- 1Departamento de Biologia e Zoologia, Universidade Federal do Mato Grosso, Cuiabá, Brazil
- 2Departamento de Biomedicina, Biotecnología y Salud Pública, Facultad de Ciencias del Mar y Ambientales, Universidad de Cádiz, Puerto Real, Spain
- 3Departamento de Geneìtica e Evolução, Universidade Federal de Sao Carlos, São Carlos, Brazil
Triportheus (Characiformes, Triportheidae) is a freshwater fish genus with 18 valid species. These fishes are widely distributed in the major river drainages of South America, having commercial importance in the fishing market, mainly in the Amazon basin. This genus has diverged recently in a complex process of speciation carried out in different river basins. The use of repetitive sequences is suitable to trace the genomic reorganizations occured along the speciation process. In this work, the 5S rDNA multigene family has been characterized at molecular and phylogenetic level. The results showed that other multigene family has been found within the non-transcribed spacer (NTS): the U1 snRNA gene. Double-FISH with 5S and U1 probes were also performed, confirming the close linkage between these two multigene families. Moreover, evidences of different transposable elements (TE) were detected within the spacer, thus suggesting a transposon-mediated mechanism of 5S-U1 evolutionary pathway in this genus. Phylogenetic analysis demonstrated a species-specific grouping, except for Triportheus pantanensis, Triportheus aff. rotundatus and Triportheus trifurcatus. The evolutionary model of the 5S rDNA in Triportheus species has been discussed. In addition, the results suggest new clues for the speciation and evolutionary trend in these species, which could be suitable to use in other Characiformes species.
Introduction
Multigene families correspond to a group of genes that have originated by duplication of a common ancestral gene and therefore have similar DNA sequences and related overlapping functions (Martins and Wasko, 2004; Nei and Rooney, 2005). The 5S rDNA array is characterized by multiple copies of one conserved transcriptional unit of about 120 base pairs, separated from each other by a non-transcribed spacer (NTS), which is variable in length and sequence between species (Rebordinos et al., 2013). The NTSs have been subjected by intense mechanisms of evolution, allowing a broader understanding of the organization and evolution of the 5S rDNA array in many organisms (Martins and Wasko, 2004). Additionally, the 5S rDNA can be found linked to other multigene families, including major ribosomal genes, histones, and small nuclear RNA (snRNA) genes (Drouin and Moniz de Sá, 1995; Manchado et al., 2006; Cabral-de-Mello et al., 2010).
The levels of intra or intergenomic divergence displayed by 5S rRNA genes have led the discussion of different evolutionary models to explain the evolution pattern found in each species or between species. A vast number of studies have pointed that the concerted evolution has driven the evolution of 5S rDNA and other multigene families (Drouin and Moniz de Sá, 1995; Eirín-López et al., 2012). However, further studies have demonstrated that the concerted evolution does not clarify the intragenomic divergence of several multigene families found in some organisms, and another model called birth-and-death was proposed (Nei and Hughes, 1992). Besides, a growing number of studies have combined these both models to illustrate the diversified scenarios regarding the 5S rDNAs evolution (Merlo et al., 2012a, 2013). Therefore, NTS regions seem to be subject to rapid evolution, which makes them important for studies concerning the organization and evolution of the 5S multigene family, and as markers for species-specific studies or tracing recent evolutionary events (Rebordinos et al., 2013).
Triportheus (Characiformes, Triportheidae) is a freshwater fish genus with 18 valid species (Froese and Pauly, 2019). These fishes are widely distributed in the major river drainages of South America, having commercial importance in the fishing market, mainly in the Amazon basin (Malabarba, 2004; Gonçalves and Batista, 2008). According to a recent phylogenetic study established for this genus, Triportheus originated at 26.2 ± 6.5 Myr and represents a monophyletic group in which T. auritus is the oldest living species within the genus (Mariguela et al., 2016). Triportheus has stood out in many cytogenetics studies, mainly because all species studied so far have a ZW sex chromosomes system well characterized (Artoni et al., 2001; Diniz et al., 2009; Yano et al., 2016, 2017a). In addition, the rDNAs (Diniz et al., 2009; Marquioni et al., 2013; Yano et al., 2017b) and the U2 snRNA genes (Yano et al., 2017b) have been also investigated, highlighting the variability in the syntenic configuration as well as in the number of sites of these multigene families, mainly regarding the 5S rDNAs (Yano et al., 2017b).
Large volumes of studies have been published on physical mapping of rDNAs in divergent taxa, especially in fish. However, the integration of cytogenetic data with molecular analysis has increasingly brought insights about the evolutionary mechanisms of multigenic families (Manchado et al., 2006; Cabral-de-Mello et al., 2012; Merlo et al., 2012b, 2013). Hence, we have investigated the 5S rRNA genes by molecular and cytogenetics analyses in eight Triportheus species in order to better understand the organization and the evolutionary pattern of this multigene family within the genus. We described here a repeat unit containing the 5S rRNA gene linked to U1 snRNA gene, and therefore, the double-FISH technique was applied to ascertain the results found in the sequences analyses. Finally, the nucleotide variability and the evolutionary divergence between species were estimated to assess if the concerted evolution or birth and death, or if both models are driving the 5S rDNAs in Triportheus.
Materials and Methods
Material Collection, Chromosome Obtaining and DNA Extraction
Individuals of Triportheus albus, Triportheus auritus, Triportheus guentheri, Triportheus nematurus, Triportheus pantanensis, Triportheus aff. rotundatus, Triportheus signatus, and Triportheus trifurcatus from different Brazilian river basins were analyzed. The collections were authorized by the Brazilian environmental agency ICMBIO/SISBIO (License number 48628-2). All species were identified and deposited in the fish museum of the Laboratory of Biology and Genetic of Fishes of the Universidade Estadual Paulista (UNESP-Botucatu, SP), with the respective deposit numbers (Table 1). The experiments followed ethical conducts, in accordance with the Ethics Committee on Animal Experimentation of the Universidade Federal de São Carlos (Process number CEUA 1853260315). Mitotic chromosomes were obtained from cell suspensions of the anterior kidney using the conventional air-drying method, according to Bertollo et al. (2015). Genomic DNA was isolated from liver, following the protocol of Sambrook and Russel (2001), and the extraction quality was validated by electrophoresis in agarose gel (1.5%), using GelRed in the loading buffer.
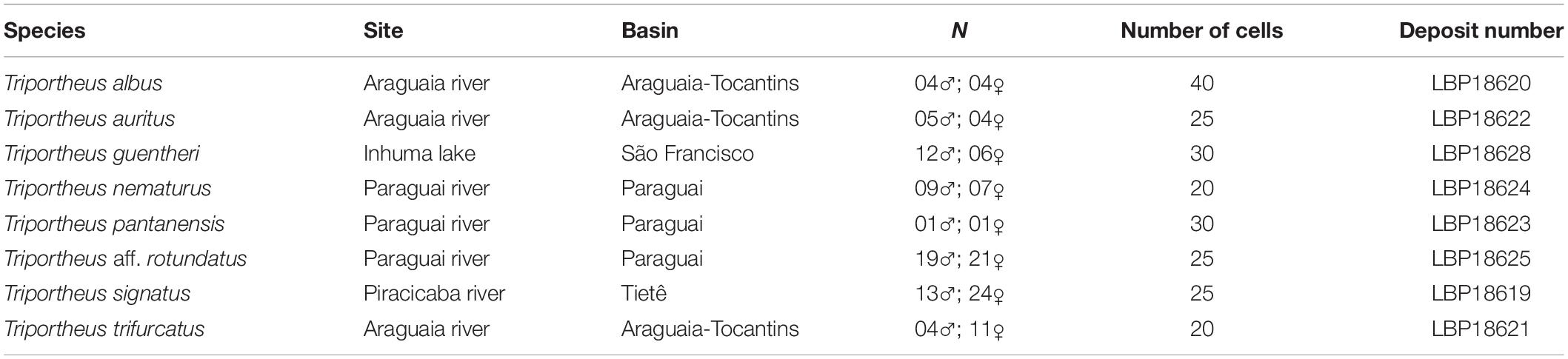
Table 1. Brazilian collection sites of the Triportheus species and number of individuals and cells examined in this study.
5S rDNA Isolation, Cloning, and Sequence Analysis
The PCR amplifications of the 5S rDNA were performed using the following primers 5SF (5′-TACGCCCGATCTCGTCCGATC-3′) and 5SR (5′-CAGGCTGGTATGGCCGTAAGC-3′), as described in Pendas et al. (1994). Reactions were carried out using 0.2 pmol of the forward and reverse primers, 100 ng of genomic DNA, 3 mM Cl2Mg, 300 μM dNTP and 3 U of Taq polymerase (Euroclone) in a final volume of 50 μl. The PCR amplification reactions were performed in a Gene Amp_PCR System 2700 (Applied Biosystems) thermal cycler, according to Cross et al. (2005). The PCR products were purified applying the NucleoSpin® Extract II kit (Macherey–Nagel), cloned into pGEM®-T Easy (Promega) and TOPO® TA Vectors, and the plasmid DNA was extracted using NucleoSpin® Plasmid (Macherey–Nagel), all of these kits were used under the manufacturer’s instructions. The positive clones were sequenced using an ABI3100 Genetic Analyzer, with fluorescence-labeled terminator (BigDye Terminator 3.1 Cycle Sequencing Kit; Applied Biosystems).
