- 1Centre for Environment, Fisheries and Aquaculture Science, Lowestoft Laboratory, Lowestoft, United Kingdom
- 2Fisheries Management, Department of Environment, Forestry and Fisheries, Cape Town, South Africa
- 3Oceans and Coasts, Department of Environment, Forestry and Fisheries, Cape Town, South Africa
- 4GRID-Arendal, Arendal, Norway
This study documented the levels of microplastics in three commercially important small pelagic fish species in South African waters, namely European anchovy (Engraulis encrasicolus), West Coast round herring (Etrumeus whiteheadi) and South African sardine (Sardinops sagax). Data suggested variation between species with a higher concentration of microplastics for S. sagax (mean of 1.58 items individual–1) compared to Et. whiteheadi (1.38 items individual–1) and En. encrasicolus (1.13 items individual–1). The occurrence of microplastics was also higher for S. sagax (72%) and Et. whiteheadi (72%) compared to En. encrasicolus (57%). Microfibers accounted for 80% of ingested microplastics (the remainder were plastic fragments) with the main ingested polymers being poly(ethylene:propylene:diene) (33% occurrence), polyethylene (20%), polyamide (20%), polyester (20%), and polypropylene (7%). The abundance of ingested items was not significantly correlated with fish caudal length or body weight, and spatial investigation indicated an increase in the abundance of ingested items from the West to the South coast. Etrumeus whiteheadi is proposed as a bio-indicator for microplastics for South Africa.
Introduction
Plastics are valuable resources with numerous societal benefits. Global plastics production was almost 360 million tonnes in 2018, of which 7% was attributed to the Middle East and Africa (PlasticsEurope, 2019). In South Africa in 2015, most plastic consumption was attributed to product packaging (53%), followed by building and construction (13%), and agriculture (9%) (Plastics SA, 2019).
Reduction of marine plastic litter through plastic waste management is the current focus of international efforts (Babayemi et al., 2019). Impacts of marine plastic litter are varied and include ingestion by biota (macro, meso, and microplastics including microfibers) or entanglement/collision [e.g., ghost fishing caused by Abandoned Lost or otherwise Discarded Fishing Gear (ALDFG)]. Plastics can also act as substrate for a wide variety of species increasing their potential for long-range transport (GESAMP, 2020). Microplastics are widespread in the environment with some known environmental and ecological impacts. Field and laboratory studies have demonstrated the ingestion of microplastics by a large range of marine organisms representing various trophic levels including seabirds, marine mammals, fish and invertebrates (GESAMP, 2015) and detrimental physical effects of microplastics have been reported following ingestion (Wright et al., 2013), including mortality (Maes et al., 2020). There is also evidence that microplastics can act as carriers for harmful sorbed co-contaminants (i.e., hydrophobic organic compounds, additives, pathogens) with the potential for transfer to biota following ingestion (Rochman et al., 2013; Tanaka et al., 2013; Bakir et al., 2014). However, the transfer of sorbed co-contaminants from microplastics to biota may be negligible compared to other routes of exposure (Bakir et al., 2016; Herzke et al., 2016; Koelmans et al., 2016; Lohmann, 2017).
Plastic litter in biota within and off South Africa has been studied on a macro scale in sharks (Cliff et al., 2002), turtles (Ryan et al., 2016a), albatrosses and Southern Ocean fur seals (Ryan et al., 2016b), on a micro scale in fish (Naidoo et al., 2015, 2020b; Ross, 2017; Naidoo and Glassom, 2019) and inland waterbirds (Reynolds and Ryan, 2018). Data on the abundance of microplastics in commercially important fish species for South Africa is, however, limited and there is a need to address this knowledge gap. Small pelagic fish are of particular importance as they occupy a vital place in marine food webs and the fishery for these species is South Africa’s largest and second-most valuable (DFFE, 2020). Whilst most of the catch is processed into agri- and aqua-feeds, small pelagic fish are an important source of food with canned sardine (pilchard) being one of the most important basic food items in the diets of South Africans (Isaacs, 2016). Investigation in microplastic contamination in small pelagic fish is therefore required to assess some related ecological impacts as well as understanding the potential for direct uptake via the human diet.
The aim of this study was to investigate the abundance of microplastics in three commercially important South African small pelagic fish species, namely European anchovy (Engraulis encrasicolus), West Coast round herring (Etrumeus whiteheadi) and South African sardine (Sardinops sagax). The main objectives were to (i) apply a proposed approach for the extraction and quantification of microplastics in small pelagic fish, (ii) to investigate interspecific differences in microplastic ingestion, (iii) to identify the main plastic and polymer types ingested by biota, (iv) to investigate spatial variations with the identification of “accumulation zones” of microplastics contamination, and (v) to identify and propose a suitable bio-indicator species for the monitoring of microplastics in South African waters.
Materials and Methods
Biota Sampling
Samples of the three small pelagic fish species were collected during the 2019 Pelagic Recruit Survey that covered the inshore shelf along the South African coastline from the Orange River mouth (West Coast) to Mossel Bay (South Coast) in seven geographical areas or strata (A–G; Figure 1, Shabangu et al., 2019). Echosounders were used to identify biomass hotspots along survey transects and midwater trawls (nylon net) were used to catch pelagic fish. Trawl composition varied from one to several species, with all three small pelagic species often being caught in a single trawl. Collected biota samples (i.e., intact individuals) were stored intact and frozen (−20°C) in labeled (date and location of capture) plastic bags until ready for dissection.
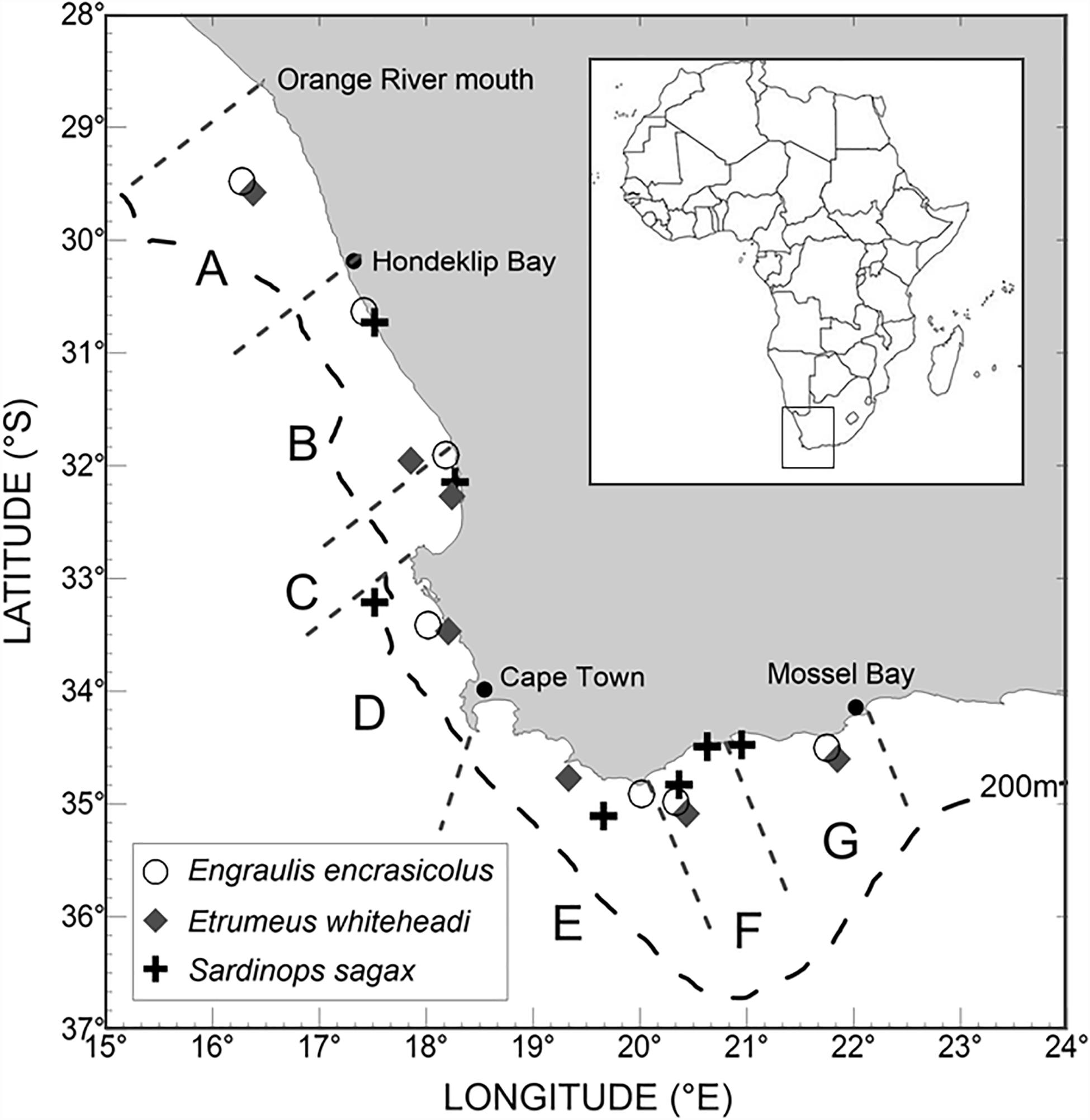
Figure 1. Locations of midwater trawls where biota (small pelagic fishes: white circles for Engraulis encrasicolus; gray diamonds for Etrumeus whiteheadi; black crosses for Sardinops sagax) samples were collected during the 2019 Pelagic Recruit Survey and processed for microplastics. The 200 m depth contour is indicated by the black dashed line and the borders of survey strata A to G by gray dashed lines. Note that sardines were collected from two trawls in stratum F in order to obtain sufficient fish for processing.
Chemicals
The chemicals used in this study are listed in Table 1.
Quantification of Contamination and Quality Control
Contamination control procedures were followed to reduce contamination of samples. Cotton lab coats were worn to avoid contamination from clothing items. Prior to use, all glassware and dissection kits were cleaned using a laboratory detergent and rinsed using reverse osmosis (RO) water and covered with RO rinsed foil until ready for use. Gastrointestinal tracts (GITs) were removed under a fume cupboard and quickly transferred to RO rinsed 120 mL glass specimen jars covered with RO rinsed foil. Jars were then transferred to a PCR workstation with laminar flow for the addition of chemical solutions. All chemical solutions used in this study were previously filtered using a 47 mm diameter, 0.2 μm regenerated cellulose membrane filter. Contamination monitoring within the laboratory was carried out by using negative and positive controls. Negative controls consisted of blank filters processed in the same way as environmental samples for each batch of samples processed. Positive controls consisted on the spiking of some filters and checking for particle recovery.
