- 1Environment, Ecology, and Energy Program, University of North Carolina at Chapel Hill, Chapel Hill, NC, United States
- 2Department of Biology, University of North Carolina at Chapel Hill, Chapel Hill, NC, United States
- 3Department of Marine Sciences, University of North Carolina at Chapel Hill, Chapel Hill, NC, United States
Ocean acidification and warming are two of the many threats to coral reefs worldwide, and Caribbean reef-building corals are especially vulnerable. However, even within the Caribbean, experimental acidification and warming studies reveal a wide array of coral calcification responses across reef systems and among species, complicating efforts to predict how corals will respond to these global-scale stressors. We conducted a meta-analysis to investigate the calcification responses of Caribbean corals to experimentally induced seawater ocean acidification, ocean warming, and the combination of both stressors. Calcification rates were reduced for corals reared under warming alone, but acidification and the combination of both stressors did not clearly reduce calcification rates. Calcification responses of corals collected from the Florida Keys and Belize were compared for regional differences since a greater number of studies were performed on corals collected from these two regions. Notably, corals from the Florida Keys did not exhibit reduced calcification under acidification, warming, or the combination of both stressors, while corals from Belize exhibited reduced calcification under warming alone. Further investigation of these regional trends suggests that the warming and acidification treatments employed dictated calcification responses, rather than collection region. Results from this meta-analysis are constrained by the very few studies that have been conducted within the Caribbean to assess ocean acidification and warming and the large variation in experimental procedure among studies. This meta-analysis reveals existing gaps in our understanding of how corals will likely respond to projected acidification and warming and highlights ways to improve comparability among experimental studies conducted on corals within the same region to better predict coral calcification response under global change.
Introduction
Reef-building corals provide the three-dimensional framework for tropical coral reef ecosystems across the globe, supporting many important ecological and economic goods and services (Costanza et al., 2014). However, coral reefs globally are experiencing declines in diversity and abundance (Pandolfi et al., 2003), raising concerns for the overall health of these essential ecosystems. Such changes in coral reef ecosystems are especially evident throughout the Caribbean Sea (Alvarez-Filip et al., 2009; Schutte et al., 2010). Declines in carbonate production rates (Perry et al., 2013) and reduced coral cover (Cote et al., 2005) on Caribbean reefs have shifted these ecosystems to less structurally complex reefs dominated by algae, sponges, and gorgonians (Norstrom et al., 2009). Over the past two decades, ocean warming and acidification have been identified as two of the primary stressors causing this shift and therefore there have been widespread efforts to better understand how coral reefs will likely respond to projected increases in these two human-induced global change stressors (Kleypas et al., 1999; Doney et al., 2009; Enochs et al., 2016; Stoltenberg et al., 2019).
The Intergovernmental Panel on Climate Change (IPCC) has projected that sea surface temperatures in the Caribbean region could rise between 0.6 and 3.0°C by the end of the twenty-first century (ocean warming), and atmospheric pCO2 will surpass 600 μatm, causing surface ocean pH to decrease by 0.1–0.3 pH units (ocean acidification) (IPCC, 2014). These projections pose significant threats to reef-building corals throughout the Caribbean, causing mass mortality events, reducing recruitment, deteriorating key physiological processes, and lowering coral calcification rates (Jokiel and Coles, 1977; Grottoli et al., 2006; Davies et al., 2016; Okazaki et al., 2017). Despite the consensus that global change will negatively affect Caribbean reef-building corals, the extent of these impacts vary widely by species, region, or measured physiological response parameter (Harvey et al., 2013). A great example of such variation in coral responses to ocean acidification and warming is seen in Okazaki et al. (2017) where some species exhibited no response to ocean acidification or warming while other corals in the same experiment exhibited reduced calcification under one or both stressors. Furthermore, a study conducted by Kenkel et al. (2015) demonstrated a variety of physiological responses (i.e., calcification and Symbiodiniaceae physiology) of corals under ocean warming even within a single species. Understanding the variability in coral responses depicted in previous studies under projected ocean acidification and warming will improve our ability to predict how coral reef ecosystems will be impacted under global change stressors.
Meta-analyses are valuable tools for combining the findings from multiple studies to summarize results across the literature (Gurevitch and Hedges, 1999). Although the use of meta-analyses to better understand biological responses to ocean acidification and warming are common (Kroeker et al., 2010; Harvey et al., 2013; Kelley and Lunden, 2017), few have focused specifically on reef-building corals (Chan and Connolly, 2013). Further, those analyzing corals have not addressed the combined effects of ocean acidification and warming (Harvey et al., 2013). Because these two stressors are not likely to act on reef-building corals independently from one another (Halpern et al., 2007), analyses that investigate the combined effects of acidification and warming provide a more realistic view of the future of tropical coral reefs. Caribbean coral reefs are experiencing region-wide declines more severely than reefs in the Red Sea and the Australian Great Barrier Reef (Pandolfi et al., 2003; Gattuso et al., 2014), highlighting the need to study coral reefs on more local scales. Because of projected further declines in Caribbean coral reefs, a better understanding of how these global stressors are impacting reef-building corals within this ocean basin is necessary for predicting the future success of these important marine ecosystems.
Here, we implement a meta-analysis approach of the peer-reviewed literature to investigate the impacts of experimentally induced ocean acidification, ocean warming, and the combination of acidification and warming on calcification responses of Caribbean corals under projected global change. This meta-analysis aims to address the following questions: (1) How do Caribbean corals respond to ocean acidification and ocean warming in isolation, and to the combined effects of acidification and warming? (2) How do coral calcification responses to these stressors vary by region within the Caribbean? In addressing these questions, this analysis will further the understanding of Caribbean-wide coral calcification responses under projected global change stressors, while providing future steps to improve this knowledge.
Methods
Study Selection
Experimental studies conducted in the Caribbean Sea were identified using Google Scholar with the following search terms: “Caribbean coral,” “ocean acidification,” “ocean warming,” “experiment,” “manipulation,” “control,” and “calcification.” Studies derived from the search were examined, and those that presented all of the following information were included in the meta-analysis: coral species, location of specimen collection, control and experimental temperature, and/or control and experimental pCO2 values, method of experimental manipulation, duration of exposure to treatment conditions, and calcification rate. Studies altering ocean acidification treatments with acid additions (i.e., hydrochloric acid) were excluded from this meta-analysis because these do not represent ecologically relevant changes in carbonate chemistry. Several other studies that met these criteria not present in the literature search were also included in the meta-analysis. Studies were collected for analysis until September 2018.
Data Extraction and Preparation
Detailed information on the location where coral colonies were collected and experimental design of each study was recorded (Table S1). Several studies included multiple species, collection sites, or experimental factors. In these cases, each species and/or collection site was considered as a separate experiment such that multiple comparisons were made against the one control treatment. Although the inclusion of all species and collection sites could reduce independence of some data points, all possible combinations were included to expand the extent of species and location responses. Additionally, studies with other experimental parameters outside of acidification and warming were only utilized in this study when these external factors were considered to be under ambient conditions to prevent confounding results (Chan and Connolly, 2013). In studies that reported calcification over time, only the final growth rate was used in this analysis.
