- 1Florida Fish Wildlife Conservation Commission, Florida Fish and Wildlife Research Institute, St. Petersburg, FL, United States
- 2School of Marine and Atmospheric Sciences, Stony Brook University, Southampton, NY, United States
- 3Dauphin Island Sea Lab, University of South Alabama, Dauphin Island, AL, United States
Introduction
Today, the best-developed coral reefs in the continental United States occur in the Florida Reef tract along some 580 km from the Dry Tortugas to the southeast coast of Florida between 24° and 26° N (Figure 1A; Morey et al., 2017; Cummings et al., 2018). These long-lived communities encrust and have built upon earlier structures deposited during the Pleistocene and Holocene epochs (Shinn and Lidz, 2018). Together, they represent the only barrier reef system in North America, and as such, have received management protection as part of the Florida Keys National Marine Sanctuary (FKNMS) since 1990. Along Florida's west coast, however, examples of actively accreting reef communities are comparatively rare, being restricted to the mesophotic reefs (40–150 m depth) of Pulley Ridge, located to the west-northwest of the FKNMS (Reed et al., 2019), and the shallower, euphotic reefs of the Florida Middle Grounds (25–45 m depth), located further north along the West Florida Shelf (WFS) at 28° N (Jaap, 2015). To a large extent, these west coast Florida ecosystems occur where they do because of warm-water delivery by the Loop Current, which brings oligotrophic water from the Caribbean into the Gulf of Mexico (GoM) (Cummings et al., 2018). Outside of this influence, actively accreting reef communities give way to more sparsely populated live bottom, characterized by cold-tolerant scleractinian and soft corals, sponges, hydrozoans, barnacles, seagrasses, and macroalgae (Jaap, 2015; Morey et al., 2017).
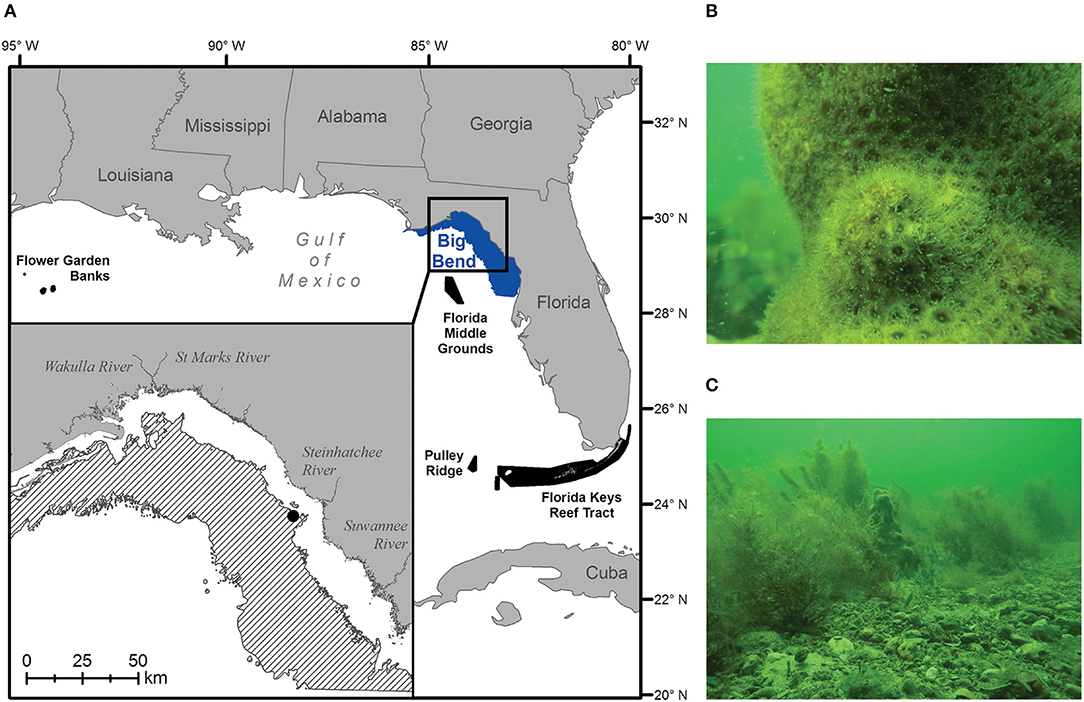
Figure 1. The 0–20 m (A: blue polygon) and 5.5–18 m (A inset: black diagonal bars) depth bands of Big Bend region of Florida, USA in the northern Gulf of Mexico. Black polygons denote potential larval sources of hermatypic coral species to the area—in decreasing order of importance: the Florida Middle Grounds, Pulley Ridge, Florida Keys Reef Tract, and the Flower Garden Banks. Scenes of current hard-bottom communities dominated by (B) cold-tolerant scleractinian corals (shown: Solenastrea sp.), and (C) soft corals and attached macroalgae (shown: Sargassum sp. and Caulerpa spp.), were taken at 6.7 m depth off Steinhatchee, Florida on April 13, 2018 (A inset; black circle).
