- 1Posgrado en Ciencias del Mar y Limnología, Universidad Nacional Autónoma de México, Mexico City, Mexico
- 2Programa de Doctorado en Ciencias y Tecnologías Marinas, Universidad de Cádiz, Cádiz, Spain
- 3Monterey Bay Aquarium Research Institute, Moss Landing, CA, United States
- 4Instituto Universitario de Investigación Marina, Universidad de Cádiz, Cádiz, Spain
- 5Instituto de Ciencias del Mar y Limnología, Universidad Nacional Autónoma de México, Mexico City, Mexico
Patterns in benthic megafauna diversity in littoral and intertidal zones in the Gulf of California have been associated with both habitat heterogeneity and substrate type. Current knowledge of invertebrate communities in hard bottom habitats at depths > 200 m in the Gulf is poor due to the methodological limitations inherent in sampling deep habitats. Using video imagery of benthic habitats coupled with environmental data from the Remotely Operated Vehicle Doc Ricketts, we documented variation in the diversity and community composition of the benthos from 849 to 990 m depth in the NW limit of the Guaymas Basin, in relation to dissolved oxygen and substrate characteristics. This depth range overlaps an oxygen minimum zone where oxygen drops to levels < 0.5 ml L–1 and strong gradients in a narrow depth range occur. Dissolved oxygen varied along our benthic survey from 0.200 to 0.135 ml L–1. We observed high taxonomic richness across an area of rocky outcrops through the lower transition zone. This megafaunal pattern differs from reports from other oxygen minimum zones characterized by a great abundance of a few species. Taxonomic richness diminished at depths with reduced dissolved oxygen in the lower boundary of the oxygen minimum zone with increasing soft sediment cover. We found that rocky outcrops and structure-forming organisms such as corals, sponges, and oyster aggregations supported a higher diversity (H’ = 0.8) than soft sediment (H′ = 0.7) as have been observed in other habitats such as seamounts. Environmental variables that explained most of the megafaunal variation were substrate type (18.4%), depth (1.14%) and temperature (0.9%). Salinity (0.45%) and dissolved oxygen (0.3%) were less important factors to explain the megafaunal composition variance. Substrate type played a key role in the diversity and composition of benthic megafauna. These results broaden our understanding concerning the potential roles of substrate characteristics in the community composition of the deep-sea benthic megafaunal assemblages in the Gulf of California and oxygen minimum zones in general.
Introduction
Habitat is defined by the space and abiotic factors in which an organism, population or species assemblage lives (Norse, 1993; Davies et al., 2004; Tapia de la Yossellin, 2018). The Gulf of California (GoC), hosts a wide variety of shallow-water habitats (e.g., coral reefs at the southern end in both margins, rocky habitats in the peninsular shore and sandy beaches in continental shore) (Lluch-Cota et al., 2007) in which biological diversity is thought to vary with substrate heterogeneity (Brusca and Hendrickx, 2010). However, biological patterns of invertebrate communities and processes are perhaps known better in littoral and intertidal zones than in hard bottom habitats at depths greater than 200 m in the GoC (Brusca and Hendrickx, 2010) due to the methodological limitations inherent in sampling deep habitats, such as the time and cost required to deploy equipment at great depths or the effectiveness of the samplers.
In the last three decades, the development of imaging methods coupled with the use of submersibles and remotely operated vehicles (ROV’s) to obtain high resolution video images of the deep seabed, has allowed a more extended overview of the habitats and faunal communities, as well as the possibility of doing long-term studies (Pilgrim et al., 2000; Méndez, 2007; Cuvelier et al., 2012; Ayma et al., 2016). ROV video surveys have been used more commonly for hard, steep, and rugged substrate and in areas of cold-water corals (CWC’s) (Du Preez and Tunnicliffe, 2012; Ayma et al., 2016). Traditional methods of sampling, such as dredge and sledges are difficult to use in hard and rugged substrate and cannot be used in areas inhabited by delicate and vulnerable structure forming-organisms (Tyler et al., 2016). Therefore, the analysis of images recorded by ROVs has become a non-invasive and complementary method (to conventional methodologies) for sampling deep water fauna in all habitats and substrate types (Cuvelier et al., 2012; Ayma et al., 2016; Tyler et al., 2016).
Preliminary data collected mainly by sledges, dredges and box cores indicate that the southeast GoC has an abundant and diverse deep-sea benthic fauna dominated by polychaetes, decapod crustaceans, echinoderms, and mollusks (Hendrickx, 2006; Lluch-Cota et al., 2007; Méndez, 2007; Zamorano et al., 2007; Zamorano and Hendrickx, 2012; Hendrickx and Serrano, 2014; Hendrickx et al., 2014; Hernández-Alcántara et al., 2014; Mejía-Mercado et al., 2014; Papiol and Hendrickx, 2016).
Many physical variables are known to be important factors associated with patterns of community composition and diversity (McArthur et al., 2010; Anderson et al., 2011). For example, substrate characteristics, structural complexity, sediment composition, organic matter, oxygen, among others, have been identified sources of heterogeneity and important descriptors of biological patterns (Gooday et al., 2010; Ramirez-Llodra et al., 2010; Anderson et al., 2011). Several studies conducted in submarine canyons, such as Schlacher et al. (2010), revealed that structural complexity enhances benthic diversity and biomass. Structural complexity refers to the physical heterogeneity of the habitat related to the rugosity of the seabed substrate and the complex architecture of biogenic aggregations (Laguionie-Marchais et al., 2015; Bartholomew et al., 2016; Ferrari et al., 2016). Increased habitat heterogeneity provides refugia, sites for settlement, feeding, predation, and parasitism of organisms (Buhl-Mortensen et al., 2010; Storlazzi et al., 2016). Consequently, substrate type and structural complexity have been identified as important descriptors of biological patterns (Anderson et al., 2011; Du Preez and Tunnicliffe, 2012). High structural complexity likely contributes to the high taxonomic wealth observed in the GoC funds is precisely a reflection of their structural complexity.
On the other hand, previous studies have documented changes in the abundance, density, and diversity of bathyal macrofauna and megafauna in habitats worldwide where the Oxygen Minimum Zone (OMZ) impinges the seafloor (Levin and Gage, 1998; Rogers, 2000; Levin et al., 2001; Levin, 2003). Macrofaunal and megafaunal densities decreased dramatically in the cores of most OMZ′s where bottom-water dissolved oxygen (DO) is < 0.15 ml l–1 (Levin, 2003). In the GoC several oceanographic processes, including wind-induced upwelling, tidal mixing, regional circulation and global thermohaline circulation (Roden, 1958; Santamaría-del-Angel et al., 1994; Álvarez-Borrego, 2010), contribute to the development of an OMZ in the southern GoC, where DO concentrations are < 0.5 ml l–1 (Levin, 2003). In the southern Gulf the OMZ has been reported from 82 to 1300 m depth and in the central Gulf from 232 to 1705 m depth, decreasing in thickness and eventually disappearing to the northern region (Hendrickx and Serrano, 2014).
In the GoC, low bottom water DO is strongly associated with the density or diversity of a range of taxa, such as Polychaeta (Méndez, 2007), Bivalvia (Zamorano et al., 2007; Zamorano and Hendrickx, 2012) and Decapoda (Hendrickx and Serrano, 2014; Papiol and Hendrickx, 2016). Nevertheless, these studies also document that other factors (e.g., temperature, depth and sediment composition) also influence deep-sea macrofaunal assemblages. The distribution and diversity of deep water polychaetes from the GoC are correlated with depth and temperature; diversity of polychaetes increased with depth and reached a maximum value at 1270 m depth, while higher density and diversity are found between 2.5 and 4.0°C (Méndez, 2007). Species richness of mollusks from the southern GoC decreases with depth, but reaches a maximum value between 1000 and 1300 m depth (Zamorano et al., 2007). Studies of decapod crustaceans in the southeastern GoC also indicate highest species richness between 1000 and 1380 m depth, suggesting that the availability of food, nature of substrate, currents, or other factors could affect their distribution (Hendrickx, 2006). While these water quality factors show strong relationships with bathyal faunal patterns, the influence of substrate type and structural complexity in a fine scale on deep-sea benthos in the GoC has received little study.
In this study, we aim to document the variation in the diversity, composition and coverage of epibenthic megafauna in relation to DO and habitat quality at bathyal depths from 849 to 990 m in the Guaymas Basin by using benthic imagery recorded on video coupled with environmental data from the ROV Doc Ricketts. These two factors in particular – substrate heterogeneity and DO concentration - are expected to play a large role in deep-sea benthic communities. Thus, we hypothesize that the taxonomic richness of megabenthos would be highest in rocky benthic habitats with the greatest structural complexity, and that soft sediment habitats will be the least diverse.
Materials and Methods
Study Area
The GoC is divided into semi-isolated basins (separated from each other by transverse ridges) which become deeper to the south (Lonsdale, 1989). The Guaymas Basin (Figure 1) in the central Gulf province is the largest depression within the Gulf (Rusnak et al., 1964; Bischoff and Henyey, 1974), with a maximum depth of 2100 m (Brusca et al., 2005; Mejía-Mercado et al., 2014). This basin is characterized by its rapid deposition of organic-matter rich sediments, particularly where the OMZ impinges on the seafloor (Bischoff and Henyey, 1974; Lonsdale, 1989; Kluesner, 2011; Kurnosov and Blinova, 2015; Teske et al., 2016). The seafloor in Guaymas Basin is mainly composed of fine-grained mud turbidites (Bischoff and Henyey, 1974), though basaltic rocks have been dredged from widespread outcrops on the southern wall of the basin (Lonsdale, 1989).
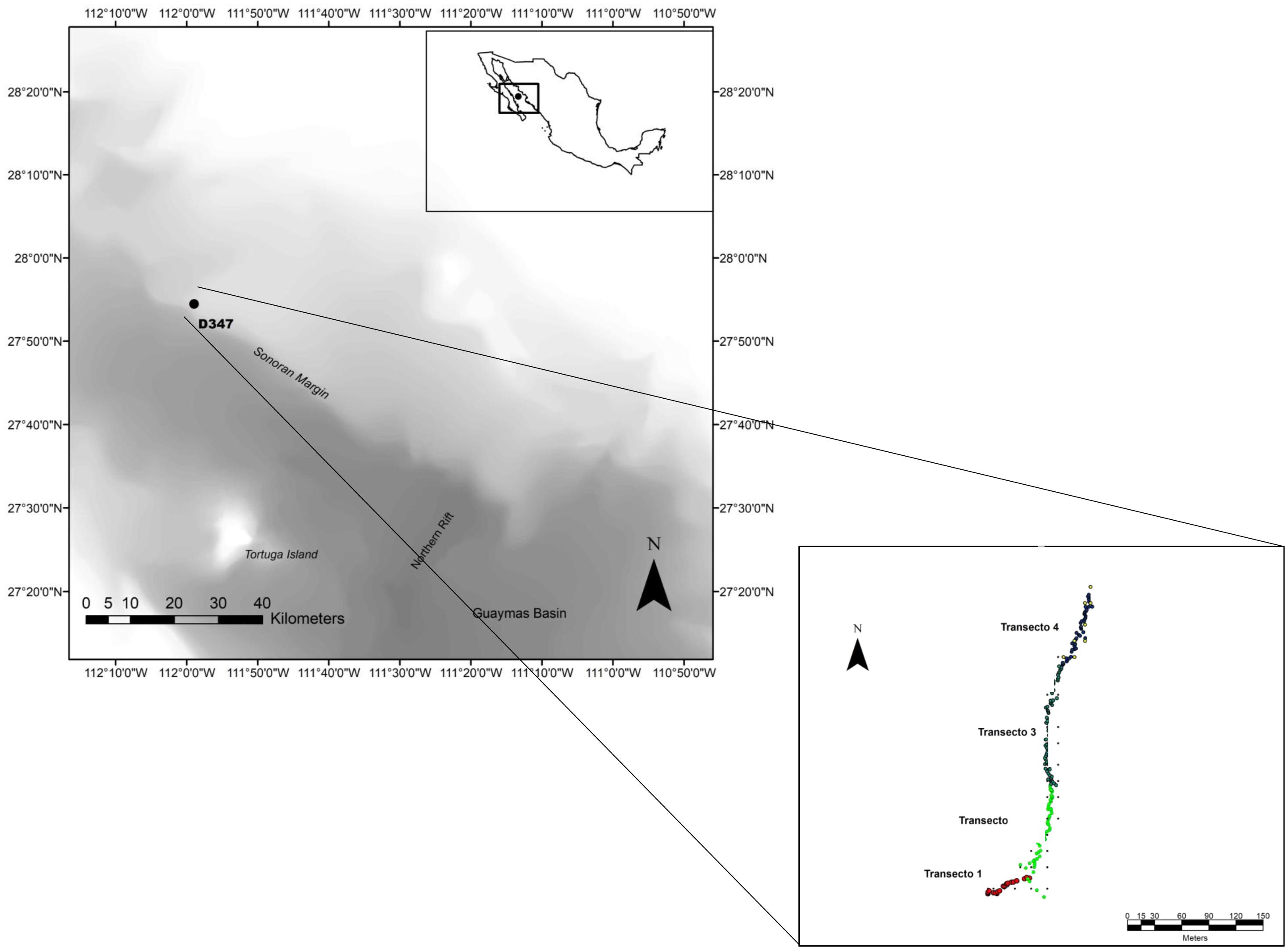
Figure 1. Location of dive D347 in the NW limit of Guaymas Basin and Sonoran Margin in the Gulf of California where four video sections for megabenthic epifauna prospection were performed at a depth range of 849–990 m.
