- 1Sorbonne Université, CNRS, Laboratoire d’Océanographie de Villefranche, Villefranche-sur-Mer, France
- 2Institut de Ciències del Mar (CSIC), Barcelona, Spain
The genus Ostreopsis includes some species that produce high biomass blooms and/or synthesize toxic compounds that can be transferred through the marine food webs or aerosolized causing ecological, human health and socio-economic impacts. Ostreopsis species are increasing their biogeographic distribution from tropical to more temperate waters and causing recurrent blooms in certain coastal areas, thus constituting an emerging concern worldwide. The proliferation capacity of Ostreopsis is due to a complex and poorly understood combination of multiple factors, and may be a paradigm of chemical ecology reviewed here. A first section summarizes the basic knowledge on the different Ostreopsis species, the toxins they produce and the described foodborne and airborne effects of Ostreopsis toxins on humans. Secondly, direct and indirect interactions between Ostreopsis species and their environment are reviewed. Mucopolysaccharide substances produced by the cells to attach to different substrates appear to be a key element on the chemical ecology and requires further study. However, this research is challenged by technical limitations to conduct ecologically realistic and harmonized studies where organisms can be in direct contact with Ostreopsis cells, their mucus and/or the released extracellular toxic compounds. Understanding the transfer mechanisms of these substances within the food web, potentially affecting humans is critical and requires further study with new analytical tools. Still, the progress in knowledge achieved in the last years, combined with experimental and field studies using cutting edge methods will facilitate to address the open questions on the chemical ecology of Ostreopsis and understand its bloom dynamics now, and under future climate and anthropogenic change scenarios.
Introduction
Marine Chemical Ecology (MCE)
Marine Chemical Ecology (MCE) is a cross-disciplinary and emergent research field which gives insights into chemical compounds that shape interactions among organisms and their environment and thus influence the structure, functioning and ecology of marine communities (Hay, 1996; McClintock and Baker, 2001; Pohnert et al., 2007). Chemical signals and cues are omnipresent in marine systems and play critical roles at different scales, by e.g., affecting cells communication, mating choices and behavior, feeding and habitats selection, food web dynamics, etc., with subsequent effects on other ecosystem-level processes (Bakus et al., 1986; Pawlik, 1992; Hay, 1996, 2009; Sieg et al., 2011; Schwartz et al., 2016). In general, chemical cues and signals occur at low concentrations in water and in air and are rapidly degraded likely to prevent miscommunication. This feature makes their sampling, isolation and structural elucidation very difficult and has limited their research. Fortunately, recent improvements in chemical technologies with the rise of high-throughput approaches have led to major advances in the understanding of the role of these compounds, notably in benthic systems (Prince and Pohnert, 2010; Kuhlisch and Pohnert, 2015). The terms “chemical signals” and “chemical cues” refer to compounds released intentionally and not intentionally, respectively. In the following, “chemical signals” comprise both concepts for simplicity.
MCE of Harmful Algal Blooms (HABs)
Chemical communication is particularly important in the life history and evolutionary strategies of eukaryotic microalgae, which live at low Reynolds numbers scale, while larger marine macro-organisms are functionally dependent on optical, acoustical and tactile sensing (Cembella, 2003; Ianora et al., 2011; Sieg et al., 2011). Chemical signals synthesized at the small cell level are dispersed by molecular diffusion assisted by small-scale turbulence and thus can operate at relatively larger scales influencing trophic interactions and life history. By fostering intra- and interspecific cooperation, or as defense mechanisms against predators, parasites or competitors (Prince et al., 2008; Poulson et al., 2009; Schwartz et al., 2016) chemical signals can play a role in the blooms of certain species. However, the information of chemical signals in the domain of HABs is very limited mainly due (as noticed above) to technical limitations. It has been suggested that allelopathy, i.e., the chemical inhibition of one organism by another due to the release into the environment of substances acting as growth inhibitors (Rizvi and Rizvi, 1992), could be an effective mechanism reinforcing high cell density blooms (Granéli and Hansen, 2006) although not the main or direct cause of HABs (Jonsson et al., 2009). Benthic marine ecosystems could be a source of great diversity of chemical signals since the competition for space and substrate is higher than in pelagic systems (Hay, 2009). Moreover, compared to the three-dimensional space experienced by planktonic cells, benthic systems are bi-dimensional, which imposes unique constraints with distinct advantages for the effectiveness of chemical ecological interactions. The importance of chemical signals is been progressively recognized as a key factor to understand the dynamics of the benthic HAB dynamics and requires complex and sophisticated experimentation and field sampling.
Objectives of the Review
Overall, 30% of the studies on Ostreopsis species focus on species identification and biogeography, 25% on toxins, 20% on the life cycle and physiology, and only 14% on ecological aspects including food web impacts, allelopathy, competition and links with the substrate. In other words, a small part of the research focuses directly on the chemical ecology of Ostreopsis species.
This work aims to review the present knowledge on the MCE concerning the biology and dynamics of blooms of the benthic dinoflagellate genus Ostreopsis. This genus has attracted scientific and social interest due to their negative impacts on certain benthic marine fauna, the production of toxins chemically close to the potent palytoxin (PLTX) associated to seldom but dramatic seafood borne poisonings in tropical areas, and the link of its blooms to mild respiratory and cutaneous irritations in beach users in temperate latitudes. The diversity, distribution and toxins produced by the Ostreopsis species are presented first, followed by a summary of the knowledge on the health impacts associated to aerosolized toxins. Next, a review of the potential chemical interactions between Ostreopsis species and their environment, from virus and bacteria to large herbivorous and carnivorous macroorganisms is presented. Overall, these aspects reveal the diverse and relevant aspects of the chemical ecology of Ostreopsis, which can constitute a model organism in this research field. The diverse produced toxins are considered until now, the main substance with a chemical ecology significance. However, very recent studies suggest that other compounds could be also at play. In the last section, some key scientific aspects and future steps to improve knowledge on MCE of Ostreopsis are suggested.
The Genus Ostreopsis
Species and Biogeographic Distribution
The genus Ostreopsis and the type species Ostreopsis siamensis were first described a century ago from the Gulf of Siam (Thailand) by Schmidt (1901). Since then, 10 other species of Ostreopsis and 7 ribotypes have been identified (Tester et al., 2020): O. ovata (Fukuyo, 1981) O. lenticularis (Fukuyo, 1981) O. heptagona (Norris et al., 1985) O. labens (Faust and Morton, 1995) O. mascarenensis (Quod, 1994) O. belizeanus, O. caribbeanus and O. marinus (Faust, 1999) and more recently, O. fattorussoi (Accoroni et al., 2016a) and O. rhodesae (Verma et al., 2016). Traditionally, the description of Ostreopsis species was based on morphological approaches combining size, shape and thecal plates pattern (as illustrated for instance in Hoppenrath et al., 2014). Nevertheless, a big part of the taxonomy of the genus remains unclear and controversial due to the high morphological and cell size variability within the different species and the fact that original descriptions lacked genetic analysis. Application of molecular approaches based on the nuclear rDNA internal transcribed spacer region (ITS1 and ITS 2), partial nuclear LSU (D1/D2, D8-D10 domains) and ITS-5.8S rDNA gene (Penna et al., 2005, 2010; Tawong et al., 2014; Chomérat et al., 2019, 2020) are nowadays key in the identification and determination of phylogeny links. These methods allowed, for example, the description of Ostreopsis sp. by Tartaglione et al. (2016) as the new species O. fattorussoi, and clarified uncertainties about former descriptions of O. lenticularis and O. mascarenensis (Chomérat et al., 2019, 2020). In particular, there is still debate regarding the taxonomy of the most widespread species, O. ovata and O. siamensis since their first description was based on morphological features only. For this reason, these two taxa are often referred to as O. cf. ovata and O. cf. siamensis. In the following, including all the tables, the species are cited as written in the corresponding published document. With the most recent progresses in chemical analysis, toxin profile could also become a taxonomic criterion.
Ostreopsis was initially considered a tropical genus, but it has been increasing its biogeographic distribution to temperate latitudes (Rhodes, 2011; Selina and Levchenko, 2011; Parsons et al., 2012; Tester et al., 2020 see Figure 5 in that paper-). In recent years, some reports have noticed the expansion of Ostreopsis cf. ovata in coastal waters of Australia, Brazil, China, Cook Islands, Caribbean Sea, French Polynesia, Hawaii, Indian Ocean, Japan, Malaysia, New Caledonia, New Zealand, Russia, Venezuela, Vietnam and specially in the Mediterranean Sea (Table 1). Other Ostreopsis species that appeared to have more confined distributions are also found in new areas. For instance, Ostreopsis fattorussoi isolated from the Eastern Mediterranean could be also growing in the Atlantic coast of the Iberia Peninsula (Portugal) although it has not been demonstrated yet (Accoroni et al., 2016a) and it was found in the Canary Islands (Fernández-Zabala et al., 2019). The wide expansion of the genus Ostreopsis is considered a potential problem for human health and wellbeing (economy, tourism, beach quality) issues due to the production of PLTX analogs which mode of action in humans is still not clear.
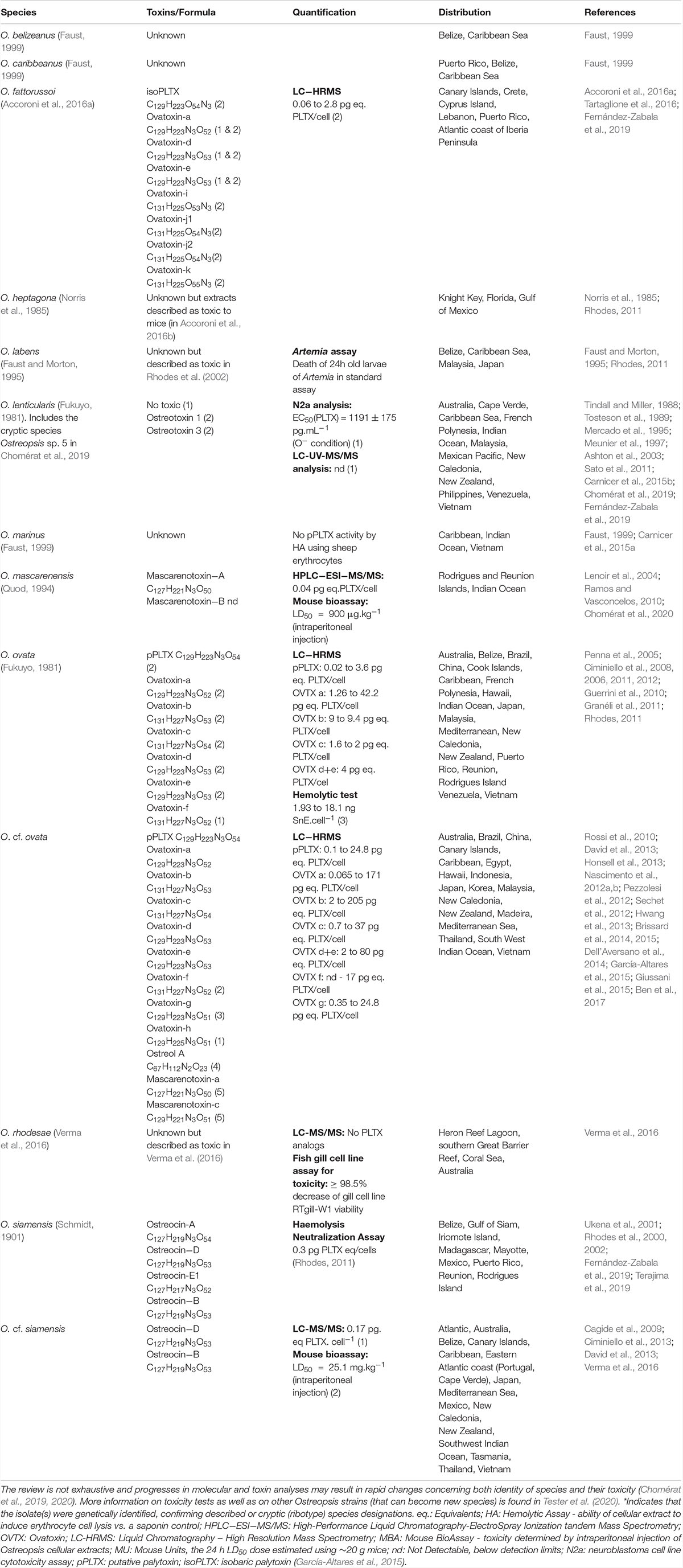
Table 1. Representative information about the range of toxin content on Ostreopsis species from different locations reported in the literature.
Toxins Synthesized by Ostreopsis Species: The Main Chemical Substances Involved in the MCE of the Genus
Several species in the Ostreopsis genus are considered potentially harmful to humans and other marine organisms due to the production of palytoxin (PLTX) analogs (Table 1). PLTX is the most potent non-bacterial toxin of biological origin, initially isolated in 1971 from the tropical soft coral genus Palythoa, and now recognized as 42-OH-PLTX (see revision by Ciminiello et al., 2011 and references in Poli et al., 2018).
Progresses in chemical analysis technology, in particular on HR LC-MS/MS and Nuclear Magnetic Resonance (NMR) have revealed the high diversity of PLTX analogs (Table 1 and Figure 1) and eight Ostreopsis species have been described as toxic. Ostreocin D (Figure 2) was the first analog isolated from O. siamensis by Usami et al. (1995) and other PLTX analogs, namely, ovatoxins, ostreocins or mascarenotoxin have been characterized for O. fattorussoi, O. mascarensis, O. cf. ovata and O. siamensis (Mercado et al., 1994; Deeds and Schwartz, 2010; Parsons et al., 2012). However, the toxicity of O. lenticularis remains uncertain. Mercado et al. (1994) reported the production of Ostreotoxins 1 and 3 by cultures of O. lenticularis isolated from the Caribbean. This study was suggested to be taken with caution in the review by Parsons et al. (2012) since subsequent research indicated that bacteria could be involved in the toxicity of O. lenticularis strains from the Caribbean (Mercado et al., 1994, 1995; Meunier et al., 1997; Pérez-Guzmán et al., 2008). Recently, Chomérat et al. (2019) have reported no toxicity of O. lenticularis strains using CBA-N2a and LC-UV-MS/MS analysis.Toxicity of O. heptagona, O. labens and O. rhodesae has only been described using biological assays and toxins involved in these effects have not been identified yet (Table 1). In the case of O. cf. ovata, up to 12 ovatoxins (OVTX), from a to l (including j1 and j2) and the isobaric PLTX (isoPLTX, García-Altares et al., 2015 corresponding to the putative PLTX in other studies) have been identified among different isolated strains, and the toxinology profile is contributing to the taxonomic characterization (Accoroni et al., 2016a). In most species and strains, OVTX-a and OVTX-b are the most common toxin forms, and isoPLTX is often absent or only accounting for no more than 1% of the total toxins (Tartaglione et al., 2017; Gémin et al., 2019). A main limitation to ascertain the health impacts risks associated to Ostreopsis blooms (and establishing legal toxin thresholds in seafood) is the lack of standards for the variety of toxins, all them complex and with high molecular weight (molecular formulas varying between C129H223N3O55 and C131H227N3O52, Table 1). As for species identification, information in Table 1 may change in the near future to rapid progresses in toxin analytical methods.
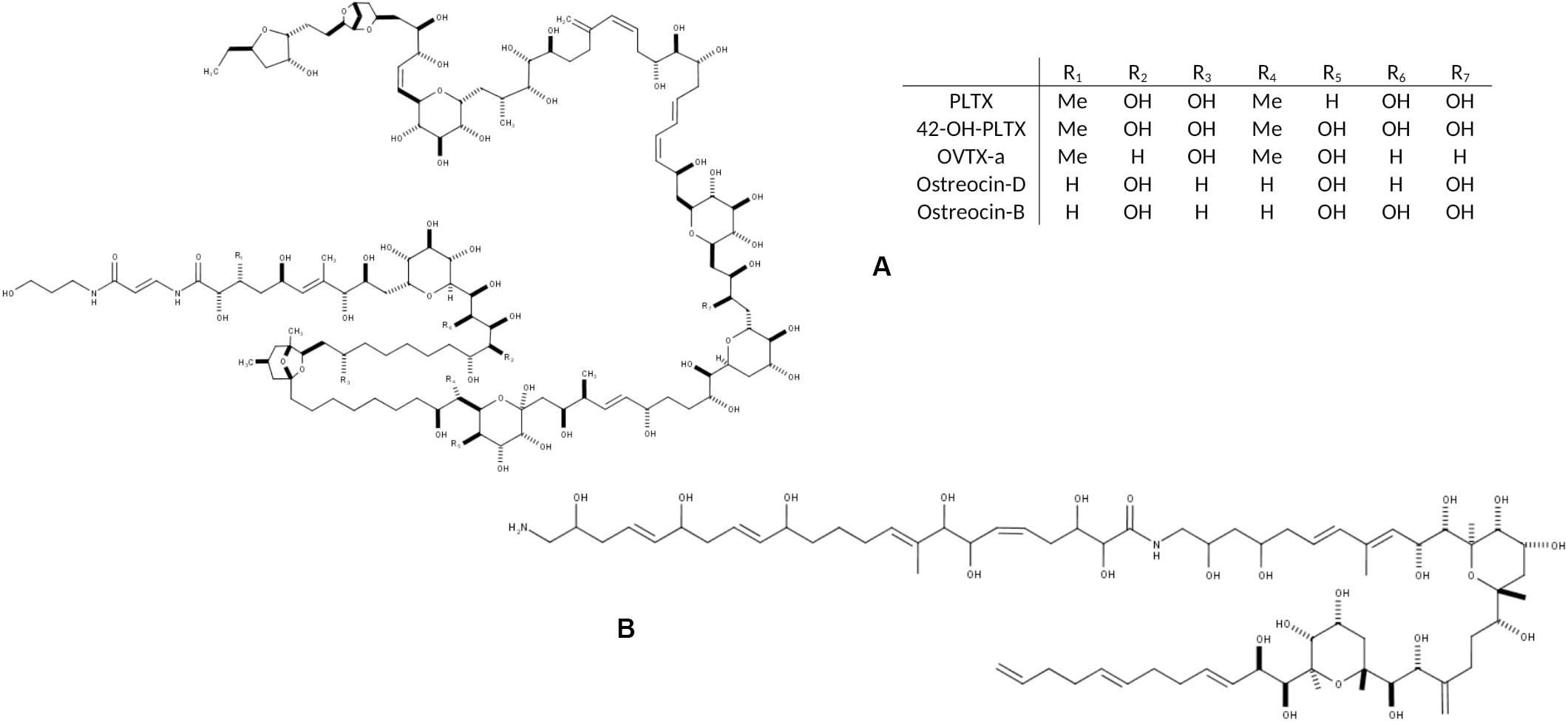
Figure 1. Chemical structure of the main toxic compounds found in Ostreopsis species: (A) Palytoxin and its main analogs, the most common; and (B) Ostreol-A, present in some Korean strains.
