- 1Institute of Parasitology, Biomedical Research Center Seltersberg (BFS), Justus Liebig University Giessen, Giessen, Germany
- 2Institute of Pathology, Faculty of Veterinary Sciences, Universidad Austral of Chile, Valdivia, Chile
- 3Biomedical Sciences Department, Ross University School of Veterinary Medicine, Basseterre, Saint Kitts and Nevis
- 4Institute of Anatomy and Cell Biology, Justus Liebig University Giessen, Giessen, Germany
- 5Institute of Parasitology, Biology Centre, Czech Academy of Sciences, Ceské Budejovice, Czechia
- 6Swiss Tropical and Public Health Institute, Basel, Switzerland
- 7Faculty of Medicine, University of Basel, Basel, Switzerland
Since late 1970s, the southern Chilean city Valdivia constitutes home for a unique bachelor group of South American sea lions (Otaria flavescens), initially descendant from colonies at the Pacific coast, but now directly living in a freshwater habitat in close proximity to human population and a vast amount of wild and domestic animal species. In the framework of a parasitological monitoring program, 115 individual fecal samples were collected from synanthropic South American sea lions between March and May 2018. For comparative reasons, 79 individual fecal samples from two free-living O. flavescens colonies at the Pacific coast were also sampled. Coproscopical analyses revealed the presence of nine different parasite taxa in individual fecal samples, including two protozoan (Cryptosporidium spp. and Giardia spp.) and seven metazoan parasites (Anisakidae gen. spp., Diphyllobothriidae gen. spp., Ogmogaster heptalineatus, Trematoda indet. type 1, Trematoda indet. type 2, Otostrongylus circumlitus, and Parafilaroides spp.), and morphological and molecular characterizations of adult helminths confirmed identification of following species: Anisakis simplex/A. pegreffi, Pseudoterranova cattani, Contracaecum ogmorhini, and Adenocephalus pacificus. For the first time, the results of the current study show the presence of zoonotic relevant Giardia- and Cryptosporidium-infections in two free-ranging colonies of South American sea lions apart from human settlement. Furthermore, a detailed literature search of previous publications on the endoparasite fauna of South American sea lions was conducted, revealing reports of at least 50 protozoan and metazoan parasite taxa including findings of the current study. Thereby, at least 25 of reported taxa (50%) have been recorded to bear zoonotic potential. The present study illustrates a successful application of non-invasive screening methods and their applicability in the field of marine mammal parasitology, bringing new insights into the endogenous parasite fauna of South American sea lions in Southern Chile, including anthropozoonotic protozoan and metazoan taxa.
Introduction
The South American sea lion (Otaria flavescens) constitutes a representative of the pinniped family Otariidae, the eared seals, and shows a broad geographical extension among Pacific and Atlantic coastlines of South America, including Peru, Chile, Argentina, Uruguay, Southern Brazil and the Falkland Islands (Vaz-Ferreira, 1982). Representing a polygynous species, O. flavescens males can lead harem structures or live together in bachelor groups, which usually occur at the edge of breeding colonies (Cárdenas-Alayza, 2017). Studies on movement patterns of O. flavescens males and females revealed a maximum travel distance of more than 850 km per trip (Campagna et al., 2001), even though South American sea lions do not show a real migratory behavior (Vaz-Ferreira, 1982). Interactions and conflicts with humans were described to mainly occur around salmon breeding farms (Sepúlveda and Oliva, 2005) or during industrial fishery, where sea lions compete with fishing activities trying to prey out of trawling nets (Szteren and Páez, 2002; Hückstädt and Antezana, 2003; De María et al., 2014). Thus, some studies documented South American sea lions as bycatches (Majluf et al., 2002; Reyes et al., 2013; Machado et al., 2015). According to International Union for Conservation of Nature (IUCN), O. flavescens is currently classified as a species of least concern (Cárdenas-Alayza et al., 2016).
Wild sea lion species have previously been reported to adapt to synanthropic lifestyles, e.g., the Galápagos sea lions (Zalophus wollebaeki) in Ecuador (Denkinger et al., 2015), or the Californian sea lions (Z. californianus) at Pier 39 in San Francisco, United States (Heath and Perrin, 2009). Colonies of free-ranging South American sea lions have been also consistently reported to adapt to human populations in the Chilean harbor cities of Valparaíso, Tomé and Talcahuano. In contrast to former colonies, the city of Valdivia harbors the only synanthropic South American sea lion colony worldwide, constantly living in a freshwater habitat 15 km east of the Pacific Ocean since late 1970s (Schlatter, 1976). Initially descendant from colonies at the Pacific coast, approximately 70 adult South American sea lion males are currently living in this anthropogenic ecosystem in closeness to humans and numerous domestic and wild endemic animals, including stray dogs (Canis lupus familiaris), cats (Felis silvestris catus), water birds [i.e., Peruvian pelicans (Pelecanus thagus), cormorants (Phalacrocorax brasilianus) and kelp gulls (Larus dominicanus)], turkey (Carthartes aura), and black vultures (Coragyps atratus).
Synanthropic South American sea lions of Valdivia differ from other free-ranging populations at Pacific coast mainly by their adaptation to anthropogenic stress, including boating-, shipping- and other sport-related activities and traffic on the river Calle-Calle. They also display altered feeding habits. Interspecific interactions occur on a daily basis and are decisive for maintenance of diverse endo- and ectoparasite life cycles in this urban colony (Sepúlveda et al., 2015; Hermosilla et al., 2016a; Cornejo-Galaz, 2017; Ebmer et al., 2019).
Most of the previous studies on the endogenous parasite fauna of free-living O. flavescens focused on helminths and were mainly based on single species reports of nematodes (Baylis, 1933; Carrara, 1952; López-Fernández, 1967; Botto and Mañé-Garzón, 1975; Cattan et al., 1976; Cattan and Carvajal, 1980; George-Nascimento et al., 1992; George-Nascimento and Llanos, 1995; George-Nascimento and Urrutia, 2000; Berón-Vera et al., 2004; Nadler et al., 2013; Timi et al., 2014; Jacobus et al., 2016; Machado-Pereira et al., 2017; González et al., 2018; Pasqualetti et al., 2018), cestodes (Markowski, 1952; Miranda et al., 1968; Cattan et al., 1977; Mondragón-Martínez, 2017), trematodes (Petrov and Chertkova, 1963; Carvajal et al., 1983; Hernández-Orts et al., 2012), and acanthocephalans (Morini and Boero, 1960; Zdzitowiecki, 1986; George-Nascimento and Marin, 1992; Aznar et al., 2006, 2012; Hernández-Orts et al., 2017, 2019). Moreover, several large-scale examinations were also published (Baylis, 1934; Holcman-Spector et al., 1977; George-Nascimento and Carvajal, 1980, 1981; Fernández, 1987; Cabrera et al., 1994; Andrade et al., 1998; Morgades et al., 2006; Pereira, 2012; Hernández-Orts et al., 2013b; Pereira et al., 2013; Calderón-Mayo, 2015; Sepúlveda et al., 2015; Seguel et al., 2018; Naupay et al., 2019) (Table 1). However, the vast majority of these studies were carried out during pathological dissections of stranded animals. Considering conservation aspects, we recently published two non-invasive fecal sample monitoring studies on parasites of South American sea lions, detecting also zoonotic relevant protozoan parasites such as Giardia, Cryptosporidium, Neobalantidium, and Entameba for first time (Hermosilla et al., 2016a, 2018b). Up to date, knowledge on protozoan parasites infecting urban and Pacific South American sea lions remains very scarce. Furthermore, detection of adult helminths and lungworms constitutes a major challenge in the field of non-invasive wildlife parasitology. Hermosilla et al. (2018b) already showed the possibility of pronounced morphological and molecular analyses of spontaneously excreted cestodes and mentioned the importance of expansion of these sampling techniques to extend knowledge on the parasite fauna of these animals.
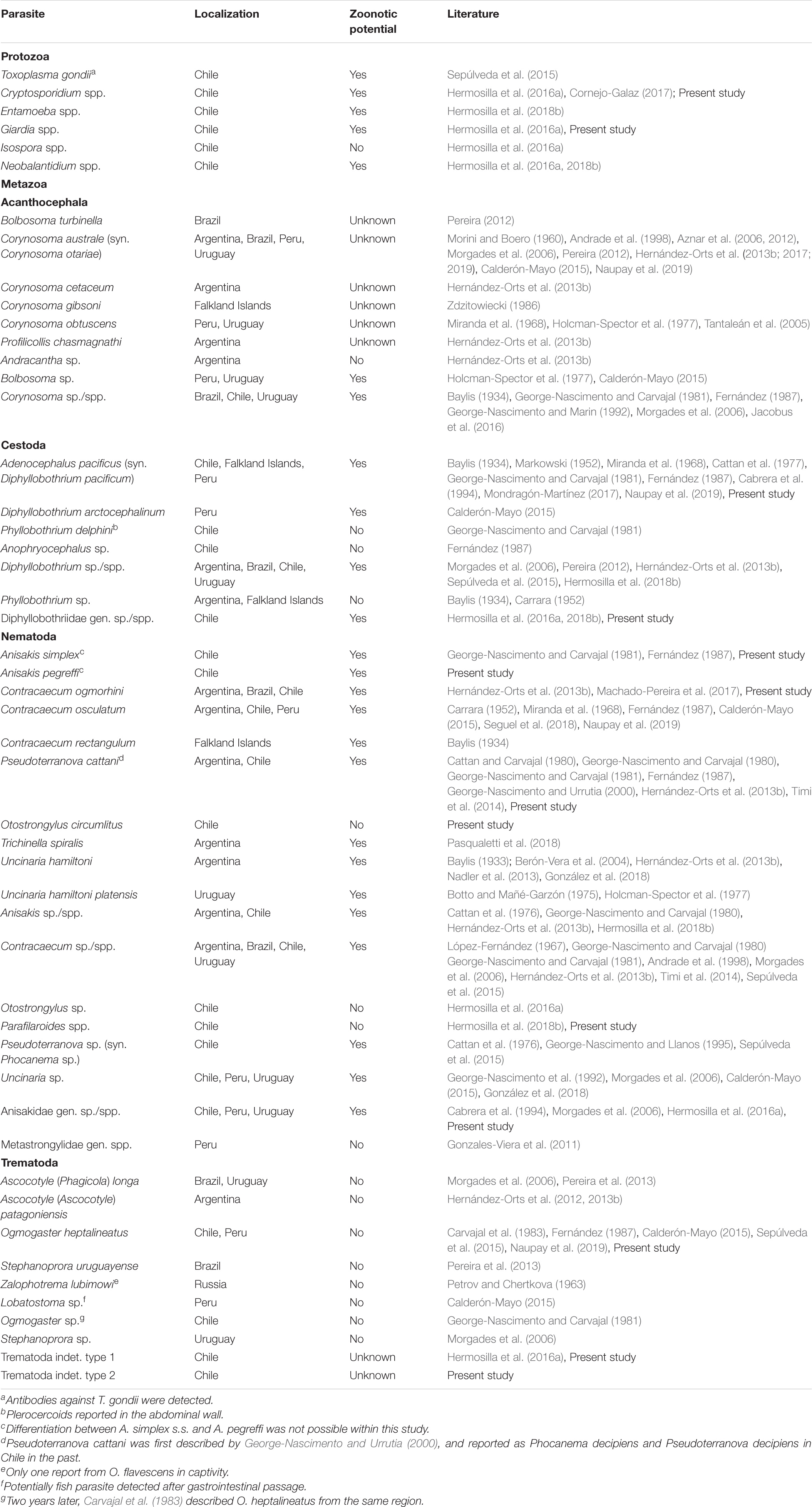
Table 1. List of endoparasites reported from South Americans sea lions (Otaria flavescens), including findings of present study.