The Sonoran Margin, the northernmost physiographic province in the basin, extends from the distal portion of the continental shelf adjacent to the state of Sonora to ca. 1300 m depth, has a gentle slope (1.7°) and is characterized by a flat and regular bathymetric relief (Figueroa Albornoz, 2013). The transitional zone from the Sonoran Margin to the floor of Guaymas Basin is affected by different tectonic processes, as well as erosion and gravitational instability (Lonsdale, 1989; Figueroa Albornoz, 2013).
The thermohaline structure of the water column in this region comprises several water masses, principally the Pacific Intermediate Water (PIW) (1200–500 m depth with salinities of 34.50 to 34.80 psu and temperature from 4 to < 9°C) and OMZ waters protruding into the southern and central regions (Álvarez-Borrego and Schwartzlose, 1979; Páez-Osuna et al., 2016).
Visual Survey of Benthic Megafauna and Data Collection
Benthic video transects were performed using the ROV Doc Ricketts operated from the R/V Western Flyer by the Monterey Bay Aquarium Research Institute. The ROV survey sites were determined during the “2012 Gulf of California Expedition” on the basis of maps created with the sonar data gathered previously by the D. Allan B. autonomous underwater vehicle (AUV) on board of the R/V Zephyr. Transects were located (dive D347, March 2012) in an unexplored area in NW limit of Guaymas Basin and the Sonoran Margin (27.91° N, 111.982° W) (Figure 1). Overall, a total of four seabed video transects (Table 1) corresponding to 51 min and 0.436 km of seabed length were performed. ROV Doc Ricketts has a maximum operation depth of 4000 m and was equipped with a high definition camera (1080 × 1920 resolution) with 10× zoom, an illumination system of six 17700 Lumen LED lights and four 250 W incandescent lights and navigation instrumentation allowing determination of ROV location to ca. 1 m of dive depth, science tools (e.g., suction sampler, swing arm and manipulators) and a CTD equipped with a transmissometer and oxygen sensor. During video transects, the ROV Doc Ricketts moved at an altitude of ∼2 m above the seafloor and a constant speed 0.14 m s–1 and the focal axis of the camera was kept tilted downward and zoomed to view a ca. 1 m wide portion of the seabed near the ROV. Visibility in seawater was appropriate enough for image analysis. Thus two-parallel lasers mounted 29 cm apart projected red dots on the seafloor to serve as a spatial scale. ROV position was determined by an ultrashort baseline acoustic tracking systems, coupled to the ship’s GPS system. Navigation information for the ROV were logged along with near-bottom environmental parameters (depth, temperature, salinity and bottom-water DO) throughout the survey.
Video and Image Analysis
Benthic images extracted from video recorded along transects during ROV dive D347 were edited using Corel Video Studio Pro X5©. Transect images of the seafloor were selected manually at 15 s intervals, equivalent to 1 image per 1.4 m along the video transect, based on the average ROV speed over the bottom and the mobility, size and behavior of the organisms related to the field of view of the camera (Bakus, 2007; Clark et al., 2012). Furthermore, this distance avoids any overlap between successive images.
For each of the images, all epibenthic megafauna > 3 cm were counted and identified to the lowest possible taxon. In cases where organisms could not be assigned to a determinate taxon, they were defined as distinct morphospecies in relation to the available taxonomic information. The identity of organisms was determined using general and specialized literature (Gage and Tyler, 1992; Ruppert and Barnes, 1996; Marshal and Richer de Forges, 2004; Solís-Marín et al., 2005, 2014; Hendrickx et al., 2007, 2014, 2016; Roberts et al., 2009; Álvarez et al., 2014; Hendrickx and Serrano, 2014; Mejía-Mercado et al., 2014; Villalobos Vazquez de la Parra, 2014), as well as specialized databases to validate the taxonomic identification (Brusca and Hendrickx, 2008; Jacobsen Stout et al., 2015; NOAA Office of Ocean Exploration and Research, 2018; WoRMS Editorial Board, 2018; OBIS, 2019). To aid the identification we supplemented these sources with a previous study that identified specimens of taxa collected during dive D347 (Villalobos Vazquez de la Parra, 2014). Additionally, to maximizes the comparability of our results with other studies, we grouped specimens and coverage data of sponges recorded in each benthic images into one of the following morphotypes categories based on the criteria proposed by Boury-Esnault and Rützler (1997) and Kazanidis et al. (2019): amorphous, tubular and clavate (“club-shaped”) (see the sponge morphotype examples provide as Supplementary Material). Taxonomic richness described the total number of morphospecies per image. Occurrences of morphospecies on each substrate type was recorded in each transect image. The cover of epibenthic megafauna (m2) was measured on-screen with Image J© software, by tracing the surfaces covered by the sessile and motile fauna visible in each transect image. We included fauna with low motility (demersal fishes, decapods, holothurians), completely passive taxa, those swimming slowly over the bottom, as well as fauna exhibiting no raction to ROV motion or lights (Lorance and Trenkel, 2006). Several studies that compared ROV observations with sampling methods, have revealed that estimations of the presence and/or abundance of motile megafauna, particularly fishes, decapod crustaceans and mollusks, are more accurate based on imagery than sampling, since the mobile fauna tend to escape during sampling (Spanier et al., 1994; Lorance and Trenkel, 2006; Cuvelier et al., 2012; Gates et al., 2012; Porteiro et al., 2013; Ayma et al., 2016). Moreover, imagery analysis has a high acccuracy in assessing surface areas, epibenthic faunal coverage and the presence of associated fauna in different habitats, such as cold-water corals (Guinan et al., 2009; Cuvelier et al., 2012; Du Preez and Tunnicliffe, 2012). Therefore, coverage (m2) of substrata also was estimated for each transect image using Image J© software.
Habitat was visually classified on the basis of substrate type (soft and hard) (Greene et al., 1999; van den Beld et al., 2017) and its structural complexity followed the rugosity criteria (Ferrari et al., 2016; Storlazzi et al., 2016; De la Torriente et al., 2018). For fine-scale habitat characteristics, we assigned seabed sub-habitats based on their composition (e.g., soft sediments and rocky outcrops), structure-forming organisms (e.g., corals, sponges and oyster aggregations that are used by other species as substrate for attachment or feeding) (Buhl-Mortensen et al., 2010) and associated biological assemblages (Greene et al., 1999). Environmental variables (depth, temperature, salinity and DO) and ROV location data (geographic coordinates) registered during dive D347 were coupled to each video transect image.
Statistical Analyses
To assess the adequacy of megabenthos sampling, we plotted species-area curves following criteria from several studies (Estacio, 1996; Krebs, 1999; Bianchi et al., 2004; Underwood, 2006; Bakus, 2007; Clark et al., 2012). Species accumulation curves included data from all extracted images from transects T1 and T2, removing all species that appeared only once among all images. We repeated species accumulation curves for an area of soft sediment (where no rocks were present) between a field of rocky outcrops on soft sediment. A minimum sampling area (with a mean of 0.7 m2/image) was defined as the number of images (selected randomly) analyzed before no new taxa were added with an additional image sampled. The minimum sampling area determined for the area of rocky outcrops was 14 m2, corresponding to 20 video images. For sediment dominated habitat, the minimum sampling area was 7 m2 or 10 benthic images. Sample size for comparisons between rocky outcrops and soft sediment were then standardized to 20 benthic images (with a mean of 0.7 m2/image) and n = 10. Images for integrate samples were selected randomly. Sample size for comparisons between OMZ zones were standardized to 20 benthic images (selected randomly and with a mean of 0.7 m2/image) and n = 3 for each OMZ zone.
Morphospecies rarefaction curves (Sanders, 1968) were obtained using the Biodiversity Professional© V.2 program and plotted per rocky outcrops and soft sediment. The cover data for each morphospecies at the two substrata (soft sediment and rocky outcrops) in each sample was used to calculate diversity indices. Diversity and related indices were estimated with the Biodiversity Professional© V.2 program. Shannon-Wiener diversity index is herein expressed as:
The evenness index is expressed as:
The dominance index was calculated as the complement of evenness:
A Shannon-Wiener diversity index was calculated for each sample and the results pooled by soft sediment and rocky outcrops. Means and standard deviations were plotted. To test for differences in Shannon-Wiener diversity index between soft sediment and rocky outcrops, we used a Kruskal–Wallis non-parametric analysis of variance. Kruskal–Wallis test was performed with software Statistica© V.8.
Redundancy analysis (RDA) was used to assessed environmental factors that were most correlated with changes in the composition of benthic assemblages. This analysis was performed in R studio software. A Hellinger transformation was applied to morphospecies cover data for images (Borcard et al., 2011; Legendre and Legendre, 2012). Environmental variables (substrate, depth temperature salinity and bottom-water dissolved oxygen) were log-transformed and then standardized to a zero mean to obtain a z-scores data matrix (Borcard et al., 2011). Water mass was interpreted on the basis of depth, temperature and salinity gathered during the survey and those from the published hydrology of the region (Álvarez-Borrego and Schwartzlose, 1979; Lavín and Marinone, 2003; Álvarez-Borrego, 2010).
Results
Benthic Megafaunal Composition
A total of 200 transect images were selected from the video transect recordings to be used for benthic megafauna and substrate analyses. The video sequences covered a depth range from 849 to 990 m and a linear distance of 0.419 km that include soft sediments with no visible rocks and a field of rocky outcrops on soft sediment. A total of 53 benthic taxa (or morphospecies) belonging to 6 phyla (Porifera, Echinodermata, Cnidaria, Mollusca, Arthropoda and Chordata) were identified in the analysis (Table 2). 12 sponges morphospecies, 7 echinoderm morphospecies, 22 cnidarian morphospecies, 2 bivalve morphospecies, 7 crustaceans morphospecies and 3 Actinopterygii morphospecies. Cnidaria was the most diverse phylum with 22 morphospecies including structure-forming cold-water corals (CWC’s) (e.g., antipatharians, zoanthids and alcyonarians) (Table 2).
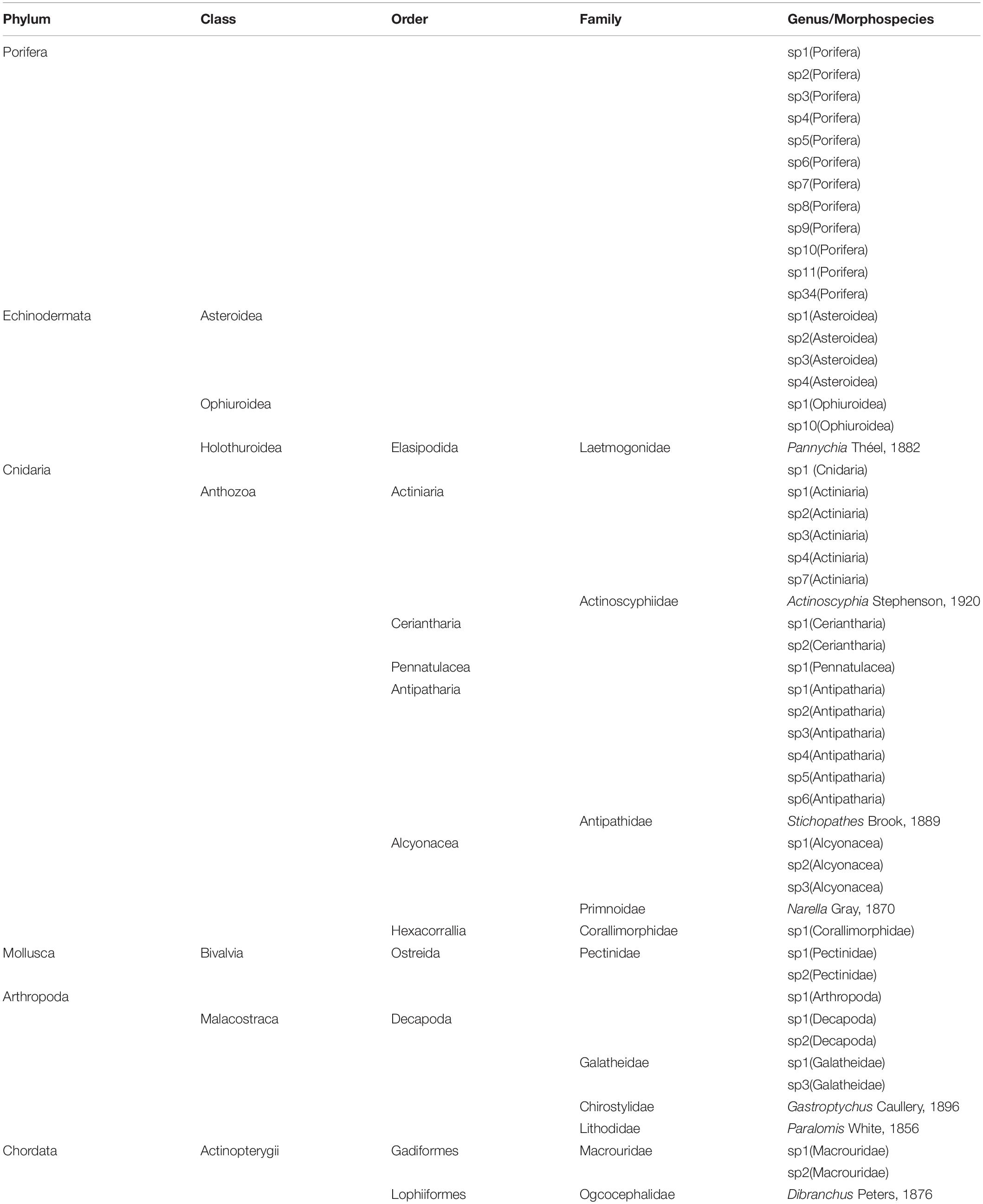
Table 2. Taxonomic list in the NW limit of Guaymas Basin and the Sonoran Margin (849–990 m) inhabiting soft and hard substrate.
