- 1Department of Zoology, Faculty of Science, Assiut University, Assiut, Egypt
- 2Department of Zoology, Faculty of Science, New Valley University, New Valley, Egypt
Heavy metals have different adverse impacts on different life stages of fish species with attempts to use natural antioxidants to counteract their effects. So, the present study investigated the potential protective effects of Amphora coffeaeformis extract against arsenic-induced hemato-biochemical alterations in African catfish, Clarias gariepinus. The fish exposed to sub-lethal concentrations of arsenic; 19.2 and 38.3 mg/L (1/8 and 1/4 of 96h-LC50 value, 153.17 mg/L) for 15 days. The main effect of arsenic was recorded in some blood parameters such as RBC’s count, hematocrit, mean corpuscular volume, mean corpuscular hemoglobin, and white blood cells. As for biochemical parameters, the main effect of arsenic was significant for alkaline phosphatase, glucose, uric acid, creatinine, albumin, globulin, and albumin/globulin. Also, the residue of arsenic in fish muscles showed significant effects. The majority of these arsenic-induced parameters were improved with dietary supplements of the diatom A. coffeaeformis. So, Amphora extract can be used as detoxification factor on fishes induced by arsenic due to its biologically active components providing protections like antioxidant, antiviral, antibacterial, and anti-inflammatory. Besides, they have excellent contents of proteins and carbohydrates which are supposed to enhance the effect of these compounds.
Introduction
Different heavy metals including Arsenic, Cadmium, Lead, Silver, and Mercury were found to be toxic to human beings, animals, and fishes with variability in doses and environmental factors (Govind and Madhuri, 2014). Arsenic is one of the most hazardous heavy metals released in the environment as a result of both natural and anthropogenic processes (Garelick et al., 2008). In nature, arsenic exists both in organic and inorganic forms; the inorganic form with trivalent arsenite or pentavalent arsenate are more toxic than organic forms (Oremland and Stolz, 2005). It became evident that the dispersal of arsenic-rich wastes generated by human activities leads to the water pollution and in turn, increased chronic arsenic poisoning of aquatic animals (Rahman et al., 2012) especially fish juveniles with a reduction in survival and growth of their populations (Erickson et al., 2011). These adverse impacts may lead to the removal of entire fish populations in polluted aquatic ecosystems (Khayatzadeh and Abbasi, 2010). Consequently, accumulation of arsenic at high concentration in fishes may lead to serious health risks for humans, causing cancer and neurological disturbances (Kapaj et al., 2006; Avigliano et al., 2015). To understand the toxicity of arsenic compounds, most studies were performed in mammalian cells (García-Esquinas et al., 2013; Selvaraj et al., 2013). However, studies on arsenic toxicity to aquatic animal species, including fish, are rare. These studies stated that exposure to arsenic led to various hematological and biochemical alterations in fishes (Sayed et al., 2015a; Singh and Srivastava, 2015; Ghaffar et al., 2016). Arsenic was found to promote apoptotic and necrotic mediated cell death in fishes according to variations in arsenic concentration and exposure time (Sayed et al., 2015a). It also leads to DNA fragmentation, alteration in mitochondrial membrane potential and formation of increased reactive oxygen species (Selvaraj et al., 2013).
And because the pollution has already become very widespread, toxicity prevention is not on; and timely mitigation is the possible solution for minimization of the environmental pollutants and their impacts by using plants and algae as the most desirable mitigation technics (Ullah et al., 2015; Mahar et al., 2016; Kumar, 2018). The algae proved to be effective in the hyperaccumulation of heavy metals as well as degradation of xenobiotics (Suresh and Ravishankar, 2004). The microalgae including Amphora, could be a source of a diverse class of bioactive compounds, especially the carotenoids (canthaxanthin and astaxanthin), polyunsaturated fatty acids, sulfated polysaccharides, β-glucans, and vitamins E and C, which are well-documented as bioactive compounds (Lee et al., 2009; El-Sayed et al., 2018). In addition, many researchers are interested in finding any natural antioxidants having safety and effectiveness, which can be substituted for current and commercial synthetic antioxidants (Taghvaei and Jafari, 2015; Kumosani et al., 2017; Glodde et al., 2018). Benthic diatoms as microalgae were considered as natural antioxidants (Lee et al., 2008; El-Sayed et al., 2018). Diatoms such as Amphora possess high metal absorption capacity and heavy high multiplication rate (Anantharaj et al., 2011) and are able to activate a definite set of biochemical and physiological processes to resist the toxic action of environmental contaminants (Gaur and Rai, 2001). Such characteristics of diatom have encouraged the application of their extract in detoxification for stress-induced fishes as well as protective and antioxidant agents (Sheikhzadeh et al., 2012) in addition to their antibacterial (Choudhury et al., 2005; Manzoor et al., 2013), antiviral (Abdel-Wahab, 2018), and anti-inflammatory factors (Lauritano et al., 2016).
Marine diatoms developed on substrate could be utilized as feed supplements in enhancing the development and survival of aquaculture species (Khatoon et al., 2009). Amphora sp. is regularly used as primary food for larvae of highly valued and praised seafood such as Crassostrea gigas (Pacific oyster), Penaeus semisulcatus (green tiger shrimp), Placopecten magellanicus (sea scallop), Crepidula onyx (limpet), and Haliotis sp. (abalone) (Daume et al., 2000; Al-Maslamani et al., 2007; Chiu et al., 2007). Recent results indicated that Amphora supplement is promising as an alternative method to antibiotics for disease prevention in Nile tilapia culture (Ayoub et al., 2019). So, the current investigation is aimed to study the supplementation of Amphora extract and whether it detoxifies and protects C. gariepinus from arsenic exposure.
Materials and Methods
Fish Collection
Ninety healthy fish of the Nile catfish, Clarias gariepinus (154.75 ± 146.9 g weight, 30.87 ± 9 cm length) were purchased from a private farm at Assiut, Egypt. Fishes immediately were transported to the Fish Biology and Pollution laboratory at the Department of Zoology, Faculty of Science, Assiut University. The experimental fishes were reared in aerated glass tanks (100 L capacity) and acclimatized for 2 weeks before being used in the experimental study. The experimental fish fed on commercial pellets (3% of fish weight) twice daily. Feces and residual food were aspirated regularly. The water temperature, pH, and dissolved oxygen concentrations were measured daily as 28.8 ± 3°C, 7.6 ± 0.34, and 3.32 ± 4.5 mg/L respectively (light cycle was 12 h light and 12 h dark).
Amphora coffeaeformis Extract Preparation
The extract of Amphora coffeaeformis was purchased from National Research Center, Cairo, Egypt. Amphora extract was sent to the Analytical Chemistry Unit at Assiut University for GC/MS analysis. The results of GC-MS analysis indicated the presence of 51 different compounds (Table 1 and Figure 1). Some of these compounds were identified according to literature (Silva et al., 2014; Salahuddin et al., 2017; El-Sayed et al., 2018) to be biologically active components such as 2,6-Dimethyl-4[3H]-quinazolinone (anticancer heterocyclic compound), Neophytadiene (Terpene; antiviral activity), Phytol (Diterpene; anti-inflammatory activity) and Hexadecanoic acid (fatty acid; antioxidant). The concentrations of total protein (24.25 g/kg) and total carbohydrate (17.92 g/kg) were estimated by using UV-VIS Double Beam Labomed, Inc. PC Scanning Spectrophotometer (Model UVD-2950) while the concentration of total lipid (56.4 g/kg) was estimated by Bligh and Dyer’s acidic extraction method (Bligh and Dyer, 1959).
