- 1Institute of Marine Biology, National Taiwan Ocean University, Keelung, Taiwan
- 2Graduate School of Fisheries and Environmental Sciences, Nagasaki University, Nagasaki, Japan
- 3Institute of Oceanography, National Taiwan University, Taipei, Taiwan
- 4Center of Excellence for the Oceans, National Taiwan Ocean University, Keelung, Taiwan
- 5Department of Environmental Biology and Fisheries Science, National Taiwan Ocean University, Keelung, Taiwan
Sepioteuthis lessoniana is a widely distributed neritic squid in the Indo-Pacific Ocean. It is an important species in fisheries, but species management is difficult because of inadequate information regarding its life history. The daily growth and δ18O values from the core to the edge of statoliths of S. lessoniana collected in northern Taiwan were analyzed to predict the experienced temperature history along with ontogenetic stages. The probability of occurrence in a given area at each life stage in three seasonal groups was determined using salinity values, deduced and measured temperatures, and the known ecology of S. lessoniana. The results showed that ontogenetic variation in the statolith δ18O values in S. lessoniana reflected the seasonal temperature fluctuation observed in Taiwanese waters, which indicated the reliability of the prediction method. Complex and diverse distribution and movement patterns were observed in the three seasonal groups. The results also indicated the importance of the waters near the coast of northeastern Taiwan as a spawning ground. Based on a model prediction, the distribution of S. lessoniana is likely associated with water temperature and current. A potential migration route from the Penghu Islands to northeastern Taiwan suggests a high level of population connectivity in S. lessoniana in Taiwan. This study provides information on the spatial and vertical distribution of S. lessoniana at various ontogenetic stages, which is essential for resource management and conservation of this commercial species.
Introduction
Planktonic paralarvae of loliginid squids are distributed widely after hatching by coastal trends and ocean currents (Young and Harman, 1988; Zeidberg and Hamner, 2002). By contrast, juveniles and adults live in coastal and inshore waters and are commonly aggregated in schools for spawning (Jereb and Roper, 2005). The distribution range of paralarvae is a critical parameter for recruitment success and gene flow in cephalopod populations (O’dor, 1998; Moreno et al., 2008), and understanding movement patterns is crucial for studies on population structure, fishery management, and marine protected area evaluation (O’dor, 1992; Semmens et al., 2007). Temperature is one of the major factors affecting the movements of squids because squids are highly vulnerable to drastic changes in temperature. Direct data of the thermal movement patterns of squids have been collected primarily through external tagging (e.g., Ueta and Jo, 1990; Jackson et al., 2005; Gilly et al., 2006; Kanamaru et al., 2007; Bazzino et al., 2010). Low recapture rates and the size of the electronic device usually limit the success of squid tagging experiments (Semmens et al., 2007). Alternatively, elemental signatures (e.g., Sr:Ca ratio) of the hard structures of squid provide a potential source of information regarding the ambient temperatures that they have been exposed to (Ikeda et al., 2003; Yamaguchi et al., 2015, 2018; Liu et al., 2016). However, studies on the relationship between the Sr:Ca ratio and temperature have provided inconsistent results in different species (Elsdon and Gillanders, 2002, 2003; Gillanders et al., 2013). A technique that is more commonly applied to teleosts should be considered as a supplementary method for improving the accuracy and reliability of predictions.
In recent years, stable oxygen isotopic ratios (expressed as δ18O) in biogenic carbonates have been used to examine the spatial distribution and movement patterns of teleosts (e.g., Steer et al., 2010; Currey et al., 2014; Shiao et al., 2017). The δ18O value in fish otoliths is in equilibrium with that in the ambient water, and δ18O value uniformly increases with a decrease in seawater temperature in various species of fish (Elsdon and Gillanders, 2002; Høie et al., 2004a). Squid statoliths are composed of calcium carbonate, and the relationships between statolith and ambient water chemistry are similar to that of fish otoliths (Arkhipkin et al., 2004; Arkhipkin, 2005; Gillanders et al., 2013). Therefore, statolith δ18O value can be analogously used as a temperature proxy for reconstructing the ontogenetic preferences of squids (Radtke, 1983; Landman et al., 2004; Trasviña-Carrillo et al., 2018). For example, Trasviña-Carrillo et al. (2018) analyzed the δ18O value in the entire statolith of the jumbo squid Dosidicus gigas and found that temperature preference did not differ between sexes but did among ontogenetic stages because of differences in living depth. Landman et al. (2004) also predicated the experienced temperature of the giant squid Architeuthis sanctipauli to be in the range of 10.5–12.9°C and average living depths to be 125–250 m by analyzing the δ18O values in one entire statolith, which are considered to provide lifelong average temperature data. However, micromill sampling for higher temporal resolution of δ18O information (Høie et al., 2004b), which was used on fish otoliths, has never been used on squid statoliths to increase the temporal resolution of their ontogenetic thermal migration history.
The bigfin reef squid, S. lessoniana, is widely distributed in the neritic waters of the Indo-Pacific Ocean, which include the waters surrounding Taiwan (Roper et al., 1984; Okutani, 2015). Field observations have revealed that adults migrate from offshore areas to shallow inshore areas for spawning (Segawa, 1987). In the waters off the northern coast of Taiwan, S. lessoniana hatching occurs almost throughout the entire year; hatching peaks in May and August–September (Chen et al., 2015). Hatching can be divided into at least two seasonal groups (spring and autumn) on the basis of hatching date and life history traits of individuals (Ching et al., 2017). This species of squid is important to fisheries because of its high economic value, however, empirical evidence concerning the movement and distribution during the ontogenetic stages is scarce. Ueta and Jo (1990) studied the migration of subadult–adult individuals of S. lessoniana around Tokushima Prefecture, Japan, by using the tag–recapture method and fishery data. Their results suggested that this species stays in inshore waters and migrates to offshore waters for overwintering. Kanamaru et al. (2007) evaluated past tagging studies for S. lessoniana migration in Japan and reported that individuals released in autumn moved farther than those released in spring. However, additional empirical evidence to support these hypotheses is required because conventional tagging studies did not provide complete information on movement patterns throughout the life history of squids. In addition, high-resolution data of seasonal and geological movement patterns of S. lessoniana populations are required for species management.
The present study investigated the ontogenetic movement patterns of different seasonal groups of S. lessoniana in northern Taiwan through statolith δ18O analyses. This study is the first attempt to generate a 3D habitat preference model, including spatial and depth preferences, and it is expected to provide new comprehensive spatial and temporal insights into migration patterns of cephalopods.
Materials and Methods
Squid Collection and Age Estimation
Twenty-two adult S. lessoniana individuals were collected by jigging from the inshore waters of northeastern Taiwan (Figure 1) between November 2017 and March 2018. The mantle length (ML; in mm) of each individual was measured. Statoliths were extracted, cleaned ultrasonically using 70% hydrogen peroxide, rinsed, oven-dried, and embedded in Epofix resin (Struers, Denmark). The left statolith of each squid was ground and polished along the posterior side to approximately 50–100 μm above the core by using a metallographic grinding and polishing machine (P20FR-HA; Top Tech Machines co. Ltd., Taiwan). This thickness ensured that a sufficient volume of milling powder was available for isotopic analysis (>40 μg per sample). Alternative formation of translucent and opaque growth zones has been previously validated as occurring on a daily basis (Jackson, 1990); thus, the growth increment at the lateral dome region of statolith was examined from the photographs recorded under a compound microscope (400×, DM-2500, Leica Microsystems GmbH, Germany) by using a digital camera (DFC-450, Leica Microsystems Lt., Switzerland) to estimate age. The growth increments were counted twice, and an average value was used as daily age. If the difference between two counts was >5%, a third count was recorded to minimize measurement error (Arkhipkin and Shcherbich, 2012). The hatching date of each individual was back-calculated from the deduced age (in days) and the date of collection. Each individual was further categorized into the nearest seasonal group according to its hatching month, namely spring (March–May), summer (June–August) and autumn (September–November). Based on the morphological and ecological changes in accordance with growth and age (Segawa, 1987), we defined four stages in S. lessoniana corresponding to age, namely the embryonic-paralarval (age: 0–20 days), juvenile (age: 20–60 days), juvenile–subadult (age: 60–110 days) and subadult–adult (age: >110 days) stages.
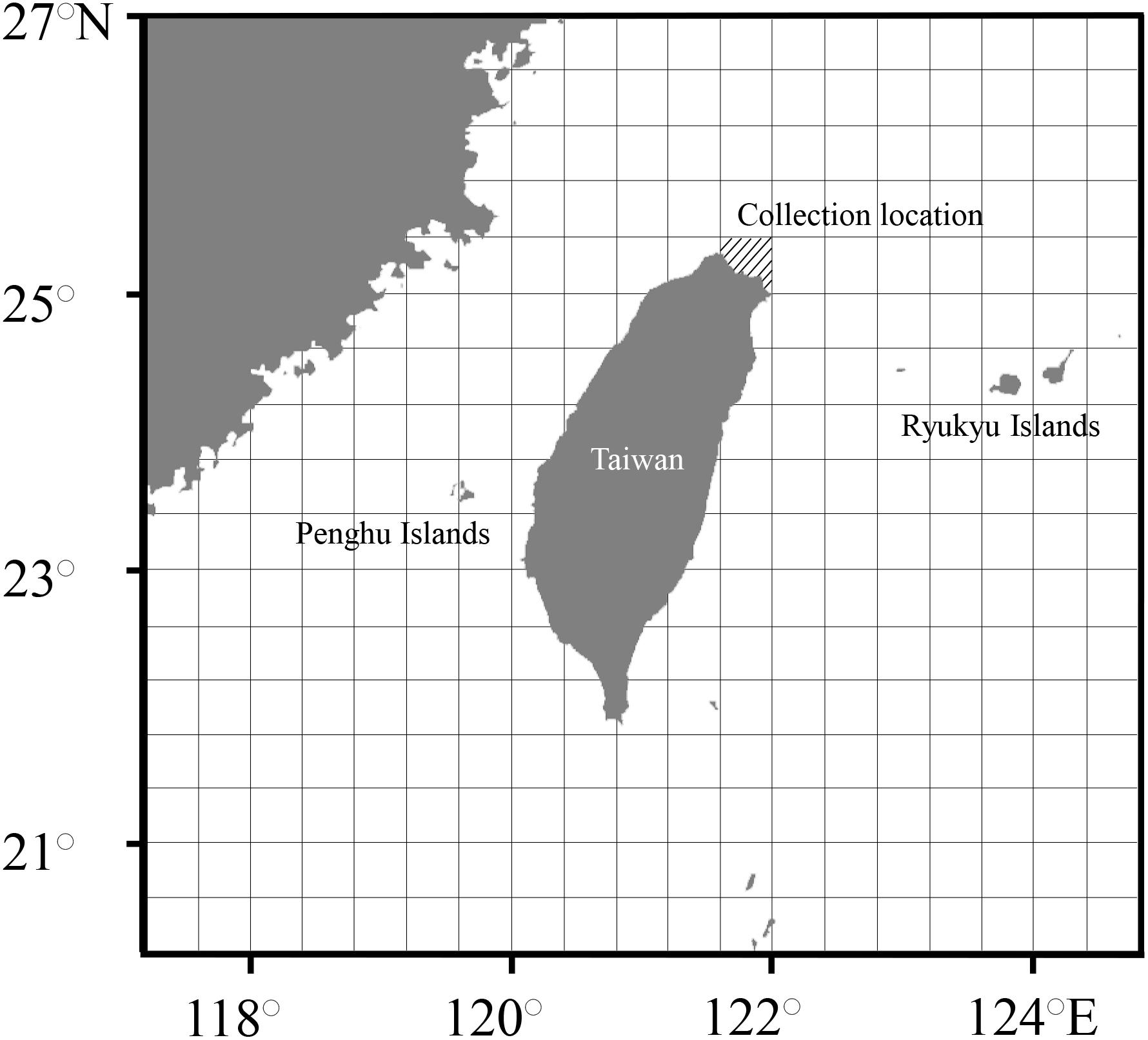
Figure 1. Map showing the collection locations (slanted lines). The unit of spatial grids was 0.4° × 0.4° for the establishment of occurrence probability.
Isotopic Analysis
After age counting, statolith slides were drilled using an ESI New Wave Research Micromill and carbonate powders were collected sequentially from the edge to the core at the lateral dome region at intervals of 150–190 μm (Figure 2). The tip of the drill was approximately 200 μm in diameter (H23RS, Comet, Germany), and milling depth was set at approximately 150 μm. Most statoliths involved four drilling paths (except a smaller statolith, K180313003, which had three paths only), and each path was recorded to estimate covered daily growth.
