- Department of Genetics and Marine Biotechnology, Institute of Oceanology, Polish Academy of Sciences, Sopot, Poland
The aim of this article is to share with fish physiologists and behaviorists our thoughts and hints regarding the involvement of nonapeptides in various physiological and behavioral processes in fishes and the advantage of measurements of bioavailable molecules. Arginine vasotocin (AVT) and isotocin (IT) are biologically active nonapeptides in teleosts which are produced in separate neurosecretory neurons in the hypothalamic nuclei. They act as neurotransmitters and/or neuromodulators in the central nervous system (CNS) or as peripheral hormones, when they are released in the neurohypophysis and distributed via the bloodstream. Biosynthesis of bioactive AVT and IT is a complicated multistage process and nonapeptides have multiple sites of action. Different analytical approaches, including determination of pro-AVT and pro-IT mRNA in the hypothalamus or AVT and IT concentration in the brain, pituitary and plasma are used depending on the aim of the study. However, the level of biologically active molecules is more straightforwardly related to their current action in the CNS and/or peripheral organs compared with indirect proxies of nonapeptides production such as the expression of pro-peptides mRNA. Therefore analysis of AVT and IT is highly recommended in many studies. Herein we compiled various works in which bioavailable AVT and IT were analyzed in order to highlight the importance of such approach. Moreover, we compared the fluctuation of nonapeptide levels in the plasma with that in the brain of round gobies in successive reproductive phases. We also showed that neither AVT nor IT level was associated with fish age.
General Facts: AVT and IT as Neurotransmitters in CNS and Peripheral Hormones (On the Basis of Kulczykowska, 2007)
Arginine vasotocin (AVT) and isotocin (IT) are biologically active nonapeptides in teleosts. They are produced in separate neurosecretory neurons in the hypothalamic nuclei, with the preoptic and lateral tuberal nuclei as the most important. Both AVT and IT are produced as a part of larger precursor molecules. The pathway of nonapeptides biosynthesis starts with the transcription of AVT and IT precursor genes into mRNAs that are translated into pro-AVT and pro-IT precursor peptides. After various modifications, including proteolysis, the peptides are packed into secretory granules and transported by axon transport to the axon terminal where they are stored in the complex with a carrier protein, neurophysin. In response to appropriate stimuli, the complex dissociates and nonapeptides are released either into the bloodstream at the neurohypophysis, at the synaptic cleft, or into the extracellular space via axon varicosities to act through volume transmission in the brain. AVT and IT are neurotransmitters and/or neuromodulators in the CNS, but when they are distributed by the bloodstream they act as peripheral hormones. Physiological action of nonapeptides is determined by their binding to the specific receptors belonging to the superfamily of guanine nucleotide binding protein (G-protein)-coupled receptors. There are many different AVT and IT receptor subtypes in fishes and binding to the specific receptor determines nonapeptides’ action (Lema, 2010; Lema et al., 2015). Widespread projections of AVT and IT immune-reactive fibers to the ventral telencephalon, diencephalon and various mesencephalic structures in the brain, and the presence of AVT and IT binding sites in various brain regions confirm nonapeptides action in the CNS. AVT and IT receptor transcripts are also recognized in different organs such as pituitary, spleen, lateral line, ovary, bladder, intestine, liver, heart, gills, kidney and muscles. There is still an unanswered question whether nonapeptides in the urophysis are synthesized locally in the Dahlgren cells or originated from the brain (Gozdowska et al., 2013).
Although the nonapeptides have been chemically identified as early as in the 1960s (Acher et al., 1961, 1962), our knowledge about their physiological role is far from satisfactory. A complex multistage process of AVT and IT biosynthesis and diverse actions of nonapeptides at various target sites are factors that make research difficult. The steps of biosynthesis, namely gene transcription to mRNA, mRNA translation into the pre-pro-peptide, maturation of the pro-peptide in axon during transporting to the terminal, dissociation of the complex and release of bioactive nonapeptides, are separated in space and time. It should be noted that only nonapeptides (composed of nine amino acids), not pro-peptides, are biologically active at the receptor level. It is accepted that different analytical approaches, for instance determination of pro-AVT and pro-IT mRNA in the hypothalamus or AVT and IT concentration in the brain, pituitary and plasma are used depending on the aim of the study. However, the measurement of bioavailable AVT and IT is highly recommended in many studies, because the concentration of biologically active molecules is more straightforwardly related to the current actions of nonapeptides in the CNS and peripheral organs compared with indirect proxies of nonapeptides production such as the expression of pro-peptides mRNA. It should be stressed that measuring of nonapeptides in combination with other approaches depends not only on the research question but also on availability of analytical material and in many cases is not possible due to small sample size. Physiologists working on AVT and IT are also faced with challenges associated with simultaneous actions of nonapeptides (central, peripheral and merged) that frequently overlap in organism (for review: Balment et al., 2006). Therefore, studying of AVT and IT biology requires careful planning to avoid traps with data interpretation.
Herein we compiled studies in which bioavailable AVT and IT were analyzed in order to highlight the importance of such methodological approach. The bioactive forms can be measured by RIA and/or HPLC techniques but our experience has shown that HPLC is a more sensitive and direct method than RIA (for comparison: Balment et al., 1993; Warne et al., 1994; Kulczykowska, 1995b; Warne and Balment, 1995; Harding et al., 1997; Bond et al., 2002; Gozdowska and Kulczykowska, 2004). In addition, using HPLC assay we can work around producing specific antibodies for each species and we can measure both nonapeptides in one sample simultaneously. The availability of AVT/IT antibodies is generally limited in fishes, at least for a large number of laboratories working with various fish species. However, it should be noted that the analysis of AVT and IT, for instance in distinct brain areas is very difficult or sometimes even impossible to do due to the very small sample size and limited sensitivity of the assay, and therefore it is omitted in many studies. In the last section, we reported our recent data that can be useful for researcher during planning and carrying out the experiments. In Figure 1, we summarized AVT and IT activities that we referred to in this paper.
