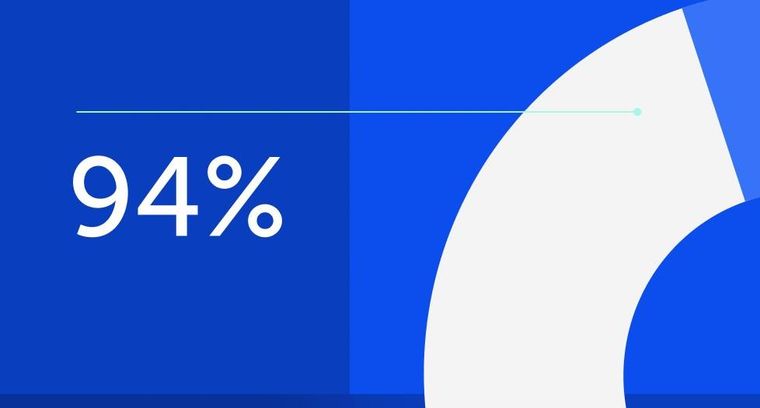
94% of researchers rate our articles as excellent or good
Learn more about the work of our research integrity team to safeguard the quality of each article we publish.
Find out more
REVIEW article
Front. Mar. Sci., 19 June 2020
Sec. Marine Conservation and Sustainability
Volume 7 - 2020 | https://doi.org/10.3389/fmars.2020.00453
Temperate Australia has extensive and diverse coast and marine habitats throughout its inshore and offshore waters. The region includes the southernmost extent of mangroves, over 500 estuaries and coastal embayments, home to extensive meadows of seagrasses and tidal saltmarsh. In areas of hard substrate, rocky reefs are abundant and productive with large forests of macroalgae. Coastal regions can be densely populated by humans and often habitats can be degraded, polluted or lost, while some remain relatively isolated and pristine. These habitats provide services to society including provision of food, regulate our climate through sequestration of carbon, treating our waste and protecting our shorelines from damage from storms. Coastal areas are culturally importantly hubs for recreation and tourism. Habitat mapping demonstrates diverse habitats throughout temperate Australia, but a formal investigation of services provided by these habitats has been lacking. This review of ecosystem services provided by coast and marine environments throughout temperate Australia reveals vast and productive ecosystems that provide multiple ecosystem services, substantial value to the Australian economy and contribute to the health and well-being of people who live in, visit of benefit from services or products from these regions. Some of these are considered within traditional economic metrics such as provision of wild catch fisheries, but this review demonstrates that regulation and maintenance services including waste treatment and protecting shorelines from extreme events are under recognized, and their value is substantial. However, consistent with many locations globally, coast and marine habitats are under threat from increasing development, sewage, agricultural, industrial discharges, urban runoff and climate change. Resultantly, temperate Australian coast and marine habitat extent and condition is generally declining in many regions, putting the provision of services and benefits to the community at risk. Continued degraded or lost habitats indicate current management frameworks are not capturing the full risk from development and there are winners and losers in trade off decision making. Incorporating ecosystem services in decision making may allow an integrated approach to management, and acknowledgment of services provided could prevent habitats from being undervalued against economic and social interests, a practice that often results in environmental degradation.
Temperate Australia has over 15,000 km of coastline and over 350 million hectares of offshore waters. The coasts have extensive rocky reefs that span almost the entire southern coastline dominated by complex and diverse macroalgal communities. Large gulfs, embayments and estuaries support mangrove forests at their southernmost distribution, coastal saltmarsh and extensive seagrass meadows, some of the largest meadows in the world (Larkum et al., 2018). Australia is known for its beaches, where people value their beauty and recreational opportunities (James, 2000).
Globally, coast and marine ecosystems provide a wealth of services to society including the provision of food and raw materials, they regulate our world though transformation and mediation of wastes, sequestering carbon and protect our shorelines from the damaging effects of storms. These systems also provide cultural services where people enjoy the natural environment for recreation and visual appreciation, which has been closely linked to good health outcomes and well-being (Bowler et al., 2010). These services provide benefit to society which have been valued at $141 trillion per year globally, of this approximately 60–68% are from marine and coastal habitats (Costanza et al., 2014).
Consistent with many locations worldwide, Australian coast and marine habitats are under threat from multiple pressures and are degrading (Clark and Johnston, 2016a). It is estimated that since European settlement of Australia from the late 18th century, 47–50% of saltmarsh has been lost (Macreadie et al., 2017), 52–78% of mangroves (Friess and Webb, 2014), and 6% of seagrass has been lost (Larkum et al., 2018), much of this in the temperate zone.
Historical pressures have been significant, and are typically increasing (Clark and Johnston, 2016a). Agricultural runoff has channeled nutrients and sediments into coastal waters impacting on seagrass and reef habitats (Kendrick et al., 2002). Urban expansion has significantly altered the ecological communities throughout south eastern Australian estuaries (Connell et al., 2008), and historical and contemporary industrial pollution is still affecting our iconic habitats (Mayer-Pinto et al., 2015).
Our oceans are being seen as new source of economic development often at the expense of sustainability resulting in degradation of the habitats and loss of species (Golden et al., 2017). Rapid improvement in technology is resulting in more efficient harvesting of fisheries (Squires and Vestergaard, 2013), exploitation of new areas for seabed mining (Mengerink et al., 2014), expansion of aquaculture (Bjelland et al., 2015) and the development of new industries such as offshore renewable energy (Esteban et al., 2011), all of which have been proposed or implemented in temperate Australia. Overlaid on these pressures are the interactive and cumulative effects of climate change, including marine heat waves driving habitat changes and impacts to biodiversity (Roberts et al., 2019). When habitats are degraded or lost, the ecosystem functions and the services they provide society also decline (Dobson et al., 2006).
Increasingly, there is acknowledgment that environmental decision-making is intertwined with economic and social outcomes including well-being, and that management must be integrated to optimize the benefits over longer timeframes (Haines-Young and Potschin, 2010). As early as 1987, sustainable development was highlighted as “development that met the needs of the current generation without compromising the ability of future generations to meet their own needs” (Brundtland, 1987). Since this time, sustainable development has been written into global policy including the United Nations Sustainable Development Goals and the Commonwealth Blue Charter. In Australia, the National Strategy for Ecological Sustainable Development, setting the broad strategic direction for sustainable development in Australia. Each State within Australia has individual legislation or policies that outline the desire for ecologically sustainable development.
In assessing whether a development is sustainable, decision makers are required to consider environmental, social and economic drivers within trade off negotiations, aiming to provide a suitable outcome for all drivers, but often with incompatible metrics (Ledoux et al., 2001). Often this leads to subjective decisions, with a lack of transparency and inconsistency. A lack of appreciation of the multiple services from coast and marine ecosystems will result in lower consequences from degradation in a risk assessment framework and trade off decisions that under value coast and marine ecosystems.
The identification and incorporation of direct and indirect interactions between ecosystems and the services that they provide, allow for an integrated policy and management approach at a landscape-scale that includes environmental, social and economic considerations (Haines-Young and Potschin, 2010). It can provide practitioners with additional and transparent lines of evidence in trade off decision-making, identify critical stakeholders, optimize benefits and value to the community and support an integrated management approach inclusive of all stakeholders (Levin et al., 2009).
The identification of ecosystem services have been published for localized areas, or specific habitats, sporadically in Australia (Pittock et al., 2012), while a broadscale review of services provided by temperate Australia is lacking. This paper identifies ecosystem services provided by coast and marine environments throughout temperate Australia for the first time. The review is structured to identify the ecosystem services common across major temperate habitat types in temperate Australia from the supra tidal zone through to the extent of Commonwealth waters. The review discusses the broad condition of habitats, the key drivers to spatial and temporal variability that influence these services and some of the pressures that may affect service delivery.
This review is aimed to be a starting point for discussion and future detailed valuation of ecosystem services from coast and marine habitats, taking into account local factors and uses.
Temperate Australia extends between the Perth in Western Australia (32° S, 115° 45′ E) eastwards through South Australia and Tasmania to the southern boundary of the Great Barrier Reef Marine Park in Queensland (23° 50′S, 151° 53′E) (Figure 1; Commonwealth of Australia, 2006). The seaward boundary extends to the Exclusive Economic Zone located 200 nautical miles from coastal low water mark (Geosciences Australia, 2020).
Figure 1. Temperate extent of major coastal habitats indicated by solid concentric lines parallel to the coast. Dashed lines indicate the extent of temperate climatic conditions included in this review, it is acknowledged that VCE exist north of the dashed line but have not been considered in this review and as such are not included in this figure. Habitat areas are outlined in Supplementary Information S1.
Searches of scientific literature databases revealed that there are large areas throughout temperate Australia where there is little to no published information describing ecosystem services or value of coast and marine habitats. Resultantly, there was insufficient literature to perform a systematic review specific to this region. In light of this, a narrative review has been undertaken looking at existing habitat information and linking this to ecosystem service delivery published in literature.
This review uses the Common International Classification of Ecosystem Services (CICES) v5.11 framework. This system provides the most up to date and common system of classifying ecosystem services consistent with the System of Environmental-Economic Accounting (SEEA) Central Framework providing the ability to cross reference categories used in other classification frameworks including the Millennium Ecosystem Assessment and The Economics of Ecosystems and Biodiversity, enabling a degree of compatibility with other systems.
The scope of CICES is orientated around the identification of ‘final services,’ but it is accepted that final services also depends on the specific context. Similar to other classification schemes, CICES has three major sections at the highest level of the hierarchy including provisioning, regulating and maintenance and cultural services (Haines-Young and Potschin, 2018).
Major contributions of ecosystem services were identified based on broad habitat types and species compositions identified through habitat maps, searches of scientific literature, government documents and discussion with local experts. Services were classified using the CICES v5.1 framework using the Group level delineation (Haines-Young and Potschin, 2018). This broadscale review of ES in temperate Australia is a starting point to identify and demonstrate the importance of coast and marine habitats at a high level across continental scales. It provides the basis for future detailed assessment using local information on habitat extent and quality, human interactions and use, enabling detailed economic valuation studies in the future.
