- 1Faculty of Science, Evolution and Ecology Research Centre, School of Biological, Earth and Environmental Sciences, University of New South Wales, Sydney, NSW, Australia
- 2Department of Earth and Environmental Sciences, Macquarie University, Sydney, NSW, Australia
In studies of habitat-forming species, those that are not spatially dominant are often considered “non-primary” habitat and may be overlooked. This is despite the fact that minority habitat formers can provide critical complexity, food, and other services that underpin ecosystem biodiversity. Octocorals and anemones are found in marine and estuarine habitats across all climate zones. Despite their potentially important ecological roles, to date there have been few studies of their specific threats and stressors or attempts at their restoration. Here we review studies of the ecology of octocorals and anemones with a focus on threats and restoration. We identify many threats including habitat damage, collection and trade, disease, predation, pollution, and the most wide-spread – climate change. While evidence suggests that some octocorals and anemone populations may be more resilient to disturbances than stony corals because they often recruit and grow quickly, resilience is not guaranteed. Instead, resilience or susceptibility within this large group is likely to be site and species specific. We find that the loss of octocorals and anemones has been difficult to quantify as most species have no hard structures that remain following a mortality event. Only through long-term monitoring efforts have researchers been able to document change in these populations. Due to the increasing extent and severity of human impacts in marine ecosystems, restoration of habitat forming species is becoming increasingly necessary after disturbance events. To illustrate the challenges ahead for octocoral and anemone restoration, we present two examples of ongoing restoration efforts assessed against the International Standards for the Practice of Ecological Restoration. Restoration planning and implementation progress are documented for the Mediterranean red coral Corallium rubrum and the temperate Australian cauliflower soft coral, Dendronephthya australis. This review and the detailed case studies demonstrate that while some octocorals and anemones can provide resilient habitat within reef systems, a greater research focus on their ecology, threats, and restoration potential is urgently required.
Introduction
In many ecosystems, studies of habitat-forming species often focus on those believed to support the greatest mobile species diversity, such as seagrass in shallow coastal water and kelp in temperate rocky reefs (Duffy, 2006; Nagelkerken et al., 2008; Teagle et al., 2017). This can lead to an unresolved understanding of the role of non-primary (or spatially sub-dominant) habitat formers and an underestimation of the ecosystem services they provide (Coleman and Wernberg, 2017). In seagrass ecosystems, mixed macroalgae-seagrass stands have significantly higher vegetative surface area than seagrass alone, leading to a proportional increase in epifaunal densities (Stoner and Lewis, 1985; Parker et al., 2001). In addition, the loss of non-primary habitat formers can negatively impact mobile species, such as in Florida Bay seagrass systems, where the loss of sponges resulted in declines of juvenile commercially important lobster species that required human intervention (Butler Iv, 1995). In temperate rocky reefs, fucoid algaes are often overlooked in favor of kelps, but fucoids support unique and abundant assemblages of macroinvertebrates (Coleman and Wernberg, 2017). In tropical coral reef ecosystems, it is often assumed that octocorals and anemones are not the primary habitat used by the majority of mobile species and as such they have tended to be overlooked relative to their stony counterparts (Norström et al., 2009; Ferrari, 2017; Epstein and Kingsford, 2019). In deeper marine ecosystems, aposymbiotic octocorals can be more prominent but these systems are less well-studied (Sánchez et al., 1998; Cerrano et al., 2009; Wareham, 2009; Baillon et al., 2012). When studied in detail, octocorals and anemones are revealed as structurally complex animals that are used as habitat, food sources, and foraging substrate by a variety of mobile fauna across the world (Fautin and Allen, 1997; Heifetz, 2002; Epstein and Kingsford, 2019; Schweitzer and Stevens, 2019).
Octocorals and anemones have a world-wide distribution in marine and estuarine habitats, with species inhabiting all climate zones and habitat types (Verselveldt and Alderslade, 1982; Dinesen, 1983; Fautin, 1992; Sánchez et al., 1998; Heifetz, 2002; Fautin et al., 2013). Reefs in the same climatic zones can also have very different patterns of octocoral distribution depending on location. For example, in tropical climates, Great Barrier Reef (GBR) octocorals are much more abundant on reef slopes than on shallow reef flats, while in the Caribbean octocorals are abundant in both shallow reefs and deep, wave exposed reef terraces (Dinesen, 1983; Sánchez et al., 1998). In the GBR, octocorals can also comprise up to 45% of coral cover on individual reefs, while in the Caribbean, octocoral abundance and density can be even higher (Sánchez et al., 1998; Australian Institute of Marine Science [AIMS], 2018; Epstein and Kingsford, 2019). Octocorals are also widely distributed in depth range, and are found in deep water reefs from temperate to arctic waters (Verselveldt and Alderslade, 1982; Heifetz, 2002). Since octocorals and anemones have varying degrees of structural complexity, they can provide important structural habitat for many species in all climate zones (Figures 1b–e; Heifetz, 2002; Poulos et al., 2013; Ferrari, 2017; Richardson et al., 2017).
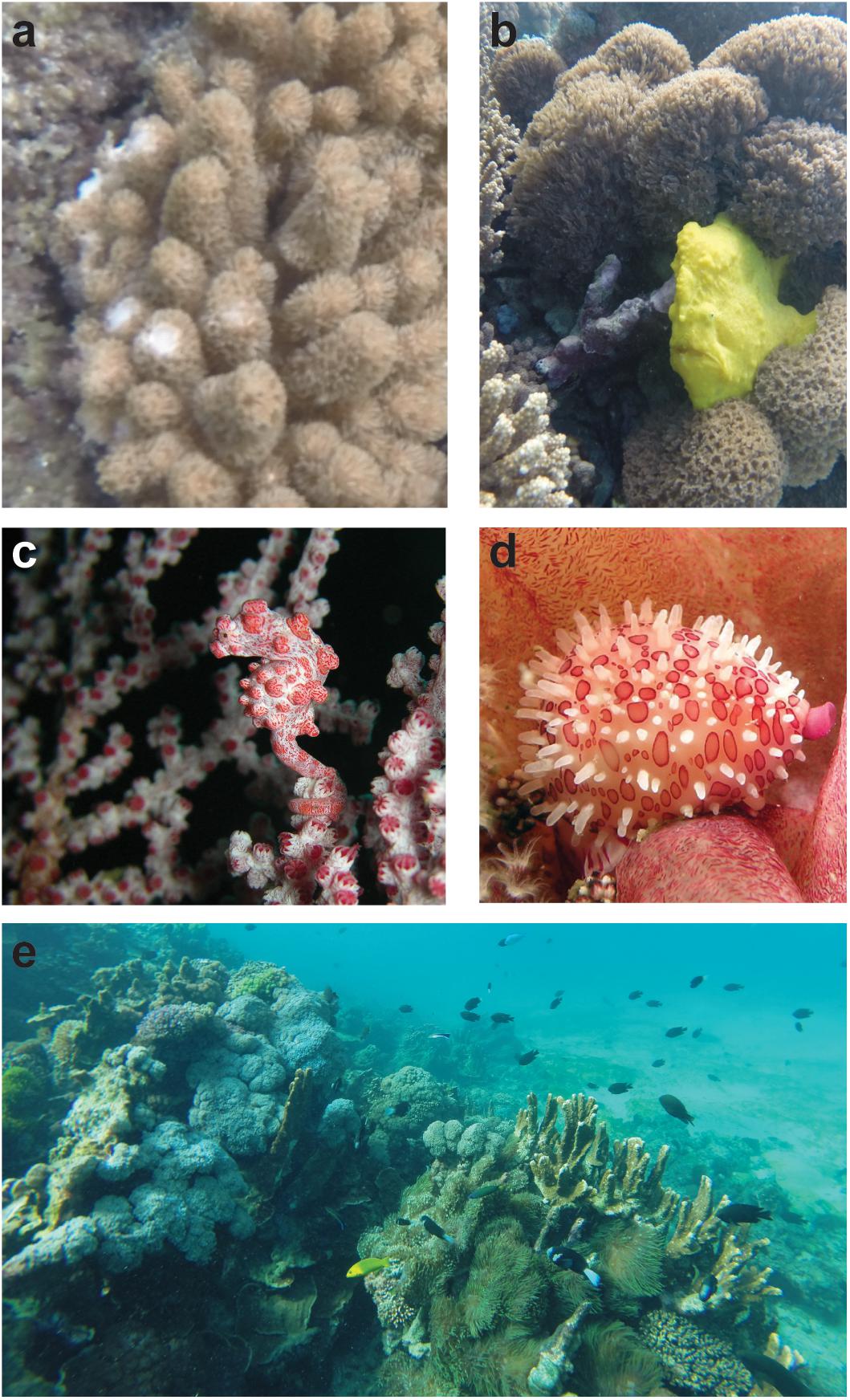
Figure 1. Use of soft corals and anemones as food and habitat. (a) Bite marks in Aldersladum sp. soft coral, (b) use of Anthelia sp. as habitat by frogfish, (c) obligate symbiont Hippocampus bargabanti in its gorgonian host, (d) corallivorous cowrie in Dendronephthya australis, and (e) use of mixed stony coral, soft coral, and anemone habitat by anemonefishes, damselfishes, wrasses, and other fish species. Photo credits – (a,b,e) Rosemary K. Steinberg, Lord Howe Island. (c) Steve Childs, https://www.flickr.com/photos/steve_childs/2643195469, d) John Turnbull, MarineExplorer.org.
Octocorals and anemones are benthic Cnidarians that share a wide variety of physical traits, most prominently that neither form solid skeletal structures, and both have strong defense mechanisms. Octocorals and anemones are soft-bodied, although octocoral tissues do contain calcified spicules and/or axes that increase tissue stiffness (Koehl, 1982; Fabricius and Alderslade, 2001; Sethmann and Wörheide, 2008; Fabricius, 2011). Octocorals primarily rely on secondary metabolites for defense, rendering them unpalatable and sometimes toxic, while anemones employ painful stings from venomous nematocysts (Changyun et al., 2008; Frazão et al., 2012). Octocorals are primarily colonial anthozoans that possess polyps with eight tentacles, often fringed with pinnules (Fabricius and Alderslade, 2001; Fabricius, 2011). Examples include photosynthetic Xeniid octocorals, also known as pompom corals, which are commonly found in tropical and subtropical shallow reefs (Figures 1b,e), and non-photosynthetic Dendronephthya sp. octocorals, also known as carnation corals, which are usually found in deep water from temperate to tropical zones and come in a wide variety of colors (Figures 1c,d; Fabricius and Alderslade, 2001). Anemones are generally solitary, though they can also form colonies, with some species employing both life histories (Francis, 1979). The most well-known group of anemones are the host anemones, which associate with anemonefish. The bubble-tip anemone Entacamaea quadricolor is particularly popular both as a tourist attraction and in home aquaria (Figure 1e; Jones et al., 2008; Scott et al., 2011; Thomas et al., 2015). In addition, octocorals and anemones have similar ecological roles as soft benthic habitat formers.
Across the world, octocorals and anemones provide shelter and food for a wide variety of mobile species, including endangered species and habitat specialists (Figures 1a–e). For example, gorgonians are famous for hosting habitat-specialist pygmy seahorses amongst other less charismatic fauna (Figure 1c; Lourie and Randall, 2003; Cerrano et al., 2009; Harasti, 2016; Epstein and Kingsford, 2019). In temperate waters, octocorals are critical habitat for the endangered White’s Seahorse and host epibenthic food sources such as amphipods for other protected sygnathids (Harasti et al., 2014; Harasti, 2016; Corry et al., 2018). In the subarctic, sea pens and other octocorals provide nursery habitat for larvae of commercially important fisheries species (Baillon et al., 2012). In tropical and subtropical systems, anemones can form aggregations that house large anemonefish populations – North Solitary Island and the surrounding region have the highest density of anemones in the world, leading to very high anemonefish densities (Richardson et al., 1997; Scott et al., 2011). Additionally, anemones support other species of macro-symbionts including Dascyllus sp. damselfishes, anemone shrimps, and anemone crabs and are food sources for other fish species, such as butterflyfishes (Ates, 1989; Fautin et al., 1995; Valdivia and Stotz, 2006). Though examples of specific mobile fauna species utilizing octocoral and anemone habitat have been recorded, few general studies exist. Recently Epstein and Kingsford (2019) reported for the first time that fish species richness in Orpheus and Pelorus Islands on the central GBR increases with octocoral – but not stony coral – cover. Similarly, in temperate waters off the eastern United States fish abundance was significantly positively correlated with sea whip coral cover, but not to any other benthic habitat group (Schweitzer and Stevens, 2019).
Hard and soft benthic cnidarians are under increasing threat from both natural and human-induced disturbances, including climate change, disease, anchor and boat damage, and commercial collection (Loya et al., 2001; Precht et al., 2001; Santangelo and Abbiati, 2001; Goldberg and Wilkinson, 2004; Garrabou et al., 2006; Bruno et al., 2007; Jones et al., 2008; Poulos et al., 2013; Thomas et al., 2015; Erni Cassola et al., 2016). Many threats facing octocorals and anemones are similar to those facing stony corals, although some responses are unique (e.g., Loya et al., 2001). Additionally, both octo- and stony corals are commercially harvested for the commercial aquarium trade, but some species of octocoral are also harvested for jewelry – a threat unique to octocorals and black corals (Grigg, 2001; Santangelo and Abbiati, 2001). Conversely, some destructive stony coral predators such as the Crown of Thorns seastar, whose populations are understood to benefit from agricultural runoff, rarely ingest octocorals, creating possible opportunities for octocoral population expansion (Endean and Stablum, 1971; Fabricius, 1997; Kayal et al., 2012).
