- 1Instituto Chico Mendes de Conservação da Biodiversidade/Centro Nacional de Pesquisa e Conservação de Mamíferos Aquáticos (ICMBio/CMA), São Paulo, Brazil
- 2U.S. Geological Survey, Wetland and Aquatic Research Center, Gainesville, FL, United States
- 3GEOMARE, A. C., Mazatlán, Mexico
- 4CIBIO-InBIO Research Center in Biodiversity and Genetic Resources, Porto, Portugal
- 5Universidade Federal de Pernambuco, Recife, Brazil
The Antillean subspecies of the West Indian manatee is classified as endangered by the International Union for the Conservation of Nature (IUCN) Red List. In Brazil, the manatee population is listed as endangered with an estimated population size of 500–1,000. Historic hunting, recent habitat degradation, and fisheries bycatch have decreased the population size. The Amazonian manatee is listed as vulnerable by the IUCN with unknown population sizes within Brazil. The Antillean manatee occurs in sympatry with the Amazonian manatee in Brazil and hybridization has been previously indicated. To provide information on the genetic structure, diversity, and degree of hybridization in the sympatric zone near the Amazon River mouth, the mitochondrial DNA control region and 13 nuclear microsatellite markers were assessed on the two species. Samples were analyzed from the Antillean subspecies across its distribution in Brazil (n = 78) and from the Amazonian species (n = 17) at the Amazon River mouth and inland mainstem river. To assess the previously defined evolutionary significant units of Antillean manatees in the area, an additional 11 samples from Venezuela and Guyana were included. The Antillean manatee was found to be a single population in Brazil and had lower than average number of alleles (3.00), expected heterozygosity (0.34), and haplotype diversity (0.15) when compared to many other manatee populations. The low values may be influenced by the small population size and extended pressures from anthropogenic threats. Gene flow was identified with Venezuela/Guyana in admixed Antillean Brazil samples, although the two populations were found to be moderately divergent. The nuclear loci in Venezuela/Guyana Antillean manatee samples indicated high differentiation from the samples collected in the Amazon River (FST = 0.35 and RST = 0.18, p = 0.0001). No indication of nuclear hybridization was found except for a single sample, “Poque” that had been identified previously. The distribution of Antillean manatees in Brazil is extensive and the areas with unique habitat and threats would benefit from independent management and conservation actions. Gene flow, resulting in genetic diversity and long-term population stability, could be improved in the southern range through habitat restoration, and the establishments of travel corridors and protected areas, which are particularly important for successful parturition and neonatal calf survival.
Introduction
Over centuries, animal populations have been under human pressures (e.g., fishing, hunting, and harvesting) causing long-term changes in abundance and in many cases extirpating the complete population (Barnosky et al., 2011; Dirzo et al., 2014; Young et al., 2016; Ceballos et al., 2020). In other cases, these threats do not result in immediate extinction, but can lead to fragmentation and potentially inbreeding depression (Brook et al., 2008). Thus, knowledge and evaluation of the genetic diversity of endangered species has become a necessary parameter in understanding population status, resilience, and viability (Willi et al., 2006; Whiteley et al., 2015). Moreover, the distribution of genetic diversity in sympatric species, hybrid zones, and small and isolated populations is relevant to management actions (Allendorf et al., 2001; Levin, 2002; Bouzat, 2010; Piett et al., 2015; Torres-Florez et al., 2018).
The Antillean subspecies of the West Indian manatee (Trichechus manatus manatus) and the Amazonian species (Trichechus inunguis) are both found within the national boundary of Brazil. The Antillean subspecies is classified as endangered by the International Union for the Conservation of Nature (IUCN) and the Brazilian Red List (IUCN, 2012; ICMBio, 2018a). In Brazil, the total population size is estimated between 500 and 1,000 individuals and the current range is from the northern state of Amapá (AP) to the state of Alagoas (AL) in the southeast, with fragmentation of the population occurring in many areas (Figure 1) (Lima, 1997; Luna, 2001; Luna et al., 2008c). Brazil is the southern limit for the distribution of Antillean manatees, with population patches along the coastline of South America (Balensiefer et al., 2017). Additionally, the Amazonian manatee is found in the mouth of the Amazon River within the northern Brazilian states of AP and Pará (PA), and upstream in other regions of South America (Luna et al., 2008b). The Antillean subspecies and Amazonian species have sympatric distributions at the mouth of the Amazon River (Luna et al., 2008a,b). Conservation efforts on the north coast of Brazil has led to higher Antillean manatee population sizes when compared to those found along the northeast, which have fewer protections (Luna et al., 2008b; Luna and Passavante, 2010). Antillean manatees are now extirpated from parts of the southern historic range in the states of Sergipe (SE), Bahia (BA), and Espírito Santo (ES) (Albuquerque and Marcovaldi, 1982; Borobia and Lodi, 1992; Lima et al., 1992; Lima, 1997; Luna et al., 2008a). Manatees are no longer established in the states of SE and BA, however, stranded and rehabilitated manatees have been reintroduced to both states with the aim of repopulation (Luna et al., 2008b).
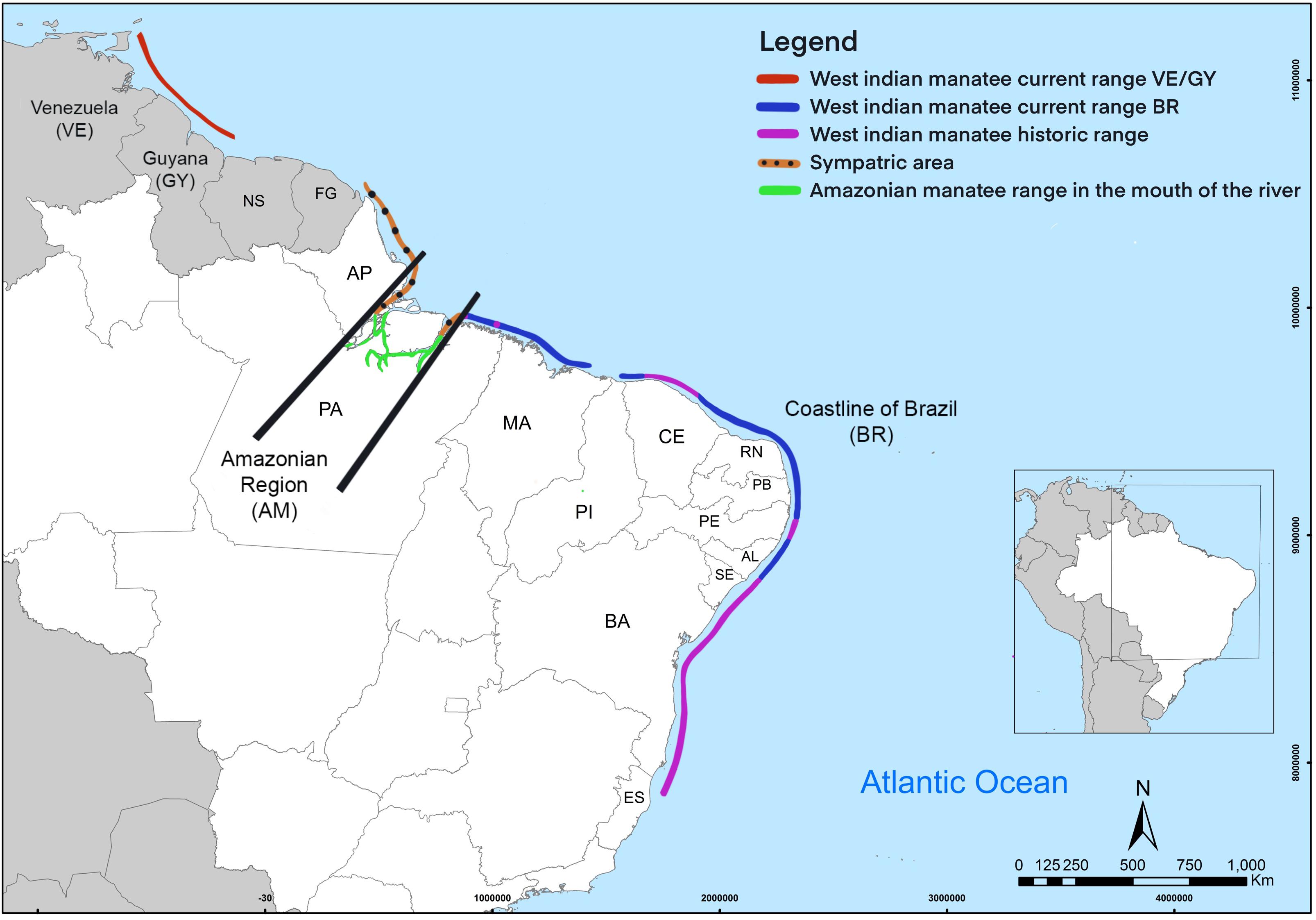
Figure 1. Map of the sample area and the geographic extent of the genetic groupings. Genetic groupings are labeled accordingly and abbreviations are identified. The black bars indicate approximate geographic separation. State and country names are abbreviated for simplicity (see main text for abbreviations). Historic areas of Brazilian distribution are also shown.
Along the Brazil coast, Amazon River Delta, and inland riverine areas, manatee habitats lack barriers to dispersal and connectivity. In the absence of conspicuous barriers, populations tend to be structured and distributed based on multiple interacting factors (e.g., demography, ecology, habitat, and anthropogenic influence) (Forcada and Würsig, 2002; Frankham et al., 2002). The manatee populations in Brazil were severely decreased by hunters during the Portuguese colonization in the 16th century and while hunting pressure has subsided, new and severe threats have emerged in this region. Current anthropogenic threats include habitat degradation caused by effluent dumping, leading to toxic water systems on coasts and estuaries; accidental death in fishing gear; and a high degree of water vessel strikes (Calleson and Frohlich, 2007; Luna et al., 2008a, 2018). The manatees’ low reproductive rate, along with a large number of stranded calves in certain areas along its distribution (especially in Ceará (CE) and Rio Grande do Norte (RN) states, Brazil), has likely contributed to a declining population trend and limited recovery (Deutsch et al., 2008; Luna et al., 2008c). The National Action Plans for West Indian Manatees and for Amazon Aquatic Mammals in Brazil correlates the loss of habitat with increased calf strandings, which further represses a population’s ability to grow in size (Luna et al., 2011; ICMBio, 2018b, 2019). Due to the historic hunting pressure, conservation status, low abundance (Luna et al., 2008a, 2018), reduced dispersal abilities at times (Normande et al., 2016), and the sympatry of the West Indian and Amazonian species, it is important to genetically characterize manatee population structure as well as document evidence of hybridization in the contact zones.
As threats continue and geographically fragmented populations persist in low numbers (Luna et al., 2018), the Brazilian Antillean manatees are vulnerable to genetic effects that can encumber recovery and population resiliency. In previously studied West Indian manatee populations, low levels of nuclear diversity and small population sizes have been generally observed (Bradley et al., 1993; García-Rodríguez et al., 1998; Vianna J. A. et al., 2006; Hunter M. E. et al., 2010; Hunter et al., 2012; Nourisson, 2011; Nourisson et al., 2011; Tucker et al., 2012; Satizábal et al., 2012). Isolated populations with these characteristics can be vulnerable to disease outbreaks or demographic and stochastic events, compounding negative genetic effects and risking local extinction (Frankham et al., 2002). Identifying and monitoring the genetic diversity in Antillean manatee populations provides a foundation for improved regional management practices across country boundaries, which can assist with the recovery and preservation of the remaining subpopulations.
Previous range-wide phylogeographic studies of West Indian and Amazonian manatees used mitochondrial DNA (mtDNA) to define the haplotype diversity in Brazil and found one clade present in each of the species’ populations (García-Rodríguez et al., 1998; Cantanhede et al., 2005; Vianna J. D. A. et al., 2006; Luna et al., 2012; Lima et al., 2019). Gene flow with other nearby populations (i.e., French Guiana, Guyana, Venezuela) could improve the long-term persistence of the Brazil population at the southern limit of distribution. However, it has been proposed that this corridor, from the mouth of the Amazon River along the coastline north to Guyana, is also a hybridization zone which could hinder effective gene flow for the Antillean manatee along the coastline (Vianna J. A. et al., 2006; Vianna J. D. A. et al., 2006; Parr et al., 2012). Hybridization of the West Indian and Amazonian species has been studied using phylogenetics, cytogenetics, and ddRAD sequencing, but limited sample sizes and low detection of hybrids have restricted interpretations (Vianna J. A. et al., 2006; Vianna J. D. A. et al., 2006; Vilaça et al., 2016; Lima et al., 2019). Detecting and characterizing hybridization is important as it can lead to genetic swamping or dilution of smaller populations and local genotypes (Bohling and Waits, 2015). Thus, this study aims to address four objectives as they build off one another: (1) assess species differentiation and evidence of hybridization between the two species, (2) investigate the population structure of the Antillean Brazil and Venezuela/Guyana samples, (3) evaluate the connectivity of the extant Antillean manatees along the Brazilian coastline, and finally (4) describe the mitochondrial and nuclear neutral genetic diversity of the identified populations.
