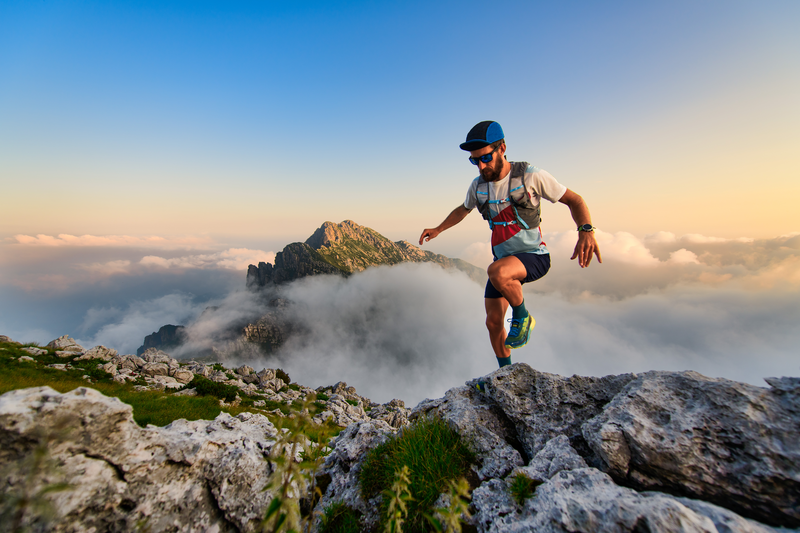
94% of researchers rate our articles as excellent or good
Learn more about the work of our research integrity team to safeguard the quality of each article we publish.
Find out more
ORIGINAL RESEARCH article
Front. Mar. Sci. , 07 August 2020
Sec. Marine Megafauna
Volume 7 - 2020 | https://doi.org/10.3389/fmars.2020.00624
Coexistence of ecologically similar species occupying the same geographic location (sympatry) poses questions regarding how their populations persist without leading to competitive exclusion. There is increasing evidence to show that micro-variations in habitat use may promote coexistence through minimizing direct competition for space and resources. We used two sympatric marine predators that show high fidelity to a small, remote coral atoll as a model to investigate how temporally dynamic partitioning of space use may promote coexistence. Using novel methods (difference network analysis and dynamic space occupancy analysis), we revealed that even though blacktip reef sharks Carcharhinus melanopterus and sicklefin lemon sharks Negaprion acutidens both show focused use of the same atoll habitats, the spatio-temporal dynamics of their use was partitioned such that they only shared the same microhabitats 26% of the time. Moreover, the degree of overlap was strongly influenced by the tidal cycle, peaking at ∼35% at higher tides as both species appear to target similar intertidal micro-habitats despite the increase in available space. Our work provides a rare example of how two marine predators with similar ecological roles and habitat preferences may coexist in the same place through dynamic segregation of habitat use in space and time, potentially reflecting adaptive behavioral traits for minimizing interactions. The strong influence of small tidal variation on species habitat use and partitioning also raises concerns over how atoll ecosystem dynamics may be influenced by sea level rises that could alter tidal dynamics.
Cyclical patterns in animal behavior are ubiquitous, the most notable being diel changes where the daily routines of most animals exhibit some change between day and night (Orsdol, 1984; Lewis and Taylor, 2009; Humphries et al., 2017). Depending on latitude, behavioral patterns can also change markedly with the seasons (Alerstam, 1990; Middleton et al., 2013; Lea et al., 2015). However, in coastal ecosystems another cycle that can significantly dictate the movements and behavior of animal species is the tidal cycle, which causes large areas of habitat to switch between terrestrial and marine with the ebb and flow of the tide (Pugh and Woodworth, 2014). For instance, there are numerous species of shorebird whose primary habitat is intertidal coastline, typically foraging on prey exposed on tidal flats as the water recedes (Recher and Recher, 1969; Burger et al., 1977) and consequently exhibit a temporally dynamic pattern of activity dictated by the tidal cycle. In contrast, many marine species exploit the shallow habitat only available when the tide is high, for example moving into complex mangrove root systems as refuge from predators (Wetherbee et al., 2007; Guttridge et al., 2011; Davy et al., 2015), or gaining access to otherwise restricted productive foraging grounds (Ackerman et al., 2000; Carlisle and Starr, 2010).
Such a productive interface between marine and terrestrial ecosystems often supports and attracts a diverse range of species (Ray, 1991). Where species are attracted to similar resources, whether it be for refuge space or prey, it may provoke competition for those resources (Zaret and Rand, 1971; Papastamatiou et al., 2006). Consequently, of interest are situations where ecologically similar species appear to coexist successfully, when by contrast it may be expected for one to exclude the other. Increasingly, however, it is becoming apparent that different species fulfilling similar ecological roles in the same habitat may segregate their use of this habitat temporally such that direct interactions are minimized (Vanak et al., 2013; Ratcliffe et al., 2014; Karanth et al., 2017; Papastamatiou et al., 2018; Heupel et al., 2019).
Understanding how sympatric species coexist in dynamic environments is important for a deeper understanding of their ecology and evolution (Papastamatiou et al., 2006, 2018; Speed et al., 2011; Humphries et al., 2016). In turn this helps identify habitat attributes that are critical for ensuring they can continue to support biodiversity, which is useful for prioritizing management initiatives, particularly for species vulnerable to commercial harvesting such as fishing (Lea et al., 2016). Here we present a case study of how novel movement analysis techniques can be used to elucidate the habitat use and partitioning of two sympatric reef shark species at a remote coral atoll in Seychelles (St Joseph). Both species, blacktip reef shark Carcharhinus melanopterus and sicklefin lemon shark Negaprion acutidens, have been demonstrated to show high fidelity to the atoll (Lea et al., 2016). In addition, both species share similar diets in Seychelles (Stevens, 1984), and it has been suggested that low growth rates of blacktip reef and sicklefin lemon sharks at St Joseph may relate to intra and interspecific competition for limited resources (Weideli et al., 2019). There could also be predator-prey dynamics between or within the two species, especially as larger lemon sharks may prey on other elasmobranchs (White and Potter, 2004; Guttridge et al., 2011).
