- HGF-MPG Group for Deep-Sea Ecology and Technology, Alfred-Wegener-Institut Helmholtz-Zentrum für Polar- und Meeresforschung, Bremerhaven, Germany
The deep sea is a major sink for debris; however, temporal changes and underlying mechanisms of litter accumulation on the seafloor remain unclear. Photographic surveys at the long-term ecological research (LTER) observatory HAUSGARTEN, in the eastern Fram Strait, have enabled the assessment of spatial and temporal variability of seafloor litter in the Arctic. Previous studies of time-series data (2002–2014) reported an increase in litter quantities from the northernmost and central stations. Here, we extended the analysis by three years until 2017 and included data from the southernmost station. A total of 16,157 images covering 60.5 km2 were analyzed and combined with previous studies, to determine litter density, type and size compositions. Moreover, the interaction of litter with epibenthic megafauna was evaluated. Indicators of local maritime traffic, fisheries activity and summer sea ice extent were examined as potential drivers. The mean annual litter density ranged between 813 ± 525 (SEM) and 6,717 ± 2,044 (SEM) items km–2. Litter density clearly increased over time, and the northernmost station experienced the strongest increase. Plastics dominated at two of the stations whereas the northern station harbored mainly glass. Small-sized items accounted for 63%. Interaction with epibenthic fauna was frequent, especially with sessile organisms. Litter densities correlated with fishing and tourism vessel abundance, but no correlation was found with summer sea ice extent. This 15-year record of marine litter shows that even secluded Arctic ecosystems become increasingly subject to plastic pollution and that it will likely continue in the face of growing global plastic production rates and ineffective waste management policies.
Introduction
Marine litter is a global problem whose lasting effects are unlikely to vanish since the leakage of debris into the oceans will likely continue to increase. Plastic accounts for ∼73% of marine debris globally (Bergmann et al., 2017b), and it has been estimated that every year ∼8 million tons of plastic waste enter the oceans from land (Jambeck et al., 2015), approximately two of which come from rivers (Lebreton et al., 2017). The global input from sea-based sources is currently unknown but may be substantial given local estimates of 46 and 99% in the North Pacific and Arctic, respectively (Bergmann et al., 2017a; Lebreton et al., 2018). Plastic pollution continues to increase (Ostle et al., 2019) along with its production, which reached 359 million tons y–1 in 2018 (PlasticsEurope, 2019) and will continue to grow given industry plans to expand production significantly as a byproduct of the shale gas boom. Plastic debris has spread to all ocean compartments, including coastlines, sea ice, surface waters, the water column, seafloor, and biota (Law, 2017). However, surface waters and coastlines have received most attention (Basurko et al., 2015; Suaria et al., 2016; Arcangeli et al., 2018; Goddijn-Murphy et al., 2018) because of their accessibility and the obviousness of deleterious effects on their fauna. While the main sources of litter have been identified (waste production on land, leakage from landfills, littering behavior, discharges from vessels and offshore platforms, derelict fishing gear) (Ryan, 2015) its ultimate fate in the ocean remains unclear. The amount of plastic debris entering the oceans does not match the estimates of global budgets by a missing fraction of 99% (van Sebille et al., 2015). Accumulation areas, hidden sinks and retention of debris are driven by hydrography and geomorphology (Schlining et al., 2013) that point to the deep ocean as a sink for marine debris (Watters et al., 2010; Woodall et al., 2014). The lightweight plastic fraction remains at the sea surface for some time before organisms colonize them or particles adhere turning them negatively buoyant such that they sink (Lobelle and Cunliffe, 2011). Over time, the integrity of plastics is compromised by sunlight, wave action, mechanical abrasion (Andrady, 2015), and biotic interaction such that it fragments into smaller pieces including microplastics (<5 mm). Recent studies highlight that plastic litter is taken up by Arctic biota and has invaded all ocean compartments in the Arctic from beaches, sea ice and snow to the sea surface, water column, and the deep ocean floor (Schulz et al., 2010; Nielsen et al., 2014; Obbard et al., 2014; Woodall et al., 2014; Lusher et al., 2015; Trevail et al., 2015; Amélineau et al., 2016; Bergmann et al., 2016; Cózar et al., 2017; Kanhai et al., 2018; Kühn et al., 2018; Morgana et al., 2018; Peeken et al., 2018; Tekman et al., 2020), which hold fragile ecosystems. These ecosystems are threatened by accelerated global warming causing a severe decrease in sea ice coverage (De Lucia, 2017) changing the ecosystem dynamics in turn (Soltwedel et al., 2016). The urge for marine litter related policy requires long-term monitoring programs capable of assessing the effectiveness of implemented measures. Accordingly, the aim of this study is to continue the long-term observations of litter on the deep Arctic seafloor at the HAUSGARTEN observatory (Bergmann and Klages, 2012; Tekman et al., 2017). In addition to already published data from the northern and central stations, we analyzed footage from the southernmost station to assess if there were latitudinal differences and if litter quantities increased over time at this station, too. Furthermore, we analyzed the spatial and temporal variability in litter material and size composition and quantified for the first time the proportion of epibenthic fauna that interact with litter. We tested possible drivers of temporal trends for correlations with maritime activities, fishing effort in the region, and summer sea ice extent.
Materials and Methods
Study Area
The stations of the HAUSGARTEN observatory are located in the Arctic at ca. 79° N along a latitudinal and bathymetric gradient, crossing the Fram Strait at water depths ranging between 250 and 5,500 m (Soltwedel et al., 2016). Previous assessments of litter covered the central station HG-IV (Bergmann and Klages, 2012; Figure 1) and the northern station N3 (Tekman et al., 2017). Here, images from the southernmost station S3 were analyzed to assess latitudinal differences of litter on the seafloor. In addition, the time series of N3 and HG-IV were extended through the analysis of new footage. The stations N3 and HG-IV are ∼300–400 m deeper than S3, which is at ∼2,300 m depth. While N3 experiences the greatest sea ice coverage as it is located close to the marginal ice zone (MIZ), HG-IV is only subject to temporary ice coverage and S3 is ice-free in most years and characterized primarily by Atlantic water masses (Taylor et al., 2017). Overall, the expeditions to the Arctic have produced data on marine litter through photographic surveys conducted in 2002, 2004, 2007, and almost annually since 2011 (Supplementary Table S1).
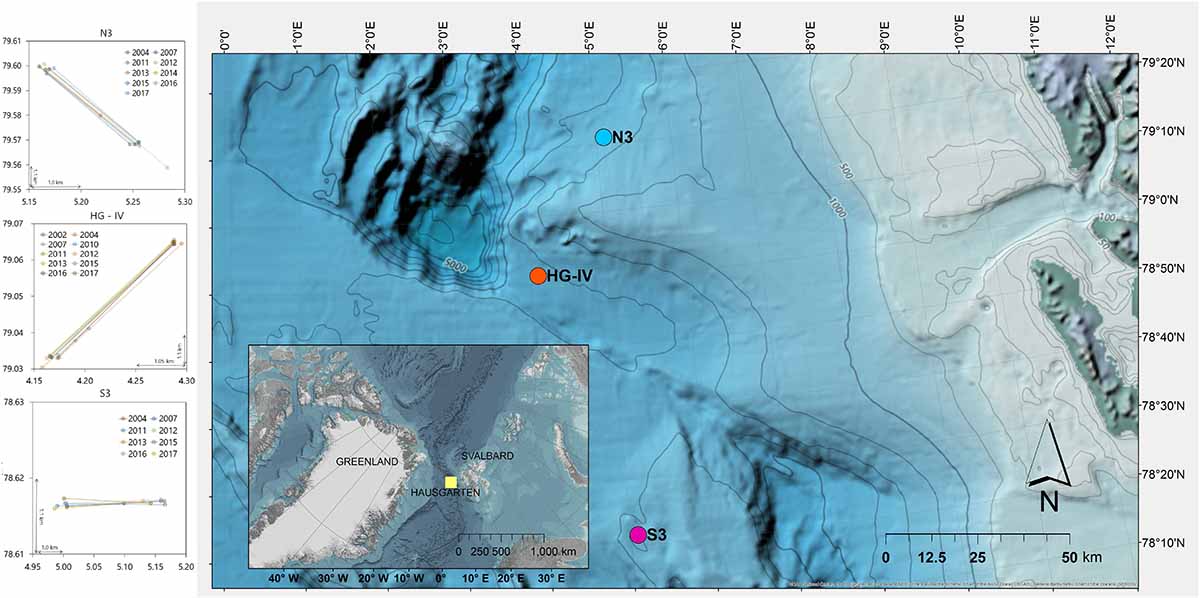
Figure 1. Location of the three stations and the HAUSGARTEN observatory and camera tracks at each site.
Photographic Surveys and OFOS Specifications
All expeditions were undertaken in summer aboard the German research icebreaker RV Polarstern, except for 2013 when RV MS Merian was used, and HG-IV could not be surveyed due to ice conditions.
Marine litter was assessed through photographic surveys of the seafloor using a towed Ocean Floor Observation System (OFOS), which is equipped with a video and still camera. The steel frame currently carries four LED lights, an altimeter, telemetry, and three red laser pointers positioned 50 cm from each other to allow accurate area calculations and scaling of objects. The OFOS has been modified and improved over time. Supplementary Table S1 gives the specifications of all deployments for each expedition. In 2002 and 2004, camera tracks were established at ∼2,500 m water depth at HG-IV, N3, and S3. The OFOS has been towed at each station along the same linear track since the beginning of the time series to assess changes in epifaunal assemblages (Figure 1). The transects were photographed for 4 h at 0.5–0.7 knots and a target altitude of 1.5 m, varying according to bottom topography and sea state. The images were triggered automatically every 20–30 s depending on speed and manually when an item of interest occurred in the field of view.
Image Analysis
The images from each year and station were treated as individual samples to allow temporal and spatial comparisons. All images were analyzed using BIIGLE version 2.0 (Bio-Image Indexing and Graphical Labeling Environment), a web-based image annotation tool (Langenkämper et al., 2017). The same computer screen was used to avoid variation from differences in resolution at a zoom of 1× using BIIGLE’s lawnmower mode, which divides an image into 36 sub-sections and scrolls through each one of them to ensure complete analysis of the whole image. For consistency with previous publications, every litter item observed was labeled and categorized according to a 13-material type classification similar to Mordecai et al. (2011), but rope and food waste were added (Table 1). Unidentifiable litter refers to items of non-mineral nor biological origin whose material could not be determined due to limitations in image resolution, but which was of anthropogenic origin judging by its color, shape, and size. Examples of the items from each category are included in Table 1. All images were screened for putative litter items, followed by a second assessment to ensure correct categorization and even out learning effects. In a third run, all remaining objects were reviewed by 1–2 further experts to exclude objects of low certainty of origin and ensure comparability with Tekman et al. (2017) and Bergmann and Klages (2012). Poor-quality images and those that showed overlap with the previous image were excluded to avoid double counts. The length of each litter item was measured using the BIIGLE rectangle tool. Items were then grouped into three size categories: small (<10 cm), medium (10–50 cm), and large (>50 cm) (Bergmann and Klages, 2012). Epibenthic fauna interactions with litter were annotated aiming for the highest possible taxonomic resolution. The form of interaction was categorized as entangled, colonized by or “in contact with.” The three laser points in each image were detected automatically through a computer algorithm (Schoening et al., 2015), enabling calculation of the area covered by each image.
Data Processing
Data from Bergmann and Klages (2012) and Tekman et al. (2017) were incorporated in the analysis to assess temporal and spatial trends in mean annual litter density, material, and size composition among and within years and stations, and at HAUSGARTEN observatory combining all three stations. The size composition of plastic items was analyzed to assess temporal and spatial trends. Litter count was converted to litter density according to:
where LD, litter density (items km–2); ni, litter count per image; Ai, area of the image (km2).