Physical Environment
The substrate along the survey was dominated by soft sediment that constituted 74.4% of the total area analyzed in all benthic images (Figure 2A). Rocky outcrops covered 25.6% of the total sampled area (Figure 2A).
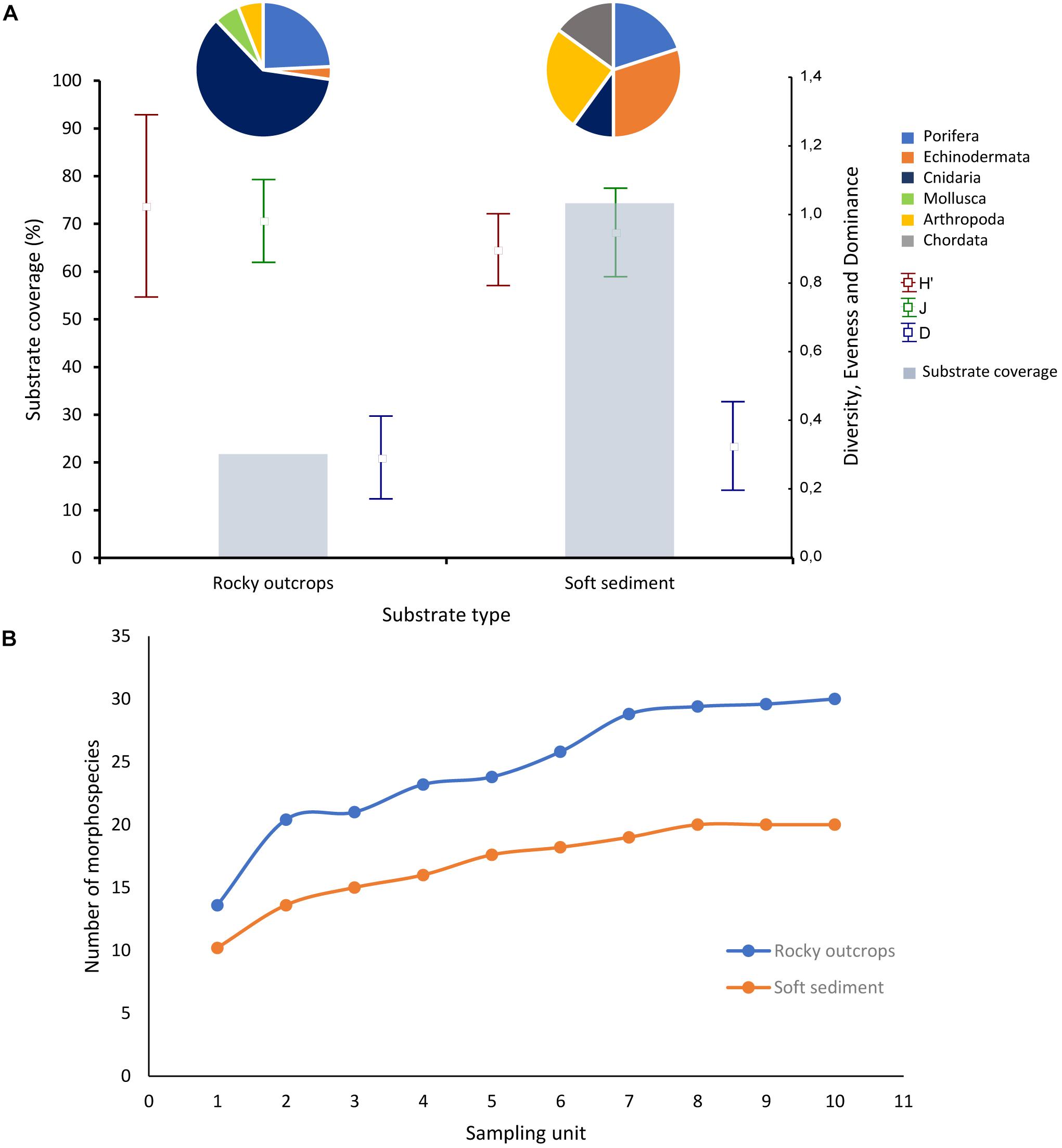
Figure 2. (A) Composition, proportional taxonomic richness and mean diversity indices by substrate type percent coverage. Pie graphs represent percent taxonomic richness contribution by phylum in each substrate type. Dots denote means of descriptors H′ = Shannon-Wiener diversity index; J = evenness index; D = dominance index. Vertical bars denote ± standard deviation. Bar graph represent substrate type percent coverage. (B) Rarefaction plots corresponding to the megafaunal assemblages on soft sediment and rocky outcrops.
Environmental sensors documented variation in near-bottom temperature (T = 4.920–4.480°C) and salinity (S = 34.536–34.547) during ROV dive D347 (Table 3). These data indicates the influence of the Pacific Intermediate Water between 1200 and 500 m depth (Álvarez-Borrego and Schwartzlose, 1979; Álvarez-Borrego, 2010; Páez-Osuna et al., 2016) in the study area. DO levels were very low (DO < 0.5 ml L–1) in the OMZ at these depths (Table 3). Changes in DO levels across this depth range allowed us to identify zonation in the OMZ, including the lower boundary of the OMZ core (849–901 m), the lower OMZ transitional zone (LTZ) (901–954 m), and the lower boundary (LB) of OMZ (955–990 m) (Figure 3).

Table 3. Environmental variables recorded near the seafloor during the ROV dive D347 in the NW limit of Guaymas Basin and the Sonoran Margin.
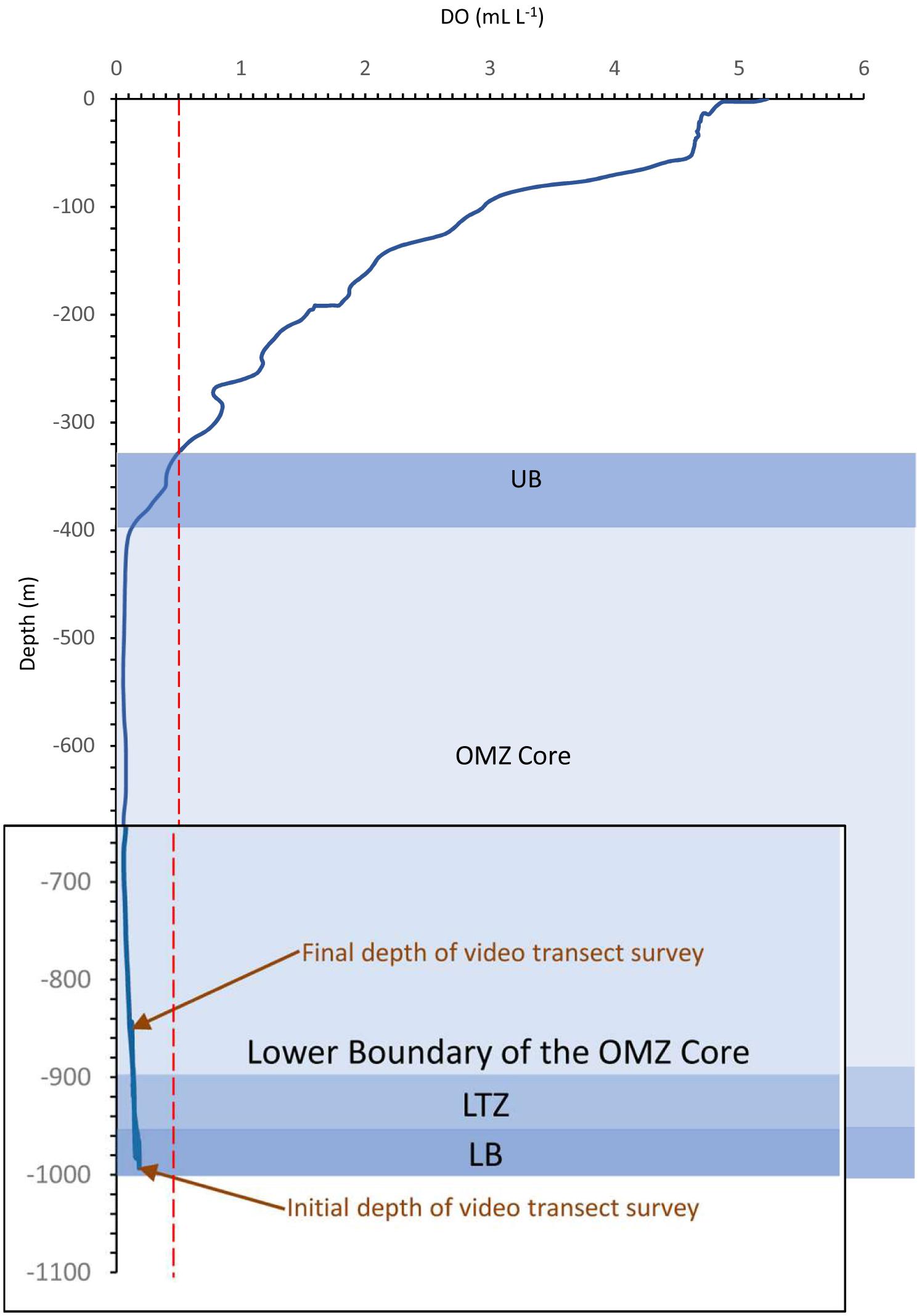
Figure 3. Dissolved oxygen profile during dive D347 in the NW limit of Guaymas Basin and the Sonoran Margin. Oxygen minimum zone (OMZ) where dissolved oxygen (DO) is < 0.5 mL L– 1; red dotted line represents the limit where DO = 0.5 mL L– 1; LB = lower OMZ boundary; LTZ = lower OMZ transitional zone; UB = upper boundary of the OMZ. Note the magnifying over OMZ survey zones.
Fine-Scale Habitat Characterization
Images analysis showed in the fine scale the presence of ten distinct sub-habitats associated to rocky outcrops and soft sediments (Figures 4, 5 and Table 4). Rocky outcrops typically had more structure-forming taxa such as CWC′s and sponges which provide a variety of habitats for decapod crustaceans (Gastroptychus) and other alcyonarians (Primnoidae). In comparison, the diversity of sub-habitats decreased considerably in soft sediment habitats (Figures 4, 5 and Table 4).
