- 1Departament de Biologia Evolutiva, Ecologia i Ciències Ambientals, Facultat de Biologia, Institut de Recerca de la Biodiversitat (IRBIO), Universitat de Barcelona, Barcelona, Spain
- 2Centro Oceanográfico de Baleares, Instituto Español de Oceanografía, Palma de Mallorca, Spain
- 3Balearic Islands Coastal Observing and Forecasting System (SOCIB), Palma de Mallorca, Spain
- 4Laboratoire Protection, Valorisation et Gestion des Ressources Marines et Littorales & Systématique Moléculaire/Département des Sciences de la Mer et de l’Aquaculture (LPVGRML), Faculté des Sciences de la Nature et de la Vie, Université Abdelhamid Ibn Badis de Mostaganem, Mostaganem, Algeria
- 5Department of Biology, Faculty of Science, Zagreb University, Zagreb, Croatia
- 6Centro de Investigación Marina, Universitat d’Alacant, Santa Pola, Spain
- 7Agencia de Medio Ambiente y Agua, Consejería de Agricultura, Pesca y Desarrollo Sostenible, Junta de Andalucía, Almería, Spain
- 8Acuario de la Universidad de Murcia, Murcia, Spain
- 9Instituto de Investigación en Medio Ambiente y Ciencia Marina (IMEDMAR-UCV), Universidad Católica de Valencia, Valencia, Spain
- 10Department of Chemical, Biological, Pharmaceutical and Environmental Sciences, ChiBioFarAm, Università degli Studi di Messina, Messina, Italy
- 11Department of Marine Science and Applied Biology, Universitat d’Alacant, Alacant, Spain
- 12Servicio de la Reserva Marina de Cabo de Gata-Níjar, Dirección General de Pesca Sostenible-Secretaría General de Pesca/MAPA, Almería, Spain
- 13Instituto de Ecología Litoral, El Campello, Spain
- 14Servicio de la Reserva Marina de Levante de Mallorca - Cala Rajada, Dirección General de Pesca Sostenible-Secretaría General de Pesca/MAPA, Palma de Mallorca, Spain
- 15Institute of Agrifood Research and Technology (IRTA)-Sant Carles de la Ràpita, Tarragona, Spain
- 16Environmental Department, Torrevieja City Hall, Torrevieja, Spain
- 17SUBMON: Awareness, Study and Conservation of the Marine Environment, Barcelona, Spain
- 18Department of Biology, Oceanographic, Valencia, Spain
- 19Laboratori d’Investigacions Marines I Aqüicultura (LIMIA), Govern de les Illes Balears, Port d’Andratx, Spain
- 20Instituto de Investigaciones Agroambientales y de la Economía del Agua (Instituto Nacional de Investigación y Tecnología Agraria y Alimentaria-Comunidad Autónoma de les Illes Balears, Universitat de les Illes Balears) [INAGEA (INIA-CAIB-UIB)], Palma de Mallorca, Spain
- 21Institut Méditerranéen de Biodiversité et d’Ecologie Marine et Continentale (IMBE) Aix-Marseille University, CNRS, IRD, Avignon University, Marseille, France
- 22Institut Océanographique Paul Ricard, Île des Embiez, France
- 23Global Change Research Group, Mediterranean Institute for Advanced Studies (CSIC-UIB), Esporles, Spain
A devastating mass mortality event (MME) very likely caused by the protozoan Haplosporidium pinnae first detected in 2016 in the Western Mediterranean Sea, is pushing the endemic bivalve Pinna nobilis to near extinction. Populations recovery, if possible, will rely on larval dispersal from unaffected sites and potential recolonization through recruitment of resistant juveniles. To assess the impact of the MME on the species’ larval recruitment, an unprecedented network of larval collector stations was implemented over several thousands of kilometers along the Western Mediterranean coasts during the 3 years after the onset of the MME. The findings of this network showed a generalized disruption in recruitment with dramatic consequences for the recovery of the species. However, there were exceptions to this pattern and recruits were recorded in a few sites where the resident population had been decimated. This hints to the importance of unaffected populations as larval exporting sources and the role of oceanographic currents in larval transport in the area, representing a beacon of hope in the current extremely worrying scenario for this emblematic species.
Introduction
The Mediterranean endemic bivalve Pinna nobilis has been pushed to an extremely worrying, near-extinction status by an unprecedented mass mortality event (MME) starting in early autumn 2016 in the western Mediterranean Sea (Vázquez-Luis et al., 2017) and rapidly spreading eastward (Cabanellas-Reboredo et al., 2019; Katsanevakis et al., 2019; Kersting et al., 2019; Panarese et al., 2019). The first histological examinations already revealed the presence of a haplosporidan-like parasite within the digestive gland of affected pen shells (Darriba, 2017; Vázquez-Luis et al., 2017) and a subsequent study described the haplosporidan parasite as Haplosporidium pinnae (Catanese et al., 2018). This parasite, but also mycobacteria and other potential pathogens have been described in several sites affected by the MME (Carella et al., 2019, 2020; Čižmek et al., 2020; Lattos et al., 2020). The implications of the presence of different pathogens in the development of the disease has still to be clarified. Most known and studied P. nobilis populations in the Western Mediterranean Sea have almost completely disappeared, with mortality rates exceeding 90% (Vázquez-Luis et al., 2017; Panarese et al., 2019; García-March et al., 2020) and populations in the Eastern Mediterranean and Adriatic Sea are suffering the same fate (Katsanevakis et al., 2019; Kersting et al., 2019; Čižmek et al., 2020; Öndes et al., 2020; Özalp and Kersting, 2020). There is the exception, however, of several sites with very specific environmental settings where P. nobilis populations remain unaffected to date: Fangar Bay (Delta del Ebro, Spain), Mar Menor (Spain), Rhône Delta (France), Etang de Thau (France), Diana and Urbino pools (Corsica, France), Venice lagoon (Italy), inner Kalloni Gulf (Greece) (Catanese et al., 2018; Cabanellas-Reboredo et al., 2019; Kersting et al., 2019; Simide et al., 2019; Prado et al., 2019; Foulquie et al., 2020; Zotou et al., 2020). This worrying scenario has led to the recent inclusion of the species as Critically Endangered on the IUCN Red List (Kersting et al., 2019). While P. nobilis populations are disappearing at an accelerating rate, it is remarkable that the congeneric species P. rudis, which shares habitat with P. nobilis in many locations (Giacobbe and Leonardi, 1987; Kersting and García-March, 2017) remains unaffected by the MME (Catanese et al., 2018).
Potential natural recovery from this dramatic situation relies on recruitment, which will depend mainly on, (1) larval supply and transport from unaffected sites, and (2) the existence and potential reproduction of resistant individuals in the affected sites. Therefore, assessing the recruitment potential of P. nobilis after the mortality outbreak is mandatory to evaluate the role of this first step toward the recovery of the species. Once settled, post-recruitment survival will of course depend on the potential existence of pathogen-resistant individuals.
Artificial recruitment (i.e., by means of larval collectors) has proved to be a useful tool for assessing recruitment potential in P. nobilis (Cabanellas-Reboredo et al., 2009; Alomar et al., 2015; Kersting and García-March, 2017; Wesselmann et al., 2018), providing insights into larval supply and recruitment previous to the exposure to pressures like predation or dislodgement (Kersting and García-March, 2017). Pinna nobilis larval collectors were specifically designed and used for the first time by De Gaulejac et al. (2003). Later on, several studies have used larval collectors to study different aspects of P. nobilis recruitment, adapting this design to the specific conditions of study sites and experimental settings (Cabanellas-Reboredo et al., 2009; Kersting and García-March, 2017; Wesselmann et al., 2018). In Cabanellas-Reboredo et al. (2009) and in Kersting and García-March (2017) larval collectors were used to assess the larval settlement period of P. nobilis in the Balearic and Columbretes islands, respectively. Both studies established the peak of the settlement period between August and September. In Kersting and García-March (2017) larval collectors were used as well to assess recruitment of the species in the long-term, showing the high interannual variability in recruitment rates of this species over a 9-year period and suggesting a positive correlation between water temperature and recruitment rates. This study also evidenced the feasibility of using larval collectors to obtain P. nobilis spat to be placed afterward in protected cages submerged in situ, where recruits can be grown to be used later for restocking or restoring actions in impacted populations. Larval collectors have been used as well to assess genetic connectivity in P. nobilis, showing the existence of source and sink populations and the connectivity potential of the species (Wesselmann et al., 2018).
