- Zoological Institute of Russian Academy of Sciences, Universitetskaya Embankment, Saint Petersburg, Russia
Biological invasions are a global trend due to globalization of the world. An important goal of invasion biology is to identify environmental characteristics that may make a region receptive to invasions. We tested the hypothesis that environmental variables have stronger effect on the biomass of the alien Cercopagis pengoi in the Neva Estuary than biotic interactions with other zooplankton species. Hierarchical cluster, principal component and Pearson correlation analyzes were used to elucidate changes within the zooplankton community along environmental gradients. The data analysis showed that the biomass of C. pengoi was higher in waters with higher salinity, low temperature, high transparency, lower chlorophyll a concentration and lower proportion of chlorophyll in particulate suspended matter. The biomass of C. pengoi showed significant positive correlations with biomasses of its potential preys. However, we did not find direct significant negative correlations between C. pengoi and larger zooplankton predators Leptodora kindtii and Bythotrephes spp. This research generally supports the hypothesis that if environmental variables are appropriate for an exotic species, then that species is likely to invade successfully, regardless of the native species already present.
Introduction
Biological invasions are a global trend due to globalization and increased traffic between different countries (Cohen and Carlton, 1998; Dirzo et al., 2014). The introduction of non-indigenous species (NIS) into ecosystems can lead to the displacement of native species and other negative consequences. Recently, this effect may be exacerbated due to climate change (Moritz and Agudo, 2013; Holopainen et al., 2016). It is believed to be conducive to invasions, and large-scale invasions of alien eurybiont species in seas can be expected in the near future (Jones and Cheung, 2014). Some non-indigenious species may have economic consequences on fisheries and the tourism industry, and can result in substantial monetary costs and/or alterations to entire ecosystems and social systems (Kumschick et al., 2012, 2015; Galil et al., 2014; Ojaveer et al., 2015). One of such species is Cercopagis pengoi. This planktonic crustacean is an important prey for fish, especially for Baltic herring (e.g., Antsulevich and Välipakka, 2000), while simultaneously appearing to compete with fish for food sources (Gorokhova et al., 2005).
Cercopagis pengoi is an invader from the Ponto-Caspian region and in a number of papers described as a highly invasive species (e.g., Telesh and Ojaveer, 2002; Jażdżewski and Grabowski, 2011; Zaiko et al., 2011). In the early 1990s, it was first recorded in the Baltic Sea and in the eastern part of the Gulf of Finland in particular, and is now spread throughout the Baltic (Telesh and Ojaveer, 2002; Katajisto et al., 2013; Bielecka et al., 2014; Rowe et al., 2016). Moreover, in 1998 it was firstly found in Lake Ontario (Laurentian Great Lakes) (MacIsaac et al., 1999), where it quickly reached high densities, has likely caused a decline in small zooplankton and may compete with the pelagic early life history stages of fish (Benoît et al., 2002; Laxson et al., 2003). The presence of C. pengoi also decreases the production of juvenile copepods both through direct predation and through a shift of copepod vertical distribution (Benoît et al., 2002). Genetic research conducted by Cristescu et al. (2001) showed that its population in the Laurentian Great Lakes most likely originated from the Neva Estuary.
After the invasion in the 1990s, C. pengoi was found in the zooplankton almost all over the Neva Estuary. It was absent only in the uppermost part, shallow and freshwater Neva Bay (Krylov et al., 1999). Studies of the seasonal dynamics of C. pengoi have shown that it is present in zooplankton from June to late October, with a peak of development in August and September (Krylov et al., 1999; Telesh and Ojaveer, 2002). It was found to a depth up to 48 m, but most of the biomass was concentrated in the upper 10-m layer, both during the day and at night (Krylov et al., 1999). Nowadays, C. pengoi has already naturalized in the Neva Estuary and formed a free-living, self-sustaining (reproducible) population (Golubkov and Litvinchuk, 2015).
An important goal of invasion biology is to identify environmental characteristics that may make a region particularly receptive to invasions (David et al., 2017). When introduced, a species may persist only if it is able to pass through environmental and biotic filters. Environmental filters include all the abiotic conditions that determine the range of physicochemical properties, often called the fundamental niche, that make a new habitat suitable for a species to complete its life cycle (David et al., 2017). While some ecologists consider abiotic conditions that are favorable for survival of exotic species (Moyle and Light, 1996; Lahdes and Karjala, 2007), the other ones focus on their biotic interactions with native biota (e.g., Marchetti et al., 2004; Ptáčníková et al., 2015; Vanderploeg et al., 2015). Moreover, some studies have shown that if abiotic factors are appropriate for an exotic species, then that species is likely to invade successfully, regardless of the native species already present (Moyle and Light, 1996; Marchetti et al., 2004). Therefore, it is very important to determine the specific abiotic and biotic environmental conditions favorable to the development of a certain alien species.
The Neva Estuary has a smooth gradient of biotic and abiotic environmental factors (Golubkov and Golubkov, 2020; Golubkov et al., 2020). The zooplankton community in this part of the Gulf of Finland develops under the conditions of a water salinity gradient, from freshwater in the upper part to brackish-water (5 PSU) in the lower. The depth increases from the delta of the Neva River, which affects the temperature profile in the water column. Starting from the middle part of the estuary, the water column in the summer period is stratified. In addition, there is a gradient of plankton primary production rate from the mouth of the Neva River toward the Gulf of Finland and from the coast to the open part of the estuary (Golubkov et al., 2017; Golubkov and Golubkov, 2020). These gradients together with climate change lead to highly variable environments (Golubkov et al., 2020; Golubkov and Golubkov, 2020, which may contribute to the extinction of native species and invasions of eurybiont species invaders (Holopainen et al., 2016). Due to of its biological features, NIS are able to more quickly and better adapt to life in such variable conditions than native species. For instance, it has recently been shown that rising temperatures in the northern part of the Baltic Sea may increase the risk of the invasions of new species (Holopainen et al., 2016). However, studies of the effects of temperature and salinity on alien species and their interaction with other zooplankton species are practically absent in the Baltic Sea (Vuorinen et al., 2015).
The purpose of this study was based on 12-year observations to assess relationships of biotic and abiotic environment variables with the biomass of C. pengoi in the Neva Estuary. We tested the hypothesis that environmental variables have stronger effect on the biomass of the alien C. pengoi in the Neva Estuary than biotic interactions with other zooplankton species. The gradient of environmental factors existing in the estuary provides a good opportunity to examine the influence of environmental variables on this alien species. We hope that this study will advance understanding of Cercopagis biology.
Materials and Methods
Study Area
The Neva Estuary receives water from the Neva River, whose catchment area exceeds 280,000 km2, and the water discharge averages 2,490 m3 s–1 (78.6 km3 yr–1), which is about a fifth of the total river discharge into the Baltic Sea. It is generally characterized by a number of features common to other major Baltic estuaries. As most of them, Neva Estuary is brackish-water, non-tidal, shallow, with horizontal and vertical gradients of salinity and a predominance of eurytopic species. The general environmental characteristics of the Neva Estuary are given in Table 1.
The Neva Estuary can be divided into three parts: the upper shallow freshwater part (Neva Bay), and two brackish water parts, the middle (the inner estuary) and the lower (the outer estuary) parts (Figure 1). At the end of 1980s, the upper part was separated from the middle part of the estuary by the Flood Protective Facility. It consists of 11 dams separated by broad water passages and ship gates in its southern and northern parts. There is no temperature stratification in this part of the estuary. Low water transparency, which does not exceed 1.8 m of Secchi depth in summer time, constrains the distribution of bottom vegetation.
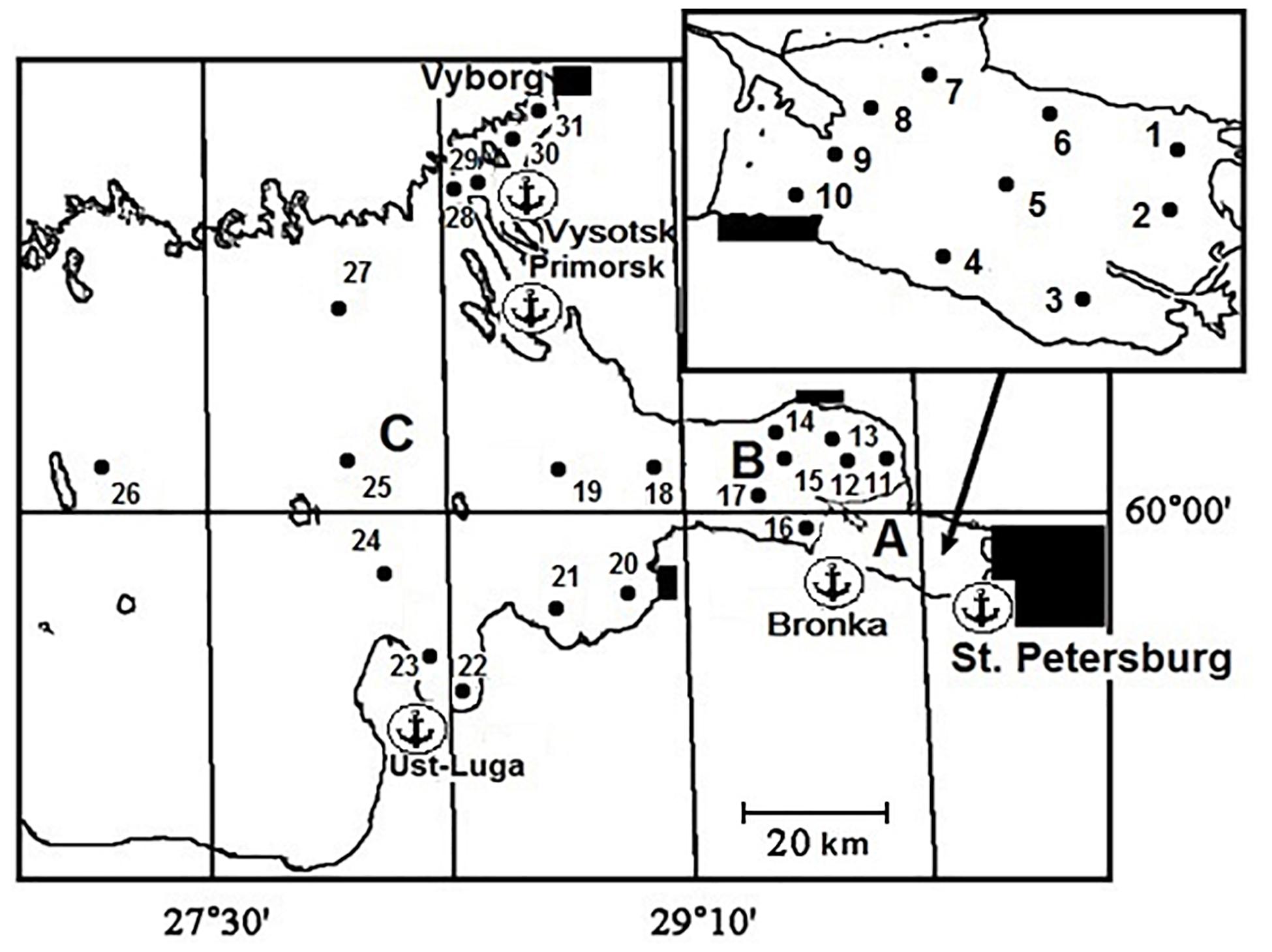
Figure 1. The Neva Estuary with indication of sampling stations (A – the upper part; B – the middle part; C – the lower part). Anchors mark passenger and cargo ports.
