- 1Leigh Marine Laboratory, Institute of Marine Science, University of Auckland, Leigh, New Zealand
- 2National Institute of Water and Atmospheric Research, Wellington, New Zealand
- 3Department of Marine Biology, Texas A&M University at Galveston, Galveston, TX, United States
- 4JASCO Applied Sciences (Australia) Pty Ltd., Capalaba, QLD, Australia
- 5National Marine Mammal Laboratory, National Oceanic and Atmospheric Administration, National Marine Fisheries Service, Alaska Fisheries Science Center, Seattle, WA, United States
- 6School of Biological Sciences, The University of Auckland, Auckland, New Zealand
Effective management of wild animal populations relies on an understanding of their spatio-temporal distributions. Passive acoustic monitoring (PAM) is a non-invasive method to investigate the distribution of free-ranging species that reliably produce sound. Critically endangered Antarctic blue whales (Balaenoptera musculus intermedia) (ABWs) co-occur with pygmy blue whales (B. m. brevicauda) (PBWs) around New Zealand. Nationally, both are listed as “data deficient” due to difficulties in access and visual sub-species identification. PAM was used to investigate the distributions of blue whales via sub-species specific song detections in central New Zealand. Propagation models, incorporating ambient noise data, enabled the comparison of detections among recording locations in different marine environments. ABW detections peaked during austral winter and spring, indicating that New Zealand, and the South Taranaki Bight (STB) in particular, is a migratory corridor for ABWs. Some ABW calls were also detected during the breeding season (September and October). PBW calls were highly concentrated in the STB, particularly between March and May, suggesting that an aggregation of PBWs may occur here. Therefore, the STB is of great importance for both sub-species of blue whale. PBW detections were absent from the STB during parts of austral spring, but PBWs were detected at east coast locations during this time. Detection area models were valuable when interpreting and comparing detections among recording locations. The results provide sub-species specific information required for management of critically endangered ABWs and highlight the relative importance of central New Zealand for both sub-species of blue whale.
Introduction
The effective management of wild animal populations relies on a foundation of knowledge regarding their distribution and movements. A thorough understanding of the areas used by a population is necessary to consider the relative importance of each area for prioritising conservation or management approaches. Distribution studies are particularly important for species that are threatened with extinction, and those that live in areas subject to increasing anthropogenic activity or global phenomena, such as climate-induced range shifts (McLellan and Shackleton, 1989; Chen et al., 2011). Passive acoustic monitoring (PAM) is a non-invasive technique that can be used to study the presence and distribution of animals that produce sound. Songbirds, primates, amphibians, and marine mammals are ideal candidates to study via PAM, as many naturally produce loud, stereotyped vocalisations (Edds-Walton, 1997; Zimmer, 2011; Sanders and Mennill, 2014; Alvarez-Berríos et al., 2016; Kalan et al., 2016). Acoustic detections act as an indicator of animal presence, from which movements and distributions can be inferred, particularly when acoustic recording devices are deployed over a large area (Hannay et al., 2013; Ross et al., 2018). Autonomous PAM can be particularly useful when collecting long-term data in environments that cannot be surveyed regularly using visual methods, such as dense forest or offshore marine areas, where access is constrained by logistical challenges and costs. An acoustic approach can also be useful for vocal species that are difficult to study visually. Such instances occur when features used for identification are cryptic, and can be magnified if the study species is difficult to observe. For example, due to their low surface profile and short surfacing periods, deep-diving beaked whales of the family Ziphiidae demonstrate visual crypsis, but species-specific signal properties of their vocalisations enable acoustic identification (Baumann-Pickering et al., 2013).
In the waters of New Zealand, two sub-species of blue whale occur (Branch et al., 2007): the Antarctic blue whale (Balaenoptera musculus intermedia) (ABW); and the pygmy blue whale (B. m. brevicauda) (PBW). Anatomical differences exist between the two sub-species (Ichihara, 1966; Olson et al., 2015), but can be difficult to recognise in the field. As such, information about blue whales in New Zealand waters has often been conflated by the inclusion of both sub-species (see Stephenson et al., 2020). Identification challenges, in addition to access constraints imposed by their offshore location, have led to both sub-species being classified as “data deficient” at a national level, although PBWs are also listed as “resident native” (Baker et al., 2019) due to focused studies on this sub-species (Torres, 2013; Olson et al., 2015). Globally, blue whales are classified as “endangered” (Cooke, 2018a). At the sub-species level, ABWs are listed as “critically endangered” (Cooke, 2018b) as a result of industrial whaling in the 1900s that greatly reduced population worldwide (Branch et al., 2007), while PBWs are listed as “unspecified” (Pollock, 2019). Accordingly, information on blue whale distributions that is not sub-species specific is not appropriate for management decisions.
Worldwide, blue whales produce low frequency, stereotyped calls, known as song when repeated regularly (Oleson et al., 2007; Lewis and Širović, 2018), which differ between sub-species, and between populations within sub-species (McDonald et al., 2006; Širovic et al., 2017). Therefore, the sub-species of a blue whale is easier to determine acoustically than visually, making PAM an ideal approach to study blue whales with confidence in sub-species identity (McDonald et al., 2006). It is straightforward to distinguish between the two blue whale sub-species found around New Zealand via song. PBW song was first recorded in New Zealand off Great Barrier Island – Aotea, northern New Zealand, in 1964 (Kibblewhite et al., 1967) and consists of four distinct units (A, B, C, and D) that form a phrase. Energy occurs between 17 and 24 Hz and overall phrase duration is 55 s, with 132 s on average between phrases (Miller et al., 2014). Conversely, ABWs produce individual song units, known as “Z” calls due to their distinctive shape when visualised as a spectrogram. ABW units commence at 26 Hz, decreasing rapidly to 18 Hz, with a duration of approximately 20 s (Širović et al., 2004; Gavrilov et al., 2012). “Z” calls are repeated every 62–65 s during song (Širović et al., 2004; McDonald et al., 2006). Female blue whales are not thought to produce song (Oleson et al., 2007), but song is produced by male blue whales year-round (Širović et al., 2004; Leroy et al., 2016; Thomisch et al., 2016). Both male and female blue whales from both sub-species also produce a non-song down-sweeping call, known as the “D” call (McDonald et al., 2001).
Blue whale song units are low frequency, have high source levels, and propagate efficiently underwater, up to hundreds, or even thousands, of kilometres (Stafford et al., 1998; Širović et al., 2007). It is presumed that blue whale song is used for long-range communication, and the acoustic properties of song units may be a result of evolutionary selection (Edds-Walton, 1997). Long propagation distances can make it difficult to interpret detections made within a PAM framework, as the location of a calling animal may be a considerable distance from the receiver. Underwater, propagation conditions vary over space and time due to parameters that include bathymetry, seabed sediment, and sound speed profile (Urick, 1983). Inferences made from call detections increase in validity when the detection area of the receiver is taken into account (Stafford et al., 2007; Širović et al., 2007; Samaran et al., 2010; Miksis-Olds et al., 2015; Širovic et al., 2015; Darras et al., 2016; Biggs et al., 2019). Moreover, it is essential to quantify effective detection areas when estimating animal abundance from acoustic detections (Marques et al., 2013; Harris et al., 2018; Nuuttila et al., 2018). The detection area of each recorder in a network should be taken into consideration to provide context to acoustic detections and to enable comparisons among recording locations (Darras et al., 2016; Biggs et al., 2019).
As implied by their “data deficient” statuses, previous investigations of blue whale distribution in New Zealand waters have been constrained by difficulties in sub-species identification, and limited access to these offshore marine mammals due to constraints arising from logistical challenges and weather and sea conditions. In this study, PAM was used to investigate the spatial and temporal distributions of PBWs and critically endangered ABWs around central New Zealand. The South Taranaki Bight (STB) in central New Zealand has been a focus for blue whale research (Barlow et al., 2018), and is predicted to be a region with high probability of occurrence of blue whales (see Stephenson et al., 2020). As acoustic recorders were deployed in a range of physical marine environments, propagation modelling was conducted to consider detections in range context and to enable comparisons among recording locations. Results from this study add sub-species specific clarity to blue whale distributions and movements in central New Zealand waters, and improve understanding on the importance of this region to the two blue whale sub-species.
Methodology
Acoustic Recordings
Autonomous multi-channel acoustic recorders (AMAR G3, JASCO Applied Science) were deployed from 4 June to 21 December 2016 at four locations (between 13 and 60 km from shore) around central New Zealand: the South Taranaki Bight (STB); Cook Strait; and off the coasts of Kaikōura and Wairarapa (henceforth referred to as Kaikōura and Wairarapa) (Figure 1). AMARs were redeployed for a second period between 21 February and 8 September 2017 at three locations: Kaikōura; Wairarapa; and a STB location 25.2 km south-east from the 2016 STB station (Figure 2; a recorder was not redeployed in Cook Strait in 2017 but is shown for reference). In the STB and Cook Strait, AMARs were bottom mounted in water depths of 111 and 252 m, respectively. At Wairarapa and Kaikōura, AMARs were moored 10 m above the sea-bed in water depths of 1480 and 1252 m, respectively. The nominal pressure sensitivity level of the hydrophones was −165 dB re 1 V/μPa (±1 dB) from 10 Hz to 100 kHz. Acoustic data were sampled over a 900 s duty cycle: 630 s at a sampling rate of 16 kHz, 125 s at a sampling rate of 250 kHz, and 145 s of sleep. Only the 16 kHz files were analysed within the scope of this study.
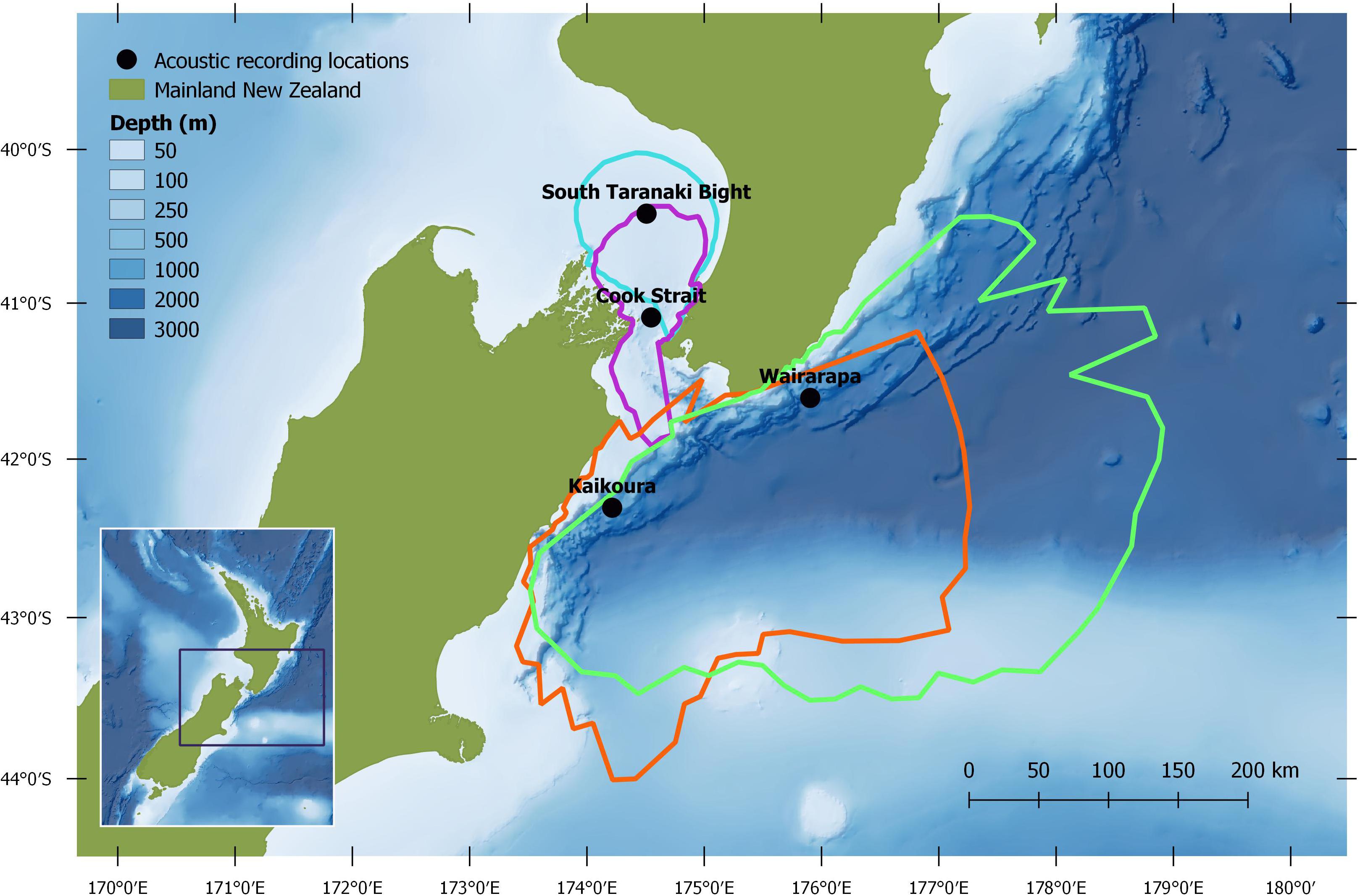
Figure 1. Study region, with median modelled Antarctic blue whale detection areas for June 2016: turquoise = South Taranaki Bight, purple = Cook Strait, green = Wairarapa, orange = Kaikōura. See Methodology for modelling configuration and assumptions.