Because of the current status of the species and the general importance of recruitment in the recovery of impacted populations, an extensive effort (both spatial and human) has been undertaken to tackle P. nobilis recruitment in larval collectors over several thousands of kilometers along the Western Mediterranean coasts, the region first impacted by the MME (Vázquez-Luis et al., 2017), and in the Adriatic Sea, where the MME was first recorded in the final year of this study (2019) but most populations still remained unaffected (Kersting et al., 2019; Čižmek et al., 2020; Kipson unpublished data). As a result, the present study aims to provide an overall interannual picture of P. nobilis recruitment during 3 years after the 2016 MME, as well as to compare that information with the health status of different populations, in order to assess potential larval connectivity and the role of recruitment in the future recovery of the species in the area.
Materials and Methods
Study Sites
Larval collectors were installed in 37 sites distributed along the coast of the Western Mediterranean Sea including north Africa, and in the northern Adriatic Sea (Figure 1) during the reproductive seasons of 2017–2019. The sites were selected according to the occurrence of P. nobilis populations previous to the mortality outbreak, with many of them subjected to periodical monitoring. However, most of the populations (with the exception of Scandola, Embiez Islands, Alfacs Bay, Mar Menor, Arzew and Kristel bays, and Brijuni MPA) were affected by the MME by the time the first collectors were installed at the beginning of the summer of 2017. The impact of the MME on each location has been assessed in previous studies (Vázquez-Luis et al., 2017; Vicente et al., 2018; Cabanellas-Reboredo et al., 2019) or during the installation of collectors in the present study.
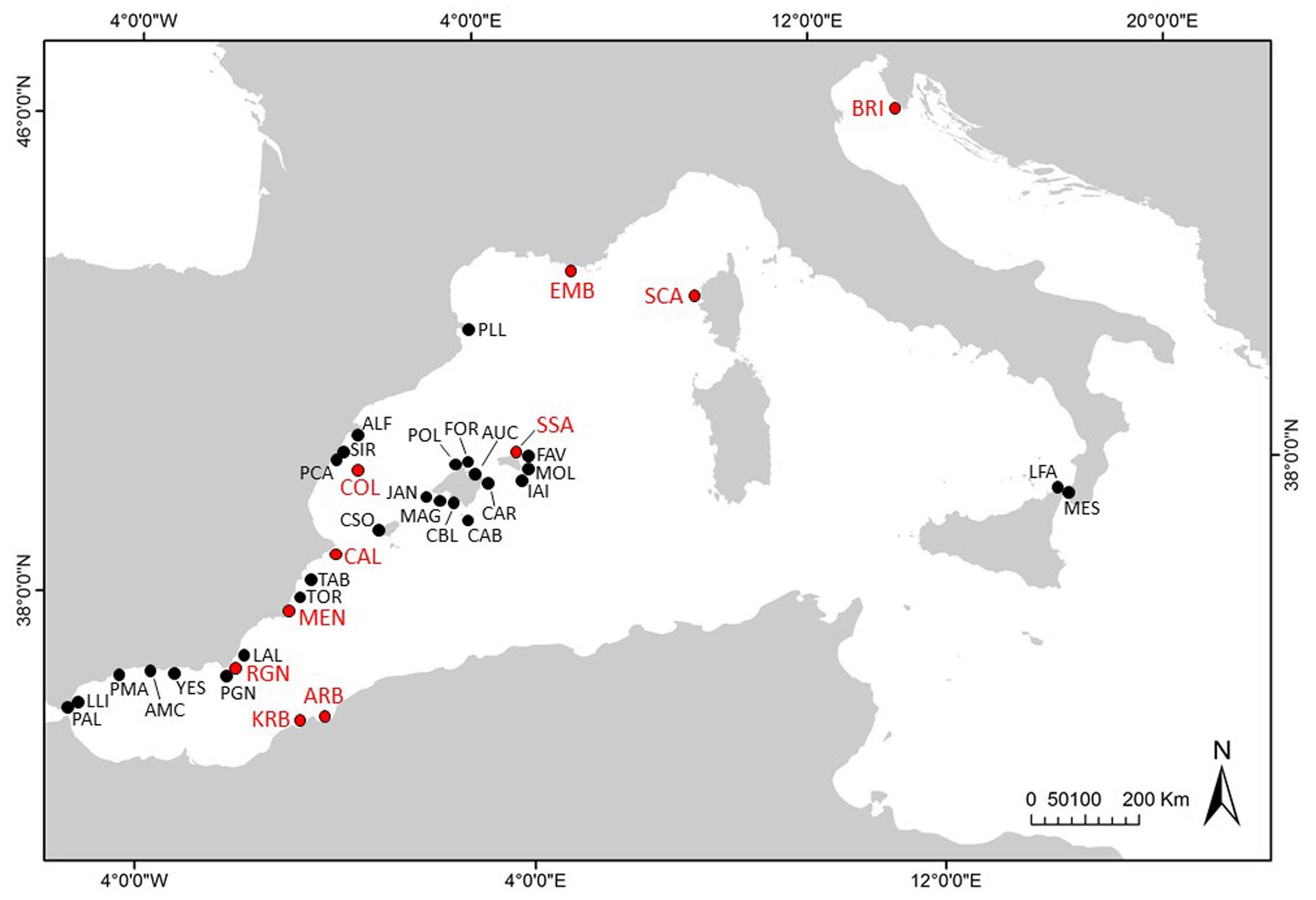
Figure 1. Larval collector sites in the Western Mediterranean and Adriatic seas. In red, sites where recruitment was recorded in, at least, one season. Full name of sites in Table 1.
Time series of P. nobilis recruitment in larval collectors previous to this study were available for some of the selected sites: the Columbretes Islands (Kersting and García-March, 2017), Embiez Islands (Vicente et al., 2017, 2018; Vicente, 2020), Calpe (García-March, unpublished data), Cabrera (Vázquez-Luis, unpublished data), and for Pollença and Magaluf (Hendriks, unpublished data).
Larval Collectors
Larval collectors consisted of a series of plastic mesh bags containing entangled nylon filament or onion bags (see De Gaulejac et al., 2003; Cabanellas-Reboredo et al., 2009; Kersting and García-March, 2017; Vicente, 2020, for more details). The bags were attached to a main rope that was fixed to a concrete mooring and kept vertical by a submerged buoy, covering a 0.5–20 m depth range (Figure 2).
