- 1Centro Oceanográfico de Santander, Instituto Español de Oceanografía, Santander, Spain
- 2Departamento de Ciencias de la Vida, Universidad de Alcalá, Madrid, Spain
- 3Department of Biological Sciences and K.G. Jebsen Centre for Deep Sea Research, University of Bergen, Bergen, Norway
- 4CIIMAR – Interdisciplinary Centre of Marine and Environmental Research, University of Porto, Porto, Portugal
- 5Centro Oceanográfico de Gijón, Instituto Español de Oceanografía, Gijón, Spain
Deep-sea sponge-dominated communities are complex habitats considered hotspots of biodiversity and ecosystem functioning. They are classified as Vulnerable Marine Ecosystem and are listed as threatened or declining as a result of anthropogenic activities. Yet, studies into the distribution, community structure and composition of these habitats are scarce, hampering the development of appropriate management measures to ensure their conservation. In this study we describe a diverse benthic community, dominated by a lithistid sponge, found in two geomorphological features of important conservation status —Le Danois Bank and El Corbiro Canyon— of the Cantabrian Sea. Based on the analyses of visual transects using a photogrammetric towed vehicle and samples collected by rock dredge, we characterize the habitat and the associated community in detail. This deep-sea sponge aggregation was found on bedrock. It is dominated by one lithistid sponge, Neoschrammeniella aff. bowerbankii (0.2 ind./m2) and further composed of various sponge species as well as of other benthic invertebrates such as cnidarians, bryozoans and crustaceans. Using a non-invasive methodology (SfM – Structure from Motion) and empirical relationships of individuals size and biomass/volume obtained in laboratory for N. aff. bowerbankii, we were able to estimate a total biomass of 41 kg and volume of 39 l of this species in the surveyed area. This approach allows a fine tune methodology for estimating biomass and volume by image-based-observed area avoiding destructive techniques for this species.
Introduction
Sponges constitute an important component of the benthic marine communities, particularly in deeper environments where they play fundamental ecological roles (Pomponi et al., 2019). In areas where they aggregate in high density and biomass they form structurally complex ecosystems (sponge aggregations or grounds) providing habitat, nursery and rearing areas for other organisms often augmenting biodiversity levels locally (Murillo et al., 2012; Beazley et al., 2013; Kazanidis et al., 2016; Hawkes et al., 2019). Due to their filter-feeding capacity, they also contribute significantly to the biogeochemical cycles of carbon, nitrogen and silica (Yahel et al., 2007; De Goeij et al., 2008, 2013; Kutti et al., 2013; Cathalot et al., 2015; Maldonado et al., 2019).
The deep-sea ecosystems of the Cantabrian Sea and particularly of the Le Danois Bank (LDB) and Avilés Canyon System (ACS) have been studied in the scope of several projects (ECOMARG, INDEMARES, SponGES, INTEMARES, among others) of the Spanish Institute of Oceanography (IEO) since 2003. Initially, the projects were focused on the characterization of sediments, bathymetry and water masses dynamics, but further developed into an integrated study of the benthic communities and the trophic ecology of these ecosystems (Cartes et al., 2007; Sánchez et al., 2008, 2017; Preciado et al., 2009).
Many of the studies, focusing on Vulnerable Marine Ecosystems (FAO, 2009) of this area have been carried out over the last years, some of which aimed to characterize the sponge-dominated habitat. Protecting and restoring sponge aggregations are considered crucial from an environmental perspective. They are recognized as singular vulnerable habitats that deserve special research attention and legal protection. Deep-water sponge aggregations are now emerging as a key component of deep-sea ecosystems, creating complex habitats hosting many other species (Hogg et al., 2010; Maldonado et al., 2017). Under particular ecological conditions sponges are able to form concentrations or beds of high abundances building habitats for other species and increasing the biodiversity with respect to surrounding areas, especially the associated fauna of other invertebrate and fish (Bett and Rice, 1992; Klitgaard, 1995; Kunzmann, 1996; Bo et al., 2012; Beazley et al., 2013; Fillinger et al., 2013). These evidences indicate that efforts to close areas dominated by deep-sea sponge aggregations to bottom-tending gears will serve to meet the conservation objectives of the UNGA Resolution 61/105, that drew attention to the importance of the benthic megafauna, in vulnerable marine ecosystems. Despite the ecological importance for the deep-sea, sponge aggregations are poorly mapped and understood (Hogg et al., 2010). This lack of knowledge is most evident in deep-sea species due to the complexity of their study.
In the El Cachucho MPA, that include Le Danois Bank and its intraslope basin, the presence of sponges habitats dominated by the hexactinellids Asconema setubalense and Pheronema carpenteri and more recently demosponges habitats for Phakellia ventilabrum, P. robusta, and Geodia barretti have been described (Sánchez et al., 2008, 2009, 2017; García-Alegre et al., 2014).
In the Avilés Canyon System the presence of habitats characterized by different sponge species was also described (Sánchez et al., 2015). These studies were focused mainly on the identification of diversity hotspots and presence of vulnerable species associated with the habitat “1170 Reefs” defined by the EU Habitat Directive (E.C, 2013), toward the creation of a coherent network of Marine Protected Areas in Spain (Rodríguez-Basalo et al., 2019). As a result of these studies, Le Danois Bank and its intraslope basin were designated as a Special Area of Conservation (SAC) category and the Avilés Canyon System as a Site of Community Importance (SCI) and integrated into the Natura 2000 network.
In the Cantabrian Sea, only one other deep-sea sponge aggregation dominated by Artemisina transiens Topsent, 1890, has been reported and characterized, although in a considerably shallower area (Ríos et al., 2018).
However, due to the need to improve the management that applies in these two areas, it is necessary to identify, map and describe other benthic communities which are in these vulnerable habitats to ensure their protection.
Understanding the community composition and the population structure of key habitat-forming species is critical to understand and quantify their ecological roles, and to assess their resilience to disturbance with the aim to develop appropriate management measures to ensure their conservation.
But nowadays, there are still few studies focused on high resolution mapping techniques to determine the distribution patterns and spatial structure of sponge aggregations in the deep-sea (Klitgaard and Tendal, 2004; Chu and Leys, 2010; Kazanidis et al., 2019; Ramiro-Sánchez et al., 2019). Remotely Operated Vehicles (ROVs) and Remotely Operated Towed Vehicles (ROTVs), which integrate video and still cameras, are increasingly used by the scientific community as a means to explore and characterize deep-sea ecosystems in a non-invasive way. In recent years, applied studies have been carried out for mapping deep-sea sponge aggregations in detail based on visual or quantitative analysis of images acquired from different platforms, i.e., ROVs and AUVs (Beazley et al., 2013; Kutti et al., 2013; McIntyre et al., 2016; Powell et al., 2018; Hawkes et al., 2019; Meyer et al., 2019). The application of quantitative image analysis to deep-sea sponge aggregations habitat characterization, in general terms, has not been used to estimate biomass and population size structure.
In order to increase our knowledge about deep-sea sponges, it is essential to know specimens volume and surface area. These data also contribute to describe the population structure and biomass of species of interest. The access to these data is very difficult in the deep-sea. Most of the available methods are invasive or require removing the organisms from their natural habitat, in order to measure these parameters. Recently, approaches using underwater photogrammetry to create digital models of deep-sea communities are providing non-invasive methods to explore morphometry of individual organisms. Techniques based on Structure-from-motion (SfM) allow the reconstruction of digital, true-scale, 3D model (James and Robson, 2012; Westoby et al., 2012). This photogrammetric approach offers the possibility of creating advanced cartographic products of the ocean floor, such as 3D models and very high spatial resolution orthomosaic, in a fast and low-cost way (Kwasnitschka et al., 2013; McCarthy and Benjamin, 2014). Structure-from-Motion (SfM) techniques have been used to determine surface area and volume of corals and marine sponges from in situ images and compare them to measurements obtained in the lab (Lavy et al., 2015).
In compliance with the EU Habitats Directive, follow-up surveys are being performed to further characterize and monitor the identified habitats including the deep-sea sponge aggregations. Such efforts are linked to the objectives of the EU-funded SponGES project which aims to develop an integrated ecosystem-based approach to preserve and sustainably use vulnerable sponge ecosystems of the North Atlantic. Through the SponGES project some of these areas (Le Danois Bank and Avilés Canyon System in the Cantabrian sea) were revisited since there was evidence that a sponge aggregation dominated by a lithistid sponge species was present. The importance of this group of sponges has been recently highlighted by Maldonado et al. (2015) which reported large aggregations of Leiodermatium pfeifferae (Carter, 1876) with extensive signs of habitat damage in the Mediterranean Sea.
Thus, the main aim of this study was to (a) characterize and describe the habitat of a benthic community dominated by a lithistid sponge, and (b) establish morphometric relationships of this species to estimate population structure parameters from underwater imagery, in the Avilés Canyon System and Le Danois Bank. This study is the first descriptive and quantitative analysis of lithistid aggregations in the Cantabrian Sea and contributes to our understanding of the population structure of this species as well as the composition of the overall benthic community in the area.
Materials and Methods
Study Area
The Cantabrian Sea central area is characterized by a complex topography, including, deep-sea canyons and seamounts, and a very narrow continental shelf. Within it, two small areas have been selected for our study for having high numbers of Neoschrammeniella aff. bowerbankii (Figure 1A).
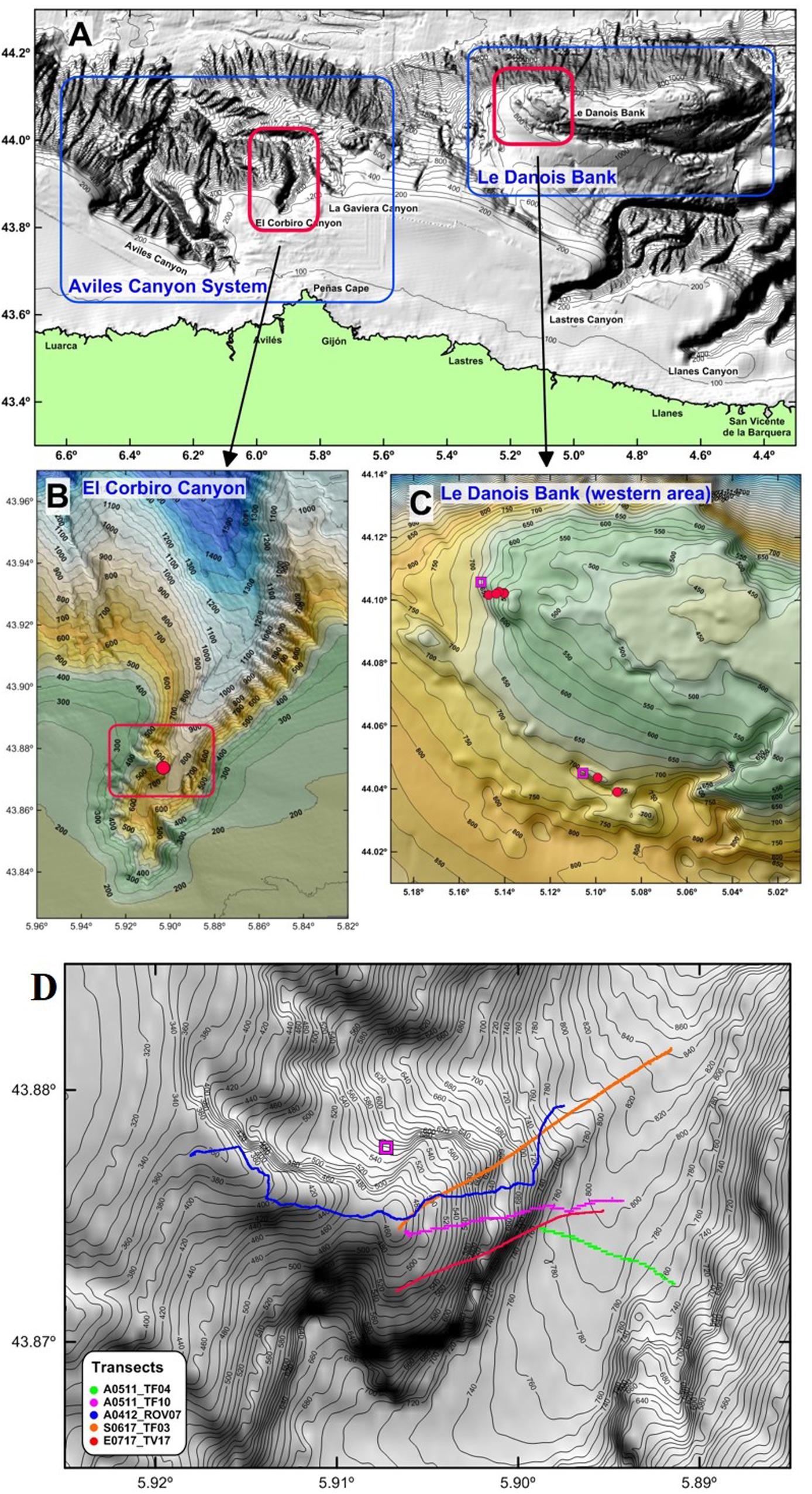
Figure 1. (A) The Cantabrian Sea central area. (B) El Corbiro Canyon and sampling zone. (C) Le Danois Bank western area and sampling stations (squares for rock dredge and dots for ROTV). (D) Video transects in El Corbiro Canyon. In red the transect analyzed with SfM (TV17, ECOMARG_2017).
The western study area corresponds to the so-called El Corbiro Canyon (ECC) (Figure 1B), which forms part of the Aviles Canyon System (ACS). The ACS is a complex region of canyons and valleys comprising three main canyons of different morpho-structural characteristics: Avilés, El Corbiro, and La Gaviera canyons. The ACS has been integrate into the Natura 2000 as a Site of Community Importance (SCI) (Boletín Oficial del Estado, 2014). The continental shelf is generally narrow; the width varies from 12 km (where the head of the Avilés Canyon is incised) to 40 km. The ACS extends from the continental shelf to the abyssal plain, at 4800 m depth, and is controlled by the tectonic regime of the area. The El Corbiro Canyon is characterized by a V-shaped profile and a pronounced axial incision at its head that starts at 176 m depth on the continental shelf. Its head is made up of several gullies, particularly on the eastern wall, that converge to form the main channel (Gomez-Ballesteros et al., 2014). In our study, we have identified a rocky outcrop located on the west side of the canyon head, suitable for the settlement of lithistids. The strong currents over the canyon and his strong slope are responsible for the scarce sedimentary cover in the area.
