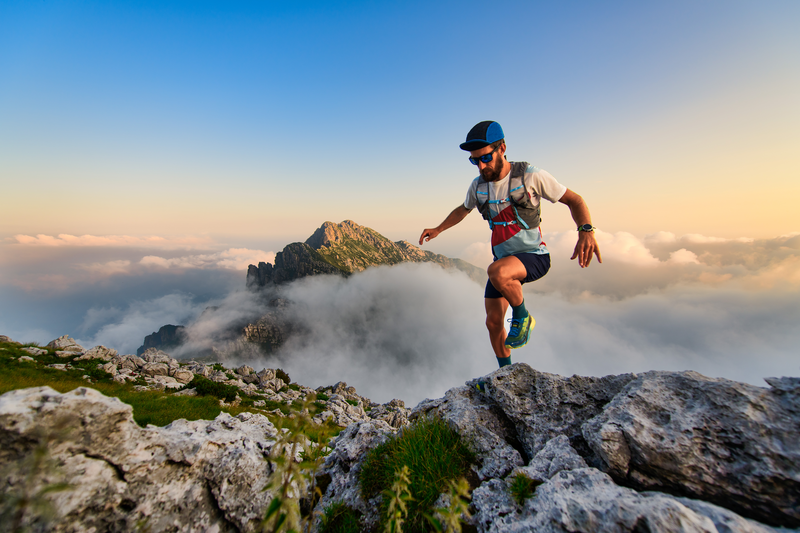
95% of researchers rate our articles as excellent or good
Learn more about the work of our research integrity team to safeguard the quality of each article we publish.
Find out more
ORIGINAL RESEARCH article
Front. Mar. Sci. , 10 September 2020
Sec. Marine Fisheries, Aquaculture and Living Resources
Volume 7 - 2020 | https://doi.org/10.3389/fmars.2020.549145
This article is part of the Research Topic Fish Nutrition, Metabolism and Physiology View all 20 articles
The economic loss of intestinal inflammation by histamine in diet has caused concern in eel culture; it is urgent to develop a natural feed additive to alleviate the negative effects of high-level dietary histamine. Oligomeric proanthocyanidins (OPCs), as the natural antioxidant to scavenge free radicals, were supplemented to alleviate the effects of dietary histamine on parameters of growth, serum biochemistry, and liver metabolites of juvenile American eels in the present study. Juvenile American eels with an initial body weight of 10.84 ± 0.16 g per fish were divided into a control group fed commercial diet, a HIS group fed commercial diet supplemented 300 mg/kg histamine, and an OPC group fed commercial diet supplemented 300 mg/kg histamine and 300 mg/kg OPC. The trial period was 11 weeks. Compared with the HIS group, the OPC group showed that dietary OPC supplementation could alleviate growth retardation; decrease activities of glutamic-pyruvic transaminase, glutamic-oxaloacetic transaminase, acid phosphatase, and alkaline phosphatase; and lower levels of immunoglobulin and complement 3 in serum. With the upregulation of L-cysteine and dihydrouracil involving cysteine and methionine metabolism, beta-alanine metabolism, sulfur metabolism, pantothenate and CoA biosynthesis, and pyrimidine metabolism in the liver, OPC supplementation could alleviate the effects of high-level dietary histamine on growth and serum biochemical parameters. There were no significant differences of growth performance and serum biochemical parameters between the HIS group and control group. These results indicated that OPC might act as a potential feed additive to effectively counteract the negative effects of dietary histamine on juvenile American eel.
The American eel (Anguilla rostrata) is a catadromous fish found in areas ranging from the Gulf of Mexico and Caribbean Sea to southern Greenland (Benchetrit and McCleave, 2016). During the past few years, this eel species has become one of the major eel species reared in southern China, especially in Fujian Province. In eel cultivation, the main ingredient of eel diet is white fish meal, which accounts for 60–70% (Tibbetts et al., 2000). However, with the global shortage and increasing price of white fish meal, brown fish meal was used to replace part of white fish meal in eel diet. It is well known that histamine content in brown fish meal is much higher than that in white fish meal (Ricque-Marie et al., 1998; Tapia-Salazar et al., 2001). The brown fish meal used in eel feed can greatly increase the level of dietary histamine, which is considered to be toxic. In previous studies, dietary histamine was found to possibly decrease growth performance of some catadromous fish species including American eel, Atlantic salmon (Salmo salar L.), Japanese seabass (Lateolabrax japonicus), and rainbow trout (Oncorhynchus mykiss) (Cowey and Cho, 1992; Opstvedt et al., 2000; Hu et al., 2013, 2019; Ma et al., 2020) and to damage some digestive organs of yellow catfish (Pelteobagrus fulvidraco) and Chinook salmon (Oncorhynchus tshawytscha, Walbaum) (Lumsden et al., 2002; Li et al., 2018). It was also found that higher levels of dietary histamine could cause oxidative stress by increasing the production of reactive oxygen species (Rada et al., 2013; Ma et al., 2020), and this might be the major factor to exert negative effects on those fish species.
Natural plant flavonoids supplemented in diet, as antioxidants, have attracted considerable attention to prevent or subdue oxidative stress and improve the health status in aquatic animals (Kao et al., 2010; Zhai and Liu, 2013; Zhai et al., 2018). Oligomeric proanthocyanidins (OPCs) are one of the commonest bioflavonoids with a special molecular structure, formed by oligomerization or polymerization of subunits catechin, epicatechin, and their gallic acid esters primarily known for their excellent antioxidant activity. It is widely reported that OPC could prevent oxidative damage in tissues by reducing lipid oxidation and/or inhibit production of free radicals (Sato et al., 2001; Bagchi et al., 2014; Gao et al., 2017) and has the ability to inhibit lipid peroxidation, platelet aggregation, capillary permeability, and fragility (Zhai et al., 2014; Nie and Stürzenbaum, 2019). In recent aquatic animal studies, dietary OPC supplementation was found to alleviate oxidative stress in juvenile tilapia (Oreochromis niloticus) and grouper (♀ Epinephelus fuscoguttatus × ♂ Epinephelus lanceolatus) under dietary cadmium stress (Wang et al., 2018; Zhai et al., 2018; Huang et al., 2019) and greenlip abalone (Haliotis laevigata Donovan) under heat stress (Duong et al., 2016; Shiel et al., 2017). There is no research about the effect of dietary OPC supplementation on growth, physiology, and metabolism of fish under stress of high-level dietary histamine. It was reported that the OPC level should be at least at 300 mg/kg supplement in diet to alleviate the retarded growth of some fish species exposed to stress condition (Wang et al., 2018; Zhai et al., 2018; Huang et al., 2019). In intensive eel culture, we observed that more than 500 mg/kg histamine in diet could cause growth retardation, intestinal inflammation, and economic loss; the same results were also found in our previous study (Ma et al., 2020). An approximate level of dietary histamine was used in the present study to induce negative effects on juvenile American eel. Therefore, the purpose of this study was conducted to evaluate the alleviation effect of 300 mg/kg OPC supplemented in diet on growth performance, serum biochemical parameters, and liver metabolites of juvenile American eels under stress of dietary histamine.