Sequence and Phylogenetic Analysis
Sixty-six 5S rDNA-bearing clones were sequenced in this study, and the number of clones for species and individual are described in Table 2. The consensus sequences were obtained with the Geneious software (Kearse et al., 2012), and were subjected to BLASTn searches at the National Center for Biotechnology Information website1 – NCBI. In addition, the CENSOR program (Kohany et al., 2006) was used to search for repeated elements inside spacers. The sequence alignments were performed using MUSCLE (Edgar, 2004). Next, the sequences obtained in this study were deposited in the NCBI database under the Accession Numbers listed in Table 2. The nucleotide variability (π) within species, the number of polymorphic sites (s) and the number of haplotypes (h) in both transcribed and spacer regions were estimated by the DnaSP version 5 program (Librado and Rozas, 2009). The evolutionary divergence among species was also inferred using DnaSP, by the average number of nucleotide differences per site using the Jukes and Cantor method [Dxy(JC)], the number of fixed differences in transcribed region and NTS was also inferred. The PhyML 3.0 program (Guindon et al., 2010) was used to determine the best-fit phylogenetic model and then to run the model. The resulting best-fit model was the generalized time-reversible (GTR) model (Tavaré, 1986), considering the proportion of invariable sites (+I) and gamma distribution (+G). The statistic used for model selection was the akaike information criterion (AIC), the value of which was 18315.92230, and the –LnL was −8784.01056. Branch support was tested by the fast likelihood-based method using aLRT SH-like (Anisimova et al., 2011). Finally, the tree was edited in the MEGA7 program (Kumar et al., 2016). Besides, 5S rDNA sequences were also subjected to a neighbor-net analysis (Bryant and Moulton, 2004) implemented in the SplitsTree 4 package (Huson and Bryant, 2006) using GTR distances.
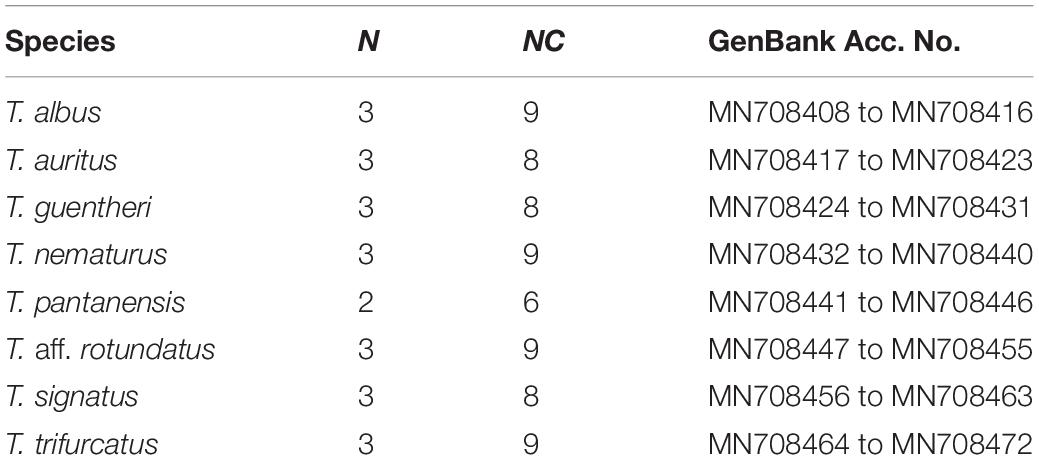
Table 2. Species, number of individuals (N), number of clones sequenced (NC), and GenBank accession number.
Probe Preparation
The PCR product of 5S rDNA was labeled with Cy5-dUTP using Nick-Translation Mix (Roche). The U1 snDNA probe was isolated using the following primers U1F (5′-GCAGTCGAGATTCCCACATT-3′) and U1R (5′-CTTACCTGGCAGGGGAGATA-3′), as described by Silva et al. (2015). The U1 snRNA probe was labeled via PCR using the same set of primers and the cycle program described in Silva et al. (2015) with Spectrum Orange-dUTP (Vysis).
Fluorescence in situ Hybridization
Chromosomal preparations of Triportheus species listed in Table 1 were used for a double-color fluorescence in situ hybridization (FISH) experiment, according to Yano et al. (2017c). The slides were first dehydrated with 70, 85, and 100% ethanol series, and incubated at 60°C for 1 h. Subsequently, they were treated with RNAse (10 mg/mL) for 1 h at 37°C. Next, a 5-min wash using 1× phosphate-buffered saline (PBS) was performed and the slides were treated with 0.005% pepsin solution in 10 mM HCl at 37°C. The slides were washed again with 1x PBS for 5 min. After further washing, the slides were dehydrated with 70, 85, and 100% ethanol series, 3 min each. The desnaturation of the chromosomal DNA was performed in 70% formamide/2× SSC for 3 min at 75°C. The slides were dehydrated in a cold ethanol 70%, and in 85 and 100% at RT, 3 min each. The hybridization mixture, containing 100 ng of each probe, 10 mg/mL dextran sulfate, 2× SSC, and 50% formamide in a final volume of 20 μL, was heated to 86°C for 10 min and then applied to the slides. Hybridization was performed during 16 h at 37°C. After hybridization, the slides were washed in 1× SSC for 5 min at 44°C, and in 4× SSCT using a shaker at room temperature. The slides were dehydrated again in an ethanol series, 3 min each. After the complete drying of the slides, the chromosomes were counterstained with 4′,6-diamidino-2-phenylindole/antifade (1.2 mg/mL; Vector Laboratories).
Microscope Analyses and Image Processing
At least 30 metaphase spreads were analyzed per individual to confirm the FISH results. Images were captured on an Olympus BX50 microscope (Olympus Corporation, Ishikawa, Japan) using CoolSNAP and the Image Pro Plus 4.1 softwares (Media Cybernetics, Silver Spring, MD, United States).
Results
5S rDNA Organization in Triportheus
Electrophoresis of PCR amplifications of 5S rDNAs revealed fragments of ∼1600 bp in length for the Triportheus species. A total of 66 clones were sequenced in the present study, as detailed in Table 2. After using BLASTn, 65 sequences matched with the 5S rRNA gene, consisting in a conservative transcribed region of 120 bp, and an adjacent and variable NTS. The Figure 1 shows an example of the 5S rDNA amplicon of T. nematurus, in which the transcribed region shows the internal control regions (ICR) for all species: Box A located at position 27–41 (positions 50–64 of the 120 bp-transcribed region); intermediate element (IE) at position 44–49 (positions 67–72 of the 120 bp-transcribed region); and Box C at position 57–74 (positions 80–97 of the 120 bp-transcribed region). The terminator region, a T-rich stretch at the end, was also verified and consists of 4–7 thymidine residues. In all sequences the TATA-like (conserved AT rich region) is located at position −30 within the NTS, the GC dinucleotide is at position −11, and the universally conserved cytosine at position −1.
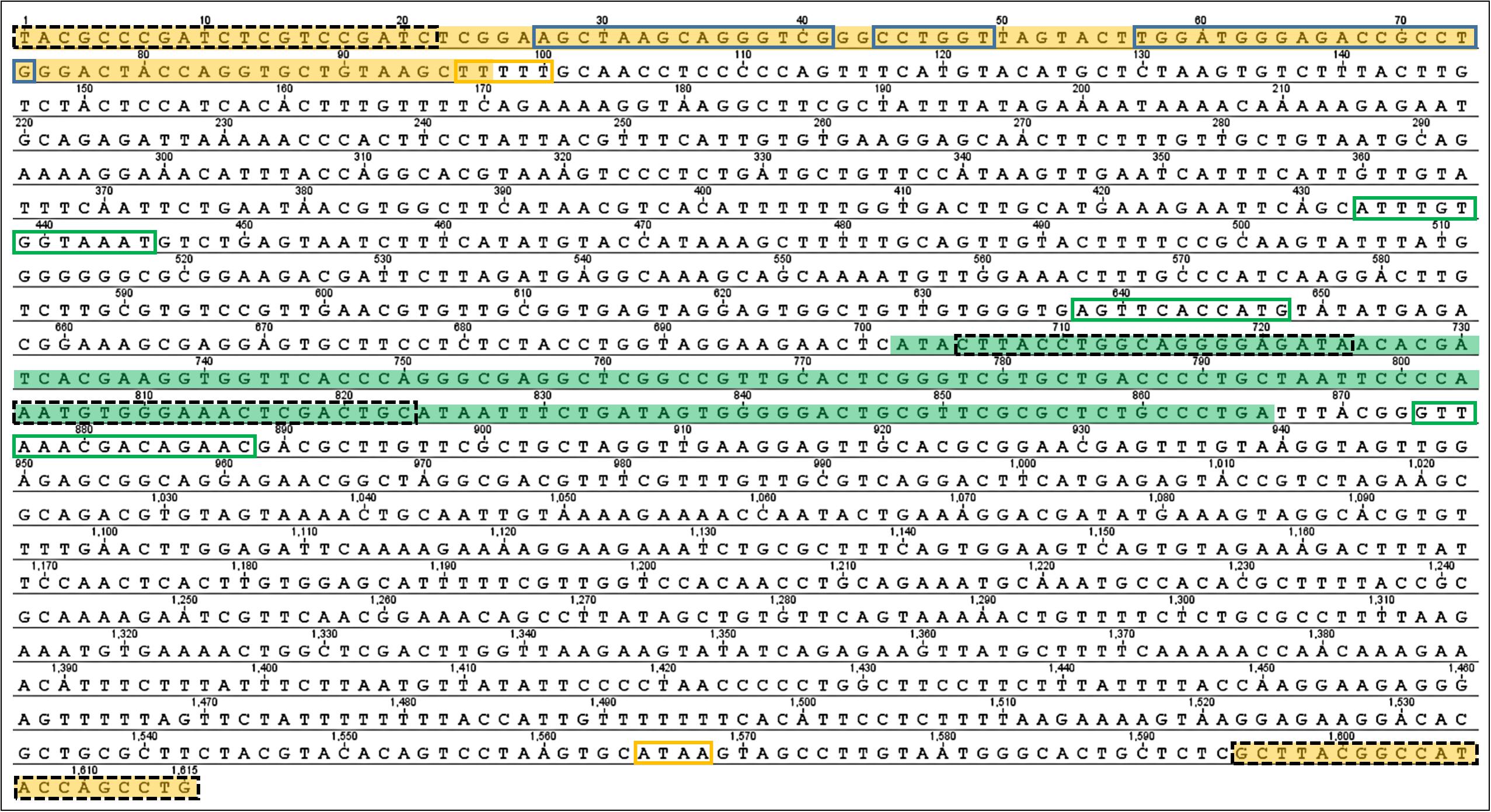
Figure 1. Nucleotide sequence of the 5S rDNA from Triportheus nematurus. The sequence corresponding to the 5S rRNA transcribed region is highlighted in yellow. The U1 snRNAs gene is highlighted in green. Dash-lined boxes indicate the primer sequences. Blue boxes denote the Internal Control Regions of 5S rRNA (box A, intermediate element; box C, from left to right). The 5S rDNA external regulatory elements are boxed in yellow (poli-T termination region and TATA box, from up to down). The U1 snDNA regulatory elements are boxed in green (DSE, PSE, and 3′ box, from up to down).
