- 1Harriet L. Wilkes Honors College, Florida Atlantic University, Jupiter, FL, United States
- 2Harbor Branch Oceanographic Institute, Florida Atlantic University, Fort Pierce, FL, United States
- 3Center for Conservation and Research, San Antonio Zoo, San Antonio, TX, United States
- 4Halmos College of Natural Sciences and Oceanography, Nova Southeastern University, Dania Beach, FL, United States
Leptocephalus larvae of elopomorph fishes are a cryptic component of fish diversity in nearshore and oceanic habitats. However, identifying those leptocephali can be important in illuminating species richness in a region. Since the Deepwater Horizon oil spill in 2010, sampling of offshore fishes in the epi-, meso-, and upper bathypelagic depth strata of the northern Gulf of Mexico resulted in 8989 identifiable specimens of leptocephalus larvae or transforming juveniles, in 118 taxa representing 83 recognized and established species and an additional 35 distinctive leptocephalus morphotypes not yet linked to a known described species. Leptocephali account for ∼13% of the total species richness of fishes collected in the offshore region. A new morphotype of Muraenidae leptocephalus is also described. We compare this study with other leptocephalus diversity studies in the western Atlantic.
Introduction
Elopomorph fishes are basal teleosts, comprising the Elopiformes (tarpon and ladyfishes), Albuliformes (bonefishes), Notacanthiformes (spiny eels and halosaurs), and Anguilliformes (true eels, including the formerly separate Saccopharyngiformes) (Forey, 1973; Arratia, 1999; Dornburg et al., 2015; Poulsen et al., 2018). One unique characteristic of elopomorph fishes is a larval stage known as the leptocephalus (Greenwood et al., 1966; Pfeiler, 1986; Wiley and Johnson, 2010). This stage features an extended planktonic phase, allowing the larva to stay in the water column from a few months to several years before metamorphosis to a juvenile, depending on the species (Hulet, 1978; Smith, 1979, 1989a; Miller, 2009). The head is small, the body is transparent (Figure 1) and laterally compressed, with thin sheets of musculature on either side of the body subdivided into V- or W-shaped myomeres (Smith, 1979, 1989a) and large amounts of gelatinous glycosaminoglycans (GAGs) as an extracellular matrix sandwiched between the sheets of musculature (Miller, 2009). Leptocephali lack hemoglobin, and most species have sparse or no pigmentation (Pfeiler, 1991; Bishop et al., 2000; Miller, 2009). Because the head is small, and therefore the gills as well, and erythrocytes with hemoglobin do not develop until the latter part of metamorphosis, much of the respiration is via cutaneous exchange across the surface of the leaf-shaped body (Pfeiler, 1991). The high surface area to volume ratio allows for gas exchange and also possibly uptake of dissolved organic matter (Pfeiler and Govoni, 1993). Besides uptake of dissolved organic matter, leptocephali are reported to feed on larvacean houses, fecal pellets, marine snow, bacteria, and protists (Mochioka and Iwamizu, 1996; Govoni, 2010; Miller et al., 2013; Liénart et al., 2016).
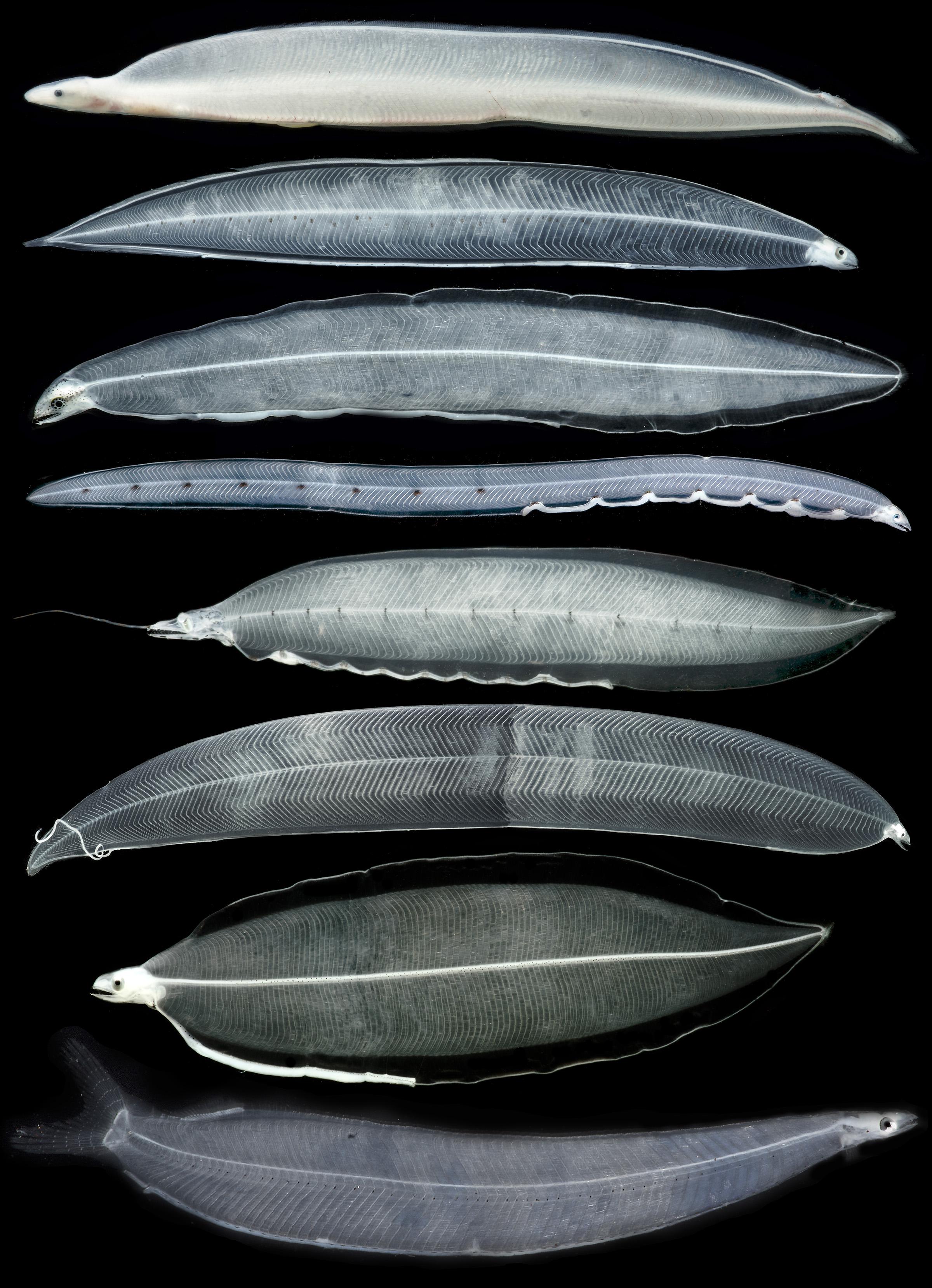
Figure 1. A sample of morphological diversity in leptocephalus larvae and a transforming juvenile. Top to bottom: transforming Congridae? juvenile eel, congrid Rhynchoconger flavus, unidentified muraenid similar to Gymnothorax miliaris, ophichthid Myrichthys breviceps, synaphobranchid Ilyophinae sp. D3 from Straits of Florida, congrid Ariosoma anale, chlopsid Chlopsis bicolor, and elopid Elops smithii. Images are not to scale and are provided to show a sample of the diversity of leptocephalus morphology and pigmentation. All photos by Dante Fenolio.
Understanding larval fishes and their ecology is very important for gaining insights into recruitment for fisheries management (Blaxter, 1984; Sammons and Bettoli, 1998; Valdez-Moreno et al., 2010). Larval fishes can also elucidate regional biodiversity (Limouzy-Paris et al., 1994; Richardson and Cowen, 2004a; Miller et al., 2006, 2016; Miller and McCleave, 2007). Distinctive larvae were recognized as representing cryptic species before the adults were distinguished; for example, Smith (1989b) recognized a unique larva of an unknown Elops sp. in the western North Atlantic and McBride et al. (2010) described adults as a new species, Elops smithi. However, many distinctive morphotypes are still not linked to the adults of described species.
While many leptocephali are smaller than 10 cm total length (TL), some are large enough to get caught in standard larger-mesh fishing nets. Some anguilliform leptocephali can reach more than 30 cm TL (Miller et al., 2013; Kurogi et al., 2016) and notacanthiform leptocephali from the families Halosauridae and Notocanthidae, in particular, grow to 1.8 m TL (Nielsen and Larsen, 1970). During various trawling expeditions to Bear Seamount, it was not unusual to find leptocephali in the cod end and wrapped around the mesh of the wings of high-speed rope trawls, Yankee 36 bottom trawls, and IGYPT midwater nets (Moore et al., 2003, 2004). Similarly, Miller et al. (2013) compared leptocephali caught with an IKMT vs. a large pelagic trawl. They show that net avoidance does occur given that the larger pelagic trawl caught more species and larger leptocephali than the IKMT. However, the larger pelagic trawl had larger mesh in the cod end and therefore failed to capture smaller leptocephali. Castonguay and McCleave (1987) indicated that net avoidance may be an issue resulting in differential day vs. night catches of at least some leptocephali. They also showed very little vertical migration in most species examined; however, there did appear to be some vertical differences in size classes of particular species. Other investigators have also noted diel vertical migrations in leptocephali on small vertical scales of 50–150 m within the epipelagic and in a few cases the upper mesopelagic zones (Kajihara et al., 1988; Smith, 1989a; Otake et al., 1998; Miller, 2015).