For consistency in treatment analysis, only pCO2 concentrations were used as the measure of acidification manipulation because of the potentially large variation in pH scale based on measurement methods (Zeebe and Wolf-Gladrow, 2001). The wide variety of reported experimental pCO2 values were categorized into one of the two categories: control or high. Below current-day or extreme pCO2 treatments from selected studies were not included in this analysis. In experiments in which more than one elevated pCO2 treatment was used to represent projected scenarios, the higher concentration treatment was selected for the meta-analysis. The pCO2 treatment considered as current-day or ambient for each experiment was categorized in the ‘control' group, despite variations in pCO2 concentrations due to experimental design (380–513 μatm). Similarly, temperature treatments were categorized as either “control” when reported to represent current-day or ambient conditions (26–30.6°C), or as “elevated” in experiments testing warming scenarios (30–32°C). No study selected analyzed the impact of multiple warming levels.
Calcification rate was chosen as the response parameter in this meta-analysis due to its common use as a stress response in manipulations experiments on Caribbean corals. Mean calcification rate, standard error, and sample size of each experiment was extracted from all included studies for the desired treatments. When raw data were not reported, values were mined from the literature using Web Plot Digitizer for analysis (https://apps.automeris.io/wpd/). Two coral species exhibited net dissolution in the control treatments and therefore were excluded from this analysis because of potential bias from additional unknown stressors.
Calculation of Effect Size
Calcification rates in response to independent ocean acidification and warming treatments were measured for each experiment using standard mean differences (SMD), or Hedge's G, to establish the proportion of change between the treatment and control. SMDs were used in this analysis because of the presence of negative calcification rates in some treatments. Analyses were performed in the R (R Core Development Team, 2016) package metafor (version 2.0–0) (Viechtbauer, 2010) to calculate effect sizes (SMD) per experiment.
The interaction strength of ocean acidification and warming was determined using methods for factorial meta-analysis (Crain et al., 2008; Harvey et al., 2013). Only studies that reported outcomes for a fully factorial experiment including acidification (YOA), warming (YOW), the combination of the two (Yboth), and a control (YC) were used to calculate the interaction effect size. The interaction strength was calculated as
where Y is the mean calcification response for the denoted treatment, and s is the pooled standard deviation. Control values are denoted by subscript C, ocean acidification is denoted by subscript OA, warming is denoted by subscript OW, and the combination treatment is represented by subscript both. The sample variance of the effect size was calculated as
where N is the sample size of the indicated treatment.
Statistical Analyses
A random effects model was implemented using the metafor package (function rma.mv) to calculate the mean effect of each treatment on calcification to account for variation in responses due to species and study design. To assess the effects of study design parameters on resulting calcification rates in either acidification or warming studies, two fully additive random effects models (function rma.mv) were fit with magnitude change between control and treatment, irradiance (μmol photons m−2 s−1), and duration (days) as continuous predictors, and seawater (natural vs. artificial) and feeding (2 times a week, 3 times a week, or no data) were assessed as discrete predictors. An additional model was used to compare effect sizes of corals by region. For this model, only corals collected from the Florida Keys or Belize were considered because of the larger sample sizes from these regions. Random intercepts of study and species within treatment were included to account for potential correlation among results due to these factors. When the 95% confidence intervals of each estimated mean do not overlap zero, the effect size is considered clear statistical evidence. A test of moderators (QM) was used to determine differences between variables (temperature and/or region). Additionally, tests for residual heterogeneity (QE) were performed, with QE signifying that additional moderators not considered may be impacting the study results (Tables S2, S3).
To better understand the qualitative differences in calcification responses of corals from Florida and Belize to ocean acidification and warming, linear mixed effects models with observations weighted by sample size divided by standard error were fit using the lme4 package (version 1.1–21) (Bates et al., 2015). Using all warming studies, we compared models with measured calcification rates as response variable and coral collection region, scaled temperature, and experiment duration as predictors with random slopes for study and species. We also fit a quadratic effect of temperature. Model selection using AICc was completed with random effects for species and study. A similar analysis was performed using reported aragonite saturation state (ΩArag) of each treatment to better assess ocean acidification on calcification rates.
Results
In total, 11 studies met the standards of this meta-analysis, including the responses of 13 Caribbean coral species collected from five different countries across the Greater Caribbean Sea (Figure 1; Table S1). Of the studies selected, only four performed fully factorial ocean acidification and warming experiments, and one performed two independent acidification and warming experiments. The most studied coral species from the Caribbean region were Porites astreoides (5 studies), Acropora cervicornis (4 studies), and Siderastrea siderea (4 studies). Finally, the Florida Keys and Belize were the two most-studied regions within the wider Caribbean fitting the criteria of this meta-analysis.
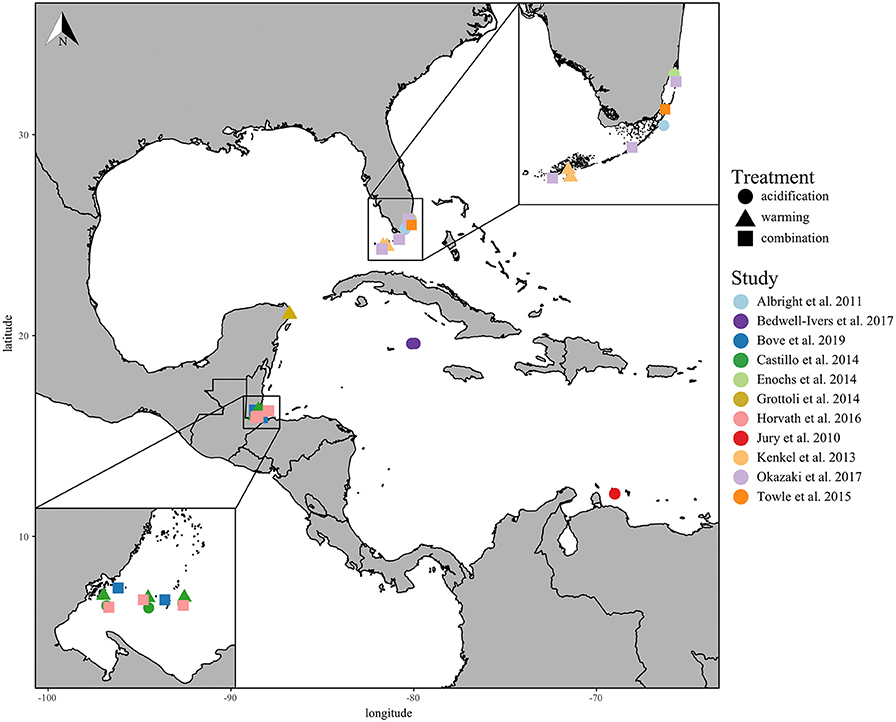
Figure 1. Coral collection sites of all included studies with experimental study represented by color and treatments represented by shape: acidification only (circle), warming only (triangle), and the combination of acidification and warming (square). The lower left insert displays close-up of the Belize collection sites and the upper right insert displays the Florida Keys collection sites.
