- 1Research and Graduate Studies Office, Texas A&M University at Galveston, Galveston, TX, United States
- 2Ocean, Coastal Environmental and Ecological Assessment Laboratory, School of Earth, Environmental, and Marine Sciences, University of Texas Rio Grande Valley, Brownsville, TX, United States
- 3The Nature Conservancy, Houston, TX, United States
- 4Centro de Investigaciones Marinas, Universidad de La Habana, Havana, Cuba
- 5Formerly NOAA Office of National Marine Sanctuaries, Galveston, TX, United States
- 6The Nature Conservancy, Big Pine Key, FL, United States
- 7Laboratorio de Ecosistemas Arrecifales, Instituto de Ciencias Marinas y Pesquerías, Universidad Veracruzana, Boca del Río, Mexico
The importance of coral reefs (CR) within marine ecosystems has become widely recognized. Although shallow CR are not as abundant in the Gulf of Mexico (GoM) as in other areas such as the Caribbean, their uniqueness, singularity, isolation, and conservation status make their conservation highly important. Corals and CR, both shallow and deep, are more widely distributed throughout the GoM than previously thought, providing new venues of research but also new challenges for their sustainable management. They are widely present in the three countries circumscribing the GoM (Cuba, Mexico, and the United States). Corals are also distributed throughout different depths, from the keys of Florida and Cuba, to the mesophotic reefs in Flower Garden Banks, Pulley Ridge, and submerged banks in the southern GoM; additional coral presence occurs even beyond mesophotic depths (∼30–150 m). Like reefs around the world, they are subject to an increased threat from anthropogenic causes, including overfishing, pollution, and climate change. But there is also hope. Some reefs in the area, such as those in Flower Garden Banks National Marine Sanctuary are probably the best-preserved reefs in the region, with coral cover greater than 50%, which is unusual in the Wider Caribbean. Others are experiencing new protections through the work of government, and local communities. The objectives of this manuscript are to summarize the overall status of corals and CR in the GoM, analyze some of the current and future threats, and explore opportunities for their conservation in the region. Aside from the above mentioned anthropogenic threats bleaching, coral diseases, and hurricanes have been identified as main contributors for CR declines not only in the GoM but abroad; some nowadays present but likely to increase threats are invasion by alien species or by Sargassum spp. Among some of the opportunities identified are to capitalize on existing and emerging multilateral agreements, and initiatives (e.g., GoM Large Marine Ecosystem, trinational sanctuaries agreement); increase financial support for conservation through international initiatives and the private sector; and a need to comprehend the inherent interconnection among corals, CR, and deeper bank ecosystems as they do not function in isolation.
Introduction
With a calculated area of >1.5 million km2 (Turner and Rabalais, 2018), the Gulf of Mexico (GoM) is considered one of the Large Marine Ecosystems (LMEs) in the World (Sherman, 1991) due to its distinctive hydrographic regimes, productivity, and biological population. Even though coral reefs (CR) are not the most abundant or representative ecosystems in the GoM, they constitute important habitats and provide significant ecosystem services for the three countries that share its waters (Mexico, Cuba, and the United States, US hereafter); among those services are commercial fisheries and recreation. Furthermore, CR protect shorelines from erosion, hurricanes, and tropical storms (Birkeland, 1997; Barbier et al., 2011). Ecologically CR provide structure that results in their high productivity; habitat contributed by corals yields a high diversity and density of marine species (Nellemann et al., 2008); while their association and interaction with other tropical marine ecosystems such as mangroves and seagrasses have shown to be of great importance for goods and services through functional linkages (Harborne et al., 2006), and their hydrological connectivity to those ecosystems is critical for completion of biological cycles (Ortiz-Lozano et al., 2013; Robertson et al., 2019), some of them remain scarcely quantified (Harborne et al., 2006).
Shallow reefs in the GoM are calculated to occupy 2,640 km2 (<0.2%) (Tunnell et al., 2007) while the extent of mesophotic corals, defined as light-dependent corals living at depths between 30–150 m (Hinderstein et al., 2010), and deep-sea corals in the LME - by comparison - are largely unknown (Brooke and Schroeder, 2007), although recent studies are helping to close this gap1. The largest distribution of shallow corals happens on the Florida coast (Florida Keys and Dry Tortugas), and Cuba, with roughly 85% of shallow corals of the GoM (Tunnell et al., 2007), but the uniqueness and singularity of reefs throughout the gulf makes them particularly important for this LME (Figure 1). The reefs within the GoM are also highly variable, having both some of the lowest (Florida Keys, just above 10%), and the highest coral cover (Flower Garden Banks, almost 60%) (Schutte et al., 2010) in the Wider Caribbean region (GoM + Caribbean) (Tunnell et al., 2007).
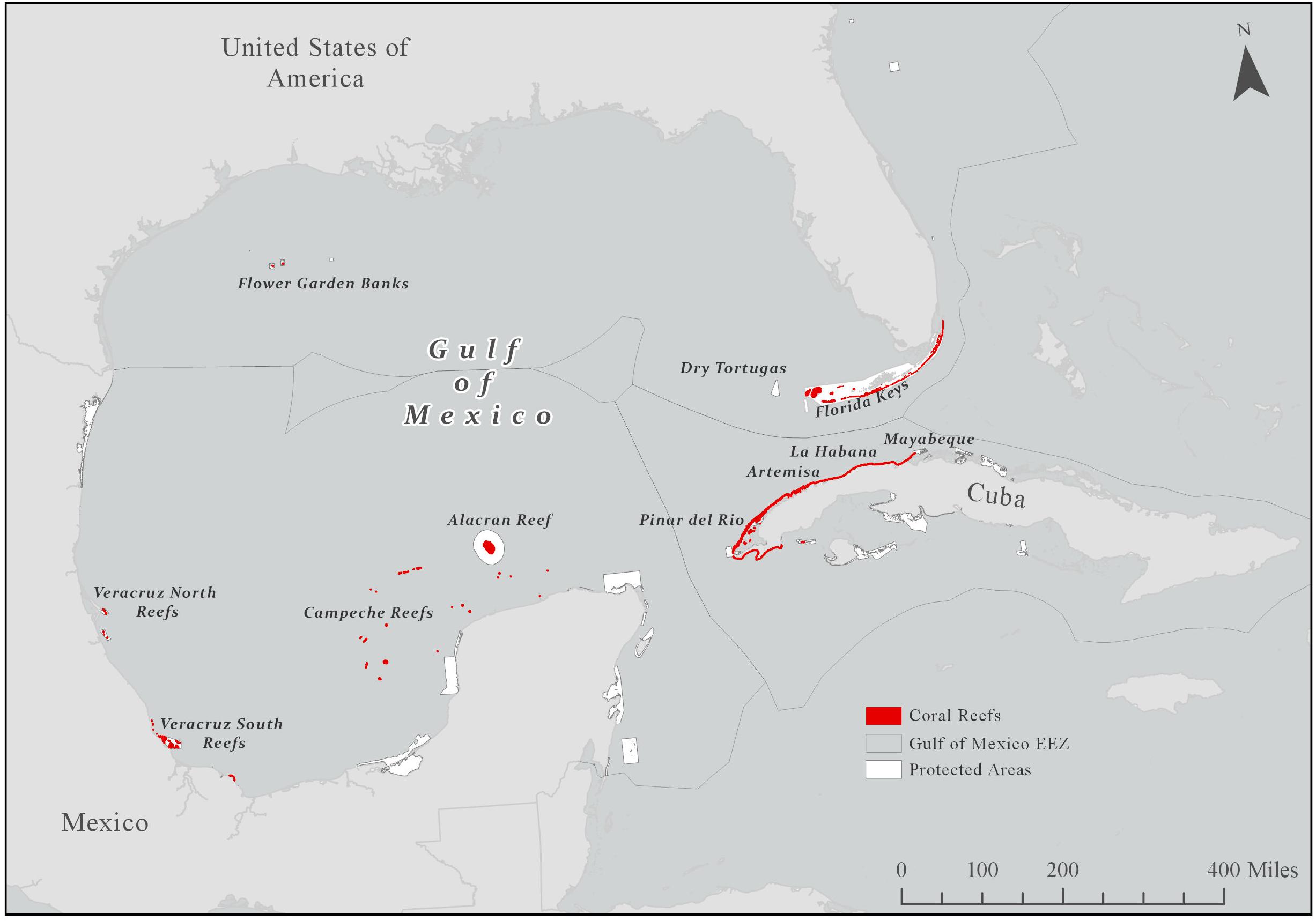
Figure 1. Distribution of shallow coral reefs (red) in the Gulf of Mexico (GoM). Exclusive Economic Zone = EEZ. Also shown are managed areas within the GoM, which tend to be focused on fisheries management.
Similar to CR all around the world, the shallow reefs of the GoM are experiencing significant declines in their overall health, expressed in loss of live coral cover due to pollution, habitat destruction, overfishing, diseases, bleaching, overgrowth by algae and sponges (Schutte et al., 2010), turbidity, and sedimentation (Jones et al., 2015) that are altering and impairing the overall function of these ecosystems, and decreasing their ecosystem services (Mumby and Steneck, 2008). The reefs of the Florida Keys have probably been one of the systems most affected by health degradation in the region, with a decrease in coral cover, and a reduction of species numbers, particularly after the bleaching event of 1997–1998, and these reefs are showing little to no recovery (Somerfield et al., 2008). In contrast, CR of the Flower Gardens Banks National Marine Sanctuary in the US have been historically relatively unaffected by coral diseases, bleaching [e.g., although corals bleaching occurred at the Flower Garden Banks every summer during 1989–1991 it was always minor (<5%), and yielded negligible mortality (Hagman and Gittings, 1992); Schmahl et al. (2008) indicated bleaching and diseases were 0–0.5% in 2004–2005], or other deleterious events, until 2016 when they were affected by a possible decrease in dissolved oxygen (DO) concentration affecting an estimated 2.6% of corals in East Flower Garden Bank, and up to 82% of corals in the area suffered partial or total mortality (Johnston et al., 2019). Moreover, and although shallow CR are by far the most affected, mesophotic and deep-sea corals have not been exempt to damages. White et al. (2012) found evidence of deterioration in one of 11 sites visited after the Deepwater Horizon oil spill in 2010, finding signs of stress that included “varying degrees of tissue loss, sclerite enlargement, excess mucous production, bleached commensal ophiuroids, and covering by brown flocculent material.”
Due to the importance of these ecosystems, several conservation initiatives are underway, from large-scale protection such as the creation and expansion of marine protected areas (MPAs), to the restoration of degraded reefs. This manuscript intends to show the current conservation status of the corals and CR of the GoM, analyze the actions taken in the three countries for their protection, and recommend future actions that managers and decision makers might need to take for their protection. Needless to say, but not surprising, the majority of the studies come from the US.
Shallow Coral Reef Distribution and Conservation Status
Coral reefs development within the ca. 360,000 km2 shallow waters (<50 m deep) of the GoM continental shelf is minimal, with <1% covered by hermatypic CR. Several unfavorable conditions in the GoM for CR development include a great riverine influence, the presence of two tidal systems (one prevalent most of the year generated by trade winds, and the second one present during winter and associated with north winds), upwelling, and perhaps freshwater inflow from groundwater (Jordán-Dahlgren, 2004). Other well-known factors affecting their distribution and abundance are turbidity, thermal stress and, particularly for zooxanthellate corals, light availability, all of which have been covered elsewhere (e.g., Veron, 1995; Birkeland, 1997; Jones et al., 2015). These authors have indicated that corals are capable of building reefs by using sunlight, which happens to be key for the existence of all modern CR; their zonation is largely due to corals adaptation to different lights levels; and aside of controlling growth and indirectly formation of calcium carbonate, light is known to be responsible for changing corals shape from mounds to plates among polymorphic coral species. It is noteworthy that some reefs in the GoM developed in spite of temperature and organic inputs, turbidity, and sedimentation produced by human activities and natural disturbances (Salas-Pérez and Granados-Barba, 2008; Gutiérrez-Ruiz et al., 2011).
United States
Although corals and CR are not the most predominant ecosystems in the US GoM, different formations that include these rich coral-based ecosystems are present throughout the area. The eastern US GoM encompasses the most developed CR formations. Meanwhile, the western US GoM is characterized by three types of banks, the south Texas Banks grow on relic carbonates while the banks east off Texas and Louisiana have carbonate reef caps, and are either midshelf or shelf-edge/outer-shelf bedrocks (Rezak et al., 1990), most of them offering habitat for mesophotic and deep-sea corals, but limited habitat for shallow corals and CR due to their depth. Finally, the central GoM is the most impoverished US zone in natural reefs covering ca. 3.3% of its area (Parker et al., 1983), probably due to the large influence of discharges from the Mississippi River.
Fishery management plans for the US GoM includes 142 scleractinian coral species under the GoM Fishery Management Council (Gulf of Mexico Fishery Management Council [GMFMC], 2011). According to Simmons et al. (2014) there are 20 sites independent of their depth distribution subjected to current management efforts in the GoM, yielding two designated sanctuaries (Flower Garden Banks and Florida Keys), five fishery reserves (Madison-Swanson, Steamboat Lumps, the Edges, Tortugas North, Tortugas South), and 18 habitat areas of particular concern (Alderdice Bank, Bouma Banks, Fathom 29, Florida Middle Grounds, East Flower Garden, West Flower Garden, Geyer Bank, Jakkula Bank, McGrail Bank, MacNeil Bank, Madison-Swanson, Pulley Ridge, Rankin Bright Bank, Rezak-Sidner Bank, Stetson Bank, Sonnier, Tortugas North, Tortugas South). Note, some of these sites are designated as more than one category, and the Florida Keys National Marine Sanctuary was not listed as such in the above reference.
The Florida Reef Tract extends ∼579.4 km (360 mi) from the St. Lucie Inlet in Martin County out to the Dry Tortugas. The most significant reef development occurs offshore of the Florida Keys, and in the Dry Tortugas, which fall within the GoM LME. Historically, the Florida Keys stands out because they host the first underwater national park or MPA (Dry Tortugas National Park, established in 1935), and the first underwater park (John Pennekamp Coral Reef State Park, established in 1963). This portion of the reef tract is also protected by the Florida Keys National Marine Sanctuary, established in 1990, which protects ∼9,603.7 km2 (2,800 nmi2) of marine habitat within Florida Bay, the GoM, and the Atlantic Ocean (Figure 2). As an addition to the Florida Keys National Marine Sanctuary, in 2001 the Tortugas Ecological Reserve was established. It was considered the largest MPA within US waters at that time with ∼514 km2 (150 nmi2), and is divided into diverse use zones (Jaap et al., 2008).