Given the local topography at St Joseph, the tidal cycle may have a particularly strong influence on the habitat use and partitioning of these species. St Joseph Atoll has a large (∼15 km2), complex expanse of flats habitat surrounding the lagoon, access to which is strictly controlled by the tides (Stoddart et al., 1979). These areas are used by an abundance of marine life, including various reef sharks, rays, bonefish Albula spp., carangids (e.g., permit Trachinotus blochii), and green Chelonia mydas and hawksbill Eretmochelys imbricata turtles (von Brandis, 2011; Lea et al., 2016; Elston et al., 2017). As such the reef flats may provide productive foraging and refuge opportunities (Carlisle and Starr, 2010; Guttridge et al., 2011), but access would be governed entirely by the tides.
Consequently, with limited resources (e.g., space, prey, refuge from predators), alongside shared ecology, there is significant potential for interaction between the two species, perhaps resulting in temporally dynamic partitioning of microhabitats for coexistence. We used a combination of difference networks and space occupancy (termed grid occupancy) analysis to detect changes in habitat use of sharks over the course of the tidal cycle, and to determine how this affected the overlap between the two species. We reveal marked, fine scale temporal partitioning of space use between blacktip reef and sicklefin sharks in a coral atoll system, with overlap between the two species increasing with the rising tide despite more habitat becoming available.
The study was performed at the coral atoll of St Joseph in the Amirantes, Seychelles, in the western Indian Ocean (Figure 1). St Joseph Atoll is unusual (and unique in Seychelles) because its large, uninterrupted reef flat (0–2 m depth, ∼15 km2) is largely exposed at low tides, thereby isolating the lagoon (3–9 m depth, ∼5 km2) from the outer reef by preventing movement across the entire perimeter of flats for extended periods (Stoddart et al., 1979). Consequently, access to flats habitats and movement into or out of the lagoon to the outer reef is controlled entirely by the semidiurnal tidal phase (see Supplementary Figure S1 for example tidal heights over time). The tidal range is narrow (∼2 m), but is sufficient to create important refuge habitats for a variety of species and the atoll supports an abundance of marine predators, in particular sharks, rays and turtles (von Brandis, 2011; Filmalter et al., 2013; Lea et al., 2016; Elston et al., 2017). While the lagoon is predominantly sandy bottomed with scattered coral outcrops, the flats have large patches of seagrass Thallasodendron sp., with stretches of Pemphis sp. and mangroves Rhizophora mucronata fringing the islands (Stoddart et al., 1979; von Brandis, 2011).
Figure 1. Habitat map of St Joseph Atoll. Gray gridlines depict the 0.25 km2 grid cells used for occupancy analysis. Habitat data created by n + p biologists (www.nplusp.ch) using supervised image classification of 2 m 8 band MS 16-bit orthorectified WorldView-2 high-resolution satellite images from LAND INFO Worldwide Mapping, LLC (Denver, CO, United States) and is Copyright Save Our Seas Foundation. The map was produced using ArcGIS (ESRI Inc., CA, United States), with the bathymetry data in the inset obtained from the United States Department of Commerce, National Oceanic and Atmospheric Administration (NOAA): 2-minute Gridded Global Relief Data (ETOPO2v2).
Very high-resolution drone imagery (1 pixel = 15 cm) of the atoll at a 0.1 m low tide and a 1.4 m high tide is available for reference in the Supplementary Figures S2, S3), courtesy and Copyright of Drone Adventures for the Save Our Seas Foundation.
Between August 2012 and April 2019, a total of 52 blacktip reef shark Carcharhinus melanopterus and 44 sicklefin lemon shark Negaprion acutidens were tagged with acoustic transmitters (either V13 180 s nominal delay or V16 120 s nominal delay, Vemco Ltd., Bedford, NS, Canada). Sharks were tracked across the Amirantes using an array of 89 acoustic receivers (VR2W, Vemco Ltd). Briefly, static, submerged receivers record detections from tagged fish, resulting in a time series of locations that can be used to determine important characteristics such as habitat use, movement and site fidelity (see Supplementary Material for a full description of acoustic data processing techniques). Tags were detected within 165 ± 33 m (SD) of a receiver (Lea et al., 2016).
This array has grown to the current total of 89 over the course of the study period. As not all receiver locations have been monitored continuously, data analyses were restricted to the period of November 2014–November 2016 to ensure continuous coverage for the receivers active within this time window. As the focus of this work was to analyze the influence of the tidal cycle on habitat use in the atoll, only detections from receivers (n = 24) in St Joseph Atoll with uninterrupted detection records were analyzed. Receivers from which detections were analyzed were categorized as either being on the flats (n = 16, 0–2 m depth, depending on the tide) or in the lagoon (n = 8, 3–9 m depth). Receivers located on the flats had been placed in depressions to ensure they remained submerged even at the lowest astronomical tide. The restriction in the study time window and receivers provided an effective sample of 59 tagged individuals for these species during the study window (Table 1).
To determine how the pattern of acoustic detections varied across the tidal cycle, it was first necessary to obtain high resolution tidal information to match each shark detection to an absolute tidal height in meters. A pressure logger (HOBO Water Level Data Logger, Onset, Bourne, MA, United States) was used to measure water pressure in the lagoon over a 6-month period. This was calibrated using the known depth of the logger to produce a depth in meters. Tidal cycles were also modeled using the Oregon State University Tidal Model Driver (Egbert and Erofeeva, 2002) based on the harmonics for St Joseph Atoll’s location, outputting predicted tidal heights in meters every 10 min. On average, the tidal heights of the logger and model differed by only 0.1 ± 0.08 m (SD) (Supplementary Figure S1). Consequently, the tidal heights from the model were deemed similar enough to actual measured heights to be used to estimate a tidal height for every detection in the database, with each detection being assigned the tidal height closest to it, temporally.
The relative proportion of time each individual blacktip reef and sicklefin lemon shark spent in the lagoon versus the flats was calculated across the tidal cycle by binning the detections into 10 cm tidal height bins. Due to the uneven distribution of tidal heights across the range (0–2 m) of the tidal cycle (resulting from the sinusoidal tidal movements), it was necessary to correct the number of detections in each bin for bias prior to calculating the proportion of detections. This was achieved by first using tidal heights from the tidal model across the whole study period to calculate the real frequency (a) that each bin of tidal heights occurred at during that period (a = frequency of tidal bin χ/total frequency of all tidal bins). Then the proportion of the cycle that each tidal height bin would occupy if they were equally represented (b) was calculated (b = 1/20 = 0.05, as with 10 cm bins a 2 m tidal cycle contains 20 height bins). A correction factor was then calculated for each tidal height bin (=b/a), which was used to multiply the number of detections in each bin and normalize the representation of each bin in the cycle prior to calculating the proportions for each individual. For each tidal bin the relative proportion of detections in the lagoon was subtracted from the relative proportion of detections on the flats for each individual, producing an index whereby positive values indicated more time spent on the flats at that tidal height, with negative values denoting more time in the lagoon at that tidal height. The mean difference across individuals was plotted for each tidal bin, along with the standard error of the mean to indicate variation between individuals. Using each individual’s relative proportion of overall detections binned by tidal height interval, instead of absolute detection rates, made their track data directly comparable with others and suitable for calculating the mean proportion for each tidal bin, despite variation in tag type, detection rates and track length.
