM during late fall and early winter, and northerly movements back to the northern GOM during late winter and spring. It was also evident from the satellite tracking data that a large portion of the sharks tagged during fall and winter (75–80%) were over wintering in the southern GOM, with most individuals utilizing western waters. Similarly, Hueter et al. (2013) reported that whale sharks move from the Yucatan Peninsula aggregation site to the SW GOM during this same time, which further suggests this region could be an important overwintering habitat and possibly represents another seasonal aggregation site in the region. Sightings data revealed that whale sharks occurred primarily in continental shelf and shelf-edge waters (81%) whereas tag data revealed the sharks primarily inhabit continental slope and open ocean waters (91%) of the GOM. Much of their time spent in open ocean waters was linked to the edge of the Loop Current and associated mesoscale features for foraging opportunities. Although several long-term satellite tracks were presented in the study, there was no evidence of any individual leaving the GOM or visiting the Yucatan Peninsula aggregation site. However, the three long-term tracked whale sharks exhibited interannual site fidelity, returning to the vicinity of the Ewing Bank aggregation site one year after tagging.
The increased habitat use of north central GOM waters by whale sharks as summer foraging grounds and potential interannual site fidelity to Ewing Bank demonstrate the importance of this region for this species. This combined with their tendency to spend a significant amount of time in surface waters makes whale sharks susceptible to ship strikes and gear entanglement from commercial shipping traffic and energy and mineral development in the region. In addition, the impacts from the Deepwater Horizon oil spill to the GOM whale shark population remain largely unknown. Therefore, establishing protections for whale sharks in the GOM and greater Atlantic region would be beneficial to their population. Additionally, the broad-scale GOM-wide movements observed in this study demonstrate multi-national, cooperative efforts are required to properly manage whale sharks in the region. Longer-term satellite telemetry and multi-year tracking data are needed to further our understanding of whale shark movement ecology in the region and will provide the foundation for future management practices.
Data Availability Statement
The datasets presented in this study can be found in online repositories. The names of the repository/repositories and accession number(s) can be found below: Movebank (https://www.movebank.org/cms/webapp?gwt_fragment=page=studies,path=study1153270750).
Ethics Statement
The animal study was reviewed and approved by the University of Southern Mississippi Institutional Animal Care and Use Committee (09031204 and 11092203), and under National Marine Fisheries Service Highly Migratory Division Exempted Fishing permits SHK-EFP-09-03, SHK-EFP-11-07, SHK-SRP-12-03, SHK-SRP-13-01, SHK-SRP-14-01, and SHK-SRP-15-01.
Author Contributions
EH, JF, JM, WD, and JH conceived the study. EH, JM, JH, WD, and BF performed the fieldwork and data collection. EH, BG, and MB analyzed and interpreted the data. EH led the writing of the manuscript. All authors read and approved the final manuscript.
Funding
Funding for this research was provided to by the International Fund for Animal Welfare, World Wildlife Fund, Defenders of Wildlife, Shared Earth Foundation, Nature Conservancy Texas Chapter, and Louisiana Department of Wildlife and Fisheries (LDWF).
Conflict of Interest
The authors declare that the research was conducted in the absence of any commercial or financial relationships that could be construed as a potential conflict of interest.
Acknowledgments
We wish to thank B. Schumaker of On Wings of Care for endless hours searching for whale sharks over the Gulf of Mexico and the associated group of experienced spotters, including T. Collins, D. Abrams, M. Sturdivant, J. Moran, G. Gray, and J. Green. We would also like to thank volunteers from the USM-Gulf Coast Research Laboratory, including W. Dempster, J. Higgs, A. Fogg, S. Ashworth, and C. Butler, the Louisiana Department of Wildlife and Fisheries, including P. Sweat, P. Kent, and C. Marshall, and colleagues E. Hickerson, M. Nuttall, and G. P. Schmahl from the Flower Gardens Banks National Marine Sanctuary. Finally, we are indebted to the following individuals who were instrumental in supporting this research including J. Levenson, S. Earle, R. Nixon, B. Groark, J. Brenner, and captains R. Underwood, W. Delabar, and K. Beach.