Experimental Design
Fishes were weighed, measured and classified randomly into nine groups (10 fish/tank) according to two concentrations of arsenic (AS1, AS2), two concentrations of amphora (AM1, AM2) and their combinations (Table 2). Exposure was continuous for 2 weeks and water was changed daily to prevent deterioration of water quality and replenish arsenic levels. Sodium arsenate (Na2HAsO4⋅7H2O) of 98% purity was purchased from Qualikemes company, India. A stock solution of sodium arsenate was prepared and stored in clean glass bottles. Three concentrations of sodium arsenate were used: zero and two sub-lethal concentrations of 19.2 and 38.3 mg/L (1/8 and 1/4 of 96h-LC50 value, 153.17 mg/L) (Abdel-Hameid, 2009). The arsenic solution was added to the water directly while the amphora extract was mixed with fish feed. This protocol and experimental design were reviewed and approved by the Committee of the Faculty of Science of Assiut University with respect to scientific content and compliance with applicable research.

Table 2. The fish groups exposed to arsenic (AS1, AS2) concentrations, amphora (AM1, AM2) percentages and their combinations.
Behavioral Assessments
The behavioral changes were recorded by observing the feeding activity, the fish equilibrium in the water beside the changes in the skin and the fins. The mortality rate was also recorded in the arsenic-exposed fishes.
Hemato-Biochemical Parameters
Two blood samples of the peripheral blood were collected from cardiac puncture. For hematological analysis, samples were freshly collected in small plastic tubes containing heparin solution (0.2 mL/mL blood) as anticoagulant. For biochemical analysis, samples were left in small plastic tubes to coagulate for 15–20 min at 4°C prior then centrifugation for 20 min at 3,000 rpm to separate serum that was used for the analysis. The red (RBCs) and white (WBCs) blood cell counts, hematocrit (HCT), and hemoglobin (HB) were estimated by using automated technical analyzer (BCC-3000B-Dirui Company). Mean corpuscular volume (MCV), mean corpuscular hemoglobin (MCH), and mean corpuscular hemoglobin concentration (MCHC) were calculated using the formulae mentioned by Dacie and Lewis (1991). Aspartic amino transferase (AST), alanine amino transferase (ALT), alkaline phosphatase (ALP), lactate dehydrogenase (LDH), glucose (GL), total protein (TP), albumin (AL), globulin (GLO), creatinine (CR), and uric acid (UA) were determined by kits of HUMAN Company, Germany.
Arsenic Residues
Tissue analyses were done according to Shaw et al. (2012) with minor variations. Muscle samples were weighed (approximately 1.0 g), dried (50°C for 48 h in the oven) and then digested in 5 mL concentrated nitric acid at 50°C in the oven until evaporating the nitric acid and become the mixture 1 mL approximately. The mixture was cooled, diluted to 10 mL using ultrapure deionized water and then filtered. The arsenic residues in the muscles were measured by iCAP 6200 Emission spectrometer in The Central Laboratory, Faculty of Agriculture, Assiut University.
Statistical Analysis
The means, standard errors and ranges of the parameters in concern were estimated. Levene’s test of equality of error variance of the parameters was applied with a wide range of variability. So, the homogeneity of variance was assumed for raw data. The pattern of variations in the variables studies was considered on the bases of arsenic and amphora concentrations and their interaction by two-way ANOVA. Moreover, in the absence of interactions, the pattern of variations was recorded by one-way ANOVA in all treatments and control group. The Tukey-HSD test was applied for multiple comparisons. The IBM-SPSS package version 22 (Spss for Windows, 2013) was used at 0.05-level of significance. The relationships between different treatments versus the control group as root group were postulated in dendrograms using R-packages (R Core Team, 2013) and Mesquites package (Maddison and Maddison, 2018) using the hematological and biochemical characters in raw form and ANOVA-based coded form (Rae and Buckley, 2009).
Results
Behavioral Changes
After exposure to arsenic, most of the fishes exhibited loss of equilibrium which was more marked with increased concentration and duration. Reduction in the feeding activity, fins hemorrhage and skin alterations were also recorded in those samples exposed to arsenic. With increased duration, arsenic- exposed fishes showed signs of tiredness and gradually lost positive rheotaxis with excessive secretion of mucus. The mortality rate (60%) was recorded in aquaria with 38.3 mg/L arsenic. The dead fishes exhibited changes and abnormality in eyes, gills, gall bladder, spleen, and liver color. On the other hand, fish groups exposed to the same doses of arsenic in combination with amphora extract did not show such abnormal behavior and did not show any mortality. Moreover, arsenic-free fishes treated with amphora extract were noticed to have a healthy status.
Hemato-Biochemical Alterations
Under treatment conditions of the present work, the uric acid was found to be significantly correlated with ALP (0.453), glucose (0.424), total protein (0.524), and globulin (0.567), whereas glucose was significantly correlated with ALP (0.575). The albumin was significantly correlated with total protein (0.554) and creatinine (0.705). The WBCs was also significantly correlated with RBCs (0.886), HB (0.875), and HCT (0.809).
The hematological and biochemical parameters of the arsenic-induced C. gariepinus versus treatment with Amphora extract are given in Tables 3, 4. The arsenic main effect was significant for RBCs, HCT, MCV, MCH, and WBCs whereas that of amphora was significant for HB and WBCs. There was no significant interaction between arsenic and amphora. As regards the biochemical parameters, the arsenic main effect was significant for ALP, glucose, uric acid, creatinine, albumin, globulin, and albumin/globulin. Also, arsenic residue in muscle showed significant effects by arsenic whereas that of Amphora was significant for ALT, glucose, uric acid, creatinine, LDH, albumin, globulin, and albumin/globulin. The arsenic amphora interaction was significant for ALP, glucose, uric acid, creatinine, LDH, albumin, globulin, albumin/globulin, and arsenic residue in muscles. The main effect of amphora recorded to be significant for glucose, uric acid, creatinine, albumin, globulin, albumin/globulin, and arsenic residue in muscles. The analysis revealed that a higher amphora dose was better than a lower one in counteracting arsenic impact.
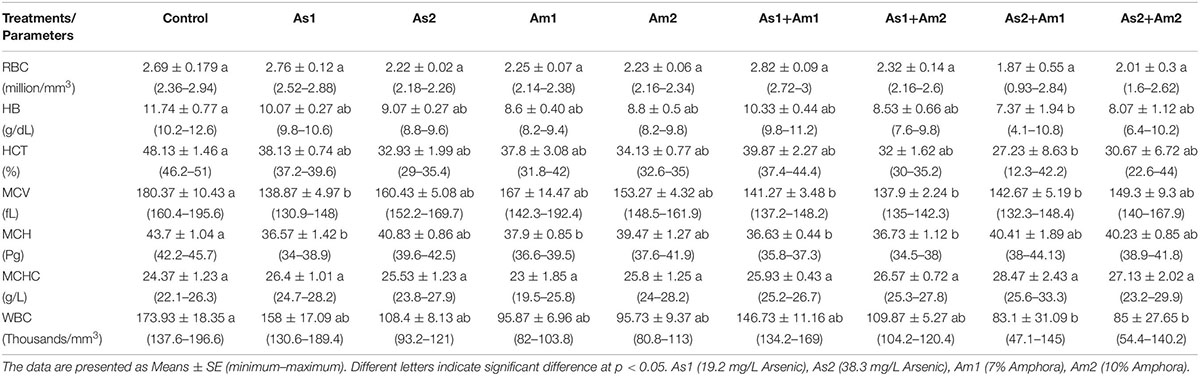
Table 3. Values of blood constituent parameters of Clarias gariepinus exposed to arsenic, amphora and their combinations.