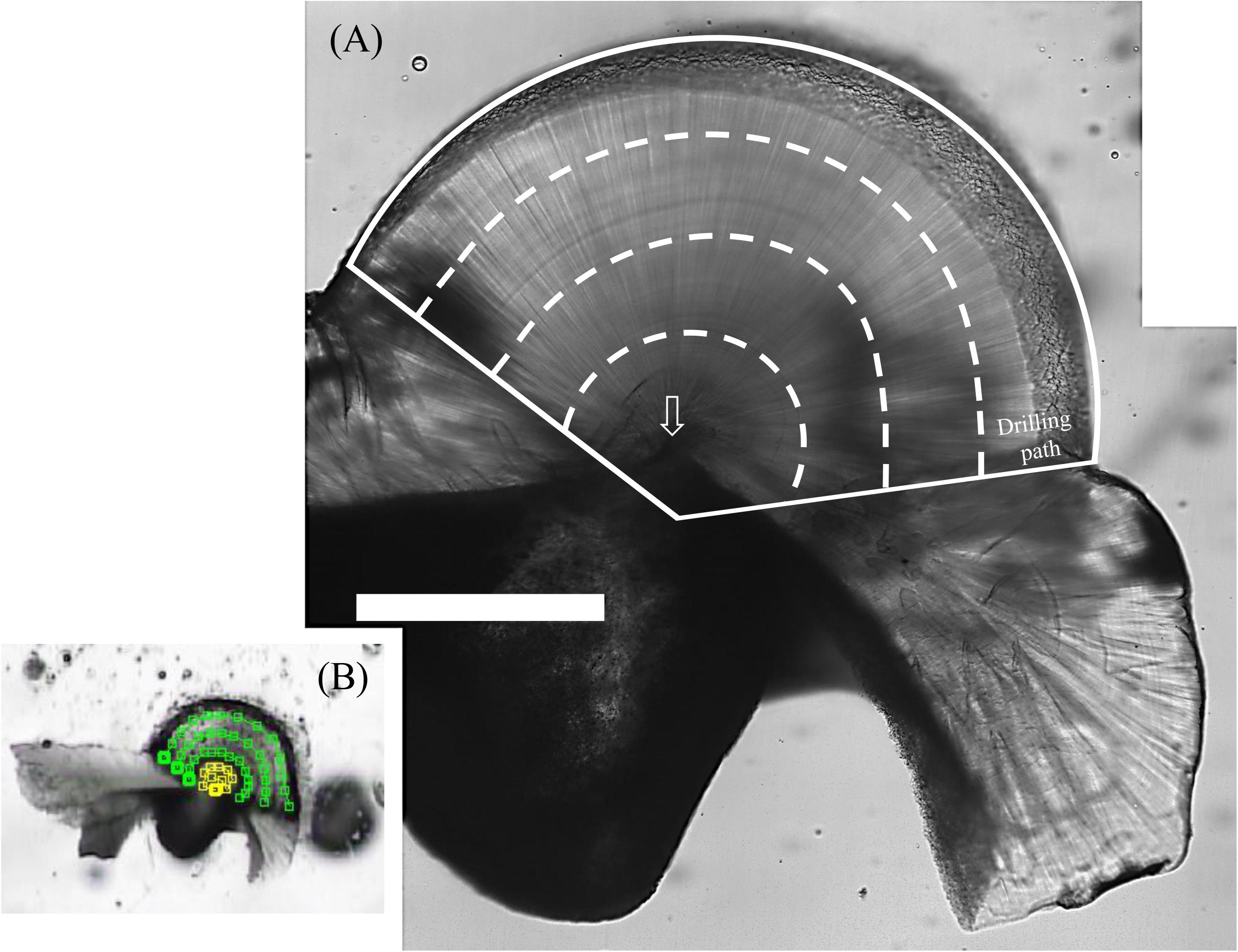
Figure 2. Polished S. lessoniana statolith (K180116012) with the drilling paths for powder collection. (A) White solid lines indicate the entire powder collection area at the lateral dome region and dashed lines indicate approximate boundaries of the drilling path. Arrow indicates the statolith core. Scale bar = 400 μm. (B) The setting of the drilling path. Yellow and green lines indicate the paths of drill tip at the core and the outers of the core, respectively, square as a turning point. The intervals between lines are 150–190 μm.
The powder samples of each drilling path were transferred to glass vials and reacted with 100% orthophosphoric acid at 70°C in an automated online system (Kiel Carbonate IV, Thermo Electron Corporation, Germany) to produce CO2. The values of δ18O were determined by analyzing the released CO2 gas by using a mass spectrometer (Finnigan MAT 253, Thermo Electron Corporation, Germany) at National Taiwan University. The long-term reproducibility of the Finnigan MAT 253 is higher than ±0.08% (one standard deviation [SD]) for δ18O, based on repeat samples of international reference standards (NBS-19, approximately 40–50 μg). The values of δ18O (%) were reported in standard notation relative to standards Vienna Pee Dee Belemnite (VPDB) after calibration against the NBS-19 standard:
The temperature-dependent relationship of δ18O values in biogenic aragonites is taxonomic and species specific (Shirai et al., 2018). However, no equation has been established for statoliths in S. lessoniana; hence, we applied the equation established for statoliths in another cephalopod species, Sepia pharaonis (Chung et al., unpublished data), to deduce experienced temperature in S. lessoniana.
where δ18Ostatolith,VPDB represents the statolith δ18O values on a VPDB scale, and δ18Owater, VSMOW represents the water δ18O values on a VSMOW (Vienna Standard Mean Ocean Water) scale. The δ18Owater, VSMOW values were derived using the relationship with salinity (S) in Taiwan Strait (Chang, 2000):
To evaluate the feasibility of the equation, the relationship of measured and deduced temperature derived from the edge of statoliths in 22 crossed-season captured individuals were analyzed to understand if it followed the 1:1 correspondence. The duration (days) which statolith δ18O values represented was considered, and the measured temperature was averaged based on the duration at the depth of 50 m.
Prediction of Movement Patterns
To accurately predict the experienced temperature and corresponding living areas of S. lessoniana, individual differences in living period and temporal and spatial variations in seawater temperature were considered in the evaluation. The individual living period and season corresponding to each data point of statolith δ18O values were established through the examination of microstructure. During the defined period, water temperature and salinity were obtained from the HYbrid Coordinate Ocean Model website (HYCOM)1 and are presented in a spatial resolution of 0.08° × 0.08° at the depths of 0, 30, 50, and 100 m, according to the living depth of this species (Roper et al., 1984; Tomano et al., 2016; Ammar and Maaroof, 2019). Next, we set the unit of the spatial grid at 0.4° × 0.4° to establish the movement pattern because this spatial grid covers the minimum range of squids captured in the adult stage in the present study (Figure 1). For setting units, the average values of temperature and salinity in each grid at each depths (0, 30, 50, and 100 m) was used to produce δ18Owater, VSMOW values based on the equation established by Chang (2000).
The deduced temperature depended on the estimated statolith δ18O value and the variation in water δ18O values among grids at different depths. Therefore, each data point of statolith δ18O values was used to deduce the experienced temperature in every grid at various depths, each of which had their specific water δ18O values. When the deduced temperature matched with the measured temperature (from the HYCOM), the grid thus obtained was considered the possible living area of the squid during a specific period; this period was estimated using the growth rings in the milling area for the statolith δ18O measurement. The extent of match between the deduced and measured temperature was based on the comparison of probability distributions between these two values determined using Student’s t test (Figure 3). The probability distribution of deduced temperature was modeled using a known living period (days) as well as an average and the uncertainty (SD) associated with the deduced temperature. The uncertainties were estimated by running Monte Carlo simulations 1,000 times and included variations in salinity, instrumental measurements, and parameters of the temperature-dependent equation. Similarly, the probability distribution of measured temperature was modeled using known living periods (days) and average values of measured temperature and associated SDs. Once the t test resulted in a p value larger than 0.05, we accepted that the two temperatures did not differ significantly and inferred a possibility of the occurrence of a living area in the grid (Figure 3). We repeated modeling to determine the matched area and depth of each statolith δ18O value from individuals at each life stage.
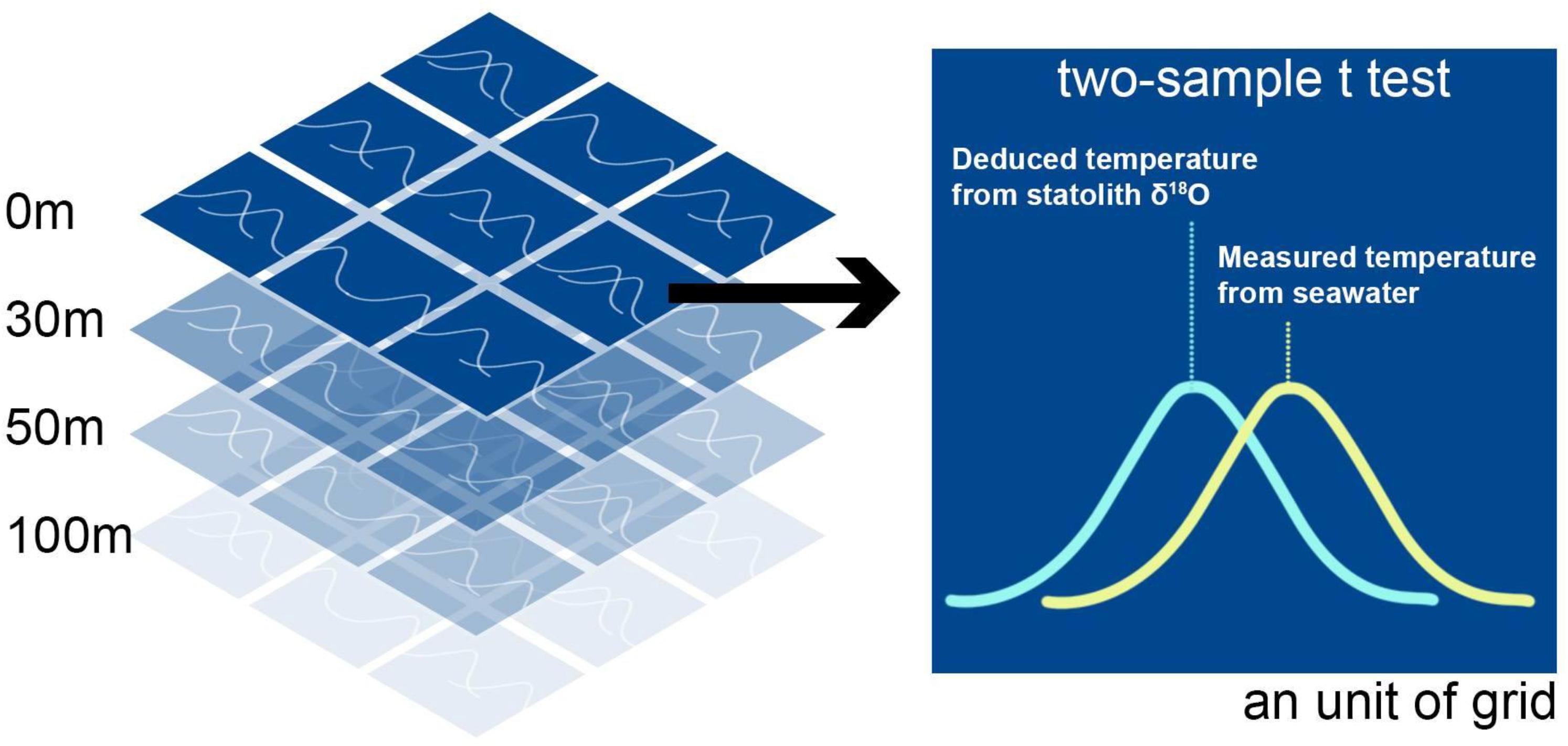
Figure 3. Determination of the extent of matching between deduced and measured temperature by using the two-sample t test. The deduced temperature was based on the statolith δ18O values, and measured temperature was the data extracted from the HYbrid Coordinate Ocean Model website. The test was conducted in each spatial grid (0.4° × 0.4°) at depths corresponding to each specific life stage of S. lessoniana. The criteria of matching were set at the p value of 0.05.
Furthermore, we considered the collection location, spawning site, and movement ability of S. lessoniana to determine the possible living areas. The δ18O value in the outermost portion of statoliths reflected the occurrence of S. lessoniana near the collection location. If this value represented the 10-day average of the signal, the maximum movement of S. lessoniana was calculated to be approximately 50 km away from the collection location because the mean swimming speed of adult S. lessoniana individuals is 5 km per day, according to a study by Kanamaru et al. (2007). Consequently, grids located farther than 50 km from the collection site were eliminated. In addition to this “backward” evaluation, based on the collection location, a “forward” method was also used to evaluate the living area at the embryonic-paralarval stage. We excluded the matched areas that were not adjacent to the coast because the egg capsules of S. lessoniana are always found in inshore waters (Segawa, 1987). Combining the results of forward and backward calculations and mobility, the possible occurrence of four life stages of individuals were further selected from the matched areas found based on the statolith δ18O value.