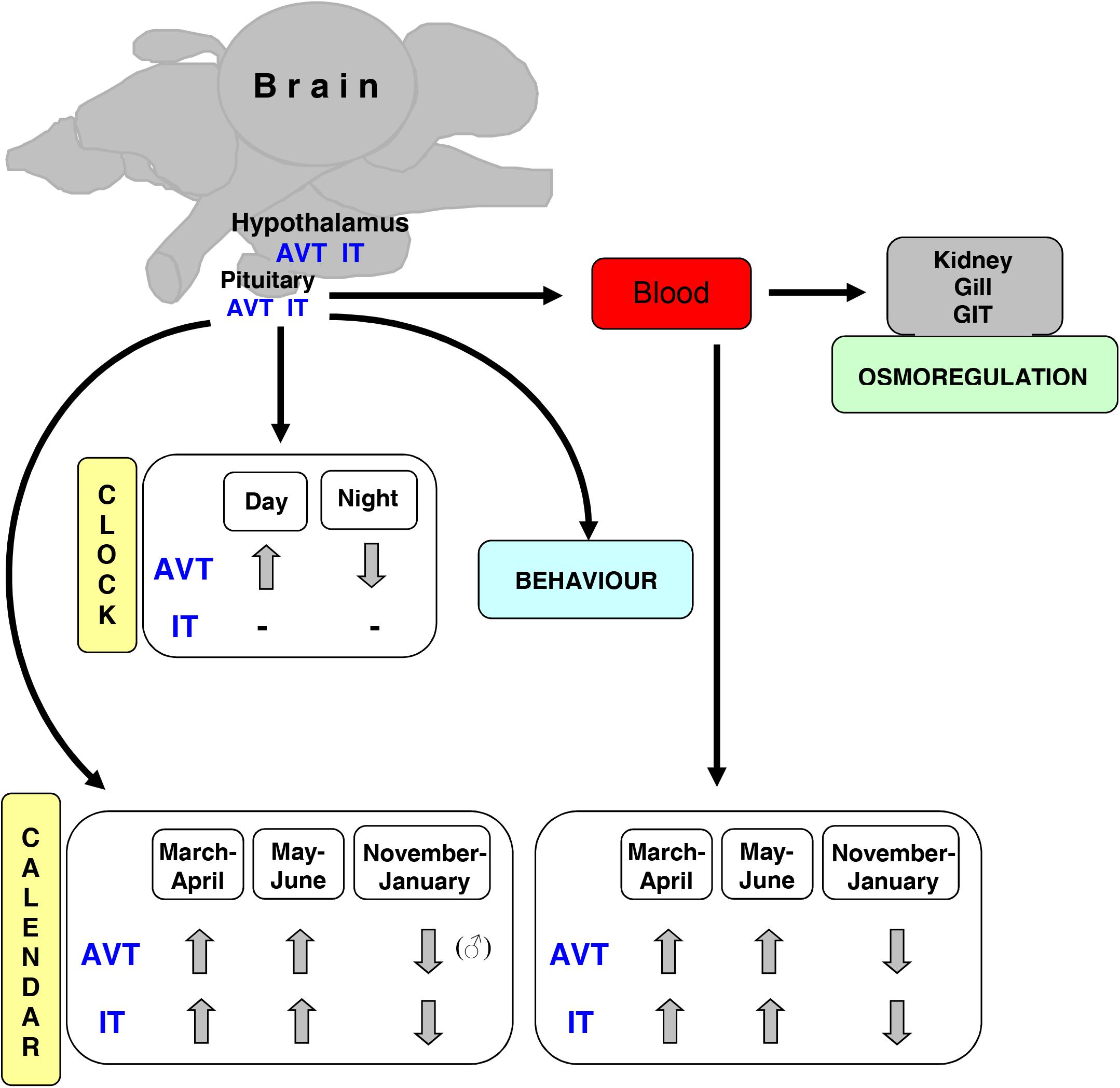
Figure 1. Scheme of AVT and IT activities in fish (AVT-arginine vasotocin, IT-isotocin, GIT-gastrointestinal tract).
Measurement of Bioavailable Nonapeptides: Why It Is Worth Doing?
Behavior, Reproduction and Timing
There are many evidences that nonapeptides play important roles in mediating social and reproductive behavior in fishes (for review: Godwin and Thompson, 2012). The first demonstration of AVT and IT as modulators of behavior connected with reproduction - the spawning reflex (spawning attitude of males and females preceding release of genital products) - was presented by Pickford and Strecker (1977) in Fundulus heteroclitus. Next, indirect measures of nonapeptide concentrations based on neuroanatomy or mRNA expression were used to examine the relationship between endogenous nonapeptide levels and behavior (for review: Godwin and Thompson, 2012). However, to date, a dozen of behavioral works have highlighted importance of measurement of bioavailable nonapeptides in fish brain (see References below). Indeed, in many studies, a relationship between AVT and IT levels (measured in various brain macro-areas or whole brain) and expression of specific behaviors was presented. For instance, in cleaner fish species (Labroides dimidiatus, L. bicolor, Labropsis australis, Labrichthys unilineatus), the assessment of cleaner-client mutualistic relationship was associated with brain AVT and IT levels independent on sex (Kulczykowska et al., 2015a; Soares et al., 2017; Abreu et al., 2018). In male three-spined sticklebacks (Gasterosteus aculeatus), providing parental care, brain AVT and IT were associated with the reproductive stage and engagement of individuals in paternal care, defense of territory and courtship (Kleszczyñska et al., 2012). On the other hand, in breeding female three-spined sticklebacks, brain AVT and IT levels depended on the presence of male and egg deposition, but were not linked with female aggressiveness (Kulczykowska and Kleszczyñska, 2014). In a cichlid fish Neolamprologus caudopunctatus, bi-parental caring species, brain AVT level was also related to the breeding phase as well as to nest maintenance and pair-bonding, and males and females AVT and IT levels differed (Cunha-Saraiva et al., 2019).
