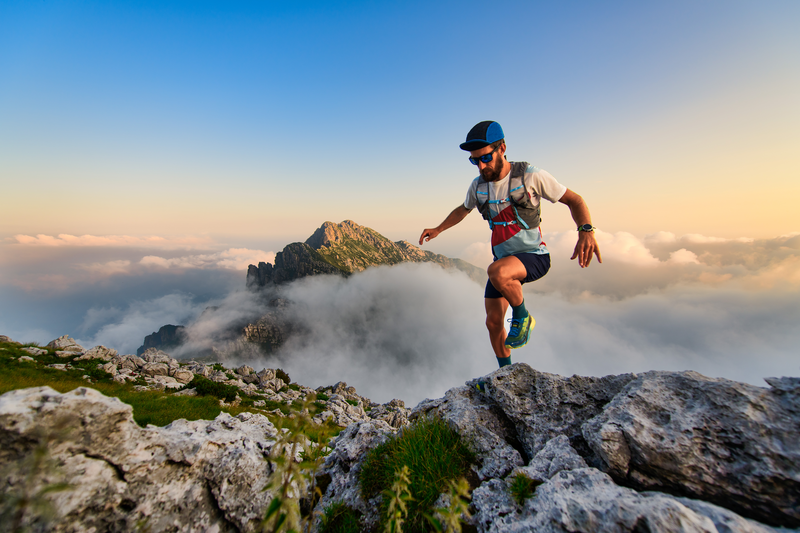
94% of researchers rate our articles as excellent or good
Learn more about the work of our research integrity team to safeguard the quality of each article we publish.
Find out more
EDITORIAL article
Front. Mar. Sci. , 14 February 2020
Sec. Coastal Ocean Processes
Volume 7 - 2020 | https://doi.org/10.3389/fmars.2020.00046
This article is part of the Research Topic Facing Marine Deoxygenation View all 15 articles
Editorial on the Research Topic
Facing Marine Deoxygenation
Marine deoxygenation is increasingly recognized as a major environmental threat (Breitburg et al., 2018).
Global warming drives a substantial part of this deoxygenation trend (Keeling et al., 2010), which is projected to continue during the next decades both in the open ocean and in the coastal waters. Future increasing anthropogenic pressures (e.g., eutrophication) are expected to further exacerbate this trend (Fennel and Testa, 2019).
Several main challenges need to be addressed by the scientific community:
1) To understand natural variability in marine oxygenation. As observations only cover the last ca. 60 years, we lack information about longer-term variability and trends in ocean oxygenation and associated drivers (Kamykowski and Zentara, 1990; Schmidtko et al., 2017; Oschlies et al., 2018).
2) To understand and predict the response of global biogeochemical cycles to deoxygenation. In particular, how lower oxygen conditions affect community respiration, the nitrogen (Zehr, 2009; Lam and Kuypers, 2011) and phosphorus cycles (Conley et al., 2002; Watson et al., 2017) across the estuarine-shelves-ocean continuum, including feed-backs on the climate system.
3) To evaluate and mitigate the threat posed by deoxygenation on valuable marine goods and services (Cooley, 2012) and on marine biodiversity (Vaquer-Sunyer and Duarte, 2008).
Open ocean (Paulmier and Ruiz-Pino, 2009) and coastal (Diaz and Rosenberg, 2008; Rabalais et al., 2009) deoxygenation differ in terms of temporal scale, morphology, driving processes, and implications. However, we gathered contributions related to both typologies in order to highlight interactions, differences and similarities, and to promote a common concern on marine deoxygenation so as to raise public awareness and facilitate mitigation strategies (Levin and Breitburg, 2015).
We provide below an overview of the contributions collected in this Research Topic, as well as emerging points of attention.
The variability in morphological expressions of low oxygen marine conditions is addressed at time scales from millenia to days, and described in relation with driving processes.
