- 1Bayworld Centre for Research and Education (BCRE), Port Elizabeth, South Africa
- 2Centre for Marine Science and Technology, Curtin University, Perth, WA, Australia
- 3KwaZulu-Natal Sharks Board, Umhlanga Rocks, South Africa
- 4University of KwaZulu-Natal, School of Life Sciences, Durban, South Africa
Bather protection nets have been in place off the coast of KwaZulu-Natal (KZN), South Africa, since the 1950’s. Besides sharks, they also catch a number of other marine vertebrates, including dolphins, the majority of which are Indo-Pacific bottlenose dolphins Tursiops aduncus. Previous analyses of dolphin bycatch in the nets indicated the potential impacts on the local populations, but a lack of information on population structure has to-date hindered a more detailed assessment. A recent re-assessment of the status and population delineations of T. aduncus off South Africa prompted a re-examination of demographic, spatial, and temporal patterns of its catches in bather protection nets over a 36-year period (January 1980 to December 2015). In total, 1169 dolphins were caught, including a slightly greater number of females and juveniles than other sex-classes, raising concern about the potential long-term effects on population demographics. More dolphins were caught off the North than the South coast. Temporal trends indicate that the bycatch during June and July every year (peak Sardine Run period) as a percentage of overall dolphin bycatch has been steadily decreasing from 39.8% in 1980 to 13% in 2015. A large inter-annual fluctuation can be seen, probably as a result of the inter-annual intensity of the Sardine Run. Although our results do not indicate a long-term decline of the individual populations as previously predicted, revised abundance estimates and data on the demographics and movement patterns of the animals are urgently required to accurately assess the impact of bycatch on the Indo-Pacific bottlenose dolphin populations off KZN and to advise conservation and management decisions going forward.
Introduction
Bycatch in fisheries poses the biggest threat to small cetaceans globally (Mannocchi et al., 2012; Brownell et al., 2019; Tulloch et al., 2019; Hines et al., 2020; Omeyer et al., 2020), including the Indian Ocean (Anderson et al., 2020), increasingly highlighting unsustainable levels (Peltier et al., 2016). Incidental bycatch of dolphins in bather protection nets off the East Coast of South Africa has been a cause for concern for a number of decades (Cockcroft and Ross, 1990b; Peddemors et al., 1990; Cockcroft, 1994). These nets were introduced in 1952 after a series of 21 human-shark interactions off the coast of KwaZulu-Natal (KZN), seven of which were fatal. For details on the bather protection program and its operation see Cliff and Dudley (1992) and Dudley and Cliff (1993). The nets also take a bycatch of large marine vertebrates other than sharks, including birds, turtles, non-target elasmobranchs, teleost fishes and several marine mammal species (Dudley and Cliff, 1993; Cliff and Dudley, 2011). The most commonly caught dolphin species are the Indo-Pacific bottlenose dolphin Tursiops aduncus (Cockcroft et al., 2016), the common dolphin Delphinus delphis (Plön and Cockroft, 2016) and the Indian Ocean humpback dolphin Sousa plumbea (Atkins et al., 2013; Plön et al., 2015, 2016). Early analyses of catches (Cockcroft, 1990, 1992, 1994; Cockcroft and Ross, 1990b) led to a concern about the potential impacts on the local populations (Peddemors, 1993, 1999; Friedmann and Daly, 2004; Natoli et al., 2008). In an effort to reduce these catches, both passive and active deterrent devices were installed on the nets of some beaches in the past, but have to-date not proven to satisfactorily reduce bycatch (Peddemors et al., 1990; Peddemors and Cockcroft, 1994; Cliff and Dudley, 2011; Erbe et al., 2016). Particularly the period of the Sardine Run, an annual phenomenon whereby migrating schools of Sardine (Sardinops sagax) move from Eastern Cape (EC) waters into KZN waters, followed by marine predators, such as sharks, dolphins and seabirds (Dudley and Cliff, 2010), has been of concern for bycatch. Although the peak season is June-July, the overall Sardine Run period may range from May to September (Dudley and Cliff, 2010). In recent years, catches have declined for D. delphis due to a removal of nets during the peak Sardine Run period (Dudley and Cliff, 2010). However, there is concern that the bycatch impact remains unchanged for T. aduncus (Cockcroft et al., 2016).
The Indo-Pacific bottlenose dolphin T. aduncus is an inshore species, occurring in groups between 20 and 50 animals in coastal, shallow waters throughout the Indian Ocean and into the south-west Pacific (Best, 2007; Braulik et al., 2019). It is distinguishable by its short, stout beak, thick teeth, large dorsal fin, and characteristic freckles on its belly and sides, which increase with age (Best, 2007). T. aduncus is known to be taxonomically distinct from T. truncatus based on concordance in molecular analyses, osteology, and external morphology (Wang et al., 1999, 2000a,b). In South African waters, its geographic extent is limited to nearshore waters less than 50 m in depth from Mossel Bay, Western Cape (WC), to Kosi Bay (KZN).
Previous research by Cockcroft (1990, 1992, 1994) and Cockcroft and Ross (1990a, c) has raised concern about population level impact of bycatch of T. aduncus in the bather protection nets. However, no bycatch statistics of the species from the nets have been analyzed since the mid 1990’s and new molecular data have emerged in the past ten years, prompting a revision of the population structure of the species in the waters of KZN and the EC. Previously, a migratory stock moving between Plettenberg Bay [Western Cape (WC)], and Durban (KZN), was assessed separately and evaluated as “endangered” (Friedmann and Daly, 2004). A recent revision of the population structure of T. aduncus as part of the South African Red List assessment (Cockcroft et al., 2016) has followed genetic data on population delineation off KZN (Natoli et al., 2008) and evaluated the different subpopulations as follows: (a) a northern resident subpopulation, stretching from Kosi Bay to Ifafa, declared as “Vulnerable,” (b) a southern resident subpopulation, occurring from Ifafa (KZN) southward toward False Bay (WC), declared as “Near Threatened” (Figure 1, Insert); and (c) a seasonal subpopulation, the role of which (i.e., whether migratory or transient) is still poorly understood (Gopal, 2013) and thus declared as “Data Deficient” (Cockcroft et al., 2016). Population size estimates for all three subpopulations are dated as no new estimates have been obtained in the past 25 years. Thus, the revised assessment indicates a population break just south of Ifafa (Cockcroft et al., 2016) and this population delineation differs significantly from previous evaluations of bycatch on these populations (Cockcroft, 1990, 1992, 1994).
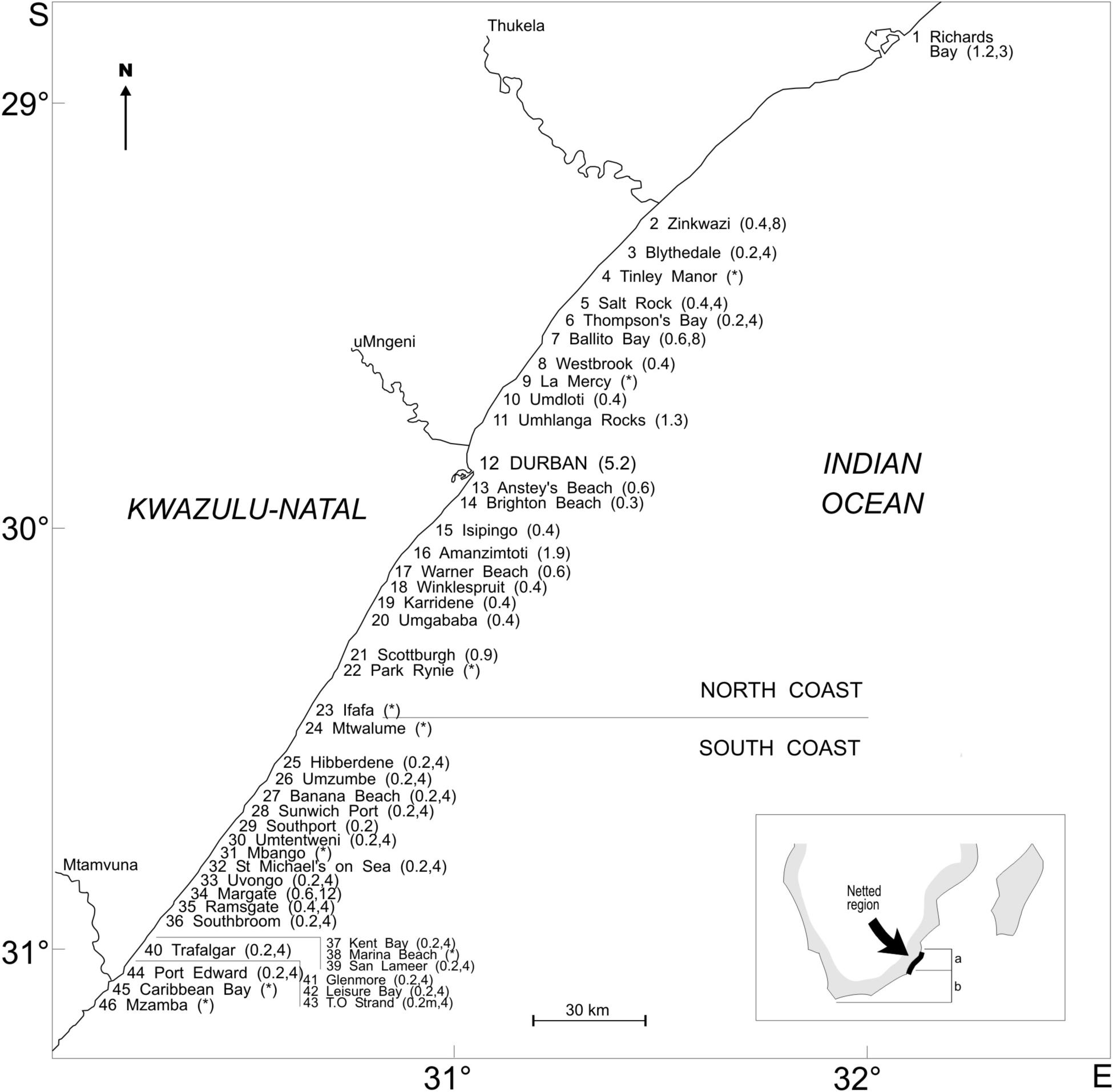
Figure 1. Locations of individual bather protection installations (numbered from North to South) along the KwaZulu-Natal coastline (numbers in parentheses indicate length of nets in km at respective beaches). The asterisk (*) indicates which nets were removed permanently during the study period. Insert: (a and b) denote the two main subpopulations of T. aduncus as indicated in text.