Materials and Methods
Sample Collection and DNA Extraction
To further assess the connectivity and genetic diversity of manatees in the southern extent of the range on the eastern Atlantic coast of South America, this study included samples from the Antillean subspecies of West Indian manatees in Brazil and a few from Venezuela/Guyana, as well as Amazonian species samples from portions of their range in Brazil. Samples from the mouth of the Amazon River and further upstream were included to investigate the degree of hybridization found between the Amazonian and eastern Antillean manatees in our samples using nuclear and mtDNA. Nuclear DNA accounts for genetic material from both parents and can provide increased resolution to address the level of introgression and temporal hybridization patterns (Vaha and Primmer, 2006). The three sampled regions included: (i) the coast of Brazil across the fragmented distribution found in the states of PA, Maranhão (MA), Piauí (PI), Ceará (CE), Rio Grande do Norte (RN), Paraíba (PB), Pernambuco (PE), and AL, n = 82; (ii) a limited assessment of the state of AP (Brazil), Venezuela (VE) and Guyana (GY), n = 12; and (iii) the Amazon River, including the river mouth and upstream in the Brazilian states of AP and PA (n = 18; Figure 1). Nine individuals were sampled from captivity at Centro Nacional de Pesquisa e Conservação de Mamíferos Aquáticos but were assigned to region (i) based on their original rescue location (ICMBio/CMA database). During collection of samples in the field, individuals were identified as T. manatus (regions i and ii) or T. inunguis (region iii) based on collection location, and these assignments were utilized until microsatellite data could provide genetic assignments. The genetic data (haplotypes and nuclear DNA) of the nine manatees released into the SE and BA states were analyzed in conjunction with the data from their original stranding locations. One individual, named “Poque,” genetically characterized in previous studies (Vianna J. A. et al., 2006; Vianna J. D. A. et al., 2006), was included and identified from region (ii) based on previous findings (Luna, 2001; Luna and Passavante, 2010).
Blood or skin tissue from wild carcasses and stranded calves was collected by manatee researchers from 2009 to 2011 for DNA analysis. Blood from wild-born captive manatees with known rescue locations was also used for this study. Blood and tissue samples were preserved in lysis or tissue buffer, respectively (Amos and Hoelzel, 1991; White and Densmore, 1992; Proebstel et al., 1993). While additional samples would increase the statistical power to detect substructure and relatedness, sample collection from endangered and elusive manatees is difficult, costly, and requires extensive efforts that would delay timely information necessary for implementation of conservation measures.
Genomic DNA extraction, amplification and fragment analysis were performed at the U.S. Geological Survey, Conservation Genetics Laboratory in Gainesville, FL, United States. DNA extractions were carried out using DNeasy Blood and Tissue Extraction Kits (QIAGEN, Valencia, CA, United States). Due to the state of decomposition, or the small physical sample size, three samples were isolated using phenol-chloroform extraction (Hillis et al., 1996). Polymerase chain reaction (PCR) amplifications were completed in a Biometra UNOII, T-Gradient thermocycler (Biometra, Gottengen, Germany) or on a PTC-100 or PTC-200 (MJ Research, Waltham, MA, United States) thermocycler.
Microsatellite DNA Analyses
Nuclear microsatellite DNA amplifications (n = 16 loci) were conducted using the following conditions: initial denaturation at 95°C for 5 min; 35 cycles consisting of a denaturation step at 95°C for 30 s, specific annealing temperature for each primer for 60 s, and extension at 72°C for 30 s; a final extension at 72°C for 10 min (Supplementary Table 1). Amplifications were performed in a total volume of 12.7 μL, with 10 ng target DNA, 1× Sigma PCR Buffer, 2.5 mM of MgCl2, 0.2 mM (each) dNTP, 0.01 U μL–1 of Sigma JumpStart Taq polymerase (Sigma–Aldrich, St. Louis, MO, United States), specific quantities of each primer (Supplementary Table 1), and 1 mg mL–1 bovine serum albumin (BSA) when indicated by García-Rodríguez et al. (2000) and Pause et al. (2007).
For fragment analysis, the forward primers were labeled with the fluorescent dyes VIC, HEX or 6-FAM for processing and visualization on an Applied Biosystems ABI 3130xl Automated DNA Analyzer (ThermoFisher Scientific Inc., Waltham, MA, United States). Fragments from the PCR products were analyzed using GENEMARKER, v. 1.5 (SoftGenetics, LLC, State College, PA, United States) to determine allele sizes. Allele sizes were standardized using previously analyzed Florida manatee samples for comparison and data binning.
Microsatellite Statistical Analyses
Nuclear microsatellite loci were tested for the presence of null alleles with MICRO-CHECKER (Van Oosterhout et al., 2004). Probability of identity (PID) and PID among siblings (P(ID)sib) was calculated in GENECAP to remove bias and GENALEX to calculate observed values following Hunter et al. (2010) methods for each species. A range of samples sizes to adequately identify individuals in a population were estimated using the unbiased 1/PID and 1/P(ID)sib for the liberal and conservative estimates, respectively. Departures from the expected genotypic frequencies in Hardy-Weinberg Equilibrium (HWE) (Jorde et al., 2007) were calculated using the Markov chain Monte Carlo (MCMC) method (dememorization 10,000, batches 1,000, and iterations per batch 10,000) in GENEPOP (Raymond and Rousset, 1995). GENEPOP was also used to test for linkage disequilibrium (LD) among loci (Raymond and Rousset, 1995). Alpha values were adjusted with Bonferroni correction for pairwise comparisons in HWE and LD tests (Rice, 1989).
Population structure was evaluated in various Bayesian and multivariate analyses. The program STRUCTURE v. 2.3.4 was used to infer populations (Falush et al., 2007). A first approach using all samples aimed to test species identification of T. manatus, T. inunguis, or potential hybrids {K = 1–8}. Hierarchical analyses were then conducted on the species individually {K = 1–5} to identify substructure in the Amazonian manatees and to assess whether the gaps at the distribution of Antillean manatees (Figure 1) act as barriers and influence structure of the population. Hierarchical analyses were conducted in which the identified sub-clusters from the initial run were each analyzed independently. This procedure was repeated consecutively until K = 1 was achieved for all sub-clusters. We used the following parameters for all simulations of the initial and consecutive hierarchical analyses: the admixture model without a priori assignment, a run-length of 100,000 MCMC repetitions following the burn-in period of 50,000 iterations, and 20 independent iterations simulated for each value of K. The most probable number of clusters was determined by calculating ΔK and the mean estimation of ln probability in STRUCTURE HARVESTER (Earl and VonHoldt, 2009). Individuals were assigned to clusters with a threshold of q ≥ 0.80, which indicates little likelihood of belonging to a different cluster. Using the GENECLASS2 software, Bayesian assignment tests were run using Rannala and Mountain (1997) methods to identify F1 and F2 hybrid individuals with a threshold of 0.05. Samples with q ≥ 0.90 in STRUCTURE were used as a reference population for each geographic grouping to evaluate the assignment of admixed individuals (q < 0.80) identified in STRUCTURE (Piry et al., 2004). With the aim to compare between two methods, we also ran BAYESASS in order to infer the proportion of recent migrants among populations (Wilson and Rannala, 2003). BAYESASS was run for 107 iterations, 106 burn-in period, and a sampling frequency of 1,000. We ran several iterations with varying delta parameters to maximize the log likelihood and to ensure the acceptance rate of each was between 40 and 60%. However, in all assessments of the various groups, the pairwise comparison of migration rates showed that the derived means and 95% confidence intervals (CIs) did not differ from the expected reference CIs. Since the two derived and reference CIs did not differ, the dataset did not have sufficient information to calculate migration rates using this method; thus, we did not include this analysis in our results.
A pairwise genetic distance matrix among all individuals was created in GENALEX v. 6.501 (Peakall and Smouse, 2006) and then used to plot a Principle Component Analysis (PCA) (adegenet, Jombart, 2008; RCoreTeam, 2019). A PCA was performed as a multivariate approach by creating synthetic variables of genetic distance to determine population structure. To assess overall genetic differentiation at the population level, GENALEX was used to calculate FST using the infinite alleles model and RST using the stepwise mutation model through an analysis of molecular variance (AMOVA). Distance values represent 10 loci since data were not complete for the eastern Antillean manatees of Venezuela and Guyana and “Poque” at loci TmaE26, TmaA02, and TmaM79.
Genetic diversity metrics were used to investigate the clusters identified in STRUCTURE and the PCA including, number of alleles (NA), effective number of alleles (EA), number of private alleles (NP), heterozygosity observed (HO), and expected (HE) for each subgroup of samples calculated in GENALEX. Kruskal–Wallis (KW) tests were performed to determine significant differences in genetic diversity among populations (stats, RCoreTeam, 2019). If significant differences were found, a Dunn’s Test was performed on pairwise comparisons to determine which pair of groupings was driving the significant difference (Dinno, 2017; RCoreTeam, 2019). The inbreeding coefficient, FIS, was calculated in FSTAT v. 2.9.3 with significance evaluated by a 95% (CI) confidence interval (Goudet, 2002). The presence of a potential bottleneck was estimated using BOTTLENECK with 13 loci and sample groups (Cornuet and Luikart, 1996). Parameters were modified based on the use of imperfect microsatellite repeat loci as suggested, with 95% of the Infinite Allele Model (IAM) in the two-phase mutation model (TPM) with a variance of 12 applied and 10,000 iterations performed (Piry et al., 1999; Cristescu et al., 2010). Since most of the 13 microsatellites were imperfect dinucleotide repeats, bottlenecks were considered to have occurred if excessive heterozygotes were found significant for the IAM and TPM sign tests (Piry et al., 1999; Cristescu et al., 2010). Effective population sizes (NE), for each genetic grouping identified, were calculated in NEESTIMATOR v. 1.3 using the single sample linkage disequilibrium method (Peel et al., 2004).
Mitochondrial DNA and Statistical Analyses
The mtDNA control region displacement loop was amplified with primers CR-4 and CR-5 (Southern et al., 1988; Palumbi et al., 1991), following the PCR techniques of Hunter et al. (2010). Briefly, the PCR reaction conditions were as follows: 10 ng DNA, 1× Sigma PCR buffer (10 mM L–1 Tris–HCl, pH 8.3, 50 mM L–1 KCl, 0.001% gelatin; Sigma-Aldrich, Inc., St. Louis MO, United States), 3 mM L–1 MgCl2, 0.8 mM L–1 dNTP, 0.24 mM L–1 of each primer, 0.04 U μL–1 of Sigma Jump Start TaqDNA polymerase. The PCR cycling profile was: initial denaturation at 94°C for 5 min; 35 cycles consisting of a denaturation step at 94°C for 1 min, annealing temperature of 55°C for 1 min, and extension at 72°C for 1 min; a final extension at 72°C for 10 min. Amplified products were purified using the QIAquick PCR purification kit (QIAGEN, Valencia, CA, United States). DNA sequencing was accomplished in the DNA Sequencing Core at the University of Florida, Gainesville, FL with the BigDye terminator protocol developed by Applied Biosystems Inc. (ThermoFisher Scientific Inc., Waltham, MA, United States) using fluorescently labeled dideoxynucleotides (ddNTPs).
Control region mtDNA sequences were cleaned and aligned with GenBank sequences using GENEIOUS v. 10.0.9 (https://www.geneious.com) and MESQUITE v. 3.51 software (Maddison and Maddison, 2018). Sequences published by Vianna J. A. et al. (2006) were obtained from GenBank (Accession numbers: AY963852-56 and AY963859-61) to compile haplotypes for the Venezuela/Guyana populations. Haplotypes of each individual were identified using reference sequences in GenBank and visualized as a Median-Joining Network (MJN) using POPART v. 1.7 software (Bandelt et al., 1999). The MJN was run for simplicity and with complexity (epsilon = 0 and 1, respectively) to visualize relationships among the three groupings but only the simplified network was reported. Variable characters within haplotypes were identified using Mesquite software (Maddison and Maddison, 2018). Samples grouped by localities were evaluated for genetic diversity through the number of haplotypes (HT), polymorphic sites (S), nucleotide diversity (π), Tajima’s D of site neutrality, and haplotype diversity (h) using DNAsp v. 4 (Rozas et al., 2003). Pairwise ΦST distance values among groupings were calculated using ARLEQUIN v. 3.5.2.2 (Excoffier and Lischer, 2010).