Overall Calcification Response
Meta-analysis of the dataset revealed that calcification rates of Caribbean corals were reduced by ocean warming but not ocean acidification (Figure 2; Figures S1–S4). However, the 95% confidence interval of the combination of ocean warming and acidification overlapped zero, indicating no statistically clear trend toward synergistic or antagonistic effects of these treatments (Figure 2; Figures S5, S6; Table S2).
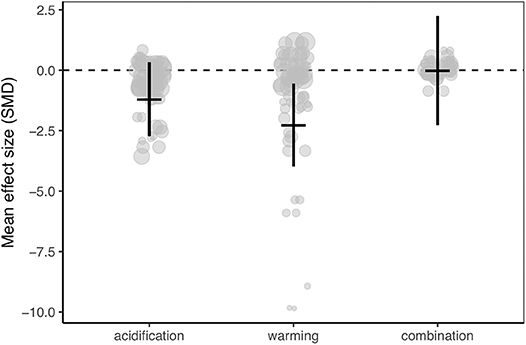
Figure 2. Mean effect (standard mean difference) and 95% confidence interval of ocean acidification, warming, and the combination of acidification and warming on calcification rate for all studies in the meta-analysis. Gray circles indicate the effect size of each individual study and the size of each circle represents the weight of each study (1/SE). Clear statistical evidence of a treatment effect is identified when the 95% confidence interval does not overlap zero.
Calcification Response of Florida Keys vs. Belize Corals
Corals from Belize only exhibited clearly reduced calcification rates under ocean warming (Figure 3A; Table S3), while acidification, warming, and the combination of both stressors did not clearly alter experimental calcification rates of corals from the Florida Keys (Figure 3B; Table S3). Further, the resulting QE suggests there is significant between-study variation (Table S3).
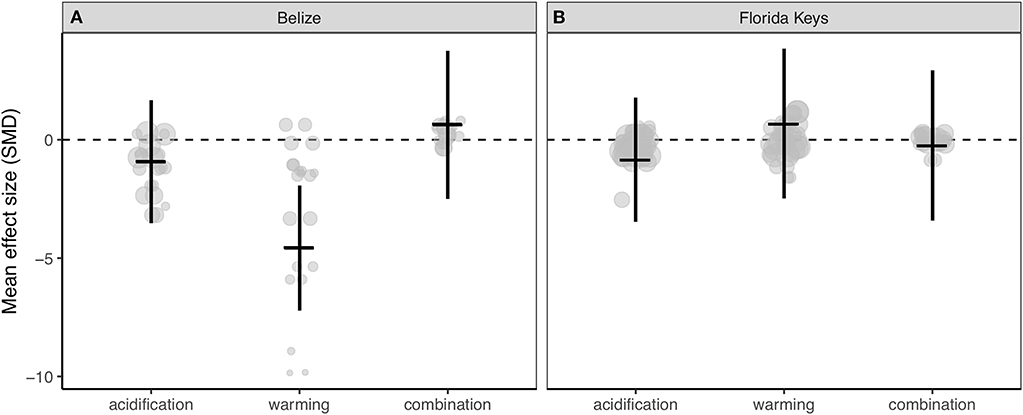
Figure 3. Mean effect (standard mean difference) and 95% confidence interval of ocean acidification, warming, and the combination of acidification and warming on calcification rate for (A) Belize corals and (B) Florida Keys corals. Gray circles indicate the effect size of each individual study and the size of each circle represents the weight of each study (1/SE). Clear statistical evidence of a treatment effect is identified when the 95% confidence interval does not overlap zero.
Temperature and Aragonite Saturation State Impacts on Calcification Rates Across Studies
Secondary analysis of mean calcification rates (mg cm−2 day−1) against treatment temperature across all Florida and Belize studies revealed a parabolic response to temperature (Figure 4; Tables S4, S5). Similarly, mean calcification rates across ΩArag resulted in a non-linear response to acidification (Figure 5; Tables S6, S7). Both non-linear trends in response to temperature and ΩArag were a result of treatment rather than region (Tables S6, S7), suggesting regional differences identified in the meta-analysis were due to experimental designs employed to represent current regional environmental differences.
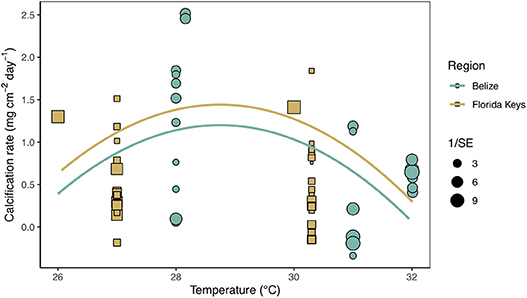
Figure 4. Mean calcification rate (mg cm−2 day−1) of corals from each warming study by treatment temperature (°C) with the linear mixed effects model quadratic fit by region and experimental duration (mean duration = 59.8 days). Shape and color of each point denotes study region (blue circle = Belize; brown square = Florida) and size of shape represents the weight (1/SE) of study.
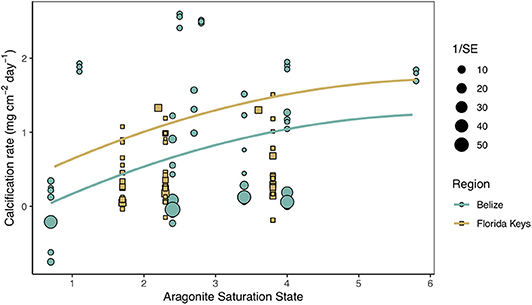
Figure 5. Mean calcification rate (mg cm−2 day−1) of corals from each acidification study by treatment aragonite saturation state (ΩArag) with the linear mixed effects model quadratic fit by region and experimental duration (mean duration = 66.5 days). Shape and color of each point denote study region (blue circle = Belize; brown square = Florida Keys) and size of shape represents the weight (1/SE) of study.
Experimental Design Impacts on Coral Calcification Rate in Studies
Quantification of experimental design parameters within warming studies identified that magnitude of treatment, irradiance, seawater type used, and feeding frequency all clearly impacted calcification rates (Table 1). Specifically, studies that utilized natural seawater and those with a larger difference between the control and treatment temperatures within a study exhibited higher effect sizes, suggesting a less negative effect of treatment. Studies that employed higher irradiance levels in their systems demonstrated more negative effects of treatment light level. Finally, studies that reported feeding their corals twice a week were less impacted by warming treatment than those feeding three times a week, however, studies with no data on feeding were the least affected by treatment. Duration of experiment was deemed redundant in the model and was thus dropped.
