- Alaska Fisheries Science Center, National Oceanic and Atmospheric Administration (NOAA), Seattle, WA, United States
In 2014–2016 an unprecedented warming event in the North Pacific Ocean triggered changes in ecosystem of the Gulf of Alaska (GOA) impacting fisheries management. The marine heatwave was noteworthy in its geographical extent, depth range, and persistence, with evidence of shifts in species distribution and reduced productivity. In 2017 a groundfish survey indicated that GOA Pacific cod (Gadus macrocephalus) had experienced a 71% decline in abundance from the previous 2015 survey. The GOA Pacific cod fishery supports a $103 million fishery which is 29% of the groundfish harvest value in the GOA. In this paper, we demonstrate that an increase in metabolic demand during this extended marine heatwave as well as a reduced prey supply can explain the decline in GOA Pacific cod biomass. Although increased mortality likely led to the decline in the Pacific cod population, historically low recruitment concurrent with the heatwave portends a slow recovery for the stock and gives a preview of impacts facing this region due to climate change. We evaluate the intersection of climate change with ecosystem-based fisheries management in the context of GOA Pacific cod with a description of the sensitivities of the ecosystem, how the changes in the ecosystem affected the Pacific cod stock, and a description of how the management system in the North Pacific handled this shock. We also provide suggestions on how fisheries management systems could be improved to better contend with the impacts of climate change such as the effects of heatwaves like that experienced in 2014–2016.
Introduction
Climate change is beginning to have a direct impact on marine resources globally (Poloczanska et al., 2013; Gattuso et al., 2015; Pecl et al., 2017; Barange et al., 2018) and current management tools must be able to adapt to future challenges that these impacts will bring (Busch et al., 2016; Holsman et al., 2019a,c; Karp et al., 2019). In 2014–2016 an unprecedented warming event occurred in the eastern North Pacific Ocean (Bond et al., 2015; Di Lorenzo and Mantua, 2016) that triggered changes in ecosystem productivity in the Gulf of Alaska (GOA, see Figure 1; Zador and Yasumiishi, 2017; Holsman et al., 2019b). The intensity and duration of this warming event has been attributed to natural drivers exacerbated by anthropogenic forcing on climate (Jacox et al., 2018; Walsh et al., 2018), and climatologists predict it is a precursor to more common occurrence of marine heatwaves for this region (Walsh et al., 2018) may be ill-equipped to address an increasing frequency of extreme heatwaves (Holsman et al., 2019c). The availability of ecosystem information provided to GOA groundfish (e.g., Pacific cod, walleye pollock, flatfish, and rockfish) fisheries managers is arguably some of the most comprehensive in the world, providing a wealth of information on ecosystem dynamics and trends to decision-makers during the management process (Zador and Yasumiishi, 2017). Even so fishery management was challenged in 2017 when an abrupt and unexpected 71% decline in GOA Pacific cod (Gadus macrocephalus Tilesius) abundance was detected following a marine heatwave (Barbeaux et al., 2017).
From 2007 to 2017 the GOA Pacific cod stock supported a $103 million-a-year (first wholesale) industry, comprising 29% of the revenue generated in the GOA groundfish fisheries (Fissel et al., 2017). Although Pacific cod has been important to GOA communities for millennia, Native coastal communities of the region have long recognized the ephemeral nature of this population; the Aleut word for Pacific cod, atxidax, literally translates to “the fish that stops” (Betts et al., 2011). Recoveries from middens on Sanak Island in the Western GOA show a long history (at least 4,500 years) of exploitation and reveal fluctuations in Pacific cod size distribution which (Betts et al., 2011) tie to changes in abundance due to climate variability. Over this period, warm climate conditions appear to have led to lower abundance with fewer small Pacific cod in the exploited population; however, zooarchaeological methods are unable to resolve the overall magnitude or timescale over which rapid population changes occur. Nevertheless, Pacific cod population dynamics appear to include periodic large-magnitude fluctuations.
In the GOA, climate-driven changes to physical oceanographic features, such as temperature and circulation structure food-web dynamics, mediate trophic interactions, and impact distribution of key predators. Gaichas et al. (2015) provide evidence for strong food-web responses to perturbations in the GOA suggesting that its dynamic ecosystem structure is more prone to dramatic reorganization and is inherently less predictable than the neighboring Bering Sea ecosystem. Further Gaichas et al. (2015) provide evidence to support predation as a major structuring pressure in the Gulf of Alaska ecosystem. Under the extreme conditions experienced during the 2014–2016 heatwave, the extent and persistence in warm temperatures caused increases in metabolic demands in ectothermic fish such that prey may have been limiting through both (1) bottom-up forced decreases in lower-trophic prey availability and quality (i.e., smaller zooplankton); and (2) through competition with fish, bird, and mammal predators with overlapping diets. This hypothesis is explored below with evidence provided to demonstrate how climate-influenced trophic interactions could explain the decline in Pacific cod relative to other groundfish during the 2014–2016 marine heatwave and its ramifications for fisheries management.
The majority of regulated fisheries worldwide are managed through single-species stock assessments with assumptions of a steady ecological state around mean conditions (Skern-Mauritzen et al., 2016). Management systems that have implemented harvest control rules which explicitly take into consideration ecosystem indicators are rare (e.g., Bering Sea northern rocksole, Lepidopsetta polyxystra; Wilderbuer et al., 2002; Wilderbauer and Nichol, 2016) and have been at times implemented on incorrect or incomplete relationships [e.g., Pacific sardines, Sardinops sagax, (Zwolinski and Demer, 2014), and Bay of Biscay anchovy, Engraulis encrarischolus (De Oliveira et al., 2005)]. Therefore, few fisheries management systems are able to explicitly take into consideration changing ecological conditions and adjust management advice through single species assessments. This highlights a global challenge for fisheries that is particularly pressing given the volatility of marine environmental conditions that are expected under likely future climate scenarios (Hollowed et al., 2013), and one that, although long recognized (Ecosystem Principles Advisory Panel, 1999; Pikitch et al., 2004), has not been well addressed.
Climate-ready fisheries management will require Ecosystem-based Fishery Management (EBFM) and robust ecosystem indicators of productivity and change (Pinsky and Mantua, 2014; Busch et al., 2016; Holsman et al., 2019c). A wide range of approaches for incorporating climate and ecosystem information into fisheries management have been proposed such as dynamic management, in-season adjustments to harvest and bycatch rates, climate-informed biological reference points, and ecological condition forecasts (Stram and Evans, 2009; Link et al., 2011; Patrick and Link, 2015; Karp et al., 2019). The NPFMC EBFM process employs components similar to those implemented throughout the world, but applied within its unique set of ecological and sociological circumstances. There are similar challenges in implementation, from determining consistent quantitative processes linking specific population dynamic parameters to ecosystem indicators, to dealing with uncertainty in model results in public decision processes where economic and social aspects are considered. In GOA groundfish management there are specific quantitative rules codified in a tier system which concerns data availability and uncertainty for single-species assessment models (DiCosimo et al., 2010; Dichmont et al., 2016), however, how to incorporate ecosystem considerations that are not integrated into the single-species models in setting annual catch limits is not prescriptive and has been largely driven by concerns of the individual assessment scientists (Ianelli et al., 2015; Zador et al., 2017).
The following provides a detailed description of the NPFMC EBFM process and a case study on how this process performed in regards to the Gulf of Alaska Pacific cod stock management in the face of a severe marine heatwave which precipitated a sudden drop in abundance of the Pacific cod stock, a key contributor to the economics of the region. With the understanding that climate change will likely have severe impacts on marine fisheries in the near future, the impact on cod was a stress test of the resilience of the NPFMC system. The resilience of fisheries management to climate change will in part depend on ecological foresight that can help coastal communities anticipate change and allocate resources and effort accordingly in order to minimize economic impacts (Allison and Bassett, 2015; Holsman et al., 2019c). Evaluating the biological and management response to the 2014–2016 heatwave event in regards to Pacific cod provides an opportunity to identify key nodes of intervention and gaps in information or tools that are needed to support successful EBFM response to future extreme events.
Materials and Methods
Marine Heatwave Index
The daily sea surface temperatures for 1981 through December 2018 were retrieved from the NOAA High-resolution Blended Analysis Data database (National Oceanic and Atmospheric Administration, 2017) and filtered to only include data from the central GOA between 145 and 160°W longitude for waters less than 300 m in depth. The overall daily mean sea surface temperature was then calculated for the entire region by averaging all points. The daily mean sea surface temperature data were processed through the R package heatwaveR (Schlegel and Smit, 2018) to obtain the marine heatwave cumulative intensity (MHCI) value (Hobday et al., 2016) where we defined a heatwave as 5 days or more with daily mean sea surface temperatures greater than the 90th percentile of the 1 January 1983 through 31 December 2012 time series. MHCI were then summed for each year to create an annual index of MHCI (Figure 2) and summed for each year for the months of January through March, November, and December to create a winter marine heatwave cumulative index (WMHCI).
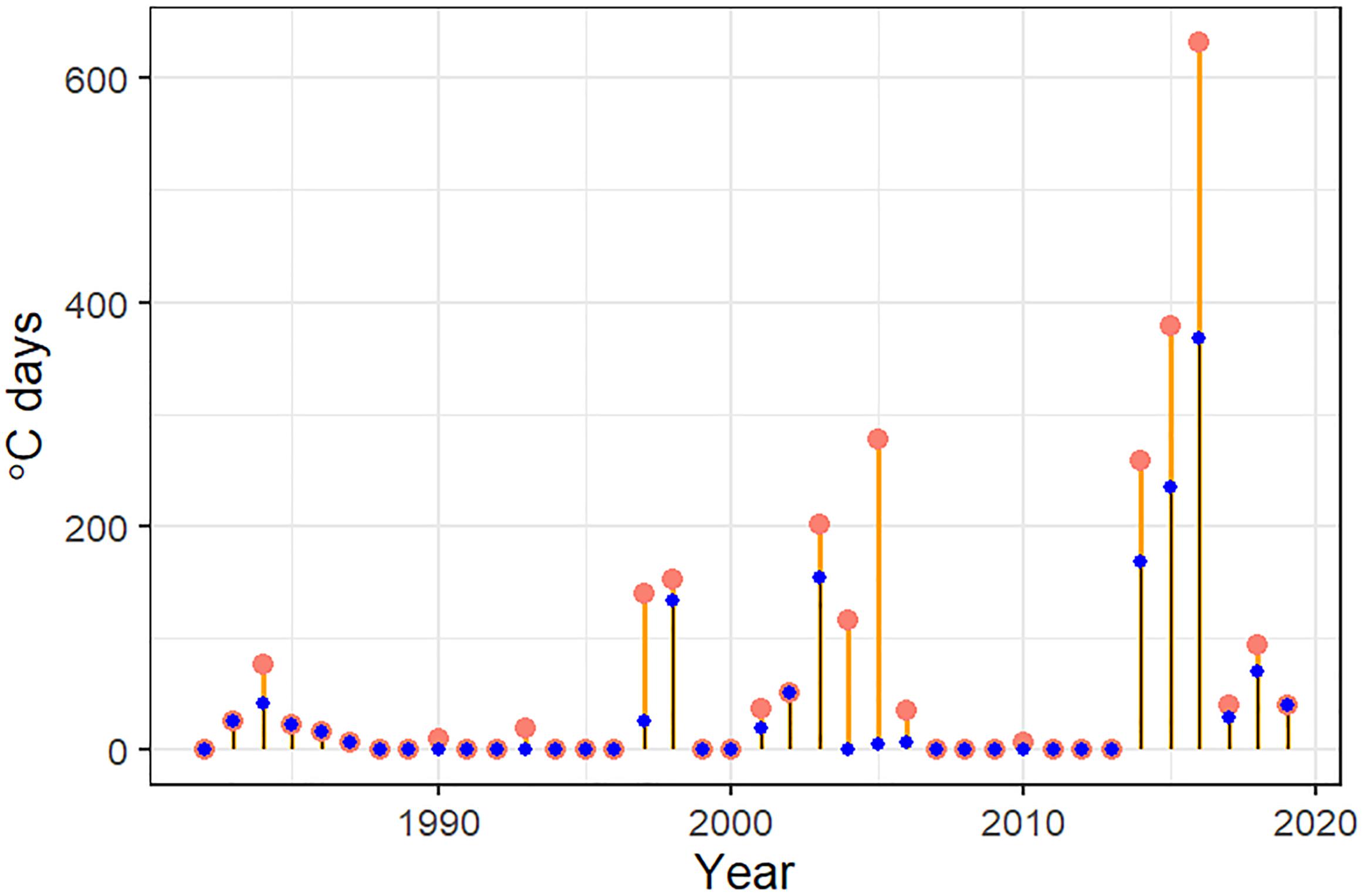
Figure 2. Index of the sum of the annual marine heatwave cumulative intensity (°C days) for 1981–2019 (larger red points) and index of the sum of the annual winter marine heatwave cumulative intensity for 1981–2019 (smaller blue points) from the daily mean sea surface temperatures NOAA high-resolution blended analysis data for the central Gulf of Alaska. The 2019 index value is the sum through 26 February 2019.
Food Habits and Bioenergetics
Stomach contents have been collected during the GOA summer bottom trawl surveys tri-annually from 1981 to 1996 and bi-annually from 1999 to 2017 (Livingston et al., 2017) to produce stomach fullness and diet composition estimates. From these and environmental data a bioenergetics model for GOA Pacific cod (Holsman et al., 2019c) was parameterized in order to evaluate potential mechanisms impacting Pacific cod growth and survival. Pacific cod samples were divided into juvenile and adult sizes classes, and their diets were analyzed for mean composition of prey items using a Dirichlet error distribution (Ainsworth et al., 2010). The relative foraging rate (RFR) was calculated as the ratio of daily rations based on synoptic stomach samples to the theoretical maximum consumption rate given a predator’s size and thermal experience. An RFR value of 0.5 indicates sampled fish foraging at 50% the rate observed in the lab, whereas a value of 1.5 indicates that fish are foraging at twice the rate observed in the lab, thus giving an indication of temperature and size-corrected foraging rate (Holsman et al., 2016, 2019c). Temperature for RFR calculations were based on the gear temperature recorded during the survey at the station where each sample was collected.
In addition to RFR, we use the bioenergetics model to estimate the scope for growth given an index of Climate Forecast System Reanalysis (CFSR) bottom temperatures for the central GOA. CFSR is the latest version of the National Centers for Environmental Prediction (NCEP) climate reanalysis (Saha et al., 2010). To make the index the CFSR reanalysis grid points were co-located with the AFSC bottom trawl survey stations in the central Gulf of Alaska between 145 and 160°W longitude. The co-located CFSR oceanic temperature profiles were linearly interpolated to obtain the temperatures at the depths centers of gravity for Pacific cod at 10–100 cm at 10 cm intervals as determined from the AFSC bottom trawl survey. All co-located grid points were then averaged to get the time series of CFSR temperatures over the period of 1979–2018. We set RFR and energy density of the prey and used the model to estimate how much energy is available for growth given a RFR of 1.0 (i.e., foraging at the laboratory-based theoretical consumption rate) and a RFR of 0.49 (mean RFR reported for GOA Pacific cod in Holsman et al., 2016).
To evaluate the condition of Pacific cod across time, length-weight residuals (Brodeur et al., 2004; Boldt et al., 2017; Holsman et al., 2019c) were calculated from the AFSC Gulf of Alaska summer bottom trawl survey data and January through April fisheries data for each gear type. The length-weight relationships for the survey and fisheries were estimated separately with linear regressions of log-transformed values overall years for each data set. Residuals for each fish were calculated by subtracting the predicted log-transformed weights from the observed log-transformed weights. Length-weight residuals were then averaged and standard deviations calculated for each data set for each year and plotted for evaluation.
Ecosystem-Based Fisheries Management in the Gulf of Alaska
The U.S. National Marine Fisheries Service (NMFS), National Oceanic and Atmospheric Administration (NOAA), defines EBFM as:
“a systematic approach to fisheries management in a geographically specified area that contributes to the resilience and sustainability of the ecosystem; recognizes the physical, biological, economic, and social interactions among the affected fishery-related components of the ecosystem, including humans; and seeks to optimize benefits among a diverse set of societal goals” (National Marine Fisheries Service, 2016).