One thousand Juvenile American eels were obtained from the Beixi eel farm in Tong’an District, Xiamen, China. Before the experiment, all fish were acclimatized in two PVC tanks with bottom center drains (110 cm diameter, 80 cm height) and about 1,000 L water volume supplied with 5 L/min of degassed and dechlorinated municipal water and were fed a commercial powder feed (Fuzhou Sea Horse Feed Co., Ltd., Fuzhou, China) two times daily (6:00 and 18:00). The powder feed was mixed with 1:1 volume water to form a dough shape, and then the dough feed was placed on a feeding table for fish. The commercial diet mainly contained white fish meal, brown fish meal, starch, yeast powder, extruded soybean, and compound premix; the ratio of white fish meal to brown fish meal was about 1:1. The common nutrient composition of the commercial diet was crude protein 46.58%, crude fat 6.70%, ash 12.35%, and moisture 7.36%. The histamine level in the commercial diet was 217 mg/kg. According to feedback from many eel farmers using this commercial diet, no intestinal inflammation and growth retardation were observed. This diet was also used in the formal trial. The following water quality parameters were maintained during the acclimation period: temperature 24–26°C, pH 7.0–7.5, dissolved oxygen 7.0–9.0 mg/L, total ammonia nitrogen <0.1 mg/L, and nitrite <0.005 mg/L. The uneaten feed was siphoned out 1 h after feeding.
After 4 weeks of acclimation, 360 American eels with similar body weight (10.84 ± 0.16 g per fish) were selected and divided randomly into three treatment groups. There were four replicates with 30 fish per replicate in each group. The three treatment groups were the control group (fed the commercial diet), HIS group (fed the commercial diet supplemented with 300 mg/kg histamine), and OPC group (fed the commercial diet supplemented with 300 mg/kg histamine and 300 mg/kg OPC). The trial period was 11 weeks. Histamine was provided by Shanghai Yuanye Biotechnology Co., Ltd., grinded to powder, and well mixed with the commercial diet. OPC was provided by Nanjing Zelang Pharmaceutical Technology Co., Ltd., extracted from grape seed with a content of 98%, powdered, and well mixed with the basal diet and histamine. The trial fish were cultured in 12 circular PVC tanks (320 L water) with a water recirculation system. An air pump was also provided for each tank. The fish management and water quality during the formal trial period were maintained the same as those in the acclimation period. The consumption of diet in each tank was recorded.
At the end of feeding trial, the feed of each tank was deprived for 24 h, and then all the fish were anesthetized with eugenol at 2 g/L. All fish in each tank were weighed and counted to calculate the growth performance parameters. The blood of nine eels from each tank were sampled, treated, and mixed as one sample prior to analysis of serum biochemical parameters according to the procedure of Zhai et al. (2014). The eels were then dissected to obtain liver samples. The liver samples were quickly frozen in lipid nitrogen and then stored at −80°C prior to further analysis.
The growth parameters of initial body weight (IBW) and final body weight (FBW) of fish, weight gain rate (WGR), feed conversion ratio (FCR), feed intake (FI), and survival rate (SR) were calculated as follows:
IBW (g/fish) = initial fish weight (g)/initial number of fish;
FBW (g/fish) = final fish weight (g)/final number of fish;
WGR (%) = 100 × [final wet weight (g) – initial wet weight (g)]/initial wet weight (g);
FCR = FI (g)/weight gain (g);
FI (g/fish) = feed consumption (g)/number of fish; and
SR (%) = 100 × (final number of fish/initial number of fish).
Serum samples from each group (four samples per tank) were selected for biochemical parameter analysis. Commercial kits produced by Nanjing Jiancheng Bioengineering Institute (Nanjing, China) were used to measure glutamic-pyruvic transaminase (GPT), glutamic-oxaloacetic transaminase (GOT), total cholesterol (TC), triglyceride (TG), high-density lipoprotein cholesterol (HDL-C), low-density lipoprotein cholesterol (LDL-C), acid phosphatase (ACP), alkaline phosphatase (AKP), immunoglobulin M (IgM), and complement 3 (C3) in serum of American eels. Spectrophotometer methods were used according to the manufacturers’ protocols.
For metabolomic analysis, eight liver samples from each group (two samples per tank) were selected for analysis of the liver metabolome; the extraction and analysis of metabolites in liver were assisted by the Beijing Allwegene Technology Co., Ltd. (Beijing, China). LC-MS/MS analyses were performed using a UHPLC system (1290, Agilent Technologies) with a UPLC BEH Amide column (1.7 μm, 2.1 mm × 100 mm, Waters) coupled to TripleTOF 6600 (Q-TOF, AB Sciex). The measurement steps, acquirement, and processing of UPLC-Q-TOF-MS data were described in the previous studies of Ma et al. (2018) and Zheng et al. (2020).
The results of growth performance and serum biochemical parameters were presented as means ± SD (n = 4). Data from each group were subjected to one-way analysis of variance (ANOVA) by SPSS 20.0 statistical software (SPSS, Chicago, IL, United States). Duncan’s multiple range test was used to compare the difference of mean values among three groups after overall differences were significant (P < 0.05). For the data expressed as percentages or ratios, square arcsine transformation was subjected prior to further statistical analysis.