The second study area is Le Danois Bank (LDB), a large seamount (marginal shelf) located on the Cantabrian Sea central area at 5°W longitude and 44°N latitude (Figure 1A). The Bank presents an elongated form about 72 km long in an E-W direction and about 15 km wide from north to south; it has an almost flat summit with a minimum depth of 424 m, and is separated 25 km from the continental shelf by a deeper intraslope basin (Van Rooij et al., 2010). This structure is a “horst” type, presenting a dissymmetry between its northern and southern flanks. The northern side of Le Danois Bank has a steep continental slope with a relief of 3600 m; its base is located at a 4400 m depth on the Biscay abyssal plain. The sedimentary cover is scarce in the bank summit and particularly in its western flank (Figure 1C), where the rocky outcrops and boulders are quite abundant. Gorgonian forests and deep-sea sponge aggregations are two of the most important habitats of ecological values in the Le Danois Bank and its intraslope basin. These types of habitats are included in the EU Habitat Directive that urges national governments to ensure the conservation and protect these vulnerable ecosystems. In this way, “El Cachucho” became the first Marine Protected Area (MPA) in Spain, and was included in the Natura 2000 network in 2011 (Boletín Oficial del Estado, 2011).
Data Collection
Oceanographic Cruises
Data were collected at 27 stations (Supplementary Table 1) during five expeditions within the framework of the different projects, namely INDEMARES AVILES_0511, ESMAREC_0514, SponGES_0617, ECOMARG_0717 and ECOMARG_2019 (see details in funding). The surveys were carried out onboard the R/V Vizconde de Eza (SGM) in May 2011, the R/V Ángeles Alvariño (IEO) in May 2014, in June and July 2017 and the R/V Ramón Margalef (IEO) in July 2019.
Sampling
Sampling on hard substrate was conducted using a rock dredge RD (80 × 30 cm; 10 mm mesh size net) towed on the seafloor during 5−15 min at a speed of 1.5 knots (Figure 2A).
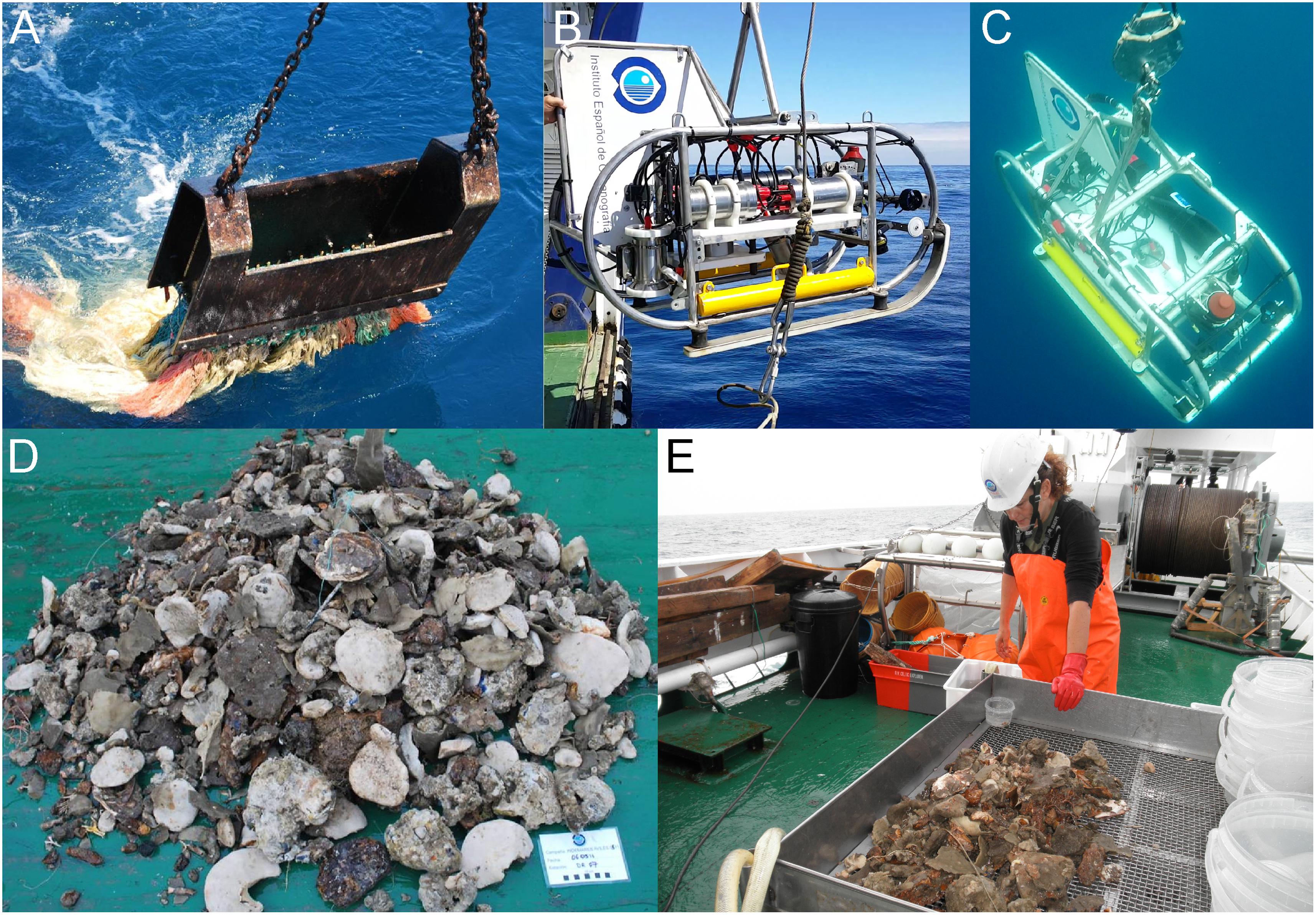
Figure 2. Sampling methods. (A) Rock dredge. (B,C) Photogrammetric towed vehicle. (D) Sample of Neoschrammeniella aff. bowerbankii agreggations on deck. (E) Seaving tower.
The sampling stations to collect sponges (2 on each study area) were selected after studying the data provided by the multibeam echosounder or after previous visualization of images from photogrammetric towed vehicle, following gradient of deep and geological structures: DR7_AVILES0511 and DR4_SponGES0617 in ECC; DR9_SponGES0617 and DR15_SponGES0617 in LDB.
Collected material was sorted onboard, and when large catches were obtained, a representative sub-sample was kept (Figures 2D,E).
The collected biological material was photographed, anesthetized and preserved in 96% ethanol for further study in the laboratory. Identifications were performed to the lowest taxonomic level possible from the analyses of taxon-specific morphological features using specialized literature such us Bowerbank (1866), Sollas (1888), Hooper and van Soest (2002), and Cárdenas et al. (2018) (Porifera); Alder (1856), Stephens and Hickson (1909), Gravier (1920), Zibrowius (1978, 1980), Zibrowius and Cairns (1992), Molodtsova (2006), Cairns and Bayer (2009), Altuna (2013), Altuna and Ríos (2014), Addamo et al. (2016), and Cairns and Taylor (2019) (Cnidaria); Thomson (1872), Kœhler (1896), Mortensen (1935), Cherbonnier (1969), Clark (1980), Paterson (1985), Clark and Downey (1992), Southward and Campbell (2006), Míguez (2009), Manjón-Cabeza et al. (2014), and Fernández-Rodríguez et al. (2019) (Echinodermata); and Jullien and Calvet (1903), Calvet (1907, 1931), Rioja (1931), Zariquiey (1968), D’Hondt (1974), Brunton and Curry (1979), Rouse and Pleijel (2001), Louisy (2002), Wisshak et al. (2009), Velasco et al. (2013), Lloris (2015), Negri and Corselli (2016), and Álvarez-Campos et al. (2018) (others).
A total of 668 organism were sampled (339 in ECC and 329 in LDB) and compared with 6321 underwater images analyzed. In ECC we collected 111 specimens of N. aff. bowerbankii and in LDB we collected 10 specimens.
Spicules on Porifera and other skeletal structures, with taxonomic value, were prepared and cleaned with bleach, distilled water and ethanol. Skeletal arrangement and spicules of Neoschrammeniella aff. bowerbankii were examined with a ZEISS SEM at Bergen University (Suplement A). Taxonomic assignments followed the classification proposed by Morrow and Cárdenas (2015) and the World Porifera Database1 for Porifera, and the World Register of Marine Species (WORMS2) for other invertebrates.
ROTV Underwater Images
The images analyzed in this study were obtained at LDB and ECC during the ESMAREC_0514, SponGES_0617, ECOMARG_0717, and ECOMARG_2019 surveys, using the Remotely Operated Towed Vehicle (ROTV) Politolana (Figures 2B,C). The vehicle can be operated up to a maximum of 2000 m in depth and transects were carried out navigating to 0.8 − 1.0 knot of speed at 2 − 4 m over the sea floor. This ROTV acquires simultaneously still pictures and HD video, and synchronizes it with environmental variables (pressure, temperature, and salinity). The Politolana uses telemetry to send and receive data in real-time from the equipment that composes the monitoring system: altimeter, CTD, positioning system and cameras (Sánchez and Rodríguez, 2013).
The acoustic positioning system Kongsberg HIPAP 502 was used to obtain the absolute position of the underwater vehicle. It is based on Super (Ultra) Short Base Line (SSBL) principle that establishes a three-dimensional position of the transponder. An SSBL system measured the horizontal and vertical angles together with the range to the ROTV. Then, OFOP (Ocean Floor Observation Protocol) software (Huetten and Greinert, 2008) processed the coordinate observation files and merges them with additional sensor data. Finally a complete data set for each ROTV trajectory deployment is obtained allowing georeferencing image data.
The photographs were revised for habitat characterization and description of associated communities. The ROTV Politolana is provided with a Nikon D90 camera with a Subtronic strobe. Four parallel laser beams spaced 25 cm apart and integrated in the still camera provided scale for photographs. Each 10 s the equipment takes a picture, obtaining representative data of the habitat and benthic communities to be characterized (Sánchez and Rodríguez, 2013; Sánchez et al., 2017).
The video-transect was used to generate an orthomosaic and to measure population of N. aff. bowerbankii sizes. It was recorded in July 2017 at ECC during the ECOMARG_2017 survey (TV17), in a range of depths going from 500 to 800 m (Figure 1D). A full-HD video-camera (Sony HD-700-CX) with two LED lights (12600 lumens/6000° Kelvin) attached to the image system. Two parallel laser beams spaced 20 cm apart provided scale for videos and constant distances to validate results. The video transect analyzed in this study was about 450 m long and ran close to steep slope in ECC. The optical sensors shows a portion of around 3 m of the seafloor, this area is referred to as the swath. This footprint varies depending of height over the seafloor and its bathymetry. The area covered and analyzed using this photogrammetric approach was 1450 m2.
Sponge Density Estimation and Faunal Identification
The pictures analyzed are scaled in the PescaWin software (Sánchez, 2015). This allows estimating the surface covered by each photograph and sponge density. In each photograph all macro and megafauna were labeled, and identified to the lowest taxonomic level possible. When the external characteristics made it impossible to identify to the species level, observed specimens were assigned to morphotypes. All records were stored in a database of the IEO that allows multiple data queries on existing species throughout the Central Cantabrian Sea, their geographical location, the type of substrate, water depth, etc.
Lithistid Morphometry
Morphometric parameters such as biomass (drained weight), surface area, perimeter and thickness were measured for 41 specimens of Neoschrammeniella aff. bowerbankii collected at ECC (N = 35) and LDB (N = 6) covering a range of individual’s sizes (Figure 3). Obtained measurements were used to establish size (perimeter) – volume – biomass (drained weight) relationships. The volume was calculated for 25 samples from ECC by measuring the liquid displacement (Jokiel et al., 1978; Hughes, 2005). Weight, surface, area, perimeter and thickness were measured in all samples.
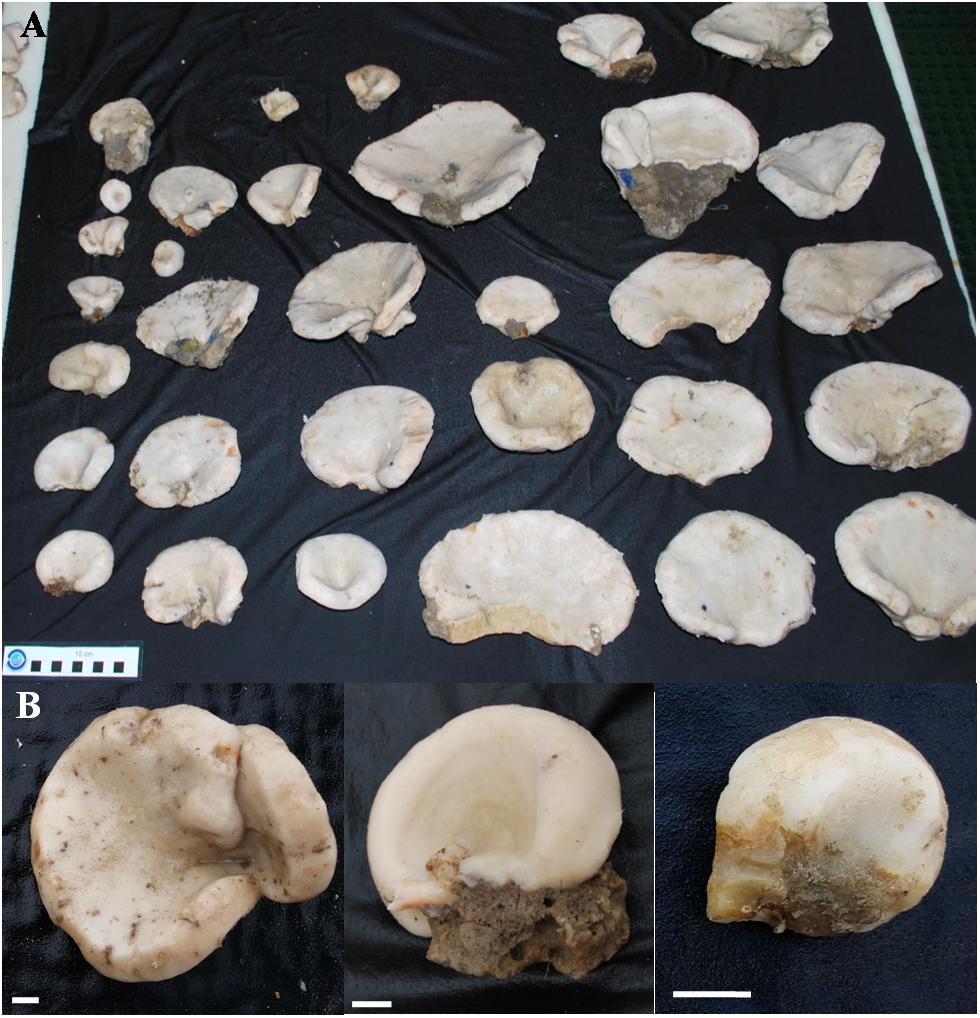
Figure 3. Samples collected with Rock Dredge. (A) In El Corbiro (INDEMARES AVILES_0511 cruise). (B) In Le Danois Bank (SponGES_0617 cruise). Scale bar 1 cm.