One issue hindering biodiversity studies in low latitude oceanic ecosystems is the difficulty in identification of some leptocephali. Even though leptocephali have a basic body plan with a transparent, compressed, leaf-like body, there is variation in many features that allow for identification to family, genus, or species for many of the elopomorphs in the western Atlantic (Figures 1, 2). Leptocephali of major clades are distinguished based on features such as tail type and presence of pelvic fins (forked tail and pelvic fins in Elopiformes and Albuliformes, long fleshy post-caudal streamer and pelvic fins in Notacanthiformes, terminal pointed or rounded caudal fin with pelvic fins absent in Anguilliformes). Other features that distinguish leptocephali include myomere counts, which correspond with vertebral counts in juveniles and adults (Fahay and Obenchain, 1978; Smith, 1989a; Miller and Tsukamoto, 2004), gut length and swellings or loops in the gut (Fahay, 2007), morphology of the liver along the gut (Leiby, 1989), location of the last vertical blood vessel coming off the posterior most portion of the larval kidney (Castle, 1970), pigmentation patterns (Smith, 1989a; Baldwin, 2013), morphology of teeth (Smith, 1989a), body and body shape (Fahay, 1983; Miller, 2009), and relative positions of the dorsal and anal fin origins (Smith, 1989a).
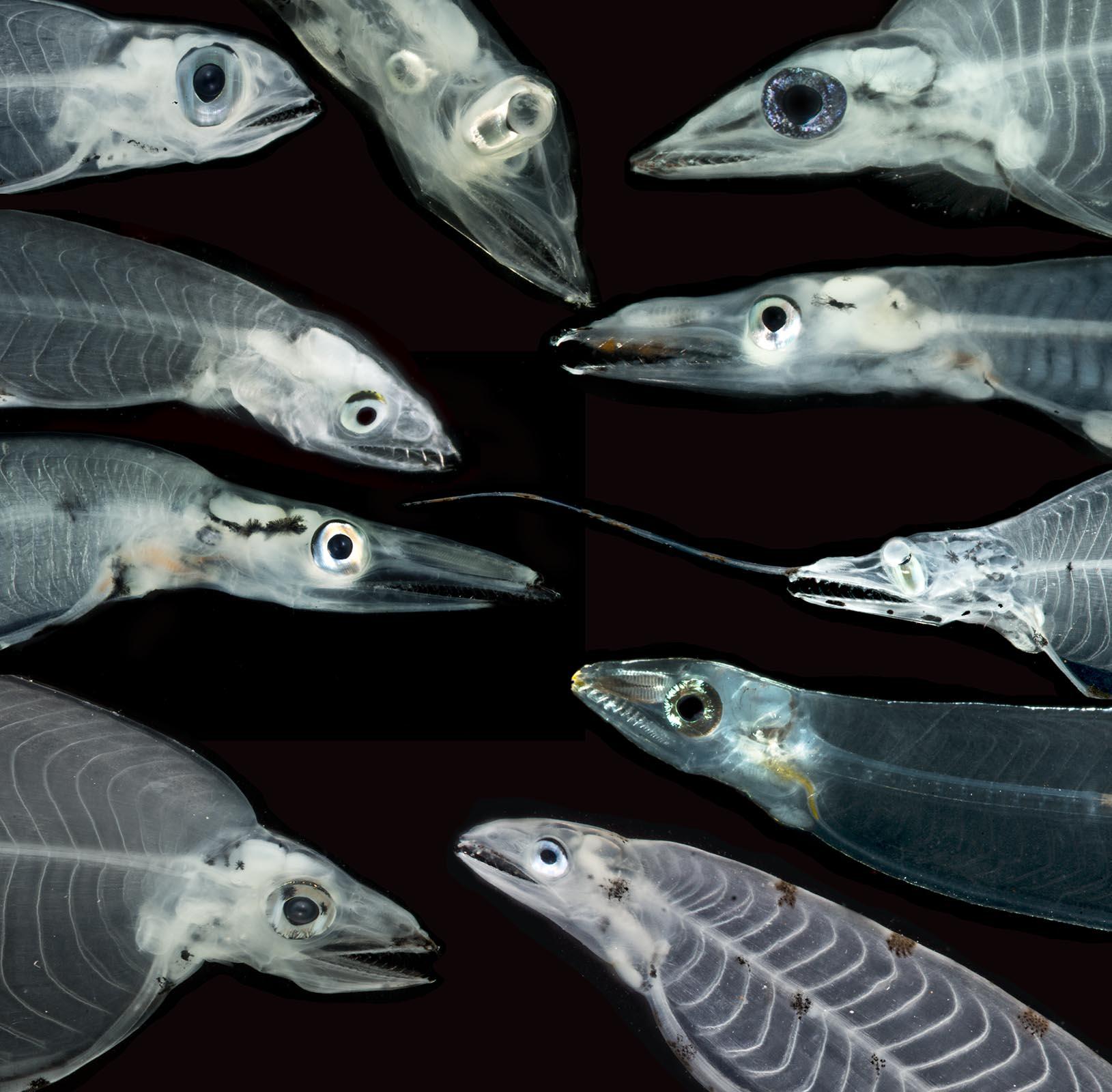
Figure 2. A sample of morphological diversity in the heads of leptocephalus larvae, showing differences in shape, dentition, pigmentation, eye shape, and rostral projections. Clockwise from top center: halosaurid “Tiluropsis,” nettastomatid Nettenchelys pygnaea, nettastomatid Facciolella sp. C FWNA, synaphobranchid Ilyophinae sp. D3 FWNA from Straits of Florida, nettastomatid Saurenchelys stylura from Straits of Florida, ophichthid Stictorhinus potamius, congrid Xenomystax congroides, nettastomatid Facciolella sp., ophichthid Ophichthus puncticeps from Straits of Florida, and congrid Bathycongrus sp. A FWNA. All photos by Dante Fenolio.
One difficulty with using these features is that they are often modified or lost during metamorphosis. Many leptocephali undergo transformations that are as profound as the metamorphosis of a tadpole to a frog. This has made it difficult to link the larva with the adult using morphological features. For example, species of the genus Ariosoma are distinguished by pigmented lines resembling angled slash marks in the myosepta along the lateral midline and both the dorsal and anal fin origins are positioned very far back, close to the caudal fin. However, before the leptocephali begin other signs of metamorphosis, such as loss of teeth, loss of transparency, and a thickening of the body, Ariosoma leptocephali initiating metamorphosis lose the lateral pigmentation and both median fins begin extending anteriorly. This can result in leptocephali with transitional morphologies that are unlike the typical leptocephalus for that species and yet distinct from juveniles and adults. Individuals caught in the midst of transformation to the juvenile stage can have a mix of features (Figure 1, top).
One traditional method for identification of leptocephali relied on growing out specimens to a point past metamorphosis so the morphology of the leptocephalus larva could be linked with the juvenile or adult morphology (Kuroki et al., 2010). Another method was assembling a series of leptocephalus specimens that bridged that same transformation (Castle, 1970). The metamorphosis of leptocephali to juveniles can occur quickly; in several species where this is known, the majority of the metamorphosis occurs over a period of several days to a very few weeks (Ochiai and Nozawa, 1980; Kuroki et al., 2010; Huang et al., 2018). This rapidity of metamorphosis means there are even fewer specimens of individuals caught in the midst of this transformation, making assembling a series of specimens difficult. These methods are even more likely to fail for rare or deep-sea species, where their numbers may be greatly reduced and their duration as epipelagic plankton may be brief or non-existent (some deep-sea eel larva may rarely rise to the epipelagic, for example, Saccopharynx spp., Monognathus spp., or cyematids; Poulsen et al., 2018).
There are more than 1058 known species of elopomorph fishes worldwide, with the true eels (Anguilliformes) making up the vast majority (1009 species; Fricke et al., 2019). Within the western Central Atlantic (e.g., the Gulf of Mexico, Caribbean Sea, and the adjacent portions of northern South America, Central America, and southern North America) there are 188 described species of elopomorphs recorded (Carpenter, 2002; McBride et al., 2010), and 127 species have been recorded from the Gulf of Mexico (Leiby, 1989; Smith, 1989c, d; McEachran and Fechhelm, 1998; McEachran, 2009). Unfortunately, the distributions of many eel species are not well known because adults may be cryptic, burrowing in soft sediments or living deep in hard structures and therefore difficult to capture. Leptocephali may prove helpful in demonstrating the wider distributions for some of these species. However, there are a number of distinctive leptocephalus larvae known from the Gulf of Mexico that have not yet been linked to a known adult species (Böhlke, 1989b). Table 1 in Miller and McCleave (1994) shows how relatively few research cruises focused on leptocephali were conducted in the Gulf of Mexico from the 1950s to the 1980s.
This paper describes the elopomorph fauna, based on leptocephali collected from the oceanic waters of the northern and eastern Gulf of Mexico following the Deepwater Horizon oil spill and subsequent surveys, and further discusses the contribution of these larvae to the overall species richness observed in the offshore Gulf environment.
Materials and Methods
Following the Deepwater Horizon oil spill, the NOAA Office of Response and Restoration provided support for the creation of the Offshore Nekton Sampling and Analysis Program (ONSAP) to generate biological information on the fauna potentially impacted by the spill (Cook et al., unpublished). The goal of this program was to provide independent data for use during the Natural Resource Damage Assessment (NRDA) process. During ONSAP, the R/V Pisces conducted four cruises in the Gulf of Mexico during late 2010 and 2011, with cruises identified as PC8 (Dec 2010), PC9 (Mar–Apr 2011), PC10 (Jun–Jul 2011), and PC12 (Aug–Sep 2011). All sampling on the Pisces used a modified Irish Herring/High-Speed Rope Trawl (HSRT). The station grid chosen for sampling aligned with SEAMAP stations, with the designation of station names retained in this study (Figure 3; modified from Eldridge, 1988), with stations occurring at every half degree latitude–longitude crossing, stations were approximately 30 nautical miles (nm) apart. Sampling at each station was conducted day and night to capture diel migration dynamics, with deployments centered around solar noon and midnight, respectively. Due to the nature of the Pisces modified trawling net, which had no closing mechanism, discrete depth bins were restricted to “shallow” and “deep,” with trawls reaching depths between 0–700 and 0–1500 m, respectively.