Partial least squares-discrimination analysis (PLS-DA) was used as a supervised method to identify important variables with discriminative power and employed with the first principal component of variable importance in projection (VIP) values (VIP > 1) combined with Student’s t-test (t-test) (P < 0.05) to determine the significantly different metabolites between the pairwise comparison groups. PLS-DA was conducted using the SIMCA-P 14.0 commercial software (Umetrics, Umea, Sweden). The metabolite pathways were searched by the Kyoto Encyclopedia of Genes and Genomes (KEGG) pathway1, and MetaboAnalyst was used for the pathway analysis2 (Hao et al., 2018; Huo et al., 2019).
The effects of dietary OPC supplementation on growth performance of juvenile American eels fed a diet with a high level of histamine are shown in Table 1. FBW, WGR, and FI of the HIS group were significantly lower than those of the control group (P < 0.05); these parameters of the OPC group were significantly higher than those of the HIS group (P < 0.05). Compared with that in the HIS group, FCR was significantly lower in the control group and OPC group (P < 0.05). No significant differences of SR were found among the three groups (P > 0.05).
Table 1. Effects of OPC supplementation on the growth performance of juvenile American eels fed a diet with a high level of histamine.
The effects of dietary OPC supplementation on serum biochemical parameters of juvenile American eels fed high-level histamine are shown in Table 2. Compared with the control group, the HIS group had higher activities of GPT and GOT (P < 0.05); these two transaminase activities of the OPC group were similar with those of the HIS group (P > 0.05). The levels of TC, TG, LDL-C, and HDL-C were not significantly affected in the HIS group and OPC group (P > 0.05). Compared with the control group, the HIS group had lower activities of ACP and AKP and lower levels of IgM and C3 (P < 0.05); these parameters of the OPC group were significantly higher in comparison with those of the HIS group (P < 0.05). There were no significant differences of all serum biochemical parameters between the OPC group and control group (P > 0.05).
Table 2. Effects of OPC supplementation on the serum biochemical parameters of juvenile American eels fed a diet with a high level of histamine.
The score plots and validation plots of PLS-DA from liver metabolite profiles of American eel between the control group and HIS group and between the HIS group and OPC group are shown in Figures 1, 2, respectively. Gross changes in the metabolic physiology were easily detectable by using PLS-DA of the entire set of measured analytes. The PLS-DA of the metabolic profiles between the control group and HIS group showed significantly separated clusters in each score scatter plot of the PLS-DA model under positive ion mode and negative ion mode (Figures 1A,C). As shown in Figures 2A,C, there were also clear separation and discrimination between the HIS group and OPC group. As shown in Figures 1B,D, 2B,D, the validation plots for the PLS-DA model showed that the permutation tests were valid with the R2 values being close to 1 (from 0.7971 to 0.9479), and lower Q2 intercepts (from −0.7005 to −0.3258) indicated the robustness of the models and showed a low risk of overfitting and reliability. These findings implied that the PLS-DA model could be utilized to identify the difference between pairwise groups.
Figure 1. The score plots and validation plots of PLS-DA from liver metabolite profiles of American eel in the control group and HIS group under positive ion mode (A,B) and negative ion mode (C,D) (n = 8). D1 = control group, D2 = HIS group.
Figure 2. The score plots and validation plots of PLS-DA from liver metabolite profiles of American eel in the HIS group and OPC group under positive ion mode (A,B) and negative ion mode (C,D) (n = 8). D2 = HIS group, D3 = OPC group.
The results of volcano plots in the HIS group compared with the control group and OPC group are shown in Figures 3, 4, respectively. The volcano plots were used to screen the differential metabolites based on the criteria of VIP values > 1 and a false discovery rate-adjusted P-value < 0.05. After summarizing the differential metabolites under positive ion mode and negative ion mode, the HIS group had 101 upregulated metabolites and 42 downregulated metabolites in comparison with the control group (Figure 3). Among all the identified metabolites, there were 24 upregulated metabolites and 13 downregulated metabolites classified mainly to aromatic compounds, nucleoside, amino acids, lipid, unsaturated fatty acids, saturated fatty acids, and vitamin (Table 3). Compared with the HIS group, the OPC group had 24 upregulated metabolites and 13 downregulated metabolites. Among all the identified metabolites, there were eight upregulated metabolites and seven downregulated metabolites classified mainly to amino acids and nucleoside (Table 4).
Figure 3. Volcano plots for the potential differential metabolites in the liver of American eels of the HIS group in comparison with the control group under positive ion mode (A) and negative ion mode (B). The point size represents the VIP value of the PLS-DA. Pink points indicate significantly upregulated metabolites, and blue points indicate significantly downregulated metabolites. D1 = control group, D2 = HIS group.
Figure 4. Volcano plots for the potential differential metabolites in the liver of American eels of the OPC group in comparison with the HIS group under positive ion mode (A) and negative ion mode (B). The point size represents the VIP value of the PLS-DA. Pink points indicate significantly upregulated metabolites, and blue points indicate significantly downregulated metabolites. D2 = HIS group, D3 = OPC group.
Table 3. Identification of significant differential metabolites between the HIS group and control group.
Table 4. Identification of significant differential metabolites between the OPC group and HIS group.
The enriched KEGG pathways of significantly differential metabolites in the HIS group compared with the control group and OPC group are shown in Figures 5, 6, respectively. The main pathways in the HIS group compared with the control group were biotin metabolism, arachidonic acid (ARA) metabolism, glutathione (GSH) metabolism, tyrosine metabolism, fatty acid biosynthesis, histidine metabolism, and alanine, aspartate, and glutamate metabolism. The main pathways in the OPC group compared with the HIS group were cysteine and methionine metabolism, beta-alanine metabolism, pantothenate and CoA biosynthesis, pyrimidine metabolism, sulfur metabolism, taurine and hypotaurine metabolism, thiamine metabolism, and GSH metabolism.
Figure 5. The scatter plot of the metabolic pathway impact and enrichment for all matching significant metabolites in the liver of juvenile American eels of the HIS group in comparison with the control group. The colored points represent different metabolic pathways. The various color levels indicate different levels of significance of metabolic pathways from low (white) to high (red). The different sizes of each point are based on the pathway impact values. Moreover, the most relevant pathways’ names of points are labeled according to the significance and impact value of the metabolic pathway.