Results
Microsatellite DNA and Statistical Analyses
Our final dataset was composed of 95 samples, containing 67 from Brazil, 11 from Venezuela and Guyana, and 17 Amazonian manatees. We originally assessed 16 loci but evidence of null alleles and HWE disequilibria across population groupings resulted in the removal of three loci: TmaH13, TmaE01, and TmaE11. Manatees from Brazil and the Amazon amplified at 13 nuclear microsatellite loci, while Venezuela and Guyana (n = 11) only amplified at 10 out of 13 loci (Table 1). Observed estimated P(ID) and P(ID)sibs were greater, but the same order of magnitude, than the unbiased estimates for both eastern Antillean groupings (Table 2). For the Amazonian species, unbiased P(ID) and P(ID)sibs estimates were at least one order of magnitude greater than observed estimates (Table 2). Individual identity values allowed us to confidently distinguish individuals separately with ten loci. MICROCHECKER found evidence of null alleles in Brazilian manatees for loci TmaSC5, TmaSC13, TmaE08, and TmaA02, but found no evidence of stutter alleles and since Hardy Weinberg disequilibrium was not found at these loci, they are not likely to influence the results. Of the 13 loci, deviations from HWE were detected for the Amazonian manatees at locus TmaF14, and Brazilian manatees at loci TmaK01 and TmaA02 after alpha was adjusted using the Bonferroni method (α = 0.0004, Table 1). Some loci for all groupings were not able to be evaluated by MICROCHECKER or for HWE due to monomorphic loci. No evidence of LD was found after alpha was adjusted using Bonferroni method (α = 0.0004).
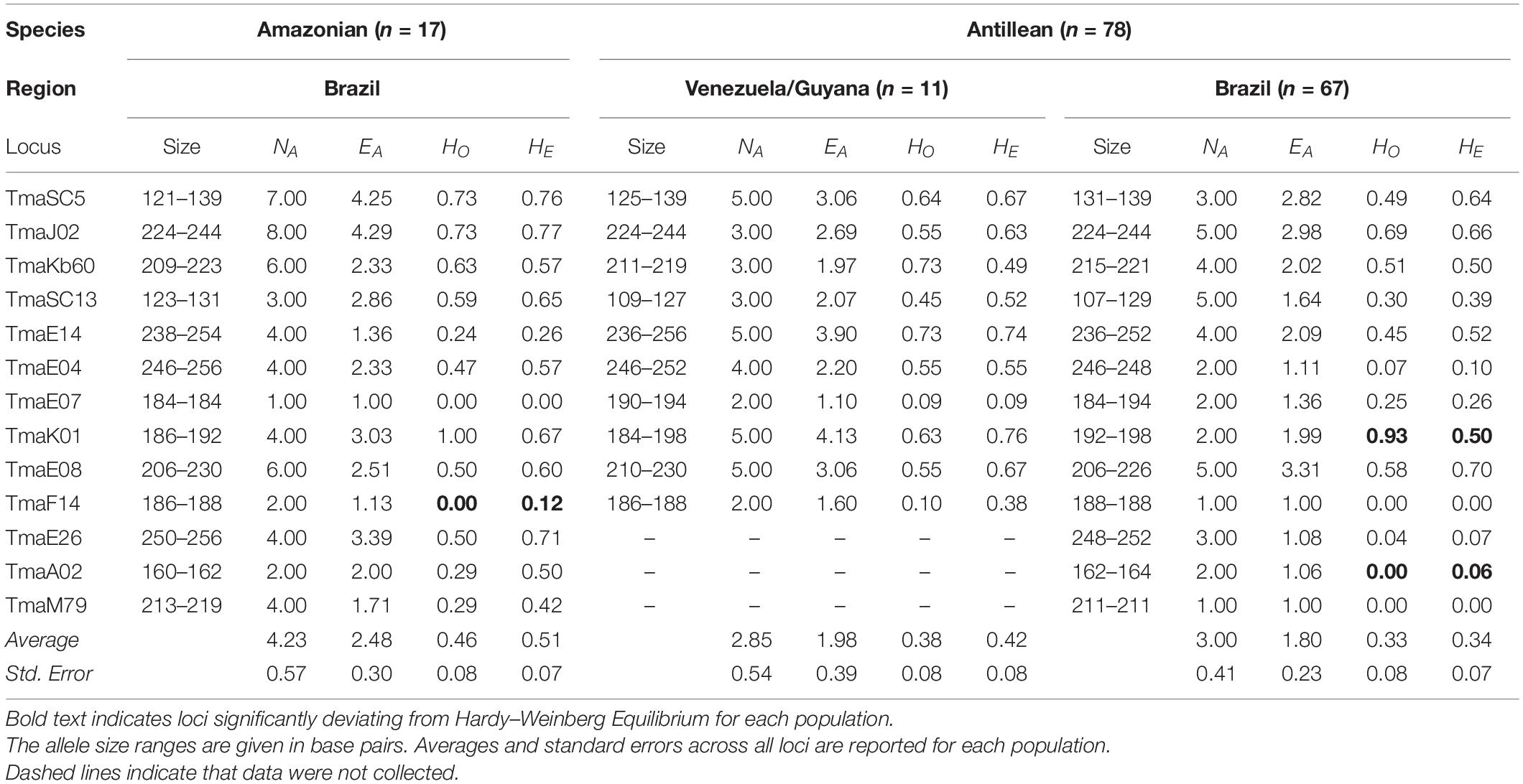
Table 1. Amazonian and Antillean manatee summary statistics for the genetically identified clusters using 13 microsatellite loci. Number of alleles (NA), effective number of alleles (EA), observed heterozygosity (HO), and expected heterozygosity (HE) are displayed.
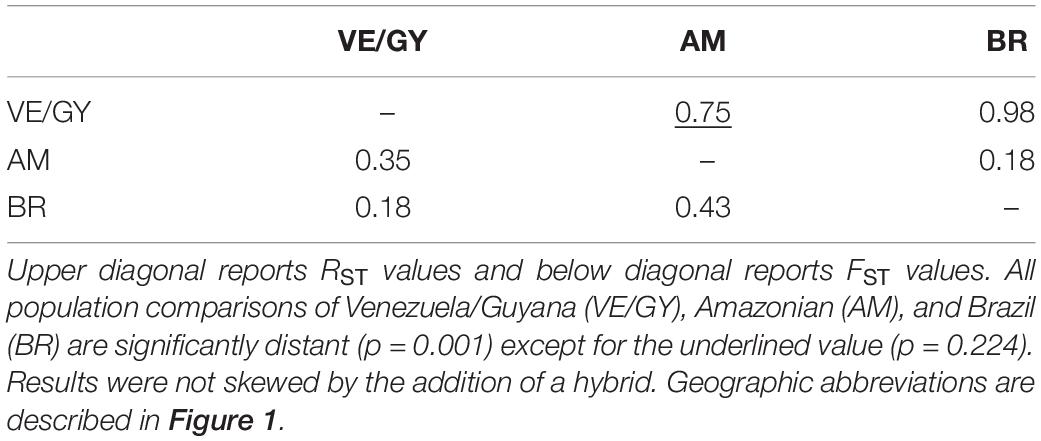
Table 2. Pairwise population matrix of RST and FST distance values for manatee genotypes across 10 microsatellite loci.
Nuclear Bayesian methods in the program STRUCTURE were used to assess the divergence of Amazonian and eastern Antillean manatees using log-likelihood and ΔK analyses (Figure 2A). In the first analysis, the two species were analyzed together, and Amazonian and West Indian samples formed K = 2 clusters, supported by the highest ΔK and lowest log-likelihood values. There, “Poque” was assigned to each cluster equally (q = 0.5; Figure 2A). Although the K = 2 hypothesis had the highest support, when the K = 3 hypothesis is considered, Amazonian, Brazil and Venezuela/Guyana populations separated into three distinct clusters. The second hierarchical analysis assessed the Amazonian sample cluster alone and identified K = 1, with no substructure in those samples (Supplementary Figure 1). There, “Poque” strongly assigned to the second cluster supported in the K = 2 hypothesis (q = 0.95; Supplementary Figure 1).
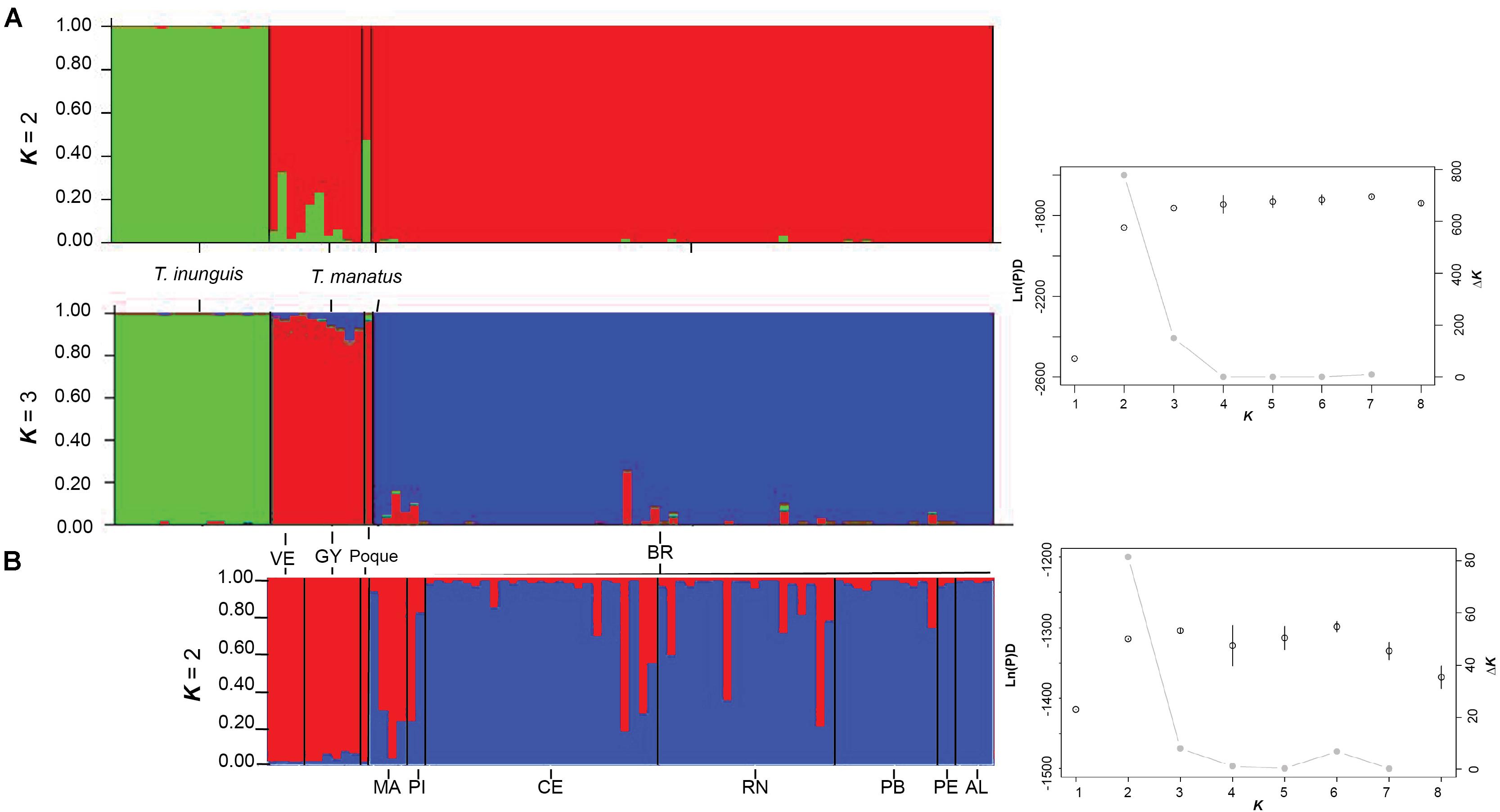
Figure 2. Hierarchical bar plots that indicate population structure found in microsatellite DNA. Populations are differentiated by color with each line indicating an individual sampled and represent Venezuela (VE), Guyana (GY), and Brazil (BR). Brazilian state abbreviations are described in the main text. Each solid black line indicates the extent for that sampling location. Bar plots represent the STRUCTURE program hypotheses of K = 2 and K = 3 populations (A), when all individuals sampled were analyzed. Substructure among the eastern Antillean manatees only is represented and hypothesized as two populations (B, K = 2). Dotted line on plot (B) displays the threshold of q > 0.8 to indicate assignment of an individual to a specific population. Each line representing “Poque” is marked with an asterisk (*) in all q plots. Results from Structure Harvester Software are presented to the right of the corresponding q plots. The Harvester plots visualize the mean estimate ln(P)D as open circles on the left y-axis. Black lines through the open circles represent standard deviation among the individual iterations for each K. The gray dot-line plot visualizes the ΔK values on the right y-axis. The value K on the x-axis indicates the number of clusters inferred.