Furthermore, fluctuations in brain AVT and IT in reproductive phases were specific for males and females in sticklebacks and round gobies (Neogobius melanostomus) (Gozdowska et al., 2006; Sokołowska et al., 2015), and pointed to the involvement of nonapeptides in timing and synchronization of breeding. Indeed, it was postulated that AVT and IT together with the pineal hormone melatonin are components of the endocrine calendar in fishes (Kulczykowska, 1995a). Besides, diurnal changes in plasma AVT in the rainbow trout (Kulczykowska and Stolarski, 1996; Kulczykowska, 1999) and flounder (Kulczykowska et al., 2001) as well as diurnal rhythms of mRNA pro-VT levels in the hypothalamus and pituitary AVT content which was demonstrated in the rainbow trout (Rodríguez-Illamola et al., 2011) indicated the involvement of AVT in biological clock system.
Many behavioral studies showed a relationship between the pattern of brain AVT and/or IT levels and aggressive behavior linked with establishing and maintaining a social status hierarchy in the group of males, for instance in the three-spined stickleback (Kleszczyñska et al., 2012) and zebrafish (Teles et al., 2016). Also in social group of males in Mozambique tilapia Oreochromis mossambicus, AVT levels in different parts of the brain were related to dominance status of individuals (Almeida et al., 2012). In cichlid fish Neolamprologus pulcher, brain AVT level in whole brain was linked with dominance rank of individuals being sex-dependent in subordinate fish (Reddon et al., 2015). Consequently, nonapeptides were proposed as specific measures of level of aggression in studies of behavioral indicators of fish welfare (Martins et al., 2012).
The roles of nonapeptides in mediating social and reproductive behavior are almost always sex-dependent, what has been shown in the three-spined stickleback (Kleszczyñska et al., 2012; Kleszczyñska and Kulczykowska, 2013; Kulczykowska and Kleszczyñska, 2014), black molly (Poecilia sphenops) (Kulczykowska et al., 2015b), N. pulcher (Reddon et al., 2015) and N. caudopunctatus (Cunha-Saraiva et al., 2019). Therefore it is recommended to analyse AVT and IT levels in males and females separately.
Osmoregulation and Response to Stress
As far back, Carlson and Holmes (1962) demonstrated in the rainbow trout (Oncorhynchus mykiss) a significant decrease in the pituitary content of “antidiuretic material” indicating the acute release of this material into circulation in response to sea water transfer of fish (osmotic stress). They identified the material as “possible vasotocin.” In the same study, an increase of “possible vasotocin” in pituitary and hypothalamus of rainbow trout after handling suggested a simultaneous increase in both the synthesis (hypothalamus) and storage (pituitary) of this hormone. These early observations indicated that “possible vasotocin” can be involved in response to both osmotic and handling stress in fishes.
Many years later, in the 90s, a positive linear relationship between plasma AVT, osmolality and ions concentrations was observed in flounder (Platichthys flesus) (Balment et al., 1993; Warne and Balment, 1995). Then a transient elevation of AVT and IT levels was reported in plasma of the rainbow trout (Kulczykowska, 1997) and that of AVT level in pituitary and plasma of flounder (Harding et al., 1997) while fish were exposed to rapid osmotic challenge. Moreover, plasma AVT and IT levels followed the changes in plasma osmolality in the rainbow trout transferred between FW (freshwater) and BW (brackish water) (Kulczykowska, 1997). Also an increase in plasma AVT level corresponded with higher plasma osmolality and ion concentration in SW (sea water) gilthead sea bream (Sparus aurata) (Kleszczyñska et al., 2006). For a comprehensive description of AVT and IT involvement in osmoregulation, see Kulczykowska (2007). However, it was also established that AVT and IT are involved in response to many different stressful situations besides osmoregulation. For instance, the disturbance stress affected plasma AVT in the rainbow trout (Kulczykowska, 2001) and high density stress altered plasma AVT and IT in the gilthead sea bream (Mancera et al., 2008). Furthermore, brain AVT and IT responded to overcrowding stress as was shown in the three-spined stickleback (Kleszczyñska and Kulczykowska, 2013) and round goby (Sokołowska et al., 2013).
In more recent studies of vasotocinergic and isotocinergic systems performed after cloning of the nucleotide sequences for pro-AVT and pro-IT precursors in the gilthead sea bream (Martos-Sitcha et al., 2013), analysis of bioactive AVT and IT allowed authors to conclude about the complex function of the vasotocinergic and isotocinergic systems. Indeed, in many studies of this species, a relationship between cortisol and AVT/IT systems during simulated chronic stress (Cádiz et al., 2015), interactions between the AVT/IT systems, and two endocrine axes, i.e., hypothalamic-pituitary-interrenal (HPI) and hypothalamic-pituitary-thyroid (HPT) (Martos-Sitcha et al., 2019), as well as an inhibition of vasotocinergic pathways by the endocrine disruptor (Aroclor 1254) during transfer of fish to hyperosmotic environment (Skrzynska et al., 2019) were presented. Hence the research on the vasotocinergic and isotocinergic systems, in which bioavailable AVT and IT were measured in the plasma, pituitary and hypothalamus provided a new insight to our knowledge on complex action of both systems in fishes.
New Facts
A Relationship Between Plasma and Brain AVT/IT Levels in Round Gobies
In our previous paper, we demonstrated seasonal changes in brain AVT and IT which were linked with the phases of reproductive cycle in wild round goby males and females (Sokołowska et al., 2015). During this experiment we also measured AVT and IT in plasma of selected individuals. Herein we present for the first time the seasonal changes in plasma AVT and IT and compare the fluctuation of nonapeptide levels in the plasma with that in the brain in successive reproductive phases in order to check whether the endocrine signal originating in the brain can provide a “seasonal” message for peripheral organs. This dataset includes those individuals in which nonapeptides were measured in both plasma and brain (males: n = 53, females: n = 19).