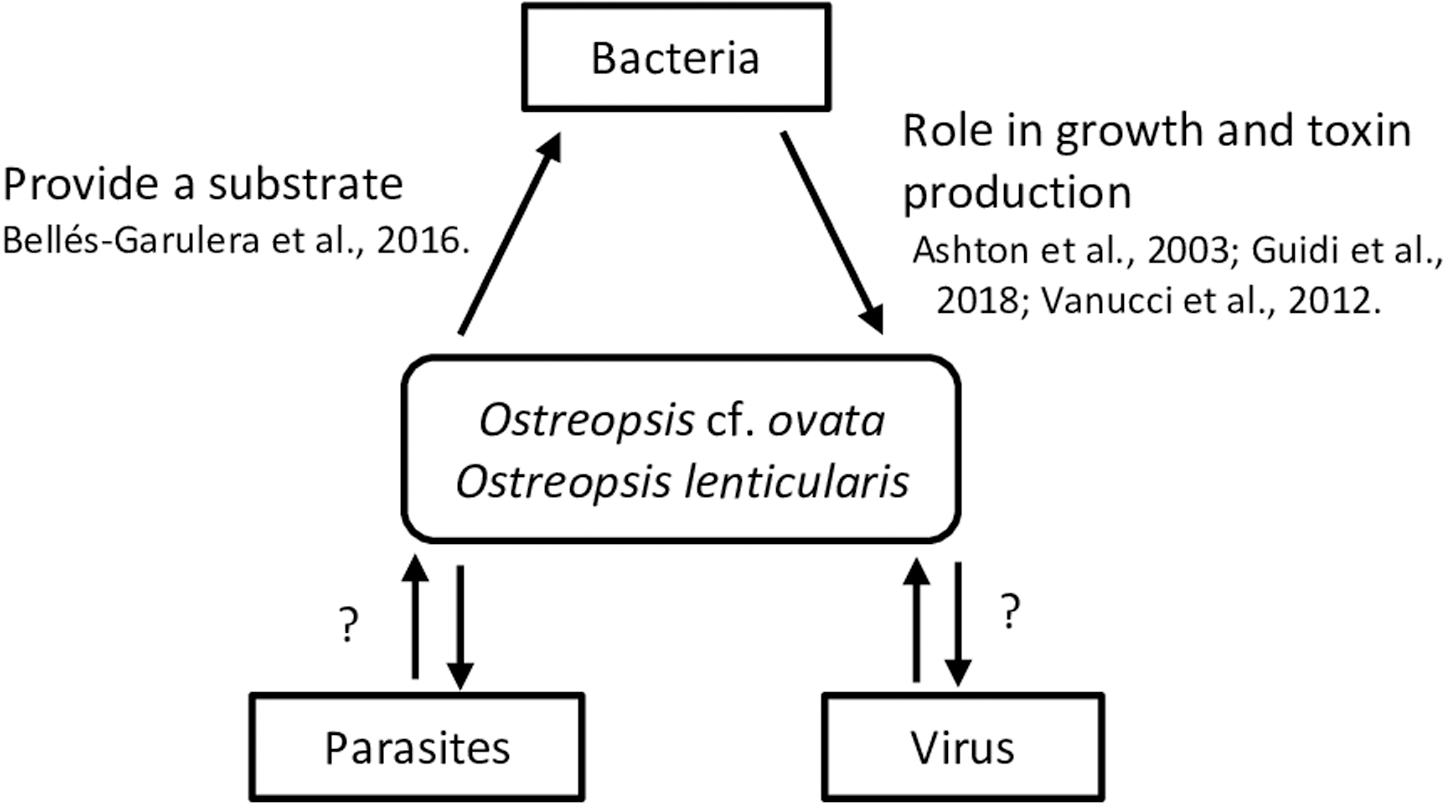
Figure 2. Summary of interactions between Ostreopsis species and virus, bacteria and parasites. Mucus could provide a surface for bacteria; bacterial metabolic activity could both provide N and P to Ostreopsis species and use excreted organic C, N and P from the microalga.
Phycotoxins in general and the PLTX group as well, are considered as secondary metabolites and their physiological role in the cells is unknown. Environmental factors such as temperature, salinity, light or nutrients, depth or macroalgal substrate, but also strain characteristics (isolation site, time kept in culture, growth phase of the culture) may influence toxin production (Granéli et al., 2011; Ciminiello et al., 2012; Pezzolesi et al., 2012, 2014; Scalco et al., 2012; Vanucci et al., 2012b; Gémin et al., 2019). However, it has been suggested that other microorganisms co-occurring along the Ostreopsis blooms could be involved in the toxin production as well. Tosteson et al. (1989) and Ashton et al. (2003) found that bacteria associated with the surfaces or the extracellular matrices of O. lenticularis were correlated with the high dinoflagellate toxicity during the stationary phase of the cultures. Biological assays and analytical chemistry analysis are both used for toxin presence detection and/or quantification. Mouse Bioassay (prohibited in Europe since 2005 for ethical reasons), Haemolysis Neutralization Assay (HNA) or Artemia bioassay have been long recognized for toxicity detection (Delaney, 1984; Bignami, 1993; Botana, 2014). Advantages provided by analytical chemistry, i.e., LC-MS/MS, are the absence of restrictions in terms of reproducibility, specificity, precision and consistency. Unfortunately, this methodology relies on very expensive technical equipment, it requires high-qualified personnel skills and it produces significant chemical waste. The variety of toxins produced by the different Ostreopsis species are considered the main substances involved in the chemical ecology of these dinoflagellates, and with this perspective are considered in this revision. However, recent studies indicate that other non-identified compounds could also be involved in the chemical ecology of Ostreopsis (sections 3.3.1. and 5.2).
Ostreopsis Marine Chemical Ecology Affecting Human Health
Health impacts in humans due to exposure to PLTX analogs synthesized by Ostreopsis constitute an indication of its related chemical ecology. Health impacts can occur: i) after consumption of seafood potentially contaminated with PLTX analogs, or ii) after direct exposure to seawater and/or aerosol during Ostreopsis blooms. The characterization of human poisonings potentially due to exposure to PLTX analogs was reviewed by Tubaro et al. (2011) and it is briefly presented here. The effects of Ostreopsis species in different marine organisms have also been reported and are presented in section 3.
Seafood Borne PLTX Poisonings
Severe illness and even lethal fatalities after consumption of seafood potentially contaminated with PLTX analogs have been rarely reported in tropical and subtropical latitudes since the 1970s (see Tables 1–3 in Tubaro et al., 2011). Among them, only a few cases were confirmed through direct PLTX analogs detection in seafood leftovers. In many other cases, screening tests on seafood samples collected or purchased after or before the poisoning episode combined or not with clinical observations and symptoms, concluded that consumption of PLTX contaminated seafood was the causative reason of the health disorders. PLTX poisoning symptoms include nausea, tiredness, diarrhea, vomiting, dizziness, numbness of the extremities, muscle cramps, paresthesia, restless-ness, respiratory distress and bradycardia. In dramatic cases, patients died after 15h to 4 days following seafood poisonings, even after hospitalization. Unusual metallic taste of the seafood is one of the common signs noted by potential PLTX intoxication.
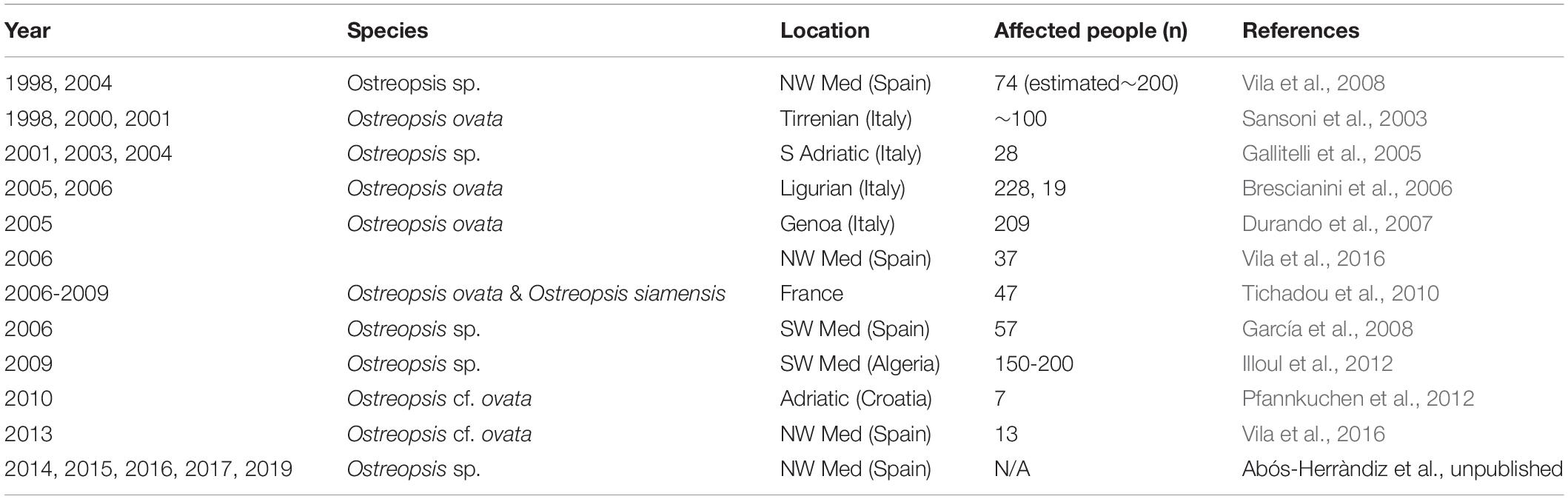
Table 2. Reports of mild respiratory, cutaneous and/or general malaise symptoms in humans after exposure to aerosols in Ostreopsis spp. blooms affected beaches.
In the Mediterranean coasts, the proliferation of O. cf. ovata and O. cf. siamensis since the end of the 21th century raised concern about the potential risk of seafood intoxication. Amzil et al. (2012), Biré et al. (2013), Biré et al. (2015), and Brissard et al. (2014) reported the presence of toxin content in macrofauna (mainly sea urchin, rock shell, crab and flathead mullet) sampled during O. cf. ovata blooms. OVTX-a was the most abundant toxin detected, and in some cases PLTX equivalents accounted on average for 223 to 392 μg⋅kg–1 of whole flesh, exceeding the threshold of 30 μg⋅kg–1 in shellfish recommended by the European Food Safety Authority (EFSA). The key questions are whether Ostreopsis was the ultimate organism synthesizing the PLTX-like compounds that contaminated the seafood and, how toxins can be transferred through the food webs. Fortunately, until now, seafood poisonings due to PLTX analogs have not been reported in the Mediterranean zone.
Mild Respiratory and Cutaneous Symptoms After Direct Exposure to Seawater and/or Aerosol During Ostreopsis Blooms
In the last 15 years, in several Mediterranean and Brazilian beaches, Ostreopsis blooms have been associated to mild skin and respiratory symptoms and general malaise in beach users, workers and inhabitants of the first coastline, after direct contact with water and/or after inhalation of marine aerosols (Table 2). Symptoms include rhinorrhea, pharyngeal pain, dry or mildly productive cough, nose irritation, general malaise, headache, fever (≤38°C), eye irritation and/or dermatitis (Durando et al., 2007; Tichadou et al., 2010). Most of the symptoms disappeared within a few hours without specific medication when people moved away from the affected area in the vicinity of the Ostreopsis bloom; only a few severe cases required hospitalization (Durando et al., 2007). A metallic taste has also been reported by people when snorkeling and windsurfing, or by researchers (including the authors of this review) when sampling Ostreopsis blooms (Pfannkuchen et al., 2012). It has been hypothesized that PLTX analogs could be the most plausible agent causing the observed symptoms, in part because the observed signs were similar, although much milder, to those experienced by people exposed to aerosols from home aquaria containing zoanthids (Deeds and Schwartz, 2010). Indeed, culturing Palythoa corals have become popular among aquarists. Unfortunately, potent irritative compounds released after pouring boiling water or bleach in aquaria with the aim to eliminate a colony of zoanthids caused within 20 min after exposure, rhinorrhoea, coughing, difficult breathing and light headedness which progressed to severe fits of coughing and chest pain 4h later. At the local hospital the patient was administered an anti-inflammatory corticosteroid and pain medication, an inhaled steroid treatment and cough suppressant for one month. A follow-up examination by a pulmonary specialist two weeks post exposure diagnosed the patient with asthma-like symptoms of bronchial inflammation and bronchoconstriction. Similar cases have been reported in the last years (see Tables 4 and 5 in Tubaro et al., 2011) one last case affecting a whole family, with three little kids in the UK in August 2019. Metallic taste was also reported by people when snorkeling and windsurfing in coral reefs containing Palithoa-like corals.
However, during Ostreopsis blooms OVTX-a and isoPLTX (putative) have been only rarely found in the aerosol and in very low concentrations (2.4 pg ovatoxins per liter of air by Ciminiello et al., 2014) and not coinciding with respiratory symptoms in humans. Several studies (Brescianini et al., 2006; Mangialajo et al., 2011) suggested that Ostreopsis fragments, mucus, and/or associated biota (including bacteria, cyanobacteria or other pico- or nanoeukaryotes) could be present in the aerosol and cause the health disorders. It should be noted here that symptoms do not occur concurrently with high cell Ostreopsis densities neither during the bloom, but only in certain periods. Vila et al. (2016) conducting a parallel epidemiology and ecology survey found that symptoms occurred at the end of the exponential phase and initial stationary phase of the bloom, at least in 2013 in a Mediterranean hot spot. This trend has been approximately repeated in the following years in that hot spot (Abós-Herràndiz et al., unpublished). Still an open question is how toxins and/or fragment of Ostreopsis can be aerosolized and whether these toxins are the causative agent of the disorders.
Toxicity on Mammalian Cells and Mode of Action
The main biological target of PLTX seems to be the Na+/K+-ATPase, a plasma membrane pump involved in the maintenance of trans-membrane ionic gradients of animal cells, essential for cellular functions and life (Habermann, 1989; Wu, 2009; Rossini and Bigiani, 2011). Impairment of the membrane pump by PLTX results in its conversion into a non-selective pore for monovalent cations, thereby destroying the transmembrane ionic gradient and triggering several adverse biological effects, some life threatening (Habermann, 1989; Artigas and Gadsby, 2003).
However, these studies have been conducted in vitro on mammalian cell tissues and the actual in vivo toxicity of the molecule is still poorly understood. In the 1970’s, semi-purified PLTX was tested in a broad range of animal species through several routes of administration. Thus, a reduced or even increased toxicity of the pure molecule are possible. More recently, high purity PLTX administration in mice showed that toxicity is dependent upon the route of administration (Munday, 2011). Poli et al. (2018) characterized the toxicity and basic histopathological effects of PLTX (derived from Japanese Palythoa tuberculosa), the analog 42-OH-PLTX (from Hawaiian P. toxica) and ovatoxin-a (isolated from a Japanese strain of Ostreopsis ovata) after intraperitoneal and aerosol administration to rats. All toxin preparations showed similar potency towards mouse erythrocytes in the erythrocyte hemolysis assay and interactions with the anti-PLTX mAb (antibody). Intraperitoneal LD50 values between 0.92 and 3.26 μg⋅kg–1 consistent with published values, and aerosol LD50 values of 0.031 to 0.063 μg⋅kg–1 confirmed the potency of PLTX suggested by the literature. Most commonly affected tissues were the lungs, liver, heart, salivary glands, and adrenal glands.
Mechanisms of Toxins Release in Sea Water and Aerosolization
It is well recognized that microorganisms including some microalgae can be dispersed by air and survive in the aerosols (e.g., Genitsaris et al., 2011; revision by Tesson et al., 2016). Microorganisms become airborne mainly due to drop formation at the water surface microlayer, which is enriched with biological material in all aquatic habitats (Schlichting, 1974; Wilson et al., 2015). Foam drops of a diameter larger than 40 μm are formed by wind friction and breaking wave crests at wind speeds exceeding 7 to 11 m⋅s–1. Bubble bursting due to waves, rainfall, boat traffic, or supersaturation of gases in the water produces film drops (1 μm to 10 μm diameter), projected in various directions, and vertically emitted jet drops (6 μm to 100 μm diameter). Recreational activities (i.e., waterskying, using personal watercraft, swimming, or wading) can also generate aerosols. Once emitted, airborne turbulent kinetic energy drags the microorganisms further up into the atmosphere.
The few available studies indicate that microalgae are found at very low concentrations (10–4 to 104 cells per m–3, Mayol et al., 2014) and with heterogeneous distributions, which requires high-volume sampling technology. High-volume sampling on filters or agar plates can be achieved by different instruments, but high-volume sampling into liquids is more complicated, as high airflows may lead to evaporation and reduce collection efficiency. Once collected, a combination of microscopy and molecular tools contribute to the identification of the airborne microalga. The main microalgal groups found in bioaerosols are chlorophytes (“green algae”), bacillariophytes (diatoms, mainly small Nitzschia) and cyanobacteria. Airborne microalgae are recognized as allergens and antigens. They are the cause of severe medical issues, including respiratory allergies (e.g., hay fevers), asthmatic attacks, dermatitis and skin lesions, rhinitis, and disturbances in lymphatic systems or vital organs (e.g., protothecosis, see review in Ashok and Gupta, 1998). In addition, certain aerosolized algal toxins, namely, microcystins produced by cyanobacteria (Backer et al., 2010) brevetoxins synthesized by Karenia brevis (Pierce and Henry, 2008; Fleming et al., 2011) and OVTX and PLTX analogs generated by Ostreopsis species, are the causes of further human illnesses.
So far, the detection of Ostreopsis cf. ovata and its related toxins in the aerosol was conducted by Casabianca et al. (2013) and Ciminiello et al. (2014). Casabianca et al. (2013) used air pump samplers (CAV-A/mb, Barcelona, Spain) equipped with 15 cm diameter QM-A quartz filters (Whatman, Maidstone, United Kingdom). Two air samplers located at 0.5 m and 3 m above sea level, respectively, pumped air continuously for 3 days during an O. cf. ovata bloom at a flow rate of 30 m3⋅h–1. Filters were replaced every 6 or 7 h and were divided in two portions to analyze, respectively, the concentration of Ostreopsis cell concentrations by qPCR and toxins by the hemolytic assay and by liquid chromatography with fluorescence detection (LC-FLD). Ostreopsis cells were detected in the aerosol by qPCR, exhibiting a marked variability during the bloom although not always directly related to the concentrations of the epiphytic Ostreopsis in the water; the authors noted that wind and other environmental factors could have affected these findings. In the seawater, epiphytic Ostreopsis contained ca. 1.2 pg total PLTX analogs per cell but toxins were not detected in the aerosol. Different technical aspects (e.g., toxin binding to glass tubes, not efficient retention by the filters) were suggested as potential technical limitation. Ciminiello et al. (2014) also employed AirCube COM2 (Analitica Strumenti srl., Italy) equipped with 47 mm diameter glass/quartz microfiber filters (Whatman, Maidstone, United Kingdom) and SAS PCR VWR International (PBI, Italy). In this case, air and a collecting fluid (50 mL distilled water in 2009, and seawater in 2010) flow together through a nozzle were poured into a vessel with a coil; the collecting fluid was circulated continuously to prolong the contact time with aerosols and to enrich itself in toxins and/or cells. Both air samplers were located 10 m from the seashore and worked continuously for 1.5-6 h. Air flow speed was 10 L⋅min–1 in 2009 and 30 L⋅min–1 in 2010. The presence of OVTX in the aerosol at levels of 2.4 pg of OVTX per liter of air was confirmed for the first time but PLTX by inhalation exposure toxicological data were not available. Overall, the presence of both cells and toxins in the aerosols provided by these studies opened new possibilities to increase the understanding of the Ostreopsis-related respiratory syndrome.
Marine Chemical Ecology of Ostreopsis in the Aquatic Environment
This section reviews the interactions of Ostreopsis cells with different marine organisms and environments as part of the MCE of Ostreopsis.
