- 1Department of Chemical, Biological, Pharmaceutical and Environmental Sciences, University of Messina, Messina, Italy
- 2Department of Veterinary Science, University of Messina, Messina, Italy
- 3Dipartimento di Scienze Biomediche, Odontoiatriche e delle Immagini Morfologiche e Funzionali, University of Messina, Messina, Italy
- 4Research Unit of Analysis and Process Applied to Environmental – APAE UR17ES32 Higher Institute of Applied Sciences and Technology Mahdia, University of Monastir, Monastir, Tunisia
The new knowledge on the bio-transformations to which the plastic material is subjected, raise concerns about their role as environmental contaminants. Microplastic have been reported to be responsible for the release and distribution of aquatic contaminants such organophosphorus esters, pesticides, polycyclic aromatic hydrocarbons, polychlorinated biphenyls, and phthalate esters. The occurrence, spatiotemporal trends, and ecological risk of phthalic acid esters (PAEs) and non-phthalate plasticizers (NPPs) released from microplastic were investigated in water and fish (Sparus aurata) from five sites along the coast of Mahdia governorate (Tunisia), during April 2018-March 2019. In seawater the most abundant and frequently detected congeners were dibutyl phthalate (DBP, 0.017 mg L–1 and 0.055 mg L–1), diisobutyl phthalate (DiBP, 0.075 mg L–1 and 0.219 mg L–1), di(2-ethylhexyl)phthalate (DEHP, 0.071 mg L–1 and 4.594 mg L–1), and di(2-ethylhexyl) terephthalate (DEHT, 0.634 mg L–1 and 2.424 mg L–1). (ΣPAEs: 1.416 mg L–1 and 5.581 mg L–1; ΣNPPs: 9.191 mg L–1 and 26.296 mg L–1), confirming that such compounds bioconcentrate through the food chain. DBP (0.389 and 0.817 mg L–1), DiBP (0.101 and 0.921 mg L–1), DEHP (0.726 and 1.771 mg L–1) and DEHT (9.191 and 23.251 mg L–1) were predominant also in S. aurata affirming that such compounds bioconcentrate through the food chain. Overall, Tunisian samples i) were much more contaminated than counterparts previously investigated for the same pollutants from other world areas, and ii) revealed NPPs at higher levels than PAEs, confirming that such plasticizers are increasingly replacing conventional PAEs.
Introduction
The environmental plastic pollution, in recent decades it has become a major worldwide problem (Barnes et al., 2009; Bonanno and Orlando-Bonaca, 2020). Moreover, the new knowledge on the bio-transformations to which the plastic material is subjected, raise concerns about their role as environmental contaminants. In fact, plastic materials can undergo different processes of transformation and degradation, such as interaction with microorganism, UV, radiation, chemical agents, mechanical abrasion by wind and ocean waves, thermal degradation, and physical weathering and aging (de Sa et al., 2018). Recently the plastic material released into the environment and especially into the aquatic environment, is classified according to their size, and in particular two important families of plastic material are distinguished which have a range of sizes <5 μm and <100 nm and are called microplastics (MPs) and nanoplastics (NPs), respectively (Frias and Nash, 2019). In recent years numerous studies have been carried out which describe the interaction of these contaminants with different compartments of marine biota such as seabirds, Barnacles (Goldstein and Goodwin, 2013), bivalves (Van Cauwenberghe and Janssen, 2014), zooplankton (Frias et al., 2014), decapod crustaceans (Devriese et al., 2015), fish (Boerger et al., 2010) and marine mammals (Besseling et al., 2015). Recently their toxic potential of MPs has also been identified, and in particular the toxic effects deriving from the ingestion by the marine biota which can occur directly or indirectly through the trophic web (Mattsson et al., 2017). MPs have been reported to be responsible for the release and distribution of aquatic contaminants such organophosphorus esters, pesticides, polycyclic aromatic hydrocarbons, polychlorinated biphenyls, and phthalate esters (Zhang et al., 2018). Phthalic acid esters (PAEs), also commonly known as phthalates, are the plasticizers most commonly employed in the world. Plasticizer such as PAEs have the characteristic, not to firmly bind the polymer chain of plastic, and therefore they can be easily released into the environment by plastic matrices containing phthalates such as MPs (Paluselli et al., 2018). Over the last half century, a variety of non-phthalate plasticizers (NPPs) PAE alternatives with lower migration rates and no use restrictions have been developed, with in some cases a similarity for PAEs and their alternatives. For example, di(2-ethylhexyl) adipate (DEHA), bis-2-ethylhexyl sebacate (DOS), and di-(2-ethylhexyl) terephthalate (DEHT) are characterized by Koct/wat, VP and water solubility close to those of di(2-ethylhexyl) phthalate (DEHP), diisononyl phthalate (DINP) and diisodecyl phthalate (DIDP). Thus, also for NPPs MPs can be an important vehicle for their release in the environment and especially in the marine environment (Andrady, 2011). These compounds also show the absence of toxic actions such as mutagenic, carcinogenic and reproductive, however, despite their great use non-standard effects, such as early developmental toxicity or endocrine disruption should also be considered and tested. Hence, the aim of the present study was to monitor and compare the spatial distribution and seasonal variation from Mahdia governorate (Tunisia), of 10 PAEs and 8 NPPs. The aim of the present study was to monitor and compare the spatial distribution and seasonal variation from Mahdia governorate (Tunisia), of 10 PAEs and 8 NPPs related compounds. Considering water and fish gilthead bream (Sparus aurata L.) that were collected in several anthropized coastal sites during 2018 and 2019 and characterized for residues of 10 PAEs and 8 NPPs. Also, an ecological risk assessment based on the Risk Quotient (RQ) methodology was carried out for the congeners most frequently detected along the coast of Mahdia. Except for a recent study conducted on individual marine matrices (i.e., fish), no study has been yet focused on the comprehensive monitoring of PAEs and their alternatives in the Tunisian coastal environment.