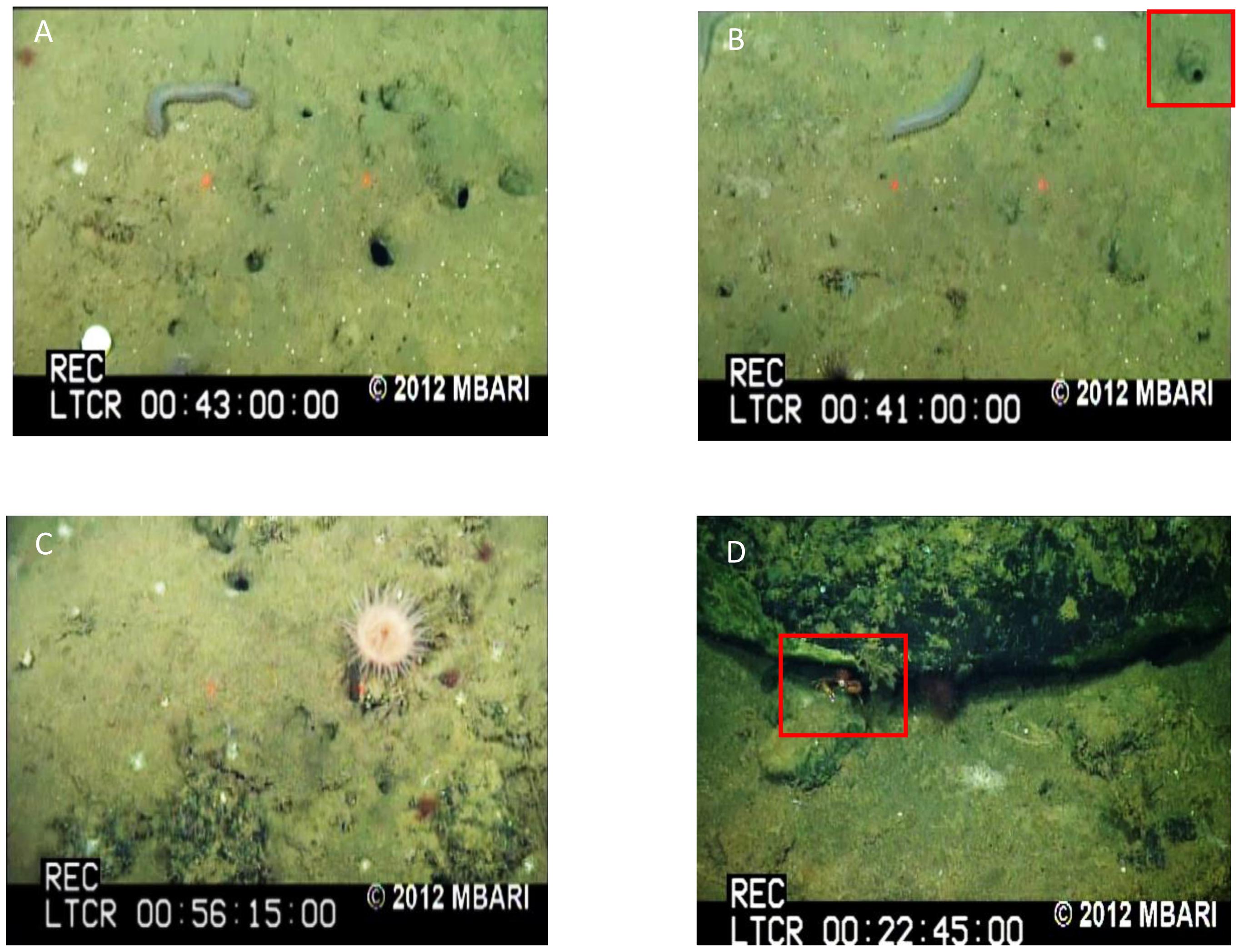
Figure 4. Seafloor images showing sub-habitats identified during the transects performed between 849 and 990 m depth in Dive D347 in the NW limit of Guaymas Basin and the Sonoran Margin within the oxygen minimum zone (OMZ). (A) Soft sediments in the lower OMZ boundary dominated by the elasipodid holothurian of the genus Pannychia, some benthopelagic fish and burrows. (B) Tubular sponges in unconsolidated sediments in the lower OMZ boundary. (C) Small rocky outcrops colonized by anemones and sponges in the lower OMZ transitional zone to the lower OMZ boundary. (D) Large rocky outcrops (> 30 cm) in the lower OMZ transitional zone to the lower OMZ boundary that provide refuge for small decapod crustacean. Images courtesy of MBARI.
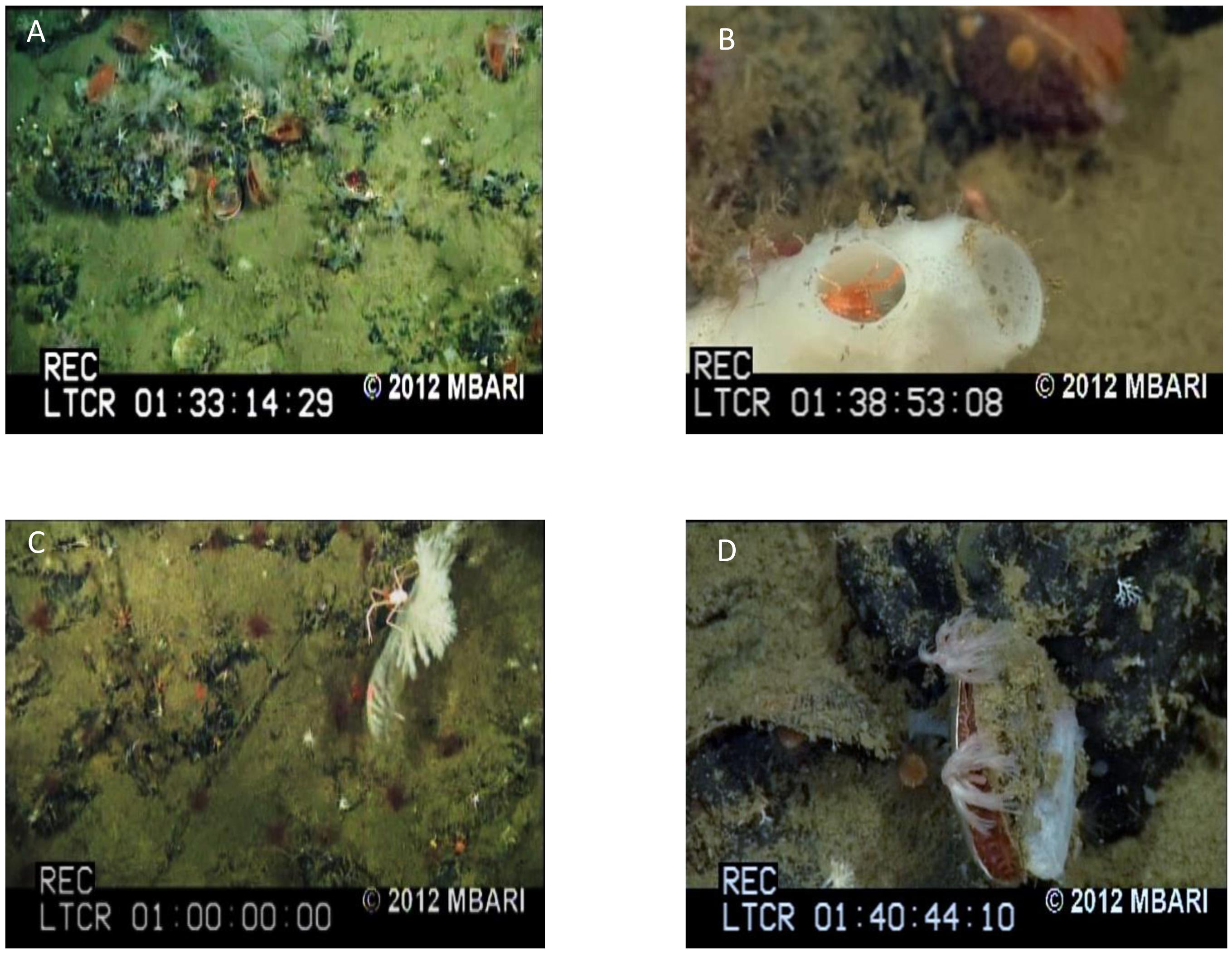
Figure 5. Seafloor images showing sub-habitats identified during the transects performed between 849 and 990 m depth in Dive D347 in the NW limit of Guaymas Basin and the Sonoran Margin within the oxygen minimum zone (OMZ). (A) Structure-forming cold-water corals (CWC′s) (antipatharians and alcyonarians) on rocky outcrops in the lower OMZ transitional zone. (B) Structure-forming sponges in the lower OMZ transitional zone that provide a refuge from predators and an enhanced food supply for diverse species. Note a decapod crustacean living in the atrium of sponge. (C) CWC′s on rocky outcrops that provided an elevated position for spider crabs (Gastroptychus) in the lower OMZ transitional zone and in the lower boundary of the OMZ core. (D) Oyster (Pectinidae) aggregations that provide with their shell a hard substrate for the attachment of solitary corals and alcyonarians. This type of habitat was observed all along the lower boundary of the OMZ core. Note that these aggregations also allow the association of solitary corals in their surroundings. Images courtesy of MBARI.
Megafaunal Patterns Among Major Habitat
Taxonomic richness was greater in rocky outcrops (33 taxa, including structure-forming organisms), than on soft sediment (20) (Table 5). The morphospecies list in table showing the occurrence of each morphospecies in each substrate is shown in Table 5. Structure-forming organisms (corals, sponges and oyster aggregations) were common on rocky outcrops and providing additional substrata for a variety of taxa such as sponges, ophiuroids, decapod crustaceans, alcyonarians, and corallimorpharians (Table 5). The variety of sponge morphotypes that occurred on rocky outcrops including amorphous, tubular and clavate, whereas sponges morphotypes occurring on soft sediments were tubular and clavate.
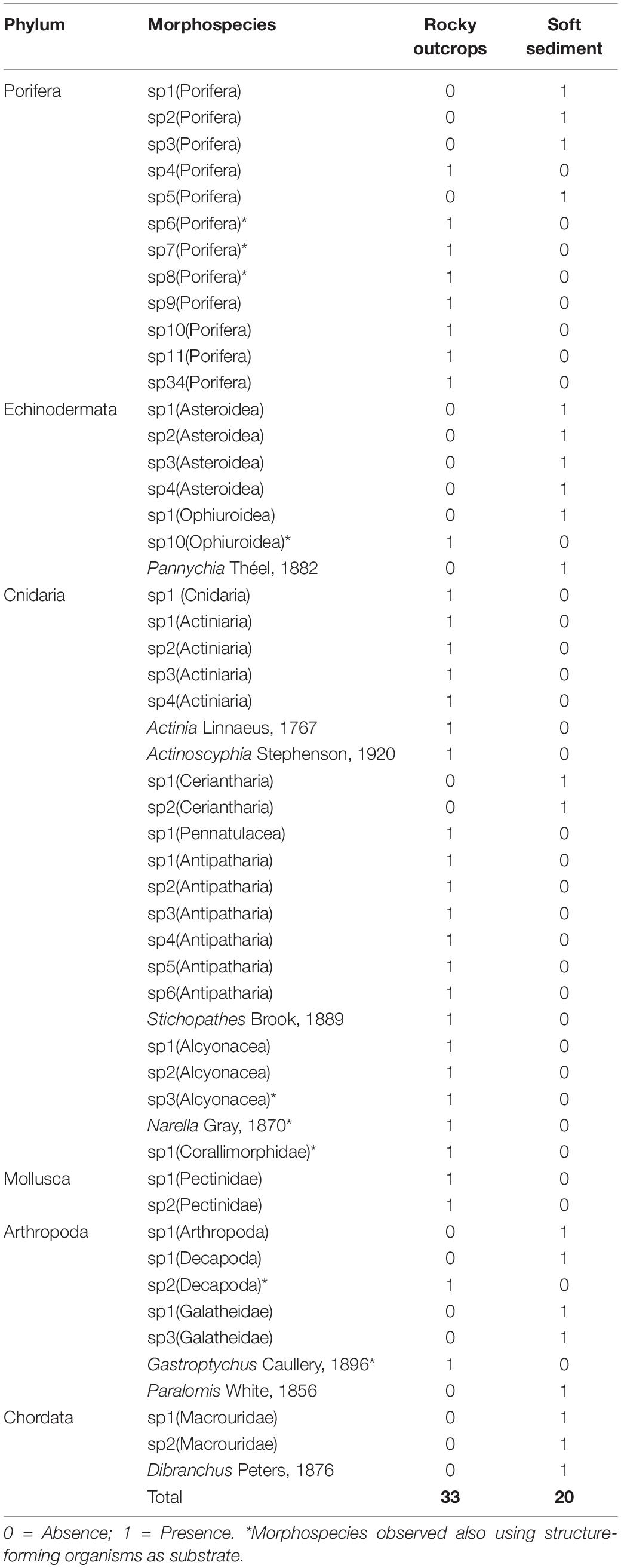
Table 5. Rocky outcrops and soft sediment morphospecies in the NW limit of Guaymas Basin and the Sonoran Margin (849–990 m).
The composition of megafaunal assemblages differed among rocky outcrops and soft sediment (Figure 2A and Table 6). Cnidaria (particularly diverse habitat-forming CWC′s species) and Porifera were far more diverse on rocky outcrops, whereas Echinodermata and Arthropoda had peak taxonomic richness on soft sediment (Figure 2A and Table 5).