Figure 6. The scatter plot of the metabolic pathway impact and enrichment for all matching significant metabolites in the liver of juvenile American eels of the OPC group in comparison with the HIS group. The colored points represent different metabolic pathways. The various color levels indicate different levels of significance of metabolic pathways from low (white) to high (red). The different sizes of each point are based on the pathway impact values. Moreover, the most relevant pathways’ names of points are labeled according to the significance and impact value of metabolic pathway.
The most relevant pathways based on the impact value and P-value and their corresponding metabolites in the HIS group compared with the control group and OPC group are shown in Tables 5, 6, respectively. The ARA metabolism, biotin metabolism, and GSH metabolism were characterized as the most relevant pathways in the HIS group compared with the control group. The 5(S)-hydroperoxyeicosatetraenoic acid (5-HPETE), biocytin, and 5-L-glutamyl-L-alanine levels were upregulated in ARA metabolism, biotin metabolism, and GSH metabolism, respectively. The 20-hydroxyeicosatetraenoic acid (20-HETE) was downregulated in ARA metabolism. The cysteine and methionine metabolism, beta-alanine metabolism, pantothenate and CoA biosynthesis, pyrimidine metabolism, and sulfur metabolism were characterized as the most relevant pathways in the OPC group compared with the HIS group. The L-cysteine was upregulated in cysteine and methionine metabolism and sulfur metabolism, and dihydrouracil was upregulated in beta-alanine metabolism, pantothenate and CoA biosynthesis, and pyrimidine metabolism. The dihydrothymine was downregulated in pyrimidine metabolism.
Table 5. The most relevant metabolic pathways and corresponding differential metabolites in the liver of juvenile American eels of the HIS group in comparison with the control group.
Table 6. The most relevant metabolic pathways and corresponding differential metabolites in the liver of juvenile American eels of the OPC group in comparison with the HIS group.
The histamine from feedstuff, one of biogenic amines, has obvious toxicity to some carnivorous fish species; it could not be removed by any methods at present (Lumsden et al., 2002; Li et al., 2018; Hu et al., 2019; Ma et al., 2020). The way it damages the digestive organs was by producing excessive reactive oxygen species to cause oxidative stress (Rada et al., 2013; Ma et al., 2020). The most common way to alleviate oxidative stress in vivo was to scavenge those excessive free radicals by supplementing natural antioxidants in fish diet (Bagchi et al., 2014; Gao et al., 2017). OPC has been widely used as a natural antioxidant in animal feed to counteract stress from different sources (Duong et al., 2016; Shiel et al., 2017; Wang et al., 2018; Zhai et al., 2018; Huang et al., 2019). In the present trial, growth depression and FI decrease were observed in juvenile American eel fed higher dietary histamine (517 mg/kg). Similar results were reported: dietary histamine levels of 414 or 534 mg/kg decreased the WGR of American eel (Hu et al., 2019; Ma et al., 2020). The WGR of rainbow trout was also decreased by supplementing 13.3 g/kg putrescine and 2 g/kg histamine in the diet (Cowey and Cho, 1992). A lower FCR was observed in Atlantic salmon fed a diet containing 169 mg/kg histamine and 195 mg/kg cadaverine (Opstvedt et al., 2000) and Japanese seabass fed a diet with common brown fish meal with a higher histamine level substituting for a high-quality fish meal with an extremely low level of histamine (Hu et al., 2013). Besides, decreased FI was found in the studies of American eel, rainbow trout, and Japanese seabass, which was consistent with the result of the present trial. The depressed growth of those fish species might be related to the damage of the gastrointestinal tract and liver caused by dietary histamine (Lumsden et al., 2002; Li et al., 2018). Compared with the HIS group, the growth performance of juvenile American eel was significantly improved by OPC supplementation. The same alleviation effects on growth performance of OPC supplementation in the diets were also observed in other studies of some stressed fish. Dietary 800 mg/kg OPC could improve the WGR, FCR, and FI in pearl gentian grouper exposed to 300 mg/kg dietary cadmium (Wang et al., 2018), and 400 mg/kg OPC supplementation in the diet could effectively improve the WGR, FCR, and FI and alleviate hepatopancreas oxidative stress of juvenile tilapia exposed to 100 mg/kg dietary cadmium (Zhai et al., 2018). In the study of greenlip abalone under heat stress, the FI was significantly increased by feeding a commercial diet supplemented with OPC (Duong et al., 2016; Shiel et al., 2017).
Blood biochemical parameters have been considered as good indicators of health status in fish (de Pedro et al., 2005; Satheeshkumar et al., 2012; Yu et al., 2019). The higher activities of blood GPT and GOT might indicate the occurrence of liver injury or dysfunction (Wells et al., 1986). In the present study, the significant increase of serum GPT and GOT activities suggested that the liver damage could be caused by histamine. Although there were no statistical differences of serum GPT and GOT activities between the OPC group and HIS group, the increasing trend of GPT and GOT activities indicated that OPC might have a protective effect on liver injury caused by dietary histamine, which was in accordance with the results of studies on tilapia and mice exposed to stress by dietary cadmium (Long et al., 2016; Huang et al., 2019). The levels of TC, TG, LDL-C, and HDL-C were relevant with lipid metabolism; there were no research to study the effects of histamine on serum lipid levels. In the present study, they were not affected by dietary histamine or OPC. Under normal conditions without obvious stress, serum lipid levels could be lowered by OPC supplementation (Bladé et al., 2010; Zhai et al., 2014). Hypolipidemic effects of OPC were not observed in this trial, the reason for which should be clarified in a future study. In comparison with the HIS group, the OPC group showed significantly increased ACP and AKP activities. These two important phosphatase enzymes might take part in several metabolic functions, uptake and transport of nutrients (Guan et al., 2019). AKP was also involved in immune defense mechanisms and immune competence. C3 and IgM played an important role in the immune response in fish (Habte-Tsion et al., 2016). In our study, levels of C3 and IgM were significantly enhanced in American eels fed with OPC supplementation in comparison with the HIS group. The higher activities of ACP and AKP and higher levels of IgM and C3 indicated that OPC might improve the non-specific immunity ability of juvenile American eels.
