- 1Yellow Sea Fisheries Research Institute, Chinese Academy of Fishery Sciences, Qingdao, China
- 2Laboratory for Marine Fisheries Science and Food Production Processes, Qingdao National Laboratory for Marine Science and Technology, Qingdao, China
Our previous studies have shown that ARA regulated the gonadal steroidogenesis and the synthesis of gonadotrophin-releasing hormone (GnRH) in the brain in a gender-dependent manner in Chinese tongue sole, a marine teleost with typical sex dimorphism. As a following up-study, the current study aimed to clone and characterize a gene responding to dietary ARA differently between males and females, cholesterol 25-hydroxylase (ch25h), which has important roles in cholesterol and lipid metabolism of mammals, but is rarely investigated in fish. The full-length cDNA of Chinese tongue sole ch25h was cloned and characterized, and its transcription in the gonads, brain and liver of both males and females in response to dietary ARA levels (0.34, 2.53, and 9.63% of TFA) was investigated. The Chinese tongue sole Ch25h, which putatively is Ch25h subtype B, shared moderate identity to its known orthologs of other teleost and lower identity to human Ch25h. It has high transcription levels in the gonads, followed by skin and muscle, but low levels in the intestine, spleen, and kidney. High ARA levels significantly increased the ch25h transcription in the gonads and brain of male fish, but did not affect the transcription in the females. However, the effect of dietary ARA on ch25h transcription in the liver showed an opposite gender-difference pattern to those in the gonads and brain. To our knowledge, this is the first study in marine teleost investigating the nutritional regulation of ch25h expression.
Introduction
The membrane-associated enzyme cholesterol 25-hydroxylase (Ch25h) catalyzes the formation of 25-hydroxycholesterol (25HC, oxysterol) from cholesterol, and thereby plays important roles in cholesterol and lipid metabolism (Lund et al., 1998; Horton et al., 2002; Joseph et al., 2002). It has been reported that 25HC is a co-repressor that blocks sterol regulatory element binding protein (SREBP) processing and ultimately leads to inhibition of gene transcription (Lund et al., 1998). 25HC also acts as a ligand of liver X receptor (LXR). The regulation of LXR/SREBP signaling pathway by 25HC reduces cholesterol synthesis and increases its efflux and elimination (Janowski et al., 1996; Accad and Farese, 1998; Radhakrishnan et al., 2007). The regulation of LXR/SREBP signaling pathway by 25HC also affects lipid metabolism in other ways depending on the roles of SREBP and LXR in lipid metabolism (Shimano, 2001; Oosterveer et al., 2010; DeBose-Boyd and Ye, 2018). In addition to the well-known metabolic role of oxysterols, some publications have also reported the function of 25HC in immune regulation and virus resistance (Yi et al., 2012; Shrivastava-Ranjan et al., 2016; Cagno et al., 2017; Doms et al., 2018; Shawli et al., 2019; Zhang et al., 2019).
Compared to mammals, the functions of Ch25h or 25HC in fish have been poorly understood. Only in zebrafish, the interferon independent antiviral role of 25HC was validated (Pereiro et al., 2017). In Chinese tongue sole, a recent study of ours with feeding trial followed by transcriptomic analysis has shown that ch25h transcription in the brain was significantly affected by dietary arachidonic acid (ARA), which plays important roles in fish reproduction (Izquierdo et al., 2001; Norberg et al., 2017), and this effect was different between male and female fish (Xu et al., 2019). Our previous studies have also shown that dietary ARA differentially regulates the gonadal steroidogenesis in Chinese tongue sole depending on fish gender (Xu et al., 2017a). Chinese tongue sole have typical characteristics of sex dimorphism. The different response of cholesterol metabolism to dietary ARA in male and female Chinese tongue sole seems interesting and is worth further investigation. As a following-up study, the present study aimed to clone and characterize the full-length mRNA of Chinese tongue sole ch25h, as well as to investigate its transcription in response to dietary ARA in different tissues of both male and female fish. The results will contribute to the general knowledge of Ch25h physiology in fish.
Materials and Methods
Feeding Trial
Three experimental diets containing different levels of ARA were used in the feeding trial (Table 1). In the control diet (Diet C), tristearin was used as the main supplemented lipid source. Diets with low (Diet ARA-L) and high (Diet ARA-H) ARA level were prepared by replacing tristearin in diet C with ARA-enriched oil. The ARA content in the three experimental diets was 0.34, 2.53, and 9.63% of total fatty acids (TFA), respectively, (Table 2). Constant levels of n-3 LC-PUFA enriched oil and soya lecithin were supplemented to all the diets to meet the requirement. The experimental diets were prepared following the routine procedures in our laboratory (Xu et al., 2016).
A 10-week feeding trial was conducted to investigate the effects of dietary ARA on the ch25h gene expressions in Chinese tongue sole. Chinese tongue sole hatched in the last autumn was used in the feeding trial. The fish have been fed a commercial diet before the experiment. Fifteen male fish with an average initial body weight of 20.3 g and eight female fish with an average initial body weight of 72.0 g were reared in each polyethylene tank (200 L). At the beginning of the feeding trial, the fish were fed the control diet for 7 days to acclimate to the experimental conditions. The feeding trial was conducted in a flow-through seawater system in Huanghai Aquaculture Co., Ltd., (Haiyang, China). Each diet was randomly assigned to triplicate tanks. Fish were hand-fed to apparent satiation twice daily (9:00 and 17:00). The tanks were cleaned daily by siphoning out residual feed and feces.
At the end of the feeding trial (late autumn), after being anesthetized with eugenol (1:10,000), the developmental status of fish gonads was determined before sampling. Most male fish were mature. The maturity of male fish was confirmed by the release of milt when handled. However, unfortunately, visual observation and microscopic examination of oocyte morphology showed that most female fish were immature, and the ovaries had not developed at all. Five mature male fish and five immature female fish per tank were dissected, and whole brain, gonad and liver samples were collected. All the tissue samples were immediately frozen with liquid nitrogen, and stored at −86°C before analysis. All sampling protocols, as well as fish rearing practices, were reviewed and approved by the Animal Care and Use Committee of Yellow Sea Fisheries Research Institute.
RNA Extraction and cDNA Synthesis
Total RNA in livers was extracted using RNAiso Plus [TaKaRa Biotechnology (Dalian) Co., Ltd., Dalian, China] and then electrophoresed on 1.5% agarose gel to test the quality and integrity. The concentration was determined with Colibri Microvolume Spectrometer (Titertek-Berthold, Germany). The RNA was then reversely transcribed with PrimeScriptTM RT reagent Kit with gDNA Eraser (Perfect Real Time) (TaKaRa) according to the user manual.
Cloning and Sequencing of ch25h
The complete CDS of ch25h can be obtained from GenBank (Accession No.: XM_008315046.3). The predicted sequence from GenBank was validated with specific PCR and sequencing of the product. The full-length cDNA of ch25h was cloned with rapid amplification of cDNA ends (RACE). Specific primers for ch25h were designed based on the known ch25h sequence to clone the 5′- and 3′-end, respectively. The SMARTerTM RACE cDNA Amplification Kit (Clontech, Mountain View, CA, United States) was used to perform the RACE cloning, and the 3′- and 5′-end cDNA templates were synthesized according to the user’s manual. The primers were synthesized by TsingKe Biological Technology, Co., Ltd., (Qingdao, China). PCR amplifications were performed on peqSTAR (PEQLAB, Erlangen, Germany). All PCR products were run on a 1.5% agarose gel, and then purified by Zymoclean Gel DNA Recovery Kit (ZYMO RESEARCH, Irvine, CA, United States). PCR products were cloned into pEASY-T1 simple cloning vector (TransGen, Beijing, China) and sequenced in TsingKe (Qingdao, China). Other details of the PCR amplification were similar to our previous studies (Xu et al., 2014).