In the region of the WFS known as “The Big Bend,” the carbonate platform is covered by a thin veneer of shifting sand, expansive seagrass meadows and abundant hard bottom (Continental Shelf Associates, Inc. and Martel Laboratories, Inc., 1985). Here, low relief rocky outcrops (~1 m) support cold-hardy corals in the genera Oculina, Siderastrea, Solenastrea, and Cladocora (Figure 1B). These non-accreting species achieve limited densities, tend to recruit and grow slowly, and are limited by cold winter temperatures, sand burial, and competition with benthic macroalgae (i.e., Sargassum; Beger et al., 2014; Tuckett et al., 2017; Figure 1C).
Tropicalization
Regional environmental conditions in the Big Bend are dominated by atmospheric forcing and watershed input, as the wide continental shelf tends to limit oceanic upwelling, waves and tides, and seasonal discharge from the Apalachicola and other rivers delivers vast freshwater plumes high in chromophoric dissolved organic matter (CDOM), nutrients, tannins and chlorophyll a (Morey et al., 2017). Presently, the subtropical latitude and frequency of winter cold fronts appear to be keeping winter minimum temperatures below the 18°C threshold critical to coral reef development (Buddemeier et al., 2004; Precht and Aronson, 2004). However, temperature minimums are becoming less severe along the northern coast of the GoM as reflected by the expansion of black mangroves into salt marsh habitats of the northern Gulf (Osland et al., 2013). Similarly, formerly rare species of tropical snappers (Lutjanidae) and parrot fishes (Labridae) are now commonly found in shallow seagrass meadows of the northern Gulf (Fodrie et al., 2010). In addition, coral species, such as Acropora palmata, have been recorded in the Flower Gardens reefs of the northwestern GoM (Precht and Aronson, 2004) at a latitude and depth previously unknown. Together, the occurrence of these taxa reflects the tropicalization of the GoM (Vergés et al., 2014; Heck et al., 2015). Tropicalization, a process by which species formerly restricted to tropical waters expand their ranges and become resident in temperate waters is a global phenomenon that follows the warming of the world's oceans (Vergés et al., 2014; Hyndes et al., 2016). This phenomenon is exemplified by global changes in coral distributions, in which coral recruitment is shifting poleward at the same time it is declining in equatorial regions (Price et al., 2019).
GoM Climate Change Predictions
Gulf surface water temperatures are predicted to rise by more than 2°C by the end of this century—this despite a 20–25% reduction in the Loop Current (Liu et al., 2015; Morey et al., 2017). In the Big Bend Area, where the shallow shelf restricts ocean mixing, the warming is expected to be particularly severe, with SST increases of nearly 4°C during the summer and fall (Liu et al., 2015). Moreover, increased frequency of drought conditions in the American Southeast (Pederson et al., 2012) coupled with groundwater withdrawal by growing human populations in the Suwannee River and adjacent basins will likely lead to reduced discharge of relatively cold water from springs and rivers (Hensley and Cohen, 2017), further alleviating cold stress to nearshore environments.
Our Prediction
It now seems reasonable to expect that the extensive hard bottom habitats in the Big Bend region of Florida will come to support the development of coral reefs in the near future. We make this assertion for a number of reasons: (1) abundant substrate is available in the form of the largest carbonate platform in the region (Morey et al., 2017), (2) low-relief outcroppings are particularly abundant in the 10–20 m isobath (Phillips et al., 1990) where light and temperature will likely be conducive for reef accretion, (3) areas of the WFS influenced by the warm Loop Current waters currently support hermatypic reefs, and (4) extant reef systems offer a supply of potential recruits. Seasonal wind patterns (Morey et al., 2003, 2017; Todd et al., 2014) and simulated drifter experiments (Johnson et al., 2017) suggest periodic connectivity to the Florida Middle Grounds, FKNMS and to a lesser extent the Flower Garden Banks, a luxuriant reef system in the northwestern GoM (Figure 1A). Thus, reefs should be seeded by a supply of coral larvae spawned by the massive and plating corals (genera: Orbicella, Agaricia, and Leptoseris) and the branching species (Acropora spp.). The extent to which these species are presently delivering ill-fated propagules to the Big Bend Area is unknown, representing a critical knowledge gap in our understanding of potential range expansion in the region.