Image Morphometry
The measurement of the morphometry of N. aff. bowerbankii specimens was made using an orthomosaic of images covering 450 m of video-transect. This orthomosaic is obtained using a photogrammetric reconstruction approach. This methodology was done decomposing video-sections in thousands of geo-positioned overlapping images processed using photogrammetric Pix4D Mapper Pro software (Pix4D SA, Switzerland). Pix4D uses the Structure-from-Motion (SfM) approach. SfM is the process of estimating the 3-D structure of a scene from a set of 2-D images. It requires point correspondences between images and finds corresponding points by matching features. Pix4D software also uses a dense image matching, an automated process based on dense image matching technology (Tola et al., 2010). Integration of the point measurements, camera calibration, and the position data given by the cameras, the software provides 3D dense point clouds (Figure 4), Digital Surface Models (DSM) and orthomosaics. Since all the information is geo-referenced in a cartographic system (UTM-WGS84), all the geographic layers obtained can be included in a GIS environment thus allowing the subsequent morphometric analysis.
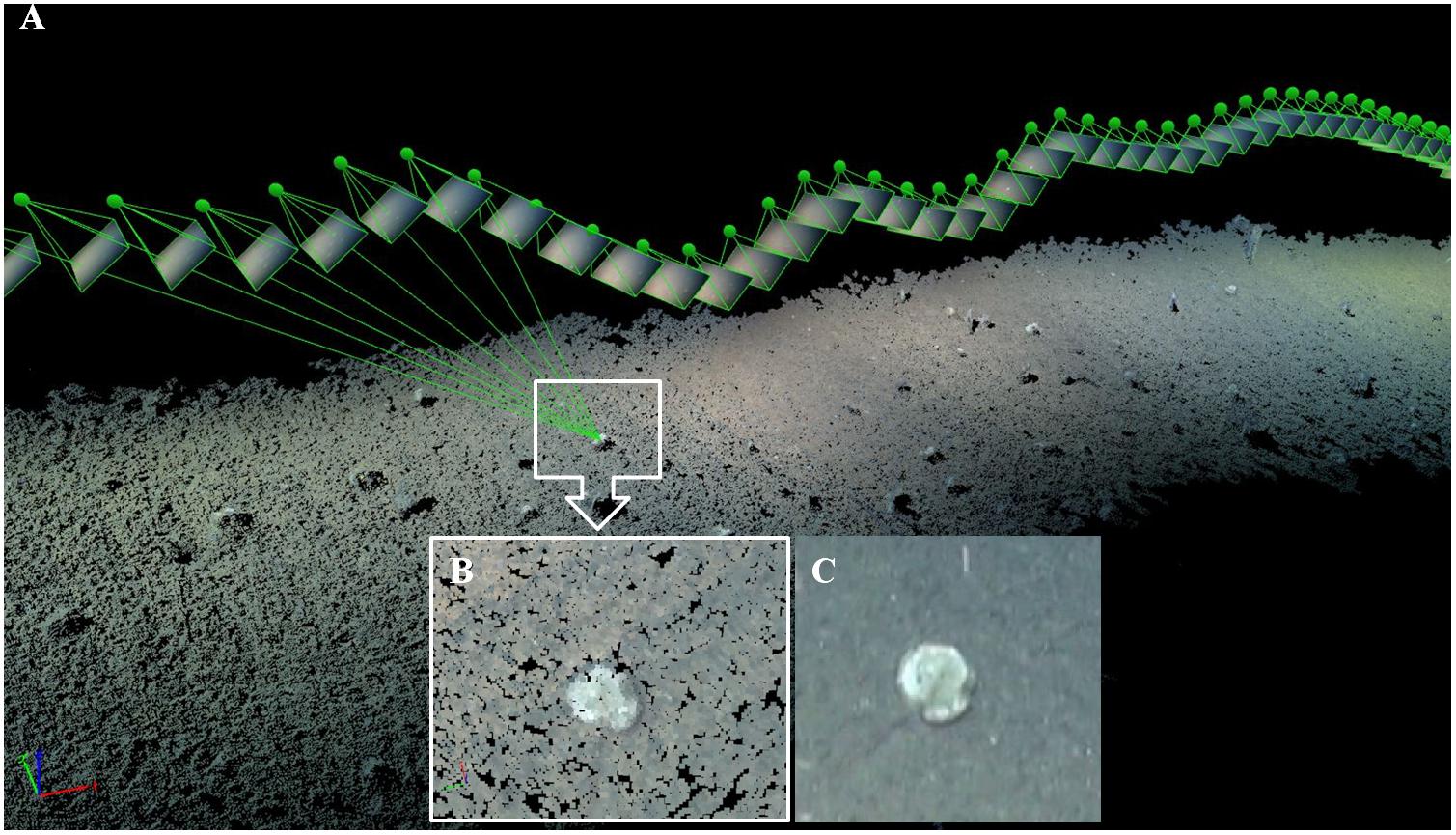
Figure 4. Sampling methods. Video Transect: 3D reconstruction of video transect using Pix4D Mapper Pro. (A) Decomposing video-sectioning thousands of geo-positioned overlapping images processed. Green points show the camera’s position. (B) Zoom 3D point cloud. (C) Zoom original video-frame.
Constant distances between laser pointers projected on the frames were used to evaluate the reconstruction of the geometric model. In this way, the geometric uncertainty of the model and errors associated to measurements over the orthomosaic were estimated.
Thousand five hundred video-frames were used as image input in the photogrammetric adjustment of the 3D block, only 11 images were discarded. The size of 203 specimens of N. aff. bowerbankii were measured; the area covered by each specimen was selected as a suitable parameter of size and the area covered by the video section was also measured. The direct measurement of this parameter is possible using a complete orthomosaic with a very high spatial resolution (0.16 cm/pixel) of the area. Using the QGis software, the area enclosed within each lithistid’s perimeter was calculated (Figure 5).
These image-based perimeter data were compared with in situ data measured in the laboratory for evaluation of the equivalence of the samples. To verify this similarity, the Mann Whitney test was used. This test can be used to investigate whether two independent samples from different populations have the same distribution. Then, empirical relationships between in situ morphometric (perimeters) and biological parameters (drained weight biomass and volume) were established for this species. This empirical relationship can be applied to infer biomass from image morphometric data.
Results
Here, we report the discovery of a benthic community dominated by a lithistid sponge in two areas of the Cantabrian Sea, providing a characterization of this community and its habitat. We also established the empirical relationship for Neoschrammeniella aff. bowerbankii surface area with drained weight biomass and volume, improving our knowledge in the relationship between 2D and 3D metrics for this species, in addition to inferring biomass data per unit of surface present in a specific area or aggregation both in weight and in volume occupied by the specimens.
We annotated 509 specimens seen in the videos at 23 stations, between 486 and 672 m depth and we collected 121 samples at 4 stations of RD, between 551 and 695 m depth (Supplementary Table 1).
Benthic Community Composition
In these study cases, 3691 animals belonging to eight Phyla were observed (Figure 6). In the El Corbiro Canyon area, 20 sponge morphotypes were identified from the video and photographs of the photogrammetric towed vehicle. These included the habitat-forming lithistid sponge Neoschrammeniella aff. bowerbankii, Axinellida (Phakellia robusta and P. hirondellei and other fun shape sponges), Tetractinellida, (Pachastrella monilifera, P. ovisternata, P. cf. nodulosa), Verongiida (Hexadella sp.), Halichondriidae (Topsentia sp.), Desmacellida (Desmacella spp.), Haplosclerida and Poecilosclerida (Hymedesmia (Hymedesmia) paupertas, and other Hymedesmia spp.) and other small or encrusting sponges (see Table 1). We also recorded other invertebrates, including the solitary Scleractinian Desmophyllum spp., the black coral Parantipathes hirondelli, the echinoids Cidaris cidaris and Araeosoma fenestratum, the echiuran Bonellia viridis, the asteroid Henricia sp. and the cnidarians Cerianthus spp. However, there were a number of species not easily distinguished and impossible to identify only by video.
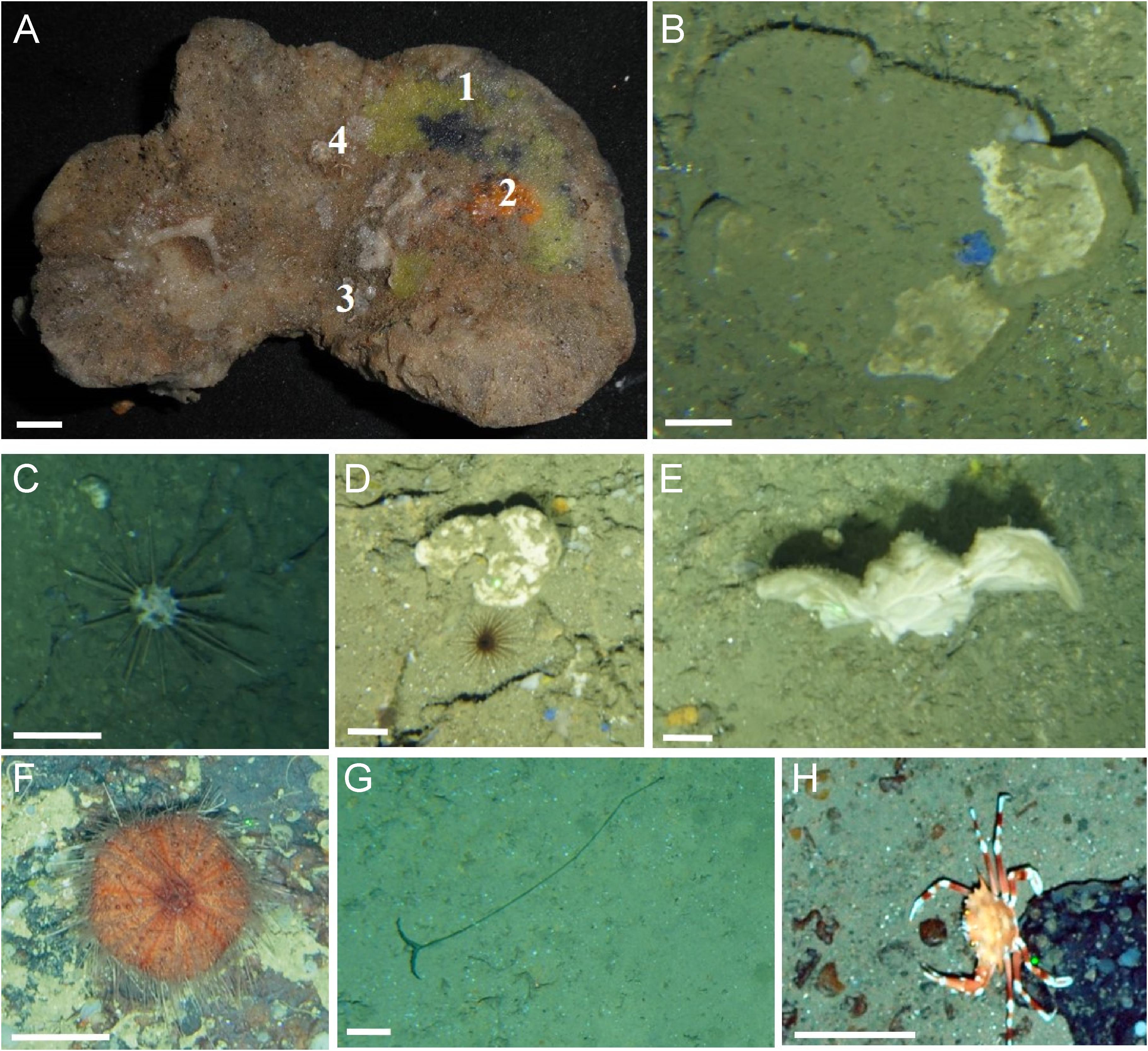
Figure 6. Associated fauna in El Corbiro (A,B) and Co-occurrent fauna, species that structure the Neoschrammeniella aff. bowerbankii community (C–H). (A) Dead specimen on which different species live, (1) Hexadella sp. (2) Hymedesmia sp. (3) Desmacella sp. (4) Disporella sp. (B) Hymedesmia (Hymedesmia) paupertas. (C) Cidaris cidaris. (D) Cerianthus sp. (E) Phakellia hirondellei. (F) Araeosoma fenestratum. (G) Bonellia viridis. (H) Bathynectes maravigna. Scale bar (A): 1 cm. (C–H): 10 cm. (B–G): 5 cm. El Corbiro (A–E). Le Danois Bank (F–H).
