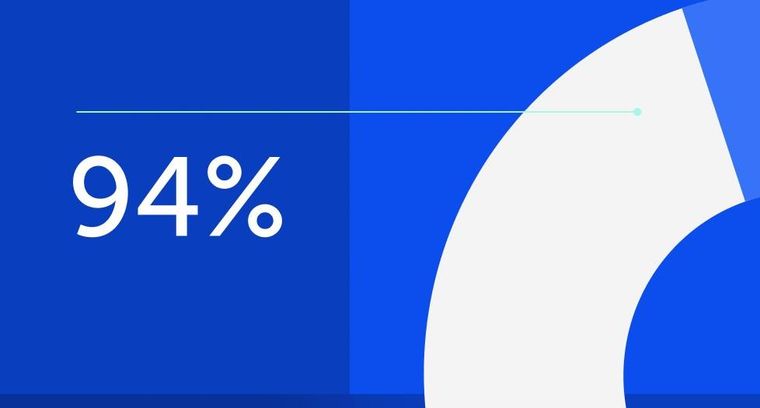
94% of researchers rate our articles as excellent or good
Learn more about the work of our research integrity team to safeguard the quality of each article we publish.
Find out more
REVIEW article
Front. Mar. Sci., 03 September 2020
Sec. Marine Fisheries, Aquaculture and Living Resources
Volume 7 - 2020 | https://doi.org/10.3389/fmars.2020.569258
This article is part of the Research TopicManaging for the Future: Understanding the Relative Roles of Climate and Fishing on Structure and Dynamics of Marine EcosystemsView all 22 articles
Harp seals are the most abundant marine mammal in the north Atlantic. As an ice obligatory predator, they reflect changes in their environment, particularly during a period of climatic change. As the focus of a commercial hunt, a large historic data set exists that can be used to quantify changes. There are three populations of harp seals: White Sea/Barents Sea, Greenland Sea and Northwest Atlantic. The objective of this paper is to review their current status and to identify the factors that are influencing population dynamics in different areas. Although important historically, recent catches have been low and do not appear to be influencing trends in either of the two northeast Atlantic populations. Massive mortalities of White Sea/Barents Sea seals occurred during the mid 1980s due to collapses in their main prey species. Between 2004 and 2006, pup production in this population declined by 2/3 and has remained low. Body condition declined during the same period, suggesting that ecosystem changes may have resulted in reduced reproductive rates, possibly due to reduced prey availability and/or competition with Atlantic cod. The most recent estimate of pup production in the Greenland Sea also suggests a possible decline during a period of reduced hunting although the trend in this population is unclear. Pupping concentrations are closer to the Greenland coast due to the reduction in ice in the traditional area and increased drift may result in young being displaced from their traditional feeding grounds leading to increased mortality. Reduced ice extent and thickness has resulted in major mortality of young in the Northwest Atlantic population in some years. After a period of increase, the population remained relatively stable between 1996 and 2013 due to increased hunting, multiple years with increased ice-related mortality of young seals, and lower reproductive rates. With a reduction in harvest and improved survival of young, the population appears to be increasing although extremely large interannual variations in body condition and fecundity have been observed which were found to be influenced by variations in capelin biomass and ice conditions. Each of these populations has been impacted differently by changes in their ecosystems and hunting practices. By identifying the factors influencing these three populations, we can gain a better understanding of how species may respond to changes that are occurring in their ecosystems.
Under marine ecosystem-based management (EBM), understanding how species are adapted to their environment and how they response to environmental change is critical for the successful management of species and their ecosystems. However, such knowledge is extremely difficult to obtain in natural systems. One way to improve our understanding of the factors that influence the distribution, habitat use and population dynamics of a species is to compare how populations that occur in similar ecosystems may be impacted by different changes (e.g., Murawski et al., 2010; Drinkwater and Pepin, 2013; Drinkwater et al., 2013; Moore et al., 2019). This technique has been successfully applied in many areas (see Drinkwater and Pepin, 2013 for references) and can provide insights into the fundamental processes impacting species.
The northern Atlantic is undergoing a period of tremendous change with adjoining Arctic areas expected to show the most rapid change with modifications in temperature, ocean circulation, pH balance, ice cover, and sea level (McCarthy et al., 2001; Walsh, 2008; Intergovernmental Panel on Climate Change [IPCC], 2014; Haug et al., 2017). The potential impact of climate change on Arctic marine mammals, both directly through ice habitat loss or indirectly through changes in foraging ecology, has been reviewed by a number of authors (e.g., Kovacs and Lydersen, 2008; Laidre et al., 2008, 2015; Kovacs et al., 2011; Hoover et al., 2013; Stenson and Hammill, 2014). The expectation that a warmer (more ice-free) ocean will lead to higher primary productivity could result in higher concentrations of zooplankton, to the benefit of some marine mammals (e.g. bowhead whales, Balaena mysticetus), but the loss of prey species that depend on ice, especially polar cod (Boreogadus saida) and amphipods, could have negative impacts on other marine mammals that feed extensively upon them, such as harp (Pagophilus groenlandicus) and ringed (Pusa hispida) seals (Eamer et al., 2013). These changes are likely to be most severe on the ice dependent marine mammals and particularly those that inhabit the southern ice edge of the Arctic pack ice which is changing rapidly (Walsh, 2008).
Given the extensive changes that have occurred, comparing ecosystems across the north Atlantic can provide important insights into the factors impacting ecosystem components. The northwest Atlantic (NWA) and northeast Atlantic (NEA) have a number of features in common. Both are transition zones between temperate and Arctic ecosystems which is reflected in both the physical and biological components. They have similar composition of species and face similar challenges including a variety of anthropomorphic activities (e.g., fishing, oil and gas exploration, contaminants, shipping) and the impact of climate change (e.g., Stenson and Hammill, 2014; CAFF, 2017). Both regions are characterized by cold water from the Arctic in the north, and warmer water from the south, experience seasonal ice cover, the most common zooplankton is Calanus finmarchicus and both generally have wasp-waist ecosystems with capelin (Mallotus villosus) as the dominant forage fish (Astthorsson et al., 2007; Drinkwater and Pepin, 2013; Buren et al., 2014). Both areas have also had major fisheries for Atlantic cod (Gadus morhua) but while the cod stock in the Barents Sea has increased significantly over the past decade, the major stocks in the NWA have collapsed and remain low (Bogstad et al., 2015; Pedersen et al., 2017, 2020). The objective of this paper is to review the information available on an abundant, wide-ranging species, the harp seal (Pagophilus groenlandicus), to improve our understanding of how anthropogenic and environmental factors can influence the population dynamics of this high trophic level predator in differing ecosystems across the north Atlantic.
Harp seals (Pagophilus groenlandicus) are the most abundant pinniped in the North Atlantic. They are a pelagic, migratory species that occurs from the coast of Canada in the west to the Barents Sea in the east and northward to Arctic waters (Figure 1). Three putative populations of harp seals, based upon their whelping (pupping) locations, have been identified. The NWA population whelps on the pack ice off Newfoundland (referred to as ‘The Front’) and in the Gulf of St. Lawrence (‘Gulf’); the Greenland Sea (‘West Ice’) population whelps off the east coast of Greenland, and the White Sea/Barents Sea (‘East Ice’) population whelps in the White Sea (Sergeant, 1991). Relationships among the three North Atlantic populations of harp seals have been examined in studies of cranial measurements (Yablokov and Sergeant, 1963), underwater vocalizations (Perry and Terhune, 1999), serum transferrins (Møller et al., 1966; Nñvdal, 1969, 1971), blood serum proteins (Borisov, 1966), allozymes (Meisfjord and Nñvdal, 1994) and DNA (Meisfjord and Sundt, 1996; Perry et al., 2000). These studies have revealed significant differences between the NEA and NWA populations, but no evidence of differences between Greenland Sea and White Sea/Barents Sea harp seals. However, a recent study (Carr et al., 2015) suggests that harp seals comprise three genetically distinguishable breeding populations in the White Sea, Greenland Sea, and Northwest Atlantic. Satellite tracking studies (Folkow et al., 2004; Nordøy et al., 2008) and tag returns (Øien and Øritsland, 1995) indicate that individuals from the Greenland Sea and White Sea/Barents Sea populations mix during the non-breeding period. Similarly, non-breeding seals from the Northwest Atlantic and Greenland Sea populations overlap, particularly in southeast Greenland (Øien and Øritsland, 1995; Stenson and Sjare, 1997). This suggests that the populations may occasionally overlap, particularly in the NEA, which would explain the lack of a clear differentiation among the populations. However, for management purposes, the three populations are considered separately (International Council for the Exploration of the Sea [ICES], 2019b).
Figure 1. Location of whelping concentrations (yellow) and range (dark shading) of harp seals in the north Atlantic.
Harp seals whelp on drifting, first-year pack ice from late February through early April, depending upon location (Sergeant, 1991). In the NEA, pupping generally takes place between late February through mid-March in the White Sea (Potelov et al., 2003), and late March through early April in the Greenland Sea (Øigård et al., 2014). The NWA population pups in late February in the southern Gulf of St. Lawrence and a little later (early to mid-March) in the northern Gulf and at the Front (i.e., the same timing as the White Sea) (Stenson et al., 2002, 2003, 2020b). Pups are nursed for approximately 12 days, after which the females wean their pups, mate and disperse to feed. Molting of adults and immatures takes place north of each whelping location approximately 3-4 weeks after weaning with immature seals and adult males molting before adult females (Sergeant, 1991). Suitable ice is necessary for pupping and during lactation, and young of the year (YOY) harp seals require ice to haul out on for a period of 4–6 weeks while they develop the physiological capability for swimming and diving (Sergeant, 1991; Burns et al., 2007; Stenson and Hammill, 2014).
As an obligatory ice-dependent species that relies on pack ice for at least part of the year and an abundant, high trophic level predator, climate change can impact harp seals directly through the loss of ice as a platform and indirectly through changes in prey availability (e.g., Stenson and Hammill, 2014; Stenson et al., 2016). Thus, harp seals are well situated to reflect changes in their environment and act as an indicator species for monitoring north Atlantic and Arctic marine biodiversity (Department of Fisheries and Oceans [DFO], 2012; CAFF, 2017). Also, harp seals have been the focus of commercial hunts across their range which has resulted in an extensive historic data set that can be used to quantify changes that may have occurred. In order to review the current status of harp seals across the north Atlantic and to identify the factors that are influencing their population dynamics in different areas, we start with an overview of the history of harvesting, abundance, environmental conditions and harp seal responses to these conditions as reflected in productivity. We then finish by discussing how anthropogenic and environmental factors appear to be affecting the dynamics of each population within the context of a changing environment. Such a review is timely and will provide us with a better understanding of how this key indicator species is being impacted by ongoing environmental change.
Following pupping and breeding in the northern portion of the White Sea, harp seals from the White Sea/Barents Sea population molt during April and May in the White Sea and south-eastern parts of the Barents Sea (Haug et al., 1994; Nordøy et al., 2008). When the molt is over, seals disperse in small herds to feed, primarily around Svalbard and in the northern Barents Sea. They follow the receding ice edge during summer, gradually moving northwards and north-eastwards in the Barents Sea throughout the summer. In November-December, White Sea/Barents Sa harp seals move southward towards the breeding areas, spending the winter in the southeastern Barents Sea and White Sea.