The dataset obtained was used to calculate the mean and standard error of the mean (SEM). The mean annual litter density was calculated as the sum of litter densities divided by the total number of images per year per station. The SEM was calculated based on the mean litter density of each transect per year. Out of the 13 material categories differentiated for statistical purposes, a summary of four categories (plastics, glass, other, unidentifiable litter) was used to illustrate the trends. The proportion of litter items that interacted with epibenthic megafauna was calculated and categorized as the percentage of items entangled, colonized or in contact with fauna. In addition, the interaction between megafauna and litter at the community and population level was determined for the most frequently affected species from 2004 to 2015. Epibenthic megafaunal density data obtained from the same OFOS surveys were used to quantify the proportion of individuals affected by litter in every year at N3 and S3 (2004–2015) and HG-IV (2002–2011) (Taylor et al., 2017; Bergmann et al., 2011). The number of interactions were presented as percentages of the mean count at the observatory. In the absence of reference data on megafaunal densities litter, data from 2016 to 2017 had to be excluded. The interaction at the population level refers to two species of sponges Cladorhiza gelida and Caulophacus arcticus, whose mean abundance was combined to assess sponge entanglement over time. The calculations were computed per station per year as follows:
(1) Number of individuals for litter study area = species density from literature × litter study area covered
(2) Proportion of individuals affected by litter =
Statistical Analysis
The litter density data were tested for significant differences among years and stations by applying a Kruskal–Wallis test performed in MINITAB (version 14.12.0). When significant overall differences were found, pairwise Mann–Whitney U tests were conducted to assess, which years and stations were different. A Bonferroni correction was applied to avoid Type-1 errors through multiple comparisons. This was done by dividing the p-value by the number of comparisons among years. For material and size composition, a one-way permutational multivariate analysis of variance (PERMANOVA) was performed in PRIMER (version 6.1.16) and PERMANOVA+ (version 1.0.6) on a Bray Curtis similarity matrix. The data were 4th-root transformed to decrease an importance of dominant categories. Station was selected as a fixed factor to assess the differences in litter composition among stations and the effect of depth and ice coverage. Year was treated as a random factor since the surveys could not be conducted every year between 2002 and 2017 due to accessibility of the sampling site, availability of ship time and equipment. When significant differences were detected, a pairwise PERMANOVA was computed to assess, which years and stations were different. We included only those years in statistical comparisons, for which data were available at all stations.
Maritime Data and Summer Sea Ice Extent
Vessel monitoring system (VMS) reports were obtained from the Norwegian Directorate of Fisheries and used as a proxy of fishing activity inside the 12-nm area west of Svalbard. VMS data refer to vessel reports of time, position, course, and speed of EU fishing vessels longer than 24 m and foreign vessels longer than 15 m every 60 min. The number of reports was provided per country of origin (Russia, Norway, and Others) for each year from 2001 to 2017. In addition, the Harbormaster of Longyearbyen provided annual figures for the number of ships calling at the harbor of Longyearbyen. The ship calls were divided in tourism activities (cruises, coastal vessels, day-tour boats, and private yachts) and other-type-of-activities (cargo, research, fishing, governor’s vessels, navy, pilot boats). The number of passengers on tourism vessels were also analyzed for temporal trends. We tested for a correlation between all the maritime data and annual mean litter density at HAUSGARTEN (Spearman’s rank correlation, for all stations combined).
Daily sea-ice concentrations over the three stations were obtained from the Center for Satellite Exploitation and Research (CERSAT) at the Institut Français de Recherche pour l’ Exploitation de la Mer (IFREMER, France) (Ezraty et al., 2007) and ice coverage was calculated based on the ARTIST Sea Ice (ASI) algorithm developed at the University of Bremen, Germany (Spreen et al., 2008) at a 12.5 × 12.5 km resolution. Mean values for summer months (May–September) during the study period were analyzed for Spearman’s rank correlation with mean litter densities at individual stations (Tekman et al., 2017).
Results
Here, we present rare time-series data for litter in the deep Arctic sea and data on interactions with epibenthic megafauna at the population level. A total of 60.5 km2 were covered in 16,157 images for this study. The mean litter densities, at all HAUSGARTEN stations combined, ranged between 813 ± 525 (SEM) and 6,717 ± 2,044 (SEM) items km–2.
Temporal and Spatial Trends in Litter Density
Mean annual litter densities were significantly different over time (K-W: H = 38.7, df = 6, p < 0.001) for all HAUSGARTEN stations combined. An initial strong increase in 2011 was followed by elevated levels above 6,000 items km–2 from 2014 and thereafter (Table 2 and Figure 2). In terms of latitudinal differences, N3 was the most polluted station with the highest litter density recorded so far in 2016 (10,358 items km–2, Table 3). It was also the only station with a clear, continuous increase over time and significant differences among years (K-W: H = 38.01, df = 6, p < 0.001). The year 2004 was significantly different from the years 2014 and thereafter (M-W: p < 0.0011). Litter densities at HG-IV reached 7,785 items km–2 in 2011 and remained elevated around 5,000 items km–2 (Table 4) with no statistical differences (K-W: H = 12.73, df = 7, p = 0.079). At the southernmost station S3, litter densities peak in 2011 followed by a decrease but remained higher than in the early surveys (Table 5). There were no significant differences among years (K-W: H = 8.62, df = 6, p < 0.196).
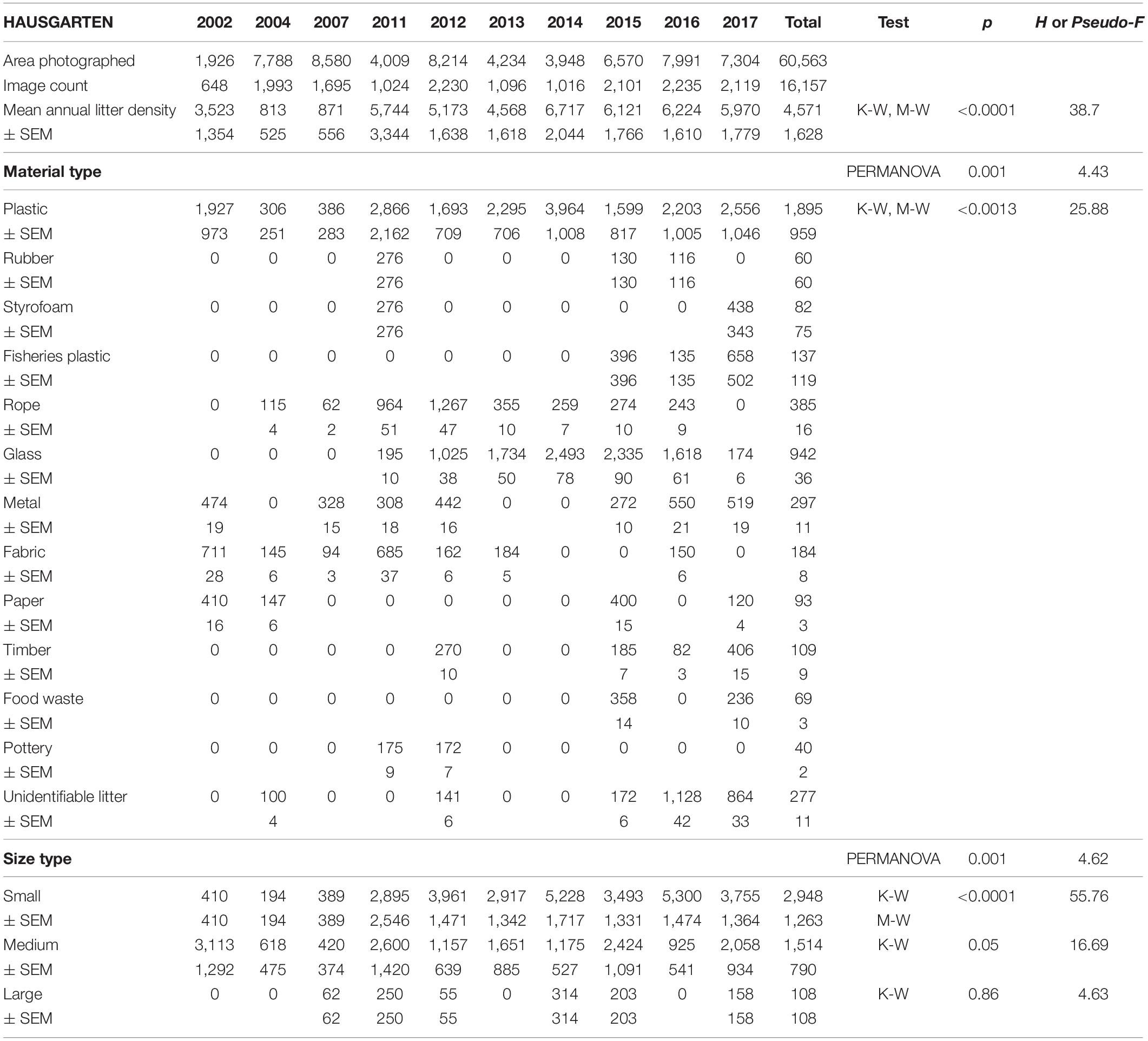
Table 2. Summary of the area covered (m2), mean annual litter densities (items km–2), material and size composition at all HAUSGARTEN stations combined.
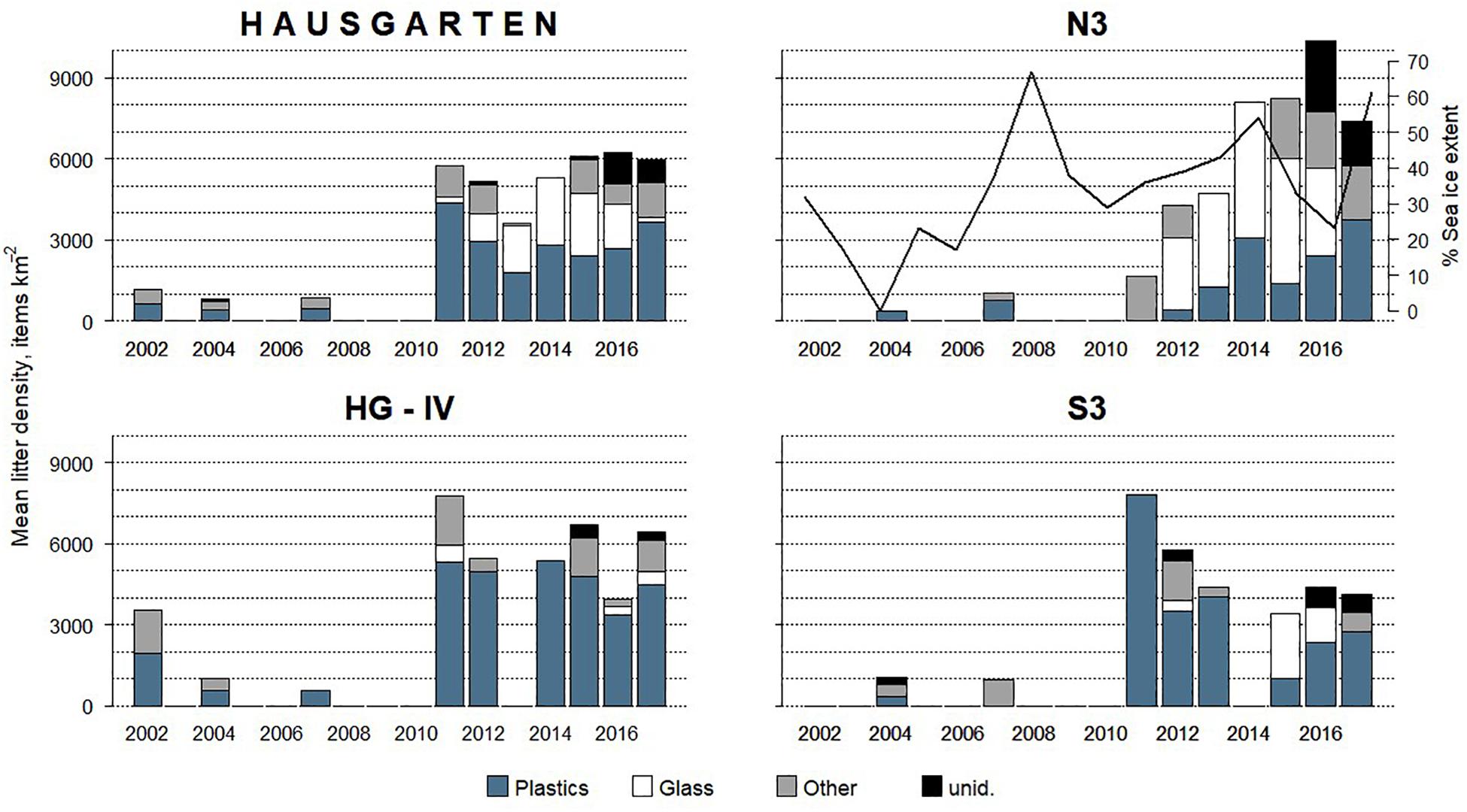
Figure 2. Mean annual litter density and composition at HAUSGARTEN (all stations combined) and individual stations between 2002 and 2017. The percentage of summer sea ice extent is shown only at N3 highlighting the inverse trend during the last 3 years of the time series.
