- Institute of Marine Sciences, University of Portsmouth, Portsmouth, United Kingdom
Behavior is a useful endpoint in ecotoxicological research; it links the biochemical effects of contamination with physiology of individuals, which can be applied to higher levels of organization with relevance to ecology. Animals exhibit species-specific and sex specific behaviors. Previous experiments within ecotoxicology using amphipods as models have either not separated by sex or have on the assumption that they may create more variability in the results. Therefore, the objectives of this study were to investigate the effect of time (which controlled light conditions), sex, and the interaction of time and sex on the swimming velocity in males and females of the marine amphipod Echinogammarus marinus. E. marinus exhibited a phototactic response to light, as is consistent with previous findings. It was determined that females swim significantly faster than males and that this response was enhanced during periods when the lights were switched off. This suggests that a greater understanding of the baseline unconditioned behaviors of experimental organisms is needed to avoid impacts of unknown variables on results. The results of this study reveal significant sexual differences in the baseline behavior of E. marinus which has implications for future research in ecotoxicology. It is recommended that in future research specimens be separated by sex prior to experimentation, in order to account for possible behavioral differences such as those observed in the present studies. This study also highlights the need for thorough observation of behavior over shorter time intervals as larger intervals may miss short-term variations.
Introduction
The use of behavior in environmental toxicology has expanded substantially over the past decade (Saaristo et al., 2018). However, there is a need to standardize protocols and understand baseline behaviors to improve the quality and use of these endpoints (Pyle and Ford, 2017). Behavior is defined as an organism-level reaction to a particular stimulus; the result of cumulative interactions between external conditions, biotic and abiotic factors with animal physiology and neurology (Boyd et al., 2002; Gerhardt, 2007; Hellou, 2011; Saaristo et al., 2018). Behavior should be adaptive (although in some situations can be maladaptive) and should enhance an organism’s fitness (Scott and Sloman, 2004). Further, although behavior is typically measured at an organism-level behavior, it is known to have ecological consequences at a population level (Saaristo et al., 2018).
As well as exhibiting species-typical behaviors, individuals will exhibit sex-typical behaviors (also referred to as heterotypical or sexually dimorphic) which can be physical, social and sexual, resulting from genetics, and mediated by endocrine and neural systems (Kelley, 1988; Brain and Haug, 1991; Dulac and Kimchi, 2007). Examples of this include behaviors specific to not only foraging and predator avoidance but also courtship, competition, communication, and care of offspring. Behaviors such as these allow individuals to survive, identify each other, and reproduce successfully which ultimately ensures continuation of the species (Boyd et al., 2002; Dulac and Kimchi, 2007). As a consequence, sexually dimorphic behaviors continue to be selected for (Kelley, 1988).
Behavior is intrinsic to survival and can therefore be a useful endpoint assess an animal’s condition, as it provides links between the biochemical effects of contamination and the physiology of individuals which can be extrapolated to population level effects (Scott and Sloman, 2004; Hellou, 2011). In recent times, the field of behavioral ecotoxicology has greatly expanded, with a growing appreciation of the utility of behavior as a toxicological endpoint, in parallel with advances in technology used to measure behavior (Noldus et al., 2001; Melvin and Wilson, 2013).
Amphipods such as those in the family Gammaridae have been used extensively in ecotoxicological research due to their ecological importance; they are ubiquitous in aquatic environments, cover a wide range of tropic levels (Sutcliffe, 1992: Kunz et al., 2010; Glazier, 2014; Conway, 2015), and provide an important food source for fish and birds (Schneider et al., 1994; McCurdy et al., 2005). They are easy to source and culture, and are sensitive to many pollutants, providing a useful bioindicator species (Kunz et al., 2010; Glazier, 2014). As well as this, cosmopolitan distribution allows for comparison of response to toxicants across environments. They have been used in many ecotoxicological studies using behavioral endpoints (Kunz et al., 2010), especially those looking at the effects of contaminants on phototactic response and mate-guarding (Boyd et al., 2002). Gammarid amphipods have shown sex differences in biochemical and physiological response generally and in response to contaminants or environmental change (see Lebrun et al., 2017 and references within), showing that sex is an important factor to consider in ecotoxicological research. There have been relatively fewer studies looking at sex differences in behavioral response.