Historically, the harvests of White Sea/Barents Sea harp seals were initially shore based and presumably small-scale, taking place along the coasts of the White Sea and around the Kanin Peninsula, and presumably of a very small magnitude (Sergeant, 1991). Norwegian vessels began hunting offshore in 1867 (Iversen, 1927), followed by Soviet vessels during the 1920s. Initially, catches quite small, perhaps a few hundred annually (Iversen, 1927), but after 1875 the total catches increased, with annual harvests of 15,000 – 60,000 animals up to around 1900. Following the turn of the century exploitation increased further with the largest catches being taken in the 1920s and 1930s (annual average of 200,000 – 300,000 animals, maximum in 1925 when nearly 470,000 seals were taken) (Iversen, 1927; Sivertsen, 1941; Nakken, 1988; Skaug et al., 2007).
While exploitation was low during World War II, hunting pressure increased from 1946 until 1955 with average catches between 150,000 and 200,000 seals (International Council for the Exploration of the Sea [ICES], 2019b; Figure 2). Quotas of 100,000 were introduced unilaterally for Soviet catches in 1955 (Yakovenko, 1963) and were gradually reduced to a total (Soviet and Norwegian) catch quota of 34,000 seals by 1965. Adult females were protected in the whelping patches starting in 1963, and Soviet catches of 1+ seals were stopped in 1965 (Kjellqwist et al., 1995). Catches increased during the late 1970s and 1980s (annual quotas increased to a maximum of 82,000 in 1983) but since the late 1980s, quotas were reduced, declining to 35,000 by 2009. Following a decline in pup production after 2003 (see below), quotas were further reduced to 7,000 during the 2010-2014 period. Since 2017, the current annual quota has been set at approximately 10,000 animals one year and older (referred to as 1+), assuming that two YOY equals one older seal. However, catches over the past decade have been well below the recommended quotas, and therefore also well below estimated sustainable levels. With the exception of 2018 and 2019, when 2,241 and 602 seals, respectively, were taken, annual catches have been below 30 animals since 2012 (International Council for the Exploration of the Sea [ICES], 2019b).
Figure 2. Reported catches of Northwest Atlantic (green), White Sea/Barents Sea (blue) and Greenland Sea (orange) harp seals, 1950–2019. Catches in the White Sea/Barents Sea from 1946-50 is a 5 year average.
Based upon a population model that incorporates data on annual age-specific reproductive rates, removals and independent estimates of pup production (e.g., Hammill et al., 2015; International Council for the Exploration of the Sea [ICES], 2019b), the high catches before and after World War II resulted in a declining harp seal population until the early 1960s when the population may have been as low as 500,000 (Sergeant, 1991; Skaug et al., 2007; International Council for the Exploration of the Sea [ICES], 2019b). Reductions in catches and protection of females allowed the population to increase until the 1980s (Figure 3; International Council for the Exploration of the Sea [ICES], 2019b). However, the collapse of forage fish (capelin, polar cod, Atlantic herring [Clupea harengus]) in the Barents Sea during the mid 1980s led to the an ‘invasion’ of seals in very poor condition along the Norwegian coast from 1986-1988 (Haug et al., 1991) and the apparent complete loss of a number of cohorts (Kjellqwist et al., 1995). A small, but similar ‘invasion’ of seals occurred again during the period of low capelin abundance in the mid 1990s (Nilssen et al., 1998).
Figure 3. Estimated abundance of Greenland Sea (orange), White Sea/Barents Sea (blue) and Northwest Atlantic (green) harp seals and 95% C.I. Data from International Council for the Exploration of the Sea [ICES], 2019b and Hammill et al., 2020.
Aerial surveys of the White Sea between 1998 and 2013 indicate that this population went through a significant and rapid decline in pup production from ca 330,000 pups from 1998-2003 to ca 130,000 since 2005 (Potelov et al., 2003; International Council for the Exploration of the Sea [ICES], 2019b). Although a variety of explanations were examined, the cause of this decline is unknown, but does not appear to be related to harvest levels or population inertia due to the loss of several cohorts in the mid 1980s. The most likely explanation is a decline in pregnancy rates (International Council for the Exploration of the Sea [ICES], 2016), potentially resulting from worsening body condition due to changes in prey (primarily capelin) abundance and distribution (Øigård et al., 2013). Thus, it appears that the population dynamics of Barents Sea/White Sea harp seals over the past eight decades have been influenced by both environmental change and changing harvest regimes.
The most recent population model indicates that the White Sea/Barents Sea population declined from the 1980s until around 2007 (Figure 3). From 2007 to present the model suggests a slow increase, but this is uncertain due to due a scarcity of data on vital rates and pup production for this population in the last decade. The current estimate for population size in 2019 is 1.5 million (95% CI 1.3 – 1. 7 million) seals (International Council for the Exploration of the Sea [ICES], 2019b).
The Barents Sea is an inflow shelf sea (mean depth ca 200 m) where Atlantic and Arctic waters meet. Warm, nutrient rich Atlantic water from the North Atlantic Current enters the Barents Sea from the Norwegian Sea primarily through its western entrance and influences the southern region, while cold Arctic water penetrates from the east and north and dominates the northern Barents Sea (see Hunt et al., 2013). Some Atlantic water also enters the Arctic Ocean through the Fram Strait and continues eastward north of Svalbard (Haug et al., 2017). Water temperature has increased within the Barents Sea as a result of increased Atlantic inflow, particularly over the past decade (Spielhagen et al., 2011; International Council for the Exploration of the Sea [ICES], 2019a). Historically, the White and Barents Seas (northern and eastern sections) have been characterized by the presence of drifting sea ice. In the Barents Sea, extensive seasonal variations were observed, particularly in the eastern areas, with maximum ice cover in March/April and minimum in August/September (Wassmann et al., 2006; Hunt et al., 2013). Increasing water temperature over recent decades has been associated with dramatic reductions in sea ice mass and coverage (see Haug et al., 2017). Laidre et al. (2015) found that the Barents Sea had the largest decline in the average annual days with sea ice among all of the circumpolar regions they examined, with an estimated three week per decade reduction in the period of ice cover between 1970 and 2013. Overall, the ice extent in the Barents Sea has decreased more than 60% over the last 200 years (Vinje, 2001; Wassmann et al., 2006), although there is high interannual variability.
Harp seals traditionally pup on relatively close pack ice, approximately 6/10 coverage or greater (Sergeant, 1991). To determine how ice cover in the White Sea has changed over the past three decades, we examined the extent of ice during the peak of pupping using data from the Norwegian Ice Service. The first surveys to estimate pup production in the White Sea were carried out in 1998. Therefore, we downloaded archived Norwegian Ice Service ice charts (Norwegian Meteorological Institute, 2020) for 10 March (+/− 1 day), 1998 to 2020, from the Norwegian Meteorological Institute Cryo web portal1 and the extent of the ice classes Open Drift Ice (4-6/10 coverage), Close Drift Ice (7-9/10), and Very Close Drift Ice (9-10/10) in the area of the White Sea (including the main basin, three inner bays, and the Gorlo) were identified (Figure 4). Because of the way in which the data were presented, we calculated the area of greater than 4/10 sea ice, which was rounded to the nearest 1000 km2 to account for digitizing error, using QGIS (QGIS Development Team, 2019). Examining the total ice extent, expressed as an anomaly from the 2000-2010 average, indicates that there is a general decline in the extent of suitable ice in the White Sea between 1998 and 2020, but ice cover was particularly low in 2003, 2015 and 2020 (Figure 5). While no pup production surveys have been carried out in the White Sea since 2013, decreases in ice cover of this magnitude have been found to be associated with increased pup mortality in the NWA (Stenson and Hammill, 2014; Hammill et al., 2015).
Figure 4. Occurrence of drift ice (4+/10 coverage) in the White Sea on 10 March 1998-2020. Data from the Norwegian Meteorological Institute Cryo web portal (https://cryo.met.no/archive/ice-service/icecharts/quicklooks/).
Figure 5. Extent of drift ice, 4/10 and greater, coverage in the White Sea 1998-2020, expressed as anomalies from the average ice coverage 2000-2010. Data obtained from the Norwegian Ice Service (https://cryo.met.no/archive/ice-service/icecharts/quicklooks/).
Due to seasonal variation in food availability, the ability to store large amounts of energy and sustain significant periods of fasting is an essential adaptation for most Arctic mammals. Harp seals exhibit a regular seasonal pattern of lipid deposition in their subcutaneous blubber layer (which also serves other purposes such as insulation and buoyancy, see Iverson et al., 2002) to take advantage of periods of high productivity to store energy required for pupping, breeding and molting. Monitoring these changes in body mass provides a metric by which changes in food availability can be measured. White Sea/Barents Sea harp seals are generally thin in spring and early summer (May – June). They improve their condition over the summer and become quite fat by September – October (Nilssen et al., 1997). The energy stores built up during the summer and autumn are maintained until February, before seals again lose mass as blubber stores are rapidly depleted during the breeding and molting period (March-June). An average adult (165 cm long) harp seal weighs ∼80 kg after in June but and increases its mass by 81.5% to ∼145 kg in October (Nilssen et al., 1997).
The diet of Barents Sea harp seals varies seasonally and geographically, but is mostly comprised of relatively few species, in particular capelin, polar cod, Atlantic herring), krill (Thysanoessa spp.) and the pelagic amphipod P. libellula (Nilssen et al., 2000; Lindstrøm et al., 2013). Polar cod and crustaceans appear to be of particular importance during summer and autumn feeding in the northern parts of the Barents Sea (May-October). As ice cover expands southwards in late autumn and winter, the diet of southward migrating seals appear to switch from mostly crustaceans to mainly fish, particularly capelin and polar cod (Nilssen et al., 1995; Lindstrøm et al., 1998). In the southernmost areas of the Barents Sea, where the seals occur during winter and early spring, herring is also an important prey (Nilssen et al., 1995). Several fish species may serve as prey for harp seals during late autumn and winter. Low capelin biomass, such as that observed in 1993-1996, was associated with a dietary switch to other fish species, in particular polar cod, other gadoids and herring (Bogstad et al., 2000).
The Barents Sea has undergone a number of shifts in the abundance of important fish species resulting from changes in environmental conditions which may also have had an impact on predator-prey relationships as well. In the mid 1980s, there was a simultaneous collapse in capelin, Atlantic herring, and polar cod stocks (Figure 6). More recently, the increase in Atlantic inflow and an overall warming trend has also resulted in a slight increase in primary productivity and a shift in community structure, particularly a reduction in the area of the Barents Sea occupied by Arctic species. Boreal species have been expanding northward with a resultant change in the structural properties and links between the boreal and the Arctic food webs (Fossheim et al., 2015; Kortsch et al., 2015). Decreases in the biomass of large mesozooplankton, particularly Themisto libellula, in Arctic waters have led to a strong decline and recruitment failure of polar cod which is currently at its lowest abundance since 1990 (International Council for the Exploration of the Sea [ICES], 2019a). Following a second collapse in the mid 1990s (1993-1996), capelin stocks recovered somewhat only to collapse again in the middle of the mid 2000s (2003-2006) and then in 2014 (Figure 6). These collapses were all primarily caused by recruitment failures (Gjøsæter et al., 2009). There is an overlap between capelin spawning areas and Norwegian spring spawning herring nursery areas in the southern Barents Sea, and the presence of strong year classes of young herring, which feed on capelin larvae, may have contributed significantly to the capelin collapses (Gjøsæter and Bogstad, 1998). In contrast, after a period of relative low abundance around 2000, the Barents Sea Atlantic cod stock has increased to the highest biomass ever observed by 2014 and extended its range northwards (International Council for the Exploration of the Sea [ICES], 2019a). This increase in cod abundance may have also contributed to the relatively low levels of two major harp seal prey species, capelin and polar cod, during the past decade.