Fish were caught in the Gulf of Gdañsk (southern Baltic Sea) and immediately transported to the laboratory and sampled. Blood samples (cardiac puncture) and brains were simultaneously collected between 10.00 and 12.00. Brains were analyzed according to Sokołowska et al. (2015). Plasma was separated by centrifugation (20,600 g, 10 min, 4°C) and stored at −80°C. Defrosted plasma samples were loaded onto equilibrated (2 ml methanol and 2 ml water) SPE columns (100 mg/3 ml; Strata-X; Phenomenex, United States). Columns were washed by water (2 × 600 μl) and 0.1% trifluoroacetic acid (TFA) in 5% acetonitryle (2 × 600 μl) and peptides were eluted using 80% acetonitrile (2 × 1,200 μl). Collected samples were dissolved and derivatized according to Sokołowska et al. (2015). Chromatographic separation was carried out on an Agilent ZORBAX Eclipse XDB-C18 column (150 mm × 4.6 mm, 5 μm). The mobile phase consisted of solvent A (0.1% TFA in water) and B [0.1% TFA in acetonitrile: water (3:1)]. A linear gradient was 45–80% of eluent B during 12 min. The detection limit was 0.34 (AVT) and 0.22 pmol/ml (IT). Intra-day repeatability expressed as relative standard deviation (RSD) was 6.9–8.0% (AVT) and 5.3–8.2% (IT), inter-day precision was 8.2–9.9% (AVT) and 5.5–8.5% (IT). An accuracy of the method was 89–93%.
Seasonal changes of AVT and IT in plasma and brain of the same individuals were presented in Figure 2. Although fish were sampled monthly throughout the year, the data on AVT and IT were grouped into six 2-month sets which reflected the successive phases of the reproductive cycle (confirmed by analysis of gonads); such grouping of data appeared to be necessary because of a small number of fish, especially females. We analyzed males and females separately, because AVT and IT levels were evidently sex-dependent. Statistically significant increase was found in plasma IT of males in pre-spawning and spawning phases and it reflected the highest brain IT level during spawning (Sokołowska et al., 2015). Thus IT seems to be a central and peripheral “signal for spawning” in male round gobies. In females, a number of fish in the groups was too small to conduct a reasonable statistical test or make any meaningful conclusion. But similar seasonal fluctuations of nonapeptide levels in the brain and plasma may suggest that plasma AVT and IT can act as peripheral synchronizing signals during breeding.
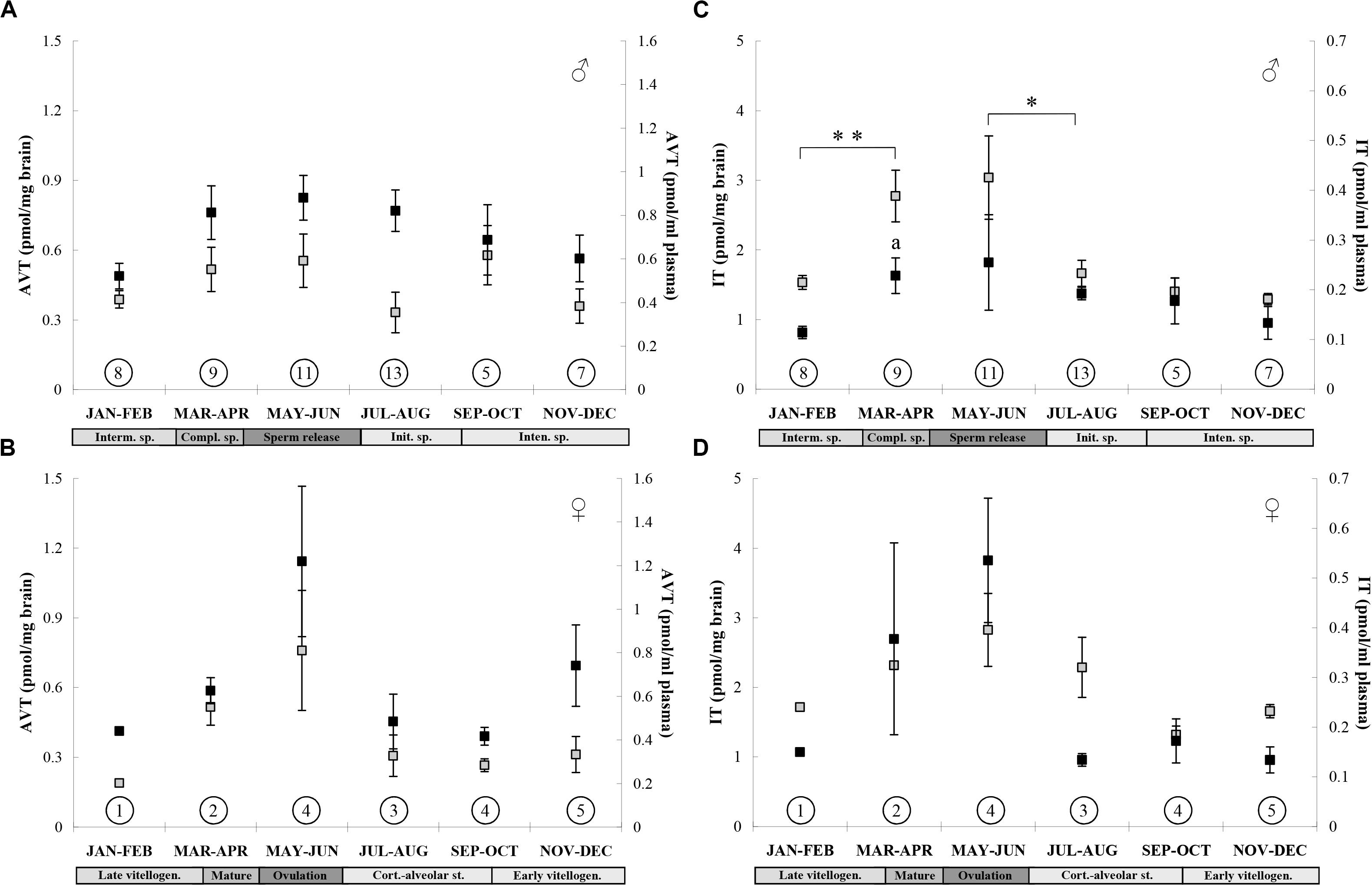
Figure 2. Seasonal changes in brain (black squares) and plasma (gray squares) AVT concentration in males (A) and females (B), and IT concentration in males (C) and females (D) of the round goby. Values are presented as means ± SEM. Number of sampled fish is given in the circles. Significant differences between brain values are indicated as a: P < 0.05 vs. Interm. sp. (JAN-FEB) and between plasma values are indicated as: *P < 0.05, **P < 0.01 (one-way ANOVA followed by Tukey’s post-hoc test). Reproductive phases are shown below months: males: Interm. sp., intermediate spermatogenesis; Compl. sp., completed spermatogenesis (pre-spawning phase), Sperm release (spawning phase); Init. sp., initial spermatogenesis; Intens. sp., intensive spermatogenesis; females: Late vitellogen., late vitellogenic stage (pre-spawning phase), Ovulation (spawning phase), Cort.-alveolar st., cortico-alveolar stage; Early vitellogen., early vitellogenic stage.