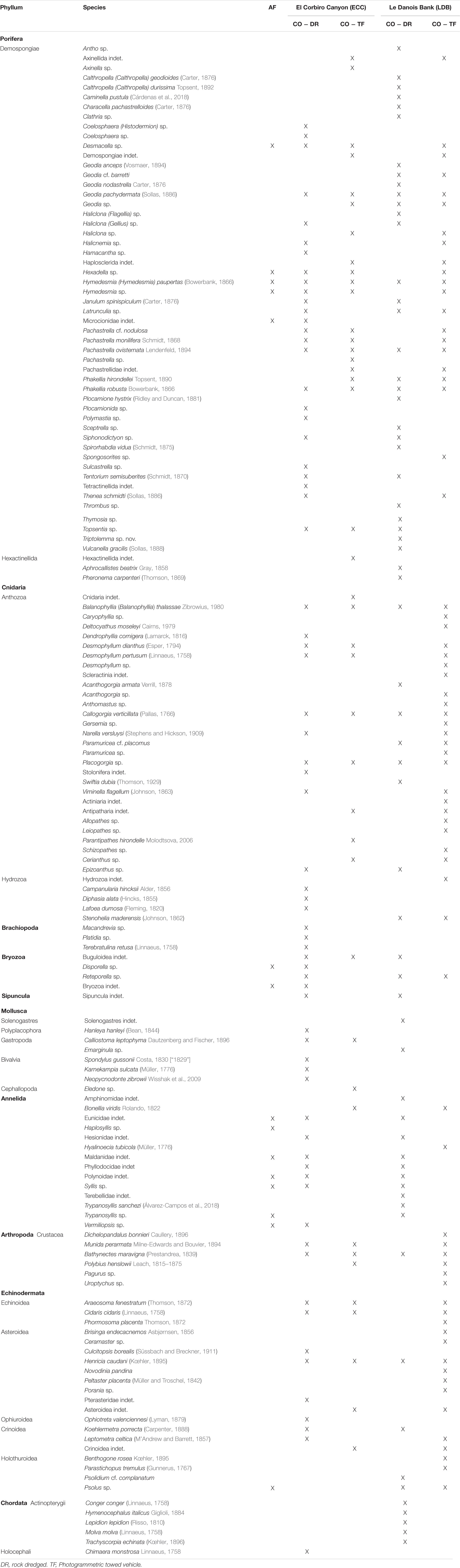
Table 1. Species in associated fauna (AF) and co-occurrence (CO) in populations of Neoschrammeniella aff. bowerbanki in El Corbiro Canyon (SCA) and Le Danois Bank (LDB).
In Le Danois Bank, several species of Demospongiae and Hexactinellida were present alongside N. aff. bowerbankii contributing to the 3D structure of the community. Analysis of the samples collected and annotation of the video transects confirmed that there are a number of erect, massive or encrusting species that play an important role because of their size, volume or abundance: erect and fan–shaped species as Phakellia hirondellei and Phakellia robusta; massive Tetractinellida, with three large Geodiid species: Geodia cf. barretti, G. pachydermata, Geodia sp. and the presence of three species of the genus Pachastrella were frequently recorded. In this area, we also collected other Tetractinellida such as Characella pachastrelloides, Calthropella (Calthropella) geodioides and Calthropella (Calthropella) durissima these last ones smaller than the previous ones. Hymedesmia (Hymedesmia) paupertas is one of the most common species recognizable using ROTV by its characteristic encrusting shape and bright blue color. The Hexactinellid Aphrocallistes beatrix was also detected not forming a biogenic framework, but as solitary individuals. Some specimens of Pheronema carpenteri were observed but in soft bottons near the N. aff. bowerbankii community.
In ECC, we collected more cnidarians (colonies or individuals) (44 samples) than in LDB (9 samples), where the most abundant are two different species of Cerianthus Anthozoa, the scleractinian Desmophyllum spp. and Balanophyllia (Balanophyllia) thalassae, the Alcyonacea Callogorgia verticillata, Paramuricea cf. placomus and Anthomastus sp., these two only present in LDB.
Including samples collected with RD and analyzed images with ROTV. The species richness is very similar in both areas (Figure 7), but slightly higher in LDB for almost all the taxonomic groups, with the phylum Porifera being dominant (35.44% ECC – 38.64% LDB), and consisting nearly entirely of species belonging to the class Demospongiae. As for Cnidarians (17.72% ECC – 20.45% LDB), they are also found in both areas, but images show that they are more frequent in the surrounding areas than in the habitat occupied by the sponges. Mollusca (6.33% ECC – 2.27% LDB), Crustacea (3.8% ECC – 5.69% LDB); Annelida (12.66% ECC – 12.5% LDB) and Echinodermata (12.66% ECC – 14.77% LDB) are other groups well represented in the area, with high density of the taxon Echinoidea.
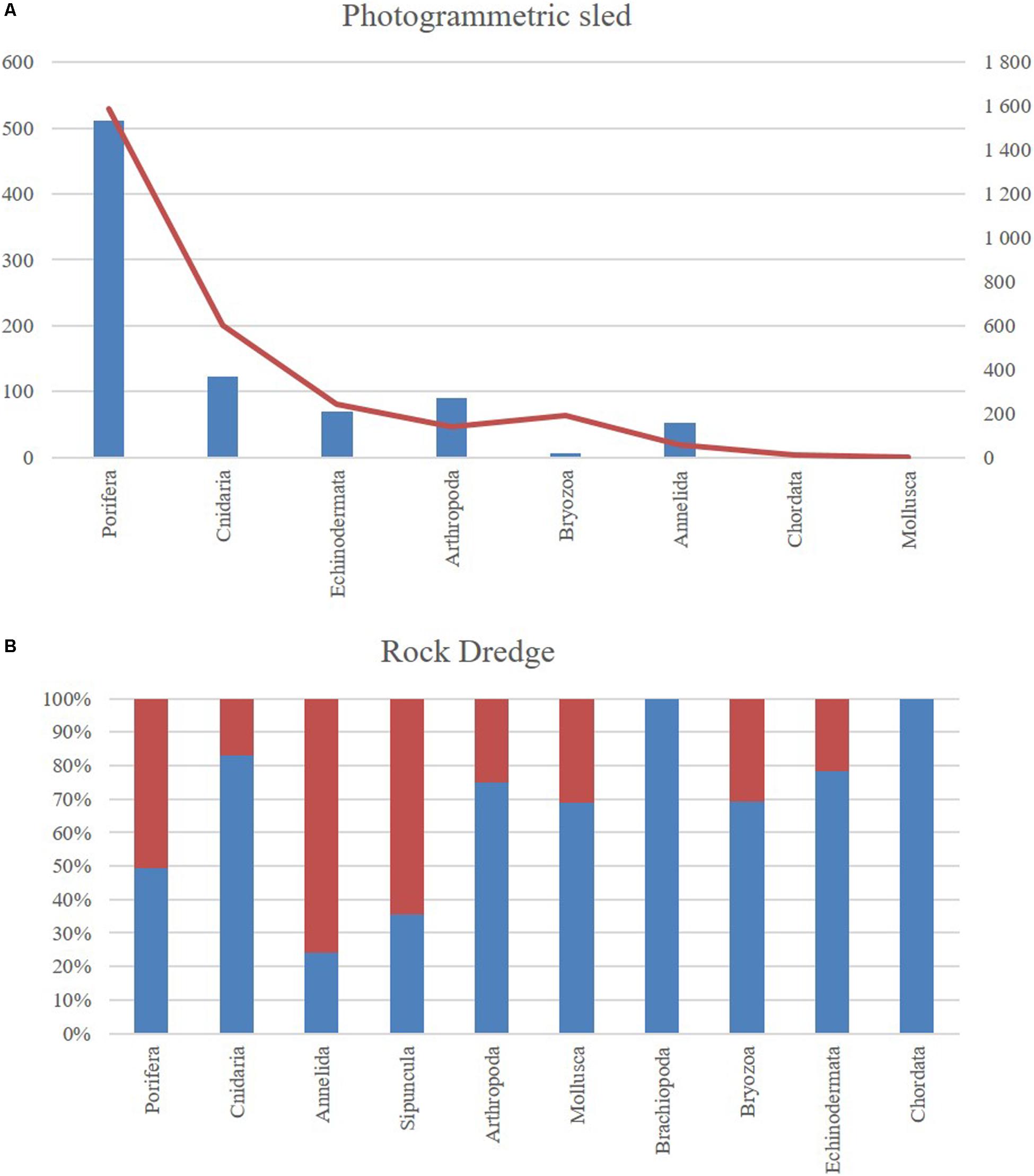
Figure 7. Richness by study area. (A) In images analyzed. (B) Samples collected by RD. In blue El Corbiro Canyon. In red Le Danois Bank.
The presence of Brachiopoda (5 ECC) and Sipuncula (5 ECC – 9 LDB) was only detected with the specimens collected by the RD.
Habitat Characterization
In the two Cantabrian Sea areas we studied, habitats have a similar structure and taxonomic composition with only slight differences. In ECC the aggregation of Neoschrammeniella aff. bowerbankii occurs on the west wall (Figures 1B,D) attached by their bases on a rocky bottom with a steep slope between 520 and 760 m in depth (Figure 8A).
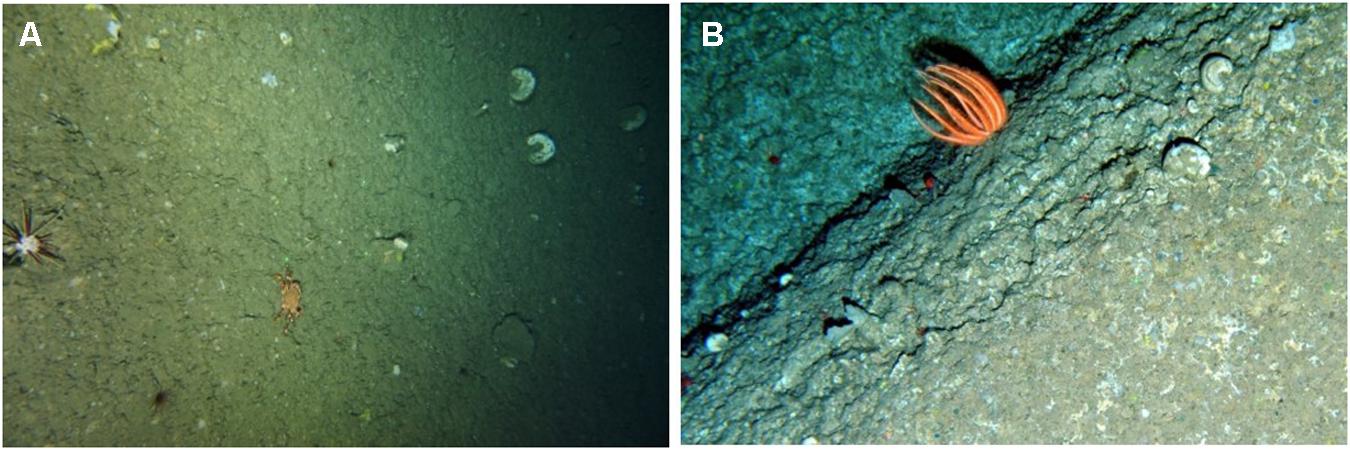
Figure 8. Population of N. aff. bowerbankii. (A) In El Corbiro Canyon (TF17, ECOMARG_0717). (B) In Le Danois Bank (TF9, ESMAREC_0514).
The massive skeletons have a very hard consistency neither disaggregate nor easily dissolved after sponge death; they persist attached to the bottom and they are an available substrate for other organisms. Sponge densities estimated by image analysis were 0.43 indiv./m2 in the TF17_ECOMARG_0717 transect (ECC) and 0.05 indiv./m2 in the TF9_ESMAREC_0514 transect (LDB). Individuals were not clustered nor densely aggregated as in other deep-sea sponge aggregations. They presented an average distance of 79.6 cm between individuals (with minimum distances of 8.30 and maximum distances of 212.40 cm).
In LDB, the aggregation occurs mainly on the rocky ridges of the western and SW flanks of the seamount (Figure 1C), at 477–760 m in depth (Figure 8B). The substrate type is quite different in both areas. In ECC, we find that the area is continuous and well defined and is formed by bedrock covered with a thin layer of mud, with a slope range o 45−60°. At LDB, facies are formed by rock ridges, large boulders and mixed sediments producing a more patchy habitat both along the seamount and inside our study area. The water temperature at the seafloor during the survey periods was 10.30−10.86°C in ECC and 10.50−11.14°C in LDB. The salinity ranged between 35.56−35.72 ppm in ECC and 35.59−35.66 ppm in LDB.
Neoschrammeniella aff. bowerbankii Morphometry
The form of the collected samples in the Cantabrian Sea is a small cup in the case of the younger specimens and the shape of a round tray with undulated margin in that of the adults. Dimensions (height, width) varied between 3.1 × 4.1 in the smallest and 19.5 × 19.6 in the largest collected specimens, with wall thickness ranging from 1.2 to 2.8 cm. The perimeter varies from 10.10 to 64.7 cm.
Morphometric parameters (perimeter, thickness, weight, and volume) obtained in the laboratory for 41 specimens allowed us to establish logarithmic morphometric relationships, with high adjustment for perimeter – drained weight (R2 = 0.93) and perimeter – volume (R2 = 0.91) for Neoschrammeniella aff. bowerbankii (Figures 9B,C).