The NTS has a peculiarity, since an U1 snRNA gene was found inside it at position 726–891 (Figure 1). The U1 snRNA sequences show identities of 95% with Argyrosomus regius and Takifugu rubripes fish species, and 92% with the frog Xenopus laevis. This gene divide the NTS in two fragments, one before the U1 gene (Spacer 1, at position 98–725) and other after U1 (Spacer 2, at position 892–1651 bp) (Figure 1). Therefore, 5S rRNA and U1 snRNA sequences are linked in the same array in all Triportheus species analyzed, and both genes displayed the same orientation. The regulatory regions were identified: (i) a proximal sequence element (PSE) is located at 56 nucleotides upstream of the transcription point; (ii) a distal sequence element (DSE) was identified at 204 nucleotides upstream of the PSE; (iii) a 3′ Box was identified at 8 nucleotides downstream of the 3′ end of the gene.
The analysis using the CENSOR software identified six types of LTR Retrotransposons, six types of non-LTR retrotransposon and seven types of DNA transposon fragments in the NTS of all sequences (Figure 2). All species, except T. albus, showed fragments of several TEs; and the LTR BEL-52_DRe-I from Danio rerio (Howe et al., 2013) was identified in five Triportheus species.
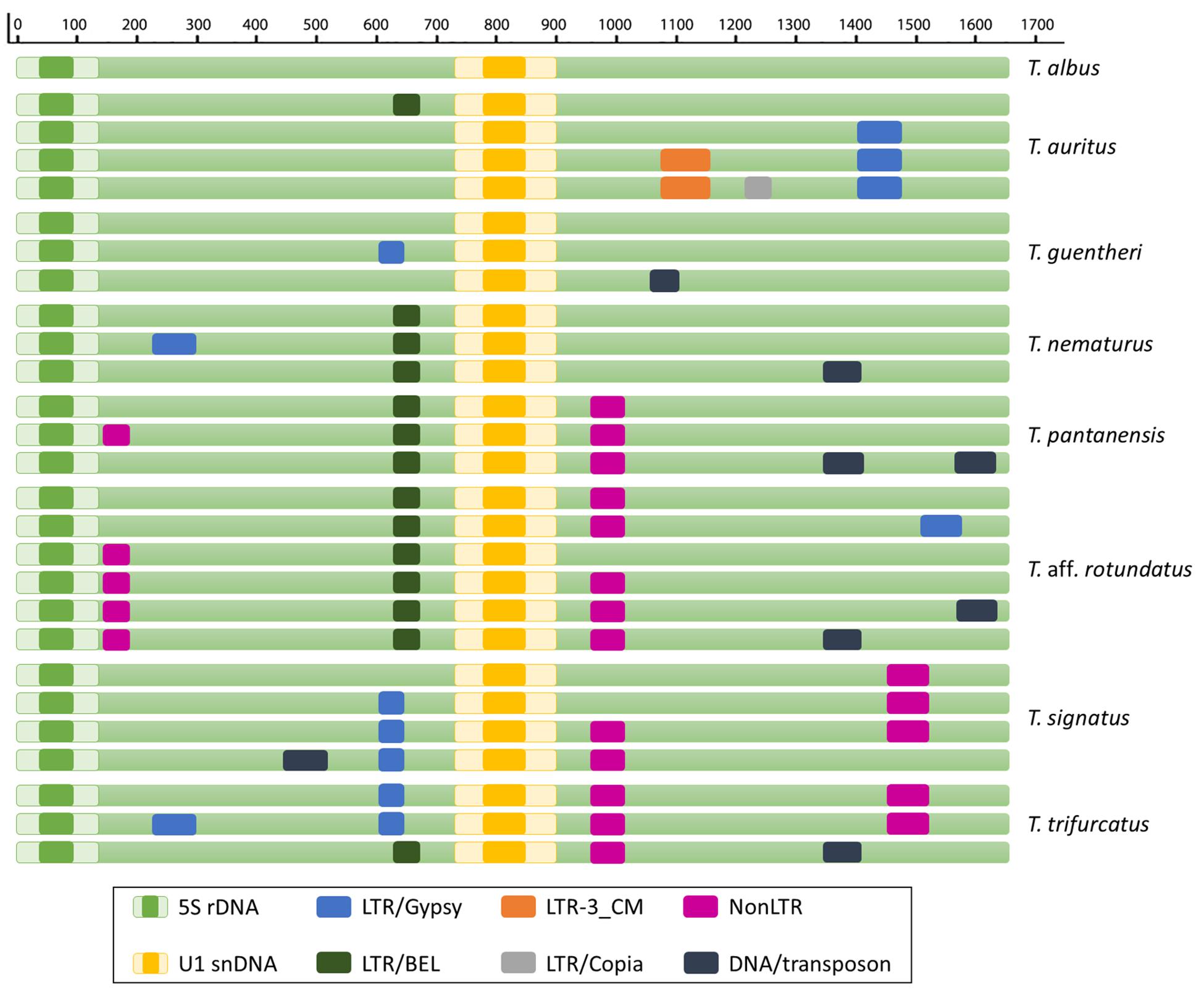
Figure 2. Schematic representation of the 5S rDNA sequences showing the different types of retrotransposons and DNA transposons found in the NTS of Triportheus species.
Variability Analysis
The transcribed region of 5S rDNA showed 22 polymorphic sites (S), 23 haplotypes (h) and 0,011 ± 0,001 nucleotide diversity (π). As expected, the NTS displayed higher values than transcribed region, with 439 polymorphic sites, 58 haplotypes and 0,065 ± 0,006 of nucleotide diversity. The nucleotide variability (π) of the two transcribed regions and the two spacers within each species was low in most species, especially in the transcribed regions (Supplementary Tables S1–S4). T. guentheri showed the highest values of nucleotide variability in the transcribed regions; while T. auritus showed the lowest values (Supplementary Tables S1, S3). In the spacers, T. trifurcatus and T. aff. rotundatus presented the highest nucleotide variability (Supplementary Tables S2, S4).
Concerning the nucleotide divergence inferred among species (K), was low in the transcribed regions, being in many species even lower than the nucleotide variability found within each species (Supplementary Tables S1, S3). However, despite the low divergence value in the transcribed regions, T. auritus was the species that showed the highest values of divergence and one and two fixed differences with all species in the 5S and U1 transcribed region, respectively. In addition, T. guentheri presented a fixed difference in the U1 transcribed region with all species, except with T. albus. Unlike the transcribed regions, the two spacers showed higher nucleotide divergence among species, especially T. auritus, since this species presented elevated values with all species analyzed (Supplementary Tables S2, S4). The number of fixed differences was much higher in the spacers, with few exceptions; T. auritus again presented the highest values (Supplementary Tables S2, S4).
Cytogenetic Analysis
All Triportheus species show 52 chromosomes, and a karyotype composed by m/sm and some st chromosomes, with a heteromorphic ZZ/ZW sex chromosome system. Concerning the double-FISH results, the 5S rDNA probe hybridized the p arms of the chromosome pair No. 9 in all species. Triportheus albus and T. guentheri showed only this site; while T. auritus, T. nematurus, T. signatus, and T. trifurcatus also showed 5S rDNA signals in the chromosome pair No. 3. Triportheus auritus also presented this cluster in chromosome pairs Nos. 4, 5, and 6, bearing a total of 10 sites in five chromosomes pairs (Figure 3). The U1 snDNA was mapped for the first time in Triportheus and in each species these sequences are clustered in the chromosome pair No. 9, being co-localized with the 5S rDNA site (Figure 3).