Microplastics in Biota
A total of 593 individual fish were processed during this study comprising of 178 En. encrasicolus, 188 Et. whiteheadi and 227 S. sagax, collected from seven stations per species split across the survey strata as shown in Figure 1. For strata with a homogeneous population size distribution, the caudal length (equivalent to standard length) and wet body weight were recorded for five individuals per species, per sampling station (thus representing each stratum), and averaged values were used for calculations. Individual fish caudal length (0.1 cm) and wet body weight (0.1 g) were recorded prior to removal of the GITs under a pre-cleaned fume cupboard to avoid ambient contamination. Stomach weight was collected for all individuals. To determine potential health effects from microplastics on sardines, the lengths and weights of all individual fish were measured in strata E and F. The wet weight of the tissues was recorded and each GIT was transferred to a 120 mL glass jar, pre-cleaned with RO water. To compensate for ambient contamination, an empty glass jar was left open inside the fume cupboard, during the time required to dissect one individual as a blank. The number of items in the blank were deducted from the total number of items in fish for the corresponding batch. As the size of En. encrasicolus varied greatly across strata, for sites where individuals were too small for dissection (∼5 cm), the outside of the individuals were rinsed with MilliQ water (18.2 M′Ωcm and TOC < 10 ppb) and the head and tail removed. The remaining body was then placed in a glass jar as previously described. A fixed volume of 40 mL of a 30% KOH:NaClO solution was added to each pot in an PCR workstation with laminar flow to avoid particle contamination and each sample was sonicated using a VWR ultrasonic cleaning bath for 5 min (Enders et al., 2017). Each sample was then incubated at 40°C for 24 h before filtration using a pre-rinsed Whatman GF/D filter (2.7 μm porosity). Identification of the extracted microplastics was carried out using the fluorescence tagging of polymers using Nile Red coupled with digital imaging and an automated particle counting method developed at Cefas based on ArcGIS (Maes et al., 2017). As a validation step, each filter was examined under a microscope (VWR Stereo microscope, VisiScope SZT360-6) to remove any false positives from the fluorescence of biological items and to differentiate suspected anthropogenic-origin items into fibers and fragments based on their morphology. For additional quality assessment, a GF/D filter was spiked with a known number of plastic particles to investigate recovery rates using both a visual (digital imaging and microscopy) and an automated particle counting method. Data were corrected from the procedural control (i.e., negative control) to compensate for ambient contamination. A summary of the methods is shown in Supplementary Figure S1.
Quality Control and Polymer Identification Using Fourier-Transform Infrared Spectroscopy
Polymer identification of particles was carried out using attenuated total reflection Fourier transform infrared spectroscopy (ATR-FT-IR) with a Thermo Fisher Scientific Nicolet iS5 ATR-FTIR with OMNIC software (version 9.9.473) and by comparison of their IR spectra to a polymer library. ATR-FT-IR has been shown to be a fast and effective tool for the identification of polymers of plastic marine debris, including those ingested by marine organisms (Jung et al., 2018). Due to size limitation using ATR-FT-IR, only particles above ∼250 μm in size were analyzed. In total, 2.3% of particles were selected for polymer identification. Spectra were collected in absorbance mode in the range 4000–400 cm–1 at a resolution of 4 cm–1. Polymer identification was verified based on the% match (>70%) against polymer libraries (HR Nicolet Sampler Library, HR Spectra IR Demo and Hummel Polymer Sample Library). Quality control was carried out with the analysis of a polystyrene (PS) and polyethylene (PE) reference material before each batch. Categorization of the extracted particles for quality control is shown in the supporting information section (Supplementary Figure S3).
Statistical Analysis
A Poisson log-linear model (Supplementary Figure S2) was applied to model the relationships between the number of items individual–1 in fish against species, geographic range (strata), and stomach weights using Eq. 1.
where μ is the Poisson mean and fn (Species, Strata, Stomach weight) is a linear function of the three potential explanatory variables. We use the link function log(μ) to constrain μ to be positive. Statistical analysis was carried out using R Core Team (2019). Once all combinations of the explanatory variables were fitted into the model, the Akaike Information Criterion (AIC) was used to judge the suitability of the models.
The effect of microplastics on fish health and fitness was investigated using the caudal length to body weight ratio. Symmetric distribution of the ratios suggested Normality and the following linear model was applied using (Eq. 2).
where f(Count,Strata)s a linear function of Count and Strata and the error is assumed to be Normally distributed with mean 0 and constant variance.
With further consideration of fish health, a linear regression model was applied to investigate the potential relationship between mean microplastic count, in each species, against the mean weight and mean caudal length of fish, within each geographical location. Finally, a modified bootstrap analysis was applied on results obtained on microplastics abundance to evaluate the optimum sampling sizes for each species for future monitoring (Manly, 2006).
Results
Interspecific Variation
This study confirmed the presence of microplastics in GITs of commercially important small pelagic fish species in South African waters, specifically En. encrasicolus (n = 178), Et. whiteheadi (n = 188), and S. sagax (n = 227). A total of 813 suspected microplastics were detected in 406 fish across all the species out of 593 individuals, representing a 68% occurrence overall. The corresponding total mean concentration of microplastics for biota across all the strata was 1.36 items individual–1. The mean occurrence of microplastics was substantially lower for En. encrasicolus (57%) as compared to Et. whiteheadi and S. sagax (each 72%) (Table 2).
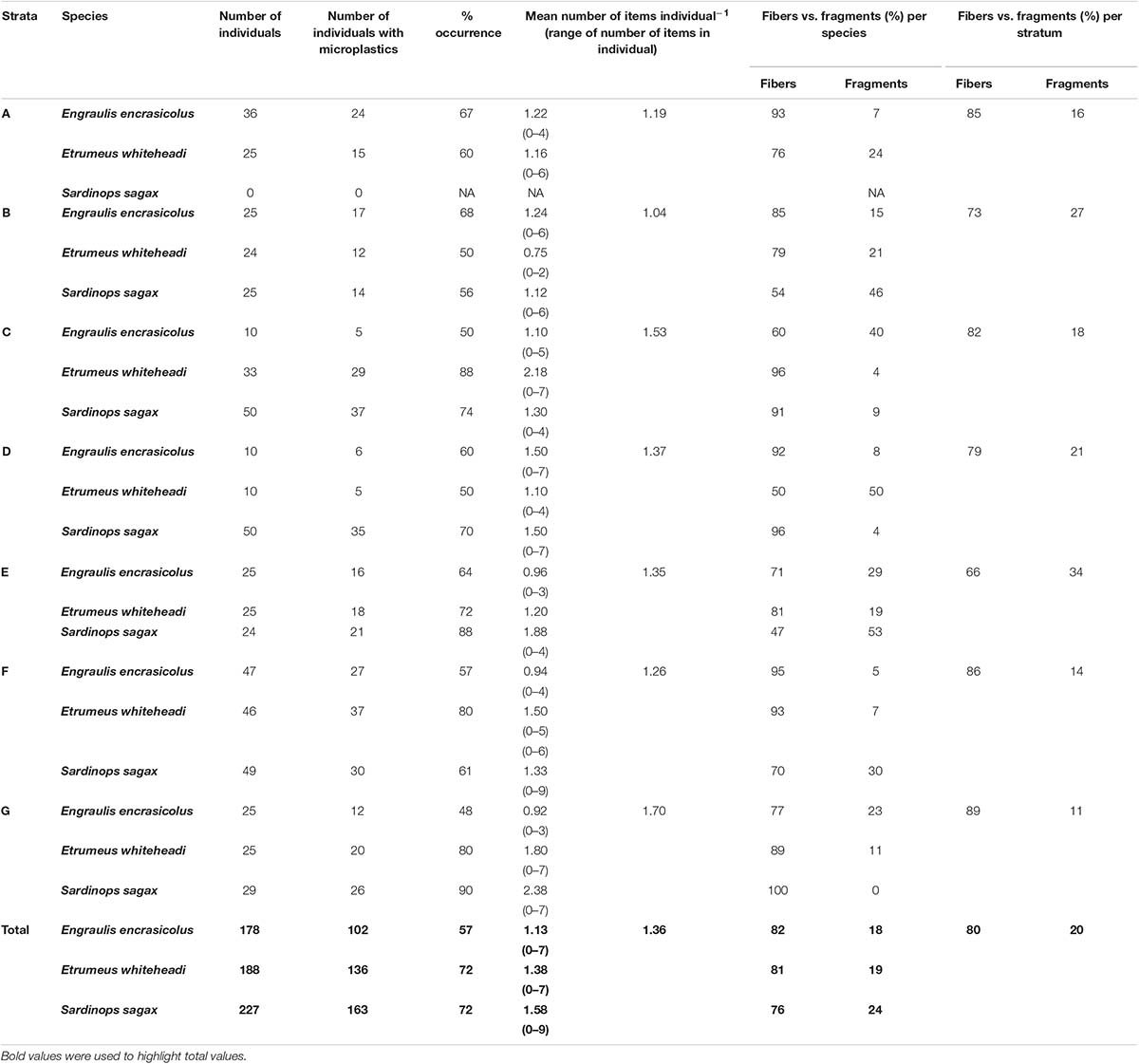
Table 2. Number of individuals studied, number of individuals with micro plastics, % occurrence per strata, and mean number of items individual−1 per species [range in ()] for anchovy (Engraulis encrasicolus), West Coast round herring (Etrumeus whiteheadi), and sardines (Sardinops sagax).
Mean number of microplastics (mean number of items individual–1) ranged from 1.13 items individual–1 for En. encrasicolus followed by Et. whiteheadi and S. sagax with 1.38 and 1.58 items individual–1, respectively (Table 2), and varied between strata (Figure 2). The results from the Poisson log-linear model (AIC values) suggested that species, strata and their interaction influence the abundance of microplastics in fish species. This makes inter-comparisons and identification of global trends difficult (Table 3). The mean number of items individual–1 per species and per stratum is shown in Figure 2, along with 95% confidence intervals derived from the fitted log-linear model with the interaction between species and strata. The overall trend, irrespective of species, indicated a slight decrease from stratum A to B followed by a distinct increase in abundance of ingested items to stratum C. The mean number of items individual–1 was then generally stable until further increasing in stratum G. For En. encrasicolus, the abundance of microplastics was generally constant and linear across strata. This trend was however different for Et. whiteheadi and S. sagax which showed a gradual increase in the abundance of ingested items from the strata B to G (Table 2 and Figure 2). This interspecific difference was particularly clear for stratum G in which Et. whitehead and S. sagax ingested substantially higher numbers of microplastic items (means of 1.80 and 2.38 items individual–1, respectively) as compared to En. encrasicolus (mean of 0.92 items individual–1) (Figure 2).