Supplementary Material
The Supplementary Material for this article can be found online at: https://www.frontiersin.org/articles/10.3389/fmars.2020.598515/full#supplementary-material
Footnotes
- ^ http://gcrl.usm.edu/whaleshark/whaleshark_survey.php
- ^ www.whaleshark.org
- ^ https://github.com/camrinbraun/HMMoce
- ^ https://www.movebank.org
- ^ http://www.marine.copernicus.eu
References
Ajemian, M. J., Marcus Drymon, J., Hammerschlag, N., David Wells, R. J., Street, G., Falterman, B., et al. (2020). Movement patterns and habitat use of tiger sharks (Galeocerdo cuvier) across ontogeny in the Gulf of Mexico. PLoS ONE 15:e0234868. doi: 10.1371/journal.pone.0234868
Andrzejaczek, S., Meeuwig, J., Pierce, S., Davies, T., Fisher, R., and Meekan, M. (2016). The ecological connectivity of whale shark aggregations in the Indian Ocean: a photoidentification approach. R. Soc. Open Sci. 3:160455. doi: 10.1098/rsos.160455
Araujo, G., Agustines, A., Tracey, B., Snow, S., Labaja, J., and Ponzo, A. (2019). Photo-ID and telemetry highlight a global whale shark hotspot in Palawan, Philippines. Sci. Rep. 9:17209. doi: 10.1038/s41598-019-53718-w
Araujo, G., Rohner, C. A., Labaja, J., Conales, S. J., Snow, S. J., Murray, R., et al. (2018). Satellite tracking of juvenile whale sharks in the Sulu and Bohol seas, Philippines. PeerJ 6:e5231. doi: 10.7717/peerj.5231
Banzon, V., Smith, T. M., Chin, T. M., Liu, C. Y., and Hankins, W. (2016). A long-term record of blended satellite and in situ sea-surface temperature for climate monitoring, modeling and environmental studies. Earth Syst. Sci. Data 8, 165–176. doi: 10.5194/essd-2015-44
Bauer, R. K., Forget, F., and Fromentin, J. M. (2015). Optimizing PAT data transmission: assessing the accuracy of temperature summary data to estimate environmental conditions. Fish. Ocean. 24, 533–539. doi: 10.1111/fog.12127
Baughman, J. T., and Springer, S. (1950). Biological and economic notes on the sharks of the Gulf of Mexico, with special reference to those of Texas, and with a key for their identification. Am. Midl. Nat. 44, 96–152. doi: 10.2307/2421758
Berumen, M. L., Braun, C. D., Cochran, J. E. M., Skomal, G. B., and Thorrold, S. R. (2014). Movement patterns of juvenile whale sharks tagged at an aggregation site in the Red Sea. PLoS One 9:e103536. doi: 10.1371/journal.pone.0103536
Bigelow, H. B., and Schroeder, W. C. (1948). Fishes of the western North Atlantic, Part I: lancelets, cyclostomes, sharks. Mem. Sears Found. Mar. Res. 1, 559–576.
Braun, C. D., Galuardi, B., and Thorrold, S. R. (2018a). HMMoce: an R package for improved geolocation of archival-tagged fishes using a hidden Markov method. Methods Ecol. Evol. 9, 1212–1220. doi: 10.1111/2041-210X.12959
Braun, C. D., Gaube, P., Sinclair-Taylor, T. H., Skomal, G. B., and Thorrold, S. R. (2019). Mesoscale eddies release pelagic sharks from thermal constraints to foraging in the ocean twilight zone. Proc. Natl. Acad. Sci. U.S.A. 116, 17187–17192. doi: 10.1073/pnas.1903067116
Braun, C. D., Skomal, G. B., and Thorrold, S. R. (2018b). Integrating archival tag data and a high-resolution oceanographic model to estimate basking shark (Cetorhinus maximus) movements in the western Atlantic. Front. Mar. Sci. 5:25. doi: 10.3389/fmars.2018.00025
Brooks, K., Rowat, D., Pierce, S. J., Jouannet, D., and Vely, M. (2010). Seeing spots: photo-identification as a regional tool for whale shark identification. West. Indian Ocean J. Mar. Sci. 9, 185–194.
Brunnschweiler, J. M., Baensch, H., Pierce, S. J., and Sims, D. W. (2009). Deep-diving behavior of a whale shark Rhincodon typus during long-distance movement in the western Indian Ocean. J. Fish Biol. 74, 706–714. doi: 10.1111/j.1095-8649.2008.02155.x
Burks, C. M., Driggers, W. B. I. I. I., and Mullin, K. D. (2006). Abundance and distribution of whale sharks (Rhincodon typus) in the northern Gulf of Mexico. Fish. Bull. 104, 579–584.
Burnham, K. P., and Anderson, D. R. (2002). Model Selection and Multimodel Inference: A Practical Information-Theoretic Approach, 2nd Edn. New York, NY: Springer.