A Relationship Between Brain AVT/IT Levels and Age in Round Gobies
Overall, some hormone levels change with age but there are no comparable data on fish nonapeptides. Here we examined age classes to test whether observed changes were due to season and not age.
In individuals in which the age was previously determined by counting the number of growth zones in otoliths according to Sokołowska and Fey (2011), we also measured brain AVT and IT (Sokołowska et al., 2015) (males: n = 169, females: n = 94). The fish were between 2 and 5 years old and thus we set up four age-groups. Herein we compared brain nonapeptide levels in male and female age-groups, for each 2-month period separately, considering that brain AVT and IT levels are sex-dependent and linked with the phases of reproductive cycle (Sokołowska et al., 2015). In males, we found no statistically significant differences in brain nonapeptides linked to the age in any period. In females, a small number of fish (below five individuals in some cases) was too small to conduct any statistical test but AVT and IT values were similar across age groups. Data were presented in Figure 3.
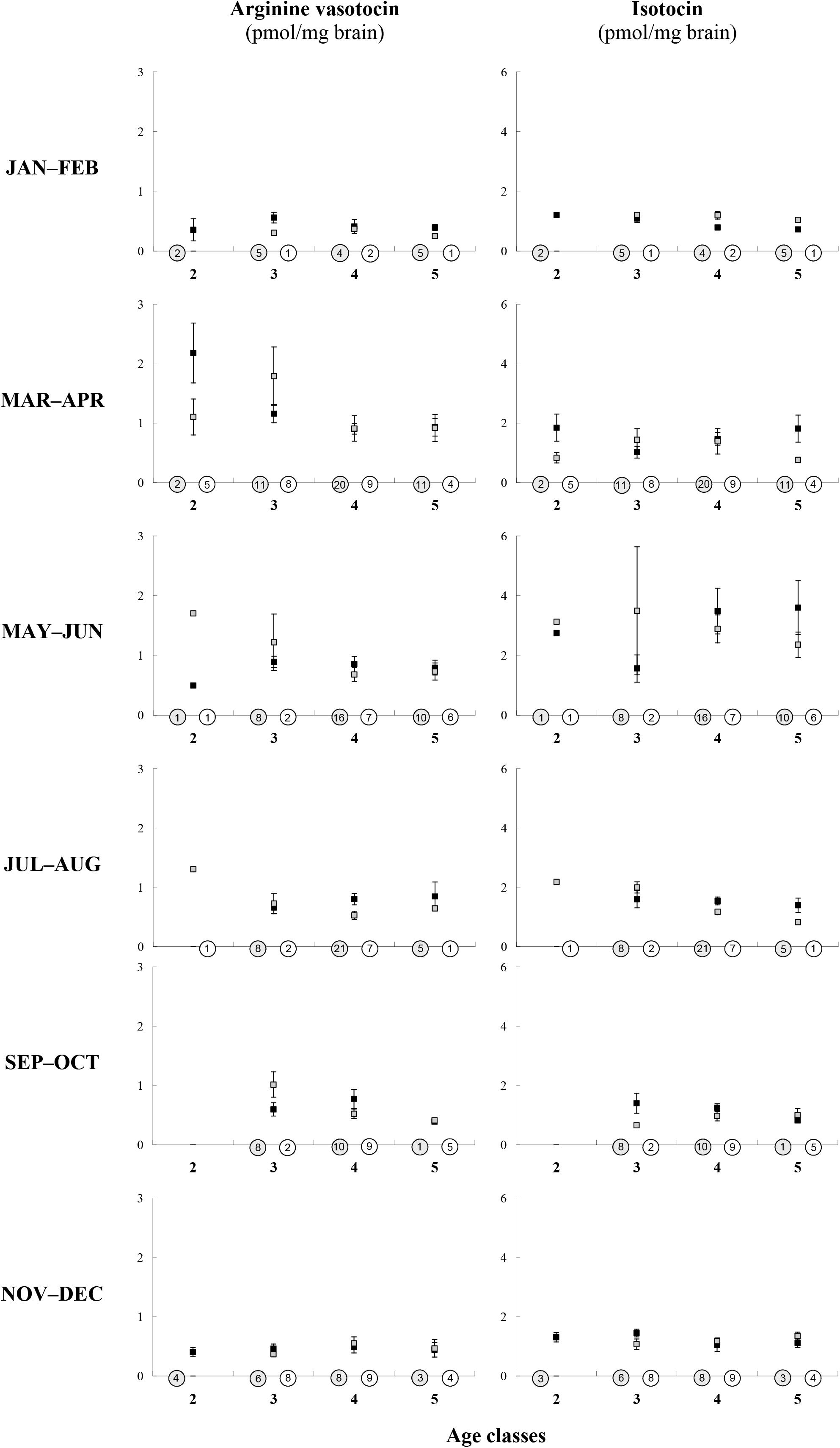
Figure 3. Age-related (x-axis: four age-classes) differences in AVT and IT brain concentration (y-axis) in males (black squares) and females (gray squares) of the round goby in various seasons. Number of fish is given in the circles (white, females; gray, males). Values are presented as means ± SEM. There are no statistically significant differences in brain AVT or IT across ages for either males or females (MANOVA, Tukey’s post-hoc test, P > 0.05).