After examining the living area at an individual level, we calculated the probability of occurrence in each setting area for each seasonal group. The probability was calculated using the following equation:
where Ckj represents the occurrence of kth squid in area j, and kj is the total number of individuals in grid j. If squid sample number 1 exists in grid 1 at a specific life stage, the Ckj value is 1; otherwise, the Ckj value is 0. Furthermore, the Pj value indicates the probability of occurrence in grid j. If the Pj value is 1, all squid samples at the same ontogenetic stage occur in grid j. If an area with a probability (p value) of >0.5 is identified, it would be considered a major residential area for S. lessoniana seasonal groups around Taiwan.
Statistical Analysis
To identify the effects of ontogenetic stage and hatching season on δ18Ostatolith value, differences in δ18Ostatolith values among all ontogenetic stages over the seasonal groups (spring, summer, and autumn) were examined using a non-parametric Scheirer–Ray–Hare extension of the Kruskal–Wallis test followed by post hoc multiple comparisons tests (Dunn’s tests). A linear regression and an Analysis of Covariance (ANCOVA) were used to test if the experienced temperature deduced by the equation (Chung et al., unpublished data) was reasonable and close to 1:1 correspondence between measured and deduced temperature. All statistical tests were conducted using SPSS (ver. 20, IBM Corp., Armonk, NY, United States). The t test used to evaluate the extent of matching between deduced and measured temperatures and the Monte Carlo simulations were used for uncertainty determination were performed using R (R Core Team, 2018).
Results
After statolith age determination, seven individuals were found to belong to the spring group with a mean (±SD) ML of 264 ± 49 mm and an age ranging from 150 to 167 days. Six individuals were found to belong to the summer group with a mean ML of 260 ± 24 mm and an age ranging from 146 to 192 days. Nine individuals were found to belong to the autumn group with a mean ML of 298 ± 45 mm and an age ranging from 140 to 177 days (Table 1). No significant difference in ML was observed between the seasonal groups [analysis of variance (ANOVA), F = 1.944, p = 0.171]. For statolith carbonate sampling, the drilling paths were averaged (±SD): 17.5 ± 8.4, 42.5 ± 7.8, 49.8 ± 7.0, and 53.3 ± 5.9 days from the core to the edge presenting the embryonic–paralarval, juvenile, juvenile–subadult, and subadult–adult stages, respectively.
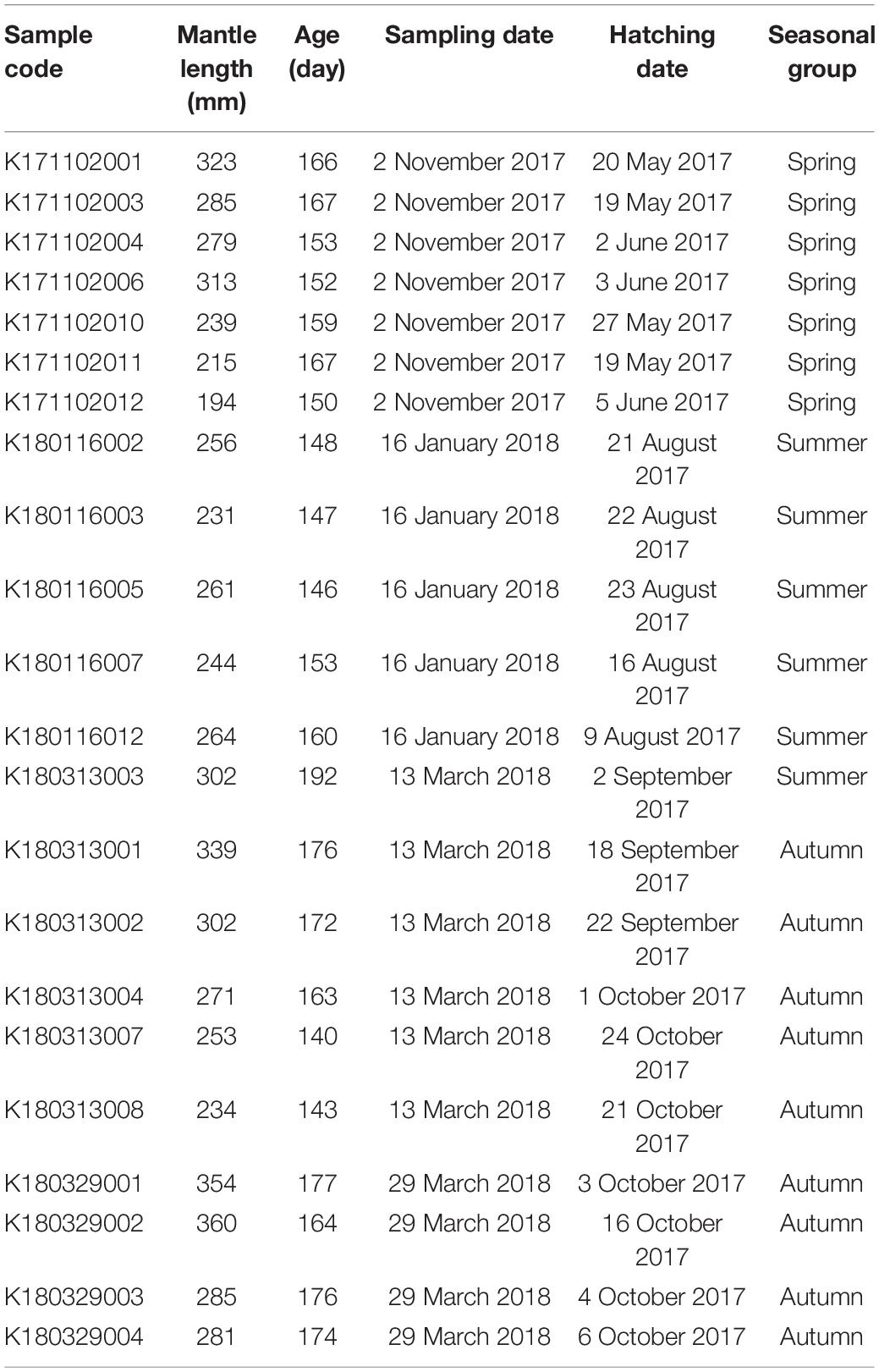
Table 1. Sampling date, mantle length, age estimation, and back-calculated hatching date and season for each S. lessoniana individual used in statolith oxygen isotopic analysis.
Oxygen Isotopic Composition of the Statolith
Overall, δ18Ostatolith values ranged from −2.93 to −0.12‰ with a mean of −1.86 ± 0.79‰. Variations in the δ18Ostatolith values were not consistent among seasonal groups (Figure 4A). Larger variations were observed in the summer and autumn groups than in the spring group, however, the δ18Ostatolith values were not significantly different among the seasonal groups (Kruskal–Wallis test, H = 1.506, p = 0.471, Table 2). Ontogenetic stage differences were observed in δ18Ostatolith values (Kruskal–Wallis test, H = 39.941, p < 0.001, Table 2). The values decreased slightly from embryonic–paralarval stage to the juvenile stage and then increased until the subadult–adult stage (Figure 4B). The values of δ18Ostatolith in embryonic–paralarval stage were significantly lower than those in the subadult–adult stage (Dunn’s tests, Z = −4.078, p < 0.001). In addition, the values in the juvenile stage were significantly different from those in the juvenile–subadult and subadult–adult stages. No interaction was observed between the seasonal group and ontogenetic stages in δ18Ostatolith values (Table 2).
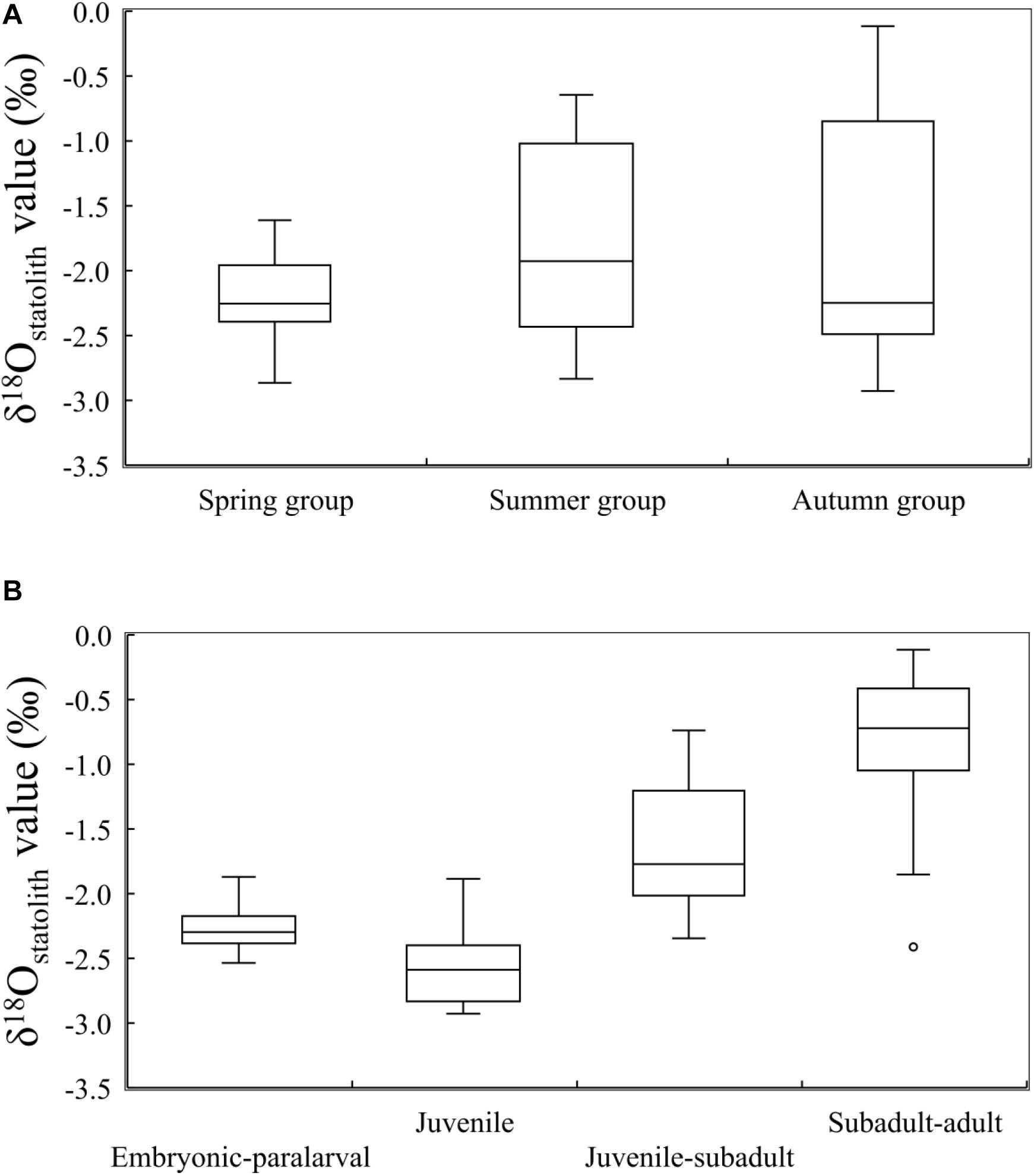
Figure 4. δ18Ostatolith values in S. lessoniana (A) in three seasonal groups and (B) at four ontogenetic stages. The box plots show the interquartile range, with the median value indicated by the horizontal line; whiskers show the range. Circles indicate outliers.
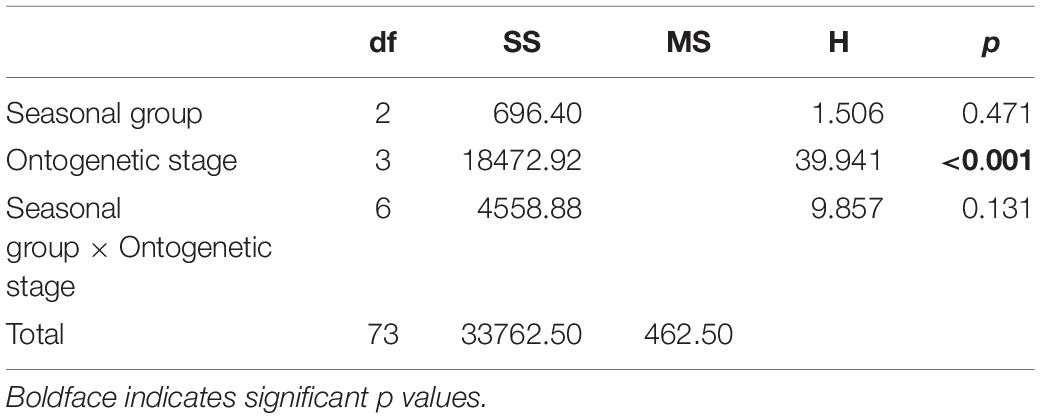
Table 2. Results of non-parametric analysis of variance to test the differences in S. lessoniana statolith oxygen isotopes ratios among seasonal groups (spring, summer, and autumn) and ontogenetic (embryonic–paralarval, juvenile, juvenile–subadult, and subadult–adult) stages.
