- 1Marine Research Department, Senckenberg am Meer, Wilhelmshaven, Germany
- 2Institute for Chemistry and Biology of the Marine Environment, Carl von Ossietzky University Oldenburg, Oldenburg, Germany
- 3J.F. Blumenbach Institute of Zoology and Anthropology, Georg August University of Göttingen, Göttingen, Germany
- 4Centre of Biodiversity and Sustainable Land Use, Georg August University of Göttingen, Göttingen, Germany
Tidal coasts are characterized by natural disturbances, forming a broad variety of habitats, which provide different niches and varying food resources for benthic foraminifera. Foraminifera occupy different trophic levels and fill key trophic positions in benthic food webs, but detailed studies on their nutrition spectrum in inter- to supratidal environments are lacking. Further, it is unknown to what extent foraminifera can switch between different feeding modes depending on resource availability in different Wadden Sea habitats. In order to shed light on these questions, we investigated potential food resources in sediments as well as the food spectrum of two dominant benthic foraminifera, Trochammina inflata and Ammonia tepida, in four characteristic habitats of the Wadden Sea: salt marsh, mixed flat, oyster reef and mud flat, using fatty acid (FA) analysis. The sediments of the four habitats provide a broad range of food sources including green algae, brown algae, diatoms and bacteria for benthic foraminifera. The FA profile of T. inflata from the salt marsh and mixed flat was dominated by green algae and bacteria, whereas the brown algae biomarker was only found in specimens from the salt marsh. In comparison, FA profiles of A. tepida suggested dominant algae feeding (green algae and diatoms), whereas brown algae and bacterial FAs were only found in specimens from the oyster reef. The results suggest that foraminifera mainly feed at low trophic levels with selective food intake closely depending on their habitat. The diverse nutritional strategies may allow efficient exploitation of resources in dynamic tidal habitats. Presumably, foraminifera serve as an important link between lower and higher trophic levels and thus fill key trophic positions in the benthic food web of the Wadden Sea.
Introduction
Intertidal flats and supratidal salt marshes are widespread along sheltered coasts with soft-sediments, distinct tidal rhythms and low hydrodynamics (van de Kam et al., 2004; Schuerch et al., 2014). The exposure to natural disturbances forms heterogeneous habitats from tidal flats, i.e., mixed flats, oyster reefs and mud flats to salt marshes (Heip et al., 1995). Salt marshes are transition areas from terrestrial to marine habitats colonized mainly by halophytic plants (Allen, 2000). Intertidal flats are dominated by mixed flats (mud content 10–50%) which are characterized by marine resources, i.e., marine detritus and microphytobenthos (Flemming and Davis, 1994; Flemming and Ziegler, 1995). Present oyster reefs are formed by the Pacific oyster (Crassostrea gigas) which invaded in the late 1990s and provide a complex habitat for epibenthic organisms (Wehrmann et al., 2000). By contrast, mud flats are characterized by a grain size <63 μm and contain high amounts of organic matter (Stock et al., 2012).
The nature of these habitats is primarily determined by sediment composition, biological activity, ecosystem engineers (e.g., reef-building oysters, in- and epifaunal species producing feces and pseudofeces), organic matter sources, nutrient inputs, environmental, and biogeochemical parameters (Scott and Medioli, 1978; Bakker and Piersma, 2006; Wright et al., 2011). The variety of habitats in the Wadden Sea with different ecosphere conditions makes them an ideal model system to study the food spectrum of benthic foraminifera in a highly dynamic environment and the variability of nutrition on a spatial scale.
Benthic foraminifera contribute about 80% to protist biomass in intertidal sediments (Lei et al., 2014) and reach high abundances primarily in surface sediments due to food and oxygen availability (Alve and Murray, 1994; Gooday and Rathburn, 1999; Jorissen, 1999; van der Zwaan et al., 1999; Fontanier et al., 2002; Hippensteel et al., 2002). The agglutinated foraminifera Trochammina inflata dominate the higher intertidal settings at 0–20 cm sediment depth (Alve and Murray, 1999; Hayward et al., 1999), whereas the calcareous species Ammonia tepida preferentially occur at lower intertidal and subtidal habitats primarily at a sediment depth of 0–5 cm (Williams, 1994; Goldstein et al., 1995; Berkeley et al., 2007). Depending on their ecological niches, foraminifera feed on different resources and therefore use diverse feeding strategies, such as selective and non-selective deposit feeding, passive suspension feeding, incorporation of dissolved organic matter, selective grazing, indiscriminate herbivory, symbiosis, parasitism, carnivore nutrition and specialized predation (Gooday et al., 1992; Suhr et al., 2008; Dupuy et al., 2010; Goldstein and Richardson, 2018). Due to their high abundance in tidal sediments and their diverse food spectra, foraminifera represent important links between lower and higher trophic levels and thus fill key trophic positions in benthic food webs (Lipps and Valentine, 1970; Gooday et al., 1992). However, whether the food spectrum of benthic foraminifera varies among different habitats of the Wadden Sea is virtually unknown.
Until to date, studies investigating the food spectrum of foraminifera were mostly performed in the laboratory under controlled conditions (Muller, 1975; Bender, 1989; Pascal et al., 2008; Wukovits et al., 2018). Few field studies focused on foraminifera nutrition in extreme and unique environments such as the deep sea (Nomaki et al., 2009; Kharlamenko et al., 2017), oxygen minimum zones (Larkin et al., 2014) or Antarctica (Suhr et al., 2003, 2008). The studies existing suggest that the calcareous foraminifera species A. tepida adopts various nutritional strategies including bacterivory, predation and deposit feeding, and feeds on bacteria, diatoms and metazoans (Goldstein and Corliss, 1994; Pascal et al., 2008; Dupuy et al., 2010; LeKieffre et al., 2017; Wukovits et al., 2018). Knowledge on the nutrition of the agglutinated foraminifera species T. inflata is based on one experimental study, which suggests preference for pennate diatoms (Salami, 1976).
In order to understand variations in the food spectrum and to uncover habitat-specific diets of foraminifera, we investigated potential resources in the sediments as well as the food spectrum of two dominant foraminifera species, T. inflata and A. tepida, using fatty acid (FA) analysis at four characteristic habitats in the central Wadden Sea of the southern North Sea: salt marshes, mixed flats, oyster reefs and mud flats. The analysis of variations in FAs is a powerful tool allowing to study dietary contribution (Kelly and Scheibling, 2012; Traugott et al., 2013), organic matter distribution and origin (Zhang et al., 2017), as well as consequences of long-term environmental changes affecting foraminifera species abundances and distribution patterns (Naeher et al., 2012). We expected that foraminifera species utilize a diverse food spectrum based on the variety of food sources available and feed mainly on low trophic levels.
Materials and Methods
Study Site
The study site comprised the sheltered backbarrier environment of the East Frisian Islands in the Wadden Sea of Lower Saxony, Germany (Figure 1). Sediments of the soft-bottom shores are characterized by a distinct zonation pattern controlled by shore height, inundation frequency and hydrodynamic energy thus forming a variety of habitats from inter- to supratidal environments. In the framework of the current study four habitats were investigated: (A) the supratidal salt marsh and the intertidal, (B) mixed flat, (C) oyster reef, and (D) mud flat (Figure 1). The sand flat habitat was excluded from the analysis due to very low density of foraminifera. Both, the salt marsh and the mixed flat stations were located in the sheltered southern part of the island of Spiekeroog. The oyster reef was situated between the mainland and the island of Norderney, whereas the mud flat was located in the sheltered coastal area of Crildumersiel (Jade Channel).