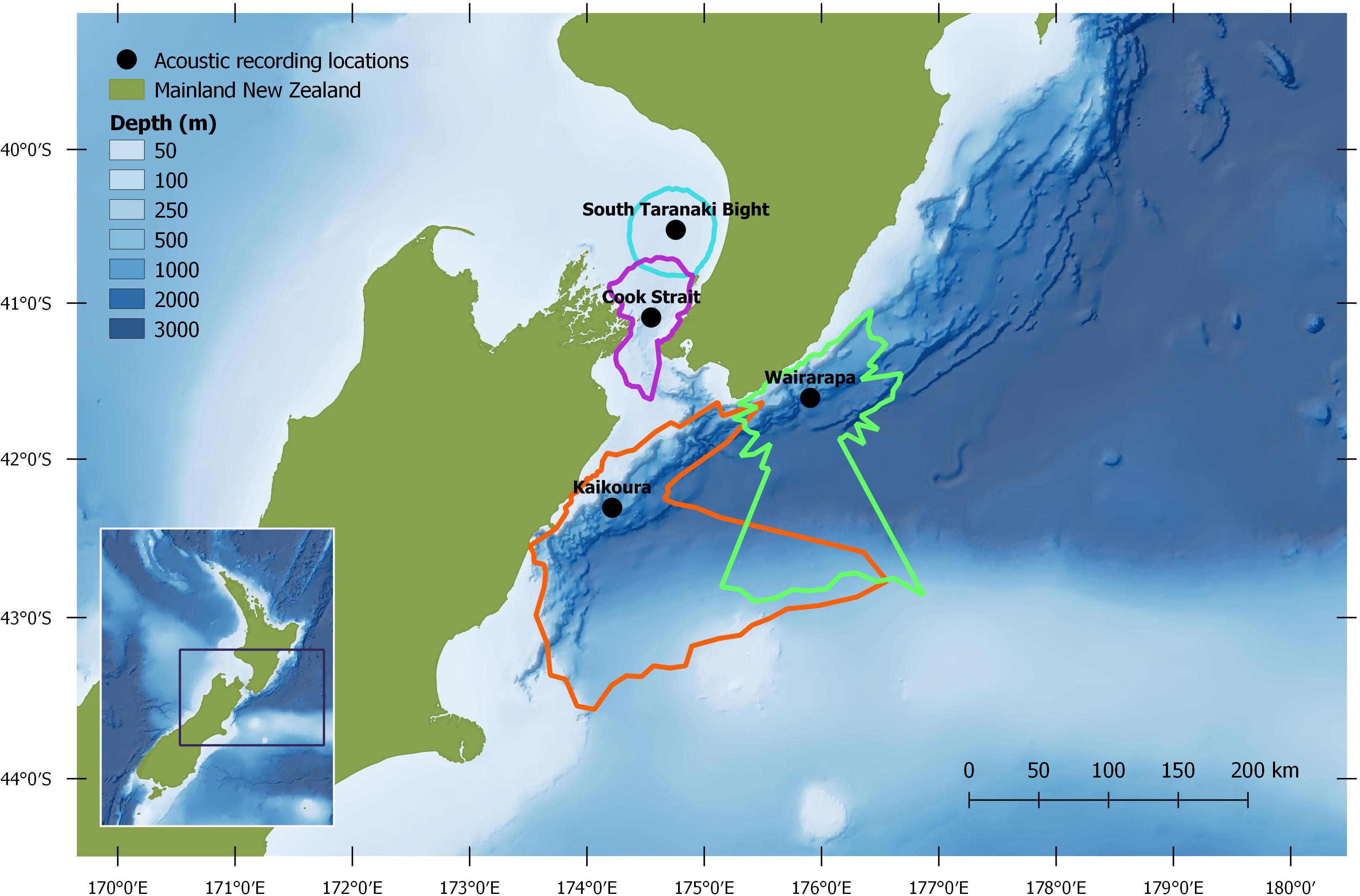
Figure 2. Study region, with median modelled pygmy blue whale detection areas for March 2017: turquoise = South Taranaki Bight, purple = Cook Strait, green = Wairarapa, orange = Kaikōura. See Methodology for modelling configuration and assumptions.
Detection of Blue Whale Calls
Data from each recording location were sub-sampled via stratified random sampling: within every 2-hour period, 630 s (10.5 min) were sampled (one randomly selected 16 kHz duty cycle, equivalent to 12.5% of all 16 kHz recordings, and 8.75% of each 2 h interval). Data were visualised using PAMlab-Lite (JASCO Applied Sciences, 2017) as spectrograms displayed over 10–100 Hz; the spectrogram frequency resolution was 0.244 Hz computed using 2 s of Hamming-windowed data (plus 2 s of zero-padding) and 75% overlap. All blue whale song units and phrases within subsamples were manually identified and marked, regardless of whether they occurred in song (with consistent inter-call intervals) or were stand-alone. The minimum and maximum fundamental frequencies, and start and end time extents of each phrase (PBW) or unit (ABW), were marked manually to “box” each call. PAMlab-Lite automatically reported time, frequency and sound level parameters for each boxed call within the stratified random subsample. Sound level information included values for the received sound pressure level (SPL) of the marked sound. For ABWs, each song unit was marked separately, while for PBWs, measurements were made across all four units (A, B, C, and D) as a single phrase. In cases where entire units or phrases were not evident in the recordings, only the evident aspects were measured. For simplicity, both ABW units and PBW phrases will henceforth be referred to as “calls.” Call annotations were imported into MATLAB (The MathWorks Inc, 2016) for analysis.
A seismic survey, the Schlumberger Pegasus Basin 3D seismic survey, was coincidentally conducted near Wairarapa between mid-November 2016 and June 2017 (Schlumberger New Zealand Limited, 2016). Data from Wairarapa were not analysed during the seismic survey period, due to raised sound levels in the blue whale call bandwidths that impeded the detection of calls. Seismic survey pulses were evident to a lesser extent in the data from Kaikōura and occurred concurrently with low frequency sound (<100 Hz) generated by earthquake activity in November and December 2016. Although sound levels in the blue whale call bandwidth were raised at Kaikōura during these periods, blue whale calls remained identifiable throughout and were marked. Blue whale calls that occurred simultaneously (in time and frequency) with tonal ship noise were annotated if their duration and/or inter-call interval were evident within the shipping noise. When ship noise dominated the blue whale call bandwidth, such that individual calls were masked, possible calls were not marked to minimise false positive detections.
Detection Areas
As central New Zealand features a variety of marine environments, sound propagation in the study area was modelled to enable comparisons to be made among recording locations, and to estimate the possible positions of calling blue whales. Transmission loss modelling was conducted for each of the four recording locations. JASCO’s Marine Operations Noise Model (MONM) (Matthews and MacGillivray, 2013) was used to estimate propagation loss within the 25 Hz-centred decidecade band [22 to 28 Hz, International Organization for Standardization (2017)]. MONM computes sound propagation via a wide-angle parabolic equation solution to the acoustic wave equation (Collins, 1993) based on a version of the U.S. Naval Research Laboratory Range-dependent Acoustic Model (RAM), which has been modified to account for a solid seabed (Zhang and Tindle, 1995). The parabolic equation method (Collins et al., 1996) has been extensively benchmarked (e.g., Racca et al., 2015; Quijano et al., 2019). The 25 Hz-centred decidecade band was chosen as it contains the upper limit of tonal energy for both blue whale sub-species calls (approximately 26 Hz for ABW “Z” call units and 23 Hz for PBW “B” call units).
Marine Operations Noise Model was used to calculate propagation losses within a 250 km radius around each recorder location. Acoustic fields in three dimensions were computed by modelling transmission loss within two-dimensional (2-D) vertical planes aligned along radials covering a 360° swath. Here, the vertical radial planes were separated by an angular step size of 5°, yielding 72 planes. Within each vertical radial plane the sound field was sampled with a horizontal step size of 50 m and across the entire water column, from 2 m to a maximum of 4000 m, with step sizes that increased with depth. Recorders were considered as either seabed receivers or 10 m above the seabed, per the AMAR deployments.
Information regarding the physical environment of the four recording locations was included in the models: bathymetry, geology, and water column sound speed profiles (SSPs). Bathymetry was derived from “Charting Around New Zealand” (CANZ, 2008). As described in McPherson et al. (2019), the seabed sediment consisted of mud in deeper waters (continental slope sediment), and dominant fine sand with sparse coarse sand in shallow waters (continental shelf sediment). SSPs were derived from temperature and salinity profiles from the U.S. Naval Oceanographic Office’s Generalized Digital Environmental Model V 3.0 (GDEM; Teague et al., 1990; Carnes, 2009). The GDEM temperature-salinity profiles were converted to sound speed profiles according to Coppens (1981). Mean monthly sound speed profiles were derived from the GDEM profiles for a 100 km radius encompassing each site.
Detection ranges were estimated for the months in which blue whale call detections were most prevalent: June 2016 for ABWs; and March 2017 for PBWs. Transmission loss for each recording location was modelled with location-specific and month-specific ambient noise data and SSPs. Median (50th percentile) ambient noise was quantified for the 25 Hz-centred decidecade band from the acoustic recordings. During March 2017, the seismic survey conducted near Wairarapa influenced ambient noise levels in the 25 Hz-centred decidecade band such that detection areas would not have been relevant to periods without seismic survey presence. As such, instead of March 2017, ambient noise was averaged over the whole duration of the first acoustic deployment at Wairarapa (June to December 2016) and incorporated into the model for PBW detection range at Wairarapa. Similarly, average ambient noise from the first deployment (June to December 2016) was used to model the detection area for PBWs at Cook Strait as no acoustic data were recorded at this location in 2017. These two models included March-specific SSP data.