Figure 6. Estimated biomass of forage species and Atlantic cod in the Barents Sea. The timing of seal ‘invasion’ along the Norwegian coast and an abrupt decline in pup production are indicated (modified from Haug et al., 2017; Protozorkevich and van der Meeren, 2020).
The impact of changes in prey availability have been observed among White Seal/Barents Sea harp seals. As outlined above, catches have declined markedly over the last several decades. However, in spite of reduced harvesting, there was a significant drop in pup production in 2004 and 2005. Øigård et al. (2013) found that harp seal body condition was significantly lower in 2011 than it was 10–15 years previous. They identified possible links between seal body condition and the abundance of several spatially overlapping potential competitors and prey including Atlantic cod, polar cod and capelin.
Changes in reproductive rates within a population are also often an indicator of their ecosystem, often reflecting changes in foraging success and body condition (e.g., Stenson et al., 2020a). The mean age at sexual maturity of White Sea/Barents Sea harp seals increased from approximately 5.5 years in the early 1960s, to 8.2 years in the early 1990s (Frie et al., 2003). In the 2000s, the mean age of maturity was estimated to be ∼7 years in 2018 which is not significantly different from a previous estimate from 2006, but still higher than observed among the other two harp seal populations (International Council for the Exploration of the Sea [ICES], 2019b). It is possible that increases in population size (see below), may have contributed to the observed changes in age at maturity. However, Frie et al. (2003) proposed that the observed changes may also be due to changes in the Barents Sea ecosystem, in particular occasional low availability of important forage fish such as capelin and polar cod during winter and early spring.
Greenland Sea harp seals form whelping concentrations along the ice edge north of the Norwegian island of Jan Mayen. Historically the Jan Mayen current creates a gyre that forms and retains a tongue shaped ice feature, known as the Odden, during the spring (Wilkinson and Wadhams, 2005). This persistent ice feature provided a suitable platform for nursing and resting during the post weaning fast. In recent years, the ice edge has shifted westward towards the Greenland coast which has resulted in a shift in the location of pupping (see below). As in the other populations, molting occurs approximately a month later at the fringe of winter ice laying seawards of the heavier Arctic ice off the east Greenland pack, located between the latitudes 69°N and 75°N (Øritsland and Øien, 1995; Haug et al., 2006). Satellite tracking studies suggest that a large proportion of the Greenland Sea stock migrates into the Barents Sea during summer and autumn, then return to spend the winter off south-east Greenland, in the Denmark Strait (Folkow et al., 2004).
The Greenland Sea (West Ice) stock of harp seals has been subject to commercial exploitation for centuries (Iversen, 1927; Nakken, 1988; Sergeant, 1991). Knowledge of the Greenland Sea catches by Dutch, British, German, and Danish ships prior to the 1870s, is poor. Norwegian sealers sailed to the Greenland Sea for the first time in 1846 and have been active ever since. Exploitation levels reached a historical maximum in the 1870s and 1880s when annual catches (pups and adults) varied between 50,000 and 120,000 (Iversen, 1927). It was evident that these catch levels were unsustainable, and regulatory measures (mainly designed to protect adult females) were introduced in 1876 (Iversen, 1927). Annual catches varied between 10,000 and 20,000 animals throughout the first decades of the 20th century, before increasing to around 40,000 seals per year by the 1930s (Iversen, 1927; Sergeant, 1991). Following a pause in sealing during World War II, total annual catches by Norway and the Soviet Union quickly rose to a postwar maximum of about 70,000 in 1948, but then followed a decreasing trend until quotas were imposed in 1971 (Sergeant, 1991; International Council for the Exploration of the Sea [ICES], 2019b). Since 1989, catches have been generally less than 10,000 seals with no Russian takes since 1995 (Figure 2; International Council for the Exploration of the Sea [ICES], 2019b).
The Greenland Sea population was heavily hunted, particularly in the late 19th Century which resulted in a population decline (Iversen, 1927). As the catches declined since the 1950s, the Greenland Sea population was thought to have been increasing slowly (Figure 3; International Council for the Exploration of the Sea [ICES], 2019b). However, due to a series of conflicting estimates from mark-recapture experiments in the 1980s and a ∼40% drop in estimated pup production from 2012 to 2018, it is difficult to determine the overall population trend. The estimate of current (2019) population size is relatively robust to a variety of model assumptions and indicates a total population size of 427,000 (95% CI 313,000 −541,000), making the Greenland Sea population the smallest of the three harp seal populations (International Council for the Exploration of the Sea [ICES], 2019b). Given the low level of catches in this population, this decline in pup production and lack of increase in the population cannot be accounted for by hunting and is most likely a result of ecological changes.
The Greenland Sea and surrounding areas are influenced by a mix of cold and warm currents. The East Greenland Current, which runs along the Greenland coast, and East Icelandic Current which is further offshore, bring colder water from the north. The North Atlantic Current and the Irminger Current bring warmer waters from the south, mixing with the colder Arctic water in the area of the Denmark Strait and north of Iceland (Astthorsson et al., 2007; Rigét et al., 2019). The East Greenland Current carries multiyear Arctic ice from the Fram Strait southward along the Greenland coast which remains ice-bound throughout much of the year. However, increasing inflow of relatively warm Atlantic waters has resulted in reduced ice coverage in recent years. In their study of historical variability of sea ice position in the Nordic Seas, Divine and Dick (2006) concluded that the spring ice edge retreated approximately 250–375 km in the Greenland Sea between the periods 1870-1920 and 1989-2002. The edge of the ice in March/April, which historically extended all the way to the island of Jan Mayen where the Odden sea ice feature regularly formed, is now situated 270–360 km west of the island (Figure 7; Wilkinson and Wadhams, 2005; Øigård et al., 2014).
Figure 7. Location of sea ice edge and the Greenland Sea harp seal whelping concentrations. Historical ice data from Divine and Dick (2006). Recent ice data and whelping locations from Øritsland and Øien (1995); Øigård et al. (2014) and International Council for the Exploration of the Sea [ICES] (2019b).
Since harp seals pup near the ice edge, this change in ice extent has resulted in a shift in the location of their whelping patches closer the Greenland coast (Figure 7). While the ice itself still appears to be suitable for nursing (International Council for the Exploration of the Sea [ICES], 2019b), the seals are now pupping on pack ice that is being carried quickly southward within the East Greenland Current, rather than remaining relatively stationary north of Jan Mayen in the Odden. These new conditions may have a negative impact on first year survival if they result in earlier loss of ice for seals to rest on or if the ecological conditions encountered by YOY during the first few months of independent feeding differ from those encountered in the traditional area of the Odden.
Pupping and breeding closer to the Greenland coast may also increase mortality of young and adults as they would be more vulnerable to predation from species such as polar bears (Ursus maritimus). Recent studies from Greenland suggest that polar bear diets are changing from being dominated by ringed seals (Phoca hispida) to include a larger proportion of hooded and harp seals (McKinney et al., 2013). Harp and hooded seal pups also appear more frequently in the diet of killer whales in East Greenland coastal waters compared to previously (Foote et al., 2013; International Council for the Exploration of the Sea [ICES], 2013).
Recent observations of the diet of harp seals in the Greenland Sea pack ice suggests a relatively narrow range of prey taxa. This is consistent with other studies that have found that food webs in Greenland waters are generally relatively simple with few species (Rigét et al., 2019). The pelagic amphipod P. libellula, polar cod, and capelin dominated the harp seal diet (63–99% of the observed diet, Haug et al., 2004; Enoksen et al., 2017). Greenland Sea and Barents Sea harp seals overlap in their feeding range during summer and autumn in Spitsbergen waters (Haug et al., 1994; Folkow et al., 2004), an area where crustaceans dominate their diet (Nilssen et al., 1995; Lindstrøm et al., 2013). Harp seals examined in coastal waters of northern Iceland during the period February-May had a varied diet which consisted mainly of sand eels (Ammodytes sp.), gadoids, capelin, and other fishes; crustaceans (amphipods and krill) and other invertebrates were also observed to a lesser extent (see Hauksson and Bogason, 1997).
The increase in water temperature in the Nordic Seas and reduced ice cover has resulted in an increase in primary productivity in Icelandic waters and a change in distribution and abundance of a number of species. Warm water species such as Atlantic mackerel (Scomber scombrus) and haddock (Melanogrammus aeglefinus) have extended their range while the abundance of migratory cetaceans, such as fin whales (Balaenoptera physalus) and humpback whales (Megaptera novaeangliae), has increased (International Council for the Exploration of the Sea [ICES], 2019c; Moore et al., 2019). Christiansen et al. (2016) reported the first occurrence of Atlantic cod, beaked redfish (Sebastes mentella), and capelin in northeast Greenland. In contrast, warming waters has led to a decline in the abundance and distribution of many cold water species such as polar cod which use sea ice for spawning and feeding (Christiansen, 2017; International Council for the Exploration of the Sea [ICES], 2019c). While capelin have moved westward from Icelandic waters into the cooler Greenland waters, Beaugrand et al. (2009) found a clear movement of warm water zooplankton species in the NEA associated with a decrease in colder water species in response to regional climate warming in the southern part of Iceland. They found that subarctic species, which tend to have a higher energy content decreased, while lower energy containing cold-temperate mixed water species increased. It is likely that the decrease in lipid content of zooplankton may affect both lipid dynamics and the trophodynamics of the entire biological and ecological system (Beaugrand et al., 2009).
Unlike the White Sea/Barents Sea harp seals, the mean age of sexual maturity in Greenland Sea seals, appears to be relatively stable at around 5–6 years, with no discernible trend over time (Frie et al., 2003; International Council for the Exploration of the Sea [ICES], 2019b). This difference between the two NEA harp seal stocks suggests that maturing animals from the two stocks have experienced different per capita food-availability in the 1980s and perhaps even before that time. This may be due to differences in overall food availability between the two ecosystems or simply a consequence of the smaller population size in the Greenland Sea. However, recent reproductive data for this population are limited and somewhat variable, so it is difficult to determine if the recent ecosystem changes are having an impact on vital parameters.
The NWA population summers in the eastern Canadian Arctic and Greenland. In the fall (November-December), most of the seals migrate southward to the Gulf of St. Lawrence and the Newfoundland Shelf. After a period of feeding, they give birth on the pack ice in the Gulf of St. Lawrence (“Gulf”) or off northern Newfoundland (“Front”) each spring. Following molting in April and May, harp seals disperse and eventually migrate northward during June (Stenson and Sjare, 1997). Small numbers of harp seals may remain in southern waters throughout the summer while others remain in the Arctic throughout the year. NWA harp seals are found primarily on the Canadian and Greenland continental shelves.
Northwest Atlantic harp seals are taken by subsistence hunters in the Canadian Arctic and Greenland, and in a commercial hunt in the Gulf of St. Lawrence and off Newfoundland. Catches in Greenland occurred primarily along the west coast. Catches in the Canadian Arctic are poorly known but are assumed to be less than 1,000 annually (Stenson and Upward, 2020). Historically they were low in comparison to the Canadian commercial catch. However, beginning in 1980 Greenland catches increased relatively steadily to a peak of approximately 100,000 in 2000. Between 2011 and 2017, catches have declined with an average of 60,000 seals reported annually which is a similar level to that currently occurring in Canada (International Council for the Exploration of the Sea [ICES], 2019b).