Monetary valuation of the environment is one method to help provide information to balance environmental factors with economic, and social metrics in trade off decision making. However consistent with other metrics, caution must be applied. Monetary valuations sometimes include broad generalizations, assumptions and transfer of benefits from one area to another, which may or may not be valid. Importantly, services such as intrinsic value are complex and often subjective and as such, difficult to provide accurate values. As a result, values should be considered as lower estimates. Notwithstanding this, valuations demonstrate the connection and importance between nature and humankind. It provides additional arguments for decision making using a common language, consistent with other metrics used in decision making. It should not replace ecological, ethical, or other non-monetary arguments but compliment them that can be used in a multiple lines of evidence framework (Schröter et al., 2014).
Where habitat ecosystem service valuations exist in the literature, values have been adjusted to 2018 International dollars (1 Int $ = 1 USD $) to allow comparison across geographic and economic regions. All data has been adjusted using World Bank gross domestic product (GDP) deflators ‘GDP deflator (base year varies by country)’ to align to a common time series and purchasing power parity (PPP) conversion factors (‘local currency per international’ series) to change to an International currency using the methods described in De Groot et al. (2012). This method takes into account the different purchasing power currencies can have in different locations according to living standards and the price of goods within each economy and adjusts the value to allow comparison (Officer, 1976). Where published monetary values for ecosystem services are quoted, local valuations have been prioritized, where local data is lacking, data within the Ecosystem Service Valuation Database (ESVD) (Van der Ploeg and de Groot, 2010) have been used. World Bank conversion factors published in 2019 were used in all cases. Summary of all data sources and currency conversions are included in the Supplementary Information S2.
Owing to their remoteness, there are large areas of temperate Australian habitats that are not mapped with a high degree of accuracy. SEAMAP Australia database2 has compiled seafloor habitat data collected from various stakeholders around Australia (Lucieer et al., 2017). As a default habitat areal extent, this review has used the SEAMAP Australia database (Seamap Australia National benthic Layer). Where more recent data was available or published data from local sources, habitat extents were compared and the default accepted where there was broad agreement. Where there is significant variability (>50% difference) between the SEAMAP database, the more recent regional estimate or the local data has been used. Geographic Information Systems (ArcGIS version 10.6) was used to delineate habitats within the temperate boundaries described in Section “Methods.” Habitat extent is described in detail in Figure 1 and Supplementary Information S1.
Relative importance of contribution of ecosystem service from each habitat has been estimated using the collated median local values, as a function of the habitat area using a simple three category scale: low, moderate, high contribution. If local values are not available, then median published values from literature have been used to provide a broadscale point of comparison.
Offshore, large oceanographic currents typically bring warm, nutrient poor waters from the north of Australia into the southern waters, resulting in oligotrophic conditions throughout the majority of the region (Pattiaratchi, 2007). Seasonally, upwellings transport cool nutrient rich water into the nearshore from off the continental shelf driving high local fisheries productivity (Table 1; Kämpf et al., 2004). Temperate Australia’s global isolation, shape and position adjoining the Southern Ocean result in distinct temperate biotas that contribute to significant biodiversity and marine endemism (Womersley, 1990). Localized terrestrial topographic features, surface and subsurface flows, and local weather conditions can also result in areas of high productivity in waters (Pattiaratchi, 2007).
Table 1. Summary of regional valuations for temperate Australia, the common threats, their current condition and trend.
Inshore waters support a range of vegetated coastal ecosystems (VCE). Coastal saltmarsh habitat occupy the intertidal to supratidal zone of low wave energy depositional environments that are regularly flooded and covered in vegetation (Saintilian, 2009). Current estimates suggest that there is almost 130,276 ha of coastal saltmarsh in temperate Australia ranging from the central eastern (and northern) coastline dominated by Suaeda arbusculiodes and Tecticornia indica julacca (Saintilian, 2009), through to small areas dominated by Chenopod scrub including Tecticornia doliformis in Western Australia, with extensive Scleroscegia arbuscula – Juncus kraussii group areas in Victoria and South Australia and throughout Tasmania (Figure 1 and Supplementary Information S1). It has been estimated that, since 1950, most estuaries in south-east Australia has lost substantial area of saltmarsh (Saintilan and Williams, 2010) and currently saltmarsh is considered in poor condition and the decline in extent continuing (Table 2; Clark and Johnston, 2016b).
Table 2. Summary of temperate Australian coastal and marine habitats, the major services provided, range of habitat specific published valuations, threats and condition.
Mangroves are trees adapted to grow with periodic saltwater inundation, growing in waterlogged soil with widely fluctuating salinity. Many species including Avicennia marina have pneumatophores to obtain oxygen for root growth and metabolism from above the water when the tide recedes (Kathiresan and Bingham, 2001). In Australia 95% of mangroves are in the tropical and subtropical zones, while only 5% inhabit temperate regions, and there are no mangroves in Tasmania. A. marina dominates the southern regions, while in northern New South Wales and temperate areas of Queensland, species including Aegiceras corniculatum inhabit upper reaches of tidal rivers and Bruguiera gymnorhiza, Rhizophora stylosa, and Excoecaria agallocha colonize north of Manning River (Macnae, 1966). Mangroves inhabit the intertidal zone along muddy shores throughout sheltered estuaries in New South Wales such as the Hunter River and Hawkesbury, and Corner Inlet, Western Port and Port Phillip Bays in Victoria, while extensive areas of temperate mangroves occur in South Australia (Jones et al., 2019). In the south of Western Australia, there is only a very small area of mangroves in Leschenault Inlet near Bunbury. It is estimated that there are over 131,000 ha of mangroves in temperate Australia (Figure 1 and Supplementary Information S1) and the area is expanding at the expense of saltmarsh (Saintilan et al., 2014).
Temperate Australia has extensive meadows of largely endemic and persistent seagrass, many with very high biomass and productivity. The high seagrass diversity reflects the variety of habitats available, including coasts, bays, estuaries, lagoons, reefs, and island systems (Macreadie et al., 2018) and it is estimated that temperate Australia has almost 4 million hectares of seagrass, potentially the largest area in the world (Figure 1 and Supplementary Information S1). In the west and south of temperate Australia, persistent species such as Posidonia spp. and Amphibolis spp. dominate coastal areas (Kilminster et al., 2018). In deeper waters, the genera Halophila and Heterozostera occur and can also be found in shallow waters colonizing from disturbance (Kirkman and Kuo, 1990). Zostera spp. and Ruppia spp. species can thrive in shallow lagoons particularly where there is a reduced salinity gradient (Moore and Short, 2007). Regionally, temperate Australia has lost over 150,000 ha of seagrass from coastal waters as a result of nutrient enrichment, sedimentation and physical destruction (Larkum et al., 2018) and Clark and Johnston (2016b) reported that the overall condition of seagrass throughout Australia is poor and declining (Table 2).
Australia is well known for its beaches. Reflective beaches are the most common wave dominated beach type in Australia often between or behind headlands while dissipative beaches occupy the high energy end of the spectrum exposed to persistent ocean swell and generally only occur in south West Australia and South Australia (Short, 2006). In areas sheltered from ocean swell, tide dominated or tide modified beaches occur when wave energy is moderate to very low and are often associated with sandy flats and in southern Australia occur in Port Phillip Bay, Spencer and St Vincent gulfs in South Australia and in northern Tasmania (Short, 2006). Clark and Johnston (2016b) indicate that sandy beaches are generally in good condition (Table 1).
Where rocky substrate dominates, intertidal and subtidal reef habitats form. Sub-tidally, macroalgal forests occur on all sunlit rocky coasts across approximately 8000 km coastline of southern Australia, known as the Great Southern Reef (Bennett et al., 2015). Algal forests are dominated by the kelp Ecklonia radiata, while fucoids dominate in moderately exposed to sheltered reefs (Connell, 2007). Algal forests are a biodiversity hotspot for a range of taxa including sponges, invertebrates and algae with the majority of species endemic to southern Australia (Womersley, 1990). Algal reef condition is likely to be stable, but at risk of declining (Table 2; Bennett et al., 2015).
Habitat mapping indicates that, the dominant coast and marine habitats were seagrass, algal forest/rocky reef, mangrove and saltmarsh (Supplementary Information S1), resultantly these feature heavily within this review. There were numerous habitat classifications which contributed to the overall habitat maps, but their area was small (<1%) and have not been included in this review. Sandy beaches are often not included in traditional biological habitat maps, but due to their value for cultural services have been included in this review, but values given only relate to part area of temperate Australia.
Provisioning services are outputs that typically direct use values, often traded in markets so are relatively easy to associate a monetary value (De Groot et al., 2002). Provisioning services include the food from fish and invertebrates from wild or cultured animals, they can include medicines derived from organisms and use of plants or animals products for fuel or materials (Haines-Young and Potschin, 2018).
Unless published in that form, this review has not standardized provisioning service values to a value per hectare as this would assume that the fishing effort is uniform across the region, where in reality the fishing effort is managed in blocks taking into account local hotspots, proximity to ports and conservation areas. Similarly, spatial heterogeneity in fish abundance can be considerable, even within similar habitats (Carnell et al., 2019).
The nursery function that VCE provide is not considered a service and as such is not valued (Liquete et al., 2016), however, this nursery function and the connectivity of various life stages of fish and invertebrates between habitats is of critical importance to maintaining fisheries. An example of the importance is the connectivity within the Penaeid prawn lifecycle, which demonstrates habitat use through various stages of its lifecycle, using inshore habitats for nursery functions, and the provisioning ecosystem service is realized in deeper waters (Figure 2). A valuation that reflected only the value for the harvest areas would not recognize the importance of the inshore systems and their connectivity in its value. As such, degradation or loss of one habitat (e.g., seagrass loss) may affect the harvest in another (Poiner et al., 1993).
Figure 2. Diagram showing connectivity between VCE for the Penaeid prawn lifecycle indicting that valuations for harvest areas may overlook critical importance within the lifecycle.
Recreational fishing is participated by an estimated 3.4 million Australians (ABS 2003). However, the majority of fishers suggest that participation is linked to cultural services including sport and relaxation, rather than provision of food (Henry and Lyle, 2003). Recreational fishing is discussed in section “Cultural Services.”