Interactions With Bacteria, Viruses and Parasites
Interactions between microalgae species and bacteria remain poorly investigated although the available studies reveal connections playing important roles in the biology and the ecology of these microorganisms. Bacteria may interact with algae within the phycosphere (Bell and Mitchell, 1972) as free-living bacteria, attached to the algal cell surface or occurring as intracellular symbionts. Algae-bacteria interactions can be synergistic and mutualistic, as commensalism or symbiotic, e.g., by producing cytokinins – phytohormones (Ramanan et al., 2016). Some vitamins and ion chelators produced by bacteria are involved in nutrient recycling facilitating nutrient incorporation by algae, while, in return, bacteria use microalgal exudates (Sarmento and Gasol, 2012). Furthermore, competition, parasitism or antagonist interactions also occur. Bacteria could interfere microalgal life cycle and growth of some HAB species. Bacterial exopolymers can increase the tendency of cells to flocculate and enhance sinking rates and degradation of decaying (harmful and innocuous) blooms (Decho, 1990). In turn, algae could also control bacterial growth by the production of antibiotic or hydroxyl radicals and other selected anti-bacterial growth substances (Bell et al., 1974). Information on these different interactions focused on HAB species can be found, e.g., in Fukami et al., 1992; Oda et al., 1992; Sawayama et al., 1993; Doucette, 1995; Hare et al., 2005; Jasti et al., 2005; Maruyama et al., 2008; Kim et al., 2009; Trick et al., 2011. These interactions exhibit spatio-temporal variability scales (Doucette, 1995) which, in the case of HABs can also involve phylogenetically and metabolically diverse bacterial groups along the bloom dynamics (Mayali et al., 2011). While some bacteria could coexist with microalgal cells during the initiation and exponential phases of the blooms, antagonist or parasitic bacteria would proliferate before the declining period and saprophytic or scavenger bacteria will occur after bloom termination when the availability of phytodetritus is high.
Bacterial communities associated to Ostreopsis lenticularis appear to be species and even clone specific (Tosteson et al., 1989) they vary with the bloom phase, and likely, with the habitat. Pérez-Guzmán et al. (2008) identified single species belonging to the Cytophaga-Flavobacter-Bacteroides complex. Vanucci et al. (2016) and Guidi et al. (2018) found Alphaproteobacteria (83 % relative abundance - genera Ruegeria, Jannaschia and Erythrobacter-), Gammaproteobacteria (13%) and Flavobacteria (7%) during the early O. cf. ovata bloom period, while Alphaproteobacteria (66%) and Flavobacteria (19%) were predominant in peak bloom phases. In the case of dinoflagellates, bacteria-algae interactions can be very strong given that some microalgae are unable to grow in axenic conditions in culture confirming the predominant role of bacteria in algal cycle. This has also been observed in Ostreopsis cultures although not always (Vanucci et al., 2012a).
Some studies indicate that bacteria could directly or indirectly modulate production and degradation of microalgal toxins (Albinsson et al., 2014) although the results appear study- and species- dependent and contradictory. While associated bacteria were linked to the production of paralytic shellfish toxin by Alexandrium spp. (Gallacher et al., 1997) higher toxin levels were found in bacteria-free than in non-axenic cultures of Alexandrium tamarense (Hold et al., 2001) and A. lusitanicum (Wang et al., 2004). Ashton et al. (2003) reported a toxicity loss of Ostreopsis lenticularis when exposed to elevated temperature and explained it by the reduction of Ostreopsis associated Pseudomonas and Alteromonas bacteria. Axenic cultures of Ostreopsis cf. ovata (Vanucci et al., 2012a) were also characterized by lower toxin content in late stationary phase compared to non-axenic cultures. Ostreopsis cf. ovata cell concentration along a bloom was correlated with the abundance of planktonic, free-living Vibrio (Bellés-Garulera et al., 2016) and a high proportion of Vibrio were found attached to particles, suggesting that Ostreopsis bloom could provide biological colonizable surfaces (fragments of thecae or mucilage) for bacteria. The study suggested that bacteria could be involved in wound infections reported by beach users during Ostreopsis blooms. In fact, Ostreopsis blooms were reported in heavily antropogenically impacted environments (Cen et al., 2019). However, the role of bacteria, potentially colonizing the mucus produced by Ostreopsis (a mucilaginous matrix, described as a network of trichocysts embedded with acidic polysaccharides filaments by Honsell et al., 2013) in the respiratory irritations associated to the microalgal bloom has not been clarified yet.
Parasite infection may play critical roles in the regulation of HABs. It has been already described for the planktonic toxic dinoflagellate Alexandrium fundyense which presented an up-regulation of genes associated to defense against the parasite Amoebophrya and thus controlling host mortality (Garcés et al., 2013a; Lu et al., 2016, 2014). Ostreopsis spp. (among other phytoplankton species) was infected by the generalistic parasitoid Parvilucifera sinerae (Garcés et al., 2013b).
To our knowledge, there has been no research on the interactions between Ostreopsis species and viruses while viruses have been proposed as natural agents to control HABs. This is the case of the virus HcRNAV, specific of the dinoflagellate Heterocapsa circularisquama (Nagasaki and Yamaguchi, 1997; Nagasaki et al., 2004; Nakayama and Hamaguchi, 2016).
Interactions With Heterotrophic Protists and Ciliates
Heterotrophic dinoflagellates (HTDs) and ciliates have been described as effective grazers on HAB species (Matsuyama et al., 1999; Tillmann, 2004; Jeong et al., 2015). However, so far, only one study has investigated feeding on Ostreopsis cf. ovata by a ciliate and various HTDs, all them potentially able to ingest it based on cell size criteria (Yoo et al., 2015). O. cf. ovata was ingested by Gyrodinium dominans, G. moestrupii, Oxyrrhis marina, and P. kofoidii by engulfment and by Pfiesteria piscicida using a peduncle. The study suggested that these HTDs can feed on O. cf. ovata by enzymes involved in the detection, capture, ingestion and digestion of toxic dinoflagellates. In contrast, lack of these enzymes in the naked ciliate Strombilidium sp. and the HTDs Gyrodinium spirale, Protoperidinium bipes, and Stoeckeria algicida would impede feeding on O. cf. ovata. Overall, information on microzooplankton grazing on Ostreopsis species in the field are lacking, and there is a major uncertainty on whether the success of this benthic microalga is caused by lack of effective predation. It has also been suggested that the mucus could be deterrent agent.
Interactions With Flora
A relatively small but recent number of studies focus on interactions between Ostreopsis species and flora, microphytobenthos and macrophytes, suggesting different allelopathic interactions during the dinoflagellate bloom dynamics. In these studies, the degree of interaction has been measured using different physiology and morphology parameters of the tested organisms, namely, growth rate (García-Portela et al., 2016; Ben et al., 2017) fluorescence (Ben et al., 2017; Ternon et al., 2018) swimming speed (Yoo et al., 2015) adherence capability (García-Portela et al., 2016) cysts formation (Accoroni et al., 2016b) cellular integrity (variation of shape, size, nucleus position using lipid and nuclear staining; Pichierri et al., 2017) and toxin production (Ben et al., 2017). The interactions with micro- and macroalgae are presented next and summarized in the Figure 3.
Microalgae
Some studies have shown that particular secondary metabolites i.e., low molecular weight compounds (as opposed to peptids or proteins) not directly involved in basic life processes, could considerably affect HABs dynamic and growth (Uchida et al., 1999; Fistarol et al., 2003; Prince et al., 2008; Yamasaki et al., 2010). These sorts of interactions have also been addressed concerning the impact of Ostreopsis cf. ovata on diatom and other dinoflagellates, as well as the retroactions of these microalgae on O. cf. ovata.
A strong inhibitory effect of O. cf. ovata on microalgae was observed in the in situ study of Accoroni et al. (2016b). In particular, it was manifested as a significant decrease in the diversity index and an increase of motile diatoms abundance during the O. cf. ovata bloom. Motile life diatom phase would be favored by the Ostreopsis mucous matrix, illustrating the importance of the mucus in the interactions between O. cf. ovata and its environment. Recently, Ternon et al. (2018) using co-cultures assays (with diatoms) suggested a contact-mediated toxicity of Ostreopsis cells, probably by the mucus, which could retain the toxins and other compounds and avoid their dilution in the surrounding environment.
Monti and Cecchin (2012) observed weak effects on the growth of the dinoflagellates Coolia monotis and Prorocentrum minimum when exposed to filtrates of O. cf. ovata cultures obtained during its exponential phase. This highlights the importance of growth stages considered in the experiments. Indeed, variation of the toxin content among the growth stage has been described in different studies (Vanucci et al., 2012a; Brissard et al., 2014; Pezzolesi et al., 2014) on Ostreopsis cf. ovata cultures, with a trend of higher concentration in the stationary phase.
García-Portela et al. (2016) using mixed cultures of different Ostreopsis strains and toxic and non-toxic dinoflagellates, demonstrated differences of toxicity among Ostreopsis strains. Indeed, O. cf. ovata was more toxic than O. sp. «Lanzarote type», highlighting the necessity to study the intraspecific variability of the interactions of Ostreopsis species with other organisms. The chemical compounds involved in the negative interaction of Ostreopsis and other microalgae (specially diatoms) are poorly known and secondary metabolites other than the OVTXs group could play a role, as suggested by Ternon et al. (2018).
In turn, it has been observed that planktonic and benthic diatoms can negatively affect O. cf. ovata physiology, morphology and growth. An inhibition of its growth rate by 57 % and 78 % was reported in cultures exposed to, respectively, filtrates of the planktonic diatoms Skeletonema marinoi and Thalassiosira sp., and similar growth inhibition, deleterious effects and genotoxic damages were also observed when exposed to filtrates of other benthic diatoms (e.g., Tabularia affinis, Proschkinia complanatoides and Navicula sp.) (Pichierri et al., 2017). These studies suggested the production of allelopathic compounds by all tested diatoms, especially released after Ostreopsis cell destruction (Pohnert et al., 2007). These diatoms are well known to produce a large family of polyunsaturated aldehydes (PUAs - Wichard et al., 2005). Pichierri et al. (2017) found that exposure to a range of PUAs (2E,4E-decadienal, 2E,4E-octadienal and 2E,4E-heptadienal) concentrations (from 3 to 36 μmol⋅L–1) caused O. cf. ovata growth inhibition, decrease in photosynthesis efficiency, increase of abnormal cells (motionless, decrease in dimensions, contraction of cytoplasm and formation of abnormal vesicle-like structure) and a decrease in cell integrity (chromatin dispersion, lack of autofluorescence of the chlorophyll and larger lipid bodies).
Macroalgae
Biotic substrate preferences of Ostreopsis species has been demonstrated in several studies (Totti et al., 2010). Substrate choice could be explained in part, by the macroalgal thallus surface and morphology: complex, three dimensional flexible and branched thalli favored Ostreopsis cell attachment (Cohu et al., 2013; Accoroni et al., 2016a; Meroni et al., 2018). These thalli would also be more suitable for O. cf. ovata (Vila et al., 2001; Accoroni et al., 2012) resistance to wave action. Higher abundances of O. cf. ovata were also found on the turf dominated community i.e., in a complex intricate matrix of small Corallinales, Ceramiales (both Florideophyceae) and other filamentous algae (Meroni et al., 2018) as reported in Vila et al. (2001) for Ellisolandia elongata and in Blanfuné et al. (2015) for Jania rubens. Lee and Park (2018) also showed that Ostreopsis sp. ingested rhodophytes because of their soft cell membrane containing microfibrillar and sulfated galactans (see section 4.2).
The production of allelopathic compounds by macroalgae could also explain Ostreopsis species substrate preferences. Macroalgae are known to produce a large number of secondary metabolites sometimes used in biotechnology as antibiofouling coatings (Hellio et al., 2002) or as a way to control HABs (Hu and Hong, 2008; Tang et al., 2015). Thus, it is reasonable to hypothesize that these metabolites could selectively determine substrate preference of Ostreopsis species. Rhodymenia pseudopalmata (Florideophyceae) had little effect on Ostreopsis growth and only in powder form (Accoroni et al., 2015). The seagrasses Zostera noltei and Cymodocea nodosa has also a low impact on O. cf. ovata growth although Z. noltei have been described as a producer of phenolic acids inhibiting the growth of Alexandrium catenella (Laabir et al., 2013). Ulva rigida (Ulvophyceae) and Dyctyota dichotoma (Phaeophyceae) had a strong effect on O. cf. ovata by decreasing its growth, physiology, morphology, toxin production and behavior with a decrease of cell adherence (Accoroni et al., 2015; Ben et al., 2017). The potential allelochemical compounds produced by this macroalgae are still poorly known but could mainly include phenols and polyunsaturated fatty acids (PUFAs - Ben et al., 2017).
Interactions With Fauna
Toxins produced by Ostreopsis have been documented in various marine organisms suggesting the potential bioaccumulation among the trophic web reaching levels above the alert threshold recommended by EFSA. The toxin transfer, which could be analogous to that of Gambierdiscus species synthesized ciguatoxins and maitotoxins (Ledreux et al., 2014), requires more research. Meanwhile, the few existing studies have reported strong differences of sensitivity (e.g., reprotoxicity potentially leading to changes in benthic communities) between organisms exposed to Ostreopsis toxins, suggesting unknown mechanisms of acclimation and detoxification.
Impacts of Ostreopsis Blooms on Fauna in Tropical and Temperate Areas
As indicated above, human intoxications have been reported in tropical areas mainly due to the consumption of fish or crustaceans contaminated with PLTX or its analogs without any indication of the effects of these toxins on the vector organisms. This suggests a direct ingestion, at least by herbivorous ingesting macroalgae supporting Ostreopsis cells. Even if no human intoxication due to the consumption of fish or crustaceans was reported in temperate areas, massive mortality of invertebrates occurred during Ostreopsis sp. bloom. Mass mortalities of sea urchins were reported in Italy (Vale and Ares, 2007) Algeria (Illoul et al., 2012) Brazil (Granéli et al., 2002) New Zealand (Shears and Ross, 2009) Spain (Vila et al., 2008) and France (Blanfuné et al., 2012). Impacts on other sessile and mobile benthic organisms such as cirripeds, mussels, limpets and cephalopods were also observed (Ciminiello et al., 2006; Totti et al., 2010). Even if the role of oxygen depletion and high seawater temperature could be involved in those mass mortalities (Shears and Ross, 2009) more and more authors rather suggest that these mortalities were a consequence of some ecotoxic effects associated to Ostreopsis sp. blooms (Sansoni et al., 2003; Simoni et al., 2003; Vila et al., 2008).
Direct Interactions Between Marine Fauna and Ostreopsis Species
Ostreopsis is an epibenthic genus, growing attached to macroalgae or rocks (Totti et al., 2010; Cohu et al., 2013, 2011; Penna et al., 2014; Carnicer et al., 2015a) thanks to the production of a mucilaginous matrix (Honsell et al., 2013; Escalera et al., 2014). Cells are also released in the water column, even if the link between planktonic and benthic abundances exhibits a high variability (Mangialajo et al., 2011) and can also aggregate on the water surface, forming what was called “sea-flowers”. Ostreopsis cells could thus have different impacts on marine ecosystems depending on whether cells are benthic, planktonic or aggregated at surface. These interactions are summarized in Figure 4.
Interactions of fauna with benthic Ostreopsis cells
When Ostreopsis cells attach on benthic substrates, they can interact with its surrounding community and notably with meiobenthic organisms. Indeed, these benthic organisms live in close contact with Ostreopsis cells and could potentially feed on this toxic dinoflagellate. To study the impact of O. cf. ovata on benthic organisms, bioassays are commonly used (Table 3). For instance, the benthic copepod Tigriopus fulvus and the amphipod Corophium insidiosum are both sensitive organisms since their Ostreopsis median lethal concentration (LC50) values after 96 hours of exposition are, respectively, 10.11 et 11.81 cell⋅mL–1 (Prato et al., 2011). Other organisms, such as the harpacticoid copepod Sarsamphiascus cf. propinquus, co-occuring with O. cf. ovata in summer, has been demonstrated as very resistant to toxins produced by O. cf. ovata (Pavaux et al., 2019) with LC50 (48h) values higher than 20,000 cells⋅mL–1 so. These numbers are at least 250 times higher than those reported for another benthic copepod, Tigriopus fulvus (Faimali et al., 2012) suggesting potential acclimation phenomenon to the presence of Ostreopsis. However, these studies are difficult to compare since they used different life stages of the targeted organism, different parameters to determine the sensitivity, and different determination of LC50 values, e.g., at 24, 48, or 96 h, the experimental conditions (e.g., temperature) during LC50 determination. As an example, Faimali et al. (2012) showed that toxicity increased with the temperature of culture: LC50 values of the barnacle Amphibalanus amphitrite were 1416.95 and 192.26 at 20 and 25°C, respectively. This overall situation suggests the need of standardizing the methods to test the toxicity of Ostreopsis species on benthic organisms.
Additionally, a reprotoxic (negative effect on reproduction) effect of O. cf. ovata has been suggested by the significant decrease of the number of copepod nauplii described in the in situ study of Guidi-Guilvard et al. (2012) and confirmed by laboratory experiments by Pavaux et al. (2019). The two studies showed a decrease of fecundity and fertility ratios of the benthic copepod Sarsamphiascus cf. propinquus. These reprotoxic effects lead to changes in benthic communities by decreasing the number of nauplii.
By forming aggregates that cover macroalgae, Ostreopsis cells are also ingested by macroherbivores such as sea urchins or herbivorous fish. Using biological assays (HNA) or toxin analysis by HPLC-MS, several studies have already quantified PLTX and analogs of these two groups in these herviborous (Milandri et al., 2010; Amzil et al., 2012; Biré et al., 2013, 2015; Brissard et al., 2014). Paracentrotus lividus (an edible and herbivorous sea urchin) and Sarpa salpa (an herbivorous fish) accumulated high concentrations of PLTX-group toxins (respectively, 423 and 361 μg⋅kg–1), making these organisms good sentinel species for the presence of PLTXs in marine organisms (Biré et al., 2015). Bioaccumulation of toxins seems to be organ-dependent: no toxins were found in sea urchin gonads although it reached up to 423 μg eq PLTX⋅kg–1 in the digestive tract (Brissard et al., 2014). However, Bauder et al. (2001) described different affinity of diarrheic shellfish toxins (DST) produced by Prorocentrum lima for tissues leading to a quicker detoxification in the gonads. This low affinity of toxins for tissues except for viscera, could explain the absence of toxins in sea urchin gonads documented in the work of Biré et al. (2015, 2013). Neves et al. (2018) also reported that the ingestion of O. cf. ovata cells by adult sea urchins had an impact on their offspring (dead and abnormal larvae, arrest of embryonic development – Table 3). These development anomalies persist at least 8 months after the exposition to O. cf. ovata and seems to be transmitted from the female gonads with the involvement of the nitric oxide pathway since the transcription of several genes directly or indirectly modulated by nitric oxide showed transcription variations (Neves et al., 2018; Tibiriçá et al., 2019).
Interactions of fauna with planktonic Ostreopsis cells
During its planktonic phase, Ostreopsis cf. ovata could reach cell concentrations in the range of 105–106 cell⋅L–1 (Mangialajo et al., 2011) and thus affect planktonic organisms but also bivalves and other benthic filter-feeders. Some monitoring of toxin concentration in bivalves is conducted to prevent sanitary impacts during these toxic events (Efsa Panel on Contaminants in the Food Chain, 2009; Accoroni et al., 2011; Amzil et al., 2012; Lemée et al., 2012). Concentrations of toxins quantified in mussels (Mytilus galloprovincialis) varied from 28 to 228 μg⋅kg–1 (Table 3). Quantities of toxins found in other filter-feeders such as the mussel Perna canaliculus or the bivalve Arca noae were well below and frequently found only in trace amounts (Rhodes et al., 2000, 2002) probably due to the use of biological assays to quantify toxins in these organisms leading to an underestimation of toxin concentration.
Mechanisms of toxicity have been addressed by exploring histological impairments on Mytilus galloprovincialis adults (Gorbi et al., 2012, 2013). These studies showed a limited role of oxidative stress and suggested that toxic effects of the compounds produced by Ostreopsis species could be rather mediated by immune response than oxidative stress. Finally, toxins produced by Ostreopsis cf. ovata had a strong impact on the planktonic ephyrae larvae of the jellyfish Aurelia sp. (Giussani et al., 2016) and embryos and eggs of the sea urchin Paracentrotus lividus (Caroppo and Pagliara, 2011; Pagliara and Caroppo, 2012; Migliaccio et al., 2016). The sea urchin Lytechinus variegatus also showed reduced fertilization success and arrest of embryonic development at the gastrula stage when exposed to Ostreopsis cf. ovata cells (Neves et al., 2018). In summary, planktonic Ostreopsis cells can affect filter feeders but also meroplanktonic larvae.