Real-Time Quantitative Polymerase Chain Reaction (qRT-PCR) Analysis
Real-time fluorescent quantitative PCR (qRT-PCR) was used to assay the relatively quantitative mRNA expression of ch25h in different tissues of Chinese tongue sole (10 3-year-old fish used, six males and four females, all at phase II of gonadal development), as well as the gene expression in fish from different dietary groups. β-actin (GenBank Accession No. KP033459.1) and β-2 microglobulin (β2M, GenBank Accession No. FJ965563.1) were used as the reference genes (Vandesompele et al., 2002). Specific primers for target genes and reference genes were designed using Primer 5.0 (Table 3) and synthesized by TsingKe Biological Technology Co., Ltd., (Qingdao, China). The amplification efficiency for all primers, which was estimated by standard curves based on a 6-step 4-fold dilution series of target template, was within 95∼105%, and the coefficients of linear regression (R2) were more than 0.99. SYBR® Premix Ex Taq TM [TaKaRa Biotechnology (Dalian) Co., Ltd., Dalian, China] and a quantitative thermal cycler (Roche LightCycler 96, Basel, Switzerland) were used for the real-time qPCR. The reaction system consists of 2 μl cDNA template, 10 μl SYBR® Premix Ex TaqTM (2×), 0.8 μl forward primer (10 μM), 0.8 μl reverse primer (10 μM), and 6.4 μl sterilized water. The program was as follows: 95°C for 5 min followed by 40 cycles of “95°C for 5 s, 55°C for 20 s, 72°C for 10 s.” Melting curve analysis (1.85°C increment/min from 58°C to 95°C) was performed after the amplification phase for confirmation of a sole product. Each sample was run in triplicate. The mRNA expression levels were calculated with qRT-PCR method: 2–ΔΔCT (Livak and Schmittgen, 2001).
Statistical Analysis
Similarity searches of the sequenced cDNA of ch25hs were done by blastn1. The multiple-sequence alignments of amino acids were performed using BioEdit. The deduced amino acid sequences were analyzed with DNAman and ExPASy Compute pI/MW2. SMART program3 and PROSITE program4 were used to predict the functional sites or domains in the amino acid sequence. Phylogenetic analyses based on amino acid sequences were carried out using the neighbor-joining method, and the trees were constructed using MEGA 4.1.
All gene expression data were subjected to one-way analysis of variance in SPSS 16.0 for Windows. Differences between means were tested by Tukey’s multiple range test. The level of significance was chosen at P < 0.05 and the results were presented as means ± standard error.
Results and Discussion
Cloning and Characterization of ch25h
The full length of ch25h cDNA from Chinese tongue sole (uploaded under GenBank Accession No. MN646884.1) was 1468 bp, including a 5′-untranslated region (UTR) of 372 bp, a 3′-UTR of 340 bp, and an open reading frame of 756 bp encoding a polypeptide of 250 amino acids with predicted molecular weight of 28.7 KDa and theoretical isoelectric point of 8.22 (Figure 1). The deduced protein sequence possessed a characteristic fatty acid hydroxylase domain, containing three transmembrane regions (20–42, 74–91, and 106–128) and clusters of histidine residues that are essential for catalytic activity (Figure 1). Unlike most other sterol hydroxylases, Ch25h is not a cytochrome P450, but rather it is a member of a small family of enzymes that utilize diiron cofactors to catalyze the hydroxylation of hydrophobic substrates (Lund et al., 1998). The deduced Chinese tongue sole Ch25h has three potential phosphorylation sites (T-7, S-18, and Y-23), but no predicted signal peptide sequence.
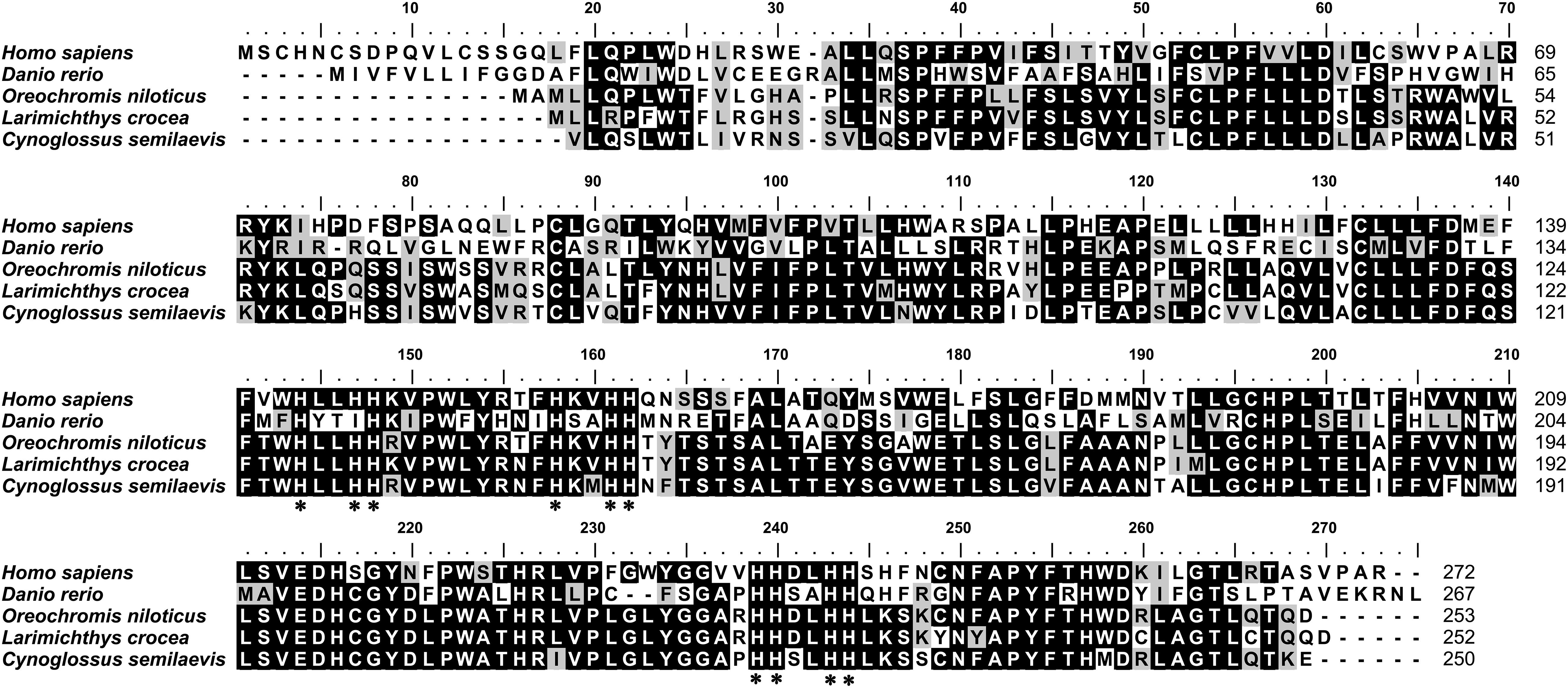
Figure 1. Comparison of the deduced Ch25h amino acid sequences from Chinese tongue sole, other fish, and human. The amino acid sequences were aligned using ClustalX. Identical residues are shaded black and similar residues are shaded gray. Gaps (-) were introduced to maximize the alignment. Asterisk indicates conserved clusters of histidine residues.
Multiple Sequences Alignment and Phylogenetic Analysis
The multi-sequence alignment (Figure 1) revealed that the Chinese tongue sole Ch25h shared moderate identity to its known orthologs of other teleost (63–76% for the listed species in Figure 1) and lower identity to human Ch25h (54.6%).
The phylogenetic analysis showed that Chinese tongue sole Ch25h clusters closer to Ch25h subtype B of zebrafish, compared to other subtypes of zebrafish Ch25h, Ch25hA, Ch25hC1, Ch25hC2, and Ch25hD (Figure 2). The zebrafish Ch25hB showed synteny conservation with its human homolog, highlighting that this gene copy is probably the original gene of the Ch25h teleost repertoire (Pereiro et al., 2017). In contrast to fish, the mammalian genomes only possess one copy of the CH25H gene.