The third hierarchical STRUCTURE analysis assessed connectivity among only the Antillean manatees and identified Brazil and Venezuela/Guyana as separate clusters with support from the ΔK and log-likelihood values (ΔK = 2; Figure 2B).
There, “Poque” grouped with Venezuela/Guyana (q = 0.98). Although the Antillean Brazil and Venezuela/Guyana samples separated into two clusters, admixture (q ≤ 0.80) was identified in 17 of the 78 Antillean samples (Figure 2B). The admixed samples identified in STRUCTURE all originated from Brazil (17/67; 25%) and were found as far south as the state of PB. GENECLASS2 assigned two samples to Venezuela/Guyana (85 and 95%) and one to Brazil at 66%, but 14 samples to Brazil at 90 and 100%. No prominent geographic patterns were observed in Brazil, or in relation to admixture between the Venezuela/Guyana and Brazilian samples. The limited samples collected in the southernmost states (PE and AL) were not admixed with the Venezuela/Guyana cluster, however, RN to the north did have admixed samples.
The Brazil and Venezuela/Guyana samples were analyzed separately in the fourth and fifth hierarchical STRUCTURE analyses. No substructure was identified in either group with K = 1 being the highest supported hypothesis. When “Poque” was assessed with the three independent STRUCTURE clusters in GENECLASS2, he was assigned to Venezuela/Guyana at 100 and 0% with the Amazon and Brazil clusters.
The PCA revealed three distinct groups: Brazilian, Venezuela/Guyana, and Amazonian (Figure 3), further supporting the K = 3 clusters identified in STRUCTURE (Figure 2A). The first two eigenvalues include 91.4% of the variation in the dataset. Overall significant genetic differentiation was found in the FST and RST distance values for all pairwise comparisons of populations after Bonferroni adjustment (α = 0.017, p = 0.001) except between the Amazonian species and Venezuela/Guyana grouping (p = 0.22; Table 3). When calculating FST distances, AMOVA results showed the highest estimated variance within individuals (61%), then among populations (35%), and least among individuals (5%). When calculating RST distances, AMOVA results showed the highest estimated variance among individuals (79%), then among populations (18%), and least within individuals (2%). Pairwise comparison of Amazonian and Venezuela/Guyana manatees was the only insignificant differentiation found in RST values (RST = 0.01, p = 0.224).
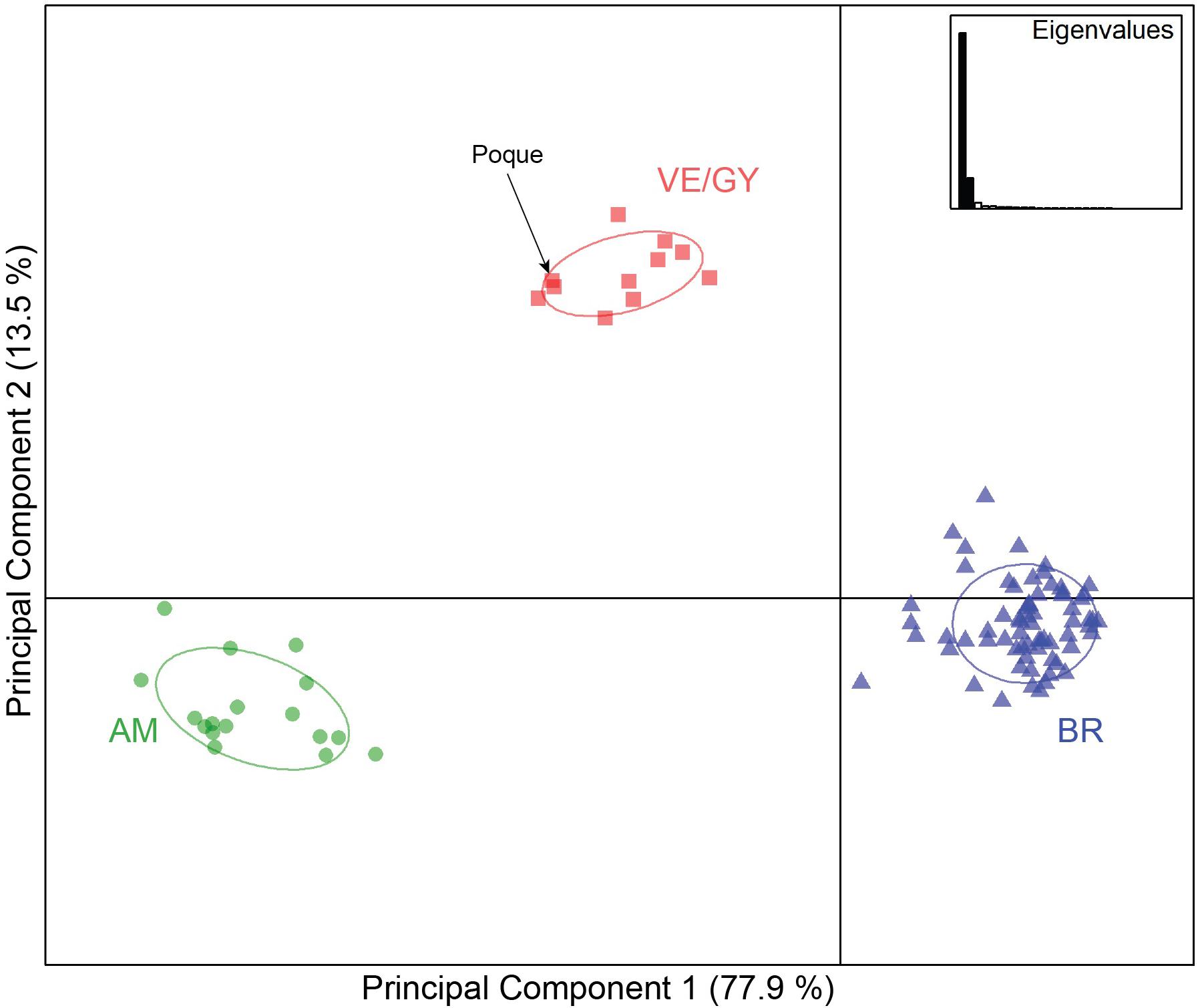
Figure 3. A multivariate Principal Component Analysis (PCA) to visualize the genetic structure using synthetic variables. Circles indicate individuals sampled, colored for the genetic grouping they assigned to, and ellipses around 95% of the variation. Genetic groupings are defined as Venezuela/Guyana (VE/GY), Amazonian (AM), and Brazil (BR).
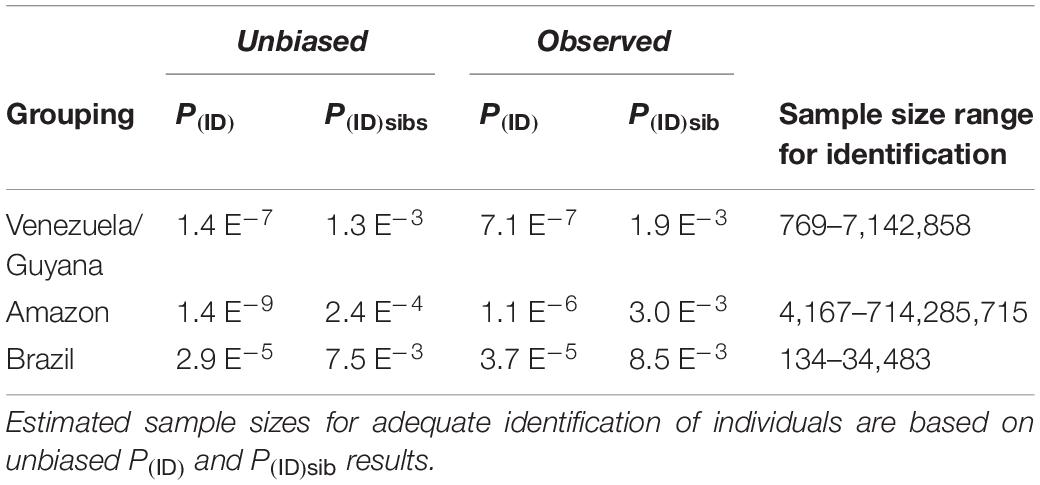
Table 3. Unbiased and observed Probability of identity (PID) and PID among siblings (P(ID)sib) for each genetically identified cluster using 10 loci.
Based on these results, three groups were identified comprising the Brazilian, Venezuela/Guyana, and Amazonian clusters separately. For nuclear DNA, the KW test only showed a significant difference in number of alleles (NA) among the three clusters. Inbreeding levels were not significant based on the 95% CI (−0.126, 0.213), but were similar for Venezuela/Guyana (FIS = 0.14) and Amazonian manatees (FIS = 0.13) and lower for Brazil manatees (FIS = 0.03). The Brazilian and the Amazonian manatees had a significant difference in NA (p = 0.04), but when the pairwise comparison results were adjusted using Bonferroni correction, no significant differences were found (α = 0.017). The number of private alleles was highest for the Amazonian species (NP = 21), then Venezuela/Guyana (NP = 11), and lowest for the Brazilian grouping (NP = 5). No evidence of a bottleneck was found for the Brazilian or Amazonian groupings. Venezuela/Guyana manatees showed evidence of a bottleneck under the IAM model (p = 0.027). Effective population size estimates were low for all three groupings. Venezuelan and Guyanese manatees had the lowest estimation (NE = 14.5, 95% CI = 8.7–32.9), then the Amazonian species (NE = 26.5, 95% CI = 17.5–48.7), and Brazilian manatees had the highest estimation (NE = 49.9, 95% CI = 35.8–74.9).
Mitochondrial DNA and Statistical Analyses
We successfully amplified mtDNA sequences from 93 individuals from the three geographic areas of this study. Sequenced samples corresponded to the three nuclear clusters: Atlantic coast of Brazil including eight captive individuals (n = 72), Venezuela and Guyana, including “Poque” (n = 5), and the Amazonian species within the Amazon River mouth (AP and PA states of Brazil) (n = 16). Control region mtDNA was trimmed to 410 bp sequences for each individual and aligned to evaluate haplotype distribution among regions. A total of eight new haplotypes was identified in Amazonian (n = 7) and West Indian species (n = 1) and were added to GenBank (Accession numbers: MW091459-1466; Table 4 and Supplementary Table 2).
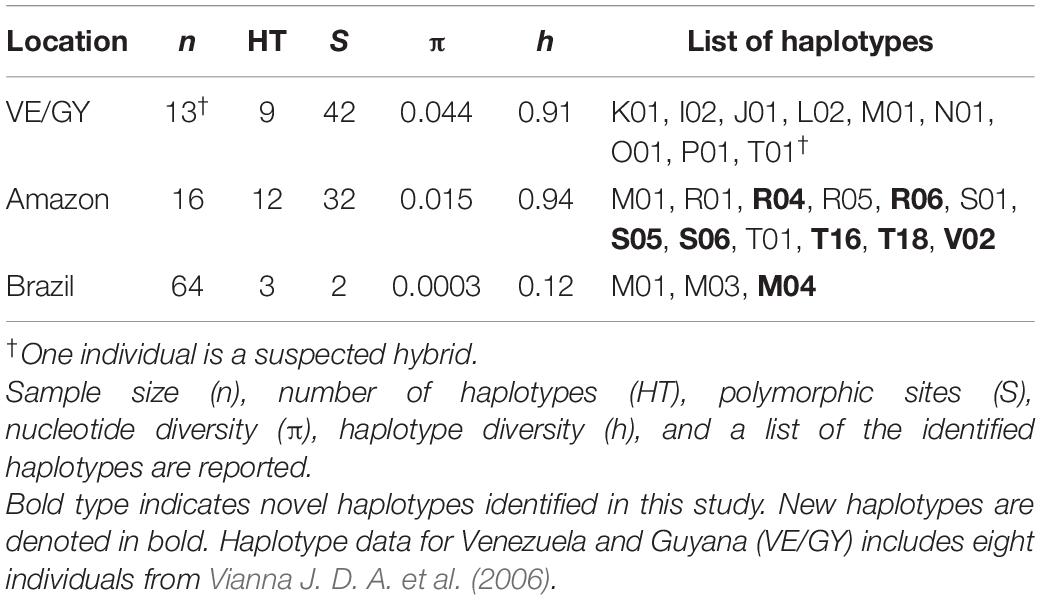
Table 4. Amazonian and Antillean manatee control region mitochondrial DNA parameters for the three genetically identified clusters.