In the GOA, groundfish fisheries management has evolved within the operations of the North Pacific Fishery Management Council (NPFMC) into a system which meets the above criteria and which in its most recent iteration (North Pacific Fishery Management Council, 2019) specifically calls for incorporation of ecosystem-based considerations. GOA groundfish fisheries management is an adaptive multi-layered process with scientific and public consultation designed to achieve a wide range of objectives which are defined in the GOA Fishery Management Plan (FMP). The management approach is stated as follows:
“As part of its policy, the Council intends to consider and adopt, as appropriate, measures that accelerate the Council’s precautionary, adaptive management approach through community-based or rights-based management, ecosystem-based management principles that protect managed species from overfishing, and where appropriate and practicable, increase habitat protection and bycatch constraints. All management measures will be based on the best scientific information available. Given this intent, the fishery management goal is to provide sound conservation of the living marine resources; provide socially and economically viable fisheries for the well-being of fishing communities; minimize human-caused threats to protected species; maintain a healthy marine resource habitat; and incorporate ecosystem-based considerations into management decisions” (North Pacific Fishery Management Council, 2019).
The process relies on the integration of single species or multi-species complex stock assessments and ecosystem status reports (ESRs) in setting annual catch limits for each managed groundfish species or groundfish complex within the 200 nautical mile (nmi) U.S. Exclusive Economic Zone off Alaska. The integration of information is overseen by the NPFMC and NOAA. To inform this decision-making process, NOAA’s Alaska Fisheries Science Center (AFSC) produces annual groundfish stock assessments. These assessments provide an evaluation of the health of each managed stock, makes recommendations on allowable catch levels, and highlights possible concerns for individual species or multi-species complexes. The AFSC also produces annual ESRs1 to provide ecosystem context for the catch level-setting process of the NPFMC (Zador et al., 2017). ESRs include assessments based on ecosystem indicators that reflect the current status and trends of ecosystem components, which range from physical oceanography to biology and human dimensions. Indicators are defined as time-series of data that represent an ecosystem component or attribute. Many indicators are based on data collected from AFSC surveys. All are developed and include contributions by scientists at NOAA, other U.S. federal and state agencies, academic institutions, and other sources. The ESRs are used to inform an assessment of factors that influence groundfish stock dynamics but are external to the single-species stock assessment models.
At the single-species or multi-species complex level, a control rule tier system is used to relate the level of uncertainty in biological reference points into consistent methods for specifying annual catch limits (DiCosimo et al., 2010). Annual catch limits are set with the intent of keeping catch below maximum sustainable yield (MSY) with the size of the buffer between the overfishing level (OFL), which is MSY or a proxy for MSY, and the allowable biological catch (ABC), which is an upper bound to the final annual catch limit, and is determined by the level of uncertainty in the reference points. There are six management tiers, from Tier 1 with the least uncertainty to Tier 6 with the most uncertainty (see North Pacific Fishery Management Council, 2019). For all managed species the annual catch levels for a stock must be set at or below the ABC which may be adjusted downward for ecosystem and other precautionary considerations.
The main integration of data for management occurs in the development of the annual single-species or species complex stock assessment models. The assessment models are developed in consideration of biology, population dynamics, and tier. Initial formal public review of newly developed single-species stock assessment models occurs at a public NPFMC Plan Team meeting. The Plan Team is comprised of scientists delegated by the NPFMC. ESRs for each ecoregion (Gulf of Alaska, Bering Sea, and Aleutian Islands Area) are presented prior to the presentation of new assessment models. During this meeting the Plan Team conducts a technical review of each new assessment model. In a separate public meeting the NPFMC Science and Statistical Committee (SSC) provides an additional technical review of the assessment models in consideration of the Plan team recommendations. The SSC is comprised of senior federal and state agency researchers and university faculty selected by the NPFMC. After the meetings the assessment models are further refined and populated with the latest data and stock assessment documents for each managed species or species complex are completed. Stock assessment documents provide a written report on the performance of the assessment models, the status of the stock, and include sections on ecosystem and economic considerations specific to the stock. The final ESRs and stock assessment documents are then presented to the Plan Team. The technical merits of each model are evaluated and the Plan Team recommends to the SSC which assessment models should be used for determining reference values for the coming year. In consideration of the advice of the Plan Team, the stock assessment documents, and ESR the SSC sets the OFL and ABC for the coming year for each managed stock. Afterward the Advisory Panel of the NPFMC (AP) recommends annual catch levels in the form of total allowable catch (TAC) for each stock and recommends allocations across a broad range of sector divisions (e.g., vessel type, gear type, management regime etc.). The AP consists of fishing industry representatives appointed by the NPFMC. The sum of the TACs for each stock must be less than or equal to the ABC set by the SSC. The NPFMC general body then sets the TACs, recommends regulatory actions, and deliberates on management objectives and research priorities for the groundfish fisheries for the coming year. Public testimony is sought formally at each stage of the NPFMC process.
Single-Species Stock Assessment Modeling and Projection
Pacific cod is a Tier 3 species meaning there is a reliable estimate of biomass, spawning biomass at 40% virgin biomass (B40%), and fishing mortality rates which reduce the stock to 35 and 40% of the virgin biomass (F35% and F40%), but no reliable probability density function for FMSY. These reference points were estimated through a single-species age-structured assessment model (Spies et al., 2017) built in Stock Synthesis version 3.30 (Methot and Wetzel, 2013). The stock assessment model description is provided in Supplementary Material – Gulf of Alaska Pacific cod assessment model documentation.
Results
Marine Heatwave Cumulative Index
The marine heatwave analysis using the daily mean central GOA sea surface temperatures indicated a prolonged period of increased temperatures in the central GOA from 2 May 2014 to 13 January 2017 with heatwave conditions persisting for 815 of the 917 days in 14 events of greater than 5 days (Figure 2). The longest stretch of uninterrupted heatwave conditions occurred between 14 December 2015 and 13 January 2017 (397 days). Using the criteria developed by Hobday et al. (2018) for marine heatwave classification the event in the central GOA reached a Category III (Severe) on 16 May 2016 with a peak intensity (Imax) of 3.02°C. The heatwave had a summed cumulative intensity (Icum) for 2016 of 635.26°C days, more than 25% of the sum of the Icum for the entire time series (1981–2018). The 14 events of this prolonged heatwave period summed to 1291.91°C days or 52% of the summed Icum for the time series.
There were three periods of increased winter heatwave activity in the central GOA, the first in 1983–1986, second in 1997–2006, and the third 2014–2016. The Imax of each event for these periods appears to have a linear increasing trend over time (Imax = 0.000006D + 1.0016 where D is the day in the timeline; R2 = 0.22) while the cumulative intensity increased exponentially (Icum = 5.82e0.0002D; R2 = 0.40). Short moderate to strong (Category I–II) winter marine heatwaves occurred every winter between 1983 and 1986 with Imax between 0.82 and 1.6, however, none of these exceeded 17 days and the total winter Icum for this period was 84.23°C days over a total of 86 days. In the winter of 1997 there were two short (7 and 12 days) moderate to strong (Category I and II) winter heatwave events with a total cumulative intensity of 17.19°C days. In 1998 there was a strong (Category II) heatwave from 3 March to the 14 June (102 days) with an Imax of 2.36°C and cumulative intensity of 146.01°C days. From 2001 through 2006 there were 6 winter heatwave events, most were moderate and less than 2 weeks in length, however, between 6 November 2002 and 4 March 2003 there were two strong (Category II) marine heatwaves that lasted in sum 141 days with a cumulative intensity of 165.94°C days and an Imax of 2.04°C. The 2014–2016 series of marine heatwave as described above was substantially longer lasting and more intense than anything experience previously in the region.
The Pacific Cod Bioenergetics
For comparison a table of life history traits for Pacific cod and other Gulf of Alaska groundfish species have been provided in Table 1. Stomach fullness of Pacific cod sampled from the AFSC summer bottom trawl survey was the lowest in the time series for 40–80 cm fish in 2015 (Figure 3), and diet composition varied from previous years, with a 47.8% drop in Tanner crab (Chionoecetes bairdi) relative to previous years and an absence of capelin which had been abundant, particularly in smaller Pacific cod, during 2011 and 2013. The proportion of Tanner crab in the diets of 40–80 cm Pacific cod dropped from the long-term mean of about 14–7% in 2015. Mean prey energy densities and annual shifts in diet composition show moderate changes in diet energy density over time, with highest cumulative diet energy densities in 2013, which occurred at the end of a 7-year cold temperature stanza in the GOA, and slightly lower values in 2015 near the long-term mean (Figure 4A).
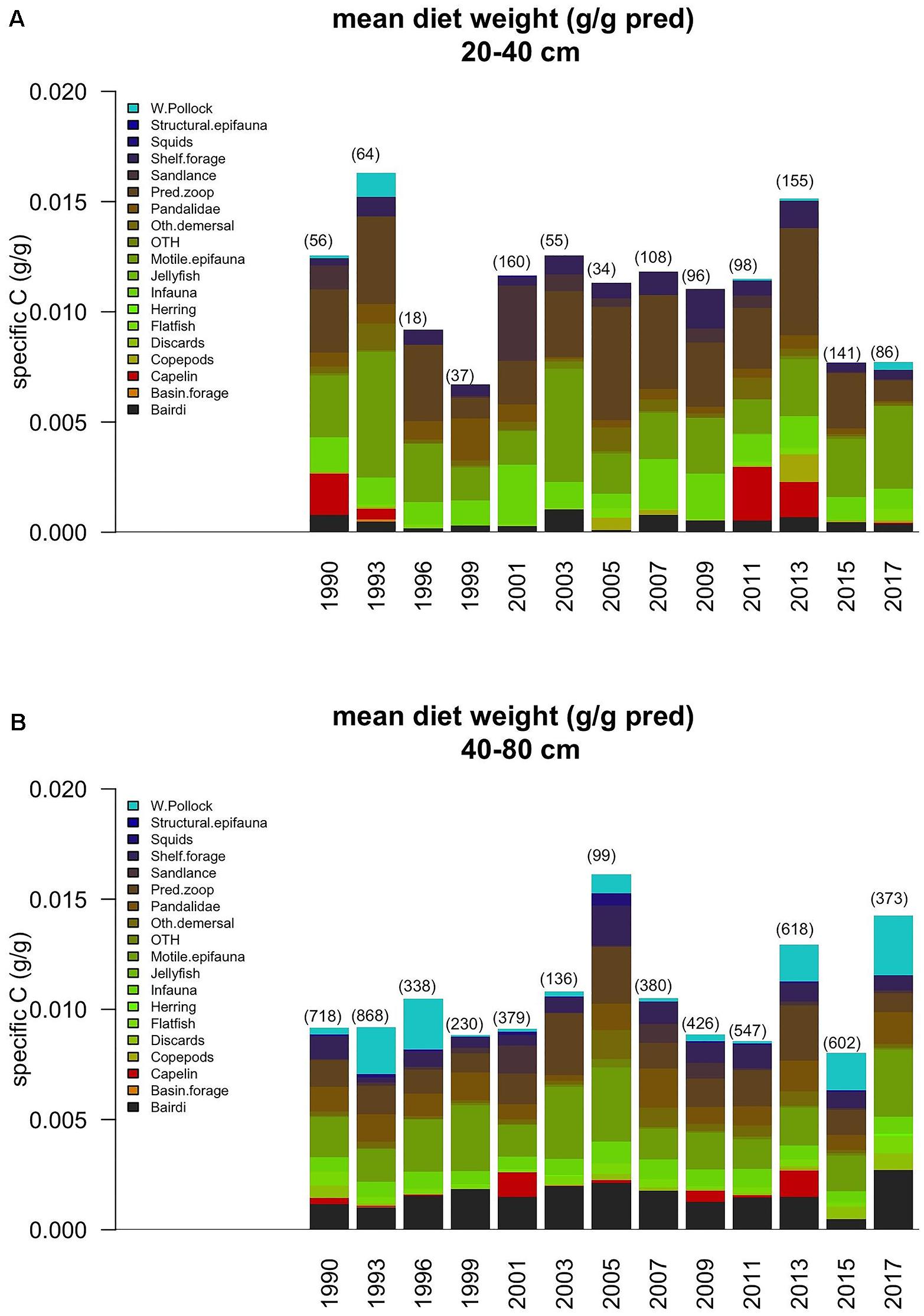
Figure 3. Specific weight (g prey/g pred) of prey in the diets of GOA Pacific cod, averaged across all survey diet samples and prey sizes for (A) 20–40 cm and (B) 40–80 cm fish. The 2019 index value is the sum through 26 February 2019.
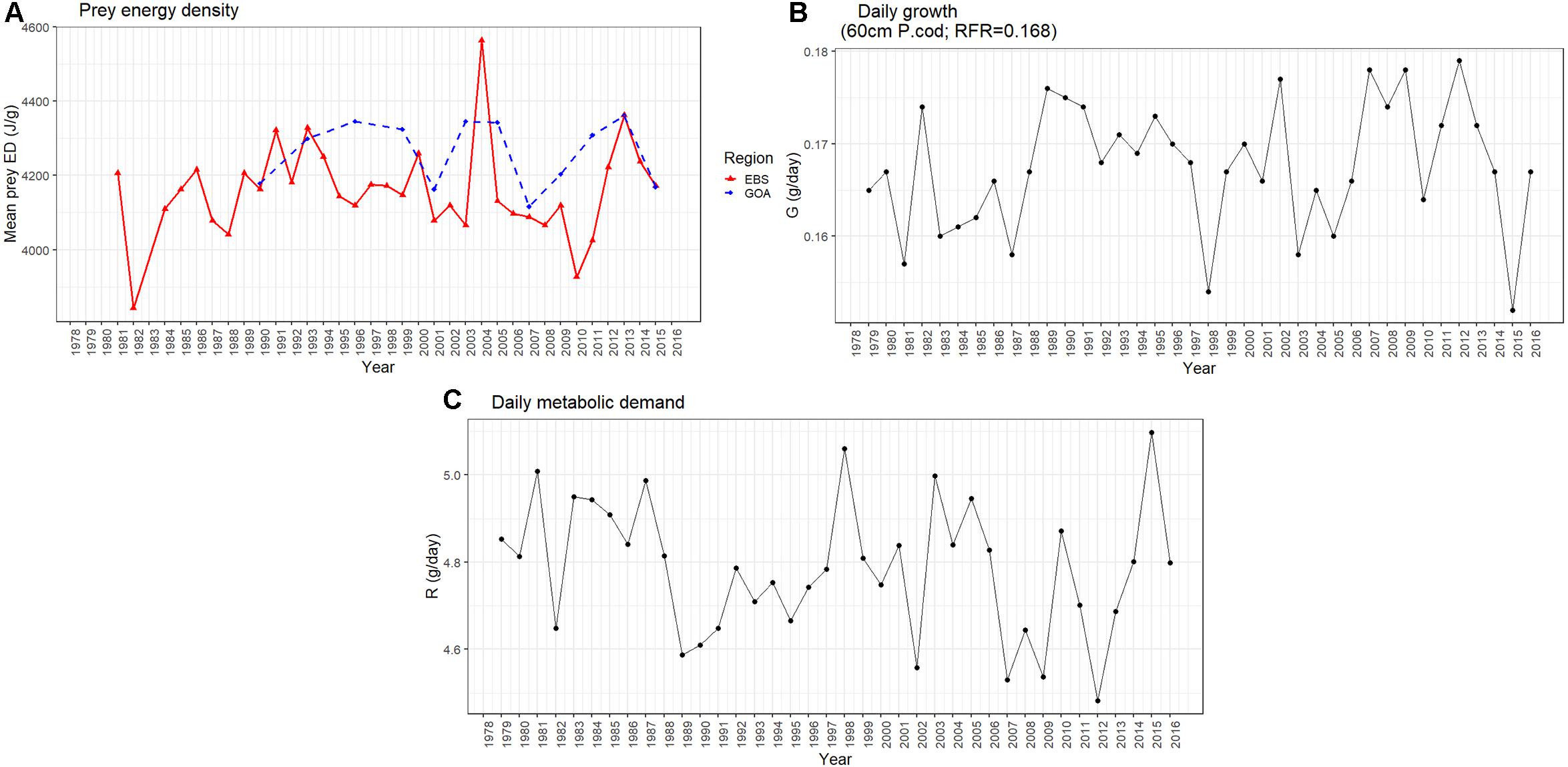
Figure 4. (A) Average prey energy density (ED) for all Alaskan samples (solid line) and for the Gulf of Alaska (red dashed line), (B) daily estimates of growth, and (C) metabolic demand. Average prey ED are based on mean energy density of prey items and diet composition from GOA Pacific cod stomach samples, growth and metabolic demand are based on the adult Pacific cod bioenergetics model (Holsman et al., 2016), a fixed relative foraging rate (RFR) = 0.65 (across years), annual indices of GOA prey energy density, and an intermediate Pacific cod energy density of 3.7 kJ/g reported in Ianelli et al. (2018).