The middle part of the Neva Estuary is a slightly brackish-water basin and is located between the Kotlin Island and a longitude of ca. 29°10′E. There is temperature stratification in summer. An even deeper the lower part of the estuary (Table 1) is located to the west of the ca. 29°10′E and to the east of the border of territorial waters of Russia. It has a depth up to 60 m, salinity 3–7 PSU and temperature stratification in summer. A more detailed description of the estuary is given in Telesh et al. (2008).
The Neva Estuary is the recipient of discharges of treated and untreated wastewaters from St. Petersburg City, which is the largest megalopolis in the Baltic region with a population of more than five million citizens (Golubkov et al., 2019). The estuary area is intensively used for recreation, sport and commercial fishing, different industries, including the nuclear power station, and shipping. Oil transportation in the eastern Gulf of Finland has increased almost five times from 1980 to 2000 (Golubkov, 2009). Four large ports and oil terminals were constructed in the late 1990s – early 2000s in addition to the port of St. Petersburg (Figure 1). As a result, there has been considerable increase in ship traffic and risk of invasions during the last decades.
Sampling
Thirty one stations were sampled in different parts of the estuary (Figure 1) in the period from 20th of July to 5th of August in 2006–2017. The temperature and salinity were determined at each station by a CTD90m probe (Sea and Sun Tech., Germany) every 20 cm from the surface to the bottom in the whole water column (three replicates). In addition, transparency (Secchi depth) was determined at each station (three replicates). Since the whole water column was mixed in the shallow upper part, we collected five water samples (2 L each): from the surface, half a meter from the bottom and from three equal depths between them. These samples were mixed to create a composite sample (10 L). The samples for chlorophyll a, suspended particulate matter and suspended particulate organic matter analysis (three replicates) were taken from the composite sample.
In the middle and the lower parts of the estuary, composite water samples were taken from the layer above the thermocline (He). The average thermocline depth in 2006–2017 is shown in Table 1. Five water samples (2 L each) were taken from He: from the surface, the thermocline and from three equal depths between them. These samples were mixed to create a composite sample (10 L). The samples for chlorophyll a (Chl), suspended particulate matter (SM), and suspended particulate organic matter (SOM) (three replicates) were taken from these composite samples.
Zooplankton samples were taken by vertical hauls (four in the upper part, two in the middle and the lower parts of the estuary) with the Juday quantitative plankton net (diameter 0.2 m, mesh size 50 μm) from bottom to surface. The samples were collected during the daytime, preserved in 4% formalin and examined in the laboratory with a light microscope.
Sample Analysis
Three hundred milliliters of water were filtered through 0.85 μm membrane filters (Millipore AAWP) to determine the Chl concentration, which was followed by 90% acetone extraction and spectrophotometric determination (Grasshoff et al., 1999). Concentrations of SM were determined in the same way by filtering water over preweighed and precombusted filters (Whatman GF/F). The determination of the SM was carried out using a standard gravimetric method (Grasshoff et al., 1999). SOM was determined by oxidation with dichromate acid after filtration through Whatman GF/F filters (Grasshoff et al., 1999).
The primary production of plankton (PP) and the mineralization of organic matter (D) in the water column were measured by the oxygen method of light and dark bottles (Hall et al., 2007; Vernet and Smith, 2007). A more detailed description of the method and experimental design was given previously (Golubkov et al., 2017).
ICES zooplankton methodology manual (ICES, 2000) and the manual for marine monitoring in COMBINE (Cooperative Monitoring in the Baltic Marine Environment (Annex C-7)) (HELCOM, 2015) were used for qualitative and quantitative zooplankton laboratory analysis. Abundances were calculated as individuals m–3 and converted to biomasses (g m–3 wet weight) using published carbon-length and length-weight regressions (Dumont et al., 1975; Bottrell et al., 1976; Ruttner-Kolisko, 1977; Winberg and Lavrentyeva, 1982). Zooplankton species have been listed in the modern nomenclature according to World Register of Marine Species (WoRMS Editorial Board, 2020).
Statistical Analysis
Since the number of stations where C. pengoi were met varied over the years, we did not analyze the interannual variability of the biomass of Cercopagis. A single survey for a year (snapshots) does not allow reliable extrapolation of data for a year or even a season. Therefore, we did not focus on temporal variability, but on environmental factors that could affect the development of C. pengoi and other zooplankton species in the estuary. In total, 51 samples collected over a 12-year period were analyzed.
Statistical analyses were performed using R software (version 3.6.0; R Development Core Team, 2020), R package “vegan” (Oksanen et al., 2020), and Microsoft Excel. Pearson’s correlation coefficients was calculated in excel.
Hierarchical cluster analysis was used to examine dissimilarity between samples in which C. pengoi was found. Hierarchic clustering was performed using function “hclust.” Function “rect.hclust” was used to visualize the cutting and function “cutree” to make a classication vector with two numbers of classes. A result was displayed by function “ordihull” R package “vegan” (Oksanen et al., 2020).
The Principal component analysis (PCA) was used to determine relationships between the biomasses of predatory cladocerans using the “rda” function in the “vegan” package (Oksanen et al., 2020). Full scripts of statistical analysis in R present in Supplementary File.
Results
Environmental Parameters
During the period of investigations, C. pengoi was found in 51 samples. The depth of the stations varied from 6.7 to 59 m and the depth of the upper warm water layer from 3.9 to 23 m. The salinity of the upper layer of warm water ranged from 0.26 to 4.3 PSU, the temperature from 13.9 to 22.4°C and the Secchi depth from 0.3 to 4.3 m. The concentration of chlorophyll a was 0.9–30 mg m–3, and the plankton primary production was 0.5–2.7 gC m–2 day–1. Concentrations of suspended particles varied between 1.7 and 45.8 g m–3, concentrations of suspended organic particles – 0.5 to 6.8 g m–3. Percent of chlorophyll in SOM was from 0.1 to 1.8. Average values of environmental parameters for each station are given in the Supplementary Table 1. Most of them correlated with each other (Table 2).
Biomass of Cercopagis pengoi and Environmental Variables
Cercopagis pengoi was distributed throughout the middle and the lower parts of the estuary, and was never found in its upper part. The highest biomass (5,558 mg m–3) was found at station 25 in the lower part in 2007, the lowest one (1.4 mg m–3) at station 18 in the middle part in 2006 (Figure 1).
Using biomass data for each zooplankton species and environmental variables for stations where C. pengoi was found, we performed cluster analysis and identified two groups of samples (Figure 2). The first group included thirty-four samples where C. pengoi biomass was low and averaged 52 mg m–3 (Figure 2 and Table 3). The second group included 17 samples, at which the biomass of C. pengoi was many times higher and averaged 1,689 mg m–3 (Figure 2 and Table 3).