Here we examine the demographic, spatial, and temporal patterns of bycatch of T. aduncus in the KZN bather protection nets over a 36-year period (January 1980 to December 2015). The results will be discussed in relation to the latest assessment of population structure for this species off the south-east coast of South Africa (Cockcroft et al., 2016) in an effort to assess population level impacts on the species.
Materials and Methods
Bather protection nets were installed at Durban beach in 1952 and subsequently at additional beaches, with a major expansion of the program taking place in the 1960s. Since 1982, all beaches have been managed by the KwaZulu-Natal Sharks Board (KZNSB). The gill nets are made of multifilament polyethylene braid with a stretched mesh size of 0.5 m. The total length of nets varies between beaches (Figure 1), but the majority of nets are 213.5 m long and 6.3 m high. The exception are 304.8 m long nets off Durban, Anstey’s Beach, and Brighton Beach, and one 320.2 m net off Richards Bay. Nets are anchored parallel to the coast, about 300–500 m from the beach (beyond the surf zone) in 10–14 m of water depth. The number of nets deployed off the KZN coastline has varied over time, reaching a maximum in the early 1990s when the bather-protection program consisted of 44 installations and 44 km of netting (Dudley and Cliff, 1993). Several installations were subsequently removed, in most cases because numbers of bathers did not warrant their retention. Between 1999 and 2004, the length of netting at the remaining installations was reduced by approximately one third with the objective to reduce catches of marine animals (Dudley and Cliff, 2010; Cliff and Dudley, 2011). Additionally, between 2005 and 2007, approximately 4.2 km of netting was replaced with baited drumlines, an alternative and more selective shark fishing device (Dudley and Cliff, 2010; Cliff and Dudley, 2011). During the study period, one dolphin was caught on a drumline and this type of fishing gear is not considered further here. By December 2015, there were 37 installations comprising a total of 22.4 km of netting along a 320-km stretch of coastline, supplemented by 107 drumlines.
In addition to this reduction of effort, nets are removed during the peak Sardine Run period (June–July), a practice which has been consistent and successful over the last 20 years. The nets are checked every weekday weather permitting (i.e., approximately 20 days/month). All animals, other than those that are released alive or are decomposed, are retained for scientific necropsy at the KZNSB laboratory where species, sex and body length are recorded, and carcasses are frozen, pending dissection at a later date. For released animals, only the length measured in the field is available. Sometimes sex could not be established in the field due to operational issues.
No reliable records on dolphin bycatch data were kept prior to 1980 (Cockcroft, 1990), thus for the present analyses we only used bycatch data over a 36-year period from January 1980 to December 2015.
Life History Parameters
To investigate potential population impacts of bycatch on T. aduncus, we followed established biological parameters (Ross, 1984; Cockcroft and Ross, 1990a). Off KZN, T. aduncus females reach sexual maturity between 2.13 and 2.3 m total length, corresponding to 9.5 and 11 years of age (Ross, 1984; Cockcroft and Ross, 1990a); here we used the average value of 2.22 m or attainment of sexual maturity in the subsequent analyses. In males, puberty is reached around 2.4 m, corresponding to between 10 and 12 years of age (Cockcroft and Ross, 1990a). Physical maturity is reached at average lengths of 2.38 m in females and 2.43 m in males (Cockcroft and Ross, 1990a).
Spatial and Seasonal Distribution of Catches
We calculated annual catch and catch per unit effort (CPUE), defined as the number of dolphins caught per km of net per year, at each beach. The area was divided south of Ifafa into North Coast (NC) and South Coast (SC) as per the molecular evidence for subpopulations (Natoli et al., 2008; Cockcroft et al., 2016). Some of the nets at some beaches were removed during some of the austral winters over the 36-year period analyzed here, making the effort variable and the CPUE non-comparable. Thus, CPUE was calculated separately for the entire year (12-month CPUE) and for the 10-month period excluding June and July (i.e., the peak of the Sardine Run; 10-month CPUE). By removing the 2 months from all years, CPUE became comparable across beaches and years. Using the 36-year time series of CPUE for each beach, zero-lag cross-correlation coefficients were computed for all 45 pairs of adjacent beaches in order to further identify any spatial patterns. We further computed the cross-correlations over the first 20 years only (i.e., prior to the installation of ADDs). In order to remove the potentially confounding factor of repeated, temporary net removals during the peak Sardine Run (June and July) along the SC, we also cross-correlated CPUE by excluding the months of June and July from all beaches.
Acoustic deterrence devices (ADDs), both active and passive, such as pingers, whale alarms, and sonar reflectors, were installed at some beaches of the KZN coast for some years (1999–2008). Previous analyses have compared bycatch between neighboring beaches with and without ADDs and, for beaches with ADDs, between exposure and control periods (immediately preceding installation of ADDs). Bycatch at beaches with pingers was sometimes more strongly correlated with bycatch at neighboring beaches without pingers than with bycatch at neighboring beaches with pingers. From control to exposure periods, bycatch decreased at only 50% of the beaches with ADDs and increased at the other 50% of beaches with ADDs. The study concluded that ADDs did not consistently reduce bycatch (Erbe et al., 2016). Therefore, nets with and without ADDs were pooled in the present analysis. In other words, 36-year time series of CPUE were computed for all beaches disregarding the fact that some beaches for some months in some years had ADDs installed.
Results
Total Bycatch and Demographics
In total, 1169 T. aduncus were incidentally caught between January 1980 and December 2015. When split into the NC and SC populations, 296 males, 316 females, and 31 animals of unidentified sex were caught on the NC (total: 643 animals or 55%), while 211 males, 278 females, and 37 animals of unidentified sex were caught on the SC (total: 526 animals or 45%; Figure 2).
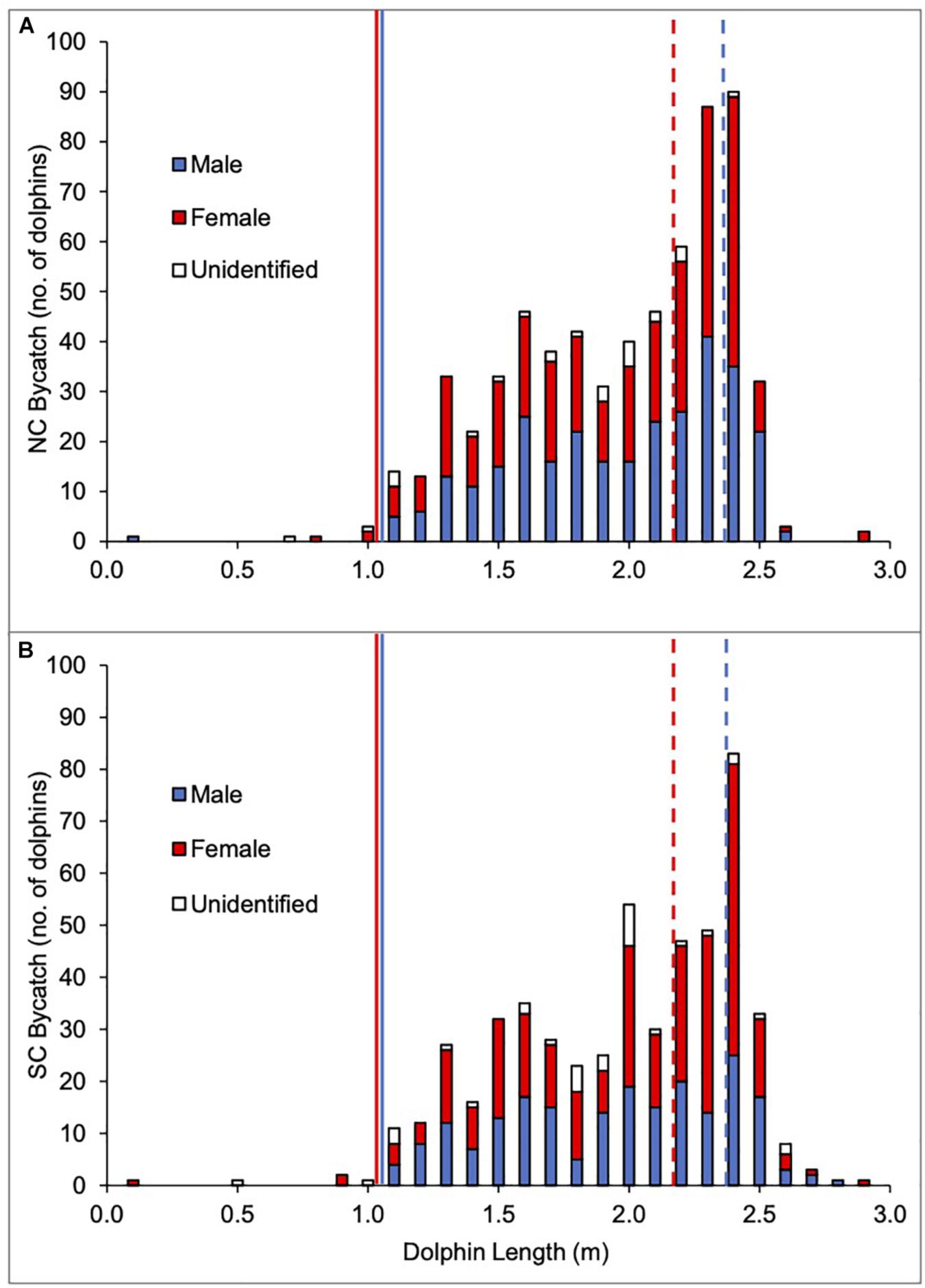
Figure 2. Length distribution by sex of Indo-Pacific bottlenose dolphin (T. aduncus) bycatch between 1980 and 2015 for the two areas: North Coast (NC; A) and South Coast (SC; B). Length at sexual maturity (as used in the analysis): 2.4 m (males) and 2.2 m (females).