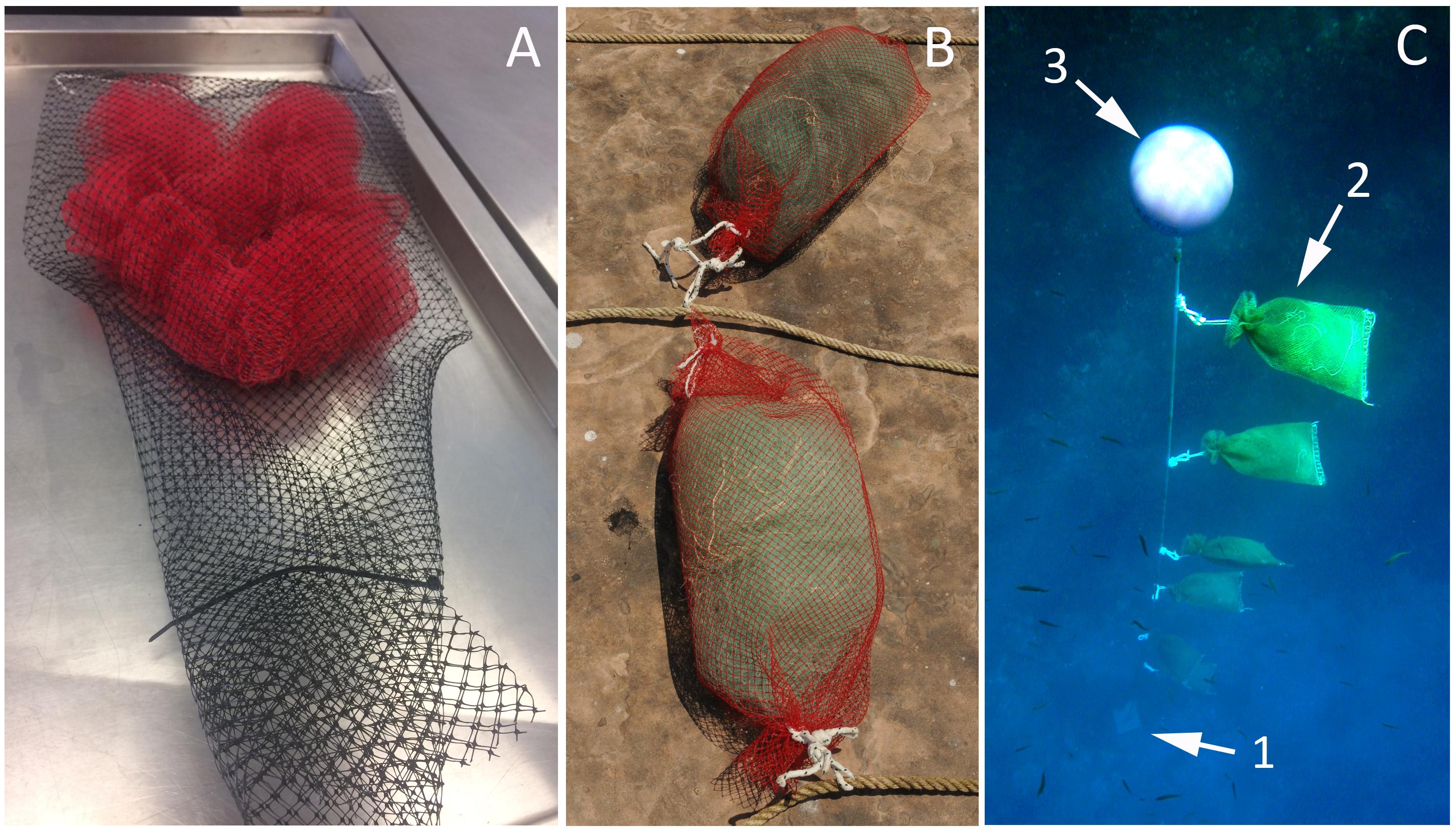
Figure 2. Larval collectors. Plastic mesh bags containing onion bags (A) and entangled nylon filament (B). (C) Components of a larval collector: concrete mooring (1), bags (2) and submerged buoy (3).
Larval collectors were moored annually in early summer (June) and removed in mid-autumn (November) from 2017 to 2019 (see Table 1 for details), thus covering the main reproduction and settlement period of the species (Cabanellas-Reboredo et al., 2009; Deudero et al., 2017; Kersting and García-March, 2017). Once removed, the bags were immediately opened, and all P. nobilis recruits were carefully collected from the nylon mesh following the procedure in Kersting and García-March (2017). Observation of P. nobilis recruits was undertaken with the naked eye, allowing the detection of recruits of sizes down to 0.3 cm antero-posterior length. Recruits extracted from the collectors were either installed in aquaria (García-March et al., 2020; Vicente, 2020) or in growth cages in the field following Kersting and García-March (2017). It must be noted that at small sizes distinguishing P. nobilis from P. rudis juveniles can be difficult. In bigger juveniles, P. rudis can be easily distinguished by the lower number of radiating ribs (4–5 ribs) and scales, which are also bigger and sturdier than in P. nobilis (Figure 3). This issue is easily solved by keeping the juveniles in growth cages or in aquaria, where they grow to a size that allows identification. In addition, genetic analyses were used to contrast with morphological identification in 5 juveniles. Nevertheless, even if small, a certain identification error must be assumed, especially if juveniles die before growing to the needed size.
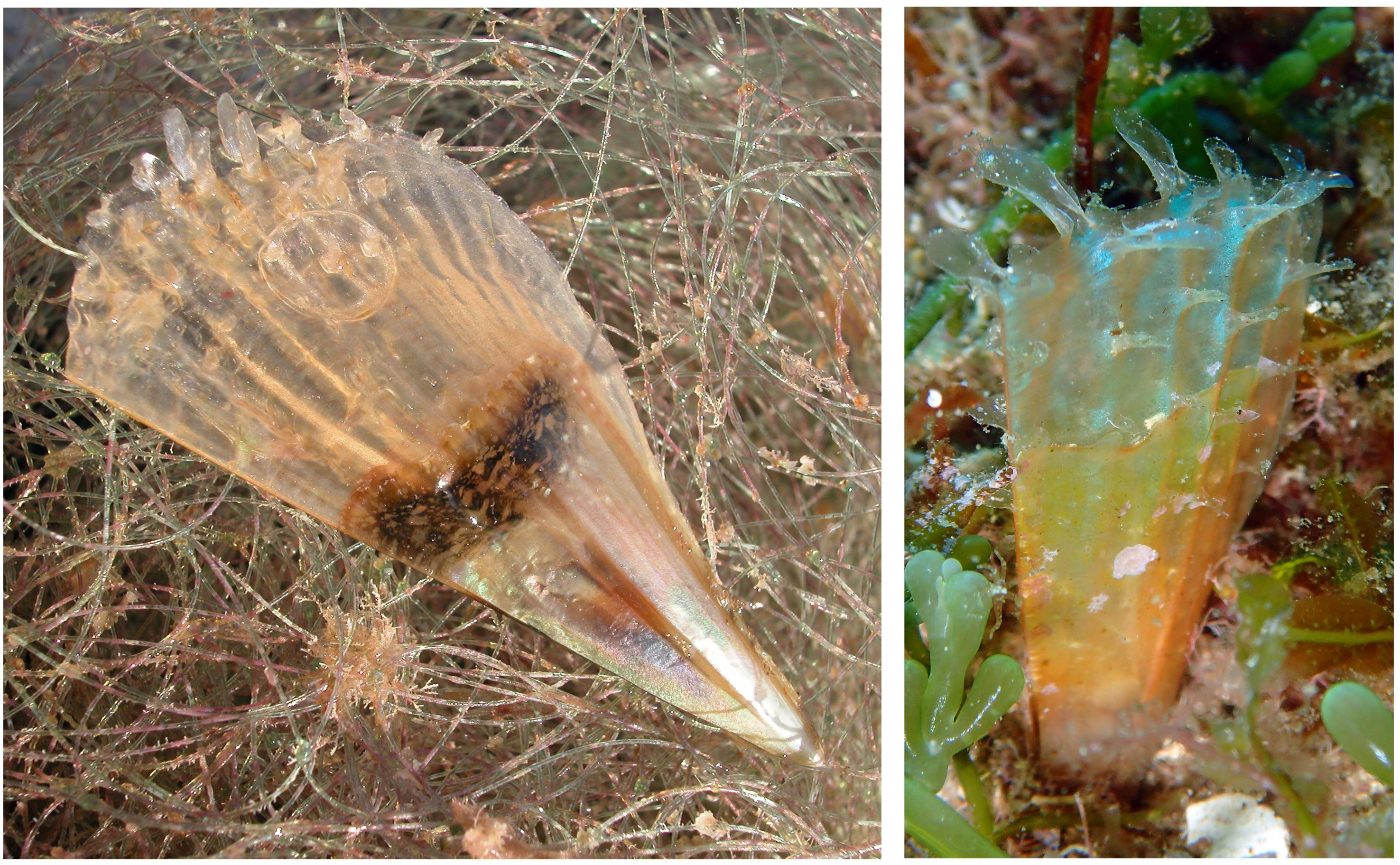
Figure 3. Pinna nobilis (left) and P. rudis (right) juveniles at a size of 5–6 cm (antero-posterior length). Notice how the number of radiating ribs and the morphology of the scales clearly differs in both species.