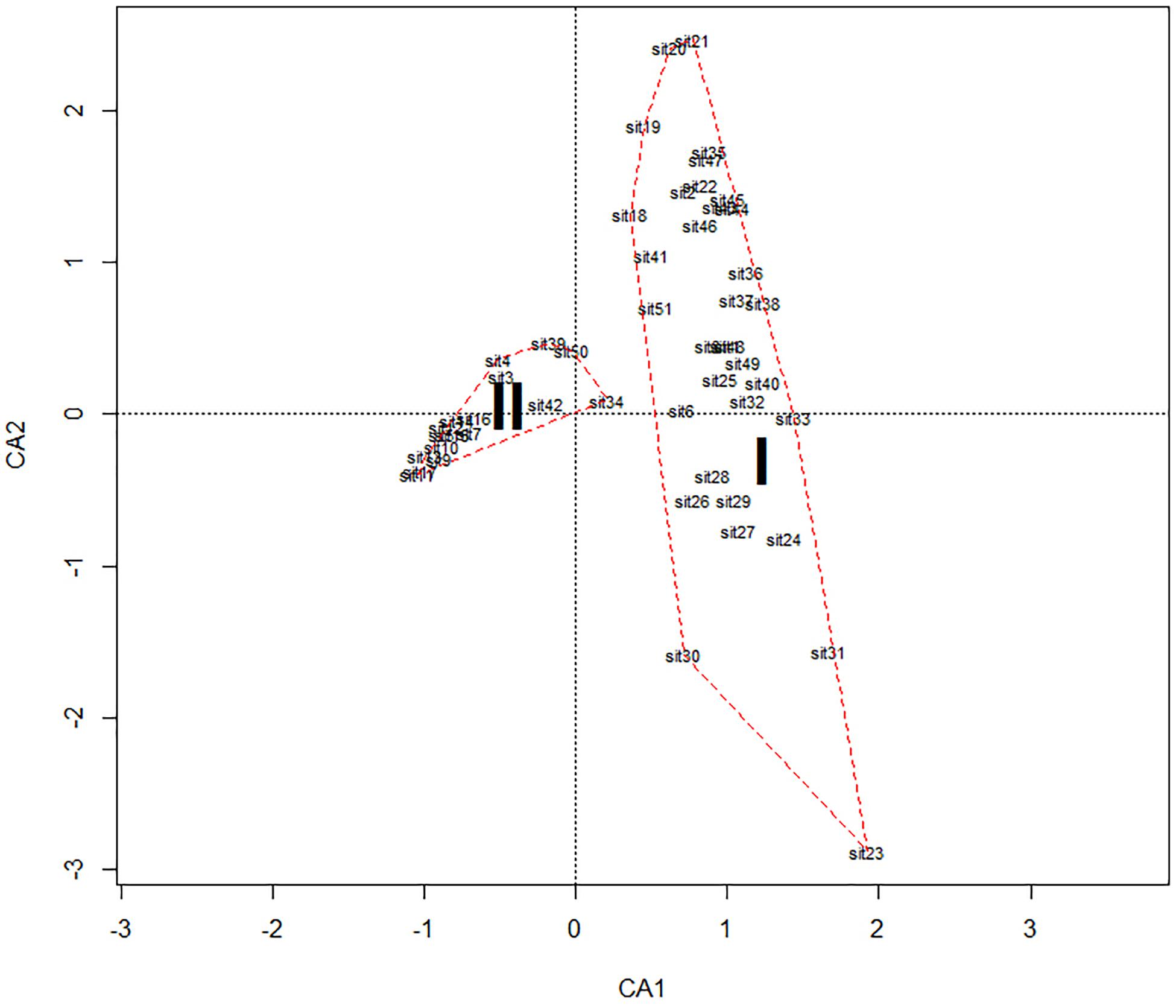
Figure 2. Two groups of samples in the Neva Estuary discriminated by biomass of C. pengoi and environmental factors. Roman numerals indicate group numbers.

Table 3. Average values of C. pengoi biomass and environmental variables in two groups of samples discriminated based on cluster analysis.
These two groups of samples, in addition to the difference in C. pengoi biomass, also differed in the average values of environmental variables (Table 3). Their values for each group of samples were used to determine the environmental conditions under which the biomass of this species in the estuary was higher, and under which it was lower.
The highest differences in environmental parameters between two groups of samples were in water salinity and chlorophyll a concentration (Figure 3). First, it turned out that the biomass of C. pengoi was higher in samples from deep-water stations with relatively high water salinity, and, accordingly, this species preferred the deeper western parts of the estuary. The Cercopagis biomass was also considerably higher in samples from the stations with low concentrations of chlorophyll a (Figure 3). In addition, these two groups of samples differed in the average depth of the warm upper layer. In the first group, where the biomass of C. pengoi was low, the depth of the warm upper layer was one and a half times less than in the second group, where this crustacean was abundant (Table 3).
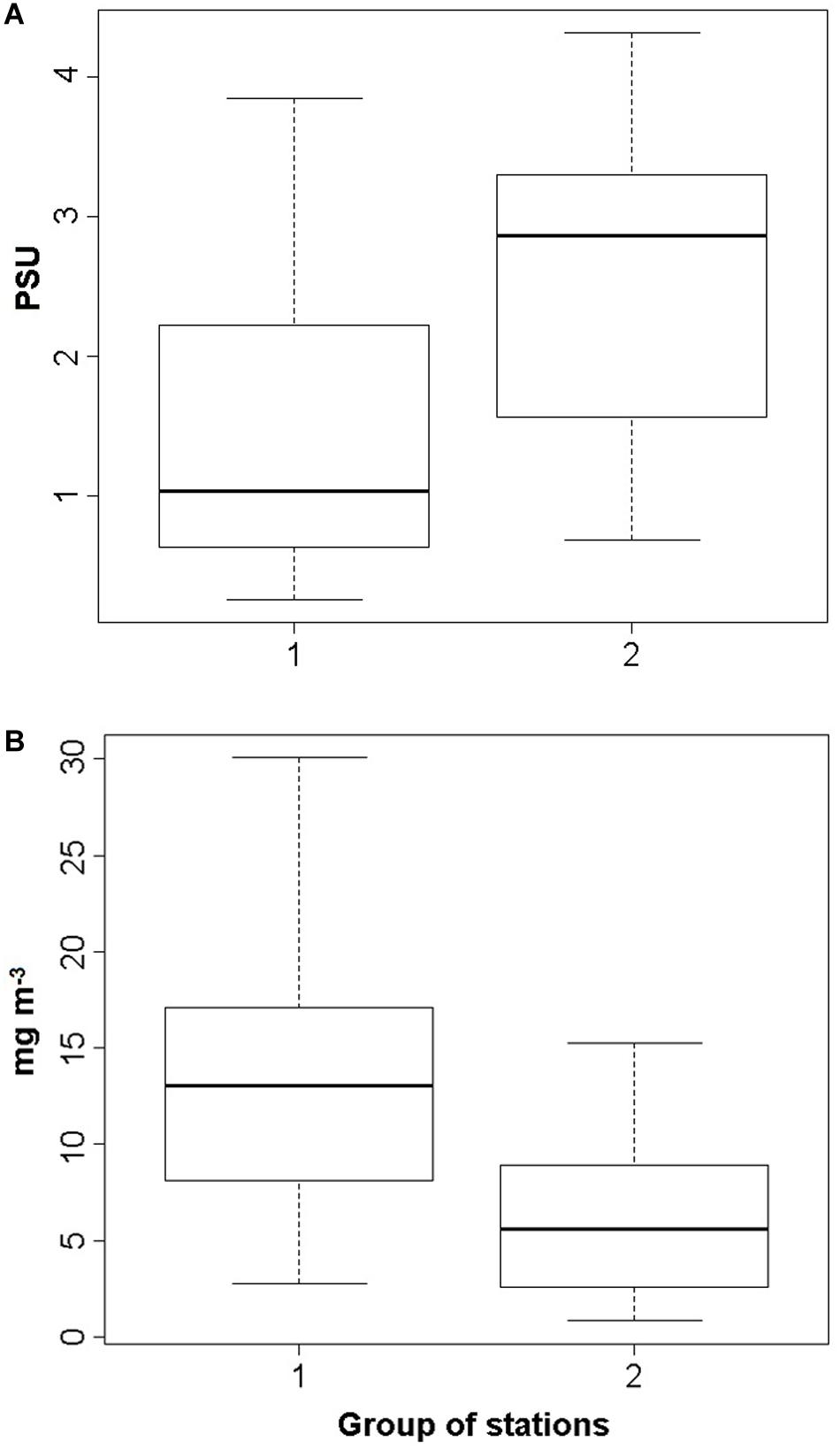
Figure 3. Median of water salinity (A) and chlorophyll a concentration (B) in two groups of stations with low (1) and high (2) biomass of C. pengoi. Bold black line, median; box, interquartile range; dotted lines, maximum and minimum values.
Composition and Biomass of Zooplankton Species Occurred Together With Cercopagis pengoi
Forty-six zooplankton species were found together with C. pengoi during the study period in the middle and the lower parts of the estuary (Supplementary Table 2). Thirteen of them belonged to Rotifera, the most abundant of which was brackish-water species Keratella cochlearis baltica. Carnivorous Cladocera were represented by eight species, the most abundant of which was the non-indigenous C. pengoi. In second place in terms of frequency and biomass was native predatory species Leptodora kindtii. Among the predatory cladocerans, another alien Ponto-Caspian species, Evadne anonyx, was common. This species hybridizes with the native Evadne nordmanni.
Non-predatory Cladocera, which belong to pelagic filter feeders, were represented by eight species, the most numerous of which was freshwater Daphnia cristata. Calanoida (Copepoda) included six species, the most abundant of which was Eurytemora affinis, and also the brackish-water alien species Acartia tonsa. The cyclopoids (Copepoda) were represented by nine predominantly freshwater species, the most common of which was Mesocyclops leuckarti. Harpacticoida (Copepoda) did not reach significant biomass, but were found in more than 50% of the samples. Veligers of Dreissena polymorpha and other mollusks were also common (Supplementary Table 2).
Pearson’s correlation analysis showed that the biomass of C. pengoi significantly correlated with several zooplankton species. The highest correlations were found with the biomass of carnivorous cladocerans, alien E. anonyx and native E. nordmanni and their hybrids. Moreover, biomass of these species like the biomass of C. pengoi negatively correlated with chlorophyll a concentration and the proportion of Chl in SOM; their biomasses positively correlated with water salinity and the depth of warm upper layer (Table 4).
