- 1Red Sea Research Center, King Abdullah University of Science and Technology, Thuwal, Saudi Arabia
- 2Beacon Development, King Abdullah University of Science and Technology, Thuwal, Saudi Arabia
Ultraviolet B (UVB) radiation has recently been recognized as a major stressor for marine vertebrates, particularly fish confined to aquaculture cages. Here, the harmful effects of UVB radiation on gilthead seabream (Sparus aurata), which is a widely cultured species, were investigated. Seabream juveniles were exposed to three UVB conditions (UVB-H – high UVB, 12 kJ m−2 d−1; UVB-M - moderate UVB, 6 kJ m−2 d−1; UVB-L – low UVB, 2.4 kJ m−2 d−1) that are representative of natural underwater UVB levels throughout the water column in the Red Sea. One experimental treatment without UVB exposure was used as a control. The adverse effects of UVB were evaluated after short- (10 days) and long-term (43 days) exposure. The results indicated that short- and long-term exposure to UVB retarded growth and decreased survival rates. UVB exposure resulted in behavioral changes, mainly in UVB-H and UVB-M exposed fish. Swimming activity was reduced; most of the fish tried to avoid exposure and showed a stationary behavior with slow caudal and dorsal fins movements (UVB-H), or a slow displacement behavior (UVB-M). Moreover, a reduction in appetite, reflected by a remarkable increase in the time required to consume the food was observed. Lesions on the skin occurred in the three UVB treatments, and the incidence and severity increased under long-term UVB exposure. Also, physiological changes were observed, including a decrease in total protein and total cholesterol concentrations (all UVB treatments). A potential modulation of the innate immune system (reduction of total anti-protease and total peroxidase activities) was observed (UVB-M, UVB-L). The present results suggest that exposure to solar underwater UVB radiation levels has the potential to interfere and affect the health of S. aurata. Indeed, aquaculture fish species growing at locations where water transparency and UVB incidence is as high as the Mediterranean in summer, and the Red Sea year-round, may be affected, and their welfare, resistance to pathogens, and survival may be compromised. Strategies should be considered to mitigate the adverse effects of UVB exposure, such as deeper and more-shaded cages, or the development of functional foods.
Introduction
The adverse effects of ultraviolet radiation (UVR) on fish have been documented since the beginning of the 20th century (reviewed by Häder et al., 2007, 2015; Lawrence et al., 2019). Increased mortality and decreased hatching success, together with higher incidence of morphological abnormalities and DNA damage, are the most common adverse effects reported in embryos and larvae (Applegate and Ley, 1988; Mitchell et al., 2009; Fukunishi et al., 2010; Aksakal and Ciltas, 2018). The early development stages appear to be very sensitive to ultraviolet A (UVA) and ultraviolet B (UVB) radiation, which both cause severe damage. The tolerance of juveniles and adult fish to the detrimental effects of UVR has also been demonstrated (Dahms and Lee, 2010). Many fish species in their later development stages are sensitive to both UVA and UVB (Jokinen et al., 2008; Rick et al., 2014; Sayed et al., 2016; Seebacher et al., 2016). UVB negatively affected the growth and body condition of Atlantic salmon (Salmo salar) and three-spined stickleback (Gasterosteus aculeatus) under long-term exposure of more than 50 days (Jokinen et al., 2008; Vitt et al., 2017). The energy allocation used by fish during the DNA repairing mechanisms may prevent the use of energy for digestion or growth (Jokinen et al., 2008, 2011; Vitt et al., 2017). A limited number of studies have reported changes in behavior due to UVB exposure. Restless behavior, an increase or decrease in swimming activity, a reduction of predatory performance, and preference for habitat selection are some of the reported behavioral changes observed in UVB-exposed fish (Alemanni et al., 2003; Pulgar et al., 2015; Seebacher et al., 2016; Valiñas and Walter Helbling, 2016).
High natural UVR levels have deleterious consequences for aquaculture fish species inhabiting shallow waters, such as tanks, ponds, and cages in offshore areas (Bullock, 1982; Rodger, 1991; Lowe and Goodman-Lowe, 1996; Zagarese and Williamson, 2001). Handinger et al. (1997) suggested that UVR can have a significant role in the infection of salmonids by Flexibacter maritimus. UVB exposure induces immunosuppression in rainbow trout (Oncorhynchus mykiss) and Mozambique tilapia (Oreochromis mossambicus), thus decreasing their resistance to infection by opportunistic agents like bacteria and parasites. Increased mortality observed in these two species most probably resulted because of the high incidence of infections (Markkula et al., 2007; Subramani et al., 2015). Losses in the production of farmed Atlantic salmon and rainbow trout exposed to ambient UVR has been reported, in which fish showed severe dorsal skin lesions (Bullock, 1988). Indeed, damage and the occurrence of lesions in the skin are the most frequently documented detrimental effects of UVB exposure in fish. Signs of sunburn, hyperpigmentation, disruption of the mucous layer (e.g., a decrease in the number of mucus-producing cells, hyperplasia of superficial mucous cells), epidermis edema, necrosis, and sloughing of the epidermis are some of the consequences of UVB overexposure on fish skin (Bullock, 1988; Blazer et al., 1997; Manek et al., 2012). In addition, UVB is also responsible for inducing melanoma in Xiphophorus hybrid fish (Mitchell et al., 1993, 2010).
UVR exposure has also been demonstrated to induce metabolic and physiologic responses in fish. For example, changes in cortisol, hematocrit, and the plasma total protein content resulted from short- and long-term UVR exposure in fathead minnow (Pimephales promelas), rainbow trout, roach (Rutilus rutilus), common carp (Cyprinus carpio), Atlantic salmon, and North African catfish (Clarias gariepinus) (Salo et al., 1998, 2000; Markkula et al., 2006; Jokinen et al., 2008; Manek et al., 2012). It has also been established that UVB exposure can induce immunological suppression and affect the susceptibility of fish to diseases. UVB-induced immunomodulation has been demonstrated in peripheral blood, spleen and head kidney (Salo et al., 1998; Jokinen et al., 2000; Markkula et al., 2009). The occurrence of lymphopenia and granulocytosis in peripheral blood is considered a stress indicator of UVB exposure in roach, common carp, rainbow trout, and three-spined stickleback, even after long-term exposure (Salo et al., 1998; Jokinen et al., 2000; Markkula et al., 2005, 2007; Vitt et al., 2017). Moreover, in Atlantic salmon, long-term exposure to UVB resulted in a decrease of both immunoglobulin M (IgM) levels and complement bacteriolytic activity in the plasma (Jokinen et al., 2008, 2011).
The impacts of UVB in marine biota are expectedly more severe in oligotrophic and highly transparent waters, mostly due to deeper UVB penetration (Morel et al., 2007; Al-Aidaroos et al., 2014; Garcia-Corral et al., 2015; Overmans and Agustí, 2019). From an economic point of view, the damage caused by UVB on sensitive species can result in a decline of harvesting in fisheries, and also significant losses in the aquaculture sector (Zagarese and Williamson, 2001; Barnes et al., 2019; Williamson et al., 2019). Gilthead seabream (Sparus aurata) is a temperate species found in the Mediterranean and along the eastern North Atlantic waters, from the British Isles to the Canary Islands. Most seabream for consumption comes from aquaculture production, with Greece, Turkey, Spain, and Italy being the major producers (FAO, 2019). The Mediterranean Sea is highly transparent in summer, allowing for deeper penetration of harmful UVB levels (Tedetti and Sempéré, 2006; Llabrés et al., 2010). Furthermore, the Kingdom of Saudi Arabia (KSA) has plans to prioritize the aquaculture sector to respond to the increasing demand for seafood by the Saudi population. However, local fish aquaculture cage production in the Red Sea is exclusively based on non-native species, namely the S. aurata (FAO, 2013; Khan et al., 2018). Thus, since the Red Sea is in a tropical region that receives high levels of solar UVR throughout the year (Overmans and Agustí, 2019), seabream growing in cages can potentially be exposed to higher UVB doses for extended periods compared to their natural habitat in the Mediterranean. To the best of our knowledge, no previous studies have evaluated the sensitivity of S. aurata to UVB radiation, and considering the aspects outlined above, many questions arise on the tolerance of S. aurata to current UVB levels in these oligotrophic waters, such as: Is the growth and survival of seabream kept in confinement affected by short- and long-term UVB exposure? What are the behavioral changes, primary physiological responses, and impacts on the immune function associated with UVB exposure? Are these UVB levels inducing damage to the skin? Finally, are the detrimental effects of UVB exposure cumulative and dose-dependent?
To this end, the aim of the present study was to assess the sensitivity of S. aurata to underwater UVB radiation levels. S. aurata juveniles were exposed to different UVB experimental treatments by simulating irradiance reduction throughout the water column (e.g., we replicate UVB levels that were recorded between 2 and 10 m depth at two fish farms in the Red Sea). The effects of short- (10 days) and long-term (43 days) UVB exposure were evaluated by measuring the following potential responses: (1) growth reduction and loss of body condition (growth and animal/tissue indexes); (2) behavioral changes; (3) presence of skin lesions/damage (sunburn); (4) stress, metabolic and physiological responses (cortisol, total protein, glucose, lactate and total cholesterol levels in the plasma); and (5) immune function suppression (anti-protease and total peroxidase activities, C reactive protein and prothrombin levels in the plasma).
Materials and Methods
Ethics Statement
The present study was carried out in the wet laboratory facilities of the Coastal and Marine Resources Core (CMOR) lab at King Abdullah University of Science and Technology (KAUST), Saudi Arabia. We performed the UVB exposure experiments during April–June 2018 with gilthead seabream (Sparus aurata) juveniles in accordance with Saudi Arabia’s National Committee of Bioethics (NCBE) code of practice and under the approval of the KAUST Institutional Animal Care and Use Committee [IACUCML(SA)#18-04-016].
Animals and Experimental Design
Sparus aurata juveniles with approximately 10–15 g of total weight were obtained from a commercial fish farm (Tharawat Seas Company, Ar Rayis, KSA), and the Fish Health and Safety Laboratory (Fisheries Research Center, Jeddah, KSA) provided the fish’s health profile. Fish were transported to the quarantine area in the CMOR laboratory facilities (following KAUST university guidelines), where the fish were kept for 30 days until the beginning of the experiment. A total of 320 specimens with similar biometric characteristics (total weight, 32.03 ± 4.17 g) were randomly and equitably distributed in eight rectangular-shaped incubating glass tanks (4 treatments × 2 replicate tanks; each tank with 300 L each of total volume, 40 specimens in each tank; Supplementary Figure S1), in a flow-through system, and kept for acclimation for 2 weeks before the UVB exposure experiments. The water flow rate was fixed to 0.3 m3 h−1 to ensure tank self-cleaning and checked the flow rate once a week. Water temperature, salinity, pH, and dissolved oxygen (DO) were daily checked using a multi-parameter measuring instrument (YSI, Professional Plus - Pro Plus, United States). The specimens were kept throughout the whole experiment under the following established conditions: water temperature (26.98 ± 0.78°C), salinity (40.48 ± 0.13‰), pH (8.09 ± 0.07), and DO (>6 mg/L, 92.90 ± 5.97%). Ammonia, nitrite and nitrate levels were weekly checked through colorimetric tests (Tropic Marin, United States), and kept below the detection limits. The photoperiod during the experiment was 12 h light and 12 h dark (12L:12D). For each tank, four daylight tubes (FH039W Reef White 10000, Aqua Medic, Germany) emitted the PAR (Photosynthetically Active Radiation) irradiance. PAR inside the tanks was also daily monitored and maintained at 36.8 ± 2.5 μE m−2 s−1. The fish were manually fed twice a day (3–5% average body weight, BW) with a commercial diet (Arasco, Saudi Arabia) based on 94.4% of dry matter, of which 52.5% was crude protein, 13.3% was crude fat, with a raw energy 21.0 MJ kg−1. After the acclimation period, fish were exposed to different UVB treatments representative of natural UVB underwater levels registered in the Mediterranean and the Red Sea (Supplementary Figure S1). We describe the methodology used to establish the UVB irradiance levels required in this study in the following section.
UVB Exposure
The UVB irradiances and daily doses applied in this study were based on the data available from the patterns of UVB attenuation and daily UVB doses in oligotrophic water, including the Mediterranean (mainly in the summer) and in the Red Sea (all year round), and based on the UVB attenuation profiles obtained in fish farms located in the central Red Sea coast of Saudi Arabia (please see Supplementary Note for detailed information). Supplementary Table S1 resumes the main published literature that showed information regarding the UVB exposure in the water column in both the Mediterranean and the Red Sea. For example, in the Mediterranean during the summer, the UVB daily doses at the water surface can vary between 11 and 41 kJ m−2 d−1, and the biologically effective depths of UVB ranged from 4.9 (Z10% – 305 nm) and 16 (Z10% – 310 nm) m, reviewed by Tedetti and Sempéré (2006). In the central Red Sea, the mean annual daily doses of UVB (280–315 nm) in 2017 at 0, 2, 4, 6, 8, and 10 m were 33.10 ± 7.84, 16.52 ± 4.46, 8.30 ± 2.57, 4.18 ± 1.48, 2.13 ± 0.85, and 1.09 ± 0.50 kJ m−2 d−1, respectively (Overmans and Agustí, 2020). Supplementary Table S2 shows the profiles of UVB attenuation obtained during March 2018 were in two fish farms located in the central Red Sea coast of Saudi Arabia, Tharawat Seas Company (Ar Rayis, KSA), and Tabuk Fisheries Company (Al Muwaileh, KSA). The maximum depth that the cages reach in the farms mentioned above is around 10–14 m.
Taking into consideration all the collected and available data described above, seabream juveniles were exposed to three different treatments representing natural underwater UVB levels from the Mediterranean and the Red Sea, simulating UVB reduction throughout the water column: (i) high UVB (UVB-H, 0.4 W m−2, 11.9 kJ m−2 d−1), (ii) moderate UVB (UVB-M, 0.21 W m−2, 6.1 kJ m−2 d−1, 50% of the irradiance of UVB-H), and (iii) low UVB (UVB-L, 0.10 W m−2, 2.5 kJ m−2 d−1, 20% of the irradiance of UVB-H). One experimental treatment without UVB exposure was used as a control (Supplementary Figure S1). The three chosen treatments are representative of the UVB irradiance/dose received by fish farms in the Red Sea: UVB-H - levels corresponding to the UVB radiation between 2 and 5 m depth; UVB-M – levels corresponding to the UVB radiation between 5 and 7 m depth; and UVB-L – levels corresponding to the UVB radiation between 7 and 10 m depth.
A lighting system using TL20W/12RS UVB Broadband lamps (Philips, Germany) was designed to simulate the required daily UVB doses. The UVB Broadband TL lamps emitted radiation in the ‘B’ bandwidth of the UV spectrum (290–315 nm). Two UVB lamps were used in the UVB-H treatment, whereas only one UVB lamp was used in the UVB-M and UVB-L treatments. The lighting systems were covered with different layers of a mosquito net with a mesh size of about 2 mm to obtain the required UVB irradiation for each experimental group. In the control tanks, the same lighting system without UVB lamps was installed to achieve the same shade as that of the UVB treatments. The distance between the tanks and the lighting system was approximately 40 cm. Fish were exposed daily for 8 h to the three different UVB conditions (from 10.00 AM to 6.00 PM). The UVR penetrating the water was daily measured in the tanks at different positions daily, using an underwater PMA2100 data-logging radiometer (Solar LightTM, United States) fitted with a UVB (280–320 nm) sensor. Before starting the experiment, adhesive tape was applied to each tank to divide it into three equal zones (top, middle, and bottom) (Supplementary Figure S1). The UVB irradiance levels were determined by measuring the irradiance at nine distinct positions within the experimental tanks, and the mean values were used to calculate the daily UVB doses for each treatment. The UVB exposure trial lasted for 43 days, and the different cumulative radiation dosages were tested by sampling fish on day 1 (short-term) and day 44 (long-term) of the experiment.