Lagrangian Trajectory Modeling
The high-resolution regional hydrodynamic model WMOP (Juza et al., 2016; Mourre et al., 2018) was used to simulate backward trajectories from the observation sites where recruitment was recorded, with the objective to identify the potential origin of larvae transported by ocean currents over the basin during the recruitment period. The WMOP model, developed at the Balearic Islands Coastal Observing and Forecasting System (SOCIB)1, provides daily predictions of the Western Mediterranean circulation from the Strait of Gibraltar to Corsica and Sardinia with a 2 km spatial resolution. The WMOP surface currents result from the effects of 3-dimensional basin-to-coastal-scale ocean processes driven by atmospheric forcing (winds, evaporation-precipitation and heat fluxes), river inflows and open boundary inputs over a realistic bathymetry. The model, based on the ROMS modeling system (Shchepetkin and McWilliams, 2005), is nested in the larger scale Mediterranean model from the Copernicus Marine Service (Clementi et al., 2017). The model air-sea fluxes are computed through bulk formulae applied to the high-resolution atmospheric fields provided by the Spanish Meteorological Agency (AEMET) Hirlam (for years 2017 and 2018) and Harmonie (for year 2019) models. The climatological runoffs of the six major rivers of the modeling domain are implemented as point sources of low saline waters. Further details of the forecasting system and model evaluations can be found in Juza et al. (2016), Mourre et al. (2018), and Aguiar et al. (2020). Operational data assimilation of sea level, sea surface temperature, Argo profiles and Ibiza Channel High-Frequency radar was implemented in November 2018, using the method described in Hernandez-Lasheras and Mourre (2018).
Moreover, the daily average wave-induced drift provided by the Copernicus Marine Service Mediterranean Sea waves model (Korres et al., 2019a, b) was added to the WMOP currents to represent the contribution of surface ocean waves.
The TRACMASS algorithm (Jönsson et al., 2015) was used to generate Lagrangian trajectories over a 1-month period to approximate larval period duration (Deudero et al., 2017; Kersting and García-March, 2017; Trigos et al., 2018). Virtual particles were released once a week during the recruitment period from July 1st to September 15th (Cabanellas-Reboredo et al., 2009; Kersting and García-March, 2017) from all sites and for all years where recruitment was recorded, with the exception of Mar Menor because of its low exchange with open waters (i.e., semi enclosed coastal lagoon). In the case of Arzew and Kristel bays in Algeria, a single location (Arzew) was used given the proximity of the two sites. Cluster of 1,000 particles were released at each location, and modeled trajectories were the result of advection by the WMOP surface currents and wave-induced drifts plus a diffusive term accounting for the effect of model uncertainties and unresolved processes.
Results
Larval Recruitment
Pinna nobilis recruits were found in the collectors installed at 10 sites (Scandola, Embiez Islands, Son Saura, Columbretes Islands, Calpe, Mar Menor, Cabo de Gata, Arzew and Kristel bays, and Brijuni); while no recruits were retrieved from the collectors in the remaining sites (Table 1). All sites had been impacted by H. pinnae, either before or during the study, apart from the sites in Algeria, Croatia and Mar Menor (Spain) (Table 1). Only one of the MME-affected sites, Columbretes Islands, recorded recruitment in the larval collectors during the three reproductive seasons (2017–2019). Besides this site, all other sites where a large number of recruits was recorded, were located in unaffected or partially unaffected regions. The congeneric species P. rudis, unaffected by the mortality, recruited in several sites during the study (Table 1).
Time series of P. nobilis recruitment in larval collectors previous to this study (i.e., Columbretes Islands, Embiez Islands, Calpe, Cabrera, Pollença, and Magaluf) showed that, although with the inherent interannual variability, recruitment in larval collectors at these sites was recorded annually before the MME.
Because larval collectors are installed in shallow waters, they are highly exposed to storms and intentional or accidental removal; collectors at 8 sites were lost due to these causes during at least 1 recruitment season (Table 1). All extracted recruits from MME affected sites that were kept in growth cages died presumably because of H. pinnae during the first months, as did the ones taken to aquaria according to García-March et al. (2020).
Larval Transport
The Lagrangian trajectory model from the sites where recruitment was recorded shows the potential geographical origin of larvae during the main reproduction and recruitment period of the species (Figure 4). After disregarding the sites where P. nobilis had disappeared because of the MME (i.e., most of the coast of Spanish mainland coast and the Balearic Islands), three main regions harboring unaffected populations remain as potential larval sources in the Western Mediterranean: French Mediterranean coast, Delta del Ebro region and the north African coasts (mainly Algeria). The northern sites showing recruitment (i.e., Son Saura, Embiez Islands, and Scandola) received larvae potentially from the French coasts, while southern locations (i.e., Calpe, Cabo de Gata, Arzew, and Kristel bays) were nourished by the north African coasts. Columbretes Islands represented an in-between situation, receiving larvae both from the south (north Africa) and the north (Delta del Ebro region) (Figure 4). According to the model, Mar Menor, which hosts unaffected populations, could be also considered as an exporting site. However, its limited water exchange with the open sea will probably hinder its potential role as a significant larval donor.
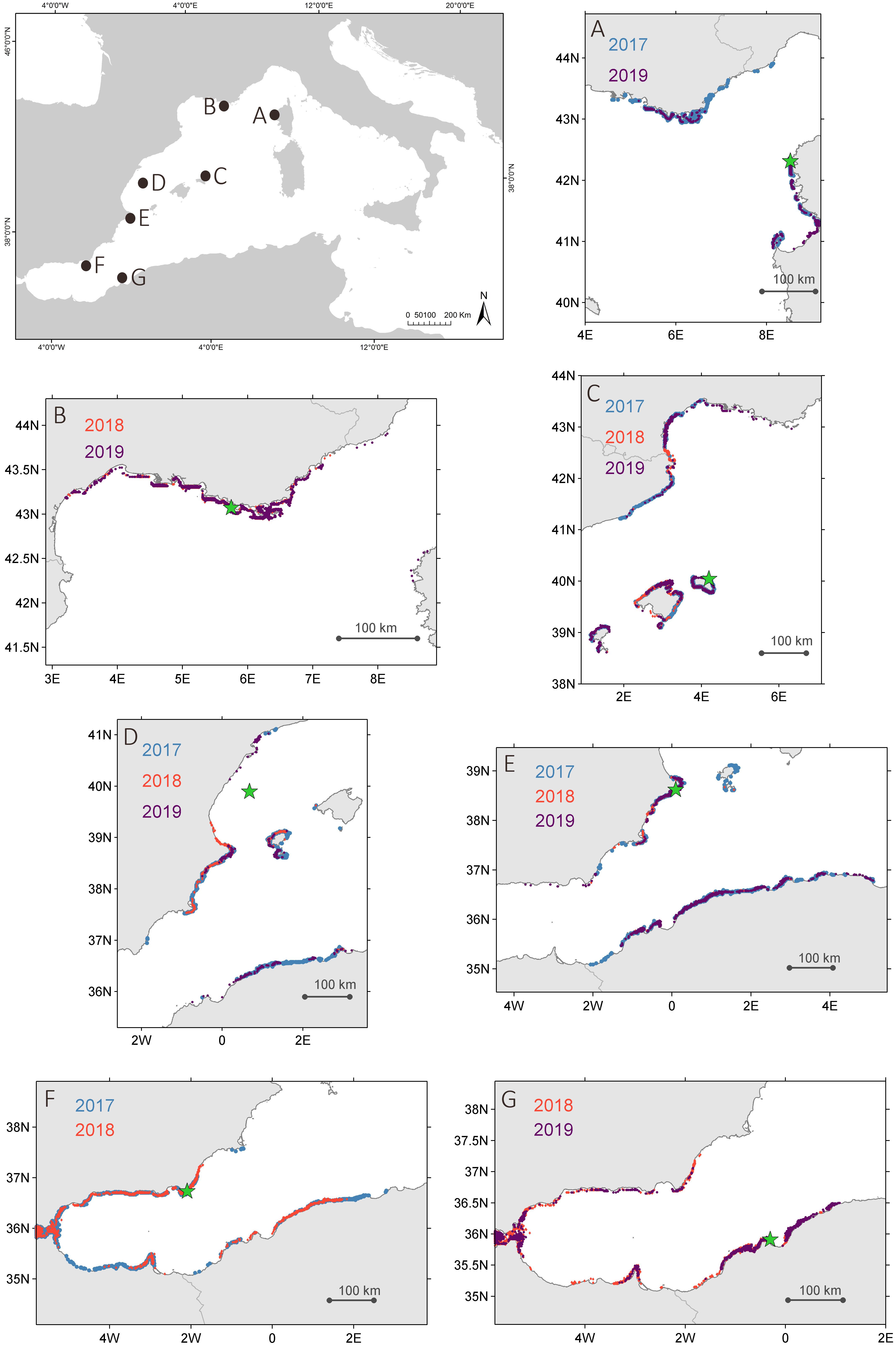
Figure 4. Results of the Lagrangian trajectory model showing the potential origin of P. nobilis larvae for each site and year, (A) Scandola, (B) Embiez, (C) Son Saura, (D) Columbretes, (E) Calpe, (F) Cabo de Gata, (G) Arzew. Sites are represented on the maps by the green star.