Materials and Methods
Study Area and Sampling
The present study was conducted between April 2018 and March 2019, along the coastline of Mahdia governorate (North-Eastern Tunisia). Such district covers an area of 2,966 km2 and borders the districts of Sfax, Kairouan, Sousse, and Monastir (Figure 1). Its coastal strip, in particular, extends for about 48 km, faces the Mediterranean Sea and comprises from North to South the municipalities of Mahdia, Ksour Essef, Chebba, and Melloulèche (35°34′48.2″N 11°01′51.5″E – 35°06′34.5″N 11°01′16.9″E, Figure 1). Five coastal sites within the Mahdia governorate were chosen as sampling stations (S1–S5, Figure 1) due to their strategic proximity to pollution sources, namely urban, industrial and touristic areas. At each site, scuba divers collected surface waters at a depth of 7 mt, respectively, by means of Niskin bottles. Adult specimens of Sparus aurata L., commonly known as gilthead bream, were monthly purchased by fish farms located in Mahdia (S1) and Chebba (S4), by experimental floating net cages (1.5 × 1.0 × 1.0, depth × length × width; water volume of 1 m3) located at the sampling stations, biometric parameters (25.56 ± 3.11 cm total length; 312.55 ± 33.20 g weight). Then, every organism was thawed and sectioned by stainless steel instruments for collecting the muscle tissue, which was subsequently stored at −20°C. No ethical approval was required for this study, in accordance with local legislation. Overall, three replicate samplings were monthly performed at each site for water (n = 165), and fish (n = 165).
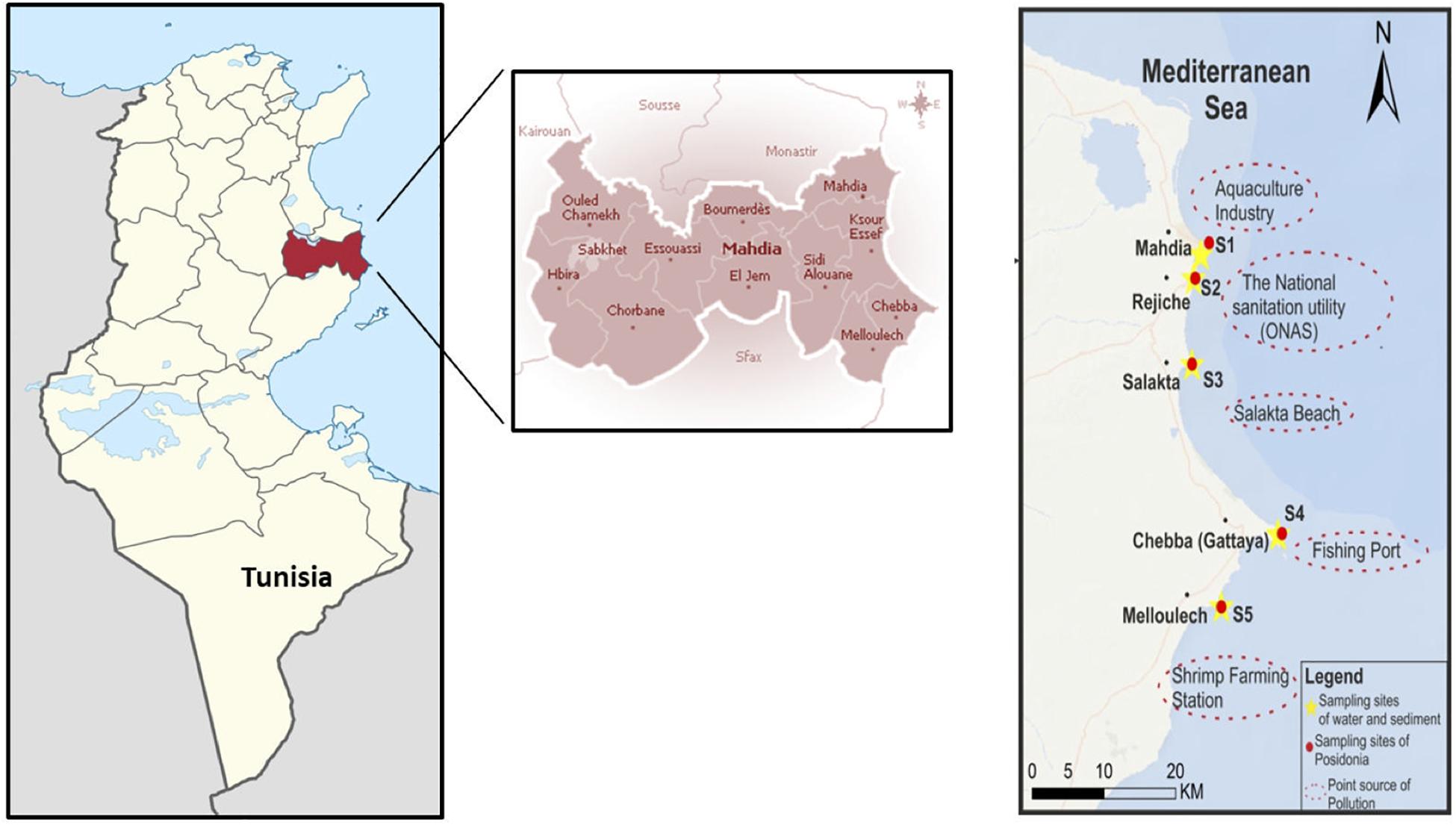
Figure 1. Map illustrating the geographical location of the Mahdia governorate (Tunisia) and of the sampling sites S1–S5 (S1, Mahdia; S2, Rejiche; S3, Salakta; S4, Chebba; S5, Melloulech) considered in the present study.