Species Interactions, Landscape Ecology, and The Challenges of Climate Change
Dispersal and autecology, however, are not the only factors constraining tropicalization of high-latitude reefs (Hoey et al., 2011; Beger et al., 2014; Tuckett et al., 2017). As recruits of tropical species begin to colonize the benthic seascape, their success will be influenced by competitive interactions among biogenic engineers and associated top-down controls. Temperate reefs dominated by persistent or ephemeral attached macroalgae and predominantly invertebrate (e.g., urchin) grazer guilds need to give way to low-algal, high-coral assemblages grazed by fishes (Beger et al., 2014). We argue that as temperature-mediated physiological constraints yield to climate change, urchin grazing rates will increase, recruiting herbivorous tropical fishes will facilitate coral settlement by grazing on resident seaweeds (Munday et al., 2008) and coral-dependent fishes will readily emigrate to nascent reefs as structural complexity increases. This succession will be aided by a number of mitigating factors, including: (1) macroalgal susceptibility to marine heat waves, which should become more common under future climate scenarios (Tuckett et al., 2017), (2) current overwintering populations of tropical herbivorous fishes (Fodrie et al., 2010; Prado and Heck, 2011), and (3) proximity of Big Bend outcroppings to typical nursery habitat, including seagrasses and mangroves. Indeed, the re-assembly of tropical seascapes in the Big Bend Area has been underway for some time, with accreting coral reefs representing possibly the last component of a migrating landscape.
We acknowledge that climate change will bring with it more than ameliorated thermal regimes. As others have noted, predictions of range expansion based on simple habitat projections can be flawed as they fail to consider the interactive effects of acidification, disease and unprecedented rate of environmental change (Pandolfi, 2011). Here, we argue that the Big Bend seascape may facilitate coral range expansion in the face of multiple stressors. For example, regarding acidification, which has been shown to be a significant threat to reef accretion, we anticipate the immense seagrass meadows surrounding the ledges and outcrops of hard substrate in the Big Bend (Continental Shelf Associates and Martel Laboratories, 1985; Iverson and Bittaker, 1986; Carlson et al., 2016; Yarbro et al., 2016) will provide a useful buffering capacity reducing the threat of acidification to coral larvae and recently settled coral recruits. These vast seagrass meadows will become increasingly autotrophic (Zimmerman et al., 1997; Palacios and Zimmerman, 2007) and that net carbon uptake within seagrass canopies will result in a localized drawdown of DIC, increasing oxygen levels, pH and the aragonite saturation state (Ωaragonite), while reducing the total CO2, effectively buffering against acidification (Manzello et al., 2012; Unsworth et al., 2012; Hendriks et al., 2013). Analyzing the effect of this seagrass mediated drawdown, Unsworth et al. (2012) determined an 18% increase in calcification of corals in the presence of seagrass. Climate fluctuations are frequently associated with disease outbreaks, either due to increased virulence of existing pathogens or the emergence and spread of novel strains to naïve populations. The degree to which climate change has underlain the recent pandemic of Stony Coral Tissue Loss (SCTL) disease in the FKNMS is unclear (Walton et al., 2018), but the prediction that future coral range expansion will need to overcome population losses due to disease appears sound. Here again, the Big Bend Area is unique, as its primary larval sources (Florida Middle Grounds) have not yet been impacted by the pandemic and its geographic isolation from the FKMS might impede transmission via a water-borne pathogen.