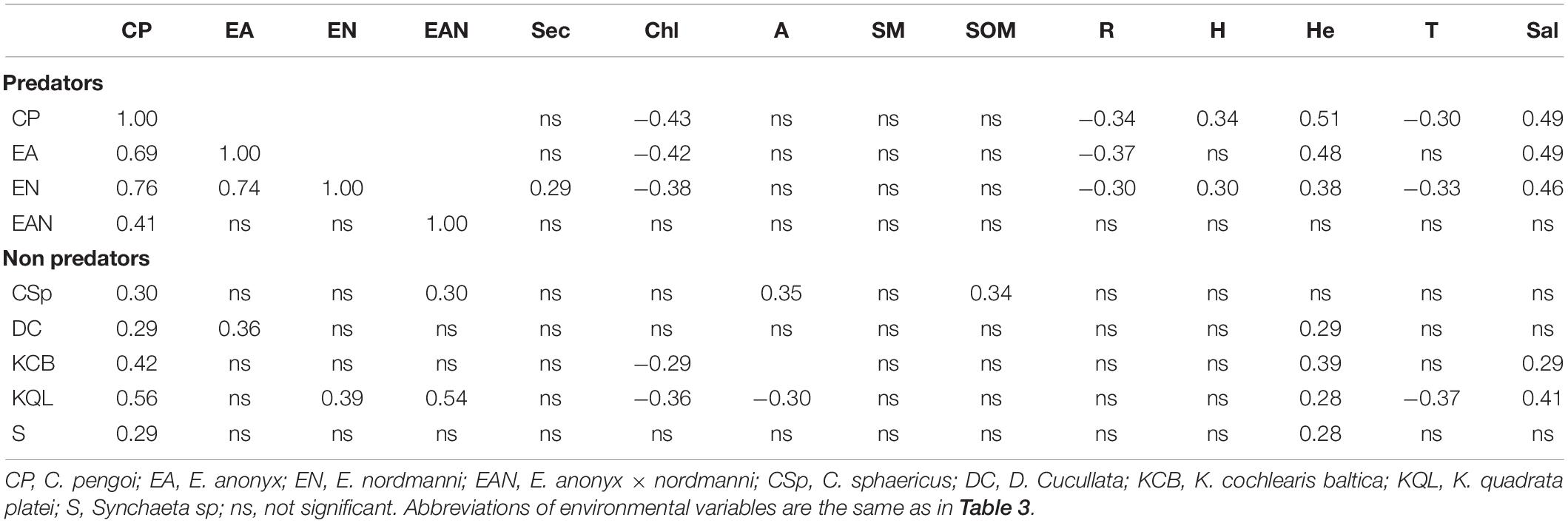
Table 4. Pearson correlation coefficients between biomass of various zooplankton species and environmental variables.
The biomass of C. pengoi positively correlated with biomass of cladocera pelagic filter feeders Chydorus sphaericus and D. cristata (Table 4). Two non-predators rotifers, K. cochlearis baltica and Keratella quadrata platei, also positively correlated with the biomass of Cercopagis. At the same time, the biomass of these rotifers negatively correlated with chlorophyll a concentration and positively with salinity. One predator rotifer, Synchaeta sp., positively correlated with C. pengoi (Table 4).
Forty-six zooplankton species were found in the samples from group 1 with a low biomass of C. pengoi, which was obtained using cluster analysis (Figure 2). In the samples from group 2, where the biomass of C. pengoi was high, several species which were found in group 1, Eudiaptomus gracilis, Eurytemora velox, Heterocope appendiculata, Bosmina coregoni, Podon intermedius, and Ploesoma hudsoni were absent. The first group of samples had also higher biomasses of predatory and filtering cladocerans, cyclopoids, and veligers of mollusks compared to the second group of samples (Figures 4A,B). However, the second group of samples had a high biomass of non-predator rotifer (Figure 4B). We calculated the Pearson’s correlation coefficient between the biomass of Cercopagis and the biomass of all non-predatory rotifers and found a significant positive correlation between them (r = 0.54, p < 0.05, n = 51).
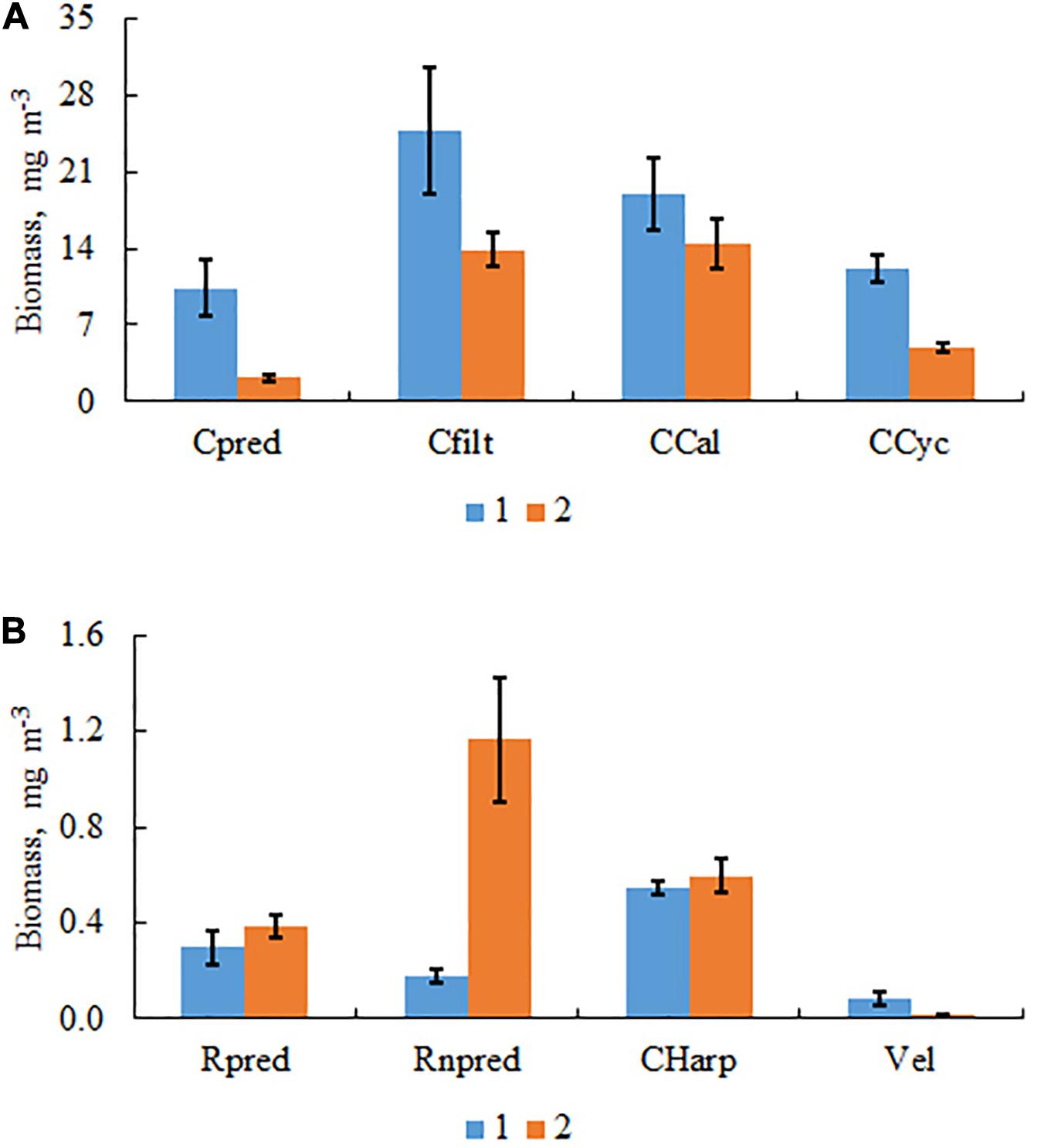
Figure 4. Biomasses of different taxonomic classes in two groups of samples differing in the biomass of C. pengoi. (A) Cpred, Cladocera predators; Cfilt, Cladocera pelagic filter feeders; CCal, Copepoda, Calanoida; CCyc, Copepoda, Cyclopoida. (B) Rpred, Rotatoria predators; Rnpred, Rotatoria non-predators; CHarp, Copepoda, Harpacticoida; Vel, veligers of Mollusca.
Relationship Between the Biomass of Cercopagis pengoi and Biomasses of Other Carnivorous Cladocerans
The PCA analysis showed that biomasses of C. pengoi, predatory cladocerans E. anonyx and E. nordmanni were positively correlated (Figure 5). Three other predatory cladocerans, L. kindtii, P. intermedilis, and Polyphemus pediculus, did not correlate with Cercopagis. The biomass of C. pengoi negatively correlated with biomasses of two species of Bythotrephes (Figure 5). However, these correlations were insignificant (Table 5). Instead, the biomasses of Cercopagis and Bythotrephes spp. were oppositely significantly correlated with salinity, depth, and chlorophyll concentration. This suggests that the negative correlation between these species is more due to the different environmental conditions they prefer than to the direct interactions of these species.