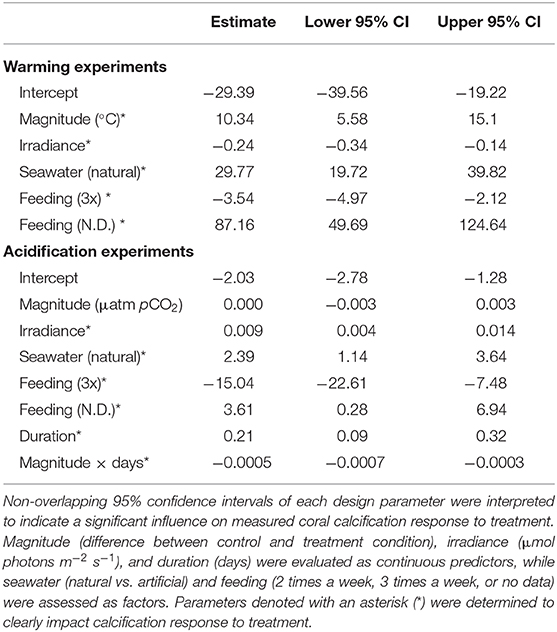
Table 1. Effect size estimate and 95% confidence intervals of experimental design parameters calculated for all warming and acidification experiments.
Within the acidification studies, irradiance, seawater type used, feeding frequency, duration, and the interaction of duration with treatment magnitude impacted effect sizes, while magnitude alone was not clearly different (Table 1). Studies using natural seawater, employing higher irradiance levels, and those with longer durations resulted in greater effect sizes, suggesting they lessened the effects of acidification treatment on calcification responses. Similar to warming studies, acidification studies in which feeding corals was conducted twice a week exhibited less negative responses to treatment than those feeding three times a week, with studies reporting no feeding data exhibiting the least negative responses to treatment. Finally, coral calcification responses were less impacted by acidification in studies with longer duration of exposure and a greater pCO2 change.
Discussion
The current study utilized a meta-analytical approach to analyze the effects of ocean acidification, warming, and the combination of the two stressors on calcification rates of Caribbean corals. The two most-studied regions within the Greater Caribbean, the Florida Keys, and Belize, were further assessed for differences in calcification responses to ocean acidification, warming, and the combination of both stressors.
Ocean Warming, but Not Acidification, Independently Impairs Calcification of Caribbean Reef-Building Corals
Ocean warming alone induced an overall adverse effect on calcification rates of the Caribbean corals analyzed in this meta-analysis, contrasting a previous meta-analysis assessing coral calcification rates from multiple ocean basins (Harvey et al., 2013). However, increased bleaching, mortality, and declines in coral growth in response to warming is well-documented throughout the Caribbean Sea in field studies and reviews (Hughes et al., 2003; Hoegh-Guldberg et al., 2007; Carricart-Ganivet et al., 2012), supporting the overall reduced calcification rates to warming observed in the current meta-analysis. While there is evidence that suggests coral calcification rates, along with other physiological processes, increase along with warming seawater temperatures (Lough and Barnes, 2000), the physiological advantages of warmer waters diminish when a thermal maxima is reached (Bahr et al., 2018; Silbiger et al., 2019). Ocean warming has also been closely associated with coral bleaching (Brown, 1997) and since corals receive a significant proportion of their energetic need from their algal endosymbionts (Muscatine et al., 1981), it is likely that the loss of algal endosymbionts diminished energy reserves utilized in calcification. Thus, a significant number of corals in warming treatments from the included studies also likely exhibited signs of bleaching (i.e., reduced algal endosymbiont density) under elevated temperature, although bleaching was not assessed as a response variable in this meta-analysis due to low reporting and the diversity of bleaching metrics reported in the studies examined.
Ocean acidification did not clearly reduce calcification rates of Caribbean corals, contradictory to the findings of previous meta-analyses that have investigated the calcification response of tropical corals to ocean acidification from various other ocean basins (Kroeker et al., 2010, 2013; Chan and Connolly, 2013; Harvey et al., 2013). Coral calcification is influenced by the ΩArag of seawater, with higher ΩArag (lower pCO2) often promoting faster calcification rates (Langdon and Atkinson, 2005). This aragonite-calcification relationship is most likely driven by corals' ability to control ΩArag at their calcification site by pumping into the calcifying fluid from the surrounding seawater, removing two protons (H+) in the process, finally converting to (Cohen and McConnaughey, 2003; Allemand et al., 2010; Ries, 2011; Von Euw et al., 2017). Thus, even under low aragonite saturation (high pCO2) corals that are able to elicit strong control over this process are likely to be more resilient against the effects of ocean acidification, although it may be energetically costly (Davies et al., 2016) and may impair other physiological processes. It is therefore likely that corals included in this meta-analysis that did not exhibit reduced calcification rates in association with simulated ocean acidification may have stronger control of their calcifying fluid to maintain comparable growth rates. Overall, corals from the studies included in the current meta-analysis exhibited variable responses under experimental seawater acidification, highlighting the diversity of responses to stress on the individual and species levels (Comeau et al., 2014; Okazaki et al., 2017; Bove et al., 2019).
Although ocean warming caused reduced calcification of Caribbean corals in the included studies, the combination of acidification with warming was not clearly different from the two independent stressors (Crain et al., 2008). Because only four studies tested the combination of acidification and warming (Towle et al., 2015; Horvath et al., 2016; Okazaki et al., 2017; Bove et al., 2019), however, the sample size for the interaction term was very low. With this small samples size in conjunction with only about 17% of Caribbean reef-building coral species analyzed in the current study, the potential for synergistic or additive effects should not be ignored. Because synergistic relationships can be unpredictable, especially when additional stressors are introduced (Harvey et al., 2013), it is important that additional combined stressor experiments be conducted to better understand the response of Caribbean reef-building corals to these interrelated stressors.
Calcification Responses to Global Change Stressors Vary by Region Within the Caribbean
Coral calcification responses to ocean acidification, warming, and the combination of the two stressors varied between corals collected from the Florida Keys and those collected from Belize. Corals from the Florida Keys did not exhibit clearly reduced calcification rates under independent ocean acidification and warming, or the combination of ocean acidification and warming. However, corals from Belize reduced calcification rates from ocean warming treatments. This regional difference is likely due in part to adaptation of the corals in each region to their local environmental conditions (Oliver and Palumbi, 2011; Putnam et al., 2017). Corals found off the coast of Belize experience a much narrower range of annual sea surface temperatures (ca 24–32°C) than corals located off the southern coast of Florida (ca 18–31°C) (Castillo and Lima, 2010; Okazaki et al., 2017), resulting in a higher annual mean temperature in Belize than the Florida Keys. This difference in annual temperature variability has likely led to the adaptation of the corals in Florida to more extreme temperatures, resulting in different thermal susceptibilities than corals in Belize (Marshall and Baird, 2000; Guest et al., 2012) and may act as a potential pathways of adaptation or acclimatization to projected global change. Although statistically clear effect sizes may be due to variations in natal environments, differences in experimental treatment levels may also confound these results. Indeed, further analysis identified that treatment level predominately drove resulting calcification rates, not specifically collection region. Further, the resulting model fit to the calcification rates across temperature exhibited a quadratic fit, similar to what would be expected for a thermal performance curve (Portner et al., 2006). Reef-building corals generally exhibit a threshold response to ocean warming when a particularly high temperature or prolonged exposure to warming water causes bleaching, reduced growth rates, and mortality (Glynn, 1996; Brown, 1997). However, the treatment temperatures used in the Florida and Belize experiments represent warming scenarios specific to the corresponding region, highlighting already-present warming patterns of these regions. Indeed, elevated temperatures employed in studies using Belize corals were 1–2°C higher than the elevated temperatures used in Florida experiments (31–32 vs. 30°C), reflecting differences in annual mean temperatures (Castillo and Lima, 2010; Okazaki et al., 2017). Additionally, the temperatures employed in the Florida Keys studies were representative of the bleaching threshold for the region (Manzello et al., 2007), while the Belize studies used end-of-century projections. Corals are known to live within a narrow thermal range (Kleypas et al., 1999) and the Florida studies tested the upper end of that threshold, while the Belize studies pushed beyond that limit. Consequently, the clear effect of temperature on Belize corals is due largely to the selected treatment temperature employed in each study, although this is likely indicative of differing warming patterns between these regions.