Burt, W. H. (1943). Territoriality and home range concepts as applied to mammals. J. Mamm. 24, 346–352. doi: 10.2307/1374834
Byrne, M. E., Vaudo, J. J., Harvey, G. C. M., Johnston, M. W., Wetherbee, B. M., and Shivji, M. (2019). Behavioral response of a mobile marine predator to environmental variables differs across ecoregion. Ecography 42, 1569–1578. doi: 10.1111/ecog.04463
Cagua, E. F., Cochran, J. E. M., Rohner, C. A., Prebble, C. E. M., Sinclair-Taylor, T. H., Pierce, S. J., et al. (2015). Acoustic telemetry reveals cryptic residency of whale sharks. Biol. Lett. 11:20150092. doi: 10.1098/rsbl.2015.0092
Calich, H., Estevanez, M., and Hammerschlag, N. (2018). Overlap between highly suitable habitats and longline gear management areas reveals vulnerable and protected regions for highly migratory sharks. Mar. Ecol. Prog. Ser. 602, 183–195. doi: 10.3354/meps12671
Cárdenas-Palomo, N., Herrera-Silveira, J., Velázquez-Abunader, I., Reyes, O., and Ordoñez, U. (2014). Distribution and feeding habitat characterization of whale sharks Rhincodon typus in a protected area in the north Caribbean Sea. J. Fish Biol. 86, 668–686. doi: 10.1111/jfb.12589
Carlson, J. K., Ribera, M. M., Conrath, C. L., Heupel, M. R., and Burgess, G. H. (2010). Habitat use and movement patterns of bull sharks Carcharhinus leucas determined using pop-up satellite archival tags. J. Fish Biol. 77, 661–675. doi: 10.1111/j.1095-8649.2010.02707.x
Castro, A. L. F., Stewart, B. S., Wilson, S. G., Hueter, R. E., Meekan, M. G., Motta, P. J., et al. (2007). Population genetic structure of Earth’s largest fish, the whale shark (Rhincodon typus). Mol. Ecol. 16, 5183–5192. doi: 10.1111/j.1365-294X.2007.03597.x
Chassignet, E. P., Hurlburt, H. E., Smedstad, O. M., Halliwell, G. R., Hogan, P. J., Wallcraft, A. J., et al. (2007). The HYCOM (HYbrid Coordinate Ocean Model) data assimilative system. J. Mar. Sys. 65, 60–83. doi: 10.1016/j.jmarsys.2005.09.016
Chen, Y. L., Chen, H. Y., Jan, S., Lin, Y. H., Kuo, T. H., and Hung, J. J. (2015). Biologically active warm-core anticyclonic eddies in the marginal seas of the western Pacific Ocean. Deep-Sea Res. I 106, 68–84. doi: 10.1016/j.dsr.2015.10.006
Chmura, H. E., Glass, T. W., and Williams, C. T. (2018). Biologging physiological and ecological responses to climatic variation: new tools for the climate change era. Front. Ecol. Evol. 6:92. doi: 10.3389/fevo.2018.00092
Cochran, J. E. M., Braun, C. D., Cagua, E. F., Campbell, M. F., Hardenstine, R. S., Kattan, A., et al. (2019). Multi-method assessment of whale shark (Rhincodon typus) residency, distribution, and dispersal behavior at an aggregation site in the Red Sea. PLoS One 14:e0222285. doi: 10.1371/journal.pone.0222285
Colman, J. G. (1997). A review of the biology and ecology of the whale shark. J. Fish. Biol. 51, 1219–1234. doi: 10.1111/j.1095-8649.1997.tb01138.x
Compagno, L. J. V. (2001). Sharks of the World: an Annotated and Illustrated Catalogue of Shark Species Known to date: Heterodontiformes, Lamniformes, Orectolobiformes. Rome: FAO Species Catalogue for Fishery.
de la Parra Venegas, R., Hueter, R., González Cano, J., Tyminski, J., Gregorio Remolina, J., Maslanka, M., et al. (2011). An unprecedented aggregation of whale sharks, Rhincodon typus, in Mexican coastal waters of the Caribbean Sea. PLoS One 6:e18994. doi: 10.1371/journal.pone.0018994
Diamant, S., Rohner, C. A., Kiszka, J. J., Guillemain, d’Echon, A., Guillemain d’Echon, T., et al. (2018). Movements and habitat use of satellite-tagged whale sharks off western Madagascar. Endanger. Species Res. 36, 49–58. doi: 10.3354/esr00889
Doherty, P. D., Baxter, J. M., Gell, F. R., Godley, B. J., Graham Hall, G., Hall, J., et al. (2017a). Long-term satellite tracking reveals variable seasonal migration strategies of basking sharks in the northeast Atlantic. Nat. Sci. Rep. 7:42837. doi: 10.1038/srep42837
Doherty, P. D., Baxter, J. M., Godley, B. J., Graham, R. T., Hall, G., Hall, J., et al. (2017b). Testing the boundaries: seasonal residency and inter-annual site fidelity of basking sharks in a proposed Marine Protected Area. Biol. Conserv. 209, 68–75. doi: 10.1016/j.biocon.2017.01.018
Dufois, F., Hardman-Mountford, N. J., Greenwood, J., Richardson, A. J., Feng, M., and Matear, R. J. (2016). Anticyclonic eddies are more productive than cyclonic eddies in subtropical gyres because of winter mixing. Sci. Adv. 2:e16000282. doi: 10.1126/sciadv.1600282
Duong, T. (2007). Ks: kernel density estimation and kernel discriminant analysis for multivariate data in R. J. Stat. Software 21, 1–16. doi: 10.18637/jss.v021.i07
Faghmous, J. H., Chamber, Y., Boriah, S., Liess, S., and Kumar, V. (2012). “A novel and scalable spatio-temporal technique for ocean eddy monitoring,” in Proceedings of the Twenty-Sixth AAAI Conference on Artificial Intelligence, (Toronto, ON: AAAI), 281–287.