Although vulnerable to anthropogenic threats (Loya et al., 2001; Santangelo and Bramanti, 2010; Harasti, 2016), there is also evidence that octocorals and anemones possess traits that may increase their ability to recover naturally and make them amendable for restoration projects. Octocorals and anemones generally do not form calcium carbonate skeletons, and therefore do not build long term reef structures. As such, soft-bodied habitat-forming organisms can often recruit and grow faster than hard-bodied ones. For example, on the GBR the octocoral Sinularia flexiblis and the fast-growing stony coral Acropora hyacinthus grow at estimated average rates of 128 ± 7 cm2y–1 and 41.22 ± 9.5 cm2y–1, respectively, following bleaching-induced population declines (Bastidas et al., 2004; Linares et al., 2011). The soft coral Efflatounaria sp. uses clonal reproduction to reach pre-disturbance abundance (but not percent cover) within 109 days of a disturbance event (Karlson et al., 1996). Additionally, in the Florida Keys, recruitment dynamics vary significantly between stony and octocorals after temperature disturbance. In recruitment surveys only three species of opportunistic or hardy stony coral were recorded, while a wide range of octocoral genera recruited (Bartlett et al., 2018). Though octocorals generally recover quickly after disturbance events, recovery times vary. For example, rocky-wall octocoral communities in the Tropical Eastern Pacific are expected to take 21–34 years to recover their full suite of octocoral species after disturbances (Gomez et al., 2018). In total, on tropical reefs octocoral communities can reach pre-disturbance densities in 10–15 years, whereas the full suite of stony corals can take up to 100 years (Rinkevich, 2005). It should be noted that stony coral can recover to pre-disturbance cover much more quickly, though community composition may not be the same (e.g., Robinson et al., 2019). After disturbances that deplete stony coral populations and cover, a phase-shift to octocoral-dominated reefs can occur, quickly increasing species and habitat diversity (Norström et al., 2009). Octocorals and anemones are also adept at colonizing novel habitats and can quickly increase both structural and species diversity in an area (Brace and Quicke, 1986; Perkol-Finkel and Benayahu, 2005; Perkol-Finkel et al., 2005; Hiscock et al., 2010). This has resulted in some species becoming particularly successful invaders (Concepcion et al., 2010; Hancock et al., 2017). Anemones grow relatively slowly, but can reproduce asexually, leading to relatively rapid local population expansion (Brace and Quicke, 1986; Veale and Lavery, 2012; Scott et al., 2014; Scott, 2017). Though the lack of skeletal structure likely facilitates rapid recovery, it also means that their initial loss can be difficult to quantify because octocorals and anemones leave no obvious traces of population declines or extirpations. Understanding soft coral ecology likely requires a finer temporal scale of sampling than necessary for habitat formers that leave a skeleton or shell. Due to certain inherent biological characteristics such as rapid growth and reproduction, benthic soft-bodied cnidarians may become an increasingly dominant component of future reefs and a focus on their biology, ecology, and restoration is dearly warranted.
In this review, we apply the international and regional frameworks provided by the Society for Ecological Restoration (SER) to assess current knowledge of octocoral restoration (McDonald et al., 2016a, b). As the first necessary step, we identify previous and/or ongoing damage and threats to octocorals and anemones and identify critical knowledge gaps. Second, we examine the information needed to successfully restore octocorals and anemones and link current knowledge gaps to potential novel restoration approaches. These goals will be different for each target species and may or may not involve returning the restoration site to pre-disturbance diversity and abundance, depending on the type and severity of the initial disturbance (McDonald et al., 2016a). Finally, we present two case studies of ongoing restoration efforts to highlight the difficulties in setting specific restoration targets and identifying successful replanting techniques.
What Are the Threats Facing Octocorals and Anemones?
The most wide-spread threat to habitat-forming cnidarians is climate change (Sokolow, 2009; Cheal et al., 2017; Hughes et al., 2017). In fact, reefs around the world are experiencing unprecedented warming-induced bleaching events (Hughes et al., 2017). Although bleaching is widely studied, most research is focused on reef-building stony corals, but zooxanthellate octocorals and anemones also bleach, which can lead to stress, disease and mortality (Loya et al., 2001; Lambo and Ormond, 2006; Hill and Scott, 2012; Hobbs et al., 2013; Scott and Hoey, 2017). For example, in the tropics the majority of recorded octocoral and anemone loss has been attributed to climate-change induced bleaching (Loya et al., 2001; Lambo and Ormond, 2006; Harrison et al., 2011; Thomas et al., 2015). These bleaching events are caused by increased sea surface temperature of as little 1°C above the physiological upper limit of the symbiosis, and coral bleaching is predicted to be the summer norm by 2050 (Harrison et al., 2011; Van Hooidonk et al., 2016).
On the GBR coral reefs have recently been subjected to repeated mass bleaching events which have caused coral mortality and significantly impacted coral species assemblages, 3D reef structure, and ecological functions, but the impact on octocorals and anemone cover has not been quantified in this period (De’ath et al., 2012; Hughes et al., 2017, 2018; Australian Institute of Marine Science [AIMS], 2019). Conversely, recent monitoring from the Australian Institute of Marine Science shows that octocoral cover is fairly stable in shallow waters of the GBR (Australian Institute of Marine Science [AIMS], 2018). In Japan after the prolonged 1998 heatwave at Sesoko Island, bleaching mortality was greater in octocorals than stony corals, with a 99% decrease in overall octocoral cover vs. 73% in stony corals (Loya et al., 2001). In the Houtman Abrolhos Islands in Western Australia, anemone cover dropped from 70% in 1992 to 0% in 2012, at least partially due to the 2011 marine heat wave (Thomas et al., 2015). Because of the loss of the obligate anemone hosts, anemonefishes were also extirpated from the area (Thomas et al., 2015). Bleaching can also have severe long-lasting, sub-lethal effects on octocorals. Laboratory experiments on Lobophytum compactum found that egg fertilization success, fecundity, and offspring viability were all significantly impacted for at least 20 months post-bleaching (Michalek-Wagner and Willis, 2001).
Ocean acidification is expected to significantly impact reef ecosystems worldwide by reducing the calcification ability of reef-building organisms (Kleypas and Yates, 2009). Though octocorals do not build reef structure, they produce calcified spicules and/or axes for tissue stiffening and structural support and can be negatively impacted by increased oceanic CO2 (Koehl, 1982; Watabe and Kingsley, 1992; Kleypas and Yates, 2009; Gómez et al., 2015). These impacts have been studied in situ using natural CO2 gradients associated with volcanic vents, and vary between study locations – For example, in Milne Bay, Papua New Guinea soft coral cover and richness were both significantly reduced in high CO2 environments, while in waters off Iwotorishima Island, Japan, community composition shifted from stony coral dominated in low CO2 environments to being dominated by a single species of octocoral in moderately acidified water (Fabricius et al., 2011; Inoue et al., 2013). At Shinkine Island in the subtropical-temperate transition zone in Japan, both octocorals and anemones were only found at the lowest concentrations of CO2 (Agostini et al., 2018). Unlike octocorals, anemones are non-calcifying, and as such, may be more resilient to ocean acidification (Doherty, 2009; Ventura et al., 2016). In fact, the host-symbiont relationship of temperate anemones shows remarkable resilience to the effects of acidification. When pH was reduced to as low as pH 7.4, zooxanthellae densities in several anemone species were either unimpacted, or zooxanthellae densities were reduced while chlorophyll concentration and photosynthetic yield per cell were increased (Doherty, 2009; Jarrold et al., 2013; Ventura et al., 2016). Though effects on tropical anemones are not as well-characterized, ability of larval anemonefish to find anemones is reduced in acidified environments and could negatively impact both anemone and anemonefish populations (Munday et al., 2009; Frisch et al., 2016).
Cyclones can have devastating effects on coral reefs that can take centuries to recover (Harmelin-Vivien, 1994), and cyclone intensity and effects on reefs are expected to rise with increasing global temperatures (Cheal et al., 2017). Cyclones can impact octocorals and anemones through wave-induced tearing or shearing and inundation with fresh water from rains (Van Woesik et al., 1995; Fabricius and De’ath, 2008). Cyclone “Joy” flooded sections of the GBR in late 1990 and early 1991, impacting several nearshore reefs. Six groups of octocorals were surveyed during this event, with only one group unimpacted, four groups partially impacted, and one group totally bleached or dead (Van Woesik et al., 1995). Although bleaching and increased cyclone intensity are major effects of climate change, elevated ocean temperatures can also contribute to other problems for corals, such as disease (Sutherland et al., 2004; Bruno et al., 2007; Sokolow, 2009).
Disease is a major threat to stony corals, especially in the Caribbean, but less is known for octocorals (Ruiz-Moreno et al., 2012; Slattery et al., 2013). Though octocorals do suffer from diseases like aspergilliosis, black and red band diseases, gorgonian labyrinthulomycosis, and at least 15 others, information on their susceptibility to and effect from these diseases is limited (Slattery et al., 2013; Weil et al., 2015). In the Caribbean, outbreaks of disease have caused mass mortality events in several species of gorgonians (Garzon-Ferreira and Zea, 1992; Geiser et al., 1998; Sutherland et al., 2004). Surveys in Arrial do Cabo, Brazil found that approximately 48% of surveyed colonies were dead, and 75% of living colonies showed signs of disease with ongoing tissue loss over time (Erni Cassola et al., 2016). In the Mediterranean, mass mortality (likely disease induced) of Eunicella singularis had long-lasting effects, with 59% of live colonies still exhibiting damage 4 years after the event (Garrabou et al., 2006). Though these examples have not been explicitly linked to climate change, they exemplify why temperature driven increases in disease prevalence could be devastating to octocoral communities. Octocorals and anemones are not only vulnerable to global disturbances such as ocean warming, they also face threats from more local sources.
Octocorals and anemones are especially vulnerable to habitat damage, both natural and anthropogenic. Mooring blocks and boat anchors can easily tear and uproot soft bodied organisms from the benthos. In Port Stephens, a single mooring block scoured over 1300 m2 of Dendronephthya australis soft coral habitat (Harasti, 2016). This species was particularly impacted because of its shallow benthic attachment and soft sediment habitat preferences (Davis et al., 2015). Other human activities, such as dredging, shift sediment and can damage corals by smothering colonies or reducing photosynthetic ability and exacerbating the effects of bleaching (Erftemeijer et al., 2012; Appeldoorn et al., 2016; Jones et al., 2016; Bessell-Browne et al., 2017). In the GBR octocorals are much less abundant in areas of high nutrients and turbidity, suggesting that dredging and industrial agricultural runoff will likely have negative effects on octocoral abundance and species richness (Fabricius and De’ath, 2001; Fabricius et al., 2005). On the other hand, some species of octocorals in South Africa were more tolerant of sedimentation than stony corals at the reef-sediment interface (Schleyer and Celliers, 2003). Additionally, one species of anemone, Mesactinia genesis, increased both its photosynthetic ability and stinging efficacy against stony corals in high nutrient and turbidity environments (Liu et al., 2015). Understanding the mechanisms behind how turbidity and sedimentation affect octocorals and anemones in different parts of the world is critical to understanding how these corals will react to threats such as dredging.
Other activities such as fishing, especially trawling, can damage benthic environments and have previously damaged both octocoral and anemone populations and their ecosystem functions (McConnaughey et al., 2000; Thrush and Dayton, 2002; Kaiser et al., 2006). In heavily trawled waters, soft bodied habitat builders can be damaged as bycatch in nets and can take years to recover (McConnaughey et al., 2000; Kaiser et al., 2006). Octocorals are especially vulnerable since many species grow in or near soft sediments and often prefer the deep water habitats also frequented by trawlers (Schleyer and Celliers, 2003; Wareham, 2009; Baillon et al., 2012; Poulos et al., 2016). Though dredging and trawling cause more widespread damage, octocoral colonies can also be destroyed by entanglement in recreational fishing gear, as the filaments and hooks can become easily entangled in soft tissue (Poulos et al., 2013; Erni Cassola et al., 2016).
Like stony corals, octocorals and anemones are commercially traded and often harvested from the wild (Dee et al., 2014). Unlike stony corals, the majority of octocorals are not listed in the Convention on International Trade in Endangered Species (UNEP-WCMC (Comps.), 2014) and therefore trade is largely unmonitored (Bruckner, 2001). In fact, no octocorals in the orders Alcyonacea, Gorgonacea, or Pennatulacea are CITES Appendix II listed, and only four species in Gorgonacea are CITES Appendix III listed (Bruckner, 2001; UNEP-WCMC (Comps.), 2014). Collection of octocorals and anemones is often unrestricted in contrast to restrictions on the scleractinian coral trade, such as in Tonga and Indonesia (Dee et al., 2014). In other areas, such as the Maldives and Australia, export of octocorals and anemones is not regulated explicitly, but is included in aggregate export limits (Dee et al., 2014). Some species of octocoral are also harvested for other commercial purposes. For example, Corallium rubrum is harvested for jewelry making, and has been significantly overharvested in the Mediterranean. This species is now considered endangered by the IUCN (Santangelo and Abbiati, 2001; Bruckner, 2009, 2016; IUCN, 2016). Like octocorals, no anemones are listed in CITES (UNEP-WCMC (Comps.), 2014). Despite this, anemones are widely traded in the aquarium industry and anemonefish host anemones are especially susceptible to overharvesting because of their popularity (Shuman et al., 2005).
There are several well-known stony coral threats that have not been well-examined in octocorals and anemones. For example, industrial runoff can have many negative impacts on coral reefs, including increased turbidity, deposition of pesticides and herbicides, and nutrient enrichment, but only the effects of turbidity have been examined in octocorals and few studies have examined both turbidity and nutrient enrichment in anemones (Fabricius, 2005; Brodie et al., 2012; Liu et al., 2015).
Although octocorals and anemones are generally more susceptible to physical disturbances than stony corals, some disturbances that strongly impact stony corals seem to have little to no impact on octocorals. For example, crown-of-thorns seastars (COTS) can devastate stony coral populations over large spatial scales, but often leave octocorals relatively unaffected (Fabricius, 1997; Kayal et al., 2012). This is likely because COTS preferentially predate on stony corals and only eat octocorals when their preferred prey are scarce (De’ath and Moran, 1998). In addition, octocorals produce a plethora of chemical defensive secondary metabolites that deter predators and protect colonies from fouling, which may give them an advantage over their stony cousins during COTS outbreaks (Coll, 1992; Changyun et al., 2008). In fact, octocorals may take advantage of the opportunity by growing on exposed coral skeleton left behind by such outbreaks, and this may result in a phase shift to octocoral dominated state (Endean and Stablum, 1971; Norström et al., 2009). In the Caribbean, octocorals are also less susceptible to population declines due to commercial diving activities and physical disturbances than stony corals, with some species seeming to prefer highly impacted sites (Tratalos and Austin, 2001). This may be due, in part, to their soft and flexible bodies; though a detriment when entangled, they will bend instead of break when kicked or touched. Thorough understanding of the unique threats facing soft corals as well as those common to soft and stony corals is needed to assist effective recovery efforts after a disturbance event.
What Information Do We Need to Successfully Restore Octocoral and Anemones?
First, we need to understand how the target species will cope with a changing climate or other large scale, unavoidable human impacts (van Oppen et al., 2017; Bellwood et al., 2019). If the species is not resilient enough for restoration to be a long-term solution, alternatives may need to be considered. For example, if a temperature-sensitive species has been extirpated from a reef that regularly experiences heat waves, restoration to this area would be risky. Instead, protection of remaining populations or restoration efforts in less impacted environments (or different temperature envelopes) may be viable alternatives. This may be considered as a form of “assisted migration” and is a contentious approach as it may be akin to deliberately introducing a species to a new area with all of the potential risks that entails (van Oppen et al., 2017; Mayer-Pinto et al., 2019). However, if long-term impacts, such as climate change or recurring port dredging cannot be avoided, then a shift in approach must be considered. Management interventions, such as genetic, reproductive, physiological, population/community, and environmental interventions, can be used to great effect in conservation and restoration efforts, though these techniques are not without complications (van Oppen et al., 2017; National Academies of Sciences Engineering and Medicine, 2019). For example, assisted evolution may be a viable method for increasing within-species resistance to ongoing pressures, and should be considered when trying to mitigate the effects of long term, unavoidable disturbances in conjunction with other short term methods (van Oppen et al., 2015, 2017; Ainsworth et al., 2019). As the topic of assisted evolution is beyond the scope of this review, please see van Oppen et al. (2015, 2017) for further discussion.