Modern Examples
While we are not alone in our prediction that corals will move poleward in response to climate change (Precht and Aronson, 2004; Greenstein and Pandolfi, 2008; Beger et al., 2014, but see Hoegh-Guldberg, 2005), we are the first to suggest a refuge for reef building species in the Big Bend Area of Florida, USA. This assertion is not without modern example as others have reported range shifts for corals during the most recent period of warming (i.e., 20–100 ybp). Precht and Aronson (2004) described species of Acropora beyond its historical northern limit in GoM in 2002 and Vargas-Ángel et al. (2003) found thickets of A. palmata along Florida's Atlantic coast in 1998 as far north as Ft. Lauderdale (26° N). We know that corals can proliferate and spread when conditions are conducive, as was seen following the introduction of the Indo-Pacific coral, Tubastrea coccinea, to the western Atlantic in the 1940s (Fenner, 2001) and Oculina patagonica to the Mediterranean Sea in 1966 (Serrano et al., 2013; Tuckett et al., 2017). Poleward shifts in native species also have been noted from Australia's east coast and South Korea (Tuckett et al., 2017). Importantly, there is mounting evidence that range extensions can be associated with phase shifts from temperate macroalgal communities to tropical coral reefs. Strong evidence for this has recently emerged from Australia's west coast (Tuckett et al., 2017) and from Japan (Yamano et al., 2011).
Paleo Examples
Modern examples mirror evidence from the paleo-record, which largely supports the controlling role that temperature plays in setting geographic limits on coral reef development (Kiessling et al., 2012). Notable range expansions, variously associated with equatorial contractions, are believed to have occurred during the Pleistocene (Pandolfi, 2011), Holocene (Kiessling et al., 2012), and Last Interglacial (Fields et al., 1993). From these, it is clear that subtropical reefs have repeatedly served as thermal refugia, and that range expansion and community reassembly has occurred repeatedly in response to climate change (Beger et al., 2014).
Management Implications
Will coral reef development occur along the WFS? As described above there are a number of factors that make coral reef development in the Big Bend Area of Florida possible over the next 30–100 years. At present, benthic monitoring programs in the region are insufficient and unable to capture such changes. To do so, more intensive work will be needed at the 5–15 m depth contours where coral recruitment will likely first be seen. Therefore, to better understand the interplay between thermal regime, larval supply and range expansions we recommend that monitoring of coral settlement (by deploying settlement tiles) and adult colonies (by benthic surveys) be coupled with detailed physicochemical monitoring of bottom-water conditions.
Throughout their geologic history coral reefs have proven to be resilient. Despite the substantial challenges of human-mediated climate change, we expect coral communities to reorganize and reassemble in a number of locations globally. Each occurrence of this process offers an opportunity to better understand how such iconic ecosystems achieved their complexity. Beyond academic inquiry, we argue that conversation should begin now regarding what active management measures could be implemented in tandem with coral reef range expansion in the GoM. For example, current paradigms do not yet include provisions for pro-active Marine Protected Area designation to secure locations for thermal refugia, or for “assisted migration,” where connectivity constraints would be overcome by active transplant of corals; however, such measures could prove essential to the long-term viability of Florida coral reefs (cf. Vergés et al., 2019).
Author Contributions
KH conceived, and BF and BP helped to develop, the central argument. BF prepared the figure. BF, BP, and KH contributed sections to the manuscript. All authors have revised, read, and approved the submitted version.
Funding
This work was conducted without explicit funding.
Conflict of Interest
The authors declare that the research was conducted in the absence of any commercial or financial relationships that could be construed as a potential conflict of interest.
Acknowledgments
We would like to thank Dottie Byron, Rob Ruzicka, Robin Jung, and Dr. Andrew Altieri for assistance in the field and for fruitful conversation, Drs. Kara Radabaugh and Penny Hall for critical reviews of early drafts, and Dauphin Island Sea Lab, Stony Brook's School of Marine and Atmospheric Sciences and the Florida Fish and Wildlife Research Institute for logistical support.
References
Beger, M., Sommer, B., Harrison, P. L., Smith, S. D., and Pandolfi, J. M. (2014). Conserving potential coral reef refuges at high latitudes. Divers. Distr. 20, 245–257. doi: 10.1111/ddi.12140
Buddemeier, R. W., Baker, A. C., Fautin, D. G., and Jacobs, J. R. (2004). “The adaptive hypothesis of bleaching,” in Coral Health and Disease, eds E. Rosenberg and Y. Loya (Berlin, Heidelberg; Springer), 427–444. doi: 10.1007/978-3-662-06414-6_24
Carlson, P. R., Yarbro, L. A., Jones, T. W., Brucker, J., Letendre, J., and Kebart, K. (2016). Summary Report for the Northern Big Bend Region. St. Petersburg, FL: Florida Fish and Wildlife ConservationCommission.