Fennell, S., and Rose, G. (2015). Oceanographic influences on deep scattering layers across the North Atlantic. Deep Sea Res. I 105, 132–141. doi: 10.1016/j.dsr.2015.09.002
Fox, S., Foisy, I., de la Parra-Venegas, R., Galvan-Pastoriza, B., Graham, R. T., Hoffmayer, E. R., et al. (2013). Population structure and residency of whale sharks Rhincodon typus at Utila, Bay Islands, Honduras. J. Fish Biol. 83, 574–587. doi: 10.1111/jfb.12195
Gaube, P., Braun, C. D., Lawson, G. L., McGillicuddy, D. J. Jr., Penna, A. D., Skomal, G. B., et al. (2018). Mesoscale eddies influence the movements of mature female white sharks in the Gulf Stream and Sargasso Sea. Sci. Rep. 8:7363. doi: 10.1038/s41598-018-25565-8
Gaube, P., McGillicuddy, D. J. Jr., Chelton, D. B., Behrenfeld, M. J., and Strutton, P. G. (2014). Regional variations in the influence of mesoscale eddies on near-surface chlorophyll. J. Geophys. Res. Oceans 119, 8195–8220. doi: 10.1002/2014JC010111
Gebco Compilation Group (2020). The GEBCO_2020 Grid - A Continuous Terrain Model of the Global Oceans and Land. Atlanta: National Oceanography Centre, NERC, doi: 10.5285/a29c5465-b138-234d-e053-6c86abc040b9
Graham, F., Rynne, P., Estevanez, M., Luo, J., Ault, J. S., and Hammerschlag, N. (2016). Use of marine protected areas and exclusive economic zones in the subtropical western North Atlantic Ocean by large highly mobile sharks. Divers. Distrib. 22, 534–546. doi: 10.1111/ddi.12425
Graham, R. T., and Roberts, C. M. (2007). Assessing the size, growth rate and structure of a seasonal population of whale sharks (Rhincodon typus Smith 1828) using conventional tagging and photo identification. Fish. Res. 84, 71–80. doi: 10.1016/j.fishres.2006.11.026
Gudger, E. (1918). Rhineodon typus, the whale shark – Further notes on its habits and distribution. Science 48, 622–627. doi: 10.1126/science.48.1251.622-a
Gudger, E. (1934). The geographical distribution of the whale shark (Rhineodon typus). Proc. Zool. Soc. 4, 863–894. doi: 10.1111/j.1096-3642.1934.tb01670.x
Hammerschlag, N., Gallagher, A. J., and Lazarre, D. M. (2011). A review of shark satellite tagging studies. J. Exp. Mar. Biol. Ecol. 398, 1–8. doi: 10.1016/j.jembe.2010.12.012
Hart, K. M., and Hyrenbach, K. D. (2009). Satellite telemetry of marine megavertebrates: the coming of age of an experimental science. Endanger. Species Res. 10, 9–20. doi: 10.3354/esr00238
Hearn, A. R., Green, J., Roman, M. H., Acuña-Marrero, D., Espinoza, E., and Klimley, A. P. (2016). Adult female whale sharks make long-distance movements past Darwin Island (Galapagos, Ecuador) in the Eastern Tropical Pacific. Mar. Biol. 163:214. doi: 10.1007/s00227-016-2991-y
Hearn, A. R., Green, J. R., Espinoza, E., Peñeherrera, C., Acuña, D., and Klimley, A. P. (2013). Simple criteria to determine detachment point of towed satellite tags provide first evidence of return migrations of whale sharks (Rhincodon typus) at the Galapagos Islands. Ecuador. Anim. Biotelemetry 1:11. doi: 10.1186/2050-3385-1-11