Next, to choose appropriate restoration sites, we must identify the preferred habitat of the target species of octocoral or anemone. Many species are associated only with certain substrates or associate strongly with other benthic species. For example, the octocoral Dendronephthya australis is strongly associated with sponge gardens and soft substrates, and restoration would likely fail outside of these habitats (Poulos et al., 2016). To develop restoration targets, it is important to try and determine previous range and densities. This can be particularly difficult for soft-bodied organisms. Ideally, historical or reference surveys should be consulted for this information, but other, more anecdotal sources may also be necessary. For example, reports from research organizations such as the AIMS long term monitoring program (Australian Institute of Marine Science [AIMS], 2018) or CSIRO South East Marine Protected Areas Seamounts project (CSIRO, 2019) can provide rigorous insights into recent distributions in Australia, while less quantitative historical accounts, such as those of Darwin (1889) may be needed to give insight into historical distributions. The state of the area to be restored should also be examined. If the previous habitat is no longer suitable for the target species (for example, there have been irreversible changes to flow or sediment regimes), then it needs to be decided whether restoring the abiotic substrate or other habitat factors is worth the time and effort involved or whether alternative areas should be considered.
The presence and effect of predators, grazers, and competitors – both native and introduced – should also be considered in the planning of restoration efforts and choice of restoration site. For example, restoration of lake trout (Selvelinus namaycush) in Lake Ontario has been hampered by predation of trout fry by alewives (Alosa pseudoharengus; Krueger et al., 1995). In plant restoration ecology, the roles of herbivory and competition are widely studied, and have significant – and often manageable – impacts on restoration of both species and habitats (e.g., Llewellyn and Shaffer, 1993; Opperman and Merenlender, 2000; Sweeney et al., 2002; Midoko-Iponga et al., 2005). In tropical coral reef systems, algae and coral compete for space which can affect the size and distribution of coral colonies (McCook et al., 2001; Sandin and McNamara, 2012). Algal competition may also affect mortality of stony coral transplants, especially in shallow waters (Yap et al., 1998). Understanding the individual roles of competition, herbivory, predation, and environmental factors in transplantation success (e.g., Johnston and Clark, 2007) will help restoration efforts focus on solutions to common setbacks and increase efficacy of restoration efforts.
Once it is decided whether restoration is feasible in the long term and where to restore, we need to decide which kind of restoration is necessary. According to the SER framework, there are three major restoration approaches – (1) natural regeneration, (2) assisted regeneration, and (3) reconstruction (McDonald et al., 2016a). These restoration approaches can be used in conjunction for multiple species occupying the same area. The restoration approach required in each case may depend on several factors, including the level of population degradation and connectivity to other sites. Given that there are many variables that may impact restoration success, treatments should be piloted before full-scale regeneration or reconstruction are implemented (McDonald et al., 2016a). This is a requirement of current restoration activities proposed for the Great Barrier Reef Marine Park (GBRMPA, 2018).
The simplest form of restoration is natural regeneration, where recolonization is allowed to happen naturally after the removal of threats and disturbances (Figure 2). This works well for species that have had limited population degradation and/or are have a strong supply of larvae. As this method is not resource intensive, it can be scaled up more easily than more direct interventions (Bellwood et al., 2019). Assisted regeneration is the support of natural regeneration without transplanting organisms to the restoration site (Figure 2). The most common type of assisted regeneration is restoration of degraded abiotic habitat to facilitate natural regeneration of populations. In stony coral restoration this technique is commonly used when rubble fields are formed due to mining, blasting, or boating incidents (e.g., Clark and Edwards, 1994; Edwards and Clark, 1999; Precht et al., 2001; Lindahl, 2003; Rinkevich, 2005). Some species of octocorals are adept at colonizing new environments, making assisted regeneration an ideal option for these species. Adding artificial structures for octocoral recruitment can increase regional diversity and improve octocoral population densities (Perkol-Finkel et al., 2006; Perkol-Finkel and Benayahu, 2007, 2009; Mayer-Pinto et al., 2019). This technique is a viable option for mitigating the impact of necessary underwater infrastructure, especially if the structures are designed to increase coral recruitment (e.g., Burt et al., 2009). Care should be taken in design and construction of artificial structures to minimize the risk of structures being dominated by invasive, non-native, or nuisance species (Dafforn et al., 2012; Geraldi et al., 2014; Dafforn, 2017).
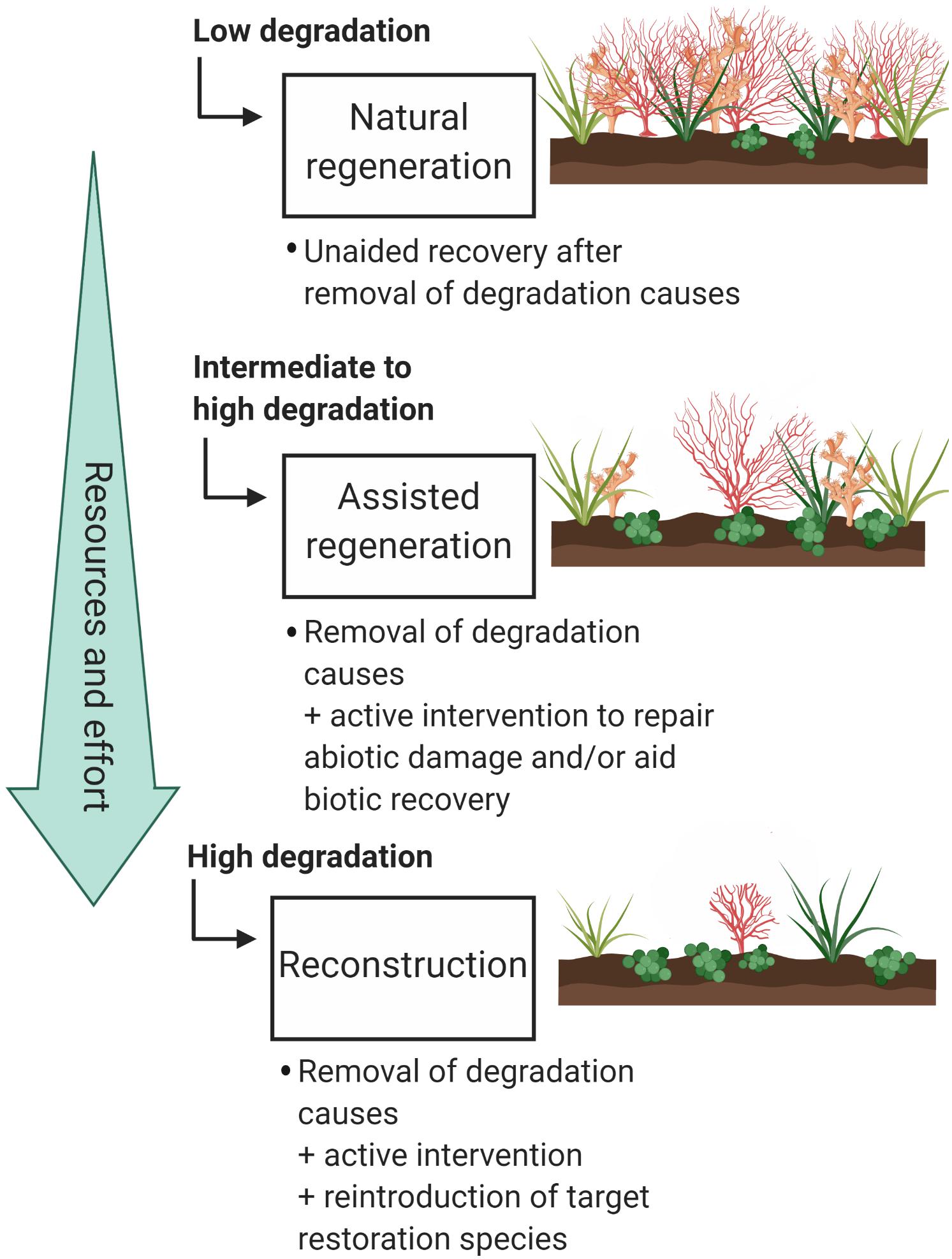
Figure 2. The three types of restoration as described by the Society for Ecological Restoration (SER). Types are listed in order of increasing resources and effort. Examples of different soft coral habitat degradation levels are illustrated on the right hand side of the figure. Figure created in BioRender (biorender.com).
Regeneration can also be facilitated by use of naturally occurring positive feedback systems (Shaver and Silliman, 2017). For example in anemones, survivorship increases when they are inhabited by anemonefish (Frisch et al., 2016). Anemone survivorship could be increased by regulating anemonefish removal by the aquarium trade or by releasing cultured juvenile anemonefishes into existing anemone populations, as aquaculture techniques are well-established (Moorhead and Chaoshu, 2010). In the Florida Keys, predation on gorgonian octocorals by predatory snails was significantly increased when the snails were released from predation pressure (Burkepile and Hay, 2007), suggesting that strategic conservation of predatory fishes can reduce predation and improve octocoral populations in this and related systems (Shaver and Silliman, 2017). Positive feedbacks can also be used to increase coral cover through natural larval settlement, as diverse herbivore populations reduce height of settlement-inhibiting turf algae and indirectly increase density of settlement-promoting crustose coralline algae (Burkepile and Hay, 2008; Shaver and Silliman, 2017). Single species facilitation dynamics can also be exploited to increase efficacy of restoration efforts. For example, saltmarsh restoration efforts in both Florida and the Netherlands exploited natural facilitation by planting Spartina sp. plugs in close proximity. This increased yields by an average of 107% compared to when they were planted further apart (Silliman et al., 2015). Understanding and exploiting positive feedbacks naturally occurring in target systems could be an effective method for increasing efficiency and impact of restoration efforts (Shaver and Silliman, 2017).
The third type of restoration, reconstruction, is the most resource intensive (Figure 2). It involves transplanting organisms to the restoration target area, either by moving them directly from one area to another or by growing them in either aquaculture or mariculture. For corals, this method is usually implemented when natural recruitment is expected to be slow or to fail, even though the water quality, substratum, and other factors are appropriate for the target species (Edwards and Clark, 1999). To simplify the decision-making process related to which types of interventions are appropriate, a simple decision tree has been provided (Figure 3).
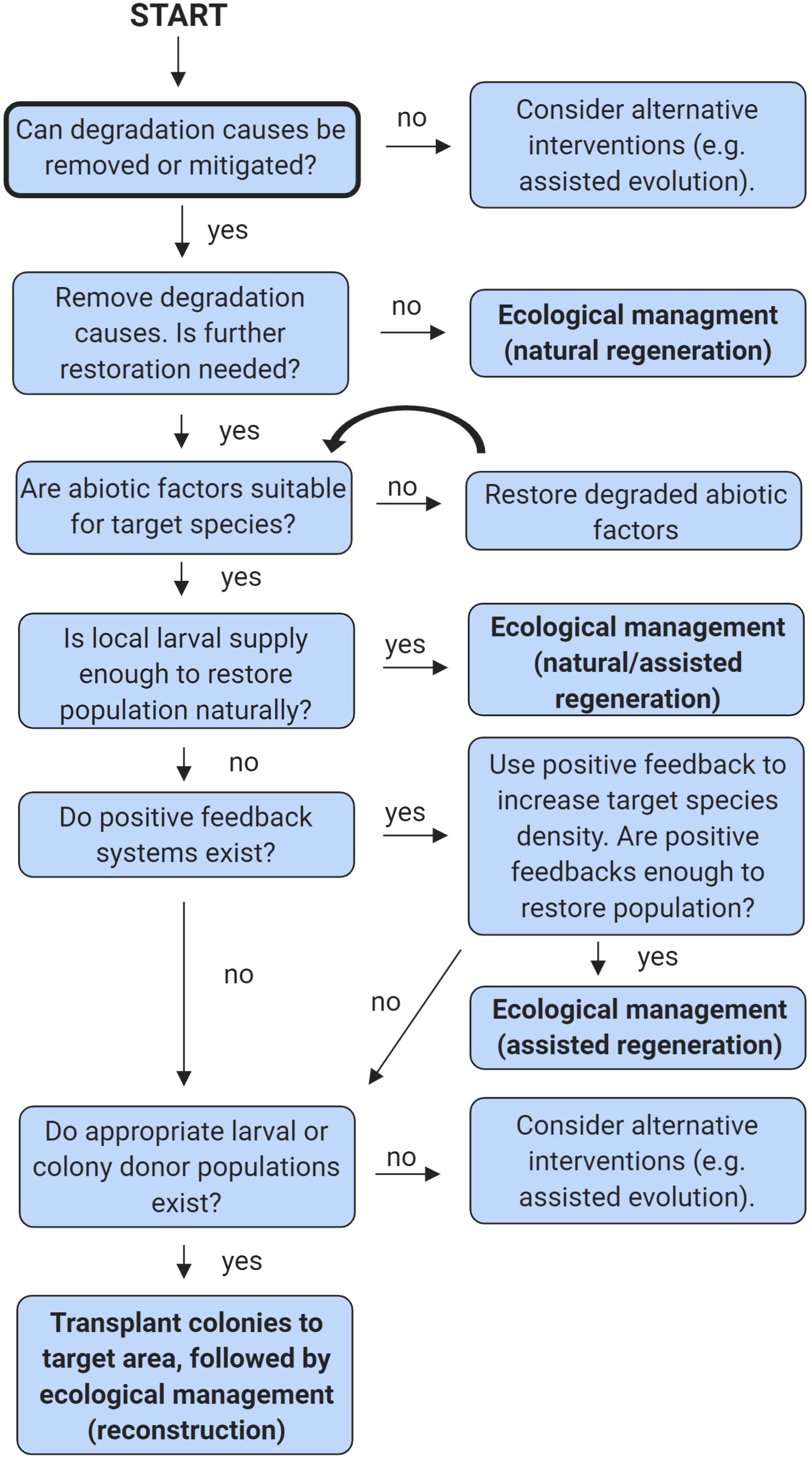
Figure 3. A simple decision tree to inform soft coral restoration efforts. For multiple species occupying the same area, begin the tree anew for each species. This tree does not consider all possible scenarios, please see the section entitled “What information do we need to successfully restore octocoral and anemones?” for further details. Figure created in BioRender (biorender.com).
If reconstruction is necessary, the first step is to decide how to procure individuals. There are two main methods used for soft corals – sexual (use of larvae) or asexual (cut nubbins) propagation (Edwards et al., 2010). In stony coral restoration, sexual propagation is generally preferred because it increases genetic diversity through recombination, though this can reduce the frequency of locally adapted genotypes (Baums, 2008; Edwards et al., 2010). Unfortunately, little is known about octocoral propagation by this method, so feasibility must be assessed species by species. For restoration via sexual propagation to be viable, we need to understand the method and timing of reproduction, settlement method, preferred settlement substrate, and growth rate of the target species (Edwards et al., 2010). Similar information would be required for anemones that have been successfully grown in captivity from fertilized eggs, but grew extremely slowly (Scott et al., 2014; Scott, 2017).