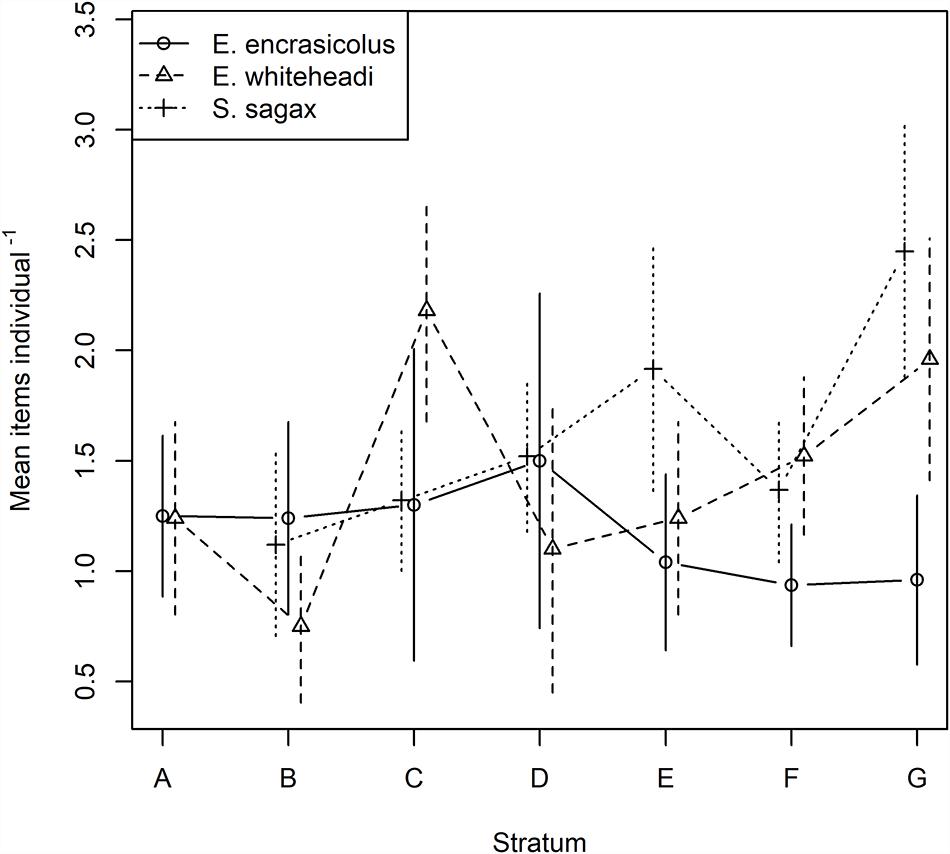
Figure 2. Mean number of items individual–1 by stratum for Engraulis encrasicolus (n = 178), Etrumeus whiteheadi (n = 188), and Sardinops sagax (n = 227). Also shown are 95% confidence intervals from the interaction Poisson log-linear model. S. sagax was not collected from Stratum A.
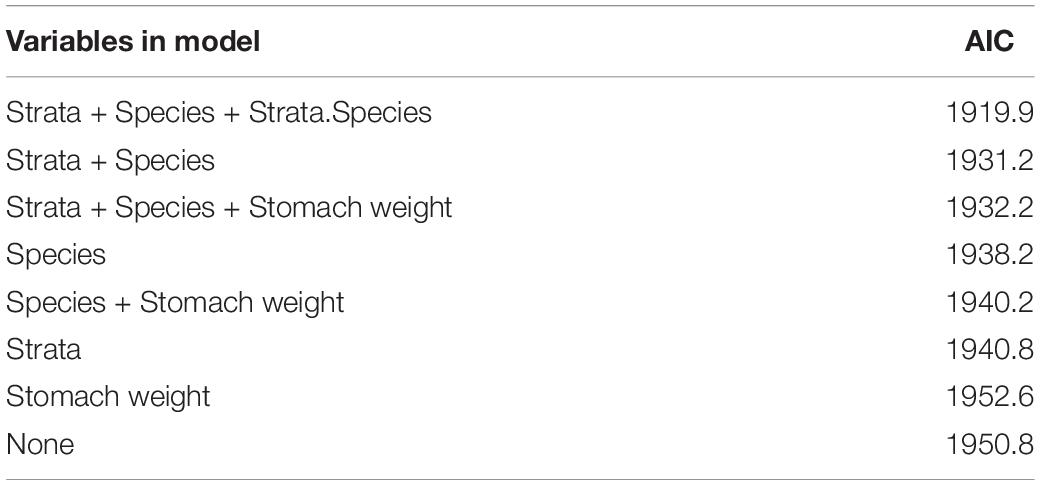
Table 3. Summary of AIC values for the Poisson log-linear models in decreasing order of model quality (low values of AIC are best).
The mean number of items per individual was also plotted against mean fish body weight (Figures 3, 4) and mean fish caudal length (Figures 3, 5) for all strata per species to assess whether larger organisms showed a higher ingestion of particles. Linear regression models were applied to derive levels of significance, but the mean number of items individual–1 was not significantly linearly related (p > 0.05) to fish body weight or to body caudal length for all species under investigation.
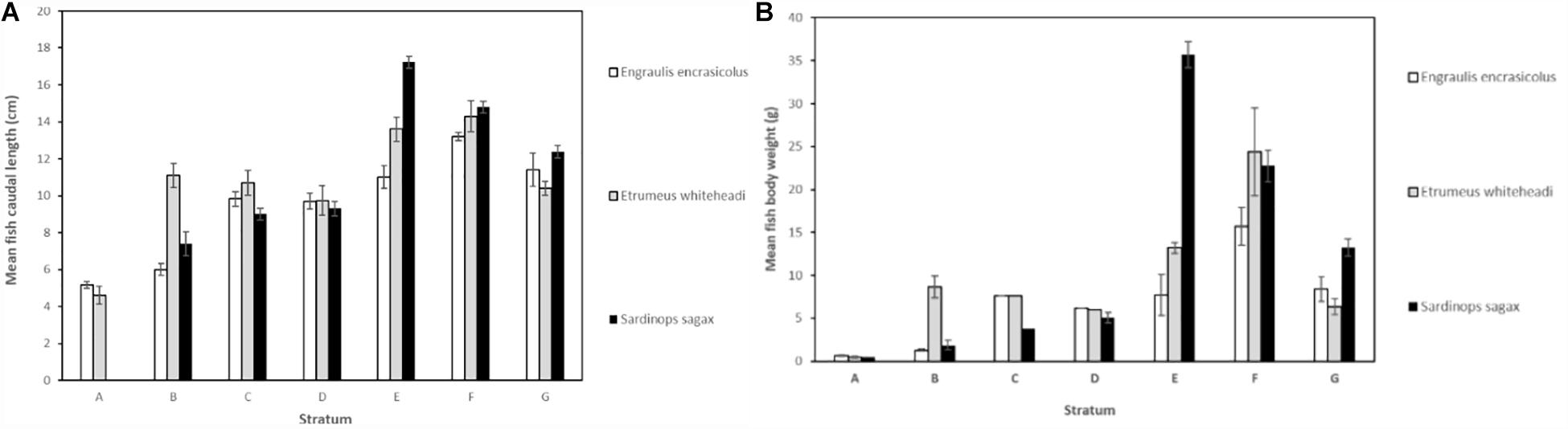
Figure 3. Mean (A) fish caudal length (in cm) and (B) mean fish body weight (g) (mean ± 95% CI) for Engraulis encrasicolus (n = 178), Etrumeus whiteheadi (n = 188), and Sardinops sagax (n = 227).
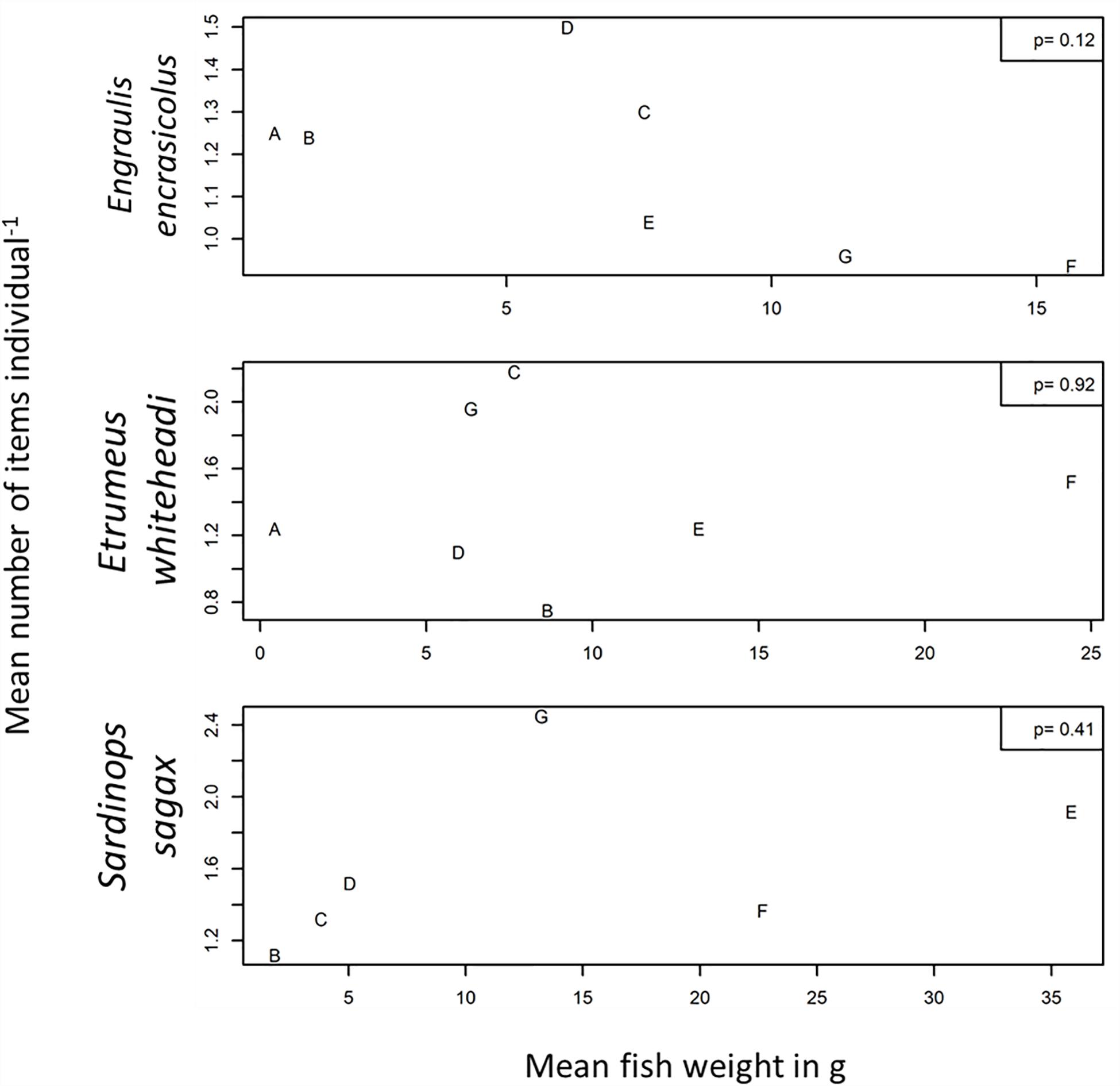
Figure 4. Mean number of items individual–1 plotted against mean weight (g–1) for Engraulis encrasicolus, Etrumeus whiteheadi and Sardinops sagax for each stratum. Also shown are p-values for a linear regression model.