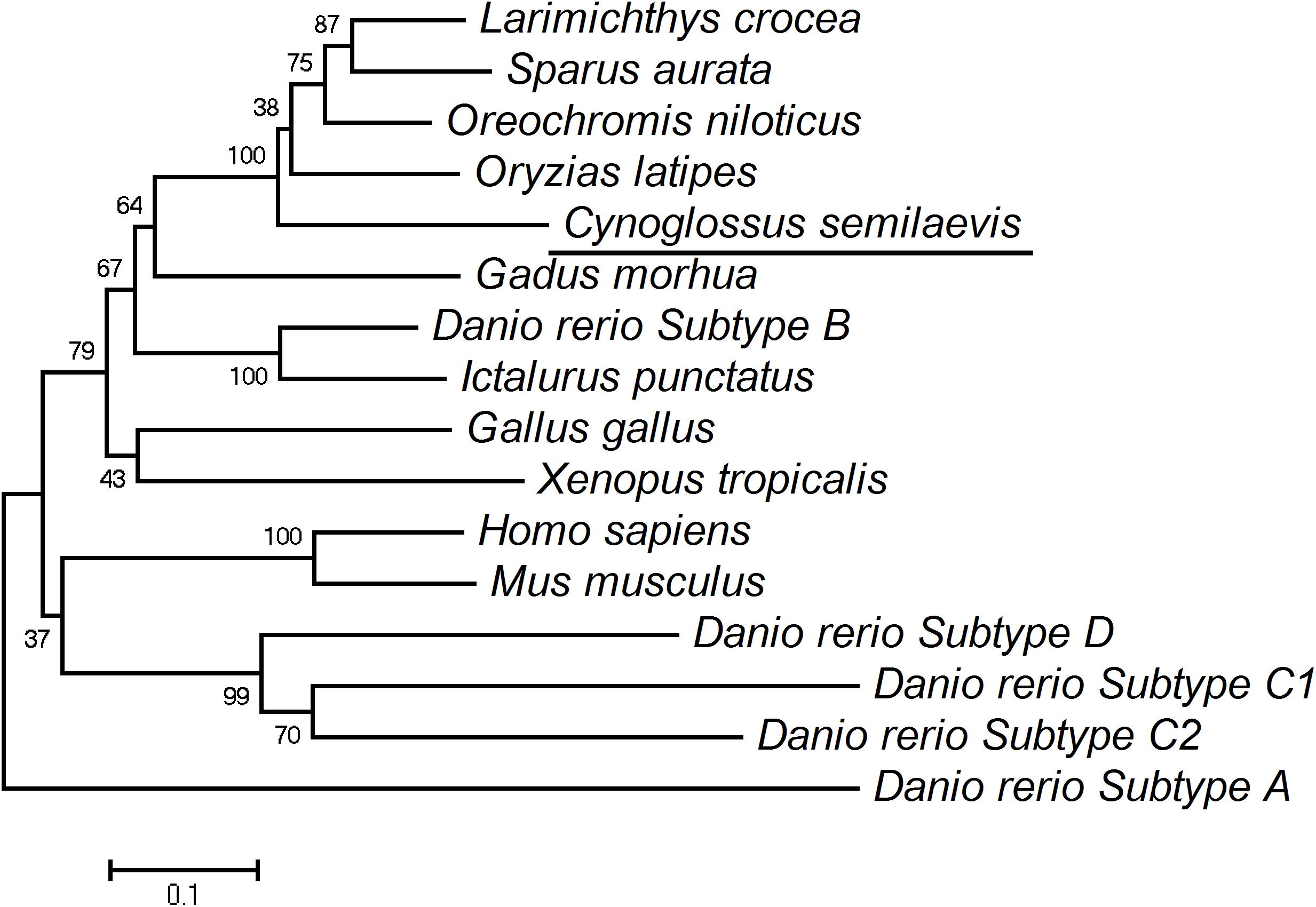
Figure 2. Phylogenetic tree of Chinese tongue sole Ch25h. The amino acid sequences used in the phylogenetic tree included those from other teleost and other animals: human (Homo sapiens) (NP_067065.1), mouse (Mus musculus) (NP_034020.1), chicken (Gallus gallus) (NP_001264283.1), frog (Xenopus tropicalis) (XP_002935478.1), zebrafish (Danio rerio) (subtype A, ASU11038.1; subtype B, ASU11039.1; subtype C1, ASU11040.1; subtype C2, ASU11041.1; subtype D, ASU11042.1), channel catfish (Ictalurus punctatus) (NP_001188084.1), Nile tilapia (Oreochromis niloticus) (XP_003449518.2), medaka (Oryzias latipes) (XP_004084712.1), large yellow croaker (Larimichthys crocea) (XP_010750904.1), Atlantic cod (Gadus morhua) (XP_030195643.1), and gilthead seabream (Sparus aurata) (XP_030255276.1). The horizontal branch length is proportional to amino acid substitution rate per site. The numbers represent the frequencies with which the tree topology presented here was replicated after 1000 bootstrap iterations.
Chinese tongue sole Ch25h clusters to Ch25h of other fish species such as Nile tilapia, large yellow croaker, and gilthead seabream, distant from Ch25h of frog, and further distant from Ch25h of mammals (Figure 2). However, Chinese tongue sole Ch25h clusters closer to human Ch25h than to other Ch25h subtypes of zebrafish. This indicated that Ch25h subtypes may have diverged from a common progenitor before the fish/mammalian divergence.
Tissue Distribution of ch25h in Chinese Tongue Sole
In mice, expression of ch25h is high in the lung, heart, and kidney (Lund et al., 1998). Another study in mice showed that ch25h is highly expressed in the liver and peritoneal macrophages (Liu et al., 2018). In Chinese tongue sole, however, ch25h is highly expressed in gonad, followed by skin and muscle, but is lowly expressed in the intestine, spleen, and kidney (Figures 3, 4). There is minor difference in tissue expression pattern of ch25h between male and female Chinese tongue sole. It seemed that the females have relatively higher ch25h expression in the brain compared to males.
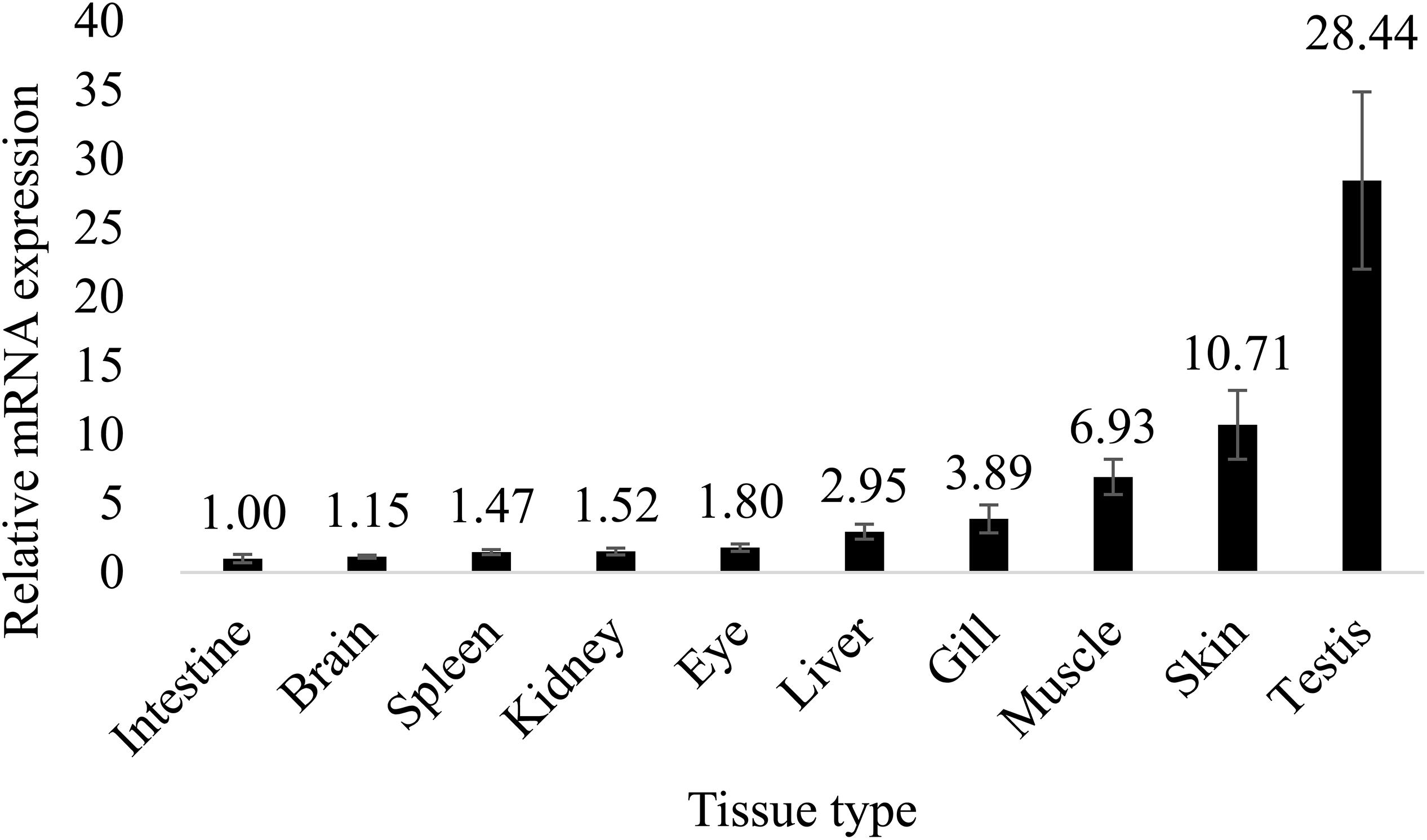
Figure 3. Tissue distribution of ch25h in male Chinese tongue sole. Results were expressed as relative mRNA expressions to the lowest expression (means ± standard error, n = 6).