The two mtDNA MJNs supported the distinction between southeastern Antillean and Amazonian manatees (Figure 4A). The complex MJN showed a more direct relationship between the Amazonian species and Venezuelan/Guyanese Antillean manatees but still aligned with the shape of the simplified network (Supplementary Figure 2). Haplotypes P01 and T01 from eastern Antillean individuals grouped in the Amazonian species complex (Figure 4A), as reported previously (Vianna J. A. et al., 2006). The haplotype network was then broken down to include only samples grouping as Brazil (Figure 4B). One other T01 haplotype was found in the state of PA matched to the hybrid “Poque” (Figure 4B). Haplotype M01 was the most common haplotype in Brazil, with all the sampled areas having at least one M01 individual, except for the state of MA. Less common haplotypes (M03 and M07), were found in MA and PI states (Figure 4B). The cluster on the left-hand side in Figure 4A, contained Antillean samples with haplotypes found in Amazonian manatees, possibly indicating past hybridization.
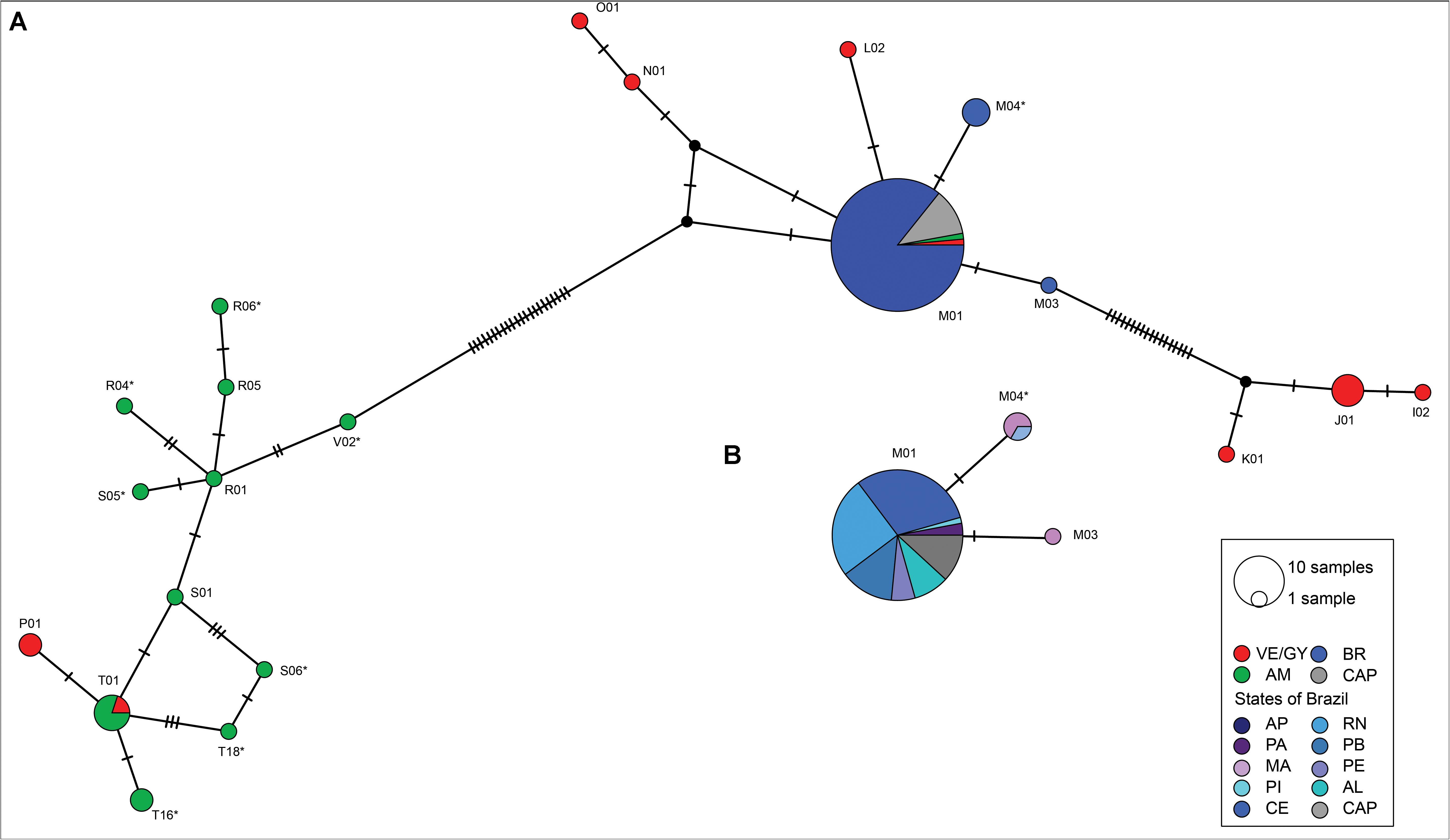
Figure 4. Control Region mtDNA simplified haplotype networks (epsilon = 0) with colors marking genetic grouping. (A) All study samples with mitochondrial haplotypes from Brazil (BR), Venezuela (VE)/Guyana (GY), and Amazonian (AM), and manatees from captivity. (B) Brazilian samples from states in the northeast coast of Brazil (see main text for abbreviations). Size of the circles represent sample size with sectors of circles indicating samples from different locations with shared haplotypes. Black tick marks indicate the number of mutations separating the various haplotypes. Haplotypes are labeled according to Vianna J. A. et al. (2006). New haplotypes found in this study are indicated with an asterisk (*). Manatees from captivity were included in the haplotype network. The identified hybrid, “Poque” (T01), was assigned to the Venezuela/Guyana group through the Bayesian analyses.
The genetic diversity and differentiation values were calculated for the three nuclear clusters (Tables 1, 4). The Brazilian manatees had the highest sample size in this study but the lowest values for every mitochondrial diversity metric calculated (Table 4). Tajima’s site neutrality D values were not significant. Distance values were highest between Brazilian and Amazonian groupings (ΦST = 0.9), lower between the two eastern Antillean groupings (ΦST = 0.7), and lowest between Amazonian and Venezuelan/Guyanese groupings (ΦST = 0.4); all statistically significant (p < 0.001).
Discussion
Our assessment of the southern range limit of the Antillean manatee identified three divergent nuclear clusters: two corresponded to the Antillean manatees of Brazil (i) and Venezuela/Guyana (ii), while the third identified the Amazonian species’ genotypes (iii). We did not detect nuclear evidence of a hybrid zone in Brazil. Only a single hybrid was indicated in our samples (the captive manatee, “Poque”) that had been previously characterized using mtDNA, one microsatellite, and chromosome karyotyping (Vianna J. A. et al., 2006). The two Antillean clusters were separated into Brazil and Venezuela/Guyana manatees by a significant FST = 0.18 and ΦST = 0.7, the PCA plot, and the STRUCTURE analysis of the full dataset (K = 3). However, admixture through more recent gene flow from Venezuela/Guyana was identified in 25% of the Brazil samples from the states of MA, PI, CE, RN, and PB. Our results indicate that the Amazon River plume is not sufficient to interrupt gene flow, or the potential of hybridization, between the north and south sides of the River. This is the first time contemporary genetic connectivity has been investigated at this geographic scale along the South American coastline.
The identified contemporary gene flow of Antillean manatees from Venezuela to Brazil does not correspond with the previous regional connectivity hypothesis containing two evolutionary significant units (ESUs; Lima et al., 2019). Additional samples from the region are needed to better assess the proposed ESUs separating Venezuela from Brazil with a hybrid zone in the middle (Lima et al., 2019). The use of single nucleotide polymorphism (SNP) data from the Florida manatee genome as the parental population could result in skewed levels of similarity in Florida and Antillean manatees in the region. The application of local samples and more than one Florida manatee genome are needed to improve the testing of ESUs in the region. Of note, no hybrids were detected in Guyana, which was proposed to be part of the hybrid zone (see next section on hybrid detection; Lima et al., 2019). The ability of manatees in Venezuela to connect genetically with their Brazilian counterparts through gene flow in a stepping-stone fashion, or directly through long-distance movements (∼1,200 km), indicates that gene flow is likely to occur westward as well (e.g., to Colombia). Because gene flow from a single migrant per generation can allow for genetic connectivity, and admixture was detected in a quarter of the Brazilian samples, it is plausible that the Antillean manatees are connected throughout the South American Caribbean and Atlantic coastlines (Mills and Allendorf, 1996; Wang, 2004; Laikre et al., 2016).
Lack of Evidence of Contemporary Antillean and Amazonian Manatee Hybridization (Beyond “Poque”)
Samples from the primary sympatric zone at the mouth of the Amazon River in Brazil, were used to comprehensively assess the degree of contemporary hybridization between the Amazonian and Antillean manatees (Luna et al., 2008b, 2018). No evidence for contemporary hybridization was detected using nuclear multi-locus genotypes in the Antillean and Amazonian manatee samples, beyond the single previously identified captive hybrid. Nuclear microsatellite markers with samples from local Amazonian and Antillean reference populations are well suited to detect hybridization considering manatees have a long generation time of 16–23 years (Vaha and Primmer, 2006). However, more in-depth genomic investigations and future genetic monitoring with additional samples would help to investigate hybridization events in the evolutionary past and those occurring more recently. In the 95 assessed samples, the single hybrid known as “Poque” was identified by a nuclear genotype equally divided between the two species (q = 0.5 probability) and also contained an Amazonian manatee haplotype of T01 (Vianna J. A. et al., 2006). “Poque” was rescued from the north coast of Brazil, but his capture location, natal origins, and prior history were not well documented – making it difficult to draw conclusions about hybridization in wild populations. Amazonian mitochondrial haplotypes were shared with two Antillean samples, likely indicating historic hybridization or incomplete lineage sorting after a speciation event. More data are needed to assess this relationship (see Scornavacca and Galtier, 2017; Wang et al., 2018).
While we did not find evidence of hybridization in our samples from the two manatee species or at the mouth of the Amazon River, previous studies recently identified a “hybrid swarm” of multigenerational hybridization in six manatees from French Guiana and Guyana (Vianna J. A. et al., 2006; Vilaça et al., 2016; Lima et al., 2019). Lima et al. (2019) detected hybrids using one nuclear locus and one mtDNA locus which, due to incomplete lineage sorting, is not a strongly reliable approach to identify hybrids in contrast to multi-locus markers (Wang et al., 2018). Vilaça et al. (2016) assessed ∼2,000 SNPs to detect hybrids but only used a single West Indian genotype obtained from the Florida manatee genome for the Antillean genotype. Florida manatees are a separate subspecies at the northern edge of the range, which could introduce challenges during population assignment in Bayesian clustering and maximum-likelihood estimators. These estimators use the proportion of alleles inherited from the parental species, which in this case would be the single Florida sample. Therefore, additional loci and additional “parental” reference samples from local populations could allow for comparisons with local genotypes. Lastly, manatees have been translocated to closed systems as weed control agents in French Guiana and Guyana, and to captive settings which could have led to admixture outside of the species standard population range (Allsopp, 1960, 1969; Haigh, 1991; de Thoisy et al., 2003; Hollowell and Reynolds, 2005).
Brazilian Manatee Genetic Diversity
The Amazonian manatees exhibited evidence of a genetically diverse, panmictic population from the mouth of the Amazon River to at least 1,000 km westward into the interior basin of the Santarém region, which supported findings from a previous mtDNA study (Cantanhede et al., 2005). Conversely, the nuclear genetic diversity of the Brazilian Antillean manatee was low, containing the lowest number of private alleles among the three clusters (NP = 5) and the largest sample sizes. Furthermore, the number of alleles in Brazilian manatees (NA = 3) was similar to those in Belize (NA = 3.4), but lower than studies of manatee populations in Puerto Rico (NA = 3.9), Florida (NA = 4.8), and Colombia (NA = 3.5 to 7) (Hunter M. E. et al., 2010; Hunter et al., 2012; Satizábal et al., 2012; Tucker et al., 2012). In fact, the Brazilian diversity values were lower than other mammalian populations which were demographically challenged through pollution, harvesting, or habitat fragmentation (Garner et al., 2005; DiBattista, 2007; Torres-Florez et al., 2014). Low genetic diversity in marine mammal populations can be common and is often related to glacial events, founder effects, bottlenecks, anthropogenic mortality, and habitat degradation (Waldick et al., 2002; Roman and Palumbi, 2003; Paster et al., 2004). The nuclear and mtDNA genetic diversity found for the Venezuelan/Guyanese samples should be used with caution as the sample size was low (N = 10 and 13, respectively).