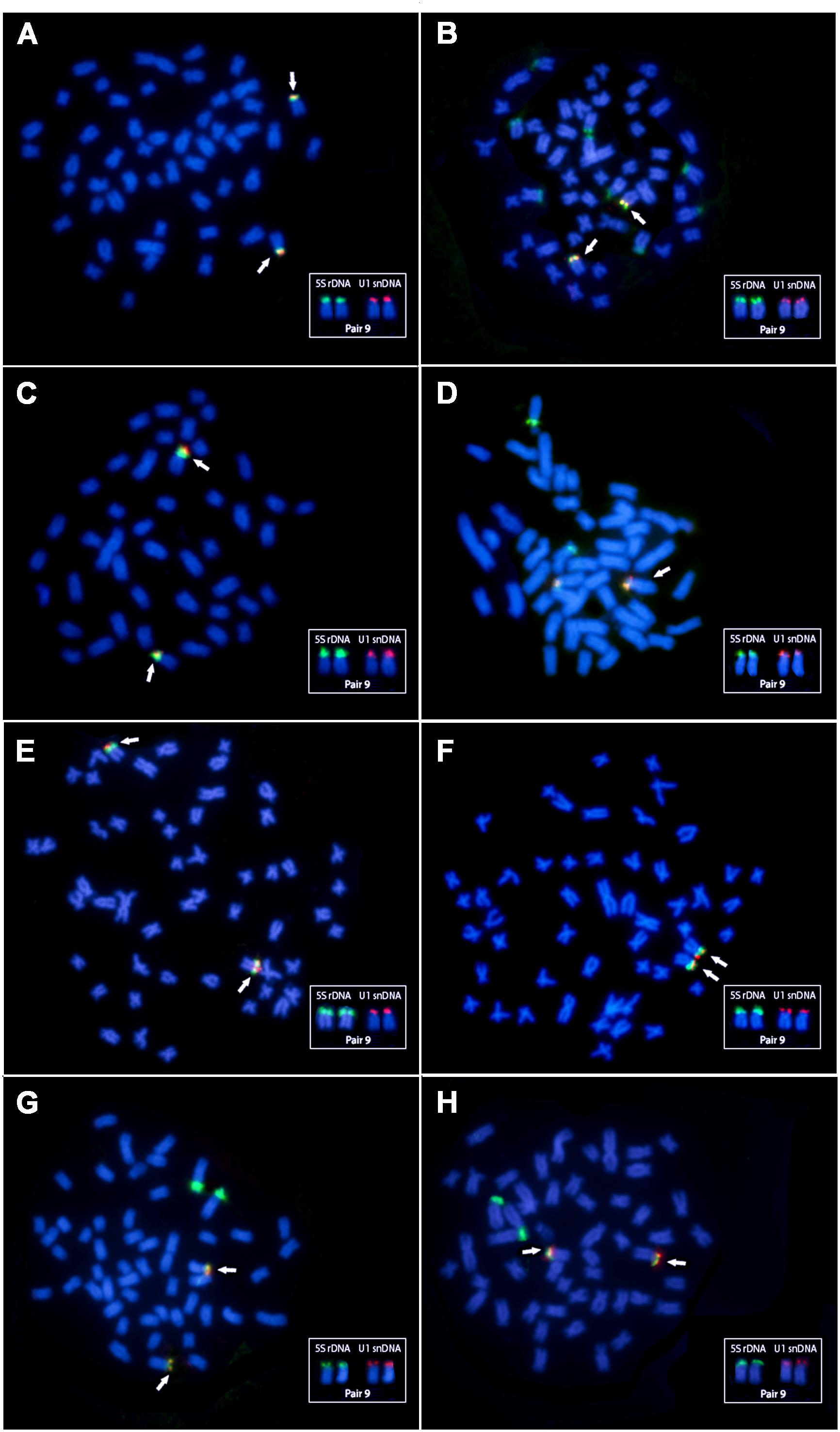
Figure 3. Karyotypes of eight Triportheus species after the FISH experiments, using the 5S rDNA (green) and U1 snDNA (red) probes on the chromosomes. (A) Triportheus albus, (B) Triportheus auritus, (C) Triportheus guentheri, (D) Triportheus nematurus, (E) Triportheus pantanensis, (F) Triportheus aff. rotundatus, (G) Triportheus signatus, and (H) Triportheus trifurcatus. The chromosomal pair No. 9 bearing syntenic sites are marked with arrows and are boxed displaying each probe separately.
Phylogenetic Analysis
The ML tree and the neighbor-network showed a clear clustering by species for T. albus, T. auritus, T. guentheri, T. nematurus, T. signatus, and T. trifurcatus, except for two sequences of T. trifurcatus. Triportheus pantanensis and T. aff. rotundatus were clustered in the same group, as two sequences of T. trifurcatus (Figure 4). Both analyses showed that, although the sequences of T. signatus and T. trifurcatus are grouped by species, they are very close to each other (Figures 4, 5). The network and the ML tree also displayed that T. auritus is notably more distant from all species. Additionally, the ML tree evidenced that the T. auritus sequences occupy the most basal position in the tree (Figure 4).
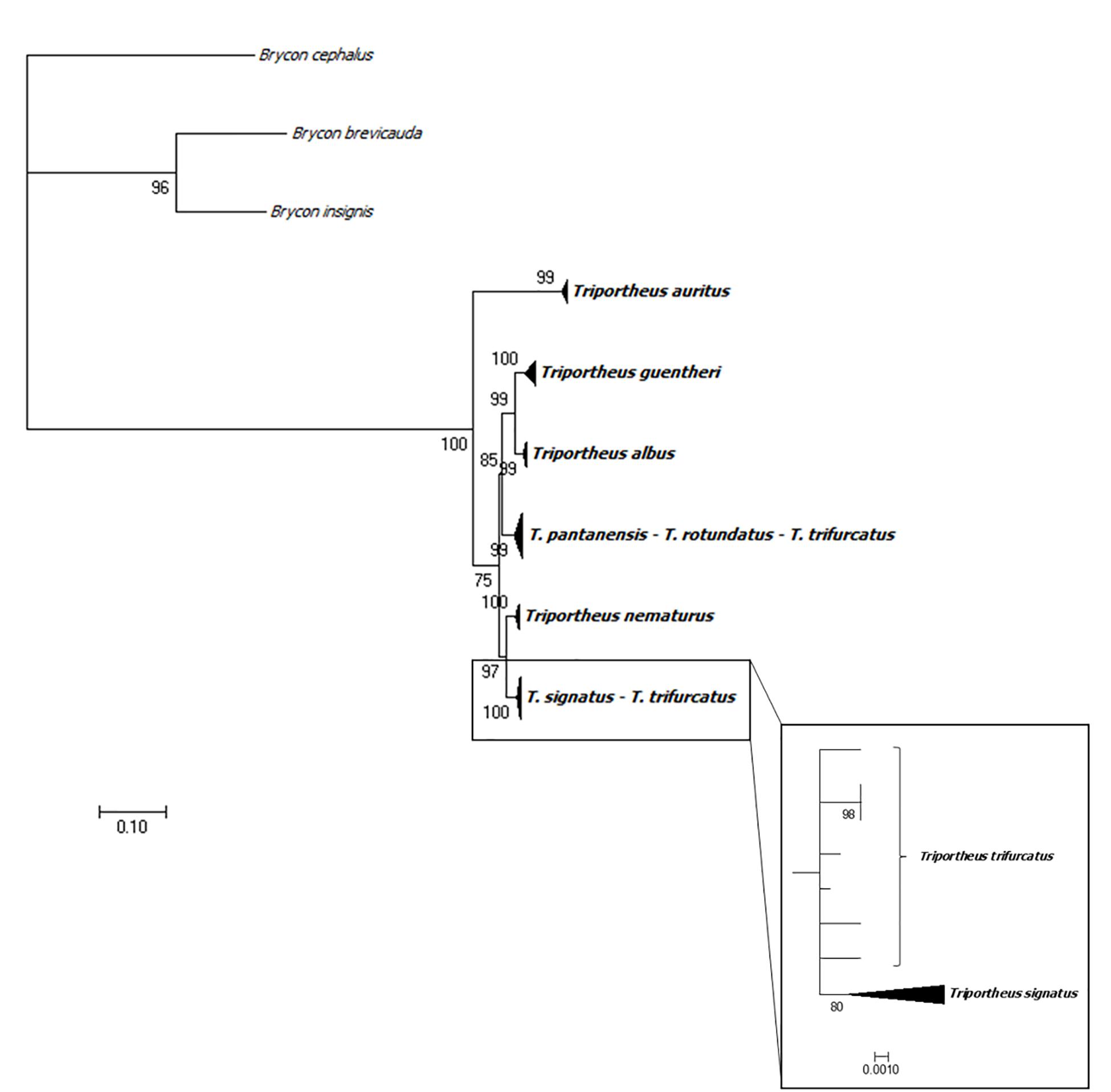
Figure 4. Phylogenetic Maximum Likelihood tree of NTS sequences based on GTR substitution model. Three sequences from Brycon brevicauda (Acc. No. AF250541), B. cephalus (Acc. No. AF250529), and B. insignis (Acc. No. AF250544) were used as outgroup. The box shows the T. signatus-T. trifurcatus clade in detail.