Sampling
Fish were sampled at two time-points to evaluate the harmful effects of short-term (10 days) and long-term (43 days) exposure to UVB radiation (Supplementary Figure S1). For each sampling point, 15 fish were randomly collected from each experimental tank (n = 30 per treatment). All the specimens were euthanized by immersion in an overdosed tricaine methanesulfonate (MS222) solution (150–200 mg/L; Sigma-Aldrich, United States) buffered with sodium bicarbonate (MS222: NaHCO3 - 1:2), sized and weighed (total length, TL, and total weight, TW). Each fish was photographed on both sides to evaluate the presence of skin lesions/damage (sunburn) due to UVB exposure. Before cervical sectioning, peripheral blood was collected by puncture of the caudal vein using a syringe previously treated with an anticoagulant (heparin ammonium salt from porcine 1,000 U mL−1 in saline solution 0.9% NaCl). The blood samples were then centrifuged (2,000 × g, 10 min, 4°C) to collect the plasma, which were stored at −80°C until further biochemical analysis. The liver, brain, and spleen were also sampled and weighted to determine the tissue fitness indexes (short- and long-term exposure/n = 30 fish from each treatment).
Behavior Assessment
The swimming/locomotor activity and feeding behavior were assessed during the whole experiment to detect any possible behavioral responses due to UVB exposure. The cameras for video recording (Sony, DCR-SX33) were mounted on a tripod and positioned it in front of the tank to document possible behavioral changes.
Swimming/Locomotor Activity
The swimming fish behavior was recorded in each tank for 10 min in the morning (daily, 1 h after starting UVB exposure) and 10 min in the afternoon (daily, 5–6 h after beginning UVB exposure) for a total of 9 days during the experiment (8th, 9th, 12th, 16th, 26th, 27th, 30th, 39th, and 40th day of UVB exposure). For each recorded video, the number of fish located in each tank zone (top, middle, bottom) were counted every 30 seconds (sec), representing a total of 20 observations. Between the 8th and 16th day of UVB exposure, a total of 160 observations for each treatment (80 per duplicate tank) was made. Between the 26th and 40th day of UVB exposure, a total of 200 observations for each treatment (100 per duplicate tank) was made. The procedure set out in Alemanni et al. (2003) was followed, where behavioral changes were classified in several categories: (a) a UVB avoidance strategy when fish remained at the bottom of the tank and at the lateral side of the tank where the UVB levels are slightly lower, (b) slow displacement behavior (“swimming smoothly”), (c) stationary behavior showing no movements other than the opercular, (d) stationary behavior with rapid caudal and dorsal fin movement; (e) stationary behavior with slow caudal and dorsal fin movement, and (f) rapid and erratic displacements.
Feeding Behavior
The behavior during feeding periods was recorded. Both feeding periods were recorded, in the morning (9 AM, 1 h before start the UVB exposure) and in the afternoon (between 2 and 3 PM, 4 h after UVB exposure commenced). The time needed to consume all the food (sec) was registered on the 8th, 9th, 10th, 23rd, 24th, 25th, 26th, 30th, 38th, and 39th day of UVB exposure. After 30 min of each feeding period, a visual confirmation of the consumption of all feeding pellets by fish was made.
Body Condition and Animal Fitness Indexes
The Weight gain rate (WGR,%) calculated according to the formula:
The Specific growth rate (SGR,%) calculated according to the formula:
The Fulton’s K index (K) was directly calculated from the biometric data to determine the fish condition, according to the formula:
where TW is the total weight, and TL is the total length (Ricker, 1975).
The relationship between fish total weight and the respective organ weight for liver (hepatosomatic index, HSI), spleen (splenosomatic index, SSI) and brain (i.e., brain to body mass ratio, BBratio) was calculated to provide information on liver and brain condition, using the following equations:
Skin Damage
The presence of superficial skin lesions (e.g., sunburn) was identified in S. aurata juveniles after short-term (10 days) and long-term (43 days) exposure to UVB radiation. Each fish was individually photographed on both sides of the body, and the presence of skin lesions/injuries (sunburn) for each UVB exposure period was monitored according to the following aspects: (a) percentage of fish showing lesions on the skin; (b) location of lesions (specific body region or whole-body); (c) severity of injuries (we defined four lesions categories after a visual inspection of all specimens: slight, moderate, severe, and extremely severe); (d) distribution of the four lesion types in affected fish. The terminology used to define the different types of skin lesions was based on the UVB-induced skin lesions described by Bullock (1988).
Plasma Biochemical and Innate Humoral Immunological Parameters
The plasma samples were used to assess the following biochemical and non-specific humoral immunological parameters: total protein, cortisol, glucose, lactate, total cholesterol, total anti-protease, and total peroxidase (PO) activities, C reactive protein (CRP) and prothrombin (PT). We analyzed total protein, cortisol, total anti-protease and total peroxidase activities, CRP and PT, in the samples from short- and long-term UVB exposure (10 and 43 days). A significant reduction in the food intake by the fish from the highest and middle UVB treatments was observed during the experiment, and thus, the glucose, lactate and total cholesterol concentrations were analyzed after 43 days of exposure.
The total protein (Bradford protein assay kit, Bio-Rad, United States), cortisol (Fish CORT ELISA Kit, MBS007869, MyBiosource, San Diego, CA, United States), glucose (MBS2540580, MyBiosource, San Diego, CA, United States), lactate (MBS169270, MyBiosource, San Diego, CA, United States), total cholesterol (MBS168109, MyBiosource, San Diego, CA, United States), C reactive protein (Fish CRP ELISA Kit, MBS016586, MyBiosource, San Diego, CA, United States) and prothrombin (Fish PT ELISA Kit, MBS037265, MyBiosource, San Diego, CA, United States) plasma concentrations were determined using commercial kits, and following the manufacturer’s instructions.
The methodology used for the total anti-protease activity was based on the ability of plasma anti-proteases to inhibit trypsin activity and is based on the method of Secombes (1990), which was modified by Hanif et al. (2004). Azocasein hydrolysis was assayed by incubating 20 μl of the plasma samples with 200 μl of phosphate buffer (0.1M, pH 7.0), 20 μl of trypsin (5 mg mL−1), and 250 μl of azocasein 2% for 2 h at 37°C. The reaction was stopped by adding 500 μl of 10% (w v−1) trichloroacetic acid (TCA) and incubated for 30 min at 22°C, followed by a centrifugation step at 10,000 × g. Hundred μl of the resultant supernatant and 100 μl 1 M NaOH were added to a 96-well plate and measured the absorbance at 450 nm. For the positive control, the phosphate buffer replaced the plasma, and for the negative control, the phosphate buffer replaced both plasma and trypsin solution. The inhibitory capacity of anti-protease was expressed in terms of percentage trypsin inhibition, using the positive control as described by Zuo and Woo (1997).
Total peroxidase activity (PO), which includes the myeloperoxidase, was measured based on the methodology described by Quade and Roth (1997). Thirty μl from each plasma sample were diluted with 120 μl of Hank’s Balanced Salt Solution (HBSS, Ca3 + 2/Mg3 + 2 -free, Thermo Fisher Scientific, United States) in a 96-well microtiter plate, and then incubated for 2 min with 50 μl of 20 mM 3,3′,5,5′-tetramethylbenzidine hydrochloride (TMB, Sigma-Aldrich, United States) and 50 μl of 5 mM H2O2. The reaction was stopped by adding 50 μl of 4 M sulfuric acid, and the absorbance was read at 450 nm in a microplate reader. The peroxidase activity (units ml−1) was determined considering that one unit of peroxidase produces an absorbance change of 1 OD.
For all the parameters, the analyses were done in duplicates, except for the total protein where triplicates were used. The total protein, cortisol, lactate, total cholesterol, total anti-protease, total PO, CRP, and PT were analyzed using the SpectraMax Paradigm Multi-Mode microplate reader (Molecular Devices, United States). Glucose was measured using a UV-1800 spectrophotometer (Shimadzu, Japan).
Statistical Analysis
Statistical analysis and data visualization were performed using JMP Pro 14 (SAS Institute Inc., Cary, United States) and SigmaPlot v10.0 (Systat Software, Inc., United States) to detect significant differences between the treatments (control, UVB-L, UVB-M, and UVB-H) and between short- and long-term exposure (within each treatment) for the following parameters: total length (TL), total weight (TW), weight gain rate (WGR), specific growth rate (SGR), Fulton’s K index (K), hepatosomatic index (HSI), splenosomatic index (SSI), brain to body mass ratio (BBratio), swimming behavior, total protein, cortisol, glucose, lactate, total cholesterol, anti-protease, total PO, CRP, and PT.
Differences between treatments and sampling points (short- and long-term exposure) for TL, TW, WGR, SGR, K, HIS, SSI, BBratio, total protein, cortisol, anti-protease, total PO, CRP, and PT were assessed using two-way ANOVA. At the long-term exposure, differences between treatments for glucose, lactate, and total cholesterol were assessed using one-way ANOVA. For the swimming behavior, a three-way ANOVA was used to assess significant differences between treatments (CTRL, UVB-L, UVB-M, UVB-H), tank zone (bottom, middle, top) and day exposure period (morning, afternoon) for sampling days 8th-16th, and 26th-40th. Before the ANOVA analysis, normality (Shapiro–Wilk) and homogeneity of variances (Levene’s test) were checked. Data were log-transformed, whenever necessary, to accomplish the assumptions of normality and homogeneity of variances required for ANOVA analysis. Tukey’s post hoc test was used for the multiple pairwise comparisons to detect for statistical significance. When normality tests failed, even for the log-transformed data, the statistical analysis was carried out using ANOVA Kruskal–Wallis on Ranks, and Dunn’s test was applied for the multiple pairwise comparisons. A value of P < 0.05 was taken as significant. Results are presented as mean ± standard deviation (SD).
Results
UVB Doses
The mean UVB irradiance, daily, and absolute UVB doses for each treatment are presented in Table 1. The irradiance values shown in Table 1 represent the mean of the irradiance measured in nine distinct positions in the tanks (three positions in each zone). The highest irradiance of UVB applied in the present experiment was 0.41 ± 0.06 W m−2 for UVB-H. UVB-H treated fish were exposed to a daily dose of 11.86 ± 1.79 kJ m−2, and the absolute dose for this treatment was 118.60 ± 17.94 kJ m−2 after 10 days of exposure. A humane endpoint for the UVB-H treatment was defined after 16 days of exposure due to the unexpectedly high mortality in the highest UVB treatment. Therefore, the fish who received the highest absolute UVB dose were the ones in the UVB-M treatment, after 43 days of exposure (260.64 ± 35.97 kJ m−2) (Table 1).
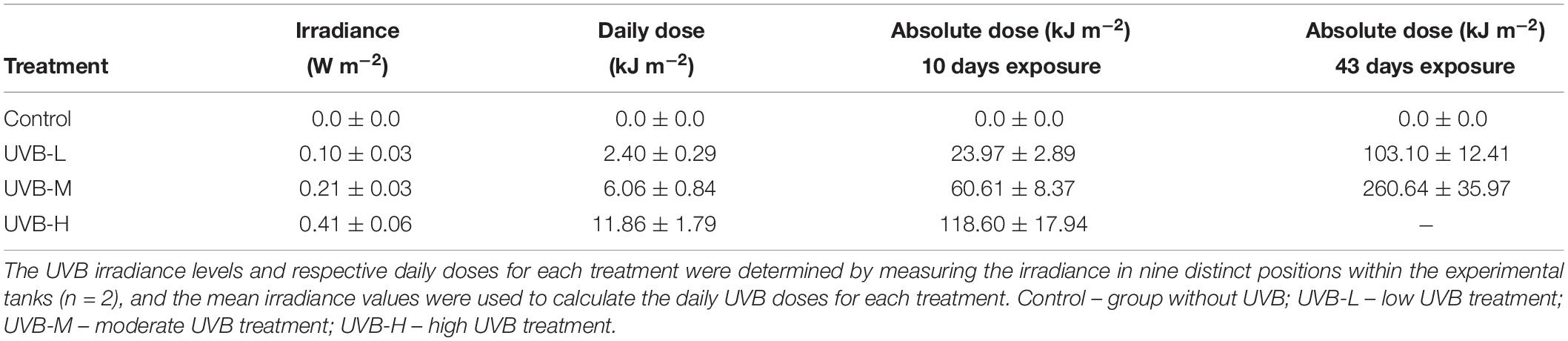
Table 1. Mean irradiance (W m−2), the daily dose (kJ m−2), and absolute dose (kJ m−2) of UVB used on the irradiated S. aurata juveniles.
For each treatment, UVB irradiance and UVB doses for each treatment (daily and absolute), in each tank zone, are presented in Supplementary Table S3. A consistent reduction in the daily UVB doses in the middle and bottom tank zones was observed across all UVB treatments when compared to the top tank zone, ranging from 34–39 and 62–72%, respectively (Supplementary Table S3).
Effects of UVB on Growth and Body Condition
In the first 10 days of exposure (short-term exposure), fish from the UVB-H and UVB-M treatments, exhibited lower total length (TL) and total weight (TW) than fish from the control treatment (Two-way ANOVA, UVB-H, P < 0.001; UVB-M, P < 0.05). However, no differences in TL or TW were observed in the UVB-L treatment (Two-way ANOVA, P > 0.05). No mortality was observed during the first 10 days of UVB exposure. After 10 days of exposure, the weight gain rate (WGR) and specific growth rate (SGR) decreased with increasing UVB doses (Two-way ANOVA, UVB-H, P < 0.001; UVB-M, P < 0.01). Similar animal conditions (Fulton’s K index – K, hepatosomatic index – HSI, and brain to body mass ratio – BBratio) were observed across all experimental groups (Two-way ANOVA P > 0.05). Fish exposed to UVB-M showed the highest SSI values (UVB-M/control, P = 0.009) (Table 2). Contrary to all other treatments, a 15% mortality was observed in UVB-H fish between 10 and 16 days of exposure.
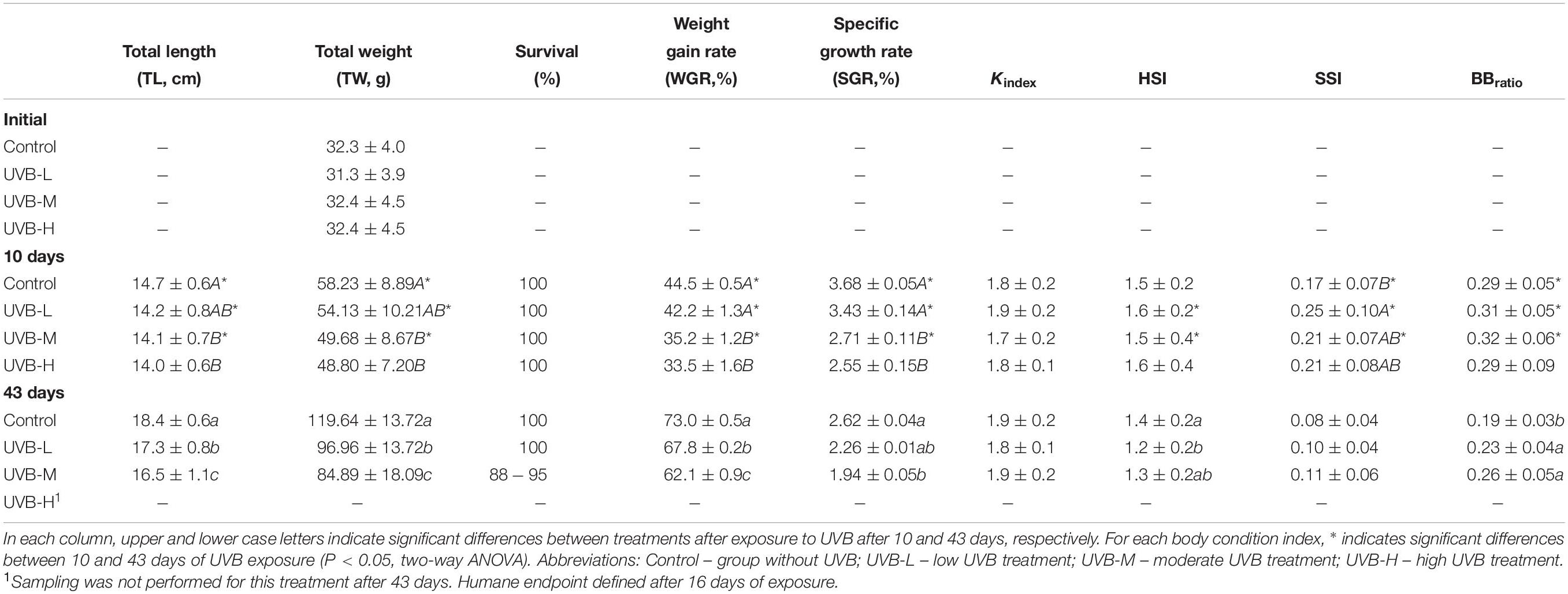
Table 2. Total length (TL, cm) and total weight (TW, g), survival (%), weight gain rate (WGR,%), specific growth rate (SGR, %), Fulton’s condition index (Kindex), hepatosomatic index (HSI), splenosomatic index (SSI) and brain-to-body mass ratio (BBratio) in S. aurata after 10 and 43 days of exposure to UVB (mean ± SD; n = 30).