Chemicals and Reagents
Analytical standards of PAEs and NPPs (certified purity ≥96%) were provided by Supelco (Bellefonte, PA, United States) and are listed in Supplementary Table 1. Di(2-ethylhexyl) phthalate-d4 (DEHP-d4) and di(2-ethylhexyl) adipate-d4 (DEHA-d4) in nonane (100 μg mL–1 each) were supplied by Cambridge Isotope Laboratories Inc. (Andover, MA, United States) and were employed as internal standards. Solid sorbent such as magnesium silicate (Florisil, 60–100 mesh, coarse powder) was purchased from Fluka (Sigma-Aldrich, AG, Switzerland); while C18 cartdriges (Supelclean C18, 3 mL, 500 mg sorbent) were from Supelco (Bellefonte, PA, United States). Solvents such as acetone, n-hexane, ethyl acetate and diethyl ether were SupraSolv grade and were provided from Merck (Darmstadt, Germany). During sample preparation and analysis, it was assured that no plastic components from laboratory equipment and materials came into contact with the different matrices. Additionally, all employed glassware was washed with acetone, then rinsed with hexane, and dried at 120°C for at least 4 h.
Sample Preparation
All marine samples and fish were treated according to the method proposed by Blair et al. (2009). Briefly, 500 mL of seawater were passed through a C18 cartridge and then conditioned with 5 mL ethyl acetate and 5 mL methanol. The cartridges were then washed with 4 mL of water, followed by 6 mL of a 35% methanol/water solution. Plasticizers were finally eluted with 5 mL of ethyl acetate and evaporated to drieness by a Büchi V700 rotating evaporator (BUCHI Labortechnik AG, Switzerland). Method blanks were prepared for every matrix by following the relative preparation procedures without sample. Before GC-MS analysis, all dried extracts were re-suspended in 950 μL of n-hexane and spiked with 50 μL of each internal standard at a final concentration level of 0.05 mg L–1. For analytical validation purposes, the described procedures were also applied to additional and representative blank samples of seawater and fish, which were previously analyzed by the GC-MS protocol reported ahead and revealed no plasticizer contamination (Di Bella et al., 2018).
GC-MS Analysis
Plasticizers were determined in all samples by a gas chromatography system (GC-2010, Shimadzu, Japan) equipped with an autosampler (HT300A, HTA, Italy) and coupled to a single quadrupole mass spectrometer (QP-2010 Plus, Shimadzu, Japan). Chromatographic separations were conducted on a SPB-5MS capillary column (30 m × 0.25 mm i.d. × 0.25 μm film thickness, Supelco, United States). The oven temperature program was: from 60°C to 190°C at 8°C min-1 (5 min hold), from 190°C to 240°C at 8°C min–1 (5 min hold), and from 240°C to 315°C at 8°C min–1. The injection port was at 260°C and was provided with a narrow inlet liner (0.75 mm ID, Agilent Technologies). Sample injection took place in splitless mode, with sampling time of 60 s, then split ratio 1:15. Injection volume was 1 μL. Carrier gas (He, 210.0 KPa, pressure control mode) was used at a linear velocity of 30 cm s–1. Concerning the MS setup, the EI source temperature was set at 200°C, ionization energy and emission current were 70 eV and 250 μA, respectively; whereas interface temperature and electron multiplier voltage were, respectively, equal to 300°C and 1.0 kV. Acquisition was performed both in full scan (mass range: 40–400 m/z) and Selected Ion Monitoring (SIM) by monitoring three characteristic mass fragments for every investigated analyte (Supplementary Table 2). Data acquisition and processing were performed by GCMS solution software version 2.50 SU3. Identification of plasticizers occurred by comparison of their retention times and mass spectra (both in full scan and SIM mode) with those of corresponding commercial standards. The quantitative procedure was carried out in SIM mode, by considering the relative base peak ions (Supplementary Table 2) and exploiting the internal standard normalization. To this purpose, five-point linear least squares calibration of the analyte peak area over the relative internal standard peak area (area ratio) was plotted versus the analyte concentration over the relative internal standard concentration (amount ratio). For the 10 PAEs the area and amount ratios were calculated considering the internal standard DEHP-d4; while for the 8 PNPPs was considered the internal standard DEHA-d4.
Statistical Analysis
Descriptive data analysis, including mean and median, standard deviation (SD), minimum and maximum concentrations was carried out. For the study of the occurrence of plasticizers, a Pearson correlation analysis was carried out to discover the relationships among investigated congeners within the representative coastal system of Mahdia governorate. A statistical comparison of every plasticizer level among different sampling sites and sampling months was carried out by one-way ANOVA, followed by Tukey’s honestly significant difference (HSD) post hoc test, for investigating, respectively, the spatial and seasonal variations. All analyses were conducted by means of the SPSS Statistics Software Version 20 (IBM, New York, United States), and their statistical significance was accepted at p ≤ 0.05.
Ecological Risk Assessment
The ecological risk assessment of PAEs, such as Diethyl phthalate (DEP), dipropyl phthalate (DPrP), DBP, DiBP, BBP, and DEHP, and NPPs, such as DEHA and DEHT, was performed by applying the Risk Quotient (RQ) methodology to the water of each sampling site considered in the present study, as already suggested by the Technical Guidance Document (TGD) of European Commission (2003). The RQ is calculated according the equation:
where MEC is the measured environmental concentration revealed at each sampling site for a given pollutant and PNEC is the predicted no effect concentration of that compound. Whereas MEC values are easily obtained from the experimental dataset, PNEC values are usually determined for a given organism based on results from single species laboratory tests or, in alternative, derived using the assessment factor (AF) approach. In this respect, the PNEC is calculated by dividing the median lethal concentration (LC50) or median effect concentration (EC50) value by an AF equal to 1000. The assessment factor reflects the degree of uncertainty in extrapolation from laboratory toxicity test data for a limited number of species to the “real” environment (European Commission, 2003). On this basis, the PNECwater, representing the PNEC for the aquatic life compartment, was calculated considering the L(E)C50 of sensitive aquatic species from different trophic levels (i.e., algae, crustaceans) divided by an AF of 1000, when short-term/acute toxicity data for a given plasticizer were available. Hence, the RQwater was calculated as follows:
Concerning the water-phase, the ecological risk was classified into 3 levels depending on the RQ value obtained, that is low risk (RQ < 0.01), medium risk (0.01 < RQ < 1), and high risk (RQ > 1) (European Commission, 2003).