Pacific cod foraging demand was elevated above long-term mean estimates, and peaked in 2015, according to adult bioenergetic model estimates of relative energetic demand (Figure 4C). During the two most recent marine heat waves (2014–2017 and the 1999) foraging demand never dropped below 70% of maximum demand, indicating a sustained demand for prey resources especially during the winter months when demand typically falls. Based on water temperatures at preferred depth, metabolic demand was greatest for 10 cm fish and > 40 cm fish but lowest for 30 cm fish. Bioenergetic model estimates of Pacific cod growth and respiration also suggest poor thermal conditions for growth in 1998 (following the record El Niño of 1997/98) and 2015 (Figure 4B) that were driven by high metabolic demand during those years (Figure 4C).
The condition of cod in the biannual AFSC summer bottom trawl surveys was the poorest in 2015 during the heatwave with a return to positive condition in 2017 (Figure 5A). For the January through April fisheries the condition of the population appeared to depended on gear with the pot (Figure 5B) and longline fisheries (Figure 5C) first seeing a decline in condition in 2015 and reaching its lowest in 2016. The pot fishery returned to positive condition in 2017, while the longline did not return to positive until 2018. The trawl fishery (Figure 5D) didn’t show negative conditions until 2016 with the poorest condition observed in 2017 and continued poor condition through 2018. The lowest condition in 2015 in the AFSC summer trawl survey matches the bioenergetics estimates for highest metabolic demand, however, the pattern of condition and metabolic demand were not strongly correlated across all years.
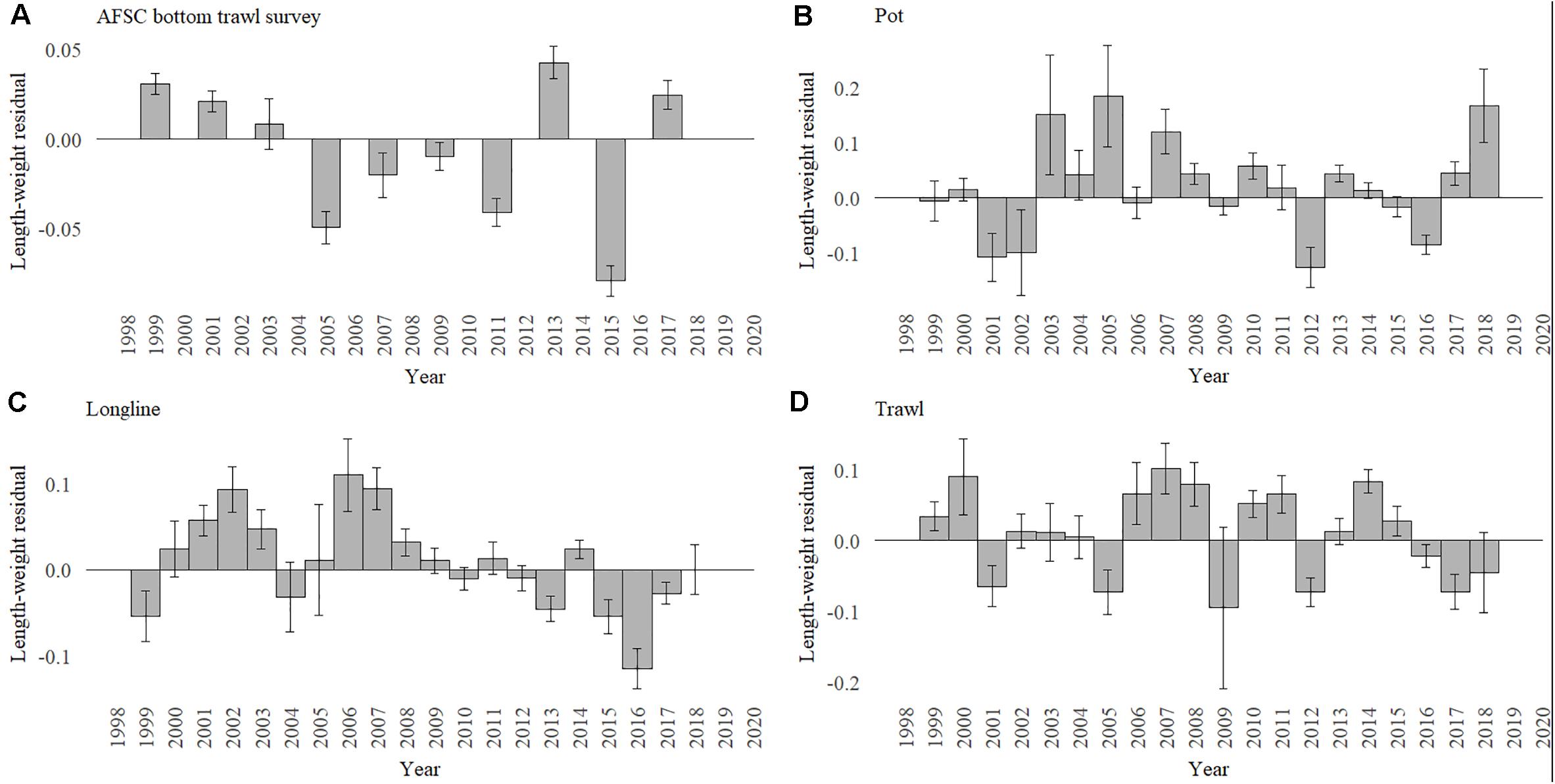
Figure 5. Gulf of Alaska Pacific cod condition calculated from length-weight regression residuals for the (A) summer AFSC bottom trawl survey and (B) pot, (C) longline, and (D) trawl fisheries for January through April for each year.
Pacific Cod Stock Assessment
In the assessment Pacific cod abundance exhibited a significant decrease during the 2014–2016 marine heatwave in both survey indices used in the stock assessment model. The 2017 AFSC Gulf of Alaska summer bottom trawl survey estimated the population of Pacific cod to be 1.96 × 108 fish and 107,324 t (0.117 CV) (Figure 6A), a 71% decline in abundance since 2015 and 83% decline since 2013. The annual AFSC longline survey (1990–2017) revealed a 53% decline in abundance from 2016 to 2017. Additional surveys not used in the assessment model corroborated declines in Pacific cod during the heatwave (Figure 6B). The annual IPHC longline survey (1997–2016; Figure 6C) observed the lowest numbers during 2016; and the ADF&G large-mesh bottom trawl survey (1988–2017; Figure 6D) recorded lowest densities in 2016 (Spalinger, 2017), which remained low in 2017 (Barbeaux et al., 2017). The Pacific cod bycatch rate in other directed fisheries was used as an independent indication of Pacific cod population trends (Spies et al., 2017). Both the pelagic walleye pollock and shallow water flatfish fisheries which regularly encounter Pacific cod showed declines in incidental catch of Pacific cod, with 2017 (walleye pollock) and 2016–2017 (shallow-water flatfish) having the lowest bycatch rates of Pacific cod on record.
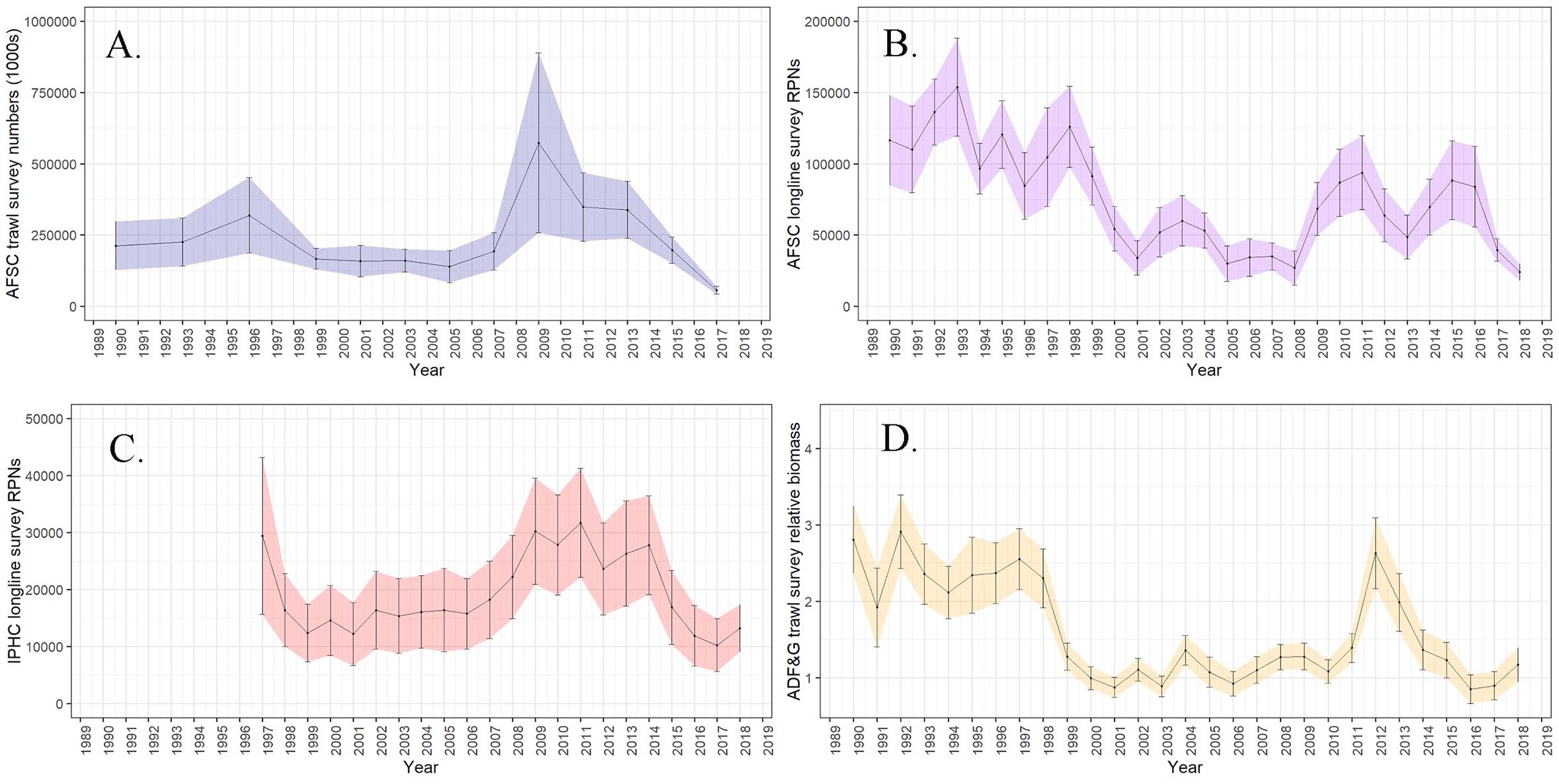
Figure 6. (A) Numbers (1 × 109) of Pacific cod from the Alaska Fisheries Science Center (AFSC) bottom trawl survey, (B) relative population numbers (RPNs 1 × 105) from the AFSC longline survey, (C) RPNs (1 × 104) from the International Pacific Halibut Commission (IPHC) longline survey, and (D) relative biomass from the Alaska Department of Fish and Game (ADF&G) bottom trawl survey with shaded 95% confidence intervals.
Total Pacific cod landings in the directed Pacific cod fisheries (longline, pot, and trawl) declined in 2016 and again in 2017. The catch in 2017 was less than 60% of the total allowable catch (TAC). All sectors had low catch per unit effort (CPUE) in 2017 in the Central GOA. In the Western GOA the pot fishery CPUE (fish per pot) declined in 2017, while the trawl and longline fishery CPUE (fish per minute towed and fish per hook) increased from 2016. However, fish weight at length was low for Pacific cod less than 80 cm from 2014 to 2017 in longline and pot fisheries in both regions indicating poor condition (Barbeaux et al., 2017).
The GOA Pacific cod stock has a long history of exploitation with an increase in fishing mortality since 1980. However exploitation rates have remained low (Figure 7) in comparison to other gadid stocks in other regions (Rose, 2019). The species is relatively short-lived (maximum age of 14) and there hasn’t been significant truncation of the age distribution (Barbeaux et al., 2018). Female spawning stock biomass (B%) and reference points based on female spawning stock biomass are the standard units on which groundfish stocks in the North Pacific are managed. The stock was at a healthy status prior to the 2014–2016 marine heatwave according to the single-species assessment model (B53% in 2013) (Barbeaux et al., 2017). In the 2016 assessments there were no substantial concerns for the health of stock as it appeared to be on an increasing trajectory through at least 2017 with a series of high abundance cohorts projected for 2005–2008 and 2012 (Barbeaux et al., 2016).
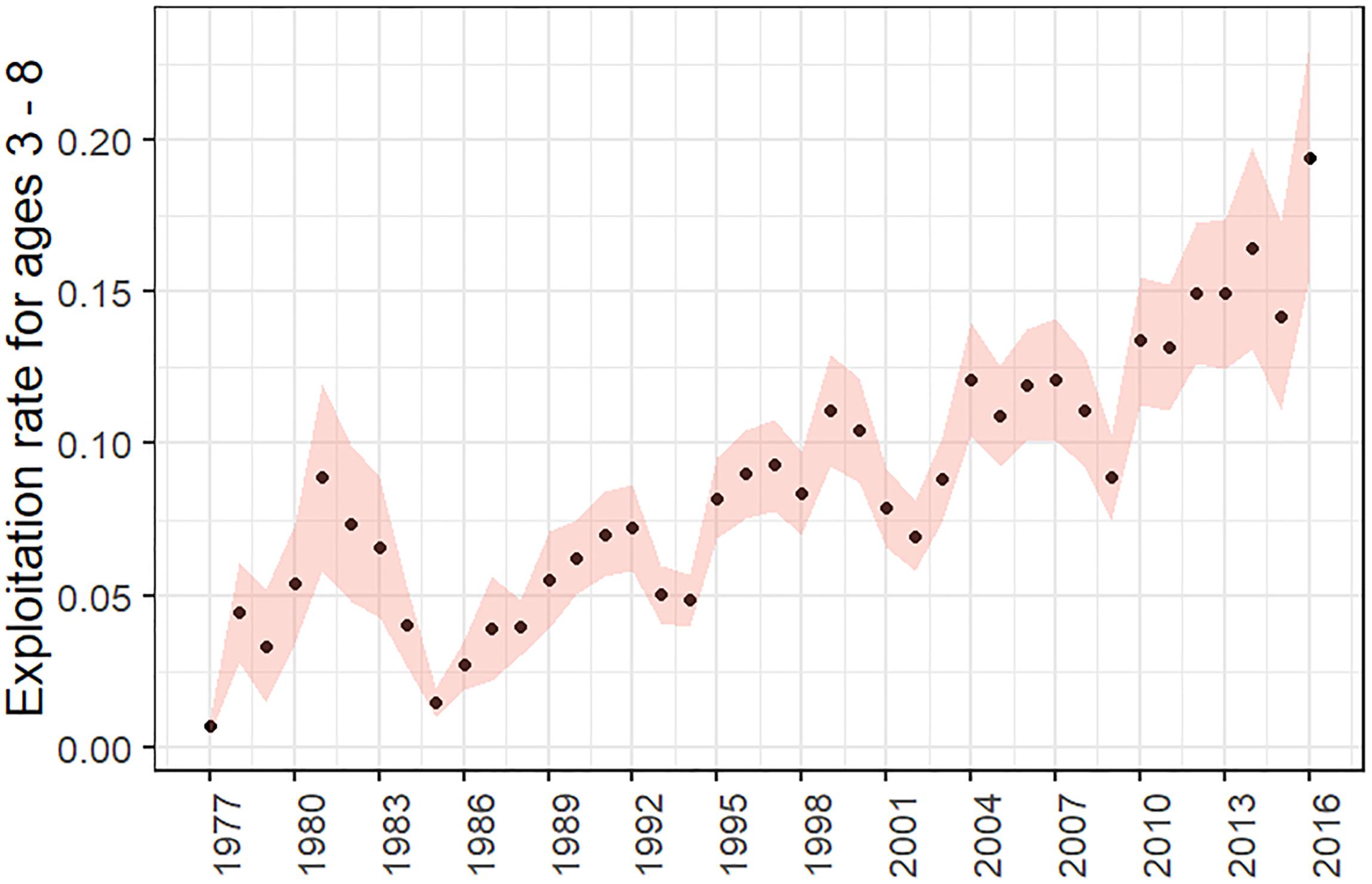
Figure 7. Exploitation rates (F) of Gulf of Alaska Pacific cod age 3–8 with 95% confidence intervals for 1977–2016 (Barbeaux et al., 2017).