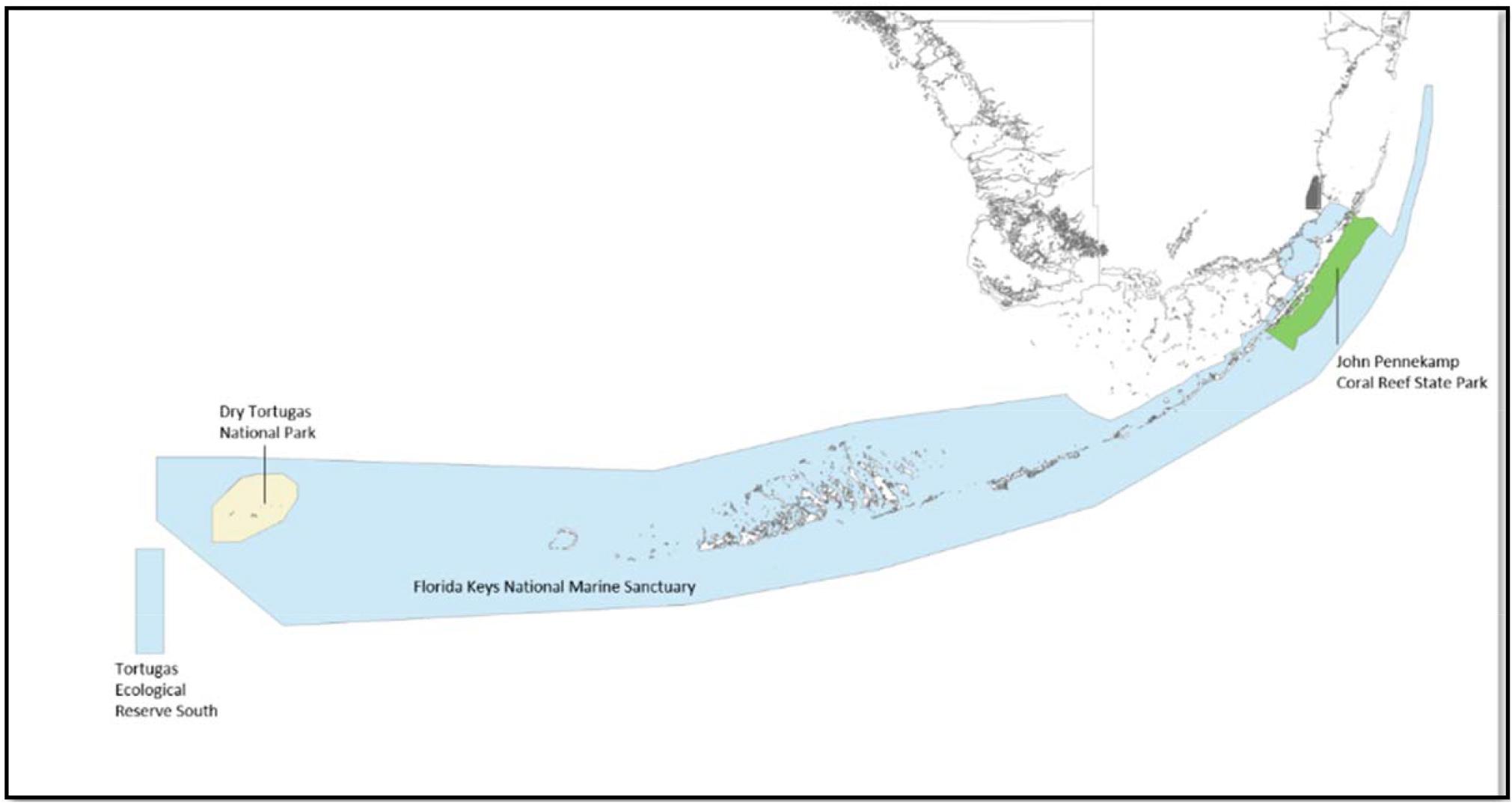
Figure 2. Florida Keys reefs are offered varying levels of protection by the Florida Keys National Marine Sanctuary, Dry Tortugas National Park, and John Pennekamp Coral Reef State Park, see text for details.
The Florida Keys reef system is a bank-barrier with shallow-water spur-and-groove formations that are connected by a linear transitional reef, and expanses that lack reef development due to the influence of Florida Bay (Shinn, 1963; Jaap, 1984). Over 6,000 patch reefs occur in the nearshore and offshore environments behind the forereef (Marszalek et al., 1977). Dry Tortugas National Park is characterized by a bank reef with spur and groove reefs, and large isolated formations, patch reefs or pinnacles, and Acropora-dominated shallow reefs (Haskell et al., 2000). To the west of the Park is Tortugas Bank, which is a deeper reef system, parts of which show very high coral cover. Just southwest of the Tortugas lies Riley’s Hump, another bank reef system that is very important for fishes and other marine life. The Dry Tortugas reefs are located ∼112.7 km (70 mi) from Key West, and at the confluence of a number of currents, so they benefit from relatively clean, clear water, and are protected from the same fishing and diving pressure experienced by reefs in the Keys both by their location, and through management actions. Moreover, throughout Florida take of any coral species is prohibited.
Zonation along the outer reef tract follows the typical Caribbean model (Goreau, 1959; Jaap, 1984), with a shallow Acropora palmata zone grading to a deeper Montastraea zone. Recent coring studies have shown that the reefs of the Florida Keys stopped accreting 3,000 years ago, and that the species assemblages have changed from the traditional reef-building species (A. palmata and Orbicella spp.) to weedier species such as Porites astreoides and Siderastrea siderea (Toth et al., 2018, 2019). Similar to elsewhere in the Caribbean, significant losses of the Acroporid species caused mainly by disease, and hurricanes in the 1980s led to a considerable reduction in coral cover on the shallow forereefs. As examples, at Carysfort Reef in the Upper Keys both species were completely lost between the summer of 1982 and April 1984, and at Looe Key in the Lower Keys snapshot monitoring revealed areal coral cover losses of ∼93% for A. palmata, and ∼98% for Acropora cervicornis (Szmant, 2005 in Acropora Biological Review Team, 2005). Similarly, the shallow reefs at Dry Tortugas once housed expansive thickets of both species but the A. palmata was almost completely lost between 1939 and 1982 based on maps published by Agassiz and Davis, and A. cervicornis declined by 95% in 1977 during a cold-water disturbance (Davis, 1982). Unfortunately, coral cover has continued to decline or remain depressed throughout the area.
The reefs of the Florida Keys support a healthy tourism economy, and both commercial and recreational fisheries. Visitors to the Keys and Dry Tortugas spend money on activities that are directly related to the reef (i.e., snorkeling and SCUBA diving), and on activities that are made possible or enjoyable because of the protection the reef provides (i.e., swimming at a beach and parasailing). Visitor expenditures on reef-related tourism range from $8,000 to 916,000 USD annually and expenditures on reef-adjacent tourism range from $8,000 to just over $2 million USD yearly for individual reefs throughout the Florida Keys (Spalding et al., 2017).
The submerged topographic features in the NW GoM supports spectacular CR communities (both shallow and mesophotic reefs) (Figure 3). Three of these features, East and West Flower Garden Banks, and Stetson Bank, are included in the National Marine Sanctuaries, and a number of other banks are under consideration to be added to the sanctuary at present2. What makes the CR of the Flower Garden Banks stand out among all the reefs in the GoM is their relative resistance to coral disease, bleaching, and other impacts that have dramatically disrupted CR communities throughout the Wider Caribbean, and the tropical world. Results of >25 years of monitoring within the sanctuary have shown that the Flower Garden Banks continue to support healthy amounts of coral cover (almost 60%), experienced few of the impacts that have been lethal to corals elsewhere (i.e., bleaching and diseases), and continue to support abundant fish assemblages (Johnston et al., 2016). As previously stated, <5% bleaching happened every summer from 1989–1991 (Hagman and Gittings, 1992), and neither bleaching nor disease were >0.5% from 2004–2005 (Schmahl et al., 2008).
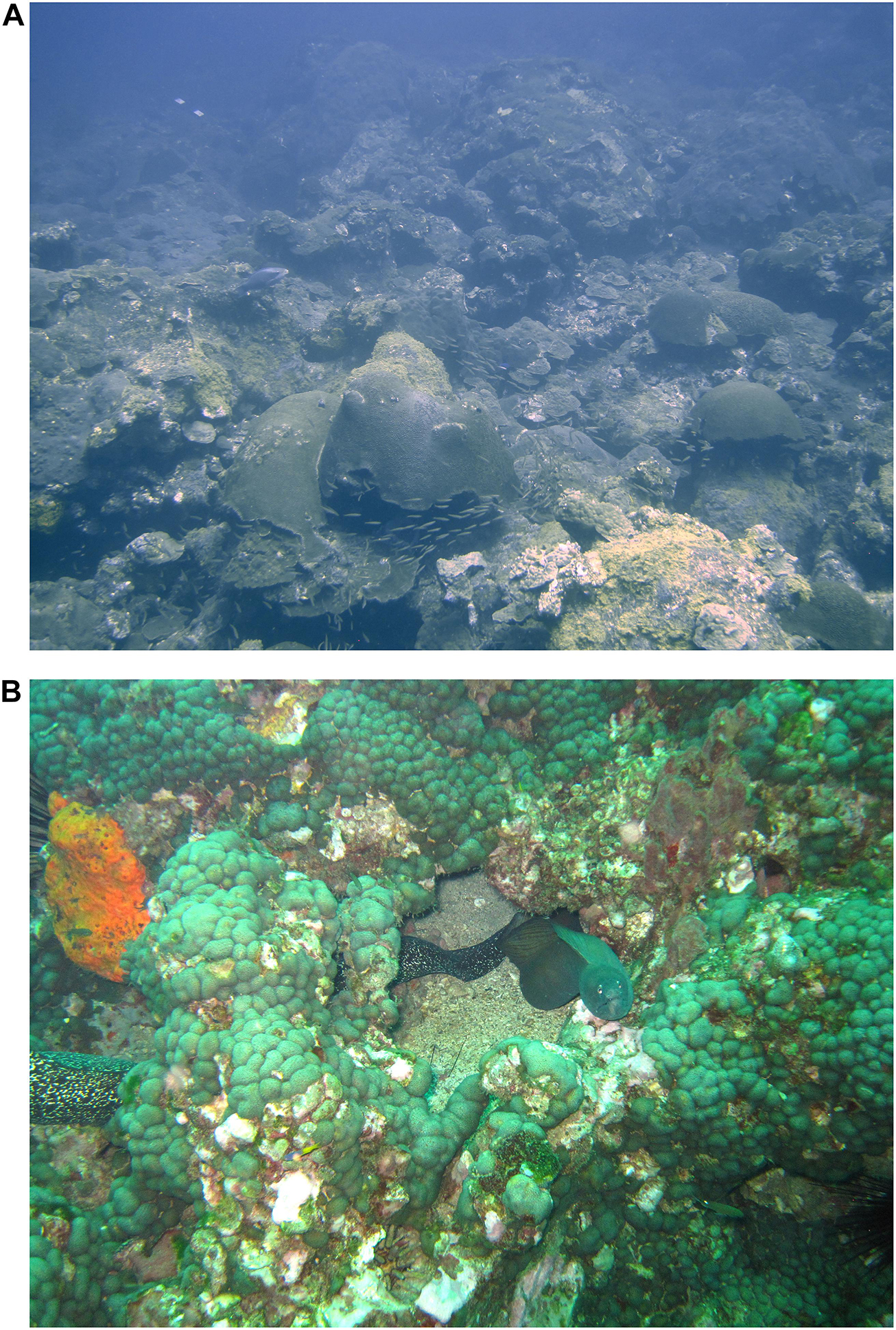
Figure 3. Coral reefs and coral communities in the Flower Garden Banks National Marine Sanctuary. (A) East Flower Garden (–18 m), and (B) Stetson Bank (–20 m). Photos by DLG-A.
The features of the NW GoM create a mosaic of biological habitats that are home to distinct biological communities distributed within several recognized biological zones (Schmahl et al., 2008). Rezak et al. (1990) showed how variations in water depth, currents, temperature minima, salinity, and turbidity combine to determine the distribution, and character of the biological communities in the NW GoM. Among the environmental factors potentially influencing the distribution of biological zones are summarized (Figure 4) based on data from Rezak et al. (1990), and descriptions by Schmahl et al. (2008).
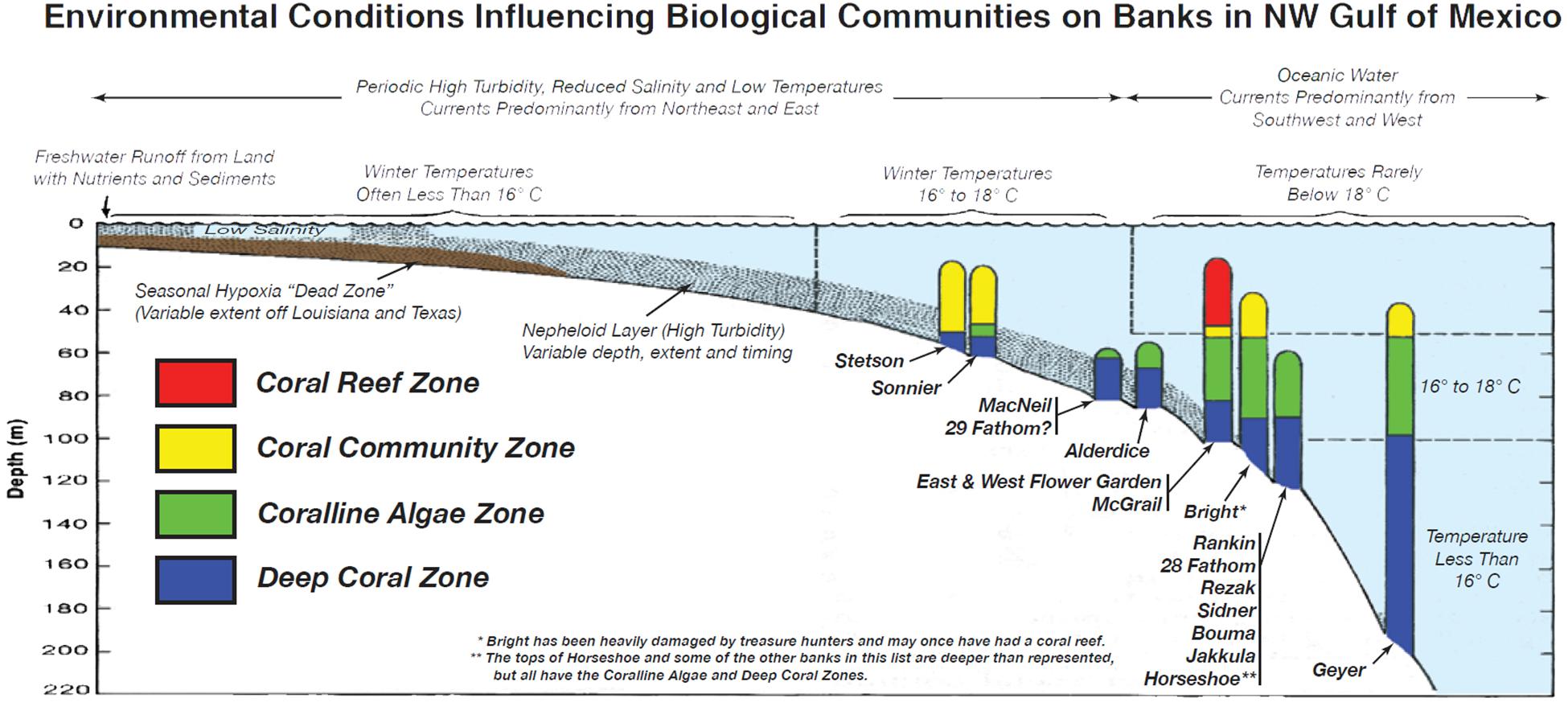
Figure 4. Environmental controls on biological communities on NW Gulf of Mexico reefs and banks using data from Rezak et al. (1990), and Schmahl et al. (2008). Some of the zones of the mentioned banks have not been fully evaluated so this diagram is only a tool for predicting the distribution of the various community zones. Evaluating how changes in the factors that control these communities will be an important basis for assessing climate impacts, and developing management responses as possible changes in the distribution, and composition of biological communities on the banks occur.
Considering these regional biological controls is an important framework for knowing how their changes may affect the distribution of biological communities on the banks in the future. Changes in the depth, and geography of water temperature, current patterns, turbidity, salinity, and nutrient levels in the region may alter the zonation patterns, and the composition of biological communities that make up the zones. These possible changes should trigger the design of monitoring programs seeking recognition of climate, and other impacts where and when they occur, and to develop resource protection strategies in response.
Mexico
When Angelo Heilprin visited Veracruz (SW GoM) in 1890, he was surprised to find a city constructed using corals (Heilprin, 1890), as scientists from that time assumed there were no CR in the western waters of the GoM. However, on its southern region the GoM presents two large zones with CR: Veracruz in the SW, and Campeche Bank to the south.
Veracruz has two well-known reefs systems: to the north, the Lobos-Tuxpan Reef System, composed of nine reefs (de la Cruz-Francisco et al., 2016), and in the south, the Veracruz Reef System, with 50 reefs (Liaño-Carrera et al., 2019), making it the largest reef system in this region (Jordán-Dahlgren, 2004). Recently, the Tuxtlas Coral Reef System, composed of 35 reef structures (Table 1) along the coast was described (Comisión Nacional de Áreas Naturales Protegidas [CONANP], 2018a). Each reef covers <10 km2 with depths between 20–45 m (Jordán-Dahlgren, 2004).