Results
Method Validation
The validation of the analytical method was performed evaluating linearity, limit of detection (LoD) and quantification (LoQ), Supplementary Table 1 reported the accuracy and precision. In particular, linearity was evaluated in terms of R2 and assessed through six-point calibration curves over a 0.002–5.00 mg L–1 range for almost all PAEs and NPPs. A satisfactory linearity (R2 ≥ 0.9865) was obtained for all the analytes in the investigated concentration ranges. Moreover, an excellent instrumental sensitivity was observed for the detection of the selected analytes, as LoD ranged from 0.033 to 0.370 μg L–1; while LoQ from 0.103 to 1.219 mg L–1 for DMA and BBP, respectively (Supplementary Table 1). Concerning the accuracy, blank samples of seawater and fish were spiked with commercial standards of PAEs and NPPs at two concentration levels (0.050 and 0.100 mg L–1), and treated according the protocol described, each concentration being replicated for five times. Precision was determined as the relative standard deviation (RSD%) of recovery at the first fortification level (0.050 mg L–1, five replicates), calculated in the same day (intra-day precision) and in five consecutive days (inter-day precision). Concerning recovery, acceptable values were obtained for all the investigated analytes, ranging from 93.34% (DiBA) to 100.98% (DEA) at the first fortification level; while they were higher at the second fortification level, namely from 95.38% (BB) to 102.00% (DEA). Precision was assessed as ≤5.78%, while intermediate precision was below 8.21% (Supplementary Table 1).
Occurrence of PAEs and NPPs in Marine Samples
For the 18 compounds investigated, in particular 6 PAE congeners (namely, DEP, DPrP, DBP, DiBP, BBP, and DEHP) and 2 NPPs (namely, DEHA, and DEHT), were identified and quantified by the GC-MS as described above. Supplementary Table 3 summarize their concentration and detection frequency in the coastal environment of Mahdia. As showed in Supplementary Table 3, the mean values of ΣPAEs was 0.177 mg L–1 in water. For the S. aurata samples the mean level was 5.581 mg L–1. Regarding NPPs, in water they amounted on average to 0.634 mg L–1 DEHT, while fish accumulated 26.296 mg L–1 of DEHA + DEHT. Among PAEs, DiBP represented the most concentrated compound in water (concentration range: <LOD-0.106 mg L–1; mean concentration: 0.075 mg L–1), instead DEHP was found at the highest levels in fish (concentration range: 1.877–4.386 mg L–1; mean concentration: 3.045 mg L–1) (Supplementary Table 3). DEHT among NPPs, was the most abundant plasticizer in water (concentration range: 0.245–0.930 mg L–1; mean concentration: 0.634 mg L–1). In Supplementary Table 5 we summarize the concentrations of the PAE most frequently founded in the marine environments (i.e., DEP, DIBP, DBP, BBP and DEHP) considering the literature of the last 20 years. Our results showed that Mahdia seawater was characterized by contents of DEP, DBP, DIBP, and DEHP, 1–9 orders of magnitude higher than other marine areas previously investigated. Interesting, ten marine fish species from the market of Hong Kong revealed lower levels of DEP, DIBP, DBP, BBP than S. aurata from Mahdia coast (respectively, 0.016 vs. 1.386, 0.98 vs. 1.771, 0.40 vs. 0.817, and 0.135 vs. 0.498 mg L–1). For DEHP we observe comparable levels (1.042 vs. 0.921 mg L–1).
Seasonal Variation
DEP, DBP, DiBP, and DEHP showed a similar temporal distribution in the seawater, and no significantly changes during the 11 sampling months (p ≥ 0.05), in particular, we observe highest levels in the summer season (Supplementary Table 3 and Figure 2). DEHP was the most abundant PAE, showing the highest concentrations in June-September (mean values: 0.066–0.107 mg L–1), and lowest concentrations in November-February (mean values: 0.039-0.032 mg L–1). While for DEHT we observed increased levels from April to January, with the highest levels between September and January (respective mean values: 0.680 mg L–1 and 1.211 mg L–1, p ≥ 0.05) (Supplementary Table 3 and Figure 2). The gilthead breams from Mahdia coast showed a seasonal PAE variation in according with seawater trend. In fact, for DEP, DBP, DiBP and DEHP the highest contents were recorded in the summer season (Supplementary Table 3 and Figure 3). Among PAEs, DEHP confirmed to be most abundant congener, but DEHA and DEHT resulted at even higher levels throughout the study period. In particular DEHA was more accumulated by S. aurata during August-January (1.098–1.160 mg L–1, p ≥ 0.05); whereas DEHT during June–September (23.153–23.983 mg L–1) (Supplementary Table 3 and Figure 3).