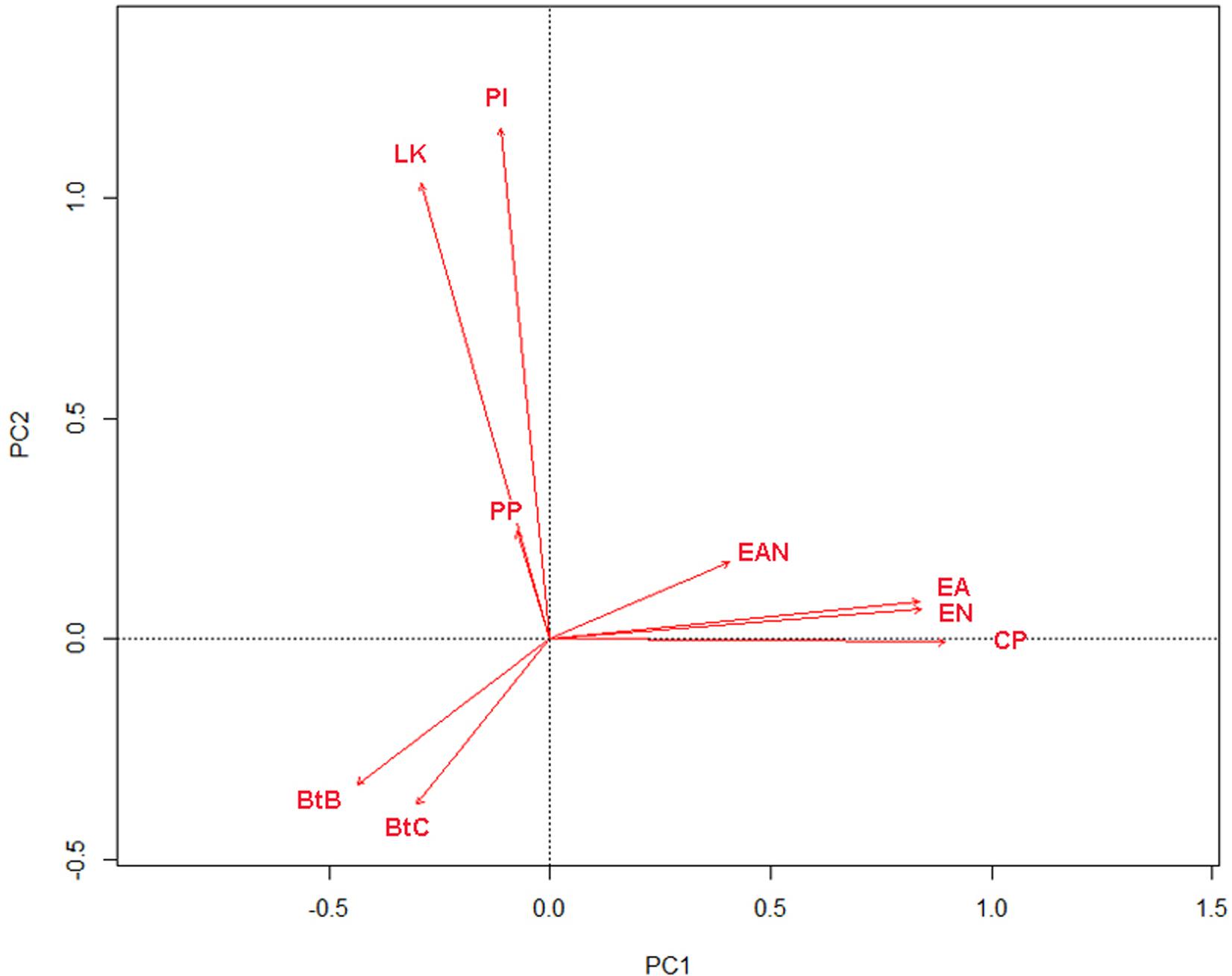
Figure 5. PCA plot of carnivorous Cladocera biomass vectors. Abbreviations of zooplankton species are given in Supplementary Table 2.
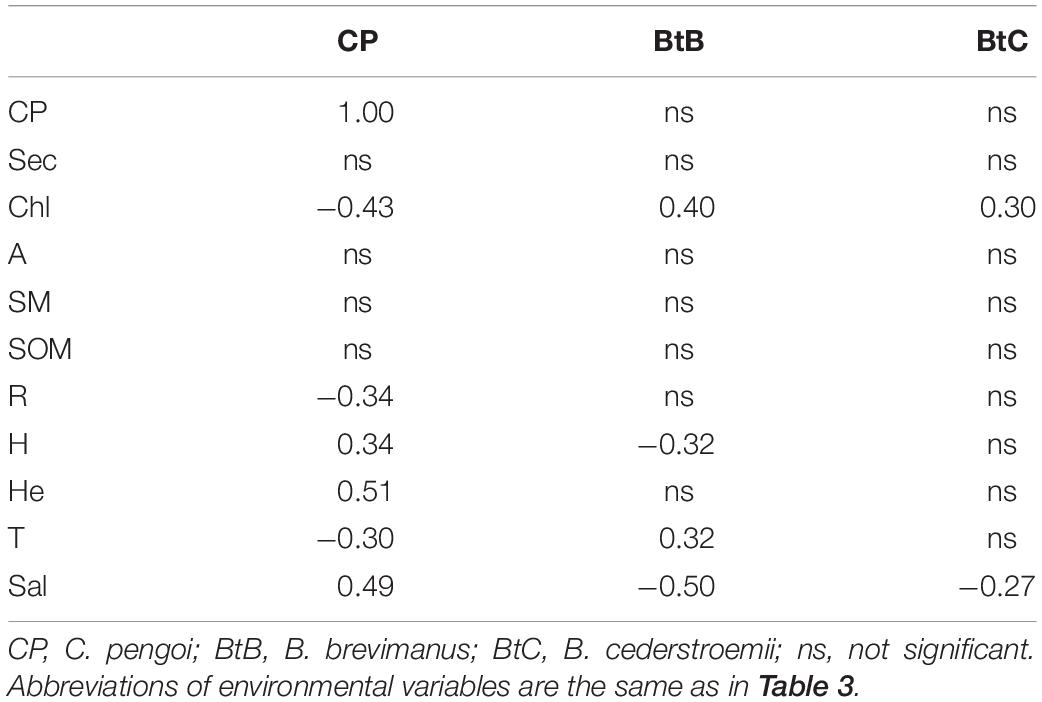
Table 5. Pearson correlation coefficients between biomasses of C. pengoi and Bythotrephes spp. and environmental variables.
Discussion
Correlation of the Biomass of Cercopagis pengoi With Abiotic Variables
In its natural habitat in the Northern Caspian Sea, C. pengoi prefers coastal water areas and bays, where the depth does not exceed 75 m and the salinity reaches 10 PSU; it is tolerant to temperature and disappears from plankton only when the water temperature drops below 10°C (Mordukhai-Boltovskoi and Rivier, 1971). In the Southern Caspian Sea, this species lived in areas with surface salinity up to 12 PSU (Bagheri et al., 2014). In terms of salinity, C. pengoi is highly tolerant and can live in wide range of salinity from completely fresh to brackish water (Mordukhai-Boltovskoi and Rivier, 1971).
A consensus of the data from the present study (Supplementary Table 1) and the literature indicates that Cercopagis is most often found at salinities of 0.3–6 PSU and temperatures of 4–24°C in the Baltic (Krylov et al., 1999; Ojaveer et al., 2004; Litvinchuk and Telesh, 2006; Bielecka et al., 2014; Rowe et al., 2016; Helenius et al., 2017; Naumenko, 2018). For instance, studies conducted in 2008–2009 in the coastal zone of the southwestern part of Finland showed that this species was found at stations with high salinity (∼6 PSU) (Helenius et al., 2017). In our study C. pengoi also preferred stations with higher salinity (Tables 3, 4). The highest abundance and biomass were observed in the lower parts of the Neva Estuary. Its biomass was significantly lower in the relatively shallow near shore habitats with low salinity (Table 3 and Figure 3). Water salinity in the Laurentian Great Lakes in which this species is now abundant is <0.5 PSU (Casties et al., 2016). As was previously shown, the composition and concentrations of ions in lake waters may form a barrier against further spread of C. pengoi to freshwater habitats of the Finnish Lake District, in which the concentration of major ions significantly lower than in the Laurentian Great Lakes (Lahdes and Karjala, 2007). In the Neva River waters, the concentrations of ions are very similar with Finnish lakes and several times lower than in the Laurentian Great Lakes (Lahdes and Karjala, 2007), so the composition and low concentrations of ions may be one of the reasons for the absence of C. pengoi in upper part the Neva Estuary – Neva Bay.
Earlier studies of seasonal changes in the abundance and biomass of C. pengoi in the Neva Estuary showed that this crustacean reached its maximum development in early August and remained in plankton until October, when the water temperature did not exceed 8°C (Krylov et al., 1999). Studies of the influence of environmental factors on the C. pengoi in the Gulf of Bothnia showed that this species was more abundant at higher temperatures (temperature range 12–16 °C) (Rowe et al., 2016). Studies conducted in the Gulf of Gdańsk also showed that the biomass of C. pengoi increased with increasing temperature from 4 up to 24°C (Bielecka et al., 2014). In shallow Vistula Lagoon of the Baltic Sea, this species appeared in plankton in May–June with warming water up to 15 °C. However, when in August the water temperature increased above 20°C, it disappeared from plankton (Naumenko, 2018). We have a similar result in the Neva Estuary, where the biomass of Cercopagis significant negatively correlated with water temperature (Table 5). This is consistent with previous investigations in the open part of the Gulf of Finland and in the Gulf of Riga, where also the density of C. pengoi negatively correlated with water temperature (Ojaveer et al., 2004; Litvinchuk and Telesh, 2006). According to Sopanen (2008) temperature influence on hatching of Cercopagis resting eggs. The best hatching success was observed at bottom water temperatures between 5 and 13°C. The hatching success was the lowest at 3°C and no hatching was detected at 18°C. This may be another reason for the absence of this species in the shallow parts of the Neva Estuary including Neva Bay, where the water temperature near the bottom in summer is usually above 18°C (Golubkov and Golubkov, 2020).
In the Neva Estuary, negative correlation of C. pengoi biomass with water temperature can also be caused by the indirect influence of this factor. With an increase in water temperature, the depth of the He decreased, but with a decrease in the water temperature due to rainy and windy weather, the stratification of waters also decreased, and He became deeper owing to active mixing of the upper water layer. Under the conditions of a deeper He the biomass of C. pengoi in the Neva Estuary was higher (Tables 4, 5), but the mechanism of this relationship requires additional investigation.