Long-term variability of Oxygen Minimum Zones (OMZ) in upwelling systems is addressed by Cardich et al. for the Peruvian system and by Choumiline et al. in the Californian system. Paleo-oceanographic and instrumental records indicate contraction and expansion periods for the Peruvian OMZ through the last 170 years, with a slight modern oxygenation trends (1960–2010) (Cardich et al.). The authors attribute centennial scales of variation to subsurface ventilation, and decadal scales to changes in local productivity. Using sediment core proxy records, Choumiline et al. highlight that expansions of the Californian OMZ during the last millennium coincide with increased productivity and upwelling during cold intervals. OMZ contractions are depicted between those intervals, pointing toward subsurface circulation and solar insolation as lead drivers of OMZ extent at a centennial scale. No evidence for recent warming effect on the OMZ extent could be depicted for the Californian area.
Long term redox history is crucial, as the closest analog for future climate and oceanographic change. However, methodological challenges remain regarding the processing of paleo proxies. Venturelli et al. question oxygenation proxies based on benthic foraminiferal assemblages, by collecting modern assemblages across spatial oxygenation gradients. A dominance of sediment-dwelling taxa over epifaunal taxa is generally considered to indicate low oxygen conditions. Here, the authors evidence that heterogeneous benthic habitat (e.g., grain size) also affects the distribution of benthic foraminifera, and thereby question the use of epifaunal foraminifera presence as an evidence for bottom oxygenated conditions.
On a closer time frame, Trucco-Pignata et al., detail the perturbation of an OMZ located in the tropical Pacific off central Mexico in response to 2015-2016 El-Nino, and describe associated changes in the carbonate system. The authors describe the role of mesoscale activity in driving the transitional regimes before and after the perturbation.
Terrestrial and atmospheric nutrient loads as drivers of coastal and shelves deoxygenation are addressed by Große et al.. As a tool to develop mitigation strategies, the authors propose a modeling approach to map the relative contributions of specific oxygen consumption terms. Targeting N-fueled oxygen consumption in the North Sea, they indicate an important contribution of Atlantic nitrogen inflow, and highlight the role of European rivers (~30–40%) in fueling O2 consumption in the southern North Sea. The authors conclude that reducing riverine N inputs would adequately mitigate low O2 levels in the southern North Sea.
Two manuscripts address microbial respiration as a lead biogeochemical term of the oxygen budget. Robinson review those processes involving or involved in variations of microbial respiration rates, which are seldom accounted for within ocean models. Mechanisms contributing to deoxygenation are reviewed, as well as common techniques for measuring respiration rates and measured ranges in different parts of the ocean. The authors describe recent advances in the field and discuss the combined effect of decreasing oxygen and increasing carbon dioxide concentrations on respiration rates. Torres-Beltrán et al. report outcomes from the Scientific Committee on Oceanographic Research (SCOR) Working Group 144 “Microbial Community Responses to Ocean Deoxygenation” workshop (Vancouver, July 2014), aiming at standardizing process rate and multi-omic data collection in marine low-oxygen environment. In particular, large differences in recovered microbial groups were identified for identical samplings following different experimental protocols, to an extent that might alter inferred metabolic rates. The authors call for standardized techniques facilitating cross-scale comparisons and accurate assessment of in situ microbial activity.
Further contributions aimed at characterizing the impacts of marine deoxygenation on biogeochemical and biological systems.
Impacts on the carbonate system were addressed by Hernandez-Ayon et al. and Trucco-Pignata et al. From a cruise across the Peruvian OMZ, Hernandez-Ayon et al. highlight that oxygenation conditions altered the carbonate system so as to affect air-sea CO2 exchanges estimates, and that the variability and spatial heterogeneity in oxygen conditions hampered clear estimates of in situ based carbon budget for the area.
Michiels et al. address deoxygenation impacts on the nitrogen cycle. They quantify pelagic denitrification and anammox in a permanently anoxic fjord (British Columbia), during and before a water renewal cycle, and set those measurements in relation with microbial communities. Denitrification accounted for two thirds of nitrogen removal, and experienced peaks related with outbursts of an Arcobacter taxa.