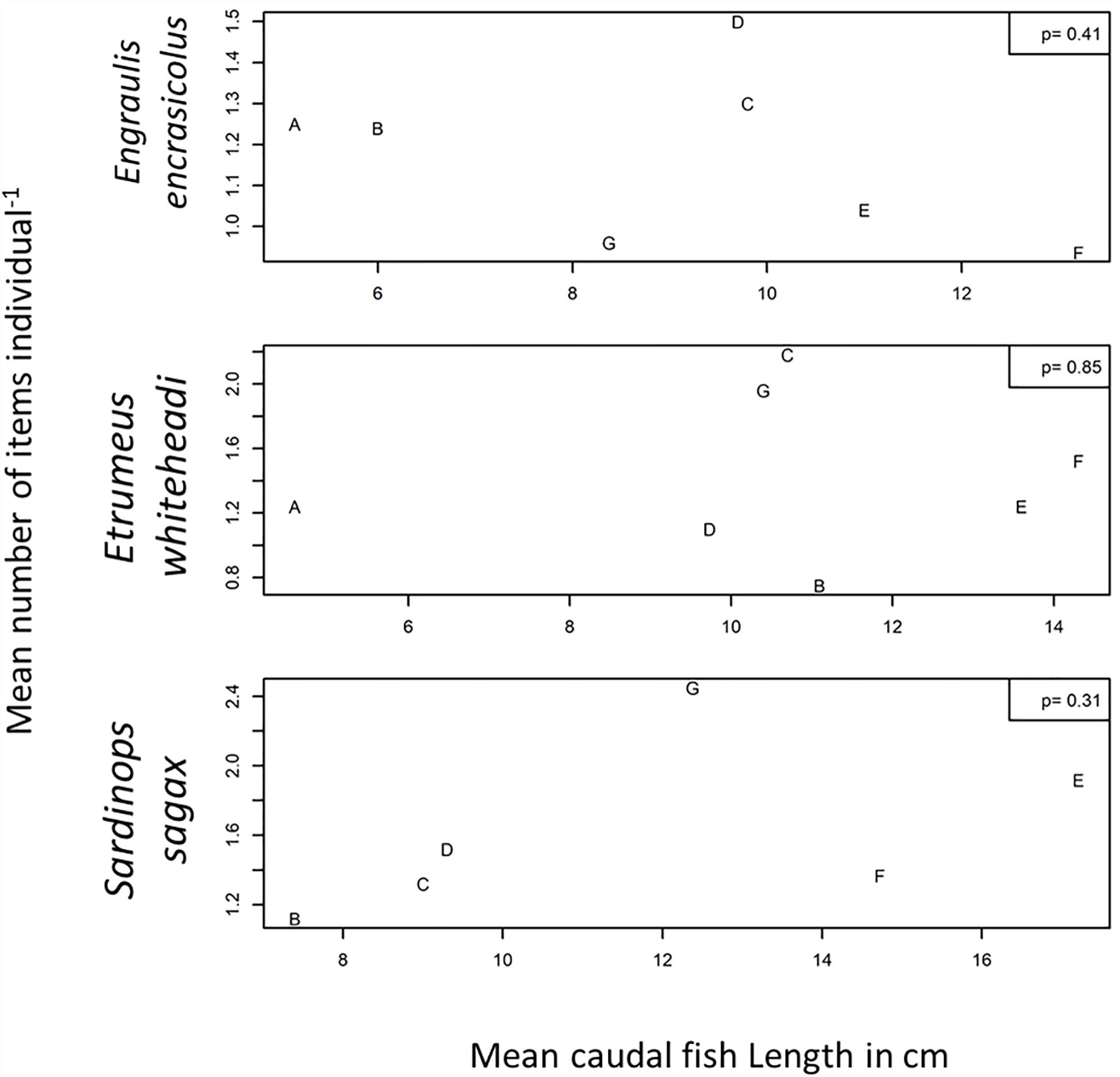
Figure 5. Mean number of items individual–1 plotted against mean caudal length (cm) for Engraulis encrasicolus, Etrumeus whiteheadi and Sardinops sagax for each stratum. Also shown are p-values for a linear regression model.
Main Microplastic Types and Sources
Fibers and fragments were the most common types of microplastics found for all the species under investigation. Overall, for all the species, fibers represented 80% of the analyzed particles and fragments 20%. This was consistent across species with fibers representing 82, 81, and 76% of the particles analyzed for En. encrasicolus, Et. whitehead, and S. sagax, respectively (Table 2). The most commonly found polymers were poly(ethylene:propylene:diene) (EPDM, 33%), polyethylene (PE, 20%), polyamide (PA, 20%), polyester (PET, 20%), and polypropylene (PP, 7%) (Supplementary Figure S3).
Discussion
Uncertainties and Evaluation of Method
The Nile red screening method for microplastics was applied for a fast and cost-effective assessment of the occurrence of microplastics in biota collected in South Africa. This method has recently been used for large-scale mapping of microplastics (Wang et al., 2018). Due to the nature of the project, only small, portable items were used during this study. This included the use of a portable ATR-FTIR for plastic particle validation and polymer identification with a validation step restricted to particles down to about 250 μm in size. No micro-FTIR or Raman spectroscopy was accessible on site and are commonly used for the identification of smaller size particles (Kniggendorf et al., 2019). The presence of false positives has been previously identified as a source of error when applying the Nile red screening method (Maes et al., 2017; Kukkola et al., 2020). Additional steps were therefore required for plastic confirmation and removal of false positives, including visual observation using digital imaging and microscopy.
Interspecific Variation
This study documented and compared levels of microplastics in three small pelagic fish species found off the coast of South Africa, namely En. encrasicolus, Et. whiteheadi, and S. sagax. All three species are planktivorous but show resource partitioning and feed primarily on different components of the plankton. Sardinops sagax are able to retain small particles so phytoplankton (diatoms and dinoflagellates) is on occasion relatively important, but the majority of the dietary intake of this species is via filter-feeding on smaller zooplankton such as poecilostomatoid and small calanoid copepods as well as fish eggs (Van Der Lingen, 2002). Phytoplankton is of less importance to En. encrasicolus which primarily particulate-feeds on larger zooplankton such as calanoid copepods and euphausiids (James, 1987). The diet of Et. whiteheadi has been reported to consist entirely of zooplankton (euphausiids, large copepods and decapods; Wallace-Fincham, 1987) but it is likely they also eat larval and early juveniles stages of fish (van der Lingen and Miller, 2011).
Given their planktivorous nature and the overlap in size between their natural prey and microplastics, the ingestion of microplastics by these small pelagic fish species is predictable. Detailed comparative analysis of diet, feeding morphology and behavior and energetics of sardine and anchovy (van der Lingen et al., 2006), as well as a comparison of nitrogen stable isotope ratios that reflect the trophic position of all three species (van der Lingen and Miller, 2011; Van Der Lingen and Miller, 2014), have demonstrated clear differences in their feeding ecologies in South African waters, suggesting that microplastic ingestion levels may vary between them. Such interspecific differences were indeed observed, with the Poisson log-linear model indicating that species, location (stratum) and their interaction all had significant effects on microplastic abundance in these small pelagic fish species. Overall, En. encrasicolus had both the lowest occurrence (57%) and lowest mean abundance (1.13 items individual–1) of microplastics, whereas Et. whiteheadi and S. sagax had the same occurrence (72%), the mean abundance of microplastics was higher in sardine (1.58 items individual–1) than in round herring (1.38 items individual–1).
The interspecific difference reported here supports the hypothesis of Collard et al. (2017) that planktivorous fish species with the most efficient filtration apparatus will be more likely to ingest microplastics. Those authors determined filtration areas and particle retention thresholds of En. encrasicolus, Clupea harengus, and Sardina pilchardus in European waters and compared these with their anthropogenic particle (including microplastics) levels, and reported that sardine, which had the largest area and closest gill raker spacing, ingested more fibers and smaller fragments than anchovy or herring. S. sagax has a markedly smaller gill raker gap than either En. encrasicolus or Et. whiteheadi in southern African waters (Vorsatz et al., 2015), which provides a plausible mechanism to explain the higher occurrence and abundance of microplastics in sardine compared to the other two species reported here. However, the hypothesis that retention of smaller particles results in increased microplastics ingestion is in contrast to findings by Lopes et al. (2020), who reported that planktivorous species from Alanto-Iberian waters that fed on larger zooplankton prey (Trachurus trachurus, Scomber scombrus, and En. encrasicolus) accumulated more microplastics than those feeding on smaller prey size (S. pilchardus, Sc. colias, and Boops boops), and recommended T. trachurus as a suitable bio-indicator species for monitoring microplastics in that ecosystem. While studies on bioaccumulation of microplastics are limited, there is indication that plastic particles can be ingested and transferred in the planktonic food web (Setälä et al., 2014), representing another potential route of uptake by biota.
Data on the occurrence and abundance of microplastics in sardines, anchovies, and round herrings from various studies of the same or similar species, including from South African waters, are listed in Table 4. It must be noted that methods used in those studies are not all standardized and that sample sizes vary substantially between them, hence inferences made from comparing those data are questionable. Nonetheless, we feel that they provide a useful contextualization of our results. Ross (2017) assessed ingestion of microplastics by the same three epipelagic species from the a similar geographical area investigated in this study and reported an overall microplastics occurrence of 67%, which was consistent with the overall 68% occurrence observed in this study using markedly larger sample sizes. In contrast to our findings, however, Ross (2017) found that En. encrasicolus had the highest (80%) and Et. whiteheadi the lowest (44%) occurrence of microplastics, whereas observations from the two studies for sardine were similar (76% from Ross, 2017 and 72% from this study; Table 4). Despite these differences, estimates from both studies for En. encrasicolus off South Africa are higher than the median occurrence of 30% calculated from available data for anchovies in the literature (Table 4). More strikingly, estimates of microplastic occurrence in S. sagax off South Africa are the highest yet reported for this species compared to a median value of 25% from the eight values reported in Table 4. We could find no other reports of microplastic occurrence in Etrumeus spp. but the median value from Ross’s (2017) and this study is 58%.