The detection range modelling approach assessed the unconditional probability of detection, accounting for the empirical probability distribution of the ambient noise and modelled distribution of received levels. The distribution of modelled received levels was computed using a normal probability distribution of source levels and modelled values of transmission loss. This analysis considered only the median ambient noise percentile to provide a general guide for the potential detection ranges under median noise conditions, although significant differences could exist across all possible noise conditions. The detection ranges associated with an unconditional probability of 0.5 and median noise conditions are hereafter referred to as “median detection areas.” A detection threshold of 5 dB re 1 μPa above the median noise level was applied to present a conservative estimate of detectability. The source level of ABW calls was assumed to be 189 dB re 1 μPa m ± 3 dB (Širović et al., 2007), and 183 dB re 1 μPa m ± 3 dB for PBW calls (McCauley et al., 2001). The transmission loss model assumed that both sub-species produced the “Z” call and “B” unit at depths between 20 and 30 m (Thode et al., 2000; Oleson et al., 2007; Širović et al., 2007; Leroy et al., 2016; Owen et al., 2016; Dziak et al., 2017; Lewis et al., 2018). A curvefit approach was used to calculate the maximum possible ranges to a calling animal along each of the 72 radial planes per location considering the aforementioned modelling configuration. Overall detection areas were calculated by generating a polygon for each recording location considering the median detection range for each modelled radial (QGIS Development Team, 2017). Blue whale detections were normalised by the respective detection areas of the recording locations in order to compare detections among sites. The normalised call counts are henceforth referred to as “call density.”
To consider the appropriateness of extrapolating detection areas beyond the specific months they were generated for, the detection ranges were also calculated for opposite scenarios i.e., detection areas of ABWs were estimated using the March-specific model, and PBW detection areas were estimated using the June-specific model. For both sub-species, March and June detection area estimates were very similar and it was therefore deemed appropriate to apply the estimated sub-species specific detection areas across both acoustic deployment periods.
Analysis of Received Levels of Blue Whale Calls
In order to consider the possible positions and movements of calling blue whales within the detection areas, the received levels of manually detected calls were examined with respect to time. To ensure relevance and comparability to the detection thresholds estimated via the propagation models, only the received levels of the upper tone of ABW calls (approximately 26 Hz) and the “B” unit of PBW phrases (23 Hz) were examined. When ABW calls were received only as the 26 Hz tone, with lower frequency aspects absent due to transmission loss, the SPL was calculated automatically by PAMlab-Lite. For ABW calls where the whole of the “Z” call was received and marked, and for PBW phrases, the marked frequency and temporal limits of each call were revised to only include the upper part of the “Z” call, or the “B” unit, respectively. SPL was recalculated for the revised temporal and frequency limits. It was not possible to revise the received levels of all calls, due to occasional interference from other sounds in the same bandwidth.
Results
Across all recording locations, a sub-sample of 106.5 days of data (14,608 files, each 630 s in length) were manually analysed. ABW calls were detected in 4.1% and PBW calls were detected in 21.2% of the subsamples. Both ABWs and PBWs were detected in 0.9% of subsamples. In total, 20,751 blue whale calls were detected; 16.0% of detected calls were from ABWs and 84.0% were from PBWs (Tables 1, 2). The median low and high frequencies of the ABW calls were 24.1 Hz (standard deviation = 3.6 Hz) and 26.8 Hz (standard deviation = 0.5 Hz), respectively. Oftentimes, only the upper frequency of the “Z” calls was detectable. The median duration of ABW calls was 14.9 s (standard deviation = 4.2 s). PBW calls featured median low frequency of 15.9 Hz (standard deviation = 3.3 Hz) and median high frequency of 25.1 Hz (standard deviation = 1.0 Hz). Median duration of PBW calls was 37.9 s (standard deviation = 12.4 s). Received levels were available for 2,848 ABW calls (85.9%) and 11,291 PBW calls (64.8%).
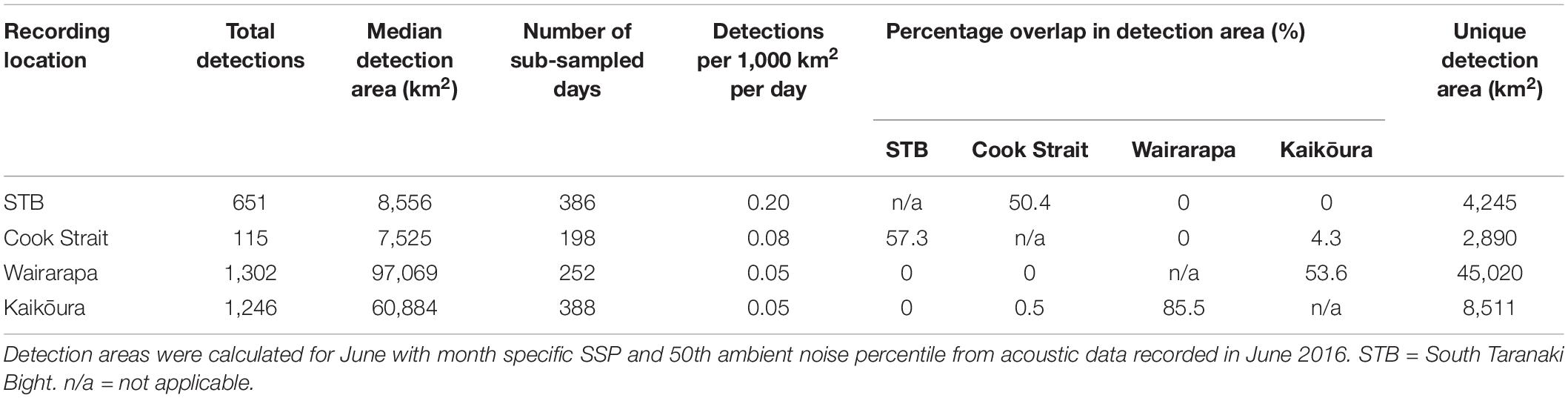
Table 1. Antarctic blue whale call detections per recording location, and detection area information.
Antarctic blue whales calls were detected at all four recording locations (Table 1 and Figure 3A). In 2016, the number of daily ABW detections peaked at the end of June into the beginning of July (austral winter), while in 2017, peak occurrence was during July and August (Figure 3A). The first winter detections of ABWs in 2016 occurred at Kaikōura (the most southerly recorder), followed by concurrent detections at Wairarapa and Cook Strait 4 days later, and lastly at STB (the most northerly recorder) an additional 3 days later (Figure 3A). There was no spatio-temporal pattern in detections during 2017 (Figure 3A). A smaller, secondary peak in ABW calls occurred during austral spring (October 2016), with detections occurring first at STB, then at Wairarapa, followed by Kaikōura, and lastly Cook Strait (Figure 3A). ABW detections were most numerous at Kaikōura and Wairarapa, particularly during 2016 (Figure 3A and Table 1), but were detected with highest density (as detections per 1000 km2) at Cook Strait and STB (Figure 4A and Table 1). The received levels of ABW calls were highest at Wairarapa and Kaikoura (Figure 5), with maximum received levels exceeding 120 dB re 1 μPa.
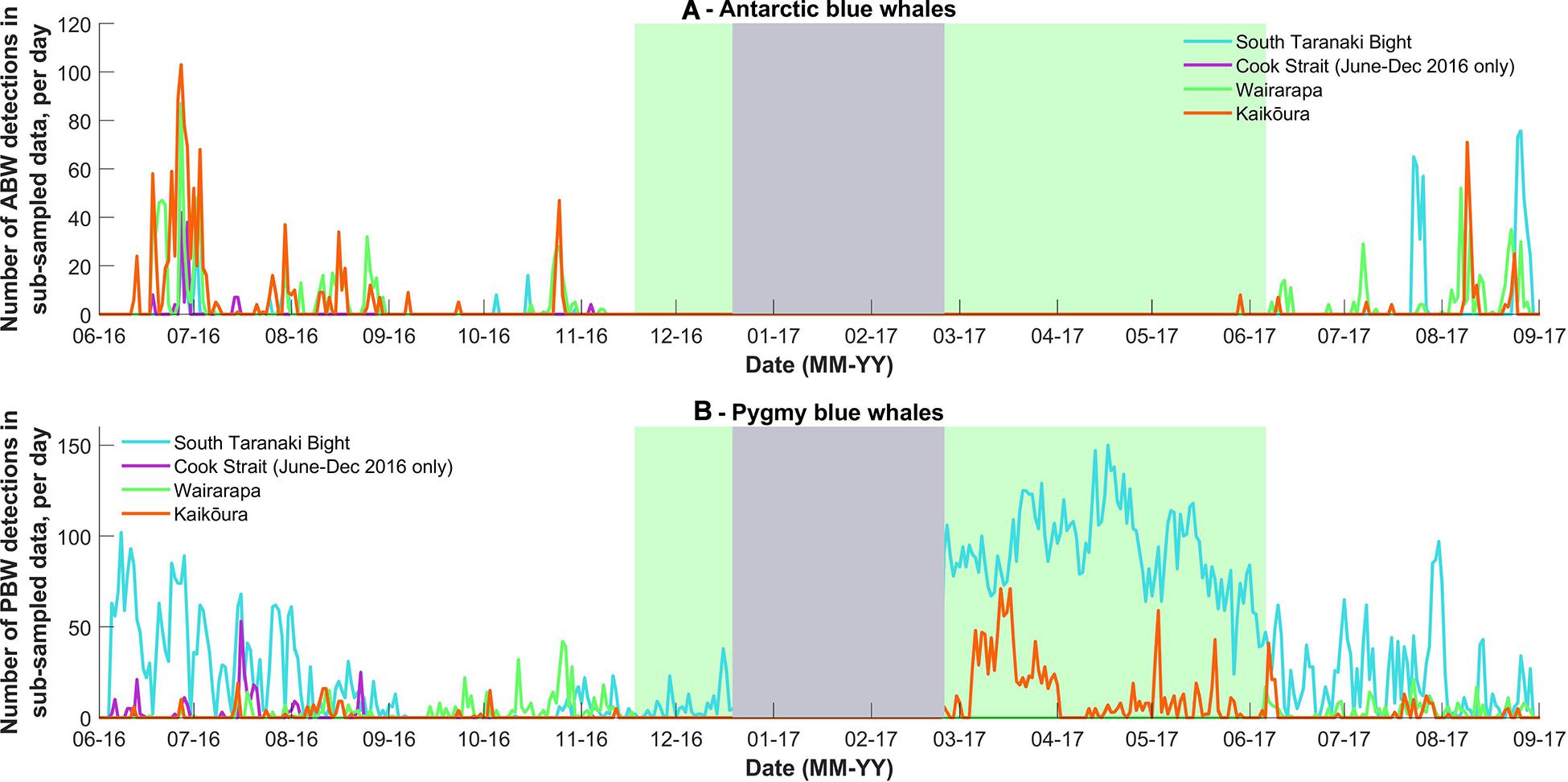
Figure 3. (A) The number of Antarctic blue whale and (B) pygmy blue whale calls detected per day in the stratified random subsample of data from 2016 and 2017. The grey block indicates when the acoustic recorders were not deployed. The green block corresponds to the period in which the Wairarapa data were not analysed due the presence of seismic survey sound in the blue whale call bandwidth. Note Y-axis scale differs between sub-species.