For both blacktip reef and sicklefin lemon sharks, differences in normalized detection frequency between the lagoon and flats for each tidal bin were tested using one-sample signed rank tests (SigmaPlot, Systat Software, San Jose, CA, United States), testing whether the difference in detection proportions between the lagoon and flats differed from 0 (no difference) for each tidal bin. As the significance was only compared directly between given tidal bins and the tests were not being used to derive an overall significance value, it was not deemed necessary to apply a Bonferroni correction. The threshold tidal height above which each species could more frequently access the atoll flats was taken to be the height at which the flats detection frequency was consistently, significantly greater than the lagoon detection frequency. Accordingly, tidal heights above this threshold were classified as “high” whereas those below the threshold were classified as “low.”
Network analysis was used to visualize movement patterns and compare space use between tidal states and species, with receivers being treated as nodes and pairs of subsequent detections between nodes treated as a connection (edge) between those nodes (Jacoby et al., 2012; Lea et al., 2016). The more detections at a receiver, the higher the node occupancy, while the more frequent a connection between two particular receivers, the stronger the connection. Due to the different ping frequencies of the V13 and V16 tags (180 s vs. 120 s nominal delays) there will on average be 1.5 V16 pings for every V13 ping. To account for this, the node and connection strengths of V13 networks were increased by 50% to account for the decreased probability of detection compared to the V16 networks [both tag types had the same transmission power (Lea et al., 2016)]. Due to contrasting detection rates and track lengths between individuals, all networks in the analyses were normalized into relative networks whereby node occupancy and connection strength were recalculated to be proportions of the overall network as opposed to absolute values. The weighted, relative networks allowed for direct comparison between relative networks, instead of the difficulty of comparing absolute networks given variation in tag type, detection rates and track length.
The tidal height threshold for greater flats use identified by the signed rank tests in the habitat switching analysis was used to construct high- vs. low-tide difference networks for each species, to determine how their habitat use changed with higher tides (with connections restricted to one hour to prevent connections between individual tidal cycles). To create a tidal difference network for a species, the association matrix and node values for the low tide network were subtracted from those for the high tide network, where positive node and connection values represent a high tide bias, whilst negative values indicate a low tide bias. Similarly, to create a difference network between species, the association matrix and node values for the blacktip reef shark network were subtracted from those for the sicklefin lemon shark network, where positive node and connection values represent a sicklefin lemon shark bias, whilst negative values indicate a blacktip reef shark bias. These species comparison networks were created for both high and low tidal states, as determined by the habitat switching analysis.
As an additional means of displaying the overall space use patterns for each species at the different tides, 50% kernel density probability plots were also determined. The method used to perform kernel density estimation used the same algorithm as ArcGIS (ESRI Inc., CA, United States), with a Quartic kernel density function (see Supplementary Material for more details). In order to account for potential bias from the uneven distribution of acoustic receivers and ping rates, each track was interpolated linearly across all gaps shorter than 3 h (longer gaps were ignored to limit erroneous interpolation between tidal phases).
A “grid” occupancy analysis was used to quantify the degree of hourly spatial overlap between the two species over the course of the tidal cycle. The study area was split into continuous grid cells of 0.25 km2 (to be conservatively greater than receiver range but still provide sufficient spatial resolution; Figure 1). A sensitivity analysis was performed using smaller grid cells to determine whether using the conservative cell size of 0.25 km2 affected any observed patterns (Supplementary Material). In order to account for potential bias from the uneven distribution of acoustic receivers and ping rates, each track was interpolated linearly across all gaps shorter than 3 h (longer gaps were ignored to limit erroneous interpolation between tidal phases). From these interpolated tracks, the presence/absence of blacktip reef and sicklefin lemon sharks within each cell was then calculated on an hourly basis. Calculating the proportion of grid cells that overlapped between the species on an hourly basis provided an hourly overlap coefficient between 0 and 1, where 0 indicated no overlap for that hour and 1 was complete overlap. To identify any significant periodicities in the overlap between the species, spectral analysis of the hourly overlap coefficients was performed using fast Fourier transformation (FFT). To identify how overlap between the two species changed over the course of the tide the hourly overlap coefficients were grouped into 10 cm tidal height bins and the mean overlap coefficient was plotted over the course of a tidal cycle. To determine whether overlap differed between the tidal threshold identified in the habitat switching analysis, a Mann Whitney U test was performed on the median overlap values of the two tidal states.
To identify whether observed patterns differed from random movements, the empirical movement data were compared to those of null model sharks that moved randomly through the network. Random networks were created according to methods established in Lea et al. (2016), whereby for a given track the first detection at the first receiver was kept, and then a swim distance was calculated based on the time between detections and an average swim speed for that individual calculated over the course of their track. Receivers were then selected at random until two were found within range of the swim distance. The closer of the two was then selected as the next receiver in the random track. If no receiver was found in range after 100 random selections then no move was deemed to occur and the current receiver was assigned (i.e., the animal was deemed not to have moved). This was repeated for the duration of the track, producing a random walk through the array with steps constrained by the observed detection intervals. To compare to the real sharks, each track was randomized and had the same overlap analyses performed, namely the FFT and plotting of mean overlap over the tidal cycle. Differences in overlap between real and random sharks were identified using a Mann Whitney U test on the median overlap values.
Over 362,000 detections across more than 23,000 tracking days were obtained from the 31 blacktip reef sharks and 28 sicklefin lemon sharks tracked between November 2014 and November 2016 (Table 1). Mean track duration for blacktip reef sharks was 392 ± 278 days (SD) and 391 ± 267 days (SD) for sicklefin lemon sharks, with both species displaying a bias toward females among tagged individuals. Tracked individuals of both species were primarily adults and sub-adults.