Commercial hunting in Newfoundland began in the early 18th Century although only low numbers were taken until the 19th Century. Peak catches occurred between 1820 and 1860 when the average annual take was over 400,000 seals. The population could not sustain this level of removals and catches declined throughout the rest of the century to an annual average of 160,000 between 1914 and 1940. Following World War II, catches increased again. By 1965 regulations were first introduce to restrict the harvest in the Gulf and to protect breeding females. An average of 280,000 seals were harvest each year between 1946 and 971 when full quotas were introduced (Figure 2). Between 1972 and the demise of the large vessel hunt in 1982, an average of 166,000 seals were taken annually. Catches decreased after 1982 and remained low, averaging approximately 52,000 per year until 1995, at which time interest in the hunt increased significantly. Annual catches consisting almost exclusively of YOY increased to an average of 272,600 between 1996 and 2006, with a maximum of 366,000 in 2004. Since then catches have declined, averaging 63,000 per year between 2009 and 2019 (Stenson and Upward, 2020).
The NWA harp seal population is one of the largest populations of pinnipeds in the world (Southwell et al., 2012; Laidre et al., 2015) and is significantly larger than the other populations (Figure 3). Following World War II, the population was subjected to high levels of hunting and by 1971, the population had declined to approximately 1.6 million (95% CI 1.5 – 1.7 million) animals (Hammill et al., 2020). With the imposition of a quota system and lower catches due to reduced demand, the population began to increase. From the mid 1990s until ∼2014, the population appeared to be relatively stable around 5.5 million due to a combination of high catches in both Canada and Greenland, reduced reproductive rates and a series of years with high ice-related mortality of YOY (see below). Since 2014, however, the population appears to be increasing again as a result of several years with high reproductive rates and low mortality of YOY, and was estimated to be 7.6 million (95% CI 6.6 – 8.8 million) in 2019 (Stenson et al., 2020a; Hammill et al., 2020). It will take 6–10 years before the impact of the high catches of YOY will work itself through the population and be reflected in pup production. Therefore, another pup production survey will be needed before this increase can be confirmed and the recent population trend understood.
The NWA is dominated by the Labrador current that brings cold Arctic water south along the Labrador and Newfoundland Shelves, the southernmost penetration of polar waters in the northern hemisphere (Rice, 2002). The Labrador current runs south along the outer edge of the shelves to the Grand Banks where it bifurcates into an ‘inshore’ and a larger, ‘offshore’ branch. This offshore branch meets the warm Gulf Stream waters coming from south, resulting in a rich mixing environment that provides an area of high productivity. Relatively warm water also enters the Labrador Sea through the West Greenland Current that runs along the Greenland shelf. Around the Davis Strait, it branches into a westward component that crosses over to Baffin Island and a northward current that continues around Baffin Bay.
During the winter, much of the Labrador Shelf, and parts of the west Greenland shelf may be covered by ice. In the west, ice can extend as far south as the northern Grand Banks (47o N) and over a 100 km outward from the coast. Some of this ice enters the northern Gulf of St Lawrence through the Strait of Belle Isle while ice in the southern Gulf is primarily formed in situ or in the St. Lawrence river. While the NWA has undergone periods of relative cooling and warming even within recent times, the overall the trend has been towards warmer temperatures and a deterioration in ice conditions, particularly in the southern Gulf of St. Lawrence (Johnston et al., 2005, 2012; Friedlaender et al., 2010; Bajzak et al., 2011; Stenson and Hammill, 2014). Laidre et al. (2015) estimated that the spring break-up in the Labrador Sea was, on average, almost 10 days per decade earlier in 2013 than in 1979.
The loss of sea ice during, or shortly after, pupping has had a direct impact on mortality of YOY harp seals in the NWA. If ice cover or thickness is not sufficient during the nursing period or while the young seals develop the capacity for diving, it can break up during storms and the YOY seals may drown (Figure 8; Stenson and Hammill, 2014). Hammill et al. (2015) found that although ice cover varied considerably among years, there was a major change in ice conditions by the late 1990s with a significant declining trend in annual ice cover in the Gulf of St. Lawrence of 1940 km2. The extent of ice at the Front also declined but the impact on harp seals was considered to be less due to the greater overall extent of ice in the area. However, they estimated that pup survival was reduced in a number of years, being as low as 50% in 2010 and 25% in 2011, as a result of less ice in both areas and therefore had to be included explicitly in the population model (Figure 9).
Figure 8. Whitecoat harp seal pups that drowned after a storm destroyed the ice on which they had been born, northern Gulf of St. Lawrence March 2010. Photo credit: G. Stenson.
Figure 9. Estimated ice-related mortality of NWA harp seal young of the year (1952-2019) based upon ice conditions in the Gulf of St. Lawrence and off Newfoundland (Hammill et al., 2020).
As in other areas NWA harp seals also rely on invertebrates and polar cod in the north, and primarily forage fish in the south (e.g., Kapel, 1995; Finley et al., 1990; Lawson and Stenson, 1997; Ogloff et al., 2019). However, there is considerable geographic and seasonal variation in the prey species consumed (e.g., see Stenson, 2012). While capelin is the primary prey overall, the proportion in the diet varies among years with importance of individual prey species generally reflecting local abundance. For example, polar cod were an important prey during the early 1990s when their abundance on the Newfoundland shelf and Grand Banks increased during a period of cold water. A variety of other species including Atlantic herring, Atlantic cod, sand eels (Ammodytes spp.), sculpins (Cottidae), redfish (Sebastes spp.), Greenland halibut (Reinhardtius hippoglossoides), amphipods (Hyperiidae), mysids, shrimp (Pandulas spp.) and squid (Teuthoidea) are also consumed (Lawson et al., 1995; Lawson and Stenson, 1997; Tucker et al., 2009; Stenson, 2012). In the NWA, harp seals appear to gain some of their energy stores during the summer feeding in the Arctic regions, but reach their maximum weight in February after feeding in southern areas during the fall (Chabot et al., 1996; Chabot and Stenson, 2002). Approximately 50% of consumption by NWA harp seals occurred on the Newfoundland Shelf south of 55°N (Stenson, 2012) so changes in prey availability in both area Arctic and southern areas can have an impact on the ability of NWA harp seals to obtain the energy needed for successful reproduction.
The NWA ecosystems have historically been dominated by demersal species, particularly Atlantic cod. However, they underwent an abrupt shift in community structure in the late 1980s and early 1990s as a result of a history of overfishing and changing environmental conditions that resulted in an extreme cold period during these years (Koen-Alonso et al., 2010; Department of Fisheries and Oceans [DFO], 2014; Koen-Alonso and Cuff, 2018). Although the collapse of the Atlantic cod stocks on the Newfoundland and Labrador shelves was the most visible change among groundfish, it was part of a synchronous decline in the biomass of a number of other commercial and non-targeted fish species, and a shift to an ecosystem dominated by shellfish such as northern shrimp (Pandalus borealis) and snow crab (Chionoecetes opilio). During the early 1990s, there was also an order of magnitude decline in biomass of capelin, the key forage fish in these ecosystems (Buren et al., 2014, 2019). These dramatic changes, recognized as a regime shift, caused a reorganization of the fish community, as well as loss of its spatial structure and functional diversity (Buren et al., 2014, 2019; Dempsey et al., 2017; Pedersen et al., 2017, 2020). Following the cold period in the late 1980s and early 1990s, the water has warmed. By the late 2000s there was some rebuilding of the demersal fish species along with signs of a modest recovery of capelin and major declines in demersal invertebrate stocks (Koen-Alonso and Cuff, 2018). In recent years, these ecosystems appear to be reverting back to a community dominated by demersal fish, but not necessarily to the same pre-collapse state, with some warmer water species such as silver hake (Merluccius bilinearis) becoming more dominant in some southern areas. If this is any indication of future changes, the Newfoundland and Labrador shelf ecosystems may never fully return to their previous state. At present, the total biomass in these ecosystems remains well below levels observed prior to the collapse in the late 1980s, and since the mid 2010s, productivity appears to be reduced (Pedersen et al., 2017, 2020; Koen-Alonso and Cuff, 2018).
While the ecosystem changes in the NWA impact harp seals directly through increased mortality of young, it has also impacted their population dynamics indirectly through changes in body condition and subsequent reproductive rates. Since the early 1980s, late-term pregnancy rates among mature females has declined while interannual variability increased, ranging from 20% to over 80%. Beginning in the late 1980s, females have been found to be aborting their foeti prematurely in some years (Stenson et al., 2016). During this same period, harp seals have undergone more than a 3-fold increase in abundance (Figure 3) and shown declines in growth rates (Chabot et al., 1996; Hammill and Sauvé, 2017; Hammill et al., 2020; Stenson and Buren, unpublished data). Stenson et al. (2016) found that while the general decline in fecundity is a reflection of density-dependent processes associated with increased population size, the large inter-annual variability is due to varying rates of late term abortions which are related to changes in capelin abundance and mid-winter ice coverage. Capelin biomass on the Newfoundland Shelf has been shown to be impacted by changes in the timing of ice retreat which influences the timing of the primary productivity bloom and, as a result, the amount of zooplankton available as prey for capelin (Buren et al., 2014; Lewis et al., 2019). This suggests that mid-winter ice extent reflects environmental conditions that influence a variety of harp seal prey species. Hammill et al. (2020) found that the fit of their population model to the observed pup production was improved by assuming that annual reproductive rates were a function of an index consisting of a variety of environmental measures (e.g., sea surface temperature, ice extent, bottom temperature, air temperature, etc.) (Colbourne et al., 2016) that reflect a changing carrying capacity in the ecosystem.
In their examination of changes in body condition since 1980, Stenson et al. (2020a) found that the average relative condition of pregnant females has remained consistently high while, that of non-pregnant and immature females varied considerably and showed a general decline since 2000. While condition did not appear to influence fecundity rates directly, it affected the rate of late term abortions; relatively small reductions in average condition resulted in much higher abortion rates. They also found that body condition was related to capelin abundance and mid-winter ice extent. They proposed that as overall condition in the population declines, females that are able to attain sufficient energy (i.e., body condition) maintained their pregnancy while those that could not attain sufficient energy reserves terminate their pregnancy prematurely.
Because of their wide range and differential movements of age classes and sexes, total abundance of harp seals is estimated using a population model that incorporates information on annual reproductive rates, known or estimated sources of mortality, and independent estimates of pup production (e.g., Øigård et al., 2014; Hammill et al., 2015, 2020). Obtaining good estimates of these parameters that are comparable among populations can often be difficult. For example, while a time series of annual late term pregnancy rates are available from the NWA, only periodic estimates based upon samples collected during the molt (i.e., shortly after weaning) are available of the NEA populations (e.g., Kjellqwist et al., 1995; Frie et al., 2003; Stenson et al., 2016; International Council for the Exploration of the Sea [ICES], 2019b). Considerable effort has been made to ensure that comparable methods are used to estimate pup production for all three populations (e.g., Stenson et al., 2002, 2003, 2014, 2020b; Haug et al., 2006; Øigård et al., 2010, 2014) but surveys can only be carried out periodically and long-term time series are difficult to maintain. As such, there can be considerable uncertainty about the population trends among some of these populations which makes interpreting the impact of ecological changes difficult to identify.