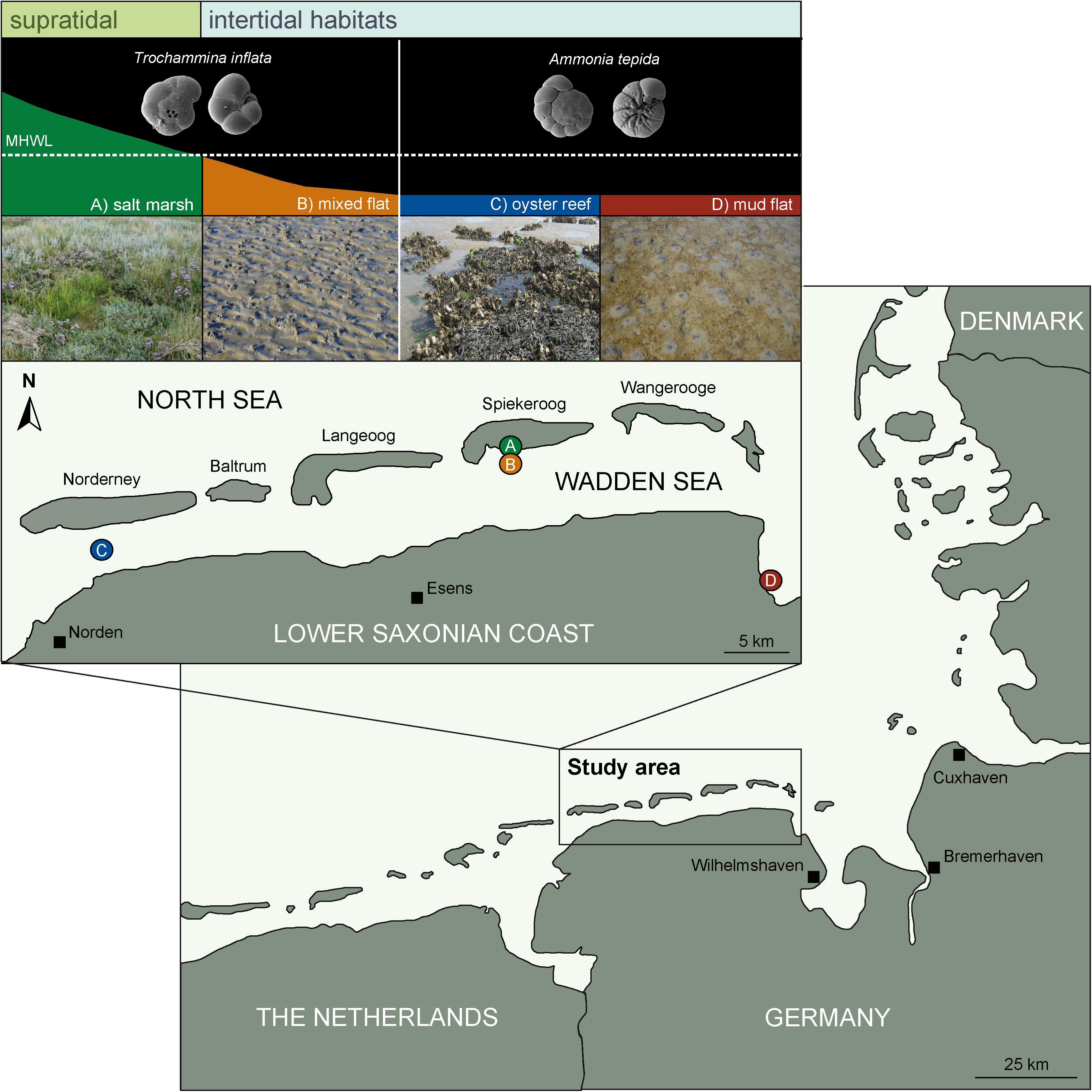
Figure 1. Map of study site including the four characteristic habitats of the Wadden Sea. Supratidal: (A) salt marsh = green, intertidal: (B) mixed flat = orange, (C) oyster reef = blue and (D) mud flat = dark red, which are located in the backbarrier environment of the East Frisian Islands of the central Wadden Sea (Germany, Southern North Sea). Small pictures represent the agglutinated species Trochammina inflata dominating in lower supratidal to higher intertidal (salt marsh and the mixed flat) and the hyaline species Ammonia tepida inhabiting the lower intertidal and subtidal (oyster reef and the mud flat).
The backbarrier environment of the East Frisian Islands is strongly influenced by inundation frequency depending on shore height. Depending on tidal level, the supratidal salt marsh reaches from >35 cm (upper salt marsh; USM) above mean high water level (MHWL) to 0–35 cm (lower salt marsh; LSM) above MHWL and is inundated 35–70 times a year in the USM and 150–250 times a year in the LSM. The salt marsh is characterized by fine-grained sediments (mainly mud, intercalated by distinct shell layers) and TOC contents <10% (Flemming and Davis, 1994; Bouillon and Boschker, 2006). Primary production in salt marshes is mainly performed by halophytic plants (Allen, 2000). However, the productivity of benthic microalgae contributes approximately 10–15 % to total ecosystem productivity and typically decreases with increasing tidal level (Tobias and Neubauer, 2009).
The investigated intertidal habitats (mixed flat, oyster reef, and mud flat) are located below the MHWL and inundated twice a day (Figure 1). Tides are semi-diurnal with a mean range of approximately 2.70 m at Spiekeroog, 2.50 m at Norderney and 3.40 m at Crildumersiel. Tidal flats of the Wadden Sea contain a TOC content <2% (Böttcher et al., 2000; Dellwig et al., 2000). Food resources in these environments, in particular microphytobenthos and detritus, play an important role as basis of the benthic food web (Flemming and Davis, 1994; Flemming and Ziegler, 1995). Especially in mud flats, high microbial activity and heterotrophic degradation of organic matter results in anoxic sediment conditions few centimeters below the sediment surface (van Oevelen et al., 2006). In contrast to tidal flats, the oyster reefs of the Pacific oyster Crassostrea gigas constitute of biogenic structures and produce biodeposits (feces and pseudofeces), thereby influencing the sediment structure (Bouchet et al., 2007; Markert et al., 2010). Consequently, the high amount of biodeposits leads to an enhanced organic enrichment and a high microphytobenthic biomass (Green et al., 2012).
Sampling and Processing
To identify the FAs of sediments and foraminifera, three replicated samples were taken with a sediment corer (30 cm length and 10 cm inner diameter) from 0 to 2 cm sediment depth at each of the four sampling stations: (A) salt marsh, (B) mixed flat, (C) oyster reef, and (D) mud flat in July 2017. This resulted in 12 samples for sediment analysis and 12 samples for the analysis of foraminifera. The sampling was only performed in the upper 2 cm of the sediment as no living individuals were observed below due to anoxic conditions.
Sediment samples were transferred with a spatula into 300 ml Kautex-containers and either frozen and stored at −15°C for sediment FA analysis or sieved with tap water through a 63 μm sieve to isolate benthic foraminifera. Two dominant foraminifera species were sampled from the sediment fraction >63 μm under a binocular microscope (Leica MZ12): Trochammina inflata (Montagu, 1808) from the salt marsh and mixed flat, and Ammonia tepida (Cushman, 1926) from the oyster reef and mud flat. Living specimens were identified by the presence of cytoplasm. Depending on their nutrition, the cytoplasm was brown to pale orange (T. inflata) or pale yellow to brown (A. tepida). The visual identification of the cytoplasm depends on the composition of the tests. Ammonia tepida tests have a smooth, but perforated surface, whereas tests of T. inflata are composed of grains of variable size and a thin organic layer. The cytoplasm of A. tepida can be seen through the calcareous tests and allows visual identification, which is not the case for the tests of the agglutinated species T. inflata. Therefore, T. inflata tests were cracked to ensure that the cytoplasm content existed. Isolated living specimens were pooled to ensure sufficient biomass for lipid extraction and to reduce individual variability in the signals of FAs (Kharlamenko et al., 2001; Nomaki et al., 2009). The number of individuals per replicate varied with test size (A. tepida: 270 ± 70 μm, T. inflata: 490 ± 130 μm); thus 300 individuals of A. tepida and 200 individuals of T. inflata were picked, respectively. Pooled specimens were transferred into Eppendorf tubes and stored at −15°C until FA analysis. Individuals of both species were photographed by scanning electron microscopy (TESCAN VEGA W-SEM Series, Czechia).