Another reason why the biomass of C. pengoi higher in the deep seaward parts of the Neva Estuary is that the water is more transparent there. We obtained a reliable positive relationship between the biomass of this crustacean and the water transparency and negative relationship with Chl concentration (Table 4). This may be explained by the fact that this predator sees worse in turbid eutrophic waters than, for example, the other planktonic predators Bythotrephes spp., because its compound eye is smaller (Armenio et al., 2017). This structure of the eyes prevents him from hunting in turbid waters. Experimental studies have shown that under all equal conditions, the level of predation of C. pengoi was higher in light flasks compared to dark ones (Pichlová-Ptáčniková and Vanderploeg, 2009). This explanation is also supported by the fact that its biomass in the Neva Estuary is mainly concentrated in the upper 10-m water layer (Krylov et al., 1999), where there is enough light to see its preys. Perhaps, in the Baltic Sea, C. pengoi requires higher water transparency and water column depth (to avoid predators) that can only be found at higher salinities (>0.5 PSU) compared to, for example, the Laurentian Great Lakes.
The Biomass of Cercopagis pengoi and Abundance of Its Preys
An important factor determining the density of a predator is the biomass of its prey. There are some papers discussing which species the C. pengoi feeds on (Benoît et al., 2002; Telesh and Ojaveer, 2002; Laxson et al., 2003; Lehtiniemi and Gorokhova, 2008; Pichlová-Ptáčniková and Vanderploeg, 2009). For example, it was shown that the appearance of C. pengoi in the zooplankton might be the cause of the reduction in the population of E. affinis in the Gulf of Finland, because laboratory studies have shown that C. pengoi actively consumes E. affinis (Lehtiniemi and Gorokhova, 2008). But when the biomass of C. pengoi increases significantly, it switches to other zooplankton species. For example, it has been experimentally shown that C. pengoi can feed on veligers of Dreissena, unlike others zooplankton species (Pichlová-Ptáčniková and Vanderploeg, 2009). However, although C. pengoi may consume Dreissena veligers, it is unlikely that it can seriously affect the biomass of Dreissena, since veligers are present in the plankton from late June up to late October (Orlova et al., 2006), while C. pengoi gives a fairly short development outbreak (Pichlová-Ptáčniková and Vanderploeg, 2009).
It was shown that C. pengoi may feed nauplii and rotifers (Laxson et al., 2003; Lehtiniemi and Lindén, 2006; Makarewicz and Lewis, 2015). We found a positive relationship between C. pengoi and brackish-water rotifers K. cochlearis baltica and K. quadrata platei (Table 4). It is difficult to say whether they are its food objects, because these rotifers are covered with a lorica, or these species prefer brackish part the estuary with similar environmental conditions (Table 4). Positive relationship between C. pengoi and freshwater K. cochlearis was found in Estonian part of the Gulf of Finland (Põllumäe and Kotta, 2007). The authors noted that this could be the result of feeding C. pengoi with a different species that eats K. cochlearis. However, although in the Neva Estuary, C. pengoi and freshwater K. cochlearis was found at the same stations, but their biomasses were higher in different environmental conditions. Hence, the reason was that these species prefer different parts of the estuary with different environmental conditions, rather than food interactions between them. In the freshwater part of the estuary, K. cochlearis prevailed, and as salinity increased, the proportion of brackish-water subspecies K. cochlearis baltica increased together with the growth of the Cercopagis biomass. In this case, apparently, the main role played by environmental factors and competition between two closely related species without the participation of C. pengoi.
In the Neva Estuary, C. pengoi was also significantly positively correlated with rotifers Synchaeta sp. We also found significant positive correlation between biomasses of C. pengoi and D. cucullata (Table 4). Different species of non-predatory Cladocera are also among the common preys of C. pengoi (e.g., Benoît et al., 2002; Laxson et al., 2003; Põllumäe and Kotta, 2007; Pichlová-Ptáčniková and Vanderploeg, 2009).
Many authors note that the predatory C. pengoi can efficiently consume small and medium-sized zooplankton and affect the abundance of its prey, Calanoida copepodites, small and medium-sized cladocerans, and non-loricated rotifers (e.g., Benoît et al., 2002; Telesh and Ojaveer, 2002; Põllumäe and Kotta, 2007; Lehtiniemi and Gorokhova, 2008; Pichlová-Ptáčniková and Vanderploeg, 2009; Makarewicz and Lewis, 2015). However, in the Neva Estuary, we did not obtain significant correlations between biomasses of these species and the biomass of Cercopagis. The reason, apparently, was the high flow of water in the estuary. As a result, the middle and lower parts of the estuary, where the biomass of Cercopagis was high, receive additional numbers of its prey from the upper part of the estuary, where this species was absent. Consequently, the peculiarities of the estuary ecosystem, which consisted of a high level of immigration processes, may be the reason for the absence of significant correlations between the biomass of C. pengoi and the biomass of its prey.
We have obtained statistically significant positive relationship between C. pengoi and predatory E. anonyx and E. nordmanni (Table 4 and Figure 5); moreover, these species prefer similar environmental conditions in the Neva Estuary (Table 4). While E. nordmanni is a native species in the Baltic Sea, E. anonyx is an invader from the Ponto-Caspian region, which was firstly found in the Neva Estuary in the early 2000s (Litvinchuk, 2005). Similar strong positive relationship was found between these species in the western part of the Gulf of Finland (Põllumäe and Kotta, 2007). Perhaps the same environmental conditions were favorable for all these species.
Effects of Zooplankton Predators on the Biomass of Cercopagis pengoi
It was noted that C. pengoi is antagonist with Bythotrephes spp. in their native habitats and, depending on the environmental conditions, either one or the other take advantage (Mordukhai-Boltovskoi and Rivier, 1971). A statistical analysis of our data showed that the biomass of Bythotrephes spp. in contrast to C. pengoi was higher in those parts of the estuary, where the waters were less saline (Table 5). Moreover, B. brevimanus and B. cederstroemii, as well as their hybrids (Litvinchuk and Litvinchuk, 2016), are common zooplankton predators in the freshwater part of the Neva Estuary, Neva Bay, where Cercopagis was never found. Similar antagonistic relationships of these predators in the Neva Estuary were shown earlier, as in the summer of 2004–2005, the biomass of C. pengoi was higher at the stations, where B. brevimanus and B. cederstroemii were few (Orlova et al., 2006). In addition, studies in Lake Michigan have shown that similar in size B. longimanus can feed on C. pengoi, the biomass of the latter species fell with an increase in the biomass of the first one (Witt and Cáceres, 2004; Cavaletto et al., 2010). Moreover, laboratory feeding experiments showed that larger B. longimanus consumed smaller C. pengoi as good as Daphnia (Ptáčníková et al., 2015). Therefore, B. brevimanus and B. cederstroemii may prey on C. pengoi, and we can suggest that press of the predatory Bythotrephes spp. may be an additional reason for the low abundance of Cercopagis in the slightly salted parts of the estuary. However, taking into account the insignificance of direct correlations between the biomasses of these species we may supposed that their antagonism is more due to the different environmental conditions they prefer than to the direct interactions of these species.
Krylov et al. (1999) indicated that the long-term presence of C. pengoi in the zooplankton community in the Neva Estuary could be caused by the disappearance of local predators Bythotrephes spp. and L. kindtii in the fall, which can compete with the Cercopagis for food. In our work, we did not get a statistically significant relationship between the biomass of L. kindtii and the biomass of C. pengoi.
Conclusion
This research generally supports the hypothesis that if environmental variables are appropriate for an exotic species, then that species is likely to successfully invade, regardless of the native species already present. The analysis of our data showed that in the Neva Estuary the biomass of Cercopagis pengoi was higher in waters with higher salinity, deeper depth of upper warm water layer, low temperature, high transparency, and lower proportion of chlorophyll in suspended particulate organic matter. There were also some positive correlations between the biomass of C. pengoi and the biomass of its potential preys. However, we did not obtain significant negative correlations between the biomass of Cercopagis and the biomasses of its preys that can be explained by the high level of zooplankton immigration from the upper parts of the estuary, where the biomass Cercopagis was low. We did not also find direct significant negative correlation between C. pengoi and larger zooplankton predators Leptodora kindtii and Bythotrephes spp. The antagonism between Cercopagis and Bythotrephes is probably related to the different environmental conditions these species prefer than to the direct interactions of these species. Nevertheless, to clarify this issue, further experimental studies on the feeding of these species are needed.
Data Availability Statement
The raw data supporting the conclusions of this article will be made available by the authors, without undue reservation.
Author Contributions
All authors drafted, contributed to, and approved the manuscript.
Funding
The study was supported by the Ministry of Education and Science of the Russian Federation (project AAAA-A19-119020690091-0) and the Federal Zoological Collection No. 96-03-16 (project AAAA-A17-117080110040-3).
Conflict of Interest
The authors declare that the research was conducted in the absence of any commercial or financial relationships that could be construed as a potential conflict of interest.
Acknowledgments
The authors thank the three reviewers for their constructive comments that significantly improved the early version of the manuscript.