Individual ontogenetic trends showed that the δ18Ostatolith values in the spring group ranged between −2.87 and –1.61‰ and were relatively stable until the statolith edge (Figures 5A,B). In the summer group, the δ18Ostatolith values in the statolith core varied from −2.54 to −1.87‰; they decreased to the lowest levels, between −2.32 and −2.83‰, at the juvenile stage and then increased to the highest levels, between −1.06 and −0.65‰, in the statolith edge (Figures 5C,D). The δ18Ostatolith values in the autumn group showed a pattern similar to that of the summer group (Figures 5E–G), but the δ18Ostatolith values were higher at the juvenile–subadult stage (−2.23 to −0.97‰) and subadult–adult stage (−0.50 to −0.12‰) than those in the summer group.
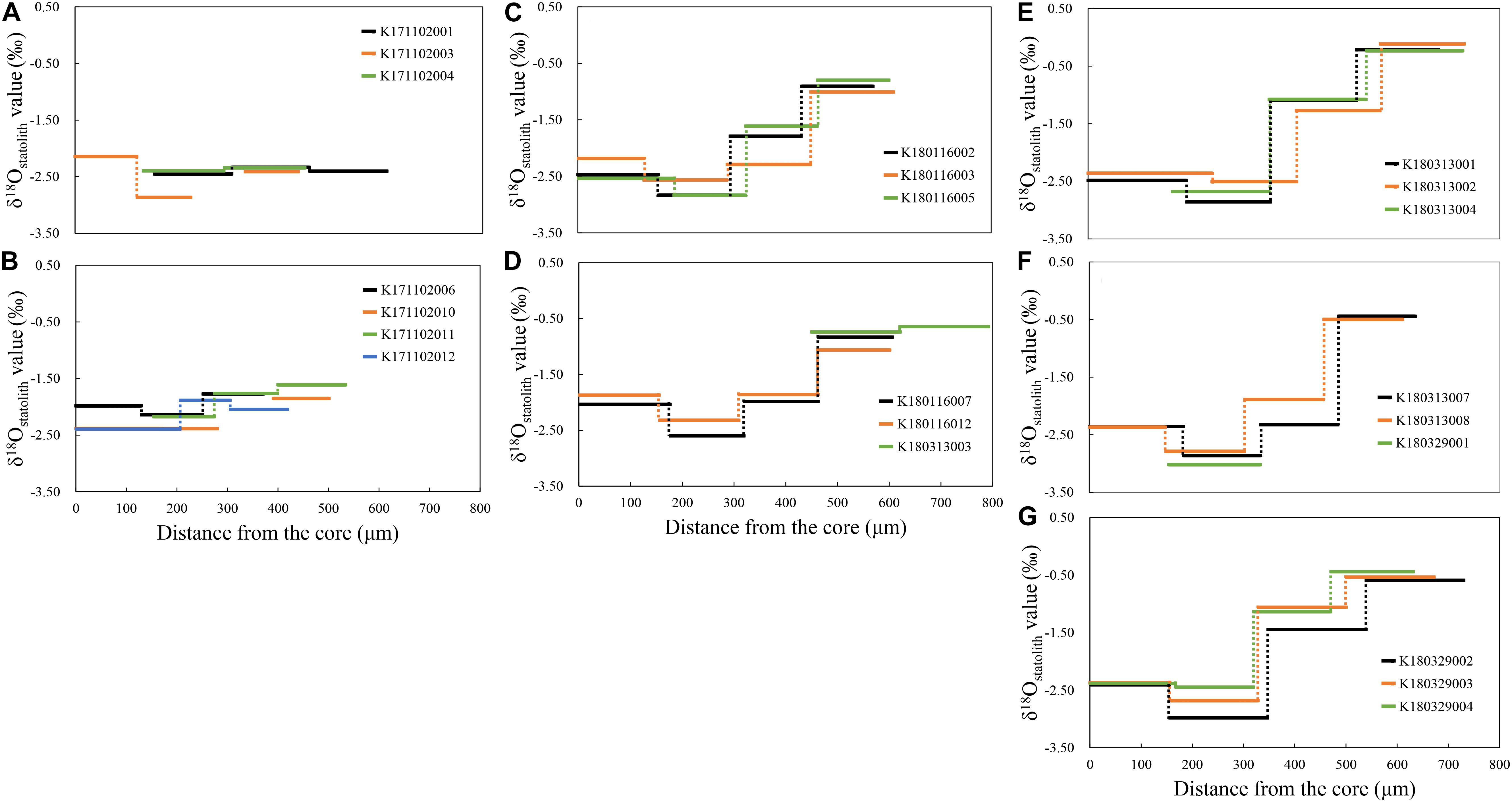
Figure 5. δ18Ostatolith profiles of S. lessoniana from the statolith core to the edge of each individual in the spring group (A,B), summer group (C,D), and autumn group (E–G).
Predicted Occurrence Area and Movement Pattern
Individual values of deduced and measured temperature were close and the linear regression showed a well correspondent (Figure 6, p < 0.001). The slope was not significantly different from the line of 1:1 (F1,34 = 0.83, p = 0.37) indicating that the deduced temperature could reflect reasonably the experienced temperature of S. lessoniana.
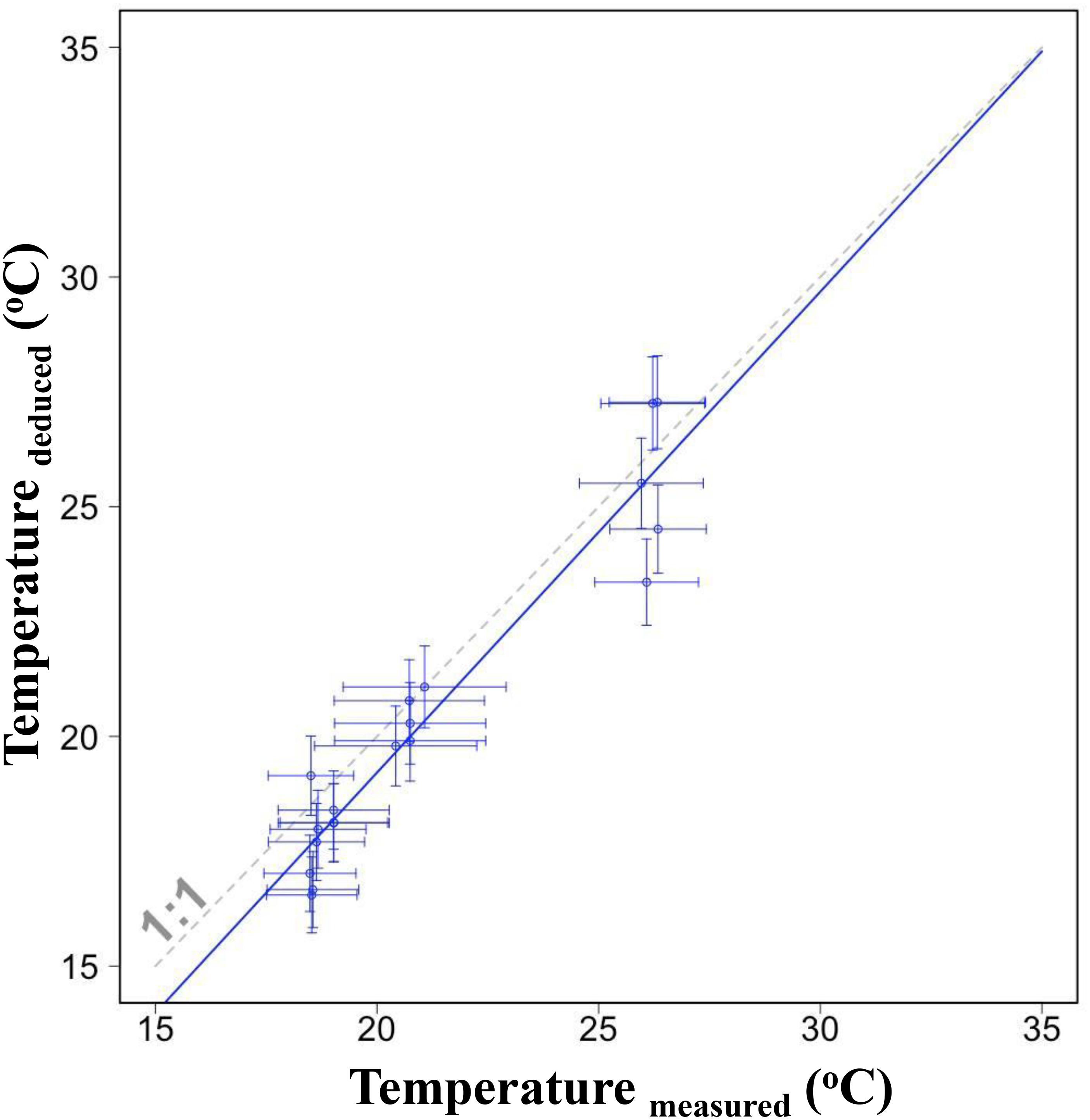
Figure 6. The linear relationship between deduced temperatures from δ18O values of individual statolith edges and corresponding measured temperatures in the same period. Horizontal error bars indicate one standard deviation of measured temperature; vertical error bars indicate one standard deviation evaluated by using Monte Carlo Simulations and considering uncertainty caused from salinity and parameter variation in the equation. Dashed line indicates the 1:1 correspondence.
Figures 7–9 present the distribution of occurrence probability of seasonal groups at different depths by comparing deduced and measured temperatures while considering collection location, spawning site, and movement ability. The probability of occurrence results indicated that the three seasonal groups of S. lessoniana in northeastern Taiwan have diverse distributions and movement patterns. The individuals in the spring group had the highest possibility of hatching in neritic waters (approximate depth: 0–50 m) near the coast, extending from northeastern to eastern Taiwan and the Ryukyu Islands (Figure 7). After hatching and reaching the paralarval stage, individuals were widely distributed in the inshore waters of northeastern Taiwan, possibly from the sea surface to an approximate depth of 50 m; these individuals may have subsequently migrated to relatively deeper waters near the coast of northeastern Taiwan or to the offshore waters of eastern Taiwan. In addition, the waters at a depth of 30–50 m along southern Taiwan were also potential hatchling grounds, and the hatched individuals moved northward as the growth proceeded. Finally, they mainly remained in the northeastern waters during the subadult–adult stages (Figure 7).