After long-term exposure (43 days), the adverse effects of UVB on fish growth and body condition were more evident. The lowest TL) and TW were observed in UVB-M exposed fish (Two-way ANOVA, P < 0.001) compared to control. Both TW and TL of fish in the UVB-L treatment were lower than in the control (P < 0.001) (Table 2). Similarly, both TL and TW of fish in the UVB-L treatment were also decreased (Two-way ANOVA, P < 0.001) (Table 2). Eight percent mortality was observed in the UVB-M treatment, with no mortality observed in the control and UVB-L treatments. A decrease in the WGR, and SGR was observed at the end of the experiment in UVB-M exposed fish (Two-way ANOVA, P < 0.01) (Table 2). No differences between treatments were observed for Kindex. A decrease in the HSI was observed in fish exposed to the UVB-L treatment (ANOVA by Ranks, P < 0.05). No significant changes were observed for SSI. In the case of BBratio, an increase in the ratio was observed in both UVB-M and UVB-L treatments (Two-way ANOVA, P < 0.05) (Table 2).
Effects of UVB on Behavior
Swimming/Locomotor Activity
Exposure day: 8th-16th
Between 8 and 16 days of exposure, the swimming/locomotor behavior changed remarkably with an increase of the UVB doses (Figure 1A).
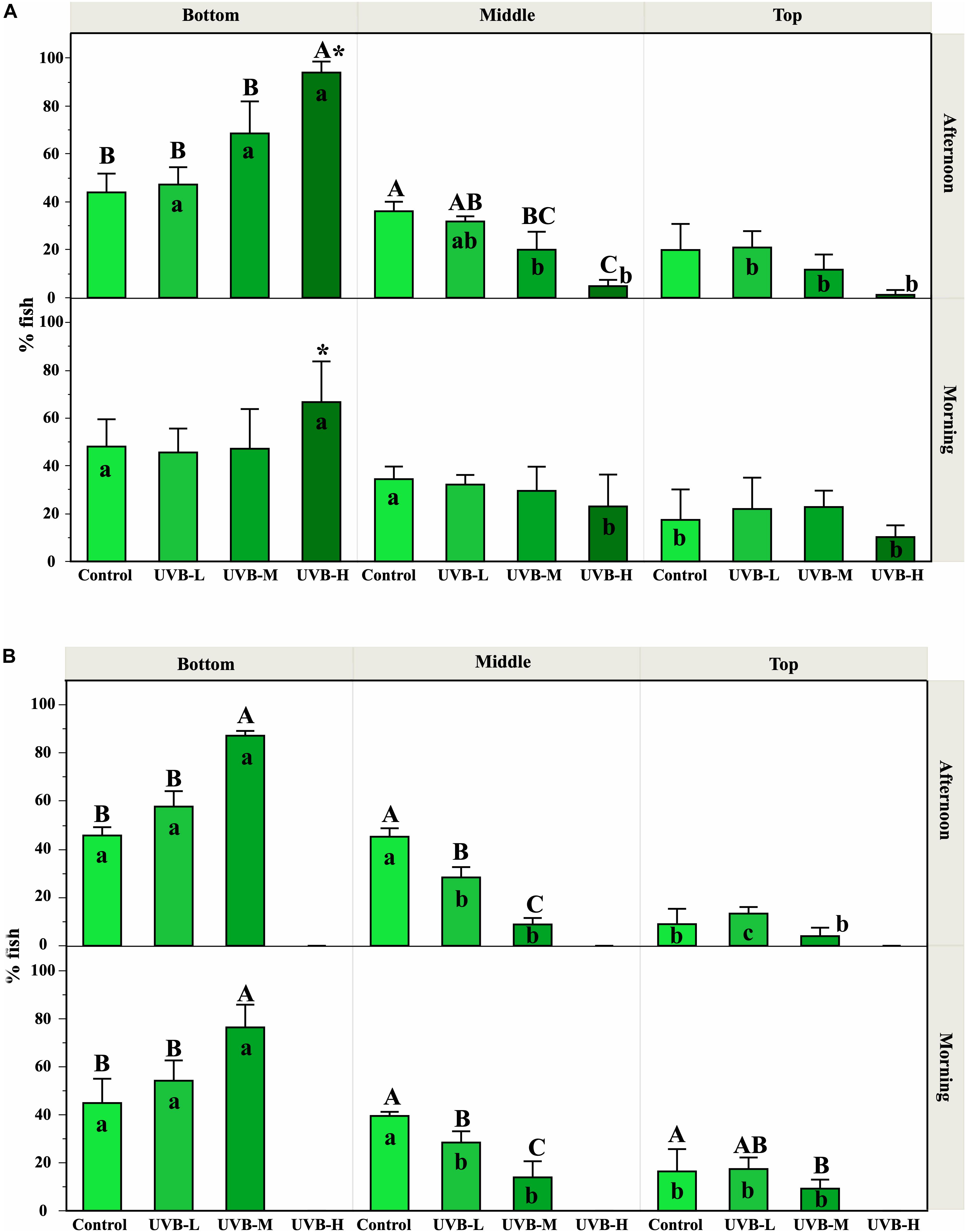
Figure 1. Effect of UVB on the S. aurata swimming behavior observed along the experimental time. The fish position inside the tanks was recorded in the morning (1 h after starting UVB exposure) and in the afternoon (after 5–6 h under UVB exposure). The percentages of fish observed (mean ± SEM) at the top, middle, and bottom positions inside the tanks represent the mean from all the observations recorded during the first 8–16 days (A) and 26–40 days (B), for each treatment and replicates. Significant differences between treatments within each tank zone and exposure period are represented by upper case letters (P < 0.05; three-way ANOVA). For each treatment (within each exposure period), significant differences between tank zones are represented by lower case letters (P < 0.05; three-way ANOVA). Within the treatment, * indicates significant differences between morning and afternoon (P < 0.05; three-way ANOVA). Supplementary Figure S2 shows the percentage of fish observed in each tank zone on each day where behavior was recorded. In general, UVB doses in the middle and bottom tank zones were reduced by about 34–40% and 62–72%, respectively, compared to UVB in the top tank zone.
During the morning exposure period, no significant differences were observed between treatments in any zone of the tank (Three-way ANOVA, P > 0.05). Regarding the preference of fish for a particular tank zone, control fish swam randomly and were evenly distributed across the three regions of the tank. Similar results were observed in fish under UVB-L and UVB-M treatments (Three-way ANOVA, P > 0.05) (Figure 1A and Table 3). However, under the UVB-H treatment, around 65% of fish remained at the bottom of the tank during the morning period, and only 10% of fish were located in the top tank zone (Three-way ANOVA, P < 0.05) (Figure 1A). Moreover, most fish from this treatment showed a slow displacement behavior (Table 3).
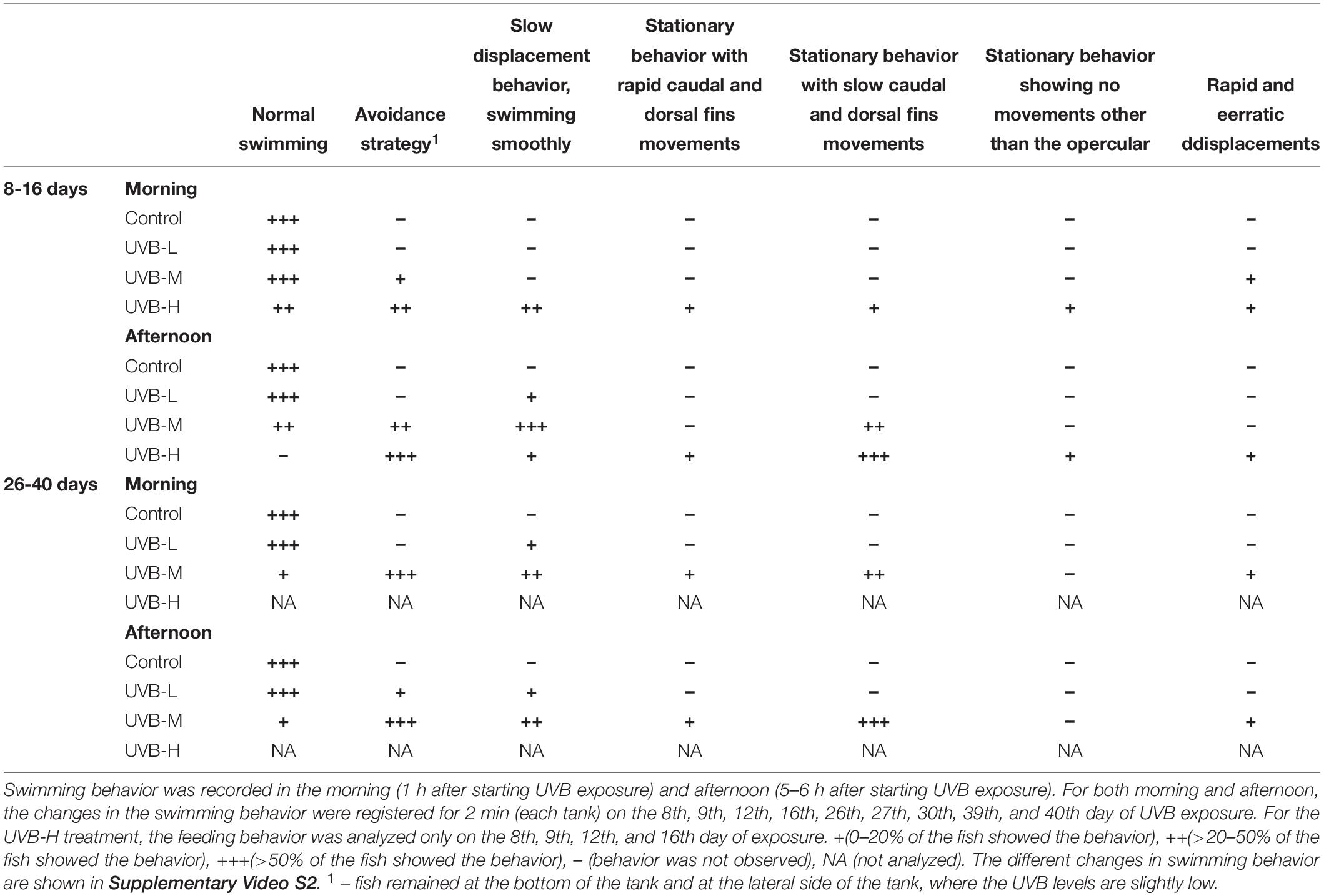
Table 3. Ethogram of the changes in swimming behavior observed in S. aurata during 43 days of exposure to UVB.
During the afternoon exposure period, in the bottom tank zone, the UVB-H treatment showed an increase in the percentage of fish when compared to all other treatments (Three-way ANOVA, UVB-M – P < 0.05; control and UVB-L – P < 0.001). In contrast, a decrease in the percentage of fish at the middle tank zone was observed with the increasing UVB doses (e.g., control – 36%, UVB-H – 5%, Three-way ANOVA, P = 0.0028) (Figure 1A).
Regarding the preference of fish for a particular tank zone, control fish showed no changes in the behavior. In the UVB-L and UVB-M treatments, the percentage of fish was significantly different between tank zones (Three-way ANOVA, P < 0.05, Figure 1A). Notably, most fish from UVB-H treatment preferred to stay in the bottom of the tank in the afternoon, representing > 90% of the UVB-H fish (Three-way ANOVA, bottom vs. middle/top, P < 0.001, Figure 1A). The daily UVB doses at the bottom of the UVB-H tanks were around 6.4 kJ m−2 (average daily UVB dose in the tank – 12 kJ m−2) (Supplementary Table S3). In the UVB-H exposed fish, the number of individuals remained at the bottom of the tank increased in the afternoon when compared with the morning period (Three-way ANOVA, P = 0.02, Figure 1A).
Most of the UVB-H exposed fish remained at the bottom of the tank and positioned themselves on the left and right sides of the tank, where the UVB irradiance was slightly lower. In this treatment, this change in behavior became evident after 8 days of exposure (UVB-H, absolute doses – 95 kJ m−2), when exposed fish started to lie at the bottom and lateral side of the tank (avoidance strategy). Supplementary Video S1 shows an example of the behavior recorded for the four experimental groups (afternoon period – 8 days of exposure). Moreover, the majority of fish during the afternoon period in the UVB-H (between 8 and 16 days of exposure) treatment showed a stationary behavior with slow caudal and dorsal fin movements (Table 3 and Supplementary Video S2). Other behavioral responses were observed between 8 and 16 days of exposure in the UVB-H treatment, which included slow displacement behavior, stationary behavior with rapid caudal and dorsal fin movements, stationary behavior showing no movements other than the opercular, and rapid and erratic displacements (Supplementary Video S2). However, less than 10% of fish showed these behavioral changes in the UVB-H during this exposure period (Table 3).
Exposure day: 26th-40th
The cumulative adverse effects in the swimming/locomotor behavior during long-term exposure to UVB were observed in UVB-L and UVB-M exposed fish (Figure 1B and Table 3). For example, the number of fish remained at the bottom of the tank was higher in the UVB-M treatment when compared with UVB-L and control (morning and afternoon, Three-way ANOVA, bottom vs. middle/top, P < 0.001). In the case of the middle tank zone (morning and afternoon), the number of fish remained in this zone decreased in UVB-L and UVB-M treatments when compared with the control (Three-way ANOVA, UVB-L – P < 0.01, UVB-M – P < 0.001). No significant differences were observed in the number of fish remained at the top tank zone between treatments at any exposure period (Three-way ANOVA, P > 0.05).
Regarding the preference of fish for a particular tank zone, significant changes were observed between treatments during the morning and afternoon exposure periods. No major changes in the swimming behavior were observed in the control fish (Figure 1B and Table 3). In the case of UVB-L treatment, the percentages of fish remaining at the bottom of the tank were 54 and 58% in the morning and the afternoon, respectively (Three-way ANOVA, bottom vs. middle/top, P < 0.01) (Figure 1B). In this treatment, behavioral changes such as avoidance strategy and slow displacement behavior were observed (Table 3).
Similar behavioral changes to those that occurred in the UVB-H exposed fish (after 10 days of exposure) were observed in the UVB-M treatment (absolute doses after 26 days of exposure, 158 kJ m−2). After 43 days of exposure, most of the UVB-M exposed fish remained at the bottom of the tank (morning – 77, afternoon – 87%), where the daily UVB doses in this tank zone were about 3 kJ m−2 (Three-way ANOVA, bottom vs. middle/top, P < 0.001) (Figure 1B and Supplementary Table S3). The majority of the UVB-M exposed fish exhibited the avoidance strategy, slow displacement behavior (morning), and stationary behavior with slow caudal and dorsal fins movements (afternoon) (Table 3).
Feeding Behavior
Throughout the 43 days of the experiment, the feeding behavior changed in UVB exposed fish, mainly during the afternoon feeding period (Figure 2 and Supplementary Videos S3, S4). Overall, a decrease in fishes’ appetite was observed during the experiment under all UVB exposure conditions.