Interactions of fauna with sea-surface Ostreopsis cells
Toxins quantified both in the digestive tract and in the whole flesh of the mullet Mugil cephalus were high and reached to 392.5 μg⋅kg–1 of flesh fresh which can be explained by the grazing behavior of this animal that feeds directly on Ostreopsis cf. ovata cells aggregates on the water surface (Biré et al., 2013). Other organism from the mediolittoral area such as the limpets Patella spp. are also exposed to surface cells of O. cf. ovata and up to now, studies have mainly focused on toxin quantification in the whole flesh that reached up to 12.7 μg⋅kg–1, well below the threshold value established by the EFSA (Efsa Panel on Contaminants in the Food Chain, 2009). The study of Blanfuné et al. (2012) reported a significant decrease of Patella spp. densities during the 2008 O. cf. ovata bloom in Genoa, attributed to the poisoning of the shellfish by ingestion of O. cf. ovata cells.
Indirect Interactions Between Ostreopsis and Marine Fauna
Toxin concentrations found in carnivorous and omnivorous organisms (Table 4) suggested transfer and bioaccumulation of toxins within the trophic web (Milandri et al., 2010; Faimali et al., 2012; Pezzolesi et al., 2012; Biré et al., 2013, 2015; Giussani et al., 2016). Toxins quantified in both the crab Eriphia verrucosa (carnivorous) and the gastropod Stramonita haemastoma (carnivorous) accounted for up to 58.6 and 26.4 μg⋅kg–1 (Fresh Weight) respectively. Octopuses also accumulated more than 446 μg⋅kg–1 (Milandri et al., 2010) suggesting a bioaccumulation of toxins among the trophic web which might have played a role in the ecosystem suffering with stranding of octopuses. However, mussels and other predators such as copepods have already been demonstrated as a vector for toxin transfer through both benthic and planktonic food webs (Furlan et al., 2013; Boisnoir et al., 2020).
Other studies reported a strong sensitivity of Dicentrarchus labrax fish juveniles to O. cf. ovata raising the question of the impact on the structure of marine ecosystems (Faimali et al., 2012; Pezzolesi et al., 2012; Giussani et al., 2016). Indeed, as mentioned by the authors, these fish are unable to eat Ostreopsis cells, the observed toxicity was exclusively due to contact with Ostreopsis cells.
Overall Perspective of the Impacts of Ostreopsis Species on Ecosystem Structure and Functioning
The studies described above allow to extract a general vision of the chemical ecology of Ostreopsis and the gaps in knowledge that are concentrated on three main levels: 1) the ecotoxic impacts, 2) the importance of the mucus in the biological interactions and 3) the regulation of the Ostreopsis blooms by biologic interactions.
Ecotoxic Impacts
The available information shows negative effects of this dinoflagellate on different trophic levels and suggests subsequent negative impacts on the structure of the ecosystems. At the level of primary producers, Ostreopsis species seem to have a strong competitive capacity as revealed by the negatively impact on diatoms and other dinoflagellates with which they co-occur, regardless of the exposure pathway (extracellular released compounds or whole living cells) leading to changes in microphytobenthic communities composition and succession. For secondary producers, a strong reprotoxic effect has also been reported. Herbivorous organisms feeding on Ostreopsis species (e.g., the benthic copepod Sarsamphiascus cf. propinquus) or organisms feeding on macroalgae supporting Ostreopsis populations (e.g., sea urchins -Paracentrotus lividus- or fish -Sarpa salpa-) could accumulate high quantity of toxins without short term lethal effects. Despite several studies reported invertebrate mass mortalities (Shears and Ross, 2009; Totti et al., 2010) research is needed to determine whether they can genuinely be attributed to toxins produced by Ostreopsis spp.
Overall, toxin transfer through the food webs poses a risk of seafood poisoning in humans; although not reported in temperate areas yet, some dramatic cases in tropical areas unfortunately occurred. However, the specific mechanisms of Ostreopsis toxin transfer through the marine foodwebs are unknown. Toxins could circulate from benthic unicellular algae to herbivorous fish as it has been demonstrated for ciguatoxins (Ledreux et al., 2014). Zooplankton, and more especially copepods or fecal pellets, could also be intermediate vectors, as described for microcystins (Smith and Haney, 2006) nodularin (Lehtiniemi et al., 2002) domoic acid (Baustian et al., 2018) okadaic acid (Maneiro et al., 2000) and paralytic shellfish toxin (Teegarden and Cembella, 1996).
Some organisms could be able to protect themselves from the presence of Ostreopsis by different mechanisms such as production of secondary metabolites or adaptation and acclimation process. Detoxification and storage mechanisms have been described in some organisms exposed to certain toxic dinoflagellates (Bricelj and Shumway, 1998; Bauder et al., 2001; Colin and Dam, 2002; Walid et al., 2010; Jauffrais et al., 2012; García-Lagunas et al., 2013). Analogous studies are lacking in the case of Ostreopsis species.
Importance of the Mucus in Ostreopsis Biological Interactions
The functional role of the mucilaginous matrix produced by Ostreopsis sp. is still unknown. Mucus production is considered as an adaptation to benthic life (Parsons et al., 2012; Escalera et al., 2014) and described as a complex structure composed by a network of long fibers, deriving from trichocysts extruded through thecal pores and by an amorphous matrix of acid polysaccharides (Honsell et al., 2013). Production of mucilaginous matrix is common in benthic dinoflagellates (MacKenzie et al., 2002) and plays a role in cellular adhesion to surfaces with the formation of a transparent film embedding many cells, providing a clear example of ecosystem engineering (Escalera et al., 2014). Its role in defense against grazers, in increasing buoyancy and metabolic self-regulation has been investigated in other microalgae (Reynolds, 2007). The role of the mucilaginous matrix as a method of defense against grazers or to capture larger organisms has also been documented for other heterotrophic (Noctiluca scintillans; KiΦrboe and Titelman, 1998) and mixotrophic dinoflagellates (Alexandrium pseudogonyaulax; Blossom et al., 2012).
For Ostreopsis, the role of the mucilage in species competition and predation has been also argued. The toxicity of the mucus was first documented by Giussani et al. (2015) with experiments of O. cf. ovata on Artemia salina and supported by images obtained by atomic force microscopy. More recently, Ternon et al. (2018) observed a toxic effect of O. cf. ovata on co-occurring diatom mediated by direct contact between cells. Based on this, the study suggested that the mucus could facilitate toxins storage, avoiding their dilution in the environment and thus contributing to the Ostreopsis competition capacity.
The role of this mucilaginous matrix in mixotrophic behavior was also suggested (Barone, 2007). Mixotrophy is a common way of nutrition in harmful algal species (Burkholder et al., 2008) and several studies have investigated this nutritional capacity in Ostreopsis, although not completely demonstrated yet. Faust and Morton (1995) observed Ostreopsis labens cells with large ingested particles within the cytoplasm. In 2012, Fraga et al. (2012) linked the presence of reddish round inclusions inside Ostreopsis cells (detected through light microscopy) to the mixotrophic behavior. Almada et al. (2017) showed evidence of phagotrophy using LysoSensor staining even if the prey inside the cells were not discernable. More recently, using molecular approaches, Lee and Park (2018) defined the red bodies as digestive vacuoles containing ingested red macroalgae (Class Florideophyceae).
The mucilage, rich in organic carbohydrates, appears also as biologically colonizable surface for the growth of some bacteria (Vanucci et al., 2016; Guidi et al., 2018) including Vibrio (Bellés-Garulera et al., 2016). This positive association for pathogenic bacteria increases the risk of health impacts posed by Ostreopsis to human populations.
Regulation of Ostreopsis Bloom by Biotic Interactions
There is a growing awareness that HABs may have been increasing globally in the last 40 years and may continue to increase under the fast-coming scenarios of global warning (Wells et al., 2019). HABs have strong impacts on human health and economy (Hoagland et al., 2002; Larkin and Adams, 2007; Adams et al., 2018). Hence the necessity to better understand factors and mechanisms favoring bloom formation and maintenance which is a complex result of biotic factors combined with chemical (salinity, nutrients,…) and physical processes (currents, upwelling, freshwater runoff,…) and, in some cases, associated to anthropogenic forcings including eutrophication and alteration of coastal habitats (Sellner et al., 2003). This review highlights the number, the importance and the complexity of interactions between Ostreopsis and its environment. The question is whether or not these biotic interactions could also regulate Ostreopsis blooms initiation, maintenance and decline. Many harmful bloom-forming species produce toxins whose adaptive significance is still unclear. Toxins could constitute a main chemical signal or cue, in the case of HAB species. It has been suggested that toxins confer some advantages in reaching very high cell densities (Anderson et al., 1998) by providing a defense against pathogens, grazers and parasites. This hypothesis is supported by the study of Selander et al. (2006) describing that toxin-producing species are less preferred by predators and that predator presence induces toxin production. In the case of O. cf. ovata, it has been noted that benthic copepods are able to actively select non-toxic diatoms in a microalgae mixture (Pavaux et al., 2019). Here, the information concerning the role of allelopathy, predation and parasitism in the Ostreopsis blooms dynamics is discussed.
It has been suggested that allelopathy could favor HABs by suppressing the growth of competitors (Smayda, 1997). Allelopathy mechanisms could include oxidative damage, loss of competitor mobility, inhibition of photosynthesis, inhibition of enzymes and membrane damage (Legrand et al., 2003). The production of allelochemicals could explain in particular the success of Karenia brevis blooms (Kubanek et al., 2005; Prince et al., 2008) but demonstrating such mechanisms in the field is difficult (Prince et al., 2008). Meta-analysis conducted by Jonsson et al. (2009) indicate that the significant effect of allelopathic mechanisms would only occur at high cell densities since at the beginning of a bloom, cell abundances are too low to concentrate enough infochemicals. Toxins could play a role as phytochemicals. However, Tillmann and John (2002) showed that toxins produced by Alexandrium spp. are not involved in allelochemical defense mechanism. In the case of Ostreopsis, Ternon et al. (2018) demonstrated the importance of the toxins in negative effects on diatoms (mediated by the mucus) and the potential involvement in the chemical control by O. cf. ovata on its competitor. These results are promising, and suggest that future studies in this line can contribute to clarify the conflicting about the role of the toxins as allelochemicals. As in many other situations, the response may be species specific.
The balance between growth- and loss-promoting factors determine the abundance of harmful species. Predation by zooplankton is the largest mortality factor for phytoplankton (Calbet and Landry, 2004). Factors such as size, shape, nutritional value are traditionally suggested to explain predation pressure. However, the production of chemical deterrents can also affect predation pressure. The few conducted studies on predation of Ostreopsis by meiofauna have shown low ingestion rate of this dinoflagellate by benthic copepods (Pavaux et al., 2019) while heterotrophic ciliates seem to be active predators of O. cf. ovata (Matsuyama et al., 1999). Thus, predation pressure mediated by heterotrophic ciliates on Ostreopsis could potentially participate in population decline, already demonstrated by Solé et al. (2006) on other HABs. However, contradictory results have been documented by the theoretical study of Mitra and Flynn (2006) describing predatory activation as a factor promoting HAB. Overall, more studies need to be conducted to clarify the role of predation as a control mechanism of Ostreopsis blooms.
Eukaryotic microparasites infecting bloom-forming dinoflagellates, belonging to Parvilucifera (Perkinsozoa) and Amoebophrya (Syndiniales) genus, have been reported in the literature (Park et al., 2004). In the case of Ostreopsis, different strains have been proved sensitive to the infection by Parvilucifera sinarae (Garcés et al., 2013a, b) but the role during natural blooms has not been described yet.
Perspectives and New Tools to Study MCE of Ostreopsis
This review summarized how blooms of Ostreopsis, that may last a relatively short time (4-6 weeks in the case of O. cf. ovata blooms in the Ligurian Sea, Mediterranean Sea), affect the different components of the marine ecosystems and this their structure and functioning. It can be envisaged that, in a context of global change leading to an intensification of Ostreopsis blooms, these effects could increase, uprising the ecosystem imbalances already posed by anthropogenic pressures in the coastal zone. A key open question is whether the ecosystems will adapt to increased Ostreopsis blooms, for instance through developing detoxification strategies and outcompetition by other microalgae. It is also important to understand the factors conducting to Ostreopsis blooms in order to minimize their occurrence and protect the environment and human health as much as possible.
To address these open questions and objectives the next studies should combine the technical approaches indicated next.
Harmonization of Ostreopsis Studies Including Ecotoxic Bio-Assays
Most field and experimental studies have been conducted on Ostreopsis cf. ovata, in part because its wide presence and recurrent blooms in the Mediterranean. However, the impacts of other Ostreopsis species need to be investigated, specially given their detection and blooms in many other areas, some of them pristine and that could constitute observatories of the climate change (Fernández-Zabala et al., 2019).
In order to better estimate the influence of Ostreopsis on marine ecosystems, experiments should be run to clarify the effect of the direct and/or indirect contact of Ostreopsis on different organisms, using realistic (i.e., naturally occurring) Ostreopsis cell concentrations, and following ecologically realistic tests are key. To understand the role of the mucus produced by Ostreopsis, it is necessary to efficiently separate it from the cells.
Several studies have documented the extreme sensitivity of Artemia franciscana and A. salina when exposed to Ostreopsis cells, cell filtrates, and toxin extracts, highlighting the relevance of Artemia as a model organism for ecotoxic studies (Table 5). In order to compare the toxicity of strains, cell filtrates and toxin extracts, a harmonized protocol is required in terms of temperature used, measured response parameters and units (i.e., median lethal concentration - LC50 - at 24/48/96 hours, lethal time -LT50 or Effect Concentration - EC50 expressed in mg⋅mL–1 or cells⋅mL–1) and selected larval stages. Indeed, Faimali et al. (2012) demonstrated strong differences of toxicity of Ostreopsis ovata strains cultivated at 20 or 25°C with a higher toxicity of strains maintained at 25°C. This bioassay presents a wide range of advantages compared to other bioassays such as the Mouse Bioassay (MBA) in terms of ethics, cost, time and simplicity. However, the responses of Artemia sp. exposed to toxins produced by Ostreopsis sp. are not specific since our studies (Pavaux et al., 2020). The Artemia bioassay can be a pre-screening test of Ostreopsis strains toxicity for OVTX analogues and other toxic metabolites produced by these dinoflagellates. Indeed, the production of other toxic compounds that do not belong to the OVTX family was suggested by Ternon et al. (2018) and confirmed by Pavaux et al. (2020) because toxicity on Artemia was caused by Ostreopsis cf. ovata’s fractions free of OVTXs. This highlights the importance of this bioassay for a pre-screening of toxic fractions before conducting the most costly and complex toxin analysis by HPLC-MS/MS. Most of the time, the quantification of toxins produced by Ostreopsis sp. is done by targeted analysis, i.e., by the quantification of ions belonging to the OVTXs family (three transitions per analog are monitored for OVTX-a to -f and isoPLTX: [M+2H]2+, [M+2H-H2O]2+ and [M+3H-H2O]3+; Brissard et al., 2014). Thanks to this bioassay, it would be more interesting to do untargeted analysis on fragments previously shown as toxic by the bioassay.
Use of Metabolomics and Other «Omics» Tools
The development of “omics” techniques, which allows the simultaneous and large-scale study of genes, transcripts, proteins and metabolites without knowing a priori their biological functions, also constitute an important tool for the study of new toxic compounds. In the field of MCE, metabolomics approaches are the most commonly used (Prince and Pohnert, 2010; Kuhlisch and Pohnert, 2015; Poulin and Pohnert, 2019). This recent technique allows the analysis of a wide range of low molecular weight metabolites of a cell, an organism in a given context (Fiehn et al., 2007). One of the distinctive features of the metabolomics approach is that, due to strong diversity of physico-chemical properties of molecules produced by an organism, it is today impossible to describe exhaustively the metabolome with the present analytic techniques. A method currently used in metabolomics combines LC-MS/MS and molecular networking and it is employed as a rapid analytical method to detect toxins (Watrous et al., 2012; Yang et al., 2013; Allard et al., 2016; Quinn et al., 2017). Mass spectrometry-based molecular networking relies on the principle that structurally similar molecules share similar MS/MS fragmentation patterns, thus giving a visual representation of molecular relatedness of compounds in a sample (Watrous et al., 2012). For instance, this technique allowed the quantitative identification of the lipopeptide malyngamide C by different strains of cyanobacteria (Winnikoff et al., 2014). More and more studies are applying metabolomic approaches to investigate biotic interactions between HAB species and their environment (Giacometti et al., 2013; Poulson-Ellestad et al., 2014; Poulin et al., 2018). The review of McLean (2013) on “omics” tools demonstrates the relevance of genomics, transcriptomics and proteomics approaches to advance our understanding on the ecology of HABs. These techniques could give insights in metabolic and regulatory pathways involved in toxins and other secondary metabolites production and the mechanisms of action of these metabolites in the target organisms. Proteomic and metabolomics coupled-analyses lead to a better understanding of allelopathy interactions between the HAB planktonic dinoflagellate Karenia brevis and co-occurring species (Poulson-Ellestad et al., 2014) and open a positive perspective to be applied to Ostreopsis spp. Indeed, it has been applied for the first time to Ostreopsis cf. ovata by Ternon et al. (2018). Using notably untargeted metabolomics approach, this study reported the production of organic compounds not linked to OVTXs family, underlining the urgent need to characterize Ostreopsis metabolome.
Isolation and Toxicity Evaluation of the Different PLTX Analogs
To date, only pure PLTX standard is commercialized and no OVTX standard is available, which restricts our knowledge on bioactivity and structure of PLTX analogs. Indeed, these studies on Ostreopsis toxins need large amounts of pure toxin standards. Poli et al. (2018) have reported differences of toxicity between PLTX and some of its analogs (42-OH-PLTX and OVTXa) by exposing rats to these toxins trough intraperitoneal routes and aerosol exposures and confirmed results already published by others colleagues (Tubaro et al., 2011; Ciminiello et al., 2013). noted huge differences of toxicity between OVTX a and PLTX: OVTX-a is 10 to 100 times less potent than the other but data obtained by Poli et al. (2018) showed only minimal differences. Such differences could explain the difficulties to compare toxin analogs concentrations from NW Mediterranean and Adriatic strains.
Conclusion
This review highlights the complexity of the interactions between Ostreopsis and its environment, summarized in Figure 5.