The Campeche Bank Reef System, which extends >200 km from the Yucatan Peninsula, is composed of 11 named emergent reefs, and four named submerged banks (Tunnell, 2007), as well as many unnamed submerged reefs, most of them yet to be described. Many reefs are grouped into a common name (for example Triángulos, which includes Triángulo Este, Triángulo Sur, and Triángulo Oeste) (Table 1). Corals in the Campeche Bank cover areas varying from 3–20 km2, except for the Alacranes Reef, which extends ∼650 km2 (Jordán-Dahlgren, 2004).
In this region, the number of scleractinian coral and octocoral species decrease from east to west (Jordán-Dahlgren, 2002; Horta-Puga et al., 2007). The conservation status of these reefs is considered good, although the only places that have been assessed with relative frequency are the CR of the Veracruz Reef System (Arguelles et al., 2019). Nonetheless, Jackson et al. (2014) established that in 1965 the coral cover of these areas was 34.1%, decreasing to 17.2% by 1999, showing a potentially important decrease in coral cover similar to the one documented in the Caribbean. In other areas such as Alacranes Reef, coral cover has been reported at 11.2% which, although lower than in the Veracruz Reef System, is still higher than some of the coral cover registered in Mexican Caribbean Reefs at <10% (Suchley and Álvarez-Filip, 2018), and very similar to the cover in Florida mentioned previously.
Due to the large number of knowledge gaps on the distribution of CR in the southern GoM, there is an imminent need to describe these “new” coral formations, in aspects such as their size, depth, and species composition, as well as their related biota, and ecosystem services.
Mexico has designated over 90 million ha of natural protected areas, with ca. 650,000 km2 of the marine coastal zones split over 37 MPAs; additionally 92% of the Mexican islands are included in the natural protected areas (CONANP, 2018b). The Lobos-Tuxpan Reef System has an area of >30,000 ha, the Veracruz-Reef System has an area of >60,000 ha, and Los Tuxtlas Reef System has an area of >177,000 ha. The first two are protected as National Parks, however the last one is not. Taking these into account, in Veracruz the protected area is roughly 30% of the reefs area, though Los Tuxtlas Reef System is under study to be included as a protected area. If this area reaches such status, all of the larger reef systems will be protected. In recent years, new reefs have been discovered in the region, and it is likely there are even more undiscovered reefs.
Close to the southwest GoM reefs there are mangroves and seagrass beds. Even when in Mexico mangroves are protected, these stands, and the coastal lagoons close to the reefs into the GoM do not form part of the same MPAs, creating management challenges. There are 10 MPAs into the GoM, three of them (Sistema Arrecifal Veracruzano, Sistema Arrecifal Lobos-Tuxpan, and Arrecife Alacranes) include CR but no mangroves; the remaining seven MPAs include mangroves but no reefs. Coastal lagoons in the GoM have an area of 5,767 km2 (Contreras and Castañeda, 2004). All lagoons are closely related to mangroves, and most of them include seagrass, especially those from the Yucatan Peninsula, requiring an integrated management that considers their connectivity. According to Contreras and Castañeda (2004), by state, from northwest to southeast, the number and area of lagoons are as follow: Tamaulipas has six lagoons with a total area of 2,171 km2, Veracruz has 20 lagoons with 1,213 km2, Tabasco has five lagoons with 3,213 km2, Campeche has three lagoons with 1,788 km2, and finally Yucatan has five lagoons with a total area of 135 km2. Tamaulipas and Tabasco, the states with the highest area of coastal lagoons, are the only states that have no CR on their continental platform. Nonetheless, it is noteworthy that in Mexico an adequate legal framework to establish networks of protected areas is lacking; mainly because connectivity is not considered an important factor in MPAs selection within the national legislation (Ortiz-Lozano et al., 2013).
Cuba
Corals and CR are distributed all around Cuba, with an estimated extension of ∼3,115 km (1,440 km of reefs in the north, and ∼1,675 km in the south). Four main reefs systems could be identified based on their geographic area: Archipiélago de Los Colorados, Archipiélago de Los Canarreos, Archipiélago Sabana-Camagüey, and Archipiélago de las Doce Leguas (Jardines de la Reina). Los Colorados, as well as the reefs of Artemisa, Havana, and Matanzas provinces, represent the southeastern portion of the GoM LME.
The biodiversity in Cuban CR has been studied for >60 years, focusing mainly in groups such as corals, algae, fishes, and mollusks (Alcolado et al., 2003; González-Ferrer, 2004; Claro, 2007; Suárez et al., 2015). Fifty-five scleractinian coral species have been identified in Cuban waters (González-Ferrer, 2004). Acropora palmata, Pseudodiploria strigosa, and Pseudodiploria clivosa are the most abundant species in healthy reef crests, while Siderastrea siderea is the most consistently-abundant coral species in all reef sites (González-Díaz et al., 2018). Other species that are abundant on Cuban reefs are Agaricia agaricites, Agaricia humilis, Montastraea cavernosa, Siderastrea radians, Stephanocoenia intersepta, Porites astreoides, Porites porites, and Porites furcata, while Agaricia lamarcki is very abundant in the fore reefs (González-Ferrer, 2004). In general, Acropora cervicornis, and Orbicella spp. are currently uncommon on Cuban CR, possibly indicating losses due to coral diseases or competition with macroalgae in recent decades (González-Díaz et al., 2018).
Between 2010 and 2013 Caballero Aragón et al. (2019) studied 199 reef sites covering 12 Cuban localities, and documented a similar number of coral species to those recorded in the 1980s; however, dominance has changed as opportunistic species (Siderastrea siderea, and Agaricia agaricites) substituted key ones (Acropora palmata, and O. annularis).
Lately, Cuban CR have been called “the crown jewels of the Caribbean Sea.” Surveys on seven of the main Cuban CR systems (Havana, Artemisa, Los Colorados, Punta Francés, Los Canarreos Archipelago, Península Ancón, and Jardines de la Reina) between 2010 and 2016, suggest differences among communities. Offshore reefs along the south-central coast at Jardines de la Reina, and Península Ancón, exhibited higher coral density, and diversity of species than those closer to populated areas, suggesting their relatively good health (González-Díaz et al., 2018).
The connectivity of CR with other ecosystems such as mangroves, and seagrass beds has required the protection of extensive areas to include these ecosystems. In the northwestern shelf of Cuba (southern border of GoM), for example, seagrass beds extend between 17–40 km from the coast, and the reefs in Archipiélago de Los Colorados are on the shelf’s border, far from the coastline. In Cuba, 105 MPAs have been proposed, covering 25% of the Cuban insular shelf; 57 of them have already been incorporated into the MPA system, and 13 more are being managed to its standards, for a total of 70 areas with some degree of implementation. About 30% of the Cuban CR, 24% of the seagrass beds, and 35% of mangroves are legally protected by the “Sistema Nacional de Areas Protegidas.” The main challenges of these areas are insufficient financial resources, and the difficulty of reinvesting profits generated within them. Prohibited fishing practices, and capture of protected species are the principal issues affecting MPAs (Perera et al., 2018).
Mesophotic and Deep-Sea Corals
The presence of mesophotic corals and CR, has been recorded in the three countries of the GoM. Locker et al. (2010) identified the potential habitat distribution for mesophotic CR in the US, finding that the area of their possible distribution in depths between 30–100 m in the northern and eastern GoM, is 20 times greater than in other areas such as in the Hawaiian Islands, and the different US jurisdictions in the Caribbean. Nonetheless, the knowledge of occurrence, and distribution of these ecosystems remains scarce, but advances continue to move forward. Probably one of the most well-known deep reef areas in the region is Pulley Ridge, located ∼250 km west of Cape Sable, Florida. Although known for its diversity, and productivity since the 1800s, the presence of corals was only documented in the 1980s after an expedition from the Department of Interior’s Mineral Management Service. Subsequent expeditions have further characterized the area, and determined the presence of coral formations composed of species such as Helioseris spp., Agaricia spp., Madracis spp., and Montastraea cavernosa. Area coverage by these corals was estimated at 1.5%, and these corals in mesophotic areas are often described as healthier than those observed on shallow reefs, although some signs of coral diseases were evident (Reed et al., 2019).
The eastern US GoM encompasses the most developed CR formations with seven offshore reefs or banks (see section “Shallow Coral Reef Distribution and Conservation Status”) with a variable area of ∼41–645 km2 (Simmons et al., 2014). The western US GoM is characterized by three types of banks, the south Texas Banks grow on relic carbonates while the banks east off Texas and Louisiana have carbonate reef caps, and are either midshelf or shelf-edge/outer-shelf bedrocks (Rezak et al., 1990). There are six midshelf banks: 32 Fathom, Claypile, Coffee Lump, Fishnet, and the two previously mentioned (Sonnier, Stetson) with areas varying from ∼0.4–18.9 km2; 18 shelf-edge banks: Applebaum, Diaphus, Elvers, Ewing, Parker, Sacket, Sweet (only listed the seven not mentioned in section “Shallow Coral Reef Distribution and Conservation Status”), with areas ranging from 0.18–71.7 km2; and 14 Texas Banks: Aransas, Baker, Big Adam, Blackfish Ridge, Dream, Harte, Hospital Rock, Mysterious, North Hospital, Seabree, Small Adam, South Baker, Southern Bank Test, Steamer covering from 0.07–∼2.4 km2 (Simmons et al., 2014).
In an attempt to locate, and chart deep-sea corals in the GoM, Schroeder et al. (2005) compiled information from different sources (i.e., peer-reviewed and unpublished material, findings from a September–October 2003 cruise in the northern GoM, and the 2003 taxonomic database from the National Museum of Natural History), and identified the presence of at least 24 sites where assemblages of Desmophyllum pertusum, and Madrepora oculata were present. Subsequently, other studies have found new geological, and man-made features in the area, and identified the presence of these and other coral species (Brooks et al., 2012). The presence of oil platforms, and programs such as “Rigs to Reefs” have created substratum appropriate for the colonization of mesophotic corals along the northern GoM, increasing the expansion of these communities (Sammarco et al., 2004; Brooks et al., 2012).
Although mesophotic corals have been identified in the southern portion of the GoM, their extension, and composition are scarcely known, requiring an imminent effort to further study these ecosystems. Ortiz-Lozano et al. (2018) studied 22 emergent, and recorded 18 submerged CR, 16 within, and two outside the Veracruz Reef System National Park in order to identify the role of submerged reefs within protection policies (e.g., port and fishing activities); noting that ∼68.8% of those submerged reefs were not officially recognized, and thus are excluded in the protection decrees. A more recent study using high-resolution bathymetry updated the recorded reef number to 27 in the Veracruz Reef System, most of them submerged (below −30 m) yielding a total of 25 submerged, and 25 emergent reefs (Liaño-Carrera et al., 2019).
Mesophotic reefs in Cuba were characterized for the first time during the joint Cuba-US expedition in 2017. Benthic macrobiota yielded 491 taxa with 149 fishes identified, and 345 specimens of benthic macroinvertebrates, and macroalgae collected to verify taxonomy, and assess population structure. The deep fore-reef escarpment had the greatest diversity, and density of macrobiota; nearly all vertical surfaces were covered with diverse sponges, algae, gorgonians, and black corals. Agaricia spp. were the most abundant scleractinian corals, particularly from 40–75 m deep. Although corals were generally in good health, some colonies (mainly Agaricia spp.) showed signs of bleaching, and one Agaricia specimen had Black Band Disease. Sites outside of MPAs generally had lower fish abundances, a possible indicator of historical overfishing.
Recent events such as the Deepwater Horizon oil spill, show the particular vulnerability of mesophotic corals, and reef communities to human impacts. Different authors, including White et al. (2012), and Silva et al. (2016), documented damages to deep-sea coral communities in the proximity of the event’s site. Characteristics of these ecosystems, such as their isolation, and slow growth, make them particularly vulnerable to long-term impacts (Fisher et al., 2016; Silva et al., 2016). Based on their importance for the ecosystem services they provide (e.g., refugia, food resources, and biological control, as well as useful biotechnological, and medicinal compounds) the study of mesophotic CR is a must.
Threats to GoM Coral Reefs
Rising ocean temperatures, and global climatic changes are among the primary threats to CR around the world, and in the GoM (Anthony et al., 2015). Coral bleaching has likely been one of the most important factors that has affected CR in the Wider Caribbean region over the last 30 years; the 2005 bleaching was recorded as the most intense event of this type in the region. At some sites it affected over 80% of shallow corals, and killed 40% (Eakin et al., 2010). Also, as in many other parts of the world, overpopulation, coastal pollution, and overfishing are considered among the top anthropogenic stressors responsible for CR decline (Jackson et al., 2014).
United States
During the last three decades, the CR of the Florida Keys have suffered a significant loss in coral cover. In the 1970s for example, Acropora palmata dominated most of the outer reef, until a series of disease outbreaks, and hurricanes in the 1980s decimated its population; in some cases, up to 98% of their baseline abundance decreased as compared to the 1970s (Bruckner, 2002). Palandro et al. (2008) found a reduction in coral cover of 37% in eight reef sites monitored by the Coral Reef Evaluation and Monitoring Project between 1996 and 2002 using satellite data, however from direct evaluation the reduction was 52%; and when 1984 Landsat images were used coral cover loss was 61%. Furthermore, taxonomic losses of corals for virtually all habitat types were between 72–73%, with a consistent decline in overall taxonomic richness for all habitats (Jaap et al., 2008).
Bleaching, coral diseases, and hurricanes have been pointed out as the main contributors to coral decline on Florida Keys’ reefs. At least six major bleaching events related to the increase in water temperature have affected the reefs of the Florida Keys since 1987 (Manzello, 2015), in some cases affecting >40% of coral colonies (Eakin et al., 2010; van Woesik and McCaffrey, 2017). In the bleaching event of 1997–1998, surface water temperatures were recorded peaking at 32°C, causing extensive bleaching to scleractinian corals, milleporids, and octocorals (Jaap et al., 2008). Similar to warm water, cold water events can cause rapid coral mortality in shallow reefs at large geographical scales (Lirman et al., 2011).
Since the first descriptions in the 1970s, coral diseases have become more widespread, and prevalent in CR throughout the region (Gil-Agudelo et al., 2009; Peters, 2015). The Wider Caribbean region seems to have been the hotspot of these threats to corals, with the Florida Keys being particularly affected. Many coral diseases known now were first identified in the Florida Keys, including White Band Disease, Yellow Band/Blotch Disease, and White Pox (Gil-Agudelo et al., 2009). A new coral disease known as Stony Coral Tissue Loss Disease was discovered in 2014, and described in the Miami area first (Precht et al., 2016). This disease has since spread to the northern limit of the reef tract, and was recently discovered on reefs west of Key West. It affects 22 species of corals, and can cause losses of as much as 30% of coral density, and 60% of living tissue area, probably becoming the most devastating coral disease to date (Walton et al., 2018) (Figure 5). Other reef organisms have also suffered disease that have decimated their population, such as gorgonian corals (Geiser et al., 1998), sea urchins (Ritchie et al., 2000).