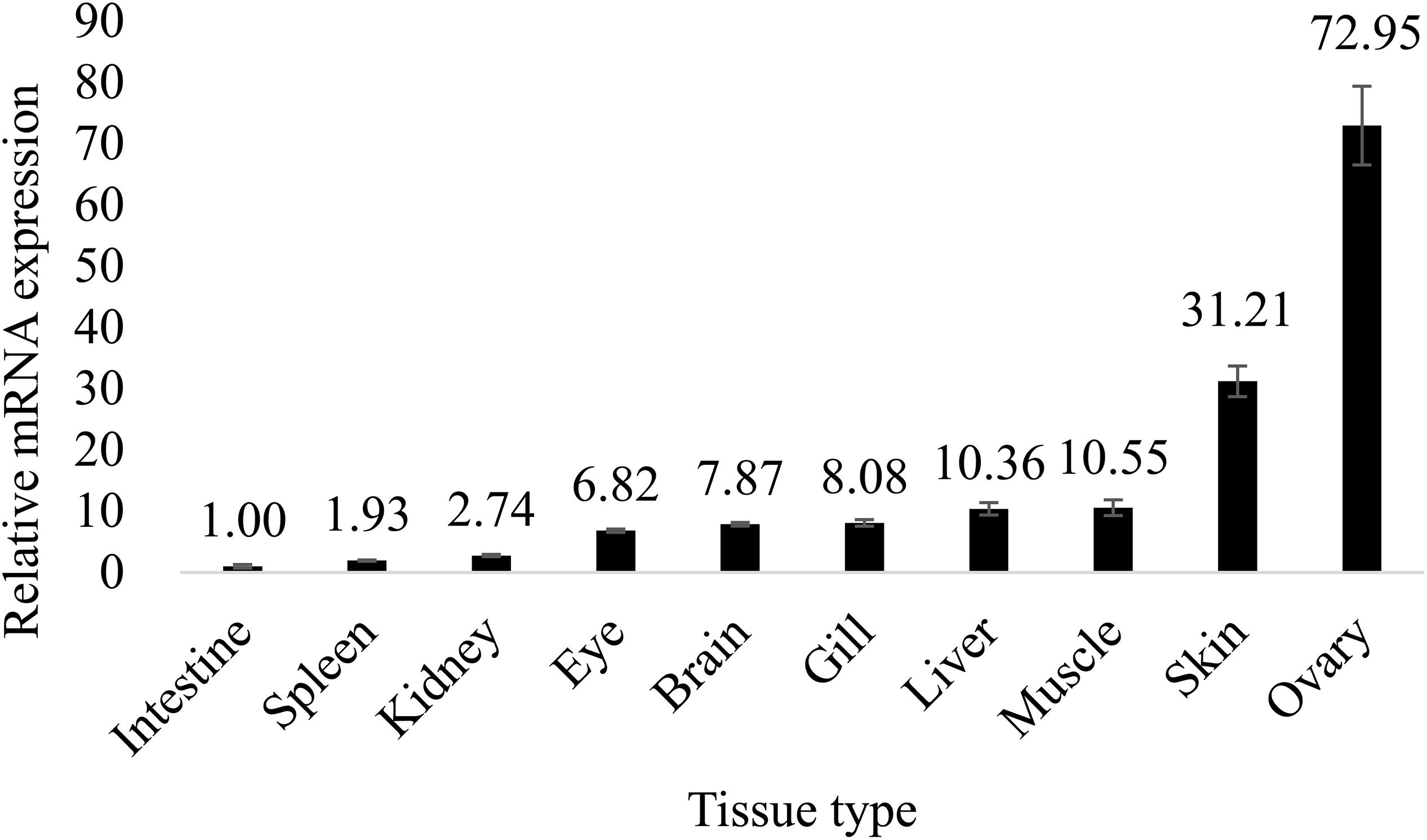
Figure 4. Tissue distribution of ch25h in female Chinese tongue sole. Results were expressed as relative mRNA expressions to the lowest expression (means ± standard error, n = 4).
High expression of ch25h in the gonads indicated that Ch25h may have important roles in reproductive physiology of Chinese tongue sole. Recent data obtained in bovine sperm showed that sperm capacitation is associated with the formation of oxysterols (Brouwers et al., 2011). Another recent study with human semen also supports a role for 25HC in sperm function (Zerbinati et al., 2017). Results of that study showed that CH25H was detected in human spermatozoa at the neck and the post acrosomal area, and that the 25HC concentration positively correlated with spermatozoa number (Zerbinati et al., 2017). High expression of ch25h in both testis and ovary of Chinese tongue sole indicated that Ch25h may have important functions in genesis of both sperm and egg.
Unexpectedly, the liver of Chinese tongue sole only has a moderate level of ch25h transcription, lower than skin and muscle, irrespective of fish gender. Liver is a very important organ for lipid and cholesterol metabolism. The present results indicate that Ch25h or 25HC may have special functions in skin and muscle of flatfish, but this speculation needs to be validated by future studies.
The Chinese Tongue Sole ch25h mRNA Expression in Response to Dietary ARA
In the present study, we analyzed the ch25h mRNA expression in the gonad, liver and brain of Chinese tongue sole fed diets with different ARA levels. We analyzed the ch25h mRNA expression in the gonads for two reasons: (1) As mentioned above, ch25h is highly expressed in the gonads; (2) Reproductive physiology is a focus of this study and its previous studies. A very interesting result of this study was that ch25h responded to dietary ARA differently between the testes and ovaries. The relative mRNA expression of ch25h in the testes significantly (P < 0.05) increased with increasing dietary ARA levels, but the transcription in the ovaries was not affected by dietary ARA (Figure 5).
