- 1Laboratory of Spatial and Movement Ecology, Centro de Investigación de Ciencias Ambientales, Facultad de Ciencias Naturales, Universidad Autónoma del Carmen, Ciudad del Carmen, Mexico
- 2Dirección de Cátedras, Consejo Nacional de Ciencia y Tecnología (CONACYT), Mexico City, Mexico
- 3LGL Ecological Research Associates Inc., Bryan, TX, United States
- 4Sea Turtle Conservation Program, Pronatura Península de Yucatán, A.C., Mérida, Mexico
- 5Sea Turtle Conservation Program, Área de Protección de Flora y Fauna Laguna de Términos, Comisión Nacional de Áreas Naturales Protegidas, Ciudad del Carmen, Mexico
- 6Graduate Program in Ecological Restoration, Centro de Investigación de Ciencias Ambientales, Facultad de Ciencias Naturales, Universidad Autónoma del Carmen, Ciudad del Carmen, Mexico
- 7Laboratory of Remote Sensing and GIS, Departamento de Recursos del Mar, Centro de Investigación y de Estudios Avanzados del Instituto Politécnico Nacional, Unidad Mérida, Mérida, Mexico
- 8Whale Shark Project, Pronatura Península de Yucatán, A.C., Mérida, Mexico
- 9Sea Turtle Conservation Program, Acuario de Veracruz, Veracruz, Mexico
- 10Acuario del Parque Xcaret, Playa del Carmen, Mexico
- 11Santuario Playa de Rancho Nuevo, Comisión Nacional de Áreas Naturales Protegidas, Rancho Nuevo, Mexico
- 12Sea Turtle Conservation Program, Parque Nacional Sistema Arrecifal Veracruzano, Comisión Nacional de Áreas Naturales Protegidas, Veracruz, Mexico
- 13Aqua Adventures Eco Divers, Isla Mujeres, Mexico
In the Gulf of Mexico, the bulk of published studies for sea turtles have focused on northern (United States) waters where economic resources are centered, with fewer studies in the southern portion of the basin, resulting in significant knowledge gaps in these underrepresented areas. Similarly, publications on adult sea turtles are dominated by research on females that come ashore to nest and can be readily studied (e.g., through the collection of biological samples and the application of satellite-telemetry devices), whereas information on adult male sea turtles is scarce. The goal of this paper is to begin filling these knowledge gaps by synthesizing available data on adult male sea turtles in the southern Gulf of Mexico. We used satellite-telemetry, boat- and drone-based surveys, and stranding records combined with ocean circulation modeling to better understand the spatial distribution of male loggerhead (Caretta caretta), green (Chelonia mydas), hawksbill (Eretmochelys imbricata), and Kemp’s ridley (Lepidochelys kempii) sea turtles in the southern Gulf of Mexico. These spatially explicit analyses will provide context for opportunistically collected data on male sea turtles and better contribute to the management and restoration of sea turtle populations that use the Gulf of Mexico. Moreover, this synthesis can serve as a launching point for directed studies on male sea turtles in this region.
Introduction
Though sea turtles have been intensively studied for decades, major knowledge gaps persist that limit the ability to prioritize conservation plans (Bjorndal et al., 2011). These gaps primarily arise from differences in the ease in which scientists can study certain parts of the sea turtle life-cycle (Hamann et al., 2010; Hays et al., 2016). Sea turtles nest on sandy beaches at tropical, subtropical, and temperate latitudes. After nesting, adult females return to the sea and their offspring incubate in egg chambers before hatching and then immediately migrate offshore (Bolten, 2003). A large portion of the juveniles returns to coastal habitats as they grow and, upon reaching maturity, return to the vicinity of their natal site to reproduce (Bowen et al., 1996). Research has focused mostly on nesting beaches (where scientists can easily access turtles on land) and is weighted toward regions where economic resources are centered (locations of relative wealth where scientific endeavors are prioritized and infrastructure exists to support research). Thus, knowledge gaps tend to exist for the demographic segments of sea turtle populations that are exclusive to marine habitats (Godley et al., 2008) and in areas where there is less economic development.
Within the Gulf of Mexico (GoM), information on sea turtles is predominantly on adult females, eggs, and hatchlings, and centered in the northern portion of the basin (Valverde and Holzwart, 2017). Thus, information on in-water life-stages of sea turtles in the southern GoM is particularly scarce. Focusing on areas associated with the reproduction of a species is important and continued work on nesting beaches is needed. However, potentially problematic knowledge gaps associated with reproduction in sea turtles involve adult males (Hamann et al., 2010). In contrast to the relative ease to access reproductive females, adult males occupy mostly offshore neritic and oceanic habitats, which are more complicated to access (Hatase et al., 2002; Plotkin, 2003; Schofield et al., 2017). Due to the complexity and cost of in-water research, and the low-profile behavior of adult males, less is known about their reproductive cycles and dynamics. Published studies have reported some behavioral features such as that males and females frequently occur at the same foraging areas, males display mating site fidelity, they likely spend less time away from their residency areas, undertake shorter migrations than females, and likely breed annually (Fitzsimmons et al., 1995; van Dam et al., 2008; Hays et al., 2010; Varo-Cruz et al., 2013). However, much more research on male demographic parameters, habitat use, and movements are needed to complete the integrative population analyses necessary for restoring sea turtle populations, particularly in underrepresented regions such as the southern GoM.
In this context, a better understanding of the spatial ecology of males would provide a foundation for designing conservation plans that explicitly account for males within a region harboring some of the largest nesting populations in the West Atlantic for at least three species (Spotila, 2004; Mortimer and Donnelly, 2008; Ceriani et al., 2019). Therefore, the objective of this study was to construct a regional panorama of this demographic segment of sea turtle populations by integrating multiple data sources including satellite telemetry, stranding records coupled with ocean/wind models, as well as boat censuses and unmanned aerial vehicle (UAV) records obtained in the southern GoM and north Mexican Caribbean.
This study represents the state of the art on male sea turtle spatial ecology in the southern GoM, provides new information for the management and restoration of sea turtle populations and serves as a launching point for other studies on male sea turtles in this region.
Materials and Methods
Study Area
The geographic scope of this study was the southern GoM and the northern Mexican Caribbean (extreme points at 29.19°N/−96.84°W – 15.09°N/−82.82°W) (Figure 1A). These areas have unique biological and oceanographic conditions that influence the spatial ecology of marine megafauna (Woolley et al., 2020). Our area of study is within the Northwest Atlantic Regional Management Unit for sea turtles (Wallace et al., 2010); and in oceanic regions delimited by the Yucatan shelf and the Yucatan Current (Wilkinson et al., 2009; Uribe-Martínez et al., 2019).