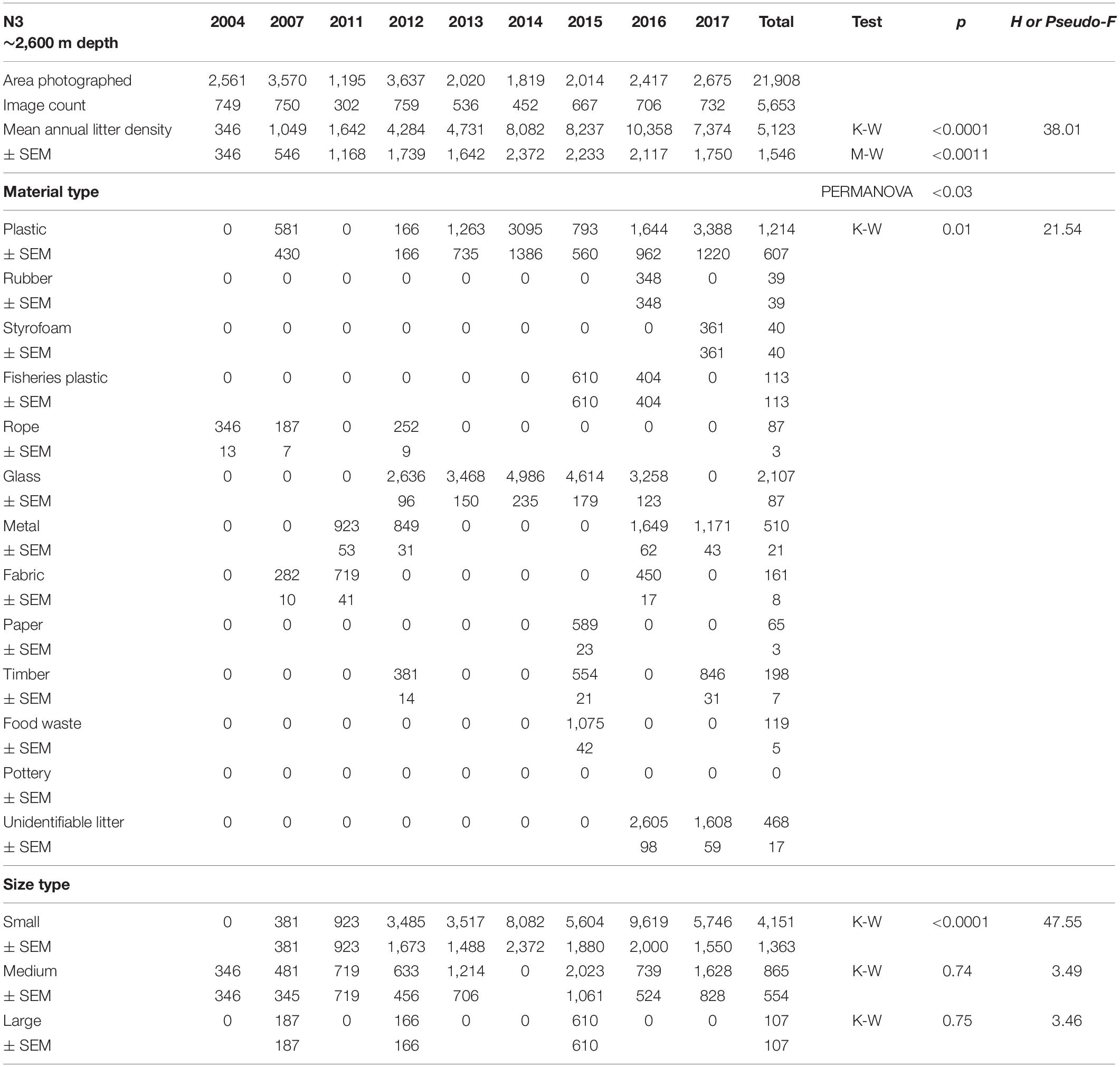
Table 3. Summary of the area covered (m2), mean annual litter densities (items km–2), material and size composition at HAUSGARTEN station N3.
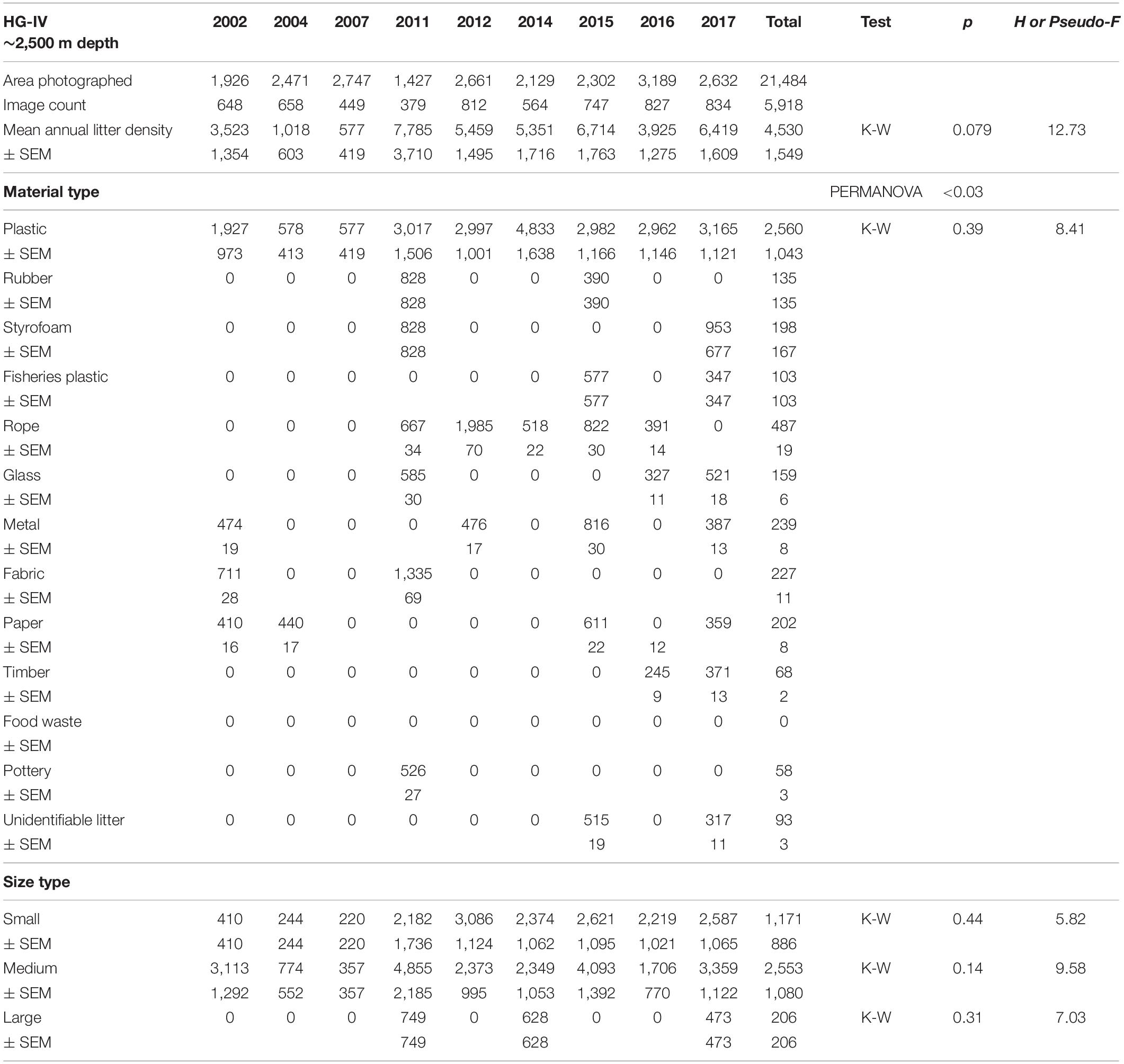
Table 4. Summary of the area covered (m2), mean annual litter densities (items km–2), material and size composition at HAUSGARTEN station HG-IV.
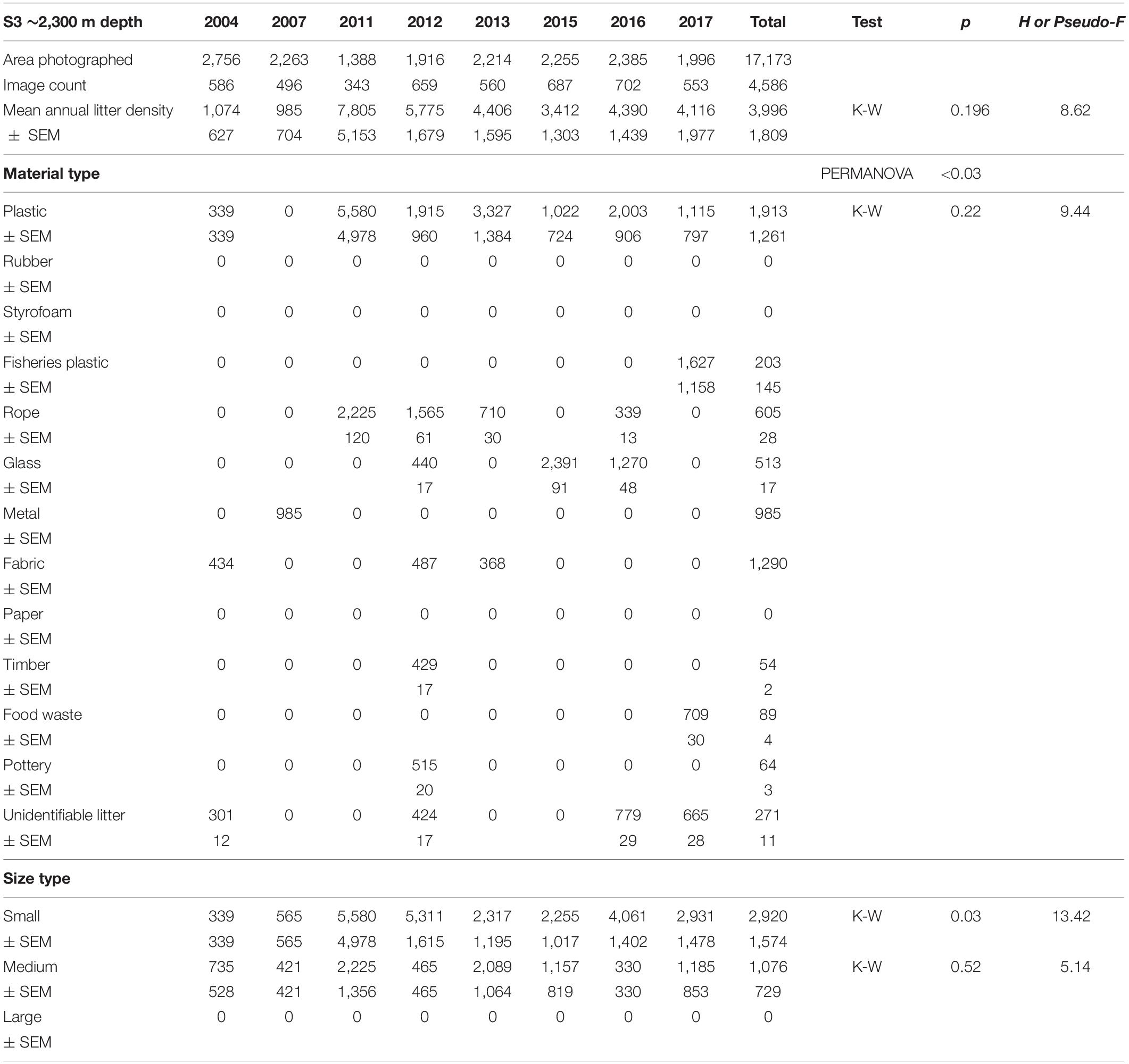
Table 5. Summary of the area covered (m2), mean litter densities (items km–2), material and size composition at HAUSGARTEN station S3.