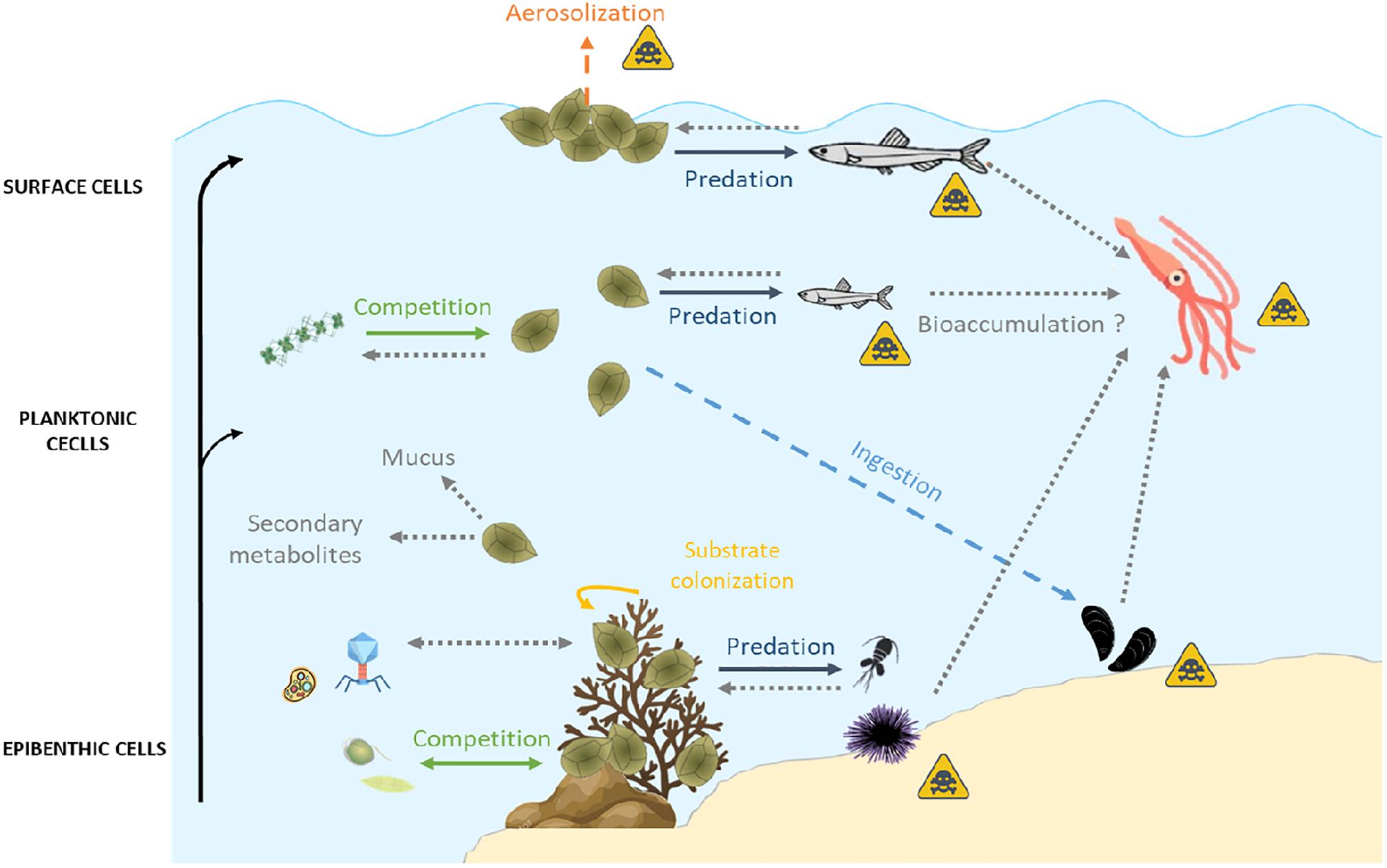
Figure 5. Summary of biotic relationships between Ostreopsis spp. and its environment. Arrows in full lines represent the direct interactions, those in dashes represent the indirect interactions. Gray dotted arrows represent unknown interactions which could provide useful guidelines for future research studies. The yellow triangular symbol represents possible human poisoning pathways by toxins produced by Ostreopsis spp. Icons on this figure are copyrighted by Flaticon.
Some potential avenues for future research on the interactions between Ostreopsis and its environment consist on:
- Increasing the research on all Ostreopsis species; so far 90% of studies documented in this review have been focused on Ostreopsis cf. ovata. This will allow to compare the potential harmful impacts on the different marine environments.
- Clarifying the role of the known toxins and other secondary metabolites in the chemical interactions of Ostreopsis and other organisms (predator deterrence, allelopathy, competition, etc.).
- Understand the factors affecting toxin production and clarify the toxicity of all Ostreopsis species (Table 1).
- Identifying the effects of ecologically realistic doses of toxic metabolites on the growth, reproduction and survival of the marine organisms exposed to Ostreopsis blooms.
- Clarifying the role of the mucus on the chemical interactions, as trap for preys, physical defence against predators, storage of toxins (or other metabolites), etc.
- Exploring the defence mechanisms of organisms exposed to Ostreopsis blooms, e.g., storage or detoxification processes, adaptation or acclimation processes.
- Elucidating the potential mechanism of toxic compounds transfer through the food web up to top predators and humans as already described for other HABs such as Gambierdiscus spp.
- Investigating the mechanisms involved in toxin aerosolization causing respiratory irritations in areas affected by Ostreopsis blooms.
Innovative approaches can contribute to address these questions and increase the knowledge of Ostreopsis spp. chemical interactions with its environment with potential consequences on human health.
Author Contributions
A-SP wrote and prepared the original draft. EB and RL wrote, reviewed, and edited the manuscript. All authors have read and agreed to the published version of the manuscript.
Conflict of Interest
The authors declare that the research was conducted in the absence of any commercial or financial relationships that could be construed as a potential conflict of interest.
Funding
This work was supported by the French ANR project (OCEAN 15: Ostreopsis Chemical Ecology and Allelopathy Network; project ANR-15-CE35-0002) and the project CoCliME [which is part of ERA4CS, an ERA-NET initiated by JPI Climate, and funded by EPA (IE), ANR (FR), BMBF (DE), UEFISCDI (RO), RCN (NO), and FORMAS (SE), with co-funding by the European, Union; Grant 6904462], endorsed to the international programme of IOC UNESCO and SCOR GlobalHAB (www.globalhab.info). French authors are members of the French National GdR PhycoTox.
Acknowledgments
We thank Dr. Rafael Abós-Herràndiz for providing information for Table 1.
References
Accoroni, S., Colombo, F., Pichierri, S., Romagnoli, T., Marini, M., Battocchi, C., et al. (2012). Ecology of Ostreopsis cf. ovata blooms in the Northwestern Adriatic Sea. Cryptogam. Algol. 33, 191–198. doi: 10.7872/crya.v33.iss2.2011.191
Accoroni, S., Percopo, I., Cerino, F., Romagnoli, T., Pichierri, S., Perrone, C., et al. (2015). Allelopathic interactions between the HAB dinoflagellate Ostreopsis cf. ovata and macroalgae. Harmful Algae 49, 147–155. doi: 10.1016/j.hal.2015.08.007
Accoroni, S., Romagnoli, T., Colombo, F., Pennesi, C., Di Camillo, C. G., Marini, M., et al. (2011). Ostreopsis cf. ovata bloom in the northern Adriatic Sea during summer 2009: ecology, molecular characterization and toxin profile. Mar. Pollut. Bull. 62, 2512–2519. doi: 10.1016/j.marpolbul.2011.08.003
Accoroni, S., Romagnoli, T., Penna, A., Capellacci, S., Ciminiello, P., Dell’Aversano, C., et al. (2016a). Ostreopsis fattorussoi sp. nov. (Dinophyceae), a new benthic toxic Ostreopsis species from the eastern Mediterranean Sea. J. Phycol. 52, 1064–1084. doi: 10.1111/jpy.12464
Accoroni, S., Romagnoli, T., Pichierri, S., and Totti, C. (2016b). Effects of the bloom of harmful benthic dinoflagellate Ostreopsis cf. ovata on the microphytobenthos community in the northern Adriatic Sea. Harmful Algae 55, 179–190. doi: 10.1016/j.hal.2016.03.003
Adams, C. M., Larkin, S. L., Hoagland, P., and Sancewich, B. (2018). “Assessing the economic consequences of harmful algal blooms: a summary of existing literature, research methods, data, and information gaps,” in Harmful Algal Blooms: A Compendium Desk Reference, Chap. 8, eds S. E. Shumway, J. M. Burkholder, and S. L. Morton (John Wiley & Sons Ltd.), 337–354.
Albinsson, M. E., Negri, A. P., Blackburn, S. I., and Bolch, C. J. S. (2014). Bacterial community affects toxin production by Gymnodinium catenatum. PLoS One 9:e104623. doi: 10.1371/journal.pone.0104623
Aligizaki, K., Katikou, P., Nikolaidis, G., and Panou, A. (2008). First episode of shellfish contamination by palytoxin-like compounds from Ostreopsis species (Aegean Sea. Greece). Toxicon 51, 418–427. doi: 10.1016/j.toxicon.2007.10.016
Allard, P.-M., Péresse, T., Bisson, J., Gindro, K., Marcourt, L., Pham, V. C., et al. (2016). Integration of molecular networking and in-silico MS/MS fragmentation for natural products dereplication. Anal. Chem. 88, 3317–3323. doi: 10.1021/acs.analchem.5b04804
Almada, E. V. C., Carvalho, W. F. D., and Nascimento, S. M. (2017). Investigation of phagotrophy in natural assemblages of the benthic dinoflagellates Ostreopsis, Prorocentrum and Coolia. Braz. J. Oceanogr. 65, 392–399. doi: 10.1590/s1679-87592017140706503
Amzil, Z., Sibat, M., Chomerat, N., Grossel, H., Marco-Miralles, F., Lemee, R., et al. (2012). Ovatoxin-a and palytoxin accumulation in seafood in relation to Ostreopsis cf. ovata blooms on the French Mediterranean coast. Mar. Drugs 10, 477–496. doi: 10.3390/md10020477
Anderson, D. M., Cembella, A. D., and Hallegraeff, G. M. (1998). Physiological Ecology of Harmful Algal Blooms. Heidelberg: Springer.
Artigas, P., and Gadsby, D. C. (2003). Na+/K+-pump ligands modulate gating of palytoxin-induced ion channels. Proc. Natl. Acad. Sci. U.S.A. 100, 501–505. doi: 10.1073/pnas.0135849100
Ashok, K. J., and Gupta, M. (1998). “Role of airborne bioparticles with special reference to algal components,” in Perspectives in Environment, eds S. K. Agarwal, J. P. Kaushik, K. K. Koul, and A. K. Jain (New Delhi: APH Publishing Corporation), 93–105.
Ashton, M., Rosado, W., Govind, N. S., and Tosteson, T. R. (2003). Culturable and nonculturable bacterial symbionts in the toxic benthic dinoflagellate Ostreopsis lenticularis. Toxicon 42, 419–424. doi: 10.1016/S0041-0101(03)00174-0
Backer, L. C., McNeel, S. V., Barber, T., Kirkpatrick, B., Williams, C., Irvin, M., et al. (2010). Recreational exposure to microcystins during algal blooms in two California lakes. Toxicon 55, 909–921. doi: 10.1016/j.toxicon.2009.07.006
Bakus, G. J., Targett, N. M., and Schulte, B. (1986). Chemical ecology of marine organisms: an overview. J. Chem. Ecol. 12, 951–987. doi: 10.1007/BF01638991
Barone, R. (2007). Behavioural trait of Ostreopsis ovata (Dinophyceae) in Mediterranean rock pools: the spider’s strategy. Harmful Algae News 33, 1–3.
Bauder, A., Cembella, A., Bricelj, V., and Quilliam, M. (2001). Uptake and fate of diarrhetic shellfish poisoning toxins from the dinoflagellate Prorocentrum lima in the bay scallop Argopecten irradians. Mar. Ecol. Prog. Ser. 213, 39–52. doi: 10.3354/meps213039
Baustian, M. M., Bargu, S., Morrison, W., Sexton, C., and Rabalais, N. N. (2018). The polychaete, Paraprionospio pinnata, is a likely vector of domoic acid to the benthic food web in the northern Gulf of Mexico. Harmful Algae 79, 44–49. doi: 10.1016/j.hal.2018.06.002
Bell, W., and Mitchell, R. (1972). Chemotactic and growth responses of marine bacteria to algal extracellular products. Biol. Bull. 143, 265–277. doi: 10.2307/1540052
Bell, W. H., Lang, J. M., and Mitchell, R. (1974). Selective stimulation of marine bacteria by algal extracellular products. Limnol. Oceanogr. 19, 833–839. doi: 10.4319/lo.1974.19.5.0833
Bellés-Garulera, J., Vila, M., Borrull, E., Riobó, P., Franco, J. M., and Sala, M. M. (2016). Variability of planktonic and epiphytic vibrios in a coastal environment affected by Ostreopsis blooms. Sci. Mar. 80, 97–106. doi: 10.3989/scimar.04405.01A
Ben, G. H., Yahia, O. K.-D., Cecchi, P., Masseret, E., Amzil, Z., Herve, F., et al. (2017). New insights on the species-specific allelopathic interactions between macrophytes and marine HAB dinoflagellates. PLoS One 12:e.0187963. doi: 10.1371/journal.pone.0187963
Bignami, G. S. (1993). A rapid and sensitive hemolysis neutralization assay for palytoxin. Toxicon 31, 817–820. doi: 10.1016/0041-0101(93)90389-Z
Biré, R., Trotereau, S., Lemée, R., Delpont, C., Chabot, B., Aumond, Y., et al. (2013). Occurrence of palytoxins in marine organisms from different trophic levels of the French Mediterranean coast harvested in 2009. Harmful Algae 28, 10–22. doi: 10.1016/j.hal.2013.04.007
Biré, R., Trotereau, S., Lemée, R., Oregioni, D., Delpont, C., Krys, S., et al. (2015). Hunt for palytoxins in a wide variety of marine organisms harvested in 2010 on the French Mediterranean coast. Mar. Drugs 13, 5425–5446. doi: 10.3390/md13085425
Blanfuné, A., Boudouresque, C. F., Grossel, H., and Thibaut, T. (2015). Distribution and abundance of Ostreopsis spp. and associated species (Dinophyceae) in the northwestern Mediterranean: the region and the macroalgal substrate matter. Environ. Sci. Pollut. Res. 22, 12332–12346. doi: 10.1007/s11356-015-4525-4
Blanfuné, A., Cohu, S., Mangialajo, L., Lemée, R., and Thibaut, T. (2012). Preliminary assessments of the impact of Ostreopsis cf. ovata (dinophyceae) development on macroinvertebrates in the north western mediterranean sea. Cryptogam. Algol. 33, 129–136. doi: 10.7872/crya.v33.iss2.2011.129
Blossom, H. E., Daugbjerg, N., and Hansen, P. J. (2012). Toxic mucus traps: a novel mechanism that mediates prey uptake in the mixotrophic dinoflagellate Alexandrium pseudogonyaulax. Harmful Algae 17, 40–53. doi: 10.1016/j.hal.2012.02.010
Boisnoir, A., Pavaux, A. S., Schizas, N. V., Marro, S., Blasco, T., Lemée, R., et al. (2020). The use of stable isotopes to measure the ingestion rate of potentially toxic benthic dinoflagellates by harpacticoid copepods. J. Exp. Mar. Biol. Ecol. 524:151285. doi: 10.1016/j.jembe.2019.151285
Botana, L. (2014). Seafood and Freshwater Toxins: Pharmacology, Physiology, and Detection. Boca Raton, FL: CRC Press, 1215. doi: 10.1201/b16662
Brescianini, C., Grillo, C., Melchiorre, N., Bertolotto, R., Ferrari, A., Vivaldi, B., et al. (2006). Ostreopsis ovata algal blooms affecting human health in Genova, Italy, 2005 and 2006. Euro Surveill. 11:E060907.3. doi: 10.2807/esw.11.36.03040-en
Bricelj, V. M., and Shumway, S. E. (1998). Paralytic shellfish toxins in bivalve molluscs: occurrence, transfer kinetics, and biotransformation. Rev. Fish. Sci. 6, 315–383. doi: 10.1080/10641269891314294
Brissard, C., Herrenknecht, C., Séchet, V., Hervé, F., Pisapia, F., Harcouet, J., et al. (2014). Complex toxin profile of French mediterranean Ostreopsis cf. ovata strains, seafood accumulation and ovatoxins prepurification. Mar. Drugs 12, 2851–2876. doi: 10.3390/md12052851
Brissard, C., Hervé, F., Sibat, M., Séchet, V., Hess, P., Amzil, Z., et al. (2015). Characterization of ovatoxin-h, a new ovatoxin analog, and evaluation of chromatographic columns for ovatoxin analysis and purification. J. Chromatogr. A 1388, 87–101. doi: 10.1016/j.chroma.2015.02.015
Burkholder, J. M., Glibert, P. M., and Skelton, H. M. (2008). Mixotrophy, a major mode of nutrition for harmful algal species in eutrophic waters. Harmful Algae 8, 77–93. doi: 10.1016/j.hal.2008.08.010
Cagide, E., Louzao, M. C., Espiña, B., Vieytes, M. R., Jaen, D., Maman, L., et al. (2009). Production of functionally active palytoxin-like compounds by Mediterranean Ostreopsis cf. siamensis. Cell. Physiol. Biochem. 23, 431–440.
Calbet, A., and Landry, M. R. (2004). Phytoplankton growth, microzooplankton grazing, and carbon cycling in marine systems. Limnol. Oceanogr. 49, 51–57. doi: 10.4319/lo.2004.49.1.0051
Carnicer, O., Guallar, C., Andree, K. B., Diogène, J., and Fernández-Tejedor, M. (2015a). Ostreopsis cf. ovata dynamics in the NW Mediterranean Sea in relation to biotic and abiotic factors. Environ. Res. 143, 89–99. doi: 10.1016/j.envres.2015.08.023
Carnicer, O., Tunin-Ley, A., Andree, K. B., Turquet, J., Diogène, J., and Fernández-Tejedor, M. (2015b). Contribution to the genus Ostreopsis in Reunion Island (Indian Ocean): molecular, morphologic and toxicity characterization. Cryptogam. Algol. 36, 101–119. doi: 10.7872/crya.v36.iss1.2015.101
Caroppo, C., and Pagliara, P. (2011). Effects of Ostreopsis cf. ovata (Dinophyceae) toxicity on Paracentrotus lividus development. Biol. Mar. Med. 18, 74–76.
Casabianca, S., Casabianca, A., Riobó, P., Franco, J. M., Vila, M., and Penna, A. (2013). Quantification of the toxic dinoflagellate Ostreopsis spp. by qPCR assay in marine aerosol. Environ. Sci. Technol. 47, 3788–3795. doi: 10.1021/es305018s
Cembella, A. D. (2003). Chemical ecology of eukaryotic microalgae in marine ecosystems. Phycologia 42, 420–447. doi: 10.2216/i0031-8884-42-4-420.1
Cen, J., Cui, L., Duan, Y., Zhang, H., Lin, Y., Zheng, J., et al. (2019). Effects of palytoxins extracted from Ostreopsis ovata on the oxidative stress and immune responses in Pacific White Shrimp Litopenaeus vannamei. Fish Shellfish Immunol. 95, 670–678. doi: 10.1016/j.fsi.2019.11.001
Chomérat, N., Bilien, G., Couté, A., and Quod, J. P. (2020). Reinvestigation of Ostreopsis mascarenensis Quod (Dinophyceae, Gonyaulacales) from Réunion Island (SW Indian Ocean): molecular phylogeny and emended description. Phycologia 59, 140–153. doi: 10.1080/00318884.2019.1710443
Chomérat, N., Bilien, G., Derrien, A., Henry, K., Ung, A., Viallon, J., et al. (2019). Ostreopsis lenticularis Y. Fukuyo (Dinophyceae, Gonyaulacales) from French Polynesia (South Pacific Ocean): a revisit of its morphology, molecular phylogeny and toxicity. Harmful Algae 84, 95–111. doi: 10.1016/j.hal.2019.02.004
Ciminiello, P., Dell’Aversano, C., Dello Iacovo, E., Fattorusso, E., Forino, M., Grauso, L., et al. (2012). Isolation and structure elucidation of ovatoxin-a, the major toxin produced by Ostreopsis ovata. J. Am. Chem. Soc. 134, 1869–1875. doi: 10.1021/ja210784u
Ciminiello, P., Dell’Aversano, C., Fattorusso, E., Forino, M., Grauso, L., and Tartaglione, L. (2011). A 4-decade-long (and still ongoing) hunt for palytoxins chemical architecture. Toxicon 57, 362–367. doi: 10.1016/j.toxicon.2010.09.005
Ciminiello, P., Dell’Aversano, C., Fattorusso, E., Forino, M., Magno, G. S., Tartaglione, L., et al. (2006). The Genoa 2005 Outbreak. Determination of putative palytoxin in mediterranean Ostreopsis ovata by a new liquid chromatography tandem mass spectrometry method. Anal. Chem. 78, 6153–6159. doi: 10.1021/ac060250j
Ciminiello, P., Dell’Aversano, C., Fattorusso, E., Forino, M., Tartaglione, L., Grillo, C., et al. (2008). Putative palytoxin and its new analogue, ovatoxin-a, in Ostreopsis ovata collected along the Ligurian coasts during the 2006 toxic outbreak. J. Am. Soc. Mass Spectrom. 19, 111–120. doi: 10.1016/j.jasms.2007.11.001
Ciminiello, P., Dell’Aversano, C., Iacovo, E. D., Fattorusso, E., Forino, M., Tartaglione, L., et al. (2013). Investigation of toxin profile of Mediterranean and Atlantic strains of Ostreopsis cf. siamensis (Dinophyceae) by liquid chromatography–high resolution mass spectrometry. Harmful Algae 23, 19–27. doi: 10.1016/j.hal.2012.12.002
Ciminiello, P., Dell’Aversano, C., Iacovo, E. D., Fattorusso, E., Forino, M., Tartaglione, L., et al. (2014). First Finding of Ostreopsis cf. ovata Toxins in Marine Aerosols. Environ. Sci. Technol. 48, 3532–3540. doi: 10.1021/es405617d
Cohu, S., Mangialajo, L., Thibaut, T., Blanfuné, A., Marro, S., and Lemée, R. (2013). Proliferation of the toxic dinoflagellate Ostreopsis cf. ovata in relation to depth, biotic substrate and environmental factors in the North West Mediterranean Sea. Harmful Algae 24, 32–44. doi: 10.1016/j.hal.2013.01.002
Cohu, S., Thibaut, T., Mangialajo, L., Labat, J.-P., Passafiume, O., Blanfuné, A., et al. (2011). Occurrence of the toxic dinoflagellate Ostreopsis cf. ovata in relation with environmental factors in Monaco (NW Mediterranean). Mar. Pollut. Bull. 62, 2681–2691. doi: 10.1016/j.marpolbul.2011.09.022
Colin, S. P., and Dam, H. G. (2002). Latitudinal differentiation in the effects of the toxic dinoflagellate Alexandrium spp. on the feeding and reproduction of populations of the copepod Acartia hudsonica. Harmful Algae 1, 113–125. doi: 10.1016/S1568-9883(02)00007-0
David, H., Laza-Martínez, A., Miguel, I., and Orive, E. (2013). Ostreopsis cf. siamensis and 9 cf. ovata from the Atlantic Iberian Peninsula: morphological and phylogenetic characterization. Harmful Algae 30, 44–55. doi: 10.1016/j.hal.2013.08.006
Decho, A. (1990). Microbial exopolymer secretions in ocean environments: their role(s) in food webs and marine processes. Oceanogr. Mar. Biol. Annu. Rev. 28, 73–154.