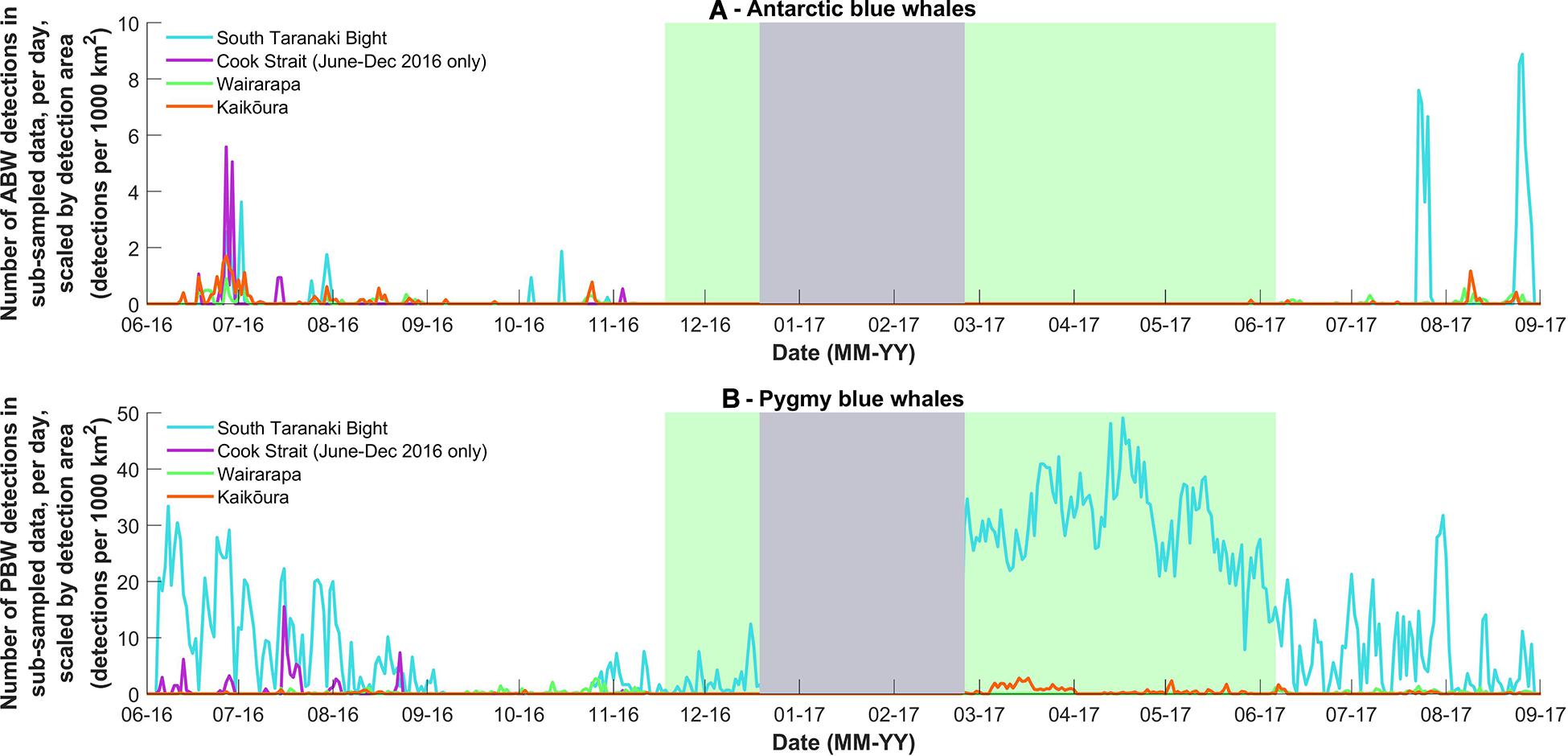
Figure 4. (A) The number of Antarctic blue whale and (B) pygmy blue whale calls detected per day in the stratified random subsample of data from 2016 and 2017, normalised by the detection area of each recording location (calculated for June 2016 or March 2017, respectively) to give daily detections per 1000 km2. The grey and green blocks are as described in Figure 3. Note Y-axis scale differs between sub-species.
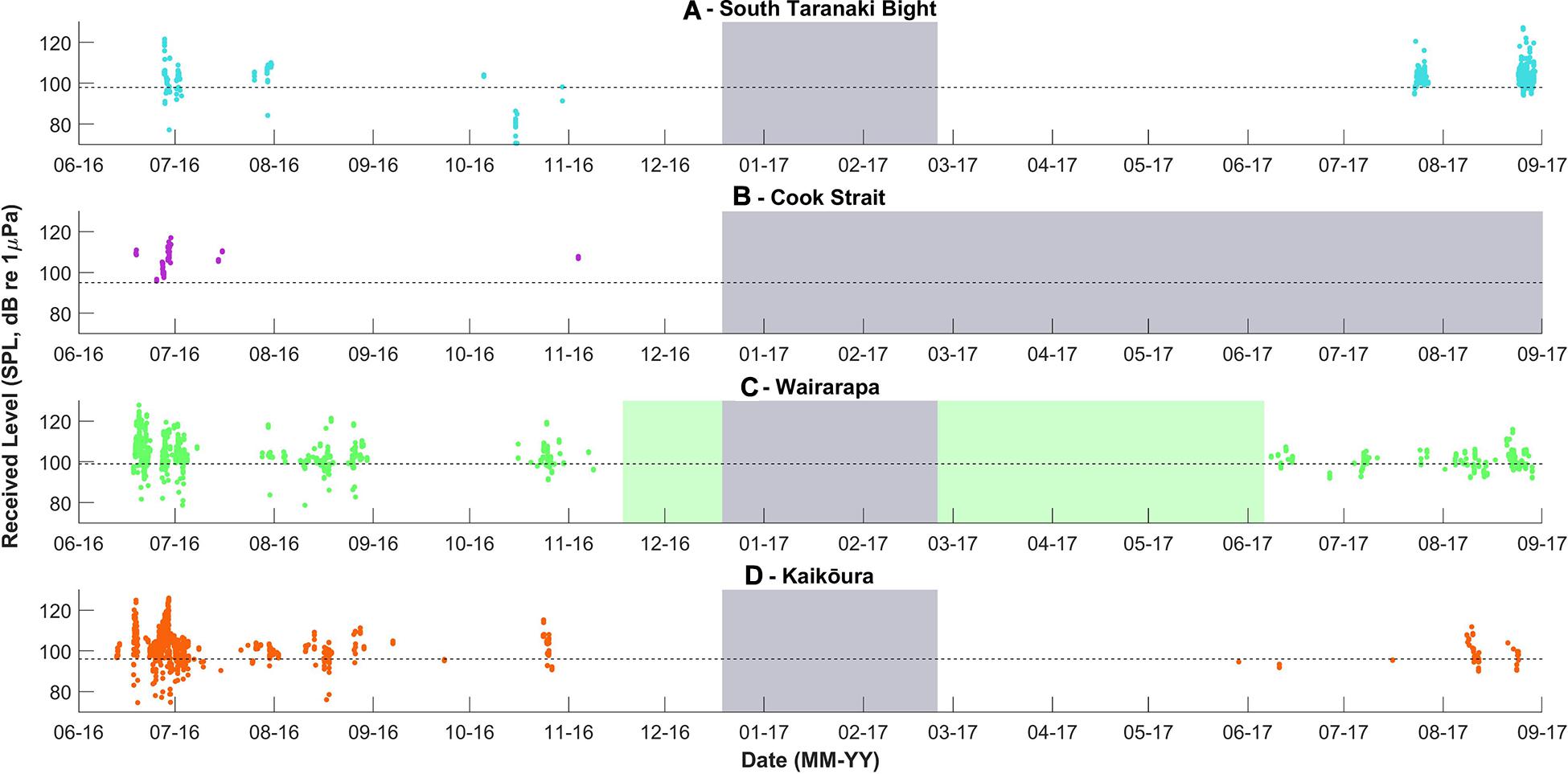
Figure 5. Received levels of Antarctic blue whale calls detected per recording location, over time. Received levels were calculated only for the upper tone (approximately 26 Hz) of each call. The dashed lines indicate the detection threshold associated with an unconditional probability of detection of 50%, the model configuration is described in the Methodology. The grey and green blocks are as described in Figure 3.
Pygmy blue whale calls were also detected at all four recording locations in central New Zealand, although 84.1% of all PBW detections were made at STB (Figure 3B and Table 2). There, PBW calls were detected with the highest density; an average of 12.43 detections were made per 1,000 km2 per day, compared to average densities of less than 1 detection per 1,000 km2 per day at the other recording locations (Table 2). Both the highest (150 dB re 1 μPa) and lowest (67 dB re 1 μPa) received intensities of PBW calls were recorded at STB (Figure 6). The presented detection area extents correspond to median detection areas, however, the received levels reported for measured calls include all annotated calls, and as such include received levels below the modelled detection threshold. At STB, PBW calls were detected throughout both recording periods, except during the majority of September and October 2016 (austral spring) (Figures 3B, 4B). While PBW calls were largely absent from STB and Cook Strait during September and October 2016, detections were made at Kaikōura and Wairarapa during this time, and even increased in number compared to prior months (Figures 3B, 4B). Call detections peaked in number and density at STB between March and May (austral autumn), and a March peak in PBW calls was also evident at Kaikōura (Figures 3B, 4B). There was no acoustic sampling at Cook Strait or Wairarapa during this period.
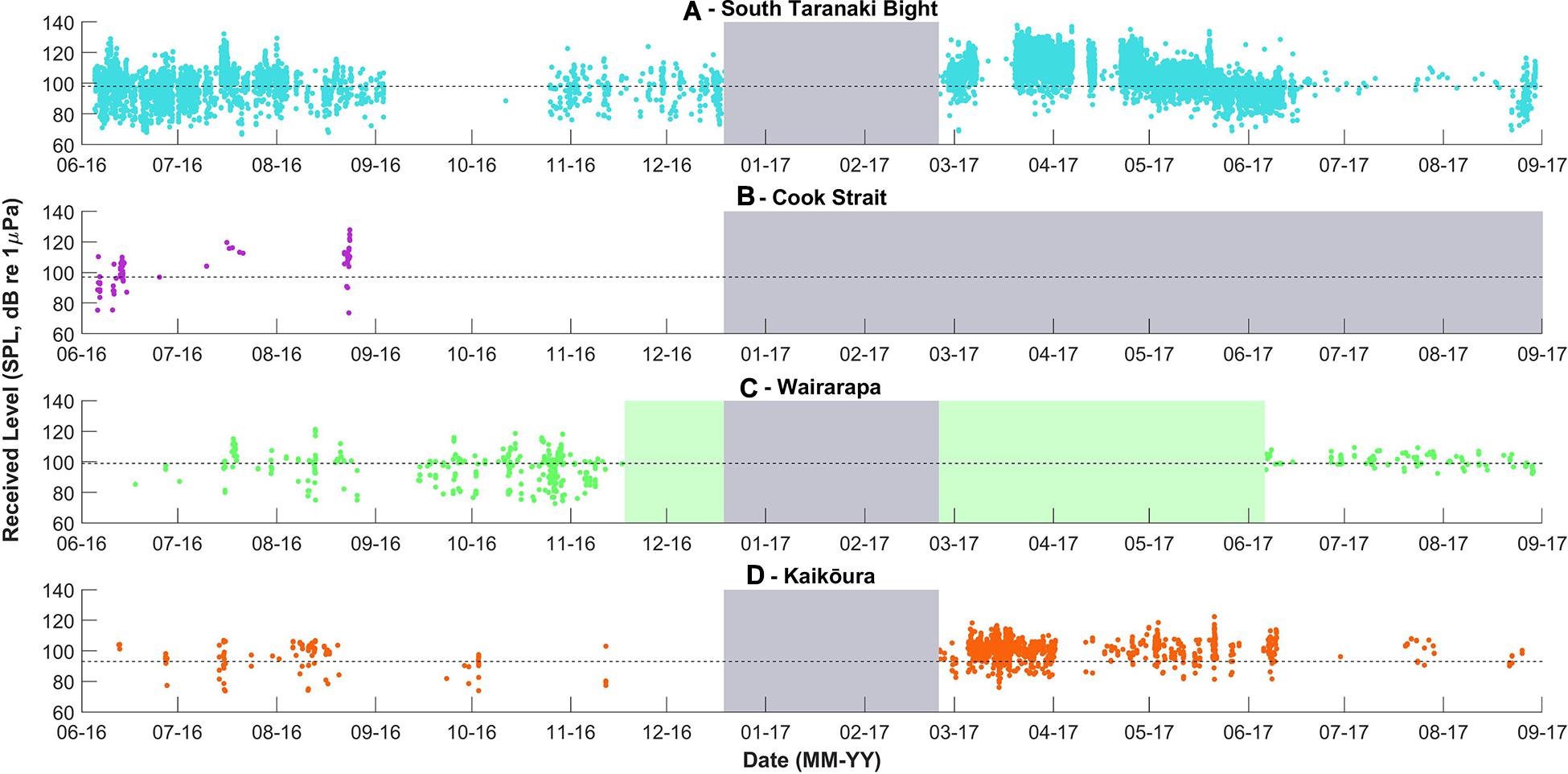
Figure 6. Received levels of pygmy blue whale calls detected per recording location, over time. Received levels were calculated only for unit “B” of each phrase. The dashed lines indicate the detection threshold associated with an unconditional probability of detection of 50%, the model configuration is described in the Methodology. The grey and green blocks are as described in Figure 3.