Deoxygenation impacts on different life forms are discussed for pelagic (Roman et al.; Limburg and Casini; Steckbauer et al.), and benthic (Sato et al.) communities, as well as for the whole benthic-pelagic ecosystem in the Namibian coast (Currie et al.).
Roman et al. review the indices used to quantify hypoxic stress on zooplankton and fish populations, both from metabolic and ecological perspectives. The authors analyze a metric (Oxygen Stress Level) integrating oxygen demand in relation to oxygen availability for a coastal copepod and compare the prediction of oxygen stress to actual copepod distributions in low-oxygen areas.
Limburg and Casini use otolith proxies to reconstruct exposure of Baltic Cod to hypoxic conditions within different periods. The authors demonstrate how cod otolith can be used to inform retrospectively about exposure to hypoxia, growth and metabolic status, thereby reflecting the worsening situation for cod in the Baltic.
Steckbauer et al. investigate how co-occurence of short-term hypoxia and acidification affects European seabass juveniles, aiming at the synergistic stress that fish populations will have to face in tomorrow's ocean. Results suggest that recovery from acidification takes longer than from short-term hypoxia, by affecting the sensorial and behavioral capacities of fish.
The combined impacts of low oxygen and low pH environment are also addressed by Sato et al. for the benthic fragile sea urchin. Although this urchin might be tolerant to foreseen evolution of oxygen and pH, differences measured across oxygen and pH gradients in critical morphological traits for reproduction and vulnerability indicate that the ecology and fitness of this dominant echinoid might be challenged on the California margin.
Finally, Currie et al. document the Namibian coastal waters as an ecosystem having evolutionarily adapted to cope with perennial anoxia and toxic hydrogen sulfide. This adaptation includes promoting sulfide-oxidizing bacteria as enablers of local detoxified habitat niches and food supply to a specific food-web.
This collection thus extends, non-exhaustively, on important aspects of the marine deoxygenation challenge: from coastal to open ocean frameworks, and from drivers to impacts. A number of overarching gaps emerge:
• Improvement of experimental design is required to isolate multi-stress impacts at different biological levels (i.e., individual metabolism, ecological functions, species interactions) while remaining consistent within realistic natural conditions.
• Driving mechanisms operating over a large range of temporal scales should be embedded within studies aiming at quantifying deoxygenation impacts on biogeochemical cycles, in particular for climatic assessment.
• Metabolic rates and microbial respiration require a strong focus in terms of sampling programs, standardized experimental protocols, and model formulation.
All authors summarized manuscripts submitted to the Research Topic to contribute to this overview. AC merged the contributions and wrote the present editorial. All authors then contributed to revision and editing.
AC was funded by a FRS-FNRS grant (Belgium). PC was supported by Australian Research Council grant DP150101281. EG-R was supported by the Poul Due Jensen Foundation (Denmark). CR was funded by INSU-EC2CO MissRhoDia project and PHC Cai Yuanpei (28001NF).
The authors declare that the research was conducted in the absence of any commercial or financial relationships that could be construed as a potential conflict of interest.