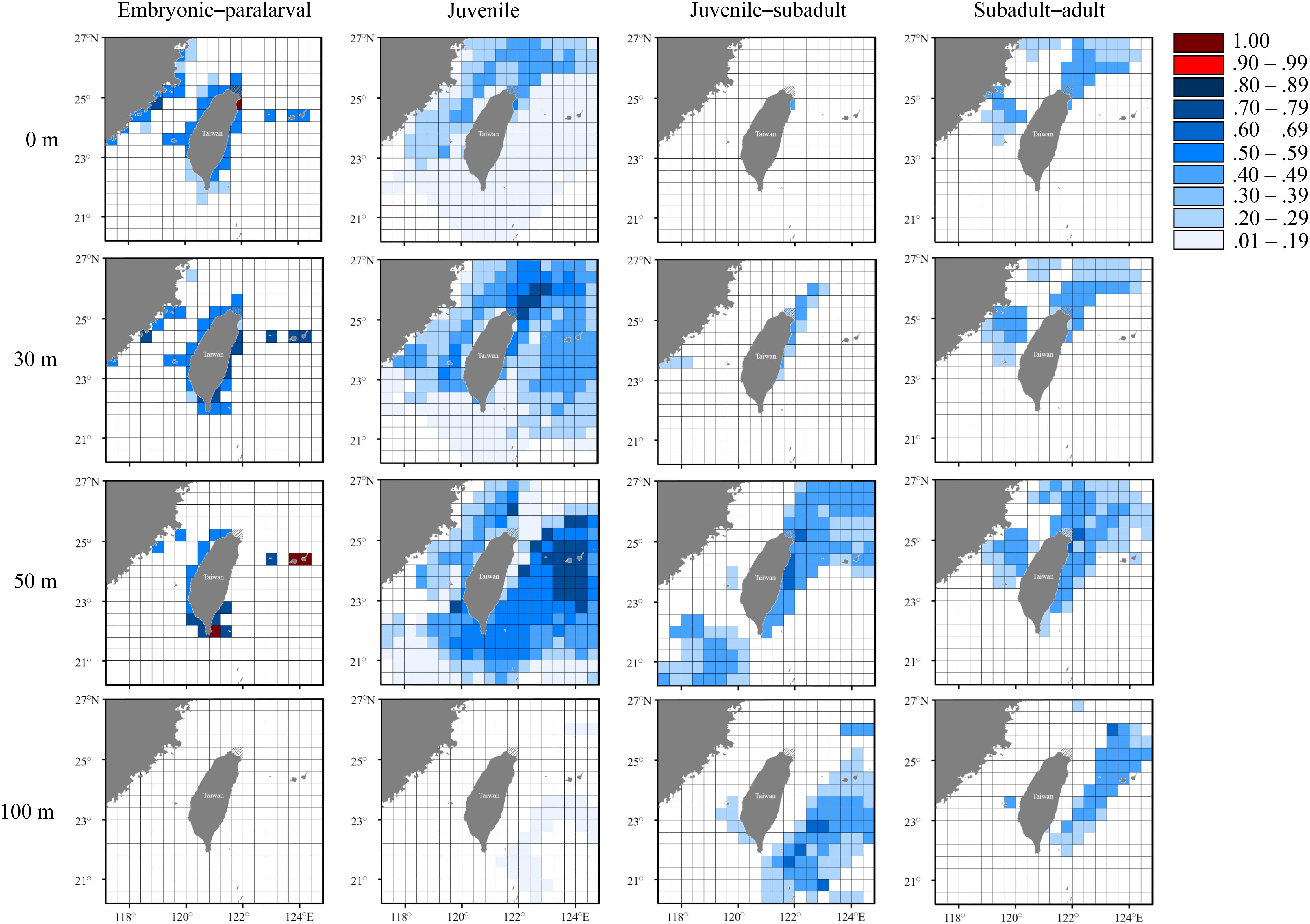
Figure 7. Probability distribution based on experienced temperatures in S. lessoniana individuals in the spring group at each life stages. The legend indicates the probability value of occurrence.
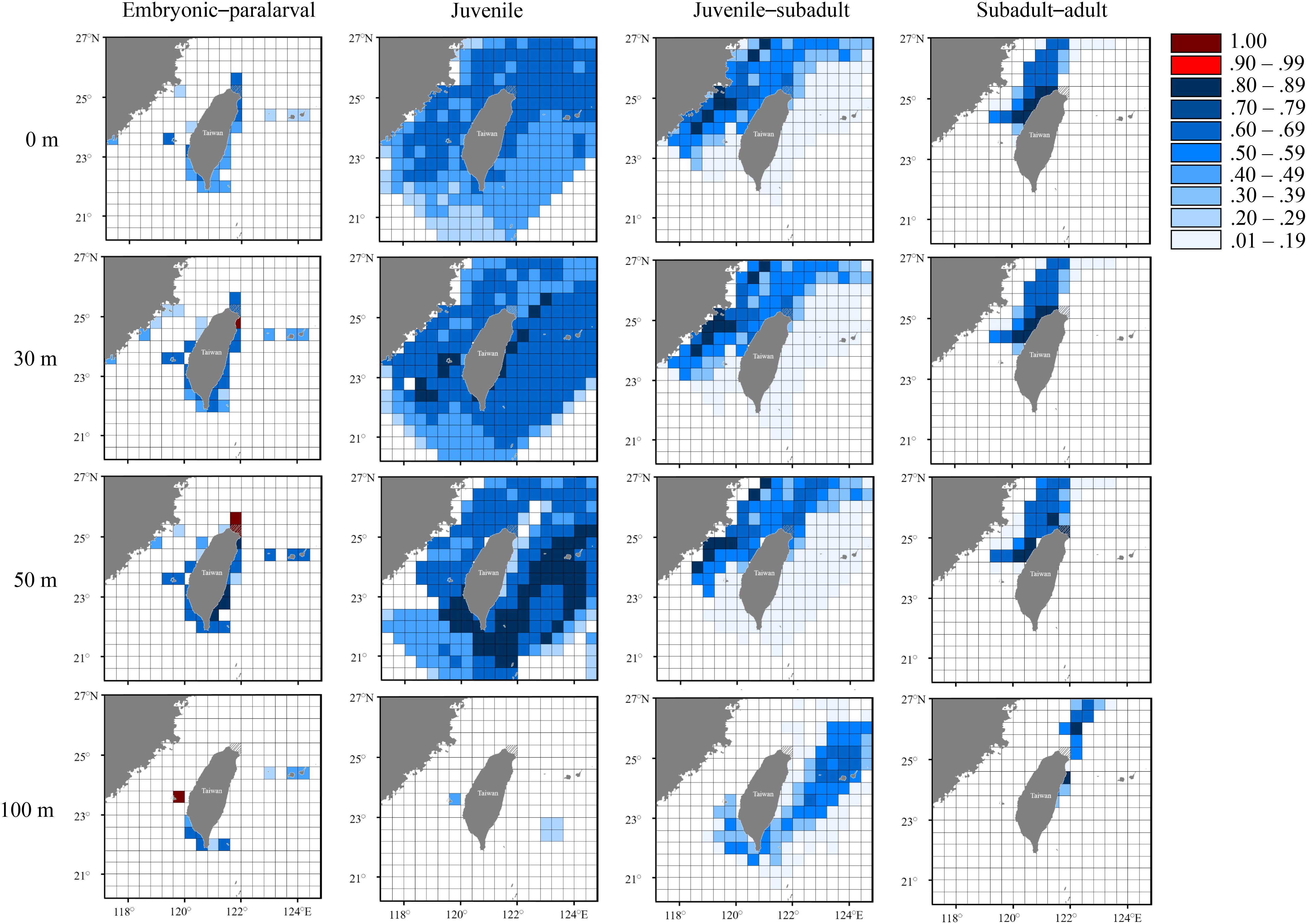
Figure 8. Probability distribution based on experienced temperatures in S. lessoniana individuals in the summer group at each life stage. The legend indicates the probability value of occurrence.
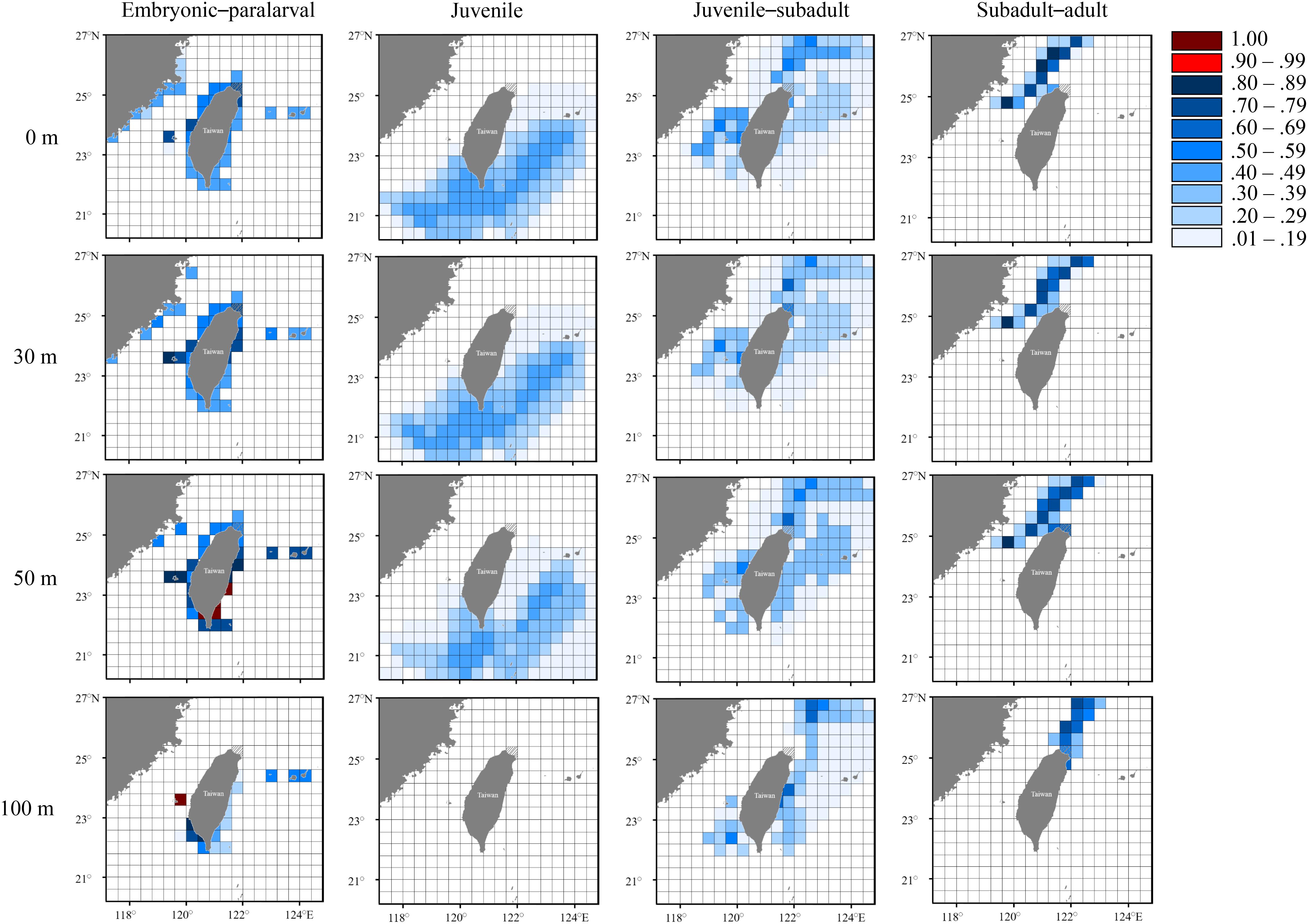
Figure 9. Probability distribution based on experienced temperatures in S. lessoniana individuals of the autumn group at each life stage. The legend indicates the probability value of occurrence.
The summer group was most likely to hatch in the areas near northeastern Taiwan, extending southward to the Penghu Islands (Figure 8). However, the predicted distribution at the juvenile stage covered a wide area, owing to constant seawater temperatures in summer. Similarly, a wide and deep living area (approximate depth: 100 m), including the inshore waters of northern Taiwan and the waters near the Ryukyu Islands (approximate depth: 100 m), was observed in the last two stages, and it differed from the pattern of the spring group.
In the autumn group, the predicted hatching sites were similar to those in the summer group (Figure 9). However, juvenile individuals might have been distributed in the southern waters (Figure 9), but they were not detected in the waters, with the probability >0.5, because the deduced temperature at this stage did not completely match the water temperature in the study area. The autumn group then spent their subadult and adult stage (approximately 3–4 months) in the inshore waters of northern Taiwan at depths between 0 and 100 m before capture.
Discussion
Stable oxygen isotope ratios recorded in statoliths provide information regarding diverse movement patterns of S. lessoniana; differences in ontogenetic distributions were observed among the three seasonal groups. Although the approach for investigating the movement of animals by using δ18O values in biogenic carbonates has been widely applied in studies on fish (Trueman et al., 2012; Currey et al., 2014; Shiao et al., 2017; Darnaude and Hunter, 2018), thus far, studies have not adapted this method to determine the movement history of cephalopods. We demonstrated the potential of using this approach in studies on cephalopod ecology. Because of the significant effects of larval dispersal and demographic population connectivity on cephalopod resources (O’dor, 1992; Boyle and Boletzky, 1996; Semmens et al., 2007), the results of the present study may improve the fishery management and conservation for the bigfin reef squid.
Variation in Statolith δ18O Values in Relation to Experienced Temperature
The variation in statolith δ18O values among individuals has been observed in several squid species and is associated with differences in experienced temperature (Radtke, 1983; Landman et al., 2004; Trasviña-Carrillo et al., 2018). The general trend of statolith δ18O values is to decrease with an increase in temperature; this trend follows the theoretical predictions and observations from other biogenic carbonates (Rexfort and Mutterlose, 2006; Trueman et al., 2012; Kitagawa et al., 2013; Linzmeier et al., 2016). However, assessing the reliability of using statolith δ18O values to reconstruct experienced temperature in cephalopods is challenging because previous studies have analyzed δ18O value from an entire statolith, which indicates the mean experienced temperature throughout its lifespan. Thus, in our study, we evaluated the deduced temperature compared with the seawater temperature and temperature preference of S. lessoniana at different life stages.