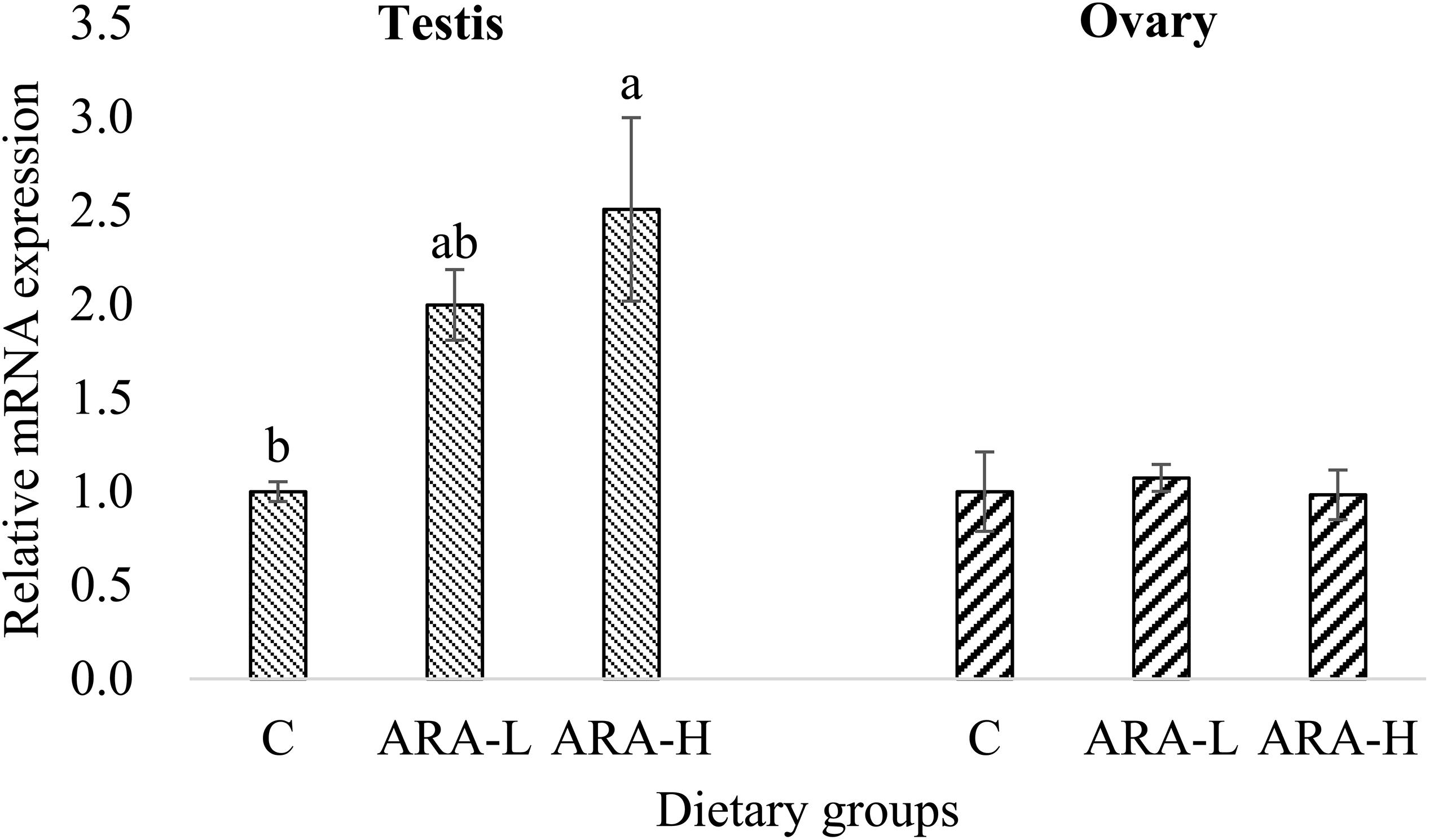
Figure 5. Effects of experimental diets on mRNA expression of ch25h in the gonad of Chinese tongue sole. Results are expressed as means ± standard error. Different letters above the bars denote significant (P < 0.05) differences among dietary groups.
This result was similar to the ch25h transcription in the brain in response to dietary ARA (Figure 6). The brain ch25h expression was significantly (P < 0.05) higher in male fish fed the high-ARA diet compared to fish fed the low-ARA diet or the control diet. However, the brain ch25h transcription in female fish was not affected by dietary ARA. This result validated the previous transcriptomic results which indicated the gender-difference in brain ch25h transcription in response to dietary ARA and inspired the current study.
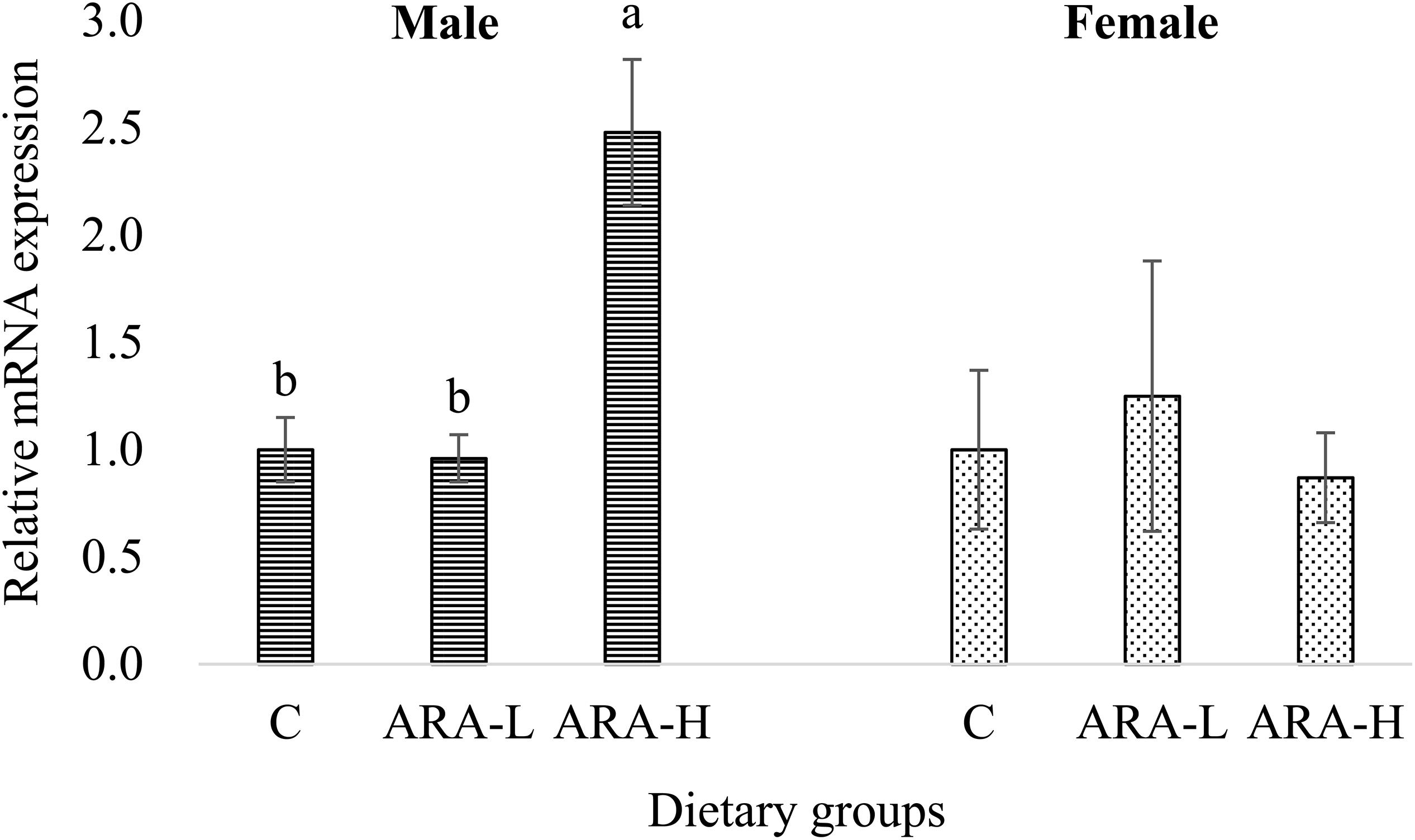
Figure 6. Effects of experimental diets on mRNA expression of ch25h in the brain of Chinese tongue sole. Results are expressed as means ± standard error. Different letters above the bars denote significant (P < 0.05) differences among dietary groups.
The differential regulation of gonadal steroidogenesis and brain GnRH synthesis by dietary ARA between male and female Chinese tongue sole has been shown in our previous studies (Xu et al., 2017a, 2019). Those results showed that dietary ARA supplementation stimulated the testosterone and GnRH production in males, but reduced the estradiol production in the females. ARA supplementation significantly reduced the mRNA expression of aromatase in ovaries but significantly increased the gene expression of 3β-hydroxysteroid dehydrogenase (3β-HSD) in testes. Moreover, this gender-dependent differential regulation was in accord with the different ARA abundance in testes and ovaries (Xu et al., 2017a, b).