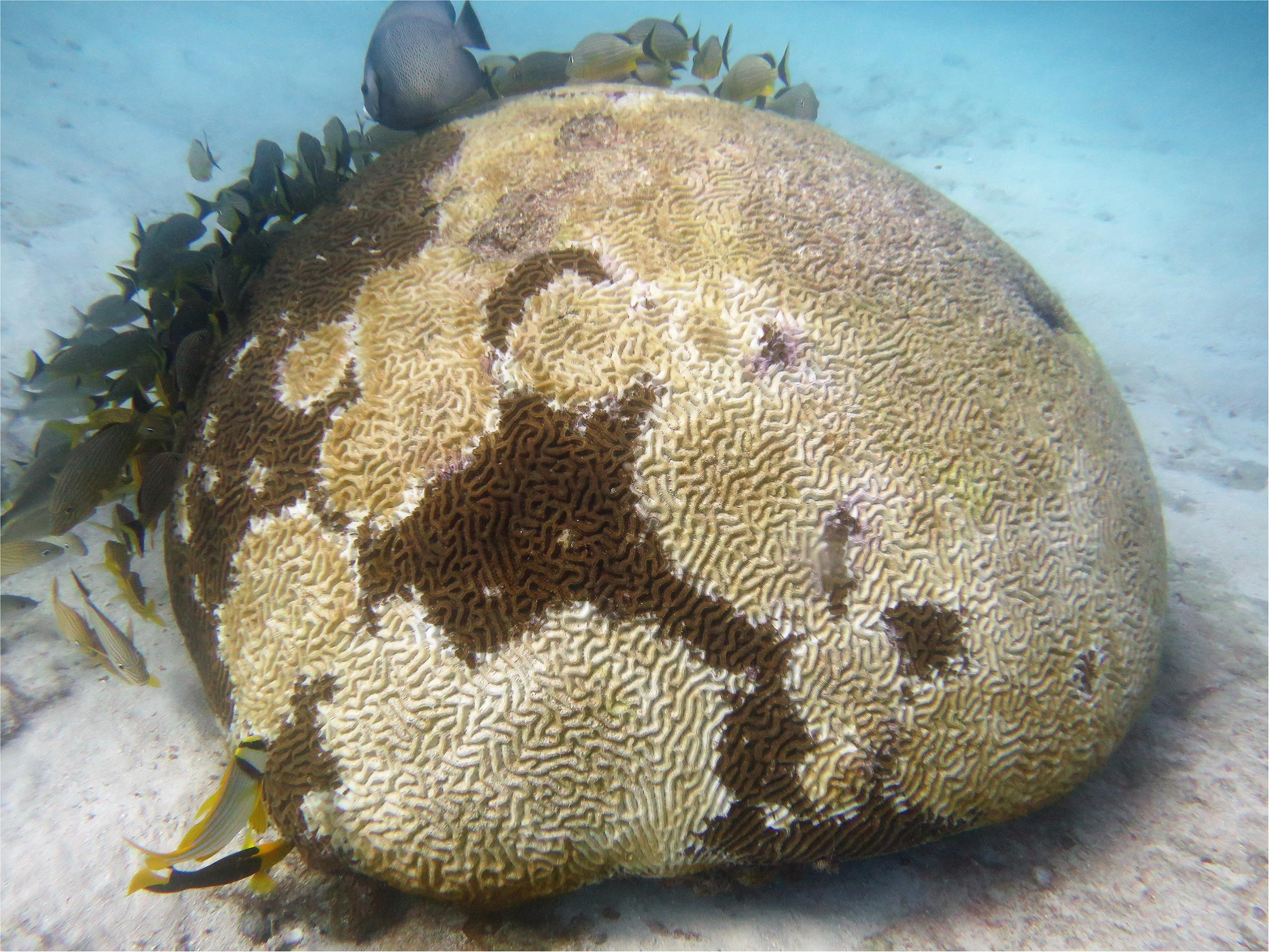
Figure 5. Stony Coral Tissue Loss Disease on a Colpophyllia natans coral head in David Reed, Upper Florida Keys. Photo by Jennifer Stein (The Nature Conservancy) in 2017.
Hurricanes have also been an important contributor to the decline of corals and CR of the Florida Keys. Although these events are part of the natural cycle of corals in the Caribbean, successive hurricane events in short periods of time over the Florida Keys, as well as the effect of other variables, seem to have had a deleterious effect on these reefs (Jaap et al., 2008). Gardner et al. (2005) showed that coral cover was reduced by ∼17%, on average after a hurricane in the Caribbean, followed by a slow recovery after; but CR have shown little recovery, particularly areas previously dominated by A. palmata, mainly due to the interaction of other stressors.
Pollution is also a determinant factor in the decline of CR in the Florida Keys. Maliao et al. (2008) found differences in the composition of the benthic biota of CR between the Lower and Upper-Middle Keys, explained by the higher nutrient concentration found in the Lower Keys, potentially due to patterns of human population, and water mixing. Furthermore, a phase shift occurred in these coral communities, changing from a coral to an algae and sponge dominated ecosystem (Maliao et al., 2008). More recently, LaPointe et al. (2019) showed correlations between increased nitrogen levels as a result of Everglades discharges, and coral stress, and decline at Looe Key reef. Over the study period (1984–2014), water temperatures exceeded the threshold for bleaching (30.5°C) repeatedly, but the three major bleaching events occurred only when nitrogen to phosphorus ratios were high following elevated rainfall or Everglades discharges (LaPointe et al., 2019).
In contrast to the Florida Keys, and other parts of the GoM and the Caribbean, corals in the Flower Garden Banks National Marine Sanctuary seems to be more protected from major bleaching events, and the mortality often associated with them (Hagman and Gittings, 1992; Precht et al., 2008; Johnston et al., 2013). The aforementioned (see section “Threats to GoM Coral Reefs”) 2005 bleaching event affecting >80% of shallow corals in the Wider Caribbean, was minute in comparison, as its effect was <0.5% for the Flower Garden Banks. Nonetheless, in 2016, a strong bleaching event affected >40% of coral colonies at different degrees but with low mortality3 (Davies et al., 2017; Kiene et al., 2017). Although most of the impacted corals have subsequently recovered from this event, its severity questions whether these CR can maintain their resistance, and resilience to changing conditions in the GoM.
Corals in the Flower Garden Banks National Marine Sanctuary also seem to be protected from coral diseases. Borneman and Wellington (2005) described the presence of several potential coral diseases in the area, finding limited incidence, and low coral mortalities, and the apparent absence of coral diseases usually present on Caribbean reefs such as Black Band Disease, and Yellow Blotch Disease. In 2019, unconfirmed signs of coral disease were found in East Garden Bank affecting diverse species, such as Pseudodiploria strigosa, and Orbicella faveolata (DLG-A personal observation; Figure 6).
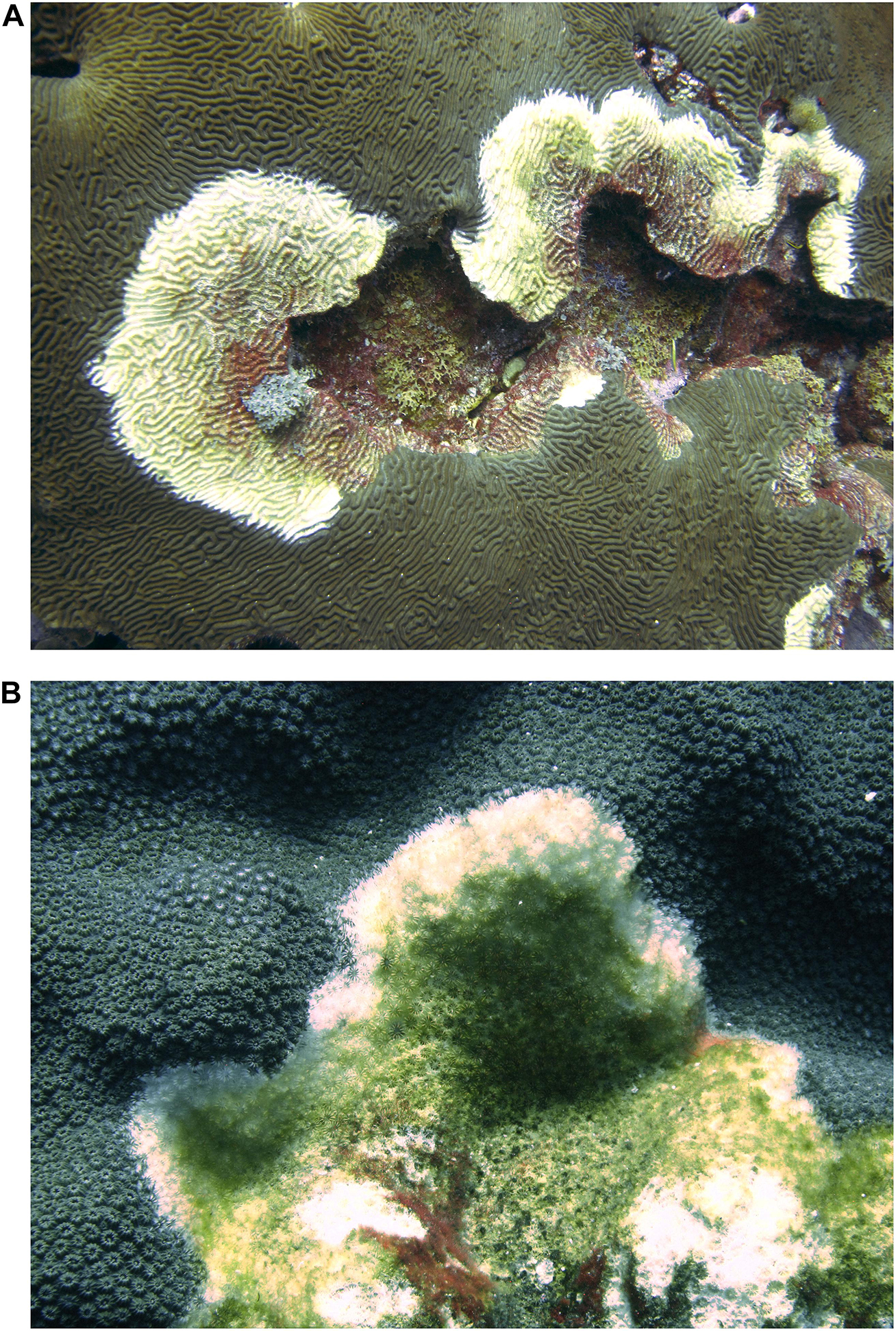
Figure 6. Corals (A) Pseudodiploria strigosa, and (B) Orbicella faveolata with signs that resemble White Band Disease in East Flower Garden Bank at –18.3 m (–60 ft). Photos by DLG-A.
The distance of these banks from the continent (∼185 km) limit the influence of land based pollution. Nonetheless, extreme events such as hurricanes can cause plumes of continental water to reach the Flower Garden Banks, potentially exposing the reef to pollutants (Schmahl et al., 2008). In 2016, an apparent decrease in DO concentration affected 5.6 ha of reef, and up to 82% of coral colonies of East Flower Garden Bank; besides corals, other organisms such as poriferans, crustaceans, mollusks, and echinoderms, also suffered extensive mortality (Johnston et al., 2019). Wright et al. (2019) also showed how the influence of runoff from storm events such as Hurricane Harvey, can produce sublethal stress in these reefs. It is unclear how these events will impact the corals in the future with the increase of nutrient pollution, and dead zones in the area.
Some direct human activities are concerning for the ecosystems of the Flower Garden Banks. Anchoring, fishing, and diving are subject to regulations in the area to minimize potentially harmful effects on the reefs, including direct damage to corals, and release of pollutants and/or debris. All corals and benthic invertebrates in the Flower Garden Banks National Marine Sanctuary are protected from any take or harm through the National Oceanic and Atmospheric Administration (NOAA) regulations. Hurricanes have also been found to cause damage to the banks corals and CR, including due to the mass movement of sand, the movement, and overturning of coral structures, and damage to sponges and other sessile organisms (Schmahl et al., 2008).
Mexico
A diverse number of threats, natural and human-associated, are affecting the reefs of the GoM coast of Mexico. Tropical storms and hurricanes, and winter “Nortes” (cold fronts with high winds that decreases sea surface temperature) increase the potential damage to CR (Jordán-Dahlgren, 2004). Similar to other areas of the GoM and the Caribbean, bleaching events have been reported along the Mexican coast, and in the Veracruz Reef System National Park, particularly in species such as Acropora spp. (Ortiz-Lozano et al., 2018), Colpophyllia natans, Orbicella spp., Siderastrea spp. (Carricart-Ganivet et al., 2011), and Porites spp. (Carricart-Ganivet, 1993). A reduction of these key species might generate catastrophic collapses of coral communities, particularly when the reproductive capacity of such taxa is reduced or eliminated (Jordán-Dahlgren, 2004).
Similar to almost every reef in the Wider Caribbean, White Band Disease affected corals from the Veracruz Reef System and Campeche Banks in the 1980s, and was the main suspect for the decline in Acroporid corals on some of these reefs. Yellow Blotch Disease, and other diseases have further impacted reef-building corals such as Orbicella spp. in this region (Jordán-Dahlgren, 2004).
As a result of their geographic location, Veracruz reefs are the most impacted CR systems in the Mexican GoM. They are not only exposed to the impact of anthropogenic development, including urban, industrial, and agricultural, but also to large riverine discharges. Over-sedimentation, pollution, overfishing, habitat destruction, and coastal development are probably the most important human-made threats to these reefs (Toledo-Ocampo, 2005), noteworthy these impacts may result in deterioration of water column transparency, with its inherent light availability reduction, and their potential consequences on zooxanthellate corals. Currently, the construction of the “Nuevo Puerto de Veracruz” is under way in an area adjacent to the Veracruz Reef System National Park4; its northern breakwater has been recently built over a fringing reef, and the southern breakwater will be built next to another reef, impacting these important ecosystems. Adverse consequences are also expected due to dredging work, anchoring, and the increase in maritime traffic (e.g., Foster et al., 2010). Meanwhile, due to their oceanic location, the reefs in the Campeche Banks experience anchoring, and overfishing as their main stressors, although pollution associated with oil platform construction, and operation has also been identified (Hudson et al., 1982; Jordán-Dahlgren, 2004). The recent massive arrivals, and accumulation of algae to Mexican shores, particularly Sargassum spp., is a concern due to the potential nutrient upload, a consequence of the decomposition of these organisms. Moreover, little is known about the effect of climate regimes on the occurrence of Sargassum spp. pelagic taxa over interannual and decadal scales (Sanchez-Rubio et al., 2018), which might be enhanced with current global climatic changes.
Cuba
The main anthropogenic threats to Cuban CR are related to overfishing by subsistence fisheries (particularly in the northern coasts of Artemisa, Havana, and Matanzas provinces due to their accessibility). Nationally, it is estimated that 20% of fishery resources are fully exploited, 74% overexploited, and 5% collapsed. Other threats such as environmental degradation, and climate change are playing a role in fishes decline, also likely affecting reefs (Baisre, 2018).
Land-based pollution is another important factor affecting the Cuban CR of the GoM. Based on their impact, three areas can be distinguished (Table 2). First, Los Colorados Reefs and Pinar del Rio Province, showing relatively healthy reefs, far from the coast, and with low population density living in small coastal communities. Second, Artemisa Province, with two bays as a major source of land based pollution, with easy accessibility to the reefs but with relatively low population density. Lastly, Havana City, with reefs highly impacted due to industrial activities, and acute and chronic land based pollution sources. Because Havana City is the capital of Cuba, it is also the most populated city.