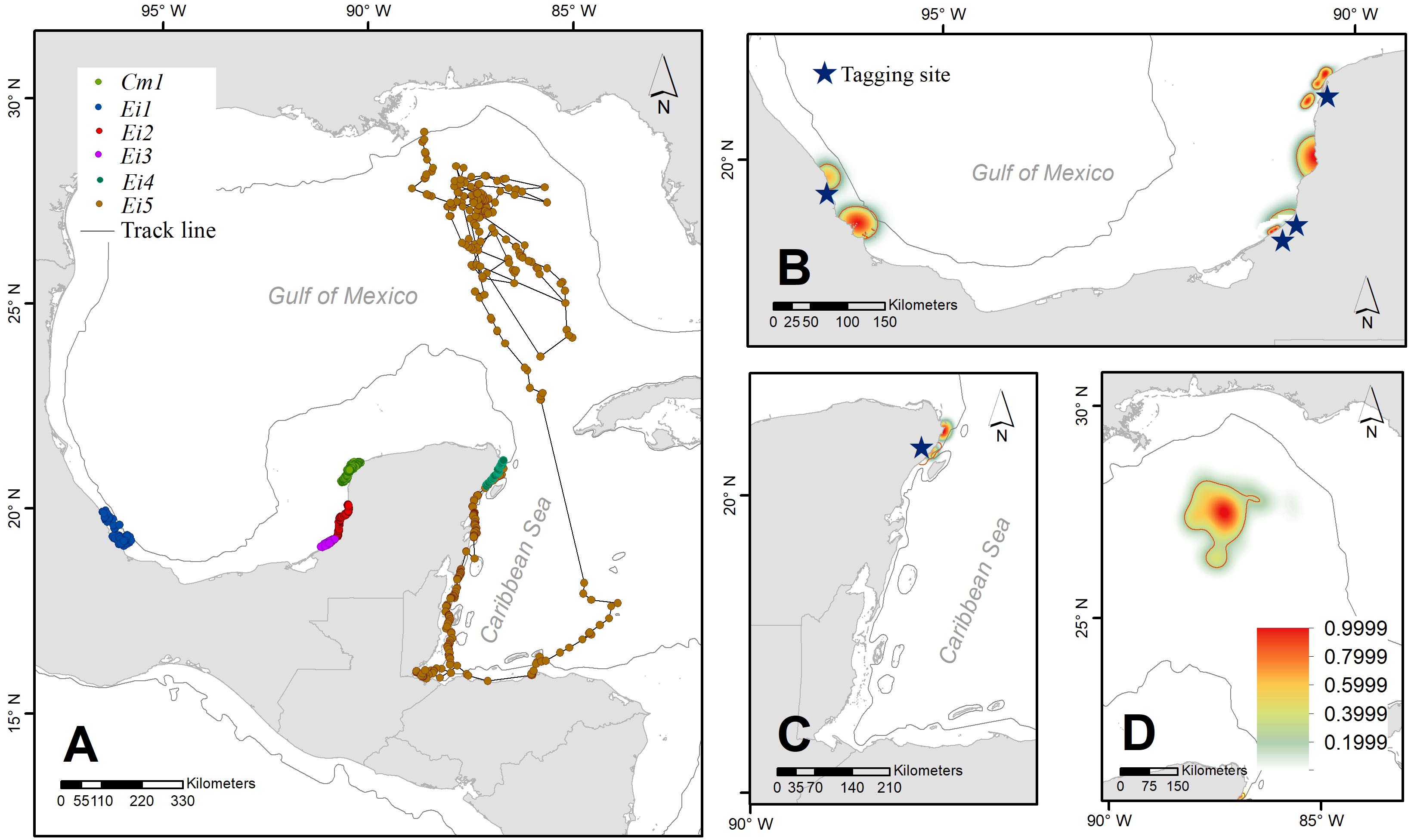
Figure 1. Pathways of six satellite-tracked male sea turtles (five hawksbills and one green) after stranding, subsequent rehabilitation, and release from capture locations on shore (blue stars) (A). Their home ranges (75%, red polygons) are presented together with space use distribution kernels for all individuals (Ei1, Ei2, Ei3, and Cm1) (B), Ei4 and Ei 5 (C), and the open ocean home range Ei 5 (D).
Satellite Telemetry
By 2008 (Godley et al., 2008), there was only one paper on satellite tracking of male hawksbill turtles (Eretmochelys imbricata, N = 8), another on male leatherback turtles (Dermochelys coriacea, N = 11) in the Wider Caribbean (James et al., 2005; van Dam et al., 2008); and one of Kemp’s ridley turtles (Lepidochelys kempii, N = 11) in the GoM (Shaver et al., 2005). In more recent years, in northwest Atlantic and Caribbean region Arendt et al. (2012) tracked male loggerheads (N = 16, b N = 29), Becking et al. (2016) tracked male hawksbills in the Lesser Antilles (N = 2), and Hughes and Landry (2016) tracked one male Kemp’s ridley in northwest GoM. Notably, most of these tracking studies have a small sample size, a reflection of the challenge to obtain information on this demographic segment of marine turtles and that research on this topic is at the “innovation and discovery” initial phase of tracking studies (Sequeira et al., 2019).
Over 8 years (2010–2017), six adult male sea turtles [five hawksbills -Eretmochelys imbricata (Ei)-, and one green turtle -Chelonia mydas (Cm)-] were tracked from different locations in the southern GoM (Supplementary Table S1). All hawksbills were tagged after stranding on the beach, three of them were kept in captivity for rehabilitation in specialized hospitals for more than 1 year, and the other two received a medical evaluation and were released the same day or 1 day later. The sixth male, a green turtle, was captured by hand in a sea turtle aggregation at the northwest corner of the Yucatan Peninsula, Mexico (Figure 1).
Though there is uncertainty regarding whether the movements of turtles that have been exposed to human interventions are representative of counterparts that remain in the wild, there are indications that even prolonged periods of captivity may not greatly alter sea turtle behavior (Cardona et al., 2012; Lyn et al., 2012; Mestre et al., 2014; Baker et al., 2015; Kuo et al., 2017; Robinson et al., 2017; Innis et al., 2019). Obtaining individuals for this study required opportunistic sampling, using incidentally caught, recovered, and rehabilitated individuals.
Satellite transmitters were deployed on turtles following the protocol recommended by Gallegos-Fernández et al. (2018). Given the tag availability, five of these individuals were tagged with TAM4510 Telonics ARGOS transmitters and one was tagged with a Wildlife Computers Mk10-AF ARGOS transmitter. The telemetry data were filtered for quality control (adehabitat filters by Freitas et al., 2008), and in cases where the male displayed a discernable migratory phase (based on speed, turning angle and increasing rate of distance to release site), the data were split into separate stages (Cuevas et al., 2008, 2019). The home range isoline polygon (75%) of the aggregation data for each individual was obtained from functions of space use distribution kernels (Worton, 1989; Schofield et al., 2013). The latter analysis was done in a lattice of 1 km2 (an area in which most of the maximum location error by ARGOS system are embedded) (Bradshaw et al., 2007; Lowther et al., 2015), and a smoothing factor (h) calculated by ad hoc methods (Calenge, 2006).
Beach Stranding Data and Probable Death Sites
Although data on sea turtle strandings may potentially present a biased view of distribution (e.g., they are more likely to occur when and where ocean/wind conditions are favorable to washing ashore), when they are derived from systematic long-term monitoring projects, their relevance for spatial inferences are robust (Koch et al., 2013; Nero et al., 2013; Putman et al., 2020). Given that recordings of sea turtle adult males are otherwise scarce, stranding data are particularly useful for building a regional panorama of the spatial ecology of this population segment.
We compiled stranding records of male individuals from the Mexican coasts along the western and southern GoM from 1994 through 2018. These data were obtained as part of 13 long-term sea turtle nesting beach monitoring programs, in which 525 km of the coastline are systematically monitored during nesting season, and from response units for stranding events throughout the year. The individuals were identified to species level, standard carapace morphometrics, date, time and geographic coordinates of each event were recorded. Stranding data were mapped, and their geographic locations and dates served as input for a numerical model that estimated probable death sites.
To determine where stranded turtles might have been before washing ashore, we simulated their transport using an ocean circulation model paired with satellite-based wind-fields, and a virtual particle tracking tool. This approach followed established methods where ocean current and wind models were applied to estimate the death sites of individual marine turtles (Nero et al., 2013; Santos et al., 2018a,b). We used surface currents from the GoM Hybrid Coordinate Ocean Model (GOM-HYCOM) experiment 50.1 (1994–2012), experiment 31.0 (2013–2014), and experiment 32.5 (2014–2018). These products provided surface current velocities at 0.04° × 0.04° grid resolution (∼4 km) at three (1994–2012) and one (2013–2018) hour time steps. GOM-HYCOM uses data assimilation of satellite and in situ measurements to produce hindcast estimates of the oceanic conditions that existed in the past (Chassignet et al., 2009).
While GOM-HYCOM represents the main features of ocean circulation relevant for the movement of marine organisms (Putman and Mansfield, 2015), objects at the ocean surface will experience additional forces that are not depicted in the model (Putman et al., 2016), such as windage (direct momentum transferred from the wind to an object at the ocean surface) and Stokes drift (residual transport due to waves) (Putman et al., 2018; Olascoaga et al., 2020). The influence of these processes can be accounted for, in part, using a “leeway model,” whereby a fraction of the wind velocity is added to the surface current velocity (Nero et al., 2013). To account for these effects, we used NOAA Blended Sea Winds to provide estimates of wind velocity at 0.25° × 0.25° resolution (∼25 km) at 6 hourly time steps (1994 – 2018) (Zhang et al., 2006). We spatially and temporally interpolated wind velocity data to the GOM-HYCOM grid and time steps and added 3% of the 10 m wind velocities to those surface velocities of GOM-HYCOM (Putman et al., 2018). The 3% windage value that we applied falls within the range of values obtained by studies that examined the drift of sea turtle carcasses [e.g., 3.5% (Nero et al., 2013), 1–4% (Santos et al., 2018a)]. The decomposition state of carcasses can be useful to infer time spent adrift (Santos et al., 2018a,b), however, this information was not available for all stranding records and was not considered in the analysis. Rather, we assessed in which oceanic locations the turtle most likely occurred during the 5 days prior to the stranding date (Nero et al., 2013).