In the 2017 assessment model presented at the NPFMC (Barbeaux et al., 2017) unfished female spawning stock biomass (B100%) was estimated at 168,583 t. The all-time high female spawning stock biomass was estimated at 190,465 t (B113%) in 1990 following a succession of large recruitment events throughout the 1980’s (Figure 8A). The 2017 female spawning stock biomass was estimated by the 2017 assessment model to be the lowest on record at 47,326 t (B28%) (Figure 8B), lower than the previous all-time low of 54,470 t (B32%) in 2008 and a drop of 47% since 2013. A brief increasing female spawning stock biomass trend observed from 2010 to 2014 was founded on large 2005 through 2008 year classes. Low recruitment was estimated for 2014 through 2016, with 2014 being the lowest and 2016 and 2015 being the second and third lowest recruitment estimates in the 1976–2016 time series. Due to these poor recruitment estimates and estimated higher natural mortality of adults during the 2014 through 2016 heatwave, the 2017 reference model projected a continued drop in Pacific cod female spawning stock biomass, reaching an all-time low in 2020 of 33,876 t (B20.1%) under the harvest levels recommended for 2018 and 2019, a drop of 62% from 2013. Assuming mean recruitment post-2016 the first increase in female spawning stock biomass will not be expected until 2021.
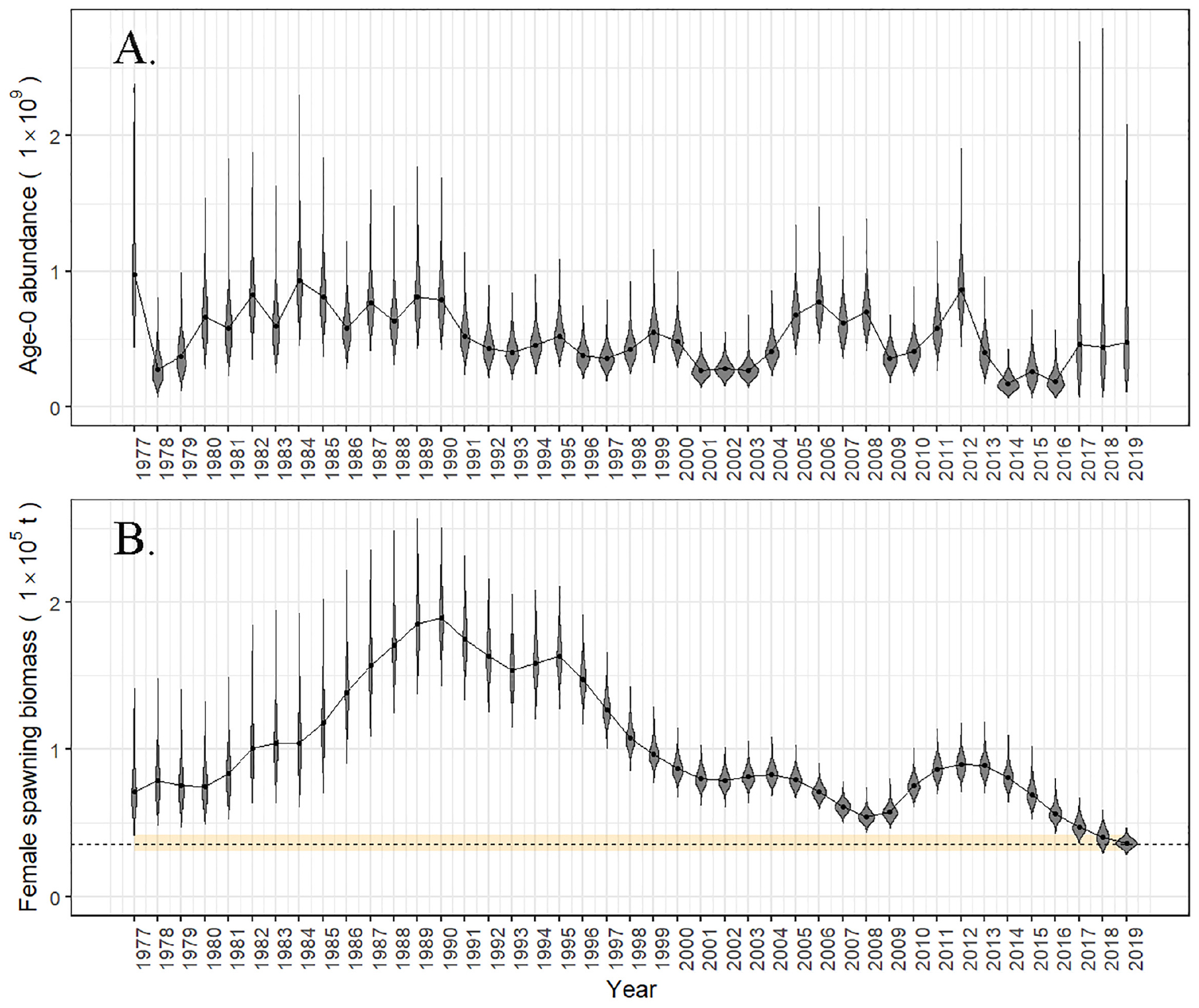
Figure 8. (A) Age-0 recruits (1 × 109) and (B) female spawning stock biomass (t) and from the 2017 Pacific cod stock assessment (Barbeaux et al., 2017) with MCMC derived posterior distributions for each year (1977–2019). The dotted line is the posterior median of B20% and the yellow shading indicates the 95% credible interval for B20%. Catch in 2018 was assumed to be at 18,000 t and recruitment for 2017 through 2019 were assumed to be at the mean.
Discussion
The 2014–2016 Marine Heatwave
Many ecosystem indicators representing physical processes such as climate and oceanography in the GOA showed strong shifts in signal direction or exhibited anomalous values beginning in early 2014 that remained relatively unchanged through the marine heatwave. Sea surface temperature and sea level pressure anomalies reflected the appearance and persistence of warm sea surface temperature anomalies described in detail by Bond et al. (2015); Di Lorenzo and Mantua (2016). At the start of the 2014–2016 marine heatwave the Pacific Decadal Oscillation (PDO; Mantua et al., 1997) shifted from a negative to positive (warm) phase where it has mostly remained through 2019 reflecting the warm sea surface temperatures in the GOA. The MHCI and WMHCI indicate a strong heatwave for this entire period persisting through the winter months (Figure 2). This was not only a surface event as warm sea temperatures deepened during the course of the heatwave (Figure 9) and remained warm throughout the winters of 2014–2015 and 2015–2016. The marine heatwave came to an end in the spring of 2017 as large portions of the GOA cooled to average sea surface temperatures, however, some warmth remained at depth. In addition to temperatures the North Pacific Gyre Oscillation (NPGO) (Di Lorenzo et al., 2008)shifted from positive to generally negative in late 2013, where it has largely remained (as of April 2019)2, indicating lower salinity and nutrients in the GOA.
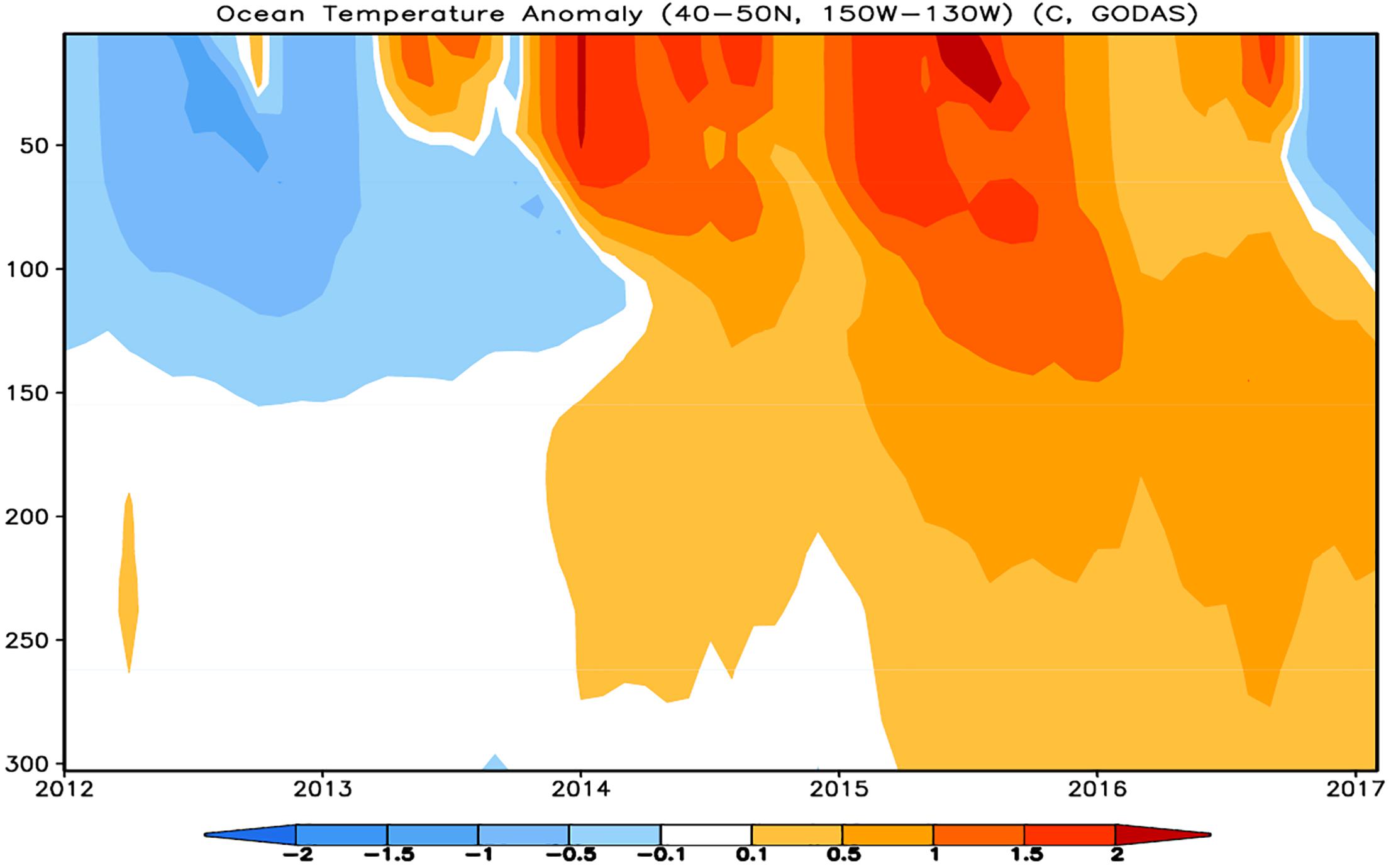
Figure 9. Subsurface temperature anomaly figure from NOAA’s Climate Prediction Center’s monthly briefing, Global Ocean Monitoring: Recent Evolution, Current Status, and Predictions, 9 March 2017 (Climate Prediction Center, 2017).
Ecosystem Response
Ecosystem indicators representing biological components varied in expression and timing of response to the marine heatwave. Mean mesozooplankton biomass appeared abundant during the heatwave although the size of copepods was smaller than average (Figure 10; Batten, 2018; Zador and Yasumiishi, 2018). Euphausiid acoustic biomass appeared moderate during summer 2015 relative to other years sampled outside the heatwave (Ressler, 2017). In general, forage fish appeared to be scarce. Age-0 walleye pollock (Gadus chalcogrammus) and Pacific cod were nearly absent from surface trawl surveys during 2015 (Dougherty and Rogers, 2017), although abundant in the year before and after the heatwave (2013 and 2017 respectively). Both capelin (Mallotus villosus) and Pacific sand lance (Ammodytes personatus) were lacking in seabird and groundfish diets in 2015 relative to years before the heatwave. Capelin all but disappeared from seabird diets at Middleton Island in the first year of the heatwave in 2014 and have remained so through 2017 (Hatch et al., 2017).
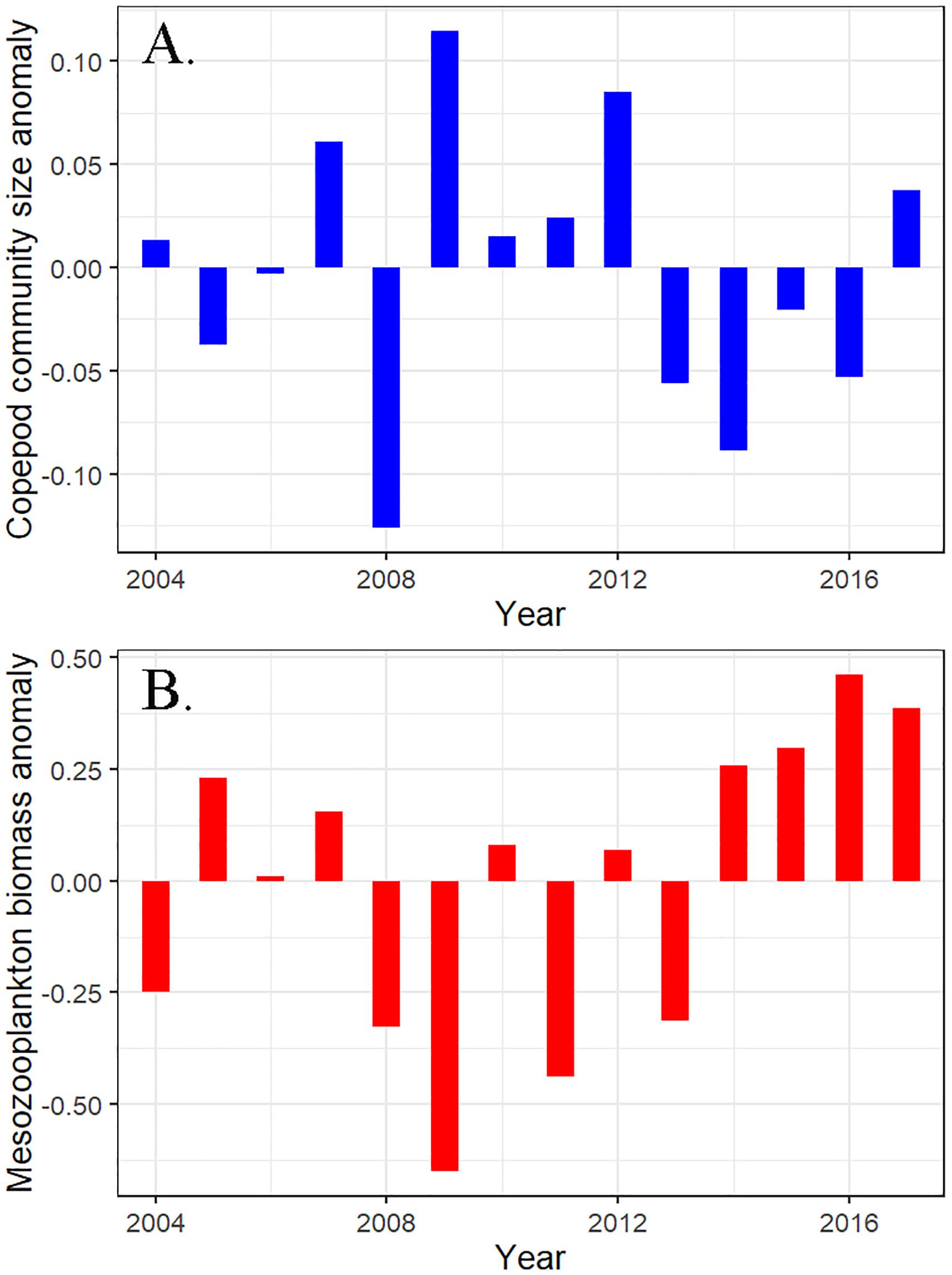
Figure 10. Gulf of Alaska (A) copepod community size anomaly and (B) mesozooplankton biomass anomaly. Data for this figure were from Batten (2018).