Supplementary Material
The Supplementary Material for this article can be found online at: https://www.frontiersin.org/articles/10.3389/fmars.2020.573289/full#supplementary-material
References
Antsulevich, A., and Välipakka, P. (2000). Cercopagis pengoi – new important food object of the Baltic herring in the Gulf of Finland. Int. Rev. Hydrobiol. 85, 609–619. doi: 10.1002/1522-2632(200011)85:5/6<609::aid-iroh609>3.0.co;2-s
Armenio, P. M., Bunnell, D. B., Adams, J. V., Watson, N. M., and Woelmer, W. (2017). It’s like night and day: Diel net-effects on Cercopagidae densities in the Laurentian Great Lakes. J. Great Lakes Res. 43, 394–398. doi: 10.1016/j.jglr.2016.12.001
Bagheri, S., Niermann, U., Mansor, M., and Yeok, F. S. (2014). Biodiversity, distribution and abundance of zooplankton in the Iranian waters of the Caspian Sea off Anzali during 1996–2010. J. Mar. Biol. Assoc. UK. 94, 129–140. doi: 10.1017/S0025315413001288
Benoît, H. P., Johannsson, O. E., Warner, D. M., Sprules, W. G., and Rudstam, L. G. (2002). Assessing the impact of a recent predatory invader: The population dynamics, vertical distribution, and potential prey of Cercopagis pengoi in Lake Ontario. Limnol. Oceanogr. 47, 626–635. doi: 10.4319/lo.2002.47.3.0626
Bielecka, L., Krajewska-Soltys, A., and Mudrak-Cegiolka, S. (2014). Spatial distribution and population characteristics of the invasive cladoceran Cercopagis pengoi in the Polish coastal zone seven years after the first record. Oceanol. Hydrobiol. St. 43, 201–210. doi: 10.2478/s13545-014-0134-y
Bottrell, H. H., Duncan, A., Gliwicz, Z. M., Grygierek, E., Herzig, A., Hillbricht-ilkowska, A., et al. (1976). A review of some problems in zooplankton production studies. Nor. J. Zool. 24, 419–456.
Casties, I., Seebens, H., and Brisk, E. (2016). Importance of geographic origin for invasion success: A case study of the North and Baltic Seas versus the Great Lakes–St. Lawrence River region. Ecol. Evol. 2016, 8318–8329. doi: 10.1002/ece3.2528
Cavaletto, J. F., Vanderploeg, H. A., Pichlová-Ptáčníková, R., Pothoven, S. A., Liebig, J. R., and Fahnenstiel, G. L. (2010). Temporal and spatial separation allow coexistence of predatory cladocerans: Leptodora kindtii, Bythotrephes longimanus and Cercopagis pengoi, in southeastern Lake Michigan. J. Great Lakes Res. 36, 65–73. doi: 10.1016/j.jglr.2010.04.006
Cohen, A. N., and Carlton, J. T. (1998). Accelerating invasion rate in a highly invaded estuary. Science 279, 555–558. doi: 10.1126/science.279.5350.555
Cristescu, M. E. A., Hebert, P. D. N., Witt, J. D. S., MacIsaac, H. J., and Grigorovich, I. A. (2001). An invasion history for Cercopagis pengoi based on mitochondrial gene sequencing. Limnol. Oceanogr. 46, 224–229. doi: 10.4319/lo.2001.46.2.0224
David, P., Thébault, E., Anneville, O., Duyck, P.-F., Chapuis, E., and Loeuille, N. (2017). Chapter One - Impacts of Invasive Species on Food Webs: A Review of Empirical Data. Adv. Ecol. Res. 56, 1–60. doi: 10.1016/bs.aecr.2016.10.001
Dirzo, R., Young, H. S., Galetti, M., Ceballo, G., Isaac, N. J. B., and Collen, B. (2014). Defaunation in the Anthropocene. Science 345, 401–406. doi: 10.1126/science.1251817
Dumont, H. J., Van de Velde, I., and Dumont, S. (1975). The dry weight estimate of biomass in a selection of Cladocera, Copepoda and Rotifera from the plankton, periphyton and benthos of continental waters. Oecologia 19, 75–97. doi: 10.1007/BF00377592
Galil, B. S., Marchini, A., Occhipinti-Ambrogi, A., Minchin, D., Narščius, A., Ojaveer, H., et al. (2014). International arrivals: widespread bioinvasions in European Seas. Ethol. Ecol Evol. 26, 152–171. doi: 10.1080/03949370.2014.897651
Golubkov, M. S. (2009). Phytoplankton primary production in the Neva Estuary at the turn of the 21st century. Inland Wat. Biol. 2, 312–318. doi: 10.1134/S199508290904004X
Golubkov, M. S., Nikulina, V. N., and Golubkov, S. M. (2020). Species-level associations of phytoplankton with environmental variability in the Neva Estuary (Baltic Sea). Oceanologia (in press). doi: 10.1016/j.oceano.2020.11.002
Golubkov, M., and Golubkov, S. (2020). Eutrophication in the Neva Estuary (Baltic Sea): response to temperature and precipitation patterns. Mar. Freshw. Res. 71, 583–595. doi: 10.1071/MF18422
Golubkov, S. M. (2009). Changes of biological communities in the eastern Gulf of Finland during the last century. Proc. Zool. Inst. RAS 313, 406–418.
Golubkov, S. M., and Litvinchuk, L. F. (2015). The role of the alien species Cercopagis pengoi in zooplankton of the Eastern Gulf of Finland of the Baltic Sea. Doklady Biol. Sci. 462, 121–123. doi: 10.1134/S0012496615030011
Golubkov, S. M., Golubkov, M. S., and Tiunov, A. V. (2019). Anthropogenic carbon as a basal resource in the benthic food webs in the Neva Estuary (Baltic Sea). Mar. Pollut. Bull. 146, 190–200. doi: 10.1016/j.marpolbul.2019.06.037
Golubkov, S., Golubkov, M., Tiunov, A., and Nikulina, L. (2017). Long-term changes in primary production and mineralization of organic matter in the Neva Estuary (Baltic Sea). J. Mar. Syst. 171, 73–80. doi: 10.1016/j.jmarsys.2016.12.009
Gorokhova, E., Hansson, S., Höglander, H., and Andersen, C. M. (2005). Stable isotopes show food web changes after invasion by the predatory cladoceran Cercopagis pengoi in a Baltic Sea bay. Oecologia 143, 251–259. doi: 10.1007/s00442-004-1791-0
Grasshoff, K., Ehrhardt, M., and Kremling, K. (1999). Methods of Seawater Analysis, 3rd, completely rev. and extended edn. New York: Wiley-VCH.
Hall, R. O. Jr., Thomas, S., and Gaiser, E. E. (2007). “Measuring freshwater primary production and respiration,” in Principles and Standards for Measuring Primary Production, eds T. J. Fahey and A. K. Knapp (Oxford: Oxford University Press), 175–203. doi: 10.1093/acprof:oso/9780195168662.003.0010
Helenius, L. K., Leskinen, E., Lehtonen, H., and Nurminen, L. (2017). Spatial patterns of littoral zooplankton assemblages along a salinity gradient in a brackish sea: A functional diversity perspective. Est. Coast Shelf Sci. 198, 400–412. doi: 10.1016/j.ecss.2016.08.031
Holopainen, R., Lehtiniemi, M., Meier, H. E. M., Albertsson, J., Gorokhova, E., Kotta, J., et al. (2016). Impacts of changing climate on the non-indigenous invertebrates in the northern Baltic Sea by end of the twenty-first century. Biol. Invas. 18, 3015–3032. doi: 10.1007/s10530-016-1197-z
Jażdżewski, K., and Grabowski, M. (2011). ““Alien Crustaceans Along the Southern and Western Baltic Sea,”,” in the Wrong Place – Alien Marine Crustaceans: Distribution, Biology and Impacts, eds B. S. Galil, P. F. Clark, and J. T. Carlton (New York: Springer), 323–344. doi: 10.1007/978-94-007-0591-3
Jones, M. C., and Cheung, W. W. L. (2014). Multi-model ensemble projections of climate change effects on global marine biodiversity. ICES J. Mar. Sci. 72, 741–752. doi: 10.1093/icesjms/fsu172
Katajisto, T., Karjala, L., and Lehtiniemi, M. (2013). Fifteen years after invasion: egg bank of the predatory cladoceran Cercopagis pengoi in the Baltic Sea. Mar. Ecol. Prog. Ser. 482, 81–92. doi: 10.3354/meps10266
Kottek, M., Grieser, J., Beck, C., Rudolf, B., and Rubel, F. (2006). World Map of the Köppen-Geiger climate classification updated. Meteorol. Z. 15, 259–263. doi: 10.1127/0941-2948/2006/0130
Krylov, P. I., Bychenkov, D. E., Panov, V. E., Rodionova, N. V., and Telesh, I. V. (1999). Distribution and seasonal dynamics of the Ponto-Caspian invader Cercopagis pengoi (Crustacea, Cladocera) in the Neva Estuary (Gulf of Finland). Hydrobiologia 393, 227–232. doi: 10.1023/A:1003558919696
Kumschick, S., Bacher, S., Dawson, W., Heikkilä, J., Sendek, A., Pluess, T., et al. (2012). A conceptual framework for prioritization of invasive alien species for management according to their impact. NeoBiota 15, 69–100. doi: 10.3897/neobiota.15.3323
Kumschick, S., Gaertner, M., Vilč, M., Essl, F., Jeschke, J. M., Pyšek, P., et al. (2015). Ecological impacts of alien species: quantification, scope, caveats, and recommendations. BioScience 65, 55–63. doi: 10.1093/biosci/biu193
Lahdes, E. O., and Karjala, L. A. (2007). Implications of water ionic composition for invasion of euryhaline species in inland waters – an experimental study with Cercopagis pengoi from the Northern Baltic Sea. Aquat Invas. 2, 422–430. doi: 10.3391/ai.2007.2.4.12
Laxson, C. L., McPhedran, K. N., Makarewicz, J. C., Telesh, I. V., and MacIsaac, H. J. (2003). Effects of the non-indigenous cladoceran Cercopagis pengoi on the lower food web of Lake Ontario. Freshwater Biol. 48, 2094–2106. doi: 10.1046/j.1365-2427.2003.01154.x
Lehtiniemi, M., and Gorokhova, E. (2008). Predation of the introduced cladoceran Cercopagis pengoi on the native copepod Eurytemora affinis in the northern Baltic Sea. Mar. Ecol. Prog. Ser. 362, 193–200. doi: 10.3354/meps07441
Lehtiniemi, M., and Lindén, E. (2006). Cercopagis pengoi and Mysis spp. alter their feeding rate and prey selection under predation risk of herring (Clupea harengus membras). Mar. Biol. 149, 845–854. doi: 10.1007/s00227-006-0243-2
Litvinchuk, L. F. (2005). Evadne anonyx Sars, 1897 (Cladocera, Polyphemoidea, Podonidae) – a new representative of the Baltic Sea fauna. Inland Wat. Biol. 1, 240–249.