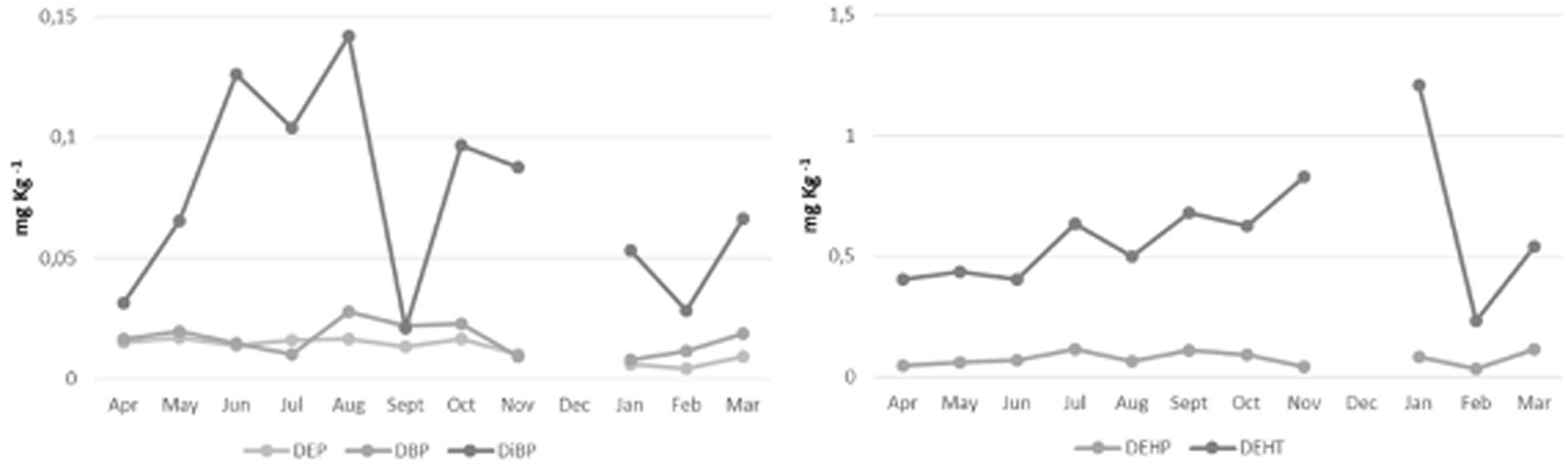
Figure 2. Seasonal variation of single PAE congeners, DEHA and DEHT in the seawater of Mahdia coast (Tunisia) from April 2018 to March 2019.
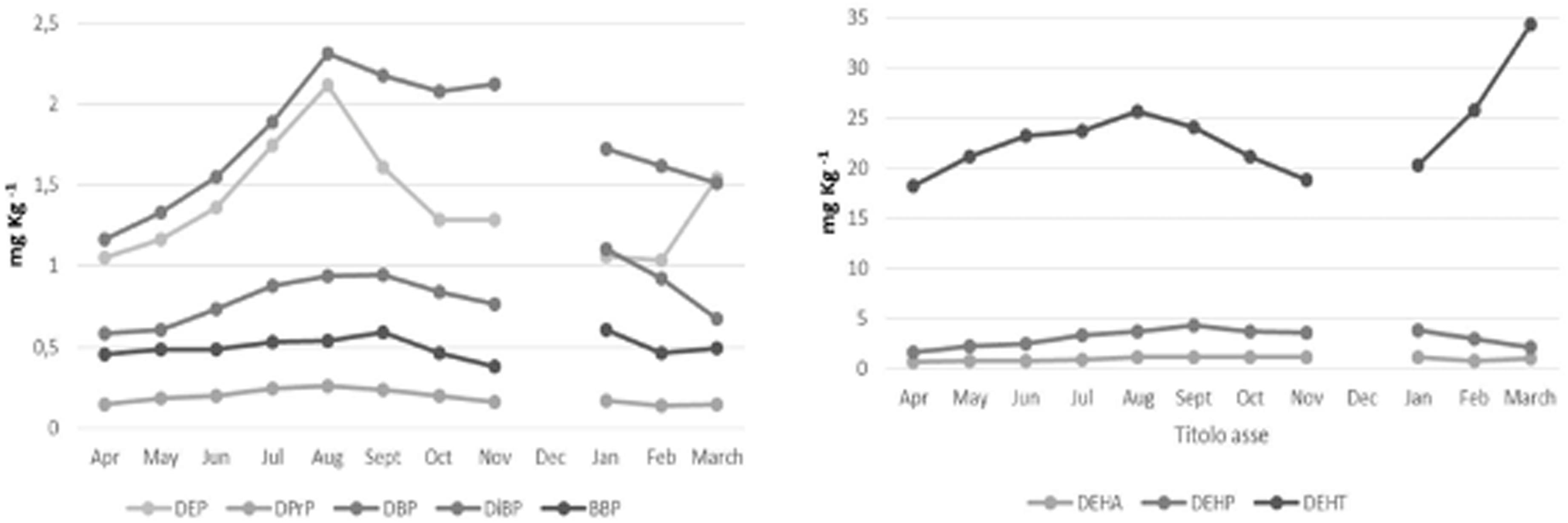
Figure 3. Seasonal variation of single PAE congeners, DEHA and DEHT in Sparus aurata from Mahdia coast (Tunisia) during the period April 2018 to March 2019.
Ecological Risk Assessment
Five PAE species (DEP, DPrP, DBP, DiBP, BBP, and DEHP) and two NPPs (DEHA and DEHT) were considered to analyze their ecological risk in the coastal environment of Mahdia according the RQ method. The RQ values obtained for seawater are reported in Supplementary Table 4. The amounts of congeners such as DEP, DBP, and DiBP revealed in waters along the coast of Mahdia posed a low risk for all test organisms, with the exception of DiBP, which may cause a medium risk for fish from all the sampling sites considered in this study (RQs from 0.164 to 0.257 (Supplementary Table 4).