Across temperate Australia, regional oceanography has a large influence on the productivity of waters, driven particularly by upwellings, suggested to contribute up to 20% of global fisheries production (Nieblas et al., 2009). Upwellings and other nutrient sources drive phytoplankton productivity, which is a key pillar of the pelagic food web. This food web spans phytoplankton, zooplankton, small pelagic fish and in turn larger fish, birds, and mammals (Goldsworthy et al., 2013). Temperate Australian offshore (Commonwealth) waters host a wealth of pelagic fisheries providing substantial final provisioning services. The fisheries include a wide variety of scalefish and shark including blue grenadier (Macruronus novaezelandiae), tiger flathead (Neoplatycephalus richardsoni), silver warehou (Seriolella punctata), gummy shark (Mustelus antarcticus), and redfish (Centroberyx gerrardi) (Tuck, 2016). In the Eastern Great Australian Bight, upwellings drive the food web influencing Australia’s largest fishery, the Australian sardine (Sardinops sagax), which is harvested largely for the southern Bluefin tuna industry (Goldsworthy et al., 2013). Other significant fisheries in the offshore waters of southern Australia include tuna and billfish including albacore (Thunnus alalunga), yellowfin tuna (Thunnus albacares) and broadbill swordfish (Xiphias gladius) (DOE, 2014).
Inshore, estuaries are spatially and temporally complex systems, an interface between the land and the sea, linking catchments and freshwater habitats to marine. Estuaries are typically mosaics of VCE that have high primary and secondary productivity (Gillanders et al., 2003), providing significant provisioning services. The close connection and structural complexity between saltmarsh, mangrove and seagrass (VCE) habitats provide shelter and protection where larval and juvenile life stages use as a nursery to grow until some move out to offshore waters (Gillanders et al., 2003). This nursery function has been shown to contribute to the fisheries production in adjacent habitats and pelagic waters and estuaries are a focal point for inshore fisheries (Pease, 1999). In south eastern Queensland and New South Wales the Eastern King Prawn (Penaeus plebejus) and school prawns (Metapenaeus macleayi) use estuaries for key stages of their life cycle and the trawl fishery is valued at over $27 million per year (O’Neill et al., 2014). The South Australian western king prawn (Melicertus latisulcatus) fishery harvests unvegetated sediments in Spencer and St Vincent Gulfs with lesser quantities caught in smaller embayments and are valued at $32 million per year (Mobsby and Koduah, 2017).
Other species targeted by commercial fishers within estuaries and coastal waters include sea mullet (Mugil cephalus), blue swimmer crab (Portunus pelagicus) and King George whiting (Sillaginodes punctata) (Hindell and Jenkins, 2004). Gillanders et al. (2003) demonstrates the movement of fish and invertebrate species between habitats into and out of estuaries. Jänes et al. (2020) reviewed fisheries enhancement value of VCE throughout south east Australia, finding an average value of $38,675 ha/yr, and the average value for seagrass across south eastern Australia of $13,240 ha/yr. Blandon and Zu Ermgassen (2014) valued seagrasses meadows at up to $155,526 ha/yr for their contribution in the production of a range of fisheries including the southern calamari (Sepioteuthis australis), southern sea garfish (Hyporhamphus melanochir) and Tarwine (Rhabdosargus sarba). While McArthur and Boland (2006) found seagrasses contribution to secondary fisheries production in Spencer Gulf, South Australia to be valued at $184 ha/yr (Supplementary Information S2) demonstrating the large variability in valuations, additionally, Carnell et al. (2019) demonstrates high spatial variability with one 11 km stretch of seagrass found to be a key nursery hotspot for S. punctata, while other areas contributed substantially less biomass. However these studies do not take into account connectivity between VCE.
Algal reefs support complex assemblages of algae, fish and invertebrates supporting fisheries throughout temperate Australia. Green and Black lip abalone (Haliotis spp.) and the highest value wild catch species caught in Australia, the rock lobster (Jasus edwardii and Panulirus cygnus) are caught on the great southern reef and these two catches alone are valued at almost $526 million per annum (Mobsby and Koduah, 2017; Supplementary Information S2).
Currently 85% of Australian fisheries are classified as sustainable or recovering (Stewardson et al., 2018), but also see Edgar et al. (2018). The total value of provisioning services from wild catches across temperate Australia has an estimated gross value of $1.0 billion each year (Mobsby and Koduah, 2017; Supplementary Information S2). This value is comprised from offshore waters making up approximately a quarter of total fisheries, while inshore waters incorporating VCE including algal reefs provide the largest value (Table 2). Using value estimates of fisheries production per area of habitat published in literature (Supplementary Information S2) produces a value estimate approximately 100 greater than actual catch data demonstrating the large variability in valuation studies.
Other provisioning services include the use of coast and marine plants and organisms for nutritional and medicinal use is relatively small in Australia. Currently, the majority of potential value is through research of bioactive compounds for medicine including compounds from marine sponges, particularly from the cosmopolitan genus Stelletta that inhabits southern Australian coasts (Goudie et al., 2013) and gastropods including Dicanthus orbita (Benkendorff, 2013). Currently, there are no valuations provisioning services for medical use, but the potential value from this service could be large.
Estuaries throughout Tasmania are extremely important habitats for the burgeoning Atlantic salmon (Salmo salar) aquaculture, with extensive sea cage salmon farms throughout the D’Entrecasteaux Channel, Huon River and through to Macquarie Harbor (Leith et al., 2014). Similarly, Spencer Gulf, an inverse estuary in South Australia, pioneered sea cage ranching of southern Bluefin tuna (SBT -Thunnus maccoyii), catching juvenile fish in the Great Australian Bight and towing them in cages to Port Lincoln where they are feed sardines and grow to harvest size (Ellis and Kiessling, 2016). These locations have the suitable biotic and abiotic conditions that allow the successful high density culture of fish. Over the last two decades Australian aquaculture has grown 6% by volume and 10% in value (Mobsby and Koduah, 2017). Salmon aquaculture is the largest aquaculture industry in Australia with farm output valued at $484 million per year (Mobsby and Koduah, 2017). While the Spencer Gulf SBT and yellowtail kingfish (Seriola lalandii) are valued at $93 million per year (Econsearch, 2018). A large proportion of these products are exported providing services at the extra-local scale indicating that the value of services is not limited to the habitat they are harvested from Drakou et al. (2017).
Estuaries and embayments throughout temperate Australia are typically sheltered, have terrestrial nutrient input and high residence times contributing to their high phytoplankton productivity (Gillanders et al., 2011). These natural phytoplankton communities facilitate the aquaculture of the Pacific oyster (Crassostrea gigas) and blue mussels (Mytilus galloprovincialis) across extensive intertidal and subtidal mudflats in South Australia and Tasmania (Mobsby and Koduah, 2017). Estuaries throughout the east coast of Australia between Hervey Bay in southern Queensland through to southern New South Wales have farmed the Sydney rock oyster (Saccostrea glomerata) for over 130 years (O’connor and Dove, 2009).
Oyster aquaculture contribute provisioning services with an estimated value of approximately $62 million, however, this value is likely to have substantially decreased with the pacific oyster mortality syndrome infecting the Tasmanian industry. In New South Wales, extensive Sydney rock oyster (Saccostrea glomerata) aquaculture is found throughout the estuaries and is valued at approximately $30 million (Mobsby and Koduah, 2017). In total, the provision of food from reared species from in situ aquaculture in coastal temperate waters is estimated to be valued at over $676 million annually and increasing over time (Mobsby and Koduah, 2017) and is concentrated within inshore waters indicating the importance of these regions (Table 1).
Regulation and maintenance services are the services that mediate key processes that maintain our marine and coastal environments and their value are often overlooked (De Groot et al., 2002). These services are often referred to as indirect or non-market values and are typically more difficult to assign a monetary value (National Research Council, 2005).
Extreme events such as storms generate large surges, coastal flooding and waves causing damage to coastal infrastructure including houses, roads and bridges (Harman et al., 2013; Muis et al., 2016). VCE form complex and dense structures that attenuate wave energy and can protect shorelines from erosion from storms and mitigate the loss of coastal land from chronic erosion and inundation (Morris et al., 2018). Recent work from Victoria suggests that coastal wetlands are currently protecting $1.7 billion in coastal infrastructure that could be at risk (Carnell et al., 2019). Additionally, seagrass loss along Adelaide’s metropolitan coast has resulted in approximately 10% higher sand accumulation on beaches (Short, 2012), effecting the cost of beach replenishment programs. Carnell et al. (2019) suggests that Victorian coastal saltmarsh, mangrove and seagrass habitats reduce wave energy by 37–71%, protecting shorelines and coastal assets.
Globally, the estimated value of the regulation and maintenance services from VCE that protect shorelines from extreme events indicates a wide range of values between $2.57 – 11,505 ha/yr with a median of $1,221 ha/yr (n = 15) (Supplementary Information S2). These values demonstrate considerable variability, affecting their usefulness. It is clear that site specific factors including infrastructure type and distance from the coast, erosion rates and habitat density are all factors that will affect the valuations and localized site specific valuations will provide the most reliable measures.
Regulating and maintenance services can often be considered as a value per unit area but need to be considered in with local characteristics, biotic and abiotic factors that may influence the provision of the service. Saltmarsh and mangroves are both important relative contributors to the overall services delivered, they present high value per unit area (Table 2). However saltmarsh extent is declining suffering from rising sea levels, encroachment by mangroves and losses from increasing urban development. Mangrove encroachment into saltmarsh may alter the shoreline protection service delivered (net positive or negative) (Kelleway et al., 2017b), but this trade off would need to be considered on a site by site basis.
VCE are typically located in close proximity to urban areas, and resultantly, coastal discharges. When anthropogenic discharges or and terrestrial runoff enters coastal habitats the plants, algae and organisms cycle and assimilate nutrients and other pollutants (e.g., metals) reducing the likelihood of an adverse effect, thereby providing regulating and maintenance services that treat wastes (Nayar et al., 2012; Lafratta et al., 2019). These wastes are taken into the biomass of VCE plants, algae and biota, cycled through growth and reproduction and can be stored in sediments or exported to different systems (e.g., offshore deep waters) (Duarte and Cebrián, 1996). VCE assimilate metals, which are trapped by habitats accumulating and storing metals as a sink (Lafratta et al., 2019), reducing their bioavailability (Marin-Guirao et al., 2005). Services provided by VCE can offset or in some cases replace the cost of traditional waste treatment processes such as technological upgrades in wastewater treatment plants or industrial facilities (Sagoff, 2011). This is not a new concept with extensive literature on the benefits and failures of using assimilative capacity to treat pollution. Nayar et al. (2012) demonstrated the 131 km2 of seagrass along Adelaide’s metropolitan coast assimilated approximately 468 tons of nitrogen in 2005, approximately 31% of anthropogenic inputs at the time, indicating substantial waste treatment service.