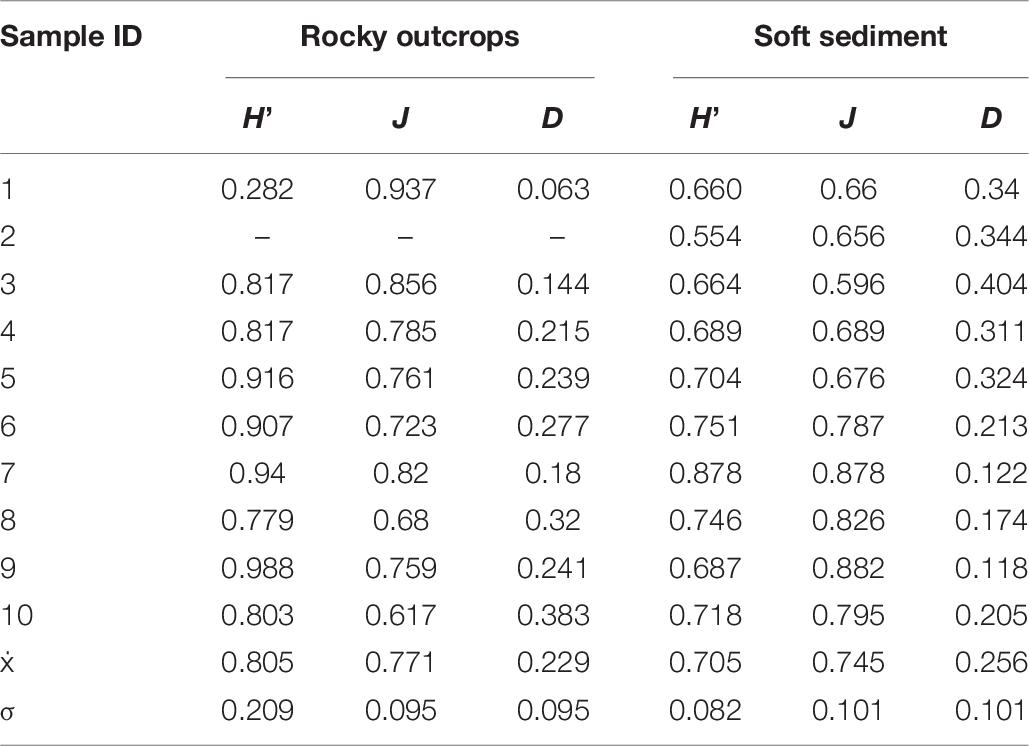
Table 6. Shannon-Wiener diversity index (H′), evenness (J) and dominance calculated for each sample and per substrate.
Relative coverage of epibenthic megafauna taxa was greater on rocky outcrops (68.8%) where Cnidaria, Mollusca and Porifera dominated, compared to soft sediment (31.2%) where Echinodermata and Arthropoda were most dominant (Figure 6A). Taxa that contributed to differences among the total coverage on rocky outcrops were structure-forming organisms such as CWC′s, oyster aggregations and sponges, whereas a sea cucumber (Pannychia sp.) and galatheids dominated soft sediment (Figure 6A). The dominant sponge morphotypes on rocky outcrops and soft sediments were clavate (Table 7).
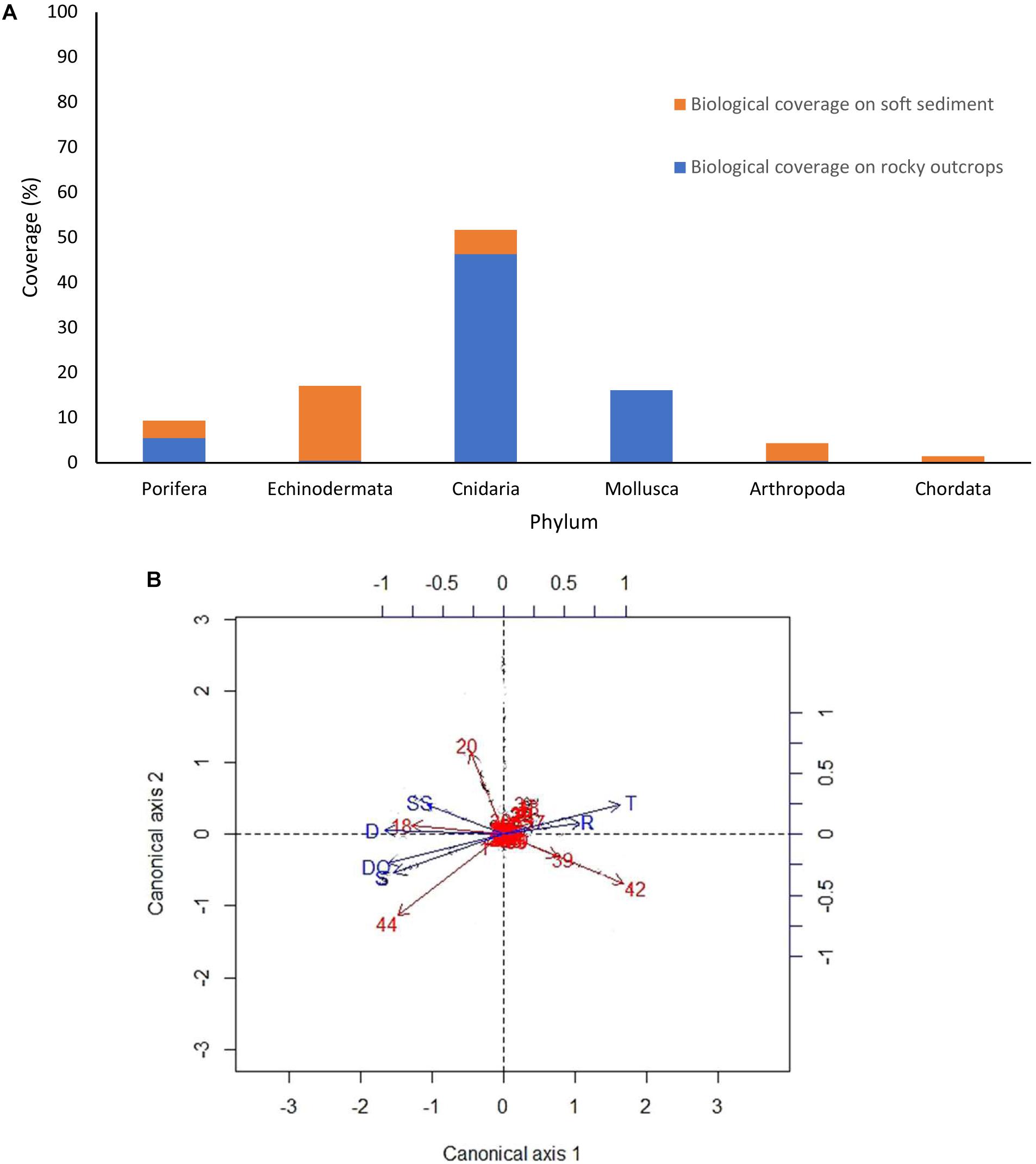
Figure 6. (A) Biological coverage of epibenthic megafauna by phylum on soft sediment and rocky outcrops at dive D347 in the NW limit of Guaymas Basin and Sonoran Margin. (B) RDA triplot of the Hellinger-transformed cover data constrained by all environmental variables. Numbers = morphospecies code; SS = soft sediment; R = rocky outcrops; T = temperature; D = depth; S = salinity; DO = dissolved oxygen.
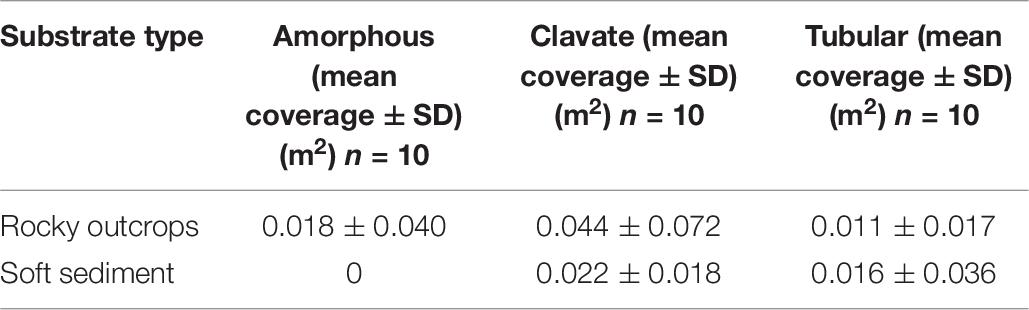
Table 7. Composition of sponge morphotypes found at the NW limit of Guaymas Basin and the Sonoran Margin (849–990 m).
Morphospecies rarefaction curves for rocky outcrops showed a general tendency to increase above the soft substrate curve (Figure 2B). These graphic results are in agreement with the significant differences (Kruskal–Wallis, H(1;19) = 6.4123; p = 0.0113) of mean Shannon-Wiener diversity index (H′) observed between rocky outcrops and soft sediment (Table 6). The mean Shannon-Wiener diversity index for rocky outcrops (H′ = 0.8) was higher than measured for the megafaunal assemblages on soft sediment (H′ = 0.7) (Figure 2A and Table 6). There were no significative differences in the mean evenness index (Kruskal–Wallis, H(1;19) = 0.1667; p = 0.6831) or mean dominance index (Kruskal–Wallis, H(1;19) = 0.1667; p = 0.6831) of megafaunal assemblages among substrata (Figure 2A).
Megafaunal Patterns and DO
Taxonomic richness in the LTZ and in the lower boundary of the OMZ core was greater than in the LB (Table 8). Taxonomic richness within the LB decreased with decreasing DO conditions and where soft sediments dominated. Although the taxonomic richness of OMZ assemblages varied with DO, their taxonomic composition showed little variation among OMZ zones (Figure 7). Cnidaria, Porifera, Echinodermata and Arthropoda were the most diverse taxa through all three OMZ zones (Figure 7).
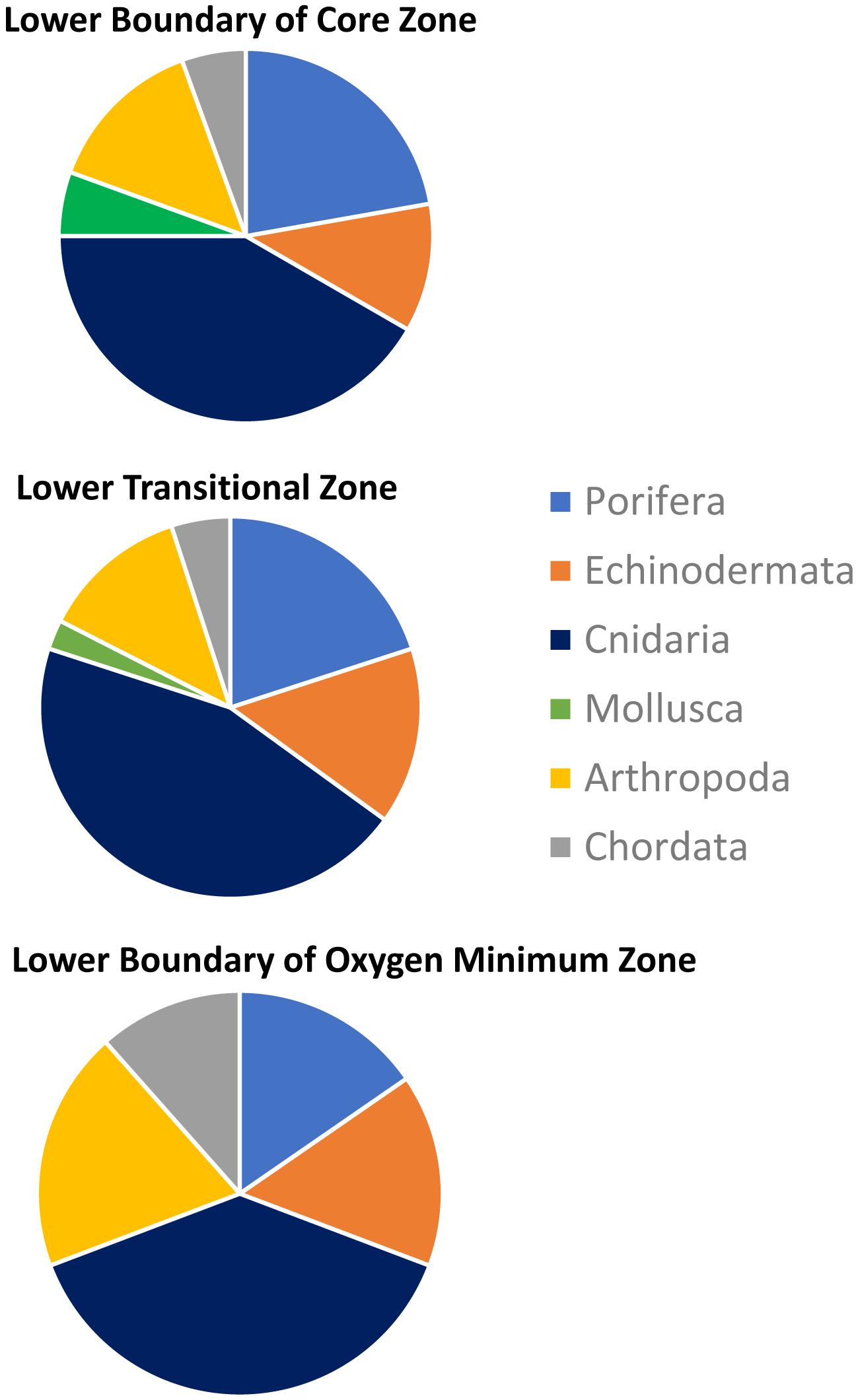
Figure 7. Composition and proportional taxonomic richness by phylum through the oxygen minimum zones during dive D347 in the NW limit of Guaymas Basin and Sonoran Margin.