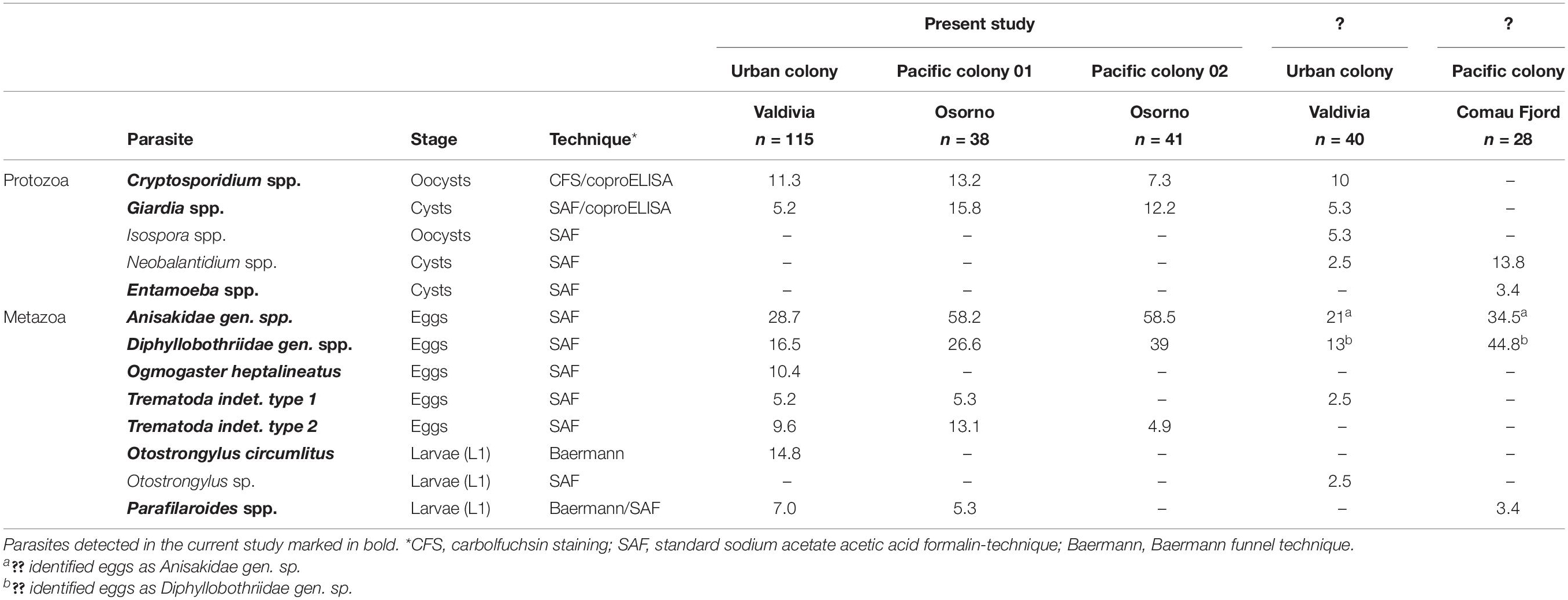
Table 2. Prevalences (%) of protozoan and metazoan endoparasites detected in Otaria flavescens scat samples within this study, including comparison with previous non-invasive fecal sample monitoring studies from Chile.
In the framework of One Health, the present study aimed to investigate the endogenous parasite fauna of a synanthropic South American sea lion colony with special emphasis on anthropozoonotic parasitoses and compared them to parasites circulating in O. flavescens colonies occurring off the Pacific coast. In order to give an overview of the current scientific knowledge of the endoparasite fauna of O. flavescens, a detailed literature search was conducted and previously published reports were listed.
Materials and Methods
Study Areas and Sample Collection
Between March and May 2018, three different free-ranging colonies of South American sea lions (O. flavescens) were investigated in Southern Chile to gain data on their endoparasite fauna. In the city of Valdivia (39° 48′ 46.84′′ S, 73° 14 54.212′′ W; 14th region of Chile: Región de Los Ríos) a special synanthropic colony of South American sea lion males living along freshwater river Calle-Calle (Figures 1, 2) was studied representing a continuation of the previous parasitological monitoring with focus on anthropozoonotic parasite species (Hermosilla et al., 2016a). Sample collection was carried out through non-invasive fecal sample monitoring following recent studies that utilized a stress-reduced methodology in parasitological research of sea lions (Hermosilla et al., 2016a, 2018b). In total, 115 individual fecal samples were collected at resting places frequented by South American sea lions ashore, such as construction areas (former riverside dock), the local fish market, and floating platforms on the river Calle-Calle.
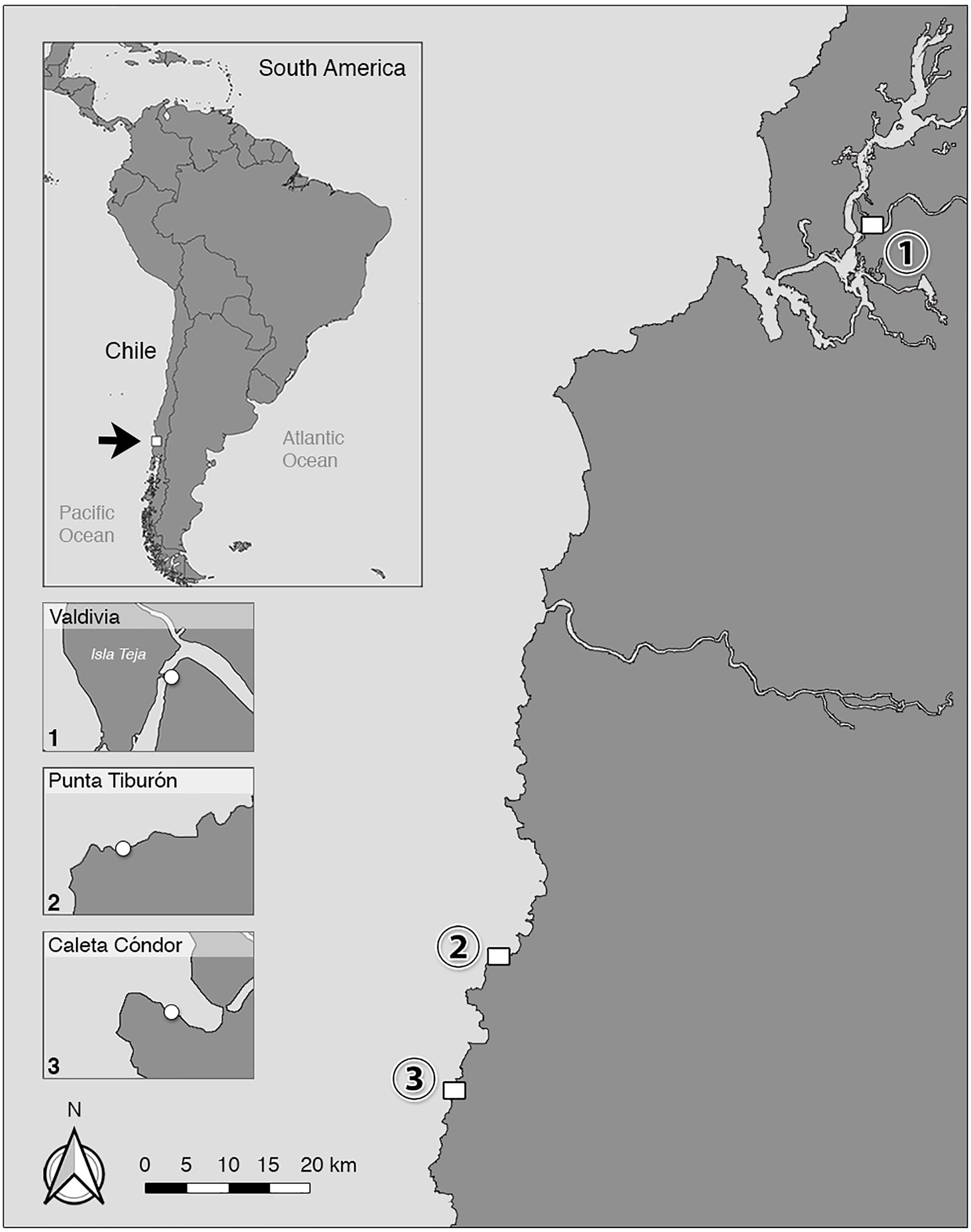
Figure 1. Locations of three different colonies of South American sea lions (Otaria flavescens) in Chile probed in this study. Fecal samples were collected from individuals of an “urban” O. flavescens colony in (1) Valdivia (39° 48′ 46.84′′ S, 73° 14′ 54.212′′ W; Región de Los Ríos), and from two colonies at the Pacific coast located in (2) Punta Tiburón (40° 37′ 58.073′′ S, 73° 47′ 43.828′′ W) and (3) Caleta Cóndor (40° 46′ 23.581′′ S, 73° 51′ 7.761′′ W; Región de Los Lagos).
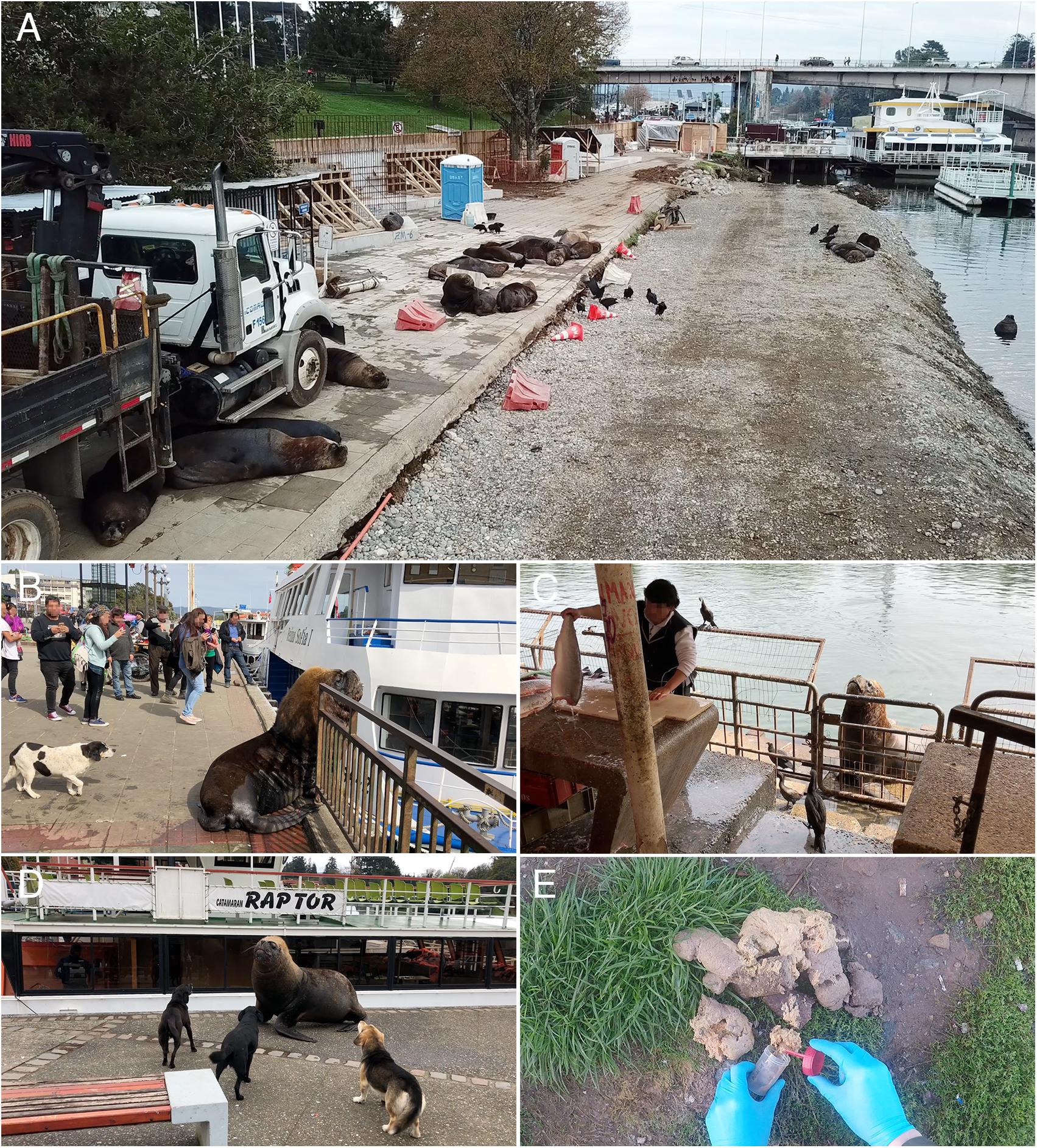
Figure 2. “Urban” colony of South American sea lions living in the city of Valdivia, Chile. (A) Individuals resting at a construction site located at river Calle-Calle in immediate proximity to construction material, a truck and a portable toilette. (B) Dominant Otaria flavescens male resting at main square in front of humans and a stray dog. (C) Sea lion waiting for fish remains infront of the local fish market of Valdivia surrounded by cormorants and black vultures. (D) Three stray dogs attacking a sea lion individual at the pier. (E) Sampling of sea lion feces within the city of Valdivia.
Wild O. flavescens colonies allocated in the Province of Osorno, Chile (10th region: Región de Los Lagos) were further sampled within this study and chosen as comparison group. Therefore, a boat trip to two different O. flavescens colonies was conducted and scat samples were collected near Punta Tiburón (n = 38 individual samples; 40° 37′ 58.073′′ S, 73° 47′43.828′′ W) and Caleta Cóndor (n = 41 individual samples; 40° 46′ 23.581′′ S, 73° 51′ 7.761′′ W), both located approximately 120 km south of the city of Valdivia. Both sea lion colonies consisted mainly of females and juveniles and were traced and observed in rugged shores using a camera drone (DJI Mavic Pro®, Shenzhen, China). When we entered the cliffs, some animals immediately escaped into the water but others (mainly adult males) remained on the rocks.