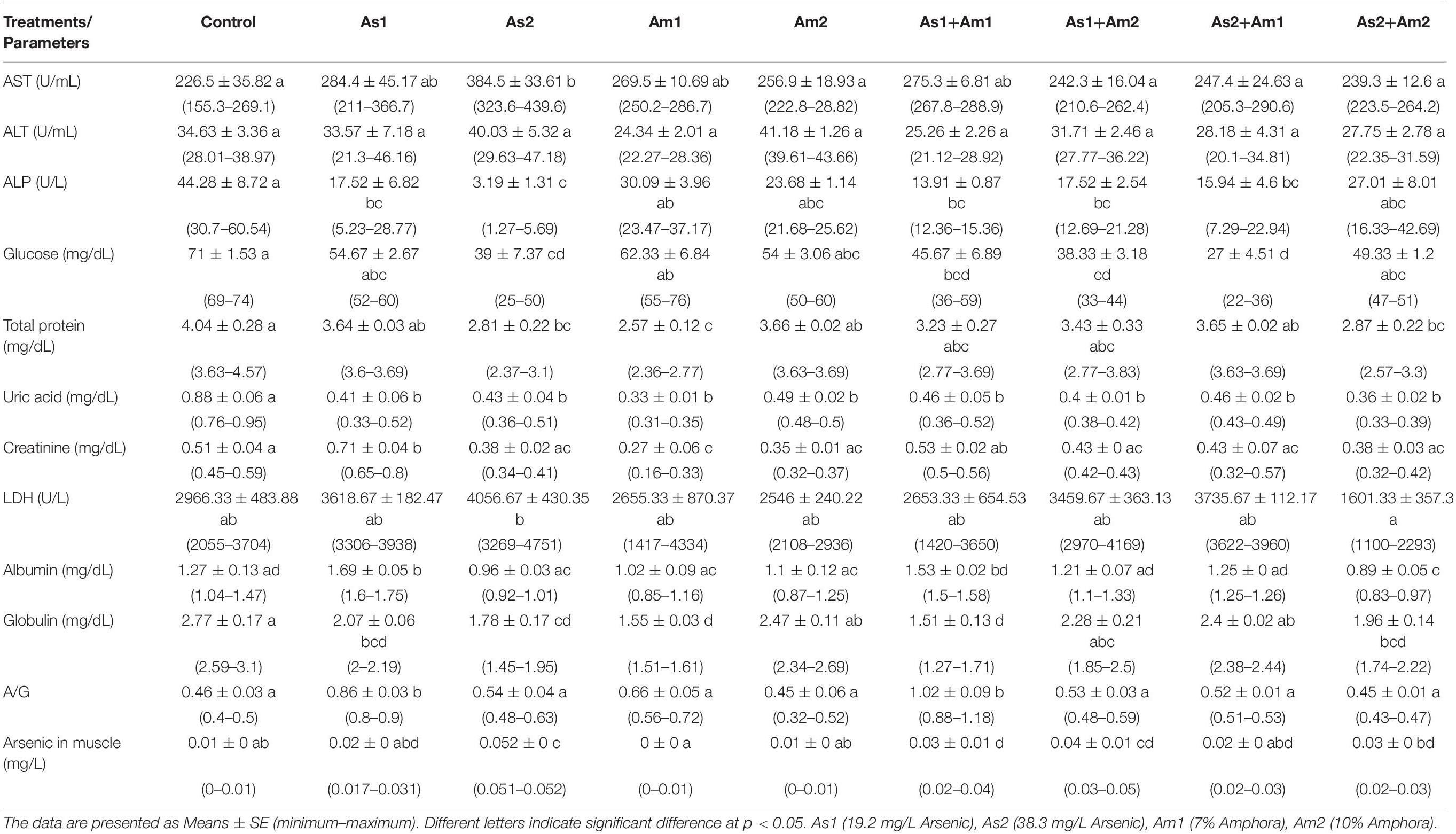
Table 4. Values of blood constituent parameters and arsenic residue in the muscle of Clarias gariepinus exposed to arsenic, amphora and their combinations.
Collecting all characters studied, all the treatments were clustered against the control using all the hematological and biochemical parameters relative to their SD units (Figure 2). These treatments are grouped into two main groups. One of these main groups included treatments with amphora only and the arsenic treatment combined with amphora reflecting the validity of amphora extracts as antioxidants. The other main group reflects a partial protective role of Amphora in counteracting the arsenic impacts. Clustering of the treatments based on the ANOVA-based coding of these parameters reflected another pattern of variation (Figure 3).
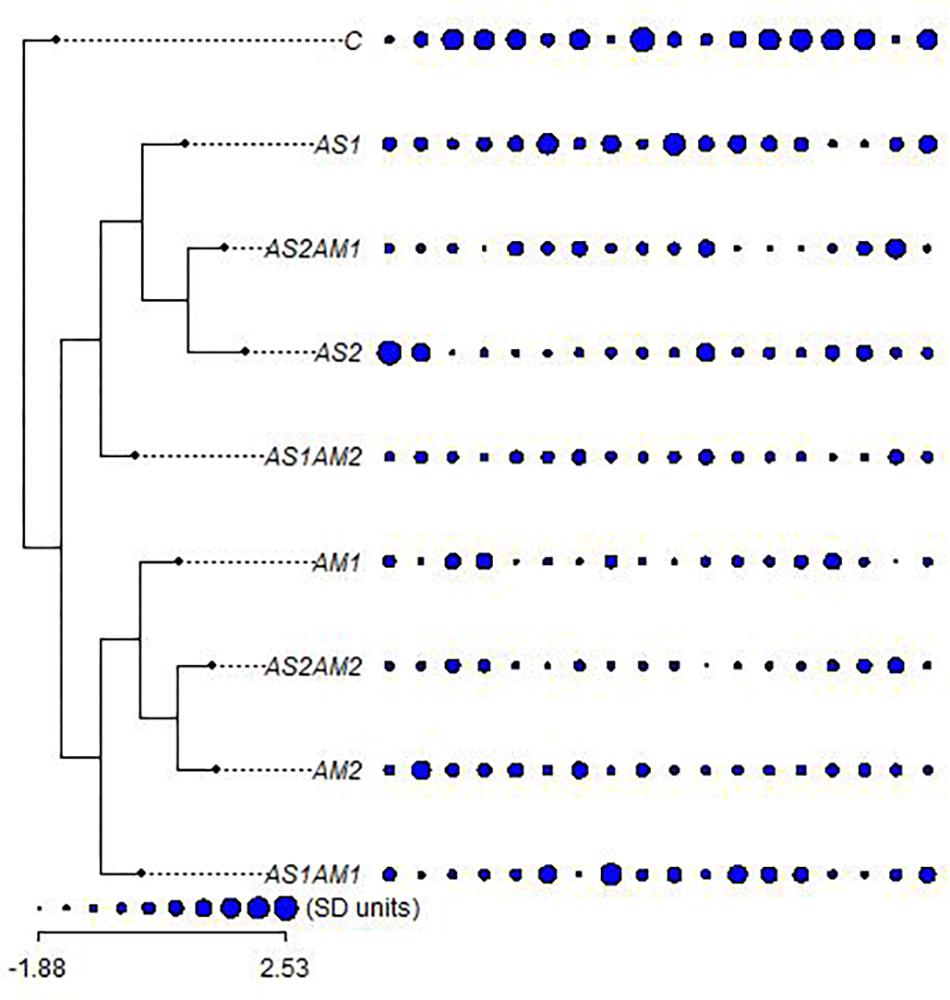
Figure 2. The relationships between arsenic-treated groups of fishes supplemented with Amphora coffeaeformis extract versus the control group with the hematological and biochemical characters (ordered as AST, ALT, ALP, GL, TP, AL, GLO, AG, UR, CR, LDH, RBCs, HB, HCT, MCV, MCH, MCHC, and WBCs) in standard deviation units (SD) (Mesquite and R packages). AS1 (19.2 mg/L arsenic), AS2 (38.3 mg/L arsenic), AM1 (7% Amphora), and AM2 (10% Amphora).