On the other hand, octocorals and anemones are usually relatively simple to propagate asexually (from cuttings) due to their soft bodies. Anemones can be cut in halves or quarters, and will close the wound within 24 h and have a fully formed mouth in as little as 1 month (Scott et al., 2014; Scott, 2017). The greatest drawback to this method is that is reduces genetic diversity due to the clonal nature of fragments, which can affect future population genetic structure and breeding success, though this can be mitigated by collecting fragments from multiple genetically distinct colonies (Baums, 2008; Shearer et al., 2009). Despite this, some octocorals and anemones naturally grow by clonal means, including budding and fission, and this method may result in naturally low diversity and an increased proportion of locally adapted genotypes and increase local adaptations (McFadden, 1991; Dahan and Benayahu, 1997; Barneah et al., 2002; Baums, 2008; Sherman and Ayre, 2008; Scott et al., 2014; Scott, 2017). Overall, the reproductive method (sexual vs. asexual), local adaptations, and genetic diversity of source populations should be considered when choosing how to source propagules for restoration (Baums, 2008; Edwards et al., 2010).
Once this has been decided, the juvenile/cut octocorals or anemones can be kept in aquaculture or mariculture systems until they are large enough for transplantation (Edwards et al., 2010; Leal et al., 2017). Fragments can be transplanted directly after collection, though mortality may be high (Weinberg, 1979; Linares et al., 2008). Similarly, some studies are now releasing stony coral larvae directly after collection (dela Cruz and Harrison, 2017). Anemones are highly sought after in the aquarium trade, and therefore aquaculture techniques are well-established for many species (Scott, 2017). Some species of octocoral are also grown for the ornamental aquarium trade, but many restoration target species are too difficult for the average home aquarist due to habitat and feeding requirements. Many species of both octocorals and anemones are non-photosynthetic, which may create difficulty for restoration projects as these need to be fed regularly (Leal et al., 2017). This leads to a more complex aquaculture technique than generally needed for stony coral restoration.
Finally, individuals must be transplanted to the restoration area, which can be challenging. Stony corals can be attached to substrate by placing adhesive material on the inner skeletal areas and using these to anchor colonies to the restoration substrate (Rinkevich, 2005; Edwards et al., 2010). Because octocorals and anemones do not have any stony, non-living surfaces, glues and epoxies do not work in the same way. Many octocorals, anemones, and sponges are sensitive to concretes, cyanoacrylates, and other materials commonly used to anchor stony corals to substrates, making attachment more difficult and labor intensive (Rinkevich, 2005; Ng et al., 2015). Therefore, alternative strategies are needed. Though clamps, clothes pegs, or other physical restraints may seem logical, these often are not strong enough to handle high-energy environments and do not encourage attachment of fragments to the benthos (Weinberg, 1979). One effective strategy that has been discovered is transplanting individuals attached to rock instead of free-living coral or coral cuttings (Weinberg, 1979; Oren and Benayahu, 1997; Ng et al., 2015). Individuals can either be collected with their attachments still in place, or can be physically attached using wire, string, or other physical material (e.g., Oren and Benayahu, 1997; Ng et al., 2015).
Because of these difficulties, attachment techniques must be developed that are appropriate for specific octocoral and anemone groups. For example, restoration of a wide range of gorgonians can be informed by trials done in the Mediterranean on Paramuricea clavata. During restoration trials, it was found that large P. clavata transplants created a lot of drag against their attachment putty media due to their surface area in the current and were easily lost, while smaller transplants remained attached (Linares et al., 2008). This team also tested different attachment methods, which included attaching fragments to putty with no support, supported within a short piece of plastic tubing, and tied to a plastic stick. The stick treatment showed the greatest survival rate despite being in direct contact with putty. These simple preliminary experiments are extremely important to long term success or failure of restoration efforts, as they inform future efforts and promote successful attachment, and can improve restoration efficacy of this and other species.
Examples of Ongoing Restoration
To place restoration of octocorals and anemones in the context of the SER framework, here we present two examples of ongoing restoration of the red coral Corallium rubrum and the cauliflower soft coral Dendronephthya australis. C. rubrum is a Mediterranean coral that grows from 10 to 300 meters depth and prefers dark environments (Figure 4a; Weinberg, 1979). Dendronepthya australis is a cold water coral found off the coast of temperate New South Wales, Australia (NSW) and is critical habitat for the endangered White’s seahorse, Hippocampus whitei (Figures 4b,d; Harasti et al., 2014; Harasti, 2016). The examples are presented against the International Standards for the Practice of Ecological Restoration Section III: Standard Practices for Planning and Implementing Ecological Restoration Projects. All headings from this section and whether information pertaining to this heading is available as presented in Table 1 and expanded upon in this section. In summary, for C. rubrum in areas where threats have been mitigated or removed but natural recolonization is expected to take decades, reconstruction is a feasible solution for speeding population recovery. On the other hand, more difficulties have been encountered in D. australis restoration efforts, and while reconstruction will likely be beneficial to recovering populations, more information is needed on feasibility for this species.
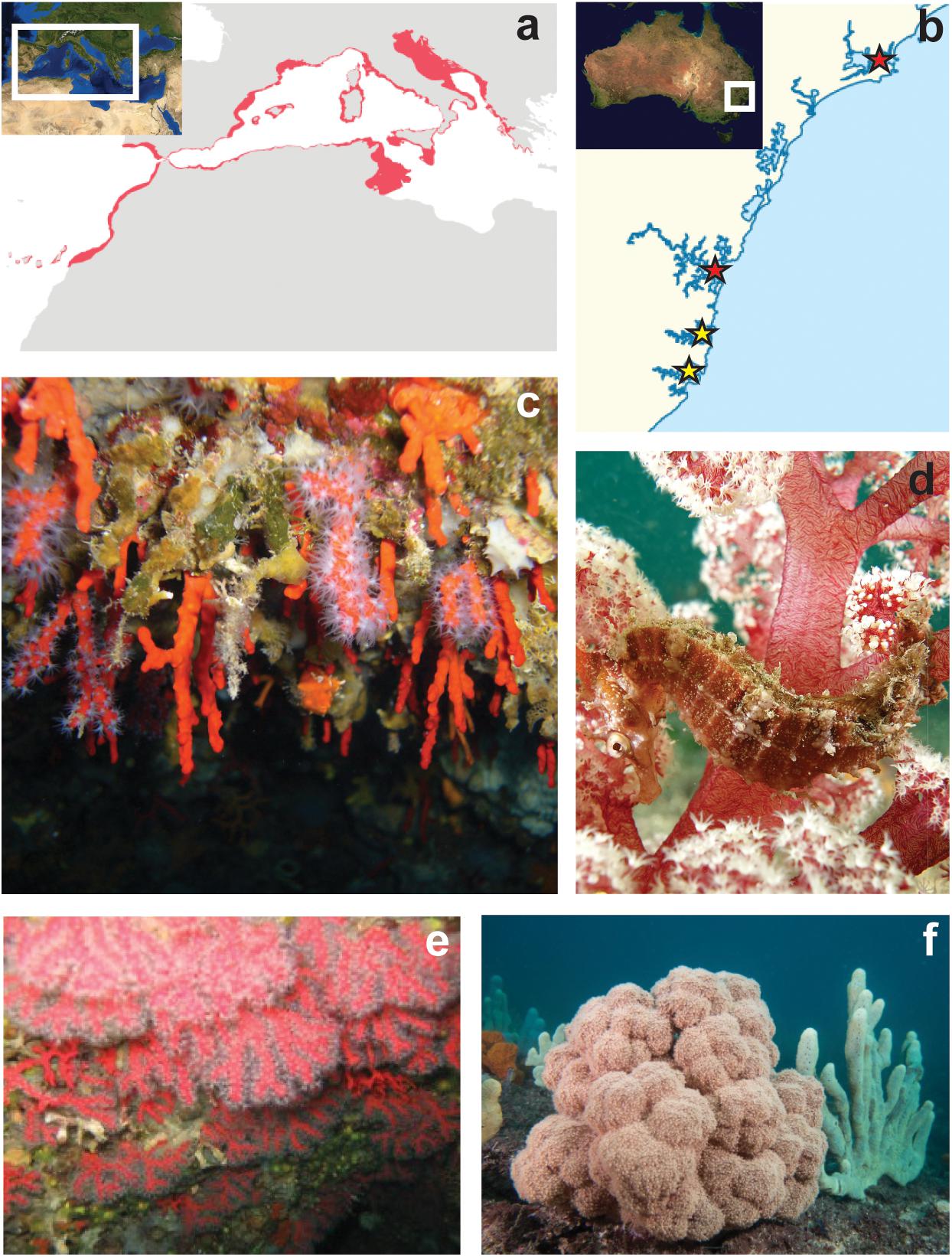
Figure 4. Distribution and identification of case study species. (a) Distribution map of Corallium rubrum, map of Mediterranean inset, (b) distribution map of Dendronephthya australis. Populations with known population declines marked in red, map of Australia inset. (c) Small, immature C. rubrum colonies in the Mediterranean, (d) the endangered White’s seahorse, Hippocampus whitei, in D. australis, (e) large, mature C. rubrum colonies, (f) mixed sponge and D. australis habitat. Photo credits – (a) modified from © FAO Fisheseries and Aquaculture Department, 2016. Aquatic Species Distribution Maps. FAO aquatic species distribution map of Corallium rubrum. In: FAO – Fisheries and Aquaculture Department (FI) (online). Rome. Updated 2016-08-24, http://www.fao.org/geonetwork/srv/en/main.home? uuid=fao-species-map-col; (b) modified from NordNordWest [CC BY-SA 3.0 de (https://creativecommons.org/licenses/by-sa/3.0/de/deed.en)]; (c) modified from Yoruno – Own work, CC BY-SA 3.0, https://commons.wikimedia.org/w/index.php?curid=2719768; (d) John Turnbull, MarineExplorer.org; (e) modified from Marco Busdraghi [CC BY-SA 3.0 (http://creativecommons.org/licenses/by-sa/3.0/)]; (f) John Turnbull, MarineExplorer.org.
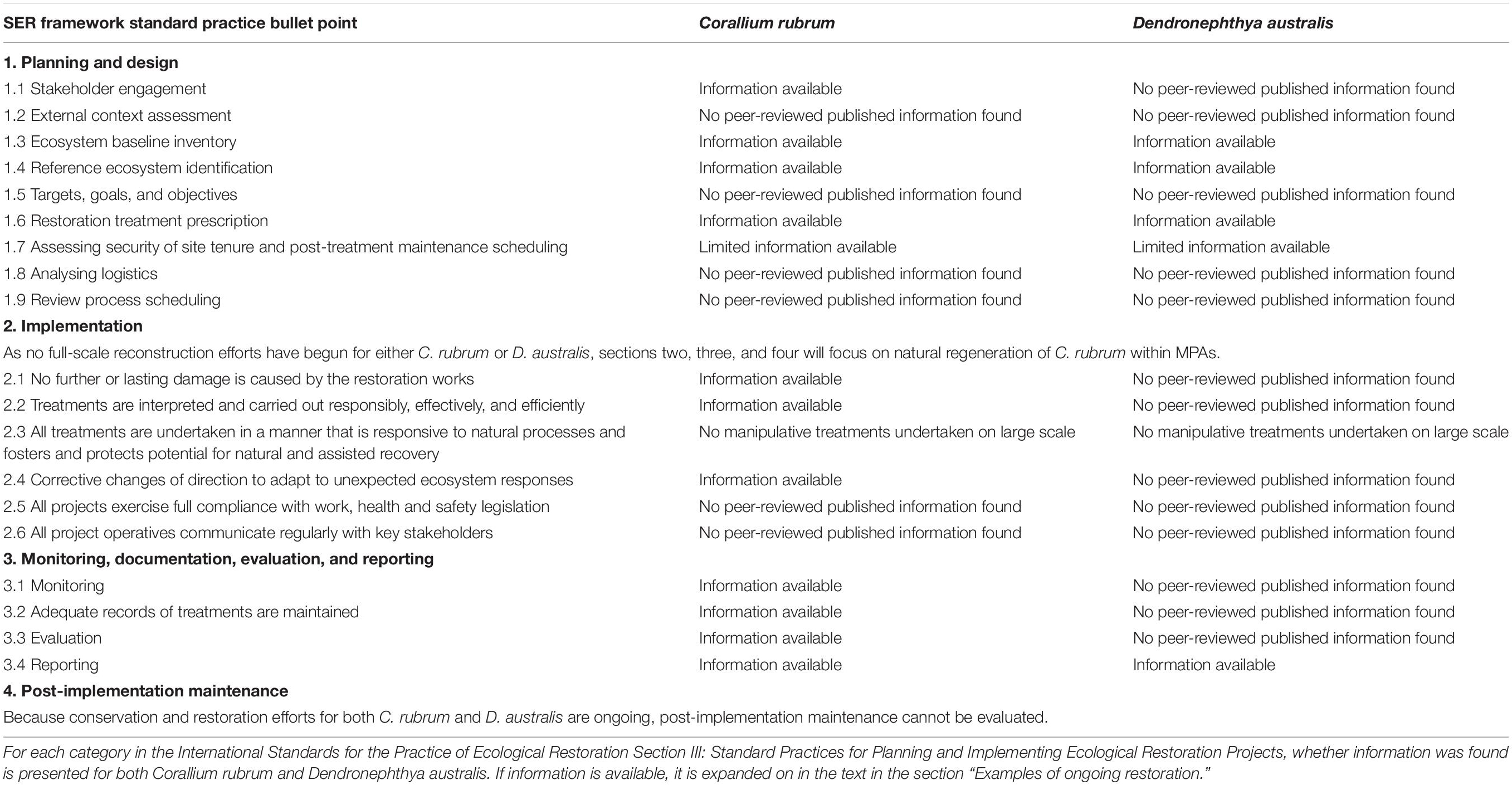
Table 1. Examples of ongoing octocoral restoration in the context of the Society for Ecological Restoration (SER) framework.
These species were chosen for several reasons. Firstly, significant literature exists against which to assess restoration efforts following the SER framework. Furthermore, the species have different life history traits, have experienced different causes and severity of declines, and are in different stages of restoration, all of which allow for a more complete picture of challenges that can arise in octocoral restoration. Finally, C. rubrum was chosen because of the extensive time frame of both impacts and protections, and breadth of literature. D. australis was chosen because of the recent nature of impacts and the apparent restricted distribution of this species which provides an urgency to any potential restoration projects. Literature was sourced using Web of Science and Google Scholar databases using the keywords “Dendronepthya australis” and “Corallium rubrum” paired with “restor∗,” “impact∗,” “declin∗,” “protect∗,” and “recover∗.” Asterisks were used to denote different forms of words, for example “restor∗” returned “restored,” “restoring,” and “restoration.” If information on a topic was not found during searches, it is reported in Table 1 as “no peer-reviewed published information found.”
Corallium rubrum
Stakeholder Engagement
Though stakeholders are identified (C. rubrum fisheries, divers, dive centers), there is little information on methods and efficacy of engagement, though when there is a second workshop on Corallium science, stakeholder participation has been identified as a priority (Bruckner and Roberts, 2009; Betti et al., 2019).