The deduced temperature of the embryonic stage and an approximate 20-day paralarval stage from the statolith core ranged from 22 to 28°C, regardless of seasonal group. This finding was consistent with those of other studies that found that S. lessoniana hatches in a warm environment of approximately 20 to 30°C (Segawa, 1987; Walsh et al., 2002; Ikeda et al., 2009). Each squid species has its optimum living temperature, which supports its growth and survival, particularly in its early life stages (Jackson and Choat, 1992; Forsythe et al., 2001). This suggests that the population of S. lessoniana in northern Taiwan shows a preference for this temperature at hatch.
The statolith δ18O values exhibited variation after the paralarval stage among seasonal groups because of seawater temperature varying with the seasons. For example, the spring group experienced warmer (summer) temperatures at the juvenile and adult stages than at the hatching stage in spring. By contrast, the summer and autumn groups reached their juvenile and adult stages in autumn and winter, respectively hence, they experienced lower temperatures in their juvenile and adult stages than in their hatching stage. The summer and autumn groups exhibited more positive δ18O values than the spring group at the juvenile and adult stages because of lower temperatures. Thus, the ontogenetic variation in statolith δ18O values in the spring group was less obvious than in the summer and autumn groups (Figure 4). The statolith δ18O values of S. lessoniana ontogenetic variation mirrored the seasonal temperature fluctuation off Taiwan waters, thus indicating the reliability of the prediction method used in the present study.
Prediction of Ontogenetic Movement and Geographical Distribution
The seasonal and vertical migration of squid species has been described in the literatures (Bazzino et al., 2010; Argüelles et al., 2012; Yamaguchi et al., 2019); this behavior was also observed in our study. Interpreting time-series data in squids is generally more difficult than interpreting data obtained from bivalves (e.g., Nakashima et al., 2004; Owen et al., 2008; Nishida et al., 2015) because the squid species move freely, unlike bivalves. We converted the measured statolith δ18O values to the deduced temperature and cross-matched them with the seasonal and depth changes in the seawater temperatures to estimate the probability of the geographical distribution of S. lessoniana by using a procedure derived from a widely used method to study fish migration (involving the use of otolith δ18O values; Thorrold et al., 1997; Weidel et al., 2007; Shiao et al., 2014). In our case, the movement patterns of S. lessoniana in four life stages exhibited diversity among the seasonal groups (Figures 7–9). These findings are comparable to the known ecology of S. lessoniana, including spawning (Segawa, 1987), migration (Ueta and Jo, 1990; Kanamaru et al., 2007) and depth distribution (Roper et al., 1984; Tomano et al., 2016).
According to statolith δ18O values, most probably the individuals of the spring and summer groups hatched near the coasts of northeastern Taiwan, with an occurrence probability of 1; these findings support empirical evidence that the waters near the coast of northeastern Taiwan are one of main spawning grounds for S. lessoniana (Chen et al., 2015; Ching et al., 2017). The dominant topography in northern Taiwan is that of an eroded coastline with complicated topographical features and structures (Song et al., 1997), forming macroalgae-rich and coral-rich environments for spawning. In addition, the quantity of nutrients supplied by the year-round upwelling of the Kuroshio Current off northeastern Taiwan supports high primary production and sustains sequential consumers in the waters (Liu et al., 1992; Chen, 1997; Gong et al., 2003). Therefore, this biomass could be a crucial factor sustaining the abundance of S. lessoniana near Taiwan (e.g., Otero et al., 2008; Rodhouse et al., 2014). However, the individuals of the autumn group had a high probability (>0.8) of hatching in the waters of southern and southwestern Taiwan (i.e., the Penghu Islands). The paralarvae were transported northward by the Kuroshio Current or the South China Warm Current into the Taiwan Strait during September and October (Tang et al., 2000; Jan et al., 2002, 2006). Although the results suggest that waters at a depth of 100 m near the coast of southern Taiwan are also potential hatching grounds, adult females are unlikely to have laid eggs at a depth of down to 100 m because the environment is unsuitable for planktonic paralarvae and no egg capsules have been observed on the seabed at the aforementioned depth.
Distribution of juvenile individuals appeared to be widespread around Taiwan, both horizontally and vertically. This distribution pattern has two possible explanations. First, the juvenile squids were passively shifted by ocean currents, thus reflecting the seawater temperatures of a larger region. Second, the consistent seawater temperatures around Taiwan in summer and autumn reduced the precision of distribution prediction. In particular, the estimated probabilities of juvenile distribution in autumn group were all less than 50%. Compared with the juvenile stage, the subadult–adult stages showed a narrower area of predicted distribution. In the spring group, the subadult individuals remained at a depth of approximately 50 m near the coast of northeastern Taiwan or at a depth of approximately 100 m in the inshore waters of eastern Taiwan. Subsequently, adult individuals migrated to the coasts of northeastern for mating and spawning. To our knowledge, larger individuals are rarely captured in the eastern waters, which suggests that the eastern waters are not a principal habitat for S. lessoniana individuals in the spring group. Nevertheless, the occurrence of S. lessoniana in the eastern water needs to be understood through additional surveys using systematic fishery records. By contrast, individuals in the juvenile–subadult stage in the summer group were mostly found in the areas between the coastal waters of China to northeastern Taiwan; furthermore, individuals in this stage in the autumn group might remain in the waters in northeastern Taiwan or around the Penghu Islands. Both the summer and autumn groups used the northern waters as their main habitat during the adult stage in winter. In addition, adult individuals in the autumn group appeared to move to further offshore waters (about 100 km). During winter months, drastic reductions in temperature occur in northern Taiwan primarily during strong northeasterly monsoon and cold surge events (Chen and Huang, 1999; Chen et al., 2002). In such a turbulent state, the individuals move offshore for overwintering and return to inshore areas for feeding and spawning when the environment becomes relatively stable. This explanation is consistent with the findings of Ueta and Jo (1990), who studied the migration of S. lessoniana subadult–adult individuals around Tokushima Prefecture.
The individuals in the seasonal groups in the present study exhibited a possible migration route from the Penghu Islands to northeastern Taiwan and considerable habitat overlap. In general, loliginid squids spawn throughout the year and consequently exhibit multicohort formation along with highly diverse dispersion patterns; hence, high levels of genetic diversity are achieved in the populations of these cephalopods (O’dor, 1998). A study reported that the elemental signatures in the entire statolith exhibited less variation between S. lessoniana samples from northern Taiwan and the Penghu Islands for the same season (Ching et al., 2017). This finding suggests a high level of population connectivity in S. lessoniana in Taiwan. In recent years, the coexistence of three cryptic lineages of S. lessoniana has been reported in the Indo-Pacific Ocean (Cheng et al., 2014; Tomano et al., 2016). These cryptic lineages of S. lessoniana exhibit similar morphology but are genetically distinct (Akasaki et al., 2006; Hsiao et al., 2016; Shen et al., 2016). Although the extent of cryptic diversity within the S. lessoniana species complex in Taiwan remains unclear, a single cryptic lineage may be predominant in northern Taiwan and the Penghu Islands, based on the migration pattern prediction. Additional studies with larger areas of geographic sampling and using a combination of molecular methods are needed to provide more knowledge regarding the population structure of the bigfin reef squid over its distribution range.
Method Improvement and Applications in Future
As the first study to determine the ontogenetic movement of squid by using statolith δ18O values, we provided information on the life history of S. lessoniana, but we acknowledge that our method can be improved considerably in the future. First, the δ18O values in the juvenile statoliths of the autumn group suggested high experienced temperatures (approximately 25–29°C), and this does not satisfactorily accord with the observed water temperature range from the sea surface to a depth of 100 m depth in autumn. We emphasize that the mechanisms of isotopic fractionation in statoliths [such as the results of fish otolith by Thorrold et al. (1997) and Høie et al. (2004a)] and other potential sources of variability (e.g., Høie et al., 2004b; Darnaude and Hunter, 2018; Linzmeier, 2019) should be carefully considered. Second, the seawater δ18O values vary by <1% across the surface in the present study area and by approximately 1% with depth (LeGrande and Schmidt, 2006), slightly biasing the prediction of experienced temperature. We used salinity to predict seawater δ18O values, based on the equation established from the waters in Taiwan Strait (Chang, 2000). The development of location-specific relationships between salinity and seawater δ18O values can minimize the bias of reconstructed temperature. Third, reducing the amount of sample powder from statolith can increase the temporal sampling resolution and enhance the precision of reconstructed temperature (Leder et al., 1996; Høie et al., 2004b). As reported by Sakamoto et al. (2019) in their otolith study, the weight of drilling powder can be as low as 0.3–11.4 μg, representing a temporal resolution of 10–30 days, which is considerably higher than that in our study (>40 μg and approximately 30–50 days). A statolith analysis with higher temporal resolution can significantly benefit the studies on stock discrimination and individual migration. Fourth, defining minimum and maximum probabilities that can indicate the existence of S. lessoniana populations requires additional statistical support. In addition, the size of the spatial grid can be reduced to match the movement behavior of S. lessoniana for a higher precision of habitat determination.
Seawater temperature directly and strongly affects cephalopod ecology and fisheries (Jackson and Moltschaniwskyj, 2002; Forsythe, 2004). Statolith δ18O is a crucial parameter that provides evidence of general patterns of distributional extent and movement. Statolith, which are involved in orientation and balance, are found in all cephalopod species; hence, they can be widely used for ecological research on cephalopods (Clarke, 1978; Arkhipkin, 2005). For example, the predicted geographical distributions based on statolith δ18O signatures are comparable to the estimated stock boundaries determined using fishery data or tagging methods. Precise geographic boundary and habitat use (e.g., spawning ground) allow managers to implement suitable management measures to conserve targeted species (Gislason et al., 2000; Hobday et al., 2010). In recent years, in response to varaitions in the behavior of oceans and difficulties in managing resources, scientists have highlighted the importance of technological improvements through use of finer spatial and temporal scales for near real-time animal tracking (Maxwell et al., 2015; Dunn et al., 2016). This study combined the deduced temperatures of the individuals over ontogenetic stages to describe continuous movement patterns of S. lessoniana lifespan. The intra- and inter-annual movement patterns also support the decisions pertaining to the establishment of fishing grounds, forecasting of catches, and dynamic fishery management for cephalopods (e.g., Yamaguchi et al., 2019). However, the extent of geographic distribution resolution using statolith δ18O may be species specific. Our results showed that accurately predicting residence waters during the periods when variations in water temperature of the region are not obvious (from summer to early autumn in Taiwan) is difficult. Thus, statolith δ18O is unlikely to serve as the main indicator of distribution for tropical cephalopod species. Species with a large diel vertical migration (e.g., Young, 1978; Hunt and Seibel, 2000) also probably biases the prediction of experienced temperature, thus increasing the risk of misinterpretation when this method is applied to a species. Establishing a temperature-dependent relationship of δ18O for specific cephalopod species is also necessary for improving the approach in the future.
Conclusion
All wild squids possess natural isotopic signatures that are incorporated into their statoliths and reflect the environmental temperature or isotopes composition. Migration between waters may produce shifts in isotopic composition. This study applied sequential isotopic sampling to statoliths, and the findings suggest the ecological features of seasonal movement strategies and population connectivity in S. lessoniana in Taiwan. As a result, the waters off northeastern Taiwan have proven to be an important spawning ground for S. lessoniana. Implementing fishery management by restrictions or closed fishing zone during spawning seasons will ensure the recruitment of S. lessoniana population in Taiwan sustainable. Flexible life history traits and a large distribution range are critical for cephalopods and support a high level of genetic diversity and ensure population abundance. These findings extend the limited knowledge about the life history of S. lessoniana within a year. Future developments can reduce the uncertainty associated with this approach and provide more accurate species-specific interpretations of the variations of statolith δ18O values within individuals and stocks of free-moving cephalopods.
Data Availability Statement
The datasets generated for this study are available on request to the corresponding author.
Ethics Statement
Ethical review and approval was not required for the animal study because we collected the squid samples from the commercial fishery and did not conduct a control or treatment experiment.
Author Contributions
C-HW led this project. C-HW, T-YC, and AY coordinated the experiment. T-YC and AY assisted in wild squid collections. C-IC wrote the manuscript and prepared the samples. C-IC and M-TC were responsible for data analysis. J-CS and P-LW contributed the isotopic analyses. All authors revised, reviewed, and finally approved the manuscript.
Funding
This study was funded by a project from the Ministry of Science and Technology, Taiwan (MOST 106-2611-M-019-004) to C-HW.
Conflict of Interest
The authors declare that the research was conducted in the absence of any commercial or financial relationships that could be construed as a potential conflict of interest.