Indicators of upper trophic organisms appeared to show negative responses to the heatwave, although the timing and expression of the responses varied. Biennial bottom trawl surveys throughout the GOA in 2015 showed slight increases in catch rates of large piscivorous groundfish such as arrowtooth flounder (Atheresthes stomas), Pacific halibut (Hippoglossus stenolepis), and sablefish (Anoplopoma fimbria), but a substantial decrease in Pacific cod catch rates, and the presence of uncommon species such as ocean sunfish (Mola mola) that are typically found south of the GOA (Zador and Yasumiishi, 2016, 2017). After the heatwave in 2017, arrowtooth flounder and Pacific halibut catch rates declined, Pacific cod declined further, but sablefish increased again (Barbeaux et al., 2017; Hanselman et al., 2017; International Pacific Halibut Commission, 2017; Zador and Yasumiishi, 2017). Groundfish condition as indicated by mean weight-length residuals showed that all measured groundfish species had average to positive condition in 2013 before the heatwave, but all had poor condition with negative mean weight-length residuals in 2015, with Pacific cod and southern rock sole (Lepidopsetta bilineata) showing record low condition that year. Pacific cod condition improved after the heatwave in 2017, but all other species’ condition remained lower than average (Zador and Yasumiishi, 2018). Alaska Department of Fish and Game annual large mesh bottom trawl surveys implemented at a smaller spatial scale also showed a decline in catch rates of Pacific cod during the heatwave, with a record low in 2016, but increasing catch rates of Pacific halibut during that time, while arrowtooth flounder remained at the lowest rates seen since 2010 (Zador and Yasumiishi, 2017).
Seabirds in the western GOA had good reproductive success in the first year of the heatwave, but showed widespread reproductive failures in 2015 (Renner et al., 2017; Zador and Yasumiishi, 2017). This included common murres, which also experienced a record die-off in the GOA during the winter of 2015–2016 (Piatt et al., 2020). In general, forage fish eating seabird fared poorly during the heatwave, while mixed fish and zooplanktivorous seabird fared better. Steller sea lions (Eumetopias jubatus) surveys showed declines in numbers of pups and adults between 2015 and 2017 throughout the GOA where recent counts had been trending upwards (Zador and Yasumiishi, 2017). Humpback whale calf production in Glacier Bay was high in 2014 but declined dramatically in 2015–2017 (Neilson et al., 2017). An unusually large number of fin, humpback and gray whale carcasses were documented throughout the western GOA in 2015–2016 (Zador and Yasumiishi, 2016).
What Happened to GOA Pacific Cod?
There are increasing lines of evidence that in temperate/subarctic ecosystems such as the GOA anomalously warm water, and in particular, consecutive years of warm water patterns, can have an overall negative effect on ecosystem productivity (Smale et al., 2019). At the population level for ectotherms, it is well understood that temperature affects consumption, recruitment, mortality, growth, and phenology (Johnston and Bennett, 2008), but it is less certain how marine heatwaves impact dynamic ecosystem components in aggregate as the interactive and cumulative effects play out among all the constituents of an ecosystem. At a global scale and over the long-term ocean warming will reduce overall marine production by as much as 20% due to changing currents and deposition of nutrients into the deep ocean where it will no longer be available for primary production (Moore et al., 2018). This will have a net negative effect on wild marine fisheries. Free et al. (2019) investigated individual stock production under warming conditions. Where they could show an impact, warming more often reduced productivity than increased, but this was not a general rule as other factors often confounded the effects. The reasons for changes in productivity for each stock varied considerably. In the absence of overfishing, their review of 154 stocks found little evidence for isolated effects of climate variability or fast population growth rates on risk of stock collapse, but cumulatively these factors interacted to increase the risk of stock collapse (Pinsky and Byler, 2015). How individual stocks perform in the face of a marine heatwave where temperatures are within the breadth of their thermal tolerance is uncertain and will likely differ depending on stock status (e.g., unfished versus overfished), biogeography, bioenergetics, genetic and phenotypic diversity and plasticity of the stock in regards to temperature, as well as the state of the species a stock is dependent upon (e.g., forage, competitors, and predators), and on the overall health of the ecosystem at the time of the heatwave (e.g., unexploited versus highly impacted or compromised) (Hollowed et al., 2013; Poloczanska et al., 2013; Pinsky and Byler, 2015).
While there are winners and losers, warm water patterns can lead to bottom-up forcing that favors smaller and less-lipid rich zooplankton which in turn increases steps in food chains and decreases trophic transfer efficiency (Hunt et al., 2011; Gaichas et al., 2015). Predator response to environmental and trophic changes can be rapid or slow and varies with natural history and adaptive capacity. For example, mobile species can shift their distributions to more favorable habitat via changes in depth or horizontal distance (Barbeaux and Hollowed, 2018; Yang et al., 2018) where biogeographical conditions allow, while sessile organisms may exhibit temperature-driven physiological changes in growth or condition (Short et al., 2015; Arias-Ortiz et al., 2018; Thomsen et al., 2019). However, changing ocean chemistry and reduced oxygen saturation under warming may limit the scope for thermal refugia (Deutsch et al., 2015). As such some predator indicators will rapidly reflect environmental change with little lag, such as within-season shifts in fish distribution (e.g., presence of southern species in surveys, Zador and Yasumiishi, 2018), while other signals may manifest over multiple years (e.g., changes in production).
Metabolic demand of Pacific cod is largely a function of thermal experience and tends to increase exponentially with increasing temperatures (Paul et al., 1988). Pacific cod can minimize metabolic costs through behaviors such as movement to thermally optimal temperatures (Claireaux et al., 1995; Hurst et al., 2009; Nichol et al., 2013), or can increase consumption of food energy to meet increasing metabolic demands. The former requires access to thermally optimal temperatures and the latter requires sufficient access to abundant or high energy prey resources. Thus, if either is limiting, metabolic costs can exceed energetic consumption and decreases in growth or increases in mortality will occur (Dutil and Lambert, 2000). For Pacific cod in the GOA during the 2014–2016 marine heatwave, prey demand was elevated above long-term mean estimates, and peaked in 2015, according to adult bioenergetic model estimates of relative energetic demand. Thus the increase in metabolic demand in 2015 has two important implications: if Pacific cod did not migrate out of the region they would have had to (1) consume an additional 6–12% of prey per day (g/1d/1) over average to maintain growth and body condition, or (2) access energetic reserves leading to net body mass loss. The protracted warm conditions from 2014 to 2016 may have exceeded both adaptive options, leading to starvation and mortality. There are several lines of evidence to support this potential mechanism for the decline in Pacific cod abundance, including poor weight-at-length condition of Pacific cod observed in 2015 (Figure 5), lowest potential growth based on mean relative foraging rates, highest recorded metabolic demands in 2015, and below average diet energy density. Also of important note is the relative absence of capelin (an important prey item) in the diets of piscivorous sea birds during the heatwave and Pacific cod from 2015, and the overall lower mean stomach fullness for Pacific cod in 2015. Large-scale northward migration of Pacific cod was unlikely given the limiting geography of the GOA (Figure 1). The geography and bathymetry of the GOA would have required the majority of Pacific cod to traverse a long southwestward corridor prior to finding an accessible northward passage. Some portion of Pacific cod in the far western GOA may have been able to move through Unimak Pass into the southeastern Bering Sea, however, concomitant declines in Pacific cod biomass there suggest this was not a significant factor (Thompson, 2017). Although there appears to have been a shift in distribution of GOA Pacific cod to deeper waters during the heatwave (Yang et al., 2018), the shift did not move fish to depths exceeding the bounds of the survey and therefore would have been included in survey estimates. In addition temperatures at the new depth during the heatwave remained up to 2°C warmer than what Pacific cod would have experienced on average over the previous decade.
Bottom-up forcing can also be tracked through factors affecting recruitment; some of which can be expected to show rapid responses (e.g., age-0 fish abundance and survival) and others longer-term lagged responses (e.g., recruitment to a fished stock). Pacific cod have a narrow temperature tolerance for egg development and larval survival (Alderdice and Forrester, 1971; Laurel et al., 2008; Laurel and Rogers, 2020) suggesting that recruitment is likely sensitive to temperature. The low abundance of larvae and juvenile Pacific cod during the heatwave were apparent in both the 2015 and 2017 AFSC bottom trawl surveys and 2015 and 2017 AFSC ichthyoplankton surveys. Although this low abundance of young fish is expected to lead to poor recruitment at age 3 into the fishery starting in 2018 and impact the recovery of the stock, it had little impact on the low survey biomass and estimated spawning stock biomass in 2017.
Considered collectively, these lines of evidence suggest that persistent anomalously warm conditions that extended from surface waters to depth may have contributed to high mortality rates for juvenile and adult Pacific cod during the marine heatwave. Thus mortality is one mechanism linking climate variability to the Pacific cod stock (Lehodey et al., 2006). Furthermore, poor recruitment during the heatwave suggests that recovery will be delayed. Numerous studies link warm temperature to poor Pacific cod larval survival (Alderdice and Forrester, 1971; Laurel et al., 2008; Hurst et al., 2009; Laurel et al., 2011; Laurel and Rogers, 2020), growth-dependent mortality due to increased prey demand, and lower quality and quantity of zooplankton prey (Gallego and Heath, 1997; Beaugrand et al., 2003). But our study is the first that we are aware of that directly links adult Pacific cod mortality to decreased abundance via insufficient prey to meet increased energetic demands during an extended warm period.
Why Pacific Cod?
Other ectothermic fish species would be expected to have similarly elevated metabolic demands during the warm conditions, increasing the potential for broad-scale prey limitations. Life history traits predict that Pacific cod would be most vulnerable (among large groundfish) to multi-year environmental warming event (Table 1). Most relevant may be the shorter lifespans and high growth rates compared to other large groundfish. King and McFarlane (2003) grouped Pacific cod as “intermediate” strategists, between opportunistic (r-selected; e.g., anchovies, sardines) and periodic (K-selected) strategists, the latter which include other groundfish such as rockfish, flatfish and sablefish. Intermediates tend to exhibit large swings in abundance, similar to opportunistic strategists, but are longer-lived than periodic strategists. This group also includes tunas, which, unlike Pacific cod, are highly migratory, thereby able to buffer the effects of poor ecosystem conditions (Polovina, 1996; McFarlane et al., 2000; King and McFarlane, 2003). These traits also allow for the rapid recovery of the Pacific cod stock biomass when ecosystem conditions are favorable (i.e., temperature are cooler and prey is abundant). In fact, the observation of good body condition for Pacific cod during 2017 surveys in contrast to the poor body condition of other species (Zador and Yasumiishi, 2017) suggests that the remaining Pacific cod were able to respond more quickly to the improved conditions post-heatwave than other species.
Specific responses to the marine heatwave appears to have varied among the other abundant groundfish in the GOA such as walleye pollock, arrowtooth flounder, and Pacific halibut. While they would be expected to have similar metabolic responses to the increased temperature, inherent differences in life history and/or adaptive capacity may have influenced how their responses manifested. For example, the relatively more pelagic and lower trophic (i.e., zooplankton) diet of walleye pollock was sufficient to maintain growth given the apparent abundance of zooplankton during the heatwave (Dorn et al., 2018). Although Pacific cod have a higher thermal optimum than walleye pollock (Laurel et al., 2016), their metabolic demand is also higher. At the same temperature gram for gram Pacific cod require 40% more forage on a daily basis than walleye pollock (Holsman et al., 2016). Arrowtooth flounder female spawning biomass also declined during the 2014–2016 heatwave (Spies et al., 2017), which may reflect similar stock-wide effects of increased metabolic demands and limiting prey resources. Additionally, as a major predator of young walleye pollock, the decline in arrowtooth flounder may have decreased predatory pressure on walleye pollock. While Pacific halibut stomachs were also lacking C. bairdi during 2015 (AFSC Groundfish Trophic Interactions Database, accessed 14 February 2019)3, their highly migratory nature, pelagic foraging capabilities, and longer lifespan may have been sufficient to buffer the immediate effects of increased metabolic demands on the population. Pacific ocean perch (Sebastes alutus) and sablefish which had increasing populations during the heatwave (Hanselman et al., 2017; Hulson et al., 2017) have higher thermal optimums than Pacific cod. Recruitment of sablefish in 2014 and 2016 was high, potentially due to the lack of predation by and competition with juvenile Pacific cod. The explanation for sablefish high recruitment is speculative as a direct mechanisms for the increase in sablefish recruitment has yet to be determined. Finally, recent evidence suggests bottom up controls on other groundfish species in the GOA and EBS over the past two decades, with declines in Pacific halibut size at age potentially driven by increasing metabolic demand and combined with declines in prey quality (Holsman et al., 2019b).
Pacific Cod Co-management and EBFM
Ecosystem indicators presented to the NPFMC in 2015 and 2016 suggested the marine heatwave induced marked changes to the GOA ecosystem, including poor groundfish condition, seabird die-offs, and large whale strandings (Zador and Yasumiishi, 2017). Nonetheless, owing to a lack of clear implications for groundfish productivity at the time, these indications of poor ecosystem performance failed to prompt precautionary change in groundfish management ahead of the severe decline of Pacific cod identified in 2017. When the decline was recognized, the fisheries management system functioned as it was designed in terms of managing stock biomass and harvest pressure, by reducing the quota until the stock recovers.
The recommended ABC for Pacific cod was 18,000 t for 2018 and 17,000 t for 2019. These values included further downward adjustments from the maximum permissible ABCs from the 2017 reference model of 19,401 t and 17,634 t in 2018 and 2019. This reduced level of catch would keep the 2019 and 2020 projections of the Pacific cod female spawning stock biomass above B20%, a threshold which triggers a fishery closure due to regulations governing minimum forage requirements for the endangered western stock of Steller sea lions. The recommended ABC represented a 77% decline from that projected for 2018 in the 2016 reference model. After acceptance of the ABC, the federal TAC for 2018 was set at 13,096 t and there were 4,904 t allocated to nearshore state water fisheries. The TAC is the number which ultimately governs the maximum amount of catch for a given year in the fisheries. The fishery sector and seasonal quotas for the federal fisheries are subsets of the TAC.