Normalisation by detection area was important as the areas of Cook Strait and STB were up to 13 times smaller than the detection areas of Wairarapa and Kaikōura (Tables 1, 2). Indeed, ABW detection areas at Wairarapa and Kaikōura extended to the limits of the 250 km modelling radius along some radials in a north-easterly direction (Figure 1). Detection areas for PBW calls were smaller than ABW detection areas at all recording locations, as a result of the lower source level for this sub-species (Tables 1, 2). Blue whale calls produced within areas where detection ranges overlapped could have been detected twice, at two recording locations. Detection areas overlapped between STB and Cook Strait, and between Kaikōura and Wairarapa (Tables 1, 2 and Figures 1, 2), and there was a small amount of overlap between the Cook Strait and Kaikōura ABW detection areas (Table 1 and Figure 1).
Discussion
In the waters of New Zealand in the western South Pacific, critically endangered Antarctic blue whales (ABWs) co-occur with pygmy blue whales (PBWs). However, due to their offshore domain and the challenge of sub-species identification, information about their movements and distributions has been limited and often conflated. Passive acoustic monitoring (PAM) proved to be a highly effective method to examine the spatial and temporal distributions of ABWs and PBWs by analysing their sub-species specific calls. The calls of both blue whale sub-species were highly stereotyped and comparable to those reported in previous studies (Širović et al., 2004; McDonald et al., 2006; Gavrilov et al., 2012; Miller et al., 2014). Modelled estimates of detection areas provided context to the detected blue whale calls, and highlighted the relative importance of the varying marine environments that exist in central New Zealand. The findings also elucidated the sympatry of ABWs and PBWs in central New Zealand. Future management and conservation of blue whales within central New Zealand waters can now be informed by sub-species-specific distribution information.
Antarctic blue whales were detected moving northward through central New Zealand waters in austral winter, and have previously been detected off northern New Zealand during this season (McDonald, 2006). A second peak in ABW calls was detected in spring, indicative of southbound movement. These data provide evidence that New Zealand waters are a migratory corridor for critically endangered ABWs, which feed in Antarctic waters during austral summer, and breed in warmer waters during austral winter (Širović et al., 2004; Branch et al., 2007). Similar bimodal blue whale detections have been reported in migratory corridors in the sub-Antarctic (Leroy et al., 2016), and off the coast of Madagascar (Cerchio et al., 2019). There is a general paucity of information regarding ABWs in the western South Pacific (Branch et al., 2007), but possible breeding areas of ABWs include Tonga and Samoa (Brodie and Dunn, 2015; Balcazar et al., 2017). Some ABW calls were detected in acoustic data from the east coast of central New Zealand (Wairarapa and Kaikōura) between the two modal peaks (September and October). These detections suggest that some ABWs remain within the detection range of the east coast recorders during the breeding season. Alternatively, the calls detected during this time could have been produced by late northbound whales or early southbound whales. Prior to 2019, ABWs were listed as “migrant” in New Zealand, implying that they occurred predictably and cyclically, but did not breed in national waters (Baker et al., 2016). The findings of the present study leave uncertainty regarding the possibility of ABWs using New Zealand waters as a breeding ground and thus support their revised national “data deficient” status (Baker et al., 2019). Further research is required to determine whether ABWs breed in New Zealand waters. The year-round acoustic presence of ABWs has been reported at both high and low latitudes (Širović et al., 2004, 2007; Samaran et al., 2010; Leroy et al., 2016; Thomisch et al., 2016), implying that their migration is complex and not obligate behaviour (Thomisch et al., 2016). However, ABW detections in central New Zealand waters were strongly indicative of migratory behaviour, and there was no evidence of their year-round acoustic presence at this mid-latitude study site.
Antarctic blue whale detections implied that the northbound migratory corridor along the east coast of the South Island of New Zealand (past Kaikōura) splits in the vicinity of Cook Strait, with some ABWs continuing along the east coast of the North Island and some ABWs travelling through Cook Strait into the STB. During migration, blue whales exhibit apparent preferences for continental shelf edges and deep water (Širović et al., 2004; Branch et al., 2007; Bailey et al., 2009; Gavrilov and McCauley, 2013; Double et al., 2014), perhaps due to the long-distance communication that is possible within such environments. These habitat features exist along the east coast of New Zealand and support the idea that some ABWs travel along the shelf edge during migration. That said, the large ABW-specific detection area of the Wairarapa recorder included the eastern entrance to Cook Strait, meaning that ABWs detected at Wairarapa could have migrated through Cook Strait. However, the received levels of some ABW calls detected at Wairarapa during northbound migration were the loudest ABW calls recorded at this location, and much louder than the threshold of the detection area. Therefore, some calling animals were likely close to the recorder, and hence travelling along the east coast of the North Island. Nonetheless, ABW detections were recorded with greatest density in the STB. Given that call density was similar between Cook Strait and Wairarapa, but substantially higher in the STB, it is plausible that only a portion of the ABWs detected in the STB had travelled through Cook Strait, with additional whales entering the STB from the western coast of New Zealand. The waters of the STB feature high productivity (Chiswell et al., 2017) and are a known hotspot for blue whale feeding (Torres, 2013; Torres et al., 2020). Opportunistic ABW feeding occurs during migration in other locations (Gill, 2002; Tripovich et al., 2015; Balcazar et al., 2017; Gill et al., 2011), and migration through the STB may therefore allow ABWs to forage before or after the breeding season. If ABWs feed opportunistically during migration, resource competition could occur between the two blue whale sub-species in New Zealand waters. A larger number of ABW calls were detected in the STB in winter 2017 than in winter 2016, although it is not known whether this was a reflection of greater productivity in the area.
In addition to the seasonal presence of ABWs, the STB proved to be an important location for PBWs, as evidenced by the high number and density of sub-species specific calls, particularly during austral autumn (March to May). Barlow et al. (2018) also reported 100% daily acoustic presence of PBWs in the STB during the 2016 austral autumn. In south-west Australia, calls of east Indian Ocean PBWs also peak during austral autumn (between February and May) (Stafford et al., 2011; Gavrilov and McCauley, 2013; McCauley et al., 2018). Unlike ABWs, PBWs do not migrate to Antarctica to feed (Branch et al., 2007) (see Attard et al., 2012 for exceptions), and therefore rely on local productive feeding areas, such as the STB (Barlow et al., 2020). An autumn peak in calling in the STB may be related to feeding activity, which has been observed in Australian waters during autumn (Gill, 2002). Male blue whales produce song year-round (Leroy et al., 2016; Thomisch et al., 2016), but song production may be mutually exclusive with feeding due to differences in energy requirements and depth between feeding and singing (Stafford et al., 2005; Wiggins et al., 2005; Oleson et al., 2007; Hazen et al., 2015). In order to explain the large number of call detections made during a presumed feeding period, the whales must have been exhibiting different behavioural states, with some singing and some feeding. Male PBWs could alternate between the two behaviours throughout the day, with song produced during times when feeding is not conducted (Oleson et al., 2007; Lewis and Širović, 2018). As female blue whales are not thought to produce song, they may spend more time feeding (Ladd et al., 2019). The apparent increase in call density during austral autumn may also have been a reflection of increased call production rates if the behavioural context in the area promoted calling.
Assuming that the number of call detections is proportional to the number of PBWs present, an aggregation of PBWs could occur in the STB, particularly during austral autumn. The variation observed in the received levels of PBW calls at STB indicated that PBWs occurred throughout the STB detection area, and likely beyond. The STB detection area overlapped somewhat with the detection area of the Cook Strait recorder, which could have resulted in double counting of some calls, but this would have scarcely altered the STB results. Aggregations of blue whales are rare in the southern hemisphere, with most animals occurring alone or in pairs (Branch et al., 2007; Garcia-Rojas et al., 2018; McCauley et al., 2018). The STB is an important industrial area in New Zealand waters. The presence of a PBW aggregation in the STB should promote the undertaking of further study in the area to determine fine-scale habitat use, their seasonal persistence of use, and whether static or dynamic protective measures should be implemented. Protective measures could include scheduling anthropogenic activities outside of key periods of PBW presence, although the seasonal movements of critically endangered ABW through this region should also be taken into consideration.
Pygmy blue whale detections were greatest during autumn at Kaikōura, and these calls were received at relatively high intensities. A satellite-tagged PBW spent several days off the Kaikōura coast in autumn 2018 (Goetz et al., in review), and also spent time in a known feeding area off the coast of Oamaru, further south on the east coast of the South Island (Olson et al., 2015; Goetz et al., in review). In the present study, PBW calls were also received at Kaikōura, and increased in number and density at Wairarapa, during austral spring (September and October), when detections declined at the STB and Cook Strait recording locations. Future research should investigate the spatial distribution and habitat use of blue whales along the east coast of the South Island, as this area may provide important resources for PBWs. Such research can now be conducted with greater confidence in the likely sub-species identity of observed blue whales, given the insights into ABW temporal presence provided here.
The decline in PBW detections in spring at Cook Strait and STB may have been a result of PBWs, particularly vocal mature males, undertaking movements away from Cook Strait and the STB. The received levels of the PBW calls at STB were lower during spring than at other times of year, suggesting PBWs had indeed moved further from the recording location. Barlow et al. (2018) also reported a decline in PBW calls in the STB during September and October 2016, although PBW calls were detected consistently year-round in deeper water to the west of the STB. Movements of individual PBW are largely unknown; two satellite tag deployments and ten inter-annual photographic resightings have been made, revealing movement between the east and west coasts of New Zealand (Olson et al., 2015; Barlow et al., 2018; Goetz et al., in review). PAM provides a population-level insight into spatial and temporal distributions, but alternative methods, such as photographic mark-recapture, genetic sampling, or satellite tag deployment would be required to ascertain individual-specific movements (Goetz et al., in review). The calls produced by PBWs in New Zealand have also been recorded in Tonga, as well as the south and east coasts of Australia (Balcazar et al., 2015; McCauley et al., 2018), and south of Tasmania (Miller et al., 2019), suggesting that the population has a larger distribution than just New Zealand national waters. Fewer call detections in the STB and Cook Strait during spring could have also resulted from vocal animals entering a behavioural state where they produced song at a reduced rate, or not at all. The proportion of mature males that produce song at a given time is largely unknown, although McCauley et al. (2001) suggested that less than 28% of PBWs call at a given time.