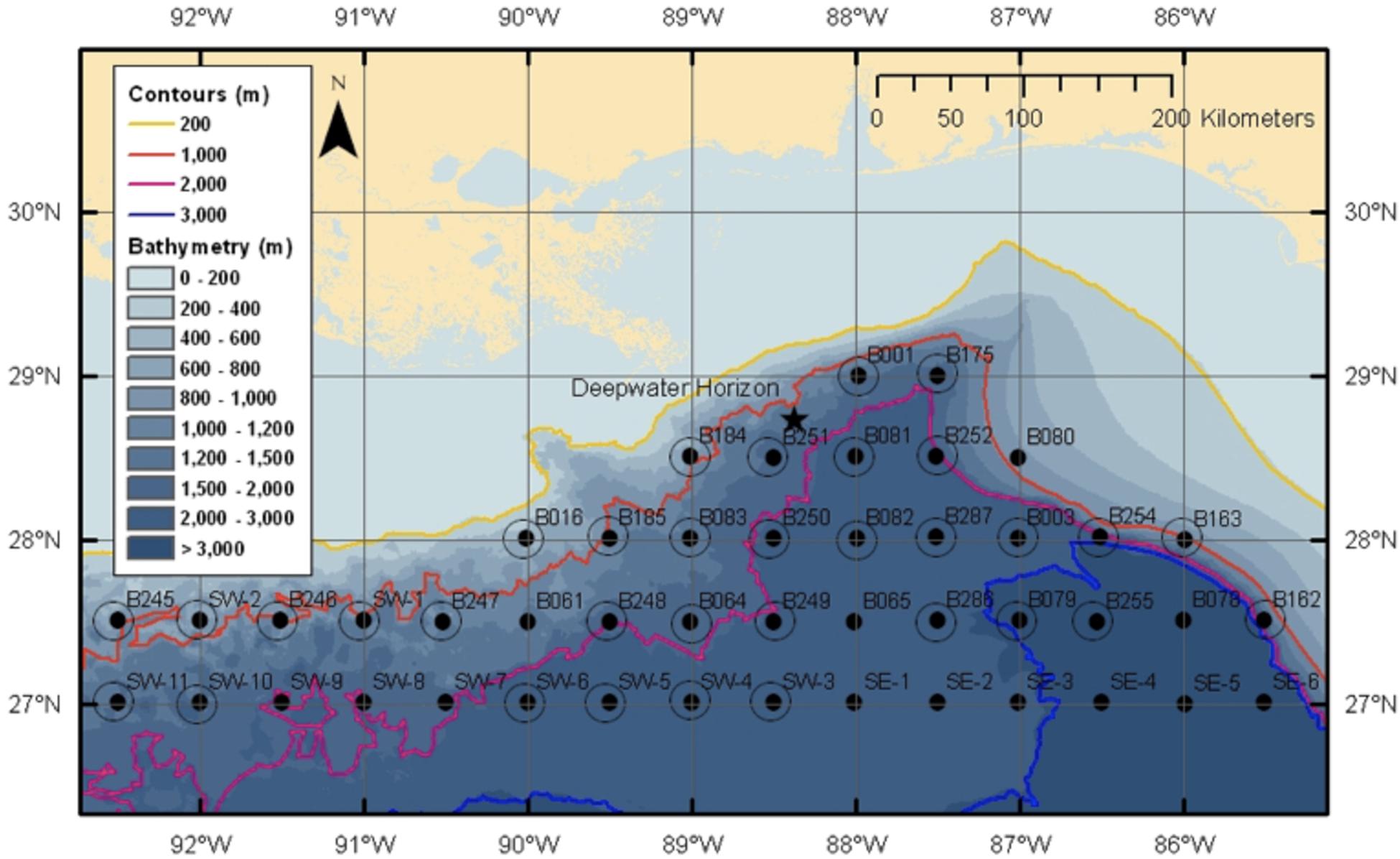
Figure 3. SEAMAP sampling grid used by both ONSAP and DEEPEND cruises (Eldridge, 1988). Note the location of the DWH marked by a star. Yellow line marks the 200 m isobath, orange the 1000 m isobath, pink the 2000 m isobath, and blue the 3000 m isobath.
Additional sampling for ONSAP was conducted in 2011 on the merchant vessel Meg Skansi, with the same sampling grid as the Pisces, but instead using a 10-m2 mouth area, 3-mm mesh, 6-net Multiple Opening Closing Net and Environmental Sensing System (MOCNESS) midwater trawl. A flowmeter attached to the frame recorded the volume filtered by each net, and a ship-board computer operated the opening and closing of the respective nets based on real-time depth information (see Weibe et al., 1985 for detailed description of unit). The MOCNESS sampling protocol was standardized at all locations: net 0 fished from the surface to the maximum depth (usually 1500 m, bottom depth permitting); net 1 fished from 1500–1200 m depth; net 2 fished from 1200–1000 m; net 3 fished from 1000–600 m; net 4 fished from 600–200 m; and net 5 fished from 200–0 m. The rationale for sampling these depth strata was to characterize the fauna below, within, and above the depth range of a large hydrocarbon plume reported at 1000–1200 m depth (Camilli et al., 2010), as well as to characterize potential trends in the vertical distributions of migrating and non-migrating taxa as reported by Sutton (2013). The M/V Meg Skansi trawling surveys were divided into three major cruise series: MS6 (25 Jan–1 Apr 2011), MS7 (19 Apr–30 Jun 2011), and MS8 (18 Jul–30 Sep 2011).
More recently, the Deep Pelagic Nekton Dynamics of the Gulf of Mexico (DEEPEND) consortium continued the MOCNESS time-series of trawl sampling at the same stations on the R/V Point Sur. There were six DEEPEND cruises: DP01 (1–8 May 2015), DP02 (8–22 Aug 2015), DP03 (1–16 May 2016), DP04 (5–20 Aug 2016) and DP05 (29 Apr–12 May 2017), and DP06 (18 Jul–2 Aug 2018). While the DEEPEND sampling cruises were shorter than those conducted during the Meg Skansi cruises, efforts were made to standardize sampling methodologies. Attention was also given to sampling mesoscale oceanographic features associated with the Loop Current and its eddies, and combined CTD, satellite, and AUV information was collated to inform and define key oceanographic features. More detailed information on the various sampling programs can be found in the paper by Cook et al. (unpublished).
The leptocephali were identified following Böhlke (1989b) and their standard lengths measured to the nearest 0.1 mm using digital calipers. Only distinctive species and morphotypes are included here; damaged leptocephalus specimens, specimens only identifiable to family, transforming juveniles that could not be identified to a species or distinctive morphotype, or juveniles/adults over 2.0 g weight were excluded from the results. Distributional information on elopomorphs used in this study came from Böhlke (1989a, b) and Carpenter (2002), with information on Gulf of Mexico occurrences from McEachran and Fechhelm (1998) and McEachran (2009).
The methodology for the vertical profiles is found in Cook et al. (unpublished). The depth profiles illustrated were calculated from just DEEPEND cruise samples.
The DEEPEND specimens were collected under the Florida Atlantic University IACUC protocols A15-06 and A18-12.
Results
A total of 8989 elopomorph leptocephali were collected, measured, weighed, identified, and included in this study from the research area in the northern Gulf of Mexico (Table 1). A total of 118 taxa representing known species or distinctive morphotypes of leptocephali were collected by the ONSAP and DEEPEND projects. Of those 118 taxa, leptocephali of 83 are currently recognized as larvae or transforming juveniles of established species. Roughly half of the taxa collected were rare, represented by four or fewer individuals. Several leptocephalus larval morphotypes represent multiple species (Paraconger sp., Gnathophis sp., Rhynchoconger gracilior/guppyi, Gymnothorax conspersus/kolpos, Gymnothorax ocellatus/nigromarginatus/saxicola) because features of the leptocephalus morphology overlap or are indistinguishable between the species. This study also found one new morphotype that is distinctive from those already known.
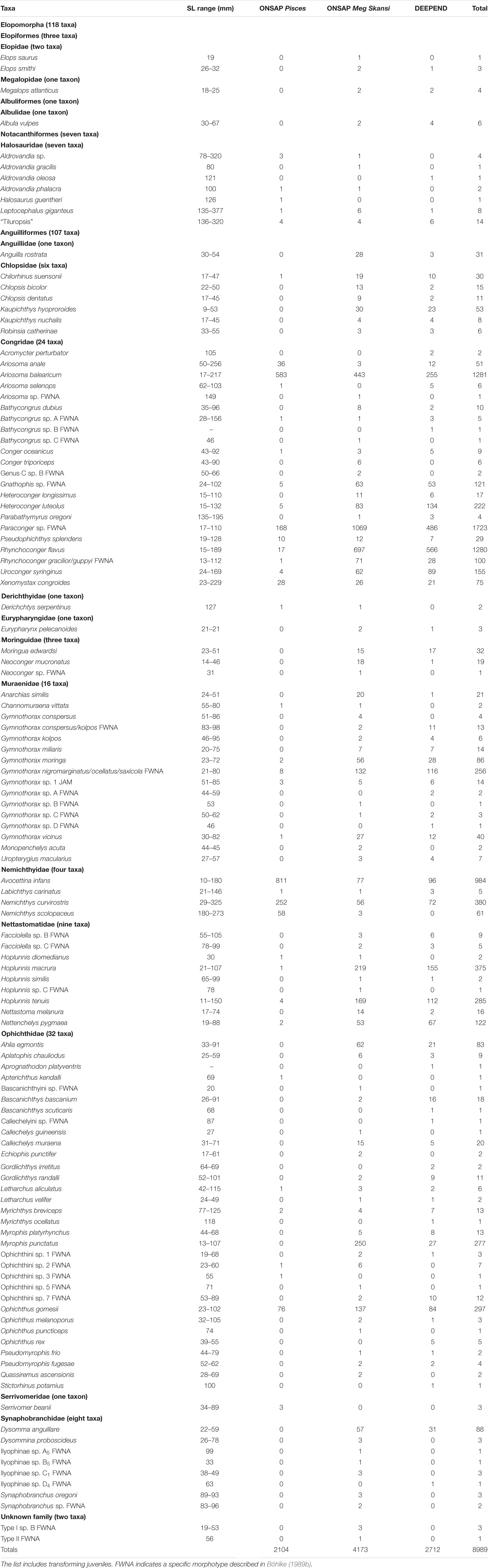
Table 1. Size range and number of specimens captured for ONSAP (2010–2011, including R/V Pisces and M/V Meg Skansi cruises) and DEEPEND (2015–2018, all six R/V Point Sur cruises) cruises by species or morphotype.