The fishery and dependent coastal communities incurred a substantial loss in catch and revenue with little time to prepare. Even so the large reduction of the GOA Pacific cod TAC was non-controversial at the NPFMC public meetings. During the public comment period at each stage there was no dissent voiced. At the December AP meeting a question on the technical aspects of the GOA bottom trawl survey was asked by a fisher. A captain of one of the commercial fishing vessels that conducted the 2017 GOA bottom trawl survey was a sitting member of AP and was able to answer the question and adroitly address the specific concerns of the fisher. Other public testimony during the Plan Team, SSC, and general NPFMC meetings primarily were of concern for the GOA ecosystem following the marine heatwave. Three fishers during public commentary at the NPFMC December meeting voiced concern for other species suggesting the NPFMC consider more conservative TACs for these species given the uncertainty of the impacts of the heatwave. The lack of a substantial negative response by fishers, as seen in other regions when large reductions in fishing quotas have been enacted (e.g., Newfoundland Atlantic cod quota reduction protests (Palmer, 2003), New England Atlantic cod quota reduction protests (Hilborn et al., 2001), France and the United Kingdom Atlantic cod quota reduction protests (BBC News, 2002; CNN, 2002; RFI (English), 2009) Newfoundland and Labrador shrimp quota reduction protests (Canada’s National Observer, 2017), South Africa quota reduction protests and riots (Isaacs and Witbooi, 2019), etc.), can be attributed in large part to the transparent cooperative co-management system (Sen and Nielsen, 1996) that has evolved in North Pacific groundfish fisheries (Stram and Evans, 2009). The NPFMC management apparatus includes both formal and informal venues for public inclusion in the decision-making process (Stram and Evans, 2009). The formal process includes representation on the AP and the NPFMC general body and public comment periods during the meetings. Stakeholders tend to be well versed in how the system functions and know their concerns will be heard and considered. Informal dialog between assessment scientists, fisheries managers, and stakeholders occurs at each stage of the management process.
EBFM in the North Pacific groundfish fisheries not only relies on the single-species assessments and aggregated ecosystem information presented in the ESR, but also on the shared experience of other stakeholders and the combined knowledge across the two advisory and two deliberative bodies of the NPFMC (Zador et al., 2017). Over time this system has evolved to increasingly include ecosystem, economic, and other social information within the process (Stram and Evans, 2009; Livingston et al., 2011). The NPFMC EBFM has developed with the increasing knowledge that management has been improved with the inclusion of ecosystem data and collaboration among stakeholders. In some cases this inclusion and collaboration has been forced with new regulatory mandates created in response to Congressional action in the Magnuson-Stevens Act (MSA), the Marine Mammal Protection Act (MMPA), the Endangered Species Act (ESA), the Migratory Bird Treaty Act, the National Environmental Policy Act (NEPA), the Regulatory Flexibility Act and litigation generated to ensure compliance with these acts.
All of the resource assessment surveys used in the GOA Pacific cod stock assessment have been conducted cooperatively with contracted commercial fishing vessels and crews (Rutecki et al., 2016; Von Szalay and Raring, 2018). Questions on the technical aspects of the surveys were answered by a fellow fisher using language and socio-cultural cues readily understood by fellow fishers. Further, Cooperation among NOAA researchers and other stakeholders including the fishing industry, non-government organizations, universities, and state of Alaska agencies in the collection and interpretation of data and development of assessment models is an integral part of the management structure. There are numerous funding sources that facilitate cooperative research in the region including federal (e.g., North Pacific Research Board4 and NOAA Fisheries Cooperative Research Program)5 and private industry (e.g., Pollock Conservation Cooperative Research Center6 and North Pacific Fisheries Foundation7). A large portion of basic groundfish research completed in the GOA is conducted cooperatively with the commercial fishing industry on commercial vessels using fishing crew, providing fishers with a first-hand understanding of where and how data were collected and by whom.
Another important component of fisheries management in the northeastern Pacific groundfish fisheries has been the long-term use of industry-funded, but NOAA administered, biological technicians (observers) to collect fishery-dependent data on board commercial fishing vessels (French et al., 1982; Cahalan et al., 2014; Alaska Fisheries Science Center, 2018). The use of onboard observers has not only provided a wealth of data on commercial catch (∼40,000 sea days per year), but it has also provided a venue for daily contact and communication among fishers and scientists and a training ground for fisheries scientists and managers. Many researchers, managers, and industry representatives working in the northeastern Pacific groundfish fisheries have previously worked as observers. This familiarity is believed to have further facilitated communication among stakeholders.
In 2017 the AFSC stock assessors made a concerted effort to warn stakeholders of the potential for a severe reduction along with a coherent story of what caused the decline in Pacific cod abundance. The warning first presented by AFSC researchers in October 2017 at the SSC and AP meetings was consistent with what the fishers had observed on the fishing grounds. In addition the ESRs identifying the heatwave and known ecological impacts had been presented in public forums since 2015 when the heatwave was first identified. The authors informed local radio and print news sources prior to the December 2017 NPFMC meeting to widen public awareness. Familiarity with the research and open communication among stakeholders has translated into a high level of trust in science-based management in this fishery. An attitude of co-responsibility for the health and management of the fish stocks has also been fostered. A cooperative political climate in the North Pacific groundfish fisheries along with a degree of co-responsibility toward the resource has facilitated the implementation of EBFM, and, in the case of GOA Pacific cod management, has made economically difficult determinations more readily accepted by stakeholders.
Lessons Learned and Suggested Improvements
Despite the identification of the GOA marine heatwave in 2015 and the negative effects on some components of the ecosystem, prior to the 2017 summer bottom trawl survey the full impact of the marine heatwave on adult GOA Pacific cod was unforeseen. Once the issue of low Pacific cod abundance was identified the management system reacted decisively. The management response, although appropriate given what was known, was too late to allow sufficient time for the fishing industry to fully prepare for the quota reduction and subsequent economic impact (Bill Walker and Byron Mallot to Wilbur Ross, Federal Fishery Disaster, 8 March 2018)8. The NPFMC process has been both cooperative and adaptive, but is generally reactionary and only rarely proactive. The question remains as to whether the drop in the GOA Pacific cod population could have been predicted and more done to prepare the industry or cushion the impacts to the Pacific cod population or was this event unpredictable. If the former then what can be done to predict the next event? If the latter, how can the management system be best adapted to reduce economic, social, and ecological impacts given unpredictable events while not unnecessarily forgoing economic opportunities?
In the assessment model, a block of years 2014–2016 with higher mortality was used as an unexplained mortality event without a predictive mechanism to allow projection of future mortality events. It is thought that the increase in adult GOA Pacific cod mortality can be tied to the marine heatwave and likely due to nutritional stress over winter as described above. However finding a relationship to an index which can be used within the single-species assessment to scale mortality is proving difficult. Temperature alone is not a consistent measure for adult Pacific cod mortality. An increase in consumptive requirements of Pacific cod with increasing temperatures within tolerance levels is only an issue if forage are not available. Available bottom trawl and longline surveys currently do a poor job of providing indices of annual forage levels for Pacific cod.
There are some measures currently reported in the GOA ESR that may be developed into useful qualitative indices but likely could not be developed into mechanistic quantitative predictors within the assessment model. Piscivorous seabird and fish stomach content samples are annually available and appear to track forage presence. Low abundance of capelin in both birds and Pacific cod and low stomach weights in Pacific cod during the heatwave suggest a possible connection. However, low abundance of capelin and light stomach contents have been observed in the past without noticeable increases in Pacific cod mortality (Barbeaux et al., 2017). Although Dutil and Lambert (2000) show a relationship between condition factors and natural mortality, their study was limited to Atlantic cod (Gadus morhua) and show considerable overlap for weight-at-length condition factors in healthy and starving fish and even more overlap between starving and dead fish. The weight-at-length condition factor for Pacific cod in the GOA summer bottom trawl survey is tracked in the ESR (Figure 5A), but this is a biennial index limited to summer collections, which is likely not the most critical time for Pacific cod survival during a marine heatwave that spans the winter.
The only available winter and spring data on Pacific cod condition are from fishery data (Figure 5). The signal in the weight-at-length condition factor has been mixed in the fishery during the 2014–2016 marine heatwave. This is likely due to how the fisheries are conducted. Trawl fisheries in the late winter and early spring target pre-spawning aggregations and the weight-at-length condition factor remains high for these fish even during the start of the marine heatwave. Weight-at-length condition in the trawl fishery may be biased toward more healthy fish, those that have enough energy reserves to partake in spawning. The longline and baited pot fishery showed a lower than average Pacific cod condition during the heatwave years for all size classes and continued poor condition for smaller fish through 2018 post-heatwave (Barbeaux et al., 2018). Unfortunately, due to the reduction in quota the winter GOA baited pot and longline fishery effort has been greatly reduced from previous years and data collection was minimal during the winter and early spring for 2018 (Ganz et al., 2018). We are therefore left without a consistent quantitative means of identifying when or how a marine heatwave will impact Pacific cod until the stock levels increase to allow a more consistent fishery. Each of these potential indices need to be assessed in aggregate and used to identify when management should be more precautionary, but a direct link through the single-species stock assessment to quantitative ABC determination is likely not an attainable goal in the short-term. Therefore, even though there is an understanding of how the 2014–2016 marine heatwave impacted the GOA Pacific cod stock, how future events will play out in this system remains unclear.
EBFM will continue to rely on adjustments to non-climate enhanced single-species assessment model with recommendations tempered by non-integrated evaluations of oceanographic conditions and other ecosystem components. However, recent developments of climate-enhanced stock assessment models in the Bering Sea (Holsman et al., 2016; Spencer et al., 2016) may provide a method for accounting for climate- and ecological-driven changes to stock biomass and survival. In the EBS the climate-enhanced multispecies assessment model has been used strategically to provide a climate-specific multispecies target for pollock, Pacific cod and arrowtooth flounder ABC and has been used to project recruitment for these species under future climate conditions (Holsman et al., 2018). As an appendix to the pollock stock assessment (Ianelli et al., 2018), the model is currently used to provide strategic contextual advice for that assessment. Such classes of climate-enhanced models may improve management advice under climate-change and extreme events.
Another lesson has been that although there is likely a mechanistic relationship with temperature that will help predict poor recruitment in the near term, a perfect understanding of recruitment processes may not improve our ability to predict the sudden impacts of marine heatwaves. For this species poor recruitment was already indicated for the 2014–2016 marine heatwave in the larval and biennial bottom trawl surveys. The low recruitment starting in 2014 had been noted in the assessment in 2016 and taken into account in the projections of future spawning biomass and management advice. The 2016 and 2017 assessments projected a drop in female spawning biomass in 2018 and later and provided a warning of probable sharp drops in ABC after 2019 (Barbeaux et al., 2016). The issue most surprising in 2017 and of most concern for the fishery was the unforeseen precipitous drop in abundance of adult fish. A better understanding of recruitment processes would have reduced the uncertainty in projections for 2019 and onward, however, this would not have led to an earlier warning for the drop in adult abundance. Where an understanding of recruitment processes may prove useful is in preparing for the increase in average temperatures with climate change and the development of climate-enhanced management strategy evaluations aimed at looking at management under climate change. A continued problem may be that the relationships and correlations we see currently will likely change as we have more marine heatwave events. Populations will die off, move, or be conditioned to warmer temperatures, advancing the rapid adaptation of ecosystems to a warmer planet. Relationships that exists now will not likely be fully applicable in the long-term (>30 years) if the International Panel on Climate Change (IPCC) projections are correct.
Although some impacts of the 2014–2016 GOA heatwave were foreseen, the increase in adult GOA Pacific cod mortality was not predictable. Climate change will result in more marine heatwaves resulting in more unforeseen consequences as we are uncertain which specific species will be impacted or how that impact will manifest resulting in management challenges both biologic and economic. Although management will likely never be proactive enough to adequately address these issues before they become crises, it will need to be robust to crises and nimble enough to adapt to emerging issues. Robust management requires that systems in place for data collection, interpretation, and dissemination, as well as the management advice process are structured in such a way that economic and social pressures created by the crises will not adversely impact them. More nimble management means that emerging crises are recognized as such as early as possible and the nature of the crises communicated within the management structure and to the public quickly. Management responses need to be decisive which requires trust by the impacted stakeholders in the management system and adequate warning given to the stakeholders to adapt. A very important lesson learned during this event is that as climate change impacts our world EBFM will require a more diverse set of expertise than traditionally employed in fisheries stock assessment.
Data Availability Statement
The data analyzed in this study is subject to the following licenses/restrictions: Stock Synthesis data files for the Pacific cod assessment can be provided upon request of the corresponding author. All of the raw data used for this study are housed at AKFIN (https://akfin.psmfc.org/). There are confidentiality rules for the raw fisheries data which cannot be provided without aggregating the data at a resolution that prevents a user from identifying a unique fisher. Otherwise the data are publicly available and can be provided at the request of the corresponding author. Requests to access these datasets should be directed to Steve.Barbeaux@noaa.gov.
Ethics Statement
Ethical review and approval was not required for the animal study because although concerning fish, this is a study on the impacts of a marine heatwave on a fish stock and fisheries. The researchers did not interact with individual animals.
Author Contributions
SB was the lead author developed the stock assessment section and brought all the pieces of the document together. KH conducted the bioenergetics analysis and wrote the section pertaining to this. SZ evaluated the ecosystem impacts and wrote the results and discussion section pertaining to ecosystem impacts. All authors reviewed the work of each other and contributed to the editing of the full document.
Funding
This project was a work product of the three authors employed by the Alaska Fisheries Science Center, National Marine Fisheries Service, NOAA and all publications fees will be paid by the same as a general budget item. No other funding was used.
Conflict of Interest
The authors declare that the research was conducted in the absence of any commercial or financial relationships that could be construed as a potential conflict of interest.
Acknowledgments
We would like to thank the observers and survey personnel that go out to sea every year and return with the data essential for the management of the North Pacific fisheries. We would like to thank Dr. Qiong Yang for providing us with the CFSR water temperature data used in this study. We would also like to thank Drs. Martin Dorn, Anne Hollowed, James Thorson, and the Gulf of Alaska Groundfish NPFMC Plan Team and Science and Statistical Committee for providing reviews and editorial comment on this research, substantially improving the final product. The scientific results and conclusions, as well as any views or opinions expressed herein, are those of the author(s) and do not necessarily reflect those of NOAA or the Department of Commerce.
Supplementary Material
The Supplementary Material for this article can be found online at: https://www.frontiersin.org/articles/10.3389/fmars.2020.00703/full#supplementary-material
Footnotes
- ^ https://access.afsc.noaa.gov/reem/ecoweb/
- ^ http://www.o3d.org/npgo/
- ^ https://access.afsc.noaa.gov/REEM/WebDietData/DietDataIntro.php
- ^ https://www.nprb.org
- ^ https://www.st.nmfs.noaa.gov/cooperative-research
- ^ https://www.pccrc.org
- ^ http://npfisheriesfoundation.org
- ^ https://www.fisheries.noaa.gov/webdam/download/76977930
References
Ainsworth, C. H., Kaplan, I. C., Levin, P. S., and Mangel, M. (2010). A statistical approach for estimating fish diet compositions from multiple data sources: gulf of California case study. Ecol. Appl. 20, 2188–2202. doi: 10.1890/09-0611.1
Alaska Fisheries Science Center (2018). 2019 Observer Sampling Manual. Seattle, WA: North Pacific Groundfish Observer Program, AFSC.
Alderdice, D. F., and Forrester, C. R. (1971). Effects of salinity, temperature, and dissolved oxygen on early development of the Pacific cod (Gadus macrocephalus). J. Fish. Board Can. 28, 883–902. doi: 10.1139/f71-130
Allison, E. H., and Bassett, H. R. (2015). Climate change in the oceans: human impacts and responses. Science 350, 778–782. doi: 10.1126/science.aac8721
Arias-Ortiz, A., Serrano, O., Masqué, P., Lavery, P. S., Mueller, U., Kendrick, G. A., et al. (2018). A marine heatwave drives massive losses from the world’s largest seagrass carbon stocks. Nat. Clim. Change 8, 338–344. doi: 10.1038/s41558-018-0096-y
Aydin, K., Gaichas, S., Ortiz, I., Kinzey, D., and Friday, N. (2007). A Comparison of the Bering Sea, Gulf of Alaska, and Aleutian Islands Large Marine Ecosystems Through Food Web Modeling. U.S. Dep. Commer., NOAA Tech. Memo. NMFS-AFSC-178, 298.