Bacterial communities are present in all habitats, but particularly sediments. They provide substantial treatment capacity and play a key role in denitrification (Sørensen, 1978), which is fundamental to export of nitrogen from coastal environments to the atmosphere. Similarly, hydrocarbon consuming bacteria are ubiquitous in the marine environment (Head et al., 2006; Yakimov et al., 2007). Whereby in the event of a maritime oil spill, indigenous bacterial communities may contain hydrocarbon degrading species, which can thrive and consume hydrocarbons (Ramsay et al., 2000). The natural breakdown of hydrocarbons may be an acceptable treatment measure, particularly when access to contaminated areas can cause substantial damage in itself (Psaraftis et al., 1986). The value of these communities for the potential treatment in an oil spill cleanup operation has not been documented but is likely to be substantial.
Bennett et al. (2015) used a benefit transfer method from values in Costanza et al. (1997) to value the 8000 km of shallow subtidal algal forests along the Great Southern reef extending throughout temperate Australia suggesting that nutrient cycling could be worth as much as $120 billion a year or $17,608 per hectare per year (Supplementary Information S2).
Globally, the median value of the waste treatment service from VCE was $10,224 ha/yr with a range of $3,832 – 748,847 ha/yr (n = 6) (Supplementary Information S2). Even with its relatively limited distribution, saltmarsh contribute substantial value to the treatment of waste (Table 2). Although it is clear that there is substantial variability in valuations, within habitats and across regions which highlights the need for fine scale local details required to undertake accurate valuation relevant for particular regions.
When nitrogen in is excess, algal growth can bloom assimilating nitrogen, but can smother habitats, block light to seagrass or other benthic vegetation causing ecosystem degradation (Shepherd et al., 1989), and cause odors as it decays and releases nutrients back into the environment (McComb and Davis, 1993). Algal and microbial consumption of carbon, results in areas of oxygen depletion, which can be responsible for fish kills and areas known as dead zones (Howarth et al., 2011). Gaining value from a waste treatment service through discharging pollutants into coast and marine habitats is a fine balance. This concept can substantially increase the value of services, such as suggested with integrated multi-trophic aquaculture (Barrington et al., 2009), but also through sustainably managing natural environments (Nayar et al., 2012). However history has demonstrated that discharges that significantly modify the structure and function of the system can degrade the environment (Shepherd et al., 1989), resulting in a loss of ecosystem service and the benefit to man.
Vegetated coastal ecosystems including mangroves, saltmarsh and seagrass have been identified as being extremely efficient as sequestering carbon (termed blue carbon) per unit area, even more than their terrestrial counterparts (Mcleod et al., 2011). Australia is home to a large proportion of the world’s blue carbon ecosystems (Macreadie et al., 2017) with extensive Posidonia sp. and Amphibolis sp. seagrass meadows particularly along the southern coastlines (Lavery et al., 2013). Intact blue carbon ecosystems typically retain stocks of carbon in the soils, with up to 90% of stock in seagrass and saltmarsh and up to 75% in mangrove systems (Kelleway et al., 2017a). While degrading habitats can be net carbon sources (Theuerkauf et al., 2015). The magnitude of this service is likely to be species- and location- dependent with differences in biomass, carbon burial capacity and export of carbon to other locations influencing carbon sequestration (Fourqurean et al., 2012; Lavery et al., 2013; Macreadie et al., 2017).
The median estimate of long term stock of carbon in the top 1 m of Australian VCE has been valued at $3,237 ha–1 (n = 10) while the annual carbon accumulation is estimated at $21 ha each year (n = 7) (Supplementary Information S2). Sequestration of carbon by seagrass meadows may be globally significant (Fourqurean et al., 2012) and within Australia, the high value is due to the large areas of intact seagrass meadows (Table 2).
Applying habitat areas throughout temperate Australia to the value per hectare for all regulating and maintenance service suggests that VCE contribute approximately $130 billion each year in value to Australians.
Cultural services are non-material benefits people gain from the environment through recreation, aesthetic and reflective appreciation, spiritual enrichment and cognitive development (Millenium Ecosystem Assessment, 2005). Cultural services such as tourism can be relatively simple to quantify but there can be wide variation depending on aspects included. However, other cultural services are often intangible including relationship between the environment and the sense of place, and human health and well-being.
Sandy beaches are iconic in Australian culture and are the most popular recreational destination in Australia supporting substantial socio-cultural activities (James, 2000; Maguire et al., 2011) providing recreation and aesthetic appreciation of nature.
Maguire et al. (2011) demonstrated that beaches are the most popular recreational destination in south eastern Australia, with walking being the most frequent past time, although a separate survey suggested passive recreation of sitting/sunbathing and picnicking were most popular along the Gold Coast of Queensland (Zhang et al., 2015). Use of VCE for fishing and bird watching can contribute significantly to recreational value (Table 2; Carnell et al., 2019). The AIMS Index of Marine Industries suggests that throughout Australia, marine based tourism and recreation in domestic and international consumption across the total environmental value (TEV) was valued at approximately $22.2 billion per year (Supplementary Information S2). Various authors have estimated the value of coastal recreation/tourism in localized areas in temperate Australia. These values include $1.3 billion per year in Victoria, $22 million per year from Adelaide beaches, South Australia, and $18 – 77 million from Gold Coast beaches, Queensland (Kirkpatrick, 2012). This demonstrates the large variability in valuations and suggests that sandy beaches are likely to be focal point in temperate regions (Table 1).
Recreational fishing is a popular interaction with coastal and marine environment. It has been demonstrated to have benefits on health and well-being (McManus et al., 2011). The majority of recreational fishers link participation to sport and relaxation, rather than provision of food (Henry and Lyle, 2003), with almost a quarter of fish caught, released (McManus et al., 2011). The inshore coastal waters including the extensive algal forests and seagrass meadows throughout temperate Australia have been linked to substantial economic value including $1.5 billion a year in recreational fishing (AIMS, 2018). More location specific estimates includes studies on recreational fishing in Victoria which estimated a direct value of $1.3 billion per year, while the value of southern blue fin tuna recreational fishing in Victoria was valued at $5.1 million (Ernst and Young, 2015). These valuations do not breakdown the value into a value per unit area as there are vast spatial differences even within small areas due to features of interest driving much of the tourism rather than the region as a whole (Casalegno et al., 2013), making area based comparisons problematic.
Lloret (2010) outlines many of the physical and psychological benefits from activities in the marine environment with physical recreation leading to improved cardio vascular condition, reducing obesity. While activities within nature have been shown to contribute to improved mood, attention, and reduce stress (Lloret, 2010). The value of these specific marine based recreational activities to reducing health care is unknown.
Australian sandy beaches represent significant economic value not only for direct use (Schlacher et al., 2008), but also for the cultural identity that Australians identify with and are internationally renowned for James (2000), Maguire et al. (2011) and has been used internationally for attraction of tourism, often with advertisements featuring beaches.
Expenditure in management and research into the marine environment is significant with Government agencies and research institutes, universities and other non-government organizations contributing substantial value for the intellectual interactions with the marine environment. Similarly, citizen science is where trained non-scientists contribute to monitoring programs in the coastal and marine environment, and participation provides emotional and mental benefits, with active learning, such as remembering names of marine biota, stimulating brain activity and memory (Koss et al., 2009).
The coastal areas of temperate Australia host a wealth of Indigenous and European spiritual and heritage values. Indigenous communities were, and in some cases still are, coastal people and environments and animals feature in stories highlighting the cultural and spiritual importance of the coastal and marine areas (McNiven, 2004). There is wide evidence of historical use of coastal and marine environments throughout the temperate region of Australia. Examples of aboriginal use include shell middens demonstrating shellfish harvesting in southern and eastern Australia (Alleway and Connell, 2015), detailed descriptions of fish traps in northern New South Wales which historically provided provisioning services as well as cultural and spiritual services.
Cultural and spiritual values have been widely acknowledged (Millenium Ecosystem Assessment, 2005; Sukhdev et al., 2010). However the identification of services for specific regions and their inclusion in valuation trade off discussions is rare (Tengberg et al., 2012), but where included have been shown to be important (Ghermandi et al., 2009).
There are other cultural services that consider the existence value of nature that is the positive feeling that people may have knowing that nature exists and is conserved, while bequest value is the knowledge that nature will be present for future generations. The relationship that people have with the marine and coastal environment is personal, subjective and typically less tangible than other services including provisioning and regulating services. The value of these cultural services will vary from person to person and therefore present significant challenges to accurately value (Small et al., 2017). This results in cultural ecosystem service valuations being difficult to value and rare (Carpenter et al., 2009).
Tourism and recreation cultural services have been estimated to be worth an approximate $22 billion per year. However, valuing cultural services is acknowledged to provide a lowest value estimate due to the difficulties in monetary valuation of other cultural services including intrinsic values.
Past history suggests that human activity often results in degradation of the natural environment, which affects ecosystem service delivery (Worm et al., 2006). Development directly landward of saltmarsh systems through reclamation and construction of roads and levees, prevents the landward retreat of saltmarsh (Creighton et al., 2015). Consequently, increases in the magnitude or frequency of tidal inundation from sea level rise or changes to flow regimes, can result in encroachment of mangroves into saltmarsh habitats ‘squeezing’ the saltmarsh and reducing its spatial extent (Table 2; Harty, 2004). Mangrove encroachment is likely to increase waste treatment and carbon sequestration functions, but potential losses in niche habitats for animals seeking open habitats such as migratory birds and bats and the associated recreational values including birdwatching (Kelleway et al., 2017b).