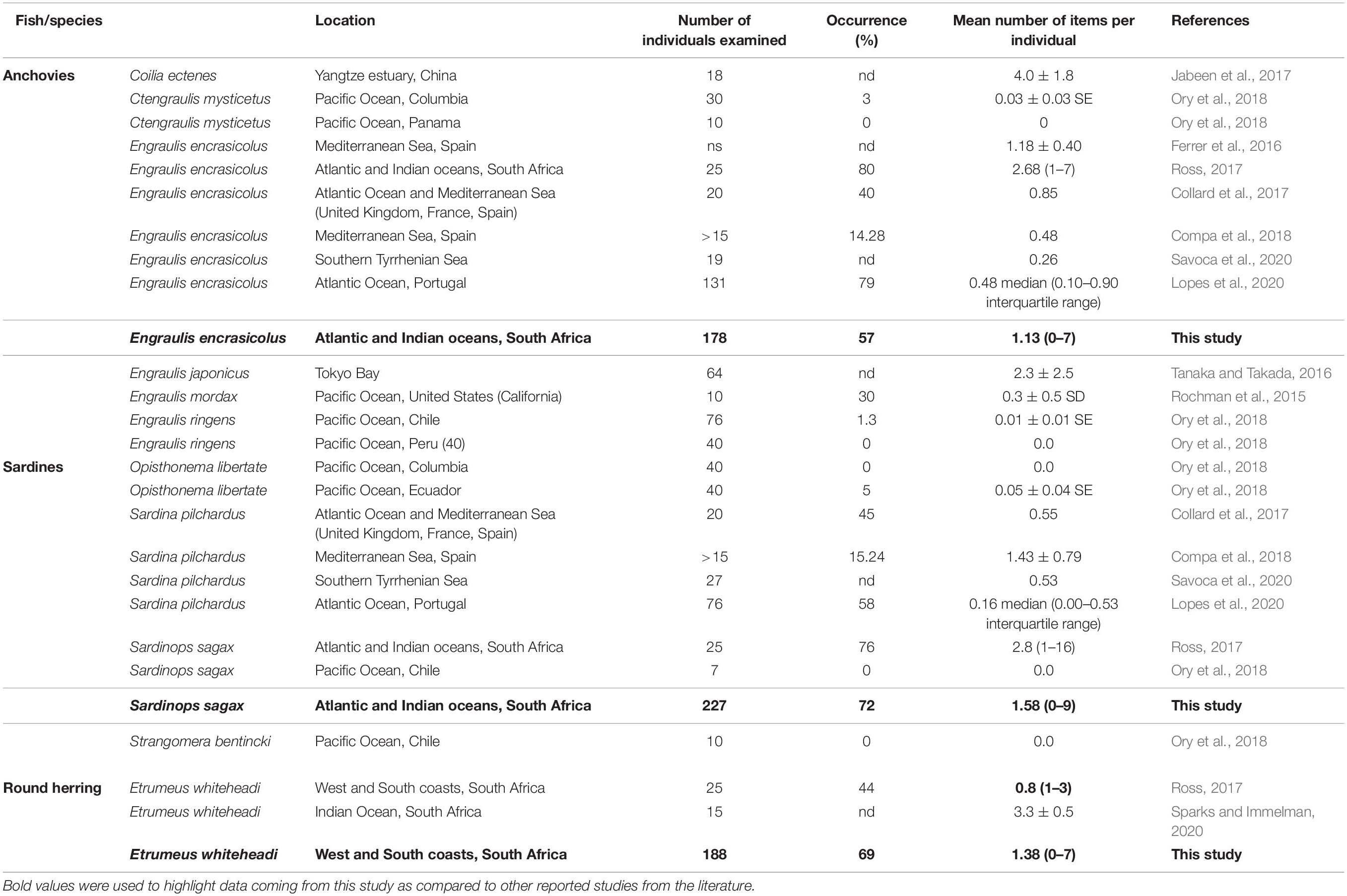
Table 4. Occurrence and number of items individual–1 for anchovies, sardine and round herring reported in the literature from several locations (number of individuals examined indicated where possible; ns: not specified; nd: not determined).
Our estimate of mean microplastics abundance for En. encrasicolus is substantially (< 50%) lower than that estimated by Ross (2017) but is above the median value of 0.48 items individual–1 for anchovies listed in Table 4, where mean abundance ranges from 0.0 to 4.0 items individual–1 across six species from three genera. Similarly, Ross (2017) reported a higher mean microplastics abundance and substantially larger range for S. sagax than observed in this study, and abundance values from both studies are the highest yet reported for this species and compare to a median of 0.35 (range 0.0–2.8 items individual–1) across the four species in four genera of sardines reported in Table 4. We could find no microplastic abundance data for any other Etrumeus species but Sparks and Immelman (2020) reported that Et. whiteheadi from a single sample collected in shelf-edge waters off South Africa’s South Coast (in Stratum G of this analysis) had a mean abundance of 3.3 (± 0.5 SE) items individual–1, which is somewhat but not substantially higher than the value of 1.80 (± 0.55 95% CI) 2.5 items individual–1 estimated for this species in Stratum G in this study.
Main Plastic Types and Sources
Fibers were the main type of ingested items in biota, representing 80% of the extracted items in this study. Microfibers are known to represent a large fraction (>85%) of microplastic debris found along shorelines globally (Carr, 2017). This was in agreement with Ross (2017) who reported that microfibers represented 99% of the items extracted from En. encrasicolus, S. sagax, Et. whiteheadi, Lampanyctodes hectoris, and Maurolicus walvisensis from the West and South coasts of South Africa. Similarly, Naidoo et al. (2016) reported that plastic fibers were ingested most commonly by Mugil cephalus in the Port of Durban off South Africa’s East Coast. Naidoo et al. (2020b) also reported that fibers represented 68% of ingested items by juvenile Oreochromis mossambicus, Terapon jarbua, Ambassis dussumieri and Mugil spp., within four mangroves also along the East Coast of South Africa, and Sparks and Immelman (2020) that filaments (i.e., fibers) comprised 67% of microplastics ingested by three species of intertidal mussel around Cape Town.
Microfibers can enter the environment from both primary sources (fibers < 5 mm in size) or resulting from the fragmentation of larger items (Henry et al., 2019). Whereas wastewater treatment plants have been previously thought to be a primary source of microfibers in the environment, recent studies have shown that direct input from shedding of fibers, from common fabric and textiles, could represent a much important source (Carr, 2017; Conley et al., 2019). De Villiers (2019) investigated the occurrence of microfibers in river sediments adjacent to South Africa’s coastline and reported a significant positive relationship between river sediment, microfiber levels, and the percentage of households in the catchment area that do not have access to piped water. That study also suggested that rivers represent direct vectors for the transport of fibers to the marine environment from rural communities, for whom rivers are the only source of accessible water, from clothes-washing activities. It is however worth noting that natural fibers (cellulose and of animal origin) represent a greater proportion of oceanic fibers (91.8%) as compared to synthetic fibers (8.2%) despite synthetic polymers currently accounting for two-thirds of global fiber production (Suaria et al., 2020).
The main commonly found polymers in biota in our study were poly(ethylene:propylene:diene) (EPDM, 33% occurrence), polyethylene (PE, 20%), polyamide (PA, 20%), polyester (PET, 20%), and polypropylene (PP, 7%) (Supplementary Figure S3). While data on the type of polymer found in biota for South Africa is limited these results are consistent with a meta-analysis by Erni-Cassola et al. (2019) that indicated the prevalence of PE, followed by PA, PET, and PP in aquatic environments globally.
Spatial Variation
Significant variation in the occurrence and abundance of microplastics in biota across the different strata were also observed in this study. The ingestion of particles throughout the inshore shelf suggested the widespread occurrence of microplastics in the pelagic environment along the South African coastline, between the Orange River mouth and Mossel Bay, with a significant increase in the number of items per individual in biota from the West to the South coast observed. This study was carried out in the southern Benguela coastal upwelling ecosystem (west of Cape Agulhas; Strata A–E) and the Agulhas Bank temperate shelf ecosystem (east of Cape Agulhas; Strata F and G), and prevailing oceanographic conditions differ between the two (Hutchings et al., 2009). The southern Benguela is characterized by seasonal upwelling that brings cool waters from the depths of the Central South Atlantic to the surface inshore, and warm Agulhas Current water offshore. The Agulhas Bank displays characteristics of both upwelling and temperate shelf ecosystems, and its dynamics are markedly impacted by the Agulhas Current that transports warm, salty water from the Indian Ocean along South Africa’s East and South coasts. Because of the differing origins and transport routes of source waters of these two systems it might be expected that microplastics load off the West Coast are lower than those off the South Coast, as suggested by the results of this study.
Along the South African coastline, macro litter has been observed in higher abundance close to urban centers (Ryan et al., 2009), whereas, micro litter on beach sediments has not been observed to be driven by population demographics, but rather by large scale ocean currents (Nel et al., 2017). Microplastic concentrations are higher in environmental matrices near or in highly populated areas, close to large coastal wastewater treatment plant discharge points, in rivers and harbors, and are affected by seasonal changes in river runoff (Naidoo et al., 2015; Nel et al., 2017; de Villiers, 2018). De Villiers (2019) noted higher microplastic levels in river sediments in KwaZulu-Natal and the Wild Coast (both along the South African East Coast) which are comparable to levels in the sediments of rivers along the Cape South Coast (ca. 20–26°E).
We also found that microplastics abundance was not significantly linearly related with individuals’ caudal length or weight, which is in agreement with other studies that reported no correlation between total number of particles per fish and fish mass (Naidoo et al., 2020b) or fish size (Vendel et al., 2017).
Selection and Proposal of a Bioindicator for Microplastics for South African Waters
One of the objectives of this study was to identify and propose a suitable and sustainable indicator species for the monitoring of microplastics in biota for South African waters, with fish considered to be appropriate bio-indicators of larger microplastics (Ryan et al., 2020). A list of criteria for the selection of a suitable bio-indicator for microplastics has been modified from GESAMP (2019) and is presented in Table 5. The species used in this study, En. encrasicolus, Et. whiteheadi and S. sagax, fulfilled most of these criteria; in particular, they are widely distributed in shelf waters along the South African coastline (Supplementary Figure S4) and are already being collected as part of annual research surveys, indicating that a broad-scale assessment of microplastics could be cost-efficiently integrated into an existing monitoring program.