Discussion
The occurrence of successful recruitment events is crucial for the potential recovery of P. nobilis populations in the Mediterranean regions impacted by the MME. However, the general picture drawn by the results of the larval collectors’ network set up for this study reflects the negative consequences of the mortality on larval recruitment, while opening a little window of hope at a few sites.
Larval Recruitment After the MME
Collapse of regional larval recruitment in many marine invertebrate species has been associated to the loss of adults after catastrophic events, seriously hindering recoveries (Miner et al., 2006; Miller et al., 2009; Lessios, 2016; Hughes et al., 2019). As expected, the high mortality rates recorded during the MME have impacted P. nobilis reproduction and are therefore impairing recruitment. In general, the results of this study show a disruption of P. nobilis larval recruitment over a vast geographical area in the Western Mediterranean Sea during 3 years after the start of the MME.
There were, however, some exceptions to this generalized absence of P. nobilis recruitment. Among the sites already affected by the MME, recruitment was recorded every year (2017–2019) in the Columbretes Islands, despite the fact that pen shell populations deceased in this area during the summer of 2017 (Cabanellas-Reboredo et al., 2019; Kersting et al., 2019). Moreover, although to a lesser extent, recruits were also recorded as well during at least one season in Scandola (Corsica, France), Son Saura (Spain), Calpe (Spain), Mar Menor (Spain), and Cabo de Gata (Spain) (Table 1). Larval recruitment was abundant in 2018 and 2019 in other Mediterranean regions like the northern Adriatic Sea (Croatia) or some sites in the southern Mediterranean like Algeria, where the mortality is still arriving (Kersting et al., 2019; Čižmek et al., 2020) and many P. nobilis populations remained unaffected. Recruitment recorded in these sites was similar to that recorded in the Columbretes Islands before the MME (Kersting and García-March, 2017). The same applies to Scandola and Embiez Islands (France), where larval recruitment was recorded during the years preceding the mortality that impacted these sites in 2018 and 2019, respectively (Vicente et al., 2020).
Larval Export and the Importance of Non-impacted Populations
Even though earlier studies proposed a larval period of 10 days for P. nobilis (Butler et al., 1993; De Gaulejac and Vicente, 1990), recent research has suggested that larval stages could last at least 1 month (Deudero et al., 2017; Kersting and García-March, 2017; Trigos et al., 2018). Populations of P. nobilis in the Western Mediterranean Sea show high genetic connectivity, functioning as a meta-population with source-sink dynamics (Wesselmann et al., 2018), which would agree with longer pelagic stages and larval transport in the area.
All known pen shell populations along the Spanish Mediterranean coast disappeared between 2016 and 2017 due to the MME, with little or no evidence of resistant individuals. There was the exception, however, of two sites with particular environmental conditions (i.e., Delta del Ebro bays -Alfacs and Fangar-, and the hypersaline coastal lagoon Mar Menor) which seem to remain at least partially pathogen-free (Vázquez-Luis et al., 2017; Catanese et al., 2018; Cabanellas-Reboredo et al., 2019; Prado et al., 2019; Giménez-Casalduero et al., in press).
The Columbretes Islands was the only location in the Western Mediterranean where recruits were found in the collectors every year (2017–2019). The pen shell mortality reached the Columbretes Islands (located between Spanish mainland and the Balearic Islands, Figure 1) in the summer of 2017 (Cabanellas-Reboredo et al., 2019). Surprisingly, during that summer, while most of the pen shells in the Columbretes Islands were sick and dying, the larval collectors registered a decadal maximum in recruitment according to the long-term recruitment data available for this site (Kersting and García-March, 2017). In 2018 and 2019 recruitment in Columbretes was much lower, however, still existent and in the range of the considerable interannual variability recorded at this site (Kersting and García-March, 2017).
The mortality context during those years forced an exclusion experiment in Columbretes, where practically all pen shells deceased during the summer of 2017. Therefore, local recruitment stopped, and most recruits recorded in the collectors must have been transported to the Columbretes area from somewhere else. Pre-mortality results on larval settlement obtained by Kersting and García-March (2017) already suggested the importance of external larval sources and the dispersion capacities of the species, as no differences were found in recruitment in the collectors among sites with and without the presence of pen shells in the Columbretes Islands. The results of the Lagrangian trajectory model for this area showed four major areas that could be acting as larval donors to the Columbretes Islands, i.e., Delta del Ebro region, Ibiza and Formentera Islands, southern Spanish mainland, and north Africa. In these regions, the only sites still holding living P. nobilis populations in 2017–2019 were Delta del Ebro, Mar Menor, and both studied sites in Algeria.
The closest unaffected site, Delta del Ebro (∼100 km north of the Columbretes Islands), hosts one of the largest P. nobilis populations in the Mediterranean Sea (Prado et al., 2014). Pinna nobilis populations in this site have survived the MME apparently due to the low salinity conditions in the area, greatly influenced by agricultural discharges from rice cultivation (Prado et al., 2019; García-March et al., 2020) and this area has been already considered as a main source of P. nobilis larvae (Wesselmann et al., 2018). Surprisingly, even though P. nobilis populations in the Delta del Ebro remain mostly unaffected, collectors installed in this location have never recorded larval settlement, and according to Prado et al. (2019) the local availability of planktonic larvae is very low. These populations seem to rely mainly on sporadic recruitment events and the absence of recruitment inside the bay has been hypothesized to be associated to processes causing larval mortality such as freshwater discharges or even pollution (Prado et al., 2019). Nevertheless, as showed by Wesselmann et al. (2018), these populations seem to be exporting larvae, which hints to the fact that even though larval mortality inside the bay might be high, larvae could be transported into the open sea. Larval transport out of the Alfacs Bay could be favored by surface currents, which are intense and flowing out of the bay in the summer (Cerralbo et al., 2018).
However, the results of the oceanographic model show a complex scenario that goes far beyond the Delta del Ebro, showing at least two other regions holding unaffected populations potentially acting as larval donors to the Columbretes Islands: north African coasts (mainly Algeria) and Mar Menor in southern Spain. As reported in this study, P. nobilis populations in the monitored sites in Algeria were unaffected by the MME and located in coastal areas well connected to the open sea, thus fully capable of exporting larvae. According to the model, this region could be the most important larval donor to the Columbretes Islands in the current MME context. The case of Mar Menor is not as clear, mainly because of the nature of the site, being a semi enclosed coastal lagoon with very little exchange with the open sea, which would impede a significant larval export. Nevertheless, hypothetically larvae of at least these three areas, could be reaching the Columbretes Islands transported by the complex and variable pattern of currents in this transition region, where the southward flowing Northern Current changes direction toward northeast to form the Balearic Current, also intermittently influenced by northward inflows flowing through the Ibiza Channel. This diversity in larval origins could be crucial in fostering potential recoveries in this area.