Allocation of detection proportion by tidal bins for both blacktip reef and sicklefin lemon sharks revealed that both species move into shallower flats habitat at higher tides, particularly over tidal heights of ∼1.4 m (Figure 2), as determined by the significance thresholds of the detection-proportion comparisons (Supplementary Table S1). The switch from lagoon to flats habitat at high tides was very clear for sicklefin lemon sharks, but the pattern for blacktip reef sharks was less clear, with some ability to exploit the flats sooner in the tidal cycle than sicklefin lemon sharks. As indicated by the moderate error bars in Figure 2 there was some variation between individuals, particularly for blacktip reef sharks, but the clear overall pattern was moving into flats habitats as tidal height increased.
Figure 2. Mean relative difference in the proportion of detections between the shallow flats and deep lagoon for blacktip reef shark Carcharhinus melanopterus and sicklefin lemon shark Negaprion acutidens across the tidal cycle. Calculated for each individual as the relative proportion of flats detections minus the relative proportion of lagoon detections, then averaged for each 10 cm tidal height bin. Positive values denote a bias toward flats usage, with negative a bias for deep lagoon usage – values around 0 suggest no difference. Error bars represent the standard error of the mean. Red asterisks by bars show where medians for that group significantly differed from 0 (no difference) as shown in Supplementary Table S1.
The tidal difference networks and kernel density plots were constructed using the thresholds for high versus low tide determined by when the difference in lagoon versus flats usage became significant for both species (≥1.4 m; Supplementary Table S1). Both blacktip reef and sicklefin lemon sharks use the shallow reef flats more at higher tides, particularly along the northern edge and in the eastern end of the atoll (Figures 3 and 4). The pattern was more pronounced for sicklefin lemon sharks, which appear to patrol the edge of the deep lagoon at lower tides, before using the flats almost exclusively at higher tides.
Figure 3. Relative 1-hour difference networks showing how space use differs between high (>1.4 m) and low (<1.4 m) tides for blacktip reef shark Carcharhinus melanopterus and sicklefin lemon shark Negaprion acutidens. Each difference has been calculated as the metrics of the high-tide network minus those for the low-tide network; red denotes more high tide use, blue more low tide use. The satellite image of St Joseph was acquired from LAND INFO Worldwide Mapping, LLC (Denver CO, United States), and includes material Copyright DigitalGlobe (Longmont, Colorado). The maps were produced using ArcGIS (ESRI Inc., CA, United States).
Figure 4. 50% kernel density plots with a 0.1 km cell size showing how space use differs between high (>1.4 m) and low (<1.4 m) tides for blacktip reef shark Carcharhinus melanopterus and sicklefin lemon shark Negaprion acutidens. Values closer to one represent prolonged occupancy. The satellite image of St Joseph at low tide was acquired from LAND INFO Worldwide Mapping, LLC (Denver, CO, United States), and includes material Copyright DigitalGlobe (Longmont, Colorado). The high-tide image of St Joseph was provided courtesy and Copyright of Drone Adventures for the Save Our Seas Foundation. The maps were produced using ArcGIS (ESRI Inc., CA, United States).
Blacktip reef and sicklefin lemons sharks displayed marked temporal partitioning in their space use of St Joseph Atoll, with low overall overlap [mean hourly overlap coefficient 0.26 ± 0.16 (SD)]. The species difference networks and kernel density plots reveal that this partitioning appears generally attributable to sicklefin lemons sharks being restricted to the deep lagoon at low tides, while blacktip reef sharks may still use certain deeper areas of the flats (Figures 4 and 5). In contrast, at high tides while both species move onto the flats (Figures 3 and 4), sicklefin lemon sharks largely abandon the deep lagoon and appear to dominate usage of the flats, with blacktip reef sharks bordering the flats and lagoon (Figures 4 and 5).
Figure 5. Relative 1-hour difference networks showing how space use differs between sicklefin lemon shark Negaprion acutidens and blacktip reef shark Carcharhinus melanopterus at low (<1.4 m) and high (>1.4 m) tidal states. Each difference has been calculated as the metrics of the sicklefin lemon shark network minus those for the blacktip reef shark network; red shows a stronger sicklefin lemon shark bias, blue shows a stronger blacktip reef shark bias. The low-tide satellite image of St Joseph was acquired from LAND INFO Worldwide Mapping, LLC (Denver CO, United States), and includes material Copyright DigitalGlobe (Longmont, Colorado). The high-tide image of St Joseph was provided courtesy and Copyright of Drone Adventures for the Save Our Seas Foundation. The maps were produced using ArcGIS (ESRI Inc., CA, United States).
Application of the FFT to the hourly overlap coefficients revealed a clear peak at 0.52 days (Figure 6), which matches the approximate 12 h 20 min duration of the tidal cycle. There is a smaller diel signal, but the tides were by far the dominant cycle in the overlap. Moreover, the tidal signal was negligible in random sharks, supporting the assertion that the tidal influence on overlap observed represents actual behavior patterns. The tidal signal was not entirely absent in random sharks, as expected, due to the randomization process incorporating certain attributes from the real shark tracks that were strongly influenced by tide.
Figure 6. Fast Fourier Transformation (FFT) analysis of peak frequency in overlap magnitude between blacktip reef sharks Carcharhinus melanopterus and sicklefin lemon sharks Negaprion acutidens, for real and random sharks.
Over the course of the tidal cycle, species overlap increased significantly at higher tides (Figure 7). During low tides (<1.4 m) the mean overlap was only 0.25 ± 0.16 (SD), whereas it increased to 0.32 ± 0.16 (SD) during high tides (significant difference according to Mann Whitney U test; U = 15094888.5, p < 0.001). The overlap changed little over the course of the tidal cycle for random sharks, with the overlap for real sharks at high tides being significantly greater than the overlap for random sharks at high tides [0.32 ± 0.16 (SD) vs. 0.24 ± 0.16 (SD), respectively, Mann Whitney U test; U = 2508605, p < 0.001].
Figure 7. Mean overlap coefficient between blacktip reef sharks Carcharhinus melanopterus and sicklefin lemon sharks Negaprion acutidens over the course of the tidal cycle for both real and random sharks.
Given that a conservative scale was used in the grid occupancy analysis to account for receiver range, it was repeated at a finer scale 250 × 250 m grid cell (0.0625 km2) as a scale sensitivity test (see Supplementary Figures S4, S5). Results were preserved at this finer scale, with the same pattern of overlap between the species and for random sharks over the tidal cycle. There was lower overall overlap [0.09 ± 0.14 (SD)], as expected with smaller grid cells, that increased at higher tides [0.149 ± 0.15 (SD)], but the absolute overlap values were inevitably lower due to the finer scale (Supplementary Figure S5). The FFT analysis at this scale also produced the same result, with a marked peak at 0.52 days for real but not random sharks (Supplementary Figure S4).