Discussion
Increasing scientific evidences suggest that microplastics (MPs) have an important role in conveying contaminants in the environment and in organisms. This has posed serious public health concerns given the rising levels of plastic pollution and MPs presence in the oceans, so it is important to better understand the impact of MPs on the ocean food web. Furthermore, numerous studies show how MPs are capable of releasing phthalate esters (PAEs) into the environment (Benson and Fred-Ahmadu, 2020). In particular a recent study showed that the MPs are able to transport and release PAEs into mouse gut (Deng et al., 2020). To Date the role of PAEs as endocrine disruptor is widely known in fact, based on the claimed concern for public health, six PAEs, namely dibutyl phthalate (DBP), diisobutyl phthalate (DIBP), DEHP, n-butyl benzyl phthalate (BBP), diisononyl phthalate (DINP), and di-isodecyl phthalate (DIDP), have been strictly regulated at European level. As confirmation of this, DEHP has been included in the list of priority substances or probably priority substances in the field of water policy (European Commission, 2001), whereas the Regulation (EC) No. 1907/2006 (European Commission, 2006. Annex XIV), controlled production and sales of DEHP, BBP, DBP, DIBP, as they have been identified as substances of very high concern, being toxic to reproduction (Category 1B chemicals). Additionally, based on proposal of the European Chemical Agency (ECHA), the European Commission has edited the Annex XIV by adding endocrine disrupting properties for above mentioned PAEs. However, the Annex XVII of the Regulation (EC) No. 1907/2006 has enabled the safe use of DINP and DIDP, classifying them not harmful for human health and saying that, just as a safety insurance, they should not be used in toys and childcare articles. Ultimately, the Directive 2007/19/EC (European Commission, 2007) imposed several restrictions for DBP, DEHP, BBP, DINP, and DIDP, by stating the permitted uses and the maximum specific migration limits (SMLs). In order to address legislations and safety issues, have been industrialized several non-phthalate plasticizers (NPPs) PAE replacements with no use limitations, and in particular they include adipates, sebacates, terephthalates, and benzoates. Evidence on the physicochemical properties of such constituents and data on human and ecological exposure are fairly limited and fragmented, thus, making risk assessment very difficult. In this study we examined and compared spatial spreading and seasonal difference of PAEs and NPPs in water samples and in Sparus aurata L. from several anthropized coastal sites during 2018 and 2019, in Mahdia governorate (Tunisia). We have studied and assessed 18 compounds, 6 PAE congeners (namely, DEP, DPrP, DBP, DiBP, BBP, and DEHP) and 2 NPPs (namely, DEHA and DEHT). Our results showed that with PAEs DiBP and DEHP are the compounds that showed the maximum levels in water and fish, respectively. Instead concerning NPPs, we detected the high levels of DEHA in water, instead the fish accumulated DEHA + DEHT. This result confirm the evidence that DEHT, an isomer of DEHP, has been used as substitute of PAEs, primarily for PVC polymers toys, childcare articles, and consumer products (Tickner, 2011). However, DEHP and DBP persist still as the most abundant PAEs found in the environment (Lo Turco et al., 2016, 2020). The contamination of ΣPAEs and ΣNPPs in S. aurata can be described by the high Koct/wat coefficients consenting such pollutants to be easily accumulated in living organisms both by the water column and indirectly through the food chain. The European Union (2013) proposed a guideline for environmental quality (NQE or Norme de Qualitè Environnementale) and established a limit of 1.3 μg L–1 for DEHP in marine waters; whereas van Wezel et al. (2000), derived the ecological risk limits (ERLs) for PAEs in marine waters and sediments using data on (eco)toxicology and environmental chemistry, and fixed limits of 10 μg L–1 and 0.19 μg L–1, respectively, for DEHP and DBP in water and of 0.7 mg L–1 and 1 mg L–1 for DEHP and DBP in sediments. Based on such recommendations and on the outcomes achieved from this study, the concentrations of DBP in water were, respectively, slightly higher than the respective ERLs. However, DEHP levels were considerably higher than the allowance values, in seawater. Based on the scientific literature of the last 20 years, on the concentrations of the PAE congeners most frequently found in marine environments around the world, our results show that the seawater of Mahdia coast was characterized by contents of DEP, DBP, DIBP, and DEHP, 1–9 orders of magnitude greater than other marine regions previously considered, with the exception of the False Creek Harbor (Vancouver, BC, Canada, and United States), which showed DEP, DBP and DEHP at levels, respectively, of 0.126, 0.110, and 0.275 mg L–1 (Mackintosh et al., 2006). However, the seawater of this Tunisian region did not show BBP contamination, differently from other sites. To the best knowledge of the authors, no comparative data on DEHA and DEHT from marine biota can be inferred from previous literature. Considering the seasonal change DEP, DBP, DiBP and DEHP exhibited a similar temporal distribution in the seawater. Among PAEs, DEHP was the most abundant with highest values in June-September. While for DEHT we observed increased levels from April to January, reporting the peak levels between September and January. Obtained results are in agreement with what previously reported by Paluselli et al. (2018) and may be due to the fact that Mahdia coast benefits from an intense recreational coastal activity and an elevated maritime traffic, reaching their maximum during the tourism season and, thus, representing primary contamination sources for the seawater (Paluselli et al., 2018). It is also probable that tourism activities specially cause DEHP increase into the coastline seawater. Similar to seawater results, the gilthead breams from Mahdia coast, concerning DEP, DBP, DiBP and DEHP showed highest contents in the summer season. Taken together our results showed that, DEHP was established as the most abundant compound among PAEs but DEHA and DEHT resulted at even higher levels during the study period. In particular DEHA was more accumulated by S. aurata during August–January whereas DEHT during June-September. Overall, the ecological risk of PAEs in seawater followed the order of DEHP > DiBP > DBP > DEP. However, species such as DiBP and BBP (logKoct/wat between 3 and 5) showed RQs > 1 for fish coming from all the considered sites. This is consistent with previous studies (Zhang et al., 2020) reporting a high risk of DiBP to sensitive fish, respectively, from the Jiulong River and the East China Sea. The incidence, spatial and seasonal trends, and environmental risk of 6 PAE congeners (DEP, DPrP, DBP, DiBP, BBP and DEHP) and 2 NPPs (DEHA and DEHT) were examined in seawater and fish from different sites along the coast of Mahdia governorate (Tunisia), during April 2018–May 2019. In conclusion our results showed that the coast of Mahdia was more contaminated by DEHA and DEHT than ΣPAEs, being coherent with the evidence that NPPs are progressively switching conventional phthalates. However, among PAEs, DEHP, DBP and DEP were the most frequently spotted and the most abundant congeners of the coastal environment. Additionally, water from Mahdia resulted much more polluted than the counterparts from other world areas. The investigated plasticizers were marked by peculiar spatial and seasonal variations. In particular, the coast in proximity of Salakta and Chebba resulted more contaminated both in terms of PAEs and NPPs. Also, PAEs, adipate and terephthalate showed their maximum concentrations between late spring and summer and their lowest levels between late autumn and winter. Results from the risk assessment proved that the levels of DEHP and DEHT found in seawater constituted a high risk for fish; whereas DEP, DBP and DiBP did not pose a risk for sensitive organisms.