Whenever possible, excrements of urban sea lions were immediately collected after spontaneous defecation and registered to individual animals. In general, only well defined feces were collected and classified as individual samples (Figure 2E), whereas indistinct zoned and greased excrements were not included within this study. Sufficient distances were kept at any time of sampling and documentation procedures by digital drone- and GoPro®-cameras.
Coproscopical Analyses
After collection, fecal samples were stored in plastic tubes at 4°C at the Institute of Pathology of the University Austral of Chile (UACH). Upon arrival to UACH, parts of collected feces were also preserved in 80% ethanol for detailed diagnosis of protozoan and helminth parasites. The ethanol-fixed scat samples were stored in 2 ml eppendorf tubes and according to official cooperation agreement transported to the Institute of Parasitology at the Justus-Liebig-University Giessen (JLU), Germany, for further coproscopical analyses including standard sodium acetate acetic acid formalin (SAF) technique for detection of protozoan cysts, trophozoites and oocysts, and also helminth eggs according to Yang and Scholten (1977). Parasite stages were determined using light microscopy (Olympus BH-52®) following diagnostic guides published before (Hermosilla et al., 2016a, 2018b). Furthermore, presence of zoonotic-relevant protozoan parasites was determined using different methods, namely carbolfuchsin fecal smears (CFS) for detection of oocysts of Cryptosporidium spp. (according to Heine, 1982), as well as coproantigen ELISAs (ProSpecT®, Oxoid) for detection of antigens of Giardia and Cryptosporidium. Fresh individual scat samples were also immediately used for Baermann funnel technique to detect first stage lungworm larvae (L1) of Otostrongylus circumlitus and Parafilaroides spp. according to Bowman (1995), as well as for sieving processes to isolate pre-adult and adult helminth specimens. For Baermann funnel technique, feces were grouped into clusters of five samples each. Thereby, in total 25 g of sea lion feces per cluster were swathed into two layers of gauze, put into a sieve (mesh size 100 μm) and added into a Baermann apparatus. Afterward, sample was spilled with tap water until half of gauze was covered with water. After 24 h at room temperature (RT), the clamp of the apparatus was carefully opened placing two to three drops on a microscope glass coverslip and analyzed microscopically for presence of lungworm L1. Positive cluster samples were re-examined individually. Morphology as well as morphometry of lungworm L1 was used to differentiate O. circumlitus from Parafilaroides species (Measures, 2001).
Using dissection needles, macroscopically observable nematodes and cestodes were immediately extracted out of fresh feces during field expeditions and preserved in 80% ethanol in order to retain good morphological condition for further morphological and molecular investigation and final species identification (Figure 3). Sieving of feces was conducted by using metal sieves (Rems, Germany) with a 50 μm pore mesh size under continuous tap water washings.
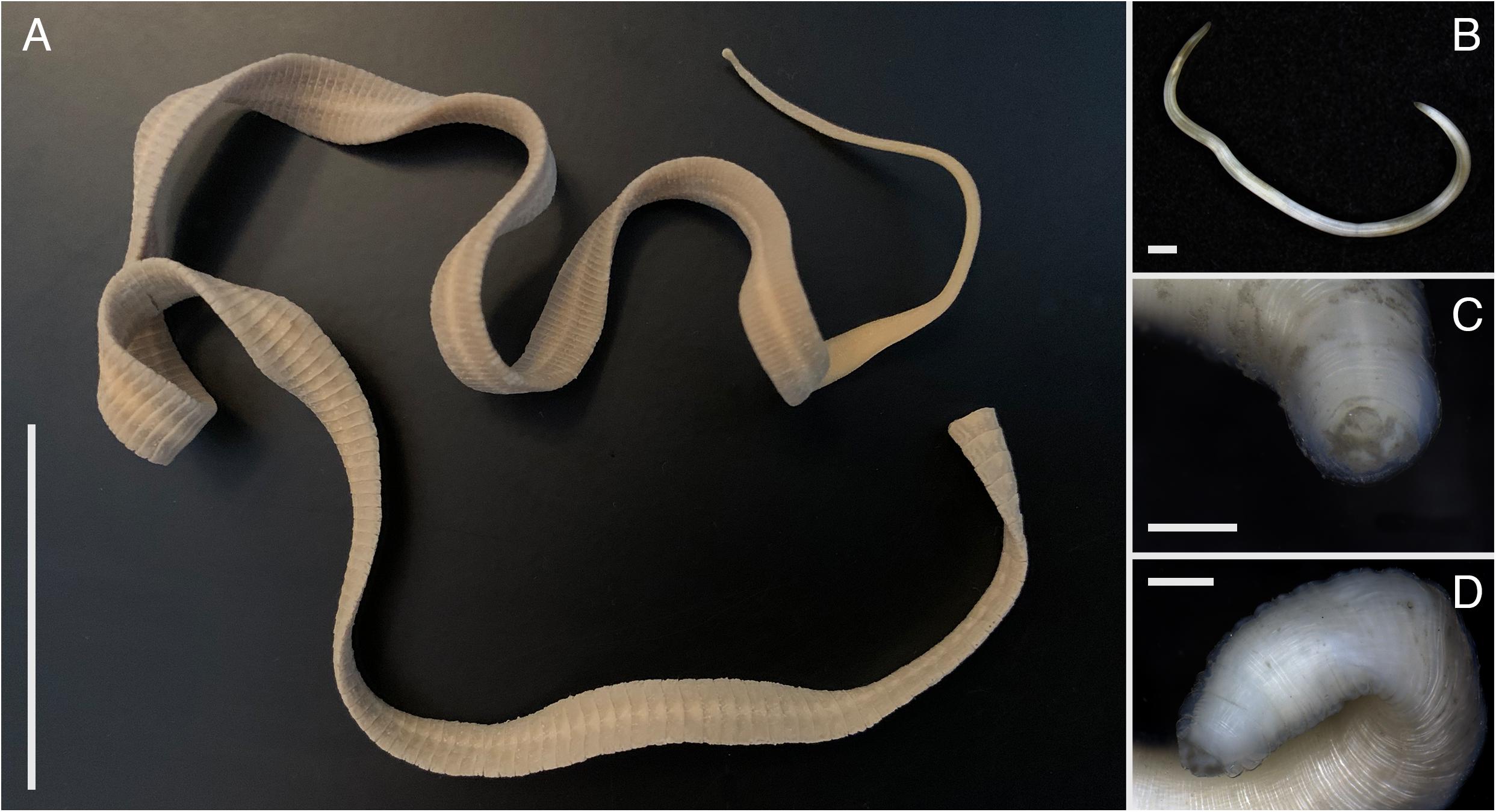
Figure 3. Helminths isolated out of Otaria flavescens feces: (A) cestode Adenocephalus pacificus, (B–D) specimes of Anisakidae nematodes. Scale bars: (A) 4 cm, (B) 1 mm, (C,D) 500 μm.
Preparation of Ogmogaster heptalineatus-Eggs for Scanning Eelectron Microscopy (SEM) Analyses
Droplets isolated out of O. heptalineatus-SAF positive scat samples were deposited on orbital glass coverslips [10 mm in diameter (Nunc) and previously pre-coated with poly-L-lysine (Sigma-Aldrich)] according to Hermosilla et al. (2018a). Thereafter, samples were fixed in glutaraldehyde (2.5%; Merck), post-fixed in osmium tetroxide (1%; Merck), washed in distilled water, dried through CO2 critical point procedure and sputter coated with gold particles. Prepared samples were then examined on Philips XL30® (Amsterdam, Netherlands) scanning electron microscope allocated at the Institute of Anatomy and Cell Biology (Justus Liebig University Giessen, Germany).
Morphological and Molecular Analyses of Adult Anisakid Nematodes and a Cestode
Anisakid nematodes and diphyllobothriid cestodes isolated through sieving were first photographed using a stereo microscope (Nikon SMZ25®, Tokyo, Japan). For molecular identification, proglottid of cestode and small pieces of nematode tissues were obtained from the middle part in order to preserve anterior and posterior parts of nematodes for morphological analyses and preservation as voucher specimens. Molecular identification of anisakids was conducted through PCR amplification and sequencing of a 464 bp sequence of the mitochondrial cytochrome c oxidase gene (COI) as described previously (Bowles et al., 1992).
Molecular characterization of the cestode parasite followed previously published protocols. Amplification of both the partial nuclear large subunit ribosomal RNA gene (lsrDNA) and the complete COI followed Hernández-Orts et al. (2015). Assembled contiguous lsrDNA and COI sequences were aligned with the original datasets from Waeschenbach et al. (2017) and Hernández-Orts et al. (2015), respectively. Maximum likelihood trees of individual gene datasets were estimated in IQ-TREE 1.6.8, employing the substitution models TIM3 + F + I + G4 (lsrDNA) and TIM2 + F + I + G4 (COI), chosen according to the corrected Akaike information criterion. Pairwise genetic p-distances were calculated from the total number of nucleotide differences from the lsrDNA alignment limited to Adenocephalus pacificus and its closely related species. Diphyllobothrium Scoticum and of the Full COI Alignment Excluding Partially Characterized Sequences.
Ethics Statement
Ethical review and approval was not required for the animal study due to the non-invasive sampling techniques applied in this study. Sample collection was conducted in accordance to the Institutional Ethic Commission and Animal Care Committee of the University Austral of Chile (Valdivia, Chile) and to current Chilean Animal Welfare Legislation.
Results
Coproscopical Analyses
Coproscopical analyses of fecal samples of different colonies of South American sea lions in Chile detected a wide spectrum of cysts, oocysts, eggs, and lungworm L1 of endogenous parasites. In total nine different taxa, including stages of two protozoan and seven helminth parasites were found. Complete list of parasite species found in the current and previous studies is given in Tables 1–3, including applied methodology and corresponding prevalences. Selected light microscopy images of parasites found in this survey are shown in Figures 3, 4.
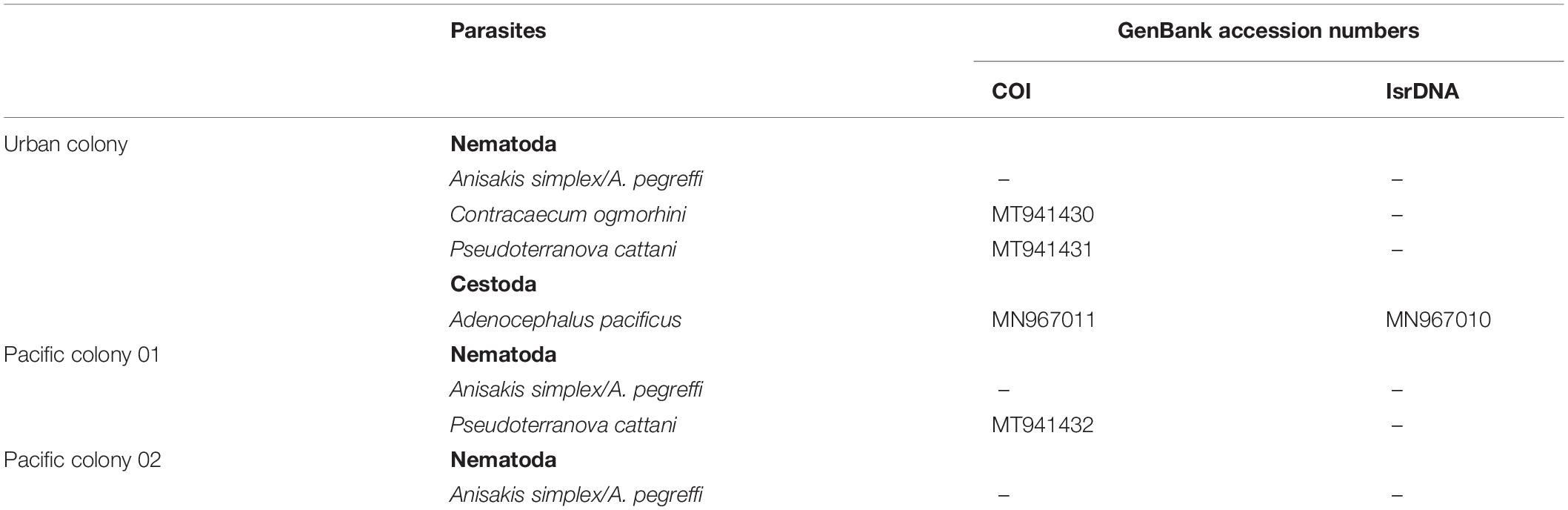
Table 3. Molecular identification of nematodes and cestodes isolated from Otaria flavescens fecal samples within this study.