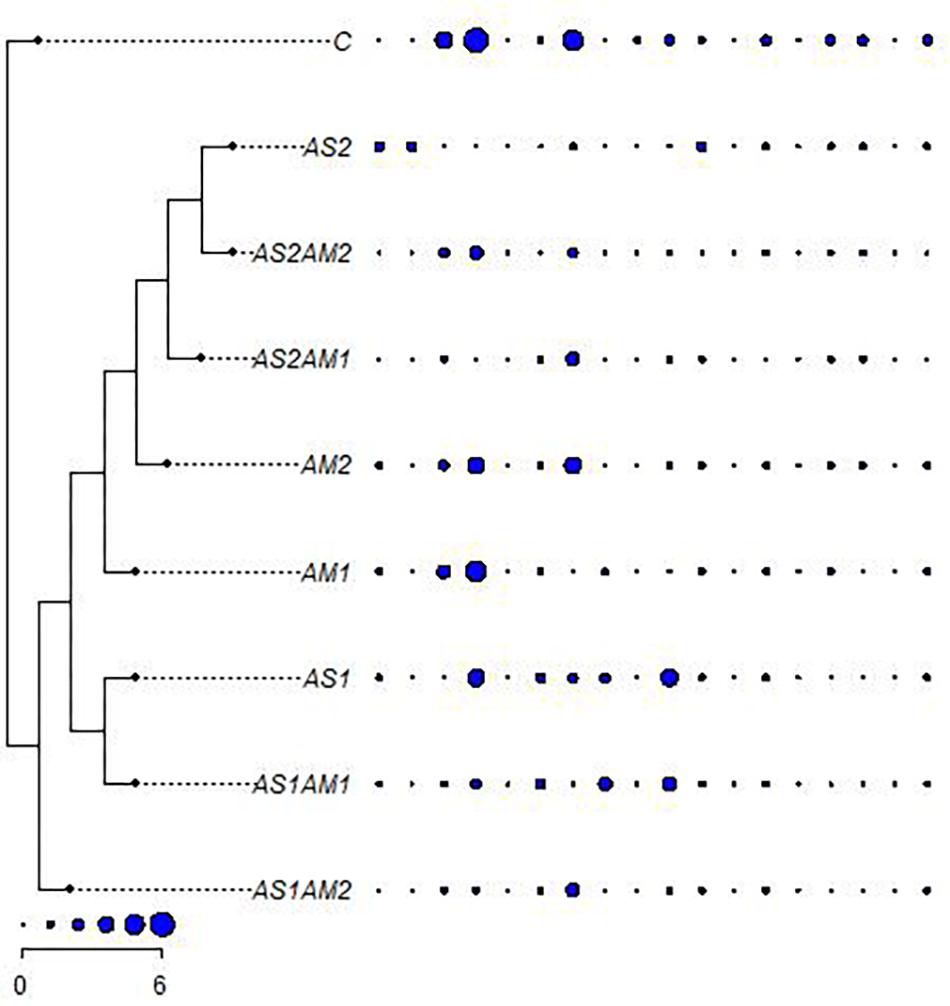
Figure 3. The relationships between arsenic-treated groups of fishes supplemented with Amphora coffeaeformis extract versus the control group with ANOVA-based coded hematological and biochemical characters (ordered as AST, ALT, ALP, GL, TP, AL, GLO, AG, UR, CR, LDH, RBCs, HB, HCT, MCV, MCH, MCHC, and WBCs) (Mesquite and R packages). AS1 (19.2 mg/L arsenic), AS2 (38.3 mg/L arsenic), AM1 (7% Amphora), and AM2 (10% Amphora).
AS1 treatment was grouped with AS1 AM1 whereas AS2 was clustered with AS2 AM2 in the main group including all treatments with amphora. AS1 AM2 represents a single cluster. Such a pattern of clustering reflects the significance of Amphora protective and antioxidant effects in a collective manner including all characteristics coded on the basis of ANOVA.
Discussion
The toxicity of arsenic highly variable within and between different fish species with respect to factors like age, sex, dose, exposure period and its organic and inorganic forms (Hallauer et al., 2016; Mahurpawar, 2017). These findings are evident with different pollutants and different fish species including C. gariepinus (Sayed and Authman, 2018; Mekkawy et al., 2019) and Oreochromis niloticus (Mekkawy et al., 2011a; Sayed et al., 2015b). The adverse impacts of these pollutants include behavioral, hematological, and biochemical characteristics (Lavanya et al., 2011; Mekkawy et al., 2013).
In the present study, C. gariepinus exhibited a lot of behavioral changes (loss of equilibrium, reduction in the feeding activity, fin hemorrhages, and skin alterations) due to exposure to sub-lethal concentrations of arsenic. Similar behavioral changes were observed in arsenic-treated fishes (Dwivedi and Trivedi, 2015; Mahurpawar, 2017). Similarly, other behavioral changes were observed by Baldissarelli et al. (2012) and Mekkawy et al. (2013) after exposure to arsenic and atrazine, respectively.
The excessive secretion of mucus which was observed especially at high doses of arsenic was probably due to skin arsenic-induced irritation and hence to protect the skin. Similar findings were recorded by Singh and Banerjee (2008). Impairments of the nervous system such as reflected in the form of swimming with the sides twisted 90 degrees and loss of equilibrium were also recorded in the present study under the stress of arsenic. Such impairments were postulated by Patro (2006) and Dwivedi and Trivedi (2015). Supplementation of Amphora to the arsenic-treated groups of C. gariepinus as well as untreated groups counteracted and improved the above behavioral changes to a great extent. These findings are suggested to be due to the multiple positive role of bioactive compounds of A. coffeaeformis working as antioxidant, antibacterial, antiviral, anti-fungal, and anti-inflammatory (Rajput and Mishra, 2012; Salahuddin et al., 2017; El-Sayed et al., 2018).
The alterations in RBC’s, HB, and HCT observed in the present study may have resulted from the disorders in hemopoietic processes due to sodium arsenate toxicity. Heath (1987) and Abo-Hegab et al. (1993) interpreted the stress-induced decrease in the hemoglobin and hematocrit values in terms of heme dilution of blood and elimination of RBCs as well as disequilibrium of the osmotic pressure inside and outside the blood cell. Similar results were observed in fish intoxicated with arsenic, pesticides, SDS, lead, and silver nanoparticles (Mekkawy et al., 2013, 2019; Amsath, 2017; Sayed et al., 2017; Sayed and Authman, 2018). Arsenic-induced changes in MCV, MCH, and WBCs of current species were evident. Some other studies reported similar results with fluctuations in some fish such as Clarias batrachus, and Catla catla (Lavanya et al., 2011; Kumar and Banerjee, 2016).
Low white blood cells of C. gariepinus were observed in the present study after arsenic exposure. According to Kotsanis et al. (2000) and Datta et al. (2009), the decrease in white blood cell counts during acute and sub-lethal treatment by arsenic may be attributed to the damage of the kidney, which is the primary site of hematopoiesis and/or due to inhibition of white blood cell maturation due to arsenic stress.
The liver is the major organ involved in the regulation of metabolic functions and most of the biotransformation of inorganic arsenic takes place in the liver (Kumar and Banerjee, 2016; Kumari et al., 2017). So, analysis of serum AST and ALT were widely used to demonstrate arsenic induced-hepatotoxicity (Dorcas and Solomon, 2014). The alterations of AST and ALT reported in the present study may be due to the rapid death of numerous liver cells (extensive hepatic necrosis) which were observed in association with liver inflammation, injury, stress, and disease (Dorcas and Solomon, 2014). Similar observations were done in C. gariepinus and other fish species exposed to arsenic (Roy and Bhattacharya, 2006; Abdel-Hameid, 2009), 4-nonylphenol (Sayed and Soliman, 2018), cadmium (Mekkawy et al., 2011b), and atrazine (Mekkawy et al., 2013).
Different authors referred to ALP alteration in C. gariepinus and different fish species under stress and environmental pollution (Ezenwaji et al., 2013; Sayed and Soliman, 2017). Garima and Himanshu (2015) postulated a decrease in ALP of C. batrachus after exposure to arsenic trioxide due to the decrease of food intake in arsenic-exposed fishes.
Different studies reported the significance of LDH alteration under stress in fishes (Mekkawy et al., 2010; Sayed and Soliman, 2018). Moreover, it was suggested that the emergency needs of increased energy demands can be met by the lactate production for gluconeogenesis in the liver to increase LDH activity after stress (Rani et al., 2017). Altered levels of LDH were recorded in the present work and confirmed by other studies on the same species (Sayed et al., 2011; Akinrotimi et al., 2018).