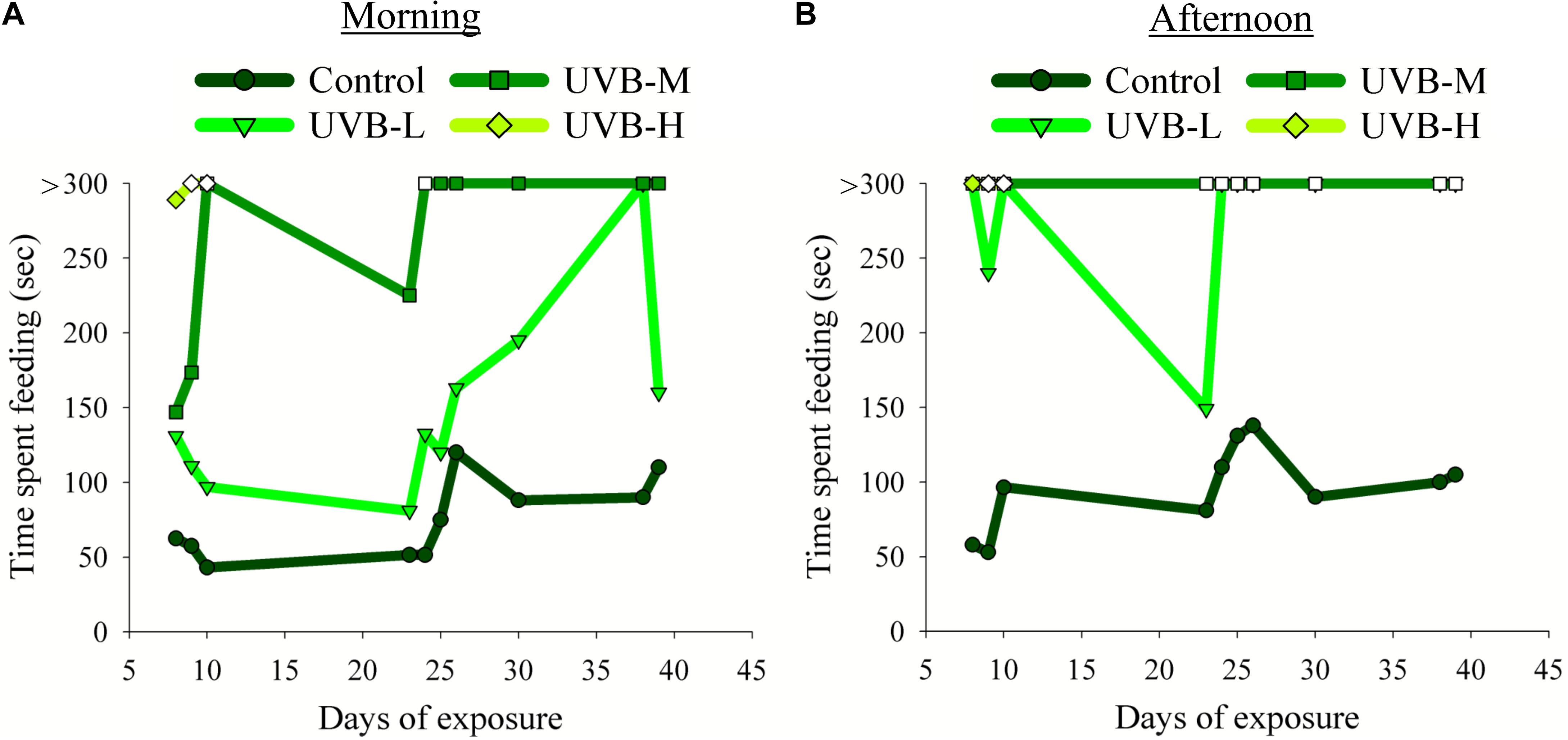
Figure 2. Effect of UVB on the S. aurata feeding behavior observed during the experimental time: (A) morning (1 h before starting the UVB exposure) and (B) afternoon (4 h after starting the UVB exposure) meals. The time spent to consume the food (seconds, sec) was recorded on the 8th, 9th, 10th, 23rd, 24th, 25th, 26th, 30th, 38th, and 39th day of UVB exposure. Due to the humane endpoint for the UVB-H experimental group, the feeding behavior analysis in this treatment was done only on the 8th, 9th, and 10th days of exposure. Filled symbols indicate that fish consumed all the feed pellets; open symbols indicate that fish did not consume all the feed pellets. Fish were fed 3–5% of their body weight per day. The feed was provided twice a day in two equal meals, one in the morning (9 AM) and the other during the afternoon (between 2 and 3 PM).
In the morning, no evident differences were observed between the control and UVB-L fish in the time required to consume all the available food. On the other hand, the time spent to consume the food increased considerably in fish from the UVB-M and UVB-H treatments (Figure 2A). For example, on the 8th day of exposure, the average time spent consuming the food for the control, UVB-L, UVB-M, and UVB-H was 63, 131, 147, and 289 s, respectively (Supplementary Video S3). On the 9th and 10th days of exposure, fish exposed to UVB-H irradiance conditions did not consume all the food available. After 10 days of exposure and until the end of UVB exposure, fish from the UVB-M treatment spent more than 5 min consuming the food (Figure 2A).
In the afternoon feeding period, the time spent consuming the food changed drastically between the control and UVB exposed fish. The increase in time to consume the food was directly proportional to the increase in UVB dose (Figure 2B and Supplementary Video S4). For example, in the UVB-L treatment, fish took more than 5 min to consume the food. For half of the days where we monitored feeding behavior, they did not consume the whole amount of food. Furthermore, fish exposed to irradiation levels of UVB-M continued to ingest some feed pellets but did not consume all of the provided feed. On the 9th and 10th day of the experiment, no food was ingested by fish exposed to the UVB-H conditions (Figure 2B and Supplementary Video S4).
Effects on Skin: Tissue Damage
On the 5th day of the experiment, the first signs of superficial skin lesions and evidence of typical sunburn from UVB exposure were observed in the UVB-H exposed fish. The percentage of fish that showed lesions on the skin after short- and long-term UVB exposure is shown in Figure 3A.
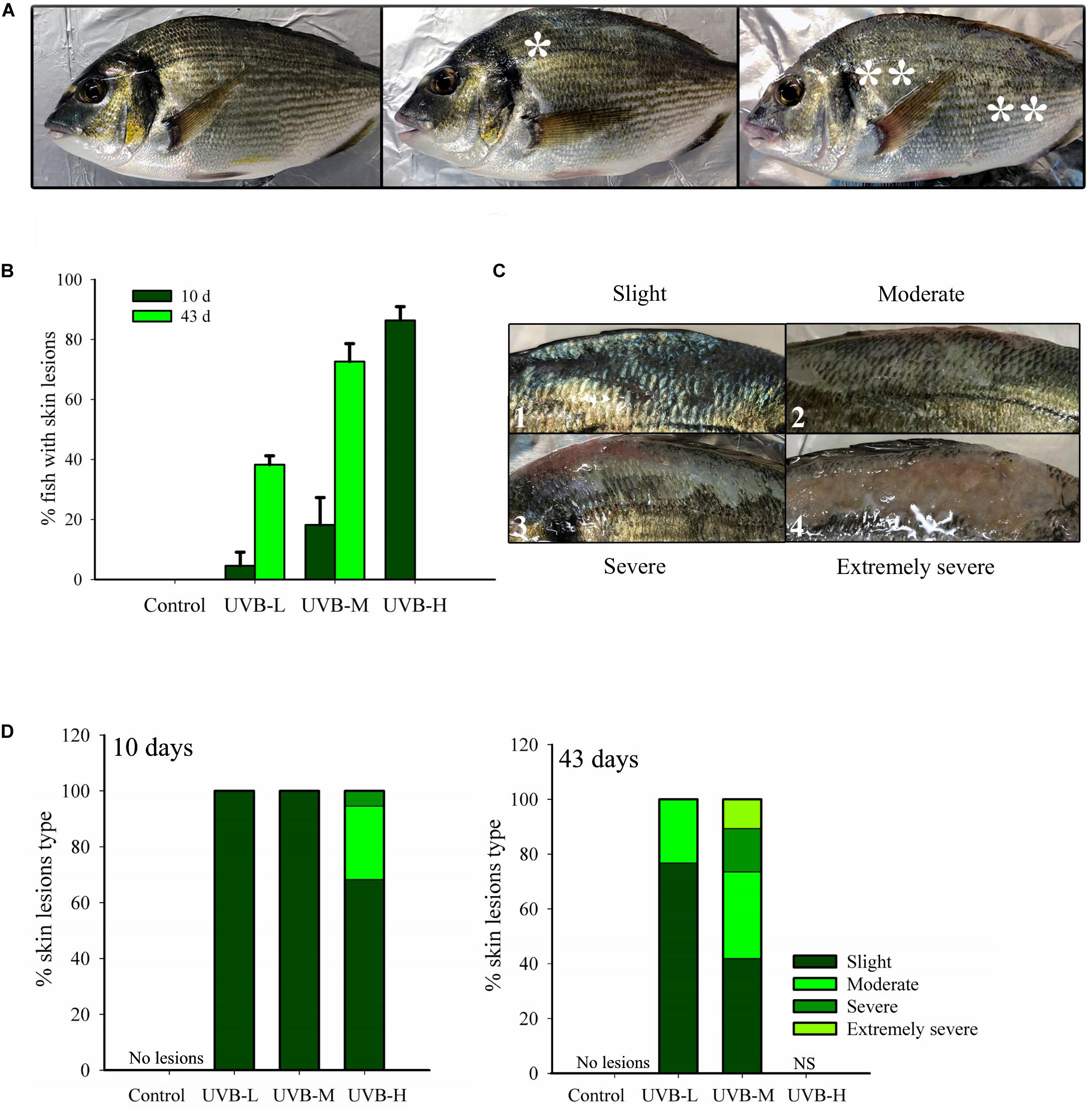
Figure 3. Superficial skin lesions (sunburn) on S. aurata after 10 and 43 days exposure to UVB. (A) Lesions location. *Lesions located only below the dorsal fin; ** lesions located on the whole body of the fish; (B) % of juveniles showing skin lesions (10 days of exposure: n = 30, 43 days of exposure: control, n = 30; UVB-L, n = 30; UVB-M, n = 28; mean ± SD); (C) Different types of skin lesions: 1 – slight, 2 – moderate, 3 – severe, 4 – extremely severe; (D) % of each type of skin lesion identified in the 10 days (n = 30) and 43 days (control, n = 30; UVB-L, n = 30; UVB-M, n = 28) UVB exposed fish.
Short- and long-term UVB irradiation dose-dependently increased the percentage of fish with skin lesions and typical signs of sunburn. The percentage of fish showing skin lesions after 10 days UVB exposure was 86, 18, 4, and 0% in UVB-H, UVB-M, UVB-L, and the control, respectively. The percentage of fish with skin lesions increased remarkably in UVB-M (73%) and UVB-L (34%) treatments by the end of the experiment (43 days) (Figure 3B). On the contrary, under qualitative inspection, the skin of the control group exhibited a normal shape with a healthy appearance (Figure 3A).
The observed skin lesions were classified into four different categories: slight (hyperpigmentation, desquamation), moderate (desquamation and emergence of epidermal sloughing), severe (desquamation, generalized epidermal sloughing), and extremely severe (advanced epidermal sloughing and necrosis) (Figure 3C). The skin lesions were mostly located on the dorsal surface of the fish between the head (after the operculum) and the caudal fin, predominantly below the dorsal fin and above the lateral line. Less than 20% of fish with skin lesions had their entire body affected (Figure 3A). By day 10, fish from the UVB-M and UVB-L treatments only showed slight skin lesions. Instead, UVB-H exposed fish showed slight (69%) moderate (26%) and severe (5%) skin lesions (Figure 3C). The cumulative damage to the skin resulting from long-term exposure to UVB was evident in the UVB-M and UVB-L treatments. For example, fish from the UVB-M irradiation group revealed all four categories of skin lesion: slight (42%), moderate (32%), severe (16%), and extremely severe (10%) (Figure 3D).
Effects of UVB on Physiological Condition
After 10 days of exposure, the highest cortisol concentration was observed in the UVB-L exposed fish (65.5 ± 3.7 ng ml−1, ANOVA by Ranks, P < 0.05), but there was no significant difference between the control and any of the UVB exposed fish after 43 days of exposure (ANOVA by Ranks, P > 0.05). Within each treatment, no significant differences in the cortisol concentration were observed 10 and 43 days of UVB exposure (ANOVA by Ranks, P > 0.05) (Figure 4A).
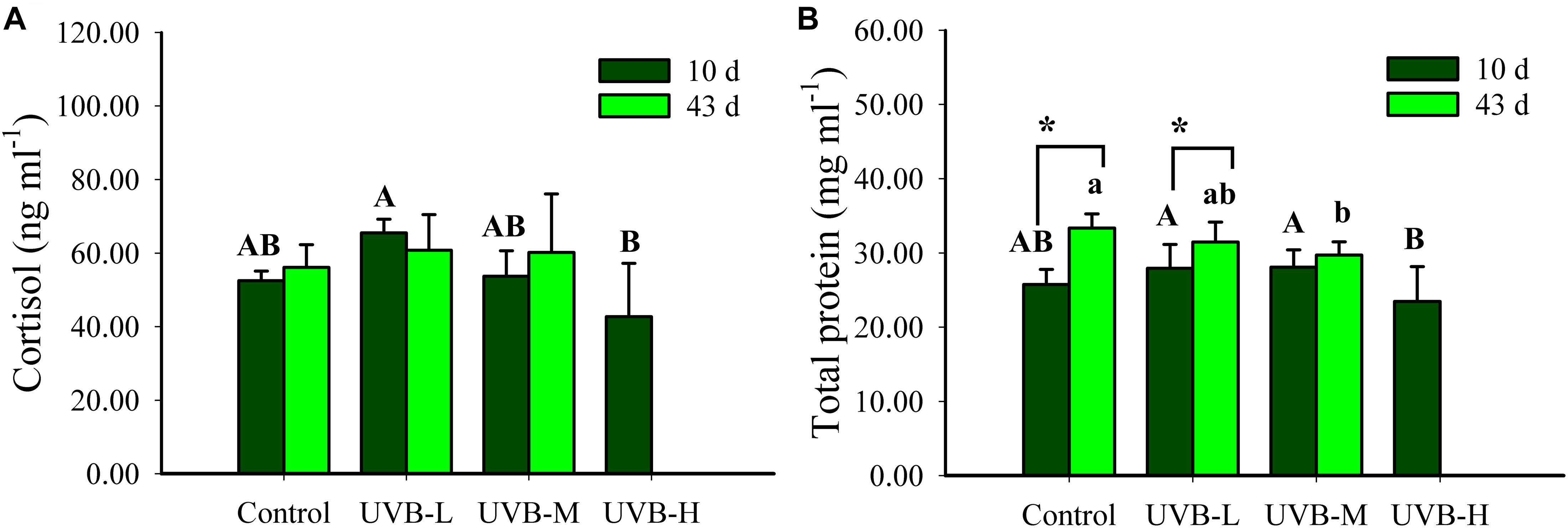
Figure 4. (A) Cortisol (ng ml−1) and (B) total protein (mg ml−1) in plasma of S. aurata after 10 and 43 days of exposure to UVB (cortisol: mean ± SD, 10 days n = 8, 43 days n = 10; total protein: mean ± SD, 10 days n = 18, 43 days n = 12). Significant differences between treatments after 10 and 43 days of UVB exposure are represented by upper and lower case letters, respectively (P < 0.05; two-way ANOVA). Within the treatment, * indicates significant differences between 10 and 43 days of UVB exposure (P < 0.05; two-way ANOVA).
Short- and long-term UVB exposure resulted in a decrease in the plasma total protein concentration (Two-way ANOVA, P < 0.05). After ten days of UVB exposure, the lowest protein concentration (23.46 ± 4.69 mg ml−1) was observed in the UVB-H exposed fish (Two-way ANOVA, P < 0.05). Furthermore, after long-term UVB exposure, the total protein concentration decreased significantly in the UVB-M treatment (29.72 ± 1.78 mg ml−1), when compared with the control (33.35 ± 1.90 mg ml−1), (Two-way ANOVA, P < 0.05) (Figure 4B). When comparing short- and long-term UVB exposure, the total protein concentration increased significantly after 43 days in the control and UVB-M (Two-way ANOVA, control – P < 0.001, UVB-L – P < 0.0195). Such increase was not observed in the UVB-M exposed fish (Two-way ANOVA, P = 0.8420) (Figure 4B).
UVB radiation did not affect the seabream plasma glucose or lactate concentrations after 43 days of exposure (One-way ANOVA, P > 0.05) (Figure 5). UVB exposure resulted in a dose-dependent significant decrease in the total cholesterol concentration, Control > UVB-L > UVB-M (One-way ANOVA, P < 0.001).