Overall coral calcification rates in response to region and treatment ΩArag across ocean acidification studies was also assessed, again resulting in a non-linear response to changing seawater chemistry. Because response of corals to ocean acidification is heavily dependent on extent of seawater chemistry change, duration, and individual colony susceptibility (Orr et al., 2005; Ries et al., 2010; Schoepf et al., 2017), it is not surprising that the non-linear response quantified in the present meta-analysis is not as dramatic as the temperature response. Although studies based their acidification treatments on projected end-of-century concentrations relevant for the environment from which the corals were collected, this resulted in a wide range of experimental pCO2 concentrations (Belize 600–900 μatm vs. 750–1,340 μatm Florida Keys). While the exact relationship between calcification and pCO2 (or ΩArag) varies, the general relationship suggests increased pCO2 reduces coral calcification (Langdon and Atkinson, 2005; Chan and Connolly, 2013). Indeed, calcification rates of Caribbean corals exhibit a general decline in response to reduced ΩArag, similar to the 15% decline in calcification reported by Chan and Connolly (2013) for every unit decrease in ΩArag. Although this is a significant decline in skeletal growth in terms of maintaining reef production, this reduction is much less dramatic than that of the measured calcification decline associated in the included ocean warming studies. This difference in threshold responses suggests that while acidification is going to be a chronic stressor continually impacting carbonate production and maintenance on coral reefs, increasing warming events will pose a more immediate and dramatic threat on the future of tropical coral reefs.
Experimental Design Considerations and Recommendations for Future Research
With such variation in study implementation and lingering gaps in the literature, results from this meta-analysis may not capture all underlying causes of calcification responses to ocean acidification, warming, and the combination of stressors. Several gaps in simulated global change studies using corals were identified in this meta-analysis including coral collection sites, variety of species included, and experimental design differences. Although corals from the Florida Keys and Belize were heavily represented in the meta-analysis, only a single study collected corals from reefs south of Belize (Jury et al., 2010) and in the Eastern Caribbean (Bedwell-Ivers et al., 2017). This scattered sampling leaves much of the Caribbean understudied in regards to how coral reefs may respond to ocean acidification and warming as the two stressors continue to co-occur. Additionally, only about 17% of Caribbean coral diversity was represented, leaving out a significant percentage of coral species from the region, including several dominant reef-building species. Without having a better understanding of the calcification responses of other species within the Caribbean, meta-analyses like this will not be able to accurately predict potential reef-wide responses to global change. Future studies should consider what regions and species within the Caribbean have been extensively studied in similar ocean acidification and warming experiments and aim to expand outside of these regions and/or species in designing experiments.
In addition to ensuring that experimental treatments (temperature and pCO2) represent ecologically relevant conditions, it is important to consider how experimental design dictates resulting coral calcification responses. For example, within all included studies in this meta-analysis experimental duration ranged from 2 h (Jury et al., 2010) up to 95 days (Castillo et al., 2014), with additional variation in seawater type used, irradiance, and how corals received nutrition throughout the experiments (see Table 1; Table S1). Upon further inspection of these design differences in both warming and acidification studies, the use of natural seawater was associated with higher effect sizes, suggesting less negative responses to treatment than when using artificial seawater, likely due to the different buffering capacity of natural seawater compared to many artificial seawater products (Atkinson and Bingman, 1997). By using natural seawater, especially taken from experimental corals' native reef environments, mesocosm studies may also be able to accommodate more difficult to rear species to expand the range of species used in such experiments. However, the benefits of using artificial seawater (i.e., potential for more consistent results between studies) should also be weighed in the planning of mesocosm experiments. Additionally, reported feeding across both acidification and warming studies played a significant role in resulting coral calcification rates, with studies feeding corals twice weekly exhibiting less negative effects of treatment than in studies feeding three times. Although increased heterotrophy may alleviate stress associated with increasing temperatures and decreasing ΩArag (Towle et al., 2015; Brown et al., 2018), the higher nutrient load on the experimental systems as a result of increased feeding may harm physiological processes in the corals, reducing overall growth rates (D'Angelo and Wiedenmann, 2014). Of the studies that did not report feeding frequency, all utilized natural seawater in their experimental systems, thus is it likely that the authors relied on natural nutrients and plankton abundances that were more beneficial for the corals. Efforts should be made to establish and report reasonable feeding practices (i.e., quantity, frequency, type of food) based on coral species, collection depth, and collection location for each study to reduce the impacts of starvation or overeating on measured coral responses.
Interestingly, the present meta-analysis identified that higher irradiance levels in warming studies were associated with more negative effects of warming on calcification rates, while higher irradiance in acidification studies potentially acted as a rescue effect in response to treatment, contrary to Chan and Connolly (2013). These results suggest that the combination of high light levels with warming seawater temperatures may exacerbate bleaching-associated reduced growth rates (Brown, 1997), while higher light levels may alleviate the negative impacts of ocean acidification by stimulating photosynthesis of the coral algal endosymbionts (Suggett et al., 2013). In addition to different responses to irradiance between the two study types, experimental duration did not play a role in warming studies while longer acidification experiments exhibited slightly less negative responses to treatment, differing from the meta-analysis performed by Chan and Connolly (2013). However, longer acidification experiments with greater treatment magnitude exhibited more negative responses to treatment, likely indicative of ocean acidification being a chronic stressor while ocean warming is generally associated with acute stress events (Hoegh-Guldberg et al., 2007). As further experiments are designed, care should be taken to use ecologically-relevant experimental durations that appropriately address questions being addressed. In order to understand how corals respond under chronic acidification and acute warming stress, future experiments should consider conducting such research on the scale of months to years as possible. The recommendations put forth in this study should be considered when designing future Caribbean coral global change studies, even those outside acidification and warming treatments, along with other previously suggested (Widdicombe et al., 2010) to improve the overall understanding of Caribbean corals under projected global change.