The low genetic diversity in the Brazilian Antillean manatee may be a combination of a founder effect and the decrease in abundance of manatees due to habitat loss and intensive historical hunting. Despite the decreased abundance due to hunting pressure, no evidence of a bottleneck within the last ∼0.2–4 NE generations (∼32–92 years) was detected using the generation time of 16–23 years (Whitehead, 1978; Vaha and Primmer, 2006). Only approximately five manatee generations (∼100 years) have occurred since indiscriminate hunting was reduced in Brazil which is likely not sufficient to produce a detectable bottleneck in our analyses (Cornuet and Luikart, 1996; Luna et al., 2008b, 2018; Luna and Passavante, 2010). Additionally, the southern and westward colonization of manatees along the coastline of Brazil may have proceeded through a series of bottlenecks, according to population size estimates and records (Whitehead, 1978). The colonization may be too distant in time to be detected with these analytical tools. The eastern Antillean manatees of Venezuela and Guyana showed evidence of a bottleneck; however, it should be carefully considered as the sample size was very low. The evidence of gene flow between the Venezuela/Guyana and Brazil Antillean clusters may also reduce the genetic effects of bottlenecks in Brazil.
Since manatees have special habitat requirements and are vulnerable to stochastic events, it is imperative to continue to monitor and reduce anthropogenic threats (Marmontel, 1995; Rathbun et al., 1995; Reynolds et al., 1995) for both species in Brazil (Luna et al., 2018). The high level of past and current manatee mortality in Brazil has resulted in a reduced population size and potentially limited the population’s genetic diversity. It is imperative that species identification – and contemporary hybridization – be tested to allow for more accurate estimates of census and effective population sizes and any subsequent changes over time. Hybridization needs to be identified through nuclear genetic data derived from appropriate reference populations and sample sizes, as opposed to potentially ambiguous morphology traits and mtDNA (Allendorf et al., 2001; Vianna J. A. et al., 2006; Bohling and Waits, 2015; Laikre et al., 2016; Lima et al., 2019).
Future Efforts to Promote Genetic Diversity Through Population Connectivity
Maintenance of population connectivity is necessary to allow for genetic diversity growth (Bouzat, 2010; Whiteley et al., 2015; Laikre et al., 2016). Based on genetic distance values, connectivity has likely been occurring within the last 40–100 years between the two Antillean clusters identified here. Of note, mtDNA sequences from these three South American countries were assigned to all three of the mtDNA clusters delimited by Vianna J. D. A. et al. (2006), potentially supporting historical geneflow. Continued gene flow could help support sharing of advantageous alleles and prevent the Brazilian population from experiencing negative genetic effects (e.g., inbreeding, the extinction vortex etc.; Frankham et al., 2002; Tigano and Friesen, 2016), assuming interspecific hybridization is low. Gene flow between small and endangered populations is important to counteract the effects of random genetic drift and inbreeding, and can be achieved primarily by increasing effective population size and minimizing potential stochastic effects (Frankham, 1995; Westemeier et al., 1998; Madsen et al., 1999; Storfer, 1999). Our study indicates that although the population has low genetic diversity, manatees are moving along the coast, connecting areas that were previously separated by human impacts (Normande et al., 2016). Reintroduction of manatees into distribution gaps and the protection of conservation units would help to continue to encourage gene flow along the species distribution in Brazil. Protecting corridors and habitat types that include mangroves, seagrass beds and available fresh water may improve broader stepping-stone movement patterns. The management of wide-range travel corridors between South American countries could facilitate gene flow and allow for augmentation of the species without direct human intervention.
Genetic mutations that become fixed over many generations can increase genetic diversity, which is more likely to occur in larger populations. Although no physiological or genetic implications of inbreeding depression have been identified to date, small manatee populations could be susceptible to detrimental genetic effects, such as limited adaptation potential during climate change (Couvet, 2002). Population sizes of NE > 50 effective breeders are recommended for short-term sustainability, while NE > 500 are needed for long-term survival and the prevention of excessive inbreeding (Wright, 1951; Jamieson and Allendorf, 2012). This implies that census population levels in the upper thousands are necessary to maintain evolutionary stability (Franklin, 1980; Lande, 1995). All three groups of manatees in this study had estimates of NE < 75, well below the 500-minimum threshold.
The rapid growth of anthropogenic activities has reduced the availability of habitat that is required for breeding and parental care (Luna et al., 2011; ICMBio, 2018b, 2019). As numerous stranded calves are rehabilitated, release locations should target natal habitats to avoid genetic swamping of locally adapted alleles (Luna et al., 2011, 2012; ICMBio, 2018a). To achieve near-term stability of the Brazilian Antillean manatee population, habitat restoration and protections, and direct anthropogenic threats (boats, oil extraction, etc.) are needed to encourage gene flow, bring NE to >50 effective breeders, and improve connectivity through the recolonization of fragmented manatee habitats (Frankham et al., 2002).
Data Availability Statement
The datasets presented in this study can be found in online repositories. The names of the repository/repositories and accession number(s) can be found in the article/ Supplementary Material.
Ethics Statement
The animal study was reviewed and approved by Instituto Chico Mendes de Conservação da Biodiversidade – Centro Nacional de Pesquisa e Conservação de Mamíferos Aquáticos (ICMBio/CMA).
Author Contributions
FL, MH, and RB developed the project and acquired funding. FL, GS, and FA completed field work to collect samples. FL, MH, and GS collected data for the project while FL, CB, CN, and MH processed data in the laboratory. MH and FL advised while CB calculated and performed data analyses. FL, CB, JT-F, and MH assisted with data interpretation, biological, and ecological relevance. FL, CB, CN, AM, JT-F, and MH contributed to preparation of the manuscript for submission. All authors contributed to the article and approved the submitted version.
Funding
This study has been funded by the Instituto Chico Mendes de Conservação da Biodiversidade – ICMBio, the Society for Marine Mammalogy and the USGS Sirenia Project. CN received funding from the project “Genomics and Evolutionary Biology” co-financed by North Portugal Regional Operational Program 2007/2013 (ON.2 – O Novo Norte), under the National Strategic Reference Framework (NSRF), through the European Regional Development Fund (ERDF). AM and JT-F are sponsored by GEF-Mar fellowships.
Conflict of Interest
The authors declare that the research was conducted in the absence of any commercial or financial relationships that could be construed as a potential conflict of interest.
Acknowledgments
We would like to thank Dr. Michelle Davis and Gaia Meigs-Friend for their generous help in the laboratory and the Sirenia Project. All the genetic analyses were performed at the U.S. Geological Survey (USGS) Sirenia Project – Conservation Genetics Laboratory in Gainesville, Florida. Samples were obtained under research permits SISBIO-ICMBio 19.204/2009 and 24.473/2010 and collected following animal welfare standards and USGS IACUC approval. Samples were exported for genetic analysis to the United States under Brazilian export CITES permit 09BR003661/DF and 10BR005242/DF and United States import CITES permits 08US808447/9 and 10US06625A/9. Any use of trade, product, or firm names is for descriptive purposes only and does not imply endorsement by the U.S. Government. This publication was approved by ethics review from ICMBio process SEI: 02034.000056/2020-54 and 7249582.
Supplementary Material
The Supplementary Material for this article can be found online at: https://www.frontiersin.org/articles/10.3389/fmars.2020.574455/full#supplementary-material
Supplementary Figure 1 | Results from STRUCTURE and Structure Harvester Software for the substructure within the Amazonian grouping and including “Poque,” a suspected hybrid. Bar plot presents STRUCTURE results as each individual as a line, assigned to inferred population clusters, K, designated by colors. “Poque” was included in the analysis and is indicated by an asterisk symbol (∗). Scatter plot presents Structure Harvest results with the mean estimate ln(P)D as open circles on the left y-axis; black lines through the open circles represent standard deviation among the individual iterations for each K. The gray dot-line plot visualizes the ΔK values on the right y-axis. The value K on the x-axis indicates the number of clusters inferred.
Supplementary Figure 2 | Control Region mtDNA complex haplotype network (epsilon = 1) for all study samples from Brazil (BR), Venezuela (VE)/Guyana (GY), Amazonian (AM), and manatees from captivity. Size of the circles represent sample size with sectors of circles indicating samples from different locations with shared haplotypes. Black tick marks indicate the number of mutations separating the various haplotypes. Black dots indicate potential haplotype missing from the dataset.
References
Albuquerque, C., and Marcovaldi, G. M. (1982). “Ocorrência e distribuição do peixe-boi marinho no litoral brasileiro (SIRENIA, Trichechidae, Trichechus manatus, Linnaeus, 1758),” in Proceedings of the First Simpósio Internacional sobre a utilizaçao de Ecossistemas Costeiros: Planejamento, Poluição e Produtividade, Cambridge.
Allendorf, F. W., Leary, R. F., Spruell, P., and Wenburg, J. K. (2001). The problems with hybrids: setting conservation guidelines. Trends Ecol. Evol. 16, 613–622. doi: 10.1016/s0169-5347(01)02290-x
Allsopp, W. H. L. (1960). The manatee: ecology and use for weed control. Nature 188:762. doi: 10.1038/188762a0
Allsopp, W. H. L. (1969). “Aquatic weed control by manatees — its prospects and problems,” in Man-Made Lakes, ed. L. E. Obeng (Accra: Ghana Universities Press), 344–351.
Amos, B., and Hoelzel, A. R. (1991). Long-term preservation of whale skin for DNA analysis. Rep. Int. Whaling Comm. Spec. Issue 13, 99–103.
Balensiefer, D. C., Attademo, F. L. N., Sousa, G. P., Freire, A. C. D. B., da Cunha, F. A. G. C., Alencar, A. E. B., et al. (2017). Three decades of Antillean manatee (Trichechus manatus manatus) stranding along the Brazilian Coast. Trop. Conserv. Sci. 10, 1–9. doi: 10.1177/1940082917728375
Bandelt, H. J., Forster, P., and Rohl, A. (1999). Median-joining networks for inferring intraspecific phylogenies. Mol. Biol. Evol. 16, 37–48. doi: 10.1093/oxfordjournals.molbev.a026036
Barnosky, A. D., Matzke, N., Tomiya, S., Wogan, G. O., Swartz, B., Quental, T. B., et al. (2011). Has the Earth’s sixth mass extinction already arrived? Nature 471, 51–57. doi: 10.1038/nature09678
Bohling, J. H., and Waits, L. P. (2015). Factors influencing red wolf–coyote hybridization in eastern North Carolina, USA. Biol. Conserv. 184, 108–116. doi: 10.1016/j.biocon.2015.01.013
Borobia, M., and Lodi, L. (1992). Recent observations and records of the West Indian manatee Trichechus manatus in northeastern Brazil. Biol. Conserv. 59, 37–43. doi: 10.1016/0006-3207(92)90711-u
Bouzat, J. L. (2010). Conservation genetics of population bottlenecks: the role of chance, selection, and history. Conserv. Gen. 11, 463–478. doi: 10.1007/s10592-010-0049-0
Bradley, J. L., Wright, S. D., and McGuire, P. M. (1993). The Florida manatee - cytochrome-b DNA sequence. Mar. Mamm. Sci. 9, 37–43.
Brook, B. W., Sodhi, N. S., and Bradshaw, C. J. A. (2008). Synergies among extinction drivers under global change. Trends Ecol. Evol. 23, 453–460. doi: 10.1016/j.tree.2008.03.011
Calleson, C. S., and Frohlich, R. K. (2007). REVIEW: slower boat speeds reduce risks to manatees. End. Sp. Res. 3, 295–304. doi: 10.3354/esr00056
Cantanhede, A. M., Da Silva, V. M. F., Farias, I. P., Hrbek, T., Lazzarini, S. M., and Alves-Gomes, J. (2005). Phylogeography and population genetics of the endangered Amazonian manatee, Trichechus inunguis Natterer, 1883 (Mammalia, Sirenia). Mol. Ecol. 14, 401–413. doi: 10.1111/j.1365-294X.2004.02413.x
Ceballos, G., Ehrlich, P. R., and Dirzo, R. (2020). Vertebrates on the brink as indicators of biological annihilation and the sixth mass extinction. Proc. Nat. Acad. Sci. U.S.A. 114, E6089–E6096. doi: 10.1073/pnas.1922686117
Cornuet, J. M., and Luikart, G. (1996). Description and power analysis of two tests for detecting recent population bottlenecks from allele frequency data. Genetics 144, 2001–2014.