Concluding Remarks
Studies of several fish species showing a relationship between AVT and IT levels measured in various brain macro-areas or whole brain and the expression of social and reproductive behavior provide new evidence for the involvement of nonapeptides in fish performance. The complex studies on the vasotocinergic and isotocinergic systems in the gilthead sea bream, with analyses of bioavailable AVT and IT in plasma, pituitary and hypothalamus, give new insight into mechanisms of nonapeptides action at a whole organism level. AVT and IT levels in the brain, and to some extent in plasma, appear to be related to the season and reproductive phase. It indicates that nonapeptides play a role in the system of endocrine calendar controlling timing of breeding and synchronizing peripheral organs activity – all that necessary for successful reproduction. AVT and IT levels are not linked with the age in round goby males, probably in females either.
There are helpful hints for researchers studying AVT and IT: (i) HPLC is a good alternative way of measuring nonapeptides in biological samples - unlike RIA, HPLC method allows researchers to work around producing specific antibodies (very difficult in case of IT) and analyze both peptides in one sample simultaneously, (ii) measurement of bioactive nonapeptides can complement other methodological approaches used in AVT/IT studies (e.g., distribution of AVT/IT pre-prohormone mRNA, measurement of transcripts abundance, including receptors’, pharmacological administration (iv or icv) of nonapeptides or receptors antagonists, etc.) and makes an important contribution to our understanding of complex action of the vasotocinergic and isotocinergic systems, (iii) combining of various methods is highly recommended if at all possible, (iv) AVT and IT should be analyzed in males and females separately, (v) the rhythmicity (daily and seasonal) of AVT/IT production and release should be taken into consideration during sampling, (vi) AVT and IT levels do not seem to be linked with the age of individuals, but given the limitation of existing studies to date, it would be advisable to do more research on AVT and IT changes with age.
Data Availability Statement
The datasets generated for this study are available on request to the corresponding author.
Ethics Statement
The animal study was reviewed and approved by the Ethics Committee for Animal Experimentation (University of Science and Technology, Bydgoszcz).
Author Contributions
ES conceived and designed the seasonal sampling and analysis, performed monthly sampling (brains, plasma, otholits), statistical and graphical data analysis. ES and MG performed SPE extractions for HPLC analysis. MG performed HPLC analysis. EK, ES, and MG wrote and edited the manuscript. All authors contributed to the article and approved the submitted version.
Funding
This study was funded by Institute of Oceanology of the Polish Academy of Sciences (statutory research task IV.2.).
Conflict of Interest
The authors declare that the research was conducted in the absence of any commercial or financial relationships that could be construed as a potential conflict of interest.
Acknowledgments
We would like to thank Marta Konieczna for her efficient help in SPE extractions of AVT and IT in plasma.
References
Abreu, M. S., Kulczykowska, E., Cardoso, S. C., André, G. I., Morais, M., Gozdowska, M., et al. (2018). Nonapeptide levels in male cleaner fish’ brains during interactions with unfamiliar intra and interspecific partners. Behav. Ecol. Sociobiol. 72:122.
Acher, R., Chauvet, J., Chauvet, M. T., and Crepy, D. (1961). Les hormones neurohypophysaires des poissons: isolement d’une vasotocine du tacaud (Gadus luscus L.). BBA 51, 419–420. doi: 10.1016/0006-3002(61)90198-6
Acher, R., Chauvet, J., Chauvet, M. T., and Crepy, D. (1962). Isolement d’une nouvelle hormone neurohypophysaire, l’isotocine presente chez les poissons osseux. BBA 58, 624–625. doi: 10.1016/0006-3002(62)90085-9
Almeida, O., Gozdowska, M., Kulczykowska, E., and Oliveira, R. F. (2012). Brain levels of arginine-vasotocin and isotocin in dominant and subordinate males of a cichlid fish. Horm. Behav. 61, 212–217. doi: 10.1016/j.yhbeh.2011.12.008
Balment, R. J., Lu, W., Weybourne, E., and Warne, J. M. (2006). Arginine vasotocin a key hormone in fish physiology and behaviour: a review with insights from mammalian models. Gen. Comp. Endocrinol. 147, 9–16. doi: 10.1016/j.ygcen.2005.12.022
Balment, R. J., Warne, J. M., Tierney, M., and Hazon, N. (1993). Arginine vasotocin and fish osmoregulation. Fish Physiol. Biochem. 11, 189–194. doi: 10.1007/bf00004566
Bond, H., Winter, M. J., Warne, J. M., McCrohan, C. R., and Balment, R. J. (2002). Plasma concentrations of arginine vasotocin and urotensin II are reduced following transfer of the euryhaline flounder (Platichthys flesus) from seawater to fresh water. Gen. Comp. Endocrinol. 125, 113–120. doi: 10.1006/gcen.2001.7736
Cádiz, L., Román-Padilla, J., Gozdowska, M., Kulczykowska, E., Martìnez-Rodrìguez, G., Mancera, J. M., et al. (2015). Cortisol modulates vasotocinergic and isotocinergic pathways in the gilthead sea bream. J. Exp. Biol. 218, 1–10.