Further drivers of habitat destruction include pollution discharges into or adjacent to VCE (Table 2). Nutrient discharges from wastewater, agricultural and urban runoff and industries has been shown to cause eutrophication, leading to degradation and loss of coastal habitats (Shepherd et al., 1989; Deegan et al., 2012). Nutrient discharges into coastal habitats can provide significant value through the mediating wastes, however, this is a fine balance which relies on maintaining discharges below an assimilative capacity to avoid impacting critical ecosystem function. If exceeded, degradation and habitat loss will affect the delivery of ecosystem services including provisioning of food through loss of fish production and loss of nursery areas, loss of historical and future carbon storage (Macreadie et al., 2013), a loss of the buffering capacity protecting shorelines from erosion (Deegan et al., 2012) and a loss in the ability for habitats to assimilate waste.
Australian fisheries catches have declined over the last 20 years (Edgar et al., 2018), coupled with increasing costs of equipment, fuel and competing with cheaper imported products, decreasing profitability may result in short term benefit from harvesting beyond a capacity, resulting in consequences for sustainable fisheries and long term value (Parker et al., 2015).
Aquaculture has been highlighted as a source of food for the growing global appetite for fish (Subasinghe et al., 2009), with fish produced from farming activities currently accounting for approximately 25% of all fish consumed (Naylor et al., 2000). However the environmental impact of pollution generated and discharged to the environment from sea cages can be significant and result in destruction of nearshore habitats, increase the risk of disease and parasite transmission, a risk to the environment and non-target species, but also to the aquaculture facility (Table 1; Pergent-Martini et al., 2006). Integrated multi-trophic aquaculture can optimize the delivery of provisioning services and limit impacts to nearshore habitats but is seldom successfully implemented (Barrington et al., 2009).
Climate change is threat to all ecosystem services (Tables 1, 2). It is likely to impact on provisioning services with up to a 35% reduction in global fisheries catch by 2050 under high CO2 discharge scenarios (Lam et al., 2016). Increased sea surface temperatures, changes in magnitudes of wind and current patterns, the loss of sea ice from polar regions and rising sea levels will all affect the physical and chemical composition of oceans and the atmosphere impacting on ecosystem productivity, habitat condition and distribution of fish (Hollowed et al., 2013). The effect of ocean acidification is less clear with potential winners and losers making predictions difficult (Narita and Rehdanz, 2017), but with potential effects on shellfish well established (Hendriks et al., 2010) and vast provisioning services value throughout temperate Australia including substantial wild catch abalone and shellfish aquaculture, the risk appears significant.
Culturally, coastal residents in the south-east of Australian value ‘natural,’ clean and uncrowded beaches most highly, and also value the opportunity to see wildlife, but these people also value beaches with facilities such as toilets, food outlets and easy access (Maguire et al., 2011). However, recreation and tourism are heavily influenced by the condition of the environment they interact with, and pressures on the habitat can impact on these services (Drius et al., 2019).
The management of coast and marine environments needs an approach that collectivity considers and optimizes the provision of services rather than exploit one over others. This would provide an integrated management framework working toward the best environmental, social and economic outcome, rather than sectoral management focusing on isolated services. This approach will benefit decision making by clearly demonstrating linkages between the environment and services important to society. It will assist in the identification of, and provide an opportunity for proactive and positive engagement with stakeholders, demonstrated to benefit ecological projects with community involvement (Druschke and Hychka, 2015). Ecosystem service approaches provide transparency and build trust in trade off discussions (Davies et al., 2015).
Developing locally relevant monetary valuations will provide a common language understandable by decision makers that can be persuasive and demonstrate the true scope of potential effects of development. The identification of ecosystem services provided by areas can demonstrate hotspots of ecological, economic and/or social importance that can be conserved or protected (Cárcamo et al., 2014). Importantly, an ecosystem services approach is not specific to one management framework, but can be adapted to existing approval process and provide critical baseline information on values of society which is fundamental in ecologically sustainable development.
This review demonstrates that consistent with other locations worldwide, temperate Australia has a diversity of coast and marine habitats that provide a wealth of final ecosystem services including provisioning, regulating and maintenance and cultural services, many of which have not been previously described. Benefits gained from coast and marine environments are a substantial contributor to Australia’s GDP, they have formed the Australian identity and continue to feature heavily in Australian culture. Provisioning services throughout temperate Australian were valued at over $1.7 billion dollars per year incorporating both wild catch and aquaculture sectors. Cultural services of recreation and tourism contributed almost $22 billion each year through traditional monetary valuations, but there are non-monetary aspects important to society that could not be valued including the intrinsic value of nature. The value of VCE for regulation and maintenance of our environment demonstrated enormous value, particularly for assimilating and treating waste, valued at over $130 billion each year. A key habitat that featured in this value was the treatment of waste by algal reefs, which also contribute almost half of the wild catch provisioning services.
The 2016 Australia State of the Environment Report demonstrates that many coast and marine habitats are in poor condition (Clark and Johnston, 2016b). It is apparent that the management of marine and coastal environments under past or existing decision making frameworks can still result in the decline in habitat structure and function, which is likely impacting on the provision of services and benefits to the community (Polasky et al., 2011). Clearly, traditional approaches to environmental management typically fail to consider the multiple uses and perspectives of all stakeholders. There are clear benefits of using integrated management approaches in environmental management (Estévez and Gelcich, 2015). Including an ecosystem services approach would further benefit projects in order to consider all ecosystem services rather than exploitation of one ES over others (Hauck et al., 2013).
Further work is recommended to understand how the provision of ES can be impacted by both natural and anthropogenic stressors including over exploitation of natural resources. This will ensure that adequate consequence is placed on decisions that affect these systems in ecological risk assessments, allowing consideration of all ES. Further to this, it’s recommended that the identification and inclusion of key indicators linked to the services they provide into long term monitoring programs will allow tracking of the benefits these habitats provide society.
Finally, tailoring processes for managers to use in decision making will ensure uptake by relevant agencies along with education of the ecological and economic concepts will ensure the implementation of balanced and sustainable decisions to protect ecosystems, economies and societal well-being. If there is no understanding of how to value ecosystems, then development cannot be managed sustainably.
SG and MW conceived and designed the review. SG wrote the first draft of the manuscript. All the authors contributed to manuscript revision, read and approved the submitted version.
This research was supported through an Australian Government Research Training Program Scholarship.
The authors declare that the research was conducted in the absence of any commercial or financial relationships that could be construed as a potential conflict of interest.
The authors would like to thank Alice Jones, Harpinder Sandhu, Heidi Alleway, and Milena Fernandes for comments and advice on the draft manuscript. Authors would also like to thank the reviewers for their valuable input to improve this manuscript.
The Supplementary Material for this article can be found online at: https://www.frontiersin.org/articles/10.3389/fmars.2020.00453/full#supplementary-material
AIMS (2018). The AIMS Index of Marine Industry: December 2018. Australian Government. Townsville: Australian Institute of Marine Science.
Alleway, H. K., and Connell, S. D. (2015). Loss of an ecological baseline through the eradication of oyster reefs from coastal ecosystems and human memory. Conserv. Biol. 29, 795–804. doi: 10.1111/cobi.12452
Barrington, K., Chopin, T., and Robinson, S. (2009). Integrated multi-trophic aquaculture (IMTA) in marine temperate waters. Integrated mariculture: a global review. FAO Fish. Aquac. Tech. Pap. 529, 7–46.
Benkendorff, K. (2013). Natural product research in the Australian marine invertebrate Dicathais orbita. Mar. Drugs 11, 1370–1398. doi: 10.3390/md11041370
Bennett, S., Wernberg, T., Connell, S. D., Hobday, A. J., Johnson, C. R., and Poloczanska, E. S. (2015). The ‘Great Southern Reef’: social, ecological and economic value of Australia’s neglected kelp forests. Mar. Freshw. Res. 67, 47–56. doi: 10.1071/MF15232
Bjelland, H. V., Føre, M., Lader, P., Kristiansen, D., Holmen, I. M., Fredheim, A., et al. (2015). “Exposed aquaculture in Norway,” in Proceedings of the OCEANS 2015-MTS/IEEE, (Washington, DC: IEEE), 1–10.
Blandon, A., and Zu Ermgassen, P. S. (2014). Quantitative estimate of commercial fish enhancement by seagrass habitat in southern Australia. Estuar. Coast. Shelf Sci. 141, 1–8. doi: 10.1016/j.ecss.2014.01.009
Bowler, D. E., Buyung-Ali, L. M., Knight, T. M., and Pullin, A. S. (2010). A systematic review of evidence for the added benefits to health of exposure to natural environments. BMC Public Health 10:456. doi: 10.1186/1471-2458-10-456
Brundtland, G. (1987). Our Common Future: Report of the 1987 World Commission on Environment and Development. Oxford: Oxford University Press.
Cárcamo, P. F., Garay-Flühmann, R., Squeo, F. A., and Gaymer, C. F. (2014). Using stakeholders’ perspective of ecosystem services and biodiversity features to plan a marine protected area. Environ. Sci. Policy 40, 116–131. doi: 10.1016/j.envsci.2014.03.003
Carnell, P. E., Reeves, S. E., Nicholson, E., Macreadie, P., Ierodiaconou, D., Young, M., et al. (2019). Mapping Ocean Wealth Australia: The Value of Coastal Wetlands to People and Nature. Melbourne: The Nature Conservancy.
Carpenter, S. R., Mooney, H. A., Agard, J., Capistrano, D., DeFries, R. S., Díaz, S., et al. (2009). Science for managing ecosystem services: beyond the millennium ecosystem assessment. Proc. Natl. Acad. Sci. U.S.A. 106, 1305–1312. doi: 10.1073/pnas.0808772106
Casalegno, S., Inger, R., DeSilvey, C., and Gaston, K. J. (2013). Spatial covariance between aesthetic value & other ecosystem services. PLoS One 8:e68437. doi: 10.1371/journal.pone.0068437
Clark, G., and Johnston, E. (2016a). Australia State of the Environment 2016 Coasts: Pressures Affecting the Coastal Environment. Canberra: Australian Government Department of the Environment and Energy.
Clark, G., and Johnston, E. (2016b). Australia State of the Environment 2016 Coasts: State and Trends of Quality of Habitat-Forming Species. Canberra: Australian Government Department of the Environment and Energy.
Commonwealth of Australia (2006). A guide to the Integrated Marine and Coastal Regionalisation of Australia Version 4.0. Canberra: Commonwealth of Australia.
Connell, S. D. (2007). “Subtidal temperate rocky habitats: habitat heterogeniety at local to continental scales,” in Marine Ecology, eds S. D. Connell and B. Gillanders (South Melbourne, VIC: Oxford University Press), 378–401.