Ecosystem Baseline Inventory
We found that historic baseline data is available for C. rubrum from several location across the Mediterranean (Garcia-Rodriguez and Mass, 1986; Garcia-Rodriguez and Massó, 1986; Garrabou and Harmelin, 2002), and has previously been used to help quantify population damage (Bruckner, 2009). C. rubrum is threatened by commercial collection for jewelry. As a consequence large, sexually mature colonies in less than 50m of water have been largely wiped out (Bruckner, 2009), though populations up to 150 m depth are also being exploited (Santangelo and Bramanti, 2010). Age structure in these exploited populations is skewed toward small and young colonies, a sign of frequent disturbance (Figures 4c,e; Tsounis et al., 2006). Exploitation has caused severe enough declines that it is now considered endangered by the IUCN (2016).
In addition to collection, C. rubrum populations are threatened by colonization by boring sponges, which can affect up to 50% of colonies in a population and are a major cause of natural coral mortality (Corriero et al., 1997; Bramanti et al., 2007). In order to mitigate threats from overharvesting strict collection quotas, size limits, and rotational harvesting plans have been implemented or are in the process of being implemented (Caddy, 1993; Santangelo and Abbiati, 2001; Bruckner, 2016). Unfortunately, these regulations have so far been unsuccessful in creating a sustainable harvest because of the slow growth and late sexual maturation of colonies (Santangelo and Abbiati, 2001; Bruckner, 2009, 2016). Additionally, marine protected areas (MPAs) have been established within the range of C. rubrum, and coral size and age has increased in these areas, though even 20–30 years of protection have not proved sufficient for full population recovery (Tsounis et al., 2006; Bruckner, 2009).
Reference Ecosystem Identification
Though protected populations have not fully recovered, study of these areas give insight into the expected recovery potential of C. rubrum regeneration or reconstruction and work well as reference ecosystems.
Restoration Treatment Prescription
Threats to C. rubrum by humans have been mitigated through collection policies as described in section “Ecosystem Baseline Inventory,” though this has not eliminated overharvesting. To mitigate natural damage by boring sponges, Bramanti et al. (2007) conducted settlement experiments using different settlement media. They concluded that using marble plates greatly reduced instances of boring sponges on colonies (Bramanti et al., 2007). This information can be used in restoration treatment prescriptions in two ways – as in-situ settlement material to reduce boring sponge mortality in assisted regeneration, or as a source of sexually derived propagules for transplantation in reconstruction efforts. This is particularly important to reconstruction as experiments with other gorgonian species in the Mediterranean show that transplanted small, attached colonies have higher survival rates than transplanted fragments (Weinberg, 1979).
In addition, A successful reconstruction pilot experiment using confiscated poached fragments of C. rubrum has shown that it is resilient to stress associated with reconstruction. Despite being harvested, kept out of water in poacher’s nets, kept in aquaria, and then transplanted back into the natural environment in direct contact with putty, over 90% of fragments survived over 4 years post-transplant. When populations were re-sampled, researchers found that fertility rates were comparable to natural populations, while fecundity was higher in transplanted populations (Montero-Serra et al., 2018). Unfortunately, models predict that recovery of populations to natural, undisturbed size distributions using this method will take upwards of 30 years due to the slow growth rate and other life history traits of C. rubrum (Montero-Serra et al., 2018).
Assessing Security of Site Tenure and Post-treatment Maintenance Scheduling
Marine Protected Areas (MPAs) exist in the range of C. rubrum, improving security of site tenure. Unfortunately, no peer-reviewed published information on post-treatment maintenance was found.
No Further or Lasting Damage Is Caused by the Restoration Works
Banning of collection within MPAs has not caused further damage to C. rubrum ecosystems, but implementation of collection quotas, size limits, and rotational harvests have not been sufficient to mitigate impacts on this species (Caddy, 1993; Santangelo and Abbiati, 2001; Bruckner, 2016).
Treatments Are Interpreted and Carried Out Responsibly, Effectively, and Efficiently
Though implementation of MPAs has increased C. rubrum population density and mean colony size, poaching is still a problem and may require additional interventions (Tsounis, 2005; Tsounis et al., 2006; Bruckner, 2009; Montero-Serra et al., 2018).
Corrective Changes of Direction to Adapt to Unexpected Ecosystem Responses
As 20–30 years of protection within MPAs have not been sufficient to completely mitigate impacts, reconstruction of populations may be required and pilot studies have been undertaken (Tsounis et al., 2006; Bruckner, 2009; Montero-Serra et al., 2018).
Monitoring
Ongoing monitoring of C. rubrum within MPAs has shown that this species requires decades without disturbance for full population recovery (Tsounis et al., 2006; Bruckner, 2009). This has been possible because of collection of pre-protection baseline data, understanding of baseline healthy population size structures, and ongoing surveys of recovering populations (Bruckner, 2009).
Adequate Records of Treatments Are Maintained
Size and date of implementation of MPAs and changes to laws regarding C. rubrum are well-documented (Caddy, 1993; Santangelo and Abbiati, 2001; Bruckner, 2009). Because of the poached nature of fragments used in the pilot reconstruction study, exact GPS coordinates of the fragments used could not be obtained (Montero-Serra et al., 2018), but should be where possible.
Evaluation
Results from monitoring, studies of life history traits, and pilot transplantations suggest that reconstruction may be an appropriate way to improve population densities in areas where impacts have been successfully mitigated (Montero-Serra et al., 2018).
Reporting
Reports and journal articles have been published and a metanalysis performed on the results of implementing MPAs (e.g., Santangelo and Abbiati, 2001; Tsounis, 2005; Tsounis et al., 2006; Bruckner, 2009), though the metanalysis has been contentious (Bruckner, 2010; Santangelo and Bramanti, 2010).
Dendronephthya australis
Ecosystem Baseline Inventory
Dendronephthya australis pre-disturbance distribution, population densities, and functional and ecosystem roles have been intensively studied in the Port Stephens estuary in central NSW, Australia (Poulos et al., 2013, 2016; Corry et al., 2018). D. australis lives in soft substrates in association with sponge gardens, and gardens with D. australis are associated with higher fish diversity than nearby sand, seagrass, and sponge habitats without D. australis (Figure 4f; Poulos et al., 2013). D. australis is non-photosynthetic, instead relying on strong currents to feed on zooplankton and detritus (Poulos et al., 2016; Corry et al., 2018). Taking all of these factors into account, models have identified appropriate unoccupied D. australis habitat within the Port Stephens estuary (Poulos et al., 2016). Unfortunately, populations are under threat from fishing line entanglement, boat anchor damage, mooring chain scour, and shifting sands (Harasti, 2016; Poulos et al., 2016). In fact populations in Port Stephens, where densities are greatest, have been reduced by up to 95% due to a catastrophic sand-shifting event (Harasti, 2016). In addition, a misplaced boat mooring scoured over 1000 m2 of D. australis and sponge garden habitat, reducing the area to bare sand (Harasti, 2016). Though this mooring block has since been moved, populations have not yet recovered in either location. Resulting population declines have been so severe that the New South Wales Fisheries Scientific Committee have recommend listing D. australis as endangered (NSW Fisheries Scientific Committee, 2019). Little is known about natural recruitment and growth rate of these corals, which makes estimates of natural recovery difficult.
Reference Ecosystem Identification
Though some populations of D. australis within the Port Stephen’s estuary were heavily impacted, others remained unaffected and are ideal reference ecosystems (Harasti, 2016).
Restoration Treatment Prescription
Little can be done to mitigate damage by large-scale, natural events such as the sand-shifting that smothered populations in 2010 (Wainwright, 2011). On the other hand, careful planning and placement of mooring blocks, and insuring that any mooring blocks near colonies are benthic friendly can help protect remnant populations (Demers et al., 2013).
Despite the wealth of demographic knowledge on this species, there is a lack of general information on non-photosynthetic octocoral transplantation and aquaculture. Many restoration techniques that work on stony corals do not necessarily work with this species. Aquaculture has proved problematic, as coral nubbins do not reliably attach to media and require labor intensive feeding.
Assessing Security of Site Tenure and Post-treatment Maintenance Scheduling
MPAs exist in the ranges D. australis, improving security of site tenure, though not all populations fall within MPA borders (Poulos et al., 2016). No peer-reviewed published information on post-treatment maintenance for D. australis.
Reporting
Results from unsuccesful transplantation trials on D. australis have been reported in an Honors thesis, but have not been published in a peer-reviewed journal. As Dr. Poulos was working with the NSW Department of Primary Industry during this research, it is available to appropriate managerial bodies.
Summary of Case Study Assessment
By reviewing restoration of C. rubrum and D. australis in the context of the SER framework we have clarified both the strengths and gaps in knowledge for both species and pinpointed areas for future research. Overall, we have found that restoration of both species has a high chance of success but understanding of restoration techniques and expected recovery times is much more advanced for C. rubrum. Though studies show that both sexually and asexually derived propagules can be used in reconstruction efforts for C. rubrum, more information is needed on the elimination/mitigation of threats, ecologically appropriate methods for triggering regeneration, choosing appropriate genetic stock for each reconstruction site, and strategies for addressing genetic stock issues (McDonald et al., 2016a). For D. australis, more information is needed on propagation, aquaculture techniques, and transplantation in addition to the knowledge gaps identified for C. rubrum (McDonald et al., 2016a). Conclusions drawn from the restoration effort of these two species can inform restoration of other octocorals and anemones. For example, creating sustainable harvesting schemes for slow-growing C. rubrum would significantly expand our knowledge of sustainable use of benthic cnidarians, such as has previously been found for the Hawai’ian black coral (Grigg, 2001). Increasing our knowledge base of sustainable harvest of benthic resources would allow for better management of other species, such as the heavily exploited anemone Entacmaea quadricolor (Frisch et al., 2019). Transplantation of D. australis has also proven to be a challenge, though not a unique one. Developing attachment techniques would greatly enhance the viability of restoration efforts for any species with soft attachment sites, including most species of anemone. Additionally, developing aquaculture techniques for the azooxanthellate octocoral D. australis would inform aquaculture of other azooxanthellate octocoral and anemone species, greatly increasing the efficacy of captive propagation. Addressing these knowledge gaps would greatly increase the chances of successful restoration of these and other species.
Conclusion and Critical Steps Forward for Effective Octocoral and Anemone Restoration
Although octocorals and anemones are often overlooked in ecological studies, our review highlights their vulnerability to climate change and other human threats, and some species may require restoration projects to maintain or rehabilitate their populations and/or ecosystem services. There are many challenges to octocoral restoration, not the least of which is the difficulty in setting restoration parameters. Octocorals and anemones don’t leave skeletons or other signs after die-off events, therefore reference surveys are needed to understand historic density and community composition. Unfortunately, in tropical environments many papers that report coral loss and bleaching either do not specify between stony corals, octocorals, or anemones or do not include them at all, instead focusing solely on stony corals, while deep-water environments are often difficult to survey. In addition, there is the added challenge that many long term studies, such as long term monitoring programs, have only recently begun reporting octocoral cover (e.g., Australian Institute of Marine Science [AIMS], 2018), so we have little understanding of historic coral cover. Temperate octocorals and anemones are often, but not always, better documented (e.g., Bruckner, 2009; Poulos et al., 2016). There are also many technical challenges to restoration of octocorals and anemones, most notably in sourcing reproductive propagules and attaching clonally sourced fragments to the benthos. Despite the challenges posed by knowledge gaps, certain characteristics of octocorals and anemones suggest they will be increasingly important components of the modern reefs of the Anthropocene across climate zones. Hence, an urgent focus on their biology, ecology, and restoration methods is needed.
Author Contributions
RS wrote the original manuscript. KD, TA, and EJ helped develop the project and helped shape the final article, including editing and directional advice. All authors contributed to the article and approved the submitted version.
Funding
This study was supported by the University of New South Wales and a Scientia Fellowship awarded to TA in 2018. RS was supported by a Research Training Scholarship provided by the Australian Government.
Conflict of Interest
The authors declare that the research was conducted in the absence of any commercial or financial relationships that could be construed as a potential conflict of interest.
Acknowledgments
Earlier versions of the manuscript were improved by helpful comments from J. Turnbull and A. Eger.
References
Agostini, S., Harvey, B. P., Wada, S., Kon, K., Milazzo, M., Inaba, K., et al. (2018). Ocean acidification drives community shifts towards simplified non-calcified habitats in a subtropical-temperate transition zone. Sci. Rep. 8, 5–10. doi: 10.1038/s41598-018-29251-7
Ainsworth, T. D., Hurd, C. L., Gates, R. D., and Boyd, P. W. (2019). How do we overcome abrupt degradation of marine ecosystems and meet the challenge of heat waves and climate extremes? Glob. Chang. Biol. 26, 343–354. doi: 10.1111/gcb.14901
Appeldoorn, R., Ballantine, D., Bejarano, I., Carlo, M., Nemeth, M., Otero, E., et al. (2016). Mesophotic coral ecosystems under anthropogenic stress: a case study at Ponce, Puerto Rico. Coral Reefs 35, 63–75. doi: 10.1007/s00338-015-1360-5
Ates, R. M. L. (1989). Fishes that eat sea anemones, a review. J. Nat. Hist. 23, 71–79. doi: 10.1080/00222938900770041
Australian Institute of Marine Science [AIMS] (2018). AIMS Long-Term Monitoring Program. Townsville, QL: AIMS.
Australian Institute of Marine Science [AIMS] (2019). Coral Bleaching Events. Data Centre. Townsville, QL: AIMS.
Baillon, S., Hamel, J.-F., Wareham, V. E., and Mercier, A. (2012). Deep cold-water corals as nurseries for fish larvae. Front. Ecol. Environ. 10, 351–356. doi: 10.1890/120022
Barneah, O., Malik, Z., and Benayahu, Y. (2002). Attachment to the substrate by soft coral fragments: desmocyte development, structure, and function. Invertebr. Biol. 121, 81–90. doi: 10.1111/j.1744-7410.2002.tb00048.x
Bartlett, L. A., Brinkhuis, V. I. P., Ruzicka, R. R., Colella, M. A., Lunz, K. S., Leone, E. H., et al. (2018). Dynamics of Stony Coral and Octocoral Juvenile Assemblages Following Disturbance on Patch Reefs of the Florida Reef Tract. London: IntechOpen, 99–120.