Trends in Material Composition
The composition of debris observed at HAUSGARTEN was significantly different throughout the years (PERMANOVA: Pseudo-F = 4.43, p = 0.001) with no clear temporal trend (Figure 2). Plastic bags, packaging material and fishing gear were the most common form of plastic. This material accounted for 41% (Figure 2) at the observatory, with a significant boost after 2014 (K-W: H = 25.88, df = 8, p < 0.001). The second most frequent type were pieces of green glass (21%). Other material types such as metallic scrap, synthetic cord, timber, fragments of ceramics, and a probably discarded fish carcass accounted for less than 10% (Table 2). There were significant differences in the litter composition of different stations (PERMANOVA: p < 0.03). N3 held a distinct composition, where glass was the single most important material type (41%) while plastic accounted only for 24%. In contrast, HG-IV and S3 harbored mainly plastic, 77 and 49%, respectively.
Temporal Trends in Litter Size Composition
Overall, 63% of the litter was small whereas medium-sized items accounted for 35% and large items were scarce (2%). The size of litter varied significantly over time (PERMANOVA: Pseudo-F = 6.08, p = 0.001) with the quantity of small items increasing after 2011 (K-W, M-W: H = 55.76, p < 0.001). These dominated (>70%) at N3 and S3 (Figure 3) although there were no significant spatial differences (PERMANOVA: p > 1.22).
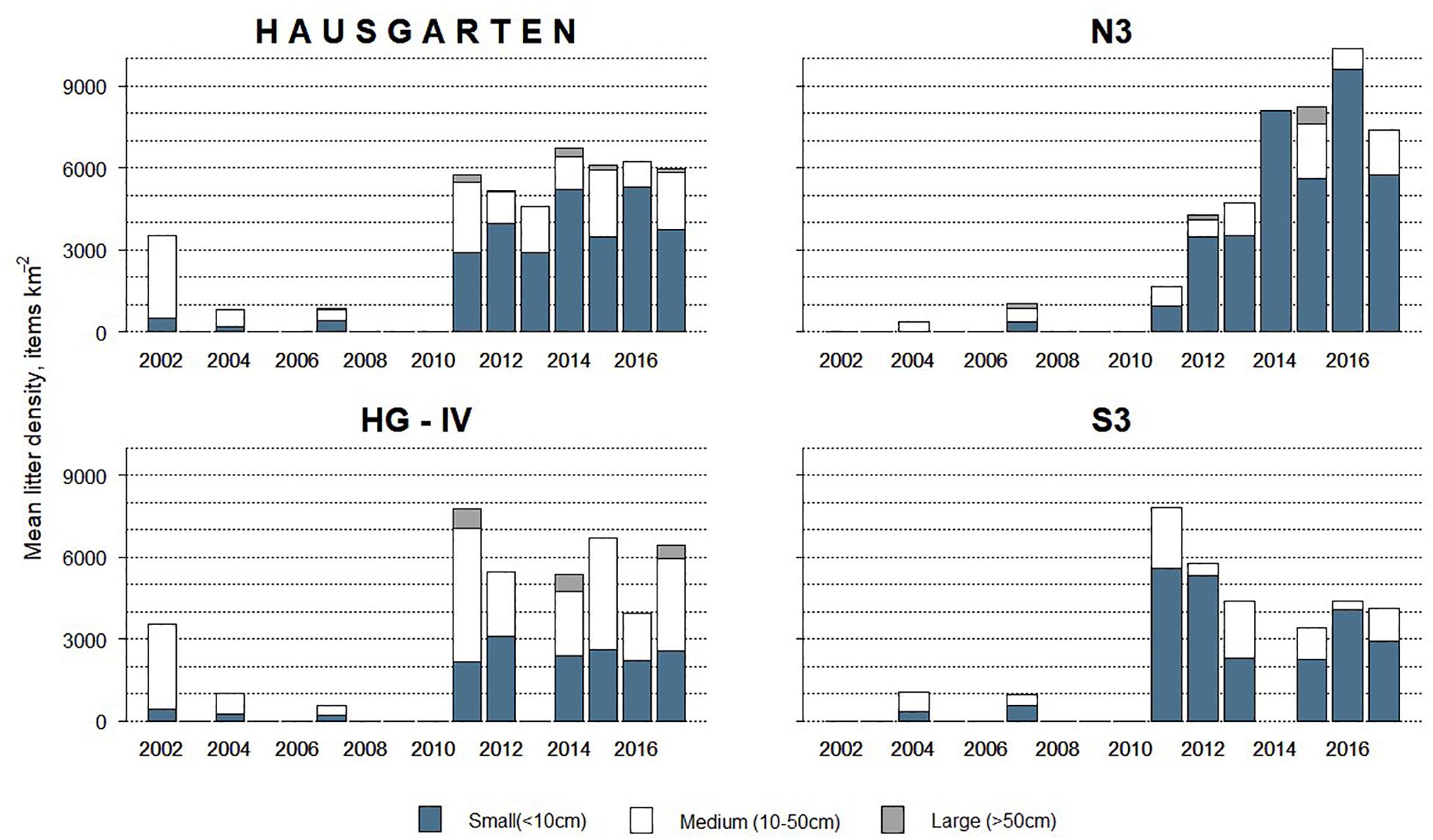
Figure 3. Size composition of litter observed at the HAUSGARTEN observatory and at each station in terms of mean annual litter density between 2002 and 2017.
Temporal Trends in Plastics Litter Size
Overall, a similar proportion of small and medium-sized plastics was observed at the observatory, 49 and 47%, respectively. Small plastics came mostly from HG-IV in 2013 and S3 in 2011. Coincidentally, both stations harbored exclusively plastics in those years (Figure 2). Plastic is the main material type and comes mostly in small size, which raises concern about further fragmentation and microplastic pollution, especially because the quantity of small plastics continued to grow after the 2014 maximum (K-W, M-W: H = 25.88, df = 8, p < 0.002).
Litter Interaction With Epibenthic Megafauna
Overall, 45% of the litter items interacted with epibenthic fauna either by entanglement, colonization or by being “in contact” (Figure 4). The most common material observed to encounter fauna was plastic (30%), other material types accounted for less than 5% each. The highest proportion of interactions was recorded at HG-IV (66%) followed by N3 (22%), and S3 (12%). Litter items interacted with 158 individuals of 12 taxa. Litter was frequently entangled in sponges, especially of the species C. gelida (26%). C. arcticus, another species of sponge, was also entangled regularly with litter (11%), its debris even more often (17%) with almost half of those interactions seen at N3. The second most common interaction entailed anemones colonizing litter, particularly of the family Hormathiidae (22%), well over half of these colonizations were observed at HG-IV. This form of interaction occurred with another anemone, Bathyphellia margaritacea (5%) and the sea lily Bathycrinus carpenterii (8%). Other interactions (<4%) included crustaceans (Bythocaris sp., Birsteiniamysis inermis), goose barnacles (Verum striolatum), soft corals (Gersemia fruticosa), other sponges (cf. Pachastrellidae) and sea cucumbers.
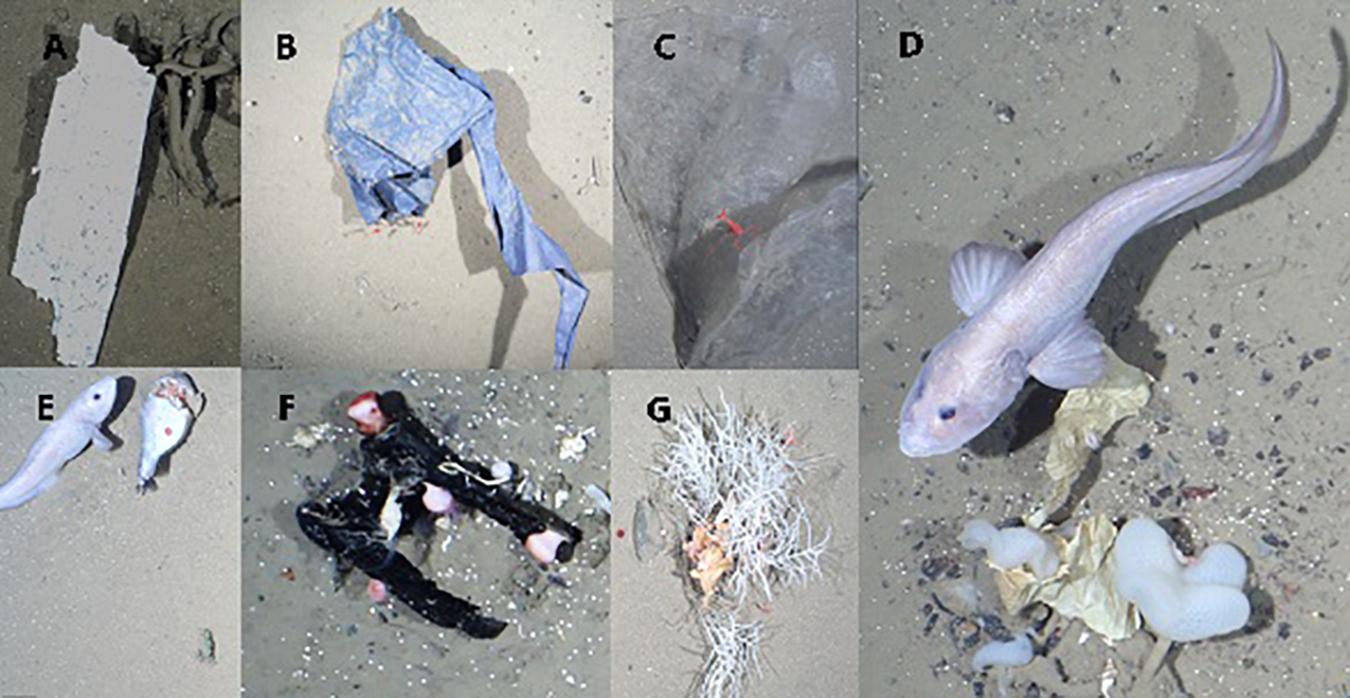
Figure 4. Examples of marine litter photographed by OFOS at HAUSGARTEN: (A) Styrofoam lying on Caulophacus debris, (B) plastic film in contact with two Bythocaris shrimps, (C) Bythocaris sp. resting on plastic bag, (D) plastic wrapping material entangled in C. arcticus and colonized by B. margaritacea, (E) fisheries plastics and probably food waste, which attracted scavenging amphipods and eelpout (Lycodes frigidus), (F) piece of rubber colonized by anemones, Verum striolatum and calcareous tube worm, (G) piece of plastic entangled in Cladorhiza gelida and colonized by sea anemones.