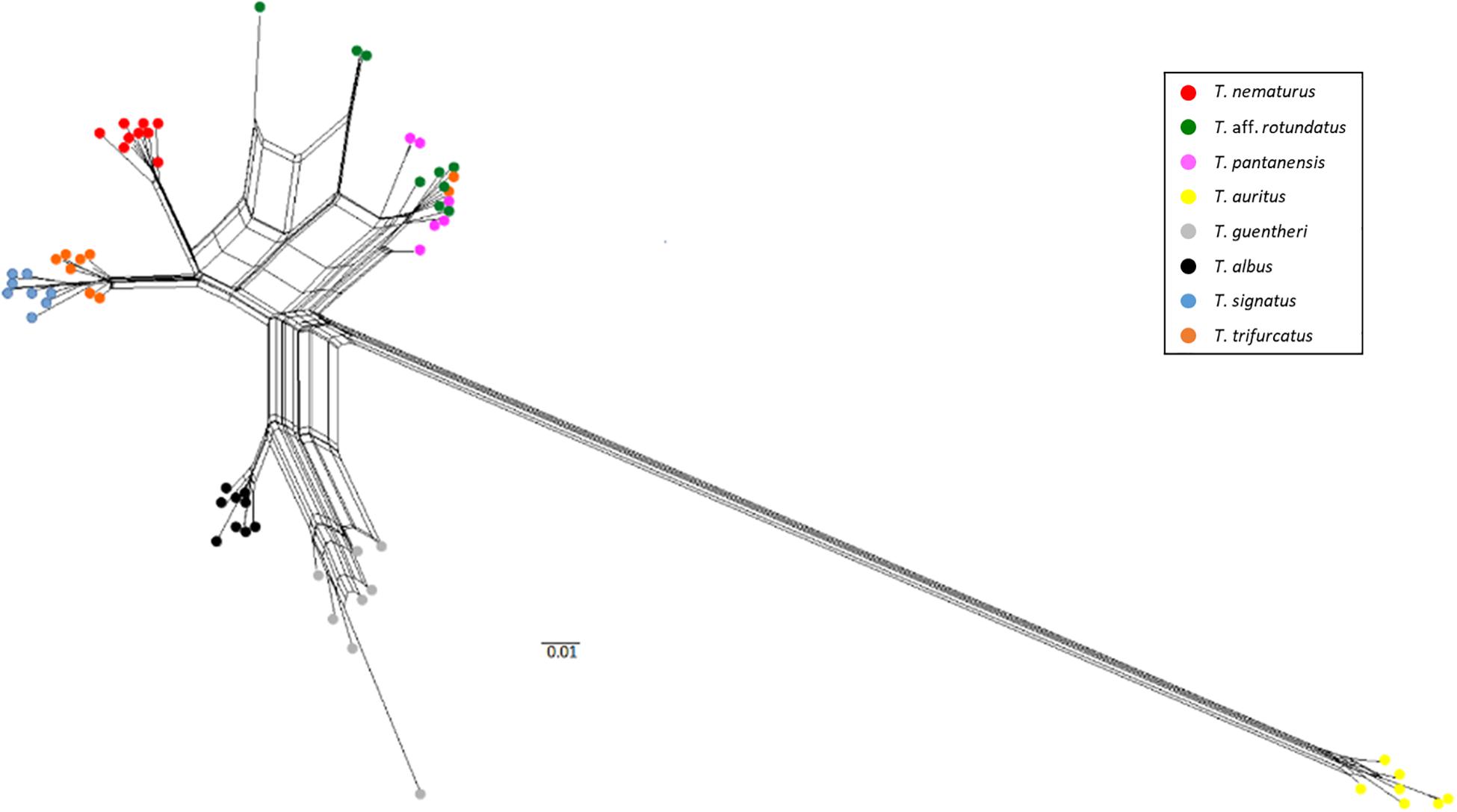
Figure 5. Phylogenetic networks of 5S rDNA constructed using the neighbor net algorithm. Triportheus species are shown in different colors.
Discussion
All species showed a main 5S rDNA amplicon that is presumably active, since both, ICR and the three external elements, are conserved along the 5S rDNA sequences. All these regions are necessary for the initiation of the transcription (Cloix et al., 2000; Volkov et al., 2017). In addition, other interesting features were observed in these sequences: (i) the U1 snRNA multigene family was detected within the NTS of all Triportheus species by sequencing and FISH technique; (ii) higher nucleotide divergence (inside spacers) was found between T. auritus and all other Triportheus species, which is supported by previously published phylogenetic data and cytogenetic data; (iii) the presence of a varied range of TE within the spacers.
The U1 snRNA multigene family was detected within the NTS of all Triportheus species. This gene presented a high interspecific similarity and there were detected the regulator regions, such as the PSE, DSE and 3′ box. The PSE has a conserved position at 50–60 bp upstream of the transcription starting point (McNamara-Schroeder et al., 2001) and determines the transcription start-site (Thomas et al., 1990). The DSE is an enhancer that, in humans, activates the transcription about 100-fold (Boyd et al., 2003). It is usually found about 200–250 bp upstream from PSE (Parry et al., 1989). The 3′ box is a region for the transcription termination and 3′ processing of the RNA (Cuello et al., 1999), and it is located about 9–19 bp downstream of the transcribed region (Barzotti et al., 2003). The conservation of these regulatory regions supports the functionality of the U1 snRNA genes.
The karyotype and 5S rDNA-FISH results agreed with previous studies (Artoni et al., 2001; Diniz et al., 2009; Marquioni et al., 2013; Yano et al., 2016, 2017b). In all species, the double-FISH results demonstrate that U1 snRNA and 5S rRNA are colocalized in the ninth chromosome pair (Figure 3), including T. auritus, which belongs to the first lineage that diverged from the ancestor of the genus (Mariguela et al., 2016). Such a co-localization is a plesiomorphic condition for the genus, so the analysis of 5S-U1 co-localization in other closely related genus should reinforce the taxonomic relationships of the Characiformes order. An exhaustive cytogenetic analysis using probes of ribosomal and spliceosomal RNA genes was carried out in five Characiformes species belonging to the Astyanax genus (Silva et al., 2015), and in four of them, 5S rDNA and U1 snDNA were localized in a same chromosome pair. These results would indicate that the linkage in a same chromosome between 5S and U1 multigene families is a common feature within the Characiformes; however, new analysis should be directed in other representative families of the order. Anyway, such a linkage is a promising marker to clarify the Characiformes taxonomy.
The linkage between 5S rRNA and U1 snRNA genes in only one of the chromosome pairs bearing the 5S sequences, indicate that this gene has frequently gone through mechanisms of transposition in the Triportheus genome, as already suggested by other authors (Drouin and Moniz de Sá, 1995). Although both genes are linked, they are transcribed by different RNA polymerases, ruling out the possibility of cotranscription (Pelliccia et al., 2001). The 5S-U1 linkage has been described in fish species such Oreochromis niloticus (Cabral-de-Mello et al., 2012), Astyanax paranae, A. bockmanni, A. fasciatus and A. jordani (Silva et al., 2015). A tandem linkage (co-localization) between these two multigene families has only described in the fish A. jordani (Silva et al., 2015) and the crustacean Asellus aquaticus (Pelliccia et al., 2001). Astyanax and Triportheus genus belong to the Characoidei suborder and both species present the 5S-U1 linkage. However, in other species of the same suborder, such as Leporinus spp. (Ferreira et al., 2007), Hoplias malabaricus (Martins et al., 2006) and Brycon spp. (Wasko et al., 2001), no 5S-U1 tandem linkage was observed, although a U1 snRNA localization by FISH has not been tested to ascertain if the two multigene family are linked. An exhaustive analysis of such linkage in other species of the same suborder (or the Characiformes) would give new insights of the evolutionary pathway of such species.
A noteworthy characteristic of the two spacer regions is the presence of different traces of TE. The presence of TE remains has been associated with an evidence for hybridization process between species, since after hybridization occurs an explosion of transpositions and a rapid genomic reorganization (Fontdevila, 2019). Similarly, in the species Diplodus sargus different TE were also described within the NTS and multiple 5S rDNA types were detected at both, electrophoretic and sequence level, probably derived from a hybridization scenario between two subspecies (Merlo et al., 2013). However, all Triportheus species showed one main electrophoretic band, thus indicating a higher level of intraspecific similarities than those observed in D. sargus. It has been postulated that D. sargus subespeciation resulted from a rapid series of colonization events (Summerer et al., 2001), leading to a parapatric speciation, in which the distribution boundaries could represent a hybridization area between two subspecies (Merlo et al., 2013). Commonly, the characiform fish diversification has been explained by allopatric speciation due to river vicariance (Seehausen and Wagner, 2014), but recent works suggest that parapatric ecological speciation have important role in this group (Cooke et al., 2012; Strecker et al., 2012). Different causes could explain the differences of 5S rDNA variability between D. sargus and the Triportheus species: (i) The transposition explosion within the Triportheus species could be lesser than that of the D. sargus due to a reduced hybridization episodes among the Triportheus species, and (ii) the 5S-U1 linkage observed in Triportheus species, leads to higher selective pressure in the spacers than in the NTS of D. sargus, since there are more regulatory elements. Moreover, a Tc1 retrotransposon located within NTS of the 5S rDNA has been proposed as the agent that causes the large number of 5S rDNA loci observed in Gymnotus mamiraua (Silva et al., 2016). Similarly, a Rex retroelement and a hAT transposon have been suggested as the causative for the 5S rDNA dispersion in Ancistrus and Rinelocaria species, respectively (Favarato et al., 2016; Glugoski et al., 2018).
Among the TE found within spacers of Triportheus species are several non-LTR retrotransposons. The short interspersed elements (SINEs) are a class of non-LTR retrotransposons that can be classified by the origin of the 5′ region. Thus, the SINE1 type has a head derived from 7SL RNA genes (Kriegs et al., 2007), SINE2 from tRNA genes (Jurka et al., 2005) and SINE3 from 5S rRNA (Kapitonov and Jurka, 2003). Recently, a new class of SINE derived from snRNAs (U1 and U2) has been described (Kojima, 2015). Marks of non-LTR transposons have been found immediately adjacent to 5S rRNA and/or U1 snRNA genes in T. pantanensis and T. aff. rotundatus, and to U1 snRNA gene in T. signatus and T. trifurcatus. Therefore, in these species, the 5S/U1 array could act as a source of 5S and U1-derived SINEs. Nevertheless, TE copies in the genome are sometimes non-functional due to distorted ORFs and are considered “molecular fossils” (Richardson et al., 2015).
The low values of nucleotide variability found in both, transcribed and spacer regions, demonstrate the high rate of homogenization exerted in such regions, as could be predicted by the concerted evolution model. It is well-accepted that the concerted model drives the evolution of the 5S rDNA multigene family within a given locus (Freire et al., 2010; Pinhal et al., 2011; Vizoso et al., 2011; Merlo et al., 2013). The mechanisms of unequal crossing over and gene conversion, in addition to the purifying selection in transcribed regions, act homogenizing the units of the multigene family (Nei and Rooney, 2005). In the Triportheus species, the repetition unit is composed by two transcribed regions and two reduced spacers with regulatory elements for two genes that are transcribed by different RNA polymerases, so the spacers also have additional selective restrictions, thus making the homogenization more effective.