Slightly more females than males were caught on the NC and SC combined (594 females [51%], 507 males [43%], 68 animals of unidentified sex [6%]) and that was reflected on both NC (49% females [316 animals], 46% males [296 animals], 5% unidentified sex [31 animals]), and SC (53% females [278 animals], 40% males [211 animals], 7% unidentified sex [37 animals], Figure 2).
When the sexes were broken down into sexual maturity classes, the majority of the males caught were immature (80% [237 animals] NC and 77% [163 animals] SC) versus mature males (20% [59 animals] NC and 23% [48 animals] SC; Figure 2). In contrast, similar numbers of both immature and mature females were caught on the NC (58% [184 animals] immature vs. 42% [132 animals] mature) and on the SC (54% [150 animals] immature vs. 46% [128 animals] mature; Figure 2).
Spatial Distribution of Bycatch
The 12-month CPUE averaged over the 36-year period is shown in Figure 3A. A lot of variability in bycatch was observed in both time and space. In general, CPUE was higher on SC than NC beaches. Beaches with high catches were (from North to South) Salt Rock (beach 5), Ballito Bay (beach 7), Umhlanga/Durban (beach 11/12), Amanzimtoti (beach 16), Park Rynie (beach 22), Hibberdene (beach 25), Banana Beach (beach 27), Umtentweni (beach 30), Margate (beach 34), Port Edward (beach 44) and Mzamba (beach 46). Port Edward, Umtentweni, and Mzamba (beaches no. 44, 30, and 46, respectively) showed the highest average annual CPUEs with 2.5, 2.25, and 2.1, respectively (Figure 3A). No catches were recorded at Marina Beach (beach no. 38) and nets at this beach were permanently removed in 1999.
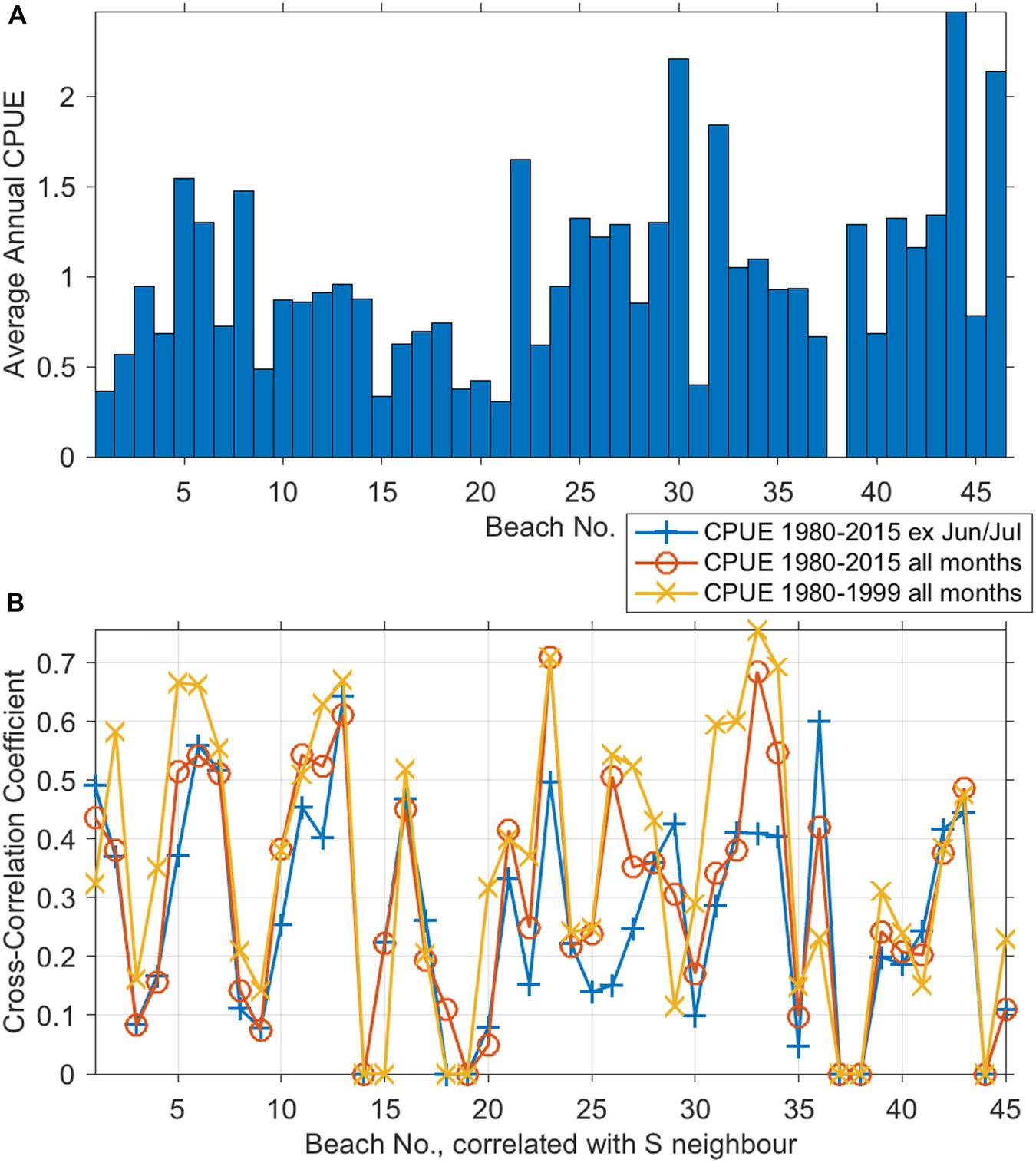
Figure 3. Average 12-month CPUE by beach of T. aduncus, 1980–2015 (A). Temporal cross-correlations of annual CPUE between beaches and their immediate neighbors to the South (B). The three correlations were: for the 36 years excluding and including the peak Sardine Run months of June and July, and for the years <1999 (i.e., prior to any deterrence devices on any beach).
Cross-correlation coefficients based on CPUE time series over 36 years and calculated for all 45 pairs of adjacent beaches ranged between 0 and 0.76 (Figure 3B). There was no group of more than five consecutive beaches that had cross-correlation coefficients amongst nearest neighbors of >0.5, indicating that there were no large-scale spatial patterns, but rather, cross-correlation varied about every 3–4 beaches. A similar grouping can be seen in the average 12-month CPUE (Figure 3A). This spatial pattern can be seen in all three cross-correlation curves: (1) based on 36-year time series (red line), (2) based on 36 years of bycatch data yet excluding catches during the Sardine Run months of June and July (blue line), and (3) based on 20-year times series up until installation of ADDs (yellow line, Figure 3B). With a few exceptions, the strongest cross-correlation was found for CPUE prior to the installation of ADDs (yellow line surpasses red and blue lines for most beaches, Figure 3B), i.e., ADDs did not consistently affect bycatch (see also Erbe et al., 2016).
Seasonal Distribution of Bycatch
The total number of T. aduncus dolphins caught at each beach from 1 January 1980 to 31 December 2015 is shown by month in Figure 4. Catch rates peaked in May on the northernmost beaches (Richards Bay to Tongaat), with Salt Rock and Ballito showing the highest catches in May, followed by Tongaat with the second highest catches in May and June (Figure 4). All beaches along this part of the coastline had catches in May, and most beaches had catches in October and December (Figure 4).
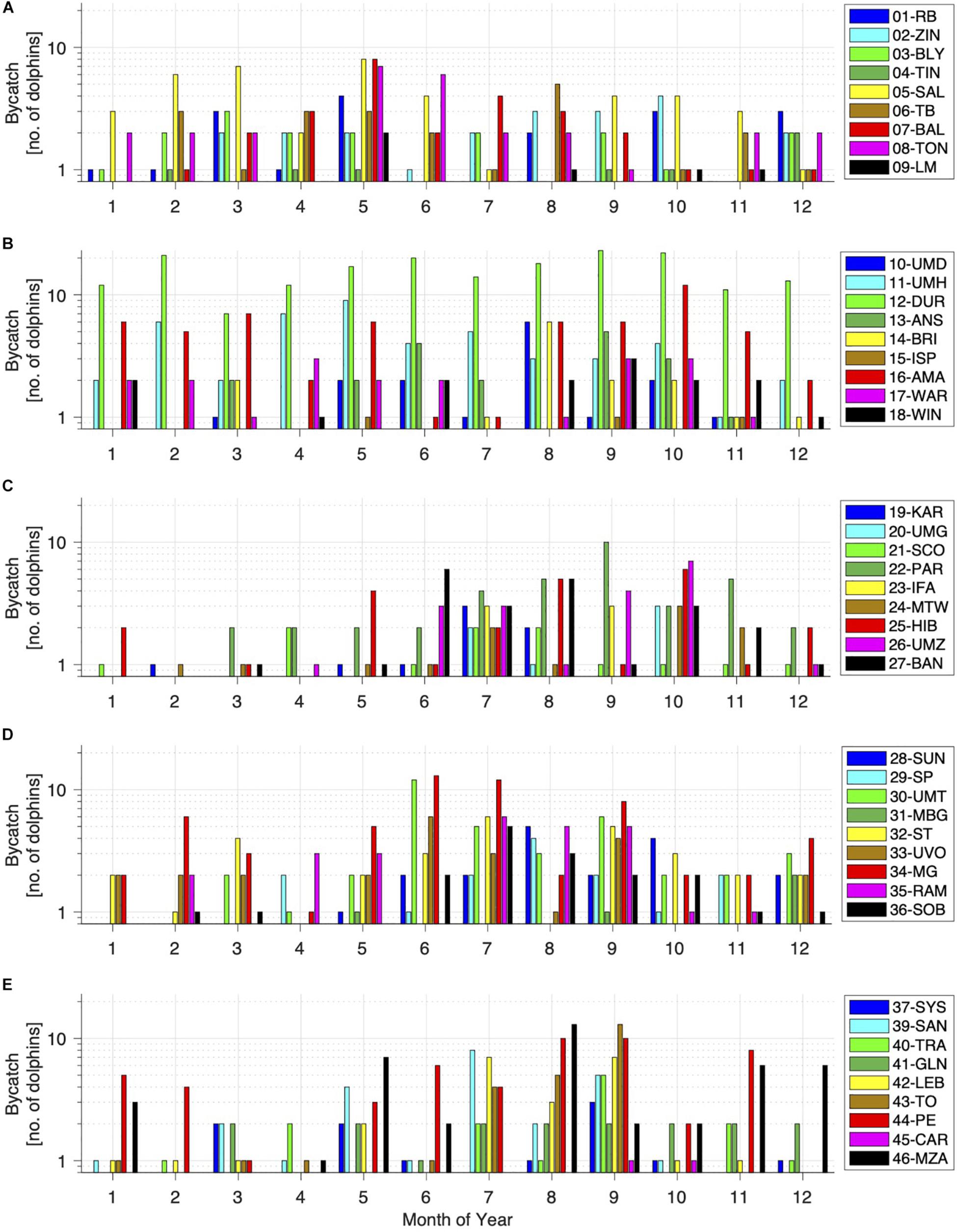
Figure 4. Monthly bycatch of T. aduncus calculated as totals across the entire period (1980–2015) per location (beach). Beaches are organized from North to South (A–E: 1–46). Note the logarithmic y-axis to better show low catches.