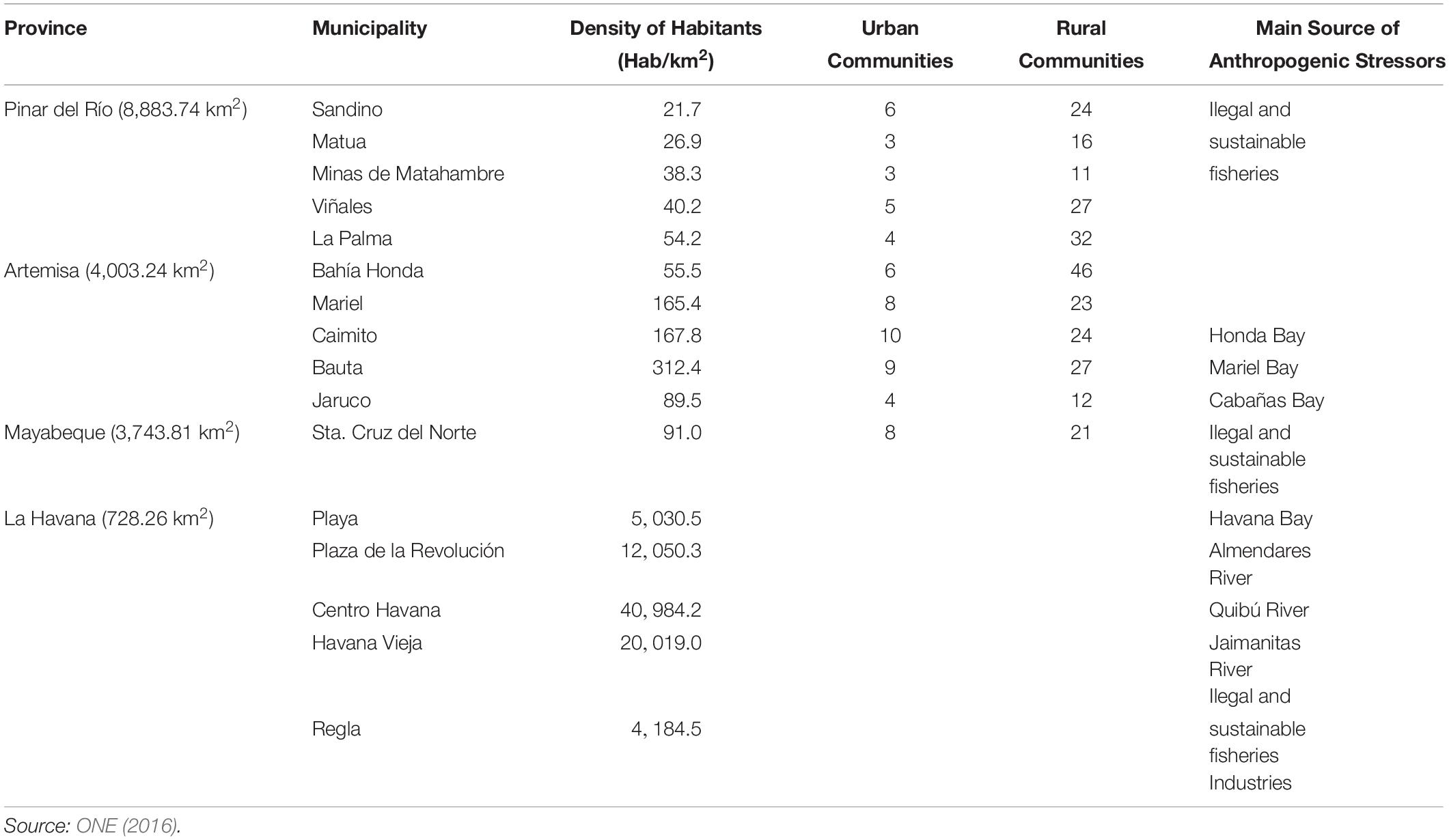
Table 2. Population density in coastal municipalities (directly related with coral reefs), and main anthropogenic stressors in the northwestern Cuban provinces facing the Gulf of Mexico.
Other Impacts
A particular area of concern in the GoM is the potential impact of oil and gas development in the region. The US GoM is one of the major areas of offshore oil and gas production in the world, producing 1.65 million barrels/day (b/d) in 2017. This is expected to rise to 1.8 million b/d by 20195. At present, more than 3,600 production platforms are used to extract oil and gas from the US GoM (Sammarco, 2013). Mexico and Cuba are also seeking to expand their offshore oil production in the GoM, which increases the need to understand the ecological implications of potential changes to the benthic ecosystems, and associated nektonic organisms.
Production platforms and other structures required for the production of offshore oil and gas are known to serve important ecological functions, including increases in secondary biomass production (Cresson et al., 2014), provision of refuge or food for diverse fishes (Nelson and Bortone, 1996; Szedlmayer and Lee, 2004), and support for diverse assemblages of epibenthic invertebrates (Lewbel et al., 1987); they also serve as stepping stones (Sammarco, 2013), which might also have deleterious impacts in natural communities by allowing the expansion of non-native species. Programs such as “Rigs to Reefs” have been designed to increase the productivity by adding available substrates for reef formation (Macreadie et al., 2011; Stephan et al., 2013).
However, there are also very real risks involved with the installation of oil and gas platforms. The risk of oil spills became evident not only after the 2010 Deepwater Horizon event, but others such as the Ixtoc in Mexico in 1979 (Jernelöv and Lindén, 1981), and many minor spills that have resulted from both natural events (such as hurricanes), and human caused incidents6. They can also serve as stepping stones (Sammarco, 2013), which might have deleterious impacts on natural communities. Since the 1930s, Tubastraea coccinea, an invasive species originally from the Pacific arrived to the Caribbean, and has been transported throughout the GoM by colonizing oil and gas platforms (Sammarco et al., 2004). Oculina patagonica is another coral species found in the Veracruz reefs, whose origin appears to be the Mediterranean Sea (Colin-García et al., 2018). Similarly invasion by lionfishes (Pterois volitans, and Pterois miles) has happened, and have reached almost all environments of the GoM (Schofield, 2010) potentially helped by these platforms. In 2013, the fish Neopomacentrus cyanomos originally from the Indo-West Pacific was reported for the first time in the SW corner of the GoM (González-Gándara and de la Cruz-Francisco, 2014). In 2017, Bennett et al. (2019) registered this species for the first time in the coasts of Alabama, northern GoM, inhabiting natural gas platforms, showing the potential effect of these structures in the rapid spread of non-native species across the GoM.
Additionally, during the extraction of oil and gas, Produced Waters (PWs, complex mix of hydrocarbons, heavy metals, and other substances both present in the reservoir, and added during the production process) are also extracted. These PWs are usually treated on site, and released to the environment, carrying substances that have been shown to be deleterious to aquatic organisms during controlled laboratory toxicology tests (Kennicutt, 1994; Fleeger et al., 2001). It is estimated that the PW: oil production ratio in the US is 7:1 (Veil, 2011), meaning that more than 12 million barrels of PWs can be potentially entering the GoM on a daily basis. The effect that these effluents can cause to marine environments such as CR is still largely understudied (Lee and Neff, 2011; Brooks et al., 2012; Cordes et al., 2016), and deserves further attention not only in the US waters, but also throughout the GoM.
Opportunities for Conservation and Sustainability
Several opportunities exist to add momentum, and strengthen conservation of CR throughout the GoM. The first involves capitalizing on existing, and emerging multi-lateral agreements and initiatives, including the GoM LME Program (Global Environment Facility, 2016), and the trinational US-Mexico-Cuba sister sanctuaries agreement. The second involves bringing greater focus onto the GoM within existing global networks of conservation practitioners, including the Reef Resilience Network, and the Coral Restoration Consortium. There is an emerging opportunity for increased financial support for conservation through international initiatives, and the private sector, including insurance policies that focus on the risk-reduction function of reefs in coastal communities, and more broadly on the emergence of corporate sustainability initiatives that emphasize the business dependency on healthy CR, and other coastal habitats.
Governments from the three countries recognize the importance of corals and CR in the GoM, and continue their efforts to protect these delicate ecosystems. In the US, the Flower Garden Banks National Marine Sanctuary is in the process of expansion, seeking to include 14 additional reefs, and banks formations of the northern GoM. Cuba has also identified the need for expansion of their MPAs network, and is working toward the designation of new areas. The Mexican government has recently signed international agreements for the protection of the environment that will help in the conservation of CR. Protection of some species has taken place in the three countries of the GoM (Table 3).
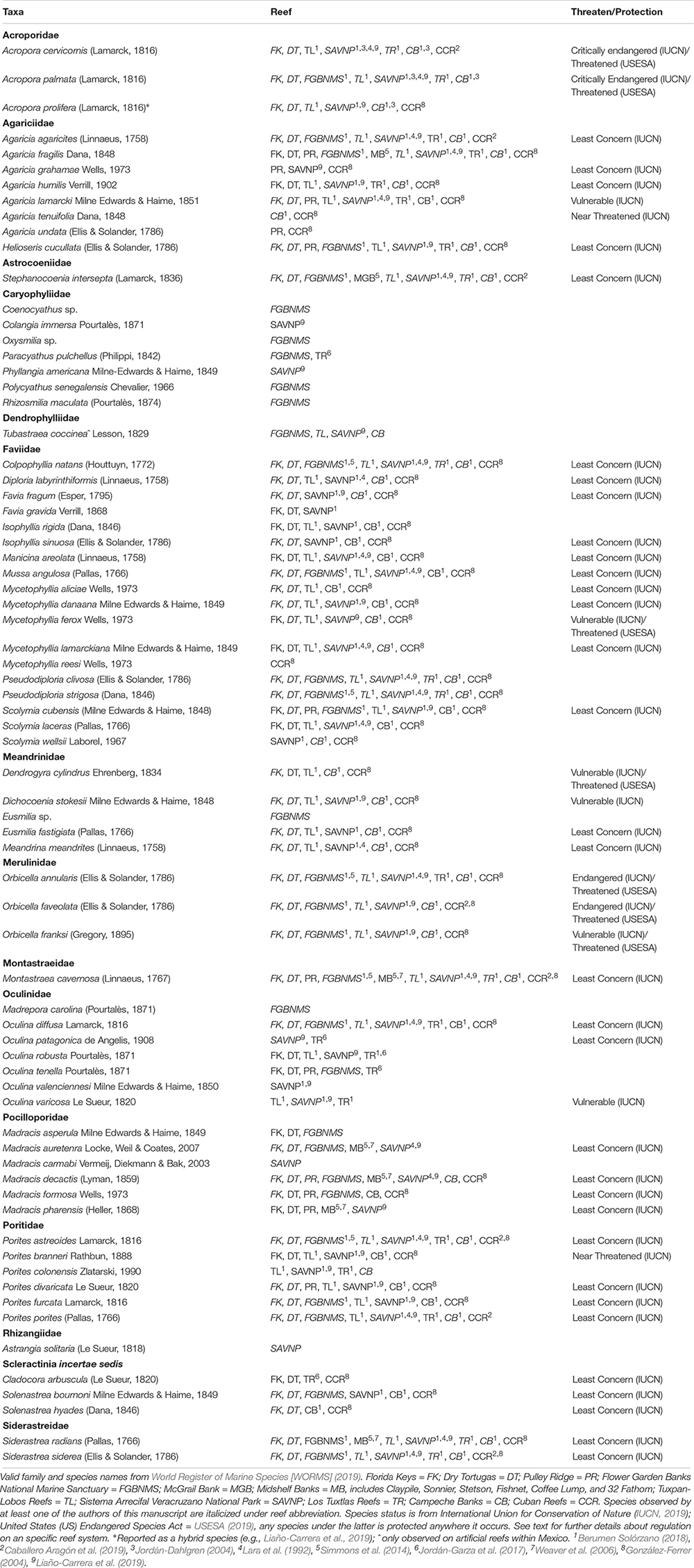
Table 3. Scleractinian coral species by reef system (Reef) in the Gulf of Mexico ordered alphabetically by Family name.
It is important to recognize that corals, CR, and coral bank ecosystems do not function in isolation from other communities, and that their condition is dependent on their ecological connectivity throughout the entire GoM (Kiene, 2018). Ocean currents that flow from the Caribbean, and into the Atlantic physically connect coral species across the Wider Caribbean region (Schill et al., 2015). Fishes, marine mammals, sea turtles, and other organisms migrate among US, Cuban, and Mexican waters, and offspring from reef associated species are transported from one country to another by the currents of the GoM (Brenner et al., 2016). Recent modeling studies on virtual larvae have allowed detection of trajectories illustrating habitat connectivity. For example, Criales et al. (2019) studied blue crab (Callinectes sapidus) showing variable larval dispersal pathways between 2015 and 2016, which were driven by the interaction of winds, the Mississippi River plume, Loop Current intrusions in the northern GoM, Loop Current eddies, and their cyclonic counterparts. Their findings supported the homogeneous genetic population structure of C. sapidus among Mid-Atlantic and GoM populations, as the Mississippi River plume provided a conduit to transport virtual larvae to the South Atlantic Bight. Virtual larvae of red grouper (Epinephelus morio), lionfishes (P. volitans, and P. miles), and a “generic” marine organism showed physical connectivity among the Campeche Banks – particularly the NE perimeter – to the NE GoM defined as locations within the gulf, and western Atlantic explained by the position, and strength of the Loop Current (Johnston and Bernard, 2017). Garavelli et al. (2018) studied the connectivity along banks in the NW GoM using virtual larvae, concluding that Montastraea cavernosa larvae, a common reef-building coral species in CR and banks in the region, is exported to the northeastern, and southwestern GoM. This same species is a key element in shallow reefs in the region, making deep banks potentially important as refugia for the changing condition that shallow reefs are experiencing (Studivan and Voss, 2018). However, see Rocha et al. (2018) for an alternative view of the refuge hypothesis associated to mesophotic reefs (basically might work as long as reefs are far away from the wise monkey’s reach), nonetheless the authors recognize inherent threats to, and prioritize protection of mesophotic reefs.
Other studies have also identified important connectivity in CR organisms that are protected or constitute commercially important resources. As an example, population dynamics of the Caribbean spiny lobster (Panulirus argus) showed the importance of reefs in Cuba and Florida for the larval export throughout the GoM, and the Caribbean (Kough et al., 2013). Similarly, Paris et al. (2005) found an important connectivity of snappers (Lutjanidae) living in CR in Cuba with other CR in the region, showing the importance, and need of transnational resource management strategies. These organisms are known to move considerable distances across shallow shelf areas to reach their spawning aggregations, and healthy habitats, particularly among those with “stepping-stone” patches of submerged reef habitats (Claro et al., 2001; Robertson et al., 2019). Organisms that use different habitats through ontogeny should benefit by provisions of proper habitat that facilitates their migration among isolated reefs.
However, the scale, and intensity of the disturbances in the GoM is threatening the biodiversity, and connectivity of the CR network among populations. For instance, new pollution or hypoxia-induced events continue to appear around the gulf, and the Caribbean. If such network fragmentation continues to increase, any given species’ ability to cope with regional extinction threats will largely depend on its dispersive capabilities (e.g., pelagic larval duration length; Cowen and Sponaugle, 2009). It is imperative then to identify, and implement “ecological corridors”, and conservation actions in order to mitigate habitat destruction, and barriers for dispersal to maintain ecological connectivity. Thus, international cooperation in ocean conservation issues is an essential part of protecting the ecological integrity of CR to preserve populations of reef-dependent species that are commercially important, and threatened throughout the GoM. Studies like those conducted by Schill et al. (2015) can help inform international collaborations like these by using connectivity mapping to inform MPA design, and siting across jurisdictional boundaries.
Recognizing the strong biophysical, and economic connectivity within the GoM, the US executed conservation agreements with Mexico (in 2012), and Cuba (in 2015) to collaborate in the creation of an international network of MPAs to conserve CR resources. This initiative was one of the first formal intergovernmental cooperative plans that came from the normalization of relations between the US, and Cuba (Wenzel et al., 2019), yielding the 2015 Memorandum of Understanding between Cuba’s Ministry of Science Technology and Environment, and the US NOAA, allowing scientific, and management cooperation between their MPAs (NOAA, 2015).
To continue benefiting marine conservation in the region, the US-Cuba relationship has been recently integrated to the ongoing transboundary GoM LME Program. This sister sanctuary initiative has provided MPA site managers in Mexico, Cuba, and the US an opportunity to share the understanding of the ecosystem protection strategies, and trends in the condition, and management of all the sites (Figure 7). Termed “RedGolfo,” the goal is to provide managers of the sites with tools to identify strengths, and weaknesses in how they address key conservation issues, and inform local, and collective decisions on how technical, and management resources can best be applied in the GoM. The ultimate goal is to make the function of the MPA network equal more than the sum of the conservation efforts at each individual site. Through this network of MPAs, and with the cooperation of resources users, Non-Government Organizations, and other stakeholders, Mexico, Cuba, and the US have the opportunity to form valuable joint marine conservation, and research programs to support the long-term management of GoM resources7.