Continental Shelf Associates, Inc., and Martel Laboratories, Inc. (1985). Florida Big Bend Seagrass Habitat Study Narrative Report. Metairie, LA.
Cummings, K. E., Ruzicka, R. R., Semon-Lunz, K., Brenner, J., Goodin, K. L., and Ames, K. W. (2018). “Ecological resilience indicators for coral ecosystems,” in Ecological Resilience Indicators for Five Northern Gulf of Mexico Ecosystems, eds K. L. Goodin, D. Faber-Langendoen, J. Breener, S. T. Allen, R. H. Day, V. M. Congdon, C. Shepard, K. E. Cummings, C. L. Stagg, C. A. Gabler, M. Osland, K. H. Dunton, R. R. Ruzicka, K. Semon-Lunz, D. Reed, and M. Love (Arlington, VA: NatureServe), 71.
Fenner, D. (2001). Biogeography of three Caribbean corals (Scleractinia) and the invasion of Tubastraea coccinea into the Gulf of Mexico. Bull. Mar. Sci. 69, 1175–1189. Available online at: https://www.ingentaconnect.com/contentone/umrsmas/bullmar/2001/00000069/00000003/art00010
Fields, P., Graham, J., Rosenblatt, R., and Somero, G. (1993). Effects of expected global climate change on marine faunas. Trends Ecol. Evol. 8, 361–367. doi: 10.1016/0169-5347(93)90220-J
Fodrie, F. J., Heck, K. L. Jr, Powers, S. P., Graham, W. M., and Robinson, K. L. (2010). Climate-related, decadal-scale assemblage changes of seagrass-associated fishes in the northern Gulf of Mexico. Global Change Biol. 16, 48–59. doi: 10.1111/j.1365-2486.2009.01889.x
Greenstein, B. J., and Pandolfi, J. M. (2008). Escaping the heat: range shifts of reef coral taxa in coastal Western Australia. Global Change Biol. 14, 513–528. doi: 10.1111/j.1365-2486.2007.01506.x
Heck, K. Jr., Fodrie, F., Madsen, S., Baillie, C., and Byron, D. (2015). Seagrass consumption by native and a tropically associated fish species: potential impacts of the tropicalization of the northern Gulf of Mexico. Mar. Ecol. Progr. Ser. 520, 165–173. doi: 10.3354/meps11104
Hendriks, I. E., Olsen, Y. S., Ramajo, L., Basso, L., Steckbauer, A., Moore, T. S., et al. (2013). Photosynthetic activity buffers ocean acidification in seagrass meadows. Biogeosci. Discuss. 10, 12313–12346. doi: 10.5194/bgd-10-12313-2013
Hensley, R. T., and Cohen, M. J. (2017). Flow reversals as a driver of ecosystem transition in Florida's springs. Freshwater Sci. 36, 14–25. doi: 10.1086/690558
Hoegh-Guldberg, O. (2005). Low coral cover in a high-CO2 world. J. Geophys. Res. 110:C09S06. doi: 10.1029/2004JC002528
Hoey, A. S., Pratchett, M. S., and Cvitanovic, C. (2011). High macroalgal cover and low coral recruitment undermines the potential resilience of the world's southernmost coral reef assemblages. PLoS ONE 6:e25824. doi: 10.1371/journal.pone.0025824
Hyndes, G. A., Heck, K. L. Jr., Vergés, A., Harvey, E. S., Kendrick, G. A., Lavery, P. S., et al. (2016). Accelerating tropicalization and the transformation of temperate seagrass meadows. Bioscience 66, 938–948. doi: 10.1093/biosci/biw111
Iverson, R. L., and Bittaker, H. F. (1986). Seagrass distribution and abundance in eastern Gulf of Mexico coastal waters. Estuarine Coastal Shelf Sci. 22, 577–602. doi: 10.1016/0272-7714(86)90015-6
Jaap, W. C. (2015). Stony coral (Milleporidae and Scleractinia) communities in the eastern Gulf of Mexico: a synopsis with insights from the Hourglass collections. Bull. Mar. Sci. 91, 207–253. doi: 10.5343/bms.2014.1049
Johnson, D. R., Perry, H., Sanchez-Rubio, G., and Grace, M. A. (2017). Loop current spin-off eddies, slope currents and dispersal of reef fish larvae from the flower gardens National Marine Sanctuary and the Florida middle grounds. Gulf Caribbean Res. 28, 29–39. doi: 10.18785/gcr.2801.10
Kiessling, W., Simpson, C., Beck, B., Mewis, H., and Pandolfi, J. M. (2012). Equatorial decline of reef corals during the last Pleistocene interglacial. Proc. Natl. Acad. Sci. U.S.A. 109, 21378–21383. doi: 10.1073/pnas.1214037110