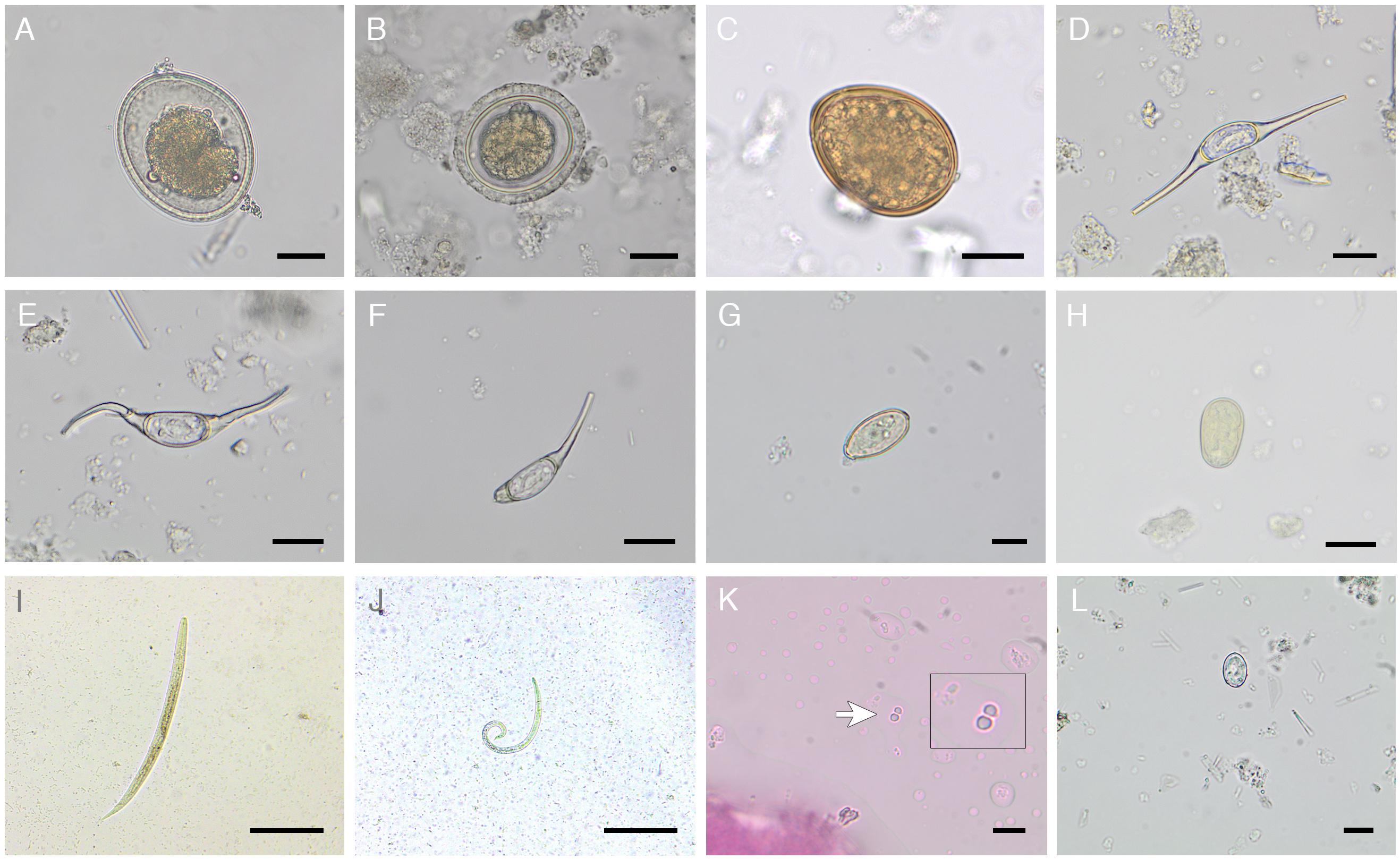
Figure 4. Illustration of metazoan and protozoan parasite stages detected within this study. Eggs of (A,B) Anisakidae gen. spp. varying in shell thickness, (C) Diphyllobothriidae gen. spp., Ogmogaster heptalineatus showing (D) intact morphology, (E) flexibility of filaments and (F) broken filament, (G) Trematoda indet. type 1, and (H) Trematoda indet. type 2. First-stage larvae of (I) Otostrongylus circumlitus and (J) Parafilaroides spp. (K) Cryptosporidium spp. oocysts, (L) Giardia spp. cyst. Scale bars: (A–H) 20 μm, (I,J) 100 μm, (K,L) 10 μm.
Within the urban South American sea lion colony, the most prevalent gastrointestinal parasite stages found were Anisakidae gen. spp.-eggs (28.7%; Figures 4A,B) and Diphyllobothriidae gen. spp.-eggs (16.5%; Figure 4C), followed by characteristical Ogmogaster heptalineatus-eggs (10.4%; Figures 4D–F). Two further trematode taxa were identified as Trematoda indet. type 1 (5.2%; Figure 4G) and Trematoda indet. type 2 (9.6%; Figure 4H). By Baermann funnel technique, pinniped lungworm L1 of Otostrongylus circumlitus (14.8%; Figure 4I) and Parafilaroides spp. (7.0%; Figure 4J) were identified based on their morphology and size. O. circumlitus-L1 presented a slightly bent shape with a conically tapering end and had a mean size of 345.97 × 15.78 μm (322.01–368.57 × 14.90–16.17). Conversely, L1 of Parafilaroides spp. were clearly smaller, exhibited a bent posterior part of the body ending in pointed shape and reaching a mean size of 243.44 × 10.82 μm (223.14–265.04 × 10.13–11.70). In two fecal samples (1.7%), a co-infection of O. circumlitus and Parafilaroides spp. was verified.
The highest protozoan parasite prevalence was detected for Cryptosporidium spp. oocysts/antigens (11.3%; Figure 4K), followed by Giardia spp. cysts/antigens (5.2%; Figure 4L). Giardia and Cryptosporidium prevalences were confirmed via SAF-technique, CFS, and coproantigen ELISAs.
Analyses of fecal samples of O. flavescens collected at Pacific coast revealed the following results: The most prevalent helminth parasites detected in Pacific colony 01 were anisakids (58.2%) and Diphyllobothriidae gen. spp. (26.6%), followed by eggs of Trematoda indet. type 2 (13.1%), Trematoda indet. type 1 (5.3%), and Parafilaroides spp.-L1 (5.3%). Regarding protozoan parasites, most prevalent genus was Giardia (15.8%), followed by Cryptosporidium (13.2%).
Helminths detected in the Pacific colony 02 were the followings: anisakids (58.5%), Diphyllobothriidae gen. spp. (39%), and Trematoda indet. type 2 (4.9%). The most prevalent protozoan parasite in Pacific colony 02 was Giardia (12.2%), followed by a lower Cryptosporidium (7.3%) prevalence. Neither O. circumlitus- nor Parafilaroides spp.-L1 were detected in the Pacific O. flavescens colony 02.
In total, four out of all parasite taxa detected bear an anthropozoonotic potential: Anisakidae gen. spp., Diphyllobothriidae gen. spp., Cryptosporidium spp., and Giardia spp.
Morphological Analysis of Ogmogaster heptalineatus-Eggs
Light microscopy of O. heptalineatus-eggs allowed parasite identification to the species level according to Carvajal et al. (1983) and showed heterogeneity of filament morphology (Figures 4D–F). SEM analysis brought first ultrastructural insights in special egg morphology and filament windings (Figures 5A,B). For measurements, eggs of O. heptalineatus were morphologically divided in a corpus section and plait-like filaments arising from each egg pole, respectively. Corpus of eggs showed an oval stretched shape (Figure 5A) exhibiting a mean size of 22.72 × 11.55 μm (21.69–23.39 × 11.26–11.80), while attached elongated filaments had a mean size of 30.29 μm (27.38–34.28) each. Filaments exhibited flexible composition and no stiff structure (Figure 4E). In some cases, eggs with broken filaments were found (Figure 4F). Both filaments were equal in length and consisted of various wisps originating broad-based from each egg pole, which were frequently entangled with increasing length of filament (Figure 5B).
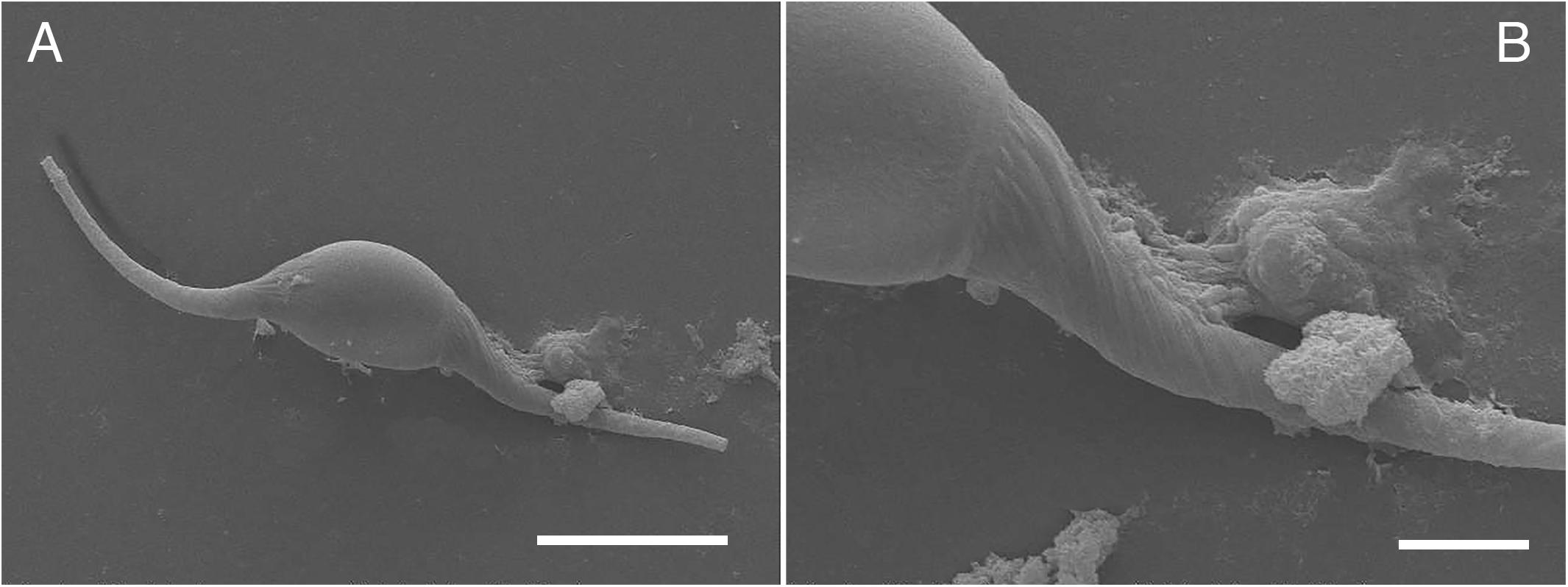
Figure 5. Scanning electron microscopic images of eggs of Ogmogaster heptalineatus. (A) Overview of closed egg exhibiting filaments on both egg poles, (B) detailed view of emanating filament composed of various intertwined wisps. Scale bars: (A) 20 μm, (B) 5 μm.
Molecular Identifications of Nematode and Cestode Species
Molecular analyses by partial sequence determination of the mitochondrial COI and BLAST of the corresponding sequences in GenBank® revealed sequences with 99% homology or more to Pseudoterranova cattani and to Contracaecum ogmorhini. In addition, sequences with a homology of 98% or more to A. simplex and A. pegreffi were found, with no higher homology to any sequences in GenBank®. Sequences belonging to A. simplex/A. pegreffi could not be further differentiated.
All species were found in the feces of urban O. flavescens, while only A. simplex/A. pegreffi and P. cattani were detected in scat samples at Pacific colonies (Table 3).
Analyses based on sequences amplified from the genomic DNA extracted from a cestode strobila fragment of “urban” O. flavescens confirmed the cestode species identity as Adenocephalus pacificus (Figure 3). The lsrDNA sequence was identical with all the other lsrDNA sequences of A. pacificus available (length of the alignment 1,415 bp), whereas it showed a 1.55% divergence from the closest related species of A. pacificus known, Diphyllobothrium scoticum. The phylogenetic analysis based on the COI alignment (1,563 bp; results not shown) unequivocally placed the Chilean specimen within the lineage of A. pacificus, sister to a specimen of A. pacificus from a human infection in Peru (GenBank® Accession KR269743). Pairwise uncorrected p-distances between the Chilean and the remaining specimens of A. pacificus in the same dataset ranged from 0.64 to 2.69%, standing in a strong contrast to the 15.4% nucleotide divergence from the closest COI sequence of the diphyllobothriidean Diphyllobothrium balaenopterae.
Literature Search
Including findings of the present study, at least 50 endoparasite taxa (six protozoan and 44 metazoan taxa) associated with O. flavescens could be detected. Thereby, 25 taxa (one protozoan and 24 metazoan taxa) could be identified to species level, while 20 parasites (five protozoan and 15 metazoan taxa) were identified to genus level. In total, at least 25 of 50 endoparasite taxa (50%) have previously been reported to bear zoonotic potential. Taxonomy and latest classification of endoparasite names and synonyms were obtained using WoRMS Editorial Board (2020) (Table 1).