For the first time, we present figures on the proportion of epibenthic megafaunal organisms interacting with litter at HAUSGARTEN. The proportion of epibenthic megafaunal organisms interacting with litter was low ranging between 1 and 31% with no clear temporal trend over time. When looking into populations of species, however, there was an increase in the number of sponges (C. gelida and C. arcticus) entangled with litter at N3, the highest percentage was observed in 2015 (10%) (Figure 5). At S3, the proportion of entangled sponges was even higher and remained elevated after an initial peak in entanglements in 2011 (20%). While a greater proportion of C. gelida suffered from entanglement at N3 (up to 28%), at S3 most litter got entangled in C. arcticus (up to 31%).
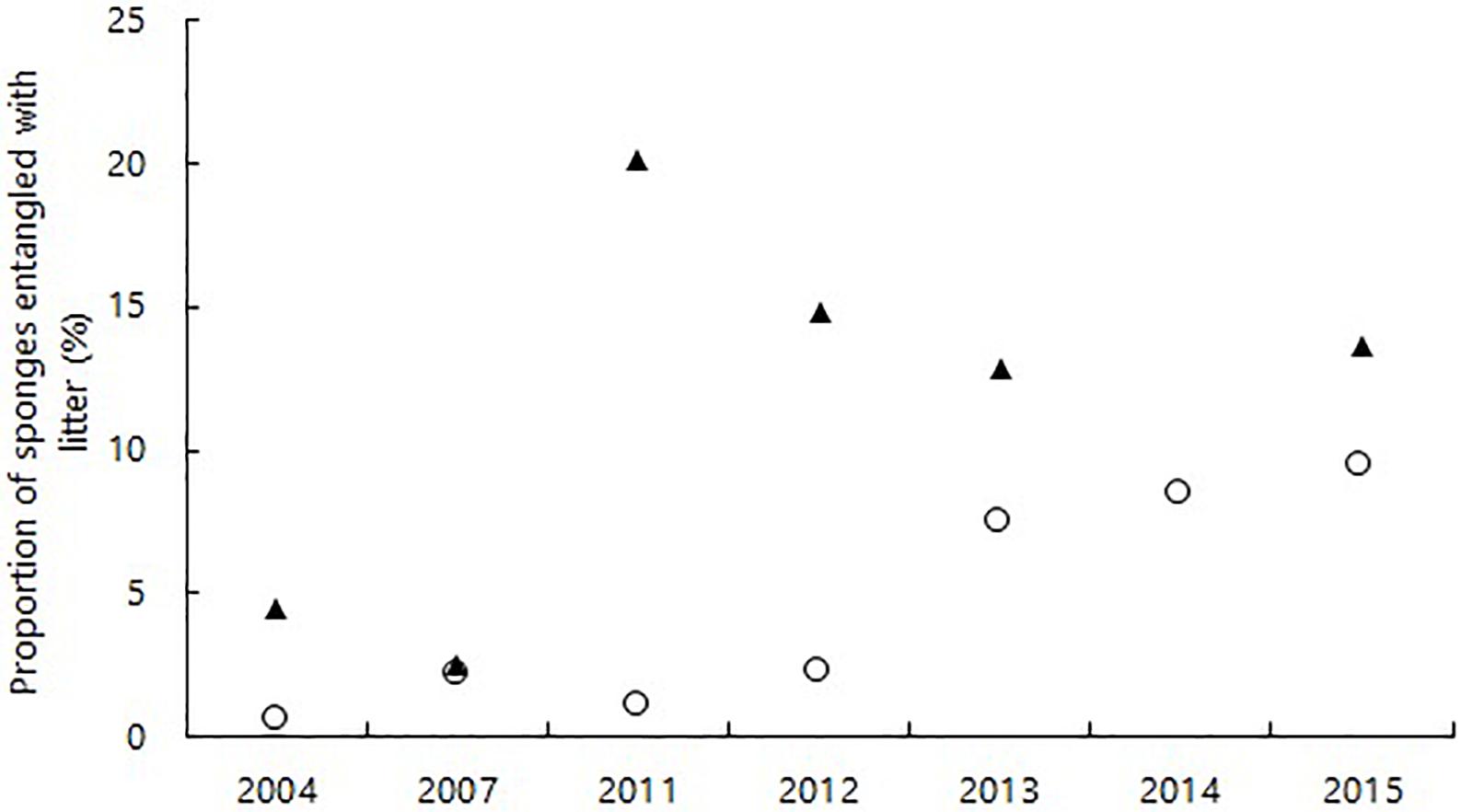
Figure 5. Proportion of the sponges Cladorhiza gelida and Caulophacus arcticus entangled with marine litter at N3 (white circles) and S3 (black triangles) between 2004 and 2015.
Maritime Traffic
The fishing activity from Russian vessels, inside the 12-nm range of Svalbard, was positively correlated with the overall litter density at HAUSGARTEN (ρ = 0.794, p = 0.006), where the number of VMS reports were particularly high in 2014 and 2017 (Figure 6). Likewise, vessels from other countries were positively correlated with litter densities (ρ = 0.656, p = 0.039) while there was no correlation with Norwegian vessels (ρ = −0.527, p = 0.117), probably because their numbers decreased over time.
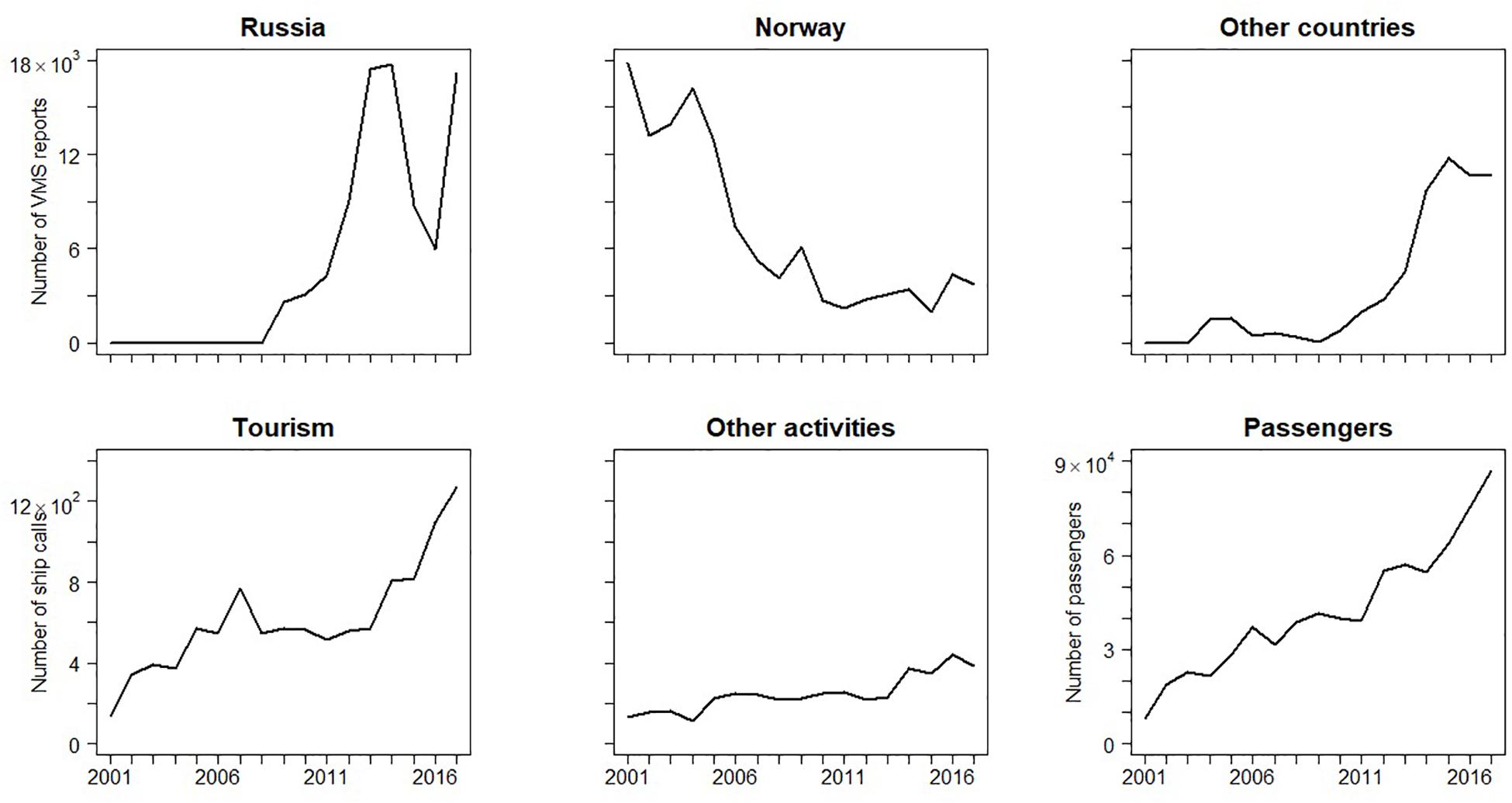
Figure 6. Temporal trend in the number of VMS reports from Russia, Norway, and other countries (upper panel, from left to right, source: Norwegian Directorate of Fisheries). Number of ships calling at the port of Longyearbyen (Svalbard) from tourism, other-type activities, and number of passengers of leisure craft (bottom panel, source: Harbormaster of Longyearbyen).
The tourist ships calling at the port of Longyearbyen as well as the number of passengers, correlated positively with litter densities at HAUSGARTEN (ρ = 0.69, p = 0.025; ρ = 0.67, p = 0.033, respectively). Tourism and the number of passengers of leisure craft have steadily increased in the time frame of almost two decades. The latter reached 87,000 passengers per year in 2017 (Figure 6). When testing tourism ship categories individually, private yachts were most strongly correlated (ρ = 0.74, p = 0.013). However, the strongest correlations were found with other-type-of-activities ships (ρ = 0.85, p = 0.002), despite a low number of calls (<400 y–1).
Summer Sea Ice Extent
There was no correlation between the mean summer sea ice extent and litter density at any station (ρ > 0.08, p > 0.556). Nonetheless, N3 experienced a reverse trend in sea ice coverage from 2015 to 2017: a decrease in sea ice cover coincided with an increase in litter density (Figure 2). As for the other two stations, HG-IV experienced a low sea ice coverage and S3 was ice-free except for the summers of 2007 and 2013.
Discussion
Temporal Trends in Litter Density
This 15-year record of observations at HAUSGARTEN shows an increase in litter pollution over time with particularly high densities in 2011, 2014, and 2016. The deep seafloor is considered a sink for marine litter (Woodall et al., 2014) and as such, a particularly suitable environment for long-term observations. However, although litter remained at an elevated level after the initial peak in 2011, there was no continuous increase across all years and stations concurring with results from other time-series studies (Law et al., 2010, 2014; Browne et al., 2015; Schulz et al., 2015; Nelms et al., 2017; Beer et al., 2018; Maes et al., 2018). Table 6 shows a comparison of litter densities and the proportion of plastics among studies on the deep seafloor. Most of the items recorded were only observed once and may have been eaten or moved by epibenthic organisms or bottom currents (on average 7.8 ± 0.9 cm s–1, Meyer-Kaiser et al., 2019). Fragmentation into smaller sizes including microplastics is another possibility although environmental conditions in the deep sea such as low temperatures, the absence of UV light and weak currents may slow down this process compared with other realms. In addition, litter items may have been hidden by a veneer of sediment over time. This suggests that litter densities at the observatory could be even higher. Assuming no transport and therefore a continuous accumulation of debris over time, cumulative litter densities could exceed our highest litter record tenfold (117,978 items km–2). Such densities have only been reported in the Mediterranean Sea (>100,000 items km–2), which has been considered the most polluted area in the world (CIESM, 2014). Although sedimentation to the deep sea is a slow process Brandon et al. (2019) reported that (micro-)plastic pollution in sediments doubled every 15 years over a multidecadal time scale, showing an exponential increase over time and clearly indicating the burial of plastics in sediments.