Couvet, D. (2002). Deleterious effects of restricted gene flow in fragmented populations. Conserv. Biol. 16, 369–376. doi: 10.1046/j.1523-1739.2002.99518.x
Cristescu, R., Sherwin, W., Handasyde, K., Cahill, V., and Cooper, D. (2010). Detecting bottlenecks using BOTTLENECK 1.2.02 in wild populations: the importance of the microsatellite structure. Conserv. Genet. 11, 1043–1049. doi: 10.1007/s10592-009-9949-2
de Thoisy, B., Spiegelberger, T., Rousseau, S., Talvy, G., Vogel, I., and Vie, J. C. (2003). Distribution, habitat, and conservation status of the West Indian manatee Trichechus manatus in French Guiana. Oryx 37, 431–436.
Deutsch, C., Self-Sullivan, C., and Mignucci-Giannoni, A. (2008). Trichechus manatus. In: 2011 IUCN Red List of Threatened Species. Version 2011.1. Available online at: www.iucnredlist.org (accessed September 2, 2011).
DiBattista, J. D. (2007). Patterns of genetic variation in anthropogenically impacted populations. Conserv. Genet. 9, 141–156. doi: 10.1007/s10592-007-9317-z
Dinno, A. (2017). Dunn’s Test of Multiple Comparisons Using Rank Sums. R package version, 1(4). Available online at: https://cran.r-project.org/web/packages/dunn.test/index.html (accessed December 12, 2019).
Dirzo, R., Young, H. S., Galetti, M., Ceballos, G., Isaac, N. J., and Collen, B. (2014). Defaunation in the Anthropocene. Science 345, 401–406. doi: 10.1126/science.1251817
Earl, D. A., and VonHoldt, B. (2009). Structure Harvester v0.3. Available online at: http://users.soe.ucsc.edu/~dearl/software/structharvest (accessed December 3, 2019).
Excoffier, L., and Lischer, H. E. (2010). Arlequin suite ver 3.5: a new series of programs to perform population genetics analyses under Linux and Windows. Mol. Ecol. Resour. 10, 564–567. doi: 10.1111/j.1755-0998.2010.02847.x
Falush, D., Stephens, M., and Pritchard, J. K. (2007). Inference of population structure using multilocus genotype data: dominant markers and null alleles. Mol. Ecol. Notes 7, 574–578. doi: 10.1111/j.1471-8286.2007.01758.x
Forcada, J., and Würsig, B. (2002). “Distribution,” in Encyclopedia of Marine Mammals, eds W. F. Perrin and J. G. M. Thewissen (San Diego, CA: Academic Press), 327–332.
Frankham, R. (1995). Inbreeding and extinction: a threshold effect. Conserv. Biol. 9, 792–799. doi: 10.1046/j.1523-1739.1995.09040792.x
Frankham, R., Ballou, J. D., and Briscoe, D. A. (2002). Introduction to Conservation Genetics. Cambridge: Cambridge University Press.
Franklin, I. R. (1980). “Evolutionary change in small populations,” in Conservation Biology: An Evolutionary-Ecological Perspective, eds M. E. Soule′ and B. A. Wilcox (Sunderland, MA: Sinauer Associations), 135.
García-Rodríguez, A. I., Bowen, B. W., Domning, D. P., Mignucci-Giannoni, A. A., Marmontel, M., Montoya-Ospina, R. A., et al. (1998). Phylogeography of the West Indian manatee (Trichechus manatus): How many populations and how many taxa? Mol. Ecol. 7, 1137–1149. doi: 10.1046/j.1365-294x.1998.00430.x
García-Rodríguez, A. I., Moraga-Amador, D., Farmerie, W. G., McGuire, P. M., and King, T. L. (2000). Isolation and characterization of microsatellite DNA markers in the Florida manatee (Trichechus manatus latirostris) and their application in selected sirenian species. Mol. Ecol. 9, 2161–2163. doi: 10.1046/j.1365-294X.2000.10534.x
Garner, A., Rachlow, J. L., and Hicks, J. F. (2005). Patterns of genetic diversity and its loss in mammalian populations. Conserv. Biol. 19, 1215–1221. doi: 10.1111/j.1523-1739.2005.00105.x
Goudet, J. (2002). FSTAT, a Program to Estimate and Test Gene Diversities and Fixation Indices. Available online at: http://www.unil.ch/izea/softwares/fstat.html (accessed May 22, 2020).
Haigh, M. D. (1991). The use of manatees for the control of aquatic weeds in Guyana. Irri. Drain. Syst. 5, 339–350. doi: 10.1007/bf01102831
Hillis, D. M., Mable, B. K., Larson, A., Davis, S. K., and Zimmer, E. A. (1996). “Nucleic Acids IV: Sequencing and Cloning,” in Molecular Systematics, 2nd Edn, eds D. M. Hillis, C. Moritz, and B. K. Mable (Sunderland, MA: Sinauer Associates, Inc).
Hollowell, T., and Reynolds, R. P. (2005). Checklist of the terrestrial vertebrates of the guiana shield. Bull. Biol. Soc. Washington 13, 1–98. doi: 10.1017/cbo9780511549717.002
Hunter, M. E., Auil-Gomez, N. E., Tucker, K. P., Bonde, R. K., Powell, J., and McGuire, P. M. (2010). Low genetic variation and evidence of limited dispersal in the regionally important Belize manatee. Anim. Conserv. 13, 592–602. doi: 10.1111/j.1469-1795.2010.00383.x
Hunter, M. K., Broderick, D., Ovenden, J. R., Tucker, K. P., Bonde, R. K., McGuire, P. M., et al. (2010). Characterization of highly informative cross-species microsatellite panels for the Australian dugong (Dugong dugon) and Florida manatee (Trichechus manatus latirostris) including five novel primers. Mol. Ecol. Res. 10, 368–377. doi: 10.1111/j.1755-0998.2009.02761.x
Hunter, M. E., Mignucci-Giannoni, A. A., Tucker, K. P., King, T. L., Bonde, R. K., Gray, B. A., et al. (2012). Puerto Rico and Florida manatees represent genetically distinct groups. Conserv. Genet. 13, 1623–1635. doi: 10.1007/s10592-012-0414-2
ICMBio (2018a). Livro Vermelho de Fauna Brasileira Ameaçada de Extinção, Vol. I. Available online at: https://www.icmbio.gov.br/portal/images/stories/comunicacao/publicacoes/publicacoes-diversas/livro_vermelho_2018_vol1.pdf (accessed July 12, 2020).
ICMBio (2018b). Portaria ICMBio n° 249, de 4 de abril de. Plano de Ação Nacional para Conservação do Peixe-boi marinho. Available online at: https://www.icmbio.gov.br/portal/images/stories/docs-plano-de-acao/pan-peixe-boi-marinho/1°_ciclo/Portaria-249-PAN-Peixe-boi-marinho.pdf (accessed July 12, 2020).
ICMBio (2019). Portaria ICMBio n° 19, de 16 de janeiro de. Plano de Ação Nacional para Conservação de Mamíferos Aquáticos Amazônicos Ameaçados de Extinção. Available online at: https://www.icmbio.gov.br/portal/faunabrasileira/planos-de-acao/10193-plano-de-acao-nacional-para-a-conservacao-mamiferos-aquaticos-amazonicos (accessed July 12, 2020).
IUCN (2012). 2012 IUCN Red List of Threatened Species. Available online at: http://www.iucnredlist.org (accessed February 19, 2012).
Jamieson, I. G., and Allendorf, F. W. (2012). How does the 50/500 rule apply to MVPs? Trends Ecol. Evol. 27, 578–584. doi: 10.1016/j.tree.2012.07.001
Jombart, T. (2008). Adegenet: a R package for the multivariate analysis of genetic markers. Bioinformatics 24, 1403–1405. doi: 10.1093/bioinformatics/btn129
Jorde, P. E., Schweder, T., Bickham, J. W., Givens, G. H., Suydam, R., Hunter, D., et al. (2007). Detecting genetic structure in migrating bowhead whales off the coast of Barrow, Alaska. Mol. Ecol. 16, 1993–2004. doi: 10.1111/j.1365-294x.2007.03268.x
Laikre, L., Olsson, F., and Jansson, E. (2016). Metapopulation effective size and conservation genetic goals for the Fennoscandian wolf (Canis lupus) population. Heredity 117, 279–289. doi: 10.1038/hdy.2016.44
Lande, R. (1995). Mutation and conservation. Conserv. Biol. 9, 782–791. doi: 10.1046/j.1523-1739.1995.09040782.x
Levin, D. A. (2002). Hybridization and extinction: in protecting rare species, conservationists should consider the dangers of interbreeding, which compound the more well-known threats to wildlife. Am. Sci. 90, 254–261.
Lima, C. S., Magalhaes, R. F., Marmontel, M., Meirelles, A. C., Carvalho, V. L., Lavergne, A., et al. (2019). A hybrid swarm of manatees along the Guianas coastline, a peculiar environment under the influence of the Amazon River plume. An. Acad. Bras. Cienc. 91, (Suppl. 3):e20190325. doi: 10.1590/0001-3765201920190325
Lima, R. P. (1997). Peixe-Boi Marinho (Trichechus manatus): Distribuição, Status de Conservação e Aspectos Tradicionais ao Longo do Litoral Nordeste do Brasil. Master’s thesis, Universidade Federal de Pernambuco, Recife.
Lima, R. P., Paludo, D., Soavinski, R. J., da Silva, K. G., and Oliveira, E. M. (1992). Levantamento da distribuição, ocorrência e status de conservação do Peixe-Boi Marinho (Trichechus manatus, Linnaeus, 1758) no litoral nordeste do Brasil. Peixe-boi 1, 47–72.
Luna, F. O. (2001). Distribuição, Status de Conservação e Aspectos Tradicionais do peixe-boi marinho (Trichechus manatus manatus) no litoral norte do Brasil. Master’s thesis, Universidade Federal de Pernambuco, Recife.
Luna, F. O., Balensiefer, D. C., Fragoso, A. B., Stephano, A., and Attademo, F. L. N. (2018). “Trichechus manatus Linnaeus, 1758,” in Livro Vermelho da Fauna Brasileira Ameaçada de Extinção: Volume II. - Mamíferos, (Brasília: ICMBio), 103–109.
Luna, F. O., Bonde, R. K., Attademo, F. L. N., Saunders, J. W., Meigs-Friend, G., Passavante, J. Z. O., et al. (2012). Phylogeographic implications for release of critically endangered manatee calves rescued in Northeast Brazil. Aquat. Conser. Mar. Freshw. Ecol. 22, 665–672. doi: 10.1002/aqc.2260
Luna, F. O., de Andrade, M. C. M., and Reis, M. L. (Org) (2011). Plano de Ação Nacional para a Conservação dos Sirênios: Peixe-boi-da-Amazônia Trichechus inunguis e Peixe-boi-marinho Trichechus manatus. (Brasília: ICMBio).
Luna, F. O., Araujo, J. P., Lima, R. P., Pessanha, M. M., Soavinski, R. J., and Passavante, J. Z. (2008a). Captura e utilização do peixe-boi marinho (Trichechus manatus manatus) no litoral Norte do Brasil. Biotemas 21, 115–123.
Luna, F. O., Araújo, J. P., Passavante, J. Z. O., Mendes, P. P., Pessanha, M. M., Soavinski, R. J., et al. (2008b). Ocorrência do peixe boi marinho (Trichechus manatus manatus) no litoral norte do Brasil. Bol. Mus. Biol. Mello Leitão 23, 37–49.
Luna, F. O., Lima, R. P., Araujo, J. P., and Passavante, J. Z. (2008c). Status de conservação do peixe-boi marinho (Trichechus manatus manatus Linnaeus, 1758) no Brasil. Zoociências 10, 145–153.
Luna, F. O., and Passavante, J. Z. (2010). Projeto Peixe-boi/ICMBio: 30 Anos de Conservação de uma Espécie Ameaçada. (Brasília: ICMBio), 108.
Maddison, W. P., and Maddison, D. R. (2018). Mesquite: A Modular System for Evolutionary Analysis. Available: http://www.mesquiteproject.org (accessed October 23, 2020).
Madsen, T., Shine, R., Olsson, M., and Wittzell, H. (1999). Restoration of an inbred adder population. Nature 404, 34–35. doi: 10.1038/46941
Marmontel, M. (1995). “Age and reproduction in female Florida manatees,” in Population Biology of the Florida Manatee, eds T. J. O’Shea, B. B. Ackerman, and H. F. Percival (Washington, DC: National Biological Service Information and Technology), 98–119.