Connell, S. D., Russell, B. D., Turner, D. J., Shepherd, S. A., Kildea, T., Miller, D., et al. (2008). Recovering a lost baseline: missing kelp forests from a metropolitan coast. Mar. Ecol. Prog. Ser. 360, 63–72. doi: 10.3354/meps07526
Costanza, R., d’Arge, R., de Groot, R., Farber, S., Grasso, M., Hannon, B., et al. (1997). The value of the world’s ecosystem services and nature capital. Nature 387, 253–260.
Costanza, R., de Groot, R., Sutton, P., van der Ploeg, S., Anderson, S. J., Kubiszewski, I., et al. (2014). Changes in the global value of ecosystem services. Glob. Environ. Change 26, 152–158.
Creighton, C., Gillies, C., and McLeod, I. (2015). Australia’s Saltmarshes: A Synopsis to Underpin the Repair and Conservation of Australia’s Environmental, Social and Economically Important Bays and Estuaries. Townsville: James Cook University.
Davies, K. K., Fisher, K. T., Dickson, M. E., Thrush, S. F., and Le Heron, R. (2015). Improving ecosystem service frameworks to address wicked problems. Ecol. Soc. 20:37. doi: 10.5751/ES-07581-200237
De Groot, R., Brander, L., Van Der Ploeg, S., Costanza, R., Bernard, F., Braat, L., et al. (2012). Global estimates of the value of ecosystems and their services in monetary units. Ecosyst. Serv. 1, 50–61. doi: 10.1016/j.ecoser.2012.07.005
De Groot, R. S., Wilson, M. A., and Boumans, R. M. (2002). A typology for the classification, description and valuation of ecosystem functions, goods and services. Ecol. Econ. 41, 393–408. doi: 10.1016/s0921-8009(02)00089-7
Deegan, L. A., Johnson, D. S., Warren, R. S., Peterson, B. J., Fleeger, J. W., Fagherazzi, S., et al. (2012). Coastal eutrophication as a driver of salt marsh loss. Nature 490, 388–392. doi: 10.1038/nature11533
Dobson, A., Lodge, D., Alder, J., Cumming, G. S., Keymer, J., McGlade, J., et al. (2006). Habitat loss, trophic collapse, and the decline of ecosystem services. Ecology 87, 1915–1924. doi: 10.1890/0012-9658(2006)87[1915:hltcat]2.0.co;2
DOE (2014). Assessment of the Eastern Tuna and Billfish Fishery. Canberra: Commonwealth of Australia.
Drakou, E. G., Pendleton, L., Effron, M., Ingram, J. C., and Teneva, L. (2017). When ecosystems and their services are not co-located: oceans and coasts. ICES J. Mar. Sci. 74, 1531–1539. doi: 10.1093/icesjms/fsx026
Drius, M., Bongiorni, L., Depellegrin, D., Menegon, S., Pugnetti, A., and Stifter, S. (2019). Tackling challenges for Mediterranean sustainable coastal tourism: an ecosystem service perspective. Sci. Total Environ. 652, 1302–1317. doi: 10.1016/j.scitotenv.2018.10.121
Druschke, C. G., and Hychka, K. C. (2015). Manager perspectives on communication and public engagement in ecological restoration project success. Ecol. Soc. 20:58.
Duarte, C. M., and Cebrián, J. (1996). The fate of marine autotrophic production. Limnol. Oceanogr. 41, 1758–1766. doi: 10.4319/lo.1996.41.8.1758
Econsearch (2018). The Economic Contribution of Aquaculture in the South Australian State and Regional Economies, 2016/17. Adelaide: EconSearch Pty Ltd.
Edgar, G. J., Ward, T. J., and Stuart-Smith, R. D. (2018). Rapid declines across Australian fishery stocks indicate global sustainability targets will not be achieved without an expanded network of ‘no-fishing’ reserves. Aquat. Conserv. Mar. Freshw. Ecosyst. 28, 1337–1350. doi: 10.1002/aqc.2934
Ellis, D., and Kiessling, I. (2016). “Ranching of southern bluefin tuna in Australia,” in Advances in Tuna Aquaculture, eds D. D. Benetti, G. J. Partridge, and A. Buentello (Tokyo: Elsevier), 217–232. doi: 10.1016/b978-0-12-411459-3.00010-2
Ernst and Young (2015). Economic Study of Recreational Fishing in Victoria. Victoria: Victorian Recreational Fishing Peak Body.
Esteban, M. D., Diez, J. J., López, J. S., and Negro, V. (2011). Why offshore wind energy? Renew. Energy 36, 444–450. doi: 10.1016/j.renene.2010.07.009
Estévez, R. A., and Gelcich, S. (2015). Participative multi-criteria decision analysis in marine management and conservation: research progress and the challenge of integrating value judgments and uncertainty. Mar. Policy 61, 1–7. doi: 10.1016/j.marpol.2015.06.022
Fourqurean, J. W., Duarte, C. M., Kennedy, H., Marbà, N., Holmer, M., Mateo, M. A., et al. (2012). Seagrass ecosystems as a globally significant carbon stock. Nat. Geosci. 5, 505–509. doi: 10.1038/ngeo1477
Friess, D. A., and Webb, E. L. (2014). Variability in mangrove change estimates and implications for the assessment of ecosystem service provision. Glob. Ecol. Biogeogr. 23, 715–725. doi: 10.1111/geb.12140
Geosciences Australia (2020). Maritime Boundary Definitions. Canberra: Commonwealth of Australia (Geoscience Australia).
Ghermandi, A., Nunes, P. A., Portela, R., Rao, N., and Teelucksingh, S. S. (eds) (2009). Recreational, Cultural and Aesthetic Services from Estuarine and Coastal Ecosystems. Burlington, MA: Elsevier.
Gillanders, B., Able, K., Brown, J., Eggleston, D., and Sheridan, P. (2003). Evidence of connectivity between juvenile and adult habitats for mobile marine fauna: an important component of nurseries. Mar. Ecol. Prog. Ser. 247, 281–295. doi: 10.3354/meps247281
Gillanders, B. M., Elsdon, T. S., Halliday, I. A., Jenkins, G. P., Robins, J. B., and Valesini, F. J. (2011). Potential effects of climate change on Australian estuaries and fish utilising estuaries: a review. Mar. Freshw. Res. 62, 1115–1131. doi: 10.1071/MF11047
Golden, J. S., Virdin, J., Nowacek, D., Halpin, P., Bennear, L., and Patil, P. G. (2017). Making sure the blue economy is green. Nat. Ecol. Evol. 1:17.
Goldsworthy, S. D., Page, B., Rogers, P. J., Bulman, C., Wiebkin, A., McLeay, L. J., et al. (2013). Trophodynamics of the eastern Great Australian Bight ecosystem: ecological change associated with the growth of Australia’s largest fishery. Ecol. Model. 255, 38–57. doi: 10.1016/j.ecolmodel.2013.01.006
Goudie, L., Norman, M. D., and Finn, J. (2013). Sponges: A Museum Victoria Field Guide. Victoria: Museum Victoria.
Haines-Young, R., and Potschin, M. (2010). “The links between biodiversity, ecosystem services and human well-being,” in Ecosystem Ecology: A New Synthesis, eds D. G. Raffaelli and C. L. Frid (Cambridge: Cambridge University Press), 110–139. doi: 10.1017/cbo9780511750458.007
Haines-Young, R., and Potschin, M. B. (2018). Common International Classification of Ecosystem Services (CICES) V5. 1 and Guidance on the Application of the Revised Structure. Nottingham: Fabis Consulting Ltd.
Harman, B. P., Heyenga, S., Taylor, B. M., and Fletcher, C. S. (2013). Global lessons for adapting coastal communities to protect against storm surge inundation. J. Coast. Res. 31, 790–801. doi: 10.2112/jcoastres-d-13-00095.1
Harty, C. (2004). Planning strategies for mangrove and saltmarsh changes in Southeast Australia. Coast. Manage. 32, 405–415. doi: 10.1080/08920750490487386
Hauck, J., Görg, C., Varjopuro, R., Ratamäki, O., and Jax, K. (2013). Benefits and limitations of the ecosystem services concept in environmental policy and decision making: some stakeholder perspectives. Environ. Sci. Policy 25, 13–21. doi: 10.1016/j.envsci.2012.08.001
Head, I. M., Jones, D. M., and Röling, W. F. (2006). Marine microorganisms make a meal of oil. Nat. Rev. Microbiol. 4, 173–182. doi: 10.1038/nrmicro1348
Hendriks, I. E., Duarte, C. M., and Álvarez, M. (2010). Vulnerability of marine biodiversity to ocean acidification: a meta-analysis. Estuar. Coast. Shelf Sci. 86, 157–164. doi: 10.1016/j.ecss.2009.11.022
Henry, G., and Lyle, J. (2003). The National Recreational and Indigenous Fishing Survey. FRDC Project No. 99/158. Australian Government Department of Agriculture, Fisheries and Forestry, Canberra.
Hindell, J., and Jenkins, G. (2004). Spatial and temporal variability in the assemblage structure of fishes associated with mangroves (Avicennia marina) and intertidal mudflats in temperate Australian embayments. Mar. Biol. 144, 385–395. doi: 10.1007/s00227-003-1201-x
Hollowed, A. B., Barange, M., Beamish, R. J., Brander, K., Cochrane, K., Drinkwater, K., et al. (2013). Projected impacts of climate change on marine fish and fisheries. ICES J. Mar. Sci. 70, 1023–1037. doi: 10.1093/icesjms/fst081
Howarth, R., Chan, F., Conley, D. J., Garnier, J., Doney, S. C., Marino, R., et al. (2011). Coupled biogeochemical cycles: eutrophication and hypoxia in temperate estuaries and coastal marine ecosystems. Front. Ecol. Environ. 9, 18–26. doi: 10.1890/100008
James, R. J. (2000). From beaches to beach environments: linking the ecology, human-use and management of beaches in Australia. Ocean Coast. Manage. 43, 495–514. doi: 10.1016/s0964-5691(00)00040-5
Jänes, H., Macreadie, P. I., Zu Ermgassen, P. S., Gair, J. R., Treby, S., Reeves, S., et al. (2020). Quantifying fisheries enhancement from coastal vegetated ecosystems. Ecosyst. Serv. 43:101105. doi: 10.1016/j.ecoser.2020.101105
Jones, A. R., Foster, N., Waycott, M., and Gillanders, B. M. (2019). Coastal Carbon Opportunities: Summary Report on Changes in the Distribution of Mangrove and Saltmarsh Across South Australia (1987 – 2015). Adelaide: Goyder Institute for Water Research.