On the other hand, the nucleotide divergence was high in the spacers between species, but with T. auritus clearly standing out, since this species showed the most elevated values when compared to other species. This high divergence between T. auritus and all other Triportheus species studied here can be perfectly associated to previous phylogenetic studies performed by Mariguela et al. (2016), since T. auritus was showed to be the oldest Triportheus species. As expected, the transcribed region showed lower divergence among species, but even in that, T. auritus was the species with higher divergence. Besides nucleotide divergence, T. auritus also shows the most divergence cytogenetic data concerning number of 5S rDNA sites (Yano et al., 2017b). While all other species show one or two chromosome pairs bearing 5S rDNA sequences, T. auritus present 5 pairs (Figure 3B). This high number of pairs could be explained by the fact that this gene is located in the terminal regions of the chromosomes, which could favor transposition events (Nakajima et al., 2012); or by the association of 5S rDNA and transposable elements, which was clearly evidenced in this study. According to Drouin (2000), the presence of TEs can be responsible for rRNA gene movements, and this process can be displayed by the cytogenetic analysis performed here and in previous studies. Moreover, it has been stated that a double strand break within the 5S array, followed by a non-homologous recombination repair mechanism during prophase, are events that lead to share 5S sequences among chromosomes (Barros et al., 2017; Glugoski et al., 2018).
Despite of the 5S-U1 co-localization found in all Triportheus species, additional not U1-linked 5S loci are present in T. signatus, T. nematurus, T. trifurcatus and, specially, in T. auritus, which has four additional loci. These additional 5S rDNA arrays could be generated during the evolutionary pathway of the Triportheus species, helped by the TE present in the main locus. These secondary 5S arrays could not be isolated in this work, so a deep analysis of the 5S rDNA sequences should be directed. However, the presence of a possible different array of 5S rDNA could give a clue to conclude that the birth-and-death evolution model is also acting in these species, since the presence of multiple loci is a typical characteristic of this model, that arise from duplication events (Nei and Rooney, 2005). A mixed model between birth-and-death and concerted has been already described for some fish species as stingrays (Pinhal et al., 2011), Plectorhinchus mediterraneus (Merlo et al., 2012a), Halobatrachus didactylus (Merlo et al., 2012b), and D. sargus (Merlo et al., 2013).
The phylogenetic tree showed a robust clustering in a species-specific manner in most species. However, three out of the eight analyzed species do not grouped in a species-specific form; these are T. pantanensis, T. aff. rotundatus, and T. trifurcatus. This data is also supported by the highest nucleotide variability observed in T. trifurcatus and T. aff. rotundatus. Nevertheless, T. pantanensis presented an intermediate nucleotide variability, despite it clustered beside T. trifurcatus and T. aff. rotundatus. The species T. pantanensis and T. aff. rotundatus belong to the Paraguay and lower Paraná basins and T. trifurcatus to the Tocatins and Araguaia Basins, a north-south adjacent basin. Some authors postulated that the fish fauna from a specific basin could be a hybrid combination of other existing in adjacent basins (Menezes, 1988; Lima and Caires, 2011). The similarity observed in the spacer regions among these three species and the close clustering in the phylogenetic analysis, especially between T. pantanensis and T. aff. rotundatus, suggest occasional introgression process during the parapatric speciation of such species. Introgression is often an integral component of species diversification and evolution that might confer an increased genetic variation (Hedrick, 2013) or exchange beneficial alleles (Arnold and Kunte, 2017). An introgression event was described between two hake species based on 5S rDNA, microsatellites and COI markers (Miralles et al., 2014).
In this work, it has been presented for the first time an integrative genetic characterization of 5S rDNAs, using extensive analysis to show the evolutionary model of these sequences in the Triportheus genus. Two main facts can be extracted as conclusions: First, a tandem linkage between 5S rDNA and U1 snDNA multigene families has been found in all Triportheus species, including T. auritus (a direct representative of the first lineage that differentiated in the genus), thus indicating that this feature emerged before the genus differentiation. Secondly, many TE have been found in the spacers of the array; this fact and the close clustering of some species indicates that the evolution of the group is driven by speciation-introgression process. In conclusion, the 5S rDNA multigene family has been revealed as a good marker for the evolutionary resolution of the Triportheus genus, which also could be tested for others closely related Characiformes species.
Data Availability Statement
All datasets generated for this study are included in the article/Supplementary Material.
Ethics Statement
The collections were authorized by the Brazilian environmental agency ICMBIO/SISBIO (License number 48628-2). The experiments followed ethical conducts, in accordance with the Ethics Committee on Animal Experimentation of the Universidade Federal de São Carlos (Process number CEUA 1853260315).
Author Contributions
CY carried out the FISH assay. CY and SP-B carried out the cloning assay. CY, CS-J, and MM carried out the molecular and phylogenetic analysis. CY drafted the manuscript. MM helped with the discussion and drafting. LR, MC, and LB conceived and coordinated the study, participated in its design, discussed the results, and corrected the manuscript. All authors read and approved the manuscript.
Funding
This study was supported by the Brazilian agencies CNPq (Conselho Nacional de Desenvolvimento Científico e Tecnológico – Proc. no 306896/2014-1), FAPESP (Fundacão de Amparo à Pesquisa do Estado de São Paulo – Proc. no 2018/22033-1), and CAPES (Coordenacão de Aperfeiçoamento de Pessoal de Nível Superior – Proc. no BEX 11744/13-8). This study was also supported by the Department of Biomedicine, Biotechnology and Public Health of the University of Cádiz and by grants of the Junta de Andalucía (Spain) to the CVI-219 group.
Conflict of Interest
The authors declare that the research was conducted in the absence of any commercial or financial relationships that could be construed as a potential conflict of interest.
Acknowledgments
We thank Luiz Henrique da Silva (Piau), Dr. Paulo Cesar Venere, and Dr. Waldo Pinheiro Troy for assistance with the collection material.
Supplementary Material
The Supplementary Material for this article can be found online at: https://www.frontiersin.org/articles/10.3389/fmars.2020.00006/full#supplementary-material
Footnotes
References
Anisimova, M., Gil, M., Dufayard, J.-F., Dessimoz, C., and Gascuel, O. (2011). Survey of branch support methods demonstrates accuracy, power, and robustness of fast likelihood-based approximation schemes. Syst. Biol. 60, 685–699. doi: 10.1093/sysbio/syr041
Arnold, M. L., and Kunte, K. (2017). Adaptive genetic exchange: a tangled history of admixture and evolutionary innovation. Trends Ecol. Evol. 32, 601–611. doi: 10.1016/j.tree.2017.05.007
Artoni, R. F., Falcão, J. D. N., Moreira-Filho, O., and Bertollo, L. A. C. (2001). An uncommon condition for a sex chromosome system in Characidae fish. Distribution and differentiation of the ZZ/ZW system in Triportheus. Chromosom. Res. 9, 449–456. doi: 10.1023/A:1011620226348
Barros, A. V., Wolski, M. A. V., Nogaroto, V., Almeida, M. C., Moreira-Filho, O., and Vicari, M. R. (2017). Fragile sites, dysfunctional telomere and chromosome fusions: What is 5S rDNA role? Gene 608, 20–27. doi: 10.1016/j.gene.2017.01.013
Barzotti, R., Pelliccia, F., and Rocchi, A. (2003). Identification and characterization of U1 small nuclear RNA genes from two crustacean isopod species. Chromosom. Res. 11, 365–373. doi: 10.1023/A:1024048108918
Bertollo, L. A. C., Cioffi, M., de, B., and Moreira-Filho, O. (2015). “Direct chromosome preparation from freshwater teleost fishes,” in Fish Cytogenetic Techniques (Chondrichthyans and Teleosts), eds C. Ozouf-Costaz, E. Pisano, F. Foresti, and L. F. De Almeida-Toledo, (Endfield: CRC Press, Inc), 21–26. doi: 10.1201/b18534-4
Boyd, D. C., Pombo, A., and Murphy, S. (2003). Interaction of proteins with promoter elements of the human U2 snRNA genes in vivo. Gene 315, 103–112. doi: 10.1016/S0378-1119(03)00717-710
Bryant, D., and Moulton, V. (2004). Neighbor-Net: An agglomerative method for the construction of phylogenetic networks. Mol. Biol. Evol. 21, 255–265. doi: 10.1093/molbev/msh018
Cabral-de-Mello, D. C., Moura, R. C., and Martins, C. (2010). Chromosomal mapping of repetitive DNAs in the beetle Dichotomius geminatus provides the first evidence for an association of 5S rRNA and histone H3 genes in insects, and repetitive DNA similarity between the B chromosome and A complement. Heredity 104, 393–400. doi: 10.1038/hdy.2009.126
Cabral-de-Mello, D. C., Valente, G. T., Nakajima, R. T., and Martins, C. (2012). Genomic organization and comparative chromosome mapping of the U1 snRNA gene in cichlid fish, with an emphasis in Oreochromis niloticus. Chromosom. Res. 20, 279–292. doi: 10.1007/s10577-011-9271-y
Cloix, C., Tutois, S., Mathieu, O., Cuvillier, C., Espagnol, M. C., Picard, G., et al. (2000). Analysis of 5S rDNA arrays in Arabidopsis thaliana: physical mapping and chromosome-specific polymorphisms. Genome Res. 10, 679–690. doi: 10.1101/gr.10.5.679
Cooke, G. M., Chao, N. L., and Beheregaray, L. B. (2012). Divergent natural selection with gene flow along major environmental gradients in Amazonia: insights from genome scans, population genetics and phylogeography of the characin fish Triportheus albus. Mol. Ecol. 21, 2410–2427. doi: 10.1111/j.1365-294X.2012.05540.x
Cross, I., Díaz, E., Sánchez, I., and Rebordinos, L. (2005). Molecular and cytogenetic characterization of Crassostrea angulata chromosomes. Aquaculture 247, 135–144. doi: 10.1016/j.aquaculture.2005.02.039
Cuello, P., Boyd, D. C., Dye, M. J., Proudfoot, N. J., and Murphy, S. (1999). Transcription of the human U2 snRNA genes continues beyond the 3′ box in vivo. EMBO J. 18, 2867–2877. doi: 10.1093/emboj/18.10.2867
Diniz, D., Laudicina, A., and Bertollo, L. A. C. (2009). Chromosomal location of 18S and 5S rDNA sites in Triportheus fish species (Characiformes, Characidae). Genet. Mol. Biol. 32, 37–41. doi: 10.1590/S1415-47572009005000017
Drouin, G. (2000). Expressed retrotransposed 5S rRNA genes in the mouse and rat genomes. Genome 43, 213–215. doi: 10.1139/g99-100
Drouin, G., and Moniz de Sá, M. (1995). The concerted evolution of 5S ribosomal genes linked to the repeat units of other multigene families. Mol. Biol. Evol. 12, 481–493.