Deeds, J. R., and Schwartz, M. D. (2010). Human risk associated with palytoxin exposure. Toxicon 56, 150–162. doi: 10.1016/j.toxicon.2009.05.035
Delaney, J. E. (1984). “Bioassay procedures for shellfish toxins,” in Laboratory Procedures for the Examination of Seawater and Shellfish, eds A. Greenburg and D. Hunt (Washington, DC: The American Public Health Association), 64–80.
Dell’Aversano, C., Tartaglione, L., Dello Iacovo, E., Forino, M., Casabianca, S., Penna, A., et al. (2014). “Ostreopsis cf. ovata from the Mediterranean Sea. Variability in toxin profiles and structural elucidation of unknowns through LC-HRMSn,” in Proceedings of the 16th International Conference on Harmful Algae: Marine and Freshwater Harmful Algae, Wellington, 70–73.
Doucette, G. J. (1995). Interactions between bacteria and harmful algae: a review. Nat. Toxins 3, 65–74. doi: 10.1002/nt.2620030202
Durando, P., Ansaldi, F., Oreste, P., Moscatelli, P., Marensi, L., Grillo, C., et al. (2007). Ostreopsis ovata and human health: epidemiological and clinical features of respiratory syndrome outbreaks from a two-year syndromic surveillance, 2005-06, in north-west Italy. Euro Surveill. 12:E070607.1. doi: 10.2807/esw.12.23.03212-en
Efsa Panel on Contaminants in the Food Chain. (2009). Scientific opinion on marine biotoxins in shellfish – palytoxin group. EFSA J. 7:1393. doi: 10.2903/j.efsa.2009.1393
Escalera, L., Benvenuto, G., Scalco, E., Zingone, A., and Montresor, M. (2014). Ultrastructural features of the benthic dinoflagellate Ostreopsis cf. ovata (Dinophyceae). Protist 165, 260–274. doi: 10.1016/j.protis.2014.03.001
Faimali, M., Giussani, V., Piazza, V., Garaventa, F., Corrà, C., Asnaghi, V., et al. (2012). Toxic effects of harmful benthic dinoflagellate Ostreopsis ovata on invertebrate and vertebrate marine organisms. Mar. Environ. Res. 76, 97–107. doi: 10.1016/j.marenvres.2011.09.010
Faust, M. A. (1999). Three new Ostreopsis species (Dinophyceae): O. marinus sp. nov. O. belizeanus sp. nov., and O. caribbeanus sp. nov. Phycologia 38, 92–99. doi: 10.2216/i0031-8884-38-2-92.1
Faust, M. A., and Morton, S. L. (1995). Morphology and ecology of the marine dinoflagellate Ostreopsis labens sp. nov. (dinophyceae). J. Phycol. 31, 456–463. doi: 10.1111/j.0022-3646.1995.00456.x
Fernández-Zabala, J., Tuya, F., Amorim, A., and Soler-Onís, E. (2019). Benthic dinoflagellates: testing the reliability of the artificial substrate method in the Macaronesian region. Harmful Algae 87:101634. doi: 10.1016/j.hal.2019.101634
Fiehn, O., Robertson, D., Griffin, J., van der Werf, M., Nikolau, B., Morrison, N., et al. (2007). The metabolomics standards initiative (MSI). Metabolomics 3, 175–178. doi: 10.1007/s11306-007-0070-6
Fistarol, G. O., Legrand, C., and Granéli, E. (2003). Allelopathic effect of Prymnesium parvum on a natural plankton community. Mar. Ecol. Prog. Ser. 255, 115–125. doi: 10.3354/meps255115
Fleming, L. E., Kirkpatrick, B., Backer, L. C., Walsh, C. J., Nierenberg, K., Clark, J., et al. (2011). Review of Florida red tide and human health effects. Harmful Algae 20, 224–233. doi: 10.1016/j.hal.2010.08.006
Fraga, S., Rodriguez, F., Bravo, I., Zapata, M., and Marañon, E. (2012). Review of the main ecological features affecting benthic dinoflagellate blooms. Cryptogam. Algol. 33, 171–179.
Fukami, K., Yuzawa, A., Toshitaka, N., and Hata, Y. (1992). Isolation and properties of a bacterium inhibiting the growth of Gymnodinium nagasakiense. Nippon Suisan Gakkaishi 58, 1073–1077.
Fukuyo, Y. (1981). Taxonomical study on benthic dinoflagellates collected in coral reefs. Bull. Jpn. Soc. Sci. Fish. 47, 967–978. doi: 10.2331/suisan.47.967
Furlan, M., Antonioli, M., Zingone, A., Sardo, A., Blason, C., Pallavicini, A., et al. (2013). Molecular identification of Ostreopsis cf. ovata in filter feeders and putative predators. Harmful Algae 21-22, 20–29. doi: 10.1016/j.hal.2012.11.004
Gallacher, S., Flynn, K. J., Franco, J. M., Brueggemann, E. E., and Hines, H. B. (1997). Evidence for production of paralytic shellfish toxins by bacteria associated with Alexandrium spp. (Dinophyta) in culture. Appl. Environ. Microbiol. 63, 239–245.
Gallitelli, M., Ungaro, N., Addante, L. M., Procacci, V., Silver, N. G., and Sabbà, C. (2005). Respiratory illness as a reaction to tropical algal blooms occurring in a temperate climate. JAMA 293, 2595–2600.
Garcés, E., Alacid, E., Bravo, I., Fraga, S., and Figueroa, R. I. (2013a). Parvilucifera sinerae (Alveolata, Myzozoa) is a generalist parasitoid of dinoflagellates. Protist 164, 245–260. doi: 10.1016/j.protis.2012.11.004
Garcés, E., Alacid, E., René, A., Petrou, K., and Simó, R. (2013b). Host-released dimethylsulphide activates the dinoflagellate parasitoid Parvilucifera sinerae. ISME J. 7, 1065–1068.
García, P. B., de la Puerta, P. R., Carreno, T. P., Martínez, P. M., and Enríquez, J. G. (2008). Brote con síntomas respiratorios en la provincia de Almería por una posible exposición a microalgas tóxicas. Gac. Sanit. 22, 578–584. doi: 10.1016/S0213-9111(08)75357-3
García-Altares, M., Tartaglione, L., Dell’Aversano, C., Carciner, O., De la Iglesia, P., Forino, M., et al. (2015). The novel ovatoxin-g and isobaric palytoxin (so far referred to as putative palytoxin) from Ostreopsis cf. ovata (NW Mediterranean Sea): structural insights by LC-high resolution MSn. Anal. Bioanal. Chem. 407, 1191–1204. doi: 10.1007/s00216-014-8338-y
García-Lagunas, N., Romero-Geraldo, R., and Hernández-Saavedra, N. Y. (2013). Genomics study of the exposure effect of Gymnodinium catenatum, a paralyzing toxin producer, on Crassostrea gigas’ defense system and detoxification genes. PLoS One 8:e72323. doi: 10.1371/journal.pone.0072323
García-Portela, M., Riobó, P., Franco, J. M., Banuelos, R. M., and Rodríguez, F. (2016). Genetic and toxinological characterization of North Atlantic strains of the dinoflagellate Ostreopsis and allelopathic interactions with toxic and non-toxic species from the genera Prorocentrum, Coolia and Gambierdiscus. Harmful Algae 60, 57–69. doi: 10.1016/j.hal.2016.10.007
Gémin, M.-P., Réveillon, D., Hervé, F., Pavaux, A.-S., Tharaud, M., Séchet, V., et al. (2019). Toxin content of Ostreopsis cf. ovata depends on bloom phases, depth and macroalgal substrate in the NW Mediterranean Sea. Harmful Algae 92:101727. doi: 10.1016/j.hal.2019.101727
Genitsaris, S., Kormas, K., and Moustaka-Gouni, M. (2011). Airborne algae and cyanobacteria: occurrence and related health effects. Front. Biosci. 3, 772–787. doi: 10.2741/e285
Giacometti, J., Tomljanoviæ, A. B., and Josiæ, D. (2013). Application of proteomics and metabolomics for investigation of food toxins. Food Res. Int. 54, 1042–1051. doi: 10.1016/j.foodres.2012.10.019
Giussani, V., Costa, E., Pecorino, D., Berdalet, E., De Giampaulis, G., Gentile, M., et al. (2016). Effects of the harmful dinoflagellate Ostreopsis cf. ovata on different life cycle stages of the common moon jellyfish Aurelia sp. Harmful Algae 57, 49–58. doi: 10.1016/j.hal.2016.05.005
Giussani, V., Sbrana, F., Asnaghi, V., Vassalli, M., Faimali, M., Casabianca, S., et al. (2015). Active role of the mucilage in the toxicity mechanism of the harmful benthic dinoflagellate Ostreopsis cf. ovata. Harmful Algae 44, 46–53. doi: 10.1016/j.hal.2015.02.006
Gorbi, S., Avio, G. C., Benedetti, M., Totti, C., Accoroni, S., Pichierri, S., et al. (2013). Effects of harmful dinoflagellate Ostreopsis cf. ovata exposure on immunological, histological and oxidative responses of mussels Mytilus galloprovincialis. Fish Shellfish Immunol. 35, 941–950. doi: 10.1016/j.fsi.2013.07.003
Gorbi, S., Bocchetti, R., Binelli, A., Bacchiocchi, S., Orletti, R., Nanetti, L., et al. (2012). Biological effects of palytoxin-like compounds from Ostreopsis cf. ovata: a multibiomarkers approach with mussels Mytilus galloprovincialis. Chemosphere 89, 623–632. doi: 10.1016/j.chemosphere.2012.05.064
Granéli, E., Ferreira, C. E. L., Yasumoto, T., Rodriguez, E., and Neves, M. H. (2002). “Sea urchins poisoning by the benthic dinoflagellate Ostreopsis ovata on the Brazilian Coast,” Proceedings of the Xth International Conference on Harmful Algae, ed. K. A. Steidinger (St. Pete Beach, FL: International Society for the Study of Harmful Algae (ISSHA)).
Granéli, E., and Hansen, P. J. (2006). “Allelopathy in harmful algae: a mechanism to compete for resources?” Ecology of Harmful Algae. Ecological Studies (Analysis and Synthesis), Vol. 189, eds E. Granéli, and J. T. Turner (Berlin: Springer), 189–201
Granéli, E., Vidyarathna, N. K., Funari, E., Cumaranatunga, P. R. T., and Scenati, R. (2011). Can increases in temperature stimulate blooms of the toxic benthic dinoflagellate Ostreopsis ovata? Harmful Algae 10, 165–172. doi: 10.1016/j.hal.2010.09.002
Guerrini, F., Pezzolesi, L., Feller, A., Riccardi, M., Ciminiello, P., Dell’Aversano, C., et al. (2010). Comparative growth and toxin profile of cultured Ostreopsis ovata from the Tyrrhenian and Adriatic Seas. Toxicon 55, 211–220. doi: 10.1016/j.toxicon.2009.07.019
Guidi, F., Pezzolesi, L., and Vanucci, S. (2018). Microbial dynamics during harmful dinoflagellate Ostreopsis cf. ovata growth: bacterial succession and viral abundance pattern. MicrobiologyOpen 7:e00584. doi: 10.1002/mbo3.584
Guidi-Guilvard, L. D., Gasparini, S., and Lemée, R. (2012). The negative impact of Ostreopsis cf. ovata on phytal meiofauna from the coastal NW Mediterranean. Cryptogam. Algol. 33, 121–128. doi: 10.7872/crya.v33.iss2.2011.121
Habermann, E. (1989). Palytoxin acts through Na+, K+-ATPase. Toxicon 27, 1171–1187. doi: 10.1016/0041-0101(89)90026-3
Hare, C. E., Demir, E., Coyne, K. J., Craig Cary, S., Kirchman, D. L., and Hutchins, D. A. (2005). A bacterium that inhibits the growth of Pfiesteria piscicida and other dinoflagellates. Harmful Algae 4, 221–234. doi: 10.1016/j.hal.2004.03.001
Hay, M. E. (1996). Marine chemical ecology: what’s known and what’s next? J. Exp. Mar. Biol. Ecol. 200, 103–134. doi: 10.1016/S0022-0981(96)02659-7
Hay, M. E. (2009). Marine chemical ecology: chemical signals and cues structure marine populations, communities, and ecosystems. Annu. Rev. Mar. Sci. 1, 193–212. doi: 10.1146/annurev.marine.010908.163708
Hellio, C., Berge, J. P., Beaupoil, C., Le Gal, Y., and Bourgougnon, N. (2002). Screening of marine algal extracts for anti-settlement activities against microalgae and macroalgae. Biofouling 18, 205–215. doi: 10.1080/08927010290010137
Hoagland, P., Anderson, D. M., Kaoru, Y., and White, A. W. (2002). The economic effects of harmful algal blooms in the United States: estimates, assessment issues, and information needs. Estuaries 25, 819–837. doi: 10.1007/BF02804908
Hold, G. L., Smith, E. A., Birkbeck, T. H., and Gallacher, S. (2001). Comparison of paralytic shellfish toxin (PST) production by the dinoflagellates Alexandrium lusitanicum NEPCC 253 and Alexandrium tamarense NEPCC 407 in the presence and absence of bacteria. FEMS Microbiol. Ecol. 36, 223–234. doi: 10.1111/j.1574-6941.2001.tb00843.x
Honsell, G., Bonifacio, A., De Bortoli, M., Penna, A., Battocchi, C., Ciminiello, P., et al. (2013). New insights on cytological and metabolic features of Ostreopsis cf. ovata Fukuyo (Dinophyceae): a multidisciplinary approach. PLoS One 8:e57291. doi: 10.1371/journal.pone.0057291
Hoppenrath, M., Murray, S. A., Chomérat, N., and Horiguchi, T. (eds). (2014). Marine Benthic Dinoflagellates - Unveiling Their Worldwide Biodiversity, Vol. 54. Kleine Senckenberg-Reihe, 276, ISBN: 978-3-510-61402-8.