Discussion
In the last years, development and application of non-invasive sampling techniques in the field of parasitology of marine mammals have impressively expanded the possibilities of examining the endo- and ectoparasite fauna of free-ranging animals in vivo, while clearly leaving the animals unmolested in their natural habitats (Kleinertz et al., 2014; Hermosilla et al., 2015; Hermosilla et al., 2016a, b, 2018a, 2018b; de Vos et al., 2018; Ebmer et al., 2019; Vélez et al., 2019). Thereby, the present study exhibits advantages and usefulness of applied non-invasive scat collection methods, showing their applicability in the field of marine mammal parasitology.
The current study presents a detailed overview of previous publications, and simultaneously extends knowledge on the (anthropozoonotic) endoparasite fauna of South American sea lions. Coproscopical analyses revealed presence of nine different parasite taxa in individual fecal samples, including two protozoan and seven metazoan parasites, while morphological and molecular characterizations of adult helminths added the diagnosis of at least four more species, covering a wide range of parasites and corresponding well to previous reports (Hernández-Orts et al., 2013b; Hermosilla et al., 2016a, 2018b). Overall, at least 50 protozoan and metazoan endoparasite taxa have been recorded for O. flavescens so far (Table 1), while at least 25 of these taxa (50%) have to be considered as zoonotic pathogens.
Current applied coproscopical techniques (i.e., SAF, carbolfuchsin-stained fecal smears, Giardia/Cryptosporidium coproantigen ELISAs) reinforced proper diagnosis of the most common parasite stages (e.g., cysts, oocysts, and eggs) in marine mammal scat samples (Kleinertz et al., 2014; Hermosilla et al., 2015, 2016a, b, 2018a, b; Vélez et al., 2018, 2019). Furthermore, sieving- and Baermann funnel-methods of freshly shed feces permitted detection of spontaneously excreted adult ascarids and cestodes (scolex and strobila) as well as detection of O. circumlitus/Parafilaroides spp.-L1. During sample-taking process, feces were carefully searched for macroscopically visible adult helminths, which were immediately extracted and preserved in 80% ethanol to protect morphological features. Together with sieving of feces in the labratory, this sample-taking technique constitutes a crucial possibility to obtain spontaneously excreted adult helminths in good morphological condition in the field of non-invasive wildlife parasitology for a broad spectrum of possible analyses (Hermosilla et al., 2018b).
Overall, the highest prevalences found in the synanthropic South American sea lion colony were recorded for eggs belonging to the helminth families Anisakidae (28.7%) and Diphyllobothriidae (16.5%), which is in good accordance to previously reported prevalences (21 and 13%, respectively) detected within the same population (Hermosilla et al., 2016a). In contrast, O. flavescens from Pacific coast colonies showed much higher prevalences for these two helminth groups [anisakids (58.2/58.5%) and diphyllobothriids (26.6/39%)]. These high helminth prevalences are in accordance to high prevalences detected in scat samples of O. flavescens collected in the Comau Fjord in Northern Patagonia, Chile (Hermosilla et al., 2018b). In this former study a prevalence of 34.5 and 44.8% were found for anisakid nematodes and diphyllobothriid cestodes. Interestingly, urban South American sea lions differ from their conspecifics in their food spectrum, and this could explain diverging parasite prevalences. Since natural foraging behavior of urban sea lions is less manifested than in animals from Pacific coastal areas, feedings of sea lions by marketeers and eating mainly fish remains and human waste at Valdivia’s fish market constitute basic food resources for members of this colony. Fish remains are mainly composed of heads and dorsal and caudal fins of various species of marine fish, which are not reported as manifestation sites of plerocercoids of Diphyllobothriidae or infective third larvae of Anisakidae within intermediate or paratenic hosts (Dezfuli et al., 2007; Moravec, 2009; Hernández-Orts et al., 2013a). Consistently, anisakid larvae have been reported to occur in different marine fish tissues sold at markets in Valdivia (Torres et al., 2000).
Irrespective of prevalence levels, both helminth parasites exhibit considerable anthropozoonotic potential worldwide, and should be considered as neglected zoonoses (Chai et al., 2005). All anisakid species found in this study (A. simplex/A. pegreffi, C. ogmorhini, and P. cattani) represent etiological agents of human anisakiasis (Acha and Szyfres, 2003). Humans constitute accidental hosts for these parasites ingesting infectious third-stage larvae (L3) via raw or insufficient cooked fishes or cephalopods (Chai et al., 2005). The A. simplex sensu lato (s.l.) complex is composed of three species, namely A. simplex sensu stricto (s.s.), A. pegreffi, and A. berlandi (Abattouy et al., 2016; Llorens et al., 2018). Since hybridization needs to be considered in areas of sympatry, differentiation between A. simplex s.s. and A. pegreffi is difficult (Abattouy et al., 2016). Focusing on molecular characterization based on mitochondrial COI, differentiation between A. simplex s.s. and A. pegreffi was not possible within the current study. The complex P. decipiens constitutes a species group composed of at least six species differing in their geographic localization (Hernández-Orts et al., 2013a). P. cattani solely occurs in the Southern Hemisphere and was recently reported as emerging infection in Chile causing oropharyngeal pseudoterranovosis in humans (Torres et al., 2007; Weitzel et al., 2015). The majority of these cases was reported to be related to consumption of raw fish dishes, e.g., ceviche, which is in accordance to other regions of South America (Menghi et al., 2020). Anisakid larvae belonging to the genus Pseudoterranova were also found in ceviche dishes sold at Valdivian fish market (Torres-Frenzel and Torres, 2014).
Diphyllobothriosis constitutes one of the most neglected fish-borne zoonosis worldwide and is caused by adult species of the genus Dibothriocephalus, Diphyllobothrium, and Adenocephalus parasitizing mainly piscivorous birds and mammal host species (Chai et al., 2005; Scholz et al., 2009; Kuchta et al., 2015). Infectious stage for final hosts (including humans), the plerocercoid, is located in different tissues of anadromous, freshwater and marine fishes, which are representing the second-intermediate- or paratenic hosts (Chai et al., 2005; Scholz et al., 2009). Thereby, Adenocephalus pacificus (syn. Diphyllobothrium pacificum) constitutes the most abundant anthropozoonotic cestode species occurring in South America (Kuchta et al., 2015) and was reported for Otaria flavescens in Chile (Cattan et al., 1977) and Peru (Mondragón-Martínez, 2017; Naupay et al., 2019), where most human cases occur (Kuchta et al., 2015). Several human infections were also described for Chile (Mercado et al., 2010).
Anthropozoonotic protozoan parasites of the genus Giardia and Cryptosporidium were already reported during the first parasitological monitoring in Valdivias “urban” colony (Hermosilla et al., 2016a). While both water-borne parasites were already described for other free-living sea lion species, e.g., the Californian sea lion (Zalophus californianus) (Deng et al., 2000) and the Australian sea lion (Neophoca cinerea) (Delport et al., 2014), the results of the current study showed the presence of Giardia and Cryptosporidium in two free-ranging colonies of South American sea lions apart from human settlement for first time. Interestingly, Giardia and Cryptosporidium were found at a higher prevalence in free-ranging colonies than in the urban colony showing that these anthropozoonotic protozoan parasites already circulate in sea lion colonies located at the Chilean pacific coast. Up to date, the “urban” sea lion colony is still staying in interchange with other O. flavescens colonies at the Pacific coast, and younger males could return to breeding colonies. Beside contamination of river Calle-Calle with environmental resistant Giardia-cysts and Cryptosporidium-oocysts, it is conceivable that these parasite stages might be transported out of the city by regular annual migration during mating season. More importantly, human studies confirmed presence of G. intestinalis not only in different age classes of patients in Valdivia but also in school children living in coastal localities nearby Valdivia (Torres et al., 1992; Navarrete and Torres, 1994) associated with inadequate sanitary installations. South American sea lions most probably become infected from cysts and/or oocysts derived from sewage waters or by livestock or vice versa. Consistently, in the city of Valdivia, direct and indirect interspecific contact between humans, sea lions and a vast amount of domestic and wildlife animal species constitutes a crucial factor for transmission of parasite stages and maintenance of zoonotic parasite life cycles within the One Health concept.
Additionally, pinniped-specific parasites were also found such as the intestinal trematode Ogmogaster heptalineatus. O. heptalineatus belongs to the family Notocotylidae and was firstly described in 1983 from South American sea lions in Chile (Carvajal et al., 1983). Considering the fact that Sepúlveda et al. (2015) already showed the presence of O. heptalineatus within the urban sea lion colony by obtaining adult specimens during dissection of a sea lion carcass, Ogmogaster eggs detected within this study were determined on species level using morphological description (Carvajal et al., 1983). Eggs of O. heptalineatus are characterized by plait-like filaments, which arise from each egg pole and are distinctly shorter when compared with eggs of other marine Ogmogaster species, e.g., O. trilineatus (Rausch and Rice, 1970). Regarding the role of similar appendices manifested by the notocotylid species Notocotylus attentuatus, it was hypothesized that these filaments might be essential for forming egg clusters and be more resistant to cecal peristalsis than single eggs (Graczyk and Shiff, 1993). Moreover, Dönges (1962) supposed that filaments of N. ralli constitute a helpful tool for floating in different depths of water thereby exhibiting a higher chance of ingestion by adequate mollusk intermediate hosts, whereas life cycle is not completely explored yet. Interestingly, O. heptalineatus eggs were solely present in feces of “urban” sea lions, although members of genus Ogmogaster have so far been reported to exclusively infect whales and pinnipeds living in marine habitats.
Up to date, trematodes of the genera Ascocotyle (Morgades et al., 2006; Hernández-Orts et al., 2012; Pereira, 2012; Pereira et al., 2013), Lobatostoma (possibly fish parasite detected after gastrointestinal passage; Calderón-Mayo, 2015), Ogmogaster (George-Nascimento and Carvajal, 1981; Carvajal et al., 1983; Sepúlveda et al., 2015), Stephanoprora (Morgades et al., 2006; Pereira et al., 2013), and Zalophotrema (only one report in captivity; Petrov and Chertkova, 1963) have been reported for South American sea lions (Table 1). In the current study, eggs of two different trematode taxa were specified on clade level determined as Trematoda indet. types 1 and 2. Eggs of Trematoda indet. type 1 were already detected by Hermosilla et al. (2016a) in synanthropic sea lion feces, and size and shape are morphologically similar to eggs of members of trematode families Opisthorchiidae and Heterophyidae (Ditrich et al., 1992), which includes the genus Ascocotyle. Members of Opistorchiidae have never been reported parasitizing O. flavescens so far, however, other pinnipeds were described to function as definitive hosts (Chai et al., 2005; Heckmann and Halajian, 2012; Neimanis et al., 2016). Depending on species and localization of adult trematodes, dimension of infections in marine mammals can range from low enteritis up to necrosis of parenchyma of affected organs (Geraci and St. Aubin, 1987).
Baermann funnel technique, a gold standard method for detection of first-stage lungworm larvae revealed two different parasites: Otostrongylus circumlitus and Parafilaroides spp. Both lungworm taxa were identified using morphological descriptions (Measures, 2001) and were detected in the synanthropic sea lion colony, whereas only Parafilaroides spp. L1 were found in Pacific sea lion colony 01. Data on lungworm infections in sea lions (subfamily Otariinae) are still scarce and exclusively caused by O. circumlitus (Kelly et al., 2005) and members of the genus Parafilaroides (Dougherty and Herman, 1947; Sweeney and Gilmartin, 1974; Greig et al., 2005), which have both been documented for South American sea lions before (Gonzales-Viera et al., 2011; Hermosilla et al., 2016a, 2018b). O. circumlitus (Metastrongyloidea: Crenosomatidae) constitutes the only described species within the genus Otostrongylus (Leidenberger and Boström, 2009) and has been reported to mainly parasitize principal airways causing distinct clinical signs especially in juvenile phocid seals and fatal cases have also been published (Gulland et al., 1997). In this study, first stage larvae of O. circumlitus reached a mean size of 345.97 × 15.78 μm and were thereby longer than maximum length published for genus Parafilaroides (304 μm; Measures, 2001). Measurements laid below references of O. circumlitus first-stage larvae, whereas length of larvae can vary according to host species (Bergeron et al., 1997). Consistently, the host immune response, nutritional status, age and body size can influence final adult parasite length and width in vivo (Morand et al., 1996). For the California sea lion, a case report of verminous pneumonia and bronchitis caused by P. decorus was published (Fleischman and Squire, 1970). Manifesting a curved and tapering posterior end, first stage larvae (L1) of Parafilaroides spp. detected within the current study exhibit a similar morphology to lungworm larvae found in Galapagós sea lions (Zalophus wollebaeki), which were identified as Parafilaroides-like nematodes (Walden et al., 2018). Measurement of our Parafilaroides spp. larvae were in good accordance to published morphometric data (Measures, 2001). As already reported by Hermosilla et al. (2016a), many synanthropic male sea lions regularly showed periods of extensive coughing and expectoration and extend rhinorrhea also during the current study indicating a possible clinical manifested otostrongylosis and/or parafilaroidosis. Sampling of expelled mucus or nasal discharge are planned for future monitoring surveys displaying a suitable collection technique to reveal either pre-adult/adult- or L1-stages of lungworms in vivo in a non-invasive and unmolested way.