Metabolomic results of American eel liver showed that high levels of dietary histamine could cause 5-L-glutamyl-L-alanine upregulation, which is a dipeptide composed of gamma-glutamate and alanine, derived from the decomposition of GSH by γ-glutamyl transpeptidase (Hanes et al., 1950; Meister and Tate, 1976). GSH is mainly produced and stored in the liver, and it also acts as a major non-enzymatic antioxidant in cells. The important role of GSH was widely documented as protecting the liver from free radical-mediated oxidative damage (Jia et al., 2015; Coutinho et al., 2017). Therefore, 5-L-glutamyl-L-alanine upregulation might promote GSH metabolism to increase GSH consumption and make the liver vulnerable to injury (Yuan et al., 2015; Chen et al., 2019; Ming et al., 2019). Biocytin was an important end product of the intraluminal digestion of dietary protein-bound biotin (Said et al., 1993). The upregulated biocytin in the HIS group might indicate that more biotin in fish might be utilized and weaken the antioxidant defense in liver by lowering the ability of scavenging free radicals and enzymatic antioxidant capacity (Feng et al., 2014). In addition, 20-HETE was generated from ARA through homolytic cleavage and removal of hydrogen on the pro-S hydrogen at carbon-7 (Radmark et al., 2015); it seemed that 20-HETE might participate in the regulation of liver metabolic activity and hemodynamics (Elshenawy et al., 2017). 5-HPETE was the intermediate from ARA and converted to biologically detrimental leukotrienes (Wang et al., 2019). The downregulated 20-HETE and upregulated 5-HPETE acid from ARA metabolism in the HIS group might disturb liver function and lower the resistance to stress and disease (Bae et al., 2010; Shahkar et al., 2016).
Compared with that in the HIS group, L-cysteine in the OPC group was one of the upregulated differential metabolites, and it could act as the precursor that might promote the synthesis of GSH, which was helpful in detoxifying toxic substances and exerting anti-inflammation effects by its antioxidant ability (Han et al., 1997; O’Donovan and Fernandes, 2000; Lee et al., 2015). In addition, L-cysteine was involved in cysteine and methionine metabolism and sulfur metabolism. Cysteine and methionine are both sulfur-containing amino acids, and sulfur metabolism mainly occurs in hepatic tissues; both have a hepatoprotective activity (Lee et al., 2013, 2015). So the liver health status of American eel in the OPC group might be improved by upregulation of L-cysteine involved in related metabolism pathways; this could be confirmed by the changes in GPT and GOT activities in the serum. A recent study on tilapia showed that the oxidative stress-mediated liver damage by dietary cadmium could be alleviated by OPC supplementation in the diet (Zhai et al., 2018). Another differential metabolite in the OPC group was dihydrouracil related to beta-alanine metabolism, pantothenate and CoA biosynthesis, and pyrimidine metabolism. Dihydrouracil is an intermediate breakdown product from pyrimidine metabolism in the liver; it could be transformed into beta-alanine and then into malonyl CoA by transamination to participate in beta-alanine metabolism and pantothenate and CoA biosynthesis (López-Sámano et al., 2020). Beta-alanine is also one of the pantothenic acid ingredients. Pantothenic acid is a precursor of CoA and could protect the liver from injury caused by oxidative stress (Wojtczak and Slyshenkov, 2003). So the upregulation of dihydrouracil might be beneficial to protect liver from the damage caused by oxidative stress of dietary histamine. Dihydrothymine is an intermediate breakdown product of pyrimidine metabolism; however, it was also believed to represent the presence of potential DNA damage (Dawidzik et al., 2004). Downregulation of dihydrothymine of the OPC group suggested that DNA damage in the liver might be alleviated by the improvements of antioxidant capacity by OPC supplementation. It was found that higher levels of dietary histamine could stimulate the generation of reactive oxygen species to induce oxidative stress (Rada et al., 2013); the changes of differential metabolites and related metabolism pathways in the OPC group indicated that the liver health status might be improved through the OPC’s role as an exogenous antioxidant to maintain oxidant/antioxidant homeostasis under the stress caused by dietary histamine (Pekkarinen et al., 1999; Zhai et al., 2018).
In conclusion, there were counteracting effects of dietary 300 mg/kg OPC supplementation on the negative changes of growth and some serum biochemical parameters caused by 517 mg/kg histamine in the diet of juvenile American eel, with the upregulation of L-cysteine and dihydrouracil involved in cysteine and methionine metabolism, beta-alanine metabolism, sulfur metabolism, pantothenate and CoA biosynthesis, and pyrimidine metabolism and the downregulation of dihydrothymine involved in pyrimidine metabolism in the liver. These results suggested that OPC could effectively counteract the negative effects of dietary histamine on American eel.
All datasets presented in this study are included in the article/supplementary material.
The animal study was reviewed and approved by the Animal Care and Use Committee of Jimei University, Xiamen, Fujian Province, China.
SZ has done the conception of the work, interpretation of the data, and revising of the manuscript. YW was responsible for metabolomics the data analysis and the manuscript draft. YH carried out the major part of practical work, acquisition of the data, and serum parameter measurement. XC instructed the eel culture trial and part of the conception of the work. All authors contributed to the article and approved the submitted version.
This work was financially supported by the China Agriculture Research System (CARS-46) and Science and Technology Key Project of Fujian Province (2018N0020).
The authors declare that the research was conducted in the absence of any commercial or financial relationships that could be construed as a potential conflict of interest.