In the present study, reduced glucose level was observed in arsenic-exposed fish. Similar observations have been reported by Lavanya et al. (2011) after exposure of fingerling C. catla to arsenic trioxide and by Garima and Himanshu (2015) after exposure of C. batracus to the same pollutant. Acute treatment by arsenic caused hypoxia which leads to an excess utilization of stored carbohydrates, since the glucose level decreases at such stress conditions (Garima and Himanshu, 2015; Kumari et al., 2017).
The arsenic-exposed fishes exhibited alterations in serum protein in the present study as well as in other studies (Garima and Himanshu, 2015; Singh and Srivastava, 2015). Such alterations are interpreted to be due to impaired protein synthesis produced by reactive oxygen species and free radicals (Lavanya et al., 2011; Carlson et al., 2013).
Urea and creatinine have been used as important indicators of renal health in toxicant-induced fish using a variety of both in vivo and in vitro methods (Davis and Beandt, 1994; Ajeniyi and Solomon, 2014). In the present study, the arsenic-exposed fish exhibited an increase in the creatinine at low arsenic concentrations which may be due to oxidative damage (Prusty et al., 2011), and a decrease under the high arsenic concentration which severely disturbs the metabolic processes in the kidney (Rana et al., 2018). A similar increase in creatinine level was observed by Kumari (2015) in Oryctolagus cuniculus exposed to arsenic. However, a decrease in creatinine level was observed by Ogamba et al. (2011) and Inyang et al. (2018) in C. gariepinus exposed to paraquat dichloride and lindane, respectively. Uric acid was found to decrease under arsenic stress on C. gariepinus of the present work and that of Ogamba et al. (2011). Different other studies revealed an increase in uric acid under arsenic stress (Kumari, 2015) and different other pollutants (Mutlu et al., 2015; Kovacik et al., 2019).
A high level of arsenic accumulation in the muscles was observed in the present study. These findings are according to the results of Tyokumbur et al. (2014) who reported that the range of arsenic levels in the organs of C. gariepinus was highest in the muscles. The studies of Kim and Kang (2015) demonstrated that arsenic exposure can induce considerable arsenic accumulation in major tissues of different fish species (Al Sayegh Petkovšek et al., 2012; Gao et al., 2018).
According to Kaparapu (2018), microalgae like Amphora, Chlorella, and Isochrysis species are utilized as live feed for all growth stages of bivalve molluscs, for larval juvenile stages of abalone, crustaceans and some fish species, and for zooplankton used in aquaculture food webs. However, little is known regarding the role of Amphora extract in ameliorating the toxic damages induced by heavy metals in fishes in spite of its bioactive compounds recorded in the present work by GC-MS analysis. These compounds show effect as antioxidant, antibacterial, antiviral, anti-fungal, and anti-inflammatory (Salahuddin et al., 2017; El-Sayed et al., 2018; Munir et al., 2018).
In the present study, amphora bioactive compounds improved most of the plasma biochemical parameters for arsenic-induced fish reflecting, in turn, cytotoxic effects. So, the modulation effect of Amphora observed in the current study was in agreement with that of Ayoub et al. (2019) who utilized Amphora supplement in improvement of the lysozyme, serum protein and increased disease resistance of Nile Tilapia to Aeromonas hydrophila infection and El-Sayed et al. (2018) who utilized Amphora supplement as detoxification factor against paracetamol stress in liver tissue of rats. Moreover, the existing concentration of carbohydrates and proteins recorded in the present study may contribute to enhance the antioxidative activities of Amphora species as postulated by Rupérez et al. (2002) studying other diatoms.
In the present work, the main effects of arsenic and amphora and their interactions were represented by cluster analysis rooted by the control in the concept of multivariate sense for whole individuals since the treated groups may represent different populations in the environment. Although, the results of Amphora against arsenic toxicity showed positive effects, more studies are required to indicate the mechanisms of those effects.
Conclusion
The supplementation of amphora extracts can be used as detoxification and protective factors for C. gariepinus induced by arsenic due to the biologically active components of Amphora with antioxidant, antiviral, antibacterial, and anti-inflammatory characteristics, besides the abundant contents of proteins and carbohydrates which enhance these components.
Data Availability Statement
The raw data supporting the conclusions of this article will be made available by the authors, without undue reservation, to any qualified researcher.
Ethics Statement
The animal study was reviewed and approved by the Faculty of Science, Assiut University.
Author Contributions
All authors listed have made a substantial, direct and intellectual contribution to the work, and approved it for publication.
Conflict of Interest
The authors declare that the research was conducted in the absence of any commercial or financial relationships that could be construed as a potential conflict of interest.
References
Abdel-Hameid, N. H. (2009). A protective effect of calcium carbonate against arsenic toxicity of the nile catfish, Clarias gariepinus. Turkish. J. Fish. Aquat. Sci. 9, 191–200. doi: 10.4194/trjfas.2009.0211
Abdel-Wahab, A. M. (2018). In-vitro studies on antiviral effects of Galaxaura elongata marine algae on white spot syndrome virus. Benha Vet. Med. J. 34, 162–171. doi: 10.21608/bvmj.2018.54231
Abo-Hegab, S., Kamel, M. G., and Labib, W. D. (1993). Some physiological and biochemical indices of the pesticide tamaron and bayluside in fresh water fish Tilapia Oreochromis niloticus. Proc. Zool. Soc. A.R.E. 24, 183–197.
Ajeniyi, S. A., and Solomon, R. J. (2014). Urea and creatinine Of Clarias gariepinus in three different commercial ponds. Nat. Sci. 12, 124–138.
Akinrotimi, O. A., Edun, O. M., and Ukwe, O. I. K. (2018). Effects of anaesthetics on metabolic enzyme activities in african catfish, Clarias gariepinus (Burchell, 1822). J. Fish. 12, 22–28. doi: 10.12691/aees-1-3-3
Al Sayegh Petkovšek, S., Mazej Grudnik, Z., and Pokorny, B. (2012). Heavy metals and arsenic concentrations in ten fish species from the Šalek lakes (Slovenia): assessment of potential human health risk due to fish consumption. Environ. Monit. Assess. 184, 2647–2662. doi: 10.1007/s10661-011-2141-4
Al-Maslamani, I., Le Vay, L., Kennedy, H., and Jones, D. A. (2007). Feeding ecology of the grooved tiger shrimp Penaeus semisulcatus De Haan (Decapoda: Penaeidae) in inshore waters of Qatar. Arabian Gulf. Mar. Biol. 150, 627–637. doi: 10.1007/s00227-006-0346-9
Amsath, A. (2017). Effect of Arsenic (As2o3) on Haemetological Parameters of Freshwater Air Breathing Fish, Channa punctatus (Bloch). Curr. Trends Biomed. Eng. Biosci 7, 555702. doi: 10.19080/ctbeb.2017.07.555702
Anantharaj, K., Govindasamy, C., Natanamurugaraj, G., and Jeyachandran, S. (2011). Effect of Heavy metals on marine diatom amphora coffeaeformis (Agardh. Kutz). Glob. J. Environ. Res. 5, 112–117.