Conclusions
Overall, results from this meta-analysis suggest the sensitivity of Caribbean corals to ocean warming, while also identifying several current knowledge gaps and potential opportunities for future research. Specifically, this analysis highlights the need for further studies including the combination of ocean acidification and warming, as well as expanding the research to additional Caribbean reef-building coral species and regions. Furthermore, although differences in calcification responses between the Florida Keys and Belize were detected in this meta-analysis, further analysis determined this difference was largely attributed to the treatments employed, highlighting the significance of experimental protocols in measured results. The studies reviewed in the current analysis exhibit a wide range of experimental designs, including variations in duration, feeding, irradiance, treatment levels, and even seawater. These variations in experimental implementation play a major role in the results of the current meta-analysis and suggest that consideration of previous experimental designs should be made when designing new experiments to make the results more comparable. Additionally, future meta-analyses of Caribbean corals should include additional parameters outside of calcification, such as bleaching, metabolism, and survival. The inclusion of additional responses will improve the overall understanding of how corals within the wider Caribbean may respond under projected ocean acidification and warming. Because ecosystem level experiments are difficult to conduct, meta-analyses are important tools for understanding the responses of a wide variety of species to projected global change stressors. This analysis provides valuable insight into the calcification response of corals throughout the Caribbean; however, further experiments must be conducted to expand understanding beyond the small selection of species and regions included in this meta-analysis.
Data Availability Statement
The datasets and code analyzed for this study can be found on GitHub (https://github.com/seabove7/Bove2020_MetaAnalysis_FrontMarSci).
Author Contributions
CB, JU, and KC conceived and designed the study. CB led and executed the meta-analysis. CB and JU completed all analyses. CB drafted the manuscript with contributions from co-authors. All authors gave final approval of this manuscript for publication.
Funding
CB was supported through a UNC Dissertation Completion Fellowship for a portion of this work.
Conflict of Interest
The authors declare that the research was conducted in the absence of any commercial or financial relationships that could be construed as a potential conflict of interest.
Acknowledgments
We are grateful to the authors of the many studies that informed this meta-analysis. We also thank J. Ries, S. Davies, and M. Piehler for reading early drafts of this manuscript and providing helpful feedback.
Supplementary Material
The Supplementary Material for this article can be found online at: https://www.frontiersin.org/articles/10.3389/fmars.2020.00127/full#supplementary-material
References
Allemand, D., Tambutté, É., Zoccola, D., and Tambutté, S. (2010). “Coral calcification, cells to reefs,” in Coral Reefs: An Ecosystem in Transition, eds Z. Dubinsky and N. Stambler (Dordrecht: Springer), 119–150. doi: 10.1007/978-94-007-0114-4_9
Alvarez-Filip, L., Dulvy, N. K., Gill, J. A., Cote, I. M., and Watkinson, A.R. (2009). Flattening of Caribbean coral reefs: region-wide declines in architectural complexity. Proc. R. Soc. B-Biol. Sci. 276, 3019–3025. doi: 10.1098/rspb.2009.0339
Atkinson, M. J., and Bingman, C. (1997). Elemental composition of commercial seasalts. J. Aqua. Aquat. Sci. 8, 39–43.
Bahr, K. D., Rodgers, K. S., and Jokiel, P. L. (2018). Ocean warming drives decline in coral metabolism while acidification highlights species-specific responses. Mar. Biol. Res. 14, 924–935. doi: 10.1080/17451000.2018.1551616
Bates, D., Machler, M., Bolker, B. M., and Walker, S. C. (2015). Fitting linear mixed-effects models using lme4. J. Stat. Softw. 67, 1–48. doi: 10.18637/jss.v067.i01
Bedwell-Ivers, H. E., Koch, M. S., Peach, K. E., Joles, L., Dutra, E., and Manfrino, C. (2017). The role of in hospite zooxanthellae photophysiology and reef chemistry on elevated pCO(2) effects in two branching Caribbean corals: acropora cervicornis and porites divaricata. Ices J. Mar. Sci. 74, 1103–1112. doi: 10.1093/icesjms/fsw026
Bove, C., Ries, J., Davies, S., Westfield, I., Umbanhowar, J., and Castillo, K. (2019). Common Caribbean corals exhibit highly variable responses to future acidification and warming. Proc. R. Soc. B-Biol. Sci. 286. doi: 10.1098/rspb.2018.2840
Brown, B. E. (1997). Coral bleaching: causes and consequences. Coral Reefs 16, S129–S138. doi: 10.1007/s003380050249
Brown, N. E. M., Bernhardt, J. R., Anderson, K. M., and Harley, C. D. G. (2018). Increased food supply mitigates ocean acidification effects on calcification but exacerbates effects on growth. Sci. Rep. 8:9800. doi: 10.1038/s41598-018-28761-8
Carricart-Ganivet, J. P., Cabanillas-Teran, N., Cruz-Ortega, I., and Blanchon, P. (2012). Sensitivity of calcification to thermal stress varies among genera of massive reef-building corals. PLoS ONE 7:e32859. doi: 10.1371/journal.pone.0032859
Castillo, K. D., and Lima, F. P. (2010). Comparison of in situ and satellite-derived (MODIS-aqua/terra) methods for assessing temperatures on coral reefs. Limnol. Oceanogr. Meth. 8, 107–117. doi: 10.4319/lom.2010.8.0107
Castillo, K. D., Ries, J. B., Bruno, J. F., and Westfield, I. T. (2014). The reef-building coral Siderastrea siderea exhibits parabolic responses to ocean acidification and warming. Proc. R. Soc. B-Biol. Sci. 281:20141856. doi: 10.1098/rspb.2014.1856
Chan, N. C. S., and Connolly, S. R. (2013). Sensitivity of coral calcification to ocean acidification: a meta-analysis. Glob. Chang. Biol. 19, 282–290. doi: 10.1111/gcb.12011
Cohen, A. L., and McConnaughey, T. A. (2003). Geochemical perspectives on coral mineralization. Biomineralization 54, 151–187. doi: 10.1515/9781501509346-011
Comeau, S., Edmunds, P. J., Spindel, N. B., and Carpenter, R. C. (2014). Fast coral reef calcifiers are more sensitive to ocean acidification in short-term laboratory incubations. Limnol. Oceanogr. 59, 1081–1091. doi: 10.4319/lo.2014.59.3.1081
Costanza, R., de Groot, R., Sutton, P., van der Ploeg, S., Anderson, S. J., Kubiszewski, I., et al. (2014). Changes in the global value of ecosystem services. Global Environ. Chang. Hum. Pol. Dimen. 26, 152–158. doi: 10.1016/j.gloenvcha.2014.04.002
Cote, I. M., Gill, J. A., Gardner, T. A., and Watkinson, A. R. (2005). Measuring coral reef decline through meta-analyses. Philos Trans R Soc Lond B Biol Sci. 360, 385–395. doi: 10.1098/rstb.2004.1591
Crain, C. M., Kroeker, K., and Halpern, B. S. (2008). Interactive and cumulative effects of multiple human stressors in marine systems. Ecol. Lett. 11, 1304–1315. doi: 10.1111/j.1461-0248.2008.01253.x
D'Angelo, C., and Wiedenmann, J. (2014). Impacts of nutrient enrichment on coral reefs: new perspectives and implications for coastal management and reef survival. Curr. Opi. Environ. Sus. 7, 82–93. doi: 10.1016/j.cosust.2013.11.029
Davies, S. W., Marchetti, A., Ries, J. B., and Castillo, K. D. (2016). Thermal and pCO2 stress elicit divergent transcriptomic responses in a resilient coral. Front. Mar. Sci. 3:112. doi: 10.3389/fmars.2016.00112
Doney, S. C., Fabry, V. J., Feely, R. A., and Kleypas, J. A. (2009). Ocean acidification: the other CO2 problem. Ann. Rev. Mar. Sci. 1, 169–192. doi: 10.1146/annurev.marine.010908.163834
Enochs, I. C., Manzello, D. P., Kolodziej, G., Noonan, S. H. C., Valentino, L., and Fabricius, K.E. (2016). Enhanced macroboring and depressed calcification drive net dissolution at high-CO2 coral reefs. Proc. R. Soc. B-Biol. Sci. 283:20161742. doi: 10.1098/rspb.2016.1742
Gattuso, J. P., Hoegh-Guldberg, O., and Pörtner, H. O. (2014). “Cross-Chapter Box on Coral Reefs,” in Climate Change 2014: Impacts, Adaptation, and Vulnerability. Part A: Global and Sectoral Aspects. Contribution of Working Group II to the Fifth Assessment Report of the Intergovernmental Panel on Climate Change, eds C. B. Field, V. R. Barros, D. J. Dokken, K. J. Mach, M. D. Mastrandrea, T. E. Bilir, et al. (Cambridge; New York, NY: Cambridge University Press), 97–100.