We have revealed marked, temporally dynamic segregation in habitat use between two sympatric species despite their high fidelity to a small, remote coral atoll. The degree of partitioning was strongly influenced by the tidal cycle, with higher tides driving increased overlap between blacktip reef and sicklefin lemon sharks despite the larger area of available intertidal habitat (∼20 km2, vs. ∼5 km2 at lower tides). Flexible partitioning of habitat use in time may represent an adaptive strategy for minimizing resource competition between the two species, especially if prey and refuge from predators are limited resources.
At St Joseph the tidal cycle dictates access to a considerable area of intertidal habitat: the flats of St Joseph (∼15 km2) are largely exposed at low tide but may provide productive foraging and refuge opportunities at higher tides. Although there is previous evidence of minor seasonal variation in the detection rates of sicklefin lemon and blacktip reef sharks in St Joseph, most still displayed perennial presence within the array, suggesting that any seasonal variation in detections may relate to changing environmental conditions and microvariations in habitat use [e.g., potential fine scale seasonal sexual segregation of blacktip reef sharks within the atoll (Lea, 2017)]. Given the most dramatic driver of habitat availability is the tidal cycle, this was the focus of the present study.
The use of difference networks greatly simplified the comparison of habitat use between tides and species, revealing that both blacktip reef and sicklefin lemon sharks exploit shallower habitats at higher tides, but that blacktip reef sharks can still exploit them to some extent at lower tides. Indeed, contrasting body size between the two species could be a major driver of which habitats are accessible to the tracked individuals and the subsequent patterns of partitioning: mean length of tracked blacktip reef sharks was 105 cm, compared to 177 cm for tracked sicklefin lemon sharks. The larger body size of sicklefin lemon sharks could mean that they are only physically able to access the lagoon flats at higher tides compared to the blacktip reef sharks. It is possible that the use of flats may have been underestimated as shallow water depth and complex topography may limit tag detection by receivers as the tide comes in, consequently the magnitude of the switch from deep lagoon to shallow flats habitats may be even greater than reported here. Although any impact should be the same for each tracked individual, additional range testing over the tidal cycle would help clarify whether this would need further consideration.
The observed tidal habitat switching is consistent with other studies showing that blacktip reef sharks move into tidal flats at high tides, and favor shallower edge habitats away from the deepest parts of the lagoon even at lower tides (Stevens, 1984; Papastamatiou et al., 2009b). Previous work on sicklefin lemon sharks at St Joseph also found that occupancy in the deep lagoon was higher at lower tides (Filmalter et al., 2013). In contrast to blacktip reef sharks, it was evident from the networks of the present study that sicklefin lemon sharks patrol the edge of the lagoon at lower tides, possibly in wait for sufficient water to access the flats. This highlights the importance of the flats habitats to the sicklefin lemon shark, as patrolling ensures they can be exploited as soon as they become available. This is consistent with sicklefin lemon shark catch data from Aldabra, where they were found to be most abundant along the lagoon perimeter (Stevens, 1984).
Movement into the flats by both species may reflect exploitation of temporally discrete foraging opportunities at St Joseph: various species of teleost, crustacean, mollusc and ray are abundant on the lagoon flats, and according to other studies all represent potential prey for blacktip reef and sicklefin lemon sharks that may be inaccessible at lower tides (Stevens, 1984; Salini et al., 1992; White and Potter, 2004; Papastamatiou et al., 2009a). Similarly, in other studies, both leopard sharks Triakis semifasciata (Carlisle and Starr, 2009, 2010) and a variety of bird species (Recher, 1969; Burger et al., 1977) have been shown to exploit the tidal cycle in estuarine habitats to target high prey abundance in intertidal mudflats.
In French Polynesia, juvenile blacktip reef and sicklefin lemon sharks display more similar trophic interactions when spatially segregated (Matich et al., 2017) – the strong, temporally dynamic partitioning of space use reported in the present work may be a mechanism that allows the two species to share dietary overlap with minimal direct interaction. Although dietary studies are required to address this further, if blacktip reef and sicklefin lemon sharks do exploit higher tides to target similar prey species, it may also explain the observed increase in overlap at higher tides (relative to lower tides and random sharks) despite the larger area of habitat available. Given the patchiness of available habitat on the flats [seagrass, sand, mangroves, Pemphis sp. (Figure 1)], although there is more available space, potential foraging patches and available refuges may not be evenly distributed but largely focused around the islands in the eastern end and northern edge of the atoll, drawing the two species to overlap more. Overlap at higher tides may also increase as sicklefin lemon sharks move through shallow habitats that blacktip reef sharks are already able to access at lower tidal heights, en route to the seemingly preferred habitats in the eastern end of the atoll, as indicated by Figures 4, 5.
Even so, their overall hourly overlap across the whole tidal cycle is still low in absolute terms (∼26%), and the tides above which overlap increases (∼1.4 m) only represent 14.5% of the study duration – essentially, although both inhabit the same small atoll habitat, the two species are rarely in the same place at the same time. The temporal partitioning may be even greater than suggested here, as indicated by the lower overlap in the scale sensitivity analysis, but the conclusions of the present work are restricted to the limitations of the passive acoustic telemetry used.
Another factor contributing to the observed overlap patterns could be the differing needs of blacktip reef and sicklefin lemon sharks to take refuge from predation risk. Mangroves at high tide have been suggested as important refuges from predation for both sharks and rays (White and Potter, 2004; Guttridge et al., 2011; Davy et al., 2015; George et al., 2019). For instance, in Australia juvenile blacktip reef sharks have been shown to track tidal movements so they remain in water shallower than 60 cm, which may be a predator avoidance strategy (George et al., 2019). It is possible that the smaller bodied blacktip reef sharks at St Joseph similarly use the Pemphis sp. and mangrove-fringed flats as a refuge from potential predators, which may include larger sicklefin lemon sharks given their diet can include other elasmobranchs (White et al., 2004). Blacktip reef sharks may be able to minimize overlap with these predators by using the flats even from relatively low tides (∼0.5 m) before they become accessible to the larger sharks (at tides ∼1.4 m). This may also help explain the higher overlap at higher tides, as larger sicklefin lemon sharks become able to access the refuges of smaller blacktip reef sharks (or possibly even both species refuging from larger shark species such as tiger sharks Galeocerdo cuvier, although the authors have yet to encounter any in the atoll).