Kämpf, J., Doubell, M., Griffin, D., Matthews, R. L., and Ward, T. M. (2004). Evidence of a large seasonal coastal upwelling system along the southern shelf of Australia. Geophys. Res. Lett. 31:L09310. doi: 10.1029/2003GL019221
Kathiresan, K., and Bingham, B. L. (2001). Biology of mangroves and mangrove ecosystems. Adv. Mar. Biol. 40, 81–251. doi: 10.1016/s0065-2881(01)40003-4
Kelleway, J. J., Serrano, O., Baldock, J., Cannard, T., Lavery, P., Lovelock, C., et al. (2017a). Technical Review of Opportunities for Including Blue Carbon in the Australian Government’s Emissions Reduction Fund. Australia: CSIRO.
Kelleway, J. J., Cavanaugh, K., Rogers, K., Feller, I. C., Ens, E., Doughty, C., et al. (2017b). Review of the ecosystem service implications of mangrove encroachment into salt marshes. Glob. Change Biol. 23, 3967–3983. doi: 10.1111/gcb.13727
Kendrick, G. A., Aylward, M. J., Hegge, B. J., Cambridge, M. L., Hillman, K., Wyllie, A., et al. (2002). Changes in seagrass coverage in Cockburn Sound, Western Australia between 1967 and 1999. Aquat. Bot. 73, 75–87. doi: 10.1016/s0304-3770(02)00005-0
Kilminster, K., Hovey, R., Waycott, M., and Kendrick, G. A. (2018). “Seagrasses of Southern and South-Western Australia,” in Seagrasses of Australia: Structure, Ecology and Conservation, eds A. Larkum, G. A. Kendrick, and P. J. E. Ralph (Cham: Springer), 61–89. doi: 10.1007/978-3-319-71354-0_3
Kirkman, H., and Kuo, J. (1990). Pattern and process in southern Western Australian seagrasses. Aquat. Bot. 37, 367–382. doi: 10.1016/0304-3770(90)90022-d
Kirkpatrick, S. (2012). The Economic Value of Natural and Built Coastal Assets: Part 1: Natural Coastal Assets in National Climate Change Adaptation Research Facility: Southport, Australia. Gold Coast: NCCARF.
Koss, R., Miller, K., Wescott, G., Bellgrove, A., Boxshall, A., McBurnie, J., et al. (2009). An evaluation of Sea Search as a citizen science programme in Marine Protected Areas. Pac. Conserv. Biol. 15, 116–127.
Lafratta, A., Serrano, O., Masqué, P., Mateo, M. A., Fernandes, M., Gaylard, S., et al. (2019). Seagrass soil archives reveal centennial-scale metal smelter contamination while acting as natural filters. Sci. Total Environ. 649, 1381–1392. doi: 10.1016/j.scitotenv.2018.08.400
Lam, V. W., Cheung, W. W., Reygondeau, G., and Sumaila, U. R. (2016). Projected change in global fisheries revenues under climate change. Sci. Rep. 6:32607.
Larkum, A. W., Kendrick, G. A., and Ralph, P. J. (2018). Seagrasses of Australia: Structure, Ecology and Conservation. Berlin: Springer.
Lavery, P. S., Mateo, M.-A., Serrano, O., and Rozaimi, M. (2013). Variability in the carbon storage of seagrass habitats and its implications for global estimates of blue carbon ecosystem service. PLoS One 8:e73748. doi: 10.1371/journal.pone.0073748
Ledoux, L., Turner, R., Mathieu, L., and Crooks, S. (2001). Valuing ocean and coastal resources: practical examples and issues for further action. Paper Presented at the Global Conference on Oceans and Coasts at Rio+ 10: Assessing Progress, Addressing Continuing and New Challenges, Paris: UNESCO.
Leith, P., Ogier, E., and Haward, M. (2014). Science and Social License: defining environmental sustainability of atlantic salmon aquaculture in South-Eastern Tasmania, Australia. Soc. Epistemol. 28, 277–296. doi: 10.1080/02691728.2014.922641
Levin, P. S., Fogarty, M. J., Murawski, S. A., and Fluharty, D. (2009). Integrated ecosystem assessments: developing the scientific basis for ecosystem-based management of the ocean. PLoS Biol. 7:e1000014. doi: 10.1371/journal.pbio.1000014
Liquete, C., Cid, N., Lanzanova, D., Grizzetti, B., and Reynaud, A. (2016). Perspectives on the link between ecosystem services and biodiversity: The assessment of the nursery function. Ecol. Indic. 63, 249–257. doi: 10.1016/j.ecolind.2015.11.058
Lloret, J. (2010). Human health benefits supplied by Mediterranean marine biodiversity. Mar. Pollut. Bull. 60, 1640–1646. doi: 10.1016/j.marpolbul.2010.07.034
Lucieer, V., Walsh, P., Flukes, E., Butler, C., Proctor, R., and Johnson, C. R. (2017). Seamap Australia - A National Seafloor Habitat Classification Scheme. Hobart: University of Tasmania.
Macnae, W. (1966). Mangroves in eastern and southern Australia. Aust. J. Bot. 14, 67–104. doi: 10.1071/BT9660067
Macreadie, P. I., Hughes, A. R., and Kimbro, D. L. (2013). Loss of ‘blue carbon’ from coastal salt marshes following habitat disturbance. PLoS One 8:e69244. doi: 10.1371/journal.pone.0069244
Macreadie, P. I., Ollivier, Q. R., Kelleway, J. J., Serrano, O., Carnell, P. E., Ewers Lewis, C. J., et al. (2017). Carbon sequestration by Australian tidal marshes. Sci. Rep. 7:44071.
Macreadie, P. I., Sullivan, B., Evans, S. M., and Smith, T. M. (2018). “Biogeography of Australian Seagrasses: NSW, Victoria, Tasmania and Temperate Queensland,” in Seagrasses of Australia: Structure, Ecology and Conservation, eds A. Larkum, G. A. Kendrick, and P. J. E. Ralph (Cham: Springer), 31–61.
Maguire, G. S., Miller, K. K., Weston, M. A., and Young, K. (2011). Being beside the seaside: beach use and preferences among coastal residents of south-eastern Australia. Ocean Coast. Manage. 54, 781–788. doi: 10.1016/j.ocecoaman.2011.07.012
Marin-Guirao, L., Atucha, A. M., Barba, J. L., Lopez, E. M., and Fernandez, A. J. G. (2005). Effects of mining wastes on a seagrass ecosystem: metal accumulation and bioavailability, seagrass dynamics and associated community structure. Mar. Environ. Res. 60, 317–337. doi: 10.1016/j.marenvres.2004.11.002
Mayer-Pinto, M., Johnston, E., Hutchings, P., Marzinelli, E., Ahyong, S., Birch, G., et al. (2015). Sydney Harbour: a review of anthropogenic impacts on the biodiversity and ecosystem function of one of the world’s largest natural harbours. Mar. Freshw. Res. 66, 1088–1105.
McArthur, L. C., and Boland, J. W. (2006). The economic contribution of seagrass to secondary production in South Australia. Ecol. Model. 196, 163–172. doi: 10.1016/j.ecolmodel.2006.02.030
McComb, A., and Davis, J. (1993). Eutrophic waters of southwestern Australia. Fertil. Res. 36, 105–114. doi: 10.1007/bf00747580
Mcleod, E., Chmura, G. L., Bouillon, S., Salm, R., Björk, M., Duarte, C. M., et al. (2011). A blueprint for blue carbon: toward an improved understanding of the role of vegetated coastal habitats in sequestering CO2. Front. Ecol. Environ. 9:552–560. doi: 10.1890/110004
McManus, A., Newton, W., Storey, J., and White, J. (2011). Identifying the Health and Well-Being Benefits of Recreational Fishing. Report No. 2011/21. (Bentley, WA: Curtin University).
McNiven, I. (2004). Saltwater People: spiritscapes, maritime rituals and the archaeology of Australian indigenous seascapes. World Archaeol. 35, 329–349. doi: 10.1080/0043824042000185757
Mengerink, K. J., Van Dover, C. L., Ardron, J., Baker, M., Escobar-Briones, E., Gjerde, K., et al. (2014). A call for deep-ocean stewardship. Science 344, 696–698. doi: 10.1126/science.1251458
Millenium Ecosystem Assessment (2005). Ecosystems and Human Well-Being: A Framework for Assessment. Washington, DC: World Resources Institute.
Mobsby, D., and Koduah, A. (2017). Australian Fisheries and Aquaculture Statistics 2017, Fisheries Research and Development Corporation Project 2017–2095. Canberra: ABARES.
Moore, K. A., and Short, F. T. (2007). “Zostera: biology, ecology, and management,” in Seagrasses: Biology, Ecology and Conservation, 361.
Morris, R. L., Konlechner, T. M., Ghisalberti, M., and Swearer, S. E. (2018). From grey to green: efficacy of eco-engineering solutions for nature-based coastal defence. Glob. Change Biol. 24, 1827–1842. doi: 10.1111/gcb.14063
Muis, S., Verlaan, M., Winsemius, H. C., Aerts, J. C., and Ward, P. J. (2016). A global reanalysis of storm surges and extreme sea levels. Nat. Commun. 7:11969.
Narita, D., and Rehdanz, K. (2017). Economic impact of ocean acidification on shellfish production in Europe. J. Environ. Plan. Manage. 60, 500–518. doi: 10.1080/09640568.2016.1162705
National Research Council (2005). Valuing Ecosystem Services: Toward Better Environmental Decision-Making. Washington, DC: National Academies Press.
Nayar, S., Collings, G., Pfennig, P., and Royal, M. (2012). Managing nitrogen inputs into seagrass meadows near a coastal city: flow-on from research to environmental improvement plans. Mar. Pollut. Bull. 64, 932–940. doi: 10.1016/j.marpolbul.2012.03.005
Naylor, R. L., Goldburg, R. J., Primavera, J. H., Kautsky, N., Beveridge, M. C., Clay, J., et al. (2000). Effect of aquaculture on world fish supplies. Nature 405, 1017.