The combined GOM-HYCOM and NOAA Blended Sea Winds velocity fields served as inputs for virtual particle tracking simulations that were run using ICHTHYOP v.3.3 (Lett et al., 2008). We created a ∼200 km buffer from the Mexican coastline across the southern GoM (18°/−98° – 24°/−86°), within which we selected 20,600 random locations to release virtual particles. We released virtual particles from these sites 5 days prior to the recorded stranding date and tracked their movement using a Runge–Kutta 4th-order time-stepping method to compute trajectories at 15-min intervals. Daily latitude and longitude were recorded for subsequent analysis. For each day of the 5-day tracking period, the distance between each virtual particle and the stranding site was measured using a custom Python script based on the pyproj module geod1. We determined the 100 virtual particles that were closest to the stranding site and each particle was weighted proportionally to the inverse of its distance to the stranding site (i.e., particles closer to the stranding site were weighted more heavily than those farther from the site). The weighting factor was applied to the initial release sites of those 100 particles to map the most likely locations of the stranded turtle each day of the previous 5-day period and to integrate with the other distribution data available for male sea turtles.
For analysis and spatial representation purposes, the probable at-sea locations for each of the 99 stranded males were averaged into a lattice of 10 km diameter hexagons, and the values were scaled 0 to 1 to have a standard variation range, so that both individual species distributions and cumulative values for all species together could be evaluated.
Point Location Data
In the northeastern corner of the Yucatan Peninsula, systematic surveys were conducted to record the number of in-water sea turtle individuals using small boats (27–30 ft.) in focal areas (hundreds of square kilometers). Between June and November 2016, and May through July 2017, an array of systematic line-transects adding 100 km in length was conducted (Buckland et al., 2012) in one of three different areas of interest (two at 51 and one at 31 km off the coast). This area was close to one of the largest sea turtle aggregations in the region and near the nesting beach of Isla Holbox (1,200 km were surveyed). Also, in 2019 in this same region, individual males and mating aggregations were recorded using drones at southeast Isla Mujeres. Given a priori knowledge of the presence of mating couples in this region, an area <10 km2 was surveyed twice in April, when mating season started, as prospective surveys using a Phantom (Dji) drone flying at 30 m and covering a 300 m transect. Sea turtle species were identified using aerial photographs based on size, carapace color and general silhouette shape.
Because of the spatial scope of these efforts, and the variability in survey consistency of the drone surveillance, the data were not included to estimate the cumulative probability of occurrence. However, in the context of multi-source data for building the regional distribution of male turtles, they were displayed as points and polygons over the probability of occurrence map, complementing the regional panorama of the distribution of males in the southern GoM.
Cumulative Probability of Occurrence of Male Sea Turtles
As an integrative and wrapping procedure of our outputs, we used the same hexagonal lattice (10 km diameter) to transfer all calculated probabilities of space use distribution kernels and scaled the values at the hexagons in the range 0–1. This allowed us to put the drift modeling and satellite telemetry kernels in the same numerical and spatial context, and conduct an arithmetic sum of both datasets (Tougaard et al., 2008; Downs et al., 2014; Domingues et al., 2016) to obtain a final cumulative probability of occurrence of males in the southern GoM.
Results
The compilation of data sets from different sources allowed us to integrate the first synthesis of the regional probability panorama of the presence of adult male sea turtles in the southern GoM. The outputs include spatially explicit information at different scales.
Satellite Telemetry
Track durations ranged from 5 to 185 days (Median = 55 days) (Supplementary Table S1). Five of the six tracked turtles moved close to the shoreline and stayed at nearshore residency areas traveling <120 km from the site of release. The shortest distance traveled by individuals of both species was displayed by Ei3, moving a net distance of 10.8 km from the coast of Campeche in 55 days. Even when some tracks were very short, that minimum time was enough to show individuals moving far from their release point, like Ei4. Cm1 traveled no more than 62 km from its capture/release site and remained in the region for 78 days, which we therefore assumed was its residency area (Figure 1).
Individual Ei5 traveled the longest distance, moving 775 km from Quintana Roo to an oceanic residency area in 185 days (Figure 1), with a home range of nearly 20,000 km2 (Figure 1C). Unlike the other individuals that stayed in neritic waters, Ei5 moved in neritic waters from June to July, and then moved to oceanic habitats. During 4 months (August–December), Ei5 moved inside an anti-cyclonic eddy linked to the Loop Current. Instead of leaving the Gulf of Mexico with the main flow of the Loop Current, the turtle remained within the eddy. The eddy detached from the Loop Current in November and the turtle traveled with it. By December Ei5 appeared to have departed from the eddy and moved northwards toward the United States continental shelf when transmissions were lost (Figure 2).
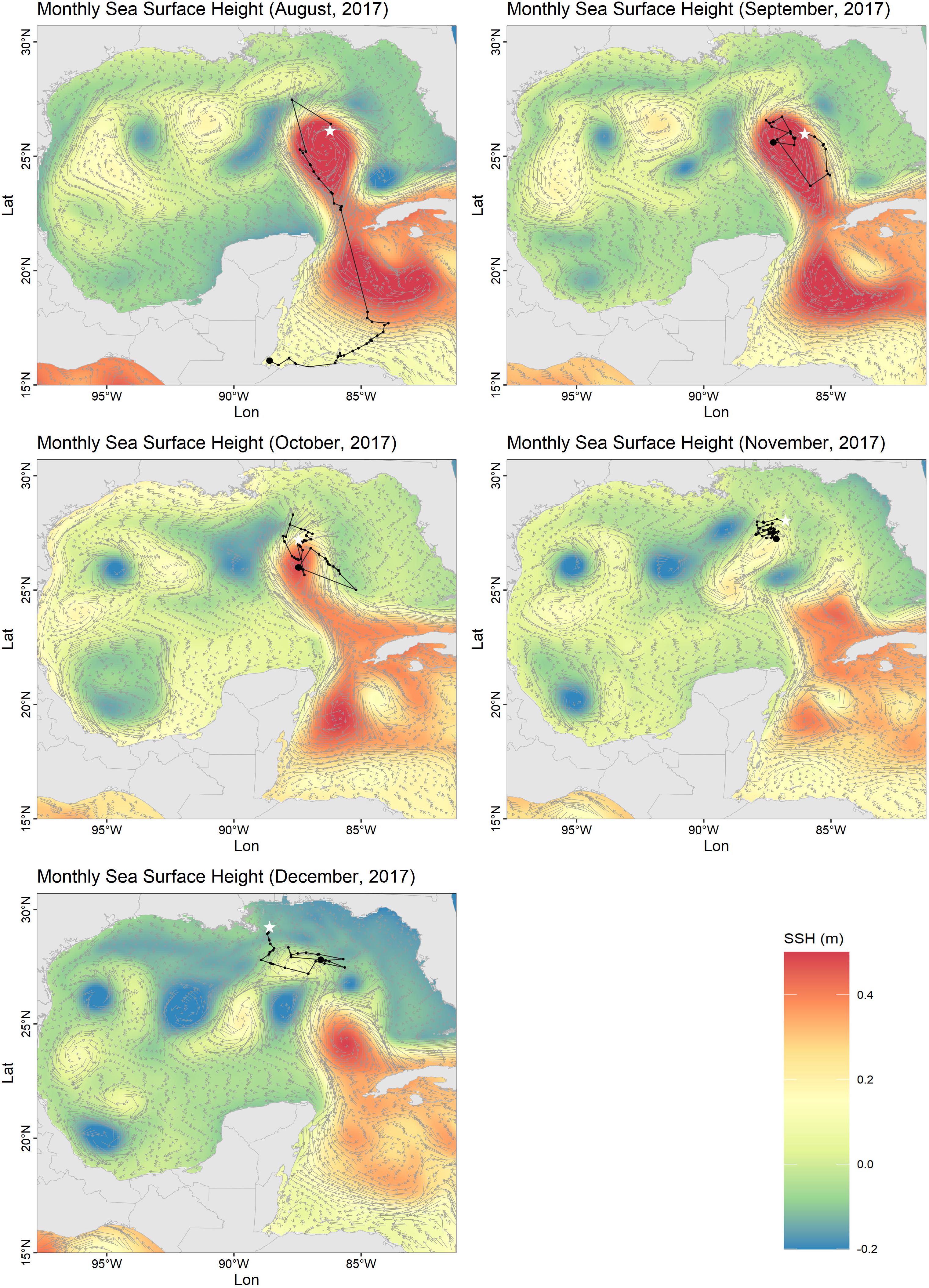
Figure 2. Monthly means of sea surface height (SSH) and ocean current velocity vectors produced by Copernicus Marine Environment Monitoring System (CMEMS) (Copernicus Marine Environment Monitoring Service [CMEMS], 2020) during the oceanic movement of a male hawksbill (Ei5) from September to December 2017. Current direction and speed are represented by gray arrows and the sea turtle tracks are plotted as black dots and lines. Largest black dots are the start of the mapped tracking period and white stars the end.