The current study delivers new data on the endogenous parasite fauna of different colonies of free-living South American sea lions and represents the continuation of a monitoring program on anthropozoonotic parasites within One Health-/Global Health-concepts. Survey revealed different parasite taxa, including parasites bearing anthropozoonotic potential, thus evidencing importance of management measures avoiding direct contact with feces or products containing infectious parasite stages as well as regular monitoring programs to avoid spillovers and human infections. By detecting a broad spectrum of parasite stages (cysts, oocysts, eggs, L1 as well as adult helminths), the current study clearly illustrates a successful application and functionality of non-invasive methods in the field of parasitology research of marine mammals.
Data Availability Statement
The original contributions presented in the study are included in the article/supplementary material, further inquiries can be directed to the corresponding author.
Ethics Statement
Ethical review and approval was not required for the animal study due to the non-invasive sampling techniques applied in this study. Sample collection was conducted in accordance to the Institutional Ethic Commission and Animal Care Committee of the University Austral of Chile (Valdivia, Chile) and to current Chilean Animal Welfare Legislation.
Author Contributions
CH designed the study. DE conducted fieldwork, coproscopical analyses, and drafted the manuscript. MN and PM provided support during laboratory work and managed organization of sampling spots. LF supported the field work. UG provided scanning electron microscopic images. SP and JB conducted molecular analyses of anisakids and the cestode. AT was responsible for organization of the study and essentially revised the manuscript. All authors read and approved the final manuscript.
Funding
This study was funded by the Institute of Parasitology (Justus Liebig University Giessen) and the Institute of Pathology (Universidad Austral de Chile).
Conflict of Interest
The authors declare that the research was conducted in the absence of any commercial or financial relationships that could be construed as a potential conflict of interest.
Acknowledgments
We gratefully acknowledge the assistance of Enrique Paredes (Institute of Pathology), Rafael Burgos (Institute of Pharmacology) and Javier Painean Peña (Institute of Parasitology) during laboratory work at the Universidad Austral de Chile, Valdivia, Chile. Further, we are deeply thankful to Esteban Reyes Lobao-Tello for the assistance during sample taking process. Special thanks go to Agnes Mohr and Birgit Reinhardt (Institute of Parasitology, Justus-Liebig-University Giessen, Germany) for technical assistance during corproscopical analyses as well as to Rahel Wampfler (Swiss Tropical and Public Health Institute, Basel, Switzerland) for great efforts during molecular analyses. Chilean National Service of Fishing and Aquaculture (SERNAPESCA) and the Municipality Service of the city of Valdivia deserves credit for sampling permission within the city of Valdivia as well as of Pacific coast colonies.
References
Abattouy, N., Valero, A., Lozano, J., Barón, S. D., Romero, C., and Martín-Sánchez, J. (2016). Population genetic analyses of Anisakis simplex s.l. and Anisakis pegreffi (Nematoda, Anisakidae) from parapatric areas and their contact zone. Parasite Epidemiol. Control 1, 169–176. doi: 10.1016/j.parepi.2016.02.003
Acha, P. N., and Szyfres, B. (2003). Zoonoses and Communicable Diseases Common to Man and Animals. Volume III. Parasitoses. Washington DC: PAHO.
Andrade, A. L., Pinedo, M. C., and Pereira, J. Jr. (1998). “Parasitos bioindicadores dos habitats dos mamìferos aquaàticos?,” in Proceedings of the Reunião de Trabalho de Especialistas em Mamiferos Aquàticos da Amèrica do Sul, Olinda.
Aznar, F. J., Hernández-Orts, J., Suárez, A. A., García-Varela, M., Raga, J. A., and Cappozzo, H. L. (2012). Assessing host-parasite specificity through coprological analysis: a case study with species of Corynosoma (Acanthocephala: Polymorphidae) from marine mammals. J. Helminthol. 86, 156–164. doi: 10.1017/S0022149X11000149
Aznar, F. J., Pérez-Ponce de León, G., and Raga, J. A. (2006). Status of Corynosoma (Acanthocephala: Polymorphidae) based on anatomical, ecological, and phylogenetic evidence, with the erection of Pseudocorynosoma n. gen. J. Parasitol. 92, 548–564. doi: 10.1645/GE-715R.1
Baylis, M. A. (1933). A new species of the nematode genus Uncinaria from sea lion, with some observations on related species. Parasitology 25, 308–319.
Baylis, M. A. (1934). “Parasites” inThe Southern sea lion, Otaria byronia (De Blainville), Vol. 8, ed. J. E. Hamilton (Discovery Rep.) 306.
Bergeron, E., Huot, J., and Measures, L. N. (1997). Experimental transmission of Otostrongylus circumlitus (Railliet, 1899) (Metastrongyloidea: Crenosomatidae), a lungworm of seals in eastern arctic Canada. Can. J. Zool. 75, 1364–1371. doi: 10.1139/z97-762
Berón-Vera, B., Crespo, E. A., Raga, J. A., and Pedraza, S. N. (2004). Uncinaria hamiltoni (Nematoda: Ancylostomatidae) in South American sea lions, Otaria flavescens, from northern Patagonia, Argentina. J. Parasitol. 90, 860–863. doi: 10.1645/GE-182R
Botto, C., and Mañé-Garzón, F. (1975). Sobre una nueva especie del género Uncinaria (Nematoda, Strongyloidea) de Otaria flavescens Shaw y la especiación en el género Uncinaria en pinnípedos americanos. Rev. Biol. Uruguay 2, 127–141.
Bowles, J., Blair, D., and McManus, D. P. (1992). Genetic variants within the genus Echinococcus identified by mitochondrial DNA sequencing. Mol. Biochem. Parasitol. 54, 165–173.
Bowman, D. D. (1995). Georgi’s Parasitology for Veterinarians, 6th Edn, Philadelphia: W. B. Saunders Company.
Cabrera, C. R., Coronado, C., and Ampuero, S. (1994). Parásitos de otáridos de la costa peruana y su importancia sanitaria. Bol. Lima 91–96, 77–80.
Calderón-Mayo, K. I. (2015). Helmintos y Ectoparásitos en Otaria flavescens “Lobo Marino Chusco” (Mammalia: Otariidae) en Punta San Juan de Marcona-ICA, Febrero-Abril 2014. Ph. D thesis, Universidad Nacional San Luis Gonzaga, Ica.
Campagna, C., Werner, R., Karesh, W., Marín, M. R., Koontz, F., Cook, R., et al. (2001). Movements and location at sea of South American sea lions (Otaria flavescens). J. Zool. 255, 205–220. doi: 10.1017/S0952836901001285
Cárdenas-Alayza, S. (2017). “South American sea lion (Otaria flavescens),” in Encyclopedia of Marine Mammals, eds B. Würsig, J. G. M. Thewissen, and K. M. Kovacs (San Diego: Academic Press), 907–910.
Cárdenas-Alayza, S., Crespo, E. A., and Oliveira, L. (2016). Otaria byronia, South American sea lion. IUCN Red List Threat. Spec. 2016:e.T41665A61948292.
Carrara, I. S. (1952). Lobos Marinos, PingüInos y Guaneras de la Costa del Litoral Marìtimo e Islas Adyacentes de la Repùblica Argentina. Publicaciòn Especial de la Facultad de Ciencias Veterinarias. Ph. D thesis, Universidad de La Plata, La Plata.
Carvajal, J., Durán, L. E., and George-Nascimento, M. (1983). Ogmogaster heptalineatus n.sp. (Trematoda: Notocotylidae) from the Chilean sea lion Otaria flavescens. Syst. Parasitol. 5, 169–173. doi: 10.1007/BF00009349
Cattan, P. E., Atias, A., Babero, B. B., and Torres, D. (1977). Helminth fauna of Chile, 5: first finding of Diphyllobothrium pacificum (Nybelin 1931) Margolis 1956 [parasitic tapeworm] in sea lions of the Chilean coast. Rev. Iber. Parasitol. 37, 285–290.
Cattan, P. E., Babero, B. B., and Torres, D. N. (1976). The helminth fauna of Chile: IV. nematodes of the genera Anisakis Dujardin, 1845 and Phocanema Myers, 1954 in relation with gastric ulcers in a South American sea lion, Otaria byronia. J. Wildlife Dis. 12, 511–515. doi: 10.7589/0090-3558-12.4.511
Cattan, P. E., and Carvajal, J. (1980). Phocanema decipiens (Krabbe 1878); nematodo parasito del lobo comun Otaria flavescens en Chile. Algunas consideraciones taxonomicas. Rev. Iber. Parasitol. 40, 1–9.
Chai, J. Y., Murrell, K. D., and Lymbery, A. J. (2005). Fish-borne parasitic zoonoses: status and issues. Int. J. Parasitol. 35, 1233–1254. doi: 10.1016/j.ijpara.2005.07.013
Cornejo-Galaz, L. A. C. (2017). Determinación de Cryptosporidium spp. en Heces de Lobo Marino Común (Otaria flavescens Shaw 1800) en Una Colonia Urbana de Valdivia, Chile. Ph. D thesis, Universidad Austral de Chile, Valdivia.
De María, M., Barboza, F. R., and Szteren, D. (2014). Predation of South American sea lions (Otaria flavescens) on artisanal fisheries in the Rio de la Plata estuary. Fish. Res. 149, 69–73. doi: 10.1016/j.fishres.2013.09.006
de Vos, A., Faux, C. E., Marthick, J., Dickinson, J., and Jarman, S. N. (2018). New determination of prey and parasite species for northern Indian Ocean Blue Whales. Front. Mar. Sci. 5:104. doi: 10.3389/fmars.2018.00104
Delport, T. C., Asher, A. J., Beaumont, L. J., Webster, K. N., Harcourt, R. G., and Power, M. L. (2014). Giardia duodenalis and Cryptosporidium occurrence in Australian sea lions (Neophoca cinerea) exposed to varied levels of human interaction. Int. J. Parasitol. Par. Wildl. 3, 269–275. doi: 10.1016/j.ijppaw.2014.09.001
Deng, M. Q., Peterson, R. P., and Cliver, D. O. (2000). First findings of Cryptosporidium and Giardia in California sea lions (Zalophus californianus). J. Parasitol. 86, 490–494. doi: 10.1645/0022-3395(2000)086[0490:FFOCAG]2.0.CO;2
Denkinger, J., Gordillo, L., Montero-Serra, I., Murillo, J. C., Guevara, N., Hirschfeld, M., et al. (2015). Urban life of Galapagos sea lions (Zalophus wollebaeki) on San Cristobal Island, Ecuador: colony trends and threats. J. Sea. Res. 105, 10–14. doi: 10.1016/j.seares.2015.07.004
Dezfuli, B. S., Pironi, F., Simoni, E., Shinn, A. P., and Giari, L. (2007). Selected pathological, immunohistochemical and ultrastructural changes associated with an infection by Diphyllobothrium dendriticum (Nitzsch, 1824) (Cestoda) plerocercoids in Coregonus lavaretus (L.) (Coregonidae). J. Fish. Dis. 30, 471–482. doi: 10.1111/j.1365-2761.2007.00833.x
Ditrich, O., Giboda, M., Scholz, T., and Beer, S. A. (1992). Comparative morphology of eggs of the Haplorchiinae (Trematoda: Heterophyidae) and some other medically important heterophyid and opisthorchiid flukes. Fol. Parasit. 39, 123–132.
Dönges, J. (1962). Entwicklungsgeschichtliche und morphologische Untersuchungen an Notocotyliden (Trematoda). Z. Parasitenk. 22, 43–67. doi: 10.1007/BF00260165
Dougherty, E. C., and Herman, C. M. (1947). New species of the genus Parafilaroides Dougherty, 1946 (Nematoda: Metastrongylidae), from sea-lions, with a list of the lungworms of the Pinnipedia. Proc. Helminth. Soc. Wash. 14, 77–87.
Ebmer, D., Navarrete, M. J., Muñoz, P., Flores, L. M., Gärtner, U., Taubert, A., et al. (2019). Antarctophthirus microchir infestation in synanthropic South American sea lion (Otaria flavescens) males diagnosed by a novel non-invasive method. Parasitol. Res. 118, 1353–1361. doi: 10.1007/s00436-019-06273-2
Fernández, J. C. (1987). Nuevos registros parasitos en mamiferos marinos chilenos. Parasitol. Al Día 11, 120–125.