The populations dynamics of North Atlantic harp seals has been heavily influenced by commercial hunts. All three populations underwent significant declines in the 19 Century and in the period since World War II (e.g., Iversen, 1927; Skaug et al., 2007; Hammill et al., 2011, 2020; International Council for the Exploration of the Sea [ICES], 2019b). However, lower catches and improved management have lessened the influence of hunting on the populations in recent years (e.g., Hammill et al., 2015, 2020; International Council for the Exploration of the Sea [ICES], 2019b). In the White Sea/Barents Sea, catches have been very low over the past decade, while catches in the Greenland Sea have remained low for the past thirty years. The primary reason for low catches in these areas is economical rather than biological as the catches have been well below the scientific advice for sustainable harvests in both populations (International Council for the Exploration of the Sea [ICES], 2019b). In spite of these low catches, neither population is recovering in a manner that would be expected. Also, the declines in pup production observed in the White Sea in 2004-2005 and in the Greenland Sea in 2018 cannot be explained by hunting. This suggests that other factors may be limiting these populations.
The situation in the NWA is slightly different. Quotas and low harvest allowed the population to increase during the 1970s and 1980s at approximately 8% per year (Hammill et al., 2015). Between 1996 and 2008, however, harvests increased significantly with combined Canadian and Greenland catches being over 400,000 in some years (Stenson and Upward, 2020). During this period, there were also a number of years with poor ice conditions that resulted in mortality of young seals. As a result of the high harvests on YOY seals and increased mortality which continued until approximately 2011, the population remained relatively stable for almost two decades (1998-2013) (Hammill et al., 2020). Stenson and Upward (2020) found very few females from the 1996 – 2012 cohorts in their reproductive samples also suggesting that there may have been low survival over these years.
NWA harp seals are now estimated to be increasing (Hammill et al., 2020) due to a reduction in the hunt, together with a series of years with good survival of young, and relatively high reproductive rates. This suggests that this population may be less limited by changes in the either environment than the other two populations. This may be because the northwest Atlantic ecosystem is still being influenced by the cold Labrador current and seasonal ice cover, while the northeast Atlantic and the Barents Sea are more strongly influenced by increased inflow of warmer Atlantic waters. The relatively stable Greenland Sea pup production during the 2000s, when the Barents Sea/White Sea population experienced a dramatic decline, may be due to Greenland Sea seals spending at least part of the year in the western margin dominated by the cold East Greenland Current, while the White Sea/Barents Sea population is more confined to the Barents Sea, which is much more strongly influenced by the Atlantic inflow. The almost 40% drop in the most recent pup production estimate in the Greenland Sea may suggest that the ecosystem associated with cold Arctic waters along the western margin are now changing, and the resilience provided to seals by having access to two oceanic regimes has disappeared. This may suggest a gradient from (1) dramatic negative population response in the Barents Sea/White sea population exposed to rapid changes in the Barents Sea, via (2) delayed and potentially less dramatic negative response in the Greenland Sea population having access to both Barents Sea and Greenland Sea regimes, to (3) continued population increase in the Northwest Atlantic population which remains in waters dominated by Arctic conditions in the Labrador Sea.
Overall, climate change has resulted in dramatic changes in ecosystems across the north Atlantic, both with respect to changes in ice conditions and community structure. Reductions in sea ice associated with climate change is impacting all three harp seal populations. Laidre et al. (2015) found that among the twelve Arctic areas they examined, the largest increase in ice free days, both in the spring and in the fall, occurred in the Barents Sea while the second largest increase occurred in the Labrador Sea, both being important pupping areas for harp seals. The shift in timing of spring breakup and fall freeze-up has not been as dramatic in the Greenland Sea, but the changes are still significant (Laidre et al., 2015). Poor ice conditions have resulted in a number of years with high mortality among YOY seals in the NWA (Stenson and Hammill, 2014; Hammill et al., 2015, 2020). The same degree of ice loss is now being observed in the White Sea, suggesting that this population may also be impacted by increased mortality of young seals. While the population may not be impacted by the occasional year with poor pup survival, repeated years with even low levels of additional mortality can have serious consequences which will not be identified for over a decade at the current frequency of pup production surveys (Hammill and Stenson, 2010; Stenson and Hammill, 2014; Hammill et al., 2020).
Shifts in the whelping locations of Greenland Sea harp seals have occurred as the ice edge recedes towards the east Greenland coast (Rosing-Asvid, 2008). A shift in the location of whelping outside of the traditional pupping areas was also observed in the NWA during a year when ice conditions were extremely poor (Stenson and Hammill, 2014). Harp seals have traditionally pupped in areas where the ice persists in the same general area during the period of nursing and the post weaning fast. This was a feature of the Odden in the Greenland Sea and off the north coast of Newfoundland where ice is often retained. When pupping occurs outside of the traditional areas, the young may be exposed to ice that can break up quicker and to different prey fields during the period when they are first learning to find food. In areas such as the Greenland Sea and off Newfoundland, they can also encounter increased predation from polar bears which travel great distances to seek out the pupping concentrations (Peacock et al., 2013).
Comparing historical locations of NWA harp seal whelping patches during periods of good and poor ice conditions led Stenson and Hammill (2014) to conclude that females pupped in areas with suitable ice outside of their traditional whelping areas if no ice was present within these traditional areas. However, if any amount of ice was present in the traditional areas, females gave birth even if the ice was too thin to sustain the pups, resulting in high pup mortality. There was no evidence to indicate that harp seals pupped on land even in areas where ice was absent and young seals that drifted to shore had high levels of abandonment and mortality. They predicted that if the current warming trends continue, ice-breeding harp seals will encounter more years with poor ice conditions and may eventually adapt by moving north. Until then, they will continue to have increased levels of mortality that could result in the disappearance of the most southern breeding component in the Gulf of St Lawrence. Such a situation occurred in 2017 when poor ice in southern Gulf resulted in an almost complete collapse of pupping with only 2% of the total NWA pup production occurring in an area that traditionally accounts for 25–30%. There was evidence that suggests that some of the females moved to the Newfoundland area to give birth but whether these females will stay there or move back to the Gulf is unknown (Department of Fisheries and Oceans [DFO], 2020; Stenson et al., 2020b).
Historically the community structure of ecosystems in the NWA and NEA, particularly the Barents Sea, shared a number of traits. They were characterized by demersal fish dominated by Atlantic cod, and had a wasp-waist ecosystem with capelin or polar cod as the dominant forage fish. All areas are influenced by seasonal sea ice cover which will influence timing and strength of primary production. However, the NWA and NEA appear to be diverging with the NWA being more influenced by the cold Labrador current, while the increased Atlantic inflow is bringing warm water and temperate species to the NEA. The unidirectional warming trend in the NEA has been conducive to increased productivity and a recovery in fish groundfish productivity to very high levels (Bogstad et al., 2015; Haug et al., 2017). In contrast, in the NWA, the climate has alternated between warm and cold periods resulting in a decline in productivity and only minimal recovery of demersal species (Pedersen et al., 2017; Koen-Alonso and Cuff, 2018).
These ecological changes have been reflected in the changes observed in body condition and vital rates of harp seals. As abundance and/or distribution of many of the key prey species has changed, body condition in both White Sea/Barents Sea and NWA harp seals has declined.
Similar declines in body condition have been observed in common minke whales (Balaenoptera acutorostrata) that inhabit the Barents Sea (Haug et al., 2002). Solvang et al. (2017) reported a negative trend in body condition over the period 1992-2013, with particular low values in 2013. Bogstad et al. (2015) suggested that the current record high cod stock in the area may have outperformed the seal and whale stocks in competition for food (mainly capelin). It is possible that collapse of the Barents Sea capelin stock that occurred around 2003 resulted in a reduction in body condition and pregnancy rates as has been seen in the NWA (Stenson et al., 2016). This may account for the decline in White Sea pup production observed in 2004 and 2005 (Øigård et al., 2013). The continued lower level of births in this population may be due to increased ice mortality and competition with the large Atlantic cod stock for food as the capelin stocks rebounded.
Northwest Atlantic capelin stocks have not recovered significantly since the collapse in the early 1990s which has led to lower body condition and reduced, but highly variable pregnancy rates in NWA harp seals (Stenson et al., 2020a). The large increase in abundance this population has undergone over the past 40 years has contributed to the lower average reproductive rates (i.e., density dependent factors), but it appears that at a high population size, females are sensitive to relatively small changes in prey availability and respond to low prey abundance by aborting their foeti (Stenson et al., 2016). It is unknown if White Sea/Barents Sea harp seals respond, similarly, as later term pregnancy data are not available. However, the very high age of sexual maturity observed in this population (Kjellqwist et al., 1995; Frie et al., 2003) suggests that they may also be near the carrying capacity of the Barents Sea. If so, they may also be responding to relatively small changes in food availability.
Unfortunately, there are no data on body condition and only limited data on reproductive rates of Greenland Sea harp seals. However, the reduction in pup production observed in 2018 in the absence of a hunt suggests that this population may also be impacted by changes in food availability. This may not be unexpected as there have been major shifts in the distribution of capelin and reduction in polar cod in the area. Also, seals from this population spend part of the year in the Barents Sea feeding and so will be influenced the same changes in prey availability that impact the Barents Sea/White Sea seals (see Folkow et al., 2004; Nordøy et al., 2008).
In summary, the current population dynamics of harp seals from the White Sea/Barents Sea population is not influenced by harvests, but the population is being limited by ecological changes in the Barents Sea including (1) reduction in sea ice possibly resulting in increased mortality of young, (2) changes in prey availability, and (3) potential competition with Atlantic cod. The latter two would result in reduced female body condition which could increase late term abortion and/or reduced energy transfer to pups thereby reducing survival. Greenland Sea harp seals are being affected by changes in ice that are not known to be associated with increase pup mortality, but may reduce survival later in the year. They may also be impacted by changes in prey both in the Barents Sea, as well as around Iceland and Greenland. Until recently, the abundance of harp seals in the NWA was limited by catches and while these catches have been reduced, the population is still influenced by changes in the age structure. The high harvest levels of young seals and reduced survival due to poor ice conditions likely resulted in the severe reduction of some cohorts that are only now coming to an age where they will be reproducing. Climate change has also been shown to impact NWA harp seals directly through ice related mortality of YOY, as well as indirectly through changes in prey availability that influence body condition and reproductive rates. Each of the three harp seal populations has been impacted differently by changes in their ecosystems and hunting practices. As their ecosystems continue to change, it is important that we continue to monitor abundance and improve our data on vital rates to further understand how ecological and anthropogenic factors influence this important indicator species.
GS and TH conceived the article. All authors contributed to the writing.
This is a contribution of the CoArc project and received some funding from the Norwegian Foreign Ministry and its Arctic 2030 program.
The authors declare that the research was conducted in the absence of any commercial or financial relationships that could be construed as a potential conflict of interest.
We would like to thank M. Koen-Alonso, L. Shepard, M. Biuw, and two reviewers for their editorial suggestions which have improved this paper considerably. We also thank J. Lawson for the map of harp seal distribution and P. Goulet for preparing the White Sea ice data.