That said, amphipods have day/night activity cycles (Holomuzki and Hoyle, 1990; Peeters et al., 2009; Navarro-Barranco and Hughes, 2015) and have been shown to exhibit negative phototaxis, or scototaxis, and increase their swimming velocity in response to light (Bossus et al., 2014; Kohler et al., 2018a, b). These are most likely behavioral adaptations for predator avoidance (Newman and Waters, 1984; De Robertis, 2002) and are examples of behavioral endpoints useful in ecotoxicology. As in all sexually dimorphic species they exhibit sex-specific behaviors, an example of which is precopulatory mate-guarding (or amplexus); males will grasp females dorsally using their modified gnathopods. They remain paired until external fertilization of eggs has occurred, which takes place after the female has molted due to the cuticle being flexible enough to release eggs to the brood pouch (Robinson and Doyle, 1985; Conlan, 1991; Boyd et al., 2002; Hyne, 2011). Borowsky and Aitken (1991) observed sexually dimorphic free-swimming behavior; female Ampelisca abdita were suggested to only enter the water column on the nights which they are able to fertilize eggs, in order to avoid predation during their most vulnerable period and to maximize success. Males on the other hand would enter the water column every night as they are readily available to reproduce for the majority of their molt cycle. A similar pattern was also observed in Corophium by Lawrie et al. (2000). Female amphipods also exhibit sex specific behaviors during amplexing attempts such as fleeing, remaining defensively curled or straightening the body either to encourage reproduction or when initiating a “kick” to break away from unsuitable mates (Borowsky and Borowsky, 1987; Sutcliffe, 1992, 1993; Hatcher and Dunn, 1997).
Previously, using the intertidal amphipod Echinogammarus marinus, Kohler et al., 2018a found species-specific behavioral responses to light, which was suggested to be a result of adaptation to differing predation between the habitats of the species (Kohler et al., 2018a). The authors also found that E. marinus presents different behaviors when recorded in different shape and sized recording arenas. For the present study it was speculated that there may also be sex-specific response within species as a result of predation, as predators such as shorebirds and benthic fish preferentially predate on male amphipods (Schneider et al., 1994; McCurdy et al., 2005). Differing predation pressure may be a selective force affecting amphipods, resulting in sexually dimorphic behavior. Sexual dimorphism in behaviors such as free-swimming (Borowsky and Aitken, 1991), aggregation and cover-seeking (Williams et al., 2017) appear to support this hypothesis.
As well as this, sexes may exhibit dimorphic behavioral response due to differences in physiology during their life cycle. For example, female amphipods invest more energy in oogenesis than males in spermatogenesis (Sornom et al., 2012), and undergo more physical changes than males, experiencing more molts in order to sexually mature (Sutcliffe, 1992; Hyne, 2011). Reproductive status may also affect behavior in females. A study by Williams et al. (2017) found that brooding females of Gammarus pseudolimnaeus were more likely to aggregate or seek cover in response to predator cues than males and non-brooding females, in order to avoid the greater energy cost of fleeing while carrying young. Cothran (2004) also suggested there may be greater risk for female amphipods when in an amplexing pair, compared to un-paired, to predation by size-selective fish.
Other studies have indicated there may be a difference between male and female behavioral response in amphipods, such as differences in locomotion (Ayari et al., 2015), and activity level (Peeters et al., 2009). Previous ecotoxicology experiments utilizing amphipods have been inconsistent with regard to separating by gender. Animals have either been assessed as a mixed gender sample or have been separated on the presumption that they would differ in behavior, and/or responses to stimuli. The implications of which are important for ecotoxicological research especially as variability in innate responses by separate sexes may add to the underlying variation in overall datasets. This is particularly important when behavioral data can have a high degree of intraspecies variability. Conversely, sorting sexes in small invertebrates can be time consuming. When trying to develop high-throughput testing protocols anything that reduces experiment time will minimize costs. Therefore, the objectives of this study were to compare the swimming velocity of the intertidal amphipod E. marinus stimulated by altering periods of light and dark.
Methodology
Specimen Collection and Husbandry
Echinogammarus marinus were collected in late November 2018 from Lock Lake, Langstone Harbor, Portsmouth, United Kingdom (50°47′22.8″N and 1°02′35.9″W), at low tide by searching through and under algae (Fucus vesiculosus and Ascophyllum nodosum). They were then transported to the Institute of Marine Sciences, Portsmouth, United Kingdom, separated by sex and placed in separate buckets.
The sex of individuals was determined by looking at specimens under a dissecting microscope, specifically for differences in secondary sex characteristics as detailed by Sexton and Spooner (1940). Males are generally larger than females and have enlarged gnathopods. Females have brood plates (oostigites) whilst males have genital papillae. Any specimens which appeared to be parasitized with behavioral altering trematodes were excluded from the analysis (Guler et al., 2015).
Specimens were acclimated for 1 week in a growth room set at 10 ± 1°C and a 24 h dark photoperiod in order to reduce any stress or physiological changes resulting from collection and transportation (Obernier and Baldwin, 2006) and to remove any variations in behavior that may result from biological rhythms. Amphipods are known to have both circadian and circatidal rhythms (Harris and Morgan, 1984; Holomuzki and Hoyle, 1990; Kohler et al., 2018a, b). Seawater was changed midway through the week. Animals were supplied with the algae F. vesiculosus and A. nodosum as a food source and substrate.