Data Availability Statement
The original contributions presented in the study are included in the article/Supplementary Material, further inquiries can be directed to the corresponding author/s.
Ethics Statement
Ethical review and approval was not required for this animal study, in accordance with the local legislation and institutional requirements. All animal specimens used in this study were purchased from commercial fish farms.
Author Contributions
EG: writing original draft. PL: data analysis and writing original draft. RC, AA, VL, and AP: data analysis and validation. HM: data analysis and supervision. SC and GD: conceptualization and supervision. All authors contributed to the article and approved the submitted version.
Conflict of Interest
The authors declare that the research was conducted in the absence of any commercial or financial relationships that could be construed as a potential conflict of interest.
Supplementary Material
The Supplementary Material for this article can be found online at: https://www.frontiersin.org/articles/10.3389/fmars.2020.589398/full#supplementary-material
References
Andrady, A. L. (2011). Microplastics in the marine environment. Mar. Pollut. Bull. 62, 1596–1605. doi: 10.1016/j.marpolbul.2011.05.030
Barnes, D. K., Galgani, F., Thompson, R. C., and Barlaz, M. (2009). Accumulation and fragmentation of plastic debris in global environments. Philos. Trans. R. Soc. Lond. B Biol. Sci. 364, 1985–1998. doi: 10.1098/rstb.2008.0205
Benson, N. U., and Fred-Ahmadu, O. H. (2020). Occurrence and distribution of microplastics-sorbed phthalic acid esters (PAEs) in coastal psammitic sediments of tropical Atlantic Ocean, Gulf of Guinea. Sci. Total Environ. 730:139013. doi: 10.1016/j.scitotenv.2020.139013
Besseling, E., Foekema, E. M., Van Franeker, J. A., Leopold, M. F., Kuhn, S., Bravo Rebolledo, E. L., et al. (2015). Microplastic in a macro filter feeder: humpback whale Megaptera novaeangliae. Mar. Pollut. Bull. 95, 248–252. doi: 10.1016/j.marpolbul.2015.04.007
Blair, J. D., Ikonomou, M. G., Kelly, B. C., Surridge, B., and Gobas, F. A. (2009). Ultra-trace determination of phthalate ester metabolites in seawater, sediments, and biota from an urbanized marine inlet by LC/ESI-MS/MS. Environ. Sci. Technol. 43, 6262–6268. doi: 10.1021/es9013135
Boerger, C. M., Lattin, G. L., Moore, S. L., and Moore, C. J. (2010). Plastic ingestion by planktivorous fishes in the North Pacific central gyre. Mar. Pollut. Bull. 60, 2275–2278. doi: 10.1016/j.marpolbul.2010.08.007
Bonanno, G., and Orlando-Bonaca, M. (2020). Marine plastics: what risks and policies exist for seagrass ecosystems in the Plasticene? Mar. Pollut. Bull. 158:111425.
de Sa, L. C., Oliveira, M., Ribeiro, F., Rocha, T. L., and Futter, M. N. (2018). Studies of the effects of microplastics on aquatic organisms: what do we know and where should we focus our efforts in the future? Sci. Total Environ. 645, 1029–1039. doi: 10.1016/j.scitotenv.2018.07.207
Deng, Y., Yan, Z., Shen, R., Wang, M., Huang, Y., Ren, H., et al. (2020). Microplastics release phthalate esters and cause aggravated adverse effects in the mouse gut. Environ. Int. 143:105916. doi: 10.1016/j.envint.2020.105916
Devriese, L. I., van der Meulen, M. D., Maes, T., Bekaert, K., Paul-Pont, I., Frere, L., et al. (2015). Microplastic contamination in brown shrimp (Crangon crangon, Linnaeus 1758) from coastal waters of the Southern North Sea and Channel area. Mar. Pollut. Bull. 98, 179–187. doi: 10.1016/j.marpolbul.2015.06.051
Di Bella, G., Ben Mansour, H., Ben Tekaya, A., Beltifa, A., Potorti, A. G., Saiya, E., et al. (2018). Plasticizers and BPA residues in tunisian and Italian culinary herbs and spices. J. Food Sci. 83, 1769–1774. doi: 10.1111/1750-3841.14171
European Commission (2001). Decision No. 2455/2001/EC of the European Parliament and of the Council of 20 November 2001 Establishing the List of Priority Substances in the Field of Water Policy and Amending Directive 2000/60/EC, Off. J. Eur. Commun. L331. Brussels: European Commission.
European Commission (2003). European Commission’s Technical Guidence Document. Technical Guidance Document on Risk Assessment in Support of Commission Directive 93/67/EEC on Risk Assessment for New Notified Substances, Commission Regulation (EC) No 1488/94 on Risk Assessment for Existing Substances, and Directive 98/8/EC of the European Parliament and of the Council Concerning the Placing of Biocidal Products on the Market. Part II. EUR, 20418. Brussels: European Commission Joint Research Centre.