Megafaunal Composition and Environmental Variables
The RDA analysis indicated that 21.15% of the variation in megafaunal abundance was explained by environmental variables (substrata type, depth, temperature, salinity and DO). A permutation test (with 999 permutations) indicated that global model (p = 0.001) was significant. Environmental variables that explained the most megafaunal variation were substrate type (18.4%), depth (1.14%) and temperature (0.9%). Salinity (0.45%) and DO (0.3%) were less important factors to explain the megafaunal composition variance. The triplot (Figure 6B) showed that substrate type, temperature and depth were correlated with megafaunal morphospecies. As illustrated by the triplot in Figure 6B, most morphospecies are bunched together with short projections at the centroid oriented toward rocky outcrops, temperature and soft sediment, but away from most of the environmental variables. We interpret this to indicate that megafaunal patterns are related to muliple explanatory variables.
Discussion
The principal aim of our study was to document variation in the diversity and composition of epibenthic megafauna in relation to DO and habitat characteristics at bathyal depths from 849 to 990 m in the Guaymas Basin in the GoC. Our data indicates a diverse fauna of Cnidaria, Porifera, Arthropoda and Echinodermata common on rocky outcrops and soft sediments in the NW limit of Guaymas Basin and the Sonoran Margin. Similarly, in the southern to central region of the Gulf (between 438 and 3747 m depth) Hinojosa-Corona (2014) found a diverse fauna of antipatharians, gorgonians and sponges on scarps and other deep-sea hard bottoms habitats. Patterns of benthic megafaunal diversity in littoral and intertidal zones in the GoC have been widely reported in relation to habitat and substrate type (Brusca and Hendrickx, 2010). These patterns are little known in the GoC at > 200 m depth, particularly for hard bottom habitats that are not easily sampled using traditional methods.
This study spans a narrow bathymetric range (849–990 m) where a strong gradient in DO levels occurred and crosses distinct OMZ zones defined on the basis of DO criteria (Hendrickx, 2006; Hendrickx and Serrano, 2014; Papiol and Hendrickx, 2016) for the southern and central regions in the GoC. We observed a great taxonomic richness through the LTZ across an area with rocky outcrops. This megafaunal pattern observed is different to that reported in other OMZs where they are characterized by a great abundance of a few species (Levin et al., 2000; Gooday et al., 2010; Papiol and Hendrickx, 2016). For instance, previous studies conducted in the southeast GoC, showed that soft sediments between 800 and 1000 m depth in the LTZ were dominated by a galatheid (Munidopsis depressa) (Papiol and Hendrickx, 2016). In Volcano 7 in the eastern tropical Pacific, the Oman margin in the Arabian Sea, and off central California, was observed high densities of crustaceans and echinoderms near the LB of the OMZ (Levin, 2003). DO appears to be an important factor that controls the diversity in the core regions of OMZs (Levin and Gage, 1998; Gooday et al., 2010). However, as DO starts to rise below the OMZ core and the potential stress related to hypoxia diminishes, other environmental factors could influence on diversity patterns (Levin and Gage, 1998; Levin, 2003; Gooday et al., 2010; Papiol and Hendrickx, 2016). Among these factors, hard substrate in the LTZ where food is not a limiting factor could allow the colonization of species (e.g., filter and suspension feeders) that already tolerate hypoxic conditions, resulting in an increase of taxonomic richness.
On the other hand, low taxonomic richness of megabenthic fauna was observed at depths with reduced DO and greater cover of soft sediment in the LB of the OMZ. Several studies in this region (Méndez, 2007; Zamorano et al., 2007; Zamorano and Hendrickx, 2012; Hendrickx and Serrano, 2014; Hinojosa-Corona, 2014; Hendrickx et al., 2016; Papiol and Hendrickx, 2016) have reported that the OMZ is coupled closely to the diversity, composition, abundance and distribution of megafauna inhabiting the water column and the benthos. Differences in the tolerances of megafauna to OMZ conditions very likely influence the shift in the species composition of communities in the LTZ (Levin, 2003; Gooday et al., 2010). However, it should be noted that in our study the distribution of a field of soft sediments with no visible rocks overlaps almost totally with the LB of the OMZ. Under these conditions, the influence of the OMZ on the composition of the megafauna is hard to interpret and our results are limited by the collinearity of substrate and DO.
We found that environmental variables under study are responsible for some of the megafaunal variation. Although, the global model explain only 21.15% of the faunal variation, this result is consistent with variation explained by environmental variables in a study realized in Seco de los Olivos seamount (De la Torriente et al., 2018). However, our model results are probably related somewhat to the spatial scale of the sampling and collection of environmental variables. We speculate that on a larger environmental scale (e.g., if the transects crossed all zones of the OMZ and more), the role of environmental factors may have been larger.
Our results indicate that variation in megafaunal composition was mostly explained by substrate type. Thus, substrate characteristics very likely play a key role in regulating community patterns. The composition of megafaunal assemblages on rocky outcrops differed from those on soft sediments. We found an abundant and diverse fauna of CWC′s (antipatharians, alcyonarians) and sponges, attached to volcanic rocky outcrops through the LTZ and LB of OMZ. Hard substrate within an OMZ are often formed by materials such as phosphorite, carbonate and volcanic materials (Levin et al., 2010). In comparison, studies realized in the seamount Volcano-7 in the eastern Pacific that protrude into the OMZ have shown a dense aggregation of sponges and serpulid polychaetes attached to basalt substrate and manganese crust (Gooday et al., 2010). Several studies of benthic megafauna have also reported higher species richness on hard substrata than on soft sediments. Canyons in the Gulf of Gioia (Pierdomenico et al., 2016; van den Beld et al., 2017; De la Torriente et al., 2018), the Bay of Biscay (800–1200 m) as well as in the seamount Seco de los Olivos (< 700 m) are sites where hard substrata (including structure-forming organisms) were shown to have higher species richness and diversity than soft sediments. Here, the higher diversity, taxonomic richness and cover of megafauna that we observed on rocky outcrops was because the species associated with hard substrate occur attached to the surface of rocky outcrops and structure-forming organisms, within or around the three-dimensional complex structures constituted by rocky outcrops and habitat-forming organisms and in crevices. More species were also recorded on soft sediment between rocks. Habitats with hard substrate and three-dimensional complex structures, generally supported a higher epibenthic megafauna diversity in comparison with more uniform substrate habitats, such as soft bottom plains (Schlacher et al., 2007; Buhl-Mortensen et al., 2012; Rowden et al., 2016; Åström et al., 2018). Structural habitat complexity was positively related to the local species diversity of sessile and mobile species (Buhl-Mortensen et al., 2010; Matias et al., 2010; Bartholomew et al., 2016; Ferrari et al., 2016; Storlazzi et al., 2016). The underlying substratum and habitat-forming organisms provide a significant proportion of three-dimensional habitat in marine communities (Ferrari et al., 2016). Numerous studies have documented that structure-forming organisms such as sponges and CWC′s play a key structural and functional role in the marine benthos, because they can modified physical properties of the sea floor and influence composition, abundance and distribution of epibenthic megafauna (Schlacher et al., 2007; Beazley et al., 2013; Howell et al., 2016; Huvenne et al., 2016; van den Beld et al., 2017; Kazanidis et al., 2019).
Taxa identified in the NW limit of Guaymas basin and Sonoran Margin span several trophic levels including filter and suspension feeders, deposit feeders and carnivorous benthophages (Sokolova, 2000). Among filter-feeding fauna we found that clavate sponge morphotypes were dominant on rocky outcrops and soft sediment habitats. These results are different to the results found by Kazanidis et al. (2019) in the northeast Atlantic (∼500 m depth), where sponges aggregations on rock and sand substrate were mainly composed of massive and flabellate sponges. Several studies have shown that environmental and anthropogenic factors, such as hydrography or trawling respectively, have an influence in the sponge morphotypes inhabiting in an specific area (Cryer et al., 2008; Kazanidis et al., 2019). For example, a study realized by Bell and Barnes (2000) showed that encrusting forms were more abundant on high flow areas. At a local scale the morphology of structure-forming organisms can also vary with local hydrodynamic patterns and create microhabitats differing in flow and the transport or settlement of particles (Buhl-Mortensen et al., 2010). Furthermore, the evolutionary origin of the fauna may account for the differences observed. Most invertebrate fauna of the Gulf of California were derived from the Caribbean Sea, the temperate shores of California and from the tropical Indo-west Pacific (Brusca and Hendrickx, 2010).
The findings of this study support the hypothesis that taxonomic richness in the NW limit of Guaymas Basin are highest in rocky benthic habitats with the greatest structural complexity in comparison with soft sediment habitats, but also, we found that diversity, composition and cover of benthic megafauna vary among soft sediment and rocky outcrops.
In our study we found that depth and temperature were also important environmental factors related to variation in the composition of benthic megafauna as reported in other studies of the southern and central regions in the GoC (Hendrickx, 1996, 2006; Méndez, 2007; Zamorano et al., 2007; Zamorano and Hendrickx, 2012). However, multiple interacting factors ranging from hydrographic (DO, water masses) to food supply and interactions act in synergy with DO and substratum type to influence the structure of these communities.
Conclusion
The present study documented that diversity, taxonomic richness, composition and cover of benthic megafauna within the OMZs from 849 to 990 m depth in the NW limit of the Guaymas Basin varied among rocky outcrops and soft sediments. Variation in megafaunal composition was highly correlated with substrate type (rocky outcrops and soft sediments) depth and temperature. Salinity and DO had a minor role in explaining variation in the composition of megafaunal assemblages. We found high taxonomic richness through the LTZ across an area with rocky outcrops, in contrast with some previous reports from other OMZ habitats where a high abundance of few species has been observed. We documented that taxonomic richness diminished at depths with reduced DO in the LB of OMZ with increasing soft sediment cover, however, the relative influence on the observed pattern is limited by the collinearity of DO and substrate. Taxonomic richness and diversity of megabenthos in the NW limit of Guaymas Basin and Sonoran Margin were positively correlated with the increase in structural habitat complexity and heterogeneity of rocky outcrops and structure-forming organisms in the lower boundary of OMZ core and LTZ of the OMZ. These results broaden our understanding concerning the potential roles of substrate characteristics in the community composition of the deep-sea benthic megafaunal assemblages in the GOC and OMZs in general. Our results also contribute to the information required by decision-makers for Marine Protected Areas and design of habitats conservation.
Data Availability Statement
The dataset analyzed for this study can be found in the UNINMAR repository at: http://metadata.icmyl.unam. mx/handle/20.500.12201/10238, http://metadata.icmyl.unam. mx/handle/20.500.12201/10239, http://metadata.icmyl.unam. mx/handle/20.500.12201/10240, http://metadata.icmyl.unam.mx/handle/20.500.12201/10241, http://metadata.icmyl.unam.mx/handle/20.500.12201/10242.
Author Contributions
PL-G coordinated the study, elaborated the manuscript, responsible for the integration of datasets, and carried out the analysis and results presented in this article. JB chief scientist of leg 3 of MBARI’s 2012 Gulf of California Expedition, and responsible for data collection and editing of the manuscript. JG-G collaborated with the design of the method for video and images analysis, as well as the results analysis, and reviewed the manuscript. EE-B collaborated with the theoretical framework of the study, contributed as the international counterpart during the 2012 MBARI Gulf of California Expedition, assisted in the results analysis, and reviewed the manuscript.
Funding
This work was possible thanks to the financial support (Beca Nacional 345620/630445) of National Council for Science and Technology (CONACYT) of Mexico, Santander Universidades through the program “Jovenes Profesores e Investigadores 2018-1” and the David and Lucile Packard Foundation.
Conflict of Interest
The authors declare that the research was conducted in the absence of any commercial or financial relationships that could be construed as a potential conflict of interest.
Acknowledgments
This work was possible thanks to the shared metadata produced during the 2012 Gulf of California Expedition performed by Monterey Bay Aquarium Research Institute, and generous support from the David and Lucile Packard Foundation. We are also grateful to CONACyT and Santander Universidades for the doctoral grant to PL-G, for technical support to the UNINMAR at Instituto de Ciencias del Mar y Limnología UNAM and the Unidad de Informática Marina and the Instituto de Investigaciones Marinas de la Universidad de Cádiz. J. Yager, Research Associate, Department of Invertebrate Zoology, Smithsonian Institution edited English language.
Supplementary Material
The Supplementary Material for this article can be found online at: https://www.frontiersin.org/articles/10.3389/fmars.2020.00154/full#supplementary-material
References
Álvarez, F., Villalobos, J. L., Hendrickx, M. E., Escobar-Briones, E., Rodríguez-Almaraz, G., and Campos, E. (2014). Biodiversidad de crustáceos decápodos (Crustacea: Decapoda) en México. Rev. Mex. Biodivers. 85, 208–219. doi: 10.7550/rmb.38758
Álvarez-Borrego, S. (2010). Physical, chemical and biological oceanography of the Gulf of California. Gulf Calif. Biodivers. Conserv. 400, 24–48.