Mills, L. S., and Allendorf, F. W. (1996). The One-Migrant-per-generation rule in conservation and management. Conserv. Biol. 10, 1509–1518. doi: 10.1046/j.1523-1739.1996.10061509.x
Normande, I. C., Malhado, A. C. M., Reid, J., Viana, P. C., Savaget, P. V. S., Correia, R. A., et al. (2016). Post-release monitoring of Antillean manatees: an assessment of the Brazilian rehabilitation and release programme. Anim. Conserv. 19, 235–246. doi: 10.1111/acv.12236
Nourisson, C. (2011). Estructura Genética de los Manatíes en México. Ph.D. thesis, El Colegio de la Fronta Sur, Mexico.
Nourisson, C., Morales-Vela, B., Padilla-Saldivar, J., Tucker, K. P., Clark, A., Olivera-Gomez, L. D., et al. (2011). Evidence of two genetic clusters of manatees with low genetic diversity in Mexico and implications for their conservation. Genetica 139, 833–842. doi: 10.1007/s10709-011-9583-z
Palumbi, S., Martin, A., Romano, S., McMillan, W. O., Stice, L., and Grabowski, G. (1991). Simple Fools Guide to PCR, version II. Honolulu, HI: University of Hawaii.
Parr, L., Santos, F. R., Waycott, M., Vianna, J. A., Mcdonald, B., Caballero, S., et al. (2012). “Sirenian genetics and demography,” in Sirenian Conservation: Issues and Strategies in Developing Countries, 1st Edn, eds E. M. Hines and J. E. Reynolds III, L. V. Aragones, A. A. Mignucci-Giannoni, and M. Marmontel (Gainesville, FL: University Press of Florida).
Paster, T., Garza, J. C., Allen, P., Amos, W., and Aguilar, A. (2004). Low genetic variability in the highly endangered Mediterranean monk seal. J. Hered. 95, 291–300. doi: 10.1093/jhered/esh055
Pause, K. C., Nourisson, C., Clark, A., Kellogg, M. E., Bonde, R. K., and McGuire, P. M. (2007). Polymorphic microsatellite DNA markers for the Florida manatee (Trichechus manatus latirostris). Mol. Ecol. Notes 7, 1073–1076. doi: 10.1111/j.1471-8286.2007.01781.x
Peakall, R., and Smouse, P. E. (2006). GENALEX 6: genetic analysis in Excel. Population genetic software for teaching and research. Mol. Ecol. Notes 6, 288–295. doi: 10.1111/j.1471-8286.2005.01155.x
Peel, D., Ovenden, J. R., and Peel, S. L. (2004). NEESTIMATOR: Software for Estimating Effective Population Size, Version 1.3. Brisbane: Department of Primary Industries and Fisheries.
Piett, S., Hager, H. A., and Gerrard, C. (2015). Characteristics for evaluating the conservation value of species hybrids. Biodivers. Conserv. 24, 1931–1955. doi: 10.1007/s10531-015-0919-3
Piry, S., Alapetite, A., Cornuet, J. M., Paetkau, D., Baudouin, L., and Estoup, A. (2004). GENECLASS2: a software for genetic assignment and first-generation migrant detection. J. Hered. 95, 536–539. doi: 10.1093/jhered/esh074
Piry, S., Luikart, G., and Cornuet, J. M. (1999). BOTTLENECK: a computer program for detecting recent reductions in the effective population size using allele frequency data. J. Hered. 90, 502–503. doi: 10.1093/jhered/90.4.502
Proebstel, D. S., Evans, R. P., Shiozawa, D. K., and Williams, R. N. (1993). Preservation of nonfrozen tissue samples from a salmonine fish Brachymystax lenok (Pallas) for DNA analysis. J. Ichthyol. 9, 9–17.
Rannala, B., and Mountain, J. L. (1997). Detecting immigration by using multilocus genotypes. Proc. Natl. Acad. Sci. U.S.A. 94, 9197–9201. doi: 10.1073/pnas.94.17.9197
Rathbun, G. B., Reid, J. P., Bonde, R. K., and Powell, J. A. (1995). “Reproduction in free-ranging Florida manatees,” in Population Biology of the Florida Manatee, eds T. J. O’Shea, B. B. Ackerman, and H. F. Percival (Washington, DC: National Biological Service Information and Technology), 135–156.
Raymond, M., and Rousset, F. (1995). GENEPOP (version 1.2): population genetics software for exact tests and ecumenicism. J. Hered. 86, 248–249. doi: 10.1093/oxfordjournals.jhered.a111573
RCoreTeam (2019). R: A Language and Environment for Statistical Computing. Vienna: R Foundation for Statistical Computing.
Reynolds, J. E., Szelistowski, W. A., and Leon, M. A. (1995). Status and conservation of manatees Trichechus manatus manatus in Costa Rica. Biol. Conserv. 71, 193–196. doi: 10.1016/0006-3207(94)00046-s
Rice, W. R. (1989). Analyzing tables of statistical tests. Evolution 43, 223–225. doi: 10.1111/j.1558-5646.1989.tb04220.x
Roman, J., and Palumbi, S. R. (2003). Whales before whaling in the North Atlantic. Science 301, 508–510. doi: 10.1126/science.1084524
Rozas, J., Sanchez-DelBarrio, J. C., Messeguer, X., and Rozas, R. (2003). DnaSP, DNA polymorphism analyses by the coalescent and other methods. Bioinformatics 19, 2496–2497. doi: 10.1093/bioinformatics/btg359
Satizábal, P., Mignucci-Giannoni, A. A., Duchêne, S., Caicedo-Herrera, D., Perea-Sicchar, C. M., García-Dávila, C. R., et al. (2012). Phylogeography and sex-biased dispersal across riverine manatee populations (Trichechus inunguis and Trichechus manatus) in South America. PLoS One 7:e52468. doi: 10.1371/journal.pone.0052468
Scornavacca, C., and Galtier, N. (2017). Incomplete lineage sorting in mammalian phylogenomics. Syst. Biol. 66, 112–120. doi: 10.1093/sysbio/syw082
Southern, S. O., Southern, P. J., and Dizon, A. E. (1988). Molecular characterization of a cloned dolphin mitochondrial genome. Jour. Mol. Evol. 28, 32–42. doi: 10.1007/bf02143495
Storfer, A. (1999). Gene flow and endangered species translocations: a topic revisited. Biol. Conserv. 87, 173–180. doi: 10.1016/s0006-3207(98)00066-4
Tigano, A., and Friesen, V. L. (2016). Genomics of local adaptation with gene flow. Mol. Ecol. 25, 2144–2164. doi: 10.1111/mec.13606
Torres-Florez, J. P., Hucke-Gaete, R., Rosenbaum, H., and Figueroa, C. C. (2014). High genetic diversity in a small population: the case of Chilean blue whales. Ecol. Evol. 4, 1398–1412. doi: 10.1002/ece3.998
Torres-Florez, J. P., Johnson, W. E., Nery, M. F., Eizirik, E., Oliveira-Miranda, M. A., and Galetti, P. M. (2018). The coming of age of conservation genetics in Latin America: what has been achieved and what needs to be done. Conserv. Genet. 19, 1–15. doi: 10.1007/s10592-017-1006-y
Tucker, K. P., Hunter, M. E., Bonde, R. K., Austin, J. D., Clark, A. M., Beck, C. A., et al. (2012). Low genetic diversity and minimal population substructure in the endangered Florida manatee: implications for conservation. J. Mamm. 93, 1504–1511. doi: 10.1644/12-mamm-a-048.1
Vaha, J. P., and Primmer, C. R. (2006). Efficiency of model-based Bayesian methods for detecting hybrid individuals under different hybridization scenarios and with different numbers of loci. Mol. Ecol. 15, 63–72. doi: 10.1111/j.1365-294X.2005.02773.x
Van Oosterhout, C., Hutchinson, W. F., Wills, D. P. M., and Shipley, P. (2004). MICRO-CHECKER: Software for identifying and correcting genotyping errors in microsatellite data. Mol. Ecol. Notes 4, 535–538. doi: 10.1111/j.1471-8286.2004.00684.x
Vianna, J. A., Bonde, R. K., Caballero, S., Giraldo, J. P., Lima, R. P., Clark, A., et al. (2006). Phylogeography, phylogeny and hybridization in trichechid sirenians: implications for manatee conservation. Mol. Ecol. 15, 433–447. doi: 10.1111/j.1365-294X.2005.02771.x
Vianna, J. D. A., Santos, F., Marmontel, M., Lima, R., Luna, F., Lazzarini, S., et al. (2006). Peixes-bois esforço de conservação no Brasil. Ciênc. Hoje 39, 32–37.
Vilaça, S. T., Lima, C. S., Mazzoni, C. J., Santos, F. R., and de Thoisy, B. (2016). Manatee genomics supports a special conservation area along the Guianas coastline under the influence of the Amazon River plume. Coast. Shelf Sci. 231:106436. doi: 10.1016/j.ecss.2019.106436
Waldick, R. C., Kraus, S. S., Brown, M., and White, B. N. (2002). Evaluating the effects of historic bottleneck events: an assessment of microsatellite variability in the endangered North Atlantic right whale. Mol. Ecol. 11, 2241–2250. doi: 10.1046/j.1365-294x.2002.01605.x
Wang, J. (2004). Application of the One-migrant-per-generation rule to conservation and management. Conserv. Biol. 18, 332–343. doi: 10.1111/j.1523-1739.2004.00440.x
Wang, K., Lenstra, J. A., Liu, L., et al. (2018). Incomplete lineage sorting rather than hybridization explains the inconsistent phylogeny of the wisent. Commun. Biol. 1:169. doi: 10.1038/s42003-018-0176-6
Westemeier, R. J., Brawn, S., Simpson, T., Esker, J., Jansen, J., Walk, J., et al. (1998). Tracking the longterm decline and recovery of an isolated population. Science 282, 1695–1698. doi: 10.1126/science.282.5394.1695
White, P. S., and Densmore, L. D. (1992). “Mitochondrial DNA isolation,” in Molecular Genetic Analysis of Populations: A Practical Approach, ed. A. R. Hoezel (New York, NY: Oxford University Press), 29–58.
Whitehead, P. J. P. (1978). Registros antigos da presença do peixe-boi do Caribe (Trichechus manatus) no Brasil. Acta Amaz. 8, 497–506. doi: 10.1590/1809-43921978083497
Whiteley, A. R., Fitzpatrick, S. W., Funk, W. C., and Tallmon, D. A. (2015). Genetic rescue to the rescue. Trends Ecol. Evol. 30, 42–49. doi: 10.1016/j.tree.2014.10.009
Willi, Y., Van Buskirk, J., and Hoffmann, A. A. (2006). Limits to the adaptive potential of small populations. Annu. Rev. Ecol. Evol. Syst. 37, 433–458. doi: 10.1146/annurev.ecolsys.37.091305.110145
Wilson, G. A., and Rannala, B. (2003). Bayesian inference of recent migration rates using multilocus genotypes. Genetics 163, 1177–1191.
Wright, S. (1951). The genetical structure of populations. Ann. Euge. 15, 323–354. doi: 10.1111/j.1469-1809.1949.tb02451.x
Keywords: Antillean manatee, conservation genetics, population structure, endangered species, sympatric species, hybrid, mitochondrial DNA, microsatellite DNA
Citation: Luna FO, Beaver CE, Nourisson C, Bonde RK, Attademo FLN, Miranda AV, Torres-Florez JP, de Sousa GP, Passavante JZ and Hunter ME (2021) Genetic Connectivity of the West Indian Manatee in the Southern Range and Limited Evidence of Hybridization With Amazonian Manatees. Front. Mar. Sci. 7:574455. doi: 10.3389/fmars.2020.574455
Received: 19 June 2020; Accepted: 19 November 2020;
Published: 06 January 2021.
Edited by:
Jeremy Kiszka, Florida International University, United StatesReviewed by:
Miriam Marmontel, Instituto de Desenvolvimento Sustentável Mamirauá, BrazilSusana Caballero, University of Los Andes, Colombia
Copyright © 2021 Luna, Beaver, Nourisson, Bonde, Attademo, Miranda, Torres-Florez, de Sousa, Passavante and Hunter. This is an open-access article distributed under the terms of the Creative Commons Attribution License (CC BY). The use, distribution or reproduction in other forums is permitted, provided the original author(s) and the copyright owner(s) are credited and that the original publication in this journal is cited, in accordance with accepted academic practice. No use, distribution or reproduction is permitted which does not comply with these terms.
*Correspondence: Fábia de Oliveira Luna, ZmFiaWFsdW5hY21hQGdtYWlsLmNvbQ==; Margaret E. Hunter, bWh1bnRlckB1c2dzLmdvdg==
†These authors have contributed equally to this work