Bae, J., Kim, D., Yoo, K., Kim, S., Lee, J., and Bai, S. C. (2010). Effects of dietary arachidonic acid (20:4n-6) levels on growth performance and fatty acid composition of juvenile eel, Anguilla japonica. Asian Austral. J. Anim. 23, 508–514. doi: 10.5713/ajas.2010.90491
Bagchi, D., Swaroop, A., Preuss, H. G., and Bagchi, M. (2014). Free radical scavenging, antioxidant and cancer chemoprevention by grape seed proanthocyanidin: an overview. Mutat. Res. 768, 69–73. doi: 10.1016/j.mrfmmm.2014.04.004
Benchetrit, J., and McCleave, J. D. (2016). Current and historical distribution of the American eel Anguilla rostrata in the countries and territories of the Wider Caribbean. ICES J. Mar. Sci. 73, 122–134. doi: 10.1093/icesjms/fsv064
Bladé, C., Arola, L., and Salvadó, M.-J. (2010). Hypolipidemic effects of proanthocyanidins and their underlying biochemical and molecular mechanisms. Mol. Nutr. Food Res. 54, 37–49. doi: 10.1002/mnfr.200900476
Chen, X., Wang, Q., Guo, Z., Zhao, Y., Gao, Y., Yu, T., et al. (2019). Effects of dietary oxidized fish oil on growth performance and antioxidant defense mechanism of juvenile Rhynchocypris lagowski Dybowskii. Aquaculture 512:734368. doi: 10.1016/j.aquaculture.2019.734368
Coutinho, F., Simões, R., Monge-Ortiz, R., Furuya, W. M., Pousão-Ferreira, P., Kaushik, S., et al. (2017). Effects of dietary methionine and taurine supplementation to low-fish meal diets on growth performance and oxidative status of European sea bass (Dicentrarchus labrax) juveniles. Aquaculture 479, 447–454. doi: 10.1016/j.aquaculture.2017.06.017
Cowey, C. B., and Cho, C. Y. (1992). Failure of dietary putrescine to enhance the growth of rainbow trout (Oncorhynchus mykiss). Can. J. Fish. Aquat. Sci. 12, 2469–2473. doi: 10.1139/f92-272
Dawidzik, J. B., Budzinski, E. E., Patrzyc, H. B., Cheng, H. C., Iijima, H., Alderfer, J. L., et al. (2004). Dihydrothymine lesion in X-irradiated DNA: characterization at the molecular level and detection in cells. Int. J. Radiat. Biol. 80, 355–361. doi: 10.1080/09553000410001695877
de Pedro, N., Guijarro, A. I., Lopez-Patino, M. A., Martinez-Alvarez, R., and Delgado, M. J. (2005). Daily and seasonal variations in haematological and blood biochemical parameters in the tench, Tinca tinca Linnaeus, 1758. Aquac. Res. 36, 1185–1196. doi: 10.1111/j.1365-2109.2005.01338.x
Duong, D. N., Qin, J. G., Harris, J. O., Hoang, T. H., Bansemer, M. S., Currie, K., et al. (2016). Effects of dietary grape seed extract, green tea extract, peanut extract and vitamin C supplementation on metabolism and survival of greenlip abalone Haliotis laevigata Donovan cultured at high temperature. Aquaculture 464, 364–373. doi: 10.1016/j.aquaculture.2016.07.011
Elshenawy, O. H., Shoieb, S. M., Mohamed, A., and El-Kadi, A. O. S. (2017). Clinical implications of 20-Hydroxyeicosatetraenoic acid in the kidney, liver, lung and brain: an emerging therapeutic target. Pharmaceutics 9:9. doi: 10.3390/pharmaceutics9010009
Feng, L., Zhao, S., Chen, G., Jiang, W., Liu, Y., Jiang, J., et al. (2014). Antioxidant status of serum, muscle, intestine and hepatopancreas for fish fed graded levels of biotin. Fish. Physiol. Biochem. 40, 499–510. doi: 10.1007/s10695-013-9861-z
Gao, J., Lv, S., Li, C., Tao, J., Jia, C., Jiang, M., et al. (2017). Bioactivity-based antioxidative components screening and evaluation in grape seed proanthocyanidin extract. J. Food Sci. Tech. 54, 2645–2652. doi: 10.1007/s13197-017-2692-7
Guan, D., Sun, H., Meng, X., Wang, J., Wan, W., Han, H., et al. (2019). Effects of different molar mass chitooligosaccharides on growth, antioxidant capacity, non-specific immune response, and resistance to Aeromonas hydrophila in GIFT tilapia Oreochromis niloticus. Fish Shellfish Immun. 93, 500–507. doi: 10.1016/j.fsi.2019.08.001
Habte-Tsion, H. M., Ren, M. C., Liu, B., Ge, X. P., Xie, J., and Chen, R. L. (2016). Threonine modulates immune response, antioxidant status and gene expressions of antioxidant enzymes and antioxidant-immune-cytokine-related signaling molecules in juvenile blunt snout bream Megalobrama amblycephala. Fish Shellfish Immun. 51, 189–199. doi: 10.1016/j.fsi.2015.11.033
Han, D., Sen, C. K., Roy, S., Kobayashi, M. S., Tritschler, H. J., and Packer, L. (1997). Protection against glutamate-induced cytotoxicity in C6 glial cells by thiol antioxidants. Am. J. Physiol. 273, R1771–R1778. doi: 10.1152/ajpregu.1997.273.5.R1771
Hanes, C., Hird, F., and Isherwood, F. (1950). Synthesis of peptides in enzymic reactions involving glutathione. Nature 166, 288–292. doi: 10.1038/166288a0
Hao, R., Wang, Z., Yang, C., Deng, Y., Zheng, Z., Wang, Q., et al. (2018). Metabolomic responses of juvenile pearl oyster Pinctada maxima to different growth performances. Aquaculture 491, 258–265. doi: 10.1016/j.aquaculture.2018.03.050
Hu, J., Ma, D., Chen, X., and Zhai, S. (2019). Effect of dietary histamine on growth performance and muscle quality of American eel (Anguilla rostrata). Feed. Res. 6, 34–37. doi: 10.13557/j.cnki.issn1002-2813.2019.06.009
Hu, L., Yun, B., Xue, M., Wang, J., Wu, X., Zheng, Y., et al. (2013). Effects of fish meal quality and fish meal substitution by animal protein blend on growth performance, flesh quality and liver histology of Japanese seabass (Lateolabrax japonicus). Aquaculture 372-375, 52–61. doi: 10.1016/j.aquaculture.2012.10.025
Huang, L., Lu, J., Chen, X., and Zhai, S. (2019). Effect of grape seed proanthocyanidins on serum biochemical indices and free radical levels in intestine of GIFT tilapia exposed to dietary cadmium stress. Feed. Res. 1, 30–33. doi: 10.13557/j.cnki.issn1002-2813.2019.01.008