Acknowledgments
The authors thank Bor-Neng Jenq and Pi-Hang Hung for their help with squid specimen collection. The authors also thank Ling-Wen Liu, Ching-Chun Cheng, and Ting-Hung Lin from National Taiwan University for assisting with statolith sample analysis and Dr. Yi-Chen Wang and Sheng-Yuan Teng from the National Taiwan Ocean University for their guidance on the temperature and salinity data capture. Comments by the two reviewers significantly improved the manuscript. This manuscript was edited by Wallace Academic Editing.
Footnotes
References
Akasaki, T., Nikaido, M., Tsuchiya, K., Segawa, S., Hasegawa, M., and Okada, N. (2006). Extensive mitochondrial gene arrangements in coleoid Cephalopoda and their phylogenetic implications. Mol. Phylogen. Evol. 38, 648–658. doi: 10.1016/j.ympev.2005.10.018
Ammar, I., and Maaroof, R. (2019). First record of the squid Sepioteuthis lessoniana Férussac, 1831 in the Syrian coastal water. SSRG Int. J. Agric. Env. Sci. 6, 52–55. doi: 10.14445/23942568/ijaes-v6i1p108
Argüelles, J., Lorrain, A., Cherel, Y., Graco, M., Tafur, R., Alegre, A., et al. (2012). Tracking habitat and resource use for the jumbo squid Dosidicus gigas: a stable isotope analysis in the Northern Humboldt current system. Mar. Biol. 159, 2105–2116. doi: 10.1007/s00227-012-1998-2
Arkhipkin, A. I. (2005). Statoliths as black boxes (life recorders) in squid. Mar. Freshwater Res. 56, 573–583.
Arkhipkin, A. I., Campana, S. E., FitzGerald, J., and Thorrold, S. R. (2004). Spatial and temporal variation in elemental signatures of statoliths from the Patagonian longfin squid (Loligo gahi). Can. J. Fish. Aquat. Sci. 61, 1212–1224. doi: 10.1139/f04-075
Arkhipkin, A. I., and Shcherbich, Z. N. (2012). Thirty years’ progress in age determination of squid using statoliths. J. Mar. Biol. Assoc. U.K. 92, 1389–1398. doi: 10.1017/s0025315411001585
Bazzino, G., Gilly, W. F., Markaida, U., Salinas-Zavala, C. A., and Ramos-Castillejos, J. (2010). Horizontal movements, vertical-habitat utilization and diet of the jumbo squid (Dosidicus gigas) in the Pacific Ocean off Baja California Sur. Mexico Prog. Oceanogr. 86, 59–71. doi: 10.1016/j.pocean.2010.04.017
Boyle, P., and Boletzky, S. (1996). Cephalopod populations: definition and dynamics. Phil. Trans. R. Soc. Lond. B 351, 985–1002. doi: 10.1111/1365-2656.12218
Chang, C. C. (2000). Spatial and Temporal Variation of 18O in the Sea Water from the Taiwan Strait. Ph.D. thesis, National Sun Yat-sen University, Taiwan.
Chen, C. S., Chen, J. Y., and Lin, C. W. (2015). Variation in life-history traits for micro-cohorts of Sepioteuthis lessoniana in the waters off northern Taiwan. Fish. Sci. 81, 53–64. doi: 10.1007/s12562-014-0831-x
Chen, C. S., and Huang, J. M. (1999). A numerical study of precipitation characteristics over Taiwan island during the winter season. Meteorol. Atmos. Phys. 70, 167–183. doi: 10.1007/s007030050032
Chen, C. T. (1997). The Kuroshio intermediate water is the major source of nutrients on the East China Sea continental shelf. Oceanogr. Lit. Rev. 44, 531.
Chen, T. C., Yen, M. C., Huang, W. R., and Gallus, W. A. Jr. (2002). An east Asian cold surge: case study. Mon. Weather Rev. 130, 2271–2290. doi: 10.1175/1520-0493(2002)130<2271:aeacsc>2.0.co;2
Cheng, S., Anderson, F. E., Bergman, A., Mahardika, G., Muchlisin, Z., Dang, B., et al. (2014). Molecular evidence for co-occurring cryptic lineages within the Sepioteuthis cf. lessoniana species complex in the Indian and Indo-West Pacific Oceans. Hydrobiologia 725, 165–188. doi: 10.1007/s10750-013-1778-0
Ching, T. Y., Chen, C. S., and Wang, C. H. (2017). Spatiotemporal variations in life-history traits and statolith trace elements of Sepioteuthis lessoniana populations around northern Taiwan. J. Mar. Biol. Assoc. U.K 99, 203–213. doi: 10.1017/s0025315417001801
Clarke, M. R. (1978). The cephalopod statolithan-introduction to its form. J. Mar. Biol. Assoc. U.K. 58, 701–712. doi: 10.1017/s0025315400041345
Currey, L. M., Heupel, M. R., Simpfendorfer, C. A., and Williams, A. J. (2014). Inferring movement patterns of a coral reef fish using oxygen and carbon isotopes in otolith carbonate. J. Exp. Mar. Biol. Ecol. 456, 18–25. doi: 10.1016/j.jembe.2014.03.004
Darnaude, A. M., and Hunter, E. (2018). Validation of otolith δ18O values as effective natural tags for shelf-scale geolocation of migrating fish. Mar. Ecol. Prog. Ser. 598, 167–185. doi: 10.3354/meps12302
Dunn, D. C., Maxwell, S. M., Boustany, A. M., and Halpin, P. N. (2016). Dynamic ocean management increases the efficiency and efficacy of fisheries management. Proc. Natl. Acad. Sci. U.S.A. 113, 668–673. doi: 10.1073/pnas.1513626113
Elsdon, T. S., and Gillanders, B. M. (2002). Interactive effects of temperature and salinity on otolith chemistry: challenges for determining environmental histories of fish. Can. J. Fish. Aquat. Sci. 59, 1796–1808. doi: 10.1139/f02-154
Elsdon, T. S., and Gillanders, B. M. (2003). Reconstructing migratory patterns of fish based on environmental influences on otolith chemistry. Rev. Fish Biol. Fish. 13, 217–235. doi: 10.1023/b:rfbf.0000033071.73952.40
Forsythe, J. (2004). Accounting for the effect of temperature on squid growth in nature: from hypothesis to practice. Mar. Freshwater Res. 55, 331–339.
Forsythe, J., Walsh, L., Turk, P., and Lee, P. (2001). Impact of temperature on juvenile growth and age at first egg-laying of the Pacific reef squid Sepioteuthis lessoniana reared in captivity. Mar. Biol. 138, 103–112. doi: 10.1007/s002270000450
Gillanders, B. M., Wilkinson, L. M., Munro, A. R., and de Vries, M. C. (2013). Statolith chemistry of two life history stages of cuttlefish: effects of temperature and seawater trace element concentration. Geochim. Cosmochim. Acta 101, 12–23. doi: 10.1016/j.gca.2012.10.005
Gilly, W., Markaida, U., Baxter, C., Block, B., Boustany, A., Zeidberg, L., et al. (2006). Vertical and horizontal migrations by the jumbo squid Dosidicus gigas revealed by electronic tagging. Mar. Ecol. Prog. Ser. 324, 1–17. doi: 10.3354/meps324001
Gislason, H., Sinclair, M., Sainsbury, K., and O’boyle, R. (2000). Symposium overview: incorporating ecosystem objectives within fisheries management. ICES J. Mar. Sci. 57, 468–475. doi: 10.1006/jmsc.2000.0741
Gong, G. C., Wen, Y. H., Wang, B. W., and Liu, G. J. (2003). Seasonal variation of chlorophyll a concentration, primary production and environmental conditions in the subtropical East China Sea. Deep Sea Res. II Top. Stud. Oceanogr. 50, 1219–1236. doi: 10.1016/s0967-0645(03)00019-5
Hobday, A. J., Hartog, J. R., Timmiss, T., and Fielding, J. (2010). Dynamic spatial zoning to manage southern bluefin tuna (Thunnus maccoyii) capture in a multi−species longline fishery. Fish. Oceanogr. 19, 243–253. doi: 10.1111/j.1365-2419.2010.00540.x
Høie, H., Andersson, C., Folkvord, A., and Karlsen, Ø (2004b). Precision and accuracy of stable isotope signals in otoliths of pen-reared cod (Gadus morhua) when sampled with a high-resolution micromill. Mar. Biol. 144, 1039–1049. doi: 10.1007/s00227-003-1275-5
Høie, H., Otterlei, E., and Folkvord, A. (2004a). Temperature-dependent fractionation of stable oxygen isotopes in otoliths of juvenile cod (Gadus morhua L.). ICES J. Mar. Sci. 61, 243–251. doi: 10.1016/j.icesjms.2003.11.006
Hsiao, C. D., Shen, K. N., Ching, T. Y., Wang, Y. H., Ye, J. J., Tsai, S. Y., et al. (2016). The complete mitochondrial genome of the cryptic “lineage A” big-fin reef squid, Sepioteuthis lessoniana (Cephalopoda: Loliginidae) in Indo-West Pacific. Mitochondrial DNA A 27, 2433–2434.
Hunt, J., and Seibel, B. (2000). Life history of Gonatus onyx (Cephalopoda: Teuthoidea): ontogenetic changes in habitat, behavior and physiology. Mar. Biol. 136, 543–552. doi: 10.1007/s002270050714
Ikeda, Y., Arai, N., Kidokoro, H., and Sakamoto, W. (2003). Strontium: calcium ratios in statoliths of Japanese common squid Todarodes pacificus (Cephalopoda: Ommastrephidae) as indicators of migratory behavior. Mar. Ecol. Prog. Ser. 251, 169–179. doi: 10.3354/meps251169
Ikeda, Y., Ueta, Y., Anderson, F., and Matsumoto, G. (2009). Reproduction and life span of the oval squid Sepioteuthis lessoniana (Cephalopoda: Loliginidae): comparison between laboratory-cultured and wild-caught squid. Mar. Biodivers. Rec. 2:E50. doi: 10.1017/S175526720900061X
Jackson, G., and Moltschaniwskyj, N. (2002). Spatial and temporal variation in growth rates and maturity in the Indo-Pacific squid Sepioteuthis lessoniana (Cephalopoda: Loliginidae). Mar. Biol. 140, 747–754. doi: 10.1007/s00227-001-0746-9
Jackson, G. D. (1990). Age and growth of the tropical nearshore loliginid squid Sepioteuthis lessoniana determined from statolith growth-ring analysis. Fish. Bull. 88, 113–118.
Jackson, G. D., and Choat, J. H. (1992). Growth in tropical cephalopods: an analysis based on statolith microstructure. Can. J. Fish. Aquat. Sci. 49, 218–228. doi: 10.1139/f92-026
Jackson, G. D., O’Dor, R. K., and Andrade, Y. (2005). First tests of hybrid acoustic/archival tags on squid and cuttlefish. Mar. Freshwater Res. 56, 425–430.
Jan, S., Sheu, D. D., and Kuo, H. M. (2006). Water mass and through flow transport variability in the Taiwan Strait. J. Geophys. Res. Oceans 111:C12. doi: 10.1029/2006JC003656
Jan, S., Wang, J., Chern, C. S., and Chao, S. Y. (2002). Seasonal variation of the circulation in the Taiwan Strait. J. Mar. Syst. 35, 249–268. doi: 10.1007/s11356-018-3093-9
Jereb, P., and Roper, C. F. (2005). “Sepioteuthis lessoniana Férussac in Lesson, 1831,” in Cephalopods of the World: An Annotated and Illustrated Catalogue of Cephalopod Species Known to Date, Volume 2, Myopisd and Oegopsid Squids, eds P. Jereb and C. F. E. Roper (Rome: FAO), 95–97.
Kanamaru, H., Umeda, T., and Morikawa, A. (2007). Migration of the oval squid, Sepioteuthis lessoniana in northern and western waters of the Kyushu. Bull. Saga Genkai Fish. Res. Dev. Cent. 4, 51–57.