Hu, H., and Hong, Y. (2008). Algal-bloom control by allelopathy of aquatic macrophytes-a review. Front. Environ. Sci. Eng. 2, 421–438. doi: 10.1007/s11783-008-0070-4
Hwang, B. S., Yoon, E. Y., Kim, H. S., Yih, W., Park, J. Y., Jeong, H. J., et al. (2013). Ostreol A: a new cytotoxic compound isolated from the epiphytic dinoflagellate Ostreopsis cf. ovata from the coastal waters of Jeju Island. Korea. Bioorg. Med. Chem. Lett. 23, 3023–3027. doi: 10.1016/j.bmcl.2013.03.020
Ianora, A., Bentley, M. G., Caldwell, G. S., Casotti, R., Cembella, A. D., Engström-Öst, J., et al. (2011). The relevance of marine chemical ecology to plankton and ecosystem function: an emerging field. Mar. Drugs 9, 1625–1648. doi: 10.3390/md9091625
Illoul, H., Hernández, F. R., Vila, M., Adjas, N., Younes, A. A., Bournissa, M., et al. (2012). The genus Ostreopsis along the Algerian coastal waters (SW Mediterranean Sea) associated with a human respiratory intoxication episode. Cryptogam. Algol. 33, 209–216. doi: 10.7872/crya.v33.iss2.2011.209
Jasti, S., Sieracki, M. E., Poulton, N. J., Giewat, M. W., and Rooney-Varga, J. N. (2005). Phylogenetic diversity and specificity of bacteria closely associated with Alexandrium spp. and Other Phytoplankton. Appl. Environ. Microbiol. 71, 3483–3494. doi: 10.1128/AEM.71.7.3483-3494.2005
Jauffrais, T., Marcaillou, C., Herrenknecht, C., Truquet, P., Séchet, V., Nicolau, E., et al. (2012). Azaspiracid accumulation, detoxification and biotransformation in blue mussels (Mytilus edulis) experimentally fed Azadinium spinosum. Toxicon 60, 582–595. doi: 10.1016/j.toxicon.2012.04.351
Jeong, H. J., Lim, A. S., Franks, P. J. S., Lee, K. H., Kim, J. H., Kang, N. S., et al. (2015). A hierarchy of conceptual models of red-tide generation: nutrition, behavior, and biological interactions. Harmful Algae 47, 97–115. doi: 10.1016/j.hal.2015.06.004
Jonsson, P. R., Pavia, H., and Toth, G. (2009). Formation of harmful algal blooms cannot be explained by allelopathic interactions. Proc. Natl. Acad. Sci. U.S.A. 106, 11177–11182. doi: 10.1073/pnas.0900964106
Kim, Y. S., Lee, D.-S., Jeong, S.-Y., Lee, W. J., and Lee, M.-S. (2009). Isolation and characterization of a marine algicidal bacterium against the harmful raphidophyceae Chattonella marina. J. Microbiol. 47, 9–18. doi: 10.1007/s12275-008-0141-z
KiΦrboe, T., and Titelman, J. (1998). Feeding, prey selection and prey encounter mechanisms in the heterotrophic dinoflagellate Noctiluca scintillans. J. Plankton Res. 20, 1615–1636. doi: 10.1093/plankt/20.8.1615
Kubanek, J., Hicks, M. K., Naar, J., and Villareal, T. A. (2005). Does the red tide dinoflagellate Karenia brevis use allelopathy to outcompete other phytoplankton? Limnol. Oceanogr. 50, 883–895. doi: 10.4319/lo.2005.50.3.0883
Kuhlisch, C., and Pohnert, G. (2015). Metabolomics in chemical ecology. Nat. Prod. Rep. 32, 937–955. doi: 10.1039/C5NP00003C
Laabir, M., Grignon-Dubois, M., Masseret, E., Rezzonico, B., Soteras, G., Rouquette, M., et al. (2013). Algicidal effects of Zostera marina L. and Zostera noltii Hornem. extracts on the neuro-toxic bloom-forming dinoflagellate Alexandrium catenella. Aquat. Bot. 111, 16–25. doi: 10.1016/j.aquabot.2013.07.010
Larkin, S. L., and Adams, C. M. (2007). Harmful algal blooms and coastal business: economic consequences in Florida. Soc. Nat. Resour. 20, 849–859. doi: 10.1080/08941920601171683
Ledreux, A., Brand, H., Chinain, M., Bottein, M.-Y. D., and Ramsdell, J. S. (2014). Dynamics of ciguatoxins from Gambierdiscus polynesiensis in the benthic herbivore Mugil cephalus: trophic transfer implications. Harmful Algae 39, 165–174. doi: 10.1016/j.hal.2014.07.009
Lee, B., and Park, M. G. (2018). Genetic analyses of the rbcL and psaA genes from single cells demonstrate a rhodophyte origin of the prey in the toxic benthic dinoflagellate Ostreopsis. Front. Mar. Sci. 5:217. doi: 10.3389/fmars.2018.00217
Legrand, C., Rengefors, K., Fistarol, G., and Granéli, E. (2003). Allelopathy in phytoplankton - biochemical, ecological and evolutionary aspects. Phycologia 42, 406–419. doi: 10.2216/i0031-8884-42-4-406.1
Lehtiniemi, M., Engström-Öst, J., Karjalainen, M., Kozlowsky-Suzuki, B., and Viitasalo, M. (2002). Fate of cyanobacterial toxins in the pelagic food web: transfer to copepods or to faecal pellets? Mar. Ecol. Prog. Ser. 241, 13–21. doi: 10.3354/meps241013
Lemée, R., Mangialajo, L., Cohu, S., Amzil, Z., Blanfune, A., Chomerat, N., et al. (2012). Interactions between scientists, managers and policy makers in the framework of the French MediOs Project on Ostreopsis (2008–2010). Cryptogam. Algol. 33, 137–142. doi: 10.7872/crya.v33.iss2.2011.137
Lenoir, S., Ten Hage, L., Turquet, J., Quod, J. P., Bernard, C., and Hennion, M. C. (2004). First evidence of palytoxin analogues from an Ostreopsis mascarenensis (dinophyceae) benthic bloom in Southwestern Indian Ocean. J. Phycol. 40, 1042–1051. doi: 10.1111/j.1529-8817.2004.04016.x
Lu, Y., Wohlrab, S., Glöckner, G., Guillou, L., and John, U. (2014). Genomic insights into processes driving the infection of Alexandrium tamarense by the parasitoid Amoebophrya sp. Eukaryot. Cell 13, 1439–1449. doi: 10.1128/EC.00139-14
Lu, Y., Wohlrab, S., Groth, M., Glöckner, G., Guillou, L., and John, U. (2016). Transcriptomic profiling of Alexandrium fundyense during physical interaction with or exposure to chemical signals from the parasite Amoebophrya. Mol. Ecol. 25, 1294–1307. doi: 10.1111/mec.13566
MacKenzie, L., Sims, I., Beuzenberg, V., and Gillespie, P. (2002). Mass accumulation of mucilage caused by dinoflagellate polysaccharide exudates in Tasman Bay, New Zealand. Harmful Algae 1, 69–83. doi: 10.1016/S1568-9883(02)00006-9
Maneiro, I., Frangópulos, M., Guisande, C., Fernández, M., Reguera, B., and Riveiro, I. (2000). Zooplankton as a potential vector of diarrhetic shellfish poisoning toxins through the food web. Mar. Ecol. Prog. Ser. 201, 155–163. doi: 10.3354/meps201155
Mangialajo, L., Ganzin, N., Accoroni, S., Asnaghi, V., Blanfuné, A., Cabrini, M., et al. (2011). Trends in Ostreopsis proliferation along the Northern Mediterranean coasts. Toxicon 57, 408–420. doi: 10.1016/j.toxicon.2010.11.019
Maruyama, A., Maeda, M., and Simidu, U. (2008). Occurrence of plant hormone (cytokinin)-producing bacteria in the sea. J. Appl. Microbiol. 61, 569–574. doi: 10.1111/j.1365-2672.1986.tb01731.x
Matsuyama, Y., Miyamoto, M., and Kotani, Y. (1999). Grazing impacts of the heterotrophic dinoflagellate Polykrikos kofoidii on a bloom of Gymnodinium catenatum. Aquat. Microb. Ecol. 17, 91–98. doi: 10.3354/ame017091
Mayali, X., Franks, P. J. S., and Burton, R. S. (2011). Temporal attachment dynamics by distinct bacterial taxa during a dinoflagellate bloom. Aquat. Microb. Ecol. 63, 111–122. doi: 10.3354/ame01483
Mayol, E., Jiménez, M. A., Herndl, G. J., Duarte, C. M., and Arrieta, J. M. (2014). Resolving the abundance and air-sea fluxes of airborne microorganisms in the North Atlantic Ocean. Front. Microbiol. 5:557. doi: 10.3389/fmicb.2014.00557
McClintock, J. B., and Baker, B. J. (eds) (2001). Marine Chemical Ecology. Boca Raton, FL: CRC Press. doi: 10.1201/9781420036602
McLean, T. I. (2013). “Eco-omics”: a review of the application of genomics, transcriptomics, and proteomics for the study of the ecology of harmful algae. Microb. Ecol. 65, 901–915. doi: 10.1007/s00248-013-0220-5
Mercado, J., Rivera-Rentas, A., González, I., Tosteson, T. R., Molgó, J., and Escalona de Motta, G. (1994). Neuro-and myo-toxicity of extracts from the benthic dinoflagellate Ostreopsis lenticularis is sensitive to μ-conotoxin. Soc. Neurosci. Abstr. 20:718.
Mercado, J. A., Viera, M., Tosteson, T. R., Gonzalez, I., Silva, W., and Escalona de Motta, G. (1995). “Differences in the toxicity and biological activity of Ostreopsis lenticularis observed using different extraction procedures harmful marine algal blooms,” in Proceedings of the Sixth International Conference on Toxic Marine Phytoplankton, eds P. Lassus, G. Arzul, E. Erard-Le Denn, P. Gentien, and C. Marcaillou-le Baut (Nantes: Lavoirsier), 321–326.
Meroni, L., Chiantore, M., Petrillo, M., and Asnaghi, V. (2018). Habitat effects on Ostreopsis cf. ovata bloom dynamics. Harmful Algae 80, 64–71. doi: 10.1016/j.hal.2018.09.006
Meunier, F. A., Mercado, J. A., Molgó, J., Tosteson, T. R., and de Motta, G. E. (1997). Selective depolarization of the muscle membrane in frog nerve-muscle preparations by a chromatographically purified extract of the dinoflagellate Ostreopsis lenticularis. Br. J. Pharmacol. 121, 1224–1230.
Migliaccio, O., Castellano, I., Di Cioccio, D., Tedeschi, G., Negri, A., Cirino, P., et al. (2016). Subtle reproductive impairment through nitric oxide-mediated mechanisms in sea urchins from an area affected by harmful algal blooms. Sci. Rep. 6:26086. doi: 10.1038/srep26086
Milandri, A., Ceredi, A., Riccardi, E., Gasperetti, L., Susini, F., Casotti, M., et al. (2010). “Impact of Ostreopsis ovata on marine benthic communities: accumulation of palytoxins in mussels, sea urchins and octopuses from Italy,” in Proceedings of the 14th International Conference on Harmful Algae. International Society for the Study of Harmful Algae and Intergovernmental Oceanographic Commission of UNESCO, eds P. Pagou and G. Hallegraeff (Crete: Intergovernmental Oceanographic Commission (IOC) of UNESCO), 23–25.
Mitra, A., and Flynn, K. J. (2006). Promotion of harmful algal blooms by zooplankton predatory activity. Biol. Lett. 2, 194–197. doi: 10.1098/rsbl.2006.0447
Monti, M., and Cecchin, E. (2012). Comparative growth of three strains of Ostreopsis ovata at different light intensities with focus on inter-specific allelopathic interactions. Cryptogam. Algol. 33, 113–119. doi: 10.7872/crya.v33.iss2.2011.113
Munday, R. (2011). Palytoxin toxicology: animal studies. Toxicon 57, 470–477. doi: 10.1016/j.toxicon.2010.10.003
Nagasaki, K., Tomaru, Y., Nakanishi, K., Hata, N., Katanozaka, N., and Yamaguchi, M. (2004). Dynamics of Heterocapsa circularisquama (Dinophyceae) and its viruses in Ago Bay, Japan. Aquat. Microb. Ecol. 34, 219–226. doi: 10.3354/ame034219
Nagasaki, K., and Yamaguchi, M. (1997). Isolation of a virus infectious to the harmful bloom causing microalga Heterosigma akashiwo (Raphidophyceae). Aquat. Microb. Ecol. 13, 135–140. doi: 10.3354/ame013135
Nakayama, N., and Hamaguchi, M. (2016). Multiplex reverse transcription quantitative PCR detection of a single-stranded RNA virus HcRNAV infecting the bloom-forming dinoflagellate Heterocapsa circularisquama. Limnol. Oceanogr. Methods 14, 370–380. doi: 10.1002/lom3.10096
Nascimento, S. M., Corrêa, E. V., Menezes, M., Varela, D., Paredes, J., and Morris, S. (2012a). Growth and toxin profile of Ostreopsis cf. ovata (Dinophyta) from Rio de Janeiro, Brazil. Harmful algae 13, 1–9. doi: 10.1016/j.hal.2011.09.008
Nascimento, S. M., França, J. V., Gonçalves, J. E., and Ferreira, C. E. (2012b). Ostreopsis cf. ovata (Dinophyta) bloom in an equatorial island of the Atlantic Ocean. Mar. Pollut. Bull. 64, 1074–1078. doi: 10.1016/j.marpolbul.2012.03.015
Neves, R. A. F., Contins, M., and Nascimento, S. M. (2018). Effects of the toxic benthic dinoflagellate Ostreopsis cf. ovata on fertilization and early development of the sea urchin Lytechinus variegatus. Mar. Environ. Res. 135, 11–17. doi: 10.1016/j.marenvres.2018.01.014
Neves, R. A. F., Fernandes, T., Santos, L. N. D., and Nascimento, S. M. (2017). Toxicity of benthic dinoflagellates on grazing, behavior and survival of the brine shrimp Artemia salina. PLoS One 12:e0175168. doi: 10.1371/journal.pone.0175168
Norris, D. R., Bomber, J. W., and Balech, E. (1985). “Benthic dinoflagellates associated with ciguatera from the Florida Keys. 1. Ostreopsis heptagona sp. nov,” in Toxic Dinoflagellates, eds D. M. Anderson, A. W. White, and D. G. Baden (New York, NY: Elsevier), 39–44.
Oda, T., Akaike, T., Sato, K., Ishimatsu, A., Takeshita, S., Muramatsu, T., et al. (1992). Hydroxyl radical generation by red tide algae. Arch. Biochem. Biophys. 294, 38–43.
Pagliara, P., and Caroppo, C. (2012). Toxicity assessment of Amphidinium carterae, Coolia cfr. monotis and Ostreopsis cfr. ovata (Dinophyta) isolated from the northern Ionian Sea (Mediterranean Sea). Toxicon 60, 1203–1214. doi: 10.1016/j.toxicon.2012.08.005
Park, M. G., Yih, W., and Coats, W. D. (2004). Parasites and phytoplankton, with special emphasis on dinoflagellate infections. J. Eukaryot. Microbiol. 51, 145–155. doi: 10.1111/j.1550-7408.2004.tb00539.x
Parsons, M. L., Aligizaki, K., Bottein, M.-Y. D., Fraga, S., Morton, S. L., Penna, A., et al. (2012). Gambierdiscus and Ostreopsis: reassessment of the state of knowledge of their taxonomy, geography, ecophysiology, and toxicology. Harmful Algae 14, 107–129. doi: 10.1016/j.hal.2011.10.017
Pavaux, A.-S., Rostan, J., Guidi-Guilvard, L., Marro, S., Ternon, E., Thomas, O. P., et al. (2019). Effects of the toxic dinoflagellate Ostreopsis cf. ovata on survival, feeding and reproduction of a phytal harpacticoid copepod. J. Exp. Mar. Biol. Ecol. 516, 103–113. doi: 10.1016/j.jembe.2019.05.004
Pavaux, A. S., Ternon, E., Dufour, L., Marro, S., Gémin, M. P., Thomas, O. P., et al. (2020). Efficient, fast and inexpensive bioassay to monitor benthic microalgae toxicity: application to Ostreopsis species. Aquat. Toxicol. 223:105485.
Pawlik, J. R. (1992). Chemical ecology of the settlement of benthic marine invertebrates. Oceanogr. Mar. Biol. Annu. Rev. 30, 273–335.
Penna, A., Battocchi, C., Capellacci, S., Fraga, S., Aligizaki, K., Lemée, R., et al. (2014). Mitochondrial, but not rDNA, genes fail to discriminate dinoflagellate species in the genus Ostreopsis. Harmful Algae 40, 40–50. doi: 10.1016/j.hal.2014.10.004
Penna, A., Fraga, S., Battocchi, C., Casabianca, S., Giacobbe, M. G., Riobó, P., et al. (2010). A phylogeographical study of the toxic benthic dinoflagellate genus Ostreopsis Schmidt. J. Biogeogr. 37, 830–841. doi: 10.1111/j.1365-2699.2009.02265.x
Penna, A., Vila, M., Fraga, S., Giacobbe, M. G., Andreoni, F., Riobó, P., et al. (2005). Characterization of Ostreopsis and Coolia (dinophyceae) isolates in the western mediterranean sea based on morphology, toxicity and internal transcribed spacer 5.8s rdna sequences1. J. Phycol. 41, 212–225. doi: 10.1111/j.1529-8817.2005.04011.x
Pérez-Guzmán, L., Pérez-Matos, A. E., Rosado, W., Tosteson, T. R., and Govind, N. S. (2008). Bacteria associated with toxic clonal cultures of the dinoflagellate Ostreopsis lenticularis. Mar. Biotechnol. 10, 492–496.
Pezzolesi, L., Guerrini, F., Ciminiello, P., Dell’Aversano, C., Iacovo, E. D., Fattorusso, E., et al. (2012). Influence of temperature and salinity on Ostreopsis cf. ovata growth and evaluation of toxin content through HR LC-MS and biological assays. Water Res. 46, 82–92. doi: 10.1016/j.watres.2011.10.029
Pezzolesi, L., Pistocchi, R., Fratangeli, F., Dell’Aversano, C., Dello Iacovo, E., and Tartaglione, L. (2014). Growth dynamics in relation to the production of the main cellular components in the toxic dinoflagellate Ostreopsis cf. ovata. Harmful Algae 36, 1–10.
Pfannkuchen, M., Godrijan, J., Pfannkuchen, D. M., Iveša, L., Kružiæ, P., Ciminiello, P., et al. (2012). Toxin-producing Ostreopsis cf. ovata are likely to bloom undetected along coastal areas. Environ. Sci. Technol. 46, 5574–5582. doi: 10.1021/es300189h
Pichierri, S., Accoroni, S., Pezzolesi, L., Guerrini, F., Romagnoli, T., Pistocchi, R., et al. (2017). Allelopathic effects of diatom filtrates on the toxic benthic dinoflagellate Ostreopsis cf. ovata. Mar. Environ. Res. 131, 116–122. doi: 10.1016/j.marenvres.2017.09.016
Pierce, R. H., and Henry, M. S. (2008). Harmful algal toxins of the Florida red tide (Karenia brevis): natural chemical stressors in South Florida coastal ecosystems. Ecotoxicology 17, 623–631. doi: 10.1007/s10646-008-0241-x
Pohnert, G., Steinke, M., and Tollrian, R. (2007). Chemical cues, defence metabolites and the shaping of pelagic interspecific interactions. Trends Ecol. Evol. 22, 198–204. doi: 10.1016/j.tree.2007.01.005
Poli, M., Ruiz-Olvera, P., Nalca, A., Ruiz, S., Livingston, V., Frick, O., et al. (2018). Toxicity and pathophysiology of palytoxin congeners after intraperitoneal and aerosol administration in rats. Toxicon 150, 235–250. doi: 10.1016/j.toxicon.2018.06.067
Poulin, R. X., and Pohnert, G. (2019). Simplifying the complex: metabolomics approaches in chemical ecology. Anal. Bioanal. Chem. 411, 13–19. doi: 10.1007/s00216-018-1470-3
Poulin, R. X., Poulson-Ellestad, K. L., Roy, J. S., and Kubanek, J. (2018). Variable allelopathy among phytoplankton reflected in red tide metabolome. Harmful Algae 71, 50–56. doi: 10.1016/j.hal.2017.12.002
Poulson, K. L., Sieg, R. D., and Kubanek, J. (2009). Chemical ecology of the marine plankton. Nat. Prod. Rep. 26, 729–745. doi: 10.1039/B806214P
Poulson-Ellestad, K. L., Jones, C. M., Roy, J., Viant, M. R., Fernandez, F. M., Kubanek, J., et al. (2014). Metabolomics and proteomics reveal impacts of chemically mediated competition on marine plankton. Proc. Natl. Acad. Sci. U.S.A. 111, 9009–9014. doi: 10.1073/pnas.1402130111
Prato, E., Biandolino, F., Bisci, A. P., and Caroppo, C. (2011). Preliminary assessment of Ostreopsis cf. ovata acute toxicity by using a battery bioassay. Chem. Ecol. 27, 117–125. doi: 10.1080/02757540.2011.625930
Prince, E. K., Myers, T. L., and Kubanek, J. (2008). Effects of harmful algal blooms on competitors: allelopathic mechanisms of the red tide dinoflagellate Karenia brevis. Limnol. Oceanogr. 53, 531–541. doi: 10.4319/lo.2008.53.2.0531
Prince, E. K., and Pohnert, G. (2010). Searching for signals in the noise: metabolomics in chemical ecology. Anal. Bioanal. Chem. 396, 193–197. doi: 10.1007/s00216-009-3162-5
Privitera, D., Giussani, V., Isola, G., Faimali, M., Piazza, V., Garaventa, F., et al. (2012). Toxic effects of Ostreopsis ovata on larvae and juveniles of Paracentrotus lividus. Harmful Algae 18, 16–23. doi: 10.1016/j.hal.2012.03.009
Quinn, R. A., Nothias, L.-F., Vining, O., Meehan, M., Esquenazi, E., and Dorrestein, P. C. (2017). Molecular networking as a drug discovery, drug metabolism, and precision medicine strategy. Trends Pharmacol. Sci. 38, 143–154. doi: 10.1016/j.tips.2016.10.011
Quod, J. P. (1994). Ostreopsis mascarenensis sp. nov. (Dinophyceae) dinoflagellé toxique associé á la ciguatéra dans l’Ocean Indien. Cryptogam. Algol. 15, 243–251.