Álvarez-Borrego, S., and Schwartzlose, R. A. (1979). Water masses of the gulf of california. Ciencias Mar. 6, 43–63. doi: 10.7773/cm.v6i1.350
Anderson, T. J., Nichol, S. L., Syms, C., Przeslawski, R., and Harris, P. T. (2011). Deep-sea bio-physical variables as surrogates for biological assemblages, an example from the Lord Howe Rise. Deep. Res. Part II Top. Stud. Oceanogr. 58, 979–991. doi: 10.1016/j.dsr2.2010.10.053
Åström, E. K. L., Carroll, M. L., Ambrose, W. G., Sen, A., Silyakova, A., and Carroll, J. L. (2018). Methane cold seeps as biological oases in the high-Arctic deep sea. Limnol. Oceanogr. 63, S209–S231. doi: 10.1002/lno.10732
Ayma, A., Aguzzi, J., Canals, M., Lastras, G., Bahamon, N., Mecho, A., et al. (2016). Comparison between ROV video and Agassiz trawl methods for sampling deep water fauna of submarine canyons in the Northwestern Mediterranean Sea with observations on behavioural reactions of target species. Deep. Res. Part I Oceanogr. Res. Pap. 114, 149–159. doi: 10.1016/j.dsr.2016.05.013
Bakus, J. G. (2007). Quantitative Analysys of Marine Biological Communities. Field Biology and Environment. Hoboken, NJ: Jhon Wiley & Sons.
Bartholomew, A., Hafezi, S. A., and Karimi, S. (2016). Effects of habitat complexity on the abundance, species richness and size of darkling beetles (Tenebrionidae) in artificial vegetation. J. Arid Environ. 129, 35–41. doi: 10.1016/j.jaridenv.2016.02.004
Beazley, L. I., Kenchington, E. L., Murillo, F. J., and Sacau, M. (2013). of epibenthic megafauna in the Northwest Atlantic. ICES J. Mar. Sci. 70, 1471–1490. doi: 10.1093/icesjms/fst124
Bell, J. J., and Barnes, D. K. A. (2000). The influences of bathymetry and flow regime upon the morphology of sublittoral sponge communities. J. Mar. Biol. Assoc. 80, 707–718. doi: 10.1017/S0025315400002538
Bianchi, C. N., Pronzato, R., Cattaneo-Vietti, R., Benedetti Cecchi, L., Morri, C., Pansini, M., et al. (2004). “Hard Bottoms,” in Mediterranean Marine Benthos: A Manual of Methods for its Sampling and Study, eds C. M. Gambi and M. Dappiano, (Cham: Springer), 185–215.
Bischoff, J. L., and Henyey, T. L. (1974). Tectonic elements of the central part of the Gulf of California. Geol. Soc. Am. Bull. 85, 1893–1904.
Borcard, D., Gillet, F., Legendre, L., and Legendre, P. (2011). Numerical Ecology with R. Berlin: Springer, doi: 10.1017/CBO9781107415324.004
Boury-Esnault, N., and Rützler, K. (1997). Thesaurus of sponge morphology. Smithson. Librar. 596, 1–55. doi: 10.5479/si.00810282.596
Brusca, R. C., Findley, L. T., Hastings, P. A., Hendrickx, M. E., Torre-Cosío, J., and Van Der Heiden, A. M. (2005). Macrofaunal Diversity in the Gulf of California. In Biodiversity, Ecosystems, and Conservation in Northern Mexico. Oxford: Oxford University Press, 179–203.
Brusca, R. C., and Hendrickx, M. E. (2008). The Gulf of California Invertebrate Database: The Invertebrate Portion of the Macrofauna Golfo Database. Available at: http://www.desertmuseum.org/center/seaofcortez/database.php (accessed September 14, 2018).
Brusca, R. C., and Hendrickx, M. E. (2010). “Invertebrate biodiversity and conservation in the Gulf of California,” in The Gulf of California Biodiversity and Conservation, ed. R. C. Brusca, (Oxford: Oxford University Press), 72–95.
Buhl-Mortensen, L., Buhl-Mortensen, P., Dolan, M. F. J., Dannheim, J., Bellec, V., and Holte, B. (2012). Habitat complexity and bottom fauna composition at different scales on the continental shelf and slope of northern Norway. Hydrobiologia 685, 191–219. doi: 10.1007/s10750-011-0988-6
Buhl-Mortensen, L., Vanreusel, A., Gooday, A. J., Levin, L. A., Priede, I. G., Buhl-Mortensen, P., et al. (2010). Biological structures as a source of habitat heterogeneity and biodiversity on the deep ocean margins. Mar. Ecol. 31, 21–50. doi: 10.1111/j.1439-0485.2010.00359.x
Clark, M. R., Consalvey, M., and Rowden, A. A. (2012). Biological Sampling in the Deep Sea. Hoboken, NJ: Wiley-Blackwell, doi: 10.1002/ejoc.201200111
Cryer, M., Hartill, B., and Shea, S. O. (2008). Modification of marine benthos by trawling?: toward a generalization for the deep ocean? Ecol. Soc. 12, 1824–1839.
Cuvelier, D., de Busserolles, F., Lavaud, R., Floc’h, E., Fabri, M. C., Sarradin, P. M., et al. (2012). Biological data extraction from imagery - How far can we go? A case study from the Mid-Atlantic Ridge. Mar. Environ. Res. 82, 15–27. doi: 10.1016/j.marenvres.2012.09.001
Davies, C. E., Moss, D., and Hill, M. O. (2004). EuNIS Habitat Classification Revised 2004. Report to the European Topic Centre on Nature Protection and Biodiversity. Denmark: European Environmental Agency.
De la Torriente, A., Serrano, A., Fernández-Salas, L. M., García, M., and Aguilar, R. (2018). Identifying epibenthic habitats on the seco de los olivos seamount: species assemblages and environmental characteristics. Deep. Res. Part I Oceanogr. Res. Pap. 135, 9–22. doi: 10.1016/j.dsr.2018.03.015
Du Preez, C., and Tunnicliffe, V. (2012). A new video survey method of microtopographic laser scanning (MiLS) to measure small-scale seafloor bottom roughness. Limnol. Oceanogr. Methods 10, 899–909. doi: 10.4319/lom.2012.10.899
Estacio, G. F. J. (1996). Distribución Espacio-Temporal de las Comunidades Macrobentónicas del Sedimento en la Bahía de Algeciras. Implicaciones en la Evolución de la Calidad Ambiental del Medio Marino. Dissertation’s thesis, University of Sevilla, Sevilla, Spain.
Ferrari, R., Bryson, M., Bridge, T., Hustache, J., Williams, S. B., Byrne, M., et al. (2016). Quantifying the response of structural complexity and community composition to environmental change in marine communities. Glob. Chang. Biol. 22, 1965–1975. doi: 10.1111/gcb.13197
Figueroa Albornoz, J. L. (2013). Estudio tectono-estratigráfico de la cuenca transtensional en el Margen de Sonora frente al rift de Guaymas, Golfo de California. 171. M.S. thesis, Universidad Nacional Autónoma de México, México.
Gage, J. D., and Tyler, P. A. (1992). Deep-Sea Biology: A Natural History of Organism at the Deep-Sea Floor. Cambridge: Cambridge University Press.
Gates, A. R., Jones, D. O. B., and Cartes, J. E. (2012). In situ video observations of benthic megafauna and fishes from the deep eastern Mediterranean Sea off Egypt. Afric. J. Mar. Sci. 34, 215–222. doi: 10.2989/1814232X.2012.675121
Gooday, A. J., Bett, B. J., Escobar, E., Ingole, B., Levin, L. A., Neira, C., et al. (2010). Habitat heterogeneity and its influence on benthic biodiversity in oxygen minimum zones. Mar. Ecol. 31, 125–147. doi: 10.1111/j.1439-0485.2009.00348.x
Greene, H. G., Yoklavich, M. M., Starr, R. M., O’Connell, V. M., Wakefield, W. W., Sullivan, D. E., et al. (1999). A classification scheme for deep seafloor habitats. Oceanol. Acta 22, 663–678. doi: 10.1016/S0399-1784(00)88957-4
Guinan, J., Grehan, A. J., Dolan, M. F. J., and Brown, C. (2009). Quantifying relationships between video observations of cold-water coral cover and seafloor features in rockall trough, west of Ireland. Mar. Ecol. Prog. Ser. 375, 125–138. doi: 10.3354/meps07739
Hendrickx, M., Brusca, R., Cordero, M., and Ramírez, R. G. (2007). Marine and brackish-water molluscan biodiversity in the Gulf of California, Mexico. Sci. Mar. 71, 637–647. doi: 10.3989/scimar.2007.71n4637
Hendrickx, M., Valentich-Scott, P., and Suárez-Mozo, N. (2016). Deep-water bivalve mollusks collected during the TALUD XV cruise off the west coast of the southern Baja California Peninsula, Mexico. Biodivers. Data J. 4, e8661. doi: 10.3897/BDJ.4.e8661
Hendrickx, M. E. (1996). Habitats and biodiversity of decapod crustaceans in the SE Gulf of California, México. Rev. Biol. Trop. 44, 603–617.
Hendrickx, M. E. (2006). Occurrence of a continental slope decapod crustacean community along the edge of the minimum oxygen zone in the south eastern Gulf of California. Mexico. Belg. J. Zool. 131, 95–110.
Hendrickx, M. E., Salgado-Barragán, J., Toledano-Granados, A., and Cordero-Ruiz, M. (2014). Los moluscos (Pelecypoda, Gastropoda, Cephalopoda, Polyplacophora y Scaphopoda) recolectados en el SE del golfo de California durante las campañas SIPCO a bordo del B/O “El Puma”. Elenco faunístico. Rev. Mex. Biodivers. 85, 682–722. doi: 10.7550/rmb.43077
Hendrickx, M. E., and Serrano, D. (2014). Effects of the oxygen minimum zone on squat lobster distributions in the Gulf of California, Mexico. Cent. Eur. J. Biol. 9, 92–103. doi: 10.2478/s11535-013-0165-6
Hernández-Alcántara, P., Salas-de León, D. A., Solís-Weiss, V., and Monreal-Gómez, M. A. (2014). Bathymetric patterns of polychaete (Annelida) species richness in the continental shelf of the Gulf of California, Eastern Pacific. J. Sea Res. 91, 79–87. doi: 10.1016/j.seares.2014.04.012
Hinojosa-Corona, A. (2014). “Explorando el mar de Cortés profundo,” in Explorando el Mar Profundo del Golfo de California 2008-2014, eds B. E. Mejía-Mercado, A. Hinojosa-Corona, and M. E. Hendrickx, (Ensenada: CICESE), 1–24.
Howell, K. L., Piechaud, N., Downie, A. L., and Kenny, A. (2016). The distribution of deep-sea sponge aggregations in the North Atlantic and implications for their effective spatial management. Deep. Res. Part I Oceanogr. Res. Pap. 115, 203–220. doi: 10.1016/j.dsr.2016.07.005
Huvenne, V. A. I., Bett, B. J., Masson, D. G., Le Bas, T. P., and Wheeler, A. J. (2016). Effectiveness of a deep-sea cold-water coral Marine protected area, following eight years of fisheries closure. Biol. Conserv. 200, 60–69. doi: 10.1016/J.BIOCON.2016.05.030
Jacobsen Stout, N., Kuhnz, L., Lundsten, L., Schlining, B., Schlining, K., and Von Thun, S. (2015). The Deep-Sea Guide (DSG). Monterey Bay Aquarium Research Institute (MBARI). Available at: http://dsg.mbari.org/dsg/home (accessed September 14, 2018).
Kazanidis, G., Vad, J., Henry, L. A., Neat, F., Berx, B., Georgoulas, K., et al. (2019). Distribution of deep-sea sponge aggregations in an area of multisectoral activities and changing oceanic conditions. Front. Mar. Sci. 6:163. doi: 10.3389/fmars.2019.00163
Kluesner, J. (2011). Marine Geophysical Study of Cyclic Sedimentation and Shallow Sill Intrusion in the Floor of the Central Gulf of California. San Diego, CA: University of California.
Kurnosov, V., and Blinova, E. (2015). Hydrothermal alteration of sediments and compositional evolution of solutions in the Guaymas basin of the Gulf of California. Dokl. Earth Sci. 461, 217–220. doi: 10.1134/S1028334X15030149
Laguionie-Marchais, C., Kuhnz, L. A., Huffard, C. L., Ruhl, H. A., and Smith, K. L. (2015). Spatial and temporal variation in sponge spicule patches at Station M, northeast Pacific. Mar. Biol. 162, 617–624. doi: 10.1007/s00227-014-2609-1
Lavín, M. F., and Marinone, S. G. (2003). “An overview of the physical oceanography of the gulf of california,” in Nonlinear Processes in Geophysical Fluid Dynamics, eds O. U. Velasco-Fuentes, J. Sheinbaum, and J. L. Ochoa de la Torre, (Berlin: Kluwer Academic Publishers), 173–204. doi: 10.1007/978-94-010-0074-1_11
Legendre, P., and Legendre, L. (2012). Numerical Ecology. Developments in Environmental Modeling, 3rd Edn. Amsterdem: Elsevier.