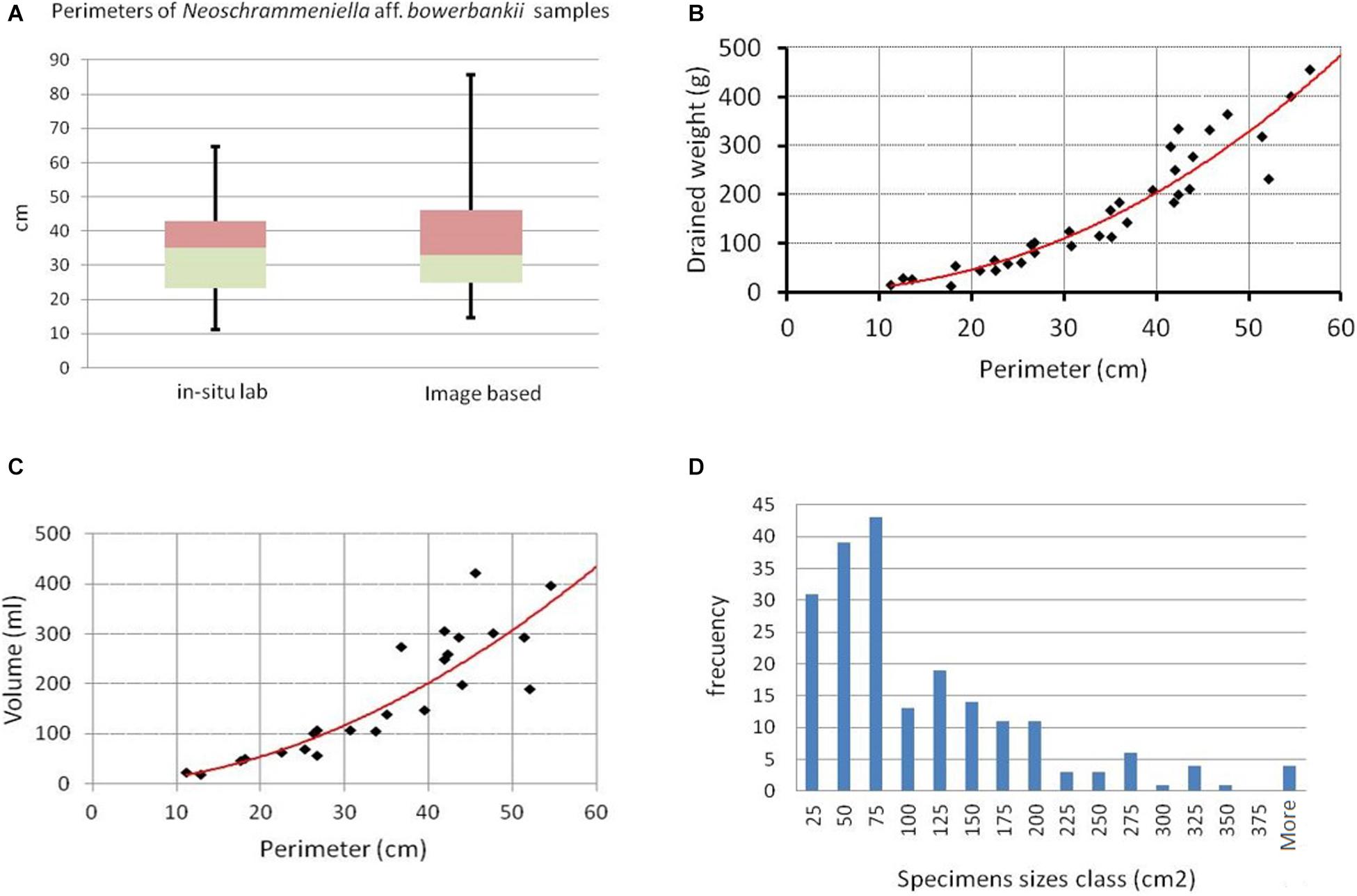
Figure 9. (A) Perimeters of samples measured (lab vs. Image). (B) Relation Perimeter-Weight. (C) Relation Perimeter-Volume. (D) Population size distribution.
The size of 203 Neoschrammeniella aff. bowerbankii specimens were obtained through manual digitalized perimeter and area enclosed measurements. Both specimens measured in the laboratory and those measured on the image orthomosaic belonged to the same study area, the El Corbiro Canyon, in ACS, at two different stations, separated at 678m.
The lithistid perimeter data of the two samples sets (measured in the lab and from the images) have been compared to verify their similarity, using Mann Whitney test (Figure 9A). The result shows that U-value is 3352; the Z-Score is 0.53167 and the p-value is 0.59612. Subsequently, we cannot reject the null hypothesis at 5% significance, indicating not significant differences between the obtained measurements.
The density of this species as assessed by the video is 0.2 indiv./m2. Using the surface data enclosed in the perimeters of the lithistids measured by digitizing on the orthomosaic image, a histogram with the population size distribution of N. aff. bowerbankii was obtained showing that specimens range from 7 to 497 cm2 (Figure 9D). Applying the relationships established with the in situ samples and empirical lab measures, we can infer the drained weight biomass and volume of each of the copies of N. aff. bowerbankii measured in the images and the total weight (41.13 kg) and volume (39.34 l) of the aggregation of lithistids present in the area, without the need for extractive sampling.
Discussion
The biology and ecology of present-day lithistid fauna remain poorly known (Carvalho et al., 2015; Maldonado et al., 2015). With this study we focus on the knowledge of lithistid aggregation in the Cantabrian Sea at two Natura 2000 sites.
Deep-sea sponge aggregations have been considered a type of habitat under the OSPAR Convention for the Protection of the Marine Environment of the North-East Atlantic (Christiansen, 2010). The document mentions grounds of Astrophorids and Hexactinellida, and in particular the genera Geodia and Pheronema, which are the predominant habitat-forming species in the NE Atlantic. Hogg et al. (2010), in the frame of United Nations Environment Programme World Conservation Monitoring Centre (UNEP-WCMC), report outlines of what is known about deep-water sponge grounds habitats and Maldonado et al. (2015), reported a distinct reef-like type from the Mediterranean, the monospecific formation built by the lithistid demosponge Leiodermatium pfeifferae. Other regions where lithistids dominate the fauna are the tropical and subtropical Atlantic (Pomponi et al., 2001; Maldonado et al., 2017) and the south of New Caledonia (Lévi, 1991) and New Zealand (Kelly, 2007; Kelly et al., 2007).
The study area is subject to some management measures in the context of the Natura 2000 Framework, the “El Cachucho” MPA, that include Le Danois Bank, and the Site of Community Importance (SCI) Avilés Canyon System. For both Natura 2000 areas the main environmental value that has conditioned its protection policies is the presence of the habitat “1170 Reefs” of the European Habitat Directive (E.C, 2013). The sponge aggregations located on hard bottoms are included in this classification of vulnerable habitats on which it is mandatory for the European countries to establish conservation measures. In this sense, the knowledge of the habitats structured by lithistids sponges is essential to provide managers with indicators for monitoring the degree of the habitat’s recovery in order to know the success of management measures and to ensure their adequate protection. Within the implementation of Marine Strategy Framework Directive (MSFD, E.C, 2008) it would be good to monitor those indicators that could help assess the environmental status of this vulnerable habitat. The methodologies described in this work will facilitate future monitoring of the MPAs using different indicators within the descriptor “Biological Diversity,” such as the species distribution, the size and condition of their populations and some indicators in relation of deep-sea sponge aggregations (as distributional range, pattern and area covered) and condition of the typical species and communities (Borja et al., 2011, 2014). Among the indicators of “Sea-floor Integrity” descriptor of MSFD (Rice et al., 2012), the most suitable are estimates of the type, abundance, biomass and the real extent of the biogenic substrate as well as all those indicators related to benthic community condition such as the presence of species on the seabed particularly sensitive and/or tolerant to human activities. Considering just those indicators in successive surveys and applying ground-truth sampling and photogrammetric techniques in representative biotopes of deep-sea sponge aggregations (using fixed sampling stations), the monitoring could be affordable and provide reliable results (Sánchez et al., 2017).
On the basis of the environmental characteristics required for the settlement of these sponges it is possible to obtain high resolution maps of their spatial distribution and identify potential impacts of anthropogenic uses. These approaches have already been used successfully in the study area by providing detailed maps needed for effective protection of gorgonians forest, deep-sea sponge aggregations and cold-water corals (Sánchez et al., 2014, 2017; Rodríguez-Basalo et al., 2019). The present study of the lithistids sponges should be taken into consideration for the design of the new management plan of the ACS.
The approach presented here to obtain the size of sponges demonstrate the consistency and reliability of SfM methodology. This is especially relevant in deep-sea environments where the access to the sizes of species is limited. The obtained results, giving a quadratic mean error of 0.10 cm in distance (using laser bean constant distance) and a retroprojection error of 0.154 pixels in image block adjustments, show very low values of geometric uncertainty, which validate the application of this approach for the measurement of parameters related to the size of sessile organism living on the sea bottom. Mean retroprojection errors values of less than 2 pixels were assumed as indicators of the effectiveness of SfM programs for creating highly accurate 3D reconstructions of underwater habitats (Burns and Delparte, 2017).
The relationship between surface area (2D) and volume (3D) metrics estimated using SfM, are particularly relevant when estimating the ecosystem services and functions performed by corals (House et al., 2018).
Surface area can be used as an indicator of structural habitat availability, which is an important ecosystem service of coral reefs and sponges (Santavy et al., 2013). The SfM technique shows a great potential for characterization and monitoring habitats and benthic communities. Thanks to the non-destructive nature it can be used in vulnerable habitats and protected areas, across multiple depths, scales, and reef types (Bythell et al., 2001; Cocito et al., 2003; Courtney et al., 2007; Burns et al., 2015a, b; Prado et al., 2019; Price et al., 2019). The high resolution cartographic outputs can increase the speed, scale and accuracy of species morphometric assessment. In addition, the methodologies based on SfM are quantitative and replicable, so these techniques, therefore allows detailed, spatially explicit observation of community change through time rather than purely typical qualitative descriptions (Ferrari et al., 2016).
“For sponges (Porifera), the amount of food available is directly proportional to the amount they can pump. All the mechanisms which could lead to induced current, depend on the flow regime around the sponge, the morphology of the sponge (mound-shaped or cylindrical) and the direction the apertures open into the flow” (Leys et al., 2011). It is, therefore key to fine-tune methodologies to evaluate the shape and size of the specimens of the different species. The relationship of these parameters to filtration rates is a complex matter in the deep-sea that has so far barely been addressed, with the exception of some experiments conducted with monitoring hoods placed on-site (Yahel et al., 2007; Maldonado et al., 2012, 2017; De Goeij et al., 2017). The progress in relating size (surface area) to volume and even biomass measurements (weight or volume) is essential to be able to understand basic processes of the biology of the species.
The accuracy of the adjustments obtained in the ratio of the size measures (perimeters) to weight and volume (R2 greater than 0.9 in both cases) make us consider a robust and logical relationship between the size of the specimens and the biomass and volume they provide in the habitat. These aspects are key not only for sponge aggregations but also for coral reefs, in this case with morphotype specific conversion parameters, the surface area and volume scale consistently with planar area (House et al., 2018) based on the SfM method. But this approach is still rarely mentioned in literature.
Photogrammetry provides accurate estimates of the surface and perimeters and the establishment of the relationship between in situ measurements and image-based measurements describing a relationship between lab conenchymal surface versus mesh surface calculation for Paramuricea clavata (Palma et al., 2018) but no known similar approximation has been made for lithistid sponges aggregations.
Common methods for measuring surface, area and volume of marine organisms include water displacement (Jokiel et al., 1978) and paraffin dipping (Stimson and Kinzie, 1991; Veal et al., 2010) and require in all cases the extraction of the specimens from seafloors. The use of extractive methodologies is usually banned for highly vulnerable species or in areas of special conservation. It is thus very important to develop and validate non-invasive methodologies to obtain morphometric parameters of the different species and subsequently, establish the empirical relationships between biological characteristics and the morphometry of the specimens. The morphometric measurements of a species can be determined using non-invasive methods and by applying obtained empirical relations, important information can be inferred from the biology of highly unknown benthic species.
Data Availability Statement
The datasets generated for this study can be found at: https://doi.pangaea.de/10.1594/PANGAEA.910127.
Author Contributions
All the authors conceived and designed the study. EP, PR, and FS analyzed the data. EP, FS, PR, and AR-B contributed to the photogrammetry and structure from motion techniques. FS and JC led the ship surveys. PR, EP, and AR-B processed the video and image material. FS, JC, and JX acquired the funding. FC, AR-B, PR, and TI identified the fauna. All the authors helped to collect process and map field data, prepared the figures and tables, reviewed drafts of the manuscript, and helped to writing the manuscript.
Funding
This research has been performed in the scope of the SponGES project, which received funding from the European Union’s Horizon 2020 Research and Innovation Programme under grant agreement no. 679849. This study was partially funded by the European Commission LIFE + “Nature and Biodiversity” call, and included in the INDEMARES (07/NAT/E/000732) and INTEMARES (LIFE15 IPE ES 012) projects. The Biodiversity Foundation, of the Ministry of Environment, was the institution responsible for coordination these projects.
Conflict of Interest
The authors declare that the research was conducted in the absence of any commercial or financial relationships that could be construed as a potential conflict of interest.
Acknowledgments
This study was made possible thanks to the invaluable work of all the participants in the five surveys involved and the crews of the R/Vs. Vizconde de Eza (SGPM), Ramón Margalef (IEO), and Angeles Alvariño (IEO). We appreciate the helpful assistance of Álvaro Altuna, Eugenia Manjón, Sergio Taboada, and Serge Gofas which helped identify associated fauna. We would like to thank Elena Isla for the careful revision of English, as well as to two reviewers for their valuable comments on the manuscript. We are especially grateful to Ines Fernández, Alejandra Calvo, and Cristina Boza for their help and technical support in the laboratory.