Nieblas, A.-E., Sloyan, B. M., Hobday, A. J., Coleman, R., and Richardsone, A. J. (2009). Variability of biological production in low wind-forced regional upwelling systems: a case study off southeastern Australia. Limnol. Oceanogr. 54, 1548–1558. doi: 10.4319/lo.2009.54.5.1548
O’connor, W. A., and Dove, M. C. (2009). The changing face of oyster culture in New South Wales, Australia. J. Shellfish Res. 28, 803–812.
Officer, L. H. (1976). The purchasing-power-parity theory of exchange rates: a review article. Staff Pap. 23, 1–60.
O’Neill, M. F., Leigh, G. M., Wang, Y.-G., Braccini, J. M., and Ives, M. C. (2014). Linking spatial stock dynamics and economics: evaluation of indicators and fishery management for the travelling eastern king prawn (Melicertus plebejus). ICES J. Mar. Sci. 71, 1818–1834. doi: 10.1093/icesjms/fst218
Parker, R. W., Hartmann, K., Green, B. S., Gardner, C., and Watson, R. A. (2015). Environmental and economic dimensions of fuel use in Australian fisheries. J. Clean. Prod. 87, 78–86. doi: 10.1016/j.jclepro.2014.09.081
Pattiaratchi, C. (2007). Understanding Areas of High Productivity within the South-West Marine Region. Canberra: Department of the Environment, Water, Heritage and the Arts.
Pease, B. C. (1999). A spatially oriented analysis of estuaries and their associated commercial fisheries in New South Wales, Australia. Fish. Res. 42, 67–86. doi: 10.1016/s0165-7836(99)00035-1
Pergent-Martini, C., Boudouresque, C. F., Pasqualini, V., and Pergent, G. (2006). Impact of fish farming facilities on Posidonia oceanica meadows: a review. Mar. Ecol. 27, 310–319. doi: 10.1111/j.1439-0485.2006.00122.x
Pittock, J., Cork, S., and Maynard, S. (2012). The state of the application of ecosystems services in Australia. Ecosyst. Serv. 1, 111–120. doi: 10.1016/j.ecoser.2012.07.010
Poiner, I., Conacher, C., Loneragan, N., and Sonters, I. (1993). Effects of cyclones on seagrass communities and penaeid prawn stocks of the Gulf of Carpentaria. CSIRO Mar. Lab. Rep. 5, 143–154.
Polasky, S., Carpenter, S. R., Folke, C., and Keeler, B. (2011). Decision-making under great uncertainty: environmental management in an era of global change. Trends Ecol. Evol. 26, 398–404. doi: 10.1016/j.tree.2011.04.007
Psaraftis, H. N., Tharakan, G. G., and Ceder, A. (1986). Optimal response to oil spills: the strategic decision case. Oper. Res. 34, 203–217. doi: 10.1287/opre.34.2.203
Ramsay, M. A., Swannell, R. P., Shipton, W. A., Duke, N. C., and Hill, R. T. (2000). Effect of bioremediation on the microbial community in oiled mangrove sediments. Mar. Pollut. Bull. 41, 413–419. doi: 10.1016/s0025-326x(00)00137-5
Roberts, S. D., Van Ruth, P. D., Wilkinson, C., Bastianello, S. S., and Bansemer, M. S. (2019). Marine Heatwave, harmful algae blooms and an extensive fish kill event during 2013 in South Australia. Front. Mar. Sci. 6:610. doi: 10.3389/fmars.2019.00610
Sagoff, M. (2011). The quantification and valuation of ecosystem services. Ecol. Econ. 70, 497–502. doi: 10.1016/j.ecolecon.2010.10.006
Saintilan, N., and Williams, R. (2010). Short note: the decline of saltmarsh in southeast Australia: results of recent surveys. Wetl. Aust. 18, 49–54.
Saintilan, N., Wilson, N. C., Rogers, K., Rajkaran, A., and Krauss, K. W. (2014). Mangrove expansion and salt marsh decline at mangrove poleward limits. Glob. Change Biol. 20, 147–157. doi: 10.1111/gcb.12341
Saintilian, N. (2009). Biogeography of Australian saltmarsh plants. Aust. Ecol. 34, 929–937. doi: 10.1111/j.1442-9993.2009.02001.x
Schlacher, T. A., Schoeman, D. S., Dugan, J., Lastra, M., Jones, A., Scapini, F., et al. (2008). Sandy beach ecosystems: key features, sampling issues, management challenges and climate change impacts. Mar. Ecol. 29, 70–90. doi: 10.1111/j.1439-0485.2007.00204.x
Schröter, M., van der Zanden, E. H., van Oudenhoven, A. P. E., Remme, R. P., Serna-Chavez, H. M., de Groot, R. S., et al. (2014). Ecosystem services as a contested concept: a synthesis of critique and counter-arguments. Conserv. Lett. 7, 514–523. doi: 10.1111/conl.12091
Shepherd, S. A., McComb, A. J., Bulthuis, D. A., Neveraskas, V., Steffensen, D. A., and West, R. (1989). “Decline of seagrasses,” in Biology of Seagrasses: A Treatise on the Biology of Seagrasses with Special Reference to the Australian Region, eds A. W. D. Larkum, A. J. McComb, and S. A. Shepherd (Amsterdam: Elsevier), 346–393.
Short, A. D. (2006). Australian beach systems—nature and distribution. J. Coast. Res. 11–27. doi: 10.2112/05a-0002.1
Short, A. D. (2012). “Adelaide Beach Management 1836–2025,” in Pitfalls of Shoreline Stabilization, eds J. A. G. Cooper and O. H. Pilkey, (Dordrecht: Springer), 15–36. doi: 10.1007/978-94-007-4123-2_2
Small, N., Munday, M., and Durance, I. (2017). The challenge of valuing ecosystem services that have no material benefits. Glob. Environ. Change 44, 57–67. doi: 10.1016/j.gloenvcha.2017.03.005
Sørensen, J. (1978). Capacity for denitrification and reduction of nitrate to ammonia in a coastal marine sediment. Appl. Environ. Microbiol. 35, 301–305. doi: 10.1128/aem.35.2.301-305.1978
Squires, D., and Vestergaard, N. (2013). Technical change in fisheries. Mar. Policy 42, 286–292. doi: 10.1016/j.marpol.2013.03.019
Stewardson, C., Andrews, J., Ashby, C., Haddon, M., Hartmann, K., Hone, P., et al. (2018). Status of Australian Fish Stocks Reports. Canberra: Fisheries Research and Development Corporation.
Subasinghe, R., Soto, D., and Jia, J. (2009). Global aquaculture and its role in sustainable development. Rev. Aquac. 1, 2–9. doi: 10.1111/j.1753-5131.2008.01002.x
Sukhdev, P., Wittmer, H., Schröter-Schlaack, C., Nesshöver, C., Bishop, J., Brink, P. T., et al. (2010). The Economics of Ecosystems and Biodiversity: Mainstreaming the Economics of Nature: A Synthesis of the Approach, Conclusions and Recommendations of TEEB. Nairobi: UNEP.
Tengberg, A., Fredholm, S., Eliasson, I., Knez, I., Saltzman, K., and Wetterberg, O. (2012). Cultural ecosystem services provided by landscapes: assessment of heritage values and identity. Ecosyst. Serv. 2, 14–26. doi: 10.1016/j.ecoser.2012.07.006
Theuerkauf, E. J., Stephens, J. D., Ridge, J. T., Fodrie, F. J., and Rodriguez, A. B. (2015). Carbon export from fringing saltmarsh shoreline erosion overwhelms carbon storage across a critical width threshold. Estuar. Coast. Shelf Sci. 164, 367–378. doi: 10.1016/j.ecss.2015.08.001
Tuck, G. N. (2016). Stock Assessment for the Southern and Eastern Scalefish and Shark Fishery 2015. Part 1. Hobart: Australian Fisheries Management Authority.
Van der Ploeg, S., and de Groot, R. S. (2010). The TEEB Valuation Database–A Searchable Database of 1310 Estimates of Monetary Values of Ecosystem Services. Wageningen: Foundation for Sustainable Development.
Worm, B., Barbier, E. B., Beaumont, N., Duffy, J. E., Folke, C., Halpern, B. S., et al. (2006). Impacts of biodiversity loss on ocean ecosystem services. Science 314, 787–790.
Yakimov, M. M., Timmis, K. N., and Golyshin, P. N. (2007). Obligate oil-degrading marine bacteria. Curr. Opin. Biotechnol. 18, 257–266. doi: 10.1016/j.copbio.2007.04.006
Keywords: ecosystem services, value, ecological sustainable development, trade off, decision-making
Citation: Gaylard S, Waycott M and Lavery P (2020) Review of Coast and Marine Ecosystems in Temperate Australia Demonstrates a Wealth of Ecosystem Services. Front. Mar. Sci. 7:453. doi: 10.3389/fmars.2020.00453
Received: 20 November 2019; Accepted: 22 May 2020;
Published: 19 June 2020.
Edited by:
Wen-Cheng Wang, National Taiwan Normal University, TaiwanReviewed by:
Amanda G. Guthrie, College of William & Mary, United StatesCopyright © 2020 Gaylard, Waycott and Lavery. This is an open-access article distributed under the terms of the Creative Commons Attribution License (CC BY). The use, distribution or reproduction in other forums is permitted, provided the original author(s) and the copyright owner(s) are credited and that the original publication in this journal is cited, in accordance with accepted academic practice. No use, distribution or reproduction is permitted which does not comply with these terms.
*Correspondence: Sam Gaylard, c2FtLmdheWxhcmRAc2EuZ292LmF1; c2FtLmdheWxhcmRAZXBhLnNhLmdvdi5hdQ==
Disclaimer: All claims expressed in this article are solely those of the authors and do not necessarily represent those of their affiliated organizations, or those of the publisher, the editors and the reviewers. Any product that may be evaluated in this article or claim that may be made by its manufacturer is not guaranteed or endorsed by the publisher.
Research integrity at Frontiers
Learn more about the work of our research integrity team to safeguard the quality of each article we publish.