Levin, L. (2003). Oxygen minimum zone benthos: adaptation and community response to hypoxia. Oceanogr. Mar. Biol. Annu. Rev. 41, 1–45.
Levin, L. A., Etter, R. J., Rex, M. A., Gooday, A. J., Smith, C. R., Pineda, J., et al. (2001). Environmental influences on regional deep-sea species diversity. Annu. Rev. Ecol. Syst. 32, 51–93. doi: 10.1146/annurev.ecolsys.32.081501.114002
Levin, L. A., and Gage, J. D. (1998). Relationships between oxygen, organic matter and the diversity of bathyal macrofauna. Deep Sea Res. Part II Top. Stud. Oceanogr. 45, 129–163. doi: 10.1016/s0967-0645(97)00085-4
Levin, L. A., Gage, J. D., Martin, C., Lamont, P. A., Levin, J. D., Gage, L. A., et al. (2000). Macrobenthic community structure within and beneath the oxygen minimum zone, NW Arabian Sea. Deep Res. II 47, 189–226. doi: 10.1016/S0967-0645(99)00103-4
Levin, L. A., Sibuet, M., Gooday, A. J., Smith, C. R., and Vanreusel, A. (2010). The roles of habitat heterogeneity in generating and maintaining biodiversity on continental margins: An introduction. Mar. Ecol. 31, 1–5. doi: 10.1111/j.1439-0485.2009.00358.x
Lluch-Cota, S. E., Aragón-Noriega, E. A., Arreguín-Sánchez, F., Aurioles-Gamboa, D., Jesús Bautista-Romero, J., Brusca, R. C., et al. (2007). The Gulf of California: review of ecosystem status and sustainability challenges. Prog. Oceanogr. 73, 1–26. doi: 10.1016/j.pocean.2007.01.013
Lonsdale, P. (1989). “Geology and tectonic history of the Gulf of California,” in The Eastern Pacific Ocean and Hawaii. The Geology of North America, eds E. L. Winterer, D. M. Hussong, and R. W. Decker, (Boulder, CO: Geological Society of America), 499–521. doi: 10.1130/dnag-gna-n.499
Lorance, P., and Trenkel, V. M. (2006). Variability in natural behaviour, and observed reactions to an ROV, by mid-slope fish species. J. Exp. Mar. Bio. Ecol. 332, 106–119. doi: 10.1016/j.jembe.2005.11.007
Marshal, B., and Richer de Forges, B. (eds) (2004). Tropical Deep-Sea Benthos. Paris: Muséum national d’Histoire naturelle.
Matias, M. G., Underwood, A. J., Hochuli, D. F., and Coleman, R. A. (2010). Independent effects of patch size and structural complexity on diversity of benthic macroinvertebrates. Ecology 91, 1908–1915. doi: 10.1890/09-1083.1
McArthur, M. A., Brooke, B. P., Przeslawski, R., Ryan, D. A., Lucieer, V. L., Nichol, S., et al. (2010). On the use of abiotic surrogates to describe marine benthic biodiversity. Estuar. Coast. Shelf Sci. 88, 21–32. doi: 10.1016/j.ecss.2010.03.003
Mejía-Mercado, B. E., Hinojosa-Corona, A., and Hendrickx, M. E. (eds) (2014). Explorando el Mar Profundo del Golfo de California 2008-2014. Mexico: CICESE, 245.
Méndez, N. (2007). Relationships between deep-water polychaete fauna and environmental factors in the southeastern Gulf of California, Mexico. Sci. Mar. 71, 605–622. doi: 10.3989/scimar.2010.74n1025
NOAA Office of Ocean Exploration and Research (2018). Benthic Deepwater Animal Identification Guide. Available online at: https://oceanexplorer.noaa.gov/okeanos/animal_guide/animal_guide.html (accessed September 14, 2018).
OBIS (2019). Ocean Biogeographic Information System. Available online at: https://obis.org/ (accessed June 2, 2019).
Páez-Osuna, F., Sanchez-Cabeza, J. A., Ruiz-Fernández, A. C., Alonso-Rodríguez, R., Piñón-Gimate, A., Cardoso-Mohedano, J. G., et al. (2016). Environmental status of the Gulf of California: a review of responses to climate change and climate variability. Earth Sci. Rev. 162, 253–268. doi: 10.1016/j.earscirev.2016.09.015
Papiol, V., and Hendrickx, M. E. (2016). Community structure of deep-water decapod crustaceans below the oxygen minimum zone in the south-east Gulf of California and analysis of environmental drivers. Mar. Freshw. Res. 67, 1862–1879. doi: 10.1071/MF15040
Pierdomenico, M., Martorelli, E., Dominguez-Carrió, C., Gili, J. M., and Chiocci, F. L. (2016). Seafloor characterization and benthic megafaunal distribution of an active submarine canyon and surrounding sectors: the case of Gioia Canyon (Southern Tyrrhenian Sea). J. Mar. Syst. 157, 101–117. doi: 10.1016/j.jmarsys.2016.01.005
Pilgrim, D. A., Parry, D. M., Jones, M. B., and Kendall, M. A. (2000). ROV image scaling with laser spot patterns. Underw. Technol. 24, 93–103. doi: 10.3723/175605400783259684
Porteiro, F. M., Gomes-Pereira, J. N., Pham, C. K., Tempera, F., and Santos, R. S. (2013). Distribution and habitat association of benthic fish on the Condor seamount (NE Atlantic, Azores) from in situ observations. Deep Res. Part II Top. Stud. Oceanogr. 98, 114–128. doi: 10.1016/j.dsr2.2013.09.015
Ramirez-Llodra, E., Company, J. B., Sardà, F., and Rotllant, G. (2010). Megabenthic diversity patterns and community structure of the Blanes submarine canyon and adjacent slope in the Northwestern Mediterranean: a human overprint? Mar. Ecol. 31, 167–182. doi: 10.1111/j.1439-0485.2009.00336.x
Roberts, J. M., Wheeler, A. J., Freiwald, A., and Cairns, S. (2009). Cold-Water Corals. Cambridge: Cambridge University Press.
Roden, G. I. (1958). Oceanographic and meteorological aspects of the Gulf of California. Pac. Sci. 12, 21–45.
Rogers, A. D. (2000). The role of the oceanic oxygen minima in generating biodiversity in the deep sea. Deep Res. Part II Top. Stud. Oceanogr. 47, 119–148. doi: 10.1016/S0967-0645(99)00107-1
Rowden, A. A., Leduc, D., Clark, M. R., and Bowden, D. A. (2016). Habitat differences in deep-sea megafaunal communities off New Zealand: implications for vulnerability to anthropogenic disturbance and management. Front. Mar. Sci. 3:241. doi: 10.3389/fmars.2016.00241
Ruppert, E. E., and Barnes, R. D. (1996). Zoología de los Invertebrados. New York, NY: McGraw-Hill Interam.
Rusnak, G. A., Fisher, R. L., and Shepard, F. P. (1964). “Bathymetry and faults of Gulf of California,” in Marine Geology of the Gulf of California, eds T. van Andel and G. G. Shor, (Wisconsin: The American Association of Petroleum Geologists), 50–75.
Sanders, H. L. (1968). Marine benthic diversity: a comparative study. Science 102, 243–282. doi: 10.1086/282541
Santamaría-del-Angel, E., Alvarez-Borrego, S., and Müller-Karger, F. E. (1994). Gulf of California biogeographic regions based on coastal zone color scanner imagery. J. Geophys. Res. 99:7411. doi: 10.1029/93JC02154
Schlacher, T., Schlacher-Hoenlinger, M., Williams, A., Althaus, F., Hooper, J., and Kloser, R. (2007). Richness and distribution of sponge megabenthos in continental margin canyons off southeastern Australia. Mar. Ecol. Prog. Ser. 340, 73–88. doi: 10.3354/meps340073
Schlacher, T. A., Williams, A., Althaus, F., and Schlacher-Hoenlinger, M. A. (2010). High-resolution seabed imagery as a tool for biodiversity conservation planning on continental margins. Mar. Ecol. 31, 200–221. doi: 10.1111/j.1439-0485.2009.00286.x
Sokolova, M. (2000). Feeding and Trophic Structure of the Deep-Sea Macrobenthos. Cambridge: Science Publishers, Inc.
Solís-Marín, F. A., Laguarda-Figueras, A., Durán-González, A., Ahearn, C. G., and Torres Vega, J. (2005). Echinoderms (Echinodermata) from the Gulf of California, Mexico. Rev. Biol. Trop. 53, (Suppl. 3), 123–137.
Solís-Marín, F. A., Laguarda-Figueras, A., and Honey-Escandón, M. (2014). Biodiversidad de equinodermos (Echinodermata) en Mexico. Rev. Mex. Biodivers. 85, 441–449. doi: 10.7550/rmb.31805
Spanier, E., Cobb, J. S., and Clancy, M. (1994). Impacts of remotely operated vehicles (ROVs) on the behavior of marine animals: an example using American lobsters. Mar. Ecol. Prog. Ser. 104, 257–266. doi: 10.3354/meps104257
Storlazzi, C. D., Dartnell, P., Hatcher, G. A., and Gibbs, A. E. (2016). End of the chain? Rugosity and fine-scale bathymetry from existing underwater digital imagery using structure-from-motion (SfM) technology. Coral Reefs 35, 889–894. doi: 10.1007/s00338-016-1462-8
Tapia de la Yossellin, O. (2018). Ofiuroideos (Echinodermata) de hábitats con características para la conservación de Cuencas hipóxicas del Golfo de California. 222. M.S. thesis, Universidad Nacional Autónoma de México, Mexico.
Teske, A., De Beer, D., McKay, L. J., Tivey, M. K., Biddle, J. F., Hoer, D., et al. (2016). The Guaymas Basin hiking guide to hydrothermal mounds, chimneys, and microbial mats: complex seafloor expressions of subsurface hydrothermal circulation. Front. Microbiol. 7:75. doi: 10.3389/fmicb.2016.00075
Tyler, P. A., Baker, M., and Ramirez-Llodra, E. (2016). “Deep-Sea benthic habitats,” in Biological Sampling in the Deep Sea, eds M. R. Clark, C. Mireille, and A. A. Rowden, (Hoboken: John Wiley & Sons, Ltd.), 1–15. doi: 10.1002/9781118332535.ch1
van den Beld, I. M. J., Bourillet, J.-F., Arnaud-Haond, S., de Chambure, L., Davies, J. S., Guillaumont, B., et al. (2017). Cold-water coral habitats in submarine canyons of the bay of biscay. Front. Mar. Sci. 4:118. doi: 10.3389/fmars.2017.00118
Villalobos Vazquez de la Parra, R. (2014). Diversidad y Distribución de la Megafauna Bentónica en el norte del Golfo de California (Isla Tortuga, Cuenca de Guaymas, Salsipuedes y Delfín). 100. M.S. thesis, Universidad Nacional Autónoma de México, Mexico.
Zamorano, P., and Hendrickx, M. E. (2012). Distribution of Lucinoma heroica (Mollusca: Bivalvia: Lucinidae) in the minimum oxygen zone in the Gulf of California, Mexico. Mar. Biodivers. Rec. 5, 1–8. doi: 10.1017/S1755267212000644
Keywords: Sonoran Margin, megafauna, bathyal, substrate, structural complexity, continental slope, Guaymas Basin, Gulf of California
Citation: Lopez-Garrido PH, Barry JP, González-Gordillo JI and Escobar-Briones E (2020) ROV’s Video Recordings as a Tool to Estimate Variation in Megabenthic Epifauna Diversity and Community Composition in the Guaymas Basin. Front. Mar. Sci. 7:154. doi: 10.3389/fmars.2020.00154
Received: 14 June 2019; Accepted: 28 February 2020;
Published: 31 March 2020.
Edited by:
Diva Amon, Natural History Museum, United KingdomReviewed by:
Lenaick Menot, Institut Français de Recherche pour l’Exploitation de la Mer (IFREMER), FranceGeorgios Kazanidis, The University of Edinburgh, United Kingdom
Copyright © 2020 Lopez-Garrido, Barry, González-Gordillo and Escobar-Briones. This is an open-access article distributed under the terms of the Creative Commons Attribution License (CC BY). The use, distribution or reproduction in other forums is permitted, provided the original author(s) and the copyright owner(s) are credited and that the original publication in this journal is cited, in accordance with accepted academic practice. No use, distribution or reproduction is permitted which does not comply with these terms.
*Correspondence: Pedro H. Lopez-Garrido, pedro_lopez@comunidad.unam.mx; Elva Escobar-Briones, escobri@cmarl.unam.mx