That new morphotype, here designated as Gymnothorax sp. 1 JAM (Figure 4), has a leptocephalus very similar to that of Gymnothorax vicinus, in that the dorsal fin origin is at midbody and there is an interrupted line of pigment spots on the ventral midline of the esophagus; however, this new morphotype has 153–169 total myomeres (131–142 in G. vicinus, Smith, 1989e), 95–105 preanal myomeres (60–68 in G. vicinus), 42–59 predorsal myomeres (53–63 in G. vicinus), and a last vertical blood vessel at 86–92 myomeres (60–67 in G. vicinus). There are no lateral pigments, no band of pigments through the eye, and very few melanophores over the brain, one melanophore lateral to the heart, and three to five at the base of the pectoral fin bud.
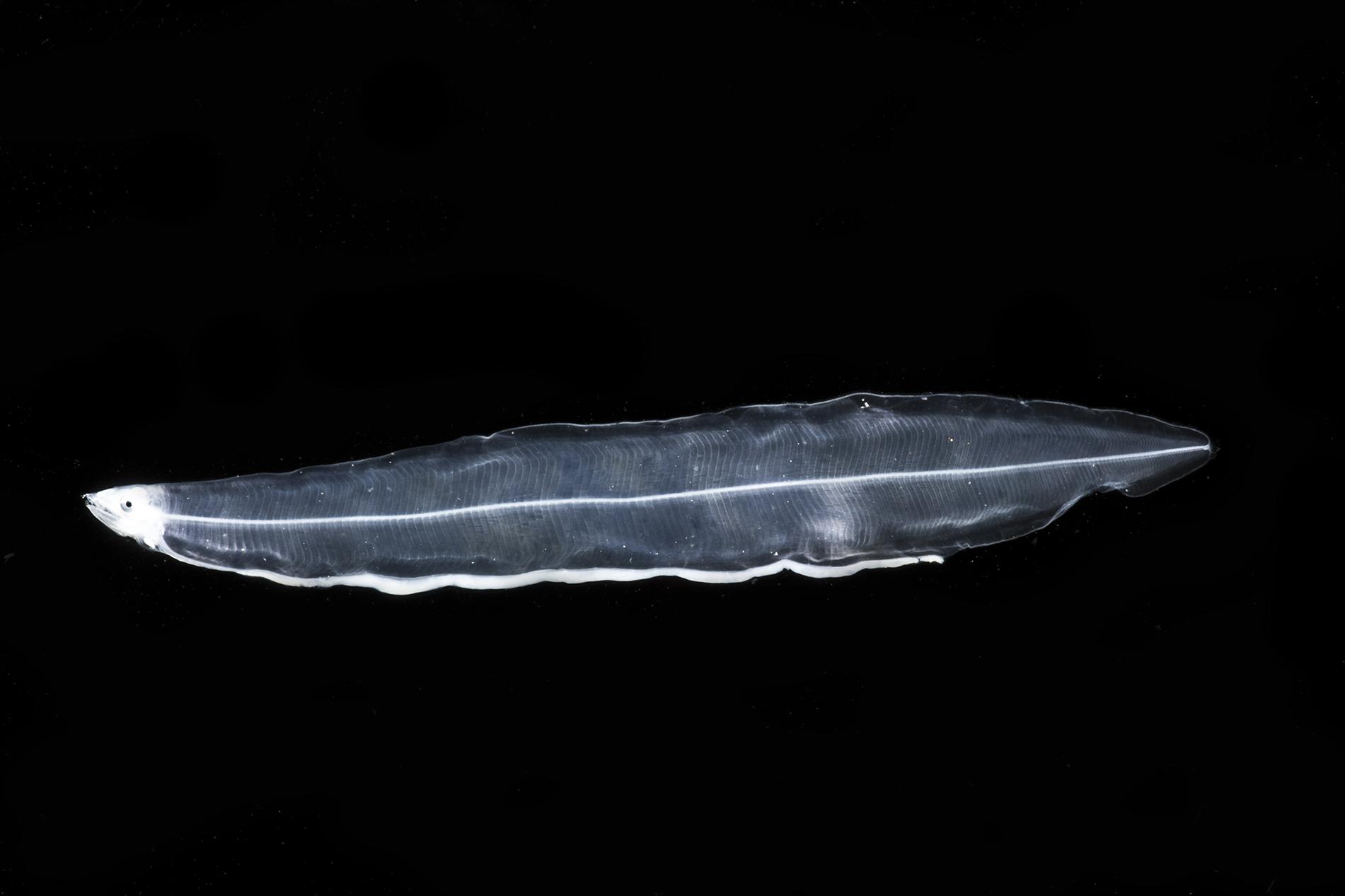
Figure 4. Muraenidae leptocephalus morphotype Gymnothorax sp.1 JAM. Collected 11 May 2017 at station B175D in the 0–200 m stratum. Specimen was 51 mm SL. Photo by Dante Fenolio.
Leptocephali account for 13% of the total species richness of fishes collected in the ONSAP and DEEPEND projects (Cook and Sutton, 2018a, b, c; Sutton et al., 2018a, b; Cook and Sutton, 2019). Leptocephali of the families Congridae (5600 specimens), Nemichthyidae (1430), Nettastomatidae (819), Ophichthidae (800), and Muraenidae (473) were especially abundant in the northern Gulf of Mexico. The most abundant species were Paraconger sp. (1698 individuals), Ariosoma balearicum (1281), Rhynchoconger flavus (1280), Avocettina infans (984), Nemichthys curvirostris (380), Hoplunnis macrura (375), Ophichthus gomesii (297), Hoplunnis tenuis (285), and Myrophis punctatus (277).
A number of the leptocephali identifiable to established species represent new records for the Gulf of Mexico based on the complete list of fishes in McEachran (2009) and chapters in Böhlke (1989a, b). Taxa that represent new records of occurrence for the Gulf of Mexico include: Dysommina proboscideus, Quassiremus ascensionis, Derichchtys serpentinus, Hoplunnis similis, and Serrivomer beanii. Several other taxa were not reported in either McEachran and Fechhelm (1998) or McEachran (2009), but were listed as leptocephali occurring in the Gulf of Mexico by Leiby (1989) and Smith (1989c) (e.g., Chilorhinus suensoni, Chlopsis bicolor, Chlopsis dentatus, Kaupichthys hyoproroides, Robinsia catherinae, Gordiichthys randalli, Pseudomyrophis frio, Pseudomyrophis fugesae, and Stictorhinus potamius).
There is some evidence for net avoidance in certain taxa, as determined by higher catches at night for species found almost exclusively in the epipelagic (Figure 5). For example, abundance estimates of Gnathophis sp. were almost four times greater at night than during the day. Likewise, Heteroconger luteolus leptocephali were collected in more than seven times greater abundance at night. Other taxa seemed to show little difference in day vs. night catch rates (Figure 6), which may indicate less ability to swim out of the way of the net. Those species that show net avoidance had firmer, more muscular leptocephali when freshly caught, compared to other taxa, such as most muraenids, which had flimsier bodies when fresh out of the net. Species caught in the high-speed rope trawl, but not in slower MOCNESS nets may also reflect taxa that are capable of net avoidance. Hoplunnis tenuis, Ahlia egmontis, and some other taxa showed evidence of diel vertical migration (Figure 7). Not all leptocephali were confined to the epipelagic; some were found in moderate abundances in the 200–600 m depth stratum and some species were collected as deep as 1500 m. A few species, particularly members of the Synaphobranchidae, spanned the water column, down to bathypelagic depths (Figure 8). These deep specimens were often still in the larval stage (not metamorphosing juveniles in the process of settling out).
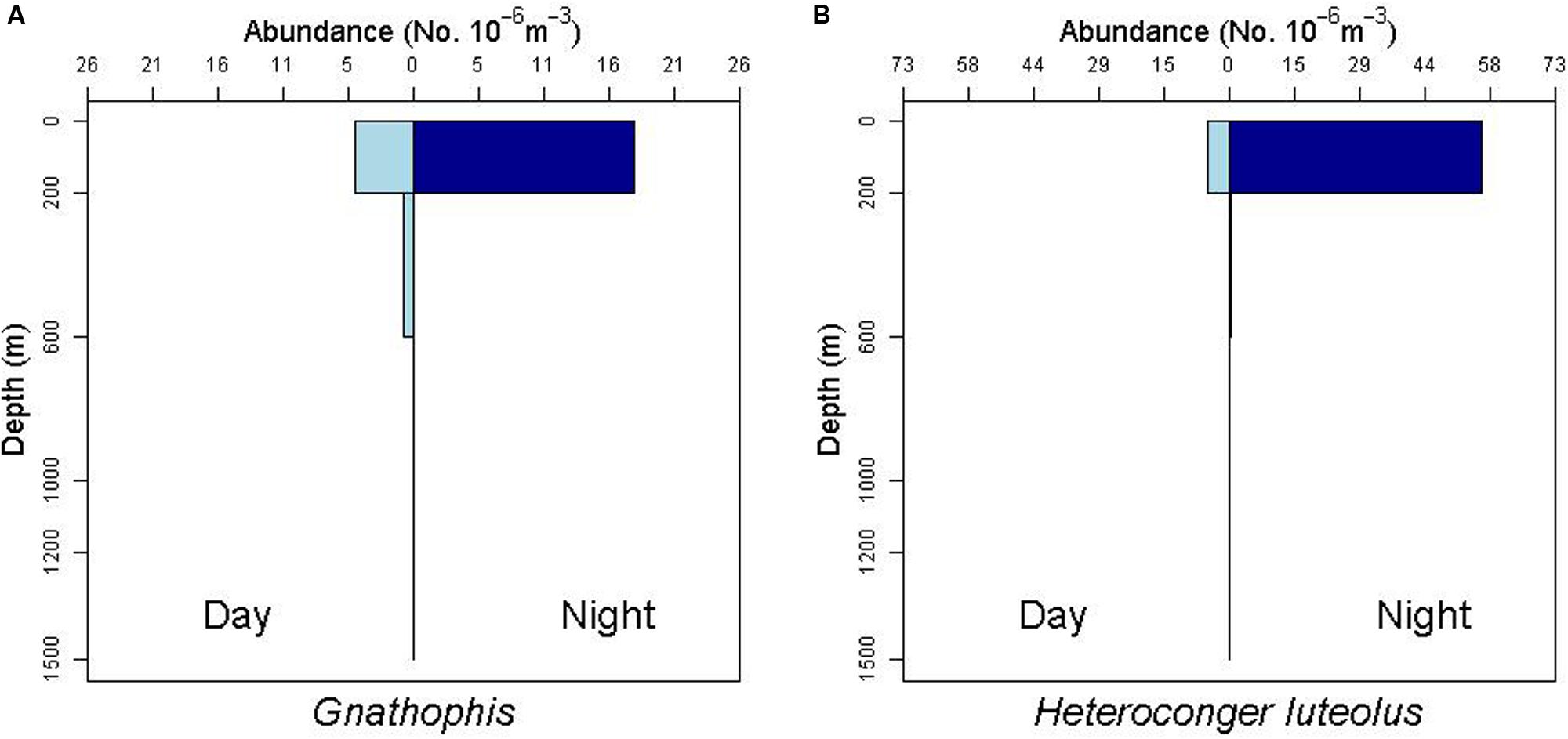
Figure 5. Depth profiles for the congrid leptocephalus morphotype Gnathophis sp. and the congrid species Heterconger luteolus. Note the greater abundances at night in the surface stratum (0–200 m) as an indication of possible net avoidance by these taxa during the day.