Avigliano, E., Schenone, N. F., Volpedo, A. V., Goessler, W., and Cirelli, A. F. (2015). The impact of water pollution on fish species in southeast region of Goiás, Brazil. Sci. Total Environ. 506, 8–16. doi: 10.1080/15287394.2015.1099484
Ayoub, H. F., Abdelghany, M. F., El-Sayed, B., and Abo El-Khair, A. (2019). Effects of Diatoms Amphora coffeaeformis on growth parameters, non specific immunity and protection of the Nile tilapia (Oreochromis niloticus) to Aeromonas hydrophila infection. Egypt. J. Aquat. Biol. Fish. 23, 413–426. doi: 10.21608/ejabf.2019.37466
Baldissarelli, L. A., Capiotti, K. M., Bogo, M. R., Ghisleni, G., and Bonan, C. D. (2012). Arsenic alters behavioral parameters and brain ectonucleotidases activities in zebrafish (Danio rerio). Comp. Biochem. Physiol. Part C Toxicol. Pharmacol. 155, 566–572. doi: 10.1016/j.cbpc.2012.01.006
Bligh, E. G., and Dyer, W. J. (1959). A rapid method of total lipid extraction and purification. Can. J. Biochem. Physiol. 37, 911–917. doi: 10.1139/o59-099
Carlson, P., Smalley, D., and Beneden, R. (2013). Proteomic analysis of arsenic-exposed zebrafish (danio rerio) identifies altered expression in proteins involved in fibrosis and lipid uptake in a gender-specific manner. Toxicol. Sci. 134, 83–91. doi: 10.1093/toxsci/kft086
Chiu, J. M.-Y., Thiyagarajan, V., Pechenik, J. A., Hung, O.-S., and Qian, P.-Y. (2007). Influence of bacteria and diatoms in biofilms on metamorphosis of the marine slipper limpet Crepidula onyx. Mar. Biol. 151, 1417–1431. doi: 10.1007/s00227-006-0580-1
Choudhury, S., Sree, A., Mukherjee, S., Pattnaik, P., and Maringanti, B. (2005). In vitro antibacterial activity of extracts of selected marine algae and mangroves against fish pathogens. Asain Fish sci 18, 287–296.
Dacie, J. V., and Lewis, S. M. (1991). Practical Haematology. 7th Edn. Edinburgh: Churchil Livingstone, 54–79.
Datta, S., Ghosh, D., Saha, D. R., Bhattacharaya, S., and Mazumder, S. (2009). Chronic exposure to low concentration of arsenic is immunotoxic to fish: role of head kidney macrophages as biomarkers of arsenic toxicity to Clarias batrachus. Aquat. Toxicol. 92, 86–94. doi: 10.1016/j.aquatox.2009.01.002
Daume, S., Krsinich, A., Farrell, S., and Gervis, M. (2000). Settlement, early growth and survival of Haliotis rubra in response to different algal species. J. Appl. Phycol. 12, 479–488.
Davis, M. E., and Beandt, W. O. (1994). “Renal methods for toxicology,” in Principles and Methods of Toxicology, ed. A. I. W. I. Hayes (New Yark, NY: Raven), 871–894.
Dorcas, I. K., and Solomon, R. J. (2014). Calculation of liver function test in Clarias gariepinus collected from three commercial fish ponds. Nat. Sci. 12:107. doi: 10.5897/ijfa15.0486
Dwivedi, S., and Trivedi, S. P. (2015). Behavioural, morphological and chromatophoric alterations induced by arsenite exposure in freshwater fish, Channa punctatus (Bloch). Int. J. Pharma Bio Sci. 6, 1328–1334.
El-Sayed, A. B., Aboulthana, W. M., El-Feky, A. M., Ibrahim, N. E., and Seif, M. M. (2018). Bio and phyto-chemical effect of Amphora coffeaeformis extract against hepatic injury induced by paracetamol in rats. Mol. Biol. Rep. 45, 2007–2023. doi: 10.1007/s11033-018-4356-8
Erickson, R. J., Mount, D. R., Highland, T. L., Hockett, J. R., and Jenson, C. T. (2011). The relative importance of waterborne and dietborne arsenic exposure on survival and growth of juvenile rainbow trout. Aquat. Toxicol. 104, 108–115. doi: 10.1016/j.aquatox.2011.04.003
Ezenwaji, N. E., Yenagoa, B., and Nwaigwe, C. (2013). Changes in liver and plasma enzymes of Clarias gariepinus exposed to sublethal concentrations of diesel. African J. Biotechnol. 12, 414–418. doi: 10.5897/ajb12.611
Gao, Y., Baisch, P., Mirlean, N., Rodrigues da Silva Júnior, F. M., Van Larebeke, N., Baeyens, W., et al. (2018). Arsenic speciation in fish and shellfish from the North Sea (Southern bight) and Açu Port area (Brazil) and health risks related to seafood consumption. Chemosphere 191, 89–96. doi: 10.1016/j.chemosphere.2017.10.031
García-Esquinas, E., Pollán, M., Umans, J. G., Francesconi, K. A., Goessler, W., and Guallar, E. (2013). Arsenic exposure and cancer mortality in a US-based prospective cohort: the Strong Heart Study. Cancer Epidemiol. Biomarkers Prev. 22, 1944–1953. doi: 10.1158/1055-9965.EPI-13-0234-T
Garelick, H., Jones, H., Dybowska, A., and Valsami-Jones, E. (2008). Arsenic pollution sources. Rev. Environ. Contam. Toxicol. 197, 17–60.
Garima, P., and Himanshu, P. (2015). Impact of Heavy metal, Arsenic trioxide on Biochemical profile of teleost, Clarias batrachus (Linn.). Int. J. Life Sci. 3, 141–146.
Gaur, J. P., and Rai, L. C. (2001). “Heavy metal tolerance in algae,” in Algal Adaptation to Environmental Stresses. Physiological, Biochemical and Molecular Mechanisms, ed. J. P. Rai Gaur (Berlin: Springer-Verlag), 363–388. doi: 10.1007/978-3-642-59491-5_12
Ghaffar, A., Hussain, R., Aslam, M., Abbas, G., and Khan, A. (2016). Arsenic and urea in combination alters the hematology, biochemistry and protoplasm in exposed rahu fish (Labeo rohita) (Hamilton, 1822). Turkish J. Fish. Aquat. Sci. 16, 289–296. doi: 10.4194/1303-2712-v16_2_09
Glodde, F., Günal, M., Kinsel, M. E., and AbuGhazaleh, A. (2018). Effects of natural antioxidants on the stability of omega-3 fatty acids in dog food. J. Vet. Res. 62, 103–108. doi: 10.1515/jvetres-2018-0014
Govind, P., and Madhuri, S. (2014). Heavy metals causing toxicity in animals and fishes. Res. J. Anim. Vet. Fish. Sci. 2, 17–23.
Hallauer, J., Geng, X., Yang, H.-C., Shen, J., Tsai, K.-J., and Liu, Z. (2016). The Effect of Chronic Arsenic Exposure in Zebrafish. Zebrafish 13, 405–412. doi: 10.1089/zeb.2016.1252
Inyang, I. R., Ayogoi, T. A., and Izah, S. C. (2018). Effect of lindane on some selected electrolytes and metabolites of Clarias gariepinus (juveniles). Adv. Plants Agric. Res 8, 394–397.
Kapaj, S., Peterson, H., Liber, K., and Bhattacharya, P. (2006). Human health effects from chronic arsenic poisoning–a review. J. Environ. Sci. Heal. Part A 41, 2399–2428. doi: 10.1080/10934520600873571
Khatoon, H., Banerjee, S., Yusoff, F., and Shariff, M. (2009). Evaluation of indigenous marine periphytic Amphora, Navicula and Cymbella grown on substrate as feed supplement in Penaeus monodon postlarval hatchery system. Aquacult. Nutr. 15, 186–193. doi: 10.1111/j.1365-2095.2008.00582.x
Khayatzadeh, J., and Abbasi, E. (2010). “The effects of heavy metals on aquatic animals,” in The 1 st International Applied Geological Congres (Iran: Islamic Azad University).