Bastidas, C., Fabricius, K. E., and Willis, B. L. (2004). Demographic aspects of the soft coral Sinularia flexibilis leading to local dominance on coral reefs. Hydrobiologia 530, 433–441. doi: 10.1007/s10750-004-2663-7
Baums, I. B. (2008). A restoration genetics guide for coral reef conservation. Mol. Ecol. 17, 2796–2811. doi: 10.1111/j.1365-294X.2008.03787.x
Bellwood, D. R., Pratchett, M. S., Morrison, T. H., Gurney, G. G., Hughes, T. P., Álvarez-Romero, J. G., et al. (2019). Coral reef conservation in the anthropocene: confronting spatial mismatches and prioritizing functions. Biol. Conserv. 236, 604–615. doi: 10.1016/j.biocon.2019.05.056
Bessell-Browne, P., Negri, A. P., Fisher, R., Clode, P. L., and Jones, R. (2017). Cumulative impacts: thermally bleached corals have reduced capacity to clear deposited sediment. Sci. Rep. 7, 1–14. doi: 10.1038/s41598-017-02810-0
Betti, F., Bavestrello, G., Fravega, L., Bo, M., Coppari, M., Enrichetti, F., et al. (2019). On the effects of recreational SCUBA diving on fragile benthic species: the Portofino MPA (NW Mediterranean Sea) case study. Ocean Coast. Manag. 182:104926. doi: 10.1016/j.ocecoaman.2019.104926
Brace, R. C., and Quicke, D. L. J. (1986). Dynamics of colonization by the beadlet anemone, actinia equina. J. Mar. Biol. Assoc. United Kingdom 66, 21–47. doi: 10.1017/S002531540003962X
Bramanti, L., Rossi, S., Tsounis, G., Gili, J. M., and Santangelo, G. (2007). Settlement and early survival of red coral on artificial substrates in different geographic areas: some clues for demography and restoration. Hydrobiologia 580, 219–224. doi: 10.1007/s10750-006-0452-1
Brodie, J. E., Kroon, F. J., Schaffelke, B., Wolanski, E. C., Lewis, S. E., Devlin, M. J., et al. (2012). Terrestrial pollutant runoff to the Great Barrier Reef: an update of issues, priorities and management responses. Mar. Pollut. Bull. 65, 81–100. doi: 10.1016/j.marpolbul.2011.12.012
Bruckner, A. (2016). “Advances in management of precious corals to address unsustainable and destructive harvest techniques,” in The Cnidaria, Past, Present and Future, eds S. Goffredo and Z. Dubinsky (Cham: Springer), 747–786.
Bruckner, A. W. (2001). Tracking the trade in ornamental coral reef organisms: the importance of CITES and its limitations. Aqu. Sci. Conserv. 3, 79–94. doi: 10.1023/A:1011369015080
Bruckner, A. W. (2009). Rate and extent of decline in Corallium (pink and red coral) populations: existing data meet the requirements for a CITES Appendix II listing. Mar. Ecol. Prog. Ser. 397, 319–332. doi: 10.3354/meps08110
Bruckner, A. W. (2010). Quantifying the decline in Corallium rubrum populations: reply to Santangelo & Bramanti (2010). Mar. Ecol. Prog. Ser. 418, 299–303. doi: 10.3354/meps08898
Bruckner, A. W., and Roberts, G. G. (eds) (2009). “Proceedings of the First International Workshop on Corallium Science, Management, and Trade (NOAA Technical Memorandum NMFS-OPR-43),” in Proceedings of the 1st International Workshop on Corallium Science, Management, and Trade Edited, (Silver Spring, MD), 153.
Bruno, J. F., Selig, E. R., Casey, K. S., Page, C. A., Willis, B. L., Harvell, C. D., et al. (2007). Thermal stress and coral cover as drivers of coral disease outbreaks. PLoS Biol. 5:e124. doi: 10.1371/journal.pbio.0050124
Burkepile, D. E., and Hay, M. E. (2007). Predator release of the gastropod Cyphoma gibbosum increases predation on gorgonian corals. Oecologia 154, 167–173. doi: 10.1007/s00442-007-0801-4
Burkepile, D. E., and Hay, M. E. (2008). Herbivore species richness and feeding complementarity affect community structure and function on a coral reef. Proc. Natl. Acad. Sci. U.S.A. 105, 16201–16206. doi: 10.1073/pnas.0801946105
Burt, J., Bartholomew, A., Bauman, A., Saif, A., and Sale, P. F. (2009). Coral recruitment and early benthic community development on several materials used in the construction of artificial reefs and breakwaters. J. Exp. Mar. Bio. Ecol. 373, 72–78. doi: 10.1016/j.jembe.2009.03.009
Butler Iv, M. J. (1995). Cascading disturbances in Florida Bay, USA: cyanobacteria blooms, sponge mortality, and implications for juvenile spiny lobsters Panulirus argus. Mar. Ecol. Prog. Ser. 129, 119–125. doi: 10.3354/meps129119
Caddy, J. F. (1993). background concepts for a rotating harvesting strategy with particular reference to the Mediterranean red coral, Corallium rubrum. Mar. Fish. Rev. 55, 10–18.
Cerrano, C., Danovaro, R., Gambi, C., Pusceddu, A., Riva, A., and Schiaparelli, S. (2009). Gold coral (Savalia savaglia) and gorgonian forests enhance benthic biodiversity and ecosystem functioning in the mesophotic zone. Biodivers. Conserv. 19, 153–167. doi: 10.1007/s10531-009-9712-5
Changyun, W., Haiyan, L., Changlun, S., Yanan, W., Liang, L., and Huashi, G. (2008). Chemical defensive substances of soft corals and gorgonians. Acta Ecol. Sin. 28, 2320–2328. doi: 10.1016/S1872-2032(08)60048-7
Cheal, A. J., MacNeil, M. A., Emslie, M. J., and Sweatman, H. (2017). The threat to coral reefs from more intense cyclones under climate change. Glob. Chang. Biol. 23, 1511–1524. doi: 10.1111/gcb.13593
Clark, S., and Edwards, A. J. (1994). Use of artificial reef structures to rehabilitate reef flats degraded by coral mining in the Maldives. Bull. Mar. Sci. 55, 724–744.
Coleman, M. A., and Wernberg, T. (2017). Forgotten underwater forests: the key role of fucoids on Australian temperate reefs. Ecol. Evol. 7, 8406–8418. doi: 10.1002/ece3.3279
Coll, J. C. (1992). The chemistry and chemical ecology of octocorals (Coelenterata, Anthozoa, Octocorallia). Chem. Rev. 92, 613–631. doi: 10.1021/cr00012a006
Concepcion, G. T., Kahng, S. E., Crepeau, M. W., Franklin, E. C., Coles, S. L., and Toonen, R. J. (2010). Resolving natural ranges and marine invasions in a globally distributed octocoral (genus Carijoa). Mar. Ecol. Prog. Ser. 401, 113–127. doi: 10.3354/meps08364
Corriero, G., Abbiati, M., and Santangelo, G. (1997). Sponges inhabiting a Mediterranean red coral population. Mar. Ecol. 18, 147–155. doi: 10.1111/j.1439-0485.1997.tb00433.x
Corry, M., Harasti, D., Gaston, T., Mazumder, D., Cresswell, T., and Moltschaniwskyj, N. (2018). Functional role of the soft coral Dendronephthya australis in the benthic food web of temperate estuaries. Mar. Ecol. Prog. Ser. 593, 61–72. doi: 10.3354/meps12498
CSIRO (2019). South East Marine Protected Areas Seamounts project. Available online at: http://www.cmar.csiro.au/research/seamounts/ (accessed January 8, 2019).
Dafforn, K. A. (2017). Eco-engineering and management strategies for marine infrastructure to reduce establishment and dispersal of non-indigenous species. Manag. Biol. Invasions 8, 153–161. doi: 10.3391/mbi.2017.8.2.03
Dafforn, K. A., Glasby, T. M., and Johnston, E. L. (2012). Comparing the invasibility of experimental “reefs” with field observations of natural reefs and artificial structures. PLoS One 7:e38124. doi: 10.1371/journal.pone.0038124
Dahan, M., and Benayahu, Y. (1997). Clonal propagation by the azooxanthellate octocoral Dendronepththya hemprichi. Coral Reefs 16, 5–12. doi: 10.1007/s003380050053
Davis, T. R., Harasti, D., and Smith, S. D. A. (2015). Extension of Dendronephthya australis soft corals in tidal current flows. Mar. Biol. 162, 2155–2159. doi: 10.1007/s00227-015-2732-7
De’ath, G., Fabricius, K. E., Sweatman, H., and Puotinen, M. (2012). The 27-year decline of coral cover on th Great Barrier Reef and its causes. PNAS 109, 17995–17999. doi: 10.1073/pnas.1208909109
De’ath, G., and Moran, P. J. (1998). Factors affecting the behaviour of crown-of-thorns starfish (Acanthaster planci L.) on the Great Barrier Reef: 2: feeding preferences. J. Exp. Mar. Bio. Ecol. 220, 107–126. doi: 10.1186/s40623-018-0820-x
Dee, L. E., Horii, S. S., and Thornhill, D. J. (2014). Conservation and management of ornamental coral reef wildlife: successes, shortcomings, and future directions. Biol. Conserv. 169, 225–237. doi: 10.1016/j.biocon.2013.11.025
dela Cruz, D. W., and Harrison, P. L. (2017). Enhanced larval supply and recruitment can replenish reef corals on degraded reefs. Sci. Rep. 7, 1–13. doi: 10.1038/s41598-017-14546-y
Demers, M. C. A., Davis, A. R., and Knott, N. A. (2013). A comparison of the impact of “seagrass-friendly” boat mooring systems on Posidonia australis. Mar. Environ. Res. 83, 54–62. doi: 10.1016/j.marenvres.2012.10.010
Dinesen, Z. D. (1983). Patterns in the distribution of soft corals across the central Great Barrier Reef. Coral Reefs 1, 229–236. doi: 10.1007/BF00304420
Doherty, M. (2009). Ocean Acidification: Comparative Impacts on the Photophysiology of a Temperate Symbiotic Sea Anemone and a Tropical Coral. MSC Thesis. New Zealand: Victoria University of Wellington.
Duffy, J. E. (2006). Biodiversity and the functioning of seagrass ecosystems. Mar. Ecol. Prog. Ser. 311, 233–250.
Edwards, A. J., and Clark, S. (1999). Coral transplantation: a useful management tool or misguided meddling? Mar. Pollut. Bull. 37, 474–487. doi: 10.1016/S0025-326X(99)00145-9
Edwards, A. J., Omori, M., Iwao, K., Dizon, R., Morse, A., Boch, C., et al. (2010). Reef Rehabilitation manual. St Lucia: The Coral Reef Targeted Research an Capacity Building for Management Program.
Endean, R., and Stablum, W. (1971). The apparent extent of recovery of reefs of Australia’s Great Barrier Reef devastated by the crown-of-thorns starfish. Atoll Res. Bull. 168, 1–26.
Epstein, H. E., and Kingsford, M. J. (2019). Are soft coral habitats unfavourable? A closer look at the association between reef fishes and their habitat. Environ. Biol. Fishes. 102, 479–497. doi: 10.1007/s10641-019-0845-4
Erftemeijer, P. L. A., Riegl, B., Hoeksema, B. W., and Todd, P. A. (2012). Environmental impacts of dredging and other sediment disturbances on corals: a review. Mar. Pollut. Bull. 64, 1737–1765. doi: 10.1016/j.marpolbul.2012.05.008
Erni Cassola, G., Pacheco, M. S. C., Barbosa, M. C., Hansen, D. M., and Ferreira, C. E. L. (2016). Decline in abundance and health state of an Atlantic subtropical gorgonian population. Mar. Pollut. Bull. 104, 329–334. doi: 10.1016/j.marpolbul.2016.01.022
Fabricius, G., and De’ath, K. (2001). “Biodiversity on the Great Barrier Reef: large-scale patterns and turbidity-related local loss of soft coral taxa,” in Oceanographic Processes of Coral Reefs: Physical and Biological Links in the Great Barrier Reef, ed. E. Wolanski (London: CRC Press), 127–144.
Fabricius, K. (2011). “Octocorallia,” in Encyclopedia of Modern Coral Reefs: Structure, Form and Process, ed. D. Hopley (Dordrecht: Springer), 740–745.
Fabricius, K., and Alderslade, P. (2001). Soft Corals and Sea Fans: a Comprehensive Guide to the Tropical Shallow Water Genera of the Central-West Pacific, the Indian Ocean and the Red Sea. Townsville, QLD: Australian Institute of Marine Science.
Fabricius, K., De’ath, G., McCook, L., Turak, E., and Williams, D. M. B. (2005). Changes in algal, coral and fish assemblages along water quality gradients on the inshore Great Barrier Reef. Mar. Pollut. Bull. 51, 384–398. doi: 10.1016/j.marpolbul.2004.10.041
Fabricius, K. E. (1997). Soft coral abundance on the central Great Barrier Reef: effects of Acanthaster planci, space availability, and aspects of the physical environment. Coral Reefs 16, 159–167. doi: 10.1007/s003380050070
Fabricius, K. E. (2005). Effects of terrestrial runoff on the ecology of corals and coral reefs: review and synthesis. Mar. Pollut. Bull. 50, 125–146. doi: 10.1016/j.marpolbul.2004.11.028
Fabricius, K. E., and De’ath, G. (2008). Photosynthetic symbionts and energy supply determine octocoral biodiversity in coral reefs. Ecology 89, 3163–3173. doi: 10.1890/08-0005.1
Fabricius, K. E., Langdon, C., Uthicke, S., Humphrey, C., Noonan, S., De’ath, G., et al. (2011). Losers and winners in coral reefs acclimatized to elevated carbon dioxide concentrations. Nat. Clim. Chang. 1, 165–169. doi: 10.1038/nclimate1122
FAO Fisheseries and Aquaculture Department (2016). FAO Aquatic Species Distribution Map of Corallium Rubrum. FAO GeoNetwork. Available online at: http://www.fao.org/geonetwork/srv/en/main.home?uuid=fao-species-map-col (accessed March 12, 2020).
Fautin, D. G. (1992). Anemonefish recruitment: the roles of order and chance. Symbiosis 14, 143–160.
Fautin, D. G., and Allen, G. R. (1997). Anemone Fishes and Their Host Sea Anemones: a Guide for Aquarists and Divers. Perth: West. Aust. Museum, 70.
Fautin, D. G., Guo, C.-C., and Hwang, J.-S. (1995). Costs and benefits of the symbiosis between the anemoneshrimp Periclimenes brevicarpalis and its host Entacmaea quadricolor. Mar. Ecol. Prog. Ser. 129, 77–84.
Fautin, D. G., Malarky, L., and Sobero, J. (2013). Latitudinal diversity of sea anemones (Cnidaria: Actiniaria). Mar. Biol. Lab. 224, 89–98.
Ferrari, R. (2017). The hidden structure in coral reefs. Coral Reefs 36:445. doi: 10.1007/s00338-017-1540-6
Francis, L. (1979). Contrast between solitary and clonal lifestyles in the sea anemone Anthopleura elegantissima. Integr. Comp. Biol. 19, 669–681. doi: 10.1093/icb/19.3.669
Frazão, B., Vasconcelos, V., and Antunes, A. (2012). Sea anemone (Cnidaria, Anthozoa, Actiniaria) toxins: an overview. Mar. Drugs 10, 1812–1851.