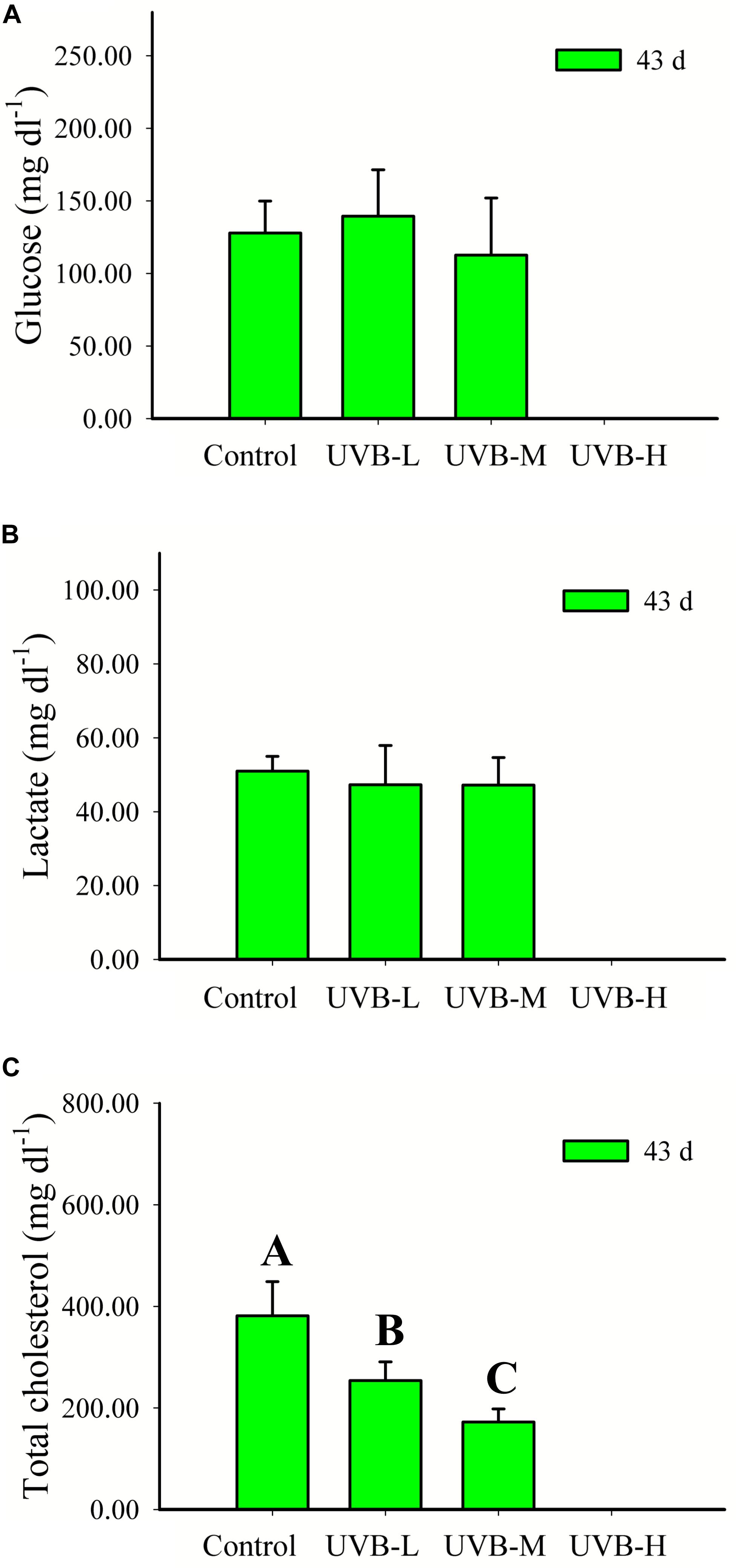
Figure 5. (A) Glucose (mg dl−1), (B) lactate (mg dl−1), and (C) total cholesterol (mg dl−1) in plasma of S. aurata after 43 days of exposure to UVB (glucose: mean ± SD, n = 16; lactate and total cholesterol: mean ± SD, n = 10). Significant differences between treatments are represented by different capital letters (P < 0.05; one-way ANOVA).
A reduction by more than 50% was observed in the UVB-M (172.41 ± 25.62 mg dl−1) treatment, when compared with the control (381.27 ± 67.32 mg dl−1), (One-way ANOVA, P < 0.001) (Figure 5).
Effects of UVB on the Innate Immune System
After 10 days of UVB exposure, there was a significant decrease in the anti-protease activity (expressed as% of trypsin inhibition) of fish exposed to UVB-M (75.0 ± 7.1%, Two-way ANOVA, P < 0.0016) and UVB-H (72.4 ± 9.9%, Two-way ANOVA, P < 0.001) treatments when compared with the control (83.4 ± 5.4%) (Figure 6A). After 43 days of exposure, the anti-protease activity significantly decreased in UVB-M exposed fish (Two-way ANOVA, P < 0.05) (Figure 6A).
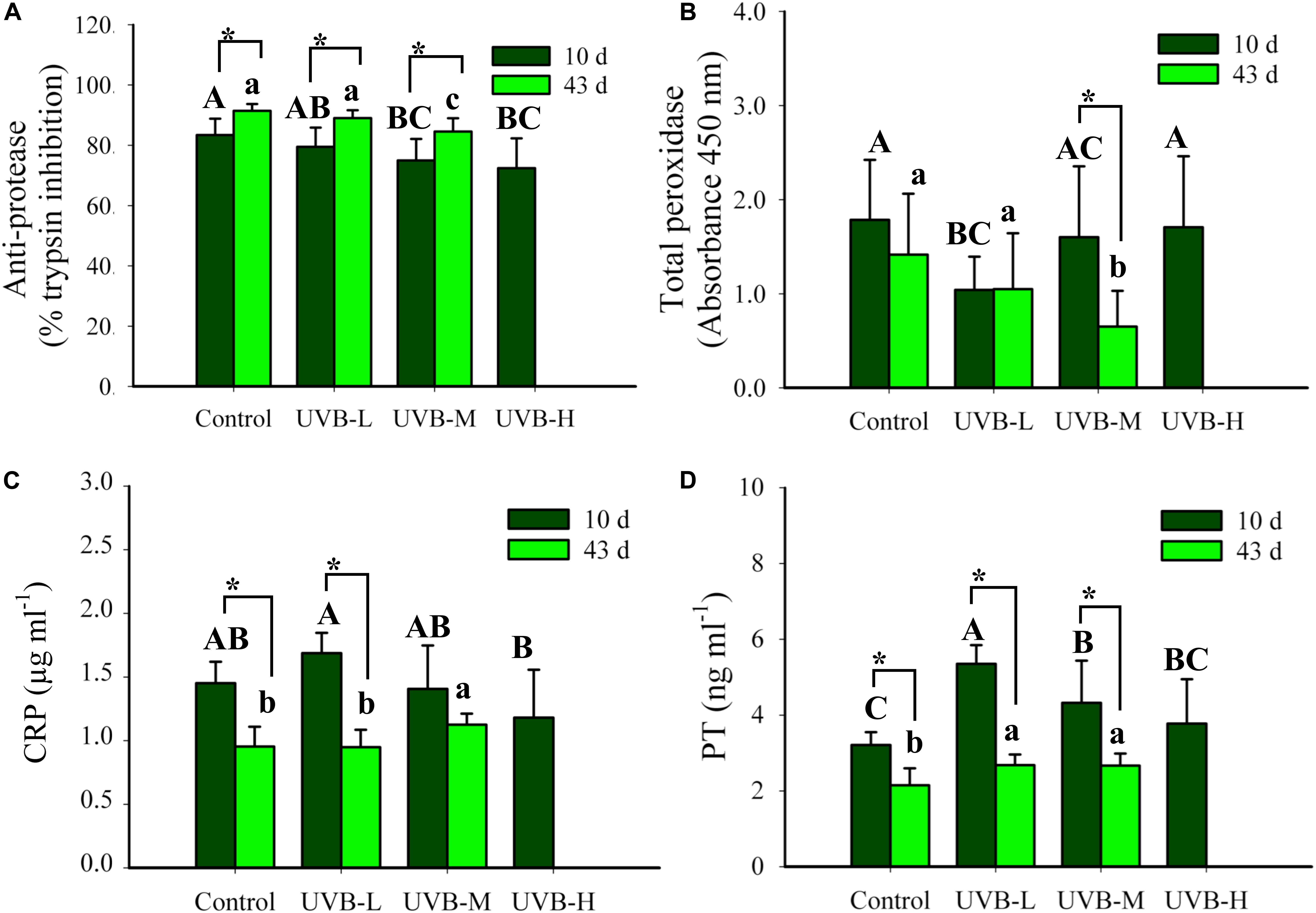
Figure 6. (A) Total anti-protease activity (% trypsin inhibition), (B) total peroxidase (TP, absorbance 450 nm), (C) C reactive protein (CRP, μg ml−1), and (D) prothrombin (PT, ng ml−1) in plasma of S. aurata after 10 and 43 days of exposure to UVB (total anti-protease: mean ± SD, 10 days n = 16, 43 days n = 14; total peroxidase: mean ± SD, 10 days n = 14, 43 days n = 12; CRP: mean ± SD, 10 days n = 8, 43 days n = 10; PT: mean ± SD, 10 days n = 9, 43 days n = 10). Significant differences between treatments after 10 and 43 days of UVB exposure are represented by upper and lower case letters, respectively (P < 0.05; two-way ANOVA). Within the treatment, * indicates significant differences between 10 and 43 days of UVB exposure (P < 0.05; two-way ANOVA).
After 10 days of UVB exposure, the lowest total peroxidase activity (PO) was observed in the UVB-L irradiation treatment (Two-way ANOVA, P < 0.05). However, none of the physiological and morphological data collected from those specimens explain this reduction. The UVB irradiation dose-dependently decreased the total PO activity after 43 days of UVB exposure, control ≥ UVB-L > UVB-M (Two-way ANOVA, P < 0.05) (Figure 6B). In UVB-M exposed fish, a significant decrease in the total PO activity was observed after 43 days when compared with 10 days of exposure (Two-way ANOVA, P = 0.004) (Figure 6B).
The C reactive protein (CRP) levels in the plasma ranged between 1.18 ± 0.38 μg/ml and 1.69 ± 0.16 μg/ml in UVB-H and UVB-L, respectively, after 10 days of UVB exposure (Two-way ANOVA, P < 0.05). Similar changes were observed in the prothrombin (PT) levels after 10 days of UVB exposure (Figure 6C). Additionally, after 43 days of UVB exposure, CRP and PT plasmatic levels increased significantly in the UVB-M fish (Two-way ANOVA, P < 0.05). In all experimental groups, a decrease in the CRP and PT plasmatic levels throughout the experiment (t-student, P < 0.05) (Figures 6C,D). Differences in the CRP and PT concentrations between 10 and 43 days of exposure may have arisen because two ELISA plates were used from the same assay, but from different lots.
Discussion
The present study is the first to describe and characterize the sensitivity of S. aurata to underwater UVB radiation. The daily UVB doses (2.40–11.86 kJ m−2) used in this experiment are of the same order of magnitude as those detected underwater in oligotrophic seas. For example, Garcia-Corral et al. (2015) reported high daily UVB doses (41 kJ m−2) during June 2013 in the surface waters of the Northwestern Mediterranean. Given that 10% of this UVB radiation (Z10%, 312 nm) can be detected at 9 m (Tedetti and Sempéré, 2006), fish at this depth can receive daily UVB doses of 4.1 kJ m−2. Another study conducted in Mediterranean waters showed daily UVB doses of 17 kJ m−2 and 1.7 kJ m−2 at the surface and at 6 m, respectively (Kouwenberg and Lantoine, 2007). Daily UVB doses of 11 kJ m−2 at the surface have also been reported during the fall in the Southwestern Mediterranean (Llabrés et al., 2010). Although S. aurata is considered to be an exotic species in the Red Sea, it has already become an established fish species in the aquaculture sector of KSA (FAO, 2013). Recent studies have reported exceptionally high daily UV doses in the Red Sea. Recent studies have reported exceptionally high daily UV doses in the Red Sea. In the central Red Sea during 2017–2018, high daily UVB doses in surface waters have been reported to range from 20 kJ m−2 in December 2017 to 45 kJ m−2 in June 2017 (Overmans and Agustí, 2020). In that study, the lowest monthly mean daily underwater dose of UVB was observed in December 2017, where the UVB doses at 1, 4, 8, and 10 m were 13.7, 4.4, 1.0, and 0.5 kJ m−2, respectively. In contrast, in July 2018, the average daily UVB dose at 4 m exceeded 14 kJ m−2, and at 10 m, UVB doses of 3 kJ m−2 were still detectable (Overmans and Agustí, 2020). The results should in the present study demonstrate that S. aurata is sensitive to the UVB doses applied in this study, which were equivalent to underwater levels above 10 m depth. These results are relevant for fish that are confined in farming cages, where UVB exposure can result in losses for the aquaculture sector, especially in oligotrophic waters with high UVB incidence. Moreover, exposure of S. aurata to UVR may increase in the future, especially in the summer and fall, if marine waters become more oligotrophic with climate change (Duarte et al., 2004; Regaudie-De-Gioux et al., 2014; Garcia-Corral et al., 2015).
UVB Exposure Resulted in Reduced Growth, Loss of Body Condition and Behavioral Changes
The present results indicate that short- and long-term UVB exposure reduces seabream juvenile’s growth in UVB-H (11.9 kJ m−2 d−1; absolute UVB doses for the experimental period of 119 kJ m−2) and UVB-M (6.1 kJ m−2 d−1; absolute UVB doses for the experimental period of 261 kJ m−2), respectively. Also, this observed reduction in growth was strongly dose-dependent. After 43 days of exposure, fish from UVB-L (2.4 kJ m−2 d−1; absolute UVB doses for the experimental period of 103 kJ m−2) and UVB-M (6.1 kJ m−2 d−1; absolute UVB doses for the experimental period of 261 kJ m−2) treatments grew 27 and 40% less, respectively, compared to the control. In agreement with findings for other teleost fish, a reduction in growth can be due to exposure to UVB radiation. For example, in Atlantic salmon, a reduction in growth was observed after exposure to enhanced UVB conditions over 52 days, simulating a stratospheric ozone loss of 20% (daily dose at the bottom of the cage, 3.5 kJ m−2 d−1; absolute UVB doses for the experimental period of 182 kJ m−2), when compared to fish kept under UVB-depleted sunlight (daily dose at the bottom of the cage, 0 kJ m−2 d−1) (Jokinen et al., 2008). Similar findings were obtained for three-spined stickleback and sea chub (Graus nigra) juveniles after 68 (6.5 kJ m−2 d−1; absolute UVB doses for the experimental period of 442 kJ m−2) and 7 (3.2 kJ m−2 d−1; absolute UVB doses for the experimental period of 22.4 kJ m−2) days of exposure to UVB, respectively. The reduction in growth due to UVB exposure was generally accompanied by poor nutritional status (García-Huidobro et al., 2017; Vitt et al., 2017).
The results obtained in the present study show a reduction in appetite and an increase in the time taken to consume food of the UVB exposed fish, mainly in the UVB-H and UVB-M treatments. These behavioral changes became more evident during the afternoon feeding period. Appetite reduction and behavioral feeding changes have been previously reported in three-spined stickleback as a consequence of UVB exposure (Vitt et al., 2017) and a decrease in food consumption often resulted in lower energy intake. This energy deficit is very detrimental because energy-demanding mechanisms, such as DNA repairing, increase upon UVB exposure due to induced DNA damage. In such cases, seabream juveniles do not allocate sufficient energy for digestion or other biological processes such as reproduction and growth (Olson et al., 2006; Groff et al., 2010; Fukunishi et al., 2013).
The animal fitness indexes also changed after UVB exposure (e.g., 10 days – UVB-L: an increase in SSI; 43 days – UVB-M: a decrease in HSI). This lower body condition may be due to cell damage caused by UVB exposure, which can result in apoptosis or activation of the (energetically costly) cell repair mechanisms, as is suggested to occur for three-spined stickleback after long-term UVB exposure (Vitt et al., 2017). Furthermore, under chronic stress conditions, metabolic changes may occur, which can interfere with the deposition of glycogen in fish liver. Subsequently, the stability of structural components such as proteins and lipids can be compromised, affecting the overall liver weight (Maulvault et al., 2018). Moreover, previous studies have shown that tissue hypertrophy and hyperplasia can also occur after UVR exposure (Blazer et al., 1997; Sayed et al., 2007, 2013).