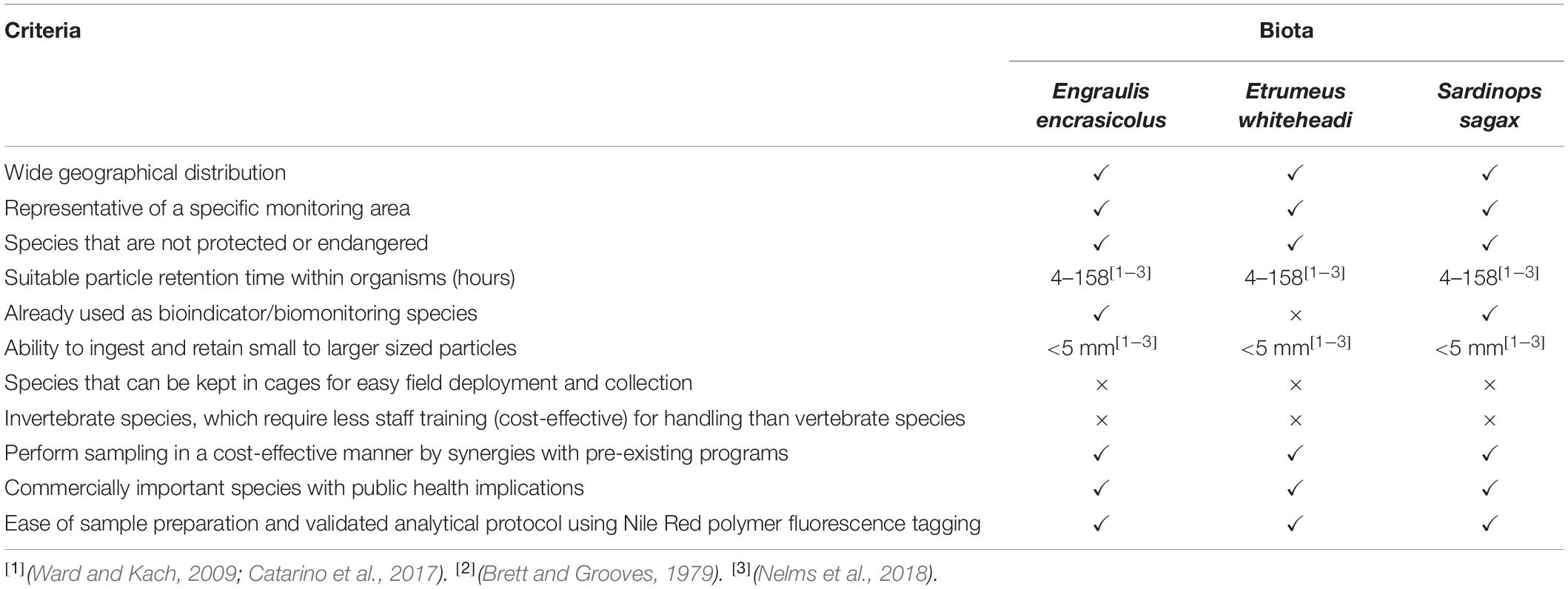
Table 5. Recommended criteria for the selection of a bioindicator species for the monitoring of microplastics in biota for SA coastal waters.
In consideration of a microplastics bio-indicator and the development of a potential monitoring plan, a bootstrap (Manly, 2006) analysis to generate confidence intervals was carried out to estimate optimum sample size for each species (Figure 6). Output from the analysis suggested a sample size of 150 individuals across all strata (22 individuals per stratum) sampled in the 2019 Pelagic Recruit survey for En. encrasicolus, and 225 individuals for both Et. whiteheadi and S. sagax across all strata (32 individuals per stratum), to obtain a precision of 0.4 items of MP per individual, as measured by a 95% confidence interval. Plots for Et. whiteheadi and S. sagax were similar due to their nearly identical standard deviations of 1.532 and 1.537, respectively. The CI widths for En. encrasicolus were also lower due to a lower standard deviation of 1.29 in this study. As mentioned previously, the Poisson log-linear model suggested that the effect of fish species was not similar across strata and the interaction between MP items in the fish species and strata was an important factor to consider (Supplementary Table S3). In other words, the pattern of MP abundance across strata was different, depending on the species. Given that the fish in this study were caught together, rather than caught per species, the interaction relationship is unlikely to have been influenced by the sampling technique.
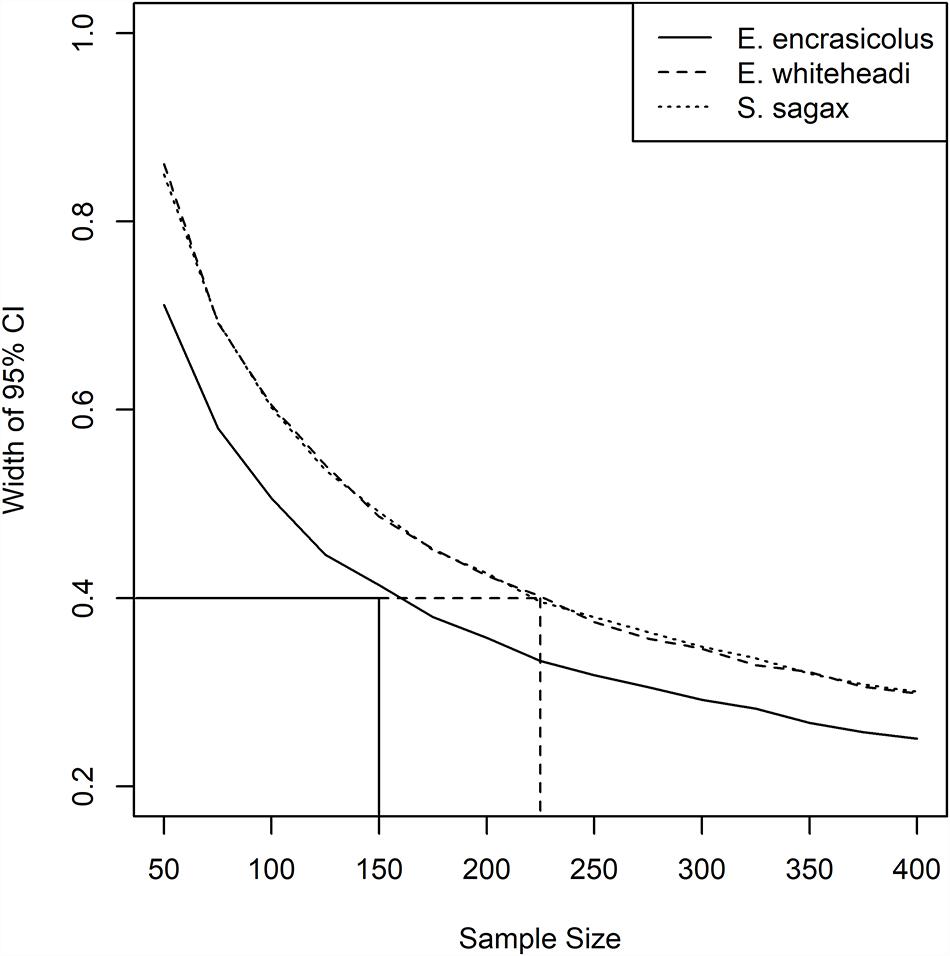
Figure 6. Widths of 95% bootstrapped confidence intervals for sample sizes between 50 and 400, for Engraulis encrasicolus, Etrumeus whiteheadi, and Sardinops sagax. Note that the lines for Etrumeus whiteheadi and Sardinops sagax are virtually identical.
Although fewer En. encrasicolus would be required for microplastics assessment to achieve the desired precision, it was clear that both Et. whiteheadi and S. sagax showed spatial trends in microplastics abundance whereas anchovy did not, suggesting that the former two species might represent more suitable candidates for monitoring programs. Sardina pilchardus has already been proposed as a bio-indicator for microplastics for the Mediterranean Sea (Fossi et al., 2018) and the selection of S. sagax would allow for global comparisons. However, its suitability in a South African context is under question as the local S. sagax population is presently in a depleted state (DFFE, 2020), which could negatively impact sample collection until it recovers. Given this, the present large population size of Et. whiteheadi, and the recommendation by Lopes et al. (2020) that planktivorous species that feed on larger zooplankton prey (which this species does) be used as bio-indicator species for monitoring microplastics, we suggest the latter as a suitable regional microplastics bio-indicator specific for South African waters. This despite the lack of knowledge of plastic ingestion by this genus elsewhere which will make global comparisons difficult. The estimate of 225 fish as a required sample size applies to the area covered in the 2019 Pelagic Recruit Survey and would need to be adjusted for other surveys that cover a larger spatial extent.
Potential Impacts on Human Health
The occurrence of microplastics in biota has caused several concerns, ranging from its effects on biodiversity and populations to potential risks to food safety and human health. As fish consumption in South Africa grew by more than 26% between 1994 and 2009, there is potential for the transfer of microplastics from fish to humans following consumption (Naidoo et al., 2020a). It has been suggested by the Food and Agriculture Organization (FAO) that the transfer of sorbed co-contaminants and additives from the ingestion of plastic particles would be negligible due to the low dietary exposure to such contaminants (Lusher et al., 2017). However, the transfer of plastics along the food chain to humans and impacts on human health has been identified as a gap in the understanding of marine plastic pollution in a South African perspective and data on levels of transferral of microplastics from edible aquatic species to humans are currently lacking (Godfrey, 2020; Naidoo et al., 2020a). This gap must be filled in order to make predictive decisions in regard to safety for consumption (Naidoo et al., 2020a).
Conclusion
The data presented in this study confirmed the occurrence of microplastics, principally microfibers, in the commercially important small pelagic fishes En. encrasicolus, Et. whiteheadi, and S. sagax in South Africa, with an overall occurrence of 68%. The abundance of microplastics in these fish was impacted by both species and location, as well as the interaction of these two factors, but the overall pattern indicated an increase from the West to the South coast. Microplastic abundance levels in South African anchovy and sardine are above median values for similar species elsewhere but were not correlated with fish biological characteristics in any of the three species examined here. We propose that Et. whiteheadi be used as a bio-indicator for microplastics in South African waters and that the collection and processing of this species be included in regular survey programs. Finally, an increase in fish consumption in South Africa suggests potential for the transfer of microplastics to human following consumption with unknown related effects on human health. However, the impacts of microplastics and associated co-contaminants on human health have been suggested to be minor by both the World Health Organization and FAO due to the low dietary exposure to such contaminants.
Data Availability Statement
The datasets presented in this study can be found in online repositories. The names of the repository/repositories and accession number(s) can be found below: 10.14466/CefasDataHub.100.