Durban, the installation with the highest number of nets, shows consistently high catches, throughout the year and over the entire study period (Figure 4). Umhlanga beach, just north of Durban, has the second highest catches along that part of the coastline, with peaks in April and May. In September, all beaches along this part of the coastline had catches, and most beaches had catches in October to November (Figure 4).
Catches are comparatively low along the next part of the coastline to the South, and are only increasing between June and November, with all beaches having catches in July and most beaches in August (Figure 4).
South of St. Michael’s on Sea the catches appear to be increasing again and more catches are seen from May to December than other times of the year, with Margate having catches in every month of the year (Figure 4). All beaches along this stretch of coast showed catches in September and most beaches had catches in July (Figure 4).
The southernmost part of the netted coastline shows a similar trend as the previous stretch. Port Edward consistently shows catches in all months of the year, except two (April and December; Figure 4). All beaches along the southernmost part of the netted area show catches in September, and most of them in August (Figure 4).
Overall, catches are spread out evenly throughout the year at the northern beaches, but a distinct seasonal pattern starts to become apparent from Park Rynie to the South, with higher catches during the austral winter.
The absolute number of dolphins caught from 1 January 1980 to 31 December 2015 is summarized in Figure 5, split into NC and SC south of Ifafa (Beach 23), clearly showing the increased bycatch during the Sardine Run period along the SC.
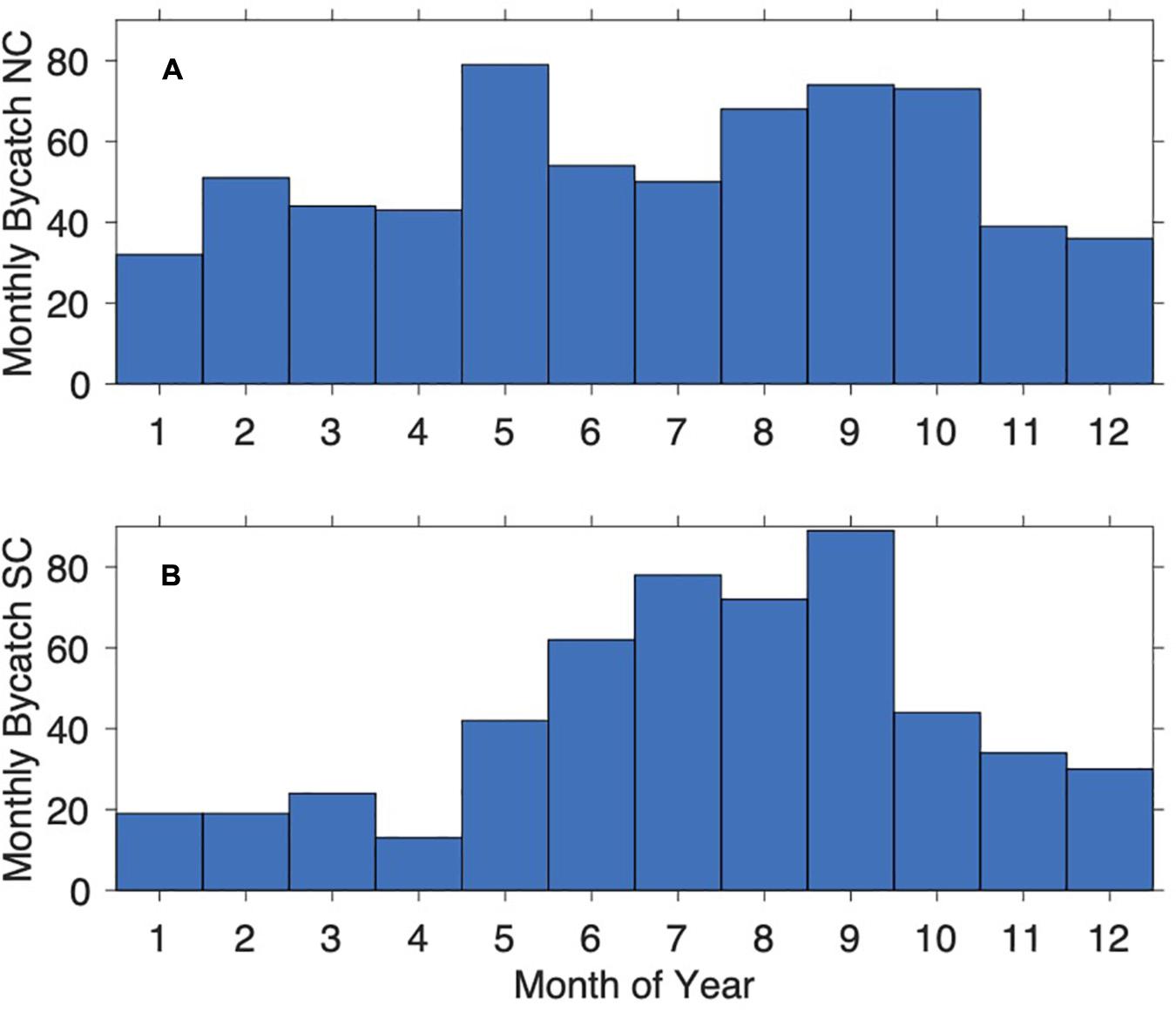
Figure 5. Monthly catch of T. aduncus calculated as totals across the entire period (1980–2015) separated into the two areas North Coast (NC; A) and South Coast (SC; B).
Long-Term Trends of Bycatch
Figures 6, 7 show the number of animals caught (per region) by sex and maturity status. In general, more females than males were caught, as were more juveniles than adults. On the North Coast, bycatch has been fairly consistent over the years in terms of numbers by age and sex (Figure 6A), with slightly more juveniles caught in recent years (Figure 6B). On the South Coast, bycatch increased up to the mid-1990s, but after that decreased and seems to be leveling off (Figure 7A).
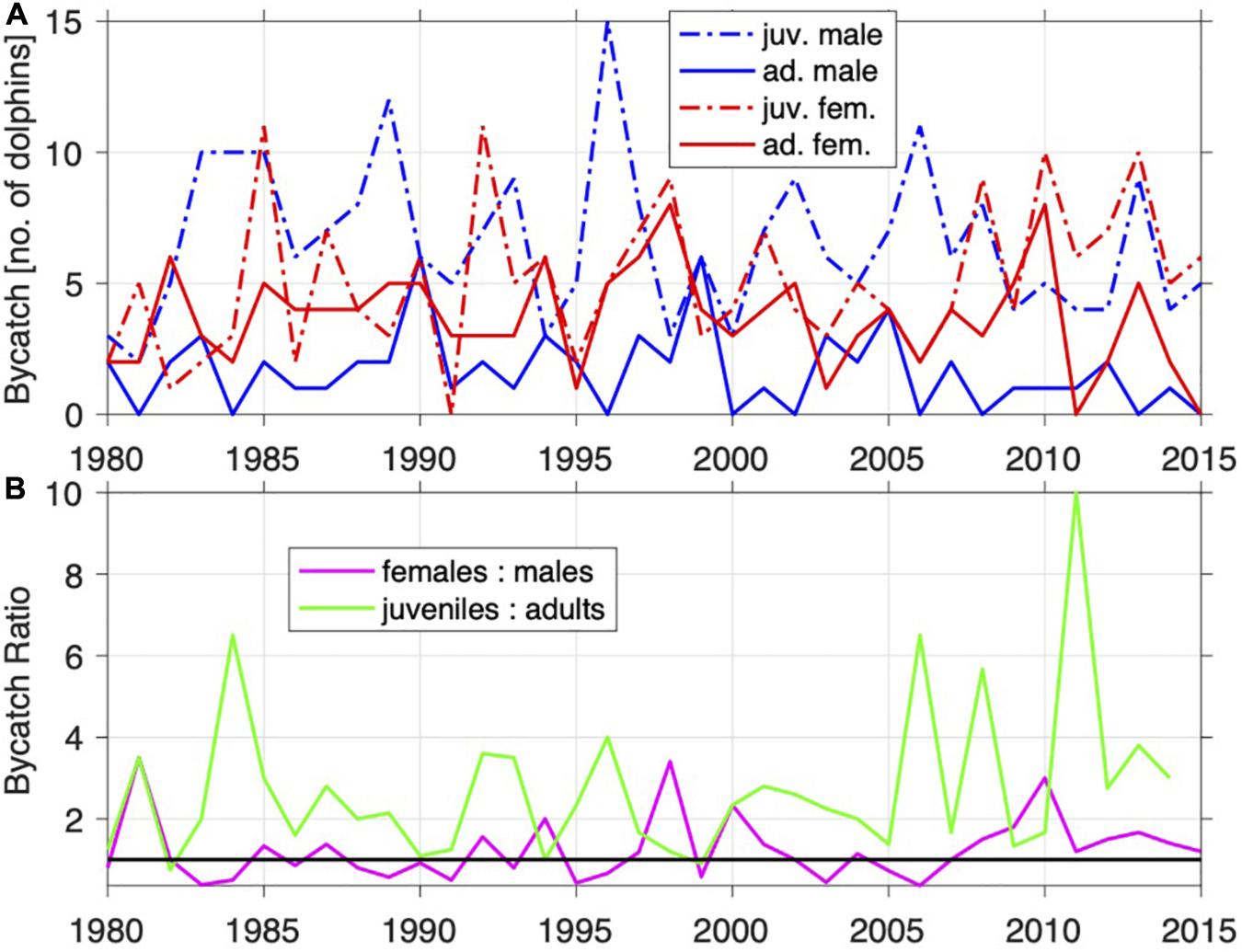
Figure 6. Annual Indo-Pacific bottlenose dolphin (T. aduncus) bycatch from the NC by sex and sexual maturity (A) and ratios of sex and sexual maturity (B).