Probable Death Sites for Stranded Individuals
The stranding dataset included 99 adult male turtles (14 loggerheads 34 greens, 33 hawksbills, and 18 Kemp’s ridley), of all the stranding records, an average of 8.8% (± 2.9) were males (Tamaulipas 9.38%, Veracruz 4.9%; Campeche 9.1%; north Yucatan 11.8%) and there were no significant differences between states (X2 (d.f = 3, n = 99) = 3.1, p = 0.3765). Most of them came from Campeche (53.33%), followed by the northern Yucatan Peninsula (17.14%), Veracruz (17.14%), and Tamaulipas (12.38%). Green turtles comprised 38.09% of stranding records, followed by hawksbills (18.86%), Kemp’s ridleys (17.14%), and loggerheads (13.21%). The average curved carapace length of the stranded individuals was 92.51 cm ± 11.27 (loggerheads), 91.43 cm ± 17.35 (green turtles), 79.07 cm ± 10.50 (hawksbills), and 65.67 cm ± 3.50 (Kemp’s ridleys). Though size is not entirely indicative of maturity, these sizes fall into the range of what are likely reproductive adults (Goshe et al., 2010; Bell and Pike, 2012; Bjorndal et al., 2014; Avens et al., 2015).
Drift modeling indicated that the most probable locations of turtles in the 5 days prior to stranding tended to be close to shore, over the continental shelf, and relatively near the points of stranding (Figure 3). Along the north and west coasts of the Yucatan Peninsula, there are high probabilities of the presence of male sea turtles of these four species. A similar scenario occurs in central and north Veracruz (Figure 3E). The standard deviation of these probabilities is mainly dictated by the combination of both high and low values near shore (Figure 3F).
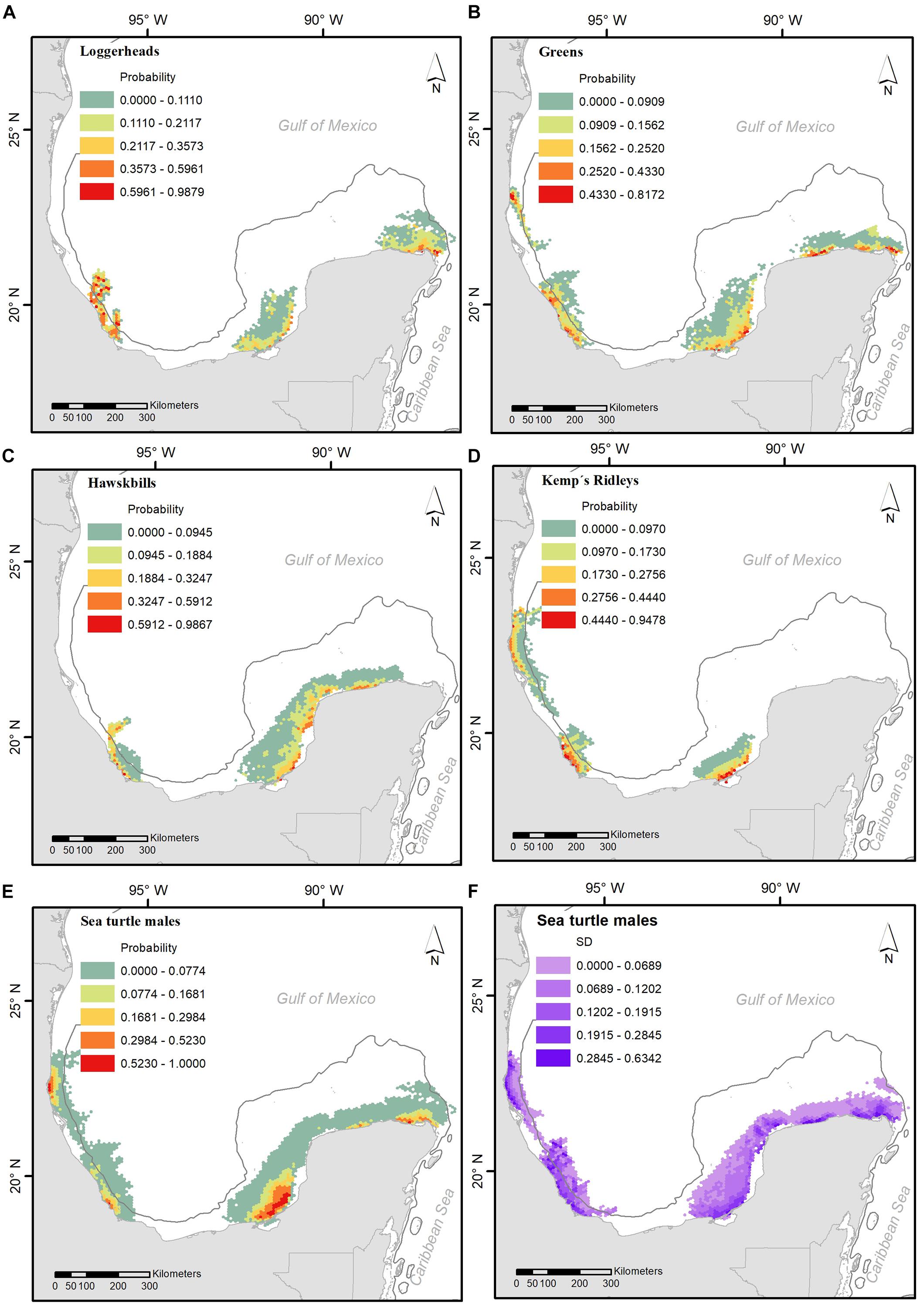
Figure 3. Average probability of death site occurrence for stranding sea turtle males along the coast, for Caretta caretta (A), Chelonia mydas (B), Eretmochelys imbricata (C) and Lepidochelys kempii (D); and an average of occurrence probability for all species (E) and its standard deviation values (F).
Cumulative Probability of Occurrence of Male Sea Turtles
We found high probabilities of space use at the northeast and west coast of the Yucatan Peninsula, together with south Veracruz and Tamaulipas (Figure 4). Locations of observed sea turtle mating occurred close to the areas identified as high cumulative probability of male sea turtle occurrence.
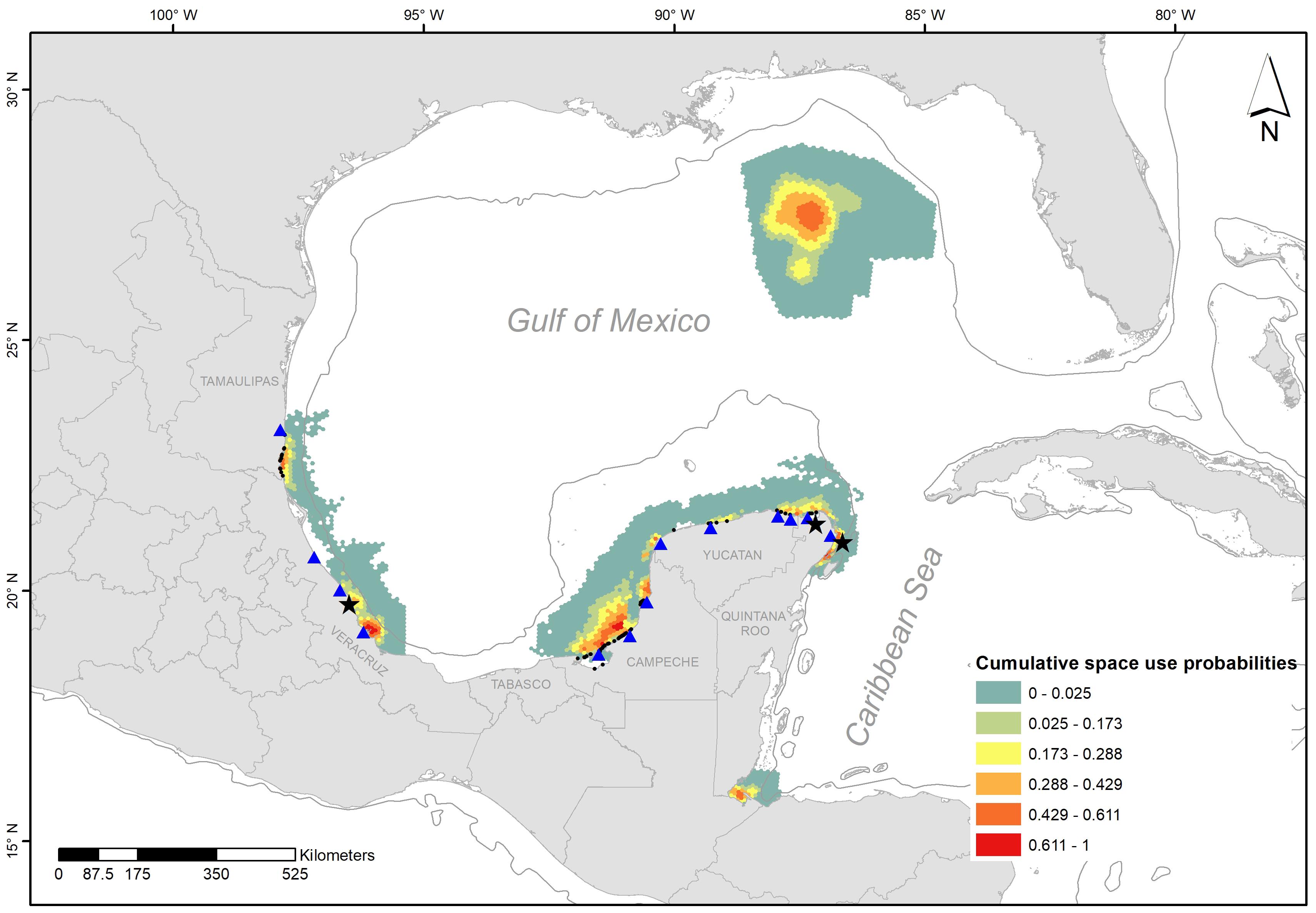
Figure 4. Cumulative space use probabilities for adult male individuals of C. caretta, C. mydas, E. imbricata, and L. kempii. The black points on the shoreline represent the sites of stranding records, the black stars are point locations where mating couples of green and loggerhead individuals have been recorded, and the blue triangles are some of the main sea turtle nesting beaches in the region.