The behavioral analysis showed that UVB exposure affects the swimming activity in gilthead seabream juveniles exposed to natural underwater UVB levels, particularly levels of UVB-M (6.1 kJ m−2 d−1) and UVB-H (11.9 kJ m−2 d−1). After the 8th day of exposure, UVB-H exposed fish began to lay in the bottom and on the lateral side of the tank. This behavioral change was evident in the UVB-M exposed fish after 26 days of exposure, and this behavior continued until the last day of the experiment. In those fish, swimming activity was significantly reduced, and most of the fish showed a slow displacement behavior and a stationary behavior with slow caudal and dorsal fins movements. Alemanni et al. (2003) described the same type of behavioral changes in juvenile rainbow trout after receiving a single UVB dose of 3.35 kJ m−2. The present study also showed that seabream juveniles tried to avoid UVB exposure, especially during the afternoon period (8–16 days – UVB-H, 26–40 days – UVB-M). Previous studies have shown that fish generally adopt a UVB avoidance strategy to escape radiation exposure (Kelly and Bothwell, 2002; Sucré et al., 2012; Pulgar et al., 2015; Vargas et al., 2018). The UVB avoidance strategy can be triggered by the early perception of skin damage, or due to UVB detection in the eyes by UV-sensitive retinal photoreceptors, as suggested by Alemanni et al. (2003) and Sharma et al. (2007). Nonetheless, the factors causing UVB-dependent behavioral changes, or how fish perceive UVB radiation, have not yet been established directly and are still poorly studied and understood.
UVB Exposure Resulted in Skin Damage
Fish skin acts as the primary barrier between the external and internal environment, and its epidermis is composed of metabolically active epithelial cells (e.g., mucous-producing cells) (Esteban, 2012; Gomez et al., 2013). Due to a lack of keratinization, fish skin is more susceptible to damage by UVR exposure than human skin (Bullock, 1982; Elliott, 2011). In the current study, the first signs of sunburn and lesions in seabream skin occurred after 5 days of exposure in the UVB-H treatment. These alterations in skin morphology increased in all fish from the three UVB exposure treatments. At the end of the experiment, the percentage of fish showing lesions on their skin was 73 and 35% in the UVB-M (6.1 kJ m−2 d−1; absolute UVB doses for the experimental period of 261 kJ m−2) and UVB-L (2.4 kJ m−2 d−1; absolute UVB doses for the experimental period of 103 kJ m−2) treatments, respectively. Evidence of sunburn and skin lesions after UVB overexposure has been reported during all fish development stages (Gevertz et al., 2012; Sucré et al., 2012). For example, Fabacher and Little (1995) studied the effect of UVB exposure over 7 days (UVB dose, 34.2 kJ m−2 d−1; absolute UVB doses after 7 days of exposure, 239.4 kJ m−2), producing sunburn and skin lesions in different fish species. In rainbow trout and Lahontan cutthroat trout (Oncorhynchus clarki henshawi), the first signs of sunburn and skin hyperpigmentation appeared after 3 days of UVB exposure and were located close to the dorsal fin just behind the head (Fabacher and Little, 1995). Similarly, in our experiment, observed skin lesions were located in the same body region. Additionally, UVB exposure (2.4 kJ m−2 d−1; absolute UVB doses after 28 days, a total of 12 irradiations – 28.8 kJ m−2) induced morphological changes in the skin of common carp (Markkula et al., 2005). Moreover, more than 50% of fish showed lesions and sunburn on their skin after 28 days of exposure (Markkula et al., 2005).
Moreover, the severe lesions (e.g., desquamation, necrosis, sloughing of the skin) observed in juveniles in this study corroborate our hypothesis that seabream is susceptible to standard underwater UVB levels from the Red Sea, and also from the Mediterranean, especially during the summer months. As mentioned in the section above, fish also avoid UVB exposure as a strategy to cope with overexposure (Fukunishi et al., 2006; Pulgar et al., 2015; Valiñas and Walter Helbling, 2016). However, when confined, such as occurs with S. aurata in aquaculture cages, fish cannot avoid UVB exposure by moving to lower depths or by sheltering. In fact, significant production losses were previously reported in several fish farms between the 1980 and 1990s, where skin lesions and sunburn in fish from outdoor tanks were indicated as a potential cause for increased mortality rates (Bullock, 1982; Bullock and Coutts, 1985; Lowe and Goodman-Lowe, 1996). Fifteen percent mortality was observed during the first 16 days of the experiment in the UVB-H (UVB doses – 11.9 kJ m−2d−1) exposed fish, and about 8% of fish from the UVB-M treatment died during the 43 days of exposure (daily UVB doses – 6.1 kJ m−2d−1). Furthermore, fish that died due to UVB exposure showed extremely severe lesions and sunburn on their skin.
UVB Exposure Resulted in Several Metabolic, Physiological and Immunological Responses
In this study, after short-term exposure, UVB-L exposed fish (2.4 kJ m−2 d−1; absolute UVB doses after 10 days of exposure – 24.0 kJ m−2) showed the highest plasma cortisol levels. Similar findings were observed in common carp (0.6 kJ m−2 d−1, absolute UVB doses after a total of 3 irradiations – 1.8 kJ m−2; Markkula et al., 2005) and rainbow trout (0.07 kJ m−2 d−1, absolute UVB doses after a total of 17 irradiations – 1.2 kJ m−2; Markkula et al., 2009) juveniles exposed to UVB. Fish exposed to the lowest UVB doses unexpectedly had the highest increase in cortisol levels. Indeed, under acute exposure to UVR, cortisol levels in plasma increased significantly in roach and fathead minnow (Jokinen et al., 2000; Manek et al., 2012). The cortisol peak in the juveniles from the UVB-H and UVB-M treatments due to UVB exposure probably occurred before the 10th day of exposure, as suggested also in Markkula et al. (2005, 2009). After 43 days of exposure, there were no significant changes in the cortisol levels between control and any of the UVB-exposed fish, probably due to adaptation to these chronic stress conditions, which can result in its reduction to basal levels (Alves et al., 2010). Similar results were obtained in common carp (absolute UVB dose, 28.8 kJ m−2) and rainbow trout (absolute dose, 10.2 kJ m−2) after long-term exposure (Markkula et al., 2005, 2009).
Low hematocrit values and a decrease in the plasma total protein levels have been used as indicators of poor nutritional status due to UVA and UVB exposure (Jokinen et al., 2000, 2008, 2011; Markkula et al., 2005, 2009). Under stress conditions, the levels of plasma circulating proteins can decrease due to the potential peripheral proteolysis or amino acid oxidation (Di Marco et al., 2008; Peres et al., 2014; Rossi et al., 2015). Our results are in agreement with previous studies, where the plasma total protein levels in seabream juveniles decreased after short- and long-term UVB exposure.
In agreement with previous findings (Osman et al., 2010; Sharma et al., 2010), the present data showed that UVR exposure can result in metabolic disturbance, as evidenced by the reduction in more than 50% of the circulating total cholesterol levels in the UVB-M exposed fish after 43 days of exposure. Also, glucose and total cholesterol concentrations decreased in adult North African catfish (Clarias gariepinus) exposed to UVA for 3 days Sayed et al. (2007). Furthermore, the decrease in the appetite observed in UVB-H and UVB-M exposed fish can also be a plausible explanation for the evidence metabolic disturbance. In fact, during starvation, lipid metabolism pathways can be affected. Total cholesterol levels decreased in some fish species due to lack of available dietary lipids and inhibition of fatty acids’ synthesis (Pérez-Jiménez et al., 2007; Peres et al., 2013). In addition, lipogenesis inhibition during food deprivation can help to maintain plasma glucose levels (Costas et al., 2011; Menezes et al., 2015). In addition, lipogenesis inhibition during food deprivation can help to maintain plasma glucose levels (Costas et al., 2011; Menezes et al., 2015).
The immunosuppressive effects of UVB exposure in fish have been previously documented (Jokinen et al., 2001, 2008, 2011; Alemanni et al., 2003; Markkula et al., 2005, 2006, 2009). The present work outlines the potential adverse effects of short- and long-term UVB exposure on the innate immune system of S. aurata juveniles, through the analysis of humoral parameters in the plasma. After 10 and 43 days of UVB exposure, both anti-protease and total peroxidase (PO) activity decreased. Furthermore, the cumulative adverse effects of UVB on the juveniles’ immune system were more evident after 43 days of exposure, as observed for total peroxidase activity in the UVB-M treatment. When circulating in plasma, anti-proteases and peroxidases (e.g., myeloperoxidase), that have anti-inflammatory and bactericidal functions, prevent pathogens from adhering and colonizing, thus preventing the spread of diseases (Magnadottir, 2010; Uribe et al., 2011). Therefore, the doses used in the present study suggest that UVB exposure can affect the seabream innate immune response against pathogens (Sitjà-Bobadilla and Pérez-Sánchez, 1999; Talpur and Ikhwanuddin, 2013). To date, no studies have provided evidence that shows the effects of UVB radiation on the activity of these two enzymes in fish. Nevertheless, some studies have demonstrated that these enzymes can be suppressed under long-term exposure to different stress factors, such as overcrowding, exposure to parasites and other pathogens, wounding on the skin, thermal stress, starvation, and exposure to pollutants (Chebaani et al., 2014; Sadhu et al., 2014; Yarahmadi et al., 2016; Eslamloo et al., 2017; Borgia et al., 2018; Ceballos-Francisco et al., 2018).
C-reactive protein is a pentraxin-like molecule that is commonly involved in the acute phase response (APR), and its levels can increase in the plasma due to tissue injury and inflammation. CRP has been identified in several fish species, and its function in the immune system, other than its role in the APR (reviewed by Whyte, 2007), includes the removal of apoptotic cells and activation of the complement pathways (Nauta et al., 2003; Tarnawska et al., 2019). The current results confirm the relationship between CRP and tissue injury and inflammation. Fish exposed to UVB-M levels during 43 days showed an increase in CRP levels, and most fish from this treatment acquired skin lesions. Moreover, 25% of these lesions were considered in the present study to be severe or extremely severe. Like CRP, prothrombin was also increased after long term exposure to UVB. PT, also known as clotting factor II, is involved in blood coagulation (Hanumanthaiah et al., 2002; Day et al., 2004; Tavares-Dias and Oliveira, 2009) but also plays a role in the immune response to tissue injury and inflammation in vertebrates (Godwin and Brockes, 2006). PT’s concentration was significantly increased in the plasma of fish from both UVB-L and UVB-M treatments after 43 days of exposure. Although the effect of long-term UVB exposure on prothrombin levels in fish is unknown, long-term UVB exposure does impact the inflammation-induced activation of coagulation. This process has been extensively described in humans (Levi et al., 2003) and the CRP levels observed in the present experiment point toward a systemic inflammation response, which may lead to the activation of coagulation due to tissue factor-mediated thrombin generation in fish with severe skin lesions.
Even though the selected markers for this study showed a possible detection of immune system suppression and inflammatory/tissue injury pathways occurring in the UVB-treated seabream, additional research to verify possible immune system suppression in fish under natural underwater UVB levels from the Red Sea is recommended. Such studies may include, for example, analysis of cellular innate/acquired immune parameters and expression of immune-related genes, and should be assessed (i) in several developmental stages, as early stages are generally more susceptible to UVB radiation and (ii) in other species that may show different resilience to UVB exposure. In addition, climate change is expected to increase the incidence of disease outbreaks and the appearance of new diseases in fish (Karvonen et al., 2010; Báez et al., 2011; Anyanwu et al., 2015), and the impact of climate change on UVR should be addressed soon in order to develop mitigation strategies to prevent the cumulative immunosuppressive effects of both stressors (Jokinen et al., 2011). A plausible mitigation strategy might be the use of immunostimulants, prebiotics, or probiotics as feed additives, promoting fish immunity, and subsequently, growth performance (Hai, 2015; Carbone and Faggio, 2016; Dawood et al., 2018).
Conclusion
The present study shows that seabream can suffer considerable stress induced by UVB radiation, being susceptible to underwater UVB levels detected in oligotrophic waters above 10 m depth. This species will be more sensitive to UVB radiation when confined in aquaculture cages in locations such as the case of the Red Sea or the Mediterranean, especially during the summer months.
In the case of confinement, short- and long-term exposure to daily UVB doses of 2.40–11.86 kJ m−2 compromised both the growth and survival of seabream juveniles, resulting in a poor nutritional status. The UVB exposure resulted in changes in normal swimming and feeding behavior, including a reduction in the swimming behavior, fish adopted an UVB avoidance strategy, and increase in the time required to consume the food (reduction in appetite).
Moreover, the reduction in the plasmatic total protein and total cholesterol levels suggest that UVB radiation has an impact on fish physiological and metabolic functions. In terms of immune system functioning, we observed a pronounced modulation of several measured parameters after long-term UVB exposure (e.g., reduction of total anti-protease and total peroxidase activities), leading to adverse effects and a potential reduction in the resistance against pathogens of seabream juveniles.
Furthermore, all these biochemical changes observed due to UVB overexposure were accompanied by the presence and development of superficial skin damage. The identified lesions included sunburn, desquamation, epidermal sloughing, and necrosis. In addition, the detrimental effects of UVB exposure observed in this study were dose-dependent and cumulative for some of the analyzed biomarkers throughout the experiment.
In a real-world scenario, fish reared in cages will, of course, not stay at the same depth throughout the whole day. Nevertheless, the information presented here can be used to adjust fish rearing conditions in offshore cages (e.g., deeper and shaded cages) to minimize the damage caused by UVB overexposure and improve aquaculture production in this species. Even though considerable uncertainty remains regarding future UVB incidence and respective doses received by fish in oligotrophic waters, recent studies indicate that climate change and stratospheric ozone depletion can interact with solar UVR, enhancing the exposure of fish to underwater UVB. This latter aspect is especially important for the Red Sea and the Mediterranean, which have both undergone remarkably fast warming recently. Further research is recommended to understand how fish growth and survival can be affected by the interactions of UVR with other environmental and atmospheric factors.
Data Availability Statement
All datasets generated for this study are included in the article/Supplementary Material.
Ethics Statement
The animal study was reviewed and approved by KAUST Institutional Animal Care and Use Committee [IACUCML(SA)#18-04-016].
Author Contributions
SA and RA conceived and designed the study. RA carried out the analysis. AM and JA assisted with the tank’s experimental setup, transport of seabream specimens from the fish farm to the lab, maintenance during the quarantine period, fish daily maintenance during the experiments, and sampling. RA, AM, and SA contributed to the critical interpretation of the data. RA and SA drafted the MS. All authors contributed to the writing of the manuscript and critically read and approved the final version.
Funding
This work was supported by King Abdullah University of Science and Technology through base-line funding to SA.
Conflict of Interest
The authors declare that the research was conducted in the absence of any commercial or financial relationships that could be construed as a potential conflict of interest.
Acknowledgments
We thank the Coastal and Marine Resources (CMOR) Core Lab at King Abdullah University of Science and Technology (KAUST). We also thank Muhammad Danial A. Nor Azli and Nurhisham Razali for their help with fish maintenance and sampling during the experiment. We also thank Sebastian Overmans for his help during sampling and for providing the UVB irradiance profile measured in the fish farms. We also thank Sara Axworthy Bacelar for her help in the editing of the videos for the behavior analysis.