In 2017, larval collectors recorded recruitment as well in a few sites south of the Columbretes Islands (i.e., Calpe and Cabo de Gata-Níjar). Altogether, according to the long-term recruitment data series in the Columbretes Islands (Kersting and García-March, 2017) and the results presented here, 2017 seems to have been a year of successful P. nobilis larval recruitment. The Lagrangian trajectory model points out to the north African coast as the main area holding unaffected populations that could be acting as a source of larvae for these sites during those years. Mar Menor is also shown here as a potential source by the model, but as already mentioned, there is a major concern about its connectivity potential due to limited seawater exchanges. Regarding the Balearic Islands, sporadic recruitment was detected in 2018 in Son Saura (Menorca). In this case, according to the model, larvae could origin from the north, i.e., the coast of France, where in fact several P. nobilis populations were still unaffected in 2018 (Kersting et al., 2019). It is remarkable that none of the other sites in the Balearic Islands holding historical larval settlement data (i.e., Cabrera, Pollença, and Magaluf) registered recruitment after the MME.
This pattern moved eastwards along the coast as other western Mediterranean sites were sequentially impacted by the mortality. Recruitment was recorded in 2018 in the Embiez Islands (France) before the mortality affected this site but ceased afterward. In Scandola (Corsica), first affected by mortality in 2018, recruitment fell from 18 in 2017 to 3 recruits in 2019. In this last case, larval origin seems more diverse, enclosing both northern sites (Occitania, France) and southern ones (southern Corsica and northern Sardinia), while in Embiez Islands recruitment seems to be more dependent on local and near-by populations and thus ceased when those were impacted by the MME.
It must be considered that P. nobilis larval recruitment has shown to have an important interannual variability (Kersting and García-March, 2017) and in pre-mortality circumstances low recruitment values were also recorded during some years. Nevertheless, the scenario presented here is different, showing an interannual absence of recruitment over great extensions of coast, including sites were recruitment was recorded annually prior to the MME. This is also showing that recruitment originating from local populations was also playing an important role before the MME, thus in many populations recruitment resulted probably from a mixed pool of local and imported larvae.
As previously reported in other studies (Catanese et al., 2018), the congeneric species P. rudis remains unaffected by the mortality and its recruits are still being recorded in many larval collectors and in the field (this study, García-March et al., 2020).
Future Consequences
Long-term follow-ups of recoveries after disease-triggered die offs of marine invertebrates have shown that recovery can be slow and geographically heterogeneous (Miner et al., 2006; Miller et al., 2009). Of course, in the case presented here it is understood that populations’ recoveries through recruitment will only occur if at least some of the settled individuals show resistance to the disease, which represents an important additional obstacle. All juveniles settled in the collectors and installed in growth cages within the MME-affected sites died before the first year of age, presumably because of H. pinnae infection. However, if resistant juveniles could establish, recovered sites could eventually export larvae to other locations and trigger a stepping stone recovery process through larval export and connectivity. Nevertheless, potential recoveries of mortality-impacted populations will presumably differ among sites and will be highly dependent on their geographical location relative to unaffected populations and the oceanographic current patterns in the area. According to Sanna et al. (2013), P. nobilis populations seem to share a common origin and a recent eastward expansion across the Mediterranean, likely facilitated by marine currents. Therefore, a similar mechanism could mediate in the recovery and, therefore, new expansion of the species after the MME if resistant recruits should occur.
In the best scenario, i.e., with resistant juveniles, successful recoveries through recruitment could take a long time and it must be regarded that natural recruitment is also limited by other factors such as predation, especially in protected sites, where predators are abundant (Kersting and García-March, 2017). In fact, in predator-rich environments, a refuge size of 45 cm has been estimated for the species, which would represent an age of about 8 years (Kersting and García-March, 2017). Therefore, it is of great importance to start assessing recruitment in the field and recruit’s survival, especially in those sites where recruits have been observed in the collectors.
Altogether, our results show a worrying scenario for the species and highlight the importance of unaffected P. nobilis populations as larval exporting sites, which could play a main role in a potential, although probably slow, recovery. This applies as well to the Adriatic and Eastern Mediterranean Seas, where unaffected populations are being described as the MME spreads eastwards (Kersting et al., 2019; Čižmek et al., 2020; Zotou et al., 2020). In addition, (1) the status “unaffected” is not static and these sites could be affected by the mortality in the near future (e.g., Alfacs Bay, Prado et al., 2019); and (2) some of these unaffected sites are highly anthropized and subjected to multiple stressors that may cause mortalities on their own (e.g., Mar Menor, García-Ayllon, 2018). As suggested by the IUCN (Kersting et al., 2019), these unaffected sites need to be protected, while P. nobilis populations along the north African coasts need to be urgently assessed, especially regarding their important role as larval donors in the region. Through this internationally joint effort, we also want to stress the importance of coordinated pan-Mediterranean research and monitoring to continue tackling the mid and long-term effects of the P. nobilis MME.
Data Availability Statement
The original contributions presented in the study are included in the article/supplementary material, further inquiries can be directed to the corresponding author/s.
Author Contributions
DK wrote the manuscript with contributions of MV-L, IH, and BM. All authors participated in the field work and contributed to the final version of the manuscript.
Funding
DK and MV-L were supported by a Juan de la Cierva-Incorporación postdoctoral contract (Spanish Ministry of Science, Innovation and Universities; IJCI-2017-31457 and IJCI-2016-29329, respectively). SK was partially supported by a postdoctoral contract (EU Horizon 2020, Project: MERCES, No. 689518). EÁ was supported by a Technical Support Staff contract (Spanish Ministry of Economy and Competiveness, PTA2015-10829-I). This study was partially funded by: EsMarEs (MITECO), SuMaEco (RTI2018-095441-B-C21, Spanish Ministry of Science, Innovation and Universities) and Prince Albert II of Monaco Foundation (Project BF/HEM 15-1662). The authors acknowledge the MEDCLIC project, funded by “La Caixa” Foundation, contributing to the development of the WMOP hydrodynamic model.
Conflict of Interest
The authors declare that the research was conducted in the absence of any commercial or financial relationships that could be construed as a potential conflict of interest.
The handling editor declared a past co-authorship with several of the authors DK, MV-L, BM, FB, EÁ, AB, SD, JG-M, SG, FG-C, SJ-G, PP, JS, AS, NV, and IH.
Acknowledgments
We want to thank the Columbretes Islands, Cala Rajada, and Cabo de Gata-Níjar Marine Reserves staff for logistic support, and Parc Natural Serra d’Irta, Cabrera National Park and Brijuni National Park for permissions. We want to thank J. Moreno (Servei de Protecció d’Espècies, Govern Balear) for his support and D. Petricioli and the staff of the Jaume Ferrer Research Station (La Mola, Menorca) for assistance in the field. AB and DM thank the managers of the Sustainable Management Program for the Marine Environment of Andalusia (F. Ortega, E. Montes, and S. Vivas) and the colleagues who have worked at sea: J. M. Remón, J. De la Rosa, M. Fernández-Casado, and M. Carmen Arroyo.
Footnotes
References
Aguiar, E., Mourre, B., Juza, M., Reyes, E., Hernández-Lasheras, J., Cutolo, E., et al. (2020). Multi-platform model assessment in the Western Mediterranean Sea: impact of downscaling on the surface circulation and mesoscale activity. Ocean. Dyn. 70, 273–288. doi: 10.1007/s10236-019-01317-8
Alomar, C., Vázquez-Luis, M., Magraner, K., Lozano, L., and Deudero, S. (2015). Evaluating stable isotopic signals at bivalve Pinna nobilis under different human pressures. J. Exp. Mar. Biol. Ecol. 467, 77–86. doi: 10.1016/j.jembe.2015.03.006
Butler, A., Vicente, N., and de Gaulejac, B. (1993). Ecology of the pterioid bivalves Pinna bicolor Gmelin and Pinna nobilis L. Mar. Life 3, 37–45.