Huo, D., Sun, L., Zhang, L., Ru, X., Liu, S., and Yang, H. (2019). Metabolome responses of the sea cucumber Apostichopus japonicus to multiple environmental stresses: heat and hypoxia. Mar. Pollut. Bull. 138, 407–420. doi: 10.1016/j.marpolbul.2018.11.063
Jia, R., Du, J., Cao, L., Liu, Y., Xu, P., and Yin, G. (2015). Hepatoprotective and antioxidant effects of phyllanthin against carbon tetrachloride-induced liver injury in Cyprinus carpio. Aquacult. Int. 23, 883–893. doi: 10.1007/s10499-014-9847-6
Kao, T., Tu, H., Chang, W., Chen, B., Shi, Y., Chang, T., et al. (2010). Grape seed extract inhibits the growth and pathogenicity of Staphylococcus aureus by interfering with dihydrofolate reductase activity and folate-mediated one-carbon metabolism. Int. J. Food Microbiol. 141, 17–27. doi: 10.1016/j.ijfoodmicro.2010.04.025
Lee, S., Han, K., Yabuki, E., Nakamura, Y., Kawakami, S., Shimada, K., et al. (2015). Dietary l-cysteine inhibits d-galactosamine-induced acute liver injury in rats. Food Sci. Biotechnol. 24, 1151–1157. doi: 10.1007/s10068-015-0147-3
Lee, S., Han, K. H., Nakamura, Y., Kawakami, S., Shimada, K., Hayakawa, T., et al. (2013). Dietary L-cysteine improves the antioxidative potential and lipid metabolism in rats fed a normal diet. Biosci. Biotech. Bioch. 77, 1430–1434. doi: 10.1271/bbb.130083
Li, W., Pan, X., Cheng, W., Cheng, Y., Yin, Y., Chen, J., et al. (2018). Serum biochemistry, histology and transcriptomic profile analysis reflect liver inflammation and damage following dietary histamine supplementation in yellow catfish (Pelteobagrus fulvidraco). Fish Shellfish Immun. 77, 83–90. doi: 10.1016/j.fsi.2018.03.036
Long, M., Yang, S., Han, J., Li, P., Zhang, Y., Dong, S., et al. (2016). The protective effect of grape-seed proanthocyanidin extract on oxidative damage induced by zearalenone in kunming mice liver. Int. J. Mol. Sci. 17:808. doi: 10.3390/ijms17060808
López-Sámano, M., Beltrán, L. F. L. A., Sánchez-Thomas, R., Dávalos, A., Villaseñor, T., García-García, J. D., et al. (2020). A novel way to synthesize pantothenate in bacteria involves β-alanine synthase present in uracil degradation pathway. MicrobiologyOpen 9:e1006. doi: 10.1002/mbo3.1006
Lumsden, J. S., Clark, P., Hawthorn, S., Minamikawa, M., Fenwick, S. G., Haycock, M., et al. (2002). Gastric dilation and air sacculitis in farmed chinook salmon, Oncorhynchus tshawytscha (Walbaum). J. Fish Dis. 25, 155–163. doi: 10.1046/j.1365-2761.2002.00346.x
Ma, D., Cai, P., Zhai, S., and Chen, X. (2020). Effect of dietary histamine on growth performance, digestive enzyme activities and antioxidant indices in intestine of juvenile American eels (Anguilla rostrata). Feed Res. 2, 42–45. doi: 10.13557/j.cnki.issn1002-2813.2020.02.012
Ma, Q., Li, P., Hua, Y., Ji, P., Yao, W., and Zhang, X. (2018). Effects of Tao-Hong-Si-Wu decoction on acute blood stasis in rats based on a LC-Q/TOF-MS metabolomics and network approach. Biomed. Chromatogr. 32:e4144. doi: 10.1002/bmc.4144
Meister, A., and Tate, S. S. (1976). Glutathione and related gamma-glutamyl compounds: biosynthesis and utilization. Annu. Rev. Biochem. 45, 559–604. doi: 10.1146/annurev.bi.45.070176.003015
Ming, J., Ye, J., Zhang, Y., Yang, X., Shao, X., Qiang, J., et al. (2019). Dietary optimal reduced glutathione improves innate immunity, oxidative stress resistance and detoxification function of grass carp (Ctenopharyngodon Idella) against microcystin-LR. Aquaculture 498, 594–605. doi: 10.1016/j.aquaculture.2018.09.014
Nie, Y., and Stürzenbaum, S. (2019). Proanthocyanidins of natural origin: molecular mechanisms and implications for lipid disorder and aging-associated diseases. Adv. Nutr. 10, 464–478. doi: 10.1093/advances/nmy118
O’Donovan, D. J., and Fernandes, C. J. (2000). Mitochondrial glutathione and oxidative stress: implications for pulmonary oxygen toxicity in premature infants. Mol. Genet. Metab. 71, 352–358. doi: 10.1006/mgme.2000.3063
Opstvedt, J., Mundheim, H., Nygard, E., Aase, H., and Pike, I. H. (2000). Reduced growth and feed consumption of Atlantic salmon Salmo salar L. fed fish meal made from stale fish is not due to increased content of biogenic amines. Aquaculture 188, 323–337. doi: 10.1016/S0044-8486(00)00343-4
Pekkarinen, S. S., Heinonen, I. M., and Hopia, A. I. (1999). Flavonoids quercetin, myricetin, kaemferol and (+)-catechin as antioxidants in methyl linoleate. J. Sci. Food Agric. 79, 499–506. doi: 10.1002/(SICI)1097-0010(19990315)79:4<499::AID-JSFA204<3.0.CO;2-U
Rada, B., Boudreau, H. E., Park, J. J., and Leto, T. L. (2013). Histamine stimulates hydrogen peroxide production by bronchial epithelial cells via histamine H1 receptor and duox. Am. J. Resp. Cell Mol. 50, 125–134. doi: 10.1165/rcmb.2013-0254OC
Radmark, O., Werz, O., Steinhilber, D., and Samuelsson, B. (2015). 5-Lipoxygenase, a key enzyme for leukotriene biosynthesis in health and disease. Biochim. Biophys. Acta. 1851, 331–339. doi: 10.1016/j.bbalip.2014.08.012
Ricque-Marie, D., Abdo-de La Parra, M. I., Cruz-Suarez, L. E., Cuzon, G., Cousin, M., and Pike, I. H. (1998). Raw material freshness, a quality criterion for fish meal fed to shrimp. Aquaculture 165, 95–109. doi: 10.1016/S0044-8486(98)00229-4
Said, H. M., Thuy, L. P., Sweetman, L., and Schatzman, B. (1993). Transport of the biotin dietary derivative biocytin (n-biotinyl-l-lysine) in rat small-intestine. Gastroenterology 104, 75–80. doi: 10.1016/0016-5085(93)90837-3