Kim, J., and Kang, J. (2015). The arsenic accumulation and its effect on oxidative stress responses in juvenile rockfish, Sebastes schlegelii, exposed to waterborne arsenic (As3+). Environ. Toxicol. Pharmacol. 39, 668–676. doi: 10.1016/j.etap.2015.01.012
Kotsanis, N., Iliopoulou-Georgudaki, J., and Kapata-Zoumbos, K. (2000). Changes in selected hematological parameters at early stages of the rainbow trout, Oncorhynchus mykiss, subjected to metal toxicants: arsenic, cadmium and mercury. J. Appl. Ichthyol. 16, 276–278. doi: 10.1046/j.1439-0426.2000.00163.x
Kovacik, A., Tvrda, E., Miskeje, M., Arvay, J., Tomka, M., Zbynovska, K., et al. (2019). Trace metals in the freshwater fish cyprinus carpio: effect to serum biochemistry and oxidative status markers. Biol. Trace Elem. Res. 188, 494–507. doi: 10.1007/s12011-018-1415-x
Kumar, R., and Banerjee, T. K. (2016). Arsenic induced hematological and biochemical responses in nutritionally important catfish Clarias batrachus (L.). Toxicol. Reports 3, 148–152. doi: 10.1016/j.toxrep.2016.01.001
Kumar, V. (2018). “Phytoremediation as a potentially promising technology: prospects and future,” in Metallic Contamination and Its Toxicity, ed. C. A. Gautam Pathak (New Delhi: Daya Publishing House A Division of Astral International Pvt. Ltd), 207–229.
Kumari, B., Kumar, V., Sinha, A. K., Ahsan, J., Ghosh, A. K., Wang, H., et al. (2017). Toxicology of arsenic in fish and aquatic systems. Environ. Chem. Lett. 15, 43–64. doi: 10.1007/s10311-016-0588-9
Kumari, M. (2015). Impacts of arsenic trioxide in some renal parameters in Oryctolagus cuniculus. An Int. Q. J. Life Sci. 2, 78–82.
Kumosani, T., Balamash, K., Ghashlan, H., Ahmed, Y., Baothman, O., Zeyadi, M., et al. (2017). Potential antioxidant and anti-proliferative activities of biologically active marine algae extracts. J. Pharm. Res. Int. 19, 1–7. doi: 10.9734/jpri/2017/38089
Lauritano, C., Andersen, J. H., Hansen, E., Albrigtsen, M., Escalera, L., Esposito, F., et al. (2016). Bioactivity screening of microalgae for antioxidant, anti-inflammatory, anticancer, anti-diabetes, and antibacterial activities. Front. Mar. Sci. 3:68. doi: 10.3389/fmars.2016.00068
Lavanya, S., Ramesh, M., Kavitha, C., and Malarvizhi, A. (2011). Hematological, biochemical and ionoregulatory responses of Indian major carp Catla catla during chronic sublethal exposure to inorganic arsenic. Chemosphere 82, 977–985. doi: 10.1016/j.chemosphere.2010.10.071
Lee, S., Karawita, R., Affan, A., Lee, J., Lee, B., and Jeon, Y. (2008). potential antioxidant activites of enzymatic digests from benthic diatoms achnanthes longipes, amphora coffeaeformis, and navicula sp. (Bacillariophyceae). Prev. Nutr. Food Sci. 13, 166–175. doi: 10.3746/jfn.2008.13.3.166
Lee, S., Karawita, R., Affan, A., Lee, J., Lee, K., Lee, B., et al. (2009). Potential of Benthic Diatoms Achnanthes longipes, Amphora coffeaeformis and Navicula sp. (Bacillariophyceae) as Antioxidant Sources. Algae 24, 47–55. doi: 10.4490/algae.2009.24.1.047
Maddison, W. P., and Maddison, D. R. (2018). Mesquite: A Modular System for Evolutionary Analysis. Version 3.51. Available at: http://www.mesquiteproject.org
Mahar, A., Wang, P., Ali, A., Awasthi, M. K., Lahori, A. H., Wang, Q., et al. (2016). Challenges and opportunities in the phytoremediation of heavy metals contaminated soils: a review. Ecotoxicol. Environ. Saf. 126, 111–121. doi: 10.1016/j.ecoenv.2015.12.023
Mahurpawar, M. (2017). Toxic effect of sodium arsenate on Clarias batrachus. Int. Res. J. Biol. Sci. 6, 15–18.
Manzoor, F., Munir, N., Sharif, N., and Naz, S. (2013). Algae: a potent antioxidant source. Sky J Microbiol. 1, 22–31.
Mekkawy, I. A., Mahmoud, U. M., Hana, M. N., and Sayed, A. E.-D. H. (2019). Cytotoxic and hemotoxic effects of silver nanoparticles on the African Catfish, Clarias gariepinus (Burchell, 1822). Ecotoxicol. Environ. Saf 171, 638–646. doi: 10.1016/j.ecoenv.2019.01.011
Mekkawy, I. A., Mahmoud, U. M., Osman, A. G., and Sayed, A. E. (2010). Effects of ultraviolet A on the activity of two metabolic enzymes, DNA damage and lipid peroxidation during early developmental stages of the African catfish, Clarias gariepinus (Burchell, 1822). Fish Physiol. Biochem. 36, 605–626. doi: 10.1007/s10695-009-9334-6
Mekkawy, I. A., Mahmoud, U. M., and Sayed Ael, D. (2011a). Effects of 4-nonylphenol on blood cells of the African catfish Clarias gariepinus (Burchell, 1822). Tissue Cell 43, 223–229. doi: 10.1016/j.tice.2011.03.006
Mekkawy, I. A., Mahmoud, U. M., Wassif, E. T., and Naguib, M. (2011b). Effects of cadmium on some haematological and biochemical characteristics of Oreochromis niloticus (Linnaeus, 1758) dietary supplemented with tomato paste and vitamin E. Fish Physiol. Biochem. 37, 71–84. doi: 10.1007/s10695-010-9418-3
Mekkawy, I. A. A., Mahmoud, U. M., and Mohammed, R. H. (2013). Protective effects of tomato paste and Vitamin E on Atrazine-Induced Hematological and biochemical characteristics of Clarias gariepinus (Burchell, 1822). Adv. Res. J. Environ. Sci. 2, 11–21.
Munir, N., Rafique, M., Altaf, I., Sharif, N., and Naz, S. (2018). Antioxidant and antimicrobial activities of extracts from selected algal species. Bangladesh J. Bot. 47, 53–61. doi: 10.1007/s00203-019-01760-7
Mutlu, E., Aydın, S., and Kutlu, B. (2015). Alterations of growth performance and blood chemistry in nile tilapia (Oreochromis niloticus) affected by copper sulfate in long-term exposure. Turkish J. Fish. Aquat. Sci. 15, 487–493.
Ogamba, E. N., Inyang, I. R., and Azuma, I. K. (2011). Effect of paraquat dichloride on some metabolic and enzyme parameters of Clarias gariepinus. Curr. Res. J. Biol. Sci. 3, 186–190.
Oremland, R. S., and Stolz, J. F. (2005). Arsenic, microbes and contaminated aquifers. Trends Microbiol. 13, 45–49. doi: 10.1016/j.tim.2004.12.002
Patro, L. (2006). Toxicological effects of cadmium chloride on acetylcholinesterase activity of a fresh water fish, Oreochromis mossambicus. Asian J. Exp. Sci. 20, 171–180.
Prusty, A. K., Kohli, M. P. S., Sahu, N. P., Pal, A. K., Saharan, N., Mohapatra, S., et al. (2011). Effect of short term exposure of fenvalerate on biochemical and haematological responses in Labeo rohita (Hamilton) fingerlings. Pestic. Biochem. Physiol 100, 124–129. doi: 10.1016/j.pestbp.2011.02.010
R Core Team (2013). R: A Language and Environment for Statistical Computing. Vienna: R Foundation for Statistical Computing.
Rae, T., and Buckley, A. (2014). TaxMan: An Online Facility for the Coding of Continuous Characters for Cladistic Analysis. Available at: arXiv:1411.4550v1 (accessed November 17, 2014).
Rahman, M. S., Molla, A. H., Saha, N., and Rahman, A. (2012). Study on heavy metals levels and its risk assessment in some edible fishes from Bangshi River, Savar, Dhaka, Bangladesh. Food Chem 134, 1847–1854. doi: 10.1016/j.foodchem.2012.03.099
Rajput, R., and Mishra, A. P. (2012). A review on biological activity of quinazolinones. Int. J. Pharm. Pharm. Sci. 4, 66–70.