Liu, Y., Lee, S. K., Enfield, D. B., Muhling, B. A., Lamkin, J. T., Muller-Karger, F. E., et al. (2015). Potential impact of climate change on the Intra-Americas Sea: part-1. A dynamic downscaling of the CMIP5 model projections. J. Mar. Syst. 148, 56–69. doi: 10.1016/j.jmarsys.2015.01.007
Manzello, D. P., Enochs, I. C., Melo, N., Gledhill, D. K., and Johns, E. M. (2012). Ocean acidification refugia of the Florida Reef Tract. PLoS ONE 7:e41715. doi: 10.1371/journal.pone.0041715
Morey, S., Koch, M., Liu, Y., and Lee, S.-K. (2017). “Florida's oceans and marine habitats in a changing climate,” in Florida's climate: Changes, variations, & impacts, eds E. P. Chassignet, J. W. Jones, V. Misra, and J. Obeysekera (Gainesville, FL: Florida Climate Institute), 391–425. doi: 10.17125/fci2017.ch13
Morey, S. L., Martin, P. J., O'Brien, J. J., Wallcraft, A. A., and Zavala Hidalgo, J. (2003). Export pathways for river discharged fresh water in the northern Gulf of Mexico. J Geophys Res. 108, 3303–3317. doi: 10.1029/2002JC001674
Munday, P. L., Jones, G. P., Pratchett, M. S., and Williams, A. J. (2008). Climate change and the future for coral reef fishes. Fish Fisher. 9, 261–285. doi: 10.1111/j.1467-2979.2008.00281.x
Osland, M. J., Enwright, N., Day, R. H., and Doyle, T. W. (2013). Winter climate change and coastal wetland foundation species: salt marshes vs. mangrove forests in the southeastern United States. Global Change Biol. 19, 1482–1494. doi: 10.1111/gcb.12126
Palacios, S. L., and Zimmerman, R. C. (2007). Response of eelgrass Zostera marina to CO2 enrichment: possible impacts of climate change and potential for remediation of coastal habitats. Mar. Ecol. Progr. Ser. 344, 1–13. doi: 10.3354/meps07084
Pandolfi, J. M. (2011). “The paleoecology of coral reefs,” in Coral Reefs: An Ecosystem in Transition, eds Z. Dubinsky and N. Stambler (Dordrecht: Springer), 13–24. doi: 10.1007/978-94-007-0114-4_2
Pederson, N., Bell, A. R., Knight, T. A., Leland, C., Malcomb, N., Anchukaitis, K. J., et al. (2012). A long-term perspective on a modern drought in the American Southeast. Environ. Res. Lett. 7:014034. doi: 10.1088/1748-9326/7/1/014034
Phillips, N. W., Gettleson, D. A., and Spring, K. D. (1990). Benthic biological studies of the southwest Florida shelf. Am. Zool. 30, 65–75. doi: 10.1093/icb/30.1.65
Prado, P., and Heck, K. L. Jr. (2011). Seagrass selection by omnivorous and herbivorous consumers: determining factors. Mar. Ecol. Progr. Ser. 429, 45–55. doi: 10.3354/meps09076
Precht, W. F., and Aronson, R. B. (2004). Climate flickers and range shifts of reef corals. Front. Ecol. Environ. 2, 307–314. doi: 10.1890/1540-9295(2004)002[0307:CFARSO]2.0.CO;2
Price, N., Muko, S., Legendre, L., Steneck, R., van Oppen, M., Albright, R., et al. (2019). Global biogeography of coral recruitment: tropical decline and subtropical increase. Mar. Ecol. Progr. Ser. 621, 1–17. doi: 10.3354/meps12980
Reed, J. K., Farrington, S., David, A., Harter, S., Pomponi, S. A., Cristina Diaz, M., et al. (2019). “Pulley Ridge, Gulf of Mexico, USA,” in Mesophotic Coral Ecosystems, eds Y. K. Loya, A. Puglise, and T. C. L. Bridge (Cham: Springer International Publishing), 57–69. doi: 10.1007/978-3-319-92735-0_4
Serrano, E., Coma, R., Ribes, M., Weitzmann, B., García, M., and Ballesteros, E. (2013). Rapid northward spread of a zooxanthellae coral enhanced by artificial structures and sea warming in the western Mediterranean. PLoS ONE 8:e52739. doi: 10.1371/journal.pone.0052739
Shinn, E. A., and Lidz, B. H. (2018). Geology of the Florida Keys. (Gainesville, FL: University Press of Florida).