This integration of data supports and strengthens local empirical knowledge on the location of mating aggregations close to shore, and together with a few directed surveys supports the hypothesis of sea turtles mating near their nesting beaches, as in other parts of the world (Miller, 1997). An apparent exception, however, is for hawksbills as no reported evidence exists on locations of consistent mating aggregations in the southern GoM.
Discussion
Satellite Telemetry
As reported in other studies, tracking rehabilitated sea turtles is a reliable alternative to the complex and often expensive enterprise of capturing in-water males (Mestre et al., 2014; Hughes and Landry, 2016; Robinson et al., 2017, 2020). To what extent subsequent behavior is influenced by exposure to humans remains an important question as it has implications for how conservation efforts for sea turtles are prioritized (Caillouet et al., 2016). The data we present can be used in future meta-analyses that address that issue. Nevertheless, our findings suggest: (1) favorable outcomes can occur for individual sea turtles rehabilitated in local specialized hospitals (i.e., Xcaret Park and Acuario de Veracruz), and (2) the tracks of rehabilitated individuals can contribute to the knowledge of the spatial ecology of male sea turtles.
Our results show that male sea turtles are prone to stay in neritic habitats nearshore of mating areas, and conduct very short migrations when these neritic habitats are located in wide, productive areas (such as those in the Yucatan Peninsula) (van Dam et al., 2008; Hughes and Landry, 2016). Another relevant result is that males occupy some of the same habitats as post-nesting females in the Yucatan Peninsula (Cuevas et al., 2008, 2019; Méndez et al., 2013), and present broadly similar movement behaviors as those observed in loggerhead males (Varo-Cruz et al., 2013; Hughes and Landry, 2016).
Male turtles in the Yucatan Peninsula may also move to oceanic habitats to feed as reported in other regions (van Dam et al., 2008; Varo-Cruz et al., 2013; Saito et al., 2015). The movement of individual Ei5 correspond to an area dominated by the Loop Current, where intensive biological activity occurs as a result of upwelling and the accumulation of drifting material along ocean current fronts, or by eddy-induced Ekman pumping (McGillicuddy, 2016). Similar broad-scale pelagic feeding areas are reported for loggerhead females in the Atlantic (Varo-Cruz et al., 2013).
Probable Death Sites for Stranded Individuals
Strandings represent a complex interaction among anthropogenic and environmental conditions that influence mortality, the probability of washing ashore and the probability of being reported (Putman et al., 2020). Further examination of the movement of turtle carcasses in response to wind and current conditions, decomposition rate, variability in survey effort, among other considerations are important for gathering more information from stranding records and numerical analyses (Nero et al., 2013; Santos et al., 2018a,b). However, even considering the present limitations of the stranding data available for synthesis here, valuable information was obtained. We showed that the peaks of stranding occurrence differed among species (April for Kemp’s ridleys, May for loggerheads and hawksbills, and June for greens), possibly because of an association with the reproductive season of each species (Xavier et al., 2006; Castro, 2016; Cuevas, 2016; Delgado, 2016; Koch et al., 2016).
Based on the latter context and their sizes, we might assume that at least the larger males were in a reproductive state when they died, suggesting that the areas with the highest probabilities of occurrence (Figure 3) may also be mating spots. Directed in situ research surveys will be needed to verify those areas are occupied by adult male turtles. We already demonstrated the feasibility of implementing these strategies using water and aerial vehicles (manned and unmanned). The areas adjacent to Isla Mujeres and Isla Holbox (17 individual sea turtles, and 16 mating aggregations of up to three turtles were recorded) are example sites for more precise, local scale, systematic in-water monitoring (Supplementary Figures S3, S4). Additionally, projects designed to encourage the participation of local communities to report bycatch of male turtles such as the one in southern Veracruz, Mexico should be prioritized.
Cumulative Probability of Occurrence of Male Sea Turtles
This study contributes to filling information gaps of basic biology and ecology of these species and expands the knowledge frontier in this region. We show a spatial overlap in important areas along the coasts of Quintana Roo, Campeche, and Veracruz, where more detailed targeted research and monitoring should be implemented. In this context, the waters adjacent to Isla Holbox and Isla Mujeres in Mexico are of significant biological relevance in this region (Cárdenas-Palomo et al., 2015; Reyes-Mendoza et al., 2016), including aggregations of under studied male individuals of endangered sea turtles.
We also identified an important in-water information gap in front of Tabasco State (Figure 4) where there is not sea turtle nesting; therefore, no systematic surveillance exists. However, there is evidence of important in-water areas that are sensitive for sea turtles in this area (Cuevas et al., 2019). Further research is needed to understand how this region functions in the ecology of sea turtles in the southern GoM.
Finally, the spatial integration of stranding datasets from long term survey projects (more than two decades), as well as the efforts of satellite-tracking individuals in this study area, are an essential contribution to the ecological knowledge of four sea turtle species in the GoM. The synthesis of this formerly dispersed data expands our knowledge on sea turtle ecology, sets new research goals, and highlights the need for long-term monitoring efforts. It also demonstrates a critical need to formally systematize the recent in-water research efforts on male sea turtles to increase the information about this underrepresented population segment in a way that can contribute to the recovery of sea turtle populations.
Data Availability Statement
Some of the datasets presented in this article are not readily available because of restrictions by funding sources. Requests to access the datasets should be directed to EC,YW1pci5jdWV2YXNAZ21haWwuY29t.
Ethics Statement
This work was carried out in the respect of Mexican regulations regarding the reporting of stranded dead sea turtles during beach patrolling (SGPA/DGVS/008368/18 and SGPA/DGVS/04900/19; No. SGPA/DGVS/05895/19); and those that were alive and went to rehabilitation processes were treated under the direct supervision of the Federal Attorney for Environmental Protection in Mexico, and the tracking study was performed with the permit SGPADGVS/SEMARNAT, Mexico, No. 09583/15. In water data at Isla Holbox were collected under permit SGPA/DGVS/06712/16. No samples were used for this study.
Author Contributions
EC, AU-M, SG-F, VG-H, MCLC, MALC, JT-C, RG-D-M, HA-S, RM-P, ML-H, PH-R, and JS collected the data. EC, NP, AU-M, SG-F, and JT-C performed the analyses. EC, NP, AU-M, SG-F, MCLC, and JT-C drafted the manuscript. NP, VG-H, MCLC, RG-D-M, AN-P, HA-S, RM-P, ML-H, PH-R, MALC, and JS reviewed and edited the manuscript. All authors contributed to the article and approved the submitted version.
Funding
The Mexican National Council for Science and Technology – Mexican Ministry of Environment and Natural Resources (project 107770), the Mexican National Council for Science and Technology – Mexican Ministry of Energy – Hydrocarbon Fund (project 201441). Alliance WWF-Carlos Slim Fund, the National Fish and Wildlife Foundation (#2006-0091-005), CALICA, Industria Salinera de Yucatán, S.A. de C.V., and funds from CONANP through different programas (PROCER, PROMOBI, PROCODES, PROMANP) allowed the collection of stranding data. The Mesoamerican Reef Fund (project A1605007 MEX008-024) supported in-water data collection in Isla Holbox. To the Secretary of Public Education in Mexico for the grant P/PFCE-2018-04MSU0238W-08 that contributed with computer equipment to UNACAR for data analysis. NP acknowledges support from the Gulf Research Program of the National Academy of Sciences under award number 2000006434. The content is the sole responsibility of the authors and does not necessarily reflect the views of the Gulf Research Program or the National Academy of Sciences. To the School of Natural Sciences (POA-2020) of the Autonomous University of Carmen that contributed for Open Access Publication payment.