Astthorsson, O. S., Gislason, A., and Jonsson, S. (2007). Climate variability and the Icelandic marine ecosystem. Deep Sea Res. II 54, 2456–2477. doi: 10.1016/j.dsr2.2007.07.030
Bajzak, C. E., Hammill, M. O., Stenson, G. B., and Prinsenberg, S. (2011). Drifting away: implications of changes in ice conditions for a pack-ice breeding phocid, the harp seal (Pagophilus groenlandicus). Can. J. Zool. 89, 1050–1062. doi: 10.1139/z11-081
Beaugrand, G., Liczak, C., and Edwards, M. (2009). Rapid biogeographical plankton shifts in the North Atlantic Ocean. Global Change Biol. 15, 1790–1803. doi: 10.1111/j.1365-2486.2009.01848.x
Bogstad, B., Gjøsæter, H., Haug, T., and Lindstrøm, U. (2015). A review of the battle for food in the Barents Sea: cod vs marine mammals. Front. Ecol. Evol. 3:29. doi: 10.3389/fevo.2015.00029
Bogstad, B., Haug, T., and Mehl, S. (2000). Who eats whom in the Barents Sea? NAMMCO Sci. Publ. 2, 98–119.
Borisov, V. I. (1966). Some data of the serological analysis of Pagophilus groenlandicus Erxleben colonies. Zool. Zh. Ukr. 45, 1890–1892.
Buren, A. D., Koen-Alonso, M., Pepin, P., Mowbry, F., Nakashima, B., Stenson, G., et al. (2014). Bottom-up regulation of capelin, a keystone forage species. PLoS One 9:e87589. doi: 10.1371/journal.pone.0087589
Buren, A. D., Murphy, H. M., Adamack, A. T., Davoren, G. K., Koen-Alonso, M., MontevDoiecchi, W. A., et al. (2019). The collapse and continued low productivity of a keystone forage fish species. Mar. Ecol. Prog. Ser. 616, 155–170. doi: 10.3354/meps12924
Burns, J. M., Lestyk, K. C., Folkow, L. P., Hammill, M. O., and Blix, A. S. (2007). Size and distribution of oxygen stores in harp and hooded seals from birth to maturity. J. Comp. Physiol. B 177, 687–700. doi: 10.1007/s00360-007-0167-2
CAFF (2017). State of the Arctic Marine Biodiversity Report. Akureyri: Conservation of Arctic Flora and Fauna International Secretariat. 978-9935-431-63–69.
Carr, S. M., Duggan, A. T., Stenson, G. B., and Marshall, H. D. (2015). Quantitative phylogenomics of within-species mitogenome variation: monte Carlo and non-parametric analysis of phylogeographic structure among discrete transatlantic breeding areas of harp seals (Pagophilus groenlandicus). PLoS One 10:e0134207. doi: 10.1371/journal.pone.0134207
Chabot, D., and Stenson, G. B. (2002). Growth and seasonal fluctuations in size and condition of male Northwest Atlantic harp seals (Phoca groenlandica): an analysis using sequential growth curves. Mar. Ecol. Prog. Ser. 227, 25–42. doi: 10.3354/meps227025
Chabot, D., Stenson, G. B., and Cadigan, N. B. (1996). Short-and long- term fluctuations in the size and condition of harp seal (Phoca groenlandica) in the northwest Atlantic. NAFO Sci. Council Stud. 26, 15–32.
Christiansen, J. S. (2017). No future for Euro-Arctic ocean fishes. Mar. Ecol. Prog. Ser. 575, 217–227. doi: 10.3354/meps12192
Christiansen, J. S., Bonsdorff, E., Byrkjedal, I., Fevolden, S. E., Karamushko, O. V., Lynghammar, A., et al. (2016). Novel biodiversity baselines outpace models of fish distribution in Arctic waters. Sci. Nat. 103, 8.
Colbourne, E., Holden, J., Senciall, D., Bailey, W., Snook, S., and Higdon, J. (2016). Physical Oceanographic Conditions on the Newfoundland and Labrador Shelf during 2015. DFO Can. Sci. Advis. Sec. Res. Doc. 2016/079. v +40 p.
Dempsey, D. P., Koen-Alonso, M., Gentleman, W. C., and Pepin, P. (2017). Compilation and discussion of driver, pressure and state indictors for the Grand Bank ecosystem. Northwest Atlantic. Ecol. Indicator. 75, 331–339. doi: 10.1016/j.ecolind.2016.12.011
Department of Fisheries, and Oceans [DFO] (2012). Science advice for identifying indicators for monitoring arctic marine biodiversity in Canada. DFO Can. Sci. Advis. Sec. Sci. Advis. Rep. 2012/053, 23.
Department of Fisheries, and Oceans [DFO] (2014). Short-term stock prospects for cod, crab and shrimp in the newfoundland and labrador region (Divisions 2J3KL). DFO Can. Sci. Adv. Sec. Sci. Resp. 2014/049, 18.
Department of Fisheries, and Oceans [DFO] (2020). 2019 status of Northwest Atlantic harp seals, Pagophilus groenlandicus. DFO Can. Sci. Advis. Sec. Sci. Advis. Rep. 2020/020, 14.
Divine, D. V., and Dick, C. (2006). Historical variability of sea ice edge position in the nordic seas. J. Geophys. Res. 111:c01001. doi: 10.1029/2004jc002851
Drinkwater, K., Colbourne, E., Loeng, H., Sundby, S., and Kristiansen, T. (2013). Comparison of the atmospheric forcing and oceanographic responses between the Labrador Sea and the Norwegian and Barents seas. Prog. Ocean 114, 11–25. doi: 10.1016/j.pocean.2013.03.007
Drinkwater, K., and Pepin, P. (2013). Comparison of climate forcing on the marine ecosystems of the Northeast and Northwest Atlantic: a synthesis of the NORCAN Project. Prog. Ocean 114, 3–10. doi: 10.1016/j.pocean.2013.05.002
Eamer, J., Donaldson, G. M., Gaston, A. J., Kosobokova, K. N., Lárusson, K. F., Melnikov, I. A., et al. (2013). Life Linked to Ice: A Guide to Sea-Ice-Associated Biodiversity ?in This Time of Rapid Change. Iceland: Conservation of Arctic Flora and Fauna. CAFF Assessment Series No. 10.
Enoksen, S., Haug, T., Lindstrøm, U., and Nilssen, K. T. (2017). Recent summer diet of hooded Cystophora cristata and harp Pagophilus groenlandicus seals in the drift ice of the Greenland Sea. Polar Biol. 40, 931–937. doi: 10.1007/s00300-016-2002-2
Finley, K. J., Bradstreet, M. S. W., and Miller, G. W. (1990). Summer feeding ecology of harp seals (Phoca groenlandica) in relation to Arctic cod (Boreogadus saida) in the Canadian high Arctic. Polar Biol. 10, 609–618. doi: 10.1007/bf00239372
Folkow, L. P., Nordøy, E. S., and Blix, A. S. (2004). Distribution and diving behaviour of harp selas (Pagophilus groenlandicus) from the Greenland Sea stock. Polar Biol. 27, 281–298. doi: 10.1007/s00300-004-0591-7
Foote, A. D., Newton, J., Avila-Arcos, M. C., Kampmann, M. L., Samaniego, J. A., Post, K., et al. (2013). Tracking niche variation over millennial timescales in sympatric killer whale lineages. Proc. R. Soc. B 280:20131481. doi: 10.1098/rspb.2013.1481
Fossheim, M., Primicerio, R., Johannesen, E., Ingvaldsen, R., Aschan, M., and Dolgov, A. (2015). Recent warming leads to a rapid borealization of fish communities in the Arctic. Nat Clim. Change 5, 673–677. doi: 10.1038/nclimate2647
Frie, A. K., Potelov, V. A., Kingsley, M. C. S., and Haug, T. (2003). Trends in age at maturity and growth parameters of female northeast Atlantic harp seals. Pagophilus groenlandicus (Erxleben, 1777). ICES J. Mar. Sci. 60, 1018–1032. doi: 10.1016/s1054-3139(03)00123-1
Friedlaender, A. S., Johnston, D. W., and Halpin, P. N. (2010). Effects of the North Atlantic Oscillation on sea ice breeding habitats of harp seals (Pagophilus groenlandicus) across the North Atlantic. Prog. Ocean 86, 261–266. doi: 10.1016/j.pocean.2010.04.002
Gjøsæter, H., and Bogstad, B. (1998). Effects of the presence of herring (Clupea harengus) on the stock-recruitment relationships of Barents Sea capelin (Mallotus villosus). Fish. Res. 38, 57–71. doi: 10.1016/s0165-7836(98)00114-3
Gjøsæter, H., Bogstad, B., and Tjelmeland, S. (2009). Ecosystem effects of the three capelin stock collapses in the Barents Sea. Mar. Biol. Res. 5, 40–53. doi: 10.1080/17451000802454866
Hammill, M. O., and Sauvé, C. (2017). Growth and condition in harp seals-evidence of density dependent and density independent influences. ICES J. Mar. Sci. 74, 1395–1407. doi: 10.1093/icesjms/fsw237
Hammill, M. O., Stenson, G. B., Doniol-Valcroze, T., and Mosnier, A. (2015). Conservation of northwest Atlantic harp seals: past success, future uncertainty? Biol. Conserv. 192, 181–191. doi: 10.1016/j.biocon.2015.09.016
Hammill, M. O., Stenson, G. B., and Kingsley, M. C. S. (2011). Historical Abundance of Northwest Atlantic harp seals (Pagophilus groenlandicus): influence of harvesting and climate. DFO Can. Sci. Advis. Sec. Res. Doc. 2011/100. iv + 16 p.
Hammill, M. O., Stenson, G. B., Mosnier, A., and Doniol-Valcroze, T. (2020). Trends in harp seal, Pagophilus groenlandicus, abundance in the Northwest Atlantic, 1952-2019. DFO Can. Sci. Advis. Sec. Res. Doc. 2020/in press. iv + 28 p.
Hammill, M. O., and Stenson, G. B. (2010). A preliminary evaluation of the performance of the Canadian management approach for harp seals using simulation studies. DFO Can. Sci. Advis. Sec. Res. Doc. 2009/093. iv + 47 p.
Haug, T., Bogstad, B., Chierici, M., Gjøsæter, H., Hallfredsson, E. H., Høines, ÅS., et al. (2017). Future harvest of living resources in the Arctic Ocean of the Nordic and Barents Seas: a review of possibilities and constraints. Fish. Res. 188, 38–57. doi: 10.11016/j.fishres.2016.12.002
Haug, T., Krøyer, A. B., Nilssen, K. T., Ugland, K. I., and Aspholm, P. E. (1991). Harp seal (Phoca groenlandica) invasions in Norwegian coastal waters: age composition and feeding habits. ICES J. Mar. Sci. 48, 363–371. doi: 10.1093/icesjms/48.3.363
Haug, T., Lindstrøm, U., and Nilssen, K. T. (2002). Variations in minke whale Balaenoptera acutorostrata diet and body condition in response to ecosystem changes in the Barents Sea. Sarsia 87, 409–422. doi: 10.1080/0036482021000155715
Haug, T., Nilssen, K. T., and Lindblom, L. (2004). Feeding habits of harp and hooded seals in drift ice waters along the east coast of Greenland in summer and winter. Polar Res. 23, 35–42. doi: 10.3402/polar.v23i1.6264
Haug, T., Nilssen, K. T., Øien, N., and Potelov, V. (1994). Seasonal distribution of harp seals (Phoca groenlandica) in the Barents Sea. Polar Res. 13, 161–172.