The strong increase in litter in 2011 may be partly due to a switch to a new camera (Canon EOS-1Ds Mark III) fitted to the OFOS. This could potentially have increased object recognition although care was taken to include objects, which could only be identified with low certainty.
Plastic was the most frequent type of litter. This is not surprising given that plastic debris has spread to the abyss in various parts of the World’s Oceans (Debrot et al., 2014; Pham et al., 2014; Woodall et al., 2014; Chiba et al., 2018; Gerigny et al., 2019; Pierdomenico et al., 2019) even reaching its deepest parts, the hadal zone (Peng et al., 2018). Some of these locations bear exceptionally high mean densities, e.g., La Fonera (15,057 items km–2) and Cap de Creus canyons (8,090 items km–2) in the Mediterranean (Tubau et al., 2015), which are in a similar range with our highest records from N3 (10,358 items km–2). Unlike those places, however, the observatory is secluded from populated and industrial regions. Although, the largest proportion of litter in the Arctic originates most likely from long-distance transport from industrialized European and Atlantic destinations and drifts to the North via the thermohaline circulation (Cózar et al., 2017) our data show that the region is subject to increasing local shipping activity, especially in terms of tourist cruises and fishing. Shipping in the tourism sector increased to more than 1,000 ships per year in 2016 coinciding with a peak in litter densities recorded in that year. Similarly, the VMS reports of vessels from Russia grew to >17,000 in 2014 and from “other countries” to >10,000 in 2016, which may also have contributed to the peaks observed. These increases in shipping could be a response to the declining sea ice and changes in the ranges of fish stocks. Merchant shipping is likely to increase in the future as the Northern Sea route represents 50% of savings over the Asia to Europe via the Suez Canal route (Aksenov et al., 2017), and sea ice is likely to decrease further given rapidly rising greenhouse gas emissions enhancing global heating. Indeed, the high quantities of glass recorded at N3 point to local emissions. Given its high density, glass can be assumed to sink to the seafloor directly. It should be noted that the disposal of glass in the Arctic was banned by MARPOL in 2013.
Material and Size Composition
Plastic materials have become a common feature of our oceans and the situation in the secluded Arctic is no exception. It accounted for 41% of the material observed on the seafloor, which is lower compared with the world’s average of ∼62% on the seafloor (LITTERBASE, 2019). The global increase in plastic production has led to a cumulative plastic production of 8.3 billion MT in 2017 (Geyer et al., 2017). Along with its extensive usage, buoyancy capacity and durability (Andrady, 2015), the inefficient recycling and waste export schemes (Brooks et al., 2018) explain the subsequent mismanaged fraction that enters the ocean (Jambeck et al., 2015).
Positively buoyant items such as polyethylene and polypropylene, are commonly found in the surface layer and shorelines and can drift for extended periods of time. It is striking that some of these (fragments of) plastic items make it to the seafloor. This is often explained with a ballasting effect of fouling organisms (Fazey and Ryan, 2016), which reduces their original buoyancy. Still, the items in our images did not show any sign of the typical rafting biota (e.g., barnacles, mussels, hydroids, algal film). While fouling biota experience changes in temperature, pressure, and food supply during their passage to the seafloor likely leading to mortality, we think it is unlikely that their remains would fall off entirely. If this was the case, the buoyancy of such items may increase such that they could rise back to the sea surface unless they were entrapped by sediments, stones, emergent fauna, or colonized by sessile benthic fauna. Indeed, 30% of the plastic items were subject to such interactions. Not only biofouling, but also hydrographic processes such as deep-water cascading and flash-flood events or eddies may promote downward transport (Lalande et al., 2011; Tubau et al., 2015; Pierdomenico et al., 2019).
As mentioned above, a large portion of glass (41%) was found at our northernmost station and to a lower extent at the other stations. This is important as it is an indicator of disposal from intensifying local maritime activity. Since glass is denser than seawater it immediately sinks. It is perceived as the least harmful material despite being very durable. Being a hard substratum, however, glass can be colonized by sessile biota and thereby alter local community structure and thus biodiversity in soft-sediment habitats.
Items smaller than 10 cm dominated at two out of the three stations (>50%), while the central station was characterized by medium-sized items (>40%). However, almost a decade ago, medium-sized items were the frequent size at HAUSGARTEN (67%) (Bergmann and Klages, 2012). These temporal changes in size composition suggest fragmentation of plastics, the prime contributor of litter. Known to break down into smaller pieces, pollution from microplastics is also of great concern as these can be taken up by a wider range of biota. Indeed, HAUSGARTEN sediments harbored very high microplastic concentrations, with highest concentrations found at the northern station (6,595 and 13,331 particles kg sediment–1) (Bergmann et al., 2017c; Tekman et al., 2020). A feedback mechanism with sea ice is suspected not only as a transport vehicle circling the Arctic basin but also as an accelerator of plastics fragmentation rates during subsequent melting and freezing seasons (Peeken et al., 2018; Tekman et al., 2020). Smaller plastics are more likely to sink due to a higher surface-to-volume ratio promoting ballasting through biofouling. In addition, small plastics can act as aggregative devices and form hetero-aggregates with algae (Fazey and Ryan, 2016; Long et al., 2017; Porter et al., 2018), also in the MIZ of polar waters with aggregates of sinking ice algae.
Litter Interactions With Epibenthic Fauna
Sixty-six percent of the organisms that encountered litter were sessile, namely anemones and sea lilies colonizing litter and entangled sponges. Like trees on land, emergent fauna such as sponges likely intercept plastic drifting above the seafloor and hold it in place as indicated by repeated observation of the same litter-sponge entanglement. The actual impact of these interactions is currently uncertain, but slow growth rates of deep-sea biota counteract the resilience of these fragile ecosystems (German et al., 2011). Colonization of debris by hormathiid anemones accounted for the greatest percentage (26%) of the observed interactions. Such associations are common since plastic provides a substrate to attach to (Watters et al., 2010). While it could be argued that plastics increases local biodiversity by providing hard substratum to sessile biota in otherwise soft-sediment environments, it could alter local food web structure and ecosystem function (Miyake et al., 2011).
The impact of entanglement in sessile organisms is uncertain but it may lead to injury, or decreased water exchange and respiration rates due to coverage of the affected body surface (Bergmann and Klages, 2012), obstruction of filter-feeding, and deleterious effects on reproduction affecting the survival of colonies. The carnivorous sponge C. gelida was the organism that encountered litter most frequently (26%), almost exclusively plastic. In food-limited environments such as the deep sea, a hindrance of prey capture could put species at particular risk. Given that up to 28% of C. gelida were entangled at N3, impacts on local populations do not seem unlikely, especially during a period when the population dropped from 0.260 to 0.049 individuals m–2 (Taylor et al., 2017). C. arcticus, a suspension-feeding sponge, was most frequently observed entangled with litter (11%), which could obstruct the aquiferous system and prevent food uptake by filter-feeding. In 2013 at S3, the number of litter items decreased by 66% compared to the previous year. However, the proportion of C. arcticus entanglements increased by 1%. In the same year, the population of C. arcticus was cut to half, changes in the environment might affect the population resilience. A more detailed study on the anthropogenic impact on this epibenthic megafaunal community is needed to further understand how reactive the species are and how much they are been affected.
Conclusion
For years, the Fram Strait has been fed with debris from lower latitudes and the Arctic basin, leading to the elevated litter densities that we have recorded over 15 years. As in other studies, plastic accounts for the majority of litter on the observatory’s seafloor and could become a continuous source of microplastics pollution in the future as larger items continue to break down. The post-2011 period of high litter density coincides with an increase in maritime activity near Svalbard from both, tourism and fishing vessels that may contribute to the pollution observed at HAUSGARTEN. Litter finds its way into the study area via local waste emissions or via pathways such as the ocean currents from the North Atlantic. In addition, sea ice may possibly play an important role in litter transportation and distribution. In order to tackle the issue more rigorous waste management systems are needed to avoid accidental litter leakages into the ocean, especially in highly populated coastal areas where most of the litter originates and travels long distances. Regulations in the maritime industry require to be strengthened to prevent discarding of litter, but also cost-efficient systems are needed to properly dispose of fishing gears and waste of ships. Beyond these measures, societal transformation is essential to better balance the necessity covered by plastics and the comfort they provide.
Data Availability Statement
All datasets generated for this study are included in the article/Supplementary Material and accessible in the data archive PANGAEA (https://doi.pangaea.de/10.1594/PANGAEA.912471).
Author Contributions
KP wrote the first draft of the manuscript. MB contributed the conception and design of the study and wrote the manuscript. MT contributed to manuscript revision. All authors organized the database and performed the statistical analysis, and read and approved the submitted version.
Funding
This work contributes to the Pollution Observatory of the Helmholtz Association-funded infrastructure program FRAM (Frontiers in Arctic Marine Research), which is funded MBT.
Conflict of Interest
The authors declare that the research was conducted in the absence of any commercial or financial relationships that could be construed as a potential conflict of interest.
Acknowledgments
The authors thank the crew of RVs Polarstern and MS Merian, T. Soltwedel, L. Dierksen, D, Langenkämper, the HGF-MPG Group for Deep-Sea Ecology and Technology of AWI for gathering the data and the support throughout the analysis of the data during this study. The authors also thank T. Krumpen for providing sea-ice data, the Harbor Master of Longyearbyen, K. Bråten, for sharing information about ship calls, P. Finne from FMC Norway Directorate of Fisheries for providing VMS and inspection data of fishing vessels. U. Hoge, B. Sablotny and J. Hagemann provided technical support. KBPM received an ERASMUS MUNDUS grant during her stay at the AWI. The authors value the comments of three reviewers inluding A. Cau and T. Vlanchogianni, who improved an earlier version of this manuscript. This publication is Eprint ID 47972 of the Alfred-Wegener-Institut Helmholtz-Zentrum für Polar- und Meeresforschung.