Cabanellas-Reboredo, M., Deudero, S., Alós, J., Valencia, J. M., March, D., Hendriks, I. E., et al. (2009). Recruitment of Pinna nobilis (Mollusca: Bivalvia) on artificial structures. Mar. Biodivers. Rec. 2:1e5.
Cabanellas-Reboredo, M., Vázquez-Luis, M., Mourre, B., Álvarez, E., Deudero, S., Amores, A., et al. (2019). Tracking the dispersion of a pathogen causing mass mortality in the pen shell Pinna nobilis: a collaborative effort of scientists and citizens. Sci. Rep. 9:13355.
Carella, F., Aceto, S., Pollaro, F., Miccio, A., Iaria, C., Carrasco, N., et al. (2019). A mycobacterial disease is associated with the silent mass mortality of the pen shell Pinna nobilis along the Tyrrhenian coastline of Italy. Sci. Rep. 9: 2725.
Carella, F., Antuonfermo, E., Farina, S., Salati, F., Mandas, D., Prado, P., et al. (2020). In the wake of the ongoing mass mortality events: co-occurrence of mycobacterium, Haplosporidium and Other Pathogens in Pinna nobilis Collected in Italy and Spain (Mediterranean Sea). Front. Mar. Sci. 7:382. doi: 10.3389/fmars.2020.00382
Catanese, G., Grau, A., Valencia, J. M., García-March, J. R., Vázquez-Luis, M., Alvarez, E., et al. (2018). Haplosporidium pinnae sp. nov., a haplosporidan parasite associated with mass mortalities of the fan mussel, Pinna nobilis, in the Western Mediterranean Sea. J. Invertebr. Pathol. 157, 9–24. doi: 10.1016/j.jip.2018.07.006
Cerralbo, P., Espino, M., Grifoll, M., and Valle-Levinson, A. (2018). Subtidal circulation in a microtidal Mediterranean bay. Sci. Mar. 82, 231–243. doi: 10.3989/scimar.04801.16a
Čižmek, H., Čolić, B., Romana, G., Grau, A., and Catanese, G. (2020). An emergency situation for pen shells in the Mediterranean: the adriatic sea, one of the last pinna nobilis redoubt, is now affected by the mass mortality event. J. Invertebr. Pathol. 173:107388. doi: 10.1016/j.jip.2020.107388
Clementi, E., Pistoia, J., Fratianni, C., Delrosso, D., Grandi, A., Drudi, M., et al. (2017). Mediterranean sea analysis and forecast (CMEMS MED-Currents 2013-2017). Copernicus Monitoring Environment Marine Service (CMEMS) doi: 10.25423/MEDSEA_ANALYSIS_FORECAST_PHYS_006_001
Darriba, S. (2017). First haplosporidan parasite reported infecting a member of the superfamily pinnoidea (Pinna nobilis) during a mortality event in Alicante (Spain. Western Mediterranean). J. Invertebr. Pathol. 148, 14–19. doi: 10.1016/j.jip.2017.05.006
De Gaulejac, B., and Vicente, N. (1990). Ecologie de Pinna nobilis (L.) mollusque bivalve sur les côtes de Corse. Essais de transplantation et experiences en milieu contrôlé. Haliotis 10, 83–100.
De Gaulejac, B., Medioni, E., and Vicente, N. (2003). “Essais de captage de Pinna nobilis et autres mollusques sur les cotes mediterraneennes francaises,” in Premier Seminaire International sur la Grande nacre de Mediterranee Pinna nobilis Ile des Embiez, (France: Mémoires de l’Institut océanographique Paul Ricard), 57–62.
Deudero, S., Grau, A., Vázquez-Luis, M., Álvarez, E., Alomar, C., and Hendriks, I. E. (2017). Reproductive investment of the pen shell Pinna nobilis Linnaeus, 1758 in Cabrera National Park (Spain). Mediterr. Mar. Sci. 18, 271–284. doi: 10.12681/mms.1645
Foulquie, M., Dupuy de la Grandrive, R., Dalias, N., and Vicente, N. (2020). Inventaire et état de santé des populations de Pinna nobilis (L.1758) dans l’étang de Thau (Hérault, France). Marinelife-revue.fr-2020 1–25.
García-Ayllon, S. (2018). The integrated territorial investment (ITI) of the Mar Menor as a model for the future in the comprehensive management of enclosed coastal seas. Ocean Coast. Manag. 166, 82–97. doi: 10.1016/j.ocecoaman.2018.05.004
García-March, J. R., Tena-Medialdea, J., Henandis-Caballero, S., Vazquez-Luis, M., Lopez, D., Tellez, C., et al. (2020). Can we save a marine species affected by a highly infective, highly lethal, waterborne disease from extinction? Biol. Cons. 243:108498. doi: 10.1016/j.biocon.2020.108498
Giacobbe, S., and Leonardi, M. (1987). Les fonds à Pinna du Détroit de Messine. Doc. Trav. IGAL 11, 253–254.
Giménez-Casalduero, F., Gomariz-Castillo, F., Alonso-Sarría, F., Cortés, E., Izquierdo-Muñoz, A., and Ramos-Esplá, A. A. (in press). Pinna nobilis in the Mar Menor coastal lagoon: a story of colonization and uncertainty. Mar. Ecol. Prog. Ser.
Hernandez-Lasheras, J., and Mourre, B. (2018). Dense CTD survey versus glider fleet sampling: comparing data assimilation performance in a regional ocean model West of Sardinia. Ocean Sci. 14, 1069–1084. doi: 10.5194/os-14-1069-2018
Hughes, T. P., Kerry, J. T., Baird, A. H., Connolly, S. R., Chase, T. J., Dietzel, A., et al. (2019). Global warming impairs stock-recruitment dynamics of corals. Nature 568, 387–390. doi: 10.1038/s41586-019-1081-y
Jönsson, B., Döös, K., and Kjellsson, J. (2015). TRACMASS: Lagrangian Trajectory Code. Geneva: Zenodo, doi: 10.5281/zenodo.34157
Juza, M., Mourre, B., Renault, L., Gómara, S., Sebastián, K., Lora, S., et al. (2016). SOCIB operational ocean forecasting system and multi-platform validation in the Western Mediterranean Sea. J. Oper. Oceanogr. 9, 155–166.
Katsanevakis, S., Tsirintanis, K., Tsaparis, D., Doukas, D., Sini, M., Athanassopoulou, F., et al. (2019). The cryptogenic parasite Haplosporidium pinnae invades the Aegean Sea and causes the collapse of Pinna nobilis populations. Aquat. Invasions 14, 150–164. doi: 10.3391/ai.2019.14.2.01
Kersting, D., Benabdi, M., Čižmek, H., Grau, A., Jimenez, C., Katsanevakis, S., et al. (2019). Pinna nobilis. The IUCN Red List of Threatened Species 2019. e.T160075998A160081499. Gland: IUCN, doi: 10.2305/IUCN.UK.2019-3.RLTS.T160075998A160081499.en
Kersting, D. K., and García-March, J. R. (2017). Long-term assessment of recruitment, early stages and population dynamics of the endangered Mediterranean fan mussel Pinna nobilis in the Columbretes Islands (NW Mediterranean). Mar. Environ. Res. 130, 282–292. doi: 10.1016/j.marenvres.2017.08.007
Korres, G., Ravdas, M., and Zacharioudaki, A. (2019a). Mediterranean sea waves analysis and forecast (CMEMS MED-Waves). Copernicus Monitoring Environment Marine Service (CMEMS) doi: 10.25423/CMCC/MEDSEA_ANALYSIS_FORECAST_WAV_006_017
Korres, G., Ravdas, M., and Zacharioudaki, A. (2019b). Mediterranean sea waves hindcast (CMEMS MED-Waves). Copernicus Monitoring Environment Marine Service (CMEMS) doi: 10.25423/CMCC/MEDSEA_HINDCAST_WAV_006_012
Lattos, A., Giantsis, I. A., Karagiannis, D., and Michaelidis, B. (2020). First detection of the invasive Haplosporidian and Mycobacteria parasites hosting the endangered bivalve Pinna nobilis in Thermaikos Gulf. North Greece. Mar. Environ. Res. 155:104889. doi: 10.1016/j.marenvres.2020.104889
Lessios, H. A. (2016). The great Diadema antillarum die-off: 30 years later. Annu. Rev. Mar. Sci. 8, 267–283.