Supplementary Material
The Supplementary Material for this article can be found online at: https://www.frontiersin.org/articles/10.3389/fmars.2020.00306/full#supplementary-material
References
Aksakal, F. I., and Ciltas, A. (2018). The impact of ultraviolet B (UV-B) radiation in combination with different temperatures in the early life stage of zebrafish (Danio rerio). Photochem. Photobiol. Sci. 17, 35–41. doi: 10.1039/c7pp00236j
Al-Aidaroos, A. M., El-Sherbiny, M. M. O., Satheesh, S., Mantha, G., Agustí, S., Carreja, B., et al. (2014). High mortality of red sea zooplankton under ambient solar radiation. PLoS One 9:108778. doi: 10.1371/journal.pone.0108778
Alemanni, M. E., Lozada, M., and Zagarese, H. E. (2003). Assessing sublethal effects of ultraviolet radiation in juvenile rainbow trout (Oncorhynchus mykiss). Photochem. Photobiol. Sci. 2:867. doi: 10.1039/b301564e
Alves, R. N., Cordeiro, O., Silva, T. S., Richard, N., de Vareilles, M., Marino, G., et al. (2010). Metabolic molecular indicators of chronic stress in gilthead seabream (Sparus aurata) using comparative proteomics. Aquaculture 299, 57–66. doi: 10.1016/j.aquaculture.2009.11.014
Anyanwu, C. N., Amadi-Eke, A. S., Nwaka, D. E., Ezeafulukwe, C. F., and Adaka, G. S. (2015). Climate change, effects and mitigation strategies on aquaculture: a review. Agric. For. Fish. 4, 70–72. doi: 10.11648/j.aff.s.2015040301.22
Applegate, L. A., and Ley, R. D. (1988). Ultraviolet radiation-induced lethality and repair of pyrimidine dimers in fish embryos. Mutat. Res. Mol. Mech. Mutagen. 198, 85–92. doi: 10.1016/0027-5107(88)90043-90047
Báez, V. H., Aigo, J., del, C., and Cussac, V. E. (2011). Climate change and fish culture in Patagonia: present situation and perspectives. Aquac. Res. 42, 787–796. doi: 10.1111/j.1365-2109.2011.02804.x
Barnes, P. W., Williamson, C. E., Lucas, R. M., Robinson, S. A., Madronich, S., Paul, N. D., et al. (2019). Ozone depletion, ultraviolet radiation, climate change and prospects for a sustainable future. Nat. Sustain. 2, 569–579. doi: 10.1038/s41893-019-0314-312
Bertoni, R., Jeffrey, W. H., Pujo-Pay, M., Oriol, L., Conan, P., and Joux, F. (2011). Influence of water mixing on the inhibitory effect of UV radiation on primary and bacterial production in mediterranean coastal water. Aquat. Sci. 73, 377–387. doi: 10.1007/s00027-011-0185-188
Blazer, V. S., Fabacher, D. L., Little, E. E., Ewing, M. S., and Kocan, K. M. (1997). Effects of Ultraviolet-B radiation on fish: histologic comparison of a UVB-Sensitive and a UVB-Tolerant Species. J. Aquat. Anim. Health 9, 132–143.
Borgia, V. J. F., Thatheyus, A. J., Murugesan, A. G., Alexander, S. C. P., and Geetha, I. (2018). Effects of effluent from electoplating industry on the immune response in the freshwater fish, Cyprinus carpio. Fish Shellfish Immunol. 79, 86–92. doi: 10.1016/j.fsi.2018.05.010
Bullock, A. M. (1982). The pathological effects of ultraviolet radiation on the epidermis of teleost fish with reference to the solar radiation effect in higher animals. Proc. R. Soc. Edinburg, Sect. B Biol. Sci. 81, 199–210.
Bullock, A. M. (1988). Solar Ultraviolet Radiation: A Potential Environmental Hazard in the Cultivation of Farmed Finfish. Dordrecht: Springer.
Bullock, A. M., and Coutts, R. R. (1985). The impact of solar ultraviolet radiation upon the skin of rainbow trout, Salmo gairdneri Richardson, farmed at high altitude in Bolivia. J. Fish Dis. 8, 263–272. doi: 10.1111/j.1365-2761.1985.tb00942.x
Carbone, D., and Faggio, C. (2016). Importance of prebiotics in aquaculture as immunostimulants. Effects on immune system of Sparus aurata and Dicentrarchus labrax. Fish Shellfish Immunol. 54, 172–178. doi: 10.1016/j.fsi.2016.04.011
Ceballos-Francisco, D., Guardiola, F. A., Cordero, H., Cuesta, A., and Esteban, M. (2018). Humoral immune parameters in serum of gilthead seabream (Sparus aurata L.) after induced skin injury. Fish Shellfish Immunol. 75, 291–294. doi: 10.1016/j.fsi.2018.02.017
Chebaani, N., Guardiola, F. A., Sihem, M., Nabil, A., Oumouna, M., Meseguer, J., et al. (2014). Innate humoral immune parameters in Tilapia zillii under acute stress by low temperature and crowding. Fish Physiol. Biochem. 40, 797–804. doi: 10.1007/s10695-013-9886-9883
Costas, B., Aragão, C., Ruiz-Jarabo, I., Vargas-Chacoff, L., Arjona, F. J., Dinis, M. T., et al. (2011). Feed deprivation in Senegalese sole (Solea senegalensis Kaup, 1858) juveniles: effects on blood plasma metabolites and free amino acid levels. Fish Physiol. Biochem. 37, 495–504. doi: 10.1007/s10695-010-9451-9452
Dahms, H. U., and Lee, J. S. (2010). UV radiation in marine ectotherms: molecular effects and responses. Aquat. Toxicol. 97, 3–14. doi: 10.1016/j.aquatox.2009.12.002
Dawood, M. A. O., Koshio, S., and Esteban, M. A. (2018). Beneficial roles of feed additives as immunostimulants in aquaculture: a review. Rev. Aquac. 10, 950–974. doi: 10.1111/raq.12209
Day, K., Krishnegowda, N., and Jagadeeswaran, P. (2004). Knockdown of prothrombin in zebrafish. Blood Cells, Mol. Dis. 32, 191–198. doi: 10.1016/j.bcmd.2003.09.004
Di Marco, P., Priori, A., Finoia, M. G., Massari, A., Mandich, A., and Marino, G. (2008). Physiological responses of European sea bass Dicentrarchus labrax to different stocking densities and acute stress challenge. Aquaculture 275, 319–328. doi: 10.1016/j.aquaculture.2007.12.012
Dishon, G., Dubinsky, Z., Fine, M., and Iluz, D. (2012). Underwater light field patterns in subtropical coastal waters: a case study from the Gulf of Eilat (Aqaba). Isr. J. Plant Sci. 60, 265–275. doi: 10.1560/IJPS.60.1-2.265
Duarte, C. M., Agustí, S., and Vaqué, D. (2004). Controls on planktonic metabolism in the Bay of Blanes, northwestern Mediterranean littoral. Limnol. Oceanogr. 49, 2162–2170. doi: 10.4319/lo.2004.49.6.2162
Elliott, D. G. (2011). “The skin | functional morphology of the integumentary system in fishes,” in Encyclopedia of Fish Physiology, ed. A. P. Farrell (Cambridge, MA: Academid Press), doi: 10.1016/B978-0-12-374553-8.00108-108
Eslamloo, K., Morshedi, V., Azodi, M., and Akhavan, S. R. (2017). Effect of starvation on some immunological and biochemical parameters in tinfoil barb (Barbonymus schwanenfeldii). J. Appl. Anim. Res. 45, 173–178. doi: 10.1080/09712119.2015.1124329
Esteban, M. A. (2012). An overview of the immunological defenses in fish skin. ISRN Immun. 2012:853470 doi: 10.5402/2012/853470
Fabacher, D. L., and Little, E. E. (1995). Skin component may protect fishes from ultraviolet-B radiation. Environ. Sci. Pollut. Res. 2, 30–32. doi: 10.1007/BF02987508
FAO (2019). Cultured Aquatic Species Information Programme. Sparus aurata. Cultured Aquatic Species Information Programme. Rome: FAO.
Fukunishi, Y., Browman, H. I., Durif, C. M. F., Bjelland, R. M., Shema, S. D., Fields, D. M., et al. (2013). Sub-lethal exposure to ultraviolet radiation reduces prey consumption by Atlantic cod larvae (Gadus morhua). Mar. Biol. 160, 2591–2596. doi: 10.1007/s00227-013-2253-2251
Fukunishi, Y., Masuda, R., and Yamashita, Y. (2006). Ontogeny of tolerance to and avoidance of ultraviolet radiation in red sea bream Pagrus major and black sea bream Acanthopagrus schlegeli. Fish. Sci. 72, 356–363. doi: 10.1111/j.1444-2906.2006.01157.x
Fukunishi, Y., Masuda, R., and Yamashita, Y. (2010). Exposure of eggs to solar UV-B leads to reduced hatching rates in two sparid fishes, red sea bream Pagrus major and black sea bream Acanthopagrus schlegeli. J. Fish Biol. 76, 734–741. doi: 10.1111/j.1095-8649.2009.02517.x
Garcia-Corral, L. S., Martinez-Ayala, J., Duarte, C. M., and Agustí, S. (2015). Experimental assessment of cumulative temperature and UV-B radiation effects on Mediterranean plankton metabolism. Front. Mar. Sci. 2:48. doi: 10.3389/fmars.2015.00048
García-Huidobro, M. R., Aldana, M., Duarte, C., Galbán-Malagón, C., and Pulgar, J. (2017). Seawater-temperature and UV-radiation interaction modifies oxygen consumption, digestive process and growth of an intertidal fish. Mar. Environ. Res. 129, 408–412. doi: 10.1016/j.marenvres.2017.06.013
Gevertz, A. K., Tucker, A. J., Bowling, A. M., Williamson, C. E., and Oris, J. T. (2012). Differential tolerance of native and nonnative fish exposed to ultraviolet radiation and fluoranthene in Lake Tahoe (California/Nevada). USA. Environ. Toxicol. Chem. 31, 1129–1135. doi: 10.1002/etc.1804
Godwin, J. W., and Brockes, J. P. (2006). Regeneration, tissue injury and the immune response. J. Anat. 209, 423–432. doi: 10.1111/j.1469-7580.2006.00626.x
Gomez, D., Sunyer, J. O., and Salinas, I. (2013). The mucosal immune system of fish: the evolution of tolerating commensals while fighting pathogens. Fish Shellfish Immunol. 35, 1729–1739. doi: 10.1016/j.fsi.2013.09.032
Groff, A. A., Silva, J., da Nunes, E. A., Ianistcki, M., Guecheva, T. N., Oliveira, A. M., et al. (2010). UVA/UVB-induced genotoxicity and lesion repair in Colossoma macropomum and Arapaima gigas Amazonian fish. J. Photochem. Photobiol. B Biol. 99, 93–99. doi: 10.1016/j.jphotobiol.2010.02.011
Häder, D. P., Kumar, H. D., Smith, R. C., and Worrest, R. C. (2007). Effects of solar UV radiation on aquatic ecosystems and interactions with climate change. Photochem. Photobiol. Sci. 6, 267–285. doi: 10.1039/b700020k
Häder, D. P., Williamson, C. E., Wängberg, S. A., Rautio, M., Rose, K. C., Gao, K., et al. (2015). Effects of UV radiation on aquatic ecosystems and interactions with other environmental factors. Photochem. Photobiol. Sci. 14, 108–126. doi: 10.1039/c4pp90035a
Hai, N. V. (2015). The use of probiotics in aquaculture. J. Appl. Microbiol. 119, 917–935. doi: 10.1111/jam.12886
Handinger, J., Soltani, M., and Percival, S. (1997). The pathology of Flexibacter maritimus in aquaculture species in Tasmania, Australia. J. Fish Dis. 20, 159–168.
Hanif, A., Bakopoulos, V., and Dimitriadis, G. J. (2004). Maternal transfer of humoral specific and non-specific immune parameters to sea bream (Sparus aurata) larvae. Fish Shellfish Immunol. 17, 411–435. doi: 10.1016/j.fsi.2004.04.013
Hanumanthaiah, R., Day, K., and Jagadeeswaran, P. (2002). Comprehensive analysis of blood coagulation pathways in teleostei: evolution of coagulation factor genes and identification of Zebrafish factor VIIi. Blood Cells Mol. Dis. 29, 57–68. doi: 10.1006/bcmd.2002.0534
Jokinen, E. I., Salo, H. M., Markkula, S. E., Aaltonen, T. M., and Immonen, A. K. (2000). Effects of ultraviolet light on immune parameters of the roach. Toxicol. Lett. 112, 303–310. doi: 10.1016/S0378-4274(99)00240-244
Jokinen, E. I., Salo, H. M., Markkula, S. E., Immonen, A. K., and Aaltonen, T. M. (2001). Ultraviolet B irradiation modulates the immune system of fish (Rutilus rutilus, cyprinidae) Part III: lymphocytes. Photochem. Photobiol. 73, 505–512. doi: 10.1562/0031-8655(2001)073<0505:UBIMTI>2.0.CO;2
Jokinen, I. E., Markkula, E. S., Salo, H. M., Kuhn, P., Nikoskelainen, S., Arts, M. T., et al. (2008). Exposure to increased ambient ultraviolet B radiation has negative effects on growth, condition and immune function of juvenile Atlantic salmon (Salmo salar). Photochem. Photobiol. 84, 1265–1271. doi: 10.1111/j.1751-1097.2008.00358.x
Jokinen, I. E., Salo, H. M., Markkula, E., Rikalainen, K., Arts, M. T., and Browman, H. I. (2011). Additive effects of enhanced ambient ultraviolet B radiation and increased temperature on immune function, growth and physiological condition of juvenile (parr) Atlantic Salmon, Salmo salar. Fish Shellfish Immunol. 30, 102–108. doi: 10.1016/j.fsi.2010.09.017
Karvonen, A., Rintamäki, P., Jokela, J., and Valtonen, E. T. (2010). Increasing water temperature and disease risks in aquatic systems: Climate change increases the risk of some, but not all, diseases. Int. J. Parasitol. 40, 1483–1488. doi: 10.1016/j.ijpara.2010.04.015
Kelly, D. J., and Bothwell, M. L. (2002). Avoidance of solar ultraviolet radiation by juvenile coho salmon (Oncorhynchus kisutch). Can. J. Fish. Aquat. Sci. 59, 474–482. doi: 10.1139/f02-023
Khan, A. Q., Aldosari, F., and Hussain, S. M. (2018). Fish consumption behavior and fish farming attitude in Kingdom of Saudi Arabia (KSA). J. Saudi Soc. Agric. Sci. 17, 195–199. doi: 10.1016/j.jssas.2016.04.003
Kouwenberg, J. H. M., and Lantoine, F. (2007). Effects of ultraviolet-B stressed diatom food on the reproductive output in Mediterranean Calanus helgolandicus (Crustacea; Copepoda). J. Exp. Mar. Bio. Ecol. 341, 239–253. doi: 10.1016/j.jembe.2006.10.047
Lawrence, K. P., Young, A. R., Diffey, B. L., and Norval, M. (2019). The impact of solar ultraviolet radiation on fish: Immunomodulation and photoprotective strategies. Fish Fish. 02, 1–16. doi: 10.1111/faf.12420
Levi, M., Keller, T. T., Van Gorp, E., and Ten Cate, H. (2003). Infection and inflammation and the coagulation system. Cardiovasc. Res. 15 60, 26–39. doi: 10.1016/s0008-6363(02)00857-x
Llabrés, M., Agustí, S., Alonso-Laita, P., and Herndl, G. J. (2010). Synechococcus and Prochlorococcus cell death induced by UV radiation and the penetration of lethal UVR in the Mediterranean Sea. Mar. Ecol. Prog. Ser. 399, 27–37. doi: 10.3354/meps08332
Lowe, C., and Goodman-Lowe, G. (1996). Suntanning in hammerhead sharks [4]. Nature 383:677. doi: 10.1038/383677a0
Magnadottir, B. (2010). Immunological control of fish diseases. Mar. Biotechnol. 12, 361–379. doi: 10.1007/s10126-010-9279-x
Manek, A. K., Ferrari, M. C. O., Sereda, J. M., Niyogi, S., and Chivers, D. P. (2012). The effects of ultraviolet radiation on a freshwater prey fish: Physiological stress response, club cell investment, and alarm cue production. Biol. J. Linn. Soc. 105, 832–841. doi: 10.1111/j.1095-8312.2011.01829.x
Markkula, E., Salo, H. M., Rikalainen, K., and Jokinen, I. E. (2009). Long-term UVB irradiation affects the immune functions of carp (Cyprinus carpio) and rainbow trout (Oncorhynchus mykiss). Photochem. Photobiol. 85, 347–352. doi: 10.1111/j.1751-1097.2008.00446.x