Analysis of Behavior
Animals were transferred from their acclimation tanks to standard six-well plates. A plastic spoon was used to transport animals in order to minimize any effect on behavior as a result of handling stress (Tran et al., 2016). Each well in the multi-well plate (diameter = 36 mm) contained seawater at a depth of 1.5 cm to limit vertical movement but still allow for free horizontal swimming. The sexes of individuals to be placed in each well were organized using a random number generator. Before trials started, it was checked and noted whether females were gravid, as their behavior may differ due to carrying eggs or offspring. As only one female was gravid, its results were omitted from the final analysis as this did not provide enough replicates to statisitcally test against males and non-gravid females.
Behavior of E. marinus was analyzed using a DanioVisionTM observation chamber following methods by Kohler et al. (2018a). The hardware consists of an infrared camera positioned above an observation chamber with a cold white light source that can be controlled via programming. There is a holder in which a petri dish or multi well plate can be secured. An external hood covers the chamber. This was connected to EthoVision® XT 11.5 video tracking software (Noldus, Netherlands) on a PC, an example of software which tracks animals using pixels resulting from frame by frame digitisation of the analog video signal, a more dependable method of recording behavior than manually (Spink et al., 2001). Experimental setup is illustrated in Figure 1. Each trial lasted 8 min, during which the light [at 50% (2000 lux)] was controlled in a dark/light cycle; off during minutes 0–2 and 4–6 and on during minutes 2–4 and 6–8. A total of 34 females and 32 males were recorded.
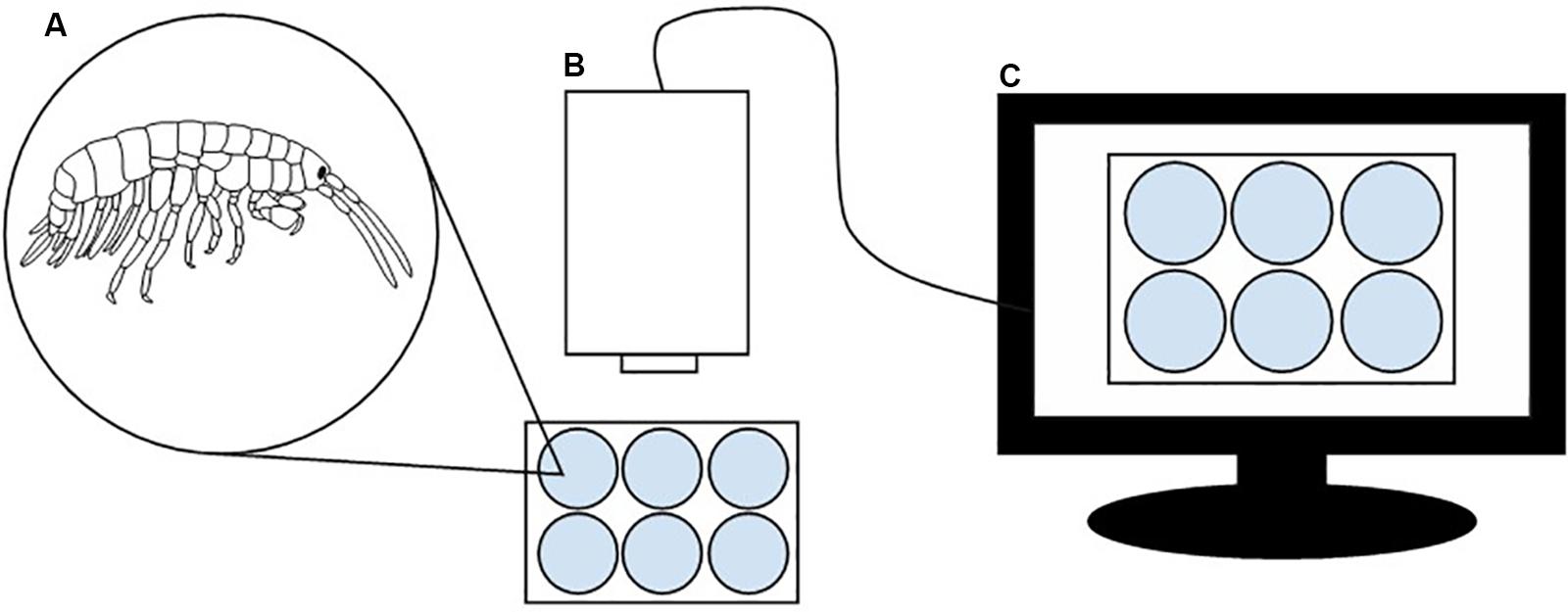
Figure 1. Experimental setup, adapted from Kohler et al. (2018a). Amphipods, one per well and in their holding seawater, in the 6-well plate (A) were tracked in the DanioVisionTM observation chamber by the infrared camera (B), which was connected to a PC with EthoVision® XT software (C).