Heyman, W., Graham, R., Kjerfve, B., and Johannes, R. E. (2001). Whale sharks Rhincodon typus aggregate to feed on fish spawn in Belize. Mar. Ecol. Prog. Ser. 251, 275–282. doi: 10.3354/meps215275
Hoffman, W., Fritts, T. H., and Reynolds, R. P. (1981). Whale sharks associated with fish schools off south Texas. Northeast Gulf Sci. 5, 55–57. doi: 10.18785/negs.0501.08
Hoffmayer, E. R., Franks, J. S., Driggers, W. B. I. I. I., McKinney, J. A., Hendon, J. M., and Quattro, J. M. (2014). Habitat, movement and environmental preferences of dusky sharks, Carcharhinus obscurus, in the northern Gulf of Mexico. Mar. Biol. 161, 911–924. doi: 10.1007/s00227-014-2391-0
Hoffmayer, E. R., Franks, J. S., Driggers, W. B. I. I. I., Oswald, K. J., and Quattro, J. M. (2007). Observations of a feeding aggregation of whale sharks, Rhincodon typus, in the north central Gulf of Mexico. Gulf Carib. Res. 19, 69–73. doi: 10.18785/gcr.1902.08
Hoffmayer, E. R., Franks, J. S., and Shelley, J. P. (2005). Recent observations of the whale shark (Rhincodon typus) in the northcentral Gulf of Mexico. Gulf Caribb. Res. 17, 117–120. doi: 10.18785/gcr.1701.11
Hsu, H. H., Joung, S. J., Liao, Y. Y., and Liu, K. M. (2007). Satellite tracking of juvenile whale sharks, Rhincodon typus, in the northwestern Pacific. Fish. Res. 84, 25–31. doi: 10.1016/j.fishres.2006.11.030
Hueter, R. E., Tyminski, J. P., and de la Parra, R. (2013). Horizontal movements, migration patterns, and population structure of whale sharks in the Gulf of Mexico and northwestern Caribbean Sea. PLoS One 8:e71883. doi: 10.1371/journal.pone.0071883
Hussey, N. E., Kessel, S. T., Aarestrup, K., Cooke, S. J., Cowley, P. D., Fisk, A. T., et al. (2015). Aquatic animal telemetry: a panoramic window into the underwater world. Science 348:1255642. doi: 10.1126/science.1255642
Jaffa, K. S., and Taher, N. A. B. A. (2007). Whale sharks in Palestinian waters: a whale shark (Rhincodon typus Smith 1828) rescued near the Tantura Beach, Carmel Coast, North Palestine. The first record from the Palestinian Mediterranean Coast. Gazelle: Palestinian. Biol. Bull. 71, 22–23. doi: 10.1007/s00227-016-2991-y
Jaine, F. R. A., Rohner, C. A., Weeks, S. J., Couturier, L. I. E., Bennett, M. B., Townsend, K. A., et al. (2014). Movements and habitat use of reef manta rays off eastern Australia: offshore excursions, deep diving and eddy affinity revealed by satellite telemetry. Mar. Eco. Prog. Ser. 510, 73–86. doi: 10.3354/meps10910
Jonsen, I. D. (2016). Joint estimation over multiple individuals improves behavioural state inference from animal movement data. Sci. Rep. 6:20625. doi: 10.1038/srep20625
Jonsen, I. D., Bestley, S., Wotherspoon, S., Sumner, M., and Flemming, J. M. (2020). bsam: Bayesian State-Space Models for Animal Movement. R Package 1.1.3. https://github.com/ianjonsen/bsam (accessed January 9, 2020).