Fatty Acid Analysis
Lipids from sediments and foraminifera were extracted according to the protocols for the Microbial Identification System (MIDI Inc., Newark, United States; Zelles, 1999). The lipid fractions were saponified, methylated, extracted and washed to obtain the total amount of FAs, i.e., glycolipid, phospholipid, and neutral lipid fractions. The extracted FA methyl esters (FAMEs) of sediments and foraminifera were transferred into gas chromatography vials, one half of the FA phase was vaporized with nitrogen to enhance the FA concentration. Afterwards, the glass vials were capped and stored at −21°C until analysis via gas chromatography (Clarus 500, PerkinElmer Corporation, Norwalk, United States). The gas chromatograph was equipped with a HP-5 capillary column (inner diameter: 30 m × 0.32 mm, film thickness: 0.25 μm, PerkinElmer, Waltham, United States) and a flame ionization detector (FID), helium was used as carrier gas (Ferlian and Scheu, 2014). FAMEs were identified by comparing chromatographic retention times of samples and standard mixtures. Standard mixtures were composed of a mix (SupelcoTM) of 37 FAMEs and 26 BAMEs (Bacterial Acid Methyl Esters; Sigma-Aldrich, St. Louis, United States).
FAs 18:2ω6, 18:3ω3, and 18:1ω7 served as specific biomarkers for green algae, 20:4ω6 for brown algae, 16:1ω7, and 20:5ω3 for diatoms, and i15:0, a15:0, 15:0, i16:0, i17:0, cy17:0, and 17:0 for bacteria (Table 1). The non-specific biomarkers (14:0, 16:0, and 18:0) were excluded from data analyses as they may originate from de novo synthesis of FAs in algae and cyanobacteria and are potentially modified (Kelly and Scheibling, 2012). Percentages of the specific biomarkers of the sediments and foraminifera are given in Supplementary Tables 1, 2.
Calculations and Statistical Analysis
Peak areas of the measured FAs were used to calculate the percentage of single FAs to total FA content. The median of the sediment and foraminifera FA percentages was calculated using the replicated raw data (Supplementary Tables 1, 2).
Data were inspected for homogeneity and normality of variances using Levene’s test and Shapiro-Wilk test using STATISTICA 7.0 (StatSoft, Inc., Tulsa, OK, 2004); the tests were not significant (p > 0.05). Differences in FA composition for sediments (salt marsh, mixed flat, oyster reef and mud flat) and for foraminifera (T. inflata and A. tepida) were analyzed by principal component analysis (PCA) using CANOCO 5 (Microcomputer Power, Ithaca, United States, 2012). The first two dimensions of the PCA were used to present foraminifera community data and FA data in a vector graph. Eigenvalues present the variance in community data explained by the axis. PCA is based on linear regression and included the different Wadden Sea habitats (Supplementary Variables) which are represented by vectors in addition to the sediment/foraminifera FAs. Therefore, vectors reflect the relative importance of certain sediment/foraminifera FAs in the different habitats. Further, FA data were reduced using non-metric multidimensional scaling (NMDS) as implemented in CANOCO 5 and the NMDS scores then were analyzed using discriminant function analysis (DFA) in STATISTICA 7.0. The DFA tested if the FAs in sediments and foraminifera differ significantly between the four habitats studied; Wilk’s lambda was used as test statistics.
Results
Fatty Acid Composition in Sediments
As indicated by FAs, the four Wadden Sea sediments (salt marsh, mixed flat, oyster reef, and mud flat) provide a broad range of food sources including green and brown algae, diatoms and bacteria (Supplementary Table 1). Marker FA composition from the salt marsh and mixed flat sediment was dominated by green algae FAs (median: 37 and 28% of total marker FAs, respectively), diatom FAs (median: 14 and 40% of total marker FAs, respectively) and bacterial FAs (median: 44 and 25% of total marker FAs, respectively). By contrast, the oyster reef and mud flat sediment FAs comprised high amounts of diatom-specific biomarkers (median: 56 and 66% of total marker FAs, respectively), followed by a moderate amount of bacterial FAs (median: 28 and 15% of total marker FAs, respectively) and green algae FAs (median: in each case 2% of total marker FAs). All sediments contained low amounts of the brown algae FA 20:4ω6 (median: <6% of total FAs). In part, sediment FA composition varied strongly between the replicated core samples from the same habitat (Supplementary Table 1).
Principal component analysis reflected the habitat-specific variations in marker FA patterns in sediments; the FA composition of the salt marsh and mixed flat sediments was separated from that of the oyster reef and mud flat sediments (Figure 2A). DFA confirmed that FAs of the four sediments (salt marsh, mixed flat, oyster reef and mud flat) varied significantly (Figure 3A; Wilks’ Lambda = 0.00, F(18,6) = 33.52, p < 0.00).
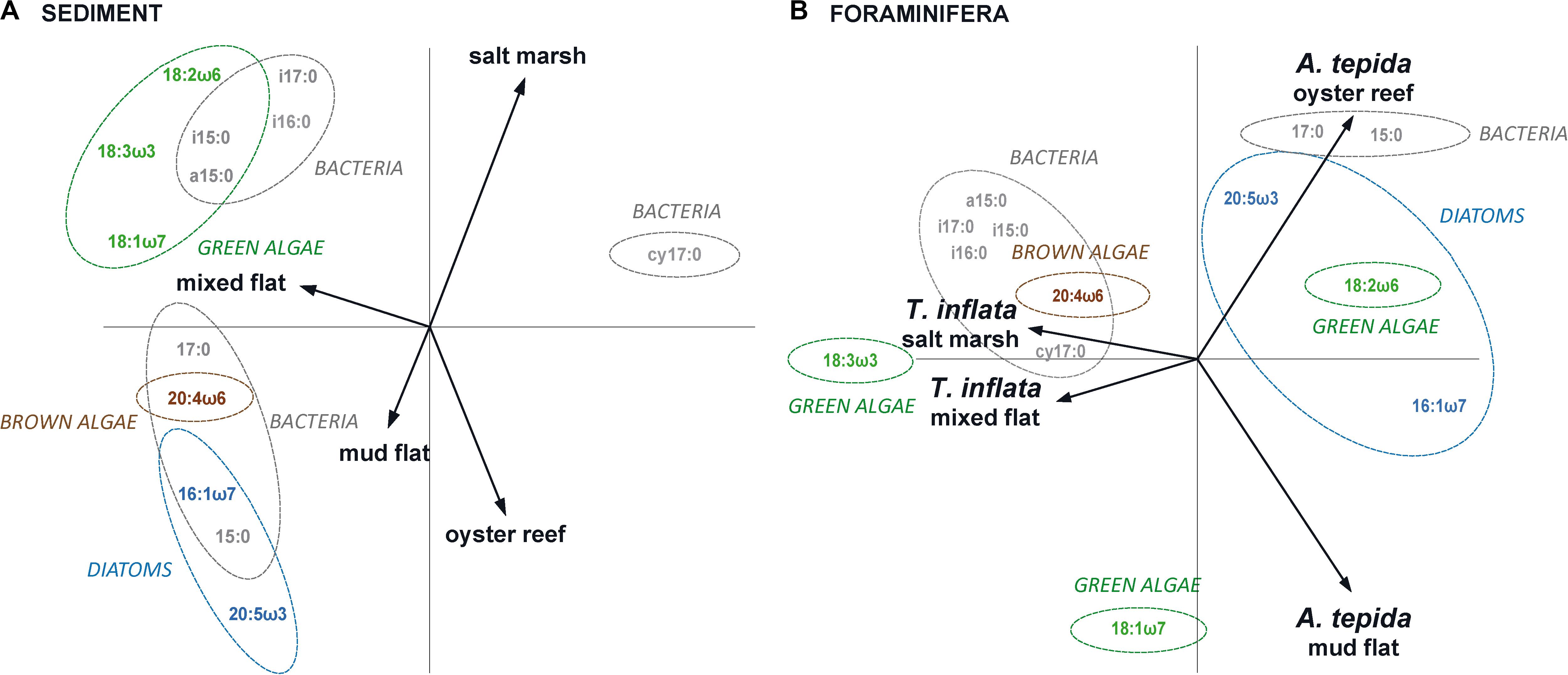
Figure 2. Fatty acid composition in sediments and foraminifera. Principal component analysis (PCA) of (A) sediment FAs and (B) foraminifera FAs of Trochammina inflata and Ammonia tepida in salt marsh, mixed flat, oyster reef and mud flat. PCA is based on percentage biomarker FAs of total FAs (logit-transformed); eigenvalues of PCA of sediment FAs: axis 1 = 0.5739 and axis 2 = 0.2533; eigenvalues of PCA of foraminifera FAs: axis 1 = 0.5770 and axis 2 = 0.1808. Dashed colored circles (drawn by eye) were added in order to visualize the fatty acid distribution between the habitats: green algae (green; 18:2ω6, 18:3ω3 and 18:1ω7), brown algae (brown; 20:4ω6), diatoms (light blue; 16:1ω7 and 20:5ω3) and bacteria (gray; i15:0, a15:0, 15:0, i16:0, i17:0, cy17:0, 17:0).