Edgar, R. C. (2004). MUSCLE: multiple sequence alignment with high accuracy and high throughput. Nucleic Acids Res. 32, 1792–1797. doi: 10.1093/nar/gkh340
Eirín-López, J. M., Rebordinos, L., Rooney, A. P., and Rozas, J. (2012). “The birth-and-death evolution of multigene families revisited,” in Repetitive DNA, ed. M. A. Garrido-Ramos, (Basel: S. Karger AG), 170–196. doi: 10.1159/000337119
Favarato, R. M., Silva, M., Oliveira, R. R., Artoni, R. F., Feldberg, E., and Matoso, D. A. (2016). Cytogenetic diversity and the evolutionary dynamics of rDNA genes and telomeric sequences in the Ancistrus genus (Loricariidae: Ancistrini). Zebrafish 13, 103–111. doi: 10.1089/zeb.2015.1140
Ferreira, I. A., Oliveira, C., Venere, P. C., Galetti, P. M., and Martins, C. (2007). 5S rDNA variation and its phylogenetic inference in the genus Leporinus (Characiformes: Anostomidae). Genetica 129, 253–257. doi: 10.1007/s10709-006-0005-6
Fontdevila, A. (2019). Hybrid genome evolution by transposition: an update. J. Hered. 110, 124–136. doi: 10.1093/jhered/esy040
Freire, R., Arias, A., Ínsua, A. M., Méndez, J., and Eirín-López, J. M. (2010). Evolutionary dynamics of the 5S rDNA gene family in the mussel Mytilus: mixed effects of birth-and-death and concerted evolution. J. Mol. Evol. 70, 413–426. doi: 10.1007/s00239-010-9341-9343
Froese, R., and Pauly, D. (eds) (2019). FishBase. World Wide Web electronic publication. www.fishbase.org, version (04/2019).
Glugoski, L., Giuliano-Caetano, L., Moreira-Filho, O., Vicari, M. R., and Nogaroto, V. (2018). Co-located hAT transposable element and 5S rDNA in an interstitial telomeric sequence suggest the formation of Robertsonian fusion in armored catfish. Gene 650, 49–54. doi: 10.1016/j.gene.2018.01.099
Gonçalves, C., and Batista, V. D. S. (2008). Avaliação do desembarque pesqueiro efetuado em Manacapuru. Amazonas, Brasil. Acta Amaz. 38, 135–144. doi: 10.1590/S0044-59672008000100015
Guindon, S., Dufayard, J.-F., Lefort, V., Anisimova, M., Hordijk, W., and Gascuel, O. (2010). New algorithms and methods to estimate Maximum-Likelihood phylogenies: Assessing the performance of PhyML 3.0. Syst. Biol. 59, 307–321. doi: 10.1093/sysbio/syq010
Hedrick, P. W. (2013). Adaptive introgression in animals: examples and comparison to new mutation and standing variation as sources of adaptive variation. Mol. Ecol. 22, 4606–4618. doi: 10.1111/mec.12415
Howe, K., Clark, M. D., Torroja, C. F., Torrance, J., Berthelot, C., Muffato, M., et al. (2013). The zebrafish reference genome sequence and its relationship to the human genome. Nature 496, 498–503. doi: 10.1038/nature12111
Huson, D. H., and Bryant, D. (2006). Application of phylogenetic networks in evolutionary studies. Mol. Biol. Evol. 23, 254–267. doi: 10.1093/molbev/msj030
Jurka, J., Kohany, O., Pavlicek, A., Kapitonov, V. V., and Jurka, M. V. (2005). Clustering, duplication and chromosomal distribution of mouse SINE retrotransposons. Cytogenet. Genome Res. 110, 117–123. doi: 10.1159/000084943
Kapitonov, V. V., and Jurka, J. (2003). A novel class of SINE elements derived from 5S rRNA. Mol. Biol. Evol. 20, 694–702. doi: 10.1093/molbev/msg075
Kearse, M., Moir, R., Wilson, A., Stones-Havas, S., Cheung, M., Sturrock, S., et al. (2012). Geneious Basic: An integrated and extendable desktop software platform for the organization and analysis of sequence data. Bioinformatics 28, 1647–1649. doi: 10.1093/bioinformatics/bts199
Kohany, O., Gentles, A. J., Hankus, L., and Jurka, J. (2006). Annotation, submission and screening of repetitive elements in Repbase: repbaseSubmitter and Censor. BMC Bioinformatics 7:474. doi: 10.1186/1471-2105-7-474
Kojima, K. K. (2015). A new class of SINEs with snRNA gene-derived heads. Genome Biol. Evol. 7, 1702–1712. doi: 10.1093/gbe/evv100
Kriegs, J. O., Churakov, G., Jurka, J., Brosius, J., and Schmitz, J. (2007). Evolutionary history of 7SL RNA-derived SINEs in Supraprimates. Trends Genet. 23, 158–161. doi: 10.1016/j.tig.2007.02.002
Kumar, S., Stecher, G., and Tamura, K. (2016). MEGA7: Molecular evolutionary genetics analysis version 7.0 for bigger datasets. Mol. Biol. Evol. 33, 1870–1874. doi: 10.1093/molbev/msw054
Librado, P., and Rozas, J. (2009). DnaSP v5: a software for comprehensive analysis of DNA polymorphism data. Bioinformatics 25, 1451–1452. doi: 10.1093/bioinformatics/btp187
Lima, F. C. T. D., and Caires, R. A. (2011). Peixes da Estação Ecológica Serra Geral do Tocantins, bacias dos Rios Tocantins e São Francisco, com observações sobre as implicações biogeográficas das “águas emendadas” dos Rios Sapão e Galheiros. Biota Neotrop. 11, 231–250. doi: 10.1590/S1676-06032011000100024
Malabarba, M. C. (2004). On the paleoichthyofauna from the Aiuruoca Tertiary Basin, Minas Gerais State, Brazil. Ameghiniana 41, 515–519.
Manchado, M., Zuasti, E., Cross, I., Merlo, A., Infante, C., and Rebordinos, L. (2006). Molecular characterization and chromosomal mapping of the 5S rRNA gene in Solea senegalensis?: a new linkage to the U1, U2, and U5 small nuclear RNA genes. Genome 49, 79–86. doi: 10.1139/g05-068
Mariguela, T. C., Roxo, F. F., Foresti, F., and Oliveira, C. (2016). Phylogeny and biogeography of Triportheidae (Teleostei: Characiformes) based on molecular data. Mol. Phylogenet. Evol. 96, 130–139. doi: 10.1016/j.ympev.2015.11.018
Marquioni, V., Bertollo, L. A. C., Diniz, D., and Cioffi, M. D. B. (2013). Comparative chromosomal mapping in Triportheus fish species. Analysis of synteny between ribosomal genes. Micron 45, 129–135. doi: 10.1016/j.micron.2012.11.008
Martins, C., Ferreira, I. A., Oliveira, C., Foresti, F., and Galetti, P. M. (2006). A tandemly repetitive centromeric DNA sequence of the fish Hoplias malabaricus (Characiformes: Erythrinidae) is derived from 5S rDNA. Genetica 127, 133–141. doi: 10.1007/s10709-005-2674-y
Martins, C., and Wasko, A. P. (2004). “Organization and evolution of 5S ribosomal DNA in the fish genome,” in Focus on Genome Research, ed. C. Williams, (New York, NY: Nova Science Publishers), 335–363.
McNamara-Schroeder, K. J., Hennessey, R. F., Harding, G. A., Jensen, R. C., and Stumph, W. E. (2001). The Drosophila U1 and U6 gene proximal sequence elements act as important determinants of the RNA polymerase specificity of small nuclear RNA gene promoters in vitro and in vivo. J. Biol. Chem. 276, 31786–31792. doi: 10.1074/jbc.M101273200
Menezes, N. A. (1988). “The Curimatidae, a lowland Neotropical fish family (Pisces: Characiformes): distribution, endemism, and phylogenetic biogeography,” in Proceedings of a Workshop on Neotropical Distribution Patterns, eds W. R. Heyer and P. E. Vanzolini, (Rio de Janeiro: Academia Brasileira de Ciencias), 343–377.