Jonsen, I. D., Flemming, J. M., and Myers, R. A. (2005). Robust state-space modeling of animal movement data. Ecol. 86, 2874–2880. doi: 10.1890/04-1852
Ketchum, J. T., Galván-Magaña, F., and Klimley, A. P. (2013). Segregation and foraging ecology of whale sharks, Rhincodon typus, in the southwestern Gulf of California. Environ. Biol. Fish 96, 779–795. doi: 10.1007/s10641-012-0071-9
Kraus, R. T., Wells, R. J. D., and Rooker, J. R. (2011). Horizontal movements of Atlantic blue marlin (Makaira nigricans) in the Gulf of Mexico. Mar. Biol. 158, 699–713. doi: 10.1007/s00227-010-1593-3
Le Fevre, J. (1986). Aspects of the biology of frontal systems. Adv. Mar. Biol. 23, 163–299. doi: 10.1016/S0065-2881(08)60109-1
Lea, J. S. E., Wetherbee, B. M., Quieroz, N., Burnie, N., Aming, C., Sousa, L. L., et al. (2015). Repeated, long-distance migrations by a philopatric predator targeting highly contrasting ecosystems. Nat. Sci. Rep. 5:11202. doi: 10.1038/srep11202
Luo, J., Ault, J. A., Ungar, B. T., Smith, S. G., Larkin, M. F., Davidson, T. N., et al. (2020). Migrations and movements of Atlantic tarpon revealed by two decades of satellite tagging. Fish Fish. 21, 290–318. doi: 10.1111/faf.12430
Luo, J., Ault, J. S., Shay, L. K., Hoolihan, J. P., Prince, E. D., Brown, C. A., et al. (2015). Ocean heat content reveals secrets of fish migrations. PLoS One 10:e0141101. doi: 10.1371/journal.pone.0141101
McGillicuddy, D. J., Anderson, L. A., Bates, N. R., Bibby, T., Buesseler, K. O., Carlson, C. A., et al. (2007). Eddy/wind interactions stimulate extraordinary mid-ocean plankton blooms. Science 316, 1021–1026. doi: 10.1126/science.1136256
McKinney, J. A., Hoffmayer, E. R., Holmberg, J., Graham, R. T., Driggers, W. B. I. I. I., de la Parra-Venegas, R., et al. (2017). Long-term assessment of whale shark population demography and connectivity using photo-identification in the western Atlantic Ocean. PLoS One 12:e0180495. doi: 10.1371/journal.pone.0180495
McKinney, J. A., Hoffmayer, E. R., Wu, W., Fulford, R., and Hendon, J. M. (2012). Feeding habitat of the whale shark Rhincodon typus in the northern Gulf of Mexico determined using species distribution modelling. Mar. Eco. Prog. Ser. 458, 199–211. doi: 10.3354/meps09777
Norman, B. M., Holmberg, J. A., Arzoumanian, Z., Reynolds, S. D., Wilson, R. P., Rob, D., et al. (2017a). Undersea constellations: the global biology of an endangered marine megavertebrate further informed through citizen science. Bioscience 67, 1029–1043. doi: 10.1093/biosci/bix127
Norman, B. M., and Stevens, J. D. (2007). Size and maturity status of the whale shark (Rhincodon typus) at Ningaloo Reef in Western Australia. Fish. Res. 84, 81–86. doi: 10.1016/j.fishres.2006.11.015
Norman, B. M., Whitty, J. M., Beatty, S. J., Reynolds, S. D., and Morgan, D. L. (2017b). Do they stay or do they go? Acoustic monitoring of whale sharks at Ningaloo Marine Park, Western Australia. J. Fish Biol. 91, 1713–1720. doi: 10.1111/jfb.13461
Nychka, D., Furrer, R., Paige, J., and Sain, S. (2015). Fields: Tools for Spatial Data. R package version 9.0. Boulder, CO: University Corporation for Atmospheric Research.
Papastamatiou, Y. P., Friedlander, A. M., Caselle, J. E., and Lowe, C. G. (2010). Long-term movement patterns and trophic ecology of blacktip reef sharks (Carcharhinus melanopterus) at Palmyra Atoll. J. Exp. Mar. Biol. Ecol. 386, 94–102. doi: 10.1016/j.jembe.2010.02.009
Pascual, A., Ruiz, S., Buongiorno Nardelli, B., Guinehut, S., Iudicone, D., and Tintoré, J. (2015). Net primary production in the Gulf Stream sustained by quasi-geostrophic vertical exchanges. Geophys. Res. Lett. 42, 441–449. doi: 10.1002/2014GL062569
Pedersen, M., Berg, C., Thygesen, U., Nielsen, A., and Madsen, H. (2011). Estimation methods for nonlinear state-space models in ecology. Ecol. Model. 222, 1394–1400. doi: 10.1016/j.ecolmodel.2011.01.007
Queiroz, N., Humphries, N. E., Mucientes, G., Hammerschlag, N., Lima, F. P., Scales, K. L., et al. (2016). Ocean-wide tracking of pelagic sharks reveals extent of overlap with longline fishing hotspots. Proc. Natl. Acad. Sci. U.S.A. 113, 1582–1587. doi: 10.1073/pnas.1510090113
R Core Team (2016). R: A Language and Environment for Statistical Computing. Vienna: R Foundation for Statistical Computing.
Ramírez-Macías, D., Queiroz, N., Pierce, S. J., Humphries, N. E., Sims, D. W., and Brunnschweiler, J. M. (2017). Oceanic adults, coastal juveniles: tracking the habitat use of whale sharks off the Pacific coast of Mexico. PeerJ 5:e3271. doi: 10.7717/peerj.3271
Reynolds, R. W., Smith, T. M., Liu, C., Chelton, D. B., Casey, K. S., and Schlax, M. G. (2007). Daily high-resolution-blended analyses for sea surface temperature. J. Clim. 20, 5473–5496. doi: 10.1175/2007JCLI1824.1
Reynolds, S. D., Norman, B. M., Beger, M., Franklin, C. E., and Dwyer, R. G. (2017). Movement, distribution and marine reserve use by an endangered migratory giant. Divers. Distrib. 23, 1268–1279. doi: 10.1111/ddi.12618
Richards, W. J., McGowen, M. F., Leming, T., Lamkin, J. T., and Kelly, S. (1993). Larval fish assemblages at the Loop Current boundary in the Gulf of Mexico. Bull. Mar. Sci. 53, 475–537.