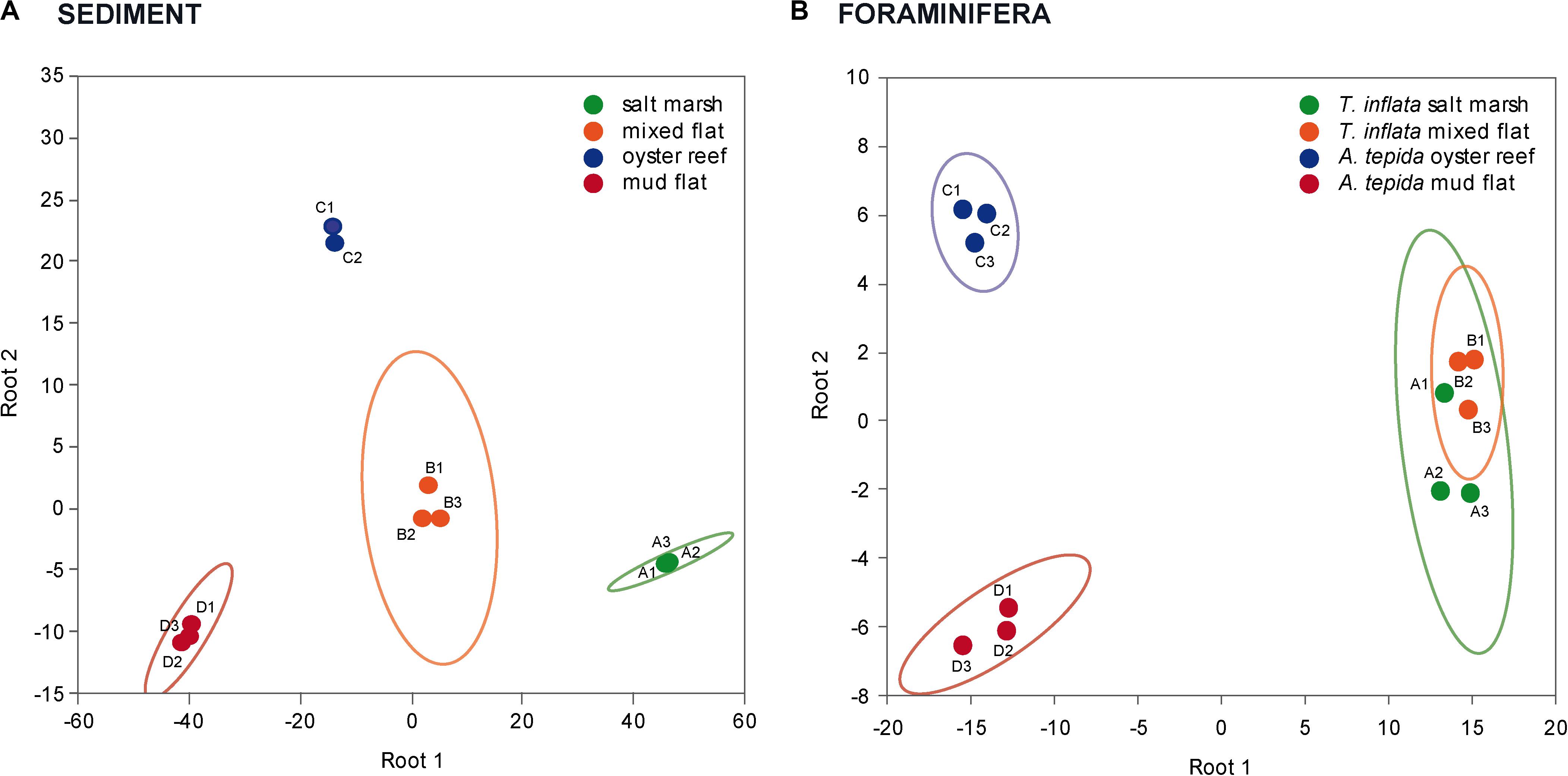
Figure 3. Separation of the four habitats studied based on variations in sediment and foraminifera FAs. Discriminant function analysis (DFA) of (A) sediment FAs and (B) foraminifera FAs of Trochammina inflata and Ammonia tepida in salt marsh (green; replicate A1-3), mixed flat (orange; replicate B1-3), oyster reef (blue; replicate C1-3) and mud flat (red; replicate D1-3); ellipses represent confidence ranges at p < 0.05.
Fatty Acid Composition in Foraminifera
As indicated by FAs, the two benthic foraminifera (T. inflata and A. tepida), living in different Wadden Sea habitats, fed on a diverse range of low trophic level food sources including green and brown algae, diatoms and bacteria (Supplementary Table 2). Marker FA composition of T. inflata from the salt marsh and mixed flat was dominated by green algae FAs (median: 47 and 68% of total FAs, respectively) and bacterial FAs (median: 21 and 31% of total FAs, respectively), whereas the brown algae FA 20:4ω6 was only found in T. inflata from the salt marsh (median: 29% of total FAs). By contrast, diatom FAs were low in A. tepida from both habitats (median: in each case 2% of total FAs). Marker FAs of A. tepida from oyster reef and mud flat sediments comprised high amounts of green algae (median: 38 and 55% of total FAs, respectively) and of diatom-specific biomarkers (median: 28 and 45% of total FAs, respectively). By contrast, brown algae and bacterial biomarkers were not found in A. tepida from the mudflat and bacterial biomarkers were only found in T. inflata from the oyster reef (median: 35% of total FAs).
Principal component analysis reflected the habitat-specific variations in marker FA patterns in foraminifera; the FA composition of T. inflata from the salt marsh and mixed flat was separated from that of A. tepida from the oyster reef and mud flat (Figure 2B). DFA confirmed that FAs of foraminifera of the four habitats (salt marsh, mixed flat, oyster reef and mud flat) varied significantly (Figure 3B; Wilks’ Lambda: 0.00, F(18,8) = 30.69, p < 0.00).
Discussion
Food Spectrum in the Sediment
Marker fatty acids in sediments suggest that the four investigated Wadden Sea habitats (salt marsh, mixed flat, oyster reef and mud flat) provide a wide range of potential food sources including green algae, brown algae, diatoms and bacteria. Green algae (e.g., Ulva sp., Chaetomorpha sp. and Cladophora sp.) occur of the sediment surface and in summer mainly in the upper and sheltered parts of the Wadden Sea habitats. Consequently, green algae are likely to form an important food source for benthic foraminifera. Similarly, diatoms are abundant in intertidal environments and play a key role as primary producers (Sullivan and Currin, 2000; Dalsgaard et al., 2003). Bacteria also may play an important role as food source in each of the studied habitats. However, bacteria are characterized by strong variations in abundance and diversity between different habitats (Decho, 2000; Dauwe et al., 2001; Musat et al., 2006) which was also true in the present study. By contrast, the brown algae biomarker occurred only in low percentages in each of the four sediments studied, although the macroalgae Fucus vesiculosus is among the most common macroalgae species in the Wadden Sea. Potentially, the low percentage of brown algae biomarkers FAs is due to the fact that brown algae live in the interface zone above the sediment and therefore do not play an important role as food source for benthic foraminifera.
The composition of marker FAs in sediments was highly variable, potentially due to the dynamic nature of Wadden Sea habitats, which may cause high local variability in sediment FA composition. In fact, other sediment characteristics (e.g., organic matter, remineralization, and chemical conditions) of the Wadden Sea habitat feature irregular distribution patterns that lead to patchy colonization by organisms (Bernstein and Meador, 1979; Swallow, 2000). The degree of patchiness varies, for instance a patchy distribution of green algae, diatoms and bacteria may reflect bloom events of microbial activity. Especially sediments of mud flats and oyster reefs are characterized by high microbial activity and remineralization of organic matter resulting in anoxic sediment conditions few millimeter to centimeters below the sediment surface. The unspecific FA 18:0 has been suggested as marker for anaerobic bacterial respiration types in Wadden Sea sediments (Langezaal, 2003). Presumably anoxic sediments are dominated by anaerobic bacteria which likely are less abundant than bacteria in oxic habitats and this might have contributed to the lack of specific sediment FAs. Finally, patchiness of microbial activity likely contributed to the observed differences in sediment FA composition within the same habitat (Supplementary Table 1).