It could be a combination of both foraging and refuging opportunities that drives use of the flats and changing overlap, as has been suggested for sea snakes Hydrophis elegans in western Australia, which only use a foraging area while the tide restricts shark access (Kerford et al., 2008). Being able to exploit the reef flats when sicklefin lemon sharks cannot gain access may also help promote habitat partitioning between the sicklefin lemon and blacktip reef sharks (Speed et al., 2011).
Such temporally dynamic partitioning of the same microhabitats by sympatric species has become recognized as behavior adopted to minimize resource competition between ecologically similar species (Wakefield et al., 2013; Papastamatiou et al., 2018). For instance, it has been suggested that several penguin species that all feed on krill Euphausea superba minimize competition through differential area and depth utilization (Wilson, 2010). Similarly, despite being sympatric and sharing prey, lions Panthera leo, leopards P. pardus, cheetah Acinonyx jubatus and African wild dogs Lycaon pictus appear to minimize competition through complex micro-segregation from partitioning their time of activity and fine-scale avoidance behaviors (Vanak et al., 2013). Although there is evidence of interference competition of this kind in provisioned sicklefin lemon sharks (Clua et al., 2010), it is ultimately difficult to conclusively determine the main drivers of observed habitat partitioning in large marine animals as it is logistically difficult to perform experimental manipulations (Papastamatiou et al., 2018). There is likely a complex web of interactions with other sympatric species (e.g., giant trevally Caranx ignobilis, great barracuda Sphyraena barracuda, and various ray species in St Joseph Atoll) that is beyond the scope of the present study. Such limitations can in part be addressed through theoretical modeling: for example it has been suggested from individual-based modeling that fine scale segregation between blacktip reef and gray reef sharks Carcharhinus amblyrhynchos at a Pacific atoll can only have arisen if there were habitat-specific competitive interactions between species (Papastamatiou et al., 2018).
A broader implication of such strong influence of small tidal fluctuations on species interactions that warrants further investigation is how such ecosystems may respond to forecast increases in sea level. As a result of the world’s changing climate it has been estimated that sea levels may rise by 30–180 cm by 2100 (Nicholls and Cazenave, 2010). If so, this could have significant and irreversible consequences for the stability of the atoll ecosystem (Woodroffe, 2008; Webb and Kench, 2010; Lovelock et al., 2015). There is risk the littoral hedge of mangroves and Pemphis sp. could be eroded or converted to open water, also removing the nutrient input from terrestrial habitats. Although there are many interacting factors that are difficult to predict, it is possible that potential refuges may be lost if they become accessible at all tides – carefully balanced competitive and predator-prey interactions could collapse, with unpredictable cascading effects on the marine community.
The present work has identified marked partitioning of space use in time between two sympatric shark species that is strongly governed by the tidal cycle. Despite their broad use of atoll habitats being very similar, at a fine temporal scale blacktip reef and sicklefin lemon sharks actually only overlapped ∼26% of the time, increasing to ∼35% as higher tides gave the larger sicklefin lemon sharks greater access to the flats habitats. This provides a rare example of how two large marine predators with similar habitat preferences and ecological roles may coexist in the same place through dynamic segregation of habitat use in time, potentially reflecting adaptive behavioral traits for minimizing interaction. Identifying habitats critical to particular species’ survival, such as those in which they take refuge from a competitor, could be particularly valuable for prioritizing habitat for protection in conservation strategies. The strong influence of only small changes in tidal height on distribution and interactions is of particular note, as it raises concerns about how these species and their associated communities may respond to sea level rises under a changing climate.
The datasets generated for this study are available on request to the corresponding author.
All fieldwork was approved by, and conducted with the knowledge of, the Ministry of Environment, Energy, and Climate Change, Seychelles. The animal handling and tagging methods were performed in accordance with the approved guidelines of the University of Plymouth, United Kingdom.
JL, CC, and DS conceived the study. JL, RD, RB, ElP, and EvP performed the field work. NH wrote the custom software for data analysis. JL, NH, and JB performed the data analysis and wrote the manuscript, with input from all authors.
We thank the Founder of the Save Our Seas Foundation for funding and providing all facilities for this work. Development of analysis tools was funded by the United Kingdom Natural Environment Research Council (to DS).
The authors declare that the research was conducted in the absence of any commercial or financial relationships that could be construed as a potential conflict of interest.
All the divers, ship crews, engineers, and volunteers at the D’Arros Research Centre provided invaluable assistance during the fieldwork and preparation. Stephen Cotterell was thanked for undertaking the tidal cycle modeling.
The Supplementary Material for this article can be found online at: https://www.frontiersin.org/articles/10.3389/fmars.2020.00624/full#supplementary-material
Ackerman, J., Kondratieff, M., and Matern, S. (2000). Tidal influence on spatial dynamics of leopard sharks, Triakis semifasciata, in Tomales Bay, California. Environ. Biol. Fishes 58, 33–43.
Alerstam, T. (1990). “Optimal bird migration: the relative importance of time, energy, and safety,” in Bird Migration, eds E. Gwinner (Berlin: Springer), 331–351. doi: 10.1007/978-3-642-74542-3_22
Burger, J., Howe, M. A., Hahn, D. C., and Chase, J. (1977). Effects of tide cycles on habitat selection and habitat partitioning by migrating shorebirds. Auk 94, 743–758. doi: 10.2307/4085271
Carlisle, A. B., and Starr, R. M. (2009). Habitat use, residency, and seasonal distribution of female leopard sharks Triakis semifasciata in Elkhorn Slough. California. Mar. Ecol. Prog. Ser. 380, 213–228. doi: 10.3354/meps07907
Carlisle, A. B., and Starr, R. M. (2010). Tidal movements of female leopard sharks (Triakis semifasciata) in Elkhorn Slough. California. Environ. Biol. Fishes 89, 31–45. doi: 10.1007/s10641-010-9667-0
Clua, E., Buray, N., Legendre, P., Mourier, J., and Planes, S. (2010). Behavioural response of sicklefin lemon sharks Negaprion acutidens to underwater feeding for ecotourism purposes. Mar. Ecol. Prog. Ser. 414, 257–266. doi: 10.3354/meps08746
Davy, L., Simpfendorfer, C., and Heupel, M. (2015). Movement patterns and habitat use of juvenile mangrove whiprays (Himantura granulata). Mar. Freshw. Res. 66, 481–492.