In the present study, it is interesting to validate another target gene, ch25h, for the differential ARA action between male and female Chinese tongue sole. The present results indicated that ch25h may have more active function in male fish. Considering the roles of ch25h in sperm mentioned previously (Brouwers et al., 2011; Zerbinati et al., 2017), the more positive roles of ARA in male Chinese tongue sole may stimulate the gene expression of ch25h in male fish by accelerating the overall reproductive performance. Also, mammal studies showed that Leydig cells can directly metabolized 25HC enzymatically produced by testicular macrophages to testosterone (Lukyanenko et al., 2001, 2002). Moreover, liver X receptors (LXRα and LXRβ), receptors of oxysterols, have been shown to present crucial activities in reproductive organs of male animals such as testis and epididymis, as well as prostate [well-reviewed by El-Hajjaji et al. (2011)]. 25HC, the product of Ch25h, may function in the testis and brain of male animals in LXR-dependent mechanisms (Liu et al., 2018).
In spite of the potential different functions of Ch25h between male and female animals, another important factor contributing to the current gender-difference in Ch25h transcription in Chinese tongue sole was the asynchronous gonadal development. In the present study, most of the male fish was mature but the females were immature. However, whether this asynchronous gonadal development resulted in gender-difference in Ch25h transcription or vice versa cannot be concluded based on the current information. In addition, considering that both ARA and Ch25h are involved in immunity (McDonald and Russell, 2010; Xu et al., 2010; Li et al., 2012; Cyster et al., 2014; Shahkar et al., 2016; Adam et al., 2017; Nayak et al., 2017; Wu et al., 2018), Ch25h-mediated immune response could be another process differently responding to dietary ARA between male and female Chinese tongue sole.
In the liver of Chinese tongue sole, a more interesting result was that the response of ch25h transcription to dietary ARA was obviously different from those in the gonad and brain. The ch25h expression in the liver of male fish was not significantly affected by dietary ARA (Figure 7). However, the hepatic ch25h expression in female fish was significantly (P < 0.05) higher in the low-ARA group compared to the control group, while the high-ARA group had an intermediate expression level (Figure 7). This result indicated that Ch25h may function differently between liver and gonad/brain of Chinese tongue sole. Liver plays a crucial role in maintaining cholesterol homeostasis by regulating absorption and synthesis (Goldstein and Brown, 1990; Goldstein and DeBose-Boyd, 2006; Lu et al., 2017). Due to the lipid nature of the ligands (oxysterol), the physiological roles of LXRs have been extensively detailed in the homeostasis of cholesterol in the gut-liver axis (D’Errico and Moschetta, 2008). However, no information is available about the difference between males and females in Ch25h-mediated hepatic cholesterol metabolism, neither in mammals nor in fish. Gender-difference in correlation between cholesterol level and liver inflammation (Comhair et al., 2011) or response of hepatic cholesterol level to dietary nutrients (Kishida et al., 2006) has been reported in rodents, but no results can be used to explain the present results. In fish, studies in freshwater fish Notopterus notopterus have shown that the hepatic cholesterol content changes differently between males and females during the gonadal development and reproductive process (Shankar and Kulkarni, 2005, 2007). Therefore, a possible explanation of the current result could be the different reproductive phases the male and female Chinese tongue sole stayed when sampled.
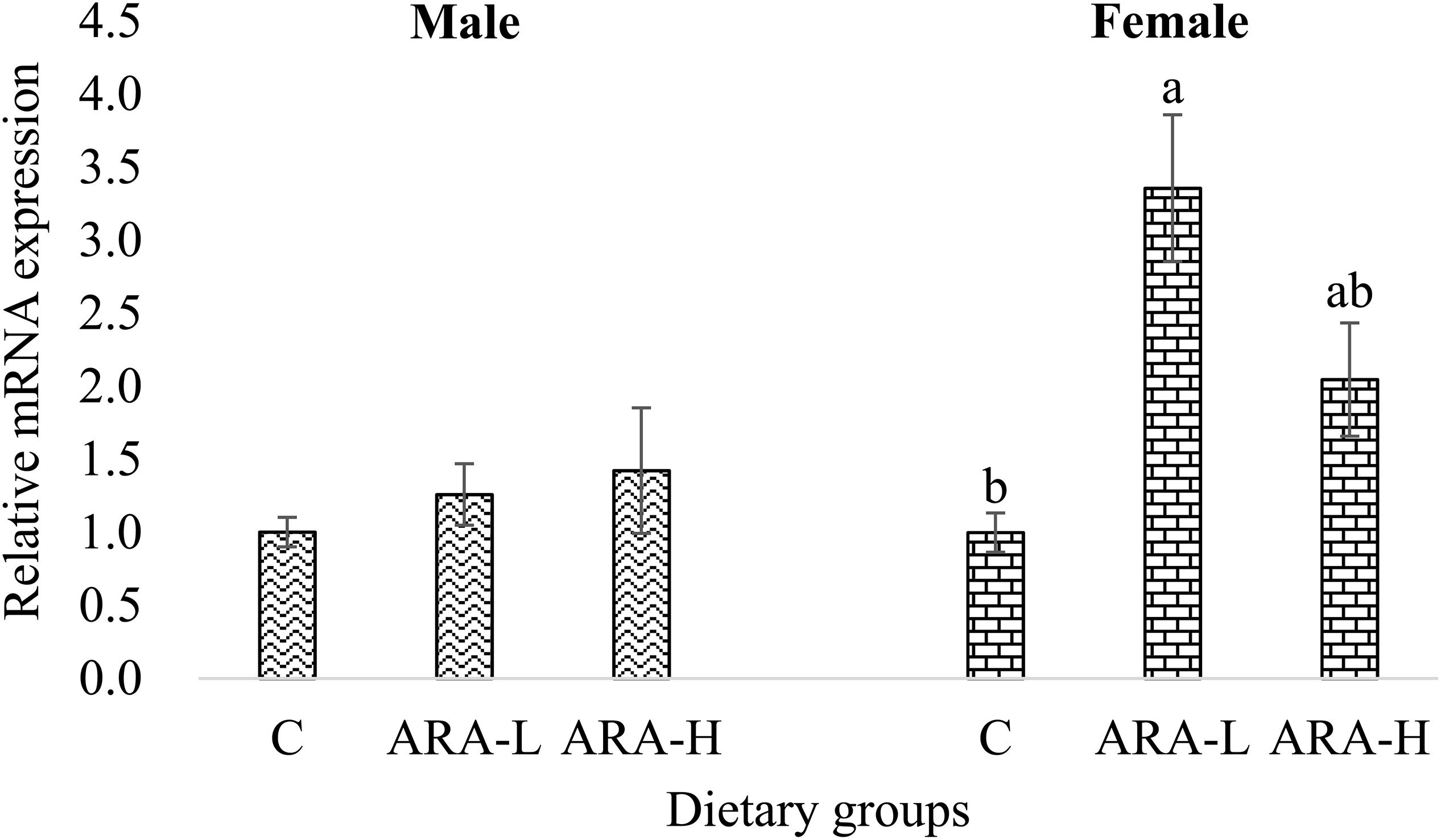
Figure 7. Effects of experimental diets on mRNA expression of ch25h in the liver of Chinese tongue sole. Results are expressed as means ± standard error. Different letters above the bars denote significant (P < 0.05) differences among dietary groups.
Conclusion
In conclusion, as a following-up study of previous studies which showed the differential function of ARA between male and female Chinese tongue sole, the current study cloned and characterized a gene responding to ARA in a gender-dependent manner, ch25h. This gene was highly expressed in gonads, followed by skin and muscle. Its mRNA expression in the gonad and brain of male fish was significantly increased by high dietary ARA levels, but the transcription in female fish was not affected by dietary ARA. The ch25h expression in the liver in response to dietary ARA showed an opposite gender-difference pattern to those in the gonad and brain. The interaction among Ch25h, ARA, and sex dimorphism of Chinese tongue sole warrants further studies.