Satheeshkumar, P., Ananthan, G., Senthilkumar, D., Khan, A. B., and Jeevanantham, K. (2012). Comparative investigation on haematological and biochemical studies on wild marine teleost fishes from Vellar estuary, southeast coast of India. Comp. Clin. Pathol. 21, 275–281. doi: 10.1007/s00580-010-1091-5
Sato, M., Bagchi, D., Tosaki, A., and Das, D. K. (2001). Grape seed proanthocyanidin reduces cardiomyocyte apoptosis by inhibiting ischemia/reperfusion-induced activation of JNK-1 and C-JUN. Free Radic. Biol. Med. 31, 729–737. doi: 10.1016/S0891-5849(01)00626-8
Shahkar, E., Yun, H., Lee, S., Kim, D., Kim, S., Lee, B. I., et al. (2016). Evaluation of the optimum dietary arachidonic acid level and its essentiality based on growth and non-specific immune responses in Japanese eel, Anguilla japonica. Aquaculture 452, 209–216. doi: 10.1016/j.aquaculture.2015.10.034
Shiel, B. P., Hall, N. E., Cooke, I. R., Robinson, N. A., Stone, D. A. J., and Strugnell, J. M. (2017). The effect of commercial, natural and grape seed extract supplemented diets on gene expression signatures and survival of greenlip abalone Haliotis laevigata during heat stress. Aquaculture 479, 798–807. doi: 10.1016/j.aquaculture.2017.07.025
Tapia-Salazar, M., Smith, T. K., Harris, A., Ricque-Marie, D., and Cruz-Suarez, L. (2001). Effect of dietary histamine supplementation on growth and tissue amine concentrations in blue shrimp Litopenaeus stylirostris. Aquaculture 193, 281–289. doi: 10.1016/S0044-8486(00)00494-4
Tibbetts, S. M., Lall, S. P., and Anderson, D. M. (2000). Dietary protein requirement of juvenile American eel (Anguilla rostrata) fed practical diets. Aquaculture 186, 145–155. doi: 10.1016/S0044-8486(99)00363-4
Wang, N., Liu, Y., Zhao, P., Zhai, S., and Ye, J. (2018). Effects of dietary oligomeric proanthocyanidins supplementation on growth and body composition of pearl gentian grouper exposed to dietary cadmium stress. Feed China 6, 37–40. doi: 10.3969/j.issn.1002-8358.2018.06.014
Wang, T., Fu, X., Chen, Q., Patra, J., Wang, D., Wang, Z., et al. (2019). Arachidonic acid metabolism and kidney inflammation. Int. J. Mol. Sci. 20:3683. doi: 10.3390/ijms20153683
Wells, R. M. G., McIntyre, R. H., Morgan, A. K., and Davie, P. S. (1986). Physiological stress responses in big gamefish after capture: observations on plasma chemistry and blood factors. Comp. Biochem. Phys. A 84, 565–571. doi: 10.1016/0300-9629(86)90366-X
Wojtczak, L., and Slyshenkov, V. S. (2003). Protection by pantothenic acid against apoptosis and cell damage by oxygen free radicals–the role of glutathione. BioFactors 17, 61–73. doi: 10.1002/biof.5520170107
Yu, H., Liang, H., Ren, M., Ji, K., Yang, Q., Ge, X., et al. (2019). Effects of dietary fenugreek seed extracts on growth performance, plasma biochemical parameters, lipid metabolism, Nrf2 antioxidant capacity and immune response of juvenile blunt snout bream (Megalobrama amblycephala). Fish Shellfish Immun. 94, 211–219. doi: 10.1016/j.fsi.2019.09.018
Yuan, X., Zhou, Y., Liang, X., Guo, X., Fang, L., Li, J., et al. (2015). Effect of dietary glutathione supplementation on the biological value of rapeseed meal to juvenile grass carp, Ctenopharyngodon idellus. Aquac. Nutr. 21, 73–84. doi: 10.1111/anu.12142
Zhai, S., and Liu, S. (2013). Effects of dietary quercetin on growth performance, serum lipids level and body composition of tilapia (Oreochromis niloticus). Ital. J. Anim. Sci. 12, 523–527. doi: 10.4081/ijas.2013.e85
Zhai, S., Lu, J., and Chen, X. (2014). Effects of dietary grape seed proanthocyanidins on growth performance, some serum biochemical parameters and body composition of tilapia (Oreochromis niloticus) fingerlings. Ital. J. Anim. Sci. 13, 536–540. doi: 10.4081/ijas.2014.3357
Zhai, S., Zhao, P., Shi, Y., Chen, X., and Liang, Y. (2018). Effects of dietary surfactin supplementation on growth performance, intestinal digestive enzymes activities, and hepatic antioxidant potential of American eel (Anguilla rostrata) elvers. Isr. J. Aquac. Bamid. 70, 1–7.
Keywords: American eel, oligomeric proanthocyanidins, liver, histamine, metabolomic
Citation: Zhai S, Wang Y, He Y and Chen X (2020) Oligomeric Proanthocyanidins Counteracts the Negative Effects of High Level of Dietary Histamine on American Eel (Anguilla rostrata). Front. Mar. Sci. 7:549145. doi: 10.3389/fmars.2020.549145
Received: 05 April 2020; Accepted: 13 August 2020;
Published: 10 September 2020.
Edited by:
Chunnuan Zhang, Henan University of Science and Technology, ChinaReviewed by:
Gabriel Cardenete, University of Granada, SpainCopyright © 2020 Zhai, Wang, He and Chen. This is an open-access article distributed under the terms of the Creative Commons Attribution License (CC BY). The use, distribution or reproduction in other forums is permitted, provided the original author(s) and the copyright owner(s) are credited and that the original publication in this journal is cited, in accordance with accepted academic practice. No use, distribution or reproduction is permitted which does not comply with these terms.
*Correspondence: Shaowei Zhai, emhhaXN3QGptdS5lZHUuY24=
†These authors share first authorship
Disclaimer: All claims expressed in this article are solely those of the authors and do not necessarily represent those of their affiliated organizations, or those of the publisher, the editors and the reviewers. Any product that may be evaluated in this article or claim that may be made by its manufacturer is not guaranteed or endorsed by the publisher.
Research integrity at Frontiers
Learn more about the work of our research integrity team to safeguard the quality of each article we publish.