Statistics
Statistical analysis was performed in IBM SPSS Statistics 25. Data were analyzed in 2-min time bins and 10-s time bins after being found to be normally distributed by Kolmogorov–Smirnov and Shapiro–Wilk tests. A linear mixed effects model was used for both the 2-min data and 10-s data in order to compare differences in amphipod swimming velocity for each time bin and sex and the interaction between times and sexes. Linear mixed effects models are useful in analysis of behavioral data as they are able to analyze categorical and hierarchical data with fixed and random effects and their interactions while correcting for repeated measures (Koerner and Zhang, 2017). In this experiment, fixed effects, or factors, were time, sex, and the interaction of time and sex (time∗sex). For Post Hoc analysis, independent samples t-tests were used for comparing differences in velocity between sexes at each time bin, for both the 2-min, and 10-s data, with manual Bonferroni correction to correct for type II error. One-way ANOVAs were used for both data sets to compare velocity of one sex between all the time bins, with Bonferroni tests. Data were considered significant where p-values were <0.05, except in the manual Bonferroni correction for the independent samples t-tests, where the critical P-value of 0.05 was divided by the number of replicates, 66, to give the new critical p-value of 0.00075.
Results
Females were significantly faster swimmers than males both when analyzing the 2 min and 10 s binned data (F = 20.738, df = 1, and p < 0.001 and F = 83.519, df = 1, p < 0.001, respectively; Figures 2A,B). The variance (±SE) for the random factor (specimen) was (0.007584 ± 0.006482) 2 min bins and (0.013064 ± 0.006752) for 10 s bins. Time was a significant factor in the velocity of the amphipods for 2 min and 10 s dataset with greater mean velocities typically occurring during periods of darkness (F = 7.080, df = 1, and p < 0.001 and F = 10.289, df = 1, p < 0.001, respectively; Figure 2). The overall patterns of swimming behavior can be interpreted differently when looking at the data binned into different time intervals (Figures 2A,B). Both males and females reacted to the lights coming on reaching their maximum speeds during the experiment before settling down. The extent to which females swam faster was greater during dark periods (∼65–85% faster) than light periods (∼25–35% faster) although no statistically significant interactions were observed between sex and time (p > 0.05).
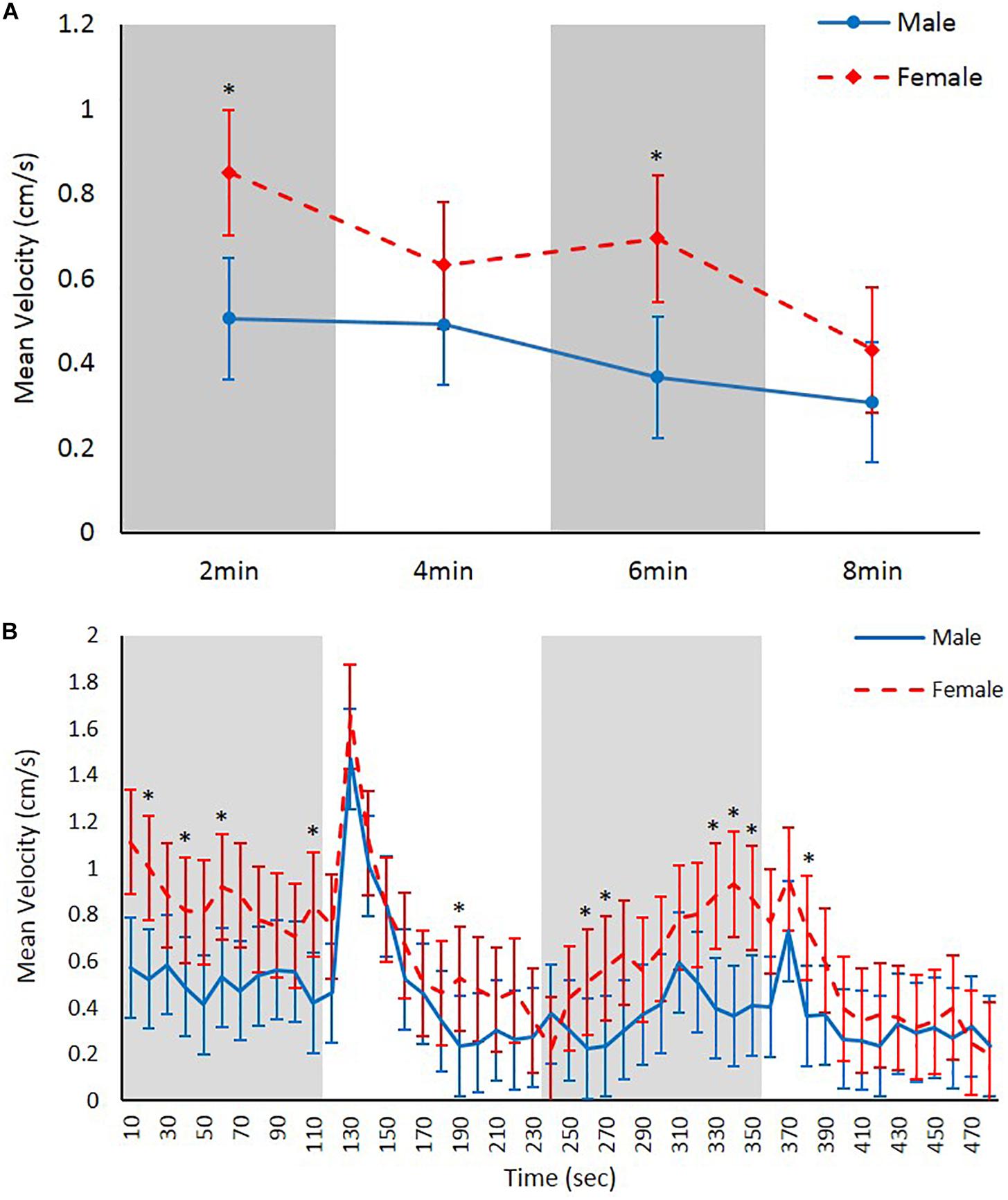
Figure 2. Mean swimming velocity (cm/s) in male and female Echinogammarus marinus recording over (A) 2 min time and (B) 10 s time bins. Error bars represent 2× SE. * ≤ 0.00075.