Egbert, G., and Erofeeva, S. (2002). Efficient inverse modeling of barotropic ocean tides. J. Atmospheric Ocean. Technol. 19, 183–204. doi: 10.1175/1520-0426(2002)019<0183:eimobo>2.0.co;2
Elston, C., von Brandis, R. G., and Cowley, P. D. (2017). Dietary composition and prey selectivity of juvenile porcupine rays Urogymnus asperrimus. J. Fish Biol. 91, 429–442. doi: 10.1111/jfb.13334
Filmalter, J. D., Dagorn, L., and Cowley, P. D. (2013). Spatial behaviour and site fidelity of the sicklefin lemon shark Negaprion acutidens in a remote Indian Ocean atoll. Mar. Biol. 160, 2425–2436. doi: 10.1007/s00227-013-2237-1
George, L., Martins, A., Heupel, M., and Simpfendorfer, C. (2019). Fine-scale movements of juvenile blacktip reef sharks Carcharhinus melanopterus in a shallow nearshore nursery. Mar. Ecol. Prorgress Ser. 623, 85–97. doi: 10.3354/meps13010
Guttridge, T. L., Gruber, S. H., Franks, B. R., Kessel, S. T., Gledhill, K. S., Uphill, J., et al. (2011). Deep danger: intra-specific predation risk influences habitat use and aggregation formation of juvenile lemon sharks Negaprion brevirostris. Mar. Ecol. Prog. Ser. 445, 279–291. doi: 10.3354/meps09423
Heupel, M., Munroe, S., Lédée, E., Chin, A., and Simpfendorfer, C. (2019). Interspecific interactions, movement patterns and habitat usein a diverse coastal shark assemblage. Mar. Biol. 166:68.
Humphries, N. E., Simpson, S. J., and Sims, D. W. (2017). Diel vertical migration and central place foraging in benthic predators. Mar. Ecol. Prog. Ser. 582, 163–180. doi: 10.3354/meps12324
Humphries, N. E., Simpson, S. J., Wearmouth, V. J., and Sims, D. W. (2016). Two’s company, three’s a crowd: fine-scale habitat partitioning by depth among sympatric species of marine mesopredator. Mar. Ecol. Prorgress Ser. 561, 173–187. doi: 10.3354/meps11937
Jacoby, D. M. P., Brooks, E. J., Croft, D. P., and Sims, D. W. (2012). Developing a deeper understanding of animal movements and spatial dynamics through novel application of network analyses. Methods Ecol. Evol. 3, 574–583. doi: 10.1111/j.2041-210X.2012.00187.x
Karanth, K., Srivathsa, A., Vasudev, D., Puri, M., Parameshwaran, R., and Kumar, N. (2017). Spatio-temporal interactions facilitate large carnivore sympatry across a resource gradient. Proc. R. Soc. B Biol. Sci. 284:20161860. doi: 10.1098/rspb.2016.1860
Kerford, M. R., Wirsing, A. J., Heithaus, M. R., and Dill, L. M. (2008). Danger on the rise: diurnal tidal state mediates an exchange of food for safety by the bar-bellied sea snake Hydrophis elegans. Mar. Ecol. Prog. Ser. 358, 289–294. doi: 10.3354/meps07346
Lea, J. S. E. (2017). Migratory Behaviour and Spatial Dynamics of Large Sharks and Their Conservation Implications. Doctoral dissertation, University of Plymouth, Plymouth.
Lea, J. S. E., Humphries, N., von Brandis, R., Clarke, C., and Sims, D. (2016). Acoustic telemetry and network analysis reveal space-use of multiple reef predators and enhance MPA design. Proc. R. Soc. Lond. B: Biol. Sci. 285:20160717. doi: 10.1098/rspb.2016.0717
Lea, J. S. E., Wetherbee, B. M., Queiroz, N., Burnie, N., Aming, C., Sousa, L. L., et al. (2015). Repeated, long-distance migrations by a philopatric predator targeting highly contrasting ecosystems. Sci. Rep. 5:11202.
Lewis, T., and Taylor, L. R. (2009). Diurnal periodicity of flight by insects. Trans. R. Entomol. Soc. Lond. 116, 393–435. doi: 10.1111/j.1365-2311.1965.tb02304.x
Lovelock, C., Cahoon, D., Freiss, D., Guntenspergen, G., Krauss, K., Reef, R., et al. (2015). The vulnerability of Indo-Pacific mangrove forests to sea-level rise. Nature 526, 559–563. doi: 10.1038/nature15538
Matich, P., Kiszka, J., Mourier, J., Planes, S., and Heithaus, M. (2017). Species co-occurrence affects the trophic interactions of two juvenile reef shark species in tropical lagoon nurseries in Moorea (French Polynesia). Mar. Environ. Res. 127, 84–91. doi: 10.1016/j.marenvres.2017.03.010
Middleton, A. D., Kauffman, M. J., McWhirter, D. E., Cook, J. G., Cook, R. C., Nelson, A. A., et al. (2013). Animal migration amid shifting patterns of phenology and predation: lessons from a Yellowstone elk herd. Ecology 94, 1245–1256. doi: 10.1890/11-2298.1
Nicholls, R. J., and Cazenave, A. (2010). Sea-level rise and its impact on coastal zones. Sci. 328, 1517–1520. doi: 10.1126/science.1185782
Orsdol, K. (1984). Foraging behaviour and hunting success of lions in Queen Elizabeth National Park. Uganda. Afr. J. Ecol. 22, 79–99. doi: 10.1111/j.1365-2028.1984.tb00682.x
Papastamatiou, Y., Wetherbee, B., Lowe, C., and Crow, G. (2006). Distribution and diet of four species of carcharhinid shark in the Hawaiian Islands: evidence for resource partitioning and competitive exclusion. Mar. Ecol. Prog. Ser. 320, 239–251. doi: 10.3354/meps320239
Papastamatiou, Y. P., Bodey, T. W., Friedlander, A. M., Lowe, C. G., Bradley, D., Weng, K., et al. (2018). Spatial separation without territoriality in shark communities. Oikos 127, 767–779. doi: 10.1111/oik.04289
Papastamatiou, Y. P., Caselle, J. E., Friedlander, A. M., and Lowe, C. G. (2009a). Distribution, size frequency, and sex ratios of blacktip reef sharks Carcharhinus melanopterus at Palmyra Atoll: a predator-dominated ecosystem. J. Fish Biol. 75, 647–654. doi: 10.1111/j.1095-8649.2009.02329.x
Papastamatiou, Y. P., Lowe, C. G., Caselle, J. E., and Friedlander, A. M. (2009b). Scale-dependent effects of habitat on movements and path structure of reef sharks at a predator-dominated atoll. Ecology 90, 996–1008. doi: 10.1890/08-0491.1
Pugh, D., and Woodworth, P. (2014). Sea-Level Science: Understanding Tides, Surges, Tsunamis and Mean Sea-Level Changes. Cambridge, MA: Cambridge University Press.