Barange, M., Bahri, T., Beveridge, M. C. M., Cochrane, K. L., Funge-Smith, S., and Poulain, F. (2018). Impacts of Climate Change on Fisheries and Aquaculture: Synthesis of Current Knowledge, Adaptation and Mitigation Options. FAO Fisheries and Aquaculture Technical Report 627. (Rome: Food and Agriculture Organization of the United Nations).
Barbeaux, S., Aydin, K., Fissel, B., Holsman, K., Palsson, W., Shotwell, K., et al. (2017). “Assessment of the walleye pollock stock in the gulf of Alaska,” in Stock Assessments and Fishery Evaluation Report for the Groundfish Resources of the Gulf of Alaska (Anchorage, AK: North Pacific Fishery Management Council).
Barbeaux, S., Aydin, K., Fissel, B., Holsman, K., Palsson, W., Shotwell, K., et al. (2018). “Assessment of the Walleye Pollock Stock in the Gulf of Alaska,” in Stock Assessments and Fishery Evaluation Report for the Groundfish Resources of the Gulf of Alaska (Anchorage, AK: North Pacific Fishery Management Council).
Barbeaux, S., D’Amar, T., and Palsson, W. (2016). “Assessment of the Walleye Pollock Stock in the Gulf of Alaska,” in Stock Assessments and Fishery Evaluation Report for the Groundfish Resources of the Gulf of Alaska (Anchorage, AK: North Pacific Fishery Management Council), 99501.
Barbeaux, S. J., and Hollowed, A. B. (2018). Ontogeny matters: climate variability and effects on fish distribution in the eastern Bering Sea. Fish. Oceanogr. 27, 1–15. doi: 10.1111/fog.12229
Batten, S. D. (2018). “Continuous Plankton Recorder Data from the Northeast Pacific through 2017,” in Ecosystem Status Report 2018: Gulf of Alaska, Stock Assessment and Fishery Evaluation Report, eds S. Zador and E. Yasumiishi (Anchorage, AK: North Pacific Fishery Management Council).
BBC News (2002). Noisy Protest Over Fishing Cuts. Available online at: http://news.bbc.co.uk/2/hi/uk_news/england/2564049.stm (accessed March 1, 2019).
Beaugrand, G., Brander, K. M., Lindley, J. A., Souissi, S., and Reid, P. C. (2003). Plankton effect on cod recruitment in the North Sea. Nature 426, 661–664. doi: 10.1038/nature02164
Betts, M. W., Maschner, H. D. G., and Clark, D. S. (2011). “Zooarchaeology of the “Fish that stops”,” in The Archaeology of North Pacific Fisheries, eds M. L. Moss and A. Cannon (Anchorage, AK: University of Alaska Press), 171–194.
Boldt, J., Rooper, C., and Hoff, J. (2017). “Gulf of Alaska groundfish condition,” in Ecosystem Considerations 2017: Status of the Gulf of Alaska Marine Ecosystem, Stock Assessment and Fishery Evaluation Report, eds S. Zador and E. Yasumiishi (Anchorage, AK: North Pacific Fishery Management Council), 99501.
Bond, N. A., Cronin, M. F., Freeland, H., and Mantua, N. (2015). Causes and impacts of the 2014 warm anomaly in the NE Pacific. Geophys. Res. Lett. 42, 3414–3420. doi: 10.1002/2015GL063306
Brodeur, R. D., Fisher, J. P., Teel, D. J., Emmett, R. L., Casillas, E., and Miller, T. W. (2004). Juvenile salmonid distribution, growth, condition, origin, and environmental and species associations in the Northern California Current. Fish. Bull. 102, 24–46.
Busch, D. S., Griffis, R., Link, J., Abrams, K., Baker, J., Brainard, R. E., et al. (2016). Climate science strategy of the US national marine fisheries service. Mar. Policy 74, 58–67. doi: 10.1016/j.marpol.2016.09.001
Cahalan, J., Gasper, J., and Mondragon, J. (2014). Catch Sampling and Estimation in the Federal Groundfish Fisheries off Alaska, 2015 edition. NOAA Tech. Memo. U.S. Department of Commerce, NMFS-AFSC-286. Available online at: http://www.afsc.noaa.gov/Publications/AFSC-TM/NOAA-TM-AFSC-286.pdf (accessed June 10, 2018).
Canada’s National Observer (2017). Fishermen Protest at Government Fisheries Offices against Lower Shrimp Quota. Available online at: https://www.nationalobserver.com/2017/04/07/news/fishermen-protest-government-fisheries-offices-against-lower-shrimp-quota (accessed March 1, 2019).
Claireaux, G., Webber, D., Kerr, S., and Boutilier, R. (1995). Physiology and behaviour of free-swimming Atlantic cod (Gadus morhua) facing fluctuating temperature conditions. J. Exp. Biol. 198, 49–60.
Climate Prediction Center (2017). Climate Prediction Center monthly briefing, Global Ocean Monitoring: Recent Evolution, Current Status, and Predictions, March 9, 2017. National Weather Service Climate Prediction Center, NCEP Global Ocean Data Assimilation System (GODAS). Available online at: http://www.cpc.ncep.noaa.gov/products/GODAS/ocean_briefing_gif/global_ocean_monitoring_2017_03.pdf (accessed September 25, 2018).
CNN (2002). Ports Blocked in Cod Quota Protest. Available online at: http://edition.cnn.com/2002/WORLD/europe/12/11/eu.fish/index.html (accessed March 1, 2019).
De Oliveira, J. A. A., Uriarte, A., and Roel, B. A. (2005). Potential improvements in the management of Bay of Biscay anchovy by incorporating environmental indices as recruitment predictors. Fish. Res. 75, 2–14. doi: 10.1016/j.fishres.2005.05.005
Deutsch, C., Ferrel, A., Seibel, B., Pörtner, H.-O., and Huey, R. B. (2015). Climate change tightens a metabolic constraint on marine habitats. Science 348, 1132–1135. doi: 10.1126/science.aaa1605
Di Lorenzo, E., and Mantua, N. (2016). Multi-year persistence of the 2014/15 North Pacific marine heatwave. Nat. Clim. Change 6, 1042–1047. doi: 10.1038/nclimate3082
Di Lorenzo, E., Schneider, N., Cobb, K. M., Franks, P. J. S., Chhak, K., Miller, A. J., et al. (2008). North Pacific Gyre Oscillation links ocean climate and ecosystem change. Geophys. Res. Lett. 35:L08607. doi: 10.1029/2007GL032838
Dichmont, C. M., Punt, A. E., Dowling, N., De Oliveira, J. A. A., Little, L. R., Sporcic, M., et al. (2016). Is risk consistent across tier-based harvest control rule management systems? A comparison of four case-studies. Fish Fish. 17, 731–747. doi: 10.1111/faf.12142
DiCosimo, J., Methot, R. D., and Ormseth, O. A. (2010). Use of annual catch limits to avoid stock depletion in the Bering Sea and Aleutian Islands management area (Northeast Pacific). ICES J. Mar. Sci. 67, 1861–1865. doi: 10.1093/icesjms/fsq060
Dorn, M., Aydin, K., Fissel, B., Palsson, W., Spalinger, K., Stienessen, S., et al. (2018). Assessment of the Walleye Pollock Stock in the Gulf of Alaska. (Anchorage, AK: North Pacific Fishery Management Council).
Dougherty, A., and Rogers, L. (2017). “Larval walleye pollock assessment in the Gulf of Alaska, Spring 2017,” in Ecosystem Status Report 2017: Gulf of Alaska, Stock Assessment and Fishery Evaluation Report, eds S. Zador and E. Yasumiishi (Anchorage, AK: North Pacific Fishery Management Council), 99501.
Dutil, J.-D., and Lambert, Y. (2000). Natural mortality from poor condition in Atlantic cod (Gadus morhua). Can. J. Fish. Aquat. Sci. 57, 826–836. doi: 10.1139/f00-023
Ecosystem Principles Advisory Panel (1999). Ecosystem-Based Fishery Management: A Report to Congress. Silver Spring, MD: National Marine Fisheries Service.
Fissel, B., Dalton, M., Garber-Yonts, B., Haynie, A., Kasperski, S., Lee, J., et al. (2017). “Economic status of the groundfish fisheries off Alaska, 2016,” in Stock Assessment and Fishery Evaluation Report for the Groundfish Fisheries of the Gulf of Alaska and Bering Sea/Aleutian Islands Area (Anchorage, AK: North Pacific Fishery Management Council) doi: 10.28966/PSESV.2018.002b.
Free, C. M., Thorson, J. T., Pinsky, M. L., Oken, K. L., Wiedenmann, J., and Jensen, O. P. (2019). Impacts of historical warming on marine fisheries production. Science 363, 979–983. doi: 10.1126/science.aau1758
French, R., Nelson, J. R. R., and Wall, J. (1982). Role of the United States observer program in management of foreign fisheries in the northeast Pacific Ocean and eastern Bering Sea. N. Am. J. Fish. Manage. 2, 122–131. doi: 10.1577/1548-8659(1982)2<122:rotuso>2.0.co;2
Gaichas, S., Aydin, K., and Francis, R. C. (2015). Wasp waist or beer belly? Modeling food web structure and energetic control in Alaskan marine ecosystems, with implications for fishing and environmental forcing. Prog. Oceanogr. 138, 1–17. doi: 10.1016/j.pocean.2015.09.010
Gallego, A., and Heath, M. (1997). The effect of growth-dependent mortality, external environment and internal dynamics on larval fish otolith growth: an individual-based modelling approach. J. Fish Biol. 51, 121–134. doi: 10.1111/j.1095-8649.1997.tb06096.x
Ganz, P., Barbeaux, S., Cahalan, J., Gasper, J., Lowe, S., Webster, R., et al. (2018). Deployment Performance Review of the 2017 North Pacific Observer Program. Washington, DC: U.S. DEPARTMENT OF COMMERCE.
Gattuso, J.-P., Magnan, A., Billé, R., Cheung, W. W. L., Howes, E. L., Joos, F., et al. (2015). Contrasting futures for ocean and society from different anthropogenic CO2 emissions scenarios. Science 349:aac4722. doi: 10.1126/science.aac4722
Hanselman, D., Rodgveller, C., Lunsford, C., and Fenske, K. (2017). “Assessment of the Sablefish Stock of Alaska,” in Stock Assessment and Fishery Evaluation Report for the Groundfish Fisheries of the Gulf of Alaska and Bering Sea/Aleutian Islands Area (Anchorage, AK: North Pacific Fishery Management Council).
Hatch, S. A., Mayumi, and Piatt, J. (2017). “Seabird-derived forage fish indicators from Middleton Island,” in Ecosystem Status Report 2017: Gulf of Alaska, Stock Assessment and Fishery Evaluation Report, eds S. Zador and E. Yasumiishi (Anchorage, AK: North Pacific Fishery Management Council).
Hilborn, R., Maguire, J.-J., Parma, A. M., and Rosenberg, A. A. (2001). The precautionary approach and risk management: can they increase the probability of successes in fishery management? Can. J. Fish. Aquat. Sci. 58, 99–107. doi: 10.1139/f00-225
Hobday, A. J., Alexander, L. V., Perkins, S. E., Smale, D. A., Straub, S. C., Oliver, E. C. J., et al. (2016). A hierarchical approach to defining marine heatwaves. Prog. Oceanogr. 141, 227–238. doi: 10.1016/j.pocean.2015.12.014
Hobday, A. J., Oliver, E. C. J., Gupta, A. S., Benthuysen, J. A., Burrows, M. T., Donat, M. G., et al. (2018). Categorizing and naming marine heatwaves. Oceanography 31, 162–173. doi: 10.5670/oceanog.2018.205
Hollowed, A. B., Barange, M., Beamish, R. J., Brander, K., Cochrane, K., Drinkwater, K., et al. (2013). Projected impacts of climate change on marine fish and fisheries. ICES J. Mar. Sci. 70, 1023–1037. doi: 10.1093/icesjms/fst081
Holsman, K., Hollowed, A., Ito, S.-I., Bograd, S., Hazen, E., King, J., et al. (2019a). Climate Change Impacts, Vulnerabilities and Adaptations: North Pacific and Pacific Arctic Marine Fisheries. (Rome: FAO), 113–138.
Holsman, K. K., Aydin, K., Sullivan, J., Hurst, T., and Kruse, G. H. (2019b). Climate effects and bottom-up controls on growth and size-at-age of Pacific halibut (Hippoglossus stenolepis) in Alaska (USA). Fish. Oceanogr. 28, 345–358. doi: 10.1111/fog.12416
Holsman, K. K., Hazen, E. L., Haynie, A., Gourguet, S., Hollowed, A., Bograd, S. J., et al. (2019c). Towards climate resiliency in fisheries management. ICES J. Mar. Sci. 76, 1368–1378. doi: 10.1093/icesjms/fsz031
Holsman, K. K., Ianelli, J., and Aydin, K. (2018). “Multi-species Stock Assessment for walleye pollock, Pacific cod, and arrowtooth flounder in the Eastern Bering Sea,” in Stock Assessment and Fishery Evaluation Report for the Groundfish Resources for the Bering Sea/Aleutian Islands Regions (Anchorage, AK: North Pacific Fishery Management Council).
Holsman, K. K., Ianelli, J., Aydin, K., Punt, A. E., and Moffitt, E. A. (2016). A comparison of fisheries biological reference points estimated from temperature-specific multi-species and single-species climate-enhanced stock assessment models. Deep Sea Res. Part II Top. Stud. Oceanogr. 134, 360–378. doi: 10.1016/j.dsr2.2015.08.001
Hulson, P., Hanselman, D., Lunsford, C., and Fissel, B. (2017). “Assessment of the Pacific ocean perch stock in the Gulf of Alaska,” in Stock Assessments and Fishery Evaluation Report for the Groundfish Resources of the Gulf of Alaska (Anchorage, AK: North Pacific Fishery Management Council).
Hunt, G. L. Jr., Coyle, K. O., Eisner, L. B., Farley, E. V., Heintz, R. A., Mueter, F., et al. (2011). Climate impacts on eastern Bering Sea foodwebs: a synthesis of new data and an assessment of the oscillating control hypothesis. ICES J. Mar. Sci. 68, 1230–1243. doi: 10.1093/icesjms/fsr036
Hurst, T. P., Cooper, D. W., Scheingross, J. S., Seale, E. M., Laurel, B. J., and Spencer, M. L. (2009). Effects of ontogeny, temperature, and light on vertical movements of larval Pacific cod (Gadus macrocephalus). Fish. Oceanogr. 18, 301–311. doi: 10.1111/j.1365-2419.2009.00512.x
Ianelli, J. N., Honkalehto, T., Barbeaux, S. J., and Kotwicki, S. (2015). “Assessment of the walleye pollock stock in the Eastern Bering Sea,” in Stock Assessment and Fishery Evaluation Report for the Groundfish Resources of the Bering Sea/Aleutian Islands Regions (Anchorage, AK: North Pacific Fishery Management Council), 53–151.
Ianelli, J. N., Kotwicki, S., Honkalehto, T., McCarthy, A., Stienessen, S., Siddon, E., et al. (2018). “Assessment of the walleye pollock stock in the Eastern Bering Sea,” in Stock Assessment and Fishery Evaluation Report for the Groundfish Resources of the Bering Sea/Aleutian Islands Regions (Anchorage, AK: North Pacific Fishery Management Council), 1–147.
International Pacific Halibut Commission (2017). Assessment of the Pacific Halibut (Hippoglossus stenolepis) Stock at the End of 2017. Seattle, WA: International Pacific Halibut Commission.
Isaacs, M., and Witbooi, E. (2019). Fisheries crime, human rights and small-scale fisheries in South Africa: a case of bigger fish to fry. Mar. Policy 105, 158–168. doi: 10.1016/j.marpol.2018.12.023
Jacox, M. G., Alexander, M. A., Mantua, N. J., Scott, J. D., Hervieux, G., Webb, R. S., et al. (2018). Forcing of multiyear extreme ocean temperatures that impacted California current living marine resources in 2016. Bull. Am. Meteorol. Soc. 99, S27–S33. doi: 10.1175/BAMS-D-17-0119.1
Johnston, I. A., and Bennett, A. F. (2008). Animals and Temperature: Phenotypic and Evolutionary Adaptation. Cambridge: Cambridge University Press.