Discussion
To aid the development of amphipods as a model for studying behavioral toxicology, this study was conducted to determine whether male and female E. marinus have detectable differences in swimming behaviors in response to a light stimulus. Ultimately there is a trade-off between increased “noise” in a dataset if variability does exist between gender vs increased resources in the form of time by separating individuals by sex. The aim of this study was to determine whether separation by gender is useful or not in behavioral experiments with E. marinus.
Both male and female E. marinus increased their swimming velocity rapidly in response to light the light switching on but then settled down as shown previously (Bossus et al., 2014; Kohler et al., 2018a, b). However, greater mean velocities were recorded during the periods when the lights were off. Females were significantly faster than males in our experiments although looking at the 2-min and 10-s time bin data, it is possible to arrive at different conclusions. The 10-s time bins give a more detailed picture of amphipod activity throughout the trials and show there is a large range in swimming velocities. While both sets of data support our hypotheses that swimming speeds differ between the sexes the extent to which they differed appeared to vary between light stimuli, with a greater differentiation of speeds during dark vs light periods. The 10-s data demonstrated a better resolution in behavioral phenotype with reaction to the light stimulus clearly visible with both sexes but lost when pooling the datasets into 2 min bins. This highlights the importance of how the data is processed and analyzed in forming conclusions as pointed out by Melvin et al. (2017) and Kohler et al. (2018a).
Our data are in contrast to another study by Williams et al. (2017) who found males had 18% higher maximum velocity than females, as well as 44% higher average velocity in the freshwater amphipod G. pseudolimnaeus. Interestingly, this study also observed that non-brooding females had 33% higher maximum velocity than brooding females. Kohler et al. (2018a) recently found species specific behaviors in amphipods suggesting this may arise from different predatory pressures. They highlighted the caution required with cross-species extrapolations, especially in behavioral ecotoxicology when “no observable effects” may arise from low or different sensitivities in some species rather than to the stimulus.
The results of our study could also have arisen through the experimental design. Another study by Kohler et al. (2018b) found that arena size and shape had an important bearing on a variety of behavioral endpoints. Interestingly, the larger the arena the greater the velocity recorded for male freshwater Gammarus pulex suggesting for some endpoints the organisms needed “space to behave.” Females (max ∼10 mm) of amphipods are typically smaller than males (max ∼15 mm; Ford et al., 2004), whether this had a bearing on the overall speeds within the 6 well plates is currently unknown. Peeters et al. (2009) observed sexual dimorphism in activity levels of G. pulex; during the first night of a 7-day, 12:12 h (light:dark) regime, whereby males were more active than females. The authors of the study emphasized that inter-individual variation in activity must have been partially linked to sex of specimens. Ayari et al. (2015) found no significant difference in locomotor rhythm patterns of male and female amphipods (Orchestia gammarellus), a semi-terrestrial species, but also noted inter-individual variations in activity patterns. Boates et al., 1995, however, found no significant difference in crawling behavior of male and female C. volutator, an intertidal species, in response to predation by birds.
This study has established that E. marinus exhibits some differential responses to light based on sex. Females were found to have greater swimming velocity than males, which is the opposite of what has been observed in other species. Irrespective of whether this represents an interspecies difference or a difference in experimental design, the consequences in generating more variation within the datasets remains the same when considering to separate sexes prior to experiments. Therefore, going forward we recommend researchers conduct baseline studies of their model species and statistically determine intra-species variation. This could be particularly important when variation between sexes maybe greater than the variation that could be induced through e.g., exposure to contaminants leaving the observer to conclude their null hypotheses.