Supplementary Material
The Supplementary Material for this article can be found online at: https://www.frontiersin.org/articles/10.3389/fmars.2020.00578/full#supplementary-material
Footnotes
References
Addamo, A. M., Vertino, A., Stolarski, J., García-Jiménez, R., Taviani, M., and Machordom, A. (2016). Merging scleractinian genera: the overwhelming genetic similarity between solitary Desmophyllum and colonial Lophelia. BMC Evolut. Biol. 16:108. doi: 10.1186/s12862-016-0654-8
Alder, J. (1856). A notice of some new genera and species of British hydroid zoophytes. Ann. Magaz. Nat. History 18, 12–14. doi: 10.1080/00222935608697652
Altuna, A. (2013). Scleractinia (Cnidaria: Anthozoa) from ECOMARG 2003, 2008 and 2009 expeditions to bathyal waters off north and northwest Spain (northeast Atlantic). Zootaxa 3641, 101–128. doi: 10.11646/zootaxa.3641.2.1
Altuna, A., and Ríos, P. (2014). Scleractinia (Cnidaria: Anthozoa) from INDEMARES 2010–2012 expeditions to the Avilés Canyon System (Bay of Biscay, Spain, northeast Atlantic). Helgol Mar. Res. 68, 399–430. doi: 10.1007/s10152-014-0398-z
Álvarez-Campos, P., Taboada, S., San Martín, G., Leiva, C., and Riesgo, A. (2018). Phylogenetic relationships and evolution of reproductive modes within flattened syllids (Annelida: Syllidae) with the description of a new genus and six new species. Inverteb. Syst. 32, 224–251. doi: 10.1071/IS17011
Asbjørnsen, P. C. (1856). Description d’un nouveau genre des Astéries in Sars, Koren, Danielssen eds. Fauna Litt. Norw. 2, 95–101.
Bean, T. C. (1844). British Marine Conchology; Being a Descriptive Catalogue, Arranged According to the Lamarckian System, of The Salt Water Shells of Great Britain. London: Edward Lumley.
Beazley, L. I., Kenchington, E. L., Murillo, F. J., and Sacau, M. (2013). Deep-sea sponge grounds enhance diversity and abundance of epibenthic megafauna in the Northwest Atlantic. ICES J. Mar. Sci. 70, 1471–1490. doi: 10.1093/icesjms/fst124
Bett, B. J., and Rice, A. L. (1992). The influence of hexactinellid sponge (Pheronema carpenteri) spicules on the patchy distribution of macrobenthos in the Porcupine Seabight (bathyal NE Atlantic). Ophelia 36, 217–226. doi: 10.1080/00785326.1992.10430372
Bo, M., Bertolino, M., Bavestrello, G., Canese, S., Giusti, M., Angiolillo, M., et al. (2012). Role of deep sponge grounds in the Mediterranean Sea: a case study in southern Italy. Hydrobiologia 687, 163–177. doi: 10.1007/s10750-011-0964-1
Boletín Oficial del Estado (2011). (295) 130084–130138. 8 de Diciembre de 2011 (BOE-A-2011-19246). Madrid: Boletín Oficial del Estado.
Boletín Oficial del Estado (2014). (293) 100065–100076. 4 de Diciembre de 2014 (BOE-A-2014-12628). Madrid: Boletín Oficial del Estado.
Borja, A., Galparsoro, I., Irigoien, X., Iriondo, A., Menchaca, I., Muxika, I., et al. (2011). Implementation of the European Marine Strategy Framework Directive: a methodological approach for the assessment of environmental status, from the Basque Country (Bay of Biscay). Mar. Pollut. Bull. 62, 889–904. doi: 10.1016/j.marpolbul.2011.03.031
Borja, A., Prins, T. C., Nomiki, S., Andersen, J. H., Torsten, B., Joao-Carlos, M., et al. (2014). Tales from a thousand and one ways to integrate marine ecosystem components when assessing the environmental status. Front. Mar. Sci. 1:72. doi: 10.3389/fmars.2014.00072
Brunton, C. H. C., and Curry, G. B. (1979). British Brachiopods. Keys and Notes for the Identification of the Species. Synopses of the British Fauna (New Series), N.S. 17. London: Academic Press.
Burns, J. H. R., and Delparte, D. (2017). “Comparison of commercial structure-from-motion photogrammety software used for underwater three-dimensional modeling of coral reef environments,” in The International Archives of the Photogrammetry, Remote Sensing and Spatial Information Sciences. Vol. XLII-2/W3, Nafplio, 127–131. doi: 10.5194/isprs-archives-XLII-2-W3-127-2017
Burns, J. H. R., Delparte, D., Gates, R. D., and Takabayashi, M. (2015a). Integrating structure-from-motion photogrammetry with geospatial software as a novel technique for quantifying 3D ecological characteristics of coral reefs. PeerJ 3:e1077. doi: 10.7717/peerj.1077
Burns, J. H. R., Delparte, D., Gates, R. D., and Takabayashi, M. (2015b). “Utilizing underwater three-dimensional modeling to enhance ecological and biological studies of coral reefs,” in The International Archives of the Photogrammetry, Remote Sensing and Spatial Information Sciences, XL-5/W5, Italy. doi: 10.5194/isprsarchives-XL-5-W5-61-2015
Bythell, J., Pan, P., and Lee, J. (2001). Three-dimensional morphometric measurements of reef corals using underwater photogrammetry techniques. Coral Reefs 20, 193–199. doi: 10.1007/s003380100157
Cairns, S. D. (1979). The deep-water Scleractinia of the Caribbean and adjacent waters. Stud. Fauna Curacao. 57:341.
Cairns, S. D., and Bayer, F. M. (2009). A generic revision and phylogenetic analysis of the Primnoidae (Cnidaria:Octocorallia). Smithsonian Contributions to Zoology, 629. Washington DC: Smithsonian Institution Press, 79. doi: 10.5479/si.00810282.629
Cairns, S. D., and Taylor, M. L. (2019). An illustrated key to the species of the genus Narella (Cnidaria, Octocorallia, Primnoidae). ZooKeys 822, 1–15. doi: 10.3897/zookeys.822.29922
Calvet, L. (1907). Bryozoaires. Expeditions scientifiques du “Travailleur” et du “Talisman” pendant les annees 1880–1883. Paris 8, 355–495.
Calvet, L. (1931). Bryozoaires provenant des campagnes scientifiques du prince albert ier de monaco. Rés. Camp. Sci. Prince Monaco 83, 1–152.
Cárdenas, P., Vacelet, J., Chevaldonné, P., Pérez, T., and Xavier, J. R. (2018). “From marine caves to the deep sea, a new look at Caminella (Demospongiae, Geodiidae) in the Atlanto-Mediterranean region,” in Deep Sea and Cave Sponges, Vol. 4466, eds M. Klautau, T. Pérez, P. Cárdenas, and N. de Voogd (Auckland: Magnolia Press), 174–196. doi: 10.11646/zootaxa.4466.1.14
Carter, H. J. (1876). Descriptions and Figures of Deep-Sea Sponges and their Spicules, from the Atlantic Ocean, dredged up on board H.M.S.‘Porcupine’, chiefly in 1869 (concluded). Ann. Mag. Natl. History 18, 226–240. doi: 10.1080/00222937608682035
Cartes, J. E., Huguet, C., Parra, S., and Sanchez, F. (2007). Trophic relationships in deep-water decapods of Le Danois bank (Cantabrian Sea, NE Atlantic): trends related with depth and seasonal changes in food quality and availability. Deep Sea Res. Part I 54, 1091–1110. doi: 10.1016/j.dsr.2007.04.012
Carvalho, F. C., Pomponi, S. A., and Xavier, J. R. (2015). Lithistid sponges of the upper bathyal of Madeira, Selvagens and Canary Islands, with description of a new species of Isabella. J. Mar. Biol. Assoc. U. K. 95, 1287–1296. doi: 10.1017/S0025315414001179
Carpenter, P. H. (1888). Report on the Crinoidea collected during the voyage of H.M.S. Challenger, during the years 1873-76. Part II. The Comatulae. Reports of the Scientific Results of the Voyage of H.M.S. Challenger. Zoology 26(Pt 60), 1–70.
Cathalot, C., van Oevelen, D., Cox, T. J. S., Kutti, T., Lavaleye, M., Duineveld, G., et al. (2015). Cold-water coral reefs and adjacent sponge grounds: hot spots of benthic respiration and organic carbon cycling in the deep sea. Front. Mar. Sci. 2:37. doi: 10.3389/fmars.2015.00037
Caullery, M. (1896). Résultats scientifiques de la Campagne du “Caudan” dans le Golfe de Gascogne. Aout-Septembre 1985. Crustacés schizopodes et décapodes. Ann. Univ. Lyon 2, 365–419.
Cherbonnier, G. (1969). Echinodermes récoltés par la Thalassa au large des côtes ouest de Bretagne et du golfe de Gascogne (3-12 aout 1967). Bull. Mus. Natl. His. Nat. Paris. Ser. 2, 343–361.
Christiansen, S. (2010). Background document for deep-sea sponge aggregations. Ospar Convent. Biodivers. Ser. 46:508.
Chu, J. W. F., and Leys, S. P. (2010). High resolution mapping of community structure in three glass sponge reefs (Porifera, Hexactinellida). Mar. Ecol. Progr. Ser. 417, 97–113. doi: 10.3354/meps08794
Clark, A. M. (1980). Crinoidea collected by the Meteor and Discovery in the NE Atlantic. Bull. Br. Mus. Nat. History (Zoology) 38, 187–210.
Clark, A. M., and Downey, M. E. (1992). Starfishes of the Atlantic. Chapman & Hall Identification Guides, 3, Vol. xxvi. London: Chapman & Hall, 794.
Cocito, S., Sgorbini, S., Peirano, A., and Valle, M. (2003). 3-D reconstruction of biological objects using underwater video technique and image processing. J. Exp. Mar. Biol. Ecol. 297, 57–70. doi: 10.1016/S0022-0981(03)00369-1
Costa, O. G. (1830 [“1829”]). Catalogo sistematico e ragionato de’ testacei delle Due Sicilie. Tipogr. Della Min. Napoli 30, 1–3.
Courtney, L. A., Fisher, W. S., Raimondo, S., Oliver, L. M., and Davis, W. P. (2007). Estimating 3-dimensional colony surface area of field corals. J. Exp. Mar. Biol. Ecol. 351, 234–242. doi: 10.1016/j.jembe.2007.06.021
De Goeij, J. M., Lesser, M. P., and Pawlik, J. R. (2017). “Nutrient fluxes and ecological functions of coral reef sponges in a changing ocean,” in Climate Change, Ocean Acidification and Sponges, eds J. L. Carballo and J. J. Bell (Berlin: Springer), 373–410. doi: 10.1007/978-3-319-59008-08
De Goeij, J. M., Moodley, L., and Houtekamer, M. (2008). Tracing 13C-enriched dissolved and particulate organic carbon in the bacteria-containing coral reef sponge Halisarca caerulea: evidence for DOM feeding. Limnol. Oceanogr. 53, 1376–1386. doi: 10.4319/lo.2008.53.4.1376
De Goeij, J. M., van Oevelen, D., and Vermeij, M. J. A. (2013). Surviving in a marine desert: the sponge loop retains resources within coral reefs. Science 342, 108–110. doi: 10.1126/science.1241981
D’Hondt, J. L. (1974). Bryozoaires récoltés par la ≪ Thalassa ≫ dans le Golfe de Gascogne. (Campagnes de 1968 à 1972). Cah. Biol. Mar. 15, 27–50.
Dautzenberg, P., and Fischer, H. (1896). Dragages effectués par l’Hirondelle et par la Princesse Alice 1888-1895. 1. Mollusques gastéropodes. Mém. Soc. Zool. France 9, 395–498.
E.C. (2008). Directive 2008/56/EC of the European Parliament and of the Council of 17 June 2008, Establishing a Framework for Community Action in the field of Marine Environmental Policy (Marine Strategy Framework Directive). Official Journal of the European Union L164. Maastricht: European Union, 19–40.
E.C. (2013). Interpretation Manual of European Union Habitats. EUR28. European Commision DG Environment. Maastricht: European Union, 144.
FAO (2009). International Guidelines for the Management of Deep-Sea Fisheries in the High Seas. Rome: FAO, 73.
Fernández-Rodríguez, I., Arias, A., Anadón, N., and Acuña, J. L. (2019). Holothurian (Echinodermata) diversity and distribution in the central cantabrian sea and the avilés canyon system (Bay of Biscay). Zootaxa 4567, 293–325. doi: 10.11646/zootaxa.4567.2.5
Ferrari, R., McKinnon, D., He, H., Smith, R. N., Corke, P., González-Rivero, M., et al. (2016). Quantifying multiscale habitat structural complexity: a cost-effective framework for underwater 3D modelling. RemoteSensing 8:113. doi: 10.3390/rs8020113
Fillinger, L., Janussen, D., Lundälv, T., and Richter, C. (2013). Rapid glass sponge expansion after climate-induced Antarctic ice shelf collapse. Curr. Biol. 23, 1–5. doi: 10.1016/j.cub.2013.05.051
Fleming, J. (1820). Observations on the natural history of Sertularia gelatinosa of Pallas. Edinbur. Philos. J. 2, 82–89.
García-Alegre, A., Sánchez, F., Gómez-Ballesteros, M., Hinz, H., Serrano, A., and Parra, S. (2014). Modelling and maping the local distribution of representative species on the Le Danois Bank, El Cachucho Marine Protected Area (Cantabrian Sea). Dep Sea Res. II 106, 151–164. doi: 10.1016/j.dsr2.2013.12.012
Giglioli, E. H. (1884). “Esplorazione talassografica del Mediterraneo eseguita sotto gli auspici del governo italiano,” in Pelagos. Saggi Sulla Vita e Sui Prodotti Del Mare. Reale Instituto de’ Sordo-Muti, eds F. Gigliogli, E. Haa, and A. Issel (Genova: Esplorazione talassografica del Mediterraneo eseguita sotto gli auspici del governo italiano), 199–291.
Gomez-Ballesteros, M., Druet, M., Muñoz, A., Arrese, B., Rivera, J., Sánchez, F., et al. (2014). Geomorphology of the avilés canyon system, cantabrian sea (Bay of Biscay). Deep Sea Res. 106, 99–117. doi: 10.1016/j.dsr2.2013.09.031
Gravier, C. (1920). Madréporaires provenant des campagnes des yachts Princesse Alice et Hirondelle II (1893-1913). Rés. Cam. Sci. Prince Monaco 55, 1–123. doi: 10.5962/bhl.title.50363
Gray, J. E. (1858). On Aphrocallistes, a new genus of Spongiadae from malacca. Proc. Zool. Soc. Lond. 26, 114–115.