Consistent acoustic monitoring over time can provide insight into movements and inter-annual variation in distribution. In this relatively short-term study, the timing of the northbound ABW migration differed between 2016 and 2017, shifting from the end of June in 2016 to the end of July and into August in 2017. Specific drivers underpinning the timing of ABW migration could include ice cover or food abundance at high latitudes (Hauser et al., 2017; Szesciorka et al., 2020). Such large inter-annual variation in ABW migration timing could lead to challenges when scheduling anthropogenic activities to occur outside of important blue whale periods. Individual-specific information would aid in determining appropriate protective measures, if these were to be considered. Marine top predators, such as blue whales, can be considered ecosystem sentinels (Hazen et al., 2019) and their movements and distributions can be directly related to prey availability, often driven by physical oceanography, both on local and global scales (Ladd et al., 2019; Barlow et al., 2020). As such, movement data from individual animals may provide insight into whether dynamic protective measures that are responsive to external drivers would be more effective than static areas of protection (Hartel et al., 2015; Dwyer et al., 2020).
Individual-specific methods, such as genetic sampling, would also be essential to elucidate the demographic information necessary to interpret call detections into population-level inferences. The results presented here are only relevant for singing blue whales, which are presumed to be mature males. Secondary information regarding the sex ratio of animals in the study area is available only for PBW (not significantly different from 1:1) (Barlow et al., 2018); non-singing female blue whales could behave differently to song-producing males, and may even have different spatial or temporal distributions. Further insight could be gained from the current dataset via an analysis of “D” calls, which are produced by males and females of all sub-species of blue whale, and have been associated with multiple behavioural contexts (Oleson et al., 2007; Lewis et al., 2018; Schall et al., 2019). As they are not sub-species specific, “D” calls were not considered within the scope of this study, but their temporal and spatial distribution in central New Zealand could reveal nuances in blue whale activity across sexes that are not demonstrated here.
Many assumptions are required in terms of estimating detection ranges through acoustic modelling; variation in source levels, calling depths, and ambient noise can have a dynamic impact on detection areas over time (Stafford et al., 2007; Samaran et al., 2010; Miksis-Olds et al., 2015). Here, estimated blue whale detection areas from one month per sub-species (June 2016 or March 2017) were extrapolated across both deployment periods. Specific acoustic events, such as the seismic survey recorded at Wairarapa during 2016 and 2017, were generally not incorporated in the ambient noise levels included in the models to avoid biased detection area estimates. Ambient noise levels that were higher than average would have resulted in smaller detection areas. Moreover, these events could have influenced call detection likelihood, and even blue whale presence or calling behaviour, as noted in other locations (Di Iorio and Clark, 2010; Melcón et al., 2012). The use of median detection thresholds was selected as an approach to balance the various sources of uncertainty. The largest contributor to variability is ambient noise, which can vary significantly. As noted during the analysis of data, periods of low ambient noise allowed very quiet calls to be annotated with confidence. The calls annotated during the quieter periods were likely to be those with received levels below the detection thresholds associated with the predicted median detection areas. Additional contributors to uncertainty in detectability, albeit with a lesser contribution than ambient noise levels, were the source levels of blue whale calls and the depths at which calls may have been produced. Regardless, the use of estimated detection areas to scale call detections provided a useful comparison across sites.
In the deep waters to the east of central New Zealand, blue whale calls and other sounds in the 25 Hz-centred decidecade band could have propagated from areas outside of the 250 km maximum modelling range. The formation of sound channels in deep water enables long distance propagation, particularly of low frequency sounds, such as blue whale calls (Stafford et al., 1998; Wille, 2005; Miller et al., 2015). The high number of ABW detections at the east coast recorders was likely in part due to the large potential detection areas. Detection areas for the Cook Strait and STB recording locations were markedly smaller; sound propagation was somewhat constrained by land masses, but sandy seabed sediments and relatively shallow water depths also limited sound transmission (Wille, 2005; McPherson et al., 2019). The modelled detection areas indicated that all blue whale calls detected at the STB recorder were produced inside the STB, enabling the importance of this region for blue whales to be truly highlighted. Estimating the effective detection area of an acoustic recorder is a necessary step to be able to estimate animal density from call detections (Marques et al., 2013; Nuuttila et al., 2018). Density estimation requires knowledge of additional multipliers, such as call production rates (Marques et al., 2013), which have yet to be estimated for blue whales, but in a New Zealand context, density estimates could be extremely valuable, providing confidence to estimates of PBW population size (Barlow et al., 2018), and an ability to investigate the recovery of the ABW population subsequent to the cessation of whaling (Branch et al., 2004, 2007; McCauley et al., 2018).
Passive acoustic monitoring is an ideal method to improve the understanding of blue whale sub-species distributions. An acoustic approach enabled consistent coverage of offshore waters that would be logistically impossible to survey via boat or air with the same resolution. In addition, PAM allowed for the sub-species identification of blue whales that is often not possible during visual observations in the field. The findings revealed that New Zealand is a migratory corridor for ABWs, and during migration, this sub-species is sympatric with PBWs in central New Zealand. PBWs were detected throughout both recording periods, and throughout the study area, supporting their “resident native” status in New Zealand (Baker et al., 2019). The study accentuated the need to interpret acoustic detections in the light of acoustic detection areas, especially when comparing detections across recording locations. In doing so, the relative importance of the STB was revealed; the migration route of critically endangered ABWs passes through the STB during winter and spring, and an apparent aggregation of PBWs occurs in the STB year-round, particularly during austral autumn. This study revealed that the east coast of the South Island may also provide important habitat or resources for PBWs, and ABWs may breed in this area; these hypotheses deserve further investigation. Blue whale information that is not sub-species specific is not useful to inform management due to the different threat classifications of ABWs and PBWs, and future conflation should be avoided as ABW and PBW distributions are markedly different over time and space. An acoustic approach to study the distribution of blue whales is highly appropriate, and findings can be used to inform management of blue whales, with confidence in sub-species identity.
Data Availability Statement
The original contributions presented in the study are included in the article/Supplementary Material, further inquiries can be directed to the corresponding author.
Ethics Statement
Ethical review and approval was not required for the animal study because free-ranging animals were studied passively using seabed acoustic recorders; animals were not interacted with directly.
Author Contributions
All authors conceived and designed the project, interpreted the data and critically revised, and approved the final manuscript. VW conducted all blue whale call analyses and wrote the manuscript. CM modelled detection areas and recalculated received levels of blue whale calls over revised limits.
Funding
VW was funded by a University of Auckland Doctoral Scholarship and the Woodside Marine Mammal Research Grant awarded by Woodside Energy. Acoustic data collection was conducted by the National Institute of Water and Atmospheric Research (NIWA), Wellington, supported by funding from OMV New Zealand Ltd., Chevron New Zealand Holdings LLC, and Marlborough District Council.
Conflict of Interest
CM was employed by the company JASCO Applied Sciences Pty Ltd.
The remaining authors declare that the research was conducted in the absence of any commercial or financial relationships that could be construed as a potential conflict of interest.
Acknowledgments
We thank the acoustic deployment and retrieval teams from the National Institute of Water and Atmospheric Research, Wellington: Sarah Searson, Mike Brewer, Olivia Price, Fiona Elliott, and the crews of the RVs Tangaroa, Kaharoa and Ikatere. We are extremely grateful to Briand Gaudet and Ildar Urazghildiiev at JASCO Applied Sciences for their input into the propagation modelling. The findings and conclusion in this manuscript are those of the authors and do not necessarily represent the views of the National Marine Fisheries Service, NOAA. Mention of trade names and commercial firms does not imply endorsement by the National Marine Fisheries Service, NOAA.
Supplementary Material
The Supplementary Material for this article can be found online at: https://www.frontiersin.org/articles/10.3389/fmars.2020.575257/full#supplementary-material
Supplementary Data Sheet 1 | Antarctic blue whale (ABW) call dataset analysed in this study: recording location, time/date, sub-species identity, and received level of each detected call. Location key: STB = South Taranaki Bight, CS = Cook Strait, WR = Wairarapa, KK = Kaikoura. Received levels of 0 are equivalent to ‘NaN’.
Supplementary Data Sheet 2 | Pygmy blue whale (PBW) call dataset analysed in this study: recording location, time/date, sub-species identity, and received level of each detected call. Location key: STB = South Taranaki Bight, CS = Cook Strait, WR = Wairarapa, KK = Kaikoura. Received levels of 0 are equivalent to ‘NaN’.
References
Alvarez-Berríos, N., Campos-Cerqueira, M., Hernández-Serna, A., Amanda Delgado, C. J., Román-Dañobeytia, F., and Aide, T. M. (2016). Impacts of small-scale gold mining on birds and anurans near the tambopata natural reserve, peru, assessed using passive acoustic monitoring. Trop. Conserv. Sci. 9, 832–851. doi: 10.1177/194008291600900216
Attard, C. R. M., Beheregaray, L. B., Jenner, K. C. S., Gill, P. C., Jenner, M. N., Morrice, M. G., et al. (2012). Hybridization of Southern hemisphere blue whale subspecies and a sympatric area off Antarctica: impacts of whaling or climate change? Mol. Ecol. 21, 5715–5727. doi: 10.1111/mec.12025
Bailey, H., Mate, B., Palacios, D., Irvine, L., Bograd, S., and Costa, D. (2009). Behavioural estimation of blue whale movements in the Northeast Pacific from state-space model analysis of satellite tracks. Endanger. Species Res. 10, 93–106. doi: 10.3354/esr00239
Baker, C. S., Boren, L. J., Childerhouse, S., Constantine, R., van Helden, A., Lundquist, D., et al. (2019). Conservation Status of New Zealand Marine Mammals, 2019. New Zealand Threat Classification Series 29. Wellington: Department of Conservation.
Baker, C. S., Chilvers, B. L., Childerhouse, S., Constantine, R., Currey, R., Mattlin, R., et al. (2016). Conservation Status of New Zealand Marine Mammals, 2013. New Zealand Threat Classification Series 14. Wellington: Department of Conservation.
Balcazar, N. E., Klinck, H., Nieukirk, S. L., Mellinger, D. K., Klinck, K., Dziak, R. P., et al. (2017). Using calls as an indicator for Antarctic blue whale occurrence and distribution across the southwest Pacific and southeast Indian Oceans. Mar. Mamm. Sci. 33, 172–186. doi: 10.1111/mms.12373
Balcazar, N. E., Tripovich, J. S., Klinck, H., Nieukirk, S. L., Mellinger, D. K., Dziak, R. R., et al. (2015). Calls reveal population structure of blue whales across the southeast Indian Ocean and the southwest Pacific Ocean. J. Mammal. 96:1184. doi: 10.1093/jmammal/gyv126
Barlow, D. R., Bernard, K. S., Escobar-Flores, P., Palacios, D. M., and Torres, L. G. (2020). Links in the trophic chain: modeling functional relationships between in situ oceanography, krill, and blue whale distribution under different oceanographic regimes. Mar. Ecol. Prog. Ser. 642, 207–225. doi: 10.3354/meps13339
Barlow, D. R., Torres, L. G., Hodge, K. B., Steel, D., Baker, C. S., Chandler, T. E., et al. (2018). Documentation of a New Zealand blue whale population based on multiple lines of evidence. Endanger. Species Res. 36, 27–40. doi: 10.3354/esr00891
Baumann-Pickering, S., McDonald, M. A., Simonis, A. E., Solsona Berga, A., Merkens, K. P. B., Oleson, E. M., et al. (2013). Species-specific beaked whale echolocation signals. J. Acoust. Soc. Am. 134, 2293–2301. doi: 10.1121/1.4817832
Biggs, C., Wilson, P. S., and Erisman, B. (2019). Propagation loss of spawning calls produced by spotted seatrout Cynoscion nebulosus and the effective detection area of passive acoustic sampling. J. Acoust. Soc. Am. 145, 1807–1807. doi: 10.1121/1.5101618
Branch, T. A., Matsuoka, K., and Miyashita, T. (2004). Evidence for increases in Antarctic blue whales based on Bayesian modelling. Mar. Mamm. Sci. 20, 726–754. doi: 10.1111/j.1748-7692.2004.tb01190.x
Branch, T. A., Stafford, K. M., Palacios, D. M., Allison, C., Bannister, J. L., Burton, C. L. K., et al. (2007). Past and present distribution, densities and movements of blue whales Balaenoptera musculus in the Southern Hemisphere and northern Indian Ocean. Mamm. Rev. 37, 116–175. doi: 10.1111/j.1365-2907.2007.00106.x
Brodie, D. C., and Dunn, R. A. (2015). Low frequency baleen whale calls detected on ocean-bottom seismometers in the Lau basin, southwest Pacific Ocean. J. Acoust. Soc. Am. 137, 53–62. doi: 10.1121/1.4904556
CANZ (2008). New Zealand Region Bathymetry, 1:4 000 000. Miscellaneous Series No. 85, 2nd Edn. Auckland: NIWA Chart.