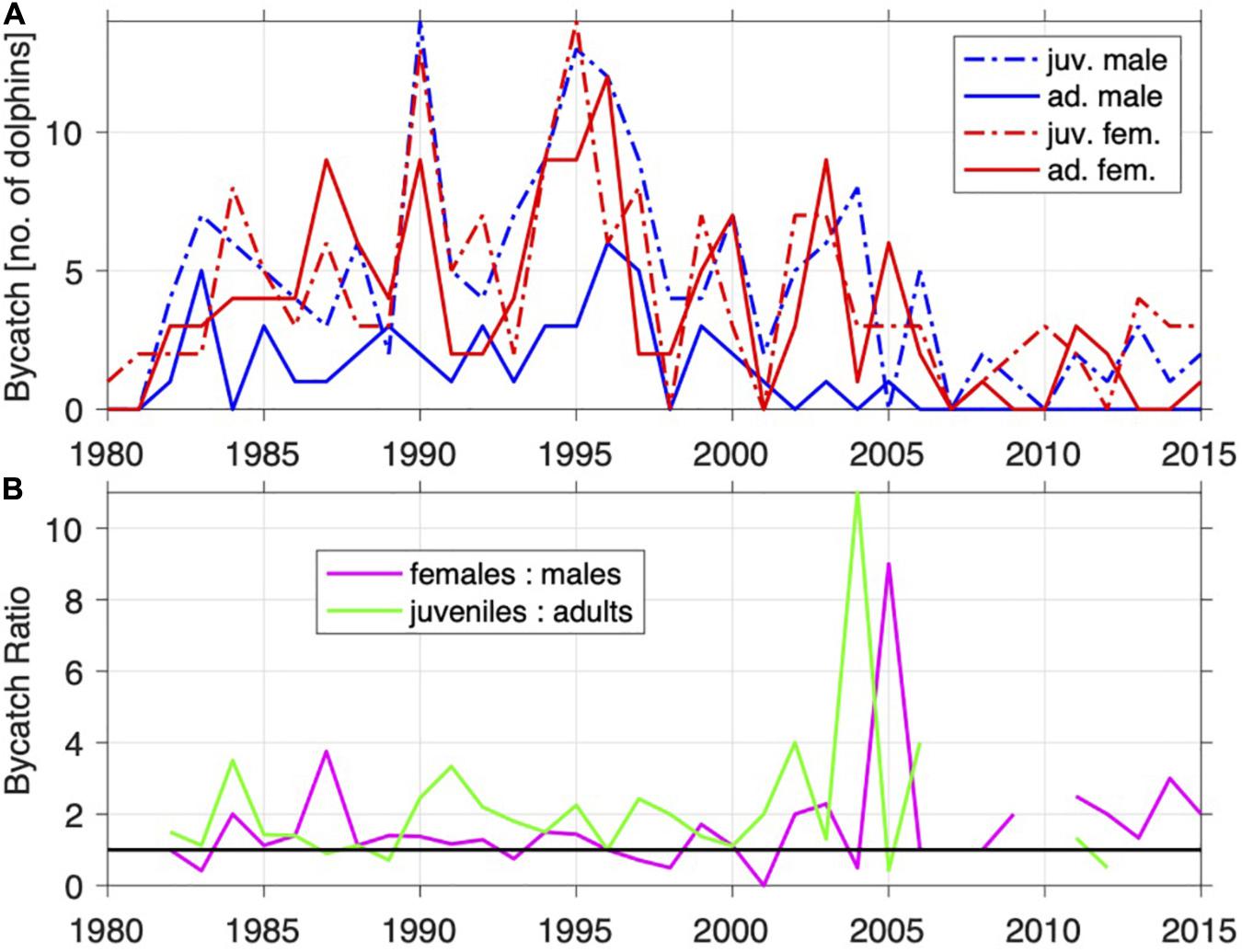
Figure 7. Annual Indo-Pacific bottlenose dolphin (T. aduncus) bycatch from the SC by sex and sexual maturity (A) and ratios of sex and sexual maturity (B).
The total annual bycatch along the KZN coast between 1980 and 2015 is shown in Figure 8A. Bycatch of dolphins peaked in 1990 and 1996, but has since decreased, averaging about 20–30 animals/year in recent years. On the NC, great interannual catch variability was observed; however, the number of animals caught does not seem to decrease over the long term, but rather averages at about 18 animals/year (Figure 8B). Due to the consistent and successful removal of nets during the peak Sardine Run period (June–July) since 1995, the SC bycatch has been decreasing, with fewer than 10 animals caught per year in recent years (Figure 8C).
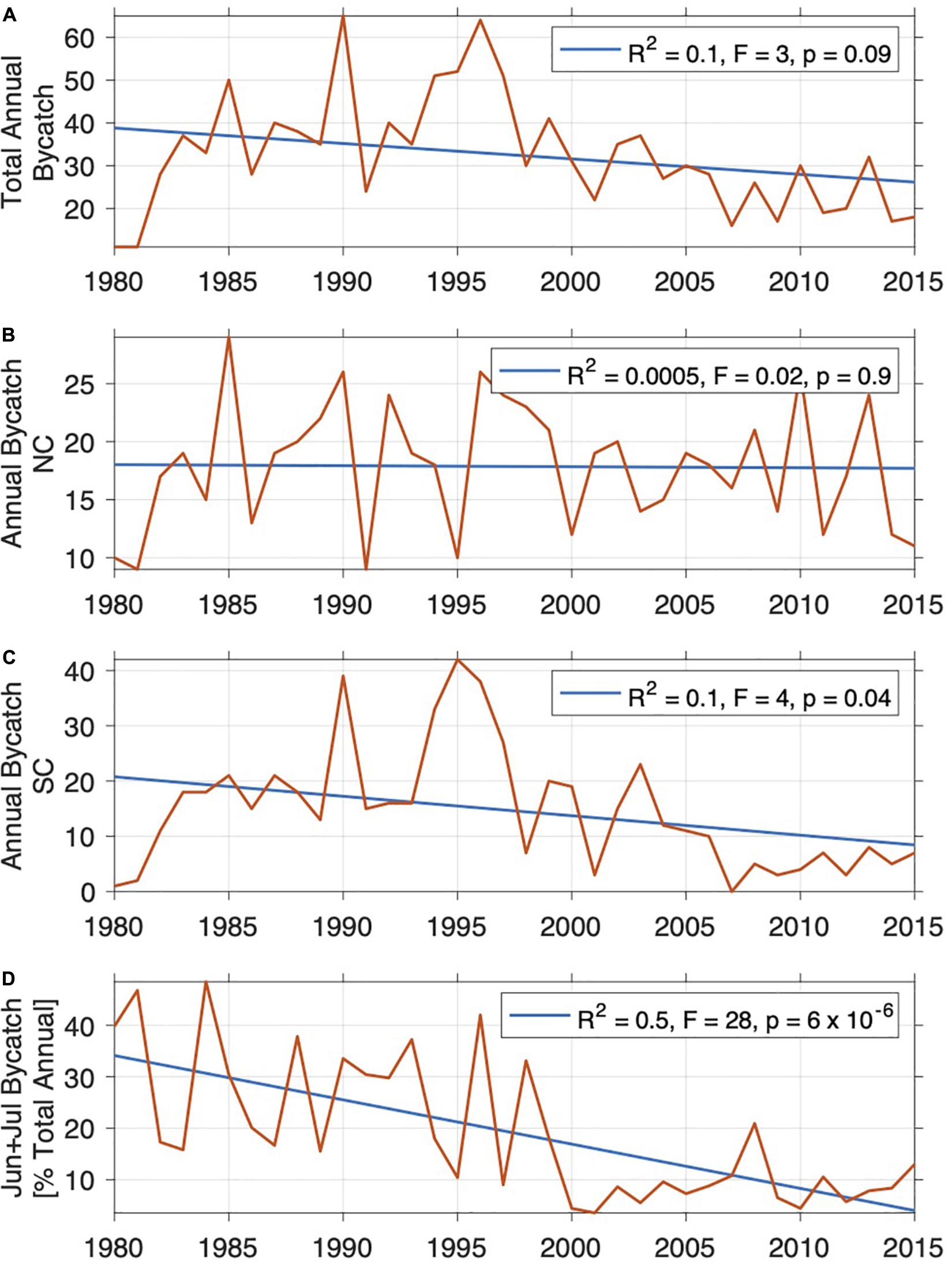
Figure 8. Total annual bycatch [numbers of animals] (A), split into NC (B) and SC (C), and bycatch during the months of June and July as a percent of the total annual bycatch (D). The R2-statistic, F-statistic, and p-value for the F-test are given.