Kitagawa, T., Ishimura, T., Uozato, R., Shirai, K., Amano, Y., Shinoda, A., et al. (2013). Otolith δ18O of Pacific bluefin tuna Thunnus orientalis as an indicator of ambient water temperature. Mar. Ecol. Prog. Ser. 481, 199–209. doi: 10.3354/meps10202
Landman, N., Cochran, J., Cerrato, R., Mak, J., Roper, C., and Lu, C. (2004). Habitat and age of the giant squid (Architeuthis sanctipauli) inferred from isotopic analyses. Mar. Biol. 144, 685–691. doi: 10.1007/s00227-003-1245-y
Leder, J., Swart, P. K., Szmant, A., and Dodge, R. (1996). The origin of variations in the isotopic record of scleractinian corals: I. Oxygen Geochim. Cosmochim. Acta 60, 2857–2870. doi: 10.1016/0016-7037(96)00118-4
LeGrande, A. N., and Schmidt, G. A. (2006). Global gridded data set of the oxygen isotopic composition in seawater. Geophys. Res. Lett. 33, L12604. doi: 10.1029/2006GL026011
Linzmeier, B. J. (2019). Refining the interpretation of oxygen isotope variability in free-swimming organisms. Swiss J. Palaeontol. 138, 109–121. doi: 10.1007/s13358-019-00187-3
Linzmeier, B. J., Kozdon, R., Peters, S. E., and Valley, J. W. (2016). Oxygen isotope variability within Nautilus shell growth bands. PLoS One 11:e0153890. doi: 10.1371/journal.pone.0153890
Liu, B. L., Cao, J., Truesdell, S. B., Chen, Y., Chen, X. J., and Tian, S. Q. (2016). Reconstructing cephalopod migration with statolith elemental signatures: a case study using Dosidicus gigas. Fish. Sci. 82, 425–433. doi: 10.1007/s12562-016-0978-8
Liu, K. K., Gong, G. C., Lin, S., Yang, C. Y., Wei, C. L., Pai, S. C., et al. (1992). The year-round upwelling at the shelf break near the northern tip of Taiwan as evidenced by chemical hydrography. Terr. Atmos. Ocean Sci. 3, 243–275.
Maxwell, S. M., Hazen, E. L., Lewison, R. L., Dunn, D. C., Bailey, H., Bograd, S. J., et al. (2015). Dynamic ocean management: defining and conceptualizing real-time management of the ocean. Mar. Policy 58, 42–50. doi: 10.1016/j.marpol.2015.03.014
Moreno, A., Dos Santos, A., Piatkowski, U., Santos, A. M. P., and Cabral, H. (2008). Distribution of cephalopod paralarvae in relation to the regional oceanography of the western Iberia. J. Plankton Res. 31, 73–91. doi: 10.1093/plankt/fbn103
Nakashima, R., Suzuki, A., and Watanabe, T. (2004). Life history of the Pliocene scallop Fortipecten, based on oxygen and carbon isotope profiles. Palaeogeogr. Palaeoclimatol. Palaeoecol. 211, 299–307. doi: 10.1016/j.palaeo.2004.05.011
Nishida, K., Suzuki, A., Isono, R., Hayashi, M., Watanabe, Y., Yamamoto, Y., et al. (2015). Thermal dependency of shell growth, microstructure, and stable isotopes in laboratory-reared Scapharca broughtonii (Mollusca: Bivalvia). Geochem. Geophy. Geosy 16, 2395–2408. doi: 10.1002/2014gc005634
O’dor, R. (1992). Big squid in big currents. S. Afr. J. Mar. Sci. 12, 225–235. doi: 10.2989/02577619209504705
O’dor, R. (1998). Can understanding squid life-history strategies and recruitment improve management? S. Afr. J. Mar. Sci. 20, 193–206. doi: 10.2989/025776198784126188
Okutani, T. (2015). “Sepioteuthis Lessoniana Férussac in Lesson, 1832,” in Cuttlefishes and Squids of the World. Tokyo, Japan: Tokai University Press, 97.
Otero, J., Álvarez-Salgado, X. A., González, ÁF., Miranda, A., Groom, S. B., Cabanas, J. M., et al. (2008). Bottom-up control of common octopus Octopus vulgaris in the Galician upwelling system, northeast Atlantic Ocean. Mar. Ecol. Prog. Ser. 362, 181–192. doi: 10.3354/meps07437
Owen, E. F., Wanamaker, A. D., Feindel, S. C., Schöne, B. R., and Rawson, P. D. (2008). Stable carbon and oxygen isotope fractionation in bivalve (Placopecten magellanicus) larval aragonite. Geochim. Cosmochim. Acta 72, 4687–4698. doi: 10.1016/j.gca.2008.06.029
R Core Team, (2018). R: A Language and Environment for Statistical Computing. Available online at: http://www.r-project.org/ (accessed January 1, 2018)Google Scholar
Radtke, R. (1983). Chemical and structural characteristics of statoliths from the short-finned squid Illex illecebrosus. Mar. Biol. 76, 47–54. doi: 10.1007/bf00393054
Rexfort, A., and Mutterlose, J. (2006). Stable isotope records from Sepia officinalis—a key to understanding the ecology of belemnites? Earth Planet Sci. Lett. 247, 212–221. doi: 10.1016/j.epsl.2006.04.025
Rodhouse, P. G., Pierce, G. J., Nichols, O. C., Sauer, W. H., Arkhipkin, A. I., Laptikhovsky, V. V., et al. (2014). “Environmental effects on cephalopod population dynamics: implications for management of fisheries,” in the Advances in Marine Biology, ed. E. A. G. Vidal (London, UK: Elsevier), 99–233. doi: 10.1016/b978-0-12-800287-2.00002-0
Roper, C. F. E., Sweeney, M. J., and Nauen, C. E. (1984). Cephalopods of the world: an annotated and illustrated catalogue of species of interest to fisheries. FAO Fish. Synop. 125, 1–277.
Sakamoto, T., Komatsu, K., Shirai, K., Higuchi, T., Ishimura, T., Setou, T., et al. (2019). Combining microvolume isotope analysis and numerical simulation to reproduce fish migration history. Methods Ecol. Evol. 10, 59–69. doi: 10.1111/2041-210x.13098
Segawa, S. (1987). Life history of the oval squid, Sepioteuthis lessoniana, in Kominato and adjacent waters central Honshu, Japan. J. Tokyo Univ. Fish 74, 67–105.
Semmens, J. M., Pecl, G. T., Gillanders, B. M., Waluda, C. M., Shea, E. K., Jouffre, D., et al. (2007). Approaches to resolving cephalopod movement and migration patterns. Rev. Fish Biol. Fish. 17, 401–423. doi: 10.1007/s11160-007-9048-8
Shen, K. N., Yen, T. C., Chen, C. H., Ye, J. J., and Hsiao, C. D. (2016). The complete mitochondrial genome of the cryptic “lineage B” big-fin reef squid, Sepioteuthis lessoniana (Cephalopoda: Loliginidae) in Indo-West Pacific. Mitochondrial DNA A 27, 2100–2101.
Shiao, J. C., Itoh, S., Yurimoto, H., Iizuka, Y., and Liao, Y. C. (2014). Oxygen isotopic distribution along the otolith growth axis by secondary ion mass spectrometry: applications for studying ontogenetic change in the depth inhabited by deep-sea fishes. Deep Sea Res. I Oceanogr. Res. Pap. 84, 50–58. doi: 10.1016/j.dsr.2013.10.006
Shiao, J. C., Sui, T. D., Chang, N. N., and Chang, C. W. (2017). Remarkable vertical shift in residence depth links pelagic larval and demersal adult jellynose fish. Deep Sea Res. I Oceanogr. Res. Pap. 121, 160–168. doi: 10.1016/j.dsr.2017.01.011
Shirai, K., Otake, T., Amano, Y., Kuroki, M., Ushikubo, T., Kita, N. T., et al. (2018). Temperature and depth distribution of Japanese eel eggs estimated using otolith oxygen stable isotopes. Geochim. Cosmochim. Acta 236, 373–383. doi: 10.1016/j.gca.2018.03.006
Song, G. S., Chang, Y. C., and Ma, C. P. (1997). Characteristics of submarine topography off northern Taiwan. Terr. Atmos. Ocean Sci. 8, 461–480.
Steer, M. A., Halverson, G. P., Fowler, A. J., and Gillanders, B. M. (2010). Stock discrimination of Southern Garfish (Hyporhamphus melanochir) by stable isotope ratio analysis of otolith aragonite. Environ. Biol. Fishes 89, 369–381. doi: 10.1007/s10641-010-9670-5
Tang, T., Tai, J., and Yang, Y. (2000). The flow pattern north of Taiwan and the migration of the Kuroshio. Cont. Shelf Res. 20, 349–371. doi: 10.1016/s0278-4343(99)00076-x
Thorrold, S. R., Campana, S. E., Jones, C. M., and Swart, P. K. (1997). Factors determining δ13C and δ18O fractionation in aragonitic otoliths of marine fish. Geochim. Cosmochim. Acta 61, 2909–2919. doi: 10.1016/s0016-7037(97)00141-5
Tomano, S., Sanchez, G., Kawai, K., Kasaoka, N., Ueta, Y., and Umino, T. (2016). Contribution of Sepioteuthis sp. 1 and Sepioteuthis sp. 2 to oval squid fishery stocks in western Japan. Fish. Sci. 82, 585–596. doi: 10.1007/s12562-016-0988-6
Trasviña-Carrillo, L. D., Hernández-Herrera, A., Torres-Rojas, Y. E., Galván-Magaña, F., Sánchez-González, A., and Aguíñiga-García, S. (2018). Spatial and trophic preferences of jumbo squid Dosidicus gigas (D’Orbigny, 1835) in the central Gulf of California: ecological inferences using stable isotopes. Rapid Commun. Mass Spectrom. 32, 1225–1236. doi: 10.1002/rcm.8147
Trueman, C. N., MacKenzie, K., and Palmer, M. (2012). Identifying migrations in marine fishes through stable−isotope analysis. J. Fish Biol. 81, 826–847. doi: 10.1111/j.1095-8649.2012.03361.x
Ueta, Y., and Jo, Y. (1990). Migration of the oval squid, Sepioteuthis lessoniana around Tokushima prefecture. Suisanzoshoku 38, 221–226.
Walsh, L., Turk, P., Forsythe, J., and Lee, P. (2002). Mariculture of the loliginid squid Sepioteuthis lessoniana through seven successive generations. Aquaculture 212, 245–262. doi: 10.1016/s0044-8486(02)00126-6
Weidel, B. C., Ushikubo, T., Carpenter, S. R., Kita, N. T., Cole, J. J., Kitchell, J. F., et al. (2007). Diary of a bluegill (Lepomis macrochirus): daily δ13C and δ18O records in otoliths by ion microprobe. Can. J. Fish. Aquat. Sci. 64, 1641–1645. doi: 10.1139/f07-157
Yamaguchi, T., Aketagawa, T., Takayama, K., Hirose, N., and Matsuyama, M. (2019). Migratory routes of different sized swordtip squid (Uroteuthis edulis) caught in the Tsushima Strait. Fish. Res. 209, 24–31. doi: 10.1016/j.fishres.2018.08.008
Yamaguchi, T., Kawakami, Y., and Matsuyama, M. (2015). Migratory routes of the swordtip squid Uroteuthis edulis inferred from statolith analysis. Aquat. Biol. 24, 53–60. doi: 10.3354/ab00635
Yamaguchi, T., Kawakami, Y., and Matsuyama, M. (2018). Analysis of the hatching site and migratory behaviour of the swordtip squid (Uroteuthis edulis) caught in the Japan Sea and Tsushima Strait in autumn estimated by statolith analysis. Mar. Biol. Res. 14, 105–112. doi: 10.1080/17451000.2017.1351616
Young, R. E. (1978). Vertical distribution and photosensitive vesicles of pelagic cephalopods from Hawaiian waters. Fish. Bull. 76, 583–615.
Young, R. E., and Harman, R. F. (1988). “ Larva”,” paralarva” and” subadult” in cephalopod terminology. Malacologia 29, 201–208.
Keywords: Sepioteuthis lessoniana, statolith oxygen isotopes, temperature, geographic distribution, ontogenetic movement
Citation: Chiang C-I, Chung M-T, Shiao J-C, Wang P-L, Chan T-Y, Yamaguchi A and Wang C-H (2020) Seasonal Movement Patterns of the Bigfin Reef Squid Sepioteuthis lessoniana Predicted Using Statolith δ18O Values. Front. Mar. Sci. 7:249. doi: 10.3389/fmars.2020.00249
Received: 14 December 2019; Accepted: 30 March 2020;
Published: 22 April 2020.
Edited by:
Alejandra Vanina Volpedo, University of Buenos Aires, ArgentinaReviewed by:
Bilin Liu, Shanghai Ocean University, ChinaKolliyil S. Mohamed, Central Marine Fisheries Research Institute (ICAR), India
Copyright © 2020 Chiang, Chung, Shiao, Wang, Chan, Yamaguchi and Wang. This is an open-access article distributed under the terms of the Creative Commons Attribution License (CC BY). The use, distribution or reproduction in other forums is permitted, provided the original author(s) and the copyright owner(s) are credited and that the original publication in this journal is cited, in accordance with accepted academic practice. No use, distribution or reproduction is permitted which does not comply with these terms.
*Correspondence: Chia-Hui Wang, Y2h3YW5nOTlAbWFpbC5udG91LmVkdS50dw==