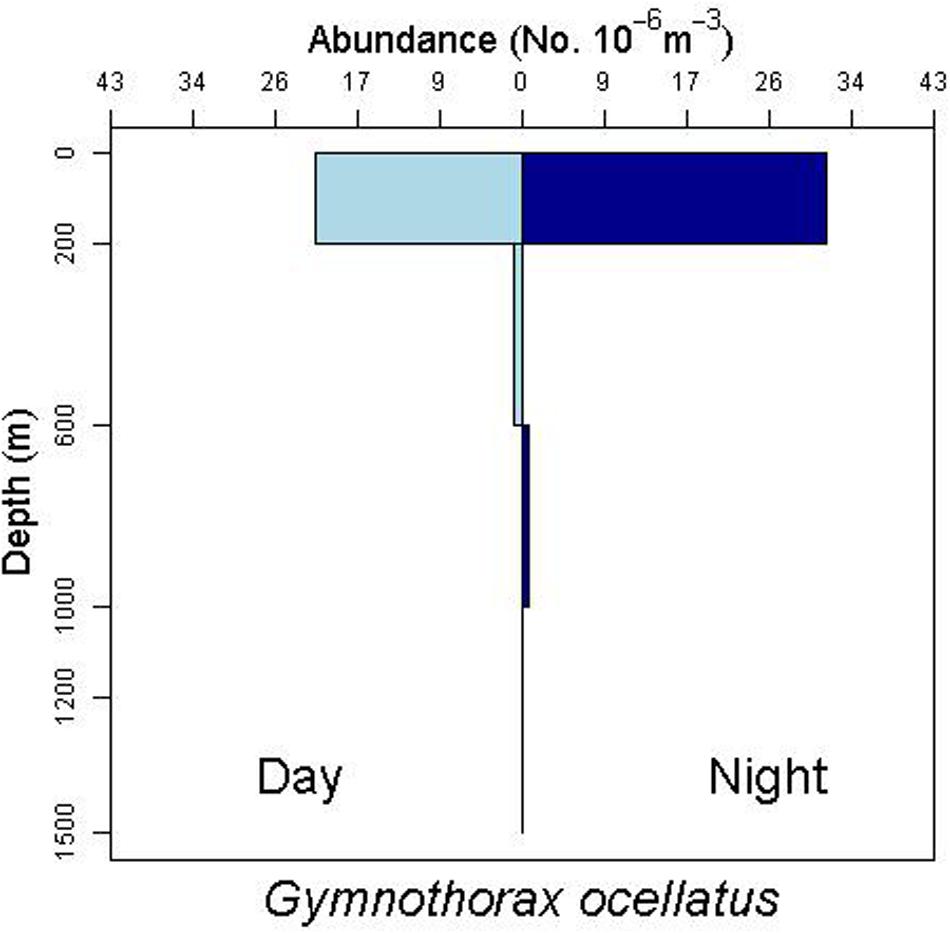
Figure 6. Depth profile for the muraenid leptocephalus species complex Gymnothorax ocellatus/nigromarginatus/saxicola, which show less difference in day vs. night abundances.
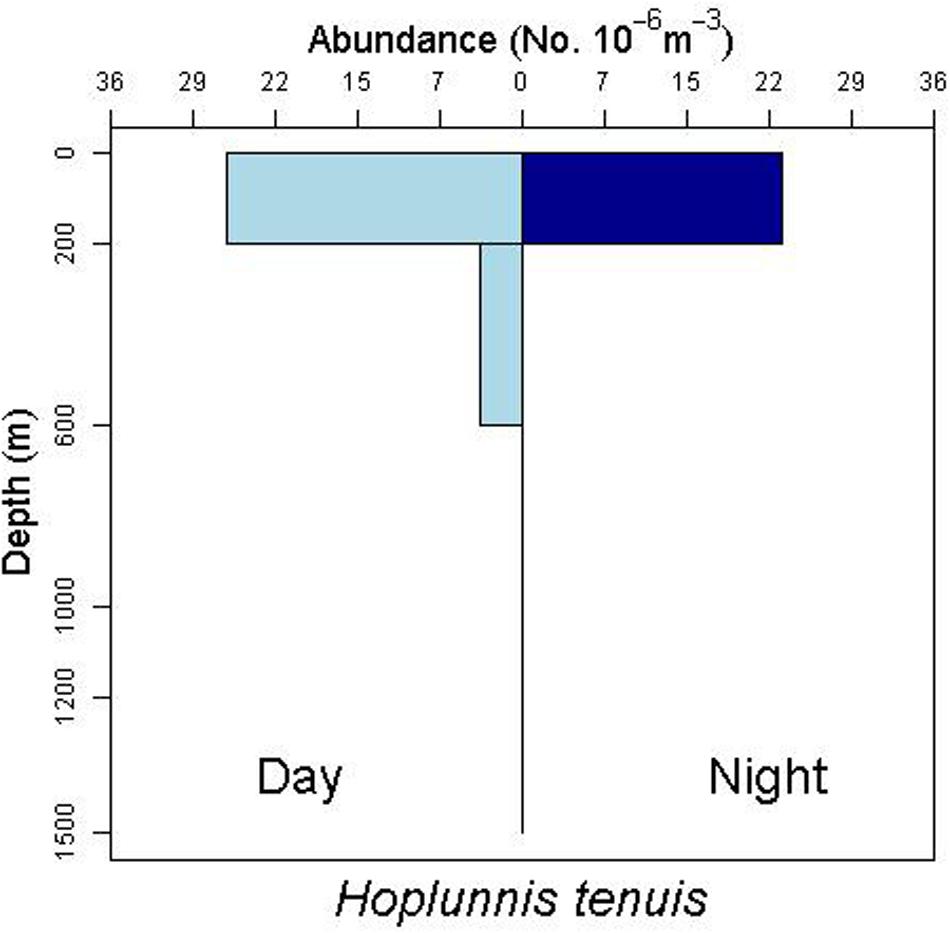
Figure 7. Depth profile for the nettastomatid leptocephalus Hoplunnis tenuis, suggesting diel vertical migration with moderate numbers of individuals below 200 m during the day.
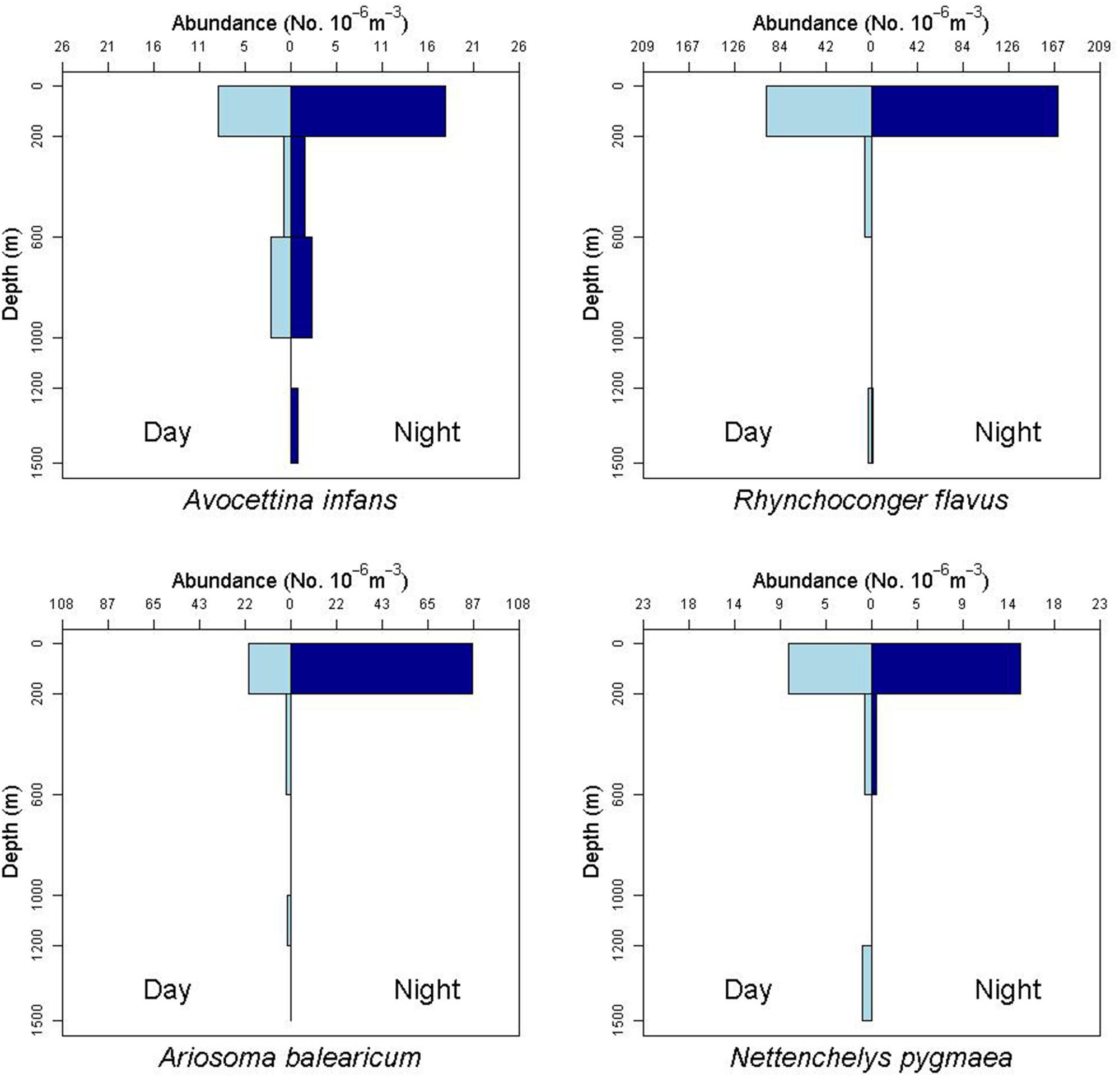
Figure 8. Depth profile of the leptocephalus for the nemichthyid species Avocettina infans, showing a distribution down to 1500 m depth and possible net avoidance at the surface. The profile of the congrid leptocephalus of Rhynchoconger flavus also shows captures at 1200–1500 m. The profile of the congrid Ariosoma balearicum shows captures down to 1200 m during the day. The profile for the synaphobranchid species Nettenchelys pygmaea also shows daytime captures down to 1500 m.