Glynn, P. W. (1996). Coral reef bleaching: facts, hypotheses and implications. Glob. Chang. Biol. 2, 495–509. doi: 10.1111/j.1365-2486.1996.tb00063.x
Grottoli, A. G., Rodrigues, L. J., and Palardy, J. E. (2006). Heterotrophic plasticity and resilience in bleached corals. Nature 440, 1186–1189. doi: 10.1038/nature04565
Guest, J. R., Baird, A. H., Maynard, J. A., Muttaqin, E., Edwards, A. J., Campbell, S. J., et al. (2012). Contrasting patterns of coral bleaching susceptibility in 2010 suggest an adaptive response to thermal stress. PLoS ONE 7:e33353. doi: 10.1371/journal.pone.0033353
Gurevitch, J., and Hedges, L. V. (1999). Statistical issues in ecological meta-analyses. Ecology 80, 1142–1149. doi: 10.1890/0012-9658(1999)080[1142:SIIEMA]2.0.CO;2
Halpern, B. S., Selkoe, K. A., Micheli, F., and Kappel, C. V. (2007). Evaluating and ranking the vulnerability of global marine ecosystems to anthropogenic threats. Conserv. Biol. 21, 1301–1315. doi: 10.1111/j.1523-1739.2007.00752.x
Harvey, B. P., Gwynn-Jones, D., and Moore, P. J. (2013). Meta-analysis reveals complex marine biological responses to the interactive effects of ocean acidification and warming. Ecol. Evol. 3, 1016–1030. doi: 10.1002/ece3.516
Hoegh-Guldberg, O., Mumby, P. J., Hooten, A. J., Steneck, R. S., Greenfield, P., Gomez, E., et al. (2007). Coral reefs under rapid climate change and ocean acidification. Science 318, 1737–1742. doi: 10.1126/science.1152509
Horvath, K. M., Castillo, K. D., Armstrong, P., Westfield, I. T., Courtney, T., and Ries, J. B. (2016). Next-century ocean acidification and warming both reduce calcification rate, but only acidification alters skeletal morphology of reef-building coral Siderastrea siderea. Sci. Rep. 6:29613. doi: 10.1038/srep29613
Hughes, T. P., Baird, A. H., Bellwood, D. R., Card, M., Connolly, S. R., Folke, C., et al. (2003). Climate change, human impacts, and the resilience of coral reefs. Science 301, 929–933. doi: 10.1126/science.1085046
IPCC (2014). “Climate change 2014: synthesis Report,” in Contribution of Working Groups I, II and III to the Fifth Assessment Report of the Intergovernmental Panel on Climate Change, ed R. K. P.a. L. A. M. Core Writing Team (Geneva: IPCC), 1–151.
Jokiel, P. L., and Coles, S. L. (1977). Effects of temperature on mortality and growth of Hawaiian reef corals. Mar. Biol. 43, 201–208. doi: 10.1007/BF00402312
Jury, C. P., Whitehead, R. F., and Szmant, A. M. (2010). Effects of variations in carbonate chemistry on the calcification rates of madracis auretenra (= madracis mirabilis sensu wells, 1973): bicarbonate concentrations best predict calcification rates. Glob. Chang. Biol. 16, 1632–1644. doi: 10.1111/j.1365-2486.2009.02057.x
Kelley, A. L., and Lunden, J. J. (2017). Meta-analysis identifies metabolic sensitivities to ocean acidification running title: ocean acidification impacts metabolic function. Aims Environ. Sci. 4, 709–729. doi: 10.3934/environsci.2017.5.709
Kenkel, C. D., Almanza, A. T., and Matz, M. V. (2015). Fine-scale environmental specialization of reef-building corals might be limiting reef recovery in the Florida keys. Ecology 96, 3197–3212. doi: 10.1890/14-2297.1
Kleypas, J. A., McManus, J. W., and Menez, L. A. B. (1999). Environmental limits to coral reef development: where do we draw the line? Am. Zool. 39, 146–159. doi: 10.1093/icb/39.1.146
Kroeker, K. J., Kordas, R. L., Crim, R., Hendriks, I. E., Ramajo, L., Singh, G. S., et al. (2013). Impacts of ocean acidification on marine organisms: quantifying sensitivities and interaction with warming. Glob. Chang. Biol. 19, 1884–1896. doi: 10.1111/gcb.12179
Kroeker, K. J., Kordas, R. L., Crim, R. N., and Singh, G. G. (2010). Meta-analysis reveals negative yet variable effects of ocean acidification on marine organisms. Ecol. Lett. 13, 1419–1434. doi: 10.1111/j.1461-0248.2010.01518.x
Langdon, C., and Atkinson, M. J. (2005). Effect of elevated pCO(2) on photosynthesis and calcification of corals and interactions with seasonal change in temperature/irradiance and nutrient enrichment. J. Geophy. Res. Oceans 110:16. doi: 10.1029/2004JC002576
Lough, J. M., and Barnes, D. J. (2000). Environmental controls on growth of the massive coral Porites. J. Exp. Mar. Biol. Ecol. 245, 225–243. doi: 10.1016/S0022-0981(99)00168-9
Manzello, D., Berkelmans, R., and Hendee, J. (2007). Coral bleaching indices and thresholds for the Florida reef tract, Bahamas, and St. Croix, US Virgin Islands. Mar. Poll. Bull. 54, 1923–1931. doi: 10.1016/j.marpolbul.2007.08.009
Marshall, P. A., and Baird, A. H. (2000). Bleaching of corals on the great barrier reef: differential susceptibilities among taxa. Coral Reefs 19, 155–163. doi: 10.1007/s003380000086
Muscatine, L., McCloskey, L. R., and Marian, R. E. (1981). Estimating the daily contribution of carbon from zooxanthellae to coral animal respiration. Limnol. Oceanogr. 26, 601–611. doi: 10.4319/lo.1981.26.4.0601
Norstrom, A. V., Nystrom, M., Lokrantz, J., and Folke, C. (2009). Alternative states on coral reefs: beyond coral-macroalgal phase shifts. Mar. Ecol. Prog. Ser. 376, 295–306. doi: 10.3354/meps07815
Okazaki, R. R., Towle, E. K., van Hooidonk, R., Mor, C., Winter, R. N., Piggot, A. M., et al. (2017). Species-specific responses to climate change and community composition determine future calcification rates of Florida keys reefs. Glob. Chang. Biol. 23, 1023–1035. doi: 10.1111/gcb.13481