Ramanan, R., Kim, B. H., Cho, D. H., Oh, H. M., and Kim, H. S. (2016). Algae–bacteria interactions: evolution, ecology and emerging applications. Biotechnol. Adv. 34, 14–29. doi: 10.1016/j.biotechadv.2015.12.003
Ramos, V., and Vasconcelos, V. (2010). Palytoxin and analogs: biological and ecological effects. Mar. Drugs 8, 2021–2037. doi: 10.3390/md8072021
Reynolds, C. S. (2007). Variability in the provision and function of mucilage in phytoplankton: facultative responses to the environment. Hydrobiologia 578, 37–45. doi: 10.1007/s10750-006-0431-6
Rhodes, L. (2011). World-wide occurrence of the toxic dinoflagellate genus Ostreopsis Schmidt. Toxicon 57, 400–407. doi: 10.1016/j.toxicon.2010.05.010
Rhodes, L., Adamson, J., Suzuki, T., Briggs, L., and Garthwaite, I. (2000). Toxic marine epiphytic dinoflagellates, Ostreopsis siamensis and Coolia monotis (Dinophyceae), in New Zealand. N. Z. J. Mar. Freshw. Res. 34, 371–383. doi: 10.1080/00288330.2000.9516939
Rhodes, L., Towers, N., Briggs, L., Munday, R., and Adamson, J. (2002). Uptake of palytoxin-like compounds by shellfish fed Ostreopsis siamensis (Dinophyceae). N. Z. J. Mar. Freshw. Res. 36, 631–636. doi: 10.1080/00288330.2002.9517118
Rizvi, S. J. H., and Rizvi, V. (1992). Allelopathy; Basic and Applied Aspects. New York, NY: Chapman and Hall, 480.
Rossi, R., Castellano, V., Scalco, E., Serpe, L., Zingone, A., and Soprano, V. (2010). New palytoxin-like molecules in Mediterranean Ostreopsis cf. ovata (dinoflagellates) and in Palythoa tuberculosa detected by liquid chromatography-electrospray ionization time-of-flight mass spectrometry. Toxicon 56, 1381–1387. doi: 10.1016/j.toxicon.2010.08.0037
Rossini, G. P., and Bigiani, A. (2011). Palytoxin action on the Na+,K+-ATPase and the disruption of ion equilibria in biological systems. Toxicon 57, 429–439. doi: 10.1016/j.toxicon.2010.09.011
Sansoni, G., Borghini, B., Camici, G., Casotti, M., Righini, P., and Rustighi, C. (2003). Fioriture algali di Ostreopsis ovata (Gonyaulacales: Dinophyceae): un problema emergente. Biol. Ambient. 17, 17–23.
Sarmento, H., and Gasol, J. M. (2012). Use of phytoplankton-derived dissolved organic carbon by different types of bacterioplankton. Environ. Microbiol. 14, 2348–2360. doi: 10.1111/j.1462-2920.2012.02787.x
Sato, S., Nishimura, T., Uehara, K., Sakanari, H., Tawong, W., Hariganeya, N., et al. (2011). Phylogeography of Ostreopsis along west Pacific coast, with special reference to a novel clade from Japan. PLoS One 6:e27983. doi: 10.1371/journal.pone.0027983
Sawayama, S., Sako, Y., and Ishida, Y. (1993). “Bacterial inhibitors for the mating reaction of Alexandrium catenella (Dinophyceae),” in Toxic Phytoplankton Blooms in the Sea. Proceedings of the Fifth International Conference on Toxic Marine Phytoplankton, eds T. J. Smayda, and Y. Shimizu (New York, NY: Elsevier), 177–181.
Scalco, E., Brunet, C., Marino, F., Rossi, R., Soprano, V., Zingone, A., et al. (2012). Growth and toxicity responses of Mediterranean Ostreopsis cf. ovata to seasonal irradiance and temperature conditions. Harmful Algae 17, 25–34. doi: 10.1016/j.hal.2012.02.008
Schlichting, H. E. (1974). Ejection of microalgae into the air via bursting bubbles. J. Allergy Clin. Immunol. 53, 185–188. doi: 10.1016/0091-6749(74)90006-2
Schmidt, J. (1901). Flora of Koh Chang: contributions to the knowledge of the vegetation in the Gulf of Siam. Peridiniales. Bot. Tidsskr. 24, 212–221.
Schwartz, E., Poulin, R. X., Mojib, N., and Kubanek, J. (2016). Chemical ecology of marine plankton. Nat. Prod. Rep. 33, 843–860. doi: 10.1039/C6NP00015K
Sechet, V., Sibat, M., Chomérat, N., Nézan, E., Grossel, H., Lehebel-Peron, J. B., et al. (2012). Ostreopsis cf. ovata in the French Mediterranean coast: molecular characterisation and toxin profile. Cryptogam. Algol. 33, 89–98. doi: 10.7872/crya.v33.iss2.2011.089
Selander, E., Thor, P., Toth, G., and Pavia, H. (2006). Copepods induce paralytic shellfish toxin production in marine dinoflagellates. Proc. R. Soc. B Biol. Sci. 273, 1673–1680. doi: 10.1098/rspb.2006.3502
Selina, M. S., and Levchenko, E. V. (2011). Species composition and morphology of dinoflagellates (Dinophyta) of epiphytic assemblages of Peter the Great Bay in the Sea of Japan. Russ. J. Mar. Biol. 37, 23–32. doi: 10.1134/S1063074011010135
Sellner, K. G., Doucette, G. J., and Kirkpatrick, G. J. (2003). Harmful algal blooms: causes, impacts and detection. J. Ind. Microbiol. Biotechnol. 30, 383–406. doi: 10.1007/s10295-003-0074-9
Shears, N. T., and Ross, P. M. (2009). Blooms of benthic dinoflagellates of the genus Ostreopsis; an increasing and ecologically important phenomenon on temperate reefs in New Zealand and worldwide. Harmful Algae 8, 916–925. doi: 10.1016/j.hal.2009.05.003
Sieg, R. D., Poulson-Ellestad, K. L., and Kubanek, J. (2011). Chemical ecology of the marine plankton. Nat. Prod. Rep. 28, 388–399. doi: 10.1039/C0NP00051E
Simoni, F., Gaddi, A., Di Paolo, C., and Lepri, L. (2003). Harmful epiphytic dinoflagellate on Tyrrhenian Sea reefs. Harmful Algae News 24, 13–14.
Simonini, R., Orlandi, M., and Abbate, M. (2011). Is the toxic dinoflagellate Ostreopsis cf. ovata harmful to Mediterranean benthic invertebrates? Evidences from ecotoxicological tests with the polychaete Dinophilus gyrociliatus. Mar. Environ. Res. 72, 230–233. doi: 10.1016/j.marenvres.2011.08.009
Smayda, T. J. (1997). Harmful algal blooms: their ecophysiology and general relevance to phytoplankton blooms in the sea. Limnol. Oceanogr. 42, 1137–1153. doi: 10.4319/lo.1997.42.5_part_2.1137
Smith, J. L., and Haney, J. F. (2006). Foodweb transfer, accumulation, and depuration of microcystins, a cyanobacterial toxin, in pumpkinseed sunfish (Lepomis gibbosus). Toxicon 48, 580–589. doi: 10.1016/j.toxicon.2006.07.009
Solé, J., Garcia-Ladona, E., and Estrada, M. (2006). The role of selective predation in harmful algal blooms. J. Mar. Syst. 62, 46–54. doi: 10.1016/j.jmarsys.2006.04.002
Tang, Y. Z., Kang, Y., Berry, D., and Gobler, C. J. (2015). The ability of the red macroalga, Porphyra purpurea (Rhodophyceae) to inhibit the proliferation of seven common harmful microalgae. J. Appl. Phycol. 27, 531–544. doi: 10.1007/s10811-014-0338-y
Tartaglione, L., Iacovo, E. D., Mazzeo, A., Casabianca, S., Ciminiello, P., Penna, A., et al. (2017). Variability in toxin profiles of the Mediterranean Ostreopsis cf. ovata and in structural features of the produced ovatoxins. Environ. Sci. Technol. 51, 13920–13928. doi: 10.1021/acs.est.7b03827
Tartaglione, L., Mazzeo, A., Dell’Aversano, C., Forino, M., Giussani, V., Capellacci, S., et al. (2016). Chemical, molecular, and eco-toxicological investigation of Ostreopsis sp. from Cyprus Island: structural insights into four new ovatoxins by LC-HRMS/MS. Anal. Bioanal. Chem. 408, 915–932. doi: 10.1007/s00216-015-9183-3
Tawong, W., Nishimura, T., Sakanari, H., Sato, S., Yamaguchi, H., and Adachi, M. (2014). Distribution and molecular phylogeny of the dinoflagellate genus Ostreopsis in Thailand. Harmful Algae 37, 160–171. doi: 10.1016/j.hal.2014.06.003
Teegarden, G. J., and Cembella, A. D. (1996). Grazing of toxic dinoflagellates, Alexandrium spp., by adult copepods of coastal Maine: implications for the fate of paralytic shellfish toxins in marine food webs. J. Exp. Mar. Biol. Ecol. 196, 145–176. doi: 10.1016/0022-0981(95)00128-X
Terajima, T., Uchida, H., Abe, N., and Yasumoto, T. (2019). Structure elucidation of ostreocin-A and ostreocin-E1, novel palytoxin analogs produced by the dinoflagellate Ostreopsis siamensis, using LC/Q-TOF MS. Biosci. Biotechnol. Biochem. 83, 381–390. doi: 10.1080/09168451.2018.1550356
Ternon, E., Pavaux, A.-S., Marro, S., Thomas, O. P., and Lemée, R. (2018). Allelopathic interactions between the benthic toxic dinoflagellate Ostreopsis cf. ovata and a co-occurring diatom. Harmful Algae 75, 35–44. doi: 10.1016/j.hal.2018.04.003
Tesson, S. V. M., Skjøth, C. A., Šantl-Temkiv, T., and Löndahl, J. (2016). Airborne microalgae: insights, opportunities, and challenges. Appl. Environ. Microbiol. 82, 1978–1991. doi: 10.1128/AEM.03333-15
Tester, P. A., Litaker, W., and Berdalet, E. (2020). Climate change and harmful benthic algae. Harmful Algae 91:101655. doi: 10.1016/j.hal.2019.101655
Tibiriçá, C. E. J. A., Leite, I. P., Batista, T. V. V., Fernandes, L. F., Nicolas Chomérat, N., Herve, F., et al. (2019). Ostreopsis cf. ovata bloom in Currais, Brazil: phylogeny, toxin profile and contamination of mussels and marine plastic litter. Toxins 11:446. doi: 10.3390/toxins11080446
Tichadou, L., Glaizal, M., Armengaud, A., Grossel, H., Lemée, R., Kantin, R., et al. (2010). Health impact of unicellular algae of the Ostreopsis genus blooms in the Mediterranean Sea: experience of the French Mediterranean coast surveillance network from 2006 to 2009. Clin. Toxicol. 48, 839–844. doi: 10.3109/15563650.2010.513687
Tillmann, U. (2004). Interactions between planktonic microalgae and protozoan grazers. J. Eukaryot. Microbiol. 51, 156–168. doi: 10.1111/j.1550-7408.2004.tb00540.x
Tillmann, U., and John, U. (2002). Toxic effects of Alexandrium spp. on heterotrophic dinoflagellates: an allelochemical defence mechanism independent of PSP-toxin content. Mar. Ecol. Prog. Ser. 230, 47–58. doi: 10.3354/meps230047
Tindall, D. R., and Miller, D. M. (1988). Two potent toxins from Ostreopsis lenticularis, a dinoflagellate common to ciguatera-endemic regions of the Caribbean and Tropical Atlantic. FASEB J. 2:ABSTRACT 458.
Tosteson, T. R., Ballantine, D. L., Tosteson, C. G., Hensley, V., and Bardales, A. T. (1989). Associated bacterial flora, growth, and toxicity of cultured benthic dinoflagellates Ostreopsis lenticularis and Gambierdiscus toxicus. Appl. Environ. Microbiol. 55, 137–141.
Totti, C., Accoroni, S., Cerino, F., Cucchiari, E., and Romagnoli, T. (2010). Ostreopsis ovata bloom along the Conero Riviera (northern Adriatic Sea): relationships with environmental conditions and substrata. Harmful Algae 9, 233–239. doi: 10.1016/j.hal.2009.10.006
Trick, C. J., Andersen, R., and Harrison, P. (2011). Environmental factors influencing the production of an antibacterial metabolite from a marine dinoflagellate, Prorocentrum minimum. Can. J. Fish. Aquat. Sci. 41, 423–432. doi: 10.1139/f84-050
Tubaro, A., Durando, P., Del Favero, G., Ansaldi, F., Icardi, G., Deeds, J. R., et al. (2011). Case definitions for human poisonings postulated to palytoxins exposure. Toxicon 57, 478–495. doi: 10.1016/j.toxicon.2011.01.005
Uchida, T., Toda, S., Matsuyama, Y., Yamaguchi, M., Kotani, Y., and Honjo, T. (1999). Interactions between the red tide dinoflagellates Heterocapsa circularisquama and Gymnodinium mikimotoi in laboratory culture. J. Exp. Mar. Biol. Ecol. 241, 285–299. doi: 10.1016/S0022-0981(99)00088-X
Ukena, T., Satake, M., Usami, M., Oshima, Y., Naoki, H., Fujita, T., et al. (2001). Structure elucidation of ostreocin D, a palytoxin analog isolated from the dinoflagellate Ostreopsis siamensis. Biosci. Biotechnol. Biochem. 65, 2585–2588. doi: 10.1271/bbb.65.2585
Usami, M., Satake, M., Ishida, S., Inoue, A., Kan, Y., and Yasumoto, T. (1995). Palytoxin analogs from the dinoflagellate Ostreopsis siamensis. J. Am. Chem. Soc. 117, 5389–5390. doi: 10.1021/ja00124a034
Vale, C., and Ares, I. R. (2007). “Biochemistry of Palytoxins and Ostreocins,” in Phycotoxins: Chemistry and Biochemistry, ed. L. Botana (Ames, IA: Blackwell Publishing), 95–118. doi: 10.1002/9780470277874.ch6
Vanucci, S., Guidi, F., Pistocchi, R., and Long, R. A. (2016). Phylogenetic structure of bacterial assemblages co-occurring with Ostreopsis cf. ovata bloom. Harmful Algae 55, 259–271. doi: 10.1016/j.hal.2016.04.003
Vanucci, S., Guerrini, F., Pezzolesi, L., Dell’Aversano, C., Ciminiello, P., and Pistocchi, R. (2012a). Cell growth and toxins’ content of Ostreopsis cf. ovata in presence and absence of associated bacteria. Cryptogam. Algol. 33, 105–112. doi: 10.7872/crya.v33.iss2.2011.105
Vanucci, S., Pezzolesi, L., Pistocchi, R., Ciminiello, P., Dell’Aversano, C., Iacovo, E. D., et al. (2012b). Nitrogen and phosphorus limitation effects on cell growth, biovolume, and toxin production in Ostreopsis cf. ovata. Harmful Algae 15, 78–90. doi: 10.1016/j.hal.2011.12.003
Verma, A., Hoppenrath, M., Dorantes-Aranda, J. J., Harwood, D. T., and Murray, S. A. (2016). Molecular and phylogenetic characterization of Ostreopsis (Dinophyceae) and the description of a new species, Ostreopsis rhodesae sp. nov., from a subtropical Australian lagoon. Harmful Algae 60, 116–130. doi: 10.1016/j.hal.2016.11.004
Vila, M., Abós-Herràndiz, R., Isern-Fontanet, J., Àlvarez, J., and Berdalet, E. (2016). Establishing the link between Ostreopsis cf. ovata blooms and human health impacts using ecology and epidemiology. Sci. Mar. 80, 107–115. doi: 10.3989/scimar.04395.08A
Vila, M., Garcés, E., and Masó, M. (2001). Potentially toxic epiphytic dinoflagellate assemblages on macroalgae in the NW Mediterranean. Aquat. Microb. Ecol. 26, 51–60. doi: 10.3354/ame026051
Vila, M., Masó, M., Sampedro, N., Illoul, H., Arin, L., Garcés, E., et al. (2008). “The genus Ostreopsis in recreational waters of the Catalan Coast and Balearic Islands (NW Mediterranean Sea): is this the origin of human respiratory difficulties,” in Proceedings of the 12th International Conference on Harmful Algae, (International Society for the Study of Harmful Algae and Intergovernmental Oceanographic Commission of UNESCO, Copenhagen, 334–336.
Walid, M., Marielle, G., Patrick, L., Michèle, B., Philippe, T., Manoella, S., et al. (2010). Detoxification enhancement in the gymnodimine-contaminated grooved carpet shell, Ruditapes decussatus (Linné). Harmful Algae 9, 200–207. doi: 10.1016/j.hal.2009.10.002
Wang, C., Ho, A. Y. T., Qian, P., Wong, P., and Hsieh, D. P. H. (2004). Antibiotic treatment enhances C2 toxin production by Alexandrium tamarense in batch cultures. Harmful Algae 3, 21–28. doi: 10.1016/j.hal.2003.08.002Get
Watrous, J., Roach, P., Alexandrov, T., Heath, B. S., Yang, J. Y., Kersten, R. D., et al. (2012). Mass spectral molecular networking of living microbial colonies. Proc. Natl. Acad. Sci. U. S. A. 109, 1743–1752.
Wells, M. L., Karlson, B., Wulff, A., Kudela, R. M., Trick, C., Asnaghi, V., et al. (2019). Future HAB science: directions and challenges in a changing climate. Harmful Algae 91:101632. doi: 10.1016/j.hal.2019.101632
Wichard, T., Poulet, S. A., Halsband-Lenk, C., Albaina, A., Harris, R., Liu, D., et al. (2005). Survey of the chemical defence potential of diatoms: screening of Fifty Species for α,β,γ,δ-unsaturated aldehydes. J. Chem. Ecol. 31, 949–958. doi: 10.1007/s10886-005-3615-z
Wilson, J. K., Kessler, A., and Woods, H. A. (2015). Noisy communication via airborne infochemicals. Bioscience 65, 667–677. doi: 10.1093/biosci/biv062
Winnikoff, J. R., Glukhov, E., Watrous, J., Dorrestein, P. C., and Gerwick, W. H. (2014). Quantitative molecular networking to profile marine cyanobacterial metabolomes. J. Antibiot. 67, 105–112. doi: 10.1038/ja.2013.120
Wu, C. H. (2009). Palytoxin: membrane mechanisms of action. Toxicon 54, 1183–1189. doi: 10.1016/j.toxicon.2009.02.030
Yamasaki, Y., Nagasoe, S., Tameishi, M., Shikata, T., Zou, Y., Jiang, Z., et al. (2010). The role of interactions between Prorocentrum minimum and Heterosigma akashiwo in bloom formation. Hydrobiologia 641, 33–44. doi: 10.1007/s10750-009-0052-y
Yang, J. Y., Sanchez, L. M., Rath, C. M., Liu, X., Boudreau, P. D., Bruns, N., et al. (2013). Molecular networking as a dereplication strategy. J. Nat. Prod. 76, 1686–1699. doi: 10.1021/np400413s
Keywords: marine chemical ecology, Ostreopsis spp., HABs, ovatoxins, dinoflagellates
Citation: Pavaux A-S, Berdalet E and Lemée R (2020) Chemical Ecology of the Benthic Dinoflagellate Genus Ostreopsis: Review of Progress and Future Directions. Front. Mar. Sci. 7:498. doi: 10.3389/fmars.2020.00498
Received: 07 April 2020; Accepted: 03 June 2020;
Published: 10 July 2020.
Edited by:
Dongyan Liu, East China Normal University, ChinaReviewed by:
Gustaaf Marinus Hallegraeff, University of Tasmania, AustraliaHaifeng Gu, Third Institute of Oceanography, Ministry of Natural Resources, China
Copyright © 2020 Pavaux, Berdalet and Lemée. This is an open-access article distributed under the terms of the Creative Commons Attribution License (CC BY). The use, distribution or reproduction in other forums is permitted, provided the original author(s) and the copyright owner(s) are credited and that the original publication in this journal is cited, in accordance with accepted academic practice. No use, distribution or reproduction is permitted which does not comply with these terms.
*Correspondence: Anne-Sophie Pavaux, YW5uZXNvcGhpZS5wYXZhdXhAZ21haWwuY29t