Supplementary Material
The Supplementary Material for this article can be found online at: https://www.frontiersin.org/articles/10.3389/fmars.2020.00321/full#supplementary-material
References
Aksenov, Y., Popova, E. E., Yool, A., Nurser, A. J. G., Williams, T. D., Bertino, L., et al. (2017). On the future navigability of Arctic sea routes: high-resolution projections of the Arctic Ocean and sea ice. Mar. Policy 75, 300–317. doi: 10.1016/J.MARPOL.2015.12.027
Amélineau, F., Bonnet, D., Heitz, O., Mortreux, V., Harding, A. M. A., Karnovsky, N., et al. (2016). Microplastic pollution in the Greenland Sea: background levels and selective contamination of planktivorous diving seabirds. Environ. Pollut. 219, 1131–1139. doi: 10.1016/j.envpol.2016.09.017
Andrady, A. L. (2015). “Persistence of plastic litter in the oceans,” in Marine Anthropogenic Litter, eds M. Bergmann, M. Gutow, and L. Klages (Cham: Springer International Publishing), 57–72. doi: 10.1007/978-3-319-16510-3_3
Arcangeli, A., Campana, I., Angeletti, D., Atzori, F., Azzolin, M., Carosso, L., et al. (2018). Amount, composition, and spatial distribution of floating macro litter along fixed trans-border transects in the Mediterranean basin. Mar. Pollut. Bull. 129, 545–554. doi: 10.1016/J.MARPOLBUL.2017.10.028
Basurko, O. C., Gabiña, G., Andrés, M., Rubio, A., Uriarte, A., and Krug, I. (2015). Fishing for floating marine litter in SE Bay of Biscay: review and feasibility study. Mar. Policy 61, 103–112. doi: 10.1016/J.MARPOL.2015.07.010
Beer, S., Garm, A., Huwer, B., Dierking, J., and Nielsen, T. G. (2018). No increase in marine microplastic concentration over the last three decades – a case study from the Baltic Sea. Sci. Total Environ. 621, 1272–1279. doi: 10.1016/j.scitotenv.2017.10.101
Bergmann, M., and Klages, M. (2012). Increase of litter at the Arctic deep-sea observatory HAUSGARTEN. Mar. Pollut. Bull. 64, 2734–2741. doi: 10.1016/J.MARPOLBUL.2012.09.018
Bergmann, M., Lutz, B., Tekman, M. B., and Gutow, L. (2017a). Citizen scientists reveal: marine litter pollutes Arctic beaches and affects wild life. Mar. Pollut. Bull. 125, 535–540. doi: 10.1016/j.marpolbul.2017.09.055
Bergmann, M., Sandhop, N., Schewe, I., and D’Hert, D. (2016). Observations of floating anthropogenic litter in the Barents Sea and Fram Strait, Arctic. Polar Biol. 39, 553–560. doi: 10.1007/s00300-015-1795-8
Bergmann, M., Soltwedel, T., and Klages, M. (2011). The interannual variability of megafaunal assemblages in the Arctic deep sea: preliminary results from the HAUSGARTEN observatory (79°N). Deep Sea Res. Part I Oceanogr. Res. Pap. 58, 711–723. doi: 10.1016/j.dsr.2011.03.007
Bergmann, M., Tekman, M. B., and Gutow, L. (2017b). Sea change for plastic pollution. Nature 544, 297–297. doi: 10.1038/544297a
Bergmann, M., Wirzberger, V., Krumpen, T., Lorenz, C., Primpke, S., Tekman, M. B., et al. (2017c). High quantities of microplastic in Arctic deep-sea sediments from the HAUSGARTEN observatory. Environ. Sci. Technol. 51, 11000–11010. doi: 10.1021/acs.est.7b03331
Brandon, J. A., Jones, W., and Ohman, M. D. (2019). Multidecadal increase in plastic particles in coastal ocean sediments. Sci. Adv. 5:eaax0587. doi: 10.1126/sciadv.aax0587
Brooks, A. L., Wang, S., and Jambeck, J. R. (2018). The Chinese import ban and its impact on global plastic waste trade. Sci. Adv. 4:eaat0131. doi: 10.1126/sciadv.aat0131
Browne, M. A., Chapman, M. G., Thompson, R. C., Amaral Zettler, L. A., Jambeck, J., and Mallos, N. J. (2015). Spatial and temporal patterns of stranded intertidal marine debris: Is there a picture of global change? Environ. Sci. Technol. 49, 7082–7094. doi: 10.1021/es5060572
Buhl-Mortensen, L., and Buhl-Mortensen, P. (2017). Marine litter in the Nordic Seas: distribution composition and abundance. Mar. Pollut. Bull. 125, 260–270. doi: 10.1016/J.MARPOLBUL.2017.08.048
Cau, A., Bellodi, A., Moccia, D., Mulas, A., Pesci, P., Cannas, R., et al. (2018). Dumping to the abyss: single-use marine litter invading bathyal plains of the Sardinian margin (Tyrrhenian Sea). Mar. Pollut. Bull. 135, 845–851. doi: 10.1016/j.marpolbul.2018.08.007
Chiba, S., Saito, H., Fletcher, R., Yogi, T., Kayo, M., Miyagi, S., et al. (2018). Human footprint in the abyss: 30 year records of deep-sea plastic debris. Mar. Policy 96, 204–212. doi: 10.1016/j.marpol.2018.03.022
CIESM (2014). “Marine litter in the Mediterranean and Black Seas,” in CIESM Workshop Monograph n° 46, ed. F. Briand (Monaco: CIESM), 180. doi: 10.1016/j.marpolbul.2014.09.044
Cózar, A., Martí, E., Duarte, C. M., García-de-Lomas, J., van Sebille, E., Ballatore, T. J., et al. (2017). The Arctic Ocean as a dead end for floating plastics in the North Atlantic branch of the Thermohaline Circulation. Sci. Adv. 3:e1600582. doi: 10.1126/sciadv.1600582
D’Onghia, G., Calculli, C., Capezzuto, F., Carlucci, R., Carluccio, A., Grehan, A., et al. (2017). Anthropogenic impact in the Santa Maria di Leuca cold-water coral province (Mediterranean Sea): observations and conservation straits. Deep Sea Res. Part II Top. Stud. Oceanogr. 145, 87–101. doi: 10.1016/J.DSR2.2016.02.012
De Lucia, V. (2017). The Arctic environment and the BBNJ negotiations. Special rules for special circumstances? Mar. Policy 86, 234–240. doi: 10.1016/J.MARPOL.2017.09.011
Debrot, A. O., Vinke, E., van der Wende, G., Hylkema, A., and Reed, J. K. (2014). Deepwater marine litter densities and composition from submersible video-transects around the ABC-islands, Dutch Caribbean. Mar. Pollut. Bull. 88, 361–365. doi: 10.1016/j.marpolbul.2014.08.016
Ezraty, R., Girard-Ardhuin, F., Piollé, J., Kaleschke, L., and Heygster, G. (2007). Arctic and Antarctic Sea Ice Concentration and Arctic Sea Ice Drift Estimated from Special Sensor Microwave Data. Brest: IFREMER.
Fazey, F. M. C., and Ryan, P. G. (2016). Biofouling on buoyant marine plastics: an experimental study into the effect of size on surface longevity. Environ. Pollut. 210, 354–360. doi: 10.1016/j.envpol.2016.01.026
Galgani, F., Leaute, J., Moguedet, P., Souplet, A., Verin, Y., Carpentier, A., et al. (2000). Litter on the sea floor along European coasts. Mar. Pollut. Bull. 40, 516–527. doi: 10.1016/S0025-326X(99)00234-9
Gerigny, O., Brun, M., Fabri, M. C., Tomasino, C., Le Moigne, M., Jadaud, A., et al. (2019). Seafloor litter from the continental shelf and canyons in French Mediterranean Water: distribution, typologies and trends. Mar. Pollut. Bull. 146, 653–666. doi: 10.1016/J.MARPOLBUL.2019.07.030
German, C. R., Ramirez-Llodra, E., Baker, M. C., and Tyler, P. A. (2011). Deep-water chemosynthetic ecosystem research during the census of marine life decade and beyond: a proposed deep-ocean road map. PLoS One 6:e23259. doi: 10.1371/journal.pone.0023259
Geyer, R., Jambeck, J. R., and Law, K. L. (2017). Production, use, and fate of all plastics ever made. Sci. Adv. 3:e1700782. doi: 10.1126/sciadv.1700782
Goddijn-Murphy, L., Peters, S., van Sebille, E., James, N. A., and Gibb, S. (2018). Concept for a hyperspectral remote sensing algorithm for floating marine macro plastics. Mar. Pollut. Bull. 126, 255–262. doi: 10.1016/J.MARPOLBUL.2017.11.011
Jambeck, J. R., Geyer, R., Wilcox, C., Siegler, T. R., Perryman, M., Andrady, A., et al. (2015). Plastic waste inputs from land into the ocean. Science 347, 768–771. doi: 10.1126/science.1260352
Kanhai, L. D. K., Gårdfeldt, K., Lyashevska, O., Hassellöv, M., Thompson, R. C., and O’Connor, I. (2018). Microplastics in sub-surface waters of the Arctic Central Basin. Mar. Pollut. Bull. 130, 8–18. doi: 10.1016/J.MARPOLBUL.2018.03.011
Keller, A. A., Fruh, E. L., Johnson, M. M., Simon, V., and McGourty, C. (2010). Distribution and abundance of anthropogenic marine debris along the shelf and slope of the US West Coast. Mar. Pollut. Bull. 60, 692–700. doi: 10.1016/j.marpolbul.2009.12.006
Kühn, S., Schaafsma, F. L., van Werven, B., Flores, H., Bergmann, M., Egelkraut-Holtus, M., et al. (2018). Plastic ingestion by juvenile polar cod (Boreogadus saida) in the Arctic Ocean. Polar Biol. 41, 1269–1278. doi: 10.1007/s00300-018-2283-8
Lalande, C., Bauerfeind, E., and Nöthig, E. (2011). Downward particulate organic carbon export at high temporal resolution in the eastern Fram Strait: influence of Atlantic Water on flux composition. Mar. Ecol. Prog. Ser. 440, 127–136. doi: 10.3354/meps09385
Langenkämper, D., Zurowietz, M., Schoening, T., and Nattkemper, T. W. (2017). BIIGLE 2.0 - browsing and annotating large marine image collections. Front. Mar. Sci. 4:83. doi: 10.3389/fmars.2017.00083
Law, K. L. (2017). Plastics in the marine environment. Ann. Rev. Mar. Sci. 9, 205–229. doi: 10.1146/annurev-marine-010816-060409
Law, K. L., Morét-Ferguson, S. E., Goodwin, D. S., Zettler, E. R., DeForce, E., Kukulka, T., et al. (2014). Distribution of surface plastic debris in the Eastern Pacific Ocean from an 11-year data Set. Environ. Sci. Technol. 48, 4732–4738. doi: 10.1021/es4053076
Law, K. L., Moret-Ferguson, S., Maximenko, N. A., Proskurowski, G., Peacock, E. E., Hafner, J., et al. (2010). Plastic accumulation in the North Atlantic Subtropical Gyre. Science 329, 1185–1188. doi: 10.1126/science.1192321
Lebreton, L. C. M., van der Zwet, J., Damsteeg, J.-W., Slat, B., Andrady, A., and Reisser, J. (2017). River plastic emissions to the world’s oceans. Nat. Commun. 8:15611. doi: 10.1038/ncomms15611
Lebreton, L., Slat, B., Ferrari, F., Sainte-Rose, B., Aitken, J., Marthouse, R., et al. (2018). Evidence that the Great Pacific Garbage Patch is rapidly accumulating plastic. Sci. Rep. 8:4666. doi: 10.1038/s41598-018-22939-w
LITTERBASE (2019). Online Portal for Marine Litter. Available online at: https://litterbase.awi.de/ (accessed September 1, 2019).
Lobelle, D., and Cunliffe, M. (2011). Early microbial biofilm formation on marine plastic debris. Mar. Pollut. Bull. 62, 197–200. doi: 10.1016/J.MARPOLBUL.2010.10.013
Long, M., Paul-Pont, I., Hégaret, H., Moriceau, B., Lambert, C., Huvet, A., et al. (2017). Interactions between polystyrene microplastics and marine phytoplankton lead to species-specific hetero-aggregation. Environ. Pollut. 228, 454–463. doi: 10.1016/j.envpol.2017.05.047
Lusher, A. L., Tirelli, V., O’Connor, I., and Officer, R. (2015). Microplastics in Arctic polar waters: the first reported values of particles in surface and sub-surface samples. Sci. Rep. 5:14947. doi: 10.1038/srep14947
Maes, T., Barry, J., Leslie, H. A., Vethaak, A. D., Nicolaus, E. E. M., Law, R. J., et al. (2018). Below the surface: twenty-five years of seafloor litter monitoring in coastal seas of North West Europe (1992–2017). Sci. Total Environ. 630, 790–798. doi: 10.1016/j.scitotenv.2018.02.245
Meyer-Kaiser, K., Bergmann, M., Soltwedel, T., and Klages, M. (2019). Recruitment of Arctic deep-sea invertebrates: results from a long-term hard-substrate colonization experiment at the Long-Term Ecological Research observatory HAUSGARTEN. Limnol. Oceanogr. 64, 1924–1938. doi: 10.1002/lno.11160
Miyake, H., Shibata, H., and Furushima, Y. (2011). “Deep-sea litter study using deep-sea observation tools,” in Interdisciplinary Studies on Environmental Chemistry—Marine Environmental Modeling & Analysis, eds E. Omori, X. Guo, N. Yoshie, N. Fujii, I. C. Handoh, A. Isobe, et al. (Tokyo: TERRAPUB), 261–269.