Fleischman, R. W., and Squire, R. A. (1970). Verminous pneumonia in the California sea lion (Zalophus californianus). Pathol. Vet. 7, 89–101. doi: 10.1177/030098587000700201
George-Nascimento, M., and Carvajal, J. (1980). Nuevos registros de nematodos anisakidos en la fauna marina chilena. Bol. Chile Parasit. 35, 15–18.
George-Nascimento, M., and Carvajal, J. (1981). Helmintos paràsitos del lobo marino comùn Otaria flavescens en el Golfo de Arauco, Chile. Bol. Chile Parasit. 36, 72–73.
George-Nascimento, M., Lima, M., and Ortiz, E. (1992). A case of parasite-mediated competition? Phenotypic differentiation among hookworms Uncinaria sp. (Nematoda: Ancylostomatidae) in sympatric and allopatric populations of South American sea lions Otaria byronia, and fur seals Arctocephalus australis (Carnivora: Otariidae). Mar. Biol. 112, 527–533. doi: 10.1007/BF00346169
George-Nascimento, M., and Llanos, A. (1995). Micro-evolutionary implications of allozymic and morphometric variations in sealworms Pseudoterranova sp.(Ascaridoidea: Anisakidae) among sympatric hosts from the Southeastern Pacific Ocean. Int. J. Parasitol. 25, 1163–1171. doi: 10.1016/0020-7519(95)00038-4
George-Nascimento, M., and Marin, S. L. (1992). Efecto de dos especies hospedadoras, el lobo fino austral Arctocephalus australis (Zimmerman) y el lobo marino común Otaria byronia (Blainville)(Carnivora; Otariidae), sobre la morfología y la fecundidad de Corynosoma sp.(Acanthocephala; Polymorphidae) en Uruguay. Rev. Chil. Hist. Nat. 65, 183–193.
George-Nascimento, M., and Urrutia, X. (2000). Pseudoterranova cattani sp. nov (Ascaridoidea: Anisakidae), un parásito del lobo marino común Otaria byronia De Blainville en Chile. Rev. Chil. Hist. Nat. 73, 93–98. doi: 10.4067/S0716-078X2000000100010
Geraci, J. R., and St. Aubin, D. J. (1987). Effects of parasites on marine mammals. Int. J. Parasitol. 17, 407–414. doi: 10.1016/0020-7519(87)90116-0
Gonzales-Viera, O., Chavera, A., Yaipén-Llanos, C., and Perales-Camacho, R. (2011). Histopathological aspects and etiology of pneumonias in stranded marine mammals from Lima, Peru. Braz. J. Vet. Pathol. 4, 23–29.
González, M. T., López, Z., Nuñez, J. J., Calderón-Mayo, K. I., Ramírez, C., Morgades, D., et al. (2018). Morphometrical and molecular evidence suggests cryptic diversity among hookworms (Nematoda: Uncinaria) that parasitize pinnipeds from the south-eastern Pacific coasts. J. Helminthol. 94:e8. doi: 10.1017/S0022149X18000950
Graczyk, T. K., and Shiff, C. J. (1993). Experimental infection of domestic ducks and rodents by Notocotylus attenuatus (Trematoda: Notocotylidae). J. Wildl. Dis. 29, 434–439. doi: 10.7589/0090-3558-29.3.434
Greig, D. J., Gulland, F. M. D., and Kreuder, C. (2005). A decade of live California sea lion (Zalophus californianus) strandings along the central California coast: causes and trends, 1991-2000. Aquat. Mamm. 31, 11–22. doi: 10.1578/AM.31.1.2005.11
Gulland, F. M. D., Beckmen, K., Burek, K., Lowenstine, L., Werner, L., Spraker, T., et al. (1997). Nematode (Otostrongylus circumlitus) infestation of northern elephant seals (Mirounga angustirostris) stranded along the central California coast. Mar. Mammal Sci. 13, 446–458. doi: 10.1111/j.1748-7692.1997.tb00651.x
Heath, C. B., and Perrin, W. F. (2009). “California, Galapagos, and Japanese sea lions: Zalophus californianus, Z. wollebaeki, and Z. japonicus,” in Encyclopedia of Marine Mammals, eds F. Perrin, B. Würsig, and J. G. M. Thewissen (New York, NY: Academic Press), 170–176.
Heckmann, R. A., and Halajian, A. (2012). Ultrastructural study of Pseudamphistomum truncatum (Rudolphi, 1819) (Opisthorchidae Trematoda) from the Caspian Seal (Pusa caspica) (Phocidae, Mammalia) using Scanning Electron Microscopy (SEM) and Energy-Dispersive X-Ray Analysis (EDXA). Sci. Parasitol. 13, 101–108.
Heine, J. (1982). An easy technique for the demonstration of cryptosporidia in faeces. Zentralb. Vet. Reihe B. 29, 324–327.
Hermosilla, C., Hirzmann, J., Silva, L. M. R., Brotons, J. M., Cerdà, M., Prenger-Berninghoff, E., et al. (2018a). Occurrence of anthropozoonotic parasitic infections and faecal microbes in free-ranging sperm whales (Physeter macrocephalus) from the Mediterranean Sea. Parasitol. Res. 117, 2531–2541. doi: 10.1007/s00436-018-5942-3
Hermosilla, C., Hirzmann, J., Silva, L. M. R., Scheufen, S., Prenger-Berninghoff, E., Ewers, C., et al. (2018b). Gastrointestinal parasites and bacteria in free-living South American sea lions (Otaria flavescens) in Chilean Comau Fjord and new host record of a Diphyllobothrium scoticum-like cestode. Front. Mar. Sci. 5:459. doi: 10.3389/fmars.2018.00459
Hermosilla, C., Silva, L. M., Navarro, M., and Taubert, A. (2016a). Anthropozoonotic endoparasites in free-ranging “urban” South American sea lions (Otaria flavescens). J. Vet. Med. 2016:7507145. doi: 10.1155/2016/7507145
Hermosilla, C., Silva, L. M. R., Kleinertz, S., Prieto, R., Silva, M. A., and Taubert, A. (2016b). Endoparasite survey of free-swimming baleen whales (Balaenoptera musculus, B. physalus, B. borealis) and sperm whales (Physeter macrocephalus) using non/minimally invasive methods. Parasitol. Res. 115, 889–896. doi: 10.1007/s00436-015-4835-y
Hermosilla, C., Silva, L. M. R., Prieto, R., Kleinertz, S., Taubert, A., and Silva, M. A. (2015). Endo- and ectoparasites of large whales (Cetartiodactyla: Balaenopteridae, Physeteridae): overcoming difficulties in obtaining appropriate samples by non-and minimally-invasive methods. Int. J. Parasitol. Parasit. Wildl. 4, 414–420. doi: 10.1016/j.ijppaw.2015.11.002
Hernández-Orts, J. S., Brandão, M., Georgieva, S., Raga, J. A., Crespo, E. A., Luque, J. L., et al. (2017). From mammals back to birds: host-switch of the acanthocephalan Corynosoma australe from pinnipeds to the Magellanic penguin Spheniscus magellanicus. PLoS One 12:e0183809. doi: 10.1371/journal.pone.0183809
Hernández-Orts, J. S., Montero, F. E., Crespo, E. A., García, N. A., Raga, J. A., and Aznar, F. J. (2012). A new species of Ascocotyle (Trematoda: Heterophyidae) from the South American sea lion, Otaria flavescens, off Patagonia, Argentina. J. Parasitol. 98, 810–816. doi: 10.1645/GE-2959.1
Hernández-Orts, J. S., Montero, F. E., García, N. A., Crespo, E. A., Raga, J. A., García-Varela, M., et al. (2019). Transmission of Corynosoma australe (Acanthocephala: Polymorphidae) from fishes to South American sea lions Otaria flavescens in Patagonia, Argentina. Parasitol. Res. 118, 433–440. doi: 10.1007/s00436-018-6177-z
Hernández-Orts, J. S., Aznar, F. J., Blasco-Costa, I., García, N. A., Víllora-Montero, M., Crespo, E. A., et al. (2013a). Description, microhabitat selection and infection patterns of sealworm larvae (Pseudoterranova decipiens species complex, nematoda: ascaridoidea) in fishes from Patagonia, Argentina. Parasite Vector. 6:252. doi: 10.1186/1756-3305-6-252
Hernández-Orts, J. S., Montero, F. E., Juan-García, A., García, N. A., Crespo, E. A., Raga, J. A., et al. (2013b). Intestinal helminth fauna of the South American sea lion Otaria flavescens and fur seal Arctocephalus australis from northern Patagonia, Argentina. J. Helminthol. 87, 336–347. doi: 10.1017/S0022149X12000454
Hernández-Orts, J. S., Scholz, T., Brabec, J., Kuzmina, T., and Kuchta, R. (2015). High morphological plasticity and global geographical distribution of the Pacific broad tapeworm Adenocephalus pacificus (syn. Diphyllobothrium pacificum). Mol. Morphol. Surv. Acta Trop. 149, 168–178. doi: 10.1016/j.actatropica.2015.05.017
Holcman-Spector, B., Botto, C., and Mañé-Garzón,, F. (1977). Estudio de la fauna parasitològica de Arctocephalus australis (Zimmermann, 1783) y Otaria flavescens (Shaw, 1800). Resùm. Sèpt. Cong. Latinoam. Zool. 198, 28–29.
Hückstädt, L. A., and Antezana, T. (2003). Behaviour of the southern sea lion (Otaria flavescens) and consumption of the catch during purse-seining for jack mackerel (Trachurus symmetricus) off central Chile. ICES J. Mar. Sci. 60, 1003–1011. doi: 10.1016/S1054-3139(03)00100-0
Jacobus, K., Marigo, J., Gastal, S. B., Taniwaki, S. A., Ruoppolo, V., Catão-Dias, J. L., et al. (2016). Identification of respiratory and gastrointestinal parasites of three species of pinnipeds (Arctocephalus australis, Arctocephalus gazella, and Otaria flavescens) in southern Brazil. J. Zoo Wildlife Med. 47, 132–140. doi: 10.1638/2015-0090.1
Kelly, T. R., Greig, D., Colegrove, K. M., Lowenstine, L. J., Dailey, M., Gulland, F. M., et al. (2005). Metastrongyloid nematode (Otostrongylus circumlitus) infection in a stranded California sea lion (Zalophus californianus)—A new host-parasite association. J. Wildl. Dis. 41, 593–598. doi: 10.7589/0090-3558-41.3.593
Kleinertz, S., Hermosilla, C., Ziltener, A., Kreicker, S., Hirzmann, J., Abdel-Ghaffar, F., et al. (2014). Gastrointestinal parasites of free-living Indo-Pacific bottlenose dolphins (Tursiops aduncus) in the Northern Red Sea, Egypt. Parasitol. Res. 113, 1405–1415. doi: 10.1007/s00436-014-3781-4
Kuchta, R., Serrano-Martínez, M. E., and Scholz, T. (2015). Pacific broad tapeworm Adenocephalus pacificus as a causative agent of globally reemerging Diphyllobothriosis. Emerg. Infect. Dis. 21, 1697–1703. doi: 10.3201/eid2110.150516
Leidenberger, S., and Boström, S. (2009). Description of the lungworm Otostrongylus circumlitus (Railliet, 1899) de Bruyn, 1933 (Metastrongyloidea: Crenosomatidae) found in the heart of harbour seals from Sweden. J. Nematode Morphol. Syst. 12, 169–175.
Llorens, C., Arcos, S. C., Robertson, L., Ramos, R., Futami, R., Soriano, B., et al. (2018). Functional insights into the infective larval stages of Anisakis simplex s.s., Anisakis pegreffi and their hybrids based on gene expression patterns. BMC Genomics 19:592. doi: 10.1186/s12864-018-4970-9
López-Fernández, J. R. (1967). Granuloma parasitario del estómago de Otaria flavescens (Shaw). Rev. Uru. Patol. Clín. 5, 20–34.
Machado, R., Oliveira, L. R., and Montealegre-Quijano, S. (2015). Incidental catch of South American sea lion in a pair trawl off southern Brazil. Neotrop. Biol. Cons. 10, 43–47. doi: 10.4013/nbc.2015.101.06
Machado-Pereira, E., Pereira, J. Jr., Secchi, E., and Müller, G. (2017). Contracaecum ogmorhini Johnston & Mawson, 1941 (Nematoda: Anisakidae) in southern sea lion, Otaria flavescens (Shaw, 1800) from southern Rio Grande do Sul State, Brazil. Pan. Am. J. Aquat. Sci. 12, 227–233.