Breitburg, D., Levin, L. A., Oschlies, A., Grégoire, M., Chavez, F. P., Conley, D. J., et al. (2018). Declining oxygen in the global ocean and coastal waters. Science 359:eaam7240. doi: 10.1126/science.aam7240
Conley, D. J., Humborg, C., Rahm, L., Savchuk, O. P., and Wulff, F. (2002). Hypoxia in the Baltic Sea and basin-scale changes in phosphorus biogeochemistry. Environ. Sci. Technol. 36, 5315–5320. doi: 10.1021/es025763w
Cooley, S. R. (2012). How human communities could “feel”changing ocean biogeochemistry. Curr. Opin. Environ. Sustain. 4, 258–263. doi: 10.1016/j.cosust.2012.04.002
Diaz, R. J., and Rosenberg, R. (2008). Spreading dead zones and consequences for marine ecosystems. Science 321,926–929. doi: 10.1126/science.1156401
Fennel, K., and Testa, J. M. (2019). Biogeochemical controls on coastal hypoxia. Annu. Rev. Mar. Sci. 11, 105–130. doi: 10.1146/annurev-marine-010318-095138
Kamykowski, D., and Zentara, S.-J. (1990). Hypoxia in the world ocean as recorded in the historical data set. Deep Sea Res. Part A Oceanogr. Res. Pap. 37,1861–1874. doi: 10.1016/0198-0149(90)90082-7
Keeling, R. E., Körtzinger, A., and Gruber, N. (2010). Ocean deoxygenation in a warming world. Annu. Rev. Mar. Sci. 2, 199–229. doi: 10.1146/annurev.marine.010908.163855
Lam, P., and Kuypers, M. M. M. (2011). Microbial nitrogen cycling processes in oxygen minimum zones. Annu. Rev. Mar. Sci. 3, 317–345. doi: 10.1146/annurev-marine-120709-142814
Levin, L. A., and Breitburg, D. L. (2015). Linking coasts and seas to address ocean deoxygenation. Nat. Clim. Change 5, 401–403. doi: 10.1038/nclimate2595
Oschlies, A., Brandt, P., Stramma, L., and Schmidtko, S. (2018). Drivers and mechanisms of ocean deoxygenation. Nat. Geosci. 11, 467–473. doi: 10.1038/s41561-018-0152-2
Paulmier, A., and Ruiz-Pino, D. (2009). Oxygen minimum zones (OMZs) in the modern ocean. Prog. Oceanogr. 80, 113–128. doi: 10.1016/j.pocean.2008.08.001
Rabalais, N. N., Díaz, R. J. and Levin, L. A. (2009). Dynamics and distribution of natural and human-caused coastal hypoxia. Biogeosciences 7, 585–619. doi: 10.5194/bg-7-585-2010
Schmidtko, S., Stramma, L., and Visbeck, M. (2017). Decline in global oceanic oxygen content during the past five decades. Nature 542, 335–339. doi: 10.1038/nature21399
Vaquer-Sunyer, R., and Duarte, C. M. (2008). Thresholds of hypoxia for marine biodiversity. Proc. Natl. Acad. Sci. U.S.A. 105, 15452–15457. doi: 10.1073/pnas.0803833105
Watson, A. J., Lenton, T. M., and Mills, B. J. W. (2017). Ocean deoxygenation, the global phosphorus cycle and the possibility of human-caused large-scale ocean anoxia. Philos. Trans. Ser. A Math. Phys. Eng. Sci. 375:20160318. doi: 10.1098/rsta.2016.0318
Keywords: marine deoxygenation, multiple stressors, coastal deoxygenation, oxygen minimum zone (OMZ), ocean dead zones, hypoxia, acidification
Citation: Capet A, Cook P, Garcia-Robledo E, Hoogakker B, Paulmier A, Rabouille C and Vaquer-Sunyer R (2020) Editorial: Facing Marine Deoxygenation. Front. Mar. Sci. 7:46. doi: 10.3389/fmars.2020.00046
Received: 09 January 2020; Accepted: 24 January 2020;
Published: 14 February 2020.
Edited and reviewed by: Marta Marcos, University of the Balearic Islands, Spain
Copyright © 2020 Capet, Cook, Garcia-Robledo, Hoogakker, Paulmier, Rabouille and Vaquer-Sunyer. This is an open-access article distributed under the terms of the Creative Commons Attribution License (CC BY). The use, distribution or reproduction in other forums is permitted, provided the original author(s) and the copyright owner(s) are credited and that the original publication in this journal is cited, in accordance with accepted academic practice. No use, distribution or reproduction is permitted which does not comply with these terms.
*Correspondence: Arthur Capet, YWNhcGV0QHVsaWVnZS5iZQ==
Disclaimer: All claims expressed in this article are solely those of the authors and do not necessarily represent those of their affiliated organizations, or those of the publisher, the editors and the reviewers. Any product that may be evaluated in this article or claim that may be made by its manufacturer is not guaranteed or endorsed by the publisher.
Research integrity at Frontiers
Learn more about the work of our research integrity team to safeguard the quality of each article we publish.