Robinson, D. P., Jaidah, M. Y., Bach, S. S., Rohner, C. A., Jabado, R. W., Ormond, R., et al. (2017). Some like it hot: repeat migration and residency of whale sharks within an extreme natural environment. PLoS One 12:e0185360. doi: 10.1371/journal.pone.0185360
Robinson, D. P., Jaidah, M. Y., Jabado, R. W., Lee-Brooks, K., Nour El-Din, N. M., Al Malki, A. A., et al. (2013). Whale sharks, Rhincodon typus, aggregate around offshore platforms in Qatari waters of the Arabian Gulf to feed on fish spawn. PLoS One 8:e58255. doi: 10.1371/journal.pone.0058255
Rohner, C. A., Armstrong, A. J., Pierce, S. J., Prebble, C. E. M., Cagua, E. F., Cochran, J. E. M., et al. (2015). Whale sharks target dense prey patches of sergestid shrimp off Tanzania. J. Plankt. Res. 37, 352–362. doi: 10.1093/plankt/fbv010
Rohner, C. A., Richardson, A. J., Jaine, F. R. A., Bennett, M. B., Weeks, S. J., Cliff, G., et al. (2018). Satellite tagging highlights the importance of productive Mozambican coastal waters to the ecology and conservation of whale sharks. Peer J. 6:e4161. doi: 10.7717/peerj.4161
Rooker, J. R., Dance, M. A., Wells, R. J. D., Ajemian, M. J., Block, B. A., Castleton, M. R., et al. (2019). Population connectivity of pelagic megafauna in the Cuba-Mexico-United States triangle. Sci. Rep. 9:1663. doi: 10.1038/s41598-018-38144-8
Rowat, D., and Brooks, K. S. (2012). A review of the biology, fisheries and conservation of the whale shark Rhincodon typus. J. Fish Biol. 80, 1019–1056. doi: 10.1111/j.1095-8649.2012.03252.x
Rowat, D., and Gore, M. (2007). Regional scale horizontal and local scale vertical movements of whale sharks in the Indian Ocean off Seychelles. Fish. Res. 84, 32–40. doi: 10.1016/j.fishres.2006.11.009
Rowat, D., Speed, C. W., Meekan, M. G., and Gore, M. A. (2009). Population abundance and apparent survival of the vulnerable whale shark Rhincodon typus in the Seychelles aggregation. Oryx 43, 591–598. doi: 10.1017/s0030605309990408
Ryan, J. P., Green, J. R., Espinoza, E., and Hearn, A. R. (2017). Association of whale sharks (Rhincodon typus) with thermos-biological frontal systems of the eastern tropical Pacific. PLoS One 12:e0182599. doi: 10.1371/journal.pone.0182599
Ryan, J. P., Yoder, J. A., and Cornillon, P. C. (1999). Enhanced chlorophyll at the shelfbreak of the Mid-Atlantic Bight and Georges Bank during the spring transition. Limnol. Oceanogr. 44, 1–11. doi: 10.4319/lo.1999.44.1.0001
Schmidt, J. V., Schmidt, C. L., Ozer, F., Ernst, R. E., Feldheim, K. A., Ashley, M. V., et al. (2009). Low genetic differentiation across three major ocean populations of the whale shark. Rhincodon typus. PLoS One 4:e4988. doi: 10.1371/journal.pone.0004988
Sequeira, A. M. M., Mellin, C., Meekan, M. G., Sims, D. W., and Bradshaw, C. J. A. (2013). Inferred global connectivity of whale shark Rhincodon typus populations. J. Fish. Biol. 82, 367–389. doi: 10.1111/jfb.12017
Silas, E. G. (1986). The whale shark (Rhiniodon typus Smith) in Indian coastal waters: is the species endangered or vulnerable? Mar. Fish. Infor. Serv. T E Ser. 66, 1–19.