Gunnerus, J. E. (1767). Beskrifning på trenne Norrska Sjö-Kråk, Sjö-Pungar kallade. Kungl. Svens. Vetenskaps. Handl. 28, 114–124.
Hawkes, N., Korabik, M., Beazley, L., Rapp, H. T., Xavier, J. R., and Kenchington, E. L. (2019). Glass sponge grounds on the Scotian Shelf and their associated biodiversity. Mar. Ecol. Prog. Ser. 614, 91–109. doi: 10.3354/meps12903
Hincks, T. (1855). Notes on British zoophytes, with description of new species. Ann. Mag. nat. Hist. 215, 127–130.
Hogg, M. M., Tendal, O. S., Conway, K. W., Pomponi, S. A., van Soest, R. W. M., Gutt, J., et al. (2010). Deep-Sea Sponge Grounds: Reservoirs of Biodiversity. UNEP-WCMC Biodiversity Series No. 32. Cambridge: UNEP-WCMC.
Hooper, J. N. A., and van Soest, R. W. M. (2002). A guide to the classification of sponges, Vol. 2. New York, NY: Kluwer Academic/Plenum Publishers, 1706.
House, J. E., Brambilla, V., Bidaut, L. M., Christie, A. P., Pizarro, O., Madin, J. S., et al. (2018). Moving to 3D: relationships between coral planar area, surface area and volume. PeerJ 6:e4280. doi: 10.7717/peerj.4280
Huetten, E., and Greinert, J. (2008). Software controlled guidance, recording and post-processing of seafloor observations by ROV and other towed devices: The software package OFOP. Geophys. Res. Abstr. 10:3088.
Hughes, S. W. (2005). Archimedes revisited: a faster, better, cheaper method of accurately measuring the volume of small objects. Phys. Educ. 40, 468–474. doi: 10.1088/0031-9120/40/5/008
James, M. R., and Robson, S. (2012). Straightforward reconstruction of 3D surfaces and topography with a camera: accuracy and geosciences application. J. Geophys. Res. 117:F03017. doi: 10.1029/2011JF002289
Johnson, J. Y. (1862). Descriptions of some new corals from Madeira. Proc. Zool. Soc. Lond. 1862, 194–197.
Johnson, J. Y. (1863). Description of a new siliceous sponge from the coast of Madeira. Proc. Zool. Soc. Lond. 1863, 257–259.
Jokiel, R. L., Maragos, J. E., and Franzisket, L. (1978). “Coral growth: buoyant weight technique,” in Coral Reef Research Methods, eds D. R. Stoddart and R. E. Johannes (Paris: United Nations Educational, Scientific and Cultural Organization), 529–542.
Jullien, J., and Calvet, L. (1903). Bryozoaires provenant des campagnes de l’Hirondelle (1886-1888). Result. Campag. Sci. Accom. Yacht Albert Prince Souver. Monaco. 23, 1–188.
Kazanidis, G., Henry, L. A., Roberts, J. M., and Witte, U. F. M. (2016). Biodiversity of Spongosorites coralliophaga (Stephens, 1915) on coral rubble at two contrasting cold-water coral reef settings. Coral Reefs 35, 193–208. doi: 10.1007/s00338-015-1355-2
Kazanidis, G., Vad, J., Henry, L. A., Neat, F., Berx, B., Georgoulas, K., et al. (2019). Seabed Images and Corresponding Environmental Data From Deep-Sea Sponge Aggregations in the Faroe-Shetland Channel Nature Conservation Marine Protected Area. Amsterdam: PANGAEA, doi: 10.1594/PANGAEA.897604
Kelly, M. (2007). The Marine Fauna of New Zealand. Porifera: Lithistid Demospongiae (Rock Sponges). The Marine Fauna of New Zealand. Wellington: National Institute of Water and Atmospheric Research (NIWA).
Kelly, M., Ellwood, M., Tubbs, L., and Buckeridge, J. (2007). “The lithistid Demospongiae in New Zealand waters: species composition and distribution,” in Porifera Research: Biodiversity, Innovation and Sustainability, eds M. R. Custódio, G. Lôbo-Hajdu, E. Hajdu, and G. Muricy (Rio de Janeiro: Museu Nacional du Rio de Janeiro, vol série livros), 393–404.
Klitgaard, A. B. (1995). The fauna associated with outer shelf and upper slope sponges (Porifera, Demospongiae) at the Faroe Islands, northeastern Atlantic. Sarsia 80, 1–22. doi: 10.1080/00364827.1995.10413574
Klitgaard, A. B., and Tendal, O. S. (2004). Distribution and species composition of mass occurrences of large-sized sponges in the northeast Atlantic. Prog. Oceanogr. 61, 57–98. doi: 10.1016/j.pocean.2004.06.002
Kœhler, R. (1895). Dragages profonds executes a bord du Caudan dans le Golfe de Gascogne. Rapport pr liminaire sur le chinodermes. Revue biologique du Nord de la France 7, 439–496.
Kœhler, R. (1896). “Échinodermes,” in Résultats scientifiques de la campagne du “Caudan” dans le Golfe de Gascogne — août-septembre 1895, Vol. 26, ed. R. Koehler (Lyon: Université de Lyon), I–IV. doi: 10.5962/bhl.title.65730
Kunzmann, K. (1996). Associated fauna of selected sponges (Hexactinellida and Demospongiae) from the Weddell Sea. Antarctica. Rep. Polar Res. 210, 1–93.
Kutti, T., Bannister, R. J., and Fossa, J. H. (2013). Community structure and ecological function of deep-water sponge grounds in the Traenadypet MPA-Northern Norwegian continental shelf. Cont. Shelf Res. 69, 21–30. doi: 10.1016/j.csr.2013.09.011
Kwasnitschka, T., Hansteen, T. H., Devey, C. W., and Kutterolf, S. (2013). Doing fieldwork on the seafloor: photogrammetric techniques to yield 3D visual models from ROV video. Comput. Geosci. 52, 218–226. doi: 10.1016/j.cageo.2012.10.008
Lamarck, J. B. M. (1816). Histoire Naturelle des Animaux sans Vertèbres. Tome Second. Paris: Verdière, 568.
Lavy, A., Eyal, G., Neal, B., Keren, R., Loya, Y., Ilan, M., et al. (2015). A quick, easy and non-intrusive method for underwater volume and surface area evaluation of benthic organisms by 3D computer modelling. Methods Ecol. Evol. 6, 521–531. doi: 10.1111/2041-210X.12331
Leach (1815-1875). Malacostraca Podophthalmata Britanniae; or Descriptions of Such British Species of the Linnean Genus Cancer as Have Their Eyes Elevated on Footstalks. London: John Sowerby, >124.
Lendenfeld, R. (1894). Eine neue pachastrella. sitzungsberichte der kaiserlichen akademie der wissenschaften. Mathem. Naturwissens. Classe 103, 439–442.
Lévi, C. (1991). “Lithistid sponges from the Norfolk Rise: recent and Mesozoic genera,” in Fossil and Recent Sponges, eds J. Reitner and H. Keupp (Berlin: Springer-Verlag Publishers), 72–82. doi: 10.1007/978-3-642-75656-6_7
Leys, S. P., Yahel, G., Reidenbach, M. A., Tunnicliffe, V., Shavit, U., and Reiswig, H. M. (2011). The sponge pump: the role of current induced flow in the design of the sponge body plan. PLoS One 6:e27787. doi: 10.1371/journal.pone.0027787
Linnaeus, C. (1758). Systema Naturae per Regna tria Naturae, Secundum Classes, Ordines, Genera, Species, cum Characteribus, Differentiis, Synonymis, Locis. Editio Decima, Reformata [10th Revised Edition], Vol. 1. Holmiae: Laurentius Salvius, 824.
Lloris, D. (2015). Ictiofauna marina. Manual de identificación de los peces marinos de la península ibérica y Baleares. Edit. Omega 15:674.
Louisy, P. (2002). Guide d’identification des Poissons Marins. Europe et Méditerranée. Edit. Ulmer 2:429.
Lyman, T. (1879). Ophiuridae and Astrophytidae of the “Challenger” Expedition. Part II, Vol. 6. Cambridge, Ma: Harvard College, 17–83.
M’Andrew, R., and Barrett, L. (1857). List of the echinodermata dredged between drontheim and the north cape. Ann. Mag. Nat. History 20, 43–44.
Maldonado, M., Aguilar, R., Bannister, R. J., Bell, J. J., Conway, K. W., Dayton, P. K., et al. (2017). “Sponge grounds as key marine habitats: a synthetic review of types, structure, functional roles, and conservation concerns,” in Marine Animal Forests: The Ecology of Benthic Biodiversity Hotspots, eds S. Rossi, L. Bramanti, A. Gori, and C. Orejas (Berlín: Springer International Publishing), 145–183. doi: 10.1007/978-3-319-17001-5_24-1
Maldonado, M., Aguilar, R., Blanco, J., García, S., Serrano, A., and Punzón, A. (2015). Aggregated clumps of lithistid sponges: a singular, reef-like bathyal habitat with relevant paleontological connections. PloS One 10:e0125378. doi: 10.1371/journal.pone.0125378
Maldonado, M., López-Acosta, M., Sitjà, C., et al. (2019). Sponge skeletons as an important sink of silicon in the global oceans.Nat. Geosci. 12, 815–822. doi: 10.1038/s41561-019-0430-7
Maldonado, M., Ribes, M., and van Duyl, F. C. (2012). Nutrient fluxes through sponges: biology, budgets, and ecological implications. Adva. Mar. Biol. 62, 114–182. doi: 10.1016/B978-0-12-394283-8.00003-5
Manjón-Cabeza, M. E., Palma-Sevilla, N., Gómez-Delgado, A. I, Andrino-Abelaira, J., and Ríos, P. (2014). “Echinoderms assemblages of Aviles canyons (preliminary results) (Biscay Bay) (INDEMARES+LIFE Project),” in XVIII Simposio Ibérico de Estudios de Biología Marina. Libro de resúmenes, eds P. Ríos, L. A. Suárez, and J. Cristobo (Gijón : Centro Oceanográfico de Gijón).
McCarthy, J., and Benjamin, J. (2014). Multi-image photogrammetry for underwater archaeological site recording: an accessible, diverbased approach. J. Mar. Archaeol. 9, 95–114. doi: 10.1007/s11457-014-9127-7
McIntyre, F. D., Drewery, J., Eerkes-Medrano, D., and Neat, F. C. (2016). Distribution and diversity of deep-sea sponge grounds on the rosemary bank seamount, NE Atlantic. Mar. Biol. 163:143. doi: 10.1007/s00227-016-2913-z
Meyer, H. K., Roberts, E. M., Rapp, H. T., and Davies, A. J. (2019). Spatial patterns of arctic sponge ground fauna and demersal fish are detectable in autonomous underwater vehicle (AUV) imagery. Deep Sea Res. Part I 19:103137 doi: 10.1016/j.dsr.2019.103137
Milne-Edwards, A., and Bouvier, E. L. (1894). Considerations génerales sur la famille des Galatheides. Ann. Sci. Nat. Zool. 16, 191–327.
Míguez, L. J. (2009). Equinodermos (Crinoideos, Equinoideos y Holothuroideos) Litorales, Batiales y Abisales de Galicia. 864. Ph.D. Thesis, Universidad de Santiago de Compostela, Santiago de Compostela.
Molodtsova, T. N. (2006). “Black corals (Antipatharia: Anthozoa: Cnidaria) of North-East Atlantic,” in Biogeography of the North Atlantic seamounts, eds A. N. Mironov, A. V. Gebruk, and A. J. Southward (Moscow: KMK Press), 141–151.
Morrow, C., and Cárdenas, P. (2015). Proposal for a revised classification of the Demospongiae (Porifera). Front. Zool. 12:7. doi: 10.1186/s12983-015-0099-8
Mortensen, T. (1935). A Monograph of the Echinoidea. II. Bothriocidaroida, Melonechinoida, Lepidocentroida, and Stirodonta, ed. C. A. Reitzel (London: Oxford University Press), 647.
Müller, O. F. (1776). Zoologiæ Danicæ Prodromus, seu Animalium Daniæ et Norvegiæ Indigenarum Characteres, Nomina, et Synonyma Imprimis Popularium. Copenhagen: Hallageri, 274.
Müller, J., and Troschel, F. H. (1842). System der Asteriden. 1. Asteriae. 2. Ophiuridae. Vieweg: Braunschweig, 12.
Murillo, F. J., Durán Muñoz, P., Cristobo, J., Ríos, P., González, C., Kenchington, E., et al. (2012). Deep-sea sponge grounds of the Flemish Cap, Flemish Pass and the Grand Banks of Newfoundland (Northwest Atlantic Ocean): Distribution and species composition. Mar. Biol. Res. 8, 842–854. doi: 10.1080/17451000.2012.682583
Negri, M. P., and Corselli, C. (2016). Bathyal Mollusca from the cold-water coral biotope of Santa Maria di Leuca (Apulian margin, southern Italy). Zootaxa 4186, 1–97. doi: 10.11646/zootaxa.4186.1.1
Pallas, P. S. (1766). Elenchus Zoophytorum Sistens Generum Adumbrationes Generaliores et Specierum Cognitarum Succintas Descriptiones, Cum Selectis Auctorum Synonymis. Hagae: Fransiscum Varrentrapp, 451.
Palma, M., Rivas, M., Pantaleo, U., Pavoni, G., Pica, D., and Cerrano, C. (2018). SfM-based method to assess gorgonian forests (Paramuricea clavata (Cnidaria, Octocorallia)). Remote Sensing 10:1154. doi: 10.3390/rs10071154
Paterson, G. L. J. (1985). The deep-sea Ophiuroidea of the north Atlantic Ocean. Bull. Br. Mus. (Natl. Hist.) Zool. 49, 1–162.
Pomponi, S. A., Diaz, M. C., Vs, R. W. M., Bell, L. J., Busutil, L., Gochfeld, D. J., et al. (2019). “Sponges,” in Mesophotic Coral Ecosystems. Coral Reefs of the World, Vol. 12, eds Y. Loya, K. Puglise, and T. Bridge (Cham: Springer).