Conflict of Interest
NP was employed by the company LGL Ecological Research Associates Inc. JS was employed by, and owns, the company Aqua Adventures Eco Divers.
The remaining authors declare that the research was conducted in the absence of any commercial or financial relationships that could be construed as a potential conflict of interest.
Acknowledgments
We sincerely thank the technical and operative personnel on the beach working every night for 25 years, and with whom the collaboration agreements were stated regarding stranding data in Campeche: Punta Xen (Quelonios, A.C.), Chenkan (APFFLT CONANP-UACAM), Isla Aguada (CRIP Carmen-APFFLT CONANP), Isla del Carmen (DECOL Ciudad del Carmen A.C.), Sabancuy (UNACAR), San Lorenzo (SEMARNYD-SECOL-SMAAS-SEMARNATCAM), Chacahito (APFFLT CONANP), and for 30 years in Yucatan: Las Coloradas (CONANP), Dzilam, Sisal, Telchac (SDS). Many volunteers at the Sea Turtle Conservation Program of Pronatura Península de Yucatán, contributed to the collection of stranding and in-water data in Yucatan and Quintana Roo. To P. A. García-Alvarado and X. Peralta-Jiménez for their contribution in collecting stranding data in Campeche. In Lechuguillas, Veracruz to the technical and Civil Protection personnel (Guardavidas de Veracruz y Boca del Río), for their contribution in stranding reports. Some of the data analysis was done in the context of the Project 028/DGIP/2019 in UNACAR.
Supplementary Material
The Supplementary Material for this article can be found online at: https://www.frontiersin.org/articles/10.3389/fmars.2020.561846/full#supplementary-material
Footnotes
References
Arendt, M. D., Segars, A. L., Byrd, J. L., Boynton, J., Schwenter, J. A., Whitaker, J. D., et al. (2012). Migration, distribution, and diving behavior of adult male loggerhead sea turtles (Caretta caretta) following dispersal from a major breeding aggregation in the Western North Atlantic. Mar. Biol. 159, 113–125. doi: 10.1007/s00227-011-1826-0
Avens, L., Goshe, L. R., Coggins, L., Snover, M. L., Pajuelo, M., Bjorndal, K. A., et al. (2015). Age and size at maturation -and adult- stage duration for loggerhead sea turtles in the western North Atlantic. Mar. Biol. 162, 1749–1767. doi: 10.1007/s00227-015-2705-x
Baker, L., Edwards, W., and Pike, D. A. (2015). Sea turtle rehabilitation success increases with body size and differs among species. Endang. Spec. Res. 29, 13–21. doi: 10.3354/esr00696
Becking, L. E., Christianen, M. J. A., Nava, M. I., Miller, N., Willis, S., and van Dam, R. P. (2016). Post-breeding migration routes of marine turtles from Bonaire and Klein Bonaire, Caribbean Netherlands. Endang. Spec. Res. 30, 117–124. doi: 10.3354/esr00733
Bell, I., and Pike, D. A. (2012). Somatic growth rates of hawksbill turtles Eretmochelys imbricata in a northern Great Barrier Reef foraging area. Mar. Ecol. Prog. Ser. 446, 275–283. doi: 10.3354/meps09481
Bjorndal, K. A., Bowen, B. W., Chaloupka, M., Crowder, L. B., Heppell, S. S., Jones, C. M., et al. (2011). Better science needed for restoration in the Gulf of Mexico. Science 331, 537–538. doi: 10.1126/science.1199935
Bjorndal, K. A., Parsons, J., Mustin, W., and Bolten, A. B. (2014). Variation in age and size at sexual maturity in Kemp’s ridley sea turtles. Endang. Spec. Res. 25, 57–67. doi: 10.3354/esr00608
Bolten, A. B. (2003). “Variation in sea turtle life story patterns: Neritic vs Oceanic developmental stages,” in The Biology of Sea Turtles, Vol. 2, eds P. L. Lutz, J. A. Musick, and J. Wyneken (Boca Ratón, FL: CRC Press), 243–258. doi: 10.1201/9781420040807.ch9
Bowen, B. W., Bass, A. L., García-Rodríguez, A., Diez, C. E., van Dam, R., Bolten, A., et al. (1996). Origin of hawksbill turtles in a Caribbean feeding area as indicated by genetic markers. Ecol. Appl. 6, 566–572. doi: 10.2307/2269392
Bradshaw, C. J. A., Sims, D. W., and Hays, G. C. (2007). Measurement error causes scale-dependent threshold erosion of biological signals in animal movement data. Ecol. Appl. 17, 628–638. doi: 10.1890/06-0964
Buckland, S. T., Anderson, D. R., Burnham, K. P., and Laake, J. L. (2012). Distance Sampling: Estimating Abundance of Biological Populations. Berlin: Springer.
Caillouet, C. W., Putman, N. F., Shaver, D. J., Valverde, R. A., Seney, E. E., Lohmann, K. J., et al. (2016). A call for evaluation of the contribution made by resuce, resuscitation, rehabilitation, and release translocations to Kemp’s ridley sea turtle (Lepidochelys Kempii) population recovery. Herpetol. Conserv. Biol. 11, 486–496.
Calenge, C. (2006). The package “adehabitat” for the R software: a tool for the analysis of space and habitat use by animals. Ecol. Model. 197, 516–519. doi: 10.1016/j.ecolmodel.2006.03.017
Cárdenas-Palomo, N., Herrera-Silveira, J., Velázquez-Abunader, I., Reyes, O., and Ordoñez, U. (2015). Distribution and feeding habitat characterization of whale sharks Rhincodon typus in a protected area in the north Caribbean Sea. J. Fish Biol. 86, 668–686. doi: 10.1111/jfb.12589
Cardona, L., Fernández, G., Revelles, M., and Aguilar, A. (2012). Readaptation to the wild of rehabilitated loggerhead sea turtles (Caretta caretta) assessed by satellite telemetry. Aquat. Conserv. Mar. Freshw. Ecosyst. 22, 104–112. doi: 10.1002/aqc.1242
Castro, M. (2016). “Tortuga lora,” in Las Tortugas Marinas en México: Logros y Perspectivas Para su Conservación, eds O. Gaona and A. Barragán (México: Soluciones Ambientales ITZENI), 119–134.
Ceriani, S. A., Casale, P., Brost, M., Leone, E. H., and Witherington, B. E. (2019). Conservation implications of sea turtle nesting trends: elusive recovery of a globally important loggerhead population. Ecosphere 10:e02936. doi: 10.1002/ecs2.2936
Chassignet, E. P., Hurlburt, H. E., Metzger, E. J., Smedstad, O. M., Cummings, J. A., Halliwell, G. R., et al. (2009). US GODAE: global ocean prediction with the HYbrid coordinate ocean model (HYCOM). Oceanography 22, 64–75. doi: 10.5670/oceanog.2009.39
Copernicus Marine Environment Monitoring Service [CMEMS] (2020). Global Ocean Physics Reanalysis. Available online at: https://resources.marine.copernicus.eu (accessed March 25, 2020).
Cuevas, E. (2016). “Tortuga carey,” in Las Tortugas Marinas en México: Logros y Perspectivas Para su Conservación, eds O. Gaona and A. Barragán (México: Soluciones Ambientales ITZENI), 59–80.
Cuevas, E., Abreu-Grobois, F. A., Guzmán-Hernández, V., Liceaga-Correa, M. A., and Van Dam, R. P. (2008). Post-nesting migratory movements of hawksbill turtles Eretmochelys imbricata in waters adjacent to the Yucatan Peninsula, Mexico. Endang. Spec. Res. 10, 123–133. doi: 10.3354/esr00128
Cuevas, E., Liceaga-Correa, M. A., and Uribe-Martínez, A. (2019). Ecological vulnerability of two sea turtle species in the Gulf of Mexico: an integrated spatial approach. Endang. Spec. Res. 40, 337–356. doi: 10.3354/esr00984
Delgado, C. (2016). “Tortuga verde,” in Las Tortugas Marinas en México: Logros y Perspectivas Para su Conservación, eds O. Gaona and A. Barragán (México: Soluciones Ambientales ITZENI), 135–158.