Habitat-Specific Diets of Foraminifera
The studied benthic foraminifera, T. inflata and A. tepida, utilized a diverse food spectrum including the variety of food resources present in the different habitats. The preferred resources were green algae, brown algae, diatoms and bacteria, indicating that both foraminifera species mainly feed on lower trophic levels.
Green Algae
The percentages of green algae FAs in T. inflata and A. tepida were high in each of the four habitats studied. Especially in the mixed flat, the FA composition of T. inflata was dominated by green algae. This is in agreement with a previous feeding experiment showing that T. inflata feeds exclusively on the green algae Chlorella sp. (Bender, 1989), which suggests T. inflata to be a selective deposit feeder preferentially feeding on green algae.
Brown Algae
The brown algae FA was only found in high percentage in T. inflata from the salt marsh. Previous studies reported the biomarker 20:4ω6 from different brown algae such as F. vesiculosus (Fleurence et al., 1994; Kelly and Scheibling, 2012), which inhabits salt marsh zones in high densities. However, the use of 20:4ω6 as biomarker for brown algae is not straightforward as it also is present in soil invertebrates such as Protozoa, Nematoda, Collembola and Lumbricidae (Ruess and Chamberlain, 2010; Kelly and Scheibling, 2012) and this may explain the predominant occurrence of this biomarker in T. inflata from the salt marsh. Soil invertebrates are highly abundant in salt marshes and play an important role as resource in benthic food webs (Haynert et al., 2017; Lange et al., 2018). Feeding on meiozoobenthos may be based on predation but also detritivory. Earlier studies suggested that T. inflata feeds on a wide range of prey including nematodes, gastropod larvae and copepods (Dupuy et al., 2010), but also juvenile crustaceans, mollusks, annelids and echinoderms (Suhr et al., 2008). Furthermore, no brown algae were found in T. inflata from the mixed flat and in A. tepida from the mudflat. Potentially, this was due to the fact that the epibenthic F. vesiculosus needs hard substrate such as stones, wood or mussel beds for anchoring. Accordingly, mixed and mud flats do not provide suitable habitat for brown alga. In addition, benthic foraminifera such as T. inflata and A. tepida colonize the surface of sediments, whereas brown algae live in the interface zone above the sediment and thus may not contribute to food resources of benthic foraminifera.
Diatoms
Whereas A. tepida from the oyster reef and mud flat utilized diatoms as important food source, diatoms played a minor role for the nutrition of T. inflata from the salt marsh and mixed flat (Bender, 1989; Kharlamenko et al., 1995; Kelly and Scheibling, 2012; LeKieffre et al., 2017). Broken chambers of A. tepida revealed an accumulation of pelagic and benthic diatoms inside their chambers (Figure 4). Presumably, food particles are transported over the surface of A. tepida and internalized by phagocytosis into the terminal chamber (Austin et al., 2005). This is supported by the fact that food vacuoles are most abundant in the terminal chamber (Goldstein and Corliss, 1994) indicating that only the terminal chamber of A. tepida is used for food intake and the others serve as storage chambers for waste products of digested food. In conclusion, the results indicate that A. tepida feeds on diatoms, but the diatom taxa cannot be specified using the applied FA analysis.
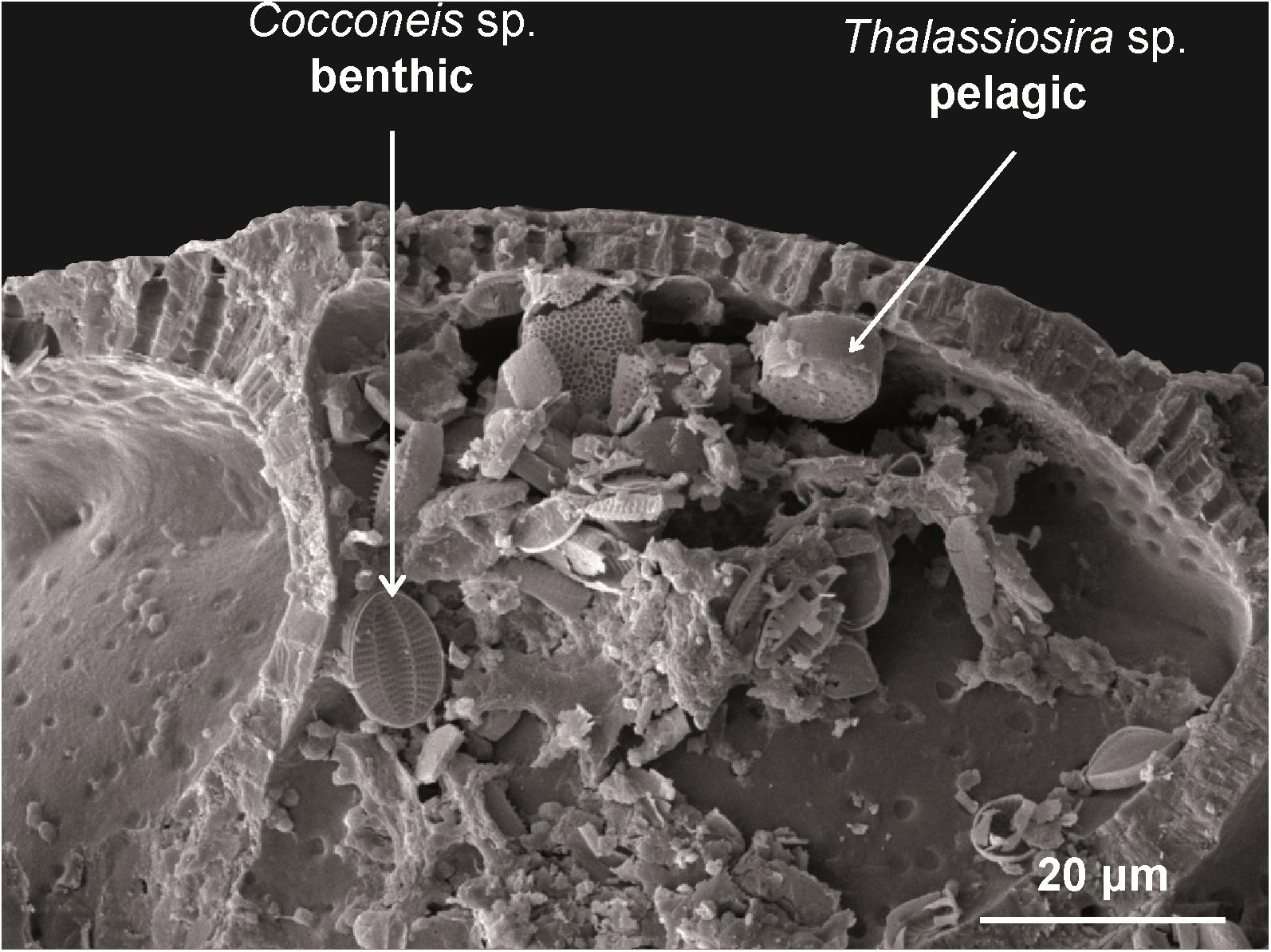
Figure 4. SEM-image. Cracked chamber of Ammonia tepida containing benthic (Cocconeis sp.) and pelagic diatoms (Thalassiosira sp.).
Bacteria
With the exception of A. tepida from the mud flat, the percentages of bacterial FAs in the studied foraminifera were high in each of the habitats. However, differences in the composition of bacterial FAs in foraminifera among habitats indicate that their contribution to the nutrition of foraminifera varies, presumably due to variations in abundance and diversity of bacteria among the Wadden Sea habitats (Decho, 2000; Dauwe et al., 2001; Musat et al., 2006). While A. tepida is known to feed on bacteria (Ward et al., 2003; Pascal et al., 2008), it is unknown to what extend bacteria are digested and contribute to the diet of T. inflata. In the mud flat, no bacterial markers were found in A. tepida, suggesting that it switched diet to other food resources such as green algae and diatoms. This may be due to higher food quality of the latter compared to bacteria (Kharlamenko et al., 1995; Hayakawa et al., 1996; Kelly and Scheibling, 2012). However, in general our results suggest that bacteria in fact play an important role in the food spectrum and nutrition of both T. inflata and A. tepida. Presumably, both foraminifera preferentially consume energetically rich bacterial cells, thereby benefitting from microbial activity (Kharlamenko et al., 1995; Hayakawa et al., 1996; Zak et al., 2003; Córdova-Kreylos et al., 2006; Kelly and Scheibling, 2012).