European Commission (2006). Regulation (EC) No. 1907/2006 of the European Parliament and of the Council of 18 December 2006 concerning the Registration. Evaluation, Authorisation and Restriction of Chemicals (REACH), establishing a European Chemicals Agency, amending Directive 1999/45/EC and repealing Council Regulation (EEC) No. 793/93 and Commission Regulation (EC) No 1488/94 as well as Council Directive 76/769/EEC and Commission Directives 91/155/EEC, 93/67/EEC, 93/105/EC and 2000/21/EC. Off. J. Eur. Un, 396. Brussels: European Commission.
European Commission (2007). Commission Directive 2007/19/EC of 2 April 2007 amending Directive 2002/72/EC Relating to Plastic Materials and Articles Intended to Come into Contact with Food and Council Directive 85/572/EEC Laying Down the List of Simulants to be Used For Testing Migration of Constituents of Plastic Materials and Articles Intended to Come into Contact With Foodstuffs. Official J. Eur. Un. L97. Brussels: European Commission.
European Union (2013). Directive 2013/39/EU of the European Parliament and of the Council of 12 August 2013 Amending Directives 2000/60/EC and 2008/105/EC as Regards Priority Substances in the Field of Water Policy. Off. J. Eur. Un. L226. Brussels: European Commission.
Frias, J., and Nash, R. (2019). Microplastics: finding a consensus on the definition. Mar. Pollut. Bull. 138, 145–147. doi: 10.1016/j.marpolbul.2018.11.022
Frias, J. P., Otero, V., and Sobral, P. (2014). Evidence of microplastics in samples of zooplankton from Portuguese coastal waters. Mar. Environ. Res. 95, 89–95. doi: 10.1016/j.marenvres.2014.01.001
Goldstein, M. C., and Goodwin, D. S. (2013). Gooseneck barnacles (Lepas spp.) ingest microplastic debris in the North Pacific Subtropical Gyre. PeerJ 1:e184. doi: 10.7717/peerj.184
Lo Turco, V., Di Bella, G., Potorti, A. G., Tropea, A., Casale, E. K., Fede, M. R., et al. (2016). Determination of plasticisers and BPA in Sicilian and Calabrian nectar honeys by selected ion monitoring GC/MS. Food Addit. Contam. Part A Chem. Anal. Control. Expo Risk Assess. 33, 1693–1699. doi: 10.1080/19440049.2016.1239030
Lo Turco, V., Potorti, A. G., Ben Mansour, H., Dugo, G., and Di Bella, G. (2020). Plasticizers and BPA in spices and aromatic herbs of Mediterranean areas. Nat. Prod. Res. 34, 87–92. doi: 10.1080/14786419.2019.1591403
Mackintosh, C. E., Maldonado, J. A., Ikonomou, M. G., and Gobas, F. A. (2006). Sorption of phthalate esters and PCBs in a marine ecosystem. Environ. Sci. Technol. 40, 3481–3488. doi: 10.1021/es0519637
Mattsson, K., Johnson, E. V., Malmendal, A., Linse, S., Hansson, L. A., and Cedervall, T. (2017). Brain damage and behavioural disorders in fish induced by plastic nanoparticles delivered through the food chain. Sci. Rep. 7:11452. doi: 10.1038/s41598-017-10813-0
Paluselli, A., Fauvelle, V., Schmidt, N., Galgani, F., Net, S., and Sempere, R. (2018). Distribution of phthalates in Marseille Bay (NW Mediterranean Sea). Sci. Total Environ. 621, 578–587. doi: 10.1016/j.scitotenv.2017.11.306
Tickner, J. (2011). Phthalates and Their Alternatives: Health and Environmental Concerns. Madison, WI: Lowell Center for Sustainable Production.
Van Cauwenberghe, L., and Janssen, C. R. (2014). Microplastics in bivalves cultured for human consumption. Environ. Pollut. 193, 65–70. doi: 10.1016/j.envpol.2014.06.010
van Wezel, A. P., van Vlaardingen, P., Posthumus, R., Crommentuijn, G. H., and Sijm, D. T. (2000). Environmental risk limits for two phthalates, with special emphasis on endocrine disruptive properties. Ecotoxicol. Environ. Saf. 46, 305–321. doi: 10.1006/eesa.2000.1930
Zhang, Z. M., Yang, G. P., Zhang, H. H., Shi, X. Z., Zou, Y. W., and Zhang, J. (2020). Phthalic acid esters in the sea-surface microlayer, seawater and sediments of the East China Sea: spatiotemporal variation and ecological risk assessment. Environ. Pollut. 259:113802. doi: 10.1016/j.envpol.2019.113802
Keywords: microplastics, phthalic acid esters, non-phthalate plasticizers, Mediterranean Sea, microplastics pollution
Citation: Gugliandolo E, Licata P, Crupi R, Albergamo A, Jebara A, Lo Turco V, Potortì AG, Mansour HB, Cuzzocrea S and Di Bella G (2020) Plasticizers as Microplastics Tracers in Tunisian Marine Environment. Front. Mar. Sci. 7:589398. doi: 10.3389/fmars.2020.589398
Received: 30 July 2020; Accepted: 12 October 2020;
Published: 03 December 2020.
Edited by:
Julian Blasco, Consejo Superior de Investigaciones Científicas (CSIC), SpainReviewed by:
Marta Sendra, Higher Council for Scientific Research (CSIC), SpainSalvatore Antonino Raccuia, National Research Council (CNR), Italy
Copyright © 2020 Gugliandolo, Licata, Crupi, Albergamo, Jebara, Lo Turco, Potortì, Mansour, Cuzzocrea and Di Bella. This is an open-access article distributed under the terms of the Creative Commons Attribution License (CC BY). The use, distribution or reproduction in other forums is permitted, provided the original author(s) and the copyright owner(s) are credited and that the original publication in this journal is cited, in accordance with accepted academic practice. No use, distribution or reproduction is permitted which does not comply with these terms.
*Correspondence: Salvatore Cuzzocrea, salvator@unime.it; Salvatore.Cuzzocrea@unime.it
†These authors have contributed equally to this work