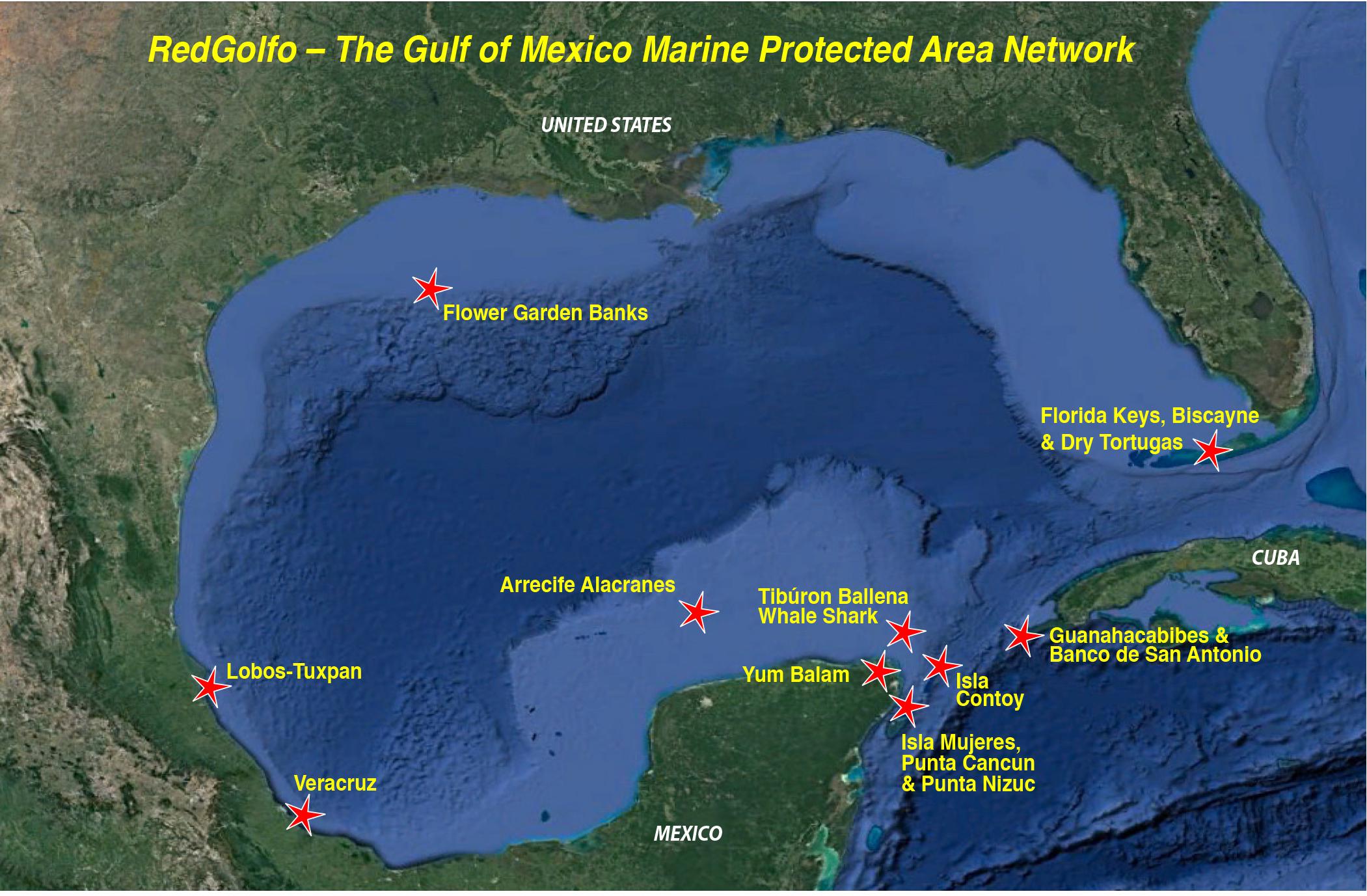
Figure 7. RedGolfo, the Gulf of Mexico Marine Protected Area Network (Source: http://www.cubamar.org).
There are several existing international communities of practice through which GoM reef managers, and scientists could collaborate more closely, and bring the attention to these important habitats. The Reef Resilience Network brings together some 1,500 members from across the globe, and is supported by over 100 experts in CR, fisheries, climate change, and science communication who act as trainers, and advisors. The network distributes pertinent information about the science, and management of CR, develops, and supports both online, and in-person training on a variety of issues, and hosts webinars on key issues, including new management techniques, current events, and publications.
The Coral Restoration Consortium is a community of practice consisting of scientists, managers, and coral restoration practitioners with the goal of encouraging collaboration, and the sharing of lessons learned to help increase the scale, and efficiency of coral restoration efforts worldwide. Working groups of the consortium are currently developing best management practices, and action plans for different types of restoration and/or issues to consider when planning, and conducting restoration (i.e., genetic diversity, how to appropriately monitor success, etc.). The consortium co-hosts webinars with the Reef Resilience Network to disseminate relevant, and timely information to practitioners.
In some places of the Wider Caribbean region, the loss of coral cover has been devastating during the last decades, followed by a slow, and sometimes null recovery. In response, active coral restoration programs have developed around the world with the goal of propagating and/or breeding corals within in-water, and land-based nurseries, and reintroducing them to degraded reef sites to help support natural recovery. Since 2012, over 60 restoration programs have focused on the Acroporid species in the Caribbean basin alone (Young et al., 2012). Coral gardening, pioneered by Rinkevich (1995), has traditionally been the most widely used method of restoration because it involves harvesting a very small amount of coral fragments from the wild, and propagating it to create a sustainable source for future transplantation efforts. More recent advances include the ability to settle sexual recruits in land-based facilities for future restoration efforts (Peterson and Tollrian, 2001), and the ability to induce spawning in corals held in land-based facilities (Craggs et al., 2017) to produce larvae more frequently. Although the restoration work began with a focus on A. cervicornis, programs have since expanded to target other branching, and boulder corals of a wide suite of species.
Finally, an effort to increase the characterization, and protection of the deeper part of the CR or mesophotic reefs in the GoM, is urgently needed. For example, in Mexico the National Commission of Protected Areas recognized only 28% of the 18 submerged reefs associated to the Sistema Arrecifal Veracruzano National Park in the management plan, but these CR were identified during consultation with reef users, and academic mapping efforts rather than through a systematic governmental effort, highlighting the need to identify, and characterize these ecosystems (Ortiz-Lozano et al., 2018). In Cuba, the Cooperative Institute for Ocean Exploration, Research and Technology of the US, and the Marine Science Institute of the University of Habana, completed in 2017 a systematic survey to characterize the extent, and health of reefs from depths of 30 to approximately 150 m (Reed et al., 2018), but there are still important gaps of information to address.
Coral reef ecosystems across the GoM have been nationally protected, and new initiatives have brought international attention to their conservation, and future. However, their sustainability is still far from being secured, as governmental, and societal commitment to their conservation still depends in the identification, and implementation of long-term strategies based in agreements in social-economic tradeoffs. Managing the large diversity, and productivity of CR in a heavily used region such as the GoM requires a shared vision that supports the provisioning of benefits to communities, but also maintains the multiple natural functions that make CR the most productive marine systems. The needed management actions to enhance the condition of CR must include punctual actions such as limiting carrying capacity, and reef visitation, as well as additional regulations that enhance fisheries, and transboundary resource management to promote the ecological connectivity beyond individual jurisdictions. At a larger scale, it is imperative to cut down on land-based pollution entering the GoM, requiring an integrative approach by the three countries that share its waters. For instance, a key management action needed is the implementation of water treatment of all anthropogenic water discharges throughout the GoM.
The present study highlights the urgent need to develop criteria that can identify impacts caused by climate factors vs. those caused by other locally produced stresses, which will provide guidance for management responses to ecosystem changes. As a starting point, the condition of the reefs needs to be characterized, mapped, and monitored in a common way across jurisdictions as a basis for comparing ecosystem responses to environmental change. On the same token, a reconstruction of the microbial communities is imperative, which will allow identification of main viral or bacterial coral diseases experienced in the region. Establishment of baseline measurements on corals, and other organisms to compare when disease or mortality events occur are also needed, thus microbial, and molecular times series on GoM reefs would be advantageous whenever disease outbreaks happen. Ideally properly designed innovative ways to identify the degree of connectivity or isolation (physical, and biological) among coral populations, and how it may threaten or sustain their persistence should be launched. Moreover, endangered, charismatic or commercially exploited species associated with any of these reefs, and preferentially inhabiting through their ontogeny more than one needs further study or verification of their migration patterns. It is important to understand how human activity can impact connectivity or create connectivity that otherwise would not exist, as this can modify the way invasive species, and disease pathogens are spread throughout the region. Thus, as suggested by Garavelli et al. (2018), future connectivity research among all mesophotic coral ecosystems, and beyond to include other important coral habitats in the region using multiple species models to assess the possibility of establishing a regional conservation plan are needed. Using large-scale mapping might help with prioritizing areas for protection and/or restoration to get a higher return on investment not just locally but regionally as well. Bearing in mind that modeling connectivity – although appealing – is always limited to source data, subjected to logical flaws, and parameterization ambiguities (Johnston and Bernard, 2017). Predictive likelihood of habitats are also an option with their own inherent data restrictions.
Coral reefs are, as stated throughout this review, relevant, structural, and functionally diverse ecosystems of the natural realm of the GoM LME. Their long-term viability is threatened by an increasing number of factors, from natural to anthropogenic. Efforts must continue not only to increase our knowledge of these ecosystems but also to implement coordinated transboundary management actions that protect them, and build the resilience needed to assure their survival in decades to come. Such efforts should not be unilateral, and require an integrative approach by Mexico, Cuba, and US to not only address and/or implement the suggested criteria, and measurements but also to foster collaborative research among the three countries sharing the GoM waters, which likely will identify further needs (e.g., response plans for disease outbreaks), and priorities.
Author Contributions
DG-A and CC-B worked equally on the manuscript and first authorship and corresponding authorship were agreed upon them upon recommendation by JB, alphabetical order was followed after the aforementioned contributors. DG-A, CC-B, and JB conceptualized the idea and provided the framework for the document. JB put together the final co-author team. All authors wrote the manuscript with major contributions by region/country as follows: PG-D (Cuba), WK (Texas), CL (Florida), and HP-E (Mexico). All authors reviewed the drafts, provided comments and suggestions, and approved the final manuscript. DG-A, CC-B, and JB wrote the final manuscript.
Funding
The open access publishing fees for this article have been partially covered by The Nature Conservancy; as well as by the Texas A&M University Open Access to Knowledge Fund (OAKFund), supported by the University Libraries and the Office of the Vice President for Research.
Conflict of Interest
The authors declare that the research was conducted in the absence of any commercial or financial relationships that could be construed as a potential conflict of interest.
Acknowledgments
The authors would like to thank Jaime L. Smyth with Dalhousie University and The Nature Conservancy for her help in producing the coral reef map (Figure 1), Jennifer Stein for providing the Colpophyllia natans picture, and the two reviewers (SE and KB), and guest editor (AC) who helped us to improve the manuscript. CL thanks Rob Brumbaugh for reviewing the sections she contributed. The authors express their special thanks to the organizers and participants of the “Gulf of Mexico Reefs: Past, Present and Future Symposium” for contributing to initial discussions that ultimately resulted in the writing of this article and others in this issue. The open access publishing fees for this article have been partially covered by The Nature Conservancy; as well as by the Texas A&M University Open Access to Knowledge Fund (OAKFund), supported by the University Libraries and the Office of the Vice President for Research.
Footnotes
- ^ https://portal.gulfcouncil.org/coralhapc.html
- ^ https://flowergarden.noaa.gov/management/expansiondeis.html
- ^ https://flowergarden.noaa.gov/newsevents/2016bleachingarticle.html
- ^ https://www.gob.mx/semarnat/articulos/sistema-arrecifal-veracruzano-y-el-desarrollo-sustentable?idiom=es
- ^ www.eia.gov
- ^ https://response.restoration.noaa.gov/oil-and-chemical-spills/oil-spills/largest-oil-spills-affecting-us-waters-1969.html
- ^ http://blueoceanproductions.com/blog/gulf-mexico-marine-protected-area-network/
References
Acropora Biological Review Team (2005). Atlantic Acropora Status Review Document. Silver Spring, MD: National Marine Fisheries Service.
Alcolado, P. M., Claro-Madruga, R., Menéndez-Macías, G., García-Parrado, P., Martínez-Daranas, B., and Sosa, M. (2003). “The Cuban coral reefs,” in Latin American Coral Reefs, ed. J. Cortés (Amsterdam: Elsevier Science), 53–71.
Anthony, K. R. N., Marshall, P. A., Abdulla, A., Beeden, R., Bergh, C., Black, R., et al. (2015). Operationalizing resilience for adaptive coral reef management under global environmental change. Glob. Change Biol. 21, 48–61. doi: 10.1111/gcb.12700
Arguelles, J., Brenner, J., and Pérez-España, H. (2019). Línea Base Para el Monitoreo de los Arrecifes del Sistema Arrecifal Veracruzano (PNSAV) a Través de la Metodología AGRRA (Atlantic and Gulf Rapid Reef Assessment). Boca del Rio: Universidad Veracruzana - The Nature Conservancy - Sea and Reef, 1–26.
Baisre, J. A. (2018). An overview of Cuban commercial marine fisheries: the last 80 years. Bull. Mar. Sci. 94, 359–375. doi: 10.5343/bms.2017.1015
Barbier, E. B., Hacker, S. D., Kennedy, C., Koch, E. W., Stier, A. C., and Silliman, B. R. (2011). The value of estuarine and coastal ecosystem services. Ecol. Monogr. 81, 169–193.
Bennett, C. T., Robertson, A., and Patterson, W. F. III (2019). First record of the non-indigenous Indo-Pacific Damselfish, Neopomacentrus cyanomos (Bleeker, 1856) in the northern Gulf of Mexico. BioInvasions Rec. 8, 154–166. doi: 10.3391/bir.2019.8.1.17
Berumen Solórzano, P. (2018). Distribución Geográfica de los Ensamblajes de Corales Escleractinios del Golfo de México y Caribe Mexicano y su Relación con las Variables Oceanográficas. Master’s thesis. Universidad Veracruzana: Boca del Río.
Borneman, E. H., and Wellington, G. M. (2005). Pathologies affecting reef corals at the flower garden banks, northwestern Gulf of Mexico. Gulf Mex. Sci. 23, 95–106.
Brenner, J., Voight, C., and Mehlman, D. (2016). Migratory Species in the Gulf of Mexico Large Marine Ecosystem: Pathways, Threats and Conservation. Arlington, VA: The Nature Conservancy.
Brooke, S., and Schroeder, W. W. (2007). “State of deep coral ecosystems in the Gulf of Mexico region: Texas to the Florida straits,” in The State of Deep Coral Ecosystems of the United States, eds S. E. Lumsden, T. F. Hourigan, A. W. Bruckner, and G. Dorr (Silver Spring, MD: NOAA Technical Memorandum), 65–108.
Brooks, J. M., Fisher, C., Roberts, H., Cordes, E., Baums, I., Bernard, B., et al. (2012). Exploration and Research of Northern Gulf of Mexico Deepwater Natural and Artificial Hard-Bottom Habitats With Emphasis on Coral Communities: Reefs, Rigs, and Wrecks - “Lophelia II” Interim Report. New Orleans, LA: U.S. Department of the Interior, Bureau of Ocean Energy Management.
Bruckner, A. W. (2002). Proceedings of the Caribbean Acropora workshop: Potential Application of the US Endangered Species Act as a Conservation Strategy. NOAA Technical Memorandum NMFS-OPR-24. Miami, FL: NOAA.
Caballero Aragón, H., Armenteros, M., Perera Valderrama, S., Rey Villiers, N., Cobián Rojas, D., Campos Verdecia, K., et al. (2019). Ecological condition of coral reef assemblages in the Cuban Archipelago. Mar. Biol. Res. 15, 61–73. doi: 10.1080/17451000.2019.1577557
Carricart-Ganivet, J. P. (1993). Blanqueamiento parcial en Porites porites (Cnidaria: Scleractinia) en el Arrecife Isla Verde, Veracruz, México. Rev. Biol. Trop. 41, 495–498.
Carricart-Ganivet, J. P., Beltrán-Torres, A. U., and Horta-Puga, G. (2011). Distribution and prevalence of coral diseases in the Veracruz Reef System, southern Gulf of Mexico. Dis. Aquat. Organ. 95, 181–187. doi: 10.3354/dao02359
Claro, R. (2007). La Biodiversidad Marina de Cuba. La Habana: Instituto de Oceanología, Ministerio de Ciencias, Tecnología y Medio Ambiente.
Claro, R., Lindeman, K. C., and Parenti, L. R. (eds) (2001). Ecology of the Marine Fishes of Cuba. Washington, DC: Smithsonian Institution.