The Biomarker Approach
The analysis of biomarker FAs proved to be a useful tool to determine the nutrition of foraminifera. However, FA biomarkers in foraminifera also left room for interpretation as one FA biomarker may originate from multiple resources. Nevertheless, the results allowed principle insight into the food resources used by benthic foraminifera, although only at high taxonomic level leaving open trophic links at the level of species or genera. Therefore, further research is required to refine the identification of food resources of foraminifera, for instance by establishing FA libraries and the application of mixing models (Iverson et al., 2004) or by combining FA and stable isotope analysis, i.e., by using compound-specific lipid analysis. However, using FA analysis the current study provided novel insight into the diet of benthic foraminifera and their variations among Wadden Sea habitats.
Conclusion
In conclusion, habitat-specific diets supported the hypothesis that foraminifera are able to utilize diverse food resources and thereby benefit from small-scale heterogeneity of resource distribution in inter- to supratidal environments. Feeding on a wide range of low trophic level taxa, foraminifera presumably function as important link between low and high trophic levels and thus fill key trophic positions in benthic food webs of a wide range of Wadden Sea habitats.
Data Availability Statement
All datasets generated for this study are included in the article/Supplementary Material.
Author Contributions
KH carried out data curation, validation, visualization, statistical analysis and drafted the manuscript, writing – original draft, review and editing. FG carried out sampling and processing of samples in the field, prepared the samples and performed the fatty acid analysis. MP assisted with the protocol for the fatty acid extraction and contributed to interpretation of results. SS assisted with conceptualization and writing – review and editing. AW assisted with conceptualization, funding acquisition, supervision, writing – review and editing. All authors contributed to the final version of the manuscript.
Funding
This study was performed within the joint research project WASA (The Wadden Sea archive of landscape evolution, climate change and settlement history: exploration – analysis – predictive modeling) funded by the Ministry for Science and Culture of Lower Saxony under ZV 3197.
Conflict of Interest
The authors declare that the research was conducted in the absence of any commercial or financial relationships that could be construed as a potential conflict of interest.
Acknowledgments
We are grateful to the Wadden Sea National Park administration for permission to perform our field work. Special thanks to Guido Humpert (J.F. Blumenbach Institute of Zoology and Anthropology, University of Göttingen, Germany) for supporting preparation and performing analyses of FAs. We thank the two reviewers for their helpful and constructive comments to improve the manuscript.
Supplementary Material
The Supplementary Material for this article can be found online at: https://www.frontiersin.org/articles/10.3389/fmars.2020.510288/full#supplementary-material
References
Allen, J. R. L. (2000). Morphodynamics of Holocene salt marshes: a review sketch from the Atlantic and southern north Sea coasts of Europe. Quat. Sci. Rev. 19, 1155–1231. doi: 10.1016/S0277-3791(99)00034-7
Alve, E., and Murray, J. W. (1994). Ecology and taphonomy of benthic foraminifera in a temperate mesotidal inlet. J. Foramin. Res. 24, 18–27. doi: 10.2113/gsjfr.24.1.18
Alve, E., and Murray, J. W. (1999). Marginal marine environments of the Skagerrak and Kattegat: a baseline study of living (stained) benthic foraminiferal ecology. Palaeogeogr. Palaeocl. 146, 171–193. doi: 10.1016/S0031-0182(98)00131-X
Austin, A., Austin, W. E. N., and Paterson, D. M. (2005). Extracellular cracking and content removal of the benthic diatom Pleurosigma angulatum (Quekett) by the benthic foraminifera Haynesina germanica (Ehrenberg). Mar. Micropaleontol. 57, 68–73. doi: 10.1016/j.marmicro.2005.07.002
Bakker, J. P., and Piersma, T. (2006). “Restoration of intertidal flats and tidal salt marshes,” in Restoration Ecology: The New Frontier, eds J. van Andel and J. Aronson (Oxford: Blackwell), 174–192.
Bender, H. (1989). Gehäuseaufbau, Gehäusegenese und Biologie agglutinierter Foraminiferen (Sarcodina, Textulariina). Geologische Bundesanstalt Wien 132, 259–347.
Berkeley, A., Perry, C. T., Smithers, S. G., Horton, B. P., and Taylor, K. G. (2007). A review of the ecological and taphonomic controls on foraminiferal assemblage development in intertidal environments. Earth Sci. Rev. 83, 205–230. doi: 10.1016/j.earscirev.2007.04.003
Bernstein, B. B., and Meador, J. P. (1979). Temporal persistence of biological patch structure in an abyssal benthic community. Mar. Biol. 51, 179–183. doi: 10.1007/BF00555197
Böttcher, M. E., Hespenheide, B., Llobet-Brossa, E., Beardsley, C., Larsen, O., Schramm, A., et al. (2000). The biogeochemistry, stable isotope geochemistry, and microbial community structure of a temperate intertidal mudflat: an integrated study. Cont. Shelf Res. 20, 1749–1769. doi: 10.1016/S0278-4343(00)00046-7
Bouchet, V. M., Debenay, J. P., Sauriau, P. G., Radford-Knoery, J., and Soletchnik, P. (2007). Effects of short-term environmental disturbances on living benthic foraminifera during the Pacific oyster summer mortality in the Marennes-Oléron Bay (France). Mar. Environ. Res. 64, 358–383. doi: 10.1016/j.marenvres.2007.02.007
Bouillon, S., and Boschker, H. T. S. (2006). Bacterial carbon sources in coastal sediments: a cross-system analysis based on stable isotope data of biomarkers. Biogeosciences 3, 175–185. doi: 10.5194/bg-3-175-2006
Córdova-Kreylos, A. L., Cao, Y., Green, P. G., Hwang, H., Kuivila, K. M., LaMontagne, M. G., et al. (2006). Diversity, composition and geographical distribution of microbial communities in California salt marsh sediments. Appl. Environ. Microbiol. 72, 3357–3366. doi: 10.1128/AEM.72.5.3357-3366.2006
Dalsgaard, J., St John, M., Kattner, G., Müller-Navarra, D., and Hagen, W. (2003). Fatty acid trophic markers in the pelagic marine environment. Adv. Mar. Biol 46, 225–340. doi: 10.1016/s0065-2881(03)46005-7
Dauwe, B., Middelburg, J. J., and Herman, P. M. J. (2001). Effect of oxygen on the degradability of organic matter in subtidal and intertidal sediments of the North Sea area. Mar. Ecol. Prog. Ser. 215, 13–22. doi: 10.3354/meps215013
Decho, A. W. (2000). Microbial biofilms in intertidal systems: an overview. Cont. Shelf Res. 20, 1257–1273. doi: 10.1016/S0278-4343(00)00022-4
Dellwig, O., Hinrichs, J., Hild, A., and Brumsack, H. J. (2000). Changing sedimentation in tidal flat sediments of the southern North Sea from the Holocene to the present: a geochemical approach. J. Sea Res. 44, 195–208. doi: 10.1016/S1385-1101(00)00051-4
Dupuy, C., Rossignol, L., Geslin, E., and Pascal, P. Y. (2010). Predation of mudflat meio-macrofaunal metazoans by a calcareous foraminifer, Ammonia tepida (Cushman, 1926). J. Foramin. Res. 40, 305–312. doi: 10.2113/gsjfr.40.4.305
Ferlian, O., and Scheu, S. (2014). Shifts in trophic interactions with forest type in soil generalist predators as indicated by complementary analyses of fatty acids and stable isotopes. Oikos 123, 1182–1191. doi: 10.1111/j.1600-0706.2013.00848.x
Flemming, B. W., and Davis, R. A. J. (1994). Holocene evolution, morphodynamics and sedimentology of the Spiekeroog barrier island system (southern North Sea). Senckenb. Marit. 24, 117–155.