Discussion
Total Bycatch and Demographics
Our analysis of the catch data from the KZN bather protection nets between January 1980 and December 2015 showed that female T. aduncus get caught more frequently than males, both overall as well as individually for the NC and SC populations (see section “Total Bycatch and Demographics”). These females are to equal parts composed of immature and mature animals, while most caught males are immature (see section “Total Bycatch and Demographics”). This is possibly a result of females with calves and young feeding closer inshore where the nets are located, while mature males often form separate groups, which may feed further offshore (Cockcroft, 1990, 1992). The impact of the catches on these demographic groups raises concern about the potential long-term effects on the overall demographics and recruitment of the resident populations and possible knock-on effects on the social system and population sizes (Cockcroft et al., 2016). Additional indirect effects of bycatch may be on the genetic diversity and life history of the populations concerned.
The fact that more young, immature T. aduncus get caught in the nets than any other age/size class is in agreement with previous studies (Cockcroft, 1990, 1992). Possible explanations include a more inquisitive nature in young animals, inexperience, the still-developing biosonar-relevant structures or the demographic composition of the population.
Research into bycatch of other delphinids has shown that a major threat for franciscana is incidental bycatch in fishery gillnets (Secchi et al., 2003) and several efforts did not assist in mitigating this (Bordino et al., 2013). Interestingly, more than half (51%) of all bycaught franciscanas were less than three years old (Ramos et al., 2000). Whether this preponderance of young individuals can be attributed to immature development of the echolocation system or is a consequence of insufficient experience is not clear. Frainer et al.’s (2015) research indicated allometric changes of the sonar-relevant head structures during the early postnatal development of the franciscana and led to the conclusion that the immature state of the topography of the biosonar relevant structures may be one of the causes of increased bycatch of young, immature animals. However, information on the demographic structure of the affected populations is usually lacking in the present dataset, the ratio of immature to mature animals caught in nets with pingers (ADD) was similar to that in nets without pingers (Erbe et al., 2016) and might simply reflect the demographic composition of this population (i.e., there may be more immature than mature animals in this population, and consequently more immature ones get caught). Neither explanation would account for a skewness toward immature males in the catch. Atkins et al. (2013) suggested a propensity for risk-taking in adolescent mammals as the cause for a bias toward immature males in the bycatch of humpback dolphins S. plumbea in the KZN bather protection nets; this may also be the case in T. aduncus.
Overall, slightly more animals get caught on the NC than on the SC (see section “Total Bycatch and Demographics”), which is consistent with the results of the aerial surveys conducted by Ross et al. (1987), during which more animals were observed on the NC. There are continued concerns about the impact of catches of T. aduncus, particularly in view of the recent re-assessment of the species and delineation into the two resident subpopulations north and south of Ifafa (Cockcroft et al., 2016). Our data indicate a steady reduction in bycatch on the SC which is likely due to improved netting management with the removal of nets during the Sardine Run. Fewer than 10 animals per year were caught in recent years (2007–2015; Figure 8). If we assume a conservative population size estimate of only 10,000 mature animals for this part of the coastline (SC, subpopulation b; Cockcroft et al., 2016), this would present 0.1% removal of the total population per year. NC catches over the same study period consistently averaged 18 dolphins per annum, i.e., 0.72% annual removal of the overall NC population (subpopulation a) if a population size of 2,500 mature individuals is assumed (Cockcroft et al., 2016). Both levels of removal are considerably less than previously reported by Cockcroft (1990); Cockcroft and Ross (1990b) and Cockcroft et al. (1991). However, at present all available population size estimates for T. aduncus populations north and south of Ifafa are dated (Ross et al., 1989; Cockcroft et al., 1990, 1992) and no new population abundance surveys have been conducted in the past 30 years. Ross et al. (1989) reported that the density of T. aduncus had declined on the KZN South Coast between 1985 and 1989/90 (Ross et al., 1989) and Cockcroft et al. (1990) indicated that sighting rates of T. aduncus had decreased between 1974 and 1978 and again between 1984 and 1988 in the same area. In addition, a recent IUCN Red List assessment indicates a decline of the species elsewhere due to fisheries bycatch (Braulik et al., 2019). This highlights the urgency of new, updated abundance estimates. In the absence of any recent, robust population size estimates, an evaluation of the impact of current catches of T. aduncus in shark nets on population sizes is not possible.
Spatial and Seasonal Distribution of Bycatch
Examination of the bycatch data over the 36-year period shows a lot of spatio-temporal variation (Figure 3). Cross-correlation of time series of CPUE between neighboring beaches was strongest in the years up to 1999; i.e., prior to installation of pingers or other ADDs, showing no significant or consistent reduction in bycatch at nets with ADDs. In fact, at half of the beaches with ADDs, bycatch increased after ADD installation (see Erbe et al., 2016). The cross-correlation coefficients between neighboring beaches clustered into groups of 3–4 beaches (Figure 3) and may correspond to the “preferred areas” noted by Ross et al. (1987, 1989) and Cockcroft et al. (1990, 1991), each extending over about 30–40 km of coastline. Ross et al. (1987) speculated that these are home ranges of local/resident populations and recent research has shown that these areas may persist over long temporal scales (Brough et al., 2019). Cockcroft et al. (1992) found that sightings of T. aduncus during aerial surveys along the KZN NC were not random, but corresponded to those identified during previous surveys conducted at the same time of year (May; Ross et al., 1987, 1989) and coincided with peaks of sightings made during boat surveys (Cockcroft et al., 1990). However, more recent genetic data indicate that T. aduncus from KZN waters do not show any genetic differentiation apart from the population break south of Ifafa (Natoli et al., 2008; Gopal, 2013). Thus, further research should focus on investigations of stock structure using biochemical markers, such as isotope and fatty acid analysis (Barros et al., 2010); in addition, external indicators, such as ectoparasite burden, may also yield further information in this regard (Urian et al., 2018). Furthermore, investigations into the levels of PCB’s and DDT’s in T. aduncus also indicated some population structuring as animals caught north of Durban showed that these were significantly greater than for animals south of Mtwalumi, suggesting little mixing of animals from the extreme North and South of KZN waters (Cockcroft et al., 1989).
The trend of animals incidentally caught in the bather protection nets appears to reflect a seasonal southward movement of animals toward more southerly beaches throughout the year on both the NC and SC (Figures 4, 5). On the NC, a peak in catches can be seen in May in the far North and another between September and November around Durban (Figures 4, 5). On the SC, a cluster can be seen in July/August between Karridene (beach 19) and Banana Beach (beach 27), with a secondary peak between Sunwich Port (beach 28) and Southbroom (beach 36) in July and between Kent Bay (beach 37) and Mzamba (beach 46) in August, with most catches occurring in September between Sunwich Port (beach 28) and Mzamba (beach 46; Figure 4). This distinct seasonal pattern may indicate the influx of animals from the south associated with the Sardine Run during the austral winter (peak: June/July; Figure 5).
Overall, catches are consistent throughout the year, showing little seasonal variation. This is in contrast to Cockcroft et al. (1992), who reported that catch rates were highly seasonal. However, no significant temporal differences in mean school sizes were observed during aerial surveys along the KZN coast (NC and SC), but varied off the former Transkei (now Eastern Cape) coastline (Ross et al., 1987). In our study, year appeared to have the biggest impact on the catch statistics (Figure 8), but it is unclear what drives the large inter-annual variability in bycatch. While environmental factors (e.g., El Nino effects) may contribute to the variability, boat-based field studies on bottlenose dolphins in KZN waters (both NC and SC) are urgently needed to understand movements of these animals. In addition, further studies to delineate populations/stock structure, such as molecular and biochemical markers (e.g., isotopes, fatty acids) are also necessary.
Long-Term Trends of Bycatch
Examination and evaluation of long-term trends in bycatch is important as patterns may be revealed that can assist in the management and conservation of populations; for example, the frequent, albeit brief, temporal removal of nets along the South Coast at times of dolphin presence associated with the Sardine Run during the austral winter has resulted in an overall decline in catches over the last 20 years (Figures 7, 8), indicating that KZNSB has become increasingly successful at reducing bycatch through net removal. This net removal appears to have been substantially more effective than active and passive acoustic mitigation devices, such as pingers or sonar reflectors (Erbe et al., 2016).
Recent data on the presence of various chemical pollutants in the animals caught in the KZN bather protection nets show alarming levels of PCB’s, DDT, flame retardants and plasticizers that are higher than most published values for small cetaceans (Gui et al., 2016; Aznar-Alemany et al., 2019). Given the potential implications for immune response and reproductive output (Jepson et al., 2016), such threats may potentially outweigh impacts from bycatch in bather protection nets.