Discussion
Given that the number of leptocephali species and morphotypes collected in the oceanic Gulf of Mexico is close to the number of adult species known from the Gulf (McEachran, 2009), leptocephali can serve as an important indicator of eel biodiversity and represent potential distributional range extensions and undiscovered species. Any ichthyofaunal biodiversity survey that does not include an analysis of leptocephali runs the risk of greatly undercounting the species richness in a given region. However, it should be acknowledged that capture of a larval species is not unequivocal evidence that the adults also occur in the Gulf of Mexico. Richardson and Cowen (2004b) stated that knowledge of both adult and larval diversity is necessary to understand the full species richness of some eel groups, such as the family Ophichthidae. Unfortunately, leptocephali are often lumped into a common “leptocephalus” category in ichthyoplankton surveys, and are also frequently omitted or minimally included in identification guides to larval fishes (Richards et al., 1993; Leis and Carson-Ewart, 2000; Richards, 2005; Lyczkowski-Shultz et al., 2013). The latter is another reason that leptocephali are “hiding in plain sight”; if students do not see them in guides and surveys, elopomorph larvae will be further overlooked.
In this study, distinctive leptocephali account for 13% of the overall species richness found in the pelagic realm of the northern and eastern Gulf of Mexico by the ONSAP and DEEPEND investigations. It is likely that some larval morphotypes will eventually be linked to adult specimens of already-known species, but in some cases, distinctive larvae could also point to undiscovered species, as was the case with E. smithi (McBride et al., 2010).
Comparing our results with those of previous investigations from other areas in the low-latitude western North Atlantic, three things become clear. First, the sampling gear used makes a big difference in results (Habtes et al., 2014). Our use of a highspeed rope trawl on the R/V Pisces added several large specimens and examples of faster swimming species not collected in our MOCNESS cruises. Limouzy-Paris et al. (1994) sampled with a 1-m2 mouth MOCNESS system for a series of trawls in the Florida Straits. Their leptocephalus diversity represents a smaller subset of what we found, and this may reflect the smaller MOCNESS gear with resulting greater net avoidance, compared to our study. Of the 28 species of elopomorphs listed in their table, our study collected 25 of those (89%). The species (Limouzy-Paris et al., 1994) captured at the greatest number of stations correspond with many of our most abundant species. Second, differences in the eel fauna in different studies also reflect smaller scale faunal differences within the tropical/subtropical western North Atlantic. Leptocephali collected from the West Florida shelf break near our sampling area shared 18 out of 21 species (86%) with our study (Crabtree et al., 1992). Miller and McCleave (2007) studied leptocephali in the Sargasso Sea and found at least 61 taxa, of those 44 (72%) were also in our collections. Interestingly, many of the dominant taxa in our study (Paraconger sp., R. flavus, A. infans, H. macrura, N. curvirostris) are relatively minor in abundance or completely absent from Miller and McCleave’s study. They also found several taxa not represented in our collections (e.g., Conger escuelentus, Mixomyrophis pusillapinna, Ichthyapus ophioneus). Richardson and Cowen (2004a) examined leptocephalus diversity around Barbados where they found 68 taxa, and 49 of those (72% of their taxa) were also collected in our study. The taxa unique to their study were either newly described by them (Richardson and Cowen, 2004b) and not found in our collections or taxa known from the southeastern Caribbean. Third, several leptocephalus larvae are very rare and only collected in studies with larger sample sizes. Our study shared many rare species/morphotypes (for example, Ophichthini sp.1, Gymnothorax sp. A and sp. C, Aprognathodon platyventris, and Ophichthus puncticeps) with that of Richardson and Cowen (2004a), who examined over 4500 specimens. To our knowledge, several of the morphotypes we report here have not been listed in the literature since Böhlke (1989b), such as Type I sp. B, Type II, Ilyophinae sp. A5, Facciolella sp. B, congrid Genus C sp. B, and Ophichthini sp. 5.
While Richards et al. (1993) did not explicitly identify many species of leptocephali in their study, instead grouping them as “leptocephali,” they did point out that the reason for the high diversity of larval fishes associated with the Loop Current in the northern Gulf of Mexico was due to mixing of tropical and warm temperate oceanic, mesopelagic, coastal demersal, and pelagic species in the region. This appears to explain the high species richness we found in our study, although we would also add bathypelagic and deep-demersal taxa to the faunal components mixed in this region.
Leptocephali show diel vertical migration in some species (Castonguay and McCleave, 1987; Kajihara et al., 1988; Otake et al., 1998; Miller, 2015). Most studies of vertical migration in leptocephali subdivide the upper 200 m of water into several distinct strata. Our collection methods integrated the entire epipelagic stratum into one net, so we were not able to discern finer-scale diel migration within that zone. However, we do have evidence of larger scale migrations, where some species move from the upper mesopelagic (200–600 m) during daytime to the epipelagic at night (Figure 7, see also Gnathophis sp. in Figure 5, and R. flavus and A. balearicum in Figure 8).
A number of leptocephali were collected in deep-water strata. For example, specimens of A. infans, R. flavus, and A. balearicum were found in reduced abundances below the epipelagic zone (Figure 8), but throughout the sampled water column to 1500 m and possibly deeper. All of the relatively rare Synaphobranchus oregoni and Synaphobranchus sp. specimens came from the 600–1200 m strata. The rarity of some leptocephalus types may be an indication that these larvae do not generally come to the surface. For example, halosaurs are ubiquitous and relatively abundant demersal fishes on the continental slope to abyssal plains, but their larvae (“Tiluropsis” and Leptocephalus giganteus) are very rarely captured, indicating that they most likely stay in the deeper layers. Leptocephalus giganteus was only collected in the MOCNESS from nets fished between 1000–1200, 600–1000, and 200–600 m depth. Leptocephali remaining at depth may help explain the absence in this study of larvae of Saccopharynx and Monognathus, and the absence or rarity of other bathypelagic species (Poulsen et al., 2018).
Data Availability Statement
The datasets generated for this study can be found in the Gulf of Mexico Research Initiative Information and Data Cooperative (GRIIDC) at https://data.gulfresearchinitiative.org (doi: 10.7266/N7R49NTN, 10.7266/N7VX0DK2, 10.7266/N70P03T, 10.7266/N7XP7385, 10.7266/N7902234, and 10.7266/n7-ac8e-0240.
Ethics Statement
The animal study was reviewed and approved by the Florida Atlantic University IACUC Committee.
Author Contributions
TS and JM conceptualized the study. JM, DF, AC, and TS participated in the research cruises and collected samples. JM identified the specimens. DF took the photographic images. AC managed the specimen database and analyzed the vertical distribution data. JM wrote the manuscript with contributions from DF, AC, and TS.
Funding
This research was funded in part by the NOAA Office of Response and Restoration and in part by a grant from The Gulf of Mexico Research Initiative (GoMRI).
Conflict of Interest
The authors declare that the research was conducted in the absence of any commercial or financial relationships that could be construed as a potential conflict of interest.
Acknowledgments
We thank the captains, crews, and other scientists who participated in the cruises of the R/V Pisces, M/V Meg Skansi, and R/V Point Sur for their assistance in collecting the leptocephalus specimens. Our heartfelt appreciation goes particularly to David Smith for his longstanding and comprehensive work on Atlantic leptocephalus larvae and their identification. Thanks also go to Rich Jones for his help in identifying some leptocephali in this study. Finally, we strongly value the feedback from four reviewers who helped improve this manuscript. The data are publicly available through the Gulf of Mexico Research Initiative Information and Data Cooperative (GRIIDC) at https://data.gulfresearchinitiative.org (doi: 10.7266/N7R49NTN, 10.7266/N7VX0DK2, 10.7266/N70P03T, 10.7266/N7XP7385, 10.7266/N7902234, and 10.7266/n7-ac8e-0240).
References
Arratia, G. (1999). “The monophyly of teleostei and stem-group teleosts,” in Mesozoic Fishes 2, Systematics and Fossil Record, eds G. Arratia and H.-P. Schultze (Munich: Verlag Dr. Friedrich Pfeil), 265–334.
Baldwin, C. C. (2013). The phylogenetic significance of colour patterns in marine teleost larvae. Zool. J. Linn. Soc. 168, 496–563. doi: 10.1111/zoj.12033
Bishop, R. E., Torres, J. J., and Crabtree, R. E. (2000). Chemical composition and growth indices in leptocephalus larvae. Mar. Biol. 137, 205–214. doi: 10.1007/s002270000362
Blaxter, J.H.S. (1984). “Ontogeny, systematics and fisheries,” in Ontogeny and Systematics of Fishes, eds H.G. Moser, W.J. Richards, D.M. Cohen, M.P. Fahay, A.W. Kendall, and S.L. Richardson, Vol. 1, (ASIH Spec. Publ.), 1–6.