Colin-García, N. A., Campos, J. E., Tello-Musi, J. L., Pérez-España, H., and Chiappa-Carrara, X. (2018). First record of the invasive coral Oculina patagonica de Angelis, 1908 (Cnidaria, Scleractinia) in the Gulf of Mexico. Check List 14, 613–617. doi: 10.15560/14.4.613
Comisión Nacional de Áreas Naturales Protegidas [CONANP] (2018a). Estudio Previo Justificativo Para la Declaratoria del Área de Protección de Flora y Fauna Arrecifes de Los Tuxtlas. México: CONABIO-SEMARNAT.
Comisión Nacional de Áreas Naturales Protegidas [CONANP] (2018b). México es Lider Mundial en la Protección de Áreas Marinas. Available at: https://www.gob.mx/conanp/es/prensa/mexico-es-lider-mundial-en-la-proteccion-de-areas-marinas?idiom=es (accessed October 14, 2019).
Contreras, F., and Castañeda, O. (2004). “Las lagunas costeras y estuarios del golfo de México: hacia el establecimiento de índices ecológicos,” in Diagnóstico Ambiental del Golfo de México, eds M. Caso, I. Pisanty, and E. Ezcurra (México: INE-SEMARNAT), 373–416.
Cordes, E. E., Jones, D. O., Schlacher, T. A., Amon, D. J., Bernardino, A. F., Brooke, S., et al. (2016). Environmental impacts of the deep-water oil and gas industry: a review to guide management strategies. Front. Environ. Sci. 4:58. doi: 10.3389/fenvs.2016.00058
Cowen, R. K., and Sponaugle, S. (2009). Larval dispersal and marine population connectivity. Annu. Rev. Mar. Sci. 1, 443–466. doi: 10.1146/annurev.marine.010908.163757
Craggs, J., Guest, J. R., Davis, M., Simmons, J., Dashti, E., and Sweet, M. (2017). Inducing broadcast coral spawning ex situ: closed system mesocosm design and husbandry protocol. Ecol. Evol. 7, 11066–11078. doi: 10.1002/ece3.3538
Cresson, P., Ruitton, S., and Harmelin-Vivien, M. (2014). Artificial reefs do increase secondary biomass production: mechanisms evidenced by stable isotopes. Mar. Ecol. Prog. Ser. 509, 15–26. doi: 10.3354/meps10866
Criales, M. M., Chérubin, L., Gandy, R., Garavelli, L., Ghannami, M. A., and Crowley, C. (2019). Blue crab larval dispersal highlights population connectivity and implications for fishery management. Mar. Ecol. Prog. Ser. 625, 53–70. doi: 10.3354/meps13049
Davies, S. W., Strader, M. E., Kool, J. T., Kenkel, C. D., and Matz, M. V. (2017). Modeled differences of coral life-history traits influence the refugium potential of a remote Caribbean reef. Coral Reefs 36, 913–925. doi: 10.1007/s00338-017-1583-8
Davis, G. E. (1982). A century of natural change in coral distribution at the Dry Tortugas: a comparison of reef maps from 1881 and 1976. Bull. Mar. Sci. 32, 608–623.
de la Cruz-Francisco, V., González-González, M., and Morales-Quijano, I. (2016). Faunística y distribución de demospongiae: porifera del arrecife enmedio sistema arrecifal lobos-tuxpan, México. Oceánides 31, 7–16.
Eakin, C. M., Morgan, J. A., Heron, S. F., Smith, T. B., Liu, G., Alvarez-Filip, L., et al. (2010). Caribbean corals in hot water: record thermal stress, bleaching, and mortality in 2005. PLoS One 5:e13969. doi: 10.1371/journal.pone.0013969
Fisher, C. R., Montagna, P. A., and Sutton, T. T. (2016). How did the deepwater horizon oil spill impact deep-sea ecosystems? Oceanography 29, 182–195. doi: 10.5670/oceanog.2016.82
Fleeger, J. W., Foltz, D. W., and Rocha-Olivares, A. (2001). How Does Produced Water Cause a Reduction in the Genetic Diversity of Harpacticoid Copepods? Final Report. New Orleans, LA: U.S. Department of the Interior.
Foster, T., Corcoran, E., Erftemeijer, P., Fletcher, C., Peirs, K., and Dolmans et al (2010). Dredging and Port Construction Around Coral Reefs Report No. 108. Belgique: PIANC.
Garavelli, L., Studivan, M. S., Voss, J. D., Kuba, A., Figueiredo, J., and Chérubin, L. M. (2018). Assessment of mesophotic coral ecosystem connectivity for proposed expansion of a marine sanctuary in the northwest Gulf of Mexico: larval dynamics. Front. Mar. Sci. 5:174. doi: 10.3389/fmars.2018.00174
Gardner, T. A., Côté, I. M., Gill, J. A., Grant, A., and Watkinson, A. R. (2005). Hurricanes and Caribbean coral reefs: impacts, recovery patterns, and role in long-term decline. Ecology 86, 174–184. doi: 10.1890/04-0141
Geiser, D. M., Taylor, J. W., Ritchie, K. B., and Smith, G. W. (1998). Cause of sea fan death in the West Indies. Nature 394, 137–138. doi: 10.1038/28079
Gil-Agudelo, D. L., Navas-Camacho, R., Rodríguez-Ramírez, A., Reyes-Nivia, M. C., Bejarano, S., Garzón-Ferreira, J., et al. (2009). Enfermedades coralinas y su investigación en los arrecifes colombianos. Bol. Invest. Mar. Cost. 38, 189–224.
Global Environment Facility (2016). Available at: https://www.thegef.org/project/integrated-assessment-and-management-gulf-mexico-large-marine-ecosystem (accessed July 18, 2019).
González-Díaz, P., González-Sansón, G., Aguilar, C., Álvarez, S., Perera, O., Hernández, L., et al. (2018). Status of Cuban coral reef. Bull. Mar. Sci. 94, 229–247. doi: 10.5343/bms.2017.1035
González-Ferrer, S. (2004). Corales Pétreos, Jardines Sumergidos de Cuba. La Habana: Editorial Academia.
González-Gándara, C., and de la Cruz-Francisco, V. (2014). Unusual record of the Indo-Pacific Pomacentrid Neopomacentrus cyanomos (Bleeker, 1856) on coral reefs of the Gulf of Mexico. BioInvasions Rec. 3, 49–52. doi: 10.3391/bit.2014.3.1.09
Goreau, T. F. (1959). The ecology of Jamaican coral reefs I. Species composition and zonation. Ecology 40, 67–90. doi: 10.2307/1929924
Gulf of Mexico Fishery Management Council [GMFMC] (2011). Final Generic Annual Catch Limits/Accountability Measures Amendment for the Gulf of Mexico Fishery Management Council’s Red Drum, Reef Fish, Shrimp, Coral and Coral Reefs, Fishery Management Plans. Silver Spring, MD: NOAA Fishery Service.
Gutiérrez-Ruiz, C. V., Román-Vives, M. A. M., Vergara, C. H., and Badano, E. I. (2011). Impacts of anthropogenic disturbances on the diversity of shallow stony corals in the Veracruz Reef System National Park. Rev. Mex. Biodiv. 82, 249–260.
Hagman, D. K., and Gittings, S. R. (1992). “Coral bleaching on high latitude reefs at the flower garden banks, NW Gulf of Mexico,” in Proceedings of the 7th International Coral Reef Symposium, Vol. 1, ed. R. H. Richmond (Guam: University of Guam), 38–43.
Harborne, A. R., Mumby, P. J., Michelli, F., Perry, C. T., Dahlgren, C. P., Holmes, K. E., et al. (2006). The functional value of Caribbean coral reef, seagrass and mangrove habitats to ecosystem processes. Adv. Mar. Biol. 50, 57–189. doi: 10.1016/S0065-2881(05)50002-6
Haskell, B. D., Leeworthy, V. R., Wiley, P. C., Beuttler, T. M., Haflich, M. R., and Franklin, E. (2000). Tortugas Ecological Reserve: Draft Supplemental Environmental Impact Statement/Draft Supplemental Management Plan. Washington, DC: U.S. Department of Commerce.
Heilprin, A. (1890). The corals and coral reefs of the western waters of the Gulf of Mexico. Proc. Acad. Nat. Sci. Phil. 42, 303–316.
Hinderstein, L. M., Marr, J. C. A., Martinez, F. A., Dowgiallo, M. J., Puglise, K. A., Pyle, R. L., et al. (2010). Theme section on “mesophotic coral ecosystems: characterization, ecology, and management”. Coral Reefs 29, 247–251. doi: 10.1007/s00338-010-0614-5
Horta-Puga, G., Vargas-Hernández, J. M., and Carricart-Ganivet, J. P. (2007). “Reef corals,” in Coral Reefs of the Southern Gulf of Mexico, eds J. W. Tunnell, Jr., E. A. Chávez, and K. Withers (College Station, TX: Texas A&M University Press), 95–101.
Hudson, J. H., Shin, E. A., and Robbin, D. M. (1982). Effects of offshore oil drilling on Philippine reef corals. Bull. Mar. Sci. 32, 890–908.
International Union for Conservation of Nature (IUCN) (2019). Available at: https://www.iucnredlist.org/ (accessed August 13, 2019).
Jaap, W. C. (1984). Ecology of the South Florida Coral Reefs: a Community Profile. St. Petersburg, FL: Departmet of Natural Resources Marine Research Laboratory.
Jaap, W. C., Szmant, A., Jaap, K., Dupont, J., Clarke, R., Somerfield, P., et al. (2008). “A perspective on the biology of Florida Keys coral reefs. Coral reefs of the world,” in Coral Reefs of the USA, Vol. 1, eds B. M. Riegl and R. E. Dodge (New York, NY: Springer), 75–125.
Jackson, J. B. C., Donovan, M. K., Cramer, K. L., and Lam, V. V. (eds) (2014). Status and Trends of Caribbean Coral Reefs: 1970-2012. Gland: IUCN.
Jernelöv, A., and Lindén, O. (1981). Ixtoc I: a case study of the world’s largest oil spill. AMBIO 10, 299–306.
Johnston, M. A., Nuttall, M. F., Eckert, R. J., Blakeway, R. D., Sterne, T. K., Hickerson, E. L., et al. (2019). Localized coral reef mortality event at East Flower Garden Bank, Gulf of Mexico. Bull. Mar. Sci. 95, 239–250. doi: 10.5343/bms.2018.0057
Johnston, M. A., Nuttall, M. F., Eckert, R. J., Embesi, J. A., Slowey, N. C., Hickerson, E. L., et al. (2013). Long-Term Monitoring at the East and West Flower Garden Banks National Marine Sanctuary, 2009-2010: Volume I: Technical Report. New Orleans, LA: U.S. Department of Interior.
Johnston, M. A., Nuttall, M. F., Eckert, R. J., Embesi, J. A., Sterne, T. K., Hickerson, E. L., et al. (2016). Persistence of coral assemblages in Flower Garden Banks National Marine Sanctuary, Gulf of Mexico. Coral Reefs 35, 821–826. doi: 10.1007/s00338-016-1452-x
Johnston, M. W., and Bernard, A. M. (2017). A bank divided: quantifying a spatial and temporal connectivity break between the Campeche Bank and the northeastern Gulf of Mexico. Mar. Biol. 164:12. doi: 10.1007/s00227-016-3038-0
Jones, R., Ricardo, G. F., and Negri, A. P. (2015). Effects of sediments on the reproductive cycle of corals. Mar. Poll. Bull. 100, 13–33. doi: 10.1016/j.marpolbul.2015.08.021
Jordán-Dahlgren, E. (2002). Gorgonian distribution patterns in coral reef environments of the Gulf of Mexico: evidence of sporadic ecological connectivity? Coral Reefs 21, 205–215. doi: 10.1007/s00338-002-0226-9
Jordán-Dahlgren, E. (2004). “Coral reefs of the Gulf of Mexico: characterization and diagnosis,” in Environmental Analysis of the Gulf of Mexico, eds K. Withers and M. Nipper (Corpus Christi, TX: Harte Research Institute for Gulf of Mexico Studies), 340–350.
Jordán-Garza, A. G., González-Gándara, C., Salas-Pérez, J. J., and Morales-Barragan, A. M. (2017). Coral assemblages are structured along a turbidity gradient on the southwestern Gulf of Mexico, Veracruz. Cont. Shelf Res. 138, 32–40. doi: 10.1016/j.csr.2017.03.002
M. C. Kennicutt II (ed.) (1994). Gulf of Mexico Offshore Operations Monitoring Experiment, Phase I: Sublethal Responses to Contaminant Exposure. Interim Report. New Orleans, LA: U.S. Department of the Interior.
Kiene, W. (2018). The Gulf of Mexico ecosystem: linking shared marine resources across international borders through the Gulf’s special places. Sea Grant Law Policy J. 9, 8–15.
Kiene, W., Johnston, M., Hickerson, E., and Schmahl, G. P. (2017). Unprecedented 2016 at Flower Garden Banks National Marine Sanctuary. Southern Climate Monitor. Available at: http://www.southernclimate.org/documents/SCIPP_Monitor_February_2017.pdf (accessed December 15, 2019).
Kough, A. S., Paris, C. B., and Butler, M. J. I. V. (2013). Larval connectivity and the international management of fisheries. PLoS One 8:e64970. doi: 10.1371/journal.pone.0064970
LaPointe, B. E., Brewton, R. A., Herren, L. W., Porter, J. W., and Hu, C. (2019). Nitrogen enrichment, altered stoichiometry, and coral reef decline at Looe Key, Florida Keys, USA: a 3-decade study. Mar. Biol. 166:108.
Lara, M., Padilla, C., García, C., and Espejel, J. J. (1992). “Coral reef of Veracruz México I. Zonation and community,” in Proceedings of the 7th International Coral Reef Symposium, Vol. 1, ed. R. H. Richmond (Guam: University of Guam Press), 535–544.
Lee, K., and Neff, J. (eds) (2011). Produced Water. Environmental Risks and Advances in Mitigation Technologies. New York, NY: Springer.
Lewbel, G. S., Howard, R. L., and Gallaway, B. J. (1987). Zonation of dominant fouling organisms on northern Gulf of Mexico petroleum platforms. Mar. Environ. Res. 21, 199–224. doi: 10.1016/0141-1136(87)90066-3
Liaño-Carrera, F., Camarena-Luhrs, T., Gómez-Barrero, A., Martos-Fernández, F. J., Ramírez-Macias, J. I., and Salas-Monreal, D. (2019). New coral reef structures in a tropical coral reef system. Lat. Am. J. Aquat. Res. 47, 270–281.
Lirman, D., Schopmeyer, S., Manzello, D., Gramer, L. J., Precht, W. F., Muller-Karger, F., et al. (2011). Severe 2010 cold-water event caused unprecedented mortality to corals of the Florida Reef Tract and reversed previous survivorship patterns. PLoS One 6:e23047. doi: 10.1371/journal.pone.0023047
Locker, S. D., Armstrong, R. A., Battista, T. A., Rooney, J. J., Sherman, C., and Zawada, D. G. (2010). Geomorphology of mesophotic coral ecosystems: current perspectives on morphology, distribution, and mapping strategies. Coral Reefs 29, 329–345. doi: 10.1007/s00338-010-0613-6
Macreadie, P., Fowler, A. M., and Booth, D. J. (2011). Rigs-to-reefs: will the deep sea benefit from artificial habitat? Front. Ecol. Environ. 9:455–461. doi: 10.1890/100112
Maliao, R. J., Turingan, R. G., and Lin, J. (2008). Phase-shift in coral reef communities in the Florida Keys National Marine Sanctuary (FKNMS). USA Mar. Biol. 154, 841–853. doi: 10.1007/s00227-008-0977-0
Manzello, D. P. (2015). Rapid recent warming of coral reefs in the Florida Keys. Sci. Rep. 5:16762. doi: 10.1038/srep16762
Marszalek, D. S., Babashoff, G., Noel, M. R., and Worley, D. R. (1977). “Reef distribution in south Florida,” in Proceedings of the 3rd International Coral Reef Symposium on Geology, Vol. 2, ed. D. L. Taylor (Miami, FL: Rosenstiel School of Marine and Atmospheric Science), 223–229.