Mordecai, G., Tyler, P. A., Masson, D. G., and Huvenne, V. A. I. (2011). Litter in submarine canyons off the west coast of Portugal. Deep. Res. Part II Top. Stud. Oceanogr. 58, 2489–2496. doi: 10.1016/j.dsr2.2011.08.009
Morgana, S., Ghigliotti, L., Estévez-Calvar, N., Stifanese, R., Wieckzorek, A., Doyle, T., et al. (2018). Microplastics in the Arctic: a case study with sub-surface water and fish samples off Northeast Greenland. Environ. Pollut. 242, 1078–1086. doi: 10.1016/j.envpol.2018.08.001
Nelms, S., Coombes, C., Foster, L., Galloway, T., Godley, B., Lindeque, P., et al. (2017). Marine anthropogenic litter on British beaches: a 10-year nationwide assessment using citizen science data. Sci. Total Environ. 579, 1399–1409. doi: 10.1016/j.scitotenv.2016.11.137
Nielsen, J., Hedeholm, R. B., Simon, M., and Steffensen, J. F. (2014). Distribution and feeding ecology of the Greenland shark (Somniosus microcephalus) in Greenland waters. Polar Biol. 37, 37–46. doi: 10.1007/s00300-013-1408-3
Obbard, R. W., Sadri, S., Wong, Y. Q., Khitun, A. A., Baker, I., and Thompson, R. C. (2014). Global warming releases microplastic legacy frozen in Arctic Sea ice. Earths Future 2, 315–320. doi: 10.1002/2014EF000240
Ostle, C., Thompson, R. C., Broughton, D., Gregory, L., Wootton, M., and Johns, D. G. (2019). The rise in ocean plastics evidenced from a 60-year time series. Nat. Commun. 10:1622. doi: 10.1038/s41467-019-09506-1
Peeken, I., Primpke, S., Beyer, B., Gütermann, J., Katlein, C., Krumpen, T., et al. (2018). Arctic sea ice is an important temporal sink and means of transport for microplastic. Nat. Commun. 9:1505. doi: 10.1038/s41467-018-038255
Peng, X., Chen, M., Chen, S., Dasgupta, S., Xu, H., Ta, K., et al. (2018). Microplastics contaminate the deepest part of the world’s ocean. Geochem. Perspect. Lett. 9, 1–5. doi: 10.7185/geochemlet.1829
Peng, X., Dasgupta, S., Zhong, G., Du, M., Xu, H., Chen, M., et al. (2019). Large debris dumps in the northern South China Sea. Mar. Pollut. Bull. 142, 164–168. doi: 10.1016/j.marpolbul.2019.03.041
Pham, C. K., Ramirez-Llodra, E., Alt, C. H. S., Amaro, T., Bergmann, M., Canals, M., et al. (2014). Marine litter distribution and density in european seas, from the shelves to deep basins. PLoS One 9:e95839. doi: 10.1371/journal.pone.0095839
Pierdomenico, M., Casalbore, D., and Chiocci, F. L. (2019). Massive benthic litter funnelled to deep sea by flash-flood generated hyperpycnal flows. Sci. Rep. 9:5330. doi: 10.1038/s41598-019-41816-8
PlasticsEurope (2019). Plastics- the Facts 2019: An Analysis of European Latest Plastics Production, Demand and Waste Data. Available online at: www.plasticseurope.org (accessed September 1, 2019).
Porter, A., Lyons, B. P., Galloway, T. S., and Lewis, C. (2018). Role of marine snows in microplastic fate and bioavailability. Environ. Sci. Technol. 52, 7111–7119. doi: 10.1021/acs.est.8b01000
Ryan, P. G. (2015). “A brief history of marine litter research,” in Marine Anthropogenic Litter, eds M. Bergmann, L. Gutow, and M. Klages (Cham: Springer International Publishing), 1–25. doi: 10.1007/978-3-319-16510-3_1
Ryan, P. G., Weideman, E. A., Perold, V., Durholtz, D., and Fairweather, T. P. (2020). A trawl survey of seafloor macrolitter on the South African continental shelf. Mar. Pollut. Bull. 150:110741. doi: 10.1016/J.MARPOLBUL.2019.110741
Schlining, K., von Thun, S., Kuhnz, L., Schlining, B., Lundsten, L., Jacobsen Stout, N., et al. (2013). Debris in the deep: using a 22-year video annotation database to survey marine litter in Monterey Canyon, central California, USA. Deep Sea Res. Part I Oceanogr. Res. Pap. 79, 96–105. doi: 10.1016/j.dsr.2013.05.006
Schoening, T., Kuhn, T., Bergmann, M., and Nattkemper, T. W. (2015). DELPHI–fast and adaptive computational laser point detection and visual footprint quantification for arbitrary underwater image collections. Front. Mar. Sci. 2:20. doi: 10.3389/fmars.2015.00020
Schulz, M., Bergmann, M., von Juterzenka, K., and Soltwedel, T. (2010). Colonisation of hard substrata along a channel system in the deep Greenland Sea. Polar Biol. 33, 1359–1369. doi: 10.1007/s00300-010-0825-9
Schulz, M., Krone, R., Dederer, G., Wätjen, K., and Matthies, M. (2015). Comparative analysis of time series of marine litter surveyed on beaches and the seafloor in the southeastern North Sea. Mar. Environ. Res. 106, 61–67. doi: 10.1016/j.marenvres.2015.03.005
Shimanaga, M., and Yanagi, K. (2016). The Ryukyu Trench may function as a “depocenter” for anthropogenic marine litter. J. Oceanogr. 72, 895–903. doi: 10.1007/s10872-016-0388-7
Soltwedel, T., Bauerfeind, E., Bergmann, M., Bracher, A., Budaeva, N., Busch, K., et al. (2016). Natural variability or anthropogenically-induced variation? Insights from 15 years of multidisciplinary observations at the arctic marine LTER site HAUSGARTEN. Ecol. Indic. 65, 89–102. doi: 10.1016/J.ECOLIND.2015.10.001
Spreen, G., Kaleschke, L., and Heygster, G. (2008). Sea ice remote sensing using AMSR-E 89-GHz channels. J. Geophys. Res. 113:C02S03. doi: 10.1029/2005JC003384
Suaria, G., Avio, C. G., Mineo, A., Lattin, G. L., Magaldi, M. G., Belmonte, G., et al. (2016). The Mediterranean Plastic Soup: synthetic polymers in Mediterranean surface waters. Sci. Rep. 6:37551. doi: 10.1038/srep37551
Taylor, J., Krumpen, T., Soltwedel, T., Gutt, J., and Bergmann, M. (2017). Dynamic benthic megafaunal communities: assessing temporal variations in structure, composition and diversity at the Arctic deep-sea observatory HAUSGARTEN between 2004 and 2015. Deep Sea Res. Part I Oceanogr. Res. Pap. 122, 81–94. doi: 10.1016/J.DSR.2017.02.008
Tekman, M. B., Krumpen, T., and Bergmann, M. (2017). Marine litter on deep Arctic seafloor continues to increase and spreads to the North at the HAUSGARTEN observatory. Deep Sea Res. Part I Oceanogr. Res. Pap. 120, 88–99. doi: 10.1016/J.DSR.2016.12.011
Tekman, M. B., Wekerle, C., Lorenz, C., Primpke, S., Hasemann, C., Gerdts, G., et al. (2020). Tying up loose ends of microplastic pollution in the Arctic: distribution from the sea surface, through the water column to deep-sea sediments at the HAUSGARTEN observatory. Environ. Sci. Technol. 54, 4079–4090. doi: 10.1021/acs.est.9b06981
Trevail, A. M., Gabrielsen, G. W., Kühn, S., and Van Franeker, J. A. (2015). Elevated levels of ingested plastic in a high Arctic seabird, the northern fulmar (Fulmarus glacialis). Polar Biol. 38, 975–981. doi: 10.1007/s00300-015-1657-4
Tubau, X., Canals, M., Lastras, G., Rayo, X., Rivera, J., and Amblas, D. (2015). Marine litter on the floor of deep submarine canyons of the Northwestern Mediterranean Sea: the role of hydrodynamic processes. Prog. Oceanogr. 134, 379–403. doi: 10.1016/J.POCEAN.2015.03.013
van den Beld, I. M. J., Guillaumont, B., Menot, L., Bayle, C., and Bourillet, J.-F. (2017). Marine litter in submarine canyons of the Bay of Biscay. Deep Sea Res. Part II Top. Stud. Oceanogr. 145, 142–152. doi: 10.1016/J.DSR2.2016.04.013
van Sebille, E., Wilcox, C., Lebreton, L., Maximenko, N., Hardesty, B. D., van Franeker, J. A., et al. (2015). A global inventory of small floating plastic debris. Environ. Res. Lett. 10:124006. doi: 10.1088/1748-9326/10/12/124006
Watters, D. L., Yoklavich, M. M., Love, M. S., and Schroeder, D. M. (2010). Assessing marine debris in deep seafloor habitats off California. Mar. Pollut. Bull. 60, 131–138. doi: 10.1016/j.marpolbul.2009.08.019
Wei, C. L., Rowe, G. T., Nunnally, C. C., and Wicksten, M. K. (2012). Anthropogenic “Litter” and macrophyte detritus in the deep Northern Gulf of Mexico. Mar. Pollut. Bull. 64, 966–973. doi: 10.1016/j.marpolbul.2012.02.015
Woodall, L. C., Robinson, L. F., Rogers, A. D., Narayanaswamy, B. E., and Paterson, G. L. J. (2015). Deep-sea litter: a comparison of seamounts, banks and a ridge in the Atlantic and Indian Oceans reveals both environmental and anthropogenic factors impact accumulation and composition. Front. Mar. Sci. 2:3. doi: 10.3389/fmars.2015.00003
Keywords: litter, seafloor, plastic, sea ice, Arctic, marine debris, deep sea, pollution
Citation: Parga Martínez KB, Tekman MB and Bergmann M (2020) Temporal Trends in Marine Litter at Three Stations of the HAUSGARTEN Observatory in the Arctic Deep Sea. Front. Mar. Sci. 7:321. doi: 10.3389/fmars.2020.00321
Received: 16 October 2019; Accepted: 21 April 2020;
Published: 19 May 2020.
Edited by:
Christopher Kim Pham, University of the Azores, PortugalReviewed by:
Alessandro Cau, University of Cagliari, ItalyThomais Vlachogianni, Mediterranean Information Office for Environment, Culture and Sustainable Development, Greece
Copyright © 2020 Parga Martínez, Tekman and Bergmann. This is an open-access article distributed under the terms of the Creative Commons Attribution License (CC BY). The use, distribution or reproduction in other forums is permitted, provided the original author(s) and the copyright owner(s) are credited and that the original publication in this journal is cited, in accordance with accepted academic practice. No use, distribution or reproduction is permitted which does not comply with these terms.
*Correspondence: Melanie Bergmann, TWVsYW5pZS5CZXJnbWFubkBhd2kuZGU=