Haug, T., Stenson, G. B., Corkeron, P. J., and Nilssen, K. T. (2006). Estimation of harp seal (Pagophilus groenlandicus) pup production in the North Atlantic completed: results from surveys in the Greenland Sea in 2002. ICES J. Mar. Sci. 63, 95–104. doi: 10.1016/j.icesjms.2005.07.005
Hauksson, E., and Bogason, V. (1997). Comparative feeding of grey (Halichoerus grypus) and common seals (Phoca vitulina) in coastal waters of Iceland, with a note on the diet of hooded (Cystophora cristata) and harp seals (Phoca groenlandica). J. Northwe. Atlant. Fish. Sci. 22, 125–135. doi: 10.2960/j.v22.a11
Hoover, C., Pitcher, T., and Christensen, V. (2013). Effects of hunting, fishing and climate change on the Hudson Bay marine ecosystem: II. Ecosystem model future projections. Ecol. Model. 264, 143–156. doi: 10.1016/j.ecolmodel.2013.01.010
Hunt, G. L. Jr., Blanchard, A. L., Boveng, P., Dalpadado, P., Drinkwater, K. F., Eisner, L., et al. (2013). The barents and chuckchi seas: comparison of two Arctic shelf ecosystems. J. Mar. Sys. 109-110, 43–68.
Intergovernmental Panel on Climate Change [IPCC] (2014). Climate Change 2014: Synthesis Report. Contribution of Working Groups I, II and III to the Fifth Assessment Report of the Intergovernmental Panel on Climate Change. Geneva: IPCC, 151.
International Council for the Exploration of the Sea [ICES] (2013). Report of the ICES Working Group on Harp and Hooded Seals, 26-30 August 2013. Murmansk: PINRO, 55. ICES CM 2013/ACOM: 20.
International Council for the Exploration of the Sea [ICES] (2016). Report of the ICES/NAFO/NAMMCO Working Group on Harp and Hooded Seals, 26-30 September 2016, ICES HQ, Copenhagen, Denmark. ICES CM 2016/ACOM: 21:1-81.
International Council for the Exploration of the Sea [ICES] (2019a). “Barents Sea Ecoregion – Ecosystem overview,” in Report of the ICES Advisory Committee, 2019, (Copenhagen: ICES), doi: 10.17895/ices.advice.5747 ICES Advice 2019, Section 5.1.
International Council for the Exploration of the Sea [ICES] (2019b). ICES/NAFO/NAMMCO Working Group on Harp and Hooded Seals (WGHARP). Copenhagen: ICES, 193. doi: 10.17895/ices.pub.5617 ICES Scientific Reports 1:72.
International Council for the Exploration of the Sea [ICES] (2019c). “Icelandic Waters ecoregion –Ecosystem overview,” in Report of the ICES Advisory Committee, 2019, (Copenhagen: ICES), doi: 10.17895/ices.advice.5746 ICES Advice 2019, Section 11.1.
Iversen, T. (1927). Drivis og selfangst. Årsberetning vedkommende Norges Fiskerier, Vol. 1927. Diamanten: Norwegian, 1–84.
Iverson, S. J., Frost, K. J., and Lang, S. (2002). Fat content and fatty acid composition of forage fish and invertebrates in Prince William Sound, Alaska: factors contributing to among and within species variability. Mar. Ecol. Prog. Ser. 241, 161–181. doi: 10.3354/meps241161
Johnston, D. W., Bowers, M. T., Friedlaender, A. S., and Lavigne, D. M. (2012). The effects of climate change on harp seals (Pagophilus groenlandicus). PLoS One 7:e29158. doi: 10.1371/journal.pone.0029158
Johnston, D. W., Friedlaender, A. S., Torres, L. G., and Lavigne, D. M. (2005). Variation in sea ice cover on the east coast of Canada from 1969 to 2002: climate variability and implications for harp and hooded seals. Clim. Res. 29, 209–222. doi: 10.3354/cr029209
Kapel, F. O. (1995). “Feeding ecology of harp and hooded seals in the Davis Strait - Baffin Bay region,” in Whales, Seals, Fish and man. Developments in Marine Biology 4, eds A. S. Blix, L. Walløe, and Ø Ulltang (Elsevier: Amsterdam), 287–304. doi: 10.1016/s0163-6995(06)80031-4
Kjellqwist, S. A., Haug, T., and Øritsland, T. (1995). Trends in age- composition, growth and reproductive parameters of Barents Sea harp seals. Phoca groenlandica. ICES J. Mar. Sci. 52, 197–208. doi: 10.1016/1054-3139(95)80035-2
Koen-Alonso, M., and Cuff, A. (2018). Status and Trends of the Fish Community in the Newfoundland Shelf (NAFO Div 2J3KL), Grand Bank (NAFO Div. 3LNO) and Southern Newfoundland Shelf (NAFO Div. 3Ps) Ecosystem Production Units. Dartmouth: NAFO. NAFO SCR Doc. 18/70.
Koen-Alonso, M., Pepin, P., and Mowbry, F. (2010). Exploring the Role of Environmental and Anthropogenic Drivers in the Trajectories of Core Fish Species in the Newfoundland-Labrador Marine Community. Dartmouth: NAFO. NAFO SCR Doc. 10/37.
Kortsch, S., Primicerio, R., Fossheim, M., Dolgov, A. V., and Aschan, M. (2015). Climate change alters the structure of arc- tic marine food webs due to poleward shifts of boreal generalists. Proc. R. Soc. Lond. B. Biol. Sci. 282:20151546. doi: 10.1098/rspb.2015.1546
Kovacs, K., and Lydersen, C. (2008). Climate change impacts on seals and whales in the North Atlantic Arctic and adjacent shelf areas. Sci. Progr. 91, 117–150. doi: 10.3184/003685008x324010
Kovacs, K., Lydersen, C., Overland, J. E., and Moore, S. E. (2011). Impacts on changing sea-ice conditions on Arctic marine mammals. Mar. Biodiv. 41, 181–194. doi: 10.1007/s12526-010-0061-0
Laidre, K. L., Stern, H., Kovacs, K. M., Lowry, L. F., Moore, S. E., Regehr, E. V., et al. (2015). Arctic marine mammal population status, sea ice habitat loss, and conservation recommendations for the 21st century. Conserv. Biol. 29, 724–737. doi: 10.1111/cobi.12474
Laidre, K. L., Stirling, I., Lowry, L. F., Wiig, Ø. Heide-Jørgensen, M. P., and Ferguson, S. H. (2008). Quantifying the sensitivity of Arctic marine mammals to climate-induced habitat change. Ecol. Appl. 18, S97–S125.
Lawson, J. W., and Stenson, G. B. (1997). Diet of northwest Atlantic harp seals (Phoca groenlandica) in offshore areas. Can J. Zool. 75, 2095–2106. doi: 10.1139/z97-844
Lawson, J. W., Stenson, G. B., and McKinnon, D. (1995). Nearshore diet of harp seals (Phoca groenlandica) in the northwest Atlantic during 1990-1993. Can. J. Zool. 75, 1805–1818. doi: 10.1139/z95-213
Lewis, K. P., Buren, A. D., Regular, P. M., Mowbray, F. K., and Murphy, H. M. (2019). Forecasting capelin Mallotus villosus biomass on the Newfoundland shelf. Mar. Ecol. Prog. Ser. 616, 171–183. doi: 10.3354/meps12930
Lindstrøm, U., Harbitz, A., Haug, T., and Nilssen, K. T. (1998). Do harp seals Phoca groenlandica exhibit particular prey preferences? ICES J. Mar. Sci. 55, 941–953. doi: 10.1006/jmsc.1998.0367
Lindstrøm, U., Nilssen, K. T., Pettersen, L. M. S., and Haug, T. (2013). Harp seal foraging behaviour during summer around Svalbard in the northern Barents Sea: diet composition and selection of prey. Polar Biol. 36, 305–320. doi: 10.1007/s00300-012-1260-x
McCarthy, J. J., Canzani, O. F., Leary, N. A., Dokken, D. J., and White, K. S. (eds) (2001). Climate change 2001: Impacts, Adaptation, and Vulnerability. Contribution of Working Group II to the Third Assessment Report of the Intergovernmental Panel on Climate Change. Cambridge: Cambridge University Press. doi: 10.1007/s00300-012-1260-x
McKinney, M. A., Iverson, S. J., Fisk, A. T., Sonne, C., Riget, F. F., Letcher, R. J., et al. (2013). Global change effects on the long-term feeding ecology and contaminant exposures of East Greenland polar bears. Global Change Biol. 19, 2360–2372. doi: 10.1111/gcb.12241
Meisfjord, J., and Nñvdal, G. (1994). Using isoelectric-focusing to discern enzyme variation in northeast Atlantic stocks of the harp seal (Phoca groelandica). Hereditas 121, 273–281. doi: 10.1111/j.1601-5223.1994.00273.x
Meisfjord, J., and Sundt, G. (1996). Genetic variation between populations of the harp seal. Phoca groenlandica. ICES J. Mar. Sci. 53, 89–95. doi: 10.1006/jmsc.1996.0008
Møller, D. G., Nñvdal, G., and Valen, A. (1966). Rapport om arbeidet med blodanalyser for populasjonsundersuÌkelser. [Report on sero- logical work in population studies]. Fisken Hav. 1966, 1–17.
Moore, S. E., Haug, T., Víkingsson, G. A., and Stenson, G. (2019). Baleen Whale Ecology in Arctic and Subarctic Seas in an Era of Rapid Habitat Alteration. Prog. Oceanog. 2019:102118. doi: 10.1016/j.pocean.2019.05.010
Murawski, S. A., Steele, J. H., Taylor, P., Fogarty, M. J., Sissenwine, M. P., Ford, M., et al. (2010). Why compare marine ecosystems? ICES J. Mar. Sci. 67, 1–9. doi: 10.1093/icesjms/fsp221
Nilssen, K. T., Haug, T., Grotnes, P. E., and Potelov, V. A. (1997). Seasonal variation in body condition of adult Barents Sea harp seals (Phoca groenlandica). J. Northw. Atl. Fish. Sci. 22, 17–25. doi: 10.2960/j.v22.a1
Nilssen, K. T., Haug, T., Øritsland, T., Lindblom, L., and Kjellqwist, S. A. (1998). Invasions of harp seals Phoca groenlandica Erxleben to coastal waters of Norway in 1995: ecological and demographic implications. Sarsia 83, 337–345. doi: 10.1080/00364827.1998.10413693
Nilssen, K. T., Haug, T., Potelov, V., and Timoshenko, Y. K. (1995). Food habits and food availability of harp seals (Phoca groenlandica) during early summer and autumn in the northern Barents Sea. Polar Biol. 15, 485–493.
Nilssen, K. T., Pedersen, O. P., Folkow, L., and Haug, T. (2000). Food consumption estimates of Barents Sea harp seals. NAMMCO Sci. Publ. 2, 9–28. doi: 10.7557/3.2968
Nordøy, E. S., Folkow, L. P., Potelov, V., Prischemikhin, V., and Blix, A. S. (2008). Seasonal distribution and dive behaviour of harp seals (Pagophilus groenlandicus) of the White Sea – Barents Sea stock. Polar Biol. 31, 1119–1135. doi: 10.1007/s00300-008-0453-9
Norwegian Meteorological Institute (2020). Updated Daily. Norwegian Ice Service Archived Ice Charts. Avaliable at: https://cryo.met.no/archive/ice-service/icecharts/quicklooks/. (accessed April 28, 2020)
Nñvdal, G. (1969). Blood protein polymorphism in harp seals in eastern Canada. J. Fish. Res. Bd. Can. 26, 1397–1399. doi: 10.1139/f69-128
Nñvdal, G. (1971). Serological studies on marine mammals. Rapp ReA∧un Cons. Int. Explor. Mer. 161, 136–138.