Todd, A. C., Morey, S. L., and Chassignet, E. P. (2014). Circulation and cross-shelf transport in the Florida Big Bend. J. Mar. Res. 72, 445–475. doi: 10.1357/002224014815540660
Tuckett, C., de Bettignies, T., Fromont, J., and Wernberg, T. (2017). Expansion of corals on temperate reefs: direct and indirect effects of marine heatwaves. Coral Reefs 36, 947–956. doi: 10.1007/s00338-017-1586-5
Unsworth, R. K., Collier, C. J., Henderson, G. M., and McKenzie, L. J. (2012). Tropical seagrass meadows modify seawater carbon chemistry: implications for coral reefs impacted by ocean acidification. Environ. Res. Lett. 7:024026. doi: 10.1088/1748-9326/7/2/024026
Vargas-Ángel, B., Thomas, J. D., and Hoke, S. M. (2003). High-latitude Acropora cervicornis thickets off Fort Lauderdale, Florida, USA. Coral Reefs 22, 465–473. doi: 10.1007/s00338-003-0336-z
Vergés, A., McCosker, E., Mayer-Pinto, M., Coleman, M. A., Wernberg, T., Ainsworth, T., et al. (2019). Tropicalisation of temperate reefs: implications for ecosystem functions and management actions. Function. Ecol. 33, 1000–1013. doi: 10.1111/1365-2435.13310
Vergés, A., Steinberg, P. D., Hay, M. E., Poore, A. G., Campbell, A. H., Ballesteros, E., et al. (2014). The tropicalization of temperate marine ecosystems: climate-mediated changes in herbivory and community phase shifts. Proc. R. Soc. B 281:20140846. doi: 10.1098/rspb.2014.0846
Walton, C., Hayes, N. K., and Gilliam, D. S. (2018). Impacts of a regional, multi-year, multi-species coral disease outbreak in Southeast Florida. Front. Mar. Sci. 5:323. doi: 10.3389/fmars.2018.00323
Yamano, H., Sugihara, K., and Nomura, K. (2011). Rapid poleward range expansion of tropical reef corals in response to rising sea surface temperatures. Geophys. Res. Lett. 38:L04601. doi: 10.1029/2010GL046474
Yarbro, L. A., Carlson, P. R., Jones, T., Brucker, J., and Letendre, J. (2016). Summary Report for the Southern Big Bend Region. St. Petersburg, FL: Florida Fish and Wildlife Conservation Commission.
Keywords: thermal refugia, assisted migration, acidification, climate change, range expansion
Citation: Furman BT, Peterson BJ and Heck KL Jr (2020) Will the Florida Big Bend Area Become the Next Gulf of Mexico Reef Tract? Front. Mar. Sci. 7:334. doi: 10.3389/fmars.2020.00334
Received: 15 July 2019; Accepted: 22 April 2020;
Published: 19 May 2020.
Edited by:
Joshua D. Voss, Florida Atlantic University, United StatesReviewed by:
Aldo Cróquer, Simón Bolívar University, VenezuelaCharles Alan Jacoby, St. Johns River Water Management District, United States
Copyright © 2020 Furman, Peterson and Heck. This is an open-access article distributed under the terms of the Creative Commons Attribution License (CC BY). The use, distribution or reproduction in other forums is permitted, provided the original author(s) and the copyright owner(s) are credited and that the original publication in this journal is cited, in accordance with accepted academic practice. No use, distribution or reproduction is permitted which does not comply with these terms.
*Correspondence: Bradley T. Furman, brad.furman@myfwc.com