Mumby, P. J., and Steneck, R. S. (2008). Coral reef management and conservation in light of rapidly evolving ecological paradigms. Trends Ecol. Evol. 23, 555–563. doi: 10.1016/j.tree.2008.06.011
Nellemann, C., Hain, S., and Alder, J. (eds) (2008). In Dead Water - Merging of Climate Change with Pollution, Over-Harvest, and Infestations in the World’s Fishing Grounds. Norway: GRID-Arendal, United Nations Environment Programme.
Nelson, B. D., and Bortone, S. A. (1996). Feeding guilds among artificial-reef fishes in the northern Gulf of Mexico. Gulf Mex. Sci. 14, 66–80.
NOAA (2015). Available at: http://sanctuaries.noaa.gov/news/nov15/us-and-cuba-to-cooperate-on-sister-sanctuaries.html (accessed July 18, 2019).
Ortiz-Lozano, L., Colmenares-Campos, C., and Gutiérrez-Velázquez, A. (2018). Submerged coral reefs in the Veracruz reef system, Mexico, and its implications for marine protected area management. Ocean Coast. Manag. 158, 11–23. doi: 10.1016/j.ocecoaman.2018.03.012
Ortiz-Lozano, L., Pérez-España, H., Granados-Barba, A., González-Gándara, C., Gutiérrez-Velázquez, A., and Matos, J. (2013). The reef corridor of the southwest Gulf of Mexico: challenges for its management and conservation. Ocean Coast. Manag. 86, 22–32. doi: 10.1016/j.ocecoaman.2013.10.006
Palandro, D. A., Andréfouët, S., Hu, C., Hallock, P., Müeller-Karger, F. E., Dustan, P., et al. (2008). Quantification of two decades of shallow-water coral reef habitat decline in the Florida Keys National Marine Sanctuary using Landsat data (1984-2002). Remote Sens. Environ. 112, 3388–3399. doi: 10.1016/j.rse.2008.02.015
Paris, C. B., Cowen, R. K., Claro, R., and Lindeman, K. C. (2005). Larval transport pathways from Cuban Snapper (Lutjanidae) spawning aggregations based on biophysical modeling. Mar. Ecol. Prog. Ser. 296, 93–106. doi: 10.3354/meps296093
Parker, R. O., Colby, D. R., and Willis, T. D. (1983). Estimated amount of reef habitat on a portion of the U.S. south Atlantic and Gulf of Mexico continental shelf. Bull. Mar. Sci. 33, 935–940.
Perera, S., Hernández, A., González, J., Moreno, O., Cobián, D., Ferro, H., et al. (2018). Marine protected areas in Cuba. Bull. Mar. Sci. 94, 423–442. doi: 10.5343/bms.2016.1129
Peters, E. C. (2015). “Diseases of coral reef organisms,” in Coral Reefs in the Anthropocene, ed. C. Birkeland (Dordrecht: Springer), 147–178. doi: 10.1007/978-94-017-7249-5_8
Peterson, D., and Tollrian, R. (2001). Methods to enhance sexual recruitment for restoration of damaged reefs. Bull. Mar. Sci. 69, 989–1000.
Precht, W. F., Aronson, R. B., Deslarzes, K. J. P., Robbart, M. L., Evans, D. J., Zimmer, B., et al. (2008). Long-Term Monitoring at the East and West Flower Garden Banks, 2004-2005 - Interim Report. Technical Report, Vol. I. New Orleans, LA: U.S. Department of the Interior.
Precht, W. F., Gintert, B. E., Robbart, M. L., Fura, R., and van Woesik, R. (2016). Unprecedented disease-related coral mortality in southeastern Florida. Sci. Rep. 6:31374. doi: 10.1038/srep31374
Reed, J. K., Farrington, S., David, A., Harter, S., Pomponi, S. A., Diaz, M. C., et al. (2019). “Pulley Ridge, Gulf of Mexico, USA,” in Mesophotic Coral Ecosystems. Coral Reefs of the World, Vol. 12, eds Y. Loya, K. A. Puglise, and T. C. L. Bridge (Cham: Springer), 57–69. doi: 10.1007/978-3-319-92735-0_4
Reed, J. K., González-Díaz, P., Busutil, L., Farrington, S., Martínez-Daranas, B., Cobián Rojas, D., et al. (2018). Cuba’s mesophotic coral reefs and associated fish communities. Cuba Rev. Invest. Mar. 38, 60–129.
Rezak, R., Gittings, S. R., and Bright, T. J. (1990). Biotic assemblages and ecological controls on reefs and banks of the northwest Gulf of Mexico. Am. Zool. 30, 23–35. doi: 10.1093/icb/30.1.23
Rinkevich, B. (1995). Restoration strategies for coral reefs damaged by recreational activities: the use of sexual and asexual recruits. Restor. Ecol. 3, 241–251. doi: 10.1111/j.1526-100x.1995.tb00091.x
Ritchie, K. B., Nagelkerken, I., James, S., and Smith, G. W. (2000). A tetrodotoxin-producing marine pathogen. Nature 404:354. doi: 10.1038/35006168
Robertson, D. R., Domínguez-Dominguez, O., López Aroyo, Y. M., Moreno Mendoza, R., and Simões, N. (2019). Reef-associated fishes from the offshore reefs of western Campeche Bank, Mexico, with a discussion of mangroves and seagrass beds as nursery habitats. ZooKeys 843, 71–115. doi: 10.3897/zookeys.843.33873
Rocha, L. A., Pinheiro, H. T., Shepherd, B., Papastamatiou, Y. P., Luiz, O. J., Pyle, R. L., et al. (2018). Mesophotic coral ecosystems are threatened and ecologically distinct from shallow water reefs. Science 361, 281–284. doi: 10.1126/science.aaq1614
Salas-Pérez, J. J., and Granados-Barba, A. (2008). Oceanographic characterization of the Veracruz reefs system. Atmósfera 21, 281–301.
Sammarco, P. W. (2013). Corals on Oil and Gas Platforms Near the Flower Garden Banks: Population Characteristics, Recruitment, and Genetic Affinity. New Orleans, LA: U.S. Department of the Interior.
Sammarco, P. W., Atchinson, A. D., and Boland, G. S. (2004). Expansion of coral communities within the northern Gulf of Mexico via offshore oil and gas platforms. Mar. Ecol. Prog. Ser. 280, 129–143. doi: 10.1371/journal.pone.0030144
Sanchez-Rubio, G., Perry, H., Franks, J. S., and Johnson, D. R. (2018). Occurrence of pelagic Sargassum in waters of the U.S. Gulf of Mexico in response to weather-related hydrographic regimes associated with decadal and interannual variability in global climate. Fish. Bull. 116, 93–106. doi: 10.7755/FB.116.1.10
Schill, S. R., Raber, G. T., Roberts, J. J., Treml, A. E., Brenner, J., and Halpin, P. N. (2015). No reef is an island: integrating coral reef connectivity data into the design of regional-scale marine protected area networks. PLoS One 10:e0144199. doi: 10.1371/journal.pone.0144199
Schmahl, G. P., Hickerson, E. L., and Precht, W. F. (2008). “Biology and ecology of coral reefs and coral communities in the Flower Garden Banks region, northwestern Gulf of Mexico,” in Coral Reefs of the USA, eds B. Riegl and R. Dodge (New York, NY: Springer), 221–261. doi: 10.1007/978-1-4020-6847-8_6
Schofield, P. J. (2010). Update on geographic spread of invasive lionfishes (Pterois volitans [Linnaeus, 1758] and P. miles [Bennett, 1828]) in the western north Atlantic Ocean, Caribbean Sea and Gulf of Mexico. Aquat. Invasions 5, S117–S119. doi: 10.3391/ai.2010.5.S1.024
Schroeder, W. W., Brooke, S. D., Olson, J. B., Phaneuf, B., McDonough, J. J. III, and Etnoyer, P. (2005). “Occurrence of deep-water Lophelia pertusa and Madrepora oculata in the Gulf of Mexico,” in Cold-Water Corals and Ecosystems, eds A. Freiwald and J. M. Roberts (Berlin: Springer-Verlag), 297–307. doi: 10.1007/3-540-27673-4_14
Schutte, V. G. W., Selig, E. R., and Bruno, J. F. (2010). Regional spatio-temporal trends in Caribbean coral reef benthic communities. Mar. Ecol. Prog. Ser. 402, 115–122. doi: 10.3354/meps08438
Sherman, K. (1991). The large marine ecosystem concept: research and management strategy for living marine resources. Ecol. Appl. 1, 349–360. doi: 10.2307/1941896
Shinn, E. A. (1963). Spur and groove formation on the Florida Reef Tract. J. Sed. Petrol. 33, 291–303.
Silva, M., Etnoyer, P. T., and MacDonald, I. R. (2016). Coral injuries observed at mesophotic reefs after the deepwater horizon oil discharge. Deep Sea Res. II 129, 96–107. doi: 10.1016/j.dsr2.2015.05.013
Simmons, C. M., Collins, A. B., and Ruzicka, R. (2014). “Distribution and diversity of coral habitat, fishes, and associated fisheries in U.S. waters of the Gulf of Mexico,” in Interrelationships Between Corals and Fisheries, ed. S. A. Bortone (Boca Raton, FL: CRC Press), 19–37.
Somerfield, P. J., Jaap, W. C., Clarke, K. R., Callahan, M., Hackett, K., Porter, J., et al. (2008). Changes in coral reef communities among the Florida Keys, 1996-2003. Coral Reefs 27, 951–965. doi: 10.1007/s00338-008-0390-7
Spalding, M., Burke, L., Wood, S. A., Ashpole, J., Hutchison, J., and zu Ermgassen, P. (2017). Mapping the global value and distribution of coral reef tourism. Mar. Policy 82, 104–113. doi: 10.1016/j.marpol.2017.05.014
Stephan, C. D., Dansby, B. G., Osburn, H. R., Matlock, G. C., Riechers, R. K., and Rayburn, R. (2013). Texas Artificial Reef Fishery Management Plan, Vol. 3. Austin, TX: RFMC, 26.
Studivan, M. S., and Voss, J. D. (2018). Population connectivity among shallow and mesophotic Montastraea cavernosa corals in the Gulf of Mexico identifies potential for refugia. Coral Reefs 37, 1183–1196. doi: 10.1007/s00338-018-1733-7
Suárez, A. M., Martínez-Daranas, B., and Alfonso, Y. (2015). Macroalgas Marinas de Cuba. La Habana: Editorial UH.
Suchley, A., and Álvarez-Filip, L. (2018). Local human activities limit marine protection efficacy on Caribbean coral reefs. Conserv. Lett. 11:e12571. doi: 10.1111/conl.12571
Szedlmayer, S. T., and Lee, J. D. (2004). Diet shifts of juvenile Red Snapper (Lutjanus campechanus) with changes in habitat and fish size. Fish. Bull. 102, 366–375.
Toledo-Ocampo, A. (2005). “Marco conceptual: caracterización ambiental del Golfo de México,” in Golfo de México Contaminación e Impacto Ambiental: Diagnóstico y Tendencias, 2nd. Edn, eds A. V. Botello, J. R. von Osten, and J. A. Benítez y Gerardo (Mexico: Instituto Nacional de Ecología).
Toth, L. T., Stathakopoulos, A., and Kuffner, I. B. (2018). Descriptive Core Logs, Core Photographs, Radiocarbon Ages, and Data on Reef Development From Cores of Holocene Reef Framework From the Florida Keys Reef Tract. Reston, VA: USGS Data Release. U.S. Geological Survey.
Toth, L. T., Stathakopoulos, A., Kuffner, I. B., Ruzicka, R. R., Colella, M. A., and Shinn, E. A. (2019). The unprecedented loss of Florida’s reef-building corals and the emergence of a novel coral-reef assemblage. Ecology 100:e02781. doi: 10.1002/ecy.2781
Tunnell, J. W. Jr. (2007). “Reef distribution,” in Coral Reefs of the Southern Gulf of Mexico, eds J. W. Tunnell, Jr., E. A. Chávez, and K. Withers (College Station, TX: Texas A&M University Press), 14–22.
Tunnell, J. W. Jr., Chavez, A. E., and Withers, K. (2007). Coral Reefs of the Southern Gulf of Mexico. College Station, TX: Texas A&M University Press.
Turner, R. E., and Rabalais, N. N. (2018). “The Gulf of Mexico,” in World Seas: An Environmental Evaluation: Volume I: Europe, The Americas and West Africa, ed. C. Sheppard (Cambridge: Academic Press), 445–464.
United States Endangered Species Act [USESA] (2019). Available at: https:// ecos.fws.gov/ecp0/profile/speciesProfile;jsessionid=57B967450DE55E4E1FFC0 802B512752C?spcode=P000 (accessed August 13, 2019).
van Woesik, R., and McCaffrey, K. R. (2017). Repeated thermal stress, shading, and directional selection in the Florida Reef Tract. Front. Mar. Sci. 4:182. doi: 10.3389/fmars.2017.00182
Veil, J. A. (2011). “Produced water management options and technologies,” in Produced Water, eds K. Lee and J. Neff (New York, NY: Springer), 537–571. doi: 10.1007/978-1-4614-0046-2_29
Veron, J. E. N. (1995). Corals in Space and Time: The Biogeography and Evolution of the Scleractinia. New York, NY: Comstock/Cornell.
Walton, C. J., Hayes, N. K., and Gilliam, D. S. (2018). Impacts of a regional, multi-year, multi-species coral disease outbreak in southeast Florida. Front. Mar. Sci. 5:323. doi: 10.3389/fmars.2018.00323
Weaver, D. C., Hickerson, E. L., and Schmahl, G. P. (2006). “Deep reef fish surveys by submersible on Alderdice, McGrail, and Sonnier Banks in the northwestern Gulf of Mexico,” in Emerging Technologies for Reef Fisheries Research and Management, ed. J. C. Tayler (Seattle, WA: NOAA Professional Paper NMFS), 69–87.
Wenzel, L., Cid, G., Haskell, B., Clark, A., Quiocho, K., Kiene, B., et al. (2019). You can choose your relatives: building MPA networks from sister sites. Aquat. Conserv. Mar. Freshw. Ecosyst. 29, 152–161. doi: 10.1002/aqc.3041
White, H. K., Hsing, P. Y., Cho, W., Shank, T. M., Cordes, E. E., Quattrini, A. M., et al. (2012). Impact of the deepwater horizon oil spill on a deep-water coral community in the Gulf of Mexico. Proc. Natl. Acad. Sci. U.S.A. 109, 20303–20308. doi: 10.1073/pnas.1118029109
World Register of Marine Species [WORMS] (2019). Available at: http://www.marinespecies.org/ (accessed May 30, 2019).
Wright, R. M., Correa, A. M. S., Quigley, L. A., Santiago-Vázquez, L. Z., Shamberger, K. E. F., and Davies, S. W. (2019). Gene expression of endangered coral (Orbicella spp.) in Flower Garden Banks National Marine Sanctuary after Hurricane Harvey. Front. Mar. Sci. 6:672. doi: 10.3389/fmars.2019.00672
Keywords: corals, coral reefs, ecosystem management, Gulf of Mexico, mesophotic reefs, resilience, threats
Citation: Gil-Agudelo DL, Cintra-Buenrostro CE, Brenner J, González-Díaz P, Kiene W, Lustic C and Pérez-España H (2020) Coral Reefs in the Gulf of Mexico Large Marine Ecosystem: Conservation Status, Challenges, and Opportunities. Front. Mar. Sci. 6:807. doi: 10.3389/fmars.2019.00807
Received: 15 August 2019; Accepted: 16 December 2019;
Published: 14 January 2020.
Edited by:
Adrienne M. S. Correa, Rice University, United StatesReviewed by:
Susana Enríquez, National Autonomous University of Mexico, MexicoKeisha D. Bahr, Texas A&M University Corpus Christi, United States
Copyright © 2020 Gil-Agudelo, Cintra-Buenrostro, Brenner, González-Díaz, Kiene, Lustic and Pérez-España. This is an open-access article distributed under the terms of the Creative Commons Attribution License (CC BY). The use, distribution or reproduction in other forums is permitted, provided the original author(s) and the copyright owner(s) are credited and that the original publication in this journal is cited, in accordance with accepted academic practice. No use, distribution or reproduction is permitted which does not comply with these terms.
*Correspondence: Carlos E. Cintra-Buenrostro, carlos.cintra@utrgv.edu