Litvinchuk, L. F., and Litvinchuk, S. N. (2016). Morphological diversity and widespread hybridization in the genus Bythotrephes Leydig, 1860 (Branchiopoda, Onychopoda, Cercopagidae). Arch. Biol. Sci. 68, 67–79. doi: 10.2298/ABS150505010L
Litvinchuk, L. F., and Telesh, I. V. (2006). Distribution, population structure and ecosystem effects of the invader Cercopagis pengoi (Polyphemoidea, Cladocera) in the Gulf of Finland and the open Baltic Sea. Oceanologia 48, 243–257.
MacIsaac, H. J., Grigorovich, I. A., Hoyle, J. A., Yan, N. D., and Panov, V. E. (1999). Invasion of Lake Ontario by the Ponto-Caspian cladoceran predator Cercopagis pengoi. Can. J. Fish Aquat Sci. 56, 1–5. doi: 10.1139/f98-210
Makarewicz, J. C., and Lewis, W. (2015). Long-term changes in Lake Ontario rotifer abundance and composition: A response to Cercopagis predation? J. Great Lakes Res. 41, 192–199. doi: 10.1016/j.jglr.2014.11.018
Marchetti, M. P., Moyle, P. B., and Levine, R. (2004). Alien fishes in California watersheds: characteristics of successful and failed invaders. Ecol. Appl. 14, 587–596. doi: 10.1890/02-5301
Mordukhai-Boltovskoi, D., and Rivier, I. K. (1971). A brief survey of the ecology and biology of the Caspian Polyphemoidea. Mar. Biol. 8, 160–169. doi: 10.1007/BF00350931
Moritz, C., and Agudo, R. (2013). The future of species under climate change: Resilience or decline? Science 341, 504–508. doi: 10.1126/science.1237190
Moyle, P. B., and Light, T. (1996). Fish invasions in California: do abiotic factors determine success? Ecology 77, 1666–1670. doi: 10.2307/2265770
Naumenko, E. N. (2018). Seasonal and long-term dynamics of population abundance of the invasive species Cercopagis pengoi (Ostroumov, 1891) in the Vistula (Kaliningrad) lagoon of the Baltic Sea. Rus. J. Biol. Invas. 1, 68–77.
Ojaveer, H., Galil, B. S., Campbell, M. L., Carlton, J. T., Canning-Clode, J., and Cook, E. J. (2015). Classification of Non-Indigenous Species Based on Their Impacts: Considerations for Application in Marine Management. PLoS Biol. 13:e1002130. doi: 10.1371/journal.pbio.1002130
Ojaveer, H., Simm, M., and Lankov, A. (2004). Population dynamics and ecological impact of the non-indigenous Cercopagis pengoi in the Gulf of Riga (Baltic Sea). Hydrobiologia 522, 261–269. doi: 10.1023/B:HYDR.0000029927.91756.41
Oksanen, J., Blanchet, F. G., Friendly, M., Kindt, R., Legendre, P., McGlinn, D., et al. (2020). Vegan: Community Ecology Package (Version 2.5-5). Available online at: https://cran.r-project.org/web/packages/vegan/index.html [Accessed on June 16, 2020].
Orlova, M. I., Telesh, I. V., Berezina, N. A., Antsulevich, A. E., Maximov, A. A., and Litvinchuk, L. F. (2006). Effects of nonindigenous species on diversity and community functioning in the eastern Gulf of Finland (Baltic Sea). Helgol. Mar. Res. 60, 98–105. doi: 10.1007/s10152-006-0026-7
Pichlová-Ptáčniková, R., and Vanderploeg, H. A. (2009). The invasive cladoceran Cercopagis pengoi is a generalist predator capable of feeding on a variety of prey species of different sizes and escape abilities. Fund. Appl. Limnol. 173, 267–279. doi: 10.1127/1863-9135/2009/0173-0267
Põllumäe, A., and Kotta, J. (2007). Factors describing the distribution of the zooplankton community in the Gulf of Finland in the context of interactions between native and introduced predatory cladocerans. Oceanologia 49, 277–290.
Ptáčníková, R., Vanderploeg, H. A., and Cavaletto, J. F. (2015). Big versus small: Does Bythotrephes longimanus predation regulate spatial distribution of another invasive predatory cladoceran, Cercopagis pengoi? J. Great Lakes Res. 41, 143–149. doi: 10.1016/j.jglr.2015.10.006
R Development Core Team (2020). The R Project for Statistical Computing (Version 3.5.3). Available online at: https://www.r-project.org [Accessed on June 16, 2020]
Rowe, O. F., Guleikova, L., Brugel, S., Byström, P., and Andersson, A. (2016). A potential barrier to the spread of the invasive cladoceran Cercopagis pengoi (Ostroumov 1891) in the Northern Baltic Sea. Reg. Stud. Mar. Sci. 3, 8–17. doi: 10.1016/j.rsma.2015.12.004
Ruttner-Kolisko, A. (1977). Suggestions for biomass calculation of planktonic rotifers. Arch. Hydrobiol. Beih. Ergebn. Limnol. 8, 71–76.
Sopanen, S. (2008). The effect of temperature on the development and hatching of resting eggs of non-indigenous predatory cladoceran Cercopagis pengoi in the Gulf of Finland, Baltic Sea. Mar. Biol. 154, 99–108. doi: 10.1007/s00227-008-0903-5
Telesh, I. V., and Ojaveer, H. (2002). “The predatory water flea Cercopagis pengoi in the Baltic Sea: invasion history, distribution and implications to ecosystem dynamics,” in Invasive aquatic species of Europe. Distribution, impacts and management, eds E. Leppäkoski, S. Gollasch, and S. Olenin (Dordrecht: Springer), 62–66. doi: 10.1007/978-94-015-9956-6
Telesh, I. V., Golubkov, S. M., and Alimov, A. F. (2008). “The Neva Estuary ecosystem,” in Ecology of Baltic Coastal Waters, ed. U. Schiewer (Berlin: Springer-Verlag), 259–284. doi: 10.1007/978-3-540-73524-3
Vanderploeg, H. A., Pothoven, S. A., Krueger, D., Mason, D. M., Liebig, J. R., Cavaletto, J. F., et al. (2015). Spatial and predatory interactions of visually preying nonindigenous zooplankton and fish in Lake Michigan during midsummer. J. Great Lakes Res. 41, 125–142. doi: 10.1016/j.jglr.2015.10.005
Vernet, M., and Smith, R. C. (2007). “Measuring and modeling primary production in marine pelagic ecosystems,” in Principles and Standards for Measuring Primary Production, eds T. J. Fahey and A. K. Knapp (Oxford: Oxford University Press), 142–174. doi: 10.1093/acprof:oso/9780195168662.003.0009
Vuorinen, I., Hänninen, J., Rajasilta, M., Laine, P., Eklund, J., Montesino-Pouzols, F., et al. (2015). Scenario simulations of future salinity and ecological consequences in the Baltic Sea and adjacent North Sea areas–implications for environmental monitoring. Ecol. Indic. 50, 196–205. doi: 10.1016/j.ecolind.2014.10.019
Winberg, G. G., and Lavrentyeva, G. M. (1982). Methodological recommendations for the collection and treatment of data in hydrobiological investigations in fresh water bodies: zooplankton and its production. Leningrad: Nauka.
Witt, A. M., and Cáceres, C. E. (2004). Potential predator–prey relationships between Bythotrephes longimanus and Cercopagis pengoi in Southwestern Lake Michigan. J. Great Lakes Res. 30, 519–527. doi: 10.1016/S0380-1330(04)70367-6
WoRMS Editorial Board (2020). World Register of Marine Species. Available online at: http://www.marinespecies.org. [Accessed on June 16, 2020].
Keywords: zooplankton, biotic interactions, Gulf of Finland, Baltic Sea, invasive species, Cercopagis pengoi, NIS
Citation: Golubkov MS, Litvinchuk LF and Golubkov SM (2020) Effects of Environmental Gradients on the Biomass of Alien Cercopagis pengoi in the Neva Estuary. Front. Mar. Sci. 7:573289. doi: 10.3389/fmars.2020.573289
Received: 18 June 2020; Accepted: 25 November 2020;
Published: 15 December 2020.
Edited by:
Alberto Basset, University of Salento, ItalyReviewed by:
Sergej Olenin, Klaipëda University, LithuaniaMatthias Obst, University of Gothenburg, Sweden
Ora Johannsson, University of British Columbia, Canada
Copyright © 2020 Golubkov, Litvinchuk and Golubkov. This is an open-access article distributed under the terms of the Creative Commons Attribution License (CC BY). The use, distribution or reproduction in other forums is permitted, provided the original author(s) and the copyright owner(s) are credited and that the original publication in this journal is cited, in accordance with accepted academic practice. No use, distribution or reproduction is permitted which does not comply with these terms.
*Correspondence: Mikhail S. Golubkov, golubkov_ms@mail.ru