Markkula, S. E., Karvonen, A., Salo, H., Tellervo Valtonen, E., and Ilmari Jokinen, E. (2007). Ultraviolet B irradiation affects resistance of rainbow trout (Oncorhynchus mykiss) against bacterium Yersinia ruckeri and trematode Diplostomum spathaceum. Photochem. Photobiol. 83, 1263–1269. doi: 10.1111/j.1751-1097.2007.00165.x
Markkula, S. E., Salo, H. M., Immonen, A. K., and Jokinen, E. I. (2005). Effects of short- and long-term ultraviolet B irradiation on the immune system of the common carp (Cyprinus carpio). Photochem. Photobiol. 81:595. doi: 10.1562/2004-07-13-ra-231.1
Markkula, S. E., Salo, H. M., Rikalainen, A. K., and Jokinen, E. I. (2006). Different sensitivity of carp (Cyprinus carpio) and rainbow trout (Oncorhynchus mykiss) to the immunomodulatory effects of UVB irradiation. Fish Shellfish Immunol. 21, 70–79. doi: 10.1016/j.fsi.2005.10.007
Maulvault, A. L., Barbosa, V., Alves, R., Anacleto, P., Camacho, C., Cunha, S., et al. (2018). Integrated multi-biomarker responses of juvenile seabass to diclofenac, warming and acidification co-exposure. Aquat. Toxicol. 202, 65–79. doi: 10.1016/j.aquatox.2018.06.016
Menezes, C., Ruiz-Jarabo, I., Martos-Sitcha, J. A., Toni, C., Salbego, J., Becker, A., et al. (2015). The influence of stocking density and food deprivation in silver catfish (Rhamdia quelen): a metabolic and endocrine approach. Aquaculture 435, 257–264. doi: 10.1016/j.aquaculture.2014.09.044
Mitchell, D. L., Adams-Deutsch, T., and Olson, M. H. (2009). Dose dependence of DNA repair in rainbow trout (Oncorhynchus mykiss) larvae exposed to UV-B radiation. Photochem. Photobiol. Sci. 8, 75–81. doi: 10.1039/B807469K
Mitchell, D. L., Fernandez, A. A., Nairn, R. S., Garcia, R., Paniker, L., Trono, D., et al. (2010). Ultraviolet A does not induce melanomas in a Xiphophorus hybrid fish model. Proc. Natl. Acad. Sci. U.S.A. 20, 9329–9334. doi: 10.1073/pnas.1000324107
Mitchell, L., Travis, J., and Morizot, C. (1993). DNA repair in the variable platyfish (Xiphophorus variatus) Irradiated in vivo With Ultraviolet B Light. Science 58, 455–459. doi: 10.1111/j.1751-1097.1993.tb09590.x
Morel, A., Gentili, B., Claustre, H., Babin, M., Bricaud, A., Ras, J., et al. (2007). Optical properties of the “clearest” natural waters. Limnol. Oceanogr. 52, 217–229. doi: 10.4319/lo.2007.52.1.0217
Nauta, A. J., Daha, M. R., Van Kooten, C., and Roos, A. (2003). Recognition and clearance of apoptotic cells: a role for complement and pentraxins. Trends Immunol. 24, 148–154. doi: 10.1016/S1471-4906(03)00030-39
Olson, M. H., Colip, M. R., Gerlach, J. S., and Mitchell, D. L. (2006). Quantifying ultraviolet radiation mortality risk in bluegill larvae: effects of nest location. Ecol. Appl. 16, 328–338. doi: 10.1890/05-0287
Osman, A. G. M., Koutb, M., and Sayed, A. E.-D. H. (2010). Use of hematological parameters to assess the efficiency of quince (Cydonia oblonga Miller) leaf extract in alleviation of the effect of ultraviolet - A radiation on African catfish Clarias gariepinus (Burchell, 1822). J. Photochem. Photobiol. B Biol. 99, 1–8. doi: 10.1016/j.jphotobiol.2010.01.002
Overmans, S., and Agustí, S. (2019). Latitudinal gradient of UV attenuation along the highly transparent red sea basin. Photochem. Photobiol. 95, 1267–1279. doi: 10.1111/php.13112
Overmans, S., and Agustí, S. (2020). Unraveling the seasonality of UV exposure in reef waters of a rapidly warming (sub-)tropical sea. Front. Mar. Sci. 7:111. doi: 10.3389/fmars.2020.00111
Peres, H., Santos, S., and Oliva-Teles, A. (2013). Selected plasma biochemistry parameters in gilthead seabream (Sparus aurata) juveniles. J. Appl. Ichthyol. 29, 630–636. doi: 10.1111/j.1439-0426.2012.02049.x
Peres, H., Santos, S., and Oliva-Teles, A. (2014). Blood chemistry profile as indicator of nutritional status in European seabass (Dicentrarchus labrax). Fish Physiol. Biochem. 40, 1339–1347. doi: 10.1007/s10695-014-9928-9925
Pérez-Jiménez, A., Guedes, M. J., Morales, A. E., and Oliva-Teles, A. (2007). Metabolic responses to short starvation and refeeding in Dicentrarchus labrax. effect of dietary composition. Aquaculture 265, 325–335. doi: 10.1016/j.aquaculture.2007.01.021
Pulgar, J., Lagos, P., Maturana, D., Valdés, M., Aldana, M., and Pulgar, V. M. (2015). Effect of UV radiation on habitat selection by Girella laevifrons and Graus nigra (Kyphosidae). J. Fish Biol. 86, 812–821. doi: 10.1111/jfb.12566
Quade, M. J., and Roth, J. A. (1997). A rapid, direct assay to measure degranulation of bovine neutrophil primary granules. Vet. Immunol. Immunopathol. 58, 239–248. doi: 10.1016/S0165-2427(97)00048-42
Regaudie-De-Gioux, A., Agustí, S., and Duarte, C. M. (2014). UV sensitivity of planktonic net community production in ocean surface waters. J. Geophys. Res. Biogeosciences 119, 929–936. doi: 10.1002/2013JG002566
Rick, I. P., Mehlis, M., Eßer, E., and Bakker, T. C. M. (2014). The influence of ambient ultraviolet light on sperm quality and sexual ornamentation in three-spined sticklebacks (Gasterosteus aculeatus). Oecologia 174, 393–402. doi: 10.1007/s00442-013-2773-x
Ricker, W. E. (1975). Computation and interpretation of biological statistics of fish populations. Fish. Res. Board Can. 1–382. doi: 10.1038/108070b0
Rodger, H. D. (1991). Summer lesion syndrome in Salmon: a retrospective study. Vet. Rec. 129, 237–239. doi: 10.1136/vr.129.11.237
Rossi, A., Cazenave, J., Bacchetta, C., Campana, M., and Parma, M. J. (2015). Physiological and metabolic adjustments of Hoplosternum littorale (Teleostei, Callichthyidae) during starvation. Ecol. Indic. 56, 161–170. doi: 10.1016/j.ecolind.2015.04.001
Ruiz-González, C., Lefort, T., Galí, M., Sala, M. M., Sommaruga, R., Sim0́, R., et al. (2012b). Seasonal patterns in the sunlight sensitivity of bacterioplankton from Mediterranean surface coastal waters. FMES Microbiol. Ecol. 79, 661–674. doi: 10.1111/j.1574-6941.2011.01247.x
Ruiz-González, C., Simó, R., Vila-Costa, M., Sommaruga, R., and Gasol, J. M. (2012a). Sunlight modulates the relative importance of heterotrophic bacteria and picophytoplankton in DMSP-sulphur uptake. ISME J. 6, 650–659. doi: 10.1038/ismej.2011.118
Sadhu, N., Sharma, S. R. K., Joseph, S., Dube, P., and Philipose, K. K. (2014). Chronic stress due to high stocking density in open sea cage farming induces variation in biochemical and immunological functions in Asian seabass (Lates calcarifer, Bloch). Fish Physiol. Biochem. 40, 1105–1113. doi: 10.1007/s10695-014-9909-9908
Salo, H. M., Aaltonen, T. M., Markkula, S. E., and Jokinen, E. I. (1998). Ultraviolet B irradiation modulates the immune system of fish (Rutilus rutilus, Cyprinidae). I. Phagocytes. Photochem. Photobiol. 67, 433–437. doi: 10.1111/j.1751-1097.1998.tb05223.x
Salo, H. M., Jokinen, E. I., Markkula, S. E., and Aaltonen, T. M. (2000). Ultraviolet B irradiation modulates the immune system of fish (Rutilus rutilus, Cyprinidae) II: Blood. Photochem. Photobiol. 71, 65–70. doi: 10.1562/0031-865520000710065UBIMTI2.0.CO2
Sayed, A. E. D. H., Abdel-Tawab, H. S., Abdel Hakeem, S. S., and Mekkawy, I. A. (2013). The protective role of quince leaf extract against the adverse impacts of ultraviolet-A radiation on some tissues of Clarias gariepinus (Burchell, 1822). J. Photochem. Photobiol. B Biol. 119, 9–14. doi: 10.1016/j.jphotobiol.2012.11.006
Sayed, A. E. D. H., Ibrahim, A. T., Mekkawy, I. A. A., and Mahmoud, U. M. (2007). Acute effects of Ultraviolet-A radiation on African Catfish Clarias gariepinus (Burchell, 1822). J. Photochem. Photobiol. B Biol. 89, 170–174. doi: 10.1016/j.jphotobiol.2007.09.010
Sayed, A. E. D. H., Watanabe-Asaka, T., Oda, S., and Mitani, H. (2016). Apoptosis and morphological alterations after UVA irradiation in red blood cells of p53 deficient Japanese medaka (Oryzias latipes). J. Photochem. Photobiol. B Biol. 161, 1–8. doi: 10.1016/j.jphotobiol.2016.05.004
Seebacher, F., Kazerouni, E. G., and Franklin, C. E. (2016). Ultraviolet B radiation alters movement and thermal selection of zebrafish (Danio rerio). Biol. Lett. 12, 2016–2019. doi: 10.1098/rsbl.2016.0258
Sharma, J., Vasudeva Rao, Y., Kumar, S., and Chakrabarti, R. (2010). Impact of UV-B radiation on the digestive enzymes and immune system of larvae of Indian major carp Catla catla. Int. J. Radiat. Biol. 86, 181–186. doi: 10.3109/09553000903419312
Sharma, J. G., Masuda, R., and Tanaka, M. (2007). Orientation behaviour of Pagrus major larvae exposed to UV-B radiation in laboratory conditions. Int. J. Radiat. Biol. 83, 49–52. doi: 10.1080/09553000601087812
Sitjà-Bobadilla, A., and Pérez-Sánchez, J. (1999). Diet related changes in non-specific immune response of European sea bass (Dicentrarchus labrax L.). Fish Shellfish Immunol. 83, 49–52. doi: 10.1006/fsim.1999.0219
Subramani, P. A., Hameed, B., and Michael, R. D. (2015). Effect of UV-B radiation on the antibody response of fish - Implication on high altitude fish culture. J. Photochem. Photobiol. B Biol. 143, 1–4. doi: 10.1016/j.jphotobiol.2014.12.021
Sucré, E., Vidussi, F., Mostajir, B., Charmantier, G., and Lorin-Nebel, C. (2012). Impact of ultraviolet-B radiation on planktonic fish larvae: Alteration of the osmoregulatory function. Aquat. Toxicol. 109, 194–201. doi: 10.1016/j.aquatox.2011.09.020
Talpur, A. D., and Ikhwanuddin, M. (2013). Azadirachta indica (neem) leaf dietary effects on the immunity response and disease resistance of Asian seabass, Lates calcarifer challenged with Vibrio harveyi. Fish Shellfish Immunol. 34, 254–264. doi: 10.1016/j.fsi.2012.11.003
Tarnawska, M., Augustyniak, M., Łaszczyca, P., Migula, P., Irnazarow, I., Krzyżowski, M., et al. (2019). Immune response of juvenile common carp (Cyprinus carpio L.) exposed to a mixture of sewage chemicals. Fish Shellfish Immunol. 88, 17–27. doi: 10.1016/j.fsi.2019.02.049
Tavares-Dias, M., and Oliveira, S. R. (2009). A review of the blood coagulation system of fish. Rev. Rev. Bras 205–224.
Tedetti, M., and Sempéré, R. (2006). Penetration of Ultraviolet radiation in the marine environment. A Review. Photochem. Photobiol. 82:389. doi: 10.1562/2005-11-09-ir-733
Uribe, C., Folch, H., Enriquez, R., and Moran, G. (2011). Innate and adaptive immunity in teleost fish: a review. Vet. Med. 56, 486–503. doi: 10.17221/3294-VETMED
Valiñas, M. S., and Walter Helbling, E. (2016). Metabolic and behavioral responses of the reef fish Patagonotothen cornucola to ultraviolet radiation: Influence of the diet. J. Exp. Mar. Bio. Ecol. 474, 180–184. doi: 10.1016/j.jembe.2015.10.011
Vargas, J., Duarte, C., Galban-Malagónac, G., García-Huidobro, M. R., Aldana, M., and Pulgar, J. (2018). Refuge quality to cope with UV radiation affects energy allocation in an intertidal fish. Mar. Pollut. Bull. 130, 268–270. doi: 10.1016/j.marpolbul.2018.03.051
Vitt, S., Rahn, A. K., Drolshagen, L., Bakker, T. C. M., Scharsack, J. P., and Rick, I. P. (2017). Enhanced ambient UVB light affects growth, body condition and the investment in innate and adaptive immunity in three-spined sticklebacks (Gasterosteus aculeatus). Aquat. Ecol. 51, 499–509. doi: 10.1007/s10452-017-9632-9635
Whyte, S. K. (2007). The innate immune response of finfish - A review of current knowledge. Fish Shellfish Immunol. 23, 1127–1151. doi: 10.1016/j.fsi.2007.06.005
Williamson, C. E., Neale, P. J., Hylander, S., Rose, K. C., Figueroa, F. L., Robinson, S. A., et al. (2019). The interactive effects of stratospheric ozone depletion, UV radiation, and climate change on aquatic ecosystems. Photochem. Photobiol. Sci. 18, 717–746. doi: 10.1039/C8PP90062K
Yarahmadi, P., Miandare, H. K., Fayaz, S., and Caipang, C. M. A. (2016). Increased stocking density causes changes in expression of selected stress- and immune-related genes, humoral innate immune parameters and stress responses of rainbow trout (Oncorhynchus mykiss). Fish Shellfish Immunol. 48, 43–53. doi: 10.1016/j.fsi.2015.11.007
Zagarese, H. E., and Williamson, C. E. (2001). The implications of solar UV radiation exposure for fish and fisheries. Fish Fish. 2, 250–260. doi: 10.1046/j.1467-2960.2001.00048.x
Zuo, X., and Woo, P. T. K. (1997). Natural anti-proteases in rainbow trout, Oncorhynchus mykiss and brook charr, Salvelinus fontinalis and the in vitro neutralization of fish α2-macroglobulin by the metalloprotease from the pathogenic haemoffagellate, Cryptobia salmositica. Parasitology 114(Pt 4), 375–381. doi: 10.1017/S0031182096008578
Keywords: Sparus aurata, aquaculture, UVB radiation, reduced growth, skin lesions, behavioral response, physiological changes, immune system modulation
Citation: Alves RN, Mahamed AH, Alarcon JF, Al Suwailem A and Agustí S (2020) Adverse Effects of Ultraviolet Radiation on Growth, Behavior, Skin Condition, Physiology, and Immune Function in Gilthead Seabream (Sparus aurata). Front. Mar. Sci. 7:306. doi: 10.3389/fmars.2020.00306
Received: 03 February 2020; Accepted: 15 April 2020;
Published: 14 May 2020.
Edited by:
JInghui Fang, Yellow Sea Fisheries Research Institute (CAFS), ChinaReviewed by:
Alberto Cuesta, University of Murcia, SpainMohsen Abdel-Tawwab, Agricultural Research Center, Egypt
Arleta Krystyna Skrzynska, University of Cádiz, Spain
Copyright © 2020 Alves, Mahamed, Alarcon, Al Suwailem and Agustí. This is an open-access article distributed under the terms of the Creative Commons Attribution License (CC BY). The use, distribution or reproduction in other forums is permitted, provided the original author(s) and the copyright owner(s) are credited and that the original publication in this journal is cited, in accordance with accepted academic practice. No use, distribution or reproduction is permitted which does not comply with these terms.
*Correspondence: Ricardo N. Alves, ricardo.alves@kaust.edu.sa