Merlo, M. A., Cross, I., Manchado, M., Cárdenas, S., and Rebordinos, L. (2013). The 5S rDNA high dynamism in Diplodus sargus is a transposon-mediated mechanism. Comparison with other multigene families and Sparidae species. J. Mol. Evol. 76, 83–97. doi: 10.1007/s00239-013-9541-9548
Merlo, M. A., Cross, I., Palazón, J. L., Úbeda-Manzanaro, M., Sarasquete, C., and Rebordinos, L. (2012a). Evidence for 5S rDNA Horizontal Transfer in the toadfish Halobatrachus didactylus (Schneider, 1801) based on the analysis of three multigene families. BMC Evol. Biol. 12:201. doi: 10.1186/1471-2148-12-201
Merlo, M. A., Pacchiarini, T., Portela-Bens, S., Cross, I., Manchado, M., and Rebordinos, L. (2012b). Genetic characterization of Plectorhinchus mediterraneus yields important clues about genome organization and evolution of multigene families. BMC Genet. 13:33. doi: 10.1186/1471-2156-13-33
Miralles, L., Machado-Schiaffino, G., and Garcia-Vazquez, E. (2014). Genetic markers reveal a gradient of hybridization between cape hakes (Merluccius capensis and Merluccius paradoxus) in their sympatric geographic distribution. J. Sea Res. 86, 69–75. doi: 10.1016/j.seares.2013.11.009
Nakajima, R. T., Cabral-de-Mello, D. C., Valente, G. T., Venere, P. C., and Martins, C. (2012). Evolutionary dynamics of rRNA gene clusters in cichlid fish. BMC Evol. Biol. 12:198. doi: 10.1186/1471-2148-12-198
Nei, M., and Hughes, A. L. (1992). “Balanced polymorphism and evolution by the birth-and-death process in the MHC loci,” in Proceedings of the 11th Histocompatibility Workshop and Conference, eds K. Tsuji, M. Aizawa, and T. Sasazuki, (Oxford: Oxford University Press), 27–38.
Nei, M., and Rooney, A. P. (2005). Concerted and birth-and-death evolution of multigene families. Annu. Rev. Genet. 39, 121–152. doi: 10.1146/annurev.genet.39.073003.112240
Parry, H. D., Tebb, G., and Mattaj, I. W. (1989). The Xenopus U2 gene PSE is a single, compact, element required for transcription initiation and 3′ end formation. Nucleic Acids Res. 17, 3633–3644. doi: 10.1093/nar/17.10.3633
Pelliccia, F., Barzotti, R., Bucciarelli, E., and Rocchi, A. (2001). 5S ribosomal and U1 small nuclear RNA genes: a new linkage type in the genome of a crustacean that has three different tandemly repeated units containing 5S ribosomal DNA sequences. Genome 44, 331–335. doi: 10.1139/gen-44-3-331
Pendas, A. M., Moran, P., Freije, J. P., and Garcia-Vazquez, E. (1994). Chromosomal mapping and nucleotide sequence of two tandem repeats of Atlantic salmon 5S rDNA. Cytogenet. Genome Res. 67, 31–36. doi: 10.1159/000133792
Pinhal, D., Yoshimura, T. S., Araki, C. S., and Martins, C. (2011). The 5S rDNA family evolves through concerted and birth-and-death evolution in fish genomes: an example from freshwater stingrays. BMC Evol. Biol. 11:151. doi: 10.1186/1471-2148-11-151
Rebordinos, L., Cross, I., and Merlo, A. (2013). High evolutionary dynamism in 5S rDNA of ish: state of the art. Cytogenet. Genome Res. 141, 103–113. doi: 10.1159/000354871
Richardson, S. R., Doucet, A. J., Kopera, H. C., Moldovan, J. B., Garcia-Perez, J. L., and Moran, J. V. (2015). “The influence of LINE-1 and SINE retrotransposons on mammalian genomes,” in Mobile DNA III, ed. M. Gellert, (Washington, DC: American Society of Microbiology), 1165–1208. doi: 10.1128/microbiolspec.MDNA3-0061-2014
Sambrook, J., and Russel, D. W. (2001). Molecular cloning: A laboratory manual. Cold Spring Harb. 3:2100.
Seehausen, O., and Wagner, C. E. (2014). Speciation in freshwater fishes. Annu. Rev. Ecol. Evol. Syst. 45, 621–651. doi: 10.1146/annurev-ecolsys-120213-191818
Silva, D. M., Utsunomia, R., Pansonato-Alves, J. C., Oliveira, C., and Foresti, F. (2015). Chromosomal mapping of repetitive DNA sequences in five species of Astyanax (Characiformes, Characidae) reveals independent location of U1 and U2 snRNA sites and association of U1 snRNA and 5S rDNA. Cytogenet. Genome Res. 146, 144–152. doi: 10.1159/000438813
Silva, M., Barbosa, P., Artoni, R. F., and Feldberg, E. (2016). Evolutionary dynamics of 5S rDNA and recurrent association of Transposable Elements in electric fish of the Family Gymnotidae (Gymnotiformes): the case of Gymnotus mamiraua. Cytogenet. Genome Res. 149, 297–303. doi: 10.1159/000449431
Strecker, U., Hausdorf, B., and Wilkens, H. (2012). Parallel speciation in Astyanax cave fish (Teleostei) in Northern Mexico. Mol. Phylogenet. Evol. 62, 62–70. doi: 10.1016/j.ympev.2011.09.005
Summerer, M., Hanel, R., and Sturmbauer, C. (2001). Mitochondrial phylogeny and biogeographic affinities of sea breams of the genus Diplodus (Sparidae). J. Fish Biol. 59, 1638–1652. doi: 10.1006/jfbi.2001.1796
Tavaré, S. (1986). Some probabilistic and statistical problems in the analysis of DNA sequences. Lect. Math. Life Sci. 17, 57–86.
Thomas, J., Lea, K., Zucker-Aprison, E., and Blumenthal, T. (1990). The spliceosomal snRNAs of Caenorhabditis elegans. Nucleic Acids Res. 18, 2633–2642. doi: 10.1093/nar/18.9.2633
Vizoso, M., Vierna, J., González-Tizón, A. M., and Martínez-Lage, A. (2011). The 5S rDNA gene family in mollusks: Characterization of transcriptional regulatory regions, prediction of secondary structures, and long-term evolution, with special attention to Mytilidae mussels. J. Hered. 102, 433–447. doi: 10.1093/jhered/esr046
Volkov, R. A., Panchuk, I. I., Borisjuk, N. V., Hosiawa-Baranska, M., Maluszynska, J., and Hemleben, V. (2017). Evolutional dynamics of 45S and 5S ribosomal DNA in ancient allohexaploid Atropa belladonna. BMC Plant Biol. 17:21. doi: 10.1186/s12870-017-0978-976
Wasko, A. P., Martins, C., Wright, J. M., and Galetti, P. M. Jr. (2001). Molecular organization of 5S rDNA in fishes of the genus Brycon. Genome 44, 893–902. doi: 10.1139/gen-44-5-893
Yano, C. F., Bertollo, L. A. C., and Cioffi, M. D. B. (2017c). “Fish-FISH: molecular cytogenetics in fish species,” in Fluorescence in situ Hybridization (FISH), ed. T. Liehr, (Berlin: Springer-Verlag), 429–443. doi: 10.1007/978-3-662-52959-1_44
Yano, C. F., Bertollo, L. A. C., Ezaz, T., Trifonov, V., Sember, A., Liehr, T., et al. (2017a). Highly conserved Z and molecularly diverged W chromosomes in the fish genus Triportheus (Characiformes, Triportheidae). Heredity 118, 276–283. doi: 10.1038/hdy.2016.83
Yano, C. F., Bertollo, L. A. C., Liehr, T., Troy, W. P., and Cioffi, M. D. B. (2016). W Chromosome dynamics in Triportheus species (Characiformes, Triportheidae): an ongoing process narrated by repetitive sequences. J. Hered. 107, 342–348. doi: 10.1093/jhered/esw021
Yano, C. F., Bertollo, L. A. C., Rebordinos, L., Merlo, M. A., Liehr, T., Portela-Bens, S., et al. (2017b). Evolutionary dynamics of rDNAs and U2 small nuclear DNAs in Triportheus (Characiformes, Triportheidae): High variability and particular syntenic organization. Zebrafish 14, 146–154. doi: 10.1089/zeb.2016.1351
Keywords: Triportheus, 5S rRNA, U1 snRNA, transposable elements, speciation
Citation: Yano CF, Merlo MA, Portela-Bens S, Cioffi MdB, Bertollo LAC, Santos-Júnior CD and Rebordinos L (2020) Evolutionary Dynamics of Multigene Families in Triportheus (Characiformes, Triportheidae): A Transposon Mediated Mechanism? Front. Mar. Sci. 7:6. doi: 10.3389/fmars.2020.00006
Received: 31 July 2019; Accepted: 08 January 2020;
Published: 24 January 2020.
Edited by:
Maria Angeles Esteban, University of Murcia, SpainReviewed by:
James S. Albert, University of Louisiana at Lafayette, United StatesClaudio Oliveira, São Paulo State University, Brazil
Marcelo Vicari, Universidade Estadual de Ponta Grossa, Brazil
Copyright © 2020 Yano, Merlo, Portela-Bens, Cioffi, Bertollo, Santos-Júnior and Rebordinos. This is an open-access article distributed under the terms of the Creative Commons Attribution License (CC BY). The use, distribution or reproduction in other forums is permitted, provided the original author(s) and the copyright owner(s) are credited and that the original publication in this journal is cited, in accordance with accepted academic practice. No use, distribution or reproduction is permitted which does not comply with these terms.
*Correspondence: Manuel A. Merlo, YWxlamFuZHJvLm1lcmxvQHVjYS5lcw==