Domingues, R., Goni, G., Bringas, F., Mauhling, B., Lindo-Atichati, D., and Walter, J. (2016). Variability of preferred environmental conditions for Atlantic bluefin tuna (Thunus thynnus) larvae in the Gulf of Mexico during 1993-2011. Fish. Oceanogr. 25, 320–336. doi: 10.1111/fog.12152
Downs, J. A., Horner, M. W., Hyzer, G., Lamb, D., and Loraam, R. (2014). Voxel-based probabilistic space-time prisms for analysing animal movements and habitat use. Intern. J. Geogr. Inform. Sci. 28, 875–890. doi: 10.1080/13658816.2013.850170
Fitzsimmons, N. N., Tucker, A. D., and Limpus, C. J. (1995). Breeding histories of male Green Turtles and fidelity to a breeding ground. Mar. Turtle Newslett. 68, 2–4.
Freitas, C., Lydersen, C., Fedak, M. A., and Kovacs, K. M. (2008). A simple new algorithm to filter marine mammal Argos locations. Mar. Mamm. Sci. 24, 315–325. doi: 10.1111/j.1748-7692.2007.00180.x
Gallegos-Fernández, S. A., Cuevas, E., and Liceaga-Correa, M. D. L. A. (2018). Procesos metodológicos para la colocación de transmisores satelitales en tortugas marinas de caparazón duro en playas de anidación. Rev. Biol. Mar. Oceanogr. 53, 147–156. doi: 10.22370/rbmo.2018.53.2.1289
Godley, B. J., Blumenthal, J. M., Broderick, A. C., Coyne, M. S., Godfrey, M. H., Hawkes, L. A., et al. (2008). Satellite tracking of sea turtles: where have we been and where do we go next? Endang. Spec. Res. 4, 3–22. doi: 10.3354/esr00060
Goshe, L. R., Avens, L., Scharf, F. S., and Sothwood, A. L. (2010). Estimation of age at maturation and growth of Atlantic green turtles (Chelonia mydas) using skeletochronology. Mar. Biol. 157, 1725–1740. doi: 10.1007/s00227-010-1446-0
Hamann, M., Godfrey, M. H., Seminoff, J. A., Arthur, K., Barata, P. C. R., Bjorndal, K. A., et al. (2010). Global research priorities for sea turtles: informing management and conservation in the 21st century. Endang. Spec. Res. 11, 245–269. doi: 10.3354/esr00279
Hatase, H., Matsuzawa, Y., Sakamoto, W., Baba, N., and Miyawaki, I. (2002). Pelagic habitat use of an adult Japanese male loggerhead turtle Caretta caretta examined by the Argos satellite system. Fish. Sci. 68, 945–947. doi: 10.1046/j.1444-2906.2002.00515.x
Hays, G. C., Ferreira, L. C., Sequeira, A. M., Meekan, M. G., Duarte, C. M., Bailey, H., et al. (2016). Key questions in marine megafauna movement ecology. Trends Ecol. Evol. 31, 463–475.
Hays, G. C., Fossette, S., Katselidis, K. A., Schofield, G., and Gravenor, M. B. (2010). Breeding periodicity for male sea turtles, operational sex ratios, and implications in the face of climate change. Conserv. Biol. 24, 1636–1643. doi: 10.1111/j.1523-1739.2010.01531.x
Hughes, C. L., and Landry, A. M. Jr. (2016). Long-term movements of an adult male Kemp’s ridley Sea Turtle (Lepidochelys kempii) in the Northwestern Gulf of Mexico. Gulf Mexico Sci. 33, 206–213. doi: 10.18785/goms.3302.07
Innis, C. J., Finn, S., Kennedy, A., Burgess, E., Norton, T., Manire, C. A., et al. (2019). A summary of sea turtles released from rescue and rehabilitation programs in the United States, with observations on re-encounters. Chelon. Conserv. Biol. 18, 3–9. doi: 10.2744/ccb-1335.1
James, M. C., Eckert, S. A., and Myers, R. A. (2005). Migratory and reproductive movements of male leatherback turtles (Dermochelys coriacea). Mar. Biol. 147, 845–853. doi: 10.1007/s00227-005-1581-1
Koch, V., Peckham, H., and Barragán, A. (2016). “Tortuga caguama,” in Las Tortugas Marinas en México: Logros y Perspectivas Para su Conservación, eds O. Gaona and A. Barragán (México: Soluciones Ambientales ITZENI), 35–58.
Koch, V., Peckham, H., Mancini, A., and Eguchi, T. (2013). Estimating at-sea mortality of marine turtles from stranding frequencies and drifter experiments. PLoS One 8:e56776. doi: 10.1371/journal.pone.0056776
Kuo, F. W., Fan, T. Y., Ka-Yan, N. C., Cai, Y., Balazs, G. H., and Li, T. H. (2017). Tale of the unlucky tags: the story of a rescued, rehabilitated, and released green sea turtle (Chelonia mydas) in southern Taiwan. Bull. Mar. Sci. 93, 689–690. doi: 10.5343/bms.2016.1108
Lett, C., Verley, P., Mullon, C., Parada, C., Brochier, T., Penven, P., et al. (2008). A Lagrangian tool for modelling ichthyoplankton dynamics. Environ. Modell. Softw. 23, 1210–1214. doi: 10.1016/j.envsoft.2008.02.005
Lowther, A. D., Lydersen, C., Fedak, M. A., Lovell, P., and Kovacs, K. M. (2015). The Argos-CLS Kalman filter: error structures and state-space modelling relative to fastloc GPS data. PLoS One 10:e0124754. doi: 10.1371/journal.pone.0124754
Lyn, H., Coleman, A., Broadway, M., Klaus, J., Finerty, S., Shannon, D., et al. (2012). Displacement and site fidelity of rehabilitated immature Kemp’s ridley sea turtles (Lepidochelys kempii). Mar. Turtle Newslett. 135, 10–13.
McGillicuddy, D. J. Jr. (2016). Mechanisms of physical-biological-biogeochemical interaction at the oceanic mesoscale. Annu. Rev. Mar. Sci. 8, 125–159. doi: 10.1146/annurev-marine-010814-015606
Méndez, D., Cuevas, E., Navarro, J., González-Garza, B. I., and Guzmán-Hernández, V. (2013). Rastreo satelital de las hembras de tortuga blanca Chelonia mydas y evaluación de sus ámbitos hogareños en la costa norte de la península de Yucatán, México. Rev. Biol. Mar. Oceanogr. 48, 497–509. doi: 10.4067/S0718-19572013000300008
Mestre, F., Bragança, M. P., Nunes, A., and dos Santos, M. E. (2014). Satellite tracking of sea turtles released after prolonged captivity periods. Mar. Biol. Res. 10, 996–1006. doi: 10.1080/17451000.2013.872801
Miller, J. D. (1997). “Reproduction in sea turtles,” in The Biology of Sea Turtles, Vol. 1, eds P. L. Lutz and J. A. Musick (Boca Raton, FL: CRC Press), 51–82.
Mortimer, J. A., and Donnelly, M. (2008). Marine Turtle Specialist Group 2007 IUCN Red List Status Assessment Hawksbill Turtle (Eretmochelys imbricata). Gland: IUCN Marine Turtle Specialist Group.