Böhlke, E. B. (eds.) (1989a). Fishes of the Western North Atlantic: Anguilliformes and Saccopharyngiformes. Mem. Sears Found. Mar. Res. 9, 1–655.
Böhlke, E. B. (eds.) (1989b). Fishes of the Western North Atlantic: Leptocephali. Mem. Sears Found. Mar. Res. 2, 656–1055.
Camilli, R., Reddy, C. M., Yoerger, D. R., Van Moov, B. A., Jakuba, M. V., Kinsey, J. C., et al. (2010). Tracking hydrocarbon plume transport and biodegradation at Deepwater Horizon. Science 330, 201–204. doi: 10.1126/science.1195223
Carpenter, K. E. (eds.) (2002). The Living Marine Resources of the Western Central Atlantic: Bony Fishes Part 1 (Acipenseridae to Grammatidae), Vol. 2. Rome: Food and Agriculture Organization of the United Nations.
Castle, P. H. J. (1970). Distribution, larval growth, and metamorphosis of the eel Derichthys serpentinus Gill, 1884 (Pisces: Derichthyidae). Copeia 1970, 444–452.
Castonguay, M., and McCleave, J. D. (1987). Vertical distributions, diel and ontogenetic vertical migrations and net avoidance of leptocephali of Anguilla and other common species in the Sargasso Sea. J. Plankton Res. 9, 195–214. doi: 10.1093/plankt/9.1.195
Cook, A., and Sutton, T. T. (2018a). Inventory of Gulf oceanic Fauna Data Including Species, Weight, and Measurements. Cruises DP01 May 1-8, 2015 and DP02 August 9-21, 2015 R/V on the Point Sur in the Northern Gulf of Mexico (Distributed by: Gulf of Mexico Research Initiative Information and Data Cooperative (GRIIDC)). Corpus Christi, TX: Harte Research Institute, Texas A&M University, doi: 10.7266/N70P0X3T
Cook, A., and Sutton, T. T. (2018b). Inventory of Gulf of Mexico Oceanic Fauna data Including Species, Weight, and Measurements from R/V Point Sur (Cruises DP03 and DP04) May-August, 2016. Distributed by: Gulf of Mexico Research Initiative Information and Data Cooperative (GRIIDC). Harte Research Institute, Texas A&M University: Corpus Christi, doi: 10.7266/N7XP7385
Cook, A., and Sutton, T. T. (2018c). Inventory of Oceanic Fauna Data Including Species, Weight, and Measurements from R/V Point Sur (Cruise DP05) in the Gulf of Mexico from 2017-05-01 to 2017-05-11. Distributed by: Gulf of Mexico Research Initiative Information and Data Cooperative (GRIIDC). Corpus Christi, TX: Harte Research Institute, Texas A&M University, doi: 10.7266/N7902234
Cook, A., and Sutton, T. T. (2019). Inventory of Oceanic Fauna Data Including Species, Weight, and Measurements from R/V Point Sur cruise PS19-04 (DP06) in the Gulf of Mexico from 2018-07-19 to 2018-08-01. Distributed by: Gulf of Mexico Research Initiative Information and Data Cooperative (GRIIDC). Corpus Christi: Harte Research Institute, Texas A&M University. doi: 10.7266/n7-ac8e-0240
Crabtree, R. E., Cyr, E. C., Bishop, R. E., Falkenstein, L. M., and Dean, J. M. (1992). Age and growth of tarpon, Megalops atlanticus, larvae in the eastern Gulf of Mexico, with notes on relative abundance and probable spawning areas. Environ. Biol. Fishes 35, 361–370. doi: 10.1007/bf00004988
Dornburg, A., Friedman, M., and Near, T. J. (2015). Phylogenetic analysis of molecular and morphological data highlights uncertainty in the relationships of fossil and living species of Elopomorpha (Actinopterygii: Teleostei). Mol. Phylog. Evol. 89, 205–218. doi: 10.1016/j.ympev.2015.04.004
Eldridge, P. J. (1988). The southeast area monitoring and assessment program (SEAMAP): a state federal university program for collection, management, and dissemination of fishery independent data and information in the southeastern United States. Mar. Fish. Rev. 50, 29–39.
Fahay, M. P. (1983). Guide to the early stages of marine fishes occurring in the western North Atlantic Ocean, Cape Hatteras to the southern Scotian Shelf. J. Northwest Atl. Fish. Sci. 4, 1–1992.
Fahay, M. P. (2007). Early Stages of Fishes in the Western North Atlantic Ocean:(Davis Strait, Southern Greenland and Flemish Cap to Cape Hatteras). Dartmouth: Northwest Atlantic Fisheries Organization.
Fahay, M. P., and Obenchain, C. L. (1978). Leptocephali of the ophichthid genera ahlia, myrophis, ophichthus, pisodonophis, callechelys, letharchus, and apterichtus on the atlantic continental shelf of the United States. Bull. Mar. Sci. 28, 442–486.
Forey, P. L. (1973). A revision of the Elopiformes fishes, fossil and recent. Bull. Br. Mus. Nat. Hist. (Geol.) 10, 1–222.
Fricke, R., Eschmeyer, W. N., and Fong, J. D. (2019). Eschmeyer’s Catalog of Fishes, Species by Family/Subfamily (Last Updated 1 July 2019). Available at http://researcharchive.calacademy.org/research/ichthyology/catalog/SpeciesByFamily.asp.
Govoni, J. J. (2010). Feeding on protists and particulates by the leptocephali of the worm eels Myrophis spp. (Teleostei: Anguilliformes: Ophichthidae), and the potential energy contribution of large aloricate protozoa. Sci. Mar. 74, 339–344. doi: 10.3989/scimar.2010.74n2339
Greenwood, P. H., Rosen, D. E., Weitzman, S. H., and Myers, G. S. (1966). Phyletic studies of teleostean fishes, with a provisional classification of living forms. Bull. Am. Mus. Nat. Hist. 131, 341–455.
Habtes, S., Muller-Karger, F. E., Roffer, M. A., Lamkin, J. T., and Muhling, B. A. (2014). A comparison of sampling methods for larvae of medium and large epipelagic fish species during spring SEAMAP ichthyoplankton surveys in the Gulf of Mexico. Limnol. Oceanogr. Methods 12, 86–101. doi: 10.4319/lom.2014.12.86
Huang, W. C., Chang, J. T., Liao, C., Tawa, A., Iizuka, Y., Liao, T. Y., et al. (2018). Pelagic larval duration, growth rate, and population genetic structure of the tidepool snake moray Uropterygius micropterus around the southern Ryukyu Islands, Taiwan, and the central Philippines. PeerJ 6:e4741. doi: 10.7717/peerj.4741
Hulet, W. H. (1978). Structure and functional development of the eel leptocephalus Ariosoma balearicum (De La Roche, 1809). Philos. Trans. Roy. Soc. Lond. B Biol. Sci. 282, 107–138. doi: 10.1098/rstb.1978.0010
Kajihara, T., Tsukamoto, K., Otake, T., Mochioka, N., Hasumoto, H., Oya, M., et al. (1988). Sampling leptocephali with reference to the diel vertical migration and the gears. Nippon Suisan Gakkaishi 54, 941–946. doi: 10.2331/suisan.54.941
Kurogi, H., Chow, S., Yanagimoto, T., Konishi, K., Nakamichi, R., Sakai, K., et al. (2016). Adult form of a giant anguilliform leptocephalus Thalassenchelys coheni Castle and Raju 1975 is Congriscus megastomus (Günther 1877). Ichthyol. Res. 63, 239–246. doi: 10.1007/s10228-015-0492-5
Kuroki, M., Fukuda, N., Yamada, Y., Okamura, A., and Tsukamoto, K. (2010). Morphological changes and otolith growth during metamorphosis of Japanese eel leptocephali in captivity. Coast. Mar. Sci. 34, 31–38.
Leiby, M. M. (1989). Family Ophichthidae. Mem. Sears. Found. Mar. Res. 9, 764–897. doi: 10.2307/j.ctvbcd0jj.10
Leis, J. M., and Carson-Ewart, B. M.(eds.) (2000). The Larvae of Indo-Pacific Coastal Fishes: An Identification Guide to Marine Fish Larvae. Leiden: Brill.
Liénart, C., Feunteun, E., Miller, M. J., Aoyama, J., Mortillaro, J. M., Hubas, C., et al. (2016). Geographic variation in stable isotopic and fatty acid composition of anguilliform leptocephali and particulate organic matter in the South Pacific. Mar. Ecol. Prog. Ser. 544, 225–241. doi: 10.3354/meps11575
Limouzy-Paris, C., McGowan, M. F., Richards, W. J., Umaran, J. P., and Cha, S. S. (1994). Diversity of fish larvae in the Florida Keys: results from SEFCAR. Bull. Mar. Sci. 54, 857–870.
Lyczkowski-Shultz, J., Hanisko, D. S., Sulak, K. J., Konieczna, M., and Bond, P. J. (2013). Characterization of ichthyoplankton in the northeastern Gulf of Mexico from SEAMAP plankton surveys, 1982-1999. Gulf Carib. Res. 25, 43–98.
McBride, R. S., Rocha, C. R., Ruiz-Carus, R., and Bowen, B. W. (2010). A new species of ladyfish, of the genus Elops (Elopiformes: Elopidae), from the western Atlantic Ocean. Zootaxa 2346, 29–41.
McEachran, J. D., and Fechhelm, J. D. (1998). Fishes of the Gulf of Mexico. Vol. 1. Austin, TX: University of Texas Press.
McEachran, J. D. (2009). “Fishes (Vertebrata: Pisces) of the Gulf of Mexico,” in Gulf of Mexico Origins, Waters, and Biota. Biodiversity, eds D. L. Felder and D. K. Camp (College Station, TX: Texas A&M Press), 1223–1316.