Pomponi, S. A., Kelly, M., Reed, J. K., and Wright, A. (2001). Diversity and bathymetric distribution of lithistid sponges in the tropical western Atlantic region. Bull. Biol. Soc. Washing. 10, 344–353.
Pouliquen, L. (1972). Les spongiaires des grottes sous-marines de la région de Marseille: Ecologie et systématique. Téthys 3, 717–758.
Powell, A., Clarke, M. E., Fruh, E., Chaytor, J. D., Reiswig, H. M., and Whitmire, C. E. (2018). Characterizing the sponge grounds of grays canyon, Washington, USA. Deep Sea Res. Part II Top. Stud. Oceanogr. 150, 146–155. doi: 10.1016/jdsr2.2018.01.004
Prado, E., Sánchez, F., Rodríguez-Basalo, A., Altuna, A., and Cobo, A. (2019). Analysis of the population structure of a gorgonian forest (Placogorgia sp.) using a photogrammetric 3D modeling approach at Le Danois Bank, Cantabrian Sea. Deep Sea Res. Part I 153:3124. doi: 10.1016/j.dsr.2019.103124
Preciado, I., Cartes, J. E., Serrano, A., Velasco, F., Olaso, I., Sánchez, F., et al. (2009). Resource utilisation by deepsea sharks at the Le Danois Bank, Cantabrian Sea, north-east Atlantic Ocean. J. Fish Biol. 75, 1331–1351. doi: 10.1111/j.1095-8649.2009.02367.x
Prestandrea, N. (1839). Descrizione di due nuovi crustacei dei mari di messina. Atti Accad. Gioe. Sci. Nat. Cat. 2, 131–136.
Price, D., Robert, K., Callaway, A., Lo lacono, C., Hall, R. A., and Huvenn, V. A. I. (2019). Using 3D photogrammetry from ROV video to quantify coldwater coral reef structural complexity and investigate its influence on biodiversity and community assemblage. Coral Reefs 38, 1007–1021. doi: 10.1007/s00338-019-01827-3
Ramiro-Sánchez, B., González-Irusta, J. M., Henry, L. A., Cleland, J., Yeo, I., Xavier, J. R., et al. (2019). Characterization and mapping of a deep-sea sponge ground on the tropic seamount (northeast tropical atlantic): implications for spatial management in the high seas. Front. Mar. Sci. 6:278. doi: 10.3389/fmars.2019.00278
Rice, J., Arvanitidis, C., Borja, A., Frid, C., Hiddink, J., Krause, J., et al. (2012). Indicators for sea-floor integrity under the european marine strategy framework directive. Ecol. Indic. 12, 174–184. doi: 10.1016/j.ecolind.2011.03.021
Ridley, S. O., and Duncan, P. M. (1881). On the genus Plocamia, schmidt, and on some other sponges of the order echinonemata. with descriptions of two additional new species of dirrhopalum. J. Linn. Soc. Zool. 15, 476–497.
Rioja, E. (1931). Estudio de los poliquetos de la Península Iberica. Memorias de la Academia de Ciencias Exactas, Físicas y Naturales de Madrid. Serie Cienc. Natur. 2, 1–471.
Ríos, P., Aguilar, R., Torriente, A., Muñoz, A., and Cristobo, J. (2018). Sponge grounds of Artemisina (Porifera, Demospongiae) in the Iberian Peninsula, ecological characterization by ROV techniques. Zootaxa 4466, 095–123. doi: 10.11646/zootaxa.4466.1.10
Risso, A. (1810). Ichthyologie de Nice, ou histoire naturelle des poissons du Département des Alpes Maritimes. F. Schoell Paris 10, 1–11.
Rodríguez-Basalo, A., Sánchez, F., Punzón, A., and Gómez-Ballesteros, M. (2019). Updating the Master Management Plan for El Cachucho MPA (Cantabrian Sea) using a spatial planning approach. Cont. Shelf Res. 19:10. doi: 10.1016/j.csr.2019.06.010
Rolando, L. (1822). (1821 volume). Description d’un animal nouveau qui appartient à la classe des Echinodermes. Mem. Della Reale Accad. Delle Sci. Torino. 26, 539–556.
Sánchez, F. (2015). Manual PescaWin 2015. Programa de Monitorización de Muestreos en Campañas de Investigación. Madrid: Instituto Español de Oceanografía, doi: 10.13140/RG.2.1.3786.5684
Sánchez, F., González-Pola, C., Druet, M., García-Alegre, A., Acosta, J., Cristobo, J., et al. (2014). Habitat characterization of deep-water coral reefs in La Gaviera canyon (Avilés Canyon System, Cantabrian Sea). Deep Sea Res. Part II. 106, 118–140. doi: 10.1016/j.dsr2.2013.12.014
Sánchez, F., Rodríguez, A., García-Alegre, A., and Gómez-Ballesteros, M. (2015). Mapping the 1170 reefs habitat of EU Directive of bathial grounds of Le Danois Bank (El Cachucho MPA, Cantabrian Sea). VIII Simposio sobre el Margen Ibérico Atlántico. Abstract Book 15, 683–686.
Sánchez, F., and Rodríguez, J. M. (2013). POLITOLANA, a new low cost towed vehicle designed for the characterization of the deep-sea floor. Instrument. Viewpoint 15:69.
Sánchez, F., Rodríguez-Basalo, A., García-Alegre, A., and Gómez-Ballesteros, M. (2017). Hard-bottom bathyal habitats and keystone epibenthic species on Le Danois Bank (Cantabrian Sea). J. Sea Res. 130, 134–153. doi: 10.1016/j.seares.2017.09.005
Sánchez, F., Serrano, A., Parra, S., Ballesteros, M., and Cartes, J. E. (2008). Habitat characteristics as determinant of the structure and spatial distribution of epibenthic and demersal communities of Le Danois Bank (Cantabrian Sea, N. Spain). J. Mar. Syst. 72, 64–86. doi: 10.1016/j.jmarsys.2007.04.008
Sánchez, F. M., Serrano, A. G., and Ballesteros, M. G. (2009). Photogrammetric quantitative study of habitat and benthic communities of deep Cantabrian Sea hard grounds. Contin. Shelf Res. 29, 1174–1188. doi: 10.1016/j.csr.2009.01.004
Santavy, D. H., Courtney, L. A., Fisher, W. S., Quarles, R. L., and Jordan, S. J. (2013). Estimating surface area of sponges and gorgonians as indicators of habitat availability on Caribbean coral reefs. Hydrobiologia 707, 1–16. doi: 10.1007/s10750-012-1359-7
Schmidt, O. (1868). Die Spongien der Küste von Algier. Mit Nachträgen zu den Spongien des Adriatischen Meeres (Drittes Supplement), Vol. i–iv. Leipzig: Wilhelm Engelmann, 1–44.
Schmidt, O. (1870). Grundzüge Einer Spongien-Fauna des Atlantischen Gebietes, Vol. iii-iv. Leipzig: Wilhelm Engelmann, 1–88.
Schmidt, O. (1875). Spongien. die expedition zur physikalisch-chemischen und biologischen untersuchung der nordsee im sommer 1872. Jahresb. Commis. Wissensch. Untersuch. Deuts. Meere Kiel 2–3, 115–120.
Sollas, W. J. (1886). Preliminary account of the Tetractinellid sponges Dredged by H.M.S. ‘Challenger’ 1872-76. Part I. The choristida. Sci. Proc. R. Dublin Soc. (New Ser.) 5, 177–199.
Sollas, W. J. (1888). Report on the Tetractinellida collected by H.M.S. Challenger, during the years 1873–1876. Zoology 25(Pt 63), 1–458.
Southward, E. C., and Campbell, A. C. (2006). Echinoderms. Linnean Society Synopses of the British Fauna (New Series), Vol. 56. Telford: FSC Publications, 272.
Stephens, J., and Hickson, S. J. (1909). Alcyonarian and madreporarian corals of the Irish coasts, with description of a new species of Stachyodes. department of agriculture and technical instruction for fisheries, ireland, fisheries branch, Scientific. Investigations. 1907, 1–18. doi: 10.5962/bhl.title.33355
Stimson, J., and Kinzie, R. A. I. I. I. (1991). The temporal pattern and rate of release of zooxanthellae from the reef coral Pocillopora damicornis(Linnaeus) under nitrogen-enrichment and control conditions. J. Exp. Mar. Biol. Ecol. 153, 63–74. doi: 10.1016/S0022-0981(05)80006-1
Süssbach, S., and Breckner, A. (1911). Die Seeigel, Seesterne und Schlangensterne der Nord- und Ostsee. Wissenschaftliche Meeresuntersuchungen, Kommission zur wissenschaftlichen Untersuchung der deutschen Meere in Kiel. Neue Folge 12, 167–300.
Topsent, E. (1892). Contribution à l’étude des Spongiaires de l’Atlantique Nord (Golfe de Gascogne, Terre-Neuve, Açores). Résultats des campagnes scientifiques accomplies par le Prince Albert I. Monaco 2, 1–165.
Thomson, C. W. (1872). “On the echinidea of the ‘porcupine’ deep-sea dredging-expeditions,” in Proceedings of the Royal Society of London, Vol. 20, London, 491–497. doi: 10.1098/rspl.1871.0095
Thomson, J. A. (1929). Alcyonaires des environs de Monaco et de localit s diverses. Bulletin de I’Institut Oc anographique 10:534
Tola, E., Lepetit, V., and Fua, P. (2010). DAISY: an efficient dense descriptor applied to wide-baseline stereo. IEEE Transact. Patt. Anal. Mach. Intell. 32, 815–830. doi: 10.1109/TPAMI.2009.77
Topsent, E. (1890). Notice préliminaire sur les spongiaires recueillis durant les campagnes de l’Hirondelle. Bull. Soc. Zool. France 15, 65–71. doi: 10.5962/bhl.part.18721
Van Rooij, D., Iglesias, J., Hernández-Molina, F. J., Ercilla, G., Gomez-Ballesteros, M., Casas, D., et al. (2010). The le danois contourite depositional system: interactions between the mediterranean outflow water and the upper cantabrian slope (north iberian margin). Mar. Geol. 274, 1–20. doi: 10.1016/j.margeo.2010.03.001
Veal, C. J., Carmi, M., Fine, M., and Hoegh-Guldberg, O. (2010). Increasing the accuracy of surface area estimation using single wax dipping of coral fragments. Coral Reefs 29, 893–897. doi: 10.1007/s00338-010-0647-9
Velasco, E. M., Amez, M. A., and Punzón, A. (2013). Especies de Interés Pesquero en Galicia, Asturias y Cantabria. Madrid: Ministerio de Economía y Competitividad, 218.
Verrill, A. E. (1878). Notice of recent additions to the marine fauna of the eastern coast aof North America, No. 2. Brief contributions to zoology from the Museum of Yale College, No. 39. Am. J. Sci. Arts Third Ser. 16, 371–378.
Vosmaer, G. C. J. (1894). Preliminary notes on some tetractinellids of the Bay of Naples. Tijdschr. Nederland. Dierkund. Vereeniging. 4, 269–286.
Westoby, M. J., Brasington, J., Glasser, N. F., Hambrey, M. J., and Reynolds, J. M. (2012). Structure-from-Motion’ photogrammetry: a low-cost, effective tool for geoscience applications. Geomorphology 179, 300–314. doi: 10.1016/j.geomorph.2012.08.021
Wisshak, M., López Correa, M., Gofas, S., Salas, C., Taviani, M., Jakobsen, J., et al. (2009). Shell architecture, element composition, and stable isotope signature of the giant deep-sea oyster Neopycnodonte zibrowii sp. n. from the NE Atlantic. Deep Sea Res. I 56, 374–404. doi: 10.1016/j.dsr.2008.10.002
Yahel, G., Whitney, F., Reiswig, H. M., Eerkes-Medrano, D. I., and Leys, S. P. (2007). In situ feeding and metabolism of glass sponges (Hexactinellida, Porifera) studied in a deep temperate fjord with a remotely operated submersible. Limnol. Oceanogr. 52, 428–440. doi: 10.4319/lo.2007.52.1.0428
Zibrowius, H. (1978). Les Scléractiniaires des grottes sous-marines en Méditerranée et dans l’Atlantique nor-oriental (Portugal, Madère, Canaries, Azores). Pubbl. Staz. Zool. Napoli 40, 516–544.
Zibrowius, H. (1980). Les Scléractiniaires de la Méditerranée et de l’Atlantique nord-oriental. Mém. Inst. Océanogr. Monaco 11, 1–284.
Keywords: sponge grounds, lithistids, demosponges, vulnerable marine ecosystems, ecology, taxonomy, structure from motion, underwater photogrammetry
Citation: Ríos P, Prado E, Carvalho FC, Sánchez F, Rodríguez-Basalo A, Xavier JR, Ibarrola TP and Cristobo J (2020) Community Composition and Habitat Characterization of a Rock Sponge Aggregation (Porifera, Corallistidae) in the Cantabrian Sea. Front. Mar. Sci. 7:578. doi: 10.3389/fmars.2020.00578
Received: 16 December 2019; Accepted: 22 June 2020;
Published: 28 July 2020.
Edited by:
Jeroen Ingels, Florida State University, United StatesReviewed by:
Georgios Kazanidis, The University of Edinburgh, United KingdomDorte Janussen, Senckenberg Museum, Germany
Copyright © 2020 Ríos, Prado, Carvalho, Sánchez, Rodríguez-Basalo, Xavier, Ibarrola and Cristobo. This is an open-access article distributed under the terms of the Creative Commons Attribution License (CC BY). The use, distribution or reproduction in other forums is permitted, provided the original author(s) and the copyright owner(s) are credited and that the original publication in this journal is cited, in accordance with accepted academic practice. No use, distribution or reproduction is permitted which does not comply with these terms.
*Correspondence: Pilar Ríos, cGlsYXIucmlvcy5sb3BlekBnbWFpbC5jb20=; Elena Prado, ZWxlbmEucHJhZG9AaWVvLmVz
†These authors have contributed equally to this work