Data Availability Statement
The datasets generated for this study can be found in the GenBank, Accession No. MN646884.1.
Ethics Statement
The animal study was reviewed and approved by the Animal Care and Use Committee of Yellow Sea Fisheries Research Institute.
Author Contributions
HX and ML designed the study and wrote the manuscript. BS and YW conducted the feeding trial. LJ and ZL did the qPCR studies. All authors read and approved the final version of the manuscript.
Funding
This work was supported by the National Natural Science Foundation of China (31772862), Central Public-Interest Scientific Institution Basal Research Fund, CAFS (2018HY-ZD0505), and China Agriculture Research System (CARS-47-G15).
Conflict of Interest
The authors declare that the research was conducted in the absence of any commercial or financial relationships that could be construed as a potential conflict of interest.
Footnotes
- ^ www.ncbi.nlm.nih.gov/blast/
- ^ http://web.expasy.org/compute_pi/
- ^ http://smart.embl-heidelberg.de/
- ^ http://kr.expasy.org/prosite/
References
Accad, M., and Farese, R. V. Jr. (1998). Cholesterol homeostasis: a role for oxysterols. Curr. Biol. 8, R601–R604. doi: 10.1016/s0960-9822(98)70386-0
Adam, A.-C., Lie, K. K., Moren, M., and Skjaerven, K. H. (2017). High dietary arachidonic acid levels induce changes in complex lipids and immune-related eicosanoids and increase levels of oxidised metabolites in zebrafish (Danio rerio). Br. J. Nutr. 117, 1075–1085. doi: 10.1017/S0007114517000903
Brouwers, J. F., Boerke, A., Silva, P. F., Garcia-Gil, N., van Gestel, R. A., Helms, J. B., et al. (2011). Mass spectrometric detection of cholesterol oxidation in bovine sperm. Biol. Reprod. 85, 128–136. doi: 10.1095/biolreprod.111.091207
Cagno, V., Civra, A., Rossin, D., Calfapietra, S., Caccia, C., Leoni, V., et al. (2017). Inhibition of herpes simplex-1 virus replication by 25-hydroxycholesterol and 27-hydroxycholesterol. Redox Biol. 12, 522–527. doi: 10.1016/j.redox.2017.03.016
Comhair, T. M., Caraballo, S. C. G., Dejong, C. H. C., Lamers, W. H., and Köhler, S. E. (2011). Dietary cholesterol, female gender and n-3 fatty acid deficiency are more important factors in the development of non-alcoholic fatty liver disease than the saturation index of the fat. Nutr. Metab. 8:4. doi: 10.1186/1743-7075-8-4
Cyster, J. G., Dang, E. V., Reboldi, A., and Yi, T. (2014). 25-hydroxycholesterols in innate and adaptive immunity. Nat. Rev. Immunol. 14, 731–743. doi: 10.1038/nri3755
DeBose-Boyd, R. A., and Ye, J. (2018). SREBPs in lipid metabolism, insulin signaling, and beyond. Trends Biochem. Sci. 43, 358–368. doi: 10.1016/j.tibs.2018.01.005
D’Errico, I., and Moschetta, A. (2008). Nuclear receptors, intestinal architecture and colon cancer: an intriguing link. Cell. Mol. Life Sci. 65, 1523–1543. doi: 10.1007/s00018-008-7552-1
Doms, A., Sanabria, T., Hansen, J. N., Altan-Bonnet, N., and Holm, G. H. (2018). 25-Hydroxycholesterol production by the cholesterol-25-hydroxylase interferon-stimulated gene restricts mammalian reovirus infection. J. Virol. 92:e1047-18. doi: 10.1128/JVI.01047-18
El-Hajjaji, F. Z., Oumeddour, A., Pommier, A. J., Ouvrier, A., Viennois, E., Dufour, J., et al. (2011). Liver X receptors, lipids and their reproductive secrets in the male. Biochim. Biophys. Acta. 1812, 974–981. doi: 10.1016/j.bbadis.2011.02.004
Goldstein, J. L., and Brown, M. S. (1990). Regulation of the mevalonate pathway. Nature 343, 425–430. doi: 10.1038/343425a0
Goldstein, J. L., and DeBose-Boyd, R. A. (2006). Brown MS. Protein sensors for membrane sterols. Cell 124, 35–46. doi: 10.1016/j.cell.2005.12.022
Horton, J. D., Goldstein, J. L., and Brown, M. S. (2002). SREBPs: activators of the complete program of cholesterol and fatty acid synthesis in the liver. J. Clin. Invest. 109, 1125–1131. doi: 10.1172/JCI200215593
Izquierdo, M. S., Fernández-Palacios, H., and Tacon, A. G. J. (2001). Effect of broodstock nutrition on reproductive performance of fish. Aquaculture 197, 25–42. doi: 10.1016/s0044-8486(01)00581-6
Janowski, B. A., Willy, P. J., Devi, T. R., Falck, J. R., and Mangelsdorf, D. J. (1996). An oxysterol signaling pathway mediated by the nuclear receptor LXR alpha. Nature 383, 728–731. doi: 10.1038/383728a0
Joseph, S. B., Laffitte, B. A., Patel, P. H., Watson, M. A., Matsukuma, K. E., Walczak, R., et al. (2002). Direct and indirect mechanisms for regulation of fatty acid synthase gene expression by liver X receptors. J. Biol. Chem. 277, 11019–11025. doi: 10.1074/jbc.m111041200
Kishida, T., Mizushige, T., Nagamoto, M., Ohtsu, Y., Izumi, T., Obata, A., et al. (2006). Lowering effect of an isoflavone-rich fermented soybean extract on the serum cholesterol concentrations in female rats, with or without ovariectomy, but not in male rats. Biosci. Biotechnol. Biochem. 70, 1547–1556. doi: 10.1271/bbb.50008
Li, Q., Ai, Q., Mai, K., Xu, W., and Zheng, Y. (2012). In vitro effects of arachidonic acid on immune functions of head kidney macrophages isolated from large yellow croaker (Larmichthys crocea). Aquaculture 33, 47–53. doi: 10.1016/j.aquaculture.2011.11.045
Liu, Y., Wei, Z., Ma, X., Yang, X., Cheng, Y., Sun, L., et al. (2018). 25-Hydroxycholesterol activates the expression of cholesterol 25-hydroxylase in an LXR-dependent mechanism. J. Lipid Res. 59, 439–451. doi: 10.1194/jlr.M080440
Livak, K. J., and Schmittgen, T. D. (2001). Analysis of relative gene expression data using realtime quantitative PCR and the 2–ΔΔCT method. Methods 25, 402–408. doi: 10.1006/meth.2001
Lu, K.-L., Wang, L.-N., Zhang, D.-D., Liu, W.-B., and Xu, W.-N. (2017). Berberine attenuates oxidative stress and hepatocytes apoptosis via protecting mitochondria in blunt snout bream Megalobrama amblycephala fed high-fat diets. Fish Physiol. Biochem. 43 65–76. doi: 10.1007/s10695-016-0268-5
Lukyanenko, Y., Chen, J. J., and Hutson, J. C. (2002). Testosterone regulates 25-hydroxycholesterol production in testicular macrophages. Biol. Reprod. 67, 1435–1438. doi: 10.1095/biolreprod.102.007575
Lukyanenko, Y. O., Chen, J. J., and Hutson, J. C. (2001). Production of 25-hydroxycholesterol by testicular macrophages and its effects on Leydig cells. Biol Reprod. 64, 790–796. doi: 10.1095/biolreprod64.3.790
Lund, E. G., Kerr, T. A., Sakai, J., Li, W. P., and Russell, D. W. (1998). cDNA cloning of mouse and human cholesterol 25-hydroxylases, polytopic membrane proteins that synthesize a potent oxysterol regulator of lipid metabolism. J. Biol. Chem. 273, 34316–34327. doi: 10.1074/jbc.273.51.34316
McDonald, J. G., and Russell, D. W. (2010). Editorial: 25-hydroxycholesterol: a new life in immunology. J. Leukoc. Biol. 88, 1071–1072. doi: 10.1189/jlb.0710418
Nayak, S., Koven, W., Meiri, I., Khozin-Goldberg, I., Isakov, N., Zibdeh, M., et al. (2017). Dietary arachidonic acid affects immune function and fatty acid composition in cultured rabbitfish, Siganus rivulatus. Fish Shellfish Immunol. 68, 46–53. doi: 10.1016/j.fsi.2017.07.003
Norberg, B., Kleppe, L., Andersson, E., Thorsen, A., Rosenlund, G., and Hamre, K. (2017). Effects of dietary arachidonic acid on the reproductive physiology of female Atlantic cod (Gadus morhua L.). Gen. Comp. Endocrinol. 250, 21–35. doi: 10.1016/j.ygcen.2017.05.020
Oosterveer, M. H., Grefhorst, A., Groen, A. K., and Kuipers, F. (2010). The liver X receptor: control of cellular lipid homeostasis and beyond: implications for drug design. Prog. Lipid Res. 49, 343–352. doi: 10.1016/j.plipres.2010.03.002
Pereiro, P., Forn-Cuní, G., Dios, S., Coll, J., Figueras, A., and Novoa, B. (2017). Interferon-independent antiviral activity of 25-hydroxycholesterol in a teleost fish. Antiviral. Res. 145, 146–159. doi: 10.1016/j.antiviral.2017.08.003
Radhakrishnan, A., Ikeda, Y., Kwon, H. J., Brown, M. S., and Goldstein, J. L. (2007). Sterolregulated transport of SREBPs from endoplasmic reticulum to golgi: oxysterols block transport by binding to Insig. Proc. Natl. Acad. Sci. U.S.A. 104, 6511–6518. doi: 10.1073/pnas.0700899104
Shahkar, E., Yun, H., Lee, S., Kim, D.-J., Kim, S.-K., Lee, B., et al. (2016). Evaluation of the optimum dietary arachidonic acid level and its essentiality based on growth and non-specific immune responses in Japanese eel. Anguilla japonica. Aquaculture 452, 209–216. doi: 10.1016/j.aquaculture.2015.10.034
Shankar, D. S., and Kulkarni, R. S. (2005). Changes in tissue cholesterol and serum cortisol level during four reproductive phases of the male freshwater fish, Notopterus notopterus. J. Environ. Biol. 26, 701–704.