Carnes, M. R. (2009). Description and Evaluation of GDEM-V 3.0. Available online at: https://apps.dtic.mil/dtic/tr/fulltext/u2/a494306.pdf (accessed December 14, 2020).
Cerchio, S., Rasoloarijao, T., and Cholewiak, D. (2019). Progress Report: Acoustic Monitoring of Blue Whales (Balaenoptera musculus) and other Baleen Whales in the Mozambique Channel off the Northwest Coast of Madagascar. (SC/67B/SH/14 Rev1). Cambridge: International Whaling Commission, 14.
Chen, I. C., Hill, J., Ohlemueller, R., Roy, D., and Thomas, C. (2011). Rapid range shifts of species associated with high levels of climate warming. Science 333, 1024–1026. doi: 10.1126/science.1206432
Chiswell, S. M., Zeldis, J. R., Hadfield, M. G., and Pinkerton, M. H. (2017). Wind-driven upwelling and surface chlorophyll blooms in Greater Cook Strait. N. Z. J. Mar. Freshwater Res. 51, 465–489. doi: 10.1080/00288330.2016.1260606
Collins, M. D. (1993). A split-step Padeì solution for the parabolic equation method. J. Acoust. Soc. Am. 93, 1736–1742. doi: 10.1121/1.406739
Collins, M. D., Cederberg, R. J., King, D. B., and Chin-Bing, S. (1996). Comparison of algorithms for solving parabolic wave equations. J. Acoust. Soc. Am. 100, 178–182. doi: 10.1121/1.415921
Cooke, J. G. (2018a). Balaenoptera Musculus. The IUCN Red List of Threatened Species 2018, e.T2477A156923585. doi: 10.2305/IUCN.UK.2018-2.RLTS.T2477A156923585.en
Cooke, J. G. (2018b). Balaenoptera Musculus ssp. Intermedia. The IUCN Red List of Threatened Species 2018, e.T41713A50226962. doi: 10.2305/IUCN.UK.2018-2.RLTS.T41713A50226962.en
Coppens, A. B. (1981). Simple equations for the speed of sound in Neptunian waters. J. Acoust. Soc. Am. 69, 862–863. doi: 10.1121/1.385486
Darras, K., Pütz, P., Fahrurrozi, Rembold, K., and Tscharntke, T. (2016). Measuring sound detection spaces for acoustic animal sampling and monitoring. Biol. Conserv. 201, 29–37. doi: 10.1016/j.biocon.2016.06.021
Di Iorio, L., and Clark, C. W. (2010). Exposure to seismic survey alters blue whale acoustic communication. Biol. Lett. 6, 51–54. doi: 10.1098/rsbl.2009.0651
Double, M. C., Andrews-Goff, V., Jenner, K. C., Jenner, M. N., Laverick, S. M., Branch, T. A., et al. (2014). Migratory movements of pygmy blue whales (Balaenoptera musculus brevicauda) between Australia and Indonesia as revealed by satellite telemetry. PLoS One 9:e93578. doi: 10.1371/journal.pone.0093578
Dwyer, S., Clement, D., and Stockin, K. (2020). Modelling habitat use suggests static spatial exclusion zones are a non-optimal management tool for a highly mobile marine mammal. Mar. Biol. 167:62. doi: 10.1007/s00227-020-3664-4
Dziak, R., Haxel, J., Lau, T. K., Heimlich, S., Caplan-Auerbach, J., Mellinger, D., et al. (2017). A pulsed-air model of blue whale B call vocalizations. Sci. Rep. 7:9122. doi: 10.1038/s41598-017-09423-7
Edds-Walton, P. L. (1997). Acoustic communication signals of Mysticete whales. Bioacoustics 8, 47–60. doi: 10.1080/09524622.1997.9753353
Garcia−Rojas, M. I., Jenner, K. C. S., Gill, P. C., Jenner, M. N. M., Sutton, A. L., and McCauley, R. D. (2018). Environmental evidence for a pygmy blue whale aggregation area in the Subtropical Convergence Zone south of Australia. Mar. Mamm. Sci. 34, 901–923. doi: 10.1111/mms.12494
Gavrilov, A. N., and McCauley, R. D. (2013). Acoustic detection and long-term monitoring of pygmy blue whales over the continental slope in southwest Australia. J. Acoust. Soc. Am. 134, 2505–2513. doi: 10.1121/1.4816576
Gavrilov, A. N., McCauley, R. D., and Gedamke, J. (2012). Steady inter and intra-annual decrease in the vocalization frequency of Antarctic blue whales. J. Acoust. Soc. Am. 131, 4476–4480. doi: 10.1121/1.4707425
Gill, P. (2002). A blue whale (Balaenoptera musculus) feeding ground in a southern Australian coastal upwelling zone. J. Cetacean Res. Manag. 4, 179–184.
Gill, P., Morrice, M., Page, B., Pirzl, R., Levings, A., and Coyne, M. (2011). Blue whale habitat selection and within-season distribution in a regional upwelling system off southern Australia. Mar. Ecol. Prog. Ser. 421, 243–263. doi: 10.3354/meps08914
Hannay, D. E., Delarue, J., Mouy, X., Martin, B. S., Leary, D., Oswald, J. N., et al. (2013). Marine mammal acoustic detections in the northeastern Chukchi Sea, September 2007–July 2011. Cont. Shelf Res. 67, 127–146. doi: 10.1016/j.csr.2013.07.009
Harris, D. V., Miksis-Olds, J. L., Vernon, J. A., and Thomas, L. (2018). Fin whale density and distribution estimation using acoustic bearings derived from sparse arrays. J. Acoust. Soc. Am. 143, 2980–2993. doi: 10.1121/1.5031111
Hartel, E. F., Constantine, R., and Torres, L. G. (2015). Changes in habitat use patterns by bottlenose dolphins over a 10−year period render static management boundaries ineffective. Aquatic Conserv. Mar. Freshwater Ecosyst. 25, 701–711. doi: 10.1002/aqc.2465
Hauser, D. D. W., Laidre, K. L., Stafford, K. M., Stern, H. L., Suydam, R. S., and Richard, P. R. (2017). Decadal shifts in autumn migration timing by Pacific Arctic beluga whales are related to delayed annual sea ice formation. Global Change Biol. 23, 2206–2217. doi: 10.1111/gcb.13564
Hazen, E. L., Abrahms, B., Brodie, S., Carroll, G., Jacox, M. G., Savoca, M. S., et al. (2019). Marine top predators as climate and ecosystem sentinels. Front. Ecol. Environ. 17, 565–574. doi: 10.1002/fee.2125
Hazen, E. L., Friedlaender, A. S., and Goldbogen, J. A. (2015). Blue whales (Balaenoptera musculus) optimize foraging efficiency by balancing oxygen use and energy gain as a function of prey density. Sci. Adv. 1:e1500469. doi: 10.1126/sciadv.1500469
Ichihara, T. (1966). “The pygmy blue whale, balaenoptera musculus brevicauda, a new subspecies from the Antarctic,” in Whales, Dolphins, and Porpoises, ed. K. S. Norris (Los Angeles, CA: University of California Press), 79–113.
International Organization for Standardization (2017). ISO 18405:2017. Underwater acoustics – Terminology. Geneva: ISO.
JASCO Applied Sciences (2017). PAMLab-Lite (Version Version 8.3.3): JASCO Applied Sciences. Halifax Regional Municipality, NS: JASCO Applied Sciences.
Kalan, A. K., Piel, A. K., Mundry, R., Wittig, R. M., Boesch, C., and Kühl, H. S. (2016). Passive acoustic monitoring reveals group ranging and territory use: a case study of wild chimpanzees (Pan troglodytes). Front. Zool. 13. doi: 10.1186/s12983-016-0167-8
Kibblewhite, A. C., Denham, R. N., and Barnes, D. J. (1967). Unusual low−frequency signals observed in New Zealand waters. J. Acoust. Soc. Am. 41, 644–655. doi: 10.1121/1.1910392
Ladd, M. I., Daniel, M. P., Barbara, A. L., and Bruce, R. M. (2019). Scales of blue and fin whale feeding behavior off California, USA, with implications for prey patchiness. Front. Ecol. Evol. 7:338. doi: 10.3389/fevo.2019.00338
Leroy, E. C., Samaran, F., Bonnel, J., and Royer, J.-Y. (2016). Seasonal and diel vocalization patterns of Antarctic blue whale (Balaenoptera musculus intermedia) in the southern Indian Ocean: a multi-year and multi-site study. PLoS One 11:e0163587. doi: 10.1371/journal.pone.0163587
Lewis, L. A., Calambokidis, J., Stimpert, A. K., Fahlbusch, J., Friedlaender, A. S., McKenna, M. F., et al. (2018). Context-dependent variability in blue whale acoustic behaviour. R. Soc. Open Sci. 5:180241. doi: 10.1098/rsos.180241
Lewis, L. A., and Širović, A. (2018). Variability in blue whale acoustic behavior off southern California. Mar. Mamm. Sci. 34, 311–329. doi: 10.1111/mms.12458
Marques, T. A., Thomas, L., Martin, S. W., Mellinger, D. K., Ward, J. A., Moretti, D. J., et al. (2013). Estimating animal population density using passive acoustics. Biol. Rev. Camb. Philos. Soc. 88, 287–309. doi: 10.1111/brv.12001
Matthews, M.-N. R., and MacGillivray, A. O. (2013). Comparing modeled and measured sound levels from a seismic survey in the Canadian Beaufort Sea. Proc. Meet. Acoust. 19, 1–8.
McCauley, R. D., Gavrilov, A. N., Jolliffe, C. D., Ward, R., and Gill, P. C. (2018). Pygmy blue and Antarctic blue whale presence, distribution and population parameters in southern Australia based on passive acoustics. Deep Sea Res. 2 157–158, 154–168. doi: 10.1016/j.dsr2.2018.09.006
McCauley, R. D., Jenner, C., Bannister, J. L., Burton, C. L. K., Cato, D. H., and Duncan, A. (2001). Blue Whale Calling in the Rottnest Trench – 2000, Western Australia. Available online at: https://www.yumpu.com/en/document/view/18827881/blue-whale-calling-in-the-rottnest-trench-2000-western-anp (accessed December 14, 2020).
McDonald, M. A. (2006). An acoustic survey of baleen whales off great barrier Island, New Zealand. N. Z. J. Mar. Freshwater Res. 40, 519–529. doi: 10.1080/00288330.2006.9517442
McDonald, M. A., Calambokidis, J., Teranishi, A. M., and Hildebrand, J. A. (2001). The acoustic calls of blue whales off California with gender data. J. Acoust. Soc. Am. 109:1728. doi: 10.1121/1.1353593
McDonald, M. A., Mesnick, S. L., and Hildebrand, J. A. (2006). Biogeographic characterisation of blue whale song worldwide: using song to identify populations. J. Cetacean Res. Manag. 8, 55–65.