Carlson, I. H., and Holmes, W. N. (1962). Changes in the hormone content of the hypothalamo-hypophysial system of the rainbow trou (Salmo gairdneri). J. Endocrinol. 24, 23–32. doi: 10.1677/joe.0.0240023
Cunha-Saraiva, F., Balshine, S., Gozdowska, M., Kulczykowska, E., Wagner, R. H., and Schaedelin, F. C. (2019). Parental care and neuropeptide dynamics in a cichlid fish Neolamprologus caudopunctatus. Horm. Behav. 116:104576. doi: 10.1016/j.yhbeh.2019.104576
Godwin, J., and Thompson, R. (2012). Nonapeptides and social behavior in fishes. Horm. Behav. 61, 230–238. doi: 10.1016/j.yhbeh.2011.12.016
Gozdowska, M., Kleszczyñska, A., Sokołowska, E., and Kulczykowska, E. (2006). Arginine vasotocin (AVT) and isotocin (IT) in fish brain: diurnal and seasonal variations. Comp. Biochem. Physiol. B 143, 330–334. doi: 10.1016/j.cbpb.2005.12.004
Gozdowska, M., and Kulczykowska, E. (2004). Determination of arginine-vasotocin and isotocin in fish plasma with solid-phase extraction and fluorescence derivatization followed by high-performance liquid chromatography. J. Chromatogr. B 807, 229–233. doi: 10.1016/j.jchromb.2004.04.014
Gozdowska, M., Ślebioda, M., and Kulczykowska, E. (2013). Neuropeptides isotocin and arginine vasotocin in urophysis of three fish species. Fish Physiol. Biochem. 39, 863–869. doi: 10.1007/s10695-012-9746-6
Harding, K. E., Warne, J. M., Hyodo, S., and Balment, R. J. (1997). Pituitary and plasma AVT content in the flounder (Platichthys flesus). Fish Physiol. Biochem. 17, 357–362.
Kleszczyñska, A., and Kulczykowska, E. (2013). Stocking density influences brain arginine vasotocin (AVT) and isotocin (IT) levels in males and females of three-spined stickleback (Gasterosteus aculeatus). Gen. Comp. Endocrinol. 183, 14–16. doi: 10.1016/j.ygcen.2012.11.024
Kleszczyñska, A., Sokołowska, E., and Kulczykowska, E. (2012). Variation in brain arginine vasotocin (AVT) and isotocin (IT) levels with reproductive stage and social status in males of three-spined stickleback (Gasterosteus aculeatus). Gen. Comp. Endocrinol. 175, 290–296. doi: 10.1016/j.ygcen.2011.11.022
Kleszczyñska, A., Vargas-Chacoff, L., Gozdowska, M., Kalamarz, H., Martìnez-Rodrìguez, G., Mancera, J. M., et al. (2006). Arginine vasotocin, isotocin and melatonin responses following acclimation of gilthead sea bream (Sparus aurata) to different environmental salinities. Comp. Biochem. Physiol. A 145, 268–273. doi: 10.1016/j.cbpa.2006.06.037
Kulczykowska, E. (1995a). Arginine vasotocin-melatonin interactions in fish: a hypothesis. Rev. Fish Biol. Fish. 5, 96–102. doi: 10.1007/bf01103367
Kulczykowska, E. (1995b). Solid-phase extraction of arginine vasotocin and isotocin in fish samples and subsequent gradient reversed-phase high-performance liquid chromatographic separation. J. Chromatogr. B 673, 289–293. doi: 10.1016/0378-4347(95)00276-3
Kulczykowska, E. (1997). Response of circulating arginine vasotocin and isotocin to rapid osmotic challenge in rainbow trout. Comp. Biochem. Physiol. A 118, 773–778. doi: 10.1016/s0300-9629(97)00033-9
Kulczykowska, E. (1999). Diel changes in plasma arginine vasotocin, isotocin, and melatonin in rainbow trout (Oncorhynchus mykiss). Fish Physiol. Biochem. 21, 141–146.
Kulczykowska, E. (2001). Responses of circulating arginine vasotocin, isotocin, and melatonin to osmotic and disturbance stress in rainbow trout (Oncorhynchus mykiss). Fish Physiol. Biochem. 24, 201–206.
Kulczykowska, E. (2007). “Arginine vasotocin and isotocin: towards their role in fish osmoregulation,” in Fish Osmoregulation, eds B. Baldisserotto, J. M. Mancera Romero, and B. G. Kapoor (Enfield, NH: Science Publishers), 151–176. doi: 10.1201/9780429063909-6
Kulczykowska, E., Cardoso, S. C., Gozdowska, M., André, G. I., Paula, J. R., Ślebioda, M., et al. (2015a). Brain levels of nonapeptides in four labrid fish species with different levels of mutualistic behaviour. Gen. Comp. Endocrinol. 222, 99–105. doi: 10.1016/j.ygcen.2015.06.005
Kulczykowska, E., Kalamarz-Kubiak, H., Nietrzeba, M., and Gozdowska, M. (2015b). Brain nonapeptide and gonadal steroid responses to deprivation of heterosexual contact in the black molly. Biol. Open 4, 69–78. doi: 10.1242/bio.20149597
Kulczykowska, E., and Kleszczyñska, A. (2014). Brain arginine vasotocin and isotocin in breeding female three-spined sticklebacks (Gasterosteus aculeatus): the presence of male and egg deposition. Gen. Comp. Endocrinol. 204, 8–12. doi: 10.1016/j.ygcen.2014.04.039
Kulczykowska, E., and Stolarski, J. (1996). Diurnal changes in plasma arginine vasotocin and isotocin in rainbow trout adapted to fresh water and brackish Baltic water. Gen. Comp. Endocrinol. 104, 197–202. doi: 10.1006/gcen.1996.0162
Kulczykowska, E., Warne, J. M., and Balment, R. J. (2001). Day-night variations in plasma melatonin and arginine vasotocin concentrations in chronically cannulated flounder (Platichthys flesus). Comp. Biochem. Physiol. A 130, 827–834. doi: 10.1016/s1095-6433(01)00444-5
Lema, S. C. (2010). Identification of multiple vasotocin receptor cDNAs in teleost fish: sequences, phylogenetic analysis, sites of expression, and regulation in the hypothalamus and gill in response to hyperosmotic. (challenge). Mol. Cell. Endocrinol. 321, 215–230. doi: 10.1016/j.mce.2010.02.015