Flemming, B. W., and Ziegler, K. (1995). High-resolution grain size distribution patterns and textural trends in the backbarrier environment of Spiekeroog Island (southern North Sea). Senckenb. Marit. 26, 1–24. doi: 10.3724/sp.j.1140.2009.00001
Fleurence, J., Gutbier, G., Mabeau, S., and Leray, C. (1994). Fatty acids from 11 marine macroalgae of the French Brittany coast. J. Appl. Phycol. 6, 527–532. doi: 10.1007/BF02182406
Fontanier, C., Jorissen, F. J., Licari, L., Alexandre, A., Anschutz, P., and Carbonel, P. (2002). Live benthic foraminiferal faunas from the Bay of Biscay: faunal density, composition, and microhabitats. Deep Sea Res. I 49, 751–785. doi: 10.1016/S0967-0637(01)00078-4
Goldstein, S. T., and Corliss, B. H. (1994). Deposit feeding in selected deep-sea and shallow-water benthic foraminifera. Deep Sea Res. I 41, 229–241. doi: 10.1016/0967-0637(94)90001-9
Goldstein, S. T., and Richardson, E. A. (2018). Fine structure of the foraminifer Haynesina germanica (Ehrenberg) and its sequestered chloroplasts. Mar. Micropaleontol. 138, 63–71. doi: 10.1016/j.marmicro.2017.10.010
Goldstein, S. T., Watkins, G. T., and Kuhn, R. M. (1995). Microhabitats of salt marsh foraminifera: St. Catherines Island, Georgia, USA. Mar. Micropaleontol. 26, 17–29. doi: 10.1016/0377-8398(95)00006-2
Gooday, A. J., Levin, L. A., Linke, P., and Heeger, T. (1992). “The role of benthic foraminifera in deep-sea food webs and carbon cycling,” in Deep-Sea Food Chains and the Global Carbon Cycle, Vol. 360, eds G. T. Rowe and V. Pariente (Dordrecht): Springer), 63–91. doi: 10.1007/978-94-011-2452-2_5
Gooday, A. J., and Rathburn, A. E. (1999). Temporal variability in living deep – sea foraminifera: a review. Earth Sci. Rev. 46, 187–212. doi: 10.1016/S0012-8252(99)00010-0
Green, D. S., Boots, B., and Crowe, T. P. (2012). Effects of non-indigenous oysters on microbial diversity and ecosystem functioning. PLoS One 7:e48410. doi: 10.1371/journal.pone.0048410
Hayakawa, K., Handa, N., Kawanobe, K., and Wong, C. S. (1996). Factors controlling the temporal variation of fatty acids in piculate matter during a phytoplankton bloom in a marine mesocosm. Mar. Chem. 52, 233–244. doi: 10.1016/0304-4203(95)00087-9
Haynert, K., Kiggen, M., Klarner, B., Maraun, M., and Scheu, S. (2017). The structure of salt marsh soil mesofauna food webs – The prevalence of disturbance. PLoS One 12:e0189645. doi: 10.1371/journal.pone.0189645
Hayward, B. W., Grenfell, H. R., and Scott, D. B. (1999). Tidal range of marsh foraminifera for determining former sea-level heights in New Zealand. New Zeal. J. Geol. Geop. 42, 395–413. doi: 10.1080/00288306.1999.9514853
Heip, C. H. R., Goosen, N. K., Herman, P. M. J., Kromkamp, J., Middelburg, J. J., and Soetaert, K. (1995). Production and Consumption of Biological Particles in Temperate Tidal Estuaries. Netherlands: Netherlands Institute of Ecology.
Hippensteel, S. P., Martin, R. E., Nikitina, D., and Pizzuto, J. E. (2002). Interannual variation of marsh foraminiferal assemblages (Bombay Hook National Wildlife Refuge, Smyrna, DE): do foraminiferal assemblages have a memory? J. Foramin. Res. 32, 97–109. doi: 10.2113/0320097
Iverson, S. J., Field, C., Bowen, W. D., and Blanchard, W. (2004). Quatitatve fatty acid signature analysis: a new method of estimating predator diets. Ecol. Monogr. 74, 211–235. doi: 10.1890/02-4105
Jorissen, F. J. (1999). “Benthic foraminiferal microhabitats,” in Modern Foraminifera, ed. B. K. S. Gupta (Dordrecht: Kluwer Academic Publishers), 161–179. doi: 10.1007/0-306-48104-9
Kelly, J. R., and Scheibling, R. E. (2012). Fatty acids as dietary tracers in benthic food webs. Mar. Ecol. Prog. Ser. 446, 1–22. doi: 10.3354/meps09559
Kharlamenko, V. I., Kiyashko, S. I., Imbs, A. B., and Vyshkvartzev, D. I. (2001). Identification of food sources of invertebrates from the seagrass Zostera marina community using carbon and sulfur stable isotope ratio and fatty acid analyses. Mar. Ecol. Prog. Ser. 220, 103–117. doi: 10.3354/meps220103
Kharlamenko, V. I., Svetashev, V. I., and Tarasova, T. S. (2017). New and uncommon fatty acids in lipids of deep-sea foraminifera. J. Lipids 52, 345–352. doi: 10.1007/s11745-017-4237-2
Kharlamenko, V. I., Zhukova, N. V., Khotimchenko, S. V., Svetashev, V. I., and Kamenev, G. M. (1995). Fatty acids as markers of food sources in a shallow-water hydrothermal ecosystem (Kraternaya Bight, Yankich Island, Kurile Islands). Mar. Ecol. Prog. Ser. 120, 231–241. doi: 10.3354/meps120231
Lange, G., Haynert, K., Dinter, T., Scheu, S., and Kröncke, I. (2018). Adaptation of benthic invertebrates to food sources along marine-terrestrial boundaries as indicated by carbon and nitrogen stable isotopes. J. Sea Res. 131, 12–21. doi: 10.1016/j.seares.2017.10.002
Langezaal, A. M. (2003). The Foraminiferal-Bacterial Connection: An Interdisciplinary Study of Meiofaunal Behaviour in the Deeper Marine Redox Zone. Utrecht: University of Utrecht.
Larkin, K. E., Gooday, A. J., Woulds, C., Jeffreys, R. M., Schwartz, M., Cowie, G., et al. (2014). Uptake of algal carbon and the likely synthesis of an “essential” fatty acid by Uvigerina ex. gr. semiornata (Foraminifera) within the Pakistan margin oxygen minimum zone: evidence from fatty acid biomarker and 13C tracer experiments. Biogeosciences 11, 3729–3738. doi: 10.5194/bg-11-3729-2014
Lei, Y. L., Stumm, K., Wickham, S. A., and Berninger, U. G. (2014). Distributions and biomass of benthic ciliates, foraminifera and amoeboid protists in marine, brackish and freshwater sediments. J. Eukaryot. Microbiol. 61, 493–508. doi: 10.1111/jeu.12129
LeKieffre, C., Spangenberg, J., Mabilleau, G., Escrig, S., Meibom, A., and Geslin, E. (2017). Surviving anoxia in marine sediments: the metabolic response of ubiquitous benthic foraminifera (Ammonia tepida). PLoS One 12:e0177604. doi: 10.1371/journal.pone.0177604
Lipps, J. H., and Valentine, J. W. (1970). The role of foraminifera in the trophic structure of marine communities. Lethaia 3, 279–286. doi: 10.1111/j.1502-3931.1970.tb01271.x
Markert, A., Wehrmann, A., and Kröncke, I. (2010). Recently established Crassostrea-reefs versus native Mytilus-beds: differences in ecosystem engineering affects the macrofaunal communities (Wadden Sea of Lower Saxony, southern German Bight). Biol. Invasions 12, 15–32. doi: 10.1007/s10530-009-9425-4
Muller, W. A. (1975). Competition for food and other niche-related studies of three species of salt-marsh foraminifera. Mar. Biol. 31, 339–351. doi: 10.1007/bf00392091
Musat, N., Werner, U., Knittel, K., Kolb, S., Dodenhof, T., van Beusekom, J. E. E., et al. (2006). Microbial community structure of sandy intertidal sediments in the North Sea, Sylt-Rømø Basin, Wadden Sea. Syst. Appl. Microbiol. 29, 333–348. doi: 10.1016/j.syapm.2005.12.006
Naeher, S., Geraga, M., Papatheodorou, G., Ferentinos, G., Kaberi, H., and Schubert, C. J. (2012). Environmental variations in a semi-enclosed embayment (Amvrakikos Gulf, Greece) – reconstructions based on benthic foraminifera abundance and lipid biomarker pattern. Biogeosciences 9, 5081–5094. doi: 10.5194/bg-9-5081-2012
Nomaki, H., Ohkouchi, N., Heinz, P., Suga, H., Chikaraishi, Y., Ogawa, N. O., et al. (2009). Degradation of algal lipids by deep-sea benthic foraminifera: an in situ tracer experiment. Deep Sea Res. I 56, 1488–1503. doi: 10.1016/j.dsr.2009.04.013
Pascal, P. Y., Dupuy, C., Richard, P., and Niquil, N. (2008). Bacterivory in the common foraminifer Ammonia tepida: isotope tracer experiment and the controlling factors. J. Exp. Mar. Biol. Ecol. 359, 55–61. doi: 10.1016/j.jembe.2008.02.018
Ruess, L., and Chamberlain, P. M. (2010). The fat that matters: soil food web analysis using fatty acids and their carbon stable isotope signature. Soil Biol. Biochem. 42, 1898–1910. doi: 10.1016/j.soilbio.2010.07.020
Salami, M. B. (1976). Biology of Trochammina cf. T. quadriloba HOGLUND (1947), an agglutinating foraminifer. J. Foramin. Res. 6, 142–153. doi: 10.2113/gsjfr.6.2.142
Schuerch, M., Dolch, T., Reise, K., and Vafeidis, A. T. (2014). Unravelling interactions between salt marsh evolution and sedimentary processes in the Wadden Sea (southeastern North Sea). Prog. Phys. Geog. 38, 691–715. doi: 10.1177/0309133314548746
Scott, D. B., and Medioli, F. S. (1978). Vertical zonations of marsh foraminifera as accurate indicators of former sea-levels. Nature 272, 528–531. doi: 10.1038/272528a0
Stock, M., Bergmann, H.-H., and Zucchi, H. (2012). WATT Lebensraum zwischen Land und Meer, 3rd Edn. Heide: Boyens Verlag.