Ratcliffe, N., Crofts, S., Brown, R., Baylis, A., Adlard, S., Horswill, C., et al. (2014). Love thy neighbour or opposites attract? Patterns of spatial segregation and association among crested penguin populations during winter. J. Biogeogr. 41, 1183–1192. doi: 10.1111/jbi.12279
Recher, H. (1969). Bird species diversity and habitat diversity in Australia and North America. Am. Nat. 103, 75–80. doi: 10.1086/282583
Recher, H. F., and Recher, J. (1969). Comparative foraging efficiency of adult and immature little blue herons (Florida caerulea). Anim. Behav. 17, 320–322. doi: 10.1016/0003-3472(69)90017-7
Salini, J., Blaber, S., and Brewer, D. (1992). Diets of sharks from estuaries and adjacent waters of the north-eastern Gulf of Carpentaria. Australia. Mar. Freshw. Res. 43, 87–96.
Speed, C. W., Meekan, M. G., Field, I. C., McMahon, C. R., Stevens, J. D., McGregor, F., et al. (2011). Spatial and temporal movement patterns of a multi-species coastal reef shark aggregation. Mar. Ecol. Prog. Ser. 429, 261–275. doi: 10.3354/meps09080
Stevens, J. D. (1984). Life-history and ecology of sharks at Aldabra Atoll. Indian Ocean. Proc. R. Soc. Lond. B Biol. Sci. 222, 79–106. doi: 10.1098/rspb.1984.0050
Stoddart, D. R., Coe, M. J., and Fosberg, F. R. (1979). D’Arros and St. Joseph, Amirante Islands. Atoll Res. Bull. 223, 1–48. doi: 10.5479/si.00775630.223.1
Vanak, A., Fortin, D., Thaker, M., Ogden, M., Owen, C., Greatwood, S., et al. (2013). Moving to stay in place: behavioral mechanisms for coexistence of African large carnivores. Ecology 94, 2619–2631. doi: 10.1890/13-0217.1
von Brandis, R. (2011). The Foraging Ecology of Hawksbill Turtles at D’Arros Island, Republic of Seychelles. Doctorate Technologiae Thesis, Tshwane University of Technology, Pretoria West.
Wakefield, E., Bodey, T., Bearhop, S., Blackburn, J., Colhoun, K., Davies, R., et al. (2013). Space partitioning without territoriality in gannets. Science 341, 68–70. doi: 10.1126/science.1236077
Webb, A. P., and Kench, P. S. (2010). The dynamic response of reef islands to sea-level rise: evidence from multi-decadal analysis of island change in the Central Pacific. Glob. Planet. Chang. 72, 234–246. doi: 10.1016/j.gloplacha.2010.05.003
Weideli, O., Papastamatiou, Y., and Planes, S. (2019). Size frequency, dispersal distances and variable growth rates ofyoung sharks in a multi-species aggregation. J. Fish Biol. 94, 789–797. doi: 10.1111/jfb.13968
Wetherbee, B. M., Gruber, S. H., and Rosa, R. S. (2007). Movement patterns of juvenile lemon sharks Negaprion brevirostris within Atol das Rocas, Brazil: a nursery characterized by tidal extremes. Mar. Ecol. Prog. Ser. 343, 283–293. doi: 10.3354/meps06920
White, W. T., Platell, M. E., and Potter, I. C. (2004). Comparisons between the diets of four abundant species of elasmobranchs in a subtropical embayment: implications for resource partitioning. Mar. Biol. 144, 439–448. doi: 10.1007/s00227-003-1218-1
White, W. T., and Potter, I. C. (2004). Habitat partitioning among four elasmobranch species in nearshore, shallow waters of a subtropical embayment in Western Australia. Mar. Biol. 145, 1023–1032. doi: 10.1007/s00227-004-1386-7
Wilson, R. P. (2010). Resource partitioning and niche hyper-volume overlap in free-living Pygoscelid penguins: competition in sympatric penguins. Funct. Ecol. 24, 646–657. doi: 10.1111/j.1365-2435.2009.01654.x
Woodroffe, C. D. (2008). Reef-island topography and the vulnerability of atolls to sea-level rise. Glob. Planet. Chang. 62, 77–96. doi: 10.1016/j.gloplacha.2007.11.001
Keywords: movement, behavior, elasmobranch, telemetry, tide
Citation: Lea JSE, Humphries NE, Bortoluzzi J, Daly R, von Brandis RG, Patel E, Patel E, Clarke CR and Sims DW (2020) At the Turn of the Tide: Space Use and Habitat Partitioning in Two Sympatric Shark Species Is Driven by Tidal Phase. Front. Mar. Sci. 7:624. doi: 10.3389/fmars.2020.00624
Received: 27 November 2019; Accepted: 08 July 2020;
Published: 07 August 2020.
Edited by:
Lyne Morissette, M – Expertise Marine, CanadaReviewed by:
Yannis Peter Papastamatiou, Florida International University, United StatesCopyright © 2020 Lea, Humphries, Bortoluzzi, Daly, von Brandis, Patel, Patel, Clarke and Sims. This is an open-access article distributed under the terms of the Creative Commons Attribution License (CC BY). The use, distribution or reproduction in other forums is permitted, provided the original author(s) and the copyright owner(s) are credited and that the original publication in this journal is cited, in accordance with accepted academic practice. No use, distribution or reproduction is permitted which does not comply with these terms.
*Correspondence: James S. E. Lea, amwyMDg2QGNhbS5hYy51aw==
Disclaimer: All claims expressed in this article are solely those of the authors and do not necessarily represent those of their affiliated organizations, or those of the publisher, the editors and the reviewers. Any product that may be evaluated in this article or claim that may be made by its manufacturer is not guaranteed or endorsed by the publisher.
Research integrity at Frontiers
Learn more about the work of our research integrity team to safeguard the quality of each article we publish.