Frisch, A. J., Hobbs, J. P. A., Hansen, S. T., Williamson, D. H., Bonin, M. C., Jones, G. P., et al. (2019). Recovery potential of mutualistic anemone and anemonefish populations. Fish. Res. 218, 1–9. doi: 10.1016/j.fishres.2019.04.018
Frisch, A. J., Rizzari, J. R., Munkres, K. P., and Hobbs, J. P. A. (2016). Anemonefish depletion reduces survival, growth, reproduction and fishery productivity of mutualistic anemone-anemonefish colonies. Coral Reefs 35, 375–386. doi: 10.1007/s00338-016-1401-8
Garcia-Rodriguez, M., and Mass, C. (1986). Estudio biometrico de poblaciones de coral rajo (Corallium rubrum L.) dellitoral de Gerona (NE de Espana). Bol. Inst. Esp. Ocean. 3, 61–64.
Garcia-Rodriguez, M., and Massó, C. (1986). Modelo de explotación por buceo del coral rojo (Corallium rubrum L.) del Mediterráneo. Bol. Inst. Esp. Ocean. 3, 75–82.
Garrabou, J., Diaz, D., Ribes, M., Ballesteros, E., Linares, C., and Coma, R. (2006). Consequences of a mass mortality in populations of Eunicella singularis (Cnidaria: Octocorallia) in Menorca (NW Mediterranean). Mar. Ecol. Prog. Ser. 327, 51–60.
Garrabou, J., and Harmelin, J.-G. (2002). A 20-year study on life-history traits of a harvested long-lived temperate coral in the NW Mediterranean: insights into conservation and management needs. J. Anim. Ecol. 71, 966–978.
Garzon-Ferreira, J., and Zea, S. (1992). A mass mortality of Gorgonia ventalina (Cnidaria: Gorgoniidae) in the Santa Marta area, Caribbean coast of Columbia. Bull. Mar. Sci. 50, 522–526.
GBRMPA (2018). Guidelines: Applications for Restoration/Adaptation Projects to Improve Resilience of Habitats in the Great Barrier Reef Marine Park. Queensland, QLD: GBRMPA, 1–15.
Geiser, D. M., Taylor, J. W., Ritchie, K. B., and Smith, G. W. (1998). Cause of sea fan death in the West Indies. Nature 394:138. doi: 10.1038/28076
Geraldi, N. R., Smyth, A. R., Piehler, M. F., and Peterson, C. H. (2014). Artificial substrates enhance non-native macroalga and N2 production. Biol. Invasions 16, 1819–1831. doi: 10.1007/s10530-013-0629-2
Goldberg, J., and Wilkinson, C. (2004). “Global threats to coral reefs: coral bleaching, global climate change, disease, predator plagues, and invasive species,” in Status Coral Reefs World 2004, Vol. I, ed. C. Wilkinson (Townsville, QL: AIMS), 67–92.
Gómez, C. E., Paul, V. J., Ritson-Williams, R., Muehllehner, N., Langdon, C., and Sánchez, J. A. (2015). Responses of the tropical gorgonian coral Eunicea fusca to ocean acidification conditions. Coral Reefs 34, 451–460. doi: 10.1007/s00338-014-1241-3
Gomez, C. G., Guzman, H. M., and Gonzalez, A. (2018). Stability and dynamic properties of octocoral communities in the Tropical Eastern Pacific. Mar. Ecol. Prog. Ser. 588, 71–84. doi: 10.3354/meps12416
Grigg, R. W. (2001). Black Coral: history of a sustainable fishery in Hawai’i. Pacific Sci. 55, 291–299. doi: 10.1353/psc.2001.0022
Hancock, Z. B., Goeke, J. A., and Wicksten, M. K. (2017). A sea anemone of many names: a review of the taxonomy and distribution of the invasive actiniarian Diadumene lineata (Diadumenidae), with records of its reappearance on the Texas coast. Zookeys 2017, 1–15. doi: 10.3897/zookeys.706.19848
Harasti, D. (2016). Declining seahorse populations linked to loss of essential marine habitats. Mar. Ecol. Prog. Ser. 546, 173–181. doi: 10.3354/meps11619
Harasti, D., Martin-Smith, K., and Gladstone, W. (2014). Ontogenetic and sex-based differences in habitat preferences and site fidelity of White’s seahorse Hippocampus whitei. J. Fish Biol. 85, 1413–1428. doi: 10.1111/jfb.12492
Harmelin-Vivien, M. L. (1994). The effects of storms and cyclones on coral Reefs: a Review. J. Coast. Res. 211–231.
Harrison, P. L., Dalton, S. J., and Carroll, A. G. (2011). Extensive coral bleaching on the world’s southernmost coral reef at Lord Howe Island, Australia. Coral Reefs 30:775. doi: 10.1007/s00338-011-0778-7
Heifetz, J. (2002). Coral in Alaska: distribution, abundance, and species associations. Hydrobiologia 471, 19–28. doi: 10.1021/jm00268a001
Hill, R., and Scott, A. (2012). The influence of irradiance on the severity of thermal bleaching in sea anemones that host anemonefish. Coral Reefs 31, 273–284. doi: 10.1007/s00338-011-0848-x
Hiscock, K., Sharrock, S., Highfield, J., and Snelling, D. (2010). Colonization of an artificial reef in south-west Englandex-HMS Scylla. J. Mar. Biol. Assoc. United Kingdom 90, 69–94. doi: 10.1017/S0025315409991457
Hobbs, J. P. A., Frisch, A. J., Ford, B. M., Thums, M., Saenz-Agudelo, P., Furby, K. A., et al. (2013). Taxonomic, spatial and temporal patterns of bleaching in anemones inhabited by anemonefishes. PLoS One 8:e70966. doi: 10.1371/journal.pone.0070966
Hughes, T. P., Kerry, J. T., Álvarez-Noriega, M., Álvarez-Romero, J. G., Anderson, K. D., Baird, A. H., et al. (2017). Global warming and recurrent mass bleaching of corals. Nature 543, 373–377. doi: 10.1038/nature21707
Hughes, T. P., Kerry, J. T., Baird, A. H., Connolly, S. R., Dietzel, A., Eakin, C. M., et al. (2018). Global warming transforms coral reef assemblages. Nature 556, 492–496. doi: 10.1038/s41586-018-0041-2
Inoue, S., Kayanne, H., Yamamoto, S., and Kurihara, H. (2013). Spatial community shift from hard to soft corals in acidified water. Nat. Clim. Chang. 3, 683–687. doi: 10.1038/nclimate1855
Jarrold, M. D., Calosi, P., Verberk, W. C. E. P., Rastrick, S. P. S., Atfield, A., and Spicer, J. I. (2013). Physiological plasticity preserves the metabolic relationship of the intertidal non-calcifying anthozoan-Symbiodinium symbiosis under ocean acidification. J. Exp. Mar. Bio. Ecol. 449, 200–206. doi: 10.1016/j.jembe.2013.09.013
Johnston, E. L., and Clark, G. F. (2007). Recipient environment more important than community composition in determining the success of an experimental sponge transplant. Restor. Ecol. 15, 638–651. doi: 10.1111/j.1526-100X.2007.00276.x
Jones, A. M., Gardner, S., and Sinclair, W. (2008). Losing “Nemo”: bleaching and collection appear to reduce inshore populations of anemonefishes. J. Fish Biol. 73, 753–761. doi: 10.1111/j.1095-8649.2008.01969.x
Jones, R., Bessell-Browne, P., Fisher, R., Klonowski, W., and Slivkoff, M. (2016). Assessing the impacts of sediments from dredging on corals. Mar. Pollut. Bull. 102, 9–29. doi: 10.1016/j.marpolbul.2015.10.049
Kaiser, M. J., Clarke, K. R., Hinz, H., Austen, M. C. V., Somerfield, P. J., and Karakassis, I. (2006). Global analysis of response and recovery of benthic biota to fishing. Mar. Ecol. Prog. Ser. 311, 1–14. doi: 10.3354/meps311001
Karlson, R. H., Hughes, T. P., and Karlson, S. R. (1996). Density-dependent dynamics of soft coral aggregations: the significance of clonal growth and form. Ecology 77, 1592–1599. doi: 10.2307/2265554
Kayal, M., Vercelloni, J., Lison de Loma, T., Bosserelle, P., Chancerelle, Y., Geoffroy, S., et al. (2012). Predator crown-of-thorns starfish (Acanthaster planci) outbreak, mass mortality of corals, and cascading effects on reef fish and benthic communities. PLoS One 7:e47363. doi: 10.1371/journal.pone.0047363
Kleypas, J. A., and Yates, K. K. (2009). Coral reefs and ocean acidification. Oceanography 22, 108–117. doi: 10.5670/oceanog.2009.101
Koehl, M. A. R. (1982). Mechanical design of spicule-reinforced connective tissue: stiffness. J. Exp. Biol. 98, 239–267.
Krueger, C. C., Perkins, D. L., Mills, E. L., and Marsden, J. E. (1995). Predation by alewives on lake trout fry in Lake Ontario: role of an exotic species in preventing restoration of a native species. J. Great Lakes Res. 21, 458–469.
Lambo, A. L., and Ormond, R. F. G. (2006). Continued post-bleaching decline and changed benthic community of a Kenyan coral reef. Mar. Pollut. Bull. 52, 1617–1624. doi: 10.1016/j.marpolbul.2006.05.028
Leal, M. C., Ferrier-Pages, C., Petersen, D., and Osinga, R. (2017). “Corals,” in Marine Ornamental Species Aquaculture, eds I. Olivotto, M. P. Oliver, and G. J. Holt (Hoboken, NJ: John Wiley and Sons), 406–436. doi: 10.1002/9781119169147.ch21a
Linares, C., Coma, R., and Zabala, M. (2008). Restoration of threatened red gorgonian populations: an experimental and modelling approach. Biol. Conserv. 141, 427–437. doi: 10.1016/j.biocon.2007.10.012
Linares, C., Pratchett, M. S., and Coker, D. J. (2011). Recolonisation of Acropora hyacinthus following climate-induced coral bleaching on the Great Barrier Reef. Mar. Ecol. Prog. Ser. 438, 97–104. doi: 10.3354/meps09272
Lindahl, U. (2003). Coral reef rehabilitation through transplantation of staghorn corals: effects of artificial stabilization and mechanical damages. Coral Reefs 22, 217–223. doi: 10.1007/s00338-003-0305-6
Liu, P., Hsin, M., Huang, Y., Fan, T., and Meng, P. (2015). Nutrient enrichment coupled with sedimentation favors sea anemones over corals. PLoS One 10:e0125175. doi: 10.1371/journal.pone.0125175
Llewellyn, D. W., and Shaffer, G. P. (1993). Marsh restoration in the presence of intense herbivory: the role of Justicia lanceolata (Chapm.) small. Wetlands 13, 176–184. doi: 10.1007/BF03160878
Lourie, S. A., and Randall, J. E. (2003). A new pygmy seahorse, Hippocampus denise (Teleostei: Sygnathidae), from the Indo-Pacific. Zool. Stud. 42, 284–291.
Loya, Y., Sakai, K., Yamazato, K., Nakano, Y., Sambali, H., and van Woesik, R. (2001). Coral bleaching: the winners and the losers. Ecol. Lett. 4, 122–131.
Mayer-Pinto, M., Dafforn, K. A., and Johnston, E. L. (2019). A decision framework for coastal infrastructure to optimize biotic resistance and resilience in a changing climate. Bioscience 69, 833–843. doi: 10.1093/biosci/biz092
McConnaughey, R. A., Mier, K. L., and Dew, C. B. (2000). An examination of chronic trawling effects on soft-bottom benthos of the eastern Bering Sea. ICES J. Mar. Sci. 57, 1377–1388. doi: 10.1006/jmsc.2000.0906
McCook, L. J., Jompa, J., and Diaz-Pulido, G. (2001). Competition between corals and algae on coral reefs: a review of evidence and mechanisms. Coral Reefs 19, 400–417. doi: 10.1007/s003380000129
McDonald, T., Gann, G. D., Jonson, J., and Dixon, K. W. (2016a). International Standards for the Practice of Ecological Restoraiton - Including Principles and Key Concepts. Washington, DC: Society for Ecological Restoration.
McDonald, T., Jonson, J., and Dixon, K. W. (2016b). National standards for the practice of ecological restoration in Australia. Restorat. Ecol. 24, S4–S32. doi: 10.1111/rec.12359
McFadden, C. S. (1991). A comparative demographic analysis of clonal reproduction in a temperate soft coral. Ecology 72, 1849–1866. doi: 10.2307/1940983
Michalek-Wagner, K., and Willis, B. L. (2001). Impacts of bleaching on the soft coral Lobophytum compactum. I. Fecundity, fertilization and offspring viability. Coral Reefs 19, 231–239. doi: 10.1007/s003380170003
Midoko-Iponga, D., Krug, C. B., and Milton, S. J. (2005). Competition and herbivory influence growth and survival of shrubs on old fields: implications for restoration of renosterveld shrubland. J. Veg. Sci. 16, 685–692. doi: 10.1111/j.1654-1103.2005.tb02411.x
Montero-Serra, I., Garrabou, J., Doak, D. F., Figuerola, L., Hereu, B., Ledoux, J. B., et al. (2018). Accounting for life-history strategies and timescales in marine restoration. Conserv. Lett. 11, 1–9. doi: 10.1111/conl.12341
Moorhead, J. A., and Chaoshu, Z. (2010). Development of captive breeding techniques for marine ornamental fish: a review. Rev. Fish. Sci. 18, 315–343. doi: 10.1080/10641262.2010.516035
Munday, P. L., Dixson, D. L., Donelson, J. M., Jones, G. P., Pratchett, M. S., Devitsina, G. V., et al. (2009). Ocean acidification impairs olfactory discrimination and homing ability of a marine fish. Proc. Natl. Acad. Sci. U.S.A. 106, 1848–1852. doi: 10.1073/pnas.0809996106
Nagelkerken, I., Blaber, S. J. M., Bouillon, S., Green, P., Haywood, M., Kirton, L. G., et al. (2008). The habitat function of mangroves for terrestrial and marine fauna: a review. Aquat. Bot. 89, 155–185. doi: 10.1016/j.aquabot.2007.12.007
National Academies of Sciences Engineering and Medicine (2019). A Decision Framework for Interventions to Increase the Persistence and Resilience of Coral Reefs. Washington, DC: National Academies Press.
Ng, C. S. L., Lim, S. C., Ong, J. Y., Teo, L. M. S., Chou, L. M., Chua, K. E., et al. (2015). Enhancing the biodiversity of coastal defence structures: transplantation of nursery-reared reef biota onto intertidal seawalls. Ecol. Eng. 82, 480–486. doi: 10.1016/j.ecoleng.2015.05.016
Norström, A. V., Nyström, M., Lokrantz, J., and Folke, C. (2009). Alternative states on coral reefs: beyond coral-macroalgal phase shifts. Mar. Ecol. Prog. Ser. 376, 293–306. doi: 10.3354/meps07815
NSW Fisheries Scientific Committee (2019). Proposed Determination / CAM Assessment for the Cauliflower Soft Coral, Dendronephthya australis. Available online at: http://www.environment.gov.au/system/files/consultations/7167fcfd-3098-4462-9452-01df13864a32/files/dendronephthya-australis.pdf (accessed February 17, 2020).