Data Availability Statement
All datasets generated for this study are included in the article/Supplementary Material.
Ethics Statement
This study was conducted and authorized through the University of Portsmouth Ethics Committee.
Author Contributions
AF led the project. T-RC and SK conducted the experiments. T-RC, SK, and AF helped writing and editing the manuscript.
Conflict of Interest
The authors declare that the research was conducted in the absence of any commercial or financial relationships that could be construed as a potential conflict of interest.
Supplementary Material
The Supplementary Material for this article can be found online at: https://www.frontiersin.org/articles/10.3389/fmars.2020.00370/full#supplementary-material
TABLE S1 | Data and statistics for Figure 2.
References
Ayari, A., Jelassi, R., Ghemari, C., and Nasri-Ammar, N. (2015). Effect of age, sex, and mutual interacton on the locomotor behaviour of Orchestia gammarellus in the supralittoral zone of Ghar El Melh lagoon (Bizerte, Tunisia). Biol. Rhythm Res. 46, 703–714. doi: 10.1080/09291016.2015.1048950
Boates, J. S., Forbes, M., Zinck, M., and McNeil, N. (1995). Male amphipods (Corophium volutator [Pallas]) show flexible behaviour in relation to risk of predation by sandpipers. Ecoscience 2, 123–128. doi: 10.1080/11956860.1995.11682276
Borowsky, B., and Aitken, P. (1991). Sexually dimorphic free-swimming behaviour in the amphipod crustacean Ampelisca abdita. J. Mar. Biol. Assoc. U. K. 71:3. doi: 10.1017/S0025315400053212
Borowsky, B., and Borowsky, R. (1987). The reproductive behaviors of the amphipod crustacean Gammarus palustris (Bousfield) and some insights into the nature of their stimuli. J. Exp. Mar. Biol. Ecol. 107, 131–144. doi: 10.1016/0022-0981(87)90191-2
Bossus, M. C., Guler, Y. Z., Short, S. J., Morrison, E. R., and Ford, A. T. (2014). Behavioural and transcriptional changes in the amphipod Echinogammarus marinus exposed to two antidepressants, fluoxetine and sertraline. Aquat. Toxicol. 151, 46–56. doi: 10.1016/j.aquatox.2013.11.025
Boyd, W. A., Brewer, S. K., and Williams, P. L. (2002). “Altered behavior of invertebrates living in polluted environments,” in Behavioral Ecotoxicology. Ecological & Environmental Toxicology Series, ed. G. Dell’Omo (Chichester: John Wiley & Sons Ltd), 293–336.
Brain, P. F., and Haug, M. (1991). “Are behaviours specific to animals of particular sex?,” in Heterotypical Behaviour in Man and Animals, eds P. F. Brain, M. Haug, and C. Aron (London: Chapman and Hall), doi: 10.1007/978-94-011-3078-3_1
Conlan, K. E. (1991). Precopulatory mating behaviour and sexual dimorphism in the amphipod Crustacea. Hydrobiologia 223, 255–282. doi: 10.1007/978-94-011-3542-9_22
Conway, D. V. P. (2015). “Marine zooplankton of southern Britain part 3: Ostracoda, Stomatopoda, Nebaliacea, Mysida, Amphipoda, Isopoda, Cumacea, Euphausiacea, Decapoda, Annelida, Tardigrada, Nematoda, Phoronida, Bryozoa, Entoprocta, Brachiopoda, Echinodermata, Chaetognatha, Hemichordata and Chordata,” in Marine Biological Association of the United Kingdom, No. 27, ed. A. W. G. John (Plymouth: Occasional Publications), 271. doi: 10.13140/RG.2.1.1216.2087
Cothran, R. D. (2004). Precopulatory mate guarding affects predation risk in two freshwater amphipod species. Anim. Behav. 68, 1133–1138. doi: 10.1016/j.anbehav.2003.09.021
De Robertis, A. (2002). Size-dependent visual predation risk and the timing of vertical migration: an optimization model. Limnol. Oceanogr. 47, 925–933. doi: 10.4319/lo.2002.47.4.0925
Dulac, C., and Kimchi, T. (2007). Neural mechanisms underlying sex-specific behaviors in vertebrates. Curr. Opin. Neurobiol. 17, 675–683. doi: 10.1016/j.conb.2008.01.009
Ford, A. T., Fernandes, T. F., Read, P. A., Robinson, C. D., and Davies, I. M. (2004). The costs of intersexuality: a crustacean perspective. Mar. Biol. 145, 951–957. doi: 10.1007/s00227-004-1390-y
Gerhardt, A. (2007). Aquatic behavioural ecotoxicology- prospects and limitations. Hum. Ecol. Risk Assess. 13, 481–491. doi: 10.1080/10807030701340839
Glazier, D. S. (2014). “Amphipoda”, in: Reference Module in Earth Systems and Environmental Sciences, ed. S. Elias (Waltham, MA: Elsevier), 1–49. doi: 10.1016/B978-0-12-409548-9.09437-9
Guler, Y., Short, S., Etxabe, A. G., Sherhod, C. M., Kille, P., and Ford, A. T. (2015). Impacts of a newly identified behaviour-altering trematode on its host amphipod: from the level of gene expression to population. Parasitology 142, 1469–1480. doi: 10.1017/S0031182015000918