Shankar, D. S., and Kulkarni, R. S. (2007). Tissue cholesterol and serum cortisol level during different reproductive phases of the female freshwater fish Notopterus notopterus (Pallas). J. Environ. Biol. 2, 137–139.
Shawli, G. T., Adeyemi, O. O., Stonehouse, N. J., and Herod, M. R. (2019). The oxysterol 25-hydroxycholesterol inhibits replication of murine norovirus. Viruses 11:E97. doi: 10.3390/v11020097
Shimano, H. (2001). Sterol regulatory element-binding proteins (SREBPs): transcriptional regulators of lipid synthetic genes. Prog. Lipid Res. 40, 439–452. doi: 10.1016/s0163-7827(01)00010-8
Shrivastava-Ranjan, P., Bergeron, É, Chakrabarti, A. K., Albariño, C. G., Flint, M., Nichol, S. T., et al. (2016). 25-hydroxycholesterol inhibition of Lassa virus infection through aberrant GP1 glycosylation. MBio 7:e1808-16. doi: 10.1128/mBio.01808-16
Vandesompele, J., De Preter, K., Pattyn, F., Poppe, B., Van Roy, N., De Paepe, A., et al. (2002). Accurate normalization of real-time quantitative RT-PCR data by geometric averaging of multiple internal control genes. Genome Biol. 3, 0034.1–0034.11. doi: 10.1186/gb-2002-3-7-research0034
Wu, T., Ma, F., Ma, X., Jia, W., Pan, E., Cheng, G., et al. (2018). Regulating innate and adaptive immunity for controlling SIV infection by 25-hydroxycholesterol. Front. Immunol. 9:2686. doi: 10.3389/fimmu.2018.02686
Xu, H., Ai, Q., Mai, K., Xu, W., Wang, J., Ma, H., et al. (2010). Effects of dietary arachidonic acid on growth performance, survival, immune response and tissue fatty acid composition of juvenile Japanese seabass, Lateolabrax japonicus. Aquaculture 307, 75–82. doi: 10.1016/j.aquaculture.2010.07.001
Xu, H., Cao, L., Zhang, Y., Johnson, R. B., Wei, Y., Zheng, K., et al. (2017a). Dietary arachidonic acid differentially regulates the gonadal steroidogenesis in the marine teleost, tongue sole (Cynoglossus semilaevis), depending on fish gender and maturation stage. Aquaculture 468, 378–385. doi: 10.1016/j.aquaculture.2016.11.002
Xu, H., Cao, L., Wei, Y., Zhang, Y., Zheng, K., and Liang, M. (2017b). Effects of different dietary DHA: EPA ratios on gonadal steroidogenesis in the marine teleost, tongue sole (Cynoglossus semilaevis). Br. J. Nutr. 118, 179–188. doi: 10.1017/S0007114517001891
Xu, H., Dong, X., Ai, Q., Mai, K., Xu, W., Zhang, Y., et al. (2014). Regulation of tissue LC-PUFA contents, Δ6 fatty acyl desaturase (FADS2) gene expression and the methylation of the putative FADS2 gene promoter by different dietary fatty acid profiles in Japanese seabass (Lateolabrax japonicus). PLoS One 9:e87726. doi: 10.1371/journal.pone.0087726
Xu, H., Mu, Y., Zhang, Y., Li, J., Liang, M., Zheng, K., et al. (2016). Graded levels of fish protein hydrolysate in high plant diets for turbot (Scophthalmus maximus): effects on growth performance and lipid accumulation. Aquaculture 454, 140–147. doi: 10.1016/j.aquaculture.2015.12.006
Xu, H., Sun, B., Liao, Z., Pribytkova, E., Zhang, Q., Wei, Y., et al. (2019). Possible involvement of PKC/MAPK pathway in the regulation of GnRH by dietary arachidonic acid in the brain of male tongue sole Cynoglossus semilaevis. Aquacult. Res. 50, 3528–3538. doi: 10.1111/are.14307
Yi, T., Wang, X., Kelly, L. M., An, J., Xu, Y., Sailer, A. W., et al. (2012). Oxysterol gradient generation by lymphoid stromal cells guides activated B cell movement during humoral responses. Immunity 37, 535–548. doi: 10.1016/j.immuni.2012.06.015
Zerbinati, C., Caponecchia, L., Puca, R., Ciacciarelli, M., Salacone, P., Sebastianelli, A., et al. (2017). Mass spectrometry profiling of oxysterols in human sperm identifies 25-hydroxycholesterol as a marker of sperm function. Redox Biol. 11, 111–117. doi: 10.1016/j.redox.2016.11.008
Keywords: Cynoglossus semilaevis, Ch25h, cloning, characterization, gene expression, arachidonic acid
Citation: Xu H, Sun B, Jia L, Wei Y, Liao Z and Liang M (2020) Cloning and Characterization of Cholesterol 25-Hydroxylase (ch25h) From a Marine Teleost, Chinese Tongue Sole (Cynoglossus semilaevis), and Its Gene Expressions in Response to Dietary Arachidonic Acid. Front. Mar. Sci. 6:800. doi: 10.3389/fmars.2019.00800
Received: 19 November 2019; Accepted: 12 December 2019;
Published: 14 January 2020.
Edited by:
Kang-le Lu, Jimei University, ChinaReviewed by:
Dizhi Xie, South China Agricultural University, ChinaMin Jin, Ningbo University, China
Copyright © 2020 Xu, Sun, Jia, Wei, Liao and Liang. This is an open-access article distributed under the terms of the Creative Commons Attribution License (CC BY). The use, distribution or reproduction in other forums is permitted, provided the original author(s) and the copyright owner(s) are credited and that the original publication in this journal is cited, in accordance with accepted academic practice. No use, distribution or reproduction is permitted which does not comply with these terms.
*Correspondence: Mengqing Liang, liangmq@ysfri.ac.cn