Nero, R. W., Cook, M., Coleman, A. T., Solangi, M., and Hardy, R. (2013). Using an ocean model to predict likely drift tracks of sea turtle carcasses in the north central Gulf of Mexico. Endang. Spec. Res. 21, 191–203. doi: 10.3354/esr00516
Olascoaga, M. J., Beron-Vera, F. J., Miron, P., Triñanes, J., Putman, N. F., Lumpkin, R., et al. (2020). Observation and quantification of inertial effects on the drift of floating objects at the ocean surface. Phys. Fluids 32:26601. doi: 10.1063/1.5139045
Plotkin, P. (2003). “Adult migrations and habitat use,” in The Biology of Sea Turtles, Vol. 2, eds P. L. Lutz, J. A. Musick, and J. Wyneken (Boca Raton, FL: CRC Press), 225–241. doi: 10.1201/9781420040807.ch8
Putman, N. F., Goni, G. J., Gramer, L. J., Hu, C., Johns, E. M., Trinanes, J., et al. (2018). Simulating transport pathways of pelagic Sargassum from the equatorial Atlantic into the Caribbean Sea. Prog. Oceanogr. 165, 205–214. doi: 10.1016/j.pocean.2018.06.009
Putman, N. F., Lumpkin, R., Sacco, A. E., and Mansfield, K. L. (2016). Passive drift or active swimming in marine organisms? Proc. R. Soc. B Biol. Sci. 283:20161689. doi: 10.1098/rspb.2016.1689
Putman, N. F., and Mansfield, K. L. (2015). Direct evidence of swimming demonstrates active dispersal in the sea turtle “lost years”. Curr. Biol. 25, 1221–1227. doi: 10.1016/j.cub.2015.03.014
Putman, N. F., Seney, E. E., Verley, P., Shaver, D. J., López-Castro, M. C., Cook, M., et al. (2020). Predicted distributions and abundances of the sea turtle ‘lost years’ in the western North Atlantic Ocean. Ecography 43, 506–517. doi: 10.1111/ecog.04929
Reyes-Mendoza, O., Mariño-Tapia, I., Herrera-Silveira, J., Ruiz-Martínez, G., Enriquez, C., and Largier, J. L. (2016). The effects of wind on upwelling off cabo catoche. J. Coast. Res. 32, 638–650. doi: 10.2112/jcoastres-d-15-00043.1
Robinson, D. P., Jabado, R. W., Rohner, C. A., Pierce, S. J., Hyland, K. P., and Baverstock, W. R. (2017). Satellite tagging of rehabilitated green sea turtles Chelonia mydas from the United Arab Emirates, including the longest tracked journey for the species. PLoS One 12:e0184286. doi: 10.1371/journal.pone.0184286
Robinson, N. J., Deguzman, K., Bonacci-Sullivan, L., DiGiovanni, R. A. Jr., and Pinou, T. (2020). Rehabilitated sea turtles tend to resume typical migratory behaviors: satellite tracking juvenile loggerhead, green, and Kemp’s ridley turtles in the Northeastern USA. Endang. Spec. Res. 43, 133–143. doi: 10.3354/esr01065
Saito, T., Kurita, M., Okamoto, H., Uchida, I., Parker, D., and Balazs, G. (2015). Tracking male loggerhead turtle migrations around southwestern Japan using satellite telemetry. Chelon. Conserv. Biol. 14, 82–87. doi: 10.2744/ccab-14-01-82-87.1
Santos, B. S., Friedrichs, M. A., Rose, S. A., Barco, S. G., and Kaplan, D. M. (2018a). Likely locations of sea turtle stranding mortality using experimentally-calibrated, time and space-specific drift models. Biol. Conserv. 226, 127–143. doi: 10.1016/j.biocon.2018.06.029
Santos, B. S., Kaplan, D. M., Friedrichs, M. A., Barco, S. G., Mansfield, K. L., and Manning, J. P. (2018b). Consequences of drift and carcass decomposition for estimating sea turtle mortality hotspots. Ecol. Indic. 84, 319–336. doi: 10.1016/j.ecolind.2017.08.064
Schofield, G., Dimadi, A., Fossette, S., Katselidis, K. A., Koutsoubas, D., Lilley, M. K. S., et al. (2013). Satellite tracking large numbers of individuals to infer population level dispersal and core areas for the protection of an endangered species. Divers. Distribut. 2013, 1–11. doi: 10.1111/ddi.12077
Schofield, G., Katselidis, K. A., Lilley, M. K. S., Reina, R. D., and Hays, G. C. (2017). Detecting elusive aspects of wildlife ecology using drones: new insights on the mating dynamics and operational sex ratios of sea turtles. Funct. Ecol. 31, 2310–2319. doi: 10.1111/1365-2435.12930
Sequeira, A. M. M., Heupel, M. R., Lea, M. A., Eguíluz, V. M., Duarte, C. M., Meekan, M. G., et al. (2019). The importance of sample size in marine megafauna tagging studies. Ecol. Appl. 29:e01947. doi: 10.1002/eap.1947
Shaver, D. J., Schroeder, B. A., Byles, R. A., Burchfield, P. M., Peña, J., Márquez, R., et al. (2005). Movements and home ranges of adult male Kemp’s ridley sea turtles (Lepidochelys kempii) in the Gulf of Mexico investigated by satellite telemetry. Chelon. Conserv. Biol. 4, 817–827.
Spotila, J. R. (2004). Sea Turtles: a Complete Guide to Their Biology, Behavior, and Conservation. Baltimore, MD: JHU Press.
Tougaard, J., Teilmann, J., and Tougaard, S. (2008). Harbour seal spatial distribution estimated from Argos satellite telemetry: overcoming positioning errors. Endang. Spec. Res. 4, 113–122. doi: 10.3354/esr00068
Uribe-Martínez, A., Aguirre-Gómez, R., Zavala-Hidalgo, J., Ressl, R., and Cuevas, E. (2019). Oceanographic units of the Gulf of Mexico and adjacent areas: the monthly integration of surface biophysical features. Geofís. Intern. 58, 295–315.
Valverde, R. A., and Holzwart, K. R. (2017). “Sea turtles of the Gulf of Mexico,” in Habitats and Biota of the Gulf of Mexico: Before the Deepwater Horizon Oil Spill, ed. C. Ward (New York, NY: Springer), doi: 10.1007/978-1-4939-3456-0_3
van Dam, R. P., Diez, C. E., Balazs, G. H., Colón-Colón, L. A., McMillan, W. O., and Schroeder, B. (2008). Sex-specific migration patterns of hawksbill turtles breeding at Mona Island, Puerto Rico. Endang. Spec. Res. 4, 85–94. doi: 10.3354/esr00044
Varo-Cruz, N., Hawkes, L. A., Cejudo, D., López, P., Coyne, M. S., Godley, B. J., et al. (2013). Satellite tracking derived insights into migration and foraging strategies of male loggerhead turtles in the Eastern Atlantic. J. Exper. Mar. Biol. Ecol. 443, 134–140. doi: 10.1016/j.jembe.2013.02.046
Wallace, B. P., DiMatteo, A., Hurley, B., Finkbeiner, E. M., Bolten, A. B., Chaloupka, M. Y., et al. (2010). Regional management units for marine turtles: a novel framework for prioritizing conservation and research across multiple scales. PLoS One 5:e15465. doi: 10.1371/journal.pone.0015465
Wilkinson, T., Wiken, E., Bezaury-Creel, J., Hourigan, T., Agardy, T., Herrmann, H., et al. (2009). Marine Ecoregions of North America. Montreal: Commission for Environmental Cooperation.
Woolley, S. N. C., Foster, S. D., Bax, N. J., Currie, J. C., Dunn, D. C., Hansen, C., et al. (2020). Bioregions in marine environments: combining biological and environmental data for management and scientific understanding. Bioscience 70, 48–59. doi: 10.1093/biosci/biz133
Worton, B. J. (1989). Kernel methods for estimating the utilization distribution in home-range studies. Ecology 70, 164–168. doi: 10.2307/1938423
Xavier, R., Palomo-Cortez, L., Cuevas, E., Barata, A., and Queiroz, N. (2006). Hawksbill turtle (Eretmochelys imbricata Linnaeus 1766) and green turtle (Chelonia mydas Linnaeus 1754) nesting activity (2002-2004) at El Cuyo Beach, Mexico. Amphib. Reptil. 27, 539–547. doi: 10.1163/156853806778877077
Keywords: numerical simulations, satellite tracking, reproductive individuals, stranding, spatial ecology
Citation: Cuevas E, Putman NF, Uribe-Martínez A, López-Castro MC, Guzmán-Hernández V, Gallegos-Fernández SA, Liceaga-Correa MÁ, Trujillo-Córdova JA, González-Díaz-Mirón RJ, Negrete-Phillipe A, Acosta-Sánchez HH, Martínez-Portugal RC, López-Hernández M, Huerta-Rodríguez P and Silver J (2020) First Spatial Distribution Analysis of Male Sea Turtles in the Southern Gulf of Mexico. Front. Mar. Sci. 7:561846. doi: 10.3389/fmars.2020.561846
Received: 13 May 2020; Accepted: 20 October 2020;
Published: 23 November 2020.
Edited by:
Margaret Lamont, United States Geological Survey (USGS), United StatesReviewed by:
Michael Arendt, South Carolina Department of Natural Resources, United StatesJeffrey Aleksandr Seminoff, Southwest Fisheries Science Center (NOAA), United States
Copyright © 2020 Cuevas, Putman, Uribe-Martínez, López-Castro, Guzmán-Hernández, Gallegos-Fernández, Liceaga-Correa, Trujillo-Córdova, González-Díaz-Mirón, Negrete-Phillipe, Acosta-Sánchez, Martínez-Portugal, López-Hernández, Huerta-Rodríguez and Silver. This is an open-access article distributed under the terms of the Creative Commons Attribution License (CC BY). The use, distribution or reproduction in other forums is permitted, provided the original author(s) and the copyright owner(s) are credited and that the original publication in this journal is cited, in accordance with accepted academic practice. No use, distribution or reproduction is permitted which does not comply with these terms.
*Correspondence: Sandra A. Gallegos-Fernández, c2FnYWZlMThAZ21haWwuY29t