Harris, G. J., and Morgan, E. (1984). Entrainment of the circatidal rhythm of the estuarine amphipod Corophium volutator (Pallas) to non-tidal cycles of inundation and exposure in the laboratory. J. Exp. Mar. Biol. Ecol. 80, 235–245. doi: 10.1016/0022-0981(84)90152-7
Hatcher, M. J., and Dunn, M. A. (1997). Size and pairing success in Gammarus duebeni: can females be too big? Anim. Behav. 54, 1301–1308. doi: 10.1006/anbe.1997.0534
Hellou, J. (2011). Behavioural ecotoxicology, an “early warning” signal to assess environmental quality. Environ. Sci. Pollut. Res. 18, 1–11. doi: 10.1007/s11356-010-0367-2
Holomuzki, J. R., and Hoyle, J. D. (1990). Effect of predatory fish on habitat use and diel movement of the stream amphipod, Gammarus minus. J. Freshw. Ecol. 24, 509–517. doi: 10.1111/j.1365-2427.1990.tb00728.x
Hyne, R. V. (2011). Review of the reproductive biology of amphipods and their endocrine regulation: identification of mechanistic pathways for reproductive toxicants. Environ. Toxicol. Chem. 30, 2647–2657. doi: 10.1002/etc.673
Kelley, D. B. (1988). Sexually dimorphic behaviors. Annu. Rev. Neurosci. 11, 225–251. doi: 10.1146/annurev.ne.11.030188.001301
Koerner, T. K., and Zhang, Y. (2017). Application of linear mixed-effects models in human neuroscience research: a comparison with Pearson correlation in two auditory electrophysiology studies. Brain Sci. 7:26. doi: 10.3390/brainsci7030026
Kohler, S. A., Parker, M. O., and Ford, A. T. (2018a). Species-specific behaviours in amphipods highlight the need for understanding baseline behaviours in ecotoxicology. Aquat. Toxicol. 202, 173–180. doi: 10.1016/j.aquatox.2018.07.013
Kohler, S. A., Parker, M. O., and Ford, A. T. (2018b). Shape and size of the arenas affect amphipod behaviours: implications for ecotoxicology. PeerJ 6:e5271. doi: 10.7717/peerj.5271
Kunz, P. Y., Kienle, C., and Gerhardt, A. (2010). Gammarus spp. in aquatic ecotoxicology and water quality assessment: toward integrated multilevel tests. Rev. Environ. Contam. Toxicol. 205, 1–76. doi: 10.1007/978-1-4419-5623-1_1
Lawrie, S. M., Raffaelli, D. G., and Ernes, C. H. (2000). Small-scale patterns in the distribution of the amphipod Corophium volutator on the Ythan estuary, Aberdeenshire, Scotland. Sarsia 85, 321–327. doi: 10.1080/00364827.2000.10414583
Lebrun, J. D., Uher, E., and Fechner, L. C. (2017). Behavioural and biochemical responses to metals tested alone or in mixture (Cd-Cu-Ni-Pb-Zn) in Gammarus fossarum: from a multi-biomarker approach to modelling metal mixture toxicity. Aquat. Toxicol. 193, 160–167. doi: 10.1016/j.aquatox.2017.10.018
McCurdy, D. G., Forbes, M. R., and Logan, S. P. (2005). Foraging and impacts by benthic fish on the intertidal amphipod Corophium volutator. J. Crustacean Biol. 25, 558–564. doi: 10.1651/C-2539.1
Melvin, S. D., Petit, M. A., Duvignacq, M. C., and Sumpter, J. P. (2017). Towards improved behavioural testing in aquatic toxicology: acclimation and observation times are important factors when designing behavioural tests with fish. Chemosphere 180, 430–436. doi: 10.1016/j.chemosphere.2017.04.058
Melvin, S. D., and Wilson, S. P. (2013). The utility of behavioural studies for aquatic toxicology testing: a meta-analysis. Chemosphere 93, 2217–2223. doi: 10.1016/j.chemosphere.2013.07.036
Navarro-Barranco, C., and Hughes, L. E. (2015). Effects of light pollution on the emergent fauna of shallow marine ecosystems: amphipods as a case study. Mar. Pollut. Bull. 94, 235–240. doi: 10.1016/j.marpolbul.2015.02.023
Newman, R. M., and Waters, T. F. (1984). Size-selective predation on Gammarus pseudolimnaeus by trout and sculpins. Ecology 65, 1535–1545. doi: 10.2307/1939133
Noldus, L. P. J. J., Spink, A. J., and Tegelenbosch, R. A. J. (2001). EthoVision: a versatile video tracking system for automation of behavioral experiments. Behav. Res. Methods Instrum. Comput. 33, 398–414. doi: 10.3758/bf03195394
Obernier, J. A., and Baldwin, R. L. (2006). Establishing an appropriate period of acclimatization following transportation of laboratory animals. Inst. Lab. Anim. Res. 47, 364–369. doi: 10.1093/ilar.47.4.364
Peeters, E. T. H. M., de Lange, H. J., and Lürling, M. (2009). Variation in the behaviour of the amphipod Gammarus pulex. Hum. Ecol. Risk Assess. 15, 41–52. doi: 10.1080/10807030802615055
Pyle, G., and Ford, A. T. (2017). Behaviour revised: contaminant effects on aquatic animal behaviour. Aquat. Toxicol. 182, 226–228. doi: 10.1016/j.aquatox.2016.11.008
Robinson, B. W., and Doyle, R. W. (1985). Trade-off between male reproduction (Amplexus) and growth in the amphipod Gammarus lawrencianus. Biol. Bull. 168, 482–488.