Rana, M. N., Tangpong, J., and Rahman, M. M. (2018). Toxicodynamics of lead, cadmium, mercury and arsenic- induced kidney toxicity and treatment strategy: a mini review. Toxicol. Rep. 5, 704–713. doi: 10.1016/j.toxrep.2018.05.012
Rani, M., Yadav, J., Kumar, S., and Gupta, R. K. (2017). Impact of organophosphates on blood serum enzymes of Indian major carps. J. Entomol. Zool. Stud. 5, 1865–1868.
Roy, S., and Bhattacharya, S. (2006). Arsenic-induced histopathology and synthesis of stress proteins in liver and kidney of Channa punctatus. Ecotoxicol. Environ. Saf. 65, 218–229. doi: 10.1016/j.ecoenv.2005.07.005
Rupérez, P., Ahrazem, O., and Leal, J. A. (2002). Potential antioxidant capacity of sulfated polysaccharides from the edible marine brown seaweed Fucus vesiculosus. J. Agric. Food Chem. 50, 840–845. doi: 10.1021/jf010908o
Salahuddin, N., Elbarbary, A. A., and Alkabes, H. A. (2017). Antibacterial and anticancer activity of loaded quinazolinone polypyrrole/chitosan silver chloride nanocomposite. Int. J. Polym. Mater. 66, 307–316. doi: 10.1080/00914037.2016.1201831
Sayed, A. H., and Authman, M. M. N. (2018). The protective role of Spirulina platensis to alleviate the Sodium dodecyl sulfate toxic effects in the catfish Clarias gariepinus (Burchell, 1822). Ecotoxicol. Environ. Saf. 163, 136–144. doi: 10.1016/j.ecoenv.2018.07.060
Sayed, A. H., Elbaghdady, H. A., and Zahran, E. (2015a). Arsenic-induced genotoxicity in Nile tilapia (Orechromis niloticus); the role of Spirulina platensis extract. Environ. Monit. Assess. 187:751. doi: 10.1007/s10661-015-4983-7
Sayed, A. H., Zaki, R. M., El-Dean, A. M. K., and Abdulrazzaq, A. Y. (2015b). The biological activity of new thieno[2,3-c]pyrazole compounds as anti-oxidants against toxicity of 4-nonylphenol in Clarias gariepinus. Toxicol Rep 2, 1445–1453. doi: 10.1016/j.toxrep.2015.10.008
Sayed, A. H., El-Sayed, Y. S., and El-Far, A. H. (2017). Hepatoprotective efficacy of Spirulina platensis against lead-induced oxidative stress and genotoxicity in catfish; Clarias gariepinus. Ecotoxicol. Environ. Saf 143, 344–350. doi: 10.1016/j.ecoenv.2017.05.013
Sayed, A. H., Mekkawy, I. A., and Mahmoud, U. M. (2011). Effects of 4-nonylphenol on metabolic enzymes, some ions and biochemical blood parameters of the African catfish Clarias gariepinus (Burchell, 1822). African J. Biochem. Res. 5, 287–297.
Sayed, A. H., and Soliman, H. A. M. (2017). Developmental toxicity and DNA damaging properties of silver nanoparticles in the catfish (Clarias gariepinus). Mutat. Res. Genet. Toxicol. Environ. Mutagen 822, 34–40. doi: 10.1016/j.mrgentox.2017.07.002
Sayed, A. H., and Soliman, H. A. M. (2018). Modulatory effects of green tea extract against the hepatotoxic effects of 4-nonylphenol in catfish (Clarias gariepinus). Ecotoxicol. Environ. Saf. 149, 159–165. doi: 10.1016/j.ecoenv.2017.11.007
Selvaraj, V., Armistead, M. Y., Cohenford, M., and Murray, E. (2013). Arsenic trioxide (As(2)O(3)) induces apoptosis and necrosis mediated cell death through mitochondrial membrane potential damage and elevated production of reactive oxygen species in PLHC-1 fish cell line. Chemosphere 90, 1201–1209. doi: 10.1016/j.chemosphere.2012.09.039
Shaw, B. J., Al-Bairuty, G., and Handy, R. D. (2012). Effects of waterborne copper nanoparticles and copper sulphate on rainbow trout, (Oncorhynchus mykiss): physiology and accumulation. Aquat. Toxicol 116–117, 90–101. doi: 10.1016/j.aquatox.2012.02.032
Sheikhzadeh, N., Tayefi-Nasrabadi, H., Khani Oushani, A., and Najafi Enferadi, M. H. (2012). Effects of Haematococcus pluvialis supplementation on antioxidant system and metabolism in rainbow trout (Oncorhynchus mykiss). Fish Physiol. Biochem. 38, 413–419. doi: 10.1007/s10695-011-9519-7
Silva, R. O., Sousa, F. B., Damasceno, S. R., Carvalho, N. S., Silva, V. G., Oliveira, F. R., et al. (2014). Phytol, a diterpene alcohol, inhibits the inflammatory response by reducing cytokine production and oxidative stress. Fundam. Clin. Pharmacol. 28, 455–464. doi: 10.1111/fcp.12049
Singh, A. K., and Banerjee, T. K. (2008). Toxic effects of sodium arsenate (Na2 HAsO4.7H2O4) on the skin epidermis of air-breathing catfish Clarias batrachus (L.). Vet. Arh. 78, 73–88.
Singh, S., and Srivastava, A. K. (2015). Sub Lethal Effects of Copper and Arsenic on Some Biochemical and Hematological Parameters of the Blood in an Air Breathing Fish Heteropneustes fossilis. J. Ecophysiol. Occup. Heal. 15, 105–114.
Spss for Windows (2013). Statistical Package for the Social Sciences, Version 22,0. Chicago, LI: SPSS Inc.
Suresh, B., and Ravishankar, G. A. (2004). Phytoremediation—A Novel and promising approach for environmental clean-up. Crit. Rev. Biotechnol. 24, 97–124. doi: 10.1080/07388550490493627
Taghvaei, M., and Jafari, S. M. (2015). Application and stability of natural antioxidants in edible oils in order to substitute synthetic additives. J. Food Sci. Technol. 52, 1272–1282. doi: 10.1007/s13197-013-1080-1
Tyokumbur, E. T., Okorie, T. G., and Umma, B. S. (2014). Bioaccumulation of arsenic and uranium in two fish species (Clarias lazera and Tilapia zilli) from Alaro stream in Ibadan. Nigeria. Heal. Sci. Res. 1, 68–71.
Keywords: arsenic, Clarias, Amphora coffeaeformis, natural product, secondary metabolite, biochemistry, detoxification
Citation: Mekkawy IA, Mahmoud UM, Moneeb RH and Sayed AE-DH (2020) Significance Assessment of Amphora coffeaeformis in Arsenic-Induced Hemato- Biochemical Alterations of African Catfish (Clarias gariepinus). Front. Mar. Sci. 7:191. doi: 10.3389/fmars.2020.00191
Received: 01 October 2019; Accepted: 11 March 2020;
Published: 31 March 2020.
Edited by:
Naser A. Anjum, Aligarh Muslim University, IndiaReviewed by:
Asta Tvarijonaviciute, University of Murcia, SpainSalvatore Antonino Raccuia, Italian National Research Council, Italy
Copyright © 2020 Mekkawy, Mahmoud, Moneeb and Sayed. This is an open-access article distributed under the terms of the Creative Commons Attribution License (CC BY). The use, distribution or reproduction in other forums is permitted, provided the original author(s) and the copyright owner(s) are credited and that the original publication in this journal is cited, in accordance with accepted academic practice. No use, distribution or reproduction is permitted which does not comply with these terms.
*Correspondence: Alaa El-Din H. Sayed, YWxhYXNheWVkQGF1bi5lZHUuZWc=; YWxhYV9oMjU0QHlhaG9vLmNvbQ==