Ogloff, W. R., Yurkowski, D. J., Davoren, G. K., and Ferguson, S. H. (2019). Diet and isotopic overlap elucidate competition potential between seasonally sympatric phocids in the Canadian Arctic. Mar. Biol. 166:103. doi: 10.1007/s00227-019-3549-6
Øien, N., and Øritsland, T. (1995). “Use of mark-recapture experiments to monitor seal populations subject to catching,” in Whales, Seals, Fish and Man. Developments in Marine Biology 4, eds A. S. Blix, L. Walløe, and Ø Ulltang (Amsterdam: Elsevier), 35–45. doi: 10.1016/s0163-6995(06)80007-7
Øigård, T. A., Haug, T., and Nilssen, K. T. (2014). From pup production to quotas: current status of harp seals in the Greenland Sea. ICES J. Mar. Sci. 71, 537–545. doi: 10.1093/icesjms/fst155
Øigård, T. A., Haug, T., Nilssen, K. T., and Salberg, A.-B. (2010). Estimation of pup production of hooded and harp seals in the Greenland Sea in 2007: reducing uncertainty using Generalized Additive Models. J. Northwest Atl. Fish. Sci. 42, 103–123. doi: 10.2960/j.v42.m642
Øigård, T. A., Lindstrøm, U., Haug, T., Nilssen, K. T., and Smout, S. (2013). Functional relationship between harp seal body condition and available prey in the Barents Sea. Mar. Ecol. Prog. Ser. 484, 287–301. doi: 10.3354/meps10272
Øritsland, T., and Øien, N. (1995). “Aerial surveys of harp and hooded seal pups in the Greenland Sea pack ice,” in Whales, Seals, Fish and Man. Developments in Marine Biology 4, eds A. S. Blix, L. Walløe, and Ø Ulltang (Amsterdam: Elsevier), 77–87. doi: 10.1016/s0163-6995(06)80012-0
Peacock, E., Taylor, M. K., Laake, J., and Stirling, I. (2013). Population ecology of polar bears in Davis Strait, Canada and Greenland. J. Wildl. Mange. 77, 463–476. doi: 10.1002/jwmg.489
Pedersen, E. J., Koen-Alonso, M., and Tunney, T. D. (2020). Detecting regime shifts in communities using estimated rates of change. ICES J. Mar. Sci. 77, 1546–1555. doi: 10.1093/icesjms/fsaa056
Pedersen, E. J., Thompson, P. L., Ball, R. A., Fortin, M. J., Gouhier, T. C., Link, H., et al. (2017). Signatures of the collapse and incipient recovery of an overexploited marine ecosystem. R. Doc. Open Sci. 4:170215. doi: 10.1098/rsos.170215
Perry, E. A., Stenson, G. B., Bartlett, S. E., Davidson, W. S., and Carr, S. M. (2000). DNA sequence analysis identifies genetically distinguishable populations of harp seals (Pagophilus groenlandicus) in the northwest and northeast Atlantic. Mar. Biol. 137, 53–58. doi: 10.1007/s002279900248
Perry, E. A., and Terhune, J. M. (1999). Variation of harp seal underwater vocalisations among three breeding locations. J. Zool. Lond. 249, 181–186. doi: 10.1111/j.1469-7998.1999.tb00756.x
Potelov, V. A., Golikov, A. P., and Bondarev, V. A. (2003). Estimated pup production of harp seals Pagophilus groenlandicus in the White Sea. Russia, in 2000. ICES J. Mar. Sci. 60, 1012–1017. doi: 10.1016/s1054-3139(03)00095-x
Protozorkevich, D., and van der Meeren, G. I. (eds) (2020). Survey Report From the Joint Norwegian/Russian Ecosystem Survey in the Barents Sea and Adjacent Waters August-October 2019. Bergen: Havforskningsinstituttet, 93. IMR/PINRO Joint Report Series, 1-2020.
QGIS Development Team (2019). QGIS Geographic Information System. Chicago: Open Source Geospatial Foundation Project.
Rice, J. (2002). “Changes to the large marine ecosystem of the Newfoundland-Labrador shelf,” in Large Marine Ecosystems of the North Atlantic, eds K. Sherman and H. R. Skjoldal (Amsterdam: Elsevier Science B.V), 51–103. doi: 10.1016/s1570-0461(02)80055-6
Rigét, F., Mosbech, A., Boertmann, D., Wegeberg, S., Merkel, F., Aastrup, P., et al. (2019). “The seas around Greenland: an environmental status and future perspective,” in World seas: An Environmental Evaluation, (Cambridge, MA: Academic Press), 45–68. doi: 10.1016/b978-0-12-805068-2.00001-2
Rosing-Asvid, A. (2008). A new harp seal whelping ground near South Greenland. Mar. Mamm. Sci. 24, 730–736. doi: 10.1111/j.1748-7692.2008.00216.x
Sivertsen, E. (1941). On the biology of the harp seal Phoca groenlandica Erxl. Investigations carried out in the White Sea 1925-1937. Hvalradets Skr. 26, 1–166.
Skaug, H. J., Frimannslund, L., and Øien, N. I. (2007). Historical population assessment of Barents Sea harp seals (Pagophilus groenlandicus). ICES J. Mari. Sci. 64, 1356–1365. doi: 10.1093/icesjms/fsm118
Solvang, H. K., Yanagihara, H., Øien, N., and Haug, T. (2017). Temporal and geographical variation in body condition of common minke whales (Balaenoptera acutorostrata acutorostrata) in the Northeast Atlantic. Polar Biol. 40, 667–683. doi: 10.1007/s00300-016-1992-0
Southwell, C., Bengston, J., Bester, M., Blix, A. S., Bornemann, H., Boveng, P., et al. (2012). A review of data on abundance, trends in abundance, habitat use and diet of ice-breeding seals in the southern ocean. CCAMLR Sci. 19, 49–74.
Spielhagen, R. F., Werner, K., Sørensen, S. A., Zamelczyk, K., Kandiano, E., Budeus, G., et al. (2011). Enhanced modern heat transfer to the Arctic by warm Atlantic water. Science 331, 450–453. doi: 10.1126/science.1197397
Stenson, G. B., Buren, A. D., and Koen-Alonso, M. (2016). The impact of changing climate and abundance on reproduction in an ice-dependent species, the Northwest Atlantic harp seal. Pagophilus groenlandicus. ICES J. Mar. Sci. 73, 250–262.
Stenson, G. B., and Hammill, M. O. (2014). Can ice breeding seals adapt to habitat loss in a time of climate change? ICES J. Mar. Sci. 71, 1977–1986. doi: 10.1093/icesjms/fsu074
Stenson, G. B., Hammill, M. O., Kingsley, M. C. S., Sjare, B., Warren, W. G., and Myers, R. A. (2002). Is there evidence of increased pup production in northwest Atlantic harp seals. Pagophilus groenlandicus? ICES J. Mar. Sci. 59, 81–92. doi: 10.1006/jmsc.2001.1129
Stenson, G. B., Hammill, M. O., Lawson, J. W., and Gosselin, J.-F. (2014). Estimating pup production of Northwest Atlantic harp seals, Pagophilus groenlandicus, in 2012. DFO Can. Sci. Advis. Sec. Res. Doc. 2014/057. doi: 10.1006/jmsc.2001.1129
Stenson, G. B., Rivest, L.-P., Hammill, M. O., Gosselin, J.-F., and Sjare, B. (2003). Estimating pup production of harp seals, Pagophilus groenlandicus, in the northwest Atlantic. Mar. Mammal. Sci. 19, 141–160. doi: 10.1111/j.1748-7692.2003.tb01098.x
Stenson, G. B. (2012). Estimating consumption of prey by harp seals, Pagophilus groenlandicus, in NAFO divisions 2J3KL. DFO Can. Sci. Advis. Sec. Res. Doc. 2012/156, 26. doi: 10.1111/j.1748-7692.2003.tb01098.x
Stenson, G. B., and Sjare, B. (1997). “Seasonal distribution of harp seals, (Phoca groenlandica),” in the Northwest Atlantic, 23. ICES C. M. 1997/CC:10, 23.
Stenson, G. B., and Upward, P. (2020). Updated estimates of harp seal bycatch and total removals in the Northwest Atlantic. DFO Can. Sci. Advis. Sec. Res. Doc. 2020/014, 19.
Stenson, G. B., Buren, A. D., and Sheppard, G. L. (2020a). Updated estimates of reproductive rates in Northwest Atlantic harp seals and the influence of body condition. DFO Can. Sci. Advis. Sec. Res. Doc. 2020/057, 21.
Stenson, G. B., Gosselin, J.-F., Lawson, J. W., Buren, A., Goulet, P., Lang, S. L. C., et al. (2020b). Estimating pup production of Northwest Atlantic Harp Seals, Pagophilus groenlandicus. DFO Can. Sci. Advis. Sec. Res. Doc. 2020/056, 34.
Tucker, S., Bowen, W. D., Iverson, S. J., Blanchard, W., and Stenson, G. B. (2009). Sources of variation in diets of harp and hooded seals estimated from Quantitative Fatty Acid Signature Analysis (QFASA). Mar. Ecol. Prog. Ser. 384, 287–302. doi: 10.3354/meps08000
Vinje, T. (2001). Anomalies and trends of sea ice extent and atmospheric circulation in the Nordic Seas during the period 1864-1998. J. Clim. 14, 255–267.
Wassmann, P., Reigstad, M., Haug, T., Rudels, B., Carroll, M. L., Hop, H., et al. (2006). Food web and carbon flux in the Barents Sea. Progr. Oceanogr. 71, 232–287. doi: 10.1016/j.pocean.2006.10.003
Wilkinson, J. P., and Wadhams, P. (2005). A method of detecting change in the ice conditions of the central Greenland Sea by the whelping locations of harp seals. J. Clim. 18, 1216–1226. doi: 10.1175/jcli3325.1
Yablokov, A. V., and Sergeant, D. E. (1963). Cranial variation in the harp seal Pagophilus groenlandicus Erxleben, 1777). Zool. Zh. Ukr. 42, 1857–1865.
Keywords: Pagophilus groenlandicus, north Atlantic, ice seal, Arctic indicator, climate change, seal hunt
Citation: Stenson GB, Haug T and Hammill MO (2020) Harp Seals: Monitors of Change in Differing Ecosystems. Front. Mar. Sci. 7:569258. doi: 10.3389/fmars.2020.569258
Received: 03 June 2020; Accepted: 13 August 2020;
Published: 03 September 2020.
Edited by:
Marta Coll, Spanish National Research Council, SpainReviewed by:
Maria Grazia Pennino, Spanish Institute of Oceanography, SpainCopyright © 2020 Stenson, Haug and Hammill. This is an open-access article distributed under the terms of the Creative Commons Attribution License (CC BY). The use, distribution or reproduction in other forums is permitted, provided the original author(s) and the copyright owner(s) are credited and that the original publication in this journal is cited, in accordance with accepted academic practice. No use, distribution or reproduction is permitted which does not comply with these terms.
*Correspondence: Garry B. Stenson, R2FycnkuU3RlbnNvbkBkZm8tbXBvLmdjLmNh
Disclaimer: All claims expressed in this article are solely those of the authors and do not necessarily represent those of their affiliated organizations, or those of the publisher, the editors and the reviewers. Any product that may be evaluated in this article or claim that may be made by its manufacturer is not guaranteed or endorsed by the publisher.
Research integrity at Frontiers
Learn more about the work of our research integrity team to safeguard the quality of each article we publish.