Saaristo, M., Brodin, T., Balshine, S., Bertram, M. G., Brooks, B. W., Ehlman, S. M., et al. (2018). Direct and indirect effects of chemical contaminants on the behaviour, ecology and evolution of wildlife. Proc. R. Soc. B Biol. Sci. 285:20181297. doi: 10.1098/rspb.2018.1297
Schneider, S. D., Boates, J. S., and Forbes, M. (1994). Sex ratios of Corophium volutator (Pallas) (Crustacea: Amphipoda) in Bay of Fundy populations. Can. J. Zool. 72, 1915–1921. doi: 10.1139/z94-260
Scott, G. R., and Sloman, K. A. (2004). The effects of environmental pollutants on complex fish behaviour: integrating behavioural and physiological indicators of toxicity. Aquat. Toxicol. 68, 369–392. doi: 10.1016/j.aquatox.2004.03.016
Sexton, E. W., and Spooner, G. M. (1940). An account of Marinogammarus marinus (Schellenberg) Gen. Nov. (Amphipoda), with a description of a new species, M. pirloti. J. Mar. Biol. Assoc. U. K. 24, 633–682. doi: 10.1017/s0025315400045501
Sornom, P., Gismondi, E., Vellinger, C., Devin, S., Férard, J. F., and Beisel, J. N. (2012). Effects of sublethal cadmium exposure on antipredator behavioural and antitoxic responses in the invasive amphipod Dikerogammarus villosus. PLoS One 7:e42435. doi: 10.1371/journal.pone.0042435
Spink, A. J., Tegelenbosch, R. A. J., Buma, M. O. S., and Noldus, L. P. J. J. (2001). The EthoVision video tracking system- A tool for behavioural phenotyping of transgenic mice. Physiol. Behav. 73, 731–744. doi: 10.1016/s0031-9384(01)00530-3
Sutcliffe, D. W. (1992). Reproduction in Gammarus (Crustacea, Amphipoda) basic processes. Freshw. Forum 2, 102–128.
Sutcliffe, D. W. (1993). Reproduction in Gammarus (Crustacea, Amphipoda): female strategies. Freshw. Forum 3, 27–64.
Tran, S., Nowicki, M., Fulcher, N., Chatterjee, D., and Gerlai, R. (2016). Interaction between handling stress and anxiolytic effects of ethanol in zebrafish: a behavioural and neurochemical analysis. Behav. Brain Res. 298, 278–285. doi: 10.1016/j.bbr.2015.10.061
Keywords: ecotoxicology, behavior, aquatic toxicology, Amphipoda, crustacea
Citation: Cherry TR, Kohler SA and Ford AT (2020) Sex Specific Differences Recorded in the Behavior of an Amphipod: Implications for Behavioral Toxicology. Front. Mar. Sci. 7:370. doi: 10.3389/fmars.2020.00370
Received: 08 November 2019; Accepted: 30 April 2020;
Published: 11 June 2020.
Edited by:
Punyasloke Bhadury, Indian Institute of Science Education and Research Kolkata, IndiaReviewed by:
Marie-jeanne Perrot-minnot, Université de Bourgogne, FranceJake Mitchell Martin, Monash University, Australia
Copyright © 2020 Cherry, Kohler and Ford. This is an open-access article distributed under the terms of the Creative Commons Attribution License (CC BY). The use, distribution or reproduction in other forums is permitted, provided the original author(s) and the copyright owner(s) are credited and that the original publication in this journal is cited, in accordance with accepted academic practice. No use, distribution or reproduction is permitted which does not comply with these terms.
*Correspondence: Alex T. Ford, YWxleC5mb3JkQHBvcnQuYWMudWs=
†These authors have contributed equally to this work