Ethics Statement
Ethical review and approval was not required for the animal study because the fish were collected as part of an already existing fisheries survey conducted by the South African Government. Research was conducted on dead fish.
Author Contributions
FP-W, TM, CL, and AdB designed and coordinated the study. CL, AsB, and YG collected the samples. AdB, YM, GM, DD, FT, and RH carried out the laboratory work. JB carried out the statistical analysis. AdB and CL wrote the manuscript. All authors contributed to the article and approved the submitted version.
Funding
Microplastic sampling and analysis were carried out as part of the Commonwealth Litter Programme (CLiP), implemented by the Centre for Environment, Fisheries and Aquaculture Science (Cefas), funded by the United Kingdom Government’s Department for Environment, Food and Rural Affairs (Defra), through Official Development Assistance (ODA) funding.
Conflict of Interest
The authors declare that the research was conducted in the absence of any commercial or financial relationships that could be construed as a potential conflict of interest.
Acknowledgments
We gratefully acknowledge the contributions of collaborators from the Department of Environment, Forestry and Fisheries [(DEFF) formerly from the Department of Agriculture, Forestry and Fisheries (DAFF)] involved in sample collection during the Pelagic Recruit Survey, including Janet C. Coetzee (Survey Co-ordinator), Dagmar Merkle, Mzwamadoda Phillips, and Fannie Shabangu (Chief Scientists), and the master, officers, and crew of the FRS Africana, as well as collaborators from the Department of Environment, Forestry and Fisheries [(DEFF) formerly from the Department of Environmental Affairs (DEA)], especially Keshnee Pillay and Marco Worship. We would also like to thank Jeroen Van Der Kooij for kindly agreeing to review the final draft of this manuscript.
Supplementary Material
The Supplementary Material for this article can be found online at: https://www.frontiersin.org/articles/10.3389/fmars.2020.574663/full#supplementary-material
References
Babayemi, J. O., Nnorom, I. C., Osibanjo, O., and Weber, R. (2019). Ensuring sustainability in plastics use in Africa: consumption, waste generation, and projections. Environ. Sci. Eur. 31:60.
Bakir, A., O’Conner, I. A., Rowland, S. J., and Hendriks, A. J. (2016). Relative importance of microplastics as a pathway for the transfer of hydrophobic organic chemicals to marine life. Env. Poll. 219, 56–65. doi: 10.1016/j.envpol.2016.09.046
Bakir, A., Rowland, S. J., and Thompson, R. C. (2014). Enhanced desorption of persistent organic pollutants from microplastics under simulated physiological conditions. Environ. Pollut. 185, 16–23. doi: 10.1016/j.envpol.2013.10.007
Brett, J. R., and Grooves, T. D. D. (1979). “Physiology Energetics,” in Fish Physiology, eds W. S. Hoar, D.J. Randall, and J. R. Brett. New York, NY: Academic Press, 279–352.
Carr, S. A. (2017). Sources and dispersive modes of micro−fibers in the environment. Integr. Environ. Assess. Manag. 13, 466–469. doi: 10.1002/ieam.1916
Catarino, A. I., Thompson, R., Sanderson, W., and Henry, T. B. (2017). Development and optimization of a standard method for extraction of microplastics in mussels by enzyme digestion of soft tissues. Environ. Toxicol. Chem. 36, 947–951. doi: 10.1002/etc.3608
Cliff, G., Dudley, S. F. J., Ryan, P. G., and Singleton, N. (2002). Large sharks and plastic debris in KwaZulu-Natal. S. Afr. Mar. Freshw. Res. 53, 575–581. doi: 10.1071/MF01146
Collard, F., Gilbert, B., Eppe, G., Roos, L., Compère, P., Das, K., et al. (2017). Morphology of the filtration apparatus of three planktivorous fishes and relation with ingested anthropogenic particles. Mar. Pollut. Bull. 116, 182–191. doi: 10.1016/j.marpolbul.2016.12.067
Compa, M., Ventero, A., Iglesias, M., and Deudero, S. (2018). Ingestion of microplastics and natural fibres in Sardina pilchardus (Walbaum, 1792) and Engraulis encrasicolus (Linnaeus, 1758) along the Spanish Mediterranean coast. Mar. Pollut. Bull. 128, 89–96. doi: 10.1016/j.marpolbul.2018.01.009
Conley, K., Clum, A., Deepe, J., Lane, H., and Beckingham, B. (2019). Wastewater treatment plants as a source of microplastics to an urban estuary: Removal efficiencies and loading per capita over one year. Water Res. X 3:100030. doi: 10.1016/j.wroa.2019.100030
R Core Team (2019). R: A language and environment for statistical computing. United States: R Core Team.
de Villiers, S. (2018). Quantification of microfibre levels in South Africa’s beach sediments, and evaluation of spatial and temporal variability from 2016 to 2017. Mar. Pollut. Bull. 135, 481–489. doi: 10.1016/j.marpolbul.2018.07.058
De Villiers, S. (2019). Short communication: Microfibre pollution hotspots in river sediments adjacent to South Africa’s coastline. Water SA 45, 97–102. doi: 10.4314/wsa.v45i1.11
DFFE (2020). Status of the South African Marine Fishery Resources 2020. Cape Town: Department of Forestry Fisheries and Environmental Affairs.
Enders, K., Lenz, R., Beer, S., and Stedmon, C. A. (2017). Extraction of microplastic from biota: Recommended acidic digestion destroys common plastic polymers. ICES J. Mar. Sci. 74, 326–331. doi: 10.1093/icesjms/fsw173
Erni-Cassola, G., Zadjelovic, V., Gibson, M. I., and Christie-Oleza, J. A. (2019). Distribution of plastic polymer types in the marine environment; A meta-analysis. J. Hazard. Mater. 369, 691–698. doi: 10.1016/j.jhazmat.2019.02.067
Ferrer, M. C., Ventero, A., Iglesias, M., and Deudero, S. (2016). Microplastic ingestion in commercial fish species Boops Boops. Sardina pilchardus and Engraulis Encrasicolus in the Western Mediterranean sea: Medias Survey. Rapp. Comm. Int. Mer. Medit. 41:218.
Fossi, M. C., Peda, C., Compa, M., Tsangaris, C., Alomar, C., Claro, F., et al. (2018). Bioindicators for monitoring marine litter ingestion and its impacts on Mediterranean biodiversity. Environ. Pollut. 237, 1023–1040. doi: 10.1016/j.envpol.2017.11.019
GESAMP (2015). Sources, fate and effects of microplastics in the marine environment: a global assessment. Rep. Stud. GESAMP 90:96. doi: 10.13140/RG.2.1.3803.7925
GESAMP (2019). Guidelines for the monitoring and assessment of plastic litter in the ocean. London: GESAMP.
GESAMP (2020). Proceedings of the GESAMP International Workshop on assessing the risks associated with plastics and microplastics in the marine environment. London: GESAMP.
Godfrey, L. (2020). Are there gaps in our understanding of marine plastic pollution? S. Afr. J. Sci. 116:sajs.2020/8170.
Henry, B., Laitala, K., and Klepp, I. G. (2019). Microfibres from apparel and home textiles: Prospects for including microplastics in environmental sustainability assessment. Sci. Total Environ. 652, 483–494. doi: 10.1016/j.scitotenv.2018.10.166
Herzke, D., Anker-Nilssen, T., Nøst, T. H., Götsch, A., Christensen-Dalsgaard, S., Langset, M., et al. (2016). Negligible Impact of Ingested Microplastics on Tissue Concentrations of Persistent Organic Pollutants in Northern Fulmars off Coastal Norway. Environ. Sci. Technol. 50, 1924–1933. doi: 10.1021/acs.est.5b04663
Hutchings, L., Van der Lingen, C. D., Shannon, L. J., Crawford, R. J. M., Verheye, H. M. S., Bartholomae, C. H., et al. (2009). The Benguela Current: An ecosystem of four components. Prog. Oceanogr. 83, 15–32. doi: 10.1016/j.pocean.2009.07.046
Isaacs, M. (2016). The humble sardine (small pelagics): fish as food or fodder. Agric. Food Secur. 5:27.
Jabeen, K., Su, L., Li, J., Yang, D., Tong, C., Mu, J., et al. (2017). Microplastics and mesoplastics in fish from coastal and fresh waters of China. Environ. Pollut. 221, 141–149. doi: 10.1016/j.envpol.2016.11.055
James, A. G. (1987). Feeding ecology, diet and field-based studies on feeding selectivity of the Cape anchovy Engraulis capensis Gilchrist. S. Afr. J. Mar. Sci. 5, 673–692. doi: 10.2989/025776187784522784
Jung, M. R., Horgen, F. D., Orski, S. V., Rodriguez C, V., Beers, K. L., Balazs, G. H., et al. (2018). Validation of ATR FT-IR to identify polymers of plastic marine debris, including those ingested by marine organisms. Mar. Pollut. Bull 127, 704–716. doi: 10.1016/j.marpolbul.2017.12.061
Kniggendorf, A.-K., Wetzel, C., and Roth, B. (2019). Microplastics detection in streaming tap water with Raman spectroscopy. Sensors 19:1839. doi: 10.3390/s19081839
Koelmans, A. A., Bakir, A., Burton, G. A., and Janssen, C. R. (2016). Microplastic as a Vector for Chemicals in the Aquatic Environment: Critical Review and Model-Supported Reinterpretation of Empirical Studies. Environ. Sci. Technol. 50, 3315–3326. doi: 10.1021/acs.est.5b06069
Kukkola, A. T., Senior, B., Maes, T., Kroger, S., and Ag, M. (2020). Digging down into the detail: a large-scale study of microplastic abundance in sediment cores from the UK continental shelf. Cambridge: Academic Press.
Lohmann, R. (2017). Microplastics are not important for the cycling and bioaccumulation of organic pollutants in the oceans—but should microplastics be considered POPs themselves? Integr. Environ. Assess. Manag. 13, 460–465. doi: 10.1002/ieam.1914
Lopes, C., Raimundo, J., Caetano, M., and Garrido, S. (2020). Microplastic ingestion and diet composition of planktivorous fish. Limnol. Oceanogr. Lett. 5, 103–112.
Lusher, A., Bråte, I. L. N., Hurley, R., Iversen, K., and Olsen, M. (2017). Testing of methodology for measuring microplastics in blue mussels (Mytilus spp) and sediments, and recommendations for future monitoring of microplastics (R & D-project). Report number: 7209-2017.
Maes, T., Jessop, R., Wellner, N., Haupt, K., and Mayes, A. G. (2017). A rapid-screening approach to detect and quantify microplastics based on fluorescent tagging with Nile Red. Sci. Rep. 7:44501.