Oliver, T. A., and Palumbi, S. R. (2011). Do fluctuating temperature environments elevate coral thermal tolerance? Coral Reefs 30, 429–440. doi: 10.1007/s00338-011-0721-y
Orr, J. C., Fabry, V. J., Aumont, O., Bopp, L., Doney, S. C., Feely, R. A., et al. (2005). Anthropogenic ocean acidification over the twenty-first century and its impact on calcifying organisms. Nature 437, 681–686. doi: 10.1038/nature04095
Pandolfi, J. M., Bradbury, R. H., Sala, E., Hughes, T. P., Bjorndal, K. A., Cooke, R. G., et al. (2003). Global trajectories of the long-term decline of coral reef ecosystems. Science 301, 955–958. doi: 10.1126/science.1085706
Perry, C. T., Murphy, G. N., Kench, P. S., Smithers, S. G., Edinger, E. N., Steneck, R. S., et al. (2013). Caribbean-wide decline in carbonate production threatens coral reef growth. Nat. Commun. 4:1402. doi: 10.1038/ncomms2409
Portner, H. O., Bennett, A. F., Bozinovic, F., Clarke, A., Lardies, M. A., Lucassen, M., et al. (2006). Trade-offs in thermal adaptation: the need for a molecular to ecological integration. Physiol. Biochem. Zoo. 79, 295–313. doi: 10.1086/499986
Putnam, H. M., Barott, K. L., Ainsworth, T. D., and Gates, R. D. (2017). The Vulnerability and resilience of reef-building corals. Curr. Biol. 27, R528–R540. doi: 10.1016/j.cub.2017.04.047
R Core Development Team (2016). R: A Language and Environment for Statistical Computing. Vienna: R Foundation for Statistical Computing.
Ries, J. B. (2011). A physicochemical framework for interpreting the biological calcification response to CO2-induced ocean acidification. Geochim. Cosmochim. Acta 75, 4053–4064. doi: 10.1016/j.gca.2011.04.025
Ries, J. B., Cohen, A. L., and McCorkle, D. C. (2010). A nonlinear calcification response to CO2-induced ocean acidification by the coral oculina arbuscula. Coral Reefs 29, 661–674. doi: 10.1007/s00338-010-0632-3
Schoepf, V., Jury, C. P., Toonen, R. J., and McCulloch, M. T. (2017). Coral calcification mechanisms facilitate adaptive responses to ocean acidification. Proc. R. Soc. B Biol. Sci. 284:20172117 doi: 10.1098/rspb.2017.2117
Schutte, V. G. W., Selig, E. R., and Bruno, J. F. (2010). Regional spatio-temporal trends in Caribbean coral reef benthic communities. Mar. Ecol. Prog. Ser. 402, 115–122. doi: 10.3354/meps08438
Silbiger, N. J., Goodbody-Gringley, G., Bruno, J. F., and Putnam, H. M. (2019). Comparative thermal performance of the reef-building coral orbicella franksi at its latitudinal range limits. Mar. Biol. 166:126. doi: 10.1007/s00227-019-3573-6
Stoltenberg, L., Schulz, K. G., Cyronak, T., and Eyre, B. D. (2019). Seasonal variability of calcium carbonate precipitation and dissolution in shallow coral reef sediments. Limnol. Ocean. 9999, 1–16. doi: 10.1002/lno.11357
Suggett, D. J., Dong, L. F., Lawson, T., Lawrenz, E., Torres, L., and Smith, D. J. (2013). Light availability determines susceptibility of reef building corals to ocean acidification. Coral Reefs 32, 327–337. doi: 10.1007/s00338-012-0996-7
Towle, E. K., Enochs, I. C., and Langdon, C. (2015). Threatened Caribbean coral is able to mitigate the adverse effects of ocean acidification on calcification by increasing feeding rate. PLoS ONE 10:e0123394. doi: 10.1371/journal.pone.0123394
Viechtbauer, W. (2010). Conducting meta-analyses in R with the metafor package. J. Stat. Softw. 36, 1–48. doi: 10.18637/jss.v036.i03
Von Euw, S., Zhang, Q. H., Manichev, V., Murali, N., Gross, J., Feldman, L. C., et al. (2017). Biological control of aragonite formation in stony corals. Science 356, 933–938. doi: 10.1126/science.aam6371
Widdicombe, S., Dupont, S., and Thorndyke, M. (2010). “Laboratory experiments and benthic mesocosm studies,” in Guide to Best Practises for Ocean Acidification Research and Data Reporting, eds U. Riebesell, V. J. Fabry, L. Hansson, and J. P. Gattuso (Luxembourg: Publications Office of the European Union).
Keywords: meta-analysis, Caribbean corals, ocean acidification, ocean warming, calcification
Citation: Bove CB, Umbanhowar J and Castillo KD (2020) Meta-Analysis Reveals Reduced Coral Calcification Under Projected Ocean Warming but Not Under Acidification Across the Caribbean Sea. Front. Mar. Sci. 7:127. doi: 10.3389/fmars.2020.00127
Received: 08 November 2019; Accepted: 17 February 2020;
Published: 03 March 2020.
Edited by:
Michelle Jillian Devlin, Centre for Environment, Fisheries and Aquaculture Science (CEFAS), United KingdomReviewed by:
Ian C. Enochs, University of Miami, United StatesDerek Manzello, National Oceanic and Atmospheric Administration (NOAA), United States
Copyright © 2020 Bove, Umbanhowar and Castillo. This is an open-access article distributed under the terms of the Creative Commons Attribution License (CC BY). The use, distribution or reproduction in other forums is permitted, provided the original author(s) and the copyright owner(s) are credited and that the original publication in this journal is cited, in accordance with accepted academic practice. No use, distribution or reproduction is permitted which does not comply with these terms.
*Correspondence: Colleen B. Bove, Y29sbGVlbmJvdmVAZ21haWwuY29t