Miller, M. J. (2009). Ecology of anguilliform leptocephali: remarkable transparent fish larvae of the ocean surface layer. Aqua-BioSci. Monogr. 2, 1–94.
Miller, M. J. (2015). Nighttime vertical distribution and regional species composition of eel larvae in the western Sargasso Sea. Region. Stud. Mar. Sci. 1, 34–46. doi: 10.1016/j.rsma.2015.03.003
Miller, M. J., Aoyama, J., Mochioka, N., Otake, T., Castle, P. H., Minagawa, G., et al. (2006). Geographic variation in the assemblages of leptocephali in the western South Pacific. Deep Sea Res. Part I: Oceanogr. Res. Pap. 53, 776–794. doi: 10.1016/j.dsr.2006.01.008
Miller, M. J., Chikaraishi, Y., Ogawa, N. O., Yamada, Y., Tsukamoto, K., and Ohkouchi, N. (2013). A low trophic position of Japanese eel larvae indicates feeding on marine snow. Biol. Lett. 9:20120826. doi: 10.1098/rsbl.2012.0826
Miller, M. J., and McCleave, J. D. (1994). Species assemblages of leptocephali in the subtropical convergence zone of the Sargasso Sea. J. Mar. Res. 52, 743–772. doi: 10.1357/0022240943076948
Miller, M. J., and McCleave, J. D. (2007). Species assemblages of leptocephali in the southwestern Sargasso Sea. Mar. Ecol. Prog. Series 344, 197–212. doi: 10.3354/meps06923
Miller, M. J., Wouthuyzen, S., Sugeha, H. Y., Kuroki, M., Tawa, A., Watanabe, S., et al. (2016). High biodiversity of leptocephali in Tomini Bay Indonesia in the center of the Coral Triangle. Reg. Stud. Mar. Sci. 8, 99–113. doi: 10.1016/j.rsma.2016.09.006
Miller, M. J., and Tsukamoto, K. (2004). An Introduction to Leptocephali: Biology and Identification, 1st Edn. Tokyo: Ocean Research Institute, University of Tokyo.
Mochioka, N., and Iwamizu, M. (1996). Diet of anguilloid larvae: leptocephali feed selectively on larvacean houses and fecal pellets. Mar. Biol. 125, 447–452.
Moore, J. A., Vecchione, M., Collette, B. B., Gibbons, R., and Hartel, K. E. (2004). Selected fauna of Bear Seamount (New England Seamount chain), and the presence of “natural invader” species. Arch. Fish. Mar. Res. 51, 241–250.
Moore, J. A., Vecchione, M., Collette, B. B., Gibbons, R., Hartel, K. E., Galbraith, J. K., et al. (2003). Biodiversity of Bear Seamount, New England seamount chain: results of exploratory trawling. J. Northwest Atl. Fish. Sci. 31, 363–372. doi: 10.2960/j.v31.a28
Nielsen, J. G., and Larsen, V. (1970). Remarks on the identity of the giant Dana eel-larva. Vidensk. Medd. Dan. Naturhist. Foren. Kbh. 133, 149–157.
Ochiai, A., and Nozawa, Y. (1980). On the metamorphosis and burrowing habits of the snake eel, Muraenichthys gymnotus. Jpn. J. Ichthyol. 27, 237–242.
Otake, T., Inagaki, T., Hasumoto, H., Mochioka, N., and Tsukamoto, K. (1998). Diel vertical distribution of Anguilla japonica leptocephali. Ichthyol. Res. 45, 208–211. doi: 10.1007/bf02678565
Pfeiler, E. (1986). Towards an explanation of the developmental strategy in leptocephalous larvae of marine teleost fishes. Environ. Biol. Fish. 15, 3–13. doi: 10.1007/bf00005385
Pfeiler, E. (1991). Glycosaminoglycan composition of anguilliform and elopiform leptocephali. J. Fish Biol. 38, 533–540. doi: 10.1111/j.1095-8649.1991.tb03140.x
Pfeiler, E., and Govoni, J. J. (1993). Metabolic rates in early life history stages of elopomorph fishes. Biol. Bull. 185, 277–283. doi: 10.2307/1542007
Poulsen, J. Y., Miller, M. J., Sado, T., Hanel, R., Tsukamoto, K., and Miya, M. (2018). Resolving deep-sea pelagic saccopharyngiform eel mysteries: identification of Neocyema and Monognathidae leptocephali and establishment of a new fish family “Neocyematidae” based on larvae, adults and mitogenomic gene orders. PLoS ONE 13:e0199982. doi: 10.1371/journal.pone.0199982
Richards, W. J., McGowan, M. F., Leming, T., Lamkin, J. T., and Kelley, S. (1993). Larval fish assemblages at the Loop Current boundary in the Gulf of Mexico. Bull. Mar. Sci. 53, 475–537.
Richards, W. J. (eds) (2005). Early Stages of Atlantic Fishes: An Identification Guide for the Western Central North Atlantic, Two Volume Set. Boca Raton, FL: CRC Press.
Richardson, D. E., and Cowen, R. K. (2004a). Diversity of leptocephalus larvae around the island of Barbados (West Indies): relevance to regional distributions. Mar. Ecol. Prog. Ser. 282, 271–284. doi: 10.3354/meps282271
Richardson, D. E., and Cowen, R. K. (2004b). New leptocephalus types collected around the island of Barbados (West Indies). Copeia 2004, 888–895. doi: 10.1643/ci-04-073r1
Sammons, S. M., and Bettoli, P. W. (1998). Larval sampling as a fisheries management tool: early detection of year-class strength. N. Am. J. Fish. Manage. 18, 137–143. doi: 10.1577/1548-8675(1998)018<0137:lsaafm>2.0.co;2
Smith, D. G. (1979). Guide to the leptocephali (Elopiformes, Anguilliformes, and Notacanthiformes). NOAA Tech. Rept. NMFS Circular 424, 1–43.
Smith, D. G. (1989a). Introduction to leptocephali. Fishes of the Western North Atlantic. Mem. Sears Found. Mar. Res. 9, 657–668.
Smith, D. G. (1989b). Order elopiformes; families Elopidae, Megalopidae, and Albulidae: leptocephali. Fishes of the Western North Atlantic. Mem. Sears Found. Mar. Res. 9, 961–972. doi: 10.2307/j.ctvbcd0jj.21
Smith, D. G. (1989c). Family Chlopsidae: leptocephali. Fishes of the Western North Atlantic. Mem. Sears Found. Mar. Res. 9, 933–942. doi: 10.2307/j.ctvbcd0jj.16
Smith, D. G. (1989d). Family Derichthyidae: leptocephali. Fishes of the Western North Atlantic. Mem. Sears Found. Mar. Res. 9, 917–920. doi: 10.2307/j.ctvbcd0jj.13
Smith, D. G. (1989e). Family Muraenidae: leptocephali. Fishes of the Western North Atlantic. Mem. Sears Found. Mar. Res. 9, 900–916. doi: 10.2307/j.ctvbcd0jj.12
Sutton, T. T., Cook, A., Moore, J. A., Frank, T., Judkins, H., Vecchione, M., et al. (2018a). Inventory of Gulf Oceanic Fauna Data Including Species, Weight, and Measurements, Pisces cruises from Dec. 1 2010 – Sept. 29, 2011 in the Northern Gulf of Mexico (Distributed by: Gulf of Mexico Research Initiative Information and Data Cooperative (GRIIDC)). Corpus Christi, TX: Harte Research Institute, Texas A&M University, doi: 10.7266/N7R49NTN
Sutton, T. T., Cook, A., Moore, J. A., Frank, T., Judkins, H., Vecchione, M., et al. (2018b). Inventory of Gulf Oceanic Fauna Data Including Species, Weight, and Measurements. Meg Skansi cruises from Jan. 25 – Sept. 30, 2011 in the Northern Gulf of Mexico (Distributed by: Gulf of Mexico Research Initiative Information and Data Cooperative (GRIIDC)). Corpus Christi, TX: Harte Research Institute, Texas A&M University, doi: 10.7266/N7VX0DK2
Sutton, T. T. (2013). Vertical ecology of the pelagic ocean: classical patterns and new perspectives. J. Fish Biol. 83, 1508–1527. doi: 10.1111/jfb.12263
Valdez-Moreno, M., Vásquez-Yeomans, L., Elías-Gutiérrez, M., Ivanova, N. V., and Hebert, P. D. (2010). Using DNA barcodes to connect adults and early life stages of marine fishes from the Yucatan Peninsula, Mexico: potential in fisheries management. Mar. Freshw. Res. 61, 655–671. doi: 10.1071/MF09222
Weibe, P. H., Morton, A. W., Bradley, A. M., Backus, R. H., Craddock, J. E., Barber, C., et al. (1985). New development in the MOCNESS, an apparatus for sampling zooplankton and micronekton. Mar. Biol. 87, 313–323. doi: 10.1007/bf00397811
Keywords: leptocephali, leptocephalus, MOCNESS, DEEPEND, ONSAP, Gulf of Mexico
Citation: Moore JA, Fenolio DB, Cook AB and Sutton TT (2020) Hiding in Plain Sight: Elopomorph Larvae Are Important Contributors to Fish Biodiversity in a Low-Latitude Oceanic Ecosystem. Front. Mar. Sci. 7:169. doi: 10.3389/fmars.2020.00169
Received: 09 August 2019; Accepted: 03 March 2020;
Published: 29 April 2020.
Edited by:
Michael Vecchione, National Oceanic and Atmospheric Administration (NOAA), United StatesReviewed by:
Mackenzie E. Gerringer, SUNY Geneseo, United StatesDave Johnson, National Museum of Natural History (SI), United States
David G. Smith, Smithsonian Institution, United States
Copyright © 2020 Moore, Fenolio, Cook and Sutton. This is an open-access article distributed under the terms of the Creative Commons Attribution License (CC BY). The use, distribution or reproduction in other forums is permitted, provided the original author(s) and the copyright owner(s) are credited and that the original publication in this journal is cited, in accordance with accepted academic practice. No use, distribution or reproduction is permitted which does not comply with these terms.
*Correspondence: Jon A. Moore, jmoore@fau.edu