Conclusion
Our analyses of the bycatch data of Indo-Pacific bottlenose dolphins in bather protection nets between January 1980 and December 2015 show no decline contrary to previous predictions about the potential severe impacts on these populations (Cockcroft, 1990; Cockcroft and Ross, 1990b). However, further work into population demographics and structure, respective abundance estimates, and movement patterns of bottlenose dolphins in the waters of KZN and the Eastern Cape is necessary to reliably assess the current impact of bycatch on the local populations and to potentially aid in reducing it. Pingers and ADDs placed in the bather protection nets in the past have proven not to be very effective (Erbe et al., 2016) and have given ambiguous results in other scenarios (Dawson et al., 1998, 2013). A recent review on marine mammal bycatch (Hamilton and Baker, 2019) indicated that it was apparent that effective bycatch mitigation strategies often comprise a suite of management measures in conjunction with technical mitigation. Being able to identify stock identity of individual Indo-Pacific bottlenose dolphins captured in the nets would add additional useful information as would novel approaches, such as biochemical markers (Barros et al., 2010) and the presence of ectoparasitic barnacles (Urian et al., 2018). In addition, surprisingly little recent information is available on both the common dolphins (D. delphis, formerly D. capensis) and Indian Ocean bottlenose dolphins (T. aduncus) following the annual Sardine Run off the Eastern Cape coast (O’Donoghue et al., 2010; Caputo et al., 2017). Thus, questions remain concerning population and stock structure as well as abundance estimates for these species throughout South African waters (Cockcroft et al., 2016; Plön and Cockroft, 2016). Given the absence of robust population size estimates for the species off the south-eastern coastline of South Africa and the continued high levels of pollutants recently reported for animals off KZN (Gui et al., 2016; Aznar-Alemany et al., 2019), there is an urgent need for updated scientific information on these populations to inform conservation and management. The present global rapid decline of the health of marine mammals in general, both on an individual as well as a population level, and T. aduncus in particular (Braulik et al., 2019), is cause for concern and can only be addressed with rigorous scientific efforts. Increasingly, triage efforts are needing to be considered in conservation and the focus with regards to the KZN populations of T. aduncus needs to urgently include further studies on the health of these animals (Lane et al., 2014), as cumulative impacts on these populations may go unnoticed at present.
Data Availability Statement
The datasets generated for this study are available on request to the corresponding author.
Ethics Statement
All activities with animals were performed under the approval and guidance with relevant permits issued by the Department of Environmental Affairs, Republic of South Africa, and the Department of Agriculture, Forestry and Fisheries, Republic of South Africa. All data were used with full permission from the KwaZulu-Natal Sharks Board (KZNSB) via a signed collaboration form between the authors.
Author Contributions
The study was jointly conceived and designed by all authors. SW contributed the data. CE and SP performed the data analysis and prepared figures. SP wrote the main manuscript text and all authors reviewed the manuscript.
Conflict of Interest
The authors declare that the research was conducted in the absence of any commercial or financial relationships that could be construed as a potential conflict of interest.
Acknowledgments
The operations staff of the KwaZulu-Natal Sharks Board (KZNSB) maintain the nets, deploy the marine mammal deterrents, and record all catches. Catch and effort data are captured by staff in the Research and Monitoring Division of the KZNSB. Graham Ross and Vic Cockcroft, previously Port Elizabeth Museum, are thanked for their early commitment to ensuring all dolphin captures were recorded and in implementing bycatch mitigation measures in this fishery. Geremy Cliff and Vic Peddemors are thanked for continuing these efforts. Sheldon Dudley provided valuable input on an earlier version of this manuscript.
References
Anderson, R. C., Herrera, M., Ilangakoon, A. D., Koya, K. M., Moazzam, M., Mustika, P. L., et al. (2020). Cetacean bycatch in Indian Ocean tuna gillnet fisheries. Endanger. Species Res. 41, 39–53. doi: 10.3354/esr01008
Atkins, S., Cliff, G., and Pillay, N. (2013). Humpback dolphin bycatch in the shark nets in KwaZulu-Natal, South Africa. Biol. Conserv. 159, 442–449. doi: 10.1016/j.biocon.2012.10.007
Aznar-Alemany, Ò, Sala, B., Plön, S., Bouwman, H., Barceló, D., and Eljarrat, E. (2019). Halogenated and organophosphorus flame retardants in cetaceans from the southwestern Indian Ocean. Chemosphere 226, 791–799. doi: 10.1016/j.chemosphere.2019.03.165
Barros, N. B., Ostrom, P. H., Stricker, C. A., and Wells, R. S. (2010). Stable isotopes differentiate bottlenose dolphins off west-central Florida. Mar. Mamm. Sci. 26, 324–336. doi: 10.1111/j.1748-7692.2009.00315.x
Best, P. B. (2007). Whales and Dolphins of the Southern African Subregion. Cape Town: Cambridge University Press.
Bordino, P., Mackay, A., Werner, T., Northridge, S., and Read, A. (2013). Franciscana bycatch is not reduced by acoustically reflective or physically stiffened gillnets. Endanger. Species Res. 21, 1–12. doi: 10.3354/esr00503
Braulik, G., Natoli, A., Kiszka, J., Parra, G., Plön, S., and Smith, B. D. (2019). Tursiops aduncus. IUCN Red List Assess. 2019:e.T41714A50381127.
Brough, T., Rayment, W., Slooten, E., and Dawson, S. (2019). Fine scale distribution for a population of New Zealand’s only endemic dolphin (Cephalorhynchus hectori) shows long-term stability of coastal hotspots. Mar. Mamm. Sci. 35, 140–163. doi: 10.1111/mms.12528
Brownell, R. L. Jr., Reeves, R. R., Read, A. J., Smith, B. D., Thomas, P. O., Ralls, K., et al. (2019). Bycatch in gillnet fisheries threatens Critically Endangered small cetaceans and other aquatic megafauna. Endang. Species Res. 40, 285–296. doi: 10.3354/esr00994
Caputo, M., Froneman, P. W., Du Preez, D., Thompson, G., and Plön, S. (2017). Long-term trends in cetacean occurrence during the annual Sardine Run off the Wild Coast, South Africa. Afr. J. Mar. Sci. 39, 83–94. doi: 10.2989/1814232x.2017.1304451
Cliff, G., and Dudley, S. F. J. (1992). Protection against shark attack in South Africa, 1952 to 1990. Aust. J. Mar. Freshw. Res. 43, 263–272. doi: 10.1071/mf9920263
Cliff, G., and Dudley, S. F. J. (2011). Reducing the environmental impact of shark-control programs: a case study from KwaZulu-Natal, South Africa. Mar. Freshw. Res. 62, 700–709. doi: 10.1071/mf10182
Cockcroft, V., Natoli, A., Reisinger, R., Elwen, S., Plön, S., Hoelzel, R., et al. (2016). “A conservation assessment of Tursiops aduncus,” in The Red List of Mammals of South Africa, Swaziland and Lesotho, eds M. F. Child, L. Roxburgh, D. Raimondo, E. D. L. San, J. Selier, and H. Davies-Mostert (Midrand: South African National Biodiversity Institute and Endangered Wildlife Trust).
Cockcroft, V. G. (1990). Dolphin catches in the Natal shark nets, 1980 to 1988. S. Afr. J. Wildl. Res. 20, 44–51.
Cockcroft, V. G. (1992). Incidental capture of bottlenose dolphins (Tursiops truncatus) in shark nets: an assessment of some possible causes. J. Zool. 226, 123–134. doi: 10.1111/j.1469-7998.1992.tb06131.x
Cockcroft, V. G. (1994). Is there common cause for dolphin capture in gillnets? A review of dolphin catches in shark nets off Natal, South Africa. Rep. Intern. Whal. Commiss. 15, 541–547.
Cockcroft, V. G., de Kock, A. C., Lord, D. A., and Ross, G. J. B. (1989). Organochlorines in bottlenose dolphins Tursiops truncatus from the east coast of South Africa. S. Afr. J. Mar. Sci. 8, 207–217.
Cockcroft, V. G., and Ross, G. J. B. (1990a). Age, growth and reproduction of bottlenose dolphins Tursiops truncatus from the east coast of Southern Africa. Fish. Bull. 88, 289–302.
Cockcroft, V. G., and Ross, G. J. B. (1990b). “Bottlenose dolphins in Natal shark nets, 1980 through 1987: catch rates and associated contributing factors,” in Cetaceans and Cetacean Research in the Indian Ocean Sanctuary, eds S. Leatherwood and G. P. Donovan (Nairobi: United Nations Environment Programme), 115–127.
Cockcroft, V. G., and Ross, G. J. B. (1990c). “Food and feeding of the Indian Ocean bottlenose dolphin off Southern Natal, South Africa,” in The Bottlenose Dolphin, eds S. Leatherwood and R. Reeves (New York, NY: Academic Press), 295–308. doi: 10.1016/b978-0-12-440280-5.50019-6
Cockcroft, V. G., Ross, G. J. B., and Peddemors, V. M. (1990). Bottlenose dolphin Tursiops truncatus distribution in Natal’s coastal waters. S. Afr. J. Mar. Sci. 9, 1–10.
Cockcroft, V. G., Ross, G. J. B., and Peddemors, V. M. (1991). Distribution and status of bottlenose dolphin Tursiops truncatus on the south coast of Natal, South Africa. S. Afr. J. Mar. Sci. 11, 203–209.
Cockcroft, V. G., Ross, G. J. B., Peddemors, V. M., and Borchers, D. L. (1992). Estimates of abundance and undercounting of bottlenose dolphins off northern Natal, South Africa. S. Afr. J. Wildl. Res. 22, 102–109.
Dawson, S., Read, A., and Slooten, E. (1998). Pingers, porpoises and power: uncertainties with using pingers to reduce bycatch of small cetaceans. Biol. Conserv. 84, 141–146. doi: 10.1016/s0006-3207(97)00127-4
Dawson, S. M., Northridge, S., Waples, D., and Read, A. J. (2013). To ping or not to ping: the use of active acoustic devices in mitigating interactions between small cetaceans and gillnet fisheries. Endanger. Species Res. 19, 201–221. doi: 10.3354/esr00464
Dudley, S. F. J., and Cliff, G. (1993). Some effects of shark nets in the nearshore environment. Environ. Biol. Fish. 36, 243–255. doi: 10.1007/bf00001720
Dudley, S. F. J., and Cliff, G. (2010). Influence of the annual sardine run on catches of large sharks in the protective gillnets off KwaZulu-Natal, South Africa, and the occurrence of sardine in shark diet. Afr. J. Mar. Sci. 32, 383–397. doi: 10.2989/1814232x.2010.502641
Erbe, C., Wintner, S., Dudley, S. F., and Plön, S. (2016). Revisiting acoustic deterrence devices: long-term bycatch data from South Africa’s bather protection nets. Proc. Meet. Acoust. 27, 1–4. doi: 10.18772/22010115164.4
Frainer, G., Huggenberger, S., and Moreno, I. B. (2015). Postnatal development of franciscana’s (Pontoporia blainvillei) biosonar relevant structures with potential implications for function, life history, and bycatch. Mar. Mamm. Sci. 31, 1193–1212. doi: 10.1111/mms.12211
Friedmann, Y., and Daly, B. (2004). Red Data Book of the Mammals of South Africa: A Conservation Assessment. Johannesburg: Endangered Wildlife Trust.