Sims, D., and Quayle, V. (1998). Selective foraging behaviour of basking sharks on zooplankton in a small-scale front. Nature 393, 460–464. doi: 10.1038/30959
Sims, D. W., Southall, E. J., Richardson, A. J., Reid, P. C., and Metcalfe, J. D. (2003). Seasonal movements and behaviour of basking sharks from archival tagging: no evidence of winter hibernation. Mar. Ecol. Prog. Ser. 248, 187–196. doi: 10.3354/meps248187
Skomal, G. B., Braun, C. D., Chisholm, J. H., and Thorrold, S. R. (2017). Movement of the white shark Carcharodon carcharias in the North Atlantic Ocean. Mar. Ecol. Prog. Ser. 580, 1–16. doi: 10.3354/meps12306
Springer, S. (1957). Some observations on the behavior of schools of fishes in the Gulf of Mexico and adjacent waters. Ecology 38, 166–171. doi: 10.2307/1932154
Stevens, J. D. (2007). Whale shark (Rhincodon typus) biology and ecology: a review of the primary literature. Fish. Res. 84, 4–9. doi: 10.1016/j.fishres.2006.11.008
Taylor, J. G. (1996). Seasonal occurrence, distribution and movements of the whale shark. Rhincodon typus, at Ningaloo Reef, Western Australia. Mar. Freshw. Res. 47, 637–642. doi: 10.1071/MF9960637
Tyminski, J. P., de la Parra-Venegas, R., González Cano, J., and Hueter, R. E. (2015). Vertical movements and patterns of diving behavior of whale sharks as revealed by pop-up satellite tags in the eastern Gulf of Mexico. PLoS One 10:e0142156. doi: 10.1371/journal.pone.0142156
van Buuren, S., and Groothuis-Oudshoorn, K. (2011). mice: multivariate imputation by chained equations in R. J. Stat. Softw. 45, 1–67. doi: 10.18637/jss.v045.i03
Vignaud, T. M., Maynard, J. A., Leblois, R., Meekan, M. G., Vázquez-Juárez, R., and Ramírez-Macías, D. (2014). Genetic structure of populations of whale sharks among ocean basins and evidence for their historic rise and recent decline. Mol. Ecol. 23, 2590–2601. doi: 10.1111/mec.12754
Wells, R. J. D., Tin-Han, T. C., Dance, M. A., Drymon, J. M., Falterman, B., Ajemian, M. J., et al. (2018). Movement, behavior, and habitat use of a marine apex predator, the scalloped hammerhead. Front. Mar. Sci. 5:321. doi: 10.3389/fmars.2018.00321
Whitford, M., and Klimley, A. P. (2019). An overview of behavioral, physiological, and environmental sensors used in animal biotelemetry and biologging studies. Anim. Biotelemetry 7:26. doi: 10.1186/s40317-019-0189-z
Wicksten, M. K., and Packard, J. M. (2005). A qualitative zoogeographic analysis of decapod crustaceans of the continental slopes and abyssal plain of the Gulf of Mexico. Deep Sea Res. Part I Oceanogr. Res. Pap. 52, 1745–1765. doi: 10.1016/j.dsr.2005.04.006
Wilson, S. G., Pauly, T., and Meekan, M. G. (2001). Daytime surface swarming by Pseudeuphausia latifrons (Crustacea, Euphausiacea) off Ningaloo Reef, Western Australia. Bull. Mar. Sci. 68, 157–162.
Wilson, S. G., Polovina, J. J., and Stewart, B. S. (2006). Movement of whale sharks (Rhincodon typus) tagged at Ningaloo Reef, Western Australia. Mar. Biol. 128, 1157–1166. doi: 10.1007/s00227-005-0153-8
Keywords: sightings, elasmobranch, satellite tags, hidden Markov model, Rhincodontidae
Citation: Hoffmayer ER, McKinney JA, Franks JS, Hendon JM, Driggers WB III, Falterman BJ, Galuardi B and Byrne ME (2021) Seasonal Occurrence, Horizontal Movements, and Habitat Use Patterns of Whale Sharks (Rhincodon typus) in the Gulf of Mexico. Front. Mar. Sci. 7:598515. doi: 10.3389/fmars.2020.598515
Received: 24 August 2020; Accepted: 16 November 2020;
Published: 07 January 2021.
Edited by:
Mark Meekan, Australian Institute of Marine Science (AIMS), AustraliaReviewed by:
Adrian C. Gleiss, Murdoch University, AustraliaPhilip D. Doherty, University of Exeter, United Kingdom
Jesse Cochran, King Abdullah University of Science and Technology, Saudi Arabia
Copyright © 2021 Hoffmayer, McKinney, Franks, Hendon, Driggers, Falterman, Galuardi and Byrne. This is an open-access article distributed under the terms of the Creative Commons Attribution License (CC BY). The use, distribution or reproduction in other forums is permitted, provided the original author(s) and the copyright owner(s) are credited and that the original publication in this journal is cited, in accordance with accepted academic practice. No use, distribution or reproduction is permitted which does not comply with these terms.
*Correspondence: Eric R. Hoffmayer, RXJpYy5ob2ZmbWF5ZXJAbm9hYS5nb3Y=