McLellan, B. N., and Shackleton, D. M. (1989). Grizzly bears and resource-extraction industries: habitat displacement in response to seismic exploration, timber harvesting and road maintenance. J. Appl. Ecol. 26, 371–380. doi: 10.2307/2404067
McPherson, C. R., Li, Z., and Quijano, J. E. (2019). Underwater Sound Propagation Modelling to Illustrate Potential Noise Exposure to Maui Dolphins from Seismic Surveys and Vessel Traffic on West Coast North Island, New Zealand. Available online at: https://mpigovtnz.cwp.govt.nz/dmsdocument/35013 (accessed December 14, 2020).
Melcón, M. L., Cummins, A. J., Kerosky, S. M., Roche, L. K., Wiggins, S. M., and Hildebrand, J. A. (2012). Blue whales respond to anthropogenic noise (response of blue whales to man-made noise). PLoS One 7:e32681. doi: 10.1371/journal.pone.0032681
Miksis-Olds, J. L., Vernon, J. A., and Heaney, K. D. (2015). The impact of ocean sound dynamics on estimates of signal detection range. Aquatic Mamm. 41:444. doi: 10.1578/AM.41.4.2015.444
Miller, B., Barlow, J., Calderan, S., Collins, K., Leaper, R., Olson, P., et al. (2015). Validating the reliability of passive acoustic localisation: a novel method for encountering rare and remote Antarctic blue whales. Endanger. Species Res. 26, 257–269. doi: 10.3354/esr00642
Miller, B. S., Calderan, S., Miller, E. J., Širović, A., Stafford, K. M., Bell, E., et al. (2019). A passive acoustic survey for marine mammals conducted during the 2019 Antarctic voyage on euphausiids and nutrient recycling in cetacean hotspots (ENRICH). Paper presented at the Acoustics 2019, Cape Schanck, VIC.
Miller, B. S., Collins, K., Barlow, J., Calderan, S., Leaper, R., McDonald, M., et al. (2014). Blue whale vocalizations recorded around New Zealand: 1964–2013. J. Acoust. Soc. Am. 135, 1616–1623. doi: 10.1121/1.4863647
Nuuttila, H., Brundiers, K., Dähne, M., Koblitz, J., Thomas, L., Evans, P., et al. (2018). Estimating effective detection area of static passive acoustic data loggers from playback experiments with cetacean vocalisations. Methods Ecol. Evol. 9, 2362–2371. doi: 10.1111/2041-210X.13097
Oleson, E. M., Calambokidis, J., Burgess, W., McDonald, M. A., Leduc, C., and Hildebrand, J. A. (2007). Behavioral context of call production by eastern North Pacific blue whales. Mar. Ecol. Prog. Ser. 330, 269–284. doi: 10.3354/meps330269
Olson, P. A., Ensor, P., Olavarría, C., Bott, N., Constantine, R., Weir, J., et al. (2015). New Zealand blue whales: residency, morphology, and feeding behavior of a little-known population. Pac. Sci. 69:477. doi: 10.2984/69.4.4
Owen, K., Jenner, C. S., Jenner, M.-N. M., and Andrews, R. D. (2016). A week in the life of a pygmy blue whale: migratory dive depth overlaps with large vessel drafts. Anim. Biotelem. 4:17. doi: 10.1186/s40317-016-0109-4
Pollock, C. M. (2019). Balaenoptera Musculus ssp. Brevicauda. The IUCN Red List of Threatened Species 2019, e.T2479A136508733.
QGIS Development Team (2017). QGIS Geographic Information System (Version 2.18.9). QGIS Association. Available online at: http://www.qgis.org
Quijano, J. E., Hannay, D. E., and Austin, M. E. (2019). Composite underwater noise footprint of a shallow arctic exploration drilling project. IEEE J. Ocean. Eng. 44, 1228–1239. doi: 10.1109/JOE.2018.2858606
Racca, R. G., Austin, M. E., Rutenko, A. N., and Bröker, K. (2015). Monitoring the gray whale sound exposure mitigation zone and estimating acoustic transmission during a 4-D seismic survey. Sakhalin Island Russia. Endanger. Species Res. 29, 131–146. doi: 10.3354/esr00703
Ross, S., Friedman, N., Dudley, K., Yoshimura, M., Yoshida, T., and Economo, E. (2018). Listening to ecosystems: data-rich acoustic monitoring through landscape-scale sensor networks. Ecol. Res. 33, 135–147. doi: 10.1007/s11284-017-1509-5
Samaran, F., Adam, O., and Guinet, C. (2010). Detection range modeling of blue whale calls in Southwestern Indian Ocean. Appl. Acoust. 71, 1099–1106. doi: 10.1016/j.apacoust.2010.05.014
Sanders, C. E., and Mennill, D. J. (2014). Acoustic monitoring of nocturnally migrating birds accurately assesses the timing and magnitude of migration through the Great Lakes. Condor 116, 371–383. doi: 10.1650/condor-13-098.1
Schall, E., Di Iorio, L., Berchok, C., Filún, D., Bedriñana-Romano, L., Buchan, S. J., et al. (2019). Visual and passive acoustic observations of blue whale trios from two distinct populations. Mar. Mamm. Sci. 36, 365–374. doi: 10.1111/mms.12643
Schlumberger New Zealand Limited (2016). Marine Mammal Impact Assessment, Schlumberger 3D Seismic Survey, Pegasus Basin (740.10032.00200). Available online at: https://www.doc.govt.nz/globalassets/documents/conservation/marine-and-coastal/mmia/schlumberger-2016-pegasus-basin-3d-seismic-survey-mmia-approved-public.pdf (accessed December 14, 2020).
Širović, A., Hildebrand, J. A., and Wiggins, S. M. (2007). Blue and fin whale call source levels and propagation range in the Southern Ocean. J. Acoust. Soc. Am. 122, 1208–1215. doi: 10.1121/1.2749452
Širović, A., Hildebrand, J. A., Wiggins, S. M., McDonald, M. A., Moore, S. E., and Thiele, D. (2004). Seasonality of blue and fin whale calls and the influence of sea ice in the Western Antarctic Peninsula. Deep Sea Res. 2 Top. Stud. Oceanogr. 51, 2327–2344. doi: 10.1016/j.dsr2.2004.08.005
Širovic, A., McDonald, M., Balcazar-Cabrera, N., Buchan, S., Cerchio, S., Clark, C., et al. (2017).Blue whale songs worldwide: an update. Paper presented at the Society for Marine Mammalogy 22nd Biennial Conference, Halifax, NS.
Širovic, A., Rice, A., Chou, E., Hildebrand, J., Wiggins, S., and Roch, M. A. (2015). Seven years of blue and fin whale call abundance in the Southern California Bight. Endanger. Species Res. 28, 61–76. doi: 10.3354/esr00676
Stafford, K., Fox, C. G., and Clark, D. (1998). Long-range acoustic detection and localization of blue whale calls in the northeast Pacific Ocean. J. Acoust. Soc. Am. 104, 3616–3625. doi: 10.1121/1.423944
Stafford, K. M., Chapp, E., Bohnenstiel, D. R., and Tolstoy, M. (2011). Seasonal detection of three types of “pygmy” blue whale calls in the Indian Ocean. Mar. Mamm. Sci. 27, 828–840. doi: 10.1111/j.1748-7692.2010.00437.x
Stafford, K. M., Mellinger, D. K., Moore, S. E., and Fox, C. G. (2007). Seasonal variability and detection range modeling of baleen whale calls in the Gulf of Alaska, 1999–2002. J. Acoust. Soc. Am. 122, 3378–3390. doi: 10.1121/1.2799905
Stafford, K. M., Moore, S. E., and Fox, C. G. (2005). Diel variation in blue whale calls recorded in the eastern tropical Pacific. Anim. Behav. 69, 951–958. doi: 10.1016/j.anbehav.2004.06.025
Stephenson, F., Goetz, K., Sharp, B. R., Mouton, T. L., Beets, F. L., Roberts, J., et al. (2020). Modelling the spatial distribution of cetaceans in New Zealand waters. Divers. Distrib. 26, 495–516. doi: 10.1111/ddi.13035
Szesciorka, A. R., Ballance, L. T., Širović, A., Rice, A., Ohman, M. D., Hildebrand, J. A., et al. (2020). Timing is everything: Drivers of interannual variability in blue whale migration. Sci. Rep. 10, 7710–7710. doi: 10.1038/s41598-020-64855-y
Teague, W. J., Carron, M. J., and Hogan, P. J. (1990). A comparison between the Generalized Digital Environmental Model and Levitus climatologies. J. Geophys. Res. 95, 7167–7183. doi: 10.1029/jc095ic05p07167
Thode, A. M., Spain, G. L., and Kuperman, W. A. (2000). Matched-field processing, geoacoustic inversion, and source signature recovery of blue whale vocalizations. J. Acoust. Soc. Am. 107:1286. doi: 10.1121/1.428417
Thomisch, K., Boebel, O., Clark, C. W., Hagen, W., Spiesecke, S., Zitterbart, D. P., et al. (2016). Spatio-temporal patterns in acoustic presence and distribution of Antarctic blue whales (Balaenoptera musculus intermedia) in the Weddell Sea. Endanger. Species Res. 30, 239–253. doi: 10.3354/esr00739
Torres, L. G. (2013). Evidence for an unrecognised blue whale foraging ground in New Zealand. N. Z. J. Mar. Freshwater Res. 47, 235–248. doi: 10.1080/00288330.2013.773919
Torres, L. G., Barlow, D. R., Chandler, T. E., and Burnett, J. D. (2020). Insight into the kinematics of blue whale surface foraging through drone observations and prey data. PeerJ 8:e8906. doi: 10.7717/peerj.8906
Tripovich, J. S., Klinck, H., Nieukirk, S. L., Adams, T., Mellinger, D. K., Balcazar, N. E., et al. (2015). Temporal segregation of the Australian and Antarctic blue whale call types (Balaenoptera musculus spp.). J. Mammal. 96, 603–610. doi: 10.1093/jmammal/gyv065
Wiggins, S., Oleson, E., McDonald, M., and Hildebrand, J. (2005). Blue whale (Balaenoptera musculus) diel call patterns offshore of Southern California. Aquatic Mamm. 31:161. doi: 10.1578/AM.31.2.2005.161
Zhang, Z. Y., and Tindle, C. T. (1995). Improved equivalent fluid approximations for a low shear speed ocean bottom. J. Acoust. Soc. Am. 98, 3391–3396. doi: 10.1121/1.413789
Keywords: blue whales, passive acoustic monitoring, distribution, sympatry, New Zealand
Citation: Warren VE, Širović A, McPherson C, Goetz KT, Radford CA and Constantine R (2021) Passive Acoustic Monitoring Reveals Spatio-Temporal Distributions of Antarctic and Pygmy Blue Whales Around Central New Zealand. Front. Mar. Sci. 7:575257. doi: 10.3389/fmars.2020.575257
Received: 23 June 2020; Accepted: 07 December 2020;
Published: 06 January 2021.
Edited by:
Eric Delory, Oceanic Platform of the Canary Islands, SpainReviewed by:
Ester Quintana, University of South Florida, United StatesRobert McCauley, Curtin University, Australia
Capri Jolliffe, Curtin University, Australia
Copyright © 2021 Warren, Širović, McPherson, Goetz, Radford and Constantine. This is an open-access article distributed under the terms of the Creative Commons Attribution License (CC BY). The use, distribution or reproduction in other forums is permitted, provided the original author(s) and the copyright owner(s) are credited and that the original publication in this journal is cited, in accordance with accepted academic practice. No use, distribution or reproduction is permitted which does not comply with these terms.
*Correspondence: Victoria E. Warren, vwar775@aucklanduni.ac.nz