Gopal, K. (2013). A Genetic Study of Two Inshore Dolphin Species (Cephalorhynchus heavisidii and Tursiops aduncus) Found Along the Coast of South Africa. Ph. D thesis, University of Pretoria, Pretoria.
Gui, D., Karczmarski, L., Yu, R.-Q., Plön, S., Chen, L., Tu, Q., et al. (2016). Profiling and spatial variation analysis of persistent organic pollutants in South African delphinids. Environ. Sci. Technol. 50, 4008–4017. doi: 10.1021/acs.est.1025b06009
Hamilton, S., and Baker, G. B. (2019). Technical mitigation to reduce marine mammal bycatch and entanglement in commercial fishing gear: lessons learnt and future directions. Rev. Fish Biol. Fish. 29, 223–247. doi: 10.1007/s11160-019-09550-6
Hines, E., Ponnampalam, L. S., Junchompoo, C., Peter, C., Vu, L., Huynh, T., et al. (2020). Getting to the bottom of bycatch: a GIS-based toolbox to assess the risk of marine mammal bycatch. Endanger. Species Res. 42, 37–57. doi: 10.3354/esr01037
Jepson, P. D., Deaville, R., Barber, J. L., Aguilar, A., Borrell, A., Murphy, S., et al. (2016). PCB pollution continues to impact populations of orcas and other dolphins in European waters. Sci. Rep. 6:18573.
Lane, E. P., de Wet, M., Thompson, P., Siebert, U., Wohlsein, P., and Plön, S. (2014). A systematic health assessment of Indian Ocean bottlenose (Tursiops aduncus) and Indo-Pacific humpback (Sousa plumbea) dolphins, incidentally caught in shark nets off the KwaZulu-Natal coast, South Africa. PLoS One 9:e0107038. doi: 10.1371/journal.pone.0107038
Mannocchi, L., Dabin, W., Augeraud-Véron, E., Dupuy, J.-F., Barbraud, C., and Ridoux, V. (2012). Assessing the impact of bycatch on dolphin populations: the case of the common dolphin in the Eastern North Atlantic. PLoS One 7:e32615. doi: 10.1371/journal.pone.032615
Natoli, A., Peddemors, V. M., and Hoelzel, A. R. (2008). Population structure of bottlenose dolphins (Tursiops aduncus) impacted by bycatch along the east coast of South Africa. Conserv. Genet. 9, 627–636. doi: 10.1007/s10592-007-9379-y
O’Donoghue, S. H., Whittington, P. A., Dyer, B. M., and Peddemors, V. M. (2010). Abundance and distribution of avian and marine mammal predators of sardine observed during the 2005 KwaZulu-Natal sardine run survey. Afr. J. Mar. Sci. 32, 361–374. doi: 10.2989/1814232x.2010.502640
Omeyer, L. C. M., Doherty, P. D., Dolman, S., Enever, R., Reese, A., Tregenza, N., et al. (2020). Assessing the effects of banana pingers as a bycatch mitigation device for harbour porpoises (Phocoena phocoena). Front. Mar. Sci. 7:285. doi: 10.3389/fmars.2020.00285
Peddemors, V., and Cockcroft, V. G. (1994). “Dolphin deterrents tested in shark nets off Natal, South Africa,” in Gillnets and Cetaceans, eds W. F. Perrin, G. P. Donovan, and J. Barlow (Impington: International Whaling Commission), 626.
Peddemors, V. M. (1993). Bottlenose dolphin Tursiops truncatus research in Natal, South Africa: a review. Lammergeyer 42, 24–34.
Peddemors, V. M. (1999). Delphinids of southern Africa: a review of their distribution, status and life history. J. Cetacean Res. Manag. 1, 157–165.
Peddemors, V. M., Cockcroft, V. G., and Wilson, R. B. (1990). “Incidental dolphin mortality in the Natal shark nets: a preliminary report on prevention measures,” in Cetaceans and Cetacean Research in the Indian Ocean Sanctuary, eds S. Leatherwood and G. Donovan (Nairobi: United Nations Environmental Programme), 129–137.
Peltier, H., Authier, M., Deaville, R., Dabin, W., Jepson, P. D., van Canneyt, O., et al. (2016). Small cetacean bycatch as estimated from stranding schemes: the common dolphin case in the northeast Atlantic. Environ. Sci. Policy 63, 7–18. doi: 10.1016/j.envsci.2016.05.004
Plön, S., Atkins, S., Conry, D., Pistorius, P., Cockcroft, V., and Child, M. (2016). “A conservation assessment of Sousa plumbea,” in The Red List of Mammals of South Africa, Swaziland and Lesotho, eds M. F. Child, D. Raimondo, E. D. L. San, L. Roxburgh, and H. Davies-Mostert (Midrand: South African National Biodiversity Institute and Endangered Wildlife Trust).
Plön, S., Cockcroft, V. G., and Froneman, P. W. (2015). The natural history and conservation of Indian Ocean humpback dolphins (Sousa plumbea) in South African waters. Adv. Mar. Biol. 72, 143–162. doi: 10.1016/bs.amb.2015.08.005
Plön, S., and Cockroft, V. (2016). “A conservation assessment of Delphinus capensis capensis,” in The Red List of Mammals of South Africa, Swaziland and Lesotho, eds M. F. Child, L. Roxburgh, E. D. L. San, D. Raimondo, and H. Davies-Mostert (Midrand: South African National Biodiversity Institute and Endangered Wildlife Trust).
Ramos, R. M. A., Di Beneditto, A. P. M., and Lima, N. R. W. (2000). Growth parameters of Pontoporia blainvillei and Sotalia fluviatilis (Cetacea) in northern Rio de Janeiro, Brazil. Aquat. Mamm. 26, 65–75.
Ross, G. J. B. (1984). The smaller cetaceans of the south east coast of Southern Africa. Ann. Cape Prov. Mus. Nat. Hist. 15:173.
Ross, G. J. B., Cockcroft, V. G., and Butterworth, D. S. (1987). Offshore distribution of bottlenosed dolphins in Natal coastal waters and Algoa Bay, Eastern Cape. S. Afr. J. Zool. 22, 50–56. doi: 10.1080/02541858.1987.11448020
Ross, G. J. B., Cockcroft, V. G., Melton, D. A., and Butterworth, D. S. (1989). Population estimates for bottlenose dolphins Tursiops truncatus in Natal and Transkei waters. S. Afr. J. Mar. Sci. 8, 119–129.
Secchi, E. R., Danilewicz, D., and Ott, P. (2003). Applying the phylogeographic concept to identify franciscana dolphin stocks: implications to meet management objectives. J. Cetacean Res. Manag. 5, 61–68.
Tulloch, V., Pirotta, V., Grech, A., Crocetti, S., Double, M., How, J., et al. (2019). Long-term trends and a risk analysis of cetacean entanglements and bycatch in fisheries gear in Australian waters. Biodivers. Conserv. 29, 251–282. doi: 10.1007/s10531-019-01881-x
Urian, K. W., Kaufman, R., Waples, D. M., and Read, A. J. (2018). The prevalence of ectoparasitic barnacles discriminates stocks of Atlantic common bottlenose dolphins (Tursiops truncatus) at risk of entanglement in coastal gill net fisheries. Mar. Mamm. Sci. 35, 290–299. doi: 10.1111/mms.12522
Wang, J. Y., Chou, L. S., and White, B. N. (1999). Mitochondrial DNA analysis of sympatric morphotypes of bottlenose dolphins (genus: Tursiops) in Chinese waters. Mol. Ecol. 8, 1603–1612. doi: 10.1046/j.1365-294x.1999.00741.x
Wang, J. Y., Chou, L. S., and White, B. N. (2000a). Differences in external morphology of two sympatric species of bottlenose dolphins (genus Tursiops) in the waters of China. J. Mamm. 81, 1157–1165. doi: 10.1644/1545-1542(2000)081<1157:ditemo>2.0.co;2
Keywords: bycatch, Indo-Pacific bottlenose dolphin, Tursiops aduncus, South Africa, sustainability
Citation: Plön S, Erbe C and Wintner S (2020) Long-Term Demographic and Spatio-Temporal Trends of Indo-Pacific Bottlenose Dolphin (Tursiops aduncus) Bycatch in Bather Protection Nets off KwaZulu-Natal, South Africa. Front. Mar. Sci. 7:542675. doi: 10.3389/fmars.2020.542675
Received: 13 March 2020; Accepted: 22 October 2020;
Published: 24 December 2020.
Edited by:
Ana M. M. Sequeira, University of Western Australia, AustraliaReviewed by:
Saana Isojunno, University of St Andrews, United KingdomVinay Udyawer, Australian Institute of Marine Science (AIMS), Australia
Copyright © 2020 Plön, Erbe and Wintner. This is an open-access article distributed under the terms of the Creative Commons Attribution License (CC BY). The use, distribution or reproduction in other forums is permitted, provided the original author(s) and the copyright owner(s) are credited and that the original publication in this journal is cited, in accordance with accepted academic practice. No use, distribution or reproduction is permitted which does not comply with these terms.
*Correspondence: Stephanie Plön, stephanie.ploen@gmail.com