Miller, M. W., Kramer, K. L., Williams, M., Johnston, L., and Szmant, A. M. (2009). Assessment of current rates of Diadema antillarum larval settlement. Coral Reefs 28, 511–515. doi: 10.1007/s00338-008-0458-4
Miner, C. M., Altstatt, J. M., Raimondi, P. T., and Minchinton, T. E. (2006). Recruitment failure and shifts in community structure following mass mortality limit recovery prospects of black abalone. Mar. Ecol. Prog. Ser. 327, 107–117. doi: 10.3354/meps327107
Mourre, B., Aguiar, E., Juza, M., Hernandez-Lasheras, J., Reyes, E., Heslop, E., et al. (2018). “Assessment of high-resolution regional ocean prediction systems using muli-platform observations: illustrations in the Western Mediterranean Sea,” in New Frontiers in Operational Oceanography, GODAE Ocean View. eds E. Chassignet, A. Pascual, J. Tintoré, and J. Verron, 663–694. doi: 10.17125/gov2018.ch24.
Öndes, F., Alan, V., Akçali, B., and Güçlüsoy, H. (2020). Mass mortality of the fan mussel, Pinna nobilis in Turkey (eastern Mediterranean). Mar. Ecol. e12607. doi: 10.1111/maec.12607
Özalp, B., and Kersting, D. K. (2020). A pan-Mediterranean extinction? Pinna nobilis mortality has reached the Turkish straits system. Mar. Biodivers 50:81. doi: 10.1007/s12526-020-01110-7
Panarese, R., Tedesco, P., Chimienti, G., Latrofa, M. S., Quaglio, F., Passantino, G., et al. (2019). Haplosporidium pinnae associated with mass mortality in endangered Pinna nobilis (Linnaeus 1758) fan mussels. J. Invertebr. Pathol. 164, 32–37. doi: 10.1016/j.jip.2019.04.005
Prado, P., Andree, K. B., Trigos, S., Carrasco, N., Caiola, N., García-March, J. R., et al. (2019). Breeding, planktonic and settlement factors shape recruitment patterns of one of the last remaining major population of Pinna nobilis within Spanish waters. Hydrobiologia 847, 771–786. doi: 10.1007/s10750-019-04137-5
Prado, P., Caiola, N., and Ibáñez, C. (2014). Habitat use by a large population of Pinna nobilis in shallow waters. Sci. Mar. 78, 555–565. doi: 10.3989/scimar.04087.03a
Sanna, D., Cossu, P., Dedola, G. L., Scarpa, F., Maltagliati, F., Castelli, A., et al. (2013). Mitochondrial DNA reveals genetic structuring of Pinna nobilis across the Mediterranean Sea. PLoS One 8:e67372. doi: 10.1371/journal.pone.0067372
Shchepetkin, A. F., and McWilliams, J. C. (2005). The regional oceanic modelling system (ROMS): a split explicit, free-surface, topography-following-coordinate oceanic model. Ocean Model. 9, 347–404. doi: 10.1016/j.ocemod.2004.08.002
Simide, R., Covray, S., and Vicente, N. (2019). Présence de Pinna nobilis (L. 1758) dans l’étang littoral de Diana (Corse). Mar. -Rev. 2019, 1–4.
Trigos, S., Vicente, N., Prado, P., and Espinos, F. J. (2018). Adult spawning and early larval development of the endangered bivalve Pinna nobilis. Aquaculture 483, 102–110. doi: 10.1016/j.aquaculture.2017.10.015
Vázquez-Luis, M., Álvarez, E., Barrajón, A., García-March, J. R., Grau, A., Hendriks, I. E., et al. (2017). S.O.S. Pinna nobilis: a mass mortality event in western Mediterranean Sea. Front. Mar. Sci. 4:220. doi: 10.3389/fmars.2017.00220
Vicente, N. (2020). La grande Nacre de Méditerranée Pinna nobilis. Un Coquillage Bivalve Plein de Noblesse. PUP, 152. Col. Marseille: Sciences Technologies Santé.
Vicente, N., Couvray, S., Simide, R., and Vion, A. (2020). Poursuite de L’inventaire de Pinna Nobilis et de Pinna rudis. Dynamique des Populations et Captage Larvaire Dans la Reserve Naturelle de Scandola. 24. Rapp. PNRC, n°168.
Vicente, N., Simide, R., Trigos, S., Cottalorda, J. M., and Dominici, J. M. (2018). Poursuite de l’inventaire de Pinna Nobilis et de Pinna Rudis. Dynamique des Populations. Nouveaux Essais de Captage Larvaire dans la Réserve Naturelles de Scandola. 30. Rapp. PNRC, n°17.
Vicente, N., Trigos, S., and Kirchhofer, D. (2017). Etat des Populations du Mollusque Bivalve Pinna Nobilis, la Grande Nacre de Méditerranée sur les côtes de Provence de 2009 à 2016. Rapp. Agence de l’eau Rhône Mediterranée Corse. 71. Convention IOPR, n° 2015-1941.
Wesselmann, M., González-Wangüemert, M., Serrão, E. A., Engelen, A. H., Renault, L., García-March, J. R., et al. (2018). Genetic and oceanographic tools reveal high population connectivity and diversity in the endangered pen shell Pinna nobilis. Sci. Rep. 8, 1–11.
Keywords: critically endangered, mass mortality, recruitment, larval connectivity, Mediterranean Sea, hydrodynamic model, Lagrangian trajectories, recovery
Citation: Kersting DK, Vázquez-Luis M, Mourre B, Belkhamssa FZ, Álvarez E, Bakran-Petricioli T, Barberá C, Barrajón A, Cortés E, Deudero S, García-March JR, Giacobbe S, Giménez-Casalduero F, González L, Jiménez-Gutiérrez S, Kipson S, Llorente J, Moreno D, Prado P, Pujol JA, Sánchez J, Spinelli A, Valencia JM, Vicente N and Hendriks IE (2020) Recruitment Disruption and the Role of Unaffected Populations for Potential Recovery After the Pinna nobilis Mass Mortality Event. Front. Mar. Sci. 7:594378. doi: 10.3389/fmars.2020.594378
Received: 13 August 2020; Accepted: 29 September 2020;
Published: 29 October 2020.
Edited by:
Stelios Katsanevakis, University of the Aegean, GreeceReviewed by:
Lotfi Rabaoui, King Fahd University of Petroleum and Minerals, Saudi ArabiaFrancesca Carella, University of Naples Federico II, Italy
Copyright © 2020 Kersting, Vázquez-Luis, Mourre, Belkhamssa, Álvarez, Bakran-Petricioli, Barberá, Barrajón, Cortés, Deudero, García-March, Giacobbe, Giménez-Casalduero, González, Jiménez-Gutiérrez, Kipson, Llorente, Moreno, Prado, Pujol, Sánchez, Spinelli, Valencia, Vicente and Hendriks. This is an open-access article distributed under the terms of the Creative Commons Attribution License (CC BY). The use, distribution or reproduction in other forums is permitted, provided the original author(s) and the copyright owner(s) are credited and that the original publication in this journal is cited, in accordance with accepted academic practice. No use, distribution or reproduction is permitted which does not comply with these terms.
*Correspondence: Diego K. Kersting, diegokersting@gmail.com