Maes, T., van Diemen, de Jel, J., Vethaak, A. D., Desender, M., Bendall, V. A., et al. (2020). You Are What You Eat, Microplastics in Porbeagle Sharks From the North East Atlantic: Method Development and Analysis in Spiral Valve Content and Tissue. Front. Mar. Sci. 7:273.
Manly, B. F. J. (2006). Randomization, bootstrap and Monte Carlo methods in biology. Florida, FL: CRC press.
Naidoo, T., and Glassom, D. (2019). Decreased growth and survival in small juvenile fish, after chronic exposure to environmentally relevant concentrations of microplastic. Mar. Pollut. Bull 145, 254–259. doi: 10.1016/j.marpolbul.2019.02.037
Naidoo, T., Glassom, D., and Smit, A. J. (2015). Plastic pollution in five urban estuaries of KwaZulu-Natal. S. Afr. Mar. Pollut. Bull. 101, 473–480. doi: 10.1016/j.marpolbul.2015.09.044
Naidoo, T., Smit, A. J., and Glassom, D. (2016). Plastic ingestion by estuarine mullet Mugil cephalus (Mugilidae) in an urban harbour, KwaZulu-Natal, South Africa. African J. Mar. Sci. 38, 145–149. doi: 10.2989/1814232X.2016.1159616
Naidoo, T., Rajkaran, A., and Sershen, N (2020a). Impacts of plastic debris on biota and implications for human health: A South African perspective. S. Afr. J. Sci. 116:sajs.2020/7693.
Naidoo, T., Sershen, Thompson, R. C., and Rajkaran, A. (2020b). Quantification and characterisation of microplastics ingested by selected juvenile fish species associated with mangroves in KwaZulu-Natal. S. Afr. Environ. Pollut. 257:113635. doi: 10.1016/J.ENVPOL.2019.113635
Nel, H. A., Hean, J. W., Noundou, X. S., and Froneman, P. W. (2017). Do microplastic loads reflect the population demographics along the southern African coastline? Mar. Pollut. Bull. 115, 115–119. doi: 10.1016/j.marpolbul.2016.11.056
Nelms, S. E., Galloway, T. S., Godley, B. J., Jarvis, D. S., and Lindeque, P. K. (2018). Investigating microplastic trophic transfer in marine top predators. Environ. Pollut. 238, 999–1007. doi: 10.1016/j.envpol.2018.02.016
Ory, N., Chagnon, C., Felix, F., Fernández, C., Ferreira, J. L., Gallardo, C., et al. (2018). Low prevalence of microplastic contamination in planktivorous fish species from the southeast Pacific Ocean. Mar. Pollut. Bull. 127, 211–216. doi: 10.1016/j.marpolbul.2017.12.016
Reynolds, C., and Ryan, P. G. (2018). Micro-plastic ingestion by waterbirds from contaminated wetlands in South Africa. Mar. Pollut. Bull. 126:330–333. doi: 10.1016/j.marpolbul.2017.11.021
Rochman, C. M., Hoh, E., Kurobe, T., and Teh, S. J. (2013). Ingested plastic transfers hazardous chemicals to fish and induces hepatic stress. Sci. Rep. 3:3263. doi: 10.1038/srep03263
Rochman, C. M., Tahir, A., Williams, S. L., Baxa, D. V., Lam, R., Miller, J. T., et al. (2015). Anthropogenic debris in seafood: Plastic debris and fibers from textiles in fish and bivalves sold for human consumption. Sci. Rep. 5:14340.
Ross, K. J. (2017). Ingestion of microplastics by epipelagic and mesopelagic fish in South African waters: A species comparison. Rondebosch: University of Cape Town.
Ryan, P. G., Cole, G., Spiby, K., Nel, R., Osborne, A., and Perold, V. (2016a). Impacts of plastic ingestion on post-hatchling loggerhead turtles off South Africa. Mar. Pollut. Bull. 107, 155–160. doi: 10.1016/j.marpolbul.2016.04.005
Ryan, P. G., de Bruyn, P. J. N., and Bester, M. N. (2016b). Regional differences in plastic ingestion among Southern Ocean fur seals and albatrosses. Mar. Pollut. Bull. 104, 207–210. doi: 10.1016/j.marpolbul.2016.01.032
Ryan, P. G., Moore, C. J., Van Franeker, J. A., and Moloney, C. L. (2009). Monitoring the abundance of plastic debris in the marine environment. Philos. Trans. R. Soc. B Biol. Sci. 364, 1999–2012. doi: 10.1098/rstb.2008.0207
Ryan, P. G., Pichegru, L., Perold, V., and Moloney, C. L. (2020). Monitoring marine plastics–will we know if we are making a difference? S. Afr. J. Sci. 116, 1–9. doi: 10.1002/9781118891230.ch1
Savoca, S., Bottari, T., Fazio, E., Bonsignore, M., Mancuso, M., Luna, G. M., et al. (2020). Plastics occurrence in juveniles of Engraulis encrasicolus and Sardina pilchardus in the Southern Tyrrhenian Sea. Sci. Total Environ. 718:137457. doi: 10.1016/j.scitotenv.2020.137457
Setälä, O., Fleming-Lehtinen, V., and Lehtiniemi, M. (2014). Ingestion and transfer of microplastics in the planktonic food web. Environ. Pollut. 185, 77–83. doi: 10.1016/j.envpol.2013.10.013
Shabangu, F., Geja, Y., Phillips, M., Merkle, D., and Coetzee, J. (2019). Results of the 2019 pelagic recruitment survey. Cape Town: Department ofAgriculture, Forestry and Fisheries.
Sparks, C., and Immelman, S. (2020). Microplastics in offshore fish from the Agulhas Bank. S. Afr. Mar. Pollut. Bull. 156:111216. doi: 10.1016/j.marpolbul.2020.111216
Suaria, G., Achtypi, A., Perold, V., Lee, J. R., Pierucci, A., Bornman, T. G., et al. (2020). Microfibers in oceanic surface waters: A global characterization. Sci. Adv. 6:eaay8493. doi: 10.1126/sciadv.aay8493
Tanaka, K., and Takada, H. (2016). Microplastic fragments and microbeads in digestive tracts of planktivorous fish from urban coastal waters. Sci. Rep. 6:34351.
Tanaka, K., Takada, H., Yamashita, R., Mizukawa, K., Fukuwaka, M., and Watanuki, Y. (2013). Accumulation of plastic-derived chemicals in tissues of seabirds ingesting marine plastics. Mar. Pollut. Bull. 69, 219–222. doi: 10.1016/j.marpolbul.2012.12.010
Van Der Lingen, C. D. (2002). Diet of sardine Sardinops sagax in the southern Benguela upwelling ecosystem. S. Afr. J. Mar. Sci. 24, 301–316.
van der Lingen, C. D., and Miller, T. W. (2011). “Trophic dynamics of pelagic nekton in the southern Benguela current ecosystem: calibrating trophic models with stable isotope analysis.” inInterdisciplinary Studies on Environmental Chemistry- Marine Environmental Modeling & Analysis, eds J. Omori, X. Guo, N. Yoshie, N. Fujii, I. Handoh, A. Isobe, et al. (Tokyo: TERRAPUB).
Van Der Lingen, C. D., and Miller, T. W. (2014). Spatial, ontogenetic and interspecific variability in stable isotope ratios of nitrogen and carbon of Merluccius capensis and Merluccius paradoxus off South Africa. J. Fish Biol. 85, 456–472. doi: 10.1111/jfb.12436
van der Lingen, C. D., Hutchings, L., and Field, J. G. (2006). Comparative trophodynamics of anchovy Engraulis encrasicolus and sardine Sardinops sagax in the southern Benguela: are species alternations between small pelagic fish trophodynamically mediated? Afr. J. Mar. Sci. 28, 465–477. doi: 10.2989/18142320609504199
Vendel, A. L., Bessa, F., Alves, V. E. N., Amorim, A. L. A., Patrício, J., and Palma, A. R. T. (2017). Widespread microplastic ingestion by fish assemblages in tropical estuaries subjected to anthropogenic pressures. Mar. Pollut. Bull. 117, 448–455. doi: 10.1016/j.marpolbul.2017.01.081
Vorsatz, L. D., van der Lingen, C. D., and Gibbons, M. J. (2015). Diet and gill morphology of the East Coast redeye round herring Etrumeus wongratanai off KwaZulu-Natal. S. Afr. J. Mar. Sci. 37, 575–581. doi: 10.2989/1814232x.2015.1108933
Wallace-Fincham, B. P. (1987). The food and feeding of Etrumeus whiteheadi Wongratana 1983, off the Cape Province of South Africa. Cape Town: University of Cape Town.
Wang, Z., Su, B., Xu, X., Di, D., Huang, H., Mei, K., et al. (2018). Preferential accumulation of small (< 300 μm) microplastics in the sediments of a coastal plain river network in eastern China. Water Res. 144, 393–401. doi: 10.1016/j.watres.2018.07.050
Ward, J. E., and Kach, D. J. (2009). Marine aggregates facilitate ingestion of nanoparticles by suspension-feeding bivalves. Mar. Environ. Res. 68, 137–142. doi: 10.1016/j.marenvres.2009.05.002
Keywords: commercial small pelagic fish species, Engraulis encrasicolus (European anchovy), Etrumeus whiteheadi (West Coast round herring), Sardinops sagax (South African sardine), marine litter, microplastics
Citation: Bakir A, van der Lingen CD, Preston-Whyte F, Bali A, Geja Y, Barry J, Mdazuka Y, Mooi G, Doran D, Tooley F, Harmer R and Maes T (2020) Microplastics in Commercially Important Small Pelagic Fish Species From South Africa. Front. Mar. Sci. 7:574663. doi: 10.3389/fmars.2020.574663
Received: 23 June 2020; Accepted: 07 October 2020;
Published: 04 November 2020.
Edited by:
Andrew Turner, University of Plymouth, United KingdomReviewed by:
Periyadan K. Krishnakumar, King Fahd University of Petroleum & Minerals, Saudi ArabiaQing Wang, Yantai Institute of Coastal Zone Research (CAS), China
Copyright © 2020 Bakir, van der Lingen, Preston-Whyte, Bali, Geja, Barry, Mdazuka, Mooi, Doran, Tooley, Harmer and Maes. This is an open-access article distributed under the terms of the Creative Commons Attribution License (CC BY). The use, distribution or reproduction in other forums is permitted, provided the original author(s) and the copyright owner(s) are credited and that the original publication in this journal is cited, in accordance with accepted academic practice. No use, distribution or reproduction is permitted which does not comply with these terms.
*Correspondence: Adil Bakir, YWRpbC5iYWtpckBjZWZhcy5jby51aw==