Majluf, P., Babcock, E. A., Riveros, J. C., Schreiber, M. A., and Alderete, W. (2002). Catch and bycatch of sea birds and marine mammals in the small-scale fishery of Punta San Juan, Peru. Conserv. Biol. 16, 1333–1343. doi: 10.1046/j.1523-1739.2002.00564.x
Markowski, S. (1952). The cestodes of pinnipeds in the Arctic and other regions. J. Helminthol. 26, 171–214. doi: 10.1017/S0022149X00032612
Measures, L. N. (2001). “Lungworms of marine mammals,” in Parasitic Diseases of Wild Animals, eds W. M. Samuel, M. J. Pybus, and A. A. Kocan (Ames: Iowa State University Press), 279–330.
Menghi, C. I., Gatta, C. L., Arias, L. E., Santoni, G., Nicola, F., Smayevsky, J., et al. (2020). Human infection with Pseudoterranova cattani by ingestion of “ceviche” in Buenos Aires, Argentina. Rev. Argent. Microbiol. 52, 118–120. doi: 10.1016/j.ram.2019.06.005
Mercado, R., Yamasaki, H., Kato, M., Muñoz, V., Sagua, H., Torres, P., et al. (2010). Molecular identification of the Diphyllobothrium species causing diphyllobothriasis in Chilean patients. Parasitol. Res. 106, 995–1000. doi: 10.1007/s00436-010-1765-6
Miranda, H., Fernandez, W., and Ibañez, N. (1968). Diphyllobothriasis. Investigaciòn de Diphyllobohrium pacificum (Nybelin, 1931) Margolis, 1956 en Otaria byronia (syn. O. flavescens) y en peces marinos. Arch. Peru. Patol. Clin. 22, 9–24.
Mondragón-Martínez, B. A. M. (2017). Identificación Molecular de los Estadios de Plerocercoide y Adulto de Diphyllobothrium sp. Obtenidos de Humanos, Lobos Marinos y Peces. Ph. D thesis, Universidad Ricardo Palma, Lima.
Morand, S., Legendre, P., Gardner, S. L., and Hugot, J. P. (1996). Body size evolution of oxyurid (Nematoda) parasites: the role of hosts. Oecologia 107, 274–282. doi: 10.1007/BF00327912
Moravec, F. (2009). Experimental studies on the development of Contracaecum rudolphii (Nematoda: Anisakidae) in copepod and fish paratenic hosts. Folia Parasit. 56, 185–193.
Morgades, D., Benítez, G., Capellino, D., Casas, L., Castro, O., Katz, H., et al. (2006). “Fauna parasitaria del lobo fino Arctocephalus australis y del león marino Otaria flavescens (Mammalia, Otariidae) en la costa uruguaya. 2006,” in Bases Para la Conservación y el Manejo de la Costa Uruguaya, eds R. Menafra, L. Rodríguez-Gallego, F. Scarabino, and D. Conde (Montevideo: Vida Silvestre), 89–96.
Morini, E. G., and Boero, J. J. (1960). Corynosoma otariae n. sp. (Acanthocephala; Polymorphidae) parasito de un lobo marino (Otaria flavescens). Actas Trabajos Primer Cong. Sudamericano Zool. 2, 229–234.
Nadler, S. A., Lyons, E. T., Pagan, C., Hyman, D., Lewis, E. E., Beckmen, K., et al. (2013). Molecular systematics of pinniped hookworms (Nematoda: Uncinaria): species delimitation, host associations and host-induced morphometric variation. Int. J. Parasitol. 43, 1119–1132. doi: 10.1016/j.ijpara.2013.08.006
Naupay, A., Castro, J., Rojas, V., and Suarez, D. (2019). Gastrointestinal helminths of the South American marine lion Otaria flavescens Shaw 1800 (Mammalia: Otariidae) from the central coast of Peru. Neotrop. Helminthol. 13, 317–333.
Navarrete, N., and Torres, P. (1994). Prevalence of infection by intestinal helminths and protozoa in school children from a coastal locality in the province of Valdivia, Chile. Bol. Chil. Parasitol. 49, 79–80.
Neimanis, A. S., Moraeus, C., Bergman, A., Bignert, A., Höglund, J., Lundström, K., et al. (2016). Emergence of the zoonotic biliary trematode Pseudamphistomum truncatum in grey seals (Halichoerus grypus) in the Baltic Sea. PLoS One 11:e0164782. doi: 10.1371/journal.pone.0164782
Pasqualetti, M. I., Fariña, F. A., Krivokapich, S. J., Gatti, G. M., Daneri, G. A., Varela, E. A., et al. (2018). Trichinella spiralis in a South American sea lion (Otaria flavescens) from Patagonia, Argentina. Parasitol. Res. 117, 4033–4036. doi: 10.1007/s00436-018-6116-z
Pereira, E. M. (2012). Identificação da Comunidade Componente de Helmintos, Gastrointestinais hepáticos, Pulmonares, Cardíacos e Renais de Otaria flavescens (Leão-Marinho-do-Sul), no Litoral sul do Brasil. Master’s thesis, Universidade Federal de Pelotas, Rio Grande Sul.
Pereira, E. M., Müller, G., Secchi, E., Pereira, J., and Valente, A. L. S. (2013). Digenetic trematodes in South American sea lions from southern Brazilian waters. J. Parasitol. 99, 910–913. doi: 10.1645/GE-3216.1
Petrov, A. M., and Chertkova, A. H. (1963). A new trematode, Zalophotrema lubimovi n. sp., of the liver of sea lions. Trudy Vsyesyuznogo Inst. Gel’mintol. 10, 26–29.
Rausch, R. L., and Rice, D. W. (1970). Ogmogaster trilineatus sp. n. (Trematoda: Notocotylidae) from the fin whale, Balaenoptera physalus. L. Proc. Helminth. Soc. Wash. 37, 196–200.
Reyes, P., Hucke-Gaete, R., and Torres-Florez, J. P. (2013). First observations of operational interactions between bottom-trawling fisheries and South American sea lion, Otaria flavescens in south-central Chile. J. Mar. Biol. Assoc. UK 93, 489–494. doi: 10.1017/S0025315412001282
Schlatter, R. P. (1976). Penetración del lobo marino común, Otaria flavescens Shaw, en el Río Valdivia y afluentes. Medio Amb. 2, 86–90.
Scholz, T., Garcia, H. H., Kuchta, R., and Wicht, B. (2009). Update on the human broad tapeworm (genus Diphyllobothrium), including clinical relevance. Clin. Microbiol. Rev. 22, 146–160. doi: 10.1128/CMR.00033-08
Seguel, M., Calderón, K., Colegrove, K., Adkesson, M., Cárdenas-Alayza, S., and Paredes, E. (2018). Helminth and respiratory mite lesions in Pinnipeds from Punta San Juan, Peru. Acta Parasitol. 63, 839–844. doi: 10.1515/ap-2018-0103
Sepúlveda, M., and Oliva, D. (2005). Interactions between South American sea lions Otaria flavescens (Shaw) and salmon farms in southern Chile. Aquac. Res. 36, 1062–1068. doi: 10.1111/j.1365-2109.2005.01320.x
Sepúlveda, M. A., Seguel, M., Alvarado-Rybak, M., Verdugo, C., Muñoz-Zanzi, C., and Tamayo, R. (2015). Postmortem findings in four South American sea lions (Otaria byronia) from an urban colony in Valdivia. Chile. J. Wildlife. Dis. 51, 279–282. doi: 10.7589/2013-07-161
Sweeney, J. C., and Gilmartin, W. G. (1974). Survey of diseases in free-living California sea lions. J. Wildl. Dis. 10, 370–376. doi: 10.7589/0090-3558-10.4.370
Szteren, D., and Páez, E. (2002). Predation by southern sea lions (Otaria flavescens) on artisanal fishing catches in Uruguay. Mar. Freshw. Res. 53, 1161–1167. doi: 10.1071/MF02006
Tantaleán, M., Sánchez, L., Gómez, L., and Huiza, A. (2005). Acantocéfalos del Perú. Rev. Peru. Biol. 12, 83–92.
Timi, J. T., Paoletti, M., Cimmaruta, R., Lanfranchi, A. L., Alarcos, A. J., Garbin, L., et al. (2014). Molecular identification, morphological characterization and new insights into the ecology of larval Pseudoterranova cattani in fishes from the Argentine coast with its differentiation from the Antarctic species, P. decipiens sp. E (Nematoda: Anisakidae). Vet. Parasitol. 199, 59–72. doi: 10.1016/j.vetpar.2013.09.033
Torres, P., Jercic, M. I., Weitz, J. C., Dobrew, E. K., and Mercado, R. A. (2007). Human pseudoterranovosis, an emerging infection in Chile. J. Parasitol. 93, 440–443. doi: 10.1645/GE-946R.1
Torres, P., Miranda, J. C., Flores, L., Riquelme, J., Franjola, R., and Perez, J. (1992). Blastocystosis and other intestinal protozoan infections in human riverside communities from Valdivia River Basin, Chile. Rev. I. Med. 34, 557–564. doi: 10.1590/S0036-46651992000600010
Torres, P., Moya, R., and Lamilla, J. (2000). Nematodos anisákidos de interés en salud pública en peces comercializados en Valdivia, Chile. Arch. Med. Vet. 32, 107–113. doi: 10.4067/S0301-732X2000000100014
Torres-Frenzel, P., and Torres, P. (2014). Anisakid parasites in commercial hake ceviche in Southern Chile. J. Food. Protec. 77, 1237–1240. doi: 10.4315/0362-028X.JFP-13-538
Vaz-Ferreira, R. (1982). “Otaria flavescens (Shaw), South American sea lion,” in Mammals in the Seas. Vol IV. Small Cetaceans, Seals, Sirenians and Otters, ed FAO (Rome: FAO Fischeries series), 477–495.
Vélez, J., Hirzmann, J., Arévalo-González, K., Lange, M. K., Seipp, A., Gärtner, U., et al. (2019). Parasite fauna of wild Antillean manatees (Trichechus manatus manatus) of the Andean Region, Colombia. Parasit. Vect. 12:183. doi: 10.1186/s13071-019-3448-1
Vélez, J., Hirzmann, J., Lange, M. K., Chaparro-Gutiérrez, J. J., Taubert, A., and Hermosilla, C. (2018). Occurrence of endoparasites in wild Antillean manatees (Trichechus manatus manatus) in Colombia. Int. J. Parasitol. Par. Wildl. 7, 54–57. doi: 10.1016/j.ijppaw.2018.01.006
Waeschenbach, A., Brabec, J., Scholz, T., Littlewood, D. T. J., and Kuchta, R. (2017). The catholic taste of broad tapeworms - multiple routes to human infection. Int. J. Parasitol. 47, 831–843. doi: 10.1016/j.ijpara.2017.06.004
Walden, H. D. S., Grijalva, C. J., Páez-Rosas, D., and Hernandez, J. A. (2018). Intestinal Parasites in Galapagos Sea Lions (Zalophus wollebaeki) Sivertsen, 1953 on San Cristóbal Island, Galapagos, Ecuador. J. Parasitol. 104, 718–721. doi: 10.1645/17-187
Weitzel, T., Sugiyama, H., Yamasaki, H., Ramirez, C., Rosas, R., and Mercado, R. (2015). Human Infections with Pseudoterranova cattani Nematodes, Chile. Emerg. Infect. Dis. 21, 1874–1875. doi: 10.3201/eid2110.141848
WoRMS Editorial Board (2020). World Register of Marine Species. Available online at: http://www.marinespecies.org at VLIZ (accessed August 31, 2020).
Yang, J., and Scholten, T. (1977). A fixative for intestinal parasites permitting the use of concentration and permanent staining procedures. Am. J. Clin. Pathol. 67, 300–304. doi: 10.1093/ajcp/67.3.300
Keywords: Anisakis, Giardia, Cryptosporidium, Pseudoterranova, Contracaecum, Adenocephalus pacificus, marine mammal parasitology
Citation: Ebmer D, Navarrete MJ, Muñoz P, Flores LM, Gärtner U, Brabec J, Poppert S, Taubert A and Hermosilla C (2020) Anthropozoonotic Parasites Circulating in Synanthropic and Pacific Colonies of South American Sea Lions (Otaria flavescens): Non-invasive Techniques Data and a Review of the Literature. Front. Mar. Sci. 7:543829. doi: 10.3389/fmars.2020.543829
Received: 18 March 2020; Accepted: 18 September 2020;
Published: 15 October 2020.
Edited by:
Leszek Rolbiecki, University of Gdańsk, PolandReviewed by:
Juan Antonio Raga, University of Valencia, SpainTetiana Kuzmina, National Academy of Sciences of Ukraine (NAN Ukraine), Ukraine
Copyright © 2020 Ebmer, Navarrete, Muñoz, Flores, Gärtner, Brabec, Poppert, Taubert and Hermosilla. This is an open-access article distributed under the terms of the Creative Commons Attribution License (CC BY). The use, distribution or reproduction in other forums is permitted, provided the original author(s) and the copyright owner(s) are credited and that the original publication in this journal is cited, in accordance with accepted academic practice. No use, distribution or reproduction is permitted which does not comply with these terms.
*Correspondence: David Ebmer, david@pinnipedia.info