Lema, S. C., Sanders, K. E., and Walti, K. A. (2015). Arginine vasotocin, isotocin and nonapeptide receptor gene expression link to social status and aggression in sex-dependent patterns. J. Neuroendocrinol. 27, 142–157. doi: 10.1111/jne.12239
Mancera, J. M., Vargas-Chacoff, L., Garcìa-López, A., Kleszczyñska, A., Kalamarz, H., Martìnez-Rodrìguez, G., et al. (2008). High density and food-deprivation affect arginine vasotocin, isotocin and melatonin in gilthead sea bream (Sparus auratus). Comp. Biochem. Physiol. A 149, 92–97. doi: 10.1016/j.cbpa.2007.10.016
Martins, C. I. M., Galhardo, L., Noble, C., Damsgard, B., Spedicato, M. T., Zupa, W., et al. (2012). Behavioural indicators of welfare in farmed fish. Fish Physiol. Biochem. 38, 17–41. doi: 10.1007/978-94-007-5383-9_3
Martos-Sitcha, J. A., Cádiz, L., Gozdowska, M., Kulczykowska, E., Martìnez-Rodrìguez, G., and Mancera, J. M. (2019). Arginine vasotocin and cortisol co-regulate vasotocinergic, isotocinergic, stress, and thyroid pathways in the gilthead sea bream (Sparus aurata). Front. Physiol. 10:261. doi: 10.3389/fphys.2019.00261
Martos-Sitcha, J. A., Wunderink, Y. S., Gozdowska, M., Kulczykowska, E., Mancera, J. M., and Martìnez-Rodrìguez, G. (2013). Vasotocinergic and isotocinergic systems in the gilthead sea bream (Sparus aurata): an osmoregulatory story. Comp. Biochem. Physiol. A 166, 571–581. doi: 10.1016/j.cbpa.2013.09.001
Pickford, G. E., and Strecker, E. L. (1977). The spawning reflex response of the killifish, Fundulus heteroclitus: isotocin is relatively inactive in comparison with arginine vasotocin. Gen. Comp. Endocrinol. 32, 132–137. doi: 10.1016/0016-6480(77)90143-5
Reddon, A. R., O’Connor, C. M., Marsh-Rollo, S. E., Balshine, S., Gozdowska, M., and Kulczykowska, E. (2015). Brain nonapeptide levels are related to social status and affiliative behaviour in a cooperatively breeding cichlid fish. R. Soc. Open Sci. 2:140072. doi: 10.1098/rsos.140072
Rodríguez-Illamola, A., López Patiño, M. A., Soengas, J. L., Ceinos, R. M., and Míguez, J. M. (2011). Diurnal rhythms in hypothalamic/pituitary AVT synthesis and secretion in rainbow trout: evidence for a circadian regulation. Gen. Comp. Endocrinol. 170, 541–549. doi: 10.1016/j.ygcen.2010.11.013
Skrzynska, A. K., Martìnez-Rodrìguez, G., Gozdowska, M., Kulczykowska, E., Mancera, J. M., and Martos-Sitcha, J. A. (2019). Aroclor 1254 inhibits vasotocinergic pathways related to osmoregulatory and stress functions in the gilthead sea bream (Sparus aurata. Linnaeus 1758). Aquat. Toxicol. 212, 98–109. doi: 10.1016/j.aquatox.2019.04.019
Soares, M. C., Cardoso, S. C., Mazzei, R., Andre, G. I., Morais, M., Gozdowska, M., et al. (2017). Region specific changes in nonapeptide levels during client fish interactions with allopatric and sympatric cleaner fish. PLoS One 12:e0180290. doi: 10.1371/journal.pone.0180290
Sokołowska, E., and Fey, D. P. (2011). Age and growth of the round goby Neogobius melanostomus in the Gulf of Gdañsk several years after invasion. Is the Baltic Sea a new Promised Land? J. Fish Biol. 78, 1993–2009.
Sokołowska, E., Kleszczyñska, A., Kalamarz-Kubiak, H., Arciszewski, B., and Kulczykowska, E. (2013). Changes in brain arginine vasotocin, isotocin, plasma 11-ketotestosterone and cortisol in round goby, Neogobius melanostomus, males subjected to overcrowding stress during the breeding season. Comp. Biochem. Physiol. A 165, 237–242. doi: 10.1016/j.cbpa.2013.03.018
Sokołowska, E., Kleszczyñska, A., Nietrzeba, M., and Kulczykowska, E. (2015). Annual changes in brain concentration of arginine vasotocin and isotocin correspond with phases of reproductive cycle in round goby, Neogobius melanostomus. Chronobiol. Int. 32, 917–924. doi: 10.3109/07420528.2015.1052142
Teles, M. C., Gozdowska, M., Kalamarz-Kubiak, H., Kulczykowska, E., and Oliveira, R. F. (2016). Agonistic interactions elicit rapid changes in brain nonapeptide levels in zebrafish. Horm. Behav. 84, 57–63. doi: 10.1016/j.yhbeh.2016.05.020
Warne, J. M., and Balment, R. J. (1995). Effect of acute manipulation of blood volume and osmolality on plasma [AVT] in seawater flounder. Am. J. Physiol. 269, R1107–R1112.
Keywords: arginine vasotocin, behavior, fish, isotocin, osmoregulation, rhythm, season, stress
Citation: Sokołowska E, Gozdowska M and Kulczykowska E (2020) Nonapeptides Arginine Vasotocin and Isotocin in Fishes: Advantage of Bioactive Molecules Measurement. Front. Mar. Sci. 7:610. doi: 10.3389/fmars.2020.00610
Received: 10 March 2020; Accepted: 02 July 2020;
Published: 17 July 2020.
Edited by:
Leonardo Julián Magnoni, University of Porto, PortugalReviewed by:
Jesús M. Míguez, University of Vigo, SpainKaren P. Maruska, Louisiana State University, United States
Copyright © 2020 Sokołowska, Gozdowska and Kulczykowska. This is an open-access article distributed under the terms of the Creative Commons Attribution License (CC BY). The use, distribution or reproduction in other forums is permitted, provided the original author(s) and the copyright owner(s) are credited and that the original publication in this journal is cited, in accordance with accepted academic practice. No use, distribution or reproduction is permitted which does not comply with these terms.
*Correspondence: Ewa Kulczykowska, ZWt1bGN6eWtvd3NrYUBpb3Bhbi5nZGEucGw=
†These authors have contributed equally to this work