Karp, M. A., Peterson, J. O., Lynch, P. D., Griffis, R. B., Adams, C. F., Arnold, W. S., et al. (2019). Accounting for shifting distributions and changing productivity in the development of scientific advice for fishery management. ICES J. Mar. Sci. 76, 1305–1315. doi: 10.1093/icesjms/fsz048
King, J. R., and McFarlane, G. A. (2003). Marine fish life history strategies: applications to fishery management. Fish. Manage. Ecol. 10, 249–264. doi: 10.1046/j.1365-2400.2003.00359.x
Laurel, B. J., Hurst, T. P., and Ciannelli, L. (2011). An experimental examination of temperature interactions in the match–mismatch hypothesis for Pacific cod larvae. Can. J. Fish. Aquat. Sci. 68, 51–61. doi: 10.1139/F10-130
Laurel, B. J., Hurst, T. P., Copeman, L. A., and Davis, M. W. (2008). The role of temperature on the growth and survival of early and late hatching Pacific cod larvae (Gadus macrocephalus). J. Plankton Res. 30, 1051–1060. doi: 10.1093/plankt/fbn057
Laurel, B. J., and Rogers, L. A. (2020). Loss of spawning habitat and prerecruits of Pacific cod during a Gulf of Alaska heatwave. Can. J. Fish. Aquat. Sci. 77, 644–650. doi: 10.1139/cjfas-2019-0238
Laurel, B. J., Spencer, M., Iseri, P., and Copeman, L. A. (2016). Temperature-dependent growth and behavior of juvenile Arctic cod (Boreogadus saida) and co-occurring North Pacific gadids. Polar Biol. 39, 1127–1135. doi: 10.1007/s00300-015-1761-5
Lehodey, P., Alheit, J., Barange, M., Baumgartner, T., Beaugrand, G., Drinkwater, K., et al. (2006). Climate variability, fish, and fisheries. J. Clim. 19, 5009–5030. doi: 10.1175/JCLI3898.1
Link, J. S., Bundy, A., Overholtz, W. J., Shackell, N., Manderson, J., Duplisea, D., et al. (2011). Ecosystem-based fisheries management in the Northwest Atlantic. Fish Fish. 12, 152–170. doi: 10.1111/j.1467-2979.2011.00411.x
Livingston, P. A., Aydin, K., Boldt, J. L., Hollowed, A. B., and Napp, J. M. (2011). “Ecosystem-Based Management for Marine Fisheries: Alaska marine fisheries management: advances and linkages to ecosystem research,” in Ecosystem-Based Management for Marine Fisheries: An Evolving Perspective, eds A. Belgrano and C. W. Fowler (London: Cambridge University Press), 113–152. doi: 10.1017/cbo9780511973956.006
Livingston, P. A., Aydin, K., Buckley, T. W., Lang, G. M., Yang, M.-S., and Miller, B. S. (2017). Quantifying food web interactions in the North Pacific–a data-based approach. Environ. Biol. Fishes 100, 443–470. doi: 10.1007/s10641-017-0587-0
Mantua, N. J., Hare, S. R., Zhang, Y., Wallace, J. M., and Francis, R. C. (1997). A Pacific interdecadal climate oscillation with impacts on salmon production. Bull. Am. Meteorol. Soc. 78, 1069–1080. doi: 10.1175/1520-0477(1997)078<1069:APICOW>2.0.CO;2
McFarlane, G. A., King, J. R., and Beamish, R. J. (2000). Have there been recent changes in climate? Ask the fish. Prog. Oceanogr. 47, 147–169. doi: 10.1016/s0079-6611(00)00034-3
Methot, R. D. Jr., and Wetzel, C. R. (2013). Stock synthesis: a biological and statistical framework for fish stock assessment and fishery management. Fish. Res. 142, 86–99. doi: 10.1016/j.fishres.2012.10.012
Moore, J. K., Fu, W., Primeau, F., Britten, G. L., Lindsay, K., Long, M., et al. (2018). Sustained climate warming drives declining marine biological productivity. Science 359, 1139–1143. doi: 10.1126/science.aao6379
National Marine Fisheries Service (2016). Ecosystem-Based Fisheries Managment Policy, May 23, 2016. (Silver Spring, MD: National Marine Fisheries Service).
National Oceanic and Atmospheric Administration (2017). ESRL : PSD : Visualize NOAA High-resolution Blended Analysis Data. (Silver Spring, MD: National Oceanic and Atmospheric Administration).
Neilson, J., Gabriele, C., and Taylor-Thomas, L. (2017). “Humpback Whale Calving and Juvenile Return Rates in Glacier Bay and Icy Strait,” in Ecosystem Status Report 2018: Gulf of Alaska, Stock Assessment and Fishery Evaluation Report, eds S. Zador and E. Yasumiishi (Anchorage, AK: North Pacific Fishery Management Council).
Nichol, D. G., Kotwicki, S., and Zimmermann, M. (2013). Diel vertical migration of adult Pacific cod Gadus macrocephalus in Alaska. J. Fish Biol. 83, 170–189. doi: 10.1111/jfb.12160
North Pacific Fishery Management Council (2019). Fishery Management Plan for the Gulf of Alaska. Anchorage, AK: North Pacific Fishery Management Council.
Palmer, C. T. (2003). “A decade of uncertainty and tenacity in northwest newfoundland,” in Retrenchment and Regeneration in Rural Newfoundland, ed. R. Byron (Toronto: University of Toronto Press), 43–64 doi: 10.3138/9781442679320-004.
Patrick, W. S., and Link, J. S. (2015). Myths that continue to impede progress in ecosystem-based fisheries management. Fisheries 40, 155–160. doi: 10.1080/03632415.2015.1024308
Paul, A. J., Paul, J. M., and Smith, R. L. (1988). Respiratory energy requirements of the cod Gadus macrocephalus Tilesius relative to body size, food intake, and temperature. J. Exp. Mar. Biol. Ecol. 122, 83–89. doi: 10.1016/0022-0981(88)90213-4
Pecl, G. T., Araújo, M. B., Bell, J. D., Blanchard, J., Bonebrake, T. C., Chen, I.-C., et al. (2017). Biodiversity redistribution under climate change: impacts on ecosystems and human well-being. Science 355:eaai9214. doi: 10.1126/science.aai9214
Piatt, J. F., Parrish, J. K., Renner, H. M., Schoen, S. K., Jones, T. T., Arimitsu, M. L., et al. (2020). Extreme mortality and reproductive failure of common murres resulting from the northeast Pacific marine heatwave of 2014–2016. PloS One 15:e0226087.
Pikitch, E. K., Santora, C., Babcock, E. A., Bakun, A., Bonfil, R., Conover, D. O., et al. (2004). Ecosystem-based fishery management. Science 305, 346–347. doi: 10.1126/science.1098222
Pinsky, M. L., and Byler, D. (2015). Fishing, fast growth and climate variability increase the risk of collapse. Proc. Biol. Sci. 282:20151053. doi: 10.1098/rspb.2015.1053
Pinsky, M. L., and Mantua, N. J. (2014). Emerging adaptation approaches for climate-ready fisheries management. Oceanography 27, 146–159. doi: 10.5670/oceanog.2014.93
Poloczanska, E. S., Brown, C. J., Sydeman, W. J., Kiessling, W., Schoeman, D. S., Moore, P. J., et al. (2013). Global imprint of climate change on marine life. Nat. Clim. Change 3, 919–925. doi: 10.1038/nclimate1958
Polovina, J. J. (1996). Decadal variation in the trans-Pacific migration of northern bluefin tuna (Thunnus thynnus) coherent with climate-induced change in prey abundance. Fish. Oceanogr. 5, 114–119. doi: 10.1111/j.1365-2419.1996.tb00110.x
Renner, H., Rojek, N., and Kettle, A. (2017). “Seabird monitoring summary for the Western Gulf of Alaska,” in Ecosystem Status Report 2018: Gulf of Alaska, Stock Assessment and Fishery Evaluation Report, eds S. Zador and E. Yasumiishi (Anchorage, AK: North Pacific Fishery Management Council).
Ressler, P. (2017). “Gulf of Alaska Euphausiid (“krill”) acoustic survey,” in Ecosystem Status Report 2017: Gulf of Alaska, Stock Assessment and Fishery Evaluation Report, eds S. Zador and E. Yasumiishi (Anchorage, AK: North Pacific Fishery Management Council).
RFI (English) (2009). French Fishing Boats Blockade Channel Ports. Available: http://www1.rfi.fr/actuen/articles/112/article_3482.asp (accessed March 1, 2019).
Rose, G. A. (2019). Atlantic Cod: A Bio-Ecology. Hoboken, NJ: John Wiley & Sons doi: 10.1002/9781119460701.
Rutecki, T. L., Rodgveller, C. J., and Lunsford, C. R. (2016). National Marine Fisheries Service Longline Survey Data Report and Survey History, 1990-2014. Silver Spring, MD: National Marine Fisheries Service. doi: 10.7289/V5/TM-AFSC-324
Saha, S., Moorthi, S., Pan, H.-L., Wu, X., Wang, J., Nadiga, S., et al. (2010). The NCEP climate forecast system reanalysis. Bull. Am. Meteorol. Soc. 91, 1015–1058. doi: 10.1175/2010BAMS3001.1
Schlegel, R. W., and Smit, A. J. (2018). heatwaveR: Detect Heatwaves and Cold-Spells. R package version 0.3. Available online at: https://CRAN.R-project.org/package=heatwaveR doi: 10.21105/joss.00821 (accessed June 10, 2018).
Sen, S., and Nielsen, J. R. (1996). Fisheries co-management: a comparative analysis. Mar. Policy 20, 405–418. doi: 10.1016/0308-597x(96)00028-0
Short, J., Foster, T., Falter, J., Kendrick, G. A., and McCulloch, M. T. (2015). Crustose coralline algal growth, calcification and mortality following a marine heatwave in Western Australia. Cont. Shelf Res. 106, 38–44. doi: 10.1016/j.csr.2015.07.003
Skern-Mauritzen, M., Ottersen, G., Handegard, N. O., Huse, G., Dingsør, G. E., Stenseth, N. C., et al. (2016). Ecosystem processes are rarely included in tactical fisheries management. Fish Fish. 17, 165–175. doi: 10.1111/faf.12111
Smale, D. A., Wernberg, T., Oliver, E. C. J., Thomsen, M., Harvey, B. P., Straub, S. C., et al. (2019). Marine heatwaves threaten global biodiversity and the provision of ecosystem services. Nat. Clim. Change 9, 306–312. doi: 10.1038/s41558-019-0412-1
Spalinger, K. (2017). Large-Mesh Bottom Trawl Survey of Crab and Groundfish: Kodiak, Chignik, South Peninsula, and Eastern Aleutian Management Districts, 2016.: Alaska Department of Fish and Game, Fishery Management Report No. 17-34. Anchorage, AK: Alaska Department of Fish and Game.
Spencer, P. D., Holsman, K. K., Zador, S., Bond, N. A., Mueter, F. J., Hollowed, A. B., et al. (2016). Modelling spatially dependent predation mortality of eastern Bering Sea walleye pollock, and its implications for stock dynamics under future climate scenarios. ICES J. Mar. Sci. 73, 1330–1342. doi: 10.1093/icesjms/fsw040
Spies, I., Aydin, K., Ianelli, J., and Palsson, W. (2017). “Assessment of the arrowtooth flounder,” in Stock Assessments and Fishery Evaluation Report for the Groundfish Resources of the Gulf of Alaska (Anchorage, AK: North Pacific Fishery Management Council).
Stram, D. L., and Evans, D. C. K. (2009). Fishery management responses to climate change in the North Pacific. ICES J. Mar. Sci. 66, 1633–1639. doi: 10.1093/icesjms/fsp138
Thompson, G. (2017). “Assessment of the Pacific cod stock in the Eastern Bering Sea,” in Stock Assessment and Fishery Evaluation Report for the Groundfish Resources of the Bering Sea/Aleutian Islands (Anchorage, AK: North Pacific Fishery Management Council).
Thomsen, M. S., Mondardini, L., Alestra, T., Gerrity, S., Tait, L., South, P., et al. (2019). Local extinction of bull kelp (Durvillaea spp.) due to a marine heatwave. Front. Mar. Sci. 6:84. doi: 10.3389/fmars.2019.00084
Von Szalay, P. G., and Raring, N. W. (2018). Data Report: 2017 Gulf of Alaska Bottom Trawl Survey. Washington, DC: U.S. DEPARTMENT OF COMMERCE.
Walsh, J. E., Thoman, R. L., Bhatt, U. S., Bieniek, P. A., Brettschneider, B., Brubaker, M., et al. (2018). The high latitude marine heat wave of 2016 and its impacts on Alaska. Bull. Am. Meteorol. Soc. 99, S39–S43. doi: 10.1175/BAMS-D-17-0105.1
Wilderbauer, T. K., and Nichol, D. G. (2016). “Assessment of the northern rock sole stock in the Bering Sea and Aleutian Islands,” in Stock Assessment and Fishery Evaluation Report for the Groundfish Resources of the Bering Sea/Aleutian Islands Regions (Anchorage, AK: North Pacific Fishery Management Council,), 1145–1228.
Wilderbuer, T. K., Hollowed, A. B., Ingraham, W. J. Jr., Spencer, P. D., Conners, M. E., Bond, N. A., et al. (2002). Flatfish recruitment response to decadal climatic variability and ocean conditions in the eastern Bering Sea. Prog. Oceanogr. 55, 235–247. doi: 10.1016/s0079-6611(02)00081-2
Yang, Q., Cokelet, E. D., Stabeno, P. J., Li, L., Hollowed, A. B., Palsson, W., et al. (2018). How “The Blob” affected groundfish distributions in the Gulf of Alaska. Fish. Oceanogr. 28, 434–453. doi: 10.1111/fog.12422
Zador, S., and Yasumiishi, E. M. (2016). Ecosystem Status Report 2016: Gulf of Alaska, Stock Assessment and Fishery Evaluation Report. Anchorage, AK: North Pacific Fishery Management Council.
Zador, S., and Yasumiishi, E. M. (2017). Ecosystem Status Report 2017: Gulf of Alaska, Stock Assessment and Fishery Evaluation Report. Anchorage, AK: North Pacific Fishery Management Council.
Zador, S., and Yasumiishi, E. M. (2018). Ecosystem Status Report 2018: Gulf of Alaska, Stock Assessment and Fishery Evaluation Report. Anchorage, AK: North Pacific Fishery Management Council.
Zador, S. G., Holsman, K. K., Aydin, K. Y., and Gaichas, S. K. (2017). Ecosystem considerations in Alaska: the value of qualitative assessments. ICES J. Mar. Sci. 74, 421–430. doi: 10.1093/icesjms/fsw144
Keywords: climate change, ecosystem-based fisheries management, fisheries, Gulf of Alaska, marine heatwave, Pacific cod
Citation: Barbeaux SJ, Holsman K and Zador S (2020) Marine Heatwave Stress Test of Ecosystem-Based Fisheries Management in the Gulf of Alaska Pacific Cod Fishery. Front. Mar. Sci. 7:703. doi: 10.3389/fmars.2020.00703
Received: 15 May 2020; Accepted: 31 July 2020;
Published: 20 August 2020.
Edited by:
Lyne Morissette, M – Expertise Marine, CanadaReviewed by:
Andrew M. Fischer, University of Tasmania, AustraliaChristian T. K.-H. Stadtlander, Independent Researcher, St. Paul Minnesota, United States
Copyright © 2020 Barbeaux, Holsman and Zador. This is an open-access article distributed under the terms of the Creative Commons Attribution License (CC BY). The use, distribution or reproduction in other forums is permitted, provided the original author(s) and the copyright owner(s) are credited and that the original publication in this journal is cited, in accordance with accepted academic practice. No use, distribution or reproduction is permitted which does not comply with these terms.
*Correspondence: Steven J. Barbeaux, Steve.Barbeaux@noaa.gov