Suhr, S. B., Alexander, S. P., Gooday, A. J., Pond, D. W., and Bowser, S. S. (2008). Trophic modes of large antarctic foraminifera: roles of carnivory, omnivory, and detritivory. Mar. Ecol. Prog. Ser. 371, 155–164. doi: 10.3354/meps07693
Suhr, S. B., Pond, D. W., Gooday, A. J., and Smith, C. R. (2003). Selective feeding by benthic foraminifera on phytodetritus on the western Antarctic Peninsula shelf: evidence from fatty acid biomarker analysis. Mar. Ecol. Prog. Ser. 262, 153–162. doi: 10.3354/meps262153
Sullivan, M. J., and Currin, C. A. (2000). “Community structure and functional dynamics of benthic microalgae in salt marshes,” in Concepts and Controversies in Tidal Marsh Ecology, eds M. P. Weinstein and D. A. Kreeger (Dordrecht: Springer), 81–106. doi: 10.1007/0-306-47534-0_6
Svenning, J. B., Dalheim, L., Eilertsen, H. C., and Vasskog, T. (2019). Temperature dependent growth rate, lipid content and fatty acid composition of the marine cold-water diatom Porosira glacialis. Algal Res. 37, 11–16. doi: 10.1016/j.algal.2018.10.009
Swallow, J. E. (2000). Intra-annual variability and patchiness in living assemblages of salt-marsh foraminifera from Mill Rythe Creek, Chichester Harbour, England. J. Micropaleontol. 19, 9–22. doi: 10.1144/jm.19.1.9
Tobias, C., and Neubauer, S. C. (2009). “Chapter 16 - Salt marsh biogeochemistry – an overview,” in Coastal Wetlands (Second Edition): An Integrated Ecosystem Approach, eds G. Perillo, E. Wolanski, D. Cahoon, and C. S. Hopkinson (Amsterdam: Elsevier), 539–596. doi: 10.1016/B978-0-444-63893-9.00016-2
Traugott, M., Kamenova, S., Ruess, L., Seeber, J., and Plantegenest, M. (2013). Empirically characterising trophic networks: what emerging DNA-based methods, stable isotope and fatty acid analyses can offer. Adv. Ecol. Res. 49, 177–224. doi: 10.1016/B978-0-12-420002-9.00003-2
van de Kam, J., Ens, B., Piersma, T., and Zwarts, L. (2004). Shorebirds: An Illustrated Behavioral Ecology. Utrecht: KNNV Publishers.
van der Zwaan, G. J., Duijnstee, I. A. P., den Dulk, M., Ernst, S. R., Jannink, N. T., and Kouwenhoven, T. J. (1999). Benthic foraminifers: proxies or problems? A review of paleocological concepts. Earth Sci. Rev. 46, 213–236. doi: 10.1016/S0012-8252(99)00011-2
van Oevelen, D., Soetaert, K., Middelburg, J. J., Herman, P. M. J., Moodley, L., Hamels, I., et al. (2006). Carbon flows through a benthic food web: integrating biomass, isotope and tracer data. J. Mar. Res. 64, 453–482. doi: 10.1357/002224006778189581
Ward, J. N., Pond, D. W., and Murray, J. W. (2003). Feeding of benthic foraminifera on diatoms and sewage-derived organic matter: an experimental application of lipid biomarker techniques. Mar. Environ. Res. 56, 515–530. doi: 10.1016/S0141-1136(03)00040-0
Wehrmann, A., Herlyn, M., Bungenstock, F., Hertweck, G., and Millat, G. (2000). The distribution gap is closed - first record of naturally settled pacific oysters Crassostrea gigas in the east frisian wadden sea, north sea. Senck. Marit. 30, 153–160. doi: 10.1007/BF03042964
Williams, H. F. L. (1994). Intertidal benthic foraminiferal biofacies on the Central Gulf Coast of Texas – modern distribution and application to sea-level reconstruction. Micropaleontology 40, 169–183. doi: 10.2307/1485774
Wright, A. J., Edwards, R. J., and van de Plassche, O. (2011). Reassessing transfer-function performance in sea-level reconstruction based on benthic salt-marsh foraminifera from the Atlantic coast of NE North America. Mar. Micropaleontol. 81, 43–62. doi: 10.1016/j.marmicro.2011.07.003
Wukovits, J., Bukenberger, P., Enge, A. J., Gerg, M., Wanek, W., Watzka, M., et al. (2018). Food supply and size class depending variations in phytodetritus intake in the benthic foraminifer Ammonia tepida. Biol. Open 7:bio030056. doi: 10.1242/bio.030056
Zak, D. R., Holmes, W. E., White, D. C., Peacock, A. D., and Tilman, D. (2003). Plant diversity, soil microbial communities, and ecosystem function: are there any links? Ecology 84, 2042–2050. doi: 10.1890/02-0433
Zelles, L. (1999). Fatty acid patterns of phospholipids and lipopolysaccharides in the characterisation of microbial communities in soil: a review. Biol. Fert. Soils 29, 111–129. doi: 10.1007/s003740050533
Keywords: protists, microorganism, benthic food webs, nutritional strategies, resources, sediment, tidal flats, North Sea
Citation: Haynert K, Gluderer F, Pollierer MM, Scheu S and Wehrmann A (2020) Food Spectrum and Habitat-Specific Diets of Benthic Foraminifera From the Wadden Sea – A Fatty Acid Biomarker Approach. Front. Mar. Sci. 7:510288. doi: 10.3389/fmars.2020.510288
Received: 05 November 2019; Accepted: 04 September 2020;
Published: 29 September 2020.
Edited by:
Stelios Katsanevakis, University of the Aegean, GreeceReviewed by:
Anna Törnroos, Åbo Akademi University, FinlandKapuli Gani Mohamed Thameemul Ansari, Indian Institute of Science Education and Research Kolkata, India
Copyright © 2020 Haynert, Gluderer, Pollierer, Scheu and Wehrmann. This is an open-access article distributed under the terms of the Creative Commons Attribution License (CC BY). The use, distribution or reproduction in other forums is permitted, provided the original author(s) and the copyright owner(s) are credited and that the original publication in this journal is cited, in accordance with accepted academic practice. No use, distribution or reproduction is permitted which does not comply with these terms.
*Correspondence: Kristin Haynert, kristin.haynert@biologie.uni-goettingen.de