Opperman, J. J., and Merenlender, A. M. (2000). Deer herbivory as an ecological constraint to restoration of degraded riparian corridors. Restor. Ecol. 8, 41–47. doi: 10.1046/j.1526-100X.2000.80006.x
Oren, U., and Benayahu, Y. (1997). Transplantation of juvenile corals: a new approach for enhancing colonization of artificial reefs. Mar. Biol. 127, 499–505. doi: 10.1007/s002270050038
Parker, J. D., Duffy, J. E., and Orth, R. J. (2001). Plant species diversity and composition: experimental effects on marine epifaunal assemblages. Mar. Ecol. Prog. Ser. 224, 55–67. doi: 10.3354/meps224055
Perkol-Finkel, S., and Benayahu, Y. (2005). Recruitment of benthic organisms onto a planned artificial reef: shifts in community structure one decade post-deployment. Mar. Environ. Res. 59, 79–99. doi: 10.1016/j.marenvres.2004.03.122
Perkol-Finkel, S., and Benayahu, Y. (2007). Differential recruitment of benthic communities on neighboring artificial and natural reefs. J. Exp. Mar. Bio. Ecol. 340, 25–39.
Perkol-Finkel, S., and Benayahu, Y. (2009). The role of differential survival patterns in shaping coral communities on neighboring artificial and natural reefs. J. Exp. Mar. Biol. Ecol. 369, 1–7. doi: 10.1016/j.jembe.2008.09.016
Perkol-Finkel, S., Shashar, N., Barneah, O., Ben-David-Zaslow, R., Oren, U., Reichart, T., et al. (2005). Fouling reefal communities on artificial reefs: does age matter? Biofouling 21, 127–140.
Perkol-Finkel, S., Shashar, N., and Benayahu, Y. (2006). Can artificial reefs mimic natural reef communities? The roles of structural features and age. Mar. Environ. Res. 61, 121–135. doi: 10.1016/j.marenvres.2005.08.001
Poulos, D. E., Gallen, C., Davis, T., Booth, D. J., and Harasti, D. (2016). Distribution and spatial modelling of a soft coral habitat in the Port Stephens-Great Lakes Marine Park: implications for management. Mar. Freshw. Res. 67, 256–265. doi: 10.1071/MF14059
Poulos, D. E., Harasti, D., Gallen, C., and Booth, D. J. (2013). Biodiversity value of a geographically restricted soft coral species within a temperate estuary. Aquat. Conserv. Mar. Freshw. Ecosyst. 23, 838–849. doi: 10.1002/aqc.2362
Precht, W. F., Aronson, R. B., and Swanson, D. W. (2001). Improving scientific decision-making in the restoration of ship-grounding sites on coral reefs. Bull. Mar. Sci. 10, 1001–1012.
Richardson, D. L., Harriott, V. J., and Harrison, P. L. (1997). Distribution and abundance of giant sea anemones (Actiniaria) in subtropical eastern Australian waters. Mar. Freshw. Res. 48, 59–66.
Richardson, L. E., Graham, N. A. J., Pratchett, M. S., and Hoey, A. S. (2017). Structural complexity mediates functional structure of reef fish assemblages among coral habitats. Environ. Biol. Fishes 100, 193–207. doi: 10.1007/s10641-016-0571-0
Rinkevich, B. (2005). Coral reef restoration handbook. Environ. Sci. Technol. 29, 317–318. doi: 10.1201/9781420003796
Robinson, J. P. W., Wilson, S. K., and Graham, N. A. J. (2019). Abiotic and biotic controls on coral recovery 16 years after mass bleaching. Coral Reefs 38, 1255–1265. doi: 10.1007/s00338-019-01831-7
Ruiz-Moreno, D., Willis, B. L., Page, A. C., Weil, E., Cróquer, A., Vargas-Angel, B., et al. (2012). Global coral disease prevalence associated with sea temperature anomalies and local factors. Dis. Aquat. Organ. 100, 249–261. doi: 10.3354/dao02488
Sánchez, J. A., Zea, S., and Díaz, J. M. (1998). Patterns of octocoral and black coral distribution in the oceanic barrier reef-complex of Providencia island, southwestern Caribbean. Caribb. J. Sci. 34, 250–264.
Sandin, S. A., and McNamara, D. E. (2012). Spatial dynamics of benthic competition on coral reefs. Oecologia 168, 1079–1090. doi: 10.1007/s00442-011-2156-0
Santangelo, G., and Abbiati, M. (2001). Red coral: conservation and management of an over-exploited Mediterranean species. Aquat. Conserv. Mar. Freshw. Ecosyst. 11, 253–259. doi: 10.1002/aqc.451
Santangelo, G., and Bramanti, L. (2010). Quantifying the decline in Corallium rubrum populations. Mar. Ecol. Prog. Ser. 418, 295–297. doi: 10.3354/meps08897
Schleyer, M. H., and Celliers, L. (2003). Coral dominance at the reef-sediment interface in marginal coral communities at Sodwana Bay, South Africa. Mar. Freshw. Res. 54, 967–972. doi: 10.1071/MF02049
Schweitzer, C. C., and Stevens, B. G. (2019). The relationship between fish abundance and benthic community structure on artificial reefs in the Mid-Atlantic Bight, and the importance of sea whip corals Leptogorgia virgulata. PeerJ 7:e7277. doi: 10.7717/peerj.7277
Scott, A. (2017). “Sea Anemones,” in Marine Ornamental Species Aquaculture, eds I. Olivotto, M. P. Oliver, and G. J. Holt (Hoboken, NJ: John Wiley and Sons), 437–456.
Scott, A., Hardefeldt, J. M., and Hall, K. C. (2014). Asexual propagation of sea anemones that host anemonefishes: implications for the marine ornamental aquarium trade and restocking programs. PLoS One 9:e109566. doi: 10.1371/journal.pone.0109566
Scott, A., and Hoey, A. S. (2017). Severe consequences for anemonefishes and their host sea anemones during the 2016 bleaching event at Lizard Island, Great Barrier Reef. Coral Reefs 36:873. doi: 10.1007/s00338-017-1577-6
Scott, A., Malcolm, H. A., Damiano, C., and Richardson, D. L. (2011). Long-term increases in abundance of anemonefish and their host sea anemones in an Australian marine protected area. Mar. Freshw. Res. 62, 187–196. doi: 10.1071/MF10323
Sethmann, I., and Wörheide, G. (2008). Structure and composition of calcareous sponge spicules: a review and comparison to structurally related biominerals. Micron 39, 209–228. doi: 10.1016/j.micron.2007.01.006
Shaver, E. C., and Silliman, B. R. (2017). Time to cash in on positive interactions for coral restoration. PeerJ 5:e3499. doi: 10.7717/peerj.3499
Shearer, T. L., Porto, I., and Zubillaga, A. L. (2009). Restoration of coral populations in light of genetic diversity estimates. Coral Reefs 28, 727–733. doi: 10.1007/s00338-009-0520-x
Sherman, C. D. H., and Ayre, D. J. (2008). Fine-scale adaptation in a clonal sea anemone. Evolution 62, 1373–1380. doi: 10.1111/j.1558-5646.2008.00375.x
Shuman, C. S., Hodgson, G., and Ambrose, R. F. (2005). Population impacts of collecting sea anemones and anemonefish for the marine aquarium trade in the Philippines. Coral Reefs 24, 564–573. doi: 10.1007/s00338-005-0027-z
Silliman, B. R., Schrack, E., He, Q., Cope, R., Santoni, A., Van Der Heide, T., et al. (2015). Facilitation shifts paradigms and can amplify coastal restoration efforts. Proc. Natl. Acad. Sci. U.S.A. 112, 14295–14300. doi: 10.1073/pnas.1515297112
Slattery, M., Renegar, D. A., and Gochfeld, D. J. (2013). Direct and indirect effects of a new disease of alcyonacean soft corals. Coral Reefs 32, 879–889. doi: 10.1007/s00338-013-1035-z
Sokolow, S. (2009). Effects of a changing climate on the dynamics of coral infectious disease: a review of the evidence. Dis. Aquat. Organ. 87, 5–18. doi: 10.3354/dao02099
Stoner, A. W., and Lewis, F. G. I. I. I. (1985). The influence of quantitative and qualitative aspects of habitat complexity in tropical sea-grass meadows. J. Exp. Mar. Bio. Ecol. 94, 19–40.
Sutherland, K. P., Porter, J. W., and Torres, C. (2004). Disease and immunity in Caribbean and Indo-Pacific zooxanthellate corals. Mar. Ecol. Prog. Ser. 266, 273–302. doi: 10.3354/meps266273
Sweeney, B. W., Czapka, S. J., and Yerkes, T. (2002). Riparian forest restoration: increasing success by reducing plant competition and herbivory. Restor. Ecol. 10, 392–400. doi: 10.1046/j.1526-100X.2002.02036.x
Teagle, H., Hawkins, S. J., Moore, P. J., and Smale, D. A. (2017). The role of kelp species as biogenic habitat formers in coastal marine ecosystems. J. Exp. Mar. Bio. Ecol. 492, 81–98. doi: 10.1016/j.jembe.2017.01.017
Thomas, L., Stat, M., Kendrick, G. A., and Hobbs, J. P. A. (2015). Severe loss of anemones and anemonefishes from a premier tourist attraction at the Houtman Abrolhos Islands, Western Australia. Mar. Biodivers. 45, 143–144. doi: 10.1007/s12526-014-0242-3
Thrush, S. F., and Dayton, P. K. (2002). Disturbance to marine benthic habitats by trawling and dredging: implications for Marine Biodiversity. Annu. Rev. Ecol. Syst. 33, 449–473. doi: 10.1146/annurev.ecolsys.33.010802.150515
Tratalos, J. A., and Austin, T. J. (2001). Impacts of recreational SCUBA diving on coral communities of the Caribbean island of Grand Cayman. Biol. Conserv. 102, 67–75. doi: 10.1016/S0006-3207(01)00085-4
Tsounis, G. (2005). Demography, Reproductive Biology and Trophic Ecology of Red Coral (Corallium rubrum L.) at the Costa Brava (NW Mediterranean): Ecological Data as a Tool for Management. Rome: Food and Agriculture Organization of the United Nations.
Tsounis, G., Rossi, S., Gili, J.-M., and Arntz, W. (2006). Population structure of an exploited benthic cnidarian: the case study of red coral (Corallium rubrum L.). Mar. Biol. 149, 1059–1070. doi: 10.1007/s00227-006-0302-8
Valdivia, N., and Stotz, W. (2006). Feeding behavior of the porcellanid crab allopetrolisthes spinifrons, symbiont of the sea anemone phymactis papillosa. J. Crustac. Biol. 26, 308–315. doi: 10.1651/c-2607.1
Van Hooidonk, R., Maynard, J., Tamelander, J., Gove, J., Ahmadia, G., Raymundo, L., et al. (2016). Local-scale projections of coral reef futures and implications of the Paris Agreement. Sci. Rep. 6, 1–8. doi: 10.1038/srep39666
van Oppen, M. J. H., Gates, R. D., Blackall, L. L., Cantin, N., Chakravarti, L. J., Chan, W. Y., et al. (2017). Shifting paradigms in restoration of the world’s coral reefs. Glob. Chang. Biol. 23, 3437–3448. doi: 10.1111/gcb.13647
van Oppen, M. J. H., Oliver, J. K., Putnam, H. M., and Gates, R. D. (2015). Building coral reef resilience through assisted evolution. Proc. Natl. Acad. Sci. U.S.A. 112, 2307–2313. doi: 10.1073/pnas.1422301112
Van Woesik, R., De Vantier, L. M., and Glazebrook, J. S. (1995). Effects of cyclone “Joy” on nearshore coral communities of the Great Barrier Reef.”. Mar. Ecol. Prog. Ser. 128, 261–270. doi: 10.3354/meps128261
Veale, A. J., and Lavery, S. D. (2012). The population genetic structure of the waratah anemone (Actinia tenebrosa) around New Zealand. New Zeal. J. Mar. Freshw. Res. 46, 523–536. doi: 10.1080/00288330.2012.730053
Ventura, P., Jarrold, M. D., Merle, P. L., Barnay-Verdier, S., Zamoum, T., Rodolfo-Metalpa, R., et al. (2016). Resilience to ocean acidification: decreased carbonic anhydrase activity in sea anemones under high pCO2 conditions. Mar. Ecol. Prog. Ser. 559, 257–263. doi: 10.3354/meps11916
Verselveldt, J., and Alderslade, P. (1982). Description of types and other alcyonacean material (Coelenterata: Octocorallia) in the Australian Museum. Sydney. Rec. Aust. Museum 34, 619–647. doi: 10.3853/j.0067-1975.34.1982.245
Wareham, V. E. (2009). “Updates on deep-sea coral distributions in the newfoundland and labrador and arctic regions, Northwest Atlantic,” in The Ecology of Deep-Sea Corals of Newfoundland and Labrador Waters: Biogeography, Life History, Biogeochemistry, and Relation to Fishes, eds K. Gilkinson and E. Edinger (Nanaimo, BC: Canadian Technical Report on Fisheries Aquatic Sciences), 2830.
Watabe, N., and Kingsley, R. J. (1992). “Calcification in octocorals,” in Hard Tissue Mineralization and Demineralization, eds S. Suga and N. Watabe (Berlin: Springer), 127–147.
Weil, E., Rogers, C. S., and Croquer, A. (2015). “Octocoral diseases in a changing ocean,” in Marine Animal Forests: the Ecology of Benthic Biodiversity Hotspots, eds S. Rossi, L. Bramanti, A. Gori, S. Orejas, and C. del Valle (Cham: Springer), 1–55.
Weinberg, S. (1979). Transplantation experiments with mediterranean gorgonians. Bijdr. tot Dierkd. 49, 31–41.
Keywords: alcyonaceae, octocoral, soft coral, sea anemone, tropical, temperate
Citation: Steinberg RK, Dafforn KA, Ainsworth T and Johnston EL (2020) Know Thy Anemone: A Review of Threats to Octocorals and Anemones and Opportunities for Their Restoration. Front. Mar. Sci. 7:590. doi: 10.3389/fmars.2020.00590
Received: 13 March 2020; Accepted: 29 June 2020;
Published: 22 July 2020.
Edited by:
Avigdor Abelson, Tel Aviv University, IsraelReviewed by:
John Nicholas Griffin, Swansea University, United KingdomMatthew David Tietbohl, King Abdullah University of Science and Technology, Saudi Arabia
Copyright © 2020 Steinberg, Dafforn, Ainsworth and Johnston. This is an open-access article distributed under the terms of the Creative Commons Attribution License (CC BY). The use, distribution or reproduction in other forums is permitted, provided the original author(s) and the copyright owner(s) are credited and that the original publication in this journal is cited, in accordance with accepted academic practice. No use, distribution or reproduction is permitted which does not comply with these terms.
*Correspondence: Rosemary Kate Steinberg, rosiekstein@gmail.com